- 1Université de Franche-Comté, UMR CNRS 6249 Chrono-Environnement, Besançon, France
- 2Université Paris-Saclay, Equipe INSERM ReSIST, Faculté de Médecine, Le Kremlin-Bicêtre, France
- 3Laboratoire Associé du Centre National de Référence de la Résistance aux Antibiotiques, Centre Hospitalier Universitaire de Besançon, Besançon, France
- 4Université Paris-Saclay, CNRS Institut de Chimie des Substances Naturelles, Gif-sur-Yvette, France
- 5Laboratoire Associé du Centre National de Référence de la Résistance aux Antibiotiques: Entérobactéries Résistantes aux Carbapénèmes, Le Kremlin-Bicêtre, France
Here, we characterized the first French NDM-9-producing Acinetobacter baumannii isolate. A. baumannii 13A297, which belonged to the STPas25 (international clone IC7), was highly resistant to β-lactams including cefiderocol (MIC >32 mg/L). Whole genome sequencing (WGS) using both Illumina and Oxford Nanopore technologies revealed a 166-kb non-conjugative plasmid harboring a blaNDM-9 gene embedded in a Tn125 composite transposon. Complementation of E. coli DH5α and A. baumannii CIP70.10 strains with the pABEC plasmid carrying the blaNDM-1 or blaNDM-9 gene, respectively, resulted in a significant increase in cefiderocol MIC values (16 to >256-fold), particularly in the NDM-9 transformants. Interestingly, steady-state kinetic parameters, measured using purified NDM-1 and NDM-9 (Glu152Lys) enzymes, revealed that the affinity for cefiderocol was 3-fold higher for NDM-9 (Km = 53 μM) than for NDM-1 (Km = 161 μM), leading to a 2-fold increase in catalytic efficiency for NDM-9 (0.13 and 0.069 μM−1.s−1, for NDM-9 and NDM-1, respectively). Finally, we showed by molecular docking experiments that the residue 152 of NDM-like enzymes plays a key role in cefiderocol binding and resistance, by allowing a strong ionic interaction between the Lys152 residue of NDM-9 with both the Asp223 residue of NDM-9 and the carboxylate group of the R1 substituent of cefiderocol.
1. Introduction
Acinetobacter baumannii is definitely one of the most successful pathogens responsible for healthcare-associated infections. Its propensity to acquire and accumulate antibiotic resistance genes has led to its classification by the WHO as one of the 12 top priority resistant bacteria (Tacconelli et al., 2018). Carbapenem resistance in A. baumannii (CRAB) is a serious threat to public health, and prevalence of CRAB, which varies widely between countries, has been increasing worldwide [Antimicrobial Resistance in the EU/EEA (EARS-Net), 2022]. Resistance of A. baumannii strains to carbapenems most commonly results from the acquisition of carbapenem-hydrolyzing class D β-lactamases (CHDL), belonging to the OXA-23-like, OXA-24/40-like, OXA-58-like, OXA-143-like and OXA-235-like groups (Rodríguez et al., 2018; Ramirez et al., 2020). However, the incidence of NDM-like class B (MBL) β-lactamases has steadily grown recently (Ramirez et al., 2020). Of the 55 NDM variants listed in the Beta-Lactamase DataBase (Naas et al., 2017), NDM-1 is the most frequently NDM-type carbapenemase identified in CRAB but strains producing other variants, such as NDM-2, NDM-5, NDM-6 or more recently NDM-42, have been sporadically reported (Kaase et al., 2011; Khalid et al., 2020; Xanthopoulou et al., 2020; Quiñones Pérez et al., 2022). While the blaNDM-9 variant was very rarely detected in A. baumannii (Poirel et al., 2021; Le Terrier et al., 2023), NDM-9 has been reported more frequently in Enterobacterales (K. pneumoniae and E. coli), especially in East Asia (South Korea, Taiwan, China and French Polynesia) and sporadically in Europe (Switzerland, Italy) (Wang et al., 2014; Di et al., 2017; Nüesch-Inderbinen et al., 2018; Lin et al., 2019; Falcone et al., 2020; Oueslati et al., 2021). More recently, it has been shown that the production of the NDM-9 enzyme in Enterobacterales and in an A. baumannii strain impairs the activity of new combinations of β-lactam-β-lactamase inhibitors (ceftazidime/avibactam, imipenem/relebactam, and meropenem/vaborbactam), including one under development (cefepime/taniborbactam) (Le Terrier et al., 2023). The most worrying threat of NDM-like producing A. baumannii is the inconsistent efficacy of cefiderocol, a recently commercialized molecule that is considered a last-resort antibiotic in the fight against CRAB. Even though some NDM-producing A. baumannii strains are fully susceptible to cefiderocol (Yamano, 2019; Mushtaq et al., 2020), cefiderocol MICs tend to be higher for NDM-producing organisms as compared to strains expressing other carbapenemases (Yamano, 2019; Mushtaq et al., 2020; Poirel et al., 2021). Undoubtedly, additional factors likely contribute to the high cefiderocol resistance of some bacteria (Yamano, 2019; Kohira et al., 2020; Simner and Patel, 2020), and in A. baumannii, downregulation or absence of specific siderophore receptors (PirA and PiuA) and alterations of PBP3 have been suggested (Malik et al., 2020). In this study, we report the genetic environment of the blaNDM-9 gene identified in a clinical strain of A. baumannii, and the comparison of steady-state kinetic parameters between the NDM-9 and NDM-1 purified enzymes for cefiderocol.
2. Materials and methods
2.1. Strains used in the study
Acinetobacter baumannii clinical strain 13A297 was isolated in 2013 from a urine sample collected from a patient hospitalized in France. Species identification was performed by MALDI-TOF mass spectrometry and confirmed by whole genome sequencing. Clinical A. baumannii strains 19A2597 and 13A297, producing the NDM-1 and NDM-9 β-lactamase, respectively, were selected for cloning of the respective genes into the pABEC vector and then transferred into E. coli DH5α and A. baumannii CIP70.10 (Vuillemenot et al., 2022). Mating out assays were carried out using the rifampicin-resistant BM4547 strain derived from CIP70.10 as the recipient strain (Potron et al., 2014). The transconjugants were selected on Mueller-Hinton agar plates supplemented with ticarcillin (100 mg/L) and rifampicin (100 mg/L). E. coli BL21 (DE3) (Novagen, Fontenay-sous-Bois, France) was used for overproduction of NDM-1 and NDM-9 enzymes.
2.2. Susceptibility testing
MICs of β-lactams (ticarcillin, piperacillin-tazobactam, ceftazidime, cefepime, imipenem, meropenem), ciprofloxacin, trimethoprim-sulfamethoxazole, amikacin, tigecycline, minocycline and colistin were determined by the broth microdilution method in cation-adjusted Mueller-Hinton broth (MHB), by using customized microplates (ThermoFisher, Illkirch-Graffenstaden, France). Cefiderocol MICs were determined using an Iron-Depleted and Cation-Adjusted Mueller-Hinton Broth (ID-CAMHB) and a titrated powder of cefiderocol (MedChem Express) according to the recommendations of the Clinical and Laboratory Standards Institute (2020). MIC values were interpreted according to the current EUCAST or CLSI (for minocycline and tigecycline) clinical breakpoints, except for cefiderocol, for which the EUCAST pharmacokinetic-pharmacodynamic (PK-PD) breakpoint (S ≤ 2; R > 2 mg/L) was applied [Clinical and Laboratory Standards Institute, 2020; The European Committee on Antimicrobial Susceptibility Testing (EUCAST), 2021].
2.3. Genomic data
Total DNA of strains 13A297 and 19A2597 was purified (NucleoSpin Microbial DNA, Macherey-Nagel, Germany), and then quantified spectrophotometrically (NanoDrop, Thermo Fisher Scientific, France). Preparation of DNA libraries and whole-genome sequencing experiments were outsourced to services using Illumina platforms generating paired-end reads (P2M platform, Institut Pasteur, Paris, France). In addition, long-reads sequencing using the Oxford Nanopore technology was performed for the strain 13A297 (MinION, R9.4.1 flow cell). Short and long reads were assembled as hybrid using SPAdes v.3.15.5 and Trycycler v.0.5.0. Quality control of reads, assembly, and genome annotation were carried out using FastQC v.0.11.9, QUAST v.5.0.2, and RASTtk on the PATRIC 3.6.9 platform. Antimicrobial resistance genes (AMR) were identified by the National Reference Center for Antimicrobial Resistance (NRC-AR) pipelines and databases. The sequence type (ST) of isolates was determined according to the MLST schemes available at PubMLST.1
2.4. Molecular experiments
From genomic DNA extracted using the QIAamp DNA mini kit (Qiagen, Courtaboeuf, France), the blaNDM-1 and blaNDM-9 genes were amplified with the specific primers NDM_fwd (5’-CAAGCTTGGTACCGAGCTCGTGGCTTTTGAAACTGTCGC-3′) and NDM_rev (5’-ACTGGCGGCCGTTACTAGTGTCGAGGTCAGGATAGGGG-3′) and subsequently cloned into the shuttle vector pABEC using the NEBuilder Hifi assembly kit according to the manufacturer’s recommendations (New England Biolabs, Evry, France) (Vuillemenot et al., 2022).
For the production of NDM-1 and NDM-9 proteins, the complete coding sequences of the β-lactamase genes were amplified by PCR using the specific primers NdeI-NDM29-270 (5′-AAAAACATATGGGTGAAATCCGCCCGACG-3′) and NDM-XhoIdelta STOP (5′-AAAAACTCGAGGCGCAGCTTGTCGGCCAT-3′). The amplicons and the plasmid pET41b were digested with the restriction enzymes NdeI and XhoI before ligation. The recombinant plasmids pET41b-NDM-1 and pET41b-NDM-9 were transferred by transformation into E. coli BL21 (DE3).
2.5. Protein purification
Two liters of brain heart infusion broth medium containing 30 mg/L kanamycin were inoculated with 2 mL overnight cultures of E. coli strains BL21 (DE3) pET41b-NDM-1 and of E. coli BL21 (DE3) pET41b-NDM-9. Production of recombinant proteins harboring the His tag was induced overnight at 22°C with 0.2 mM IPTG. Then, the cultures were centrifuged at 6,000 g for 15 min and the pellets were resuspended with the binding buffer (10 mM imidazole, 25 mM sodium phosphate pH 7.4 and 300 mM NaCl). Bacterial cells were lysed by sonication and the pellet was removed by two consecutive centrifugation steps at 10,000 g for 1 h at 4°C; the supernatant was then centrifuged at 96,000 g for 1 h at 4°C. The soluble fractions were filtered, passed through a HisTrapTM HP column (GE Healthcare) and proteins were eluted with the elution buffer (500 mM imidazole, 25 mM sodium phosphate pH 7.4 and 300 mM NaCl). Finally, gel filtration was performed with 50 mM sodium phosphate buffer pH 7 and 150 mM NaCl with a Superdex 75 column (GE Healthcare). The fractions were pooled according to purity by SDS–PAGE, dialyzed in 100 mM sodium phosphate pH 7, 50 μM ZnSO4, and concentrated using Vivaspin columns. The concentration was determined by measuring the OD at 280 nm and with the extinction coefficients obtained from the ProtParamtool (Swiss Institute of Bioinformatics online resource portal).
2.6. Steady-state kinetic determinations
Kinetic parameters were determined using purified NDM-1 and NDM-9 β-lactamases in 20 mM Hepes pH 7.5 supplemented with 50 μM ZnSO4. The kcat and Km values were determined by analyzing β-lactam hydrolysis under initial-rate conditions with an ULTROSPEC 2000 UV spectrophotometer with the SWIFT II software (GE Healthcare, Velizy-Villacoublay, France) and the Eadie-Hofstee linearization of the Michaelis–Menten equation.
2.7. Molecular modeling
Three-dimensional structure of NDM-9 was generated by in silico mutagenesis from the structure of NDM-1 (PDB code 4RL0) (Feng et al., 2014) using UCSF Chimera (version 1.14) (Pettersen et al., 2004). Molecular docking of cefiderocol (open form) into the active sites of both NDM-1 and NDM-9 was performed using CCDC Gold (part of CSD 2021 software) (Verdonk et al., 2003) with the GoldScore scoring function and the binding site defined as a sphere with 20 Å radius around Zn301.
2.8. Nucleotide sequence accession number
The whole genome sequences generated in the study have been submitted to the Genbank nucleotide sequence database under the accession number PRJNA989410.
3. Results and discussion
3.1. Phenotypic and genomic characterization of Acinetobacter baumannii 13A297 clinical isolate
Acinetobacter baumannii 13A297 strain was resistant to all β-lactams, including cefiderocol (MIC >32 mg/L), aminoglycosides, fluoroquinolones, trimethoprim-sulfamethoxazole and remained susceptible to colistin and tetracyclines (Table 1). WGS sequencing of A. baumannii 13A297 revealed a genome of 4,229,816 bp with a mean coverage of 280 X. Analysis of WGS data revealed that this isolate possessed the naturally-occurring blaOXA-64 and blaADC-26 genes. Its resistance profile to β-lactams was the result of acquisition of the carbapenemase NDM-9, that differs from NDM-1 by a single amino-acid substitution (Glu152Lys). NDM-9 was first reported in a clinical Klebsiella pneumoniae isolate from China in 2013 (Wang et al., 2014). This enzyme was also identified in a mcr-1-harboring Escherichia coli strain, in three environmental Klebsiella variicola isolates in South Korea (Yao et al., 2016; Di et al., 2017) and in a K. pneumoniae strain isolated from wastewater in Switzerland in 2015 (Nüesch-Inderbinen et al., 2018). Since then, NDM-9 was sporadically detected in clinical, environmental or animal strains belonging to various Enterobacterales species including Salmonella sp. in Asia and Europe (Italy), but also in Africa (Tunisia) and in French Polynesia (Falcone et al., 2020; Li et al., 2021; Oueslati et al., 2021; Song et al., 2022; Sun et al., 2023; Zouaoui et al., 2023). MLST assigned A. baumannii 13A297 strain to STPas25, and STOx2280, according to the MLST Pasteur and Oxford schemes, respectively. In addition to the globally disseminated multidrug-resistant STPas1 and STPas2, STPas25, which belongs to international clone 7 (IC7), has emerged worldwide as epidemic, endemic or sporadic cases in the last two decades (Sahl et al., 2015; Rodríguez et al., 2018; Rafei et al., 2022). A hallmark of this clone is its evolution toward multidrug resistance and a high capacity for biofilm formation (Rafei et al., 2022). In addition, it has been associated with high mortality rates among infected patients (da Silva et al., 2018).
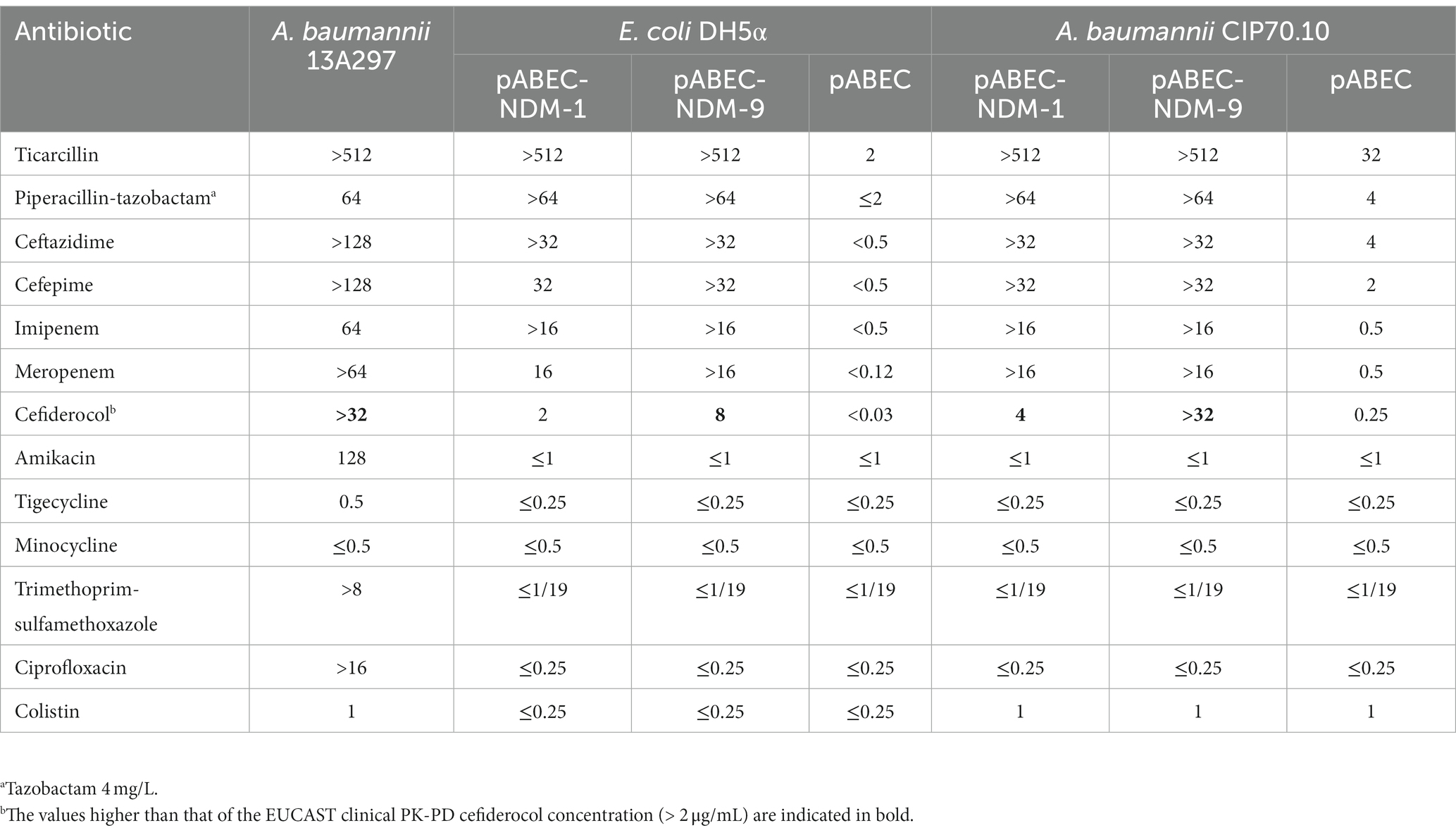
Table 1. MICs (mg/L) of β–lactams and other antibiotics for A. baumannii 13A297, A. baumannii CIP70.10 (pABEC), A. baumannii CIP70.10 (pABEC-NDM-1), A. baumannii CIP70.10 (pABEC-NDM-9), E. coli DH5α (pABEC), E. coli DH5α (pABEC-NDM-1), and E. coli DH5α (pABEC-NDM-9).
3.2. Genetic support and environment of blaNDM-9 gene
Analysis of Nanopore sequencing data showed that the blaNDM-9 gene was located on a full-length Tn125 composite transposon, carried by a 166,254-bp long plasmid named pNDM-9. A 3-bp target site duplication (TTT) identified on both sides of Tn125 was indicative of a transposition event. Interestingly, the genetic environment of blaNDM-9 in A. baumannii 13A297 corresponded to the original Tn125 and differed from that identified in Enterobacterales. Indeed, IS26 was associated with blaNDM-9 in all but one of the plasmids identified in Enterobacterales, highlighting that IS26 may play a key role in the transfer of the blaNDM-9 gene between Enterobacterales (Li et al., 2021). Plasmid pNDM-9 encoded 165 open reading frames and possessed a GC content of 39.1%. It exhibited significant similarity (99.9%) to 75% of the sequence of plasmid pIOMTU433 (accession number: AP014650) (Figure 1). Plasmid pIOMTU433 is a 189,354 bp long plasmid identified in an A. baumannii clinical strain isolated in Nepal in 2013. Interestingly, pIOMTU433 did not carry a blaNDM-like gene but a blaPER-7 ESBL-encoding gene. In addition to blaNDM-9, pNDM-9 also carried other resistance genes, such as sul2, aph(3″)-Ib and aph(6)-Id, which confer resistance to sulfonamides and aminoglycosides, respectively. Furthermore, the pNDM-9 plasmid shared 99.9% nucleotide identity with 90% of the sequence of the blaNDM-1 positive plasmid pPM194122 (150,385 bp-long, accession number CP050426), which was sequenced from an A. baumannii strain from India (Figure 1). Consistent with the identification of an incomplete tra operon, attempts to transfer the pNDM-9 plasmid by mating-out assays were unsuccessful.
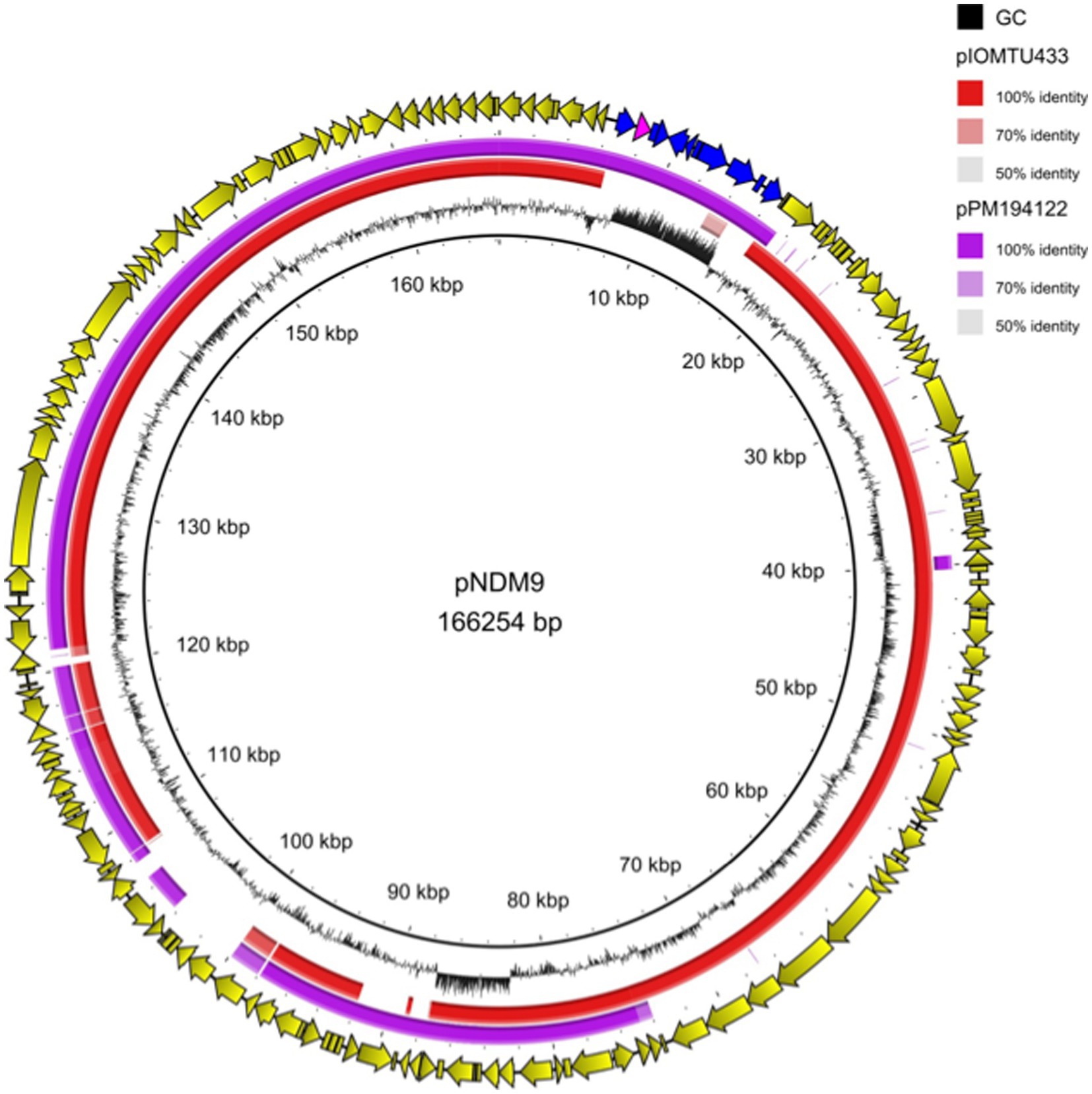
Figure 1. Linear sequence alignment of plasmids pNDM-9, pIOMTU433 (accession number: AP014650) and pPM194122 (accession number: CP050426). Yellow arrows indicate the open reading frames of pNDM-9 plasmid; blue arrows indicate the Tn125 transposon and pink arrow indicates the blaNDM-9 gene.
3.3. Antibiotic susceptibilities of transformants with NDM-1 and NDM-9
To clarify the impact of NDM-9 on cefiderocol susceptibility and to compare it to that of NDM-1, both corresponding blaNDM-1 and blaNDM-9 genes were cloned and expressed in E. coli and A. baumannii reference strains. NDM expression from the plasmid pABEC in E. coli DH5α conferred resistance to ceftazidime, imipenem, meropenem, and cefiderocol for NDM-9 (8 mg/L) and a decrease in susceptibility to cefiderocol (at least 64-fold) for NDM-1 (2 mg/L), using the pharmacokinetic-pharmacodynamic (PK-PD) cut-off of 2 mg/L (S ≤ 2 mg/L; R > 2 mg/L) (Table 1). Interestingly, DH5α cells transformed with blaNDM-9-carrying vector pABEC-NDM-9 were 4-fold more resistant to cefiderocol and at least 2-fold to meropenem than those containing pABEC-NDM-1. In agreement with this result, the transfer of the vector pABEC-NDM-9 into the A. baumannii CIP70.10 strain led to a higher level of resistance to cefiderocol (MIC >32 mg/L) than that of pABEC-NDM-1 (MIC = 4 mg/L) (Table 1).
3.4. Biochemical properties of NDM-1 and NDM-9 against ceftazidime and cefiderocol and molecular modeling
NDM-9 was previously shown to possess slightly increased hydrolytic activity toward meropenem, imipenem and cefotaxime as compared to NDM-1 (Wang et al., 2014). To gain insight into the structural features that may explain the different levels of resistance of NDM-1 and NDM-9 to cefiderocol, both enzymes were purified and steady-state kinetic parameters of ceftazidime and cefiderocol were determined. NDM-9 exhibited a 7-fold higher hydrolytic efficiency (kcat/Km) toward ceftazidime, which is linked to a higher turnover rate (kcat) of this antibiotic by NDM-9 (Table 2). Although weak, a slight hydrolysis of cefiderocol by NDM-1 was evidenced. As compared to NDM-1, NDM-9 possessed a 3-fold higher affinity for cefiderocol, which was associated with a 2-fold increase in its catalytic efficiency (Table 2).

Table 2. Steady-state kinetic parameters for hydrolysis of ceftazidime and cefiderocol by NDM-1 and NDM-9.
Molecular modeling revealed stabilizing interactions between cefiderocol and residues Lys211, Lys216, Asn220 of NDM-9, and a strong ionic interaction of Lys152 with both Asp223 and the carboxylate group of R1 substituent in cefiderocol (Figure 2). In NDM-1, this latter favorable interaction is replaced by a repulsive interaction with Glu152, leading to a lower affinity for cefiderocol. Altogether, these results explain the higher cefiderocol MIC and catalytic efficiency observed for NDM-9 as compared to NDM-1.
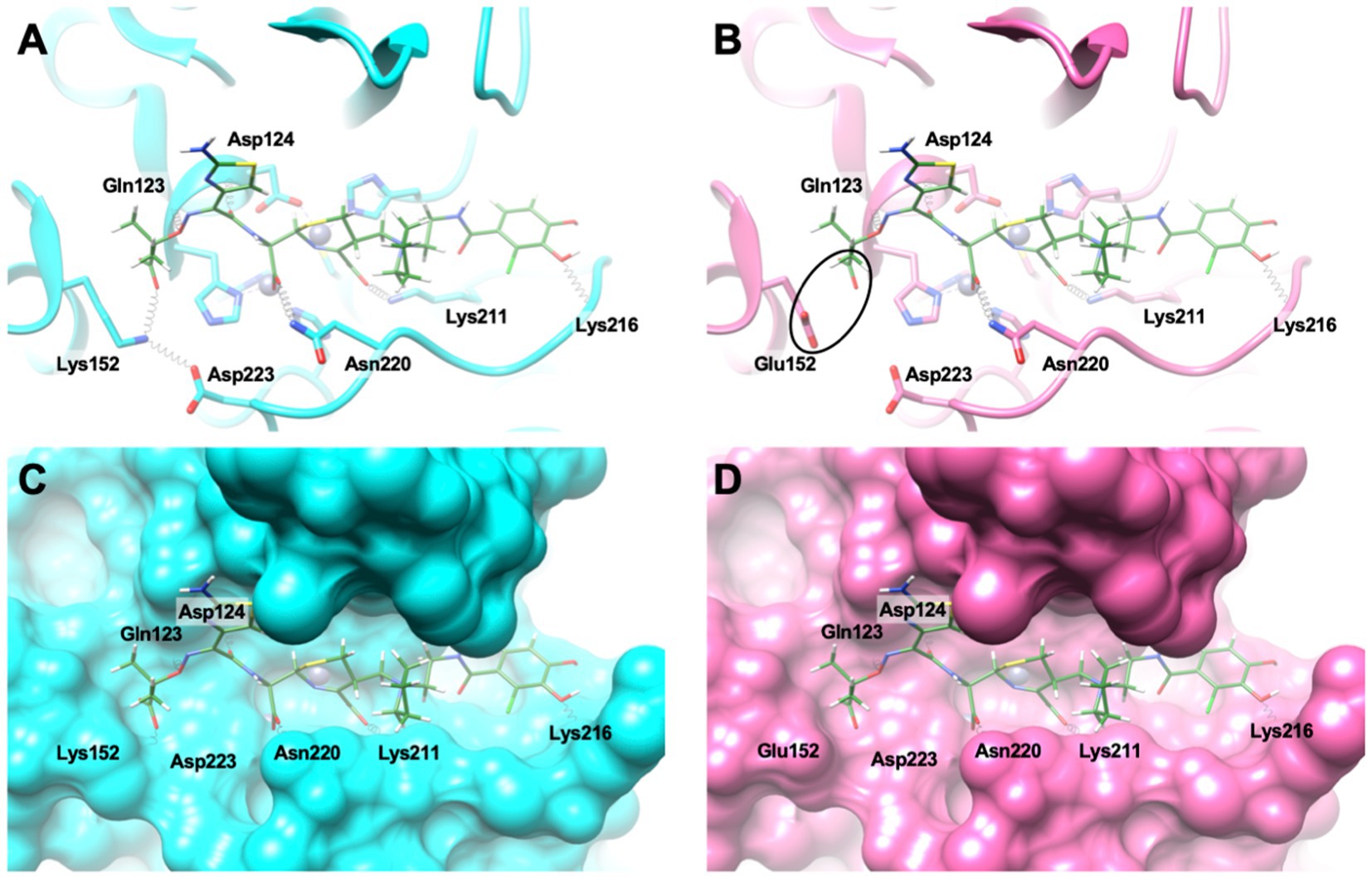
Figure 2. (A,C) Docking conformation of cefiderocol (represented as green sticks) in the active site of NDM-9 (represented as cyan cartoon and surface, respectively). (B,D) The same docking conformation superposed with the active site of NDM-1 (represented as pink cartoon and surface, respectively). The black ellipse highlights the repulsive interaction between the side chain of Glu152 and the carboxylate group from the R1 substituent in cefiderocol. Hydrogen bonds are represented as black springs.
4. Conclusion
To the best of our knowledge, this is the first characterization of an NDM-9 A. baumannii clinical isolate, assigned to STPas25, which belongs to the emerging international clone 7 (IC7). The high level of resistance to cefiderocol of this clinical isolate is at least partly due to the Glu152Lys substitution of NDM-9, but further studies are needed to investigate the contribution of other genetic determinants. The substitution of residue 152 in NDM-9 is unique among NDM variants and it remains to be elucidated whether other substitutions in other NDM variants may have impacts on cefiderocol hydrolysis. Since cefiderocol is the only new active molecule against CRAB strains, the emergence of the NDM-9 variant, which may contribute to high levels of resistance to cefiderocol, is a major threat to human health, given the subsequent therapeutic dead end. As the rates of NDM-positive isolates among carbapenemase-producing A. baumannii isolates is increasing in France (1.7% in 2012, 15.9% in 2016 and 32.2% in 2021, French National Reference Centre for Antimicrobial Resistance) and in other countries (Adams et al., 2020; Fam et al., 2020; Kindu et al., 2020; Lukovic et al., 2020) it may seriously compromise the efficacy of cefiderocol for this highly drug resistant pathogen that is among the WHO list of critical bacteria,2 for which new antibiotics are urgently needed.
Data availability statement
The datasets presented in this study can be found in online repositories. The names of the repository/repositories and accession number(s) can be found in the article/supplementary material.
Author contributions
KJ, RAB, TN, and AP contributed to conception and design of the study. SG, PT, J-BV, SO, and BII performed the experiments. SG, BII, AP, and MB analyzed the data. SG and AP wrote the first draft of the manuscript. AP, PP, KJ, RAB, TN, and BII reviewed and edited the manuscript. All authors read and approved the version.
Funding
The French National Reference Centre for Antimicrobial Resistance is funded by the French Ministry of Health through the Santé publique France agency.
Acknowledgments
The authors thank medical microbiologists for referring the carbapenem resistant A. baumannii strains to the French NRC-AR.
Conflict of interest
The authors declare that the research was conducted in the absence of any commercial or financial relationships that could be construed as a potential conflict of interest.
Publisher’s note
All claims expressed in this article are solely those of the authors and do not necessarily represent those of their affiliated organizations, or those of the publisher, the editors and the reviewers. Any product that may be evaluated in this article, or claim that may be made by its manufacturer, is not guaranteed or endorsed by the publisher.
Footnotes
2. ^https://www.who.int/news/item/27-02-2017s-who-publishes-list-of-bacteria-for-which-new-antibiotics-are-urgently-needed
References
Adams, M. D., Pasteran, F., Traglia, G. M., Martinez, J., Huang, F., Liu, C., et al. (2020). Distinct mechanisms of dissemination of NDM-1 metallo-β-lactamase in Acinetobacter species in Argentina. Antimicrob. Agents Chemother. 64:20. doi: 10.1128/aac.00324-20
Antimicrobial Resistance in the EU/EEA (EARS-Net). (2022). Annual Epidemiological Report for 2021. Available at: https://www.ecdc.europa.eu/sites/default/files/documents/AER-EARS-Net-2021_2022-final.pdf
Clinical and Laboratory Standards Institute. (2020). Performance standards for antimicrobial susceptibility testing M100 S, 33th Edn; Clinical and Laboratory Standards Institute: Wayne, PA. 2023.
da Silva, K. E., Maciel, W. G., Croda, J., Cayô, R., Ramos, A. C., de Sales, R. O., et al. (2018). A high mortality rate associated with multidrug-resistant Acinetobacter baumannii ST79 and ST25 carrying OXA-23 in a Brazilian intensive care unit. PLoS One 13:e0209367. doi: 10.1371/journal.pone.0209367
Di, D. Y. W., Jang, J., Unno, T., and Hur, H.-G. (2017). Emergence of Klebsiella variicola positive for NDM-9, a variant of New Delhi metallo-β-lactamase, in an urban river in South Korea. J. Antimicrob. Chemother. 72, 1063–1067. doi: 10.1093/jac/dkw547
Falcone, M., Giordano, C., Barnini, S., Tiseo, G., Leonildi, A., Malacarne, P., et al. (2020). Extremely drug-resistant NDM-9-producing ST147 Klebsiella pneumoniae causing infections in Italy, May 2020. Euro Surveill. 25:2001779. doi: 10.2807/1560-7917.ES.2020.25.48.2001779
Fam, N. S., Gamal, D., Mohamed, S. H., Wasfy, R. M., Soliman, M. S., El-Kholy, A. A., et al. (2020). Molecular characterization of carbapenem/colistin-resistant Acinetobacter baumannii clinical isolates from Egypt by whole-genome sequencing. Infect. Drug Resist. 13, 4487–4493. doi: 10.2147/IDR.S288865
Feng, H., Ding, J., Zhu, D., Liu, X., Xu, X., Zhang, Y., et al. (2014). Structural and mechanistic insights into NDM-1 catalyzed hydrolysis of cephalosporins. J. Am. Chem. Soc. 136, 14694–14697. doi: 10.1021/ja508388e
Kaase, M., Nordmann, P., Wichelhaus, T. A., Gatermann, S. G., Bonnin, R. A., and Poirel, L. (2011). NDM-2 carbapenemase in Acinetobacter baumannii from Egypt. J. Antimicrob. Chemother. 66, 1260–1262. doi: 10.1093/jac/dkr135
Khalid, S., Ahmad, N., Ali, S. M., and Khan, A. U. (2020). Outbreak of efficiently transferred carbapenem-resistant blaNDM-producing gram-negative bacilli isolated from neonatal intensive care unit of an Indian hospital. Microb. Drug Resist. 26, 284–289. doi: 10.1089/mdr.2019.0092
Kindu, M., Derseh, L., Gelaw, B., and Moges, F. (2020). Carbapenemase-producing non-glucose-fermenting gram-negative bacilli in Africa, Pseudomonas aeruginosa and Acinetobacter baumannii: a systematic review and meta-analysis. Int. J. Microbiol. 2020:e9461901. doi: 10.1155/2020/9461901
Kohira, N., Hackel, M. A., Ishioka, Y., Kuroiwa, M., Sahm, D. F., Sato, T., et al. (2020). Reduced susceptibility mechanism to cefiderocol, a siderophore cephalosporin, among clinical isolates from a global surveillance programme (SIDERO-WT-2014). J. Glob. Antimicrob. Resist. 22, 738–741. doi: 10.1016/j.jgar.2020.07.009
Le Terrier, C., Nordmann, P., Buchs, C., Di, D. Y. W., Rossolini, G. M., Stephan, R., et al. (2023). Wide dissemination of gram-negative bacteria producing the taniborbactam-resistant NDM-9 variant: a one health concern. J. Antimicrob. Chemother. doi: 10.1093/jac/dkad210. Online ahead of print
Li, Y., Wang, Q., Xiao, X., Li, R., and Wang, Z. (2021). Emergence of blaNDM-9-bearing tigecycline-resistant Klebsiella aerogenes of chicken origin. J. Glob. Antimicrob. Resist. 26, 66–68. doi: 10.1016/j.jgar.2021.04.028
Lin, Y. C., Kuroda, M., Suzuki, S., and Mu, J. J. (2019). Emergence of an Escherichia coli strain so-harbouring mcr-1 and blaNDM-9 from a urinary tract infection in Taiwan. J. Glob. Antimicrob. Resist. 16, 286–290. doi: 10.1016/j.jgar.2018.10.003
Lukovic, B., Gajic, I., Dimkic, I., Kekic, D., Zornic, S., Pozder, T., et al. (2020). The first nationwide multicenter study of Acinetobacter baumannii recovered in Serbia: emergence of OXA-72, OXA-23 and NDM-1-producing isolates. Antimicrob. Resist. Infect. Control 9:101. doi: 10.1186/s13756-020-00769-8
Malik, S., Kaminski, M., Landman, D., and Quale, J. (2020). Cefiderocol resistance in Acinetobacter baumannii: roles of β-lactamases, siderophore receptors, and penicillin binding protein 3. Antimicrob. Agents Chemother. 64:1221. doi: 10.1128/AAC.01221-20
Mushtaq, S., Sadouki, Z., Vickers, A., Livermore, D. M., and Woodford, N. (2020). In vitro activity of cefiderocol, a siderophore cephalosporin, against multidrug-resistant gram-negative bacteria. Antimicrob. Agents Chemother. 64:20. doi: 10.1128/aac.01582-20
Naas, T., Oueslati, S., Bonnin, R. A., Dabos, M. L., Zavala, A., Dortet, L., et al. (2017). Beta-lactamase database (BLDB) – structure and function. J. Enzyme Inhib. Med. Chem. 32, 917–919. doi: 10.1080/14756366.2017.1344235
Nüesch-Inderbinen, M., Zurfluh, K., Stevens, M. J. A., and Stephan, R. (2018). Complete and assembled genome sequence of an NDM-9- and CTX-M-15-producing Klebsiella pneumoniae ST147 wastewater isolate from Switzerland. J. Glob. Antimicrob. Resist. 13, 53–54. doi: 10.1016/j.jgar.2018.03.001
Oueslati, S., Emeraud, C., Grosperrin, V., Levy, M., Cotellon, G., Creton, E., et al. (2021). Polyclonal dissemination of NDM-1- and NDM-9-producing Escherichia coli and Klebsiella pneumoniae in French Polynesia. Antimicrob. Agents Chemother. 65:20. doi: 10.1128/aac.02437-20
Pettersen, E. F., Goddard, T. D., Huang, C. C., Couch, G. S., Greenblatt, D. M., Meng, E. C., et al. (2004). UCSF chimera—a visualization system for exploratory research and analysis. J. Comput. Chem. 25, 1605–1612. doi: 10.1002/jcc.20084
Poirel, L., Sadek, M., and Nordmann, P. (2021). Contribution of PER-type and NDM-type β-lactamases to cefiderocol resistance in Acinetobacter baumannii. Antimicrob. Agents Chemother. 65:e0087721. doi: 10.1128/AAC.00877-21
Potron, A., Poirel, L., and Nordmann, P. (2014). Derepressed transfer properties leading to the efficient spread of the plasmid encoding carbapenemase OXA-48. Antimicrob. Agents Chemother. 58, 467–471. doi: 10.1128/aac.01344-13
Quiñones Pérez, D., Aung, M. S., Carmona Cartaya, Y., González Molina, M. K., Pereda Novales, N., and Kobayashi, N. (2022). Clonal diversity of Acinetobacter clinical isolates producing NDM-type carbapenemase in Cuba, 2013–19. IJID Reg. 5, 93–96. doi: 10.1016/j.ijregi.2022.08.008
Rafei, R., Koong, J., Osman, M., Al Atrouni, A., Hamze, M., and Hamidian, M. (2022). Analysis of pCl107 a large plasmid carried by an ST25 Acinetobacter baumannii strain reveals a complex evolutionary history and links to multiple antibiotic resistance and metabolic pathways. FEMS Microbes 3:xtac027. doi: 10.1093/femsmc/xtac027
Ramirez, M. S., Bonomo, R. A., and Tolmasky, M. E. (2020). Carbapenemases: transforming Acinetobacter baumannii into a yet more dangerous menace. Biomol. Ther. 10:720. doi: 10.3390/biom10050720
Rodríguez, C. H., Nastro, M., and Famiglietti, A. (2018). Carbapenemases in Acinetobacter baumannii. Review of their dissemination in Latin America. Rev. Argent. Microbiol. 50, 327–333. doi: 10.1016/j.ram.2017.10.006
Sahl, J. W., Del Franco, M., Pournaras, S., Colman, R. E., Karah, N., Dijkshoorn, L., et al. (2015). Phylogenetic and genomic diversity in isolates from the globally distributed Acinetobacter baumannii ST25 lineage. Sci. Rep. 5:15188. doi: 10.1038/srep15188
Simner, P. J., and Patel, R. (2020). Cefiderocol antimicrobial susceptibility testing considerations: the Achilles’ heel of the Trojan horse? J. Clin. Microbiol. 59, e00951–e00920. doi: 10.1128/JCM.00951-20
Song, Y., Wang, Z., Wang, C., Zhang, G., Li, J., and Feng, J. (2022). An urban soil environmental E. coli ST354 isolate co-harbouring mcr-1 and blaNDM-9. J. Glob. Antimicrob. Resist. 29, 173–175. doi: 10.1016/j.jgar.2022.03.011
Sun, Y., Han, Y., Qian, C., Zhang, Q., Yao, Z., Zeng, W., et al. (2023). A novel transposon Tn7540 carrying blaNDM-9 and fosA3 in chromosome of a pathogenic multidrug-resistant Salmonella enterica serovar Indiana isolated from human faeces. J. Glob. Antimicrob. Resist. 33, 72–77. doi: 10.1016/j.jgar.2023.01.013
Tacconelli, E., Carrara, E., Savoldi, A., Harbarth, S., Mendelson, M., Monnet, D. L., et al. (2018). Discovery, research, and development of new antibiotics: the WHO priority list of antibiotic-resistant bacteria and tuberculosis. Lancet Infect. Dis. 18, 318–327. doi: 10.1016/S1473-3099(17)30753-3
The European Committee on Antimicrobial Susceptibility Testing (EUCAST). (2021). Breakpoints tables for interpretation of MICs and zone diameters. Version 11.0, 2021. Available at: https://www.eucast.org/fileadmin/src/media/PDFs/EUCAST_files/Breakpoint_tables/v_11.0_Breakpoint_Tables.pdf
Verdonk, M. L., Cole, J. C., Hartshorn, M. J., Murray, C. W., and Taylor, R. D. (2003). Improved protein–ligand docking using GOLD. Proteins 52, 609–623. doi: 10.1002/prot.10465
Vuillemenot, J.-B., Bour, M., Beyrouthy, R., Bonnet, R., Laaberki, M.-H., Charpentier, X., et al. (2022). Genomic analysis of CTX-M-115 and OXA-23/−72 co-producing Acinetobacter baumannii, and their potential to spread resistance genes by natural transformation. J. Antimicrob. Chemother. 77, 1542–1552. doi: 10.1093/jac/dkac099
Wang, X., Li, H., Zhao, C., Chen, H., Liu, J., Wang, Z., et al. (2014). Novel NDM-9 metallo-β-lactamase identified from a ST107 Klebsiella pneumoniae strain isolated in China. Int. J. Antimicrob. Agents 44, 90–91. doi: 10.1016/j.ijantimicag.2014.04.010
Xanthopoulou, K., Urrutikoetxea-Gutiérrez, M., Vidal-Garcia, M., de Tuesta, D., del Arco, J.-L., Sánchez-Urtaza, S., et al. (2020). First report of New Delhi Metallo-β-lactamase-6 (NDM-6) in a clinical Acinetobacter baumannii isolate from northern Spain. Front. Microbiol. 11:589253. doi: 10.3389/fmicb.2020.589253
Yamano, Y. (2019). In vitro activity of cefiderocol against a broad range of clinically important gram-negative bacteria. Clin. Infect. Dis. 69, S544–S551. doi: 10.1093/cid/ciz827
Yao, X., Doi, Y., Zeng, L., Lv, L., and Liu, J.-H. (2016). Carbapenem-resistant and colistin-resistant Escherichia coli co-producing NDM-9 and MCR-1. Lancet Infect. Dis. 16, 288–289. doi: 10.1016/S1473-3099(16)00057-8
Zouaoui, E., Mercuri, P. S., Radaoui, A., Ben Salah, N., Galleni, M., Ben-Mahrez, K., et al. (2023). High prevalence of blaNDM among carbapenem non-susceptible Klebsiella pneumoniae in a Tunisian hospital first report of blaNDM-9, blaKPC-20, and blaKPC-26 genes. Curr. Microbiol. 80:152. doi: 10.1007/s00284-023-03268-2
Keywords: Acinetobacter baumannii, cefiderocol, carbapenemases, NDM, resistance
Citation: Gaillot S, Oueslati S, Vuillemenot J-B, Bour M, Iorga BI, Triponney P, Plésiat P, Bonnin RA, Naas T, Jeannot K and Potron A (2023) Genomic characterization of an NDM-9-producing Acinetobacter baumannii clinical isolate and role of Glu152Lys substitution in the enhanced cefiderocol hydrolysis of NDM-9. Front. Microbiol. 14:1253160. doi: 10.3389/fmicb.2023.1253160
Edited by:
Benjamin Andrew Evans, University of East Anglia, United KingdomReviewed by:
Abid Ali, Texas A&M University, United StatesMariagrazia Perilli, University of L’Aquila, Italy
Copyright © 2023 Gaillot, Oueslati, Vuillemenot, Bour, Iorga, Triponney, Plésiat, Bonnin, Naas, Jeannot and Potron. This is an open-access article distributed under the terms of the Creative Commons Attribution License (CC BY). The use, distribution or reproduction in other forums is permitted, provided the original author(s) and the copyright owner(s) are credited and that the original publication in this journal is cited, in accordance with accepted academic practice. No use, distribution or reproduction is permitted which does not comply with these terms.
*Correspondence: Anaïs Potron, YW5haXMucG90cm9uQHVuaXYtZmNvbXRlLmZy