- 1Key Laboratory of Vegetation Ecology, Ministry of Education, Jilin Songnen Grassland Ecosystem National Observation and Research Station, Northeast Normal University, Changchun, China
- 2College of Animal Science and Veterinary Medicine, Heilongjiang Bayi Agricultural University, Daqing, China
The interaction between poisonous weeds and neighboring plants is complex. Poisonous weeds frequently have a competitive advantage in the interaction between poisonous weeds and neighboring plants. Arbuscular mycorrhizal fungi (AMF) and plant pathogenic fungi (PPF) are closely related to the interspecific relationships of plants. However, the role of AMF and PPF between poisonous weeds and neighboring grasses remains unclear. Here, we designed a pot experiment to determine the interspecific relationship between Leymus chinensis and Stellera chamaejasme and the regulation of AMF and PPF. The results showed that interactive effects between L. chinensis and S. chamaejasme significantly inhibited the aboveground growth of both but promoted the underground growth of L. chinensis. As the proportions of S. chamaejasme increased, the total nitrogen content and pH in the rhizosphere soil of L. chinensis were reduced, the soil pH of S. chamaejasme was reduced, and the relative abundance of AMF in the rhizosphere soil of L. chinensis significantly increased and that of S. chamaejasme decreased considerably. The relative abundances of PPF in the rhizosphere soil of both in the mono-cultures were significantly higher than those in the mixed cultures. Structural equation modeling indicated that soil abiotic (pH and N availability) and biotic (AMF and PPF) factors are major drivers explaining the interactive effects between L. chinensis and S. chamaejasme. We provided new evidence for the interspecific interactions between poisonous weeds and neighboring grasses and revealed the regulatory role of AMF and PPF in the interactive effects of both plants. This study will provide a scientific basis for the prevention and control of poisonous weeds and the vegetation restoration of degraded grasslands in the future.
Introduction
Grassland degradation is a major threat to global ecosystem function and regional ecological security (Lu et al., 2015). With the severe degradation of natural grasslands, the proportion of poisonous weeds in grassland communities is gradually increasing. The interspecific relationship between poisonous weeds and neighboring plants is one of the research hotspots in grassland ecology. In addition, compared to forage plants, poisonous weeds have a stronger ability to compete for resources such as water and mineral nutrients (Jandová et al., 2014), leading to the formation of different spatial patterns in plant populations. On the other hand, root exudates of poisonous weeds may comprise a diversity of chemical compounds that can differentially affect the growth of neighboring plants, which further affects the interspecific relationships of plants (Bertin et al., 2003).
Studies have shown that the poisonous weed Ligularia virgaura has a significant competitive effect with Elymus nutans. It competes with E. nutans for essential elements for growth, water, light, and other external environmental resources and encroaches on the aboveground and underground ecological niche spaces (Jin et al., 2011). In addition, the growth of poisonous weeds such as Tibet lancea, Oxytropis ochrocephala, and Astragalus polycladus has reduced the number of dominant plant species in the grassland due to its strong competitive advantage. They gradually formed a single population of poisonous weeds in local grassland areas (Li et al., 2014). The presence of poisonous weeds could significantly reduce soil nutrient availability and enzyme activity (Wu et al., 2014), which might be an important reason for the inhibition of adjacent plant growth. These studies indicated a competitive relationship between poisonous weeds and neighboring plants, but these studies mainly focused on the aboveground growth of poisonous weeds and neighboring plants. Poisonous weeds affect the growth of their own and neighboring plants through plant–soil-microorganism interactions; however, some studies have indicated that soil pH can directly or indirectly affect the availability of soil nutrients and soil microbial composition, which indicates an inseparable relationship between soil pH and the interactions between plants (Trouvelot et al., 1986; Staddon et al., 1998; Kobayashi, 2004; Turner and Haygarth, 2005). There are also some studies that have shown that the acidity and alkalinity of root exudates secreted by poisonous weeds can affect the growth of themselves and neighboring plants by changing the content of soil elements (Zhu et al., 2020). However, there is insufficient evidence that pH and soil nutrient availability can jointly regulate the interaction between poisonous weeds and adjacent plants. In addition, the changes in soil microecology are of great significance for their interspecific interactions. The changes in soil microecology might affect their underground growth first (He et al., 2019). Their underground growth also responded most quickly to changes in soil microecology. However, current research has ignored their underground growth and changes in rhizosphere soil microorganisms.
S. chamaejasme is a common invasive weed that has solid toxicity to livestock in the degraded grasslands of northern China (Lu et al., 2012) and has even become the dominant species in some significantly degraded grasslands (Zhang et al., 2015; Xing, 2016). Research has shown that carbon and nitrogen cycling between S. chamaejasme patches and adjacent patch soils are different; the patches have higher soil organic contents, microbial biomass and respiration, and nitrate levels (Sun et al., 2009). An increasing number of studies have shown that S. chamaejasme can exert allelochemicals to inhibit the germination of other plant seeds and the growth of seedlings (Cao et al., 2007; Zhou and Wang, 2010; Guo et al., 2015). The nutrient absorption characteristics of S. chamaejasme, the influence on the effectiveness of soil nutrients, and allelopathic effects might be the main reasons for its successful invasion and spread. However, little is known about whether and how S. chamaejasme affects the growth of neighboring grasses and their soil characteristics, and there is not yet a sufficient theoretical basis for the interaction between S. chamaejasme and adjacent plants.
In addition to poisonous weeds, in general, AMF play a role in plant-interspecific relationships. AMF can expand the nutrient absorption area of plant roots, form a symbiotic relationship with plants, further promote plant nutrient absorption and stress resistance, and thus affect plant interspecific interactions (Smith and Read, 2008). Studies have shown that AMF can regulate the growth differences of plant populations, help weaker plants absorb more nutrients, promote their growth, or enhance the growth of larger plants, expanding the differences in plant population structure (Scheublin et al., 2007). Furthermore, AMF influence the nutrient absorption capacity within species (Facelli et al., 1999) or between species (Martins and Cruz, 1998) and mediate interspecific competition among adjacent plants (Sylvia et al., 2001). Interestingly, a study found that AMF infection in the roots of S. chamaejasme is zero in the grasslands of Inner Mongolia, China (Bao and Yan, 2004). Studies have detected that the relative abundance of AMF in the rhizosphere soils of S. chamaejasme is very low (He et al., 2019). These discoveries indicated that S. chamaejasme may be able to inhibit AMF in rhizosphere soil. Nevertheless, little is known about whether AMF can regulate the interspecific relationship between S. chamaejasme and adjacent plants.
In addition, pathogenic fungi also play an important role in plant interactions and in regulating plant interspecific relationships. Studies have shown that some pathogenic fungi could even produce toxic metabolites to the host, which might lead to plant diseases (Li et al., 2022). The interactions between plants and pathogenic fungi can be divided into incompatibility and affinity. In noncompatible interactions, local necrotic points with significant differences will appear at the infected site and form adjacent healthy tissues, known as a hypersensitive reaction (HR) (Dang et al., 1996). In affinity interactions, some fungi use stomata or trauma on the host surface to invade, usually resulting in infection structures formed by specialized hyphae, which then invade and infect plants (Goyet et al., 2017). Pathogenic fungi can directly inhibit growth and reproduction and/or increase the mortality of host plants through two interactions, thus affecting interactions between plant species (Mitchell, 2003; Borer et al., 2007; Mordecai, 2011; Creissen et al., 2016). There was evidence to prove that chemical substances from S. chamaejasme have activity against plant pathogenic fungi (Shi et al., 2013), but there is no theoretical basis for whether pathogenic fungi have a certain role in the interspecific interaction between S. chamaejasme and adjacent plants.
L. chinensis is a perennial rhizomatous grass and is also a dominant plant in arid to semiarid grasslands in northern China (Gao et al., 2012). This species can coexist with S. chamaejasme for a long time in typical and meadow steppes (Guo et al., 2019). In this study, we performed mono- and mixed-cultures of L. chinensis and S. chamaejasme in different combinations of the initial individual ratios of both plants to detect plant growth, soil properties, and the relative abundance of fungi in rhizosphere soil. We hypothesized that (1) the aboveground growth of L. chinensis and S. chamaejasme has a significant competitive effect, while the underground development of both plants has a significant reciprocal impact; (2) soil pH and nitrogen availability in the rhizosphere may be important factors in regulating the aboveground growth of L. chinensis and S. chamaejasme, and AMF and pathogenic fungi in the rhizosphere soil may regulate the underground development of L. chinensis.
Materials and methods
Study site
The experiment was conducted at the Jilin Songnen Grassland Ecosystem National Observation and Research Station in western Jilin Province, China (44°40′-44°44′N, 123°44′-123°47′E). This site experiences a northern temperate continental monsoon climate. The mean annual temperature is 4.9°C, the mean annual precipitation is 470 mm, and more than 70% of the rainfall is concentrated during the growing season (from May to August) (Song et al., 2017). The vegetation is meadow steppe dominated by Leymus chinensis, and the soil types include meadow soil, sandy soil, and saline-alkaline soil (Liu et al., 2008; Song et al., 2017).
Experimental design
PVC boxes (length 65 cm × width 40 cm × height 70 cm) were used to plant L. chinensis and S. chamaejasme. We set up five combinations of the initial individual ratios of L. chinensis (L) and S. chamaejasme (S). Specifically, the individual ratios of the two plant species were L:S = 12:0 (C1) (i.e., L. chinensis was in monoculture), L: S = 8:4 (C2), L: S = 6:6 (C3), L: S = 4:8 (C4), and L: S = 0:12 (C5) (i.e., S. chamaejasme was in monoculture), respectively. A total of 12 individuals were transplanted into each box. There were eight replicates in each combination and a total of 40 boxes. When we took samples, we randomly selected 5 boxes for each treatment.
The seeds of L. chinensis were collected from natural grassland near the field station in the fall of 2016. In early May 2017, after disinfection with 0.5% potassium permanganate solution, L. chinensis seeds were sown in a nursery with sterilized soil and germinated in a greenhouse. After the seedlings grew to 20 cm in height, they were transplanted into PVC boxes in early June to construct initial individual ratios of L. chinensis and S. chamaejasme. S. chamaejasme individuals were also obtained from the natural grassland. We dug up live S. chamaejasme and brought them back to the field station. Before transplantation, we performed “standardization” to ensure that the sizes of different individuals of L. chinensis or S. chamaejasme were the same (Xing et al., 2004; Chen et al., 2023). In addition, the roots were rinsed repeatedly with distilled water to remove soil sediment attached to the surface of the root system of S. chamaejasme. The soil substrates in the boxes were meadow soil collected from the same area where plant materials were collected. The weight of soil substrates in each box was identical. The soil depth was 40 cm inside each box. The initial physicochemical properties of the experimental soil substrates are shown in Supplementary Table S1.
The boxes were randomly placed outdoors, allowing the plants to grow naturally. Weeds should be removed at any time while the plant is growing. At the end of the growing season in mid-September, the upper part of the boxes was wrapped with double layers of nylon window mesh (the aperture was 0.5 mm) to prevent debris from mixing during overwintering. When the plants turned green the next spring, the window mesh was removed, and the plants were allowed to grow naturally. This experiment was continued until July 2020. The experiment lasted for nearly 4 years.
Plant sample collection and analyses and competition index calculation
We collected plant samples during the full-bloom stage of S. chamaejasme in the middle of June 2020. The whole plant tissues in each mesocosm were harvested to measure plant biomass. The plant samples were then dried to a constant at 65°C, and the plant materials were weighed after oven-drying to calculate plant biomass. In this study, L. chinensis-AGB: the aboveground biomass of L. chinensis; L. chinensis-UGB: the underground biomass of L. chinensis; the aboveground biomass/underground biomass of L. chinensis is the total biomass of the average number of per plant (including increased ramets); S. chamaejasme-AGB: the aboveground biomass of S. chamaejasme; S. chamaejasme-UGB: the underground biomass of S. chamaejasme; the aboveground biomass/underground biomass of S. chamaejasme is the average biomass per plant. We used the relative competition index (RCI) to represent the strength of the interaction between two plants (Wilson and Keddy, 1986).
where Bw represents the individual biomass of the target plant with neighbors and B 0 represents the individual biomass of the target plant without neighbors. For the relative competition index (RCI), the positive value is larger, and the competition between plants is more intense. Conversely, the negative value is larger, and the promoting effect between plants is greater.
Soil sample collection and analyses
After sampling the ground parts of the plants, soil samples were taken from the deep 0–30 cm at five random points close to individuals of S. chamaejasme or L. chinensis in each box. In detail, we used a drill twice at the same point to collect soil samples. The first drill goes 15 cm deep. After soil collection, we drilled another 15 cm deep and collected the soil again. For L. chinensis, each drill contained a large number of L. chinensis roots. During this process, we carefully selected the root system of L. chinensis and sampled its rhizosphere soil through a brush at a location close to the root system. For S. chamaejasme, we adopted the same method. Due to the large number of fibrous roots, during our sampling process, we brushed the S. chamaejasme fibrous roots in the soil drill using a brush to remove the soil near the root system as the rhizosphere soil of S. chamaejasme. Finally, we thoroughly mixed soil samples from the five points to form one rhizosphere soil sample. Each composite soil sample was passed through a 2 mm sieve to remove any roots and stones. Once collected, the samples were brought back to the laboratory with dry ice. Each sample was divided into three subsamples for analysis. One sample was stored at −20°C and was used for the analysis of the soil water content (SWC), available nitrogen (NO3−-N and NH4+-N), and available phosphorus (AP). The second sample was air-dried for analysis of the soil pH, soil electrical conductivity (EC), total carbon (TC), total nitrogen (TN), and total phosphorus (TP). Soil electrical conductivity (EC) was measured using a DDS-307 conductivity meter (Shanghai Lei Ci Instrument Factory, Shanghai, China), and soil pH was measured using a PHS-3C pH meter (Shanghai Lei Ci Instrument Factory, Shanghai, China) (soil sample: ultrapure water = 1:5). The soil SWC was measured as the weight loss recorded after the fresh soils had been oven-dried to constant weight at 105°C. Soil available nitrogen (AN) was measured using an AMS France-A Hiance Instruments Flow Analyzer, and soil available phosphorus (AP) was measured using the Olsen method. We extracted soils with 2 M KCl (soil: water suspension = 1:5, w:v), and the values of soil NO3−-N and NH4+-N were measured with a continuous flow analyzer (Futura, Alliance-AMS, France). Soil total carbon and nitrogen were measured with an elemental analyzer (Isoprime 100, Isoprime Ltd., Manchester, UK). Soil phosphorus was measured using the digestive molybdenum-antimony-resist method. The third sample was stored at −80°C and was used to extract soil DNA and subsequent high-throughput sequencing.
Rhizosphere soil DNA extraction, amplification, and sequencing
Genomic DNA from L. chinensis and S. chamaejasme in the rhizosphere soil was extracted using the CTAB/SDS method. The fungal ITS1 genes were amplified by the primer pair ITS1F (5′-CTTGGTCATTTAGAGGAAGTAA-3′)/ITS4F (5′-TCCTCCGCTTATTGATATGC-3′). The PCR contained 15 μL Phusion High-Fidelity PCR Master Mix (New England Biolabs), 0.2 μM forward and reverse primers, and approximately 10 ng template DNA. All amplifications were conducted using the following PCR procedure. Initial DNA denaturation at 98°C for 1 min, followed by 30 cycles of 10 s at 98°C for, 30 s at 50°C for, and 1 min at 72°C and final extension for 5 min at 72°C. Then, the PCR product from each sample was mixed in equal density ratios for purification with the Gene JET Gel Extraction Kit (Thermo Scientific) and sequenced on the Illumina HiSeq 2500 system. After sequencing, we used Usearch software (Edgar, 2013) and performed taxonomic annotation for operational taxonomic units (OTUs) on the basis of the UNITE taxonomic database. The OTU count of each sample was obtained at a 97% similarity level. Finally, we clustered the optimized sequences (clean tags) to obtain OTUs and then obtained the species classification according to the sequence composition of the OTUs. The ecological function of the fungi was assigned to each taxon (where identified) at the species, genus, or family level using the FUNGuild database (Nguyen et al., 2016). The specific steps and preliminary results of our microbial diversity sequencing are detailed in the Supplementary material.
Statistical analyses
First, we used one-way ANOVA to test the changes in RCI and aboveground and underground biomass of L. chinensis and S. chamaejasme to determine the interspecific relationship between the two plants. p < 0.05 was considered to identify statistically significant differences. Then, we used one-way ANOVA to test the changes in the relative abundance of AMF and pathogenic fungi in the rhizosphere soil, AMF characteristics, and soil characteristics of L. chinensis and S. chamaejasme. Finally, we used Spearman correlation analysis to study the relative abundance of AMF and pathogenic fungi in L. chinensis and S. chamaejasme, the characteristics of rhizosphere soil, and the relationship between aboveground and underground biomass of L. chinensis and S. chamaejasme. To explore the indirect effects of different combinations of ramets of L. chinensis and S. chamaejasme on the aboveground and underground growth of L. chinensis and S. chamaejasme, we conducted structural equation modeling (SEM) using the “piecineSEM” package. According to Fisher’s C statistics and AIC (Akaike information criterion), we selected appropriate variables and ultimately determined the optimal mode. We used R (http://www.r-project.org/) for all statistical analyses.
Results
Interspecific relationship between Leymus chinensis and Stellera chamaejasme
The RCI in the aboveground biomass of L. chinensis and S. chamaejasme was greater than zero. However, the RCI in the underground biomass of L. chinensis and S. chamaejasme was less than zero. With the increase in the initial individual ratios of S. chamaejasme and the decrease in the initial individual ratios of L. chinensis, the RCI in the aboveground biomass of L. chinensis increased, and the RCI in the underground biomass of L. chinensis decreased (p < 0.05, Figure 1A) (p < 0.05, Figure 1B). Meanwhile, the RCI in the aboveground biomass and underground biomass of S. chamaejasme decreased (p < 0.05, Figures 1C,D).
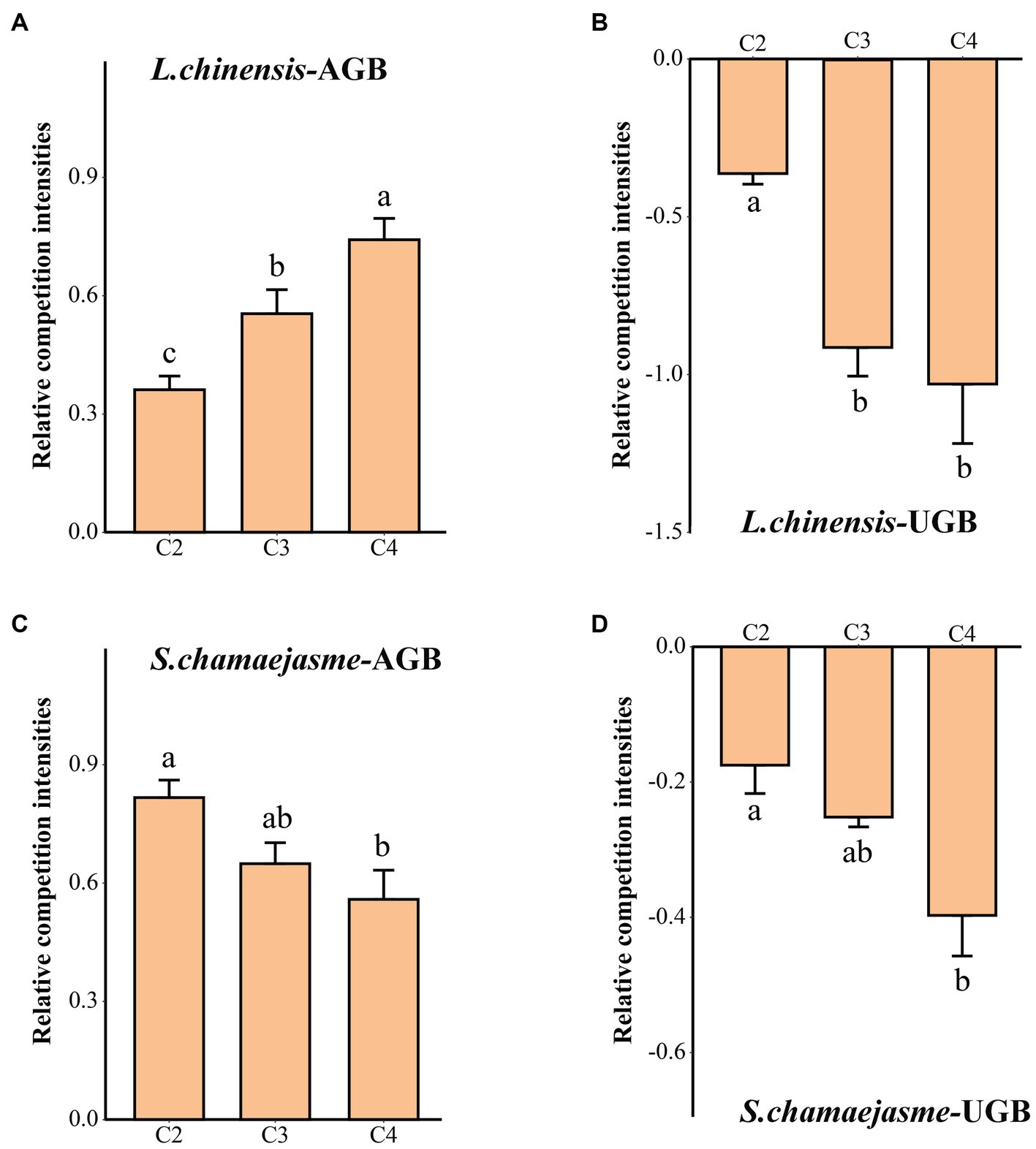
Figure 1. The differences in the relative competition intensities of L. chinensis (A,B) and S. chamaejasme (C,D) among the different combinations of the initial individual ratios (mean ± SE). C1, C2, C3, C4, and C5 indicate that the respective proportions of L. chinensis and S. chamaejasme were 12:0, 8:4, 6:6, 4:8, and 0:12, respectively. L. chinensis-AGB: aboveground biomass of L. chinensis; L. chinensis-UGB: underground biomass of L. chinensis; S. chamaejasme-AGB: aboveground biomass of S. chamaejasme; S. chamaejasme-UGB: underground biomass of S. chamaejasme. The different lowercase letters indicate significant differences among the different combinations (p < 0.05).
With the increase in the initial individual ratios of S. chamaejasme and the decrease in the initial individual ratios of L. chinensis, the aboveground biomass of L. chinensis decreased, and the underground biomass of L. chinensis increased (p < 0.05, Figures 2A,B). The aboveground biomass of S. chamaejasme increased, and there was no significant difference in the underground biomass of S. chamaejasme (p < 0.05, Figures 2D,E). The root/shoot ratio of L. chinensis decreased, and the root/shoot ratio of S. chamaejasme declined (p < 0.05, Figures 2C,F).
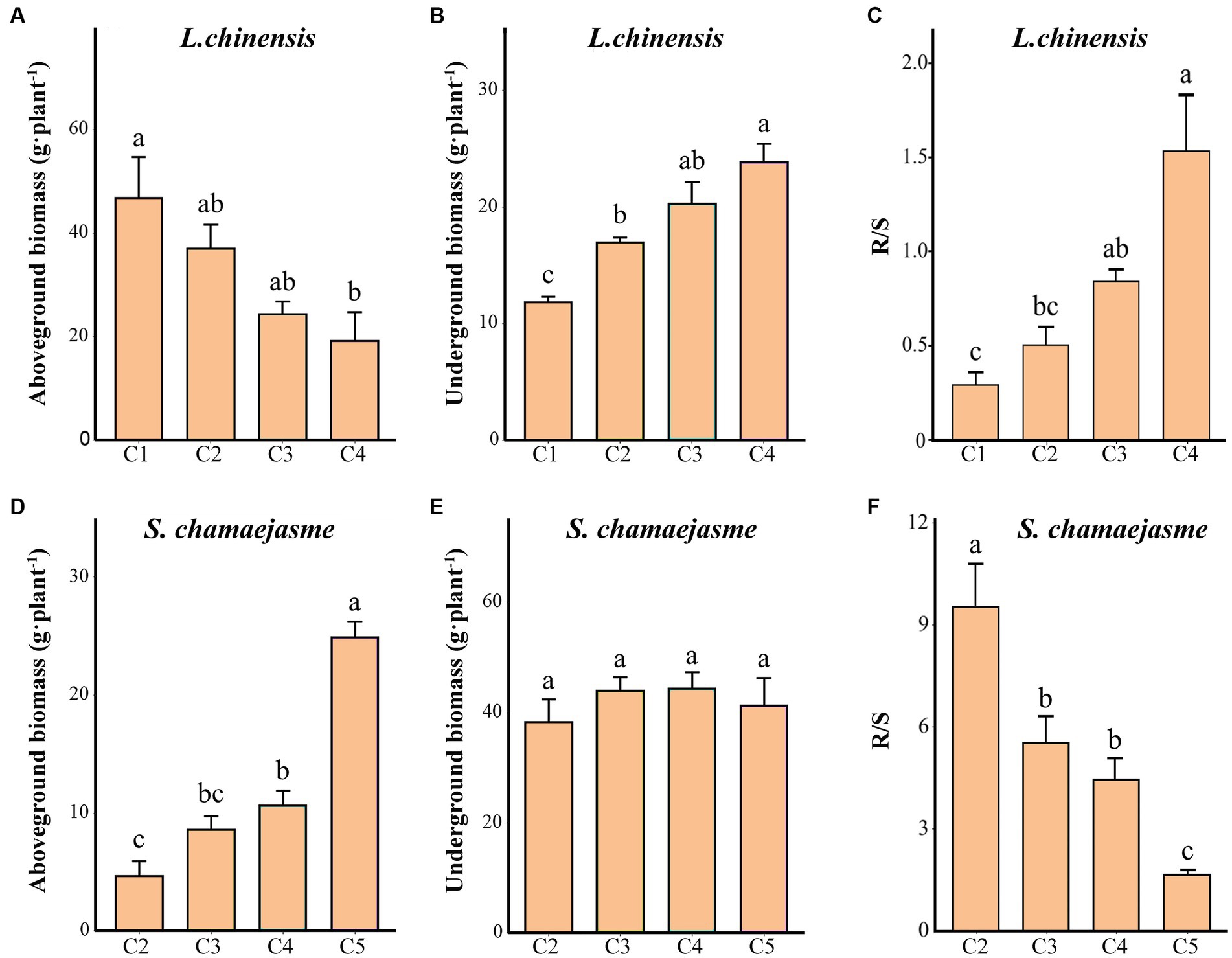
Figure 2. The differences in the growth characteristics of L. chinensis (A–C) and S. chamaejasme (D–F) among the different combinations of the initial individual ratios (mean ± SE). C1, C2, C3, C4, and C5 indicate that the respective proportions of L. chinensis and S. chamaejasme were 12:0, 8:4, 6:6, 4:8, and 0:12, respectively. R/S: root/shoot ratio. The different lowercase letters indicate significant differences among the different combinations (p < 0.05).
The variations in AMF and PPF in rhizosphere soil of Leymus chinensis and Stellera chamaejasme
After sequencing, there were 3,200,605 pairs of reads among 40 samples and 3,129,756 clean tags after paired-end read alignment and filtering. Each sample has at least 74,290 clean tags and 78,244 clean tags on average.
Further analysis by FUNGuild showed that the relative abundance of fungi belonging to different functional groups was different combinations of the initial individual ratios of L. chinensis and S. chamaejasme (Supplementary Figure S1). According to the functional prediction results, AMF mainly include Glomeraceae and Glomus_indicum (species), and PPF mainly include Coniothyrium_sidae and Penicillium_oxalicum (species). With the changes in the initial individual ratios (from C1 to C4), the relative abundance of AMF increased, and the relative abundance of the PPF decreased in the rhizosphere soil of L. chinensis (p < 0.05, Figures 3A,B). However, the abundance variation of AMF and PPF in the rhizosphere soil of S. chamaejasme showed the opposite patterns (Figures 3C,D). Interestingly, both L. chinensis (Figure 3C) and S. chamaejasme (Figure 3D) had higher abundances of PPF under mono-culture than under mixed culture. In addition, under the same combinations of the initial individual ratios, the relative abundance of AMF in the rhizosphere soil of L. chinensis gradually increased as the initial individual ratios of S. chamaejasme increased, while the relative abundance of AMF in the rhizosphere soil of S. chamaejasme gradually decreased (p < 0.05, Supplementary Figure S2A). However, there was no significant difference in the relative abundance of the PPF in the rhizosphere soil of both mixtures (Supplementary Figure S2B).
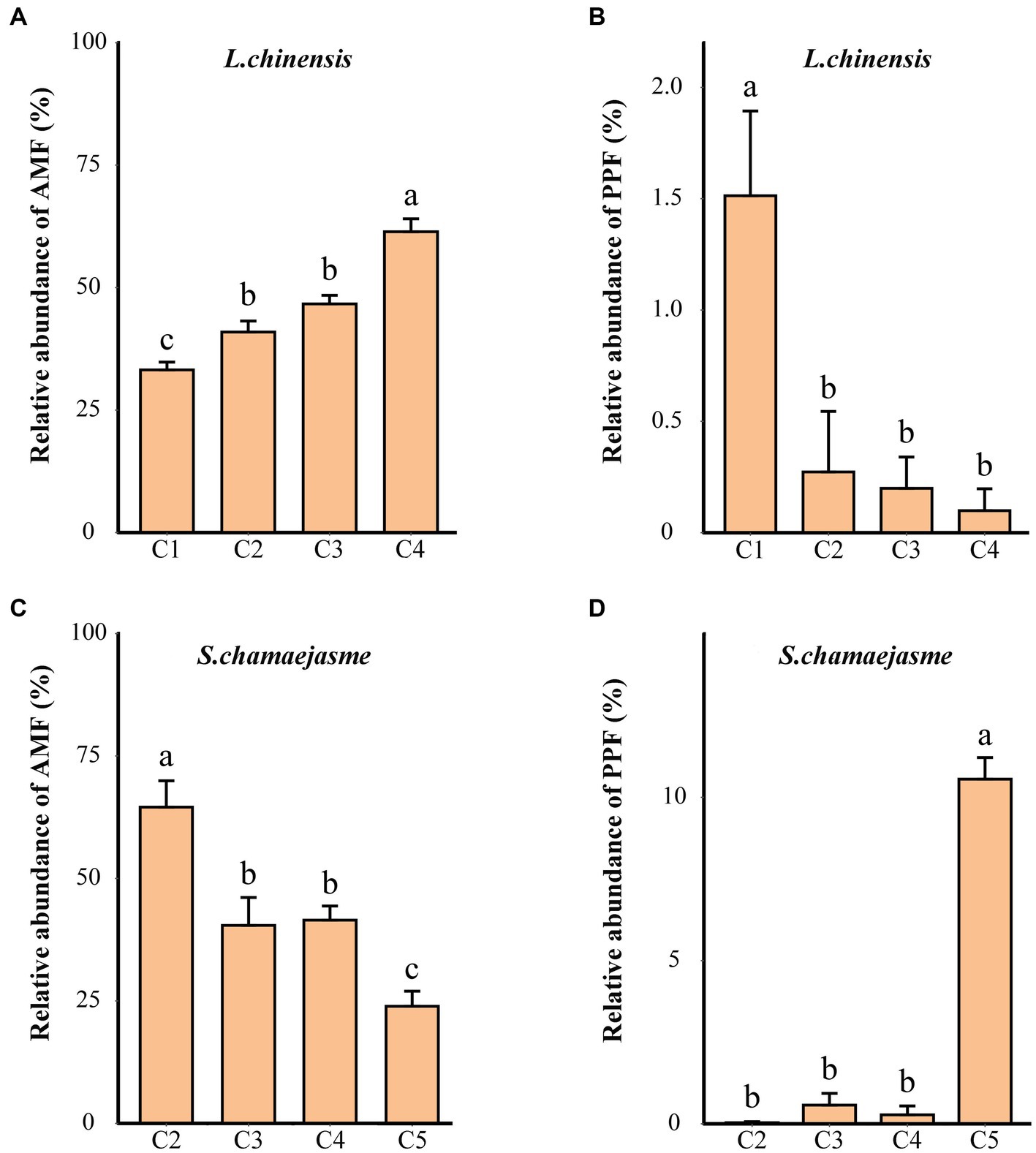
Figure 3. The relative abundances of the predicted arbuscular mycorrhizal fungi (AMF) and plant pathogenic fungi (PPF) in the rhizosphere soil of L. chinensis (A,B) and S. chamaejasme (C,D) among the different combinations of the initial individual ratios (mean ± SE). C1, C2, C3, C4, and C5 indicate that the respective proportions of L. chinensis and S. chamaejasme were 12:0, 8:4, 6:6, 4:8, and 0:12, respectively. The different lowercase letters indicate significant differences among the different combinations (p < 0.05).
Relationship between aboveground and underground biomass, soil parameters and the relative abundance of AMF and PPF
The results of SEM analysis showed that soil TN, pH, the abundance of AMF, and PPF in rhizosphere soil were key factors affecting the aboveground and underground growth of L. chinensis (Figure 4A) and S. chamaejasme (Figure 4B). Different combinations of the initial individual ratios of both plants could change the pH and TN content of the rhizosphere soil of L. chinensis (Figure 5A; Table 1) and thereby affect its aboveground growth, but it could affect its underground growth by changing the soil TN and the relative abundance of AMF and PPF (Figure 5A). Different combinations of the initial individual ratios of L. chinensis and S. chamaejasme could significantly change the pH of the rhizosphere soil of S. chamaejasme and thereby affect the aboveground growth of S. chamaejasme (Figure 5B; Table 2), while the underground growth of S. chamaejasme was almost not affected by factors such as pH and the relative abundance of AMF and PPF (Figure 5B).
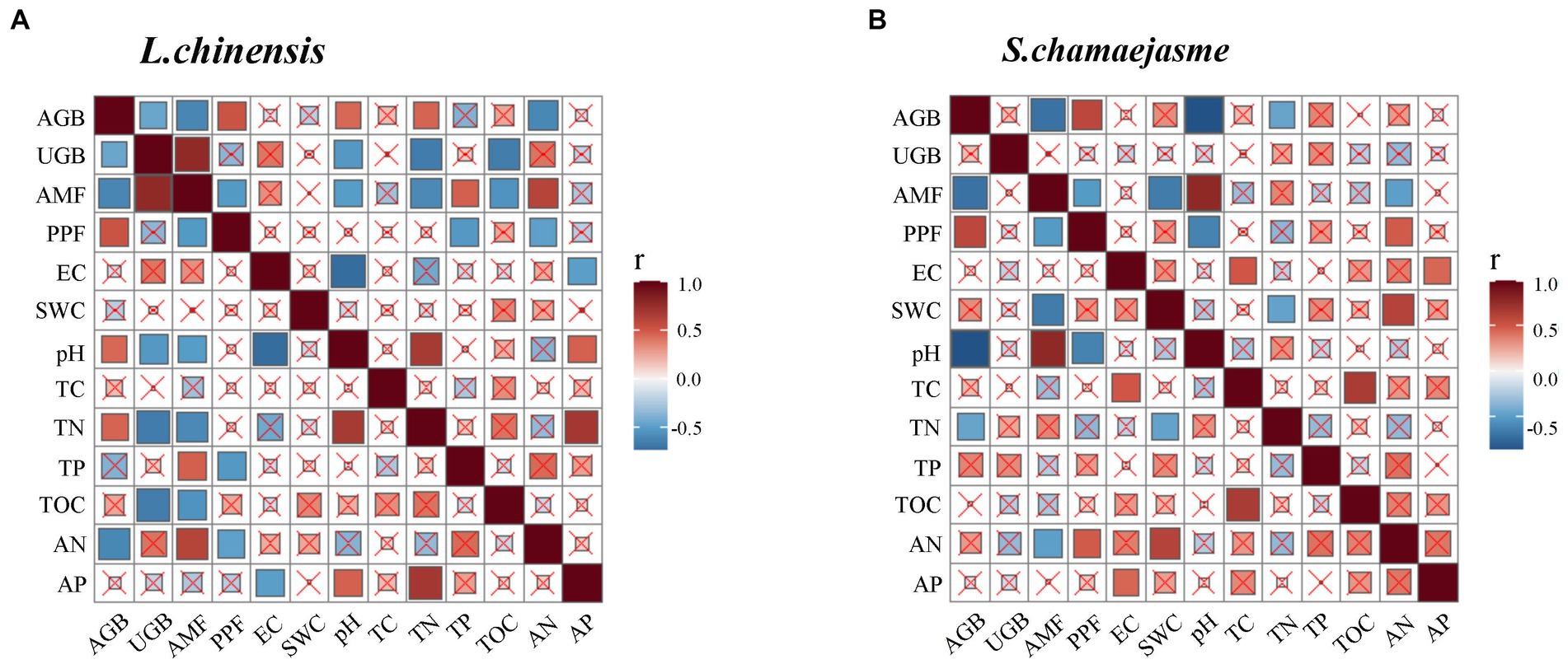
Figure 4. The Pearson correlation analyses among the aboveground and underground biomass, the relative abundance of arbuscular mycorrhizal fungi (AMF) and plant pathogenic fungi (PPF), and soil properties in the rhizosphere of L. chinensis and S. chamaejasme. r, correlation index; AGB, aboveground biomass; UGB, underground biomass; EC, electrical conductivity; SWC, soil water content; TC, total carbon; TN, total nitrogen; TP, total phosphorus; TOC, total organic carbon; AN, available nitrogen; AP, available phosphorus.
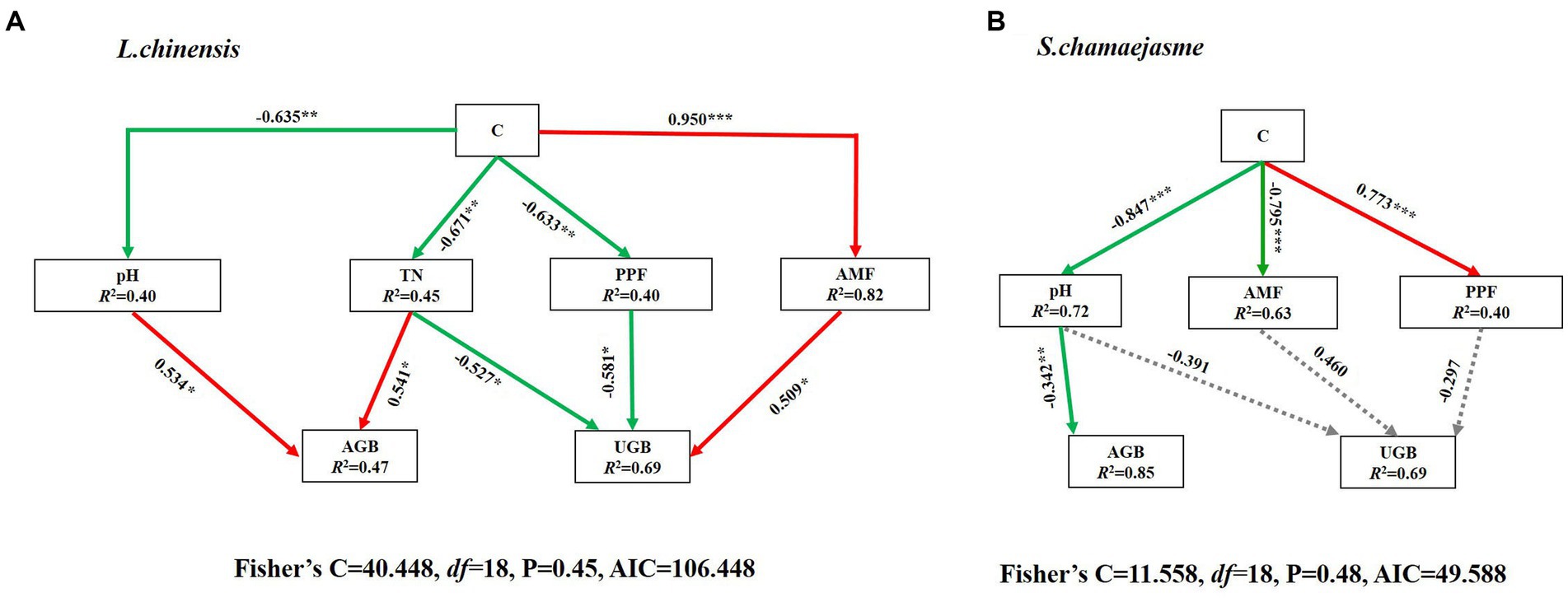
Figure 5. Piecewise structural equation model (SEM) analysis depicting the direct and indirect effects of different combinations of the initial individual ratios (C) of L. chinensis and S. chamaejasme on the aboveground and underground growth of L. chinensis (A) and S. chamaejasme (B). The continuous arrows indicate positive (red color) and negative (green color) effects, and gray dashed arrows represent nonsignificant effects. For each model, the proportion of variance explained (R2) and the various goodness-of-fit statistics are shown below the response variables. Significance levels are as follows: *p < 0.05, **p < 0.01 and ***p < 0.001. AGB, aboveground biomass; UGB, underground biomass; AMF, arbuscular mycorrhizal fungi; PPF, Plant pathogenic fungi; TN, Soil total nitrogen. AIC, Akaike information criterion.

Table 1. The differences in the soil properties in the rhizosphere soil of L. chinensis among the different combinations of the initial individual ratios (mean ± SE).

Table 2. The differences in the soil properties in the rhizosphere soil of S. chamaejasme among the different combinations of the initial individual ratios (mean ± SE).
Discussion
Determination of interspecific relationships between Leymus chinensis and Stellera chamaejasme and main soil influencing factors
Our study revealed that there is a significant competitive effect between the aboveground growth of L. chinensis and S. chamaejasme (Figures 1A,C). As the initial individual ratios of L. chinensis or S. chamaejasme increase, the aboveground biomass of the other side will significantly decrease (Figures 2A,D). The soil total nitrogen content in the rhizosphere of L. chinensis decreased significantly as the initial individual ratios of S. chamaejasme increased (Table 1), which ultimately inhibited the aboveground growth of L. chinensis (Figure 5A). As an invasive species, S. chamaejasme interferes with the nutrient accumulation of the local species L. chinensis. This was consistent with the results of previous studies, and Solidago Canada L. could form a single species and replace local species because of its strong ability to compete with local species (Szymura and Szymura, 2016; Wang et al., 2018; Adomako et al., 2020). In addition, the high nitrogen use efficiency of invasive Sporobolus alterniflorus was also the main reason for its nutrient absorption advantage in competition (Li et al., 2021). Compared with local plants, invasive plants may have faster root growth and higher growth rates, and they also have higher nutrient acquisition efficiency and the ability to efficiently and widely absorb nutrients in the soil (Dawson, 2015; Jo et al., 2015; Phillips et al., 2019; Jelincic et al., 2021). These functional characteristics give invaders the advantage of obtaining nutrients, thereby reducing the nutrient availability of local species. This can precisely explain our result that due to the reduced nutrient supply of L. chinensis, the aboveground growth of L. chinensis was inhibited. In addition, the pH of the rhizosphere soil of L. chinensis and S. chamaejasme significantly decreased as the initial individual ratios of S. chamaejasme increased (Tables 1, 2). The decrease in pH could inhibit the aboveground growth of L. chinensis. Phenolic substances in the roots of S. chamaejasme may lead to a decrease in pH in the rhizosphere soil of L. chinensis (Batish et al., 2002; Yan et al., 2014). The decrease in soil pH (increase in phenolic compounds) can also significantly inhibit the absorption of mineral elements by plants, thus inhibiting the aboveground growth of plants (Harper and Balke, 1981). However, in our results, there was a significant decrease in pH when treated with C4 (Table 1). This indicated that the inhibition of aboveground growth of L. chinensis from the C1 to C3 treatments was mainly due to the inhibition of soil nitrogen absorption by S. chamaejasme, while the inhibition of aboveground growth of L. chinensis from the C4 treatment was mainly due to the combined effect of soil total nitrogen absorption inhibition and the decrease in pH.
Although the aboveground growth of L. chinensis was significantly inhibited (Figure 2A), the aboveground growth of S. chamaejasme was also significantly inhibited (Figure 2D) and the increase in pH would inhibit the aboveground growth of S. chamaejasme. From C2 to C5, there is a significant decrease in pH in the rhizosphere soil of S. chamaejasme. S. chamaejasme is more suitable for growth in environments with low pH, which might be related to its ability to secrete allelochemicals. However, even small changes in pH could have a significant impact on the metabolism of allelochemicals, nutrient transport, and enzyme activity in the body of S. chamaejasme. There is evidence to suggest that soil pH can affect the availability of soil nutrients, soil microbial community structure, soil enzyme activity, and the metabolism of plant allelochemicals (Staddon et al., 1998; Kobayashi, 2004; Turner and Haygarth, 2005). This could partially explain the reasons for the aboveground growth changes in S. chamaejasme in our results. In addition, competition theory predicts that when plants compete for resources that limit their growth, such as space, light, water, and nutrients, yield will decrease (Sylvia et al., 2001; Fynn et al., 2005; Andersen et al., 2007). Although the absorption of water and nutrients by S. chamaejasme might not have a significant impact on its growth, space or light might have a significant inhibitory effect on the aboveground growth of S. chamaejasme, as L. chinensis can continuously tiller. Compared to L. chinensis, the space occupied by S. chamaejasme might be reduced to some extent. The increase in the number of branches of L. chinensis might impact the photosynthesis of S. chamaejasme. Of course, we will also clarify these speculations in the future.
Our results indicated that the interactive effects between L. chinensis and S. chamaejasme were reciprocal and only had a significant promoting effect on the underground growth of L. chinensis (Figure 2B). The results showed that the decrease in total nitrogen content in the rhizosphere soil of L. chinensis had a significant promoting effect on the underground growth of L. chinensis (Figure 5A). Although the total nitrogen content in the rhizosphere soil of L. chinensis significantly decreased (Table 1), L. chinensis could expand the scope of resource acquisition through clonal reproduction and asexual propagation and amplification, which was also possible to avoid stress interference (Sun et al., 2021; Wang et al., 2021). In this study, S. chamaejasme was considered a disturbance to L. chinensis. This characteristic of L. chinensis could improve its root system’s absorption and utilization of soil nitrogen under conditions of insufficient soil nutrients, leading to a certain increase in its underground biomass.
There was an interesting result, i.e., no significant changes in the underground biomass of S. chamaejasme (Figure 2B), which might be related to its unique root characteristics. S. chamaejasme is a perennial axial root-type plant with deeper taproots than L. chinensis, S. chamaejasme can absorb water and nutrients from deeper soil (Leng et al., 2013), so L. chinensis, with a relatively shallow root system, may have less competitive inhibition against S. chamaejasme. In addition, the slow growth of the root system of S. chamaejasme may also be a reason that its underground parts were not significantly changed.
The regulatory role of AMF and PPF
Different combinations of the initial individual ratios of L. chinensis and S. chamaejasme can promote the underground growth of L. chinensis by increasing the relative abundance of AMF in the rhizosphere soil and inhibiting the relative abundance of PPF in the rhizosphere soil (Figure 5A). With the increase in the initial individual ratios of S. chamaejasme, the relative abundance of AMF in the rhizosphere soil of L. chinensis significantly increased (Figure 3A) because most allelochemicals contain carbon/nitrogen elements and can serve as carbon and nitrogen sources for microorganisms, which can to some extent promote their growth (Kong et al., 2008; Zuo et al., 2014). Some studies have shown that AMF promote the growth of the grass E. nutans by suppressing that of L. virgaurea, a native poisonous weed spread in grasslands in China (Jin et al., 2011). Some studies have also shown that AMF slow the competition between the high-quality forage grasses E. nutans and Poa pratensis and the poisonous weed Saussurea japonica, promoting the growth of the poisonous weed S. japonica (Wang, 2016). This is not consistent with our research results, which found that the underground biomass of L. chinensis increased while the aboveground biomass decreased. Although our research results did not directly prove that AMF have a significant impact on the aboveground growth of L. chinensis, we found that the root to shoot ratio of L. chinensis gradually decreased from the C1 to C4 treatment, and we believed that AMF may regulate the nutrient allocation strategy of L. chinensis, leading to more photosynthetic products being distributed to the roots (Selosse et al., 2006). S. chamaejasme could secrete allelochemicals to inhibit the growth of neighboring plants. Whether the aboveground or underground growth of plants should have been subjected to certain inhibitory effects, studies have shown that AMF may play a key role in regulating the movement of allelochemicals in soil and affecting their bioactive zones (Barto et al., 2012). The mycelia of AMF can mediate the transport of allelochemicals, such as juglone (Achatz and Rillig, 2014). This indicated that AMF have the ability to regulate allelopathic substances. We infer that AMF alleviated the allelopathic effects produced by S. chamaejasme to some extent, which could also explain why the underground biomass of L. chinensis increased. In addition, AMF could increase the root area of plants by promoting the formation of a large number of extracellular mycelia. At the same time, due to the ammonium transporter of AMF, extracellular mycelium can promote the absorption of NH4+ and NO3− from the soil in plant roots (Tian et al., 2010; Pérez-Tienda et al., 2011). The increase in the relative abundance of AMF to some extent enhances the ability of the root system of L. chinensis to absorb nutrients, leading to an increase in the underground biomass of L. chinensis. In summary, we speculated that AMF alleviated the competition that should have occurred in the underground growth of L. chinensis and S. chamaejasme and then promoted the underground growth of L. chinensis.
In addition, our results also indicated that the abundance of PPF in the rhizosphere soil of L chinensis was significantly higher under the mono-cultures than under the mixed cultures of the two plants (Figure 3B; Supplementary Figure S2B). Compared with mono-cultures of L. chinensis, mixed cultures of L. chinensis and S. chamaejasme can promote the underground growth of L. chinensis by reducing the relative abundance of PPF in the rhizosphere soil of L. chinensis. PPF can prey on living host plant cells (biotrophic pathogens) or kill cells to obtain nutrition (necrotrophic pathogens) (Reinhart et al., 2005; Bell et al., 2006). It can alter plant interspecific relationships by affecting plant fitness, reducing the growth and competitive ability of plants (Maron et al., 2011; Latz et al., 2012). The PPF in the rhizosphere soil of L. chinensis have no significant impact on its aboveground growth. Due to the relatively low abundance of PPF in the rhizosphere soil of L. chinensis, we speculated that it could not reach the threshold that can affect the aboveground growth of L. chinensis, and it might also have a time lag that the PPF impact the aboveground growth of L. chinensis. The underground biomass of the mono-culture of L. chinensis and S. chamaejasme was the lowest, which may be due to the high abundance of pathogenic fungi, which killed normal cells in the root system of L. chinensis and further reduced root growth and competitiveness. When L. chinensis and S. chamaejasme were mixed, the root exudates of S. chamaejasme inhibited the relative abundance of PPF in the rhizosphere soil of L. chinensis to a certain extent, probably improving the adaptability and competitiveness of the roots of L. chinensis, which may be a reason for promoting the underground growth of L. chinensis. Some studies have shown that the flavonoids in S. chamaejasme help plants resist PPF, which might provide a competitive advantage for the underground growth of L. chinensis (Yan et al., 2015). In addition, AMF may also be the key reason for inhibiting PPF, which can provide a competitive advantage for the underground growth of L. chinensis. AMF are involved in the defense against PPF (Hooker et al., 1994; Herre et al., 2007), possibly through modification of the infection site (Vigo et al., 2000) or the host defense system (Liang et al., 2015; Pérez-de-Luque et al., 2017). This indicates that the relative abundance of AMF and the relative abundance of PPF are inherently interactive processes. Therefore, we boldly speculate that AMF and PPF can synergistically regulate the interspecific interaction between L. chinensis and S. chamaejasme. They mainly regulate the underground growth of L. chinensis. Further research is needed on the accumulation or specific mode of action of PPF in the interspecific interaction between L. chinensis and S. chamaejasme.
Our results indicated that the relative abundance of AMF significantly decreased with an increase in the initial individual ratios of S. chamaejasme and a decrease in the initial individual ratios of L. chinensis (Figure 3C). Compared with the mixed culture, the relative abundance of PPF in the monoculture of S. chamaejasme was also much higher (Figure 3D). In this experiment, L. chinensis and S. chamaejasme always interacted, so the relative abundance changes of AMF and PPF in the rhizosphere soil of both also changed together. This is basically consistent with the relative abundance changes in AMF and PPF in the rhizosphere soil of L. chinensis. The change in AMF was mainly due to the inhibition of allelopathy provided by S. chamaejasme, while the change in PPF was mainly caused by the amount of AMF and the secretion of allelopathic substances. Due to the root characteristics of S. chamaejasme, the relative abundance changes of AMF and PPF in the rhizosphere soil were also insufficient to regulate the underground growth of S. chamaejasme.
In our research, planting L. chinensis and S. chamaejasme at different initial individual ratios can better simulate the natural processes in grassland ecosystems, the relative abundance changes between AMF and PPF have regulatory effects on interspecific competition between L. chinensis and S. chamaejasme and mainly regulate the underground growth of L. chinensis. It has provided some theoretical support for the regulatory role of AMF and PPF in the interactive effects between L. chinensis and S. chamaejasme. However, this study did not provide direct evidence that the root exudates of S. chamaejasme affect the interspecific interaction between L. chinensis and S. chamaejasme. In the future, we will complete research in this area.
Conclusion
In this study, we analyzed the effects of different combinations of the initial individual ratios of L. chinensis and S. chamaejasme on their growth, soil nutrients, and specific rhizosphere microorganisms, including AMF and PPF. Our results showed a significant competitive effect on the aboveground growth of L. chinensis and S. chamaejasme, while there was a significant reciprocal effect on their underground growth. Different combinations of the initial individual ratios of both plants can indirectly affect soil total nitrogen and pH in their rhizosphere soil and further affect their growth. Therefore, soil nutrient availability and pH changes may be potential mechanisms for their competitive effects on plant aboveground growth. The soil total nitrogen and relative abundance of AMF and PPF in the rhizosphere soil of L. chinensis were critical factors in promoting its underground growth. AMF and PPF in the rhizosphere soil of L. chinensis can regulate the interspecific interaction between L. chinensis and S. chamaejasme. Overall, this study revealed the regulatory roles of AMF and PPF in the interaction between L. chinensis and S. chamaejasme and deepened our understanding of the relationship between poisonous weeds and palatable grasses in degraded grasslands. In the future, artificial regulation of AMF and pathogenic fungi in rhizosphere soil may be a possible way to control poisonous weeds in degraded grasslands.
Data availability statement
The datasets presented in this study can be found in online repositories. The names of the repository/repositories and accession number(s) can be found below: https://www.ncbi.nlm.nih.gov/, PRJNA1000115.
Author contributions
FX: conceptualization, writing—review and editing. FX and RZ: methodology. RZ: validation, formal analysis, data curation, writing—original draft preparation, and visualization. BZ, SQ, and YG: investigation. All authors contributed to the article and approved the submitted version.
Funding
This work was supported by the National Natural Science Foundation of China (31570452).
Acknowledgments
The authors are grateful to Chen Chen and Xiaowei Liu for their support and assistance in the field work.
Conflict of interest
The authors declare that the research was conducted in the absence of any commercial or financial relationships that could be construed as a potential conflict of interest.
Publisher’s note
All claims expressed in this article are solely those of the authors and do not necessarily represent those of their affiliated organizations, or those of the publisher, the editors and the reviewers. Any product that may be evaluated in this article, or claim that may be made by its manufacturer, is not guaranteed or endorsed by the publisher.
Supplementary material
The Supplementary material for this article can be found online at: https://www.frontiersin.org/articles/10.3389/fmicb.2023.1236891/full#supplementary-material
References
Achatz, M., and Rillig, M. C. (2014). Arbuscular mycorrhizal fungal hyphae enhance transport of the allelochemical juglone in the field. Soil Biol. Biochem. 78, 76–82. doi: 10.1016/j.soilbio.2014.07.008
Adomako, M. O., Xue, W., Tang, M., Du, D. L., and Yu, F. H. (2020). Synergistic effects of soil microbes on Solidago canadensis depend on water and nutrient availability. Microb. Ecol. 80, 837–845. doi: 10.1007/s00248-020-01537-2
Andersen, M. K., Hauggaard-Nielsen, H., Weiner, J., and Jensen, E. S. (2007). Competitive dynamics in two- and three-component intercrops. J. Appl. Ecol. 44, 545–551. doi: 10.1111/j.1365-2664.2007.01289.x
Bao, Y. Y., and Yan, W. (2004). Arbuscular mycorrhizae and their structural types on common plants in grasslands of mid-western Inner Mongolia. Chinese Biodivers. 12, 501–508. doi: 10.17520/biods.2004062
Barto, E. K., Weidenhamer, J. D., Cipollini, D., and Rillig, M. C. (2012). Fungal superhighways: do common mycorrhizal networks enhance below ground communication? Trends Plant Sci. 17, 633–637. doi: 10.1016/j.tplants.2012.06.007
Batish, D. R., Singh, H. P., Pandher, J. K., Arora, V., and Kohli, R. K. (2002). Phytotoxic effect of Parthenium residues on the selected soil properties and growth of chickpea and radish. Weed Biol. Manag. 2, 73–78. doi: 10.1046/j.1445-6664.2002.00050.x
Bell, T., Freckleton, R. P., and Lewis, O. T. (2006). Plant pathogens drive density-dependent seedling mortality in a tropical tree. Ecol. Lett. 9, 569–574. doi: 10.1111/j.1461-0248.2006.00905.x
Bertin, C., Yang, X. H., and Weston, L. A. (2003). The role of root exudates and allelochemicals in the rhizosphere. Plant Soil 256, 67–83. doi: 10.1023/A:1026290508166
Borer, E. T., Hosseini, P. R., Seabloom, E. W., and Dobson, A. P. (2007). Pathogen-induced reversal of native dominance in a grassland community. PNAS 104, 5473–5478. doi: 10.1073/pnas.0608573104
Cao, C. Y., Fu, Y., Wang, W. X., and Gao, F. F. (2007). Inhibition influence of extraction liquids from Stellera chamaejasme root on seed germination. J. Northeast. Univ. 28, 29–32.
Chen, C., Xing, F., and Li, Z. (2023). Nitrogen addition changes the Allelopathic effects of the root leachate from the invasive weed Stellera chamaejasme L. on a dominant grass in the Songnen grassland. J. Plant Biol. 66, 243–255. doi: 10.1007/s12374-023-09389-2
Creissen, H. E., Jorgensen, T. H., and Brown, J. K. (2016). Impact of disease on diversity and productivity of plant populations. Funct. Ecol. 30, 649–657. doi: 10.1111/1365-2435.12552
Dang, I. J. L., Dietrich, R. A., and Richberg, M. H. (1996). Death Don’t have no mercy: cell death programs in plant–microbe interactions. Plant Cell 8, 1793–1807. doi: 10.1105/tpc.8.10.1793
Dawson, W. (2015). Release from belowground enemies and shifts in root traits as interrelated drivers of alien plant invasion success: a hypothesis. Ecol. Evol. 5, 4505–4516. doi: 10.1002/ece3.1725
Edgar, R. C. (2013). UPARSE: highly accurate OTU sequences from microbial amplicon reads. Nat. Methods 10, 996–998. doi: 10.1038/nmeth.2604
Facelli, E., Facelli, J. M., Smith, S. E., and Mclaughlin, M. J. (1999). Interactive effects of arbuscular mycorrhizal symbiosis, intraspecific competition and resource availability on Trifolium subterraneum cv. Mt. Barker. New Phytol. 141, 535–547. doi: 10.1046/j.1469-8137.1999.00367.x
Fynn, R. W. S., Morris, C. D., and Kirkman, K. P. (2005). Plant strategies and trait trade-offs influence trends in competitive ability along gradients of soil fertility and disturbance. J. Ecol. 93, 384–394. doi: 10.1111/j.0022-0477.2005.00993.x
Gao, Y., Xing, F., Jin, Y., Nie, D., and Wang, Y. (2012). Foraging responses of clonal plants to multipatch environmental heterogeneity: spatial preference and temporal reversibility. Plant Soil 359, 137–147. doi: 10.1007/s11104-012-1148-0
Goyet, V., Billard, E., Pouvreau, J. B., Lechat, M. M., Pelletier, S., Bahut, M., et al. (2017). Haustorium initiation in the obligate parasitic plant Phelipanche ramosa involves a host-exudated cytokinin signal. J. Exp. Bot. 68, 5539–5552. doi: 10.1093/jxb/erx359
Guo, H. R., Cui, H. Y., Jin, H., Yan, Z. Q., Ding, L., and Qin, B. (2015). Potential allelochemicals in root zone soils of Stellera chamaejasme L. and variations at different geographical growing sites. Plant Growth Regul. 77, 335–342. doi: 10.1007/s10725-015-0068-4
Guo, L., Li, J., He, W., Liu, L., Huang, D., and Wang, K. (2019). High nutrient uptake efficiency and high water use efficiency facilitate the spread of Stellera chamaejasme L. in degraded grasslands. BMC Ecol. 19:50. doi: 10.1186/s12898-019-0267-3
Harper, J. R., and Balke, N. E. (1981). Characterization of the inhibition of K+ absorption in oat roots by salicylic acid. Plant Physiol. 68, 1349–1353. doi: 10.1104/pp.68.6.1349
Herre, E. A., Mejía, L. C., Kyllo, D. A., Rojas, E., Maynard, Z., Butler, A., et al. (2007). Ecological implications of anti-pathogen effects of tropical fungal endophytes and mycorrhizae. Ecology 88, 550–558. doi: 10.1890/05-1606
He, W., Detheridge, A., Liu, Y. M., Wang, L., Wei, H. C., and Griffith, G. W. (2019). Variation in soil fungal composition associated with the invasion of Stellera chamaejasme L. in Qinghai–Tibet plateau grassland. Microorganisms 7:587. doi: 10.3390/microorganisms7120587
Hooker, J., Jaizme-Vega, M., and Atkinson, D. (1994). “Biocontrol of plant pathogens using arbuscular mycorrhizal fungi.” Impact of Arbuscular Mycorrhizas on Sustainable Agriculture and Natural Ecosystems. ALS Advances in Life Sciences. eds. S. Gianinazzi and H. Schüepp (Basel:Birkhäuser).
Jandová, K., Klinerová, T., Müllerová, J., Pyšek, P., Pergl, J. C., Cajthaml, T., et al. (2014). Long-term impact of Heracleum mantegazzianum invasion on soil chemical and biological characteristics. Soil Biol. Biochem. 68, 270–278. doi: 10.1016/j.soilbio.2013.10.014
Jelincic, A., Sajna, N., Zgorelec, Z., and Perci, A. (2021). The bracken-induced increase in soil P availability, along with its high P acquisition efficiency, enables it to invade Pdeficient meadows. J. Plant Ecol. 15, 783–794. doi: 10.1093/jpe/rtab114
Jin, L., Zhang, G. Q., Wang, X. J., Dou, C. Y., Chen, M., Lin, S. S., et al. (2011). Arbuscular mycorrhiza regulate inter-specific competition between a poisonous plant, Ligularia virgaurea, and a co-existing grazing grass, Elymus nutans, in Tibetan plateau alpine meadow ecosystem. Symbiosis (Philadelphia, PA) 55, 29–38. doi: 10.1007/s13199-011-0141-3
Jo, I., Fridley, J. D., and Frank, D. A. (2015). Linking above- and belowground resource use strategies for native and invasive species of temperate deciduous forests. Biol. Invasions 17, 1545–1554. doi: 10.1007/s10530-014-0814-y
Kobayashi, K. (2004). Factors affecting phytotoxic activity of allelochemicals in soil. Weed Biol. Manag. 4, 1–7. doi: 10.1111/j.1445-6664.2003.00112.x
Kong, C. H., Wang, P., Zhao, H., Xu, X. H., and Zhu, Y. D. (2008). Impact of allelochemicals exuded from allelopathic rice on the soil microbial community. Soil Biol. Biochem. 40, 1862–1869. doi: 10.1016/j.soilbio.2008.03.009
Latz, E., Eisenhauer, N., Rall, B. C., Allan, E., Roscher, C., Scheu, S., et al. (2012). Plant diversity improves protection against soil-borne pathogens by fostering antagonistic bacterial communities. J. Ecol. 100, 597–604. doi: 10.1111/j.1365-2745.2011.01940.x
Leng, X., Cui, J., Zhang, S. T., Zhang, W. G., Liu, Y. H., Liu, S. R., et al. (2013). Differential water uptake among plant species in humid alpine meadows. J. Veg. Sci. 24, 138–147. doi: 10.1111/j.1654-1103.2012.01439.x
Liang, M., Liu, X., Etienne, R. S., Huang, F., Wang, Y., and Yu, S. (2015). Arbuscular mycorrhizal fungi counteract the Janzen-Connell effect of soil pathogens. Ecology 96, 562–574. doi: 10.1890/14-0871.1
Li, L., Zhu, X. M., Zhang, Y. R., Cai, Y. Y., Wang, J. Y., Liu, M. Y., et al. (2022). Research on the molecular interaction mechanism between plants and pathogenic Fungi. Int. J. Mol. Sci. 23, 46–58. doi: 10.3390/ijms23094658
Li, Q. W., Zhang, X. Y., Liang, J. F., Gao, J. Q., Xu, X. L., and Yu, F. H. (2021). High nitrogen uptake and utilization contribute to the dominance of invasive Spartina alterniflora over native Phragmites australis. Biol. Fert. Soils 57, 1007–1013. doi: 10.1007/s00374-021-01575-z
Liu, H., Wang, B., and Fu, C. (2008). Relationships between surface albedo, soil thermal parameters and soil moisture in the semiarid area of Tongyu, northeastern China. Adv. Atmos. Sci. 25, 757–764. doi: 10.1007/s00376-008-0757-2
Li, Y. Y., Dong, S. K., Liu, S. L., Wang, X. X., Wen, L., and Wu, Y. (2014). The interaction between poisonous weed and soil quality in response to grassland degradation in the alpine region of the Qinghai-Tibetan plateau. Plant Ecol. 215, 809–819. doi: 10.1007/s11258-014-0333-z
Lu, H., Wang, S. S., Zhou, Q. W., Zhao, Y. N., and Zhao, B. Y. (2012). Damage and control of major poisonous weed in the western grasslands of China—a review. Rangel. J. 34, 329–339. doi: 10.1071/RJ12057
Lu, X., Yan, Y., Sun, J., Zhang, X., Chen, Y., Wang, X., et al. (2015). Short-term grazing exclusion has no impact on soil properties and nutrients of degraded alpine grassland in Tibet, China. Solid Earth 6, 1195–1205. doi: 10.5194/se-6-1195-2015
Maron, J. L., Marler, M., Klironomos, J. N., and Cleveland, C. C. (2011). Soil fungal pathogens and the relationship between plant diversity and productivity. Email Lett. 14, 36–41. doi: 10.1111/j.1461-0248.2010.01547.x
Martins, M. A., and Cruz, A. F. (1998). The role of the external mycelialn network of arbuscular mycorrhizal fungi: III. Study of nitrogen transfer between plants interconnected by a common mycelium. Annu. Rev. Microbiol. 29, 289–294. doi: 10.1590/S0001-37141998000400011
Mitchell, C. E. (2003). Trophic control of grassland production and biomass by pathogens. Email Lett. 6, 147–155. doi: 10.1046/j.1461-0248.2003.00408.x
Mordecai, E. A. (2011). Pathogen impacts on plant communities: unifying theory, concepts, and empirical work. Ecol. Monogr. 81, 429–441. doi: 10.1890/10-2241.1
Nguyen, N. H., Song, Z., Bates, S. T., Branco, S., Tedersoo, L., Menke, J., et al. (2016). FUNGuild: An open annotation tool for parsing fungal community datasets by ecological guild. Fungal Ecol. 20, 241–248. doi: 10.1016/j.funeco.2015.06.006
Pérez-de-Luque, A., Tille, S., Johnson, I., Pascual-Pardo, D., Ton, J., and Cameron, D. D. (2017). The interactive effects of arbuscular mycorrhiza and plant growth-promoting rhizobacteria synergistically enhance host plant defenses against pathogens. Sci. Rep. 7:16409. doi: 10.1038/s41598-017-16697-4
Pérez-Tienda, J., Testillano, P. S., Balestrini, R., Fiorilli, V., Azcón-Aguilar, C., and Ferrol, N. (2011). GintAMT2, a new member of the ammonium transporter family in the arbuscular mycorrhizal fungus Glomus intraradices. Fungal Genet. Biol. 48, 1044–1055. doi: 10.1016/j.fgb.2011.08.003
Phillips, M. L., McNellis, B. E., Allen, M. F., and Allen, E. B. (2019). Differences in root phenology and water depletion by an invasive grass explains persistence in a Mediterranean ecosystem. Am. J. Bot. 106, 1210–1218. doi: 10.1002/ajb2.1344
Reinhart, K. O., Royo, A. A., Van der Putten, W. H., and Clay, K. (2005). Soil feedback and pathogen activity associated with Prunus serotina throughout its native range. J. Ecol. 93, 890–898. doi: 10.1111/j.1365-2745.2005.01028.x
Scheublin, T. R., van Logtestijn, R., and van der Heijden, M. (2007). Presence and identity of arbuscular mycorrhizal fungi influence competitive interactions between plant species. J. Ecol. 95, 631–638. doi: 10.1111/j.1365-2745.2007.01244.x
Selosse, M. A., Richard, F., He, X. H., and Simard, S. W. (2006). Mycorrhizal networks: des liaisons dangereuses? Trends Ecol. Evol. 21, 621–628. doi: 10.1016/j.tree.2006.07.003
Shi, G., Tao, K., Zhou, W., and Hou, T. (2013). Isolation of antifungal compound against phytophthora infestans from Stellera chamaejasme L. Chem Asian J. 25, 4058–4060. doi: 10.14233/ajchem.2013.13982
Smith, S. E., and Read, D. J. (2008). Mycorrhizal symbiosis. Q. Rev. Biol. 137:204. doi: 10.1097/00010694-198403000-00011
Song, X., Wang, L., Zhao, X., Liu, C., Chang, Q., Wang, Y., et al. (2017). Sheep grazing and local community diversity interact to control the litter decomposition of dominant species in grassland ecosystems. Soil Biol. Biochem. 115, 364–370. doi: 10.1016/j.soilbio.2017.09.003
Staddon, W. J., Trevors, J. T., and Duchesne, L. C. (1998). Soil microbial diversity and community structure across a climatic gradient in western Canada. Biodivers. Conserv. 7, 1081–1092. doi: 10.1023/A:1008813232395
Sun, G., Luo, P., Wu, N., Qiu, P., Gao, Y., Chen, H., et al. (2009). Stellera chamaejasme L. increases soil N availability, turnover rates and microbial biomass in an alpine meadow ecosystem on the eastern Tibetan plateau of China. Soil Biol. Biochem. 41, 86–91. doi: 10.1016/j.soilbio.2008.09.022
Sun, H., Zheng, C. C., Chen, T. P., Postma, J. A., and Gao, Y. (2021). Motherly care: how Leymus chinensis ramets support their offspring exposed to saline-alkali and clipping stresses. Sci. Total Environ. 801:149675. doi: 10.1016/j.scitotenv.2021.149675
Sylvia, D. M., Alagely, A. K., Chellemi, D. O., and Demchenko, L. W. (2001). Arbuscular mycorrhizal fungi influence tomato competition with bahiagrass. Biol. Fertil. Soils 34, 448–452. doi: 10.1007/s00374-001-0429-1
Szymura, M., and Szymura, T. H. (2016). Interactions between alien goldenrods (Solidago and Euthamia species) and comparison with native species in Central Europe. Flora 218, 51–61. doi: 10.1016/j.flora.2015.11.009
Tian, C., Kasiborski, B., Koul, R., Lammers, P. J., Bücking, H., and Shachar-Hill, Y. (2010). Regulation of the nitrogen transfer pathway in the arbuscular mycorrhizal symbiosis: gene characterization and the coordination of expression with nitrogen flux. Plant Physiol. 153, 1175–1187. doi: 10.1104/pp.110.156430
Trouvelot, A., Kough, J. L., and Gianiazzi-Pearson, V. (1986). “Mesure du taux de mycorhization VA d’unsystème radiculaire. Recherche de methods d’estimation ayant une signification fonctionnelle” in Physiological and genetical aspects of mycorrhizae. eds. V. Gianiazzi-Pearson and S. Gianiazzi (Paris: INRA press), 217–221.
Turner, B. L., and Haygarth, P. M. (2005). Phosphatase activity in temperate pasture soils: potential regulation of labile organic phosphorus turnover by phosphodiesterase activity. Sci. Total Environ. 344, 27–36. doi: 10.1016/j.scitotenv.2005.02.003
Vigo, C., Norman, J., and Hooker, J. (2000). Biocontrol of the pathogen Phytophthora parasitica by arbuscular mycorrhizal fungi is a consequence of effects on infection loci. Plant Pathol. 49, 509–514. doi: 10.1046/j.1365-3059.2000.00473.x
Wang, C. Y., Jiang, K., Liu, J., Zhou, J. W., and Wu, B. D. (2018). Moderate and heavy Solidago canadensis L. invasion are associated with decreased taxonomic diversity but increased functional diversity of plant communities in East China. Ecol. Eng. 112, 55–64. doi: 10.1016/j.ecoleng.2017.12.025
Wang, J. Y., Xu, T. T., Wang, Y., Li, G. Y., Abdullah, I., Zhong, Z. W., et al. (2021). A meta-analysis of effects of physiological integration in clonal plants under homogeneous vs. heterogeneous environments. Funct. Ecol. 35, 578–589. doi: 10.1111/1365-2435.13732
Wang, Q. (2016). Identification of AM fungal spores in the rhizosphere of toxic plants on the Qinghai Tibet plateau and their regulation of plant interspecific relationships.
Wilson, S. D., and Keddy, P. A. (1986). Measuring diffuse competition along an environmental gradient - results from a shoreline plant community. Am. Nat. 127, 862–869. doi: 10.1086/284530
Wu, G. L., Ren, G. H., Dong, Q. M., Shi, J. J., and Wang, Y. L. (2014). Above- and belowground response along degradation gradient in an alpine grassland of the Qinghai-Tibetan plateau. Clean Soil Air Water 42, 319–323. doi: 10.1002/clen.201200084
Xing, F. (2016). Ecological study on poisonous weed in grassland ; Academy of Sciences Press: Beijing.
Xing, F., Guo, J., and Wei, C. (2004). Judging method of individual age and age structure of Stellera chamaejasme population in degraded steppe. Chin. J. Appl. Ecol. 6, 2485–2488. doi: 10.1088/1009-0630/6/5/011
Yan, L. F., Xu, C., Liu, Q., Gu, A. H., and Jiang, Z. Y. (2015). Altered profile of gut microbiota after subchronic exposure to neochamaejasmin An in rats. Environ. Toxicol. Phar. 39, 927–933. doi: 10.1016/j.etap.2015.03.005
Yan, Z. Q., Guo, H. G., Yang, J. Y., Liu, Q., Jin, H., Xu, R., et al. (2014). Phytotoxic flavonoids from roots of Stellera chamaejasme L. (Thymelaeaceae). Phytochemistry 106, 61–68. doi: 10.1016/j.phytochem.2014.07.013
Zhang, Y. H., Yue, J. P., and Sun, H. (2015). Identification of twelve novel polymorphic microsatellite loci in the severe weed, Stellera chamaejasme L. (Thymelaeaceae). J. Genet. 94, 24–26. doi: 10.1007/s12041-015-0516-y
Zhou, S. Q., and Wang, H. (2010). Allelopathy of S. chamaejasme on Elymus dahuricus. Grassl Turf. 30, 63–65.
Zhu, X., Li, X., Xing, F., Chen, C., Huang, G., and Gao, Y. (2020). Interaction between root exudates of the poisonous plant Stellera chamaejasme L. and arbuscular mycorrhizal Fungi on the growth of Leymus chinensis (Trin.) Tzvel. Microorganisms 8:364. doi: 10.3390/microorganisms8030364
Keywords: poisonous weed, rhizosphere interaction, arbuscular mycorrhizal fungi, plant pathogenic fungi, interspecific competition
Citation: Zhang R, Qu S, Zhang B, Gao Y and Xing F (2023) Interactive effects between the invasive weed Stellera chamaejasme and grass: can arbuscular mycorrhizal fungi and fungal pathogens coregulate interspecific relationships? Front. Microbiol. 14:1236891. doi: 10.3389/fmicb.2023.1236891
Edited by:
Jingping Ge, Heilongjiang University, ChinaReviewed by:
Geetika Sirhindi, Punjabi University, IndiaNitika Kapoor, Guru Nanak Dev University, India
Rodica Pena, University of Reading, United Kingdom
Copyright © 2023 Zhang, Qu, Zhang, Gao and Xing. This is an open-access article distributed under the terms of the Creative Commons Attribution License (CC BY). The use, distribution or reproduction in other forums is permitted, provided the original author(s) and the copyright owner(s) are credited and that the original publication in this journal is cited, in accordance with accepted academic practice. No use, distribution or reproduction is permitted which does not comply with these terms.
*Correspondence: Fu Xing, eGluZ2Y1MjJAMTI2LmNvbQ==