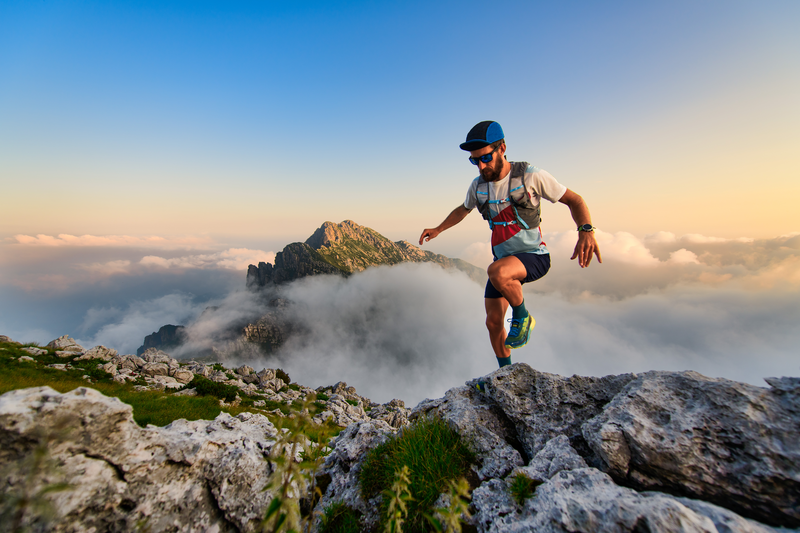
95% of researchers rate our articles as excellent or good
Learn more about the work of our research integrity team to safeguard the quality of each article we publish.
Find out more
ORIGINAL RESEARCH article
Front. Microbiol. , 21 September 2023
Sec. Microbial Physiology and Metabolism
Volume 14 - 2023 | https://doi.org/10.3389/fmicb.2023.1257905
This article is part of the Research Topic Morels: Physiology, Genetics, and Interactions With the Environment View all 9 articles
The successful large-scale cultivation of morel mushrooms (Morchella sextelata) requires a comprehensive understanding of the soil bacterial communities associated with morel-farming beds, as the interactions between fungi and bacteria play a crucial role in shaping the soil microbiome. In this study, we investigated the temporal distribution and ecological characteristics of soil bacteria associated with morel fruiting bodies at different stages, specifically the conidial and primordial stages, under two cropping regimes, non-continuous cropping (NCC) and continuous cropping (CC). Our findings revealed a significant reduction in the yield of morel primordia during the third year following 2 years of CC (0.29 ± 0.25 primordia/grid), in comparison to the NCC regime (12.39 ± 6.09 primordia/grid). Furthermore, inoculation with morel mycelia had a notable impact on soil bacterial diversity, decreasing it in the NCC regime and increasing the number of generalist bacterial members in the CC regime. The latter regime also led to the accumulation of nutrients in the soil beds, resulting in a shift from a stochastic to a deterministic process in the composition of the bacterial community, which differed from the NCC regime. Additionally, mycelial inoculation had a positive effect on the abundance of potential copiotrophic/denitrifying and N-fixing bacteria while decreasing the abundance of oligotrophic/nitrifying bacteria. Interestingly, this effect was more pronounced in the NCC regime than in the CC regime. These results suggest that the increase in potential copiotrophic/denitrifying and N-fixing bacteria facilitated the decomposition of nutrients in exogenous nutrient bags by morel mushrooms, thereby maintaining nitrogen balance in the soil. Overall, our study provides valuable insights into the interactions between morel mycelia and the associated soil bacteriome as well as the influence of different cultivation regimes on these interactions. These findings contribute to our understanding of the complex dynamics of the soil microbiome and can inform strategies for optimizing morel mushroom cultivation.
Morels (Morchella spp.) are highly prized mushrooms known for their exceptional taste and nutritional composition, which includes amino acids, polysaccharides, and trace elements (Liu et al., 2017, 2018; Tietel and Masaphy, 2018). With the growing demand for morel mushrooms that exceeds the availability of wild resources, the cultivation of black morel species (such as M. sextelata, M. importuna, and M. exima) in China has expanded rapidly in recent decades (Zhang et al., 2023a) because of the widespread application of exogenous nutrient bag (ENB) technology, a special type of organic substrate enriched in plant polysaccharides (Tan et al., 2019). Previous studies have demonstrated the presence of complex microbiota associated with morels under natural conditions and production systems (Orlofsky et al., 2021). Changes in the structure and composition of bacterial communities have been observed in the presence of morels and during their development, indicating that morels can modify soil microbiota. These changes seem to coincide with successful morel fruiting, regardless of substrate, cultivation method, greenhouse, or natural habitat (Orlofsky et al., 2021; Tan et al., 2021; Liu et al., 2022; Zhang et al., 2023a). Functional inference analyses have highlighted the important roles of specific bacterial communities associated with nitrogen fixation, nitrification, and nutrient mobilization during the cultivation of morels (Pion et al., 2013; Orlofsky et al., 2021; Yu et al., 2022).
To achieve high yields, it is a common practice to cultivate the same species or isolates of morels continuously on the same farm beds without interruption. However, this intensive cultivation often leads to diminishing yields over time and heightened vulnerability to soil-borne diseases. Similar challenges have been noted in the production systems of other edible fungi, such as Agaricus bisporus and Ganoderma lingzhi, wherein declines in yield or quality deterioration have been documented (Chen et al., 2015; Yuan et al., 2021). The practice of continuous cropping presents significant hurdles to the advancement of the black morel agroindustry. Previous investigations have revealed that the continuous cultivation of M. sextelata is associated with elevated soil nutrient levels, including organic matter, total nitrogen, alkali-hydrolyzable nitrogen, and available phosphorus. This increase in nutrient content is attributed to the decomposition of the raw substrate within ENB. Additionally, imbalances within the soil mycobiome have been observed in systems characterized by continuous cropping (Zhang et al., 2023b).
The patterns of community composition, assembly mechanisms, and ecological functions of soil bacteria, especially within the context of continuous cultivation throughout the entire morel production cycle, remain poorly understood. We postulated that the success or failure of M. sextelata establishment and fruiting, alongside the consecutive soil nutrient changes, would influence distinct responses of the soil bacterial community across various developmental stages and cropping regimes. Furthermore, we hypothesized that bacteria exert a pivotal role in facilitating the growth and maturation of morel fruiting bodies. To test these hypotheses, a controlled indoor experiment was set up to investigate the dynamic progression of soil bacterial communities, indicators linked to specific developmental stages and cropping strategies, ecological processes, and functional attributes during morel cultivation under two cropping regimes: non-continuous cropping (NCC) and continuous cropping (CC). Our findings will enhance our understanding of the complex interactions between morels and soil bacteria, the role of the bacterial community in supporting morel growth and development, and contribute to the development of sustainable morel cultivation practices.
The field experiment was conducted in Hu County, Shaanxi Province, north-western China (34°6′31.05’ N, 108°36′18.04′ E). Black morels (Morchella sextelata isolate HX13) were cultivated in a monoculture for two consecutive years (2019–2020). Soils from the NCC and CC regimes were collected, sieved (2 mm), and placed in crates (Figure 1). These crates were then placed in a walk-in chamber at Xi’an University, sown with the HX13 isolate, and managed according to the established morel cultivation procedures. Samples were collected at three stages: bare soil (BS), conidial (CD), and fruiting body primordium (PD). All soil preparation, inoculation procedures, and sample collection procedures were performed as previously described by Zhang et al. (2023b). The primordium yield was estimated by counting the number of morel primordia per 6 × 6 cm grid. For molecular analysis, soil samples were obtained from both NCC and CC regimes. Four replicates were collected for each stage (BS, CD, and PD), resulting in 24 samples. Soil cores, with a diameter of 2.5 cm and spanning the full thickness of the soil, were collected from each crate and mixed thoroughly. Subsequently, 15 g of the mixed soil was used for further DNA extraction and related analyses. All soil samples were immediately frozen in liquid N and stored at −80°C until further processing. Soil physicochemical characteristics, including soil pH, organic matter content, total N, alkali-hydro N, total phosphorus, available phosphorus, and total potassium, were measured according to previously established protocols (Zhang et al., 2023b).
Figure 1. Schematic of experimental design. (A) The soil preparation of continuous cropping (CC) and non-continuous cropping (NCC) regimes. (B) The crates preparation. (C) Cultivation process.
Soil DNA was extracted using the TIANamp Soil DNA Kit (TIANGEN, Beijing, China). For amplification of 16S rDNA, specific primers targeting the V4 hypervariable regions, namely 515F-806R primers (5′- GTGCCAGCMGCCGCGGTAA-3′ and 5’-GGACTACHV GGGTWTCTAAT-3′), were used (Edwards et al., 2015). The PCR products were purified using a Qiagen Gel Extraction Kit (QIAGEN, Hilden, Germany) and subjected to paired-end sequencing on an Illumina NovaSeq sequencer at Novogene Biotechnology Co., Ltd (Tianjin, China). Sequencing data were processed using the QIIME pipeline software (Caporaso et al., 2010). Bacterial sequences were trimmed and assigned to respective samples based on their barcodes. Subsequently, cluster analysis was performed to group the sequences into Operational Taxonomic Units (OTUs) at a 97% identity threshold (Blaxter et al., 2005). Representative sequence alignments for each OTU were generated using MUSCLE (Edgar, 2004). Taxonomic assignment of representative sequences was performed using the SILVA 132 SSU database (Quast et al., 2012). Relative abundance data for taxa were generated by scaling the read count for each taxon across samples using the total-sum scaling method. Archaea, chloroplasts, and mitochondrial 16S rRNA genes were excluded from further analysis. Raw sequencing data were deposited in the NCBI Sequence Read Archive (SRA) database under the accession number PRJNA993383.
Statistical analyses were performed using R v4.2.2 (R Core Team, 2021) and SPSS version 23.0 software (SPSS Inc., Chicago, IL). Chao1 (Chao, 1984) and phylogenetic diversity (Faith, 1992) indices were used to assess differences in alpha diversity at the OTU level between the three stages in the NCC and CC regimes, providing comprehensive measures of species richness and evolutionary distance. Bacterial beta diversity was visualized using principal coordinate analysis (PCoA) plots (Minchin, 1987) and evaluated by permutational multivariate analysis of variance (PERMANOVA), using the adonis function of the vegan package (Oksanen et al., 2013). Community-level niche breadth, which indicates habitat specialization, was calculated using the spaa package (Zhang et al., 2016). The distributions of generalist, specialist, and neutral taxa were determined using the EcolUtils R package (Salazar, 2018). To quantify the contributions of different ecological processes to the microbial community structure and succession in different treatments, a null-modeling-based statistical framework (Zhou and Ning, 2017) was employed. The beta nearest taxon index (βNTI) was calculated using 999 randomizations. A value of |βNTI| ≥ 2 indicates dominant deterministic processes, whereas a |βNTI| < 2 indicates dominant stochastic processes (Liu et al., 2022). Based on both βNTI and Bray-Curtis-based Raup-Crick Index (RCBray) values, deterministic and stochastic processes were classified into five ecological processes: heterogeneous selection (βNTI< −2), homogeneous selection (βNTI> +2), dispersal limitation (|βNTI| < 2 and RCBray>0.95), homogenizing dispersal (|βNTI| < 2 and RCBray< − 0.95), and ecological drift (|βNTI| < 2 and |RCBray| < 0.95) (Xiong et al., 2021b). Indicator OTUs, identified through indicator species analysis and likelihood ratio tests (LRT) (Hartman et al., 2018), were determined based on collective abundances exceeding 0.03. The correlations between bacterial communities, soil physicochemical variables, relative abundance, and primordium yield in NCC and CC soils were explored using Mantel tests (Xiong et al., 2021a), and taxonomic differences in indicator OTUs among different regimes and stages were evaluated using stacked bar charts and heatmaps and analyzed using the R package dplyr (Yarberry and Yarberry, 2021; Xiong et al., 2021b). Potential trophic behavior (i.e., oligotrophs vs. copiotrophs) and roles in the N cycle (i.e., nitrifying, denitrifying, and N fixation) were established based on published studies. Data are presented as mean ± SEM, with the number of samples (n) indicated in each group. Differences between samples were analyzed using one-way analysis of variance (ANOVA) with Duncan’s multiple range test and an independent sample t-test. Results were considered significant at p < 0.05.
The primordium yields were significantly higher in the NCC regime, with an average of 12.39 ± 6.09 per grid, compared to the CC regime, where the yield per grid was much lower at 0.29 ± 0.25 per grid. This stark contrast in primordium production corresponds to a significant reduction of 99.7% in the CC regime compared to the NCC regime. rDNA metabarcoding analyses also revealed a notable decrease in the relative abundance of M. sextelata in the soil under the CC regime compared to that under the NCC regime, further supporting the decline in morel abundance under continuous cropping (Figure 2A). Furthermore, the CC regime of M. sextelata had a profound effect on the soil properties, particularly in terms of nutrient content. The content of organic matter, total N, alkali-hydrolyzable N, and available P increased throughout the course of morel cultivation (Figure 2B and Supplementary Table S1). This suggests that the successive cultivation of M. sextelata has a significant influence on soil properties and specifically affects its nutritive content.
Figure 2. Soil morel mycelium abundance and soil properties. (A) Relative abundance (%) of M. sextelata and other main taxa/genera detected at different stages [i.e., bare soil (BS), conidial (CD), and primordial (PD)] under both non-continuous cropping (NCC) and continuous cropping (CC). (B) Ratio line chart of soil physicochemical characteristics over time.
After removing contaminating plant and mitochondrial sequences, the remaining high-quality sequences used for analysis totaled 1,558,026 (Supplementary Table S2), corresponding to 9,155 bacterial OTUs. Alpha diversity analysis revealed significant decreases (p < 0.05) in both Chao1 and Faith phylogenetic diversity indices under the NCC regime after morel spawn inoculation at the CD and PD stages (Figure 3A and Supplementary Table S3). The highest values of Chao1 (3549.50 ± 299.18) and Faith’s Phylogenetic diversity (203.73 ± 15.83) were observed in the pre-inoculation baseline samples of the NCC regime (BS_NCC). However, at the CD and PD stages, both Chao1 (Chao1CD_NCC: 2887.25 ± 301.79 and Chao1PD_NCC: 2857.75 ± 270.60) and Faith’s Phylogenetic diversity (FaithCD_NCC: 171.26 ± 14.31 and FaithPD_NCC: 174.97 ± 14.96) decreased and fell within a similar range of values. In contrast, no significant differences were observed in Chao1 and Faith’s phylogenetic diversity indices under the CC regime at any of the developmental stages (BS, CD, and PD) (Figure 3A).
Figure 3. Temporal dynamics of diversity and distribution patterns of crop-associated bacteriomes under the NCC and CC regimes at stages BS, CD, and PD. (A) Diversity of the bacterial community of Morchella sextelata, as indicated by Chao1 and Faith’s phylogenetic diversity. All values are presented as mean ± SE. Different lowercase letters indicate (p < 0.05, Duncan’s test). (B) Principal coordinate analysis of the soil bacterial community of M. sextelata based on the ray-distance metric. The percentage value for each axis represents the proportion of the total variation explained. The samples collected from NCC and CC are shown in red and blue, respectively. Cycles, squares, and triangles represent the bare soil (BS), conidial stage (CD), and primordial stage (PD), respectively. (C) Box plots showing the mean niche breadth of the soil bacterial communities. Lower letters represent p < 0.05; (D) Relative contributions of habitat generalists and specialists in soil bacterial communities.
Potential variations in the bacterial community composition associated with different cropping regimes and stages were assessed using PERMANOVA and PCoA (Figure 3B). These analyses revealed that the structure of the bacterial community was primarily influenced by the developmental stage (R2 = 30.5%, p < 0.001), followed by the cropping regime (R2 = 29.4%, p < 0.001). The niche breadth of the soil bacterial community was the highest in the BS samples of the CC regime (Figure 3C). Following morel spawn inoculation, niche breadth exhibited a decreasing trend in the CC regime, whereas no significant differences were observed among the three developmental stages in the NCC regime (Figure 3C). Furthermore, the proportion of both generalist and specialist bacterial taxa increased in the CC regime at the CD and PD stages, with generalists showing a greater increase than at the BS stage (Figure 3D).
Taxonomic composition analysis showed that the dominant bacterial taxa belonged to the phyla Proteobacteria, Bacteroidetes, and Acidobacteria (Supplementary Figure S1), and the families Comamonadaceae, Flavobacteriaceae, and Oxalobacteraceae (Supplementary Figure S2). Notably, the relative abundance of all three dominant families was higher in the NCC regime than in the CC regime.
To gain insights into the ecological processes driving the structure of bacterial communities, we performed a βNTI analysis. The results indicated that in the NCC regime, stochastic processes played a prominent role in the structure of the soil bacterial community (Figure 4A). The assembly of the bacterial community in the BS stage was mainly influenced by dispersal limitation, whereas ecological drift was the predominant process in the CD and PD stages (Figure 4B). In contrast, deterministic processes significantly contributed to the assembly of the bacterial community in the CC regime. Heterogeneous selection emerged as the main ecological process shaping the bacterial community assembly (Figure 4B). A comparison of the βNTI patterns revealed how the cropping regime influenced the ecological processes governing bacterial community assembly, with CC leading to a shift from stochasticity to determinism.
Figure 4. Relative influence of the ecological assembly process in the NCC and CC cropping systems. (A) βNTI values for different communities and cropping systems. The percentages above and below the violin plot represent the relative contributions of deterministic and stochastic processes, respectively, and (B) the assembly processes of different communities and cropping systems using the null model.
To examine the relationships between bacterial community composition and various factors, such as soil properties, relative abundance of morels, and yield of morel primordia, we conducted a Mantel test (Figure 5 and Table 1). Stronger correlations were observed in the NCC soils compared to the CC soils between the bacterial community and soil properties such as pH, organic matter, total N, and total P. Specifically, organic matter and total P were significantly correlated with bacterial community composition but only in the NCC regime. Conversely, alkali-hydro N and available P content in the CC soils exhibited higher R values and more significant differences in p values compared with the NCC soils. Regarding the potential association between morel abundance and yield, strong correlations were found between the bacterial community composition and morel abundance under the NCC regime. However, no correlation was observed in the CC regime. These results suggest that the successful establishment of M. sextelata in the soil affects the bacterial community. No relationship was found between the bacterial community composition and morel primordium yield in either the NCC or CC regimes.
Figure 5. Correlations between bacterial communities and soil physicochemical characteristics, relative abundance of morel soil, and primordial yield in the NCC and CC regimes. Different bacterial communities (in the NCC and CC regimes) were related to each physicochemical variable, the relative abundance of morel soil, and primordial yield by Mantel tests. The proportion of pie indicates correlation strength, with a higher proportion representing a higher correlation strength.
Table 1. Spearman’s correlations between bacterial community and soil physicochemical characteristics, morel abundance, and yield based on Mantel tests.
To identify specific bacterial OTUs that exhibited varying abundances across cropping regimes and developmental stages, we conducted indicator species analysis (Hartman et al., 2018). This analysis identified 1,216 indicator OTUs (p < 0.05), with indicator OTUs having a relative abundance exceeding 3% considered as abundant indicator OTUs (Figure 6 and Supplementary Table S4). These abundant indicator OTUs were affiliated with nine phyla (at the class level for Proteobacteria): Acidobacteria, Actinobacteria, Alphaproteobacteria, Bacteroidetes, Betaproteobacteria, Firmicutes, Gammaproteobacteria, Gemmatimonadota, and Nitrospirota (Figure 6).
Figure 6. Taxonomic composition of bacterial indicator OTUs present at >3% relative abundance in all treatments. (A) Stacked bar chart of the relative percentages of the abundant indicator OTUs at the phylum level (Proteobacteria at the class level). (B) Heatmap of bacterial abundance indicator OTUs for each treatment. Colors in the left column indicate the same taxa as A. The numbers on the right represent the R value of Spearman’s correlation between the relative abundance of each bacterial family and the abundance of Morel fungi in the soil. Significant correlation coefficients are noted in bold font (p < 0.05).
Spearman’s correlation analysis was performed to examine the correlation between the abundance of the indicator OTUs and M. sextelata across all stages in the NCC and CC regimes. The results revealed that all abundant indicator OTUs belonging to Alphaproteobacteria, Bacteroidetes, Betaproteobacteria, and Gammaproteobacteria exhibited a positive relationship with morel abundance in the soil (Supplementary Table S5). In contrast, Acidobacteria, Actinobacteria, Firmicutes, Nitrospirota, and Gemmatimonadota were negatively correlated with Morel abundance (Supplementary Table S5).
In terms of lifestyle, the taxa positively associated with morel abundance were mainly classified as potential copiotrophic bacteria (Goldfarb et al., 2011; Spring et al., 2015; Bennett et al., 2020). Conversely, taxa negatively related to morel abundance correspond to potential oligotrophic bacteria (Bledsoe et al., 2020; Lu et al., 2022).
Among the taxa that were positively correlated with morel abundance, several families have been identified as N-fixing bacteria, including Rhizobiaceae, Devosiaceae, Rhodobacteraceae, and Xanthomonadaceae (Rivas et al., 2002; Altshuler et al., 2019; Rosselli et al., 2021). In addition, families such as Cellvibrionacea, Comamonadaceae, Sphingomonadaceae, Methylophilaceae, Sphingobacteriaceae, and Flavobacteriaceae have been identified as denitrifying bacteria (Khan et al., 2002; Li et al., 2019; Zhou et al., 2019, 2021; Vishnyakova et al., 2022). In contrast, taxa that showed a negative correlation with morel abundance, including Actinobacteria, Firmicutes, Gemmatimonadota, and Nitrospirota, at the phylum level, were identified as potential nitrifying bacteria (Zhou et al., 2020; Lu et al., 2022). Notably, one specific OTU associated with the phylum Actinobacteria (OTU 7) exhibited both nitrifying and denitrifying capabilities (Wendeborn, 2020). Furthermore, following the inoculation of M. sextelata in the soil under both NCC and CC regimes, the population of oligotrophic/nitrifying bacteria decreased, whereas copiotrophic/denitrifying and nitrogen-fixing bacteria showed a more pronounced increase in the NCC regime (Figure 7).
The assembly of bacterial communities in morel cultivation under different cropping regimes, namely NCC and CC, displayed distinct patterns of stochasticity and determinism across the BS, CD, and PD stages. Stochastic processes, primarily driven by dispersal limitations and ecological drift, played a prominent role in the NCC regime, particularly in the BS stage, whereas deterministic processes, dominated by heterogeneous selection, were prevalent throughout all stages in the CC regime. The prevalence of stochasticity at the BS stage in the NCC regime was expected because of the prior state of the soil as an untilled wild field with a low nutrient content. Previous studies have indicated that stochastic processes are more pronounced in wild bacterial communities due to limited nutrient availability, which hampers microbial dispersal (Wang et al., 2013; Yu et al., 2021). Furthermore, the introduction of morel spawns in the NCC regime can be seen as an invasion of a highly abundant alien species, resulting in the rapid and widespread growth of M. sextelata and intense competition with other soil microbes. This competitive asymmetry accounts for the significant reduction in bacterial diversity within the soil communities. Because competitive asymmetries often lead to smaller species populations, ecological drift has become a more influential factor in shaping communities (Gilbert and Levine, 2017). Consequently, random ecological drift likely governed the community shifts after spawn inoculation at the CD and PD stages in the NCC regime.
In contrast, deterministic processes played a prominent role in shaping bacterial community composition at all stages of the CC regime. This shift from stochasticity in the NCC regime to determinism in the CC regime can be attributed to the changes in nutritional balance in the soil after the CC regime because the expansion of the morel exhausts some specific nutrients, resulting in the accumulation of unutilized nutrients, which confers a selective advantage to certain bacterial taxa that are better adapted to new nutritional conditions. Heterogeneous selection, driven by specific environmental conditions and nutrient gradients in the CC regime, has become the primary ecological process influencing bacterial community assembly.
These findings indicate that the choice of cropping regime has a significant impact on the ecological processes governing the bacterial community dynamics during morel cultivation. The transition from stochasticity to determinism in the CC regime underscores the importance of managing nutrient levels and understanding the ecological interactions between morels and soil bacteria to implement sustainable cultivation practices. Moreover, these findings contribute to a broader understanding of the underlying mechanisms governing the bacterial community assembly in agricultural systems.
In addition to the impact of mycelial proliferation and invasion, the cultivation of M. sextelata resulted in the enrichment of many fungal decomposers (Zhang et al., 2023a), further leading to an increase in soil organic matter (SOM), total N, alkali-hydrolyzable N, and available P content under the NCC regime. This increase was attributed to the decomposition of ENB, which is a specialized culture substrate enriched in plant polysaccharides, by M. sextelata and other fungal decomposers present in soil beds (Zhang et al., 2023b). Fungal decomposers decompose complex organic matter, such as the substrate in ENB, into simpler compounds, releasing essential nutrients, such as nitrogen, phosphorus, and carbon back into the soil. This is the basic function of saprophytic fungi as decomposers in the ecosystem. Experienced morel growers measure the N and P content in the soil before inoculation with spawn because of the adverse effects of high soil nutrition on the differentiation of morels (Zhang et al., 2023a). Changes in soil nutrient concentrations can significantly influence the selective environment and drive turnover in bacterial community composition across different successional stages (Jorgensen et al., 2012). In cases where the initial SOM levels are low or there is a substantial increase in SOM due to nutrient addition, elevated nutrient content can induce divergence in community composition through variable selection, known as a heterogeneous process (Feng et al., 2018). Therefore, in the NCC regime, deterministic heterogeneous selection was evident in the CD and PD stages, although it was not the dominant process. In contrast, in the CC regime, the deterministic process was strongest at the BS stage because of the nutrient increase resulting from two years of continuous cropping of M. sextelata.
However, after two years of successful fruiting production, imbalances emerged in the CC regime, resulting in a significant decline in M. sextelata primordium formation. Soil colonization by M. sextelata mycelia was unsuccessful, as indicated by low soil morel abundance and poor fruiting body primordium production. Although deterministic processes remained dominant in the CD and PD stages in the CC regime, a stochastic process of homogenizing dispersal emerged. This process involves a high rate of dispersal among communities, which results in similar community structures (Stegen et al., 2012). The decreasing trend in niche breadth after morel inoculation in the CC regime further supported this finding. The dispersed bacterial community displayed generalist characteristics and was capable of utilizing a wide range of soil resources and habitats. This broad habitat colonization ability facilitates effective stochastic dispersion (Xu et al., 2020) and narrows niche breadth (Zhang et al., 2018). Overall, our results underscore the intricate interplay between stochastic and deterministic processes in shaping the assembly of bacterial communities during morel cultivation under different cropping regimes. The NCC regime exhibited a stronger influence of stochastic processes driven by initial soil conditions and morel inoculation. In contrast, the CC regime demonstrated a predominance of deterministic processes, particularly heterogeneous selection at earlier stages and the emergence of homogeneous dispersal at later stages. These findings enhance our understanding of the ecological mechanisms underlying bacterial community dynamics and succession in morel cultivation, emphasizing the significance of both deterministic and stochastic processes in shaping microbial communities in agricultural systems.
Our findings revealed a distinct difference between the characteristics of bacterial OTUs positively associated with morel abundance and those negatively associated with morel abundance. Morel-abundance-positive OTUs were predominantly composed of copiotrophic bacteria, which are known for their rapid growth and reproduction in nutrient-rich environments. These bacteria have high metabolic rates and rely on a continuous supply of organic matter for their growth. Copiotrophic bacteria are commonly found in environments with a high organic matter content, such as recently fertilized soils (Leff et al., 2015). In contrast, the majority of morel-abundance-negative-related OTUs were oligotrophic bacteria. Oligotrophic bacteria have adapted to survive in nutrient-poor environments. They have slower growth rates and lower metabolic rates than copiotrophic bacteria. However, oligotrophic bacteria are efficient at extracting nutrients from their surroundings, allowing them to thrive under nutrient-limited conditions (Leff et al., 2015).
The enrichment of copiotrophic bacteria in morel-abundance-positive OTUs aligned with the increase in soil nutrients following morel inoculation. Copiotrophs thrive in nutrient-enriched environments (Goldfarb et al., 2011; Leff et al., 2015). However, it is worth noting that in the CC regime, despite the higher SOM content compared to the NCC regime, the abundance of copiotrophic bacteria was lower. Furthermore, no significant correlation was found between SOM content and community patterns in the CC regime. These findings suggest that while soil nutrient levels may contribute to the enrichment of copiotrophic taxa, the establishment of morel mycelial networks plays a more crucial role. It is plausible that the presence of morels and their associated activities, rather than nutrient levels alone, shaped the abundance patterns of the copiotrophic bacteria. This indicated that morel establishment influences the ecological function of copiotrophic bacteria during morel cultivation. The mechanisms underlying the relationship between the microbes and copiotrophic bacteria require further investigation. Our results highlighted a clear distinction between morel-abundance-positive copiotrophic bacteria and morel-abundance-negative oligotrophic bacteria. These findings enhance our understanding of microbial dynamics and functional interactions in morel cultivation, and provide insights into the role of copiotrophic bacteria and their ecological functions in this agricultural system.
The family Pseudomonadaceae, particularly Pseudomonas spp., is particularly important for morel cultivation. Although no OTUs belonging to the Pseudomonadaceae family were identified as abundant indicator OTUs, they were ranked among the top 10 abundant families and showed a positive relationship with the abundance of M. sextelata mycelia in the soil. Previous studies have emphasized the significance of Pseudomonas putida, a member of the Pseudomonadaceae family, as a bacterium farmed by the saprotrophic and ectomycorrhizal soil fungus M. crassipes (Pion et al., 2013). Similar associations between Pseudomonas spp. and M. sextelata fruiting have also been observed in outdoor greenhouses (Benucci et al., 2019). Pseudomonas has also been found to be accompanied by the induction of fruiting body formation and an increased yield of fruiting bodies during cultivation of other mushrooms, such as Agaricus bisporus and Pleurotus ostreatus (Cho et al., 2008; Vieira and Pecchia, 2022). These findings underscore the widespread occurrence of bacterial-fungal interactions (BFI) in various ecological contexts (Steffan et al., 2020).
Denitrifying bacteria are responsible for the conversion of nitrate to N2 (Vishnyakova et al., 2022). This denitrification process is closely linked to SOM, because heterotrophic bacteria utilize organic carbon sources for their metabolism during denitrification (Burford and Bremner, 1975). For example, the Comamonadaceae family is known for its ability to perform heterotrophic denitrification when supplied with organic compounds as electron donors (Khan et al., 2002). This finding aligns with the significant correlation observed between the community patterns in the NCC regime and SOM content. The reduced organic N content likely acts as a key factor in triggering bacterial farming by M. crassipes (Pion et al., 2013; Lohberger et al., 2019).
This study revealed distinct responses of the bacterial community in terms of community patterns, assembly processes, and ecological functions under NCC and CC regimes (Figure 8). The successful establishment of morels in the soil resulted in a decrease in bacterial diversity, whereas failure of morel invasion in the CC regime led to the dispersal of generalist bacterial members and a reduction in niche breadth. Continuous cultivation of M. sextelata influences the assembly of the bacterial community, shifting it from a stochastic process to a deterministic regime. Furthermore, the inoculation of morels had a more pronounced effect on enriching copiotrophic bacteria with denitrifying and N-fixing functions while simultaneously reducing the abundance of oligotrophic bacteria with nitrifying functions. This effect was particularly evident in the NCC regime compared to the CC regime. These findings provide valuable insights into the potential use of bacteria in soil improvement strategies to address challenges associated with continuous cropping in the agricultural industry.
Figure 8. Responses of bacterial community pattern, assembly, and ecological function in NCC and CC regimes during morel cultivation.
The datasets presented in this study can be found in online repositories. The names of the repository/repositories and accession number(s) can be found at: https://www.ncbi.nlm.nih.gov/bioproject/PRJNA993383.
YZ: Conceptualization, Data curation, Formal analysis, Investigation, Methodology, Project administration, Resources, Software, Writing – original draft, Writing – review & editing. QZ: Data curation, Formal analysis, Investigation, Methodology, Writing – original draft. SU: Supervision, Writing – review & editing. TG: Methodology, Formal analysis, Funding acquisition, Writing – original draft. JL: Data curation, Investigation, Writing – original draft. FH: Data curation, Formal analysis, Funding acquisition. RR: Formal analysis, Methodology, Writing – original draft, Writing – review & editing. FM: Funding acquisition, Project administration, Supervision, Writing – review & editing, Investigation, Writing – original draft. LX: Data curation, Formal analysis, Funding acquisition, Investigation, Supervision, Writing – original draft, Writing – review & editing, Methodology, Project administration, Resources, Software, Validation.
The author(s) declare financial support was received for the research, authorship, and/or publication of this article. This research was funded by the National Natural Science Foundation of China (32270530) and the Special Plan of the Education Department of the Shaanxi Provincial Government (Grant No. 21JC028), the Key Research and Development Project of the Department of Science and Technology of Shaanxi Province (Grant No. 2021NY-068), the Innovation Foundation of Science and Technology Bureau of Xi’an (Grant Nos. 2020KJWL05 and 2020KJWL21), and Science and Technology Project of the Science and Technology Bureau of Xi’an (Grant Nos. 20193016YF004NS004 and 21NYYF0023), and the French National Research Agency (ANR) as part of the “Initiative for the Future’ program (ANR-11-LABX-0002-01, Lab of Excellence ARBRE).
The authors thank the Key Laboratory for Ecological Restoration and High Quality Development of the Qinling Mountains in the upper and middle reaches of the Yellow River and the State Scholarship Fund (File No. 202008610105) from the China Scholarship Council (CSC).
The authors declare that the research was conducted in the absence of any commercial or financial relationships that could be construed as a potential conflict of interest.
All claims expressed in this article are solely those of the authors and do not necessarily represent those of their affiliated organizations, or those of the publisher, the editors and the reviewers. Any product that may be evaluated in this article, or claim that may be made by its manufacturer, is not guaranteed or endorsed by the publisher.
The Supplementary material for this article can be found online at: https://www.frontiersin.org/articles/10.3389/fmicb.2023.1257905/full#supplementary-material
Altshuler, I., Ronholm, J., Layton, A., Onstott, T. C., and Whyte, L. G. (2019). Denitrifiers, nitrogen-fixing bacteria and N2O soil gas flux in high Arctic ice-wedge polygon cryosols. FEMS Microbiol. Ecol. 95:fiz049. doi: 10.1093/femsec/fiz049
Bennett, A. C., Murugapiran, S. K., and Hamilton, T. L. (2020). Temperature impacts community structure and function of phototrophic Chloroflexi and Cyanobacteria in two alkaline hot springs in Yellowstone National Park. Environ. Microbiol. Rep. 12, 503–513. doi: 10.1111/1758-2229.12863
Benucci, G. M. N., Longley, R., Zhang, P., Zhao, Q., Bonito, G., and Yu, F. (2019). Microbial communities associated with the black morel Morchella sextelata cultivated in greenhouses. PeerJ 7:e7744. doi: 10.7717/peerj.7744
Blaxter, M., Mann, J., Chapman, T., Thomas, F., Whitton, C., Floyd, R., et al. (2005). Defining operational taxonomic units using DNA barcode data. Phil. Trans. Royal Soc. B 360, 1935–1943. doi: 10.1098/rstb.2005.1725
Bledsoe, R. B., Goodwillie, C., and Peralta, A. L. (2020). Long-term nutrient enrichment of an oligotroph-dominated wetland increases bacterial diversity in bulk soils and plant rhizospheres. Msphere 5, e00035–e00020. doi: 10.1128/msphere.00035-20
Burford, J. R., and Bremner, J. M. (1975). Relationships between the denitrification capacities of soils and total, water-soluble and readily decomposable soil organic matter. Soil Biol. Biochem. 7, 389–394. doi: 10.1016/0038-0717(75)90055-3
Caporaso, J. G., Kuczynski, J., Stombaugh, J., Bittinger, K., Bushman, F. D., Costello, E. K., et al. (2010). QIIME allows analysis of high-throughput community sequencing data. Nat. Methods 7, 335–336. doi: 10.1038/nmeth.f.303
Chao, A. (1984). Nonparametric estimation of the number of classes in a population. Scand. J. Stat., 11, 265–270.
Chen, M., Wang, J. T., Feng, Y. Z., Li, J., Wang, J. H., and Lin, X. G. (2015). Changes in soil microbial community in response to tomato-Agaricus bisporus interplanting. Acta Pedol. Sin. 52, 145–153. doi: 10.11766/trxb201312160594
Cho, Y.S., Weon, H.Y., Joh, J.H., Lim, J.H., Kim, K.Y., et al., (2008). Effect of casing layer on growth promotion of the edible mushroom Pleurotus ostreatus. Mycobiology 36(1), 40–44., doi: 10.4489/MYCO.2008.36.1.040
Edgar, R. C. (2004). MUSCLE: multiple sequence alignment with high accuracy and high throughput. Nucleic Acids Res. 32, 1792–1797. doi: 10.1073/pnas.1414592112
Edwards, J., Johnson, C., Santos-Medellín, C., Lurie, E., Podishetty, N. K., Bhatnagar, S., et al. (2015). Structure, variation, and assembly of the root-associated microbiomes of rice. Proc. Natl. Acad. Sci. 112, E911–E920.
Faith, D. P. (1992). Conservation evaluation and phylogenetic diversity. Biol. Conserv. 61, 1–10. doi: 10.1016/0006-3207(92)91201-3
Feng, Y., Chen, R., Stegen, J. C., Guo, Z., Zhang, J., Li, Z., et al. (2018). Two key features influencing community assembly processes at regional scale: initial state and degree of change in environmental conditions. Mol. Ecol. 27, 5238–5251. doi: 10.1111/mec.14914
Gilbert, B., and Levine, J. M. (2017). Ecological drift and the distribution of species diversity. Proc. R. Soc. B Biol. Sci. 284:20170507. doi: 10.1098/rspb.2017.0507
Goldfarb, K. C., Karaoz, U., Hanson, C. A., Santee, C. A., Bradford, M. A., Treseder, K. K., et al. (2011). Differential growth responses of soil bacterial taxa to carbon substrates of varying chemical recalcitrance. Front. Microbiol. 2:94. doi: 10.3389/fmicb.2011.00094
Hartman, K., van der Heijden, M. G., Wittwer, R. A., Banerjee, S., Walser, J. C., and Schlaeppi, K. (2018). Cropping practices manipulate abundance patterns of root and soil microbiome members paving the way to smart farming. Microbiome 6:14. doi: 10.1186/s40168-017-0389-9
Jorgensen, S. L., Hannisdal, B., Lanzén, A., Baumberger, T., Flesland, K., Fonseca, R., et al. (2012). Correlating microbial community profiles with geochemical data in highly stratified sediments from the Arctic Mid-Ocean ridge. Proc. Natl. Acad. Sci. 109, E2846–E2855. doi: 10.1073/pnas.1207574109
Khan, S. T., Horiba, Y., Yamamoto, M., and Hiraishi, A. (2002). Members of the family Comamonadaceae as primary poly (3-hydroxybutyrate-co-3-hydroxyvalerate)-degrading denitrifiers in activated sludge as revealed by a polyphasic approach. Appl. Environ. Microbiol. 68, 3206–3214. doi: 10.1128/AEM.68.7.3206-3214.2002
Leff, J. W., Jones, S. E., Prober, S. M., Barberán, A., Borer, E. T., Firn, J. L., et al. (2015). Consistent responses of soil microbial communities to elevated nutrient inputs in grasslands across the globe. Proc. Natl. Acad. Sci. 112, 10967–10972. doi: 10.1073/pnas.1508382112
Li, J., Wang, J. T., Hu, H. W., Cai, Z. J., Lei, Y. R., Li, W., et al. (2019). Changes of the denitrifying communities in a multi-stage free water surface constructed wetland. Sci. Total Environ. 650, 1419–1425. doi: 10.1016/j.scitotenv.2018.09.123
Liu, W. Y., Guo, H. B., Bi, K. X., Sibirina, A. L., Qi, X. J., and Yu, X. D. (2022). Determining why continuous cropping reduces the production of the morel Morchella sextelata. Front. Microbiol. 13: 903983 doi: 10.3389/fmicb.2022.903983
Liu, Q., Liu, H., Chen, C., Wang, J., Han, Y., and Long, Z. (2017). Effects of element complexes containing Fe, Zn and Mn on artificial morel’s biological characteristics and soil bacterial community structures. PLoS One 12:e0174618. doi: 10.1371/journal.pone.0174618
Liu, Q., Ma, H., Zhang, Y., and Dong, C. (2018). Artificial cultivation of true morels: current state, issues and perspectives. Crit. Rev. Biotechnol. 38, 259–271. doi: 10.1080/07388551.2017.1333082
Liu, J., Wang, X., Liu, J., Liu, X., Zhang, X. H., and Liu, J. (2022). Comparison of assembly process and co-occurrence pattern between planktonic and benthic microbial communities in the Bohai Sea. Front. Microbiol. 13:3623. doi: 10.3389/fmicb.2022.1003623
Lohberger, A., Spangenberg, J. E., Ventura, Y., Bindschedler, S., Verrecchia, E. P., Bshary, R., et al. (2019). Effect of organic carbon and nitrogen on the interactions of Morchella spp. and bacteria dispersing on their mycelium. Front. Microbiol. 10:124. doi: 10.3389/fmicb.2019.00124
Lu, L., Chen, C., Ke, T., Wang, M., Sima, M., and Huang, S. (2022). Long-term metal pollution shifts microbial functional profiles of nitrification and denitrification in agricultural soils. Sci. Total Environ. 830:154732. doi: 10.1016/j.scitotenv.2022.154732
Minchin, P.R. (1987). An evaluation of the relative robustness of techniques for ecological ordination, in Theory and models in vegetation science: proceedings of symposium, Uppsala, July 8–13, 1985. Springer, Netherlands, 89–107.
Oksanen, J., Blanchet, F. G., Kindt, R., Legendre, P., Minchin, P. R., Ohara, R., et al. (2013). Community ecology package. R package version 2, 321–326. Available at: http://CRAN.R-project.org/package=vegan
Orlofsky, E., Zabari, L., Bonito, G., and Masaphy, S. (2021). Changes in soil bacteria functional ecology associated with Morchella rufobrunnea fruiting in a natural habitat. Environ. Microbiol. 23, 6651–6662. doi: 10.1111/1462-2920.15692
Pion, M., Spangenberg, J. E., Simon, A., Bindschedler, S., Flury, C., Chatelain, A., et al. (2013). Bacterial farming by the fungus Morchella crassipes. Proc. R. Soc. B Biol. Sci. 280:20132242. doi: 10.1098/rspb.2013.2242
Quast, C., Pruesse, E., Yilmaz, P., Gerken, J., Schweer, T., Yarza, P., et al. (2012). The SILVA ribosomal RNA gene database project: improved data processing and web-based tools. Nucleic Acids Res. 41, D590–D596. doi: 10.1093/nar/gks1219
R Core Team. (2021). R: A language and environment for statistical computing. R foundation for statistical computing, Vienna, Austria.
Rivas, R., Velázquez, E., Willems, A., Vizcaíno, N., Subba-Rao, N. S., Mateos, P. F., et al. (2002). A new species of Devosia that forms a unique nitrogen-fixing root-nodule symbiosis with the aquatic legume Neptunia natans (lf) Druce. Appl. Environ. Microbiol. 68, 5217–5222. doi: 10.1128/AEM.68.11.5217-5222.2002
Rosselli, R., La Porta, N., Muresu, R., Stevanato, P., Concheri, G., and Squartini, A. (2021). Pangenomics of the symbiotic Rhizobiales. Core and accessory functions across a group endowed with high levels of genomic plasticity. Microorganisms 9:407. doi: 10.3390/microorganisms9020407
Salazar, G. (2018). EcolUtils: utilities for community ecology analysis. R package version 0.1. Available at: https://github.com/GuillemSalazar/EcolUtils
Spring, S., Scheuner, C., Göker, M., and Klenk, H. P. (2015). A taxonomic framework for emerging groups of ecologically important marine gammaproteobacteria based on the reconstruction of evolutionary relationships using genome-scale data. Front. Microbiol. 6:281. doi: 10.3389/fmicb.2015.00281
Steffan, B. N., Venkatesh, N., and Keller, N. P. (2020). Let’s get physical: bacterial-fungal interactions and their consequences in agriculture and health. J. Fungi 6:243. doi: 10.3390/jof6040243
Stegen, J. C., Lin, X. J., Konopka, A. E., and Fredrickson, J. K. (2012). Stochastic and deterministic assembly processes in subsurface microbial communities. ISME J. 6, 1653–1664. doi: 10.1038/ismej.2012.22
Tan, H., Kohler, A., Miao, R. Y., Liu, T. H., Zhang, Q., Zhang, B., et al. (2019). Multi-omic analyses of exogenous nutrient bag decomposition by the black morel Morchella importuna reveal sustained carbon acquisition and transferring. Environ. Microbiol. 21, 3909–3926. doi: 10.1111/1462-2920.14741
Tan, H., Yu, Y., Tang, J., Liu, T. H., Miao, R. Y., Huang, Z. Q., et al. (2021). Build your own mushroom soil: microbiota succession and nutritional accumulation in semi-synthetic substratum drive the fructification of a soil-saprotrophic morel. Front. Microbiol. 12:656656. doi: 10.3389/fmicb.2021.656656
Tietel, Z., and Masaphy, S. (2018). True morels (Morchella)—nutritional and phytochemical composition, health benefits and flavor: a review. Crit. Rev. Food Sci. Nutr. 58, 1888–1901. doi: 10.1080/10408398.2017.1285269
Vieira, F. R., and Pecchia, J. A. (2022). Bacterial community patterns in the Agaricus bisporus cultivation system, from compost raw materials to mushroom caps. Microb. Ecol. 84, 20–32. doi: 10.1007/s00248-021-01833-5
Vishnyakova, A., Popova, N., Artemiev, G., Botchkova, E., Litti, Y., and Safonov, A. (2022). Effect of mineral carriers on biofilm formation and nitrogen removal activity by an indigenous Anammox community from cold groundwater ecosystem alone and bioaugmented with biomass from a “warm” Anammox reactor. Biology 11:1421. doi: 10.3390/biology11101421
Wang, J., Shen, J., Wu, Y., Tu, C., Soininen, J., Stegen, J. C., et al. (2013). Phylogenetic beta diversity in bacterial assemblages across ecosystems: deterministic versus stochastic processes. ISME J. 7, 1310–1321. doi: 10.1038/ismej.2013.30
Wendeborn, S. (2020). The chemistry, biology, and modulation of ammonium nitrification in soil. Angew. Chem. Int. Ed. 59, 2182–2202. doi: 10.1002/anie.201903014
Xiong, C., He, J. Z., Singh, B. K., Zhu, Y. G., Wang, J. T., Li, P. P., et al. (2021a). Rare taxa maintain the stability of crop mycobiomes and ecosystem functions. Environ. Microbiol. 23, 1907–1924. doi: 10.1111/1462-2920.15262
Xiong, C., Singh, B. K., He, J. Z., Han, Y. L., Li, P. P., Wan, L. H., et al. (2021b). Plant developmental stage drives the differentiation in ecological role of the maize microbiome. Microbiome 9, 1–15. doi: 10.1186/s40168-021-01118-6
Xu, Q., Ling, N., Chen, H., Duan, Y., Wang, S., Shen, Q., et al. (2020). Long-term chemical-only fertilization induces a diversity decline and deep selection on the soil bacteria. Msystems 5, e00337–e00320. doi: 10.1128/msystems.00337-20
Yarberry, W. (2021). “Dplyr”. in CRAN recipes: DPLYR, Stringr, Lubridate, and RegEx in R, (Berkeley, CA: Apress ), 1–58. doi: 10.1007/978-1-4842-6876-6
Yu, F. M., Jayawardena, R. S., Thongklang, N., Lv, M. L., Zhu, X. T., and Zhao, Q. (2022). Morel production associated with soil nitrogen-fixing and nitrifying microorganisms. J. Fungi 8:299. doi: 10.3390/jof8030299
Yu, Y., Cui, M., Xiao, Y., Chang, M., Wang, C., Zhao, L., et al. (2021). Quantitative estimation of stochastic and deterministic processes for soil prokaryotic community assembly in the Yellow River floodplain. Eur. J. Soil Sci. 72, 1462–1477. doi: 10.1111/ejss.13056
Yuan, Y., Huang, H. C., Li, L., Liu, G. H., Fu, J. S., and Wu, X. P. (2021). Effect of lime on preventing and controlling continuous cropping obstacle of ganoderma lingzhi and analysis of its microbial community. Biotechnol. Bull. 37:70. doi: 10.13560/j.cnki.biotech.bull.1985.2020-0786
Zhang, C., Shi, X., Zhang, J., Zhang, Y., and Wang, W. (2023a). Dynamics of soil microbiome throughout the cultivation life cycle of morel (Morchella sextelata). Front. Microbiol. 14:979835. doi: 10.3389/fmicb.2023.979835
Zhang, J., Ding, Q., and Huang, J. (2016). Spaa: species association analysis. R package version 0.2 2. Available at: https://cran.r-project.org/web/packages/spaa/
Zhang, J., Zhang, B., Liu, Y., Guo, Y., Shi, P., and Wei, G. (2018). Distinct large-scale biogeographic patterns of fungal communities in bulk soil and soybean rhizosphere in China. Sci. Total Environ. 644, 791–800. doi: 10.1016/j.scitotenv.2018.07.016
Zhang, Y., Sun, S., Luo, D., Mao, P., Rosazlina, R., Martin, F., et al. (2023b). Decline in Morel production upon continuous cropping is related to changes in soil Mycobiome. J. Fungi 9:492. doi: 10.3390/jof9040492
Zhou, B., Duan, J., Xue, L., Zhang, J., and Yang, L. (2019). Effect of plant-based carbon source supplements on denitrification of synthetic wastewater: focus on the microbiology. Environ. Sci. Pollut. Res. 26, 24683–24694. doi: 10.1007/s11356-019-05454-x
Zhou, J., and Ning, D. (2017). Stochastic community assembly: does it matter in microbial ecology? Microbiol. Mol. Biol. Rev. 81, e00002–e00017. doi: 10.1128/mmbr.00002-17
Zhou, X., Zhang, C., and Li, Y. (2021). Time-delayed photocatalysis enhanced microbial nitrate reduction via solar energy storage in carbon nitrides. Chem. Eng. J. 417:127904. doi: 10.1016/j.cej.2020.127904
Keywords: Morchella sextelata, community assemblage, bacteriome, continuous cropping, ecological traits
Citation: Zhang Y, Zhao Q, Uroz S, Gao T, Li J, He F, Rosazlina R, Martin F and Xu L (2023) The cultivation regimes of Morchella sextelata trigger shifts in the community assemblage and ecological traits of soil bacteria. Front. Microbiol. 14:1257905. doi: 10.3389/fmicb.2023.1257905
Received: 13 July 2023; Accepted: 05 September 2023;
Published: 21 September 2023.
Edited by:
Hao Tan, Sichuan Academy of Agricultural Sciences, ChinaReviewed by:
Xingzhong Liu, Nankai University, ChinaCopyright © 2023 Zhang, Zhao, Uroz, Gao, Li, He, Rosazlina, Martin and Xu. This is an open-access article distributed under the terms of the Creative Commons Attribution License (CC BY). The use, distribution or reproduction in other forums is permitted, provided the original author(s) and the copyright owner(s) are credited and that the original publication in this journal is cited, in accordance with accepted academic practice. No use, distribution or reproduction is permitted which does not comply with these terms.
*Correspondence: Rusly Rosazlina, cm9zYXpsaW5hcnVzbHlAdXNtLm15; Francis Martin, ZnJhbmNpcy5tYXJ0aW5AaW5yYWUuZnI=; Lingling Xu, bWlzc2lsZXhsbEAxNjMuY29t
Disclaimer: All claims expressed in this article are solely those of the authors and do not necessarily represent those of their affiliated organizations, or those of the publisher, the editors and the reviewers. Any product that may be evaluated in this article or claim that may be made by its manufacturer is not guaranteed or endorsed by the publisher.
Research integrity at Frontiers
Learn more about the work of our research integrity team to safeguard the quality of each article we publish.