- 1Department of Plant Sciences, Faculty of Biosciences (BIOVIT), Norwegian University of Life Sciences (NMBU), Ås, Norway
- 2Division of Biotechnology and Plant Health, Norwegian Institute of Bioeconomy Research (NIBIO), Ås, Norway
Phytophthora cactorum has two distinct pathotypes that cause crown rot and leather rot in strawberry (Fragaria × ananassa). Strains of the crown rot pathotype can infect both the rhizome (crown) and fruit tissues, while strains of the leather rot pathotype can only infect the fruits of strawberry. The genome of a highly virulent crown rot strain, a low virulent crown rot strain, and three leather rot strains were sequenced using PacBio high fidelity (HiFi) long read sequencing. The reads were de novo assembled to 66.4–67.6 megabases genomes in 178–204 contigs, with N50 values ranging from 892 to 1,036 kilobases. The total number of predicted complete genes in the five P. cactorum genomes ranged from 17,286 to 17,398. Orthology analysis identified a core secretome of 8,238 genes. Comparative genomic analysis revealed differences in the composition of potential virulence effectors, such as putative RxLR and Crinklers, between the crown rot and the leather rot pathotypes. Insertions, deletions, and amino acid substitutions were detected in genes encoding putative elicitors such as beta elicitin and cellulose-binding domain proteins from the leather rot strains compared to the highly virulent crown rot strain, suggesting a potential mechanism for the crown rot strain to escape host recognition during compatible interaction with strawberry. The results presented here highlight several effectors that may facilitate the tissue-specific colonization of P. cactorum in strawberry.
1. Introduction
Phytophthora cactorum is an economically important soil-borne oomycete pathogen that infects more than 200 plant species, a majority of which are herbaceous and woody plants (Erwin and Ribeiro, 1996; Chen et al., 2023). The pathogen has two distinct pathotypes that causes crown rot and/or leather rot in strawberry (Fragaria × ananassa Duch)(van der Scheer, 1971; Harris and Stickels, 1981; Eikemo et al., 2004). Both diseases cause substantial yield losses in strawberry production; in addition the leather rot affects post-harvest processing of strawberry fruits (Harris and Stickels, 1981; Ellis and Grove, 1998; Stensvand et al., 1999; Pánek et al., 2022). The crown rot pathotype can cause disease in both crown and fruit tissues, while the leather rot pathotype causes disease only in the strawberry fruit. Strains of P. cactorum from other hosts such as almond, apple, peach, rhododendron and silver birch can cause leather rot in strawberry, but not crown rot (Eikemo et al., 2004). The use of fungicides such as methyl bromide, mefenoxam, and metalaxyl are effective against P. cactorum (Ellis et al., 1998; De Cal et al., 2004; Maas, 2014); however, the phasing-out of methyl bromide due to its impact on stratospheric ozone, development of fungicide resistance, and strict regulation on the use of metalaxyl in agriculture due its potential high risk on human and animal health have provided challenges to control both the diseases in strawberry (Hrelia et al., 1996; European Food Safety Authority, 2015; Marin et al., 2021; Ali et al., 2022).
The pathogenicity of P. cactorum on strawberry crowns varies among strains and depends on host cultivars (Pettitt and Pegg, 1994; Eikemo et al., 2003; Nellist et al., 2021). For instance, several strawberry cultivars and their wild relatives F. vesca, F. chiloensis, and F. virginiana show varying degrees of susceptibility to both crown rot and leather rot (Parikka, 2003; Shaw et al., 2006; Eikemo and Stensvand, 2015). In a previous study, no correlation was observed between resistance to crown rot and resistance to leather rot among a selected group of strawberry genotypes (Eikemo and Stensvand, 2015).
Phytophthora cactorum reproduces with asexual sporangia that release zoospores upon physical contact with water and with oospores resulting from sexual reproduction. The pathogen is homothallic which limits the possibility of crossing between strains and among pathotypes (Blackwell, 1943; Goodwin, 1997). The genetic distinction between crown rot and leather rot pathotypes have mainly been studied through the use of polymorphic markers (Hantula et al., 2000; Eikemo et al., 2004). Based on random amplified microsatellite markers, no genetic differentiation was observed between strains from leather rot and crown rot of strawberry. However, using amplified fragment length polymorphism markers, Eikemo et al. (2004) showed that P. cactorum strains designated as crown rot and leather rot were genetically different, and that crown rot strains had low genetic diversity. This was later confirmed in a study investigating evolutionary relationships within the Phytophthora cactorum species complex in Europe (Pánek et al., 2016). The genetic difference between crown rot and leather rot pathotypes of P. cactorum could be explained by different sexual behavior in various mating population (Pánek et al., 2021).
The feasibility and cost-effectiveness of sequencing whole genomes have provided a framework to understand disease effector repertoires in several Phytophthora species (Armitage et al., 2018; Zhang et al., 2019; Lee et al., 2020). Species within Phytophthora are known to secret an arsenal of effectors, which play diverse roles in plant colonization (McGowan and Fitzpatrick, 2017; Wang et al., 2019). These effectors may localize to the intercellular spaces of the host (apoplastic effectors) or translocate into different cytoplasmic compartments of the host cell (cytoplasmic effectors). Apoplastic effectors include enzyme inhibitors such as serine and cysteine protease inhibitors, carbohydrate-active enzymes (CAZymes) and cutinases that have been proposed to degrade plant cell wall components, elicitins (sterol-binding proteins), cellulose-binding elicitor lectin (CBEL), Nep1-like proteins, phytotoxins, and PcF (Phytophthora cactorum-Fragaria)-like small cysteine-rich proteins (SCRs) (Orsomando et al., 2001; Kamoun, 2006; Raaymakers and Van den Ackerveken, 2016). Two key groups of cytoplasmic effectors, RxLRs and Crinklers (CRN for crinkling and necrosis) have been identified in Phytophthora spp. The canonical motifs, RxLR-dEER of RxLR effectors and LFLAK-HVLV of CRN effectors that are present downstream of a signal peptide in the N-terminus are known to be involved in translocation into the host. The highly variable C-terminal domain of these effectors is dedicated to diversifying host targets and helps in modulating plant defense responses (Win et al., 2007; Haas et al., 2009; Armitage et al., 2018).
Plants employ diverse immune receptors to sense microbe- or pathogen- associated molecular patterns (MAMPs or PAMPs) and virulence effectors of pathogens, to trigger host immunity (Jones and Dangl, 2006). These includes plasma membrane localized receptor-like kinases (RLKs) and receptor-like proteins (RLPs), also known as pattern recognition receptors (PRRs) and intracellular immune receptors such as nucleotide-binding and leucine-rich repeat proteins (NLRs). The recognition of molecular patterns (MAMPs or PAMPs) by host PRRs activates pattern-triggered immunity (PTI), whereas recognition of effectors by host NLRs activates effector-triggered immunity (ETI) in plants (Dodds and Rathjen, 2010; Boutrot and Zipfel, 2017). Several elicitors from Phytophthora species such as CBEL, elicitins, transglutaminases, and a secreted protein OPEL from P. parasitica act as PAMPs that are sensed by PRRs, and activate PTI (Raaymakers and Van den Ackerveken, 2016; Boutrot and Zipfel, 2017). PTI responses in plants can mediate a broad spectrum and durable resistance against several Phytophthora species (Du et al., 2015). Similarly, the recognition of Phytophthora effectors such as RxLRs by NLRs activate ETI, resulting in localized cell death to restrict growth of the pathogen (Wu et al., 2017). However, the virulent strains of a pathogen species can evade the recognition capabilities of host proteins during a so-called compatible plant-pathogen interaction. This can be accomplished through sequence variations, gene losses and/or transcriptional polymorphisms of the target ligand (Gilroy et al., 2011; Na and Gijzen, 2016). In addition, changes in copy number of effector genes have been proposed as a contributing factor for pathogen fitness (Qutob et al., 2009; Knaus et al., 2019).
Previously sequenced genomes of P. cactorum have been reported with different genome sizes and compositions (Grenville-Briggs et al., 2017; Armitage et al., 2018; Yang et al., 2018a). The Illumina-based genome assemblies of P. cactorum strains 10300 and LV007 were based on strains from infected strawberry (Armitage et al., 2018) and European beech (Fagus sylvatica; Grenville-Briggs et al., 2017), while the PacBio-based genome assembly of P. cactorum was of strain from infected Chinese ginseng (Panax notoginseng; Yang et al., 2018a), designated as “Yunnan” in this work. Recently, a PacBio RS-II-based and several Illumina-based genome assemblies of P. cactorum from infected strawberry and apple (Malus × domestica) have been reported (Nellist et al., 2021). The latter study showed that P. cactorum from strawberry and apple hosts comprises distinct phylogenetic lineages. They identified several candidate RxLR effectors proposed to be involved in host specialization on strawberry crown and apple tissues. The available transcriptome datasets of P. cactorum are also valuable resources for understanding the role of effectors during infection in hosts (Chen et al., 2014, 2018; Nellist et al., 2021).
In the study presented here, we aimed to detect specific genetic differences between the P. cactorum crown rot pathotype and the leather rot pathotype, and to understand the mechanisms of tissue specific colonization of the P. cactorum pathotypes. Hence, we sequenced the genomes of crown rot and leather rot strains using the latest PacBio Sequel II system, which provides single molecule high-fidelity (HiFi) long reads from the circular consensus sequencing (CCS) mode and a base-resolution with >99.8% single molecule read accuracy (Wenger et al., 2019). The use of long HiFi reads can facilitate de novo assembly of repetitive genomes and accurate representation of structural variants, small insertions/deletions and single nucleotide polymorphisms (Hon et al., 2020). We report five new genome assemblies of P. cactorum with high contiguity and accuracy. In addition, we report several new candidate genes that have a potential role in tissue-specific colonization of P. cactorum in strawberry.
2. Materials and methods
2.1. Isolation of Phytophthora cactorum
Plants with visible crown rot or leather rot symptoms were collected from different strawberry and apple plantations in Norway. Infected rhizomes (crowns) or fruits were rinsed in tap water for 20 min to remove superficial soil and debris. The samples were surface sterilized with 70% ethanol for 10 s, followed by treatment with 0.5% sodium hypochlorite for 90 s, and finally rinsed three times with distilled water. Infected plant parts were sliced into rectangular cubes using a sterilized scalpel and were placed on solid PARP medium (Jeffers and Martin, 1986). The plates were incubated in the dark at room temperature (20°C) for 5–7 days and were examined for mycelium growth and typical sporulation patterns (sporangia and oospores) of P. cactorum under light microscopy.
2.2. Plant material and pathogen inoculation
Eleven P. cactorum strains were tested for their ability to cause (1) crown rot in the rhizome of the susceptible strawberry cultivars Polka and Korona; and (2) leather rot on unripe fruits of Korona. Five of the P. cactorum strains from naturally infected strawberry fruits (252360, 252362, 252363, 252364, 252365), and one strain from apple fruit (251539) were tested for their ability to cause crown rot in the highly susceptible cultivar Polka, to identify the leather rot pathotype. The four strains from naturally infected strawberry rhizomes (251171, 251615, 251616, 251683) were tested for their ability to cause crown rot and leather rot in “Korona” (moderately resistant to P. cactorum; Eikemo and Stensvand, 2015), together with previously isolated crown rot strains 10300 (Armitage et al., 2018) and P414 (Nellist et al., 2021). A moderately resistant cultivar was used in this case to study the aggressiveness of different P. cactorum strains based on the degree of necrosis in the rhizome.
Strawberry plants were grown in a greenhouse at 18°C with a 16/8 h light/dark regime. For pathogen inoculation, each P. cactorum strain was grown on 10% V8 (vegetable juice) agar plates (Jeffers, 2015) and incubated at room temperature (20°C) for 2 weeks to promote sporangia formation. Zoospore suspensions were prepared using autoclaved pond water as previously described by Eikemo et al. (2000). Plant tissue was gently wounded using a sterilized scalpel (for rhizome inoculation) or a pipette tip (for fruit inoculation) and inoculated with 2 × 105 zoospores of P. cactorum. Subsequently, the rhizome infection was followed for 4 weeks, and disease symptoms were scored 8-1, where scores 8, 7, 6, 5 represent plants that died due to wilting during the first, second, third, and fourth week after inoculation with P. cactorum, respectively. Plants that survived 4 weeks after inoculation were bisected longitudinally using a sterile scalpel and scored based on the degree of necrosis in the rhizome (4 = clear necrosis covering at least 50% of the rhizome area, 3 = small patches of necrosis, 2 = minor dark brown speckles, 1 = no symptoms) as described by Bell et al. (1997). Inoculated fruits were given a binary score (0 = no infection and 1 = infection).
2.3. Isolation of genomic DNA from Phytophthora cactorum
Genomic DNA was isolated from the mycelium of five P. cactorum strains 251539, 251616, 251683, 252360, and 252365 using a modified phenol-chloroform extraction protocol for Phytophthora (Joint Genome Institute, 2021). Briefly, 5 g of mycelial mat grown in 100 mL V8 broth was collected using a Miracloth (EMD Millipore, Merck, Germany) and ground using a pre-cooled mortar and pestle, plus liquid N2. Ten mL of extraction buffer (0.2 M of tris(hydroxymethyl)aminomethane, pH 8.5, 0.25 M sodium chloride, 25 mM ethylenediaminetetraacetic acid, and 0.5% sodium dodecyl sulfate) was added to the mycelial powder and mixed well using a pipette. Subsequently, 20 μL of 100 μg/mL RNase A (Qiagen, Germany) was added and the lysate was incubated at 65°C for 10 min. Following the incubation, 7 mL of tris-equilibrated phenol, pH 8.0 and 3 mL of chloroform was added, gently mixed, and incubated at room temperature (20°C) for 1 h. Samples were centrifuged at 6,000 g for 15 min at 4°C. The aqueous phase was added to an equal volume of chloroform, gently mixed, and centrifuged at 6,000 g for 5 min at 4°C. After centrifugation, the aqueous phase was transferred to a pre-cooled 50 mL centrifuge tube (VWR, United States), gently mixed with 0.6 volume of chilled isopropanol, incubated on ice for 30 min, and centrifuged at 6,000 g for 30 min at 4°C to recover the precipitated DNA. The DNA pellet was washed three times with 70% ethanol, air dried and dissolved in 300 μL of nuclease free water. The DNA concentration was measured using the NanoDrop™ 2000 Spectrophotometer (ThermoFisher Scientific, United States). An aliquot of the isolated DNA was analyzed using agarose gel electrophoresis run at 30 V for 16 h to assess the quality of the intact genomic DNA for sequencing.
2.4. Genome sequencing
Twenty micrograms (50 ng/μL) of the isolated DNA for each sample was sent to the Norwegian Sequencing Centre, Oslo, for library preparation and sequencing. All five P. cactorum genomic libraries were prepared according to the manufacturer’s protocol for Multiplexing SMRTbell libraries using the SMRTbell Express Template Prep Kit 2.0 (Pacific Biosciences, United States). DNA was fragmented to 20 kb fragments using Megaruptor® 3 (Diagenode s.a., Belgium) and size selected using Bluepippin with 15 kb cut-off. The libraries were sequenced on one 8 M SMRT cell using the Sequel II Binding Kit 2.0 with the sequencing chemistry v2.0 from the PacBio Sequel II (Pacific Biosciences, United States). Reads were demultiplexed using Demultiplex Barcodes pipeline on SMRT Link v 8.0.0.80529 (SMRT Tools v 8.0.0.80502).
2.5. De novo genome assembly, gene prediction, and genome completeness
Raw subreads were trimmed for adapters and low-quality regions and processed to obtain error corrected CCS (circular consensus sequencing) reads. The high-quality CCS (HiFi) reads (Phred quality score ≥ Q20) with a minimum predicted accuracy 0.9 were used for de novo genome assembly using the automated Flye v2.8.2 pipeline (Kolmogorov et al., 2019) in OmicsBox (2019). Because of the high-quality of the sequenced reads and to increase the possibility of detecting true sequence diversity among the five strains of P. cactorum, de novo genome assembly was performed. Assembly statistics were generated using QUAST v5.0.2 (Gurevich et al., 2013) and BUSCO statistics were obtained using BUSCO v4.1.2 (Simão et al., 2015) with the eukaryota_odb10 database (updated on 2020-03-06). The repeat contents of the assembled genomes were obtained using RepeatMasker Open-4.0 (Smit et al., 2015) and RepeatMasker combined library of Dfam_Consensus and RepBase v20170127 (Bao et al., 2015; Hubley et al., 2016). Genes were predicted using the assembled contigs of each P. cactorum genomes in the ab initio mode of Augustus v3.3.3 (Stanke et al., 2008). Augustus was trained using predicted protein sequences from P. cactorum strain 10300 (Armitage et al., 2018).
2.6. Functional annotations and secretome prediction
The predicted proteome of the P. cactorum strains were annotated using a combination of BLASTP (Altschul et al., 1990) against the NCBI non-redundant (Nr) database and InterProScan (Zdobnov and Apweiler, 2001) to assign individual sequences with gene ontology (GO) terms (Ashburner et al., 2000) using Blast2GO (Götz et al., 2008).
Secreted proteins of P. cactorum (secretome) were predicted using a combination of tools that included SignalP v5.0 (Armenteros et al., 2019b) to detect signal peptide cleavage sites, Phobius (Käll et al., 2007) to predict transmembrane domains and signal peptide cleavage sites, TargetP v2.0 (Armenteros et al., 2019a) to predict the presence of mitochondrial transit peptides and signal peptide cleavage sites. The presence of endoplasmic reticulum retention signals (KDEL or HDEL motifs) were predicted as previously described by Evangelisti et al. (2017) using PROSITE-Scan (de Castro et al., 2006). No subcellular localization of “extracellular” as criterion for predicting secretion was considered, as recommended for oomycetes (Sperschneider et al., 2015). A protein was defined as “secreted” if it contained a signal peptide cleavage site within the first 70 amino acids in the N-terminus, had no more than one transmembrane domain, as recommended for oomycetes (Sperschneider et al., 2015), and had no mitochondrial localization or endoplasmic reticulum retention signals. The predicted secretome was used to predict the apoplastic proteins using ApoplastP (Sperschneider et al., 2018).
Genes encoding RxLR and CRN effectors were predicted using the six-frame translation of open reading frames (ORFs) with a minimum cutoff of 100 aa using getorf from EMBOSS (Rice et al., 2000). The translated ORFs were used to search for canonical RxLR-EER motifs (for RxLR effectors) and the LFLAK-HVLV motifs (for CRN effectors) in R using the effectR package (Tabima and Grünwald, 2019). Predicted RxLR and CRN effector proteins were searched for signal peptides in the N-termini using SignalP v5 and annotated using BLASTP (Altschul et al., 1990) against the NCBI non-redundant (Nr) database. Candidate effectors with a predicted signal peptide in the N-terminus and no more than one transmembrane domain were considered high-confidence effectors.
2.7. Gene orthology analysis
Orthologues of the predicted proteome of the P. cactorum strains sequenced in this study (251539, 251616, 251683, 252360, and 252365), the P. cactorum 10300 strain (Armitage et al., 2018), the Yunnan strain (Yang et al., 2018a), and predicted proteomes of publicly available P. capsici LT1534 v11.0 (Lamour et al., 2012), P. infestans T30-4 (Haas et al., 2009), P. palmivora ZC01 v1 (Gil et al., 2020), P. parasitica INRA-310 (NCBI dataset, GCA_000247585.2 PP_INRA-310_V2, unpublished), and P. sojae P6497 v3.0 (Tyler et al., 2006) were identified using OrthoFinder v2.4.0 (Emms and Kelly, 2019). The concatenated orthologues (one ortholog per orthogroup per genome) shared by the P. cactorum strains and other Phytophthora spp. were used to construct a phylogenomic tree using the maximum-likelihood method implemented in the phangorn package (Schliep, 2011), with the Le Gascuel (LG) model for amino acid substitutions (Le and Gascuel, 2008). Predicted secreted proteins of the sequenced P. cactorum strains were compared using Orthovenn2 with expectation (E) value equal to 1e-10 and inflation value equal to 1.5 (default) to obtain higher resolution between groups (Xu et al., 2019). Predicted RxLR and CRN proteins were clustered using the OrthoFinder v2.4.0 and visualized in R using the Tidyverse package collection (Wickham et al., 2019).
2.8. Read mapping and variant calling
The HiFi reads were aligned to the de novo assembled genome of P. cactorum crown rot strain 251616 (used as reference) using pbmm2 v1.3.01 wrapper for minimap2 aligner (Li, 2018). The output bam files were indexed using samtools v1.10 (Danecek et al., 2021), and variants were called in the HiFi reads using DeepVariant v1.0.0 with a pre-defined model (--model_type = PACBIO; Poplin et al., 2018). Phasing of DNA variants was performed using WhatsHap v1.0 (Patterson et al., 2015). The phased variants (SNPs) were filtered and sorted using VCFtools v0.1.15 (Danecek et al., 2011). The resulting phased SNPs and indels (unphased) were annotated, and variant effects were predicted using SnpEff (Cingolani et al., 2012). To perform variant annotations, a manual database was built using the general feature format (GFF3) file of the predicted genes of strain 251616. High-quality SNPs and indels with Phred quality score ≥ 20 were used for downstream analysis. Pairwise alignment of selected protein-coding genes from strain 251616 and a representative leather rot strain 251539 was done using LASTZ v1.04.032 (Harris, 2007). The rate of nonsynonymous and synonymous substitutions (dN/dS or ω) was calculated using KaKs_Calculator 2.0 (Wang et al., 2010). Genes with dN/dS ratio greater than one and p < 0.05 (calculated by the Fisher’s extract test) were considered under positive selection.
3. Results
3.1. Pathogenicity of Phytophthora cactorum strains in strawberry
Seven P. cactorum strains that were isolated from strawberry fruits and apple fruit were tested for virulence in the rhizome (crown) by inoculation in the highly susceptible cultivar Polka. Four of these (252361, 252362, 252363, 252364) developed brown necrotic lesions in the rhizome, and were therefore classified as crown rot (CR) pathotype. In contrast, the strain from apple fruit 251539, and two other strawberry fruit strains 252360 and 252365, did not develop crown rot symptoms (Table 1; Figure 1A). Consequently, the latter three P. cactorum strains were classified as leather rot (LR) pathotype.
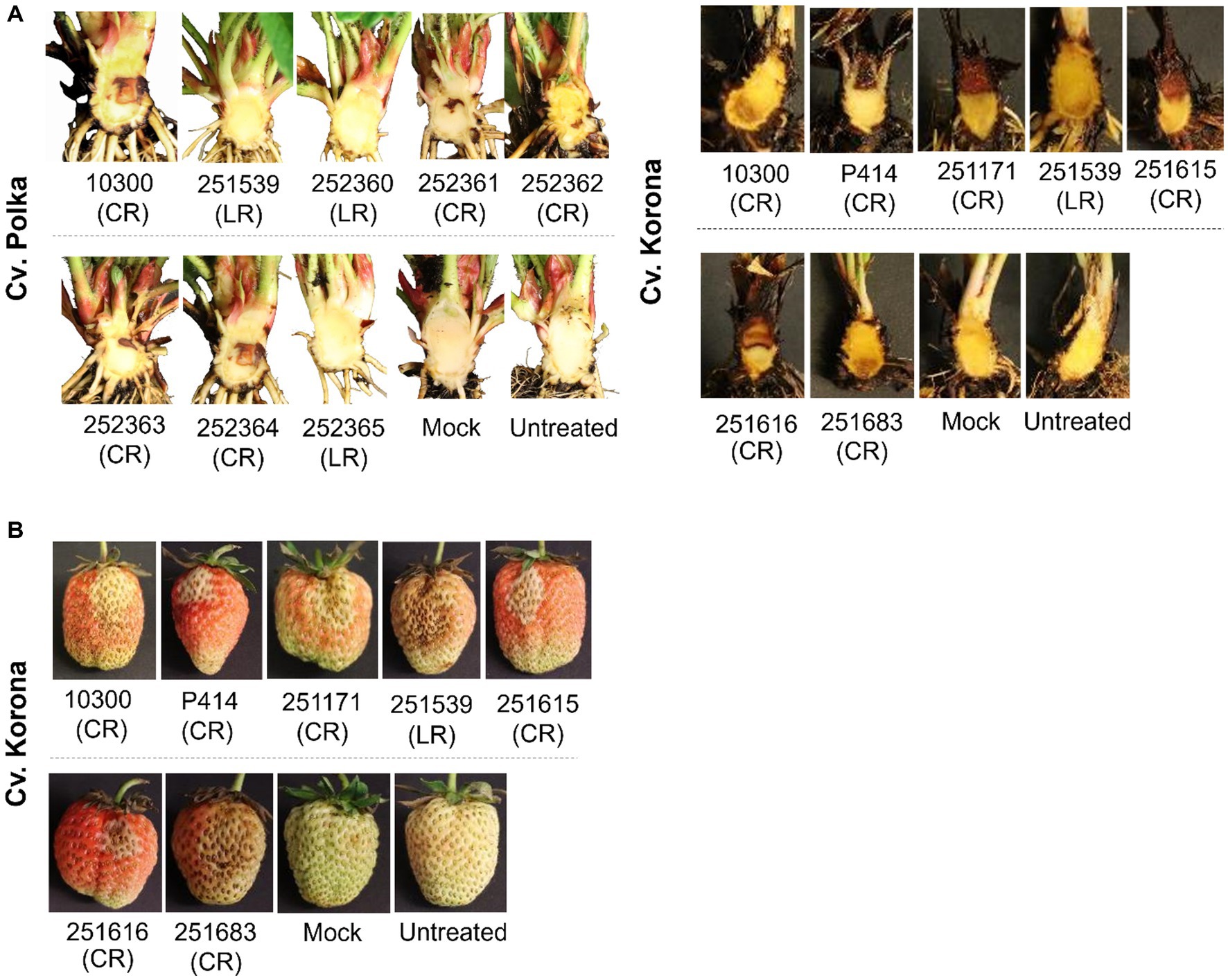
Figure 1. Phytophthora cactorum strains show tissue-specific colonization in strawberry. (A) Crown rot symptoms after inoculation with different strains of P. cactorum into the rhizome of a highly susceptible cultivar “Polka” (left panel) and into the rhizome of a moderately resistant cultivar “Korona” (right panel). Photos were taken 4 weeks after inoculation with P. cactorum zoospores. (B) Leather rot symptoms after inoculation with different strains of P. cactorum into strawberry fruits. A typical fruit discoloration on the infected fruits was observed within 4–6 days after inoculation (dai) with P. cactorum. Each photo is a representative of 5 biological replicates at 4 dai. Negative controls tissues were wounded and inoculated with autoclaved pond water (mock). “CR” indicate crown rot pathotype, whereas “LR” indicate leather rot pathotype.
The four P. cactorum strains isolated from rhizomes with symptoms of crown rot, 251171, 251615, 251616, and 251683, were tested for virulence by inoculation into the rhizome of the moderately resistant cultivar Korona, together with two control crown rot strains (10300 and P414). As expected, all strains developed necrosis in the rhizome, but symptoms varied in severity (Table 1; Figure 1A). Strain 251616 developed a severe necrosis in the strawberry rhizome and is hereafter referred to as high virulence crown rot strain, while the strain 251683 developed few necrotic lesions and is therefore referred to as low virulence crown rot strain (Table 1; Figure 1A). All these strains also developed leather rot on strawberry fruit 4 days after inoculation of berries (Figure 1B).
3.2. Highly contiguous genome assemblies of Phytophthora cactorum strains
The genomes of two CR strains and three LR strains were sequenced. A total of approximately 3.9 million raw reads were obtained with an average read length of 73 kilobases (kb). High-quality CCS (HiFi) reads used for de novo genome assemblies of the five P. cactorum strains resulted in genome sizes of 66.4 to 67.6 Mb, each in 178 to 203 contigs with N50 values ranging from 892.1 to 1036.2 kb. The repeat content of the de novo assembled P. cactorum genomes ranged from 14.5 to 16.1 Mb (mean 15.7 Mb), which constituted a mean of 23.3% of the total genome size. The CR strains of P. cactorum, 251616 and 251683, contained slightly more repetitive elements than the LR strains, 251539, 252360, and 252365 (Table 2).
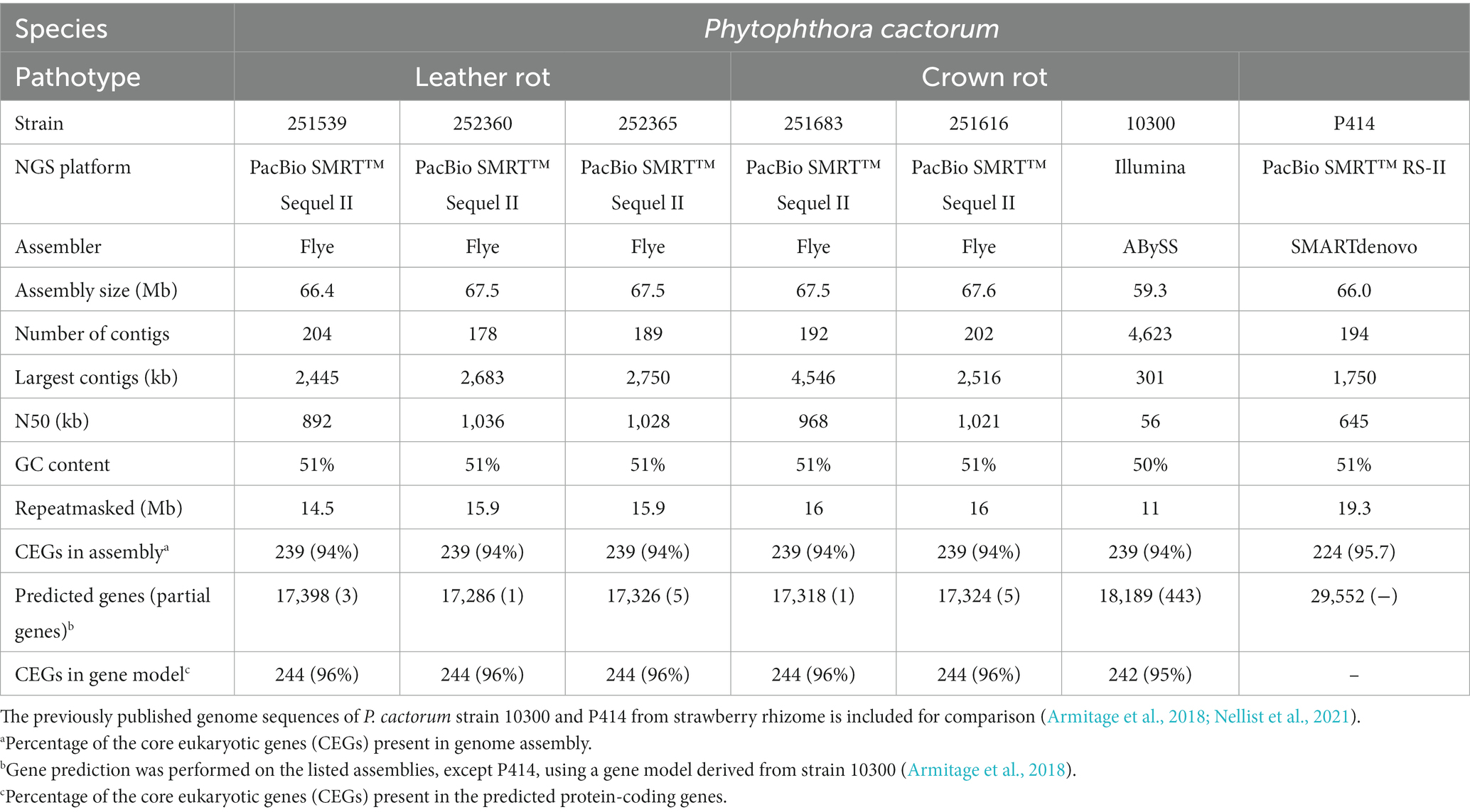
Table 2. Overview of the genome assemblies and gene prediction statistics for Phytophthora cactorum strains used in this study.
The total number of predicted complete genes in the assembled genomes of the five P. cactorum strains ranged from 17286 to 17398 (Supplementary Table S1). All five P. cactorum assemblies contained 239 of 255 core eukaryotic genes (CEGs), representing a genome completeness of 94%, which was comparable to the percentage of CEGs (96%) in the predicted proteomes. The near complete set of CEGs suggested overall accurate gene prediction and support the validity of the used model. The percentage of CEGs was similar in the assembly (94–96%) to those of the previously published CR strains 10300 and P414 assembly (Table 2).
3.3. Comparative genomic analysis of Phytophthora cactorum strains
3.3.1. Phylogenomic analysis
The predicted proteomes of the P. cactorum strains sequenced in this study were compared to the proteomes of P. capsici, P. infestans, P. palmivora, P. parasitica, and P. sojae, to identify orthologous protein sequences. A total of 253,402 protein sequences from 12 genomes were assigned to 21,031 orthogroups, which accounted for 96.2% of all predicted proteomes from all investigated Phytophthora species. For phylogenomic analysis, 2031 single-copy orthologs that were present in all investigated genomes (one copy per orthogroup) were concatenated into one sequence per genome and used to construct a phylogenomic tree using a maximum-likelihood model and 10,000 bootstraps (Figure 2). The P. cactorum strains were highly similar to each other when compared to those of other Phytophthora species (Figure 2A). For finer resolution of the differences between CR and LR strains, a phylogenomic tree containing only the P. cactorum strains sequenced in this study and the previously published crown rot strain 10300 was constructed (Figure 2B). The two LR strains from strawberry (252360 and 252365) clustered, while the LR strain from apple (251539) was phylogenetically distinct from the strawberry strains. The CR strains 251616 (high virulence) and 251683 (low virulence) were closely related but did not form a stable cluster that was distinct from the strawberry LR strains. Instead, 251616 was closer to the strawberry LR strains than to 251683 in 76% of the bootstraps. The previously sequenced CR strain 10300 (medium virulence) was in a separate clade (Figure 2B).
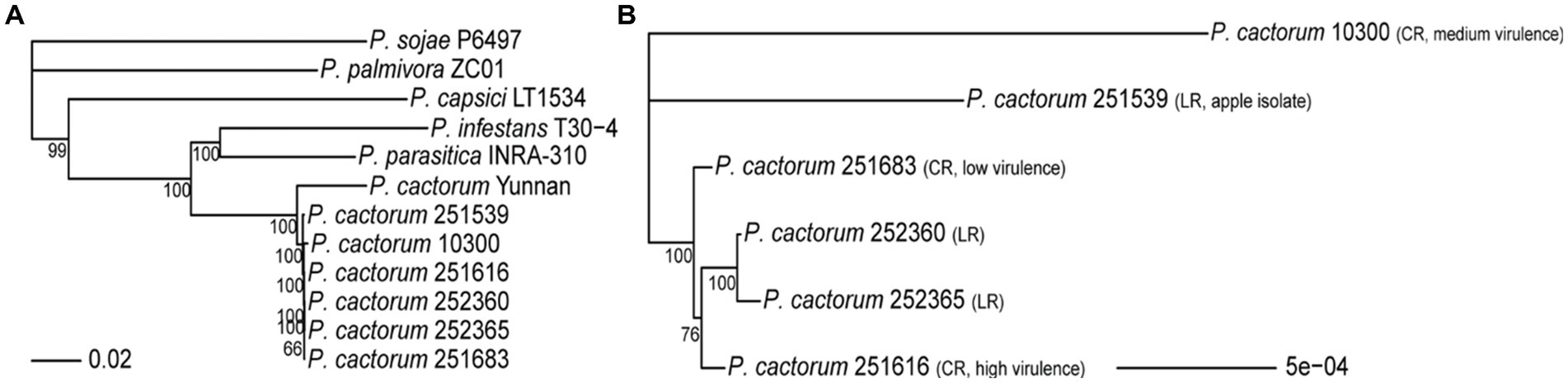
Figure 2. Phylogenomic tree of Phytophthora cactorum based on 2031 single-copy orthologous protein sequences. (A) Maximum-likelihood phylogenomic tree based on 2031 concatenated single-copy orthologues per genome of the sequenced P. cactorum strains (251539, 252360, 252365, 251683, and 251616), the previously published crown rot strain 10300, the “Yunnan” strain as well as other publicly available Phytophthora species. (B) Maximum-likelihood phylogenomic tree containing only the sequenced crown rot (CR) and leather rot (LR) strains of P. cactorum. Nodes were labeled with the percentage of bootstraps (n = 10,000) that supported a given node, values lower than 50% are not shown.
3.3.2. Orthologous clustering of secreted proteins from Phytophthora cactorum strains
To identify the secretome associated with the crown- and leather-rot pathotypes of P. cactorum, genes encoding putatively secreted proteins in the sequenced genomes were predicted and compared using Orthovenn2 (Table 3; Figure 3).
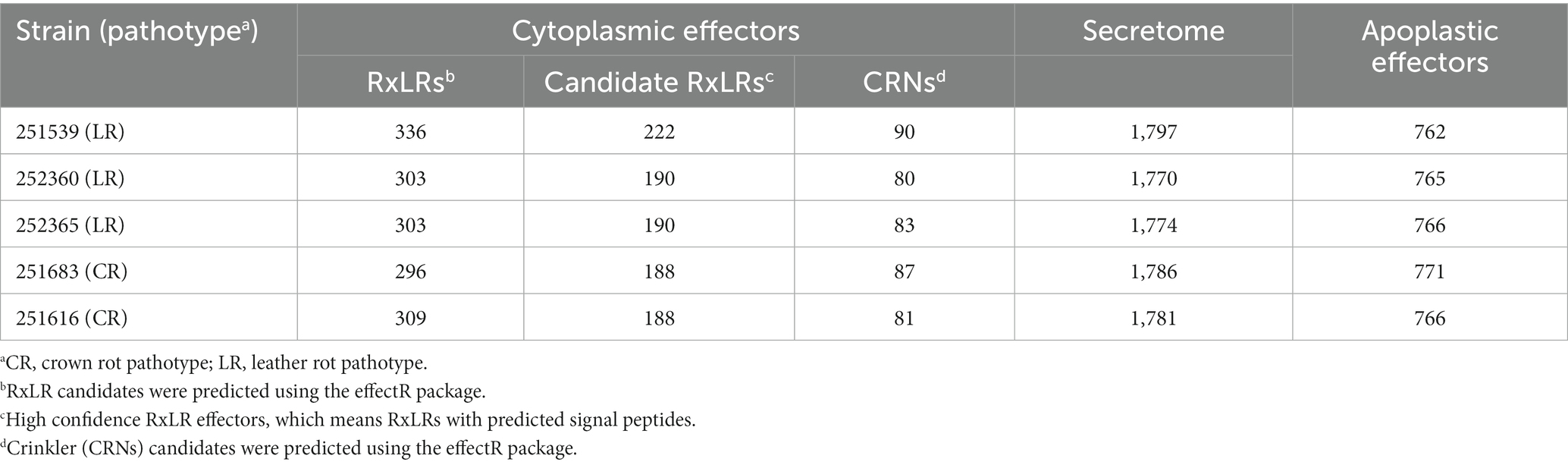
Table 3. The number of predicted cytoplasmic effectors (RxLRs and CRNs), total predicted secretome and apoplastic effectors of the five Phytophthora cactorum strains from strawberry.
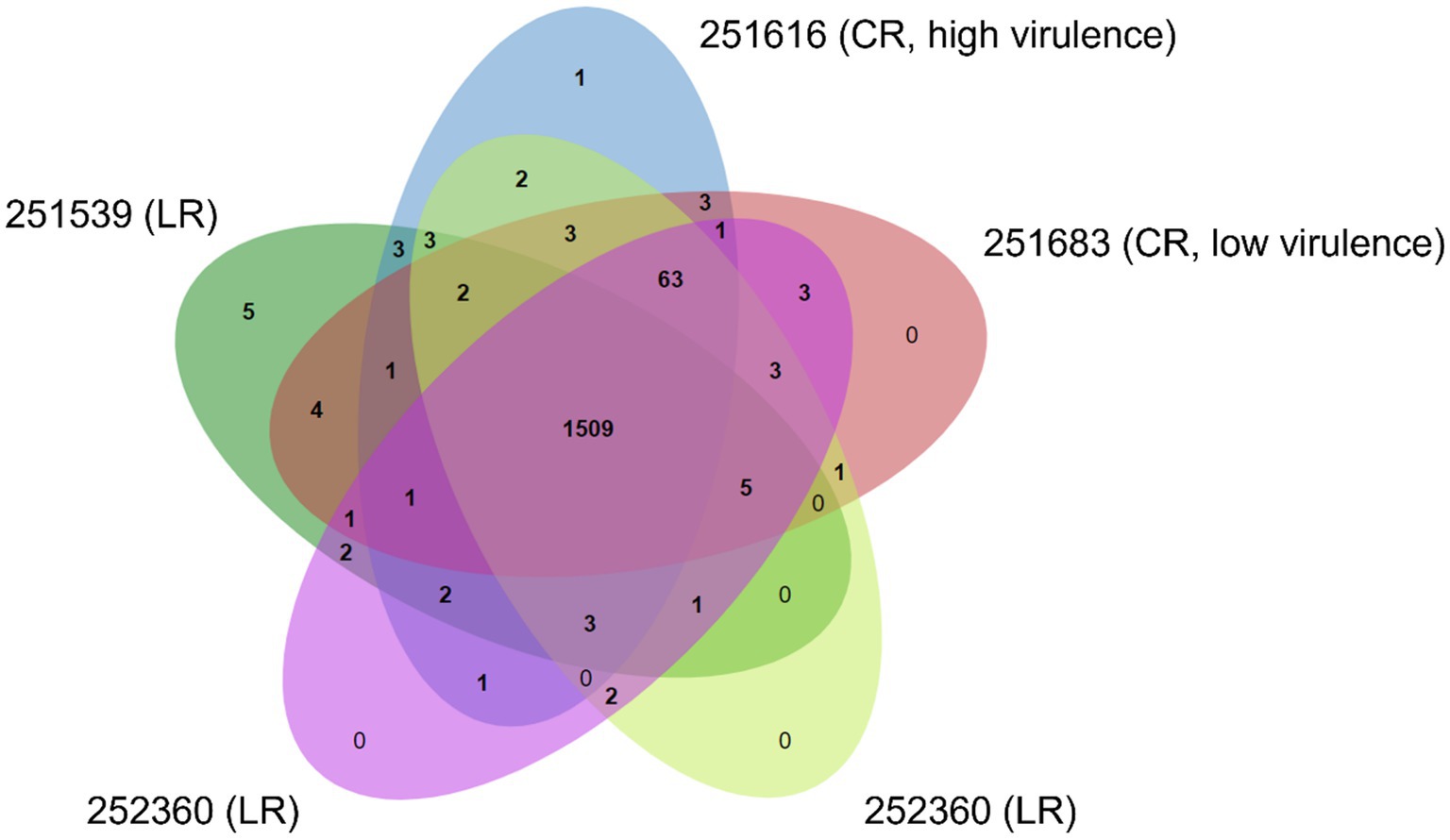
Figure 3. Comparative genomics of the crown- and leather rot pathotypes of Phytophthora cactorum. The Venn diagram shows number of shared and unique ortholog clusters of predicted secreted proteins in strawberry crown rot (CR) strains (251616—high virulence; 251683—low virulence) and leather rot (LR) strains (251539, 252360, and 252365).
The predicted secreted proteins from the two CR strains (251616 and 251683) and the three LR strains (251539, 252360, and 252365) of P. cactorum formed 1,625 clusters. A total of 1,509 clusters containing 8,238 secreted proteins were common to all P. cactorum strains, hereafter referred to as the core P. cactorum secretome (Figure 3; Supplementary Table S2). These included genes potentially involved in pathogenesis such as glucan, lignin, pectin, polysaccharide, xylan and xyloglucan catabolic processes (GO:0045490, GO:0000272, GO:0046274, GO:0009251, and GO:2000899, respectively); response to oxidative stress (GO:0006979); pathogenesis (GO:0009405); serine-type endopeptidase and carboxypeptidase activity (GO:0004252); protein with serine/threonine kinase activity (GO:0004674); proteolysis (GO:0006508, GO:0051603); metal ion binding (GO:0046872); cutinase activity (GO:0050525); and cell redox homeostasis (GO:0045454).
To identify genes specific to CR or LR pathotypes, ortholog clusters unique to the LR strains (251539, 252360, and 252365) and the CR strains (251616 and 251683) were analyzed for their potential role in pathogenesis. In the high virulence CR strain 251616, one unique cluster containing two paralogous genes (251616_g6270 and 251616_g6271) encoding pectate lyases was detected.
The LR strains and the low virulence CR strain (251683) all contain orthologous genes (251539_g13496, 252360_g4306, 252365_g5402, and 251683_g8771) encoding proteins with a fungal-type cellulose-binding domains (CBDs) that formed a cluster. The CBD protein orthologs have sequence similarity to a putative lectin from P. palmivora (accession nr. POM66073.1, sequence identity 70%).
The LR strains and the low virulence CR strain (251683) also contain orthologous genes (251539_g17366, 252360_g7426, 252365_g11583, 251683_g7046) encoding beta-elicitin proteins that formed a cluster. Using manual BLASTP similar protein sequences were identified in the high virulence CR strain 251616 (251616_g15069, 251616_g15070) and also in the previously sequenced CR strains (10300, P414, 15-13, 17-21) and in the apple strains (62471 and R36-14; Armitage et al., 2018; Nellist et al., 2021). Sequence alignment of the beta elicitin protein sequences from the P. cactorum strains revealed two single amino acid polymorphisms in the C-terminus, at positions 154 and 170 (Supplementary Figure S1). All the strains contained two alleles of the beta-elicitin gene. In the CR strains 251616 (251616_g15069, 251616_g15070), P414 (Pcac1_g22870, Pcac1_g22871), and 10300 (10300_g5489, 10300_g17965), both alleles (or gene copies) encoded proteins with threonine residues at these positions, T154 and T169. In the LR strains and the low virulence CR strain one or both alleles encoded proteins with methionine and isoleucine at the same positions, M154 and I169 (Supplementary Figure S1; Supplementary Table S3).
In addition, three genes (251539_g673, 252360_g9838, 252365_g11353) encoding identical protein sequences annotated as small secreted protein with phosphorylation and kinase activity (GO:0016310, GO:0016301) were only detected in the LR strains (Supplementary Table S2).
Comparison of the secretomes of the P. cactorum strains showed 63 clusters containing 299 proteins that did not occur in the strain 251539 isolated from apple. Based on the functional annotations, including protein family domain classifications (InterPro) and GO terms, these proteins are involved in several biological processes and molecular functions such as polysaccharide catabolic processes (GO:0000272, GO:0045490, GO:0030245), e.g., 1,4-beta-D-glucan cellobiohydrolase B (IPR001722), pectate and pectin lyases (IPR002022, IPR011050), pectin esterases (IPR000070), polygalacturonases (IPR000743); proteolysis (GO:0006508) including serine-type endopeptidase activity (GO:0004252), e.g., serine proteases with a trypsin domain and chymotrypsin BII (IPR001254) and cysteine-type peptidase activity (GO:0008234), e.g., cysteine peptidase (IPR000668); proteins with serine/threonine kinase activity (GO:0004674), e.g., serine/threonine-protein kinase (IPR008271); pathogenesis (GO:0009405), e.g., avr4-like secreted RxLR effector protein; carbohydrate metabolic process (GO:0005975), e.g., fructose-1,6-bisphosphatase class 1 (IPR044015; IPR033391); lipid catabolism (GO:0016042), e.g., GDSL (Gly-Asp-Ser-Leu) esterase/lipase (IPR036514); fungal-type cell wall organization (GO:0031505), e.g., glycoside hydrolase (IPR004886;); cutinase activity (GO:0050525), e.g., cutin hydrolase (IPR000675) and several proteins with no GO terms (Supplementary Table S2). Of particular interest was a cluster of 20 small cysteine-rich secretory proteins (cluster 9) with 95% sequence similarity to small cysteine-rich secretory protein SCR99 (accession nr. ALC04449.1), which was not detected in the predicted secretome of the apple strain 251539 (Supplementary Table S2).
3.3.3. Genomic variation in Phytophthora cactorum strains
The genome sequence comparisons of the P. cactorum LR strains (251539, 252360, 252365) and the low virulence CR strain (251683) with the high virulence CR strain (251616; reference) revealed sequence variations, including single-nucleotide polymorphisms (SNPs) and small insertions or deletions (indels). Most of the SNP and indel calls were biallelic, suggesting that the mycelial stage of P. cactorum is diploid. The apple strain 251539 has more genetic variants than the strains isolated from the strawberry host (Figure 4). The total number of high-quality phased SNPs detected in this strain was 5458, which is nearly double the number of SNPs in the LR and low virulence CR strains (Supplementary Table S4). Similarly, the number of high-quality indels in the apple strain 251539 was 15185, which is more than 6-fold the number in the LR and low virulence CR strains (Supplementary Table S5). No phased indels were detected in the P. cactorum strains, and thus, haplotype information could not be inferred for the indels.
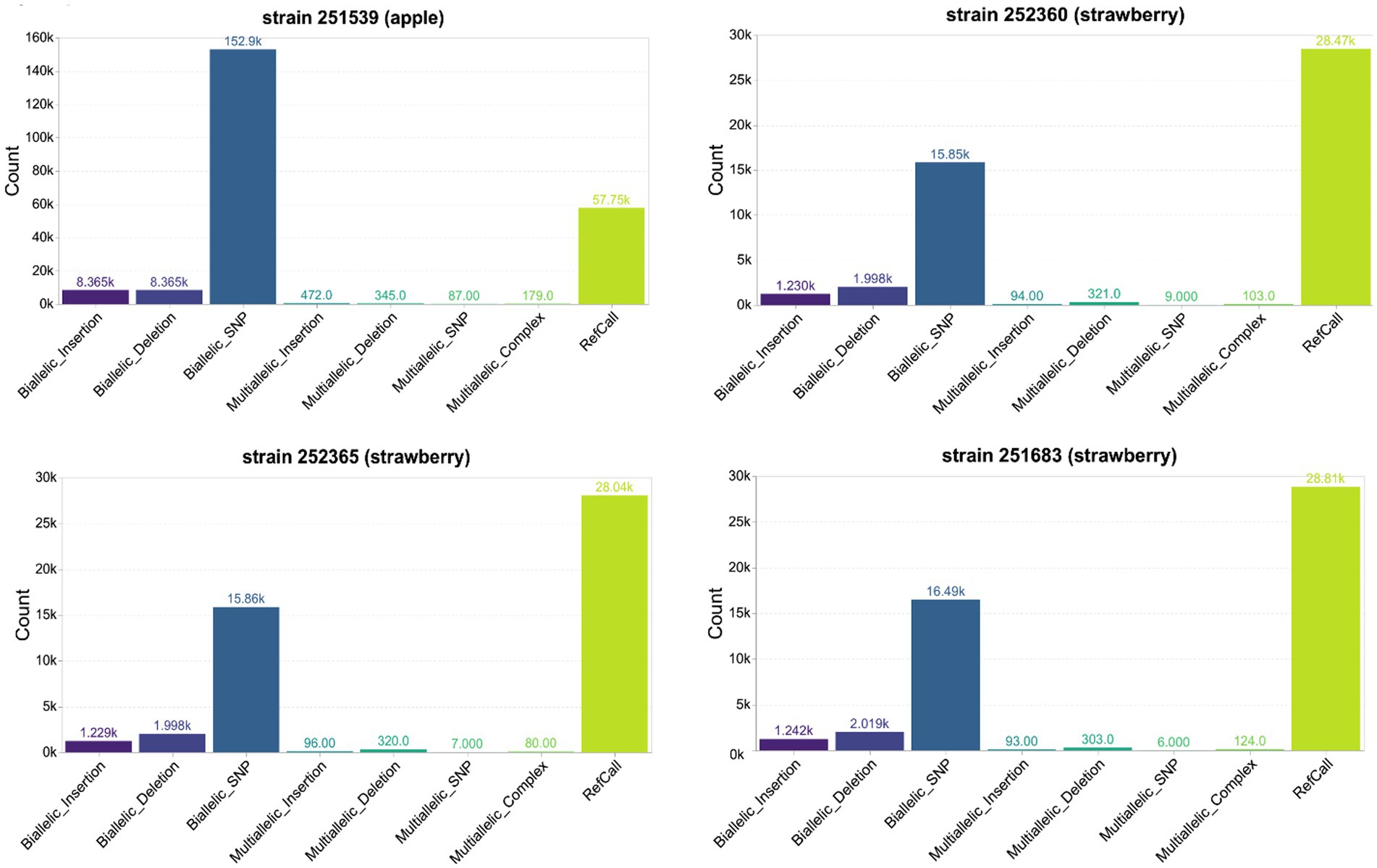
Figure 4. An overview of the variant types detected in the genome of Phytophthora cactorum strains from apple and strawberry hosts. Biallelic variants such as single nucleotide polymorphisms (SNPs) and insertions or deletions (Indels) represent genomic variants with one alternate allele detected at a particular site compared to the corresponding site in the reference genome of strain 251616. Multiallelic variants such as multiallelic insertion/deletion/SNP represent more than one alternate allele, while multiallelic complex indicates variants with multiple alternate alleles. Reference call (RefCall) represent candidates that were determined to match the reference. The y-axis shows the count of variants in thousands (k = 1,000).
To identify genomic variants associated with virulence of P. cactorum on strawberry crown, the SNPs and indels detected in the LR strains, 251539, 252360, and 252365, were compared and assigned to the genomic locations in the reference CR strain 251616. Most of the SNPs and indels shared by all LR strains were detected upstream of predicted genes (Figures 5A,B). Forty-six synonymous, 68 nonsynonymous (missense), and two nonsense mutations were detected in the coding sequences (Supplementary Table S4). Nonsynonymous mutations were detected in the genes encoding an ankyrin repeat domain-containing protein (g10239), an electron transfer flavoprotein beta subunit (g344), a fungal-type cellulose-binding domain-containing protein (g13858), a hemolysin-type calcium-binding protein (g15182), a homeobox protein Wariai (g15156), a hypothetical protein PC110g22478 (g14092), a maleylacetoacetate isomerase (g15372), a mucin protein (g87), an RNA methylase (g16315), a RING finger protein (g16013), an SNQ2 protein (g14515; Figure 5C). More nonsynonymous than synonymous mutations were observed in the genes encoding a membrane protein, a cyclic AMP-specific 3′ protein, a Harbinger transposase-derived nuclease domain protein and a hypothetical protein PC110_g23417 (Figure 5C). To investigate whether these genes were under selective pressure, the rate of nonsynonymous and synonymous mutations per substitution sites (dN/dS or ω) was calculated. The ω was greater than 1 in all the above-tested genes, which signifies positive selection; however, the p-values were greater than 0.05 (Supplementary Table S4). Thus, no evolutionary inference could be drawn for the selected genes.
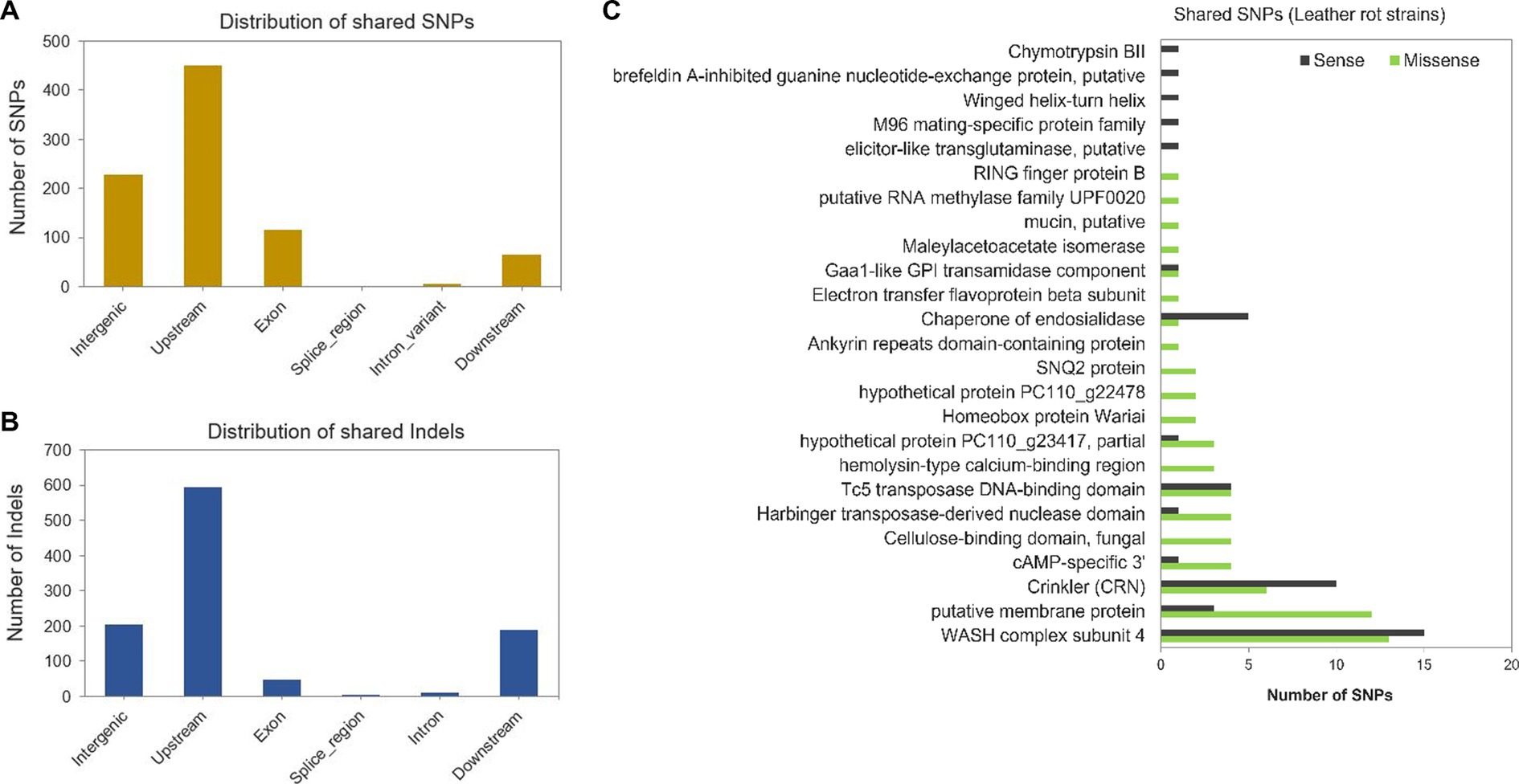
Figure 5. Genomic variants detected in Phytophthora cactorum strains. (A) Distribution of shared SNPs and (B) Indels present in the HiFi reads of leather rot strains 251539, 252360, and 252365 compared to the assembled genome of high virulence crown rot strain 251616 (reference). (C) The number of shared synonymous (sense) and nonsynonymous (missense) variants detected in genes belonging to different annotated classes.
Most of the indels in the coding regions caused frameshift mutations (29%). These were in genes encoding an ankyrin repeat protein, a Crinkler, a Harbinger transposase-derived nuclease domain protein, an RNA methylase, a ribonuclease H-like domain protein, a RING finger protein, a WASH complex subunit 4 protein, a voltage-gated potassium channel subunit beta protein, and in three hypothetical proteins (Supplementary Table S5). In addition, in-frame indels were detected in several genes encoding an annexin family protein, a cellulose-binding domain protein, a Crinkler protein, a cysteine-rich secretory protein, a mucin protein, and a transmembrane protein (Supplementary Table S5).
3.3.4. Predicted RxLR and Crinkler effectors in Phytophthora cactorum
The initial prediction of candidate RxLR effectors with the RxLR-ERR motif and Crinklers (CRNs) with the LFLAK-HVLV motif using regular expressions (regex) and a Hidden Markov Model (HMM) resulted in varied numbers of candidate effectors in the five sequenced P. cactorum strains (Table 3). Most of the predicted CRN effectors lacked a conventional signal peptide in the N-terminus. The prediction tool Phobius predicted more CRNs with a signal peptide than SignalP5 (Supplementary Table S6). Thus, all CRN effectors with or without a signal peptide were considered for further analysis. To identify candidate effectors involved in the infection of the strawberry rhizome, predicted high-confidence RxLR and CRN effectors from the CR and LR strains were compared. The comparison of RxLR protein sequences revealed several orthogroups that contained multiple copies of RxLRs, and notably differences in copy number between the CR and LR strains (Figure 6; Supplementary Figure S2). A higher number of RxLRs in orthogroup OG0000414 was observed in the LR strains than in the CR strains. This orthogroup, contained a gene encoding a protein with similarity to the P. palmivora avirulence-like protein Avr1b-1 (accession nr. POM73746.1, 74.5% sequence identity). By contrast, the orthogroup OG0001375 contained more RxLR copies in the CR strains than the LR strains.
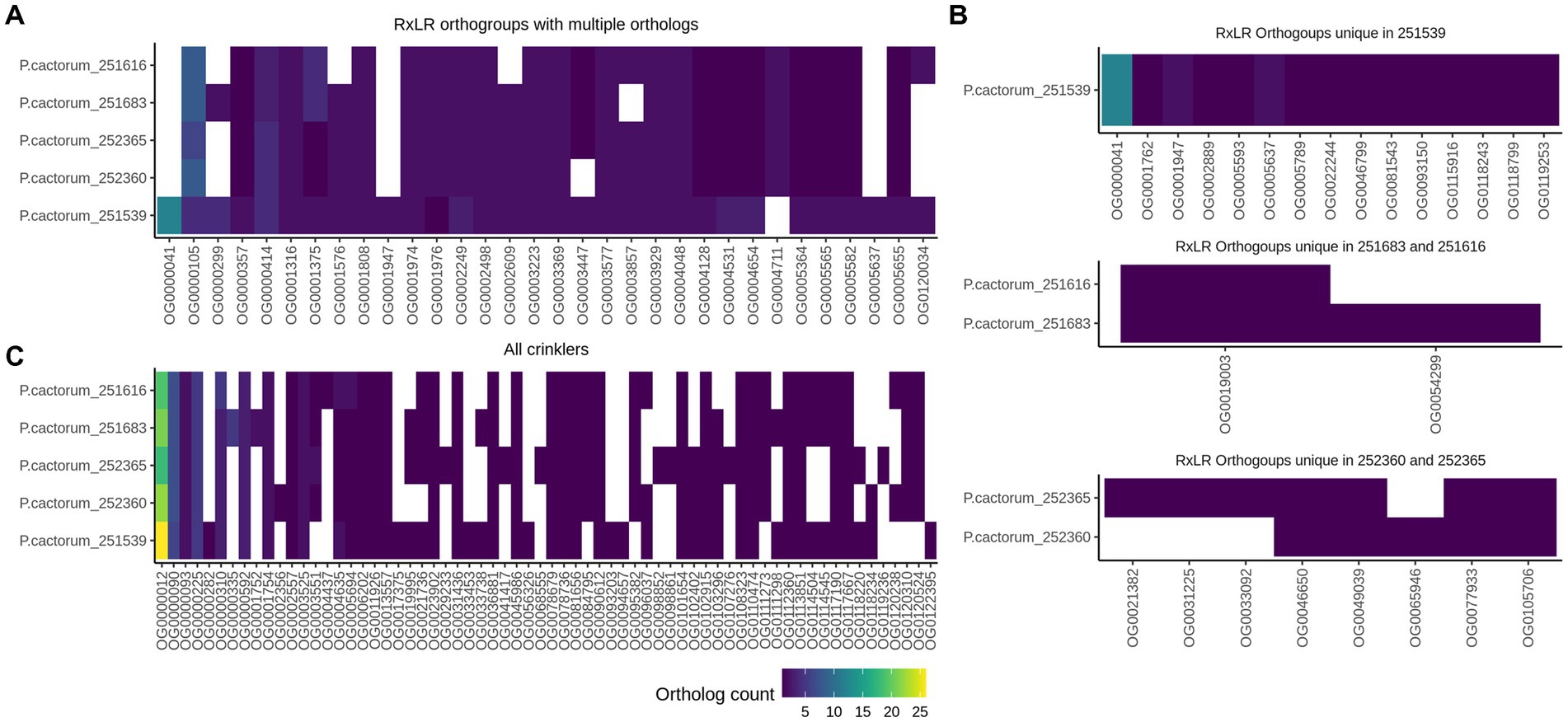
Figure 6. Shared and unique predicted RxLR and Crinkler effector genes in five Phytophthora cactorum strains. (A) High-confidence candidate RxLRs with multiple gene clusters per orthogroup in the genome of crown rot strains (251616—high virulence and 251683—low virulence) and the leather rot (LR) strains (251539, 252360, and 252365). (B) Unique RxLR orthogroups in the apple strain 251539, unique orthogroups in the crown rot and leather rot strains. (C) Orthogroups containing predicted Crinkler effectors in the genome of P. cactorum strains. Each column represents an orthogroup. The color gradient represents number of RxLR and Crinkler genes per genome.
No RxLR candidates were identified that were unique to the CR strains. Conversely, the LR strains contained an orthogroup OG0024976 with a single gene, which was not found in the CR strains. In addition, all LR strains and the low virulence CR strain (251683) contained orthogroups OG0001576, OG0002609, OG0005599, and OG0057065, which was not detected in the high virulence CR strain 251616 (Figure 6A; Supplementary Table S6). Several unique orthogroups of RxLRs were also identified in the apple strain 251539 (Figure 6B).
The comparison of CRN effectors of P. cactorum strains showed a similar pattern of expansion and contraction of genes in certain orthogroups as the RxLRs (Figure 6C). CR strains contained a higher number of CRN genes in orthogroups OG0000310 and OG0005694 than in the LR strains. Furthermore, the high virulence crown rot strain contained an orthogroup OG0004437 with a single CRN gene 251616_g2371 that was unique to this strain (Figure 6C; Supplementary Table S6).
4. Discussion
4.1. Highly contiguous and accurate genome assemblies of Phytophthora cactorum
Highly contiguous genome assemblies provide a better framework to understand the biology of the studied organisms by offering better information on the genomic context of traits of interest. In this work, long HiFi reads facilitated high-quality genome assemblies of the five sequenced P. cactorum strains. The total number of contigs ranged from 178 to 203, which was a major improvement from the 4,623 contigs of our previously sequenced P. cactorum 10300 genome assembly and 3644-20136 contigs of other publicly available P. cactorum genome assemblies (Grenville-Briggs et al., 2017; Armitage et al., 2018; Nellist et al., 2021). The genome sizes of the P. cactorum strains sequenced here were similar to the 10300 assembly and several other P. cactorum assemblies recently reported (Nellist et al., 2021), and therefore, according to expectations. The high number of contigs and low N50 value in the Yunnan assembly might help to explain the unexpectedly large genome size of 121.5 Mb and a distinct clade in the phylogenomic analysis in this work (Figure 2A). The previously sequenced genome of strain P414 (deposited as a reference genome in NCBI) assembled into 194 contigs (Nellist et al., 2021), which is in the range of our five newly sequenced genomes reported here. However, the new genome assemblies have 38–60% higher (and better) N50 value than the P414 assembly, which likely improves genome quality, particularly in regions of repetitive sequences (Table 2).
4.2. Genomic variations between crown rot and leather rot pathotypes of Phytophthora cactorum
The whole genome phylogenomic study showed that P. cactorum strains from the strawberry rhizome or fruit tissues were genetically more similar than the apple strain 251539. The result was consistent with the variant analysis that identified a higher number of SNPs and small indels in the apple strain than the P. cactorum strains from strawberry host. A similar study showed that P. cactorum from strawberry and apple hosts were genetically two distinct population that formed two non-recombining clades for strawberry CR and apple strains (Nellist et al., 2021). The present study supports the previous findings that describe P. cactorum as species complex and should not be considered as generalist pathogen.
Understanding the mechanisms of plant recognition of pathogen molecules (PAMPs/MAMPs) and identifying virulence determinants of the pathogen is essential to develop effective strategies to control strawberry diseases. The comparative genomic analyses of the crown- and leather-rot strains revealed genes encoding proteins potentially involved in pathogenesis, with sequence variations and presence or absence polymorphisms. The presence of two unique pectate lyase genes (251616_g6270 and 251616_g6271) in the CR strain 251616 might contribute to the virulence in the strawberry crown. Interestingly, the homologous gene Pcac1_g14072 of the previously sequenced CR strain P414 was shown to be upregulated during early infection in strawberry (Nellist et al., 2021). Pectate lyases secreted by plant pathogens are known to degrade networks of pectin present in the plant cell wall. They cleave the glycosidic bonds of two saccharide units via a β-elimination mechanism (Collmer and Keen, 1986; Herron et al., 2000). Studies have shown that pectate lyases contribute to virulence of bacteria, fungi, nematodes, and oomycetes (Wegener, 2002; Fu et al., 2013; Cho et al., 2015; Chen et al., 2021). A study of pectate lyase genes PL1, PL15, PL16, and PL20 from P. capsici showed that overexpression of these genes in a mildly virulent strain transformed it to a highly aggressive strain (Fu et al., 2015). Another study demonstrated that deletion of a pectate lyase gene PEL1 in the fungal pathogen Verticillium dahliae compromised virulence in Nicotiana benthamiana and cotton plants (Yang et al., 2018b). The two pectate lyases genes in the high virulence CR strain (251616_g6270 and 251616_g6271) are promising candidates to further study P. cactorum virulence in strawberry.
A unique cluster of putative CBD proteins was observed in all the LR strains. Previous studies suggested that CBD proteins are involved in oomycetes cell wall development and interacts with plant cell wall components (Gaulin et al., 2006; Jones and Ospina-Giraldo, 2011). The presence of CBD proteins at the cellular surface of Phytophthora facilitates binding to cellulosic substrates (Gaulin et al., 2002). In plants, alteration of cellulose in the plant cell wall is a warning signal to activate defense responses (Dumas et al., 2008). Although, an increased accumulation of cytosolic calcium was reported in response to CBEL in tobacco cells (Dumas et al., 2008), the exact mechanism of plant defense is unknown. Studies have shown that Phytophthora CBD proteins with lectin-like activities such as CBELs act as potent elicitors of plant defense (Mateos et al., 1997; Gaulin et al., 2006). In this study, the CBD protein-coding genes (251539_g13496, 252360_g4306, 252365_g5402, and 251683_g8771) was of particular interest as these were identified in a cluster unique to the LR strains and the low virulence CR strain. A similar CBD gene (251616_g13858) with four nonsynonymous but no synonymous SNPs was detected in the high virulence CR strain, reflecting signs of positive selection or result of functionally neutral mutation fixed due to drift.
In addition to the variations in the CBD proteins between the high virulence CR strain and the LR strains (including the low virulence CR strain), two single amino acid polymorphisms (T154M and T169I) were observed in the C-terminus of a beta elicitin protein. Interestingly, threonine residues were conserved in both the alleles of the beta elicitin in the high virulence CR strain 251616 and in the previously sequenced CR strains 10300 and P414, except in the strains 15–13 (M154, I169) and 17–21 (M154, T169; Supplementary Table S3). All LR strains from strawberry fruit and apple including the low virulence CR strain 251683 contained one or both alleles with methionine and isoleucine at these positions. Many Phytophthora elicitins are rich in threonine, serine and proline residues at their C-terminal domain and these residues are suggested to be involved in association with the cell wall through extensive glycosylation (Jiang et al., 2006). Elicitins are often recognized as PAMPs by the host defense machinery, possibly by immune receptors such as BRI1-associated receptor kinase 1 (BAK1) and cell wall-associated receptor-like kinase 1 (WAK1) (Chaparro-Garcia et al., 2011; Raaymakers and Van den Ackerveken, 2016). Pernollet (1993) studied the role of threonine residues in α-elicitin from P. cactorum and proposed that the replacement of a lysine residue by threonine is partly responsible for reduced necrotic activity of the α-elicitin, possibly by avoiding the host defense machinery (Pernollet, 1993). Similar peculiarities were observed in a beta elicitin protein sequence from P. megasperma that was less toxic than related proteins from other Phytophthora spp. (Huet and Pernollet, 1993). Furthermore, cysteine-rich secretory proteins encoded by the SCR-108-like gene cluster detected in the LR strains are interesting candidates for host recognition as similar SCR (e.g., SCR96) of P. cactorum was reported to induce cell death responses in other host plants (Chen et al., 2016). Further study is needed to examine if the putative elicitor genes such as CBD, beta elicitin and SCR-108-like from the LR strains are expressed during interaction with strawberry. It is worth mentioning that the expression of the beta elicitin genes (Pcac1_g22870, Pcac1_g22871) from the CR strain P414 were upregulated during strawberry infection in the transcriptome dataset previously reported by Nellist et al. (2021).
Several genes encoding annexin family members, Crinklers, cysteine-rich secretory protein, a mucin protein, a putative transmembrane protein had sequence variation in the LR strains compared to the high virulence CR strain. Some of the gene products from these, such as an annexin family protein and mucin have been reported to be associated with the cell surface in the close relative P. infestans (Grenville-Briggs et al., 2010). Larousse et al. (2014) studied the role of mucin-like genes in P. parasitica and found that their gene products accumulated on the surface of biofilms, which favor attachment and promote virulence through aggregation. Thus, sequence variations in these genes might affect P. cactorum tissue specific interaction or pathotype.
The high virulence CR strain may possess altered recognition domains or have lost potential elicitors as a mechanism to escape host defense. Since the LR strains were able to cause disease in strawberry fruits after inoculation, but not in the rhizome of the same cultivar, led us to question whether activation of host defense machinery was tissue specific. Eikemo and Stensvand (2015) studied the resistance level of both the tissues (rhizome and fruit) of the same strawberry cultivars against crown rot and leather rot. They reported that cultivars most robust to leather rot was susceptible to crown rot and vice versa. Thus, the mechanisms for resistance against the two diseases seem to be different on the two tissues types. Casado-Díaz et al. (2006) studied the spatial expression pattern of defense-related genes in strawberry during infection with Colletotrichum acutatum, a hemibiotrophic fungal pathogen. They reported that expression of a leucine rich repeat receptor-like protein-coding gene Falrrk-1 and a chitinase gene Fachit-1 was strongly downregulated in infected fruit tissue, while their expression increased several folds higher in the infected rhizome (crown) tissue. They also found that strawberry fruit was more susceptible to C. acutatum infection than crown tissues. Thus, host-pathogen interactions may be influence by tissue architecture and/or differential expression of defense-related genes. The identification of potential elicitor genes of P. cactorum warrants further investigation to understand the virulence mechanisms of the CR and LR pathotypes in strawberry. In addition, several secreted protein-coding genes were detected only in the genome of P. cactorum strains from strawberry host and not present in the genome of apple strain. Most of these genes encode proteins that have a putative role in pathogenesis, suggesting co-evolution and acquisition of pathogenesis-related genes during host adaptation as observed in other pathosystems (Gaulin et al., 2018; Jangir et al., 2021).
The new genome assemblies of P. cactorum identified a greater number of predicted RxLRs via the REGEX and HMM models than previously reported for other strains of the species (Armitage et al., 2018; Yang et al., 2018a; Nellist et al., 2021). Remarkably, most of the predicted CRNs lacked a conventional signal peptide in the N-terminus. It has been proposed that CRN effectors that lack a conventional signal peptide might translocate into host cells via an unconventional secretion pathway (Meijer et al., 2014; Amaro et al., 2017; Voß et al., 2018). The number of predicted CRN effectors was in agreement with the previously published genomes from P. cactorum strains (Armitage et al., 2018; Nellist et al., 2021) and P. palmivora P16830 (Evangelisti et al., 2017).
The analysis of the predicted RxLR and CRN effectors provided evidence for expansion and contraction of certain gene clusters in the investigated P. cactorum strains (Figure 6). In the high virulence CR strain, the unique CRN gene 251616_g2371 and the expansion of CRNs genes in the orthogroups OG0000310 and OG0005694 need further investigation for their virulence function in the P. cactorum-strawberry interaction. In addition, the loss of RxLR genes in orthogroup OG0000414 in this strain should be considered for future functional studies, if they are expressed during infection of strawberry. Among the reduced effector genes in this strain was an RxLR gene with sequence similarity to Avr1b-1 from P. palmivora. Gene loss has been recognized as an important mechanism for emergence of virulence in bacterial, fungal, oomycete pathogens (Maurelli, 2007; Qutob et al., 2009; Bliven and Maurelli, 2012; Rouxel and Balesdent, 2017; Siscar-Lewin et al., 2019). For instance, loss of Avr-Co39 in rice-infecting haplotypes of Magnaporthe oryzae has been proposed as an adaptive mechanism to escape recognition by the R protein Pi-Co39 (Couch et al., 2005). However, loss or mutated effectors may impose a fitness penalty on the pathogen (Leach et al., 2001; Montarry et al., 2010). The functional redundancy of paralogous genes of RxLRs of P. cactorum could play a potential role in conserving virulence function and fitness, as addressed for bacterial and oomycete effectors during pathogenesis (Birch et al., 2008; Ghosh and O’Connor, 2017). Nellist et al. reported that over half of the candidate effectors were not expressed or showed low expression in planta (Nellist et al., 2021). Thus, further expression analysis is required to pinpoint candidate effectors involved in tissue-specific interactions of P. cactorum in strawberry.
5. Conclusion
The comparative genome analysis identified sequence variations between the studied CR and LR strains, which might contribute to the tissue-specific interactions of P. cactorum in strawberry. The presence of two unique pectate lyase genes, and expansion of potential virulence effector genes such as RxLRs and CRNs in orthogroups OG0001375, OG0000310, and OG0005694 or loss of avirulence determinants (e.g., Avr1b-1-like gene) in the orthogroup OG0000414 may play a role in increased virulence of the CR strain 251616 in the strawberry rhizome. However, functional analysis is required to validate these genes as pathogenicity determinants of P. cactorum in strawberry tissues. The non-virulence of the LR strains in the rhizome tissues might be an event of recognition of elicitors by host proteins. The data presented here can be used as a basis for future functional analysis of potential effector genes of P. cactorum during interaction with strawberry.
Data availability statement
The data presented in the study are deposited in the DDBJ/ENA/GenBank repository under the BioProject PRJNA885305, and under the accessions JAPDOL000000000, JAPDOM000000000, JAPDON000000000, JAPDOO000000000, JAPDOP000000000. The raw sequence reads are deposited in the NCBI Sequence Read Archive (SRA) under the accessions SRR21764265, SRR21764266, SRR21764267, SRR21764268, SRR21764269.
Author contributions
AG and AS performed the inoculation experiment and data analysis. AG, SR, and EL analyzed the sequencing data. MB and AS formulated the research idea and designed the experimental setup. AG interpreted the results and wrote the first draft of the manuscript. All authors contributed to the article and approved the submitted version.
Funding
AG was funded by a PhD scholarship from the Norwegian University of Life Sciences, Ås, Norway. The work was supported by NIBIO (basic funding) and the Research Council of Norway, grant number 326212.
Acknowledgments
Bikal Ghimire, PhD candidate at the Department of Plant Sciences, Norwegian University of Life Sciences, Ås, Norway is acknowledged for his technical help during pathogen inoculation and disease scoring.
Conflict of interest
The authors declare that the research was conducted in the absence of any commercial or financial relationships that could be construed as a potential conflict of interest.
Publisher’s note
All claims expressed in this article are solely those of the authors and do not necessarily represent those of their affiliated organizations, or those of the publisher, the editors and the reviewers. Any product that may be evaluated in this article, or claim that may be made by its manufacturer, is not guaranteed or endorsed by the publisher.
Supplementary material
The Supplementary material for this article can be found online at: https://www.frontiersin.org/articles/10.3389/fmicb.2023.1214924/full#supplementary-material
Footnotes
References
Ali, A., Kumar, R., Mazákova, J., Maňasová, M., Zouhar, M., and Pánek, M. (2022). Evaluation of the ability of seven active ingredients of fungicides to suppress Phytophthora cactorum at diverse life stages, and variability in resistance found among isolates. J. Fungi 8:1039. doi: 10.3390/jof8101039
Altschul, S. F., Gish, W., Miller, W., Myers, E. W., and Lipman, D. J. (1990). Basic local alignment search tool. J. Mol. Biol. 215, 403–410. doi: 10.1016/S0022-2836(05)80360-2
Amaro, T. M. M. M., Thilliez, G. J. A., Motion, G. B., and Huitema, E. (2017). A perspective on CRN proteins in the genomics age: evolution, classification, delivery and function revisited. Front. Plant Sci. 8:99. doi: 10.3389/fpls.2017.00099
Armenteros, A. J. J., Salvatore, M., Emanuelsson, O., Winther, O., von Heijne, G., Elofsson, A., et al. (2019a). Detecting sequence signals in targeting peptides using deep learning. Life Sci. Alliance 2:e201900429. doi: 10.26508/lsa.201900429
Armenteros, A. J. J., Tsirigos, K. D., Sønderby, C. K., Petersen, T. N., Winther, O., Brunak, S., et al. (2019b). SignalP 5.0 improves signal peptide predictions using deep neural networks. Nat. Biotechnol. 37, 420–423. doi: 10.1038/s41587-019-0036-z
Armitage, A. D., Lysøe, E., Nellist, C. F., Lewis, L. A., Cano, L. M., Harrison, R. J., et al. (2018). Bioinformatic characterisation of the effector repertoire of the strawberry pathogen Phytophthora cactorum. PLoS One 13, e0202305–e0202324. doi: 10.1371/journal.pone.0202305
Ashburner, M., Ball, C. A., Blake, J. A., Botstein, D., Butler, H., Cherry, J. M., et al. (2000). Gene ontology: tool for the unification of biology. Nat. Genet. 25, 25–29. doi: 10.1038/75556
Bao, W., Kojima, K. K., and Kohany, O. (2015). Repbase update, a database of repetitive elements in eukaryotic genomes. Mob. DNA 6:11. doi: 10.1186/s13100-015-0041-9
Bell, J. A., Simpson, D. W., and Harris, D. C. (1997). Development of a method for screening strawberry germplasm for resistance to Phytophthora cactorum. Acta Hortic. 439, 175–180. doi: 10.17660/ActaHortic.1997.439.23
Birch, P. R. J., Boevink, P. C., Gilroy, E. M., Hein, I., Pritchard, L., and Whisson, S. C. (2008). Oomycete RXLR effectors: delivery; functional redundancy and durable disease resistance. Curr. Opin. Plant Biol. 11, 373–379. doi: 10.1016/j.pbi.2008.04.005
Blackwell, E. (1943). The life history of Phytophthora cactorum (Leb. & Cohn) Schroet. Trans. Br. Mycol. Soc. 26, 71–89. doi: 10.1016/s0007-1536(43)80013-9
Bliven, K. A., and Maurelli, A. T. (2012). Antivirulence genes: insights into pathogen evolution through gene loss. Infect. Immun. 80, 4061–4070. doi: 10.1128/IAI.00740-12
Boutrot, F., and Zipfel, C. (2017). Function, discovery, and exploitation of plant pattern recognition receptors for broad-spectrum disease resistance. Annu. Rev. Phytopathol. 55, 257–286. doi: 10.1146/annurev-phyto-080614-120106
Casado-Díaz, A., Encinas-Villarejo, S., Santos, B. D. L., Schilirò, E., Yubero-Serrano, E. M., Amil-Ruíz, F., et al. (2006). Analysis of strawberry genes differentially expressed in response to Colletotrichum infection. Physiol. Plant. 128, 633–650. doi: 10.1111/j.1399-3054.2006.00798.x
Chaparro-Garcia, A., Wilkinson, R. C., Gimenez-Ibanez, S., Findlay, K., Coffey, M. D., Zipfel, C., et al. (2011). The receptor-like kinase SERK3/BAK1 is required for basal resistance against the late blight pathogen Phytophthora infestans in Nicotiana benthamiana. PLoS One 6, –e16608. doi: 10.1371/journal.pone.0016608
Chen, X. R., Huang, S. X., Zhang, Y., Sheng, G. L., Zhang, B. Y., Li, Q. Y., et al. (2018). Transcription profiling and identification of infection-related genes in Phytophthora cactorum. Mol. Gen. Genomics. 293, 541–555. doi: 10.1007/s00438-017-1400-7
Chen, X. R., Li, Y. P., Li, Q. Y., Xing, Y. P., Liu, B. B., Tong, Y. H., et al. (2016). SCR96, a small cysteine-rich secretory protein of Phytophthora cactorum, can trigger cell death in the Solanaceae and is important for pathogenicity and oxidative stress tolerance. Mol. Plant Pathol. 17, 577–587. doi: 10.1111/mpp.12303
Chen, J., Li, Z., Lin, B., Liao, J., and Zhuo, K. (2021). A Meloidogyne graminicola pectate lyase is involved in virulence and activation of host defense responses. Front. Plant Sci. 12:651627. doi: 10.3389/fpls.2021.651627
Chen, X. R., Wen, K., Zhou, X., Zhu, M. Y., Liu, Y., Jin, J. H., et al. (2023). The devastating oomycete phytopathogen Phytophthora cactorum: insights into its biology and molecular features. Mol. Plant Pathol, 1–16. doi: 10.1111/mpp.13345
Chen, X.-R., Zhang, B.-Y., Xing, Y.-P., Li, Q.-Y., Li, Y.-P., Tong, Y.-H., et al. (2014). Transcriptomic analysis of the phytopathogenic oomycete Phytophthora cactorum provides insights into infection-related effectors. BMC Genomics 15:980. doi: 10.1186/1471-2164-15-980
Cho, Y., Jang, M., Srivastava, A., Jang, J.-H., Soung, N.-K., Ko, S.-K., et al. (2015). A pectate lyase-coding gene abundantly expressed during early stages of infection is required for full virulence in Alternaria brassicicola. PLoS One 10, –e0127140. doi: 10.1371/journal.pone.0127140
Cingolani, P., Platts, A., Wang, L. L., Coon, M., Nguyen, T., Wang, L., et al. (2012). A program for annotating and predicting the effects of single nucleotide polymorphisms. SnpEff. Fly (Austin). 6, 80–92. doi: 10.4161/fly.19695
Collmer, A., and Keen, N. T. (1986). The role of pectic enzymes in plant pathogenesis. Annu. Rev. Phytopathol. 24, 383–409. doi: 10.1146/annurev.py.24.090186.002123
Couch, B. C., Fudal, I., Lebrun, M.-H., Tharreau, D., Valent, B., van Kim, P., et al. (2005). Origins of host-specific populations of the blast pathogen Magnaporthe oryzae in crop domestication with subsequent expansion of pandemic clones on rice and weeds of rice. Genetics 170, 613–630. doi: 10.1534/genetics.105.041780
Danecek, P., Auton, A., Abecasis, G., Albers, C. A., Banks, E., DePristo, M. A., et al. (2011). The variant call format and VCFtools. Bioinformatics 27, 2156–2158. doi: 10.1093/bioinformatics/btr330
Danecek, P., Bonfield, J. K., Liddle, J., Marshall, J., Ohan, V., Pollard, M. O., et al. (2021). Twelve years of SAMtools and BCFtools. Gigascience 10:giab008. doi: 10.1093/gigascience/giab008
De Cal, A., Martinez-Treceño, A., Lopez-Aranda, J. M., and Melgarejo, P. (2004). Chemical alternatives to methyl bromide in spanish strawberry nurseries. Plant Dis. 88, 210–214. doi: 10.1094/pdis.2004.88.2.210
De Castro, E., Sigrist, C. J. A., Gattiker, A., Bulliard, V., Langendijk-Genevaux, P. S., Gasteiger, E., et al. (2006). ScanProsite: detection of PROSITE signature matches and ProRule-associated functional and structural residues in proteins. Nucleic Acids Res. 34, W362–W365. doi: 10.1093/nar/gkl124
Dodds, P. N., and Rathjen, J. P. (2010). Plant immunity: towards an integrated view of plant–pathogen interactions. Nat. Rev. Genet. 11, 539–548. doi: 10.1038/nrg2812
Du, J., Verzaux, E., Chaparro-Garcia, A., Bijsterbosch, G., Keizer, L. C. P., Zhou, J., et al. (2015). Elicitin recognition confers enhanced resistance to Phytophthora infestans in potato. Nat. Plants 1:15034. doi: 10.1038/nplants.2015.34
Dumas, B., Bottin, A., Gaulin, E., and Esquerré-Tugayé, M. T. (2008). Cellulose-binding domains: cellulose associated-defensive sensing partners? Trends Plant Sci. 13, 160–164. doi: 10.1016/j.tplants.2008.02.004
Eikemo, H., Klemsdal, S. S., Riisberg, I., Bonants, P., Stensvand, A., and Tronsmo, A. M. (2004). Genetic variation between Phytophthora cactorum isolates differing in their ability to cause crown rot in strawberry. Mycol. Res. 108, 317–324. doi: 10.1017/S0953756204009244
Eikemo, H., and Stensvand, A. (2015). Resistance of strawberry genotypes to leather rot and crown rot caused by Phytophthora cactorum. Eur. J. Plant Pathol. 143, 407–413. doi: 10.1007/s10658-015-0685-9
Eikemo, H., Stensvand, A., Davik, J., and Tronsmo, A. M. (2003). Resistance to crown rot (Phytophthora cactorum) in strawberry cultivars and in offspring from crosses between cultivars differing in susceptibility to the disease. Ann. Appl. Biol. 142, 83–89. doi: 10.1111/j.1744-7348.2003.tb00232.x
Eikemo, H., Stensvand, A., and Tronsmo, A. M. (2000). Evaluation of methods of screening strawberry cultivars for resistance to crown rot caused by Phytophthora cactorum. Ann. Appl. Biol. 137, 237–244. doi: 10.1111/j.1744-7348.2000.tb00064.x
Ellis, M. A., and Grove, G. G. (1998). Leather rot in Ohio strawberries. Plant Dis. 67:549. doi: 10.1094/PD-67-549
Ellis, M. A., Wilcox, W. F., and Madden, L. V. (1998). Efficacy of metalaxyl, fosetyl-aluminum, and straw mulch for control of strawberry leather rot caused by Phytophthora cactorum. Plant Dis. 82, 329–332. doi: 10.1094/PDIS.1998.82.3.329
Emms, D. M., and Kelly, S. (2019). OrthoFinder: phylogenetic orthology inference for comparative genomics. Genome Biol. 20:238. doi: 10.1186/s13059-019-1832-y
European Food Safety Authority (2015). Conclusion on the peer review of the pesticide risk assessment of the active substance metalaxyl-M. EFSA J. 13:3999. doi: 10.2903/j.efsa.2015.3999
Evangelisti, E., Gogleva, A., Hainaux, T., Doumane, M., Tulin, F., Quan, C., et al. (2017). Time-resolved dual transcriptomics reveal early induced Nicotiana benthamiana root genes and conserved infection-promoting Phytophthora palmivora effectors. BMC Biol. 15:39. doi: 10.1186/s12915-017-0379-1
Fu, L., Wang, H. Z., Feng, B. Z., and Zhang, X. G. (2013). Cloning, expression, purification and initial analysis of a novel pectate lyase Pcpel1 from Phytophthora capsici. J. Phytopathol. 161, 230–238. doi: 10.1111/jph.12059
Fu, L., Zhu, C., Ding, X., Yang, X., Morris, P. F., Tyler, B. M., et al. (2015). Characterization of cell-death-inducing members of the pectate lyase gene family in Phytophthora capsici and their contributions to infection of pepper. Mol. Plant-Microbe Interact. 28, 766–775. doi: 10.1094/MPMI-11-14-0352-R
Gaulin, E., Dramé, N., Lafitte, C., Torto-Alalibo, T., Martinez, Y., Ameline-Torregrosa, C., et al. (2006). Cellulose binding domains of a Phytophthora cell wall protein are novel pathogen-associated molecular patterns. Plant Cell 18, 1766–1777. doi: 10.1105/tpc.105.038687
Gaulin, E., Jauneau, A., Villalba, F., Rickauer, M., and Bottin, A. (2002). The CBEL glycoprotein of Phytophthora parasitica var. nicotianae is involved in cell wall deposition and adhesion to cellulosic substrates. J. Cell Sci. 115, 4565–4575. doi: 10.1242/jcs.00138
Gaulin, E., Pel, M. J. C., Camborde, L., San-Clemente, H., Courbier, S., Dupouy, M. A., et al. (2018). Genomics analysis of Aphanomyces spp. identifies a new class of oomycete effector associated with host adaptation. BMC Biol. 16:43. doi: 10.1186/s12915-018-0508-5
Ghosh, S., and O’Connor, T. J. (2017). Beyond paralogs: the multiple layers of redundancy in bacterial pathogenesis. Front. Cell. Infect. Microbiol. 7:467. doi: 10.3389/fcimb.2017.00467
Gil, J., Herrera, M., Duitama, J., Sarria, G., Restrepo, S., and Romero, H. M. (2020). Genomic variability of Phytophthora palmivora isolates from different oil palm cultivation regions in Colombia. Phytopathology 110, 1553–1564. doi: 10.1094/PHYTO-06-19-0209-R
Gilroy, E. M., Breen, S., Whisson, S. C., Squires, J., Hein, I., Kaczmarek, M., et al. (2011). Presence/absence, differential expression and sequence polymorphisms between PiAVR2 and PiAVR2-like in Phytophthora infestans determine virulence on R2 plants. New Phytol. 191, 763–776. doi: 10.1111/j.1469-8137.2011.03736.x
Goodwin, S. B. (1997). The population genetics of Phytophthora. Phytopathology 87, 446–447. doi: 10.1094/phyto.1997.87.4.446
Götz, S., García-Gómez, J. M., Terol, J., Williams, T. D., Nagaraj, S. H., Nueda, M. J., et al. (2008). High-throughput functional annotation and data mining with the Blast2GO suite. Nucleic Acids Res. 36, 3420–3435. doi: 10.1093/nar/gkn176
Grenville-Briggs, L. J., Avrova, A. O., Hay, R. J., Bruce, C. R., Whisson, S. C., and van West, P. (2010). Identification of appressorial and mycelial cell wall proteins and a survey of the membrane proteome of Phytophthora infestans. Fungal Biol. 114, 702–723. doi: 10.1016/j.funbio.2010.06.003
Grenville-Briggs, L. J., Kushwaha, S. K., Cleary, M. R., Witzell, J., Savenkov, E. I., Whisson, S. C., et al. (2017). Draft genome of the oomycete pathogen Phytophthora cactorum strain LV007 isolated from European beech (Fagus sylvatica). Genom. Data 12, 155–156. doi: 10.1016/j.gdata.2017.05.010
Gurevich, A., Saveliev, V., Vyahhi, N., and Tesler, G. (2013). QUAST: quality assessment tool for genome assemblies. Bioinformatics 29, 1072–1075. doi: 10.1093/bioinformatics/btt086
Haas, B. J., Kamoun, S., Zody, M. C., Jiang, R. H. Y., Handsaker, R. E., Cano, L. M., et al. (2009). Genome sequence and analysis of the Irish potato famine pathogen Phytophthora infestans. Nature 461, 393–398. doi: 10.1038/nature08358
Hantula, J., Lilja, A., Nuorteva, H., Parikka, P., and Werres, S. (2000). Pathogenicity, morphology and genetic variation of Phytophthora cactorum from strawberry, apple, rhododendron, and silver birch. Mycol. Res. 104, 1062–1068. doi: 10.1017/S0953756200002999
Harris, R. S. (2007). Improved pairwise alignment of genomic DNA. Ph.D. Dissertation, Pennsylvania State University, University Park, PA, United States.
Harris, D. C., and Stickels, J. E. (1981). Crown rot (Phytophthora cactorum) in glasshouse-grown strawberries at east Malling Research Station. Plant Pathol. 30, 205–212. doi: 10.1111/j.1365-3059.1981.tb01258.x
Herron, S. R., Benen, J. A. E., Scavetta, R. D., Visser, J., and Jurnak, F. (2000). Structure and function of pectic enzymes: virulence factors of plant pathogens. Proc. Natl. Acad. Sci. 97:8762. doi: 10.1073/pnas.97.16.8762
Hon, T., Mars, K., Young, G., Tsai, Y.-C., Karalius, J. W., Landolin, J. M., et al. (2020). Highly accurate long-read HiFi sequencing data for five complex genomes. Sci. Data 7:399. doi: 10.1038/s41597-020-00743-4
Hrelia, P., Maffei, F., Fimognari, C., Vigagni, F., and Cantelli-Forti, G. (1996). Cytogenetic effects of Metalaxyl on human and animal chromosomes. Mutat. Res. Genet. Toxicol. 369, 81–86. doi: 10.1016/S0165-1218(96)90051-8
Hubley, R., Finn, R. D., Clements, J., Eddy, S. R., Jones, T. A., Bao, W., et al. (2016). The Dfam database of repetitive DNA families. Nucleic Acids Res. 44, D81–D89. doi: 10.1093/nar/gkv1272
Huet, J. C., and Pernollet, J. C. (1993). Sequences of acidic and basic elicitin isoforms secreted by Phytophthora megasperma megasperma. Phytochemistry 33, 797–805. doi: 10.1016/0031-9422(93)85277-X
Jangir, P., Mehra, N., Sharma, K., Singh, N., Rani, M., and Kapoor, R. (2021). Secreted in xylem genes: drivers of host adaptation in Fusarium oxysporum. Front. Plant Sci. 12:628611. doi: 10.3389/fpls.2021.628611
Jeffers, S. (2015). “PROTOCOL 07-11.1: V8 agar (V8A) or broth,” in Laboratory protocols for Phytophthora species protocols (St. Paul, MN, USA: The American Phytopathological Society), 1–2. doi: 10.3389/fpls.2021.628611
Jeffers, S. N., and Martin, S. B. (1986). Comparison of two media selective for Phytophthora and Pythium species. Plant Dis. 70:1038. doi: 10.1094/pd-70-1038
Jiang, R. H. Y., Tyler, B. M., Whisson, S. C., Hardham, A. R., and Govers, F. (2006). Ancient origin of elicitin gene clusters in Phytophthora genomes. Mol. Biol. Evol. 23, 338–351. doi: 10.1093/molbev/msj039
Joint Genome Institute (2021). Isolation of genomic DNA from Phytophthora. Available at: https://jgi.doe.gov/user-programs/pmo-overview/protocols-sample-preparation-information/ (Accessed April 25, 2021).
Jones, J. D. L., and Dangl, J. L. (2006). The plant immune system. Nature 444, 323–329. doi: 10.1038/nature05286
Jones, R. W., and Ospina-Giraldo, M. (2011). Novel cellulose-binding-domain protein in Phytophthora is cell wall localized. PLoS One 6, 2–7. doi: 10.1371/journal.pone.0023555
Käll, L., Krogh, A., and Sonnhammer, E. L. L. (2007). Advantages of combined transmembrane topology and signal peptide prediction--the Phobius web server. Nucleic Acids Res. 35, W429–W432. doi: 10.1093/nar/gkm256
Kamoun, S. (2006). A catalogue of the effector secretome of plant pathogenic oomycetes. Annu. Rev. Phytopathol. 44, 41–60. doi: 10.1146/annurev.phyto.44.070505.143436
Knaus, B. J., Tabima, J. F., Shakya, S. K., Judelson, H. S., and Grünwald, N. J. (2019). Genome-wide increased copy number is associated with emergence of super-fit clones of the Irish potato famine pathogen Phytophthora infestans. bioRxiv 11, 1–13. doi: 10.1101/633701
Kolmogorov, M., Yuan, J., Lin, Y., and Pevzner, P. A. (2019). Assembly of long, error-prone reads using repeat graphs. Nat. Biotechnol. 37, 540–546. doi: 10.1038/s41587-019-0072-8
Lamour, K. H., Mudge, J., Gobena, D., Hurtado-Gonzales, O. P., Schmutz, J., Kuo, A., et al. (2012). Genome sequencing and mapping reveal loss of heterozygosity as a mechanism for rapid adaptation in the vegetable pathogen Phytophthora capsici. Mol. Plant-Microbe Interact. 25, 1350–1360. doi: 10.1094/MPMI-02-12-0028-R
Larousse, M., Govetto, B., Séassau, A., Etienne, C., Industri, B., Theodorakopoulos, N., et al. (2014). Characterization of PPMUCL1/2/3, three members of a new oomycete-specific mucin-like protein family residing in Phytophthora parasitica biofilm. Protist 165, 275–292. doi: 10.1016/j.protis.2014.03.003
Le, S. Q., and Gascuel, O. (2008). An improved general amino acid replacement matrix. Mol. Biol. Evol. 25, 1307–1320. doi: 10.1093/molbev/msn067
Leach, J. E., Cruz, C. M. V., Bai, J., and Leung, H. (2001). Pathogen fitness penalty as a predictor of durab 10.1094/9780890544969.07.11.1 ility of disease resistance genes. Annu. Rev. Phytopathol. 39, 187–224. doi: 10.1146/annurev.phyto.39.1.187
Lee, Y., Cho, K. S., Seo, J. H., Sohn, K. H., and Prokchorchik, M. (2020). Improved genome sequence and gene annotation resource for the potato late blight pathogen Phytophthora infestans. Mol. Plant-Microbe Interact. 33, 1025–1028. doi: 10.1094/MPMI-02-20-0023-A
Li, H. (2018). Minimap2: pairwise alignment for nucleotide sequences. Bioinformatics 34, 3094–3100. doi: 10.1093/bioinformatics/bty191
Maas, J. L. (2014). Strawberry diseases and pests - progress and problems. Acta Hortic. 1049, 133–142. doi: 10.17660/ActaHortic.2014.1049.11
Marin, M. V., Seijo, T. E., Zuchelli, E., and Peres, N. A. (2021). Resistance to mefenoxam of Phytophthora cactorum and Phytophthora nicotianae causing crown and leather rot in Florida strawberry. Plant Dis. 105, 3490–3495. doi: 10.1094/PDIS-11-20-2474-RE
Mateos, F. V., Rickauer, M., Végétale, C. D. P., Ups-cnrs, U. M. R., Sabatier, U. P., and Narbonne, D. (1997). Cloning and characterization of a cDNA encoding an elicitor of Phytophthora parasitica var. nicotianae that shows cellulose-binding and lectin-like activities. Mol. Plant-Microbe Interact. 10, 1045–1053. doi: 10.1094/MPMI.1997.10.9.1045
Maurelli, A. T. (2007). Black holes, antivirulence genes, and gene inactivation in the evolution of bacterial pathogens. FEMS Microbiol. Lett. 267, 1–8. doi: 10.1111/j.1574-6968.2006.00526.x
McGowan, J., and Fitzpatrick, D. A. (2017). Genomic, network, and phylogenetic analysis of the oomycete effector arsenal. mSphere 2, e00408–e00417. doi: 10.1128/msphere.00408
Meijer, H. J. G., Mancuso, F. M., Espadas, G., Seidl, M. F., Chiva, C., Govers, F., et al. (2014). Profiling the secretome and extracellular proteome of the potato late blight pathogen Phytophthora infestans. Mol. Cell. Proteomics 13, 2101–2113. doi: 10.1074/mcp.M113.035873
Montarry, J., Hamelin, F. M., Glais, I., Corbière, R., and Andrivon, D. (2010). Fitness costs associated with unnecessary virulence factors and life history traits: evolutionary insights from the potato late blight pathogen Phytophthora infestans. BMC Evol. Biol. 10:283. doi: 10.1186/1471-2148-10-283
Na, R., and Gijzen, M. (2016). Escaping host immunity: new tricks for plant pathogens. PLoS Pathog. 12, e1005631–e1005636. doi: 10.1371/journal.ppat.1005631
Nellist, C. F., Armitage, A. D., Bates, H. J., and Sobczyk, M. K. (2021). Comparative analysis of host-associated variation in Phytophthora cactorum. Front. Microbiol. 12, 1–23. doi: 10.3389/fmicb.2021.679936
OmicsBox (2019). Bioinformatics made easy BioBam bioinformatics Available at: https://www.biobam.com/omicsbox.
Orsomando, G., Lorenzi, M., Raffaelli, N., Dalla Rizza, M., Mezzetti, B., and Ruggieri, S. (2001). Phytotoxic protein PcF, purification, characterization, and cDNA sequencing of a novel hydroxyproline-containing factor secreted by the strawberry pathogen Phytophthora cactorum. J. Biol. Chem. 276, 21578–21584. doi: 10.1074/jbc.M101377200
Pánek, M., Fér, T., Mráček, J., and Tomšovský, M. (2016). Evolutionary relationships within the Phytophthora cactorum species complex in Europe. Fungal Biol. 120, 836–851. doi: 10.1016/j.funbio.2016.03.006
Pánek, M., Maňasová, M., Wenzlová, J., Zouhar, M., and Mazáková, J. (2022). Peronosporales species associated with strawberry crown rot in the Czech Republic. J. Fungi 8:346. doi: 10.3390/jof8040346
Pánek, M., Střížková, I., Zouhar, M., Kudláček, T., and Tomšovský, M. (2021). Mixed-mating model of reproduction revealed in european Phytophthora cactorum by ddRADseq and effector gene sequence data. Microorganisms 9, 1–22. doi: 10.3390/microorganisms9020345
Parikka, P. (2003). Susceptibility of strawberry varieties to crown rot (Phytophthora cactorum) in greenhouse tests. Acta Hortic. 626, 183–189. doi: 10.17660/ActaHortic.2003.626.24
Patterson, M., Marschall, T., Pisanti, N., van Iersel, L., Stougie, L., Klau, G. W., et al. (2015). WhatsHap: weighted haplotype assembly for future-generation sequencing reads. J. Comput. Biol. 22, 498–509. doi: 10.1089/cmb.2014.0157
Pernollet, J. (1993). Amino acid sequence of the α-elictin secreted by Phytophthora cactorum. Phytochemistry 34, 1261–1264. doi: 10.1016/0031-9422(91)80012-P
Pettitt, T. R., and Pegg, G. F. (1994). Sources of crown rot (Phytophthora cactorum) infection in strawberry and the effect of cold storage on susceptibility to the disease. Ann. Appl. Biol. 125, 279–292. doi: 10.1111/j.1744-7348.1994.tb04969.x
Poplin, R., Chang, P. C., Alexander, D., Schwartz, S., Colthurst, T., Ku, A., et al. (2018). A universal SNP and small-indel variant caller using deep neural networks. Nat. Biotechnol. 36, 983–987. doi: 10.1038/nbt.4235
Qutob, D., Tedman-Jones, J., Dong, S., Kuflu, K., Pham, H., Wang, Y., et al. (2009). Copy number variation and transcriptional polymorphisms of Phytophthora sojae RXLR effector genes Avr1a and Avr3a. PLoS One 4:e5066. doi: 10.1371/journal.pone.0005066
Raaymakers, T. M., and Van den Ackerveken, G. (2016). Extracellular recognition of oomycetes during biotrophic infection of plants. Front. Plant Sci. 7:906. doi: 10.3389/fpls.2016.00906
Rice, P., Longden, I., and Bleasby, A. (2000). EMBOSS: the European molecular biology open software suite. Trends Genet. 16, 276–277. doi: 10.1016/S0168-9525(00)02024-2
Rouxel, T., and Balesdent, M.-H. (2017). Life, death and rebirth of avirulence effectors in a fungal pathogen of Brassica crops, Leptosphaeria maculans. New Phytol. 214, 526–532. doi: 10.1111/nph.14411
Schliep, K. P. (2011). Phangorn: phylogenetic analysis in R. Bioinformatics 27, 592–593. doi: 10.1093/bioinformatics/btq706
Shaw, D. V., Hansen, J., and Browne, G. T. (2006). Genotypic variation for resistance to Phytophthora cactorum in a California strawberry breeding population. J. Am. Soc. Hortic. Sci. 131, 687–690. doi: 10.21273/jashs.131.5.687
Simão, F. A., Waterhouse, R. M., Ioannidis, P., Kriventseva, E. V., and Zdobnov, E. M. (2015). BUSCO: assessing genome assembly and annotation completeness with single-copy orthologs. Bioinformatics 31, 3210–3212. doi: 10.1093/bioinformatics/btv351
Siscar-Lewin, S., Hube, B., and Brunke, S. (2019). Antivirulence and avirulence genes in human pathogenic fungi. Virulence 10, 935–947. doi: 10.1080/21505594.2019.1688753
Smit, A. F. A., Hubley, R., and Green, P. (2015). RepeatMasker Open-4.0. Available at: http://www.repeatmasker.org/.
Sperschneider, J., Dodds, P. N., Singh, K. B., and Taylor, J. M. (2018). ApoplastP: prediction of effectors and plant proteins in the apoplast using machine learning. New Phytol. 217, 1764–1778. doi: 10.1111/nph.14946
Sperschneider, J., Williams, A. H., Hane, J. K., Singh, K. B., and Taylor, J. M. (2015). Evaluation of secretion prediction highlights differing approaches needed for oomycete and fungal effectors. Front. Plant Sci. 6:1168. doi: 10.3389/fpls.2015.01168
Stanke, M., Diekhans, M., Baertsch, R., and Haussler, D. (2008). Using native and syntenically mapped cDNA alignments to improve de novo gene finding. Bioinformatics 24, 637–644. doi: 10.1093/bioinformatics/btn013
Stensvand, A., Herrero, M. L., and Talgø, V. (1999). Crown rot caused by Phytophthora cactorum in Norwegian strawberry production. EPPO Bull. 29, 155–158. doi: 10.1111/j.1365-2338.1999.tb00809.x
Tabima, J. F., and Grünwald, N. J. (2019). effectR: an expandable R package to predict candidate RxLR and CRN effectors in oomycetes using motif searches. Mol. Plant-Microbe Interact. 32, 1067–1076. doi: 10.1094/MPMI-10-18-0279-TA
Tyler, B. M., Tripathy, S., Zhang, X., Dehal, P., Jiang, R. H. Y., Aerts, A., et al. (2006). Phytophthora genome sequences uncover evolutionary origins and mechanisms of pathogenesis. Science 313, 1261–1266. doi: 10.1126/science.1128796
van der Scheer, H. A. T. (1971). Isolation of Phytophthora cactorum from soil in orchards and strawberry fields and differences in pathogenicity to apple. Netherlands J. Plant Pathol. 77, 65–72. doi: 10.1007/BF01981494
Voß, S., Betz, R., Heidt, S., Corradi, N., and Requena, N. (2018). RiCRN1; a crinkler effector from the arbuscular mycorrhizal fungus Rhizophagus irregularis; functions in arbuscule development. Front. Microbiol. 9:2068. doi: 10.3389/fmicb.2018.02068
Wang, S., McLellan, H., Bukharova, T., He, Q., Murphy, F., Shi, J., et al. (2019). Phytophthora infestans RXLR effectors act in concert at diverse subcellular locations to enhance host colonization. J. Exp. Bot. 70, 343–356. doi: 10.1093/jxb/ery360
Wang, D., Zhang, Y., Zhang, Z., Zhu, J., and Yu, J. (2010). KaKs_Calculator 2.0: a toolkit incorporating gamma-series methods and sliding window strategies. Genomics Proteomics Bioinformatics 8, 77–80. doi: 10.1016/S1672-0229(10)60008-3
Wegener, C. B. (2002). Induction of defence responses against Erwinia soft rot by an endogenous pectate lyase in potatoes. Physiol. Mol. Plant Pathol. 60, 91–100. doi: 10.1006/pmpp.2002.0377
Wenger, A. M., Peluso, P., Rowell, W. J., Chang, P. C., Hall, R. J., Concepcion, G. T., et al. (2019). Accurate circular consensus long-read sequencing improves variant detection and assembly of a human genome. Nat. Biotechnol. 37, 1155–1162. doi: 10.1038/s41587-019-0217-9
Wickham, H., Averick, M., Bryan, J., Chang, W., Mcgowan, L. D. A., François, R., et al. (2019). Welcome to the Tidyverse. J. Open Source Softw. 4:1686. doi: 10.21105/joss.01686
Win, J., Morgan, W., Bos, J., Krasileva, K. V., Cano, L. M., Chaparro-Garcia, A., et al. (2007). Adaptive evolution has targeted the C-terminal domain of the RXLR effectors of plant pathogenic oomycetes. Plant Cell 19, 2349–2369. doi: 10.1105/tpc.107.051037
Wu, C.-H., Abd-El-Haliem, A., Bozkurt, T. O., Belhaj, K., Terauchi, R., Vossen, J. H., et al. (2017). NLR network mediates immunity to diverse plant pathogens. Proc. Natl. Acad. Sci. U. S. A. 114, 8113–8118. doi: 10.1073/pnas.1702041114
Xu, L., Dong, Z., Fang, L., Luo, Y., Wei, Z., Guo, H., et al. (2019). OrthoVenn2: a web server for whole-genome comparison and annotation of orthologous clusters across multiple species. Nucleic Acids Res. 47, W52–W58. doi: 10.1093/nar/gkz333
Yang, M., Duan, S., Mei, X., Huang, H., Chen, W., Liu, Y., et al. (2018a). The Phytophthora cactorum genome provides insights into the adaptation to host defense compounds and fungicides. Sci. Rep. 8, 6534–6511. doi: 10.1038/s41598-018-24939-2
Yang, Y., Zhang, Y., Li, B., Yang, X., Dong, Y., and Qiu, D. (2018b). A Verticillium dahliae pectate lyase induces plant immune responses and contributes to virulence. Front. Plant Sci. 9:1271. doi: 10.3389/fpls.2018.01271
Zdobnov, E. M., and Apweiler, R. (2001). InterProScan – an integration platform for the signature-recognition methods in InterPro. Bioinformatics 17, 847–848. doi: 10.1093/bioinformatics/17.9.847
Keywords: oomycete, PacBio sequel II sequencing, elicitors, RxLRs, Crinklers, effectors
Citation: Gogoi A, Rossmann SL, Lysøe E, Stensvand A and Brurberg MB (2023) Genome analysis of Phytophthora cactorum strains associated with crown- and leather-rot in strawberry. Front. Microbiol. 14:1214924. doi: 10.3389/fmicb.2023.1214924
Edited by:
Guillaume J. Bilodeau, Canadian Food Inspection Agency (CFIA), CanadaReviewed by:
Ahmed H. El-Sappah, Zagazig University, EgyptMalkhan Singh Gurjar, Indian Agricultural Research Institute (ICAR), India
Copyright © 2023 Gogoi, Rossmann, Lysøe, Stensvand and Brurberg. This is an open-access article distributed under the terms of the Creative Commons Attribution License (CC BY). The use, distribution or reproduction in other forums is permitted, provided the original author(s) and the copyright owner(s) are credited and that the original publication in this journal is cited, in accordance with accepted academic practice. No use, distribution or reproduction is permitted which does not comply with these terms.
*Correspondence: May Bente Brurberg, bWF5LmJydXJiZXJnQG5pYmlvLm5v