- 1UMR Procédés Alimentaires et Microbiologiques, Institut Agro Dijon, Université de Bourgogne Franche-Comté, Équipe Vin Alimentation Micro-Organismes Stress (VAlMiS), Dijon, France
- 2Changins, Viticulture and Enology, HES-SO University of Applied Sciences and Arts Western Switzerland, Nyon, Switzerland
Finding alternatives to the use of chemical inputs to preserve the sanitary and organoleptic quality of food and beverages is essential to meet public health requirements and consumer preferences. In oenology, numerous manufacturers already offer a diverse range of bio-protection yeasts to protect must against microbiological alterations and therefore limit or eliminate sulphites during winemaking. Bio-protection involves selecting non-Saccharomyces yeasts belonging to different genera and species to induce negative interactions with indigenous microorganisms, thereby limiting their development and their impact on the matrix. Although the effectiveness of bio-protection in the winemaking industry has been reported in numerous journals, the underlying mechanisms are not yet well understood.
The aim of this review is to examine the current state of the art of field trials and laboratory studies that demonstrate the effects of using yeasts for bio-protection, as well as the interaction mechanisms that may be responsible for these effects. It focuses on the yeast Metschnikowia pulcherrima, particularly recommended for the bio-protection of grape musts.
1. Introduction
Current concerns to reduce chemical pesticides, fungicides and bactericides in the agri-food industry have encouraged researchers and industries to develop new strategies over the last 20 years. One of them consists in adding microorganisms after harvest to control spoilage microorganisms: this is the biocontrol concept mainly used in agriculture for the protection of fruits and vegetables (Spadaro and Gullino, 2004; Droby et al., 2009; Sharma et al., 2009). Non-Saccharomyces (NS) yeasts have been given great attention due to their microbial antagonisms that biologically inhibit potential post-harvest moulds (Comitini et al., 2023). The development of this approach has more recently been extended to the field of oenology, as an alternative to the use of sulphites to protect musts, mainly from potential alteration by indigenous microbiota at the pre-fermentative step. This is the strategy of bio-protection (García et al., 2016), corresponding to the implementation of non-Saccharomyces (NS) yeasts as in biocontrol but in winemaking framework. However, the function of a strain used in biocontrol differs from that expected of a strain recommended for bio-protection. Indeed, a bioprotective strain in oenology has to be effective against specific microorganisms considered as potential spoilage agents (such as Hanseniaspora and Brettanomyces yeasts, and lactic acid bacteria) in specific environmental conditions linked to the first steps of transforming grapes into must (acidity of must, low temperature and levels of oxygen).
In winemaking, NS yeasts have long been considered as undesirable microorganisms, responsible for sluggish or incomplete fermentations and the production of unpleasant aromas (Bisson, 1999), thus leading to economic losses for winemakers. However, the focus on these yeasts which are largely predominant on grape berry and must at the beginning of alcoholic fermentation (Albertin et al., 2014; Garofalo et al., 2016; Mateus et al., 2020) has changed over the last few decades, and NS yeasts including Metschnikowia pulcherrima are now marketed in Active Dry Yeast form (ADY) (Roudil et al., 2020). Metschnikowia pulcherrima strains were first recommended in winemaking for their contribution to the aromatic development of wine through their enzymatic activities (β-D-glucosidase, cysteine β-lyase) (Charoenchai et al., 1997; Morata et al., 2019) and the production of a wide range of metabolites (esters, higher alcohols) resulting from alcoholic fermentation. An extensive bibliography highlighted the positive effects of M. pulcherrima, tested on different grape varieties (Sauvignon blanc, Chenin blanc, Muscat, Merlot) in sequential inoculation with Saccharomyces cerevisiae, on the organoleptic qualities of wines (Zohre and Erten, 2002; Jolly et al., 2003; Rodríguez et al., 2010; Comitini et al., 2011; Zott et al., 2011; Sadoudi et al., 2012; Morales et al., 2015; Zhang et al., 2020; Aplin et al., 2021; Lin et al., 2022). However, some strains have been described as producing off-flavour, such as ethyl acetate leading to unpleasant banana or glue flavours in wine (Cosme et al., 2018), underlining the importance of strain selection for oenological application. More recently, the use of M. pulcherrima for the bio-protection of grape must has been proposed to winemakers as an alternative to using sulphites. A new positive function of M. pulcherrima is now being highlighted: an antagonistic role against indigenous grape microbiota during the pre-fermentation phases without necessarily impacting on the final organoleptic properties of the wines.
This review presents the state of the art in the application and effectiveness of bio-protection by M. pulcherrima in cellar conditions. An investigation into the mechanisms potentially involved in the bioprotective effect of this yeast, such as the production of antimicrobial compounds and enzymatic activities is proposed. It also focuses on data relating to M. pulcherrima’s nutritional needs which could be implicated in its bioprotective effect through nutrient competition.
2. Bio-protection by Metschnikowia pulcherrima yeast: effectiveness in field trials
The strains available on the market have been phenotypically characterised under oenological conditions and meet the criteria imposed by the specifications allowing their use in winemaking (Roudil et al., 2020).
Metschnikowia pulcherrima strains are those most frequently tested for bio-protection, pure or associated with Torulaspora delbrueckii (Table 1). Not only do these yeasts have antagonistic effects on other indigenous yeast species, they also appear to be cold-resistant, making them particularly effective in the pre-fermentation phases conducted at low temperatures. Furthermore, Grazia et al. (2019) showed that M. pulcherrima could tolerate SO2 concentration ranging from 125 to 200 mg/L, which is higher than the concentration used in standard winemaking process.
The implementation protocols for bio-protectants containing Metschnikowia strains are consistent across different companies, with all products available in dry form. The rehydration protocol is similar to that used to prepare S. cerevisiae. Regardless of the strain used, seeding rates typically range from 5 g/qt on grapes to 3–5 g/hL on juice, resulting in values between 5.105 and 106 Colony Forming Unit (CFU)/mL. It is recommended to apply the product as early as possible, either in the bin or during vatting, or even by spraying during mechanical harvesting. In the case of white grapes, the product can be applied just after pressing.
Although many technical reports have underlined the positive results obtained by the bio-protection strategy, the first scientific evidence highlighting the efficiency of bio-protective strains have become available only recently. Figure 1 provides some examples of experiments that demonstrate the efficacy of bio-protection under cellar conditions.
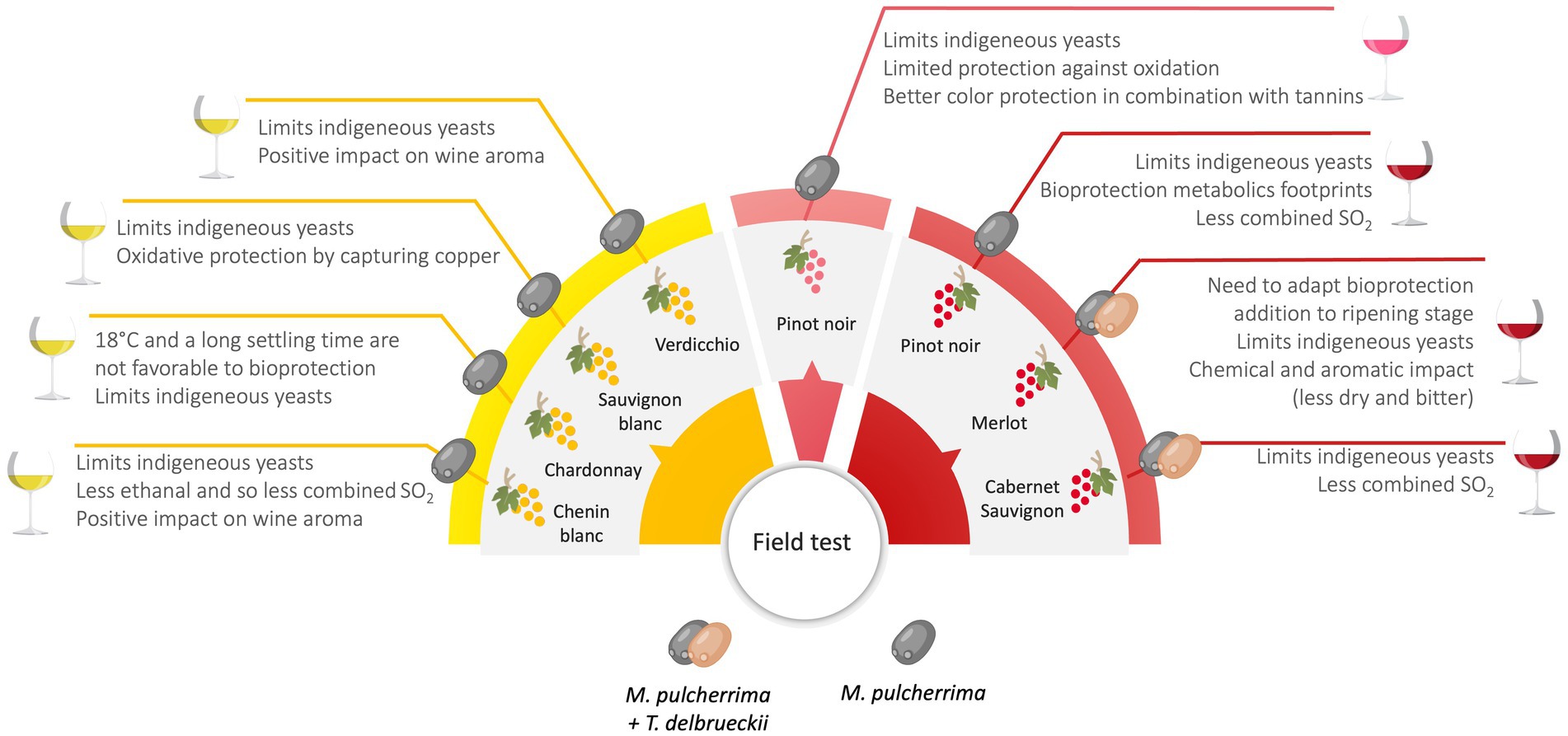
Figure 1. Main results on bio-protection obtained in field trials (From data published in journals for professionals and in Chacon-Rodriguez et al., 2020; Simonin et al., 2020, 2022; Windholtz et al., 2021a,b,c; Agarbati et al., 2023; Puyo et al., 2023).
In white winemaking, a M. pulcherrima strain was tested on Chardonnay must using three temperatures (7°C, 12°C and 18°C) and two settling times (36 and 72 h) in order to determine the optimal conditions for a bioprotective effect (Simonin et al., 2022). The results showed a considerable impact of temperature on the bioprotectant implantation. On must with a settling time of 72 h at 7°C, M. pulcherrima represented 99% of the total yeast concentration, with only 1% of Hanseniaspora uvarum, and the inhibition of Brettanomyces bruxellensis which became undetectable. The implantation of M. pulcherrima during a cold static clarification of a Verdicchio must at low temperature (10°C, 24 h) and its control on indigenous wild yeast populations were confirmed by the work of Agarbati et al. (2023). However, an increase in settling temperature to 18°C did not allow the implantation of the bio-protection strain and therefore the control of indigenous flora (Simonin et al., 2022). The impact of bio-protection on the organoleptic qualities of wines gave contradictory results, depending on the grape varieties tested. Tested on Chardonnay, the bio-protection of must did not have a significant influence on the chemical and sensory characteristics of wines (Simonin et al., 2022), whilst on Verdicchio must, the application of bio-protection induced a specific aromatic imprint in the wine due to volatile compounds and confirmed by sensorial analyses (Agarbati et al., 2023).
The bio-protection strategy was also used during red winemaking on different grape varieties. On Pinot noir, the addition of a M. pulcherrima strain during the cold pre-fermentative stage limited the growth of indigenous flora, like for white musts (Simonin et al., 2020). On Cabernet Sauvignon, the inoculation of a combination of the bioprotective strains M. pulcherrima and T. delbrueckii after grape vatting and maceration at 13°C for 3 days, induced an antagonistic effect on microorganisms responsible for wine spoilage, like Zygosaccharomyces, Lactobacillus kunkeei, H. uvarum, and acetic acid bacteria (Chacon-Rodriguez et al., 2020). The work of Windholtz et al. (2021a) confirmed the implantation of the mixture of M. pulcherrima and T. delbrueckii on Merlot with its capacity to occupy the microbiological niche, leading to the decrease of fungal communities and the cultivable H. uvarum population. Chemical analysis of the wines obtained from Pinot noir underlined that bio-protection had no influence on the level of phenolic compounds and the volatile content of wines, suggesting that the bio-protection of must could also protect must and wines from oxidation (Simonin et al., 2020). Concerning the first available data on the application of bio-protection in the production of Rosé wines (grape variety Pinot noir), the results underlined the predominance of the bioprotective strain M. pulcherrima after pressing (representing more than 70% of the total yeast population), despite the high concentration of indigenous yeasts in the must. However, only the combination of the bio-protection and the addition of an antioxidant such as oenological tannins made it possible to preserve the colour of the wines (Puyo et al., 2023).
In many cases, field trials have proven the ability of bio-protection to reduce the development of spoilage flora, with the same effectiveness as adding sulfites. However, a number of recommendations have been suggested to ensure the effectiveness of this strategy: the maintenance of low temperatures during the pre-fermentation phases (Simonin et al., 2022), and considering the level of indigenous populations in grape must (Windholtz et al., 2021b). Indeed, a high wild microorganisms concentration (above 105 CFU/mL) linked to advanced grape maturity can compromise the implantation of the bioprotective strain.
These limitations highlight the importance of investigating the mechanisms linked to the antagonistic effects of M. pulcherrima on the indigenous flora initially present in the must, in order to adapt and improve protocols for the winemaker’s benefit.
3. What specific mechanisms of Metschnikowia pulcherrima are involved in bio-protection?
Oenological conditions influence complex microbial ecosystems including yeasts and bacteria. The study of interactions inside these ecosystems present on must and during fermentation has become essential. A deeper understanding of these interactions is crucial to ensure better control of bio-protection in winemaking.
Interactions can be indirect, such as nutrient competition or the production of antimicrobial compounds, or direct interactions like cell–cell contact (Bordet et al., 2020; Zilelidou and Nisiotou, 2021) (Figure 2). Interactions are referred to as positive, neutral or negative. All populations involved can benefit from the interaction, or on the contrary be negatively affected. The effect of the interaction can also be asymmetric: one population can benefit from the interaction whilst the other population is negatively or not impacted. The effects of interaction impact the populations at different levels. Their growth parameters can be modified (latency phase, μmax value, maximal population, etc.), as can their metabolism (Sadoudi et al., 2017; Bordet et al., 2023).
Only few studies have focused on the investigation of direct interaction “cell–cell contact” in winemaking context (Kemsawasd et al., 2015a; Petitgonnet et al., 2019; Hu et al., 2022), and there is no evidence of such an interaction within the Metschnikowia genus. The only information suggesting a possible cell–cell contact interaction in Metschnikowia was given by Oztekin and Karbancioglu-Guler (2021) who showed the ability of some M. pulcherrima and M. fructicola strains to produce biofilm on polystyrene. Today, to explain the bio-protective action of NS yeasts in oenology, research focuses mainly on the potential mechanisms of indirect interaction.
3.1. Killer toxins
The production of antimicrobial peptides or proteins, such as killer toxins, was first reported in Saccharomyces cerevisiae (Bevan and Makover, 1963), and has been widely characterised since. Three main killer toxins have been reported in S. cerevisiae: K1, K2, and K28 (Novotna et al., 2004; Orentaite et al., 2016). Yeast killer toxin producers exhibit antimicrobial properties against other S. cerevisiae sensitive strains and are immune to their own killer toxin family (K1, K2, or K28), but could be sensitive to another killer family (Orentaite et al., 2016).
A killer phenotype has been reported in NS yeasts found in grape must and other environments (Santos and Marquina, 2004; Liu et al., 2012) (Table 2). Amongst these yeasts, some of them were reported to be killer toxin producers with antimicrobial activity on the main wine spoilage yeasts, Brettanomyces and Hanseniaspora genera (Mannazzu et al., 2019).
Recently, the production of a killer toxin by a M. pulcherrima strain (TB26) isolated from grape vine was demonstrated (Büyüksırıt-Bedir and Kuleaşan, 2022). The application of the purified killer toxin was tested in ready to cook meatballs in order to extended their shelf life (Büyüksırıt Bedir and Kuleaşan, 2021). The same authors purified, characterised and tested different growth conditions for killer activity (Büyüksırıt-Bedir and Kuleaşan, 2022). Incubation at 20°C at a pH value of 7 showed the highest inhibition diameter on agar plates. An analysis by MALDI-TOF mass spectrometry gave a molecular weight of 10.3 kDa and provided the amino acid sequence of this toxin. The sequence comparison underlined that part of the sequence obtained (amino acids 31 to 50) showed a 100% correspondence score with the KHR killer toxin of S. cerevisiae characterised by Goto et al. (1990). Molecular weights of killer toxins are extremely variable. The size range reported in the literature can vary from 1.8 to 300 kDa (Kagiyama et al., 1988). The M. pulcherrima TB26 killer toxin seems to be in the low molecular weight range of the killer toxins characterised until now. These results were supported by Hicks et al. (2021) who tested the killer phenotypes of 11 M. pulcherrima strains. The authors showed that some strains exhibit a killer activity against Escherichia coli, Salmonella enterica, and Staphylococcus aureus on agar, an effect also achieved by adding the culture supernatant. They also analysed the protein extract of Metschnikowia culture and found a considerable amount of proteins with a size around 10 kDa, which corresponds to the killer toxin size identified by Büyüksırıt-Bedir and Kuleaşan (2022).
This approach remains to be explored with more strains of M. pulcherrima used for bio-protection in oenology. Farris et al. (1991) and Lopes and Sangorrín (2010) shed light on the killer phenotype (i.e., antagonist effect on agar plate by visualising a halo of inhibition) of M. pulcherrima strains isolated from grape, must, and the wine matrix. However, the killer phenomenon observed in those studies were not proved to be linked to the production of a peptidic killer toxin.
3.2. Pulcherriminic acid regulation and production
Microorganisms can produce non-peptidic compounds with antimicrobial properties. In the particular case of M. pulcherrima, the non-peptidic antimicrobial compound composed of four genes: PUL1, PUL2, PUL3 is pulcherriminic acid. The first reports in the literature on pulcherriminic acid production date from the last century and concern yeasts and Bacillus bacteria (Kluyver et al., 1953; MacDonald, 1965; Uffen and Canale-Parola, 1972). The metabolic pathway involved in pulcherriminic acid synthesis has been well characterised since, especially in Bacillus subtilis and Bacillus licheniformis. Pulcherriminic acid is produced from two leucyl-tRNA that are cyclised by cyclodileucine synthase, encoded by the yvmC gene, which leads to the production of cyclo-(L-Leu-L-Leu). The latter is then oxidised in pulcherriminic acids by pulcherriminic acid synthase, encoded by the cypX gene (coding for a P450 cytochrome family protein). After its production in the cell, pulcherriminic acid is excreted into the extracellular medium by transporters encoded by the yvmA gene, where it can chelate iron ion (Fe3+) by a non-enzymatic reaction to form the red pigment named pulcherrimin (Wang et al., 2018; Yuan et al., 2020). The production of pulcherriminic acid is down-regulated by three main genes in Bacillus: yvnA, yvmB and abrB. Moreover, Wang et al. (2018) showed that the iron concentration of the medium impacts pulcherriminic acid production. These authors have shown that an iron-limited medium inhibited the yvmC-cypX cluster and thus inhibited pulcherriminic acid production.
In M. pulcherrima, as well as in other pulcherrimin-producer Metschnikowia species, the metabolic pathway of pulcherriminic acid production is not yet as well-known as in Bacillus. Having prior knowledge of the genes involved in the synthesis of this pigment in Bacillus, Piombo et al. (2018) searched for homologous genes by sequence alignment in two newly sequenced M. fructicola strains. But the results were negative. Genes that may be involved in the production of this antimicrobial pigment in Metschnikowia were identified by Sipiczki (2020). The PUL (for PULcherrimin) gene cluster was identified as being involved in the production of pulcherriminic acid in Metschnikowia, Kluyveromyces, and Zygotorulaspora genera (Krause et al., 2018). This cluster is supposedly composed of four genes: PUL1, PUL2, PUL3 and PUL4 (Figure 3). Krause et al. (2018) were able to assign potential functions to each of these genes. The PUL1 gene is found to be potentially responsible for the production of cyclo-(L-Leu-L-Leu) from two leucyl-tRNA (corresponding to yvnB in Bacillus), followed by PUL2 acting to oxidise the cyclodipeptide into pulcherriminic acid (corresponding to cpyX in Bacillus). The PUL4 gene encodes a transcriptional regulator of PUL1, PUL2 and PUL3 genes. The PUL3 gene appears to be involved in the transport of iron in pulcherrimin, from outside to inside the cell for its reuse in cell metabolism. Studies conducted on Bacillus seem to indicate that iron once chelated by pulcherriminic acid becomes unavailable for the other microorganisms as well as for the bacteria producing pulcherrimin, unlike for yeasts producing this pigment which seem to possess a mechanism allowing them to reuse the iron in the medium even after complexation with pulcherriminic acid. The work of Krause et al. (2018) also showed the presence of genes homologous to PUL3 and PUL4 in other genera and species, such as S. cerevisiae, Lachancea thermotolerans, and M. bicuspidata. Some of them have both genes, and others have only PUL4. Species with only PUL4 are not able to reuse iron once chelated by pulcherriminic acid, unlike strains that have both genes (PUL3 and PUL4). Amongst them we find S. cerevisiae which has PUL3 and PUL4 and seems to be able to reuse iron despite the presence of pulcherriminic acid in the medium. It is hypothesised that PUL3 encodes the transporter that allows the internalisation of pulcherrimin and that PUL4 regulates the transcription of PUL3 by binding DNA (Krause et al., 2018).
The genes of the PUL cluster do not appear to be the only ones involved in the production of pulcherriminic acid. Using the PacBio method, Gore-Lloyd et al. (2019) sequenced a new strain of Metschnikowia, identified as a Metschnikowia aff. pulcherrima strain, as well as three spontaneous pigmentless mutants of this strain. Genome comparison between the wild-type strain and the pigmentless mutants showed a single mutation in the gene homologous to the SNF2 gene in S. cerevisiae. This mutation introduces a premature stop codon in the sequence, leading to the production of a truncated and therefore non-functional protein. In S. cerevisiae, SNF2 codes for a transcriptional regulator by chromatin remodelling (Snf2) (Hirschhorn et al., 1992). This mutation seems to impact the transcription of many genes including genes of the PUL cluster. Indeed, strains mutated on the SNF2 gene show a strong decrease of PUL cluster gene transcription. Since Snf2 is involved in chromatin remodelling, it is possible that the non-functional Snf2 prevents the remodelling of chromatin at the PUL cluster loci, and thus decreases the transcription of these genes (Gore-Lloyd et al., 2019).
Iron chelation by pulcherriminic acid makes this resource unavailable for microorganisms in the environment, and thus negatively impacts the growth of microorganisms needing Fe3+. The secretion of pulcherriminic acid into the medium by pulcherrimin producing yeasts, such as M. pulcherrima, could partially explain the antagonistic effect of this species on some microorganisms. The antagonistic effect of pulcherrimin was therefore studied with different species of yeasts producing this pigment. These studies were conducted in vitro (mostly with agar plates) and in vivo. Different species of Metschnikowia (citrensis, fructicola, pulcherrima) have shown an inhibitory effect on microorganisms that can alter the fruit or the resulting food products (Türkel and Ener, 2009; Hicks et al., 2021; Wang et al., 2021; Zhang et al., 2023). It was shown that the intensity of the pigmented halo around Metschnikowia on agar increases with the iron concentration in the medium, contrary to the inhibition zone which decreases when increasing the iron concentration. Indeed, in a medium with excess iron, Fe3+ chelation by pulcherriminic acid will not be sufficient to cause a deficiency in the environment, and thus not be deleterious for the surrounding microorganisms (Sipiczki, 2006; Saravanakumar et al., 2008; Türkel and Ener, 2009; Wang et al., 2021). Furthermore, Kregiel et al. (2022) showed that pulcherrimin has no antimicrobial effect. Their results support the hypothesis that it is iron chelation and not the pigment itself that has an inhibitory effect. In the oenological context, Oro et al. (2014) showed that the production of pulcherriminic acid by M. pulcherrima had an inhibitory effect on non-Saccharomyces yeasts found in oenology, including spoilage yeasts such as apiculate yeasts (Hanseniaspora guillermondii) and Brettanomyces bruxellensis.
Under the conditions tested, the production of pulcherriminic acid seems to explain most of the antagonistic effect of Metschnikowia observed. However, the production of this compound does not seem to be the only explanation for its antimicrobial effect. Indeed, in studies that sought to confirm the involvement of iron chelation in inhibitory effects, the authors supplemented the medium with iron or induced the knock-out or down-regulation of genes involved in pulcherriminic acid production. In most cases, the inhibitory effect is strongly reduced (by 80%) (Gore-Lloyd et al., 2019; Wang et al., 2021), but not always completely eliminated (Gore-Lloyd et al., 2019). This suggests that iron chelation is not the only negative interaction that M. pulcherrima establishes in playing its bioprotective role.
3.3. Quorum sensing
Quorum Sensing (QS) is intercellular communication mediated by the excretion of a density-dependent signal molecule. Once the target cell density is reached, the signal molecule concentration also reaches a threshold value, inducing signal transduction pathways that coordinate a response at the population level rather than at the cell level. This communication mechanism was first discovered in Vibrio fischeri for bioluminescence production (Nealson et al., 1970; Dunlap, 1999). In bacteria, QS has been found to be involved in different cellular mechanisms such as biofilm formation and the production of enzymes and other compounds required for pathogenicity or growth regulation (Miller and Bassler, 2001).
Bacteria are not the only microorganisms able to regulate their gene expression at the population scale to adapt to their environment. QS is also an interaction phenomenon investigated in yeasts, and more extensively in the pathogenic yeast Candida albicans, as it controls various cellular transformations such as biofilms, transitions between cellular growth and stationary phases, hyphal production, and many others (Kügler et al., 2000; Chen and Fink, 2006; Hogan, 2006; Sprague and Winans, 2006). In yeasts, it seems that QS is induced by aromatic alcohols in response to environmental factors such as a low nitrogen level in the medium. In the model yeast S. cerevisiae, the Quorum Sensing Molecules (QSMs) found are 2-phenylethanol and tryptophol. Tyrosol has been found in C. albicans but its role as a QSM in S. cerevisiae is still controversial (Chen and Fink, 2006; Wuster and Babu, 2009; Zupan et al., 2013; Avbelj et al., 2016; Padder et al., 2018; Mehmood et al., 2019; Jagtap et al., 2020). These molecules are produced from phenylalanine, tryptophan and tyrosine respectively, through the Ehrlich pathways. In order to produce these QSMs, amino acids must undergo three phases of transformation: transamination, decarboxylation and oxidation, involving the genes ARO8/ARO9, ADH, and ARO10, respectively (Sprague and Winans, 2006; Hazelwood et al., 2008; Wuster and Babu, 2009; Avbelj et al., 2015; Jagtap et al., 2020). Saccharomyces cerevisiae and C. albicans are not alone in being assumed to be capable of communicating through QS. Pu et al. (2014) showed that the bioprotective effect of H. uvarum on lemon through biofilm formation in fruit wounds was induced by phenylethanol secretion and thus under the potential control of QS. Furthermore, other yeasts and fungi are suspected of being able to use QS for their intercellular communication, mainly through aromatic alcohol production (Kügler et al., 2000; Sprague and Winans, 2006; Gori et al., 2011; Padder et al., 2018).
Except for C. albicans, this type of interaction remains less studied and understood in yeasts. The existence of QS as well as the role of aromatic alcohols as signalling molecules remains controversial and requires further research (Winters et al., 2019, 2022). Nevertheless, it has been shown that some non-Saccharomyces wine yeasts, including M. pulcherrima, are able to produce aromatic alcohols suspected of playing the role of QSMs in S. cerevisiae (González et al., 2018; Petitgonnet et al., 2019). It could therefore be interesting to extend research in M. pulcherrima to the involvement of these molecules as potential QSMs and to their control of gene expression amongst non-Saccharomyces yeasts mainly present on a grape must.
3.4. Enzymatic activities in Metschnikowia pulcherrima: a potential role in bio-protection?
Amongst the NS yeasts, Metschnikowia pulcherrima stands out due to its substantial production of a very diverse range of enzymatic activities, such as amylase, lichenase, cellulase, lipase, and glucanase (Charoenchai et al., 1997; Strauss et al., 2001; Oztekin and Karbancioglu-Guler, 2021). Saravanakumar et al. (2008) showed that a M. pulcherrima strain isolated from an apple carposphere is able to secrete chitinase in the medium. This enzymatic production was found to be involved in its biocontrol activity on Botrytis cinerea. Other studies have also reported chitinase (Banani et al., 2015; Pretscher et al., 2018; Freimoser et al., 2019; Morata et al., 2019) and β-1,3-glucanase productions (Oztekin and Karbancioglu-Guler, 2021) by yeasts belonging to the Metschnikowia genus, justifying their potential use as biocontrol agents on vegetables and fruits, as well as grapevine. To our knowledge, enzyme production by M. pulcherrima and potential enzymatic activity levels have never been tested in relation to the acidity associated with oenological conditions, in order to verify the possible implication of extracellular enzymes synthesised by the yeast in a bio-protection context.
3.5. Oxygen needs and possible competition
Oxygen plays a key role in the metabolism of NS yeasts. At the pre-fermentative stage, the level of dissolved oxygen (DO) in must is about 8 mg/L (at 20°C) and decreases during alcoholic fermentation. Depending on oenological practices, the concentration of DO added to the must can vary and practices such as punching down and pumping over to the vat incorporate significative quantities of oxygen into the medium (Moenne et al., 2014).
Many NS yeasts are high oxygen consumers (Visser et al., 1990). Quirós et al. (2014) studied the respiratory quotient (RQ) of S. cerevisiae and many NS yeasts. Amongst the yeasts studied, they showed that at the beginning of alcoholic fermentation when oxygen is available in the must, M. pulcherrima has an RQ of 1. This RQ means that all the sugar metabolised by the yeast is respired and not fermented, in contrast to S. cerevisiae which has an RQ of 4 (meaning that only about 10% of the sugar consumed is respired). The authors also showed that the RQ of M. pulcherrima could reach a maximum fermentative capacity of 2.6, which indicates that the yeast may consume at least 17% of the sugar by respiration.
Several studies have investigated the role of oxygen in the persistence and survival of yeasts in co-cultures with S. cerevisiae. Most of the time, NS yeasts do not persist in must, especially after inoculation with a S. cerevisiae strain. In the literature, the addition of oxygen has been found to improve the persistence of NS yeasts in the medium (Holm Hansen et al., 2001; Shekhawat et al., 2016, 2018; Englezos et al., 2018; Yan et al., 2020). Morales et al. (2015) and Shekhawat et al. (2016) showed that the decline of M. pulcherrima in co-culture with S. cerevisiae is delayed by increasing O2 supply in white grape juice and synthetic must. Although these studies focused on oxygen requirements during co-cultures combining S. cerevisiae/non-Saccharomyces yeast, they nonetheless highlighted the importance of oxygen needs for NS yeasts. In order to better understand and control the bioprotective effect of M. pulcherrima, it appears essential to quantify its oxygen requirement, as well as those of the indigenous flora, such as Hanseniaspora yeasts, with the aim of optimising the implantation of the bioprotective strain in relation to its efficiency. High oxygen consumption exhibited by M. pulcherrima yeasts could induce competition between bioprotective and indigenous yeasts, leading to the inhibition of potential spoilage yeasts.
3.6. Nutrient requirement: a path to competition
Oxygen is not the only resource consumed by yeast, which could lead to competitive phenomena. Amongst these nutrients, requirements for nitrogenous resources (ammonium and amino acids) are those studied most, playing a central role in yeast metabolism synthesising protein and nitrogenous bases, and in the production of aromatic compounds by the Ehrlich pathway (Hazelwood et al., 2008). As for oxygen, competition for nitrogen compounds could be involved in the negative interactions triggered by the bioprotective yeast.
Nitrogen sources are classified as “preferential” and “non-preferential” resources. Preferential resources are consumed in priority by the yeasts and support their growth more efficiently, contrary to non-preferential resources which are consumed once the preferred resources have been entirely consumed or have become limited in quantity in the medium (Crépin et al., 2012). The regulation of nitrogen consumption has been extensively studied in S. cerevisiae and appears to be regulated by two main systems: the Nitrogen Catabolic System (NCR) and regulation involving the Ssy1-Ptr3-Ssy5 (SPS) sensor of the plasma membrane (Crépin et al., 2012).
In NS yeasts, the regulatory pathways involved remain poorly investigated. Moreover, nitrogen requirements were found to be highly variable between yeast species but also strain-dependent (Kemsawasd et al., 2015a; Gobert et al., 2017; Prior et al., 2019; Roca-Mesa et al., 2020; Seguinot et al., 2020). The data heterogeneity can be explained by the great diversity of experimental conditions: variable temperatures, different strains analysed as well as different growth media (grape juice, synthetic must or synthetic medium). According to Gobert et al. (2017), the preferential sources of M. pulcherrima in grape juice at 28°C are Ile, Leu, Lys, Met, Glu and Cys, whilst at 20°C the preferential resources are Ala, Cys, Glu, His, Lys and Thr. In the synthetic medium YNBMAF at 25°C, the resources consumed are mainly Ala and Asn (Kemsawasd et al., 2015b). In synthetic must at 22°C Lys is found mainly consumed with Glu, Gln, His and Val in medium with amino acids and ammonium, or with Phe in medium with only amino acids as nitrogen sources (Roca-Mesa et al., 2020). Furthermore, Seguinot et al. (2020) showed that consumption also varies according to the initial nitrogen concentration, with an increase of the consumption of resources with the concentration of nitrogen in the environment.
To the best of our knowledge, there are still no studies that have attempted to demonstrate competition for nitrogen resources in a bio-protection strategy. Better understanding of the nitrogen requirements of M. pulcherrima in relation with the environmental conditions of growth is essential in order to investigate whether potential competition for nitrogen compounds may be involved in the negative effect on the growth of indigenous yeasts.
In must, other compounds could lead to competition, such as lipids or vitamins. Lipids are necessary for the maintenance of cell membrane integrity and they improve resistance to the stresses induced by ethanol. Moreover a medium limited in lipids can lead to languishing fermentation (Tesnière, 2019; Mbuyane et al., 2022). Vitamins are also an important factor for yeast growth, and a medium limited in vitamins could also lead to sluggish fermentation. Also, vitamin consumption appears to be species- and strain-dependent (Evers et al., 2021). The lipid and vitamin requirements of NS yeasts are still poorly explored and deserve further investigation to determine if they are involved in the bioprotective phenomenon.
4. Conclusion and perspectives
Non-Saccharomyces yeasts are found to be predominant on grapes and must during the early stages of fermentation, before being replaced by S. cerevisiae which will complete alcoholic fermentation. NS yeasts have long been criticised for their negative effects on the organoleptic qualities of wine, but numerous studies have subsequently highlighted many properties of considerable oenological interest amongst NS yeasts. Metschnikowia pulcherrima presents numerous advantages for the wine industry and is now distinguished by its effectiveness in the bio-protection of musts, making it a possible alternative to the use of sulphites to protect against indigenous yeasts, potential spoilage agents.
Although much research has highlighted the physiological characteristics of this yeast that may play an anti-microbial role, it remains difficult to predict and ensure perfect efficiency under cellar conditions. Indeed, Windholtz et al. (2021b) showed that on must from grapes with advanced maturity, bio-protection was not sufficient to limit the proliferation of indigenous flora. It is important to study in greater detail the resource requirements of bioprotective yeasts as well as those of indigenous flora to better understand the mechanisms and the limits of bio-protection. But many other interactions can lead to the inhibition of spoilage microorganisms in the must. Indeed, many questions remain concerning the production of toxic compounds by M. pulcherrima, such as potential killer toxins, and the existence of quorum sensing in this yeast. In addition, other non-Saccharomyces yeasts have shown the ability to make cell–cell contact interactions. Metschnikowia pulcherrima was found to be able to adhere to a polystyrene surface (Parafati et al., 2015) which could suggest its capacity to induce adhesion between cells and surfaces. Cell–cell interaction has never been demonstrated in the context of bio-protection with M. pulcherrima, but given its surface adhesion properties, this appears to be an important topic to investigate. The genetic diversity of M. pulcherrima is still little known and a recent study tended to indicate that the genus Metschnikowia deserve more detailed study to better understand strain diversity and distribution within the different species of this genus (Sipiczki, 2020).
In addition, the perfect protection of a grape must also includes protection against oxidation. As reported in this review, M. pulcherrima is described in the literature to be a strong consumer of oxygen. The high consumption of this resource could make O2 rapidly unavailable in the grape must and thus prevents oxygen from entering the redox pathways that leads to wine browning and to the production of undesirable aromas. In addition, M. pulcherrima is known to secrete pulcherriminic acid, which once in the medium chelates Fe3+. This ion is also involved in redox mechanisms through the Fenton reaction. The depletion of iron in the medium by pulcherriminic acid could also contribute, to a lesser degree, to protecting the matrix against oxidation. The literature has also highlighted interaction phenomena between the yeast cell wall and anthocyanins. These interactions are species-dependent and impact the final colour of the wine (Morata et al., 2019; Vicente et al., 2020). More information on the effect of M. pulcherrima on must and wine colour, and on its combination with other antioxidant compounds, could also be crucial for professionals to optimise its application in the wine sector.
Author contributions
All authors listed have made a substantial, direct, and intellectual contribution to the work and approved it for publication.
Acknowledgments
We would like to thank the Society AEB for their financial support for this scientific work. We thank Biorender website for the access to scientific illustration.
Conflict of interest
The authors declare that the research was conducted in the absence of any commercial or financial relationships that could be construed as a potential conflict of interest.
Publisher’s note
All claims expressed in this article are solely those of the authors and do not necessarily represent those of their affiliated organizations, or those of the publisher, the editors and the reviewers. Any product that may be evaluated in this article, or claim that may be made by its manufacturer, is not guaranteed or endorsed by the publisher.
References
Abu-Mejdad, N. M. J. A., Al-Badran, A. I., and Al-Saadoon, A. H. (2020). Purification and characterization of two killer toxins originated from Torulaspora delbrueckii (Lindner) and Wickerhamomyces anomalus (E.C.Hansen) Kurtzman, Robnett, and Basehoar-powers. Bull Natl Res Cent 44:48. doi: 10.1186/s42269-020-00308-w
Agarbati, A., Canonico, L., Ciani, M., and Comitini, F. (2023). Metschnikowia pulcherrima in cold clarification: biocontrol activity and aroma enhancement in Verdicchio wine. Fermentation 9:302. doi: 10.3390/fermentation9030302
Albertin, W., Miot-Sertier, C., Bely, M., Marullo, P., Coulon, J., Moine, V., et al. (2014). Oenological prefermentation practices strongly impact yeast population dynamics and alcoholic fermentation kinetics in chardonnay grape must. Int. J. Food Microbiol. 178, 87–97. doi: 10.1016/j.ijfoodmicro.2014.03.009
Aplin, J. J., Paup, V. D., Ross, C. F., and Edwards, C. G. (2021). Chemical and sensory profiles of merlot wines produced by sequential inoculation of Metschnikowia pulcherrima or Meyerzyma guilliermondii. Fermentation 7:126. doi: 10.3390/fermentation7030126
Avbelj, M., Zupan, J., Kranjc, L., and Raspor, P. (2015). Quorum-sensing kinetics in Saccharomyces cerevisiae: a symphony of ARO genes and aromatic alcohols. J. Agric. Food Chem. 63, 8544–8550. doi: 10.1021/acs.jafc.5b03400
Avbelj, M., Zupan, J., and Raspor, P. (2016). Quorum-sensing in yeast and its potential in wine making. Appl. Microbiol. Biotechnol. 100, 7841–7852. doi: 10.1007/s00253-016-7758-3
Banani, H., Spadaro, D., Zhang, D., Matic, S., Garibaldi, A., and Gullino, M. L. (2015). Postharvest application of a novel chitinase cloned from Metschnikowia fructicola and overexpressed in Pichia pastoris to control brown rot of peaches. Int. J. Food Microbiol. 199, 54–61. doi: 10.1016/j.ijfoodmicro.2015.01.002
Belda, I., Ruiz, J., Alonso, A., Marquina, D., and Santos, A. (2017). The biology of Pichia membranifaciens killer toxins. Toxins 9:112. doi: 10.3390/toxins9040112
Bevan, A., and Makover, M. (1963) in The physiological basis of the killer character in yeast. ed. S. G. Geers (Oxford: Pergamon Press), 202–203.
Bisson, L. F. (1999). Stuck and sluggish fermentations. Am. J. Enol. Vitic. 50, 107–119. doi: 10.5344/ajev.1999.50.1.107
Bordet, F., Joran, A., Klein, G., Roullier-Gall, C., and Alexandre, H. (2020). Yeast-yeast interactions: mechanisms, methodologies and impact on composition. Microorganisms 8:600. doi: 10.3390/microorganisms8040600
Bordet, F., Romanet, R., Bahut, F., Ballester, J., Eicher, C., Peña, C., et al. (2023). Expanding the diversity of chardonnay aroma through the metabolic interactions of Saccharomyces cerevisiae cocultures. Front. Microbiol. 13:1032842. doi: 10.3389/fmicb.2022.1032842
Büyüksırıt Bedir, T., and Kuleaşan, H. (2021). A natural approach, the use of killer toxin produced by Metschnikowia pulcherrima in fresh ground beef patties for shelf life extention. Int. J. Food Microbiol. 345:109154. doi: 10.1016/j.ijfoodmicro.2021.109154
Büyüksırıt-Bedir, T., and Kuleaşan, H. (2022). Purification and characterization of a Metschnikowia pulcherrima killer toxin with antagonistic activity against pathogenic microorganisms. Arch. Microbiol. 204:337. doi: 10.1007/s00203-022-02940-8
Chacon-Rodriguez, L., Joseph, C. M. L., Nazaris, B., Coulon, J., Richardson, S., and Dycus, D. A. (2020). Innovative use of non-Saccharomyces in bio-protection: T. delbrueckii and M. pulcherrima applied to a machine harvester. Catalyst Discov Pract 4, 82–90. doi: 10.5344/catalyst.2020.20003
Charoenchai, C., Fleet, G. H., Henschke, P. A., and Todd, B. E. N. T. (1997). Screening of non-Saccharomyces wine yeasts for the presence of extracellular hydrolytic enzymes. Aust. J. Grape Wine Res. 3, 2–8. doi: 10.1111/j.1755-0238.1997.tb00109.x
Chasseriaud, L. (2015). Interactions entre levures Saccharomyces cerevisiae et non-Saccharomyces en vinification.: Incidence de facteurs de l’environnement.
Chen, H., and Fink, G. R. (2006). Feedback control of morphogenesis in fungi by aromatic alcohols. Genes Dev. 20, 1150–1161. doi: 10.1101/gad.1411806
Ciani, M., and Fatichenti, F. (2001). Killer toxin of Kluyveromyces phaffii DBVPG 6076 as a biopreservative agent to control apiculate wine yeasts. Appl. Environ. Microbiol. 67, 3058–3063. doi: 10.1128/AEM.67.7.3058-3063.2001
Comitini, F., Canonico, L., Agarbati, A., and Ciani, M. (2023). Biocontrol and probiotic function of non-Saccharomyces yeasts: new insights in Agri-food industry. Microorganisms 11:1450. doi: 10.3390/microorganisms11061450
Comitini, F., Gobbi, M., Domizio, P., Romani, C., Lencioni, L., Mannazzu, I., et al. (2011). Selected non-Saccharomyces wine yeasts in controlled multistarter fermentations with Saccharomyces cerevisiae. Food Microbiol. 28, 873–882. doi: 10.1016/j.fm.2010.12.001
Comitini, F., Ingeniis De, J., Pepe, L., Mannazzu, I., and Ciani, M. (2004a). Pichia anomala and Kluyveromyces wickerhamii killer toxins as new tools against Dekkera/Brettanomyces spoilage yeasts. FEMS Microbiol. Lett. 238, 235–240. doi: 10.1111/j.1574-6968.2004.tb09761.x
Comitini, F., Pietro, N. D., Zacchi, L., Mannazzu, I., and Ciani, M. (2004b). Kluyveromyces phaffii killer toxin active against wine spoilage yeasts: purification and characterization. Microbiology 150, 2535–2541. doi: 10.1099/mic.0.27145-0
Cosme, F., Vilela, A., Filipe-Ribeiro, L., António, I., and Nunes, F. M. (2018). “Chapter 9–wine microbial spoilage: advances in defects remediation” in Microbial contamination and food degradation. Academic press. 271–314.
Crépin, L., Nidelet, T., Sanchez, I., Dequin, S., and Camarasa, C. (2012). Sequential use of nitrogen compounds by Saccharomyces cerevisiae during wine fermentation: a model based on kinetic and regulation characteristics of nitrogen permeases. Appl. Environ. Microbiol. 78, 8102–8111. doi: 10.1128/AEM.02294-12
De Ingeniis, J., Raffaelli, N., Ciani, M., and Mannazzu, I. (2009). Pichia anomala DBVPG 3003 secretes a ubiquitin-like protein that has antimicrobial activity. Appl. Environ. Microbiol. 75, 1129–1134. doi: 10.1128/AEM.01837-08
Droby, S., Wisniewski, M., Macarisin, D., and Wilson, C. (2009). Twenty years of postharvest biocontrol research: is it time for a new paradigm? Postharvest Biol. Technol. 52, 137–145. doi: 10.1016/j.postharvbio.2008.11.009
Dunlap, P. V. (1999). Quorum regulation of luminescence in Vibrio fischeri. J. Mol. Microbiol. Biotechnol. 1, 5–12.
Englezos, V., Cravero, F., Torchio, F., Rantsiou, K., Ortiz-Julien, A., Lambri, M., et al. (2018). Oxygen availability and strain combination modulate yeast growth dynamics in mixed culture fermentations of grape must with Starmerella bacillaris and Saccharomyces cerevisiae. Food Microbiol. 69, 179–188. doi: 10.1016/j.fm.2017.08.007
Evers, M. S., Roullier-Gall, C., Morge, C., Sparrow, C., Gobert, A., and Alexandre, H. (2021). Vitamins in wine: which, what for, and how much? Compr. Rev. Food Sci. Food Saf. 20, 2991–3035. doi: 10.1111/1541-4337.12743
Farris, G. A., Mannazzu, I., and Budroni, M. (1991). Identification of killer factor in the yeast genus Metschnikowia. Biotechnol. Lett. 13, 297–298. doi: 10.1007/BF01041488
Fernández de Ullivarri, M., Mendoza, L. M., and Raya, R. R. (2014). Killer yeasts as biocontrol agents of spoilage yeasts and bacteria isolated from wine. BIO Web Conf 3:02001. doi: 10.1051/bioconf/20140302001
Fleet, G. H. (2003). Yeast interactions and wine flavour. Int. J. Food Microbiol. 86, 11–22. doi: 10.1016/S0168-1605(03)00245-9
Freimoser, F. M., Rueda-Mejia, M. P., Tilocca, B., and Migheli, Q. (2019). Biocontrol yeasts: mechanisms and applications. World J. Microbiol. Biotechnol. 35:154. doi: 10.1007/s11274-019-2728-4
García, M., Esteve-Zarzoso, B., and Arroyo, T. (2016). “Non-Saccharomyces yeasts: biotechnological role for wine production” in Grape and wine biotechnology. eds. A. Morata and I. Loira (Rijeka: InTech).
Garofalo, C., Russo, P., Beneduce, L., Massa, S., Spano, G., and Capozzi, V. (2016). Non-Saccharomyces biodiversity in wine and the ‘microbial terroir’: a survey on Nero di Troia wine from the Apulian region, Italy. Ann. Microbiol. 66, 143–150. doi: 10.1007/s13213-015-1090-5
Gobert, A., Tourdot-Maréchal, R., Morge, C., Sparrow, C., Liu, Y., Quintanilla-Casas, B., et al. (2017). Non-Saccharomyces yeasts nitrogen source preferences: impact on sequential fermentation and wine volatile compounds profile. Front. Microbiol. 8:2175. doi: 10.3389/fmicb.2017.02175
González, B., Vázquez, J., Morcillo-Parra, M. Á., Mas, A., Torija, M. J., and Beltran, G. (2018). The production of aromatic alcohols in non-Saccharomyces wine yeast is modulated by nutrient availability. Food Microbiol. 74, 64–74. doi: 10.1016/j.fm.2018.03.003
Gore-Lloyd, D., Sumann, I., Brachmann, A. O., Schneeberger, K., Ortiz-Merino, R. A., Moreno-Beltrán, M., et al. (2019). Snf2 controls pulcherriminic acid biosynthesis and antifungal activity of the biocontrol yeast Metschnikowia pulcherrima. Mol. Microbiol. 112, 317–332. doi: 10.1111/mmi.14272
Gori, K., Knudsen, P. B., Nielsen, K. F., Arneborg, N., and Jespersen, L. (2011). Alcohol-based quorum sensing plays a role in adhesion and sliding motility of the yeast Debaryomyces hansenii. FEMS Yeast Res. 11, 643–652. doi: 10.1111/j.1567-1364.2011.00755.x
Goto, K., Iwatuki, Y., Kitano, K., Obata, T., and Hara, S. (1990). Cloning and nucleotide sequence of the KHR killer gene of Saccharomyces cerevisiae. Agric. Biol. Chem. 54, 979–984. doi: 10.1271/bbb1961.54.979
Grazia, A., Pietrafesa, A., Capece, A., Pietrafesa, R., Siesto, G., and Romano, P. (2019). Exploitation of technological variability among wild non-Saccharomyces yeasts to select mixed starters for the production of low alcohol wines. BIO Web Conf. 15:02031. doi: 10.1051/bioconf/20191502031
Hazelwood, L. A., Daran, J.-M., van Maris, A. J. A., Pronk, J. T., and Dickinson, J. R. (2008). The Ehrlich pathway for fusel alcohol production: a century of research on Saccharomyces cerevisiae metabolism. Appl. Environ. Microbiol. 74, 2259–2266. doi: 10.1128/AEM.02625-07
Hicks, R. H., Moreno-Beltrán, M., Gore-Lloyd, D., Chuck, C. J., and Henk, D. A. (2021). The oleaginous yeast Metschnikowia pulcherrima displays killer activity against avian-derived pathogenic Bacteria. Biology 10:1227. doi: 10.3390/biology10121227
Hirschhorn, J. N., Brown, S. A., Clark, C. D., and Winston, F. (1992). Evidence that SNF2/SWI2 and SNF5 activate transcription in yeast by altering chromatin structure. Genes Dev. 6, 2288–2298. doi: 10.1101/gad.6.12a.2288
Hogan, D. A. (2006). Talking to themselves: autoregulation and quorum sensing in Fungi. Eukaryot. Cell 5, 613–619. doi: 10.1128/EC.5.4.613-619.2006
Holm Hansen, E., Nissen, P., Sommer, P., Nielsen, J. C., and Arneborg, N. (2001). The effect of oxygen on the survival of non-Saccharomyces yeasts during mixed culture fermentations of grape juice with Saccharomyces cerevisiae. J. Appl. Microbiol. 91, 541–547. doi: 10.1046/j.1365-2672.2001.01426.x
Hu, K., Zhao, H., Edwards, N., Peyer, L., Tao, Y., and Arneborg, N. (2022). The effects of cell-cell contact between Pichia kluyveri and Saccharomyces cerevisiae on amino acids and volatiles in mixed culture alcoholic fermentations. Food Microbiol. 103:103960. doi: 10.1016/j.fm.2021.103960
Jagtap, S. S., Bedekar, A. A., and Rao, C. V. (2020). “Quorum sensing in yeast” in ACS symposium series. ed. S. S. Dhiman (Washington, DC: American Chemical Society), 235–250.
Jolly, N. P., Augustyn, O. P. H., and Pretorius, I. S. (2003). The effect of non-Saccharomyces yeasts on fermentation and wine quality. South Afric J Enol Viticult 24:2638. doi: 10.21548/24-2-2638
Kagiyama, S., Aiba, T., Kadowaki, K., and Mogi, K. (1988). New killer toxins of halophilic Hansenula anomala. Agric. Biol. Chem. 52, 1–7. doi: 10.1080/00021369.1988.10868638
Kemsawasd, V., Branco, P., Almeida, M. G., Caldeira, J., Albergaria, H., and Arneborg, N. (2015a). Cell-to-cell contact and antimicrobial peptides play a combined role in the death of Lachanchea thermotolerans during mixed-culture alcoholic fermentation with Saccharomyces cerevisiae. FEMS Microbiol. Lett. 362:fnv103. doi: 10.1093/femsle/fnv103
Kemsawasd, V., Viana, T., Ardö, Y., and Arneborg, N. (2015b). Influence of nitrogen sources on growth and fermentation performance of different wine yeast species during alcoholic fermentation. Appl. Microbiol. Biotechnol. 99, 10191–10207. doi: 10.1007/s00253-015-6835-3
Kluyver, A. J., van der Walt, J. P., and van Triet, A. J. (1953). Pulcherrimin, the pigment of Candida Pulcherrima. PNAS 39, 583–593. doi: 10.1073/pnas.39.7.583
Krause, D. J., Kominek, J., Opulente, D. A., Shen, X.-X., Zhou, X., Langdon, Q. K., et al. (2018). Functional and evolutionary characterization of a secondary metabolite gene cluster in budding yeasts. Proc. Natl. Acad. Sci. U. S. A. 115, 11030–11035. doi: 10.1073/pnas.1806268115
Kregiel, D., Nowacka, M., Rygala, A., and Vadkertiová, R. (2022). Biological activity of Pulcherrimin from the Meschnikowia pulcherrima clade. Molecules 27:1855. doi: 10.3390/molecules27061855
Kügler, S., Sebghati, T. S., Eissenberg, L. G., and Goldman, W. E. (2000). Phenotypic variation and intracellular parasitism by Histoplasma capsulatum. Proc. Natl. Acad. Sci. U. S. A. 97, 8794–8798. doi: 10.1073/pnas.97.16.8794
Lin, X., Tang, X., Han, X., He, X., Han, N., Ding, Y., et al. (2022). Effect of Metschnikowia pulcherrima on Saccharomyces cerevisiae PDH by-pass in mixed fermentation with varied sugar concentrations of synthetic grape juice and inoculation ratios. Fermentation 8:480. doi: 10.3390/fermentation8100480
Liu, J., Sui, Y., Wisniewski, M., Droby, S., and Liu, Y. (2013). Review: utilization of antagonistic yeasts to manage postharvest fungal diseases of fruit. Int. J. Food Microbiol. 167, 153–160. doi: 10.1016/j.ijfoodmicro.2013.09.004
Liu, G.-L., Wang, K., Hua, M.-X., Buzdar, M. A., and Chi, Z.-M. (2012). Purification and characterization of the cold-active killer toxin from the psychrotolerant yeast Mrakia frigida isolated from sea sediments in Antarctica. Process Biochem. 47, 822–827. doi: 10.1016/j.procbio.2012.02.016
Lopes, C. A., and Sangorrín, M. P. (2010). Optimization of killer assays for yeast selection protocols. Rev. Argent. Microbiol. 42, 298–306. doi: 10.1590/S0325-75412010000400011
MacDonald, J. (1965). Biosynthesis of pulcherriminic acid. Biochem. J. 96, 533–538. doi: 10.1042/bj0960533
Mannazzu, I., Domizio, P., Carboni, G., Zara, S., Zara, G., Comitini, F., et al. (2019). Yeast killer toxins: from ecological significance to application. Crit. Rev. Biotechnol. 39, 603–617. doi: 10.1080/07388551.2019.1601679
Mateus, D., Sousa, S., Coimbra, C., Rogerson, S., and Simões, J. (2020). Identification and characterization of non-Saccharomyces species isolated from port wine spontaneous fermentations. Foods 9:120. doi: 10.3390/foods9020120
Mbuyane, L. L., Bauer, F. F., Bloem, A., Camarasa, C., Ortiz-Julien, A., and Divol, B. (2022). Species-dependent metabolic response to lipid mixtures in wine yeasts. Front. Microbiol. 13:823581. doi: 10.3389/fmicb.2022.823581
Mehlomakulu, N. N., Prior, K. J., Setati, M. E., and Divol, B. (2017). Candida pyralidae killer toxin disrupts the cell wall of Brettanomyces bruxellensis in red grape juice. J. Appl. Microbiol. 122, 747–758. doi: 10.1111/jam.13383
Mehlomakulu, N. N., Setati, M. E., and Divol, B. (2014). Characterization of novel killer toxins secreted by wine-related non-Saccharomyces yeasts and their action on Brettanomyces spp. Int. J. Food Microbiol. 188, 83–91. doi: 10.1016/j.ijfoodmicro.2014.07.015
Mehmood, A., Liu, G., Wang, X., Meng, G., Wang, C., and Liu, Y. (2019). Fungal quorum-sensing molecules and inhibitors with potential antifungal activity: a review. Molecules 24:1950. doi: 10.3390/molecules24101950
Miller, M. B., and Bassler, B. L. (2001). Quorum sensing in bacteria. Annu. Rev. Microbiol. 55, 165–199. doi: 10.1146/annurev.micro.55.1.165
Moenne, M. I., Saa, P., Laurie, V. F., Pérez-Correa, J. R., and Agosin, E. (2014). Oxygen incorporation and dissolution during industrial-scale red wine fermentations. Food Bioproc. Tech. 7, 2627–2636. doi: 10.1007/s11947-014-1257-2
Morales, P., Rojas, V., Quirós, M., and Gonzalez, R. (2015). The impact of oxygen on the final alcohol content of wine fermented by a mixed starter culture. Appl. Microbiol. Biotechnol. 99, 3993–4003. doi: 10.1007/s00253-014-6321-3
Morata, A., Loira, I., Escott, C., del Fresno, J. M., Bañuelos, M. A., and Suárez-Lepe, J. A. (2019). Applications of Metschnikowia pulcherrima in wine biotechnology. Fermentation 5:63. doi: 10.3390/fermentation5030063
Nealson, K. H., Platt, T., and Hastings, J. W. (1970). Cellular control of the synthesis and activity of the bacterial luminescent system. J. Bacteriol. 104, 313–322. doi: 10.1128/jb.104.1.313-322.1970
Nissen, P., Nielsen, D., and Arneborg, N. (2003). Viable Saccharomyces cerevisiae cells at high concentrations cause early growth arrest of non-Saccharomyces yeasts in mixed cultures by a cell-cell contact-mediated mechanism. Yeast 20, 331–341. doi: 10.1002/yea.965
Novotna, D., Flegelova, H., and Janderova, B. (2004). Different action of killer toxins K1 and K2 on the plasma membrane and the cell wall of Saccharomyces cerevisiae. FEMS Yeast Res. 4, 803–813. doi: 10.1016/j.femsyr.2004.04.007
Orentaite, I., Poranen, M. M., Oksanen, H. M., Daugelavicius, R., and Bamford, D. H. (2016). K2 killer toxin-induced physiological changes in the yeast Saccharomyces cerevisiae. FEMS Yeast Res. 16:fow003. doi: 10.1093/femsyr/fow003
Oro, L., Ciani, M., and Comitini, F. (2014). Antimicrobial activity of Metschnikowia pulcherrima on wine yeasts. J. Appl. Microbiol. 116, 1209–1217. doi: 10.1111/jam.12446
Oztekin, S., and Karbancioglu-Guler, F. (2021). Bioprospection of Metschnikowia sp. isolates as biocontrol agents against postharvest fungal decays on lemons with their potential modes of action. Postharvest Biol. Technol. 181:111634. doi: 10.1016/j.postharvbio.2021.111634
Padder, S. A., Prasad, R., and Shah, A. H. (2018). Quorum sensing: a less known mode of communication among fungi. Microbiol. Res. 210, 51–58. doi: 10.1016/j.micres.2018.03.007
Parafati, L., Vitale, A., Restuccia, C., and Cirvilleri, G. (2015). Biocontrol ability and action mechanism of food-isolated yeast strains against Botrytis cinerea causing post-harvest bunch rot of table grape. Food Microbiol. 47, 85–92. doi: 10.1016/j.fm.2014.11.013
Petitgonnet, C., Klein, G. L., Roullier-Gall, C., Schmitt-Kopplin, P., Quintanilla-Casas, B., Vichi, S., et al. (2019). Influence of cell-cell contact between L. thermotolerans and S. cerevisiae on yeast interactions and the exo-metabolome. Food Microbiol. 83, 122–133. doi: 10.1016/j.fm.2019.05.005
Piombo, E., Sela, N., Wisniewski, M., Hoffmann, M., Gullino, M. L., Allard, M. W., et al. (2018). Genome sequence, assembly and characterization of two Metschnikowia fructicola strains used as biocontrol agents of postharvest diseases. Front. Microbiol. 9:593. doi: 10.3389/fmicb.2018.00593
Pretscher, J., Fischkal, T., Branscheidt, S., Jäger, L., Kahl, S., Schlander, M., et al. (2018). Yeasts from different habitats and their potential as biocontrol agents. Fermentation 4:31. doi: 10.3390/fermentation4020031
Prior, K. J., Bauer, F. F., and Divol, B. (2019). The utilisation of nitrogenous compounds by commercial non-Saccharomyces yeasts associated with wine. Food Microbiol. 79, 75–84. doi: 10.1016/j.fm.2018.12.002
Pu, L., Jingfan, F., Kai, C., Chao-an, L., and Yunjiang, C. (2014). Phenylethanol promotes adhesion and biofilm formation of the antagonistic yeast Kloeckera apiculata for the control of blue mold on citrus. FEMS Yeast Res. 14, 536–546. doi: 10.1111/1567-1364.12139
Puyo, M., Simonin, S., Klein, G., David-Vaizant, V., Quijada-Morín, N., Alexandre, H., et al. (2023). Use of oenological tannins to protect the colour of Rosé wine in a bioprotection strategy with Metschnikowia pulcherrima. Foods 12:735. doi: 10.3390/foods12040735
Quirós, M., Rojas, V., Gonzalez, R., and Morales, P. (2014). Selection of non-Saccharomyces yeast strains for reducing alcohol levels in wine by sugar respiration. Int. J. Food Microbiol. 181, 85–91. doi: 10.1016/j.ijfoodmicro.2014.04.024
Roca-Mesa, H., Sendra, S., Mas, A., Beltran, G., and Torija, M.-J. (2020). Nitrogen preferences during alcoholic fermentation of different non-Saccharomyces yeasts of oenological interest. Microorganisms 8:157. doi: 10.3390/microorganisms8020157
Rodríguez, M. E., Lopes, C. A., Barbagelata, R. J., Barda, N. B., and Caballero, A. C. (2010). Influence of Candida pulcherrimapatagonian strain on alcoholic fermentation behaviour and wine aroma. Int. J. Food Microbiol. 138, 19–25. doi: 10.1016/j.ijfoodmicro.2009.12.025
Roudil, L., Russo, P., Berbegal, C., Albertin, W., Spano, G., and Capozzi, V. (2020). Non-Saccharomyces commercial starter cultures: scientific trends, recent patents and innovation in the wine sector. Recent Pat. Food Nutr. Agric. 11, 27–39. doi: 10.2174/2212798410666190131103713
Sadoudi, M., Rousseaux, S., David, V., Alexandre, H., and Tourdot-Maréchal, R. (2017). Metschnikowia pulcherrima influences the expression of genes involved in PDH bypass and Glyceropyruvic fermentation in Saccharomyces cerevisiae. Front. Microbiol. 8:1137. doi: 10.3389/fmicb.2017.01137
Sadoudi, M., Tourdot-Maréchal, R., Rousseaux, S., Steyer, D., Gallardo-Chacón, J.-J., Ballester, J., et al. (2012). Yeast–yeast interactions revealed by aromatic profile analysis of sauvignon blanc wine fermented by single or co-culture of non-Saccharomyces and Saccharomyces yeasts. Food Microbiol. 32, 243–253. doi: 10.1016/j.fm.2012.06.006
Santos, A., and Marquina, D. (2004). Ion channel activity by Pichia membranifaciens killer toxin. Yeast 21, 151–162. doi: 10.1002/yea.1069
Saravanakumar, D., Ciavorella, A., Spadaro, D., Garibaldi, A., and Gullino, M. L. (2008). Metschnikowia pulcherrima strain MACH1 outcompetes Botrytis cinerea, Alternaria alternata and Penicillium expansum in apples through iron depletion. Postharvest Biol. Technol. 49, 121–128. doi: 10.1016/j.postharvbio.2007.11.006
Seguinot, P., Ortiz-Julien, A., and Camarasa, C. (2020). Impact of nutrient availability on the fermentation and production of aroma compounds under sequential inoculation with M. pulcherrima and S. cerevisiae. Front. Microbiol. 11:305. doi: 10.3389/fmicb.2020.00305
Sharma, R. R., Singh, D., and Singh, R. (2009). Biological control of postharvest diseases of fruits and vegetables by microbial antagonists: a review. Biol. Control 50, 205–221. doi: 10.1016/j.biocontrol.2009.05.001
Shekhawat, K., Bauer, F. F., and Setati, M. E. (2016). Impact of oxygenation on the performance of three non-Saccharomyces yeasts in co-fermentation with Saccharomyces cerevisiae. Appl. Microbiol. Biotechnol. 101, 2479–2491. doi: 10.1007/s00253-016-8001-y
Shekhawat, K., Porter, T. J., Bauer, F. F., and Setati, M. E. (2018). Employing oxygen pulses to modulate Lachancea thermotolerans–Saccharomyces cerevisiae chardonnay fermentations. Ann. Microbiol. 68, 93–102. doi: 10.1007/s13213-017-1319-6
Simonin, S., Honoré-Chedozeau, C., Monnin, L., David-Vaizant, V., Bach, B., Alexandre, H., et al. (2022). Bioprotection on chardonnay grape: limits and impacts of settling parameters. Aust. J. Grape Wine Res. 2022, 1–13. doi: 10.1155/2022/1489094
Simonin, S., Roullier-Gall, C., Ballester, J., Schmitt-Kopplin, P., Quintanilla-Casas, B., Vichi, S., et al. (2020). Bio-protection as an alternative to Sulphites: impact on chemical and microbial characteristics of red wines. Front. Microbiol. 11:1308. doi: 10.3389/fmicb.2020.01308
Sipiczki, M. (2006). Metschnikowia strains isolated from Botrytized grapes antagonize fungal and bacterial growth by Iron depletion. Appl. Environ. Microbiol. 72, 6716–6724. doi: 10.1128/AEM.01275-06
Sipiczki, M. (2020). Metschnikowia pulcherrima and related Pulcherrimin-producing yeasts: fuzzy species boundaries and complex antimicrobial antagonism. Microorganisms 8:1029. doi: 10.3390/microorganisms8071029
Spadaro, D., and Gullino, M. L. (2004). State of the art and future prospects of the biological control of postharvest fruit diseases. Int. J. Food Microbiol. 91, 185–194. doi: 10.1016/S0168-1605(03)00380-5
Sprague, G. F., and Winans, S. C. (2006). Eukaryotes learn how to count: quorum sensing by yeast. Genes Dev. 20, 1045–1049. doi: 10.1101/gad.1432906
Strauss, M. L. A., Jolly, N. P., Lambrechts, M. G., and van Rensburg, P. (2001). Screening for the production of extracellular hydrolytic enzymes by non-Saccharomyces wine yeasts. J. Appl. Microbiol. 91, 182–190. doi: 10.1046/j.1365-2672.2001.01379.x
Taillandier, P., Lai, Q. P., Julien-Ortiz, A., and Brandam, C. (2014). Interactions between Torulaspora delbrueckii and Saccharomyces cerevisiae in wine fermentation: influence of inoculation and nitrogen content. World J. Microbiol. Biotechnol. 30, 1959–1967. doi: 10.1007/s11274-014-1618-z
Tesnière, C. (2019). Importance and role of lipids in wine yeast fermentation. Appl. Microbiol. Biotechnol. 103, 8293–8300. doi: 10.1007/s00253-019-10029-4
Türkel, S., and Ener, B. (2009). Isolation and characterization of new Metschnikowia pulcherrima strains as producers of the antimicrobial pigment Pulcherrimin. Z. Naturforsch. C 64, 405–410. doi: 10.1515/znc-2009-5-618
Uffen, R. L., and Canale-Parola, E. (1972). Synthesis of Pulcherriminic acid by Bacillus subtilis. J. Bacteriol. 111, 86–93. doi: 10.1128/jb.111.1.86-93.1972
Vicente, J., Ruiz, J., Belda, I., Benito-Vázquez, I., Marquina, D., Calderón, F., et al. (2020). The genus Metschnikowia in enology. Microorganisms 8:1038. doi: 10.3390/microorganisms8071038
Villalba, M. L., Susana Sáez, J., del Monaco, S., Lopes, C. A., and Sangorrín, M. P. (2016). TdKT, a new killer toxin produced by Torulaspora delbrueckii effective against wine spoilage yeasts. Int. J. Food Microbiol. 217, 94–100. doi: 10.1016/j.ijfoodmicro.2015.10.006
Visser, W., Scheffers, W. A., Batenburg-van der Vegte, W. H., and van Dijken, J. P. (1990). Oxygen requirements of yeasts. Appl. Environ. Microbiol. 56, 3785–3792. doi: 10.1128/aem.56.12.3785-3792.1990
Wang, D., Zhan, Y., Cai, D., Li, X., Wang, Q., and Chen, S. (2018). Regulation of the synthesis and secretion of the Iron Chelator Cyclodipeptide Pulcherriminic acid in Bacillus licheniformis. Appl. Environ. Microbiol. 84:e00262-18, /aem/84/13/e00262-18.atom. doi: 10.1128/AEM.00262-18
Wang, S., Zhang, H., Ruan, C., Yi, L., Deng, L., and Zeng, K. (2021). Metschnikowia citriensis FL01 antagonize Geotrichum citri-aurantii in citrus fruit through key action of iron depletion. Int. J. Food Microbiol. 357:109384. doi: 10.1016/j.ijfoodmicro.2021.109384
Windholtz, S., Dutilh, L., Lucas, M., Maupeu, J., Vallet-Courbin, A., Farris, L., et al. (2021a). Population dynamics and yeast diversity in early winemaking stages without sulfites revealed by three complementary approaches. Appl. Sci. 11:2494. doi: 10.3390/app11062494
Windholtz, S., Redon, P., Lacampagne, S., Farris, L., Lytra, G., Cameleyre, M., et al. (2021c). Non-Saccharomyces yeasts as bioprotection in the composition of red wine and in the reduction of sulfur dioxide. LWT 149, 111781. doi: 10.1016/j.lwt.2021.111781
Windholtz, S., Vinsonneau, E., Farris, L., Thibon, C., and Masneuf-Pomarède, I. (2021b). Yeast and filamentous Fungi microbial communities in organic red grape juice: effect of vintage, maturity stage, SO2, and bioprotection. Front. Microbiol. 12:748416. doi: 10.3389/fmicb.2021.748416
Winters, M., Arneborg, N., Appels, R., and Howell, K. (2019). Can community-based signalling behaviour in Saccharomyces cerevisiae be called quorum sensing? A critical review of the literature. FEMS Yeast Res. 19:foz046. doi: 10.1093/femsyr/foz046
Winters, M., Aru, V., Howell, K., and Arneborg, N. (2022). Saccharomyces cerevisiae does not undergo a quorum sensing-dependent switch of budding pattern. Sci. Rep. 12:8738. doi: 10.1038/s41598-022-12308-z
Wuster, A., and Babu, M. M. (2009). Transcriptional control of the quorum sensing response in yeast. Mol. Biosyst. 6, 134–141. doi: 10.1039/B913579K
Yan, G., Zhang, B., Joseph, L., and Waterhouse, A. L. (2020). Effects of initial oxygenation on chemical and aromatic composition of wine in mixed starters of Hanseniaspora vineae and Saccharomyces cerevisiae. Food Microbiol. 90:103460. doi: 10.1016/j.fm.2020.103460
Yuan, S., Yong, X., Zhao, T., Li, Y., and Liu, J. (2020). Research Progress of the biosynthesis of natural bio-antibacterial agent Pulcherriminic acid in Bacillus. Molecules 25:5611. doi: 10.3390/molecules25235611
Zhang, H., Wang, S., Yi, L., and Zeng, K. (2023). Tryptophan enhances biocontrol efficacy of Metschnikowia citriensis FL01 against postharvest fungal diseases of citrus fruit by increasing pulcherriminic acid production. Int. J. Food Microbiol. 386:110013. doi: 10.1016/j.ijfoodmicro.2022.110013
Zhang, W., Zhuo, X., Hu, L., and Zhang, X. (2020). Effects of crude β-glucosidases from Issatchenkia terricola, Pichia kudriavzevii, Metschnikowia pulcherrima on the flavor complexity and characteristics of wines. Microorganisms 8:953. doi: 10.3390/microorganisms8060953
Zilelidou, E. A., and Nisiotou, A. (2021). Understanding wine through yeast interactions. Microorganisms 9:1620. doi: 10.3390/microorganisms9081620
Zohre, D. E., and Erten, H. (2002). The influence of Kloeckera apiculata and Candida pulcherrima yeasts on wine fermentation. Process Biochem. 38, 319–324. doi: 10.1016/S0032-9592(02)00086-9
Zott, K., Thibon, C., Bely, M., Lonvaud-Funel, A., Dubourdieu, D., and Masneuf-Pomarede, I. (2011). The grape must non-Saccharomyces microbial community: impact on volatile thiol release. Int. J. Food Microbiol. 151, 210–215. doi: 10.1016/j.ijfoodmicro.2011.08.026
Keywords: bio-protection, Metschnikowia pulcherrima, oenology, microbial interactions, non-Saccharomyces
Citation: Puyo M, Simonin S, Bach B, Klein G, Alexandre H and Tourdot-Maréchal R (2023) Bio-protection in oenology by Metschnikowia pulcherrima: from field results to scientific inquiry. Front. Microbiol. 14:1252973. doi: 10.3389/fmicb.2023.1252973
Edited by:
Alice Vilela, University of Trás-os-Montes and Alto Douro, PortugalReviewed by:
Maurizio Ciani, Marche Polytechnic University, ItalyIlaria Mannazzu, University of Sassari, Italy
Maret Du Toit, Stellenbosch University, South Africa
Roberto Foschino, University of Milan, Italy
Giacomo Zara, University of Sassari, Italy
Copyright © 2023 Puyo, Simonin, Bach, Klein, Alexandre and Tourdot-Maréchal. This is an open-access article distributed under the terms of the Creative Commons Attribution License (CC BY). The use, distribution or reproduction in other forums is permitted, provided the original author(s) and the copyright owner(s) are credited and that the original publication in this journal is cited, in accordance with accepted academic practice. No use, distribution or reproduction is permitted which does not comply with these terms.
*Correspondence: Raphaëlle Tourdot-Maréchal, tourdot@u-bourgogne.fr
†These authors have contributed equally to this work and share first authorship