- 1Department of Parasitology, College of Veterinary Medicine, Sichuan Agricultural University, Chengdu, China
- 2College of Science, Sichuan Agricultural University, Ya'an, China
Sarcoptes scabiei (S. scabiei) endangers human and other mammalian health. There has been limited research into S. scabiei pathogenic mechanisms and the immunological interaction between S. scabiei and hosts. Galectins have critical roles in biological processes such as cell adhesion, signal transduction, and immune response mediation. Galectins of S. scabiei (SsGalectins) were cloned, expressed, and identified, and their transcriptional levels in S. scabiei were measured at various developmental stages. Fluorescent tissue localization was performed on SsGalectins of S. scabiei and scabies skin. A mouse AD model was constructed to evaluate the effect of rSsGalectins on skin pathogenic changes. Quantitative polymerase chain reaction and enzyme-linked immunoassay were used to identify macrophage polarization-related components and investigate the immunoregulatory effect of rSsGalectins on mouse macrophages. The results demonstrated that the S. scabiei infection causes macrophage infiltration in the scabies skin. The rSsGalectins displayed strong reactogenicity, and distinct genes of the SsGalectins were differently expressed in different developmental stages of S. scabiei. Fluorescence tissue localization revealed that the SsGalectins were mainly in the mouthparts, intestines, and body surface. Additionally, S. scabiei could secrete SsGalectins into the infected skin, proving that SsGalectins were excretion and secretion proteins of S. scabiei. In the mouse atopic dermatitis model, cutaneous macrophage infiltration and inflammation increase after rSsGalectins injection. Simultaneously, when rSsGalectins acted on bone marrow-derived macrophages, M1 macrophage-related polarization factors IL-1β, IL-6, and inducible nitric oxide synthase all increased, demonstrating that rSsGalectins can induce M1 polarization and produce pro-inflammatory cytokines. In conclusion, the SsGalectins are involved in the pathogenic process of S. scabiei by regulating the polarization of host macrophages to the M1 type when S. scabiei invade the host and promoting the incidence and development of the host's inflammatory response. This study offers fresh light on the pathogenic process of scabies mites, investigates the immunological interaction mechanism between S. scabiei and the host, and offers new insights into S. scabiei prevention and therapy.
Background
In 2021, the World Health Organization included scabies in its report on “Ending the neglect to attain the Sustainable Development Goals: a road map for neglected tropical diseases 2021–2030” (World Health Organization, 2022). Scabies is an infectious disease caused by Sarcoptes scabiei parasitizing the epidermis of humans and other mammals. S. scabiei mites are transmitted by contact and can infect more than 100 host species (El-Moamly, 2021). Scabies is mainly caused by host infection with scabies mites, which can lead to severe itching, scabbing, hair loss, and skin thickening and is generally followed by a bacterial skin infection, namely impetigo (Taiaroa et al., 2021). Improper handling can easily lead to severe complications, such as sepsis, kidney disease, rheumatic heart disease, and even death. Approximately 300 million people worldwide are affected by scabies every year (Reynolds et al., 2014). Similar to other neglected tropical diseases, the infection and morbidity of the disease are more pronounced in developing countries, especially in poor areas (Romani et al., 2015). S. scabiei mite infestation has a significant negative impact on the performance of economic animals in terms of output and quality of livestock products, in addition to negatively affecting their growth and development, causing severe global public health problems and substantial financial losses (Fischer and Walton, 2014; Bernigaud et al., 2019). At present, there is no vaccine against scabies. Therefore, developing new drugs and vaccines against scabies is an urgent problem to be solved. Exploring the interaction between SsGalectins and host may provide new insights for developing novel anti-scabies drugs and prevention strategies.
Galectins are a family in the lectin superfamily that have 1 or 2 sugar recognition domains in their molecular structure. Galectin-1 (GAL-1) is a powerful anti-inflammatory and immunoregulatory factor that is involved in the pathophysiology of inflammatory disorders (Astorgues-Xerri et al., 2014). Galectin-3 (GAL-3) is an essential regulator of biological processes, and it has recently been found that it is closely related to the pathogenesis of host immune and inflammatory diseases (Nangia-Makker et al., 2000; Dumic et al., 2006). Galectin-2 (GAL-2) is mainly expressed in the gastrointestinal tract and can promote the healing of gastrointestinal epithelial wounds. Galectin-5 (GAL-5) is expressed in rat erythrocytes and may play a role in erythropoiesis (Barrès et al., 2010). In the study of parasite–host interactions, Galectins are engaged in a range of immune-related host life functions, including pathogen and host cell adhesion, activation of host innate and adaptive immunity, immune cell proliferation and death, and inflammatory modulation (Farhadi and Hudalla, 2016; Hafidi et al., 2021; Loghry et al., 2022). Macrophages are thought to play a crucial role in the innate immune response to scabies, and macrophage infiltration was observed in skin samples from several species of domestic and wild mammals (Martínez et al., 2020; Nwufoh et al., 2020). S. scabiei mite infection can elicit an innate immune response in the host. Although it has been shown that S. scabiei mites are able to inhibit host macrophages from participating in the inflammatory response by expressing macrophage migration inhibitory factors (Cote et al., 2013), skin biopsies from dogs with mange mites show the presence of macrophage infiltration within the skin (Nwufoh et al., 2020). Activation of macrophages leads to the production of prostaglandins, leukotrienes, and IL-31, which exacerbate host skin itching (Hashimoto et al., 2019; Arora et al., 2020). Galectins family is involved in the regulation of macrophages (Zangbede et al., 2018; Shi et al., 2020; Loghry et al., 2022). Thus, exploring the role of members of the Galectins family in the invasion of S. scabiei mites is of great significance for the study of the pathogenic mechanism of S. scabiei mites.
Methods
Mites and animals
S. scabiei mites and New Zealand white rabbits were provided by the Department of Parasitology at Sichuan Agricultural University. Mites (adults, nymphs, and larvae) were collected first, then live adult mites (male and female mites) were separated from the sample after morphological identification, and larvae and nymphs were left and collected together. Eggs are laid in the epidermis of the host skin and cannot be collected. Eight female Sprague-Dawley rats (6 weeks old, 150–200 g), 6-week-old female BALB/c mice (6 weeks old, 20–22 g), and 6-week-old male C57BL/6J mice (6 weeks old, 20–22 g) were purchased from SPF Biotechnology Company (Beijing).
Macrophages in the scabies skin
Healthy and naturally infected New Zealand rabbit toe skins were fixed overnight with a 4% paraformaldehyde fixative (Biosharp, Guangzhou, China). The paraffin-embedded skin samples were sectioned to 5 μm, dried in an oven at 37°C for 24 h, deparaffinized, immersed in 0.1 M sodium citrate for 15 min for antigen retrieval, and washed three times with phosphate-buffered saline (PBS). The 5% goat serum was used to block at room temperature for 2 h, and then the primary antibody, F4/80 Rabbit pAb (1:200) (ABclonal, Wuhan, China), was incubated overnight at 4°C and washed three times with PBS, followed by the secondary antibody, FITC Goat Anti-Rabbit IgG (H+L) (1:400) (ABclonal, Wuhan, China), incubated for 45 min at room temperature. After washing four times, DAPI (Solarbio, Beijing, China) was used to incubate for 5 min at room temperature. Following mounting, tissue sections were examined and captured on a camera using a digital slice microscope [OLYMPUS, VS120-S6-W (BX61VS), Japan].
Galectin mRNA levels in S. scabiei mites
Real-time quantitative polymerase chain reaction (RT-qPCR) analysis was performed to validate the mRNA expression levels of SsGAL-1, SsGAL-2, SsGAL-3, and SsGAL-5 in different developmental stages of scabies mites, and β-actin was used as a housekeeping gene. The collected mites of different stages were ground in a pre-cooled mortar. Total RNAs of larvae/nymph and adult mites were extracted using the RNAprep Pure Tissue Kit (Tiangen, Beijing, China) according to the manufacturer's instructions. RNA quality and quantity were assessed using the 1.5% agarose gel electrophoresis and the NanoDrop 2000 spectrophotometer. An amount of 1 μg of total RNA was reverse-transcribed to cDNA using the RevertAi™ First Strand cDNA Synthesis Kit (Thermo Fisher Scientific, Waltham, MA, USA) according to the manufacturer's instructions. The qPCR primers (Supplementary Table S1) were designed using the nucleic acid sequences of Galectins in NCBI (SsGAL-1: JXLN01009935.1, SsGAL-2: JXLN01010345.1, SsGAL-3: JXLN01010568.1, SsGAL-5: JXLN01013773.1) as templates, and the qPCR was carried out using the LightCycler480 System (Roche Diagnostics) after the primers were synthesized. The reaction mix (20 μl) includes SYBR Permix Ex TaqII (FOREGENE, Chengdu, China) 10 μl, upstream primer 0.8 μl, downstream primer 0.8 μl, cDNA 2 μl, and ddH2O 6.4 μl. The cycling conditions were as follows: an initial denaturation at 95 °C for 30 s, followed by 40 cycles at 95°C for 5 s and 60°C for 30 s, and then melting curve analysis at 95°C for 5 s, 60°C for 60 s, and 95°C for 1 s. qPCR was conducted in triplicate, and the relative gene expression levels were calculated using 2−ΔΔCt method (Livak and Schmittgen, 2001).
Expression and identification of SsGalectins
Total RNA from S. scabiei mite (larvae+nymphs, and adults) was isolated and reverse-transcribed. Primers were created using the nucleic acid sequence of Galectins from NCBI as a guide (Supplementary Table S2), and PCR amplification was performed using scabies mite cDNA as a template. PCR products were separated and cloned into the PMD19-T vector (TaKaRa, Beijing, China) and subcloned into the pET32a (+) vector (Novagen, USA) using restriction sites (underlined in the above primers); then, the recombinant plasmids were sequenced (Sango, Shanghai, China). The identified recombinant plasmid was transformed into E. coli BL21 (DE3) cells (Invitrogen, USA), and 1 mM isopropyl β-D-1-thiogalactopyranoside was used to induce the expression of cultured cells. The recombinant protein was purified by chromatography with a Ni-NTA His-tag affinity kit (Bio-Rad, USA) according to the manufacturer's instructions. Recombinant protein was analyzed for purity by SDS-PAGE using a 12% gel and subsequently with a bicinchoninic acid protein assay kit (Pierce, USA) to estimate protein concentration. A Western blot was used to identify recombinant Galectins. Protein samples were boiled for 10 min in electrophoresis sample buffer before being separated by 12% SDS-PAGE. An electrophoretic transfer cell (Bio-Rad, USA) was used to transfer the protein onto a PVDF membrane, then the membranes were incubated with 5% skim milk for 2 h after washing three times with TBST, followed by 1:100 diluted serum samples [S. scabiei negative rabbit serum, positive rabbit serum, rat negative serum, and rat anti-Ss-GAL (1,2,3,5) IgG, respectively] at 4°C overnight after washing three times with TBST. Then, the membranes were incubated with horseradish peroxidase (HRP)-conjugated goat anti-rabbit IgG antibody or horseradish peroxidase (HRP)-conjugated goat anti-rat IgG antibody (1:3,000; Boster, China) for 2 h. After washing four times with TBST, the Enhanced HRP-DAB Chromogenic Substrate Kit (Tiangen, China) was used to visualize the protein signal according to the manufacturer's instructions.
Immunohistochemical analysis of SsGalectins
Paraffin sections of scabies mite skin and scabies mite bodies were produced in the same way as shown in macrophage localization, and the immunofluorescence procedure was performed as previously described. Eight healthy rats were prepared and randomly divided into four groups of two rats each. To prepare murine anti-rSsGalectins-IgG, the purified rSsGAL-1, rSsGAL-2, rSsGAL-3, and rSsGAL-5 were used as antigens for immunization for each group by subcutaneous injection, respectively. Purified rat anti-rSsGAL-1-IgG, rSsGAL-2-IgG, rSsGAL-3-IgG, rSsGAL-5-IgG, and rat negative serum were used as primary antibodies (diluted in 1:400), and FITC Goat Anti-Rat IgG (H+L) (1:400) (ABclonal, Wuhan, China) was used as secondary antibody to conduct immunofluorescence localization experiments on scabies skin and scabies mites, respectively, to determine the distribution of SsGAL-1, SsGAL-2, SsGAL-3, and SsGAL-5 in scabies mites and secretion in scabies skin. H&E staining was done as a control to make the fluorescent immunohistochemistry results more understandable.
Establishment of AD model and stimulation of recombinant protein
On day 0, a 3 cm by 3 cm portion of the mice's backs had their hair removed. Then, 200 μl of 1% 1-chloro-2,4-dinitrobenzene (DNCB) (olive oil:acetone = 4:1) (Sigma-Aldrich, St. Louis, MO) was evenly applied to the region that has been shaved on days 1–3. An amount of 100 μg of dialyzed and purified rSsGAL-1, rSsGAL-2, rSsGAL-3, and rSsGAL-5 was administered subcutaneously at the same place on days 8 through 14. The control group received the same dose of tacrolimus (MedChemExpress, USA), histamine (VETEC, USA), recombinant pET32a protein, and PBS injections, respectively (10 BALB/c mice for each group), and on days 8, 11, and 14, 4% SDS was applied for 2 h, and then 0.5% DNCB was applied to keep the dermatitis condition. Tacrolimus (Tac) is a macrolide immunosuppressant with anti-inflammatory, antipruritic, and immunomodulatory properties that are extensively used in the treatment of AD skin disorders. Histamine (His) can elicit allergy symptoms such as skin irritation, itching, erythema, and wheal and can be utilized as a positive control in AD models. The tissue samples from the skin lesions were collected on the 15th day, the skin thickness was measured with a micrometer (DeQing, Zhejiang, China), and the samples were kept in a −80°C refrigerator or in a 4% paraformaldehyde fixative solution.
H&E and macrophages in the AD model
Paraffin sections of mouse AD model skin were prepared as previously described. Stained with hematoxylin and eosin (H&E) to determine the thickening and cellular infiltration of the skin. Furthermore, the immunofluorescence procedure was performed in the same way as shown in macrophage localization. F4/80 Rabbit pAb (1:200) (ABclonal, Wuhan, China) was employed as the primary antibody and FITC Goat Anti-Rabbit IgG (H+L) (1:400) (ABclonal, Wuhan, China) as the secondary antibody to observe macrophage infiltration in experimental mice. A digital section microscope was used to monitor and photograph both H&E and F4/80 practical sections.
Preparation and culture of bone marrow-derived macrophages from mice
The collection of bone marrow cells and differentiation into bone marrow-derived macrophages were performed according to Toda et al. (2021). Cells were resuspended in 400 μl PBS and evenly divided into four tubes. CD11b+ (Elabscience, Wuhan, China) and F4/80+ (BioLegend, Beijing, China) fluorescent dyes were used for staining at 4°C for 30 min. Before flow cytometry, cells were resuspended in 400 μl PBS. The Kaluza 2.1 program was used to examine the data. More than 85% of the cells bearing CD11b+ and F4/80+ double-labeled antibodies could be recognized as macrophages.
Effects of recombinant SsGalectins on BMDMs
The working concentration range of recombinant Galectins (0, 0.1, 1, 10, 50, and 100 μg) was established using the CCK-8 (Solarbio, Beijing, China) assay. Based on the results of the CCK-8 cytotoxicity assay, 20 μg rSsGAL-1, rSsGAL-2, rSsGAL-3, and rSsGAL-5 proteins were added to each well and incubated for 24 h, while 20 μg of pET32a protein and 20 μl PBS were added to each well and incubated for 24 h as a blank control group. A volume of 1 ml of BMDM suspension was added to each well of a 6-well plate (the number of cells was approximately 10*6), and every group received three samples that were identical. Cell supernatant was gathered and kept at −80°C in storage. The cells were washed two times with PBS, and the protein was immediately removed in preparation for further research.
qPCR analysis of inflammatory factors
The impact of recombinant galectins on IL-4, IL-12, TGF-β, IL-6, IL-10, IL-1β, inducible nitric oxide synthase (iNOS), and Arg1 sections was examined using real-time PCR analysis (Na, 2019; Feng et al., 2020). The BMDM cells were collected, the total RNA was extracted as directed, and then the RNA obtained was reverse-transcribed into cDNA. The reaction mixture and cycling conditions were maintained as described previously. The qPCR was carried out using the LightCycler480 System (Roche Diagnostics) after primers were synthesized according to the reported primer sequences (Supplementary Table S3). The β-actin gene was simultaneously used as a reference to normalize the data. qPCR was conducted in triplicate, and the relative gene expression levels were calculated using the 2−ΔΔCt method (Livak and Schmittgen, 2001).
Enzyme-linked immunosorbent assay
The above cell supernatant was collected, and then, IL-1β, IL-6, TGF-β, IL-10, iNOS, and Arg1 were detected according to the ELISA kit instructions (4A BIOTECH/CUSABIO, China).
Statistical analysis
Statistics are expressed as mean ± standard deviation (X ± SD, n = 3) and were determined using one-way analysis of variance (ANOVA) using the GraphPad Prism version 8.0 software (GraphPad Software, USA). Probabilities (p) less than 0.05, 0.01, or 0.001 were considered significant (*p < 0.05, **p < 0.01, or ***p < 0.001), and a p-value of above 0.05 means no significant difference (p > 0.05). Graphs were created using the GraphPad Prism version 8.0 software.
Results
Macrophages in the skin of scabies
The results showed that the green fluorescence intensity of scabies mite skin was higher than that of healthy skin, indicating that scabies mite disease can cause an increased infiltration of macrophages in rabbit skin. F4/80 was used as a macrophage surface marker to label macrophages for immunofluorescence staining (Figure 1).
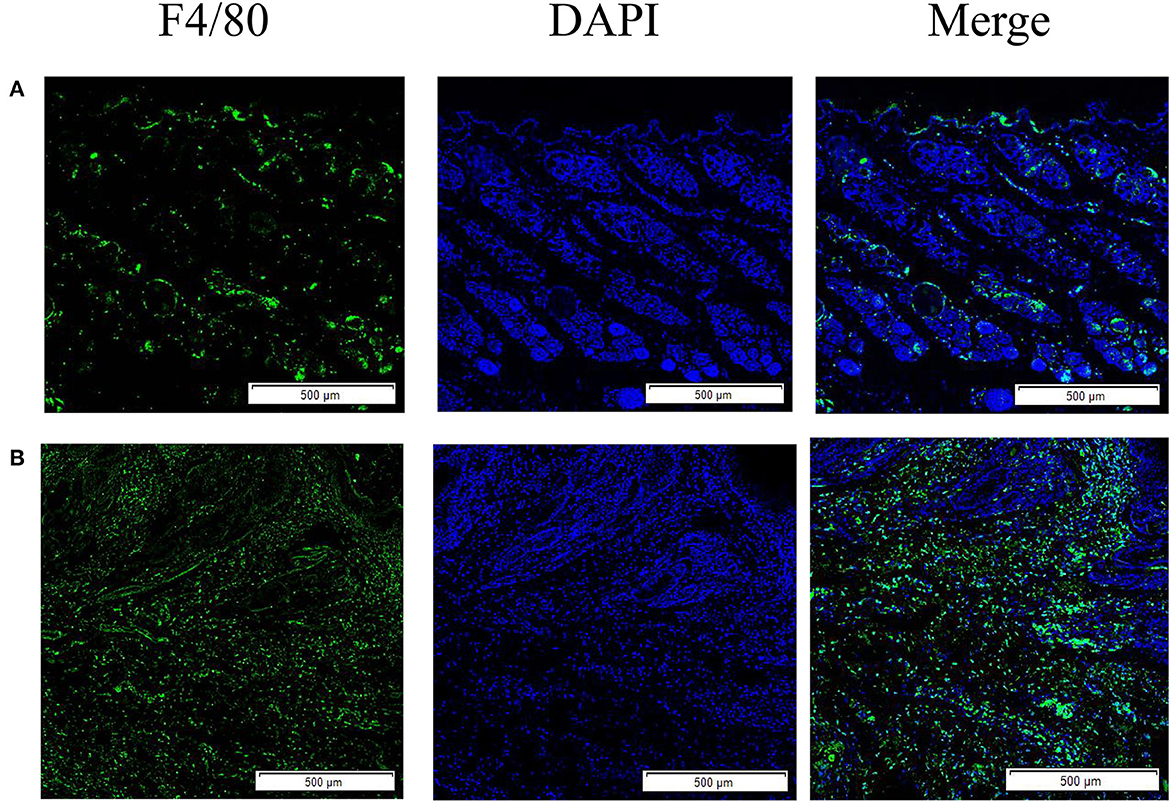
Figure 1. Skin macrophage immunofluorescence staining. (A) Immunofluorescence staining of healthy rabbit skin; (B) immunofluorescence staining of skin tissue infection with S. scabiei. Scale bar = 500 μm.
mRNA expression profiles of Galectins
The SsGAL-1, SsGAL-2, SsGAL-3, and SsGAL-5 were expressed in adult mites and in the mixed sample of larvae and nymphs. The expression levels of SsGAL-2 and SsGAL-5 in adult mites were higher than in mixed samples; however, the expression levels of SsGAL-1 and SsGAL-3 in adult mites were slightly lower than those in mixed samples (Figure 2).
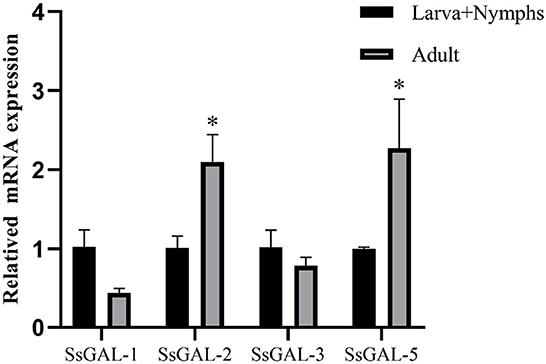
Figure 2. Transcriptional levels of SsGalectins in different developmental stages. Data are represented as the mean ± standard deviation (M ± SD) of three replicates per groups. A one-way ANOVA analysis was used to analyze the variances between the groups. “*” is the secretion level of the treatment group compared with the control group (*p < 0.05).
Expression and identification of rSsGalectins
The SsGAL-1, SsGAL-2, SsGAL-3, and SsGAL-5 genes were successfully cloned from the cDNA of the mixed sample (larvae, nymph, and adult mites) with specific primers. The size was all around 1,000 bp (1,131, 1,080, 1,008, and 1,020 bp, respectively). The rSsGAL-1 and rSsGAL-5 presented as soluble proteins, while the rSsGAL-2 and rSsGAL-3 presented in inclusion bodies. SDS-PAGE analysis revealed that galectins were purified as clear and single bands at locations 59, 57, 54, and 55 kDa, respectively (the His-tag protein of the expression vector was 18 kDa). Four rSsGalectins were specifically recognized by S. scabiei-positive rabbit serum and anti-Ss-GAL (1,2,3,5) IgG, but no band was observed after incubation with S. scabiei-naive rabbit serum (Figure 3).
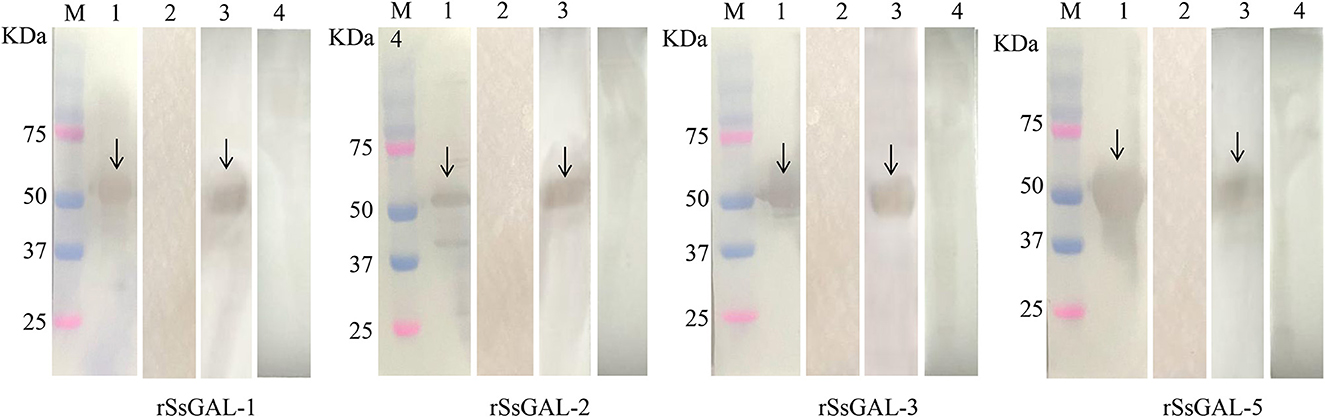
Figure 3. rSsGalectins immunogenicity analysis. M: Protein marker; 1: Rat polyclonal antibody reacts with recombinant protein; 2: Rat negative serum reacts with recombinant protein; 3: Rabbit sera infected with scabies mites react with recombinant protein; 4: Healthy rabbit serum reacts with recombinant protein.
Immunohistochemical analysis of SsGalectins
No green fluorescence was detected in the scabies skin or scabies mites using negative serum from rats. On the body surface of scabies mites, SsGAL-1 and SsGAL-2 were discovered to include the mouthpiece, limbs, and epidermis, whereas SsGAL-3 and SsGAL-5 were widely distributed within and on the bodies of scabies mites and also included the gut and stomach (Figure 4). Furthermore, green fluorescence was discovered not only on the mites but also on the scabies skin (Figures 5, 6).
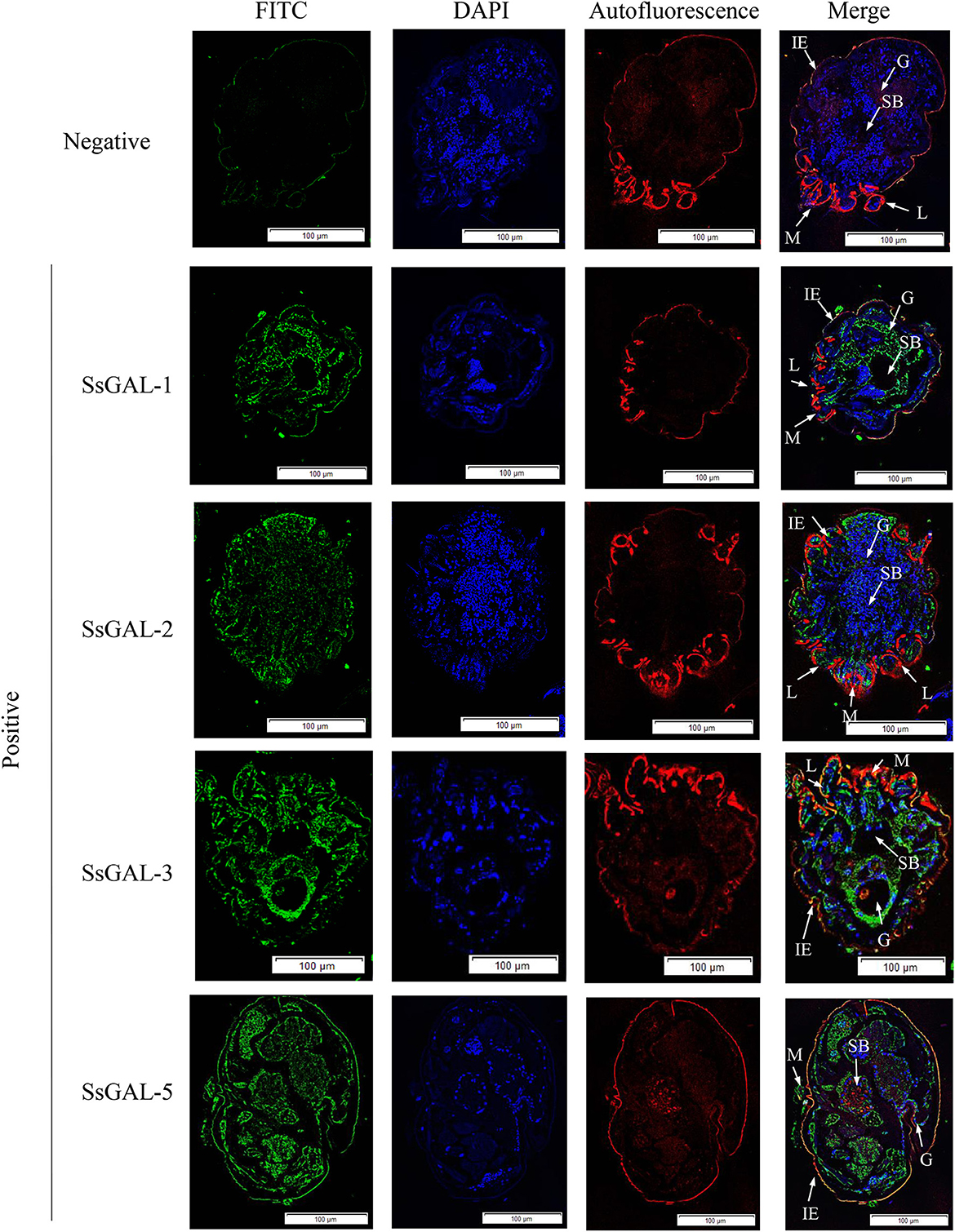
Figure 4. Immunofluorescence of SsGalectins. Positive: Incubated with rat anti-rSsGalectins antibody as the primary antibody; Negative: Incubate with rat negative serum as the primary antibody. M, mouthpart; L, leg; G, gut; SB, stomach blocks; IE, epidermal integument. Green fluorescence indicates F4/80 as an antibody to recognize macrophages, blue fluorescence indicates DAPI-labeled nuclei, and red fluorescence is skin autofluorescence. Scale bar = 100 μm.
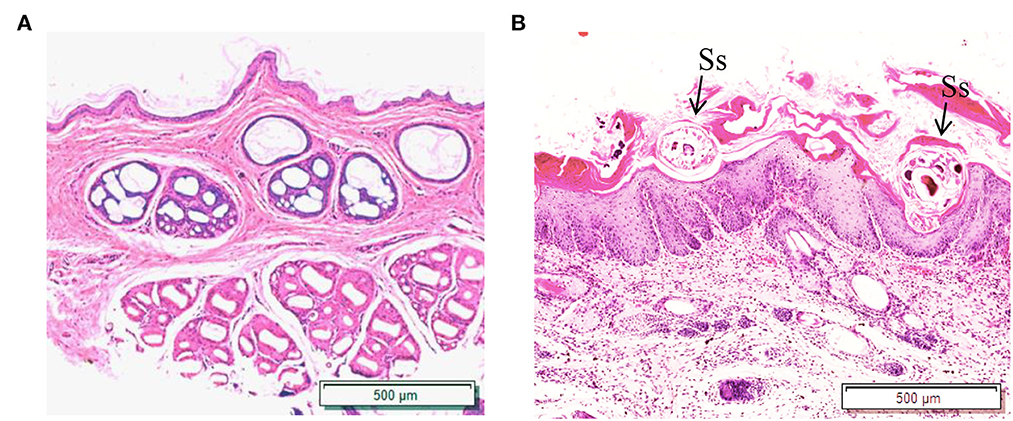
Figure 5. Hematoxylin and eosin (HandE) staining of rabbit skin. (A) Healthy skin; (B) Scabies skin. The arrows point to the S. scabiei. Scale bar = 500 μm.
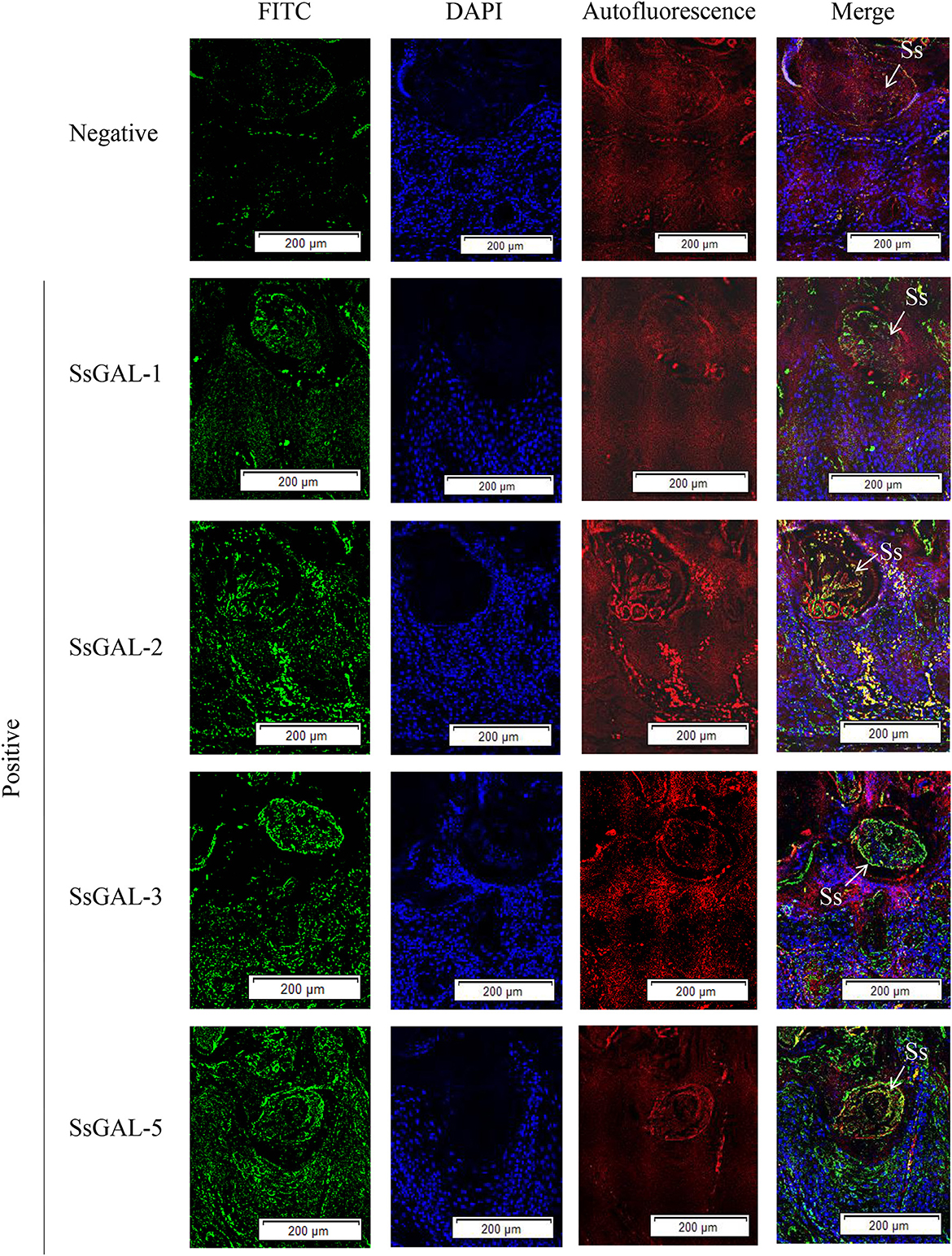
Figure 6. Immunofluorescence of SsGalectins in the skin of rabbits infected with scabies. Positive: Incubated with rat anti-rSsGalectins antibody as the primary antibody, Negative: Incubated with negative rat serum as the primary antibody. Green fluorescence indicates F4/80-labeled macrophages, blue fluorescence indicates DAPI-labeled nuclei, and red fluorescence is skin autofluorescence. The arrows point to the S. scabiei. Scale bar = 500 μm.
Clinical symptoms of AD model mice
On the 5th day, after 3 days of continuous application of 1% DNCB, erythema, edema, and a minor number of scabs occurred on the back skin of mice. With continued application of 0.5% DNCB, the mice exhibited severe scratching, dry skin, scab thickness, erosion, and bleeding. There was no significant difference in clinical symptoms between the rSsGAL-1 and rSsGAL-5 protein groups compared to the AD group after 8–14 days of recombinant protein stimulation. Compared to the AD group, the rSsGAL-2 and rSsGAL-3 protein groups experienced more severe crusting and exudation on the skin surface, similar to histamine group symptoms. The Tacrolimus group had reduced erythema on the skin's surface, no significant scabs, and more hair. The pET32a (+) group exhibited slightly thicker crusts than the AD group, but there was no difference in symptoms between the PBS and AD groups (Figure 7).
H&E of mice skin
It was clear that the mouse AD model was developed successfully because the stratum corneum of the back skin of the mice in the AD group had been damaged, showing hyperkeratosis, parakeratosis, thickness of the spinous layer, and infiltration of inflammatory cells in the dermis. Furthermore, inflammatory cell infiltration in the dorsal skin of mice was higher in the rSsGAL-1, rSsGAL-2, rSsGAL-3, and rSsGAL-5 groups than in the AD group, and the pathological alterations in the skin tissue were more severe. In the rSsGAL-2 group, some necrotic subcutaneous tissues were discovered. The His group had the most severe pathological abnormalities as compared to the positive control group. In contrast, the Tac group had typical stratum corneum structure, thickening of the spinous layer, and spinous layer edema. Inflammatory cells infiltrated more in the pET32a group than in the AD group, but there was no significant difference in the PBS group (Figure 8).
Immunofluorescence of mice skin
The green fluorescence of the AD group was higher than that of the healthy group. The fluorescence intensity of the rSsGAL-1, rSsGAL-2, rSsGAL-3, and rSsGAL-5 groups rose compared to the AD group, particularly the rSsGAL-3 group, which was similar to the His group. The Tac group's fluorescence intensity was reduced; there was no significant difference between the PBS group, the pET32a group, and the AD group (Figure 9).
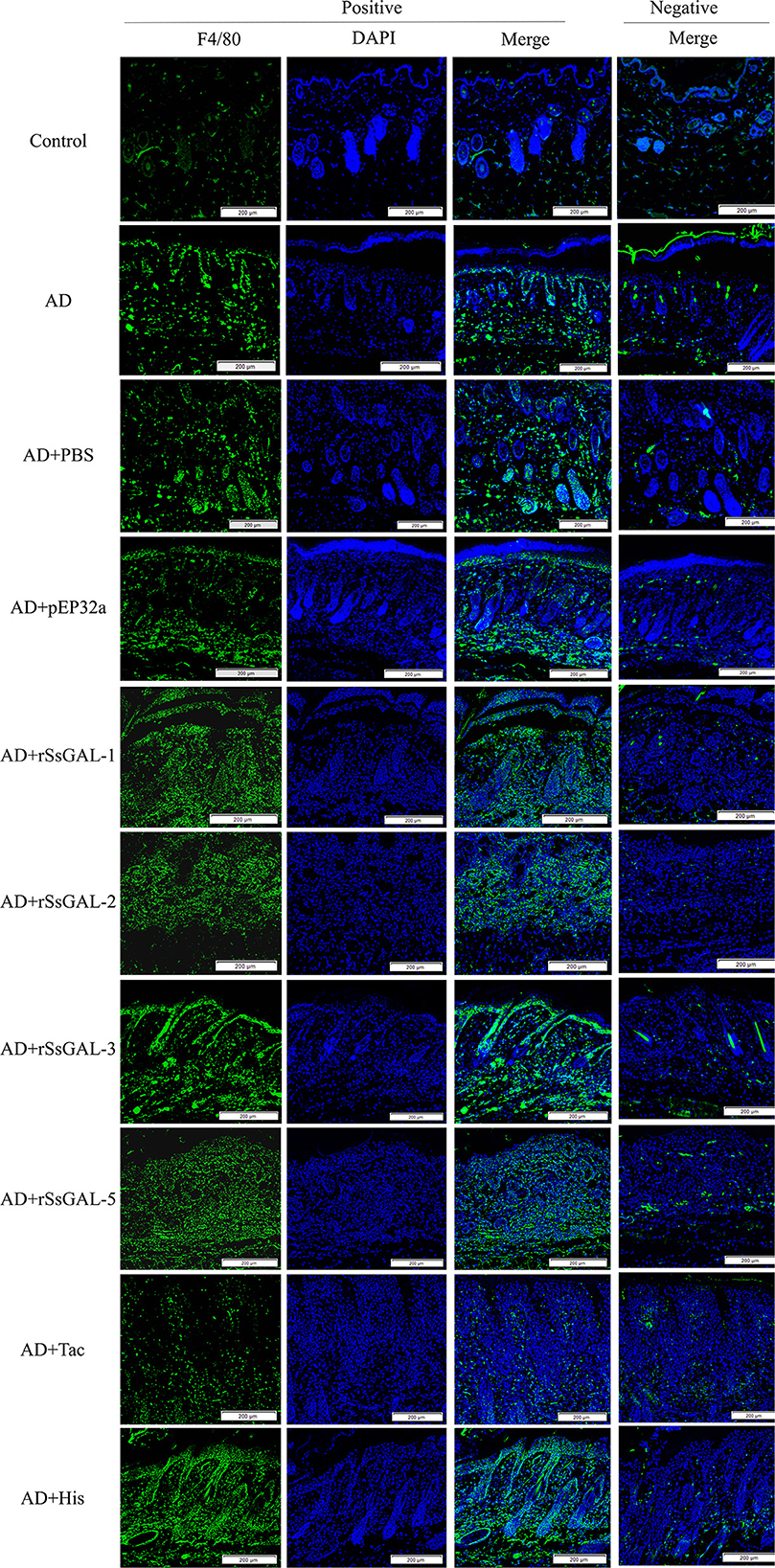
Figure 9. Immunofluorescence staining of skin lesions. Green fluorescence indicates F4/80-labeled macrophages and blue fluorescence indicates DAPI-labeled nuclei. Scale bar = 200 μm.
Flow cytometry and CCK-8 assay
Flow cytometry revealed that 99.7% of F4/80+ cells were present, 97.2% of CD11b+ cells were present, and 97% of F4/80+ CD11b+ cells were present (Figure 10), showing that this approach successfully converted bone marrow cells into BMDM. The CCK8 test findings indicated that the cell survival rates of rSsGAL-1, rSsGAL-2, rSsGAL-3, rSsGAL-5, and recombinant pET32a proteins were all over 90% at 0.1–100 μg, showing that the above recombinant proteins were effective at 0.1–100 μg. The viability of BMDM has no influence, and further tests can be conducted within this dose range.
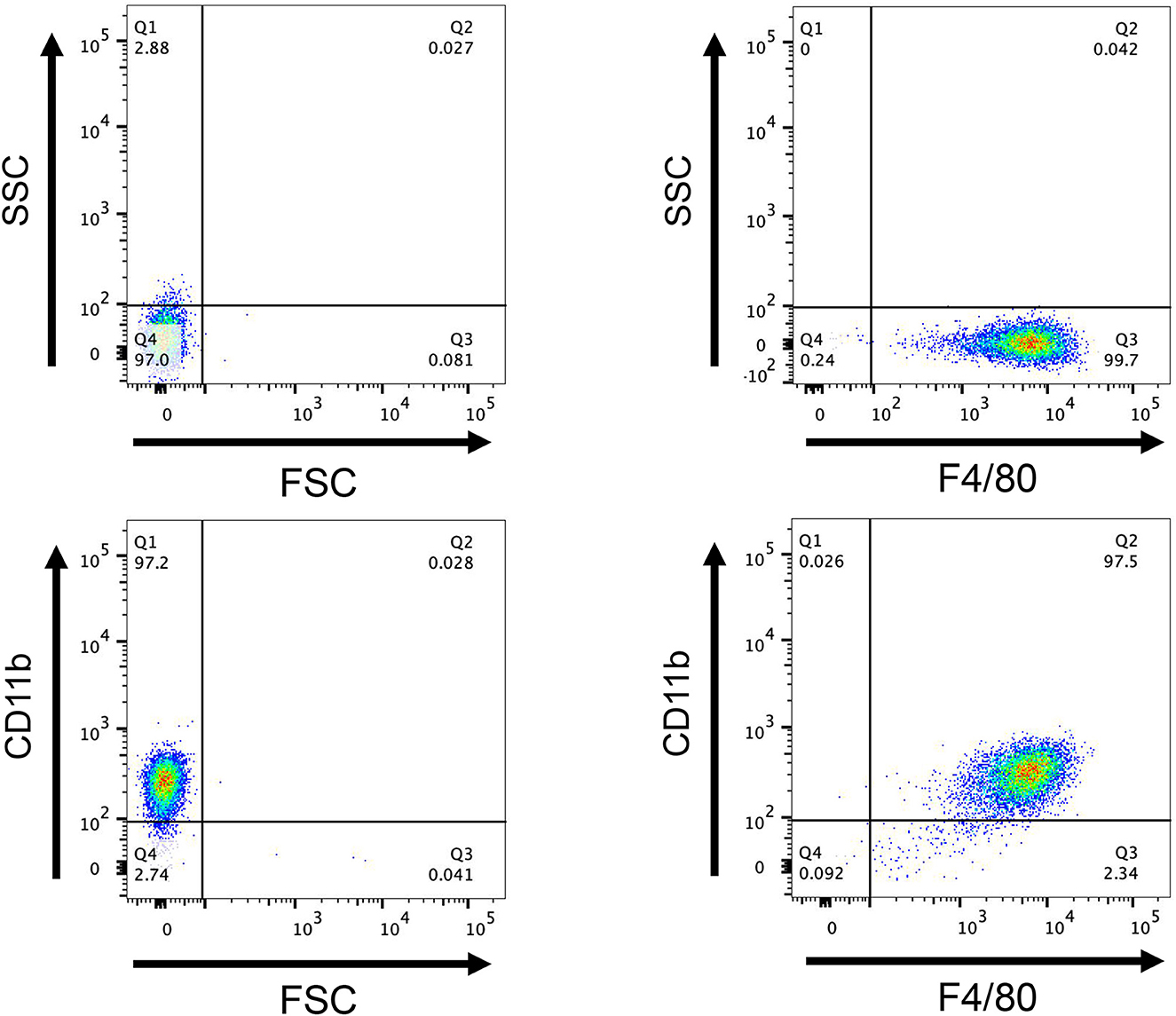
Figure 10. Flow cytometry detection of macrophages. After induction of mouse bone marrow-derived cells for 7 days, the expression of CD11b+ and F4/80+, a marker molecule for macrophages, was detected by flow cytometry. FCS, Reactive cell volume; SSC, Reflects cell granularity.
qPCR of inflammatory factors
The mRNA transcription levels of IL-1β, IL-6, IL-12, iNOS, and Arg1 were higher in the rSsGAL-1, rSsGAL-2, rSsGAL-3, rSsGAL-5, and recombinant pET32a groups than in the control group (p < 0.01). Simultaneously, the levels of IL-4 and IL-10 mRNA transcription were increased (p < 0.05) in the rSsGAL-1 group, while the transcription of IL-10 mRNA was increased (p < 0.05) in the rSsGAL-3 and rSsGAL-5 groups. The PBS group and the blank control group had no significant change in the relative transcription levels of inflammatory-related factors (p > 0.05) (Figure 11).
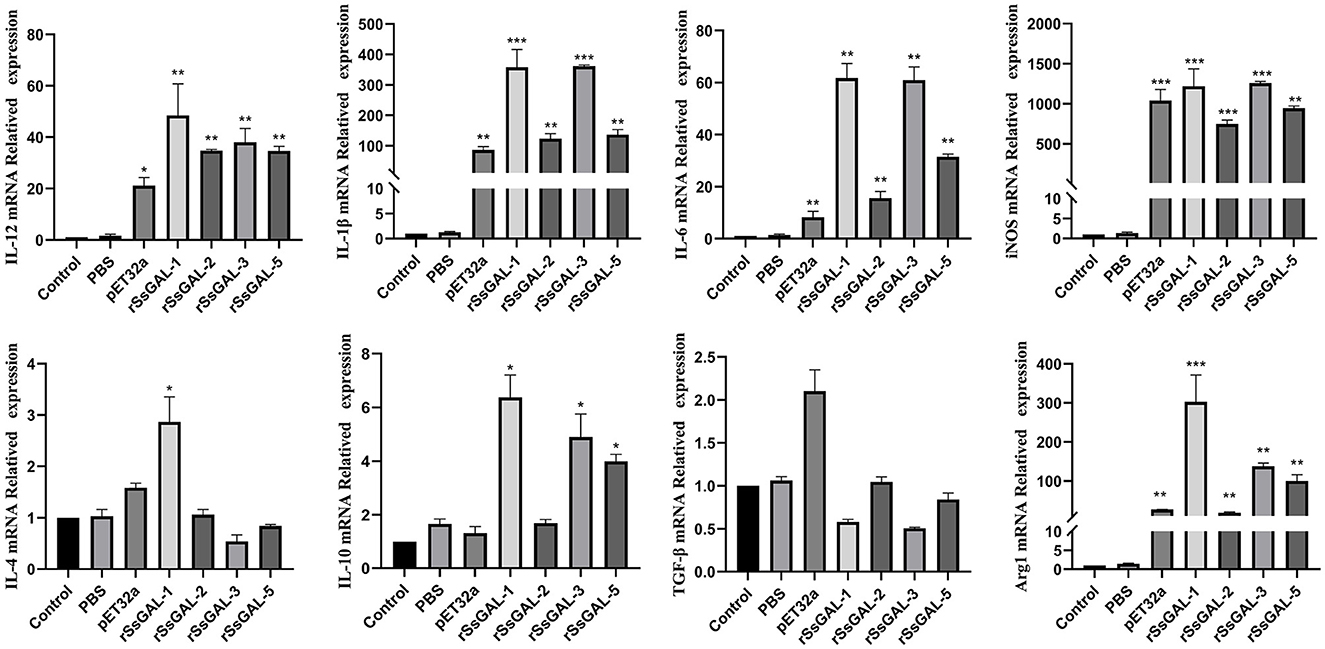
Figure 11. Relative transcript levels of inflammatory-related factors in BMDM. Data are represented as the mean ± standard deviation (M ± SD) of three replicates per groups. A one-way ANOVA analysis was used to analyze the variances between the groups. “*” is the secretion level of the treatment group compared with the control group (*p < 0.05, **p < 0.01, ***p < 0.001).
ELISA of inflammatory factors
ELISA detection of inflammatory cytokines in BMDM cell supernatant revealed that rSsGAL-1, rSsGAL-2, rSsGAL-3, and rSsGAL-5 increased IL-10, IL-1β, IL-6, and iNOS (p < 0.01), but Arg1 reduced (p < 0.01). TGF-β increased in the rSsGAL-3 group, but there was no significant difference between the rSsGAL-1, rSsGAL-2, and rSsGAL-5 groups (p > 0.05). Meanwhile, IL-10, IL-1β, and iNOS levels were higher in the rSsGAL-1 and rSsGAL-3 groups than in the rSsGAL-2 and rSsGAL-5 groups. Only Arg1 rose considerably in the PBS group (p > 0.05) (Figure 12).
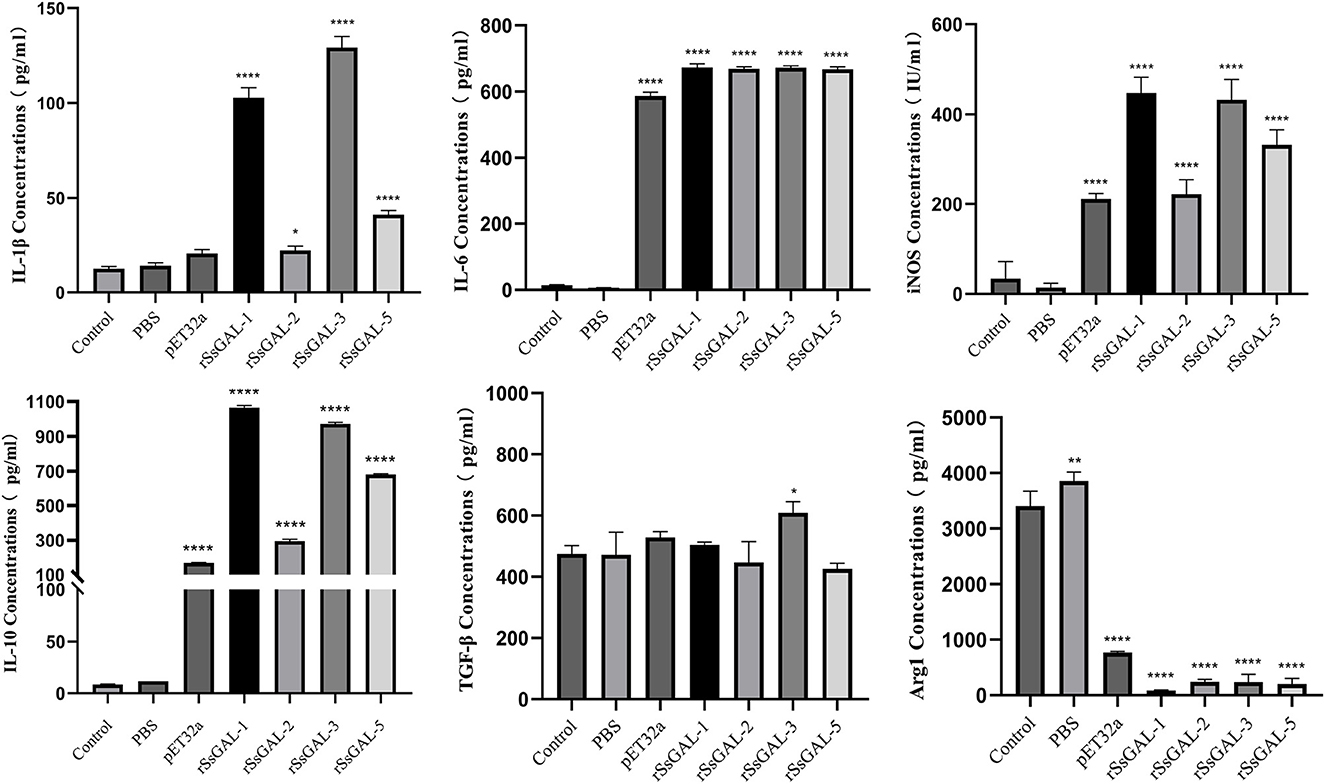
Figure 12. Secretion levels of inflammatory-related factors in BMDM. Data are represented as the mean ± standard deviation (M ± SD) of three replicates per groups. A one-way ANOVA analysis was used to analyze the variances between the groups, “*” is the secretion level of the treatment group compared with the control group (*p < 0.05, **p < 0.01, ****p < 0.0001).
Discussion
The parasite body surface contains a variety of complex carbohydrates, including glycolipids, glycoproteins, and glycosylated phosphatidylinositol glycolipids, and these glycoconjugates play an important role in host invasion. Specific interactions between parasite glycoconjugates and host galectins are required for pathogen recognition. Glycoconjugates cause pro-inflammatory effects in the host, antigen presentation, and innate immune response (Mascanfroni et al., 2011). Galectins have a high affinity for β-galactoside and are involved in various physiological and pathological processes, such as cell adhesion, apoptosis, and inflammatory response.
F4/80 is a surface marker of macrophages and shows green fluorescence, and the green fluorescence was stronger in the scabies skin, demonstrating that S. scabiei invasion causes macrophage infiltration in the host skin. S. scabiei has a life cycle that includes eggs, larvae, nymphs, and adults. Eggs are typically found in the meandering tunnels of the skin epidermis. By microscopy, the larvae are tiny and difficult to distinguish from the nymphs. As a result, the larvae and nymphs are gathered together, while the adult mites are collected separately in this study. SsGAL-1, SsGAL-2, SsGAL-3, and SsGAL-5 were expressed at each developmental stage of S. scabiei, with SsGAL-2 and SsGAL-5 having higher transcript levels in adults, indicating that SsGalectins play a crucial role in the development of S. scabiei, especially during scabies mite pathogenesis. It has been observed that the transcriptional level of galectins in Haemonchus contortus is discovered in distinct developmental stages, and HcoGAL-1 is significantly expressed in each developmental stage, HcoGAL-3 is exclusively expressed in adults, and HcoGAL-4 is expressed in L3 larvae (Greenhalgh et al., 2000). It implies that different galectins may participate in the parasite's life activities at various stages.
Based on the genomic sequence of S. scabiei, we cloned, expressed, and discovered Galectins. The Western blot analysis revealed that rSsGalectins were specifically recognized by S. scabiei-positive rabbit serum and anti-Ss-GAL (1,2,3,5) IgG. Immunohistochemical analysis of SsGalectins in scabies skin revealed that SsGalectins could be secreted and diffused into the host skin, confirming that rSsGalectins were an essential component of ES antigens. SsGalectins may play a significant role in parasite-induced inflammation in the host. As a result, understanding the mechanism of scabies mites on host inflammation is the primary goal for scabies mite prevention and therapy.
By generating different cytokines and growth factors, macrophages execute antigen presentation, phagocytosis, and immunological control (Fujiwara and Kobayashi, 2005; Chazaud, 2020). Macrophages are crucial for the initiation, continuation, and resolution of inflammation (Fujiwara and Kobayashi, 2005). Macrophages can secrete some pro-inflammatory factors to exacerbate inflammation and microbial clearance and can also produce anti-inflammatory factors to inhibit inflammation (Hofmann et al., 2010; Mounsey et al., 2015). This is due to a process called macrophage polarization, which allows macrophages to divide into cells with different functions in response to various stimuli. According to classical immunology, they are categorized as either classically activated macrophages (M1 macrophages) or alternatively activated macrophages (M2 macrophages), depending on the type of stimulus and the polarized cell phenotype. M1 macrophages primarily secrete pro-inflammatory factors, perform immune defense and pro-inflammatory functions, and could significantly increase iNOS and TNF-α levels. Thus, IL-6, IL-12, iNOS, and TNF-α can be used as molecular markers to identify M1 macrophages. On the contrary, M2 macrophages primarily release anti-inflammatory factors and exhibit anti-inflammatory effects, and their marker molecules include IL-10, Arginase 1 (Arg1), and TGF-β (Funes et al., 2018; Reichel et al., 2019). Macrophage polarization is a dynamic process, and under specific conditions, M1 and M2 types can be interconverted (Yang et al., 2021). Early in local inflammation, the afflicted macrophages exhibit the M1 type, which has a strong potential to deliver antigens. On the contrary, persistent M1-type macrophages cause excessive tissue damage and impair wound healing (Hu et al., 2012). When the inflammation is severe enough to interfere with the body's normal physiological operations, M2 macrophages expand, removing debris and apoptotic cells with great phagocytic capabilities and secreting anti-inflammatory substances to assist wound healing.
Scabies mite migratory activity, excreta production, and scabies mite breakdown products after death can induce allergic dermatitis in the host (Gazi et al., 2022). Scabies is classified into two types of clinical manifestations, namely, ordinary scabies (OS), and crusted scabies (CS). OS is produced mainly by a limited number of mites and appears as papules, erythema, and allergic skin responses with intense itching. CS has a significant number of mites and eggs in the skin epidermis, and the epidermis is thick and scaly (Arlian and Morgan, 2017; Stienstra et al., 2019). Atopic dermatitis (AD) is a chronic inflammatory skin condition marked by intense itching, recurring eczema, erythema, and dry skin. Currently, AD models are often used in fundamental research on allergic dermatitis. Many studies have employed 1-chloro-2,4-dinitroenzene (DNCB) to induce a persistent clinical AD model (Lee et al., 2022). Tacrolimus is a macrolide immunosuppressant that is widely used to treat AD. It has anti-inflammatory, antipruritic, and immunomodulatory characteristics (Bajgai et al., 2017). Histamine (His) can elicit allergy symptoms such as skin irritation, itching, erythema, and wheal, and it can be utilized as a positive control (Tachibana et al., 1990; Kim et al., 2013). In addition, an AD mouse model that is equivalent to the etiology and clinical indications of scabies was selected for future study due to a lack of secondary antibodies for rabbit cytokines.
It was found that the host GAL-3 and GAL-9 can attach to the parasite and cause it to bind to macrophages by detecting and binding to the β-galactosyl epitope on the lipopolysaccharide on the surface of Leishmania, potentially assisting the parasite in invading the body and causing infection (Pelletier et al., 2003; Turner et al., 2008). By attaching to annexin (Annexin A2) and activating JNK in the apoptotic signaling cascade, Angiostrongylus cantonensis GAL-1 can cause macrophage apoptosis (Shi et al., 2020). Exogenous galectins have been demonstrated in several disease models to have either anti-inflammatory or pro-inflammatory effects (Bastón et al., 2014; Martínez-Bosch et al., 2014). In this study, an AD model was constructed to investigate the involvement of rSsGalectins in scabies. Observing changes in skin lesions and macrophage infiltration suggested that rSsGalectin was implicated in the incidence and progression of scabies, primarily through pro-inflammatory effects. Additionally, alterations in BMDM inflammation-related variables following the stimulation with rSsGalectins were discovered, and the impact of rSsGalectins on macrophages was studied using cultured BMDM cells.
The mRNA transcription levels of IL-1β, IL-6, IL-12, iNOS, and Arg1 were significantly higher in the rSsGAL-1, rSsGAL-2, rSsGAL-3, rSsGAL-5, and pET32a (+) groups compared to those in the blank control group, according to qPCR data, indicating that the relative transcription level of M1 polarization marker factors is mainly increased by rSsGAL-1, rSsGAL-2, rSsGAL-3, and rSsGAL-5. It is hypothesized that rSsGalectins triggers the polarization of BMDM to the M1 type, which enhances the inflammatory response. Among these, the transcription levels of both iNOS and Arg1 increased at the same time, with iNOS increasing significantly more than Arg1. It is possible that the rSsGalectins family can drive BMDM polarization to the M1 and M2 types. However, M1-type polarization is predominant, with only a few cells polarized to the M2 type. According to ELISA data, rSsGAL-1, rSsGAL-2, rSsGAL-3, and rSsGAL-5 increased IL-10, IL-1β, IL-6, and iNOS inflammatory components while decreasing Arg1 levels. It indicated that the inflammatory response might be induced by rSsGAL-1, rSsGAL-2, rSsGAL-3, and rSsGAL-5 by encouraging BMDM cells to secrete the M1 polarization regulators IL-1β, IL-6, and iNOS.
IL-10 is an endogenous anti-inflammatory molecule that also acts as a maker for M2 macrophages (Gao et al., 2014). However, when BMDM was mainly polarized to M1, the expression level of IL-10 was dramatically raised in this research. IL-10 is an endogenous anti-inflammatory substance that also serves as a marker for M2-type macrophage polarization (Gao et al., 2014). M2 macrophages are classified into four subtypes, namely, M2a, M2b, M2c, and M2d. Although all M2 isoforms express IL-10, the cytokines produced and their roles vary. In this study, IL-10, IL-1β, and IL-6 expression levels increased when rSsGalectins acted on BMDM; however, TGF-β did not change significantly. It is possible that the polarization type of a small number of M2 macrophages mediated by rSsGalectins is M2b. M2b macrophages, also known as “type II” macrophages, are polarized states between M1 and M2a, with both pro-inflammatory and anti-inflammatory effects involved in the regulation of late cellular inflammation (Wang et al., 2019; Gharavi et al., 2022). This indicates that macrophages are in the process of changing from the MI type to the M2 type. Arg1 is essential in the anti-infection mechanism of several parasitic diseases. If the host is infected with Schistosoma mansoni, Arg1 can effectively suppress Th2-mediated fibrosis, Th1/Th17-mediated intestinal damage, iNOS production, and endotoxemia (Pesce et al., 2009; Herbert et al., 2010). The mRNA transcription level of Arg1 was dramatically enhanced after rSsGalectins were treated with BMDM in this investigation; however, the protein level was lowered. This could be connected to microRNAs' post-transcriptional control of macrophages. MicroRNAs are tiny noncoding RNAs that bind to the 3′ untranslated region of mRNA and govern post-transcriptional gene silencing (Iwakawa and Tomari, 2015). MicroRNAs have been implicated in the regulation of macrophage polarization, inflammation, and cancer progression (Li et al., 2018). For instance, miR-155 inhibits M2-type polarization and decreases Arg1 by targeting C/EBP, promoting the production of M1-type cell-related marker factors, such as TNF-α, IL-1β, and IL-6, and increasing the levels of mRNA and post-transcriptional translation of Arg1 (Arranz et al., 2012). In conclusion, by controlling macrophage polarization, rSsGalectins play a role in the occurrence and progression of host inflammation. rSsGAL-1, rSsGAL-2, rSsGAL-3, and rSsGAL-5 all contributed to the pathogenic process of scabies by fostering inflammation in the host. This study offers new information on the immune relationship between the scabies mite and the host as well as a different approach to the investigation of the scabies pathogenic mechanism.
Conclusion
The SsGalectins are involved in the pathogenic process of S. scabiei by regulating the polarization of host macrophages to M1 type when S. scabiei invade the host and promoting the incidence and development of the host's inflammatory response. This study offers new insights into S. scabiei prevention and therapy.
Data availability statement
The original contributions presented in the study are included in the article/Supplementary material, further inquiries can be directed to the corresponding author.
Ethics statement
The animal study was approved by the Sichuan Agricultural University Animal Care and Use Committee (SYXK2019-189). The study was conducted in accordance with the local legislation and institutional requirements.
Author contributions
RH conceived and designed the experiments. RH and QZ performed the experiments and analyzed the data. RH and ZS wrote the manuscript. LX, MG, XG, YX, and JX provided material. ZS improved the paragraphs and English grammar and made constructive suggestions for revisions.
Funding
This work was funded by the Sichuan Province Science and Technology Support Program (Grant No. 2020YJ0114), the Sichuan Science and Technology Program (2023NSFSC1136), and the Two-Way Support Programs of Sichuan Agricultural University (Grant No. 035/2021993079). The funder had no role in study design, sample collection, data analysis, preparation, or publication of the manuscript.
Acknowledgments
The authors thank Jiabo Zhang, Guangyou Yang, Xuerong Peng, Lang Xiong, Ruiqi Hua, Jie Xiao, Ge Hao, Yanting Xu, and Ziyi Xu for their technical help and suggestions on this study.
Conflict of interest
The authors declare that the research was conducted in the absence of any commercial or financial relationships that could be construed as a potential conflict of interest.
Publisher's note
All claims expressed in this article are solely those of the authors and do not necessarily represent those of their affiliated organizations, or those of the publisher, the editors and the reviewers. Any product that may be evaluated in this article, or claim that may be made by its manufacturer, is not guaranteed or endorsed by the publisher.
Supplementary material
The Supplementary Material for this article can be found online at: https://www.frontiersin.org/articles/10.3389/fmicb.2023.1251475/full#supplementary-material
References
Arlian, L. G., and Morgan, M. S. (2017). A. review of Sarcoptes scabiei: past, present and future. Parasit. Vectors 10, 1–22. doi: 10.1186/s13071-017-2234-1
Arora, P., Rudnicka, L., Sar-Pomian, M., Wollina, U., Jafferany, M., Lotti, T., et al. (2020). Scabies: a comprehensive review and current perspectives. Dermatol. Ther. 33, e13746. doi: 10.1111/dth.13746
Arranz, A., Doxaki, C., Vergadi, E., Torre, Y. M., Vaporidi, K., Lagoudaki, E. D., et al. (2012). Akt1 and Akt2 protein kinases differentially contribute to macrophage polarization. Proc. Nat. Acad. Sci. 109, 9517–9522. doi: 10.1073/pnas.1119038109
Astorgues-Xerri, L., Riveiro, M. E., Tijeras-Raballand, A., Serova, M., Neuzillet, C., Albert, S., et al. (2014). Unraveling galectin-1 as a novel therapeutic target for cancer. Cancer Treat Rev. 40, 307–319. doi: 10.1016/j.ctrv.2013.07.007
Bajgai, J., Fadriquela, A., Ara, J., Begum, R., Ahmed, M. F., Kim, C., et al. (2017). Balneotherapeutic effects of high mineral spring water on the atopic dermatitis-like inflammation in hairless mice via immunomodulation and redox balance. BMC Compl. Altern. Med. 17, 1–9. doi: 10.1186/s12906-017-1985-8
Barrès, C., Blanc, L., Bette-Bobillo, P., André, S., Mamoun, R., Gabius, H., et al. (2010). Galectin-5 is bound onto the surface of rat reticulocyte exosomes and modulates vesicle uptake by macrophages. Blood J. Am. Soc. Hematol. 115, 696–705. doi: 10.1182/blood-2009-07-231449
Bastón, J. I., Barañao, R. I., Ricci, A. G., Bilotas, M. A., Olivares, C. N., Singla, J. J., et al. (2014). Targeting galectin-1-induced angiogenesis mitigates the severity of endometriosis. J. Pathol. 234, 329–337. doi: 10.1002/path.4397
Bernigaud, C., Samarawickrama, G. R., Jones, M. K., Gasser, R. B., and Fischer, K. (2019). The challenge of developing a single-dose treatment for scabies. Trends Parasitol. 35, 931–943. doi: 10.1016/j.pt.2019.08.002
Chazaud, B. (2020). Inflammation and skeletal muscle regeneration: leave it to the macrophages! Trends Immunol. 41, 481–492. doi: 10.1016/j.it.2020.04.006
Cote, N. M., Jaworski, D. C., Wasala, N. B., Morgan, M. S., and Arlian, L. G. (2013). Identification and expression of macrophage migration inhibitory factor in Sarcoptes scabiei. Exp. Parasitol. 135, 175–181. doi: 10.1016/j.exppara.2013.06.012
Dumic, J., Dabelic, S., and Flögel, M. (2006). Galectin-3: an open-ended story. Biochim. Biophys. Acta (BBA)-General Sub. 1760, 616–635. doi: 10.1016/j.bbagen.2005.12.020
El-Moamly, A. A. (2021). Scabies as a part of the World Health Organization roadmap for neglected tropical diseases 2021–2030: what we know and what we need to do for global control. Trop. Med. Health 49, 1–11. doi: 10.1186/s41182-021-00348-6
Farhadi, S. A., and Hudalla, G. A. (2016). Engineering galectin–glycan interactions for immunotherapy and immunomodulation. Exp. Biol. Med. 241, 1074–1083. doi: 10.1177/1535370216650055
Feng, H., Yin, Y., Ren, Y., Li, M., Zhang, D., Xu, M., et al. (2020). Effect of CSE on M1/M2 polarization in alveolar and peritoneal macrophages at different concentrations and exposure in vitro. In Vitro Cell Dev. Biol. Anim. 56, 154–164. doi: 10.1007/s11626-019-00426-4
Fischer, K., and Walton, S. (2014). Parasitic mites of medical and veterinary importance–is there a common research agenda? Int. J. Parasitol. 44, 955–967. doi: 10.1016/j.ijpara.2014.08.003
Fujiwara, N., and Kobayashi, K. (2005). Macrophages in inflammation. Curr. Drug Targets-Inflam. Aller. 4, 281–286. doi: 10.2174/1568010054022024
Funes, S. C., Rios, M., Escobar-Vera, J., and Kalergis, A. M. (2018). Implications of macrophage polarization in autoimmunity. Immunology 154, 186–195. doi: 10.1111/imm.12910
Gao, S., Mao, F., Zhang, B., Zhang, L., Zhang, X., Wang, M., et al. (2014). Mouse bone marrow-derived mesenchymal stem cells induce macrophage M2 polarization through the nuclear factor-κB and signal transducer and activator of transcription 3 pathways. Exp. Biol. Med. 239, 366–375. doi: 10.1177/1535370213518169
Gazi, U., Taylan-Ozkan, A., and Mumcuoglu, K. Y. (2022). Immune mechanisms in human Sarcoptes scabiei (Acari: Sarcoptidae) infestations. Paras. Immunol. 44, e12900. doi: 10.1111/pim.12900
Gharavi, A. T., Hanjani, N. A., Movahed, E., and Doroudian, M. (2022). The role of macrophage subtypes and exosomes in immunomodulation. Cell Mol. Biol. Lett. 27, 83. doi: 10.1186/s11658-022-00384-y
Greenhalgh, C. J., Loukas, A., Donald, D., Nikolaou, S., and Newton, S. E. (2000). A family of galectins from Haemonchus contortus. Mol. Biochem. Parasitol. 107, 117–121. doi: 10.1016/S0166-6851(99)00230-3
Hafidi, N. N., Swan, J., Faou, P., Lowe, R., Rajapaksha, H., Cairns, C., et al. (2021). Teladorsagia circumcincta galectin-mucosal interactome in sheep. Veter. Sci. 8, 216. doi: 10.3390/vetsci8100216
Hashimoto, T., Satoh, T., and Yokozeki, H. (2019). Pruritus in ordinary scabies: IL-31 from macrophages induced by overexpression of thymic stromal lymphopoietin and periostin. Allergy. 74, 1727–1737. doi: 10.1111/all.13870
Herbert, D. B. R., Orekov, T., Roloson, A., Ilies, M., Perkins, C., O'Brien, W., et al. (2010). Arginase I suppresses IL-12/IL-23p40–driven intestinal inflammation during acute schistosomiasis. J. Immunol. 184, 6438–6446. doi: 10.4049/jimmunol.0902009
Hofmann, A., Osman, A., Leow, C. Y., Driguez, P., McManus, D. P., Jones, M. K., et al. (2010). Parasite annexins–new molecules with potential for drug and vaccine development. Bioessays 32, 967–976. doi: 10.1002/bies.200900195
Hu, X., Li, P., Guo, Y., Wang, H., Leak, R. K., Chen, S., et al. (2012). Microglia/macrophage polarization dynamics reveal novel mechanism of injury expansion after focal cerebral ischemia. Stroke 43, 3063–3070. doi: 10.1161/STROKEAHA.112.659656
Iwakawa, H., and Tomari, Y. (2015). The functions of microRNAs: mRNA decay and translational repression. Trends Cell Biol. 25, 651–665. doi: 10.1016/j.tcb.2015.07.011
Kim, S-. J., Lee, G-. Y., Jung, J-. W., Oh, S-. R., Ahn, E-. M., Kim, S-. H., et al. (2013). The ameliorative effect of sophoricoside on mast cell-mediated allergic inflammation in vivo and in vitro. Molecules 18, 6113–6127. doi: 10.3390/molecules18056113
Lee, J. H., Dong, L., Noh, H. M., Park, S-. G., Kim, S-. H., Jo, E. H., et al. (2022). Inhibitory effects of donkey hide gelatin on DNCB-induced atopic dermatitis in NC/Nga mice. Front. Pharmacol. 13, 896450. doi: 10.3389/fphar.2022.896450
Li, H., Jiang, T., Li, M. Q., Zheng, X. L., and Zhao, G. (2018). Transcriptional regulation of macrophages polarization by MicroRNAs. Front Immunol. 9, 1175. doi: 10.3389/fimmu.2018.01175
Livak, K. J., and Schmittgen, T. D. (2001). Analysis of relative gene expression data using real-time quantitative PCR and the 2– ΔΔCT method. Methods 25, 402–408. doi: 10.1006/meth.2001.1262
Loghry, H. J., Sondjaja, N. A., Minkler, S. J., and Kimber, M. J. (2022). Secreted filarial nematode galectins modulate host immune cells. Front. Immunol. 13, 952104. doi: 10.3389/fimmu.2022.952104
Martínez, I. Z., Oleaga, Á., Sojo, I., García-Iglesias, M. J., Pérez-Martínez, C., Marín, J. F. G., et al. (2020). Immunohistochemical assessment of immune response in the dermis of Sarcoptes scabiei-infested wild carnivores (wolf and fox) and ruminants (chamois and red deer). Animals 10, 1146. doi: 10.3390/ani10071146
Martínez-Bosch, N., Fernández-Barrena, M. G., Moreno, M., Ortiz-Zapater, E., Munné-Collado, J., Iglesias, M., et al. (2014). Galectin-1 drives pancreatic carcinogenesis through stroma remodeling and Hedgehog signaling activation. Cancer Res. 74, 3512–3524. doi: 10.1158/0008-5472.CAN-13-3013
Mascanfroni, I. D., Cerliani, J. P., Dergan-Dylon, S., Croci, D. O., Ilarregui, J. M., Rabinovich, G. A., et al. (2011). Endogenous lectins shape the function of dendritic cells and tailor adaptive immunity: mechanisms and biomedical applications. Int. Immunopharmacol. 11, 833–841. doi: 10.1016/j.intimp.2011.01.021
Mounsey, K. E., Murray, H. C., Bielefeldt-Ohmann, H., Pasay, C., Holt, D. C., Currie, B. J., et al. (2015). Prospective study in a porcine model of Sarcoptes scabiei indicates the association of Th2 and Th17 pathways with the clinical severity of scabies. PLoS Negl. Trop. Dis. 9, e0003498. doi: 10.1371/journal.pntd.0003498
Na, T. (2019). Molecular mechanism of ZLW regulating SmAP intervention in host macrophage polarization. NanHua University.
Nangia-Makker, P., Honjo, Y., Sarvis, R., Akahani, S., Hogan, V., Pienta, K. J., et al. (2000). Galectin-3 induces endothelial cell morphogenesis and angiogenesis. Am. J. Pathol. 156, 899–909. doi: 10.1016/S0002-9440(10)64959-0
Nwufoh, O. C., Sadiq, N. A., Adediran, O. A., Jarikre, T. A., and Emikpe, B. O. (2020). Sequential histopathological changes and cytokine expressions in dogs naturally infested with sarcoptes scabiei mites. Acta Parasitol. 65, 452–461. doi: 10.2478/s11686-020-00181-7
Pelletier, I., Hashidate, T., Urashima, T., Nishi, N., Nakamura, T., Futai, M., et al. (2003). Specific recognition of Leishmania major poly-β-galactosyl epitopes by galectin-9: possible implication of galectin-9 in interaction between L. major and host cells. J. Biol. Chem. 278, 22223–22230. doi: 10.1074/jbc.M302693200
Pesce, J. T., Ramalingam, T. R., Mentink-Kane, M. M., Wilson, M. S., El Kasmi, K. C., Smith, A. M., et al. (2009). Arginase-1 expressing macrophages suppress Th2 cytokine–driven inflammation and fibrosis. PLoS Pathog. 5, e1000371. doi: 10.1371/journal.ppat.1000371
Reichel, D., Tripathi, M., and Perez, J. M. (2019). Biological effects of nanoparticles on macrophage polarization in the tumor microenvironment. Nanotheranostics. 3, 66. doi: 10.7150/ntno.30052
Reynolds, S. L., Pike, R. N., Mika, A., Blom, A. M., Hofmann, A., Wijeyewickrema, L. C., et al. (2014). Scabies mite inactive serine proteases are potent inhibitors of the human complement lectin pathway. PLoS Negl. Trop. Dis. 8, e2872. doi: 10.1371/journal.pntd.0002872
Romani, L., Steer, A. C., Whitfeld, M. J., and Kaldor, J. M. (2015). Prevalence of scabies and impetigo worldwide: a systematic review. Lancet Infect. Dis. 15, 960–967. doi: 10.1016/S1473-3099(15)00132-2
Shi, X., Xiao, M., Xie, Z., Shi, Q., Zhang, Y., Leavenworth, J. W., et al. (2020). Angiostrongylus cantonensis Galectin-1 interacts with Annexin A2 to impair the viability of macrophages via activating JNK pathway. Paras. Vectors. 13, 183. doi: 10.1186/s13071-020-04038-w
Stienstra, Y., Beeres, D. T., Phillips, R., Vonk, M., and Ravensbergen, S. J. (2019). The public health control of scabies: priorities for research and action. Lancet. 394, 81–92. doi: 10.1016/S0140-6736(19)32479-1
Tachibana, T., Toda, K. I., Furukawa, F., Taniguchi, S., and Imamura, S. (1990). Histamine metabolism in delayed type hypersensitivity-Comparative analysis with cellular infiltrates. Arch Dermatol Res. 282, 217–222. doi: 10.1007/BF00371639
Taiaroa, G., Matalavea, B., Tafuna'i, M., Lacey, J. A., Price, D. J., Isaia, L., et al. (2021). Scabies and impetigo in Samoa: a school-based clinical and molecular epidemiological study. Lancet Reg. Health-Western Pacif. 6, 100081. doi: 10.1016/j.lanwpc.2020.100081
Toda, G., Yamauchi, T., Kadowaki, T., and Ueki, K. (2021). Preparation and culture of bone marrow-derived macrophages from mice for functional analysis. STAR Protoc. 2, 100246. doi: 10.1016/j.xpro.2020.100246
Turner, D. G., Wildblood, L. A., Inglis, N. F., and Jones, D. G. (2008). Characterization of a galectin-like activity from the parasitic nematode, Haemonchus contortus, which modulates ovine eosinophil migration in vitro. Vet. Immunol. Immunopathol. 122, 138–145. doi: 10.1016/j.vetimm.2007.11.002
Wang, L-. X., Zhang, S-. X., Wu, H-. J., Rong, X-. L., and Guo, J. (2019). M2b macrophage polarization and its roles in diseases. J. Leukoc. Biol. 106, 345–358. doi: 10.1002/JLB.3RU1018-378RR
World Health Organization. (2022). Ending the Neglect to Attain the Sustainable Development Goals: A Strategic Framework For Integrated Control and Management of Skin-Related Neglected Tropical Diseases. Geneva: WHO.
Yang, D., Yang, L., Cai, J., Hu, X., Li, H., Zhang, X., et al. (2021). A sweet spot for macrophages: Focusing on polarization. Pharmacol. Res. 167, 105576. doi: 10.1016/j.phrs.2021.105576
Keywords: Sarcoptes scabiei, galectins, macrophage, inflammation, mouse AD model
Citation: He R, Zhang Q, Xu L, Guo M, Gu X, Xie Y, Xu J and Shen Z (2023) Characterization of a novel galectin in Sarcoptes scabiei and its role in regulating macrophage functions. Front. Microbiol. 14:1251475. doi: 10.3389/fmicb.2023.1251475
Received: 07 July 2023; Accepted: 03 August 2023;
Published: 24 August 2023.
Edited by:
Guang-hui Zhao, Northwest A&F University, ChinaCopyright © 2023 He, Zhang, Xu, Guo, Gu, Xie, Xu and Shen. This is an open-access article distributed under the terms of the Creative Commons Attribution License (CC BY). The use, distribution or reproduction in other forums is permitted, provided the original author(s) and the copyright owner(s) are credited and that the original publication in this journal is cited, in accordance with accepted academic practice. No use, distribution or reproduction is permitted which does not comply with these terms.
*Correspondence: Ran He, ranhe1991@sicau.edu.cn; ranhe1991@hotmail.com
†These authors have contributed equally to this work