- 1Department of Microbiology, Graphic Era (Deemed to be University), Dehradun, Uttarakhand, India
- 2National Centre for Cell Science, Pune, Maharashtra, India
- 3Department of Biotechnology, Graphic Era (Deemed to be University), Dehradun, Uttarakhand, India
The cold adapted microorganisms, psychrophiles/psychrotolerants, go through several modifications at cellular and biochemical levels to alleviate the influence of low temperature stress conditions. The low temperature environments depend on these cold adapted microorganisms for various ecological processes. The ability of the microorganisms to function in cold environments depends on the strategies directly associated with cell metabolism, physicochemical constrains, and stress factors. Pseudomonas is one among such group of microorganisms which is predominant in cold environments with a wide range of ecological and biotechnological applications. Bioformulations of Pseudomonas spp., possessing plant growth promotion and biocontrol abilities for application under low temperature environments, are well documented. Further, recent advances in high throughput sequencing provide essential information regarding the prevalence of Pseudomonas in rhizospheres and their role in plant health. Cold adapted species of Pseudomonas are also getting recognition for their potential in biodegradation and bioremediation of environmental contaminants. Production of enzymes and bioactive compounds (primarily as an adaptation mechanism) gives way to their applications in various industries. Exopolysaccharides and various biotechnologically important enzymes, produced by cold adapted species of Pseudomonas, are making their way in food, textiles, and pharmaceuticals. The present review, therefore, aims to summarize the functional versatility of Pseudomonas with particular reference to its peculiarities along with the ecological and biotechnological applications.
Introduction
The earth is dominated by a large portion of both terrestrial as well as aquatic low temperature environments. The Polar and Alpine region occupies 25% of the world’s terrestrial area. While 30% of the Earth’s landscape is covered with glaciers, permafrost, snow and ice (Deming, 2002; Yadav et al., 2015c), making them biodiversity hotspots and constitute a separate discipline known as “cryosphere.” The oceans are one of the essential microbiomes consisting of unique microflora with interesting traits (Cavicchioli, 2015). One of the biggest hurdles in such places is the energy equity generated by various essential drivers such as sunlight cycles, water, and nutrients, oceanic limnology influencing the biological activities. Bioprospection of such unique ecological niches has revealed a plethora of microorganisms (psychrophiles/psychrotolerants) with intriguing survival methods (Prasad et al., 2014). Both psychrophiles (cold-loving) and psychrotolerants (cold-tolerant) possess a major difference in the range of their growth temperatures; former has the ideal growth around 15°C reaching to the maximum temperature around 20°C and a minimum temperature of 0°C or below while latter is adapted to thermal fluctuations as well (Margesin and Feller, 2010; Dhakar and Pandey, 2020; Kanekar and Kanekar, 2022). A few examples of psychrophilic Pseudomonas spp., reported are P. helmanticensis, P. antarctica sp. nov., P. meridian sp. nov., P. proteolytica sp. nov., Pseudomonas sp. LSK25 (Reddy et al., 2004; Salwoom et al., 2019; Kumar et al., 2020) and psychrotolerant Pseudomonas spp., are P. palleroniana (GBPI_508), P. syringae, Pseudomonas sp. NB-1, Pseudomonas sp. MPC6 (Kumar et al., 2002; Selvakumar et al., 2009; Orellana-Saez et al., 2019; Jain et al., 2020; Cui et al., 2022; Thathola et al., 2022).
Microorganisms, as the simplest yet most unique life forms, extend tremendous applications in various sectors. They are the most numerous and diversified organisms present in almost all the extreme conditions on earth. The implication that microbes are necessary for life has attracted attention in microbial diversity research as they perform a variety of functions, critical to the biosphere’s survival. Prominent examples are the biogeochemical cycles and environmental biotransformation involving processes such as nutrient augmentation, recycling, and supplementation which are critical to environmental sustainability (Deming, 2002; Yadav et al., 2015a).
The metabolism of psychrophilic microorganisms is influenced by abiotic factors such as temperature, pH, salinity, organic content, and inorganic nutrients. These factors are also responsible for the composition of various groups of microorganisms. Based on a comprehensive literature survey, major inhabiting psychrophilic groups comprise of Proteobacteria, Bacteroidetes, Cyanobacteria, Actinobacteria, Firmicutes and Chlamydiae (Annapure and Pratisha, 2022). Psychrophiles belonging to Bacillus such as B. megaterium, B. amyloliquefaciens (Verma et al., 2015a), Bacillus-derived genera (BBDG), Pseudomonas gessardii (Kaur et al., 2021) have been observed as pigmented organisms that synthesize exopolysaccharides, antibiotics, antifreezing compounds, antagonistic amalgams, and cold active enzymes (Yadav et al., 2018; Silva et al., 2021). The hypothesis stating whether a microbiome trapped in a cryosphere for an extended period of time results in effective growth stimulation has been proven correct. In this context, numerous examples support the fact that species of Pseudomonas inhabit a diverse variety of niches due to physiological flexibility. They can adapt to high and low temperatures, oxygen, moisture and nutrients (Sherpa et al., 2019; Snopková et al., 2020; Cui et al., 2022; Suyal et al., 2022). Pseudomonas can use a wide range of organic substances as carbon and energy sources. Some species are capable of utilizing a variety of toxic compounds via production of a myriad of secondary metabolites and are being recognized in agriculture, medicine, and other industries (Orellana-Saez et al., 2019; Yadav et al., 2019; Craig et al., 2021; Thathola et al., 2021, 2022).
The microbial communities have been the focus of chosen scenario, not just in respect of biotechnological prospects, but also in terms of understanding how primitive counterparts of biomolecules were used in early Earth habitats. The field of bioprospecting research is quickly expanding using various approaches, technologies, and methodologies as well as the scientific rationales and hypotheses. The concerned areas in the current review majorly include the Indian Himalayan region (IHR) – that forms a large part of Himalayan global biodiversity hotspot along with reports from the neighbor countries; Tropical Andes- a huge region in South America stretching along Andes mountains from Southwestern Venezuela to Northern Chile and Argentina, regarded as the origin of agriculture in America; and Antarctica the fifth largest southernmost continent consisting of 95% of the ice coverage. The critical factor differentiating Antarctica from the rest is the detrimental solar radiations and variable photoperiod (Chettri et al., 2008; Pandey and Yarzábal, 2019; Sharma et al., 2019; Rizzo et al., 2021; Yarzábal, 2021).
Factors regulating microbial diversity in cold environments
Most studies utilize temperature dependency criteria to designate psychrophiles, however soil nutrition also plays a significant role in growth determination. As a result, the available substrate regulates metabolism and changes the enzymatic rate. Owing to the mineral absorption tactics, certain strains of Pseudomonas intensively invade roots and begin rapid root colonization and develop host-microorganism interaction and a tactical defensive pathway to regulate neighboring microorganisms. These properties allow Pseudomonas to inhabit most of the nutrient deficient locations. Pseudomonas tends to degrade a wide range of compatible solutes such as heterosides, aminoacids and sugars (Craig et al., 2021) and organic compounds adopting various metabolic pathways such as Entner-Doudoroff pathway, β-ketoadipate pathway (Cabrera et al., 2022) etc. The psychrophiles have been considered to possess the ability of tolerating far higher temperature ranges than the real ambient temperature from which they were isolated, when cultivated in-vivo (Cavicchioli, 2016); for example, P. psychrophila MTCC12324 (Abraham et al., 2020) and P. frederiksbergensis (Cui et al., 2022) etc., were observed to grow between 4–37°C, Pseudomonas sp. 30–3 with a survival range of 0–35°C retrieved from oil-affected soil, Wright Valley, Antarctica (Panicker et al., 2002); P. lundensis, isolated from Antarctic meltwater pond was also reported to survive at 0°C (Ravi et al., 2022). Similarly, pH is another important factor regulating the survival and growth of microorganisms in extreme climatic conditions. Microorganisms, isolated from extreme temperature environments revealed the ability to grow at wide pH range, extreme acidic to extreme alkaline (Dhakar and Pandey, 2016). Some of the examples include P. stutzeri, P. frederiksbergensis, P. simiae, P. brenneri, P. azotoformans from Kongsfjorden and Ny-Alesund, Svalbard, Arctic (Srinivas et al., 2009); and P. corrugata, P. PGERs17, P. putida B0, P. chlororaphis GBPI_507 (MCC2693) from different regions in Indian Himalaya (Pandey and Palni, 1998; Pandey et al., 2006; Mishra et al., 2008; Jain and Pandey, 2016). It was connected to the concept that the genome is a probable repository of hidden variants. While extremophilic microorganisms have the potential to adapt to the changing environmental conditions, this is most likely accomplished through the expression/regulation of certain genes originally existing in the genome (Dhakar and Pandey, 2016).
Elevation is another important factor affecting the growth of microorganisms in a given set of climate. In the Colorado Rocky Mountains, Bryant et al. (2008) reported a linear decline in soil microbiome diversity as elevation climbed. Bhattacharya et al. (2022a) strongly suggested the influence of edaphic factors on microbial diversity using meta-barcoding based analyses. The report indicated an almost similar diversity at high as well as low elevation; however at mid-elevational gradient the fluctuation was recorded due to the soil moisture, organic carbon, and total nitrogen content. The authors also reported no significant microbial diversity variation in annual and seasonal patterns. The importance of edaphic factors on microbial community composition and their metabolism was studied in Gangotri glacier’s post-deglaciation patterns during different time span (Bhattacharya et al., 2022b). Another notable finding from Indian Himalayan region (IHR) research is the influence of mountain slope orientation on several bioactivities displayed by plant growth promoting microorganisms colonizing these habitats (Selvakumar et al., 2009). Nottingham et al. (2018) also noted the coordination pattern of elevation, temperature, and species (plants and microbial) diversity in Andes (Peru). They considered temperature as the major diversity determinant with less influence of edaphic factors (soil pH). They also suggested that the enzymes responsible for organic matter degradation were accompanied in microbial transition patterns taking place along the elevation. As a result, in mountain ecosystems, climate conditions work together to favour the colonization and dominance of specific microbial populations. Dominance of cold adapted microbial communities has been documented to colonize tree species of IHR namely Abies, Rhododendron and Betula in alpine and glacier environments along an altitudinal gradient (Pandey and Palni, 2007).
Pseudomonas: A diverse and important genus
Pseudomonas is ubiquitous, inhabiting the atmosphere, terrain, and hydrosphere while surviving a broad range of topographical characteristics. While Parte (2018) refers to the List of Prokaryotic Names withstanding in Nomenclature with 122 validly published species of Pseudomonas, Gomila et al. (2015) describes the major species groupings as P. aeruginosa, P. syringae, P. chlororaphis, P. putida, and P. fluorescens.
Low temperature environments have been ideal sites for colonization of cold adapted Pseudomonas species, both psychrophilic and psychrotolerant. Novel psychrophilic Pseudomonads namely P. antarctica sp. nov., P. prosekii, P. meridiana sp. nov., P. proteolytica sp. nov., Pseudomonas sp. ID1 have been isolated from low temperature environments of Antartica (Reddy et al., 2004; Carrión et al., 2015; Snopková et al., 2020). Diversity of the genus Pseudomonas including P. azotoformans, P. poae, P. fluorescens, P. reactants, P. hibiscicola and P. synxantha has been reported from Kanchengayao glacier, North Sikkim Himalaya, India, as one of the most dominant genera in the glacier ice samples (Sherpa et al., 2019). Other psychrophilic species namely Pseudomonas sp. BGI-2 from Batura glacier, Pakistan (Ali et al., 2020), and P. frederiksbergensis ERDD5:01 isolated from the East Rathong glacier in the Sikkim Himalaya have been reported for their resistance to high altitude stress conditions such as prevalent freeze–thaw patterns and UV radiation (Kumar et al., 2019). Pseudomonas species namely P. azotoformans, P. corrugata, P. granadensis, P. paralactis, P. proteolytica, P. palleroniana, P. helmanticensis and P. putida have been isolated from various locations including cold deserts and glacial sites in Indian Himalaya (Pandey et al., 2019; Kumar et al., 2020). P. psychrophila MTCC12324, a cold adapted strain has been reported for its specificities for inhabiting the Arctic region (Abraham et al., 2020).
Cold adapted Pseudomonas species, with plant growth promotion (PGP) activities, have been identified from Himalayan and Andean mountain environments (Balcázar et al., 2015; Pandey and Yarzábal, 2019). Pseudomonads have been identified as the most physiologically varied bacterial immigrants to alpine soils, with the ability to thrive across a wide temperature range while resisting a variety of biotic and abiotic challenges. As a consequence, it exhibits its use as a bioinoculant in overcoming hill agricultural challenges. The low temperature environment will have an influence on cellular structure due to osmotic imbalance, water content, membrane fluidity, enzyme kinetics, and diffusion rate. Some traits must be different in order to maintain the critical metabolism (De Meeyer et al., 2014).
Adaptation strategies – A facet of versatility
Diverse topographical, ecological, and biological factors form the basis of physiological and molecular acclimatization (Figure 1). Pseudomonas are known to acclimatize to low temperature via physiological changes (Table 1) like membrane integrity, production of anti-freeze proteins, carotenoid pigments, the release of cryoprotectants, basic metabolic adjustment such as suppression of glycolysis and TCA cycle during cold and the acquisition of energy generation by secondary or intermediate molecules via alternate routes to circumvent the whole system emerge as significant aspects for energy production (Tribelli et al., 2015; Abraham et al., 2020).
The capacity of the cell to control the fluidity of the membrane is one key tactic that is essential to the cell’s survival at low temperatures. A robust hydrophobic lipid membrane is required for all biological membranes. Pseudomonas genera generally modify membrane fluidity by changing the lipid polar head, charge, constituents, size and location, length and arrangement of hydrophobic fatty acid chain, peptidoglycan biosynthesis, membrane’s protein composition, amino acid stocking, kind of carotenoids generated, and ratio of cis to trans fatty acids (De Meeyer et al., 2014). Certain reports suggested that Pseudomonas may have a two-part signal transduction system that consists of a membrane confined sensor and a dissolvable cytoplasmic response regulator that is involved in the perception and transmission of low temperature signals (Chintalapati et al., 2004; De Meeyer et al., 2014; Raymond-Bouchard et al., 2018; Weinstein et al., 2019; Abraham et al., 2020). P. syringae, P. aeruginosa, and P. extremaustralis are a few examples reported from Antarctic, Arctic, and IHR, known to exhibit similar phenomenon (Kumar et al., 2002; Benforte et al., 2018). The breakdown of amino acids behaves as one of the pathways that resulted in the production of the cytoprotective polyamines as observed in P. helmanticensis (L-arginine degradation leads to the formation of spermidine and putrescine) and P. putida (Tribelli and López, 2018; Kumar et al., 2020).
According to proteomic research, transport proteins discovered in the membrane have been reported to compensate for poor diffusion rates by increasing the absorption of suitable solutes, nutrients, and peptides for peptidoglycan formation (Cacace et al., 2010). Chevalier et al. (2017) supported the fact and observed similar outcomes in P. aeruginosa; they express specific channel proteins for nutrient uptake. Exopolymeric compounds are known to be produced in large quantities by bacteria living in cold aquatic environments (e.g., Antarctic and Arctic Sea ice). These are hypothesized to help cells attach to wet surfaces and create the extracellular matrix structure of biofilms (owing to the development of a large quantity of alginate; main ingredient of glycocalyx), which absorbs nutrients, protects the cell from adverse ecological parameters, and facilitates metabolic interactions (Palleroni, 2010; Yadav et al., 2015c; Ciofu and Tolker-Nielsen, 2019; Abraham et al., 2020). P. mandelii, a novel strain isolated from Antarctic environment, has been reported for overproduction of alginate in comparison to other species like P. aeruginosa and P. fluorescens. According to this study, the expression levels of the alginate operon were recorded highest at 4°C (Vasquez-Ponce et al., 2017). The high concentration of EPS lowers water’s freezing point and ice nucleation temperature. They must also account for increased pore density and disorganized ice crystals, which reduce permeability and salt retention (De Meeyer et al., 2014).
The evolution of cryoprotective carbohydrates is one of the significant features of pschryophilic microorganisms. Numerous examples belonging to Pseudomonas genera, such as Pseudomonas sp. ID1 and Pseudomonas sp. BGI-2 have been reported to form such exopolysacchrides conferring significant cold adaptation (Carrión et al., 2015; Ali et al., 2020). Furthermore, the culture media, growth substrates and environmental factors can be optimized for enhanced yield (Ali et al., 2020; Nair et al., 2021).
Lowering the temperature has an inverse effect on enzyme kinetics, with a 10°C drop, the enzyme activity decreases by two fold. Psychrophilic enzymes (phospholipases, proteases) maximise low temperature activity by destabilizing the structures containing the active site as well as the whole molecule. This attributes to a decrease in the strength of intramolecular connections (subunit, electrostatic interactions, disulfide linkages) (Rapuano and Graziano, 2022) and the exclusion of stability factors, resulting in better active site dynamics (Feller, 2013). Other factors responsible for the flexibility of such enzymes include enhanced surface, core hydrophobicity and the arrangement of aminoacids. Some of the latest reports strongly support that the presence and orientation of specific amino acids within the structure of enzyme affects the stability and activity, for example, the catalytic triads Ser, His and Asp in lipase encoding genes found in P. marinensis were observed to be necessary for preserving enzyme functions (Guo et al., 2021). Öten et al. (2022) observed a boost in the adaptation of enzymes to cold conditions by proline (Pro) and glycine (Gly) residues modifying conformational changes in the three-dimensional (3-D) structure of proteins. The study demonstrated the mechanism of Gly and Pro in the cold-adaptive model, the inclusion of Gly residue enhances the flexibility of the peptide backbone, allowing conformational changes (Raymond-Bouchard et al., 2018). Gly is entirely made of hydrogen which provides the property of being achiral, and the inability to participate in intramolecular interactions promoting the destabilization of helix structures. On the other hand, the bulky N-CH2 groups of Pro, successive residues have limited conformations, affecting helix formation. If Pro appears on the first turn of the helix and aligns closely to the backbone shape, it causes a significant influence on protein stabilization (Öten et al., 2022). Lipase, protease, amylase, cellulose, urease and beta-glucosidase are some of the reported enzymes from Pseudomonas sp. (Choo et al., 1998; Salwan et al., 2010; Jain et al., 2017; Salwoom et al., 2019; Salwan et al., 2020; Rubiano-Labrador et al., 2022). The stability of secondary structures in cold temperature were studied and determined by Gianese et al. (2001). The authors suggested the following substitutions of charged residues such as Val (omission in beta strands), Ala (presence in coiled region), and Glu, Lys and Arg (at the alpha helix and coil regions) to favor psychrophilic adaptation. Extracellular metalloprotease extracted from Pseudomonas sp. (Yang et al., 2010) and protease (Martínez-Rosales and Castro-Sowinski, 2011) correspond to diverse industrial applications.
The synthesis of polyhydroxyalkanoates (PHAs), such as, polyhydroxybutyrate (PHBs) was discovered to be critical for microbial growth, tolerance and survival to a number of environmental stressors while possessing environmental significance. PHB accumulation maintains cellular redox homeostatis, cope oxidative stress, boosted motility and survival in P. extremaustralis, indicating that the capacity to accumulate PHB might be an adaptive benefit for colonizing new biological niches in such circumstances (Tribelli and López, 2011).
Cold temperatures, as encountered in higher altitudes and polar region, disrupt nucleic acid structure hampering protein structures and basic molecular processes. In order to deal with these, certain cold adapted microorganisms produce specialized proteins known as ice-nucleating proteins (INPs) and antifreeze proteins (AFPs). The role of each differs; AFPs prevent the production of large ice crystals within the cell which can damage organelles further resulting in cell death whereas INPs are linked with the cell membrane, they induce extracellular ice generation preventing the freezing of cytosolic water (Białkowska et al., 2020; Craig et al., 2021). P. putida GR12-2 was reported to release an AFP (AfpA protein) that sustain life at subzero temperature and exhibit both ice nucleation as well as antifreeze abilities (Muryoi et al., 2004). The INPs from P. syringae and P. borealis were taken as model to study their binding mechanisms (Hudait et al., 2018). These species play a pivotal role in food preservation, cryopreservation, material technology etc. P. helmanticensis (Kumar et al., 2020), P. psychrophila MTCC12324 (Abraham et al., 2020), P. prosekii (Snopková et al., 2020) demonstrated the potential of genes encoding for cold shock proteins serving as nucleic acid chaperons and prevents the misfolding of mRNA to withstand freezing patterns.
Another ecologically as well as biotechnologically important adaptation possessed by psychrophiles is pigment formation in order to confront ultraviolet and oxidative stress conditions. Pigments are one of the characteristic properties of such cold adapted organisms that are being researched for numerous industrial uses. They are largely produced inside the cytoplasm as a response agent to harsh ecological circumstances, serving a variety of ecological as well as physiological purposes including membrane fluidity regulation, cell differentiation, and photo-oxidative injuries (Sajjad et al., 2020). A few pigments regulate iron concentration and metabolism (Dhakar and Pandey, 2020). P. aeruginosa, P. chloraphis, P. reptilivora, P. stutzeri, P. corrugata, P. mendocina and P. fluorescens are some of the most widely known species to release blue-green pyocyanin or yellow-green fluorescein (pyoveridine), orange chlororaphin, reddish brown pyorubin, and brownish black pyomelanin pigments (Dhakar and Pandey, 2020). The pigments possess a variety of industrial applications and have a good market potential (Venil et al., 2020), thus has been an interesting objective for researchers.
On account of genetic transformation, certain modifications include chaperone formation responsible for efficient protein folding and nucleic acid machinery in order to generate a stable secondary structure at low temperature. Phosphorus mobilization is aided by the expression of mineral solubilizing genes such as pyrroloquinoline quinine (pqq) and glucose dehydrogenase (gcd) (Rawat et al., 2021). The specialized porin genes (OrpF, OprB, OprH, OprP etc.) help regulate adherence, relay signals, nutrient propagation, and anatomical functions reported in P. aeruginosa (Chevalier et al., 2017).
Multiples of cold associated genes encoding cold active chaperons, for example, HscA/HscB, CspD, GroES, GroEL, HtpG etc., carbon storage/starvation, overall stress response (Ctc, UspA, LexA etc.), oxidative stress, membrane/cell wall modification, osmotic pressure, and DNA repair mechanisms (recombinase RecA and RecQ) aid in the resistance of P. frederiksbergensis ERDD5:01 and P. prosekii to extreme cold and radiations correlating with bacterial physiological observations (Kumar et al., 2019; Snopková et al., 2020). Genes encoding cold shock domain such as capB was suggested by Panicker et al. (2002) as a cold survival promoter which was later proven due to its presence in all the Pseudomonas spp., isolated from Antarctica (Panicker et al., 2010). UV-radiation has a significant impact on higher altitude and cryospheric areas, and Pseudomonas has several photoreactivation genes, such as cyclobutane pyrimidine dimers (CPDs), to assist against radiation stress (Kumar et al., 2019). Plasmid (for example, P. fluorescence – E. coli shuttle plasmid, P. chlororaphis PA23) genes usually contribute in organisms’ quick adaptation processes by enhancing the phenotypic flexibility of host strains via horizontal gene transfer, hence assisting them in cold tolerance (Dziewit and Bartosik, 2014; Ghergab et al., 2021; Wu et al., 2021). Figure 2 depicts a diagrammatic description of the aforementioned adaptations. The distinguishing characteristics of psychrophilic and mesophilic Pseudomonas are summarized in Figure 3.
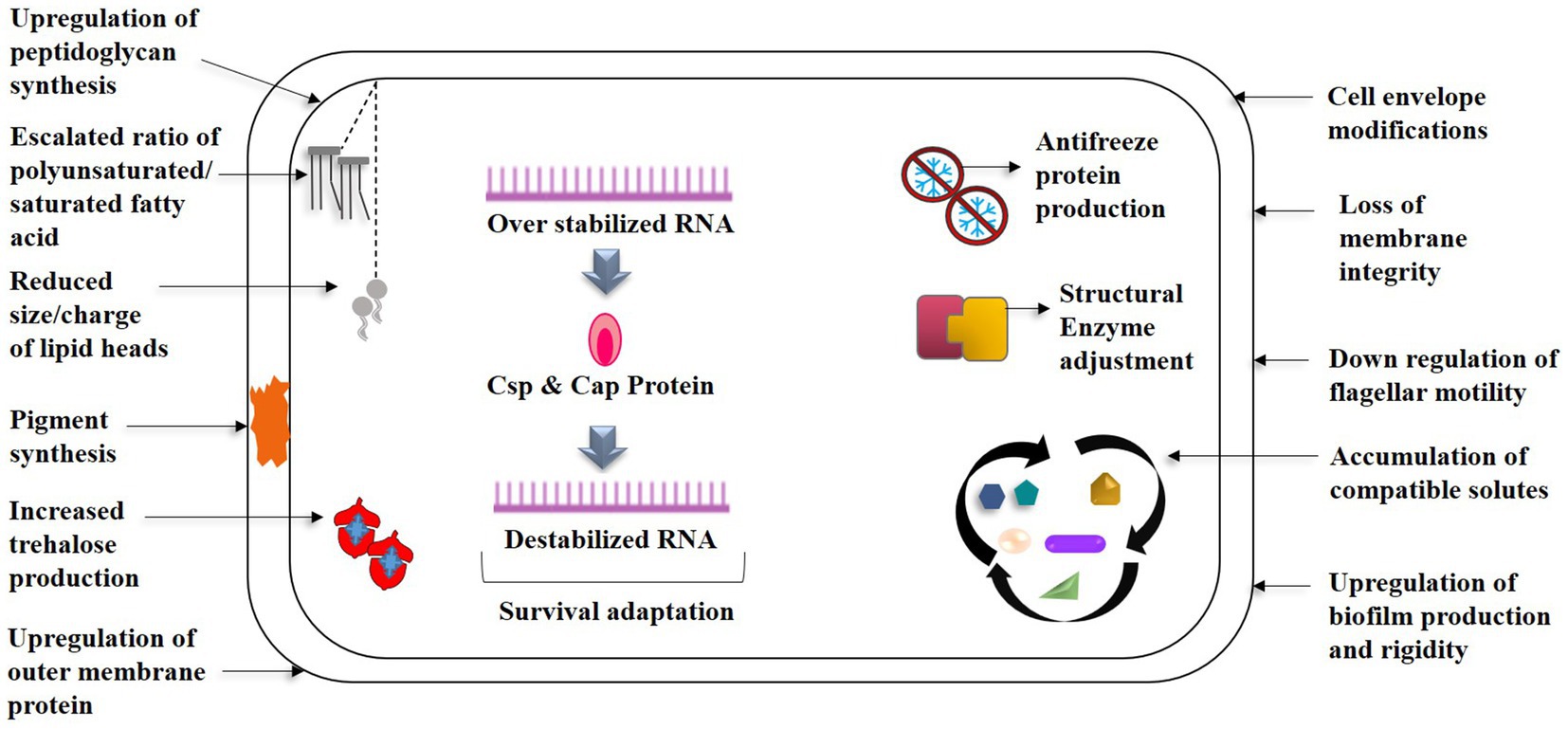
Figure 2. Cellular mechanisms of Pseudomonas spp. towards the survival strategies in low temperature- An overview.
Potential applications of Pseudomonas
Aboveground and belowground microorganisms have been shown to have substantial ability in dealing with diverse ecological stressors and nutritional deficit. Microorganisms and plants interact through associative, endophytic, or symbiotic processes with varying degrees of proximity to the roots and rhizosphere (Kaur et al., 2021). Agricultural sustainability is supported through microbial interactions. Among cold adapted microorganisms, dominance and biotechnological applications of Pseudomonas species have been investigated for their contribution mainly in agriculture, and industries (Table 2).
Plant growth promotion
Plant growth promotion (PGP) responsibilities can be mediated by indirect or direct or both kinds of mechanisms. The former is closely related to biological management of plant diseases, and relies on the manufacture of antibiotics, lysozymes, siderophores, and biocidal metabolites. Some microbial secondary metabolites, such as the generation of phytohormones, release of bioavailable nitrogen to the host, and phosphate solubilization, also contribute to support the plant’s defense responses (Pandey and Yarzábal, 2019). Plant growth promoting properties mainly consisted of production of phytohormones, IAA, HCN, siderophore, ACC deaminase activity, mineralization of K, P, Zn, and ethylene biosynthesis inhibition (Balcázar et al., 2015; Ortiz-Ojeda et al., 2017; Kaur et al., 2021). Psychrophiles also produce phytohormones, including gibberellins, cytokinins, and auxins; the most significant modulators of physiological and molecular responses.
Several research groups have expressed interest in developing cold active biofertilizers and biopesticides to promote sustainable agriculture in mountainous regions (Pandey and Yarzábal, 2019; Yadav et al., 2019; Torracchi et al., 2020). Numerous field trials, directed at determining the impact of PGP microorganisms on vegetation, have declined due to exogenous microorganism’s low survival rates when introduced to soils from another location due to the fact that a 10°C temperature decrease may result in around two-fold reduction in metabolism (Feller and Gerday, 2003). Thus, it was proposed that microorganisms intended for use as bioinoculants be prospected and selected from their own inhabiting microbial communities (Velázquez et al., 2016).
The development of microbial formulations for boosting agricultural productivity in cold conditions was considered to be reliant on two crucial factors: the microorganisms’ environmental resilience and rhizosphere proficiency. Psychrophiles are known to have significant ecological traits in addition to acquired survival skills. A field research done at various elevations in the Mamlay watershed of the Sikkim Himalaya (Pandey et al., 1998) demonstrated the relevance of local microorganisms and the necessity for cold adapted PGP microorganisms to be employed in mountain ecosystems. The study also demonstrated the feasibility of cold adapted Pseudomonas (P. corrugata) for development as bioinoculants for maize grown in higher altitudes (Kumar et al., 2007). Later, PGP features of a number of Pseudomonas species, namely P. corrugata, P. fragi, P. putida, P. vancouverensis, and P. libanensis EU-LWNA-33, isolated from various mountain locations were documented (Negi et al., 2011; Kour et al., 2020). A polyextremophilic P. chlororaphis (MCC2693) strain from the Indian Himalaya, that produced phenazine-1-carboxylic acid in culture conditions, was shown to promote plant growth in bioassays (Jain and Pandey, 2016).
Pseudomonas strains isolated from the rhizosphere of potato cultivated at various elevations in Peru and Bolivia’s Central Andean Highlands were shown to have a variety of PGP activities (Ghyselinck et al., 2013). Psychrotolerant PGP bacteria were isolated from the rhizosphere of a tuber crop, Lepidium meyenii Walp. (maca), of the Andean region (Ortiz-Ojeda et al., 2017). The diversity of rhizosphere bacteria colonizing tubers, one of the most important staple crops in the Andean highlands, has been reported for the dominance of Pseudomonas and Bacillus strains with PGP activities (Chica et al., 2019). ILQ215 (P. silesiensis) and JUQ307 (P. plecoglossicida), isolated from Chenopodium quinoa rhizosphere with considerably benefitting plant growth impacts and the potential for producing bioinoculants, have been investigated from the Peruvian Andean Plateau region (Chumpitaz-Segovia et al., 2020).
Investigation on the genetic diversity and PGP activities of wheat rhizosphere related bacteria from the northern hills of India was undertaken and reported the dominance of Pseudomonas (Verma et al., 2015b). The analysis revealed the importance of cold adapted microorganisms in producing bioformulations for use in winter crops cultivated in high altitude prevailed by low temperature climatic conditions (Verma et al., 2015a). PGP positive Pseudomonas isolates (next to Bacillus) have been reported to predominate the frigid desert of Leh Ladakh in Indian Himalaya (Yadav et al., 2015a).
Several Pseudomonas species are reported to have PGP activities such as mineral phosphate solubilization (da Silva et al., 2021) and the generation of chemicals that contributes to plant development or suppress plant pathogens; indole acetic acid (IAA), 1-aminocyclopropane-1-carboxylate (ACC) deaminase, ammonia, and HCN. Furthermore, these cold adapted Pseudomonas species have been shown to relieve plant stress induced by several environmental conditions (Mishra et al., 2011; Ma et al., 2017). Many biostimulants, regardless of the nutrients they contain, enhance nutrition. Subramanian et al. (2015) investigated the biostimulant potential of cold adapted endophytic P. vancouverensis OB155-gfp and P. frederiksbergensis OS261-gfp in Solanum lycopersicum Mill. da Silva et al. (2021) isolated Pseudomonas sp. 11. LB15 and Pseudomonas sp. 1.LB34 from Antarctic lichen that was capable of phosphate solubilization and also released tartaric and fumaric acids during the process.
Pseudomonas genus has been successfully reported for antagonistic behaviour against diverse phytopathogens. For instance, P. corrugata isolated from Sikkim (maize field) with an ability of growing at 4°C was tested to protect Cedrus plants from cutworms and Fusarium wilt at nursery stage (Bisht et al., 2003). P. putida B0, an isolate from IHR, was found to inhibit the growth of Phytophthora parasitica (Pandey et al., 2006). PGP activities of Pseudomonas spp. obtained from glacier samples in Venezuela’s tropical Andes were investigated by Balcázar et al. (2015). While some of the bacterial isolates were psychrophilic, others grew at a wide range of temperature. Pseudomonas spp. collected from Greenwich Island soil, Antarctica thrived well at temperatures between 4 to 30°C and screened positive for PGP characteristics. Furthermore, the bacteria suppressed phytopathogens such as Fusarium oxysporum, Phytophthora infestans and Pythium ultimum and promoted germination of wheat seeds. The researchers concluded that the selected Antarctic Pseudomonas spp. can be developed as cold active biofertilizers (Yarzábal et al., 2018). A few Pseudomonas spp., retrieved from Venezuelan glaciers, have been demonstrated for their antagonism behaviour against P. ultimum in order to induce growth in Triticum (Rondón et al., 2019).
In the coming years, agriculture is likely to confront the combined task of feeding a growing global population while also reducing the environmental effect of cropping systems. Pseudomonas retrieved from the cryosphere has long been employed as potent inoculants for carrier-based formulations; they were thoroughly investigated for their plant growth boosting properties at low temperatures. Trivedi et al. (2005) demonstrated this point with a successful formulation of P. corrugata covered with alginate beads. Moreover, it has been shown that the creation of biostimulants acquires a potent mechanism that boosts plant development, improves nutrient use efficiency, and generates novel mechanisms for plant nutrient acquisition and abiotic stress tolerance (Du Jardin, 2015), resulting in minimizing the need of chemical fertilizers. Advancement in research with respect to development of carrier based formulations, applicable to cold environments retaining the desirable PGP traits, will be necessary for large scale production and commercialization of this technology.
Bioremediation and biodegradation
Cold adapted microorganisms have been investigated for their potential use in the environmental remediation of oil-polluted mountainous soils (Margesin, 2000). Many components of petroleum hydrocarbons have been observed to possess biodegrading potential in Arctic, Alpine, and Antarctic soils, saltwater and Alaskan groundwater as well as in sub soils where temperatures persist up to 10°C (Margesin and Schinner, 1999; Margesin, 2000). P. frigusceleri MPC6 from Antarctica can convert a wide spectrum of chemicals, including phenols, benzene, toluene, and styrene (Orellana-Saez et al., 2019). The phenanthrene degradation potential of P. frederiksbergensis JAJ28T (DSM 13022 T) obtained from a gasification station in Frederiksberg, Denmark has been described (Andersen et al., 2000). P. aeruginosa holds significance in iron-rich sludge hydrothermal degradation and release phosphates (Yu et al., 2022) suggesting their surviving potential to a wide range of temperature.
Pseudomonas sp. MC1, which can thrive at temperatures ranging from 5 to 30°C, has been shown to decompose naphthalene. This property was attributed to the occurrence of thermolabile NahH enzyme which may be connected to the cold adaptation process of the bacteria with respect to the survival of MC1 strain on salicylate and naphthalene at low temperature conditions (Ahn et al., 2017). Degradation of polyhydroxyalkanoates (PHAs; Orellana-Saez et al., 2019), nitrobenzene, TNT (2,4,6-trinitrotoluene) were observed by Pseudomonas sp. MPC6, P. frederiksbergensis (NB-1; Cui et al., 2022) and Pseudomonas sp., isolated from Deception Island (Cabrera et al., 2022), respectively. While the bacterium was thought to be a good model strain for studying adaptation mechanisms in cold conditions, the possibility of its use as a bioremediator and biopolymer factory was also suggested (Orellana-Saez et al., 2019).
Pseudomonas species from the Himalaya are also being investigated for their ability to degrade chemicals present in medicines and personal care items (PPCP). For example, Pseudomonas sp. GBPI Hb5 and P. palleroniana GBPI 508 have been examined for caffeine and bisphenol A degradation, respectively (Thathola et al., 2021, 2022). These investigations will most likely have an impact on the bioremediation of PPCP from the contaminated settings.
Industrial applications
The global demand for microorganisms and their products is constantly increasing in the last decade, from approximately USD 144 billion in 2010 to be an expected USD 303 billion by 2023 (McWilliams, 2018; Staff, 2018). Thus, they have been the prime focus for the industrially relevant materials such as enzymes, biofertilizers, etc. The products, prominently cryoenzymes extracted from psychrophiles have attained milestones in various disciplines. Pseudomonas species, isolated from cold environments, have been considered suitable for the production of cold-active enzymes (Li et al., 2014; Ganasen et al., 2016), for instance, P. proteolytica (GBPI_Hb61) isolated from cold Himalayan desert produce cold active lipase (Jain et al., 2017). Pseudomonas sp. LSK25 obtained from Signy Station, South Orkney Islands, maritime Antarctic reported for the production of recombinant lipase that is tolerant to organic solvents (Salwoom et al., 2019). Zhang and Zeng (2011) reported the identification of the amylase gene from the psychrotolerant P. stutzeri strain 7,193. Numerous other Pseudomonas species isolated from the regions like Indian Himalaya (Salwan et al., 2010; Jain and Pandey, 2016; Pandey et al., 2019; Jain et al., 2020), Ny-Alesund, Svalbard, Arctic (Srinivas et al., 2009), Humboldt Glacier, Mount Humboldt, Andean region of Venezuela (Ball et al., 2014) have been studied for production of industrially important enzymes such as amylases, proteases, RNA polymerase and lipases (Uma et al., 1999; Zhang and Zeng, 2011; Jain et al., 2017; Salwoom et al., 2019; Guo et al., 2021). Beside mountains, the oceans represent an untapped potential that exists in the microbiome to produce enzymes with distinctive properties. For example P. marinensis studied by Guo et al. (2021) possess two novel genes encoding for cold-active lipases. Such enzymes are also reported to survive and remain active at alkaline pH (Salwoom et al., 2019; Salwan et al., 2020).
Based on the global market data, the lipase demand was estimated as USD 420–430 million in 2018 with an approximate rise to USD 590–595 million by 2023 (Chandra et al., 2020). Certain intra and extracellular cold adapting enzymes namely phospholipase, protease, catalase, alcohol dehydrogenase, glycosyltransferase, alkaline phosphatase and translocase hold major applications in food and textile industries as well as biopharmaceuticals (Yang et al., 2010; Kumar et al., 2019). Farooq et al. (2022) suggested the efficiency of the gene amplification techniques employed to psychrotrophic Pseudomonas sp. CRBC14 isolated from the Himalayan glacier to induce the yield and purity of enzymes producing microorganisms. Being cold tolerant, these enzymes may also act as a food additive and preservative in food processing (Jin et al., 2022). Sah et al. (2021) highlighted various properties of Pseudomonas in the arena of bioaugmentation and biocontrol. Besides, they are also considered as good biocatalysts due to their strong catalytic activity which eliminates the need for heating operations that affects the efficiency, sustainability, and quality of industrial production (Santiago et al., 2016). As the enzymes must be used sequentially in processes and must be rendered inactive after serving their purpose, cold adapted enzymes are advantageous for molecular biosciences. When used in precise chemical synthesis, cold adapted esterases and lipases have been discovered to have a high degree of stereospecificity. Cold adapted enzymes’ stereospecificity may be helpful for the synthesis of chiral drugs (Cavicchioli et al., 2011). A possible substitute for the effective biofuel generation might be cold active enzymes (Wang et al., 2020). The serine alkaline proteases extracted from Pseudomonas spp., were demonstrated to remain active at a wide temperature and pH range (Zeng et al., 2003; Hao and Sun, 2015; Salwoom et al., 2019; Salwan et al., 2020), thus, behaves as efficient substrates for detergent preparation. Beside enzymes, the cold adapted microorganisms produce a range of bioactive compounds, pigments, polysaccharides, mainly with the purpose of their survival under low temperature stress; these compounds in turn have a range of applications in various industries (Sah et al., 2021).
Antarctic and Arctic environments are increasingly becoming the key habitats for the colonization of cold adapted microbial resources of biotechnological and industrial applications. Cold adapted species of Pseudomonas are receiving attention for their potential to produce exopolysaccharides that can be used as alternative source of commercial polysaccharides in biotechnology and various industries. A cold adapted Antarctic strain Pseudomonas sp. ID1 has been studied for the production of a new exopolysaccharide, mainly composed of glucose, fructose and galactose. Further, its biotechnological applications, as an emulsifier and cryoprotectant, have been highlighted; the emulsifying activity being higher as compared to the other commercial emulsifiers (Carrión et al., 2015). Pseudomonas sp. PAMC 28620, a psychrotrophic Arctic soil bacterium, has been reported for a distinct structural composition and metal removal and reduction potential in Arctic environment. The purified exopolysaccharide was recorded with remarkable flocculating and emulsification abilities leading to biotechnological applications such as metal adsorption (99%) potential showing the complexities and immobilization of metal ions and their further reduction to nanoparticles (Sathiyanarayanan et al., 2016). Various strategies such as optimization of culture media, secondary metabolites, physiological parameters, chemical derivatives etc., can be employed for enhanced production of the desired compound (Nair et al., 2021).
Human health
The recent area of microbiome research has revealed the important role microorganisms are performing in our body; therefore, it is essential to explore microorganisms for the benefit of humankind. Microorganisms since their origin have played an important role in addressing the health issues right from the discovery of Penicillin which has save millions of lives. Species belonging to Pseudomonas were studied by Onbasli and Aslim (2008) for the synthesis of rhamnolipids, pyocins (bacteriocin), alginate, and exopolysaccharides. Rhamnolipids are also employed as biosurfactants from Pseudomonas sp. (Onbasli and Aslim, 2009; Braz et al., 2023), which have the potential to degrade oil and an efficient biocontrol molecule for long-term agricultural sustainability (Onlamool et al., 2022). Microorganisms may enhance the yield of secondary metabolites when a suitable substrate is added, for instance, Pseudomonas sp. was evaluated for an increase in rhamnolipid synthesis when supplemented with sugar beet molasses (Onbasli and Aslim, 2008; Braz et al., 2023). Further, it has been reported that the pyocins (F-and R-type) secreted by Pseudomonas sp. are a significant narrow spectrum antibiotic (Ge et al., 2020; Blasco et al., 2023). Domröse et al. (2015) worked on the development of PK/NRP hybrid compound which is similar to prodigiosin- a known immunosuppressant and anticancer agent. Methicillin-resistant Staphylococcus aureus (MRSA) can be treated alternatively using phenazines, a compound produced by Pseudomonas sp. (Cardozo et al., 2013). The same compound showed beneficial effects when combined with nanoparticles described by Cardozo et al., 2013. Afonso et al. (2022) examined the organocupric Fluopsin C (FlpC) isolated from Pseudomonas spp., with inhibitory activity against human and phytopathogens such as Klebsiella pneumonia (Navarro et al., 2019), Xanthomonas citri pv. citri (de Oliveira et al., 2011), Xanthomonas citri subsp. citri (de Oliveira et al., 2016), Xanthomonas axonopodis (Lopes et al., 2012) etc. Tyrosol was studied by Allouche et al. (2004) and proven to boost the production of hydroxytyrosol, a powerful antioxidant that can also be generated by immobilization (Bouallagui and Sayadi, 2006). P. putida was observed for de novo production of geranic acid (terpenoid), carotenoids, polyketides, mcl- PHA (polyhydroxyalkanoates) with reported antibiotic potential and uses as fragrance and flavor component (Weimer et al., 2020).
Pseudomonas is also known as a source of exopolysaccharides such as pellicle loci (Pel), polysaccharide synthesis loci (Psl) and alginate (Franklin et al., 2011), which are important structural elements of biofilm formation and are believed to be essential for a variety of purposes such as coherence, adherence, enzyme binding, resistance to abiotic stress, and antimicrobial agents (Jennings et al., 2015; Heredia-Ponce et al., 2021; Fleming et al., 2022). Psl and Pel are also reported for the synthesis of mannose and glucose-rich matrix, respectively (Friedman and Kolter, 2004). These characteristic features of the biofilm matrix of Pseudomonas spp. may help in the early diagnosis of Pseudomonas related infections. Alginate is a major component with many medical applications, for instance, advanced wound care dressings employ alginate hydrogels to absorb fluid creating a moist environment that lowers the risk of infection (Paul and Sharma, 2004) and a popular bio-ink used to create biocompatible, non-immunogenic frameworks for the 3D printing of tissues and organs (Lee and Mooney, 2012).
Antibiotic resistance in Pseudomonas is a rising problem in both clinical and community settings, limiting the efficacy of many traditional treatment approaches. P. aeruginosa strains possess resistance-nodulation-division efflux pumps that physically sequester incoming drugs (Hwang and Yoon, 2019) and disturb the action mechanism of the drugs; also the genetic mutations or horizontal gene transfer exhibit intrinsic antibiotic resistance (Pestrak et al., 2019). Furthermore, biofilms serve as a barrier against antibiotic penetration. In this context, researchers have developed recombinants formed by the omission of virulence genes present within the Pseudomonas genome (Valentine et al., 2020; Farooq et al., 2022). Rezzoagli et al. (2020) identified antibiotic-antivirulence combinations as a potentially powerful tool to efficiently treat infections of nosocomial pathogens caused by P. aeruginosa. Horcajada et al. (2019) reported ceftolozane-tazobactam combination to possess significant antimicrobial activity against an extensively drug-resistant and multidrug-resistant Pseudomonas.
Cosmetics
Over the last few decades, it has been observed that the cosmetic industries are developing interest in the bio-based products. Due to the rising health issues from chemical based products, various developed and developing countries are now focusing on the microbe based products for health benefits. Several microorganism-derived products have shown huge potential in cosmetic industry. Specifically talking about Pseudomonas, alginate produced by P. aeruginosa possesses good water holding ability and is thus utilized in the manufacture of skin moisturizer and gelling agents (Valentine et al., 2020). Pseudomonas spp., however, are well known opportunistic microorganisms reported worldwide for causing various fatal infections. Thus, the concept of recombinants development plays a significant role and allows the physiology and genetic makeup of the organism. The in-vivo observations noted by Valentine et al. (2020) supported the mentioned fact. In this study, five important pathogenicity genes were omitted from the P. aeruginosa genome using a homologous recombination technique that decreased the systemic virulence and also enhanced the yield of alginate.
A SEM and TEM analysis suggested the presence of melanin in P. koreensis UIS 19 with high tyrosinase activity. The strain demonstrated a promising sun protection factor (SPF) value of more than 60 for 20 mg/mL and more than 92% effective in scavenging DPPH radicals (Eskandari and Etemadifar, 2021). Pseudomonas sp., is known to produce another important compound, Vanillin with a wide application in cosmetic industry. It is basically used to provide a pleasant aroma and flavor while also enhances the texture of the products. Wang et al. (2021) developed a recombinant isoeugenol enzyme extracted from P. nitroreducens Jin1 by optimizing the culture conditions in order to obtain vanillin, which further increased the yield by 82% with more than 95% purity.
Conclusion and future prospects
Cold adapted microorganisms, inhabiting low temperature environments, address the growing concerns related to ecosystem protection in the present climate change scenario. In this context, Pseudomonads are seen as one of the most metabolically diversified bacterial colonizers of cold environments. Their capacity to grow in a wide range of temperatures (as psychrophiles or psychrotolerants) and pH (extreme acidic to alkaline) makes them ideal candidates to withstand the extreme industrial processes. Their abilities to produce bioactive chemicals, enzymes, and pigments allow them for their utilization in a variety of ecological and biotechnological applications. Utilizing the recently developed technologies and methodologies for cultivation of majority of the microorganisms, which are yet to be cultured, will be an important step to explore the biodiversity and metabolic potentials of Pseudomonas. Given their multifarious uses, conservation of microorganism from such ecosystems in National and International Microbial Resource Centers is essential for a sustainable future.
Author contributions
AP and MC: conceptualization and draft preparation. AK and MC: figures and tables. AP and AS: reviewing and editing. All authors contributed to the article and approved the submitted version.
Conflict of interest
The authors declare that the research was conducted in the absence of any commercial or financial relationships that could be construed as a potential conflict of interest.
Publisher’s note
All claims expressed in this article are solely those of the authors and do not necessarily represent those of their affiliated organizations, or those of the publisher, the editors and the reviewers. Any product that may be evaluated in this article, or claim that may be made by its manufacturer, is not guaranteed or endorsed by the publisher.
References
Abraham, W. P., Raghunandanan, S., Gopinath, V., Suryaletha, K., and Thomas, S. (2020). Deciphering the cold adaptive mechanisms in Pseudomonas psychrophila MTCC12324 isolated from the Arctic at 79° N. Curr. Microbiol. 77, 2345–2355. doi: 10.1007/s00284-020-02006-2
Afonso, L., de Lima Andreata, M. F., Chryssafidis, A. L., Alarcon, S. F., das Neves, A. P., da Silva, J. V. F. R., et al. (2022). Fluopsin C: a review of the antimicrobial activity against phytopathogens. Agronomy 12:2997. doi: 10.3390/agronomy12122997
Ahn, E., Choi, K. Y., Kang, B. S., Zylstra, G. J., Kim, D., and Kim, E. (2017). Salicylate degradation by a cold-adapted Pseudomonas sp. Ann. Microbiol. 67, 417–424. doi: 10.1007/s13213-017-1273-3
Ali, P., Shah, A. A., Hasan, F., Hertkorn, N., Gonsior, M., Sajjad, W., et al. (2020). A glacier bacterium produces high yield of cryoprotective exopolysaccharide. Front. Microbiol. 10:3096. doi: 10.3389/fmicb.2019.03096
Allen, C. C. R., Boyd, D. R., and Dalton, H. (2000). Process for the resolution in cis 1,2- indane diols using Pseudomonas putida. US Patent No. 6165777. London: Zeneca limited.
Allouche, N., Damak, M., Ellouz, R., and Sayadi, S. (2004). Use of whole cells of Pseudomonas aeruginosa for synthesis of the antioxidant hydroxytyrosol via conversion of tyrosol. Appl. Environ. Microbiol. 70, 2105–2109. doi: 10.1128/AEM.70.4.2105-2109.2004
Andersen, S. M., Johnsen, K., Sørensen, J., Nielsen, P., and Jacobsen, C. S. (2000). Pseudomonas frederiksbergensis sp. nov., isolated from soil at a coal gasification site. Int. J. Sys. Evol. Microbiol. 50, 1957–1964. doi: 10.1099/00207713-50-6-1957
Annapure, U. S., and Pratisha, N. (2022). “Psychrozymes: a novel and promising resource for industrial applications” in Microbial Extremozymes. ed. M. Kuddus (New York: Academic Press), 185–195.
Balcázar, W., Rondón, J., Rengifo, M., Ball, M. M., Melfo, A., Gómez, W., et al. (2015). Bioprospecting glacial ice for plant growth promoting bacteria. Microbiol. Res. 177, 1–7. doi: 10.1016/j.micres.2015.05.001
Ball, M. M., Go’mez, W., Magallanes, X., Rosales, R., Melfo, A., and Yarzábal, L. A. (2014). Bacteria recovered from a high-altitude, tropical glacier in Venezuelan Andes. World J. Microbiol.Biotechnol. 30, 931–941. doi: 10.1007/s11274-013-1511-1
Benforte, F. C., Colonnella, M. A., Ricardi, M. M., Venero, E. C. S., Lizarraga, L., López, N. I., et al. (2018). Novel role of the LPS core glycosyltransferase WapH for cold adaptation in the Antarctic bacterium Pseudomonas extremaustralis. PLoS One 13:e0192559. doi: 10.1371/journal.pone.0192559
Bhattacharya, P., Tiwari, P., Rai, I. D., Talukdar, G., and Rawat, G. S. (2022a). Edaphic factors override temperature in shaping soil bacterial diversity across an elevation-vegetation gradient in Himalaya. Appl. Soil Ecol. 170:104306. doi: 10.1016/j.apsoil.2021.104306
Bhattacharya, P., Tiwari, P., Talukdar, G., and Rawat, G. S. (2022b). Shifts in bacterial community composition and functional traits at different time periods post-deglaciation of Gangotri Glacier. Himalaya. Curr.Microbiol. 79:91. doi: 10.1007/s00284-022-02779-8
Białkowska, A., Majewska, E., Olczak, A., and Twarda-Clapa, A. (2020). Ice binding proteins: diverse biological roles and applications in different types of industry. Biomol.Ther. 10:274. doi: 10.3390/biom10020274
Bisht, D., Pandey, A., and Palni, L. M. S. (2003). Influence of microbial inoculations on Cedrus deodara in relation to survival, growth promotion and nutrient uptake of seedlings and general soil microflora. J. Sustain. For. 17, 37–54. doi: 10.1300/J091v17n03_03
Blasco, L., de Aledo, M. G., Ortiz-Cartagena, C., Blériot, I., Pacios, O., López, M., et al. (2023). Study of 32 new phage tail-like bacteriocins (pyocins) from a clinical collection of Pseudomonas aeruginosa and of their potential use as typing markers and antimicrobial agents. Sci. Rep. 13:117. doi: 10.1038/s41598-022-27341-1
Bouallagui, Z., and Sayadi, S. (2006). Production of high hydroxytyrosol yields via tyrosol conversion by Pseudomonas aeruginosa immobilized resting cells. J. Agric. Food Chem. 54, 9906–9911. doi: 10.1021/jf062145g
Braz, L. M., Salazar-Bryam, A. M., Andrade, G. S. S., and Tambourgi, E. B. (2023). Optimization and characterization of rhamnolipids produced by Pseudomonas aeruginosa ATCC 9027 using molasses as a substrate. World J. Microbiol. Biotechnol. 39:51. doi: 10.1007/s11274-022-03494-z
Bryant, J. A., Lamanna, C., Morlon, H., Kerkhoff, A. J., Enquist, B. J., and Green, J. L. (2008). Microbes on mountainsides: contrasting elevational patterns of bacterial and plant diversity. PNAS 105, 11505–11511. doi: 10.1073/pnas.0801920105
Cabrera, M. Á., Márquez, S. L., and Pérez-Donoso, J. M. (2022). Comparative genomic analysis of Antarctic Pseudomonas isolates with 2,4,6-trinitrotoluene transformation capabilities reveals their unique features for xenobiotics degradation. Genes 13:1354. doi: 10.3390/genes13081354
Cacace, G., Mazzeo, M. F., Sorrentino, A., Spada, V., Malorni, A., and Siciliano, R. A. (2010). Proteomics for the elucidation of cold adaptation mechanisms in Listeria monocytogenes. J. Proteome 73, 2021–2030. doi: 10.1016/j.jprot.2010.06.011
Cardozo, V. F., Oliveira, A. G., Nishio, E. K., Perugini, M. R., Andrade, C. G., Silveira, W. D., et al. (2013). Antibacterial activity of extracellular compounds produced by a Pseudomonas strain against methicillin-resistant Staphylococcus aureus (MRSA) strains. Ann. Clin. Microbiol. Antimicrob. 12, 1–8. doi: 10.1186/1476-0711-12-12
Carrión, O., Delgado, L., and Mercade, E. (2015). New emulsifying and cryoprotective exopolysaccharide from Antarctic Pseudomonas sp. ID1. Carbohy. Poly. 117, 1028–1034. doi: 10.1016/j.carbpol.2014.08.060
Cavicchioli, R. (2015). Microbial ecology of Antarctic aquatic systems. Nat. Rev. Microbiol. 13, 691–706. doi: 10.1038/nrmicro3549
Cavicchioli, R. (2016). On the concept of psychrophiles. ISME J. 10, 793–795. doi: 10.1038/ismej.2015.160
Cavicchioli, R., Charlton, T., Ertan, H., Mohd Omar, S., Siddiqui, K. S., and Williams, T. J. (2011). Biotechnological uses of enzymes from psychrophiles. Microb. Biotechnol. 4, 449–460. doi: 10.1111/j.1751-7915.2011.00258.x
Chandra, P., Singh, R. E., and Arora, P. K. (2020). Microbial lipases and their industrial applications: a comprehensive review. Microb. Cell Factories 19:169. doi: 10.1186/s12934-020-01428-8
Chettri, N., Shakya, B., Thapa, R., and Sharma, E. (2008). Status of a protected area system in the Hindu Kush-Himalayas: an analysis of PA coverage. Int. J. Biodivers. Sci. Manag. 4, 164–178. doi: 10.3843/Biodiv.4.3:4
Chevalier, S., Bouffartigues, E., Bodilis, J., Maillot, O., Lesouhaitier, L., Feuilloley, M. G. J., et al. (2017). Structure, function and regulation of Pseudomonas aeruginosa porins. FEMS Microbiol. Rev. 41, 698–722. doi: 10.1093/femsre/fux020
Chica, E., Buela, L., Valdez, A., Villena, P., Peña, D., and Yarzábal, L. A. (2019). Metagenomic survey of the bacterial communities in the rhizosphere of three Andean tuber crops. Symbiosis 79, 141–150. doi: 10.1007/s13199-019-00631-5
Chintalapati, S., Kiran, M. D., and Shivaji, S. (2004). Role of membrane lipid fatty acids in cold adaptation. Cell. Mol. Biol. 50, 631–642.
Choo, D. W., Kurihara, T., Suzuki, T., Soda, K., and Esaki, N. (1998). A cold-adapted lipase of an Alaskan psychrotroph, Pseudomonas sp. strain B11-1: gene cloning and enzyme purification and characterization. Appl. Environ. Microbiol. 64, 486–491. doi: 10.1128/AEM.64.2.486-491.1998
Chumpitaz-Segovia, C., Alvarado, D., Ogata-Gutiérrez, K., and Zúñiga-Dávila, D. (2020). Bioprospection of native psychrotolerant plant-growth-promoting rhizobacteria from Peruvian Andean Plateau soils associated with Chenopodium quinoa. Can. J. Microbiol. 66, 641–652. doi: 10.1139/cjm-2020-0036
Ciofu, O., and Tolker-Nielsen, T. (2019). Tolerance and resistance of Pseudomonas aeruginosa biofilms to antimicrobial agents— how P. aeruginosa can escape antibiotics. Front. Microbiol. 10:913. doi: 10.3389/fmicb.2019.00913
Craig, K., Johnson, B. R., and Grunden, A. (2021). Leveraging Pseudomonas stress response mechanisms for industrial applications. Front. Microbiol. 12:660134. doi: 10.3389/fmicb.2021.660134
Cui, T., Wang, P., Li, J., Su, Y. M., Liu, N., and Hong, M. (2022). Effects of temperature, pH, and salinity on the growth kinetics of Pseudomonas sp. NB-1, a newly isolated cold-tolerant, alkali-resistant, and high-efficiency nitrobenzene-degrading bacterium. Environ. Technol. 44, 1–13, 2171–2183. doi: 10.1080/09593330.2021.2024886
da Silva, A. V., de Oliveira, A. J., Tanabe, I. S. B., Silva, J. V., da Silva Barros, T. W., da Silva, M. K., et al. (2021). Antarctic lichens as a source of phosphate-solubilizing bacteria. Extremophiles 25, 181–191. doi: 10.1007/s00792-021-01220-5
De Meeyer, P., Anderson, D., Cary, C., and Cowan, D. A. (2014). Some like it cold: understanding the survival strategies of psychrophiles. EMBO Rep. 15, 508–517. doi: 10.1002/embr.201338170
de Oliveira, A. G., Murate, L. S., Spago, F. R., Lopes, L. P., Beranger, J. P. O., Martin, J. A. B. S., et al. (2011). Evaluation of the antibiotic activity of extracellular compounds produced by the Pseudomonas strain against the Xanthomonas citri pv. citri 306 strain. Biol. Control 56, 125–131. doi: 10.1016/j.biocontrol.2010.10.008
de Oliveira, A. G., Spago, F. R., Simionato, A. S., Navarro, M. O. P., da Silva, C. S., Barazetti, A. R., et al. (2016). Bioactive organocopper compound from Pseudomonas aeruginosa inhibits the growth of Xanthomonas citri subsp. citri. Front. Microbiol. 7:113. doi: 10.3389/fmicb.2016.00113
Deming, J. W. (2002). Psychrophiles and polar regions. Curr. Opin. Microbiol. 5, 301–309. doi: 10.1016/s1369-5274(02)00329-6
Dhakar, K., and Pandey, A. (2016). Wide pH range tolerance in extremophiles: towards understanding an important phenomenon for future biotechnology. Appl. Microbiol. Biotechnol. 100, 2499–2510. doi: 10.1007/s00253-016-7285-2
Dhakar, K., and Pandey, A. (2020). Microbial ecology from the himalayan cryosphere perspective. Microorganisms 8:257. doi: 10.3390/microorganisms8020257
Domröse, A., Klein, A., Hage-Hülsmann, J., Thies, S., Svensson, V., Classen, T., et al. (2015). Efficient recombinant production of prodigiosin in Pseudomonas putida. Front. Microbiol. 6:972. doi: 10.3389/fmicb.2015.00972
Du Jardin, P. (2015). Plant biostimulants: definition, concept, main categories and regulation. Sci. Hortic. 196, 3–14. doi: 10.1016/j.scienta.2015.09.021
Dziewit, L., and Bartosik, D. (2014). Plasmids of psychrophilic and psychrotolerant bacteria and their role in adaptation to cold environments. Front. Microbiol. 5:596. doi: 10.3389/fmicb.2014.00596
Eskandari, S., and Etemadifar, Z. (2021). Melanin biopolymers from newly isolated Pseudomonas koreensis strain UIS 19 with potential for cosmetics application, and optimization on molasses waste medium. J. Appl. Microbiol. 131, 1331–1343. doi: 10.1111/jam.15046
Farooq, S., Ganai, S. A., Ganai, B. A., Mohan, S., Uqab, B., and Nazir, R. (2022). Molecular characterization of lipase from a psychrotrophic bacterium Pseudomonas sp. CRBC14. Curr. Genet. 68, 243–251. doi: 10.1007/s00294-021-01224-w
Feller, G. (2013). Psychrophilic enzymes: from folding to function and biotechnology. Scientifica 2013:28. doi: 10.1155/2013/512840
Feller, G., and Gerday, C. (2003). Psychrophilic enzymes: hot topics in cold adaptation. Nat. Rev. Microbiol. 1, 200–208. doi: 10.1038/nrmicro773
Fleming, D., Niese, B., Redman, W., Vanderpool, E., Gordon, V., and Rumbaugh, K. P. (2022). Contribution of Pseudomonas aeruginosa exopolysaccharides Pel and Psl to wound infections. Front. Cell. Infect. Microbiol. 12:835754. doi: 10.3389/fcimb.2022.835754
Franklin, M. J., Nivens, D. E., Weadge, J. T., and Howell, P. L. (2011). Biosynthesis of the Pseudomonas aeruginosa extracellular polysaccharides, alginate, Pel, and Psl. Front. Microbiol. 2:167. doi: 10.3389/fmicb.2011.00167
Friedman, L., and Kolter, R. (2004). Two genetic loci produce distinct carbohydraterich structural components of the Pseudomonas aeruginosa biofilm matrix. J. Bacteriol. 186, 4457–4465. doi: 10.1128/jb.186.14.4457-4465.2004
Friedrich, T, and Stürmer, R. (2003). Immobilizing lipase by adsorption from a crude solution onto nonpolar polyolefin particles. US patent No. 6596520. Ludwigshafen: BASF Aktiengesellschaft
Ganasen, M., Yaacob, N., Rahman, R. N. Z. R. A., Leow, A. T. C., Basri, M., Salleh, A. B., et al. (2016). Cold-adapted organic solvent tolerant alkalophilic family I.3 lipase from an Antarctic Pseudomonas. Int. J. Biol. Macromol. 92, 1266–1276. doi: 10.1016/j.ijbiomac.2016.06.095
Ge, P., Scholl, D., Prokhorov, N. S., Avaylon, J., Shneider, M. M., Browning, C., et al. (2020). Action of a minimal contractile bactericidal nanomachine. Nature 580, 658–662. doi: 10.1038/s41586-020-2186-z
Ghergab, A., Mohanan, N., Saliga, G., Brassinga, A. K. C., Levin, D., and de Kievit, T. (2021). The effect of polyhydroxyalkanoates in Pseudomonas chlororaphis PA23 biofilm formation, stress endurance, and interaction with the protozoan predator Acanthamoeba castellanii. Can. J. Microbiol. 67, 476–490. doi: 10.1139/cjm-2020-0497
Ghyselinck, J., Velivelli, S. L., Heylen, K., O’Herlihy, E., Franco, J., Rojas, M., et al. (2013). Bioprospecting in potato fields in the Central Andean Highlands: screening of rhizobacteria for plant growth-promoting properties. Syst. Appl. Microbiol. 36, 116–127. doi: 10.1016/j.syapm.2012.11.007
Gianese, G., Argos, P., and Pascarella, S. (2001). Structural adaptation of enzymes to low temperatures. Protein Eng. Des. Sel. 14, 141–148. doi: 10.1093/protein/14.3.141
Gomila, M., Peña, A., Mulet, M., Lalucat, J., and García-Valdés, E. (2015). Phylogenomics and systematics in Pseudomonas. Front. Microbiol. 6:214. doi: 10.3389/fmicb.2015.00214
Guo, C., Zheng, R., Cai, R., Sun, C., and Wu, S. (2021). Characterization of two unique cold-active lipases derived from a novel deep-sea cold seep bacterium. Microorganisms 9:802. doi: 10.3390/microorganisms9040802
Hao, J. H., and Sun, M. (2015). Purification and characterization of a cold alkaline protease from a psychrophilic Pseudomonas aeruginosa HY1215. Appl. Biochem. Biotechnol. 175, 715–722. doi: 10.1007/s12010-014-1315-2
Heredia-Ponce, Z., de Vicente, A., Cazorla, F. M., and Gutiérrez-Barranquero, J. A. (2021). Beyond the wall: exopolysaccharides in the biofilm lifestyle of pathogenic and beneficial plant-associated Pseudomonas. Microorganisms 9:445. doi: 10.3390/microorganisms9020445
Hilton, M. D., Strobel, R. J. Jr., Millar, P. J. B., Thomas, D. N., Cockshott, A. R., Brian, G. G., et al. (2009). Pseudomycin production by Pseudomonas syringae. US Patent No. 7556960. Indianapolis: Eli Lilly and Company.
Horcajada, J. P., Montero, M., Oliver, A., Sorlí, L., Luque, S., Gómez-Zorrilla, S., et al. (2019). Epidemiology and treatment of multidrug-resistant and extensively drug-resistant Pseudomonas aeruginosa infections. Clin. Microbiol. Rev. 32, e00031–e00019. doi: 10.1128/CMR.00031-19
Huang, S. L., Chen, H., Hu, A., Tuan, N. N., and Yu, C. P. (2014). Draft genome sequence of Pseudomonas nitroreducens strain TX1, which degrades nonionic surfactants and estrogen-like alkylphenols. Genome Announc. 2:e01262-13. doi: 10.1128/genomea.01262-13
Huang, S., Tseng, D., and Chen, H. (2004). Biological method for removing organic polymers.US Patent No. 7332325. Taoyuan: National Central University.
Hudait, A., Odendahl, N., Qiu, Y., Paesani, F., and Molinero, V. (2018). Ice-nucleating and antifreeze proteins recognize ice through a diversity of anchored clathrate and icelike motifs. J. Am. Chem. Soc. 140, 4905–4912. doi: 10.1021/jacs.8b01246
Hwang, W., and Yoon, S. S. (2019). Virulence characteristics and an action mode of antibiotic resistance in multidrug-resistant Pseudomonas aeruginosa. Sci. Rep. 9, 1–15. doi: 10.1038/s41598-018-37422-9
Imamura, T., Yano, T., Kobayashi, S., Suda, S., and Honma, T. (2002).Method for producing microbial polyester. US patent No. 6492147. Tokyo: Canon Kabushiki Kiasha.
Jain, R., and Pandey, A. (2016). A phenazine-1-carboxylic acid producing polyextremophilic Pseudomonas chlororaphis (MCC2693) strain, isolated from mountain ecosystem, possesses biocontrol and plant growth promotion abilities. Microbiol. Res. 190, 63–71. doi: 10.1016/j.micres.2016.04.017
Jain, R., Pandey, N., and Pandey, A. (2020). Aggregation properties of cold-active lipase produced by a psychrotolerant strain of Pseudomonas palleroniana (GBPI_508). Biocatal. Biotransform. 38, 1–11. doi: 10.1080/10242422.2019.1666829
Jain, R., Pandey, A., Pasupuleti, M., and Pande, V. (2017). Prolonged production and aggregation complexity of cold active lipase from Pseudomonas proteolytica (GBPI_ Hb61) isolated from cold desert Himalaya. Mol. Biotechnol. 59, 34–45. doi: 10.1007/s12033-016-9989-z
Jennings, L. K., Storek, K. M., Ledvina, H. E., Coulon, C., Marmont, L. S., Sadovskaya, I., et al. (2015). Pel is a cationic exopolysaccharide that cross-links extracellular DNA in the Pseudomonas aeruginosa biofilm matrix. Proc. Natl. Acad. Sci. 112, 11353–11358. doi: 10.1073/pnas.1503058112
Jin, S., Wang, Y., and Zhao, X. (2022). Cold-adaptive mechanism of psychrophilic bacteria in food and its application, 105652. Microb. Pathog 169. doi: 10.1016/j.micpath.2022.105652
John, M. S., Nagoth, J. A., Ramasamy, K. P., Mancini, A., Giuli, G., Natalello, A., et al. (2020). Synthesis of bioactive silver nanoparticles by a Pseudomonas strain associated with the antarctic psychrophilic protozoon Euplotes focardii. Mar. Drugs 18:38. doi: 10.3390/md18010038
Kanekar, P. P., and Kanekar, S. P. (2022). Psychrophilic, psychrotrophic, and psychrotolerant microorganisms. In: Kanekar, P.P., and Kanekar, S.P., Diversity and biotechnology of extremophilic microorganisms from India. Microorganisms for sustainability. Springer, Singapore
Kaur, T., Devi, R., Kour, D., Yadav, A., and Yadav, A. N. (2021). Plant growth promotion of barley (Hordeum vulgare L.) by potassium solubilizing bacteria with multifarious plant growth promoting attributes. Plant Sci. Today 8, 17–24. doi: 10.14719/pst.1377
Keeler, S.J., Fallon, R.D., Hendrickson, E.R., Hnatow, L.L., Jackson, S.C., and Perry, M.P. (2008). Identification, characterization, and application of (LH4:15), useful in microbially enhanced oil release. US Patent No. 8357526. Wilmington, DE: E.l. du Pont de Nemours and company.
Kour, D., Rana, K. L., Sheikh, I., Kumar, V., Yadav, A. N., Dhaliwal, H. S., et al. (2020). Alleviation of drought stress and plant growth promotion by Pseudomonas libanensis EU-LWNA-33, a drought-adaptive phosphorus-solubilizing bacterium. Proc. Natl. Acad. Sci. India Sect. B Biol. Sci. 90, 785–795. doi: 10.1007/s40011-019-01151-4
Kumar, R., Acharya, V., Mukhia, S., Singh, D., and Kumar, S. (2019). Complete genome sequence of Pseudomonas frederiksbergensis ERDD5:01 revealed genetic bases for survivability at high altitude ecosystem and bioprospection potential. Genomics 111, 492–499. doi: 10.1016/j.ygeno.2018.03.008
Kumar, G. S., Jagannadham, M. V., and Ray, M. K. (2002). Low-temperature-induced changes in composition and fluidity of lipopolysaccharides in the antarctic psychrotrophic bacterium Pseudomonas syringae. J. Bacteriol. 184, 6746–6749. doi: 10.1128/JB.184.23.6746-6749.2002
Kumar, S., Suyal, D. C., Yadav, A., Shouche, Y., and Goel, R. (2020). Psychrophilic Pseudomonas helmanticensis proteome under simulated cold stress. Cell Stress Chaperones 25, 1025–1032. doi: 10.1007/s12192-020-01139-4
Kumar, B., Trivedi, P., and Pandey, A. (2007). Pseudomonas corrugata: a suitable bacterial inoculant for maize grown under rainfed conditions of Himalayan region. Soil Biol. Biochem. 39, 3093–3100. doi: 10.1016/j.soilbio.2007.07.003
Lee, K. Y., and Mooney, D. J. (2012). Alginate: properties and biomedical applications. Prog. Polym. Sci. 37, 106–126.
Li, X. L., Zhang, W. H., Wang, Y. D., Dai, Y. J., Zhang, H. T., Wang, Y., et al. (2014). A high-detergent-performance, cold-adapted lipase from Pseudomonas stutzeri PS59 suitable for detergent formulation. J. Mol. Catal. B Enzym. 102, 16–24. doi: 10.1016/j.molcatb.2014.01.006
Liu, L., Gao, J., Huang, Z., Li, Y., Shang, N., Gao, J., et al. (2021). Potential application of a Pseudomonas geniculata ATCC 19374 and Bacillus cereus EC3 mixture in livestock wastewater treatment. Waste Biomass Valorization 12, 3927–3938. doi: 10.1007/s12649-020-01286-w
Lopes, L. P., Oliveira Jr, A. G., Beranger, J. P. O., Góis, C. G., Vasconcellos, F. C. S., Martin, J. A. B. S., et al. (2012). Activity of extracellular compounds of Pseudomonas sp. against Xanthomonas axonopodis in vitro and bacterial leaf blight in eucalyptus. Trop. Plant Pathol. 37, 233–238. doi: 10.1590/s1982-56762012000400001
Ma, Y., Rajkumar, M., Moreno, A., Zhang, C., and Freitas, H. (2017). Serpentine endophytic bacterium Pseudomonas azotoformans ASS1 accelerates phytoremediation of soil metals under drought stress. Chemosphere 185, 75–85. doi: 10.1016/j.chemosphere.2017.06.135
Margesin, R. (2000). Potential of cold-adapted microorganisms for bioremediation of oil-polluted alpine soils. Int. Biodeterior. Biodegrad. 46, 3–10. doi: 10.1016/S0964-8305(00)00049-4
Margesin, R., and Feller, G. (2010). Biotechnological applications of psychrophiles. Environ. Technol. 31, 835–844. doi: 10.1080/09593331003663328
Margesin, R., and Schinner, F. (1999). Biological decontamination of oil spills in cold environments. J. Chem. Technol. Biotechnol. 74, 381–389. doi: 10.1002/(SICI)1097-4660(199905)74:53.0.CO;2-0
Martin, R., Hilaire, P., Pineau, N., and Breton, L. (2002). Use of bacterial extracts of the Pseudomonadaceae family as cosmetic agents. US Patent No. 6806070. Paris: L'Oreal.
Martínez-Rosales, C., and Castro-Sowinski, S. (2011). Antarctic bacterial isolates that produce cold-active extracellular proteases at low temperature but are active and stable at high temperature. Polar Res. 30:7123. doi: 10.3402/polar.v30i0.7123
Mazolla, M. (1999). Control of replant disease of tree fruits with Pseudomonas putida. US patent number 5948671. Washington, DC.
McWilliams, A. (2018). Microbial products: Technologies, applications and global markets, BCC research LLC, Wellesley, MA, USA.
Mishra, P. K., Bisht, S. C., Ruwari, P., Selvakumar, G., Joshi, G. K., Bisht, J. K., et al. (2011). Alleviation of cold stress in inoculated wheat (Triticum aestivum L.) seedlings with psychrotolerant pseudomonads from NW Himalayas. Arch. Microbiol. 193, 497–513. doi: 10.1007/s00203-011-0693-x
Mishra, P. K., Mishra, S., Selvakumar, G., Bisht, S. C., Bisht, J. K., Kundu, S., et al. (2008). Characterisation of a psychrotolerant plant growth promoting Pseudomonas sp. strain PGERs17 (MTCC 9000) isolated from North Western Indian Himalayas. Ann. Microbiol. 58, 561–568. doi: 10.1007/BF03175558
Mizusawa, E.A., Anderson, S. A., EL-Sayed, M.Y., Leiske, D.R., Wiersema, R.J., and Yang, C. (2001). Immobilization of Pseudomonas lipase on surface for oil removal. US Patent No. 6265191. Okland, CA: The Clorox Company.
Mukhia, S., Kumar, A., Kumari, P., Kumar, R., and Kumar, S. (2022). Multilocus sequence based identification and adaptational strategies of Pseudomonas sp. from the supraglacial site of Sikkim Himalaya. PLoS One 17:e0261178. doi: 10.1371/journal.pone.0261178
Muryoi, N., Sato, M., Kaneko, S., Kawahara, H., Obata, H., Yaish, M. W., et al. (2004). Cloning and expression of afpA, a gene encoding an antifreeze protein from the Arctic plant growth-promoting rhizobacterium Pseudomonas putida GR12-2. J. Bacteriol. 186, 5661–5671. doi: 10.1128/JB.186.17.5661-5671.2004
Nair, A. V., Praveen, N. K., Leo-Antony, M., and Vijayan, K. K. (2021). Antibacterial assay guided isolation of a novel hydroxy-substituted pentacyclo ketonic compound from Pseudomonas aeruginosa MBTDCMFRI Ps04. Braz. J. Microbiol. 52, 335–347. doi: 10.1007/s42770-020-00404-z
Narbad, A., Rhodes, M.J.C., Gasson, M.J, and Walton, N.J. (2003). Production of vanillin. US patent No. 6664088. Norwich: Plant Bioscience Limited.
Navarro, M. O. P., Simionato, A. S., Pérez, J. C. B., Barazetti, A. R., Emiliano, J., Niekawa, E. T. G., et al. (2019). Fluopsin C for treating multidrug-resistant infections: in vitro activity against clinically important strains and in vivo efficacy against carbapenemase-producing Klebsiella pneumoniae. Front. Microbiol. 10:2431. doi: 10.3389/fmicb.2019.02431
Negi, Y. K., Prabha, D., Garg, S. K., and Kumar, J. (2011). Genetic diversity among cold-tolerant fluorescent Pseudomonas isolates from Indian Himalayas and their characterization for biocontrol and plant growth-promoting activities. J. Plant Growth Regul. 30, 128–143. doi: 10.1007/s00344-010-9175-7
Nottingham, A. T., Fierer, N., Turner, B. L., Whitaker, J., Ostle, N. J., McNamara, N. P., et al. (2018). Microbes follow Humboldt: temperature drives plant and soil microbial diversity patterns from the Amazon to the Andes. Ecology 99, 2455–2466. doi: 10.1002/ecy.2482
Onbasli, D., and Aslim, B. (2008). Determination of antibiotic activity and production of some metabolites by Pseudomonas aeruginosa B1 and B2 in sugar beet molasses. Afr. J. Biotechnol. 7, 4814–4819.
Onbasli, D., and Aslim, B. (2009). Biosurfactant production in sugar beet molasses by some Pseudomonas spp. J. Environ. Biol. 30, 161–163. doi: 10.5897/AJB08.691
Onlamool, T., Saimmai, A., and Maneerat, S. (2022). Antifungal activity of rhamnolipid biosurfactant produced by Pseudomonas aeruginosa A4 against plant pathogenic fungi. Trends Sci. 20:6524. doi: 10.48048/tis.2023.6524
Orellana-Saez, M., Pacheco, N., Costa, J. I., Mendez, K. N., Miossec, M. J., Meneses, C., et al. (2019). In-depth genomic and phenotypic characterization of the Antarctic psychrotolerant strain Pseudomonas sp. MPC6 reveals unique metabolic features, plasticity, and biotechnological potential. Front. Microbiol. 10:1154. doi: 10.3389/fmicb.2019.01154
Ortiz-Ojeda, P., Ogata-Gutiérrez, K., and Zúñiga-Dávila, D. (2017). Evaluation of plant growth promoting activity and heavy metal tolerance of psychrotrophic bacteria associated with maca (Lepidium meyenii Walp.) rhizosphere. AIMS Microbiol. 3, 279–292. doi: 10.3934/microbiol.2017.2.279
Öten, A. M., Atak, E., Taktak Karaca, B., Fırtına, S., and Kutlu, A. (2022). Discussing the roles of proline and glycine from the perspective of cold adaptation in lipases and cellulases. Biocatal. Biotransformat. 41, 243–260. doi: 10.1080/10242422.2022.2124111
Palleroni, N. J. (2010). The Pseudomonas story. Environ. Microbiol. 12, 1377–1383. doi: 10.1111/j.1462-2920.2009.02041.x
Pandey, A., Jain, R., Sharma, A., Dhakar, K., Kaira, G. S., Rahi, P., et al. (2019). 16S rRNA gene sequencing and MALDI-TOF mass spectrometry based comparative assessment and bioprospection of psychrotolerant bacteria isolated from high altitudes under mountain ecosystem. SN Appl. Sci. 1:278. doi: 10.1007/s42452-019-0273-2
Pandey, A., and Palni, L. M. S. (1998). Isolation of Pseudomonas corrugata from Sikkim Himalaya. World J. Microbiol.Biotechnol. 14, 411–413. doi: 10.1023/A:1008825514148
Pandey, A., and Palni, L. M. S. (2007). The rhizosphere effect in trees of the Indian central Himalaya with special reference to altitude. Appl. Ecol. Environ. Res. 5, 93–102. doi: 10.15666/aeer/0501_093102
Pandey, A., Sharma, E., and Palni, L. M. S. (1998). Influence of bacterial inoculation on maize in upland farming systems of the Sikkim Himalaya. Soil Biol. Biochem. 30, 379–384. doi: 10.1016/s0038-0717(97)00121-1
Pandey, A., Singh, S., Kumar, A., Malviya, M. K., and Rinu, K. (2009). Isolation of an endophytic plant growth promoting bacterium Pseudomonas sp. strain gb3 (MTCC 9476) from Ginkgo biloba L., growing in temperate Himalaya. Natl. Acad. Sci. Lett. 32, 83–88.
Pandey, A., Trivedi, P., Kumar, B., and Palni, L. M. S. (2006). Characterization of a phosphate solubilizing and antagonistic strain of Pseudomonas putida (B0) isolated from a sub-alpine location in the Indian Central Himalaya. Curr. Microbiol. 53, 102–107. doi: 10.1007/s00284-006-4590-5
Pandey, A., and Yarzábal, L. A. (2019). Bioprospecting cold-adapted plant growth promoting microorganisms from mountain environments. Appl. Microbiol. Biotechnol. 103, 643–657. doi: 10.1007/s00253-018-9515-2
Panicker, G., Aislabie, J., Saul, D., and Bej, A. K. (2002). Cold tolerance of Pseudomonas sp. 30-3 isolated from oil-contaminated soil. Antarctica. Polar Biol. 25, 5–11. doi: 10.1007/s003000100304
Panicker, G., Mojib, N., Nakatsuji, T., Aislabie, J., and Bej, A. K. (2010). Occurrence and distribution of capB in Antarctic microorganisms and study of its structure and regulation in the Antarctic biodegradative Pseudomonas sp. 30/3. Extremophiles 14, 171–183. doi: 10.1007/s00792-009-0296-5
Parte, A. C. (2018). LPSN — list of prokaryotic names with standing in nomenclature (bacterio.net), 20 years on. Int. J. Syst. Evol. Microbiol. 68, 1825–1829. doi: 10.1099/ijsem.0.002786
Paul, W., and Sharma, C. P. (2004). Chitosan and alginate wound dressings: a short review. Trends Biomater. Artif. Organs 18, 18–23.
Pestrak, M. J., Baker, P., Dellos-Nolan, S., Hill, P. J., Passos da Silva, D., Silver, H., et al. (2019). Treatment with the Pseudomonas aeruginosa glycoside hydrolase PslG combats wound infection by improving antibiotic efficacy and host innate immune activity. Antimicrob. Agents Chemother. 63, e00234–e00219. doi: 10.1128/AAC.00234-19
Prasad, S., Manasa, P., Buddhi, S., Tirunagari, P., Begum, Z., Rajan, S., et al. (2014). Diversity and bioprospective potential (cold-active enzymes) of cultivable marine bacteria from the subarctic glacial Fjord, Kongsfjorden. Curr. Microbiol. 68, 233–238. doi: 10.1007/s00284-013-0467-6
Rapuano, R., and Graziano, G. (2022). Some clues about enzymes from psychrophilic microorganisms. Microorganisms 10:1161. doi: 10.3390/microorganisms10061161
Ravi, K., Falkowski, N. R., Scales, B. S., Akulava, V. D., Valentovich, L. N., and Huffnagle, G. B. (2022). The psychrotrophic Pseudomonas lundensis, a non- aeruginosa pseudomonad, has a type III secretion system of the Ysc family, which is transcriptionally active at 37°C. MBio 13, e03869–e03821. doi: 10.1128/mbio.03869-21
Rawat, P., Das, S., Shankhdhar, D., and Shankhdhar, S. C. (2021). Phosphatesolubilizing microorganisms: mechanism and their role in phosphate solubilization and uptake. J. Soil Sci. Plant Nutr. 21, 49–68. doi: 10.1007/s42729-020-00342-7
Raymond-Bouchard, I., Julien, T., Ianina, A., Greer, C. W., and Whyte, L. G. (2018). Comparative transcriptomics of cold growth and adaptive features of a eury- and stenopsychrophile. Front. Microbiol. 9:1565. doi: 10.3389/fmicb.2018.01565
Reddy, G. S. N., Matsumoto, G. I., Schumann, P. I., Stackebrandt, E., and Shivaji, S. (2004). Psychrophilic pseudomonads from Antarctica: Pseudomonas antarctica sp. nov., Pseudomonas meridiana sp. nov. and Pseudomonas proteolytica sp. nov. Int. J. Syst. Evol. Microbiol. 54, 713–719. doi: 10.1099/ijs.0.02827-0
Rezzoagli, C., Archetti, M., Mignot, I., Baumgartner, M., and Kümmerli, R. (2020). Combining antibiotics with antivirulence compounds can have synergistic effects and reverse selection for antibiotic resistance in Pseudomonas aeruginosa. PLoS Biol. 18:e3000805. doi: 10.1371/journal.pbio.3000805
Rizzo, C., Papale, M., and Giudice, A. L. (2021). “New trends in Antartic bioprospecting: the case of cold adapted bacteria” in Extreme environments: unique ecosystems- amazing microbes. eds. A. Pandey and A. Sharma (Boca Raton, Florida: CRC Press), 117–139.
Rondón, J. R., Ball, M. M., Castro, L. T., and Yarzába, L. A. (2019). Eurypsychrophilic Pseudomonas spp. isolated from Venezuelan tropical glaciers as promoters of wheat growth and biocontrol agents of plant pathogens at low temperatures. Environ. Sustain. 2, 265–275. doi: 10.1007/s42398-019-00072-2
Rubiano-Labrador, C., Acevedo-Barrios, R., Lazaro, A. G., Bowie, L. W., Acosta, A. K. T., and Molina, B. M. (2022). “Pseudomonas strains from the Livingston Island, Antarctica: a source of cold-active hydrolytic enzymes” in Proceedings of the 20th LACCEI international multi-conference for engineering, education and technology: “education, research and leadership in post-pandemic engineering: resilient, inclusive and sustainable actions”
Sah, S., Krishnani, S., and Singh, R. (2021). Pseudomonas mediated nutritional and growth promotional activities for sustainable food security. Curr. Res. Microbial. Sci. 2:100084. doi: 10.1016/j.crmicr.2021.100084
Sajjad, W., Din, G., Rafiq, M., Iqbal, A., Khan, S., Zada, S., et al. (2020). Pigment production by cold-adapted bacteria and fungi: colorful tale of cryosphere with wide range applications. Extremophiles 24, 447–473. doi: 10.1007/s00792-020-01180-2
Salwan, R., Gulati, A., and Kasana, R. C. (2010). Phylogenetic diversity of alkaline protease-producing psychrotrophic bacteria from glacier and cold environments of Lahaul and Spiti, India. J. Basic Microbiol. 50, 150–159. doi: 10.1002/jobm.200800400
Salwan, R., Sharma, V., Kasana, R. C., and Gulati, A. (2020). Bioprospecting psychrotrophic bacteria for serine-type proteases from the cold areas of Western Himalayas. Curr. Microbiol. 77, 795–806. doi: 10.1007/s00284-020-01876-w
Salwoom, L., Rahman, R. R. A., Salleh, A., Shariff, F. M., Convey, P., and Ali, M. M. (2019). New recombinant cold-adapted and organic solvent tolerant lipase from psychrophilic Pseudomonas sp. LSK25, isolated from Signy Island Antarctica. Int. J. Mol. Sci. 20:1264. doi: 10.3390/ijms20061264
Santiago, M., Ramírez-Sarmiento, C. A., Zamora, R. A., and Parra, L. P. (2016). Discovery, molecular mechanisms, and industrial applications of cold-active enzymes. Front. Microbiol. 7:1408. doi: 10.3389/fmicb.2016.01408
Sathiyanarayanan, G., Bhatia, S. K., Kim, H. J., Kim, J. H., Jeon, J. M., Kim, Y. G., et al. (2016). Metal removal and reduction potential of an exopolysaccharide produced by Arctic psychrotrophic bacterium Pseudomonas sp. PAMC 28620. RSC Adv. 6, 96870–96881. doi: 10.1039/c6ra17450g
Selvakumar, G., Joshi, P., Mishra, P. K., Bisht, J. K., and Gupta, H. S. (2009). Mountain aspect influences the genetic clustering of psychrotolerant phosphate solubilizing pseudomonads in the Uttarakhand Himalayas. Curr. Microbiol. 59, 432–438. doi: 10.1007/s00284-009-9456-1
Sharma, E., Molden, D., Rahman, A., Khatiwada, Y. R., Zhang, L., Singh, S. P., et al. (2019). “Introduction to the Hindu Kush Himalaya assessment” in The Hindu Kush Himalaya assessment: mountains, climate change, sustainability and people. eds. P. Wester, A. Mishra, A. Mukherji, and A. B. Shrestha (Cham: Springer International Publishing), 1–16. doi: 10.1007/978-3-319-92288-1_1
Sherpa, M. T., Najar, I. N., Das, S., and Thakur, N. (2019). The diversity of Pseudomonas species from the accumulation zone of Kanchengayao Glacier, North Sikkim, India. J. Pure. Appl. Microbiol. 13, 339–348. doi: 10.22207/JPAM.13.1.37
Silva, T. R. E., Silva, L. C. F., de Queiroz, A. C., Alexandre Moreira, M. S., de Carvalho Fraga, C. A., de Menezes, G. C. A., et al. (2021). Pigments from Antarctic bacteria and their biotechnological applications. Crit. Rev. Biotechnol. 41, 809–826. doi: 10.1080/07388551.2021.1888068
Snopková, K., Čejková, D., Dufková, K., Sedláček, I., and Šmajs, D. (2020). Genome sequences of two Antarctic strains of Pseudomonas prosekii: insights into adaptation to extreme conditions. Arch. Microbiol. 202, 447–454. doi: 10.1007/s00203-019-01755-4
Srinivas, T. N., Nageswara Rao, S. S., Vishnu Vardhan Reddy, P., Pratibha, M. S., Sailaja, B., Kavya, B., et al. (2009). Bacterial diversity and bioprospecting for cold-active lipases, amylases and proteases, from culturable bacteria of Kongsfjorden and Ny-Ålesund, Svalbard, Arctic. Curr. Microbiol. 59, 537–547. doi: 10.1007/s00284-009-9473-0
Staff, B.R. (2018). Global Markets for Enzymes in industrial applications. BCC Research LLC: Wellesley, MA, USA
Subramanian, P., Mageswari, A., Kim, K., Lee, Y., and Sa, T. (2015). Psychrotolerant endophytic Pseudomonas sp. strains OB155 and OS261 induced chilling resistance in tomato plants (Solanum lycopersicum mill.) by activation of their antioxidant capacity. Mol. Plant-Microbe Interact. 28, 1073–1081. doi: 10.1094/mpmi-01-15-0021-r
Suyal, D. C., Joshi, D., Kumar, S., Bhatt, P., Narayan, A., Giri, K., et al. (2022). Himalayan microbiomes for agro-environmental sustainability: current perspectives and future challenges. Microb. Ecol. 84, 643–675. doi: 10.1007/s00248-021-01849-x
Szell, V., Lang, I., Barta, I., Tedges, A., Albrecht, K., Suto, J.M.N., et al. (2001). Process for the preparation of pseudomonic acid an antibiotic by microbiological method. US Patent Grant No. 6506591.
Thathola, P., Aghnihotri, V., and Pandey, A. (2021). Microbial degradation of caffeine using Himalayan psychrotolerant Pseudomonas sp.GBPI_Hb5 (MCC 3295). Curr. Microbiol. 78, 3924–3935. doi: 10.1007/s00284-021-02644-0
Thathola, P., Aghnihotri, V., Pandey, A., and Upadhyaya, S. K. (2022). Biodegradation of bisphenol a using psychrotolerant bacterial strain Pseudomonas palleroniana GBPI_508. Arch. Microbiol. 204:272. doi: 10.1007/s00203-022-02885-y
Torracchi, J. C., Morel, M. A., Tapia-Vázquez, I., Castro-Sowinsk, S., Batista-García, R. A., and Yarzábal, L. A. (2020). Fighting plant pathogens with coldactive microorganisms: biopesticide development and agriculture intensification in cold climates. Appl. Microbiol. Biotechnol. 104, 8243–8256. doi: 10.1007/s00253-020-10812-8
Tribelli, P. M., and López, N. I. (2011). Poly (3-hydroxybutyrate) influences biofilm formation and motility in the novel Antarctic species Pseudomonas extremaustralis under cold conditions. Extremophiles 15, 541–547. doi: 10.1007/s00792-011-0384-1
Tribelli, P. M., and López, N. I. (2018). Reporting key features in cold-adapted bacteria. Life 8:8. doi: 10.3390/life8010008
Tribelli, P. M., Solar Venero, E. C., Ricardi, M. M., Gómez-Lozano, M., Raiger Iustman, L. J., Molin, S., et al. (2015). Novel essential role of ethanol oxidation genes at low temperature revealed by transcriptome analysis in the Antarctic bacterium Pseudomonas extremaustralis. PLoS One 10:e0145353. doi: 10.1371/journal.pone.0145353
Trivedi, P., Pandey, A., and Palni, L. M. S. (2005). Carrier-based preparations of plant growth-promoting bacterial inoculants suitable for use in cooler regions.World. J. Microbiol. Biotechnol. 21, 941–945. doi: 10.1007/s11274-004-6820-y
Uma, S., Jadhav, R. S., Seshu-Kumar, G., Shivaji, S., and Ray, M. K. (1999). A RNA polymerase with transcriptional activity at 0°C from the Antarctic bacterium. FEBS Lett. 453, 313–317. doi: 10.1016/s0014-5793(99)00660-2
Valentine, M. E., Kirby, B. D., Withers, T. R., Johnson, S. L., Long, T. E., Hao, Y., et al. (2020). Generation of a highly attenuated strain of Pseudomonas aeruginosa for commercial production of alginate. Microb. Biotechnol. 13, 162–175. doi: 10.1111/1751-7915.13411
Vasquez-Ponce, F., Higuera-Llantén, S., Pavlov, M. S., Ramírez-Orellana, R., Marshall, S. H., and Olivares-Pacheco, J. (2017). Alginate overproduction and biofilm formation by psychrotolerant Pseudomonas mandelii depend on temperature in Antarctic marine sediments. Electron. J. Biotechnol. 28, 27–34. doi: 10.1016/j.ejbt.2017.05.001
Velázquez, E., Silva, L. R., Ramírez-Bahena, M. H., and Peix, A. (2016) in “Diversity of potassium-solubilizing microorganisms and their interactions with plants” in potassium solubilizing microorganisms for sustainable agriculture. eds. V. S. Meena, B. R. Maurya, J. P. Verma, and R. S. Meena (New Delhi: Springer), 99–110.
Velivelli, S. L. S., Kromann, P., Lojan, P., Rojas, M., Franco, J., Suarez, J. P., et al. (2015). Identification of mVOCs from Andean Rhizobacteria and field evaluation of bacterial and mycorrhizal inoculants on growth of potato in its Center of Origin. Microb. Ecol. 69, 652–667. doi: 10.1007/s00248-014-0514-2
Venil, C. K., Dufossé, L., and Devi, P. R. (2020). Bacterial pigments: sustainable compounds with market potential for pharma and food industry. Front. Sustain. Food Syst. 21:100. doi: 10.3389/fsufs.2020.00100
Verma, P., Yadav, A. N., Khannam, K. S., Kumar, S., Saxena, A. K., and Suman, A. (2015a). Alleviating of cold stress in wheat seedlings by Bacillus amyloliquefaciens IARIHHS2-30, an endophytic psychrotolerant K-solubilizing bacterium from NW Indian Himalayas. Nat. J. Life Sci. 12, 105–110.
Verma, P., Yadav, A. N., Khannam, K. S., Panjiar, N., Kumar, S., Saxena, A. K., et al. (2015b). Assessment of genetic diversity and plant growth promoting attributes of psychrotolerant bacteria allied with wheat (Triticum aestivum) from the northern hills zone of India. Ann. Microbiol. 65, 1885–1899. doi: 10.1007/s13213-014-1027-4
Wang, S., Cui, J., Bilal, M., Hu, H., Wang, W., and Zhang, X. (2020). Pseudomonas spp. as cell factories (MCFs) for value-added products: from rational design to industrial applications. Crit. Rev. Biotechnol. 40, 1232–1249. doi: 10.1080/07388551.2020.1809990
Wang, Q., Wu, X., Lu, X., He, Y., Ma, B., and Xu, Y. (2021). Efficient biosynthesis of vanillin from isoeugenol by recombinant isoeugenol monooxygenase from Pseudomonas nitroreducens Jin1. Appl. Biochem. Biotechnol. 193, 1116–1128. doi: 10.1007/s12010-020-03478-5
Weimer, A., Kohlstedt, M., Volke, D. C., Nikel, P. I., and Wittmann, C. (2020). Industrial biotechnology of Pseudomonas putida: advances and prospects. Appl. Microbiol. Biotechnol. 104, 7745–7766. doi: 10.1007/s00253-020-10811-9
Weinstein, R. N., Montiel, P. O., and Johnstone, K. (2019). Influence of growth temperature on lipid and soluble carbohydrate synthesis by fungi isolated from fellfield soil in the maritime Antarctic. Mycologia 92, 222–229. doi: 10.1080/00275514.2000.12061148
Wu, P., Wang, Z., Zhu, Q., Xie, Z., Mei, Y., Liang, Y., et al. (2021). Stress preadaptation and overexpression of rpoS and hfq genes increase stress resistance of Pseudomonas fluorescens ATCC13525. Microbiol. Res. 250:126804. doi: 10.1016/j.micres.2021.126804
Yadav, A. N., Sachan, S. G., Verma, P., and Saxena, A. K. (2015a). Prospecting cold deserts of north western Himalayas for microbial diversity and plant growth promoting attributes. J. Biosci. Bioeng. 119, 683–693. doi: 10.1016/j.jbiosc.2014.11.006
Yadav, A. N., Sachan, S. G., Verma, P., Tyagi, S. P., Kaushik, R., and Saxena, A. K. (2015b). Culturable diversity and functional annotation of psychrotrophic bacteria from cold desert of Leh Ladakh (India). World J. Microbiol. Biotechnol. 31, 95–108. doi: 10.1007/s11274-014-1768-z
Yadav, A. N., Verma, P., Kumar, M., Pal, K. K., Dey, R., Gupta, A., et al. (2015c). Diversity and phylogenetic profiling of niche-specific bacilli from extreme environments of India. Ann. Microbiol. 65, 611–629. doi: 10.1007/s13213-014-0897-9
Yadav, A. N., Verma, P., Sachan, S. G., Kaushik, R., and Saxena, A. K. (2018). “Psychrotrophic microbiomes: molecular diversity and beneficial role in plant growth promotion and soil health” in Microorganisms for green revolution. Microorganisms for sustainability. eds. D. Panpatte, Y. Jhala, H. Shelat, and R. Vyas, vol. 7 (Singapore: Springer), 197–240.
Yadav, A. N., Yadav, N., Sachan, S. G., and Saxena, A. K. (2019). Biodiversity of psychrotrophic microbes and their biotechnological applications.J. App. Biol. Biotech. 7, 99–108. doi: 10.7324/JABB.2019.70415
Yang, C., Wang, F., Hao, J., Zhang, K., Yuan, N., and Sun, M. (2010). Identification of a proteolytic bacterium, HW08, and characterization of its extracellular cold-active alkaline metalloprotease Ps5. Biosci. Biotechnol. Biochem. 74, 1220–1225. doi: 10.1271/bbb.100011
Yarzábal, L. A. (2021). “Bioprospecting extreme ecosystems before they vanish: the (poorly studied) microbiology of tropical glaciers” in Extreme environments: unique ecosystems- amazing microbes. eds. A. Pandey, A. Sharma, and C. R. C. Press (Boca Raton: Florida), 1–17.
Yarzábal, L. A., Monserrate, L., Buela, L., and Chica, E. (2018). Antarctic Pseudomonas spp. promote wheat germination and growth at low temperatures. Polar Biol. 41, 2343–2354. doi: 10.1007/s00300-018-2374-6
Yu, Z., Sun, M., Xiao, K., Ou, B., Liang, S., Hou, H., et al. (2022). Changes of phosphorus species during (hydro) thermal treatments of iron-rich sludge and their solubilization mediated by a phosphate solubilizing microorganism. Sci. Total Environ. 838:156612. doi: 10.1016/j.scitotenv.2022.156612
Zeng, R., Zhang, R., Zhao, J., and Lin, N. (2003). Cold-active serine alkaline protease from the psychrophilic bacterium Pseudomonas strain DY-A: enzyme purification and characterization. Extremophiles 7, 335–337. doi: 10.1007/s00792-003-0323-x
Keywords: Pseudomonas , psychrophiles, adaptation, ecological processes, biotechnological applications
Citation: Chauhan M, Kimothi A, Sharma A and Pandey A (2023) Cold adapted Pseudomonas: ecology to biotechnology. Front. Microbiol. 14:1218708. doi: 10.3389/fmicb.2023.1218708
Edited by:
Ram Karan, King Abdullah University of Science and Technology, Saudi ArabiaReviewed by:
Alysson Wagner Fernandes Duarte, Federal University of Alagoas, BrazilHitarth B. Bhatt, Atmiya University, India
Copyright © 2023 Chauhan, Kimothi, Sharma and Pandey. This is an open-access article distributed under the terms of the Creative Commons Attribution License (CC BY). The use, distribution or reproduction in other forums is permitted, provided the original author(s) and the copyright owner(s) are credited and that the original publication in this journal is cited, in accordance with accepted academic practice. No use, distribution or reproduction is permitted which does not comply with these terms.
*Correspondence: Anita Pandey, anitapandey333@gmail.com; anita.pandey@geu.ac.in