- Department of Infectious Diseases, Wuhan Jinyintan Hospital, Tongji Medical College of Huazhong University of Science and Technology, Hubei Clinical Research Center for Infectious Diseases, Wuhan Research Center for Communicable Disease Diagnosis and Treatment, Chinese Academy of Medical Sciences, Joint Laboratory of Infectious Diseases and Health, Wuhan Institute of Virology and Wuhan Jinyintan Hospital, Chinese Academy of Sciences, Wuhan, Hubei, China
Combination antiretroviral therapy has demonstrated proved effectiveness in suppressing viral replication and significantly recovering CD4+ T cell count in HIV type-1 (HIV-1)-infected patients, contributing to a dramatic reduction in AIDS morbidity and mortality. However, the factors affecting immune reconstitution are extremely complex. Demographic factors, co-infection, baseline CD4 cell level, abnormal immune activation, and cytokine dysregulation may all affect immune reconstitution. According to report, 10–40% of HIV-1-infected patients fail to restore the normalization of CD4+ T cell count and function. They are referred to as immunological non-responders (INRs) who fail to achieve complete immune reconstitution and have a higher mortality rate and higher risk of developing other non-AIDS diseases compared with those who achieve complete immune reconstitution. Heretofore, the mechanisms underlying incomplete immune reconstitution in HIV remain elusive, and INRs are not effectively treated or mitigated. This review discusses the recent progress of mechanisms and factors responsible for incomplete immune reconstitution in AIDS and summarizes the corresponding therapeutic strategies according to different mechanisms to improve the individual therapy.
1. Introduction
The hallmark of infection with the human immunodeficiency virus (HIV) is the progressive destruction of CD4+ T cells in both count and function, particularly the activated CD4+ T cells. Loss of CD4+ T cells can cause opportunistic infections, non-AIDS-defining events (nADEs) and death in those who develop acquired immunodeficiency syndrome (AIDS; Deeks et al., 2013; Maartens et al., 2014). The development of combination antiretroviral therapy (cART) has achieved the suppression of viral replication and the increase in CD4+ T cell count in the majority of patients, leading to a significant decrease in morbidity of AIDS and mortality (Vieillard et al., 2016; Saag et al., 2020). Yet, 10–40% of people living with HIV (PLHIV) fail to achieve CD4 cell count reconstitution with cART, despite achieving suppression of HIV-1 viral load in the blood, and are referred to as immunological non-responders (INRs). Compared with immune responders (IRs) and sub-optimal immunologic responders (ISRs), INRs still have a higher incidence of AIDS-related fatalities and morbidity (Piconi et al., 2010; Lederman et al., 2011; Briceno et al., 2020; Shive et al., 2021; Wang L. et al., 2021; Zhao et al., 2021). In addition, chronic immune activation has been reported among PLHIV, elevating the risk of cardiovascular disease (Shah et al., 2018), metabolic syndrome (Dragović et al., 2021), kidney abnormalities (Swanepoel et al., 2018), and non-AIDS-related malignancies (Chammartin et al., 2021) compared with non-HIV infected individuals, causing significant negative effects on quality of life as well as mortality (Wilson and Sereti, 2013; Ipp et al., 2014; Sims et al., 2018). As an important global public health issue, incomplete immune reconstitution continues to adversely affect the survival quality of PLHIV. In this review, we highlight areas of recent advances in the mechanisms and risk factors of incomplete immune reconstitution and explore new targeted therapeutic interventions to improve immune restoration.
2. Complete and incomplete immune reconstitution
Immune reconstitution is broadly divided into two phases. First, after cART is initiated, HIV viral load declines, and lymphocytes are soon redistributed, leading to a rise in peripheral blood memory CD4+ T cells (CD45RO+). Naive CD4+ T cell (CD4+ CD45RA+CD62L+) regeneration enhances after treatment for 2–3 months which leads a slow and steady increase in CD4+ T cells without normal immune function. Second, after 3–6 months of late treatment, CD4+ T cells gradually recall their antigen-specific response to various antigens, such as cytomegalovirus (CMV) and tuberculin, except for HIV (Autran et al., 1997; Macdonald et al., 1998). Studies have reported that as viral load decreases, T lymphocyte activation markers like CXCL13, HLA-DR, and CD38 decline, promoting the immune system return to homeostasis (Autran et al., 1997; Li et al., 1998; Mehraj et al., 2019).
However, immune restoration is not always successful. Although viral load can be controlled at low or even undetectable levels with cART, there is considerable variability in terms of CD4+ T cell recovery. And this inconsistency between the decline in plasma viral load and the rise in CD4 count is influenced by multiple factors such as immune-related or pathogenic host-related which like abnormal immune activation and generally characteristics. People failing to completely restore CD4 T cell count are called immunological non-responders. To date, there is no consensus on the definition of “immunological non-responders.” Studies and guidelines generally define them in regard to confine CD4+ T cell count, increased CD4+ T cell counts from baseline, or confine percentage of CD4+ T cell increase over baseline (Yang et al., 2020; Table 1). In 2008, the Department of Health and Human Services (DHHS) defined “immunological non-responders” as patients whose CD4+ T cell counts had not reached 350–500 cells/μl after 4–7 years of effective antiretroviral therapy. In 2021, the World Health Organization (WHO) defined adult immunological failure as a CD4 cell count of 250 cells/μl or a CD4 cell count consistently below 100 cells/μl after 6 months of effective treatment (WHO, 2021). In 2021, DHHS defined “immunological responders” as patients with an increase in CD4 count of 50–150 cells/μl in the first year of cART treatment. In the first 3 months, the response of treatment was rapid, followed by an average annual increase of approximately 50–100/μl until steady state (Adolescents., P.O.A.G.F.A.A, 2021).
INRs are unable to maintain the basic normal immune function of the body due to low CD4+ T cell counts and exhibit severe immune dysfunction. Under the influence of lifestyle, drug toxicities, chronic inflammation, immune activation, and many other factors, nADEs still account for an increasing proportion of PLHIV, despite viral load being controlled to undetectable levels after cART (Elvstam et al., 2021). However, the normal CD4 counts could not always reflect complete restore, though it be extensively applied in evaluating immune reconstitution (Ron et al., 2023). Recently, CD4/CD8 ratio was demonstrated having an amazing performance in immune reconstitution. Some studies insisted that a low CD4/CD8 ratio reflects increased immune activation and immune senescence, also associating with an higher risk of severe nADEs (Gibellini et al., 2017; Serrano-Villar et al., 2022). Therefore, we suggested incomplete immune reconstitution an independent risk factor for increased risk of SNAEs under sustained viral suppression (Prabhu et al., 2019; Noiman et al., 2022).
3. Potential mechanisms of incomplete immune reconstitution
Failure to normalize the reduced CD4+ T cell population after treatment is regard as incomplete immune reconstitution. This outcome results from decreased production, increased destruction, and increased senescence of CD4 cells. The mechanisms that cause these abnormal changes in CD4 cells are currently the subject of much speculation. Such as damage and hypofunction of lymphoid organs, residual viral replication, disruption of T cell subpopulation homeostasis, dysregulation of cytokine secretion, and translocation of microorganisms might reduce CD4+ T cell count directly and induce inflammation and immune activation. It is worth to mention that excessive activation of CD4+ T cells during cART has been suggested to upregulate the expression of the HIV-1-binding target CCR5 on CD4+ T cells, thereby accelerating the depletion of CD4+ T cells (Gandhi et al., 2017; Xia et al., 2018). Therefore, long-term chronic abnormal immune activation can also affect immune reconstitution. In addition, factors associated with the host can also affect CD4 cell recovery (Figure 1).
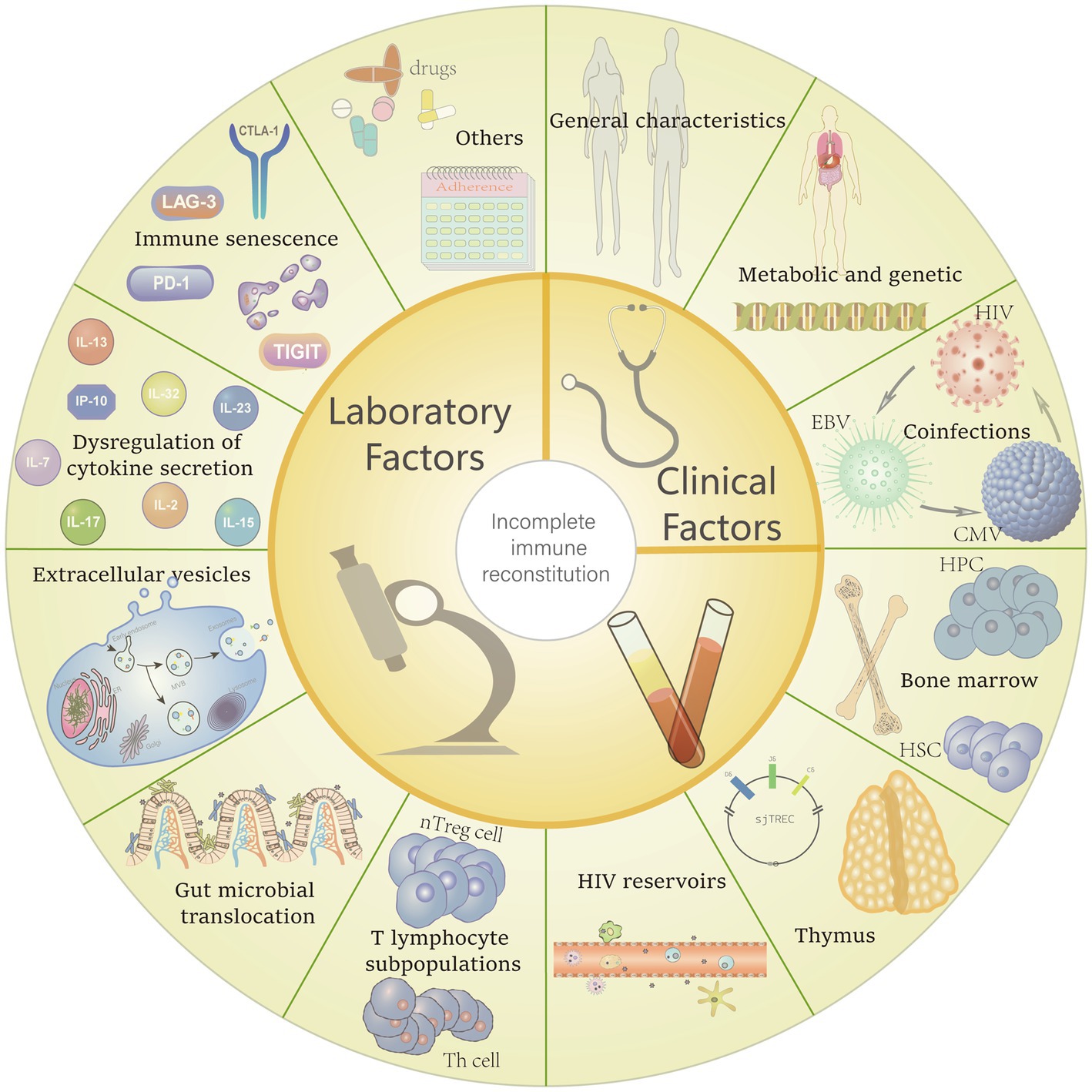
Figure 1. Potential risk factors for incomplete immune reconstitution. Including general characteristics (e.g., age, gender, CD4+ T-cell counts, body mass index (BMI) and the underlying diseases), metabolic and genetic factors also be confirmed can make some difference in immune recovery between different people living with HIV (PLHIV). Furthermore, coinfections with other virus, poor adherence and the regimens which with severe side effects also can interfere the immune restore. Factors that contribute to the reduction and destruction of CD4+ T cells also affect immune reconstitution, including: reduced haematopoiesis of bone marrow, thymic insufficiency, replication of HIV reservoirs, alteration of CD4+ T cell subpopulations, gut microbial translocation, transport effects of extracellular vesicles, dysregulation of cytokine and, immune senescence.
To this end, we discuss herein the possibilities affecting incomplete immune reconstitution and its mechanisms (Figure 2).
3.1. Dysfunction of the lymphoid organs
T cells are produced from hematopoietic progenitor cells (HPCs) and hematopoietic stem cells (HSCs) in the bone marrow, educated on thymic tissue for development and maturation. Hematopoietic stem and progenitor cells (HSPCs) have the capacity for lifelong survival, self-renewal, and daughter cell production. HIV-1 infection can lead to impaired hematopoietic function of HSPCs in several ways. First, a large body of evidence indicates that low levels of CD4 receptors expressed by HSPCs can be targeted by HIV thereby leading to impaired function (Sebastian et al., 2017; Chen, 2019; Karuppusamy et al., 2022). Second, HIV-1-produced Nef proteins expressed in HSPCs impeding the development and production of myeloid–erythroid cells and inducing apoptosis (Zou et al., 2021). In addition, HIV-1 is capable of causing alterations to the bone marrow hematopoietic microenvironment. HIV-1 may complete with stromal cell-derived factor-1 (SDF-1, also known as CXCL12) for the site of binding to CXCR4, affecting the homing of HSCs (Tsukamoto, 2020). However, efficient homing is a prerequisite for the successful re-establishment of hematopoiesis in the bone marrow hematopoietic microenvironment. Furthermore, Yuan et al. (2019) found that HIV-Tat reduced the ability of bone marrow mesenchymal stem cells (BMSCs) to support HSC expansion in vitro and reduced the expression levels of a series of key hematopoietic factors produced by BMSCs. These studies suggest that incomplete immune reconstitution in HIV-1-infected patients may be associated with reduced bone marrow hematopoietic function and HSPCs numbers.
The thymus is the site of T cell development and maturation, and good thymus function ensures the stability of CD4+ T cell count and function. As a marker of thymic function, CD31+CD4+ T cells (CD31%) reflects the kinetics of the recent thymic immigrant CD4+ T cells. In a research, the results revealed a lesser percentage of CD31% in the INR group than in the IR group, and both of them exhibited significantly lesser percentages than the healthy volunteer group (Li et al., 2011). Ferrando-Martinez et al. (2017) applied sj/β-TREC (accurate technique for measuring thymic function) to 774 PLHIV of different types and found that patients with thymic failure (sj/β-TREC ratio < 10) had significantly lower levels of CD4+ T cells, suggesting that thymic function influences AIDS progression. Recent thymus emigrants (RTE) is one of the most established markers to evaluate recent thymic output function, representing a high sj-TRECs content CD4+ T cell subpopulation (Kimmig et al., 2002). Briceno et al. (2020) identified that HIV-positive subjects had fewer RTEs in absolute terms compared with the HIV-negative group. The application of cART resulted in increased levels of circulating RTEs in IRs compared with those in INRs. A further study (Rb-Silva et al., 2019) reported that IRs demonstrated a significant increase in thymus volume at 12 months of cART treatment, were prone to higher sj-TREC and sj/β-TREC, and had higher proportions and absolute numbers of RTEs in peripheral blood CD4+ T cells than INRs. These studies suggest that the thymus influences HIV progression by affecting CD4+ T cell levels, and impaired thymic output and reduced function are one of important mechanisms leading to incomplete reconstitution.
3.2. Replication of residual virus in HIV reservoirs
HIV-1 invades the body and preferentially attacks activated CD4+ T cells, most of which die rapidly after infection, whereas a small proportion, called latent cells, tend to enter a resting state and stay dormant for a long time. HIV-1 proviruses have the capacity for stable integration and high replication and can persist in vivo in cells (Wacleche et al., 2018; Zaikos et al., 2018; Kruize and Kootstra, 2019; Wallet et al., 2019; Lutgen et al., 2020; Wiche Salinas et al., 2021; Chen et al., 2022b) and tissues (Damouche et al., 2015; Cantero-Pérez et al., 2019; Ganor et al., 2019; Wallet et al., 2019; Chaillon et al., 2020a) of PLHIV receiving cART. Reactivation of these latent proviruses is regarded as the leading cause of rebound viremia after cART cessation (Vanhamel et al., 2019; Cohn et al., 2020). We found a majority of people on suppressive cART have residual virus production, which may cause immunological activation as a result of the anti-HIV immune response. Therefore, the increased immune activation and inflammation in those using cART appears to be mostly caused by HIV persistence (Scherpenisse et al., 2021). Mavigner et al. (2009) investigated the connection between residual virus and T-cell activation in both groups of good immunological responders and incomplete immunological responders. In individuals with incomplete immunological responses, there was a strong correlation only between the degree of residual viremia and the frequency of CD4 T-cell activation. Furthermore, they also found the prevalence in CD31+ naive CD4 T cells of RTEs seemed to be inversely linked with the residual viremia (Mavigner et al., 2009). The findings of these studies imply that the presence of prolonged HIV-1 replication in vivo provides a basis for viral rebound while leading to a long-term chronic reduction in CD4 T cells.
3.3. Breakdown of CD4 T cell subsets
During chronic HIV infection, a slow and sustained decline in circulating CD4 T cells is accompanied by changes in major CD4 cell subsets. Regulatory T cells (Treg) are one of the most important elements in suppressing immune responses, which can both control aberrant immune activation and suppress specific T cell responses to a variety of pathogens for causing immunodeficiency, critical in maintaining immune homeostasis (Chen Y. et al., 2020). As they express the CCR5 receptor, Tregs are vulnerable to HIV infection, and the interaction of gp120 and CD4 inhibits Treg apoptosis and improves its survival. Therefore, Treg is also a potential reservoir for the HIV-1 virus (Nilsson et al., 2006; Sivanandham et al., 2020). In addition, Tregs are capable of controlling low residual immune activation in virologically suppressed patients under cART. A previous study (Chevalier and Weiss, 2013) found that the proportion of Tregs under cART treatment was negatively correlated with CD 4 and CD8 T cell activation. Thus, under viral control, Treg frequency is negatively correlated with residual immune activation. While the early initiation of cART restored CD4+ T cell count, it was unable to control the massive expansion and intestinal migration of Treg, which may eventually cause HIV disease progression and intestinal tissue fibrosis (Yero et al., 2019, 2021).
Helper T cells (Th) are the most numerous subsets of T cells and work by amplifying the immune response function of other immune cells. HIV preferentially infects Th1 and Th17 cells. Among them, Th17 cells are enriched within the mucosal tissue and promote protective immune responses against extracellular bacteria and fungi, preserving the integrity of the mucosal barrier and maintaining immune homeostasis at mucosal sites (Planas et al., 2019; Wiche Salinas et al., 2021). PLHIV commonly exhibits a progressive decline in intestinal CD4+ T cells, with a preferential absence and significant depletion of Th17 cells, throughout infection (Le Hingrat et al., 2021). Depletion of Th17 cells has important implications for disease progression and viral persistence and is a major cause of microbial translocation and inflammatory progression (Ortiz et al., 2016). At the same time, Th17 cells are closely associated with Treg and altered Th17/Treg balance is associated with a persistent chronic inflammatory state in HIV-1 disease (Chevalier and Weiss, 2013). Chronic inflammation has long been known to be harmful in conditions when the immune system is unable to successfully remove infectious germs. Therefore, chronic HIV-1 infection activated the host immune system and attacked the virus through multiple mediators. Then, it led to deprivation of T-cell function and ultimately to immunosuppression (Favre et al., 2010). The alterations in T cell subsets can affect immune activation through multiple pathways leading to incomplete control of viral replication and facilitating the progression of immune activation. And now, how to detect changes in each subpopulation and discover their role is crucial to our next step of targeted preventive and control for this progression.
3.4. Gut microbial translocation
The gut-associated lymphoid tissue contains at least 80% of the body’s lymphocytes, including approximately 60% of the body’s CD4+ T lymphocytes, making the intestinal mucosal immune system a major target of HIV-1 attack, and the body’s immune function is closely related to the intestinal microenvironment (Brenchley and Douek, 2008). Microbial translocation can lead to changes in species diversity and composition, affecting systemic inflammation and thus CD4 T cell recovery.
In healthy individuals, a wide variety of microorganisms reside in the gut, including beneficial and pathogenic bacteria, which together participate in various host physiological functions and maintain normal physiological activities. Lee et al. (2018) found that Lactobacillus is involved in maintaining the integrity of the intestinal mucosal barrier. It reduces microbial translocation, has a role in reducing systemic immune activation, and its abundance is positively correlated with CD4+ T cell count. Another study confirmed that Bacteroides has LPS or LOS molecules capable of signaling through TLR4 to induce IFN-β to initiate antiviral responses and resistance to viral infection (Stefan et al., 2020).
However, HIV-1 infection leads to a disruption in the bacterial community structure and function of the intestinal microbiota, primarily characterized by an overall decreased α diversity, a significant increase in relative abundance of Prevotella and reduced abundances of Bacteroides and Faecalibacterium (Vujkovic-Cvijin et al., 2013; Dillon et al., 2014; Mak et al., 2021). These changes in the gut microbiota can stimulate the activation of T cells and chronic inflammation directly or indirectly, leading to systemic immune activation (Vujkovic-Cvijin and Somsouk, 2019; Geng et al., 2020; Serrano-Villar et al., 2021). Ishizaka et al. (2021) observed that compared with healthy controls, PLHIV with low CD4 counts had reduced gut microbiome α diversity and a higher abundance of Prevotella, and the relative abundance of it was positively correlated with inflammatory cytokines and negatively correlated with anti-inflammatory cytokines. A recent study concluded that Prevotella increased the susceptibility to gut inflammation through the suppression of IL-18 production indirectly (Iljazovic et al., 2021). The findings of Pinacchio et al. (2020) suggest that Prevotella levels were negatively correlated with IFN-I gene expression and Th17 cell counts. It is further hypothesized that Prevotella enrichment may affect intestinal mucosal IFN-I pathways and T cell response in HIV-1-infected patients, therefore leading to immune dysfunction. Another study (Pickard et al., 2017) suggested that gut microbes can induce Th17 and Treg production by modulating immune cell differentiation. In addition, some studies have demonstrated that gut microbes can regulate myeloid hematopoietic cell development and maturation by modulating local metabolites and tissue-specific mediators as well as by driving Toll-like receptor (TLR) and myeloid differentiation factor 88 (MyD88)-mediated signaling pathways (Khosravi et al., 2014; Serrano-Villar et al., 2016; Thaiss et al., 2016). The results of these studies suggest that gut microbes have a role in the regulation of T-cell subsets and bone marrow hematopoiesis. The above findings suggest that alterations in the gut microbiota may be associated with abnormal immune activation and chronic inflammation during chronic HIV-1 infection. We suggest that after HIV-1 invasion, intestinal mucosal lymphocytes, the primary target of attack, are depleted by direct viral action and post-activation apoptosis, and the intestinal mucosal barrier is compromised, indirectly leading to dysbiosis/microenvironmental changes in the intestinal flora. These changes in turn further exacerbate the damage to the intestinal mucosa, leading to an increased risk of inflammation and promoting abnormal immune activation.
3.5. HIV-1 infected extracellular vesicles changed structure and function, interfering immune reconstitution
Extracellular vesicles (EVs) are heterogeneous phospholipid bilayer vesicles released from cells into the external environment and act as specific carriers and mediate intercellular communication in the immune system (Théry et al., 2018; Gudbergsson et al., 2019). Furthermore, they are involved in the regulation of immune responses in different physiologic and pathogenic settings, by delivering nucleic acids, lipids, and proteins from their surface or lumen to target cells (Cheruiyot et al., 2018; Witwer and Théry, 2019).
First, EVs can contribute to HIV transmission and HIV reservoirs in direct and indirect ways. Several studies have demonstrated that large numbers of EVs released from HIV-1-infected cells are involved in HIV-1 transmission under their carrier properties, and cART is unable to reduce their concentration levels (Lee et al., 2016; Patters and Kumar, 2018). Weber et al. (2020) treated cultured monocytes with HIV-Tat in vitro and found that monocyte-derived microvesicles (MDMVs) released by Tat-treated monocytes into the culture medium were significantly increased. In addition, another HIV protein, Nef, can promote HIV infection by decreasing the expression of CD4 in exosomes to improve the ability for neutralize with CD4-bearing cells (Navarrete-Muñoz et al., 2021). Another study (Perez et al., 2019) found that EVs secreted by infected cells carry pathogen-associated molecular patterns, which that interact with immune cells to produce inflammation and also evade recognition by the immune system. EVs can also expand the number of susceptible target cells by mediating the transfer of CXCR4 and CCR5 to target cells that do not express or low express these molecules.
Secondly, EV can promote immune cell activation and secondary inflammatory responses leading to failure of immune reconstitution. Okoye et al. (2021) noted that plasma extracellular vesicles from HIV-1 patients could induce miR-139-5p expression to promote CD4+ T cell activation. Gabriel et al.(Duette et al., 2018) observed that T cells in the HIV-1 replication cycle can enhance viral replication by inducing hypoxia-inducible factor 1α (HIF-1α) activity. They also noted that HIF-1α induced the release of EVs, causing associated inflammation to stimulate the secretion of inflammatory mediators IL-6 and IL-1β by uninfected lymphocytes and macrophages, further promoting viral replication and inflammation. EVs are involved in HIV-1 and intercellular communication, contributing to the spread of the virus between cells. The presence of this modality greatly increases HIV-1 latency and also results in the inability of cART to completely clear the virus, increasing the risk of inducing an inflammatory response.
3.6. Dysregulation of cytokine secretion
Interleukin-2 (IL-2) promotes the activation and proliferation of T lymphocytes and NK cells, stimulates the secretion of various cytokines, and provides important common signals for the specific immune response. As a result of HIV-1 infection, the production of IL-2-expressing memory CD4+ T cells is reduced, leading to decreased IL-2 levels. This is one of the earliest functional defects observed in chronic PLHIV and predicts the loss of CD4+ T cells and the progression of AIDS (Xia et al., 2018).
IL-7 and IL-15 are the primary stimulators for the production of naive and memory T cells during chronic infection, maintaining T cell function and internal environmental stability (Nasi and Chiodi, 2018; Harwood and O'Connor, 2021; Simonetti et al., 2021). However, the persistent low proliferative response of CD8+ T cells and Th17 CD4+ T cells to IL-7 after cART affects immune dysfunction among PLHIV (Côté et al., 2020). However, by observing IRs and ISRs after cART, Pino et al. (2021) found that ISRs had higher plasma levels of IL-7 and IL-15 before cART compared with IRs, which in turn supported homeostatic proliferation of T cells. This paradox led us to speculate that elevated levels of IL-7 and IL-15 in ISRs might also promote the persistence of HIV-1 viral hosts by enhancing cell survival. This has the same results as chemokine IP-10, which exerts a pro-inflammatory effect by triggering the activation of cofilin and actin to enhance the kinetic activity of quiescent CD4+ T cells and induce viral entry, thereby promoting latent HIV infection (Wang Z. et al., 2021).
IL-17 is mainly produced by Th17 and Tc17 cells, and can encourage the integrity of the intestinal mucosal barrier by promoting tight junctions of intestinal epithelial cells, secreting antimicrobial peptides, and summoning neutrophils to the sites of mucosal injury (Liang et al., 2006; Lee et al., 2015). During HIV-1 infection, the number and function of IL-17-producing Th17 cells and Tc17 cells are impaired in the peripheral and intestinal mucosa due to direct viral infection and impaired IL-23 signaling pathways that maintain Th17 cell production and stability, and IL-17 levels are reduced, exacerbating impaired intestinal barrier integrity and thus causing microbial translocation and immune activation (Fernandes et al., 2017; Perdomo-Celis et al., 2018). Furthermore, Moreira Gabriel et al. (2021) found that overexpression of IL-32b mRNA in the colon of HIV-1 patients receiving cART had a negative correlation with IL-17A mRNA levels, suggesting that overexpression of IL-32 may also affect the integrity of the intestinal epithelial barrier.
Studies have reported that PLHIV has higher levels of pro-inflammatory cytokines such as IL-1β, IL-6, IL-8, and IL-18 compared with healthy individuals, which are associated with reduced CD4+ T cell recovery at month 12 after cART treatment (Vanpouille et al., 2020; Nganou-Makamdop et al., 2021). In addition, Wang et al. (2020) found that the persistent inflammatory response caused by HIV-1-induced cytokine production leads to a reduction in the intestinal mucosa in ILCs. ILCs do not express markers or receptors such as CD4 or CCR5, even with the application of cART, ILCs in blood and intestinal were depleted. That also led to a loss of intestinal epithelial integrity and microbial translocation, further exacerbating chronic inflammation.
Cytokines regulate cell growth and differentiation, immune response, and inflammatory response. Excessive immune activation leads to persistent chronic inflammation, causing loss of T-cell function and ultimately immunosuppression.
3.7. Premature immune senescence due to increased T cell apoptosis and senescence
T-cell-mediated cellular immunity is the primary mode of defense against foreign pathogens. However, with prolonged and sustained exposure to chronic stimuli such as chronic infections and cancer, T cells can enter a state of dysfunctional failure if the immune response is unable to fully clear foreign antigens. This failure is first manifested in PLHIV by a progressive decline in the killing function of CD8+ T cells (Barber et al., 2006; Okoye and Picker, 2013). Without CD8+ T cell suppression, viral load rises rapidly, and as antigen levels continue to rise, T cells, which are the target cells of HIV-1, are depleted and gradually failed to kill infected cells effectively (Collins et al., 2021). Meantime, the body’s negative regulatory factors are highly expressed in CD4+ and CD8+ memory T cells, including a variety of inhibitory receptors associated with T cell failure, also known as immune checkpoint inhibitors (ICIs), such as programmed cell death 1 (PD-1), lymphocyte-activation gene 3 (LAG-3), cytotoxic T lymphocyte antigen 4 (CTLA-4), and the newly identified T-cell immunoglobulin and ITIM domains (TIGIT) and T cell immunoglobulin and mucin-domain containing protein 3 (TIM3; Minnie et al., 2018; Dixon et al., 2021; Kubli et al., 2021; Tawbi et al., 2022). These ICIs negatively regulate T cell function by binding to their respective ligands to generate-T cell-suppressive signals, which associate with viral load and CD4+ and CD8+ T cell counts and dysfunction, reflecting to some extent the state of T cell failure (Fenwick et al., 2019).
3.8. Coinfection
In cART, HIV is often combined with other types of viral infections due to low immunity, and studies have found that hepatitis B virus (HBV), hepatitis C virus (HCV), cytomegalovirus (CMV), Epstein–Barr virus (EBV), and other viruses may affect CD4+ T cell recovery and immune reconstitution. Plasma sCD163 and sCD14 are sensitive indicators of the level of response to chronic immune activation and have a significant relation with the progression of HIV and HBV (Wilson et al., 2014; Shive et al., 2015). He et al. (2021) found both sCD163 and sCD14 presented elevated expressions in groups with coinfected and single-infected patients compared with those in the healthy group. However, compared with HIV-1 single-infected patients, sCD14 expression remained at a high level after reduction in HIV-1/HBV-coinfected patients, and the Treg cell frequency was significantly higher than the former, at week 24 of cART. It has been reported that PLHIV (Garcia-Broncano et al., 2018; Gobran et al., 2021) were noted to be six folds more likely to be exposed to HCV and get infected compared normal people. The infection affects the stability of CD4+ T cell count negatively while promoting HIV-1 replication and viral reservoir persistence. Moreover, several studies have affirmed that HIV-1/HCV-coinfected individuals presented a remarkable reduction in the median expression of HLA-DR and CD38 on CD4 and CD8 T cells, as HCV is controlled. Meanwhile, after HCV eradication, significantly lower levels of innate immune activation markers (sCD163, sCD14), HIV-1 proviral load and LPS (López-Cortés et al., 2018; French et al., 2021). Furthermore, the coexistence of CMV or EBV with HIV-1 in PLHIV also contributes to elevated plasma inflammatory factors and promotes chronic immune activation, which is a key element for T cell activation and incomplete immune reconstitution after cART (Hernandez et al., 2018). Chaillon et al. (2020b) observed a significant increase in the diversity of HIV-1 DNA molecules among partitions with high CMV and EBV transmission, thus suggesting that sustained CMV and EBV replication and associated inflammation may maintain the persistence of HIV-1 hosts. These studies suggest that HIV-1 in combination with other viral infections leads to disruption of T cell subpopulation homeostasis, increased HIV-1 replication, and abnormal activation of CD4+ T and CD8+ T cells, further causing massive CD4+ T cell destruction and a chronic inflammatory response.
3.9. Host-associated factors
In addition, some of the risk factors for immune reconstitution recovery include older age (Kroeze et al., 2018; Ahn et al., 2019; Chen et al., 2022a), males (Boatman et al., 1999; Fiseha et al., 2022), low CD4+ T cell baseline level (Davy-Mendez et al., 2019; Handoko et al., 2020),delay in cART initiation (Sharma et al., 2019; Saluzzo et al., 2021; Jesson et al., 2022),inappropriate cART regimen (Veil et al., 2020; Lu et al., 2022), adherence, and the underlying diseases.
In addition, host metabolic level and genetic factors play a role in immune reconstitution. Studies (Zhu et al., 2022) have demonstrated that PLHIV had better immune reconstitution with a higher baseline BMI. This may be due to the fact that leptin has higher levels in people with a higher BMI. As an adipokine regulatory protein, leptin play an essential role in acquired immunity (de Candia et al., 2021). Many researches pointed that abnormalities in leptin synthesis contribute to the dysregulation of T-cell immunity (Batra et al., 2010). Therefore, we speculate that a lower BMI may predicts an incomplete immune reconstitution. Furthermore, hereditary variables of the organism have been suggested to influence immune reconstitution. A previous study (Pereira et al., 2022) HLA class 1 B*13, B*35 and B*39 alleles were linked to influence recovery failure. And even while cART is being used, HLA can facilitate the loss of CD4 T cells in non-responders due to the uncontrolled immunological activation in HIV infection.
4. Interventions for poor HIV immune reconstitution
Since the residual virus cannot be completely removed from the body, once cART is started it is a lifelong treatment. However, some patients do not recover well after treatment, and at the same time, long-term adherence problems and adverse effects lead to poor outcomes in an increasing number of patients, which affects the progression of the disease. Heretofore, there is no definitive treatment for patients with incomplete immune reconstitution, but there are many attempts and trials to improve immune reconstitution and slow down disease progression (Figure 3).
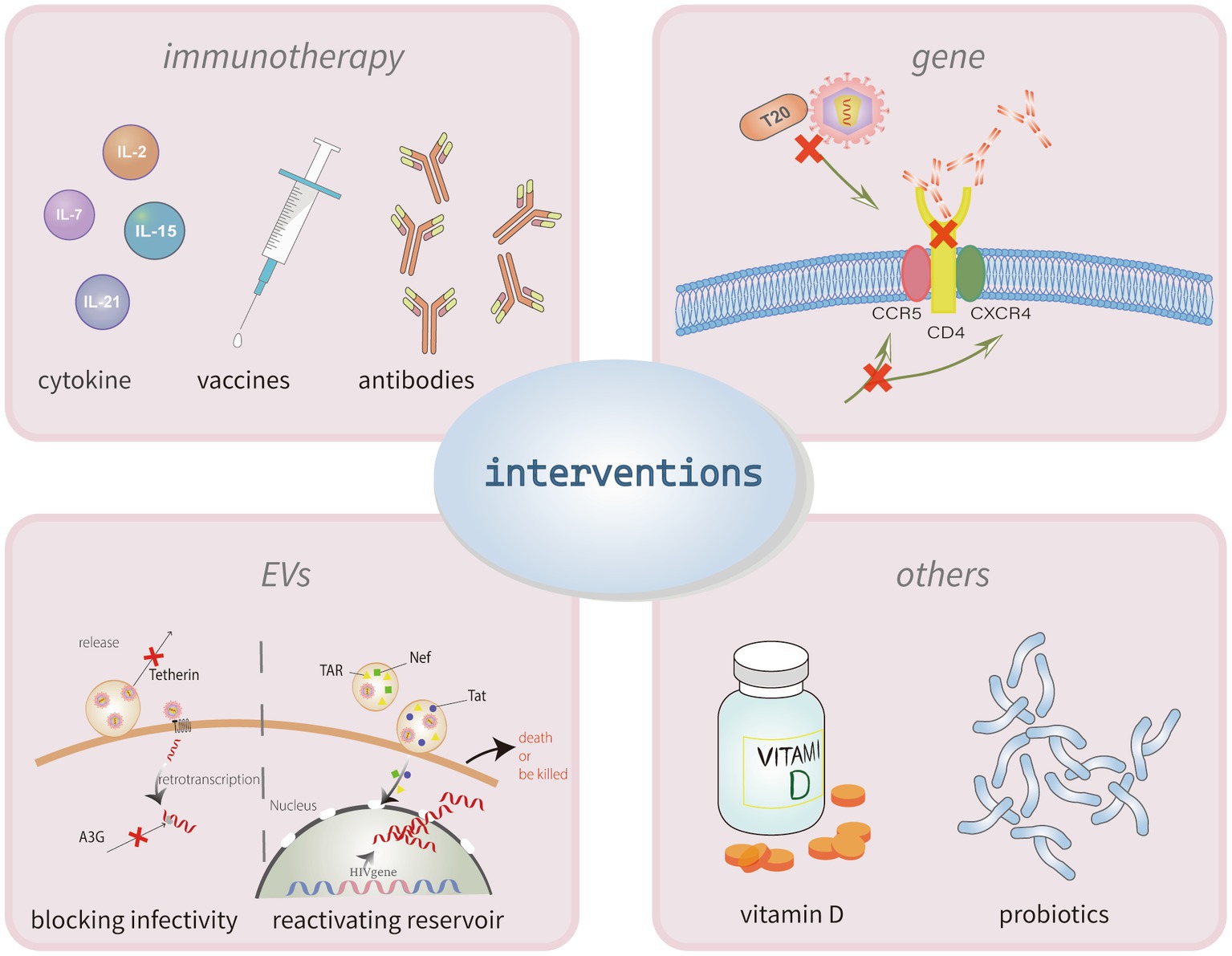
Figure 3. Interventions for poor HIV immune reconstitution. The mechanisms of incomplete immune reconstitution in PLHIV have not yet been clarified. The current intervention strategies for recover the CD4+ T cells mainly through two methods, immunotherapy and gene therapy. Immunotherapy can use the cytokines, vaccines or antibodies to activate latent cells and enhancing immune killing capacity. Similarly, treatments of targeting the anti-HIV-1 gene could make target cells resistant to viral infection. Other treatments to modulate immune also have some effect, like the application of vitamin D and probiotics.
4.1. Immunotherapy
The prime goal of immunotherapy, a novel alternative strategy, is to induce immune recovery, reduce pathogenicity, reduce HIV-1 inflammation and immune activation, promote a direct and effective specific immune response, and normalize the immune system. The main approaches currently available include cytokine therapy, therapeutic vaccines, and targeted antibody therapy.
Some cytokines appear to improve CD4+ T cell count in modulating immunity. Many studies have found that IL-7 does not cause significant changes in plasma viral load while amplifying CD4+ and CD8+ T cells (Vassena et al., 2012; Thiébaut et al., 2016; Steele et al., 2017). Thus, the application of recombinant human interleukin-7 stimulates the proliferation of CD4+ T cells in circulating blood and helps INRs to re-establish immune function (Logerot et al., 2018; Wang et al., 2018). Moreover, Goshu et al. (2020) and Chen P. et al. (2020) found that rhIL-15/PD-L1 antibody combination therapy resulted in a transient increase in Ki67+ NK cells and CD8+ T cells as well as enhanced CD8+ T cell function safely and endurably, with no significant increase in plasma viral load after cART interruption (Okoye et al., 2019). In rhesus monkeys infected with the Simian immunodeficiency virus, Harper et al. (2021) found that combination therapy with IL-21 and IFNα during cART was effective in promoting NK cell terminal differentiation maturation and production of IFN-γ, which contributed to the clearance of virus from tissue cells.
Presently, HIV-1 vaccines can be categorized into therapeutic and preventive vaccines. In 2009, a DNA vaccine, ALVAC-HIV+, was reported to prevent HIV-1 infection by eliciting antibody-dependent cytotoxic effects, and numerous clinical trials have shown efficacy rates of up to 31.1% (Rerks-Ngarm et al., 2009). However, data from a recent phase III clinical replication trial (HVTN702) showed no protective effect in the vaccination group compared with the control group (Gray et al., 2021). Furthermore, the main peptide vaccines currently available are C4-V3 polyvalent peptide vaccine (Vieillard et al., 2016), Vacc-4x (Pollard et al., 2014), and VAC-3S (Vieillard et al., 2019), and there are also several prophylactic or therapeutic dendritic cell vaccines, such as Dec205 (Hossain and Wall, 2019; Matsuo et al., 2021) and Clec9A (Canton et al., 2021), Ags-004 (Gay et al., 2018), and DCV-2 (Espinar-Buitrago and Muñoz-Fernández, 2021).
Tat proteins can influence the activation and latency of the HIV-1 reservoir, thereby determining disease progression. A few studies have reported that anti-Tat immunization during infection or cART may inhibit potential HIV reactivation, thereby reducing persistent low-level viremia and T cell activation and promoting CD4+ T cell recovery, with positive effects on the long-term kinetics of immune reconstitution in individuals on long-term cART therapy (Sgadari et al., 2019; Tripiciano et al., 2021). A study found that HIV-1 gp120 envelope V1V2 region epitope-specific V2p and V2i antibodies failed to neutralize HIV-1 in plasma effectively but could mediate Fc segment-dependent antiviral activity, thereby inducing a more focused functional V2p and V2i-specific antibody response such as antibody-dependent cell-mediated cytotoxicity and antibody-dependent cell-mediated phagocytosis (Weiss et al., 2022). It has been reported frequently that anti-HIV-1 broadly neutralizing antibodies (bNAbs), such as 3BNC117 (Gaebler et al., 2022), 10-1074 (Gaebler et al., 2022), and VRC01 (Bar et al., 2016; Corey et al., 2021), are safe and effective for maintaining viral suppression in a short period and can be used as an adjunctive therapy to cART.
The main immunotherapeutic strategies currently available are targeting the inducible activation of latently infected cells and enhancing their own immune killing capacity. Although numerous researchers have been fruitful in this field over the years, whether the desired effect on HIV clearance mechanisms can be achieved remains unanswered.
4.2. Gene therapy
With two HIV-infected patients successfully achieving a “functional cure” after receiving CCR5Δ32D allele-pure HSC transplants (Hütter et al., 2009; Gupta et al., 2019; Claireaux et al., 2022), scientists believe that HIV-1 target cells can be made resistant to viral infection by targeting the anti-HIV-1 gene. The three main types of gene therapy currently under investigation are blocking co-receptor binding, blocking CD4 binding, and blocking membrane fusion.
Co-receptors play a critical role in the entry of HIV-1 into CD4+ cells. Scientists have conducted numerous trials and found that T cells infused with zinc finger nucleases disrupted the CCR5 gene giving transgenic cells a survival advantage and a good safety profile (Tebas et al., 2014, 2021).
Ibalizumab, a humanized IgG4 monoclonal antibody, blocks HIV-1 binding to chemokine receptors to inhibit viral entry by binding non-competitively to the CD4 receptor and causing deformation of the CD4-gp120 complex (Jacobson et al., 2009; Beccari et al., 2019; Kufel, 2020). Several clinical trials (Gathe et al., 1999; Emu et al., 2018) have shown that significant antiviral activity of Ibalizumab in advanced disease and multidrug-resistant PLHIV with limited treatment options.
Enfuvirtide (T20) is a synthetic peptide antiretroviral drug and the only entry inhibitor approved by the Food and Drug Administration (FDA) for the treatment of HIV-1. As a mimic of the HIV-1 glycoprotein gp41, enfuvirtide binds to the viral envelope and interferes with membrane fusion by interfering with the conformation required for viral invasion into the host cell, blocking viral entry into the host cell (Carr, 2003; Lalezari et al., 2003).
Although the application of gene therapy in HIV treatment still faces some limitations, more strategies need to be developed in order to improve the therapeutic power. Effective gene modification options, smart targeting combined with CAR T-cell therapy, and novel genome editing strategies, are novel future research directions (Sheykhhasan et al., 2021).
4.3. Extracellular vesicles therapy
At present, EVs control HIV-1 infection mainly via two aspects: blocking HIV infection and reactivating the HIV reservoir. Tetherin proteins that mediate MVs transport and contact with receptor membranes can keep microvesicles arrested on the cell surface prevent the release and transmission of HIV-1-carrying extracellular vesicles and, to a certain extent, alleviate the associated inflammatory disease (Weber et al., 2020). A3G is a human cytidine deaminase that can potentially hypermutate viral genomes, causing impairment of reverse transcriptase activity during the retrotranscription process (Kaake et al., 2021; Chesarino and Emerman, 2022). Tetherin and A3G expressions might be increased by EVs at the mRNA level of cellular restriction factors (Navarrete-Muñoz et al., 2021).
Current research has demonstrated that EVs can act as the latency reversal agents (LRAs) delivered to target HIV-1 infection cells. Activation of latent viruses and their elimination by cART therapy is currently a hot topic of research for functional cures. Tang et al. (2018) showed that after exogenous Tat protein targeting, the virus reactivated and produced replication-competent HIV-1 in three of the six subjects. Nef and TAR have also been shown to activate the Akt–mTOR and NF-kβ pathways by triggering immunity leading to persistent inflammation (Arenaccio et al., 2014, 2015; Navarrete-Muñoz et al., 2021; Sviridov et al., 2022).
4.4. Other treatments
Vitamin D can modulate innate and adaptive immune (Al-Tarrah et al., 2018; Eckard et al., 2018) and maintain intestinal flora balance (Dimitrov and White, 2017). Appropriate exogenous Vitamin D supplementation can promote CD4+ T cell proliferation, reduce inflammation and immune activation, prevent PLHIV from developing immune reconstitution inflammatory syndrome, tuberculosis, and mortality, and delay HIV disease progression (Jimenez-Sousa et al., 2018; Currò et al., 2020).
Other studies have demonstrated that probiotics/prebiotics can improve microbial translocation, regulate intestinal microbes, help increase Th17 frequency, and promote CD4 T cell rebuilding (Ortiz et al., 2016; Geng et al., 2020).
5. Summary
In conclusion, the process of incomplete immune reconstitution in AIDS is extremely complex and is the result of a multiplicity of factors which can reinforce each other and continuously advance the disease. The most obvious manifestations include a reduction in the number and function of CD4+ cells and chronic and sustained immune activation. Different INRs and ISRs may behave in the same way but may have different pathogenic mechanisms, and therefore, individualized treatment for each of these mechanisms is necessary to achieve good outcomes and restore immune reconstitution. Although the mechanisms of incomplete immune reconstitution are currently incompletely elucidated and its application in targeted therapy still faces some difficulties, extensive and ongoing research can help conquer many of these challenges. Therefore, in addition to early cART where possible, clarifying the mechanisms of incomplete immune reconstitution and developing targeted individualized treatment regimens is currently a daunting task in the field of AIDS.
Author contributions
LR conceived and designed the subject. WZ wrote the article. All authors contributed to the article and approved the submitted version.
Funding
This work was supported by National Health and Health Commission of Hubei province, China (No. ZY2021M039).
Acknowledgments
The authors acknowledge the guidance of HIV professors of Wuhan Jinyintan Hospital. The Figure was partly generated using Servier Medical Art and Reactome, provided by Servier, licensed under a Creative Commons Attribution 3.0 unported License and reactome.org/icon-lib.
Conflict of interest
The authors declare that the research was conducted in the absence of any commercial or financial relationships that could be construed as a potential conflict of interest.
Publisher’s note
All claims expressed in this article are solely those of the authors and do not necessarily represent those of their affiliated organizations, or those of the publisher, the editors and the reviewers. Any product that may be evaluated in this article, or claim that may be made by its manufacturer, is not guaranteed or endorsed by the publisher.
References
Adolescents., P.O.A.G.F.A.A (2021). Guidelines for the use of antiretroviral agents in adults and adolescents with HIV. Department of Health and Human Services.
Ahn, M. Y., Jiamsakul, A., Khusuwan, S., Khol, V., Pham, T. T., Chaiwarith, R., et al. (2019). The influence of age-associated comorbidities on responses to combination antiretroviral therapy in older people living with HIV. J. Int. AIDS Soc. 22:e25228. doi: 10.1002/jia2.25228
Al-Tarrah, K., Hewison, M., Moiemen, N., and Lord, J. M. (2018). Vitamin D status and its influence on outcomes following major burn injury and critical illness. Burns Trauma 6:11. doi: 10.1186/s41038-018-0113-4
Arenaccio, C., Anticoli, S., Manfredi, F., Chiozzini, C., Olivetta, E., and Federico, M. (2015). Latent HIV-1 is activated by exosomes from cells infected with either replication-competent or defective HIV-1. Retrovirology 12:87. doi: 10.1186/s12977-015-0216-y
Arenaccio, C., Chiozzini, C., Columba-Cabezas, S., Manfredi, F., Affabris, E., Baur, A., et al. (2014). Exosomes from human immunodeficiency virus type 1 (HIV-1)-infected cells license quiescent CD4+ T lymphocytes to replicate HIV-1 through a Nef- and ADAM17-dependent mechanism. J. Virol. 88, 11529–11539. doi: 10.1128/JVI.01712-14
Autran, B., Carcelain, G., Li, T. S., Blanc, C., Mathez, D., Tubiana, R., et al. (1997). Positive effects of combined antiretroviral therapy on CD4+ T cell homeostasis and function in advanced HIV disease. Science 277, 112–116. doi: 10.1126/science.277.5322.112
Bandera, A., Masetti, M., Fabbiani, M., Biasin, M., Muscatello, A., Squillace, N., et al. (2018). The NLRP3 Inflammasome is upregulated in HIV-infected antiretroviral therapy-treated individuals with defective immune recovery. Front. Immunol. 9:214. doi: 10.3389/fimmu.2018.00214
Bar, K. J., Sneller, M. C., Harrison, L. J., Justement, J. S., Overton, E. T., Petrone, M. E., et al. (2016). Effect of HIV antibody VRC01 on viral rebound after treatment interruption. N. Engl. J. Med. 375, 2037–2050. doi: 10.1056/NEJMoa1608243
Barber, D. L., Wherry, E. J., Masopust, D., Zhu, B., Allison, J. P., Sharpe, A. H., et al. (2006). Restoring function in exhausted CD8 T cells during chronic viral infection. Nature 439, 682–687. doi: 10.1038/nature04444
Batra, A., Okur, B., Glauben, R., Erben, U., Ihbe, J., Stroh, T., et al. (2010). Leptin: a critical regulator of CD4+ T-cell polarization in vitro and in vivo. Endocrinology 151, 56–62. doi: 10.1210/en.2009-0565
Beccari, M. V., Mogle, B. T., Sidman, E. F., Mastro, K. A., Asiago-Reddy, E., and Kufel, W. D. (2019). Ibalizumab, a novel monoclonal antibody for the Management of Multidrug-Resistant HIV-1 infection. Antimicrob. Agents Chemother. 63:e00110–19. doi: 10.1128/AAC.00110-19
Boatman, J. A., Baker, J. V., Emery, S., Furrer, H., Mushatt, D. M., Sedláček, D., et al. (1999). Risk factors for low CD4+ count recovery despite viral suppression among participants initiating antiretroviral treatment with CD4+ counts > 500 cells/mm3: findings from the strategic timing of AntiRetroviral therapy (START) trial. J. Acquir. Immune Defic. Syndr. 2019, 10–17. doi: 10.1097/QAI.0000000000001967
Brenchley, J. M., and Douek, D. C. (2008). HIV infection and the gastrointestinal immune system. Mucosal Immunol. 1, 23–30. doi: 10.1038/mi.2007.1
Briceno, O., Chavez-Torres, M., Peralta-Prado, A., Garrido-Rodriguez, D., Romero-Mora, K., Pinto-Cardoso, S., et al. (2020). Associations between recent thymic emigrants and CD4+ T-cell recovery after short-term antiretroviral therapy initiation. AIDS 34, 501–511. doi: 10.1097/QAD.0000000000002458
Cantero-Pérez, J., Grau-Expósito, J., Serra-Peinado, C., Rosero, D. A., Luque-Ballesteros, L., Astorga-Gamaza, A., et al. (2019). Resident memory T cells are a cellular reservoir for HIV in the cervical mucosa. Nat. Commun. 10:4739. doi: 10.1038/s41467-019-12732-2
Canton, J., Blees, H., Henry, C. M., Buck, M. D., Schulz, O., Rogers, N. C., et al. (2021). The receptor DNGR-1 signals for phagosomal rupture to promote cross-presentation of dead-cell-associated antigens. Nat. Immunol. 22, 140–153. doi: 10.1038/s41590-020-00824-x
Carr, A. (2003). Enfuvirtide, an HIV-1 fusion inhibitor. N. Engl. J. Med. 349, 1770–1771; author reply 1770-1771. doi: 10.1056/NEJM200310303491815
Chaillon, A., Gianella, S., Dellicour, S., Rawlings, S. A., Schlub, T. E., De Oliveira, M. F., et al. (2020a). HIV persists throughout deep tissues with repopulation from multiple anatomical sources. J. Clin. Invest. 130, 1699–1712. doi: 10.1172/JCI134815
Chaillon, A., Nakazawa, M., Rawlings, S. A., Curtin, G., Caballero, G., Scott, B., et al. (2020b). Subclinical cytomegalovirus and Epstein-Barr virus shedding is associated with increasing HIV DNA molecular diversity in peripheral blood during suppressive antiretroviral therapy. J. Virol. 94:e00927–20. doi: 10.1128/JVI.00927-20
Chammartin, F., Lodi, S., Logan, R., Ryom, L., Mocroft, A., Kirk, O., et al. (2021). Risk for non-AIDS-defining and AIDS-defining Cancer of early versus delayed initiation of antiretroviral therapy: a multinational prospective cohort study. Ann. Intern. Med. 174, 768–776. doi: 10.7326/M20-5226
Chen, B. (2019). Molecular mechanism of HIV-1 entry. Trends Microbiol. 27, 878–891. doi: 10.1016/j.tim.2019.06.002
Chen, P., Chen, H., Moussa, M., Cheng, J., Li, T., Qin, J., et al. (2020). Recombinant human Interleukin-15 and anti-PD-L1 combination therapy expands a CXCR3+PD1−/low CD8 T-cell subset in simian immunodeficiency virus-infected Rhesus macaques. J. Infect. Dis. 221, 523–533. doi: 10.1093/infdis/jiz485
Chen, J., Titanji, K., Sheth, A. N., Gandhi, R., McMahon, D., Ofotokun, I., et al. (2022a). The effect of age on CD4+ T-cell recovery in HIV-suppressed adult participants: a sub-study from AIDS clinical trial group (ACTG) A5321 and the bone loss and immune reconstitution (BLIR) study. Immun. Ageing 19:4. doi: 10.1186/s12979-021-00260-x
Chen, Y., Xu, Z., Liang, R., Wang, J., Xu, A., Na, N., et al. (2020). CD4CD126 Foxp3 cell population represents a superior subset of regulatory T cells in treating autoimmune diseases. Mol. Therapy 28, 2406–2416. doi: 10.1016/j.ymthe.2020.07.020
Chen, J., Zhou, T., Zhang, Y., Luo, S., Chen, H., Chen, D., et al. (2022b). The reservoir of latent HIV. Front. Cell. Infect. Microbiol. 12:945956. doi: 10.3389/fcimb.2022.945956
Cheruiyot, C., Pataki, Z., Ramratnam, B., and Li, M. (2018). Proteomic analysis of exosomes and its application in HIV-1 infection. Proteomics Clin. Appl. 12:e1700142. doi: 10.1002/prca.201700142
Chesarino, N. M., and Emerman, M. (2022). HIV-1 Vif gained breadth in APOBEC3G specificity after cross-species transmission of its precursors. J. Virol. 96:e0207121. doi: 10.1128/jvi.02071-21
Chevalier, M. F., and Weiss, L. (2013). The split personality of regulatory T cells in HIV infection. Blood 121, 29–37. doi: 10.1182/blood-2012-07-409755
Claireaux, M., Robinot, R., Kervevan, J., Patgaonkar, M., Staropoli, I., Brelot, A., et al. (2022). Low CCR5 expression protects HIV-specific CD4+ T cells of elite controllers from viral entry. Nat. Commun. 13:521. doi: 10.1038/s41467-022-28130-0
Cohn, L. B., Chomont, N., and Deeks, S. G. (2020). The biology of the HIV-1 latent reservoir and implications for cure strategies. Cell Host Microbe 27, 519–530. doi: 10.1016/j.chom.2020.03.014
Collins, D. R., Urbach, J. M., Racenet, Z. J., Arshad, U., Power, K. A., Newman, R. M., et al. (2021). Functional impairment of HIV-specific CD8 T cells precedes aborted spontaneous control of viremia. Immunity 54, 2372–2384.e7. doi: 10.1016/j.immuni.2021.08.007
Corey, L., Gilbert, P. B., Juraska, M., Montefiori, D. C., Morris, L., Karuna, S. T., et al. (2021). Two randomized trials of neutralizing antibodies to prevent HIV-1 acquisition. N. Engl. J. Med. 384, 1003–1014. doi: 10.1056/NEJMoa2031738
Côté, S. C., Burke Schinkel, S. C., Berthoud, T. K., Barros, P. O., Sanchez-Vidales, M., Davidson, A. M., et al. (2020). IL-7 induces sCD127 release and mCD127 downregulation in human CD8 T cells by distinct yet overlapping mechanisms, both of which are impaired in HIV infection. Eur. J. Immunol. 50, 1537–1549. doi: 10.1002/eji.201948453
Currò, M., Visalli, G., Pellicanò, G. F., Ferlazzo, N., Costanzo, M. G., D'Andrea, F., et al. (2020). Vitamin D status modulates inflammatory response in HIV+ subjects: evidence for involvement of autophagy and TG2 expression in PBMC. Int. J. Mol. Sci. 21:7558. doi: 10.3390/ijms21207558
Dai, X.-P., Wu, F.-Y., Cui, C., Liao, X.-J., Jiao, Y.-M., Zhang, C., et al. (2021). Increased platelet-CD4 T cell aggregates are correlated with HIV-1 permissiveness and CD4 T cell loss. Front. Immunol. 12:799124. doi: 10.3389/fimmu.2021.799124
Damouche, A., Lazure, T., Avettand-Fènoël, V., Huot, N., Dejucq-Rainsford, N., Satie, A.-P., et al. (2015). Adipose tissue is a neglected viral reservoir and an inflammatory site during chronic HIV and SIV infection. PLoS Pathog. 11:e1005153. doi: 10.1371/journal.ppat.1005153
Davy-Mendez, T., Napravnik, S., Zakharova, O., Wohl, D. A., Farel, C. E., and Eron, J. J. (2019). Effectiveness of integrase strand transfer inhibitors among treatment-experienced patients in a clinical setting. AIDS (London, England) 33, 1187–1195. doi: 10.1097/QAD.0000000000002194
de Candia, P., Prattichizzo, F., Garavelli, S., Alviggi, C., La Cava, A., and Matarese, G. (2021). The pleiotropic roles of leptin in metabolism, immunity, and cancer. J. Exp. Med. 218:e20191593. doi: 10.1084/jem.20191593
Deeks, S. G., Lewin, S. R., and Havlir, D. V. (2013). The end of AIDS: HIV infection as a chronic disease. Lancet 382, 1525–1533. doi: 10.1016/S0140-6736(13)61809-7
Dillon, S. M., Lee, E. J., Kotter, C. V., Austin, G. L., Dong, Z., Hecht, D. K., et al. (2014). An altered intestinal mucosal microbiome in HIV-1 infection is associated with mucosal and systemic immune activation and endotoxemia. Mucosal Immunol. 7, 983–994. doi: 10.1038/mi.2013.116
Dimitrov, V., and White, J. H. (2017). Vitamin D signaling in intestinal innate immunity and homeostasis. Mol. Cell. Endocrinol. 453, 68–78. doi: 10.1016/j.mce.2017.04.010
Dixon, K. O., Tabaka, M., Schramm, M. A., Xiao, S., Tang, R., Dionne, D., et al. (2021). TIM-3 restrains anti-tumour immunity by regulating inflammasome activation. Nature 595, 101–106. doi: 10.1038/s41586-021-03626-9
Dragović, G., Andjić, M., Toljić, B., Jevtović, D., Lukić, R., de Luka, S., et al. (2021). Correlation between metabolic syndrome and relative telomere length shortening in HIV/AIDS patients on combined antiretroviral therapy. Exp. Gerontol. 147:111269. doi: 10.1016/j.exger.2021.111269
Duette, G., Pereyra Gerber, P., Rubione, J., Perez, P. S., Landay, A. L., Crowe, S. M., et al. (2018). Induction of HIF-1α by HIV-1 infection in CD4 T cells promotes viral replication and drives extracellular vesicle-mediated inflammation. MBio 9:e00757–18. doi: 10.1128/mBio.00757-18
Eckard, A. R., O'Riordan, M. A., Rosebush, J. C., Lee, S. T., Habib, J. G., Ruff, J. H., et al. (2018). Vitamin D supplementation decreases immune activation and exhaustion in HIV-1-infected youth. Antivir. Ther. 23, 315–324. doi: 10.3851/IMP3199
Elvstam, O., Marrone, G., Medstrand, P., Treutiger, C. J., Sönnerborg, A., Gisslén, M., et al. (2021). All-cause mortality and serious non-AIDS events in adults with low-level human immunodeficiency virus viremia during combination antiretroviral therapy: results from a Swedish Nationwide observational study. Clin. Infect. Dis. 72, 2079–2086. doi: 10.1093/cid/ciaa413
Emu, B., Fessel, J., Schrader, S., Kumar, P., Richmond, G., Win, S., et al. (2018). Phase 3 study of Ibalizumab for multidrug-resistant HIV-1. N. Engl. J. Med. 379, 645–654. doi: 10.1056/NEJMoa1711460
Espinar-Buitrago, M., and Muñoz-Fernández, M. A. (2021). New approaches to dendritic cell-based therapeutic vaccines against HIV-1 infection. Front. Immunol. 12:719664. doi: 10.3389/fimmu.2021.719664
Favre, D., Mold, J., Hunt, P. W., Kanwar, B., Loke, P. N., Seu, L., et al. (2010). Tryptophan catabolism by indoleamine 2,3-dioxygenase 1 alters the balance of TH17 to regulatory T cells in HIV disease. Sci. Transl. Med. 2:32ra36. doi: 10.1126/scitranslmed.3000632
Fenwick, C., Joo, V., Jacquier, P., Noto, A., Banga, R., Perreau, M., et al. (2019). T-cell exhaustion in HIV infection. Immunol. Rev. 292, 149–163. doi: 10.1111/imr.12823
Fernandes, J. R., Berthoud, T. K., Kumar, A., and Angel, J. B. (2017). IL-23 signaling in Th17 cells is inhibited by HIV infection and is not restored by HAART: implications for persistent immune activation. PLoS One 12:e0186823. doi: 10.1371/journal.pone.0186823
Ferrando-Martinez, S., De Pablo-Bernal, R. S., De Luna-Romero, M., De Ory, S. J., Genebat, M., Pacheco, Y. M., et al. (2017). Thymic function failure is associated with human immunodeficiency virus disease progression. Clin. Infect. Dis. 64, 1191–1197. doi: 10.1093/cid/cix095
Fiseha, T., Ebrahim, H., Ebrahim, E., and Gebreweld, A. (2022). CD4+ cell count recovery after initiation of antiretroviral therapy in HIV-infected Ethiopian adults. PLoS One 17:e0265740. doi: 10.1371/journal.pone.0265740
French, A. L., Grennan, D., Daubert, E., Seaberg, E. C., Peters, M., Augenbraun, M., et al. (2021). Decreases in markers of monocyte/macrophage activation after hepatitis C eradication in HIV/hepatitis C virus coinfected women. AIDS 35, 1433–1438. doi: 10.1097/QAD.0000000000002869
Gaebler, C., Nogueira, L., Stoffel, E., Oliveira, T. Y., Breton, G., Millard, K. G., et al. (2022). Prolonged viral suppression with anti-HIV-1 antibody therapy. Nature 606, 368–374. doi: 10.1038/s41586-022-04597-1
Gandhi, R. T., McMahon, D. K., Bosch, R. J., Lalama, C. M., Cyktor, J. C., Macatangay, B. J., et al. (2017). Levels of HIV-1 persistence on antiretroviral therapy are not associated with markers of inflammation or activation. PLoS Pathog. 13:e1006285. doi: 10.1371/journal.ppat.1006285
Ganor, Y., Real, F., Sennepin, A., Dutertre, C.-A., Prevedel, L., Xu, L., et al. (2019). HIV-1 reservoirs in urethral macrophages of patients under suppressive antiretroviral therapy. Nat. Microbiol. 4, 633–644. doi: 10.1038/s41564-018-0335-z
García, M., Jiménez-Sousa, M. A., Blanco, J., Restrepo, C., Pacheco, Y. M., Brochado-Kith, Ó., et al. (2019). CD4 recovery is associated with genetic variation in IFNγ and IL19 genes. Antivir. Res. 170:104577. doi: 10.1016/j.antiviral.2019.104577
Garcia-Broncano, P., Medrano, L. M., Berenguer, J., González-García, J., Jiménez-Sousa, M. Á., Carrero, A., et al. (2018). Dysregulation of the immune system in HIV/HCV-Coinfected patients according to liver stiffness status. Cells 7:196. doi: 10.3390/cells7110196
Gathe, J. C., Hardwicke, R. L., Garcia, F., Weinheimer, S., Lewis, S. T., and Cash, R. B. (1999). Efficacy, pharmacokinetics, and safety over 48 weeks with Ibalizumab-based therapy in treatment-experienced adults infected with HIV-1: a phase 2a study. J. Acquir. Immune Defic. Syndr. 2021, 482–489. doi: 10.1097/QAI.0000000000002591
Gay, C. L., DeBenedette, M. A., Tcherepanova, I. Y., Gamble, A., Lewis, W. E., Cope, A. B., et al. (2018). Immunogenicity of AGS-004 dendritic cell therapy in patients treated during acute HIV infection. AIDS Res. Hum. Retrovir. 34, 111–122. doi: 10.1089/aid.2017.0071
Geng, S. T., Zhang, Z. Y., Wang, Y. X., Lu, D., Yu, J., Zhang, J. B., et al. (2020). Regulation of gut microbiota on immune reconstitution in patients with acquired immunodeficiency syndrome. Front. Microbiol. 11:594820. doi: 10.3389/fmicb.2020.594820
Geng, S.-T., Zhang, J.-B., Wang, Y.-X., Xu, Y., Lu, D., Zhang, Z., et al. (2021). Pre-digested protein enteral nutritional supplementation enhances recovery of CD4 T cells and repair of intestinal barrier in HIV-infected immunological non-responders. Front. Immunol. 12:757935. doi: 10.3389/fimmu.2021.757935
Gibellini, L., Pecorini, S., De Biasi, S., Bianchini, E., Digaetano, M., Pinti, M., et al. (2017). HIV-DNA content in different CD4+ T-cell subsets correlates with CD4+ cell: CD8+ cell ratio or length of efficient treatment. AIDS (London, England) 31, 1387–1392. doi: 10.1097/QAD.0000000000001510
Gobran, S. T., Ancuta, P., and Shoukry, N. H. (2021). A tale of two viruses: immunological insights into HCV/HIV coinfection. Front. Immunol. 12:726419. doi: 10.3389/fimmu.2021.726419
Goshu, B. A., Chen, H., Moussa, M., Cheng, J., and Catalfamo, M. (2020). Combination rhIL-15 and anti-PD-L1 (Avelumab) enhances HIVGag-specific CD8 T-cell function. J. Infect. Dis. 222, 1540–1549. doi: 10.1093/infdis/jiaa269
Gray, G. E., Bekker, L.-G., Laher, F., Malahleha, M., Allen, M., Moodie, Z., et al. (2021). Vaccine efficacy of ALVAC-HIV and bivalent subtype C gp120-MF59 in adults. N. Engl. J. Med. 384, 1089–1100. doi: 10.1056/NEJMoa2031499
Gudbergsson, J. M., Jønsson, K., Simonsen, J. B., and Johnsen, K. B. (2019). Systematic review of targeted extracellular vesicles for drug delivery - considerations on methodological and biological heterogeneity. J. Control. Release 306, 108–120. doi: 10.1016/j.jconrel.2019.06.006
Gupta, R. K., Abdul-Jawad, S., McCoy, L. E., Mok, H. P., Peppa, D., Salgado, M., et al. (2019). HIV-1 remission following CCR5Δ32/Δ32 haematopoietic stem-cell transplantation. Nature 568, 244–248. doi: 10.1038/s41586-019-1027-4
Handoko, R., Colby, D. J., Kroon, E., Sacdalan, C., de Souza, M., Pinyakorn, S., et al. (2020). Determinants of suboptimal CD4 T cell recovery after antiretroviral therapy initiation in a prospective cohort of acute HIV-1 infection. J. Int. AIDS Soc. 23:e25585. doi: 10.1002/jia2.25585
Harper, J., Huot, N., Micci, L., Tharp, G., King, C., Rascle, P., et al. (2021). IL-21 and IFNα therapy rescues terminally differentiated NK cells and limits SIV reservoir in ART-treated macaques. Nat. Commun. 12:2866. doi: 10.1038/s41467-021-23189-7
Harwood, O., and O'Connor, S. (2021). Therapeutic potential of IL-15 and N-803 in HIV/SIV infection. Viruses 13:1750. doi: 10.3390/v13091750
He, Y., Cai, W., Chen, J., Hu, F., Li, F., Lin, W., et al. (2021). Persistent chronic immune activation in HIV/HBV-coinfected patients after antiretroviral therapy. J. Viral Hepat. 28, 1355–1361. doi: 10.1111/jvh.13559
Hernandez, D. M., Valderrama, S., Gualtero, S., Hernandez, C., Lopez, M., Herrera, M. V., et al. (2018). Loss of T-cell multifunctionality and TCR-Vbeta repertoire against Epstein-Barr virus is associated with worse prognosis and clinical parameters in HIV(+) patients. Front. Immunol. 9:2291. doi: 10.3389/fimmu.2018.02291
Hossain, M. K., and Wall, K. A. (2019). Use of dendritic cell receptors as targets for enhancing anti-Cancer immune responses. Cancers (Basel) 11:418. doi: 10.3390/cancers11030418
Hütter, G., Nowak, D., Mossner, M., Ganepola, S., Müssig, A., Allers, K., et al. (2009). Long-term control of HIV by CCR5 Delta32/Delta32 stem-cell transplantation. N. Engl. J. Med. 360, 692–698. doi: 10.1056/NEJMoa0802905
Iljazovic, A., Roy, U., Gálvez, E. J. C., Lesker, T. R., Zhao, B., Gronow, A., et al. (2021). Perturbation of the gut microbiome by Prevotella spp. enhances host susceptibility to mucosal inflammation. Mucosal Immunol. 14, 113–124. doi: 10.1038/s41385-020-0296-4
Ipp, H., Zemlin, A. E., Erasmus, R. T., and Glashoff, R. H. (2014). Role of inflammation in HIV-1 disease progression and prognosis. Crit. Rev. Clin. Lab. Sci. 51, 98–111. doi: 10.3109/10408363.2013.865702
Ishizaka, A., Koga, M., Mizutani, T., Parbie, P. K., Prawisuda, D., Yusa, N., et al. (2021). Unique gut microbiome in HIV patients on antiretroviral therapy (ART) suggests association with chronic inflammation. Microbiol. Spectr. 9:e0070821. doi: 10.1128/Spectrum.00708-21
Jacobson, J. M., Kuritzkes, D. R., Godofsky, E., DeJesus, E., Larson, J. A., Weinheimer, S. P., et al. (2009). Safety, pharmacokinetics, and antiretroviral activity of multiple doses of ibalizumab (formerly TNX-355), an anti-CD4 monoclonal antibody, in human immunodeficiency virus type 1-infected adults. Antimicrob. Agents Chemother. 53, 450–457. doi: 10.1128/AAC.00942-08
Jesson, J., Crichton, S., Quartagno, M., Yotebieng, M., Abrams, E. J., Chokephaibulkit, K., et al. (2022). Growth and CD4 patterns of adolescents living with perinatally acquired HIV worldwide, a CIPHER cohort collaboration analysis. J. Int. AIDS Soc. 25:e25871. doi: 10.1002/jia2.25871
Jimenez-Sousa, M. A., Martinez, I., Medrano, L. M., Fernandez-Rodriguez, A., and Resino, S. (2018). Vitamin D in human immunodeficiency virus infection: influence on immunity and disease. Front. Immunol. 9:458. doi: 10.3389/fimmu.2018.00458
Kaake, R. M., Echeverria, I., Kim, S. J., Von Dollen, J., Chesarino, N. M., Feng, Y., et al. (2021). Characterization of an A3G-VifHIV-1-CRL5-CBFβ structure using a cross-linking mass spectrometry pipeline for integrative modeling of host-pathogen complexes. Mol. Cell. Proteomics 20:100132. doi: 10.1016/j.mcpro.2021.100132
Karuppusamy, K. V., Demosthenes, J. P., Venkatesan, V., Christopher, A. C., Babu, P., Azhagiri, M. K., et al. (2022). The CCR5 gene edited CD34CD90 hematopoietic stem cell population serves as an optimal graft source for HIV gene therapy. Front. Immunol. 13:792684. doi: 10.3389/fimmu.2022.792684
Khosravi, A., Yáñez, A., Price, J. G., Chow, A., Merad, M., Goodridge, H. S., et al. (2014). Gut microbiota promote hematopoiesis to control bacterial infection. Cell Host Microbe 15, 374–381. doi: 10.1016/j.chom.2014.02.006
Kimmig, S., Przybylski, G. K., Schmidt, C. A., Laurisch, K., Möwes, B., Radbruch, A., et al. (2002). Two subsets of naive T helper cells with distinct T cell receptor excision circle content in human adult peripheral blood. J. Exp. Med. 195, 789–794. doi: 10.1084/jem.20011756
Kroeze, S., Ondoa, P., Kityo, C. M., Siwale, M., Akanmu, S., Wellington, M., et al. (2018). Suboptimal immune recovery during antiretroviral therapy with sustained HIV suppression in sub-Saharan Africa. AIDS (London, England) 32, 1043–1051. doi: 10.1097/QAD.0000000000001801
Kruize, Z., and Kootstra, N. A. (2019). The role of macrophages in HIV-1 persistence and pathogenesis. Front. Microbiol. 10:2828. doi: 10.3389/fmicb.2019.02828
Kubli, S. P., Berger, T., Araujo, D. V., Siu, L. L., and Mak, T. W. (2021). Beyond immune checkpoint blockade: emerging immunological strategies. Nat. Rev. Drug Discov. 20, 899–919. doi: 10.1038/s41573-021-00155-y
Kufel, W. D. (2020). Antibody-based strategies in HIV therapy. Int. J. Antimicrob. Agents 56:106186. doi: 10.1016/j.ijantimicag.2020.106186
Lalezari, J. P., Henry, K., O'Hearn, M., Montaner, J. S. G., Piliero, P. J., Trottier, B., et al. (2003). Enfuvirtide, an HIV-1 fusion inhibitor, for drug-resistant HIV infection in north and South America. N. Engl. J. Med. 348, 2175–2185. doi: 10.1056/NEJMoa035026
Le Hingrat, Q., Sereti, I., Landay, A. L., Pandrea, I., and Apetrei, C. (2021). The hitchhiker guide to CD4 T-cell depletion in lentiviral infection. A critical review of the dynamics of the CD4 T cells in SIV and HIV infection. Front. Immunol. 12:695674. doi: 10.3389/fimmu.2021.695674
Lederman, M. M., Calabrese, L., Funderburg, N. T., Clagett, B., Medvik, K., Bonilla, H., et al. (2011). Immunologic failure despite suppressive antiretroviral therapy is related to activation and turnover of memory CD4 cells. J. Infect. Dis. 204, 1217–1226. doi: 10.1093/infdis/jir507
Lee, S. C., Chua, L. L., Yap, S. H., Khang, T. F., Leng, C. Y., Raja Azwa, R. I., et al. (2018). Enrichment of gut-derived Fusobacterium is associated with suboptimal immune recovery in HIV-infected individuals. Sci. Rep. 8:14277. doi: 10.1038/s41598-018-32585-x
Lee, J.-H., Schierer, S., Blume, K., Dindorf, J., Wittki, S., Xiang, W., et al. (2016). HIV-Nef and ADAM17-containing plasma extracellular vesicles induce and correlate with immune pathogenesis in chronic HIV infection. EBioMedicine 6, 103–113. doi: 10.1016/j.ebiom.2016.03.004
Lee, J. S., Tato, C. M., Joyce-Shaikh, B., Gulen, M. F., Gulan, F., Cayatte, C., et al. (2015). Interleukin-23-independent IL-17 production regulates intestinal epithelial permeability. Immunity 43, 727–738. doi: 10.1016/j.immuni.2015.09.003
Li, T. S., Tubiana, R., Katlama, C., Calvez, V., Mohand, H. A., and Autran, B. (1998). Long-lasting recovery in CD4 T-cell function and viral-load reduction after highly active antiretroviral therapy in advanced HIV-1 disease. Lancet 351, 1682–1686. doi: 10.1016/s0140-6736(97)10291-4
Li, T., Wu, N., Dai, Y., Qiu, Z., Han, Y., Xie, J., et al. (2011). Reduced thymic output is a major mechanism of immune reconstitution failure in HIV-infected patients after long-term antiretroviral therapy. Clin. Infect. Dis. 53, 944–951. doi: 10.1093/cid/cir552
Liang, S. C., Tan, X.-Y., Luxenberg, D. P., Karim, R., Dunussi-Joannopoulos, K., Collins, M., et al. (2006). Interleukin (IL)-22 and IL-17 are coexpressed by Th17 cells and cooperatively enhance expression of antimicrobial peptides. J. Exp. Med. 203, 2271–2279. doi: 10.1084/jem.20061308
Logerot, S., Rancez, M., Charmeteau-de Muylder, B., Figueiredo-Morgado, S., Rozlan, S., Tambussi, G., et al. (2018). HIV reservoir dynamics in HAART-treated poor immunological responder patients under IL-7 therapy. AIDS 32, 715–720. doi: 10.1097/QAD.0000000000001752
López-Cortés, L. F., Trujillo-Rodríguez, M., Báez-Palomo, A., Benmarzouk-Hidalgo, O. J., Dominguez-Molina, B., Milanés-Guisado, Y., et al. (2018). Eradication of hepatitis C virus (HCV) reduces immune activation, microbial translocation, and the HIV DNA level in HIV/HCV-Coinfected patients. J. Infect. Dis. 218, 624–632. doi: 10.1093/infdis/jiy136
Lu, H., Cole, S. R., Westreich, D., Hudgens, M. G., Adimora, A. A., Althoff, K. N., et al. (2022). Virologic outcomes among adults with HIV using integrase inhibitor-based antiretroviral therapy. AIDS (London, England) 36, 277–286. doi: 10.1097/QAD.0000000000003069
Luo, Z., Health, S. L., Li, M., Yang, H., Wu, Y., Collins, M., et al. (2022). Variation in blood microbial lipopolysaccharide (LPS) contributes to immune reconstitution in response to suppressive antiretroviral therapy in HIV. EBioMedicine 80:104037. doi: 10.1016/j.ebiom.2022.104037
Lutgen, V., Narasipura, S. D., Barbian, H. J., Richards, M., Wallace, J., Razmpour, R., et al. (2020). HIV infects astrocytes in vivo and egresses from the brain to the periphery. PLoS Pathog. 16:e1008381. doi: 10.1371/journal.ppat.1008381
Lv, J.-N., Li, J.-Q., Cui, Y.-B., Ren, Y.-Y., Fu, Y.-J., Jiang, Y.-J., et al. (2021). Plasma MicroRNA signature panel predicts the immune response after antiretroviral therapy in HIV-infected patients. Front. Immunol. 12:753044. doi: 10.3389/fimmu.2021.753044
Maartens, G., Celum, C., and Lewin, S. R. (2014). HIV infection: epidemiology, pathogenesis, treatment, and prevention. Lancet 384, 258–271. doi: 10.1016/s0140-6736(14)60164-1
Macdonald, J. C., Torriani, F. J., Morse, L. S., Karavellas, M. P., Reed, J. B., and Freeman, W. R. (1998). Lack of reactivation of cytomegalovirus (CMV) retinitis after stopping CMV maintenance therapy in AIDS patients with sustained elevations in CD4 T cells in response to highly active antiretroviral therapy. J. Infect. Dis. 177, 1182–1187. doi: 10.1086/515281
Mak, G., Zaunders, J. J., Bailey, M., Seddiki, N., Rogers, G., Leong, L., et al. (2021). Preservation of gastrointestinal mucosal barrier function and microbiome in patients with controlled HIV infection. Front. Immunol. 12:688886. doi: 10.3389/fimmu.2021.688886
Malazogu, F., Rousseau, R. K., Shivappa, N., Huibner, S., Walmsley, S. L., Kovacs, C. M., et al. (2021). The dietary inflammatory index is not associated with gut permeability or biomarkers of systemic inflammation in HIV immunologic non-responders. Front. Nutr. 8:736816. doi: 10.3389/fnut.2021.736816
Matsuo, K., Yoshie, O., Kitahata, K., Kamei, M., Hara, Y., and Nakayama, T. (2021). Recent Progress in dendritic cell-based Cancer immunotherapy. Cancers (Basel) 13:2495. doi: 10.3390/cancers13102495
Mavigner, M., Delobel, P., Cazabat, M., Dubois, M., L'Faqihi-Olive, F.-E., Raymond, S., et al. (2009). HIV-1 residual viremia correlates with persistent T-cell activation in poor immunological responders to combination antiretroviral therapy. PLoS One 4:e7658. doi: 10.1371/journal.pone.0007658
Mehraj, V., Ramendra, R., Isnard, S., Dupuy, F. P., Lebouché, B., Costiniuk, C., et al. (2019). CXCL13 as a biomarker of immune activation during early and chronic HIV infection. Front. Immunol. 10:289. doi: 10.3389/fimmu.2019.00289
Minnie, S. A., Kuns, R. D., Gartlan, K. H., Zhang, P., Wilkinson, A. N., Samson, L., et al. (2018). Myeloma escape after stem cell transplantation is a consequence of T-cell exhaustion and is prevented by TIGIT blockade. Blood 132, 1675–1688. doi: 10.1182/blood-2018-01-825240
Moreira Gabriel, E., Wiche Salinas, T. R., Gosselin, A., Larouche-Anctil, E., Durand, M., Landay, A. L., et al. (2021). Overt IL-32 isoform expression at intestinal level during HIV-1 infection is negatively regulated by IL-17A. AIDS 35, 1881–1894. doi: 10.1097/QAD.0000000000002972
Nasi, A., and Chiodi, F. (2018). Mechanisms regulating expansion of CD8+ T cells during HIV-1 infection. J. Intern. Med. 283, 257–267. doi: 10.1111/joim.12722
Navarrete-Muñoz, M. A., Llorens, C., Benito, J. M., and Rallón, N. (2021). Extracellular vesicles as a new promising therapy in HIV infection. Front. Immunol. 12:811471. doi: 10.3389/fimmu.2021.811471
Nganou-Makamdop, K., Talla, A., Sharma, A. A., Darko, S., Ransier, A., Laboune, F., et al. (2021). Translocated microbiome composition determines immunological outcome in treated HIV infection. Cells 184, 3899–3914 e3816. doi: 10.1016/j.cell.2021.05.023
Nilsson, J., Boasso, A., Velilla, P. A., Zhang, R., Vaccari, M., Franchini, G., et al. (2006). HIV-1-driven regulatory T-cell accumulation in lymphoid tissues is associated with disease progression in HIV/AIDS. Blood 108, 3808–3817. doi: 10.1182/blood-2006-05-021576
Noiman, A., Esber, A., Wang, X., Bahemana, E., Adamu, Y., Iroezindu, M., et al. (2022). Clinical factors and outcomes associated with immune non-response among virally suppressed adults with HIV from Africa and the United States. Sci. Rep. 12:1196. doi: 10.1038/s41598-022-04866-z
Okoye, A. A., DeGottardi, M. Q., Fukazawa, Y., Vaidya, M., Abana, C. O., Konfe, A. L., et al. (2019). Role of IL-15 signaling in the pathogenesis of simian immunodeficiency virus infection in Rhesus macaques. J. Immunol. 203, 2928–2943. doi: 10.4049/jimmunol.1900792
Okoye, A. A., and Picker, L. J. (2013). CD4(+) T-cell depletion in HIV infection: mechanisms of immunological failure. Immunol. Rev. 254, 54–64. doi: 10.1111/imr.12066
Okoye, I., Xu, L., Oyegbami, O., Shahbaz, S., Pink, D., Gao, P., et al. (2021). Plasma extracellular vesicles enhance HIV-1 infection of activated CD4(+) T cells and promote the activation of latently infected J-Lat10.6 cells via miR-139-5p transfer. Front. Immunol. 12:697604. doi: 10.3389/fimmu.2021.697604
Ortiz, A. M., Klase, Z. A., DiNapoli, S. R., Vujkovic-Cvijin, I., Carmack, K., Perkins, M. R., et al. (2016). IL-21 and probiotic therapy improve Th17 frequencies, microbial translocation, and microbiome in ARV-treated, SIV-infected macaques. Mucosal Immunol. 9, 458–467. doi: 10.1038/mi.2015.75
Patters, B. J., and Kumar, S. (2018). The role of exosomal transport of viral agents in persistent HIV pathogenesis. Retrovirology 15:79. doi: 10.1186/s12977-018-0462-x
Perdomo-Celis, F., Feria, M. G., Taborda, N. A., and Rugeles, M. T. (2018). A low frequency of IL-17-producing CD8(+) T-cells is associated with persistent immune activation in people living with HIV despite HAART-induced viral suppression. Front. Immunol. 9:2502. doi: 10.3389/fimmu.2018.02502
Pereira, L. M. S., França, E. D. S., Costa, I. B., Jorge, E. V. O., Mattos, P. J. D. S. M., Freire, A. B. C., et al. (2022). HLA-B*13, B*35 and B*39 alleles are closely associated with the lack of response to ART in HIV infection: a cohort study in a population of northern Brazil. Front. Immunol. 13:829126. doi: 10.3389/fimmu.2022.829126
Perez, P. S., Romaniuk, M. A., Duette, G. A., Zhao, Z., Huang, Y., Martin-Jaular, L., et al. (2019). Extracellular vesicles and chronic inflammation during HIV infection. J. Extracell. Vesicl. 8:1687275. doi: 10.1080/20013078.2019.1687275
Pickard, J. M., Zeng, M. Y., Caruso, R., and Núñez, G. (2017). Gut microbiota: role in pathogen colonization, immune responses, and inflammatory disease. Immunol. Rev. 279, 70–89. doi: 10.1111/imr.12567
Piconi, S., Trabattoni, D., Gori, A., Parisotto, S., Magni, C., Meraviglia, P., et al. (2010). Immune activation, apoptosis, and Treg activity are associated with persistently reduced CD4+ T-cell counts during antiretroviral therapy. AIDS (London, England) 24, 1991–2000. doi: 10.1097/QAD.0b013e32833c93ce
Pinacchio, C., Scagnolari, C., Iebba, V., Santinelli, L., Innocenti, G. P., Frasca, F., et al. (2020). High abundance of genus Prevotella is associated with dysregulation of IFN-I and T cell response in HIV-1-infected patients. AIDS (London, England) 34, 1467–1473. doi: 10.1097/QAD.0000000000002574
Pino, M., Pereira Ribeiro, S., Pagliuzza, A., Ghneim, K., Khan, A., Ryan, E., et al. (2021). Increased homeostatic cytokines and stability of HIV-infected memory CD4 T-cells identify individuals with suboptimal CD4 T-cell recovery on-ART. PLoS Pathog. 17:e1009825. doi: 10.1371/journal.ppat.1009825
Planas, D., Routy, J.-P., and Ancuta, P. (2019). New Th17-specific therapeutic strategies for HIV remission. Curr. Opin. HIV AIDS 14, 85–92. doi: 10.1097/COH.0000000000000522
Pollard, R. B., Rockstroh, J. K., Pantaleo, G., Asmuth, D. M., Peters, B., Lazzarin, A., et al. (2014). Safety and efficacy of the peptide-based therapeutic vaccine for HIV-1, Vacc-4x: a phase 2 randomised, double-blind, placebo-controlled trial. Lancet Infect. Dis. 14, 291–300. doi: 10.1016/S1473-3099(13)70343-8
Prabhu, V. M., Singh, A. K., Padwal, V., Nagar, V., Patil, P., and Patel, V. (2019). Monocyte based correlates of immune activation and viremia in HIV-infected long-term non-Progressors. Front. Immunol. 10:2849. doi: 10.3389/fimmu.2019.02849
Rb-Silva, R., Nobrega, C., Azevedo, C., Athayde, E., Canto-Gomes, J., Ferreira, I., et al. (2019). Thymic function as a predictor of immune recovery in chronically HIV-infected patients initiating antiretroviral therapy. Front. Immunol. 10:25. doi: 10.3389/fimmu.2019.00025
Rerks-Ngarm, S., Pitisuttithum, P., Nitayaphan, S., Kaewkungwal, J., Chiu, J., Paris, R., et al. (2009). Vaccination with ALVAC and AIDSVAX to prevent HIV-1 infection in Thailand. N. Engl. J. Med. 361, 2209–2220. doi: 10.1056/NEJMoa0908492
Ron, R., Moreno, E., Martínez-Sanz, J., Brañas, F., Sainz, T., Moreno, S., et al. (2023). CD4/CD8 ratio during human immunodeficiency virus treatment: time for routine monitoring? Clin. Infect. Dis. 76, 1688–1696. doi: 10.1093/cid/ciad136
Rousseau, R. K., Szadkowski, L., Kovacs, C. M., Saikali, M. F., Nadeem, R., Malazogu, F., et al. (2021). Activation and gut-homing of peripheral T cells in HIV immunologic non-responders despite long term viral suppression. PLoS One 16:e0254149. doi: 10.1371/journal.pone.0254149
Russo, E., Nannini, G., Sterrantino, G., Kiros, S. T., Pilato, V. D., Coppi, M., et al. (2022). Effects of viremia and CD4 recovery on gut "microbiome-immunity" axis in treatment-naïve HIV-1-infected patients undergoing antiretroviral therapy. World J. Gastroenterol. 28, 635–652. doi: 10.3748/wjg.v28.i6.635
Saag, M. S., Gandhi, R. T., Hoy, J. F., Landovitz, R. J., Thompson, M. A., Sax, P. E., et al. (2020). Antiretroviral drugs for treatment and prevention of HIV infection in adults: 2020 recommendations of the international antiviral society-USA panel. JAMA 324, 1651–1669. doi: 10.1001/jama.2020.17025
Saluzzo, S., Pandey, R. V., Gail, L. M., Dingelmaier-Hovorka, R., Kleissl, L., Shaw, L., et al. (2021). Delayed antiretroviral therapy in HIV-infected individuals leads to irreversible depletion of skin- and mucosa-resident memory T cells. Immunity 54, 2842–2858 e2845. doi: 10.1016/j.immuni.2021.10.021
Scherpenisse, M., Kootstra, N. A., Bakker, M., Berkhout, B., and Pasternak, A. O. (2021). Cell-associated HIV-1 Unspliced-to-multiply-spliced RNA ratio at 12 weeks of ART predicts immune reconstitution on therapy. MBio 12:e00099–21. doi: 10.1128/mBio.00099-21
Sebastian, N. T., Zaikos, T. D., Terry, V., Taschuk, F., McNamara, L. A., Onafuwa-Nuga, A., et al. (2017). CD4 is expressed on a heterogeneous subset of hematopoietic progenitors, which persistently harbor CXCR4 and CCR5-tropic HIV proviral genomes in vivo. PLoS Pathog. 13:e1006509. doi: 10.1371/journal.ppat.1006509
Serrano-Villar, S., Rojo, D., Martínez-Martínez, M., Deusch, S., Vázquez-Castellanos, J. F., Bargiela, R., et al. (2016). Gut Bacteria metabolism impacts immune recovery in HIV-infected individuals. EBioMedicine 8, 203–216. doi: 10.1016/j.ebiom.2016.04.033
Serrano-Villar, S., Talavera-Rodríguez, A., Gosalbes, M. J., Madrid, N., Pérez-Molina, J. A., Elliott, R. J., et al. (2021). Fecal microbiota transplantation in HIV: a pilot placebo-controlled study. Nat. Commun. 12:1139. doi: 10.1038/s41467-021-21472-1
Serrano-Villar, S., Wu, K., Hunt, P. W., Lok, J. J., Ron, R., Sainz, T., et al. (2022). Predictive value of CD8+ T cell and CD4/CD8 ratio at two years of successful ART in the risk of AIDS and non-AIDS events. EBioMedicine 80:104072. doi: 10.1016/j.ebiom.2022.104072
Sgadari, C., Monini, P., Tripiciano, A., Picconi, O., Casabianca, A., Orlandi, C., et al. (2019). Continued decay of HIV Proviral DNA upon vaccination with HIV-1 tat of subjects on long-term ART: an 8-year follow-up study. Front. Immunol. 10:233. doi: 10.3389/fimmu.2019.00233
Shah, A. S. V., Stelzle, D., Lee, K. K., Beck, E. J., Alam, S., Clifford, S., et al. (2018). Global burden of atherosclerotic cardiovascular disease in people living with HIV: systematic review and Meta-analysis. Circulation 138, 1100–1112. doi: 10.1161/CIRCULATIONAHA.117.033369
Sharma, S., Schlusser, K. E., de la Torre, P., Tambussi, G., Draenert, R., Pinto, A. N., et al. (2019). The benefit of immediate compared with deferred antiretroviral therapy on CD4+ cell count recovery in early HIV infection. AIDS 33, 1335–1344. doi: 10.1097/QAD.0000000000002219
Sheykhhasan, M., Foroutan, A., Manoochehri, H., Khoei, S. G., Poondla, N., and Saidijam, M. (2021). Could gene therapy cure HIV? Life Sci. 277:119451. doi: 10.1016/j.lfs.2021.119451
Shive, C. L., Freeman, M. L., Younes, S.-A., Kowal, C. M., Canaday, D. H., Rodriguez, B., et al. (2021). Markers of T cell exhaustion and senescence and their relationship to plasma TGF-β levels in treated HIV+ immune non-responders. Front. Immunol. 12:638010. doi: 10.3389/fimmu.2021.638010
Shive, C. L., Jiang, W., Anthony, D. D., and Lederman, M. M. (2015). Soluble CD14 is a nonspecific marker of monocyte activation. AIDS (London, England) 29, 1263–1265. doi: 10.1097/QAD.0000000000000735
Simonetti, F. R., Zhang, H., Soroosh, G. P., Duan, J., Rhodehouse, K., Hill, A. L., et al. (2021). Antigen-driven clonal selection shapes the persistence of HIV-1-infected CD4+ T cells in vivo. J. Clin. Invest. 131:e145254. doi: 10.1172/JCI145254
Sims, E. K., Park, G., Mather, K. J., Mirmira, R. G., Liu, Z., and Gupta, S. K. (2018). Immune reconstitution in ART treated, but not untreated HIV infection, is associated with abnormal beta cell function. PLoS One 13:e0197080. doi: 10.1371/journal.pone.0197080
Sivanandham, R., Kleinman, A. J., Sette, P., Brocca-Cofano, E., Kilapandal Venkatraman, S. M., Policicchio, B. B., et al. (2020). Nonhuman primate testing of the impact of different regulatory T cell depletion strategies on reactivation and clearance of latent simian immunodeficiency virus. J. Virol. 94:e00533–20. doi: 10.1128/JVI.00533-20
Steele, A. K., Carrasco-Medina, L., Sodora, D. L., and Crawley, A. M. (2017). Increased soluble IL-7 receptor concentrations associate with improved IL-7 therapy outcomes in SIV-infected ART-treated Rhesus macaques. PLoS One 12:e0188427. doi: 10.1371/journal.pone.0188427
Stefan, K. L., Kim, M. V., Iwasaki, A., and Kasper, D. L. (2020). Commensal microbiota modulation of natural resistance to virus infection. Cells 183, 1312–1324.e10. doi: 10.1016/j.cell.2020.10.047
Sviridov, D., Miller, Y. I., and Bukrinsky, M. I. (2022). Trained immunity and HIV infection. Front. Immunol. 13:903884. doi: 10.3389/fimmu.2022.903884
Swanepoel, C. R., Atta, M. G., D'Agati, V. D., Estrella, M. M., Fogo, A. B., Naicker, S., et al. (2018). Kidney disease in the setting of HIV infection: conclusions from a kidney disease: improving global outcomes (KDIGO) controversies conference. Kidney Int. 93, 545–559. doi: 10.1016/j.kint.2017.11.007
Tang, X., Lu, H., Dooner, M., Chapman, S., Quesenberry, P. J., and Ramratnam, B. (2018). Exosomal tat protein activates latent HIV-1 in primary, resting CD4+ T lymphocytes. JCI Insight 3:e95676. doi: 10.1172/jci.insight.95676
Tawbi, H. A., Schadendorf, D., Lipson, E. J., Ascierto, P. A., Matamala, L., Castillo Gutiérrez, E., et al. (2022). Relatlimab and Nivolumab versus Nivolumab in untreated advanced melanoma. N. Engl. J. Med. 386, 24–34. doi: 10.1056/NEJMoa2109970
Tebas, P., Jadlowsky, J. K., Shaw, P. A., Tian, L., Esparza, E., Brennan, A. L., et al. (2021). CCR5-edited CD4+ T cells augment HIV-specific immunity to enable post-rebound control of HIV replication. J. Clin. Invest. 131:e144486. doi: 10.1172/JCI144486
Tebas, P., Stein, D., Tang, W. W., Frank, I., Wang, S. Q., Lee, G., et al. (2014). Gene editing of CCR5 in autologous CD4 T cells of persons infected with HIV. N. Engl. J. Med. 370, 901–910. doi: 10.1056/NEJMoa1300662
Thaiss, C. A., Zmora, N., Levy, M., and Elinav, E. (2016). The microbiome and innate immunity. Nature 535, 65–74. doi: 10.1038/nature18847
Théry, C., Witwer, K. W., Aikawa, E., Alcaraz, M. J., Anderson, J. D., Andriantsitohaina, R., et al. (2018). Minimal information for studies of extracellular vesicles 2018 (MISEV2018): a position statement of the International Society for Extracellular Vesicles and update of the MISEV2014 guidelines. J. Extracell. Vesicl. 7:1535750. doi: 10.1080/20013078.2018.1535750
Thiébaut, R., Jarne, A., Routy, J.-P., Sereti, I., Fischl, M., Ive, P., et al. (2016). Repeated cycles of recombinant human interleukin 7 in HIV-infected patients with low CD4 T-cell reconstitution on antiretroviral therapy: results of 2 phase II multicenter studies. Clin. Infect. Dis. 62, 1178–1185. doi: 10.1093/cid/ciw065
Tripiciano, A., Picconi, O., Moretti, S., Sgadari, C., Cafaro, A., Francavilla, V., et al. (2021). Anti-tat immunity defines CD4(+) T-cell dynamics in people living with HIV on long-term cART. EBioMedicine 66:103306. doi: 10.1016/j.ebiom.2021.103306
Tsukamoto, T. (2020). Hematopoietic stem/progenitor cells and the pathogenesis of HIV/AIDS. Front. Cell. Infect. Microbiol. 10:60. doi: 10.3389/fcimb.2020.00060
Vanhamel, J., Bruggemans, A., and Debyser, Z. (2019). Establishment of latent HIV-1 reservoirs: what do we really know? J. Virus Erad. 5, 3–9. doi: 10.1016/S2055-6640(20)30275-2
Vanpouille, C., Frick, A., Rawlings, S. A., Hoenigl, M., Lisco, A., Margolis, L., et al. (2020). Cytokine network and sexual human immunodeficiency virus transmission in men who have sex with men. Clin. Infect. Dis. 71, 2655–2662. doi: 10.1093/cid/ciz1150
Vassena, L., Miao, H., Cimbro, R., Malnati, M. S., Cassina, G., Proschan, M. A., et al. (2012). Treatment with IL-7 prevents the decline of circulating CD4+ T cells during the acute phase of SIV infection in rhesus macaques. PLoS Pathog. 8:e1002636. doi: 10.1371/journal.ppat.1002636
Veil, R., Poizot-Martin, I., Reynes, J., Goujard, C., Seng, R., Delobel, P., et al. (2020). Virological and immunological impact of integrase inhibitor-based regimens initiated during primary HIV-1 infection. AIDS (London, England) 34, 493–500. doi: 10.1097/QAD.0000000000002447
Vieillard, V., Combadière, B., Tubiana, R., Launay, O., Pialoux, G., Cotte, L., et al. (2019). HIV therapeutic vaccine enhances non-exhausted CD4 T cells in a randomised phase 2 trial. NPJ Vaccines 4:25. doi: 10.1038/s41541-019-0117-5
Vieillard, V., Gharakhanian, S., Lucar, O., Katlama, C., Launay, O., Autran, B., et al. (2016). Perspectives for immunotherapy: which applications might achieve an HIV functional cure? Oncotarget 7, 38946–38958. doi: 10.18632/oncotarget.7793
Vlasova, V. V., Saidakova, E. V., Korolevskaya, L. B., Shmagel, N. G., Chereshnev, V. A., and Shmagel, K. V. (2021). Metabolic features of activated memory CD4 T-cells derived from HIV-infected immunological non-responders to highly active antiretroviral therapy. Dokl. Biol. Sci. 501, 206–209. doi: 10.1134/S0012496621060090
Vujkovic-Cvijin, I., Dunham, R. M., Iwai, S., Maher, M. C., Albright, R. G., Broadhurst, M. J., et al. (2013). Dysbiosis of the gut microbiota is associated with HIV disease progression and tryptophan catabolism. Sci. Transl. Med. 5:193ra191. doi: 10.1126/scitranslmed.3006438
Vujkovic-Cvijin, I., and Somsouk, M. (2019). HIV and the gut microbiota: composition, consequences, and avenues for amelioration. Curr. HIV/AIDS Rep. 16, 204–213. doi: 10.1007/s11904-019-00441-w
Wacleche, V. S., Tremblay, C. L., Routy, J.-P., and Ancuta, P. (2018). The biology of monocytes and dendritic cells: contribution to HIV pathogenesis. Viruses 10:65. doi: 10.3390/v10020065
Wallet, C., De Rovere, M., Van Assche, J., Daouad, F., De Wit, S., Gautier, V., et al. (2019). Microglial cells: the Main HIV-1 reservoir in the brain. Front. Cell. Infect. Microbiol. 9:362. doi: 10.3389/fcimb.2019.00362
Wang, C., Edilova, M. I., Wagar, L. E., Mujib, S., Singer, M., Bernard, N. F., et al. (2018). Effect of IL-7 therapy on Phospho-ribosomal protein S6 and TRAF1 expression in HIV-specific CD8 T cells in patients receiving antiretroviral therapy. J. Immunol. 200, 558–564. doi: 10.4049/jimmunol.1601254
Wang, Y., Lifshitz, L., Gellatly, K., Vinton, C.L., Busman-Sahay, K., McCauley, S., et al. (2020). HIV-1-induced cytokines deplete homeostatic innate lymphoid cells and expand TCF7-dependent memory NK cells. Nat. Immunol. 21, 274–286. doi: 10.1038/s41590-020-0593-9
Wang, Z., Yin, X., Ma, M., Ge, H., Lang, B., Sun, H., et al. (2021). IP-10 promotes latent HIV infection in resting memory CD4 T cells LIMK-Cofilin pathway. Front. Immunol. 12:656663. doi: 10.3389/fimmu.2021.656663
Wang, L., Zhang, Z., Xu, R., Wang, X., Shu, Z., Chen, X., et al. (2021). Human umbilical cord mesenchymal stem cell transfusion in immune non-responders with AIDS: a multicenter randomized controlled trial. Signal Transduct. Target. Ther. 6:217. doi: 10.1038/s41392-021-00607-2
Weber, E. A., Singh, M. V., Singh, V. B., Jackson, J. W., Ture, S. K., Suwunnakorn, S., et al. (2020). Novel mechanism of microvesicle regulation by the antiviral protein Tetherin during HIV infection. J. Am. Heart Assoc. 9:e015998. doi: 10.1161/JAHA.120.015998
Weiss, S., Itri, V., Pan, R., Jiang, X., Luo, C. C., Morris, L., et al. (2022). Differential V2-directed antibody responses in non-human primates infected with SHIVs or immunized with diverse HIV vaccines. Nat. Commun. 13:903. doi: 10.1038/s41467-022-28450-1
WHO (2021). Consolidated guidelines on HIV prevention, testing, treatment, service delivery and monitoring: recommendations for a public Health approach [internet]. World Health Organization.
Wiche Salinas, T. R., Zhang, Y., Sarnello, D., Zhyvoloup, A., Marchand, L. R., Fert, A., et al. (2021). Th17 cell master transcription factor RORC2 regulates HIV-1 gene expression and viral outgrowth. Proc. Natl. Acad. Sci. U. S. A. 118:e2105927118. doi: 10.1073/pnas.2105927118
Wilson, E. M., and Sereti, I. (2013). Immune restoration after antiretroviral therapy: the pitfalls of hasty or incomplete repairs. Immunol. Rev. 254, 343–354. doi: 10.1111/imr.12064
Wilson, E. M. P., Singh, A., Hullsiek, K. H., Gibson, D., Henry, W. K., Lichtenstein, K., et al. (2014). Monocyte-activation phenotypes are associated with biomarkers of inflammation and coagulation in chronic HIV infection. J. Infect. Dis. 210, 1396–1406. doi: 10.1093/infdis/jiu275
Witwer, K. W., and Théry, C. (2019). Extracellular vesicles or exosomes? On primacy, precision, and popularity influencing a choice of nomenclature. J. Extracell. Vesicl. 8:1648167. doi: 10.1080/20013078.2019.1648167
Xia, H., Jiang, W., Zhang, X., Qin, L., Su, B., Li, Z., et al. (2018). Elevated level of CD4(+) T cell immune activation in acutely HIV-1-infected stage associates with increased IL-2 production and cycling expression, and subsequent CD4(+) T cell preservation. Front. Immunol. 9:616. doi: 10.3389/fimmu.2018.00616
Xie, Y., Sun, J., Hu, C., Ruan, B., and Zhu, B. (2021). Oral microbiota is associated with immune recovery in human immunodeficiency virus-infected individuals. Front. Microbiol. 12:794746. doi: 10.3389/fmicb.2021.794746
Yang, X., Su, B., Zhang, X., Liu, Y., Wu, H., and Zhang, T. (2020). Incomplete immune reconstitution in HIV/AIDS patients on antiretroviral therapy: challenges of immunological non-responders. J. Leukoc. Biol. 107, 597–612. doi: 10.1002/JLB.4MR1019-189R
Yero, A., Farnos, O., Rabezanahary, H., Racine, G., Estaquier, J., and Jenabian, M.-A. (2019). Differential dynamics of regulatory T-cell and Th17 cell balance in mesenteric lymph nodes and blood following early antiretroviral initiation during acute simian immunodeficiency virus infection. J. Virol. 93. doi: 10.1128/JVI.00371-19
Yero, A., Shi, T., Farnos, O., Routy, J.-P., Tremblay, C., Durand, M., et al. (2021). Dynamics and epigenetic signature of regulatory T-cells following antiretroviral therapy initiation in acute HIV infection. EBioMedicine 71:103570. doi: 10.1016/j.ebiom.2021.103570
Yuan, Y., Zhou, C., Yang, Q., Ma, S., Wang, X., Guo, X., et al. (2019). HIV-1 tat protein inhibits the hematopoietic support function of human bone marrow mesenchymal stem cells. Virus Res. 273:197756. doi: 10.1016/j.virusres.2019.197756
Zaikos, T. D., Terry, V. H., Sebastian Kettinger, N. T., Lubow, J., Painter, M. M., Virgilio, M. C., et al. (2018). Hematopoietic stem and progenitor cells are a distinct HIV reservoir that contributes to persistent viremia in suppressed patients. Cell Rep. 25, 3759–3773.e9. doi: 10.1016/j.celrep.2018.11.104
Zhao, J., Schank, M., Wang, L., Li, Z., Nguyen, L. N., Dang, X., et al. (2021). Mitochondrial functions are compromised in CD4 T cells from ART-controlled PLHIV. Front. Immunol. 12:658420. doi: 10.3389/fimmu.2021.658420
Zhu, J., Huang, H., Wang, M., Zhang, Y., Mo, J., Tian, W., et al. (2022). High baseline body mass index predicts recovery of CD4+ T lymphocytes for HIV/AIDS patients receiving long-term antiviral therapy. PLoS One 17:e0279731. doi: 10.1371/journal.pone.0279731
Keywords: HIV-1 infection, immunological non-responders, CD4+ T cells, immune reconstitution, therapeutic interventions
Citation: Zhang W and Ruan L (2023) Recent advances in poor HIV immune reconstitution: what will the future look like? Front. Microbiol. 14:1236460. doi: 10.3389/fmicb.2023.1236460
Edited by:
Alejandro Vallejo, Ramón y Cajal Institute for Health Research, SpainReviewed by:
Hang Su, Albert Einstein College of Medicine, United StatesMaria Jesus Perez Elias, Ramón y Cajal University Hospital, Spain
Copyright © 2023 Zhang and Ruan. This is an open-access article distributed under the terms of the Creative Commons Attribution License (CC BY). The use, distribution or reproduction in other forums is permitted, provided the original author(s) and the copyright owner(s) are credited and that the original publication in this journal is cited, in accordance with accepted academic practice. No use, distribution or reproduction is permitted which does not comply with these terms.
*Correspondence: Lianguo Ruan, MjAyMGp5MDAwNEBodXN0LmVkdS5jbg==