- 1Laboratory of Industrial Biotechnology, Department of Food Science, Centro de Investigación en Alimentación y Desarrollo, Hermosillo, Mexico
- 2Laboratory of Nematology, Centro de Investigación en Alimentación y Desarrollo, Culiacán, Mexico
- 3Innovak Global, Chihuahua, Mexico
Phytopathogenic nematodes (PPNs) are responsible for substantial damages within agricultural crops worldwide which can be controlled employing beneficial microorganisms and/or their metabolites in an ecofriendly way. Nevertheless, the success of the control regards not only on the virulence of the strains or the toxicity of their metabolites but also on their ability to colonize and remain in the rhizospheric environment, particularly in those crops affected by abiotic stresses promoted by the climate change. Consequently, the bioprospection of beneficial microorganisms able to control PPNs and to thrive in adverse conditions has attracted attention. On this way, deserts are perfect ecological niches to isolate microorganisms adapted to harsh enviroments. The purpose of this research was to isolate and characterize bacteria from rhizospheric soil samples collected in the Northwestern Desert of Mexico with potential for PPNs control. As first screening, secretomes of each isolate were tested in vitro for nematicidal activity (NA). Then, activities from secretomes and endospores from the selected isolate were confirmed in vivo assays. From 100 thermotolerant isolates, the secretome of the isolate identified as Bacillus paralicheniformis TB197 showed the highest NA (>95%) against Meloidogyne incognita, both in vitro and in vivo tests, suppressing infections caused by M. enterolobii in tomato crops, too. In open field tests, the endospores of TB197 strain showed a reduction of 81% in the infection severity caused by M. enterolobii (p ≤ 0.05), while the galling index (GI) was reduced 84% (p ≤ 0.05) in tomato greenhouse-tests. Also, a reduction of the root necrosis (81%) caused by Radopholus similis in banana plantations (p ≤ 0.05), compared to the control was observed. Owing to their efficacy in controlling PPNs infections, the endospores and secondary metabolites of B. paralicheniformis TB197 strain could be used in bionematicidal formulations.
1. Introduction
Phytopathogenic nematodes (PPNs) are one of the greatest threats to agricultural, ornamental and forestry activities (Archidona-Yuste et al., 2019). According to the report of the American Society of Phytopathology (APS), losses in agricultural production caused by PPNs are approximately 14%, which translates into almost 125 billion dollars annually (Mesa-Valle et al., 2020). A common feature of PPNs is the stylet located in the stoma or in the mouth that allows them to cross the host cell wall and inject enzymes that partially digest the cellular content before the nematode sucks it into its digestive system (Kimenju et al., 2009). According to their life cycle and feeding behavior, PPNs are classified into three groups: endoparasites, ectoparasites and semiendoparasites (Gullino et al., 2020). Endoparasites penetrate inside the plant, where all or part of their life cycle occurs; they are mainly root-knot nematodes (e.g., Meloidogyne spp.), encysted nematodes (e.g., Heterodera spp., Globodera spp.), and root-injuring nematodes (e.g., Pratylenchus spp., Hirschmanniella spp., Radopholus spp.), among others (Pires et al., 2022). In contrast, the ectoparasite life cycle occurs entirely outside the plant, piercing the epidermis or the superficial layers of the root cortex of the host plant with the stylet (e.g., Xiphinema spp., Trichodorus spp., Paratrichodorus spp.); therefore, they can also be found in seeds, stems and the aerial part of the plant (leaves and flowers; Pires et al., 2022). Finally, semiendoparasites can partially penetrate the plant, leaving part of the nematode inside the plant and another part outside, laying eggs toward the outside (e.g., Sphaeronema spp., Hoplolaimus spp., and Helycotylenchus spp.; Mesa-Valle et al., 2020).
Any agricultural crop can be affected by the action of some PPNs, and their effect is often underestimated as they produce nonspecific symptoms that are often confused with situations of water stress, nutritional disorders, soil fertility problems, or other secondary fungal or bacterial infections (Pires et al., 2022). Additionally, PPNs have fast generation times, high reproductive rates, and cryptic behaviors, contributing to the difficulties experienced in effectively controlling PPNs infections (Naz and Khan, 2013; Horak et al., 2019). Several strategies have been reported for the control of PPNs infections, such as nonhost crop rotation, soil solarization and the use of chemical nematicides, which include halogenated aliphatic hydrocarbons (e.g., 1,3-dichloropropene), methyl isothiocyanate, oxamyl, thionazine, and carbofuran (Engelbrecht et al., 2018; Naz et al., 2021). Although the use of chemical nematicides is the most successful strategy for the control of PPNs, their long-term repeated use leads to increased nematode resistance, exacerbating the difficulty of nematode management (Chen et al., 2020). In addition, there is evidence of their negative impact on the environment, for example, the groundwater contamination, disturbance of soil fertility, and the accumulation of chemical residues within the trophic levels of soil ecosystems (Engelbrecht et al., 2018). As eco-friendly alternatives, the use of microorganisms and/or their metabolites as biological control agents has attracted scientific interest due to their minimal environmental side effects (He et al., 2020).
Previous studies have shown that certain plant-associated rhizosphere microorganisms belonging to the Pasteuria, Pseudomonas, Streptomyces, and Bacillus genera control and antagonize PPNs through their ability to colonize the rhizosphere and secrete enzymes and/or secondary metabolites that are toxic to PPNs and/or elicit an induced systemic response (ISR) in plants (Berini et al., 2018; Zhao et al., 2018; Horak et al., 2019). However, in addition to their nematode control capabilities, this kind of microorganism must thrive under adverse conditions such as droughts, high temperatures and/or soil salinity promoted by climate change, which has been dramatically accelerated in recent years (Bokhari et al., 2020). Indeed, recent research has focused on the bioprospecting of plant-associated beneficial microorganisms capable of adapting to adverse conditions (Engelbrecht et al., 2018; Bokhari et al., 2020).
Desert soils are characterized by extreme conditions, such as high temperatures, water scarcity, high soil salinity, low nutrient levels and high UV radiation (Alsharif et al., 2020; Franklin et al., 2021). In addition, the survival of plants in desert areas is intrinsically related to their microbial communities (Sayed et al., 2019; Bokhari et al., 2020). Alsharif et al. (2020), Bokhari et al. (2020), and Franklin et al. (2021) showed that desert microorganisms not only relieve water stress in plants and promote growth but also protect the plant against pathogens, such as PPNs. Moreover, Viljoen and Labuschagne (2019) and Abd-Elgawad and Mahfouz (2020) found that Streptomyces and Bacillus strains isolated from the Egyptian desert are capable of effectively controlling PPNs and surviving in extreme conditions of drought and water stress. In this way, the plant-associated microbiota from deserts is a perfect ecological niche to isolate new microorganisms that are well adapted to extreme conditions and have potential for the biological control of phytopathogens, including PPNs.
The Sonoran Desert is one of the warmest regions in North America and in the world, where temperatures above 50°C have been recorded and cover a territory of 260,000 km2, including southern Arizona and southeastern California, EUA; northeast, north and south-central Baja California; and north-central and western Sonora, Mexico (Zolotokrylin et al., 2020; Flower et al., 2021). However, unlike other deserts, the Sonoran Desert is the most biodiverse; this is the case for flora, with approximately 2,500 species identified (Andrew et al., 2012; del Conagua, 2020; Franklin et al., 2021). This is relevant under the premise that the microbial communities are important for the survival of these plants, constituting a diverse and untapped pool of microorganisms with the potential to control phytopathogenic agents, such as PPNs, and to support extreme biotic and abiotic conditions or to synthesize thermostable or operatively stable metabolites. For example, Galaviz et al. (2018) and Bashan et al. (2012) found that the Sonoran Desert is home to plant growth-promoting rhizobacteria (PGPRs), such as Azospirillum brasilense and Bacillus pumilus. However, research on the potential of microorganisms isolated from this ecological niche for biological control of PPNs is scarce. Hence, the objectives of this research were to isolate, characterize and apply bacteria from the rhizosphere of plants growing in the Sonoran Desert with the potential for PPNs control.
2. Materials and methods
2.1. Reagents
Malt extract, yeast extract, LB broth and bacteriological agar were purchased from BD Difco (Sparks, MD, United States). Methanol and chloroform were provided by J.T. Baker (Xalostoc, Edo. Mex., Mexico). Glucose was provided by Faga Lab (Guamúchil, Sin., México). Verango® [fluopyram, 41.7% (w/v)], Lila Plus® [Paecilomyces lilacinus 3% (w/w)] and Nemacem® [Aqueous extract of Tagetes erecta, Alpha terthienyl 10% (w/w)] were provided by a local supplier.
2.2. Rhizospheric soil sample collection from the Sonoran Desert
Three zones of the Sonoran Desert were chosen for soil sampling: The Pinacate Biosphere Reserve and the Great Desert Altar (latitude: 31°31′55.01′′N, longitude: 113°25′40.05′′ W), La Primavera (latitude: 28°48′09.70′′ N, longitude: 111°12′13.20′′ W) and El Apache (28°18′59.60′′ N, 111°14′40.60′′ W). Two kilograms of rhizospheric soil were collected from at least 10 randomly selected points within each zone. The samples were collected with a small shovel from depths 0–30 cm, immediately placed in sterile plastic bags, refrigerated, and transported to the lab for processing on the same day.
2.3. Isolation of bacteria
Ten grams of soil samples were suspended in 150 mL Erlenmeyer flasks containing 90 mL of sterile distilled water and were incubated at 200 rpm and 27°C for 1 h (Innova 44, New Brunswick Scientific). Then, the soil sample suspensions were diluted in sterile distilled water (up to 10−4). After that, 0.5 mL of the diluted suspensions were inoculated into Petri dishes containing ISP2 agar (10 g/L malt extract, 4 g/L yeast extract, 4 g/L glucose and, 20 g/L bacteriological agar) and incubated at 40°C for 72 h. Isolated colonies were transferred to new Petri dishes with fresh ISP2 agar and incubated again at 40°C for 72 h. Axenic cultures were stored at 4°C for further analysis.
2.4. Submerged culture to obtain secretomes
Submerged cultures of isolates were established in 250 mL Erlenmeyer flasks containing 50 mL of ISP2 broth. The culture media were inoculated with a single colony of the axenic isolates and incubated at 37°C at 180 rpm for 72 h (Innova 44, New Brunswick Scientific). Then, the culture media were centrifuged at 10,034 x g and 4°C for 20 min (Allegra 64R Centrifuge, Beckman Coulter), and supernatants (cell-free) containing secretomes were stored at −20°C for further chemical analysis or bioassays.
2.5. Chemical characterization of secretomes by thin-layer chromatography
The selected secretomes with the highest nematicidal activities were freeze-dried (Yamato DC401 freeze dryer), and metabolites contained in the lyophilized powder were further extracted with methanol. 300 μL of solvent were added to 8 mg of lyophilized secretome and sonicated for 15 min in an ultrasonic bath (Branson 2510). Then, the samples were centrifuged (Eppendorf Centrifuge 5417R) at 9,279 × g and 4°C for 10 min, and the supernatants were collected for TLC analysis. Eight microliters of the extracts were spotted on silica gel plates (10 × 10 cm, TLC Silica gel 60 F254, Merck) and eluted with a mobile phase containing chloroform: methanol: distilled water (65:25:4 v/v). After elution, compounds were visualized with UV light (A: 254, and C: 365 nm), ninhydrin (sprayed with 0.1% w/v ethanol and heated at 60°C for 15 min), or iodine vapors.
2.6. Molecular identification of bacterial isolates
2.6.1. Genomic DNA extraction
Genomic DNA was extracted from a liquid culture of isolate A81 (further TB197 strain) grown aerobically in LB broth for 24 h at 30°C using the PowerSoil® DNA isolation kit (MO BIO Laboratories Inc.) according to the standard protocol provided by the manufacturer.
2.6.2. PCR amplification of the 16S rRNA, gyrA, and groEL genes
PCR amplification of marker genes was performed using OnePCR™ Ultra Supermix with Fluorescent Dye (Bio-Helix) according to the methodology described by Ben Gharsa et al. (2021) with some modifications. The primer pairs used for the 16S rRNA, gyrA, and groEL genes are detailed in Table 1. For all markers, a Studio™ 5 Real-Time PCR System was used (Applied Biosystem by Thermo Fisher Scientific), with a unified PCR program that consisted of (1) an initial denaturation phase at 95°C for 5 min; (2) 30 cycles of denaturation at 95°C for 40 s, annealing at 56°C (for the 16S region) or 55°C (for the other genes) for 1 min and elongation at 72°C for 30 s; and (3) final elongation at 72°C for 2 min.
The amplified 16S rRNA, gyrA and groEL products were confirmed by horizontal agarose gel electrophoresis with 1Kb Plus DNA ladder RTU (Bio-Helix). The gels were visualized on a UVP® High-Performance UV Transilluminator (Thermo Fisher Scientific).
2.6.3. Sequencing and phylogenetic analysis of the 16S rRNA, gyrA, and groEL genes
The PCR products were purified and analyzed by PSOMAGEN. Raw sequence data were combined into a single consensus sequence for each marker gene using the MEGA program version 10.0.05 (Kumar et al., 2018). The consensus sequences obtained were used as queries in GenBank database searches using the BlastN algorithm (NCBI GenBank database). Phylogenetic analysis was performed using MEGA software version 10.0.05 (Kumar et al., 2018). Evolutionary distances were calculated by Kimura’s two-parameter model (Kimura, 1980). Phylogenetic trees based on the 16S rRNA, gyrA, and groEL sequences of the isolates and different isolates retrieved from NCBI GenBank1 were constructed using the neighbor-joining method with bootstrap values based on 1,000 replicates (Saitou and Nei, 1987). The obtained 16S rRNA, gyrA, and groEL gene sequences of the isolates were submitted to the GenBank database.
2.7. Collection and synchronization of larval and egg stages of phytopathogenic nematodes
Nematode synchronization was performed based on the Zhao et al. (2020) methodology with some modifications. Tomato roots highly infested with Meloidogyne incognita, M. enterolobii or Radopholus similis were collected and vigorously washed with water to remove any adhered soil. The roots were carefully cut into 2–4 cm long pieces and placed into a blender containing a NaClO solution (2% w/v) to grind for 30 s. The crushed roots were rinsed with tap water and passed through 100-, 325-, and 500-mesh sieves. Second-stage (J2) juveniles of PPNs retained on the 325-mesh sieves and eggs retained in the 500-mesh sieves were suspended in sterile tap water and stored at 15°C for further analysis. On average, approximately 200 J2s or eggs per milliliter were collected by this procedure (five counts visualized at 10X, MOTIC, AE 2000, inverted microscope).
2.8. In vitro screening for nematicidal activity
In vitro nematicidal activity bioassays employing M. incognita J2 larvae in aqueous suspension were performed according to the methodology of Ala et al. (2020) with some modifications. Twenty microliters of secretomes obtained from submerged cultures were added to 96-well flat-bottom sterile polystyrene microplates (Corning® Costar® 3,595) containing 40–60 J2 larvae suspended in 200 μL of sterile tap water per well. Subsequently, the plates were sealed with parafilm and incubated at 25°C in the dark for 48 h, and motile and immotile larvae (considered death) were counted at 10X amplification (MOTIC, AE 2000, inverted microscope).
All experiments were performed considering three biological replicates (with three technical repeats each). Nematicidal activity was estimated according to Equation (1).
2.9. Nematicidal activity evaluation of secretomes in field assays
The evaluation was performed on a tomato (Solanum lycopersicum L.) crop within the presence of a shade mesh (∼50% reduction of sunlight radiation) at Agroindustrias Tombell (Culiacan, Sinaloa-Mexico). The experimental area was selected based on previous analyses of the nematode populations, and areas with the highest level of M. enterolobii infestation were selected. Tomato seedlings of the commercial Dionysus® hybrid (Ahern Seeds) were transplanted into cultivation plots, and the following three treatments were applied: (1) conventional management (undisclosed by the farmer), (2) secretome of the selected isolate from in vitro screening, and (3) Verango® (fluopyram, 41.7%), as a positive control. The secretome (cell free supernatant) of the selected isolate was applied at a concentration of 8 L/ha, while Verango® was applied at 1 L/ha. A total of 12 applications were made using a drench system with intervals of 8 days between them for a period of 90 days. Each experimental unit consisted of three plots (1.80 m between them) and was 50 m long (270 m2 per treatment). Only the central plot of each treatment was evaluated to avoid the influence of adjacent treatments.
To evaluate the root damage produced by nematodes, root washings were performed at 30, 60, and 90 days after transplantation, for which 10 plants were selected randomly from the central plot of each treatment. The galling index (GI) in tomato roots was determined based on the visual scale proposed by Baker (1978) (Supplementary Table S1; Supplementary Figure S1) in a range of 0–5, where 0 represents 0% galling and 5 represents greater than 80%.
2.10. Endospores production
Five hundred milliliter Erlenmeyer flasks, containing 200 mL of sterile Soy based medium (15 g/L soybean flour, 5 g/L dextrose, 5 g/L cornstarch, 1 g/L K2HPO4, 1 g/L of KH2PO4, 0.3 g/L MgSO4*7H2O, 0.02 g/L FeSO4*7H2O, 0.02 g/L ZnSO4*7H2O and 1 g/L CaCO3; pH: 7) were aseptically inoculated with a single bacterial colony obtained from a Petri dish. The flasks were incubated at 37°C, 200 rpm, during 72 h. Finishing the incubation period, a heat treatment was employed by subjecting the culture to 80°C for 15 min to eliminate selectively vegetative cells, following the procedure described by Vignesh et al. (2022).
Subsequently, the endospore suspension was added with 10% (w/v) of maltodextrin as a carrier (Bhagwat et al., 2020; Vignesh et al., 2022) and dried using a laboratory spray dryer (Yamato ADL3115) at 110°C and 75°C as inlet and outlet air temperature, respectively, obtaining a fine powder containing around 1 × 108 endospores/g. Endospores were quantified, resuspending the powder in water and employing two methods: (a) using a Newbauer chamber (40X); (b) by plate count, according to Bolanos-Carriel and Vazquez Soto (2022) method. Endospores morphological and wall characteristics were verified through the application of the Endospore Stain Protocol, recommended by the American Society for Microbiology (Hussey and Zayaitz, 2016). The endospores powder was employed further in greenhouse and open field bioassays.
2.11. Biological control in greenhouse assays
The assays were performed at Centro de Investigación en Alimentación y Desarrollo (Culiacán, Sinaloa, Mexico) in the spring of 2020 (March and April). Taureg hybrid tomato seeds were sown directly in plastic pots under aseptic conditions at a greenhouse temperature of ~25°C, relative humidity of ~60% and 16 h of daylight. A cluster of 4 to 6 seeds was sown approximately 1.5 inches deep in a 15-cm-wide pot, which was filled with 1.0 kg of a high-quality well-drained potting mix. The plants were watered every 3–4 days, and when they were 30 days old, a suspension of M. enterolobii eggs (approximately 1,500 eggs per pot) was inoculated using a sterile micropipette following standard inoculation procedures (Naz and Khan, 2013).
To evaluate the biological control in greenhouse assays, 400 mL of (a) water (negative control), (b) selected strain endospores suspension (1 × 106 endospores/mL; prepared resuspending 1 g of dried spores (1 × 108) in 100 mL of water), and (c) Verango® (fluopyram, 41.7%, as a positive control, 1 L/ha) were applied by irrigation to 20 plants postinoculation of the M. enterolobii eggs. After 30 and 60 days of nematode inoculation, five plants from each treatment were picked randomly, and the roots were carefully washed to remove soil remnants. The galling index was measured to evaluate the biological efficacy of the treatments applied according to the aforementioned visual scale proposed by Baker (1978) (Supplementary Table S1; Supplementary Figure S1). The number of eggs per pot was also recorded by counting under an inverted microscope to determine the reproduction factor (RF) of M. enterolobii, according to Equation (2).
2.12. Biological control in open field assays
2.12.1. Root-knot nematodes
Biological control of the root-knot nematode M. incognita was conducted in Calvillo, Aguascalientes, Mexico, using tomato plants (Solanum licopersicum) of the Optimax variety during May and June 2022. A randomized complete block design was employed, where 100 mL (per plant) of (a) water as a negative control, (b) selected isolate endospores (1 × 108 endospores/g; 4 kg/ha) and (3) Lila Plus® as a positive control (Purpureocillium lilacinum, 0.48 kg/ha) were applied by uniform drenching after 4 weeks of tomato crop growth in four replicate plots (1.8 × 4 m) per treatment. At 15 and 30 days after the treatments, five plants were extracted from each plot (20 plants per treatment), and the galling index was determined by the visual scale described previously (Supplementary Table S1; Supplementary Figure S1). Additionally, to determine the population density of M. incognita J2 at the beginning, middle, and end of the assay, 100 g of roots of 4 different plants for each treatment were obtained by 4 zigzag samplings and processed for the extraction and quantification of M. incognita (J2, five counts visualized at 10X, MOTIC, AE 2000, inverted microscope) as described previously.
2.12.2. Burrowing nematodes
The biological control of the burrowing nematode Radopholus similis was conducted in Cihuatlan, Manzanillo-Mexico using banana plants (Musa sp.) of the gran nain cultivar during May and June 2022. A randomized complete block design was employed, where 100 mL of (a) water (negative control), (b) B. paralicheniformis TB197 isolate (1 × 108 endospores/g; 4 kg/ha), and (c) Nemacem® (6 L/ha, positive control) were applied by uniform drenching in four replicate plots (1.8 × 4 m) per treatment. At 15 and 30 days of the assay, five plants were extracted from each plot (20 plants per treatment), and the necrosis index was determined based on a visual scale of 0 to 5 proposed by Coyne et al. (2007) (Supplementary Table S2). To determine the population density of R. similis J2 at the beginning, middle and end of the assay, 100 g of roots of 4 different plants for each treatment were obtained by 4 zigzag samplings and processed for the extraction and quantification of R. similis J2 as described previously.
2.13. Statistical analysis
The results of all experiments are reported as the mean ± standard deviation. One-way analysis of variance (ANOVA) and Tukey–Kramer (95% confidence limit) tests were performed to establish significant differences among treatments using NCSS software (Number Cruncher Statistical System or Windows, Kaysville, UT, United States, version 7.0).
3. Results
3.1. In vitro screening for nematicidal activity from secretomes
A total of 100 bacterial isolates were isolated from rhizospheric soil samples collected at the Sonoran Desert. Among them, 43 from “La Primavera,” 30 from “Apache,” and 27 from “El Pinacate.” From all isolates, only 27 secretomes showed some nematicidal activity (3–96% mortality M. incognita J2 larvae) (Figure 1). Secretomes from the A106, A136, A137, A138, A144, A21, A22, A44, A51, A58, A62, and A73 isolates showed low nematicidal activities (≤50%), those from the A12, A31, A39, A52, and A6 isolates presented moderate activities (50–70%), those from the A101, A124, A131, A132, A133, A134, A82, A95, A74, and A81 isolates were highly effective (70–96%). Among these, A81 isolate showed the highest and consistent nematicidal activity (96%), it was chosen as the most promising microorganism for PPNs control; thus, a comprehensive characterization of this isolate, including its molecular identification, in vivo effectiveness assays (both endospores and secretome), and chemical characterization of its metabolites, were performed.
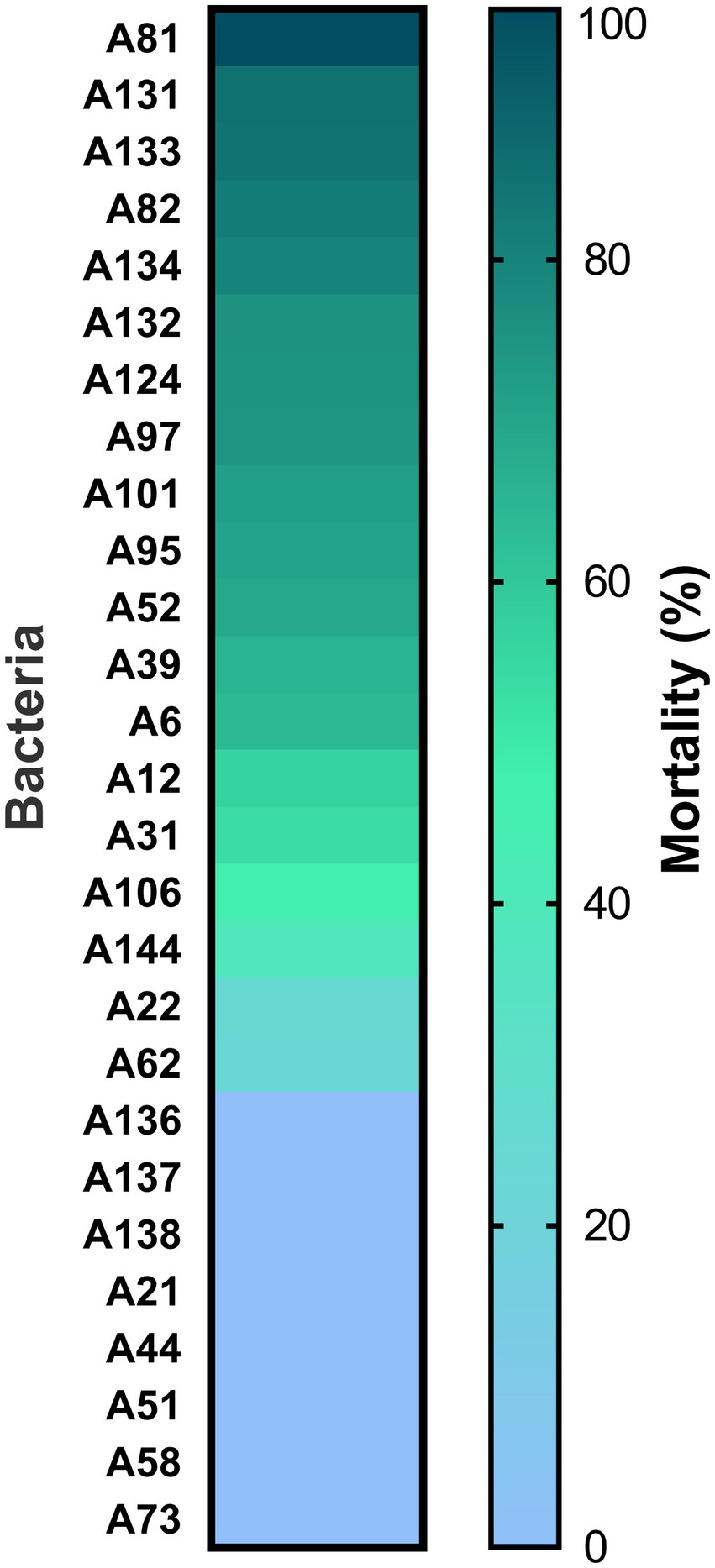
Figure 1. Toxicity of secretomes from bacteria isolated from rhizospheric soil samples from the Sonoran Desert against M. incognita. Color bars represent the means of three replicates.
3.2. Molecular identification of the selected isolate
According to the 16S rRNA (Figure 2) and the housekeeping gyrA and groEL gene sequences (Supplementary Figures S2, S3), the A81 isolate showed 99.27–100% identity to Bacillus paralicheniformis. The percent identity values and the accession numbers of the sequences are listed in Table 2. The A81 isolate was deposited at the National Genetic Resources of Mexico, CNRG, and renamed as Bacillus paralicheniformis TB197 strain.
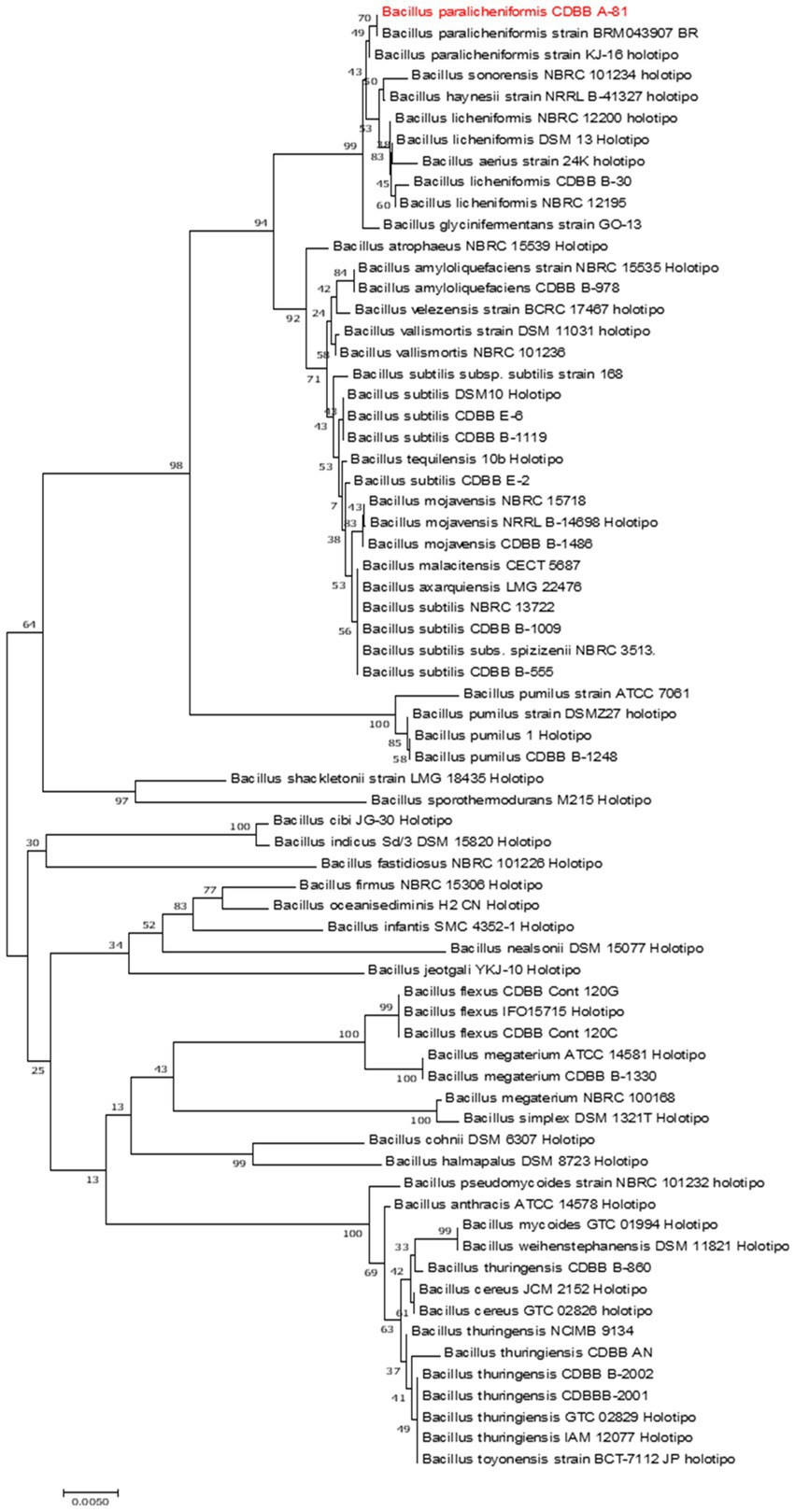
Figure 2. Phylogenetic tree of the representative TB197 isolate and Bacillus species from NCBI GenBank based on the 16S rRNA gene. The sequence was constructed using the neighbor-joining method with 1,000 replicates. The bar indicates 0.005 substitutions per nucleotide position.
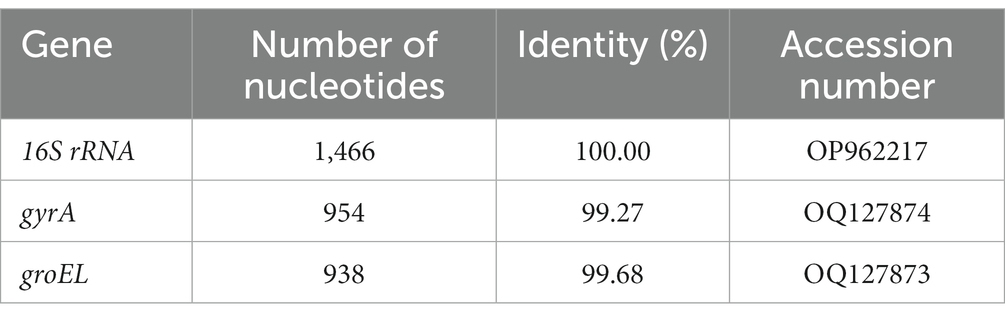
Table 2. Molecular identification and genotyping of Bacillus paralicheniformis TB197 based on 16S rRNA, gyrA, and groEl gene sequences.
3.3. Chemical characterization of secretomes by thin-layer chromatography
The secretome of the B. paralicheniformis TB197 strain was analyzed by thin-layer chromatography (TLC) employing UV light (to reveal conjugated pi bonds, such as aromatic compounds), ninhydrin (to reveal peptides and free amino groups) and iodine vapors (to reveal unsaturated compounds, particularly fatty acids), as shown in Figure 3. TLC plates demonstrated the presence of high-polarity compounds with low distance traveled (Rf from 0 to 0.4), active to UV light (Figures 3A,B), and high reactivity to ninhydrin and iodine vapors (Figures 3C,D, respectively). On the other hand, at least one compound of medium polarity (Rf near 0.5) was revealed with ninhydrin and iodine vapors (Figures 3C,D) but was inactive or slightly active to UV light (Figures 3A,B). Finally, two compounds of low polarity with an Rf of 0.8 and 0.9 were visualized employing UVC and UVA, respectively (Figures 3A,B), and both were reactive to ninhydrin and iodine vapors (Figures 3C,D, respectively).
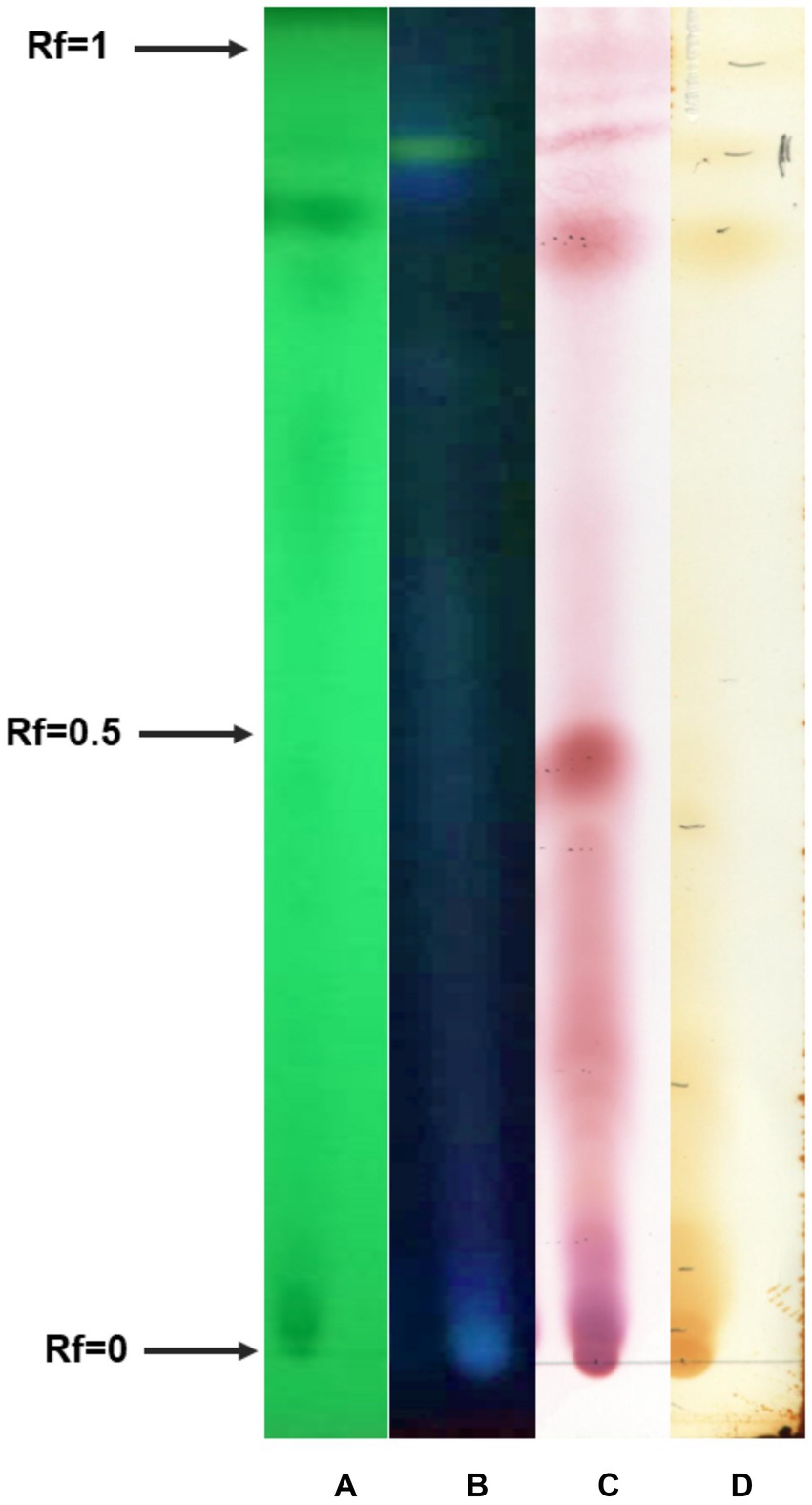
Figure 3. Thin-layer chromatogram of the B. paralicheniformis TB197 secretome visualized with (A) UVA light, 254 nm; (B) UVC light, 365 nm; (C) ninhydrin (0.1%); and (D) iodine vapors. Rf, retard factor.
3.4. Secretome nematicidal activity in field assays
The effect of the secretome of the TB197 strain on the galling index (GI) and the percentage of infestation severity on tomato roots caused by M. enterolobii was evaluated in field experiments and compared with conventional management (employed by the owner of the field and kept confidential) and the application of a chemical nematicide as a positive control (fluopyram, 41.7% w/v). The three treatments suppressed the rate of galling (0) caused by M. enterolobii during the 60 days of application. At 90 days of the experiment, no differences were observed (p ≥ 0.05) in the treatments, where the secretome of the TB197 strain (GI: 0.2; Severity (%): 4) and the conventional management (GI: 0.2; severity (%): 4) were applied. In both cases, the progress of the infection was slight, with a GI and severity percentage less than 0.5 and 5%, respectively, while the positive control (fluopyram) completely suppressed the galling and damage caused by M. enterolobii.
3.5. Biological control in greenhouse assays
A higher and progressive nematode infestation was observed in the control (water) at the first (30 days) and second evaluation (60 days) (Figures 4A,D), resulting in a galling index (Table 3) up to 10-fold higher than the application of B. paralicheniformis TB197 endospores and the chemical nematicide (p ≥ 0.05). Bacillus paralicheniformis TB197 endospores effectively suppressed the infestation of M. enterolobii in tomato roots (Figures 4B,E) in a similar manner (p ≤ 0.05) as the chemical nematicide (Figures 4C,F) at 30 and 60 days of application. The reproduction factor of M. enterolobii in tomato roots (Table 3) treated with the chemical nematicide and TB197 endospores were similar between them (p ≤ 0.05) but 116-fold lower than that of the negative control (p ≥ 0.05).
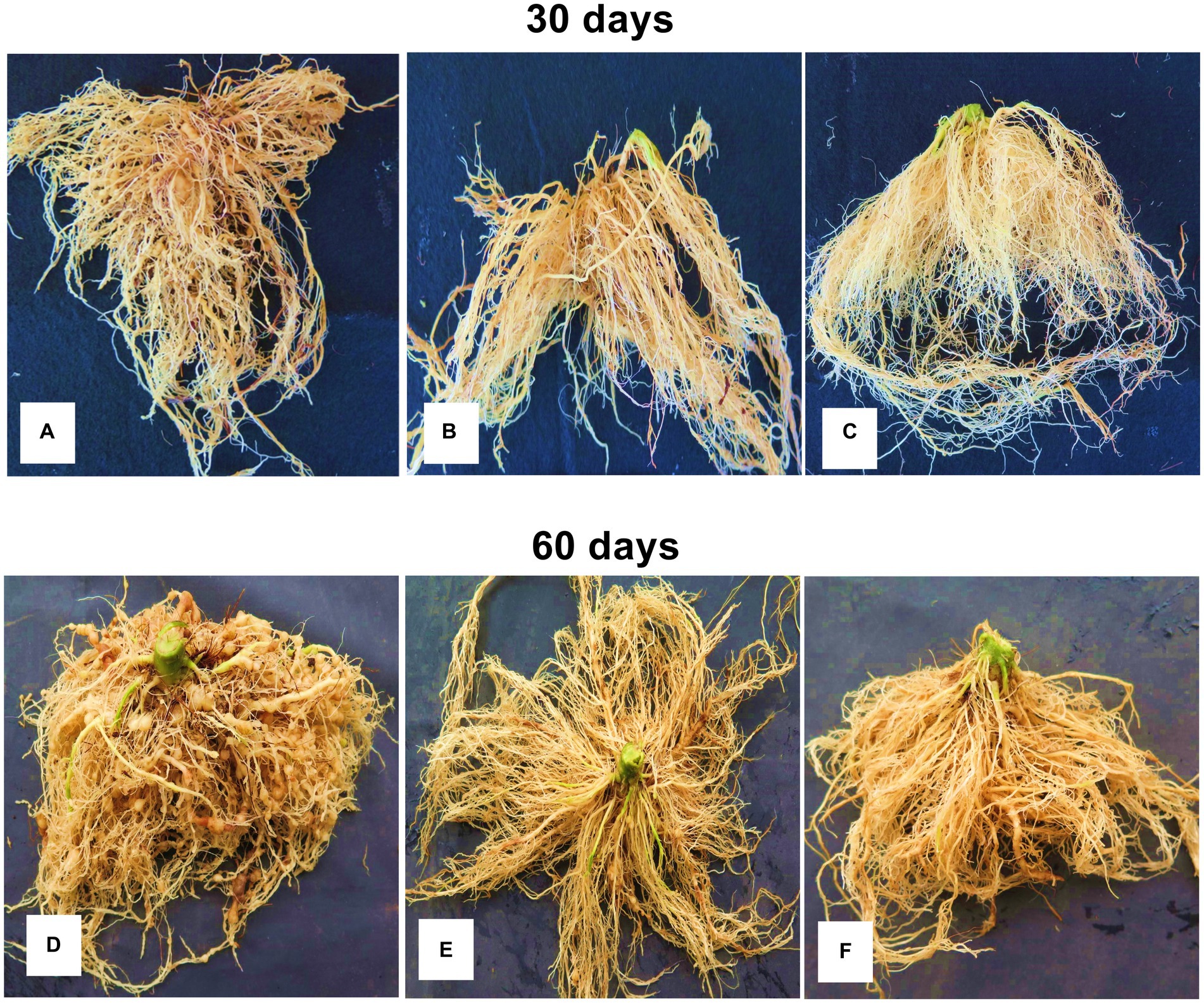
Figure 4. Gall formation on tomato roots caused by M. enterolobii in a greenhouse assay after 60 days of treatment. (A,D) Negative control (water); (B,E) B. paralicheniformis TB197 endospores; (C,F) positive control (fluopyram).
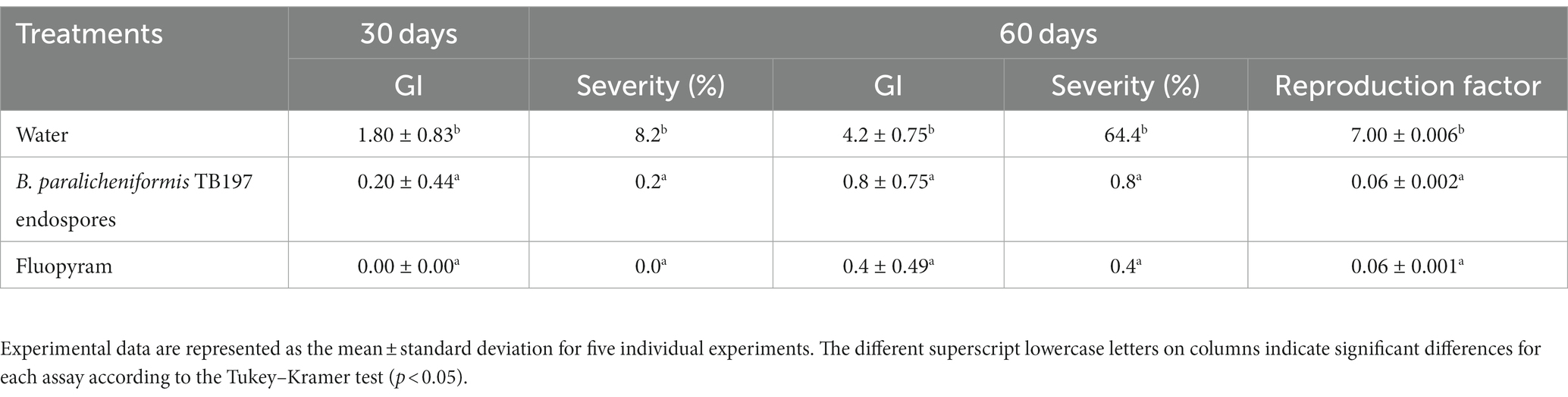
Table 3. Effect of treatments on M. enterolobii (greenhouse test) infestation of tomato plants measured through the galling index (GI), infestation severity, population density and reproduction factor.
3.6. Biological control in open-field assays
Like greenhouse assay, a higher and rapid infestation of the root-knot nematode (M. incognita) was observed in the control (water) during the open-field assay (15 and 30 days), while Bacillus paralicheniformis TB197 endospores and Lila-Plus® (Paecilomyces lilacinus 3% w/w) effectively reduced the infection parameters evaluated in tomato plants (p ≤ 0.05) without significant differences between them (Table 4). With both treatments, the GI, severity percentage and, population density of M. incognita were up to 2-, 5-, and 7-fold lower than those of the control, respectively (Table 4). Additionally, based on the reduction in galling severity, the efficacy of TB197 and the positive control in controlling M. incognita was 83.7 and 81.3%, respectively (Figure 5B).
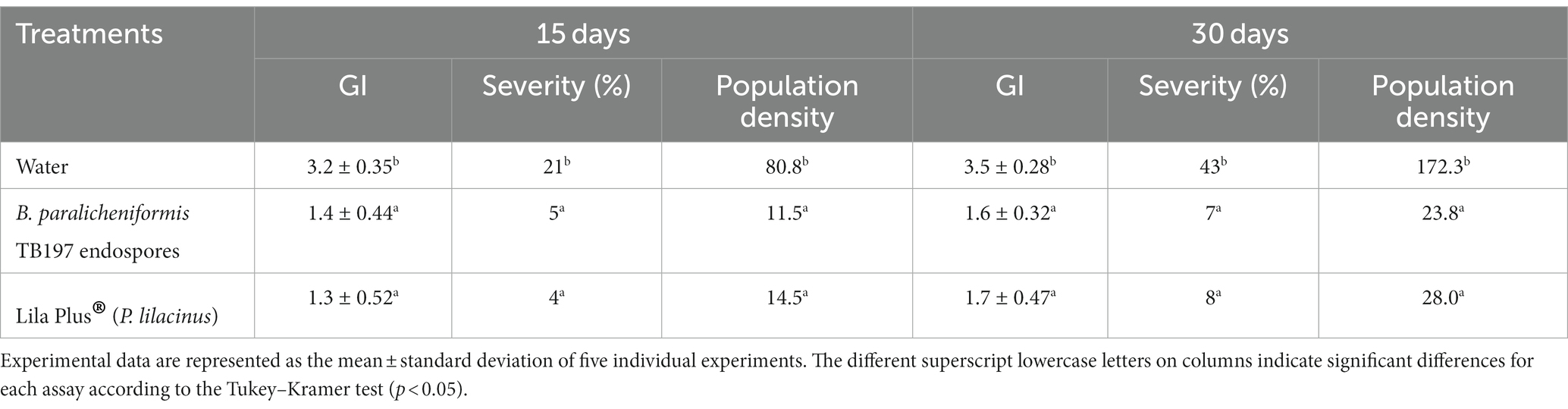
Table 4. Effect of treatments on M. incognita infestation of tomato plants (field test) measured through the galling index (GI), infestation severity and population density.
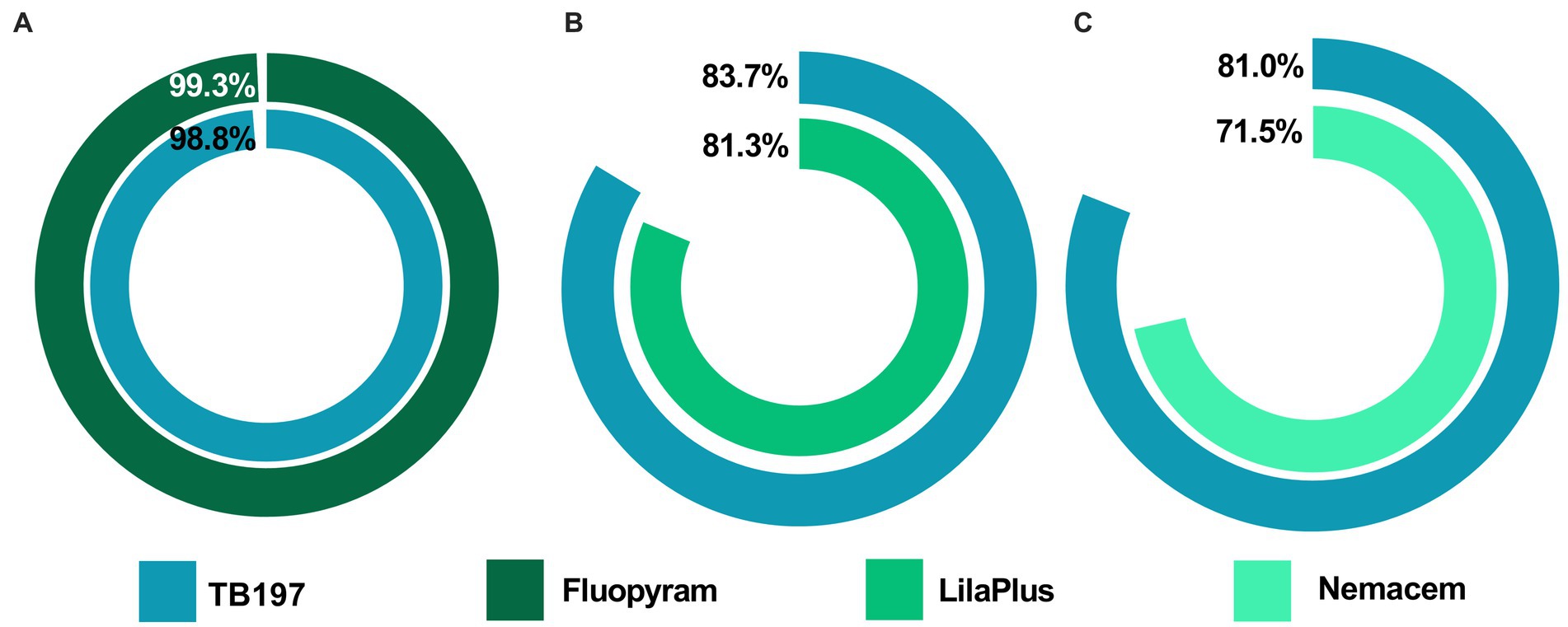
Figure 5. Control efficacy on phytopathogenic nematodes using endospores of the B. paralicheniformis TB197 strain and commercial nematicides. (A) Reduction in the galling severity (%) produced by M. enterolobii (greenhouse test); (B) reduction in the galling severity (%) produced by M. incognita (field test) and (C) reduction in the necrosis percentage produced by R. similis (field test). The reduction percentages are based on the negative control (water).
The B. paralicheniformis TB197 endospores also showed consistent nematicidal activity against the burrowing nematode R. similis in banana crops. TB197 strain and Nemacem® (aqueous extract from Tagetes erecta, alpha terthienyl 10% w/w) effectively reduced the infection caused by R. similis (Table 5) without significant differences between them (p ≥ 0.05) during the open-field assay (15 and 30 days). Both reduced necrosis of roots and nematode population density up to 4.4- and 2.6-fold, respectively, compared with the control (water), with which banana crops showed a faster R. similis infestation. Additionally, based on the reduction in the necrosis percentage, the efficacy of TB197 and the positive control in controlling R. similis was 81 and 71.5%, respectively (Figure 5C).
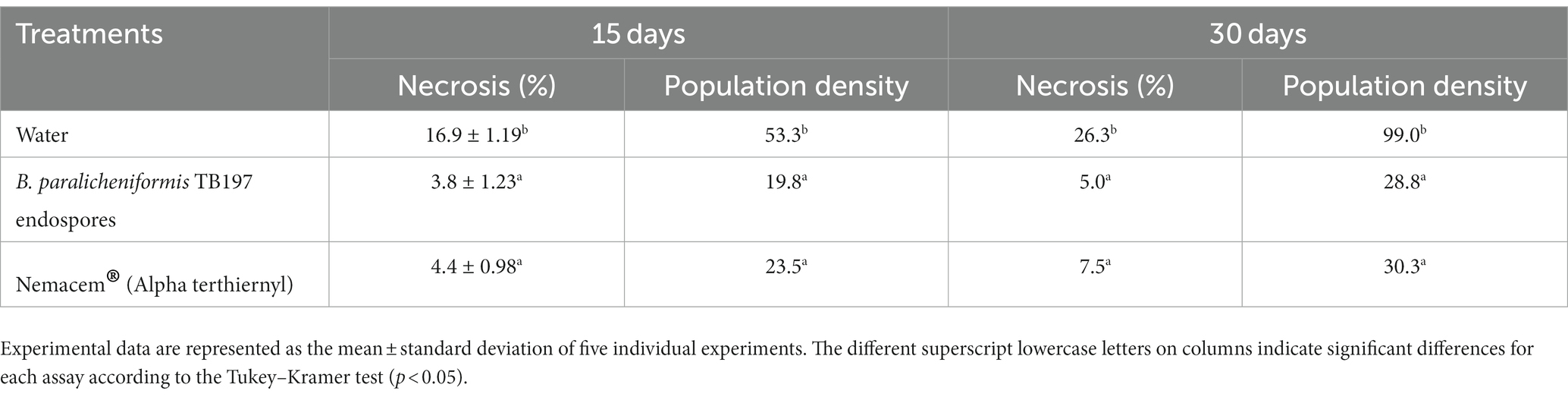
Table 5. Effect of treatments on Radopholus similis infestation of banana plants (field test) measured through the necrosis percentage and population density.
4. Discussion
Harsh abiotic conditions existing in desert environments (e.g., extreme temperatures and radiation, water scarcity, erosion, low organic matter content, and sedimentation, among others) negatively impact living organism development especially in plants and microorganisms (Alsharif et al., 2020). However, several species have developed adaptations to remain metabolically active (Schultz and Soares, 2020) and to be able to proliferate under these adverse conditions. Such is the case for the Sonoran Desert, which possesses the greatest biodiversity of flora and fauna among deserts worldwide, although the temperatures in summer can exceed 45–50°C (Zolotokrylin et al., 2020). Desert soils worldwide possess a huge diversity of microorganisms dominated by Actinobacteria (36.8%), Proteobacteria (23.8%), Firmicutes (8.6%), and Acidobacteria (5.5%) (Dean et al., 2020). According to this microbial diversity distribution and the well-known potential of Actinobacteria as control agents (Bhatti et al., 2017; Cruz Silva et al., 2022); ISP2 medium was employed in the first screening, looking for isolates belonging to this phylum or to Firmicutes (Tian et al., 2022). Since, although ISP2 is considered as a selective medium for actinomycetes, it allows the growth of some other bacterial genera, such as Bacillus, too (Paz et al., 2016; Zhou et al., 2019).
Specifically, regarding the Sonoran Desert, there is scarce information about rhizospheric and soil microbiota composition and the biotechnological potential from their microorganisms. Sutto-Ortiz et al. (2017) and Bacame-Valenzuela et al. (2015) reported the isolation of thermotolerant actinomycetes and fungal strains capable of synthesizing enzymes of industrial interest, such as phospholipase A and feruloyl esterases, respectively. Additionally, Camacho-Ruiz et al. (2020) reported the isolation from Sonoran Desert soils of Streptomyces, Bacillus, Aspergillus, and Aureobasidium strains able to produce gastrointestinal lipase inhibitors. Moreover, Baqueiro et al. (2019) reported the biotechnological potential of Bacillus strains capable of producing stable proteases. Regardless of agricultural applications, some plant growth-promoting rhizobacteria (PGPRs), such as Azospirilum brasilense and Bacillus pumilus, have been isolated (Bashan et al., 2012; Galaviz et al., 2018), but there are no reports focusing on PPNs control. Indeed, this is the first work to explore and demonstrate the potential of microorganisms from the Sonoran Desert for the control of PPNs.
In the current work, from 100 thermotolerant bacteria isolated from rhizospheric soils collected in the Sonoran Desert, the secretomes from 27 of them showed some nematicidal activity in vitro (3–96%) against M. incognita. Of these isolates, 10 produced high mortality in M. incognita larvae (70–90%), but the secretome from the TB197 strain produced the highest (>95%) (Figure 1). Consequently, it was selected for identification and evaluation in greenhouse and open-field tests. Sequence analysis of the 16S rRNA gene had the most significant impact on the identification and taxonomic classification of the TB197 strain. Even so, analyses of the housekeeping gene sequences gyrA and groEL were also included because previous studies mention difficulties in the differentiation and phylogenetic relationships of certain groups of Bacillus based on 16S rRNA gene sequences due to their similarities (Table 2). Additionally, the sequencing of housekeeping genes has been shown to be more appropriate than 16S rRNA sequencing for discriminating certain species of Bacillus (Ozdemir and Arslan, 2022). Therefore, the use of these housekeeping genes provides a high resolution between species closely related to groups of Bacillus, as they are generally more conserved between bacterial species than the 16S rRNA gene (Liu et al., 2013; Franco-sierra et al., 2020). This information allowed the robust identification of the TB197 strain as Bacillus paralicheniformis.
In agriculture, many strains from the Bacillus genus have been employed in biofungicides and biofertilizer formulations due to their biological activities, including antagonism against phytopathogenic agents, such as bacteria, fungi and nematodes; induction of plant systemic resistance against phytopathogens; and promotion of plant growth and development (Villarreal-delgado et al., 2018; Valenzuela-Ruiz et al., 2019). Furthermore, Bacillus is one of the three most important genera for PPNs control by several mechanisms: direct parasitism, antibiosis, reduction in plant root penetration and competition for essential nutrients (Engelbrecht et al., 2018). Antibiosis has been identified as the main control mechanism of PPNs through the production of antibiotics and other secondary metabolites (lipopeptides, siderophores, and endotoxins, among others) and lytic enzymes secreted during growth and the stationary phase (Xiong et al., 2015; Gao et al., 2016; Engelbrecht et al., 2018; Liu et al., 2020a). Nevertheless, information on secondary metabolites produced by B. paralicheniformis with nematicidal activity is almost nonexistent. Valenzuela-Ruiz et al. (2019) reported that the draft genome of B. paralicheniformis TRQ65, isolated from wheat fields in the Yaqui Valley, Mexico, revealed the presence of putative genes related to osmotic and oxidative stress responses and auxin, lipopeptides, siderophores and antibiotic biosynthesis.
For preliminary chemical characterization, extracts obtained by lixiviation of freeze-dried supernatants with methanol were employed. TLC plates from samples obtained by methanolic extraction are shown in Figure 3, revealing the presence of several fractions with different Rf values. The spots from TLC plates containing the methanolic extract can be visualized by different methods (UV and stains). Almost all of them were reactive to ninhydrin and iodine vapors (Figures 3C,D), but only some of them were active to UVA and/or UVC (Figures 3A,B) with slight activity for UV light but high activity for iodine vapors and ninhydrin (Figure 3). The reactivity to ninhydrin suggests the presence of peptide-natural compounds (Dlamini et al., 2020) and probably molecules that contain unsaturated fatty acids due to reaction with iodine vapors (Meyers and Meyers, 2008). In the case of some UV-active spots, the molecules could include aromatic compounds, such as aromatic amino acids.
The information provided by TLC analyses fits with the description of siderophores and cyclic lipopeptides usually produced by Bacillus species and the presence of putative genes to produce them, according to the draft genome of B. paralicheniformis TRQ65 (Horak et al., 2019; Valenzuela-Ruiz et al., 2019). In general, lipopeptides contain a hydrophobic fatty acid chain bonded to a hydrophilic cyclic peptide. According to the nature of the cyclic peptide, lipopeptides produced by Bacillus species, are generally classified into three families: surfactin, fengycin, and iturin (Falardeau et al., 2013; Yang et al., 2015). Members from the fengycin and iturin families usually contain aromatic amino acids in their peptide ring, such as tyrosine (Zhao et al., 2017), which would explain the UV activity of some compounds (Figure 3). Molecules with Rf values between 0 and 0.3 or 0.2 and 0.4 could belong to the fengycin and iturin families, respectively, as mentioned by Geissler et al. (2016). On the other hand, low-molecular-weight metal-chelating compounds of the hydroxamate and catecholate types, which are involved in the uptake of iron (siderophores) by microorganisms, have been reported as metabolites produced by several Bacillus species (Sansinenea and Ortiz, 2011). The results of the TLC analysis strongly suggest that secondary metabolites synthesized by the B. paralicheniformis TB197 strain could be siderophores and/or cyclic lipopeptides. However, an exhaustive chemical characterization of its secretome is currently ongoing to verify this hypothesis.
This study demonstrated the potential of the secondary metabolites contained in the secretome from B. paralicheniformis TB197 to effectively control infections caused by M. enterolobii in open-field assays. The GI and the infection severity percentage observed in tomato roots were very low and similar to the conventional management performed by the farmer. In this sense, there are few studies evaluating the effectiveness of secretomes of Bacillus species for the control of PPNs in agricultural fields. For example, Liu et al. (2020b) showed that the application of 16 mL/m2 of various secretomes of Bacillus strains in tomato roots resulted in a GI of 1, while the GI reported in the present work by employing the secretome of the TB197 strain was 0.2. Therefore, the secretome of B. paralicheniformis TB197 appears to be more effective as a lower concentration (0.8 mL/m2) was applied and obtained better GI results.
However, additional work over the application of secretomes regarding biosafety, doses, application times, optimization of culture medium, culture conditions and the exhaustive characterization of their bioactive metabolites is required. Although the chemical nematicide fluopyram (pyridinyl-ethyl-benzamide) was highly effective in suppressing infection by Meloidogyne species, there are reports that this chemical shows a reversible inhibition phenomenon that could lead to possible crop infestation even after application (Watson, 2022). In addition, fluopyram is reported to be a recalcitrant compound that modifies the general soil structure and the activity and function of beneficial soil microorganisms (Zhang et al., 2014). Consequently, as an advancement toward sustainable agriculture, the secondary metabolites contained in the secretome of the TB197 strain appear to be a promising alternative to chemical nematicides.
Although bacterial metabolites look promising for PPNs control, the use of cells or endospores from Bacillus species is the most common way for formulating commercial products (Zhou et al., 2016; Engelbrecht et al., 2018). The use of live cells and/or spores offers several additional advantages over the use of metabolites, such as the ability to combine multiple mechanisms, like interference in nematode-host recognition, competition for nutrients, promotion of plant growth, and induction of systemic resistance (Chinheya et al., 2017; Engelbrecht et al., 2018; Ramírez-Cariño et al., 2020). Additionally, endospores are preferred to prepare commercial formulations because they are highly resistant to adverse environmental conditions, making them suitable for long-term storage and convenient for formulating powdered products for agricultural application (Zhou et al., 2019). In this study, the capacity of B. paralicheniformis TB197 to control PPNs was evaluated for the first time. In the greenhouse assay, both the TB197 strain and fluopyram demonstrated a control efficacy of M. incognita greater than 98% based on the reduction in galling severity (Figure 5A).
On the other hand, several studies mention that success of controlling PPNs at the greenhouse level does not guarantee bacterial performance at the field level (Tian et al., 2007; Abdel-Salam et al., 2018; Zhao et al., 2020; Wang et al., 2021), usually because strains are affected by unfavorable factors, such as climatic changes, physical and chemical properties of soil and the competition of native microorganisms (Wang et al., 2021). However, our results show the consistent ability of B. paralicheniformis TB197 to control PPNs even at the field level. One assay was performed against root-knot PPN comparing the bacterial effectiveness with a probed commercial biopesticide LilaPlus® (P. lilacinum). Both treatments were highly effective and showed consistent results for the control and reduction of the severity of M. incognita infection (~84% compared with the control, Figure 5B). Several studies and patents report Bacillus cells or endospores as biological control agents, particularly against root-knot nematodes from the Meloidogyne genus; however, none of them surpassed the effectiveness reported here for B. paralicheniformis TB197. For example, the percent reduction in GI in field assays through the use of Bacillus altitudinis AMCC1040 (Wang et al., 2021), B. subtilis Bs-1, B. cereus Bc-cm103 (Cao et al., 2019), B. aryabhattai KMT-4 (Yin et al., 2021), B. velezensis Bv-25 (Antil et al., 2021), B. marisflavi CRB2 (Tian et al., 2022), B. subtilis CRB7 and B. methylotrophicus (Gowda et al., 2022) was <70%.
A second assay was performed against the burrowing nematode R. similis, a migratory, polyphagous endoparasite with a wide global distribution mainly found in tropical and subtropical regions that infects banana crops. The control effectiveness of the TB197 strain was similar to that of Nemacem®, an effective commercial botanical extract from Tagetes erecta, employed as a positive control. In both cases, the percentage of root necrosis and the nematode population density were reduced by 81.0 and 71.5% (Figure 5C), respectively, compared to the control (water). The main active metabolite from the botanical extract (Nemacem®) is alpha terthienyl, a well-known molecule acting on the central and peripheral nervous systems of nematodes, causing their immobilization and subsequent death (Plantoria, 2022). Although this extract is considered an environmentally friendly alternative, its half-life is very short (̴ 4 h, according to the National Institute of Ecology and Climate Change of Mexico2), which could be a disadvantage compared with bacterial-based nematicides, that in the case of molecules synthetized by the Bacillus genus, are stable at extreme soil pH, temperature and salinity conditions for more than 25 days (Raaijmakers et al., 2010; Engelbrecht et al., 2018). Our results demonstrate that both the bioactive metabolites contained in the secretome of the B. paralicheniformis TB197 strain and their endospores can effectively control PPNs infections. They provide an ecological alternative to chemically synthesized nematicides, and can compete successfully with commercial botanical and microbiological bionematicides.
Despite the fact that the TB197 strain was isolated from the desert rhizosphere and showed an optimum growth temperature range between 40 and 45°C (data not shown), its ability to growth in different environmental conditions is highlighted. For example, the greenhouse trials were developed under controlled conditions of temperature and humidity (25°C and 60%, respectively), while the field trials on tomato plants were performed in Aguascalientes, Mexico, where the temperature ranged from 13 to 30°C with a relative humidity of 6.7%. By the contrary, the trials on banana crops were carried out in Colima, Mexico, where the temperature varies from 23 to 31°C with a relative humidity of 81%, higher than greenhouse and field trials conditions. These facts suggest that the TB197 strain has the ability to colonize different crop roots under distinct environmental conditions, possibly due to a unique adaptation mechanism inherent to its niche of isolation. However, further studies are required to establish more precisely the colonization capacity of the TB197 strain under extreme conditions (ongoing studies).
5. Conclusion
Plant-associated bacteria from the Sonoran Desert show potential for controlling PPNs. In particular, the new Bacillus paralicheniformis TB197 strain exhibited a high capacity for PPNs control in vitro, greenhouse and agricultural field tests. Moreover, the TB197 strain successfully proliferated in various rhizospheric soils with different climate, soil conditions and crops, indicating its high adaptability to colonize environments different from its niche of isolation. The effective reduction of root infection by PPNs and the resulting damage, achieved through the application of the secretome or the endospores of TB197 strain, are comparable to those obtained with commercial chemically synthesized and natural nematicides. These results support B. paralicheniformis TB197 as a potential control agent for the development of commercial bionematicidal formulations.
Data availability statement
The datasets generated during and/or analyzed during the current study are available from the corresponding author on reasonable request.
Author contributions
EC-Q, VC-J, AC-F, FD, and AA-T contributed to the study conception and design. AC-F performed the secretome field and the greenhouse assays. VC-J obtained the secretomes for the in vitro nematicidal assays. FD performed the molecular characterization. EC-Q performed the chemical characterization of the secretome, analyzed the data, and wrote the original manuscript. AA-T and VC-J supervised the research and reviewed the manuscript. All authors contributed to the article and approved the submitted version.
Funding
This work was supported by Grant N° 20624 Innovak Global-CIAD A.C.
Acknowledgments
The author EC-Q acknowledges the scholarship number 867046 granted by the National Council on Science and Technology (CONACYT) for PhD studies. We are grateful to Innovak Global for the financial support and the performance of the in vitro tests by Noemí Rivera-Zabala.
Conflict of interest
The authors declare that the research was conducted in the absence of any commercial or financial relationships that could be construed as a potential conflict of interest.
Publisher’s note
All claims expressed in this article are solely those of the authors and do not necessarily represent those of their affiliated organizations, or those of the publisher, the editors and the reviewers. Any product that may be evaluated in this article, or claim that may be made by its manufacturer, is not guaranteed or endorsed by the publisher.
Supplementary material
The Supplementary material for this article can be found online at: https://www.frontiersin.org/articles/10.3389/fmicb.2023.1213306/full#supplementary-material
Footnotes
1. ^https://blast.ncbi.nlm.nih.gov/Blast.cgi
2. ^http://www2.inecc.gob.mx/sistemas/plaguicidas/pdf/Alfa%20terthienil.pdf
References
Abd-Elgawad, M., and Mahfouz, M. (2020). Optimizing biological control agents for controlling nematodes of tomato in Egypt. Egypt. J. Biol. Pest Control 30, 3–10. doi: 10.1186/s41938-020-00252-x
Abdel-Salam, M. S., Ameen, H. H., Soliman, G. M., Elkelany, U. S., and Asar, A. M. (2018). Improving the nematicidal potential of Bacillus amyloliquefaciens and Lysinibacillus sphaericus against the root-knot nematode Meloidogyne incognita using protoplast fusion technique. Egypt. J. Biol. Pest Control 28:31. doi: 10.1186/s41938-018-0034-3
Ala, S., Zaidat, E., Mouhouche, F., Babaali, D., Abdessemed, N., and De Cara, M. (2020). Nematicidal activity of aqueous and organic extracts of local plants against Meloidogyne incognita (Kofoid and white) Chitwood in Algeria under laboratory and greenhouse conditions. Egypt. J. Biol. Pest Control 30, 1–8. doi: 10.1186/s41938-020-00242-z
Alsharif, W., Saad, M. M., Hirt, H., and Fuentes-ramírez, L. E. (2020). Desert microbes for boosting sustainable agriculture in extreme environments. Front. Microbiol. 11:1666. doi: 10.3389/fmicb.2020.01666
Andrew, D. R., Fitak, R. R., Munguia-vega, A., Racolta, A., Martinson, V. G., and Dontsova, K. (2012). Abiotic factors shape microbial diversity in Sonoran Desert soils. ASM journals on CD 78, 7527–7537. doi: 10.1128/AEM.01459-12
Antil, S., Kumar, R., Pathak, D. V., Kumar, A., Panwar, A., Kumari, A., et al. (2021). On the potential of Bacillus aryabhattai KMT-4 against Meloidogyne javanica. Egypt. J. Biol. Pest Control 31:67. doi: 10.1186/s41938-021-00417-2
Archidona-Yuste, A., Cantalapiedra-Navarrete, C., Liébanas, G., Rapoport, H. F., Castillo, P., and Palomares-Rius, J. E. (2019). Diversity of root-knot nematodes of the genus Meloidogyne Göeldi, 1892 (Nematoda: Meloidogynidae) associated with olive plants and environmental cues regarding their distribution in southern Spain. PLoS One 13, e0198236–e0198240. doi: 10.1371/journal.pone.0198236
Baker, R. (1978). “Inoculum potential; How Disease Develops in Populations,” in Plant disease: Advance treatise. eds. J. G. Horsfall and E. B. Cowling (Academic Press). 197–158.
Bacame-Valenzuela, F. J., Rodriguez, J. A., Mateos-Díaz, J. C., Kirchmayr, M., Valenzuela-Soto, E. M., Reyes-Vidal, Y., et al. (2015). Screening of sonoran desert fungal strains for feruloyl esterase activity. J. Pure Appl. Microbiol. 9, 131–138. Available at: https://microbiologyjournal.org/screening-of-sonoran-desert-fungal-strains-for-feruloyl-esterase-activity/.
Baqueiro, I., Ali, P., Torres, A., Kirchmayr, M. R., Valenzuela, E. M., and Arturo, S. (2019). Biotechnological potential of bacteria isolated from cattle environments of desert soils in Sonora Mexico. World J. Microbiol. Biotechnol. 35, 4–13. doi: 10.1007/s11274-018-2574-9
Bashan, Y., Salazar, B., Moreno, M., Lopez, B., and Linderman, R. (2012). Restoration of eroded soil in the Sonoran Desert with native leguminous trees using plant growth-promoting microorganisms and limited amounts of compost and water. J. Environ. Manag. 102, 26–36. doi: 10.1016/j.jenvman.2011.12.032
Ben Gharsa, H., Bouri, M., Hamdane, A. M., Schuster, C., Leclerque, A., and Rhouma, A. (2021). Bacillus velezensis strain MBY2, a potential agent for the management of crown gall disease. PLoS One 16, e0252823–e0252818. doi: 10.1371/journal.pone.0252823
Berini, F., Katz, C., Gruzdev, N., Casartelli, M., Tettamanti, G., and Marinelli, F. (2018). Microbial and viral chitinases: attractive biopesticides for integrated pest management. Biotechnol. Adv. 36, 818–838. doi: 10.1016/j.biotechadv.2018.01.002
Bhagwat, A., Bhushette, P., and Annapure, U. S. (2020). Spray drying studies of probiotic enterococcus strains encapsulated with whey protein and maltodextrin. Beni-Suef Univ. J. Basic Appl. Sci. 9:33. doi: 10.1186/s43088-020-00061-z
Bhatti, A. A., Haq, S., and Bhat, R. A. (2017). Microbial pathogenesis actinomycetes benefaction role in soil and plant health. Microb. Pathog. 111, 458–467. doi: 10.1016/j.micpath.2017.09.036
Bokhari, A., Essack, M., Lafi, F. F., Andres-barrao, C., Jalal, R., Alamoudi, S., et al. (2020). Bioprospecting desert plant Bacillus endophytic strains for their potential to enhance plant stress tolerance. Sci. Rep. 9:18154. doi: 10.1038/s41598-019-54685-y
Bolanos-carriel, C., and Vazquez Soto, D. V. (2022). Persistence of Bacillus thuringiensis and Bacillus pumilus potential biological control agents of the coffee berry borer under field conditions of Puerto Rico. Ciencia Agricult. 19, 45–56. doi: 10.19053/01228420.v19.n3.2022.14685
Camacho-Ruiz, M. A., Ordaz, E., Kirchmayr, M. R., Esquivel-Solís, H., Asaff-Torres, A., Mateos-Díaz, J. C., et al. (2020). Screening of gastrointestinal lipase inhibitors produced by microorganisms isolated from soil and Lake sediments. Int. Microbiol. 23, 335–343. doi: 10.1007/s10123-019-00107-y
Cao, H., Jiao, Y., Yin, N., Li, Y., Ling, J., Mao, Z., et al. (2019). Analysis of the activity and biological control efficacy of the Bacillus subtilis strain Bs-1 against Meloidogyne incognita. Crop Prot. 122, 125–135. doi: 10.1016/j.cropro.2019.04.021
Chen, J., Li, Q. X., and Song, B. (2020). Chemical nematicides: recent research Progress and outlook. J. Agric. Food Chem. 68, 12175–12188. doi: 10.1021/acs.jafc.0c02871
Chinheya, C. C., Yobo, K. S., and Laing, M. D. (2017). Biological control of the rootknot nematode, Meloidogyne javanica (Chitwood) using Bacillus isolates, on soybean. Biol. Control 109, 37–41. doi: 10.1016/j.biocontrol.2017.03.009
Coyne, D. L., Nicol, J. M., and Claudius-Cole, B. (2007). Practical plant nematology: A field and laboratory guide, “Appendix 3”, SP-IPM Secretariat. International Institute of Tropical Agriculture (IITA). 75–82.
Cruz Silva, G., Takahashi Kitano, I., Figueiredo Ribeiro, I. A., and Teixeira Lacava, P. (2022). The potential use of actinomycetes as microbial inoculants and biopesticides in agriculture. Front. Soil Sci. 2, 1–20. doi: 10.3389/fsoil.2022.833181
Dean, V., Res, B., Dean, J. V., Maza, F., Morel, I., Pulgar, R., et al. (2020). Microbial communities from arid environments on a global scale. A systematic review. Biol. Res. 53:29. doi: 10.1186/s40659-020-00296-1
Dlamini, B., Rangarajan, V., and Clarke, K. G. (2020). A simple thin layer chromatography based method for the quantitative analysis of biosurfactant surfactin Vis-a-Vis the presence of lipid and protein impurities in the processing liquid. Biocatal. Agric. Biotechnol. 25:101587. doi: 10.1016/j.bcab.2020.101587
Engelbrecht, G., Horak, I., Jansen van Rensburg, P. J., and Claassens, S. (2018). Bacillus-based bionematicides: development, modes of action and commercialisation. Biocontrol Sci. Tech. 28, 629–653. doi: 10.1080/09583157.2018.1469000
Falardeau, J., Wise, C., Novitsky, L., and Avis, T. J. (2013). Ecological and mechanistic insights into the direct and indirect antimicrobial properties of Bacillus subtilis lipopeptides on plant pathogens. J. Chem. Ecol. 39, 869–878. doi: 10.1007/s10886-013-0319-7
Flower, C., Hodgson, W. C., Salywon, A. M., Maitner, B. S., Enquist, B. J., Peeples, M. A., et al. (2021). Human food use increases plant geographical ranges in the Sonoran Desert. Glob. Ecol. Biogeogr. 30, 1461–1473. doi: 10.1111/geb.13311
Franco-sierra, N. D., Posada, L. F., Santa-maría, G., and Romero-tabarez, M. (2020). Bacillus subtilis EA-CB0575 genome reveals clues for plant growth promotion and potential for sustainable agriculture. Funct. Integr. Genomics 20, 575–589. doi: 10.1007/s10142-020-00736-x
Franklin, K. A., Sommers, N., Aslan, C. E., López, B. R., Bronstein, J. L., Bustamante, E., et al. (2021). Plant biotic onteractions in the Sonoran Desert: current knowlege and future research perspectives. Int. J. Plant Sci. 177, 217–234. doi: 10.1086/684261
Galaviz, C., Lopez, B. R., Maymon, M., Bashan, Y., and Hirsch, A. M. (2018). Root growth improvement of mesquite seedlings and bacterial rhizosphere and soil community changes are induced by inoculation with plant growth - promoting bacteria and promote restoration of eroded desert soil. LDD 29, 1453–1466. doi: 10.1002/ldr.2904
Gao, H., Qi, G., Yin, R., Zhang, H., Li, C., and Zhao, X. (2016). Bacillus cereus strain S2 shows high nematicidal activity against Meloidogyne incognita by producing sphingosine. Sci. Rep. 6:28756. doi: 10.1038/srep28756
Geissler, M., Oellig, C., Moss, K., Schwack, W., Henkel, M., and Hausmann, R. (2016). High-performance thin-layer chromatography (HPTLC) for the simultaneous quantification of the cyclic lipopeptides Surfactin, Iturin a and Fengycin in culture samples of Bacillus species. J. Chromatogr. B 1044-1045, 214–224. doi: 10.1016/j.jchromb.2016.11.013
Gowda, M. T., Meena, B. R., Krishnan, N., Manjunath, M., Sellaperumal, C., Rai, A. B., et al. (2022). Antimicrobial peptides producing native Bacillus spp. for the management of root-knot nematode Meloidogyne incognita infecting okra (Abelmoschus esculentus L. Moench). Biol. Control 171:104951. doi: 10.1016/j.biocontrol.2022.104951
Gullino, M. L., Albajes, R., and Nicot, P. C. (2020). Integrated Pest and disease Management in Greenhouse Crops. Switzerland: Springer Cham.
He, Q., Wang, D., Li, B., and Maqsood, A. (2020). Nematicidal evaluation and active compounds isolation of aspergillus japonicus ZW1 against root-knot nematodes Meloidogyne incognita. Agronomy 10, 1–16. doi: 10.3390/agronomy10091222
Horak, I., Engelbrecht, G., van Rensburg, P. J. J., and Claassens, S. (2019). Microbial metabolomics: essential definitions and the importance of cultivation conditions for utilizing Bacillus species as bionematicides. J. Appl. Microbiol. 127, 326–343. doi: 10.1111/jam.14218
Hussey, M. A., and Zayaitz, A. (2016). Endospore stain protocol Am. Soc. Microbiol., 1–11. Available at: https://asm.org/ (Accessed June 28, 1BC).
Kimenju, J., Sibanda, Z., Talwana, H., and W, W. (2009). “Nematology training manual” in Niesa, 1–128.
Kimura, M. (1980). A simple method for estimating evolutionary rates of base substitutions through comparative studies of nucleotide sequences. J. Mol. Evol. 16, 111–120. doi: 10.1007/BF01731581
Kumar, S., Stecher, G., Li, M., Knyaz, C., and Tamura, K. (2018). MEGA X: molecular evolutionary genetics analysis across computing platforms. Mol. Biol. Evol. 35, 1547–1549. doi: 10.1093/molbev/msy096
Liu, Y., Dong, C., Lai, Q., Sun, F., Wang, L., Li, G., et al. (2013). Phylogenetic diversity of the Bacillus pumilus group and the marine ecotype revealed by multilocus sequence analysis. PLoS ONE 8:e80097:e80097. doi: 10.1371/journal.pone.0080097
Liu, G., Lin, X., Xu, S., Liu, G., Liu, F., and Mu, W. (2020a). Screening, identification and application of soil bacteria with nematicidal activity against root-knot nematode (Meloidogyne incognita) on tomato. Pest Manag. Sci. 28, 629–653. doi: 10.1080/09583157.2018.1469000
Liu, G., Lin, X., Xu, S., Liu, G., Liu, F., and Mu, W. (2020b). Screening, identification and application of soil bacteria with nematicidal activity against root-knot nematode (Meloidogyne incognita) on tomato. Pest Manag. Sci. 76, 2217–2224. doi: 10.1002/ps.5759
Mesa-Valle, C. M., Garrido-Cardenas, J. A., Cebrian-Carmona, J., Talavera, M., and Manzano-Agugliaro, F. (2020). Global research on plant nematodes. Agronomy 10:1148. doi: 10.3390/agronomy10081148
Meyers, C. L. F., and Meyers, D. J. (2008). “Thin-layer chromatography” in Current protocols in nucleic acid chemistry, vol. 34 (Baltimore: Wiley Interscience), 1–13.
Naz, I., and Khan, M. R. (2013). Nematicidal activity of Nonacosane-10-ol and 23a-Homostigmast-5-en-3β-ol isolated from the roots of Fumaria parviflora (Fumariaceae). J. Agric. Food Chem. 61, 5689–5695. doi: 10.1021/jf401309r
Naz, I., Khan, R. A. A., Masood, T., Baig, A., Siddique, I., and Haq, S. (2021). Biological control of root knot nematode, Meloidogyne incognita, in vitro, greenhouse and field in cucumber. Biol. Control 152:104429. doi: 10.1016/j.biocontrol.2020.104429
Ozdemir, F., and Arslan, S. (2022). Molecular characterization and toxin profiles of Bacillus spp. isolated from retail fish and ground beef. J. Food Sci. 84, 548–556. doi: 10.1111/1750-3841.14445
Paz, A., Jiulia, C., Perez, M. J., and Dominguez, J. M. (2016). Bacillus aryabhattai BA03: a novel approach to the production of natural value-added compounds. World J. Microbiol. Biotechnol. 32, 1–11. doi: 10.1007/s11274-016-2113-5
Pires, D., Vicente, S. L., Men, E., Faria, J. M. S., Rusinque, L., Camacho, M. J., et al. (2022). The fight against plant-parasitic nematodes: current status of bacterial and fungal biocontrol agents. Patogens 11, 1–22. doi: 10.3390/pathogens11101178Academic
Raaijmakers, J. M., de Bruijn, I., Nybroe, O., and Ongena, M. (2010). Natural functions of lipopeptides from Bacillus and pseudomonas: more than surfactants and antibiotics. FEMS Microbiol. Rev. 34, 1037–1062. doi: 10.1111/j.1574-6976.2010.00221.x
Ramírez-Cariño, H. F., Guadarrama-Mendoza, P. C., Sánchez-López, V., Cuervo-Parra, J. A., Ramírez-Reyes, T., Dunlap, C. A., et al. (2020). Biocontrol of Alternaria alternata and fusarium oxysporum by Trichoderma asperelloides and Bacillus paralicheniformis in tomato plants. Antonie van Leeuwenhoek. Int. J. Gen. Mol. Microbiol. 113, 1247–1261. doi: 10.1007/s10482-020-01433-2
Saitou, N., and Nei, M. (1987). The neighbor-joining method: a new method for reconstructing phylogenetic trees. Mol. Biol. Evol. 4, 406–425. doi: 10.1093/oxfordjournals.molbev.a040454
Sansinenea, E., and Ortiz, A. (2011). Secondary metabolites of soil Bacillus spp. Biotechnol. Lett. 33, 1523–1538. doi: 10.1007/s10529-011-0617-5
Sayed, A. M., Rateb, M. E., Alhadrami, H. A., Hassan, H. M., and Goodfellow, M. (2019). Extreme environments: microbiology leading to specialized metabolites. J. Appl. Microbiol. 128, 630–657. doi: 10.1111/jam.14386
Schultz, J., and Soares, A. (2020). Extreme environments: a source of biosurfactants for biotechnological applications. Extremophiles 24, 189–206. doi: 10.1007/s00792-019-01151-2
Sutto-Ortiz, P., de los Angeles Camacho-Ruiz, M., Kirchmayr, M. R., Camacho-Ruiz, R. M., Mateos-Díaz, J. C., Noiriel, A., et al. (2017). Screening of phospholipase a activity and its production by new actinomycete strains cultivated by solid-state fermentation. PeerJ 5:e3524. doi: 10.7717/peerj.3524
Tian, B., Yang, J., and Zhang, K. Q. (2007). Bacteria used in the biological control of plant-parasitic nematodes: populations, mechanisms of action, and future prospects. FEMS Microbiol. Ecol. 61, 197–213. doi: 10.1111/j.1574-6941.2007.00349.x
Tian, X. L., Zhao, X. M., Zhao, S. Y., Zhao, J. L., and Mao, Z. C. (2022). The biocontrol functions of Bacillus velezensis strain Bv-25 against Meloidogyne incognita. Front. Microbiol. 13, 1–11. doi: 10.3389/fmicb.2022.843041
Valenzuela-Ruiz, V., Robles-Montoya, R. I., Parra-Cota, F. I., Santoyo, G., del Carmen Orozco-Mosqueda, M., Rodríguez-Ramírez, R., et al. (2019). Draft genome sequence of Bacillus paralicheniformis TRQ65, a biological control agent and plant growth-promoting bacterium isolated from wheat (Triticum turgidum subsp. durum) rhizosphere in the Yaqui Valley, Mexico. 3 Biotech. 9:436. doi: 10.1007/s13205-019-1972-5
Vignesh, M., Madhan, S., Subramani, N., Narayanan, B., and Ramyadevi, D. (2022). Study on spray-drying of Bacillus velezensis NKMV-3 strain, its formulation and bio efficacy against early blight of tomato. Biocatal. Agric. Biotechnol. 45:102483. doi: 10.1016/j.bcab.2022.102483
Viljoen, J. J. F., and Labuschagne, N. (2019). Biological control of the root-knot nematode Meloidogyne incognita on tomatoes and carrots by plant growth-promoting rhizobacteria. Trop. Plant Pathol. 44, 284–291. doi: 10.1007/s40858-019-00283-2
Villarreal-delgado, M. F., Villa-rodríguez, E. D., Cira-chávez, L. A., Isa-, M., De Sonora, I. T., Sur, D. F., et al. (2018). The genus Bacillus as a biological control agent and its implications in the agricultural biosecurity. Mex. J. Phytopathol. 36, 95–130. doi: 10.18781/R.MEX.FIT.1706-5
Wang, J., Guo, C., Zhao, P., Yu, F., Su, Y., Qu, J., et al. (2021). Biocontrol potential of Bacillus altitudinis AMCC1040 against root-knot nematode disease of ginger and its impact on rhizosphere microbial community. Biol. Control 158:104598. doi: 10.1016/j.biocontrol.2021.104598
Watson, T. T. (2022). Sensitivity of Meloidogyne enterolobii and M. Incognita to flurinated nematicides. Pest Manag. Sci. 78, 1398–1406. doi: 10.1002/ps.6756
Xiong, J., Zhou, Q., Luo, H., Xia, L., Li, L., Sun, M., et al. (2015). Systemic nematicidal activity and biocontrol efficacy of Bacillus firmus against the root-knot nematode Meloidogyne incognita. World J. Microbiol. Biotechnol. 31, 661–667. doi: 10.1007/s11274-015-1820-7
Yang, H., Li, X., Li, X., Yu, H., and Shen, Z. (2015). Identification of lipopeptide isoforms by MALDI-TOF-MS/MS based on the simultaneous purification of iturin, fengycin, and surfactin by RP-HPLC. Anal. Bioanal. Chem. 407, 2529–2542. doi: 10.1007/s00216-015-8486-8
Yin, N., Zhao, J. L., Liu, R., Li, Y., Ling, J., Yang, Y. H., et al. (2021). Biocontrol efficacy of Bacillus cereus strain Bc-cm103 against Meloidogyne incognita. Plant Dis. 105, 2061–2070. doi: 10.1094/PDIS-03-20-0648-RE
Zhang, Y., Xu, J., Dong, F., Liu, X., Wu, X., and Zheng, Y. (2014). Ecotoxicology and environmental safety response of microbial community to a new fungicide fluopyram in the silty-loam agricultural soil. Ecotoxicol. Environ. Saf. 108, 273–280. doi: 10.1016/j.ecoenv.2014.07.018
Zhao, J., Liu, D., Wang, Y., Zhu, X., Chen, L., and Duan, Y. (2020). Evaluation of Bacillus aryabhattai Sneb517 for control of Heterodera glycines in soybean. Biol. Control 142:104147. doi: 10.1016/j.biocontrol.2019.104147
Zhao, H., Shao, D., Jiang, C., Shi, J., Li, Q., Huang, Q., et al. (2017). Biological activity of lipopeptides from Bacillus. Appl. Microbiol. Biotechnol. 101, 5951–5960. doi: 10.1007/s00253-017-8396-0
Zhao, D., Zhao, H., Zhao, D., Zhu, X., Wang, Y., Duan, Y., et al. (2018). Isolation and identifi cation of bacteria from rhizosphere soil and their effect on plant growth promotion and root-knot nematode disease. Biol. Control 119, 12–19. doi: 10.1016/j.biocontrol.2018.01.004
Zhou, Y., Wang, J., Gao, X., Wang, K., Wang, W., and Wang, Q. (2019). Isolation of a novel deep-sea Bacillus circulus strain and uniform design for optimization of its anti- aflatoxigenic bioactive metabolites production. Bioengineered 10, 13–22. doi: 10.1080/21655979.2019.1586055
Zhou, L., Yuen, G., Wang, Y., Wei, L., and Ji, G. (2016). Evaluation of bacterial biological control agents for control of root- knot nematode disease on tomato. Crop Prot. 84, 8–13. doi: 10.1016/j.cropro.2015.12.009
Keywords: bacterial screening, biocontrol, nematicidal secretomes, bionematicides, Bacillus
Citation: Chavarria-Quicaño E, Contreras-Jácquez V, Carrillo-Fasio A, De la Torre-González F and Asaff-Torres A (2023) Native Bacillus paralicheniformis isolate as a potential agent for phytopathogenic nematodes control. Front. Microbiol. 14:1213306. doi: 10.3389/fmicb.2023.1213306
Edited by:
Stanislav Trdan, University of Ljubljana, SloveniaReviewed by:
Anandham Rangasamy, Tamil Nadu Agricultural University, IndiaMirjana Brmez, Josip Juraj Strossmayer University of Osijek, Croatia
Copyright © 2023 Chavarria-Quicaño, Contreras-Jácquez, Carrillo-Fasio, De la Torre-González and Asaff-Torres. This is an open-access article distributed under the terms of the Creative Commons Attribution License (CC BY). The use, distribution or reproduction in other forums is permitted, provided the original author(s) and the copyright owner(s) are credited and that the original publication in this journal is cited, in accordance with accepted academic practice. No use, distribution or reproduction is permitted which does not comply with these terms.
*Correspondence: Francisco De la Torre-González, fdelatorre@innovakglobal.com; Ali Asaff-Torres, asaff@ciad.mx