- 1Univ Brest, CNRS, IRD, Ifremer, LEMAR, Plouzané, France
- 2EPHE, PSL Research University, UPVD, CNRS, USR CRIOBE, Perpignan, France
- 3CNRS, UMR7144 Adaptation et Diversité en Milieu Marin, Ecology of Marine Plankton (ECOMAP), Station Biologique de Roscoff SBR, Sorbonne University, Roscoff, France
- 4Univ. Bordeaux, CNRS, Bordeaux INP, EPOC, UMR, Station Marine d’Arcachon, Arcachon, France
- 5Ifremer, ASIM Adaptation et Santé des Invertébrés Marins, La Tremblade, France
The parasitic species Perkinsus olseni (= atlanticus) (Perkinsea, Alveolata) infects a wide range of mollusc species and is responsible for mortality events and economic losses in the aquaculture industry and fisheries worldwide. Thus far, most studies conducted in this field have approached the problem from a “one parasite-one disease” perspective, notably with regards to commercially relevant clam species, while the impact of other Perkinsus species should also be considered as it could play a key role in the disease phenotype and dynamics. Co-infection of P. olseni and P. chesapeaki has already been sporadically described in Manila clam populations in Europe. Here, we describe for the first time the parasitic distribution of two Perkinsus species, P. olseni and P. chesapeaki, in individual clam organs and in five different locations across Arcachon Bay (France), using simultaneous in situ detection by quantitative PCR (qPCR) duplex methodology. We show that P. olseni single-infection largely dominated prevalence (46–84%) with high intensities of infection (7.2 to 8.5 log-nb of copies. g−1of wet tissue of Manila clam) depending on location, suggesting that infection is driven by the abiotic characteristics of stations and physiological states of the host. Conversely, single P. chesapeaki infections were observed in only two sampling stations, Ile aux Oiseaux and Gujan, with low prevalences 2 and 14%, respectively. Interestingly, the co-infection by both Perkinsus spp., ranging in prevalence from 12 to 34%, was distributed across four stations of Arcachon Bay, and was detected in one or two organs maximum. Within these co-infected organs, P. olseni largely dominated the global parasitic load. Hence, the co-infection dynamics between P. olseni and P. chesapeaki may rely on a facilitating role of P. olseni in developing a primary infection which in turn may help P. chesapeaki infect R. philippinarum as a reservoir for a preferred host. This ecological study demonstrates that the detection and quantification of both parasitic species, P. olseni and P. chesapeaki, is essential and timely in resolving cryptic infections and their consequences on individual hosts and clam populations.
1 Introduction
Co-infection by multiple parasites has become the rule rather than the exception since the revision of the gold-standard – but outdated – paradigm “one parasite-one disease” (Petney and Andrews, 1998). Deciphering these interactions is essential for understanding the dynamics and phenotypic outcomes of diseases (Paul-Pont et al., 2010; Vayssier-Taussat et al., 2014; Bass et al., 2019). Indeed, the prediction of co-infection outcomes is tricky because heterogeneous disease phenotypes can emerge with the amplification or the suppression of the weight of one or more parasite species (Johnson and Hoverman, 2012) by altering direct competition for resources or space (ecological interactions) or infectivity towards the host immune system (immunological interactions) (Cox, 2001; Graham, 2008). In medical and veterinary fields, co-occurrence of multiple pathogens has gained significant interest with reported studies on human (e.g., between different Plasmodium spp.: see McKenzie and Bossert, 1999) and animal health (e.g., multiple Borrelia species in ticks: Raileanu et al., 2017). However, in the field of marine protistology and microbial parasites, this concept represents a Pandora’s box that needs to be opened. One explanation for this lasting knowledge gap is the difficulty inherent in characterising these micro-parasitic organisms, which has often been limited to culture and microscopy techniques. The advent of molecular biology and environmental sequencing has revolutionised the concept of microbial diversity with the (re)-discovery and (re)-characterisation of many eukaryotic parasite species (Moon-van der Staay et al., 2001; López-García et al., 2003; Chambouvet et al., 2008). This has brought new insights in species definition and unravelled many cases of cryptic infectious agents (Putaporntip et al., 2009; Chambouvet et al., 2015), but the prevalence and severity of mortality events associated with these pathogens continue to increase worldwide due to global change, making it all the more urgent to tackle the fundamental questions regarding the role of multiple infections in the dynamics of aquatic disease in marine ecosystems (Harvell, 1999; Burge et al., 2014).
The marine parasite Perkinsus olseni (Perkinsea, Alveolata) infects commercially important bivalve (e.g., clams and oysters) and gastropod species (abalone) and is the aetiological agent of Perkinsosis disease (see review by Ruano et al., 2015). Heavily infected hosts might show the presence of milky white nodules in the gills, mantle, and foot, a disruption of connective tissue and epithelial cells, pale appearance of the digestive gland, reduction of the condition index, and severe emaciation, all of which could contribute to host death (Ruano et al., 2015). In Europe, P. olseni is regularly detected along the Atlantic and Mediterranean coasts and has been associated with high mortality rates, resulting in severe economic loss mainly in Portugal, Spain, and along the Mediterranean coast (Da Ros and Canzonier, 1985; Ruano and Cachola, 1986; Azevedo, 1989; Pretto et al., 2014). It has been hypothesised that P. olseni was accidentally co-introduced into European waters with its host the Manila clam (Ruditapes philippinarum), which was imported from Asia in the 1970s for aquaculture purposes (de Montaudouin et al., 2016a). This hypothesis is supported by the low genetic diversity observed in P. olseni in Europe compared to those collected in Japan and in New Zealand (Vilas et al., 2011). However, the situation may not be so clear-cut given recent findings that revealed P. olseni is not the only Perkinsus parasite detected in Europe. Indeed, along the Atlantic coast, this parasite co-infects in sympatry with a congeneric species, P. chesapeaki, in Ruditapes decussatus from Leucate lagoon, France (Arzul et al., 2012) and Manila clams, R. philippinarum, from Arcachon Bay (France) (Itoïz et al., 2021) and in Galicia (Spain) (Ramilo et al., 2016). The parasite P. chesapeaki was firstly detected in the soft-shell clam Mya arenaria from the Chesapeake Bay, U.S.A. (McLaughlin and Faisal, 2000). Since then, it has been detected in many other bivalves, e.g., Mercenaria mercenaria (Reece et al., 2008), Tagelus plebeius (Bushek et al., 2008) or Crassostrea rhizophorae (Dantas Neto et al., 2016), in South America, North America, Asia, and Europe (see review by Itoïz et al., 2022). This parasitic species has also been found in association with Perkinsus marinus in oysters and clams from the Chesapeake Bay (U.S.A.) (Reece et al., 2008). One hypothesis suggests that, much like P. olseni, P. chesapeaki was putatively and accidentally introduced to Europe through its hosts the soft-shell clam, Mya arenaria, or the hard clam, Mercenaria mercenaria from the U.S.A (Arzul et al., 2012). While no mortality event has thus far been associated with P. chesapeaki (e.g., Bushek et al., 2008; Carrasco et al., 2014), co-infection in sympatry by these congeneric species, P. olseni and P. chesapeaki, remains enigmatic according to the competitive exclusion principle (Gause, 1934) and warrants further explanations. Furthermore, Perkinsus species are not the only pathogens present in Arcachon Bay. Manila clams may also become infected by a virus-like agent Brown Muscle Disease (BMD) (Dang et al., 2008) and by Vibrio tapetis, the bacterial agent of Brown Ring Disease (BRD) (Paillard, 2004). Although these two infectious agents were regularly detected in Arcachon Bay, their prevalence remains quite low compared to those of Perkinsus spp. (de Montaudouin et al., 2010).
The aim of this study was to evaluate (1) the distribution of P. olseni and P. chesapeaki parasites across five contrasted sampling areas in Arcachon Bay, a lagoon where Perkinsus spp. is particularly prevalent and abundant (Dang et al., 2008; de Montaudouin et al., 2010); and (2) the occurrence of these two parasitic protists across each organ type of individual clams from the five sampling stations.
2 Materials and methods
2.1 Sampling site and strategy
Arcachon Bay (South West of France, Atlantic coast, 44°41′60” N; 1°10’W) is a mesotidal semi-sheltered lagoon of 180 km2. This marine system is under oceanic (Atlantic tidal regime) and freshwater influences (Leyre River flow) encompassed by a 280 km2 drainage basin. The exotic Manila clam, Ruditapes philippinarum was introduced in France in 1972 for aquaculture purpose (de Montaudouin et al., 2016a). Since then, Arcachon Bay clam populations ranked first in France in terms of biomass and exploitation, despite a significant lack of spat recruitment and growth (de Montaudouin et al., 2016b). Perkinsosis disease is regularly detected in local Manila clam populations in Arcachon Bay, but no associated mortality events have been reported so far (Dang et al., 2010a,b; de Montaudouin et al., 2016b). Sampling station localisation within the lagoon and some environmental parameters were determined for Andernos station exclusively by de Montaudouin et al. (2010) and at a higher scale by Binias et al. (2014). Here, we sampled 50 Manila clams on the 7th to 8th of November 2018 in five contrasted stations for which some environmental data were available (Dang et al., 2010a; Binias et al., 2014; Figure 1). Two stations (IAO and Piquey) are in “group 2” as defined by Binias et al. (2014), and qualified herein as “external.” This group gathered stations in oceanic position with high grain size median, high mean salinity, low organic matter and silt & clay contents (Supplementary Table S1). Piquey exhibits higher sediment grain size median than IAO. Andernos, Lanton and Gujan belong to “group 4” as defined by Binias et al. (2014), and qualified herein as “internal.” This group gathered stations undergoing lower mean salinity. In this group, Andernos differs from Gujan and Lanton by its coarser sediments (Table 1). All these stations are situated at a rather high tidal level (Table 1), and Piquey is the only one close to oyster parks and oyster reefs.
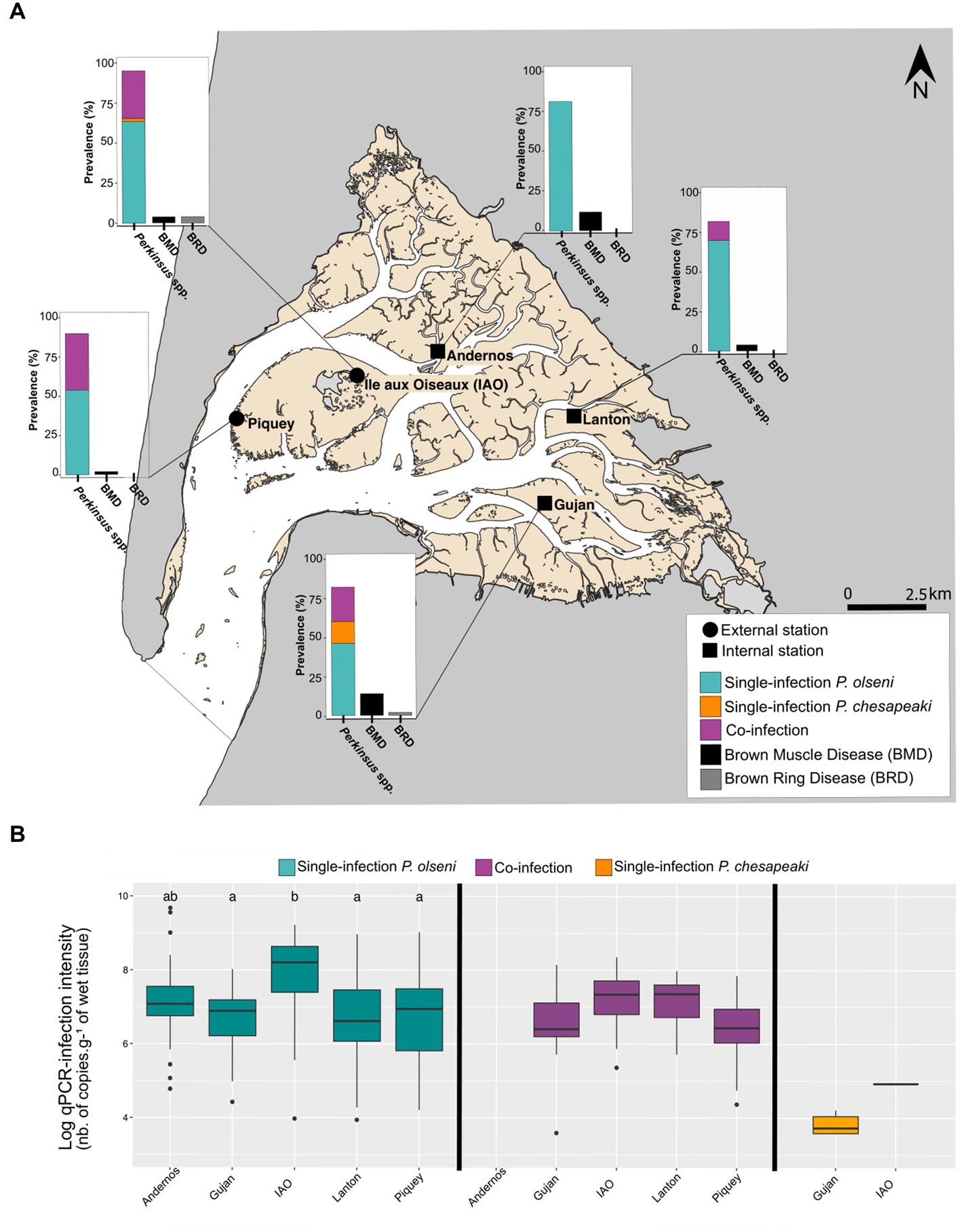
Figure 1. Distribution of infection and co-infection in whole clam body from five stations sampled in November 2018 in the Arcachon bay. (A) Prevalences of P. olseni single-infection, P. chesapeaki single-infection and (co-)infections determined by duplex qPCR with prevalences of Brown muscle disease (BMD) and Brown ring disease (BRD). Each prevalence was determined on a total of 50 clams per station. (B) Log-qPCR infection intensities estimated on the whole-body clam are represented for each type of infection: P. olseni single-infection, P. chesapeaki single-infection and co-infection. Differences are estimated by a pairwise Wilcoxon rank sum test. Differences between each parameter are represented by a and b.
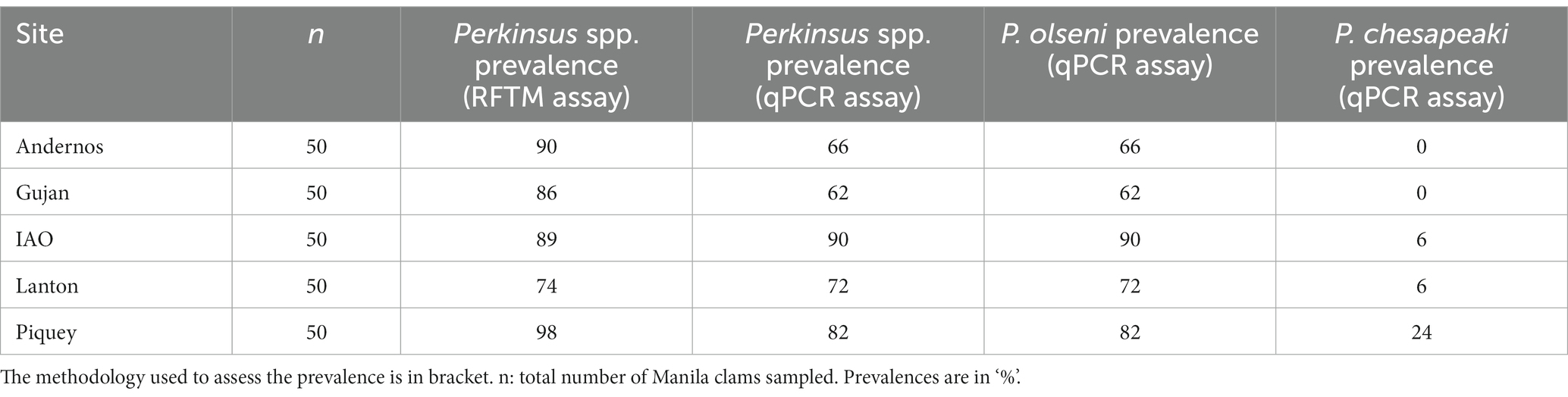
Table 1. Prevalence of Perkinsus spp., Perkinsus olseni and Perkinsus chesapeaki in gill tissue samples for each sampling site.
2.1.1 Manila clam biometry
The shell length of all clams was measured individually using a vernier calliper. Normality, homogeneity of variance (Shapiro–Wilk normality test) and one-way analysis of variance (ANOVA) were conducted in order to compare size distribution between sites, before multiple comparisons of means by Tukey test.
2.1.2 Sample preparation
Each clam was dissected on ice and each organ was weighted independently. For each individual, one gill was incubated in RFTM (Ray’s Fluid Thioglycolate Medium) supplemented with antibiotics (penicillin G, 1x105U.L−1 and streptomycin sulphate 0.1 g.L−1, Sigma) and antimycotic (nystatin 4 g.L−1) for Perkinsus spp. cells counting. The six other organs, including the second gill, the digestive gland, the mantle, the adductor muscle, the foot and the remaining tissue were fixed separately in 80% ethanol and stored at 4°C for molecular analysis.
2.1.3 Visual inspection of BRD and BMD infectious agents
During the dissection, other disease phenotypes associated with commonly detected infectious agents in Manila clams such as Brown Muscle Disease (BMD) or the Brown Ring Disease (BRD) were considered. Briefly, we checked the presence of necropsies of the posterior adductor muscle for the virus-like agent of BMD (Dang et al., 2008; Pierron et al., 2019) and the presence of a brown organic conchiolin deposit in the inner face of the valves for the Vibrio tapetis agent of BRD (see Paillard et al., 2006). Prevalence (in %) for both diseases was determined for each sampling station. Their distributions between stations were tested by Fisher’s exact test.
2.1.4 Perkinsus spp. intensity of infection in gill tissue samples
Infection intensity in gill tissue was determined using the Ray’s Fluid Thioglycollate Medium (RFTM) as recommended by the O.I.E. (Ray, 1952; Choi et al., 1989; OIE-Listed Diseases 2021: OIE – World Organisation for Animal Health, 2021). Briefly, gills were incubated in RFTM medium supplemented with antibiotics (penicillin G, 1×105 U.L−1 and streptomycin sulphate 0.1 g.L−1, Sigma) for 5 days in the dark at room temperature. This step allows for the transformation of trophozoites into enlarged and more visible hypnospores (Ray, 1952). To lyse the gill tissue, the RFTM was discarded following a 1,000 × g centrifugation and the remaining gills were digested with 2 M NaOH solution for 3 h at 60°C preserving the cell structure of Perkinsus hypnospores (Choi et al., 1989). Hypnospores were then recovered using 1,000 × g centrifugation for 10 min. The remaining pellet was washed twice in PBS and stained with a Lugol’s iodine solution (4%). The number of Perkinsus hypnospores per individual gill was assessed using an aliquot of 100 μL in a Nageotte chamber (10 lines counted in triplicate) under an optical microscope (Leica DM-IRB; x10 magnification). The counting results were expressed as the number of hypnospores per gram of wet tissue.
2.2 DNA extraction of clam tissue samples
The genomic DNA (gDNA) of the six organs (gill, digestive gland, mantle, adductor muscle, foot and the remaining tissue samples) was extracted using the CTAB-based DNA extraction method adapted from Winnepenninckx et al. (1993) described by Itoïz et al., 2021. Clam tissue samples were transferred in bead beating tubes containing three different sizes of beads (2.8 mm, 1.4 mm and 0.1 mm of diameter, Ozyme) with 1 mL of CTAB extraction buffer (2% CTAB, 100 mM TrisHCl pH = 8.0, 20 mM EDTA, 1.4 mM NaCl). Tissues were ground and homogenised following two bead beating cycles (45 s of bead beating at 6 m.s−1 followed by 20 s stop) in a cooling rack of the FastPrep-24 5G benchtop homogeniser (MP Biomedicals). β-mercaptoethanol (0.2%) and proteinase K (1 g.L−1) were then added to each tube and samples were incubated for 30 min at 60°C. Foot tissue samples were specifically incubated 12 h due to the tougher tissue structure. Lysates were mixed in chloroform/isoamylalcohol (24:1, v/v) and emulsified, then centrifuged at 18,000 × g for 10 min at 4°C. This step is repeated twice for foot tissue samples. Aqueous phases were treated with RNAse solution (10 g.L−1) (Sigma-Aldrich) for 30 min at 37°C prior to DNA precipitation with cold isopropanol overnight at 4°C. DNA was pelleted, rinsed twice with cold 70% ethanol, dried at room temperature and resuspended in 300 μL of pure molecular grade water (Corning). gDNA samples were quantified using the Qubit dsDNA HS assay kit (Invitrogen) and stored at −20°C until further processing.
2.3 Real-time qPCR duplex diagnostic assay for Perkinsus olseni and Perkinsus chesapeaki
Real-time qPCR duplex assays were carried out as described by Itoïz et al. (2021). Briefly, P. olseni and P. chesapeaki plasmidic-standards from 2.5×101 to 2.5×106 total copy number were used to calculate infection intensity. This standard-plasmidic material was previously used in Itoïz et al. (2021) allowing for identical qPCR parameters in this study (P. olseni: y = −3.33x + 39.49, efficiency (E) = 99.8%, limit of detection (LOD) = 2.5×101 total copies; P. chesapeaki: y = −3.38x + 40.29, E = 97.8%, LOD = 2.5×101 total copies).
Each organ gDNA was diluted following specific concentrations recommended in Itoïz et al. (2021) to avoid PCR inhibitors. Gill and digestive gland tissue samples were diluted to 20 ng.μL−1; adductor muscle and foot to 5 ng.μL−1; and, mantle and remaining tissue to 2 ng.μL−1. Real-time PCR was performed using the LightCycler 480 II (Roche) thermocycler using LightCycler 480 Probes Master (Roche) kit following the manufacturer’s recommendations. All probes and primers used in this study were described in Itoïz et al. (2021). Briefly, the reaction volume for duplex qPCR contained 1X LightCycler 480 Probes Master premixed, 0.2 μM of primer PolsITS2-F, 0.2 μM of PolsITS2-R, 0.2 μM of PchesITS2-F, 0.2 μM of PchesITS2-R, 0.5 μM of PolsITS2-probe (FAM), 0.5 μM of PchesITS2-probe (LC640), and 5 μL of DNA sample with adjusted concentration. The reaction volume for simplex qPCR contained 1X LightCycler 480 Probes Master premixed, 0.2 μM of primer PolsITS2-F or PchesITS2-F, 0.2 μM of PolsITS2-R or PchesITS2-R, 0.5 μM of PolsITS2-probe or PchesITS2-probe, 5 μL of DNA sample with adjusted concentration. The thermal cycling conditions were described in Itoïz et al. (2021). In each qPCR run, diluted gDNA samples, plasmidic-standards and two negative controls (non-infected Manila clam DNA and pure molecular grade water) were run. Ct value triplicates were averaged for downstream statistical analysis. If one or two of the triplicates were outliers, the sample was reprocessed to eliminate potential manipulation error. Each gDNA per organ was processed independently, allowing an estimation of concentrations of both parasites per organ. The whole-body concentration was calculated by summing the parasite concentrations for all organs per clam.
2.4 Statistical analysis of prevalence and infection intensities
2.4.1 Comparison of RFTM and qPCR methodologies on gill tissue samples
To confirm congruence between RFTM and qPCR methodologies, gill tissue samples were chosen to calculate the indicative parameters of prevalence, concordance, and infection intensity following the O.I.E. recommendations for Perkinsus spp. diagnosis (OIE-Listed Diseases 2021: OIE – World Organisation for Animal Health, 2021). Perkinsus prevalence (% of infected individuals out of the total number of hosts sampled) were plotted for both methods and single-or co-infection was represented when possible. The concordance and the discordance parameters, adapted from Langton et al. (2002), were calculated. The concordance, calculated by counting paired positive samples and paired negative samples, is the percentage of chance that an identical sample analysed by two different methodologies will yield the same result. The discordance, calculated by counting the unpaired samples (i.e., positive in RFTM and negative in qPCR assay, and vice versa), is the percentage of chance that an identical sample analysed by two different methodologies will yield different results. The Cohen’s Kappa (κ) coefficient was estimated to compare the level of agreement obtained between the real-time PCR and the RFTM assays (Pfeiffer, 2010). Finally, the relationship between both methods was tested using a linear model and the Spearman correlation coefficient on infection intensities established by qPCR and RFTM from paired-positive individuals.
The global prevalence and infection intensities of the Perkinsus genus were calculated on gill samples using standard RFTM methodology. The infection intensity means (nb of hypnospores. g−1 of wet gill) measured by the two methods were compared to each other by a non-parametric Kruskal-Wallis test followed by Dunn post-hoc test.
2.4.2 Distribution and infection intensity of two Perkinsus species in host tissue samples
Infection intensity, prevalence and tissue distribution of P. olseni and P. chesapeaki were estimated using the duplex qPCR methodology across six Manila clam organs: gills, digestive gland, adductor muscle, foot, mantle and the remaining tissue. Prevalences in each station were compared by Fisher’s exact test, adapted for small samples. Infection intensity means were evaluated in each station for each type of infection (P. olseni single-infection, P. chesapeaki single-infection, co-infection) and compared by a pairwise Wilcoxon rank sum test.
The distribution of single-and co-infected individuals in the Arcachon Bay was investigated using multivariate analysis on P. olseni and P. chesapeaki infection intensities in each organ. A principal component analysis (PCA) was performed using the FactomineR and Factoextra packages on R (Lê et al., 2008; Kassambara and Mundt, 2017). Extracted coordinates (axis 1; 2) of each individual were checked for significant differences among stations by non-parametric Kruskal-Wallis test in combination with multiple pairwise comparison using Dunn post-hoc test.
Repartition of both Perkinsus species across co-infected host organs (gills, digestive gland, adductor muscle, foot, mantle and the remaining tissue) was detailed in a visual matrix table. Global infection intensities (copy number.g−1 of wet tissue) for P. olseni and P. chesapeaki were log-transformed and classified in four levels represented with a colour gradient. Infection intensity data follows a Gaussian distribution, thus, the boundaries of the four categories were delimited by: minimum, 1st quartile, median, 3rd quartile and maximum values of infection intensity. Mean occupation of P. olseni and P. chesapeaki cells (in %) was represented for each type of co-infected organ sample from each station. Finally, P. olseni and P. chesapeaki log-transformed infection intensities from co-infected organs were plotted to investigate potential negative or positive influence of one species on the other and vice versa. Based on a reduced dataset restricted to co-infected organ samples, the relationship was tested using a linear model and Spearman correlation coefficient.
3 Results
3.1 Comparison of RFTM and qPCR assays for the detection of Perkinsus spp. in gills
The duplex qPCR assay developed by Itoïz et al. (2021) allowed simultaneous detection and quantification of both parasitic species, P. olseni and P. chesapeaki in the Manila clam (Table 1). We estimated the concordance and correlation between RFTM and duplex qPCR assays on gill tissue samples across all sampling stations (Supplementary Table S2). To guarantee an accurate representation of the qPCR detection, only Ct values within the standard range from 2.5 × 101 to 2.5 × 106 total copy number were considered as positive, as recommended by Itoïz et al. (2021).
Using RFTM and qPCR assays in gill tissue samples, the overall prevalence of Perkinsus spp. showed no significant differences between sampling stations (Chi-squared test: X2 = 4.14, df = 4, value of p > 0.05). Using RFTM assays, the prevalence of Perkinsus spp. ranged from 74% (Lanton) to 98% (Piquey) (Table 1). Using qPCR assays, prevalence for both Perkinsus species ranged from 62% (Gujan) to 90% (IAO). Concordances between RFTM and qPCR methodologies across all stations varied from 76% (Andernos and Gujan) to 86% (IAO and Lanton) (Supplementary Table S2). Overall, the mean concordance was 81.6 ± 5.2% (n = 5 stations).
Additionally, the κ-value was calculated to determine the level of agreement between each methodology for the gill samples (Supplementary Table S2). The κ values ranged from a slight agreement, 0.17 for Piquey, through moderate agreement, 0.35, 0.41 and 0.42 for Andernos, IAO and Gujan respectively, to substantial agreement, 0.62 for Lanton. The overall κ-value for all sampling stations was 0.42 indicating moderate agreement.
A linear regression was determined between for the qPCR-infection intensity and the RFTM-infection intensity (y = 0.56x + 4.34, y: log of qPCR-infection intensity in copy number.g of wet gill−1; x: log of RFTM-infection intensity in nb of cells.g of wet gill−1; adjusted-R2 = 0.41; n = 178) (Supplementary Figure S1). A significant positive correlation was determined using Pearson’s coefficient between the qPCR-infection intensity and the RFTM-infection intensity (r = 0.64, value of p < 0.001).
3.2 Prevalence and infection intensity of Perkinsus olseni and Perkinsus chesapeaki at the spatial scale: single-and co-infections
3.2.1 Diagnosis of Perkinsus in whole body samples
Global prevalence and infection intensities of single- and co-infected individuals across the Arcachon Bay were assessed by analysing the whole body of Manila clams using duplex qPCR methodology. In this study, a clam is qualified as “co-infected” when P. olseni and P. chesapeaki infect at least one of the six organs analysed.
The global prevalence of Perkinsus spp. in the whole body was 82% for Lanton and Gujan, 84% for Andernos, 90% for Piquey, and 96% for IAO (Figure 1A). Single-infection by P. olseni was detected in every station with prevalence ranging from 46% in Gujan (n = 23/50 clams) to 84% (n = 42/50 clams) in Andernos. Co-infection was detected in all stations except Andernos, with prevalence ranging from 12% (n = 6/50 clams) in Lanton to 36% (n = 17/50 clams) in Piquey. Surprisingly, single-infection by P. chesapeaki was rarely detected, with prevalence ranging from 2% (= 1/50 clams) in IAO to 14% (= 7/50 clams) in Gujan.
Infection intensities detected in the whole body showed differences particularly in P. olseni-single infections (Figure 1B). Samples from IAO had the highest mean intensity of infection with 8.5 log-nb. of copies. g−1 of wet tissue, whereas four other stations had mean intensity of infection varying from 7.2 log-nb. of copies. g−1 of wet tissue in Gujan to 8.4 log-nb. of copies. g−1 of wet tissue in Andernos (Wilcoxon rank sum test: value of p < 0.05; groups a and b; Figure 1B). For co-infected hosts, infection intensities between stations showed no difference (Wilcoxon rank sum test: value of p > 0.05; Figure 1B). The P. chesapeaki-single infection was of very low infection intensity with a mean of 3.8 log-nb. of copies. g−1 of wet tissue (i.e., 7.92 × 103 copy number.g−1 of wet tissue) in Gujan (Supplementary Table S3).
3.2.2 Diagnosis of BRD and BMD in Arcachon Bay
The clams were visually inspected during dissection to record the presence of symptoms associated with other diseases potentially detected in Arcachon Bay, such as BRD and BMD (Figure 1A). Brown organic conchiolin deposits classically identified as a BRD phenotype were only detected in Gujan and IAO with very low prevalence, 2 and 4%, respectively. The presence of necropsies of the posterior adductor muscle identified as a BMD phenotype was retrieved in all sampling sites with low to moderate prevalence ranging from 2% in Piquey to 14% in Gujan.
3.2.3 Spatial patterns of Perkinsus spp. infection
The results of the principal component analysis (PCA) performed on intensity of infection of Perkinsus species are presented in Figure 2. The axis 1, representing 24.6% of the total variance, was mainly explained by P. olseni infection intensities within the different organs, with contribution ranging from ~3.7% for foot to ~21.3% for mantle and gill (Figure 2A). The axis 2, representing 11.1% of the total variance, was mainly explained by P. chesapeaki infection intensities in all organs except gills. Indeed, the contribution of each organ ranged from ~0.1% to ~40.8% for foot to adductor muscle, respectively (Figure 2A). The ellipses of all sampling stations were not well separated along both axes (Figure 2A). Along axis 1, the intensity of infection by P. olseni in IAO sampling station was significantly different from those at Gujan, Lanton and Piquey stations. In addition, along the axis 2, the intensity of infection by P. chesapeaki in IAO sampling station was significantly different from those of other sampling stations (Figure 2B; Dunn post-hoc test: value of p < 0.01). Individual clams from Piquey station were characterised by high shell length (35.3 ± 2.7 mm; Tukey multiple comparison means test: value of p < 0.001) and the occurrence of P. chesapeaki in gill tissues (Figure 3 and Supplementary Figure S2). Other stations were characterised by smaller individual clam shell lengths (IAO 28.2 ± 2.1 mm and Lanton 29.3 ± 2.3 mm, compared to Andernos 31.9 ± 2.6 mm and Gujan 31.8 ± 5.27 mm; Tukey multiple comparison means test: value of p < 0.001) and P. chesapeaki being mainly localised in the adductor muscle (Figure 3 and Supplementary Figure S2).
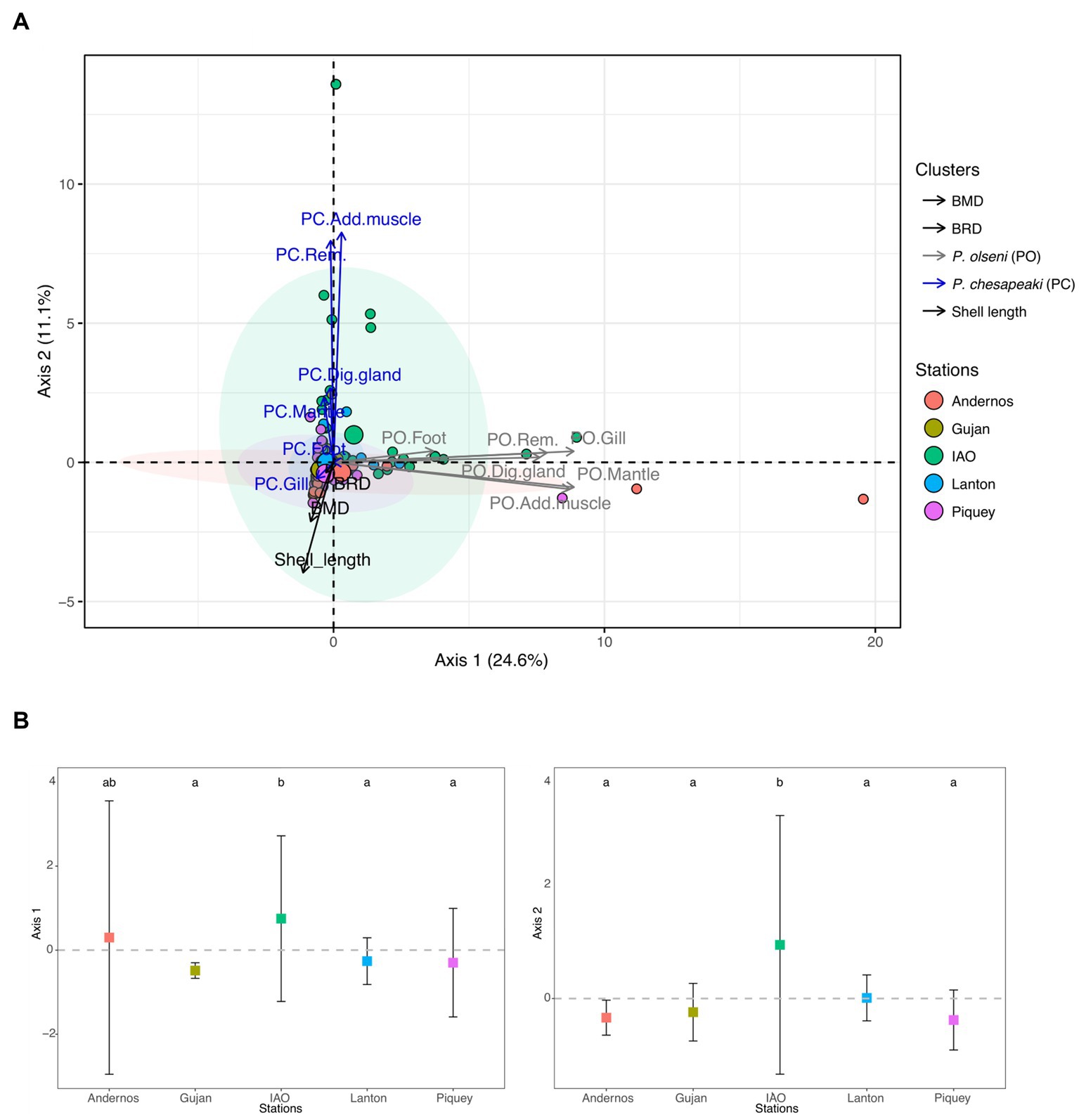
Figure 2. Principal component analysis (PCA) of P. olseni and P. chesapeaki infection intensities in different Manila clam organs from Arcachon Bay in November 2018. The first axis explains 24.6% of total variance of infection intensities while axis 2 explains 11.1%. (A) PCA including 250 individuals coloured by station. Ellipses included at least 50% of the individuals in a station. Variables implied in the PCA are the shell length, BMD, BRD and infection intensities specified by ‘PO’ for P. olseni and ‘PC’ for P. chesapeaki followed by the type of organ. Dig. gland, digestive gland; Add. muscle, adductor muscle; Rem. tissue, remaining tissue. (B) Individual values grouped by station on the Axis 1 and 2 of the PCA represented by infection intensities. Differences between stations were indicated by letters a, b and c according to multiple pairwise comparison of Dunn post-hoc test (value of p < 0.05).
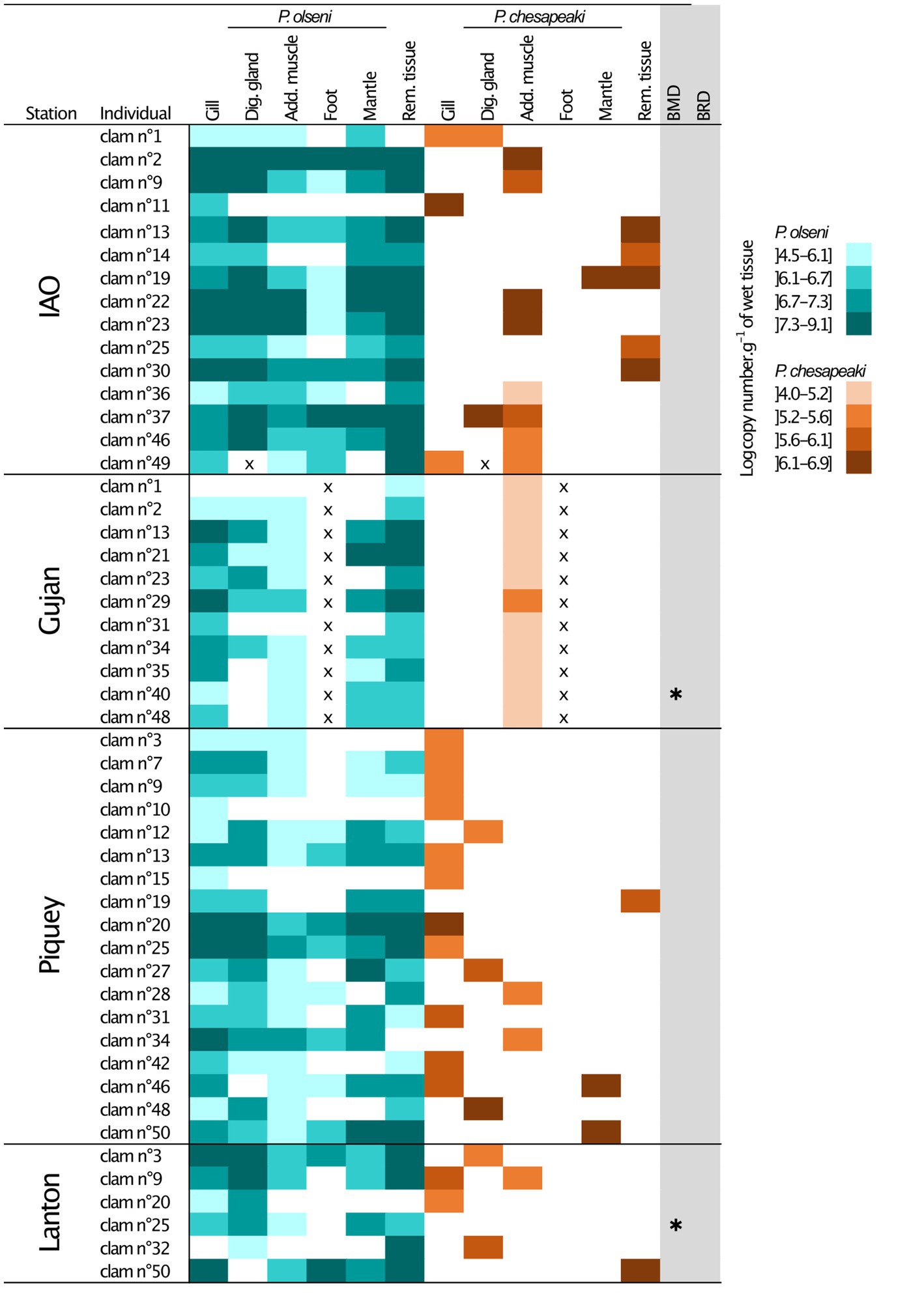
Figure 3. Infection intensities of co-infected individual clam per organs determined by qPCR duplex assays. The P. olseni repartition is represented in blue and the P. chesapeaki repartition in orange. Four levels of infection intensities were determined. x, missing value; Dig. gland, Digestive gland; Add. muscle, Adductor muscle; Rem. tissue, Remaining tissue; IAO, Ile aux Oiseaux; *, occurrence of Brown Muscle Disease (BMD) or Brown Ring Disease (BRD).
3.3 Patterns of co-infection between Perkinsus species
Distribution of P. olseni and P. chesapeaki in each Manila clam organ was investigated to more accurately evaluate potential patterns in co-infected clams. In this study, clams were considered to be co-infected when both parasites are detected in at least one of the six organs (n = 50 co-infected individuals dispatched in four stations).
Perkinsus olseni appeared to be distributed in all organs tested with high infection intensity reaching up to 9.1 log-nb. of copies. g−1 of wet tissue (Figures 1B, 3). In co-infected hosts, P. olseni reached a maximum of 8.3 log-nb. of copies. g−1 of wet tissue (Figure 3). Conversely, P. chesapeaki was more heterogeneously distributed across organs, with weaker infection intensity reaching 6.9 log-nb. of copies. g−1 of wet tissue. Our results highlighted four different profiles between the five sampling stations: (1) co-infection was often detected in gill tissue and characterised by medium P. chesapeaki infection intensity as, for example, in Piquey (between 5.2 and 5.6 log-nb. of copies. g−1 of wet tissue, n = 11/18 clams); (2) co-infection was more frequently detected in the adductor muscle (53% of co-infected Manila clams; n = 8/15 clams in IAO) with moderate infection intensity (5.6 to 6.9 log-nb. of copies. g−1 of wet tissue, n = 8/15 clams); (3) co-infection exclusively detected in the adductor muscle with low infection intensity (4.0 to 5.6 log-nb. of copies. g−1 of wet tissue, n = 11/11 clams in Gujan); and (4) co-infection was sporadically detected in the digestive gland, mantle or remaining tissue, dispatched across stations.
Proportions of P. olseni and P. chesapeaki in different compartments (gills, digestive gland, adductor muscle, mantle and remaining tissue) were assessed based on mean infection intensity (nb of copy number. g−1 of wet tissue) in order to estimate their spatial distribution within host tissues (Figure 4A and Table 2). We showed that P. olseni dominated all co-infected organs with a mean proportion of 92 ± 6%. For P. chesapeaki, the mean proportion was estimated at 8 ± 6%. In gill tissues, the weakest proportions of P. olseni were detected mainly in IAO (n = 3), with 77% of occupation while it was up to 96% for Piquey (n = 12) and Lanton (n = 3). In adductor muscle, the lowest proportions of P. olseni were detected in Gujan (n = 9) and Lanton (n = 1), with 84 and 85%, respectively. In every other organ (digestive gland, mantle and remaining tissue), P. olseni distribution took up over 90% of the total space (n = 18).
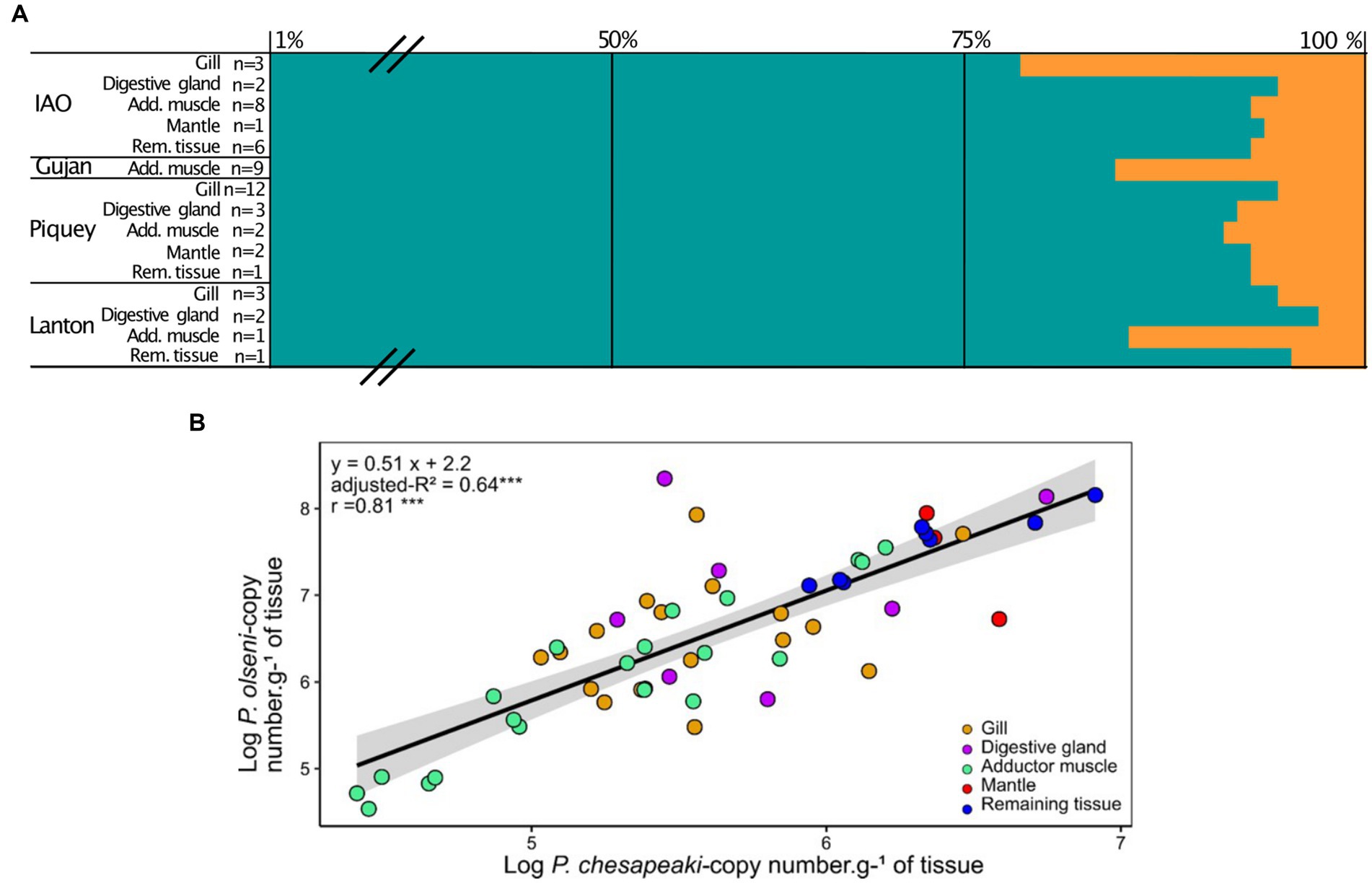
Figure 4. (A) Mean proportions of P. olseni (blue) and P. chesapeaki (orange) in common co-infected organ. Proportions are represented by the percentage of the mean of each parasitic species related to the total of copies of parasites in the organ. n: the number of clams co-infected by P. olseni and P. chesapeaki. Means and standard-deviation are detailed in Supplementary Table S4. Add. muscle, Adductor muscle; Rem. tissue, Remaining tissue. Means and standard deviations are detailed in Supplementary Table S5. (B) Linear regression between P. olseni and P. chesapeaki infection intensities (copy number.g−1 of wet tissue) from co-infected organ samples (n = 50). The linear relationship corresponds to the equation model: y = 0.51 x + 2.2 and explains 64% (adjusted-R2) of the variability. The relationship Organs (gill, digestive gland, adductor muscle, mantle and remaining tissue) were represented with coloured circles. r, Pearson’s coefficient. ‘***’ value of p < 0.001.
At the Gujan sampling station, P. chesapeaki was only detected in the adductor muscle (Figure 3). Due to technical issues, we were not able to weigh foot samples before molecular analysis. The intensity of infection for these samples is thus missing only for this station. However, no co-infection has been detected in foot samples in other individual clams from other stations, we can therefore assume that this trend might be similar for P. chesapeaki in Gujan.
To understand the distribution of co-infection across tissues, infection intensities of P. olseni and P. chesapeaki in co-infected organ samples were plotted (Figure 4B). A linear regression associated both parasitic species (y = 0.51x + 2.2, adjusted-R2 = 0.64, n = 50 of co-infected organs). A significant Pearson’s coefficient showed a strong positive correlation between P. olseni and P. chesapeaki infection intensities (r = 0.81, value of p < 0.001). Mantle (n = 3) and remaining tissue (n = 8) samples showed the highest infection intensities of P. olseni (> 6.7 log-nb. of copies. g−1 of wet tissue. g−1 of wet tissue) and P. chesapeaki (> 5.6 log-nb. of copies. g−1 of wet tissue). Gill, digestive gland and adductor muscle samples did not aggregate following infection level. However, low values of P. olseni (< 6.1 log-nb. of copies. g−1 of wet tissue) and P. chesapeaki (< 5.2 log-nb. of copies. g−1 of wet tissue) were mainly found in the adductor muscle (n = 8) (Figure 4B). This relationship revealed that co-occurrence of both parasitic species within the same organ, concerning 96% of co-infected hosts (n = 48/50 co-infected clam), occurred in a restricted range of infection intensity from 4.5 and 6.9 log-nb. of copies. g−1 of wet tissue. Above this threshold, only P. olseni single-infection and co-infection in different organs (n = 2) were observed.
4 Discussion
The development of the duplex qPCR methodology allows the quantification of infection intensity and prevalence of both parasitic species, P. olseni and P. chesapeaki, in each organ of Manila clams from populations of the Arcachon Bay, as described by Itoïz et al. (2021) (Figure 1 and Supplementary Figure S1). The concordance parameter (81.6 ± 5.2%) and the linear regression (y = 0.61x + 4.34, R2 = 0.41; Spearman correlation coefficient: r = 0.64, value of p < 0.001) strengthen the reliability of this molecular method to assess prevalence of Perkinsus species from in situ samples. In this study, we decided to discard samples with Ct values below the quantifiable range (2.1×101 total copies), thus underestimating the number of low infection intensities that could however be quantified using the RFTM methodology. Indeed, we observed that 14% of samples (n = 36 / 250 gill samples) were negative to qPCR assays but positive in RFTM assays, and conversely 4% of samples were positive to qPCR assays but negative in RFTM assays (n = 10/250 gill samples). One explanation could be the asymmetric distribution of the parasite within the two gills. Hence, these results lead to a slight to substantial agreement between both methodologies depending of sampling sites. That said, only qPCR assays allow for a distinction between P. olseni and P. chesapeaki within a tissue sample.
4.1 Single-infection and co-infection in Arcachon Bay
4.1.1 At the spatial scale
The monitoring of single-infections and co-infections provides a better understanding of Perkinsosis in Arcachon Bay. In this study, stations are mainly differentiated following P. olseni infection intensities with: medium-low values in Gujan (0.13×108 ± 0,28×108 nb. of copies.g−1 of wet tissue) to high values in IAO and Andernos (3.06×108 ± 3.91×108 and 2.60×108 ± 9.10×108 nb. of copies.g−1 of wet tissue respectively) (Supplementary Table S3). Clams with high infection intensity are retrieved from the external station IAO and the internal station Andernos (Figure 1B). This distribution could be explained by environmental abiotic factors, such as lower salinity and muddy sediment in internal stations due to the stronger influence of continental freshwater input, or the higher salinity and medium sand composition found in external stations (Dang et al., 2010a; Binias et al., 2014). Indeed, Arcachon Bay is a peculiar ecosystem where marine and continental water inputs mix, resulting in varying temperature and salinity gradients between stations (Plus et al., 2006). Dang et al. (2013) demonstrated lower infection intensity close to the Leyre river mouth located between Gujan and Lanton sampling stations, which is coherent with our observations. Conversely, higher infection intensity and prevalence of P. olseni are expected to be correlated with size, the age of the clam or higher salinity levels with the optimal salinity range for P. olseni is between 25 and 35 (Auzoux-Bordenave et al., 1996; Park and Choi, 2001; Dang et al., 2010a). Hence, the higher salinity observed in oceanic stations may explain such dichotomy in our observations except for Piquey sampling station (Dang et al., 2010a; Binias et al., 2014). This station is influenced by oceanic currents but harboured lower infection intensities compared to IAO and Andernos which could be explained by its proximity to the mouth of the lagoon with a better accessibility to fresh input of oceanic phytoplankton (Dang et al., 2009, 2010a,b). Moreover, there is no clear distribution of co-infection between stations except for Piquey. Significant occurrences of P. chesapeaki in gills appear to depend on clam shell size, suggesting a higher primary infection of the parasite due to higher clearance activity. Despite this exception, P. chesapeaki seems here to be a secondary infection in Manila clams.
In this study, the prevalence of Brown muscle disease (BMD) and Brown ring disease (BRD) phenotypes were quite low compared to previous monitoring results in Arcachon Bay (Lassalle et al., 2007; Dang et al., 2008; Binias et al., 2014). However, our results show here that BMD and BRD, both of which can be responsible for Manila clam mortality (Dang et al., 2008), are not correlated with Perkinsosis distribution across sampling sites.
4.1.2 At the individual scale
If we consider the analysis carried out on the whole Manila clam body, cases of single-infection by P. olseni are dominant in all stations and represented in all cases of infection detected at the Andernos station. Co-infection is regularly detected across Gujan, IAO, Lanton and Piquey, ranging from 12 to 36% of total infections. Conversely, P. chesapeaki single-infections are restricted in Gujan and IAO stations with very low prevalence, 14 and 2% respectively, combined with low infection intensities. It is common for one pathogen to influence the acquisition of and/or the infection dynamics of a second opportunistic pathogen (Susi et al., 2015). Two hypotheses could be formed: (1) P. olseni may play a facilitating role in the development of P. chesapeaki infections in the Manila clam. The settlement of a first parasite can be beneficial to a secondary parasite as the host is already immune-compromised by the initial infection; a gross probability calculation supports this hypothesis. Taking the example of IAO (Supplementary Table S4), the percentage of P. olseni single-infection is 64% and the percentage of P. chesapeaki single infection is 2%. In a case of random co-infection, the prevalence of double infection should be (64 × 2)/100 = 1.3%. However, the observed prevalence of co-infection was 30%. A similar trend was observed in the other stations, suggesting a facilitation process, but without knowing which parasite facilitates the infection process for the other. (2) P. chesapeaki infection may be independent of P. olseni infection. Indeed, in Europe, along the Galician coast (Spain), Ramilo et al. (2016), recorded a low number of co-infections between P. chesapeaki and P. olseni. They hypothesised an accidental or punctual introduction as well as a poor adaptation of P. chesapeaki to a “new” host. However, co-infection by multiple Perkinsus species is a recurrent phenomenon as described, for example, along East coast of United States, P. marinus, P. chesapeaki are detected co-infecting sympatric host bivalves (e.g., the baltic clam Macoma balthica, the soft-shell clam Mya arenaria, the oyster Crassostrea virginica, and the hard clams Mercenaria mercenaria) (Coss et al., 2001; Pecher et al., 2008; Reece et al., 2008; Marquis et al., 2020).
4.2 Co-infection: a patchy distribution at the individual scale
Among Perkinsus species, P. marinus is known to be phagocytosed and divided by oyster hemocytes, a process that plays a significant role in parasitic infection (La Peyre et al., 1995a,b; Villalba et al., 2004; Bassem et al., 2013; Lau et al., 2018). In contrast, in clams, while P. olseni and P. chesapeaki cells have been observed to be occasionally phagocytosed by hemocytes (e.g., Burreson et al., 2005), the question of their viability after phagocytosis remains unclear. Therefore, it is still uncertain whether these parasites propagate extracellularly or within hemocytes or both.
Among co-infected clams, P. olseni infection appears to be homogeneously distributed across all tissue samples, while the detection of P. chesapeaki is often restricted to one or two organs. We identified here three major detection patterns of co-infected clams: P. chesapeaki detection (1) is mainly prevalent in gill tissues in the Piquey station clams (n = 11/18 clams) ranging from 5.0 to 6.4 log-nb. of copies. g−1 of wet tissue; (2) is present in the adductor muscle mainly in Gujan (n = 11/11) and IAO (n = 8/15); (3) displays a moderate level of infection in the digestive gland, mantle and remaining tissue (5.9 to 6.9 log-nb. of copies. g−1 of wet tissue) distributed homogeneously across stations.
The high variability of co-infection groups, in terms of organ(s) or stations, suggests that P. chesapeaki may be more affected by local biotic or abiotic factors than P. olseni. Hence co-infection patterns may be the result of a complex overlap between individual infection level of the host and the presence of other micro or macro parasites or environmental factors affecting both the host and the parasites (e.g., sediment type, localisation in the basin, temperature, salinity). Finally, 98% of co-infected hosts are associated with moderate P. olseni infection intensities. A positive relationship links P. olseni and P. chesapeaki infection intensities when they are detected in sympatry within the same organ. However, this relationship theoretically demonstrates that we should not detect P. chesapeaki when the infection intensity of P. olseni falls below 2.2 log-nb. of copies. g−1 of wet tissue. Here, the recurrent observation that P. olseni dominates the co-infected organs of R. philippinarum is in accordance with the results obtained by Arzul et al. (2012) regarding Ruditapes decussatus in the Leucate lagoon (Mediterranean Sea). However, in the same study, P. chesapeaki was predominating in R. philippinarum at Bonne Anse (Atlantic coast). Such results support the previous hypothesis that P. chesapeaki infections may be facilitated by moderate infection of P. olseni which could increase transepithelial migration of hemocytes, as described for P. marinus in C. virginica (Lau et al., 2018), or reduce the efficiency of host defence mechanisms similarly to secondary infections by bacteria or virus (Montes et al., 2001). Hence, our results suggest a competitive exclusion of P. chesapeaki infection in cases of high P. olseni infection intensity due to direct or indirect interspecific competition for resource or space within host tissues.
Interestingly, in all sampling stations, P. chesapeaki was never detected in the foot. The possibility of a PCR amplification bias was excluded because P. chesapeaki was detected in artificial infection with a plasmid mix containing P. chesapeaki ITS sequence and host foot gDNA (Itoïz et al., 2021). The foot is one of the last organs to be infected by P. olseni when the infection intensity is heavy (Wang et al., 2018). Thus, if P. chesapeaki were a secondary invader as previously hypothesised, the detection of P. chesapeaki cells may correspond either to an early infection or to the parasite’s inability to infect the foot compartment the way P. olseni does. Sporadic detections of P. chesapeaki have also been confirmed by histology techniques in different in situ studies on co-infection (Reece et al., 2008; Arzul et al., 2012; Ramilo et al., 2016). P. chesapeaki was described in the digestive gland of two R. philippinarum individuals by Ramilo et al. (2016), while detection in the digestive gland and in gonadal tissue were also reported in R. decussatus (Arzul et al., 2012). In other bivalves, such as Mya arenaria or Cyrtopleura costata, infection in the gills was also detected but appeared localised and concentrated into compact cell clusters (Reece et al., 2008). Low throughout-tissue spreading and infrequent detection of infection in Ruditapes species maintains the hypothesis that these clams are a “sub-optimal” host for P. chesapeaki.
The variability in the kinds of organ infected by P. chesapeaki may confirm that local conditions can influence the co-infection process at a small scale. Surprisingly, Gujan station showed an unexpected P. chesapeaki infection pattern with only one organ infected, the adductor muscle. Dang et al. (2008) and Binias et al. (2014) hypothesised, in the case of BMD, that the posterior muscle, being located nearest to the sediment surface, was more vulnerable to certain pathogenic agents and environmental variations. Even if, in this study, BMD and BRD distributions were not correlated to Perkinsus distributions, the sediment may still represent a potential reservoir of Perkinsus spp. (Choi, 2002; Park et al., 2010). Thus, muddy sediments may better retain Perkinsus hypnospores (Binias et al., 2014) and favour contamination by this life stage.
5 Conclusion
The recent detection of two Perkinsus species highlights recurrent cryptic infections in host clam populations. In this study, Perkinsus infections are dominated by P. olseni and appear along an environmental gradient corresponding to its optimal proliferation conditions. Conversely, P. chesapeaki is recurrent in Manila clams across five sampling stations with moderate and low prevalence in co-infection and in single-infection, respectively, suggesting a more secondary role of P. chesapeaki infection. Moreover, the organ-targeted infection pattern seen with P. chesapeaki compared to more widespread whole-body infections by P. olseni supports the hypothesis that R. philippinarum may not be the preferred host of P. chesapeaki. To date, it is not possible to conclude whether P. olseni facilitates the opportunistic infection of P. chesapeaki or whether this secondary infection is independent. Taken all together, these data support the hypothesis that P. chesapeaki was introduced in Europe alongside other bivalves’ species, Mya arenaria or Mercenaria mercenaria, and confirms the need to monitor these parasites, which are able to spill over to new indigenous host populations, using a combination of histological and molecular analyses. It is now urgent and timely to expand our view of the host range of Perkinsus species and investigate the sympatric benthic fauna for hot-spots of co-infections. In the light of these results, the co-infection process is a Pandora’s box, justifying the need to shift from the study of isolated pathogens to a more integrated approach.
Data availability statement
The original contributions presented in the study are included in the article/Supplementary material, further inquiries can be directed to the corresponding authors.
Author contributions
SI, PS, and AC designed the study. SI, CM, and MP performed the analyses. AC provided the funds. All authors drafted the article and approved the final version of the manuscript.
Funding
The author(s) declare financial support was received for the research, authorship, and/or publication of this article. SI was funded by a French doctoral research grant from Ecole Doctorale des Sciences de la Mer (EDSM) and Region Bretagne. SM was founded by the SAD region Bretagne (No PLASPERK 948348) and received funding from the European Union’s Horizon 2020 research and innovation program under the Marie Sklodowska-Curie grant agreement No BIENVENÜE 899546. EC was funded by a French doctoral research grant from Ecole Doctorale 227 “Sciences de la nature et de l’Homme: évolution et écologie.” This research was funded by the ANR—Agence Nationale de la Recherche, Grant PANIC ANR-21-CE02-0025—and the French National program EC2CO (Ecosphère Continentale et côtière) project N°13046. The funders had no role in study design, data collection and analysis, decision to publish, or preparation of the manuscript.
Acknowledgments
Samples were performed with Planula 4 vessel (CNRS-INSU, Flotte Océanographique Française). Thank you to N. Lavesque for his significant help during the sampling campaigns.
Conflict of interest
The authors declare that the research was conducted in the absence of any commercial or financial relationships that could be construed as a potential conflict of interest.
Publisher’s note
All claims expressed in this article are solely those of the authors and do not necessarily represent those of their affiliated organizations, or those of the publisher, the editors and the reviewers. Any product that may be evaluated in this article, or claim that may be made by its manufacturer, is not guaranteed or endorsed by the publisher.
Supplementary material
The Supplementary material for this article can be found online at: https://www.frontiersin.org/articles/10.3389/fmicb.2023.1250947/full#supplementary-material
References
Arzul, I., Chollet, B., Michel, J., Robert, M., Garcia, C., Joly, J.-P., et al. (2012). One Perkinsus species may hide another: characterization of Perkinsus species present in clam production areas of France. Parasitology 139, 1757–1771. doi: 10.1017/S0031182012001047
Auzoux-Bordenave, S., Vigario, A. M., Ruano, F., Domart-Coulon, I., and Doumenc, D. (1996). In vitro sporulation of the clam pathogen Perkinsus atlanticus (Apicomplexa, Perkinsea) under various environmental conditions. Oceanogr. Lit. Rev. 9:926.
Azevedo, C. (1989). Fine structure of Perkinsus atlanticus n. sp. (Apicomplexa, Perkinsea) parasite of the clam Ruditapes decussatus from Portugal. J. Parasitol. 75, 627–635. doi: 10.2307/3282915
Bass, D., Stentiford, G. D., Wang, H.-C., Koskella, B., and Tyler, C. R. (2019). The pathobiome in animal and plant diseases. Trends Ecol. Evol. 34, 996–1008. doi: 10.1016/j.tree.2019.07.012
Bassem, A., Carden, W. E., Ward, J. E., Ralph, G., Winnicki, S., and Pales Espinosa, E. (2013). Early host-pathogen interactions in marine bivalves: evidence that the alveolate parasite Perkinsus marinus infects through the oyster mantle during rejection of pseudofeces. J. Invertebr. Pathol. 113, 26–34. doi: 10.1016/j.jip.2012.12.011
Binias, C., Tu Do, V., Jude-Lemeilleur, F., Plus, M., Froidefond, J.-M., and de Montaudouin, X. (2014). Environmental factors contributing to the development of brown muscle disease and perkinsosis in Manila clams (Ruditapes philippinarum) and trematodiasis in cockles (Cerastoderma edule) of Arcachon Bay. Mar. Ecol. 35, 67–77. doi: 10.1111/maec.12087
Burge, C. A., Mark Eakin, C., Friedman, C. S., Froelich, B., Hershberger, P. K., Hofmann, E. E., et al. (2014). Climate change influences on marine infectious diseases: implications for management and society. Annu. Rev. Mar. Sci. 6, 249–277. doi: 10.1146/annurev-marine-010213-135029
Burreson, E. M., Reece, K. S., and Dungan, C. F. (2005). Molecular, morphological, and experimental evidence support the synonymy of Perkinsus chesapeaki and Perkinsus andrewsi. J. Eukaryot. Microbiol. 52, 258–270. doi: 10.1111/j.1550-7408.2005.05-00035.x
Bushek, D., Landau, B., and Scarpa, E. (2008). Perkinsus chesapeaki in stout razor clams Tagelus plebeius from Delaware Bay. Dis. Aquat. Org. 78, 243–247. doi: 10.3354/dao01871
Carrasco, N., Rojas, M., Aceituno, P., Andree, K. B., Lacuesta, B., and Furones, M. D. (2014). Perkinsus chesapeaki observed in a new host, the European common edible cockle Cerastoderma edule, in the Spanish Mediterranean coast. J. Invertebr. Pathol. 117, 56–60. doi: 10.1016/j.jip.2014.01.009
Chambouvet, A., Gower, D. J., Jirků, M., Yabsley, M. J., Davis, A. K., Leonard, G., et al. (2015). Cryptic infection of a broad taxonomic and geographic diversity of tadpoles by Perkinsea protists. Proc. Natl. Acad. Sci. U. S. A. 112, E4743–E4751. doi: 10.1073/pnas.1500163112
Chambouvet, A., Morin, P., Marie, D., and Guillou, L. (2008). Control of toxic marine dinoflagellate blooms by serial parasitic killers. Science 322, 1254–1257. doi: 10.1126/science.1164387
Choi, K.-S. (2002). Infection intensity, prevalence and histology of Perkinsus sp. in the Manila clam, Ruditapes philippinarum, in Isahaya Bay, Japan. J. Shellfish Res. 21, 119–125.
Choi, K.-S., Wilson, E. A., Lewis, D. H., Powell, E. N., and Ray, S. M. (1989). The energetic cost of Perkinsus marinus parasitism in oysters: quantification of the thioglycollate method. J. Shellfish Res. 8, 125–131.
Coss, C. A., Robledo, J. A. F., Ruiz, G. M., and Vasta, G. R. (2001). Description of Perkinsus andrewsi n. sp. isolated from the Baltic clam (Macoma balthica) by characterization of the ribosomal RNA locus, and development of a species-specific PCR-based diagnostic assay. J. Eukaryot. Microbiol. 48, 52–61. doi: 10.1111/j.1550-7408.2001.tb00415.x
Cox, F. E. G. (2001). Concomitant infections, parasites and immune responses. Parasitology 122, S23–S38. doi: 10.1017/S003118200001698X
Da Ros, L., and Canzonier, W. J. (1985). Perkinsus, a protistan threat to bivalve culture in the Mediterranean basin. Bull. Eur. Assoc. Fish Pathol. 5, 23–27.
Dang, C., de Montaudouin, X., Binias, C., Salvo, F., Caill-Milly, N., Bald, J., et al. (2013). Correlation between perkinsosis and growth in clams Ruditapes spp. Dis. Aquat. Org. 106, 255–265. doi: 10.3354/dao02640
Dang, C., de Montaudouin, X., Caill-Milly, N., and Trumbiç, Æ. (2010a). Spatio-temporal patterns of perkinsosis in the Manila clam Ruditapes philippinarum from Arcachon Bay (SW France). Dis. Aquat. Org. 91, 151–159. doi: 10.3354/dao02243
Dang, C., de Montaudouin, X., Gam, M., Paroissin, C., Bru, N., and Caill-Milly, N. (2010b). The Manila clam population in Arcachon Bay (SW France): can it be kept sustainable? J. Sea Res. 63, 108–118. doi: 10.1016/j.seares.2009.11.003
Dang, C., de Montaudouin, X., Gonzalez, P., Mesmer-Dudons, N., and Caill-Milly, N. (2008). Brown muscle disease (BMD), an emergent pathology affecting Manila clam Ruditapes philippinarum in Arcachon Bay (SW France). Dis. Aquat. Org. 80, 219–228. doi: 10.3354/dao01941
Dang, C., Sauriau, P., Savoye, N., Caill-Milly, N., Martinez, P., Millaret, C., et al. (2009). Determination of diet in Manila clams by spatial analysis of stable isotopes. Mar. Ecol. Prog. Ser. 387, 167–177. doi: 10.3354/meps08100
Dantas Neto, M. P., Gesteira, T. C. V., Sabry, R. C., Feijó, R. G., Forte, J. M., Boehs, G., et al. (2016). First record of Perkinsus chesapeaki infecting Crassostrea rhizophorae in South America. J. Invertebr. Pathol. 141, 53–56. doi: 10.1016/j.jip.2016.10.007
de Montaudouin, X., Arzul, I., Caill-Milly, N., Khayati, A., Labrousse, J.-M., Lafitte, C., et al. (2016a). Asari clam (Ruditapes philippinarum) in France: history of an exotic species 1972 – 2015. Bulletin, 42, 35–42.
de Montaudouin, X., Lucia, M., Binias, C., Lassudrie, M., Baudrimont, M., Legeay, A., et al. (2016b). Why is Asari (=Manila) clam Ruditapes philippinarum fitness poor in Arcachon Bay: a meta-analysis to answer? Estuar. Coast. Shelf Sci. 179, 226–235. doi: 10.1016/j.ecss.2015.09.009
de Montaudouin, X., Paul-Pont, I., Lambert, C., Gonzalez, P., Raymond, N., Jude, F., et al. (2010). Bivalve population health: multistress to identify hot spots. Mar. Pollut. Bull. 60, 1307–1318. doi: 10.1016/j.marpolbul.2010.03.011
Gause, G. F. (1934). Experimental analysis of Vito Volterra’s mathematical theory of the struggle for existence. Science 79, 16–17. doi: 10.1126/science.79.2036.16.b
Graham, A. L. (2008). Ecological rules governing helminth microparasite coinfection. Proc. Natl. Acad. Sci. 105, 566–570. doi: 10.1073/pnas.0707221105
Harvell, C. D. (1999). Emerging marine diseases--climate links and anthropogenic factors. Science 285, 1505–1510. doi: 10.1126/science.285.5433.1505
Itoïz, S., Metz, S., Derelle, E., Reñé, A., Garcés, E., Bass, D., et al. (2022). Emerging parasitic protists: the case of Perkinsea. Front. Microbiol. 12:735815. doi: 10.3389/fmicb.2021.735815
Itoïz, S., Perennou, M., Mouronvalle, C., Derelle, E., Le Goïc, N., Bidault, A., et al. (2021). Development of duplex TaqMan-based real-time PCR assay for the simultaneous detection of Perkinsus olseni and P. chesapeaki in host Manila clam tissue samples. J. Invertebr. Pathol. 184:107603. doi: 10.1016/j.jip.2021.107603
Johnson, P. T. J., and Hoverman, J. T. (2012). Parasite diversity and coinfection determine pathogen infection success and host fitness. Proc. Natl. Acad. Sci. 109, 9006–9011. doi: 10.1073/pnas.1201790109
Kassambara, A., and Mundt, F. (2017). Draw Surviv Curves using ‘ggplot2’ (R Packag version 0.3. 1). Package ‘survminer’.
La Peyre, J. F., Chu, F.-L. E., and Meyers, J. M. (1995b). Haemocytic and humoral activities of eastern and Pacific oysters following challenge by the protozoan Perkinsus marinus. Fish Shellfish Immunol. 5, 179–190. doi: 10.1016/S1050-4648(05)80012-9
La Peyre, J. F., Chu, F. E., and Vogelbein, W. K. (1995a). In vitro interaction of Perkinsus marinus merozoites with eastern and pacific oyster hemocytes. Dev. Comp. Immunol. 19, 291–304. doi: 10.1016/0145-305X(95)00017-N
Langton, S. D., Chevennement, R., Nagelkerke, N., and Lombard, B. (2002). Analysing collaborative trials for qualitative microbiological methods: accordance and concordance. Int. J. Food Microbiol. 79, 175–181. doi: 10.1016/S0168-1605(02)00107-1
Lassalle, G., de Montaudouin, X., Soudant, P., and Paillard, C. (2007). Parasite co-infection of two sympatric bivalves, the Manila clam (Ruditapes philippinarum) and the cockle (Cerastoderma edule) along a latitudinal gradient. Aquat. Living Ressour. 20, 33–42. doi: 10.1051/alr:2007013
Lau, Y.-T., Gambino, L., Santos, B., Pales Espinosa, E., and Allam, B. (2018). Transepithelial migration of mucosal hemocytes in Crassostrea virginica and potential role in Perkinsus marinus pathogenesis. J. Invertebr. Pathol. 153, 122–129. doi: 10.1016/j.jip.2018.03.004
Lê, S., Josse, J., and Husson, F. (2008). FactoMineR: an R package for multivariate analysis. J. Stat. Softw. 25, 1–18. doi: 10.18637/jss.v025.i01
López-García, P., Philippe, H., Gail, F., and Moreira, D. (2003). Autochthonous eukaryotic diversity in hydrothermal sediment and experimental microcolonizers at the mid-Atlantic ridge. Proc. Natl. Acad. Sci. 100, 697–702. doi: 10.1073/pnas.0235779100
Marquis, N. D., Bishop, T. J., Record, N. R., Countway, P. D., and Fernández Robledo, J. A. (2020). A qPCR-based survey of Haplosporidium nelsoni and Perkinsus spp. in the eastern oyster, Crassostrea virginica in Maine, USA. Pathogens 9:256. doi: 10.3390/pathogens9040256
McKenzie, F. E., and Bossert, W. H. (1999). Multispecies Plasmodium infections of humans. J. Parasitol. 85, 12–18. doi: 10.2307/3285692
McLaughlin, S., and Faisal, M. (2000). Prevalence of Perkinsus spp. in Chesapeake Bay soft-shell clams, Mya arenaria Linnaeus, 1758 during 1990-1998. J. Shellfish Res. 19, 349–352.
Montes, J., Durfort, M., and García-Valero, J. (2001). Parasitism by the protozoan Perkinsus atlanticus favours the development of opportunistic infections. Dis. Aquat. Org. 46, 57–66. doi: 10.3354/dao046057
Moon-van der Staay, S. Y., De Wachter, R., and Vaulot, D. (2001). Oceanic 18S rDNA sequences from picoplankton reveal unsuspected eukaryotic diversity. Nature 409, 607–610. doi: 10.1038/35054541
OIE-Listed Diseases 2021: OIE – World Organisation for Animal Health. (2021). Available at: https://www.oie.int/en/animal-health-in-the-world/oie-listed-diseases-2021/ (Accessed February 10, 2021).
Paillard, C. (2004). A short-review of brown ring disease, a vibriosis affecting clams, Ruditapes philippinarum and Ruditapes decussatus. Aquat. Living Resour. 17, 467–475. doi: 10.1051/alr:2004053
Paillard, C., Gausson, S., Nicolas, J. L., le Pennec, J. P., and Haras, D. (2006). Molecular identification of Vibrio tapetis, the causative agent of the brown ring disease of Ruditapes philippinarum. Aquaculture 253, 25–38. doi: 10.1016/j.aquaculture.2005.03.047
Park, K.-I., and Choi, K.-S. (2001). Spatial distribution of the protozoan parasite Perkinsus sp. found in the Manila clams, Ruditapes philippinarum, in Korea. Aquaculture 203, 9–22. doi: 10.1016/S0044-8486(01)00619-6
Park, K.-I., Yang, H.-S., Kang, H.-S., Cho, M., Park, K.-J., and Choi, K.-S. (2010). Isolation and identification of Perkinsus olseni from feces and marine sediment using immunological and molecular techniques. J. Invertebr. Pathol. 105, 261–269. doi: 10.1016/j.jip.2010.07.006
Paul-Pont, I., Gonzalez, P., Baudrimont, M., Jude, F., Raymond, N., Bourrasseau, L., et al. (2010). Interactive effects of metal contamination and pathogenic organisms on the marine bivalve Cerastoderma edule. Mar. Pollut. Bull. 60, 515–525. doi: 10.1016/j.marpolbul.2009.11.013
Pecher, W. T., Alavi, M. R., Schott, E. J., Fernandez-Robledo, J. A., Roth, L., Berg, S. T., et al. (2008). Assessment of the northern distribution range of selected Perkinsus species in eastern oysters (Crassostrea virginica) and hard clams (Mercenaria mercenaria) with the use of PCR-based detection assays. J. Parasitol. 94, 410–422. doi: 10.1645/GE-1282.1
Petney, T. N., and Andrews, R. H. (1998). Multiparasite communities in animals and humans: frequency, structure and pathogenic significance. Int. J. Parasitol. 28, 377–393. doi: 10.1016/S0020-7519(97)00189-6
Pfeiffer, D. (2010). Veterinary epidemiology: An introduction, Chichester, United Kingdom: John Wiley & Sons.
Pierron, F., Gonzalez, P., Bertucci, A., Binias, C., Mérour, E., Brémont, M., et al. (2019). Transcriptome-wide analysis of wild Asari (=Manila) clams affected by the Brown muscle disease: etiology and impacts of the disease. Fish Shellfish Immunol 86, 179–185. doi: 10.1016/j.fsi.2018.11.043
Plus, M., Maurer, D., Stanisiere, J.-Y., and Dumas, F. (2006). Caractérisation des composantes hydrodynamiques d’une lagune mésotidale, le Bassin d’Arcachon. RST/LER/AR/06.007. Available at: https://archimer.ifremer.fr/doc/00000/2352/.
Pretto, T., Zambon, M., Civettini, M., Caburlotto, G., Boffo, L., Rossetti, E., et al. (2014). Massive mortality in Manila clams (Ruditapes philippinarum) farmed in the lagoon of Venice, caused by Perkinsus olseni. Bull. Eur. Assoc. Fish Pathol. 34:43.
Putaporntip, C., Hongsrimuang, T., Seethamchai, S., Kobasa, T., Limkittikul, K., Cui, L., et al. (2009). Differential prevalence of Plasmodium infections and cryptic Plasmodium knowlesi malaria in humans in Thailand. J. Infect. Dis. 199, 1143–1150. doi: 10.1086/597414
Raileanu, C., Moutailler, S., Pavel, I., Porea, D., Mihalca, A. D., Savuta, G., et al. (2017). Borrelia diversity and co-infection with other tick borne pathogens in ticks. Front. Cell. Infect. Microbiol. 7:36. doi: 10.3389/fcimb.2017.00036
Ramilo, A., Pintado, J., Villalba, A., and Abollo, E. (2016). Perkinsus olseni and P. chesapeaki detected in a survey of perkinsosis of various clam species in Galicia (NW Spain) using PCR–DGGE as a screening tool. J. Invertebr. Pathol. 133, 50–58. doi: 10.1016/j.jip.2015.11.012
Ray, S. M. (1952). A culture technique for the diagnosis of infection with Dermocystidium marinum Mackin, Owell and Collier in oysters. Science 116, 360–361. doi: 10.1126/science.116.3014.360
Reece, K., Dungan, C., and Burreson, E. (2008). Molecular epizootiology of Perkinsus marinus and P. chesapeaki infections among wild oysters and clams in Chesapeake Bay, USA. Dis. Aquat. Organ. 82, 237–248. doi: 10.3354/dao01997
Ruano, F., Batista, F. M., and Arcangeli, G. (2015). Perkinsosis in the clams Ruditapes decussatus and R. Philippinarum in the northeastern Atlantic and Mediterranean Sea: a review. J. Invertebr. Pathol. 131, 58–67. doi: 10.1016/j.jip.2015.07.015
Ruano, F., and Cachola, R. (1986). Outbreak of a severe epizootic of Perkinsus marinus (LEVIN-87) at Ria de Faro clam’s culture beds. Proccedings of the 2nd international colloquium on pathology in marine aquaculture (Porto), Portugal. 41–43.
Susi, H., Barrès, B., Vale, P. F., and Laine, A.-L. (2015). Co-infection alters population dynamics of infectious disease. Nat. Commun. 6:5975. doi: 10.1038/ncomms6975
Vayssier-Taussat, M., Albina, E., Citti, C., Cosson, J.-F., Jacques, M.-A., Lebrun, M.-H., et al. (2014). Shifting the paradigm from pathogens to pathobiome: new concepts in the light of meta-omics. Front. Cell. Infect. Microbiol. 4:29. doi: 10.3389/fcimb.2014.00029
Vilas, R., Cao, A., Pardo, B. G., Fernández, S., Villalba, A., and Martínez, P. (2011). Very low microsatellite polymorphism and large heterozygote deficits suggest founder effects and cryptic structure in the parasite Perkinsus olseni. Infect. Genet. Evol. 11, 904–911. doi: 10.1016/j.meegid.2011.02.015
Villalba, A., Reece, K. S., Camino Ordás, M., Casas, S. M., and Figueras, A. (2004). Perkinsosis in molluscs: a review. Aquat. Living Resour. 17, 411–432. doi: 10.1051/alr:2004050
Wang, Y., Yoshinaga, T., and Itoh, N. (2018). New insights into the entrance of Perkinsus olseni in the Manila clam, Ruditapes philippinarum. J. Invertebr. Pathol. 153, 117–121. doi: 10.1016/j.jip.2018.03.005
Keywords: Perkinsosis, parasite, multiple-infection, co-infection, Manila clam, organ distribution
Citation: Itoïz S, Mouronvalle C, Perennou M, Chailler E, Smits M, Derelle E, Metz S, Le Goïc N, Bidault A, de Montaudouin X, Arzul I, Soudant P and Chambouvet A (2024) Co-infection of two eukaryotic pathogens within clam populations in Arcachon Bay. Front. Microbiol. 14:1250947. doi: 10.3389/fmicb.2023.1250947
Edited by:
Wei Zhu, Chinese Academy of Sciences (CAS), ChinaReviewed by:
Jose A. Fernandez Robledo, Bigelow Laboratory for Ocean Sciences, United StatesLuísa Magalhães, University of Aveiro, Portugal
Copyright © 2024 Itoïz, Mouronvalle, Perennou, Chailler, Smits, Derelle, Metz, Le Goïc, Bidault, de Montaudouin, Arzul, Soudant and Chambouvet. This is an open-access article distributed under the terms of the Creative Commons Attribution License (CC BY). The use, distribution or reproduction in other forums is permitted, provided the original author(s) and the copyright owner(s) are credited and that the original publication in this journal is cited, in accordance with accepted academic practice. No use, distribution or reproduction is permitted which does not comply with these terms.
*Correspondence: Philippe Soudant, philippe.soudant@univ-brest.fr; Aurélie Chambouvet, auchambouvet@sb-roscoff.fr
†Present address: Sebastian Metz, Department of Archaeology, University of York, York, United Kingdom