- 1Chengdu Medical College, Chengdu, Sichuan, China
- 2Department of Gastroenterology, The First Affiliated Hospital of Chengdu Medical College, Chengdu, Sichuan, China
Gastric cancer (GC) is the leading cause of cancer-related death worldwide, and reducing its mortality has become an urgent public health issue. Gastric microecological dysbiosis (including bacteria, fungi, viruses, acid suppressants, antibiotics, and surgery) can lead to gastric immune dysfunction or result in a decrease in dominant bacteria and an increase in the number and virulence of pathogenic microorganisms, which in turn promotes development of GC. This review analyzes the relationship between gastric microecological dysbiosis and GC, elucidates dynamic alterations of the microbiota in Correa’s cascade, and identifies certain specific microorganisms as potential biomarkers of GC to aid in early screening and diagnosis. In addition, this paper presents the potential of gastric microbiota transplantation as a therapeutic target for gastric cancer, providing a new direction for future research in this field.
1. Introduction
Gastric cancer (GC) is the fifth most common cancer and the third most common cause of cancer death globally (Smyth et al., 2020). Although treatment strategies for gastric cancer have been continuously updated over centuries, from surgery, chemotherapy, radiotherapy, molecular targeted therapy to immunotherapy, the mortality rate remains high. Addressing the issue of reducing mortality from GC has become an urgent public health concern (Etemadi et al., 2020; Thrift and el-Serag, 2020). GC development involves a multifactorial and dynamic process that results from interaction of various genetic and environmental factors in the host. GC is a cancer type characterized by high heterogeneity. The Cancer Genome Atlas (TCGA) initiated an exhaustive examination that molecularly characterized four subtypes of gastric cancer through genomic analysis, including (i) tumors positive for Epstein–Barr virus (EBV), (ii) tumors with microsatellite instability (MSI), (iii) genomically stable (GS) tumors, and (iv) tumors with chromosomally instability (CIN) (Cancer Genome Atlas Research Network, 2014). GC can be classified into two distinct types, intestinal and diffuse, based on Lauren’s classification (Lauren, 1965). Intestinal GC is well defined by Correa’s cascade alterations that involve a sequence of changes starting from a normal gastric mucosa, erosive gastritis, atrophic gastritis (AG), and intestinal metaplasia (IM), leading to heterogeneous hyperplasia and eventually progressing to in situ gastric cancer and invasive carcinoma (Correa et al., 1975).
In recent years, the role of gastric microecological dysbiosis in gastric carcinogenesis has received increasing attention. The gastric microbiota is the most important subset of the gastric microecology. The microbial community in the stomach is predominantly composed of the phyla Firmicutes, Actinobacteria, Bacteroidetes, and Proteobacteria, as well as the genera Lactobacillus, Streptococcus, and Propionibacterium. It has been established that H. pylori is a major risk factor for development of GC (Plummer et al., 2016; Alarcón et al., 2017; Yang et al., 2022). In addition to H. pylori, other microorganisms (e.g., fungi and viruses) are present in the stomach. The composition of the gastric microbiota can be influenced by various external factors, such as diet, proton pump inhibitors, antibiotics, Helicobacter pylori infection, gastric mucosal inflammation, and the mode of delivery at birth. A growing body of research suggests a strong correlation between the gastric microbiota and the occurrence and progression of GC. The gastric microbiota can produce carcinogenic substances, trigger inflammatory reactions, and affect the functionality of immune cells within the immunosuppressive microenvironment, thereby promoting the development of GC. However, the specific composition of the microbiota in gastric tissues remains unclear, and our understanding of how the gastric microbiota changes throughout the different stages of gastric cancer development is still limited. This review provides a detailed analysis of the relationship between gastric microecological dysbiosis and gastric carcinogenesis, along with the potential diagnostic value of the gastric microbiota as a biomarker for GC, which may culminate in the discovery of new diagnostic modalities for gastric cancer. Furthermore, we discuss the potential of gastric/fecal microbiota transplantation as a therapeutic target for gastric cancer, providing a new direction for future research in this area.
2. Composition and diversity of gastric microecology
The human gastric microbiota constitutes a distinct microecosystem that participates in maturation and regulation of host metabolism and immunity, as well as inhibition of pathogen colonization. The stomach, with its unique physiological structure characterized by acidic conditions, digestive enzyme secretion, bile reflux, bicarbonate mucus barrier, and gastrointestinal peristalsis, forms a natural screening barrier. Owing to these features, the stomach was once regarded as a sterile organ, and the microbiota was thought to be isolated in the intestine. However, advancements in microbial culture and sequencing techniques have led to the discovery of Veronococcus, Lactobacillus, Clostridium, Propionibacterium, Streptococcus, and Staphylococcus in the human stomach, in addition to H. pylori (Marshall et al., 1984; Zilberstein et al., 2007; Delgado et al., 2013). The microbial density of the stomach is approximately 101–103 colony forming units (CFU)/ml, which is substantially lower than that of the intestine (1010–1012 CFU/ml). The most abundant phyla in the normal gastric microbiota are mainly Bacteroides, Actinomycetes, Firmicutes, Proteobacteria, and Fusobacteria (Bik, 2006; Li et al., 2009). Nevertheless, current techniques far underestimate the diversity of bacteria, as a large proportion of them remain undiscovered by culture (Vartoukian et al., 2010). The microbiota present in the gastric fluid consists mainly of microorganisms from the respiratory tract, oral cavity, and those that retrograde through the intestine into the pylorus (Sanduleanu et al., 2001; Yu et al., 2017). There is significant heterogeneity in the microbiota composition of the gastric mucosa and gastric fluid. Compared to gastric fluid, the gastric mucosa has a greater richness of flora but lower flora diversity (Sung et al., 2016). Many studies have found that the dominant phyla in the normal gastric mucosa are Firmicutes (42%), Bacteroidetes (24%), Proteobacteria (17%), Actinobacteria (7%), and Fusobacteria (6%) (Bik et al., 2006; Delgado et al., 2013; Liu et al., 2019; Pereira-Marques et al., 2019; Ndegwa et al., 2020). In contrast, gastric fluid is mainly composed of the phyla Proteobacteria and Firmicutes (Nardone and Compare, 2015; Sung et al., 2016). These studies revealed a previously unnoticed abundance of the gastric flora and found a heterogeneous community abundance among individuals. Microorganisms in gastric fluid may only reside transiently, without colonizing the gastric mucosa; thus, their diversity is spurious and altered by various factors (Alarcón et al., 2017). Bacterial overgrowth in the stomach has been found in a variety of precancerous conditions, such as hypoacidity and mucosal atrophy. Gastritis caused by chronic H. pylori may lead to glandular atrophy and reduced acid production, resulting in a increase in gastric pH. Reduced gastric acid promotes colonization of the gastric mucosa by other bacteria, viruses, and/or fungi (Schulz et al., 2015). These microbes can promote production of nitrite, which in turn leads to accumulation of carcinogenic nitroso compounds, promoting development of gastric cancer.
3. Gastric microecological dysbiosis and gastric cancer
In recent years, a growing number of studies have highlighted the role of ecological dysbiosis in cancer development. In a broad sense, ecological dysbiosis refers to changes in the composition and function of the host’s resident microbiota, which can lead to a microecosystem that is conducive to growth and proliferation of cancer cells. The microecosystem comprises a host, a microbiota, and an external environment that can affect the microbiota. The composition of the microbiota is highly dynamic and influenced by several factors, including age, sex, dietary habits, lifestyle, geographic location, H. pylori infection, gastric mucosal inflammation, mode of delivery during birth, and use of medications such as antimicrobial agents and proton pump inhibitors (Tsuda et al., 2015; Bokulich et al., 2016; Lloyd-Price et al., 2016; Figure 1). Ecological dysbiosis can manifest in several ways, including loss of beneficial microorganisms, expansion of potentially harmful microorganisms, and reduction in overall microbial diversity (Petersen et al., 2014). These changes create an environment that is favorable for development and progression of cancer, including gastric cancer. In this context, understanding the role of ecological dysbiosis in the pathogenesis of gastric cancer provides important insight into the development of effective prevention and treatment strategies.
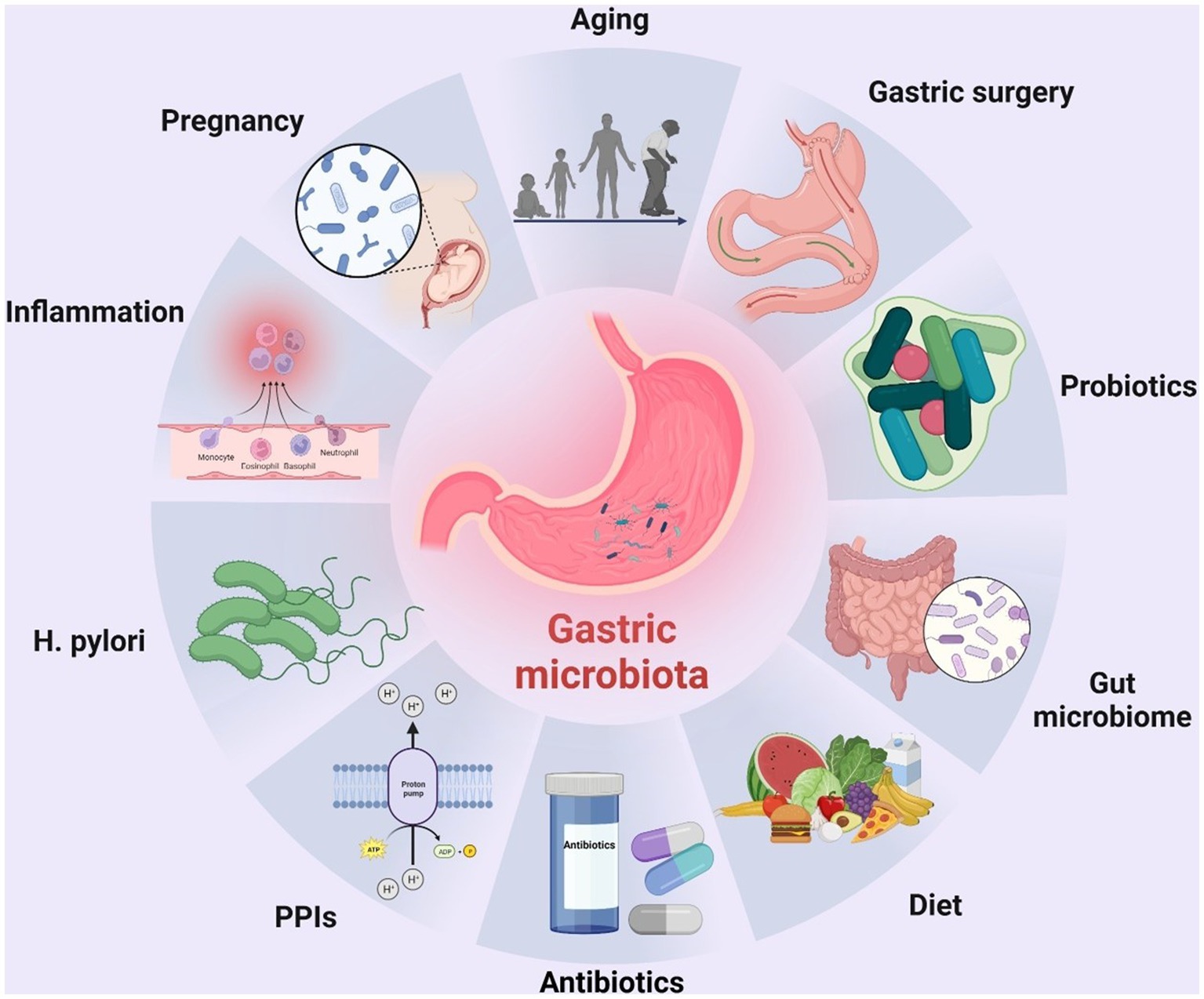
Figure 1. Factors altering the composition of the human gastric microbiota. To date, many factors have been identified that can alter the composition of the human gastric microbiota, including age, surgery, probiotics, gut microbiota, diet, H. pylori infection, inflammation of the gastric mucosa, mode of delivery at birth, and use of drugs such as antibiotics and proton pump inhibitors (PPIs). The figure was drawn by BioRender.
3.1. Gastric microbiota dysbiosis
3.1.1. Helicobacter pylori
Helicobacter pylori has been widely recognized as a crucial risk factor for developing gastric cancer (Touati et al., 2003; Graham, 2015; Hartung et al., 2015; Mégraud et al., 2015), as highlighted by the World Health Organization (WHO), which classifies it as a Group I carcinogen (IARC, 2003; Bouvard et al., 2009). The prevalence of H. pylori infection is over 50% worldwide. In China, over 80% of non-cardia gastric cancer cases and over 60% of cardia gastric cancer cases each year can be attributed to H. pylori infection (Yang et al., 2021). The bacterium achieves successful colonization in the human gastric mucosal layer through various mechanisms, as follows: (1) using urease and α-carbonic anhydrase to produce ammonia and HCO32− to neutralize gastric acid and raise the pH of local tissues, creating a favorable environment for overproliferation of itself and other bacteria in the stomach (Scott et al., 1998; Celli et al., 2009); (2) enhancing its penetration into the gastric mucosal layer through flagella (Suerbaum, 1995; Martínez et al., 2016); and (3) through bacterial virulence proteins, such as vacuolar cytotoxin (VacA) protein and cytotoxin-associated gene A (CagA) protein. The structure and function of immune cells are regulated in various ways to suppress the body’s immune response to H. pylori (Bakhti et al., 2020; Xi et al., 2023). Moreover, Helicobacter pylori has the ability to manipulate the physiological functions of gastric epithelial cells by expressing mucins such as Muc1, Muc4, and Muc5b, resulting in loss of cell polarity and release of nutrients and chemokines, including interleukin-8 (IL-8) (Navabi et al., 2013).
The virulence factors CagA and VacA of H. pylori are closely associated with GC occurrence, and the carcinogenesis mechanisms among them have been extensively explored. It has been demonstrated that individuals infected with CagA-positive strains are at a higher risk of developing gastric cancer (Blaser et al., 1995; Parsonnet et al., 1997; Huang et al., 2003; Nell et al., 2018). CagA is an oncoprotein that activates multiple signaling pathways, including RAS/ERK, WNT/β-linked protein, JAK/STAT, and PI3K/AKT, and inhibits tumor suppressors, promoting GC (Palrasu et al., 2021). CagA can act as an anti-apoptotic protein that inhibits pro-apoptotic factors such as SIVA1, BIM, and BAD; it also regulates autophagy and induces inflammation (Vallejo-Flores et al., 2015; Palrasu et al., 2020). Humans infected with CagA-positive H. pylori strains are known to exhibit an intense inflammatory response and severe damage to gastric tissues (Yamaoka et al., 1997; Figura et al., 1998). The interaction of CagA with Lactobacillus enhances maturation of human monocyte-derived dendritic cells (DCs) and induction of inflammatory mediators other than H. pylori (Castaño-Rodríguez et al., 2017). This suggests that bacteria capable of producing lactic acid may increase the inflammatory response induced by H. pylori, thus promoting gastric carcinogenesis. CagA was also found to promote the Ye s-Associated-Protein (YAP) signaling pathway, thereby promoting the epithelial-mesenchymal transition (EMT) and gastric carcinogenesis (Li et al., 2018). The EMT causes epithelial cells to lose their characteristic cell–cell contact and become more migratory and invasive by a process that may contribute to the ability of H. pylori to penetrate deeper into the gastric mucosa. VacA, another virulence factor of H. pylori, is associated with a variety of functions including disruption of the gastric mucosal barrier, interference with antigen presentation pathways, and downregulation of autophagy and phagocytosis, which contribute to bacterial inhibition of immune cells and establishment of persistent infection (Atherton et al., 1995; Boncristiano et al., 2003; Sundrud et al., 2004). The ability of VacA to downregulate autophagy and lysosomal degradation contributes to accumulation of CagA in gastric epithelial cells (Abdullah et al., 2019).
Moreover, H. pylori significantly inhibits colonization of the stomach by other bacteria, resulting in lower gastric microbiota diversity (Gao et al., 2018). In female BALB/c mice without specific pathogens, colonization by H. pylori leads to an increase in the gastric microbiota of Clostridia, Bacteroides/Prevotella spp., Eubacterium spp., Ruminococcus spp., Streptococci, and Escherichia coli, with decreases in lactobacilli (Aebischer et al., 2006). An early study used 16S rRNA microarrays to analyze gastric biopsies from subjects infected or not with H. pylori (Maldonado-Contreras et al., 2011). This study found that H. pylori-positive patients had a higher abundance of Proteobacteria, Firmicutes, Bacteroides and Actinobacteria in the stomach and a lower abundance of Actinobacteria, Bacteroidetes, and Firmicutes. All the above studies reveal a significant effect of H. pylori colonization on the gastric microbial community. However, other studies have clearly shown that the gastric microbiota is not altered by chronic H. pylori infection (Tan et al., 2007; Khosravi et al., 2014; Coker et al., 2018). Thus, the relationship between H. pylori and other flora remains controversial (Table 1).
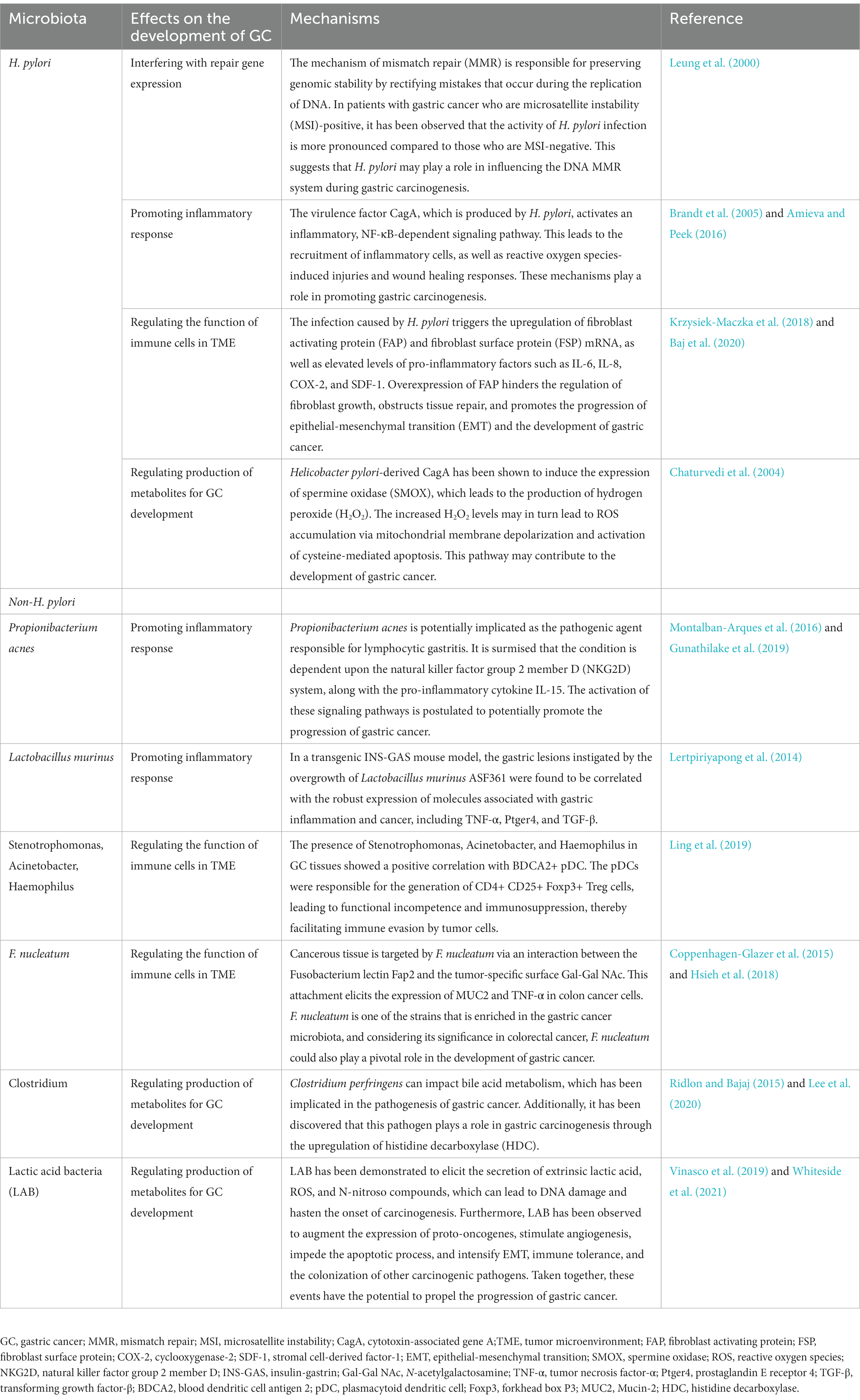
Table 1. Possible roles of Helicobacter pylori and non-Helicobacter pylori bacteria in the development of gastric cancer.
3.1.2. The bacteria beyond Helicobacter pylori
Recent research has suggested that the presence of microbes other than Hp may also contribute to the occurrence of GC. Insulin-gastrin-secreting (INS-GAS) mice are genetically susceptible to GC and are therefore commonly used in developing mouse gastric cancer models. Tumorigenesis is relatively delayed in mice infected with H. pylori alone compared to INS-GAS mice infected with H. pylori and other gastric microbiota (Lee et al., 2008). Furthermore, H. pylori-infected germ-free (GF) INS-GAS mice exhibit less severe gastric mucosal lesions and slower tumor progression than H. pylori-infected specific pathogen-free (SPF) INS-GAS mice (Lofgren et al., 2011). These findings suggest that GC may be promoted by microorganisms in addition to H. pylori, with other gastric microbial communities also playing a potential role in the carcinogenesis and progression of GC in mice.
In another study, the stomachs of H. pylori-infected INS-GAS mice were colonized with different types of intestinal microorganisms, including restricted altered Schaedler flora (rASF; consisting of Clostridum species ASF356, Bacteroides species ASF519 and Lactobacillus murinus ASF361) and pathogen-free (complex IF) mice (Lertpiriyapong et al., 2014). The results showed that the mice colonized by either rASF Hp or IF Hp exhibited severe pathology. The IF Hp-colonized mice showed the strongest inflammatory response, with 40% developing invasive gastrointestinal intraepithelial neoplasia (GIN). The same phenomenon was observed in 23% of the rASF Hp mice. Moreover, it was found that ASF and H. pylori coinfection leads to gastric mucosal changes in mice; gastritis in mice was accompanied by reduced colonization of Clostridium perfringens ASF356 and Bacillus mimicus ASF519 and overgrowth of Lactobacillus murinus ASF361.
A recent study conducted by Kwon et al. (2022) inoculated gastric tissue and gastric fluid from patients with chronic superficial gastritis (CSG), intestinal metaplasia (IM) or gastric cancer (GC) into GF C57BL/6 mice. The gastric microbiota of the mice was analyzed by amplicon sequencing and immunohistochemical analysis of the histopathological features of the stomach of the mice. The results revealed that when microbiomes from IM or GC patients were transplanted into the stomachs of GF mice, precancerous features were induced, including increased inflammation, decreased mural cells, and increased cell proliferation. In addition, long-term observations of mice inoculated with the microbiota from IM or GC patients led to a relatively high incidence of features of gastric dysplasia in mice. From the above evidence, it appears that commensal microorganisms in the stomach other than H. pylori are associated with development of GC.
3.1.3. Fungi
Fungal species can be detected in the gastrointestinal tract of approximately 70% of healthy adults. The number of fungi in the human stomach is 0–102 cfu/ml, and Candida is the most common species (Schulze and Sonnenborn, 2009; Zwolinska-Wcisło et al., 2009). A study examined gastric fluid from 25 patients undergoing clinical indications using PCR amplification of the internal transcribed spacer region, with Candida and Phaalemonium found to be the only two genera present in all gastric fluid samples (von Rosenvinge et al., 2013). Candida albicans can survive under acidic conditions of pH 1.4 and above, and specific genotypes such as DST1593 may exacerbate the severity of gastric mucosal lesions (Gong et al., 2012). Candida albicans was detected in 54.2% of gastric ulcer cases and in 10.3% of chronic gastritis cases in a fungal analysis of biopsies from 293 patients with clinical manifestations of dyspepsia or ulcer disease (Karczewska et al., 2009). Gastric fungal infections and colonization are common in patients with GC, and chronic ingestion of exogenous mycotoxins from spoiled foods is a common cause of GC due to fungi. Candida infection is present in 20% of patients with gastric cancer (Scott and Jenkins, 1982). A study performed ITS rDNA genetic analysis of GC-associated fungal composition in cancerous lesions and paracancerous and noncancerous tissues from GC patients, reporting identification of 17 fungal species with significant differences between the two groups at the family level. In the GC group, Pseudeurotiaceae, Trimorphomycetaceae, Chaetomiaceae, and Aspergillaceae were significantly decreased and Saccharomycetales_fam_Incertae_sedis and Pleosporaceae increased compared to the control group. In addition, at the genus level, there were 15 different fungi between the two groups, and two fungal genera were enriched in the GC group, including Candida and Alternaria, whereas Saitozyma and Thermomyces were depleted (Zhong et al., 2021).
3.1.4. Viruses
In addition to the microorganisms mentioned above, EBV can also cause an imbalance in gastric microecology and promote gastric cancer (Martínez-López et al., 2014). More than 90% of adults are infected with EBV, and EBV-associated gastric cancer (EBVaGC) accounts for 5–20% of all gastric cancer cases worldwide (Takada, 2000; Iizasa et al., 2012). Recent studies have described a synergistic role between Helicobacter pylori and EBV in gastric carcinogenesis. Individuals coinfected with H. pylori and EBV exhibit more severe inflammatory lesions than those infected with H. pylori alone (Cárdenas-Mondragón et al., 2013). In addition, some studies have shown that colonization by H. pylori induces reactivation of latent EBV in gastric epithelial cells (Minoura-Etoh et al., 2006; Shukla et al., 2012). Proliferation of lymphocytes after EBV infection and their ability to interact with immune effects may be directly influenced by the presence of bacterial or other microbial components at the site of infection (Iskra et al., 2010). These reports suggest that infections caused by dysbiosis may activate latent EBV, thereby increasing risk of developing cancers associated with EBV infection (Matsusaka et al., 2014; Nishikawa et al., 2014; Rickinson, 2014). Although EBV has been suspected of causing several upper gastrointestinal diseases, most studies to date have been case reports, and large-scale studies to support a causal relationship between EBV and these diseases are lacking (Hisamatsu et al., 2010; Owens et al., 2011). In addition to EBV, various other viruses have been found to contribute to tumorigenic changes in the stomach. These viruses include John Cunningham virus (JCV) (Murai et al., 2007), human cytomegalovirus (HCMV) (Jin et al., 2014; Fattahi et al., 2018; Kosari-Monfared et al., 2019), hepatitis B (HBV) or C (HCV) viruses (Song et al., 2019; Cui et al., 2020; Huang et al., 2020), human immunodeficiency virus (HIV) or human T-cell lymphophilic virus (HTLV) (Matsumoto et al., 2018; Kang et al., 2019; Schierhout et al., 2020), and papillomavirus (HPV) (Zeng et al., 2016; Bozdayi et al., 2019).
3.2. Other clinical conditions
3.2.1. Acid inhibitors
The presence of hydrochloric acid within the gastric fluid serves as a barrier to many microorganisms, protecting the stomach against potential infections (Yoshiyama and Nakazawa, 2000; Beasley et al., 2015). Over the past decade, acid-suppressing drugs such as proton pump inhibitors (PPIs) and H2 receptor antagonists (H2RAs) have been frequently used to treat gastrointestinal disorders, which have led to significant changes in the microbial diversity of the stomach (Fisher and Fisher, 2017). This shift can trigger hypochlorhydria, a condition that reduces microbial diversity, fosters proliferation of genotoxic microorganisms, and heightens activity of bacterial nitrate/nitrite reductases, ultimately leading to conversion of nitrite and other nitrogenous compounds in gastric fluid to cancer-associated N-nitroso compounds (Correa, 1992; Ahn et al., 2013; Ferreira et al., 2018). Some bacteria have been isolated from the stomachs of patients with hypochlorhydria, including Lactobacillus, Streptococcus, Pseudomonas, Xanthomonas, Proteus, Klebsiella, Neisseria, E. coli, and Campylobacter jejuni (Williams and McColl, 2006).
Acid-suppressing drugs have also been shown to impact progression of H. pylori, thereby promoting gastric carcinogenesis. In the case of reduced gastric acid secretion (caused by acid-suppressive drugs or chronic atrophy), H. pylori leads to a shift from sinusoidal to gastric body-dominated gastritis (Malfertheiner et al., 2017), reducing the lining cells and potentially enhancing RONS-mediated gastric mucosal damage (Suzuki et al., 1992), all of which are associated with increased risk of gastric cancer (Moayyedi et al., 2000; Sanduleanu et al., 2001). Acid-suppressing drugs have been shown to have negative effects on gastric function and host defense mechanisms, ultimately leading to delayed gastric emptying, decreased gastric mucus viscosity, increased gastric pH, increased bacterial load, and increased bacterial translocation (Wandall, 1992; Scarpignato et al., 2016). Several studies have documented changes in the gastric microbiota of patients treated with PPIs versus those not treated, with reduced bacterial clearance noted in the former (Tsuda et al., 2015; Paroni Sterbini et al., 2016). As the duration of PPI treatment increases, there is a gradual rise in the number of culturable bacteria in the gastric lumen and mucosa (Sanduleanu et al., 2001; del Piano et al., 2012), with maintenance treatment for more than 1 year resulting in a 106-fold increase in CFU counts (del Piano et al., 2012). Other studies have reported an increased risk of gastric cancer in long-term PPI users, with or without H. pylori eradication, up to 2.4 times higher than in nonusers (Ahn et al., 2013; Brusselaers et al., 2017; Cheung et al., 2018).
Interestingly, two recent meta-analyses suggest that current evidence does not support that maintenance PPI can cause or accelerate development or progression of gastric precancerous lesions such as gastric atrophic changes, intestinal chemosis, intestinal chromophobic (ECL) cell hyperplasia and heterogeneous hyperplasia (Eslami and Nasseri-Moghaddam, 2013; Song et al., 2018). Therefore, further prospective studies are needed to elucidate how maintenance antacids lead to alterations in the microbiota and whether such alterations ultimately increase risk of GC.
3.2.2. Antibiotics
In general, application of antibiotics fundamentally alters the normal microbial community of the body, and gastric microecology is no exception. For example, treatment with cefoperazone sodium/sulbactam sodium disrupts gastric microecology, resulting in overproliferation of enterococci and a marked decrease in lactobacilli. A meticulous investigation of bacterial and fungal microbiota conducted in 25 dyspeptic patients demonstrated that antibiotics lead to reduced bacterial colonization while having a negligible effect on fungal diversity (Eslami and Nasseri-Moghaddam, 2013). Nevertheless, exposure to penicillin causes yeast overgrowth in gastric epithelial cells in mice (Nardone and Compare, 2015), whereas germ-free mice exposed to cefoperazone develop gastritis due to an increase in Candida albicans (Mason et al., 2012). A 15-year long-term study comprising 3,365 individuals diagnosed with H. pylori infection revealed that short-term treatment with amoxicillin and omeprazole reduces the incidence of gastric cancer by 39% overall (OR = 0.61, 95% CI = 0.38–0.96, p = 0.032) (Ma et al., 2012). Interestingly, eradication of H. pylori failed in more than half of the individuals treated with antibiotics at the 15-year follow-up, suggesting that antibiotic therapy may curb development of gastric cancer by instigating alterations in the non-H. pylori microbiota. It is worth noting that the eradication rate of H. pylori during the follow-up period was only 47%, highlighting that antibiotic intervention can possibly mitigate progression of gastric cancer by inducing modifications in the non-H. pylori microbiota.
3.2.3. Gastric surgery
Alterations in the anatomy of the gastric environment can also affect its microbiota composition. Roux-en-Y gastric bypass surgery, a common treatment for morbid obesity, involves division of the stomach into two parts, a small proximal pouch and a larger bypass chamber. Postoperative comparison of pH, microbial counts, and mucosal cytokine levels between these two gastric pouches revealed a neutral pH in the proximal gastric capsule (pH 7.0 ± 0.2), with a significant increase in the number of aerobic and anaerobic bacteria, whereas the pH of the anastomotic capsule decreased to 3.3 ± 2.2, with a clear decrease in the total number of bacteria (Faintuch et al., 2007; Ishida et al., 2007). Although no differences in microbiota composition were detected between the two pouches, this is likely due to the limited number of isolates obtained. Residual gastric cancer frequently occurs after distal gastrectomy of benign lesions, with an incidence rate of 1–7% among all gastric cancers (Lagergren et al., 2012). EBV infection has been associated with residual gastric cancer, and atrophic changes in residual gastritis often accompany EBV-positive residual gastric cancer. Moreover, Billroth-II anastomosis (Nishikawa et al., 2002; Kaneda et al., 2012) can significantly alter the gastric microbiota by reducing levels of nitrate and nitrite reductase and expression of nitrosation-related genes, which greatly increases risk of gastric cancer. The diversity of the microbiota in the stomach also becomes richer after subtotal gastrectomy (Clyne and May, 2019). Preoperatively, Ralstonia and Helicobacter were the two dominant genera identified in gastric cancer, and Streptococcus and Prevotella were the two most abundant genera in the gastric mucosal microbiome after gastric lesion resection. Overall, these findings suggest that gastric surgery is a key factor in determining the composition of the gastric microbiota.
4. Gastric microbial dysbiosis in Correa’s cascade
Correa’s cascade refers to a sequence of pathological changes that occur in the gastric mucosa over time, including chronic atrophic gastritis (CAG), intestinal metaplasia (IM), and dysplasia, eventually progressing to invasive carcinoma (Figure 2). The network of characteristics, functions and interactions of the gastric microbiota varies according to the stage of Correa’s cascade (Ferreira et al., 2018; Zhang et al., 2021; Table 2). The current challenge in studying the gastric microbiota is the lack of standardized methods for sample collection, processing and analysis. Different sequencing platforms, sample types (e.g., biopsy vs. aspirate), and analytical methods (e.g., OTU clustering vs. exact sequence variants) can all impact the results obtained. Additionally, differences in the regions of the 16S rRNA gene sequenced also affect the accuracy of the results. This lack of standardization makes it difficult to compare results across different studies, which can lead to conflicting conclusions.
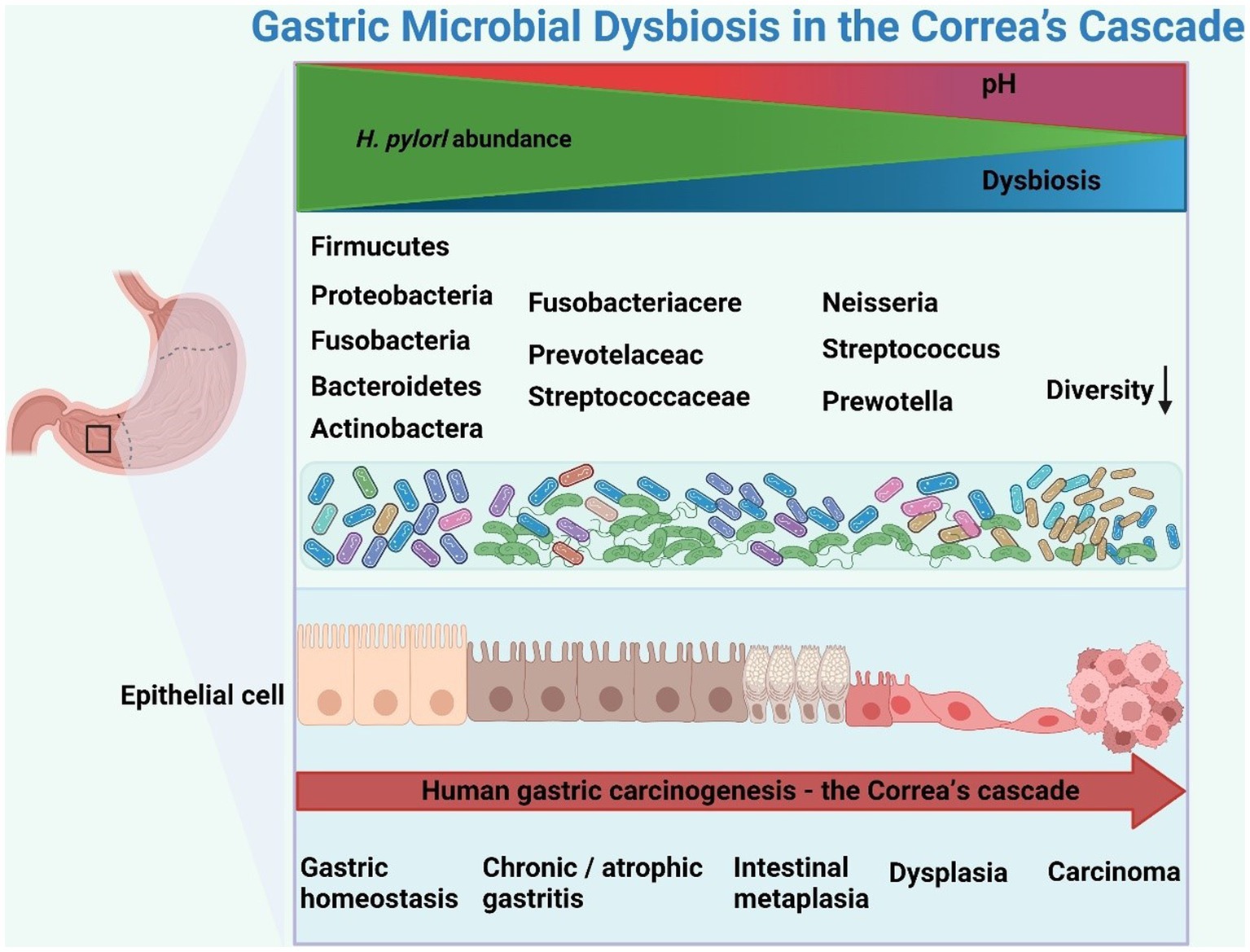
Figure 2. Schematic representation of gastric microbial dysbiosis in Correa’s cascade. Helicobacter pylori infection triggers an inflammatory cascade, altering the gastric microbiota and promoting gastric cancer through dysbiosis and inflammation. The figure was drawn by BioRender.
4.1. Precancerous state
The precancerous state of chronic atrophic gastritis (CAG) and intestinal metaplasia (IM) is a recognized high-risk factor for gastric cancer (Watabe et al., 2005; Naylor et al., 2006; Rugge et al., 2010; Sugano et al., 2015). In Correa’s cascade reaction phase, H. pylori dominance was the main finding for the microbiota, with a decreasing trend in alpha diversity and gastric microbiota interactions that remained relatively stable after onset of GC (Wang et al., 2020; Yu et al., 2020). Furthermore, the relative abundance of H. pylori during chronic gastritis correlated negatively with other phyla, such as Proteobacteria, Firmicutes and Bacteroidetes.
Microarray G3PhyloChip™ analysis of changes in the gastric mucosal microbiota from NAG to IM to GC patients revealed significant microbiota segregation between NAG and GC, with 44 taxa showing changes in abundance (Aviles-Jimenez et al., 2014). Decreases in Porphyromonas, Neisseria, TM7 group, and S. sinensis, as well as increases in L. coleohominis and Lachnospiraceae, were observed and may have contributed to the development of gastric cancer. A study revealed changes in gastrointestinal microbial diversity and interactions at various stages of gastric precancerous lesions, particularly at the GIN stage (Liu et al., 2021), and network analysis showed that the intensity of intergeneric symbiotic interactions increased in IM and decreased in GIN with gastritis progression. It was also found that the interaction is stronger in IM than in SG (Coker et al., 2018). Moreover, a higher degree of centrality and strong cooccurrence interactions among the genera Gemella, Veillonella, Streptococcus, Actinobacillus, and Haemophilus were observed in gastric biopsies of gastric precancerous lesions. These results suggest that bacteria tend to interact with each other simultaneously to form specific microecological networks prior to gastric carcinogenesis.
In a recent study, the changes in the microbiota of GM and GF at different disease stages were evaluated in 180 patients with superficial gastritis, intestinal chemosis (IM), and GC (He et al., 2022). The Shannon index and observed species were lower in GC patients than in SG patients in the GF sample. In contrast, the Shannon index of GC was higher than that of SG in the GM samples, with no significant differences observed between the different stages. Further examination of gastric acid at different disease stages revealed elevated pH in GC compared to SG, suggesting that neutralization of the gastric microenvironment promotes diversity in the mucosal microbiota. Microscopic interactions between GM and GF in GC were significant compared to SG, with some genera in GF showing significant positive correlations with their counterparts in GM, including Helicobacter, Streptococcus, and Haemophilus. These results suggest that H. pylori plays a crucial role in development of Correa’s cascade and hypogastric acidity, which creates a favorable environment for growth of other microbiota, promoting progression of gastric cancer. Correa’s cascade responds to different stages of gastric microbiota composition, and targeting specific gastric microbiota and manipulating their composition may be a new strategy for GC prevention and treatment.
In addition, the research on the gastric microbiome faces challenges due to a lack of standardized methods for sample collection, processing, and analysis. Different sequencing platforms, sample types, and analysis methods can all affect the comparability of results. Additionally, the choice of 16S rRNA gene sequencing region can impact result accuracy. To mitigate these differences, several measures can be taken. Firstly, standardized sample processing methods should be established to ensure consistent procedures are followed during sample collection and handling. Secondly, a uniform selection of reliable sequencing platforms and analysis methods should be implemented, allowing for similar technical approaches across different studies. Simultaneously, it is important to choose the same region of the 16S rRNA gene for analysis during the sequencing process to ensure result consistency. Moreover, conducting large-scale and multicenter studies that encompass diverse geographical regions and population samples can reduce the impact of geographic and population factors. Encouraging data sharing and promoting collaborative research efforts can facilitate result validation and reproducibility. Finally, the development of consistent standards and guidelines to regulate the methods and reporting in gastric microbiome research will enhance the comparability of research findings.
4.2. Gastric cancer
Helicobacter pylori is the strongest known risk factor for intestinal and diffuse GC. However, little is known about the sequence of events in diffuse gastric cancer (DGC). Only a few studies have reported that H. pylori and/or EBV infection play an important role in development of sporadic diffuse gastric cancer (Uemura et al., 2001; Kwak et al., 2014). In one study, patients with current H. pylori infection were more likely to develop DGC than those with previous infection (Misra et al., 2007). Serological studies have shown that patients with high H. pylori IgG titers are at greater risk of developing DGC than intestinal gastric adenocarcinoma (Tatemichi et al., 2009; Lee et al., 2017). Several studies have indicated that as H. pylori decreases during the gastric cancer stage, there is a corresponding increase in non-H. pylori microbiota that promote cancer (Ferreira et al., 2018; Hsieh et al., 2018). This indicates that the effect of H. pylori should be fully considered when studying these GC-associated microbiota. The greatest benefit was obtained by eradicating H. pylori in patients before development of gastric mucosal atrophy and IM (Ford et al., 2014; Chen et al., 2018). In contrast, no significant benefit was obtained by eradicating H. pylori in patients with malignant progression of gastric cancer after IM (Wong et al., 2004; Chen et al., 2016; Malfertheiner et al., 2017; Mera et al., 2018). Hence, cancer may occur even after H. pylori eradication therapy (de Vries et al., 2009).
Loss of GC tissue-specific glands and reduced acid secretion lead to depletion of H. pylori and significant enrichment of intestinal commensal bacteria, including Lactobacillus, Enterococci, Carnobacterium, Parvimonas, Citrobacter, Clostridium, Achromobacter, and Rhodococcus (Amieva and Peek, 2016; Hsieh et al., 2018; Gantuya et al., 2020). Interestingly, certain strains of lactic acid bacteria used as probiotics have a complicated interplay with H. pylori, inhibiting its adherence to epithelial cells, bacteriocin or organic acid production, and releasing associated inflammatory factors (Kabir et al., 1997; Yang and Sheu, 2012; Sakarya and Gunay, 2014). Nevertheless, some lactobacilli can contribute to the development of gastric cancer. LAB have been shown to stimulate production of exogenous lactic acid, reactive oxygen species (ROS), and N-nitroso compounds, which cause DNA damage and accelerate carcinogenesis, and to increase expression of proto-oncogenes, induce angiogenesis, inhibit the apoptotic process, and enhance the epithelial-mesenchymal transition (EMT), immune tolerance, and colonization by other carcinogenic pathogens (Vinasco et al., 2019; Whiteside et al., 2021). Regardless, there is still a dearth of research that explains the specific mechanisms by which these symbiotic microorganisms promote gastric carcinogenesis.
Several studies have shown an association between specific microorganisms and gastric cancer. Enrichment of the microbial flora from oral or intestinal origin has frequently been observed in the gastric microbiota of GC patients (Hsieh et al., 2018; Hu et al., 2018; Castaño-Rodríguez et al., 2017; Yu et al., 2017). Oral microbiota such as Fusobacterium, Veillonella, Peptostreptococcus, Streptococcus, Slackia, Parvimonas, and Haemophilus have been identified in patients diagnosed with gastric cancer (Hu et al., 2018; Chen et al., 2019; Wang et al., 2020). Different combinations of oral microorganisms can be used to differentiate the stages of Correa’s cascade (Coker et al., 2018; Zhang et al., 2021). Liu et al. (2019) investigated the diversity, composition, and bacterial symbiotic correlates and predicted functional profiles of the gastric microbiota in three microenvironments, including 230 normal, 247 perineural, and 229 tumor tissues. They reported that P. melaninogenica, S. anginosus, and P. acnes are enriched in tumor microhabitats but that P. copri and B. uniformis are significantly reduced, with B. fragilis and A. muciniphila showing similar patterns of variation between peritumor and tumor tissues. Most notably, bacterial abundance was found to be reduced in peritumor and tumor microhabitats, and the network associated with enrichment of gastric bacteria in tumor microhabitats was simplified. Moreover, we compared differences in the gastric microbiota between three microhabitats in patients with intestinal, diffuse and mixed gastric cancer. Within the same gastric microhabitat, several nondominant bacterial phylotypes differed between intestinal and diffuse GC types, though the composition of the overall gastric microbiota did not differ significantly. This suggests that the gastric microhabitat of GC, rather than its stage, type or cellular differentiation, determines the overall structure of the gastric microbiota.
Recent clinical studies have also revealed dysregulation of the fungal flora between a tumorigenic gastric mucosa group and normal gastric mucosa in GC (Zhang et al., 2022), with a lower abundance of Pezizomycetes, Sordariales, Chaetomiaceae, and Rozellomycota in the GC group than in the normal group in terms of taxonomic classification. Solicoccozyma was found to be more abundant and differentially enriched. Additionally, a significant increase in Candida albicans, which promotes gastric cancer development by decreasing the diversity and abundance of microorganisms in the stomach, in GC was reported (Zhong et al., 2021). These two studies reveal altered GC-associated fungal composition and ecology and demonstrated that Candida albicans and Solicoccozyma may be used as fungal biomarkers for GC.
4.3. Mechanisms of gastric microbiota in gastric carcinogenesis
Microorganisms in the precancerous state interact with each other through complex mechanisms. The microbial community within the affected tissue undergoes dynamic changes, with certain microorganisms promoting the growth and survival of others. For example, pathogenic bacteria can create an environment conducive to the growth of other harmful microorganisms by producing virulence factors and altering the local pH levels. This alteration in the microbial composition and diversity can lead to dysbiosis, where the balance between beneficial and harmful microorganisms is disrupted. Dysbiosis, in turn, triggers inflammatory responses and compromises the integrity of the host tissue. Additionally, the interplay between microorganisms can result in the production of harmful metabolites, such as genotoxic compounds or reactive oxygen species, which further contribute to tissue damage and genomic instability. The cumulative effect of these adverse mechanisms can drive the progression of the precancerous state toward malignancy.
From current preclinical studies, gastric microbiota has been shown to induce mechanisms such as DNA damage, inflammation, and immune suppression, thereby promoting the development of gastric cancer. In some studies, Propionibacterium acnes, associated with acne, has been found to induce DNA damage and inflammatory reactions to promote the development of GC. Propionibacterium acnes and microbial metabolites such as short-chain fatty acids can induce the expression of the NKG2D ligand. These bacteria activate the NKG2D system, leading to a significant upregulation of the pro-inflammatory IL-15, and resulting in autoimmune lymphocytic gastritis (LyG) (Montalban-Arques et al., 2016). Prevotella can promote various inflammatory reactions, including GC, by enhancing resistance to host-derived reactive oxygen species and producing redox proteins (Hofer, 2014; Irfan et al., 2020). Additionally, some studies suggest that nitrate-reducing bacteria may contribute to the development of gastric cancer by increasing the concentration of carcinogenic N-nitroso compounds in the stomach. Several bacteria, including Clostridium, Haemophilus, Staphylococcus, Neisseria, Lactobacillus, and Nitrospirae, have been implicated in promoting gastric cancer through the stimulation of N-nitroso compounds (NOC) production (Kaźmierczak-Siedlecka et al., 2022). Moreover, the dysbiosis of the microbiota can modulate the components of the tumor microenvironment. In the GC microenvironment, relevant analyses have indicated that an abundance of Stenotrophomonas and Selenomonas are positively correlated with plasmacytoid dendritic cells (pDC) and regulatory T cells (Tregs), respectively. On the other hand, the abundance of Comamonas and Gaiella is negatively correlated with pDC and Tregs, respectively (Ling et al., 2019).
Research on the role of fungal and viral microbiota in tumors is relatively limited compared to bacteria (Papon et al., 2021). However, studies have identified a significant elevation of Candida albicans in GC patients (Zhong et al., 2021). Candida albicans may contribute to carcinogenesis through multiple mechanisms, particularly by triggering inflammation and inducing Th17 response. The activation of NF-κB and Wnt pathways, facilitated by IL-17, can create a pro-inflammatory environment that promotes tumor development (Ramirez-Garcia et al., 2016; Dai et al., 2019). EBV can inhibit the proliferation of CD8+ T cells and reduce the cytotoxicity of NK cells, thereby contributing to the development of both acute and chronic gastritis and increasing the risk of tumor formation (Polakovicova et al., 2018; Nie and Yuan, 2020). Additionally, specific EBV miRNAs have been identified that can impact the proliferation of infected cells, raising the risk of malignant tumor formation. For instance, one study discovered that Epstein–Barr virus miR-BART17-5p directly downregulates KLF2, thereby promoting migration and growth of gastric cancer cells (Kim et al., 2015).
These findings highlight the potential involvement of alterations in the gastric microbiota in regulating multiple mechanisms that drive the occurrence of gastric cancer. Nevertheless, further research is necessary to fully understand the intricate mechanisms through which these microbial changes influence tumor development.
5. Potential clinical value of the gastric microbiota for gastric cancer diagnosis
Microbiota-based biomarkers for gastric cancer screening, diagnosis, and therapy can be developed through identification of specific gastric microorganisms that are enriched or depleted in GC patients. Currently, studies focusing on gastric microbial models to predict the performance of PLGC or GC have largely centered on H. pylori-positive patients. However, few studies have reported significantly altered non-H. pylori species for use as potential microbial biomarkers for GC (Coker et al., 2018; Wang et al., 2020; Kadeerhan et al., 2021). Analysis of the microbial characteristics associated with gastric cancer have revealed enrichment of Proteobacteria, Citrobacter, Lactobacillus, Clostridium, and Rhodococcus in gastric cancer (Ferreira et al., 2018).
Ferreira et al. integrated enriched and deficient taxa in GC as the Microbial Dysbiosis Index (MDI), a gastric microbiota-based diagnostic measure, to differentiate patients with GC from those with chronic gastritis. The superior sensitivity and specificity of MDI for detecting gastric cancer compared to using a single taxonomic unit suggests that changes in the microbial community rather than individual taxa contribute to gastric cancer development. In receiver operating characteristic (ROC) analysis, MDI performed well in identifying gastric cancer, with the highest area under the curve (AUC) of 0.91 for the gastric cancer cohort. Another study constructed a random forest classifier model between GC and SG to assess the diagnostic value of gastric mucosa (GM) and gastric fluid (GF) microbial markers for GC (He et al., 2022). The model was validated using seven GMs (Lactobacillus, Gemella, Enterococcus, Helicobacter, etc.) genera and 13 GF genera (Lactobacillus, Filifactor, Staphylococcus, Dialister, etc.) as the best marker set. The probability of disease (POD) index was found to be significantly higher for GC than for SG. In ROC analysis, the gastric microbial markers had AUCs of 83–94%. In the validation cohort (including 60 SG and 60 GC patients), the AUCs of the GM markers and GF markers were 84 and 89%, respectively, revealing that the gastric microbial-based classifier was able to accurately differentiate between GC and SG.
To determine whether specific bacterial signatures may be used as a diagnostic tool for gastric cancer, one study analyzed gastric epithelium-associated bacterial species in patients with gastritis, IM and GC and found a sensitivity of 73% and specificity of 100% when using a combination of five species. In contrast, the combination of C. colicanis, F. canifelinum, and F. nucleatum showed 100% sensitivity and approximately 70% specificity. These data indicate that C. colicanis and F. nucleatum are enriched in cancer specimens and might identify gastric cancer with 100% sensitivity. This study validated that C. colicanis and F. nucleatum represent diagnostic markers for detection of gastric cancer (Hsieh et al., 2018).
Although gastric microbes can be used as potential diagnostic markers for GC, use of gastric microbe prediction as an alternative to traditional screening or diagnostic methods for gastric cancer seems remote. Indeed, gastric microbes are influenced by multiple factors, and the accuracy of predictive models as potential microbial biomarkers for GC requires further evaluation and validation in subsequent experimental studies in a broader population to examine whether these specific bacteria contribute to progression of GC.
6. Microbiome-based therapeutic approaches for gastric cancer
6.1. Probiotics
In the contemporary medical landscape, probiotics and their metabolites have become widely employed for treating human conditions linked to dysbiosis of the gastrointestinal microbiota. Probiotics are defined as live microbial agents that coexist symbiotically within the human host, and when ingested in sufficient amounts, they can have beneficial effects on the host (Hill et al., 2014; Cunningham et al., 2021). Probiotic supplementation is an emerging therapy for H. pylori eradication (Francavilla et al., 2014; Goderska et al., 2018; Ji and Yang, 2020). Probiotics comprising the genera Lactobacillus and Bifidobacterium have been shown to reduce adverse effects (e.g., nausea, vomiting, diarrhea, abdominal pain) caused by antibiotic therapy and may improve eradication efficiency while supporting balance in the intestinal microbiota (Javanmard et al., 2018). Over the past decade, immune checkpoint inhibitors such as PD-1/PD-L1, CTLA-4, and LAG-3 have radically transformed management of advanced cancer. The microbiota is intricately involved in regulating the host immune system via diverse signaling pathways, thereby influencing the body’s response to cancer immunotherapy (Ma et al., 2019). In preclinical studies, specific microorganisms have been found to contribute to immunotherapy in conjunction with immune checkpoint inhibitors. In animal models, controlling tumor growth with Bacteroidales alone is comparable to treatment with PD-L1 monoclonal antibody therapy (Weng et al., 2019). Bacteroidales can also boost CTLA-4 blockers to enhance the efficacy of cancer immunotherapy (Vétizou et al., 2015). Several probiotic strains, including Lactobacillus acidophilus, Lactobacillus bulgaricus, and Lactobacillus salivarius, have been shown to downregulate IL-8 levels, which correspondingly reduces expression of the bacterial tumor protein CagA (Zhou et al., 2008; Yang et al., 2012). Furthermore, probiotics can be utilized to mitigate the side effects of perioperative enteral nutrition in patients with gastric cancer. Clinical trials have established that enteral nutrition supplemented with probiotics not only decreases the incidence of postoperative diarrhea in gastric cancer patients but also augments the immune response (Zhao et al., 2017; Xie et al., 2018). Probiotics can interact with dendritic cells (DCs), activating them and promoting favorable immune responses while suppressing Th1, Th2, and Th17-mediated inflammatory responses. In enterocytes, secretion of TNF-α inhibitory metabolites and blocking NF-κB signaling can reduce production of TLR, leading to a further reduction in the inflammatory response (Raheem et al., 2021). Research has found that Lactobacillus acidophilus and Bifidobacterium longum can exhibit anti-gastric cancer proliferation and anti-angiogenesis effects by downregulating the expression of COX2 (Nada et al., 2020). In a clinical trial, probiotics can be used as a probiotic supplement in combination with antibiotics and proton pump inhibitors to assist in eradicating Helicobacter pylori infection. The results show that the eradication rate of Helicobacter pylori in the combination therapy group (88.5%) is significantly higher than that in the monotherapy group (63.3%) (Rafiei et al., 2021).
6.2. Fecal microbiota transplantation
Fecal microbiota transplantation (FMT) is transplantation of beneficial flora from the feces of healthy individuals into patients via enema, oral, gastric tube or capsule to restore or improve the patient’s gastrointestinal microecology. FMT has been shown to be effective in treatment of refractory C. difficile infections, inflammatory bowel disease and antibiotic-associated diarrhea, as well as other intestinal disorders (Ademe, 2020). To date, numerous studies have identified that FMT modulates the gut microbiome and immune system associated with tumors and may represent a major therapy for advanced cancers (Frankel et al., 2017; Chandra and McAllister, 2021). FMT has yielded encouraging results in animal models and clinical trials. A comprehensive analysis was conducted on the gut microbiota of gastrointestinal (GI) cancer patients (19 colorectal cancer, 23 gastric cancer, 14 esophageal cancer, and 18 other GI cancer types) undergoing PD-1/PD-L1 therapy (Peng et al., 2020). The cohort included 45 responders and 29 non-responders. Regardless of their clinical response, the gut microbiota in the cohort was primarily composed of the phyla Bacteroidetes and Firmicutes. In patients with favorable outcomes, there was a higher proportion of Prevotella/Bacteroides, and the responder subgroup exhibited a higher abundance of Prevotella. Research by Routy et al. (2018) established that an abnormal gut microbiota composition is a primary cause of resistance to ICIs. In patients with advanced cancer, administration of oral antibiotics during immunotherapy inhibited the clinical benefit of ICIs. Transplanting fecal microbiota from ICI-responsive cancer patients into germ-free or antibiotic-treated mice improved the antitumor effects of PD-1 blockade (Routy et al., 2018). Currently, a clinical trial (NCT04130763) is underway to investigate the use of FMT to enhance the efficacy of PD-1 therapy in gastrointestinal cancer patients.
6.3. Gastric microbiota transplantation
Similar to FMT, it is postulated that gastric microbiota transplantation (GMT) has potential benefits in treatment of cancer. Nevertheless, research on GMT is still in its infancy and presents multiple challenges, as described below.
6.3.1. Microbiota complexity
The gastric environment is characterized by an acidic and hypoxic setting, accompanied by robust gastric acid secretion and peristaltic motility. The composition and abundance of the gastric microbiota are influenced by various factors, including dietary patterns, lifestyle habits, and medication usage. These factors may impede the survival and colonization of transplanted microbial communities, thereby increasing the challenges associated with establishing a stable microbial population within the stomach. Moreover, unlike the intestinal microbiota, the gastric microbiota exhibits lower diversity and abundance, making it challenging to precisely regulate and restructure the microbial community. Introducing the donor microbiota into the recipient’s gastric environment necessitates ensuring the survival of microorganisms under adverse conditions such as gastric acid, while adapting to the ecological milieu within the stomach. The viability and colonization capacity of the gastric microbiota pose significant challenges.
6.3.2. High technical difficulty
The transplantation techniques associated with the gastric microbial community are comparatively intricate, requiring a high level of technical expertise and precise execution. In contrast, fecal transplantation can be prepared through simple procedures such as centrifugation and filtration, with relatively accessible sample sources. However, the acquisition of gastric microbiota necessitates invasive procedures such as endoscopic biopsy or other specialized collection methods, which are intrusive operations involving patient fasting and sedation or anesthesia. During the process of preparing gastric samples, stringent quality control is imperative to ensure the purity, activity, and stability of the microbiota, thereby rendering the attainment of microbiota that meets the required standards a challenging endeavor. The transplantation modality may encompass the introduction of the donor microbiota into the recipient’s stomach through means like oral capsules, nasogastric tubes, or gastroscopy. Different transplantation methods may influence the survival rate and colonization efficacy of microorganisms. Additionally, determining the appropriate dosage of microbiota for transplantation presents a complex issue that necessitates consideration of the gastric capacity for accommodation and the survival rate of the microbiota.
6.3.3. Lack of standardized operating procedures
The research on GMT remains in its nascent stages, lacking standardized operational procedures and therapeutic protocols. Optimal practices concerning the sourcing and quality control of transplant material, transplantation dosage, frequency, and routes are yet to be definitively established. Currently, there is a dearth of standardized methods for preserving microbial samples to ensure their viability and efficacy during transplantation. The preservation and freezing processes of microorganisms require precise control of temperature, oxygen levels, and other environmental factors to prevent microbial inactivation or damage. Furthermore, the lack of standardization has led to variations in transplantation methods among different medical institutions and practitioners, compromising the comparability and reproducibility of outcomes.
6.3.4. Safety
Due to the transplantation of diverse microorganisms involved in GMT, potential safety concerns such as infection, allergic reactions, and rejection responses may arise. Additionally, GMT may give rise to adverse reactions and side effects. These reactions can include allergic responses, gastrointestinal discomfort, and digestive disturbances. Further research is needed to evaluate and understand the specific occurrence rates and severity of adverse reactions and side effects. Currently, limited knowledge exists regarding the long-term safety of GMT. Long-term follow-up and monitoring studies are crucial for assessing the long-term safety of GMT, enabling the identification and resolution of any potential long-term safety issues.
6.3.5. Ethical
GMT is still in its preliminary research stage, and ensuring that the experimental process aligns with ethical principles and safeguards the rights and safety of participants is a crucial challenge. Additionally, acquiring transplant material involves obtaining gastric microbiota samples from donors. When selecting donors, careful consideration must be given to their health status, screening for infectious diseases, and ethical considerations. GMT may potentially benefit the health of recipients, but it also entails inherent risks and uncertainties. Ethical considerations require a balance between the benefits and risks of transplantation, ensuring that the process is based on scientific data and clinical practice.
6.3.6. Cost
Gastric microbiota transplantation technology is still in the research stage, and the cost of treatment is high. More economic investment and research are needed to reduce the cost of treatment and increase the penetration rate.
6.3.7. Evaluation of treatment outcomes
Currently, there is a lack of standardized evaluation criteria and methods to assess the therapeutic efficacy of GMT. Microbiome analysis can provide information about the composition of microbial communities, but further research is needed to determine how to interpret this data and relate it to treatment outcomes. Additionally, quantifying improvements in patient symptoms, gastric mucosal histopathological changes, and other relevant indicators poses a challenge. There are significant variations in the composition and functionality of microbiota among individuals. Individual factors, including genotype, lifestyle, and dietary habits, contribute to potential variations in the therapeutic effects of GMT among different patients. Therefore, predicting the long-term effects of GMT and the duration of microbial community stability post-transplantation remain challenging.
Recently, only one study on GMT has been reported by a Korean researcher. This study entailed transplantation of the gastric microbiota from individuals with diverse gastric states into germ-free (GF) mice, whereby it was discovered that the gastric microbiota from individuals with intestinal chemosis or gastric cancer (GC) selectively colonized the mouse stomach and induced precancerous lesions (Kwon et al., 2022). Conversely, if healthy human gastric microbiota is transplanted into the stomachs of mice with distinct gastric diseases, what alterations would be made to the disease status of the mice? This is an issue that requires investigation. To understand this issue, we are conducting a clinical study (ChiCTR2200066339) on the effect of GMT for eradicating H. pylori infection. Probiotics and FMT/GMT represent current areas of focus in the field of microecological therapy, with these microbial transplantation techniques projected to serve as effective therapies for gastric cancer and other diseases in the future. To enhance the success of probiotic or FMT/GMT therapy, it is vital for researchers to gain a comprehensive understanding of the systemic impact of the microbiota on the immune system, as well as the relationship of the microbiota with cancer treatment.
7. Conclusion
Gastric cancer is one of the most common cancers in the world, and the mortality rate remains high. The discovery of gastric microbiota characteristics in various clinical conditions, from normal stomach to precancerous lesions and GC development, may significantly impact our understanding of the carcinogenic process of GC. The presence or absence of specific microbial communities is closely associated with the occurrence and progression of GC. The existence of these microorganisms may impact gastric cancer development through various mechanisms, including chronic inflammation, immune modulation, and metabolic changes. Therefore, by targeting the dysbiosis of the gastric microbiota, we may be able to develop novel treatment approaches, such as microbiota transplantation, probiotics, and antibiotics, to reduce the risk of GC or slow down disease progression. Although some progress has been made in studying the human gastric microbiota in recent years, the causal relationship between the gastric microbiota and gastric cancer has not been fully established, and the research in this field is still limited. Future investigations should focus on delving deeper into the mechanisms underlying the dysbiosis of the gastric microecology and its role in gastric cancer development. Thus, there is an urgent need for large-scale, multicenter, prospective studies to elucidate the dynamic changes of the microbiota within the Correa’s cascade and identify specific microorganisms that could serve as potential biomarkers for GC and alternative indicators for monitoring disease progression. This provides new avenues for early screening and diagnosis of GC, while also offering guidance for determining optimal treatment strategies and timing.
In conclusion, the gastric microbiota holds significant potential in the diagnosis and treatment of GC. Through in-depth research on the changes in the gastric microbiota and its association with GC, we can provide new insights and approaches for early screening, diagnosis, and treatment of GC. This will contribute to improving patient outcomes and providing personalized and precise management strategies for GC.
Author contributions
HH, WZ, XW, YY, TW, RC, and YL drafted the preliminary manuscript. FH and JL refined and approved the final manuscript. All authors contributed to the article and approved the submitted version.
Funding
This research was supported by the Sichuan Provincial Department of Science and Technology, under Grant number 22NSFCS1601. The funding organization had no role in the design of the study, data collection, analysis, or in the writing of the manuscript.
Acknowledgments
We would like to express our special thanks to the members of the Department of Gastroenterology at the First Affiliated Hospital of Chengdu Medical College.
Conflict of interest
The authors declare that the research was conducted in the absence of any commercial or financial relationships that could be construed as a potential conflict of interest.
Publisher’s note
All claims expressed in this article are solely those of the authors and do not necessarily represent those of their affiliated organizations, or those of the publisher, the editors and the reviewers. Any product that may be evaluated in this article, or claim that may be made by its manufacturer, is not guaranteed or endorsed by the publisher.
References
Abdullah, M., Greenfield, L. K., Bronte-Tinkew, D., Capurro, M. I., Rizzuti, D., and Jones, N. L. (2019). VacA promotes CagA accumulation in gastric epithelial cells during Helicobacter pylori infection. Sci. Rep. 9:38. doi: 10.1038/s41598-018-37095-4
Ademe, M. (2020). Benefits of fecal microbiota transplantation: a comprehensive review. J. Infect. Dev. Ctries. 14, 1074–1080. doi: 10.3855/jidc.12780
Aebischer, T., Fischer, A.Ã., Walduck, A., SchlÃtelburg, C., Lindig, M., Schreiber, S.Ã., et al. Vaccination prevents Helicobacter pylori-induced alterations of the gastric flora in mice. FEMS Immunol. Med. Microbiol., (2006). 46, 221–229, doi: 10.1111/rp10.1016-j.femsim.2004.05.008
Ahn, J. S., Eom, C. S., Jeon, C. Y., and Park, S. M. (2013). Acid suppressive drugs and gastric cancer: a meta-analysis of observational studies. World J. Gastroenterol. 19, 2560–2568. doi: 10.3748/wjg.v19.i16.2560
Alarcón, T., Jeon, C. Y., and Park, S. M. (2017). Impact of the microbiota and gastric disease development by Helicobacter pylori. Curr. Top. Microbiol. Immunol., 253–275. doi: 10.1007/978-3-319-50520-6_11
Amieva, M., and Peek, R. M. Jr. (2016). Pathobiology of Helicobacter pylori-induced gastric Cancer. Gastroenterology 150, 64–78. doi: 10.1053/j.gastro.2015.09.004
Atherton, J. C., Cao, P., Peek, R. M., Tummuru, M. K. R., Blaser, M. J., and Cover, T. L. (1995). Mosaicism in Vacuolating Cytotoxin alleles of Helicobacter pylori. J. Biol. Chem. 270, 17771–17777. doi: 10.1074/jbc.270.30.17771
Aviles-Jimenez, F., Vazquez-Jimenez, F., Medrano-Guzman, R., Mantilla, A., and Torres, J. (2014). Stomach microbiota composition varies between patients with non-atrophic gastritis and patients with intestinal type of gastric cancer. Sci. Rep. 4:4202. doi: 10.1038/srep04202
Baj, J., Korona-Głowniak, I., Forma, A., Maani, A., Sitarz, E., Rahnama-Hezavah, M., et al. (2020). Mechanisms of the epithelial-mesenchymal transition and tumor microenvironment in Helicobacter pylori-induced gastric Cancer. Cells 9:1055. doi: 10.3390/cells9041055
Bakhti, S. Z., Latifi-Navid, S., and Zahri, S. (2020). Unique constellations of five polymorphic sites of Helicobacter pylori vacA and cagA status associated with risk of gastric cancer. Infect. Genet. Evol. 79:104167. doi: 10.1016/j.meegid.2019.104167
Beasley, D. E., Koltz, A. M., Lambert, J. E., Fierer, N., and Dunn, R. R. (2015). The evolution of stomach acidity and its relevance to the human microbiome. PLoS One 10:e0134116. doi: 10.1371/journal.pone.0134116
Bik, E. M., Eckburg, P. B., Gill, S. R., Nelson, K. E., Purdom, E. A., Francois, F., et al. (2006). Molecular analysis of the bacterial microbiota in the human stomach. Proc. Natl. Acad. Sci. U. S. A. 103, 732–737. doi: 10.1073/pnas.0506655103
Blaser, M. J., Perez-Perez, G. I., Kleanthous, H., Cover, T. L., Peek, R. M., Chyou, P. H., et al. (1995). Infection with Helicobacter pylori strains possessing cagA is associated with an increased risk of developing adenocarcinoma of the stomach. Cancer Res. 55, 2111–2115.
Bokulich, N. A., Chung, J., Battaglia, T., Henderson, N., Jay, M., Li, H., et al. (2016). Antibiotics, birth mode, and diet shape microbiome maturation during early life. Sci. Transl. Med. 8:343ra82. doi: 10.1126/scitranslmed.aad7121
Boncristiano, M., Paccani, S. R., Barone, S., Ulivieri, C., Patrussi, L., Ilver, D., et al. (2003). The Helicobacter pylori vacuolating toxin inhibits T cell activation by two independent mechanisms. J. Exp. Med. 198, 1887–1897. doi: 10.1084/jem.20030621
Bouvard, V., Baan, R., Straif, K., Grosse, Y., Secretan, B., el Ghissassi, F., et al. (2009). A review of human carcinogens--part B: biological agents. Lancet Oncol. 10, 321–322. doi: 10.1016/S1470-2045(09)70096-8
Bozdayi, G., Dinc, B., Avcikucuk, H., Turhan, N., Altay-Kocak, A., Ozkan, S., et al. (2019). Is human papillomavirus and Helicobacter pylori related in gastric lesions? Clin. Lab. 65. doi: 10.7754/Clin.Lab.2019.181244
Brandt, S., Kwok, T., Hartig, R., König, W., and Backert, S. (2005). NF-kappaB activation and potentiation of proinflammatory responses by the Helicobacter pylori CagA protein. Proc. Natl. Acad. Sci. U. S. A. 102, 9300–9305. doi: 10.1073/pnas.0409873102
Brusselaers, N., Wahlin, K., Engstrand, L., and Lagergren, J. (2017). Maintenance therapy with proton pump inhibitors and risk of gastric cancer: a nationwide population-based cohort study in Sweden. BMJ Open 7:e017739. doi: 10.1136/bmjopen-2017-017739
Cancer Genome Atlas Research Network (2014). Comprehensive molecular characterization of gastric adenocarcinoma. Nature 513, 202–209. doi: 10.1038/nature13480
Cárdenas-Mondragón, M. G., Carreón-Talavera, R., Camorlinga-Ponce, M., Gomez-Delgado, A., Torres, J., and Fuentes-Pananá, E. M. (2013). Epstein Barr virus and Helicobacter pylori co-infection are positively associated with severe gastritis in pediatric patients. PLoS One 8:e62850. doi: 10.1371/journal.pone.0062850
Castaño-Rodríguez, N., Kaakoush, N. O., Lee, W. S., and Mitchell, H. M. (2017). Dual role of Helicobacter and Campylobacter species in IBD: a systematic review and meta-analysis. Gut 66, 235–249. doi: 10.1136/gutjnl-2015-310545
Celli, J. P., Turner, B. S., Afdhal, N. H., Keates, S., Ghiran, I., Kelly, C. P., et al. (2009). Helicobacter pylori moves through mucus by reducing mucin viscoelasticity. Proc. Natl. Acad. Sci. U.S.A. 106, 14321–14326. doi: 10.1073/pnas.0903438106
Chandra, V., and McAllister, F. (2021). Therapeutic potential of microbial modulation in pancreatic cancer. Gut 70, 1419–1425. doi: 10.1136/gutjnl-2019-319807
Chaturvedi, R., Cheng, Y., Asim, M., Bussière, F. I., Xu, H., Gobert, A. P., et al. (2004). Induction of polyamine oxidase 1 by Helicobacter pylori causes macrophage apoptosis by hydrogen peroxide release and mitochondrial membrane depolarization. J. Biol. Chem. 279, 40161–40173. doi: 10.1074/jbc.M401370200
Chen, X.-H., Wang, A., Chu, A. N., Gong, Y. H., and Yuan, Y. (2019). Mucosa-associated microbiota in gastric cancer tissues compared with non-cancer tissues. Front. Microbiol. 10:1261. doi: 10.3389/fmicb.2019.01261
Chen, H.-N., Wang, Z., Li, X., and Zhou, Z. G. (2016). Helicobacter pylori eradication cannot reduce the risk of gastric cancer in patients with intestinal metaplasia and dysplasia: evidence from a meta-analysis. Gastric Cancer 19, 166–175. doi: 10.1007/s10120-015-0462-7
Chen, L., Xu, W., Lee, A., He, J., Huang, B., Zheng, W., et al. (2018). The impact of Helicobacter pylori infection, eradication therapy and probiotic supplementation on gut microenvironment homeostasis: an open-label, randomized clinical trial. EBioMedicine 35, 87–96. doi: 10.1016/j.ebiom.2018.08.028
Cheung, K. S., Chan, E. W., Wong, A. Y. S., Chen, L., Wong, I. C. K., and Leung, W. K. (2018). Long-term proton pump inhibitors and risk of gastric cancer development after treatment for Helicobacter pylori: a population-based study. Gut 67, 28–35. doi: 10.1136/gutjnl-2017-314605
Clyne, M., and May, F. E. B. (2019). The interaction of Helicobacter pylori with TFF1 and its role in mediating the tropism of the Bacteria within the stomach. Int. J. Mol. Sci. 20:4400. doi: 10.3390/ijms20184400
Coker, O. O., Dai, Z., Nie, Y., Zhao, G., Cao, L., Nakatsu, G., et al. (2018). Mucosal microbiome dysbiosis in gastric carcinogenesis. Gut 67, 1024–1032. doi: 10.1136/gutjnl-2017-314281
Coppenhagen-Glazer, S., Sol, A., Abed, J., Naor, R., Zhang, X., Han, Y. W., et al. (2015). Fap2 of Fusobacterium nucleatum is a galactose-Inhibitable Adhesin involved in Coaggregation, cell adhesion, and preterm birth. Infect. Immun. 83, 1104–1113. doi: 10.1128/IAI.02838-14
Correa, P., Haenszel, W., Cuello, C., Tannenbaum, S., and Archer, M. (1975). A model for gastric cancer epidemiology. J. Investig. Med. 306, 58–60.
Correa, P. (1992). Human gastric carcinogenesis: a multistep and multifactorial process-- first American Cancer Society award lecture on Cancer epidemiology and prevention. Cancer Res. 52, 6735–6740.
Cui, H., Jin, Y., Chen, F., Ni, H., Hu, C., Xu, Y., et al. (2020). Clinicopathological evidence of hepatitis B virus infection in the development of gastric adenocarcinoma. J. Med. Virol. 92, 71–77. doi: 10.1002/jmv.25584
Cunningham, M., Azcarate-Peril, M. A., Barnard, A., Benoit, V., Grimaldi, R., Guyonnet, D., et al. (2021). Shaping the future of probiotics and prebiotics. Trends Microbiol. 29, 667–685. doi: 10.1016/j.tim.2021.01.003
Dai, Z., Zhang, J., Wu, Q., Chen, J., Liu, J., Wang, L., et al. (2019). The role of microbiota in the development of colorectal cancer. Int. J. Cancer 145, 2032–2041. doi: 10.1002/ijc.32017
de Vries, A. C., Kuipers, E. J., and Rauws, E. A. J. (2009). Helicobacter pylori eradication and gastric cancer: when is the horse out of the barn? Am. J. Gastroenterol. 104, 1342–1345. doi: 10.1038/ajg.2008.15
del Piano, M., Anderloni, A., Balzarini, M., Ballarè, M., Carmagnola, S., Montino, F., et al. (2012). The innovative potential of Lactobacillus rhamnosus LR06, Lactobacillus pentosus LPS01, Lactobacillus plantarum LP01, and Lactobacillus delbrueckii Subsp. delbrueckii LDD01 to restore the “gastric barrier effect” in patients chronically treated with PPI. J. Clin. Gastroenterol. 46 Suppl, S18–S26. doi: 10.1097/MCG.0b013e318267b55d
Delgado, S., Cabrera-Rubio, R., Mira, A., Suárez, A., and Mayo, B. (2013). Microbiological survey of the human gastric ecosystem using culturing and pyrosequencing methods. Microb. Ecol. 65, 763–772. doi: 10.1007/s00248-013-0192-5
Deng, Y., Ding, X., Song, Q., Zhao, G., Han, L., Ding, B., et al. (2021). Alterations in mucosa-associated microbiota in the stomach of patients with gastric cancer. Cell. Oncol. (Dordr.) 44, 701–714. doi: 10.1007/s13402-021-00596-y
Dicksved, J., Lindberg, M., Rosenquist, M., Enroth, H., Jansson, J. K., and Engstrand, L. (2009). Molecular characterization of the stomach microbiota in patients with gastric cancer and in controls. J. Med. Microbiol. 58, 509–516. doi: 10.1099/jmm.0.007302-0
Eslami, L., and Nasseri-Moghaddam, S. (2013). Meta-analyses: does long-term PPI use increase the risk of gastric premalignant lesions? Arch. Iran. Med. 16, 449–458.
Etemadi, A., Safiri, S., Sepanlou, S. G., Ikuta, K. S., Bisignano, C., Shakeri, R., et al. (2020). The global, regional, and national burden of stomach cancer in 195 countries, 1990–2017: a systematic analysis for the global burden of disease study 2017. Lancet Gastroenterol Hepatol 5, 42–54. doi: 10.1016/S2468-1253(19)30328-0
Eun, C. S., Kim, B. K., Han, D. S., Kim, S. Y., Kim, K. M., Choi, B. Y., et al. (2014). Differences in gastric mucosal microbiota profiling in patients with chronic gastritis, intestinal metaplasia, and gastric cancer using pyrosequencing methods. Helicobacter 19, 407–416. doi: 10.1111/hel.12145
Faintuch, J., Ishida, R. K., Jacabi, M., Ribeiro, A. S., Kuga, R., Sakai, P., et al. (2007). Increased gastric cytokine production after Roux-en-Y gastric bypass for morbid obesity. Arch. Surg. 142, 962–968. doi: 10.1001/archsurg.142.10.962
Fattahi, S., Nikbakhsh, N., Taheri, H., Ghadami, E., Kosari-Monfared, M., Amirbozorgi, G., et al. (2018). Prevalence of multiple infections and the risk of gastric adenocarcinoma development at earlier age. Diagn. Microbiol. Infect. Dis. 92, 62–68. doi: 10.1016/j.diagmicrobio.2018.04.015
Ferreira, R. M., Pereira-Marques, J., Pinto-Ribeiro, I., Costa, J. L., Carneiro, F., Machado, J. C., et al. (2018). Gastric microbial community profiling reveals a dysbiotic cancer-associated microbiota. Gut 67, 226–236. doi: 10.1136/gutjnl-2017-314205
Figura, N., Vindigni, C., Covacci, A., Presenti, L., Burroni, D., Vernillo, R., et al. (1998). cagA positive and Negative Helicobacter pylori strains are simultaneously present in the stomach of most patients with non-ulcer dyspepsia: relevance to histological damage. Gut 42, 772–778. doi: 10.1136/gut.42.6.772
Fisher, L., and Fisher, A. (2017). Acid-suppressive therapy and risk of infections: pros and cons. Clin. Drug Investig. 37, 587–624. doi: 10.1007/s40261-017-0519-y
Ford, A. C., Forman, D., Hunt, R. H., Yuan, Y., and Moayyedi, P. (2014). Helicobacter pylori eradication therapy to prevent gastric cancer in healthy asymptomatic infected individuals: systematic review and meta-analysis of randomised controlled trials. BMJ 348:g3174. doi: 10.1136/bmj.g3174
Francavilla, R., Polimeno, L., Demichina, A., Maurogiovanni, G., Principi, B., Scaccianoce, G., et al. (2014). Lactobacillus reuteri strain combination in Helicobacter pylori infect ion: a randomized, double-blind, placebo-controlled study. J. Clin. Gastroenterol. 48, 407–413. doi: 10.1097/MCG.0000000000000007
Frankel, A. E., Coughlin, L. A., Kim, J., Froehlich, T. W., Xie, Y., Frenkel, E. P., et al. (2017). Metagenomic shotgun sequencing and unbiased Metabolomic profiling identify specific human gut microbiota and metabolites associated with immune checkpoint therapy efficacy in melanoma patients. Neoplasia 19, 848–855. doi: 10.1016/j.neo.2017.08.004
Gantuya, B., el Serag, H. B., Matsumoto, T., Ajami, N. J., Uchida, T., Oyuntsetseg, K., et al. (2020). Gastric mucosal microbiota in a Mongolian population with gastric cancer and precursor conditions. Aliment. Pharmacol. Ther. 51, 770–780. doi: 10.1111/apt.15675
Gao, J.-J., Zhang, Y., Gerhard, M., Mejias-Luque, R., Zhang, L., Vieth, M., et al. (2018). Association between gut microbiota and Helicobacter pylori Rela ted gastric lesions in a high-risk population of gastric Cancer. Front. Cell. Infect. Microbiol. 8:202. doi: 10.3389/fcimb.2018.00202
Goderska, K., Agudo Pena, S., and Alarcon, T. (2018). Helicobacter pylori treatment: antibiotics or probiotics. Appl. Microbiol. Biotechnol. 102, 1–7. doi: 10.1007/s00253-017-8535-7
Gong, Y.-B., Zheng, J. L., Jin, B., Zhuo, D. X., Huang, Z. Q., Qi, H., et al. (2012). Particular Candida albicans strains in the digestive tract of dyspeptic patients, identified by multilocus sequence typing. PLoS One 7:e35311. doi: 10.1371/journal.pone.0035311
Graham, D. Y. (2005). Helicobacter pylori update: gastric cancer, reliable therapy, and possible benefits. Gastroenterology 148, 719–731. doi: 10.1053/j.gastro.2015.01.040
Gunathilake, M. N., Lee, J., Choi, I. J., Kim, Y. I., Ahn, Y., Park, C., et al. (2019). Association between the relative abundance of gastric microbiota and the risk of gastric cancer: a case-control study. Sci. Rep. 9:13589. doi: 10.1038/s41598-019-50054-x
Hartung, M. L., Gruber, D. C., Koch, K. N., Grüter, L., Rehrauer, H., Tegtmeyer, N., et al. (2015). H. pylori-induced DNA Strand breaks are introduced by nucleotide excision repair endonucleases and promote NF-κB target gene expression. Cell Rep. 13, 70–79. doi: 10.1016/j.celrep.2015.08.074
He, C., Peng, C., Shu, X., Wang, H., Zhu, Z., Ouyang, Y., et al. (2022). Convergent dysbiosis of gastric mucosa and fluid microbiome during stomach carcinogenesis. Gastric Cancer 25, 837–849. doi: 10.1007/s10120-022-01302-z
Hill, C., Guarner, F., Reid, G., Gibson, G. R., Merenstein, D. J., Pot, B., et al. (2014). The international scientific association for probiotics and prebiotics consensus statement on the scope and appropriate use of the term probiotic. Nat. Rev. Gastroenterol. Hepatol. 11, 506–514. doi: 10.1038/nrgastro.2014.66
Hisamatsu, A., Nagai, T., Okawara, H., Nakashima, H., Tasaki, T., Nakagawa, Y., et al. (2010). Gastritis associated with Epstein-Barr virus infection. Int. Med. 49, 2101–2105. doi: 10.2169/internalmedicine.49.3789
Hsieh, Y.-Y., Tung, S. Y., Pan, H. Y., Yen, C. W., Xu, H. W., Lin, Y. J., et al. (2018). Increased abundance of Clostridium and Fusobacterium in gastric microbiota of patients with gastric Cancer in Taiwan. Sci. Rep. 8:158. doi: 10.1038/s41598-017-18596-0
Hu, Y.-L., Pang, W., Huang, Y., Zhang, Y., and Zhang, C. J. (2018). The gastric microbiome is perturbed in advanced gastric adenocarcinoma identified through shotgun metagenomics. Front. Cell. Infect. Microbiol. 8:433. doi: 10.3389/fcimb.2018.00433
Huang, C.-F., Lai, H. C., Chen, C. Y., Tseng, K. C., Kuo, H. T., Hung, C. H., et al. (2020). Extrahepatic malignancy among patients with chronic hepatitis C after antiviral therapy: a real-world Nationwide study on Taiwanese chronic hepatitis C cohort (T-COACH). Am. J. Gastroenterol. 115, 1226–1235. doi: 10.14309/ajg.0000000000000606
Huang, J. Q., Zheng, G. F., Sumanac, K., Irvine, E. J., and Hunt, R. H. (2003). Meta-analysis of the relationship between cagA seropositivity and gastric cancer. Gastroenterology 125, 1636–1644. doi: 10.1053/j.gastro.2003.08.033
IARC (2003). Infection with Helicobacter pylori. IARC monographs on the evaluation of carcinogenic risks to humans. Environ. Health Perspect. 61, 177–240.
Iizasa, H., Nanbo, A., Nishikawa, J., Jinushi, M., and Yoshiyama, H. (2012). Epstein-Barr virus (EBV)-associated gastric carcinoma. Viruses 4, 3420–3439. doi: 10.3390/v4123420
Irfan, M., Delgado, R. Z. R., and Frias-Lopez, J. (2020). The oral microbiome and cancer. Front. Immunol. 11:591088. doi: 10.3389/fimmu.2020.591088
Ishida, R. K., Faintuch, J., Paula, A. M. R., Risttori, C. A., Silva, S. N., Gomes, E. S., et al. (2007). Microbial flora of the stomach after gastric bypass for morbid obesity. Obes. Surg. 17, 752–758. doi: 10.1007/s11695-007-9139-6
Iskra, S., Kalla, M., Delecluse, H. J., Hammerschmidt, W., and Moosmann, A. (2010). Toll-like receptor agonists synergistically increase proliferation and activation of B cells by Epstein-Barr virus. J. Virol. 84, 3612–3623. doi: 10.1128/JVI.01400-09
Javanmard, A., Ashtari, S., Sabet, B., Davoodi, S. H., Rostami-Nejad, M., Esmaeil Akbari, M., et al. (2018). Probiotics and their role in gastrointestinal cancers prevention and treatment; an overview. Gastroenterol. Hepatol. 11, 284–295.
Ji, J., and Yang, H. (2020). Using probiotics as supplementation for Helicobacter pylori antibiotic therapy. Int. J. Mol. Sci. 21:1136. doi: 10.3390/ijms21031136
Jin, J., Hu, C., Wang, P., Chen, J., Wu, T., Chen, W., et al. (2014). Latent infection of human cytomegalovirus is associated with the development of gastric cancer. Oncol. Lett. 8, 898–904. doi: 10.3892/ol.2014.2148
Kabir, A. M., Aiba, Y., Takagi, A., Kamiya, S., Miwa, T., and Koga, Y. (1997). Prevention of Helicobacter pylori infection by lactobacilli in a gnotobiotic murine model. Gut 41, 49–55. doi: 10.1136/gut.41.1.49
Kadeerhan, G., Gerhard, M., Gao, J. J., Mejías-Luque, R., Zhang, L., Vieth, M., et al. (2021). Microbiota alteration at different stages in gastric lesion progression: a population-based study in Linqu, China. Am. J. Cancer Res. 11, 561–575.
Kaneda, A., Matsusaka, K., Aburatani, H., and Fukayama, M. (2012). Epstein-Barr virus infection as an epigenetic driver of tumorigenesis. Cancer Res. 72, 3445–3450. doi: 10.1158/0008-5472.CAN-11-3919
Kang, J. S., Lee, S. H., Lee, S., Kim, G. H., Park, Y. J., Han, I. S., et al. (2019). Role of upper gastrointestinal endoscopy in patients with human immunodeficiency virus infection in the era of combination antiretroviral therapy. Infect. Chemother. 51, 35–44. doi: 10.3947/ic.2019.51.1.35
Karczewska, E., Wojtas, I, Sito, E., Trojanowska, D., Budak, A., Zwolinska-Wcislo, M., et al. (2009). Assessment of co-existence of Helicobacter pylori and Candida fungi in diseases of the upper gastrointestinal tract. J. Physiol. Pharmacol. 60, 33–39.
Kaźmierczak-Siedlecka, K., Daca, A., Roviello, G., Catalano, M., and Połom, K. (2022). Interdisciplinary insights into the link between gut microbiome and gastric carcinogenesis-what is currently known? Gastric Cancer 25, 1–10. doi: 10.1007/s10120-021-01260-y
Khosravi, Y., Dieye, Y., Poh, B. H., Ng, C. G., Loke, M. F., Goh, K. L., et al. (2014). Culturable bacterial microbiota of the stomach of Helicobacter pylori positive and negative gastric disease patients. Sci. World J. 2014, 1–10, doi: 10.1155/2014/610421
Kim, H., Choi, H., and Lee, S. K. (2015). Epstein-Barr virus miR-BART20-5p regulates cell proliferation and apoptosis by targeting BAD. Cancer Lett. 356, 733–742. doi: 10.1016/j.canlet.2014.10.023
Kosari-Monfared, M., Nikbakhsh, N., Fattahi, S., Ghadami, E., Ranaei, M., Taheri, H., et al. (2019). CTNNBIP1downregulation is associated with tumor grade and viral infections in gastric adenocarcinoma. J. Cell. Physiol. 234, 2895–2904. doi: 10.1002/jcp.27106
Krzysiek-Maczka, G., Targosz, A., Szczyrk, U., Strzałka, M., Sliwowski, Z., Brzozowski, T., et al. (2018). Role of Helicobacter pylori infection in cancer‐associated fibroblast‐induced epithelial‐mesenchymal transition in vitro. Helicobacter 23:e12538. doi: 10.1111/hel.12538
Kwak, H. W., Choi, I. J., Cho, S. J., Lee, J. Y., Kim, C. G., Kook, M. C., et al. (2014). Characteristics of gastric cancer according to Helicobacter pylori infection status. J. Gastroenterol. Hepatol. 29, 1671–1677. doi: 10.1111/jgh.12605
Kwon, S.-K., Park, J. C., Kim, K. H., Yoon, J., Cho, Y., Lee, B., et al. (2022). Human gastric microbiota transplantation recapitulates premalignant lesions in germ-free mice. Gut 71, 1266–1276. doi: 10.1136/gutjnl-2021-324489
Lagergren, J., Lindam, A., and Mason, R. M. (2012). Gastric stump cancer after distal gastrectomy for benign gastric ulcer in a population-based study. Int. J. Cancer 131, E1048–E1052. doi: 10.1002/ijc.27614
Lauren, P. (1965). The two histological main types of gastric carcinoma: diffuse and so-called intestinal-type carcinoma. Acta Pathol. Microbiol. Scand. 64, 31–49. doi: 10.1111/apm.1965.64.1.31
Lee, J. Y., Gong, E. J., Chung, E. J., Park, H. W., Bae, S. E., Kim, E. H., et al. (2017). The characteristics and prognosis of diffuse-type early gastric Cancer diagnosed during health check-ups. Gut Liver 11, 807–812. doi: 10.5009/gnl17033
Lee, C.-W., Rickman, B., Rogers, A. B., Ge, Z., Wang, T. C., and Fox, J. G. (2008). Helicobacter pylori eradication prevents progression of gastric cancer in hypergastrinemic INS-GAS mice. Cancer Res. 68, 3540–3548. doi: 10.1158/0008-5472.CAN-07-6786
Lee, W., Um, J., Hwang, B., Lee, Y. C., Chung, B. C., and Hong, J. (2020). Assessing the progression of gastric cancer via profiling of histamine, histidine, and bile acids in gastric juice using LC-MS/MS. J. Steroid Biochem. Mol. Biol. 197:105539. doi: 10.1016/j.jsbmb.2019.105539
Lertpiriyapong, K., Whary, M. T., Muthupalani, S., Lofgren, J. L., Gamazon, E. R., Feng, Y., et al. (2014). Gastric colonisation with a restricted commensal microbiota replicates the promotion of neoplastic lesions by diverse intestinal microbiota in the Helicobacter pylori ins-GAS mouse model of gastric carcinogenesis. Gut 63, 54–63. doi: 10.1136/gutjnl-2013-305178
Leung, W. K., Kim, J. J., Kim, J. G., Graham, D. Y., and Sepulveda, A. R. (2000). Microsatellite instability in gastric intestinal metaplasia in patients with and without gastric cancer. Am. J. Pathol. 156, 537–543. doi: 10.1016/S0002-9440(10)64758-X
Li, N., Feng, Y., Hu, Y., He, C., Xie, C., Ouyang, Y., et al. (2018). Helicobacter pylori CagA promotes epithelial mesenchymal transition in gastric carcinogenesis via triggering oncogenic YAP pathway. J. Exp. Clin. Cancer Res. 37:280. doi: 10.1186/s13046-018-0962-5
Li, X.-X., Wong, G. L. H., To, K. F., Wong, V. W. S., Lai, L. H., Chow, D. K. L., et al. (2009). Bacterial microbiota profiling in gastritis without Helicobacter pylori infection or non-steroidal anti-inflammatory drug use. PLoS One 4:e7985. doi: 10.1371/journal.pone.0007985
Ling, Z., Shao, L., Liu, X., Cheng, Y., Yan, C., Mei, Y., et al. (2019). Regulatory T cells and plasmacytoid dendritic cells within the tumor microenvironment in gastric Cancer are correlated with gastric microbiota dysbiosis: a preliminary study. Front. Immunol. 10:533. doi: 10.3389/fimmu.2019.00533
Liu, X., Shao, L., Liu, X., Ji, F., Mei, Y., Cheng, Y., et al. (2019). Alterations of gastric mucosal microbiota across different stomach microhabitats in a cohort of 276 patients with gastric cancer. EBioMedicine 40, 336–348. doi: 10.1016/j.ebiom.2018.12.034
Liu, D., Chen, S., Gou, Y., Yu, W., Zhou, H., Zhang, R., et al. (2021). Gastrointestinal microbiota changes in patients with gastric precancerous lesions Front. Cell. Infect. Microbiol. 11:749207. doi: 10.3389/fcimb.2021.749207
Lloyd-Price, J., Abu-Ali, G., and Huttenhower, C. (2016). The healthy human microbiome. Genome Med. 8:51. doi: 10.1186/s13073-016-0307-y
Lofgren, J. L., Whary, M. T., Ge, Z., Muthupalani, S., Taylor, N. S., Mobley, M., et al. (2011). Lack of commensal Flora in Helicobacter pylori–infected INS-GAS mice reduces gastritis and delays intraepithelial neoplasia. Gastroenterology 140, 210–220.e4. doi: 10.1053/j.gastro.2010.09.048
Ma, W., Mao, Q., Xia, W., Dong, G., Yu, C., and Jiang, F. (2019). Gut microbiota shapes the efficiency of cancer therapy. Front. Microbiol. 10:1050. doi: 10.3389/fmicb.2019.01050
Ma, J.-L., Zhang, L., Brown, L. M., Li, J. Y., Shen, L., Pan, K. F., et al. (2012). Fifteen-year effects of Helicobacter pylori, garlic, and vitamin treatments on gastric Cancer incidence and mortality. JNCI 104, 488–492. doi: 10.1093/jnci/djs003
Maldonado-Contreras, A., Goldfarb, K. C., Godoy-Vitorino, F., Karaoz, U., Contreras, M., Blaser, M. J., et al. (2011). Structure of the human gastric bacterial community in relation to Helicobacter pylori status. ISME J. 5, 574–579. doi: 10.1038/ismej.2010.149
Malfertheiner, P., Kandulski, A., and Venerito, M. (2017). Proton-pump inhibitors: understanding the complications and risks. Gastroenterol. Hepatol. 14, 697–710. doi: 10.1038/nrgastro.2017.117
Malfertheiner, P., Megraud, F., O’Morain, C. A., Gisbert, J. P., Kuipers, E. J., Axon, A. T., et al. (2017). Management of Helicobacter pylori infection-the Maastricht V/Florence consensus report. Gut 66, 6–30. doi: 10.1136/gutjnl-2016-312288
Marshall, B., and Warren, J. R. (1984). Unidentified curved BACILLI in the stomach of patients with gastritis and peptic ulceration. Lancet 323, 1311–1315. doi: 10.1016/S0140-6736(84)91816-6
Martínez, L. E., Hardcastle, J. M., Wang, J., Pincus, Z., Tsang, J., Hoover, T. R., et al. (2016). Helicobacter pylori strains vary cell shape and flagellum number to maintain robust motility in viscous environments. Mol. Microbiol. 99, 88–110. doi: 10.1111/mmi.13218
Martínez-López, J. L. E., Torres, J., Camorlinga-Ponce, M., Mantilla, A., Leal, Y., and Fuentes-Pananá, E. (2014). Evidence of Epstein-Barr virus association with gastric cancer and non-atrophic gastritis. Viruses 6, 301–318. doi: 10.3390/v6010301
Mason, K. L., Erb Downward, J. R., Falkowski, N. R., Young, V. B., Kao, J. Y., and Huffnagle, G. B. (2012) Interplay between the gastric bacterial microbiota and Candida albicans during postantibiotic recolonization and gastritis. Infect. Immun. 80, 150–158.
Matsumoto, S., Yamasaki, K., Tsuji, K., and Shirahama, S. (2008). Human T lymphotropic virus type 1 infection and gastric cancer development in Japan. J. Infect. Dis. 198, 10–15. doi: 10.1086/588733
Matsusaka, K., Funata, S., Fukayama, M., and Kaneda, A. (2014). DNA methylation in gastric cancer, related to Helicobacter pylori and Epstein-Barr virus. World J. Gastroenterol. 20, 3916–3926. doi: 10.3748/wjg.v20.i14.3916
Mégraud, F., Bessède, E., and Varon, C. (2015). Helicobacter pylori infection and gastric carcinoma. Clin. Microbiol. Infect. 21, 984–990. doi: 10.1016/j.cmi.2015.06.004
Mera, R. M., Bravo, L. E., Camargo, M. C., Bravo, J. C., Delgado, A. G., Romero-Gallo, J., et al. (2018). Dynamics of Helicobacter pylori infection as a determinant of progression of gastric precancerous lesions: 16-year follow-up of an eradication trial. Gut 67, 1239–1246. doi: 10.1136/gutjnl-2016-311685
Minoura-Etoh, J., Gotoh, K., Sato, R., Ogata, M., Kaku, N., Fujioka, T., et al. (2006). Helicobacter pylori-associated oxidant monochloramine induces reactivation of Epstein–Barr virus (EBV) in gastric epithelial cells latently infected with EBV. J. Med. Microbiol. 55, 905–911. doi: 10.1099/jmm.0.46580-0
Misra, V., Misra, S. P., Singh, M. K., Singh, P. A., and Dwivedi, M. (2007). Prevalence of H. pylori in patients with gastric cancer. Indian J. Pathol. Microbiol. 50, 702–707.
Moayyedi, P., Wason, C., Peacock, R., Walan, A., Bardhan, K., Axon, A. T. R., et al. (2000). Changing patterns of Helicobacter pylori gastritis in long‐standing acid suppression. Helicobacter 5, 206–214. doi: 10.1046/j.1523-5378.2000.00032.x
Montalban-Arques, A., Wurm, P., Trajanoski, S., Schauer, S., Kienesberger, S., Halwachs, B., et al. (2016). Propionibacterium acnes overabundance and natural killer group 2 member D system activation in corpus-dominant lymphocytic gastritis. J. Pathol. 240, 425–436. doi: 10.1002/path.4782
Murai, Y., Zheng, H. C., Aziz, H. O. A., Mei, H., Kutsuna, T., Nakanishi, Y., et al. (2007). High JC virus load in gastric cancer and adjacent non-cancerous mucosa. Cancer Sci. 98, 25–31. doi: 10.1111/j.1349-7006.2006.00354.x
Nada, H. G., Sudha, T., Darwish, N. H. E., and Mousa, S. A. (2020). Lactobacillus acidophilus and Bifidobacterium longum exhibit antiproliferation, anti-angiogenesis of gastric and bladder cancer: impact of COX2 inhibition. Pharma Nutr. 14:100219. doi: 10.1016/j.phanu.2020.100219
Nardone, G., and Compare, D. J. U. E. G. J. (2015). The human gastric microbiota: is it time to rethink the pathogenesis of stomach diseases? Gastroenterol. J. 3, 255–260. doi: 10.1177/2050640614566846
Navabi, N., Johansson, M. E. V., Raghavan, S., and Lindén, S. K. (2013). Helicobacter pylori infection impairs the mucin production rate and turnover in the murine gastric mucosa. Infect. Immun. 81, 829–837. doi: 10.1128/IAI.01000-12
Naylor, G. M., Gotoda, T., Dixon, M., Shimoda, T., Gatta, L., Owen, R., et al. (2006). Why does Japan have a high incidence of gastric cancer? Comparison of gastritis between UK and Japanese patients. Gut 55, 1545–1552. doi: 10.1136/gut.2005.080358
Ndegwa, N., Ploner, A., Andersson, A. F., Zagai, U., Andreasson, A., Vieth, M., et al. (2020). Gastric microbiota in a low–Helicobacter pylori prevalence general population and their associations with gastric lesions. Clin. Transl. Gastroenterol. 11:e00191. doi: 10.14309/ctg.0000000000000191
Nell, S., Estibariz, I., Krebes, J., Bunk, B., Graham, D. Y., Overmann, J., et al. (2018). Genome and Methylome variation in Helicobacter pylori with a cag pathogenicity Island during early stages of human infection. Gastroenterology 154, 612–623.e7. doi: 10.1053/j.gastro.2017.10.014
Nie, S., and Yuan, Y. (2020). The role of gastric mucosal immunity in gastric diseases. J Immunol Res 2020, 1–8. doi: 10.1155/2020/7927054
Nishikawa, J., Yanai, H., Hirano, A., Okamoto, T., Nakamura, H., Matsusaki, K., et al. (2002). GI Cancer high prevalence of Epstein-Barr virus in gastric remnant carcinoma after Billroth-II reconstruction. Scand. J. Gastroenterol. 37, 825–829. doi: 10.1080/gas.37.7.825.829
Nishikawa, J., Yoshiyama, H., Iizasa, H., Kanehiro, Y., Nakamura, M., Nishimura, J., et al. (2014). Epstein-Barr virus in gastric carcinoma. Cancers 6, 2259–2274. doi: 10.3390/cancers6042259
Owens, S. R., Walls, A., Krasinskas, A. M., and Rund, C. R. (2011). Epstein-Barr virus gastritis: rare or rarely sampled? A case report. Int. J. Surg. Pathol. 19, 196–198. doi: 10.1177/1066896908316905
Palrasu, M., Zaika, E., el-Rifai, W., Garcia-Buitrago, M., Piazuelo, M. B., Wilson, K. T., et al. (2020). Bacterial CagA protein compromises tumor suppressor mechanisms in gastric epithelial cells. J. Clin. Invest. 130, 2422–2434. doi: 10.1172/JCI130015
Palrasu, M., Zaika, E., El-Rifai, W., Que, J., and Zaika, A. I. (2021). Role of bacterial and viral pathogens in gastric carcinogenesis. Cancers (Basel). 13:1878. doi: 10.3390/cancers13081878
Papon, N., Hohl, T. M., and Zhai, B. (2021). Mycobiota dysbiosis and gastric tumorigenesis. Theranostics 11, 7488–7490. doi: 10.7150/thno.61480
Paroni Sterbini, F., Palladini, A., Masucci, L., Cannistraci, C. V., Pastorino, R., Ianiro, G., et al. (2016). Effects of proton pump inhibitors on the gastric mucosa-associated microbiota in dyspeptic patients. Appl. Environ. Microbiol. 82, 6633–6644. doi: 10.1128/AEM.01437-16
Parsonnet, J., Friedman, G. D., Orentreich, N., and Vogelman, H. (1997). Risk for gastric cancer in people with CagA positive or CagA negative Helicobacter pylori infection. Gut 40, 297–301. doi: 10.1136/gut.40.3.297
Peng, Z., Cheng, S., Kou, Y., Wang, Z., Jin, R., Hu, H., et al. (2020). The gut microbiome is associated with clinical response to anti–PD-1/PD-L1 immunotherapy in gastrointestinal cancer. Cancer Immunol. Res. 8, 1251–1261. doi: 10.1158/2326-6066.CIR-19-1014
Pereira-Marques, J., Ferreira, R. M., Pinto-Ribeiro, I., and Figueiredo, C. (2019). Helicobacter pylori infection, the gastric microbiome and gastric cancer. Adv. Exp. Med. Biol. 1149, 195–210. doi: 10.1007/5584_2019_366
Petersen, C., and Round, J. L. (2014). Defining dysbiosis and its influence on host immunity and disease. Cell. Microbiol. 16, 1024–1033. doi: 10.1111/cmi.12308
Plummer, M., de Martel, C., Vignat, J., Ferlay, J., Bray,, and Franceschi, S. (2016). Global burden of cancers attributable to infections in 2012: a synthetic analysis. Lancet Glob. Health 4, e609–e616. doi: 10.1016/S2214-109X(16)30143-7
Polakovicova, I., Jerez, S., Wichmann, I. A., Sandoval-Bórquez, A., Carrasco-Véliz, N., and Corvalán, A. H. (2018). Role of microRNAs and exosomes in Helicobacter pylori and Epstein-Barr virus associated gastric cancers. Front. Microbiol. 9:636. doi: 10.3389/fmicb.2018.00636
Rafiei, R., Ebrahimi, A., Bahrami, M., Nazari, M., Torabi, Z., Ebrahimi, A., et al. (2021). Sequential therapy for Helicobacter pylori infection with and without probiotics. Gastroenterol Hepatol (Bartlesville) 11, 231–234. doi: 10.15406/ghoa.2020.11.00444
Raheem, A., Liang, L., Zhang, G., and Cui, S. (2021). Modulatory effects of probiotics during pathogenic infections with emphasis on immune regulation. Front. Immunol. 12:616713. doi: 10.3389/fimmu.2021.616713
Ramirez-Garcia, A., Rementeria, A., Aguirre-Urizar, J. M., Moragues, M. D., Antoran, A., Pellon, A., et al. (2016). Candida albicans and cancer: can this yeast induce cancer development or progression? Crit. Rev. Microbiol. 42, 181–193. doi: 10.3109/1040841X.2014.913004
Ravegnini, G., Fosso, B., Saverio, V. D., Sammarini, G., Zanotti, F., Rossi, G., et al. (2020). Gastric Adenocarcinomas and signet-ring cell carcinoma: unraveling gastric Cancer complexity through microbiome analysis-deepening heterogeneity for a personalized therapy. Int. J. Mol. Sci. 21:9735. doi: 10.3390/ijms21249735
Rickinson, A. B. (2014). Co-infections, inflammation and oncogenesis: future directions for EBV research. Semin. Cancer Biol. 26, 99–115. doi: 10.1016/j.semcancer.2014.04.004
Ridlon, J. M., and Bajaj, J. S. (2015). The human gut sterolbiome: bile acid-microbiome endocrine aspects and therapeutics. Acta Pharm. Sin. B 5, 99–105. doi: 10.1016/j.apsb.2015.01.006
Routy, B., le Chatelier, E., Derosa, L., Duong, C. P. M., Alou, M. T., Daillère, R., et al. (2018). Gut microbiome influences efficacy of PD-1–based immunotherapy against epithelial tumors. Science 359, 91–97. doi: 10.1126/science.aan3706
Rugge, M., de Boni, M., Pennelli, G., de Bona, M., Giacomelli, L., Fassan, M., et al. (2010). Gastritis OLGA-staging and gastric cancer risk: a twelve-year clinico- pathological follow-up study. Aliment. Pharmacol. Ther. 31, 1104–1111. doi: 10.1111/j.1365-2036.2010.04277.x
Sakarya, S., and Gunay, N. (2014). Saccharomyces boulardii expresses neuraminidase activity selective for α2,3-linked sialic acid that decreases Helicobacter pylori adhesion to host cells 122, 941–950.
Sanduleanu, S., Jonkers, D. M. A. E., De Bruine, A., Hameeteman, W., and Stockbrügger, R. W. (2001). Non-Helicobacter pylori bacterial flora during acid-suppressive therapy: differential findings in gastric juice and gastric mucosa. Aliment. Pharmacol. Ther. 15, 379–388. doi: 10.1016/s1590-8658(01)80050-5
Sanduleanu, S., Jonkers, D., de Bruïne, A., Hameeteman, W., and Stockbrügger, R. W. (2001). Changes in gastric mucosa and luminal environment during acid-suppressive therapy: a review in depth. Dig Liver Dis. 33, 707–719. doi: 10.1016/S1590-8658(01)80050-5
Scarpignato, C., Gatta, L., Zullo, A., and Blandizzi, C. (2016). Effective and safe proton pump inhibitor therapy in acid-related diseases – a position paper addressing benefits and potential harms of acid suppression. BMC Med. 14:179. doi: 10.1186/s12916-016-0718-z
Schierhout, G., McGregor, S., Gessain, A., Einsiedel, L., Martinello, M., and Kaldor, J. (2020). Association between HTLV-1 infection and adverse health outcomes: a systematic review and meta-analysis of epidemiological studies. Lancet Infect. Dis. 20, 133–143. doi: 10.1016/S1473-3099(19)30402-5
Schulz, C., Koch, N., Schütte, K., Pieper, D. H., and Malfertheiner, P. (2015). H. pylori and its modulation of gastrointestinal microbiota. J. Dig. Dis. 16, 109–117. doi: 10.1111/1751-2980.12233
Schulze, J., and Sonnenborn, U. (2009). Yeasts in the gut: from commensals to infectious agents. Dtsch. Arztebl. Int.l 106, 837–842. doi: 10.3238/arztebl.2009.0837
Scott, B. B., and Jenkins, D. (1982). Gastro-oesophageal candidiasis. Gut 23, 137–139. doi: 10.1136/gut.23.2.137
Scott, D. R., Weeks, D., Hong, C., Postius, S., Melchers, K., and Sachs, G. (1998). The role of internal urease in acid resistance of Helicobacter pylori. Gastroenterology 114, 58–70. doi: 10.1016/S0016-5085(98)70633-X
Shao, D., Vogtmann, E., Liu, A., Qin, J., Chen, W., Abnet, C. C., et al. (2019). Microbial characterization of esophageal squamous cell carcinoma and gastric cardia adenocarcinoma from a high‐risk region of China. Cancer 125, 3993–4002. doi: 10.1002/cncr.32403
Shukla, S. K., Prasad, K. N., Tripathi, A., Ghoshal, U. C., Krishnani, N., and Husain, N. (2012). Expression profile of latent and lytic transcripts of Epstein-Barr virus in patients with gastroduodenal diseases: a study from northern India. J. Med. Virol. 84, 1289–1297. doi: 10.1002/jmv.23322
Smyth, E. C., Nilsson, M., Grabsch, H. I., van Grieken, N. C. T., and Lordick, F. (2020). Gastric cancer. Lancet 396, 635–648. doi: 10.1016/S0140-6736(20)31288-5
Song, C., Lv, J., Liu, Y., Chen, J. G., Ge, Z., Zhu, J., et al. (2019). Associations between hepatitis B virus infection and risk of all Cancer types. JAMA Netw. Open 2:e195718. doi: 10.1001/jamanetworkopen.2019.5718
Song, H., Zhu, J., and Lu, D. (2018). Long-term proton pump inhibitor (PPI) use and the development of gastric pre-malignant lesions. Cochrane Database Syst. Rev. :CD010623. doi: 10.1002/14651858.CD010623.pub2
Suerbaum, S. (1995). The complex flagella of gastric Helicobacter species. Trends Microbiol. 3, 168–170. doi: 10.1016/S0966-842X(00)88913-1
Sugano, K., Tack, J., Kuipers, E. J., Graham, D. Y., el-Omar, E. M., Miura, S., et al. (2015). Kyoto global consensus report on Helicobacter pylori gastritis. Gut 64, 1353–1367. doi: 10.1136/gutjnl-2015-309252
Sundrud, M. S., Torres, V. J., Unutmaz, D., and Cover, T. L. (2004). Inhibition of primary human T cell proliferation by Helicobacter pylori vacuolating toxin (VacA) is independent of VacA effects on IL-2 secretion. Proceed. Nat. Acad. Sci. U. S. A. 101, 7727–7732. doi: 10.1073/pnas.0401528101
Sung, J., Kim, N., Kim, J., Jo, H. J., Park, J. H., Nam, R. H., et al. (2016). Comparison of gastric microbiota between gastric juice and mucosa by next generation sequencing method. J Cancer Prev. 21, 60–65. doi: 10.15430/JCP.2016.21.1.60
Suzuki, M., Miura, S., Suematsu, M., Fukumura, D., Kurose, I., Suzuki, H., et al. (1992). Helicobacter pylori-associated ammonia production enhances neutrophil- dependent gastric mucosal cell injury. Am. J. Phys. 263, G719–G725. doi: 10.1152/ajpgi.1992.263.5.G719
Takada, K. (2000). Epstein-Barr virus and gastric carcinoma. Molec. Pathol. 53, 255–261. doi: 10.1136/mp.53.5.255
Tan, M. P., Kaparakis, M., Galic, M., Pedersen, J., Pearse, M., Wijburg, O. L. C., et al. (2007). Chronic Helicobacter pylori infection does not significantly alter the microbiota of the murine stomach. Appl. Environ. Microbiol. 73, 1010–1013. doi: 10.1128/AEM.01675-06
Tatemichi, M., Sasazuki, S., Inoue, M., and Tsugane, S., for the JPHC Study Group (2009). Clinical significance of IgG antibody titer against Helicobacter pylori. Helicobacter 14, 231–236. doi: 10.1111/j.1523-5378.2009.00681.x
Thrift, A. P., and el-Serag, H. B. (2020). Burden of gastric Cancer. Clin. Gastroenterol. Hepatol. 18, 534–542. doi: 10.1016/j.cgh.2019.07.045
Touati, E., Michel, V., Thiberge, J. M., Wuscher, N., Huerre, M., and Labigne, A. (2003). Chronic Helicobacter pylori infections induce gastric mutations in mice1 1This report is dedicated to the memory of Prof. Maurice Hofnung. Gastroenterology 124, 1408–1419. doi: 10.1016/S0016-5085(03)00266-X
Tsuda, A., Suda, W., Morita, H., Takanashi, K., Takagi, A., Koga, Y., et al. (2015). Influence of proton-pump inhibitors on the luminal microbiota in the gastrointestinal tract. Minerva Gastroenterol. Dietol. 6:e89. doi: 10.1038/ctg.2015.20
Uemura, N., Okamoto, S., Yamamoto, S., Matsumura, N., Yamaguchi, S., Yamakido, M., et al. (2001). Helicobacter pylori infection and the development of gastric Cancer. N. Engl. J. Med. 345, 784–789. doi: 10.1056/NEJMoa001999
Vallejo-Flores, G., Torres, J., Sandoval-Montes, C., Arévalo-Romero, H., Meza, I., Camorlinga-Ponce, M., et al. (2015). Helicobacter pylori CagA suppresses apoptosis through activation of AKT in a nontransformed epithelial cell model of glandular acini formation. Biomed. Res. Int.. 2015, 1–12, doi: 10.1155/2015/761501
Vartoukian, S. R., Palmer, R. M., and Wade, W. G. (2010). Strategies for culture of ‘unculturable’ bacteria. FEMS Microbiol. Lett. 309, 1–7. doi: 10.1111/j.1574-6968.2010.02000.x
Vétizou, M., Pitt, J. M., Daillère, R., Lepage, P., Waldschmitt, N., Flament, C., et al. (2015). Anticancer immunotherapy by CTLA-4 blockade relies on the gut microbiota. Science 350, 6264, 1079–1084. doi: 10.1126/science.aad1329
Vinasco, K., Mitchell, H. M., Kaakoush, N. O., and Castaño-Rodríguez, N. (2019). Microbial carcinogenesis: lactic acid bacteria in gastric cancer. Biochim. Biophys. Acta Rev. Cancer 1872:188309. doi: 10.1016/j.bbcan.2019.07.004
von Rosenvinge, E. C., Song, Y., White, J. R., Maddox, C., Blanchard, T., and Fricke, W. F. (2013). Immune status, antibiotic medication and pH are associated with changes in the stomach fluid microbiota. ISME J. 7, 1354–1366. doi: 10.1038/ismej.2013.33
Wandall, J. H. (1992). Effects of omeprazole on neutrophil chemotaxis, super oxide production, degranulation, and translocation of cytochrome b-245. Gut 33, 617–621. doi: 10.1136/gut.33.5.617
Wang, Z., Gao, X., Zeng, R., Wu, Q., Sun, H., Wu, W., et al. (2020). Changes of the gastric mucosal microbiome associated with histological stages of gastric carcinogenesis. Front. Microbiol. 11:997. doi: 10.3389/fmicb.2020.00997
Wang, L., Zhou, J., Xin, Y., Geng, C., Tian, Z., Yu, X., et al. (2016). Bacterial overgrowth and diversification of microbiota in gastric cancer. Eur. J. Gastroenterol. Hepatol. 28, 261–266. doi: 10.1097/MEG.0000000000000542
Watabe, H., Mitsushima, T., Yamaji, Y., Okamoto, M., Wada, R., Kokubo, T., et al. (2005). Predicting the development of gastric cancer from combining Helicobacter pylori antibodies and serum pepsinogen status: a prospective endoscopic cohort study. Gut 54, 764–768. doi: 10.1136/gut.2004.055400
Weng, M.-T., Chiu, Y. T., Wei, P. Y., Chiang, C. W., Fang, H. L., and Wei, S. C. (2019). Microbiota and gastrointestinal cancer. J. Formos. Med. Assoc. 118, S32–S41. doi: 10.1016/j.jfma.2019.01.002
Whiteside, S. A., Mohiuddin, M. M., Shlimon, S., Chahal, J., MacPherson, C. W., Jass, J., et al. (2021). In vitro framework to assess the anti-Helicobacter pylori potential of lactic acid Bacteria secretions as alternatives to antibiotics. Int. J. Mol. Sci. 22:5650. doi: 10.3390/ijms22115650
Williams, C., and McColl, K. E. L. (2006). Review article: proton pump inhibitors and bacterial overgrowth. Aliment. Pharmacol. Ther. 23, 3–10. doi: 10.1111/j.1365-2036.2006.02707.x
Wong, B. C.-Y., Lam, S. K., Wong, W. M., Chen, J. S., Zheng, T. T., Feng, R. E., et al. (2004). Helicobacter pylori eradication to prevent gastric Cancer in a high-risk region of China. JAMA 291, 187–194. doi: 10.1001/jama.291.2.187
Xi, J., Li, Y., Zhang, H., and Bai, Z. (2023). Dynamic variations of the gastric microbiota: key therapeutic points in the reversal of Correa’s cascade. Environ. Health Perspect. 152, 1069–1084. doi: 10.1002/ijc.34264
Xie, H., Lu, Q., Wang, H., Zhu, X., and Guan, Z. (2018). Effects of probiotics combined with enteral nutrition on immune function and inflammatory response in postoperative patients with gastric cancer. J. BUON 23, 678–683.
Yamaoka, Y., Kita, M., Kodama, T., Sawai, N., Kashima, K., and Imanishi, J. (1997). Induction of various cytokines and development of severe mucosal inflammation by CagA gene Positive Helicobacter pylori strains. Gut 41, 442–451. doi: 10.1136/gut.41.4.442
Yang, Y.-J., Chuang, C. C., Yang, H. B., Lu, C. C., and Sheu, B. S. (2012). Lactobacillus acidophilus ameliorates H. pylori-induced gastric inflammation by inactivating the Smad7 and NFκB pathways. BMC Microbiol. 12:38. doi: 10.1186/1471-2180-12-38
Yang, L., Kartsonaki, C., Yao, P., de Martel, C., Plummer, M., Chapman, D., et al. (2021). The relative and attributable risks of cardia and non-cardia gastric cancer associated with Helicobacter pylori infection in China: a case-cohort study. Lancet Public Health 6, e888–e896. doi: 10.1016/S2468-2667(21)00164-X
Yang, Y. J., and Sheu, B. S. (2012). Probiotics-containing yogurts suppress Helicobacter pylori load and modify immune response and intestinal microbiota in the Helicobacter pylori-infected children Helicobacter pylori 17, 297–304.
Yang, Y., Huang, Y., Lin, W., Liu, J., Chen, X., Chen, C., et al. (2022). Host miRNAs-microbiota interactions in gastric cancer. J. Transl. Med. 20, 1–8. doi: 10.1186/s12967-022-03264-3
Yoshiyama, H., and Nakazawa, T. (2000). Unique mechanism of Helicobacter pylori for colonizing the gastric mucus. Microbes Infect. 2, 55–60. doi: 10.1016/s1286-4579(00)00285-9
Yu, C., Su, Z., Li, Y., Li, Y., Liu, K., Chu, F., et al. (2020). Dysbiosis of gut microbiota is associated with gastric carcinogenesis in rats. Biomed. Express 126:110036. doi: 10.1016/j.biopha.2020.110036
Yu, G., Torres, J., Hu, N., Medrano-Guzman, R., Herrera-Goepfert, R., Humphrys, M. S., et al. (2017). Molecular characterization of the human stomach microbiota in gastric cancer patients. Front. Cell. Infect. Microbiol. 7:302. doi: 10.3389/fcimb.2017.00302
Zeng, Z.-M., Luo, F. F., Zou, L. X., He, R. Q., Pan, D. H., Chen, X., et al. (2016). Human papillomavirus as a potential risk factor for gastric cancer: a meta-analysis of 1,917 cases. Onco. Targets. Ther. 9, 7105–7114. doi: 10.2147/OTT.S115053
Zhang, X., Li, C., Cao, W., and Zhang, Z. (2021). Alterations of gastric microbiota in gastric Cancer and precancerous stages. Front. Cell. Infect. Microbiol. 11, –559148. doi: 10.3389/fcimb.2021.559148
Zhang, Z., Feng, H., Qiu, Y., Xu, Z., Xie, Q., Ding, W., et al. (2022). Dysbiosis of gastric mucosal fungal microbiota in the gastric Cancer microenvironment. J Immunol Res 2022:6011632. doi: 10.1155/2022/6011632
Zhao, R., Wang, Y., Huang, Y., Cui, Y., Xia, L., Rao, Z., et al. (2017). Effects of fiber and probiotics on diarrhea associated with enteral nutrition in gastric cancer patients. Medicine 96:e8418. doi: 10.1097/MD.0000000000008418
Zhong, M., Xiong, Y., Zhao, J., Gao, Z., Ma, J., Wu, Z., et al. (2021). Candida albicans disorder is associated with gastric carcinogenesis. Theranostics 11, 4945–4956. doi: 10.7150/thno.55209
Zhong, M., Xiong, Y., Zhao, J., Gao, Z., Ma, J., Wu, Z., et al. (2021). Candida albicans disorder is associated with gastric carcinogenesis. Theranostics 11, 4945–4956. doi: 10.7150/thno.55209
Zhou, C., Ma, F. Z., Deng, X. J., Yuan, H., and Ma, H. S. (2008). Lactobacilli inhibit interleukin-8 production induced by Helicobacter pylori lipopolysaccharide-activated toll-like receptor 4. World J. Gastroenterol. 14, 5090–5095. doi: 10.3748/wjg.14.5090
Zilberstein, B., Budak, A., Bogdał, J, Zagai, U., Trojanowska, D., Stachura, J., et al. (2007). Digestive tract microbiota in healthy volunteers. Clinics (Sao Paulo) 62, 47–56. doi: 10.1590/s1807-59322007000100008
Keywords: Helicobacter pylori, dysbiosis, gastric microbiota, microbial transplantation, gastric cancer
Citation: Huang H, Zhong W, Wang X, Yang Y, Wu T, Chen R, Liu Y, He F and Li J (2023) The role of gastric microecological dysbiosis in gastric carcinogenesis. Front. Microbiol. 14:1218395. doi: 10.3389/fmicb.2023.1218395
Edited by:
Dongdong Wang, McMaster University, CanadaReviewed by:
Chao Li, Shandong University of Traditional Chinese Medicine, ChinaChenggui Miao, Anhui University of Chinese Medicine, China
Copyright © 2023 Huang, Zhong, Wang, Yang, Wu, Chen, Liu, He and Li. This is an open-access article distributed under the terms of the Creative Commons Attribution License (CC BY). The use, distribution or reproduction in other forums is permitted, provided the original author(s) and the copyright owner(s) are credited and that the original publication in this journal is cited, in accordance with accepted academic practice. No use, distribution or reproduction is permitted which does not comply with these terms.
*Correspondence: Feng He, ODU0NTMwMDFAcXEuY29t; Jun Li, ODQxODM5NjdAcXEuY29t