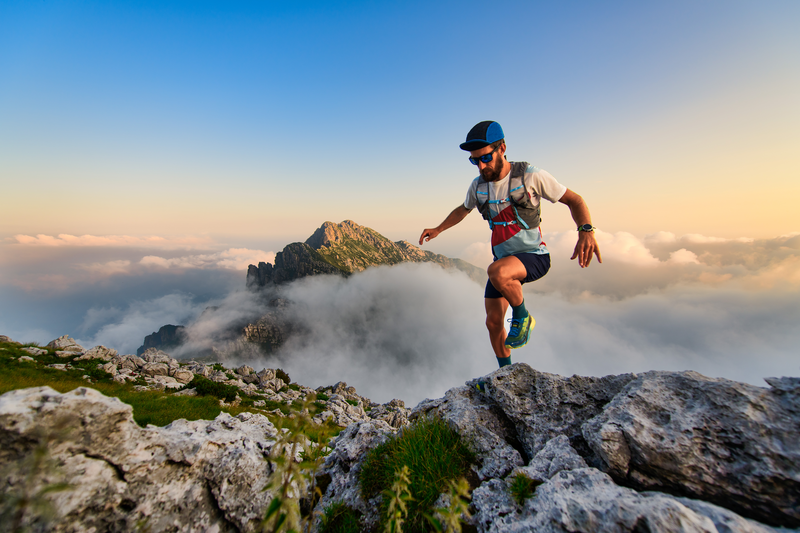
94% of researchers rate our articles as excellent or good
Learn more about the work of our research integrity team to safeguard the quality of each article we publish.
Find out more
REVIEW article
Front. Microbiol. , 13 July 2023
Sec. Food Microbiology
Volume 14 - 2023 | https://doi.org/10.3389/fmicb.2023.1229838
Effective control of foodborne pathogen contamination is a significant challenge to the food industry, but the development of new antibacterial nanotechnologies offers new opportunities. Notably, selenium nanoparticles have been extensively studied and successfully applied in various food fields. Selenium nanoparticles act as food antibacterial agents with a number of benefits, including selenium as an essential trace element in food, prevention of drug resistance induction in foodborne pathogens, and improvement of shelf life and food storage conditions. Compared to physical and chemical methods, biogenic selenium nanoparticles (Bio-SeNPs) are safer and more multifunctional due to the bioactive molecules in Bio-SeNPs. This review includes a summarization of (1) biosynthesized of Bio-SeNPs from different sources (plant extracts, fungi and bacteria) and their antibacterial activity against various foodborne bacteria; (2) the antibacterial mechanisms of Bio-SeNPs, including penetration of cell wall, damage to cell membrane and contents leakage, inhibition of biofilm formation, and induction of oxidative stress; (3) the potential antibacterial applications of Bio-SeNPs as food packaging materials, food additives and fertilizers/feeds for crops and animals in the food industry; and (4) the cytotoxicity and animal toxicity of Bio-SeNPs. The related knowledge contributes to enhancing our understanding of Bio-SeNP applications and makes a valuable contribution to ensuring food safety.
Foodborne pathogens are a main cause of foodborne illness and food poisoning, which are food safety issues with serious implications for human health and economic development. According to the World Health Report, millions of hospital cases occur due to food contamination, and hundreds of thousands die due to foodborne diseases every year (Wei and Zhao, 2021). Globally, foodborne illnesses are normally caused by 31 major pathogens (Riley, 2020), most of which are bacterial pathogens, which can result in intoxication, infection and toxicoinfections (Abebe et al., 2020). Usually, the clinical syndromes of foodborne bacterial infection are fever, mild diarrhea, headaches, vomiting, muscle cramps, abdominal pain and even more complex illnesses (Iwu and Okoh, 2019). The potential risk of foodborne bacteria is commonly present in various foods during production, packaging, and transportation (Xing et al., 2022). The ingestion of foodborne pathogenic bacteria contaminated foods, such as seafoods (Ali et al., 2020), milk and dairy products (Keba et al., 2020), meat and meat products (Zhao et al., 2022), raw and ready-to-eat green leafy vegetables (Azimirad et al., 2021) and grains (such as rice, noodles, and rice noodles) (Li et al., 2020), might lead to serious foodborne diseases. The increasing incidences of foodborne diseases (Hoffmann and Scallan Walter, 2020) and product corruption (Gurtler and Gibson, 2022) cause serious economic losses and significantly hinder social development, which has brought food safety to the forefront of public health concerns.
Currently, as standards of life have improved, one of the most serious challenges for the food industry is to ensure food safety while also ensuring food quality (Yan et al., 2021). To ensure food safety, thermal sterilization is the most common method for inactivating foodborne pathogens, but high temperatures also diminish the quality of food products (Guo et al., 2022). Although nonthermal physical technologies have emerged in recent years to improve the quality of food products, their application has been severely limited by their high cost and technical threshold (Chacha et al., 2021; Khouryieh, 2021). Chemical bacteriostatic agents, including antibiotics, are the most common method for inhibiting the growth of bacteria in livestock, aquaculture and agriculture (Chen et al., 2020; Shao et al., 2021; Tadic et al., 2021). However, this method leads to the emergence of residual antibiotics and multiple foodborne drug-resistant bacteria in the food chain, posing a serious threat to food safety (Liao et al., 2020; Thapa et al., 2020). Various foodborne bacteria, such as Escherichia coli (MDR) (Liu et al., 2022), Staphylococcus aureus (MRSA) (Algammal et al., 2020), Salmonella enterica serovar Rissen (Xu et al., 2020) and Listeria monocytogenes (Baquero et al., 2020), have been reported to exhibit drug resistance. These bacteria have evolved multiple mechanisms, including reduced cell membrane permeability, efflux pump mechanisms, target site mutation mechanisms, and enzymatic hydrolysis, to cope with antibiotics (Ge et al., 2022). Consequently, innovative technological approaches are urgently required to combat foodborne pathogens.
In recent years, nano antimicrobial agents have attracted researchers’ attention (Fatima et al., 2021). In the food industry, selenium nanoparticles, as an alternative antimicrobial agent, have many benefits over other nanomaterials, such as (1) selenium as a trace element in food (Kieliszek, 2019), (2) prevention of drug resistance induction in foodborne pathogens (Truong et al., 2021), and (3) improvement of shelf life and food storage conditions (Ndwandwe et al., 2020; Salem M. F. et al., 2022). Selenium nanoparticles are normally synthesized by physical, chemical and biological methods (Nayak et al., 2021) in which Se(IV) can be reduced to Se(0) and then form SeNPs (Zambonino et al., 2021). However, compared to other conventional physical and chemical methods, microbial and plant-mediated synthesis of biogenic selenium nanoparticles (Bio-SeNPs) with various bioactive substances have extensive biological applications (Vijayakumar et al., 2022). In addition, Bio-SeNPs also have the advantages of high biocompatibility, eco-friendliness and low toxicity (Ikram et al., 2021). At the same time, numerous studies have also demonstrated the excellent antibacterial activity of Bio-SeNPs against food-borne pathogens (Abu-Elghait et al., 2021; Zhang et al., 2021; Salem S. S. et al., 2022). Additionally, numerous studies have demonstrated the low/nontoxicity of Bio-SeNPs at the cellular and animal levels (Majeed et al., 2020; Perumal et al., 2021; Souza et al., 2022). Overall, Bio-SeNPs have value in improving food safety against food pathogens in the food industry.
In this review, the target references were searched using Google Scholar database and the selected keywords were “biosynthesis + selenium nanoparticles + antibacterial/food/toxicity.” We focus on the new research breakthroughs of Bio-SeNPs, including (1) the biosynthesis methods and antibacterial activity of Bio-SeNPs; (2) the mechanisms of Bio-SeNPs against foodborne pathogens; (3) the potential application of Bio-SeNPs in the food industry; and (4) the toxicity of Bio-SeNPs.
Biological approaches to the synthesis of selenium nanoparticles arose from the need to develop new and environmentally friendly antibacterial agents. Numerous studies have shown that Bio-SeNPs have excellent antibacterial capacity and inhibit foodborne pathogens by various antibacterial mechanisms. Usually, Bio-SeNPs are synthesized from bacterial, fungal, and plant extracts (Shoeibi et al., 2017). Figure 1 illustrates the various sources of synthetic Bio-SeNPs against foodborne pathogens. These bioderived selenium nanoparticles have more potential applications in the food industry due to their excellent antibacterial activity and safety (Ndwandwe et al., 2020). Table 1 summarizes the reported Bio-SeNPs and their antibacterial properties against foodborne pathogens, such as S. aureus, E. coli, L. monocytogenes, Salmonella, Bacillus cereus, and Alicyclobacillus acidoterrestris.
Figure 1. The technical routes for Bio-SeNPs synthesis based on plants extracts, bacteria, and fungi (inner ring) and some representative plants, bacteria and fungi used in the synthesis of Bio-SeNPs (outer ring).
Research on green synthetic functional nanomaterials based on plants has attracted the attention of an increasing number of researchers (Naikoo et al., 2021). Plant extract-based Bio-SeNPs have the advantages of mild reaction, low cost, and easy operation (Bao et al., 2021). Furthermore, natural compounds are abundant in all parts of the plant, which provides suitable conditions for the synthesis of Bio-SeNPs (Jadoun et al., 2020; Ikram et al., 2021). In the synthesis of Bio-SeNPs, plant extracts provide three major groups of substances, including reducing agents, stabilizers, and capping agents (Abadi et al., 2022; Khan et al., 2022). In addition, plant extracts are abundant in antibacterial substances such as phenols, phenolic acids, terpenoids, and alkaloids (Alibi et al., 2021), which contribute to the synthesis and bioactivity of antibacterial nanomaterials.
Edible medicinal plants and agricultural waste are good choices among the plant sources for Bio-SeNP synthesis (Kumari et al., 2019; Jeevanandam et al., 2022). In particular, edible medicinal plants, combined with nanotechnology, have produced a number of extremely excellent nano antibacterial agents (Ghosh et al., 2021). Costus root extract was used as a reducing agent for the synthesis of antibacterial Bio-SeNPs against Salmonella enterica serotype Typhimurium, E. coli, and S. aureus with MICs of 17.5, 20.0, and 25.0 μg/mL, respectively (Al-Saggaf et al., 2020). Similarly, Ephedra aphylla aqueous extract was also used to synthesize Bio-SeNPs that inhibited S. enterica serotype Typhimurium, E. coli, B. cereus, L. monocytogenes, and S. aureus, containing phenolic, flavonoid and tannin compounds from Ephedra aphylla (El-Zayat et al., 2021). Bio-SeNPs produced by tarragon leaf extract could act against B. cereus, E. coli, L. monocytogenes, S. aureus and Salmonella spp. (Yilmaz et al., 2021). Additionally, Bio-SeNPs synthesized by ascorbic acid and Cirsium setidens extract could control B. cereus, E. coli, Salmonella enterica, and S. aureus (Shin et al., 2021).
Compared to edible-medicinal extract-mediated Bio-SeNPs, Bio-SeNPs based on agricultural waste took advantage of economy, which showed promising antibacterial results (Krishnani et al., 2022). Bio-SeNPs based on extracts of prickly pear peel waste (PPPW) demonstrated great antibacterial activity against S. aureus and E. coli (Hashem et al., 2022). Similarly, Bio-SeNPs from orange peel waste were resistant to S. aureus, MDR S. aureus and MDR E. coli, most sensitive to S. aureus with an MIC of 25 μg/mL and exhibited noticeable antibiofilm activity (Salem S. S et al., 2022). Additionally, Bio-SeNPs based on green orange peel could work against methicillin-resistant S. aureus with an MIC of 0.00494 μg/mL, mainly attributed to the higher polyphenol content of the orange peel extract (Dang-Bao et al., 2022). Both edible medicinal plants and agricultural waste-synthesized Bio-SeNPs have special features of their own, and edible medicinal plants probably have better antibacterial properties and safety, while agricultural waste is more affordable. Therefore, rational selection is essential to balance antibacterial activity and economic cost.
Bacteria are considered biofactory for the synthesis of nanomaterials because they can efficiently transform toxic metals/nonmetals into useful nanomaterials (Mohanta et al., 2020; Spivak et al., 2020). Selenium-resistant bacteria can convert highly toxic selenite and selenate oxyanions into nontoxic Bio-SeNPs through a cellular detoxification mechanism (Ojeda et al., 2020). Meanwhile, bacterial synthesis of Bio-SeNPs is also a process of self-detoxification, and there are many proteins in the cell involved in this process (Tugarova and Kamnev, 2017). The mechanism of Bio-SeNPs synthesis by bacteria is complex, and further exploration is necessary for the specific synthesis mechanism (Escobar-Ramírez et al., 2021; Ullah et al., 2022). At present, many bacteria have been reported to synthesize Bio-SeNPs, such as Bacillus licheniformis (Khiralla and El-Deeb, 2015), Bacillus subtilis (Abdel-Moneim et al., 2022), Streptomyces enissocaesilis (Shaaban and El-Mahdy, 2018), Providencia sp. (Zhang et al., 2021), Streptomyces sp. (Ramya et al., 2019), and Ralstonia eutropha (Srivastava and Mukhopadhyay, 2015), which could inhibit various foodborne pathogens. However, some bacteria might carry toxins or other harmful factors (Abebe et al., 2020), so it is necessary to select harmless selenium-resistant bacteria to synthesize safer Bio-SeNPs.
Fortunately, probiotics possess multiple benefits to human health and are considered factories for the production of Bio-SeNPs (Kerry et al., 2018; Yang and Yang, 2023), which is advantageous to the food industry. Bio-SeNPs synthesized by Lactobacillus pentosus ADET MW861694 were used to control foodborne pathogens such as Salmonella enterica subsp. arizonae, E. coli, S. enterica serotype Typhimurium, and S. aureus (Christianah Adebayo-Tayo et al., 2021). Similarly, Bio-SeNPs synthesized by Lactobacillus sporogenes were used to inhibit S. aureus and E. coli (Kaur et al., 2018). Furthermore, in Lactobacillus acidophilus, extracellularly synthesized Bio-SeNPs were reported against the drug-resistant bacteria S. aureus and E. coli to inhibit biofilms (Alam et al., 2019). Currently, research on probiotic bacteria-synthesized Bio-SeNPs against foodborne microorganisms is relatively scarce and requires further study.
Fungi possess high metal tolerance and abundant metabolites, which are powerful tools for the synthesis of biogenic nanomaterials (Adebayo et al., 2021; Sonawane et al., 2022). Recently, some fungi have been used to synthesize Bio-SeNPs, such as Mariannaea sp. HJ (Zhang et al., 2019), Aureobasidium pullulans, Mortierella humilis, Trichoderma harzianum and Phoma glomerata (Liang et al., 2019), and Aspergillus quadrilineatus, Aspergillus ochraceus, Aspergillus terreus, and Fusarium equiseti (Hussein et al., 2022). Furthermore, fungal synthesis of Bio-SeNPs has great antibacterial potential. Bio-SeNPs synthesized by Monascus purpureus could perform against S. aureus and E. coli with an MIC of 100 μg/mL (El-Sayed et al., 2020) and against A. acidoterrestris with an MIC of 3,000 μg/mL (Sun et al., 2021). In particular, the Bio-SeNPs synthesized by some Penicillium spp. demonstrated formidable antibacterial abilities. Bio-SeNPs synthesis from Penicillium chrysogenum PTCC 5031 could inhibit S. aureus and L. monocytogenes (Vahidi et al., 2020). Bio-SeNPs produced by Penicillium corylophilum could operate against E. coli and S. aureus with MICs of 9.37 μg/mL and 37.5 μg/mL, respectively (Salem et al., 2020). Bio-SeNPs synthesized by Penicillium expansum ATTC 36200 could control S. aureus and E. coli (Hashem et al., 2021b). However, Penicillium spp. might produce antibiotics such as penicillin (Kumar et al., 2018), resulting in limited applications within the food industry.
Bio-SeNPs produced by fermentation of edible mycelium and yeast were safer and may be more promising in the food industry. Edible Lentinula edodes could be used to synthesize Bio-SeNPs, and mycelium reddening (Tsivileva et al., 2012) and accumulation of Bio-SeNPs (Vetchinkina et al., 2013) were observed during mycelial growth. Additionally, Saccharomyces cerevisiae extract was used to synthesize Bio-SeNPs, and it showed excellent antibacterial activity against S. aureus and E. coli (Salem, 2022). However, there are fewer studies on the synthesis of antibacterial Bio-SeNPs from edible mycelium and yeast, which may have better applications in the food industry.
The antibacterial mechanism of nanomaterials is complex due to various attributes (Matai et al., 2020). Some general mechanisms are summarized as follows: (1) penetration of the cell wall, (2) cell membrane damage and contents leakage, (3) inhibiting the formation of biofilm, and (4) inducing oxidative stress (Figure 2).
Figure 2. Antibacterial mechanisms of Bio-SeNPs. (A) Penetration of the cell wall (Alam et al., 2019; Cittrarasu et al., 2021; Zhang et al., 2021). (B) Cell membrane damage and contents leakage (Tareq et al., 2018; Sun et al., 2021; Prasathkumar et al., 2022). (C) Inhibiting the formation of biofilm (Ramya et al., 2015; Shakibaie et al., 2015; Miglani and Tani-Ishii, 2021; Haddadian et al., 2022; Ullah et al., 2023). (D) Oxidative stress (Cremonini et al., 2018; Alam et al., 2019; Prasathkumar et al., 2022).
Bio-SeNPs bind to the cell wall and further affect the integrity of cell membranes and cell morphology. Nanoparticles can anchor to Singh et al. (2014) and/or burrow into bacterial cell walls (Galbadage et al., 2019), causing structural changes in cell membrane permeability and leading to bacterial death. Compared to gram-negative bacteria, the thicker peptidoglycan structure of the gram-positive bacterial cell wall might make it more resistant to drugs (Reygaert, 2018; Impey et al., 2020; Pasquina-Lemonche et al., 2020). Bio-SeNPs synthesized by Providencia sp. DCX exhibited concentration-dependent inhibition against five pathogenic bacteria, including G+ (S. aureus and B. cereus) and G− (Pseudomonas aeruginosa, Vibrio parahemolyticus and E. coli). Bio-SeNPs were more lethal to gram-negative bacteria, probably due to the thin peptidoglycan of G− bacteria, and selenium nano could more easily penetrate their cell walls and disrupt the integrity of cell membranes (Zhang et al., 2021). Bio-SeNPs produced by L. acidophilus inhibited pathogens such as Klebsiella pneumoniae and P. aeruginosa, with much lower MIC values compared to gentamicin. The lower MIC values of Bio-SeNPs might be due to the electrostatic interactions responsible for Bio-SeNPs adhesion to the bacterial cell wall, causing bacterial death (Alam et al., 2019). Meanwhile, Ceropegia bulbosa Roxb extract-based Bio-SeNPs could inhibit bacteria such as B. subtilis and E. coli. It is possible that ionic interactions caused the negatively charged Bio-SeNPs to bind to the bacterial surface, blocking the synthesis of bacterial cell walls (Cittrarasu et al., 2021).
Bio-SeNPs may disrupt cell membrane integrity and cause leakage of cytoplasmic contents. A biophysical model for the interaction of nanomaterials with bacterial cell membranes has been proposed, which suggests that adsorption of NPs leads to membrane stretching and squeezing, causing cell rupture and death (Linklater et al., 2020). The use of Bio-SeNPs to disrupt bacterial cell wall integrity and cause leakage of contents was considered an effective strategy (Makabenta et al., 2021). Bio-SeNPs synthesized in M. purpureus were used to assess the inhibition of A. acidoterrestris (Sun et al., 2021). The SEM results showed that 3,000 μg/mL Bio-SeNPs caused the bacterial cells to shrink slightly, and the surface became rough with holes and wrinkles, while the bacterial cells were damaged with severe distortion and irregularity when the concentration was increased to 5,000 μg/mL. Meanwhile, further determination of the leakage of cellular contents was performed. Bacteria treated with Bio-SeNPs showed a significant amount of leakage of protein, DNA and RNA. Azadirachta indica leaf aqueous extract was used to synthesize Bio-SeNPs against Clostridium botulinum (Tareq et al., 2018). The SEM results showed that the bacteria treated with 100 μg/mL Bio-SeNPs were severely damaged, misshapen and fragmentary. Moreover, after 4 h of Bio-SeNPs treatment, the bacteria leaked more reducing sugars and proteins. It was revealed that Bio-SeNPs can disrupt cell membranes and accelerate the leakage of reducing sugars and proteins from bacteria. The Senna auriculata flower and leaf aqueous extract was used to synthesize Bio-SeNPs against B. subtilis, MRSA, E. coli, and P. aeruginosa (Souza et al., 2022). Optical microscopy results showed that Bio-SeNPs inhibited pathogenic bacteria, and FESEM results also showed the deposition of Bio-SeNPs on the cell surface, causing bacterial rupture. Furthermore, protein and reducing sugar leakage was detected after treatment with 250 μg/mL Bio-SeNPs.
The inhibition of biofilm formation and subsequent growth inhibition is another antibacterial mechanism of Bio-SeNPs. Bio-SeNPs synthesized by Lysinibacillus sp. NOSK effectively inhibited P. aeruginosa biofilm formation, and its large surface area, small size and spherical shape may be an important factor (San Keskin et al., 2020). Bio-SeNPs produced by Bacillus subtilis BSN313 were strongly bound to bacterial surfaces and destroyed bacterial cells by disintegrating the membranes of P. aeruginosa, S. enterica serotype Typhimurium and S. aureus (Ullah et al., 2023). In addition, Bio-SeNPs (2 μg/mL) generated by Bacillus sp. MSh-1 had strong adhesion to biofilm-producing bacteria and inhibited the biofilm formation of S. aureus, P. aeruginosa, and Proteus mirabilis (Shakibaie et al., 2015). Bio-SeNPs (1,000 μg/mL) synthesized by fresh guava leaves inhibited the growth of biofilm formation, and the carbohydrate and protein concentrations of the treated Enterococcus faecalis biofilm decreased by approximately 73 and 71%, respectively (Miglani and Tani-Ishii, 2021). Bio-SeNPs also have a better inhibitory effect on many biofilms forming multidrug resistant bacteria. For instance, Bio-SeNPs produced by Streptomyces minutiscleroticus M10A62 could effectively inhibit biofilm formation of six biofilm-forming multidrug-resistant strains of Acinetobacter (4,117, 1,677, 2,030, 674, 2,020, and 1,370) (Ramya et al., 2015). In addition, the Trifolium cherleri aerial aqueous extract was used to synthesize Bio-SeNPs for anti-biofilm of S. aureus, E. faecalis, E. coli, and P. aeruginosa (Souza et al., 2022). Further analysis of the expression levels of biofilm-related genes such as icaD, Ace, fmH, and pelf revealed that the expression levels of related genes were significantly reduced in bacteria treated with Bio-SeNPs (Souza et al., 2022). This result suggested that Bio-SeNPs might bind to transcription factors and repress the expression of biofilm-related genes.
Bio-SeNPs induce high ROS production, break ROS homeostasis and cause oxidative stress. Many nanomaterials produce excess ROS, leading to various injuries, such as membrane disabilities, mitochondrial damage, and destruction of nucleic acids and proteins (Sadoq et al., 2023). In addition, the large amount of ROS disrupted the antioxidant system of bacteria and severely limited their viability (Mourenza et al., 2020). Many studies have shown that ROS produced by selenium nano exhibited effective antibacterial ability (Sakr et al., 2018; Kondaparthi et al., 2019; Bisht et al., 2022). The ROS assay results indicated that Senna auriculata extract-produced Bio-SeNPs entered the bacterial cell, causing a rapid increase in fluorescence intensity from intracellular ROS production, resulting in oxidative stress damage and contributing to bacterial death (Souza et al., 2022). Similarly, Bio-SeNPs synthesized by Stenotrophomonas maltophilia SeITE02 might kill bacteria by producing ROS (Cremonini et al., 2018). The intracellular ROS production of P. aeruginosa PAO1, S. aureus Mu50 and Burkholderia cenocepacia LMG16656 increased after treatment with Bio-SeNPs, while the survival of these strains was significantly limited (Cremonini et al., 2018). In L. acidophilus, the synthesized Bio-SeNPs could also control bacteria by producing ROS (Alam et al., 2019). The expression levels of superoxide dismutase (SOD) and catalase were substantially induced by ROS in E. coli, S. aureus, B. subtilis, P. aeruginosa, and K. pneumoniae after treatment with Bio-SeNPs (Alam et al., 2019).
Food packaging and food additives are used to solve the contamination of food-borne pathogens. Nanomaterial-based food packaging and additives exhibit great potential in food antibacterial applications. Although various nanomaterial-based food packaging and additives have shown excellent antibacterial ability, their application might be limited by toxicity or nonedible components, which could result in food safety problems (Chaudhry et al., 2010). Fortunately, selenium is an essential trace element for the human body (Yang et al., 2022), with promising application prospects in the Bio-SeNPs form as depicted (Figure 3).
Figure 3. The potential application forms of antibacterial Bio-SeNPs in the food industry. (A) Bio-SeNPs were combined with membrane materials to form composite food packaging film (Jamróz et al., 2019a,b; Lu et al., 2020; Alghuthaymi et al., 2021; Ndwandwe et al., 2022). (B) Bio-SeNPs were used as food additives (Sun et al., 2021; Ao et al., 2022; Saad et al., 2022). (C) Bio-SeNPs were used as fertilizers/feeds for crops and animals (Hu et al., 2019; Ayoub et al., 2021; El-Saadony et al., 2021; Hashem et al., 2021a; Sarkar and Kalita, 2022; Shahbaz et al., 2022; Taha et al., 2023).
Several studies have proven that Bio-SeNPs can be used as food packaging material to extend shelf life. Jamróz and group developed furcellaran-gelatin films with SeNPs and AgNPs, that possessed great antibacterial activity against S. aureus, MRSA and E. coli (Jamróz et al., 2019a). The packaging system could extend the shelf life of mini kiwi (Jamróz et al., 2019a). Similarly, the SeNPs and natural extract-modified furcellaran film showed excellent antibacterial activity against S. aureus, MRSA and E. coli and showed great potential applications in fish products shelf life (Jamróz et al., 2019b). Selenium microparticles and polylactic acid–based films also showed noticeable inhibition of S. aureus and E. coli (Lu et al., 2020). Alghuthaymi et al. (2021) developed coatings based on chitosan and cinnamon extract synthesized Bio-SeNPs that had antibacterial activities against E. coli, S. enterica serotype Typhimurium, S. aureus, and L. monocytogenes, which are potential edible coating (EC) basements (Alghuthaymi et al., 2021). Bio-SeNPs were also found to enhance the activity of potato starch films. SeNPs/potato starch nanofilm exhibited an inhibitory effect on S. enterica serotype Typhimurium, E. coli and B. cereus (Souza et al., 2022). All these studies indicated that Bio-SeNPs could be used as active food packaging material in replacement of the traditional material.
At present, Bio-SeNPs are rarely reported to be added directly to foods as antibacterial agents. Bio-SeNPs produced by M. purpureus showed the ability to inhibit A. acidoterrestris, which is an acid-resistant and heat-resistant bacterium that causes fruit juice spoilage (Sun et al., 2021). In our previous study, Bio-SeNPs generated by Moringa oleifera could efficiently clear L. monocytogenes on raw salmon (Ao et al., 2022). More interestingly, Bio-SeNPs synthesized by Bacillus subtilis AS12 could decrease the accumulation of heavy metals and pathogenic microbes in fish organs while improving growth performance (Souza et al., 2022). These reports suggested that Bio-SeNPs have the potential to be used as food additives or additives for food-derived animal culture for better antibacterial activity and extended shelf life.
In addition, Bio-SeNPs were used to control the crop and animal pathogens and supply the selenium element in foods. Bio-SeNPs (100 μg/mL) synthesized by Bacillus cereus showed an 85.1% reduction on mycelial growth of Alternaria alternata, which could effectively control leaf spot disease caused by Alternaia alternata in common beans and also improve plant growth and yield (Taha et al., 2023). Similarly, Bio-SeNPs produced by Bacillus megaterium ATCC 55000 could effectively inhibit the growth of Rhizoctonia solani RCMB 031001 to reduce root rot, improve morphological and metabolic indicators, and increase yield (Hashem et al., 2021a). Trichoderma harzianum-derived Bio-SeNPs (200 μg/mL) could significantly inhibit Alternaria alternata XJa1, Fusarium verticillioide BJ6 and Fusarium graminearum PH1 to protect corn and pears (Hu et al., 2019). Additionally, Bio-SeNPs were also used to suppress Triticum aestivum L. crown and root rot diseases induced by Fusarium species (El-Saadony et al., 2021), control stripe rust disease on Triticum aestivum L. (Souza et al., 2022), and promote the growth of mustard (Sarkar and Kalita, 2022). Likewise, Bio-SeNPs were exhibited excellent antibacterial against animal pathogens (Gad et al., 2022). Bio-SeNPs synthesized by Citrullus colocynthis extract could reduce mortality after Aeromonas sobria infection and improved immune function, antioxidant capacity and disease resistance in Oreochromis niloticus (Ayoub et al., 2021). Bio-SeNPs produced by Lactobacillus delbrueckii subsp. bulgaricus (NCAIM B 02206) were also used as feed additives for effective supplementation in O. niloticus diets to improve growth, oxidative status and immune-related gene expression (Dawood et al., 2020). Additionally, Bio-SeNPs were reported to promote the growth of Macrobrachium rosenbergii (Satgurunathan et al., 2023), and improve broiler performance and intestinal integrity (Ali et al., 2022). Overall, Bio-SeNPs may be a promising material to antibacterial against crop and animal pathogens, as well as contribute to the growth of crops and animals, and supply selenium element.
As a result of their great biological activities and potential applications, the toxicity of Bio-SeNPs has attracted increasing attention (Figure 4).
Figure 4. Toxicity of Bio-SeNPs in cells and animals. Cytotoxicity of Bio-SeNPs on normal cells (Prasad et al., 2013; Anu et al., 2016; Xu et al., 2019; Yazhiniprabha and Vaseeharan, 2019; Abbas et al., 2021; Fouda et al., 2022; Olawale et al., 2022) and tumor cells (Ranjitha and Ravishankar, 2018; Rajkumar et al., 2020; Salem et al., 2020; Shin et al., 2021; Hashem et al., 2021b; Al-Otaibi et al., 2022; Fouda et al., 2022). Toxicity of Bio-SeNPs on zebrafish (Chandramohan et al., 2018, 2019; Fan et al., 2022), rats (Al-Brakati et al., 2021), Artemia nauplii (Yazhiniprabha and Vaseeharan, 2019), and brine shrimp (Nagalingam et al., 2022).
Cytotoxicity assessment provides an essential foundation for the usage of Bio-SeNPs in the food industry. Various cells were used to test the toxicity of different biogenic selenium nanoparticles (Table 2). Bio-SeNPs produced by Spirulina platensis exhibited minimal cytotoxicity to normal kidney (Vero) cells and transformed human liver epithelial-2 (THLE-2) cell lines at concentrations of 0.39–100 μg/mL (Abbas et al., 2021). Similarly, 31.25–62.5 μg/mL Bio-SeNPs synthesized by Portulaca oleracea were almost nontoxic to Vero normal cells and human normal lung fibroblast (WI-38) lines (Fouda et al., 2022). Interestingly, Bio-SeNPs synthesized by Lactococcus lactis NZ9000 were not only nontoxic to intestinal porcine enterocytes jejunum (IPEC-J2) cells but could also alleviate enterotoxigenic E. coli K88-induced cell injury (Xu et al., 2019). In addition, Bio-SeNPs synthesized by lemon leaf extract protected lymphocytes, prevented DNA damage and reduced reactive oxygen species toxicity under UVB irradiation (Prasad et al., 2013). On the other hand, Bio-SeNPs also showed low cytotoxicity in some studies. Bio-SeNPs produced by Ocimum tenuiflorum revealed low toxicity to human embryonic kidney (HEK293) cells (Olawale et al., 2022). Bio-SeNPs (10–50 μg/mL) synthesized from M. koenigii berries exhibited low cytotoxicity on mouse mononuclear macrophages cells (RAW 264.7 macrophages), and minor cell destruction was observed at 50 μg/mL (Yazhiniprabha and Vaseeharan, 2019). The CC50 of Bio-SeNPs synthesized from Allium sativum pulp extract was 31.8 ± 0.6 μg/mL for Vero cells, while the CC50 of chemically synthesized SeNPs was 18.8 ± 0.8 μg/mL (Anu et al., 2016). These results suggested that the toxicity of Bio-SeNPs was lower than that of chemically synthesized SeNPs. Bio-SeNPs from different sources exhibited varied thresholds of toxicities depending on the dosage and constituents of the Bio-SeNPs.
Interestingly, Bio-SeNPs seem to exhibit higher toxicity to cancer cells than to normal cells. Bio-SeNPs synthesized by Cirsium setidens extracts were nontoxic to a normal mouse fibroblast cell line (NIH3T3) in the low concentration range (3.1–100 μg/mL) but significantly toxic to human non-small cell lung cancer (A549) cells (Shin et al., 2021). Likewise, Bio-SeNPs synthesized using P. corylophilum were less toxic to human normal lung fibroblasts (WI-38) than to human cancer colorectal adenocarcinoma epithelial cells (Caco-2) (Salem et al., 2020). Bio-SeNPs (31.25–1,000 μg/mL) from P. expansum ATTC 36200 also showed low toxicity to the Vero cell line CCL-81 but high toxicity to the human prostate cancer (PC3) cell line (Hashem et al., 2021b). Similar anticancer activity was also observed in human hepatocellular carcinomas HepG2 cells (Souza et al., 2022), human mammary tumor MCF-7 cells (Souza et al., 2022), human cervical carcinoma HeLa cells (Rajkumar et al., 2020) and human colorectal adenocarcinoma HT-29 cells (Ranjitha and Ravishankar, 2018). Compared to normal cells, Bio-SeNPs may be more inclined to counteract the rapid tumor cell proliferation and release more ROS to suppress tumor cells (Cui et al., 2018; Menon and Shanmugam, 2019). Accordingly, Bio-SeNPs might be great tumor agents.
It is critical to carry out animal toxicity tests before using Bio-SeNPs in the food industry. Researchers have used zebrafish, Artemia nauplii, shrimp and rats to test the toxicity of Bio-SeNPs. Zebrafish embryos treated with Bio-SeNPs synthesized by potato extract showed less toxicity at concentrations of 10–20 μg/mL, but exhibited improper heartbeat and edema of the embryonic sac, eye and head at concentrations of 30–50 μg/mL (Chandramohan et al., 2019). Similarly, Bio-SeNPs produced by B. subtilis MTCC441 were nontoxic to zebrafish embryos at 5 μg/mL, with low mortality at 10 μg/mL, but caused low heart rate, delayed hatching and low survival at 15–25 μg/mL (Chandramohan et al., 2018). Bio-SeNPs synthesized from Providencia sp. DXC had lower toxicity with an LC50 of 1.668 μg/mL at 96 h, whereas the chem-SeNPs caused more significant injury to liver and gill cells of zebrafish (Souza et al., 2022). Meanwhile, the LC50 and LC90 values of 68.27 μg/mL and 121.75 μg/mL for A. nauplii treated with Bio-SeNPs based on M. koenigii berry extracts, also showed slight toxicity while Bio-SeNPs accumulation was observed in the region of the median eye and food groove/gut, but damage to appendages and carapace was not evident (Yazhiniprabha and Vaseeharan, 2019). In addition, the survival ratio of brine shrimp treated with Morinda citrifolia-mediated Bio-SeNPs (5, 10, and 25 μg/mL) was 70, 80 and 30% within 2 days, respectively (Nagalingam et al., 2022). Interestingly, biosynthesized Lycopene-coated Bio-SeNPs (0.5 mg/kg) showed no significant toxicity to the liver and kidney organs and hematological parameters of rats, and even exhibited nephroprotective activity against AKI (glycerol-treated)-caused tissue damage in rat models (Al-Brakati et al., 2021). Different sources of biological selenium nanoparticles showed different toxic effects on different animals. Overall, the toxicity of Bio-SeNPs to animals is low, but it is essential to perform toxicity evaluation before any Bio-SeNPs are applied in food.
In this review, we summarize the great potential of Bio-SeNPs for the control of foodborne pathogens and analyze the antibacterial application and safety in the food industry. Currently, microbes and plant extracts are being explored on large scale for the synthesis of Bio-SeNPs. Microbes and plants contribute various bioactive substances which are thought to confer higher antibacterial potential to these Bio-SeNPs. The Bio-SeNPs were applied in food additives, food packaging and fertilizers/feeds for crop and animal. In addition, some cellular and animal toxicity assessment experiments have shown that Bio-SeNPs are non-toxic/low toxicity at low antibacterial concentrations. It implied that Bio-SeNPs showed great potential in the application of food industry.
Even though Bio-SeNPs exhibit excellent application prospect, there is still lots of work to do before its application. (1) Due to the diversity of Bio-SeNPs synthesis processes and the complexity components, there are some uncontrollable factors in the actual production. So, it is more essential to choose a safe biological system to synthesize Bio-SeNPs with excellent antibacterial properties and higher economic value. Probiotics and edible fungi may be good choices. (2) The antibacterial mechanisms of Bio-SeNPs are not very deep yet, mainly focusing on the description of antibacterial phenomena. Further researches should be paid to the genetic level and focus on the relationship between the properties of Bio-SeNPs and their mechanisms and pathways of antibacterial activity. (3) At present, Bio-SeNPs are mainly used in food packaging materials or fertilizers for crop in some cases. However, the application forms of Bio-SeNPs need to be further developed for maximum benefits. (4) The toxicity analysis showed that Bio-SeNPs were either low toxic or nontoxic at low concentrations while their antibacterial activities were evident at high concentrations. Accordingly, the activities of Bio-SeNPs need to be further strengthened.
BA, QD, and DL prepared the draft manuscript and the figures. XX, JT, and XS revised the manuscript. All authors contributed to the article and approved the submitted version.
This study was supported by the Hubei Province Key R&D Program Project (2022BCE010), the National Natural Science Foundation of China (32000066), Hubei Province Central Government Guides Local Project, Natural Science Foundation of Hubei Province (2022CFB503), the Innovation Team Project of Hubei Education Department (T2022010), and the Open Foundation of the Hubei Key Laboratory of Edible Wild Plants Conservation and Utilization (EWPL202209).
The authors declare that the research was conducted in the absence of any commercial or financial relationships that could be construed as a potential conflict of interest.
All claims expressed in this article are solely those of the authors and do not necessarily represent those of their affiliated organizations, or those of the publisher, the editors and the reviewers. Any product that may be evaluated in this article, or claim that may be made by its manufacturer, is not guaranteed or endorsed by the publisher.
Abadi, B., Hosseinalipour, S., Nikzad, S., Pourshaikhali, S., Fathalipour-Rayeni, H., Shafiei, G., et al. (2022). Capping agents for selenium nanoparticles in biomedical applications. J. Clust. Sci. 34, 1669–1690. doi: 10.1007/s10876-022-02341-3
Abbas, H. S., Abou Baker, D. H., and Ahmed, E. A. (2021). Cytotoxicity and antimicrobial efficiency of selenium nanoparticles biosynthesized by Spirulina platensis. Arch. Microbiol. 203, 523–532. doi: 10.1007/s00203-020-02042-3
Abdel-Moneim, A. E., El-Saadony, M. T., Shehata, A. M., Saad, A. M., Aldhumri, S. A., Ouda, S. M., et al. (2022). Antioxidant and antimicrobial activities of Spirulina platensis extracts and biogenic selenium nanoparticles against selected pathogenic bacteria and fungi. Saudi J. Biol. Sci. 29, 1197–1209. doi: 10.1016/j.sjbs.2021.09.046
Abebe, E., Gugsa, G., and Ahmed, M. (2020). Review on major food-borne zoonotic bacterial pathogens. J. Trop. Med. 2020:4674235. doi: 10.1155/2020/4674235
Abu-Elghait, M., Hasanin, M., Hashem, A. H., and Salem, S. S. (2021). Ecofriendly novel synthesis of tertiary composite based on cellulose and myco-synthesized selenium nanoparticles: characterization, antibiofilm and biocompatibility. Int. J. Biol. Macromol. 175, 294–303. doi: 10.1016/j.ijbiomac.2021.02.040
Adebayo, E. A., Azeez, M. A., Alao, M. B., Oke, A. M., and Aina, D. A. (2021). Fungi as veritable tool in current advances in nanobiotechnology. Heliyon 7:e08480. doi: 10.1016/j.heliyon.2021.e08480
Alam, H., Khatoon, N., Khan, M. A., Husain, S. A., Saravanan, M., and Sardar, M. (2019). Synthesis of selenium nanoparticles using probiotic bacteria Lactobacillus acidophilus and their enhanced antimicrobial activity against resistant bacteria. J. Clust. Sci. 31, 1003–1011. doi: 10.1007/s10876-019-01705-6
Alam, H., Khatoon, N., Raza, M., Ghosh, P. C., and Sardar, M. (2018). Synthesis and characterization of nano selenium using plant biomolecules and their potential applications. BioNanoScience 9, 96–104. doi: 10.1007/s12668-018-0569-5
Al-Brakati, A., Alsharif, K. F., Alzahrani, K. J., Kabrah, S., Al-Amer, O., Oyouni, A. A., et al. (2021). Using green biosynthesized lycopene-coated selenium nanoparticles to rescue renal damage in glycerol-induced acute kidney injury in rats. Int. J. Nanomedicine 16, 4335–4349. doi: 10.2147/IJN.S306186
Algammal, A. M., Hetta, H. F., Elkelish, A., Alkhalifah, D. H. H., Hozzein, W. N., Batiha, G. E., et al. (2020). Methicillin-resistant Staphylococcus aureus (MRSA): one health perspective approach to the bacterium epidemiology, virulence factors, antibiotic-resistance, and zoonotic impact. Infect. Drug. Resist. 13, 3255–3265. doi: 10.2147/IDR.S272733
Alghuthaymi, M. A., Diab, A. M., Elzahy, A. F., Mazrou, K. E., Tayel, A. A., Moussa, S. H., et al. (2021). Green biosynthesized selenium nanoparticles by cinnamon extract and their antimicrobial activity and application as edible coatings with nano-chitosan. J. Food Quality 2021, 1–10. doi: 10.1155/2021/6670709
Ali, A., Parisi, A., Conversano, M. C., Iannacci, A., D’Emilio, F., Mercurio, V., et al. (2020). Food-borne bacteria associated with seafoods: a brief review. J. Food Qual. HazardsControl 7, 4–10. doi: 10.18502/jfqhc.7.1.2446
Ali, F., Saeed, K., and Fatemeh, H. (2022). Nano-bio selenium synthesized by Bacillus subtilis modulates broiler performance, intestinal morphology and microbiota, and expression of tight junction’s proteins. Biol. Trace Elem. Res. 200, 1811–1825. doi: 10.1007/s12011-021-02767-2
Alibi, S., Crespo, D., and Navas, J. (2021). Plant-derivatives small molecules with antibacterial activity. Antibiotics 10:231. doi: 10.3390/antibiotics10030231
Al-Otaibi, A. M., Al-Gebaly, A. S., Almeer, R., Albasher, G., Al-Qahtani, W. S., and Abdel Moneim, A. E. (2022). Potential of green-synthesized selenium nanoparticles using apigenin in human breast cancer MCF-7 cells. Environ. Sci. Pollut. Res. Int. 29, 47539–47548. doi: 10.1007/s11356-022-19166-2
Al-Saggaf, M. S., Tayel, A. A., Ghobashy, M. O. I., Alotaibi, M. A., Alghuthaymi, M. A., and Moussa, S. H. (2020). Phytosynthesis of selenium nanoparticles using the costus extract for bactericidal application against foodborne pathogens. Green Process. Synth. 9, 477–487. doi: 10.1515/gps-2020-0038
Anu, K., Singaravelu, G., Murugan, K., and Benelli, G. (2016). Green-synthesis of selenium nanoparticles using garlic cloves (Allium sativum): biophysical characterization and cytotoxicity on Vero cells. J. Clust. Sci. 28, 551–563. doi: 10.1007/s10876-016-1123-7
Ao, B., Lv, J., Yang, H., He, F., Hu, Y., Hu, B., et al. (2022). Moringa oleifera extract mediated the synthesis of Bio-SeNPs with antibacterial activity against Listeria monocytogenes and Corynebacterium diphtheriae. LWT 165:113751. doi: 10.1016/j.lwt.2022.113751
Ayoub, H. F., Tohamy, E. Y., Salama, H. M., and Mohamed, S. S. (2021). Citrullus colocynthis extract and synthesized selenium nanoparticles enhance non-specific response and resistance against Aeromonas sobria in Nile tilapia (Oreochromis niloticus). Aquac. Res. 52, 4969–4982. doi: 10.1111/are.15366
Azimirad, M., Nadalian, B., Alavifard, H., Negahdar Panirani, S., Mahdigholi Vand Bonab, S., Azimirad, F., et al. (2021). Microbiological survey and occurrence of bacterial foodborne pathogens in raw and ready-to-eat green leafy vegetables marketed in Tehran, Iran. Int. J. Hyg. Envir. Heal. 237:113824. doi: 10.1016/j.ijheh.2021.113824
Bao, Y., He, J., Song, K., Guo, J., Zhou, X., Liu, S., et al. (2021). Plant-extract-mediated synthesis of metal nanoparticles. J. Chem. 2021, 1–14. doi: 10.1155/2021/6562687
Baquero, F., F Lanza, V., Duval, M., and Coque, T. M. (2020). Ecogenetics of antibiotic resistance in Listeria monocytogenes. Mol. Microbiol. 113, 570–579. doi: 10.1111/mmi.14454
Bisht, N., Phalswal, P., and Khanna, P. K. (2022). Selenium nanoparticles: a review on synthesis and biomedical applications. Mater. Adv. 3, 1415–1431. doi: 10.1039/d1ma00639h
Chacha, J. S., Zhang, L., Ofoedu, C. E., Suleiman, R. A., Dotto, J. M., Roobab, U., et al. (2021). Revisiting non-thermal food processing and preservation methods-action mechanisms, pros and cons: a technological update (2016-2021). Foods 10:1430. doi: 10.3390/foods10061430
Chandramohan, S., Sundar, K., and Muthukumaran, A. (2018). Monodispersed spherical shaped selenium nanoparticles (SeNPs) synthesized by Bacillus subtilisand its toxicity evaluation in zebrafish embryos. Mater. Res. Express. 5:025020. doi: 10.1088/2053-1591/aaabeb
Chandramohan, S., Sundar, K., and Muthukumaran, A. (2019). Hollow selenium nanoparticles from potato extract and investigation of its biological properties and developmental toxicity in zebrafish embryos. IET Nanobiotechnol. 13, 275–281. doi: 10.1049/iet-nbt.2018.5228
Chaudhry, Q., Watkins, R., and Castle, L. (2010). Nanotechnologies in the food arena: new opportunities, new questions, new concerns. J. Nanotechnol. Food 14, 1–17. doi: 10.1039/9781847559883-00001
Chen, J., Sun, R., Pan, C., Sun, Y., Mai, B., and Li, Q. X. (2020). Antibiotics and food safety in aquaculture. J. Agric. Food Chem. 68, 11908–11919. doi: 10.1021/acs.jafc.0c03996
Christianah Adebayo-Tayo, B., Olawunmi Yusuf, B., and Omoniyi Alao, S. (2021). Antibacterial activity of intracellular greenly fabricated selenium nanoparticle of Lactobacillus pentosus ADET MW861694 against selected food pathogens. Indian J. Biochem. Bio. 10, 39–51. doi: 10.18488/journal.57.2021.101.39.51
Cittrarasu, V., Kaliannan, D., Dharman, K., Maluventhen, V., Easwaran, M., Liu, W. C., et al. (2021). Green synthesis of selenium nanoparticles mediated from Ceropegia bulbosa Roxb extract and its cytotoxicity, antimicrobial, mosquitocidal and photocatalytic activities. Sci. Rep. 11:1032. doi: 10.1038/s41598-020-80327-9
Cremonini, E., Boaretti, M., Vandecandelaere, I., Zonaro, E., Coenye, T., Lleo, M. M., et al. (2018). Biogenic selenium nanoparticles synthesized by Stenotrophomonas maltophilia SeITE02 loose antibacterial and antibiofilm efficacy as a result of the progressive alteration of their organic coating layer. Microb. Biotechnol. 11, 1037–1047. doi: 10.1111/1751-7915.13260
Cui, D., Liang, T., Sun, L., Meng, L., Yang, C., Wang, L., et al. (2018). Green synthesis of selenium nanoparticles with extract of hawthorn fruit induced HepG2 cells apoptosis. Pharm. Biol. 56, 528–534. doi: 10.1080/13880209.2018.1510974
Dang-Bao, T., Ho, T. G., Do, B. L., Phung Anh, N., Phan, T. D. T., Tran, T. B. Y., et al. (2022). Green orange peel-mediated bioinspired synthesis of nanoselenium and its antibacterial activity against methicillin-resistant Staphylococcus aureus. ACS Omega 7, 36037–36046. doi: 10.1021/acsomega.2c05469
Dawood, M. A. O., Zommara, M., Eweedah, N. M., and Helal, A. I. (2020). The evaluation of growth performance, blood health, oxidative status and immune-related gene expression in Nile tilapia (Oreochromis niloticus) fed dietary nanoselenium spheres produced by lactic acid bacteria. Aquaculture 515:734571. doi: 10.1016/j.aquaculture.2019.734571
El-Saadony, M. T., Saad, A. M., Najjar, A. A., Alzahrani, S. O., Alkhatib, F. M., Shafi, M. E., et al. (2021). The use of biological selenium nanoparticles to suppress Triticum aestivum L. crown and root rot diseases induced by Fusarium species and improve yield under drought and heat stress. Saudi J. Biol. Sci. 28, 4461–4471. doi: 10.1016/j.sjbs.2021.04.043
El-Sayed, E. R., Abdelhakim, H. K., and Ahmed, A. S. (2020). Solid-state fermentation for enhanced production of selenium nanoparticles by gamma-irradiated Monascus purpureus and their biological evaluation and photocatalytic activities. Bioprocess Biosyst. Eng. 43, 797–809. doi: 10.1007/s00449-019-02275-7
El-Zayat, M. M., Eraqi, M. M., Alrefai, H., El-Khateeb, A. Y., Ibrahim, M. A., Aljohani, H. M., et al. (2021). The antimicrobial, antioxidant, and anticancer activity of greenly synthesized selenium and zinc composite nanoparticles using Ephedra aphylla extract. Biomol. Ther. 11:470. doi: 10.3390/biom11030470
Escobar-Ramírez, M. C., Castañeda-Ovando, A., Pérez-Escalante, E., Rodríguez-Serrano, G. M., Ramírez-Moreno, E., Quintero-Lira, A., et al. (2021). Antimicrobial activity of se-nanoparticles from bacterial biotransformation. Fermentation 7:130. doi: 10.3390/fermentation7030130
Fan, S., Yang, Y., Sun, L., Yu, B., Dai, C., and Qu, Y. (2022). Different toxicity to liver and gill of zebrafish by selenium nanoparticles derived from bio/chemical methods. Environ. Sci. Pollut. Res. Int. 29, 61512–61521. doi: 10.1007/s11356-022-20295-x
Fatima, F., Siddiqui, S., and Khan, W. A. (2021). Nanoparticles as novel emerging therapeutic antibacterial agents in the antibiotics resistant era. Biol. Trace Elem. Res. 199, 2552–2564. doi: 10.1007/s12011-020-02394-3
Fouda, A., Al-Otaibi, W. A., Saber, T., AlMotwaa, S. M., Alshallash, K. S., Elhady, M., et al. (2022). Antimicrobial, antiviral, and in-vitro cytotoxicity and mosquitocidal activities of Portulaca oleracea-based green synthesis of selenium nanoparticles. J. Funct. Biomater. 13:157. doi: 10.3390/jfb13030157
Gad, H. A., Alshubaily, F. A., Alsieni, M. A., Tayel, A. A., and Diab, A. M. (2022). Biosynthesis of nano-curcumin/nano-selenium composite and their potentialities as bactericides against fish-borne pathogens. Green Process. Synth. 11, 1098–1107. doi: 10.1515/gps-2022-0095
Galbadage, T., Liu, D., Alemany, L. B., Pal, R., Tour, J. M., Gunasekera, R. S., et al. (2019). Molecular nanomachines disrupt bacterial cell wall, increasing sensitivity of extensively drug-resistant Klebsiella pneumoniae to meropenem. ACS Nano 13, 14377–14387. doi: 10.1021/acsnano.9b07836
Ge, H., Wang, Y., and Zhao, X. (2022). Research on the drug resistance mechanism of foodborne pathogens. Microb. Pathog. 162:105306. doi: 10.1016/j.micpath.2021.105306
Ghosh, S., Nandi, S., and Basu, T. (2021). Nano-antibacterials using medicinal plant components: an overview. Front. Microbiol. 12:768739. doi: 10.3389/fmicb.2021.768739
Gunti, L., Dass, R. S., and Kalagatur, N. K. (2019). Phytofabrication of selenium nanoparticles from Emblica officinalis fruit extract and exploring its biopotential applications: antioxidant, antimicrobial, and biocompatibility. Front. Microbiol. 10:931. doi: 10.3389/fmicb.2019.00931
Guo, L., Azam, S. M. R., Guo, Y., Liu, D., and Ma, H. (2022). Germicidal efficacy of the pulsed magnetic field against pathogens and spoilage microorganisms in food processing: an overview. Food Control 136:108496. doi: 10.1016/j.foodcont.2021.108496
Gurtler, J. B., and Gibson, K. E. (2022). Irrigation water and contamination of fresh produce with bacterial foodborne pathogens. Curr. Opin. Food Sci. 47:100889. doi: 10.1016/j.cofs.2022.100889
Haddadian, A., Robattorki, F. F., Dibah, H., Soheili, A., Ghanbarzadeh, E., Sartipnia, N., et al. (2022). Niosomes-loaded selenium nanoparticles as a new approach for enhanced antibacterial, anti-biofilm, and anticancer activities. Sci. Rep. 12:21938. doi: 10.1038/s41598-022-26400-x
Hashem, A. H., Abdelaziz, A. M., Askar, A. A., Fouda, H. M., Khalil, A. M. A., Abd-Elsalam, K. A., et al. (2021a). Bacillus megaterium-mediated synthesis of selenium nanoparticles and their antifungal activity against Rhizoctonia solani in faba bean plants. J. Fungi 7:195. doi: 10.3390/jof7030195
Hashem, A. H., Khalil, A. M. A., Reyad, A. M., and Salem, S. S. (2021b). Biomedical applications of mycosynthesized selenium nanoparticles using Penicillium expansum ATTC 36200. Biol. Trace Elem. Res. 199, 3998–4008. doi: 10.1007/s12011-020-02506-z
Hashem, A. H., and Salem, S. S. (2022). Green and ecofriendly biosynthesis of selenium nanoparticles using Urtica dioica (stinging nettle) leaf extract: antimicrobial and anticancer activity. Biotechnol. J. 17:e2100432. doi: 10.1002/biot.202100432
Hashem, A. H., Selim, T. A., Alruhaili, M. H., Selim, S., Alkhalifah, D. H. M., Al Jaouni, S. K., et al. (2022). Unveiling antimicrobial and insecticidal activities of biosynthesized selenium nanoparticles using prickly pear peel waste. J. Funct. Biomater. 13:112. doi: 10.3390/jfb13030112
Hoffmann, S., and Scallan Walter, E. (2020). Acute complications and sequelae from foodborne infections: informing priorities for cost of foodborne illness estimates. Foodborne Pathog. Dis. 17, 172–177. doi: 10.1089/fpd.2019.2664
Hu, D., Yu, S., Yu, D., Liu, N., Tang, Y., Fan, Y., et al. (2019). Biogenic Trichoderma harzianum-derived selenium nanoparticles with control functionalities originating from diverse recognition metabolites against phytopathogens and mycotoxins. Food Control 106:106748. doi: 10.1016/j.foodcont.2019.106748
Hussein, H. G., El-Sayed, E. R., Younis, N. A., Hamdy, A., and Easa, S. M. (2022). Harnessing endophytic fungi for biosynthesis of selenium nanoparticles and exploring their bioactivities. AMB Express 12:68. doi: 10.1186/s13568-022-01408-8
Ikram, M., Javed, B., Raja, N. I., and Mashwani, Z. U. (2021). Biomedical potential of plant-based selenium nanoparticles: a comprehensive review on therapeutic and mechanistic aspects. Int. J. Nanomedicine 16, 249–268. doi: 10.2147/IJN.S295053
Impey, R. E., Hawkins, D. A., Sutton, J. M., and Soares da Costa, T. P. (2020). Overcoming intrinsic and acquired resistance mechanisms associated with the cell wall of gram-negative bacteria. Antibiotics 9:623. doi: 10.3390/antibiotics9090623
Iwu, C. D., and Okoh, A. I. (2019). Preharvest transmission routes of fresh produce associated bacterial pathogens with outbreak potentials: a review. Int. J. Environ. Res. Public Health 16:4407. doi: 10.3390/ijerph16224407
Jadoun, S., Arif, R., Jangid, N. K., and Meena, R. K. (2020). Green synthesis of nanoparticles using plant extracts: a review. Environ. Chem. Lett. 19, 355–374. doi: 10.1007/s10311-020-01074-x
Jamróz, E., Kopel, P., Juszczak, L., Kawecka, A., Bytesnikova, Z., Milosavljevic, V., et al. (2019a). Development of furcellaran-gelatin films with Se-AgNPs as an active packaging system for extension of mini kiwi shelf life. Food Packag. Shelf 21:100339. doi: 10.1016/j.fpsl.2019.100339
Jamróz, E., Kulawik, P., Kopel, P., Balková, R., Hynek, D., Bytesnikova, Z., et al. (2019b). Intelligent and active composite films based on furcellaran: structural characterization, antioxidant and antimicrobial activities. Food Packag. Shelf 22:10040. doi: 10.1016/j.fpsl.2019.100405
Jeevanandam, J., Kiew, S. F., Boakye-Ansah, S., Lau, S. Y., Barhoum, A., Danquah, M. K., et al. (2022). Green approaches for the synthesis of metal and metal oxide nanoparticles using microbial and plant extracts. Nanoscale 14, 2534–2571. doi: 10.1039/d1nr08144f
Kaur, R., Kaudal, T., and Sharma, A. (2018). Probiotic mediated synthesis of selenium nanoparticles: characterization and biofilm scavenging analysis. RJLBPCS 4:291. doi: 10.26479/2018.0403.26
Keba, A., Rolon, M. L., Tamene, A., Dessie, K., Vipham, J., Kovac, J., et al. (2020). Review of the prevalence of foodborne pathogens in milk and dairy products in Ethiopia. Int. Dairy J. 109:104762. doi: 10.1016/j.idairyj.2020.104762
Kerry, R. G., Patra, J. K., Gouda, S., Park, Y., Shin, H. S., and Das, G. (2018). Benefaction of probiotics for human health: a review. J. Food Drug Anal. 26, 927–939. doi: 10.1016/j.jfda.2018.01.002
Khan, F., Shariq, M., Asif, M., Siddiqui, M. A., Malan, P., and Ahmad, F. (2022). Green nanotechnology: plant-mediated nanoparticle synthesis and application. Nanomaterials 12:673. doi: 10.3390/nano12040673
Khiralla, G. M., and El-Deeb, B. A. (2015). Antimicrobial and antibiofilm effects of selenium nanoparticles on some foodborne pathogens. LWT 63, 1001–1007. doi: 10.1016/j.lwt.2015.03.086
Khouryieh, H. A. (2021). Novel and emerging technologies used by the U.S. food processing industry. Innov. Food Sci. Emerg. 67:102559. doi: 10.1016/j.ifset.2020.102559
Kieliszek, M. (2019). Selenium-fascinating microelement, properties and sources in food. Molecules 24:1298. doi: 10.3390/molecules24071298
Kondaparthi, P., Flora, S. J. S., and Naqvi, S. (2019). Selenium nanoparticles: an insight on its pro-oxidant and antioxidant properties. Front. Nanosci. Nanotechnol. 6, 1, –5. doi: 10.15761/fnn.1000189
Krishnan, M., Ranganathan, K., Maadhu, P., Thangavelu, P., Kundan, S., and Arjunan, N. (2020). Leaf extract of Dillenia indica as a source of selenium nanoparticles with larvicidal and antimicrobial potential toward vector mosquitoes and pathogenic microbes. Coatings 10:626. doi: 10.3390/coatings10070626
Krishnani, K. K., Boddu, V. M., Chadha, N. K., Chakraborty, P., Kumar, J., Krishna, G., et al. (2022). Metallic and non-metallic nanoparticles from plant, animal, and fisheries wastes: potential and valorization for application in agriculture. Environ. Sci. Pollut. Res. Int. 29, 81130–81165. doi: 10.1007/s11356-022-23301-4
Kumar, A., Asthana, M., Gupta, A., Nigam, D., and Mahajan, S. (2018). Chapter3-secondary metabolism and antimicrobial metabolites of Penicillium. New Fut. Dev. Microb. Biotechnol. Bioeng. 2018, 47–68. doi: 10.1016/B978-0-444-63501-3.00003-X
Kumari, P., Luqman, S., and Meena, A. (2019). Application of the combinatorial approaches of medicinal and aromatic plants with nanotechnology and its impacts on healthcare. Daru 27, 475–489. doi: 10.1007/s40199-019-00271-6
Lashin, I., Hasanin, M., Hassan, S. A. M., and Hashem, A. H. (2021). Green biosynthesis of zinc and selenium oxide nanoparticles using callus extract of Ziziphus spina-christi: characterization, antimicrobial, and antioxidant activity. Biomass Convers. Bior 2021, 1–14. doi: 10.1007/s13399-021-01873-4
Li, W., Pires, S. M., Liu, Z., Ma, X., Liang, J., Jiang, Y., et al. (2020). Surveillance of foodborne disease outbreaks in China, 2003–2017. Food Control 118:107359. doi: 10.1016/j.foodcont.2020.107359
Liang, X., Perez, M. A. M., Nwoko, K. C., Egbers, P., Feldmann, J., Csetenyi, L., et al. (2019). Fungal formation of selenium and tellurium nanoparticles. Appl. Microbiol. Biot. 103, 7241–7259. doi: 10.1007/s00253-019-09995-6
Liao, X., Ma, Y., Daliri, E. B.-M., Koseki, S., Wei, S., Liu, D., et al. (2020). Interplay of antibiotic resistance and food-associated stress tolerance in foodborne pathogens. Trends Food Sci. Tech. 95, 97–106. doi: 10.1016/j.tifs.2019.11.006
Linklater, D. P., Baulin, V. A., Le Guével, X., Fleury, J. B., Hanssen, E., Nguyen, T. H. P., et al. (2020). Antibacterial action of nanoparticles by lethal stretching of bacterial cell membranes. Adv. Mater. 32:e2005679. doi: 10.1002/adma.202005679
Liu, Y., Wu, Y., Wu, J., Li, X., Yu, L., Xie, K., et al. (2022). Exposure to veterinary antibiotics via food chain disrupts gut microbiota and drives increased Escherichia coli virulence and drug resistance in young adults. Pathogens 11:1062. doi: 10.3390/pathogens11091062
Lu, R., Sameen, D. E., Qin, W., Wu, D., Dai, J., Li, S., et al. (2020). Development of polylactic acid films with selenium microparticles and its application for food packaging. Coatings 10:280. doi: 10.3390/coatings10030280
Majeed, A., Javed, F., Akhtar, S., Saleem, U., Anwar, F., Ahmad, B., et al. (2020). Green synthesized selenium doped zinc oxide nano-antibiotic: synthesis, characterization and evaluation of antimicrobial, nanotoxicity and teratogenicity potential. J. Mater. Chem. B 8, 8444–8458. doi: 10.1039/d0tb01553a
Makabenta, J. M. V., Nabawy, A., Li, C. H., Schmidt-Malan, S., Patel, R., and Rotello, V. M. (2021). Nanomaterial-based therapeutics for antibiotic-resistant bacterial infections. Nat. Rev. Microbiol. 19, 23–36. doi: 10.1038/s41579-020-0420-1
Matai, I., Pandey, S. K., Garg, D., Rani, K., and Sachdev, A. (2020). Phytogreen synthesis of multifunctional nano selenium with antibacterial and antioxidant implications. Nano Express 1:010031. doi: 10.1088/2632-959X/ab8bea
Menon, S., and Shanmugam, V. (2019). Cytotoxicity analysis of biosynthesized selenium nanoparticles towards A549 lung cancer cell line. J. Inorg. Organomet. P. 30, 1852–1864. doi: 10.1007/s10904-019-01409-4
Menon, S., Shrudhi Devi, K. S., Agarwal, H., and Shanmugam, V. K. (2019). Efficacy of biogenic selenium nanoparticles from an extract of ginger towards evaluation on anti-microbial and anti-oxidant activities. Colloid Interfac Sci. 29, 1–8. doi: 10.1016/j.colcom.2018.12.004
Miglani, S., and Tani-Ishii, N. (2021). Biosynthesized selenium nanoparticles: characterization, antimicrobial, and antibiofilm activity against Enterococcus faecalis. PeerJ 9:e11653. doi: 10.7717/peerj.11653
Mohanta, Y. K., Hashem, A., Abd Allah, E. F., Jena, S. K., and Mohanta, T. K. (2020). Bacterial synthesized metal and metal salt nanoparticles in biomedical applications: an up and coming approach. Appl. Organomet. Chem. 34:e5810. doi: 10.1002/aoc.5810
Mourenza, A., Gil, J. A., Mateos, L. M., and Letek, M. (2020). Oxidative stress-generating antimicrobials, a novel strategy to overcome antibacterial resistance. Antioxidants 9:361. doi: 10.3390/antiox9050361
Nagalingam, M., Rajeshkumar, S., Balu, S. K., Tharani, M., Arunachalam, K., and Roy, A. (2022). Anticancer and antioxidant activity of Morinda Citrifolia leaf mediated selenium nanoparticles. J. Nanomater. 2022, 1–7. doi: 10.1155/2022/2155772
Naikoo, G. A., Mustaqeem, M., Hassan, I. U., Awan, T., Arshad, F., Salim, H., et al. (2021). Bioinspired and green synthesis of nanoparticles from plant extracts with antiviral and antimicrobial properties: a critical review. J. Saudi Chem. Soc. 25:101304. doi: 10.1016/j.jscs.2021.101304
Nayak, V., Singh, K. R. B., Singh, A. K., and Singh, R. P. (2021). Potentialities of selenium nanoparticles in biomedical science. New J. Chem. 45, 2849–2878. doi: 10.1039/d0nj05884j
Ndwandwe, B. K., Malinga, S. P., Kayitesi, E., and Dlamini, B. C. (2020). Advances in green synthesis of selenium nanoparticles and their application in food packaging. Int. J. Food Sci. Tech. 56, 2640–2650. doi: 10.1111/ijfs.14916
Ndwandwe, B. K., Malinga, S. P., Kayitesi, E., and Dlamini, B. C. (2022). Selenium nanoparticles–enhanced potato starch film for active food packaging application. Int. J. Food Sci. Tech. 57, 6512–6521. doi: 10.1111/ijfs.15990
Ojeda, J. J., Merroun, M. L., Tugarova, A. V., Lampis, S., Kamnev, A. A., and Gardiner, P. H. E. (2020). Developments in the study and applications of bacterial transformations of selenium species. Crit. Rev. Biotechnol. 40, 1250–1264. doi: 10.1080/07388551.2020.1811199
Olawale, F., Ariatti, M., and Singh, M. (2022). Ocimum tenuiflorum L mediated green synthesis of silver and selenium nanoparticles: antioxidant activity, cytotoxicity and density functional theory studies. Adv. Nat. Sci. Nanosci. 13:015015. doi: 10.1088/2043-6262/ac5d4a
Pasquina-Lemonche, L., Burns, J., Turner, R. D., Kumar, S., Tank, R., Mullin, N., et al. (2020). The architecture of the gram-positive bacterial cell wall. Nature 582, 294–297. doi: 10.1038/s41586-020-2236-6
Perumal, S., Gopal Samy, M. V., and Subramanian, D. (2021). Selenium nanoparticle synthesis from endangered medicinal herb (Enicostema axillare). Bioprocess Biosyst. Eng. 44, 1853–1863. doi: 10.1007/s00449-021-02565-z
Prasad, K. S., Patel, H., Patel, T., Patel, K., and Selvaraj, K. (2013). Biosynthesis of se nanoparticles and its effect on UV-induced DNA damage. Colloid. Surface. B 103, 261–266. doi: 10.1016/j.colsurfb.2012.10.029
Prasathkumar, M., Sakthivel, C., Becky, R., Dhrisya, C., Prabha, I., and Sadhasivam, S. (2022). Phytofabrication of cost-effective selenium nanoparticles from edible and non-edible plant materials of Senna auriculata: characterization, antioxidant, antidiabetic, antimicrobial, biocompatibility, and wound healing. J. Mol. Liq. 367:120337. doi: 10.1016/j.molliq.2022.120337
Rajkumar, K., Mvs, S., Koganti, S., and Burgula, S. (2020). Selenium nanoparticles synthesized using Pseudomonas stutzeri (MH191156) show antiproliferative and anti-angiogenic activity against cervical cancer cells. Int. J. Nanomedicine 15, 4523–4540. doi: 10.2147/IJN.S247426
Ramya, S., Shanmugasundaram, T., and Balagurunathan, R. (2015). Biomedical potential of actinobacterially synthesized selenium nanoparticles with special reference to anti-biofilm, anti-oxidant, wound healing, cytotoxic and anti-viral activities. J. Trace Elem. Med. Biol. 32, 30–39. doi: 10.1016/j.jtemb.2015.05.005
Ramya, S., Shanmugasundaram, T., and Balagurunathan, R. (2019). Actinobacterial enzyme mediated synthesis of selenium nanoparticles for antibacterial, mosquito larvicidal and anthelminthic applications. Particul. Sci. Technol. 38, 63–72. doi: 10.1080/02726351.2018.1508098
Ranjitha, V. R., and Ravishankar, V. R. (2018). Extracellular synthesis of selenium nanoparticles from an actinomycetes Streptomyces griseoruber and evaluation of its cytotoxicity on HT-29 cell line. Pharm. Nanotechnol. 6, 61–68. doi: 10.2174/2211738505666171113141010
Reygaert, W. C. (2018). An overview of the antimicrobial resistance mechanisms of bacteria. AIMS Microbiol. 4, 482–501. doi: 10.3934/microbiol.2018.3.482
Riley, L. W. (2020). Extraintestinal foodborne pathogens. Annu. Rev. Food Sci. Technol. 11, 275–294. doi: 10.1146/annurev-food-032519-051618
Saad, A. M., Sitohy, M. Z., Sultan-Alolama, M. I., El-Tarabily, K. A., and El-Saadony, M. T. (2022). Green nanotechnology for controlling bacterial load and heavy metal accumulation in Nile tilapia fish using biological selenium nanoparticles biosynthesized by Bacillus subtilis AS12. Front. Microbiol. 13:1015613. doi: 10.3389/fmicb.2022.1015613
Sadoq, B.-E., Britel, M. R., Bouajaj, A., Maâlej, R., Touhami, A., Abid, M., et al. (2023). A review on antibacterial activity of nanoparticles. Biointerface. Res. App. 13:405. doi: 10.33263/BRIAC135.405
Sakr, T. M., Korany, M., and Katti, K. V. (2018). Selenium nanomaterials in biomedicine—an overview of new opportunities in nanomedicine of selenium. J. Drug Deliv. Sci. Tec. 46, 223–233. doi: 10.1016/j.jddst.2018.05.023
Salem, S. S. (2022). Bio-fabrication of selenium nanoparticles using Baker’s yeast extract and its antimicrobial efficacy on food borne pathogens. Appl. Biochem. Biotechnol. 194, 1898–1910. doi: 10.1007/s12010-022-03809-8
Salem, M. F., Abd-Elraoof, W. A., Tayel, A. A., Alzuaibr, F. M., and Abonama, O. M. (2022). Antifungal application of biosynthesized selenium nanoparticles with pomegranate peels and nanochitosan as edible coatings for citrus green mold protection. J. Nanobiotechnol. 20:182. doi: 10.1186/s12951-022-01393-x
Salem, S. S., Badawy, M., Al-Askar, A. A., Arishi, A. A., Elkady, F. M., and Hashem, A. H. (2022). Green biosynthesis of selenium nanoparticles using orange peel waste: characterization, antibacterial and antibiofilm activities against multidrug-resistant bacteria. Life 12:893. doi: 10.3390/life12060893
Salem, S. S., Fouda, M. M. G., Fouda, A., Awad, M. A., Al-Olayan, E. M., Allam, A. A., et al. (2020). Antibacterial, cytotoxicity and larvicidal activity of green synthesized selenium nanoparticles using Penicillium corylophilum. J. Clust. Sci. 32, 351–361. doi: 10.1007/s10876-020-01794-8
San Keskin, N. O., Akbal Vural, O., and Abaci, S. (2020). Biosynthesis of noble selenium nanoparticles from Lysinibacillus sp. NOSK for antimicrobial, antibiofilm activity, and biocompatibility. Geomicrobiol J. 37, 919–928. doi: 10.1080/01490451.2020.1799264
Sarkar, R. D., and Kalita, M. C. (2022). Se nanoparticles stabilized with Allamanda cathartica L. flower extract inhibited phytopathogens and promoted mustard growth under salt stress. Heliyon 8:e09076. doi: 10.1016/j.heliyon.2022.e09076
Satgurunathan, T., Bhavan, P. S., Kalpana, R., Jayakumar, T., Sheu, J. R., and Manjunath, M. (2023). Influence of garlic (Allium sativum) clove-based selenium nanoparticles on status of nutritional, biochemical, enzymological, and gene expressions in the freshwater prawn Macrobrachium rosenbergii (De Man, 1879). Biol. Trace Elem. Res. 201, 2036–2057. doi: 10.1007/s12011-022-03300-9
Shaaban, M., and El-Mahdy, A. M. (2018). Biosynthesis of Ag, Se, and ZnO nanoparticles with antimicrobial activities against resistant pathogens using waste isolate Streptomyces enissocaesilis. IET Nanobiotechnol. 12, 741–747. doi: 10.1049/iet-nbt.2017.0213
Shah, V., Medina-Cruz, D., Vernet-Crua, A., Truong, L. B., Sotelo, E., Mostafavi, E., et al. (2022). Pepper-mediated green synthesis of selenium and tellurium nanoparticles with antibacterial and anticancer potential. J. Funct. Biomater. 14:24. doi: 10.3390/jfb14010024
Shahbaz, M., Fatima, N., Mashwani, Z. U. R., Akram, A., Haq, E. U., Mehak, A., et al. (2022). Effect of phytosynthesized selenium and cerium oxide nanoparticles on wheat (Triticum aestivum L.) against stripe rust disease. Molecules 27:8149. doi: 10.3390/molecules27238149
Shakibaie, M., Forootanfar, H., Golkari, Y., Mohammadi-Khorsand, T., and Shakibaie, M. R. (2015). Anti-biofilm activity of biogenic selenium nanoparticles and selenium dioxide against clinical isolates of Staphylococcus aureus, Pseudomonas aeruginosa, and Proteus mirabilis. J. Trace Elem. Med. Biol. 29, 235–241. doi: 10.1016/j.jtemb.2014.07.020
Shao, Y., Wang, Y., Yuan, Y., and Xie, Y. (2021). A systematic review on antibiotics misuse in livestock and aquaculture and regulation implications in China. Sci. Total Environ. 798:149205. doi: 10.1016/j.scitotenv.2021.149205
Shin, S., Saravanakumar, K., Mariadoss, A. V. A., Hu, X., Sathiyaseelan, A., and Wang, M.-H. (2021). Functionalization of selenium nanoparticles using the methanolic extract of Cirsium setidens and its antibacterial, antioxidant, and cytotoxicity activities. J. Nanostructure Chem. 12, 23–32. doi: 10.1007/s40097-021-00397-7
Shoeibi, S., Mozdziak, P., and Golkar-Narenji, A. (2017). Biogenesis of selenium nanoparticles using green chemistry. Top. Curr. Chem. (Cham) 375:88. doi: 10.1007/s41061-017-0176-x
Singh, R., Smitha, M. S., and Singh, S. P. (2014). The role of nanotechnology in combating multi-drug resistant bacteria. J. Nanosci. Nanotechnol. 14, 4745–4756. doi: 10.1166/jnn.2014.9527
Sonawane, H., Shelke, D., Chambhare, M., Dixit, N., Math, S., Sen, S., et al. (2022). Fungi-derived agriculturally important nanoparticles and their application in crop stress management - prospects and environmental risks. Environ. Res. 212:113543. doi: 10.1016/j.envres.2022.113543
Souza, L. M., Dibo, M., Sarmiento, J. J. P., Seabra, A. B., Medeiros, L. P., Lourenço, I. M., et al. (2022). Biosynthesis of selenium nanoparticles using combinations of plant extracts and their antibacterial activity. Curr. Res. Green Sustain. Chem. 5:100303. doi: 10.1016/j.crgsc.2022.100303
Spivak, M. Y., Tymoshok, N. O., Horalskyi, L. P., Tsekhmistrenko, O. S., Bityutskyy, V. S., and Tsekhmistrenko, S. I. (2020). Bacterial synthesis of nanoparticles: a green approach. Biosyst. Diversity 28, 9–17. doi: 10.15421/012002
Srivastava, N., and Mukhopadhyay, M. (2015). Green synthesis and structural characterization of selenium nanoparticles and assessment of their antimicrobial property. Bioprocess Biosyst. Eng. 38, 1723–1730. doi: 10.1007/s00449-015-1413-8
Sun, Y., Shi, Y., Jia, H., Ding, H., Yue, T., and Yuan, Y. (2021). Biosynthesis of selenium nanoparticles of Monascus purpureus and their inhibition to Alicyclobacillus acidoterrestris. Food Control 130:108366. doi: 10.1016/j.foodcont.2021.108366
Tadic, D., Bleda Hernandez, M. J., Cerqueira, F., Matamoros, V., Pina, B., and Bayona, J. M. (2021). Occurrence and human health risk assessment of antibiotics and their metabolites in vegetables grown in field-scale agricultural systems. J. Hazard. Mater. 401:123424. doi: 10.1016/j.jhazmat.2020.123424
Taha, N. A., Hamden, S., Bayoumi, Y. A., Elsakhawy, T., El-Ramady, H., and Solberg, S. O. (2023). Nanofungicides with selenium and silicon can boost the growth and yield of common bean (Phaseolus vulgaris L.) and control alternaria leaf spot disease. Microorganisms 11:728. doi: 10.3390/microorganisms11030728
Tareq, F. K., Fayzunnesa, M., Kabir, M. S., and Nuzat, M. (2018). Mechanism of bio molecule stabilized selenium nanoparticles against oxidation process and Clostridium botulinum. Microb. Pathog. 115, 68–73. doi: 10.1016/j.micpath.2017.12.042
Thapa, S. P., Shrestha, S., and Anal, A. K. (2020). Addressing the antibiotic resistance and improving the food safety in food supply chain (farm-to-fork) in Southeast Asia. Food Control 108:106809. doi: 10.1016/j.foodcont.2019.106809
Truong, L. B., Medina-Cruz, D., Mostafavi, E., and Rabiee, N. (2021). Selenium nanomaterials to combat antimicrobial resistance. Molecules 26:3611. doi: 10.3390/molecules26123611
Tsivileva, O. M., Loshchinina, E. A., Pankratov, A. N., Burashnikova, M. M., Yurasov, N. A., Bylinkina, N. N., et al. (2012). Biodegradation of an organoselenium compound to elemental selenium by Lentinula edodes (shiitake) mushroom. Biol. Trace Elem. Res. 149, 97–101. doi: 10.1007/s12011-012-9399-4
Tugarova, A. V., and Kamnev, A. A. (2017). Proteins in microbial synthesis of selenium nanoparticles. Talanta 174, 539–547. doi: 10.1016/j.talanta.2017.06.013
Ullah, A., Mirani, Z. A., Binbin, S., Wang, F., Chan, M. W. H., Aslam, S., et al. (2023). An elucidative study of the anti-biofilm effect of selenium nanoparticles (SeNPs) on selected biofilm producing pathogenic bacteria: a disintegrating effect of SeNPs on bacteria. Process Biochem. 126, 98–107. doi: 10.1016/j.procbio.2022.12.031
Ullah, A., Mu, J., Wang, F., Chan, M. W. H., Yin, X., Liao, Y., et al. (2022). Biogenic selenium nanoparticles and their anticancer effects pertaining to probiotic bacteria-a review. Antioxidants 11:1916. doi: 10.3390/antiox11101916
Vahidi, H., Kobarfard, F., Kosar, Z., Mahjoub, M. A., Saravanan, M., and Barabadi, H. (2020). Mycosynthesis and characterization of selenium nanoparticles using standard Penicillium chrysogenum PTCC 5031 and their antibacterial activity: a novel approach in microbial nanotechnology. Nanomed. J. 7, 315–323. doi: 10.22038/nmj.2020.07.00008
Vetchinkina, E., Loshchinina, E., Kursky, V., and Nikitina, V. (2013). Reduction of organic and inorganic selenium compounds by the edible medicinal basidiomycete Lentinula edodes and the accumulation of elemental selenium nanoparticles in its mycelium. J. Microbiol. 51, 829–835. doi: 10.1007/s12275-013-2689-5
Vijayakumar, S., Chen, J., Divya, M., Durán-Lara, E. F., Prasannakumar, M., and Vaseeharan, B. (2022). A review on biogenic synthesis of selenium nanoparticles and its biological applications. J. Inorg. Organomet. P. 32, 2355–2370. doi: 10.1007/s10904-022-02366-1
Wei, X., and Zhao, X. (2021). Advances in typing and identification of foodborne pathogens. Curr. Opin. Food Sci. 37, 52–57. doi: 10.1016/j.cofs.2020.09.002
Xing, G., Li, N., Lin, H., Shang, Y., Pu, Q., and Lin, J. M. (2022). Microfluidic biosensor for one-step detection of multiplex foodborne bacteria ssDNA simultaneously by smartphone. Talanta 253:123980. doi: 10.1016/j.talanta.2022.123980
Xu, X., Biswas, S., Gu, G., Elbediwi, M., Li, Y., and Yue, M. (2020). Characterization of multidrug resistance patterns of emerging Salmonella enterica serovar Rissen along the food chain in China. Antibiotics 9:660. doi: 10.3390/antibiotics9100660
Xu, C., Qiao, L., Ma, L., Yan, S., Guo, Y., Dou, X., et al. (2019). Biosynthesis of polysaccharides-capped selenium nanoparticles using Lactococcus lactis NZ9000 and their antioxidant and anti-inflammatory activities. Front. Microbiol. 10:1632. doi: 10.3389/fmicb.2019.01632
Yan, H., Cui, Z., Manoli, T., and Zhang, H. (2021). Recent advances in non-thermal disinfection technologies in the food industry. Curr. Opin. Food Sci. 27, 695–710. doi: 10.3136/fstr.27.695
Yang, J., and Yang, H. (2023). Recent development in Se-enriched yeast, lactic acid bacteria and bifidobacteria. Crit. Rev. Food Sci. 63, 411–425. doi: 10.1080/10408398.2021.1948818
Yang, H., Yang, X., Ning, Z., Kwon, S. Y., Li, M.-L., Tack, F. M., et al. (2022). The beneficial and hazardous effects of selenium on the health of the soil-plant-human system: an overview. J. Hazard. Mater. 422:126876. doi: 10.1016/j.jhazmat.2021.126876
Yazhiniprabha, M., and Vaseeharan, B. (2019). In vitro and in vivo toxicity assessment of selenium nanoparticles with significant larvicidal and bacteriostatic properties. Mat. Sci. Eng. C Mater. 103:109763. doi: 10.1016/j.msec.2019.109763
Yilmaz, M. T., İspirli, H., Taylan, O., and Dertli, E. (2021). A green nano-biosynthesis of selenium nanoparticles with Tarragon extract: structural, thermal, and antimicrobial characterization. LWT 141:110969. doi: 10.1016/j.lwt.2021.110969
Zambonino, M. C., Quizhpe, E. M., Jaramillo, F. E., Rahman, A., Santiago Vispo, N., Jeffryes, C., et al. (2021). Green synthesis of selenium and tellurium nanoparticles: current trends, biological properties and biomedical applications. Int. J. Mol. Sci. 22:989. doi: 10.3390/ijms22030989
Zhang, H., Li, Z., Dai, C., Wang, P., Fan, S., Yu, B., et al. (2021). Antibacterial properties and mechanism of selenium nanoparticles synthesized by Providencia sp. DCX. Environ. Res. 194:110630. doi: 10.1016/j.envres.2020.110630
Zhang, H., Zhou, H., Bai, J., Li, Y., Yang, J., Ma, Q., et al. (2019). Biosynthesis of selenium nanoparticles mediated by fungus Mariannaea sp. HJ and their characterization. Colloid. Surface. A 571, 9–16. doi: 10.1016/j.colsurfa.2019.02.070
Keywords: Bio-SeNPs, synthesis, antibacterial, foodborne pathogens, toxicity
Citation: Ao B, Du Q, Liu D, Shi X, Tu J and Xia X (2023) A review on synthesis and antibacterial potential of bio-selenium nanoparticles in the food industry. Front. Microbiol. 14:1229838. doi: 10.3389/fmicb.2023.1229838
Received: 27 May 2023; Accepted: 29 June 2023;
Published: 13 July 2023.
Edited by:
Mehran Moradi, Urmia University, IranReviewed by:
Seema Sachin Borgave, Sangamner College, IndiaCopyright © 2023 Ao, Du, Liu, Shi, Tu and Xia. This is an open-access article distributed under the terms of the Creative Commons Attribution License (CC BY). The use, distribution or reproduction in other forums is permitted, provided the original author(s) and the copyright owner(s) are credited and that the original publication in this journal is cited, in accordance with accepted academic practice. No use, distribution or reproduction is permitted which does not comply with these terms.
*Correspondence: Xian Xia, eGlhbnhpYUBoYm51LmVkdS5jbg==
Disclaimer: All claims expressed in this article are solely those of the authors and do not necessarily represent those of their affiliated organizations, or those of the publisher, the editors and the reviewers. Any product that may be evaluated in this article or claim that may be made by its manufacturer is not guaranteed or endorsed by the publisher.
Research integrity at Frontiers
Learn more about the work of our research integrity team to safeguard the quality of each article we publish.