- Department of Gastroenterology and Hepatology, Sichuan Provincial People's Hospital, School of Medicine, University of Electronic Science and Technology of China, Chengdu, China
Background: Portal vein thrombosis (PVT) is a serious complication of liver cirrhosis (LC) and is closely related to gut homeostasis. The study aimed to investigate the composition of gut microbiota and its putative role in PVT development in LC.
Methods: 33 patients with LC admitted between January 2022 and December 2022 were enrolled in this study. Based on imaging findings, they were categorized into LC without PVT (n = 21) and LC with PVT (n = 12) groups. Fecal samples were collected from each participant and underwent 16S rDNA sequencing.
Results: D-Dimer and platelet elevations were the main clinical features of LC with PVT. The alpha and beta diversity of the gut microbiota in LC with PVT group was found to be significantly higher compared to the control group. The structure of the gut microbiota was significantly different between the two groups. Based on LEfSe data, the genera Akkermansia, Eubacterium hallii group, Fusicatenibacter, and Anaerostipes were enriched in the LC with PVT, while Enterococcus, Weissella, Bacteroides, and Subdoligranulum were enriched in those of the LC subjects. Changes in microbiota structure result in significant differences in gut microbiota metabolism between the two groups. Altered levels of the microbiota genera were shown to be correlated with coagulation factor parameters. In animal experiments, the addition of Bacteroides reversed the CCl4-induced PVT.
Conclusion: Liver cirrhosis with PVT led to a disorder in the gut microbiota, which was characterized by an increase in pathogenic bacteria and a decrease in beneficial bacteria. Furthermore, modulating the gut microbiota, especially Bacteroides, may be a promising therapeutic approach to reduce the progression of PVT in LC.
Introduction
Portal vein thrombosis (PVT) is an increasingly recognized complication of liver cirrhosis (LC). The prevalence of PVT has been found to increase in parallel with the severity of cirrhosis. It is observed to be 10% in patients with compensated cirrhosis (Tsochatzis et al., 2010), 7.4–17% in those with advanced cirrhosis (Child-Pugh B/C; Violi et al., 2019), and even up to 26% in liver transplant (LT) candidates (Francoz et al., 2012; Senzolo et al., 2021). PVT reportedly exerts a further increase in resistance to portal blood flow, leading to worsening portal hypertension, which results in an increased risk of gastrointestinal bleeding (Amitrano et al., 2002) and decompensation associated with portal hypertension (Senzolo et al., 2019), especially in complete PVT.
The development of PVT in cirrhosis is believed to have a multifactorial pathogenesis and is mainly due to changes in the different components of Virchow’s triad, including hypercoagulability, decreased portal vein flow, and damage to the vessel wall (Intagliata et al., 2019). The gut microbiota represents the most extensive population of microorganisms within the human body, and it directly interacts with the liver through the hepatointestinal axis (Baffy, 2019). The central role of the microbiota in a variety of chronic liver diseases has been established. Dysregulation of the gut microbiota may influence the intensity of liver inflammation and fibrosis by engaging in multiple interactions with the host immune system (Baffy, 2019). Bacterial translocation and bacterial products (such as endotoxins) lead to hepatic encephalopathy and spontaneous bacterial peritonitis (Bellot et al., 2013). Microflora imbalance may be involved in portal hypertension (Violi et al., 2023); however, a correlation between PVT occurrence and gut microflora has not been reported. Theoretically, PVT is closely related to dysbiosis of gut microbiota. We hypothesized that the presence of PVT in patients with cirrhosis can lead to disturbances in the gut microbiota, which aggravate the progression of cirrhosis and PVT.
Thus, we utilized 16S rRNA gene sequencing to compare and characterize the microbial community composition in patients with cirrhosis with and without PVT. Additionally, we conducted an analysis of the potential correlation between gut microbiota and coagulation function. The purpose of this study was to investigate the relationship between PVT development and gut microbiota and to provide a theoretical basis for improving gut dysbiosis in the treatment of PVT.
Methods
Study design and patients
Consecutive patients with LC were recruited for this prospective observational study, which was conducted between January 2022 and December 2022. The diagnosis of LC was established by evaluating clinical, laboratory, and radiological data, and/or conducting liver biopsies. The diagnosis of PVT followed the consensus guidelines for managing PVT in LC patients (2020, Shanghai), in brief the diagnosis of PVT relies mainly on imaging (liver vascular ultrasound, CT or MRI; Hepatobiliary Disease Study Group et al., 2021). The inclusion criteria required patients to be between 18 and 70 years of age, with well-characterized cirrhosis that was confirmed by compatible clinical, imaging, liver transient elastography, and laboratory values. Patients were excluded from the study if they were on anticoagulation therapy at the time of enrollment, had hepatocellular carcinoma (HCC), pregnant, prior trans jugular intrahepatic portosystemic shunt (TIPS), prior liver transplant, or systemic neoplasia.
Ethic statement
This research was conducted in compliance with the tenets of the Declaration of Helsinki. The Ethics Committee of Sichuan Provincial People’s Hospital approved the study (No. SCCT2021-525), and all patients provided informed consent prior to participation.
Fecal samples
A standardized method was used to collect fecal samples. Samples were transported to the laboratory within 2 h of collection along with an ice pack. The samples were promptly frozen and reserved at −80°C until the time of analysis.
DNA extraction and microbiome profiling
The QIAamp Fast DNA Stool Mini Kit (Qiagen, Germany) was used to extract microbial DNA from fecal samples, following the manufacturer’s instructions. For PCR amplification of the bacterial 16S ribosomal RNA genes, primers 341F (5′-CCTACGGGRSGCAGCAG-3′) and 806R (5′-GGACTACVVGGGTATCTAATC-3′) were used to target the V3-V4 regions of the genes. Amplicons were extracted from 2% agarose gels and purified using the AxyPrep DNA Gel Extraction Kit (Axygen Biosciences, Union City, CA, United States) according to the manufacturer’s instructions and quantified using Qubit®2.0 (Invitrogen, United States). All quantified amplicons were pooled to equalize concentrations for sequencing using Illumina NovaSeq (Illumina, Inc., CA, United States). Using cutadapt in Qiime2 (v2022.2) to remove primer sequences from the data. DNA extraction, Library construction, and sequencing were conducted at Realbio Genomics Institute (Shanghai, China).
Bioinformatics analysis
The DADA2 plug-in in Qiime2 (v2022.2) software was used to filter, denoise, merged and nonchimeric the sequence after the removal of primes, and the Amplicon Sequence Variant or Feature sequence (ASV) was formed. The Feature representative sequences were compared with the Silva database (v138) to obtain the species classification of each feature, and then subsequent analysis was conducted according to the species abundance table.
Alpha-diversity metrics, such as Chao1 richness estimator, observed species, Faith’s PD, and Good’s coverage were calculated using the ASV table in QIIME2, and visualized as box plots. Beta diversity analysis was performed to investigate the structural variation of microbial communities across samples using Jaccard metrics, Bray–Curtis metrics, and UniFrac distance metrics, and visualized via principal coordinate analysis, non-metric multidimensional scaling, and unweighted pair-group method with arithmetic means hierarchical clustering. We employed linear discriminant analysis (LDA) of effect size (LEfSe) to identify significantly different taxa between the two groups (the default screening condition was LDA ≥ 2). Microbial functions were predicted by PICRUSt2 upon MetaCyc and KEGG databases.
Animal studies
Male Wistar rats were purchased from Yangzhou University Animal Medicine Center (SCXK 2022-0009). Rats were randomly divided into three groups (five rats per group): Control, CCl4, and CCl4+ Bacteroides group. Animals in all groups were provided a standard commercial rodent diet and were maintained on a 12-h light/dark cycle at constant temperature and humidity. All experimental procedures were approved by the Ethical Committee of Sichuan Provincial People’s Hospital. In addition, the National Institutes of Health guide for the care and use of laboratory animals was strictly followed.
Rats in the CCl4 group or CCl4+ Bacteroides group were gavaged with CCl4 dissolved (MACKLIN, C805329) in olive oil (40% v/v, 3 mL/kg) twice per week for 12 weeks. During this 12-week treatment course, rats in the CCl4+ Bacteroides group were gavaged with 1*106 pieces/mL of bacterial (BeNa Culture, BNCC336948) solution (5 mL/day). At weeks 8, 9, 10, 11, and 12, portal blood flow and thrombosis were detected in rats using small animal ultrasound. The animals were euthanized at 12 weeks under deep anesthesia by intraperitoneal injection with an overdose of pentobarbital sodium (200 mg/kg) to obtain their samples for biochemical and molecular analyses.
Statistical analysis
For bioinformatics statistical analysis, an independent sample t-test was used to analyze normally distributed variables. Non-normally distributed variables were analyzed using the Mann–Whitney U test. A value of p < 0.05 was considered statistically significant. The α-diversity index analysis was performed using Qiime 2 software. Each index of Alpha diversity was analyzed by rank sum test. If two sets of samples are compared, wilcox.test function in R is used, and if more than two sets of samples are compared, the kruskal.test function in R is used.
For clinical data analysis, the statistical analysis was conducted with SPSS software. Continuous variables that exhibited normal distribution were presented as mean ± SD, and the t-test was utilized to compare the differences. We utilized nonparametric tests to compare continuous variables that were not normally distributed. Categorical variables were represented using percentages and integers, and groups were compared using Fisher’s exact test. Statistical significance was determined at a p value of <0.05.
Results
Clinical characteristics
Twelve patients with liver cirrhosis with PVT and 21 patients without PVT as controls were included in this cross-sectional study. The demographic characteristics and clinical parameters of the participants are presented in Table 1. The LC with PVT group had higher levels of platelet and D-Dimer compared to the control group. However, there were no statistically significant differences in body mass index, sex, other laboratory indicators, primary etiology of cirrhosis, or Child-Pugh grade between the two groups (Table 1).
Dynamics of gut microbiota in LC patients with PVT
Initially, we examined the species composition and abundance of gut microorganisms in both groups at both the phylum and genus levels. Firmicutes, Proteobacteria, Actinobacteria, and Bacteroidetes collectively accounted for >95% of the relative abundance in both groups. Compared to the control group, the abundances of Firmicutes (66.8 vs. 66.0%) and Proteobacteria (16.9 vs. 14.8%) in the LC with PVT group were relatively low, whereas the abundances of Actinobacteria (9.0 vs. 10.0%) and Bacteroidetes (6.1 vs. 2.9%) were higher (Figure 1A). At the genus level, the five most abundant bacterial taxa in the LC group were Escherichia-Shigella (14.9%), Blautia (13.4%), Streptococcus (10.7%), Lactobacillus (7.9%), and Enterococcus (7.2%). However, in the LC with PVT group, Blautia (20.2%), Lactobacillus (11.4%), Streptococcus (11.2%), Escherichia-Shigella (8.9%), and Bifidobacterium (8.0%) was detected (Figure 1B). When comparing the alpha diversity of the gut microbiota between the two groups, we found that the Chao1, observed species, and PD diversity indices were significantly higher in the group with LC and PVT than in the control group. The PCoA of beta diversity based on unweighted UniFrac revealed significant differences in community composition between the two groups (Figures 2A–D).
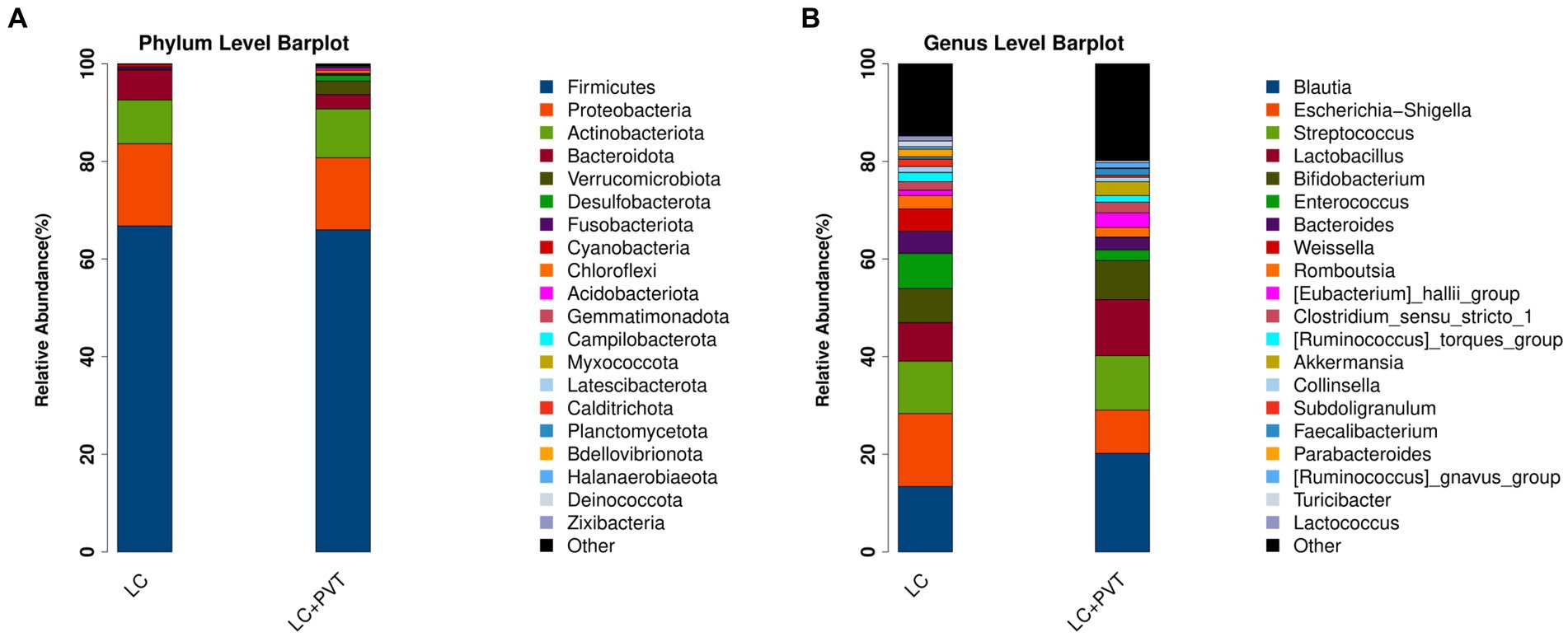
Figure 1. Different profiles of the gut microbiota between the LC with PVT and LC controls. (A) Top 20 abundant phyla. (B) Top 20 abundant genera.
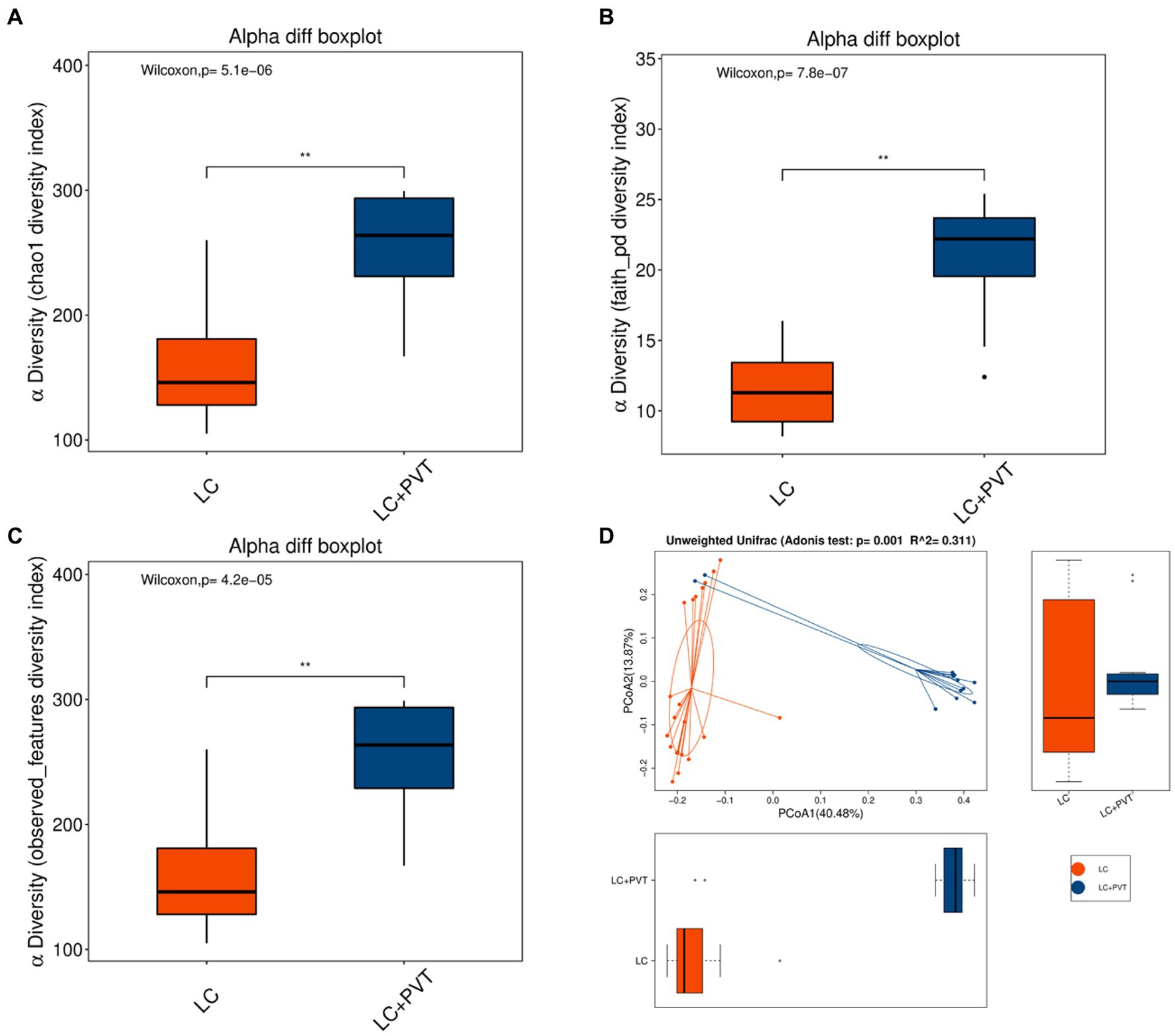
Figure 2. Gut microbiota alpha and beta diversity indices in LC with PVT and LC controls. The box plots depict differences in microbiome diversity indices between two groups using the (A) Chao1, (B) Phylogenetic Diversity (PD) whole-tree distance index, and (C) Observed species. (D) The level of similarity between the detected gut microbiota communities was assessed via an unweighted analysis of similarity (ANOSIMs).
To identify differences in gut microbiota between the two groups, LDA and LEfSe analyses were conducted. Using a logarithmic LDA score with a cutoff value of 2.0, we identified important taxonomic differences and presented the top 30 differential gut microbiota. Our results suggest that there was a remarkable difference in gut microbiota between the two groups. The highest relative abundances in LC with PVT were for Akkermansia, Eubacterium hallii group, Fusicatenibacter, Anaerostipes, and Pseudomonas. In contrast, Enterococcus, Weissella, Bacteroides, Subdoligranulum, Lactococcus, Collinsella, RF39, and Hungatella were enriched in LC controls (Figures 3A,B).
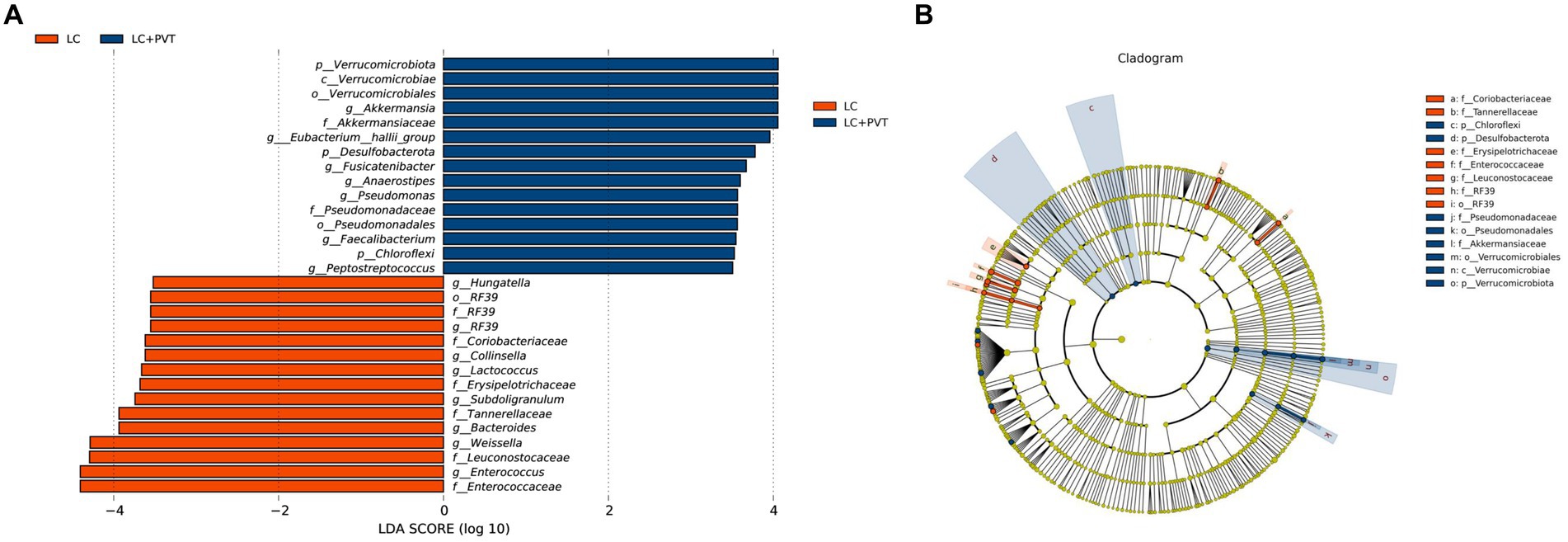
Figure 3. Taxonomic differences in the gut microbiota exhibited by LC patients with and without PVT. (A) The histogram of the LDA scores presented the relative abundance of the main bacterial in the two groups. (B) LEfSe results in a cladogram of gut microbiota between the two groups.
Gut microbiota metabolic pathway analysis
Kyoto Encyclopedia of Genes and Genomes (KEGG) pathways were analyzed using linear discriminant analysis (LDA) effect size (LEfSe) to predict the potential association between PVT and gut microbial metabolism. There were significant differences in gut microbial metabolism between the two groups; the top five candidate metabolic pathways in the LC group were other glycan degradation, pentose phosphate pathway, pentose and glucuronate interconversions, glycosaminoglycan degradation, and glycolysis/gluconeogenesis. The top five metabolic pathways in the LC with PVT group were bisphenol degradation, limonene and pinene degradation, linoleic acid metabolism, toluene degradation, and atrazine degradation (Figure 4A). We further analyzed the associations between the microbes and the derived metabolites (Figure 4B).
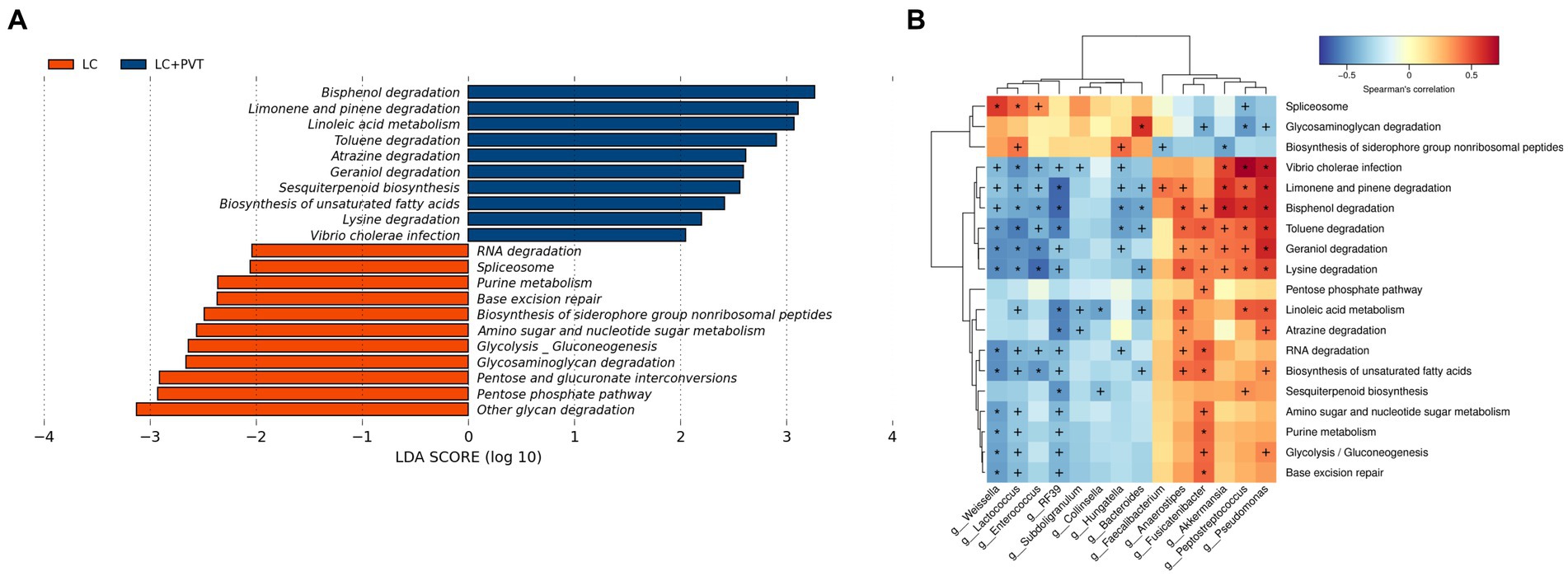
Figure 4. Gut microbiota metabolic pathway analysis. (A) KEGG metabolic pathway analysis. (B) Microbe-derived metabolite enrichment analysis results.
Associations among gut microbiota and coagulation function
We analyzed the potential correlations between differentially abundant gut microbiota and coagulation function parameters (PLT, PT, INR, FIB, and D-Dimer). PLT and D-Dimer were correlated with a partial abundant of gut microbiota. Vibrio, Fusicatenibacter, Halomonas, Ralstonia, Thiomicrorhabdus, Peptostreptococcus, Guyparkeria, and Subgroup-22 significantly and actively correlated with D-Dimer levels, whereas Bacteroides, Adlercreutzia, Incertae-Sedis, Weissella, CAG-352, Lactococcus, Kurthia, and Pediococcus negatively correlated. Monoglobus, Bacteroides, and Adlercreutzia were significantly and negatively correlated with PLT (Figure 5).
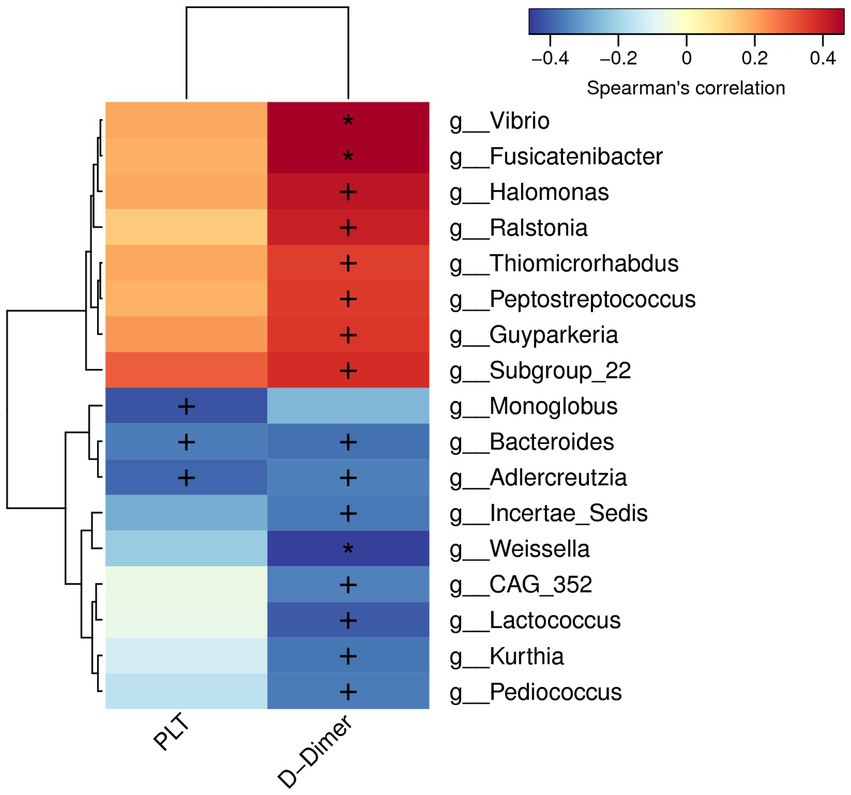
Figure 5. Correlations between differentially abundant microbiota genera and coagulation factor parameters.
Bacteroides improve CCl4-induced PVT in Wistar rats
The Bacteroides, a type of intestinal probiotic, is widely considered as source of novel beneficial candidates for attenuating inflammation (Tan et al., 2019; Guo et al., 2021). In our results, Bacteroides was significantly decreased in the PVT group, so we explored its effect on PVT by supplementing with Bacteroides. Initially, we successfully induced a cirrhosis model using CCL4 and confirmed PVT formation using ultrasound. Supplementation with Bacteroides significantly reduced PVT volume as well as increased blood flow (Figures 6A,B). We examined fibrinogen, D-Dimer, and P-Selectin in plasma by ELISA and showed that they were significantly elevated in the CCL4 group and decreased after Bacteroides treatment (Figures 6C-E). These data indicate that Bacteroides supplementation ameliorates CCl4-induced PVT in Wistar rats.
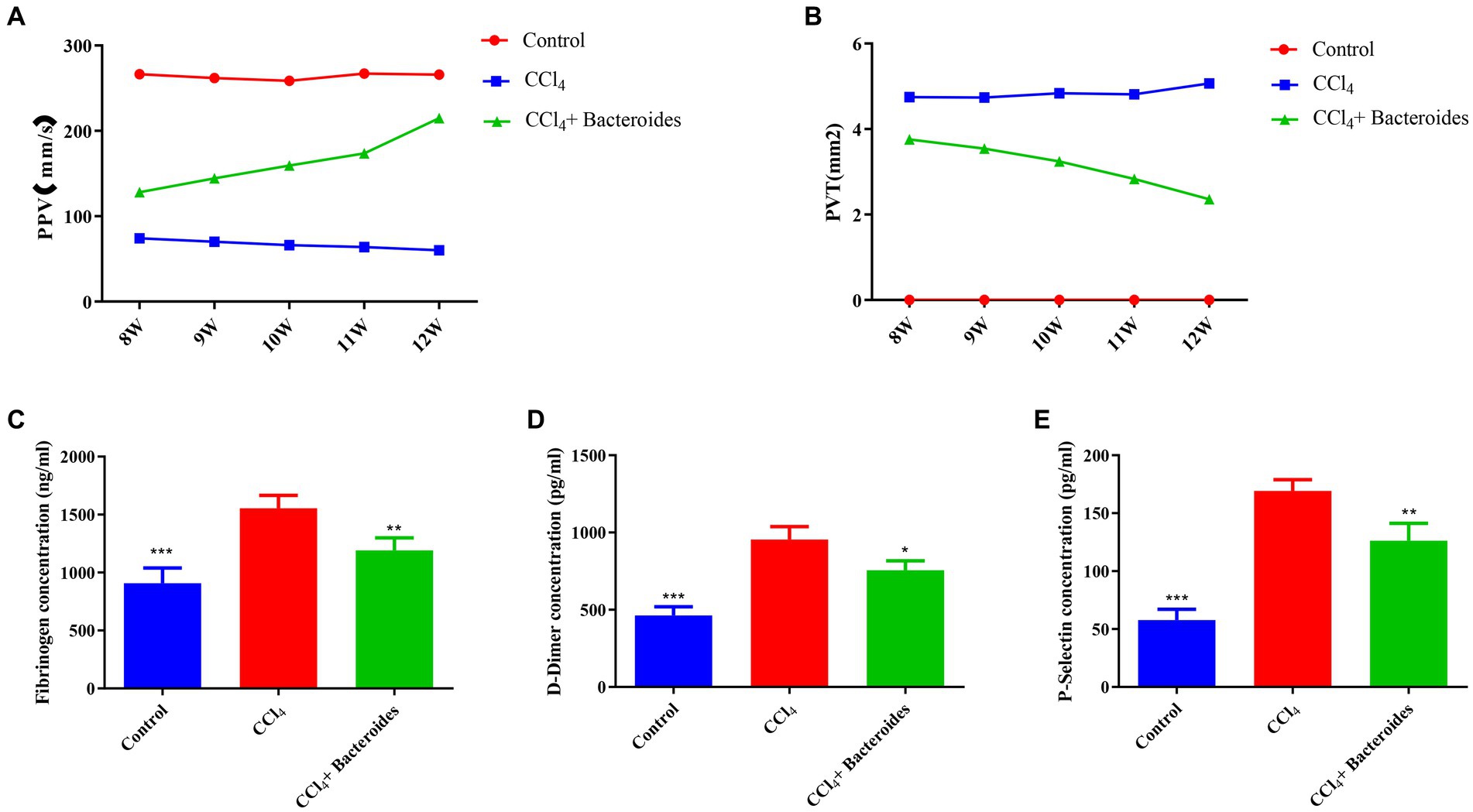
Figure 6. Bacteroides improve CCl4-induced PVT in Wistar rats. Using a small animal ultrasound instrument to detect (A) the blood flow velocity of portal vein, (B) the size of portal vein thrombosis. ELISA analysis of the fibrinogen (C), D-Dimer (D), and P-Selectin (E) in plasma.
Discussion
One of the characteristics of cirrhosis is gut dysbiosis, which is a reduction in gut microbiota diversity with a relative or absolute increase in opportunistic species (Trebicka et al., 2021). PVT is a serious complication of late-stage cirrhosis, and our data suggest that a correlation between the gut microbiota and PVT in LC, gut dysbiosis may be involved in its occurrence, which can affect PVT progression through the following mechanisms: First, translocation of bacteria or bacterial products (such as LPS) into the portal vein accounts for endotoxemia (Fukui, 2015). Hyperendotoxemia aggravates the inflammatory response in the liver (Baffy, 2019) and also leads to abnormal coagulation and PVT formation (Violi et al., 1995). The vasoactive substances released by some gut bacteria may promote visceral vasodilation, resulting in arteriolar vasodilation, reduced systemic vascular resistance, and increased portal inflow, further contributing to portal hypertension, which is a key factor in PVT formation (Wiest and Groszmann, 2002).
Our study focused on changes at the genus level in the gut microbiota and identified distinctive differences in the proportions of several bacterial taxa in fecal samples collected from patients with LC and PVT. Patients with LC typically exhibit gut dysbiosis, which is characterized by an overgrowth of gram-negative bacterial taxa (Chen et al., 2011; Wang et al., 2021). Similarly, in this study, we found that the gut microbiota of patients with cirrhosis mainly comprised gram-negative bacteria. However, in cirrhosis with PVT, the diversity of the gut microbiota increased significantly, and the abundance of pathogenic bacteria such as Anaerostipes and Pseudomonas increased. The abundance of probiotic microorganisms such as Bacteroides and Weissella decreased significantly in cirrhosis patients with PVT. This difference in microbiota also led to significant differences in metabolism, which could lead to large differences in metabolites; however, relevant tests were not performed in this study.
The abundance of Anaerostipes is increased in a variety of diseases, such as endometrial cancer (Walther-António et al., 2016), encephalitis (Gong et al., 2021), and depression (Barandouzi et al., 2020), and it is involved in disease progression, mainly through immune regulation. Pseudomonas is a typical pathogenic bacterium, especially Pseudomonas aeruginosa, which causes many infectious diseases. Similarly, our data also confirms that their abundance increases in the PVT group, these pathogenic bacteria induce an inflammatory response that aggravates liver damage by releasing inflammatory cytokines and LPS.
The Bacteroides, a type of intestinal probiotic, our research confirms that was significantly less abundant in the LC with PVT. We effectively reversed PVT formation by supplementing Carbon tetrachloride-constructed rat models of cirrhosis with Bacteroides, which surfaced that it may be a potential treatment for PVT. Although the specific mechanisms we have not explored in depth, some studies have reported that Bacteroides involved in several functions within the human gut bacteriome, such as the metabolism of polysaccharides and oligosaccharides. Additionally, they aid in the provision of nutrition and vitamins to both the host and other gut microbial residents (Zafar and Saier, 2021). More importantly, it can play an important role in tumors and inflammatory diseases by regulating inflammatory responses (Tan et al., 2019). The other important probiotics microorganism, such as Weissella, plays a beneficial role by anti-inflammatory and immunomodulatory properties (Ahmed et al., 2022). The Weissella supplementation has been shown to have a reversal effect on hepatic fibrosis (Jantararussamee et al., 2021) and fatty liver (Choi et al., 2021). Our results suggest that these probiotics are significantly decreased in LC with PVT, which we speculate plays an important role in the occurrence of PVT.
Coagulation disorders are integral components of liver cirrhosis and are more pronounced in PVT. Consistent with previous reports, D-Dimer levels were significantly elevated in PVT in our study, which promoted thrombus formation (O'Leary et al., 2019; Pan et al., 2022). Interestingly, our results demonstrated a clear correlation between the presence of various microbiome genera and coagulation disorders. The imbalance of inflammatory states and metabolites caused by a large number of gram-negative bacteria and anaerobic bacteria leads to activation of the coagulation cascade (Hasan et al., 2020), which may mediate PVT pathogenesis.
Conclusion
The composition of gut microbiome shows significant variation between LC patients with and without PVT, and certain genera exhibit differing levels of abundance that are correlated with the coagulation function’s clinical characteristics. We emphasizing modulating the gut microbiota, especially Bacteroides, may be a promising therapeutic approach to reduce the progression of PVT in LC.
Data availability statement
The data presented in the study are deposited in the NCBI repository, accession number PRJNA967488.
Ethics statement
The studies involving humans and animal study were approved by The Ethics Committee of Sichuan Provincial People’s Hospital. The studies were conducted in accordance with the local legislation and institutional requirements. The participants provided their written informed consent to participate in this study.
Author contributions
X-yH, Y-hZ, and DZ contributed to conception and design of the study. S-yY, LL, and TM organized the database. S-yY and X-yH performed the statistical analysis. X-yH wrote the first draft of the manuscript. RH, LY, Z-mL, and DZ wrote sections of the manuscript. All authors contributed to the article and approved the submitted version.
Funding
This study was supported by Department of Science and Technology of Sichuan Province (Key R&D Projects, No. 2023YFS0288) and the Sichuan Provincial People’s Hospital Item (2022QN36).
Conflict of interest
The authors declare that the research was conducted in the absence of any commercial or financial relationships that could be construed as a potential conflict of interest.
Publisher’s note
All claims expressed in this article are solely those of the authors and do not necessarily represent those of their affiliated organizations, or those of the publisher, the editors and the reviewers. Any product that may be evaluated in this article, or claim that may be made by its manufacturer, is not guaranteed or endorsed by the publisher.
Abbreviations
PVT, Portal vein thrombosis; LC, Liver cirrhosis.
References
Ahmed, S., Singh, S., Singh, V., Roberts, K. D., Zaidi, A., and Rodriguez-Palacios, A. (2022). The Weissella genus: clinically treatable bacteria with antimicrobial/probiotic effects on inflammation and cancer. Microorganisms 10:2427. doi: 10.3390/microorganisms10122427
Amitrano, L., Guardascione, M. A., Brancaccio, V., Iannaccone, L., Ames, P. R., and Balzano, A. (2002). Portal and mesenteric venous thrombosis in cirrhotic patients. Gastroenterology 123, 1409–1410. doi: 10.1053/gast.2002.36391
Baffy, G. (2019). Potential mechanisms linking gut microbiota and portal hypertension. Liver Int. 39, 598–609. doi: 10.1111/liv.13986
Barandouzi, Z. A., Starkweather, A. R., Henderson, W. A., Gyamfi, A., and Cong, X. S. (2020). Altered composition of gut microbiota in depression: a systematic review. Front. Psychol. 11:541. doi: 10.3389/fpsyt.2020.00541
Bellot, P., Francés, R., and Such, J. (2013). Pathological bacterial translocation in cirrhosis: pathophysiology, diagnosis and clinical implications. Liver Int. 33, 31–39. doi: 10.1111/liv.12021
Chen, Y., Yang, F., Lu, H., Wang, B., Chen, Y., Lei, D., et al. (2011). Characterization of fecal microbial communities in patients with liver cirrhosis. Hepatology 54, 562–572. doi: 10.1002/hep.24423
Choi, S. I., You, S., Kim, S., Won, G., Kang, C. H., and Kim, G. H. (2021). Weissella cibaria MG5285 and Lactobacillus reuteri MG5149 attenuated fat accumulation in adipose and hepatic steatosis in high-fat diet-induced C57BL/6J obese mice. Food Nutr. Res. :65.
Francoz, C., Valla, D., and Durand, F. (2012). Portal vein thrombosis, cirrhosis, and liver transplantation. J. Hepatol. 57, 203–212. doi: 10.1016/j.jhep.2011.12.034
Fukui, H. (2015). Gut-liver axis in liver cirrhosis: how to manage leaky gut and endotoxemia. World J. Hepatol. 7, 425–442. doi: 10.4254/wjh.v7.i3.425
Gong, X., Liu, Y., Liu, X., Li, A., Guo, K., Zhou, D., et al. (2021). Disturbance of gut Bacteria and metabolites are associated with disease severity and predict outcome of NMDAR encephalitis: a prospective case-control study. Front. Immunol. 12:791780. doi: 10.3389/fimmu.2021.791780
Guo, C., Wang, Y., Zhang, S., Zhang, X., Du, Z., Li, M., et al. (2021). Crataegus pinnatifida polysaccharide alleviates colitis via modulation of gut microbiota and SCFAs metabolism. Int. J. Biol. Macromol. 181, 357–368. doi: 10.1016/j.ijbiomac.2021.03.137
Hasan, R. A., Koh, A. Y., and Zia, A. (2020). The gut microbiome and thromboembolism. Thromb. Res. 189, 77–87. doi: 10.1016/j.thromres.2020.03.003
Intagliata, N. M., Caldwell, S. H., and Tripodi, A. (2019). Diagnosis, development, and treatment of portal vein thrombosis in patients with and without cirrhosis. Gastroenterology 156, 1582–1599.e1. doi: 10.1053/j.gastro.2019.01.265
Hepatobiliary Disease Study Group, Chinese Society of Gastroenterology, and Chinese Medical Association (2021). Consensus for management of portal vein thrombosis in liver cirrhosis (2020, Shanghai). J. Dig. Dis. 22, 176–186. doi: 10.1111/1751-2980.12970
Jantararussamee, C., Rodniem, S., Taweechotipatr, M., Showpittapornchai, U., and Pradidarcheep, W. (2021). Hepatoprotective effect of probiotic lactic acid Bacteria on Thioacetamide-induced liver fibrosis in rats. Probiot. Antimicrob. Proteins 13, 40–50. doi: 10.1007/s12602-020-09663-6
O'Leary, J. G., Greenberg, C. S., Patton, H. M., and Caldwell, S. H. (2019). AGA clinical practice update: coagulation in cirrhosis. Gastroenterology 157, 34–43.e1. doi: 10.1053/j.gastro.2019.03.070
Pan, J., Wang, L., Gao, F., An, Y., Yin, Y., Guo, X., et al. (2022). Epidemiology of portal vein thrombosis in liver cirrhosis: a systematic review and meta-analysis. Eur. J. Intern. Med. 104, 21–32. doi: 10.1016/j.ejim.2022.05.032
Senzolo, M., Garcia-Tsao, G., and García-Pagán, J. C. (2021). Current knowledge and management of portal vein thrombosis in cirrhosis. J. Hepatol. 75, 442–453. doi: 10.1016/j.jhep.2021.04.029
Senzolo, M., Riva, N., Dentali, F., Sartori, M. T., and Ageno, W. (2019). Portal vein thrombosis in decompensated cirrhosis: rationale for treatment. Clin. Transl. Gastroenterol. 10:e00026. doi: 10.14309/ctg.0000000000000026
Tan, H., Zhai, Q., and Chen, W. (2019). Investigations of Bacteroides spp. towards next-generation probiotics. Food Res. Int. 116, 637–644. doi: 10.1016/j.foodres.2018.08.088
Trebicka, J., Bork, P., Krag, A., and Arumugam, M. (2021). Utilizing the gut microbiome in decompensated cirrhosis and acute-on-chronic liver failure. Nat. Rev. Gastroenterol. Hepatol. 18, 167–180. doi: 10.1038/s41575-020-00376-3
Tsochatzis, E. A., Senzolo, M., Germani, G., Gatt, A., and Burroughs, A. K. (2010). Systematic review: portal vein thrombosis in cirrhosis. Aliment. Pharmacol. Ther. 31, 366–374. doi: 10.1111/j.1365-2036.2009.04182.x
Violi, F., Corazza, G. R., Caldwell, S. H., Talerico, G., Romiti, G. F., Napoleone, L., et al. (2019). Incidence and recurrence of portal vein thrombosis in cirrhotic patients. Thromb. Haemost. 119, 496–499. doi: 10.1055/s-0038-1676981
Violi, F., Ferro, D., Basili, S., Saliola, M., Quintarelli, C., Alessandri, C., et al. (1995). Association between low-grade disseminated intravascular coagulation and endotoxemia in patients with liver cirrhosis. Gastroenterology 109, 531–539. doi: 10.1016/0016-5085(95)90342-9
Violi, F., Pignatelli, P., Castellani, V., Carnevale, R., and Cammisotto, V. (2023). Gut dysbiosis, endotoxemia and clotting activation: a dangerous trio for portal vein thrombosis in cirrhosis. Blood Rev. 57:100998. doi: 10.1016/j.blre.2022.100998
Walther-António, M. R., Chen, J., Multinu, F., Hokenstad, A., Distad, T. J., Cheek, E. H., et al. (2016). Potential contribution of the uterine microbiome in the development of endometrial cancer. Genome Med. 8:122.
Wang, R., Tang, R., Li, B., Ma, X., Schnabl, B., and Tilg, H. (2021). Gut microbiome, liver immunology, and liver diseases. Cell. Mol. Immunol. 18, 4–17. doi: 10.1038/s41423-020-00592-6
Wiest, R., and Groszmann, R. J. (2002). The paradox of nitric oxide in cirrhosis and portal hypertension: too much, not enough. Hepatology 35, 478–491. doi: 10.1053/jhep.2002.31432
Keywords: liver cirrhosis, portal vein thrombosis, gut microbiota, coagulation disorders, Bacteroides
Citation: Huang X-y, Zhang Y-h, Yi S-y, Lei L, Ma T, Huang R, Yang L, Li Z-m and Zhang D (2023) Potential contribution of the gut microbiota to the development of portal vein thrombosis in liver cirrhosis. Front. Microbiol. 14:1217338. doi: 10.3389/fmicb.2023.1217338
Edited by:
Tianyi Qiu, Fudan University, ChinaReviewed by:
Jinyan Feng, Tianjin Medical University, ChinaDong Wu, Chinese Academy of Medical Sciences, China
Na Jiao, Zhejiang University, China
Copyright © 2023 Huang, Zhang, Yi, Lei, Ma, Huang, Yang, Li and Zhang. This is an open-access article distributed under the terms of the Creative Commons Attribution License (CC BY). The use, distribution or reproduction in other forums is permitted, provided the original author(s) and the copyright owner(s) are credited and that the original publication in this journal is cited, in accordance with accepted academic practice. No use, distribution or reproduction is permitted which does not comply with these terms.
*Correspondence: Di Zhang, Wendy602@sina.com
†These authors have contributed equally to this work