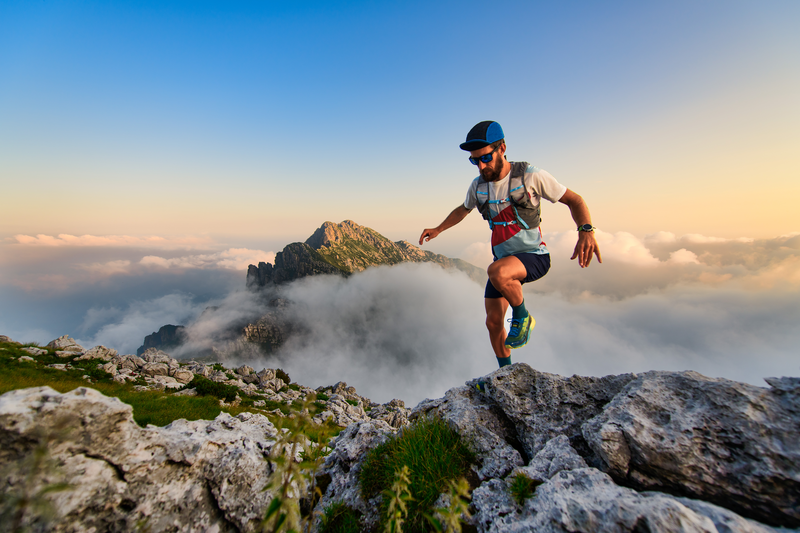
95% of researchers rate our articles as excellent or good
Learn more about the work of our research integrity team to safeguard the quality of each article we publish.
Find out more
ORIGINAL RESEARCH article
Front. Microbiol. , 10 October 2023
Sec. Microbiological Chemistry and Geomicrobiology
Volume 14 - 2023 | https://doi.org/10.3389/fmicb.2023.1251346
Geobacter sulfurreducens mediates extracellular electron transfer (EET) reactions with different substrates, such as solid-phase Fe(III)-containing minerals, anodes and the cells of Geobacter metallireducens. To compare their roles in EET, the pilA-N, omcE, omcS, omcT and omcZ genes of G. sulfurreducens were systematically deleted. All mutants showed impaired and varied ability to form biofilms on nonconductive surface. Deletion of omcE also impaired bacterial ability to reduce ferrihydrite, but its impacts on the ability for anode reduction and the co-culture of G. metallireducens-G. sulfurreducens were minimal. The mutant without omcS showed diminished ability to reduce ferrihydrite and to form the co-culture, but was able to regain its ability to reduce anodes. Deletion of omcT, omcZ or pilA-N alone impaired bacterial ability to reduce ferrihydrite and anodes and to form the co-culture. Deletion of all tested genes abolished bacterial ability to reduce ferrihydrite and anodes. Triple-deletion of all omcS, omcT and omcZ abolished the ability of G. sulfurreducens to co-culture with G. metallireducens. However, deletion of only omcZ or pilA-N or both omcS and omcT abolished the ability of G. sulfurreducens without hydrogenase gene hybL to co-culture with G. metallireducens, which show their indispensable roles in direct electron transfer from G. metallireducens to G. sulfurreducens. Thus, the roles of pilA-N, omcE, omcS, omcT and omcZ for G. sulfurreducens in EET vary substantially, which also suggest that possession of PilA-N and multiple cytochromes of different structures enables G. sulfurreducens to mediate EET reactions efficiently with substrates of different properties.
The dissimilatory Fe(III)-reducing bacterium Geobacter sulfurreducens possesses extracellular electron transfer (EET) capability, through which G. sulfurreducens can respire on solid-phase Fe(III) oxides and anodes and form syntrophic co-culture with Geobacter metallireducens (Caccavo et al., 1994; Bond and Lovley, 2003; Summers et al., 2010). In latter, G. metallireducens oxidizes ethanol and then directly transfers the electrons released from ethanol oxidation to G. sulfurreducens (i.e., direct interspecies electron transfer or DIET). G. sulfurreducens subsequently uses the received electrons to reduce fumarate (Summers et al., 2010). The pilin protein PilA-N is crucial to the EET capability of G. sulfurreducens and was believed to form the conductive nanowire to mediate EET directly (Reguera et al., 2005; Richter et al., 2009; Summers et al., 2010). In addition to DIET, H2-mediated interspecies electron transfer also occurs from G. metallireducens to G. sulfurreducens during their co-culture (Summers et al., 2010).
Recent structural analyses, however, revealed that the PilA-N and PilA-C of G. sulfurreducens formed a filamentous structure that was unstable extracellularly and electrically nonconductive (Gu et al., 2021; Wang et al., 2022b,c). Instead, the nanowire made of PilA-N and PilA-C was proposed to be responsible for extracellular secretion of multiheme c-type cytochromes (c-Cyts) OmcS and OmcZ that formed conductive filaments, respectively (Filman et al., 2019; Wang et al., 2019; Yalcin et al., 2020; Yalcin and Malvankar, 2020; Gu et al., 2021; Wang et al., 2022a,b; Gu et al., 2023). Similarly, the multiheme c-Cyt OmcE of G. sulfurreducens also formed the conductive nanowire extracellularly (Wang et al., 2022c). Notably, the structures of these proteins vary substantially (Filman et al., 2019; Wang et al., 2019; Yalcin et al., 2020; Yalcin and Malvankar, 2020; Gu et al., 2021; Wang et al., 2022a,b,c; Gu et al., 2023).
Similar to PilA-N, OmcS, OmcT (an OmcS homolog) and OmcE are involved in extracellular reduction of metal oxides by G. sulfurreducens (Lovley et al., 2004; Mehta et al., 2005; Reguera et al., 2005; Shi et al., 2007), OmcZ is involved in extracellular reduction of anodes (Nevin et al., 2009; Richter et al., 2009; Inoue et al., 2011; Richter et al., 2012) and OmcS is involved in the co-culture of G. metallireducens-G. sulfurreducens (Summers et al., 2010). Compared to that of PilA-N and OmcZ, the roles of OmcE and OmcS in extracellular reduction of anodes are trivial (Nevin et al., 2009; Richter et al., 2009). In addition to their roles in EET, PilA-N and OmcZ also play non-conductive roles in biofilm formation (Reguera et al., 2006; Richter et al., 2009). However, our current understanding of the roles of PilA-N, OmcE, OmcS, OmcT and OmcZ for G. sulfurreducens in EET is still far from complete. For instance, the roles of OmcT, OmcE and OmcZ in formation of co-culture with G. metallireducens are unknown.
In this investigation, we systemically compared the roles of pilA-N, omcE, omcS, omcT and omcZ genes of G. sulfurreducens in EET as well as biofilm formation. Our results showed that their roles in biofilm formation, extracellular reductions of Fe(III) oxides and anodes and the co-culture of G. metallireducens-G. sulfurreducens varied substantially.
Both Geobacter metallireducens GS-15 (ATCC® 53774™) and Geobacter sulfurreducens PCA (ATCC® 51573™) were purchased from American Type Culture Collection (Manassas, VA, United States) (Supplementary Table S1). G. metallireducens was routinely cultured in the anaerobic NB medium (0.38 g/L KCl, 0.2 g/L NH4Cl, 0.069 g/L NaH2PO4·H2O, 0.04 g/L CaCl2·2H2O, 0.2 g/L MgSO4· 7H2O, 1% [vol/vol] trace mineral mix) with 20 mM acetate as the electron donor and 55 mM Fe(III)-citrate as the electron acceptor. The pH was adjusted to 6.8 with 2 g/L NaHCO3 and degassed with 80% N2 and 20% CO2 (Levar et al., 2017). G. sulfurreducens was also routinely cultured in the anaerobic NB medium with 20 mM acetate and 40 mM fumarate as the sole electron donor and receptor, respectively.
The gene-deletion mutants were constructed by following the procedures described previously (Coppi et al., 2001; Marx and Lidstrom, 2002; Lloyd et al., 2003; Liu et al., 2014, 2015). All constructed mutants were verified by PCR and DNA sequencing. To complement the mutants, the respective genes were cloned separately into the plasmid pBBR1MCS-5 that was maintained in Escherichia coli DH5α (Kovach et al., 1995) (Supplementary Tables S1, S2). The constructs were verified by DNA sequencing and then transformed into their respective mutants. As controls, the mutants were also transformed with empty vector pBBR1MCS-5. All gene-deletion mutants and plasmid constructs made and the oligonucleotide primers used in this investigation are listed in Supplementary Tables S1–S3, respectively.
All constructed mutants were tested for their ability to grow with fumarate as the terminal electron acceptor and to reduce Fe(III)-citrate and ferrihydrite. Fumarate and Fe(III)-citrate were purchased from Sinopharm Chemical Reagent Co., Ltd. (Shanghai, China). Ferrihydrite was prepared and characterized as described before (Schwertmann and Cornell, 2000; Guo et al., 2022). Growth with fumarate and reductions of Fe(III)-citrate and ferrihydrite were carried out by following the previously established procedures (Liu et al., 2014, 2015).
All constructed mutants were also tested for their ability to form biofilms and to reduce anodes. The procedures for measuring biofilm formation with crystal violet staining and electricity production by monitoring output voltages from microbial fuel cells (MFC) were described before (Wang et al., 2023). Briefly, MFCs of dual-chambers were used in this study. PS2024V multi-channel data acquisition unit (SMACQ, Beijing, China) was used to monitor bacterial growth on the anodes and electricity outputs. At the end of experiments, a Leica TCS SP8 MP multiphoton confocal microscope (Wetzlar, Germany) was used to examine the biofilms formed on the anodes.
All experimental procedures were carried in an anaerobic chamber (Coy Laboratory Products, Inc., Grass Lake, MI, United States) with 80% N2 and 20% CO2.
Co-culture. The wild-type (WT) of G. sulfurreducens and its mutants were co-cultured with G. metallireducens in the anaerobic NB medium with 20 mM ethanol as the electron donor and 40 mM fumarate as the terminal electron acceptor (Summers et al., 2010). At the beginning of the first generation of co-culture, the cell densities used were 3.9 × 105 cells/mL for G. metallireducens and 7.7 × 105 cells/mL for the WT and mutants of G. sulfurreducens. Exponential phase of the cells from the first generation of co-cultures were used for the second generation of co-culture at initial cell densities of ~2.5 × 106 copies/mL Geobacter 16S RNA genes. The levels of ethanol, fumarate, malate and succinate during co-culture were monitored with a LC-20A high performance liquid chromatography (HPLC) that was equipped with a SPD-M20A UV detector and a RID-20A high-sensitivity refractive index detector (Shimadzu, Kyoto, Japan). Supplementary Figure S1 shows metabolisms of ethanol, fumarate, malate and succinate between these Geobacter species during co-culture. The chemical compounds were separated with an Aminex NPX-87H column (Bio-Rad Laboratories, Shanghai, China) (Summers et al., 2010; Shi et al., 2022). Ethanol, malate and succinate were purchased from Sinopharm Chemical Reagent Co., Ltd. Quantitative PCR (qPCR) was used to monitor bacterial growth during co-culture (QuantStudio3, Thermo Fisher Scientific China Co., Ltd., Shanghai, China) (Summers et al., 2010). The plasmids and the oligonucleotide primers used in qPCR are listed in Supplementary Tables S1, S2.
All values are expressed as means ± standard deviations. Student’s t test was used for comparing groups.
To investigate their roles in EET, the genes for PilA-N, OmcE, OmcS, OmcT and OmcZ of G. sulfurreducens were systematically deleted. A total of nine gene-deletion mutants were constructed in this investigation, which included five single-gene-deletion mutants ΔomcS, ΔomcT, ΔomcZ, ΔomcE and ΔpilA-N; a double-gene-deletion mutant ΔomcSΔomcT; a triple-gene-deletion mutant ΔomcSΔomcTΔomcZ; a quadruple-gene-deletion mutant ΔomcSΔomcTΔomcZΔomcE and a mutant defective in all investigated genes (i.e., ΔomcSΔomcTΔomcZΔomcEΔpilA-N) (Supplementary Table S1).
All constructed mutants were tested for their ability to grow with fumarate and to reduce Fe(III)-citrate and ferrihydrite. Similar to the WT of G. sulfurreducens, all mutants showed no apparent deficiency in growth on fumarate (Figure 1A) or Fe(III)-citrate reduction (Figure 1B). However, all mutant displayed diminished ability to reduce ferrihydrite under the condition tested, as compared to that of WT (Figure 1C). At 15 days after reduction of ferrihydrite, the amounts of Fe(II) formed by all tested mutants were significantly lower than that by WT (Figure 1C; Supplementary Table S4). Furthermore, the more gene deleted, the less Fe(II) formed (Figure 1C; Supplementary Table S4).
Figure 1. Growth with fumarate and reductions of Fe(III)-citrate and ferrihydrite by the constructed mutants of Geobacter sulfurreducens. (A) Growth with fumarate as the sole terminal electron acceptor. (B) Fe(III)-citrate reduction. (C) Ferrihydrite reduction over 15 days. (D) Complement of single-gene-deletion mutants for ferrihydrite reduction. The reductions were carried over 15 days. The shown are those at 15 days after reduction. WT, wild-type of G. sulfurreducens; Δ1, ΔomcS; Δ2, ΔomcT; Δ3, ΔomcE; Δ4, ΔomcZ; Δ5, ΔpilA-N; Δ6, ΔomcSΔomcT; Δ7, ΔomcSΔomcTΔomcZ; Δ8, ΔomcSΔomcTΔomcZΔomcE; Δ9, ΔomcSΔomcTΔomcZΔomcEΔpilA-N; NC, no cell control. The values plotted at each time point are the average OD600 (A) and 0.5 N HCl extractable Fe(II) (B,D) measured for each strain (n = 3), respectively, and error bars are standard deviations. For points with no error bar, the error was smaller than the size of the symbol. In (D), the results are reported as the percentage of activity relative to that observed with PCA + empty vector and standard error of the mean (n = 3). Student’s t test was used for comparing complement strains and their respective control groups. ***, p ≤ 0.001.
The single-gene-deletion mutants were complemented with their respective genes. Empty vector was also introduced into these mutants and WT as controls. The complement and control strains were all tested for ferrihydrite reduction over 15 days. Figure 1D shows the results at 15 days after reduction. Compared to those with empty vector, the single-gene-deletion mutants complemented with their respective gene all showed improved ability to reduce ferrihydrite, which indicated that the gene deletions were nonpolar.
Compared to WT, all mutants exhibited reduced ability to form biofilms on the cell culture plates that were not electrically conductive over 72 h after incubation (Figure 2A). At 72 h after incubation, the absorbance of crystal violet (OD565) extracted from stained biofilms grown on non-conductive surfaces by the WT was higher than that of all tested mutants. Furthermore, the OD565 of ΔpilA-N was lower than that of ΔomcS, ΔomcT, ΔomcZ, ΔomcE or ΔomcSΔomcT, but was similar to that of ΔomcSΔomcTΔomcZ or ΔomcSΔomcTΔomcZΔomcE (Figure 2A; Supplementary Table S5). All mutants also exhibited reduced ability to grow on the anode surfaces, as compared to WT. Notably, ΔomcS grew very slowly at beginning, as measured by its output voltage on anodes. At 60 h after growth, its output voltage increased substantially and then plateaued at 85 h after growth (Figure 2B). At 110 h after growth, the measured maximum output currents of ΔomcS and ΔomcE on anodes were similar to that of WT, but significantly higher than that of the rest of mutants (Figure 2C; Supplementary Table S6). Additionally, the maximum output current of ΔpilA-N was lower than that of ΔomcT, ΔomcZ and ΔomcSΔomcT, but was similar to that of other mutants deficient in >2 genes (Figure 2C; Supplementary Table S6). Similar phenomena were also observed on the ability of WT and tested mutants to form biofilms on the anodes (Figure 2D; Supplementary Figure S2).
Figure 2. Biofilm formation and anode reduction by the constructed mutants of Geobacter sulfurreducens. (A) Absorbance of crystal violet (OD565) extracted from stained biofilms grown on non-conductive surfaces (n = 3). (B). Output voltage of bacterial strains grown on anodes over 110 h. The values plotted at each time point are the average output voltage measured for each strain from triplicate assays and error bars are standard deviations. For points with no error bar, the error was smaller than the size of the symbol. (C) Maximum output current of bacterial strains grown on anodes at 110 h (n = 3). (D). Bacterial protein contents on anodes at 110 h after growth (n = 3). WT, wild-type of G. sulfurreducens; Δ1, ΔomcS; Δ2, ΔomcT; Δ3, ΔomcE; Δ4, ΔomcZ; Δ5, ΔpilA-N; Δ6, ΔomcSΔomcT; Δ7, ΔomcSΔomcTΔomcZ; Δ8, ΔomcSΔomcTΔomcZΔomcE; Δ9, ΔomcSΔomcTΔomcZΔomcEΔpilA-N. In (A,C,D), Student’s t test was used for comparing WT and the mutants. ns, p > 0.05; *, p ≤ 0.05; **, p ≤ 0.01; ***, p ≤ 0.001.
During the first and second generations of the co-cultures between G. metallireducens and the WT or gene-deletion mutants of G.sulfurreducens, substantial differences in the metabolisms of ethanol, fumarate, malate and succinate as well as the copy numbers of combined bacterial 16S rRNA genes were detected between WT and other mutants, with the exception of ΔomcE. Compared to that of the first generation (Supplementary Figures S3A–E), these differences between that of WT and ΔomcE and that of other mutants were much more pronounced in the second generation of the co-cultures (Figures 3A–E). Furthermore, these differences in decreases of ethanol (Figure 3A) and fumarate (Figure 3B), changes of malate (Figure 3C) and increases of succinate (Figure 3D) and the copy numbers of combined 16S rRNA genes (Figure 3E) were similar with each other. The maximum copy numbers of combined 16S rRNA genes detected at 35 days after the second generation of co-cultures between G. metallireducens and the WT were similar to that between G. metallireducens and ΔomcE, but were much higher than that between G. metallireducens and the rest of mutants (Figure 3E; Supplementary Table S7). In latter, the more genes deleted; the less copy numbers of combined 16S rRNA genes detected (Figure 3E; Supplementary Table S6).Similar phenomena were also observed in the levels of succinate detected in 35 days after the second generation of co-cultures (Figure 3D).
Figure 3. The second generation of co-cultures in the presence of hybL gene of Geobacter sulfurreducens over 35 days. The values plotted at each time point are the average ethanol (A), fumarate (B), malate (C) and succinate (D) measured for each strain (n = 3), respectively, and error bars are standard deviations. For points with no error bar, the error was smaller than the size of the symbol. (E) The copies of combined 16S rRNA genes of Geobacter metallireducens (Gme) and G. sulfurreducens (Gsu) (n = 3). The predetermined time points for sample collections in (E) are the same to those in (A–D). WT, wild-type of G. sulfurreducens or G. metallireducens; Δ1, ΔomcS of G. sulfurreducens; Δ2, ΔomcT of G. sulfurreducens; Δ3, ΔomcE of G. sulfurreducens; Δ4, ΔomcZ of G. sulfurreducens; Δ5, ΔpilA-N of G. sulfurreducens; Δ6, ΔomcSΔomcT of G. sulfurreducens; Δ7, ΔomcSΔomcTΔomcZ of G. sulfurreducens; Δ8, ΔomcSΔomcTΔomcZΔomcE of G. sulfurreducens; Δ9, ΔomcSΔomcTΔomcZΔomcEΔpilA-N of G. sulfurreducens. In (E), Student’s t test was used for comparing the maximum copies of combined 16S rRNA genes of Gme and Gus from WT and that of the mutants. ns, p > 0.05; ***, p ≤ 0.001.
Previous results suggested the involvement of H2 in the interspecies electron transfer during the co-culture of G. metallireducens and G. sulfurreducens as deletion of the hydrogenase gene hybL of G. sulfurreducens improved the bacterial ability to form culture with G. metallireducens (Summers et al., 2010). To investigate the roles of pilA-N, omcE, omcS, omcT and omcZ of G. sulfurreducens in the interspecies electron transfer during the co-culture with G. metallireducens in the absence of H2-mediated interspecies electron transfer, we further deleted the hybL gene in the mutants described above (Supplementary Table S1). No apparent differences between the ΔhybL and the mutants without hybL were observed in their ability to grow with fumarate and to reduce Fe(III)-citrate (Supplementary Figures S4A,B). Similar to previous observations (Summers et al., 2010), deletion of hybL increased the growth of the first and second generations of co-culture between ΔhybL of G. sulfurreducens and G. metallireducens (Supplementary Figures S5A–E; Figures 4A–E). In the absence of hybL, differences between ΔhybL and all the mutants tested were observed in the metabolisms of ethanol, fumarate, malate and succinate as well as the maximum copy numbers of combined bacterial 16S rRNA genes during the first and second generations of the co-culture (Supplementary Figures S5A–E; Figures 4A–E). In 35 days after the second generation of co-culture, no or little growth was observed between ΔomcZ/ΔhybL, ΔpilA-N/ΔhybL, ΔomcSΔomcT/ΔhybL, ΔomcSΔomcTΔomcZ/ΔhybL, ΔomcSΔomcTΔomcZΔomcE/ΔhybL or ΔomcSΔomcTΔomcZΔomcEΔpilA-N/ΔhybL and G. metallireducens. Furthermore, the maximum copy numbers of combined 16S rRNA genes detected in the second generation of co-cultures decreased in the order of ΔhybL > omcE/ΔhybL > ΔomcT/ΔhybL > ΔomcS /ΔhybL, which showed that omcE/ΔhybL displayed a modest decrease (p < 0.001) in its ability to form co-culture with G. metallireducens as compared to that of ΔhybL (Figure 4E; Supplementary Table S8).
Figure 4. The second generation of co-cultures in the absence of hybL gene of Geobacter sulfurreducens over 35 days. The values plotted at each time point are the average ethanol (A), fumarate (B), malate (C) and succinate (D) measured for each strain (n = 3), respectively, and error bars are standard deviations. For points with no error bar, the error was smaller than the size of the symbol. (E) The copies of combined 16S rRNA genes of Geobacter metallireducens (Gme) and G. sulfurreducens (Gsu) (n = 3). The predetermined time points for sample collections in (E) are the same to those in (A–D). WT, wild-type of G. metallireducens; ΔhybL, ΔhybL of G. sulfurreducens; Δ1/ΔhybL, ΔomcS of ΔhybL; Δ2/ΔhybL, ΔomcT of ΔhybL; Δ3/ΔhybL, ΔomcE of ΔhybL; Δ4/ΔhybL, ΔomcZ of ΔhybL; Δ5/ΔhybL, ΔpilA-N of ΔhybL; Δ6/ΔhybL, ΔomcSΔomcT of ΔhybL; Δ7/ΔhybL, ΔomcSΔomcTΔomcZ of ΔhybL; Δ8/ΔhybL, ΔomcSΔomcTΔomcZΔomcE of ΔhybL; Δ9/ΔhybL, ΔomcSΔomcTΔomcZΔomcEΔpilA-N of ΔhybL. In (A,D,E) student’s t tests were used for comparing the ethanol and succinate levels at 21 days after growth between the co-culture of Gme and Gus from ΔhybL and that of ΔomcE/ΔhybL (A,D) and the maximum copies of combined 16S rRNA genes of Gme and Gus from ΔhybL and that of other mutants without hybL (E). **, p ≤ 0.01; ***, p ≤ 0.001.
Deletions of pilA-N, omcE, omcS, omcT or/and omcZ of G. sulfurreducens had no impact on the bacterial growth on fumarate and reduction of Fe(III)-citrate, which are consistent with previous observations (Mehta et al., 2005; Reguera et al., 2005; Nevin et al., 2009; Summers et al., 2010). Previous results also showed the involvements of the pilA-N, omcE, omcS and omcT in reduction of solid-phase Fe(III) oxide (Mehta et al., 2005; Reguera et al., 2005). Consistent with these previous results, the ΔpilA-N, ΔomcE, ΔomcS, and ΔomcT prepared in this investigation showed the impaired ability to reduce solid-phase ferrihydrite under the condition tested, as compared to the WT. However, it should be noted that omcE was only involved in the initial stage of Fe(III) oxide reduction and the role of omcT in Fe(III) oxide reduction was via omcS (Mehta et al., 2005). Furthermore, our results also showed the diminished ability of omcZ in ferrihydrite reduction under the conditions tested. This was different from previous results showing no involvement of omcZ in reduction of Fe(III) oxides (Nevin et al., 2009). Although it remains unclear, this apparent discrepancy maybe attributed to the different bacterial strains and experimental conditions used. Compared to that of single-gene-deletion mutants, the abilities to reduce ferrihydrite of the mutants deficient in more than one genes were lower. Deletion of all these genes nearly abolished the bacterial ability to reduce ferrihydrite, which demonstrate the essential roles of these proteins in extracellular reduction of ferrihydrite by G. sulfurreducens.
Involvement of pilA-N in biofilm formation on nonconductive surface by G. sulfurreducens was demonstrated previously (Reguera et al., 2007; Richter et al., 2009, 2012). Over expressions of pilA-N, omcS, omcT, omcZ or omcS and omcT also increased biofilm formation on non-conductive surface (Wang et al., 2023). Our results were consistent with these previous observations. They also showed the involvements of OmcE in biofilm formation on non-conductive surface. Thus, PilA-N and all tested c-Cyts have roles in biofilm formation on nonconductive surface. Notably, the ability to form biofilm on non-conductive surface by ΔpilA-N was lower than that of ΔomcE, ΔomcS, ΔomcT, ΔomcZ and ΔomcSΔomcT, which is consistent with the proposed roles of PilA-N in extracellular secretions of OmcS and OmcZ (Gu et al., 2021).
Previous results showed that deletions of pilA-N or omcZ of G. sulfurreducens severely diminished bacterial ability to produce electricity on anodes, while deletions of omcS or omcE only slightly decreased bacterial ability to produce electricity (Nevin et al., 2009; Richter et al., 2009). Over expressions of pilA-N, omcS, omcT, omcZ or omcS and omcT also increased electricity production on anodes (Wang et al., 2023). Our results not only were consistent with these observations, but also showed that the output voltage on anodes by ΔomcS during first 60-h growth on anodes was trivial, which show the importance of OmcS in the early stage of growth on anodes. After that, the output voltage on anodes by ΔomcS increased rapidly and then plateaued at 85-h after growth, which suggest that ΔomcS may regain its ability to grow on anodes though compensatory roles of other multiheme c-Cyts, such as OmcZ (Yalcin et al., 2020; Yalcin and Malvankar, 2020). Deletion of omcT also severely diminished bacterial ability to produce electricity. Notably, the electricity production by ΔpilA-N was similar to that of ΔomcSΔomcTΔomcZ, but lower than that of ΔomcT, ΔomcZ and ΔomcSΔomcT, which demonstrate a more dominant role of PilA-N in electricity production than that OmcZ as well as that of OmcS and OmcT combined. The observed dominant roles of PilA-N in electricity production and biofilm formation on nonconductive surface are consistent with its proposed function in extracellular translocation of OmcS and OmcZ across the outer membrane (Gu et al., 2021). Finally, deletion of pilA-N and all tested c-Cyt genes nearly abolished bacterial ability to produce electricity and to form biofilm on anodes, which also show the essential roles of PilA-N and tested c-Cyts in extracellular respiration of anodes.
Previous results showed the involvements of pilA-N and omcS of G. sulfurreducens in the co-culture of G. metallireducens and G. sulfurreducens and growth improvements of the co-culture by deleting hybL gene G. sulfurreducens (Summers et al., 2010). Our results are consistent with these results. Additionally, our results also showed that compared to that of WT and ΔhybL, ability of the ΔomcT and ΔomcZ with or without hybL to form the co-culture with G. metallireducens was impaired, which show for the first time the involvements of OmcT and OmcZ in the DIET from G. metallireducens to G. sulfurreducens. Although ΔomcE exhibited similar ability of co-culture with G. metallireducens to that of WT, ΔomcEΔhybL showed slightly decreased ability (p ≤ 0.001) to co-culture with G. metallireducens as compared to that ΔhybL, which also demonstrate for the first time the involvement of OmcE in the DIET from G. metallireducens to G. sulfurreducens.
In the presence of hybL, deletions of any single c-Cyt gene or both omcS and omcT did not abolish bacterial ability to co-culture with G. metallireducens. Deletion of all omcS, omcT and omcZ nearly abolished the ability to co-culture. However, in the absence of hybL, deletion of pilA-N, omcZ or both omcS and omcT abolished the ability to co-culture, which clearly demonstrate the essential role of PilA-N, OmcZ and both OmcS and OmcT in the DIET from G. metallireducens to G. sulfurreducens. Requirement of both OmcS and OmcT in the DIET from G. metallireducens to G. sulfurreducens suggests an overlapping role of these two multiheme c-Cyts in the DIET. Finally, our results also show that H2-mediated indirect electron transfer may overshadow the roles of c-Cyts in the DIET from G. metallireducens to G. sulfurreducens.
Our results show the importance of PilA-N, OmcE, OmcS, OmcT and OmcZ of G. sulfurreducens in biofilm formation on nonconductive surface, extracellular reduction of ferrihydrite and anodes as well as the DIET from G. metallireducens to G. sulfurreducens. However, substantial differences were observed in the contributing roles of these extracellular proteins for G. sulfurreducens in these processes. Although it plays significant roles in ferrihydrite reduction and biofilm formation on nonconductive surface, OmcE only plays minor roles in anode reduction and the DIET from G. metallireducens to G. sulfurreducens. Different from OmcE, OmcS plays a crucial role in ferrihydrite reduction, the early stage of anode reduction, biofilm formation on nonconductive surface and the DIET from G. metallireducens to G. sulfurreducens. But, its role in the late stage of anode reduction is dispensable. PilA-N, OmcT and OmcZ, however, all play critical roles in reduction of ferrihydrite and anodes, biofilm formation on nonconductive surface and the DIET from G. metallireducens to G. sulfurreducens. The results from this investigation suggest that possession of PilA-N and multiple c-Cyts of different structures enables G. sulfurreducens to mediate EET efficiently with substrates of different properties. One of future researches should focus on whether these tested extracellular proteins form conductive filaments under the conditions investigated in this study.
The original contributions presented in the study are included in the article/Supplementary material, further inquiries can be directed to the corresponding author.
LS designed the experiment and acquired funding. JJ, PH, and YL performed the experiment. ZP and LQ developed experimental method. YJ, YH, XD, YD, and LS analyzed the data and prepared manuscript. All authors contributed to the article and approved the submitted version.
This research was supported by The National Key Research and Development Program of China (2018YFA0901303), National Natural Science Foundation of China (NSFC91851211, 42272353) and the Fundamental Research Funds for the Universities of Chinese Central Government, China University of Geosciences-Wuhan (122-G1323533057).
The authors declare that the research was conducted in the absence of any commercial or financial relationships that could be construed as a potential conflict of interest.
All claims expressed in this article are solely those of the authors and do not necessarily represent those of their affiliated organizations, or those of the publisher, the editors and the reviewers. Any product that may be evaluated in this article, or claim that may be made by its manufacturer, is not guaranteed or endorsed by the publisher.
The Supplementary material for this article can be found online at: https://www.frontiersin.org/articles/10.3389/fmicb.2023.1251346/full#supplementary-material
Bond, D. R., and Lovley, D. R. (2003). Electricity production by Geobacter sulfurreducens attached to electrodes. Appl. Environ. Microbiol. 69, 1548–1555. doi: 10.1128/AEM.69.3.1548-1555.2003
Caccavo, F., Lonergan, D. J., Lovley, D. R., Davis, M., Stolz, J. F., and Mcinerney, M. J. (1994). Geobacter sulfurreducens sp. nov., a hydrogen-and acetate-oxidizing dissimilatory metal-reducing microorganism. Appl. Environ. Microbiol. 60, 3752–3759. doi: 10.1128/aem.60.10.3752-3759.1994
Coppi, M. V., Leang, C., Sandler, S. J., and Lovley, D. R. (2001). Development of a genetic system for Geobacter sulfurreducens. Appl. Environ. Microbiol. 67, 3180–3187. doi: 10.1128/AEM.67.7.3180-3187.2001
Filman, D. J., Marino, S. F., Ward, J. E., Yang, L., Mester, Z., Bullitt, E., et al. (2019). Cryo-EM reveals the structural basis of long-range electron transport in a cytochrome-based bacterial nanowire. Commun. Biol. 2:219. doi: 10.1038/s42003-019-0448-9
Gu, Y., Guberman-Pfeffer, M. J., Srikanth, V., Shen, C., Giska, F., Gupta, K., et al. (2023). Structure of Geobacter cytochrome OmcZ identifies mechanism of nanowire assembly and conductivity. Nat. Microbiol. 8, 284–298. doi: 10.1038/s41564-022-01315-5
Gu, Y., Srikanth, V., Salazar-Morales, A. I., Jain, R., O’brien, J. P., Yi, S. M., et al. (2021). Structure of Geobacter pili reveals secretory rather than nanowire behaviour. Nature 597, 430–434. doi: 10.1038/s41586-021-03857-w
Guo, J., Jiang, Y., Hu, Y., Jiang, Z., Dong, Y., and Shi, L. (2022). The roles of DmsEFAB and MtrCAB in extracellular reduction of iodate by Shewanella oneidensis MR-1 with lactate as the sole electron donor. Environ. Microbiol. 24, 5039–5050. doi: 10.1111/1462-2920.16130
Inoue, K., Leang, C., Franks, A. E., Woodard, T. L., Nevin, K. P., and Lovley, D. R. (2011). Specific localization of the c-type cytochrome OmcZ at the anode surface in current-producing biofilms of Geobacter sulfurreducens. Environ. Microbiol. Rep. 3, 211–217. doi: 10.1111/j.1758-2229.2010.00210.x
Kovach, M. E., Elzer, P. H., Hill, D. S., Robertson, G. T., Farris, M. A., Roop, R. M. 2nd, et al. (1995). Four new derivatives of the broad-host-range cloning vector pBBR1MCS, carrying different antibiotic-resistance cassettes. Gene 166, 175–176. doi: 10.1016/0378-1119(95)00584-1
Levar, C. E., Hoffman, C. L., Dunshee, A. J., Toner, B. M., and Bond, D. R. (2017). Redox potential as a master variable controlling pathways of metal reduction by Geobacter sulfurreducens. ISME J. 11, 741–752. doi: 10.1038/ismej.2016.146
Liu, Y., Fredrickson, J. K., Zachara, J. M., and Shi, L. (2015). Direct involvement of ombB, omaB, and omcB genes in extracellular reduction of Fe(III) by Geobacter sulfurreducens PCA. Front. Microbiol. 6:1075. doi: 10.3389/fmicb.2015.01075
Liu, Y., Wang, Z., Liu, J., Levar, C., Edwards, M. J., Babauta, J. T., et al. (2014). A trans-outer membrane porin-cytochrome protein complex for extracellular electron transfer by Geobacter sulfurreducens PCA. Environ. Microbiol. Rep. 6, 776–785. doi: 10.1111/1758-2229.12204
Lloyd, J. R., Leang, C., Hodges Myerson, A. L., Coppi, M. V., Cuifo, S., Methe, B., et al. (2003). Biochemical and genetic characterization of PpcA, a periplasmic c-type cytochrome in Geobacter sulfurreducens. Biochem. J. 369, 153–161. doi: 10.1042/bj20020597
Lovley, D. R., Holmes, D. E., and Nevin, K. P. (2004). Dissimilatory Fe(III) and Mn(IV) reduction. Adv. Microb. Physiol. 49, 219–286. doi: 10.1016/S0065-2911(04)49005-5
Marx, C. J., and Lidstrom, M. E. (2002). Broad-host-range cre-lox system for antibiotic marker recycling in gram-negative bacteria. BioTechniques 33, 1062–1067. doi: 10.2144/02335rr01
Mehta, T., Coppi, M. V., Childers, S. E., and Lovley, D. R. (2005). Outer membrane c-type cytochromes required for Fe(III) and Mn(IV) oxide reduction in Geobacter sulfurreducens. Appl. Environ. Microbiol. 71, 8634–8641. doi: 10.1128/AEM.71.12.8634-8641.2005
Nevin, K. P., Kim, B. C., Glaven, R. H., Johnson, J. P., Woodard, T. L., Methe, B. A., et al. (2009). Anode biofilm transcriptomics reveals outer surface components essential for high density current production in Geobacter sulfurreducens fuel cells. PLoS One 4:e5628. doi: 10.1371/journal.pone.0005628
Reguera, G., Mccarthy, K. D., Mehta, T., Nicoll, J. S., Tuominen, M. T., and Lovley, D. R. (2005). Extracellular electron transfer via microbial nanowires. Nature 435, 1098–1101. doi: 10.1038/nature03661
Reguera, G., Nevin, K. P., Nicoll, J. S., Covalla, S. F., Woodard, T. L., and Lovley, D. R. (2006). Biofilm and nanowire production leads to increased current in Geobacter sulfurreducens fuel cells. Appl. Environ. Microbiol. 72, 7345–7348. doi: 10.1128/AEM.01444-06
Reguera, G., Pollina, R. B., Nicoll, J. S., and Lovley, D. R. (2007). Possible nonconductive role of Geobacter sulfurreducens pilus nanowires in biofilm formation. J. Bacteriol. 189, 2125–2127. doi: 10.1128/JB.01284-06
Richter, H., Nevin, K. P., Jia, H., Lowy, D. A., Lovley, D., and Tender, L. (2009). Cyclic voltammetry of biofilms of wild type and mutant Geobacter sulfurreducens on fuel cell anodes indicates possible roles of OmcB, OmcZ, type IV pili, and protons in extracellular electron transfer. Energy Environ. Sci. 2, 506–516. doi: 10.1039/b816647a
Richter, L. V., Sandler, S. J., and Weis, R. M. (2012). Two isoforms of Geobacter sulfurreducens PilA have distinct roles in pilus biogenesis, cytochrome localization, extracellular electron transfer, and biofilm formation. J. Bacteriol. 194, 2551–2563. doi: 10.1128/JB.06366-11
Schwertmann, U., and Cornell, R. M. (2000). Iron oxides in the laboratory: preparation and characterization : Wiley-VCH: Weinheim.
Shi, L., Squier, T. C., Zachara, J. M., and Fredrickson, J. K. (2007). Respiration of metal (hydr) oxides by Shewanella and Geobacter: a key role for multihaem c-type cytochromes. Mol. Microbiol. 65, 12–20. doi: 10.1111/j.1365-2958.2007.05783.x
Shi, X., Tremblay, P. L., Xue, M., Song, X., and Zhang, T. (2022). Fumarate disproportionation by Geobacter sulfurreducens and its involvement in biocorrosion and interspecies electron transfer. Sci. Total Environ. 827:154251. doi: 10.1016/j.scitotenv.2022.154251
Summers, Z. M., Fogarty, H. E., Leang, C., Franks, A. E., Malvankar, N. S., and Lovley, D. R. (2010). Direct exchange of electrons within aggregates of an evolved syntrophic coculture of anaerobic bacteria. Science 330, 1413–1415. doi: 10.1126/science.1196526
Wang, F., Chan, C. H., Suciu, V., Mustafa, K., Ammend, M., Si, D., et al. (2022a). Structure of Geobacter OmcZ filaments suggests extracellular cytochrome polymers evolved independently multiple times. elife 11:e81551. doi: 10.7554/eLife.81551
Wang, F., Craig, L., Liu, X., Rensing, C., and Egelman, E. H. (2022b). Microbial nanowires: type IV pili or cytochrome filaments? Trends Microbiol. 31, 384–392. doi: 10.1016/j.tim.2022.11.004
Wang, F., Gu, Y., O’brien, J. P., Yi, S. M., Yalcin, S. E., Srikanth, V., et al. (2019). Structure of microbial nanowires reveals stacked hemes that transport electrons over micrometers. Cells 177, 361–369 e310. doi: 10.1016/j.cell.2019.03.029
Wang, Z., Hu, Y., Dong, Y., Shi, L., and Jiang, Y. (2023). Enhancing eletrical outputs of the fuel cells with Geobacter sulferreducens by overexpressing nanowire proteins. Microb. Biotechnol. 16, 534–545. doi: 10.1111/1751-7915.14128
Wang, F., Mustafa, K., Suciu, V., Joshi, K., Chan, C. H., Choi, S., et al. (2022c). Cryo-EM structure of an extracellular Geobacter OmcE cytochrome filament reveals tetrahaem packing. Nat. Microbiol. 7, 1291–1300. doi: 10.1038/s41564-022-01159-z
Yalcin, S. E., and Malvankar, N. S. (2020). The blind men and the filament: understanding structures and functions of microbial nanowires. Curr. Opin. Chem. Biol. 59, 193–201. doi: 10.1016/j.cbpa.2020.08.004
Keywords: pilA-N , multiheme c-type cytochromes, extracellular electron transfer, biofilms, Geobacter metallireducens , Geobacter sulfurreducens
Citation: Jiang J, He P, Luo Y, Peng Z, Jiang Y, Hu Y, Qi L, Dong X, Dong Y and Shi L (2023) The varied roles of pilA-N, omcE, omcS, omcT, and omcZ in extracellular electron transfer by Geobacter sulfurreducens. Front. Microbiol. 14:1251346. doi: 10.3389/fmicb.2023.1251346
Received: 01 July 2023; Accepted: 25 September 2023;
Published: 10 October 2023.
Edited by:
Catarina M. Paquete, Universidade Nova de Lisboa, PortugalReviewed by:
Carlos Salgueiro, New University of Lisbon, PortugalCopyright © 2023 Jiang, He, Luo, Peng, Jiang, Hu, Qi, Dong, Dong and Shi. This is an open-access article distributed under the terms of the Creative Commons Attribution License (CC BY). The use, distribution or reproduction in other forums is permitted, provided the original author(s) and the copyright owner(s) are credited and that the original publication in this journal is cited, in accordance with accepted academic practice. No use, distribution or reproduction is permitted which does not comply with these terms.
*Correspondence: Liang Shi, bGlhbmcuc2hpQGN1Zy5lZHUuY24=
†These authors have contributed equally to this work
Disclaimer: All claims expressed in this article are solely those of the authors and do not necessarily represent those of their affiliated organizations, or those of the publisher, the editors and the reviewers. Any product that may be evaluated in this article or claim that may be made by its manufacturer is not guaranteed or endorsed by the publisher.
Research integrity at Frontiers
Learn more about the work of our research integrity team to safeguard the quality of each article we publish.