- 1Key Laboratory of Tropical Biological Resources of Ministry of Education, School of Pharmaceutical Sciences, Collaborative Innovation Center of One Health, Hainan University, Haikou, China
- 2College of Life Sciences, Hainan University, Haikou, China
- 3School of Science, Hainan University, Haikou, China
- 4Department of Neurology, The First Affiliated Hospital of Hainan Medical University, Haikou, China
- 5School of Animal Science and Technology, Hainan University, Haikou, China
Introduction: Temporin-GHa obtained from the frog Hylarana guentheri showed bactericidal efficacy against Streptococcus mutans. To enhance its antibacterial activity, the derived peptides GHaR and GHa11R were designed, and their antibacterial performance, antibiofilm efficacy and potential in the inhibition of dental caries were evaluated.
Methods: Bacterial survival assay, fluorescent staining assay and transmission electron microscopy observation were applied to explore how the peptides inhibited and killed S. mutans. The antibiofilm efficacy was assayed by examining exopolysaccharide (EPS) and lactic acid production, bacterial adhesion and cell surface hydrophobicity. The gene expression level of virulence factors of S. mutans was detected by qRT-PCR. Finally, the impact of the peptides on the caries induced ability of S. mutans was measured using a rat caries model.
Results: It has been shown that the peptides inhibited biofilm rapid accumulation by weakening the initial adhesion of S. mutans and reducing the production of EPS. Meanwhile, they also decreased bacterial acidogenicity and aciduricity, and ultimately prevented caries development in vivo.
Conclusion: GHaR and GHa11R might be promising candidates for controlling S. mutans infections.
Introduction
Dental caries adversely affects more than 60% of school-aged children and most adults worldwide and has been identified as the third major disease endangering human health by the World Health Organization (WHO) after cardiovascular diseases and malignant tumors (Peres et al., 2019). When oral microorganisms attach to tooth surfaces, an acquired biofilm is formed, mainly composed of salivary glycoproteins. Subsequently, the initially established weak interaction between bacterial adhesins and cell membrane glycoprotein receptors became stronger, followed by more bacteria aggregating to the attached bacteria, forming multispecies communities, known as dental plaque biofilms. Biofilms are constructed by highly organized oral microorganisms and are rich in proteins, extracellular polysaccharides (EPSs), carbohydrates, and nucleic acids, providing a perfect extracellular polymer matrix for the subsequent adhesion of oral cariogenic bacteria, in some cases leading to the progression of oral biofilms into cariogenic biofilms (Mosaddad et al., 2019). Among the oral bacteria, Streptococcus, Actinomyces, and Lactobacilli contribute more to secreting glucan to cell surfaces, thereby strengthening the adhesion of cariogenic microorganisms to teeth. At the same time, they ferment carbohydrates into lactic acid, which continuously demineralizes teeth and leads to the tooth decay (Ma et al., 2015; Lemos et al., 2019).
Streptococcus mutans is considered to be the dominant etiologic bacteria in dental caries (Lemos et al., 2019) and a potential risk factor in cardiovascular diseases, as it has been detected on cardiac valves and atherosclerotic plaques in patients (Nakano et al., 2006). Streptococcus mutans secretes a large amount of lactic acid synthesized by lactate dehydrogenase (LDH) in the presence of dietary sugars in oral cavity, causing serious damage to dental hard tissues (Ma et al., 2022). Most bacteria have difficulty growing in a low pH environment created by lactic acid, but S. mutans actively pumps protons out of the cells by consuming ATP to maintain intracellular pH and ensure growth, which leads to remarkable aciduricity (Harper and Loesche, 1983). In addition, S. mutans can synthesize extracellular polysaccharides (EPS) using dietary sucrose by its glucosyltransferases (GTFs) and fructosyltransferases (FTFs) (Zhang et al., 2021) and secrete them onto cell surfaces, enhancing the adhesion ability of S. mutans; meanwhile, they also provide a matrix for colonization of other cariogenic bacteria, leading the dental plaque biofilm formation (Klein et al., 2015). The microorganisms embedded in biofilms exhibit low metabolic activities and strong tolerance to antimicrobial reagents (Yue et al., 2018). Therefore, targeting S. mutans has become an effective way to prevent the onset of dental caries or treat early caries.
Antimicrobial peptides (AMPs) with broad-spectrum activities against pathogenic microorganisms have widely found in natural organisms, which are important components in the body’s innate immune system and produced by almost all tissues and cells that are regularly exposed to microorganisms (Wiesner and Vilcinskas, 2010). The oral cavity is an open environment, in which the microbiome is acquired in childhood and will fluctuate in the proportion of species by such as diet and age. Once the homeostasis is broken, the pathogenic microorganisms might be predominant and cause oral diseases (Diamond et al., 2008). Several antimicrobial peptides, including statin, defensin, and LL-37, were founded in the oral epithelium and saliva and were considered to be the core defense system in the oral cavity (Guncu et al., 2015). Additionally, known as host defense peptides, these naturally occurring AMPs in the oral cavity are involved in regulating the innate immune response of host cells and exhibit resistance to oral pathogenic microorganisms (Griffith et al., 2022).
Temporin peptides, first found in amphibians, are one of the shortest naturally occurring peptide families, consisting of 10–14 amino acid residues. Because of their low manufacturing cost and diverse activities, these peptides are ideal candidates for therapeutic drug development (Wang et al., 2016). Through previous studies, we cloned natural antimicrobial peptide temporin-GHa (GHa) (FLQHIIGALGHLF-NH2) (Dong et al., 2017). In this article, we designed temporin-GHa11R (GHa11R) (FLQHIIGALGRLF-NH2) and temporin-GHaR (GHaR) (FLQRIIGALGRLF-NH2) to improve the antimicrobial and antibiofilm activities under physiological conditions by enhancing the net positive charges of GHa. The bacteriostatic effects and mechanism of action of the derived peptides were measured in vitro. The anti-caries properties of GHaR and GHa11R were studied in the caries rat model induced by S. mutans.
Materials and methods
Peptides, bacterial strains, and growth conditions
The peptides were synthesized by Ji’er Biochemical (Shanghai, China) with a purity greater than 95%. Before use, the peptides were dissolved in sterile deionized water at the concentration of 2 mM as stock solution, and diluted with phosphate buffered saline (PBS) to work concentration. Streptococcus mutans UA159 was purchased from Guangdong Microbial Culture Collection Center (Guangzhou, China) and cultured anaerobically in brain heart infusion broth (BHI; Thermo Fisher, CN) at 37°C under mixed gas conditions of 5% CO2, 10% H2, and 85% N2 to logarithmic growth phase before experiments. The colony forming units (CFUs) and the optical density at 600 nm (OD600) of the bacteria were plotted. OD600 = 0.1 is equal to the density of S. mutans at 2 × 106 CFU/mL in BHI.
Streptococcus sanguis ATCC 10556 and Porphyromonas gingivalis ATCC 33277 were cultured anaerobically in BHI. Bifidobacterium adolescentis ATCC 15703 and B. breve ATCC 15700 were cultured anaerobically in MRS broth (MRS; Thermo Fisher, CN) containing 0.05% L-cysteine hydrochloride. Lactobacillus acidophilus ATCC 4356 was cultured anaerobically in MRS broth.
Peptide structure determination by circular dichroism (CD) spectroscopy
The peptides (1 mM) were dissolved in 10 mM PBS, 30 mM sodium dodecyl sulfate (SDS) and 50% (v/v) trifluoroethanol (TFE), respectively. The peptide spectrum was scanned by a CD spectrometer (Brighttime Chirascan, Applied Photophysics Limited, UK) at wavelengths of 190 nm to 260 nm with a speed of 10 nm/min.
Antibacterial activity assay
Equal volumes (50 μL) of bacterial suspension (2 × 106 CFU/mL) and the peptide solution were mixed in wells on polystyrene plates (Corning, USA). After the plates were incubated anaerobically for 24 h, the OD600 was measured. In negative control group, the peptides were replaced with PBS only, and chlorhexidine (CHX) was used as a positive control. The minimum inhibitory concentration (MIC) is defined as the minimum concentration that inhibits the visible growth of bacteria within 24 h. The minimum bactericidal concentration (MBC) is the minimum concentration that kills 99% of bacterial cells determined by colony counting (Cao et al., 2020).
Time killing assay
The bacterial suspension (2 × 106 CFU/mL, 100 μL) in BHI was mixed with the same volume of the peptide solution (3.1–12.5 μM). The peptides were replaced with PBS only in the negative control. Aliquots of the mixtures were pipetted at 0, 15, 30, 45, 60, 90, 120, and 180 min, and plated on BHI agar after proper dilution. The plates were incubated anaerobically for 48 h, followed by colony counting.
Observation of bacteria by transmission electron microscopy (TEM)
After exposing to the peptides (4 × MIC) for 1 h, the bacteria (1 × 109 CFU/mL) were collected, fixed with 2.5% glutaraldehyde and dehydrated in serially diluted ethanol solutions at room temperature. The peptides were replaced with PBS only in the negative control. After embedding in resin for 48 h at 60°C, the bacteria were sliced into 60–80 nm sections and loaded on 150 mesh copper mesh. After staining with 2% uranium acetate, the sections were washed with 70% ethanol solution and ultra-pure water, respectively, then re-stained with 2.6% lead citrate solution. After washing and drying overnight, the sections were observed by TEM (HT7800, Hitachi, Japan) (Zhang et al., 2022).
Cell membrane integrity assay
The cell membrane integrity was studied by fluorescent staining (Zhang et al., 2020). Streptococcus mutans (1 × 109CFU/mL) pre-treated with the peptides (1 × MIC) for 1 h was centrifuged and collected to remove the peptides. The bacteria were fixed with 4% paraformaldehyde for 1 h, followed by staining with PI solution (10 μg/mL) for 15 min in the dark. Then the bacteria were collected by centrifugation and stained with DAPI solution (10 μg/mL) for 15 min. The bacteria were washed, collected, and observed under an inverted fluorescence microscope with S. mutans treated with PBS as a negative control.
To confirm the impact of the peptides on S. mutans cell membranes, S. mutans treated with the peptides and stained with PI as described above were detected by flow cytometry (BF32366, Beckman Coulter, Brea, CA, USA).
Glycolytic pH drops and acid tolerance assay
Influence of the peptides on acid-producing capacity of S. mutans has been assessed (Wang et al., 2018). The bacterial suspension was adjusted to 1 × 108 CFU/mL in BHI broth (the initial pH was 7.2) containing the peptides (1/2 × MIC, at which the bacterial growth was not affected), and 1% (w/v) glucose was added to trigger glycolysis. In negative control, the peptides were replaced with PBS only. After incubating for 180 min, an aliquot of the suspension was sampled every 30 min for detecting the pH value using a glass electrode pH meter.
Acid tolerance of S. mutans was assessed (Svensater et al., 1997). Bacteria (1 × 106 CFU/mL) grown in tryptone-yeast extract medium (TYEM) broth (pH 7.5, containing 20 mM glucose) were transferred to BHI culture medium (pH 5.5) with or without the peptides (1/2 × MIC). The same volume of suspension was diluted and applied to BHI agar for determining the number of viable bacteria. After the remaining suspension was incubated for 2 h, the pH was immediately reduced to the killing pH (3.0) for S. mutans by adding 20% hydrochloric acid and further incubated for 3 h. The suspensions were also plated. After incubating all plates anaerobically for 48 h, the total CFUs were calculated.
Lactic acid production assay
The bacterial suspensions (1 × 106 CFU/mL, 2 mL) were added to each well containing a coverslip on a 24-well microtiter plate, and incubated anaerobically for 24 h. After discarding planktonic bacteria, the coverslips covered by biofilms were removed to a new 24-well plate, and treated by the peptides (1/2 × MIC, containing 2% sucrose) in buffered peptone water (BPW). In negative control, the peptides were replaced with PBS only. After incubating anaerobically for 180 min, the supernatant was collected, and the production of lactic acid was measured by lactic acid measurement kit (Solarbio, China). OD570 was recorded and the concentration of lactic acid was calculated (Chen et al., 2020).
Cell membrane permeabilization and F1F0-ATPase assay
The permeabilized cells were prepared and the activity of F1-F0 ATPase was determined (Xu et al., 2011). In general, S. mutans was collected and dispersed in Tris–HCl buffer (pH 7.0) containing 10 mM MgSO4 and 10% (v/v) toluene. After swirling for 1 min, the bacterial suspension was incubated for 5 min at 37°C and rapidly frozen in liquid nitrogen. The frozen cells were quickly thawed and re-frozen twice. After collecting and exposing to the peptides (1/2 × MIC) for 120 min, the bacteria were collected by centrifugation and resuscitated in Tris-maleate buffer (pH 7.0, containing 10 mM MgCl2). Finally, 5 mM ATP was added to trigger F1-F0 ATPase activity. In negative control, the peptides were replaced with PBS only. The released phosphate was identified (Bencini et al., 1983).
The assay of inhibition biofilm formation
The effect of the derived peptides on the biofilm formation of S. mutans was evaluated (de Breij et al., 2018). Briefly, an equal volume of bacterial suspension (2 × 106 CFU/mL) in BHI broth containing 1% sucrose (BHIs) was mixed with the peptide solution (0.8 to 25 μM) on a 96-well polystyrene plate (Corning, USA), and incubated anaerobically for 24 h. In negative control, the peptides were replaced with PBS only. After discarding the supernatant, the remaining biofilm was rinsed twice with PBS, fixed by methanol solution and thoroughly dried. CV solution (0.1%) was added to stain the biofilm for 15 min. After the excess dye was thoroughly washed with PBS, 200 μL of ethanol was added and OD595 was determined.
Bacterial adhesion assay
The activity of the derived peptides on the bacterial adhesion ability was evaluated both on 96-well polystyrene plate surfaces and saliva-coated surfaces (Zhang et al., 2020). Bacterial suspension (1 × 109 CFU/mL) was treated with the peptides (4 × MIC) for 15 min and the unbound peptides were removed by centrifugation (3,000 × g, 3 min). In negative control, the peptides were replaced with PBS only. After resuspending in BHI broth, an aliquot of the suspension was pipetted, plated on BHI agar, and cultured anaerobically for 48 h. The CFUs were calculated to determine the number of viable bacteria. A 96-well polystyrene plate was precoated with 100 μL of artificial saliva at 37°C for 15 min. After the saliva was removed, the saliva-coated plate was prepared. The remaining suspension (100 μL) was added to a polystyrene plate or a saliva-coated plate and incubated for 1 h under static conditions. After discarding the supernatant, the plates were gently washed twice with PBS to remove unadhered bacteria. After adding 200 μL of PBS to each well, all the plates were sonicated three times at a power of 120 W and a frequency of 40 kHz on ice for 90 s with an interval of 30 s. The sonication fluid was plated and the CFUs were numbered. The bacterial adhesion rate was expressed as the CFUs of adhesive bacteria divided by the CFUs of the viable bacteria.
Cell surface hydrophobicity assay
The bacterial suspension (1 × 109 CFU/mL) was incubated with the derived peptides (0.8–25 μM) in Phosphate urea magnesium (PUM) buffer for 20 min, and the OD550 of the bacterial suspension was measured. Cetane (200 μL) was added to the remaining bacterial suspension, vortexed, and settled for 30 min. In negative control, the peptides were replaced with PUM only. The supernatant was pipetted to determine the OD550. The degree of changes in surface hydrophobicity of S. mutans was shown as a percentage decrease of the OD550 (Kim et al., 2019).
Water-insoluble EPS measurement
The production of water-insoluble EPS in biofilms was evaluated by phenol-sulfuric acid method (Abdel-Aziz et al., 2020). Briefly, equal volumes of bacterial suspension (1 × 106 CFU/mL) and the peptides (1 × MIC) were mixed in a 24-well polystyrene plate and incubated anaerobically for 24 h to generate biofilms. In negative control, the peptides were replaced with PBS only. After discarding planktonic bacteria, the biofilms were collected and washed twice to clean out water-soluble EPS. After centrifugation, the pellet was re-suspended in 1 M NaOH solution (200 μL) for 2 h to extract water-insoluble EPS. Finally, 5% frozen phenol and sulfuric acid were added to the mixture at 1:1:5 (volume ratio), incubated for 1 h, followed by measuring OD625.
Confocal laser scanning microscope (CLSM) observation
The EPS distribution in biofilms was observed by CLSM (Deng et al., 2021). Equal volumes (1 mL) of the bacterial suspension (2 × 106 CFU/mL) in BHIs broth and the peptides solution (1 × MIC) were mixed in each well containing a coverslip on a 24-well polystyrene plate. In negative control, the peptides were replaced with PBS only. Alexa Fluor 647 glucan (1 μmol/L) was used to label the dextran conjugate for 24 h. After that, S. mutans nucleic acids were labeled with SYTO 9 (2.5 μmol/L). CLSM (TCS SP8, LEICA, Germany) was used to observe.
The expression of virulence genes by quantitative real-time reverse transcription PCR (qRT-PCR)
The expression levels of virulence genes in S. mutans were measured by qRT-PCR with 16S rRNA serving as a quantified internal control (Xiong et al., 2020; Aqawi et al., 2021). Streptococcus mutans in the logarithmic growth phase was diluted to 1 × 106 CFU/mL in BHIs broth, pretreated with the peptides (1/2 × MIC, diluted with BHIs) for 8 h and total RNA was purified. In negative control, the peptides were replaced with PBS only. The first-strand cDNA was synthesized using RT kit (TES201, Tsingke, China). The tested genes and primers are listed (Table 1). The reaction was performed on a CFX96 real-time system (CFX Connect, Bio-Rad, USA). Gene expression was presented by using the 2–Δ Δ Ct method and normalized to the 16S rRNA level.
Cytotoxicity assay on human oral keratinocytes (HOK)
The cytotoxicity of the peptides was measured by the Cell Counting Kit-8 (CCK-8) (Utheim et al., 2016). Briefly, HOK cells (5,000 cells/well) were inoculated in a 96-well plate. After reaching 80% confluence, the cells were exposed to the peptides (3.1, 6.2, 12.5, 25, 50, and 100 μM) for 2 h. In negative control, the peptides were replaced with PBS only. Then CCK-8 solution (10 μL) was added and incubated for 2 h. OD450 was measured.
Anti-caries assay on a caries rat model
The anti-caries effect of GHaR and GHa11R was detected in a caries rat model infected by S. mutans (Wang et al., 2018; Tian et al., 2022). Twenty-seven Sprague Dawley (SD) male rats aged 18 days (Slyke Jingda Experimental Animal Co., Ltd., China) were fed food (supplemented with 0.1% ampicillin) and water (containing 4,000 U/mL penicillin) for 3 days to sterilize the oral endogenous microorganisms. At the age of 22–24 days, 24 rats were anesthetized by isoflurane and infected orally with 200 μL of S. mutant (1 × 109CFU/mL) once daily. Twenty-four hours after the last infection, a sterile cotton swab was used to scratch the oral cavity of the rats, which was then rinsed with PBS. The solution was plated on Mitis Salivarius agar (MSA) containing 20 U/mL bacitracin to confirm colonization. The infected rats were randomly divided into 4 groups with 6 rats in each, including the negative control group (UA159 + PBS), the positive control group (UA159 + CHX), and two peptide-treated groups (UA159 + GHaR and UA159 + GHa11R). Uninfected rats served as the blank group. The rat teeth were evenly brushed with a sterilized cotton swab soaked with 200 μL of PBS, CHX, GHaR or GHa11R three times a week for 8 weeks. All rats were fed Keyes 2,000 # cariogenic diet and purified water containing 5% sucrose. The health status of the rats was recorded. At the age of 81 days, after suffocation by CO2, the left and right mandibles were removed. After autoclaving at 121°C for 10 min, the mandibles were cleaned by peeling off the remaining tissue, washed with PBS, and stained overnight with 0.4% murexide. The main groove of the tooth was exposed by a proximal sagittal semi-section and observed under a stereo microscope (M205 FA, LEICA, Germany) to assess the caries level according to a modified Keyes score. An “E” score of 1 indicates that the caries involved only the enamel. A “D” score of 2 indicates that the caries did not exceed 1/4 of the dentin thickness. A “Dm” score of 3 indicates that the range of caries was 1/4–3/4 of the dentin thickness. A “Dx” score of 4 indicates that the caries was more than 3/4 of the dentin thickness. The score for each mandible was the sum of the scores for the first and second molars.
Statistical analysis
The experiments were performed independently three times in triplicates. The data in the tables and graphs was a representation of one biological replicate and presented as the mean ± standard deviation (SD). The data were analyzed statistically using t-test with the GraphPad Prism, with a P-value of less than 0.05 considered statistically significant when comparing the treated groups to the control groups.
Results
The characteristics and structures of GHa and its derived peptides
In our previous study, we performed sequence alignment on different AMPs and found that the positively charged amino acid lysine (Lys, K) in temporin peptides occurs more frequently than arginine (Arg, R) and histidine (His, H), while in the antibiofilm peptides, R and K are similarly chosen over H (Xie et al., 2019). In order to obtain derived peptides with antibiofilm efficacy, we used R to replace the H at GHa to design GHaR and GHa11R. Compared with GHa, the charge, amphiphilic index (AI) and Boman index (BI) of GHaR and GHa11R increased, and the grand average of hydropathy value (GRAVY) decreased (Table 2). Generally, α-helical AMPs with high amphiphilicity show strong activity; meanwhile their GRAVY is positive, and BI is negative or close to 0 (Saporito et al., 2018). The changes in the physiochemical characteristics of the derived peptides may enhance their antibacterial activities.
The structures of the peptides are shown in Figure 1. The spiral wheel of the peptides presented that on the one side the hydrophilic amino acid residues were located, while the hydrophobic residues were arranged on the opposite side, forming the hydrophilic and hydrophobic surfaces, respectively. The CD spectrum revealed that the peptides showed a random-coiled structure in aqueous conditions, while dissolved in a solvent of SDS (30 mM) or TFE (50%), they shown typical α-helical structures, indicating that GHa and the derived peptides had an amphiphile α-helical structure in simulated bacterial membranes. The predicted 3D structure of the peptides is also an α-helix structure. Meanwhile, the increased amphiphilicity of the derived peptides indicated that they have a more stable amphipathic α-helix structure than that of the parent peptide (Abbassi et al., 2013).
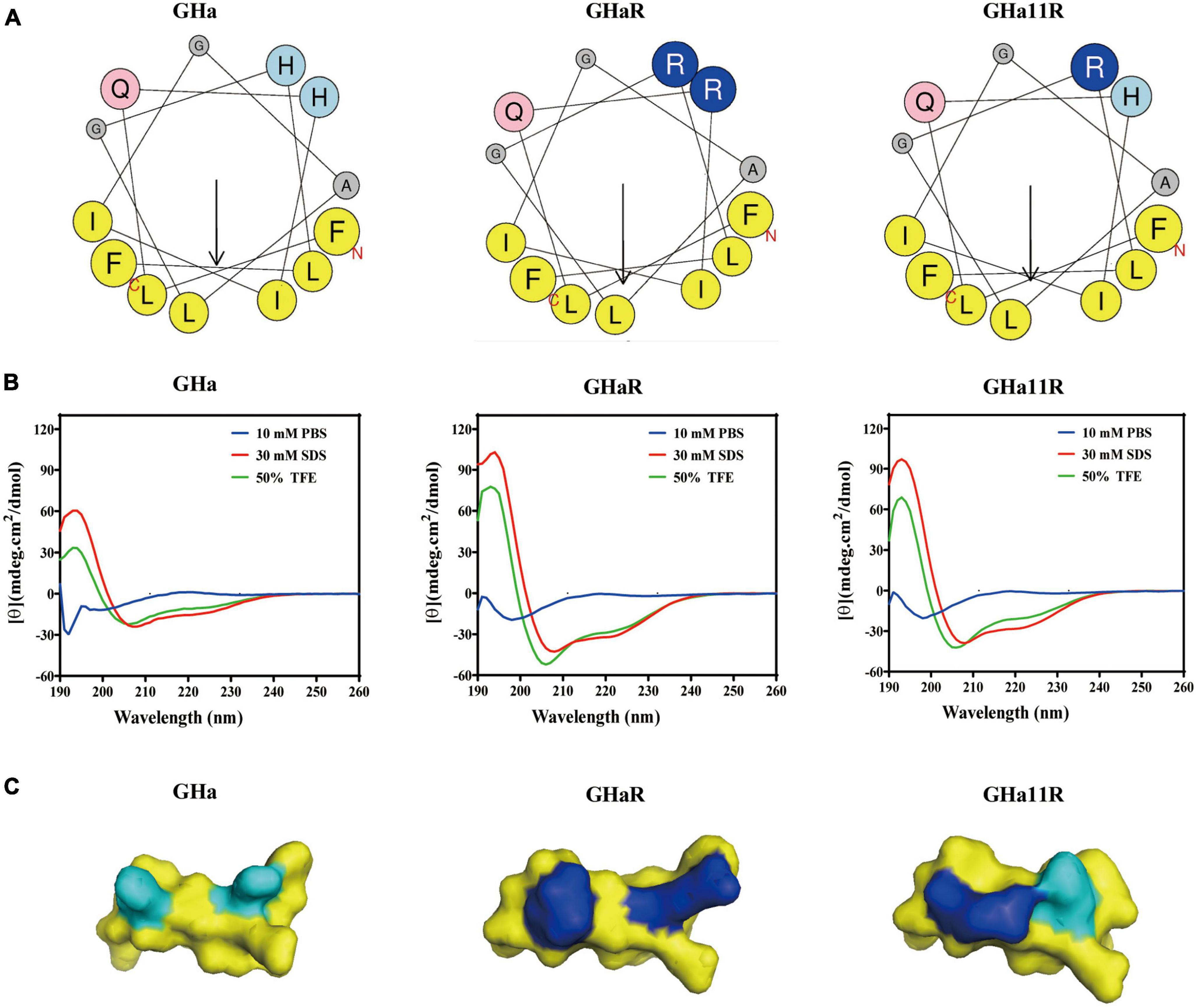
Figure 1. The structures of temporin-GHa, GHaR, and GH11R. (A) Heliquest (https://heliquest.ipmc.cnrs.fr/) was used to draw helical wheel projections. The arrows show the hydrophobic moment direction. (B) The secondary structures were detected by CD spectroscopy. (C) The 3D structures were predicted by PEP-FOLD (http://bioserv.rpbs.univ-paris-diderot.fr/services/PEP-FOLD/) and displayed by PyMol. The light and dark blue represent histidine and arginine residues, respectively.
The peptides show stronger antibacterial activity against S. mutans than against the tested probiotics
GHa has mild antibacterial activity against S. mutans with MIC of 12.5 μM and MBC of 25 μM. By mutating the amino acid residues of GHa, the ability of the derived peptides GHa11R and GHaR to inhibit S. mutans growth was improved, with the MIC decreased by 4 times and 8 times, respectively. The antibacterial efficacy of the peptides against several oral etiologic bacteria and probiotics was detected. As shown in Table 3, the peptides also showed good inhibitory activity against the oral pathogenic bacteria S. sanguinis and P. gingivalis, which are associated with oral infections and cardiovascular diseases. Comparatively, GHa and its derived peptides against pathogenic bacteria had lower MIC/MBC values; however, they showed higher antibacterial concentrations against the tested probiotics. CHX exhibits strong antibacterial efficacy against both pathogenic bacteria and probiotics.
The peptides exerted antibacterial activity by damaging cell membrane integrity
The bacteriostatic activity of GHa, GHaR, and GHa11R against planktonic S. mutans was investigated (Figure 2A). GHaR inhibited S. mutans growth at 3.1 μM and GHa11R at 6.2 μM, similar to CHX. The time-killing kinetics demonstrated that the killing effect of these peptides on S. mutans was concentration- and time-dependent. After treatment with 6.2 μM GHaR and GHa11R, all bacteria were killed within 90 ∼ 120 min (Figure 2B). The microscopic morphology of S. mutans was observed under TEM. As shown in Figure 2C, the bacterial cell membrane was destroyed, resulting in leakage of cellular contents.
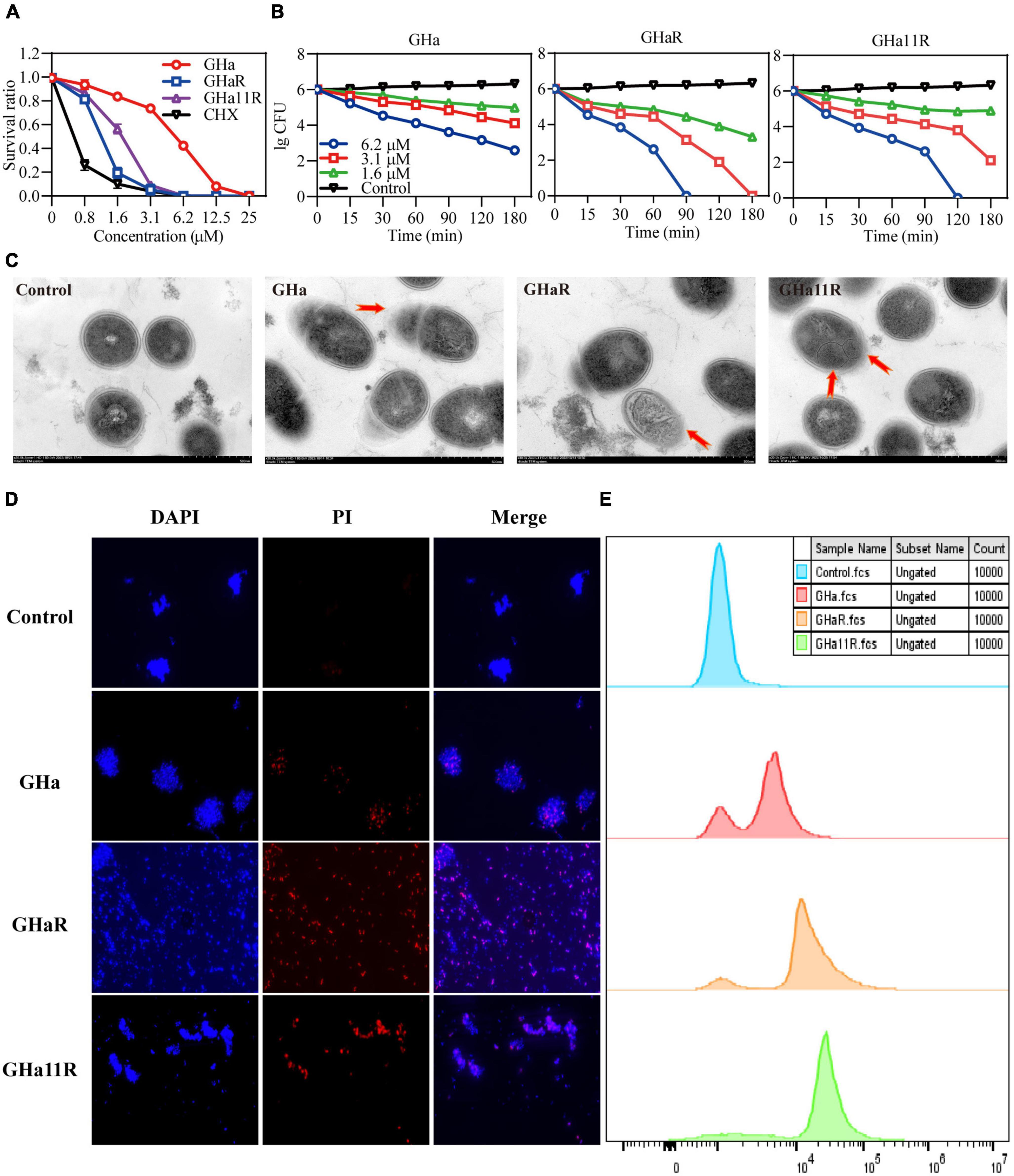
Figure 2. Antibacterial effect of GHa, GHaR, and GHa11R against S. mutans. (A) The antibacterial activity of GHa, GHaR, and GHa11R against planktonic S. mutans. The survival rate was defined as the OD600 ratio of S. mutans treated with or without the peptides or CHX. (B) The time-killing kinetics of GHa, GHaR and GHa11R against S. mutans were determined. (C) Streptococcus mutans exposed to the peptides (12.5 μM ) for 1 h were observed by TEM. (D) The cell membrane integrity of S. mutans was detected by fluorescence staining. Streptococcus mutans treated with GHa, GHaR, or GHa11R were double stained with DAPI and PI. The images were photographed under a fluorescence microscope at a magnification of 40 ×. (E) The bacteria treated with GHa, GHaR, or GHa11R (1 × MIC) were stained with PI and analyzed by flow cytometry. Bacteria treated with PBS were the negative control.
Fluorescence staining revealed the membrane destruction mechanism of the peptides
As shown in Figure 2D, after the bacteria were treated with the peptides (1 × MIC), PI penetrated cell membranes, showing red fluorescence, which indicated that the structure of S. mutans cell membranes was destroyed. Flow cytometry was used to further detect the membrane destruction efficiency of the peptides on S. mutans. In comparison with the untreated bacteria, after 1 h of treatment with the peptides (1 × MIC), a large number of S. mutans stained by PI was detected (Figure 2E).
The peptides decreased the acidogenicity and aciduricity of S. mutans
As shown in Figure 3A, the pH value of the suspension in the control group declined sharply from 7.4 to 4.5 within 180 min. After exposure to the peptides, the drop in pH was alleviated and decreased to 5.4, indicating that the peptides inhibited the acidogenicity of S. mutans, especially GHaR and GHa11R. The effect of the peptides on the acid production of S. mutans was further determined (Figure 3B). In comparison with GHa, GHaR and GHa11R reduced lactic acid production by 50% within 180 min. After 3 h incubation under killing pH conditions, 10% of the S. mutans bacteria without the peptide treatment still survived, while the treated bacteria decreased to less than 1% of their original population (Figure 3C). Meanwhile, the activity of F1-F0-ATPase related to acid tolerance of S. mutans decreased by 50–70% after being treated with 1/2 × MIC of the peptides for 120 min (Figure 3D).
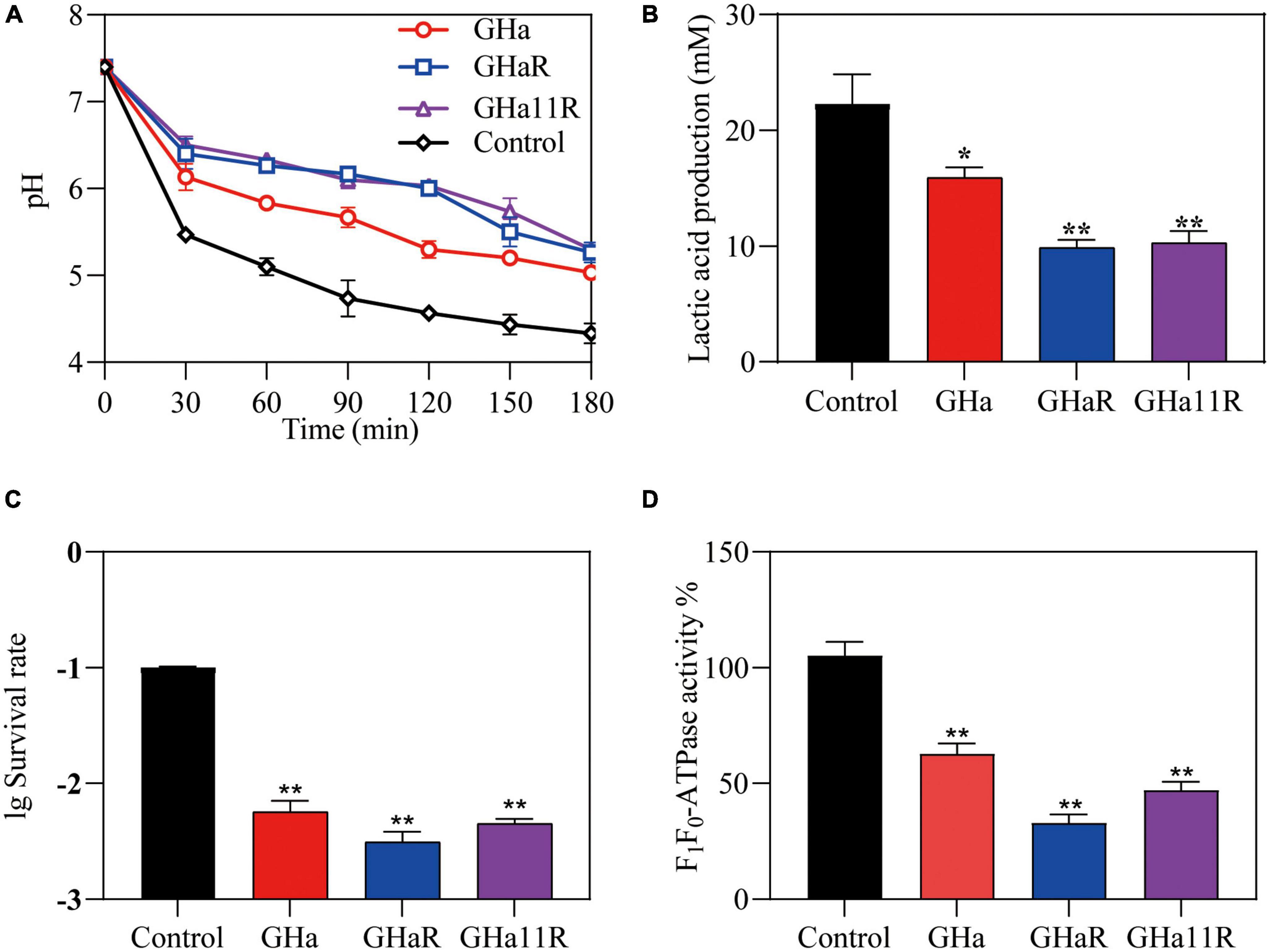
Figure 3. Effects of GHa and its derived peptides on the acidogenicity and aciduricity of S. mutans. (A) Effects of the peptides on glycolytic pH, (B) lactic acid production, and (C) aciduricity of S. mutans were assayed. (D) The F1-F0 ATPase activity of S. mutans after 120 min of treatment with GHa, GHaR and GHa11R was determined. The final concentration of the peptides was 1/2 × MIC. *Represents comparison with the control. *P < 0.05 and **P < 0.01.
The peptides inhibited the attachment of S. mutans biofilms
As shown in Figure 4A, when S. mutans was incubated with sub-MICs of GHaR and GHa11R for 24 h, more than 25–30% of the bacterial biomass in the S. mutans biofilms has been inhibited. Furthermore, we explored the attachment ability of the bacteria to polystyrene plates and saliva-coated polystyrene plates (Figure 4B). After treatment with GHaR and GHa11R, more than 60% of the S. mutans attached to polystyrene plates was reduced, indicating that the peptides disturbed the initial adhesion of S. mutans, thus inhibiting biofilm formation. Applying saliva to the surface of the polystyrene plate significantly enhanced bacterial attachment, with doubling biofilm formation in the negative control. However, both GHaR and GHa11R still showed effective efficacy in preventing the initial attachment of biofilms.
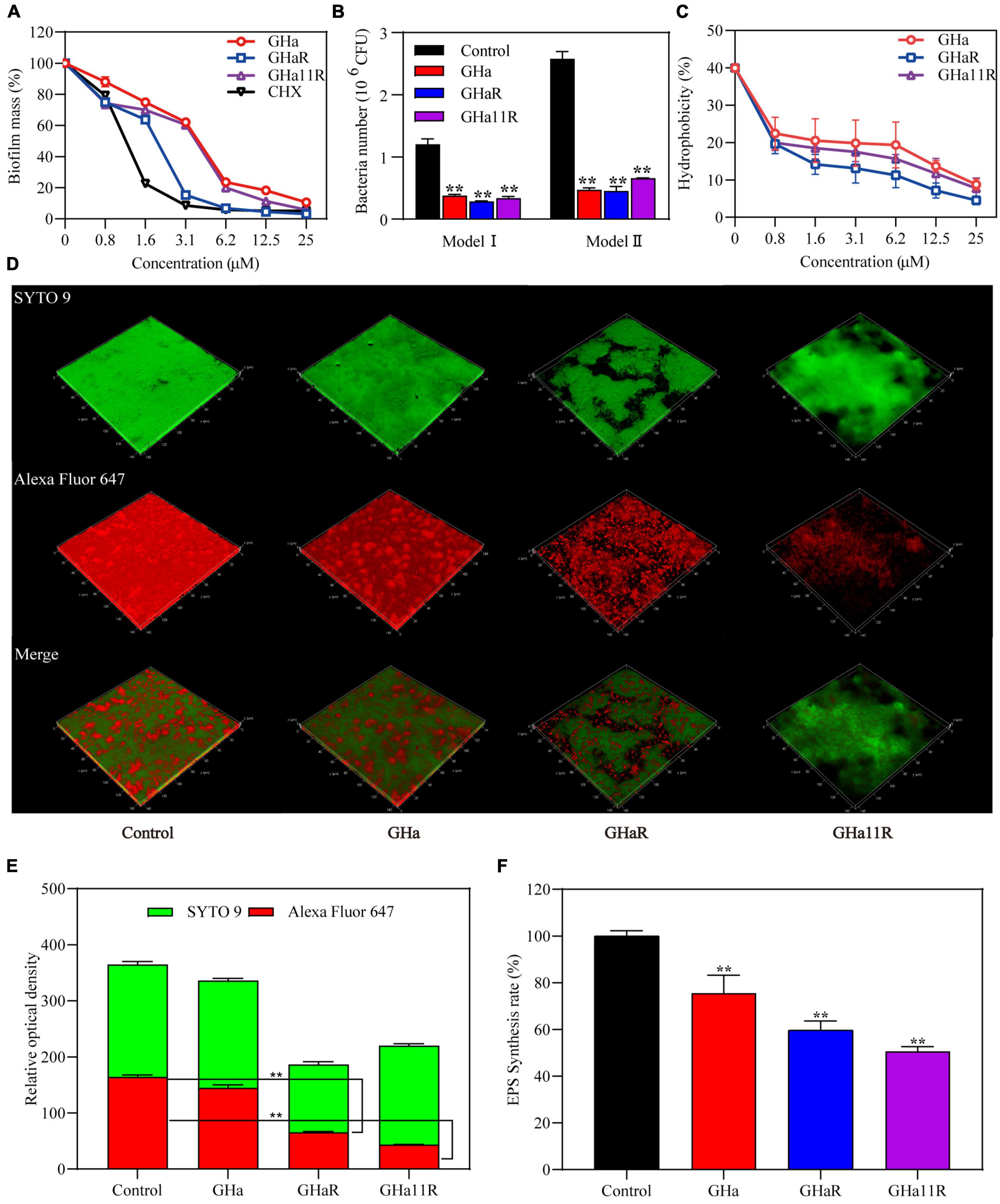
Figure 4. The peptides inhibited S. mutans biofilm formation. (A) The peptides inhibited the formation of biofilms. After incubation with the peptides for 24 h, the biomass in the biofilms was measured. (B) The peptides inhibited the initial bacterial adhesion. Model I represents S. mutans adhesion on polyethylene plates, and Model II represents S. mutans adhesion on polyethylene plates precoated with artificial saliva. (C) The peptides reduced the hydrophobicity of S. mutans. (D) Observation of S. mutans biofilms by CLSM. Representative images of glucan distribution and biofilms are presented. EPS marked with Alexa Fluor 647 are in red; the bacteria labeled with SYTO 9 are in green. Images were taken at 64 × magnification. (E) Quantitative data of bacteria and EPS biomass. (F) The peptides reduced EPS production in biofilms of S. mutans. *Represents comparison with the control. **P < 0.01.
To explain how the peptides impact the adhesion ability of S. mutans, the hydrophobicity of bacterial surfaces exposed to the peptides was investigated. Nearly 40% of S. mutans in the negative control was transferred from the aqueous phase to the organic phase. After treatment, approximately 20% of S. mutans was transferred, and the percentage decreased dose-dependently (Figure 4C), confirming that the peptides decreased the hydrophobicity of S. mutans.
The peptides reduced the EPS production in biofilms
The glucan labeled by Alexa Fluor 647 can be embedded into EPS during the synthesis of the S. mutans biofilm matrix, showing red fluorescence under CLSM. The biofilms in the blank control were tightly packed, and EPS was highly expressed (Figure 4D). Although GHa (1/2 × MIC) showed no significant efficacy on the S. mutans biofilms, GHaR and GHa11R destroyed the confluent, tightly packed layers of the biofilms, resulting in scattered clusters of bacteria in the biofilms. As shown in Figure 4E, the EPS in biofilms decreased significantly after exposure to the derived peptides. The inhibitory activity of the peptides on EPS production has been explored (Figure 4F). The water-insoluble EPS production had been decreased by 40–50% after the bacteria were exposed to GHaR and GHa11R.
The peptides downregulated virulence gene expression in S. mutans
As shown in Figure 5A, the tested genes were downregulated after treatment with sub-MICs of the peptides, especially the gtfB and gtfC, which encode water-insoluble EPS, and the ldh. GHaR and GHa11R reduced the expression of the gtfB and gtfC genes by 70 to 80%. GHa11R reduced the expression of the gtfD gene by more than 99%. For the ldh gene, both GHaR and GHa11R reduced the expression of the gtfD gene by more than 95%.
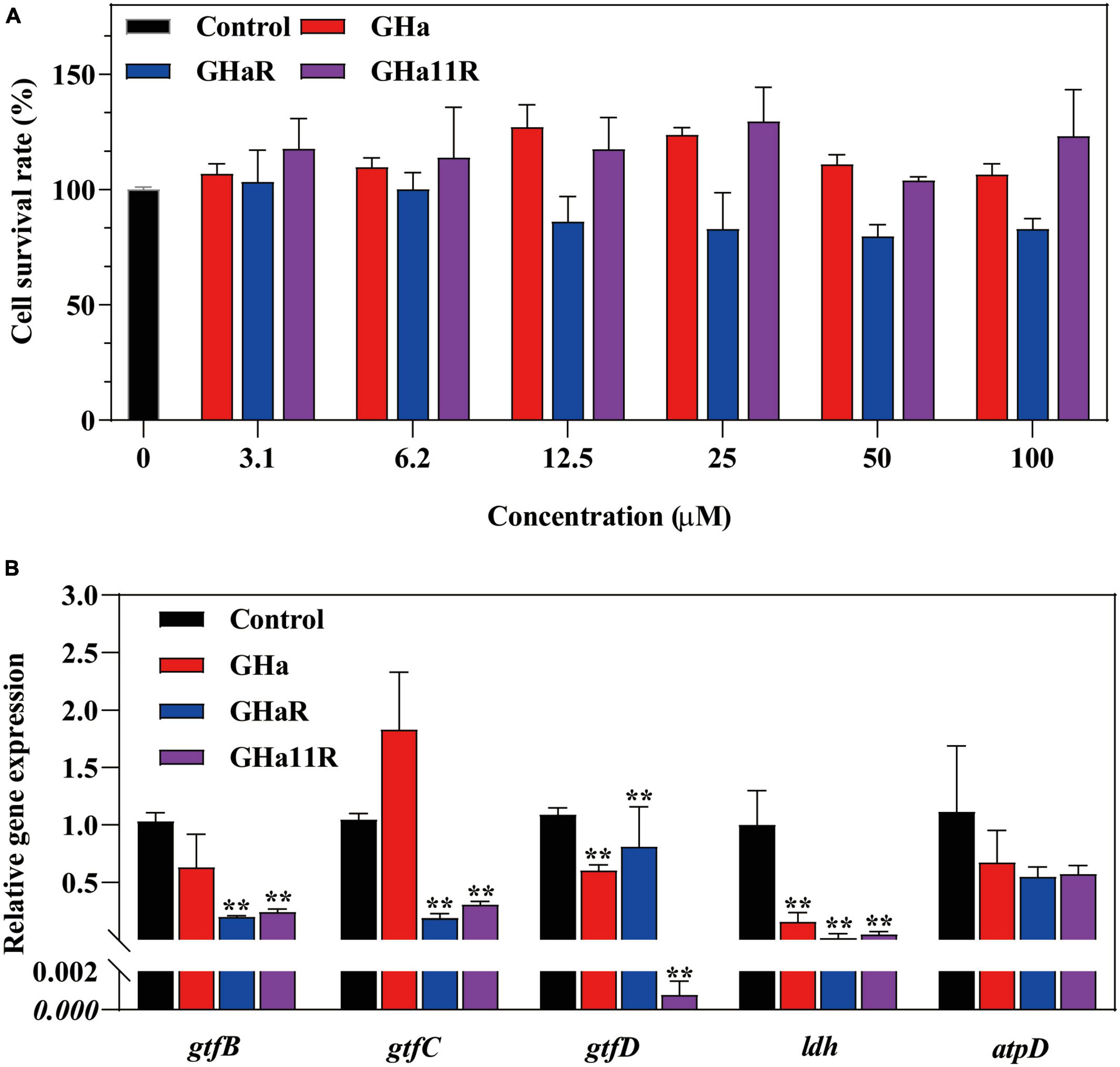
Figure 5. (A) The cytotoxicity of GHa, GHaR and GHa11R was tested by CCK-8 assay on human oral keratinocytes. *Represents comparison with the control. (B) The effect of GHa, GHaR, and GHa11R on virulence gene expression in S. mutans biofilms was tested by qRT-PCR. Gene expression in the peptide-treated S. mutans in comparison with the untreated control was quantified. **P < 0.01.
The peptides showed less cytotoxicity
After HOK cells were cocultured with GHa and its derived peptides, cell viability was detected. The results showed that GHa and GHa11R had no toxic effect on HOK cells at a concentration of 100 μM (Figure 5B). GHaR showed a weak inhibitory effect on cell viability at concentrations greater than 12.5 μM. However, the cytotoxicity was not dose dependent, and at least 80% of HOK cells treated with 100 μM GHaR were still alive.
The peptides showed anti-caries activity in rats
The derived peptides exhibited better antibacterial efficacy than that of GHa, so we investigated whether GHaR and GHa11R reduce the incidence and severity of tooth decay in a rat caries model (Figure 6A). As shown in Figure 6B, all molars of the rats inoculated with S. mutans showed progressive development of dental caries. As indicated by the arrows, the lesions on the sulci surface were significantly more extensive in the control group. The total Keyes scores in mandibular first molar and second molar of the rats were evaluated. The severity of dental caries was decreased by treated with 25 μM GHaR and GHa11R (Figure 6C). GHaR showed effective anti-caries activity as good as CHX.
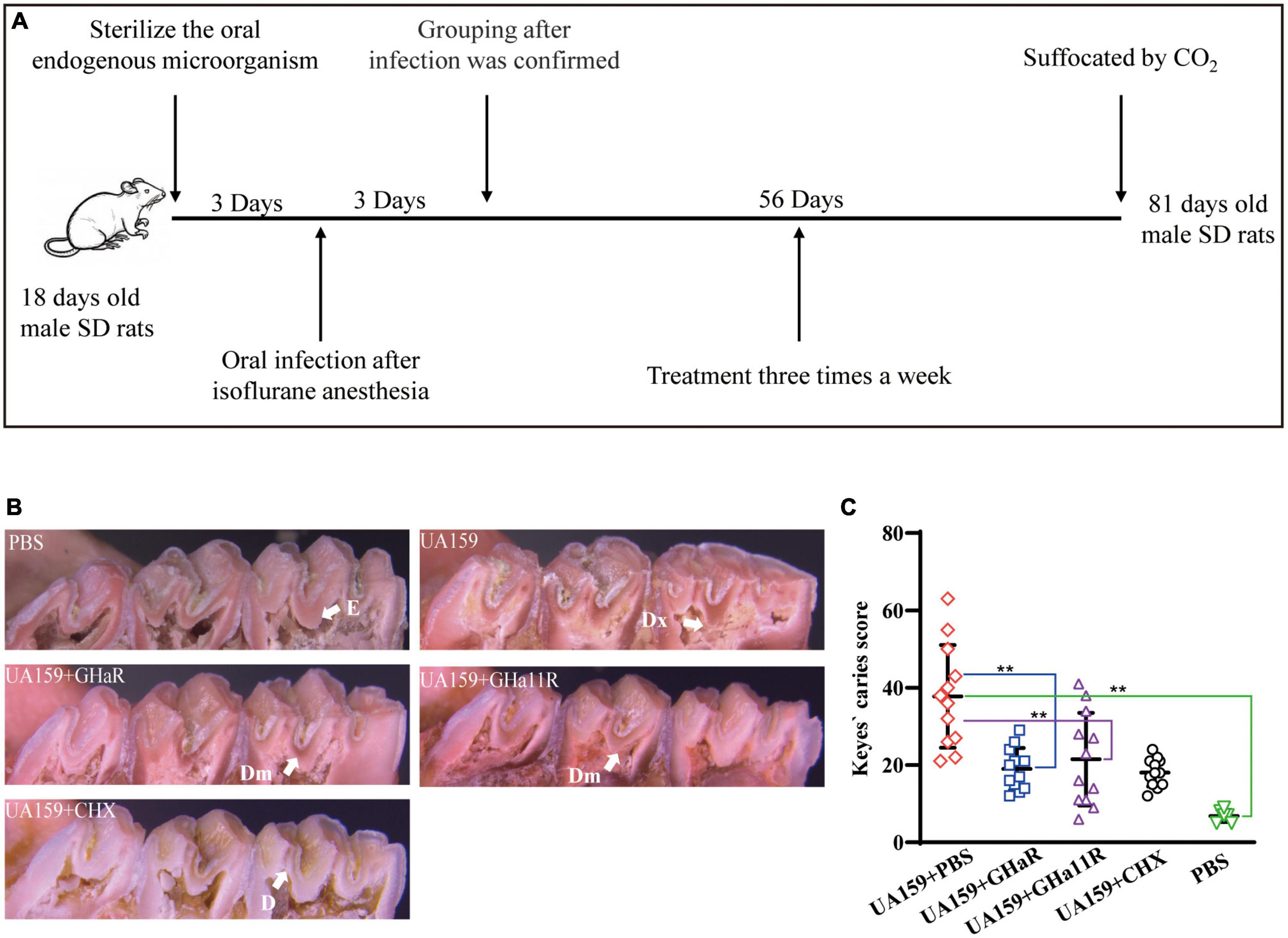
Figure 6. Anti-caries efficacy of the peptides. (A) Schematic diagram of the experimental scheme for establishment of the rat caries model. (B) Pit and fissure caries lesions on the mandibular molars were observed by stereoscopic microscopy. The arrows indicate the lesions on the sulci surface. E indicates that the caries involved only the enamel. D indicates that the caries did not exceed 1/4 of the dentin thickness. Dm indicates that the range of caries was 1/4–3/4 of the dentin thickness. Dx indicates that the caries was more than 3/4 of the dentin thickness. (C) Total Keyes scores of the caries on the first and second mandibular molars were evaluated. *Represents comparison with the control. *P < 0.05 and **P < 0.01.
Discussion
The oral microbiome consisting of bacteria, fungi, and viruses, is a complicated microbial ecosystem in living organisms (Jiang et al., 2021). The balance of the microbiome is crucial for maintaining oral health; if lost, predominant pathogens may cause oral diseases, such as dental caries, periodontitis and oral mucositis (Lamont et al., 2018). AMPs, the important molecules in the innate immune system, participate in defensing oral microbial infection and controlling resident microbial populations with other innate defense molecules in the oral cavity (Devine, 2003). The cell walls of bacteria, both Gram-positive and Gram-negative, are rich in teichoic acid and lipopolysaccharide, respectively, resulting in negatively charged surfaces, whereas most AMPs have net positive charges due to the basic amino acid residues in their amino acid sequences (Savini et al., 2020). When bacteria are exposed to AMPs, the positive charges of AMPs interact with the negative charges on the bacterial cell surfaces through electrostatic forces, which results in AMPs penetrating the bacterial cells or damaging the cell membranes to exert bactericidal efficacy (Ho et al., 2013).
Histatins are the main AMPs in saliva and are rich in histidine (Griffith et al., 2022), aggregating and integrating into the lipid bilayer of cell membranes and ultimately causing microbial cell death (Lynge Pedersen and Belstrom, 2019). Inspired by the role of histatins in oral defense, we applied the same histidine-rich cationic AMP temporin-GHa to S. mutans, and it showed antibacterial activity. It was calculated that the average frequency of occurrence of the positively charged amino acid residues H, R, and K are 2.14%, 5.97%, and 9.72% in 3,569 AMPs in APD3 (Wang et al., 2016), indicating that K and R are the most abundant positively charged amino acids in AMPs. Comparatively, H (pKa 6.5) carries no charge at physiological pH and only a positive charge at low pH (Kacprzyk et al., 2007). The amino acid sequence was changed by substituting R for H, at position 11 to produce the derived peptide GHa11R, which improved the interaction of GHa with the anionic membranes of S. mutans. The antibacterial activity of GHa11R (MIC of 3.1 μM) was 4 times higher than that of GHa, and the efficacy of inhibiting biofilm formation was also significantly improved, while the cytotoxicity was similar to that of GHa, without showing a toxic effect on HOK cells at a concentration of 100 μM. To further explore the contribution of the positive charges on the antimicrobial activity and cytotoxicity of GHa, two H amino acid residues on GHa were substituted by R, leading to GHaR, whose antibacterial performance was also enhanced with an MIC of 1.6 μM, and the bactericidal efficacy on S. mutans was faster than that of GHa11R. Although the cytotoxicity of GHaR to HOK cells was increased, at least 80% of HOK cells survived at a concentration of 100 μM. We confirmed that the increase of the net positive charges in peptides improves the activity of AMPs and correspondingly enhances their toxicity to cells (Ramezanzadeh et al., 2021).
The mechanism of action of the peptides on S. mutans was determined. Both DAPI and PI can bind to DNA, while DAPI passes through intact cell membranes and PI penetrates damaged cell membranes. S. mutans treated with GHa and its derived peptides showed bright red fluorescence after double staining with PI and DAPI, indicating that the membrane permeability was increased and the membrane integrity was damaged. The results were further confirmed by flow cytometry assay. The clear and smooth cell surface edge of the peptide-treated bacteria was lost; the large-scale damage of the membranes and the rupture of the membrane surfaces were clearly observed through SEM. Therefore, GHa and its derived peptides exerted their killing effect on S. mutans by increasing the cell membrane permeability, leading to the destruction of their integrity and leakage of intracellular components.
The cariogenic potential of S. mutans is regulated mainly by its virulence factor related genes (Shanmugam et al., 2020). Through our research, S. mutans carotenoid virulence factors were blocked by GHa and its derived peptides, which reduced the bacteria’s capacity for acidogenicity and aciduricity, decreased the production of EPS, and prevented the growth of biofilms. One of the main pathogenic features of S. mutans is lactic acid production, in which LDH encoded by the ldh gene, plays a key role (Allen et al., 2021). GHa, GHaR, and GHa11R significantly inhibited the expression of the ldh gene, thereby reducing lactic acid production and alleviating the pH drop in S. mutans. Acid resistance and production of S. mutans are highly correlated, which determines whether S. mutans can survive in the acidic environment created by itself. F1-F0 ATPase regulates and maintains the acid-base characteristics by participating in proton transport, which reflects the acid resistance level of S. mutans (Sekiya et al., 2019). The expression of the atpD gene encoding the α subunit of the F1-F0 ATPase was downregulated by the peptides, resulting in a reduction in the aciduricity of S. mutans. In general, GHa and its derived peptides showed the ability to reduce cariogenic virulence of S. mutans by inhibiting the expression of acidogenicity genes and aciduricity genes of S. mutans.
During biofilm formation, S. mutans initially attaches to tooth surfaces, followed by the production of EPS, which can be utilized as a matrix to encourage the aggregation of additional pathogen, ultimately leading to the creation of multispecies biofilms (Zhang et al., 2021). We found that the adhesion ability of S. mutans exposed to the peptides was weakened, which reduced the initial bacterial attachment and blocked or delayed the biofilm formation at the initial stage. S. mutans synthesizes EPS by Gtfs converting sucrose into sticky gelatinous extracellular glucan, providing a perfect matrix for other oral microorganisms to attach (Ma et al., 2015). There are three Gtfs in S. mutans, namely, GtfB, GtfC, and GtfD (Bowen and Koo, 2011). Water-insoluble glucans are produced by GtfB, and soluble glucans synthesized by GtfD mainly; while GtfC catalyzes both soluble and insoluble glucans (Ma et al., 2021). Therefore, downregulating the expression of Gtfs is a significant way to inhibit the initial biofilm formation. The expression of the gtfB, gtfC and gtfD genes in S. mutans was downregulated significantly by GHa, GHaR, and GHa11R, especially that of gtfB and gtfC, which decreased by nearly 80%. GHa and its derived peptides reduced EPS production by 20–40% at sub-MICs. Downregulation of Gtfs expression by the peptides reduced the production of EPS and impaired subsequent adhesion and biofilm formation. We further observed EPS in the biofilms using CLSM and found that in the blank control, S. mutans formed dense and thick biofilms, and many bacteria clustered together in it; EPS expression was highly coincident with the biofilms by labeling with Alexa Fluor 647 glucan in situ. In comparison with GHa, the derived peptides GHaR and GHa11R showed better inhibitory efficacy of EPS synthesis, especially GHaR, which significantly reduced the accumulation of S. mutans biofilms and decreased EPS production; although GHa11R showed mild inhibitory activity on S. mutans aggregation, the production of EPS was reduced significantly. In conclusion, GHaR and GHa11R exhibited different performances in inhibiting biofilm formation. GHaR showed a stronger effect in reducing the initial adhesion and hydrophobicity of S. mutans and subsequently inhibited the accumulation of biofilms. GHa11R reduced the expression of EPS-related genes, showing a stronger effect in reducing the EPS synthesis.
The safety of AMPs has been a concern in their application. GHa and GHa11R were not toxic to HOK cells at a concentration of 100 μM; although GHaR showed slight cytotoxicity, the IC50 was more than 100 μM, indicating that GHa and its derived peptides have excellent biocompatibility. We tested the effect of the peptides on other oral pathogenic bacteria and found that they showed strong antibacterial activity against S. sanguinis, which plays an important role in the formation of upper and subgingival plaques, and P. gingivalis, the main pathogenic bacteria of periodontal disease. In addition, we tested the inhibitory ability of GHa and its derived peptides against several common probiotic bacteria, such as Bifidobacterium and Lactobacillus, and the results demonstrated that the peptides showed much higher inhibitory concentrations for probiotic bacteria than oral pathogenic bacteria. These results indicated that the peptides at the therapeutic concentration selectively exerted bactericidal efficacy on oral pathogenic bacteria, which has positive significance for the application of GHa and its derivatives to oral diseases. We evaluated the in vivo effects of GHaR and GHa11R in a rat caries model. The results of Keyes scores showed that compared with the model group, caries development significantly slowed in the GHaR and GHa11R groups, and the severity of caries was controlled. The results demonstrated that GHaR and GHa11R had the potential to relieve caries related to S. mutans in the oral cavity of rats.
Here, we designed temporin-GHa-derived peptides GHaR and GHa11R, which showed selectively strong antibacterial activity against oral etiologic bacteria, including S. mutans, S. sanguinis, and P. gingivalis. The derived peptides exerted bactericidal efficacy on S. mutans plankton by interrupting the integrity and permeability of cell membranes, resulting in intracellular component leakage. They also showed antibiofilm activity against S. mutans by reducing surface hydrophobicity, disturbing the initial adhesion, and inhibiting virulence factor production, including lactic acid and EPS. GHaR and GHa11R delay the development of dental caries associated with S. mutans in the oral cavity of rats, showing promising application in the treatment of S. mutans infections.
Data availability statement
The original contributions presented in this study are included in the article/supplementary material, further inquiries can be directed to the corresponding author.
Ethics statement
The animal study was approved by the Animal Ethics Committee of Hainan University. The study was conducted in accordance with the local legislation and institutional requirements.
Author contributions
SJ: Writing–original draft, Writing–review and editing, Conceptualization. YZ: Methodology, Writing–review and editing. TZ: Methodology, Writing–review and editing. XJ: Methodology, Writing–review and editing. RZ: Methodology, Writing–review and editing. SW: Writing–review and editing. RW: Writing–review and editing. YS: Writing–review and editing. LL: Writing–review and editing. JL: Writing–review and editing. WH: Writing–review and editing. DZ: Writing–review and editing. MW: Writing–review and editing. YZ: Writing–original draft, Writing–review and editing.
Funding
The author(s) declare financial support was received for the research, authorship, and/or publication of this article. This work was supported by the Hainan Science and Technology Project under Grant ZDYF2021SHFZ042, the National Natural Science Foundation of China under Grants 32060130 and 82104129, the Natural Science Foundation of Hainan Province under Grant 820MS140, and the Foundation of Collaborative Innovation Center of One Health of Hainan University under Grant XTCX2022JKB05.
Conflict of interest
The authors declare that the research was conducted in the absence of any commercial or financial relationships that could be construed as a potential conflict of interest.
Publisher’s note
All claims expressed in this article are solely those of the authors and do not necessarily represent those of their affiliated organizations, or those of the publisher, the editors and the reviewers. Any product that may be evaluated in this article, or claim that may be made by its manufacturer, is not guaranteed or endorsed by the publisher.
References
Abbassi, F., Raja, Z., Oury, B., Gazanion, E., Piesse, C., Sereno, D., et al. (2013). Antibacterial and leishmanicidal activities of temporin-SHd, a 17-residue long membrane-damaging peptide. Biochimie 95, 388–399. doi: 10.1016/j.biochi.2012.10.015
Abdel-Aziz, M. M., Emam, T. M., and Raafat, M. M. (2020). Hindering of cariogenic Streptococcus mutans biofilm by fatty acid array derived from an endophytic Arthrographis kalrae strain. Biomolecules 10:811. doi: 10.3390/biom10050811
Allen, L. L., Heng, N. C. K., and Tompkins, G. R. (2021). Streptococcus salivarius isolates of varying acid tolerance exhibit F1F0-ATPase conservation. Caries Res. 55, 288–291. doi: 10.1159/000516175
Aqawi, M., Sionov, R. V., Gallily, R., Friedman, M., and Steinberg, D. (2021). Anti-biofilm activity of cannabigerol against Streptococcus mutans. Microorganisms 9:10. doi: 10.3390/microorganisms9102031
Bencini, D. A., Shanley, M. S., Wild, J. R., and O’Donovan, G. A. (1983). New assay for enzymatic phosphate release: Application to aspartate transcarbamylase and other enzymes. Anal. Biochem. 132, 259–264. doi: 10.1016/0003-2697(83)90005-2
Bowen, W. H., and Koo, H. (2011). Biology of Streptococcus mutans-derived glucosyltransferases: Role in extracellular matrix formation of cariogenic biofilms. Caries Res. 45, 69–86. doi: 10.1159/000324598
Cao, Y., Yin, H., Wang, W., Pei, P., Wang, Y., Wang, X., et al. (2020). Killing Streptococcus mutans in mature biofilm with a combination of antimicrobial and antibiofilm peptides. Amino Acids 52, 1–14. doi: 10.1007/s00726-019-02804-4
Chen, H., Tang, Y., Weir, M. D., Gao, J., Imazato, S., Oates, T. W., et al. (2020). Effects of S. mutans gene-modification and antibacterial monomer dimethylaminohexadecyl methacrylate on biofilm growth and acid production. Dent. Mater. 36, 296–309. doi: 10.1016/j.dental.2019.12.001
de Breij, A., Riool, M., Cordfunke, R. A., Malanovic, N., de Boer, L., Koning, R. I., et al. (2018). The antimicrobial peptide SAAP-148 combats drug-resistant bacteria and biofilms. Sci. Transl. Med. 10:eaan4044. doi: 10.1126/scitranslmed.aan4044
Deng, Y., Yang, Y., Zhang, B., Chen, H., Lu, Y., Ren, S., et al. (2021). The vicK gene of Streptococcus mutans mediates its cariogenicity via exopolysaccharides metabolism. Int. J. Oral Sci. 13:45. doi: 10.1038/s41368-021-00149-x
Devine, D. A. (2003). Antimicrobial peptides in defence of the oral and respiratory tracts. Mol. Immunol. 40, 431–443. doi: 10.1016/s0161-5890(03)00162-7
Diamond, G., Beckloff, N., and Ryan, L. K. (2008). Host defense peptides in the oral cavity and the lung: Similarities and differences. J. Dent. Res. 87, 915–927. doi: 10.1177/154405910808701011
Dong, Z., Luo, W., Zhong, H., Wang, M., Song, Y., Deng, S., et al. (2017). Molecular cloning and characterization of antimicrobial peptides from skin of Hylarana guentheri. Acta Biochim. Biophys. Sin. 49, 450–457. doi: 10.1093/abbs/gmx023
Griffith, A., Mateen, A., Markowitz, K., Singer, S. R., Cugini, C., Shimizu, E., et al. (2022). Alternative antibiotics in dentistry: Antimicrobial peptides. Pharmaceutics 14:1679. doi: 10.3390/pharmaceutics14081679
Guncu, G. N., Yilmaz, D., Kononen, E., and Gursoy, U. K. (2015). Salivary antimicrobial peptides in early detection of periodontitis. Front. Cell. Infect. Microbiol. 5:99. doi: 10.3389/fcimb.2015.00099
Harper, D. S., and Loesche, W. J. (1983). Effect of pH upon sucrose and glucose catabolism by the various genogroups of Streptococcus mutans. J. Dent. Res. 62, 526–531. doi: 10.1177/00220345830620050101
Ho, S., Pothoulakis, C., and Koon, H. W. (2013). Antimicrobial peptides and colitis. Curr. Pharm. Des. 19, 40–47. doi: 10.2174/13816128130108
Jiang, W., Deng, Z., Dai, X., and Zhao, W. (2021). PANoptosis: A new insight into oral infectious diseases. Front. Immunol. 12:789610. doi: 10.3389/fimmu.2021.789610
Kacprzyk, L., Rydengard, V., Morgelin, M., Davoudi, M., Pasupuleti, M., Malmsten, M., et al. (2007). Antimicrobial activity of histidine-rich peptides is dependent on acidic conditions. Biochim. Biophys. Acta 1768, 2667–2680. doi: 10.1016/j.bbamem.2007.06.020
Kim, K., An, J. S., Lim, B. S., and Ahn, S. J. (2019). Effect of bisphenol a glycol methacrylate on virulent properties of Streptococcus mutans UA159. Caries Res. 53, 84–95. doi: 10.1159/000490197
Klein, M. I., Hwang, G., Santos, P. H., Campanella, O. H., and Koo, H. (2015). Streptococcus mutans-derived extracellular matrix in cariogenic oral biofilms. Front. Cell. Infect. Microbiol. 5:10. doi: 10.3389/fcimb.2015.00010
Lamont, R. J., Koo, H., and Hajishengallis, G. (2018). The oral microbiota: Dynamic communities and host interactions. Nat. Rev. Microbiol. 16, 745–759. doi: 10.1038/s41579-018-0089-x
Lemos, J. A., Palmer, S. R., Zeng, L., Wen, Z. T., Kajfasz, J. K., Freires, I. A., et al. (2019). The biology of Streptococcus mutans. Microbiol. Spectr. 7:1. doi: 10.1128/microbiolspec.GPP3-0051-2018
Lynge Pedersen, A. M., and Belstrom, D. (2019). The role of natural salivary defences in maintaining a healthy oral microbiota. J. Dent. 80(Suppl. 1), S3–S12. doi: 10.1016/j.jdent.2018.08.010
Ma, C., Chen, F., Zhang, Y., Sun, X., Tong, P., Si, Y., et al. (2015). Comparison of oral microbial profiles between children with severe early childhood caries and caries-free children using the human oral microbe identification microarray. PLoS One 10:e0122075. doi: 10.1371/journal.pone.0122075
Ma, Q., Pan, Y., Chen, Y., Yu, S., Huang, J., Liu, Y., et al. (2022). Acetylation of lactate dehydrogenase negatively regulates the acidogenicity of Streptococcus mutans. mBio 13:e0201322. doi: 10.1128/mbio.02013-22
Ma, Q., Pan, Y., Chen, Y., Yu, S., Huang, J., Liu, Y., et al. (2021). Acetylation of glucosyltransferases regulates Streptococcus mutans biofilm formation and virulence. PLoS Pathog. 17:e1010134. doi: 10.1371/journal.ppat.1010134
Mosaddad, S. A., Tahmasebi, E., Yazdanian, A., Rezvani, M. B., Seifalian, A., Yazdanian, M., et al. (2019). Oral microbial biofilms: An update. Eur. J. Clin. Microbiol. Infect. Dis. 38, 2005–2019. doi: 10.1007/s10096-019-03641-9
Nakano, K., Inaba, H., Nomura, R., Nemoto, H., Takeda, M., Yoshioka, H., et al. (2006). Detection of cariogenic Streptococcus mutans in extirpated heart valve and atheromatous plaque specimens. J. Clin. Microbiol. 44, 3313–3317. doi: 10.1128/JCM.00377-06
Peres, M. A., Macpherson, L. M. D., Weyant, R. J., Daly, B., Venturelli, R., Mathur, M. R., et al. (2019). Oral diseases: A global public health challenge. Lancet 394, 249–260. doi: 10.1016/S0140-6736(19)31146-8
Ramezanzadeh, M., Saeedi, N., Mesbahfar, E., Farrokh, P., Salimi, F., and Rezaei, A. (2021). Design and characterization of new antimicrobial peptides derived from aurein 1.2 with enhanced antibacterial activity. Biochimie 181, 42–51. doi: 10.1016/j.biochi.2020.11.020
Saporito, P., Vang Mouritzen, M., Lobner-Olesen, A., and Jenssen, H. (2018). LL-37 fragments have antimicrobial activity against Staphylococcus epidermidis biofilms and wound healing potential in HaCaT cell line. J. Pept. Sci. 24:e3080. doi: 10.1002/psc.3080
Savini, F., Loffredo, M. R., Troiano, C., Bobone, S., Malanovic, N., Eichmann, T. O., et al. (2020). Binding of an antimicrobial peptide to bacterial cells: Interaction with different species, strains and cellular components. Biochim. Biophys. Acta Biomembr. 1862:183291. doi: 10.1016/j.bbamem.2020.183291
Sekiya, M., Izumisawa, S., Iwamoto-Kihara, A., Fan, Y., Shimoyama, Y., Sasaki, M., et al. (2019). Proton-pumping F-ATPase plays an important role in Streptococcus mutans under acidic conditions. Arch. Biochem. Biophys. 666, 46–51. doi: 10.1016/j.abb.2019.03.014
Shanmugam, K., Sarveswari, H. B., Udayashankar, A., Swamy, S. S., Pudipeddi, A., Shanmugam, T., et al. (2020). Guardian genes ensuring subsistence of oral Streptococcus mutans. Crit. Rev. Microbiol. 46, 475–491. doi: 10.1080/1040841X.2020.1796579
Svensater, G., Larsson, U. B., Greif, E. C., Cvitkovitch, D. G., and Hamilton, I. R. (1997). Acid tolerance response and survival by oral bacteria. Oral Microbiol. Immunol. 12, 266–273. doi: 10.1111/j.1399-302x.1997.tb00390.x
Tian, Y., Zhang, Y., Zhang, M., Chen, X., Lei, L., and Hu, T. (2022). Antisense vicR-loaded dendritic mesoporous silica nanoparticles regulate the biofilm organization and cariogenicity of Streptococcus mutans. Int. J. Nanomedicine 17, 1255–1272. doi: 10.2147/IJN.S334785
Utheim, T. P., Islam, R., Fostad, I. G., Eidet, J. R., Sehic, A., Olstad, O. K., et al. (2016). Storage temperature alters the expression of differentiation-related genes in cultured oral keratinocytes. PLoS One 11:e0152526. doi: 10.1371/journal.pone.0152526
Wang, G., Li, X., and Wang, Z. (2016). APD3: The antimicrobial peptide database as a tool for research and education. Nucleic Acids Res. 44, D1087–D1093. doi: 10.1093/nar/gkv1278
Wang, Y., Wang, X., Jiang, W., Wang, K., Luo, J., Li, W., et al. (2018). Antimicrobial peptide GH12 suppresses cariogenic virulence factors of Streptococcus mutans. J. Oral Microbiol. 10:1442089. doi: 10.1080/20002297.2018.1442089
Wiesner, J., and Vilcinskas, A. (2010). Antimicrobial peptides: The ancient arm of the human immune system. Virulence 1, 440–464. doi: 10.4161/viru.1.5.12983
Xie, Z., Wei, H., Meng, J., Cheng, T., Song, Y., Wang, M., et al. (2019). The analogs of Temporin-GHa exhibit a broader spectrum of antimicrobial activity and a stronger antibiofilm potential against Staphylococcus aureus. Molecules 24:4173. doi: 10.3390/molecules24224173
Xiong, K., Chen, X., Hu, H., Hou, H., Gao, P., and Zou, L. (2020). Antimicrobial effect of a peptide containing novel oral spray on Streptococcus mutans. Biomed. Res. Int. 2020:6853652. doi: 10.1155/2020/6853652
Xu, X., Zhou, X. D., and Wu, C. D. (2011). The tea catechin epigallocatechin gallate suppresses cariogenic virulence factors of Streptococcus mutans. Antimicrob. Agents Chemother. 55, 1229–1236. doi: 10.1128/AAC.01016-10
Yue, J., Yang, H., Liu, S., Song, F., Guo, J., and Huang, C. (2018). Influence of naringenin on the biofilm formation of Streptococcus mutans. J. Dent. 76, 24–31. doi: 10.1016/j.jdent.2018.04.013
Zhang, J., Chen, C., Chen, J., Zhou, S., Zhao, Y., Xu, M., et al. (2020). Dual mode of anti-biofilm action of G3 against Streptococcus mutans. ACS Appl. Mater. Interfaces 12, 27866–27875. doi: 10.1021/acsami.0c00771
Zhang, Q., Ma, Q., Wang, Y., Wu, H., and Zou, J. (2021). Molecular mechanisms of inhibiting glucosyltransferases for biofilm formation in Streptococcus mutans. Int. J. Oral Sci. 13:30. doi: 10.1038/s41368-021-00137-1
Keywords: antimicrobial peptides, temporin, Streptococcus mutans, antibiofilm, anti-caries
Citation: Jiang S, Zha Y, Zhao T, Jin X, Zhu R, Wei S, Wang R, Song Y, Li L, Lyu J, Hu W, Zhang D, Wang M and Zhang Y (2023) Antimicrobial peptide temporin derivatives inhibit biofilm formation and virulence factor expression of Streptococcus mutans. Front. Microbiol. 14:1267389. doi: 10.3389/fmicb.2023.1267389
Received: 26 July 2023; Accepted: 12 September 2023;
Published: 26 September 2023.
Edited by:
Octavio Luiz Franco, Catholic University of Brasília (UCB), BrazilReviewed by:
Carla Cugini, The State University of New Jersey, United StatesLucia Lombardi, Imperial College London, United Kingdom
Copyright © 2023 Jiang, Zha, Zhao, Jin, Zhu, Wei, Wang, Song, Li, Lyu, Hu, Zhang, Wang and Zhang. This is an open-access article distributed under the terms of the Creative Commons Attribution License (CC BY). The use, distribution or reproduction in other forums is permitted, provided the original author(s) and the copyright owner(s) are credited and that the original publication in this journal is cited, in accordance with accepted academic practice. No use, distribution or reproduction is permitted which does not comply with these terms.
*Correspondence: Yingxia Zhang, emhhbmd5aW5neGlhQGhhaW5hbnUuZWR1LmNu