- Fujian Key Laboratory of Innate Immune Biology, College of Life Science, Biomedical Research Center of South China, Fujian Normal University, Fuzhou, China
Kombucha is a customary tea-based beverage that is produced through the process of fermenting a mixture of tea and sugar water with symbiotic culture of bacteria and yeast (SCOBY). Traditional kombucha has various beneficial effects and can improve immunity. The significant market share of Kombucha can be attributed to the growing consumer inclination towards healthy foods within the functional beverage industry. The research focus has recently expanded from the probiotics of traditional black tea kombucha to encompass other teas, Chinese herbs, plant materials, and alternative substrates. There is a lack of comprehensive literature reviews focusing on substance transformation, functional, active substances, and efficacy mechanisms of alternative kombucha substrates. This article aimed to bridge this gap by providing an in-depth review of the biological transformation pathways of kombucha metabolites and alternative substrates. The review offers valuable insights into kombucha research, including substance metabolism and transformation, efficacy, pharmacological mechanism, and the purification of active components, offering direction and focus for further studies in this field.
1. Introduction
Kombucha, a traditional fermented tea beverage rich in probiotics and bio-active factors, is generally prepared from sugary tea water fermented with a symbiotic culture of bacteria and yeast (SCOBY) (Sinir et al., 2019). The composition of the symbiotic flora of kombucha is complex, and the dominant flora are composed of Acetobacter, Saccharomyces, and Lactobacillus. Previous studies on the composition of kombucha have predominantly focused on kombucha fermented with acetic acid bacteria, such as Acetobacter xylinum, Gluconobacter liquefaciens, and Komagataeibacter intermedius, yeast species, such as Saccharomyces cerevisiae, Candida tropicalis, and Schizosaccharomyces pombe, and lactic acid bacteria, for instance Lactobacillus bulgaricus and Lactobacillus nagelii (Antolak et al., 2021). Kombucha can influence various physiological functions, such as lowering blood pressure, reducing inflammation, promoting liver function, and enhancing immune resistance. Kombucha products have many metabolites, such as organic acids, polyphenols, vitamins, amino acids, protein/enzymes, and minerals (Bishop et al., 2022; Kitwetcharoen et al., 2023). The main metabolic components of kombucha are organic acids, D-saccharic acid-1,4-lactone (DSL), and tea polyphenols (Jayabalan et al., 2014). However, the probiotic effects of kombucha are independent of its unique metabolites.
Kombucha is composed of a diverse array of organic acids, including acetic acid, which can eliminate fatigue, lower blood pressure, and improve gastrointestinal health (Sinir et al., 2019). The tea polyphenols in kombucha can stabilise blood sugar, promote fat oxidation, scavenge free radicals, and delay ageing (Jayabalan et al., 2014). DSL has been found to be an effective inhibitor of β-glucosidase, an enzyme that is closely linked to the process of carcinogenesis and plays a crucial role in the prevention of cancer (Taha et al., 2020). The selection of different fermentation substrates also produces substances different from those produced using traditional kombucha. In addition to the fermentation of traditional tea leaves (black and green tea), the effects of using new fermentation substrates on the probiotic efficacy of kombucha are of growing interest (Wang et al., 2021).
Analysis of the active ingredients of kombucha and the study of the probiotic mechanisms are essential prerequisites for the industrial manufacturing and commercialization of kombucha. They are also the basis for clinical research on probiotic functional flora to prevent and treat diseases.
Herein, we reviewed recent studies on kombucha, including fermentation conditions, the composition of raw materials before and after fermentation, and the efficacy of active substances after fermentation in alternative matrices. This review will facilitate further research into the metabolism of kombucha, its efficacy mechanisms, and the development of specific kombucha-based products.
2. Alternative kombucha substrates
In recent years, research into alternative substrates for kombucha production has increased as most scholars have become dissatisfied with the use of tea as a substrate for traditional kombucha fermentation. The use of raw materials, such as plant leaves, fruit juices, herbs, spices, and flowers, as substitutes for tea or as co-fermentation ingredients along with black tea have been reported. These alternative substrates can produce novel fermented functional products, yield kombucha with improved organoleptic value or health properties (Table 1) (Sinir et al., 2019; Emiljanowicz and Malinowska-Pańczyk, 2020), provide carbon and nitrogen sources for the fermentation of kombucha, and alter its fermentation products to produce more beneficial and healthy bioactive beverages.
3. Composition of kombucha and analogous kombucha
3.1. Composition of traditional kombucha
The chemical composition of kombucha is dependent on different substrate compositions, source and microbial community in the SCOBY, fermentation methods, and detection methods.
Kombucha is composed of sugars (including sucrose, glucose, and fructose), organic acids [including acetic acid, gluconic acid, glucuronic acid, lactic acid, DSL, citric acid, oxalic acid, and pyruvic acid (Jayabalan et al., 2007)], B-vitamins and vitamin C (Bauer-Petrovska and Petrushevska-Tozi, 2000), theophyllines, tea polyphenols, flavonoids, various amino acids and proteins, ethanol, biogenic amines, purine bases, hydrolytic enzymes, minerals (primarily Cu, Fe, Zn, Ni, and Mn), and metabolites secreted by yeast and bacteria (Table 2) (Malbaša et al., 2011).
3.2. Material transformation of the main metabolites of traditional kombucha
Traditional kombucha fermentation utilises tea and white sugar as the main substrates. These substrates are transformed through fermentation by microorganisms, such as yeast and bacteria, which involves the gradual breakdown of sugar and a metabolic cascade that produces CO2 and an acidic, slightly alcoholic beverage (Chakravorty et al., 2016). In a bacteriophage fermentation environment, yeasts are the primary ethanol producers, as they produce hydrolytic enzymes (of the fructosidase class) that hydrolyse sucrose to glucose and fructose, which subsequently undergo metabolic processes leading to the production of ethanol, glycerol, and carbon dioxide. The involvement of different bacterial strains during fermentation leads to metabolic differentiation. The yeast genus Saccharomyces spp. can utilise glucose and produce ethanol through the glycolytic pathway, whereas the jointed yeast Zygosaccharomyces spp. can efficiently ferment fructose to produce ethanol. Furthermore, specific yeast strains, such as the corn wine fission yeast (Schizosaccharomyces pombe), have the capability to generate ethanol from malic acid or Brettanomyces bruxellensis in the presence of elevated levels of acetic acid in aerobic environments (Villarreal-Soto et al., 2018).
During fermentation, microorganisms interact with each other, and the ethanol produced by yeast fermentation can be used by Acetobacter as a metabolic substrate for oxidation to acetic acid. In addition to acetic acid, Acetobacter can further metabolise glucose in the fermentation broth to produce glucuronic acid, which is later metabolised to gluconic acid and converted to glucuronic acid (Villarreal-Soto et al., 2019; Martínez-Leal et al., 2020). In addition, B. gluconii strains have the capability to enzymatically produce L-ascorbic acid, commonly known as vitamin C, utilizing D-sorbitol as a precursor compound, which is itself derived from glucose (Mamlouk and Gullo, 2013). Depending on the specific strain, certain lactic acid bacteria have the capability to utilize glucose in either the glycolytic pathway, resulting in the production of lactic acid as the primary metabolite, or the pentose phosphate pathway, leading to the synthesis of lactic acid, ethanol, and carbon dioxide. However, when fructose is present, the production of acetic acid occurs instead of ethanol (Laureys et al., 2020).
Bacterial cellulose film is a distinctive byproduct of kombucha fermentation, which is generated by acetate bacteria through alcohol metabolism and can be removed as waste. Komagataeibacter spp. use glucose to synthesise bacterial cellulose, and this anabolic process involves sucrose, ethanol, and glycerol (Chawla et al., 2009; Villarreal-Soto et al., 2019). The fermentation and metabolic processes of kombucha are accomplished through mutual facilitation or constraints within each flora. The synergistic effects of the flora allow for the synthesis of certain antimicrobial metabolites, the accumulation of organic acids leading to low pH, and the production of physical barriers (cellulose membranes), and other factors contributed to the hindrance of bacterial growth among competitors (Villarreal-Soto et al., 2019).
As depicted in Figure 1, the intricate phenolic compounds present in kombucha have the potential to undergo degradation or conversion into smaller biological molecules through the process of fermentation occurring within an acidic milieu, or through the enzymatic activities released by bacteria and yeast. For example, the observed elevation in overall catechin content in green and black tea kombucha subsequent to fermentation can be ascribed to the biotransformation process wherein epigallocatechin-3-gallate (EGCG) undergoes conversion into epigallocatechin gallate (ECG) and epicatechin (EC), by enzymes released by microbial communities in an acidic environment. EGCG is hydrolysed into smaller molecules and converted into epigallocatechin (EGC), EGCG, and EC (Jayabalan et al., 2008). Theaflavins and thearubigin are complex polyphenol derivatives found in black tea and are associated with colour changes in tea (Martínez-Leal et al., 2018). The lighter colour of a fully fermented kombucha may be attributable to the conversion of theaflavins to theobromine (Jayabalan et al., 2007).
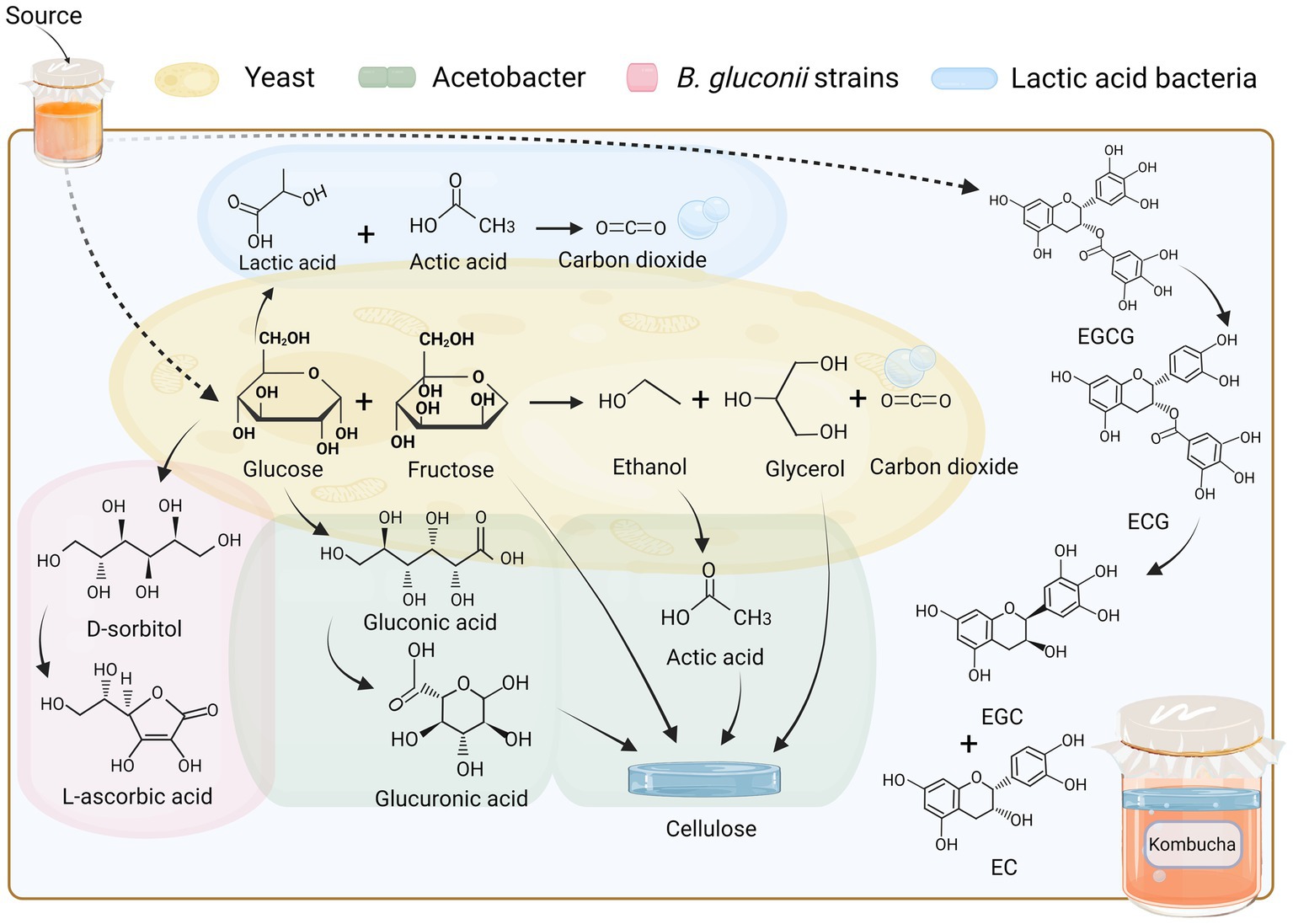
Figure 1. Material transformation relationship of raw materials and microbiota. Fermentation in an acidic environment or enzyme releases by bacteria and yeast may degrade or convert the complex phenolic compounds in kombucha into small biological molecules. EGCG, epigallocatechin-3-gallate; ECG, epigallocatechin gallate; EGC, epigallocatechin; EC, epicatechin.
3.3. Substance transformation of alternative raw materials after fermentation by kombucha
Traditional kombucha is prepared from black tea and white sugar, which are the primary raw materials used in microbial fermentation. However, in the last three decades, considerable research has resulted in the replacement of black tea and white sugar with other teas, herbs, plant materials, and sugars. The pharmacological products of fermentation depend on the active substances and their amount in the broth. The metabolites generated during kombucha fermentation of alternative substrates exhibit a strong correlation with the characteristics of the initial raw materials (Table 3).
3.4. Antibacterial property
Kombucha beverages produced through the fermentation of alternative raw material extracts are known to have antibacterial potential. The antibacterial profile of various substrates after fermentation with kombucha (Table 4) is influenced by different factors, including fermentation time, raw materials, temperature, and kombucha strains. The fermentation of kombucha is often considered a key factor contributing to its antibacterial activity. The competitive growth advantage of the dominant flora of traditional kombucha and the production of secondary metabolites, such as tea polyphenols, organic acids, and ethanol, can impede the growth and proliferation of pathogenic bacteria and fungi. Ayed et al. found that the growth of certain microbes, such as Staphylococcus aureus, Bacillus cereus, and Staphylococcus epidermidis, was found to be inhibited by fermented cactus pear juice; whereas unfermented pear fruit cactus juice had no antibacterial effect, and this inhibitory property was lost when the samples were neutralized (Ayed and Hamdi, 2015). The organic acids, specifically acetic acid, were identified as the primary contributors to the observed antimicrobial activity (Ayed and Hamdi, 2015). Četojević-Simin et al. employed an agar diffusion method to investigate the antimicrobial properties of lemon balm tea. The results indicated that unfermented lemon balm tea, with a dry weight concentration of 5 g/L, did not demonstrate any antimicrobial activity. However, the fermented lemon balm tea kombucha exhibited significant antimicrobial activity against prokaryotic microorganisms (G+ bacteria and G− bacteria) (Četojević-Simin et al., 2012). While no inhibitory activity was observed against fungi, yeast, or mould, the observed inhibition had similar activity to an acetic acid solution, which inferred that acetic acid served as the primary inhibitory agent within the kombucha beverage. The presence of other heat-resistant antibacterial components was verified by neutralizing with heating (Četojević-Simin et al., 2012). Vitas et al. (2018) identified significant antimicrobial properties in subcritical aqueous extracts of yarrow after fermentation; the potential antimicrobial substances were likely organic acids, phenolic compounds of plant origin, enzymes, proteins, and bacteriocins produced through fermentation.
The primary antimicrobial agent found in kombucha is acetic acid. The phenomenon encompasses the capacity of the undissociated acid to undergo unhindered diffusion across the lipid bilayer and subsequently release protons from the cytoplasm, thereby reducing the pH of the cytoplasm. This process also involves the integration of the undissociated acid within the lipid bilayer under conditions of low external pH, ultimately resulting in the accumulation of anions. The antimicrobial activity of kombucha is primarily attributed to two mechanisms: cytoplasmic acidification and the accumulation of free acid anions at toxic concentrations (Jayabalan et al., 2008). The acidification of the bacterial cytoplasm can impede bacterial growth through the inhibition of glycolysis, hindrance of active transport, or disruption of signal transduction (Roe et al., 2002).
In addition to organic acids, other components introduced by alternative raw materials cannot be ignored in bacterial inhibition. Some phenolic compounds with antimicrobial activity can affect the hyperacidification of the plasma membrane interface.
The integrity of this membrane can be compromised either by the H+-ATPase enzyme necessary for ATP production or through interaction with bacterial DNA, resulting in modifications to bacterial physiology and impeding cellular proliferation. The presence of Lycium barbarum polysaccharides in the fermentation broth exerted a pronounced inhibitory impact on both gram-positive and gram-negative bacteria. This effect was attributed to the rapid disruption of cellular membranes, thereby impeding the passage of essential nutrients and metabolites across the bacterial cells, ultimately leading to their inhibition (Zhang et al., 2022). Zubaidah et al. (2021c) revealed that the antibacterial activity of turmeric kombucha increased with the turmeric concentration and decreased after reaching a certain level. The antibacterial components of turmeric kombucha differ from turmeric concentrations; the curcuminoid compounds in turmeric and its essential oil act as antimicrobial agents by inhibiting the metabolism of microorganisms. In addition, terpene derivatives (sesquiterpenes and monoterpenes) in essential oils can disrupt the structure of bacterial cell membranes (Zubaidah et al., 2021b). Shahbazi et al. (2018) observed a notable reduction in the minimal inhibitory concentration (MIC) of cinnamon fermentation broth with decreasing pH; cinnamon components also disrupted the cytoplasmic membranes of gram-positive and gram-negative bacteria and reduced intracellular ATP concentrations. Pure and Pure (2016b) reported that gram-positive bacteria were more sensitive to garlic extracts than gram-negative bacteria. This phenomenon can be attributed to the influence of the lipid constituents present in the cell wall, which hinder the penetration of garlic’s active compounds into the cells.
In summary, fermented broth often combines the antibacterial properties of the raw material and the microbial strain, with organic acids being the active components. Fermentation creates an acidic environment for drugs and influences the antibacterial properties of raw materials, and the active substances of the raw materials also contribute to the antibacterial power. However, owing to the differences in strains, the intricate composition of strains, and diverse culture environments, it is difficult to evaluate the chemical composition and core flora associated with the inhibitory effects reported in each study, making it difficult to achieve consistent inhibitory activity across different studies. Pure and Pure (2016b) tested various samples using the disk diffusion method against S. typhimurium, S. aureus, E. coli, S. saprophytic, B. saprophyticus, and Pseudomonas aeruginosa. The authors found that the infused and fermented samples of common nettles, banana peel, and black tea did not demonstrate any antibacterial efficacy against the tested bacteria; this was thought to be attributed to the differences in the concentration of the aqueous extracts and the assay method (Pure and Pure, 2016b). Therefore, the development of kombucha fermentation broth as a natural biological preservative requires further work. More in-depth research on the antibacterial mechanisms of kombucha is required to optimise the strain composition, regulate the fermentation process, and actively develop kombucha products that are suitable for consumption and have clear health benefits.
3.5. Antioxidant effects
The fermentation process can enhance the antioxidant properties of Kombucha, thereby rendering it an excellent source of antioxidants (Table 5). Gamboa-Gómez et al. (2016) evaluated the effect of kombucha fermentation on the antioxidant activities of Litsea glaucescens and Eucalyptus camaldulensis, using three antioxidant activity indicators, namely thiobarbituric acid reactive substances (TBARS), α, α-diphenyl-ß-picrylhydrazyl (DPPH), and nitric oxide (NO). The researchers discovered that the process of kombucha fermentation enhanced the capacity of natural herbal infusions to effectively scavenge free radicals and inhibit lipid peroxidation (Gamboa-Gómez et al., 2016). Sun et al. used traditional kombucha as a control to test against a 1:1 mixture of wheatgrass juice and brown sugar tea and found that the modified fermentation broth exhibited higher levels of phenolic acid content and oxidative radical absorption capacity compared to the traditional kombucha. Notably, the DPPH scavenging rate reached 90% (Sun et al., 2015).
Polyphenols are the main antioxidants in the kombucha fermentation broth, and these entities possess the ability to readily donate hydroxyl hydrogen due to their resonance stabilization (Miller et al., 1993). This hydrogen supply enhances the DPPH-scavenging ability of the phenolic compounds. The increase in antioxidant properties of alternative raw materials for fermentation is usually associated with increased total phenol content, which is attributable to enzymatic synthesis and acid hydrolysis (Ayed et al., 2017). During the process of fermentation, microorganisms, specifically Acetobacter and Saccharomyces, present in kombucha, secrete enzymes that break down polyphenols into smaller compounds possessing potent antioxidant properties. Enzymes secreted by the kombucha SCOBY cleave flavonoid ring structures and/or phenolic conjugation sites, leading to structural rearrangements. Common rearrangements include the depolymerisation of theaflavins and theobromine and the cleavage of gallic acid into gallic acid (Chen and Liu, 2000). The potential outcome of these rearrangements is an enhancement in the bioavailability of phenolic compounds, specifically gallic acid, EC, and ECG 32.
The antioxidant properties of alternative raw materials stem from the differences in their total phenol contents and the specific substrates that generate antioxidant components during fermentation. Ayed et al. (2017) used red grape juice as an alternative substrate, and the fermentation process generated beneficial compounds that enhanced the antioxidant properties of the beverage. Ulusoy and Tamer (2019) discovered that the predominant contributors to the antioxidant activity of raspberries were anthocyanins and ellagitannins, accounting for 25% and 52% of the overall antioxidant activity, respectively. In addition, Zhang et al. (2022) found that the kombucha SCOBY has the potential to alter the composition of polysaccharides and the ratio of monosaccharides to polysaccharides after fermentation with Lycium barbarum, which affected its antioxidant activity.
3.6. Anti-inflammatory effects
As depicted in Figure 2, the anti-inflammatory effect of kombucha can be attributed to the beneficial components, including flavonoids and phenolic acids (gallic acid, catechins, and theaflavins), produced by the biotransformation and metabolism of the flora. The ameliorating effect of kombucha (15 mg/kg) on gastric ulcers was comparable to that of the positive control drug, omeprazole (3 mg/kg). Theaflavin was the main anti-inflammatory component, and the healing rate of theaflavin (1 mg/kg) in mice over 7 days was 81.4% (Banerjee et al., 2010). Many kombucha fermentation broths prepared using alternative raw materials exhibit anti-inflammatory properties. Kanno et al. (2006) used tea made from oak leaves as a substrate for preparing kombucha fermentation broth, and the broth effectively downregulated NO production and exhibited a substantial decrease in the expression of tumour necrosis factor-alpha (TNF-α) and interleukin-6 (IL-6) in lipopolysaccharide (LPS)-stimulated macrophages (THP-1). The presence of naringin in oak demonstrated a significant inhibition of LPS-induced nitric oxide (NO) production, as well as the expression of inflammatory gene products, including inducible nitric oxide synthase (iNOS), TNF-α, and IL-6 (Kanno et al., 2006); moreover, (+)-catechin present in oak inhibited LPS-stimulated NO and TNF-α production in macrophages (Guruvayoorappan and Kuttan, 2008). The phenolic content of Kombucha, which is produced through the fermentation of oak leaf tea, contributes to its notable anti-inflammatory and antioxidant properties (Vázquez-Cabral et al., 2017). Ziemlewska et al. (2021) found that yerba mate extracts used as an alternative raw fermentation material showed potent inhibition of lipoxygenase (LOX) after 14 and 21 days of fermentation. The enzyme LOX plays a crucial role in the synthesis of leukotrienes, which are known as mediators of the pro-inflammatory response. Therefore, the regulation of LOX activity holds significant importance in the treatment of inflammation (Nworu and Akah, 2015; Oguntibeju, 2018).
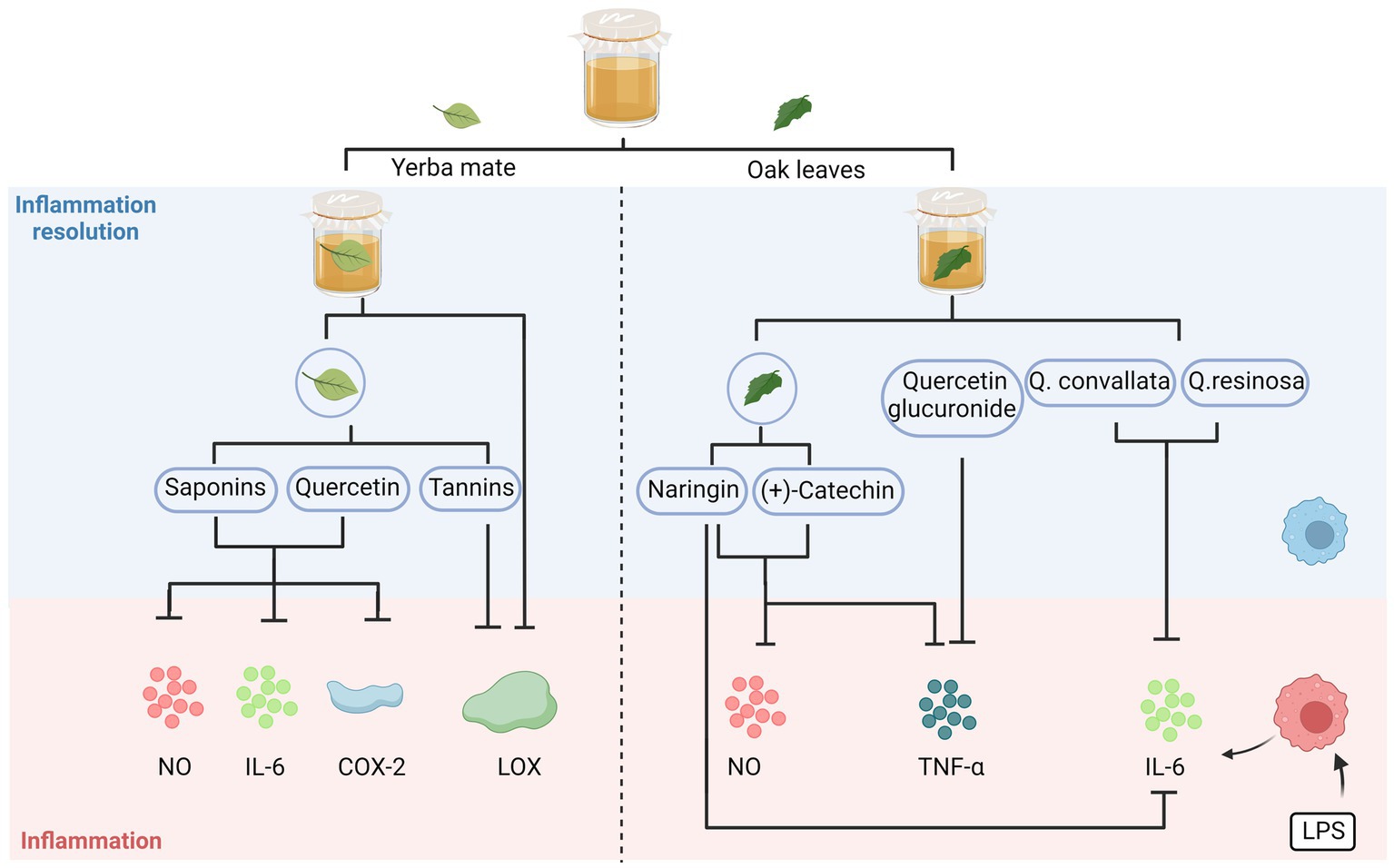
Figure 2. Anti-inflammatory activities of alternative substrates after fermentation. The anti-inflammatory properties of kombucha are attributed to a combination of beneficial compounds generated by the flora’s biotransformation and metabolism. NO, nitric oxide; IL-6, interleukin-6; COX-2, cyclooxygenase-2; LOX, lipoxygenase; TNF-α, tumour necrosis factor alpha; LPS, lipopolysaccharides.
Quercetin and saponins from yerba mate extract are the main components responsible for their anti-inflammatory effects, and these substances can reduce the production of IL-6, cyclooxygenase-2 (COX-2), and NO—the main mediators of the inflammatory process. Additionally, the tannins in yerba mate extracts have an inhibitory effect on LOX activity (Ziemlewska et al., 2021).
3.7. Anti-diabetic effects
Diabetes mellitus is a metabolic disorder characterized by inadequate insulin secretion or islet insufficiency. As shown in Figure 3, studies on the hypoglycaemic effects of kombucha have mainly focused on the analysis and histological observations of the biological activities of key enzymes in the glucose metabolic pathway; only a few in-depth studies have been reported on the associated molecular mechanisms. Zubaidah et al. (2019) demonstrated that the fermentation process of snake fruit extracts into kombucha effectively mitigated oxidative stress and provided stability to fluctuations in fasting blood glucose levels within a streptozotocin-induced diabetic model. This glucose-lowering effect was comparable to that of metformin hydrochloride—the “king” glucose-lowering drug (Zubaidah et al., 2019). Watawana et al. (2016) found that king coconut water fermented into kombucha resulted in the enhanced inhibition of starch hydrolases. The inhibitory activity of both α-amylase and glucosidase was enhanced by the fermentation process, with a greater enhancement observed in α-amylase inhibitory activity compared to α-glucosidase inhibitory activity. The enzyme α-amylase exerts its action prior to α-glucosidase, thus hindering the reaction rate of α-glucosidase and impeding the release of glucose into the physiological system. This outcome proves advantageous as it demonstrates a beneficial anti-hyperglycemic effect (Watawana et al., 2016). Aung and Eun (2021) showed that the fermentation broth of laver, as an alternative raw material, inhibited amylase activity in vitro. Fermented laver kombucha, which contained important flavonoid compounds, enhanced α-amylase inhibitory activity at 25°C (Aung and Eun, 2021). Al-Dulaimi et al. (2018) explored the effects of kombucha fermentation with skim milk as a substrate on serum glucose concentrations, total lipid profiles, and body weight in male rats. Skim milk fermentation reduced blood glucose, total lipid, alanine aminotransferase (ALT), aspartate transaminase (AST), and alkaline phosphatase (ALP) in the rats and exerted beneficial effects on the human liver and overall health. This was attributable to the combined effects of polyphenols (such as flavonoids and catechins), vitamin E, and organic acids in the fermentation broth (Al-Dulaimi et al., 2018). Permatasari et al. (2022) found that butterfly pea flower kombucha (KBPF) inhibited α-amylase and α-glucosidase activities, achieving similar levels of inhibition to the acarbose control at concentrations of 50–250 μg/mL. In vivo, KBPF administration (130 mg/kg BW) significantly alleviated the metabolic disturbances induced by a high-fat diet through the modulation of glucose level, oxidative stress markers (superoxide dismutase), metabolic enzymes (lipases and amylases), inflammatory markers (PGC-1α, TNF-α, and IL-10), and lipids, such as total cholesterol (TC), triglyceride (TG), low-density lipoprotein cholesterol (LDL), and high-density lipoprotein cholesterol (HDL). In addition, KBPF had a positive effect on the phyla Bacteroidetes and Firmicutes (Permatasari et al., 2022).
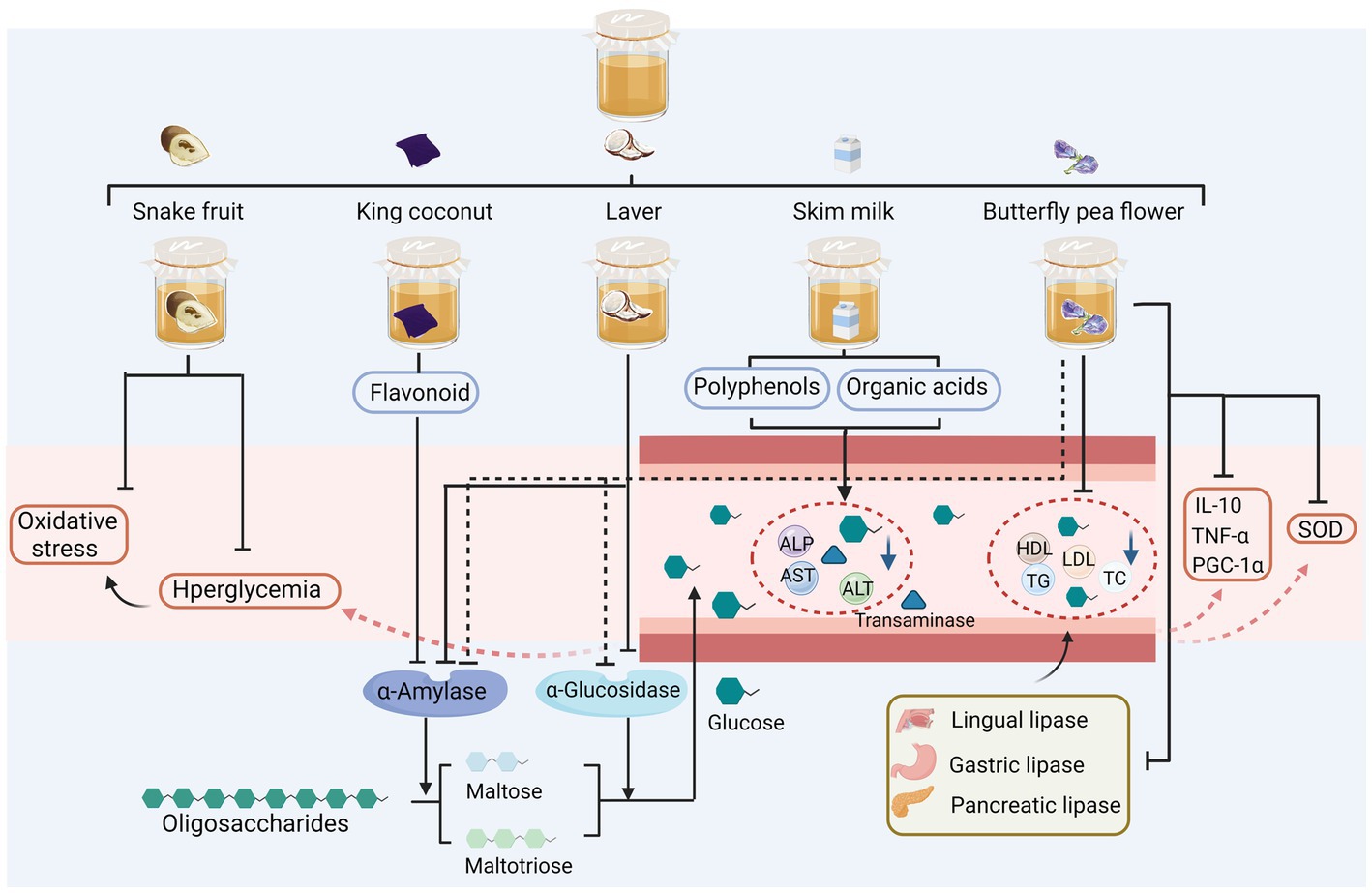
Figure 3. Sugar-reducing activity of alternative substrates after fermentation. ALP, alkaline phosphatase; ALT, alanine aminotransferase; AST, aspartate transaminase; HDL, high-density lipoprotein cholesterol; LDL, low-density lipoprotein cholesterol; TC, total cholesterol, TG, triglyceride; PGC-1α, peroxisome proliferator-activated receptor-γ coactivator 1-α; TNF-α, tumour necrosis factor alpha; IL-10, interleukin-10.
3.8. Skincare applications
As shown in Figure 4, Ziemlewska et al. (2021) determined the skin care effects of fermented yerba mate kombucha on keratinocytes and fibroblast cell lines. They found that fermentation strongly inhibited lipoxygenase, collagenase, and elastase activities. Furthermore, the researchers noted a sustained moisturizing effect subsequent to the topical administration. The fermentation solution comprised of phenolic acids, methylxanthines, and flavonoids, which actively contributed to the inhibition of enzymatic activity associated with skin aging. The primary compounds possessing moisturizing properties are antioxidants (polyphenols and flavonoids), proteins, amino acids, and carbohydrates, which feature hydroxyl groups capable of forming hydrogen bonds with water (Ziemlewska et al., 2021). Zofia et al. (2020) showed that kombucha with coffee beans as a substrate could inhibit collagenase and elastase activities, improve skin hydration, and exert sunscreen effects. The concentrations of polyphenols, flavonoids, and caffeine in the fermentation broth considerably increased the inhibition of collagenase and elastase activities. The active ingredients in the coffee fermentation product, such as monosaccharides, amino acids, vitamins, polyphenols, and flavonoids, showed nourishing and soothing effects. The sunscreen properties of fermentates are mainly attributable to substances derived from flavonoids, polyphenols, anthocyanins, proteins, amino acids, and vitamins (Zofia et al., 2020).
3.9. Anti-cancer effects
The consumption of kombucha can improve the immune system and enhance the ability of the body to fight cancer (Figure 5). Salafzoon et al. (2017) obtained samples from a 10-day kombucha fermentation of ginger infusion containing ginger bioactive components, such as gingerol and shogaol, which have anti-inflammatory and anti-tumour activities. These samples could inhibit tumour proliferation and stimulate apoptosis. Fermented ginger infusions reduced the activities of peroxidase, glutathione, and malondialdehyde in tumours, liver, and kidney homogenates (Salafzoon et al., 2017). Vitas et al. (2018) found that the fermentation broth of kombucha with yarrow as a substrate has antiproliferative activity against human rhabdomyosarcoma cells, human cervical cancer Hep2 cells (HeLa), murine fibroblasts (L2OB), and other tumour cells. Uțoiu et al. found that the fermentation broth of kombucha with bee pollen as a substrate exerted different levels of antitumour activity in Hep-2 and Caco-2 cells (Uțoiu et al., 2018). The fermented pollen exhibited elevated concentrations of bioactive compounds, including polyphenols, soluble silica substances, and short-chain fatty acids, thereby augmenting the health-promoting properties of pollen through kombucha fermentation (Uțoiu et al., 2018). Furthermore, the parameters for Kombucha fermentation were optimized to enhance the cytotoxic efficacy of the n-hexane fruit extract derived from Solanum nigrum L. against MCF-7 breast cancer cells (Ziska et al., 2019).
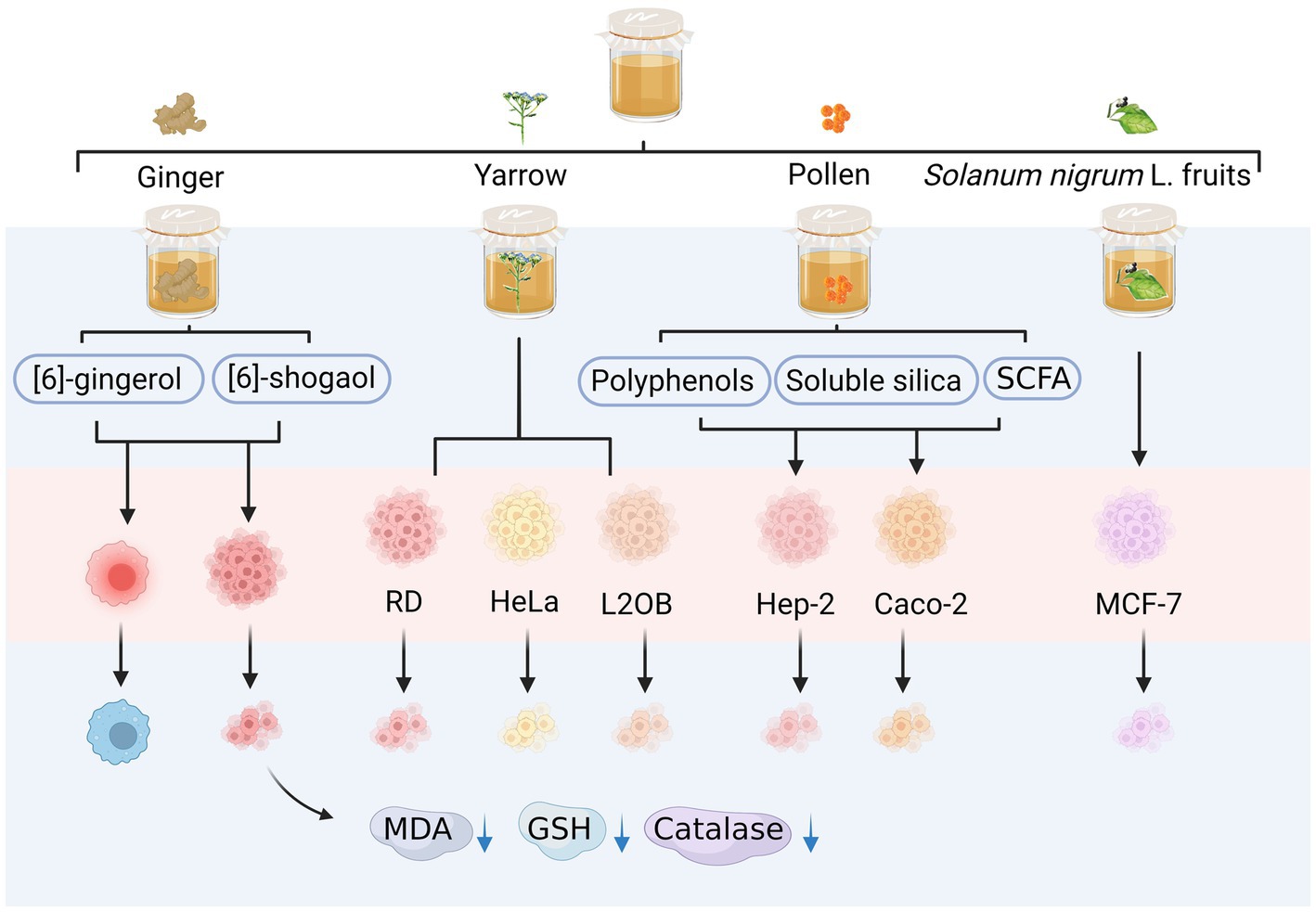
Figure 5. Anti-cancer activity of alternative substrates after fermentation. RD, rhabdomyosarcoma; HeLa, human cervical cancer Hep2c cells; L2OB, murine fibroblasts cancer cells; GSH, glutathione; MDA, malondialdehyde.
3.10. Other effects
The fermentation process of African mustard leaves by kombucha resulted in a notable enhancement in the overall phenolic and ethyl acetate levels, as well as the antioxidant capacity, when compared to the unfermented samples (Rahmani et al., 2019). In addition, kombucha fermentation increased anti-acetylcholinesterase activity; moreover, it reduced the cytotoxicity of Brassica tournefortii leaves and its inhibitory effect on xanthine oxidase (Rahmani et al., 2019). Zubaidah et al. conducted a study to examine the immunomodulatory properties of turmeric and black tea kombucha on BALB/c mice. The results of their investigation revealed that turmeric kombucha exhibited a significant enhancement in the adaptive immune response, mainly in the form of increased expression levels of CD4+, TNF-α, and IFN-γ, while enhancing the innate immune response, mainly in the form of decreased expression levels of CD68 and IL-6 (Zubaidah et al., 2021c). L. barbarum (Zhang et al., 2022), rose, and jujube kernels (Zhang et al., 2020) can contribute to cellulase activity when used as alternative fermentation substrates. With the use of winter savoury peppermint, stinging nettle, wild thyme, elderberry, quince (Vitas et al., 2020), and milk (Elkhtab et al., 2017), kombucha fermentation broth showed inhibitory activity against angiotensin-converting enzyme, and the resulting beverage showed antihypertensive potential.
As shown in Figure 6, there have been numerous studies on the functions of kombucha. However, most have focused on functional efficacy, with minimal investigation into the underlying fermentation process.
3.11. Adverse effects
Instances of health issues resulting from the consumption of kombucha have been documented in scholarly literature, encompassing symptoms such as dizziness, headaches, allergic reactions, acidosis, and gastrointestinal ailments (Villarreal-Soto et al., 2018; Batista et al., 2022). These complications primarily arise from various factors, including excessive consumption, inadequate sanitation practices at home, contamination by pathogenic microorganisms, and susceptibility of immunocompromised individuals. For instance, pregnant women are advised against consuming kombucha due to its ethanol content, substantial acetic acid concentration, and potential presence of substances like heparin (Martínez-Leal et al., 2018; de Miranda et al., 2022). The excessive ingestion of kombuella in individuals with a history of excessive alcohol consumption has the potential to result in significant liver necrosis. Findings from pathological examination suggest a correlation between kombuella and liver damage in these patients, as evidenced by markedly elevated serum levels of aspartate aminotransferase and alanine aminotransferase, surpassing what would be expected solely from alcohol consumption (Sannapaneni et al., 2023).
4. Concluding remarks
We reviewed recent studies on the functional components of kombucha produced using alternative raw materials. The antibacterial and antioxidant effects of kombucha have been extensively studied. Kombucha contains diverse bioactive constituents, and the microorganisms responsible for the fermentation process exhibit intricate characteristics. At present, alternative raw materials for kombucha fermentation are mostly limited to foodstuffs, mainly for the development of beverages. This post-fermentation efficacy investigation identified the basic functions of kombucha, such as its antibacterial and antioxidant activities and focused on assessing the mixture of total acids, total phenols, and total flavonoids that could be beneficial.
Despite the numerous studies on kombucha, several unresolved issues remain. First, few studies have reported the inhibitory effects of fermentation broths produced from different substrates on drug-resistant, lethal, pathogenic, and typical inflammatory microbiota. Moreover, limited research has been conducted to investigate the constituent elements of distinct antibacterial and antioxidant compounds subsequent to the process of fermenting alternative raw materials. The refinement of its antimicrobial spectrum as well as the characterisation and purification of specific antimicrobial and antioxidant substances, are of great research significance.
Second, although kombucha has good anti-inflammatory properties, the experimental models used have been relatively homogeneous; clinical value assessments have not yet been carried out, and exploration of the anti-inflammatory mechanisms is still in the preliminary stages. Thus, more in vivo and ex vivo experiments are required to further elucidate the anti-inflammatory components, molecular mechanisms of action, and key functional strains to lay a solid theoretical foundation for subsequent clinical trials on the effects of kombucha on human health.
Third, insufficient scholarly investigation has been undertaken regarding the qualitative and quantitative analyses of particular substances, such as polyphenols and terpenoids, or the reaction processes of specific substances in mixed strains. The identification and purification of active ingredients after the fermentation of alternative raw materials, the metabolic pathways in kombucha, and the specific pharmacological mechanisms of action have not yet been extensively studied. It may be possible to broaden the range of raw materials for kombucha fermentation to traditional Chinese medicines or pure monomeric compounds, similar to the development of specific pharmaceutical fermenters. The metabolic reaction pathways and products of specific substances during strain fermentation could be supplemented. Alternatively, controlling the process to increase the yield of the main drug could retain the advantages of kombucha, such as containing many beneficial ingredients and natural antibacterial properties, which have promising applications in cosmetics and pharmaceuticals.
Future research should focus on the predominant fermentation strains and their interactions to develop improved and consistent artificial agents that can aid in standardising kombucha production.
Author contributions
JS: Conceptualization, Data curation, Formal analysis, Funding acquisition, Investigation, Methodology, Project administration, Resources, Supervision, Validation, Visualization, Writing – original draft, Writing – review & editing. QTan: Data curation, Formal analysis, Investigation, Methodology, Software, Validation, Visualization, Writing – original draft. QTang: Data curation, Formal analysis, Investigation, Methodology, Software, Writing – original draft. ZT: Data curation, Formal analysis, Investigation, Methodology, Software, Writing – original draft. MY: Conceptualization, Data curation, Formal analysis, Funding acquisition, Investigation, Project administration, Supervision, Validation, Visualization, Writing – review & editing.
Funding
The author(s) declare financial support was received for the research, authorship, and/or publication of this article. This work was supported by the National Natural Science Foundation of China (81901243), the Fujian Provincial Regional Development Project (2021N3005), and the Natural Science Foundation of Fujian Province, China (2021J01204).
Acknowledgments
We would like to thank Songying Ouyang for providing suggestions regarding the manuscript.
Conflict of interest
The authors declare that the research was conducted in the absence of any commercial or financial relationships that could be construed as a potential conflict of interest.
Publisher’s note
All claims expressed in this article are solely those of the authors and do not necessarily represent those of their affiliated organizations, or those of the publisher, the editors and the reviewers. Any product that may be evaluated in this article, or claim that may be made by its manufacturer, is not guaranteed or endorsed by the publisher.
References
Abuduaibifu, A., and Tamer, C. E. (2019). Evaluation of physicochemical and bioaccessibility properties of goji berry kombucha. J. Food Process. Preserv. 43:e14077. doi: 10.1111/jfpp.14077
Ahmed, R. F., Hikal, M. S., and Abou-Taleb, K. A. (2020). Biological, chemical and antioxidant activities of different types Kombucha. Ann. Agri. Sci. 65, 35–41. doi: 10.1016/j.aoas.2020.04.001
Akbarirad, H., Assadi, M. M., Pourahmad, R., and Khaneghah, A. M. (2017). Employing of the different fruit juices substrates in vinegar kombucha preparation. Curr. Nutr. Food Sci. 13, 303–308. doi: 10.2174/1573401313666170214165641
Al-Dulaimi, F. K., Abd-Alwahab, W. I., and Hasan, A. S. L. (2018). Bioactivity study of kombucha black tea and kombucha with skim milk on some of physiological and biochemical parameters in male albino rats. Int. J. Pharmacol. Res. 10, 301–306. doi: 10.13140/RG.2.2.25181.87527
Antolak, H., Piechota, D., and Kucharska, A. (2021). Kombucha tea-a double power of bioactive compounds from tea and symbiotic culture of bacteria and yeasts (SCOBY). Antioxidants (Basel). 10:1541. doi: 10.3390/antiox10101541
Aung, T., and Eun, J. B. (2021). Production and characterization of a novel beverage from laver (Porphyra dentata) through fermentation with kombucha consortium. Food Chem. 350:129274. doi: 10.1016/j.foodchem.2021.129274
Aung, T., and Eun, J.-B. (2022). Impact of time and temperature on the physicochemical, microbiological, and nutraceutical properties of laver kombucha (Porphyra dentata) during fermentation. LWT 154:112643. doi: 10.1016/j.lwt.2021.112643
Ayed, L., Ben Abid, S. B., and Hamdi, M. (2017). Development of a beverage from red grape juice fermented with the kombucha consortium. Ann. Microbiol. 67, 111–121. doi: 10.1007/s13213-016-1242-2
Ayed, L., and Hamdi, M. (2015). Manufacture of a beverage from cactus pear juice using ‘tea fungus’ fermentation. Ann. Microbiol. 65, 2293–2299. doi: 10.1007/s13213-015-1071-8
Banerjee, D., Hassarajani, S. A., Maity, B., Narayan, G., Bandyopadhyay, S. K., and Chattopadhyay, S. (2010). Comparative healing property of kombucha tea and black tea against indomethacin-induced gastric ulceration in mice: possible mechanism of action. Food Funct. 1, 284–293. doi: 10.1039/c0fo00025f
Batista, P., Penas, M. R., Pintado, M., and Oliveira-Silva, P. (2022). Kombucha: perceptions and future prospects. Foods 11:1977. doi: 10.3390/foods11131977
Battikh, H., Bakhrouf, A., and Ammar, E. (2012). Antimicrobial effect of kombucha analogues. LWT-Food Sci. Technol. 47, 71–77. doi: 10.1016/j.lwt.2011.12.033
Batubara, S. (2022). Study on kombucha tea from dragon fruit peel potential as a high-antioxidant functional drink. Curr. Dev. Nutr. 6:506. doi: 10.1093/cdn/nzac077.009
Bauer-Petrovska, B., and Petrushevska-Tozi, L. (2000). Mineral and water soluble vitamin content in the kombucha drink. Int. J. Food Sci. Tech. 35, 201–205. doi: 10.1046/j.1365-2621.2000.00342.x
Bishop, P., Pitts, E. R., Budner, D., and Thompson-Witrick, K. A. (2022). Chemical composition of kombucha. Beverages 8:45. doi: 10.3390/beverages8030045
Candra, A., Prasetyo, B. E., and Tarigan, J. B. (2021). Study of vitamin C level of soursop leaves (Annona muricata L.) and galactomannan utilization in kombucha during fermentation. AIP Conf. Proc. 2342:100007. doi: 10.1063/5.0045669
Cetojevic-Simin, D. D., Bogdanovic, G. M., Cvetkovic, D. D., and Velicanski, A. S. (2008). Antiproliferative and antimicrobial activity of traditional kombucha and Satureja montana L. Kombucha. J. Buon. 13, 395–401. doi: 10.2527/jas.2007-0702
Četojević-Simin, D. D., Velićanski, A. S., Cvetković, D. D., Markov, S. L., Mrđanović, J. Ž., Bogdanović, V. V., et al. (2012). Bioactivity of lemon balm kombucha. Food Bioprocess Technol. 5, 1756–1765. doi: 10.1007/s11947-010-0458-6
Chakravorty, S., Bhattacharya, S., Chatzinotas, A., Chakraborty, W., Bhattacharya, D., and Gachhui, R. (2016). Kombucha tea fermentation: microbial and biochemical dynamics. Int. J. Food Microbiol. 220, 63–72. doi: 10.1016/j.ijfoodmicro.2015.12.015
Chawla, P. R., Bajaj, I. B., Survase, S. A., and Singhal, R. S. (2009). Microbial cellulose: fermentative production and applications. Food Technol. Biotechnol. 47, 107–124. doi: 10.1016/S0141-3910(97)00197-3
Chen, C., and Liu, B. Y. (2000). Changes in major components of tea fungus metabolites during prolonged fermentation. J. Appl. Microbiol. 89, 834–839. doi: 10.1046/j.1365-2672.2000.01188.x
Christiani Dwiputri, M., and Lauda Feroniasanti, Y. M. (2019). Effect of fermentation to total titrable acids, flavonoid and antioxidant activity of butterfly pea kombucha. J. Phys.: Conf. Ser. 1241:012014. doi: 10.1088/1742-6596/1241/1/012014
Coelho, R. M. D., De Almeida, A. L., Do Amaral, R. Q. G., da Mota, R. N., and de Sousa, P. H. M. (2020). Kombucha: review. Int. J. Gastron. Food Sci. 22:100272. doi: 10.1016/j.ijgfs.2020.100272
de Miranda, J. F., Ruiz, L. F., Silva, C. B., Uekane, T. M., Silva, K. A., Gonzalez, A. G. M., et al. (2022). Kombucha: a review of substrates, regulations, composition, and biological properties. J. Food Sci. 87, 503–527. doi: 10.1111/1750-3841.16029
Elkhtab, E., El-Alfy, M., Shenana, M., Mohamed, A., and Yousef, A. E. (2017). New potentially antihypertensive peptides liberated in milk during fermentation with selected lactic acid bacteria and kombucha cultures. J. Dairy Sci. 100, 9508–9520. doi: 10.3168/jds.2017-13150
Emiljanowicz, K. E., and Malinowska-Pańczyk, E. (2020). Kombucha from alternative raw materials – the review. Crit. Rev. Food Sci. Nutr. 60, 3185–3194. doi: 10.1080/10408398.2019.1679714
Francisco, Á. R., José, R. M., and Igor, H. (2021). Development of a no added sugar kombucha beverage based on germinated corn. Int. J. Gastron. Food Sci. 24:100355. doi: 10.1016/j.ijgfs.2021.100355
Gaggìa, F., Baffoni, L., Galiano, M., Nielsen, D. S., Jakobsen, R. R., Castro-Mejía, J. L., et al. (2018). Kombucha beverage from green, black and rooibos teas: a comparative study looking at microbiology, chemistry and antioxidant activity. Nutrients 11:1. doi: 10.3390/nu11010001
Gamboa-Gómez, C. I., González-Laredo, R. F., Gallegos-Infante, J. A., Pérez, M. D., Moreno-Jiménez, M. R., Flores-Rueda, A. G., et al. (2016). Antioxidant and angiotensin-converting enzyme inhibitory activity of Eucalyptus camaldulensis and Litsea glaucescens infusions fermented with kombucha consortium. Food Technol. Biotechnol. 54, 367–374. doi: 10.17113/ftb.54.03.16.4622
Guruvayoorappan, C., and Kuttan, G. (2008). (+)-Catechin inhibits tumour angiogenesis and regulates the production of nitric oxide and TNF-alpha in LPS-stimulated macrophages. Innate Immun. 14, 160–174. doi: 10.1177/1753425908093295
Jakubczyk, K., Kałduńska, J., Kochman, J., and Janda, K. (2020). Chemical profile and antioxidant activity of the kombucha beverage derived from white, green, black and red tea. Antioxidants 9:447. doi: 10.3390/antiox9050447
Jayabalan, R., Malbaša, R. V., Lončar, E. S., Vitas, J. S., and Sathishkumar, M. (2014). A review on kombucha tea-microbiology, composition, fermentation, beneficial effects, toxicity, and tea fungus. Compr. Rev. Food Sci. Food Saf. 13, 538–550. doi: 10.1111/1541-4337.12073
Jayabalan, R., Marimuthu, S., and Swaminathan, K. (2007). Changes in content of organic acids and tea polyphenols during kombucha tea fermentation. Food Chem. 102, 392–398. doi: 10.1016/j.foodchem.2006.05.032
Jayabalan, R., Subathradevi, P., Marimuthu, S., Sathishkumar, M., and Swaminathan, K. (2008). Changes in free-radical scavenging ability of kombucha tea during fermentation. Food Chem. 109, 227–234. doi: 10.1016/j.foodchem.2007.12.037
Kaewkod, T., Bovonsombut, S., and Tragoolpua, Y. (2019). Efficacy of kombucha obtained from green, oolong, and black teas on inhibition of pathogenic bacteria, antioxidation, and toxicity on colorectal cancer cell line. Microorganisms 7:700. doi: 10.3390/microorganisms7120700
Kanno, S.-I., Shouji, A., Tomizawa, A., Hiura, T., Osanai, Y., Ujibe, M., et al. (2006). Inhibitory effect of naringin on lipopolysaccharide (LPS)-induced endotoxin shock in mice and nitric oxide production in RAW 264.7 macrophages. Life Sci. 78, 673–681. doi: 10.1016/j.lfs.2005.04.051
Kitwetcharoen, H., Phung, L. T., Klanrit, P., Thanonkeo, S., Tippayawat, P., Yamada, M., et al. (2023). Kombucha healthy drink—recent advances in production, chemical composition and health benefits. Fermentation 9:48. doi: 10.3390/fermentation9010048
Klawpiyapamornkun, T., Uttarotai, T., Wangkarn, S., Sirisa-ard, P., Kiatkarun, S., Tragoolpua, Y., et al. (2023). Enhancing the chemical composition of kombucha fermentation by adding Indian gooseberry as a substrate. Fermentation 9:291. doi: 10.3390/fermentation9030291
Laureys, D., Britton, S. J., and De Clippeleer, J. (2020). Kombucha tea fermentation: a review. J. Am. Soc. Brew. Chem. 78, 165–174. doi: 10.1080/03610470.2020.1734150
Leonarski, E., Cesca, K., Zanella, E., Stambuk, B. U., de Oliveira, D., and Poletto, P. (2021). Production of kombucha-like beverage and bacterial cellulose by acerola by-product as raw material. LWT 135:110075. doi: 10.1016/j.lwt.2020.110075
Li, S., Zhang, Y., Gao, J., Li, T., Li, H., Mastroyannis, A., et al. (2022). Effect of fermentation time on physiochemical properties of kombucha produced from different teas and fruits: comparative study. J. Food Qual. 2022, 1–10. doi: 10.1155/2022/2342954
Lopes, D. R., Santos, L. O., and Prentice-Hernández, C. (2021). Antioxidant and antibacterial activity of a beverage obtained by fermentation of yerba-maté (Ilex paraguariensis) with symbiotic kombucha culture. J. Food Process. Preserv. 45:e15101. doi: 10.1111/jfpp.15101
Malbaša, R., Lončar, E., and Djurić, M. (2008). Comparison of the products of kombucha fermentation on sucrose and molasses. Food Chem. 106, 1039–1045. doi: 10.1016/j.foodchem.2007.07.020
Malbaša, R. V., Lončar, E. S., Vitas, J. S., and Čanadanović-Brunet, J. M. (2011). Influence of starter cultures on the antioxidant activity of kombucha beverage. Food Chem. 127, 1727–1731. doi: 10.1016/j.foodchem.2011.02.048
Mamlouk, D., and Gullo, M. (2013). Acetic acid bacteria: physiology and carbon sources oxidation. Indian J. Microbiol. 53, 377–384. doi: 10.1007/s12088-013-0414-z
Martínez-Leal, J., Ponce-García, N., and Escalante-Aburto, A. (2020). Recent evidence of the beneficial effects associated with glucuronic acid contained in kombucha beverages. Curr. Nutr. Rep. 9, 163–170. doi: 10.1007/s13668-020-00312-6
Martínez-Leal, J., Valenzuela Suárez, L., Jayabalan, R., Huerta Oros, J. H., and Escalante-Aburto, A. (2018). A review on health benefits of kombucha nutritional compounds and metabolites. CyTA-J. Food 16, 390–399. doi: 10.1080/19476337.2017.1410499
Miller, N. J., Rice-Evans, C., Davies, M. J., Gopinathan, V., and Milner, A. (1993). A novel method for measuring antioxidant capacity and its application to monitoring the antioxidant status in premature neonates. Clin. Sci. (Lond.) 84, 407–412. doi: 10.1042/cs0840407
Neffe-Skocińska, K., Sionek, B., Ścibisz, I., and Kołożyn-Krajewska, D. (2017). Acid contents and the effect of fermentation condition of kombucha tea beverages on physicochemical, microbiological and sensory properties. CyTA-J. Food 15, 601–607. doi: 10.1080/19476337.2017.1321588
Nworu, C. S., and Akah, P. A. (2015). Anti-inflammatory medicinal plants and the molecular mechanisms underlying their activities. Afr. J. Trad. Compl. Alt. Med. 12, 52–61. doi: 10.4314/ajtcam.v12i6.3S
Oguntibeju, O. O. (2018). Medicinal plants with anti-inflammatory activities from selected countries and regions of Africa. J. Inflamm. Res. 11, 307–317. doi: 10.2147/JIR.S167789
Permatasari, H. K., Nurkolis, F., Gunawan, W. B., Yusuf, V. M., Yusuf, M., Kusuma, R. J., et al. (2022). Modulation of gut microbiota and markers of metabolic syndrome in mice on cholesterol and fat enriched diet by butterfly pea flower kombucha. Curr. Res Food Sci. 5, 1251–1265. doi: 10.1016/j.crfs.2022.08.005
Petrović, S. E., Petrović, S. E., Malbaša, R. V., and Verac, R. M. (2000). Biosynthesis of glucuronic acid by means of tea fungus. Nahrung 44, 138–139. doi: 10.1002/(SICI)1521-3803(20000301)44:2<138::AID-FOOD138>3.0.CO;2-#
Phung, L. T., Kitwetcharoen, H., Chamnipa, N., Boonchot, N., Thanonkeo, S., Tippayawat, P., et al. (2023). Changes in the chemical compositions and biological properties of kombucha beverages made from black teas and pineapple peels and cores. Sci. Rep. 13:7859. doi: 10.1038/s41598-023-34954-7
Pure, A. E., and Pure, M. E. (2016a). Antioxidant, antibacterial and color analysis of garlic fermented in kombucha and red grape vinegar. Appl. Food Biotechnol. 3, 246–252. doi: 10.22037/afb.v3i4.12312
Pure, A. E., and Pure, M. E. (2016b). Antioxidant and antibacterial activity of kombucha beverages prepared using banana peel, common nettles and black tea infusions. Appl. Food Biotechnol. 3, 125–130. doi: 10.22037/afb.v3i2.11138
Rahmani, R., Beaufort, S., Villarreal-Soto, S. A., Taillandier, P., Bouajila, J., and Debouba, M. (2019). Kombucha fermentation of African mustard (Brassica tournefortii) leaves: chemical composition and bioactivity. Food Biosci. 30:100414. doi: 10.1016/j.fbio.2019.100414
Roe, A. J., O’Byrne, C., Mclaggan, D., and Booth, I. R. (2002). Inhibition of Escherichia coli growth by acetic acid: a problem with methionine biosynthesis and homocysteine toxicity. Microbiology (Reading). 148, 2215–2222. doi: 10.1099/00221287-148-7-2215
Salafzoon, S., Mahmoodzadeh Hosseini, H., and Halabian, R. (2017). Evaluation of the antioxidant impact of ginger-based kombucha on the murine breast cancer model. J. Complement. Integr. Med. 15, 1–8. doi: 10.1515/jcim-2017-0071
Sannapaneni, S., Philip, S., Desai, A., Mitchell, J., and Feldman, M. (2023). Kombucha-induced massive hepatic necrosis: a case report and a review of literature. Gastro Hep Advances 2, 196–198. doi: 10.1016/j.gastha.2022.09.014
Shahbazi, H., Hashemi Gahruie, H., Golmakani, M. T., Eskandari, M. H., and Movahedi, M. (2018). Effect of medicinal plant type and concentration on physicochemical, antioxidant, antimicrobial, and sensorial properties of kombucha. Food Sci. Nutr. 6, 2568–2577. doi: 10.1002/fsn3.873
Shin, S.-S., Ko, H.-M., Kim, C.-I., and Park, S.-S. (2016). Bioactivity and cytotoxicity of kombucha fermented with extracts from green tea and citrus peel. J. Korean Soc. Food Sci. Nutr. 45, 1838–1842. doi: 10.3746/jkfn.2016.45.12.1838
Sinir, G. Ö., Tamer, C. E., and Suna, S. (2019). “Kombucha tea: a promising fermented functional beverage” in Fermented beverages (Elsevier). eds. A. M. Grumezescu and A. M. Holban (Sawston: Woodhead Publishing), 401–432.
Sun, T. Y., Li, J. S., and Chen, C. (2015). Effects of blending wheatgrass juice on enhancing phenolic compounds and antioxidant activities of traditional kombucha beverage. J. Food Drug Anal. 23, 709–718. doi: 10.1016/j.jfda.2015.01.009
Taha, M., Uddin, N., Ali, M., Anouar, E. H., Rahim, F., Khan, G., et al. (2020). Inhibition potential of phenyl linked benzimidazole-triazolothiadiazole modular hybrids against β-glucuronidase and their interactions thereof. Int. J. Biol. Macromol. 161, 355–363. doi: 10.1016/j.ijbiomac.2020.06.006
Tan, W. C., Muhialdin, B. J., and Meor Hussin, A. S. (2020). Influence of storage conditions on the quality, metabolites, and biological activity of soursop (Annona muricata. L.) Kombucha. Front. Microbiol. 11:603481. doi: 10.3389/fmicb.2020.603481
Tanticharakunsiri, W., Mangmool, S., Wongsariya, K., and Ochaikul, D. (2021). Characteristics and upregulation of antioxidant enzymes of kitchen mint and oolong tea kombucha beverages. J. Food Biochem. 45:e13574. doi: 10.1111/jfbc.13574
Tu, C., Tang, S., Azi, F., Hu, W., and Dong, M. (2019). Use of kombucha consortium to transform soy whey into a novel functional beverage. J. Funct. Foods 52, 81–89. doi: 10.1016/j.jff.2018.10.024
Ulusoy, A., and Tamer, C. E. (2019). Determination of suitability of black carrot (Daucus carota L. spp. sativus var. atrorubens Alef.) juice concentrate, cherry laurel (Prunus laurocerasus), blackthorn (Prunus spinosa) and red raspberry (Rubus ideaus) for kombucha beverage production. Food Measure. 13, 1524–1536. doi: 10.1007/s11694-019-00068-w
Uțoiu, E., Matei, F., Toma, A., Diguță, C. F., Ștefan, L. M., Mănoiu, S., et al. (2018). Bee collected pollen with enhanced health benefits, produced by fermentation with a kombucha consortium. Nutrients 10:1365. doi: 10.3390/nu10101365
Vázquez-Cabral, B. D., Larrosa-Pérez, M., Gallegos-Infante, J. A., Moreno-Jiménez, M. R., González-Laredo, R. F., Rutiaga-Quiñones, J. G., et al. (2017). Oak kombucha protects against oxidative stress and inflammatory processes. Chem. Biol. Interact. 272, 1–9. doi: 10.1016/j.cbi.2017.05.001
Villarreal-Soto, S. A., Beaufort, S., Bouajila, J., Souchard, J.-P., Renard, T., Rollan, S., et al. (2019). Impact of fermentation conditions on the production of bioactive compounds with anticancer, anti-inflammatory and antioxidant properties in kombucha tea extracts. Process Biochem. 83, 44–54. doi: 10.1016/j.procbio.2019.05.004
Villarreal-Soto, S. A., Beaufort, S., Bouajila, J., Souchard, J. P., and Taillandier, P. (2018). Understanding kombucha tea fermentation: a review. J. Food Sci. 83, 580–588. doi: 10.1111/1750-3841.14068
Vitas, J. S., Cvetanović, A. D., Mašković, P. Z., Švarc-Gajić, J. V., and Malbaša, R. V. (2018). Chemical composition and biological activity of novel types of kombucha beverages with yarrow. J. Funct. Foods 44, 95–102. doi: 10.1016/j.jff.2018.02.019
Vitas, J., Vukmanović, S., Čakarević, J., Popovic, L., and Malbasa, R. (2020). Kombucha fermentation of six medicinal herbs: chemical profile and biological activity. Chem. Ind. Chem. Eng. Q. 26, 157–170. doi: 10.2298/CICEQ190708034V
Wang, P.-H., Feng, Z.-H., Sang, X., Chen, W.-Z., Zhang, X.-N., Xiao, J.-B., et al. (2021). Kombucha ameliorates LPS-induced sepsis in a mouse model. Food Funct. 12, 10263–10280. doi: 10.1039/d1fo01839f
Watawana, M. I., Jayawardena, N., Gunawardhana, C. B., and Waisundara, V. Y. (2016). Enhancement of the antioxidant and starch hydrolase inhibitory activities of king coconut water (Cocos nucifera var. aurantiaca) by fermentation with kombucha ‘tea fungus’. Int. J. Food Sci. Technol. 51, 490–498. doi: 10.1111/ijfs.13006
Xiao, J., Ni, X., Kai, G., and Chen, X. (2013). A review on structure–activity relationship of dietary polyphenols inhibiting α-amylase. Crit. Rev. Food Sci. Nutr. 53, 497–506. doi: 10.1080/10408398.2010.548108
Yavari, N., Mazaheri-Assadi, M., Mazhari, Z. H., Moghadam, M. B., and Larijani, K. (2018). Glucuronic acid rich kombucha-fermented pomegranate juice. J. Food Res. 7, 61–69. doi: 10.5539/jfr.v7n1p61
Yıkmış, S., and Tuğgüm, S. (2019). Evaluation of microbiological, physicochemical and sensorial properties of purple basil Kombucha beverage. Turkish JAF Sci. Tech. 7, 1321–1327. doi: 10.24925/turjaf.v7i9.1321-1327.2550
Zhang, S., Cheng, M., Li, Z., Guan, S., Cai, B., Li, Q., et al. (2020). Composition and biological activity of rose and jujube kernel after fermentation with kombucha SCOBY. J. Food Process. Preserv. 44:e14758. doi: 10.1111/jfpp.14758
Zhang, S., Tang, Y., and Chen, J. (2022). Changes in functional components and biological activity of Lycium barbarum after fermentation with kombucha SCOBY. J. Food Process. Preserv. 46:e16758. doi: 10.1111/jfpp.16758
Zhiwen, W., Yijiao, S., Xianhua, M., Kai, L., and Fahu, Y. (2021). Production and characterization of a novel beverage from maize silk through fermentation with kombucha consortium. IOP Conf. Ser.: Earth Environ. Sci. 792:012053. doi: 10.1088/1755-1315/792/1/012053
Ziemlewska, A., Nizioł-Łukaszewska, Z., Bujak, T., Zagórska-Dziok, M., Wójciak, M., and Sowa, I. (2021). Effect of fermentation time on the content of bioactive compounds with cosmetic and dermatological properties in kombucha yerba mate extracts. Sci. Rep. 11:18792. doi: 10.1038/s41598-021-98191-6
Ziska, R., and Agustina, A., Supriadi (2019). Cytotoxic activity assay of n-hexane extract of Solanum nigrum L. fruits fermented by kombucha against MCF-7 breast cancer cell line. J. Phys. Conf. Ser. 1338:012027. doi: 10.1088/1742-6596/1338/1/012027
Zubaidah, E., Afgani, C. A., Kalsum, U., Srianta, I., and Blanc, P. J. (2019). Comparison of in vivo antidiabetes activity of snake fruit kombucha, black tea kombucha and metformin. Biocatal. Agricul. Biotechnol. 17, 465–469. doi: 10.1016/j.bcab.2018.12.026
Zubaidah, E., Dewantari, F. J., Novitasari, F. R., Srianta, I., and Blanc, P. J. (2018). Potential of snake fruit (Salacca zalacca (Gaerth.) Voss) for the development of a beverage through fermentation with the kombucha consortium. Biocatal. Agric. Biotechnol. 13, 198–203. doi: 10.1016/j.bcab.2017.12.012
Zubaidah, E., Iastika, A. R., Widyaningsih, T. D., and Febrianto, K. (2021a). Immunomodulatory activity of black tea kombucha (Camellia sinensis) and Arabica coffee leaves tea kombucha (coffee Arabica) for Salmonella typhi-infected mice. IOP Conf. Series Earth Environ. 733:012128. doi: 10.1088/1755-1315/733/1/012128
Zubaidah, E., Nisak, Y. K., Susanti, I., Widyaningsih, T. D., Srianta, I., and Tewfik, I. (2021c). Turmeric Kombucha as effective immunomodulator in Salmonella typhi-infected experimental animals. Biocatal. Agri. Biotechnol. 37:102181. doi: 10.1016/j.bcab.2021.102181
Zubaidah, E., Nisak, Y. K., Wijayanti, S. A., and Christianty, R. A. (2021b). Characteristic of microbiological, chemical, and antibacterial activity of turmeric (Curcuma longa) kombucha. IOP Conf. Series Earth Environ. 924:012080. doi: 10.1088/1755-1315/924/1/012080
Keywords: kombucha, functional active substance, efficacy mechanism, tea beverage, probiotics
Citation: Su J, Tan Q, Tang Q, Tong Z and Yang M (2023) Research progress on alternative kombucha substrate transformation and the resulting active components. Front. Microbiol. 14:1254014. doi: 10.3389/fmicb.2023.1254014
Edited by:
Christian Ariel Lopes, Institute for Research and Development in Process Engineering, Biotechnology and Alternative Energies (CONICET PROBIEN), ArgentinaReviewed by:
Pornthap Thanonkeo, Khon Kaen University, ThailandGeorgi Kostov, University of Food Technologies, Bulgaria
Copyright © 2023 Su, Tan, Tang, Tong and Yang. This is an open-access article distributed under the terms of the Creative Commons Attribution License (CC BY). The use, distribution or reproduction in other forums is permitted, provided the original author(s) and the copyright owner(s) are credited and that the original publication in this journal is cited, in accordance with accepted academic practice. No use, distribution or reproduction is permitted which does not comply with these terms.
*Correspondence: Jingqian Su, c2pxMDI3QGZqbnUuZWR1LmNu; Minhe Yang, bWluaGUyMTRAZmpudS5lZHUuY24=
†These authors have contributed equally to this work