- 1College of Animal Science and Technology, China Agricultural University, Beijing, China
- 2College of Agriculture and Animal Husbandry, Qinghai University, Xining, China
Yak (Bos grunniens) is an important economic animal species on the Qinghai-Tibet Plateau. Yaks grazed in the cold season often suffer from nutritional stress, resulting in low production performance. This situation can be improved by properly feeding the grazing yaks in the cold season; however, there is still little information about the effect of different feeding levels on the intestinal microflora and metabolites of yaks. Therefore, this study aimed to explore the effect of feeding different doses of concentrate supplements on rumen bacterial communities and metabolites in grazing yaks during the cold season. Feed concentrate supplementation significantly improved the production performance and rumen fermentation status of grazing yaks during the cold season, and switched the type of ruminal fermentation from acetic acid fermentation to propionic acid fermentation. Ruminal fermentation parameters and ruminal bacterial abundance correlated strongly. At the phylum level, the abundance of Firmicutes increased with increasing concentrate supplementation, while the opposite was true for Bacteroidota. At the genus level, the abundance of Christensenellaceae_R-7_group, NK4A214_group, Ruminococcus, norank_f__Eubacterium_coprostanoligenes_group, norank_f__norank_o__ Clostridia_UCG-014, Lachnospiraceae_NK3A20_group, Acetitomaculum, and Family_XIII_AD3011_group increased with increasing concentrate supplementation, while the abundance of Rikenellaceae_RC9_gut_ group decreased. Dietary concentrate supplementation altered the concentration and metabolic mode of metabolites in the rumen, significantly affecting the concentration of metabolites involved in amino acid and derivative metabolism (e.g., L-aspartic acid, L-glutamate, and L-histidine), purine metabolism (e.g., guanine, guanosine, and hypoxanthine), and glycerophospholipid metabolism (e.g., phosphatidate, phosphatidylcholine, and phosphocholine), and other metabolic pathways. The strong correlation between yak rumen microorganisms and metabolites provided a more comprehensive understanding of microbial community composition and function. This study showed significant changes in the composition and abundance of bacteria and metabolites in the rumen of cool season grazing yaks fed with concentrate supplements. Changes in ruminal fermentation parameters and metabolite concentration also showed a strong correlation with ruminal bacterial communities. These findings will be helpful to formulate supplementary feeding strategies for grazing yaks in the cold season from the perspective of intestinal microorganisms.
1. Introduction
Yaks (Bos grunniens) live mainly in plateau areas above 3,000 m and are an important economic resource for plateau herders (Sapkota et al., 2022). Most yaks are raised using year-round grazing, which rarely involves hand-feeding (Long et al., 2005). The cold season on the Tibetan Plateau runs from October to May, and because of the low temperatures, the pasture does not provide enough nutrition for yak growth. This results in low productivity and a long growth cycle for yaks, with the standard age for slaughter often being over 5 years old (Long et al., 1999). However, with the improvement of economic conditions in the modern Tibetan plateau pastoral areas, yak production has also been subject to human intervention. Timely supplemental feeding of yaks during winter when forage resources are scarce is an effective means to alleviate nutritional stress in yaks (Zou et al., 2019). Bacteria in the rumen can carry out complex metabolic activities by fermenting feed, and use the resultant metabolites to reproduce, contributing to the homeostasis of the rumen environment (Clemmons et al., 2019; Xue et al., 2020). However, supplemental feeding disrupts this homeostasis because it changes the structure of the diet fed to the yak. Studies have shown that changes in diet (including the type of diet and the ratio of diet concentrate to forage) can have a dramatic effect on the ruminal bacterial community (Granja-Salcedo et al., 2016; Chen et al., 2021). Most studies on supplemental feeding of grazing yaks have focused on the effects on yak production performance. Fewer studies related to changes in the rumen microbiota and metabolome responsiveness of yaks grazing in the cool season after supplemental feeding have been published. Studies have confirmed that seasonal differences in the nutritional value of forage in pastures are an important reason for differences in the ruminal microbial communities of yaks between seasons (Ahmad et al., 2021; Huang et al., 2022). In addition, feed type and dietary concentrate ratio significantly affected the diversity and abundance of bacterial communities and the concentration of metabolites in the rumen of yaks (Liu et al., 2019; Yi et al., 2022). Therefore, it is necessary to study rumen bacteria and metabolites in cold-season supplemented grazing yaks to determine the environmental adaptation mechanisms of the yak rumen during grazing in the cold season.
Herein, we hypothesized that fermentation products, microbial communities, and metabolites in the rumen would change according to the amount of supplementation. To test this hypothesis, we characterized the yak rumen bacterial communities and metabolites using multi-omics techniques. In addition, we focused on correlations between rumen bacterial communities and metabolites, which could provide a more comprehensive knowledge base to manage cool-season grazing yaks.
2. Materials and methods
2.1. Animal feeding and management
To achieve the objective of studying the environmental adaptation mechanisms of yaks during the cold season, we chose 3 months of the cold season on the Tibetan plateau to conduct the experiment (October to December). Forty-eight 30-month-old male yaks of similar weight (204.90 ± 10.65 kg) were randomly divided into four groups of 12 yaks each. The control group was grazed only, and the remaining three groups were divided into the low supplementation group (LCS, 0.5 kg/d), the medium supplementation group (MCS, 2.5 kg/d), and the high supplementation group (HCS, 4.5 kg/d) according to the daily amount of concentrate supplementation fed to each yak. Table 1 shows the ingredient composition and chemical composition of the concentrate supplements. Supplementary Table 1 demonstrates the quality of forage during the trial period. The trial period was 105 days, divided into a pre-feeding period of 15 days and a formal feeding period of 90 days. Grazing took place at 8:00 a. m. and ended at 6:00 p. m. each day during the trial. Grass species in the pasture included Artemisia subulata Nakai, Poa crymophila Keng, and Elymus nutans Griseb. The three supplementation groups were fed half an hour before the start and half an hour after the end of grazing to ensure that each yak in the same supplementation group received the same weight of the concentrate supplement and that it was consumed in full. The yaks were allowed to drink freely throughout the experiment.
2.2. Sample collection and storage
All yaks were weighed before the first day of supplementation in the official period, which was recorded as the initial weight. All yaks were weighed before morning feeding on the last day of the experiment, which was recorded as the final weight. Growth performance was calculated based on final and initial body weights. One-way ANOVA model analysis of variance (ANOVA) was performed using SPSS software, and multiple comparisons were performed using Duncan’s method, with P < 0.05 being considered significant and 0.05 < P < 0.01 being highly significant.
All yaks were numbered (1 to 48). Random sampling was performed using SPSS software, and six numbers were randomly selected from each group of 12. The rumen fluid from the yaks represented by the six numbers was selected for all subsequent analyses. Select the yak rumen fluid represented by six numbers for all subsequent analyses. Six yaks in each group were randomly selected for sampling. Before morning feeding on the last day of the experiment, rumen fluid was collected transorally through a rumen cannula with an initial filter in the front port using a syringe with a volume of 50 mL. The aspirated rumen fluid was filtered through four layers of sterile gauze and its pH determined. The filtered rumen fluid was dispensed into centrifuge tubes and frozen.
2.3. Determination and screening of rumen fermentation parameters
Determination of the volatile fatty acid (VFA) concentration was carried out with reference to a previously published method (Erwin et al., 1961). The NH3-N concentration of rumen fluid was measured using the phenol sodium hypochlorite colorimetric method (Broderick and Kang, 1980). Ruminal fermentation parameters with strong covariance (variance inflation factor (VIF) > 10) were screened out by VIF analysis and subjected to redundancy analysis (RDA). The final rumen fermentation parameters that could be used for subsequent correlation analysis were obtained (Supplementary Table 2).
2.4. DNA Extraction, PCR amplification, and sequencing using Illumina Miseq
Total DNA from the microbial community in the ruminal fluid from 24 yaks was extracted according to the instructions of the FastDNA® Spin Kit for Soil (MP Biomedicals, Solon, OH, United States). The determination of DNA concentration and purity, primer selection for PCR amplification, and PCR amplification procedures were performed according to our previous study (Yi et al., 2022). The PCR products of the same sample were mixed, subjected to 2% agarose gel to recover the PCR products, and extracted using an AxyPrep DNA Gel Extraction Kit (Axygen Biosciences, Union City, CA, USA). A Quantum Fluorometer (Promega, Madison, WI, USA) was used to detect and quantify the recovered products. The sequencing library was constructed and sequenced using the Illumina Miseq technique (Illumina, San Diego, CA, USA) in a manner consistent with our previous study (Yi et al., 2022). All raw sequences have been uploaded to the NCBI database with the accession number: PRJNA978645.
2.5. Sequencing data processing and analysis
Raw sequence quality control was carried out using fastp software (Chen et al., 2018), and splicing was performed using FLASH software (Magoc and Salzberg, 2011). The splicing process was carried out according our previously published methods (Yi et al., 2022). Based on the default parameters, after QC splicing, the optimized sequences were noise-reduced using the DADA2 (Callahan et al., 2016) plug-in in the Qiime2 process (Bolyen et al., 2019). Thereafter, the sequences were commonly referred to as amplicon sequence variants (ASVs). The number of sequences per sample after noise reduction was estimated according to the minimum value of the number of sample sequences, and the average coverage of each sample after leveling could reach 97.90%. Species taxonomy analysis of ASVs was performed based on the Silva 16S rRNA database (v 138) using the Naive bayes (or Vsearch, or Blast) classifier in Qiime2. The Majorbio cloud platform (a Qiime2 process) was used for the subsequent analysis.1
2.6. Metabolomics, data processing, and analysis
The extraction of rumen fluid metabolites, the analysis of extracted samples, and the analytical conditions for liquid chromatography and mass spectrometry (LC-MS) were the same as those in our previous study (Yi et al., 2022).
The LC-MS data were processed to obtain a data matrix (containing retention time, mass-to-charge ratio, and peak intensity). This data matrix was filled with vacant values using the 80% rule to remove missing values, i.e., variables with at least 80% of non-zero values were retained in at least one set of samples. Variables from quality control samples with a relative standard deviation (RSD) greater than 30% were removed and subjected to log10 logarithmic processing to obtain the final data matrix for subsequent analysis. The R package ropls (Version1.6.2) was used for principal component analysis (PCA). Partial least squares discriminant analysis (PLS-DA) and model stability were assessed using validation of the 7-cycle interaction. In addition, Student’s t-test analysis was performed. Student’s t-test p-values and the variable importance in the projection (VIP) values were used to select significantly differentially abundant metabolites, which were screened according to the criteria: VIP > 1 and P < 0.05. The above analyses were performed on the Majorbio Cloud Platform.seetextfootnote1 Metabolic pathway enrichment analysis and topological analysis were performed on the identified significantly differentially abundant metabolites using MetabolAnalyst 5.02 (Pang et al., 2022). The correlation network between rumen metabolites and the microbiota was visualized using the software Gephi 0.10.1.3
3. Results
3.1. Effect of supplemental feeding on growth performance and rumen fermentation parameters
Table 2 shows that final body weight (FBW) of the HCS group was higher than that of the LCS and CON groups. The average daily gain (ADG) in the four groups increased with the increase in supplementation. Table 2 demonstrates the effect of concentrate supplement feeding on rumen pH, NH3-N and VFAs. Rumen pH was lower in the MCS and HCS groups than in the CON and LCS groups (P < 0.01), while the NH3-N and total VFA concentrations were higher in the MCS and HCS groups than in the CON and LCS groups. Meanwhile, acetate concentrations were significantly lower in the MCS and HCS groups than in the CON and LCS groups. The propionate concentration was higher in the HCS group than in the other three groups, while the acetate:propionate ratio was lower in the HCS group than in the other three groups (P < 0.01). Valerate concentrations in the MCS and HCS groups were higher than those in the CON and LCS groups, while the valerate concentration in the HCS group were higher than that in the MCS group. Besides, isovalerate concentrations in the MCS and HCS groups were significantly higher than those in the CON group (P < 0.05).
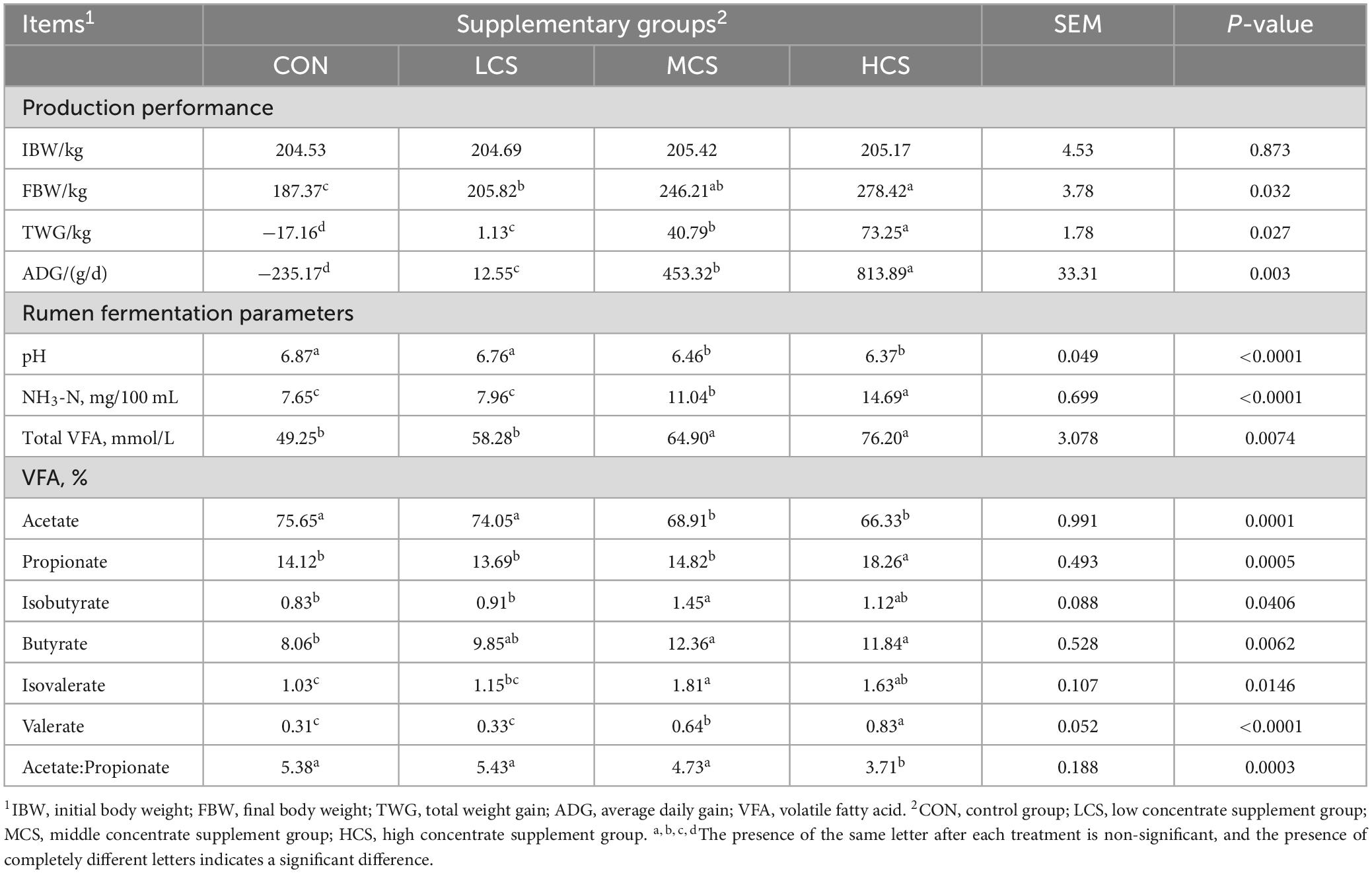
Table 2. Concentrate supplementation affects performance and rumen fermentation parameters of cool-season grazing yaks.
3.2. Ruminal bacterial diversity and composition
Ruminal fluid from 24 yaks was subjected to 16S rRNA high-throughput sequencing, which produced 1,430,293 optimized sequences, from which a total of 9,681 ASVs were obtained after noise reduction. After sampling the minimum number of sequences, 9,647 ASVs remained. Supplementary Figures 1A, B shows the bacterial communities’ α-diversity indices in the rumen of 24 yaks, including the Chao1 and Shannon indices. There was no significant difference in the α-diversity indices between the four groups. PCoA of the rumen bacterial communities showed that they were clearly differentiated between the four treatment groups (Supplementary Figure 1C). Figures 1, 2 depict the composition of the rumen at the bacterial phylum and genus levels, respectively. Twenty-one bacterial phyla and 281 bacterial genera were identified in the rumen bacterial flora, with Firmicutes (48.64%) and Bacteroidota (46.5%) as the dominant phyla (Figure 1A), and Rikenellaceae_RC9_gut_group (14.74%), Prevotella (11.17%), Christensenellaceae_R-7_group (5.88%), NK4A214_group (5.23%), norank_f__F082 (4.77%), Prevotellaceae_UCG-003 (3.75%), norank_f__Muribaculaceae (3.53%), and Ruminococcus (3.39%) as the dominant genera (Figure 2A).
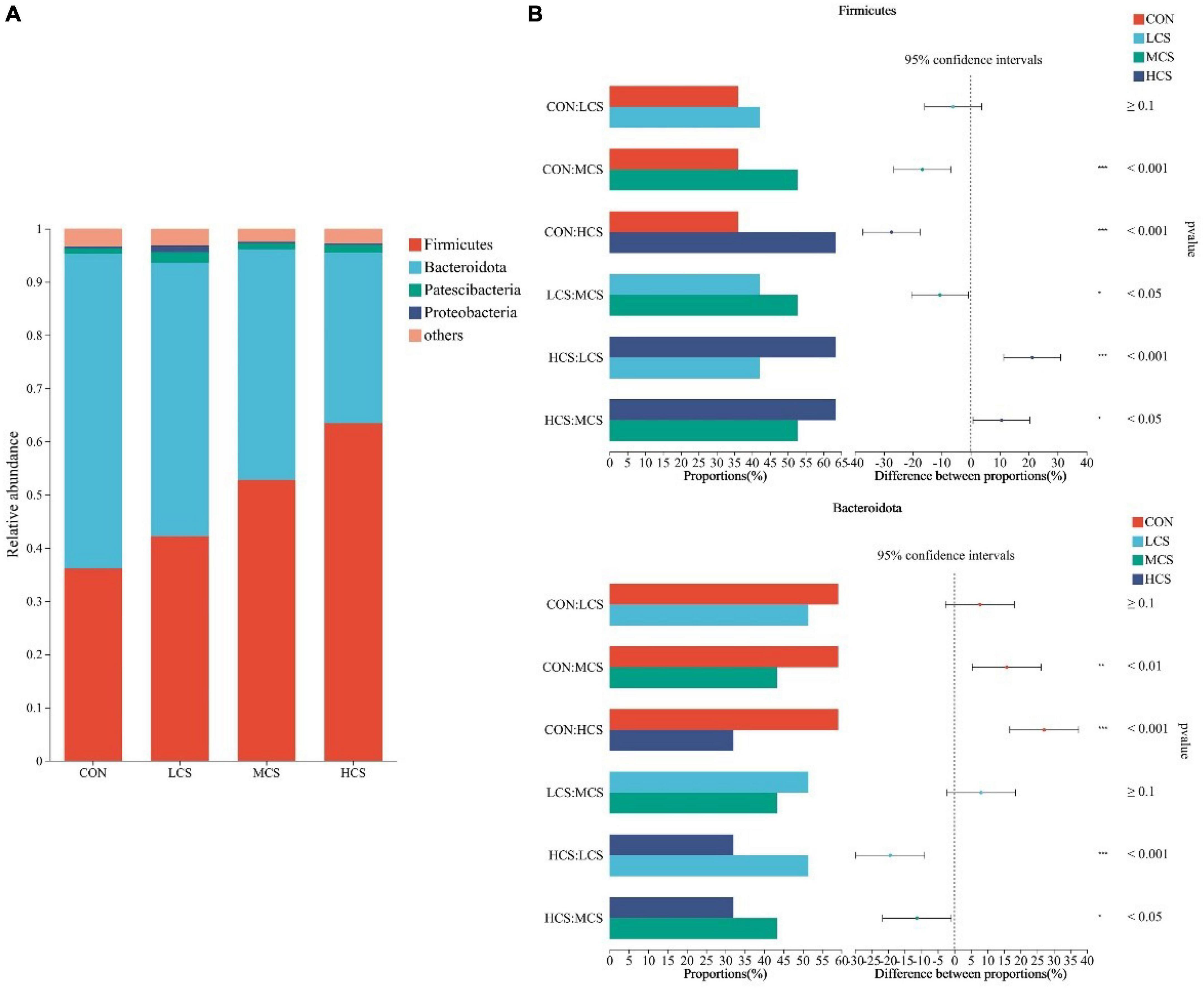
Figure 1. Composition of the rumen microbiota at bacterial phylum level (A) and differences between groups for major bacterial phyla (B). CON, control group; LCS, low concentrate supplement group; MCS, middle concentrate supplement group; HCS, high concentrate supplement group. *0.01 ≤ P ≤ 0.05, **0.001 ≤ P ≤ 0.01, ***P ≤ 0.001.
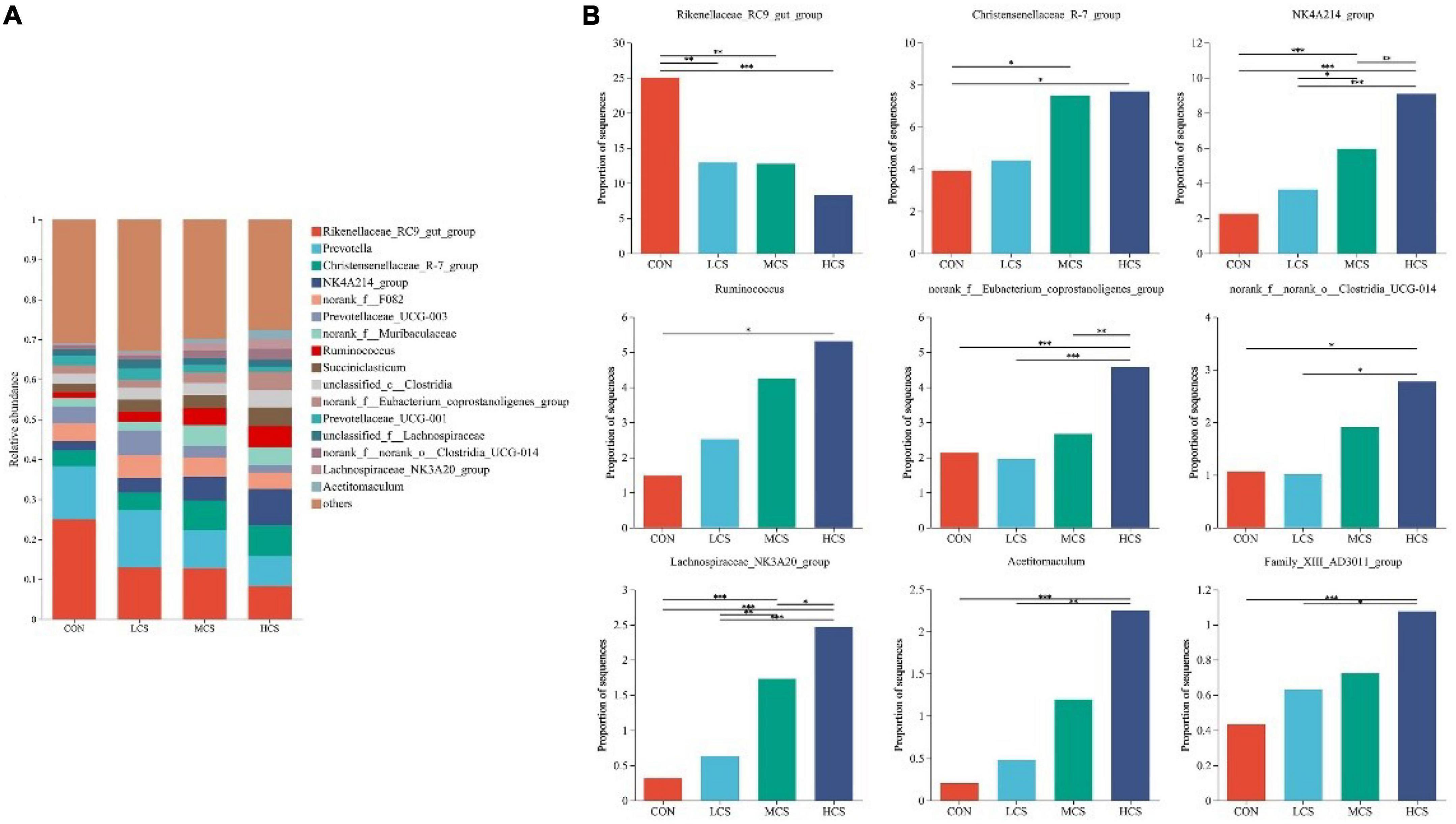
Figure 2. Composition of the rumen microbiota at bacterial genus level (A) and differences between groups for major bacterial genera (B). *0.01 ≤ P ≤ 0.05, **0.001 ≤ P ≤ 0.01, ***P ≤ 0.001.
3.3. Differences in ruminal bacterial species
Figures 1, 2 also demonstrate the differences in the relative abundance of bacterial communities in the rumen fluid samples among the four treatment groups. The abundance of Firmicutes in the rumen increased with the increasing supplementation level, while Bacteroidota showed the opposite trend. Among the four groups, the abundance of Firmicutes was highest in the HCS group and was higher in the MCS group than in the CON and LCS groups; meanwhile, the relative abundance of Bacteroidota in the HCS group was significantly lower than that in the other three groups, and that of Bacteroidota in the MCS group was significantly lower than that of the CON group (Figure 1B). Concentrate feeding significantly decreased the relative abundance of Rikenellaceae_RC9_gut_group at the genus level, while it increased the relative abundance of Christensenellaceae_R-7_group, NK4A214_group, Ruminococcus, norank_f__ Eubacterium_coprostanoligenes_group, norank_f__norank_o__Clostridia_UCG-014, Lachnospiraceae_NK3A20_group, Acetitomaculum, and Family_ XIII_AD3011_group. The abundance of Rikenellaceae_RC9_gut_group was lower than that of the CON group in all concentrate supplementation groups. The relative abundance of norank_f__Eubacterium_coprostanoligenes_group in the HCS group was significantly higher than that in the CON group, while the relative abundance of norank_f__norank_o__Clostridia_UCG-014 in the HCS group was significantly higher than that in the other three groups. The abundance of Acetitomaculum and Family_XIII_AD3011_group in the HCS group was higher than that in the CON and LCS groups. Meanwhile, Ruminococcus abundance was higher in the HCS group than that in the CON group (Figure 2B).
3.4. Correlation of rumen differentially abundant genera with rumen fermentation parameters
Supplementary Table 2 shows the significance of rumen fermentation parameters after VIF and RDA analysis. Among them, there were significant correlations (P < 0.05) between pH, NH3-N, propionate, and butyrate for subsequent analysis. Analysis of the species variability results showed that nine of the top 20 bacterial genera in the abundance ranking at the genus level of the rumen bacterial community differed between groups. Therefore, the above four rumen fermentation parameters were correlated with the nine differential bacterial genera based on Spearman’s correlation coefficient. The Christensenellaceae_R-7_group showed a positive correlation with NH3-N and butyrate levels, and a negative correlation with pH. The norank_f__Eubacterium_coprostanoligenes_group showed a significant positive correlation with both NH3-N and propionate levels, and a significant negative correlation with pH. In addition, NH3-N and propionate levels correlated positively with the abundances of NK4A214_group, Acetitomaculum, Lachnospiraceae_NK3A20_group, and norank_f__Eubacterium_coprostanoligenes_group, whereas pH correlated negatively correlated with the levels of these three bacterial genera. In contrast, Rikenellaceae_RC9_gut_group showed a significant negative correlation with NH3-N, propionate and butyrate levels, and a significant positive correlation with pH. In addition, Ruminococcus showed a positive correlation with NH3-N levels. Both norank_f__norank_o__Clostridia_UCG-014 and Family_XIII_AD3011_group showed positive correlations with Butyrate levels, and the latter also showed a negative correlation with pH (Figure 3).
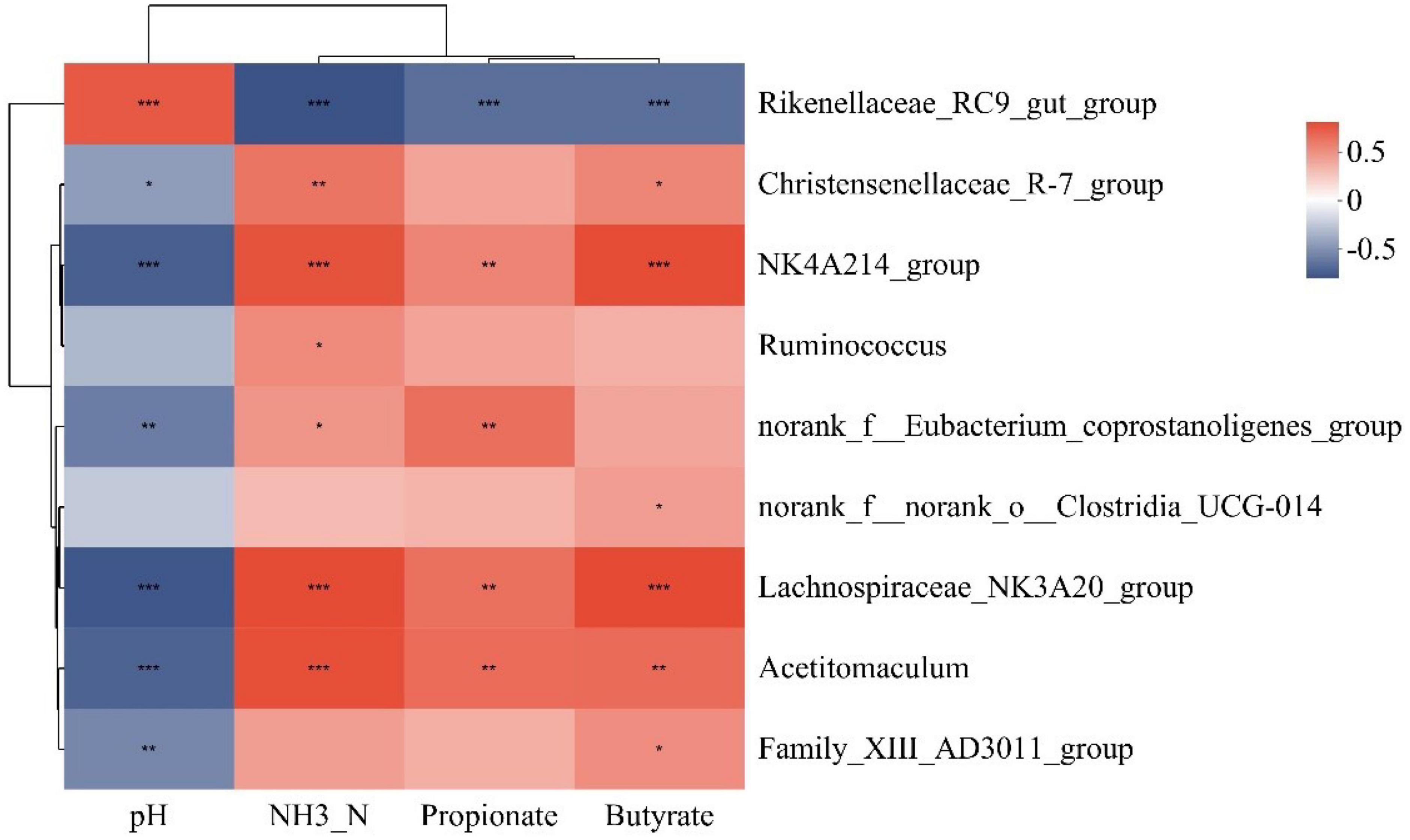
Figure 3. Heat map of correlation between rumen differential bacterial genus and screened rumen fermentation parameters. The color of the squares represents the magnitude of the correlation coefficients. *0.01 ≤ P ≤ 0.05, **0.001 ≤ P ≤ 0.01, ***P ≤ 0.001.
3.5. Comparative analysis of rumen metabolite samples
Supplementary Figure 2 depicts the intra-group similarity and inter-group variability of metabolites among the four groups of rumen fluid samples. PCA and PLS-DA analysis showed good intra-group aggregation and significant inter-group separation among the four groups of rumen fluid samples (Supplementary Figures 2A, B).
The correlation heat map of rumen fluid samples can indicate the degree of variability in metabolite composition and abundance between samples. Quantitative analysis can be performed by correlation analysis between samples, with correlations closer to 1 indicating a higher similarity in metabolic composition and abundance between samples (Supplementary Figure 2C). The heat map showed that the CON group showed a positive correlation with the LCS group and a significant negative correlation with the MCS and HCS groups. Meanwhile, the LCS group also showed a negative correlation with the MCS and HCS groups. With the increase of concentrate feeding, the degree of variation in metabolite composition and abundance in the MCS and HCS groups appeared to be significantly different from those in the CON and LCS groups, and there was a clear distinction.
3.6. Ruminal metabolite identification and differential metabolite analysis
Using LC-MS analysis of the four groups of rumen fluid samples, 1,453 metabolites were identified and screened by VIP > 1 and P < 0.05 (Supplementary Table 3). Table 3 lists the main metabolites that differed among the four treatment groups, and the metabolite concentrations of all six Metabolic classes in the table were significantly upregulated with increasing concentrate supplementation feeding (p < 0.05, fold-change (FC) > 1). The metabolite concentrations of all six metabolic classes in the table were significantly upregulated (p < 0.05, FC > 1) with the increase in concentrate supplementation feeding. In the HCS group, the concentrations of L-histidine, L-isoleucine, L-phenylalanine, L-tryptophan, L-tyrosine, L-valine, phosphocholine, deoxyadenosine monophosphate deoxyguanosine, deoxyinosine, xanthosine, guanosine, hypoxanthine, xanthine, histamine, uric acid, urocanic acid, choline, and phenylacetaldehyde were higher than in the other three groups. The concentrations of L-aspartic Acid, L-glutamate, L-histidine, L-isoleucine, L-phenylalanine, L-tryptophan, L-tyrosine, L-valine, N-acetyl-DL-glutamic acid, N-acetyl-L-ornithine, deoxyinosine, xanthosine, inosine, hypoxanthine, xanthine and urocanic acid were higher in MCS group than in CON and LCS groups. The concentrations of N-Acetyl-DL-Glutamic acid, Deoxyadenosine monophosphate, Deoxyguanosine, Guanine, Guanosine, Histamine, Uric acid, Choline Pantothenic Acid, and Phenylacetaldehyde were higher in the LCS group than in the CON group (Table 3).
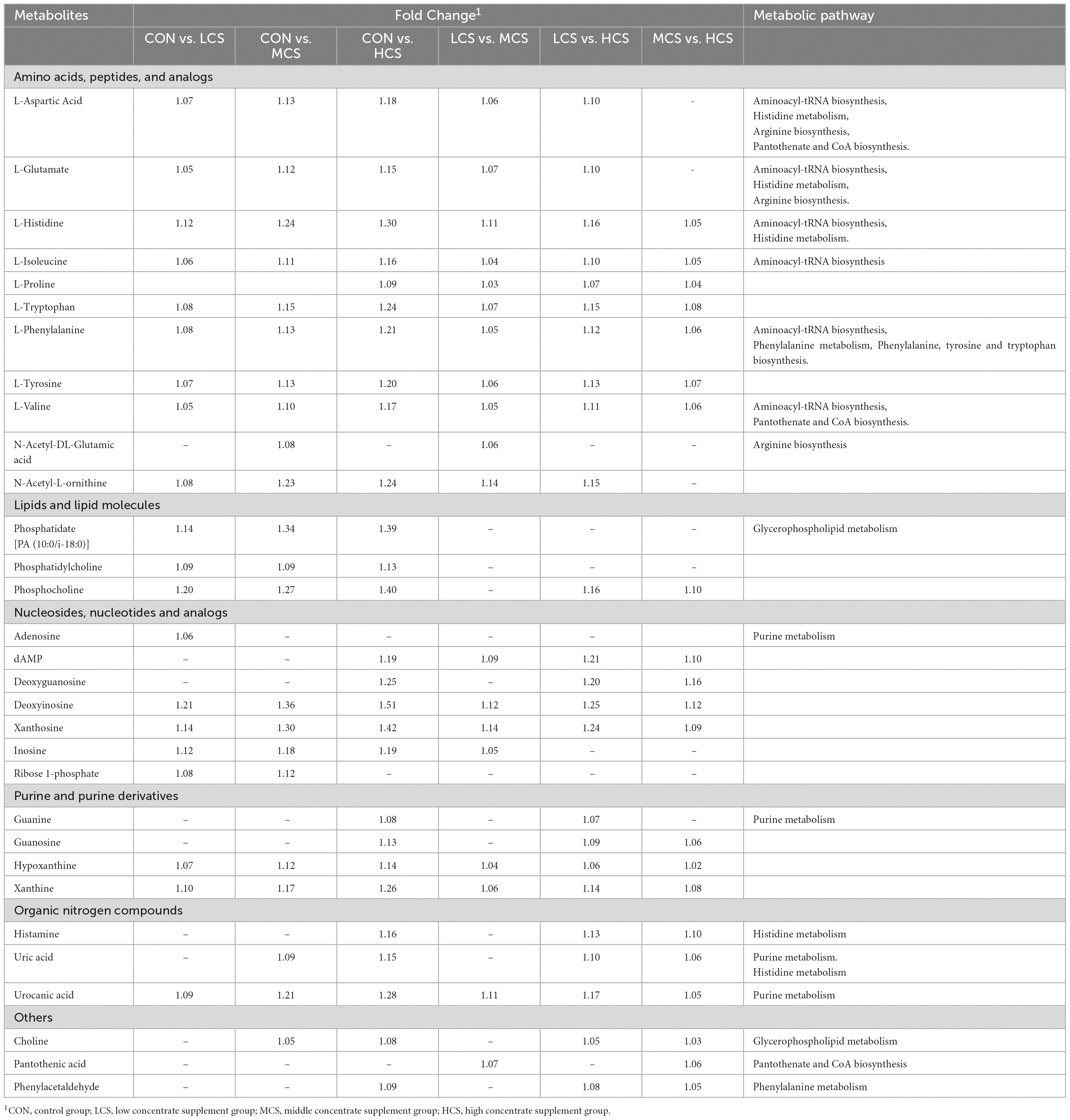
Table 3. The main differentially abundant metabolites and their metabolic pathways among the four groups (VIP > 1, p < 0.01).
Topological analysis of metabolites showed that the main enrichment pathways of the four treatment groups were aminoacyl-tRNA biosynthesis; purine metabolism; histidine metabolism; arginine biosynthesis; phenylalanine, tyrosine and tryptophan biosynthesis; glycerophospholipid metabolism; phenylalanine metabolism; and arginine and proline metabolism (Figure 4). The enrichment pathways in the CON, LCS, and MCS groups were aminoacyl-tRNA biosynthesis, purine metabolism, histidine metabolism, arginine biosynthesis, glycerophospholipid metabolism and phenylalanine, tyrosine and tryptophan biosynthesis (Figures 4A, B). Similarly, the HCS group was enriched with the CON and LCS groups for phenylalanine, tyrosine and tryptophan biosynthesis; purine metabolism; histidine metabolism; glycerophospholipid metabolism; phenylalanine metabolism; arginine biosynthesis; arginine and proline metabolism; and aminoacyl-tRNA biosynthesis (Figures 4C, E). Figure 4D demonstrates the enrichment pathways of the LCS and MCS groups, including aminoacyl-tRNA biosynthesis, Arginine biosynthesis, histidine metabolism, and phenylalanine, tyrosine and tryptophan biosynthesis. The enrichment pathways of the LCS and MCS groups are shown in Figure 4F, including purine metabolism, aminoacyl-tRNA biosynthesis, phenylalanine metabolism, histidine metabolism, glycerophospholipid metabolism, and phenylalanine, tyrosine and tryptophan biosynthesis.
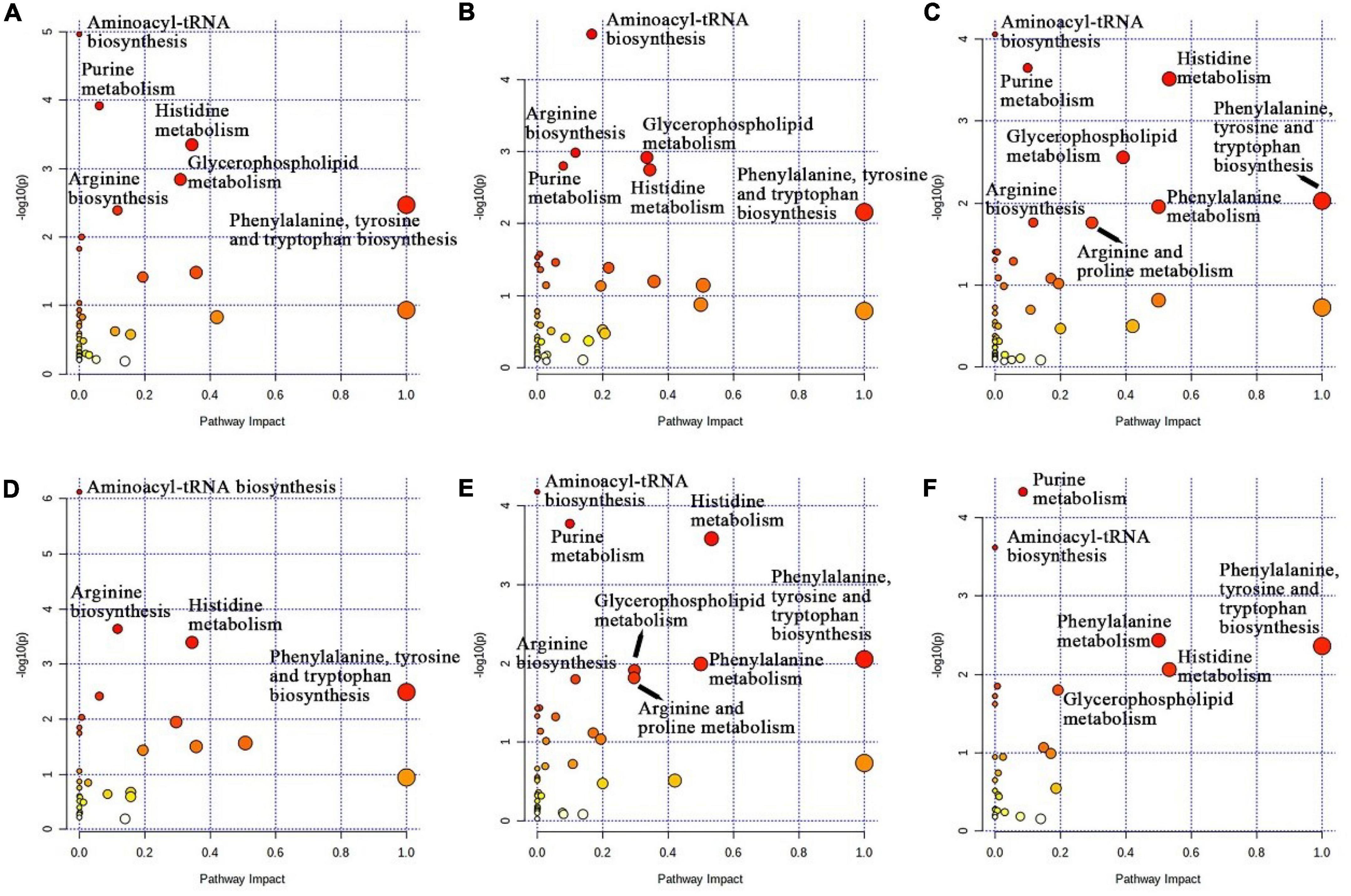
Figure 4. KEGG enrichment map of metabolic pathways. The X-axis indicates the pathway impact and the Y-axis indicates the degree of pathway enrichment. Larger circles represent higher pathway enrichment, and darker circles represent higher pathway impact values. (A) CON vs. LCM; (B) CON vs. MCS; (C) CON vs. HCS; (D) LCS vs. MCS; (E) LCS vs. HCS; (F) MCS vs. HCS. CON, control group; LCS, low concentrate supplement group; MCS, middle concentrate supplement group; HCS, high concentrate supplement group.
3.7. Correlation of rumen metabolites with rumen bacteria
To explore the potential relationships between rules bacterial communities and metals affected by concentrated feeding, we assessed correlations between nine affected rules bacterial general and major metals by Spearman correlation analysis, based on which we further mapped correlation networks (Supplementary Figure 3). Forty nodes and one hundred and eighty-nine edges formed a correlation network of rumen metabolites and microbial communities (Figure 5), of which 158 correlated positively and 31 correlated negatively (| r| >0.5, P < 0.05). The size of a node represents the degree of connectivity; the larger the node, the more nodes connected to it and the higher the degree of connection; by contrast, the fewer connected nodes, the lower the degree of connection. Among the nine bacterial genera, Lachnospiraceae_NK3A20_group and Rikenellaceae_RC9_gut_group were the most connected bacterial genera. The former belongs to the Firmicutes and had significant positive correlations with 31 metabolites, while the latter belongs to the Bacteroidota and also had significant negative correlations with 31 metabolites.
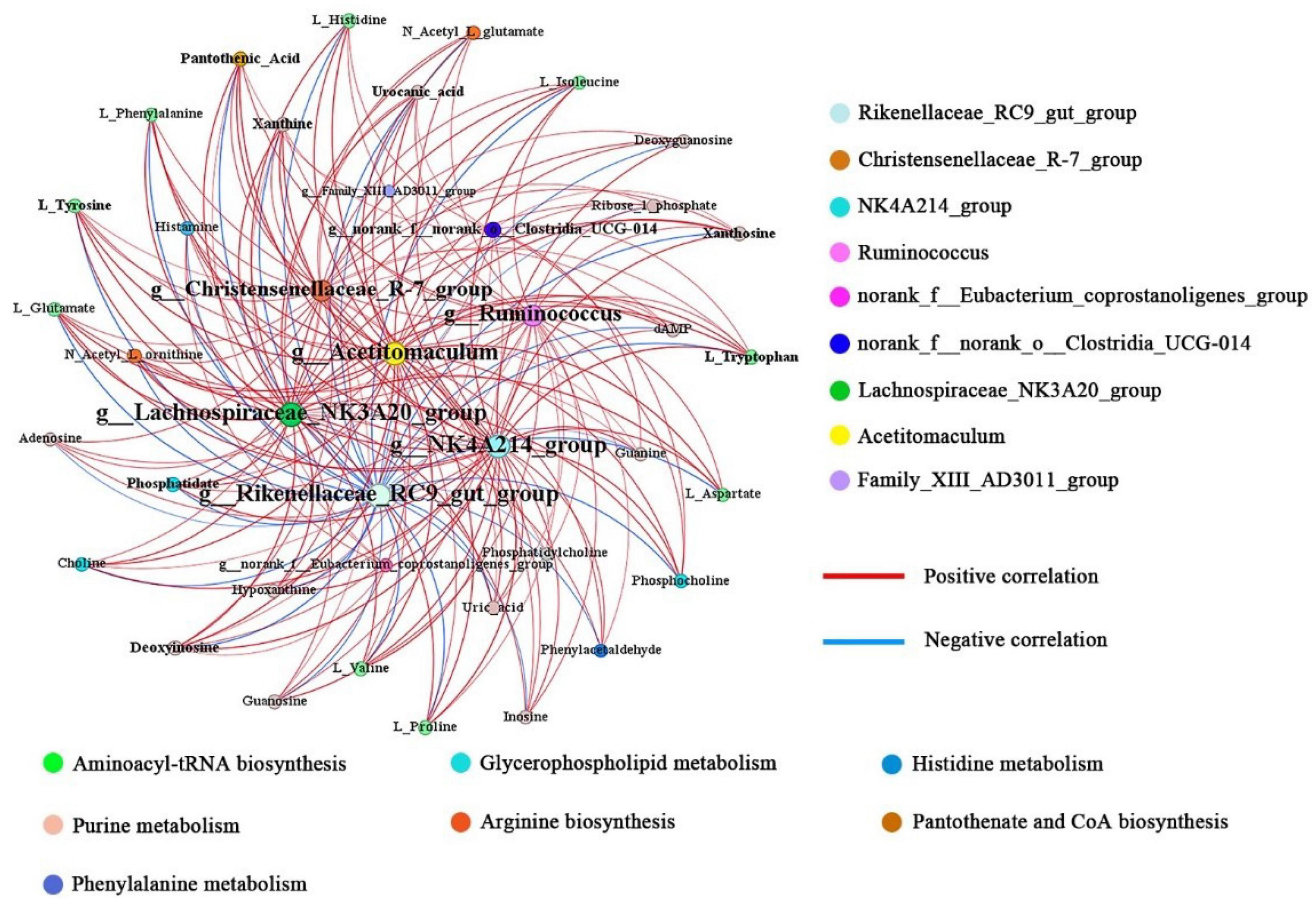
Figure 5. Networks associated with differentially abundant bacterial genera and differentially abundant metabolites. Node size represents the size of the degree; more edges connected, the larger the node.
In addition, the four bacterial genera NK4A214_group, Acetitomaculum, Ruminococcus, and Christensenellaceae_R-7_group were also highly connected, with significant positive correlations with 30, 30, 26, and 25 metabolites, respectively. All four bacterial genera belong to the Firmicutes. By contrast, norank_f__norank_o__Clostridia _UCG-014, Family_XIII_AD3011_group and norank_f__ Eubacterium_coprostanoligenes_group had fewer positive correlations with metabolites, with significant positive correlations with 11, 1, and 4 metabolites, respectively. Therefore, based on the above results, we suggest that the abundance of Firmicutes in the rumen might have an important influence on the changes in rumen metabolite levels.
4. Discussion
In the cold season on the Tibetan plateau, the nutrient content of forage grasses is low and cannot provide yaks with the nutrients they need to grow properly (Sapkota et al., 2022). In this study, the CON group did not gain weight; however, the growth performance of the yaks improved significantly with higher concentrate supplement feeding, which is consistent with the results of a previous study (Long et al., 2005). This suggests that supplemental feeding can help yaks resist nutritional stress and improve their ability to adapt to their environment.
Ruminal fermentation parameters can reflect the reasons for the differences in growth performance of yaks. Among them, VFAs are fermentation products of microorganisms in the yak rumen, and their production depends on diet and the rumen microbiota (Sha et al., 2021). Total VFAs increased significantly and the pH decreased significantly with higher concentrate feeding. This is because of the high content of fermentable carbohydrates, such as starch, in the concentrate supplements. Their rapid degradation increases the concentration of total VFAs and lowers the pH. Insufficient availability of forage in the cold season and low quality of forage can lead to lower dry matter intake of grazing yaks (Sapkota et al., 2022), which might be responsible for the lower concentration of total VFAs in the CON group relative to the other groups. Previous studies found that an increase in concentrate supplement feeding increased the concentration of VFAs other than acetate, but decreased the concentration of acetate and the ratio of acetate to propionate (Liu et al., 2019; Yi et al., 2022). The acetate:propionate ratio reflects the efficiency of feed energy use by animals, and a decrease in the ratio represents an increase in the efficiency of feed energy use by yaks (Chen et al., 2021), which is consistent with the significant increase in growth performance of yaks fed concentrate supplements. This change in the acetate:propionate ratio could provide guidance for future cold-season grazing yak production. In addition, isobutyrate and isovalerate in the rumen originate from feed protein degradation and amino acid deamination (Eugène et al., 2004), and their increase reflected the increased protein content in the rumen of the yaks after supplementation, which also led to an increase in the ruminal NH3-N content. Meanwhile, elevation of NH3-N, the main source of nitrogen for rumen microbial protein synthesis, was accompanied by an increase in microbial protein production in the rumen (Zhang et al., 2017a). Therefore, we concluded that on the one hand, feeding of concentrate supplements can alter the available fermentation substrates in the yak rumen, resulting in significant fluctuations in rumen fermentation parameters. On the other hand, changes in fermentation substrates can affect ruminal homeostasis, thereby affecting rumen microorganisms and their metabolic pathways (Ghimire et al., 2017). Therefore, changes in rumen pH, VFAs, and NH3-N might be related to changes in the rumen bacterial community of yaks.
In the present study, the most dominant bacterial phyla in the rumen of grazing yaks were Firmicutes and Bacteroides, accounting for approximately 95% of all bacterial phyla, which agreed with the results of previous studies (Liu et al., 2019; Yi et al., 2022). The abundance of Firmicutes increased with increasing concentrate supplementation, while the opposite was true for Bacteroidota. This change in Firmicutes can be explained by the fact that concentrate supplementation added a large amount of energy, and Firmicutes have the effect of absorbing a large amount of energy from a small amount of food (Liao et al., 2023). The decrease in abundance of Bacteroidota was attributed to the decrease in structural polysaccharides in the rumen caused by the increase in concentrate supplementation. Corroboratively, Bacteroidota are the main degraders of structural polysaccharides in the rumen (Lu et al., 2023).
At the genus level, we detected nine bacterial genera with significant differences (proportion of sequences > 1%). The Rikenellaceae_RC9_gut_group is a member of the Rikenellaceae family, which is involved in the degradation of fibrous polysaccharides (Zhang et al., 2022b,2023). Feeding of concentrate supplements led to a decrease in the concentration of fibrous polysaccharides in the rumen, which could also explain the correlation between Rikenellaceae_RC9_gut_group and rumen fermentation parameters. The results of a study on the differences in rumen microbial composition between house-fed yaks (high protein diet) and grazing yaks showed that Christensenellaceae_R-7_group was significantly more abundant in the rumen of the house-fed yaks than in in the grazing yaks (Yao et al., 2022), which is consistent with the results of the present study. Christensenellaceae_R-7_group, as a probiotic in the rumen, promotes host digestion and their absorption of nutrients or improves feed utilization (Ghimire et al., 2017), which was confirmed by its positive correlation with NH3-N and butyrate. The relative abundance of NK4A214_group, Acetitomaculum, and Lachnospiraceae_NK3A20_group all increased with increasing addition of concentrate supplementation, which is generally consistent with the results of previous studies (Liu et al., 2019; Sha et al., 2021; Yi et al., 2022). Among them, the NK4A214_group belongs to the Ruminococcaceae family, which plays an important role in the digestion of resistant starch (Anderson et al., 2016; Ferrario et al., 2017), and the feeding of concentrate supplements would deliver more resistant starch to the rumen. This might also be the main reason for the positive correlation between the NK4A214_ group and the concentrations of propionate and butyrate. Like the NK4A214_ group, Ruminococcus belongs to the Ruminococcaceae family. The abundance of Ruminococcus was highest in the HCS group, correlated positively with NH3-N levels, which is consistent with a previous report (Ahmad et al., 2021). The reason for this phenomenon might be that the difference in abundance of Ruminococcus was not significant among the groups other than in the HCS group. Lachnospiraceae_NK3A20_group and Acetitomaculum both belong to the Lachnospiraceae family, which is involved in the metabolism of many carbohydrates (e.g., starch) in the rumen (Ravelo et al., 2022, 2023). This is the reason why the abundance of Lachnospiraceae_NK3A20_group and Acetitomaculum increased significantly with the increase of concentrate supplement feeding. In addition, they can produce butyrate and other short-chain fatty acids by hydrolyzing carbohydrates such as starch (Reichardt et al., 2014; Chen et al., 2017), which is also consistent with the result that Lachnospiraceae_NK3A20_group and Acetitomaculum were significantly and positively correlated with propionate and butyrate. The norank_f__Eubacterium_coprostanoligenes_group can breakdown cholesterol into fecal sterols, thus playing an important role in lowering intestinal cholesterol levels in animals (Macdonald et al., 1983). The abundance of norank_f__Eubacterium_coprostanoligenes_group was higher in the HCS group than in the CON and LCS groups, presumably because the increased concentrate supplementation introduced a large amount of cholesterol into the rumen of the yaks in the HCS group. Both norank_f__norank_o__Clostridia_UCG-014 and Family_XIII_AD3011_group belong to Clostridia Class in the Firmicutes phylum, and their changes in abundance tended to be consistent with those of the Firmicutes phylum. Meanwhile, their significant positive correlation with butyrate suggested that they might be butyrate-producing bacteria.
In this study, we used metabolomics to fully reveal the effects of supplemental feeding on rumen metabolites in grazing yaks. Our previous study found that changes in diet structure altered the rumen environment and had a greater impact on the rumen microbial community (Yi et al., 2022). In the present study, based on the results of rumen fermentation parameters and microbial diversity, we suggest that changes in rumen metabolites might occur. The amino acids in the rumen are mainly derived from the degradation of feed proteins and microproteins by resident microorganisms. We observed that L-phenylalanine and L-tyrosine levels were significantly upregulated with increased concentrate supplement feeding. These two amino acids could be used as markers of protein metabolism (Liu et al., 2019; Xu et al., 2021), suggesting that feeding concentrate supplements brought sufficient amino acid reserves to yaks and enhanced their protein synthesis. This represents a new insight into yak production, in which supplemental feeding in the cool season mobilizes protein synthesis and metabolism in grazing yaks, which might promote muscle growth. In addition, L-valine and L-glutamic acid were also significantly upregulated with higher supplementation concentrations, and the former can be metabolized in the rumen to produce isobutyrate and isovalerate, which act as potential ketogenic and glucogenic substances (Menahan and Schultz, 1964). This also explains the significant increase in isobutyrate and isovalerate concentrations in previous studies of rumen fermentation parameters (Liu et al., 2019; Yi et al., 2022). Meanwhile, L-isoleucine, which is a branched chain amino acid (BCAA), together with L-valine, was also significantly upregulated in the supplemented group. BCAAs can repair muscles, control blood glucose, and provide energy to body tissues (Buse and Weigand, 1976; Freund and Fischer, 1978), indicating that supplementation could help repair muscle damage caused by antinutritional stress to some extent. L-histidine has been reported to be the third limiting amino acid in ruminants after L-lysine and L-methionine (Van den Bossche et al., 2023), and its increased content helps to regulate ruminant nitrogen utilization and improve counterproductive performance (Morris and Kononoff, 2020). L-proline is a functional amino acid (Wu et al., 2011) whose levels in the yak rumen have been reported to increase with increasing levels of dietary concentrates (Zhang et al., 2017b), which is in agreement with the results of the present study. The concentration of L-aspartate increased significantly in the supplemented groups, probably because the supplementation provided a large source of nitrogen to the yaks. L-aspartic acid participates in the ornithine cycle and promotes the production of urea from oxygen and carbon dioxide, thereby reducing the amount of nitrogen and carbon dioxide in the blood (Peng et al., 2019). In addition, L-aspartic acid, N-acetyl-DL-glutamic acid and N-acetyl-L-ornithine are all involved in the biosynthesis of L-arginine, which is also an important player in the urea cycle (Lerzynski et al., 2006). L-tryptophan can regulate the immune function of ruminant organism (Patil et al., 2013), and its concentration was significantly upregulated in the supplemented group, indicating that supplementation contributes to improving the immunity of the organism. All of the above amino acids are involved in the aminoacyl-tRNA biosynthesis process, which is a step that all amino acids must undergo before they can be incorporated into the peptide chain. Amino acids undergo an activation reaction catalyzed by the synthesis of aminoacyl-tRNA enzymes for energy, and the amino acids then bind to tRNAs to form aminoacyl-tRNA, which provides the material basis for protein synthesis (Li et al., 1997). The enrichment of this process once again proves that feeding concentrate supplements enhances protein synthesis and metabolism in yaks.
Glycerophospholipids participate in protein recognition and signal transduction in animal cell membranes (Hayashi and Dennis, 2023). Supplemental feeding of concentrate increased the levels of metabolites associated with glycerophospholipid metabolism (phosphatidate, phosphatidylcholine, phosphocholine, and choline) in the yak rumen, which might be associated with enhanced protein metabolism in yaks organism. The concentration of pantothenic acid was upregulated in the concentrate supplemented, probably because the concentrate supplement enhanced fatty acid and pyruvate metabolism in yaks (Tahiliani and Beinlich, 1991; Abo Alrob and Lopaschuk, 2014). Biogenic amines (e.g., spermine, tyramine, and histamine) regulate the synthesis of DNA, RNA, and proteins, as well as maintain the stability of biological membranes (Wojcik et al., 2021). In the present study, histamine levels were significantly upregulated in the HCS group compared with those in the CON group, which is consistent with the results of a previous study (Humer et al., 2018).
Previous studies have found that increased biogenic amine production in ruminants is closely associated with an acidic rumen environment (Zhang et al., 2022a), suggesting that our supplementation of concentrates might lead to an overly acidic ruminal environment in yaks, reminding us to take steps to ensure animal health during production.
Notably, a number of metabolites associated with purine metabolism showed changes in their concentrations. Nucleic acids are synthesized by microbial populations in the rumen by degrading nitrogen from the feed (Fujihara and Shem, 2011). These metabolites were found in higher levels in three concentrate supplementation groups in the present study. Xanthine and hypoxanthine, products of nucleic acid degradation by gram-negative bacteria (Zhang et al., 2022a), were elevated in the high concentrate diet. Additionally, purines are converted into hypoxanthine by intermediates, and hypoxanthine is transformed into xanthine by secondary metabolism. Thus, xanthine and hypoxanthine are capable of acting as biomarkers of microbial protein synthesis (Ametaj et al., 2010; Zhang et al., 2017b). According to these findings, concentrate supplements are beneficial for purine metabolism in cool-season grazing yaks. Moreover, both Lachnospiraceae_NK3A20_group and Acetitomaculum are gram-negative bacteria that correlate positively with purine metabolites. Their relative abundance was upregulated in the concentrate supplement groups. This suggested that the feeding with the concentrate supplement accelerated the microbial passaging process in the rumen, which might be responsible for the increase in the number of bacterial degradation products. The NK4A214_ group, Acetitomaculum, and Lachnospiraceae_NK3A20_ group all correlated positively with the levels of differentially abundant metabolites. Similarly, Christensenellaceae_R-7_group and Ruminococcus also showed a positive correlation with the levels of most differentially abundant metabolites. This indicated that these five bacterial genera are more active in the degradation of the concentrate supplements. In addition, Rikenellaceae_RC9_gut_group showed a negative correlation with the levels of all differentially abundant metabolites, which was associated with a decrease in their relative abundance with increasing supplementation levels.
5. Conclusion
This study used multi-omics techniques to explore the dynamics of rumen bacterial communities and metabolites in cool season grazing yaks that received supplemental feeding. Changes in rumen bacterial communities and metabolites, and correlations among them, might affect the productivity of cool-season grazing yaks. Our comprehensive analysis revealed the association between the rumen environment and bacterial genera. These findings showed that diet influences the function of the rumen microbial community in a complex manner, and suggest that high-dose supplementation of yaks during the cold season can avoid nutritional stress and achieve good economic returns. This study provides a theoretical basis for developing an appropriate cold season supplemental feeding program for yaks.
Data availability statement
The datasets presented in this study can be found in online repositories. The names of the repository/repositories and accession number(s) can be found in this article/Supplementary material.
Ethics statement
The animal study was approved by the Experimental Animal Welfare and Animal Experimentation Ethics Committee of China Agricultural University. The study was conducted in accordance with the local legislation and institutional requirements.
Author contributions
HW, SL, SC, and ZZ designed the study. SY and DD performed the sample process. SY performed the data analysis and wrote the manuscript. HW and ZZ revised the manuscript. All authors have read and approved the final manuscript.
Conflict of interest
The authors declare that the research was conducted in the absence of any commercial or financial relationships that could be construed as a potential conflict of interest.
Publisher’s note
All claims expressed in this article are solely those of the authors and do not necessarily represent those of their affiliated organizations, or those of the publisher, the editors and the reviewers. Any product that may be evaluated in this article, or claim that may be made by its manufacturer, is not guaranteed or endorsed by the publisher.
Supplementary material
The Supplementary Material for this article can be found online at: https://www.frontiersin.org/articles/10.3389/fmicb.2023.1247251/full#supplementary-material
Supplementary Figure 1 | Alpha diversity and beta diversity values for the four treatment groups.
Supplementary Figure 2 | PCA plots, PLS-DA plots, and sample correlation heat maps for the four treatment groups.
Supplementary Figure 3 | Spearman’s correlation coefficient between differential bacterial genera and rumen metabolites.
Supplementary Table 1 | Nutritional value of forage grasses during the experiment.
Supplementary Table 2 | Ruminal fermentation parameters after variance inflation factor (VIF) analysis and RDA analysis.
Supplementary Table 3 | List of identified metabolites.
Footnotes
References
Abo Alrob, O., and Lopaschuk, G. D. (2014). Role of CoA and acetyl-CoA in regulating cardiac fatty acid and glucose oxidation. Biochem. Soc. Trans 42, 1043–1051. doi: 10.1042/BST20140094
Ahmad, A. A., Zhang, J. B., Liang, Z., Yang, C., Kalwar, Q., Shah, T., et al. (2021). Dynamics of rumen bacterial composition of yak (Bos grunniens) in response to dietary supplements during the cold season. PeerJ 9:e11520. doi: 10.7717/peerj.11520
Ametaj, B. N., Zebeli, Q., Saleem, F., Psychogios, N., Lewis, M. J., Dunn, S. M., et al. (2010). Metabolomics reveals unhealthy alterations in rumen metabolism with increased proportion of cereal grain in the diet of dairy cows. Metabolomics 6, 583–594. doi: 10.1007/s11306-010-0227-6
Anderson, C. L., Schneider, C. J., Erickson, G. E., MacDonald, J. C., and Fernando, S. C. (2016). Rumen bacterial communities can be acclimated faster to high concentrate diets than currently implemented feedlot programs. J. Appl. Microbiol. 120, 588–599. doi: 10.1111/jam.13039
Bolyen, E., Rideout, J. R., Dillon, M. R., Bokulich, N. A., Abnet, C. C., Al-Ghalith, G. A., et al. (2019). Reproducible, interactive, scalable and extensible microbiome data science using QIIME 2. Nat. Biotechnol. 37, 852–857. doi: 10.1038/s41587-019-0209-9
Broderick, G. A., and Kang, J. H. (1980). Automated simultaneous determination of ammonia and total amino-acids in ruminal fluid and in vitro media. J. Dairy Sci. 63, 64–75. doi: 10.3168/jds.S0022-0302(80)82888-8
Buse, M. G., and Weigand, D. A. (1976). Effect of branched-chain amino-acids on protein turnover of muscles - role of leucine. Federation Proc. 35, 1516–1516.
Callahan, B. J., McMurdie, P. J., Rosen, M. J., Han, A. W., Johnson, A. J., and Holmes, S. P. (2016). DADA2: High-resolution sample inference from illumina amplicon data. Nat. Methods 13, 581–583. doi: 10.1038/nmeth.3869
Chen, H., Wang, C., Huasai, S., and Chen, A. (2021). Effects of dietary forage to concentrate ratio on nutrient digestibility, ruminal fermentation and rumen bacterial composition in Angus cows. Sci. Rep. 11:17023. doi: 10.1038/s41598-021-96580-5
Chen, L., Wilson, J. E., Koenigsknecht, M. J., Chou, W. C., Montgomery, S. A., Truax, A. D., et al. (2017). NLRP12 attenuates colon inflammation by maintaining colonic microbial diversity and promoting protective commensal bacterial growth. Nat. Immunol. 18, 541–551. doi: 10.1038/ni.3690
Chen, S., Zhou, Y., Chen, Y., and Gu, J. (2018). fastp: An ultra-fast all-in-one FASTQ preprocessor. Bioinformatics 34, i884–i890. doi: 10.1093/bioinformatics/bty560
Clemmons, B. A., Martino, C., Powers, J. B., Campagna, S. R., Voy, B. H., Donohoe, D. R., et al. (2019). Rumen bacteria and serum metabolites predictive of feed efficiency phenotypes in beef cattle. Sci. Rep. 9:19265. doi: 10.1038/s41598-019-55978-y
Erwin, E. S., Marco, G. J., and Emery, E. M. (1961). Volatile fatty acid analyses of blood and rumeen fluid by gas chromatography. J. Dairy Sci. 44:1768. doi: 10.3168/jds.S0022-0302(61)89956-6
Eugène, M., Archimède, H., and Sauvant, D. (2004). Quantitative meta-analysis on the effects of defaunation of the rumen on growth, intake and digestion in ruminants. Livestock Prod. Sci. 85, 81–97. doi: 10.1016/s0301-6226(03)00117-9
Ferrario, C., Statello, R., Carnevali, L., Mancabelli, L., Milani, C., Mangifesta, M., et al. (2017). How to feed the mammalian gut microbiota: Bacterial and metabolic modulation by dietary fibers. Front. Microbiol. 8:1749. doi: 10.3389/fmicb.2017.01749
Freund, H., and Fischer, J. E. (1978). Nitrogen-conserving quality of branched-chain amino-acids – possible regulator effect of valine in postinjury muscle catabolism. Surgical Forum 29, 69–71.
Fujihara, T., and Shem, M. N. (2011). Metabolism of microbial nitrogen in ruminants with special reference to nucleic acids. Anim. Sci. J. 82, 198–208. doi: 10.1111/j.1740-0929.2010.00871.x
Ghimire, S., Kohn, R. A., Gregorini, P., White, R. R., and Hanigan, M. D. (2017). Representing interconversions among volatile fatty acids in the Molly cow model. J. Dairy Sci. 100, 3658–3671. doi: 10.3168/jds.2016-11858
Granja-Salcedo, Y. T., Ribeiro Junior, C. S., de Jesus, R. B., Gomez-Insuasti, A. S., Rivera, A. R., Messana, J. D., et al. (2016). Effect of different levels of concentrate on ruminal microorganisms and rumen fermentation in Nellore steers. Arch. Anim. Nutr. 70, 17–32. doi: 10.1080/1745039X.2015.1117562
Hayashi, D., and Dennis, E. A. (2023). Molecular basis of unique specificity and regulation of group VIA calcium-independent phospholipase A(2) (PNPLA9) and its role in neurodegenerative diseases. Pharmacol. Ther. 2023:108395. doi: 10.1016/j.pharmthera.2023.108395
Huang, X., Mi, J., Denman, S. E., Basangwangdui, P., Zhang, Q., et al. (2022). Changes in rumen microbial community composition in yak in response to seasonal variations. J. Appl. Microbiol. 132, 1652–1665. doi: 10.1111/jam.15322
Humer, E., Kroger, I., Neubauer, V., Schedle, K., Reisinger, N., and Zebeli, Q. (2018). Supplementing phytogenic compounds or autolyzed yeast modulates ruminal biogenic amines and plasma metabolome in dry cows experiencing subacute ruminal acidosis. J. Dairy Sci. 101, 9559–9574. doi: 10.3168/jds.2018-14744
Lerzynski, G., Suschek, C. V., and Kolb-Bachofen, V. (2006). In hepatocytes the regulation of NOS-2 activity at physiological L-arginine levels suggests a close link to the urea cycle. Nitric Oxide 14, 300–308. doi: 10.1016/j.niox.2005.11.009
Li, Y., Li, T., and Wang, E. D. (1997). Recognition of tRNAs by aminoacyl-tRNA synthetases. Prog. Biochem. Biophys. 24, 2–7.
Liao, Y., Wang, C., Gao, Z., Pan, Z., Peng, M., Ma, J., et al. (2023). Anti-obesity mechanism of Ganpu tea revealed by microbiome, metabolome and transcriptome analyses. Food Chem. 412:135048. doi: 10.1016/j.foodchem.2022.135048
Liu, C., Wu, H., Liu, S., Chai, S., Meng, Q., and Zhou, Z. (2019). Dynamic alterations in yak rumen bacteria community and metabolome characteristics in response to feed type. Front. Microbiol. 10:1116. doi: 10.3389/fmicb.2019.01116
Long, R. J., Dong, S. K., Wei, X. H., and Pu, X. P. (2005). The effect of supplementary feeds on the bodyweight of yaks in cold season. Livestock Prod. Sci. 93, 197–204. doi: 10.1016/j.livprodsci.2004.08.016
Long, R. J., Zhang, D. G., Wang, X., Hu, Z. Z., and Dong, S. K. (1999). Effect of strategic feed supplementation on productive and reproductive performance in yak cows. Prev. Vet. Med. 38, 195–206. doi: 10.1016/s0167-5877(98)00125-1
Lu, X., Xu, H., Fang, F., Liu, J., Wu, K., Zhang, Y., et al. (2023). In vitro effects of two polysaccharide fractions from Laminaria japonica on gut microbiota and metabolome. Food Funct. doi: 10.1039/d2fo04085a [Epub ahead of print].
Macdonald, I. A., Bokkenheuser, V. D., Winter, J., McLernon, A. M., and Mosbach, E. H. (1983). Degradation of steroids in the human gut. J. Lipid Res. 24, 675–700.
Magoc, T., and Salzberg, S. L. (2011). FLASH: Fast length adjustment of short reads to improve genome assemblies. Bioinformatics 27, 2957–2963. doi: 10.1093/bioinformatics/btr507
Menahan, L. A., and Schultz, L. H. (1964). Metabolism of leucine + valine within rumen. J. Dairy Sci. 47:1080. doi: 10.3168/jds.S0022-0302(64)88849-4
Morris, D. L., and Kononoff, P. J. (2020). Effects of rumen-protected lysine and histidine on milk production and energy and nitrogen utilization in diets containing hydrolyzed feather meal fed to lactating Jersey cows. J. Dairy Sci. 103, 7110–7123. doi: 10.3168/jds.2020-18368
Pang, Z., Zhou, G., Ewald, J., Chang, L., Hacariz, O., Basu, N., et al. (2022). Using MetaboAnalyst 5.0 for LC-HRMS spectra processing, multi-omics integration and covariate adjustment of global metabolomics data. Nat. Protoc. 17, 1735–1761. doi: 10.1038/s41596-022-00710-w
Patil, R. J., Tyagi, J. S., Sirajudeen, M., Singh, R., Moudgal, R. P., and Mohan, J. (2013). Effect of dietary melatonin and L-tryptophan on growth performance and immune responses of broiler chicken under experimental aflatoxicosis. Iran. J. Appl. Anim. Sci. 3, 139–144.
Peng, M. Z., Cai, Y. N., Shao, Y. X., Zhao, L., Jiang, M. Y., Lin, Y. T., et al. (2019). Simultaneous quantification of 48 plasma amino acids by liquid chromatography-tandem mass spectrometry to investigate urea cycle disorders. Clin. Chim. Acta 495, 406–416. doi: 10.1016/j.cca.2019.05.011
Ravelo, A. D., Arce-Cordero, J. A., Lobo, R. R., Liu, T., Jeong, K. C., and Faciola, A. (2023). Effects of partially replacing dietary corn with sugars in a dual-flow continuous culture system on the ruminal microbiome. Transl. Anim. Sci. 7, txad011. doi: 10.1093/tas/txad011
Ravelo, A. D., Calvo Agustinho, B., Arce-Cordero, J., Monterio, H. F., Bennet, S. L., Sarmikasoglou, E., et al. (2022). Effects of partially replacing dietary corn with molasses, condensed whey permeate, or treated condensed whey permeate on ruminal microbial fermentation. J. Dairy Sci. 105, 2215–2227. doi: 10.3168/jds.2021-20818
Reichardt, N., Duncan, S. H., Young, P., Belenguer, A., McWilliam Leitch, C., Scott, K. P., et al. (2014). Phylogenetic distribution of three pathways for propionate production within the human gut microbiota. ISME J. 8, 1323–1335. doi: 10.1038/ismej.2014.14
Sapkota, S., Acharya, K. P., Laven, R., and Acharya, N. (2022). Possible consequences of climate change on survival, productivity and reproductive performance, and welfare of Himalayan Yak (Bos grunniens). Vet. Sci. 9:449. doi: 10.3390/vetsci9080449
Sha, Y., Hu, J., Shi, B., Dingkao, R., Wang, J., Li, S., et al. (2021). Supplementary feeding of cattle-yak in the cold season alters rumen microbes, volatile fatty acids, and expression of SGLT1 in the rumen epithelium. PeerJ 9:e11048. doi: 10.7717/peerj.11048
Tahiliani, A. G., and Beinlich, C. J. (1991). Pantothenic acid in health and disease. Vitam. Horm. 46, 165–228. doi: 10.1016/s0083-6729(08)60684-6
Van den Bossche, T., Goossens, K., Ampe, B., Haesaert, G., De Sutter, J., De Boever, J. L., et al. (2023). Effect of supplementing rumen-protected methionine, lysine, and histidine to low-protein diets on the performance and nitrogen balance of dairy cows. J. Dairy Sci. 106, 1790–1802. doi: 10.3168/jds.2022-22041
Wojcik, W., Lukasiewicz, M., and Puppel, K. (2021). Biogenic amines: Formation, action and toxicity - a review. J. Sci. Food Agric. 101, 2634–2640. doi: 10.1002/jsfa.10928
Wu, G., Bazer, F. W., Burghardt, R. C., Johnson, G. A., Kim, S. W., Knabe, D. A., et al. (2011). Proline and hydroxyproline metabolism: Implications for animal and human nutrition. Amino Acids 40, 1053–1063. doi: 10.1007/s00726-010-0715-z
Xu, C., Liu, W., Sun, B., Zhang, S., Zhang, S., Yang, Y., et al. (2021). Multi-omics analysis reveals a dependent relationship between rumen bacteria and diet of grass- and grain-fed yaks. Front. Microbiol. 12:642959. doi: 10.3389/fmicb.2021.642959
Xue, M. Y., Sun, H. Z., Wu, X. H., Liu, J. X., and Guan, L. L. (2020). Multi-omics reveals that the rumen microbiome and its metabolome together with the host metabolome contribute to individualized dairy cow performance. Microbiome 8:64. doi: 10.1186/s40168-020-00819-8
Yao, X., Wang, W., Xu, C., Zhang, R., Chen, Y., Niu, Y., et al. (2022). Effects of feeding pattern on rumen tissue structure and rumen bacterial community diversity and composition of Datong yaks (Bos grunniens). Chin. J. Anim. Nutr. 34, 3777–3787. doi: 10.3969/j.issn.1006-267x.2022.06.038
Yi, S., Dai, D., Wu, H., Chai, S., Liu, S., Meng, Q., et al. (2022). Dietary concentrate-to-forage ratio affects rumen bacterial community composition and metabolome of yaks. Front. Nutr. 9:927206. doi: 10.3389/fnut.2022.927206
Zhang, J., Shi, H., Wang, Y., Li, S., Cao, Z., Ji, S., et al. (2017a). Effect of dietary forage to concentrate ratios on dynamic profile changes and interactions of ruminal microbiota and metabolites in holstein heifers. Front. Microbiol. 8:2206. doi: 10.3389/fmicb.2017.02206
Zhang, R. Y., Zhu, W. Y., Jiang, L. S., and Mao, S. Y. (2017b). Comparative metabolome analysis of ruminal changes in Holstein dairy cows fed low- or high-concentrate diets. Metabolomics 13:74. doi: 10.1007/s11306-017-1204-0
Zhang, Q., Zhao, Y., Li, Y., Guo, X., Guo, Y., Ma, G., et al. (2023). Dietary polysaccharide-rich extract from noni (Morinda citrifolia L.) fruit modified ruminal fermentation, ruminal bacterial community and nutrient digestion in cashmere goats. Animals 13:221. doi: 10.3390/ani13020221
Zhang, T., Mu, Y., Zhang, R., Xue, Y., Guo, C., Qi, W., et al. (2022a). Responsive changes of rumen microbiome and metabolome in dairy cows with different susceptibility to subacute ruminal acidosis. Anim. Nutr. 8, 331–340. doi: 10.1016/j.aninu.2021.10.009
Zhang, X., Liu, X., Chang, S., Zhang, C., Du, W., and Hou, F. (2022b). Effect of Cistanche deserticola on rumen microbiota and rumen function in grazing sheep. Front. Microbiol. 13:840725. doi: 10.3389/fmicb.2022.840725
Keywords: yak, rumen, microbiota, metabolomics, concentrate supplementation
Citation: Yi S, Wu H, Liu Y, Dai D, Meng Q, Chai S, Liu S and Zhou Z (2023) Concentrate supplementation improves cold-season environmental fitness of grazing yaks: responsive changes in the rumen microbiota and metabolome. Front. Microbiol. 14:1247251. doi: 10.3389/fmicb.2023.1247251
Received: 25 June 2023; Accepted: 08 August 2023;
Published: 28 August 2023.
Edited by:
Ignacio Badiola, Institute of Agrifood Research and Technology (IRTA), SpainReviewed by:
Yangchun Cao, Northwest A&F University, ChinaYu Pi, Chinese Academy of Agricultural Sciences, China
Copyright © 2023 Yi, Wu, Liu, Dai, Meng, Chai, Liu and Zhou. This is an open-access article distributed under the terms of the Creative Commons Attribution License (CC BY). The use, distribution or reproduction in other forums is permitted, provided the original author(s) and the copyright owner(s) are credited and that the original publication in this journal is cited, in accordance with accepted academic practice. No use, distribution or reproduction is permitted which does not comply with these terms.
*Correspondence: Zhenming Zhou, emhvdXptQGNhdS5lZHUuY24=