- 1Institute of Forestry and Grassland Ecology, Ningxia Academy of Agricultural and Forestry Sciences, Yinchuan, China
- 2Chengdu Institute of Biology, Chinese Academy of Sciences, Chengdu, China
- 3State Key Laboratory for Quality Ensurance and Sustainable Use of Dao-di Herbs, National Resource Center for Chinese Materia Medica, China Academy of Chinese Medical Sciences, Beijing, China
- 4Ottawa Research and Development Centre, Agriculture and Agri-Food Canada, Ottawa, ON, Canada
Gravel-mulched land in China suffers from poor natural resources and fragile ecological environment, posing a challenge to effective restoration of ecological function. Lonicera japonica, a traditional Chinese herb used for treating human diseases, is a highly adaptable and resilient plant species, can effectively improve the soil properties, and may have important implications for the ecology and economy of gravel-mulched land. A study was conducted in a gravel-mulched field to measure the impact of planting the L. japonica (including control (CK), 1-year, 2-year, and 4-year cultivation of plants) on (i) dynamic changes in soil nutrient and enzyme activity properties, and (ii) soil rhizosphere microbial community structure characteristics. We found that the concentration of soil organic carbon, available nitrogen, available phosphorus and available potassium in L. japonica soil after cultivation for 1–4 years increased by 11–409%. The urease, phosphatase and catalase activities were increased by 11–560%, with the highest nutrient concentration and enzyme activity in 4-year plants. The pH value gradually decreased after cultivation. The improved soil environments increased soil bacterial community diversity. Planting L. japonica significantly increased the bacterial ACE, Chao1 index, Simpson index, and Shannon-Wiener index. The Firmicutes, Proteobacteria and Bacteroidetes were observed in dominant phyla. The relative abundance of eight genera, including Streptococcus, Veillonella and Rothia, was significantly reduced by more than 1%. Taken together, these soil indicators suggest that planting L. japonica in the short term would be a cost-effective strategy to combat soil degradation in a gravel-mulched ecosystem.
1 Introduction
It is well-established that soil nutrients, microorganisms and enzymes are important components of soil ecosystems (Na et al., 2021; Li Y. et al., 2023). In soil ecosystems, the rhizosphere is closely related to plants and interacts with them throughout their life cycle (Liu et al., 2019). An increasing body of evidence suggests that the diversity of rhizosphere soil microorganisms and the presence of specific genera can ensure proper soil function and promote plant health (Chen et al., 2019; Zhang et al., 2019). Soil enzymes act as biocatalysts, and their activity level reflects soil biological activity and fertility (Gregorich et al., 1994; Mi et al., 2018). Changes in soil microbial and enzyme activities are important indicators of the effectiveness of soil nutrient fertility utilization and respond rapidly to changes in soil environmental conditions, providing an early indication of changes in soil health (Li J. et al., 2023).
The arid and semi-arid areas of north-west China have a poor natural resource endowment and a fragile ecological environment, and are subject to serious soil erosion, accounting for their poor economic status. The past few years have witnessed a burgeoning interest in restoring the natural habitat of this region. Gravel mulching which began in the early Kangxi period of the Qing Dynasty (1,644 to 1911) dating back to about 300 years ago, is a unique form of drought-resistant farming in arid and semi-arid areas of northwest China (Zhao et al., 2017). Gravel mulching involves spreading a mixture of sand and gravel washed down from alluvial fans to form a 10 to 20 cm thick surface layer on farmland (Zhao et al., 2016, 2020). This method can effectively inhibit evaporation, and improve water storage and moisture conservation. Gravel-mulched land in China is mainly distributed in the arid and semi-arid climate transition zone of Gansu and Ningxia provinces. The vegetation types are mostly desert or desert steppe in this region. Vegetation degradation and land desertification have seriously affected the socio-economic development of the region. Since 2004, the Chinese government has widely promoted this technology by means of subsidies and encouraged planting watermelon (Citrullus lanatus (Thunb.) Matsum. & Nakai) on gravel-mulched fields. Because of the long sunshine hours and the large temperature difference between day and night during the growth period, the watermelon grown from the stone crevices is crisp., fleshy and high in sugar (Ren et al., 2023). At the same time, it absorbs the trace element selenium in the sand and gravel, and is actively sought after in the market, which in turn has given birth to a unique selenium sand melon industry. However, long-term watermelon continuous cropping leads to drastic shifts in soil bacterial community composition (Gu et al., 2022), soil mineral deficiencies, and the ecological environment was severely negatively impacted. Watermelon production has declined (Wu et al., 2022), and people began to look for other crops to grow.
L. japonica commonly known as “Ren Dong,” has been widely used in traditional medicine in East Asian countries for thousands of years. Its dry flower buds, leaves, and stems are rich in flavonoids, polysaccharides and other components, which have a broad spectrum of antioxidant, anti-carcinogenic, anti-inflammatory, and anti- bacterial effects (Yang et al., 2017; Tang et al., 2021). L. japonica extract is widely used in cosmetics, pharmacological preparations, food, and animal husbandry (Li et al., 2020; Zhang X. et al., 2022; Yang et al., 2023). L. japonica has strong adaptability (Thanabhorn et al., 2006; Yan et al., 2016), is tolerant of severe cold and drought, and does not require high soil nutrient conditions. Cultivation of L. japonica can effectively improve soil nutrients, prevent soil erosion, and fertilize the soil, and has great potential for improvement of the ecological environment and economic development of the gravel-mulched land (Thanabhorn et al., 2006; Yang et al., 2017). Because of its good economic benefits and the emergence of obstacles associated with continuous watermelon production, local enterprises began planting 33.3 ha of L. japonica in 2017, and the sales amounted to more than 960,000 yuan in 2018, reaching a gratifying achievement of one-year investment recovery; in 2019, the planting area was expanded to 66.7 ha. The local government began to encourage the people living locally to plant L. japonica. By 2023, the cropped area of L. japonica was 2000 ha, with a yield of more than 600 kg•ha−1 of high-quality dried flowers, a total output value of nearly 192 million yuan. L. japonica has truly become the ‘rich flower’ of the masses in Zhongwei City, Ningxia, China.
Currently, research on gravel-mulched land has primarily centered on the effects of soil water infiltration and evaporation (Xie et al., 2006), soil nutrients (Qiu et al., 2015), fertilization (Bao et al., 2022) and sand mulching (Qiu et al., 2023) on crops. Our hypothesis was that planting L. japonica will lead to longer-term positive effect on soil microbes, soil enzyme activity and nutrient cycling. To test this hypothesis, a field experiment was conducted in a typical gravel-mulched land to compare growing L. japonica for 1 year, 2 years and 4 years with a control with natural shrub vegetation. The specific objectives of the current study were to determine the effects of different years of growing L. japonica on (i) the soil properties, and (ii) the composition of the bacterial community of L. japonica rhizosphere soil in a gravel-mulched land.
2 Materials and methods
2.1 Site description
The research site is in the Xiangshan gravel-sand area (E105°15′, N36°06′) of Zhongwei City, Ningxia, China, which has a classic continental weather pattern with 189.5 mm precipitation and 2,400 mm potential evapotranspiration. In this field, the soil structure is loose, water is easily infiltrated and susceptible to evaporation, leading to droughts, sand storms, soil desertification and other natural disasters. The natural vegetation coverage is generally less than 20%, and the vegetation types are dominated by herbs and dry shrubs with low and highly unstable productivity. Land in the Xiangshan gravel-sand area has been used to for monoculture production of watermelon. Since 2017, local enterprises have gradually switched to planting L. japonica. The variety of L. japonica in the test site was “Beihua No. 2,” provided by Zhongwei Sunshine Muchang Agriculture and Pasture Company Ltd.
2.2 Experimental design and field management
The experimental design was a randomized complete block (RCB) design with 3 replicates and four treatments; each plot had dimensions of 5.8 m × 4.4 m. The treatments were control or check with no L. japonica cuttings planted (CK), and L. japonica cuttings planted in different years: 2020 (T1), 2019 (T2) and 2017 (T3) (Figure 1). Plots were covered by about 20-cm-deep layer of gravel (5–8 cm diameter) mulch. L. japonica cuttings (30 cm height) were manually transplanted each year at the end of March to the field after cultivation. Transplanting consisted of digging a hole depth approximately 20 cm, putting the cuttings into hole vertically, backfilling with loose fine soil and compacting, and then covering with a thin layer of gravel-sand. Because of extreme water shortage, limited or no irrigation was applied throughout the growing season, watering was only done 24 h and 1 week after transplanting. Row spacing was 2 m, plant spacing was 1 m, and the planting density was 5,000 plants•ha−1. The experiment followed local recommended management practices for weed control and other agronomic operations.
2.3 Sample collection
In May 2021, the plant residue and stones were removed from the soil surface and soil samples were collected from 0–20 cm soil layers using a soil auger (5 cm internal diameter) and three sampling points were randomly chosen between two adjacent rows within each plot (Ma et al., 2022), The collected soil samples were passed through a 2 mm mesh sieve and divided into two parts. One part was frozen at −80°C to determine the soil microbial microbiome, while the other part was air-dried indoors to determine other soil factors.
2.4 Determination of soil characteristics
The soil pH was measured by PHS-3C (Leici, China). The SOC (soil organic carbon) concentration was measured by an Elemental rapid CS cube analyzer (Elementar, Germany). The AN (available nitrogen) concentration in soil was measured by the alkaline diffusion method. The AP (available phosphorous) concentration was measured by NaHCO3 leaching and an AA3 flow analyzer (Seal, Germany). Finally, the AK (available potassium) concentration was measured by NaOH fusion-flame photometry (Pansu and Gautheyrou, 2007). Soil phosphatase, catalase and urease activities were measured by disodium phenyl phosphate colorimetry, potassium permanganate titration and indophenol-blue colorimetry, respectively (Guan et al., 1986).
2.5 Soil microbial community analysis
The genomic DNA was extracted by the CTAB or SDS method, and then the purity and concentration of DNA were detected by agarose gel electrophoresis. The diluted genomic DNA was used as a template. Primers were 515F and 806R. Phusion ® High-Fidelity PCR Master Mix with GC Buffer and high-efficiency high-fidelity enzyme (New England Biolabs, United States) were used for PCR. PCR products were detected by 2% agarose gel electrophoresis. The target bands were recovered using the gel recovery kit provided by QIAGEN. The library was constructed using the TruSeq ® DNA PCR-Free Sample Preparation Kit. The constructed library was quantified by a Qubit fluorometer and qPCR. Illumina NovaSeq 6,000 platform (Biomarker Technologies, Beijing, China) was used for sequencing.
2.6 Data analysis and processing
The data presented in this study were expressed as mean ± standard deviation, and statistical analyses were conducted in R software. Differences in soil properties, enzyme activities and bacterial diversity were calculated with a one-way analysis of variance (ANOVA). The alpha-diversity was evaluated using the ACE, Shannon, Simpson and Chao1 diversity indices. To examine the correlation between soil characteristics and the diversity of the soil microorganism community and the abundance of dominant phyla, Canoco5.0 and “vegan” packages were utilized for redundancy analysis (RDA) and Mantel test. Throughout this paper, probability levels of p < 0.05 and < 0.01 were considered to be statistically significant and highly significant, respectively.
3 Results
3.1 Chemical indicators and enzyme activities
With an increase in cultivation years, there was a significant decrease in soil pH, while the other soil characteristics showed an annual increase (Table 1). The soil pH in CK and T1 (both 9.70) was significantly higher than that in T2 and T3. The SOC in T3 and T2 increased by 70 and 65% compared with CK, respectively. All of AN, AK and AP concentrations were highest in T3, while CK exhibited the lowest levels. Moreover, both urease and phosphatase activities were highest in T3, and were significantly higher by 560 and 37% compared with CK. In contrast, catalase activity was comparable in T3, T2, and T1, and were significantly higher by 31–35% compared with CK.
3.2 OTUs analysis of the microbial community
To identify the dominant OTUs in different treatments, both the overlap and the distribution of the 5,910 most abundant OTUs across all samples were determined (Figure 2). Venn diagram analysis identified 1,566, 1,579, 1,609 and 1,156 OTUs in the T3, T2, T1 and CK groups, respectively. There were 1,034 common OTUs and 17, 33, 20, and 26 unique OTUs, accounting for 1.66, 1.27, 2.05, and 1.47% of total OTUs in T3, T2, T1 and CK groups, respectively. The number of OTUs in T3, T2 and T1 increased significantly compared with CK.
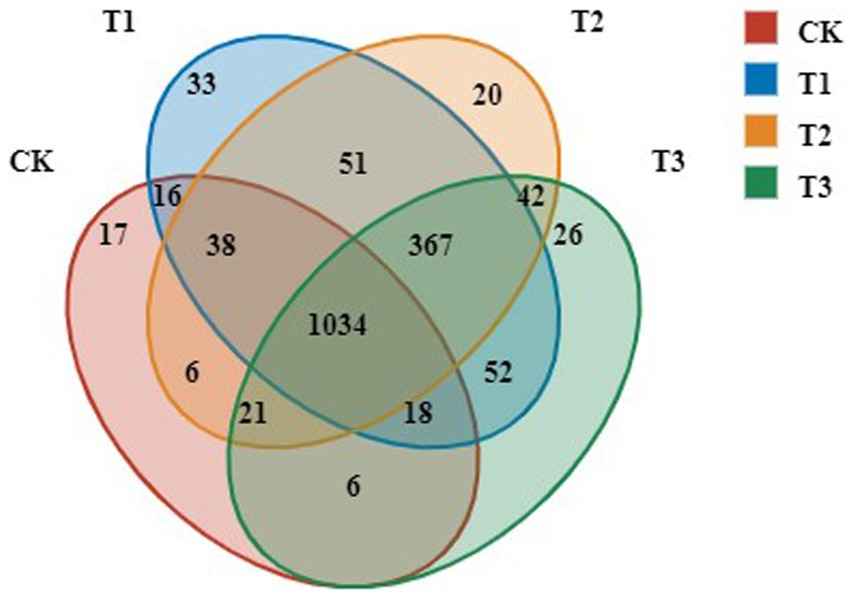
Figure 2. OTUs analysis of bacteria in rhizosphere soil following different years of L. japonica cultivation.
3.3 Composition of the bacterial community
To study the differences in soil microbial communities following different years of L. japonica cultivation, the dominant bacteria with a relative abundance of more than 1% were plotted (Figure 3). The CK, T1, T2 and T3 soil bacteria comprised 8 phyla, including Firmicutes, Proteobacteria, Bacteroidetes, Actinobacteria, Fusobacteria, Acidobacteria, Epsilonbacteraeota and Gemmatimonadetes, accounting for 94.73–96.72% of the relative abundance. Among them, the relative abundance of Firmicutes, Bacteroidetes and Fusobacteria was highest in CK (36.24, 13.52 and 8.55%) and lowest in T2 (32.07, 12.48 and 7.16%, respectively). The abundance in CK was significantly higher than in T2. The relative abundance of Acidobacteria was highest in T2 (4.82%), and was significantly higher than CK (1.30%) (Table 2).
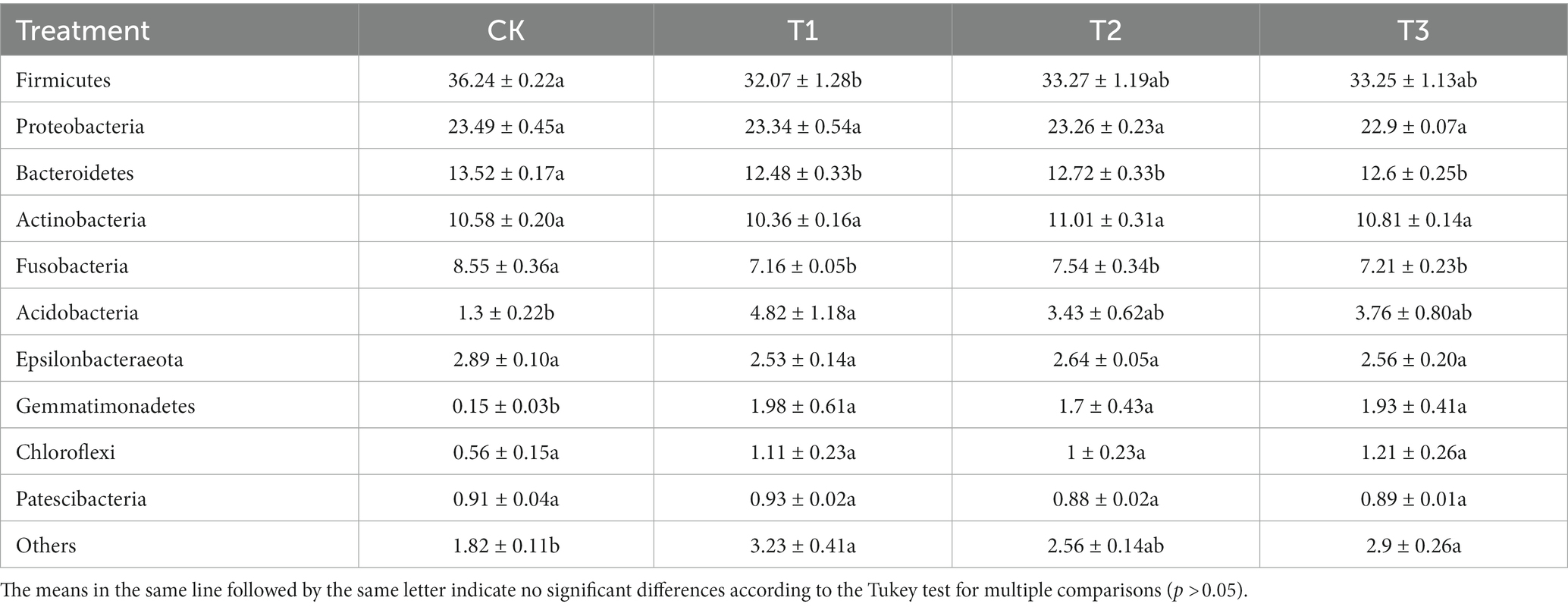
Table 2. Relative abundance of bacteria at phylum levels in rhizosphere soil as affected by different years of L. japonica cultivation.
The relative abundances of the bacterial population in the rhizosphere of L. japonica which were greater than 1% at the genus level, were classified and were composed of 16 genera (Figure 3). In comparison with the control group (68.44%), the relative abundance of T1 (62.94%), T2 (63.38%), and T3 (62.42%) decreased by 5.06–6.02%, indicating that the relative abundance of other soil bacterial genera increased in T1, T2 and T3. The relative abundances of Streptococcus, Veillonella, Rothia, Fusobacterium, Neisseria, Leptotrichia, Corynebacterium and Actinomyces were highest in CK (13.32, 7.11, 6.80, 5.80, 5.04, 2.61, 1.55 and 1.39%, respectively). The relative abundances of Fusobacterium, Corynebacterium and Actinomyces were significantly higher in CK than T1 and T2. The relative abundance of the remaining bacteria was significantly higher in CK than in T1, T2 and T3 (Table 3).
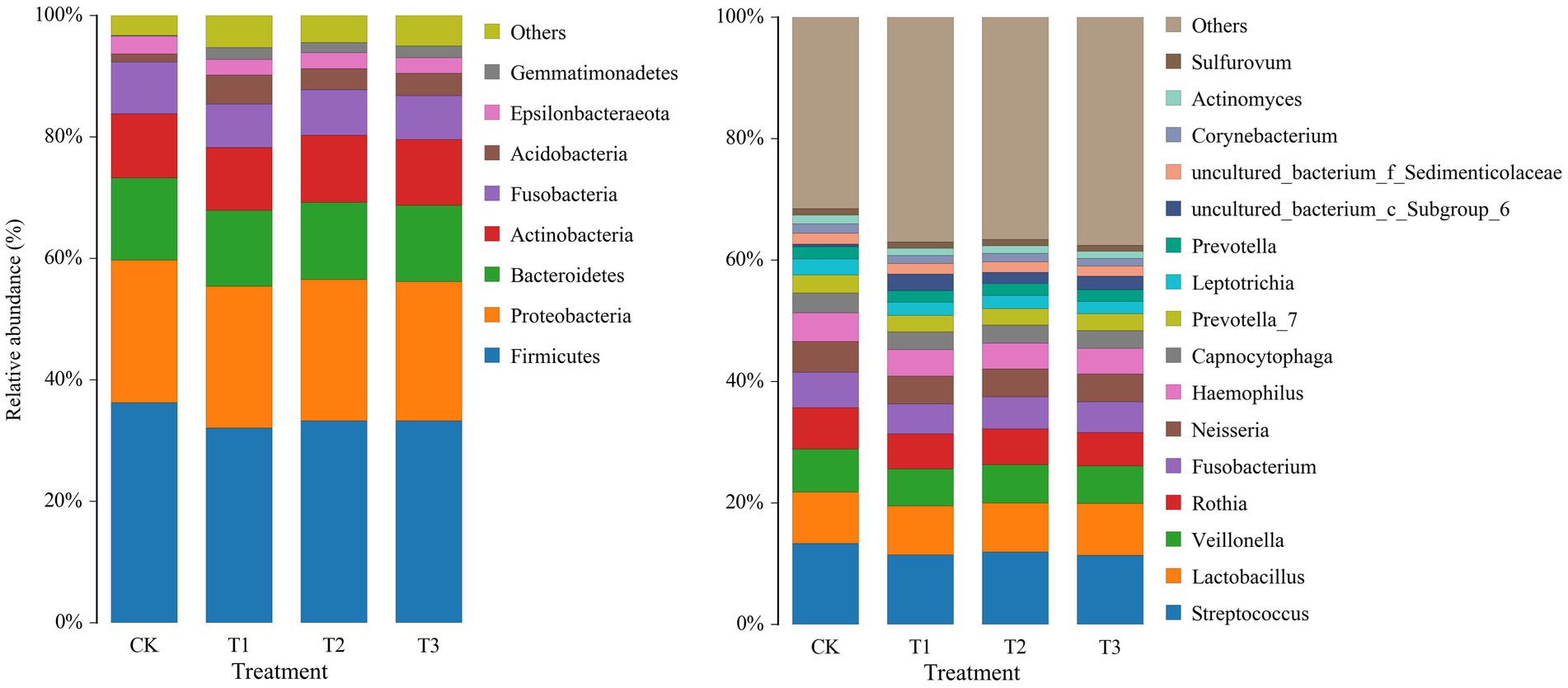
Figure 3. Relative abundance of bacteria at phylum (left) and genus (right) levels in rhizosphere soil following different years of L. japonica cultivation.
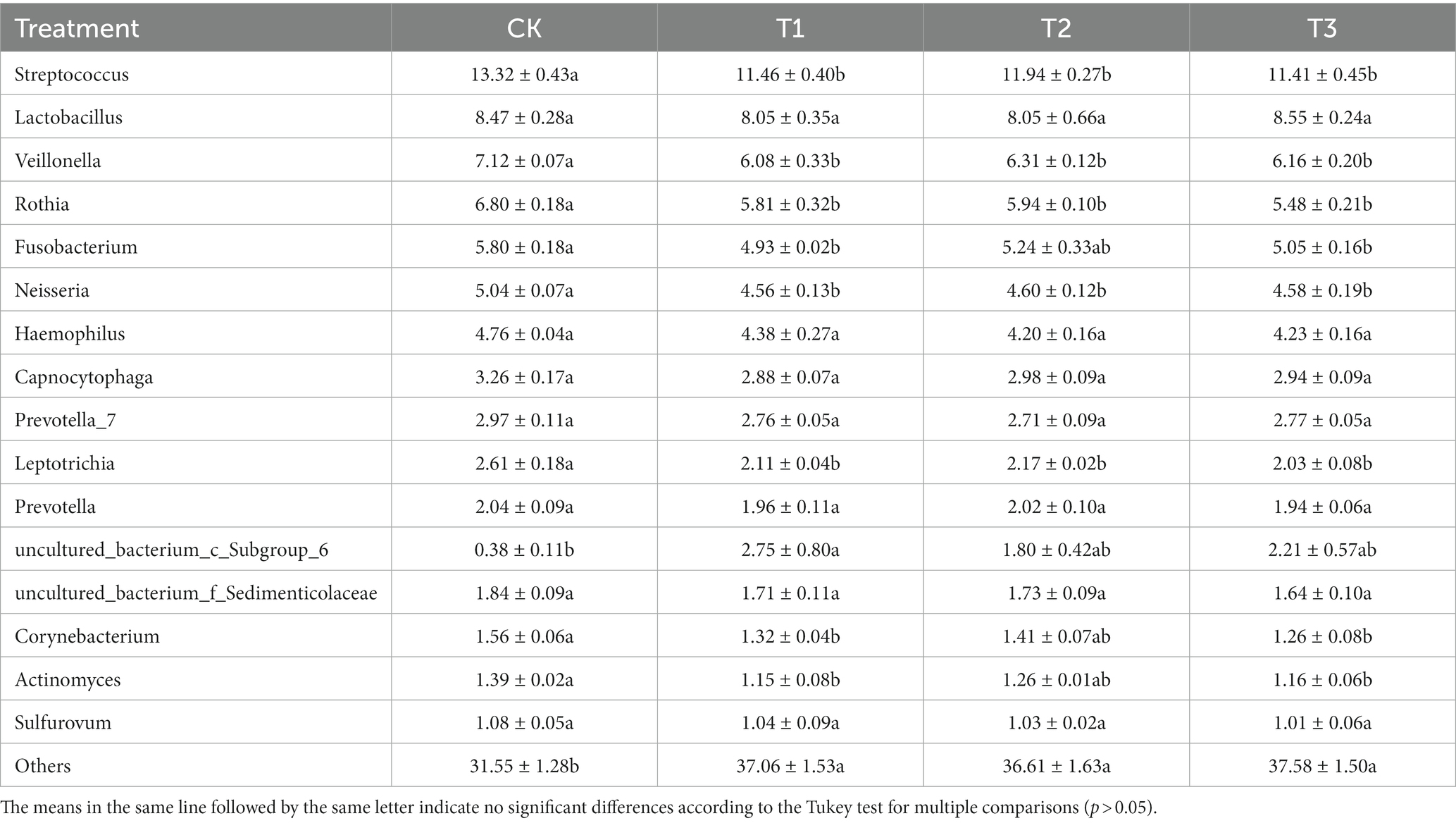
Table 3. Relative abundance of bacteria at genus levels in rhizosphere soil as affected by different years of L. japonica cultivation.
3.4 Bacterial community diversity
The soil bacterial diversity indices for different years of cultivation showed the same trend (Table 4). In T1, T2 and T3, the soil bacteria ACE index (1,503, 1,465 and 1,487), the Chao1 index (1,520, 1,495 and 1,505), the Simpson index (all 0.97), and the Shannon-Wiener index (all 7.0) were significantly higher than CK. The planting L. japonica formed a cohesive group, which was significantly separated from the treatment with no L. japonica cuttings planted (CK) (Figure 4).
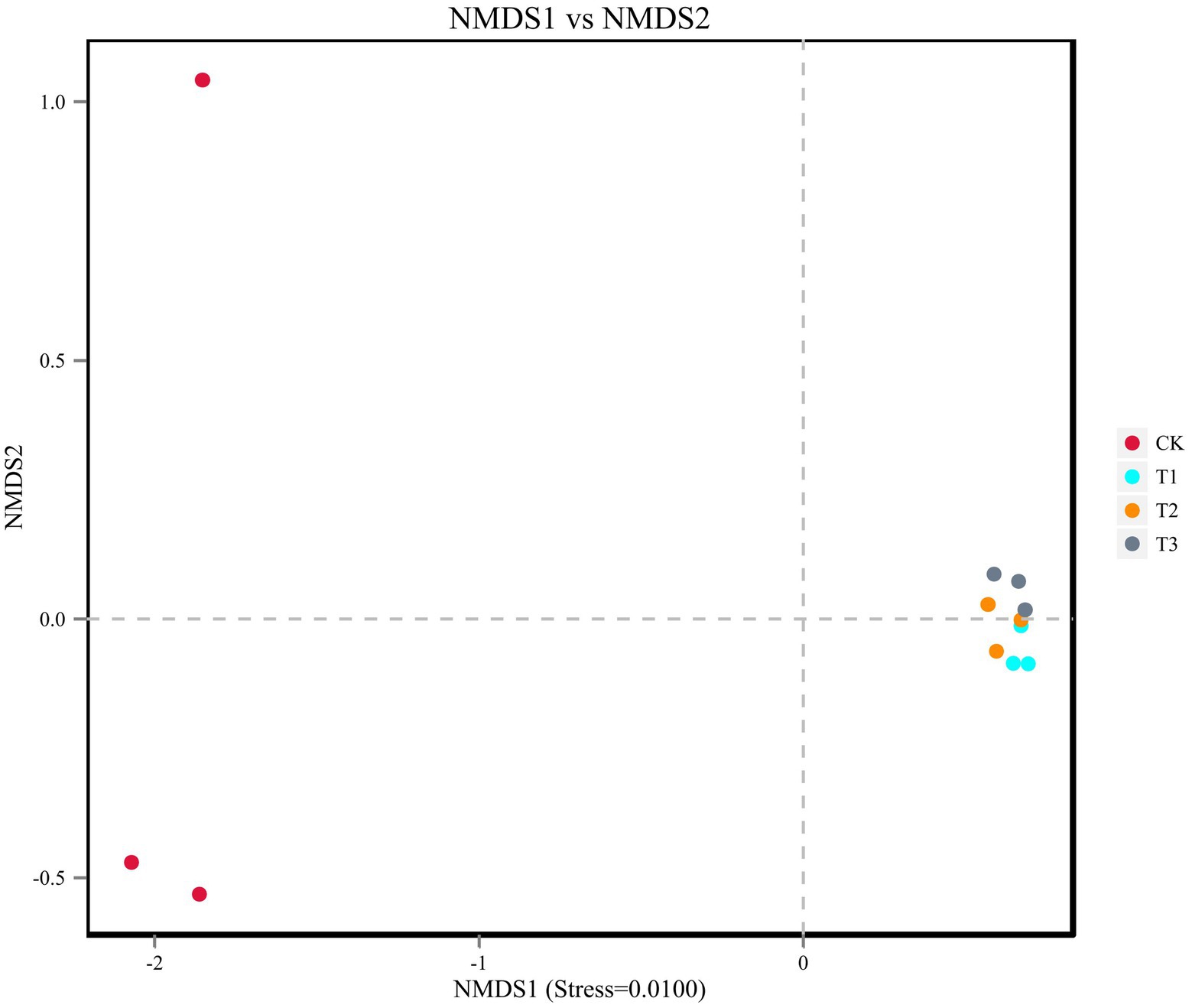
Figure 4. Beta diversity was analyzed using NMDS ordinations of Binary_Jaccard similarities calculated based on relative OTU abundances of the four different treatments.
3.5 Redundancy analysis of bacteria and soil factors
The redundancy analysis showed significant relationships among soil organic carbon, available nitrogen, available phosphorus, available potassium, urease activity, phosphatase activity, catalase activity, and bacterial community diversity (Figure 5). All soil parameters were positively correlated with each other, except pH which was negatively correlated with the other parameters. The phyla Actinobacteria, Acidobacteria and Gemmatimonadetes were positively correlated with soil catalase, phosphatase and urease activity and SOC, AK and AP, and were negatively correlated with pH. Conversely, the relative abundance of Firmicutes, Proteobacteria, Bacteroidetes, Fusobacteria and Epsilonbacteraeota showed a decreasing trend with increasing of soil parameters except pH. The Monte Carlo test showed that AK, urease and catalase activity all significantly affected soil bacterial diversity and relative abundance at the phylum level (Figure 5).
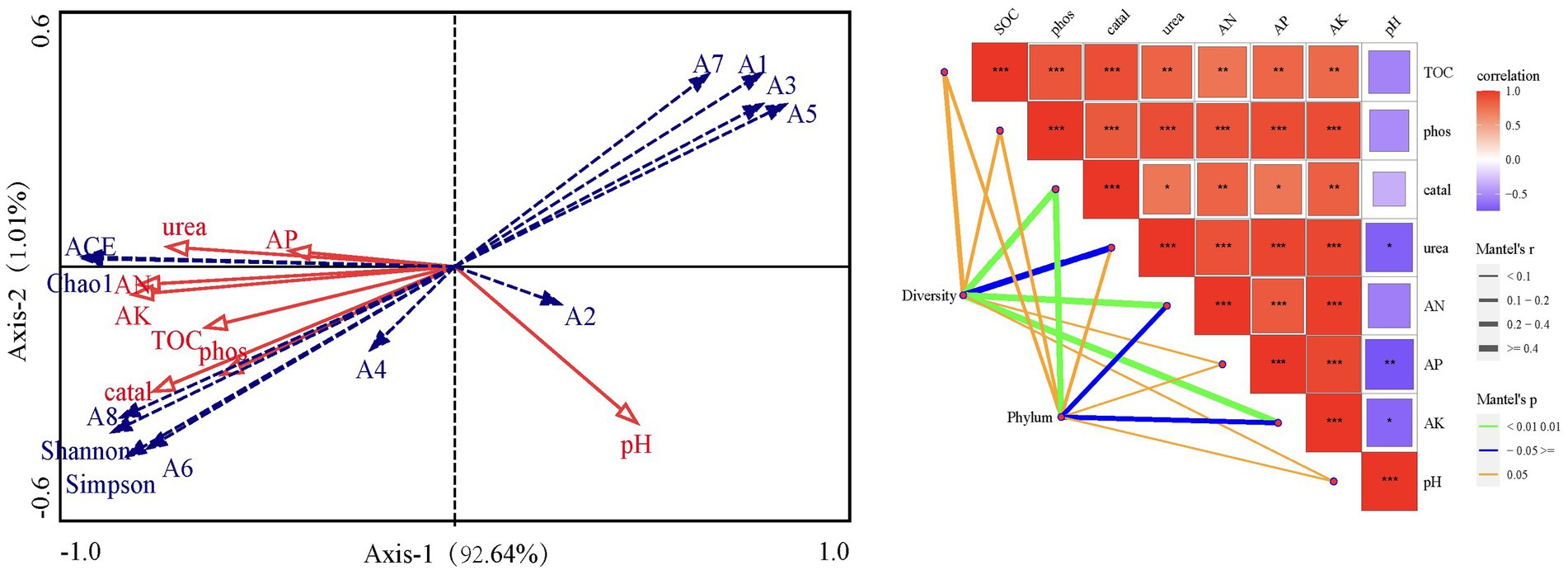
Figure 5. Redundancy analysis and mantel test plot of dominant bacterial community and environmental factors. SOC, Soil organic carbon; AN, Available nitrogen; AP, Available phosphorus; AK, Available potassium; Urea, Urease activity; Phos: Phosphatase activity; Catal, Catalase activity; A1 ~ A8, Firmicute, Proteobacteria, Bacteroidetes, Actinobacteria, Fusobacteria, Acidobacteria, Epsilonbacteraeota, Gemmatimonadetes; ACE, ACE index; Shannon, Shannon-Wiener index; Simpson, Simpson index; Chao1, Chao1 index.
4 Discussion
Our study found that planting L. japonica significantly increased SOC, AN, AP, AK and enzyme activity (Table 1). The improved soil quality parameters might be related to improved soil conditions resulting from greater input of plant residues, especially roots (Abbott et al., 2018; Upadhyay et al., 2022). The accumulation of these nutrients activated soil urease, phosphatase and catalase, boosting microbial activity, metabolism, and activity after planting L. japonica in the gravel-mulched land, with faster cycling and transformation of soil nutrients. Planting L. japonica increased the plant biomass and subsequent accumulation of the SOC, which could have affected pH (Table 1). It is well-known that soils rich in SOC have a better buffer capacity to adjust the soil pH (Ma et al., 2022). Moreover, the soil quality can be improved relatively quickly, alleviating the problems related to aeolian sandy soil and crop production in the region (Scheibe et al., 2015). There were little to no statistically significant differences in any of those measured soil properties between plots planted 1, 2 or 4 years ago.
Soil properties are among the most important factors shaping microbial communities (Xi et al., 2022). Rhizosphere microbial populations are constantly exposed to both low and high molecular weight organic compounds exuded from roots, which may be affected by specific physical, chemical, and biological conditions and microbial activities (Xie et al., 2019). Current evidence suggests that soil physical and chemical variables have a substantial impact on the microbial abundance and community diversity during land degradation (Li J. et al., 2023). Research on red soil in southern China showed that pH, AP and AN significantly affected soil bacterial community structure (Muneer et al., 2022). Moreover, in the alpine plateau of northern Tibet, variations in soil pH and SOC significantly affected composition of arbuscular mycorrhizal fungal communities (Chen et al., 2022; Zhou et al., 2022; Zhang M. et al., 2022). These studies overlap in their assertion that the change in soil microbial diversity composition and structure may be caused by change in soil characteristics. In the present study, soil nutrients and enzyme activities accounted for 93.63% of the changes in soil bacterial communities, among which available nitrogen, potassium, urease and catalase were significantly affected.
In this study, the most dominant bacterial phyla observed were Firmicutes, Proteobacteria and Bacteroidetes (Figure 3). There is a growing consensus that Bacteroidetes, as R-strategy flora, occupy a dominant position in the early stage of microbial community succession, and with the gradual maturity of microbial community succession, the relative abundance of K-strategy flora such as Acidobacteria gradually increases (Chen et al., 2016). L. japonica treatment resulted in a significant improvement in the relative abundance of Acidobacteria, and resulted in a reduction in Bacteroidetes and Fusobacteria (Figure 3). In this respect, our study revealed that after L. japonica planting, the bacterial community transitioned from a rapidly growing copiotrophic group to a slowly growing oligotrophic group. After the cultivation of L. japonica in the gravel-mulched field, more plant residues are produced, and a large amount of cellulose is decomposed, creating a favorable environment for the Acidobacteria that can use cellulose. Acidobacteria have been shown to be closely related to soil pH regulation and the degradation of plant residue polymers (Xu et al., 2020). This finding also accounts for the decrease in pH value to a certain extent (Xi et al., 2022). In addition, L. japonica planting in the previous year significantly decreased the relative abundance of Firmicute. This finding indicates that L. japonica planting for a short-term would reduce the pathogenic bacteria belonging to Firmicute (Ehrich et al., 1995).
After L. japonica planting, some bacteria at the genus level with relative abundance greater than 1.0% decreased, and those with less than 1.0% abundance increased significantly, which may be related to the significant improvement of soil physicochemical properties and increased bacterial abundance (Urbanová et al., 2015). Growing crops could provide more C and N to the soil for bacterial utilization through residual roots and litter, increase the number of microorganisms, change the bacterial community structure, and promote a change in the soil bacterial community composition.
Soil microbial diversity is a commonly used index to describe the stability of the microbial community, and is informative to fully reflect the significance of the soil environment on the microbial community and assess the health of terrestrial ecosystem (Coyte et al., 2015). Our study showed that L. japonica planting increased the number of bacterial OTU (Figure 2) and diversity index (Table 4) in the rhizosphere soil. This occurrence might be ascribed to the fact that rhizosphere soil bacteria can use the nutrients secreted by roots to grow and reproduce in the short term, and the diversity and richness of abundant communities gradually increase after long-term continuous cropping (Zhang H. et al., 2022).
The effect observed in the soils might be mediated primarily via a change in the composition and structure of the vegetation present on both planted and non-planted plots (due to L. japonica becoming dominant) (in ‘t Zandt et al., 2023). After planting L. japonica, the nutrient substrates such as C, N and P in the soil were affected by roots and litter, and increased significantly (Table 1), promoting bacterial growth in symbiotic groups. As a result of the impact of changes in land use on soil structure and nutrient availability, the configuration of the soil microbial community undergoes alterations (Gschwend et al., 2021; Xi et al., 2022; Labouyrie et al., 2023). A study by Jiang et al. (2022) on the rhizosphere soil of Torreya grandis cv. Merrillii showed that the effects of pH, organic matter, water-soluble SOC and AN and AP were significant on the dominant soil bacteria.
Overall, L. japonica planting significantly changed the soil microbial structure and diversity of the gravel-mulched land by affecting the soil characteristics and contributing significantly in shaping the diversity of microbial communities.
5 Conclusion
We found that growing L. japonica decreased soil pH, and increased SOC, AN and other nutrients and enzyme activities, with the highest levels observed after 4 years. The improved soil environments gave rise to an increased soil bacterial community diversity. The main dominant phyla of soil bacteria were Firmicutes, Proteobacteria and Bacteroidetes. The relative abundance of Acidobacteria increased after planting L. japonica, and Firmicutes, Bacteroidetes and Fusobacteria decreased. Collectively, the planting L. japonica in the short term could be a low-cost strategy to improve soil nutrients availability and soil bacterial community diversity, and effectively restore degraded land while promoting the local economy in the gravel-mulched area.
Data availability statement
The authors acknowledge that the data presented in this study must be deposited and made publicly available in an acceptable repository, prior to publication. The names of the repository/repositories and accession number(s) can be found at: NCBI – PRJNA1044469.
Author contributions
XW and BM developed the concept of this study and are main contributors to writing the manuscript, data analysis, and preparing figures. HL and YB performed all experiments. ML, NM, and LG contributed to the manuscript edit and review. All authors contributed to the article and approved the submitted version.
Funding
This study was financially supported by Ningxia Hui Autonomous Region Agricultural Science and Technology Independent Innovation Funding Project (NGSB-2021-16), Natural Science Foundation of Ningxia Hui Autonomous Region (2022AAC03421), the sixth batch of autonomous region youth science and technology talents lift project, and ministry of Finance-Ministry of Agriculture and Rural Affairs: National Modern Agricultural Industry Technology System Funding (CARS-21).
Acknowledgments
We want to thank all the members of our team who have contributed by involvement in the experimental process.
Conflict of interest
The authors declare that the research was conducted in the absence of any commercial or financial relationships that could be construed as a potential conflict of interest.
Publisher’s note
All claims expressed in this article are solely those of the authors and do not necessarily represent those of their affiliated organizations, or those of the publisher, the editors and the reviewers. Any product that may be evaluated in this article, or claim that may be made by its manufacturer, is not guaranteed or endorsed by the publisher.
References
Abbott, L. K., Macdonald, L. M., Wong, M. T. F., Webb, M. J., Jenkins, S. N., and Farrell, M. (2018). Potential roles of biological amendments for profitable grain production – a review. Agric. Ecosyst. Environ. 256, 34–50. doi: 10.1016/j.agee.2017.12.021
Bao, Y., Liu, H., Li, M., and Ma, B. (2022). Effects of boron fertilizer on the yield and chlorogenic acid content of Lonicera japonica thumb in Ningxia gravel-sand mulched field. Northern Hortic. 15, 113–120. Available at: https://kns.cnki.net/kcms2/article/abstract?v=vCcGnC-OR22cPEJ0r2qJ7rKPgFPnMbPlfgxu2Xdt3kpAfXnLK_68uPHMhtWJgF8qpu_1ii_HKe1i25hwhdTwZbCa7uGycir4sznKbU3s2xZvsyUUc0LgELg0o4le3QW7obcOUUU7a8pkW851AOE8lg==&uniplatform=NZKPT&language=CHS
Chen, Y., Chen, G., Robinson, D., Yang, Z., Guo, J., Xie, J., et al. (2016). Large amounts of easily decomposable carbon stored in subtropical forest subsoil are associated with r-strategy-dominated soil microbes. Soil Biol. Biochem. 95, 233–242. doi: 10.1016/j.soilbio.2016.01.004
Chen, J., Li, J., Yang, Y., Wang, Y., Zhang, Y., and Wang, P. (2022). Effects of conventional and organic agriculture on soil arbuscular mycorrhizal fungal community in low-quality farmland. Front. Microbiol. 13:914627. doi: 10.3389/fmicb.2022.914627
Chen, S., Waghmode, T. R., Sun, R., Kuramae, E. E., Hu, C., and Liu, B. (2019). Root-associated microbiomes of wheat under the combined effect of plant development and nitrogen fertilization. Microbiome 7, 136–149. doi: 10.1186/s40168-019-0750-2
Coyte, K. Z., Schluter, J., and Foster, K. R. (2015). The ecology of the microbiome: networks, competition, and stability. Science 350, 663–666. doi: 10.1126/science.aad2602
Ehrich, S., Behrens, D., Lebedeva, E., Ludwig, W., and Bock, E. (1995). A new obligately chemolithoautotrophic, nitrite-oxidizing bacterium, Nitrospira moscoviensis sp. nov. and its phylogenetic relationship. Arch. Microbiol. 164, 16–23. doi: 10.1007/BF02568729
Gregorich, E., Carter, M., Angers, D., Monreal, C., and Ellert, B. (1994). Towards a minimum data set to assess soil organic matter quality in agricultural soils. Can. J. Soil Sci. 74, 367–385. doi: 10.4141/cjss94-051
Gschwend, F., Hartmann, M., Hug, A. S., Enkerli, J., Gubler, A., Frey, B., et al. (2021). Long-term stability of soil bacterial and fungal community structures revealed in their abundant and rare fractions. Mol. Ecol. 30, 4305–4320. doi: 10.1111/mec.16036
Gu, X., Yang, N., Zhao, Y., Liu, W., and Li, T. (2022). Long-term watermelon continuous cropping leads to drastic shifts in soil bacterial and fungal community composition across gravel mulch fields. BMC Microbiol. 22:189. doi: 10.1186/s12866-022-02601-2
Guan, S., Zhang, D., and Zhang, Z. (1986). Soil enzyme and its research methods. Chinese Agricultural Press, Beijing, China.
in ‘t Zandt, D., Kolaříková, Z., Cajthaml, T., and Münzbergová, Z. (2023). Plant community stability is associated with a decoupling of prokaryote and fungal soil networks. Nat. Commun. 14:3736. doi: 10.1038/s41467-023-39464-8
Jiang, N., Liang, C., Zhang, Y., Jiang, Z., Dong, J., Wu, J., et al. (2022). Microbial composition and diversity in soil of Torreya grandis cv. Merrillii relative to different cultivation years after land use conversion. Environ. Sci. 43, 530–539. Available at: https://kns.cnki.net/kcms2/article/abstract?v=vCcGnC-OR20E1RZPX5Ukxq4nPHIr5m5C1eLSJt-lbsTv9KAaXqXYN3BrcYoXY6OUefnyqreXfO9AZBD9fFA3_ZCvgkroL5di7pA2qNhVPvivXHkVI8wY2oylrDZQYmr33xo5UdgnGrlhGo-7Ki-FDw==&uniplatform=NZKPT&language=CHS
Labouyrie, M., Ballabio, C., Romero, F., Panagos, P., Jones, A., Schmid, M. W., et al. (2023). Patterns in soil microbial diversity across Europe. Nat. Commun. 14:3311. doi: 10.1038/s41467-023-37937-4
Li, Y., Li, W., Fu, C., Song, Y., and Fu, Q. (2020). Lonicerae japonicae flos and Lonicerae flos: a systematic review of ethnopharmacology, phytochemistry and pharmacology. Phytochem. Rev. 19, 1–61. doi: 10.1007/s11101-019-09655-7
Li, J., Niu, X., Wang, P., Yang, J., Liu, J., Wu, D., et al. (2023). Soil degradation regulates the effects of litter decomposition on soil microbial nutrient limitation: evidence from soil enzymatic activity and stoichiometry. Front. Plant Sci. 13:1090954. doi: 10.3389/fpls.2022.1090954
Li, Y., Zou, N., Liang, X., Zhou, X., Guo, S., Wang, Y., et al. (2023). Effects of nitrogen input on soil bacterial community structure and soil nitrogen cycling in the rhizosphere soil of Lycium barbarum L. Front. Microbiol. 13:1070817. doi: 10.3389/fmicb.2022.1070817
Liu, Y.-X., Qin, Y., and Bai, Y. (2019). Reductionist synthetic community approaches in root microbiome research. Curr. Opin. Microbiol. 49, 97–102. doi: 10.1016/j.mib.2019.10.010
Ma, B., Ma, B.-L., McLaughlin, N. B., Li, M., and Liu, J. (2022). Improvement in dryland crop performance and soil properties with multiple annual applications of lignite-derived humic amendment. Soil Tillage Res. 218:105306. doi: 10.1016/j.still.2021.105306
Mi, J. Z., Gregorich, E. G., Xu, S., McLaughlin, N. B., and Liu, J. H. (2018). Effects of a one-time application of bentonite on soil enzymes in a semi-arid region. Can. J. Soil Sci. 98, 542–555. doi: 10.1139/cjss-2018-0011
Muneer, M. A., Hou, W., Li, J., Huang, X., ur Rehman Kayani, M., Cai, Y., et al. (2022). Soil pH: a key edaphic factor regulating distribution and functions of bacterial community along vertical soil profiles in red soil of pomelo orchard. BMC Microbiol. 22:38. doi: 10.1186/s12866-022-02452-x
Na, X., Ma, S., Ma, C., Liu, Z., Xu, P., Zhu, H., et al. (2021). Lycium barbarum L. (goji berry) monocropping causes microbial diversity loss and induces fusarium spp. enrichment at distinct soil layers. Appl. Soil Ecol. 168:104107. doi: 10.1016/j.apsoil.2021.104107
Pansu, M., and Gautheyrou, J. (2007). Handbook of soil analysis: Mineralogical, organic and inorganic methods. Springer-Verlag, Heidelberg, New York.
Qiu, Y., Chen, X., Wang, Y., Zhang, Y., and Xie, Z. (2023). What drives soil degradation after gravel mulching for 6 years in Northwest China? Front. Microbiol. 14:1224195. doi: 10.3389/fmicb.2023.1224195
Qiu, Y., Xie, Z., Wang, Y., Malhi, S. S., and Ren, J. (2015). Long-term effects of gravel—sand mulch on soil organic carbon and nitrogen in the loess plateau of northwestern China. J. Arid. Land 7, 46–53. doi: 10.1007/s40333-014-0076-7
Ren, K. L., Su, Y. Q., Zhang, H. H., Kong, W. P., Tang, T. X., Cheng, H., et al. (2023). Analysis of volatile aroma components of dry Sandy soil-grown watermelon from Jingyuan, Gansu Province. Food Sci. 44, 320–326. Available at: https://kns.cnki.net/kcms2/article/abstract?v=vCcGnC-OR22gAEReCxK6SLGRoaPhejFEr0PFJM_Au4rH6uagx7F6PSBs-Lhxwzp_pMCXdysy9In6xxAZ-1xWriBzW3HMscOuyiYxaOk_E5dZ-drzZ-bCsriJnwAkwIKeyFKSC1PdvDYvT7suD9sGNw==&uniplatform=NZKPT&language=CHS
Scheibe, A., Steffens, C., Seven, J., Jacob, A., Hertel, D., Leuschner, C., et al. (2015). Effects of tree identity dominate over tree diversity on the soil microbial community structure. Soil Biol. Biochem. 81, 219–227. doi: 10.1016/j.soilbio.2014.11.020
Tang, X., Liu, X., Zhong, J., and Fang, R. (2021). Potential application of Lonicera japonica extracts in animal production: from the perspective of intestinal health. Front. Microbiol. 12:719877. doi: 10.3389/fmicb.2021.719877
Thanabhorn, S., Jaijoy, K., Thamaree, S., Ingkaninan, K., and Panthong, A. (2006). Acute and subacute toxicity study of the ethanol extract from Lonicera japonica Thunb. J. Ethnopharmacol. 107, 370–373. doi: 10.1016/j.jep.2006.03.023
Upadhyay, S. K., Srivastava, A. K., Rajput, V. D., Chauhan, P. K., Bhojiya, A. A., Jain, D., et al. (2022). Root exudates: mechanistic insight of plant growth promoting rhizobacteria for sustainable crop production. Front. Microbiol. 13:916488. doi: 10.3389/fmicb.2022.916488
Urbanová, M., Šnajdr, J., and Baldrian, P. (2015). Composition of fungal and bacterial communities in forest litter and soil is largely determined by dominant trees. Soil Biol. Biochem. 84, 53–64. doi: 10.1016/j.soilbio.2015.02.011
Wu, X., Wu, C., Lu, D., Wu, Y., Ye, Z., Xia, L., et al. (2022). Variation of soil microbial community and sterilization to fusarium oxysporum f. sp. niveum play roles in slightly acidic electrolyzed water-alleviated watermelon continuous cropping obstacle. Front. Microbiol. 13:837121. doi: 10.3389/fmicb.2022.837121
Xi, D., Jin, S., and Wu, J. (2022). Soil bacterial community is more sensitive than fungal community to canopy nitrogen deposition and understory removal in a Chinese fir plantation. Front. Microbiol. 13:1015936. doi: 10.3389/fmicb.2022.1015936
Xie, Z., Wang, Y., Jiang, W., and Wei, X. (2006). Evaporation and evapotranspiration in a watermelon field mulched with gravel of different sizes in Northwest China. Agric. Water Manag. 81, 173–184. doi: 10.1016/j.agwat.2005.04.004
Xie, X. G., Zhang, F. M., Yang, T., Chen, Y., Li, X. G., and Dai, C. C. (2019). Endophytic fungus drives nodulation and N(2) fixation attributable to specific root exudates. MBio 10:e00728-19. doi: 10.1128/mBio.00728-19
Xu, Y., Zhang, G., Ding, H., Ci, D., Dai, L., and Zhang, Z. (2020). Influence of salt stress on the rhizosphere soil bacterial community structure and growth performance of groundnut (Arachis hypogaea L.). Int. Microbiol. 23, 453–465. doi: 10.1007/s10123-020-00118-0
Yan, K., Cui, M., Zhao, S., Chen, X., and Tang, X. (2016). Salinity stress is beneficial to the accumulation of chlorogenic acids in honeysuckle (Lonicera japonica Thunb.). Front. Plant Sci. 7:1563. doi: 10.3389/fpls.2016.01563
Yang, X., Liu, Y., Hou, A., Yang, Y., Tian, X., and He, L. (2017). Systematic review for geo-authentic Lonicerae Japonicae Flos. Front. Med. 11, 203–213. doi: 10.1007/s11684-017-0504-0
Yang, X., Yu, A., Hu, W., Zhang, Z., Ruan, Y., Kuang, H., et al. (2023). Extraction, purification, structural characteristics, health benefits, and application of the polysaccharides from Lonicera japonica Thunb.: a review. Molecules 28:4828. doi: 10.3390/molecules28124828
Zhang, M., Liu, S., Cao, X., Chen, M., Chen, J., Xu, G., et al. (2022). The effects of ectomycorrhizal and saprotropic fungi on soil nitrogen mineralization differ from those of arbuscular and ericoid mycorrhizal fungi on the eastern Qinghai-Tibetan plateau. Front. Plant Sci. 13:1069730. doi: 10.3389/fpls.2022.1069730
Zhang, X., Qian, H., Hua, K., Chen, H., Deng, A., Song, Z., et al. (2022). Organic amendments increase crop yield while mitigating greenhouse gas emissions from the perspective of carbon fees in a soybean-wheat system. Agric. Ecosyst. Environ. 325:107736. doi: 10.1016/j.agee.2021.107736
Zhang, H., Shi, Y., Dong, Y., Lapen, D. R., Liu, J., and Chen, W. (2022). Subsoiling and conversion to conservation tillage enriched nitrogen cycling bacterial communities in sandy soils under long-term maize monoculture. Soil Tillage Res. 215:105197. doi: 10.1016/j.still.2021.105197
Zhang, C., Zhang, Y., Ding, Z., and Bai, Y. (2019). Contribution of microbial inter-kingdom balance to plant health. Mol. Plant 12, 148–149. doi: 10.1016/j.molp.2019.01.016
Zhao, W., Cui, Z., and Li, N. (2016). Scale dependency of spatial variability of soil moisture in gravel-mulched field. Environ. Earth Sci. 75:1455. doi: 10.1007/s12665-016-6263-4
Zhao, W., Cui, Z., Zhang, J., and Jin, J. (2017). Temporal stability and variability of soil-water content in a gravel-mulched field in northwestern China. J. Hydrol. 552, 249–257. doi: 10.1016/j.jhydrol.2017.06.031
Zhao, W., Cui, Z., and Zhou, C. (2020). Spatiotemporal variability of soil–water content at different depths in fields mulched with gravel for different planting years. J. Hydrol. 590:125253. doi: 10.1016/j.jhydrol.2020.125253
Keywords: gravel-mulched land, Lonicera japonica, soil physical and chemical indicators, enzyme activity, bacterial community
Citation: Wang X, Ma B, Liu H, Bao Y, Li M, McLaughlin NB and Guo L (2023) Improvement in gravel-mulched land soil nutrient and bacterial community diversity with Lonicera japonica. Front. Microbiol. 14:1225503. doi: 10.3389/fmicb.2023.1225503
Edited by:
Anna Gałązka, Institute of Soil Science and Plant Cultivation, PolandReviewed by:
Gwen-Aelle Grelet, Manaaki Whenua Landcare Research, New ZealandXiaopeng Tang, Guizhou Normal University, China
Copyright © 2023 Wang, Ma, Liu, Bao, Li, McLaughlin and Guo. This is an open-access article distributed under the terms of the Creative Commons Attribution License (CC BY). The use, distribution or reproduction in other forums is permitted, provided the original author(s) and the copyright owner(s) are credited and that the original publication in this journal is cited, in accordance with accepted academic practice. No use, distribution or reproduction is permitted which does not comply with these terms.
*Correspondence: Bin Ma, bWJpbjg5QDE2My5jb20=