- 1Department of Botany and Microbiology, Faculty of Science, Zagazig University, Zagazig, Egypt
- 2Department of Chemistry, Faculty of Science, Zagazig University, Zagazig, Egypt
- 3Department of Biochemistry, Faculty of Agriculture, Zagazig University, Zagazig, Egypt
- 4Department of Clinical Microbiology, Infection and Immunology, Umeå University, Umeå, Sweden
- 5Department of Radiation Sciences, Oncology, Umeå University, Umeå, Sweden
The antibacterial and anti-biofilm activities of the 11S globulins isolated from lupin seeds (Lupinus termis), and its methylated derivative (M11S), were investigated against seven pathogenic gram-positive and gram-negative bacteria. The MIC of 11S ranged from 0.1 to 4.0 μg/ml against 0.025 to 0.50 μg/ml for M11S, excelling some specific antibiotics. The MICs of M11S were 40–80 times lower than some specific antibiotics against gram-positive bacteria and 2–60 times lower than some specific antibiotics against gram-negative bacteria. One MIC of 11S and M11S highly reduced the liquid growth of all tested bacteria during 24 h at 37°C. They also inhibited biofilm formation by 80%−86% and 85%−94%, respectively (gram-positive), and 29%−44% and 43%−50%, respectively (gram-negative). M11S prevented biofilm formation by gram-positive bacteria at minimum biofilm inhibitory concentration (MBIC), 0.025–0.1 μg/ml against 0.1–0.5 μg/ml for gram-negative bacteria, i.e., 4–20 times and 4–7 times anti-biofilm inhibitory action compared with 11S, respectively. Biofilm formation of two bacteria revealed no adhered cells on glass slides for 24 h at 37°C, i.e., was entirely prevented by one MBIC of 11S and M11S. Scanning electron microscopy indicated microbial biofilm deformation under the action of 11S and M11S, indicating their broad specificity and cell membrane-targeted action.
1. Introduction
Antibiotic resistance has reached world records, dangerously threatening public health (Gonzalez-Zorn, 2021). Biofilm-forming bacteria, e.g., Pseudomonas aeruginosa and Staphylococcus aureus, are among the most virulent and problematic pathogens, causing life-threatening hazards. Biofilm can protect bacterial species from environmental challenges, e.g., nutrient depletion and the threat of antibiotics (McLaughlin et al., 2018), as a consequence of forming dormant permanent cells resisting conventional antibiotics. Therefore, there is a crucial demand for novel treatment strategies affecting resistant strains and persisting bacterial biofilms. Antimicrobial peptides (AMPs) have successfully recorded inhibitory actions against bacterial biofilm before or after its formation (Dawgul et al., 2014).
AMPs appeared as promising antiplaque agents preventing bacterial colonization at early biofilm developmental stages (Hancock and Lehrer, 1998). AMPs are already ancient in origin and ubiquitous. They are naturally produced by bacteria, plants, vertebrates, and invertebrates (Altman et al., 2006) and are essential to human innate immunity. Recently, AMPs have drawn attention as an alternative class of antimicrobials with high selectivity for prokaryotes while exhibiting minimal emergence of microbial resistance (Concannon et al., 2003). In addition, AMPs were reported to reduce biofilm formation at later stages (Gilmore et al., 2009).
For several years, basic proteins, spices, herbs, and herbal extracts with antimicrobial activities have been thoroughly and widely discussed (Abdel-Shafi et al., 2019b; Imbabi et al., 2021; Osman et al., 2021; Ebrahim et al., 2022; Mahmoud et al., 2023). Plant biodiversity provides an essential source of chemical compounds with variable and diversified multiple therapeutic applications, including antiviral, antibacterial, antifungal, and anticancer activities (Osman et al., 2013). Legumes represent an essential component of the traditional diets of many regions worldwide (Ebert, 2014). Principally, lupin seeds are distinctly characterized by a virtually absent starch and high protein content compared with other legumes such as beans and peas (Torres et al., 2005). Globulins and albumins are the two main protein fractions in lupin seeds, present at a 9:1 quantitative ratio. Lupin globulin consists of two major subunits called α-conglutin (11S and “legumin-like”) and β-conglutin (7S and “vicilin-like”), accounting for ~33 and 45% of the total protein content in Lupinus albus, respectively (Dourado et al., 2019).
Several strategies have been suggested to enhance the antimicrobial activities of proteins, including chemical modification to obtain new cationic proteins. Esterification is an effective and easy tool for protein modification that blocks free carboxyl groups, thus elevating the net positive charge and rendering the modified protein that is more basic and biologically active, especially as an antimicrobial agent (Sitohy et al., 2001; Sitohy and Osman, 2010). However, these modified cationic proteins have never been tested for their potential as anti-biofilm agents. It is hypothesized that these positively charged esterified products may have two mechanistic pathways in counteracting bacterial biofilm formation. The first pathway is through their direct attack on bacterial viability, affecting consequently their ability to form biofilms. The second pathway may act by competing for the negatively charged solid surfaces, either glass or metallic, by their positively charged chemical characteristics, thus affecting bacterial adhesion and biofilm formation. Therefore, this study aimed to test this hypothesis and evaluate the esterified lupin seed globulin (M11S) capacity to counteract bacterial biofilm formation compared with its native form (11S).
2. Materials and methods
2.1. Isolation, chemical characterization, and esterification of lupine proteins
2.1.1. Sample preparation, protein isolation, and 11s globulin from lupin seed isolation
Lupine seeds (Lupinus termis), obtained from a local market in Zagazig City, Sharkia, Egypt, were thoroughly cleaned and finely ground at the maximum speed with a Moulinex mixer for 3 min (Type 716, France), to pass through a 1 mm2 sieve. Then, the powder was defatted with n-hexane for 8 h, and the defatted meal was dried and stored at 4°C till analysis.
Lupin protein isolate was prepared from defatted lupin flour by acid–base precipitation as described by Johnson and Brekke (1983). Defatted lupin flour was dispersed in water at a concentration of 5% (w/v). Then, the pH was corrected to 9 using 0.1 N NaOH at room temperature before the mixture was agitated for 1 h and centrifuged for 15 min at 2,000 × g. The residue was subjected to a second round of extraction and centrifugation to boost the yield. Protein was precipitated by mixing the extracts and adjusting the pH to 4.5 with 1 N HCl and centrifuged at 2,000 × g for 15 min, followed by decantation of the supernatant and extraction of the proteins. After dialysis overnight and lyophilization, crude protein was dispersed in a small volume of distilled water at pH 7.5.
The 11S globulin was isolated from a defatted meal of lupine seeds following the procedure outlined by Abdel-Shafi et al. (2019a). To isolate the soluble components from the defatted lupin seed meal, 10 g were dissolved in 150 ml of buffer [0.03 mol/L Tris HCl at pH 8.5, 0.4 M NaCl, 10 mM mercaptoethanol, 1 mM EDTA, and 0.02% (w/v) NaN3] and centrifuged at 5,000 × g for 10 min. The solution was agitated for 1 h in a water bath at 45°C. Then, ammonium sulfate (65%−85%) precipitated 11S globulin. Following dispersing and solubilizing the precipitate, the salts were removed from the solution by dialysis using the same buffer for 48 h.
2.1.2. Esterification of 11S globulin
The procedure mentioned by Sitohy et al. (2000, 2001) was employed to esterify lupine seed 11S globulin. Esterification of 11S globulin was performed by dispersing the protein in more than 99.5% pure methanol at a concentration of 5% by weight. Hydrochloric acid was injected dropwise at the beginning of the process at a sufficient concentration to induce the protonation of carboxylates at a 50-molar ratio (mole acid/mol carboxyl group). For 10 h, we stirred each reaction mixture at 4°C. The samples were centrifuged at 10,000 × g for 10 min, following the completion of the 10 h reaction time. The resulting precipitate was dialyzed for 3 days at 4°C against distilled water and then lyophilized after being dissolved in the necessary volume of distilled water at pH 7.5. The extent of esterification of proteins was quantified according to Bertrand-Harb et al. (1991).
2.1.3. Chemical characterization
Protein pH–solubility curves were assayed using lyophilized 11S globulins and M11S from lupine seeds in the pH range of 2–10 (Chobert et al., 1991). Urea-PAGE of 11S globulins and M11S was performed following the procedure by Osman et al. (2014).
The potassium bromide (KBr) pellet method (Souillac et al., 2002) estimated the infrared spectra of protein samples at 25°C using an FT-IR spectrometer (Nicolet Nexus 470, DTGS, Thermo Scientific, Waltham, MS, USA). The 1H NMR spectra were assayed on a Bruker Avance III 400 MHZ High-Performance Digital FT-NMR spectrometer using dimethyl sulfoxide (DMSO-d6) as the solvent. Tetramethylsilane (TMS) was used as an internal standard to report the chemical shifts in δ (ppm).
2.2. Antibacterial activities against gram-negative and gram-positive bacteria
The antibacterial activities of lupine seed 11S and M11S were bio-assayed against seven tested pathogenic bacteria through disk diffusion assay (Abdel-Hamid et al., 2016) and agar well diffusion assay (Abdel-Shafi et al., 2019b).
2.2.1. Minimum inhibitory concentration
Minimum inhibitory concentration (MIC) was defined using agar well diffusion and disk diffusion methods. The MIC of an antimicrobial agent was taken as the lowest concentration (μg/ml) inhibiting the visible growth of a microorganism after overnight incubation.
2.2.2. Agar well diffusion assay
The conventional well diffusion assay was used to determine the antibacterial activity against 11S globulin and the M11S against seven tested bacteria (Nanda and Saravanan, 2009). Pure cultures of the bacterial strains were subcultured on brain heart infusion broth (BHIB) at 37°C on a rotary shaker at 200 rpm. The exponential phase culture of each strain was adjusted to 1.05 × 109 CFU ml−1 before spreading uniformly onto individual plates using sterile cotton swabs. Wells of 6 mm in diameter were created on BHI agar employing a gel-puncturing tool. Aliquots (40 μl) of tested 11S solutions (0, 0.1, 0.3, 0.5, 1, 2, 4, and 8 μg/ml) and M11S solutions (0, 0.0125, 0.025, 0.05, 0.1, 0.3, and 0.5 μg/ml) were transferred into each well. A transparent ruler measured the developed inhibition zone diameters after 24-h incubation at 37°C.
2.2.3. Disk diffusion assay
The antibacterial activities of 11S and the M11S globulins were also tested against the seven experimental pathogenic bacteria utilizing the Kirby–Bauer disk diffusion method (Hudzicki, 2009). Bacterial suspensions were evenly spread over the surfaces of nutrient agar plates. Then, sterilized 6-mm paper disks were soaked in different dilutions of 11S globulin (0, 0.1, 0.3, 0.5, 1, 2, 4, and 8 μg/ml) and various dilutions of M11S globulin (0, 0.0125, 0.025, 0.05, 0.1, 0.3, and 0.5 μg/ml) and placed on the top of nutrient agar medium allowing appropriate distances separating the samples. The agar plates were incubated at 37°C for 24 h before the diameters of inhibition zones (mm) were measured using a millimeter ruler. Finally, the diameter of the original disks (6 mm) was subtracted from the total zone diameters, giving the net inhibition zone.
2.2.4. Comparison of MICs of lupine 11S and M11S with specific antibiotics
The antibiotic sensitivity test was carried out on seven tested pathogenic bacteria using four antibiotics (Abdel-Shafi et al., 2016). The MICs of antibiotics, such as chloramphenicol, gentamycin, ciprofloxacin, ciprofloxacin, vancomycin, ciprofloxacin, and ciprofloxacin antibiotics, were determined against Listeria ivanovii, Klebsiella oxytoca, Salmonella typhimurium, Listeria monocytogenes, S. aureus, P. aeruginosa, and Proteus mirabilis, respectively.
2.2.5. Bacterial growth curve (turbidity test)
An aliquot (50 μl) of 1 MIC of each tested substance (11S and M11S) was added to 4-h-old bacterial cultures in BHIB, incubated at 37°C, and dispensed into the wells of a 96-well plate. The negative control was BHIB only, whereas the positive control contained cell cultures without adding treatment. Growth was determined by measuring turbidity (optical density) at 600 nm for 24 h using a microplate reader (Bio-Rad 680XR, Hertfordshire, U.K.).
2.2.6. Biofilm inhibition activity
The anti-biofilm activity of 11S and M11S was assessed according to the protocol mentioned by Saporito et al. (2018). In brief, overnight cultures of the seven tested bacteria were 100 times diluted before inoculating 90 μl of bacterial suspension into wells prefilled with 10 μl of the tested substances at concentrations equivalent to 1 MIC, 0.1 MIC, and 0.01 MIC in a microtiter plate. In the control wells, 10 μl of MQ-water was added instead of the sample. After 24-h incubation at 37°C, the supernatants were removed, and the wells were gently washed twice with 150 μl/well of phosphate-buffered saline (PBS) to remove non-adhered planktonic bacteria and cellular debris. Next, the attached biofilms were stained by adding 125 μl/well of crystal violet (0.1% w/v in water) and incubated for 10 min at room temperature. Then, the excess dye was washed out with PBS while the stained biofilm was dissolved by adding 200 μl ethanol (96%) to each well for 10 min. Eventually, 100 μl of each well was transferred to a clean flat bottom microtiter plate, and the absorbance was recorded at 595 nm in a microplate reader (Synergy HT, BioTek). The biofilm inhibition (%) was estimated by comparing the optical density values for the treated samples with the untreated control according to the following formula:
2.2.7. Minimum biofilm inhibitory concentration
Inhibition of biofilm formation was assessed according to Nostro et al. (2007). Aliquots (100 μl) of an overnight culture (108 CFU ml−1) in BHIB supplemented with 1% (w/v) glucose were dispensed into each well of a 96-well plate and combined with another 100 μl of 11S (0.05, 0.1, 0.3, 0.5, 1, 2, and 4 μg/ml) or 100 μl of M11S (0.006, 0.0125, 0.05, 0.1, 0.3, and 0.5 μg/ml) for M11S. The negative control was BHIB only, whereas the positive control contained cell cultures without 11S or M11S. Following 24-h incubation at 37°C, the liquid layer of each well was decanted and gently rinsed twice with 300 μl of PBS (pH: 73 ± 03). The plates were air-dried for 30 min, stained with 0.1% (w/v) crystal violet for 30 min at room temperature (Wijman et al., 2007), washed three times with PBS (200 μl per well), and dried. The crystal violet was, then, solubilized using 10% (v/v) glacial acetic acid, and the OD was measured at 595 nm using a microplate reader (Bio-Rad 680XR). The minimum biofilm inhibitory concentration (MBIC) was defined as the lowest level of the tested substance causing at least 90% inhibition in biofilm biomass compared with the untreated control using the following formula:
2.2.8. Quantitative biofilm formation assay
The quantitative biofilm formation by both S. aureus and K. oxytoca was assayed using the protocol according to Jeyasekaran et al. (2003). Clean, grease-free glass slides were placed in 100 ml screw-cap bottles containing 48 ml of BHIB and autoclaved. Then, the medium was inoculated with 2 ml of cultures grown in BHIB, combined with 10 μl of 11S and M11S at concentrations equivalent to MBIC, and incubated for 24 h at 37°C under shaking conditions at 150 rpm. The glass slides were aseptically removed and washed in sterile PBS to remove unattached cells. The cells were removed by rubbing with a sterile cotton swab (Hi-Media). The swab was then transferred to 10 ml PBS and shaken vigorously, and serial 10-fold dilutions of each strain were plated on BHI agar. Multiple swabs were used for the same area and inoculated in PBS to limit the variations in the data resulting in the incomplete removal of the cells from the glass slides. Colony count was performed and calculated for cells in biofilm/cm2. The experiment was repeated thrice.
2.2.9. Scanning electron microscopy of bacterial biofilm
Two bacteria, S. aureus (gram-positive) and K. oxytoca (gram-negative), were selected to compare the morphological appearance of the bacterial biofilm developed in the absence or presence of 11S and M11S using Jeol JSM-6510 L.V. Scanning electron microscopy (SEM) and following the standard protocols (Adukwu et al., 2012). Two-cm diameter sterile stainless-steel disks (Goodfellow Cambridge Ltd, Huntingdon, U.K.) were immersed in the wells of six-well plates (Nunclon Surface, Roskilde, Denmark) containing each 5 ml of BHIB and 100 μl of an overnight culture (108 CFU ml−1). Then, the two tested bacteria were treated with 1 MIC of 11S globulin and M11S, i.e., 0.5 and 0.025 μg/ml in the case of S. aureus and 0.5 and 0.1 μg/ml in the case of K. oxytoca, respectively. The control for each bacterium did not receive any treatment. All plates were placed in a shaking incubator for 24 h of incubation period, after which the disks were removed and gently washed three times with sterile PBS to eliminate the loosely attached bacterial cells, followed by a 2-h fixation with 2.5% (v/v) glutaraldehyde in PBS solution at 4°C. Finally, the plates were washed twice with PBS for 10 min before dehydration with graded ethanol concentrations: 30%, 50%, 70%, 90%, and 100% (v/v), followed by a 10-min subjection to serial dilutions of acetone (30%, 50%, and 100%) at 25°C. The samples were then dried to a critical point using an automated critical point dryer (Leica EM CPD300, GmbH, Mannheim, Germany), before coating with gold–palladium and observation at 30 K.V.
2.2.10. Statistical analysis
SPSS program version 23 statistically analyzed the mean and standard deviation data. Two-way ANOVA tests were performed to compare the different microorganisms (i.e., L. monocytogenes, K. oxytoca, L. ivanovii, S. aureus, P. mirabilis, P. aeruginosa, and S. typhimurium), as well as the different concentrations, i.e., 0.05, 0.1, 0.3, 0.5, 1, 2, 4, and 8 μg/ml for 11S and 0.0125, 0.025, 0.05, 0.1, 0.3, and 0.5 μg/ml for M11S, and the interactions between them for inhibition zone diameter. The null hypothesis was rejected if the p-value of the ANOVA was <0.05, referring to the absence of significant differences between the means. P-values higher than 0.05 indicated the absence of significant differences. The two-way ANOVA test with a post-hoc test using Duncan's test was applied to make multiple comparisons between the averages of different groups. Means followed by the same letter were not significantly different at the 5% probability level (Duncan's multiple range tests). The results were presented as the means of three replicates ± SD.
3. Results
3.1. Chemical characterization of 11S and M11S
The data in Figure 1A confirm that esterifying lupine 11S globulins accelerated its migration into the cathode in Urea-PAGE. This change was further confirmed by tracing the pH–solubility curves of both the native (11S) and esterified protein (M11S), indicating that the isoelectric point of the methylated form (M11S) was relatively higher (pH 8.8) than that of the native form (11S), i.e., pH 7.3.
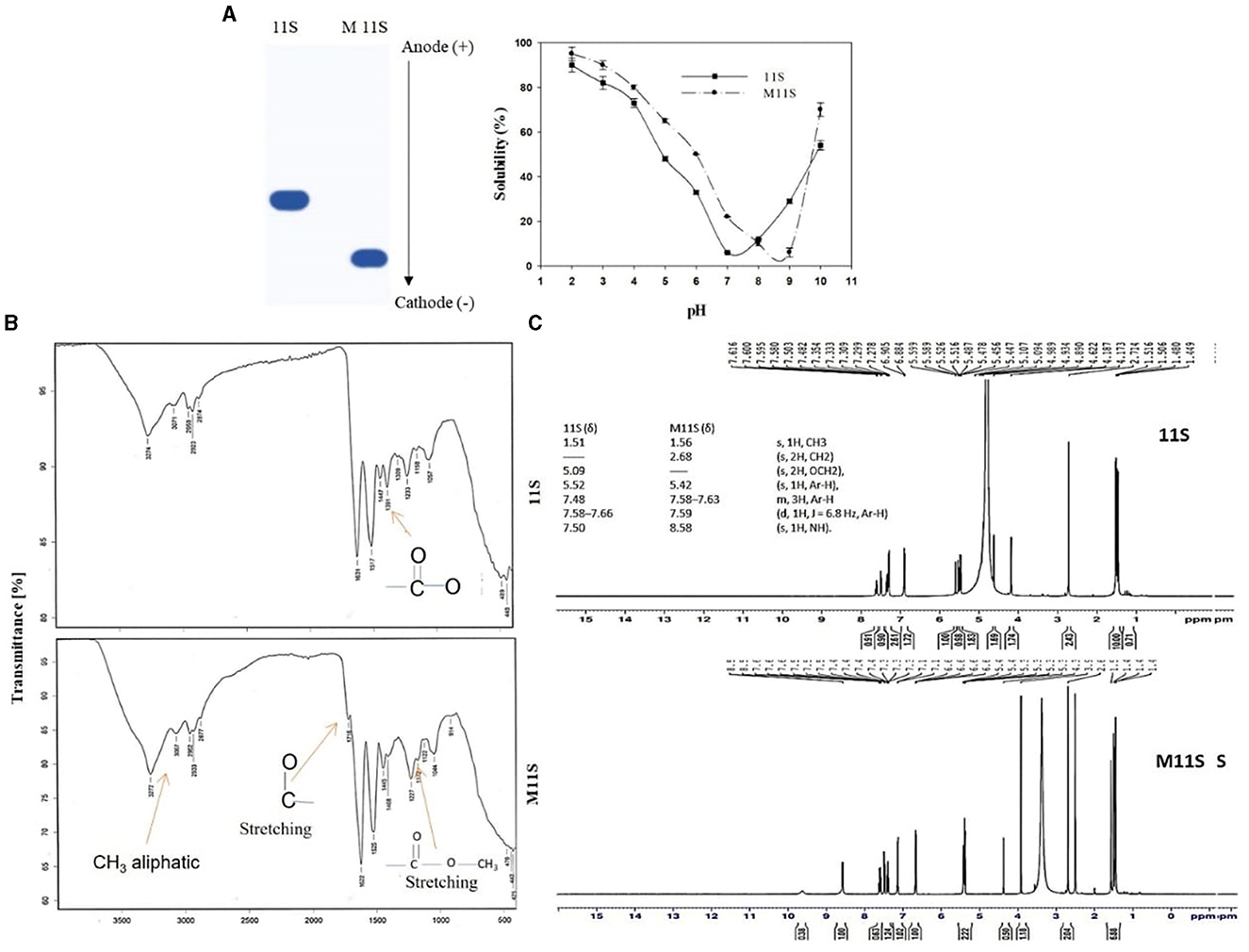
Figure 1. (A) UREA-PAGE electropherogram and pH–solubility curves of native (11S) and methylated lupine seed globulin (M11S), (B) FT-IR spectra of lupine seed 11S and its methylated derivative M11S globulins, and (C) 1HNMR spectroscopy of native (11S) and its methylated 11S globulin (M11S).
The FTIR data in Figure 1B show that the modified lupine globulin (M11S) contains three specific absorption peaks at 3,067, 1716, and 1,172 cm−1, which are absent in the native form (11S), referring to the presence of aliphatic CH3 group, C=O stretching, and C=O stretching ester, respectively, according to I.R. Spectrum Table (sigmaaldrich.com). Alternatively, the native form (11S) showed an I.R. absorption peak at 1,391 cm−1, absent from the modified form (M11S), referring to the free carboxylate groups. 1H NMR of native 11S globulin indicates the presence of main six δ signals at 1.51 (s, 1H, CH3), 5.09 (s, 2H, OCH2), 5.52 (s, 1H, Ar-H), 7.48 (d, 1H, J = 6.8 Hz, Ar-H), 7.50 (s, 1H, N.H.), and 7.58–7.66 (m, 3H, Ar-H). The esterified product (M11S) indicated other 6 signals, 4 of them are shared with the native form (11S), while the other 2 are different (Figure 1C), precisely the signal 5.09 which is present in the native structure (11S) and the signal 2.68 which is present only in the modified state (M11S). Additionally, the signal appearing at 7.5 Hz in the native protein (11S) shifted to 8.56 Hz in the modified protein (M11S).
3.2. Antibacterial activity of 11S and M11S
Initially, the antibacterial activities of 11S and M11S were tested at a high concentration (1000 μg/ml) by the disk diffusion method, demonstrating antibacterial activity against all the tested bacteria, while the whole lupine seed protein isolate (LPI) did not produce any inhibition zones (data not shown).
3.2.1. Minimum inhibitory concentration
The data in Table 1 and Supplementary Figure 1 show that lupine 11S affected the gram-positive and gram-negative bacteria (S. aureus and K. oxytoca, respectively) equally in terms of the specific MIC, detected at 0.5 μg/ml for both. However, the inhibition zone area data indicated that the gram-positive bacteria were more susceptible than the gram-negative ones. Chemically modifying 11S into the methylated form (M11S) enhanced the antibacterial action, where the effect on the gram-positive bacteria was much more pronounced than on the gram-negative bacteria. Regarding MIC, M11S recorded 0.025 and 0.100 μg/ml against the gram-positive and the gram-negative bacteria, respectively. Expanding the analysis to the whole bacterial collection (3 gram-positive and 4 gram-negative) confirmed that 11S and M11S were more inhibitory on gram-positive than gram-negative bacteria (Table 1). At the same time, the antibacterial action of M11S was more effective on the two bacterial types (gram-positive and gram-negative bacteria) than on 11S. M11S achieved the lowest MIC against both gram-positive and gram-negative bacteria, i.e., 0.025, 0.025, and 0.1 μg/ml in the first case (L. monocytogenes, S. aureus, and L. ivanovii), respectively, and 0.1, 0.3, 0.5, and 0.1 μg/ml in the second case (K. oxytoca, P. mirabilis, P. aeruginosa, and S. typhimurium), respectively. The MIC of M11S was 4, 20, and 10 times less than the corresponding values of 11S against the three tested gram-positive bacteria, respectively, and 5, 3, 8, and 20 times lower than the corresponding values of 11S against the gram-negative bacteria, respectively.
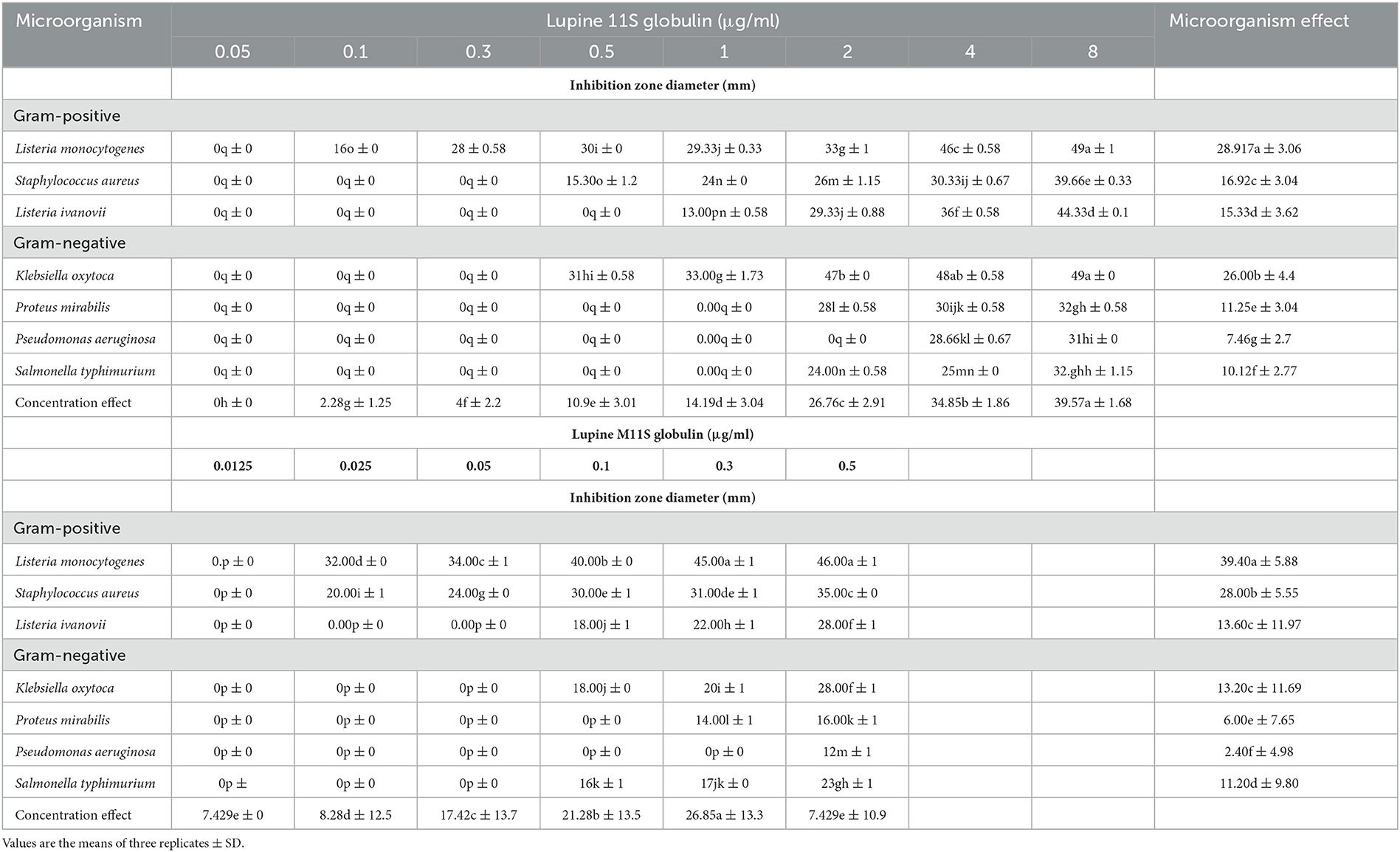
Table 1. Bacterial inhibitory action of graded concentrations of lupines 11S and M11S using agar well diffusion assay as displayed by the Inhibition zone diameter (mm /11S μg ml−1).
3.2.2. Inhibition of liquid bacterial growth
The data in Figure 2, representing the 24-h growth curves of seven pathogenic bacteria subjected to one MIC of 11S and M11S, show general substance-based growth inhibition. In the liquid media, 1.0 MIC of both agents was nearly sufficient to completely prevent the 24-h liquid bacterial growth of all the tested microorganisms. Listeria monocytogenes and K. oxytoca were the most inhibited organisms.
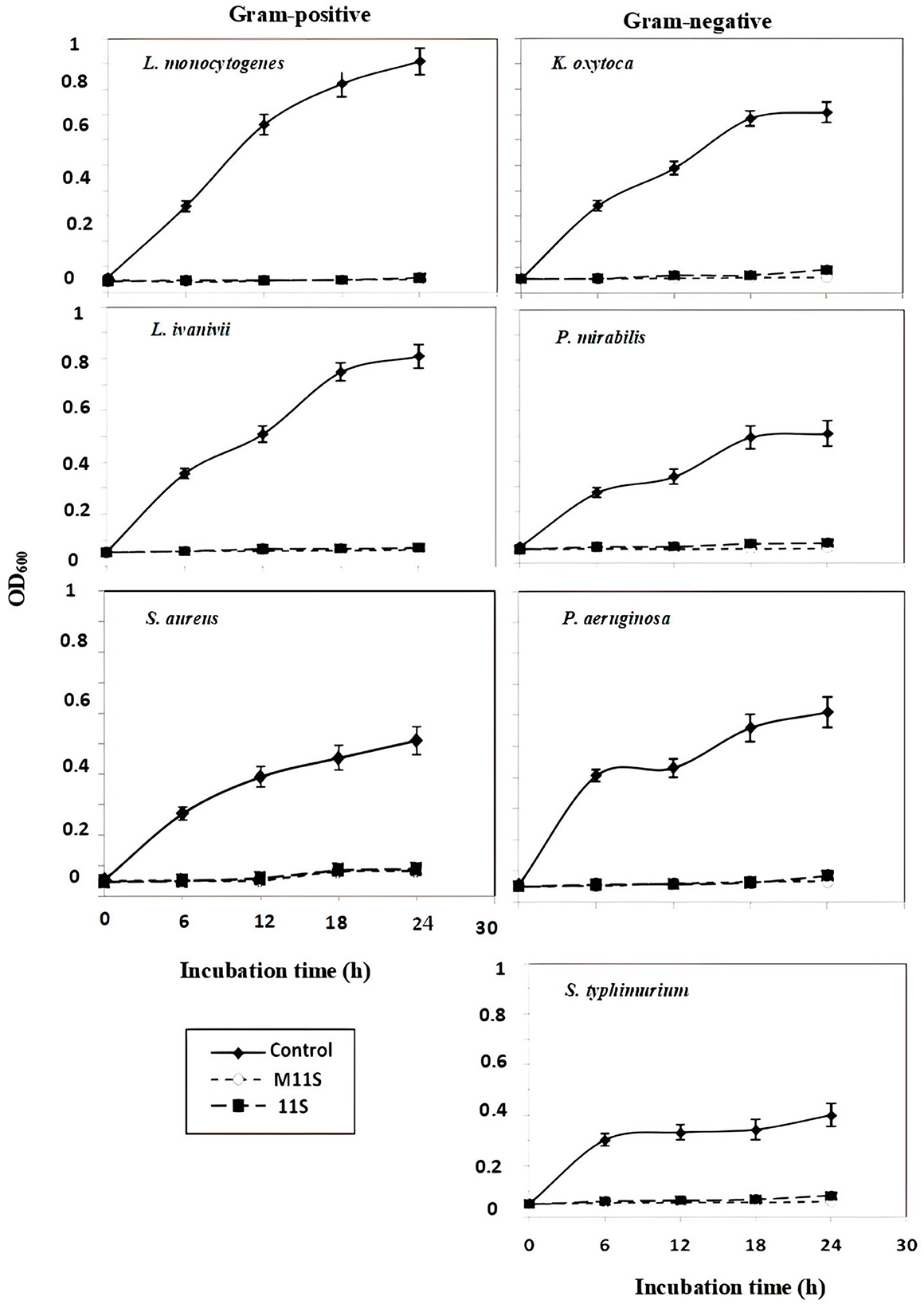
Figure 2. Twenty-four-hour growth curves of seven pathogenic bacteria (three gram-positive and four gram-negative) in the presence of 1.0 MIC of 11S and M11S compared with control (untreated bacteria). All the values were the means of three replicates ± SD.
3.2.3. Comparison of the MIC of 11s and M11S with specific antibiotics
Figure 3 presents comparative measurements of MIC of the 11S and M11S against seven pathogenic bacteria as compared with the action of specific antibiotics. The antibiotics such as chloramphenicol, ciprofloxacin, and vancomycin were considered as typically specific against the three investigated gram-positive bacteria, namely L. monocytogenes, L. ivanovii, and S. aureus, exhibiting MIC at 2, 7.5, and 1 μg/ml, respectively, while the four investigated gram-negative bacteria were explicitly susceptible to gentamycin and ciprofloxacin. The first bacterium (K. oxytoca) showed MIC at 6 μg/ml. In contrast, the three other bacteria (P. mirabilis, P. aeruginosa, and S. typhimurium) were susceptible to ciprofloxacin at 2, 1, and 4 μg/ml, respectively. Comparatively, the MICs of M11S were much lower than the specific antibiotics but slightly lower than 11S.
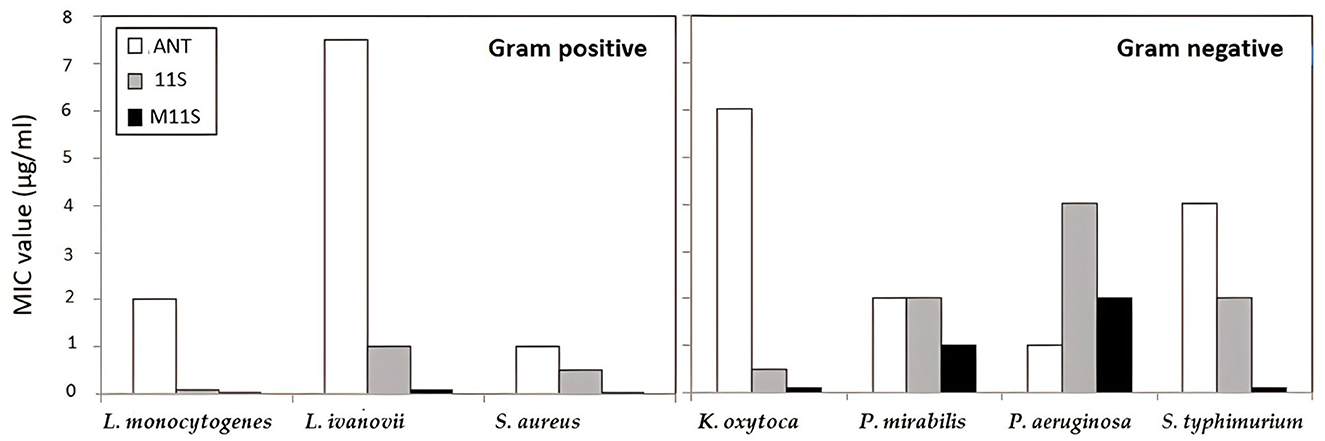
Figure 3. Comparison of MIC of the tested lupine 11S globulins and its methylated form M11S. Chloramphenicol, ciprofloxacin, and vancomycin were used as specific against Listeria ivanovii, Listeria monocytogenes, and Staphylococcus aureus, (MIC at 7.5, 2, and 1 μg/ml, respectively). The four investigated gram-negative bacteria (Klebsiella oxytoca, Salmonella typhimurium, Pseudomonas aeruginosa, and Proteus mirabilis) were specifically susceptible to gentamycin, ciprofloxacin, ciprofloxacin, and ciprofloxacin at MIC 6, 4, 1, and 2 μg/ml, respectively.
In the case of the three tested gram-positive bacteria, the MICs of 11S and M11S were 0.1 and 0.025 μg/ml against L. monocytogenes while 1 and 0.1 μg/ml against L. ivanovii, respectively. Compared with the specific antibiotics, the MICs of M11S were 80, 75, and 40 times lower than those of the specific antibiotics against L. monocytogenes, L. ivanovii, and S. aureus, respectively. At the same time, they were 60, 2, 0.5, and 40 times lower than the respective values against the gram-negative bacteria, such as K. oxytoca, P. mirabilis, P. aeruginosa, and S. typhimurium.
3.2.4. Anti-biofilm activity of 11S and M11S
The data in Figure 4 show that the inhibitory action of 11S and M11S applied at 0.01, 0.1, and 1.0 MIC levels on the bacterial biofilm formation was considerably concentration-dependent. One MIC of 11S and M11S inhibited the gram-positive film formation by 80–86% and 85–94%, respectively. Lower anti-biofilm inhibitory actions occurred against the gram-negative bacteria, amounting to 29%−44% and 43%−50% by 11S and M11S (1 MIC), respectively. M11S achieved 9%, 6%, and 4% increases in the biofilm inhibitory action in the case of the gram-positive bacteria (L. monocytogenes, L. ivanovii, and S. aureus). The anti-biofilm formation activity exerted by M11S against the gram-negative bacteria such as K. oxytoca, P. aeruginosa, P. mirabilis, and S. typhimurium registering 4%, 14%, 12%, and 48% increases over 11S.
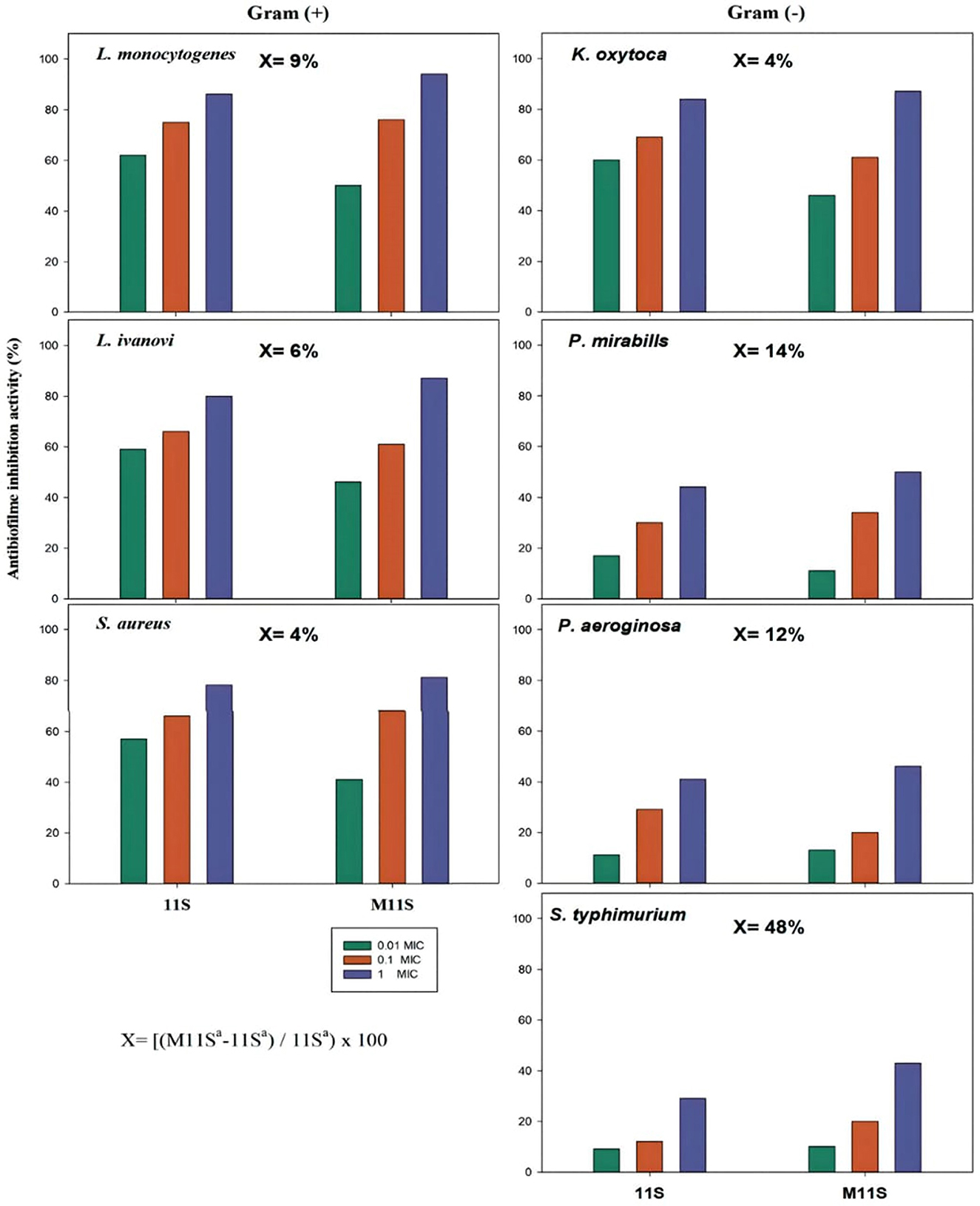
Figure 4. Inhibitory activity of native (11S) and methylated (M11S) lupine seed globulin on the bacterial biofilm formation by gram-positive and gram-negative bacteria at 0.01, 0.1, and 1.0 MIC levels. The increase in anti-biofilm activity (x) of M11S over 11S was calculated at 1 MIC according to the equation under the figure, where a is the anti-biofilm activity.
3.2.5. Minimum biofilm inhibitory concentration
Matching the inhibitory action of 11S and M11S with bacterial film formation by gram-positive and gram-negative bacteria in terms of the minimum biofilm inhibitory concentration (MBIC), with their corresponding MICs (Table 2), revealed close low values for each. Lupine 11S globulin prevented biofilm formation by gram-positive bacteria (L. monocytogenes, S. aureus, and L. ivanovii) at MBIC of 0.1, 0.5, and 1 μg/ml against 0.025, 0.025, and 0.1 μg/ml for M11S. Similarly, the MBIC against the gram-negative bacteria, such as K. oxytoca, P. mirabilis, P. aeruginosa, and S. typhimurium, recorded 0.5, 2, 4, and 2 μg/ml in the case of 11S against 0.1, 0.3, 0.5, and 0.1 μg/ml in the case of M11S. The MBIC and MIC of 11S and M11S (Table 2) revealed very close values.
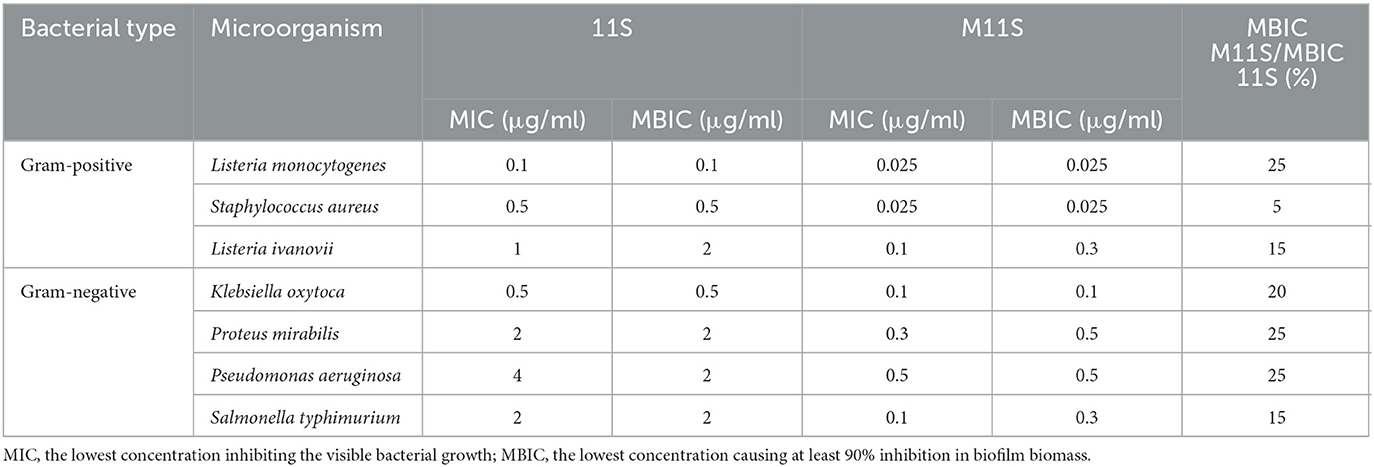
Table 2. Minimum biofilm inhibitory concentration (MBIC) of 11S and M11S assayed by microtiter plate technique as compared to the minimum inhibitory concentration (MIC).
3.2.6. Quantitative biofilm formation assay
The quantitative biofilm formation assay was conducted to enumerate both S. aureus and K. oxytoca after overnight growth on BHIB in the absence or presence of 11S and M11S at 1 MBIC at 37°C under 150 rpm shaking condition followed by 24 h of incubation. Colony count was performed and calculated as cells per 1 cm2 biofilm. The data in Supplementary Figure 2 revealed the actual number of cells present in the biofilms formed by S. aureus (gram-positive) and K. oxytoca (gram-negative) as 7.23 and 6.24 log CFU/cm2 in the absence of the two antibacterial agents. Both 11S and M11S, at 1 MBIC at 37°C under 150 rpm shaking condition followed by 24-h incubation, revealed no attached cells, confirming that they have equal potencies to completely counteract the formation of microbial biofilms developed by either gram-positive or gram-negative bacteria. This test indicated complete prevention of the biofilm formation in each case under low concentrations of the two antibacterial agents, i.e., 0.5 and 0.5 μg/ml in the case of 11S and 0.025 and 0.1 μg/ml in the case of M11S against S. aureus and K. oxytoca, respectively.
3.2.7. SEM image analysis
The SEM images in Figure 5 represent the bacterial biofilms formed by two bacteria, namely S. aureus (gram-positive) and K. oxytoca (gram-negative), on stainless-steel disks immersed in the wells of 6-well plates containing BHIB and 100 μl of an overnight culture (108 CFU ml−1) in the absence or presence of 11S and M11S (1 MIC) after 24-h incubation at 37°C. The SEM image of the control S. aureus (gram-positive) formed biofilm presents spherical bacterial cells dispersed on the adhered biofilm matrix. The bacterial cells lay on the extracellular polymer surface without fragmented or indented cells. The cells seem adherent to each other and confluent. The presence of 1.0 MBIC concentrations of 11S globulin (0.5 μg/ml) and M11S (0.025μg/ml) in BHIB media containing S. aureus and K. oxytoca reduced the relative content of the intact cells, thus destroying the biofilm after 2.5 h of incubation at 37°C. After treatment with 11S or M11S (1 MBIC), a few regular cells appeared, while most cells were either moderately or entirely deformed. The resulting apparent biofilm deformation was more observed in the case of M11S.
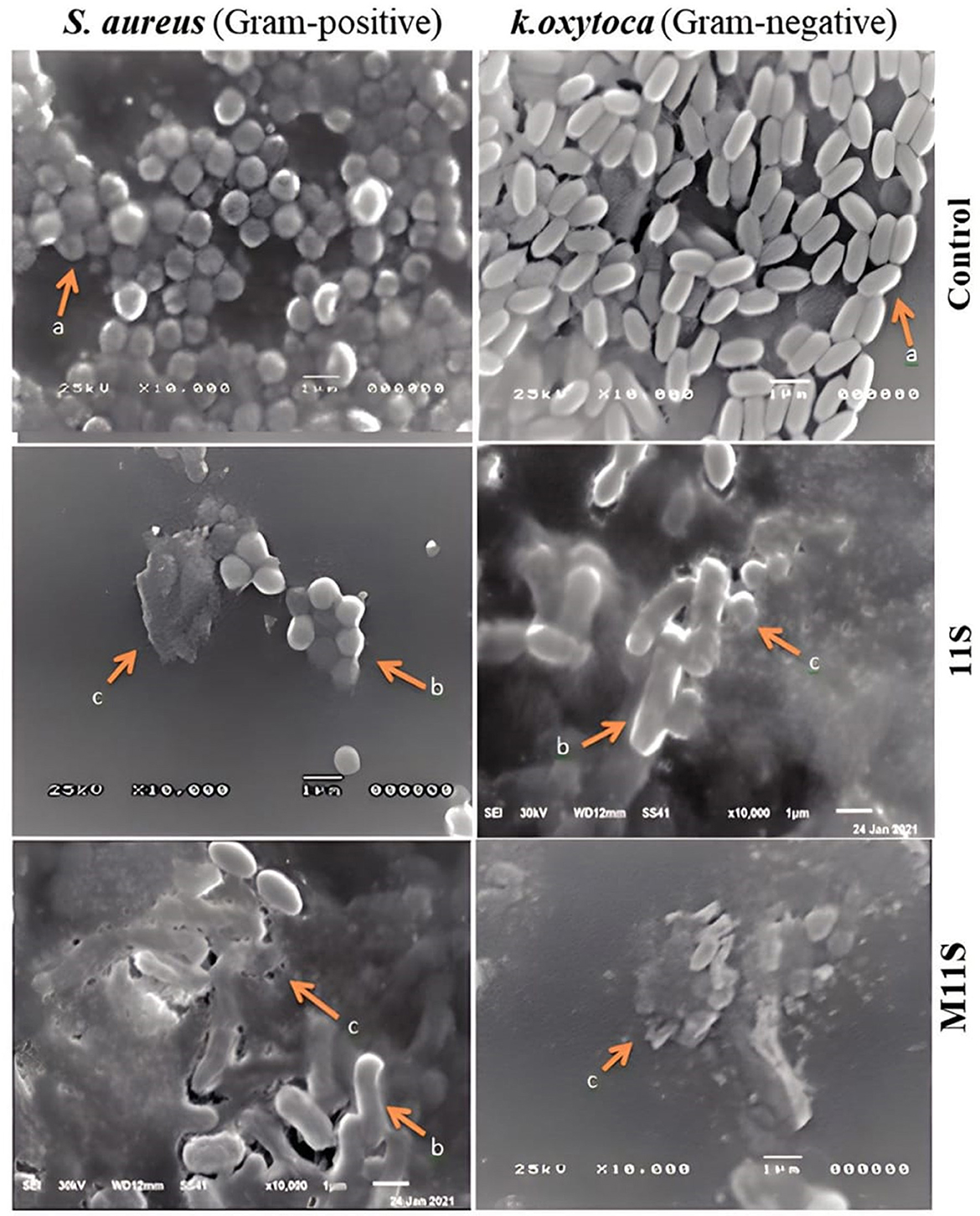
Figure 5. SEM images (10,000×) of biofilm formation by Staphylococcus aureus (gram-positive) and Klebsiella oxytoca (gram-negative) as influenced by the presence of 1 MIC of 11S and M11S, where (a) = intact or none deformed, (b) = moderately deformed, and (c) = completely deformed.
4. Discussion
The enhanced migration of the methylated form (M11S) toward the cathode proves its bigger net positive charges than the native globulin (11S), apparently due to the neutralization of the side-chain negative carboxylate groups on the protein molecule following the study by Mahgoub et al. (2011). Elevating the isoelectric points confirms the more basic nature and higher positive charge of the modified protein, agreeing with Sitohy and Osman (2010). The value of the isoelectric point (IEP) of lupine 11S is higher than other previously studied similar fractions isolated from legumes, e.g., soy protein (Osman et al., 2016) and chickpea protein (Sitohy et al., 2011), explaining the difference in the antibacterial activity according to legume sources. Consequently, the IEP of the methylated (M11S) excelled over the corresponding methylated fractions from other legume sources (Sitohy et al., 2021).
Comparing the FTIR spectrum data of M11S with the native form (11S) confirmed the formation of the ester groups and the disappearance of the free carboxylic groups on the modified protein molecules. The similarity of the other absorption peaks indicates that the modification reaction was mainly restricted to the regions rich in free carboxylic groups arising primarily from residual aspartate and glutamate groups on the surface protein molecules.
The presence of two δ signals in the HNMR profile of M11S (2.68) is different from those of 11S (5.09), whereas the other four signals are similar, confirming that the esterification reaction induced only a few structural changes while maintaining most of the original structural features. Additionally, the shift of the signal appearing at 7.5 Hz in the native protein (11S) referring to the imine groups (s, 1H, N.H.) to be at 8.56 Hz in the modified protein (M11S) may be due to the incorporated electron-donating methyl groups which enhance the electron intensity around the imine groups transforming them into a de-shielded status. Alternatively, the lower levels of proton resonance for most groups in the modified protein (M11S) may refer to a general shielding process by the incorporated methyl groups. Initially, these chemical changes predicted and supported outstanding biological activities of the methylated products as antibacterial and anti-biofilm formation agents.
The values of both MIC and inhibition zones indicated that protein modification generally enhanced the antibacterial activity against the two bacterial types (gram-positive and gram-negative), following similar proteins (Abdel-Shafi et al., 2019b). Both modified plant proteins (Osman et al., 2014) and animal proteins (Chobert et al., 2007) were reported for higher antimicrobial activities. The more effective antibacterial action of M11S than 11S on the two bacterial types (gram-positive and gram-negative bacteria) follows previous results (Sitohy et al., 2013). It can be calculated that the MIC of M11S was 4, 20, and 10 times less than the corresponding values of 11S against the three tested gram-positive bacteria, respectively, and 5, 3, 8, and 20 times lower than the corresponding values of 11S against the gram-negative bacteria, respectively. Thus, M11S can be preferred to 11S as an antibacterial. However, both of them can be classified as broad-specific and highly effective due to their relative richness in hydrophobicity and alkalinity (Sitohy et al., 2012), initiating the electrostatic interactions between the antimicrobial agent's positive charges and the bacterial cell walls or membranes' negatives charges.
The 24-h growth of the tested gram-positive and gram-negative bacteria subjected to one MIC of 11S and M11S was nearly entirely prevented by both agents in agreement with previous results (Osman et al., 2018). However, the antibacterial inhibition recorded in this study seems considerably higher, probably due to some chemical structural differences of the original proteins. The lower levels of MIC of M11S than the specific antibiotic may refer to higher antibacterial action, following previous reports (Sitohy and Osman, 2010). This is the first time to record such minimal MIC values, resulting probably from the specific chemical characteristics of the original globulin (11S) and the esterification process. This enhanced antibacterial activity of M11S is expected to reflect considerable anti-biofilm formation activity.
In the current study, the exhibited high capacity of 11S and M11S to inhibit biofilm formation is evidently due to their antimicrobial effect on the viable bacteria preventing biofilm forming. This association between antimicrobial and anti-biofilm formation was previously observed as treating the surface of a medical appliance with a cationic antimicrobial protein (lysozyme), inhibiting bacterial colonization and preventing biofilm formation (Song et al., 2023).
Alternatively, the cationic M11S and 11S could have affected biofilm formation by disrupting the gel formed by the extracellular polymeric substances in agreement with Zhang et al. (2022), evidencing that two cationic dextrans and polyethyleneimine (PEI) destructed P. aeruginosa biofilms. In addition, the cationic conjugates of phthalocyanine derivatives with the nanosphere “NH2-ZnONPy” showed great eradication potential on the mixed microbial biofilms of S. aureus and Escherichia coli and fungus (Candida albicans) (Sindelo et al., 2022). The positively charged phthalocyanine analogs showed potential anti-biofilm agents against S. aureus and E. coli (Openda and Nyokong, 2023). Thus, the current results confirm that the previous studies on the impact of the protein-positive charge on antibacterial efficiency (Abdel-Shafi et al., 2023; Mahmoud et al., 2023) can predict a potential anti-biofilm formation activity.
The observed close values of MBIC and MIC of 11S and M11S suggest that the tested substances have affected the bacteria in their free state before forming the bacterial biofilm. However, the always smaller value of MBIC of M11S than 11S (i.e., only 5%−25%) may refer to a higher anti-biofilm potential. Calculative comparisons substantiated this conclusion as the anti-biofilm inhibitory action of M11S represented 4–20 times that of 11S against the gram-positive bacteria and 4–7 times against the gram-negative bacteria. The recorded lower values of the MBICs of M11S than 11S confirm its higher anti-biofilm capacity, probably due to the higher cationic nature following Sindelo et al. (2022) and Openda and Nyokong (2023).
The quantitative biofilm formation assay of S. aureus and K. oxytoca revealing no attached cells when subjected to 1 MBIC of 11S and M11S at 37°C indicated equal potencies to completely counteract biofilm formation by low concentrations of the two agents (0.5 and 0.5 μg/ ml). This action resulted probably from a quick adhesion of the tested proteins (11S and M11S) on the surface of Petri dishes due to their cationic nature, preventing the bacterial adhesion agreeing with Singh et al. (2011) and stating that increased protein adsorption on the solid surface reduced the bacterial adhesion. Since different bacterial proteins are involved in biofilm formation (Abdel-Hamid et al., 2020), the applied agents (11S and M11S) might compete with the bacterial proteins for the adhesion sites on the solid surface, affecting the biofilm formation. The multifunctional soy protein adhesives with antibacterial properties could adhere to solid surfaces (Hsu et al., 2023). Accordingly, the positively charged esterified M11S might have electrostatically interacted with the negative charges of the solid glass surface and thus prevented bacterial biofilm formation. Both glass and metallic surfaces have negatively charged surfaces, giving an advantage to the M11S to attach and prevent bacterial biofilm formation. This fact may create different applications of these positively charged proteins in preventing bacterial biofilm formation.
The manifested SEM bacterial ultrastructures revealing a higher degree of damage by M11S than 11S may be due to more vigorous interactions with the bacterial membranes based on its higher net positive charges (Abdel-Shafi et al., 2019b). The evidenced anti-biofilm formation of 11S and M11S against gram-negative (particularly K. oxytoca) and gram-positive bacteria (S. aureus) is a significant result since the first one is known as an opportunistic and clinically dangerous pathogen (Savino et al., 2011). The second one has multiple public health concerns, is responsible for food poisoning, and can produce several virulence factors (Enan et al., 2020). The SEM-evidenced action of 11S and M11S against the two bacteria followed previously published studies on other similar antibacterials (Mahmoud et al., 2023).
5. Conclusion
Both 11S and M11S lupine seed globulins can be employed as effective antibacterial and anti-biofilm formation agents, both broad-specific and effective. The antibacterial activities of 11S and M11S considerably affected the growth of the pathogenic bacteria either on the solid agar or in liquid media. On the solid agar media, very minimal MIC values marked both 11S (0.1–4.0 μg/ml) and M11S (0,025–0.50 μg/ml), where the MICs of M11S were 4, 20, and 10 times lower than the corresponding values of 11S against the three tested gram-positive bacteria, L. monocytogenes, S. aureus, and L. ivanovii, respectively, and 5, 3, 8, and 20 times lower than the corresponding values of 11S against the gram-negative bacteria, K. oxytoca, P. mirabilis, P. aeruginosa, and S. typhimurium, respectively. There was an association between antimicrobial and anti-biofilm formation activities of 11S and M11S as the general antimicrobial effect on viable bacterial cells may prevent biofilm formation. The values of MBIC and MIC of the two substances (11S and M11S) were nearly close, and the MBIC of M11S is always smaller than that of 11S. Both 11S and M11S excelled specific antibiotics in antibacterial efficiency and can be nominated as proper antibacterial substitutes. The more positively charged esterified product (M11S) is highly efficient as an anti-biofilm agent since its more considerable positive charges facilitate its attachment to the negatively charged solid surfaces, either metallic, glass, or plastic, thus preventing bacteria from forming biofilms. Moreover, M11S can be highly recommended as an anti-biofilm agent.
Data availability statement
The original contributions presented in the study are included in the article/Supplementary material, further inquiries can be directed to the corresponding authors.
Author contributions
GE: Resources, Supervision, Writing—original draft. SA-S: Resources, Supervision, Writing—review and editing. ME-N: Investigation, Methodology, Software, Writing—original draft. WS: Investigation, Methodology, Software, Writing—review and editing. AO: Methodology, Software, Conceptualization, Writing—original draft, Writing—review and editing. MS: Conceptualization, Writing—original draft, Writing—review and editing, Supervision. BS: Writing—review and editing, Funding acquisition, Validation.
Funding
The author(s) declare financial support was received for the research, authorship, and/or publication of this article. This study received funding from the University of Zagazig and the County Council of Västerbotten (BS), Lions Cancer Research Fund (BS), and Kempestiftelserna (BS), Sweden.
Acknowledgments
The authors would like to thank the University of Zagazig and the County Council of Västerbotten (BS), Lions Cancer Research Fund (BS) and Kempestiftelserna (BS), Sweden.
Conflict of interest
The authors declare that the research was conducted in the absence of any commercial or financial relationships that could be construed as a potential conflict of interest.
Publisher's note
All claims expressed in this article are solely those of the authors and do not necessarily represent those of their affiliated organizations, or those of the publisher, the editors and the reviewers. Any product that may be evaluated in this article, or claim that may be made by its manufacturer, is not guaranteed or endorsed by the publisher.
Supplementary material
The Supplementary Material for this article can be found online at: https://www.frontiersin.org/articles/10.3389/fmicb.2023.1259334/full#supplementary-material
References
Abdel-Hamid, M., Goda, H. A., De Gobba, C., Jenssen, H., and Osman, A. (2016). Antibacterial activity of papain hydrolysed camel whey and its fractions. Int. Dairy J. 61, 91–98. doi: 10.1016/j.idairyj.2016.04.004
Abdel-Hamid, M., Romeih, E., Saporito, P., Osman, A., Mateiu, R. V., Mojsoska, B., et al. (2020). Camel milk whey hydrolysate inhibits growth and biofilm formation of Pseudomonas aeruginosa PAO1 and methicillin-resistant Staphylococcus aureus. Food Control 111, 107056. doi: 10.1016/j.foodcont.2019.107056
Abdel-Shafi, S., Al-Mohammadi, A.-R., Osman, A., Enan, G., Abdel-Hameid, S., Sitohy, M., et al. (2019a). Characterization and antibacterial activity of 7s and 11s globulins isolated from cowpea seed protein. Molecules 24 1082. doi: 10.3390/molecules24061082
Abdel-Shafi, S., El-Nemr, M., Enan, G., Osman, A., Sitohy, B., Sitohy, M., et al. (2023). Isolation and characterization of antibacterial conglutinins from lupine seeds. Molecules 28, 35. doi: 10.3390/molecules28010035
Abdel-Shafi, S., Osman, A., Al-Mohammadi, A.-R., Enan, G., Kamal, N., Sitohy, M., et al. (2019b). Biochemical, biological characteristics and antibacterial activity of glycoprotein extracted from the epidermal mucus of African catfish (Clarias gariepinus). Int. J. Biol. Macromol. 138, 773–780. doi: 10.1016/j.ijbiomac.2019.07.150
Abdel-Shafi, S., Osman, A., Enan, G., El-Nemer, M., and Sitohy, M. (2016). Antibacterial activity of methylated egg white proteins against pathogenic G+ and G– bacteria matching antibiotics. Springerplus 5, 1–13. doi: 10.1186/s40064-016-2625-3
Adukwu, E., Allen, S. C., and Phillips, C. (2012). The anti-biofilm activity of lemongrass (Cymbopogon flexuosus) and grapefruit (Citrus paradisi) essential oils against five strains of Staphylococcus aureus. J. Appl. Microbiol. 113, 1217–1227. doi: 10.1111/j.1365-2672.2012.05418.x
Altman, H., Steinberg, D., Porat, Y., Mor, A., Fridman, D., Friedman, M., et al. (2006). In vitro assessment of antimicrobial peptides as potential agents against several oral bacteria. J. Antimicrob. Chemother. 58, 198–201. doi: 10.1093/jac/dkl181
Bertrand-Harb, C., Chobert, J., Dufour, E., and Haertlé, T. (1991). Esterification of food proteins: characterization of the derivatives by a colorimetric method and by electrophoresis. Sci. Aliments 11, 641–652.
Chobert, J.-M., Sitohy, M., Billaudel, S., Dalgalarrondo, M., and Haertlé, T. (2007). Anticytomegaloviral activity of esterified milk proteins and L-polylysines. Microb. Physiol. 13, 255–258. doi: 10.1159/000104755
Chobert, J.-M., Touati, A., Bertrand-Harb, C., Dalgalarrondo, M., Nicolas, M.-G., Haertle, T., et al. (1991). In vitro proteolysis and functional properties of reductively alkylated β-casein derivatives. J. Dairy Res. 58, 285–298. doi: 10.1017/S0022029900029861
Concannon, S. P., Crowe, T., Abercrombie, J., Molina, C., Hou, P., Sukumaran, D., et al. (2003). Susceptibility of oral bacteria to an antimicrobial decapeptide. J. Med. Microbiol. 52, 1083–1093. doi: 10.1099/jmm.0.05286-0
Dawgul, M., Maciejewska, M., Jaskiewicz, M., Karafova, A., and Kamysz, W. (2014). Antimicrobial peptides as potential tool to fight bacterial biofilm. Acta Pol. Pharm. 71, 39–47.
Dourado, C., Pinto, C., Barba, F. J., Lorenzo, J. M., Delgadillo, I., Saraiva, J. A., et al. (2019). Innovative non-thermal technologies affecting potato tuber and fried potato quality. Trends Food Sci. Technol. 88, 274–289. doi: 10.1016/j.tifs.2019.03.015
Ebert, A. (2014). Potential of underutilized traditional vegetables and legume crops to contribute to food and nutritional security, income and more sustainable production systems. Sustainability 6, 319–335. doi: 10.3390/su6010319
Ebrahim, A. E., Abd El-Aziz, N. K., Elariny, E. Y., Shindia, A., Osman, A., Hozzein, W. N., et al. (2022). Antibacterial activity of bioactive compounds extracted from red kidney bean (Phaseolus vulgaris L.) seeds against multidrug-resistant Enterobacterales. Front. Microbiol. 13, 1035586. doi: 10.3389/fmicb.2022.1035586
Enan, G., Al-Mohammadi, A.-R., Mahgoub, S., Abdel-Shafi, S., Askar, E., Ghaly, M. F., et al. (2020). Inhibition of Staphylococcus aureus LC 554891 by Moringa oleifera seed extract either singly or in combination with antibiotics. Molecules 25, 4583. doi: 10.3390/molecules25194583
Gilmore, K., Chen, P., and Leung, K. (2009). Antimicrobial peptides for plaque control and beyond. J. Calif. Dent. Assoc. 37, 779–788. doi: 10.1080/19424396.2009.12223032
Gonzalez-Zorn, B. (2021). Antibiotic use in the COVID-19 crisis in Spain. Clin. Microbiol. Infect. 27, 646–647. doi: 10.1016/j.cmi.2020.09.055
Hancock, R. E., and Lehrer, R. (1998). Cationic peptides: a new source of antibiotics. Trends Biotechnol. 16, 82–88. doi: 10.1016/S0167-7799(97)01156-6
Hsu, H.-M., Yang, Y.-Y., Huang, Y.-H., Chu, C.-H., Tu, T.-J., Wu, Y.-T., et al. (2023). Distinct features of the host-parasite interactions between nonadherent and adherent Trichomonas vaginalis isolates. PLoS Negl. Trop. Dis. 17, e0011016. doi: 10.1371/journal.pntd.0011016
Hudzicki, J. (2009). Kirby-Bauer disk diffusion susceptibility test protocol. Am. Soc. Microbiol. 15, 55–63.
Imbabi, T., Sabeq, I., Osman, A., Mahmoud, K., Amer, S. A., Hassan, A. M., et al. (2021). Impact of fennel essential oil as an antibiotic alternative in rabbit diet on antioxidant enzymes levels, growth performance, and meat quality. Antioxidants 10, 1797. doi: 10.3390/antiox10111797
Jeyasekaran, G., Karunasagar, I., and Karunasagar, I. (2003). Occurrence of Listeria spp. in seafood handling environments. Indian J. Fish. 50, 211–214.
Johnson, E. A., and Brekke, C. (1983). Functional properties of acylated pea protein isolates. J. Food Sci. 48, 722–725. doi: 10.1111/j.1365-2621.1983.tb14883.x
Mahgoub, S., Osman, A., and Sitohy, M. (2011). Inhibition of growth of pathogenic bacteria in raw milk by legume protein esters. J. Food Prot. 74, 1475–1481. doi: 10.4315/0362-028X.JFP-11-065
Mahmoud, S. Y., Ghaly, M. F., Essa, S. M., Abdel-Shafi, S., Abdel-Monaem, A.-S., Osman, A., et al. (2023). Antimicrobial activity of whey milk and their fractions against Staphylococcus pasteuri clinical strain. Food Biosci. 53, 102741. doi: 10.1016/j.fbio.2023.102741
McLaughlin, M. M., Guerrero, A. J., and Merker, A. (2018). Renal effects of non-tenofovir antiretroviral therapy in patients living with HIV. Drugs Context 7, 212519. doi: 10.7573/dic.212519
Nanda, A., and Saravanan, M. (2009). Biosynthesis of silver nanoparticles from Staphylococcus aureus and its antimicrobial activity against MRSA and MRSE. Nanomedicine 5, 452–456. doi: 10.1016/j.nano.2009.01.012
Nostro, A., Roccaro, A. S., Bisignano, G., Marino, A., Cannatelli, M. A., Pizzimenti, F. C., et al. (2007). Effects of oregano, carvacrol and thymol on Staphylococcus aureus and Staphylococcus epidermidis biofilms. J. Med. Microbiol. 56, 519–523. doi: 10.1099/jmm.0.46804-0
Openda, Y. I., and Nyokong, T. (2023). Combination of photodynamic antimicrobial chemotherapy and ciprofloxacin to combat S. aureus and E. coli resistant biofilms. Photodiagnosis Photodyn. Ther. 42, 103142. doi: 10.1016/j.pdpdt.2022.103142
Osman, A., Abbas, E., Mahgoub, S., and Sitohy, M. (2016). Inhibition of Penicillium digitatum in vitro and in postharvest orange fruit by a soy protein fraction containing mainly β-conglycinin. J. Gen. Plant Pathol. 82, 293–301. doi: 10.1007/s10327-016-0686-3
Osman, A., Enan, G., Al-Mohammadi, A.-R., Abdel-Shafi, S., Abdel-Hameid, S., Sitohy, M. Z., et al. (2021). Antibacterial peptides produced by Alcalase from cowpea seed proteins. Antibiotics 10, 870. doi: 10.3390/antibiotics10070870
Osman, A., Goda, H. A., and Sitohy, M. (2018). Storage stability of minced beef supplemented with chickpea legumin at 4 C as a potential substitute for nisin. LWT Food Sci. Technol. 93, 434–441. doi: 10.1016/j.lwt.2018.03.071
Osman, A., Mahgoub, S., and Sitohy, M. (2014). Hindering milk quality storage deterioration by mild thermization combined with methylated chickpea protein. Int. Food Res. J. 21, 693–701.
Osman, A. O., Mahgoub, S. A., and Sitohy, M. (2013). Preservative action of 11S (glycinin) and 7S (β-conglycinin) soy globulin on bovine raw milk stored either at 4 or 25 C. J. Dairy Res. 80, 174–183. doi: 10.1017/S0022029913000095
Saporito, P., Vang Mouritzen, M. Løbner-Olesen, A., and Jenssen, H. (2018). LL-37 fragments have antimicrobial activity against Staphylococcus epidermidis biofilms and wound healing potential in HaCaT cell line. J. Peptide Sci. 24, e3080. doi: 10.1002/psc.3080
Savino, F., Roana, J., Mandras, N., Tarasco, V., Locatelli, E., Tullio, V., et al. (2011). Faecal microbiota in breast-fed infants after antibiotic therapy. Acta Paediatr. 100, 75–78. doi: 10.1111/j.1651-2227.2010.01988.x
Sindelo, A., Nene, L., and Nyokong, T. (2022). Photodynamic antimicrobial chemotherapy with asymmetrical cationic or neutral metallophthalocyanines conjugated to amino-functionalized zinc oxide nanoparticles (spherical or pyramidal) against planktonic and biofilm microbial cultures. Photodiagnosis Photodyn. Ther. 40, 103160. doi: 10.1016/j.pdpdt.2022.103160
Singh, B. R., Singh, V., Singh, R. K., and Ebibeni, N. (2011). Antimicrobial activity of lemongrass (Cymbopogon citratus) oil against microbes of environmental, clinical and food origin. Int. Res. Pharm. Pharmacol. 1, 228–236.
Sitohy, M., Chobert, J. M., and Haertlé, T. (2000). Study of factors influencing protein esterification using β-lactoglobulin as a model. J. Food Biochem. 24, 381–398. doi: 10.1111/j.1745-4514.2000.tb00708.x
Sitohy, M., Chobert, J. M., and Haertlé, T. (2001). Improvement of solubility and of emulsifying properties of milk proteins at acid pHs by esterification. Nahrung 45, 87–93. doi: 10.1002/1521-3803(20010401)45:2<87::AID-FOOD87>3.0.CO;2-Z
Sitohy, M., Mahgoub, S., and Osman, A. (2011). Controlling psychrotrophic bacteria in raw buffalo milk preserved at 4 C with esterified legume proteins. LWT - Food Sci. Technol. 44, 1697–1702. doi: 10.1016/j.lwt.2011.03.008
Sitohy, M., Mahgoub, S., Osman, A., El-Masry, R., and Al-Gaby, A. (2013). Extent and mode of action of cationic legume proteins against Listeria monocytogenes and Salmonella enteritidis. Probiotics Antimicrob. Proteins 5, 195–205. doi: 10.1007/s12602-013-9134-2
Sitohy, M., and Osman, A. (2010). Antimicrobial activity of native and esterified legume proteins against Gram-negative and Gram-positive bacteria. LWT - Food Sci. Technol. 120, 66–73. doi: 10.1016/j.foodchem.2009.09.071
Sitohy, M., Taha, S., Abdel-Hamid, M., Abdelbacki, A., Hamed, A., Osman, A., et al. (2021). Protecting potato plants against PVX and PVY viral infections by the application of native and chemically modified legume proteins. J. Plant Dis. Protect. N. Series 128, 1101–1114. doi: 10.1007/s41348-021-00448-9
Sitohy, M. Z., Mahgoub, S. A., and Osman, A. O. (2012). In vitro and in situ antimicrobial action and mechanism of glycinin and its basic subunit. Int. J. Food Microbiol. 154, 19–29. doi: 10.1016/j.ijfoodmicro.2011.12.004
Song, L., Yang, H., Meng, X., Su, R., Cheng, S., Wang, H., et al. (2023). Inhibitory effects of trans-cinnamaldehyde against Pseudomonas aeruginosa biofilm formation. Foodborne Pathog. Dis. 20, 47–58. doi: 10.1089/fpd.2022.0073
Souillac, P. O., Middaugh, C. R., and Rytting, J. H. (2002). Investigation of protein/carbohydrate interactions in the dried state. 2. Diffuse reflectance FTIR studies. Int. J. Pharm. 235, 207–218. doi: 10.1016/S0378-5173(01)00987-5
Torres, A., Frias, J., and Vidal-Valverde, C. (2005). Changes in chemical composition of lupin seeds (Lupinus angustifolius) after selective α-galactoside extraction. Sci. Food Agric. 85, 2468–2474. doi: 10.1002/jsfa.2278
Wijman, J. G., de Leeuw, P. P., Moezelaar, R., Zwietering, M. H., and Abee, T. J. (2007). Air-liquid interface biofilms of Bacillus cereus: formation, sporulation, and dispersion. Appl. Environ. Microbiol. 73, 1481–1488. doi: 10.1128/AEM.01781-06
Zhang, H., Wu, J., Li, R., Kim, D.-H., Bi, X., Zhang, G., et al. (2022). Novel intertidal wetland sediment-inoculated moving bed biofilm reactor treating high-salinity wastewater: metagenomic sequencing revealing key functional microorganisms. Bioresour. Technol. 348, 126817. doi: 10.1016/j.biortech.2022.126817
Keywords: 11S globulin, methylated, antibiofilm activity, pathogenic bacteria, FTIR
Citation: Enan G, Abdel-Shafi S, El-Nemr M, Shehab W, Osman A, Sitohy M and Sitohy B (2023) Controlling bacterial biofilm formation by native and methylated lupine 11S globulins. Front. Microbiol. 14:1259334. doi: 10.3389/fmicb.2023.1259334
Received: 15 July 2023; Accepted: 01 September 2023;
Published: 26 September 2023.
Edited by:
Amr H. Hashem, Al-Azhar University, EgyptReviewed by:
Pantu Kumar Roy, Gyeongsang National University, Republic of KoreaEbrahim Saied, Al Azhar University, Egypt
Copyright © 2023 Enan, Abdel-Shafi, El-Nemr, Shehab, Osman, Sitohy and Sitohy. This is an open-access article distributed under the terms of the Creative Commons Attribution License (CC BY). The use, distribution or reproduction in other forums is permitted, provided the original author(s) and the copyright owner(s) are credited and that the original publication in this journal is cited, in accordance with accepted academic practice. No use, distribution or reproduction is permitted which does not comply with these terms.
*Correspondence: Mahmoud Sitohy, bXpzaXRvaHlAaG90bWFpbC5jb20=; Basel Sitohy, YmFzZWwuc2l0b2h5QHVtdS5zZQ==