- 1Univ. Lille, CNRS, INRAE, Centrale Lille, UMR 8207 - UMET - Unité Matériaux et Transformations, Lille, France
- 2Univ. Lille, CNRS, UMR 8576 - UGSF - Unité de Glycobiologie Structurale et Fonctionnelle, Lille, France
Spore-forming bacteria of the Bacillus subtilis group are responsible for recurrent contamination of processing lines in the food industry which can lead to food spoilage. The persistence of B. subtilis would be due to the high resistance of spores to extreme environmental condition and their propensity to contaminate surfaces. While it is well known that sporulation conditions modulate spore resistance properties, little is known about their effect on surface and adhesion properties. Here, we studied the impact of 13 sporulation conditions on the surface and adhesion properties of B. subtilis 168 spores. We showed that Ca2+ or Mg2+ depletion, lower oxygen availability, acidic pH as well as oxidative stresses during sporulation lead to the release of more hydrophobic and adherent spores. The consequences of these sporulation conditions on crust composition in carbohydrates and proteins were also evaluated. The crust glycans of spores produced in a sporulation medium depleted in Ca2+ or Mg2+ or oxygen-limited conditions were impaired and contained lower amounts of rhamnose and legionaminic acid. In addition, we showed that lower oxygen availability or addition of hydrogen peroxide during sporulation decreases the relative amount of two crust proteins (CgeA and CotY) and the changes observed in these conditions could be due to transcriptional repression of genes involved in crust synthesis in late stationary phase. The fact that sporulation conditions affect the ease with which spores can contaminate surfaces could explain the frequent and recurrent presence of B. subtilis spores in food processing lines.
1. Introduction
Spore-forming bacteria of the Bacillus subtilis group, including B. subtilis sensu stricto, B. amyloliquefaciens, and B. licheniformis, are a major economic concern in the food industry. Indeed, these bacteria are among the most commonly identified species in spoiled heat-treated food. For example, B. amyloliquefaciens is commonly responsible for the contamination of bakery products, causing notably ropey bread spoilage while B. licheniformis is often involved in the contamination of dairy products (André et al., 2017). The raw materials (food, ingredients) but also the surfaces of the food processing lines would be the major sources of contamination by these spore-forming bacteria. Bacillus spores are known to be highly adherent to all types of surfaces and resistant to most transformation and hygiene procedures encountered in food processing plants (André et al., 2017). Therefore, after cleaning and disinfection procedures, viable adherent Bacillus spores are still commonly found on equipment surfaces and thus potentially initiate new contaminations in subsequent processing cycles by germinating, multiplying, and then forming biofilms in which sporulation is often observed (Soni et al., 2016).
Bacillus subtilis spores are oval-shaped structures formed by concentric layers surrounding the core (McKenney et al., 2013; Driks and Eichenberger, 2016) that contain the chromosomal DNA wrapped around Small Acid Soluble Proteins. The core is surrounded, from innermost to outermost, by the inner membrane, the germ cell wall, the cortex, the outer spore membrane, and the coat. The coat contains more than 80 proteins and represents around 25% of total spore proteins. The coat is subdivided into three parts, the basement layer, the inner coat, and the outer coat. The coat assembly is guided by five morphogenetic proteins (SpoIVA, SafA, CotE, SpoIVM, and SpoVID) that play a key role in coat morphogenesis. Most of the spores of strains of the B. subtilis group are also surrounded by an outermost layer, called the crust (Zhang et al., 1994; McKenney et al., 2010; Faille et al., 2014). Faille and colleagues removed the crust of B. subtilis spores by a mechanical treatment with a French press. The removal of the crust changed these spores from hydrophilic to hydrophobic and increased their adhesion to stainless steel demonstrating that the crust gives B. subtilis spores their specific surface properties, notably a marked hydrophilic character. It was deduced that the crust could be the reason for the relatively low capacity of B. subtilis spores to contaminate surfaces, compared to other Bacillus species such as B. cereus (Faille et al., 2014).
The crust is composed of proteins and carbohydrates (Faille et al., 2014; Bartels et al., 2019; Shuster et al., 2019a,b; Dubois et al., 2020). So far, six proteins have been identified in the crust: CotV, CotW, CotX, CotY, CotZ, and CgeA (Bartels et al., 2019; Shuster et al., 2019a). CotX, CotY, and CotZ are the morphogenetic proteins of the crust and CotZ could be the major one, as the localization of all crust proteins, except CotW, depends on it (Bartels et al., 2019; Shuster et al., 2019a). These proteins are rich in cysteine residues and form an insoluble protein fraction suggesting the presence of disulfide cross-link between these proteins (Ursem et al., 2021). The protein backbone of the crust is predominantly formed by CotY and to a less extent by CotV and CotX (Bartels et al., 2019). When overexpressed in Escherichia coli, CotY self-assembles into crystal structures that tend to form multilayered stacks (Jiang et al., 2015). How the crust is anchored to the spore surface remains to be investigated. However, it was suggested that CotW is a linker protein at the interface of the outer coat and the crust (Bartels et al., 2019). Accordingly, it was shown by transmission electron microscopy that the crust of a ∆cotW mutant is disorganized and separated from the coat (Shuster et al., 2019a). In a heterologous host, CotW and CotV were self-organized into fibrous assemblies of consistent diameter (Jiang et al., 2015). Therefore, it is likely that the fibrous assembly formed by the CotV-CotW complex participates in the anchoring of the crust to the spore surface. Finally, the function of CgeA is still to be determined but CgeA might be a target of glycosylation or a glycosylation hub playing a role in coordinating the glycosylation of crust proteins (Bartels et al., 2019; Shuster et al., 2019a). Carbohydrates are the other key components of the crust. The crust contains rhamnose (Rha), quinovose (Qui), glucose (Glc), glucosamine (GlcN), and muramic lactam which would play a significant role in spore surface properties since mutations in genes expressed in late sporulation and encoding putative glycosyltransferase modify crust structure and/or spore surface hydrophilicity (Roels and Losick, 1995; Cangiano et al., 2014; Faille et al., 2014; Shuster et al., 2019b). Finally, the spore surface of B. subtilis is covered with legionaminic acid (Leg), a nine-carbon backbone nonulosonic acid, whose biosynthesis pathway is required for proper crust assembly (Dubois et al., 2020). Nevertheless, the structure and localization of crust carbohydrates remain poorly described.
Previous studies demonstrated that environmental conditions during sporulation can modulate spore resistance properties (Bressuire-Isoard et al., 2018). For example, it has been shown that supplementation of the sporulation medium with divalent cations such as Ca2+, Mg2+, or Fe2+ increases the resistance to wet heat of B. subtilis spores while the addition of some amino acids such as thioproline or cysteine improves B. subtilis spore resistance to UV-A, UV-B and peracetic acid (Cazemier et al., 2001; Moeller et al., 2011). Other parameters such as pH and temperature during sporulation can also affect spore resistance to wet heat or chemical denaturants (Melly et al., 2002; Baweja et al., 2008). In B. anthracis, spores produced at pH 5 are more resistant to wet heat, and sporulation at pH 9 leads to spores more resistant to sodium hydroxide, compared to spores produced at pH 7 (Baweja et al., 2008). Lastly, B. subtilis produced spores that are more resistant to wet heat when sporulation occurs at 37°C rather than 30°C (Melly et al., 2002). As illustrated by these few examples, most of the works on sporulation conditions have focused on spore resistance, while little is known about the effects of sporulation conditions on the surface and adhesion properties of spores. On B. subtilis spores, a study conducted in 1990 showed minor changes in the hydrophobic character of spores produced in different culture media (Wiencek et al., 1990). More recently, studies on B. subtilis spores have shown that pH and temperature during sporulation affect spore surface properties. Indeed, B. subtilis spores produced at 25°C are more hydrophobic than those produced at 37°C (Isticato et al., 2020). The hydrophobic character of the B. subtilis spores is also affected by the pH of the sporulation medium since it increases with pH for values between 7.0 and 8.5 (Eschlbeck et al., 2017).
In this study, we investigated the influence of environmental conditions encountered by B. subtilis 168 during sporulation on spore surface and adhesion properties. For sporulation conditions leading to an increase in spore adhesion, we investigated the crust structure and composition in carbohydrates and proteins to identify the changes responsible for the increased adhesion. Finally, we performed qRT-PCR experiments to determine whether the changes in crust composition can be explained by transcriptional regulation of genes involved in crust synthesis during the late sporulation phase.
2. Materials and methods
2.1. Bacterial strains and growth conditions
Sporulation experiment were performed with the B. subtilis 168 strain (BGSC, 1A1). Escherichia coli K-12 strain TG1 was used as a host for the construction of plasmids and cloning experiments. E. coli strains were grown in Lysogeny Broth (LB, Sigma-Aldrich, L3022) at 37°C. Bacillus strains were grown at 30 or 37°C in LB, Nutrient Broth (NB, Biokar, BK003HA) or Spo8, a sporulation medium composed of 12.3 g/L NB, 0.51 g/L MgSO4 7H2O, 0.97 g/L KCl, 0.2 g/L CaCl2 2H2O, 3 mg/L MnCl2 4H2O and 0.55 mg/L FeSO4 7H2O at pH 6.9 (Faille et al., 2013). The following concentrations of antibiotic were used for bacterial selection: 100 μg/mL ampicillin or 20 μg/mL chloramphenicol for E. coli and 5 μg/mL chloramphenicol for Bacillus.
For sporulation experiments in the Spo8 condition (control), the following procedure was achieved. Sterile Spo8 medium was inoculated at an optical density at 600 nm of 0.05 with an overnight preculture of the B. subtilis 168 strain. Then, the culture was incubated at 30°C under agitation (220 rpm). After 5 days, spores were harvested by centrifugation (2500 g for 15 min, 4°C), washed three times in chilled sterile water, and stored at 4°C. After 1 week, two additional washes were performed to remove the residual vegetative cell debris and spores were stored at 4°C in sterile water until use for up to 3 months. Spores were checked under microscope and spore preparations with more than 98% of spores were kept for further experiments. The other sporulation conditions are listed in Table 1. Most of the changes in sporulation conditions (addition of chemicals, UV treatment) were implemented 2 h after the transition to the stationary phase. Only the sporulation phase was therefore affected. The chemicals used are listed in Supplementary Table 1. UV treatment was performed by exposing the culture to 254 nm UV radiations (Kingrate, UVC 6WT5, China) for 30 min while maintaining agitation and temperature. In -CaCl2 and -MgSO4 conditions, Spo8 medium depleted in CaCl2 or MgSO4 was inoculated and incubated as described above. Therefore, the bacteria were subjected to these depletions from the beginning of the growth. Ca2+ and Mg2+ concentrations in Spo8 and Spo8 media depleted in CaCl2 or MgSO4 were determined using the Varian SpectrAA-55B atomic absorption spectrophotometer with a Varian SpectrAA Ca/Mg lamp. A standard range was used for each cation. Ca2+ was detected at λ = 422.7 nm and Mg2+ at λ = 285.2 nm. Before analysis, the three Spo8 growth media were diluted in a solution of lanthanum (III) chloride hexahydrate (10 g/L) and hydrochloric acid (84 mL/L of 37% solution).
2.2. Construction of recombinant strains
The plasmids pBS1CΩamyE::cgeA-GFP and pBS1CΩamyE::cotY-GFP were constructed as follows. The gfp-λter DNA fragment containing the gfp gene and the terminator of the λ prophage was obtained by SOE-PCR using the primers gfp-1 / gfp-2 and λter-1 / λter-2. The plasmids pSB1C3-mGFPmut1-orginal and pMUTIN2 were respectively, used as templates (Vagner et al., 1998; Popp et al., 2017). Then, the cgeA or cotY genes with their respective promoters and gfp-λter fragment were amplified by SOE-PCR using primers cgeA-1 / cgeA-2 or cotY-1 / cotY-2 and gfp-1 / λter-2 (Supplementary Table 2). The B. subtilis 168 chromosomal DNA and the gfp-λter fragment were respectively, used as templates. The latter amplification also introduced a sequence encoding 5 glycines that act as a linker between CotY or CgeA and the GFP. The resulting fragments (cgeA-gfp-λter and cotY-gfp-λter) were purified as XbaI-PstI fragments and were inserted between the XbaI and PstI sites of pBS1C (Radeck et al., 2013). The nucleotide sequences of the fragments inserted into pBS1C were verified by Sanger sequencing (Eurofins Genomics, Germany). B. subtilis 168 was then transformed with the plasmids pBS1CΩamyE:: cgeA-GFP or pBS1CΩamyE:: cotY-GFP and homologous recombination at the amyE locus was performed as described previously (Radeck et al., 2013). Nucleotide sequences of the introduced chromosomal modifications were verified by Sanger sequencing (Eurofins Genomics, Germany). The B. subtilis 168 pHT304-18 P sspA -sspA-GFP strain was obtained by transforming the B. subtilis 168 strain with the plasmid pHT304-18 P sspA -sspA-GFP (Richard et al., 2020).
2.3. Heat resistance and germination of spores
The percentage of heat-resistant spores was defined as the ratio of the number of colony-forming units (CFU) after heating to the number of CFU before heating. The number of CFU before heating was evaluated after plating the spore suspensions on NB-agar and incubation for 24 h at 37°C. The number of CFU after heating was evaluated by the same procedure after heat treatment of the spore suspensions for 12 min at 80°C. The ability of spores to germinate was assessed by monitoring the decrease in optical density at 580 nm (OD580) of spore suspensions which reflects the transition of spores from phase-bright to phase-dark during germination (Xing and Harper, 2020). Spores were heat activated at 70°C for 30 min and chilled on ice for 5 min. Then, the spore suspension was adjusted to an initial OD580 of 1 in a 10 mM L-alanine solution in Tris buffer (Tris base 10 mM, NaCl 10 mM, pH 7.4) and the OD580 was monitored with a microplate reader (Biotek, synergy HTX) for 2 h at 30°C with a measurement every 2 min following 5 s of stirring.
2.4. Adhesion, surface properties, and structure of spores
The adhesion of spores to polypropylene was measured as described previously with the following modifications (Abe et al., 2014). The spore suspension was adjusted to an OD600 of about 0.6 in physiological water and 1 mL of the spore suspension was added to a polypropylene tube (2.0 mL Safe-lock tube, Eppendorf). The spore suspension was mixed with a vortex for 10 s to promote spore adhesion to the tube wall, then transferred to a new polypropylene tube. This step was repeated 9 times. The OD600 of the spore suspension was measured after 2, 5, 8, and 10 adhesion steps. The adhesion percentage was calculated by following the formula: 100 × [ODi – ODn]/ODi, where ODi is the initial OD600 and ODn is the OD600 after n steps.
The hydrophilic/hydrophobic property of spores was characterized by MATH, a partitioning method based on the affinity of spores to the apolar solvent hexadecane (Sigma-Aldrich, H6703), as described previously (Faille et al., 2019). The higher the affinity of the spores for hexadecane, the more hydrophobic the spores. Quickly, spores were suspended in physiological water at an OD600 of 0.6 (A0). Three-milliliter aliquots of the spore suspension and 500 μL of hexadecane were placed in glass tubes (10 mm × 75 mm), vortexed for times ranging from 5 s to 240 s, and left to settle for 30 min to allow the two phases to separate. The OD600 of the aqueous phase was then measured (At). Aeq is the asymptotic or lowest absorbance value obtained. The Gibbs energy of partitioning (ΔGpar) is obtained from the equilibrium constant K (ΔGpar = log K), which expresses the partitioning of bacteria between the aqueous and hexadecane phases. It was calculated from the Equation K = [6(A0–Aeq)/Aeq].
To measure the global charge of the spore surface, zetametry experiments were performed as previously described (Faille et al., 2014).
Microscopic observations of spores were carried out as follows. B. subtilis spores were observed on agarose pads (1% Agarose in H2O) using a Zeiss Axioskop 2 microscope (Zeiss, Germany) configurated for phase-contrast observation with a 100x immersion objective (Plan-NEOFLUAR, 100x/1,30 oil, Ph3). Spores were negatively stained with India ink as described previously (Abe et al., 2014) and they were observed in the configuration described above. Fluorescence and localization of CotY-GFP and CgeA-GFP fusion proteins on the spore surface were observed using the Zeiss Axioskop 2 microscope with the appropriate filter set (Filter set 09, BP 450–490 FT510 LP515) and a 100x immersion objective (αPlan-APOCHROMAT, 100x/1,46 Oil).
2.5. Observation of sporulating cells by confocal microscopy
Sporulating cells were collected 8 and 24 h (t8 and t24) after the transition to the stationary phase. They were fixed in a 4% formaldehyde solution in phosphate buffer saline (PBS) for 7 min and washed in PBS before being suspended in GTE buffer (50 mM glucose, 10 mM EDTA at pH 8, and 20 mM tris–HCl at pH 8). After fixation, the cells were kept at 4°C until observations. The membranes of fixated cells were stained by adding FM 4–64 (SynaptoRed C2, Sigma-Aldrich, S6689) at a working concentration of 10 μM for 30 min at room temperature in the dark. Then, the cells were washed, concentrated by centrifugation, and transferred on 1% agarose pads. Microscopic observations were performed by using the inverted confocal laser scanning microscope LSM780 (Zeiss, Germany) with an excitation wavelength of 514 nm, and by detecting the signal at 650–740 nm using a 63x immersion objective (αPlan-APOCHROMAT, 63x/1,4 Oil).
2.6. Experiments on mature spores
To verify that the conditions tested affected the sporulating bacteria and not the mature spores, the same conditions were tested on mature spores. For this purpose, spores were first produced in optimal condition (Spo8) and then subjected to the -CaCl2, -MgSO4, -O2, -O2 + CO2, peracetic acid, H2O2 or pH 5 conditions, except that Spo8 was modified by removing the NB to prevent spore germination. Then, mature spores were incubated at 30°C under agitation (220 rpm). After 3 days, spores were harvested by centrifugation (2,500 g for 15 min, 4°C) and washed five times in chilled sterile water (2,500 g for 15 min, 4°C) before being stored at 4°C in sterile water until use. Spores were then tested for their surface hydrophilic/hydrophobic properties.
2.7. Crust extraction and analysis
The crust was removed from the spore surface by three successive passages of spore suspensions through a French press (SLM instruments, Urbana, IL) at 20000 psi. Spores were separated from the crust by centrifugation (6,000 g, 15 min, 4°C) and the supernatant (the crust extract) was kept at −20°C until use.
Release and derivation of the crust monosaccharides were adapted from previous studies (Anumula, 1994; Windwarder et al., 2016). Crust extracts were dried by lyophilization and hydrolyzed at 100°C for 4 h in 4 M trifluoroacetic acid (TFA) to release the monosaccharides. Samples were dried under a nitrogen flow at 40°C in an evaporator (puriVap-6, Interchim) and the dried pellets were suspended in a 50:50 (v/v) mix of a fresh 1% sodium acetate solution in methanol and a fresh solution containing 30 mg of anthranilic acid (AA) and 20 mg of sodium cyanoborohydride in a solution of 2% boric acid and 4% sodium acetate in methanol. The monosaccharides were then coupled to the anthranilic acid by heating the samples at 80°C for 1 h. Release and derivation of Leg were performed as described previously (Dubois et al., 2020). Crust extracts were dried by lyophilization and hydrolyzed at 80°C for 2 h in 0.1 M TFA to release the nonulosonic acids. Nonulosonic acids were subsequently coupled to 1,2-diamino-4,5-methylenedioxybenzene (DMB) by heating the samples at 50°C for 2 h in the dark in 7 mM DMB, 1 M β-mercaptoethanol, 18 mM sodium hydrosulfite in 0.02 mM trifluoroacetic acid.
The monosaccharide derivatives were separated on a C18 reverse-phase HPLC column (Thermo Scientific, ODS Hypersil, 5 μm, 4.6 × 250 mm) at a flow rate of 1 mL/min following a protocol adapted from Windwarder et al. (2016). The solvent A was 80 mM formic acid adjusted to pH 3.4 with ammonia and the solvent B was a mixture of acetonitrile:solvent A (80:20, v/v). The elution program was a gradient from 9.5 to 16% of solvent B for 20 min followed by a second gradient from 16 to 36% for 20 min. Fluorescence was measured with a Waters 2,475 fluorimeter (λexc = 230 nm, λem = 425 nm). A standard containing GlcN, galactose (Gal), Glc, Rha, and Qui was used to estimate the amount of the different monosaccharides. The nonulosonic acid derivatives were separated isocratically at a flow rate of 0.8 mL/min on a C18 reverse phase HPLC column (Waters, 3.5 μm, 4.6 × 150 mm) using a solvent mixture of acetonitrile:methanol:water (7:9:84, v/v/v) and they were detected by a fluorimeter (Waters 2,475, λexc = 373 nm, λem = 448 nm). The Leg amount was estimated by measuring the area of peak 1 and by reporting this area to a standard range of Neu5Ac.
Gas chromatography–mass spectrometry (GC–MS) experiments were performed as previously described (Faille et al., 2014).
The relative amount of CotY or CgeA in the crust was evaluated by using B. subtilis 168 amyE::cotY-GFP strain or B. subtilis 168 amyE::cgeA-GFP strain in the sporulation conditions described above. After washing in chilled water, spores were diluted in 1X PBS buffer and gently vortexed. Then, the fluorescence of spores was evaluated by flow-cytometry with the BD Acuri C6 Plus flow-cytometer (BD biosciences, USA) using a 488 nm laser and 585/40 bandpass filter to detect FITC fluorescence. The following voltage values were used: 200 V for the forward scatter, 250 V for the side scatter, and 400 V for the FITC channel according to the manufacturer’s recommendations. The cytometer was programmed to count 40,000 events and the spore suspension was diluted to obtain less than 2,500 events per second.
2.8. Transcriptional analysis of genes involved in crust biosynthesis
The sporulating cells were collected and harvested by centrifugation (5,000 g, 15 min at 4°C) 8 h after the transition to the stationary phase. RNA extraction, cDNA synthesis, amplification, and detection were performed as previously described (Dubois et al., 2016) except that the cell lysis was performed using screw tubes containing 150 mg of glass beads (acid-washed, Sigma-Aldrich, G1277-500G,) and a MiniBeadBeater-16 (Biospec Products). The tubes were shaken for 2 min and cooled on ice for 2 min. The previous step was repeated once and the lysates were centrifuged at 12,000 g for 10 min at 4°C before transferring to a new 2 mL tube. The quantity of each cDNA was normalized to the quantity of the cDNA of the DNA polymerase III gene (polC, BSU16580) (Supplementary Table 2). The relative change in gene expression was recorded as the ratio to normalized target concentrations (threshold cycle [∆∆CT]) (Livak and Schmittgen, 2001).
3. Results
To investigate the influence of the environmental conditions encountered by B. subtilis during sporulation on spore surface and adhesion properties, sporulation conditions were modified before bacteria inoculation or 2 h after the transition to the stationary phase (Table 1; Supplementary Figure 1). These 13 conditions were tested to evaluate the influence of depletion in divalent cations (-CaCl2 or -MgSO4), oxidative stresses (peracetic acid or H2O2), atmospheres (-O2 or -O2 + CO2), pH (pH 9 or pH 5), preservatives (benzoic acid or nitrites), UV as well as cleaning and disinfecting agents (RBS or Benzalkonium Chloride [BAC]). The chemicals added were used at the maximum concentration that did not reduce the total number of CFU at the end of sporulation to avoid major structural changes in the internal layers of spores.
3.1. Influence of sporulation conditions on refractivity, heat resistance, and germination rate of Bacillus subtilis 168 spores
It was first investigated whether the sporulation conditions affected the total number of CFU as well as some key characteristics of spores, i.e., their refractivity, heat resistance, and ability to germinate. The total number of CFU at the end of sporulation was increased in the -O2, -O2 + CO2, peracetic acid, and pH 5 conditions while it was reduced by a logarithmic factor of 0.45 in the -CaCl2 condition compared to the Spo8 condition (Supplementary Table 3). None of the sporulation conditions changed the spore characteristics except for those obtained in the -CaCl2 condition (Supplementary Table 3; Supplementary Figure 2). In this latter condition, the percentage of heat resistant spores was unchanged but 35% of spores were non-refractive and the ability of spores to germinate was reduced by 40% compared to the Spo8 condition. These results suggest that in the -CaCl2 condition, a part of the cells that engage in the sporulation process do not release viable spores. However, the viable spores produced in this condition keep their heat resistance and most of them can germinate. Therefore, this condition was kept for subsequent experiments.
3.2. Divalent cations depletion, oxidative stresses, lower oxygen availability and acidic pH during sporulation promote spore adhesion
To evaluate the consequences of the sporulation conditions on spore adhesion, an adhesion test on polypropylene was performed. B. subtilis 168 spores produced in 7 of the 13 conditions tested were significantly more adherent to polypropylene than those produced in Spo8 (Figure 1; Supplementary Figure 3). This is particularly true for spores produced in -CaCl2 or -MgSO4 conditions. Replacement of CaCl2 or MgSO4 by NaCl or Na2SO4 in the sporulation medium also led to the production of more adherent spores indicating that the low concentrations of Mg2+ or Ca2+ rather than the associated anions (SO42− or Cl−) were responsible for the increased adhesion of spores. Bacteria that sporulated in a modified atmosphere (-O2 and -O2 + CO2), at acidic pH (pH 5) and to a lesser extent those that were exposed to oxidative stress (H2O2, peracetic acid) also produced spores that were more adherent than spores produced in Spo8. Interestingly, there was no significant difference in adhesion between spores produced in -O2 and -O2 + CO2 conditions, which would indicate that the decrease in O2 and not the increase in CO2 concentration during sporulation is responsible for this increase in adhesion. To verify that the increase in adhesion was not due to a delay in the sporulation process, sporulating cells were observed by confocal microscopy 8 and 24 h after the transition to the stationary phase. No delay in the sporulation process was observed (Supplementary Figure 4).
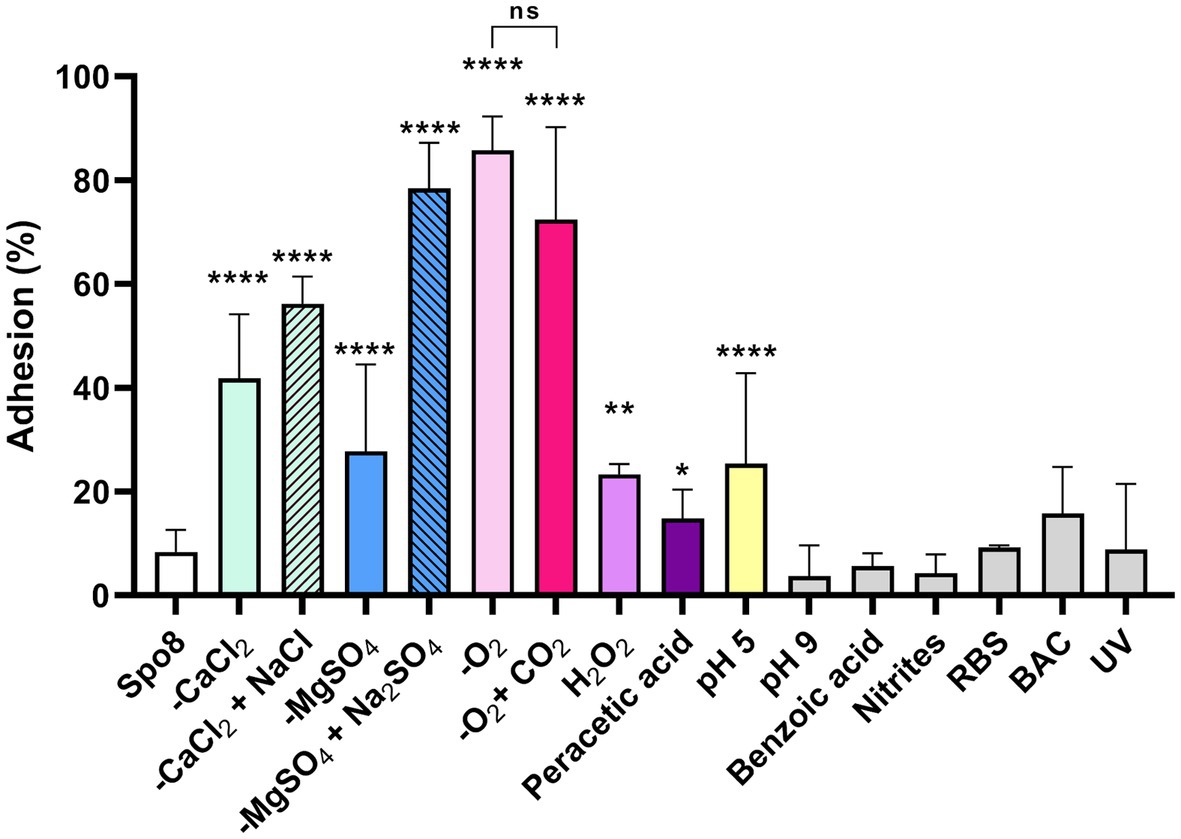
Figure 1. Influence of sporulation conditions on spore adhesion to polypropylene. Adhesion (%) was determined after 10 successive adhesion steps of the spores to polypropylene tubes. The whole adhesion kinetics are presented in Supplementary Figure 3. Error bars represent the SDs of the means. ns, not significant. *p ≤ 0.05; **p ≤ 0.01; ***p ≤ 0.001; ****p ≤ 0.0001 for each condition versus Spo8 by Mann–Whitney.
3.3. Increased spore adhesion is correlated with an increase of spore hydrophobicity
Since the spores are metabolically dormant, the increase in spore adhesion can only be explained by modifications of the spore surface properties including hydrophobicity and charge. Thus, the surface hydrophilic/hydrophobic property and the surface charge of spores were evaluated by MATH and zetametry (Table 2; Supplementary Figure 5). All the spores obtained under the sporulation conditions that led to an increase in spore adhesion were more hydrophobic, whereas no modification of this character could be demonstrated for spores whose adhesion was not affected. The correlation factor between spore hydrophobicity and spore adhesion to polypropylene was 0.71 indicating that the increased adhesion of spores is at least in part due to an increase of spore’s hydrophobicity (Supplementary Figures 6A,C). By contrast, whatever the sporulation condition, the global charge of spores at a neutral pH was similar (around −50 mV), except for spores produced at pH 5 and in the presence of benzoic acid (Table 2). However, the spore electronegativity would not play a significant role in their ability to adhere to polypropylene (correlation factor = −0.14) (Supplementary Figures 6B,C). It is now well documented that alteration of the crust generally leads to an increased hydrophobicity and adhesion of spores (Faille et al., 2014; Shuster et al., 2019a,b; Dubois et al., 2020). Therefore, our results suggest that environmental conditions encountered during sporulation affect crust composition and/or structure.
3.4. The modification of the spore surface properties occurs during sporulation
We investigated whether the same environmental conditions applied to mature spores affected their surface properties, i.e., whether the changes in spore surface observed above were due to phenomena occurring during sporulation or after the release of the mature spores. For this purpose, mature spores produced in Spo8 condition were subjected to the seven conditions shown to modify the adhesion and hydrophobicity of spores when applied during sporulation. Then, the hydrophobicity of spores was evaluated by MATH (Table 3). The seven conditions applied to the mature spores did not alter their hydrophobic character, which confirmed that the changes observed above were indeed the result of phenomena occurring during sporulation.
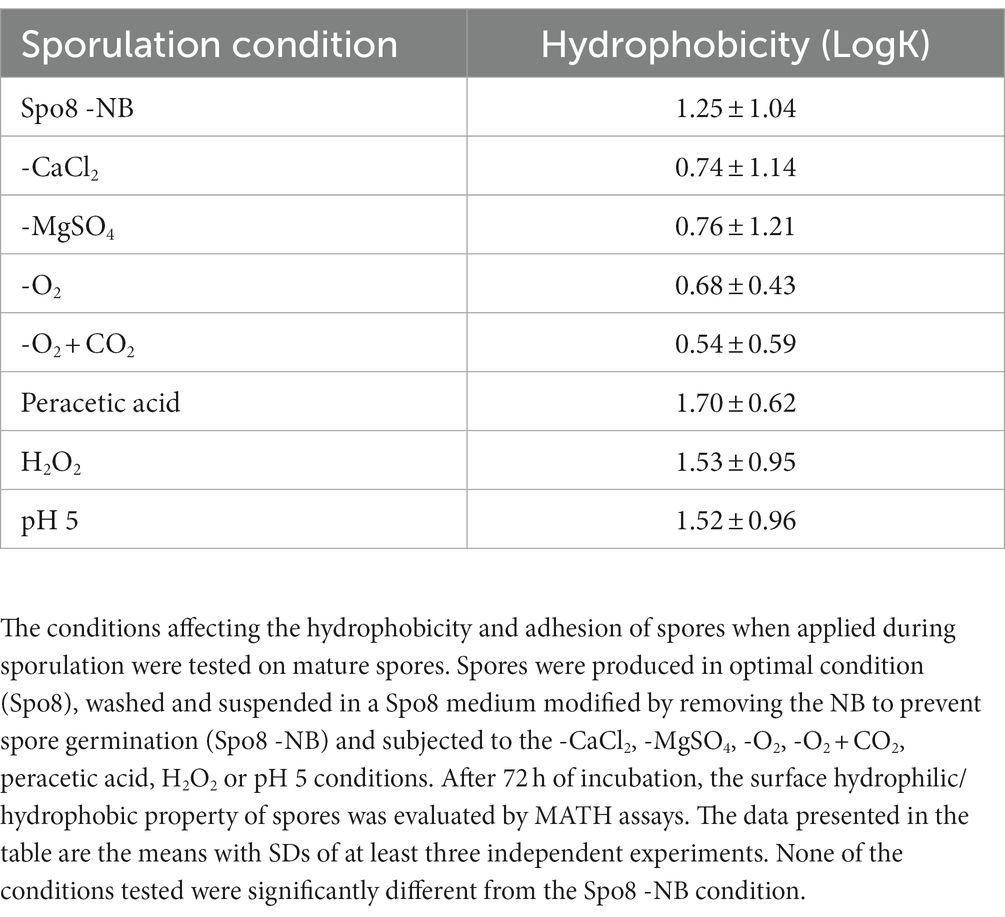
Table 3. Influence of environmental conditions on surface hydrophilic/hydrophobic property of mature spores.
3.5. The crust glycans of spores produced in divalent cations depletion or oxygen-limited conditions are impaired
To evaluate the impact of the sporulation conditions on crust structure, spores were observed in phase-contrast microscopy after India ink staining (Figure 2). Indeed, the India ink staining enables the observation of the crust glycans around B. subtilis spores. Spores produced in Spo8 (positive control) showed a white halo surrounding the outer coat of spores (white arrow), which reflects the presence of the crust glycans. Conversely, no white halo was observed when spores produced in Spo8 were passed through a French press, this treatment resulting in the removal of the crust without damaging the coat (negative control) (Faille et al., 2014). A white halo was observed around spores produced in the H2O2, peracetic acid, pH 5, pH 9, benzoic acid, nitrites, BAC, RBS, and UV conditions. In contrast, no halo was observed around spores produced in the -CaCl2, -MgSO4, -O2, and -O2 + CO2 conditions indicating that the crust glycans are deeply impaired in these conditions.
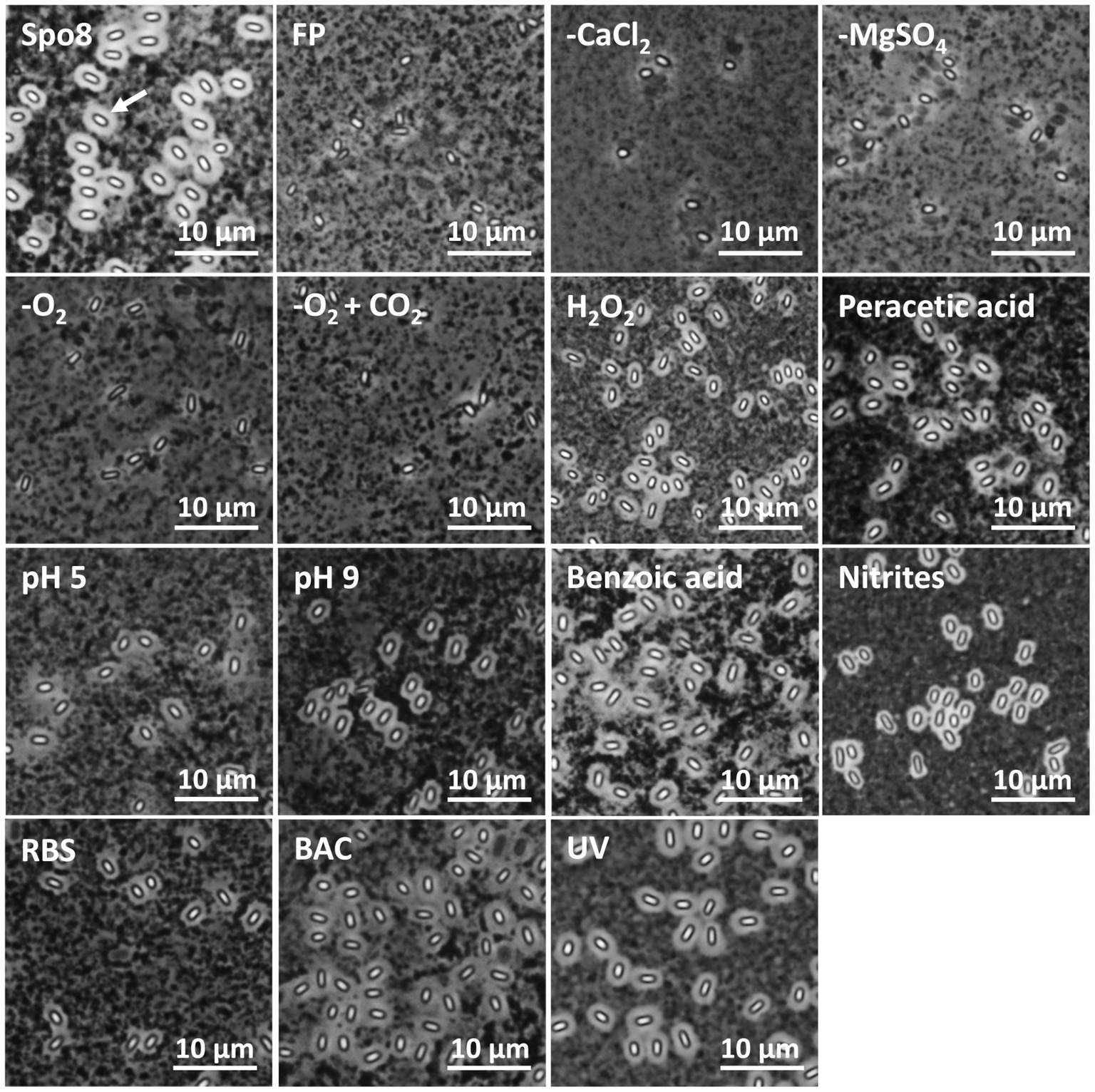
Figure 2. Observation of spores by phase contrast microscopy after India ink staining. FP: Spores after a French press treatment (negative control). A white halo around the spores (white arrow) indicates the presence of crust glycans.
3.6. Divalent cation depletion or lower oxygen availability during sporulation change the crust composition in monosaccharides
The monosaccharide composition of the crust was further investigated. Spores were mechanically treated with a French press to remove the crust. Then, the crust glycans were hydrolyzed and the released monosaccharides were derivatized by AA, a fluorogenic reagent that shows high specificity for monosaccharides. The AA-complexed monosaccharides were then analyzed by reverse-phase high-performance liquid chromatography coupled to a fluorescence detector (RP-HPLC-FL) (Anumula, 1994; Windwarder et al., 2016). In the Spo8 condition, the chromatogram of RP-HPLC-FL showed 12 major peaks (Supplementary Figure 7A). By performing co-injections with standards four peaks were associated with monosaccharides previously identified in the crust: GlcN with a retention time of about 11.8 min, Glc with a retention time of about 16.7 min, Rha with a retention time of about 20.8 min and Qui with a retention time of about 25.4 min (Supplementary Figure 7B; Faille et al., 2014). The peak with a retention of 15.9 min co-eluted with the Gal standard and GC–MS experiments confirmed the presence of Gal in the crust of B. subtilis spores (Supplementary Figures 7A–F). Using RP-HPLC-FL, the influence of the conditions shown to increase spore adhesion on the amount of the five monosaccharides identified in the crust was evaluated. In all the conditions tested, there was no significant change in the amounts of GlcN, Gal, and Glc compared to the Spo8 condition (Supplementary Figures 8A–G). The composition of Rha and Qui was also not modified in the crust of spores produced in the H2O2, peracetic acid, and pH 5 conditions (Figures 3E–G). Conversely, the amount of Rha was lowered in the crust of spores produced in the -CaCl2, -MgSO4, -O2 and -O2 + CO2 conditions, and the amount of Qui was lowered in the crust of spores produced in the -CaCl2 and -O2 + CO2 conditions (Figures 3A–D).
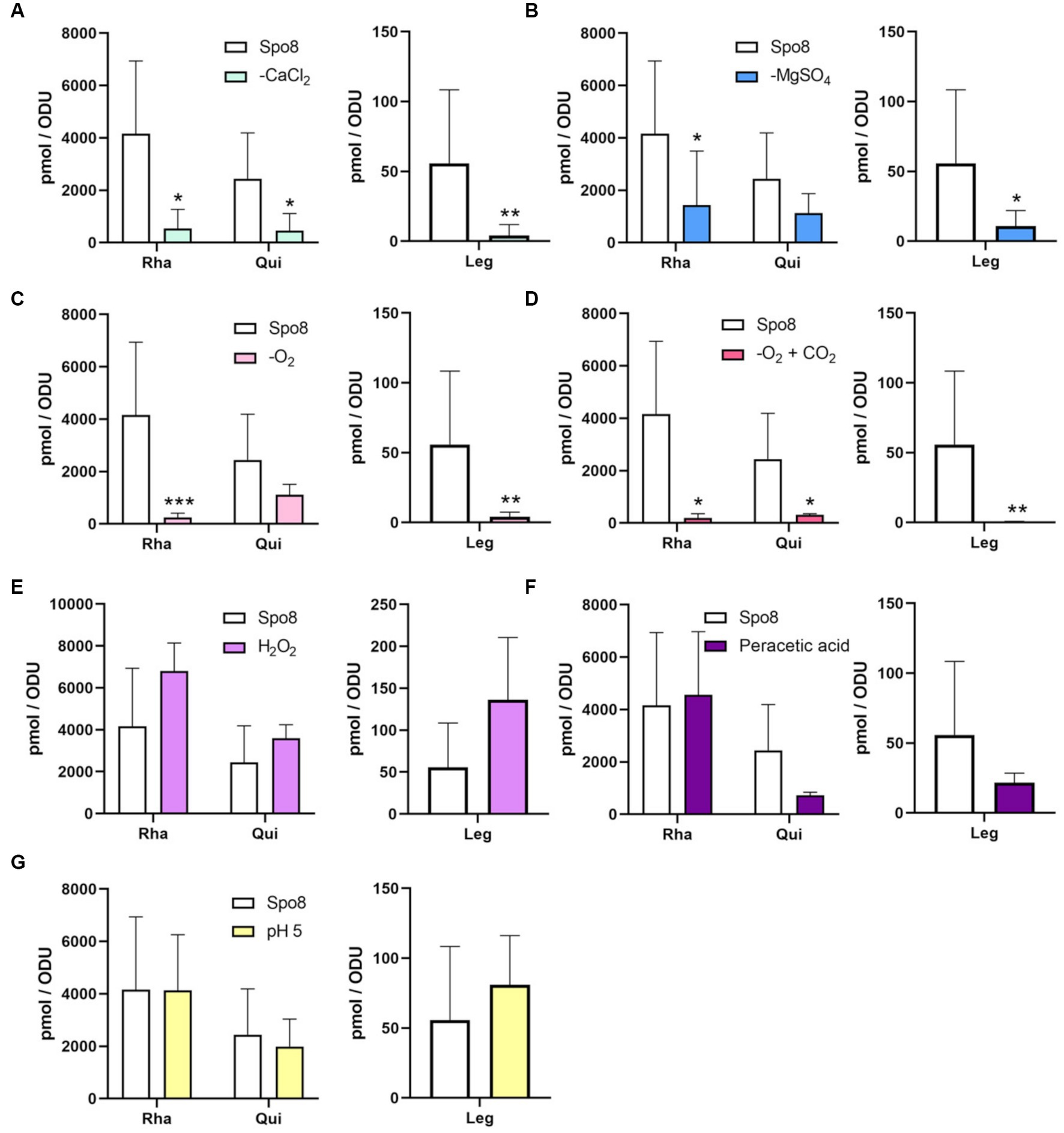
Figure 3. Influence of sporulation conditions on the monosaccharide composition of the crust. The relative amounts of Rha, Qui, and Leg in the crust were evaluated by RP-HPLC-FL. The experiments were performed on the crust of spores released from B. subtilis 168 cells produced in Spo8 and in the sporulation conditions shown to affect spore surface properties: -CaCl2 (A), -MgSO4 (B), -O2 (C), -O2 + CO2 (D), H2O2 (E), peracetic acid (F), and pH 5 (G). The results were standardized by the OD600nm of the spore preparations. Error bars represent the SDs of the means. *p ≤ 0.05; **p ≤ 0.01; ***p ≤ 0.001 for each condition versus Spo8 by Mann–Whitney.
3.7. Sporulating cells exposed to divalent cations depletion or lower oxygen availability produce spores with a lower amount of leg in the crust
To evaluate the impact of the sporulation conditions on the amount of Leg on the spore surface, crust samples were hydrolyzed, and nonulosonic acids were derivatized by DMB before being analyzed by RP-HPLC-FL. The chromatogram obtained in the Spo8 condition showed three major peaks with retention times of ~8.3 min (peak 1), 14.5 min (peak 2), and 20.4 min (peak 3). The area of peak 1, which was previously identified as Leg, was used to estimate the Leg amount in the crust of B. subtilis spores (Supplementary Figure 9; Dubois et al., 2020). The amount of Leg was significantly reduced in the -CaCl2, -MgSO4, -O2, and -O2 + CO2 conditions compared to the Spo8 condition (Figures 3A–D). In contrast, it was not significantly different in the H2O2, peracetic acid, and pH 5 conditions (Figures 3E–G).
3.8. The protein backbone of the crust of spores produced in the oxygen-limited and H2O2 conditions is impaired
To assess the effect of the sporulation conditions on crust proteins, the cotY and cgeA genes with their respective promoter were fused to the gene encoding the green fluorescent protein (GFP). Fusions were then integrated at the amyE locus of the B. subtilis 168 strain to obtain the B. subtilis 168 amyE::cotY-GFP and B. subtilis 168 amyE::cgeA-GFP. These two strains produced CotY-GFP and CgeA-GFP fusion proteins, respectively. The CotY-GFP fusion protein was used here as a marker of the integrity of the protein backbone of the crust while the CgeA-GFP fusion protein was used to detect any change in the composition and/or structure of the crust proteins. Spores of the B. subtilis 168, B. subtilis 168 amyE::cotY-GFP, and B. subtilis 168 amyE::cgeA-GFP strains were produced in the Spo8 condition and the fluorescence of spores was evaluated by fluorescence microscopy. Spores of the B. subtilis 168 strain were slightly auto-fluorescent at the excitation wavelength of GFP and a strong fluorescence signal was observed on the spore surface of the B. subtilis 168 amyE::cotY-GFP and B. subtilis 168 amyE::cgeA-GFP strains indicating that CotY-GFP and CgeA-GFP fusion proteins were properly expressed and localized (Supplementary Figure 10). Then, spores of the B. subtilis 168 amyE::cotY-GFP and B. subtilis 168 amyE::cgeA-GFP strains were produced in the seven sporulation conditions affecting surface properties and fluorescence of spores was measured by flow-cytometry (Figure 4; Supplementary Figures 11A,B). In the -O2, -O2 + CO2, and H2O2 sporulation conditions, the fluorescence of spores of B. subtilis 168 amyE::cotY-GFP strain was significantly lower than that of spores of the same strain obtained in the Spo8 condition. These results indicate that these sporulation conditions lead to a decrease in the relative amount of CotY in the crust. The decrease in spore fluorescence in the -O2 and -O2 + CO2 conditions was not due to a decrease in GFP fluorescence caused by a lower oxygen availability during sporulation (Supplementary Figure 11C). In the -CaCl2, -O2, -O2 + CO2, H2O2, and pH 5 sporulation conditions, fluorescence of spores of the B. subtilis 168 amyE::cgeA-GFP strain was lower than that of spores of the same strain obtained in the Spo8 condition indicating a decrease in the relative amount of CgeA in the crust of spores produced in these conditions.
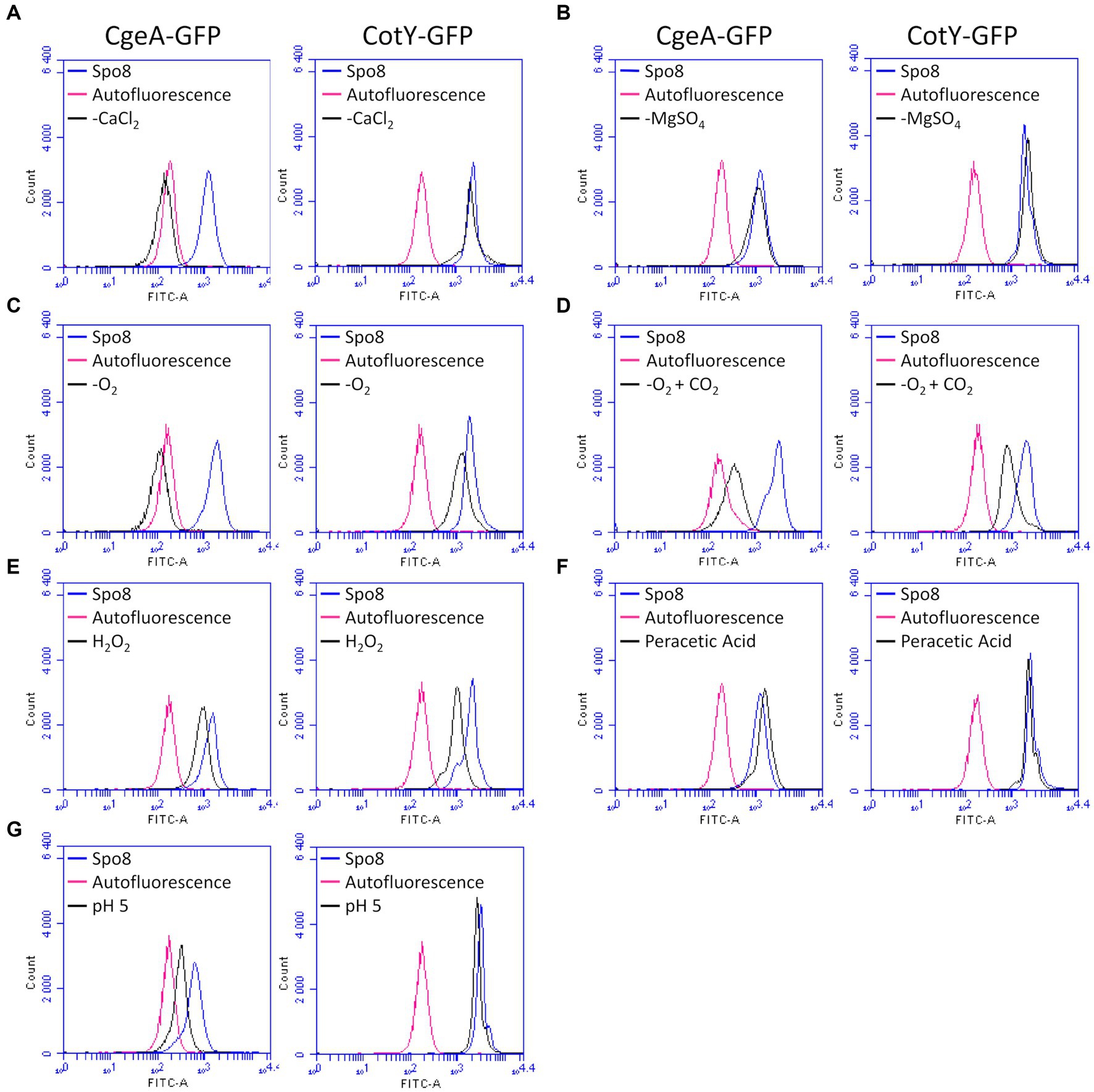
Figure 4. Influence of sporulation conditions on the relative amount of CgeA and CotY in the crust. Fluorescence of spores of the B. subtilis 168 amyE::cgeA-GFP and B. subtilis 168 amyE::cotY-GFP strains was measured by flow cytometry after sporulation of both strains in Spo8 and in the sporulation conditions shown to affect spore surface properties: -CaCl2 (A), -MgSO4 (B), -O2 (C), -O2 + CO2 (D), H2O2 (E), peracetic acid (F) and pH 5 (G). The fluorescence of spores is proportional to the amount of CgeA-GFP and CotY-GFP fusion proteins on the spore surface. Autofluorescence of the B. subtilis 168 strain is represented in red in all the panels (negative control). The data are presented as overlaid histograms that represent the distribution of fluorescence per cell of the same number of events.
3.9. Transcription of genes involved in crust biosynthesis is decreased during late sporulation in oxygen-limited and H2O2 conditions
We have shown above that -CaCl2, -MgSO4, -O2, -O2 + CO2, H2O2, and pH 5 sporulation conditions modify the glycan and/or protein composition of the crust of the released spores. To determine whether these crust modifications are due to transcriptional regulation of genes involved in crust synthesis, qRT-PCR experiments were performed on RNA extracted from sporulating cells in the late stationary phase (8 h after the transition to the stationary phase). The genes encoding crust proteins (cotY and cgeA) and the genes encoding the first enzyme of the Rha and Leg biosynthesis pathway (spsI and spsM, respectively) were targeted (Figure 5A). In addition, a primer pair flanking the SPβ prophage was tested to evaluate the influence of sporulation conditions on SPβ prophage excision. In the -CaCl2, -MgSO4, and pH 5 conditions, transcription of the four genes was unchanged compared to the Spo8 condition (Figures 5B–D). In contrast, transcription of the cgeA and spsM genes was decreased in the -O2 condition, and transcription of all the genes tested was reduced in the -O2 + CO2 condition (Figures 5E,F). Finally, in the H2O2 condition, transcription of cotY and cgeA genes was decreased compared to the Spo8 condition (Figure 5G). Our results also showed that prophage excision is not changed whatever the condition tested. These results suggest that the changes in spore surface properties obtained in the -O2, -O2 + CO2, and H2O2 conditions are, at least in part due to a decrease in the transcription of genes involved in crust synthesis during late sporulation.
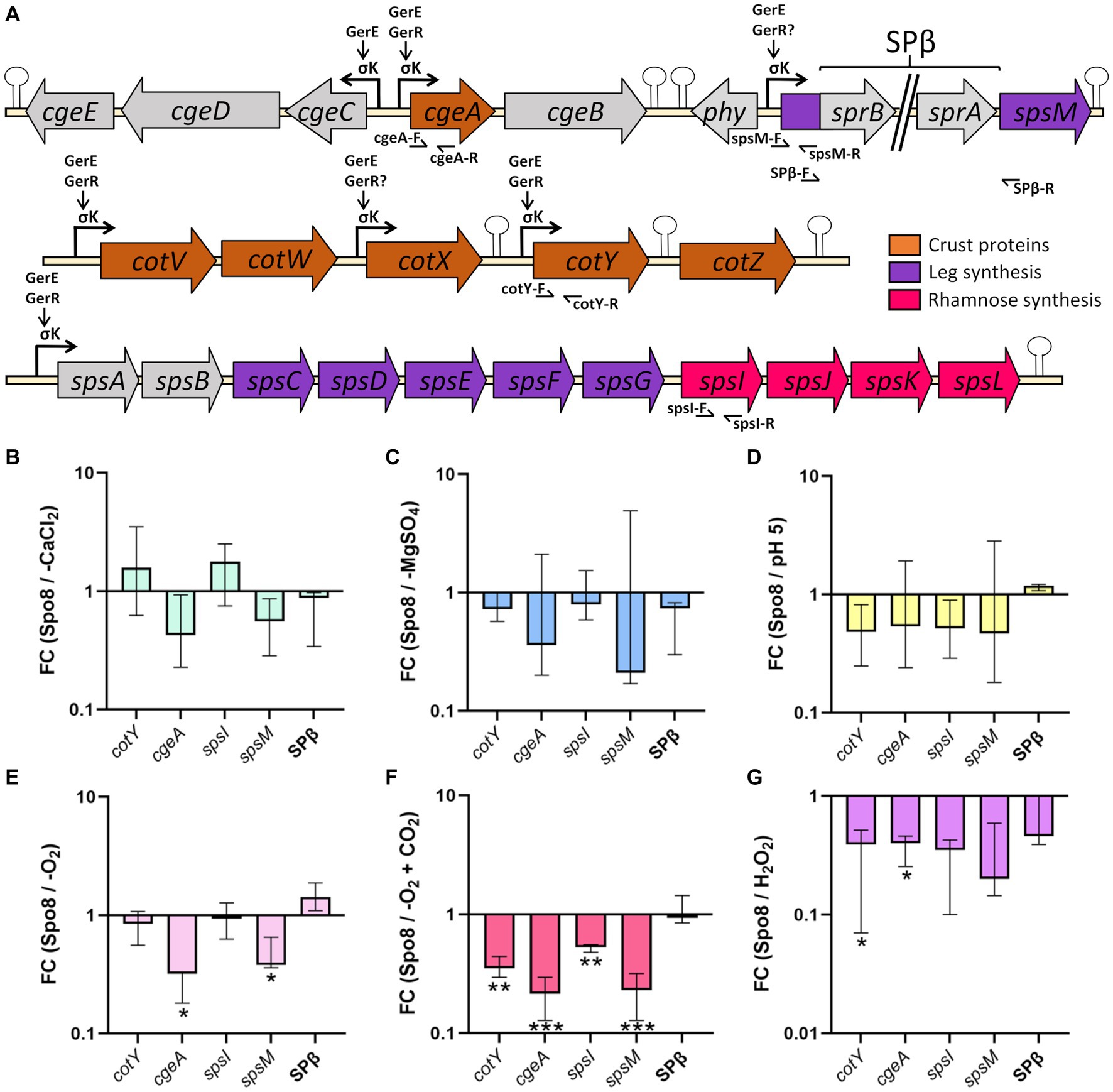
Figure 5. Influence of sporulation conditions on the transcription of genes involved in crust synthesis. (A) Schematic representation of the genetic organization of the cge, cot, and sps genes involved in crust synthesis. Broken arrows, arrows and, stem-loop, respectively, represent the σK dependent promoters, direct or indirect activation of transcription by a transcriptional regulator and transcriptional terminators defined previously (Zhang et al., 1994; Roels and Losick, 1995; Eichenberger et al., 2004; Kuwana et al., 2005; Nicolas et al., 2012; Cangiano et al., 2014; Arrieta-Ortiz et al., 2015). Primers used for qRT-PCR are indicated by half arrows. Relative transcript levels of the cotY, cgeA, spsI, and spsM genes and SPβ prophage excision were evaluated by qRT-PCR in the late stationary phase (t8). SPβ prophage excision was assessed using primers flanking the prophage (SPβ-F/SPβ-R). Relative transcript levels were calculated as the ratio of the mRNA level (arbitrary units) of each gene in the sporulation conditions shown to affect spore surface properties: -CaCl2 (B), -MgSO4 (C), pH 5 (D), -O2 (E), -O2 + CO2 (F) and H2O2 (G), compared to that obtained in the Spo8 condition. The fold change (FC) was calculated by the ΔΔCT method using the polC gene for normalization. Error bars represent the median with the interquartile range. *p ≤ 0.05; **p ≤ 0.01; ***p ≤ 0.001 for each condition versus Spo8 by t-test.
4. Discussion
In optimized growth conditions, e.g., in laboratory conditions, sporulation of B. subtilis is triggered when the cells enter in the transition to the stationary phase, and it takes 8–10 h to complete with the release of a mature spore. Therefore, sporulation is a long process and its duration can be further extended in suboptimal growth conditions such as those found in natural and industrial environments. In these environments, spore-forming bacteria often encounter unfavorable conditions for growth and sporulation, such as low or high temperatures and pH, which can modulate sporulation rate and length, but also the properties of mature spores (Bressuire-Isoard et al., 2018). Many works have been reported in the literature on the influence of sporulation conditions on spore resistance, notably to extreme temperatures and UV radiations. Other works have concerned the structure of the spores, e.g., the water or dipicolinic acid content, the cortex peptidoglycan structure, the fatty acid composition, or the coat protein profile, which are probably at least partly related to changes in spore resistance (Abhyankar et al., 2016; Setlow and Christie, 2023). Conversely, only a few works have been reported on the surface properties of spores produced in different environmental conditions, despite their major role in surface contamination and therefore their possible role in the risk of further dissemination, e.g., in agri-food industries or medical environments.
This study evaluated the impact of 13 sporulation conditions likely to be encountered in real environments, on B. subtilis spore surface and adhesion properties. Some of these conditions, such as reduced cation availability, or acidic pH, have previously been shown to affect spore resistance (Cazemier et al., 2001; Baweja et al., 2008). In contrast, the conditions used to limit the growth and/or survival of bacteria, such as preservatives, detergents, and disinfectants seem not to have been investigated in the literature. Out of the 13 sporulation conditions, 7 led to the release of spores that are more hydrophobic and adherent, while the other conditions did not affect these spore properties. Concerning the lack of effect of the detergent and antibacterial treatments, it can be assumed that this would be due to the selected relatively mild conditions, so as not to impede sporulation. Six of these seven sporulation conditions leading to more adherent spores can be categorized into three groups according to the nature of the stresses (Table 4): depletion in divalent cation (-CaCl2 and -MgSO4), lower oxygen availability (-O2 and -O2 + CO2) and oxidative stress (H2O2 and peracetic acid). These results suggest that in their ecological niches and industrial environments, where growth and sporulation conditions are not optimal, the bacteria of the B. subtilis group probably produce spores that are often more hydrophobic and adherent than spores obtained in optimized laboratory conditions.
The surface and adhesion properties of spores depend on their outermost layer which is in contact with surfaces in the environment. In most strains of the B. subtilis group, the crust is the outermost layer of spores and it has been shown that its mechanical removal makes B. subtilis spores hydrophobic and more adherent to stainless steel (Faille et al., 2014; Dubois et al., 2020). Therefore, we investigated the impact of the sporulation conditions on the crust structure and composition. Spores produced in the different conditions shown to affect their surface properties were observed under microscope after staining with India ink, a stain that does not penetrate the mucous layer formed by the crust glycans, producing a white halo around the spores (Abe et al., 2014; Shuster et al., 2019b). In the divalent cation depletion (-CaCl2 or -MgSO4) and oxygen-limited (-O2 or -O2 + CO2) conditions, the crust glycans were impaired confirming the negative impact of these sporulation conditions on crust glycans synthesis and/or assembly on the spore surface. In contrast, the crust glycans of spores obtained from sporulating cells exposed to oxidative stresses (H2O2 and peracetic acid) or low pH (pH 5) seemed unaffected.
To determine if these observations were related to a decrease in Leg or one or more monosaccharides, the composition of the crust of these spores was implemented. We first confirmed that the crust contains Rha, Glc, Qui, GlcN, and Leg (Faille et al., 2014; Dubois et al., 2020) and we also demonstrated the presence of Gal (Supplementary Figure 7). A reduced amount of Rha, Qui, and Leg was observed on some spores, the alteration of the crust of which was revealed by staining with India ink, while no changes could be evidenced on the spores still surrounded with a clear halo. Interestingly, the relative amounts of Rha and Leg decreased concomitantly in divalent cations depletion as well as oxygen-limited conditions. This result is consistent with a previous study that showed that inactivation of the Leg biosynthesis pathway abolishes Leg production but also decreases the amount of Rha in the crust (Dubois et al., 2020). These data suggest that Rha and Leg are part of a same glycan. It was shown previously that the Leg biosynthesis pathway is required for crust assembly and spores from mutants of this pathway are more hydrophobic and adherent to stainless steel (Dubois et al., 2020). Similarly, it was shown by transmission electron microscopy that the crust of a rhamnose-deficient mutant (∆spsI) is present around B. subtilis spores but it is barely detectable and spores of this mutant were more hydrophobic than spores of a rhamnose producing strain (Shuster et al., 2019b). These data strongly suggest that Rha and Leg play an important role in spore surface and adhesion properties. Therefore, it seems likely that the increased adhesion of spores obtained after sporulation in divalent cations depletion or oxygen-limited conditions is due to a decrease in the amount of a glycan containing Rha and Leg in the crust. On the other hand, the increased adhesion of spores produced under oxidizing conditions cannot be explained by the modification of the crust glycans.
We also evaluated the impact of the sporulation conditions on the relative amount of the CotY and CgeA proteins in the crust. CotY is one of the morphogenetic proteins and the main structural protein of the crust of B. subtilis spores (Bartels et al., 2019). Indeed, expressed in E. coli, CotY can arrange intracellularly into highly stable honeycomb-like structures through processes of self-assembly (Jiang et al., 2015). Therefore, the CotY-GFP fusion protein was used as a marker of the integrity of the protein backbone of the crust. In contrast, CgeA is not required for the assembly or the correct localization of the other crust proteins and it relies on all other crust proteins for proper localization (Bartels et al., 2019; Shuster et al., 2019a). It was deduced that CgeA is at the bottom of the crust assembly hierarchy (Bartels et al., 2019; Shuster et al., 2019a). Therefore, the CgeA-GFP fusion protein was used to detect any change in the composition and/or structure of the crust proteins. Our results showed that all the sporulation conditions tested changed the protein composition of the crust except for -MgSO4 and peracetic acid conditions. In the -CaCl2 and pH 5 conditions, the changes in the crust appear to be moderate, as only the relative amount of CgeA decreased. In contrast, when sporulation was perturbed by hydrogen peroxide stress or oxygen-limited conditions, the relative amount of both CgeA and CotY decreased suggesting that the protein backbone is impaired. The underlying molecular mechanisms that explain the changes in crust composition and structure will have to be studied but they probably interfere with crust synthesis and/or assembly. Nevertheless, it is also possible that defects in crust assembly were the indirect consequence of defects in the inner layers of spores, especially the outer coat.
To determine whether environmental conditions regulate the transcription of genes involved in crust synthesis during sporulation we performed qRT-PCR experiments. Four genes were targeted: cotY, cgeA, spsI, and spsM. spsI is the first gene of the spsIJKL locus that encode the enzymes of a pathway converting D-glucose-1-phosphate to dTDP-L-rhamnose. The spsM gene encodes the first enzyme of the pathway that converts UDP-GlcNAc to Leg and it was shown that this pathway is required for proper crust synthesis and/or anchoring (Dubois et al., 2020). In the B. subtilis 168 strain, the spsM gene is interrupted by the prophage SPβ. Excision of SPβ occurs in the mother cell of sporulating cells during the middle-to-late stages of sporulation, thus reconstituting a functional spsM gene (Abe et al., 2014, 2017). Therefore, any change in prophage excision potentially causes a change in crust synthesis and spore surface properties. Depletion in calcium or magnesium during sporulation did not impact the transcription of the targeted genes indicating that these sporulation conditions prevent crust assembly or negatively regulate crust synthesis at a post-transcriptional level. Post-transcriptional regulation of crust synthesis by divalent cations remains unknown but it might be mediated by glycosyltransferases that participate in the assembly of crust glycans. Indeed, nine glycosyltransferases are potentially involved in the synthesis and/or the anchoring of crust glycans on the spore surface: SpsA, SpsB, YfnF, YfnE, YfnD, YtcC, CgeD, CgeB and CotSA (Shuster et al., 2019a,b; Dubois et al., 2020). At least three of them (SpsA, YfnE, and CgeD) are putative GT-A type glycosyltransferases, a class of enzymes that are dependent on divalent metal ions. The metal ion is coordinated by a highly conserved DXD motif of the active site and it aids leaving group departure by stabilizing the charged phosphate groups in the nucleotide sugar donor (Gloster, 2014). Therefore, a depletion in divalent cations during sporulation might inhibit the activity of the GT-A glycosyltransferases and ultimately the assembly of glycans on the spore surface. When sporulation occurs in oxygen-limited conditions, transcription of genes involved in crust protein and glycans synthesis is decreased indicating that a lower availability in oxygen during sporulation represses crust synthesis at a transcriptional level. Transcription of the spsM gene was decreased in the -O2 and -O2 + CO2 conditions and transcription of spsI was also decreased in the -O2 + CO2 condition. Therefore, it is likely that the decrease in the relative amount of Leg and Rha in the crust of spores obtained in the oxygen-limited conditions is a consequence of the downregulation of the sps genes transcription during sporulation. Similarly, the decreased transcription of the cotY and/or cgeA genes might also explain the decrease in the relative amount of CotY and/or CgeA in the crust of spores obtained in oxygen-limited conditions. In contrast, the acidic pH did not modify transcription of the cotY, cgeA, spsI, and spsM genes suggesting that an acidic pH disrupts crust synthesis or assembly at a post-transcriptional level. It has been shown in Bacillus that acid stresses increase the intracellular amount of reactive oxygen species inducing secondary oxidative stress (Mols and Abee, 2011; Desriac et al., 2013). Therefore, the physiological changes and stress responses caused by acidic pH to sporulating cells may be similar to those induced by oxidizing molecules. It is well documented that oxidizing molecules can have a devastating effect on the structure and activity of proteins, especially those rich in sulfur amino acids (Ezraty et al., 2017). Moreover, it has been shown that the structural and morphogenetic proteins CotY and CotZ are rich in cysteines (9.3 and 6.7%, respectively) (Zhang et al., 1993). Therefore, an acidic pH during sporulation could induce a misfolding of CotY and/or CotZ, leading to the loss of their morphogenetic activity and ultimately to a defect in the crust proteins assembly. Transcription of all the genes targeted in qRT-PCR (cotY, cgeA, spsI, and spsM) is under the control of SigK and GerE and cotY, cgeA, and spsI genes transcription is also regulated by GerR (Figure 5A). Therefore, these transcriptional factors are potentially involved in the transcriptional regulation of these genes in response to sporulation conditions. Nevertheless, in the -O2 and -H2O2 conditions, only two out of the four genes were significantly less transcribed, suggesting a more complex regulation involving other regulators.
The crust is quite well conserved within the Bacillus subtilis group, although it is polymorphic from one species to another. For example, long flexuous filaments were observed on the surface of B. licheniformis spores, whereas the filaments were assembled into spikes on spores of B. clausii (Faille et al., 2014). However, the differences in crust composition and structure that explain these differences have not yet been identified. In clostridia and bacteria of the B. cereus group, the outermost layer of spores is the exosporium (Bozue et al., 2015; Stewart, 2015). Across species, the basic design of the exosporium consists of a thin continuous proteinaceous layer. In B. cereus, this basal layer is mainly composed of ExsY and CotY which are orthologs of CotZ and CotY in B. subtilis. The external face of the basal layer may be surrounded by a hairy nap made of collagen-like glycoproteins. In B. cereus, the main collagen-like glycoprotein is BclA (Sylvestre et al., 2002; Lequette et al., 2011). There is no collagen-like glycoprotein in the crust of B. subtilis suggesting major structural differences between crust and exosporium, particularly about glycans localization and anchoring. However, it seems that sporulation conditions also influence the structure of the exosporium of B. cereus spores. Indeed, when produced at high temperature or in anaerobic conditions B. cereus spores showed a damaged exosporium (Faille et al., 2007; Abbas et al., 2014). Therefore, interesting perspectives of this study would be to better characterize the diversity of the outermost layers of spores and further investigate the consequences of sporulation conditions on these layers, which are so crucial to the adhesion properties of spores.
To summarize, in B. subtilis a wide range of sporulation conditions can result in the production of spores with surface and adhesion properties very different from those produced under optimal conditions (Table 4). These sporulation conditions include divalent cations depletion, reduced oxygenation, oxidative stress, and acidic pH. Despite a similar effect on spore hydrophobicity and adhesion, the effect on the crust composition differs depending on the type of stress. Divalent cations depletion during sporulation results in a decrease in the amount of glycans on the spore surface and this decrease would be due to a crust assembly defect or a post-transcriptional regulation of glycan synthesis. By contrast, sporulation in oxygen-limited condition causes a decrease in the amount of glycans and proteins in the crust. This decrease is probably due to a repression of the transcription of genes involved in the synthesis of these compounds during sporulation. On the other hand, there is no clear trend in the consequences of oxidative stress and acidic pH during sporulation on the composition of the crust. Nevertheless, our results suggest that these categories of stresses affect the composition and/or structure of crust proteins rather than glycans.
The fact that sporulation conditions affect the ease with which spores can contaminate surfaces is therefore probably a major consideration in estimating the risk posed by B. subtilis spores in real environments. It could explain the frequent and recurrent presence of B. subtilis spores in food processing lines, despite laboratory results suggesting their low ability to contaminate surfaces. In view of these results, it could also be interesting to investigate the influence of environmental conditions encountered in the food industry during processing or cleaning operations on the surface properties of mature spores and their ability to adhere to all types of materials.
Data availability statement
The raw data supporting the conclusions of this article will be made available by the authors, without undue reservation.
Author contributions
AH: investigation, methodology, data collection, analysis, and writing. CL: investigation, methodology, data collection, and analysis. FK: investigation, data collection, and analysis. CF: supervision and writing – review and editing. TD: project administration, funding acquisition, conceptualization, supervision, investigation, methodology, and writing–review and editing. All authors contributed to the article and approved the submitted version.
Funding
This work was supported by the Agence Nationale de la Recherche (SOGLOSSI project; ANR-21-CE21-0012). The Hauts-de-France region provided a Ph.D. grant to AH.
Acknowledgments
The authors thank the Plateforme d’Analyse des Glycoconjugués (PAGés, http://plateforme-pages.univ-lille1.fr/) and the Bio Imaging Center Lille (BICeL) of the US 41 – UAR 2014 – PLBS for providing access to the instrumental facilities for carbohydrate analysis and confocal microscopy.
Conflict of interest
The authors declare that the research was conducted in the absence of any commercial or financial relationships that could be construed as a potential conflict of interest.
Publisher’s note
All claims expressed in this article are solely those of the authors and do not necessarily represent those of their affiliated organizations, or those of the publisher, the editors and the reviewers. Any product that may be evaluated in this article, or claim that may be made by its manufacturer, is not guaranteed or endorsed by the publisher.
Supplementary material
The Supplementary material for this article can be found online at: https://www.frontiersin.org/articles/10.3389/fmicb.2023.1219581/full#supplementary-material
References
Abbas, A. A., Planchon, S., Jobin, M., and Schmitt, P. (2014). Absence of oxygen affects the capacity to sporulate and the spore properties of Bacillus cereus. Food Microbiol. 42, 122–131. doi: 10.1016/j.fm.2014.03.004
Abe, K., Kawano, Y., Iwamoto, K., Arai, K., Maruyama, Y., Eichenberger, P., et al. (2014). Developmentally-regulated excision of the SPβ prophage reconstitutes a gene required for spore envelope maturation in Bacillus subtilis. PLoS Genet. 10:e1004636. doi: 10.1371/journal.pgen.1004636
Abe, K., Takamatsu, T., and Sato, T. (2017). Mechanism of bacterial gene rearrangement: SprA-catalyzed precise DNA recombination and its directionality control by SprB ensure the gene rearrangement and stable expression of spsM during sporulation in Bacillus subtilis. Nucleic Acids Res. 45, 6669–6683. doi: 10.1093/nar/gkx466
Abhyankar, W. R., Kamphorst, K., Swarge, B. N., van Veen, H., van der Wel, N. N., Brul, S., et al. (2016). The influence of sporulation conditions on the spore coat protein composition of Bacillus subtilis spores. Front. Microbiol. 7:1636. doi: 10.3389/fmicb.2016.01636
André, S., Vallaeys, T., and Planchon, S. (2017). Spore-forming bacteria responsible for food spoilage. Res. Microbiol. 168, 379–387. doi: 10.1016/j.resmic.2016.10.003
Anumula, K. R. (1994). Quantitative determination of monosaccharides in glycoproteins by high-performance liquid chromatography with highly sensitive fluorescence detection. Anal. Biochem. 220, 275–283. doi: 10.1006/abio.1994.1338
Arrieta-Ortiz, M. L., Hafemeister, C., Bate, A. R., Chu, T., Greenfield, A., Shuster, B., et al. (2015). An experimentally supported model of the Bacillus subtilis global transcriptional regulatory network. Mol. Syst. Biol. 11:839. doi: 10.15252/MSB.20156236
Bartels, J., Blüher, A., López Castellanos, S., Richter, M., Günther, M., and Mascher, T. (2019). The Bacillus subtilis endospore crust: protein interaction network, architecture and glycosylation state of a potential glycoprotein layer. Mol. Microbiol. 112, 1576–1592. doi: 10.1111/mmi.14381
Baweja, R. B., Zaman, M. S., Mattoo, A. R., Sharma, K., Tripathi, V., Aggarwal, A., et al. (2008). Properties of Bacillus anthracis spores prepared under various environmental conditions. Arch. Microbiol. 189, 71–79. doi: 10.1007/s00203-007-0295-9
Bozue, J. A., Welkos, S., and Cote, C. K. (2015). The Bacillus anthracis exosporium: What’s the big “hairy” Deal? Microbiol. Spectr. 3. doi: 10.1128/microbiolspec.tbs-0021-2015
Bressuire-Isoard, C., Broussolle, V., and Carlin, F. (2018). Sporulation environment influences spore properties in bacillus: evidence and insights on underlying molecular and physiological mechanisms. FEMS Microbiol. Rev. 42, 614–626. doi: 10.1093/femsre/fuy021
Cangiano, G., Sirec, T., Panarella, C., Isticato, R., Baccigalupi, L., De Felice, M., et al. (2014). The sps gene products affect the germination, hydrophobicity, and protein adsorption of Bacillus subtilis spores. Appl. Environ. Microbiol. 80, 7293–7302. doi: 10.1128/AEM.02893-14
Cazemier, A. E., Wagenaars, S. F. M., and Ter Steeg, P. F. (2001). Effect of sporulation and recovery medium on the heat resistance and amount of injury of spores from spoilage bacilli. J. Appl. Microbiol. 90, 761–770. doi: 10.1046/j.1365-2672.2001.01302.x
Desriac, N., Broussolle, V., Postollec, F., Mathot, A. G., Sohier, D., Coroller, L., et al. (2013). Bacillus cereus cell response upon exposure to acid environment: toward the identification of potential biomarkers. Front. Microbiol. 4:284. doi: 10.3389/fmicb.2013.00284
Driks, A., and Eichenberger, P. (2016). The spore coat. Microbiol. Spectr. 4, 179–200. doi: 10.1128/microbiolspec.TBS-0023-2016
Dubois, T., Dancer-Thibonnier, M., Monot, M., Hamiot, A., Bouillaut, L., Soutourina, O., et al. (2016). Control of Clostridium difficile physiopathology in response to cysteine availability. Infect. Immun. 84, 2389–2405. doi: 10.1128/IAI.00121-16
Dubois, T., Krzewinski, F., Yamakawa, N., Lemy, C., Hamiot, A., Brunet, L., et al. (2020). The sps genes encode an original legionaminic acid pathway required for crust assembly in Bacillus subtilis. mBio 11, 1–17. doi: 10.1128/mBio.01153-20
Eichenberger, P., Fujita, M., Jensen, S. T., Conlon, E. M., Rudner, D. Z., Wang, S. T., et al. (2004). The program of gene transcription for a single differentiating cell type during sporulation in Bacillus subtilis. PLoS Biol. 2:e328. doi: 10.1371/journal.pbio.0020328
Eschlbeck, E., Bauer, S. A. W., and Kulozik, U. (2017). Effect of cultivation pH on the surface hydrophobicity of Bacillus subtilis spores. AMB Express 7:157. doi: 10.1186/s13568-017-0458-2
Ezraty, B., Gennaris, A., Barras, F., and Collet, J. F. (2017). Oxidative stress, protein damage and repair in bacteria. Nat. Rev. Microbiol. 157, 385–396. doi: 10.1038/nrmicro.2017.26
Faille, C., Bénézech, T., Blel, W., Ronse, A., Ronse, G., Clarisse, M., et al. (2013). Role of mechanical vs. chemical action in the removal of adherent bacillus spores during CIP procedures. Food Microbiol. 33, 149–157. doi: 10.1016/j.fm.2012.09.010
Faille, C., Lemy, C., Allion-Maurer, A., and Zoueshtiagh, F. (2019). Evaluation of the hydrophobic properties of latex microspheres and bacillus spores. Influence of the particle size on the data obtained by the MATH method (microbial adhesion to hydrocarbons). Colloids Surf. B. Biointerfaces 182:110398. doi: 10.1016/j.colsurfb.2019.110398
Faille, C., Ronse, A., Dewailly, E., Slomianny, C., Maes, E., Krzewinski, F., et al. (2014). Presence and function of a thick mucous layer rich in polysaccharides around Bacillus subtilis spores. Biofouling 30, 845–858. doi: 10.1080/08927014.2014.939073
Faille, C., Tauveron, G., Le Gentil-Lelievre, C., and Slomianny, C. (2007). Occurrence of Bacillus cereus spores with a damaged exosporium: consequences on the spore adhesion on surfaces of food processing lines. J. Food Prot. 70, 2346–2353. doi: 10.4315/0362-028X-70.10.2346
Gloster, T. M. (2014). Advances in understanding glycosyltransferases from a structural perspective. Curr. Opin. Struct. Biol. 28, 131–141. doi: 10.1016/J.SBI.2014.08.012
Isticato, R., Lanzilli, M., Petrillo, C., Donadio, G., Baccigalupi, L., and Ricca, E. (2020). Bacillus subtilis builds structurally and functionally different spores in response to the temperature of growth. Environ. Microbiol. 22, 170–182. doi: 10.1111/1462-2920.14835
Jiang, S., Wan, Q., Krajcikova, D., Tang, J., Tzokov, S. B., Barak, I., et al. (2015). Diverse supramolecular structures formed by self-assembling proteins of the Bacillus subtilis spore coat. Mol. Microbiol. 97, 347–359. doi: 10.1111/MMI.13030
Kuwana, R., Okumura, T., Takamatsu, H., and Watabe, K. (2005). The ylbO gene product of Bacillus subtilis is involved in the coat development and lysozyme resistance of spore. FEMS Microbiol. Lett. 242, 51–57. doi: 10.1016/j.femsle.2004.10.038
Lequette, Y., Garénaux, E., Tauveron, G., Dumez, S., Perchat, S., Slomianny, C., et al. (2011). Role played by exosporium glycoproteins in the surface properties of Bacillus cereus spores and in their adhesion to stainless steel. Appl. Environ. Microbiol. 77, 4905–4911. doi: 10.1128/AEM.02872-10
Livak, K. J., and Schmittgen, T. D. (2001). Analysis of relative gene expression data using real-time quantitative PCR and the 2-ΔΔCT method. Methods 25, 402–408. doi: 10.1006/meth.2001.1262
McKenney, P. T., Driks, A., and Eichenberger, P. (2013). The Bacillus subtilis endospore: assembly and functions of the multilayered coat. Nat. Rev. Microbiol. 11, 33–44. doi: 10.1038/nrmicro2921
McKenney, P. T., Driks, A., Eskandarian, H. A., Grabowski, P., Guberman, J., Wang, K. H., et al. (2010). A distance-weighted interaction map reveals a previously uncharacterized layer of the Bacillus subtilis spore coat. Curr. Biol. 20, 934–938. doi: 10.1016/J.CUB.2010.03.060
Melly, E., Genest, P. C., Gilmore, M. E., Little, S., Popham, D. L., Driks, A., et al. (2002). Analysis of the properties of spores of Bacillus subtilis prepared at different temperatures. J. Appl. Microbiol. 92, 1105–1115. doi: 10.1046/j.1365-2672.2002.01644.x
Moeller, R., Wassmann, M., Reitz, G., and Setlow, P. (2011). Effect of radioprotective agents in sporulation medium on Bacillus subtilis spore resistance to hydrogen peroxide, wet heat and germicidal and environmentally relevant UV radiation. J. Appl. Microbiol. 110, 1485–1494. doi: 10.1111/j.1365-2672.2011.05004.x
Mols, M., and Abee, T. (2011). Bacillus cereus responses to acid stress. Environ. Microbiol. 13, 2835–2843. doi: 10.1111/j.1462-2920.2011.02490.x
Nicolas, P., Mäder, U., Dervyn, E., Rochat, T., Leduc, A., Pigeonneau, N., et al. (2012). Condition-dependent transcriptome reveals high-level regulatory architecture in Bacillus subtilis. Science 335, 1103–1106. doi: 10.1126/SCIENCE.1206848
Popp, P. F., Dotzler, M., Radeck, J., Bartels, J., and Mascher, T. (2017). The bacillus BioBrick box 2.0: expanding the genetic toolbox for the standardized work with Bacillus subtilis. Sci. Rep. 71, 1–13. doi: 10.1038/s41598-017-15107-z
Radeck, J., Kraft, K., Bartels, J., Cikovic, T., Dürr, F., Emenegger, J., et al. (2013). The bacillus BioBrick box: generation and evaluation of essential genetic building blocks for standardized work with Bacillus subtilis. J. Biol. Eng. 7, 1–17. doi: 10.1186/1754-1611-7-29
Richard, E., Dubois, T., Allion-Maurer, A., Jha, P. K., and Faille, C. (2020). Hydrophobicity of abiotic surfaces governs droplets deposition and evaporation patterns. Food Microbiol. 91:103538. doi: 10.1016/J.FM.2020.103538
Roels, S., and Losick, R. (1995). Adjacent and divergently oriented operons under the control of the sporulation regulatory protein GerE in Bacillus subtilis. J. Bacteriol. 177, 6263–6275. doi: 10.1128/jb.177.21.6263-6275.1995
Setlow, P., and Christie, G. (2023). New thoughts on an old topic: secrets of bacterial spore resistance slowly being revealed. Microbiol. Mol. Biol. Rev. 87:e0008022. doi: 10.1128/mmbr.00080-22
Shuster, B., Khemmani, M., Abe, K., Huang, X., Nakaya, Y., Maryn, N., et al. (2019a). Contributions of crust proteins to spore surface properties in Bacillus subtilis. Mol. Microbiol. 111, 825–843. doi: 10.1111/mmi.14194
Shuster, B., Khemmani, M., Nakaya, Y., Holland, G., Iwamoto, K., Abe, K., et al. (2019b). Expansion of the spore surface polysaccharide layer in Bacillus subtilis by deletion of genes encoding glycosyltransferases and glucose modification enzymes. J. Bacteriol. 201:e00321. doi: 10.1128/JB.00321-19
Soni, A., Oey, I., Silcock, P., and Bremer, P. (2016). Bacillus spores in the food industry: a review on resistance and response to novel inactivation technologies. Compr. Rev. Food Sci. Food Saf. 15, 1139–1148. doi: 10.1111/1541-4337.12231
Stewart, G. C. (2015). The Exosporium layer of bacterial spores: a connection to the environment and the infected host. Microbiol. Mol. Biol. Rev. 79, 437–457. doi: 10.1128/mmbr.00050-15
Sylvestre, P., Couture-Tosi, E., and Mock, M. (2002). A collagen-like surface glycoprotein is a structural component of the Bacillus anthracis exosporium. Mol. Microbiol. 45, 169–178. doi: 10.1046/j.1365-2958.2000.03000.x
Ursem, R., Swarge, B., Abhyankar, W. R., Buncherd, H., De Koning, L. J., Setlow, P., et al. (2021). Identification of native cross-links in Bacillus subtilis spore coat proteins. J. Proteome Res. 20, 1809–1816. doi: 10.1021/acs.jproteome.1c00025
Vagner, V., Dervyn, E., and Ehrlich, S. D. (1998). A vector for systematic gene inactivation in Bacillus subtilis. Microbiology 144, 3097–3104. doi: 10.1099/00221287-144-11-3097
Wiencek, K. M., Klapes, N. A., and Foegeding, P. M. (1990). Hydrophobicity of bacillus and clostridium spores. Appl. Environ. Microbiol. 56, 2600–2605. doi: 10.1128/AEM.56.9.2600-2605.1990
Windwarder, M., Figl, R., Svehla, E., Mócsai, R. T., Farcet, J. B., Staudacher, E., et al. (2016). “Hypermethylation” of anthranilic acid-labeled sugars confers the selectivity required for liquid chromatography-mass spectrometry. Anal. Biochem. 514, 24–31. doi: 10.1016/j.ab.2016.09.008
Xing, Y., and Harper, W. F. (2020). Bacillus spore awakening: recent discoveries and technological developments. Curr. Opin. Biotechnol. 64, 110–115. doi: 10.1016/j.copbio.2019.12.024
Zhang, J., Fitz-James, P. C., and Aronson, A. I. (1993). Cloning and characterization of a cluster of genes encoding polypeptides present in the insoluble fraction of the spore coat of Bacillus subtilis. J. Bacteriol. 175, 3757–3766. doi: 10.1128/JB.175.12.3757-3766.1993
Keywords: Bacillus subtilis, sporulation, spores, crust, adhesion, hydrophobicity, glycans
Citation: Hamiot A, Lemy C, Krzewinski F, Faille C and Dubois T (2023) Sporulation conditions influence the surface and adhesion properties of Bacillus subtilis spores. Front. Microbiol. 14:1219581. doi: 10.3389/fmicb.2023.1219581
Edited by:
Beatrix Stessl, University of Veterinary Medicine Vienna, AustriaReviewed by:
Ezio Ricca, University of Naples Federico II, ItalyLei Rao, China Agricultural University, China
Daniel R. Zeigler, The Ohio State University, United States
Eduardo Robleto, University of Nevada, Las Vegas, United States
Copyright © 2023 Hamiot, Lemy, Krzewinski, Faille and Dubois. This is an open-access article distributed under the terms of the Creative Commons Attribution License (CC BY). The use, distribution or reproduction in other forums is permitted, provided the original author(s) and the copyright owner(s) are credited and that the original publication in this journal is cited, in accordance with accepted academic practice. No use, distribution or reproduction is permitted which does not comply with these terms.
*Correspondence: Thomas Dubois, thomas.dubois@inrae.fr