- 1Key Laboratory of Environment Correlative Dietology, College of Food Science and Technology, Huazhong Agricultural University, Wuhan, China
- 2Center for Cancer Immunology, Institute of Biomedicine and Biotechnology, Shenzhen Institute of Advanced Technology, Chinese Academy of Sciences, Shenzhen, China
Salmonella including drug-resistant strains are major foodborne pathogens causing serious illness and pose a great threat to the prevention and control for food safety. Phages can naturally defect the bacterium, is considered as a new and promising biological antimicrobial agent in the post-antibiotic era. A poultry facility in Wuhan, China provided wastewater samples from which a collection of 29 phages were isolated and purified. A broad host spectrum phage ISTP3, which capable of infecting all tested Salmonella, including drug-resistant Salmonella enterica, were examined. Additionally, the effectiveness of this phage ISTP3 in reducing drug-resistant S. enterica was assessed in diverse food samples. Transmission electron microscopy (TEM) and whole genome sequencing demonstrated that ISTP3 was found to belong to family Ackermannviridae. The one-step growth experiment and assays of stability demonstrated that ISTP3 exhibited short periods of inactivity before replicating, produced a significant number of viral progeny during infection, and remained high stable under varying pH and temperature conditions. We evaluated the efficacy of phage ISTP3 against drug-resistant Salmonella on chicken breast and lettuce samples at different temperatures. When applying phage ISTP3 in food matrices, the drug resistant Salmonella count significantly reduced at 4°C and 25°C at an MOI of 100 or 1,000 within a timescale of 12 h. Overall, the results, such as broad host ranges, strictly lytic lifestyles, absence of lysogenic related genes, toxin genes, or virulence genes in the genome, demonstrate that the application of phage ISTP3 as a biocontrol agent has promising potential for preventing and controlling drug-resistant S. typhimurium in the context of food safety, processing, and production.
1 Introduction
Salmonella bacteria, which are members of the Enterobacteriaceae family, have a rod-shaped morphology with a diameter ranging from 0.2 to 1.5 μm, and they are facultative anaerobes. They can be classified as gram-negative bacilli (Ali et al., 2018). Salmonella genus can be classified into two main species, S. enterica and S. bongori, which are distinguished by variations in their 16S rRNA sequence. S. enterica encompasses a total of 2,600 distinct serovars and a many of these are responsible to cause diseases for humans and animals (Yang et al., 2019). In the world Salmonella is described as the most common foodborne pathogen, mainly infect to humans through contaminated food matrices (Jiang et al., 2021). Infected animals and their faeces act as reservoirs for the contamination of Salmonella and this contamination can then spread through products like eggs, meat, milk, and other agricultural goods that have been fertilized or grown in feces contaminated with Salmonella (Finn et al., 2013). It is estimated that globally approximately 500,000 deaths are caused by 33 million Salmonella infections (Zhou et al., 2023). While Salmonella infection is highly suspected in age groups <20 years and >70 years, the highest incidence is observed in infants, with 130 cases per 100,000 individuals (Zaki and Karande, 2011). Approximately 25 to 33% of children diagnosed with typhoid fever are below the age of 5, with a subset of 6 to 21% being younger than 2 years old (Owais et al., 2010). A group of scientist from USA reported that the chance of hospitalization due to 47–80% for infected people aged ≥65 years because of non-typhoidal Salmonella infection (Chen et al., 2012).
Freshly prepared vegetables provide an enjoyable way to incorporate essential dietary fiber, vitamins, and minerals which are vital components of a healthy diet. Hence, these globally renowned food items pose a significant risk to public health if consumed raw or subjected to insufficient heat treatment, as their fresh form may potentially contain harmful bacterial contaminants (Pem and Jeewon, 2015; Moye et al., 2018). In the past 15 years during the years of 1990 to 2005, there were 768 outbreaks associated with fresh produce, leading to 35,060 reported cases of illness (Sharma, 2013). Lettuce, a highly popular leafy vegetable worldwide, is typically consumed raw and has been frequently associated with outbreaks of foodborne diseases such as salmonellosis. The prevalence of Salmonella in lettuce was found to be 0.064 in developing countries and 0.028 in developed countries, respectively (De Oliveira Elias et al., 2019). In China, the prevalence of Salmonella in raw vegetables was found to be 3.4% (14 out of 406 samples). Among the different vegetables tested, coriander exhibited the highest contamination rate at 7.8% (90 out of the samples), followed by lettuce at 6.0% (83 out of the samples) (Yang et al., 2020). Furthermore, Salmonella is commonly present in the intestinal tract of warm-blooded animals, particularly in chickens. This facilitates the easy transfer of Salmonella to meat during processes such as slaughter, handling, processing, and distribution. Chicken meat has been identified as a significant source of Salmonella infections in humans, contributing to global outbreaks, including those involving drug-resistant strains (Mori et al., 2018; Duc et al., 2019; Wessels et al., 2021). In 2019, an extensive investigation was carried out on 3,508 samples collected from poultry breeding farms across nine provinces in China. The study identified 126 strains of Salmonella, indicating a positivity rate of 3.59% for the samples (Song et al., 2020). During the period from April to November 2011, a study conducted by Alali et al. (2012) reported a Salmonella prevalence of 31.5% (n = 698) in chicken samples collected from Russia. In Malaysia the prevalence of Salmonella was found 39.4% (n = 156) in the year of 2017 (Thung et al., 2017). Salmonella was responsible for over 50% of the reported cases of foodborne illnesses in the European Union (Ehuwa et al., 2021). The economic impact of foodborne Salmonella in the United States has been substantial. According to the USDA’s Economic Research Service (ERS), the total cost for foodborne Salmonella infections in the United States was estimated at $4.1 billion annually (Ford et al., 2023).
The estimated annual cost for Salmonella control program is increasing in some countries (Niemi et al., 2019). Various industrialized systems have been developed for addressing bacterial contamination, including Salmonella, in food. However, these methods still have specific disadvantages, such as potential harm or toxicity to food, inconsistency in quality, and high practical application costs (Muncke et al., 2020; Lebelo et al., 2021; Wessels et al., 2021). One instance is the use of non-thermal methods like washing food in chlorinated water, which fails to completely eliminate enteric bacterial pathogens. This is primarily due to challenges such as biofilm formation, inaccessible attachment sites, the strength of attachment, or internalization of the pathogen (Gil Prieto et al., 2009). However, the use of chemicals has also led to the emergence of drug-resistant bacteria, posing a significant public health risk (Racine and Gualtieri, 2019). Drug-resistant poses a major threat to global human health, as it makes the treatment of pathogen infections challenging due to their high prevalence rate, associated mortality, and treatment costs. The potential transmission link to humans from antibiotic-resistant Salmonella at the farm level can occur through direct contact or consumption of contaminated meat (Dolejska et al., 2012). Globally, antimicrobial-resistant Salmonella strains have become increasingly prevalent over the past decade (Pelyuntha et al., 2021). Therefore, it is imperative to search for control agents that enable the production of food with minimal or no harm to human consumption, without compromising the organoleptic and nutritional quality of the food.
Bacteriophages, also known as phages, are naturally existing viruses that vary in size from 20 to 200 nm and act as predators of bacteria (D'accolti and Soffritti, 2021). Phages, which are present everywhere in the environment, pose no harm to humans and animals. Phages have gained recognition as promising antimicrobial agents that can assist in controlling specific bacterial pathogens in food processing or production, potentially saving millions of lives and revolutionizing the field of medicine since their discovery (Hyla et al., 2022). On the contrary, phages are considered one of the most crucial, cost-effective, and straightforward approaches to combat microbes in both planktonic and biofilm environments (Totten and Patel, 2022). Consequently, lytic phages are increasingly being employed as alternative tools to eliminate pathogenic bacteria in food products and combat drug-resistant bacteria. Due to their ability to offer a safe, natural, and effective solution against pathogenic bacterial contamination in fresh produce items and chicken meat, various national and international regulatory authorities are progressively granting approval for the utilization of phages (García et al., 2019). The application of targeted phage treatment in the food industry can prevent product degradation and the transmission of bacterial diseases. As a result, this will ultimately enhance safety in the production, processing, and handling of animal and plant-based food, creating safe environments. From a food safety standpoint, phages have been suggested as a novel, efficient, stable, and safe antibacterial method, considered one of the least harmful approaches (Lee et al., 2022). In this study, we isolated, screened, and fully characterized phage ISTP3, which demonstrated sustained activity in reducing drug-resistant S. enterica specifically in food applications, particularly for lettuce and chicken meat.
2 Materials and methods
2.1 Bacteria strains and growth conditions
Drug-resistant S. typhimurium SA32 was used as a host for isolation of phage. For the host range activity, we employed a collection of 43 distinct bacterial strains, including 31 strains of Salmonella and 12 strains of bacteria that were not of the Salmonella type (Table 1). All bacterial strains were properly preserved at −80°C with a 20% (v/v) glycerol solution. Prior to the experiment, the strains were cultured on Luria-Bertani agar (LA) agar plates at 37°C using the streak plate method. A single colony was selected and inoculated into Luria-Bertani broth (LB) broth medium at 37°C overnight to validate the purification of the bacteria.
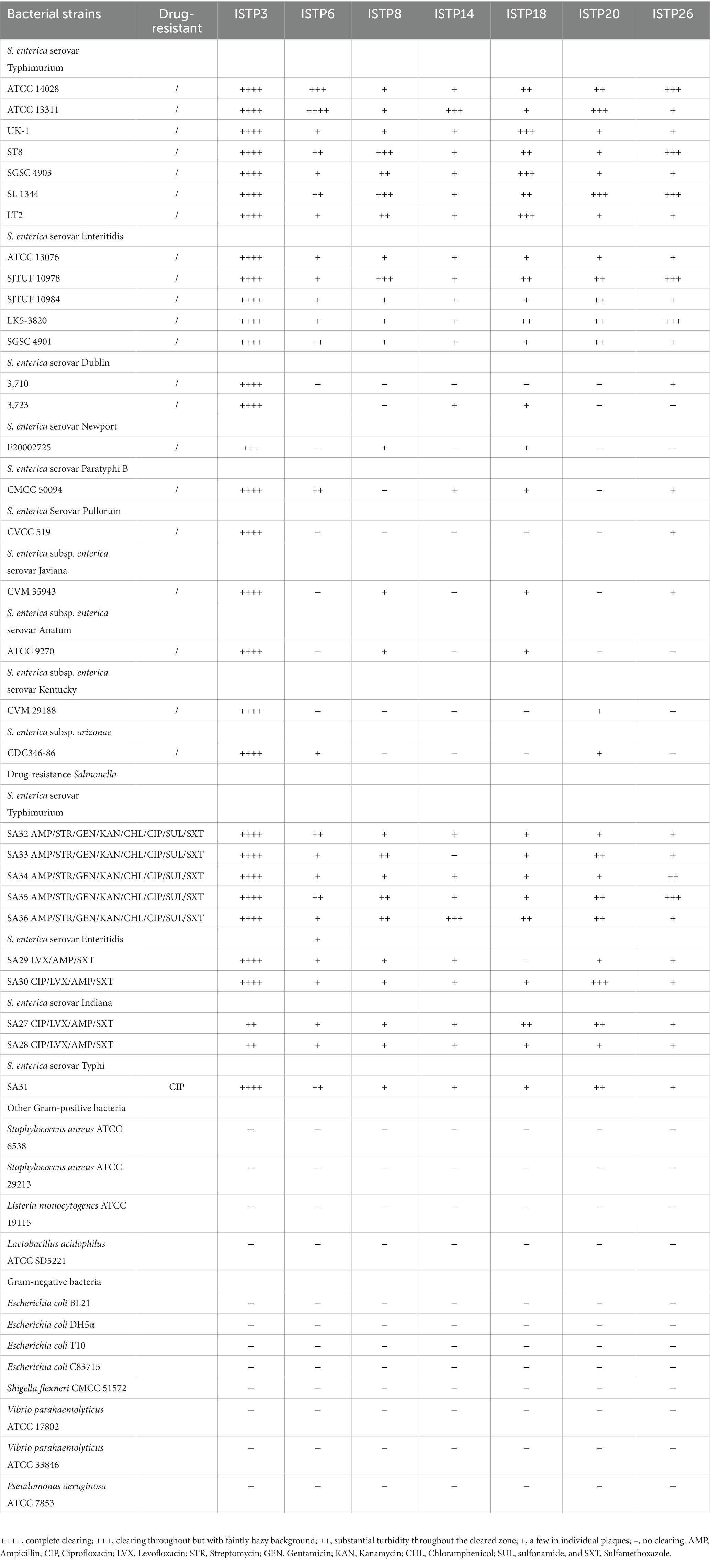
Table 1. Host range of phage ISTP3, ISTP6, ISTP8, ISTP14, ISTP18, ISTP20, and ISTP26 against different Salmonella serovars and other bacterial strains determined by spot testing.
2.2 Isolation, propagation, and purification of phage
According to established protocols (Islam et al., 2021), a collection of 29 putative distinct phages were collected from wastewater samples taken from a chicken farm located in Wuhan, China. The enrichment process for phage isolation was altered based on a previous study conducted by Guo et al. (2021). A mixture was created by combining 5 mL of a sample filtered through a 0.22 μm filter with 10 mL of LB and 2.5 mL of the host bacterial strain. The mixture was left to incubate overnight at a temperature of 37°C. After the incubation period, the culture was collected and subjected to centrifugation at 11,200 × g for 10 min at 4°C. The resulting supernatants were then filtered using a 0.22 μm pore size filter. The phage lysate, which is the liquid obtained after removing the sediment, was collected and utilized in spot tests to identify the presence of phages. To purify the phage plaques, a single plaque was chosen and cultured in 1 mL of LB medium along with the host bacteria. The propagation process was repeated at least four times to ensure the purification of the phage as a single entity. The purified phages were stored at a temperature of 4°C until they were needed for various experiments.
2.3 Spot test
The phages capability for lysis diverse strains was identified using spot test. In brief, 100 μL of target exponential growth phase cultured bacteria with 4 mL 0.7% agar was poured on LA plates then mixed and wait for 15 min at room temperature to solidify. Subsequently, 5 μL of phage lysates were spotted onto the lawns formed by different bacterial strains. The Petri dishes were then incubated at 37°C for 24 h. Following incubation, the formation of clear zones on the bacterial lawn was determined as an indication of phage sensitivity.
2.4 Efficiency of plating
To assess the host range, we performed an Efficiency of Plating (EOP) assay with modifications based on previous studies (Islam et al., 2019b). The phage was serially diluted and triplicates were tested on the designated bacterial host. The bacterial strains were cultivated to the exponential phase at 37°C. After incubation, 100 μL of the bacterial culture was applied in double-layer plate assays along with 100 μL of the diluted phage lysate. Dilution factors ranging from 106 to 109 were applied in this study. Subsequently, the plates were incubated at 37°C for 12 h, and the number of plaque-forming units (PFUs) on the plate was enumerated. The relative EOP was then calculated by dividing the average PFUs on the test bacteria by the average PFUs on the host bacteria. The EOP assessment was categorized as follows: high efficiency (EOP 0.5 to 1.0), moderate efficiency (EOP 0.1 to 0.49), low efficiency (0.001 to 0.9), and inefficient (0 to 0.009).
2.5 Transmission electron microscopy
Freshly prepared phage suspension (1010 PFU/mL) was re-suspended in SM buffer after ultracentrifugation at 50,000 × g for 2 h at 4°C. Negative staining method (2% phosphotungstic acid) was used for preparation of phages to observe their morphology under transmission electron microscopy. The TEM images were analyzed using the software Digital Micrograph Demo 3.9.1.1
2.6 Absorption rate
Assays for phage adsorption were conducted following the methodology previously described by Fister et al. (2016), with slight modifications. In brief, a fresh phage solution and a host bacterial solution were combined in a sterile tube at an optimal MOI value (MOI 0.01), followed by incubation at 37°C for 20 min on a shaker. The mixture was subsequently centrifuged at 12,000 × g for 5 min, and the resulting supernatants were immediately filtered using 0.22 μm filters (Millipore, Ireland). The filtered supernatants, which contained unabsorbed phages, were then quantified using a double-layered agar plate method. The adsorption percentage was calculated by comparing the phage titer in the supernatant with control samples with no bacteria.
2.7 One-step growth curves of phage ISTP3
The methods used for the one-step growth tests were based on Park et al.’s study (Park et al., 2012) with minor modifications. Briefly, a phage suspension containing 4 log PFU/mL of 500 μL was combined with a host bacterial culture containing 6 log CFU/mL of 500 μL. The mixture was then incubated at 37°C for 10 min to allow phage adsorption to the host, followed by centrifugation at 8000 × g for 5 min. The supernatant was discarded to remove free phage, and the phage attached to the bacterial pellet was gently washed three times with LB. The pellet was subsequently resuspended in 5 mL of LB and cultured at 37°C for 180 min in a shaker at 150 rpm. Two sets of samples were prepared, each with a different duration. In the second set, 1% chloroform was added to the samples before titration to effectively lyse the host bacteria and precisely determine the initial infectious phage count for detecting the eclipse time. The one-step curve was determined by evaluating the phage titers using the double-layered agar plate method for each set of samples.
2.8 Thermal and pH stability of phage
To assess the thermal and pH stability of the phage, a method similar to previous studies (Zhang et al., 2023) was employed with some minor modifications. In order to evaluate the thermal stability, the LB medium was heated beforehand, and various temperatures ranging from 30°C to 80°C were maintained for 1 h. Subsequently, the phage lysate (at a concentration of 108 PFU/mL) was mixed into the preheated medium and incubated for 30 and 60 min, respectively. To determine the pH stability of phage ISTP3, the phage lysate (108 PFU/mL) was mixed with LB medium adjusted to different pH levels ranging from 2 to 13, followed by incubation at 37°C for 1 h. At the end of the incubation period, the number of PFU/mL recovered from the test samples was recorded.
2.9 Phage sequencing, genome annotation, and comparison
The DNA of phage ISTP3 was isolated and purified based on a previous study conducted by Islam et al. (2020a). Sequencing of ISTP3 was performed using the HiSeq platform from Illumina. The resulting reads were assembled using MicrobeTrakr plus (v0.9.1) software. To predict the open reading frames (ORFs) of ISTP3, three website tools, namely RAST, GeneMarkS, and ORF finder, were applied. Gene annotation was carried out using BLASTP, Pfam, and CD searching against the NCBI nonredundant database and the conserved domain database (CDD). To identify antimicrobial resistant genes (ARGs) within the phage genome, the ResFinder 3.1 database from CGE2 was used. Additionally, the VFDB database3 was used to identify any potential virulence factors present in the phage genome. The sequences of various phages were compared for whole genome similarities using BLASTN analysis at NCBI. A comparative circular genome map of the phage genome was created using the BRIG comparison tool. The terminase large subunit and the main capsid protein sequence of ISTP3 were compared with the corresponding sequences of related phages using MEGA7. The resulting tree was then enhanced using ITOL.
2.10 Biological control of drug resistant S. enterica in foods
Iceberg lettuce (Lactuca sativa) and raw chicken breast were purchased from a grocery store located at Huzuan Agriculture University in Wuhan, China. The plastic packaging of the lettuce heads was thoroughly rinsed with 70% ethanol before being removed. Outer leaves of the lettuce were discarded, and using a sterile stainless-steel scalpel, inner leaves were cut. Subsequently, 2 cm × 2 cm pieces of lettuce were cut on a sterile wooden tray. The outer surface of chicken breast samples was pull out by the sterilized knife, then cut meat into 2 cm3 cubes. Lettuce and chicken samples were confirming any bacteria absence before going for biocontrol test procedure inoculating on LA agar (Guo et al., 2021). Then all the samples were added by 10 μL of drug-resistant S. typhimurium SA32 the final concentration 4 log10 CFU/sample and keep at room temperature in the laminar air flow hood for 10 min for bacteria to attach on the surface of food. Then, 10 μL of the phage aliquots were added using a micropipette at a final concentration of 6 log (MOI 100) and 7 log (MOI 1000) PFU/sample. The control groups consisted of only bacteria and PBS administration. All samples were incubated for 12 h at either 4°C or 25°C. After the incubation period, bacterial samples were processed for enumeration at 1, 3, 6, and 12 h. All experiments were conducted in triplicate.
2.11 Statistical analysis
The food model assays were conducted in triplicates, with two samples per treatment analyzed in each replicate. The mean values of the three replicates were reported, along with error bars indicating the standard deviation of the mean. Both bacterial and phage data were logarithmically transformed. To assess the effectiveness of phage treatment in reducing the number of viable drug-resistant Salmonella in the tested foods, the data from the phage treated samples were compared to the control samples treated with PBS. Statistical analysis was performed using Prism 9 for Windows (GraphPad software, San Diego, CA, United States), utilizing a two-way analysis of variance (ANOVA) followed by Bonferroni’s test with a 95% confidence interval. Statistical significance was determined at a significance level of p < 0.05.
3 Results
3.1 Phage isolation and characterization
A total of 29 phages were obtained from wastewater samples taken from a Chinese chicken farm, using drug-resistant S. typhimurium SA32 as the host bacterium. All the phages were subsequently tested to determine their efficacy in lysing the different Salmonella strains including drug-resistant strains. The results of host range showed that, among those phages, 7 out of 29 is about 24% of the isolates were able to lyse 100% of the Salmonella strains tested in this study (Table 2), while the rest were capable to lyse <80% tested strains. Among of them, one phage which showed high lytic activity against different Salmonella were selected and named as ISTP3 (Table 1). Spot tests results showed that phage ISTP3 had the highest host range compared to others 6 phages, this specific phage has demonstrated the ability to lyse every tested Salmonella strain in the study, encompassing a comprehensive representation. Nevertheless, none of the isolated phages exhibited the ability to cause the lysis of E. coli or any other tested gram-positive and gram-negative bacteria. Transmission electron microscope revealed that ISTP3 possessed an icosahedral head with 77 ± 3 nm in diameter, and accompanied by a contractile tail with a 112 ± 4 nm long (Figure 1B). The morphology suggested that ISTP3 belonged to family Myoviridae. Host range of the phage was studied using diverse Salmonella strains collected by our research group.
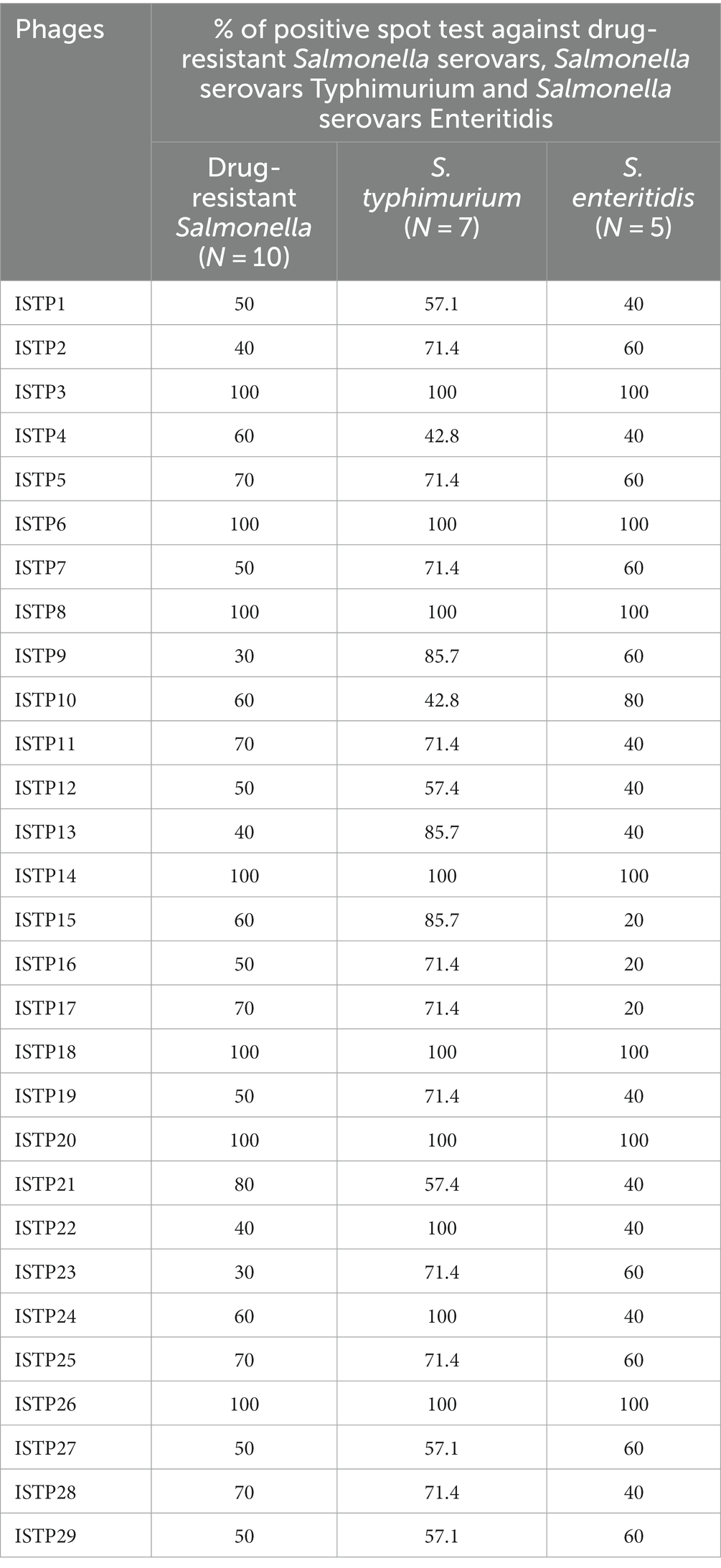
Table 2. Sensitivity of different Salmonella serovars and other bacterial strains to lyse by selected phages determined by spot testing.
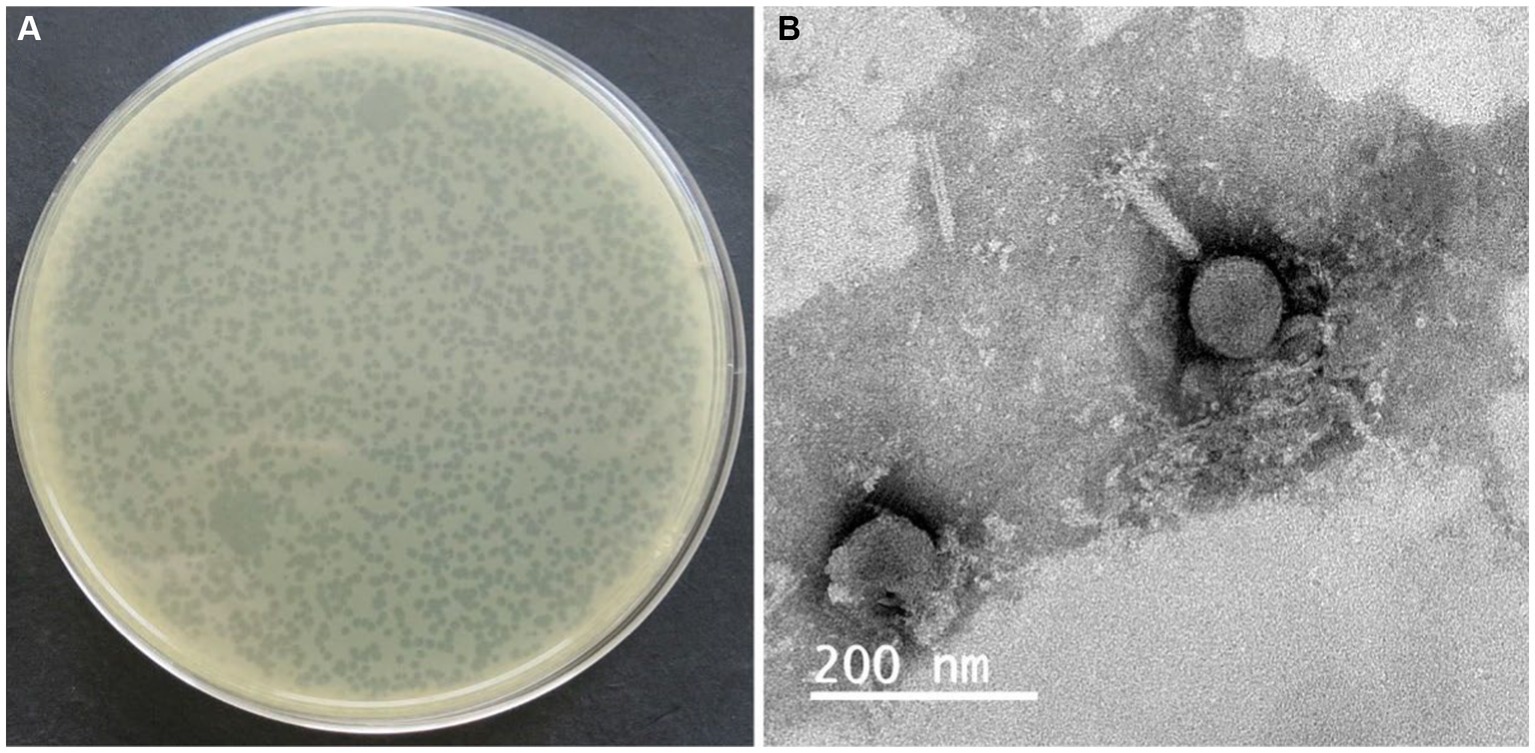
Figure 1. Plaque morphology (A) and electron micrographs of phage ISTP3 (B). The bars represent 200 nm.
3.2 Characteristics of phage ISTP3
The characteristics of phage ISTP3 are shown in Figure 2. The results of adsorption rate of this phage was show in Figure 2A. About 52% of the phage ISTP3 was adsorbed with host bacteria after 5 min, and accelerated to 97% after 15 min. The one-step growth curve analysis of phage ISTP3 revealed a latent period of around 30 min, an eclipse period of approximately 20 min, and an average burst size of 137 ± 7 PFU (plaque-forming units) per cell (Figure 2B). Using the phage ISTP3 as a biocontrol agent in different food processing environment, the stability and feasibility required confirming during diverse stress conditions including pH and temperature. Stability of pH challenging of the ISTP3 exhibited that it is extremely stable between pH 4 to 12. Nonetheless, the phage titers exhibited a slight decline when the pH was less than 4. However, a reduction was observed at pH 13, with levels dropping to less than 1 log10 PFU/mL (Figure 2C). Interestingly, there was no significant reduce of titer of phage ISTP3 when this phage was kept at 30°C to 60°C for 1 h. The optimum temperature activity of phage was designate between 30°C and 50°C. The phage titers were lost by about 40 and 70% during incubated at 70°C and 80°C, respectively (Figure 2D). The capability of phage ISTP3 for survival in the extensive range of pH and temperatures conditions supports its ability for using in different processing environments and applications as a biocontrol agent for Salmonella.
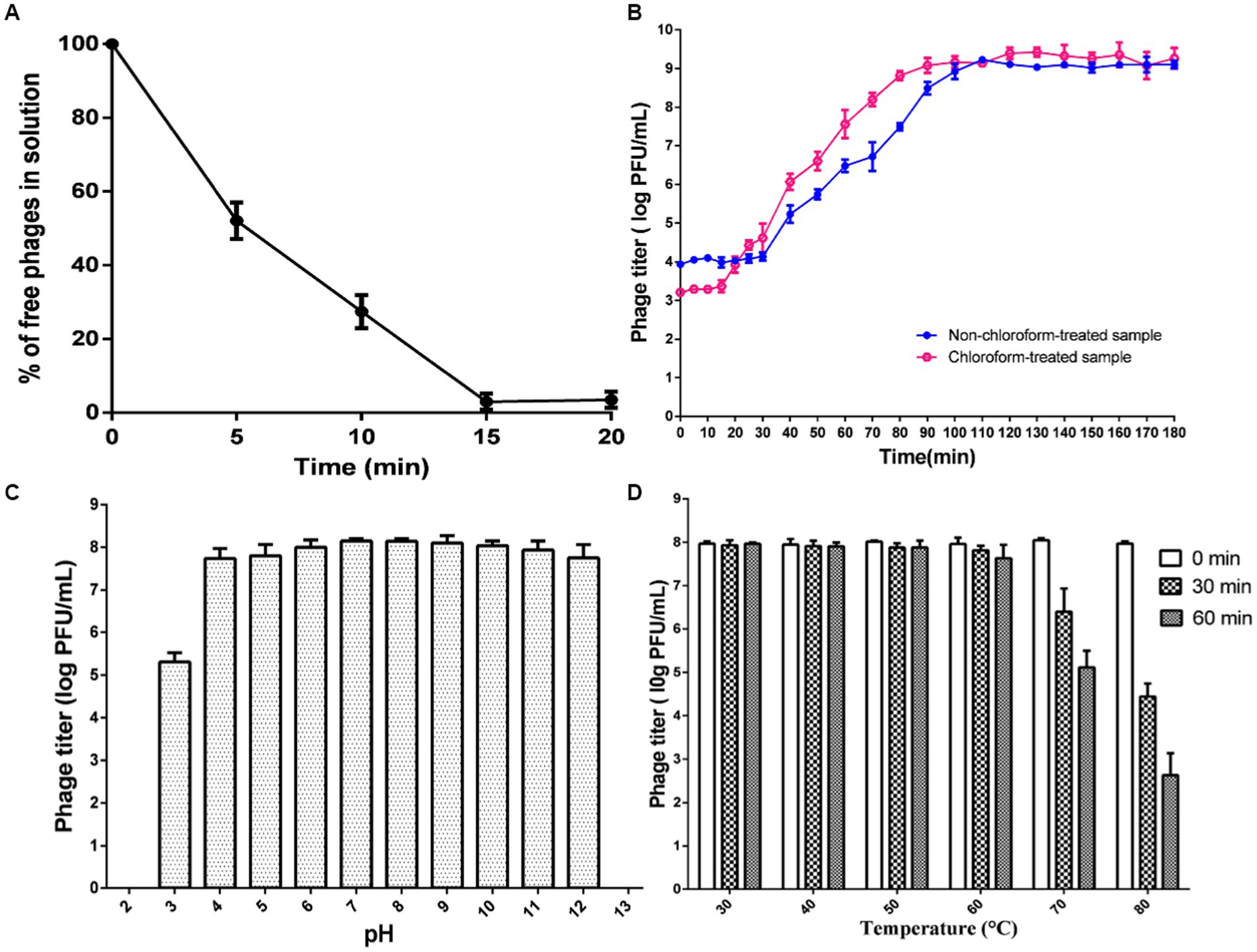
Figure 2. Characteristics of ISTP3. (A) Absorption rate. (B) One-step growth curve. (C) Effect of pH. (D) Effect of temperature.
3.3 Relative replication efficiency of phage ISTP3
According to Mirzaei et al., single dilution spot tests can yield misleading positive outcomes due to factors such as lysis from outside, presence of bacteriocins in the phage lysate, or the presence of prophages (Islam et al., 2020b). We conducted the EOP test to verify the host range of phage ISTP3 (Table 3). This particular phage demonstrated a significant ability, ranging from 0.5 to less than 1.0, to infect most strains of S. typhimurium. However, its effectiveness, as measured by the efficiency of plating (EOP) values, was moderate, ranging from 0.2 to less than 0.5, against certain S. typhimurium strains and all S. enteritidis strains. The phage demonstrates equal effectiveness against both drug-resistant and non-resistant Salmonella strains. The EOP of the ISTP3 phage was examined on seven Salmonella strains that are resistant to drugs in our collection. We observed distinct plaques for all seven strains, with relative EOP values varying between 0.2 and 1. The findings indicate that the phage ISTP3 can cause the lysis of various zoonotic Salmonella strains.
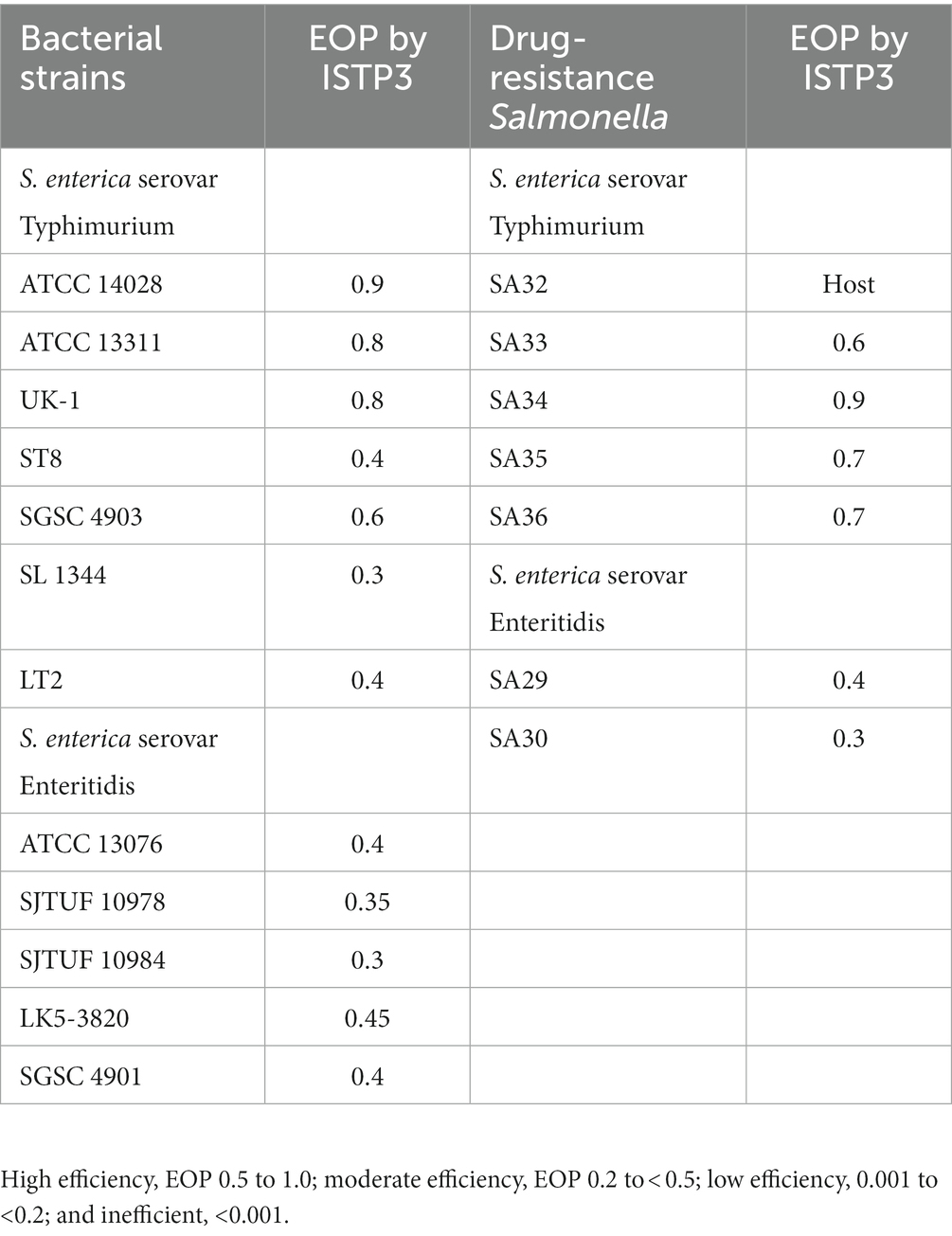
Table 3. Efficiency of plating (EOP) by phage ISTP3 against different Salmonella serovars including drug-resistant Salmonella.
3.4 Genome sequencing and bioinformatics analysis
The entire genetic material of phage ISTP3 consists of a 156,121 bp long double-stranded DNA, with a G + C content of 44.78% (Figure 3). The genome sequence of ISTP3 exhibited significant similarity to Salmonella phage bering, with a coverage of 90% and an identification rate of 97.28% (Supplementary Table S1). In the ISTP3 genome, a total of 204 open reading frames (ORFs) were found, as indicated in Supplementary Table S2. Out of these, 91 ORFs were determined to be responsible for encoding functional proteins, while the remaining 113 ORFs were found to encode proteins with unidentified functions. Within the group of 91 functional genes, 60 of them were associated with nucleic acid metabolism and DNA packaging, 14 were related to structural proteins other than tails, 4 were cleavage-related genes, and 13 were associated with tail-related proteins, as shown in Figure 3. In the gene annotation of phage ISTP3, we have identified a protein that possesses an Ig-like domain and functions as a tail-related tail fiber protein. This protein has been reported to have associations with phages and has the ability to bind to the human intestinal mucosa, aiding the body in its defense against infections. However, the precise role of the Ig-like protein in the interaction between the phage and the human immune system remains uncertain. The ISTP3 phage does not contain any known virulence or antibiotic resistance genes, indicating that it is a safe phage. Consequently, ISTP3 can be employed in various applications for the control of drug-resistant Salmonella in a safe manner.
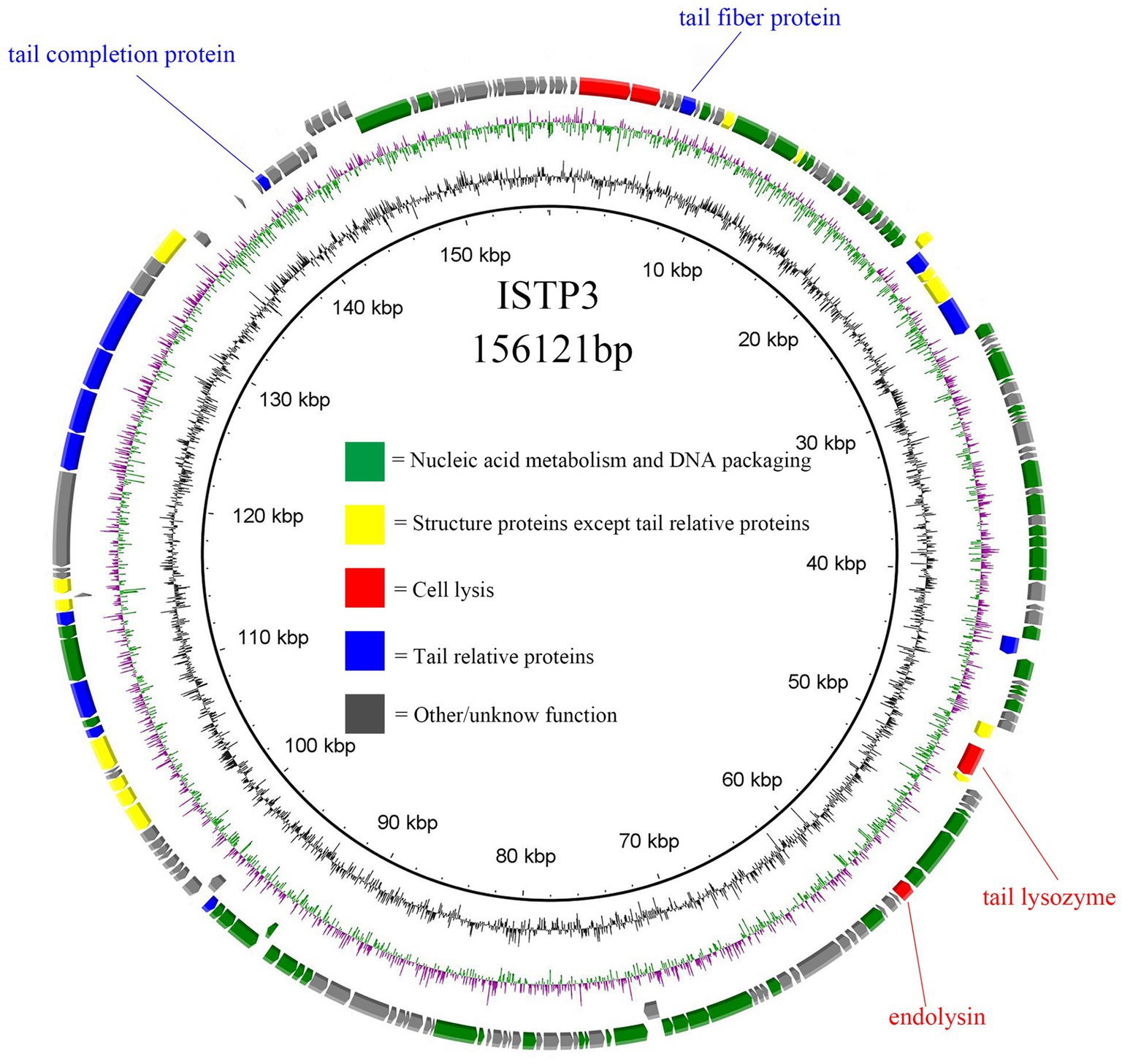
Figure 3. Genome map of phage ISTP3. ORFs set down in the clockwise or the counterclockwise direction. Structural proteins of phage, nucleic acid metabolism, cell lysis, and tail relative protein marked in yellow, green, red, and blue, respectively.
Given that the structure and fundamental features of the large terminal enzyme subunit tend to be conserved in tail phages, it is expected that phages of the same genus will have clusters of their terminal large subunits. Due to the relative conservation, the major capsid proteins are also very suitable, and there is almost no evidence of horizontal exchange in the head gene region of the phage. The main capsid protein and the terminase large subunit of the phage ISTP3 were compared by BLASTP, and 100 sequences with higher homology were downloaded, and the MEGA7 software was used to select the branches with higher reliability for phylogenetic analysis. ISTP3 forms a same cluster with the main capsid protein and the terminase large subunit of Salmonella phage S117, GG32 and E. coli phage 4HA1 (Figure 4). According to International Committee on Taxonomy of Viruses (ICTV) classification these four phages belonged to Ackermannviridae, which are very closely relationship in cluster.
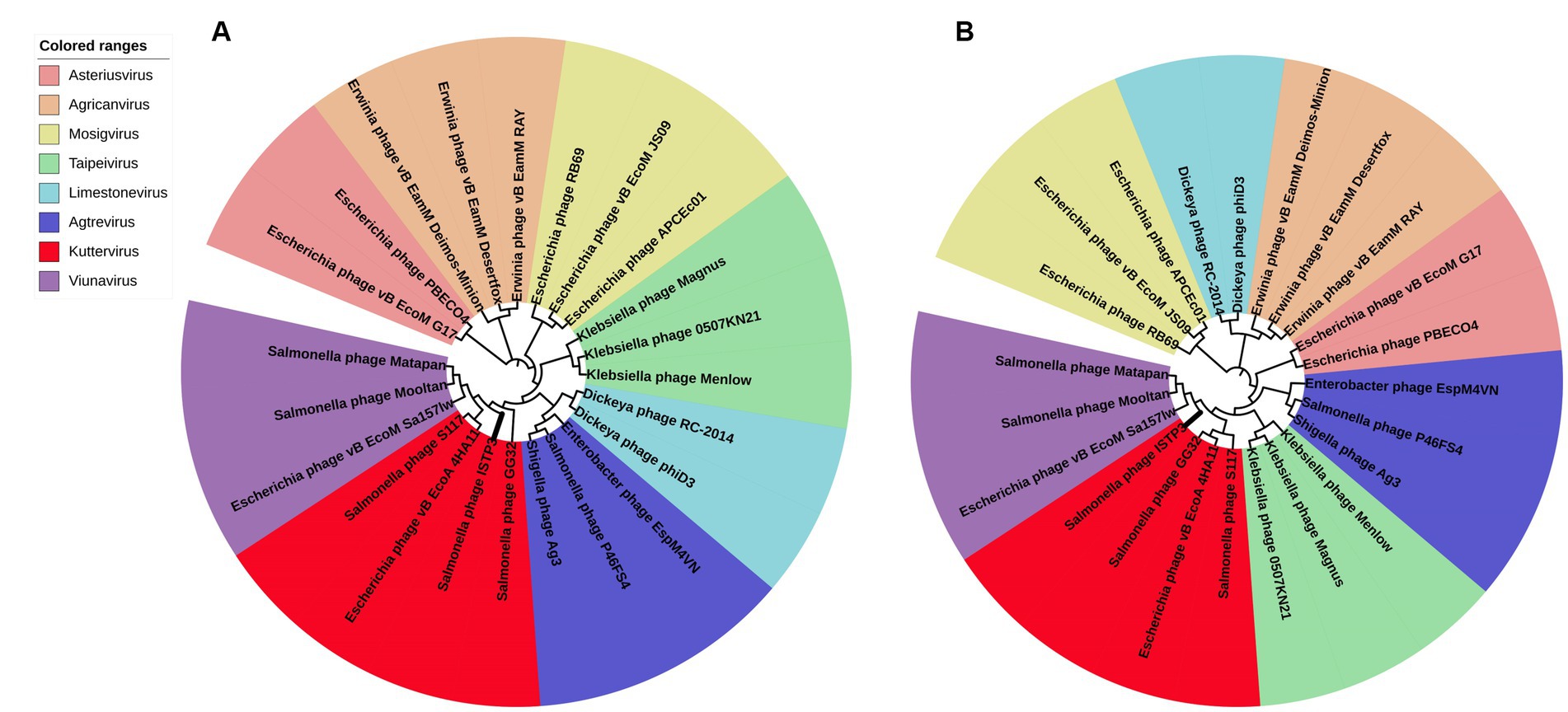
Figure 4. Phylogenetic analysis of phage ISTP3 and related phages based on protein sequence of (A) major capsid and (B) terminase large subunit.
3.5 Evaluating the efficacy of phage ISTP3 to reduce the drug resistant S. typhimurium in foods
It has been found that the phage ISTP3 could lyse the drug-resistant Salmonella during the evaluation of host spectrum, so foods have been contaminated with drug-resistant S. typhimurium in the application experiments. The experimental findings regarding chicken breast are represented in Figures 5A,B. When compared to the control group, a notable decrease of 1.6 log CFU/cm2 and 1.8 log CFU/cm2 in viable drug-resistant S. typhimurium was observed after 12 h at 4°C, with MOIs of 100 and 1,000, respectively (Figure 5A). At a temperature of 25°C, the population of viable S. typhimurium decreased by 2.1 log CFU/cm2 and 2.4 log CFU/cm2 when exposed to MOIs of 100 and 1,000 respectively, following a 12 h incubation period (as shown in Figure 5B).
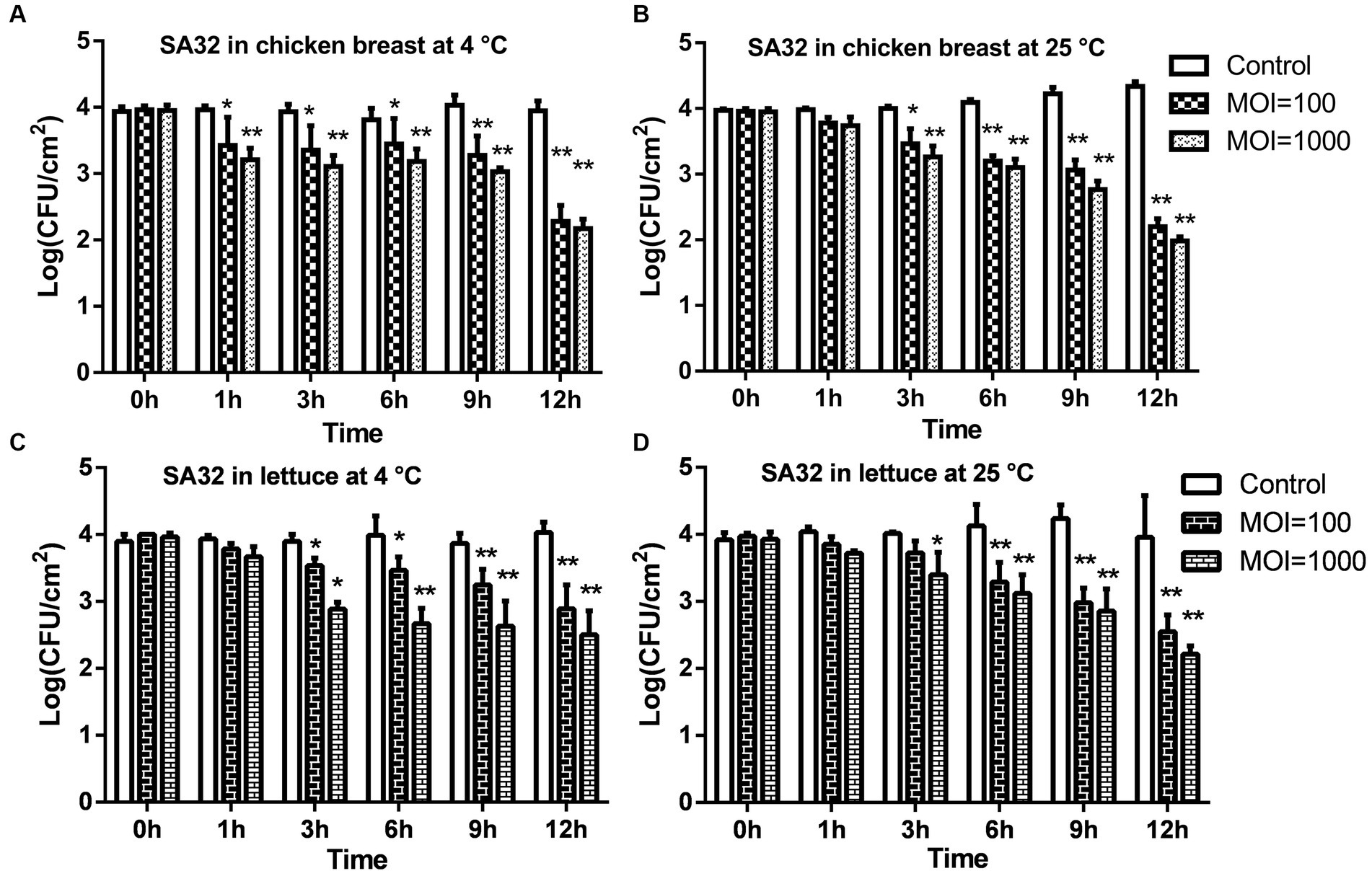
Figure 5. The application of phage ISTP3 to reduce the S. typhimurium on food matrices. The efficacy of phage was tested chicken breast and lettuce with bacteria using phage treated. Viable bacteria were counted at 0, 1, 3, 6, 9, 12 h. (A) Viable S. typhimurium on the chicken breast at 4°C. (B) Viable S. typhimurium on the chicken breast at 25°C. (C) Viable S. typhimurium on lettuce at 4°C. (D) Viable S. typhimurium on lettuce at 25°C. Control samples; without phage treated; MOI = 100, samples were treated with 6 log PFU of ISTP3 and 4 log CFU bacteria; MOI = 1,000, samples were treated with 7 log PFU of ISTP3 and 4 log CFU bacteria. *p < 0.05 and **p < 0.01.
The antibacterial effectiveness of the ISTP3 phage in reducing drug-resistant S. typhimurium was investigated through artificial contamination of bacteria on the surface of lettuce. Phage ISTP3 demonstrated its effectiveness in reducing bacterial counts on lettuce samples when incubated at 4°C for 12 h. At MOIs of 100 and 1,000, viable cell counts decreased by at least 1.1 log CFU/cm2 and 1.5 log CFU/cm2 respectively, as shown in Figure 5C. The application of a MOI of 100 and 1,000 resulted in a reduction of viable bacteria at 25°C after 12 h. Specifically, there was a decrease of 1.4 log 10 CFU/cm2 and 1.7 log CFU/cm2, respectively, at the end of the 12 h incubation period (as shown in Figure 5D).
4 Discussion
Salmonella poses a significant challenge in terms of outbreaks of zoonotic pathogens within the food industry, impacting safety measures. While phage usage offers the key advantage of specificity towards its target bacteria, allowing non-target bacterial populations to remain undisturbed, phages are still capable of lysing the majority of strains belonging to a particular bacterial species (Almeida et al., 2009). Phage has been intended as a promising green technology for biocontrol of different foodborne pathogenic bacteria. We isolated 29 phages capable of lysing various strains of Salmonella, and during screening for effectiveness against different Salmonella serovars, phage ISTP3 demonstrated a broad host range activity by successfully lysing all tested Salmonella serovars and achieving complete clearing. Furthermore, bacteriophages exhibiting a broad host range, capable of lysing various serovars of pathogenic bacteria, including diverse strains, prove highly effective in the context of food and food processing environments. In our study, we assessed the host range of phage ISTP3 against various Salmonella serovars, including drug-resistant strains. The results demonstrated that phage ISTP3 was able to lyse all 31 tested Salmonella strains, including 10 drug-resistant strains. These findings provide evidence of the potential of phage therapy in controlling drug-resistant zoonotic Salmonella, offering a promising solution for combating antibiotic-resistant strains. The significance of having a broad host range, which enables the phage to interact and replicate efficiently with various bacterial hosts, has been well established. This criterion is considered crucial in determining the suitability of a phage as a leading candidate in terms of its dynamics with host bacteria (Li et al., 2021). The categorical differentiation between various stages of the broad host range is not clearly explained. Recent studies by Dekel-Bird et al. (2015), Kauffman et al. (2018) focusing on newly isolated marine broad-host-range phage, as well as the examination of extreme environments through community-wide single-cell metagenomics by Munson-McGee et al. (2018), have provided evidence of the widespread presence of broad host range phages in natural environments, as supported by Brum et al. (2016), Paez-Espino et al. (2016). Host range analysis is a crucial factor when choosing lytic phages for potential utilization in the biocontrol purposes. Additionally, the EOP results indicate that phage ISTP3 demonstrated the capability to lyse not only its host but also all the tested Salmonella strains. In our research, we demonstrated that the tailed phage ISTP3 displayed a broad range of hosts, which may be attributed to the abundance or superior quality of Salmonella strains, including those resistant to drugs, coexisting with phage ISTP3 in various environments.
In this study, we selected the most virulent phage with the broadest host range, which is capable of lysing diverse zoonotic Salmonella strains within our collection, for potential biocontrol applications. Upon TEM examination, it was determined that phage ISTP3 belongs to the Caudovirales order and Ackermannviridae family. Other studies (Hooton et al., 2011; Yele et al., 2012; Sylwia et al., 2014; Novacek et al., 2016; Sunderland et al., 2017; Islam et al., 2019a) have suggested that phages within this family possess the potential to be used as biocontrol candidates against Salmonella. The process of phage adsorption, which involves the attachment of phage particles to host cells, is influenced by multiple factors such as the characteristics of the receptor, its position within the cell wall, ease of access, arrangement in space, as well as the abundance and concentration at different sites. Phage ISTP3 exhibits a shorter latent period and a larger burst during its one-step growth curve. These characteristics make it more advantageous for biocontrol purposes as it can effectively and quickly propagate, leading to the rapid elimination of the targeted bacterial population (Duc et al., 2020). The capacity of phages to serve as biocontrol agents across diverse environmental conditions is enhanced by their stability. In this study, the Salmonella phage ISTP3, isolated for evaluation, underwent incubation at various pH levels and temperatures to assess its ability to maintain infectivity. Phage ISTP3 demonstrated tolerated within a pH range of 4 to 12 for a duration of 60 min, as its viral titers remained largely unaffected. Previous research indicates that many phages have an optimal pH range of 6 to 8, although certain phages like T2 phage experience a 50% decline in infectivity between pH levels of 5 and 9 (Jończyk et al., 2011). On the other hand, the present study revealed that phage ISTP3 maintained its titer without any notable decrease when exposed to temperatures ranging from 30°C to 60°C for a period of 60 min.
Further genomic sequencing and bioinformatics analysis of phage ISTP3 confirmed that it is a member of the Ackermannviridae family, classified as a double-stranded DNA virus. However, upon examination, it was observed that the ISTP3 genome lacks any recognized lysogenic genes, which are vital criteria for a lytic phage to effectively target and eliminate pathogens. Additionally, no ORFs were detected that were linked to pathogenicity or the production of toxins, rendering the phage unsuitable for therapeutic purposes. These findings substantiate the potential of the ISTP3 phage for applications in bio-control and ensuring safety (Alves et al., 2020).
In the realm of managing Enterobacteriaceae, particularly S. enterica, which stands out as the most frequently encountered zoonotic microorganism, phages are commonly employed as a means of control. Several studies have documented the identification and usage of lytic phages to combat prevalent foodborne pathogens, such as E. coli O157:H7 (Hudson et al., 2013), Salmonella (Guenther et al., 2012), Listeria monocytogenes (Bigot et al., 2011), Campylobacter (Loc Carrillo et al., 2005), Enterobacter sakazaki (Zuber et al., 2008), and Staphylococcus aureus (García et al., 2009). To our knowledge, there are very few studies to evaluate efficacy of phage to control drug-resistant Salmonella in foods. In this study we used phage ISTP3 for efficiently reducing the drug resistant S. typhimurium SA32 in food matrices at 4°C or 25°C. When applied to chicken breast samples, phage ISTP3 exhibited a reduction of Salmonella by over 1.5 log at 4°C and over 2 log at 25°C within a 12 h incubation period. Diverse studies have provided evidence of employing individual phages, such as LPST153, ZCSE2, ST-W77, BSP101, SI1, and S144, specifically to mitigate Salmonella contamination in food items (Fong et al., 2017; Bai et al., 2019; Gambino et al., 2020; Mohamed et al., 2020; Phothaworn et al., 2020; Islam et al., 2020a). Typically, increasing the MOI (multiplicity of infection) on food samples leads to a greater decrease in pathogens. Theoretically, using high phage titers would enhance the chances of phages interacting with the target bacteria. Numerous studies have shown the effectiveness of applying phages to meat under low-temperature conditions (Carter et al., 2012; Hudson et al., 2013). The successful inactivation of Salmonella at low temperatures and with high MOI values may be attributed to the mechanism known as lysis from without (LO), as proposed in previous research (David Tomat et al., 2018).
Additionally, the application of ISTP3 phage treatment has demonstrated a significant reduction in drug-resistant S. enterica on lettuce. After 12 h of incubation at both 4°C and 25°C, viable cell counts decreased by approximately 1 to 1.8 log. The findings of the current study align with previous research evaluating the effectiveness of ECP-100 phage on fresh-cut lettuce. In a study conducted by Li et al. (2021), it was observed that the application of phage ECP-100 resulted in a reduction of bacterial populations by approximately 1.82 log CFU/cm2. Complete eradication of bacteria through phage application may not be achieved. However, the significant reduction in viable bacterial counts following phage therapy suggests that it may lower the bacterial load below the infectious dose for individuals.
5 Conclusion
In this study, the phage ISTP3 was isolated and it is fully active at 60°C for 1 h which is defined as thermostable. This particular phage exhibited a wide host range and demonstrated the capacity to effectively lyse drug-resistant strains of S. enterica. ISTP3 did not contain any virulent genes, lysogenic, or drug-resistance which are make it safe for application on food matrices. Additionally, the phage ISTP3 achieved reductions of drug-resistant S. enterica on chicken breast and lettuce, making it an efficient biocontrol agent for food and food processing chain which may offer one of the safe and environmentally friendly approach. The phage treatment is optimizing novel strategies for the control of drug-resistant Salmonella contamination on chicken and fresh produce and it could reduce the economic loss and burden of health associated with diverse outbreaks.
Data availability statement
The original contributions presented in the study are included in the article/Supplementary material, further inquiries can be directed to the corresponding authors.
Author contributions
MSI: Conceptualization, Data curation, Formal analysis, Investigation, Methodology, Project administration, Resources, Software, Validation, Visualization, Writing – original draft, Writing – review & editing. IN: Conceptualization, Data curation, Formal analysis, Methodology, Software, Writing – original draft. FP: Conceptualization, Funding acquisition, Investigation, Project administration, Resources, Supervision, Validation, Writing – review & editing. XW: Conceptualization, Funding acquisition, Investigation, Project administration, Resources, Supervision, Validation, Writing – review & editing.
Funding
The author(s) declare financial support was received for the research, authorship, and/or publication of this article. This work was supported by the Key R&D Program of Hubei Province (2022BBA0057-02), the National Key R&D Program of China (2019YFC1606001), National Key R&D Program of China (2021YFC2400500), the National Natural Science Foundation of China (grant 32170925), Shenzhen Science and Technology Program (KQTD20210811090115019), the Shenzhen Science and Technology Program (JCYJ2022081800807016), the startup fund of SIAT and CAS.
Conflict of interest
The authors declare that the research was conducted in the absence of any commercial or financial relationships that could be construed as a potential conflict of interest.
Publisher’s note
All claims expressed in this article are solely those of the authors and do not necessarily represent those of their affiliated organizations, or those of the publisher, the editors and the reviewers. Any product that may be evaluated in this article, or claim that may be made by its manufacturer, is not guaranteed or endorsed by the publisher.
Supplementary material
The Supplementary material for this article can be found online at: https://www.frontiersin.org/articles/10.3389/fmicb.2023.1260181/full#supplementary-material
Footnotes
1. ^http://www.gatan.com/products/tem-analysis/gatan-microscopy-suite-software
References
Alali, W. Q., Gaydashov, R., Petrova, E., Panin, A., Tugarinov, O., Kulikovskii, A., et al. (2012). Prevalence of salmonella on retail chicken meat in Russian Federation. J. Food Prot. 75, 1469–1473. doi: 10.4315/0362-028X.JFP-12-080
Ali, S., Hassan, A., Hassan, G., Eun, C.-H., Bae, J., Lee, C. H., et al. (2018). Disposable all-printed electronic biosensor for instantaneous detection and classification of pathogens. Sci. Rep. 8:5920. doi: 10.1038/s41598-018-24208-2
Almeida, A., Cunha, A., Gomes, N. C. M., Alves, E., Costa, L., et al. (2009). Phage therapy and photodynamic therapy: low environmental impact approaches to inactivate microorganisms in fish farming plants. Mar. Drugs 7, 268–313. doi: 10.3390/md7030268
Alves, D., Cerqueira, M. A., Pastrana, L. M., and Sillankorva, S. (2020). Entrapment of a phage cocktail and cinnamaldehyde on sodium alginate emulsion-based films to fight food contamination by Escherichia coli and Salmonella Enteritidis. Food Res. Int. 128:108791. doi: 10.1016/j.foodres.2019.108791
Bai, J., Jeon, B., and Ryu, S. (2019). Effective inhibition of Salmonella Typhimurium in fresh produce by a phage cocktail targeting multiple host receptors. Food Microbiol. 77, 52–60. doi: 10.1016/j.fm.2018.08.011
Bigot, B., Lee, W. J., Mcintyre, L., Wilson, T., Hudson, J. A., Billington, C., et al. (2011). Control of Listeria monocytogenes growth in a ready-to-eat poultry product using a bacteriophage. Food Microbiol. 28, 1448–1452. doi: 10.1016/j.fm.2011.07.001
Brum, J. R., Ignacio-Espinoza, J. C., Kim, E. H., Trubl, G., Jones, R. M., Roux, S., et al. (2016). Illuminating structural proteins in viral dark matter with metaproteomics. Proc. Natl. Acad. Sci. U. S. A. 113, 2436–2441. doi: 10.1073/pnas.1525139113
Carter, C. D., Parks, A., Abuladze, T., Li, M., Woolston, J., Magnone, J., et al. (2012). Bacteriophage cocktail significantly reduces Escherichia coli O157: H7 contamination of lettuce and beef, but does not protect against recontamination. Bacteriophage 2, 178–185. doi: 10.4161/bact.22825
Chen, P. L., Li, C. Y., Hsieh, T. H., Chang, C. M., Lee, H. C., Lee, N. Y., et al. (2012). Epidemiology, disease spectrum and economic burden of non-typhoidal salmonella infections in Taiwan, 2006–2008. Epidemiol. Infect. 140, 2256–2263. doi: 10.1017/S0950268812000088
D'accolti, M., and Soffritti, I. (2021). Bacteriophages as a potential 360-degree pathogen control strategy. Microorganisms 9:261. doi: 10.3390/microorganisms9020261
David Tomat, C. C., Aquili, V., Balagué, C., and Quiberoni, A. (2018). Evaluation of a novel cocktail of six lytic bacteriophages against Shiga toxin-producing Escherichia coli in broth, milk and meat. Food Microbiol. 76, 434–442. doi: 10.1016/j.fm.2018.07.006
De Oliveira Elias, S., Noronha, T. B., and Tondo, E. C. (2019). Salmonella spp. and Escherichia coli O157:H7 prevalence and levels on lettuce: a systematic review and meta-analysis. Food Microbiol. 84:103217. doi: 10.1016/j.fm.2019.05.001
Dekel-Bird, N. P., Sabehi, G., Mosevitzky, B., and Lindell, D. (2015). Host-dependent differences in abundance, composition and host range of cyanophages from the Red Sea. Environ. Microbiol. 17, 1286–1299. doi: 10.1111/1462-2920.12569
Dolejska, M., Villa, L., Hasman, H., Hansen, L., and Carattoli, A. (2012). Characterization of Inc N plasmids carrying Bla CTX-M-1 and qnr genes in Escherichia coli and salmonella from animals, the environment and humans. J. Antimicrob. Chemother. 68, 333–339. doi: 10.1093/jac/dks387
Duc, V. M., Nakamoto, Y., Fujiwara, A., Toyofuku, H., Obi, T., and Chuma, T. (2019). Prevalence of salmonella in broiler chickens in Kagoshima, Japan in 2009 to 2012 and the relationship between serovars changing and antimicrobial resistance. BMC Vet. Res. 15:108. doi: 10.1186/s12917-019-1836-6
Duc, H. M., Son, H. M., Yi, H. P. S., Sato, J., Ngan, P. H., Masuda, Y., et al. (2020). Isolation, characterization and application of a polyvalent phage capable of controlling salmonella and Escherichia coli O157:H7 in different food matrices. Food Res. Int. 131:108977. doi: 10.1016/j.foodres.2020.108977
Ehuwa, O., Jaiswal, A. K., and Jaiswal, S. (2021). Salmonella, food safety and food handling practices. Foods 10:907. doi: 10.3390/foods10050907
Finn, S., Condell, O., Mcclure, P., Amézquita, A., and Fanning, S. (2013). Mechanisms of survival, responses and sources of salmonella in low-moisture environments. Front. Microbiol. 4:331. doi: 10.3389/fmicb.2013.00331
Fister, S., Fuchs, S., Stessl, B., Schoder, D., Wagner, M., and Rossmanith, P. (2016). Screening and characterisation of bacteriophage P100 insensitive Listeria monocytogenes isolates in Austrian dairy plants. Food Control 59, 108–117. doi: 10.1016/j.foodcont.2015.05.026
Fong, K., Labossiere, B., Switt, A. I. M., Delaquis, P., Goodridge, L., Levesque, R. C., et al. (2017). Characterization of four novel bacteriophages isolated from British Columbia for control of non-typhoidal salmonella in vitro and on sprouting alfalfa seeds. Front. Microbiol. 8:2193. doi: 10.3389/fmicb.2017.02193
Ford, L., Buuck, S., Eisenstein, T., Cote, A., Mccormic, Z. D., Kremer-Caldwell, S., et al. (2023). Salmonella outbreaks associated with not ready-to-eat breaded, stuffed chicken products – United States, 1998–2022. MMWR Morb. Mortal. Wkly Rep. 72, 484–487. doi: 10.15585/mmwr.mm7218a2
Gambino, M., Nørgaard Sørensen, A., Ahern, S., Smyrlis, G., and Gencay, Y. E. (2020). Phage S144, a new polyvalent phage infecting salmonella spp. and Cronobacter sakazakii. Int. J. Mol. Sci. 21:5196. doi: 10.3390/ijms21155196
García, R., Latz, S., Romero, J., Higuera, G., García, K., et al. (2019). Bacteriophage production models: an overview. Front. Microbiol. 10:1187. doi: 10.3389/fmicb.2019.01187
García, P., Madera, C., Martínez, B., Rodríguez, A., and Evaristo Suárez, J. (2009). Prevalence of bacteriophages infecting Staphylococcus aureus in dairy samples and their potential as biocontrol agents. J. Dairy Sci. 92, 3019–3026. doi: 10.3168/jds.2008-1744
Gil Prieto, R., Alejandre, C. G., Meca, A. A., Barrera, V. H., and De Miguel, A. G. (2009). Epidemiology of hospital-treated salmonella infection; data from a national cohort over a 10-year period. J. Infect. 58, 175–181. doi: 10.1016/j.jinf.2009.01.002
Guenther, S., Herzig, O., Fieseler, L., Klumpp, J., and Loessner, M. J. (2012). Biocontrol of Salmonella Typhimurium in RTE foods with the virulent bacteriophage FO1-E2. Int. J. Food Microbiol. 154, 66–72. doi: 10.1016/j.ijfoodmicro.2011.12.023
Guo, Y., Li, J., Islam, M. S., Yan, T., Zhou, Y., Liang, L., et al. (2021). Application of a novel phage vB_SalS-LPSTLL for the biological control of salmonella in foods. Food Res. Int. 147:110492. doi: 10.1016/j.foodres.2021.110492
Hooton, S. P., Timms, A. R., Rowsell, J., Wilson, R., and Connerton, I. F. (2011). Salmonella Typhimurium-specific bacteriophage ΦSH19 and the origins of species specificity in the Vi01-like phage family. Virol. J. 8, 1–14. doi: 10.1186/1743-422X-8-498
Hudson, J. A., Billington, C., Cornelius, A. J., Wilson, T., On, S. L., et al. (2013). Use of a bacteriophage to inactivate Escherichia coli O157:H7 on beef. Food Microbiol. 36, 14–21. doi: 10.1016/j.fm.2013.03.006
Hyla, K., Dusza, I., and Skaradzińska, A. (2022). Recent advances in the application of bacteriophages against common foodborne pathogens. Antibiotics 11:1536. doi: 10.3390/antibiotics11111536
Islam, M. S., Hu, Y., Mizan, M. F. R., Yan, T., Nime, I., Zhou, Y., et al. (2020a). Characterization of salmonella phage LPST153 that effectively targets Most prevalent salmonella Serovars. Microorganisms 8:1089. doi: 10.3390/microorganisms8071089
Islam, M. S., Raz, A., Liu, Y., Elbassiony, K. R. A., Dong, X., Zhou, P., et al. (2019a). Complete genome sequence of Aeromonas phage ZPAH7 with halo zones, isolated in China. Microbiol. Resour. Announc. 8:e01678. doi: 10.1128/MRA.01678-18
Islam, M. S., Yang, X., Euler, C. W., Han, X., Liu, J., Hossen, M. I., et al. (2021). Application of a novel phage ZPAH7 for controlling multidrug-resistant Aeromonas hydrophila on lettuce and reducing biofilms. Food Control 122:107785. doi: 10.1016/j.foodcont.2020.107785
Islam, M. S., Zhou, Y., Liang, L., Nime, I., Liu, K., Yan, T., et al. (2019b). Application of a phage cocktail for control of salmonella in foods and reducing biofilms. Viruses 11:841. doi: 10.3390/v11090841
Islam, M. S., Zhou, Y., Liang, L., Nime, I., Yan, T., Willias, S. P., et al. (2020b). Application of a broad range lytic phage LPST94 for biological control of salmonella in foods. Microorganisms 8:247. doi: 10.3390/microorganisms8020247
Jiang, Z., Anwar, T. M., Peng, X., Biswas, S., Elbediwi, M., Li, Y., et al. (2021). Prevalence and antimicrobial resistance of salmonella recovered from pig-borne food products in Henan. China. Food Control 121:107535. doi: 10.1016/j.foodcont.2020.107535
Jończyk, E., Kłak, M., Międzybrodzki, R., and Górski, A. (2011). The influence of external factors on bacteriophages – review. Folia Microbiol. 56, 191–200. doi: 10.1007/s12223-011-0039-8
Kauffman, K. M., Hussain, F. A., Yang, J., Arevalo, P., Brown, J. M., Chang, W. K., et al. (2018). A major lineage of non-tailed dsDNA viruses as unrecognized killers of marine bacteria. Nature 554, 118–122. doi: 10.1038/nature25474
Lebelo, K., Malebo, N., Mochane, M. J., and Masinde, M. (2021). Chemical contamination pathways and the food safety implications along the various stages of food production: a review. Int. J. Environ. Res. Public Health 18:5795. doi: 10.3390/ijerph18115795
Lee, C., Kim, H., and Ryu, S. (2022). Bacteriophage and endolysin engineering for biocontrol of food pathogens/pathogens in the food: recent advances and future trends. Crit. Rev. Food Sci. Nutr. 63, 8919–8938. doi: 10.1080/10408398.2022.2059442
Li, J., Li, Y., Ding, Y., Huang, C., Zhang, Y., Wang, J., et al. (2021). Characterization of a novel Siphoviridae salmonella bacteriophage T156 and its microencapsulation application in food matrix. Food Res. Int. 140:110004. doi: 10.1016/j.foodres.2020.110004
Loc Carrillo, C., Atterbury, R. J., El-Shibiny, A., Connerton, P. L., Dillon, E., et al. (2005). Bacteriophage therapy to reduce Campylobacter jejuni colonization of broiler chickens. Appl. Environ. Microbiol. 71, 6554–6563. doi: 10.1128/AEM.71.11.6554-6563.2005
Mohamed, A., Taha, O., and El-Sherif, H. M. (2020). Bacteriophage ZCSE2 is a potent antimicrobial against Salmonella enterica Serovars: ultrastructure. Genomics Efficacy. Viruses 12:424. doi: 10.3390/v12040424
Mori, T., Okamura, N., Kishino, K., Wada, S., Zou, B., Nanba, T., et al. (2018). Prevalence and antimicrobial resistance of salmonella serotypes isolated from poultry meat in Japan. Food Saf. 6, 126–129. doi: 10.14252/foodsafetyfscj.2017019
Moye, Z. D., Woolston, J., and Sulakvelidze, A. (2018). Bacteriophage applications for food production and processing. Viruses 10:205. doi: 10.3390/v10040205
Muncke, J., Andersson, A.-M., Backhaus, T., Boucher, J. M., Carney Almroth, B., Castillo Castillo, A., et al. (2020). Impacts of food contact chemicals on human health: a consensus statement. Environ. Health 19:25. doi: 10.1186/s12940-020-0572-5
Munson-Mcgee, J. H., Peng, S., Dewerff, S., and Stepanauskas, R. (2018). A virus or more in (nearly) every cell: ubiquitous networks of virus-host interactions in extreme environments. SME J. 12, 1706–1714. doi: 10.1038/s41396-018-0071-7
Niemi, J. K., Heinola, K., Simola, M., and Tuominen, P. (2019). Salmonella control programme of pig feeds is financially beneficial in Finland. Front. Vet. Sci. 6:200. doi: 10.3389/fvets.2019.00200
Novacek, J. M. Š., Benešík, M., Pantůček, R., Doškař, J., and Plevka, P. (2016). Structure and genome release of Twort-like Myoviridae phage with a double-layered baseplate. Proc. Natl. Acad. Sci. U. S. A. 113, 9351–9356. doi: 10.1073/pnas.1605883113
Owais, A., Sultana, S., Zaman, U., Rizvi, A., and Zaidi, A. K. (2010). Incidence of typhoid bacteremia in infants and young children in southern coastal Pakistan. Pediatr. Infect. Dis. J. 29, 1035–1039. doi: 10.1097/INF.0b013e3181e39f8b
Paez-Espino, D., Eloe-Fadrosh, E. A., Pavlopoulos, G. A., Thomas, A. D., Huntemann, M., Mikhailova, N., et al. (2016). Uncovering Earth's virome. Nature 536, 425–430. doi: 10.1038/nature19094
Park, M., Lee, J. H., Shin, H., Kim, M., Choi, J., Kang, D. H., et al. (2012). Characterization and comparative genomic analysis of a novel bacteriophage, SFP10, simultaneously inhibiting both and O157:H7. Appl. Environ. Microbiol. 78, 58–69. doi: 10.1128/AEM.06231-11
Pelyuntha, W., Ngasaman, R., Yingkajorn, M., Chukiatsiri, K., Benjakul, S., and Vongkamjan, K. (2021). Isolation and characterization of potential salmonella Phages targeting multidrug-resistant and major Serovars of salmonella derived from broiler production chain in Thailand. Front. Microbiol. 12:662461. doi: 10.3389/fmicb.2021.662461
Pem, D., and Jeewon, R. (2015). Fruit and vegetable intake: benefits and Progress of nutrition education interventions- narrative review article. Iran. J. Public Health 44, 1309–1321.
Phothaworn, P., Supokaivanich, R., Lim, J., Klumpp, J., Imam, M., Kutter, E., et al. (2020). Development of a broad-spectrum salmonella phage cocktail containing Viunalike and Jerseylike viruses isolated from Thailand. Food Microbiol. 92:103586. doi: 10.1016/j.fm.2020.103586
Racine, E., and Gualtieri, M. (2019). From Worms to drug candidate: the story of Odilorhabdins, a new class of antimicrobial agents. Front. Microbiol. 10:2893. doi: 10.3389/fmicb.2019.02893
Sharma, M. (2013). Lytic bacteriophages: potential interventions against enteric bacterial pathogens on produce. Bacteriophage 3:e25518. doi: 10.4161/bact.25518
Song, Y., Wang, F., Liu, Y., Song, Y., Zhang, L., Zhang, F., et al. (2020). Occurrence and characterization of salmonella isolated from chicken breeder flocks in nine Chinese provinces. Front. Vet. Sci. 7:479. doi: 10.3389/fvets.2020.00479
Sunderland, K., Yang, M., and Mao, C. (2017). Phage-enabled nanomedicine: from probes to therapeutics in precision medicine. Angew. Chem. Int. Ed. Engl. 56, 1964–1992. doi: 10.1002/anie.201606181
Sylwia, P., Magdalena, K., Romuald, G., Lidia, M., and Anna, M. (2014). Bacteriophages as an alternative strategy for fighting biofilm development. Pol. J. Microbiol. 63, 137–145. doi: 10.33073/pjm-2014-019
Thung, T. Y., Radu, S., Mahyudin, N. A., Rukayadi, Y., Zakaria, Z., Mazlan, N., et al. (2017). Prevalence, virulence genes and antimicrobial resistance profiles of salmonella Serovars from retail beef in Selangor. Malaysia. Front. Microbiol. 8:2697. doi: 10.3389/fmicb.2017.02697
Totten, K. M. C., and Patel, R. (2022). Phage activity against planktonic and biofilm Staphylococcus aureus Periprosthetic joint infection isolates. Antimicrob. Agents Chemother. 66:e0187921. doi: 10.1128/AAC.01879-21
Wessels, K., Rip, D., and Gouws, P. (2021). Salmonella in chicken meat: consumption, outbreaks, characteristics, current control methods and the potential of bacteriophage use. Foods 10:1742. doi: 10.3390/foods10081742
Yang, X., Wu, Q., Huang, J., Wu, S., Zhang, J., Chen, L., et al. (2020). Prevalence and characterization of salmonella isolated from raw vegetables in China. Food Control 109:106915. doi: 10.1016/j.foodcont.2019.106915
Yang, X., Wu, Q., Zhang, J., Huang, J., Chen, L., Wu, S., et al. (2019). Prevalence, bacterial load, and antimicrobial resistance of salmonella serovars isolated from retail meat and meat products in China. Front. Microbiol. 10:2121. doi: 10.3389/fmicb.2019.02121
Yele, A. B., Thawal, N. D., Sahu, P. K., and Chopade, B. A. (2012). Novel lytic bacteriophage AB7-IBB1 of Acinetobacter baumannii: isolation, characterization and its effect on biofilm. Arch. Virol. 157, 1441–1450. doi: 10.1007/s00705-012-1320-0
Zaki, S. A., and Karande, S. (2011). Multidrug-resistant typhoid fever: a review. J. Infect. Dev. Ctries. 5, 324–337. doi: 10.3855/jidc.1405
Zhang, Y., Zou, G., Islam, M. S., Liu, K., Xue, S., Song, Z., et al. (2023). Combine thermal processing with polyvalent phage LPEK22 to prevent the Escherichia coli and Salmonella enterica contamination in food. Food Res. Int. 165:112454. doi: 10.1016/j.foodres.2022.112454
Zhou, K., Sun, L., Zhang, X., Xu, X., Mi, K., Ma, W., et al. (2023). Salmonella antimicrobials inherited and the non-inherited resistance: mechanisms and alternative therapeutic strategies. Front. Microbiol. 14:1176317. doi: 10.3389/fmicb.2023.1176317
Keywords: phage, drug-resistant; salmonella, characterization, biological control, foods
Citation: Islam MS, Nime I, Pan F and Wang X (2023) Isolation and characterization of phage ISTP3 for bio-control application against drug-resistant Salmonella. Front. Microbiol. 14:1260181. doi: 10.3389/fmicb.2023.1260181
Edited by:
Robert Czajkowski, University of Gdansk, PolandReviewed by:
Chunlei Shi, Shanghai Jiao Tong University, ChinaSandra Sevilla-Navarro, Centro de Calidad Avícola y Alimentación Animal de la Comunidad Valenciana, Spain
Bukola Adenike Onarinde, University of Lincoln, United Kingdom
Copyright © 2023 Islam, Nime, Pan and Wang. This is an open-access article distributed under the terms of the Creative Commons Attribution License (CC BY). The use, distribution or reproduction in other forums is permitted, provided the original author(s) and the copyright owner(s) are credited and that the original publication in this journal is cited, in accordance with accepted academic practice. No use, distribution or reproduction is permitted which does not comply with these terms.
*Correspondence: Fan Pan, fan.pan@siat.ac.cn; Xiaohong Wang, wxh@mail.hzau.edu.cn
†These authors have contributed equally to this work