- 1Department of Plant Protection, Faculty of Agricultural Sciences, Aligarh Muslim University, Aligarh, India
- 2Plant-Microbe Interaction and Rhizosphere Biology, ICAR-NBAIM, Kushmaur, India
- 3Research Centre, Future University in Egypt, New Cairo, Egypt
- 4Kumaraguru Institute of Agriculture, Tamil Nadu Agricultural University (TNAU), Coimbatore, India
Global food security is a critical challenge to fulfill the demands of an exponentially growing population. To date, growers rely on chemicals; the broad-spectrum application of synthetic molecules leads to environmental contamination, resistance development, residual toxicity, pest resurgence, and a detrimental effect on human health and cattle. Crop production needs to be improved considering environmental and human health concerns to ensure food security. Furthermore, economically important crops are prone to attack by insect pests, causing considerable yield losses. Microbes are an eco-friendly, versatile alternative, and a potential candidate for combatting destructive pests below the economic injury level and improving the plant's health and productivity. Several microbial pathogens, including parasites, predators, parasitoids, pollinators, and many beneficial microorganisms, possess toxic properties against target organisms but do not cause harm to the non-target organisms. Entomopathogens (ENMs) have great potential for pest suppression due to their remarkable properties. Bacteria are host-specific, but fungi have a broader host range and can be significantly affected by both soil-dwelling and terrestrial insect pests. Virulent pathogens cause mortality in target insect pests known as ENMs and can penetrate through natural openings, ingestions, and integuments to cause a possible effect on target insect pests. The objective of using ENMs is to sustain productivity, improve environmental health, reduce pesticides, and conserve natural resources. Moreover, research is ongoing to discover other possible aspects, especially exploring potential ENMs. Therefore, there is a need for identification, isolation, and bioformulation to overcome the existing issues. This study is mainly focused on the status of bio-formulations, pathogenicity, their mode of action, and the potential application of different types of microbial formulations for sustainable pest management.
1. Introduction
Insects require adequate nutritious food for survival and metabolism and search for their food through migration or distribution. They are considered successful creatures because of their wide distribution and tremendous climatic adaptability. Food scarcity impacts insect growth and reproduction and can even result in mortality (Zhang et al., 2019). Crops are prone to attack by various insect pests, and it has been recorded that insect pests have a significant impact on the nutritional and economic values of agricultural produce. There is an urgent need to overcome pest infestation through the potential application of crop protection measures.
Several insecticides are commercially available and extensively applied to suppress pest density, but these synthetic agricultural chemicals negatively impact agro-ecosystem, crop productivity (Shahid and Khan, 2017, 2018, 2022; Shahid et al., 2017, 2018, 2019, 2021a,b; Khan et al., 2020), human health, and diverse beneficial non-target organisms. Moreover, the repeated application of the same group of pesticides leads to resistance development and pest resurgence of the insect pest population (Abrol and Shankar, 2016; Islam, 2017). Several successful attempts to manage insect pests have been made to reduce pesticide exposure by conserving the environment and preventing pollution (Haase et al., 2015). As a result, an alternative and ecologically sound strategy to improve the nutritional quality and quantity of agricultural produce for long-term crop protection has been adopted extensively (Yadav et al., 2018; Kiran Kumar et al., 2019; Hesham et al., 2021). Due to the adverse effects of insecticides, biopesticides are needed because of their eco-friendly, safe, highly effective, and quickly decomposable properties. Biopesticides manufactured from microbiomes have a non-toxic approach to controlling the insect pest population. Many agents, such as viruses, fungi, and bacteria, are used to manage the insect pest population (Rastegari et al., 2020). More than 100 pathogenic bacteria species are being extensively used in managing the insect pest population, with the entomopathogenic bacteria Bacillus thuringiensis most widely used. Despite the fact that B. thuringiensis has been demonstrated to be insightful for its entomopathogenic actions.
Apart from bacteria, fungi have also been recognized as potential candidates and are successfully employed in pest management programs. The most common entomopathogenic fungi (EPFs) are Beauveria, Metarhizium, Hirsutella, Verticillium, Lecanicillium, and Paecilomyces.
Entomopathogenic fungi have a wide host range, toxicity, and ability to suppress chewing and sucking insect pests, and have gained a significant position as a biocontrol agent (Khan et al., 2012). Entomopathogenic microbiomes are generally more eco-friendly, more suitable, specific, and less expensive. The effect of ENMs has been tested on non-target organisms, and human health demonstrated satisfactory results (Yadav et al., 2018; Kumari et al., 2020). Their use is justified, and they can survive in the natural ecosystem. Soil microbes, including bacteria and fungi, also help in the decomposition of organic matter and the reutilization of dead plant materials; plants quickly absorb nutrients from decomposing organic matter (Schmeisser et al., 2007). Fungal biocontrol agents are far more specific and have a distinct mechanism of infection. They penetrate their hosts directly through the cuticle, in contrast to bacteria and viruses, which require ingestion to thrive (Mejía et al., 2008; St. Leger and Wang, 2010). Fungi cause damage to the host by producing spores that germinate on the host's surface and subsequently proliferate inside the host's body, resulting in infection (Brivio and Mastore, 2020). The infection rate is determined by the species of fungus and the number of infectious spores (Lu and Leger, 2016). The fungus continues to develop new spores on the deceased host body after infection and death of the target organism. These spores will disperse and persist on new hosts (Jaber and Ownley, 2018). Several researchers have highlighted the various insect-pathogenic fungal species as natural colonizers/endophytes of a diverse variety of economically valuable crops, such as maize (Zea mays), coffee (Coffea arabica), potato (Solanum tuberosum), cotton (Gossypium spp.), tomato (Solanum lyocpersicum), and chickpea (Cicer arietinum) (Arnold and Lewis, 2005; Qayyum et al., 2015).
Entomopathogenic fungi are a diverse and systematized heterogeneous category with varying biology. The majority of EPF are pathogenic to insects (Shah and Pell, 2003; Scholte et al., 2004; Vega et al., 2009; Dash et al., 2018), with a high degree of efficacy in infecting their host and acting as a regulator for reducing the population of detrimental insects (Ortiz-Urquiza and Keyhani, 2013; Vidal and Jaber, 2015; Lu and Leger, 2016). Several bacterial species belonging to the family Bacillaceae have been investigated and recorded as pathogenic to invertebrates, especially insects (Castagnola and Stock, 2014; Ruiu, 2015). The most studied and extensively used bacterial species is B. thuringiensis. In bacteria, some crystal toxins (Cry and Cyt) are produced during the sporulation phase, and other toxins are also produced and released during the vegetative stage of growth. It is made by the genetic modification of bacteria, fungi, algae, viruses, protozoans, and entomopathogenic nematodes. These are produced toxins and damage the integument and gut of the insect. This review highlights the potential application of microbial formulations to reduce or suppress the pest population and enhance sustainable crop production. It is an innovative and ecological tactic, significantly employed to tackle the insect pest population, with the environment, ecosystem, and human health concerns regarded as biopesticides (Fang et al., 2014). The degree of acceptability and adoption of entomopathogens is growing substantially due to the overall performance in laboratory and field conditions; thus, investigation into their biology, ecology, and mechanism of action is gaining more scientific attention (Dong et al., 2016). Several microbial biopesticides have been discovered, developed, and commercialized in recent decades as a result of several academic and corporate research efforts. Despite their enormous potential in biocontrol operations, the application of ENMs has been neglected due to a lack of awareness among growers. In this context, this review analyzes the current state of knowledge on ENMs utilization and mechanisms as biological agents for plant growth promotion and pest suppression, consequently addressing the prospects and limitations of their adoption as alternatives to synthetic pesticides (Figure 1).
2. Entomopathogenic bacteria
Most bacterial species belonging to the family Bacillaceae and their pathogenicity have been tested in invertebrates, especially insects (Ruiu, 2015). The most commercially used bacterial species are B. thuringiensis, a gram-positive and spore-forming bacterium. The sporulation process produces proteins with insecticidal properties in a parasporal crystal, comprising Cry and Cyt toxins, known as delta-endotoxin. Sometimes, cells release some compounds during vegetative development (De Maagd et al., 2001). Entomopathogenic bacteria are employed to suppress insect pests.
2.1. Bacillus thuringiensis subsp. kurstaki (Btk)
Government research institutes collaborate with business enterprises to ensure that Btk products are readily accessible in India. The IIOR has popularized manufacturing indigenous Btk isolates (DORBt-1 and DORBt-5) using solid-state fermenter technology for distribution and sale to suppliers. In addition, the NBAIR has industrialized liquid fermentation technology for the indigenous Btk isolates PDBCBT1 and NBAIIBTG4 (Ramanujam et al., 2014), which are also licensed for manufacture and sale. In India, numerous Btk formulations are used to combat bollworms, loopers, and other lepidopteran pests. In Andhra Pradesh (Kumara et al., 2016), Telangana (Vimala Devi and Vineela, 2015), and Punjab (Kumar and Kaur, 2017), for example, liquid Btk formulations have been effectively tested and used to manage Helicoverpa armigera and noctuid pests in pigeon pea. In sugarcane in Tamilnadu, commercial formulations (Delfin, Biobit, Dipel, and Halt) decreased the shoot borer, Chilo infuscatellus, below economic limits (Kesavan et al., 2003). Btk has proven to be effective against a variety of citrus pests. A study conducted in Andhra Pradesh, India (Rao et al., 2015) observed up to 100% mortality of the citrus leaf miner, Phyllocnistis citrella, for up to 10 days after spraying. The application of Dipel (1 kg/ha) resulted in a 90% decrease in the larvae population of the citrus butterfly, Papilio demoleus, in the fruits of sweet orange (Gopalakrishnan and Gangavisalakshy, 2005).
These and other studies showed that Btk is widely employed in various crops. Different control approaches, such as biological control agents, are compatible with these, although they are most successful when applied on the first and second instars of immature larvae. On the other hand, residues of Btk decay quickly in the sun and rain (Van Frankenhuyzen, 2009), necessitating reapplication, especially when insect numbers are high.
The Indian subcontinent has many Bt strains, some containing new assumed genes. Reyaz et al. (2017) discovered 68 Bt strains from the Kashmir Valley with four crystalline inclusion types. Mishra et al. (2017) identified 45 Bt strains from a new ecological niche in the Uttarakhand Himalayas, several of which (UKBt3, UKBt11, UkBt13, and UKBt18) exhibited promising approaches against H. armigera, Pieris brassicae, Plutella xylostella, and Spodoptera litura under laboratory conditions and are good candidates for commercialization. A number of strains have been tested and found effective against a wide range of lepidopteran, coleopteran, dipteran, and homopteran pests, gaining considerable attention.
2.2. Bacillus thuringiensis subsp. israelensis (Bti)
In India, 12 Bt-based products, including mosquito, black fly, and fungus gnat larvae, are registered to be used against dipteran pests. In many parts of India, Bt plays a vital role in controlling a variety of human disease vectors (Amalraj et al., 2000; Poopathi et al., 2014). The efficiency of these products usually depends on the strain and target, although they are effective under specific circumstances. Bactoculicide sprayed at 0.5 g/m2 (5 kg/ha) reduces Aedes aegypti and Aedes albopictus mosquito larvae by >90% inbreeding habitats and Culex quinquefasciatus in drains (Mittal, 2003). Despite up to the 150-fold field resistance recorded in treated field populations of the Culex pipiens complex, commercial formulations of the related species Lysinibacillus sphaericus are used to a limited extent in mosquito control programs in India (Poopathi et al., 2015). These products are being applied to manage the dipterans, especially hematophagous mosquitoes.
2.3. Mode of action
Bacillus contains Cry toxin, which primarily affects lepidopteran insects. The insect gut with high pH or alkalic conditions activates the delta-endotoxin and becomes attached to the midgut receptors. Furthermore, it generates pores in midgut cells, due to which the cell loses its fluids; consequently, the midgut epithelial cell lyses after the midgut paralyzes. The cell fluids become mixed with the hemocoel and hemolymph of the insect. The pH level become imbalanced, resulting in septicemia and insect mortality. This is a direct action of the cry toxin (De Maagd et al., 2001) (Figure 2).
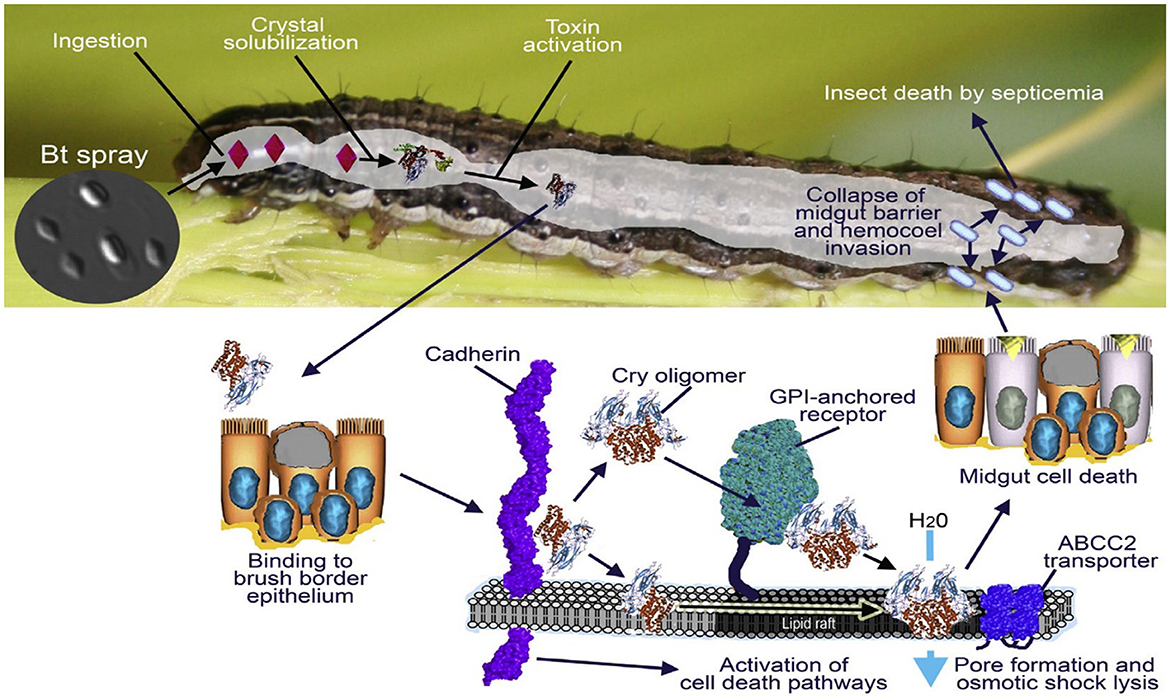
Figure 2. Graphical illustration of Bt Cry toxin and its impact on the host, after the ingestion crystal reaches the midgut and the toxin becomes activated, causing mortality; the figure was adapted with permission from Glare et al. (2017). Copyright © 2017, All rights are reserved.
Nowadays, several researchers are focusing on entomopathogenic bacteria that can be effectively used in different integrated pest management strategies (Table 1).
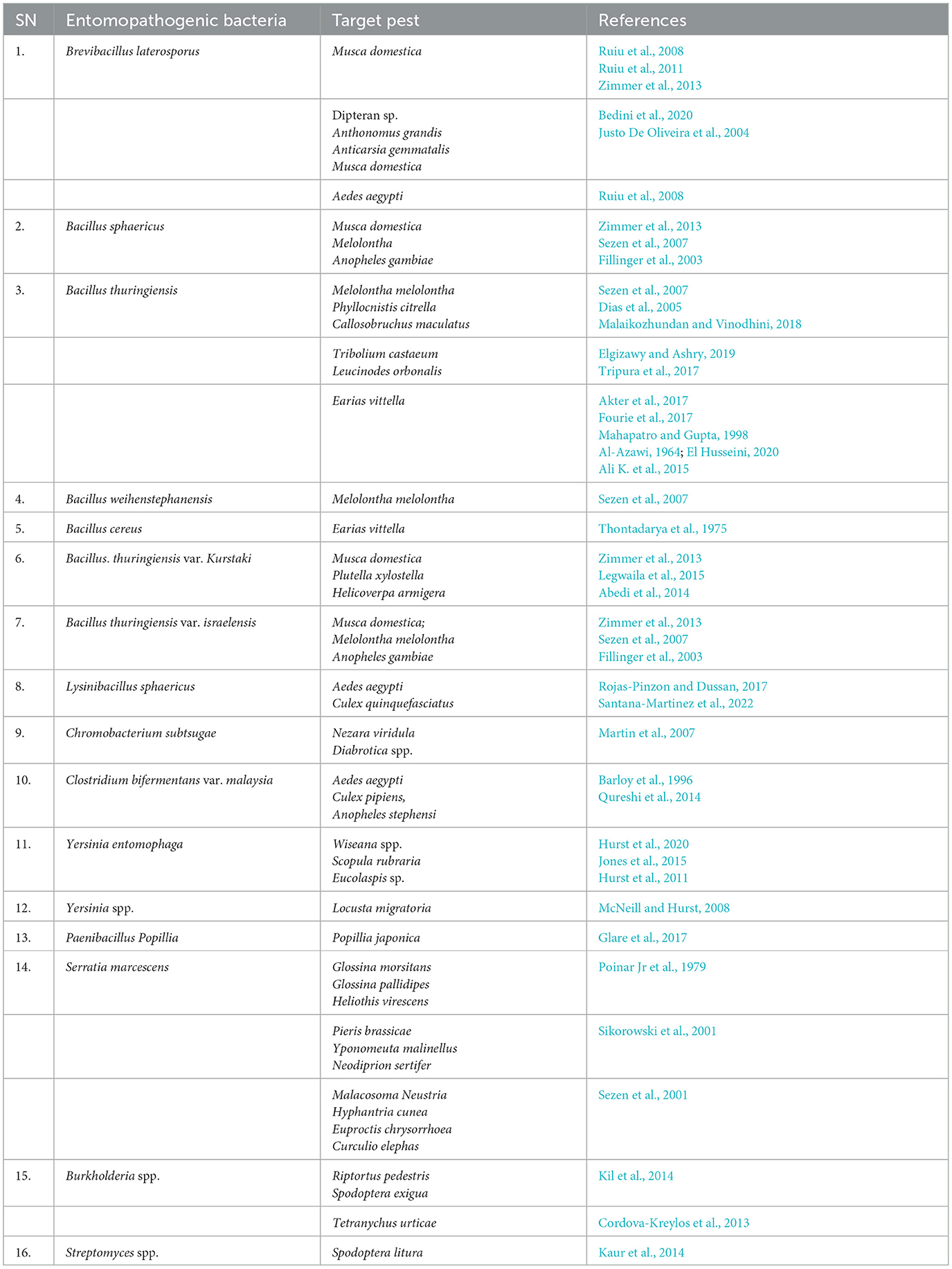
Table 1. Different strains of bacterial products are commercially available worldwide against target species.
2.4. Mode of action of Bt in causing insect mortality
Insect mortality caused by Bt cotton has been intensively investigated over the last few decades, and several recent studies offer new insights into the mechanism of action.
The mechanism of action of the B. thuringiensis toxin Cry1Ac in the cotton bollworm (H. armigera) was examined by Zhang et al. (2021). They determined that the toxin alters the proportion of amino acids in the insect's midgut, increasing the number of deadly dipeptides that ultimately result in the death of gut cells and insect mortality. In addition to generating oxidative stress in cotton bollworm larvae, a study by Yao et al. (2020) showed that the Cry1Ac toxin may also cause mitochondrial malfunction, which leads to insect mortality.
Zheng et al. (2019) discuss how the insect gut microbiota affects the toxicity of Bt toxins such as Cry1Ac. They suggest that interactions between Bt toxins and the gut microbiota can reduce the toxin's toxicity and enable certain insects to develop resistance to it.
These investigations demonstrate the intricacy of Bt cotton's method of producing insect mortality and conclude that the Cry protein may kill insects through various channels. Researchers can create measures to maximize the efficacy of Bt cotton and reduce the possibility that resistance may emerge over time by comprehending these mechanisms.
3. Efficient entomopathogenic fungi against devastating pests
Entomopathogenic fungi are parasitic microorganisms that can infect and kill insects. They are mainly employed as biopesticides in ecological farming as a non-toxic alternative to toxic chemical insecticides, while some are used in biotechnological processes (Jaihan et al., 2016; Ríos-Moreno et al., 2016; Lovett and St. Leger, 2017). Generally, they have immobile chitinized cells (Badii and Abreu, 2006). Entomopathogenic fungi do not belong to a single phylogenetic group. To date, 12 Oomycetes species, 65 Chytridiomycota species, 339 Microsporidia species, 474 Entomophtoromycota species, 238 Basidiomycota species, and 476 Ascomycota species have been reported (Araújo and Hughes, 2016). Several biopesticides based on Metarhizium, Beauveria, Paecilomyces, Isaria, and Lecanicillium species are being applied worldwide. Because all these fungi have a broad range of action, they can infect a large range of arthropod species (Khan et al., 2012; Castro et al., 2016; Ríos-Moreno et al., 2016). They attack many insect and mite species, but particular species and fungal strains are distinct. Depending on the species of fungus and the number of infecting spores, host death might take 4–10 days. When the host dies, the fungus generates thousands of new spores on the corpse, spreading and completing the life cycle of new victims.
Beauveria bassiana was identified as the source of the destructive muscardine infection of silkworms by Agostino Bassi in 1835. It was essential in establishing the germ theory of disease. Elie Metchnikoff was among the first to suggest feasible microbial management of an insect crop pest in 1880 when he began testing the fungus Metarhizium anisopliae against grain beetles (Lord, 2005). In India, several research institutes are conducting experiments to determine entomopathogens' efficacy, including NBAIR in Bengaluru, Indian Institute of Oilseed Research (IIOR), Hyderabad, Central Plantation Crops Research Institute (CPCRI), Kayamkulam, and University of Agricultural Sciences, Dharwad. To date, studies have been conducted on species B. bassiana, Metarhizum anisoplie, Lecanicillium lecanii, Nomuraea rileyi, and Hirsutella spp. (Ramanujam et al., 2014).
3.1. Beauveria species
Beauveria bassiana has been extensively studied on crops against a wide range of lepidopteran, coleopteran, and hemipteran pests. Commercial formulations are available in aqueous suspensions, wettable powder (WP), and talc, with recommended application rates ranging from 107 to 108 conidia/ml and 400 to 750 lit/ha, depending on the crop. Sahayaraj and Namachivayam (2011) observed that an oil-based formulation of B. bassiana revealed 72% mortality of the larval population of S. litura, and a significantly higher yield was found in Tamilnadu. The suspension of commercial product (Daman 1% WP) demonstrated a potential impact on reducing H. armigera and enhanced sunflower seed yield (Kumar and Kaur, 2017). Recent studies indicated the potential efficacy of B. bassiana against the sugarcane internode borer, Chilo Sacchariphagus indices, and do not cause adverse effects on natural enemies.
The application of B. bassiana demonstrated the preservation of parasitoids of Chilosacchariphagus indicus (Ramasubramanian et al., 2014; Srikanth et al., 2016). Another study found that an isolate of B. bassiana at 4 gm/l from the Indian Institute of Horticultural Research (IIHR) had shown 81% reduction in fruit infestation caused by the tea mosquito bug, Helopeltis antonii on guava (NBAIR, 2017). Beauveria-based commercial products have been widely used to suppress white grubs (scarabids) in sugarcane for several years; their application facilitated the semi-perennial crop environment and limited the efficacy of conventional pesticides. Visalakshi et al. (2015) found that applying commercial talc-based B. bassiana formulations (5 × 1013) treated with FYM to the soil diminished the white grub Holotrichia consanguinea and damaged the sugarcane by 88% as compared with the control. Due to its long-term durability in treated fields and effectiveness against scarab, Holotrichia serrata, a related species, B. brongniartii, has emerged as a possible biocontrol agent in sugarcane (Srikanth et al., 2010). Another study advocated that the soil-based application of formulation B. brongniartii (2.5 kg/ha) improved yield under field conditions in Tamilnadu, India (Chelvi et al., 2011).
3.2. Metarhizium anisopliae
In India, ~30 products based on M. anisopliae are used to combat foliar and soil-inhabiting pests, primarily in areca nut, coconut, coffee, corn, potato, pigeon pea, and sugarcane crops. These formulations have offered a remarkable suppression of devastating insect pests in several cases. Previous experimental results revealed that a talc-based formulation of M. anisopliae (5 × 1013 spores/ha) supplemented with FYM suppressed white grub damage to sugarcane by 93% and grub population by 77% over 2 years (Visalakshi et al., 2015). Treatment applied at the time of planting revealed spectacular success compared to late application. A fungal formulation combined with FYM and sprayed to the root zone at 4 × 109 conidia/ha reduced grubs by 92%. Metarhizium substances are utilized to keep lepidopteran pests away from tuber crops. Pandey (2013) noted the efficacy of fungal formulations and recommended applying with compost (5 × 10 spores/gm) in potatoes to protect the tuber from cutworm, Agrotis ipsilon attack.
3.3. Lecanicillium lecanii
More than 60 products based on L. lecanii (still registered under the species Verticillium) are made in liquid and dry formulations in India to regulate aphids, scales, and other soft-bodied sucking pests on a range of crops. Several recent studies attest to their efficiency against pests, including thrips and mealybugs, especially when treated at 108 CFU/ml and in combination with other biopesticides (Annamalai et al., 2016; Halder et al., 2018). Lecanicillium lecanii is one of the most potent fungal products against aphids. According to Ramanujam et al. (2017), among 10 isolates of entomopathogenic fungi evaluated in field plots in Karnataka, L. lecanii VI-8 proved to be highly effective against cowpea aphid, Aphis craccivora (78% decrease at 108 CFU/ml) and boosted yields by 32%. Similar investigations were done and observed in field conditions that L. lecanii at 109 resulted in 72% mortality of cowpea aphids (Suresh et al., 2012).
3.4. Isaria fumosorosea
The entomopathogenic fungi, Isaria farinosa and Isaria fumosorosea, were known as Paecilomyces farinosus and Paecilomyces fumosoroseus, respectively, for more than 30 years. Both fungi have a worldwide distribution and a relatively wide host range. While I. farinosa currently is of minor importance in research and as a biocontrol agent, I. fumosorosea is regarded as a species complex, and various strains are successfully used for the biocontrol of several pest insects, mainly whiteflies (Luangsa-Ard et al., 2005). They can initiate epizootics under natural field conditions. This fungus is used as a potential biocontrol agent against invasive pests of India viz., Aleurodicus rugioperculatus and Bondar's nesting whitefly, Paraleyrodes bondari on coconut (Ali A. D. et al., 2015; Kumar et al., 2016), Ficus whitefly, Singhiella simplex on Ficus (Avery et al., 2019). Isaria fumosorosea also showed high pathogenicity (80.65%) on fourth-instar nymphs (pupae), leading to a drastic reduction of adult emergence that may result in less perpetuation of the pest in the coconut ecosystem. In addition, high mycosis was also observed in newly emerged adults. The percentage mortality of different stages of A. rugioperculatus significantly increased with increased spore concentrations. Findings indicate that a higher concentration of fungal spores resulted in faster control of the targeted pest (Kumar et al., 2018).
3.5. Nomuraea rileyi
Nomuraea rileyi infects many lepidopteran larvae, such as S. litura, Spodoptera exigua, H. armigera, Helicoverpa zea, Trichoplusia ni, Plusia sp., and various noctuid defoliators. When a favorable condition of humidity (>70%) and temperature (20–30°C) exist for a long time, N. rileyi is known to cause natural epizootic in larval populations of S. litura, H. armigera, and Plusia sp. on castor, cotton, groundnut, red gram, and niger in Andhra Pradesh. Colonies of the fungus are white initially and later turn pale green to malachite green. Hyphae are 2–3 μm in diameter, smooth, septate, hyaline, and slightly pigmented. Conidiophores are long (160 μm) and consist of dense compacted clusters of phialides and branches in whorls on the upper section. The branches are short and swollen. Phialides are short and cylindrical to globose, with a very swollen base tapering abruptly to a narrow neck. Conidia are produced in divergent dry chains on phialides, elliptical to cylindrical, measure 3–4 × 2–2.5μm, and are pale green. The fungus sporulates well only on specific media such as Sabouraud Maltose Agar with yeast (SMYA). Crushed sorghum grain supplemented with 1% yeast extract was used to mass-produce N. rileyi. A maximum of 1.4 × 109 conidia/g of N. rileyi was obtained after 8–9 days at 25°C on this medium (Devi, 1994). Carrot agar supplemented with 1% yeast extract was found suitable for culturing and producing N. rileyi. Compatibility tests of N. rileyi with plant extracts and vegetable oils were conducted against S. litura. None of the vegetable oils were detrimental to the N. rileyi grown on crushed sorghum, but they were found effective in the field control of S. litura on castor and groundnut when applied as a foliar spray (2 × 1011 conidia/l) or as soil treatment at 10g/m2 (2 × 1011 conidia/g). It controls pod borers, cutworms, and cabbage borers.
3.6. Hirsutella thompsonii
These fungi control different hoppers, bug pests, whiteflies, and red mites. It is a promising candidate for the control of citrus red mites and coconut eriophyid mites. It is a synnamatous fungus with bulbous phialides arising laterally from synnema or the hyphae. The conidia are one-celled and hyaline. In India, the fungus has been isolated from the eriophyid mite, Aceria guerreronis, and tested for control of same (Table 2).
3.7. Mode of action on the target insect
The fungus develops specialized structures to penetrate into the host integument, such as appressoria, which allows the developing hyphae to enter into the host integument (Ortiz-Urquiza and Keyhani, 2013). Some enzymes, such as metalloid proteases and aminopeptidases, perform cuticle degradation through germ tubes (Bidochka and Small, 2005). The fungus hyphae disseminate inside the insect hemocoel and attack various muscle tissues, fatty bodies, Malpighian tubes, mitochondria, and hemocytes; as a result, infected insects die after some days (Kachhawa, 2017). The Hyphomycetes genus kills insects through nutritional deprivation, tissue damage, and producing toxins in the insect body. Entomopathogenic fungus and cuticle degrading enzymes, such as chitinase, protease, and lipase, play a vital role in the pathogenicity in insects because they dissolve the cuticle to enter the germ tube insect's body (Figure 3).
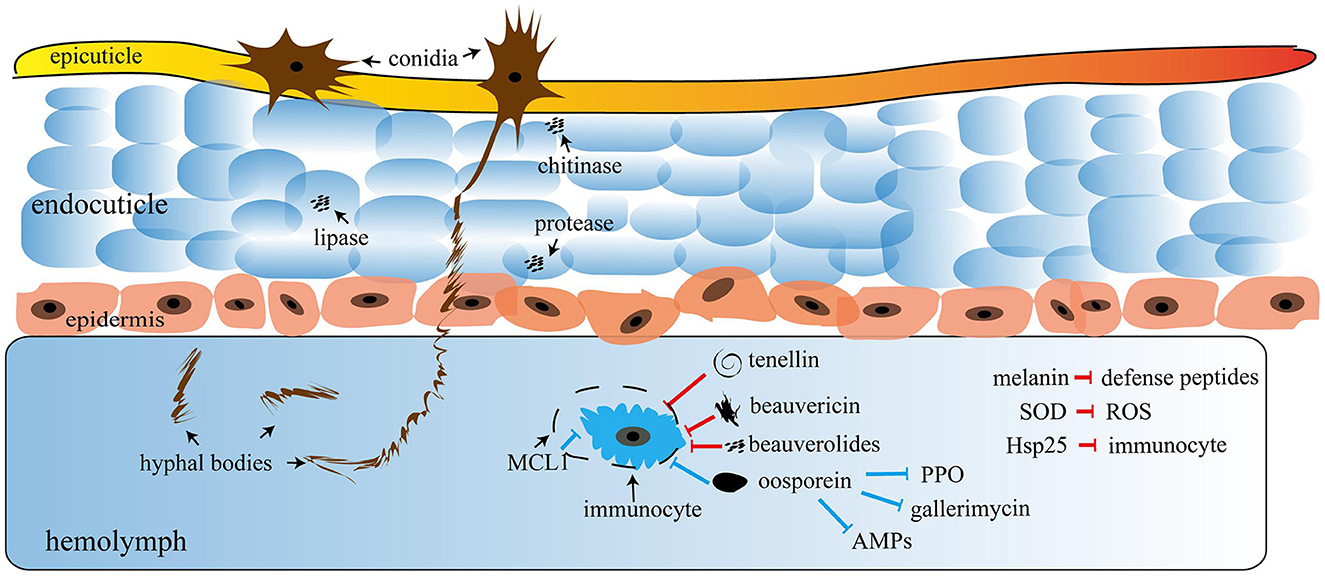
Figure 3. Mechanism of entomopathogenic fungi (EPF); the figure was adapted with permission from Wang H. et al. (2021). Copyright © 2021 Wang, Peng, Li, Cheng and Gong.
Entomopathogenic fungi enter insects through two processes: the first is mechanical pressure on the cuticle, and the second is enzymatic degradation. At the time of pathogenesis, several mycotoxins are produced, including Beauvericin (B. bassiana), Beauverolides (Verticillium lecanii), Bassianolide (Paecilomyces spp.), and Destruxins A, B, C, D, E, F (M. anisopliae), which are poisonous to the insects. After the insect's death, fungus emerges from the integument, produces aerial mycelia, and sporulates on the cadavers. Some Hypoclealean fungi, such as B. bassiana, I. fumosorosea, Hirsutella thompsonii, L. lecanii, Metarhizium acridum, M. anisopliae, and M. brunneum, are commercially available as biopesticides in a variety of formulations all over the world. These are effective against piercing and sucking mouthparts and pests with chewing mouthparts. The entomopathogenic fungus obligates insects that kill their hosts by colonizing their tissues without toxins (Pell et al., 2001). Natural epizootics of entomophthoralean fungi, such as Entomophaga maimaiga, Entomophthora muscae, Neozygites fresenii, Neozygites floridana, and Pandora neoaphidis, are known to cause a massive decrease in the host population. Even though such delicate fungi are challenging to subculture under artificial conditions, they may be crucial in the natural control of particular pest species (Table 3).
4. Compatibility of entomopathogenic fungi with other biological agents
As part of integrated pest management (IPM) techniques, there is increased interest in combining entomopathogenic fungi with other biological control agents. The compatibility of entomopathogenic fungi with other biological agents, including parasitoids, predators, and other microbes, has been the subject of some recent investigations. Here are a few current study examples: Wei et al. (2021) investigated the compatibility between the parasitoid wasp, Habrobracon hebetor, employed to manage several moth pests, and the entomopathogenic fungus, B. bassiana. They observed that the two plants, B. bassiana and H. hebetor, worked better together than alone to combat the moth pest, Plodia interpunctella. In research by Dubovskiy et al. (2020), the predatory mite Amblyseius swirskii and the entomopathogenic fungus Metarhizium robertsii were used to suppress the tomato russet mite (Aculops lycopersici). The scientists discovered that M. robertsii and A. swirskii worked synergistically to reduce the tomato russet mite population significantly. To manage the autumn armyworm (Spodoptera frugiperda), Wang B. et al. (2021) studied the compatibility of the entomopathogenic fungus I. fumosorosea with the bacterium B. thuringiensis. They determined that I. fumosorosea and B. thuringiensis worked together to suppress the autumn armyworm more effectively than each of them did by itself.
These studies highlight how entomopathogenic fungus may be applied with other biological pest control agents to boost pest control effectiveness and decrease dependency on chemical pesticides. However, the compatibility of various biological control agents in contexts of several pest management needs additional investigation.
5. Entomopathogenic viruses
An entomopathogenic virus is a tiny, essential infectious agent of nucleic acid (RNA or DNA) encased in a protective protein coat known as the capsid, which can multiply in a susceptible host cell. If a lipid bilayer envelops the nucleocapsid, it is a virion. The word virus means “poison,” derived from a Latin term.
Mathews and Shenk (1991) defined a virus as “a set of one or more nucleic acid template molecules, generally encased in a protective coat or coats of protein or lipoprotein, that can organize its replication only within suitable host cells.” Within such cells, virus replication is (i) dependent on the host's protein-synthesizing machinery, (ii) organized from pools of the required materials rather than by binary fission, (iii) located at sites that are not separated from the host cell contents by a lipoprotein bilayer membrane, and (iv) continually giving rise to variants through various kinds of change in the viral nucleic acid.
The virus nucleic acid (DNA or RNA) is crucial to the infection of the host cell and drives viral replication. These are classified into two genera, nucleopolyhedroviruses (NPVs) and granuloviruses (GVs). Both genera have a circular double-stranded DNA genome of ~80–180 kb packed within rod-shaped nucleocapsids and anticipated to encode 90–180 genes (Okano et al., 2006). Nucleopolyhedroviruses have polyhedral OBs, made of crystalline polyhedrin protein, which occludes massive virions. In contrast, granuloviruses have smaller granule-like OBs, made up of granulin protein. Every granulovirus OB contains a single virion. The Baltimore classification used to classify viruses is based on nucleic acids and capsid function (Sparks et al., 2010). When a virus enters a compatible host cell, its nucleic acid takes control of the metabolic process. It multiplies rapidly, producing new virus particles until the cell is deprived of its contents and dies; thus, a virus is classified as an obligatory parasite since it consumes the cell's material and its metabolic processes. Viruses cannot multiply “in vitro.” Although all viruses have the same fundamental structure and require host cells to reproduce, viruses in nature come in various shapes and sizes. All viruses have different morphologies, genomes, infectivities, and host ranges. Entomopathogenic viruses are both a potential and essential part of an integrated pest control approach. The few families of viruses show infectivity against the insects.
Moreover, entomopathogenic viruses belong to the Baculoviridae family. That family infests some orders, such as lepidoptera, hymenoptera, and diptera, as a natural host. These entomopathogenic viruses are managed by various agricultural and forest insect pest populations worldwide as biopesticides (Sun and Peng, 2007). Viruses are being used as a promising tool for managing economically important insect pests; various viruses, viz. nucleopolyhedrovirus (NPV), granulosis viruses (GVs), and cytoplasmic polyhedrosis viruses, are used for the management of insect pests throughout the world. The insects ingest the host-specific viral particles, and the virions infect the gut wall cells, fat body, and hemolymph, leading to the death of the insects. As a result, their application is innovative and environmentally friendly. They are non-hazardous to human health and the environment, offering novel industrial products and field uses (Sumathy et al., 1996). Baculoviruses have a single circular genome surrounded by rod-shaped nucleocapsids. To produce virions, enveloped by membrane singly or in groups, occluded in a protein matrix, forming the occlusion body (OB) (Williams et al., 2017).
5.1. General features of insect viruses
Insect viruses belong to many different virus families, some of which occur exclusively in arthropods and some of which include representatives in vertebrates and/or plants. A feature of many insect viruses, which does not occur in viruses infecting plants or vertebrates, is that they are occluded, i.e., the virions are embedded within a proteinaceous body. Occlusion bodies (OBs) vary in size from about 0.5 to over 20 μm across but are all visible under the light microscope.
5.2. Application of NPV on the target pest
Helicoverpa armigera granulosis virus is known as HearGV, while the Autographa californica multiple nucleopolyhedroviral is known as AcMNPV. As a result, all nuclopolyhedrovirus is known as NPV, whereas granulosis viruses are GV. In managing H. armigera and H. zea populations that have gained resistance to chemical pesticides, NPV-based products are an important alternative to chemical pesticides (Kranthi et al., 2002). HaNPV is applied at 250 LE/ha (1.5 × 1012 POBs/ha) according to the author's recommendation (Saxena and Ahmad, 2005; Srinivasa et al., 2008). In the field trials, the HaNPV CBE-1 isolate reduces the H. armigera by 64 and 62% in cotton chickpeas, respectively, compared to untreated plots (Jeyarani et al., 2010). While we are uninformed of any granuloviruses (GV) available on the market in India, research conducted with native isolates has shown that GV might be employed to effectively tackle the sugarcane shoot borer C. infuscatellus (Rabindra, 2005; Rao and Babu, 2005). It is also noticed that it effectively suppresses the diamondback moth, P. xylostella (Jayanth, 2002).
The table shows the entomopathogenic virus and its target insect host, tested and found effective in causing insect mortality (Table 4).
5.3. Mechanism of NPV
The baculovirus is transmitted orally. The entomopathogenic virus spreads by ingesting infested food from insects, such as bacterial infection in insects. Because these are suitable for controlling insects. The virus enters the body of insects by infesting food. The occlusion bodies get dissolved in the midgut; virus particles invade the midgut epithelium, fat body, and other tissue cells, damaging the tissue integrity and dissolving the cadavers. Before death, infected larvae climb the upper portion of the plant, allowing virus particles from the cadavers to the lower parts of the plant. This behavior allows the virus to spread to cause infection in healthy larvae. Viruses are very host-specific and may cause a significant reduction in host populations (Figure 4).
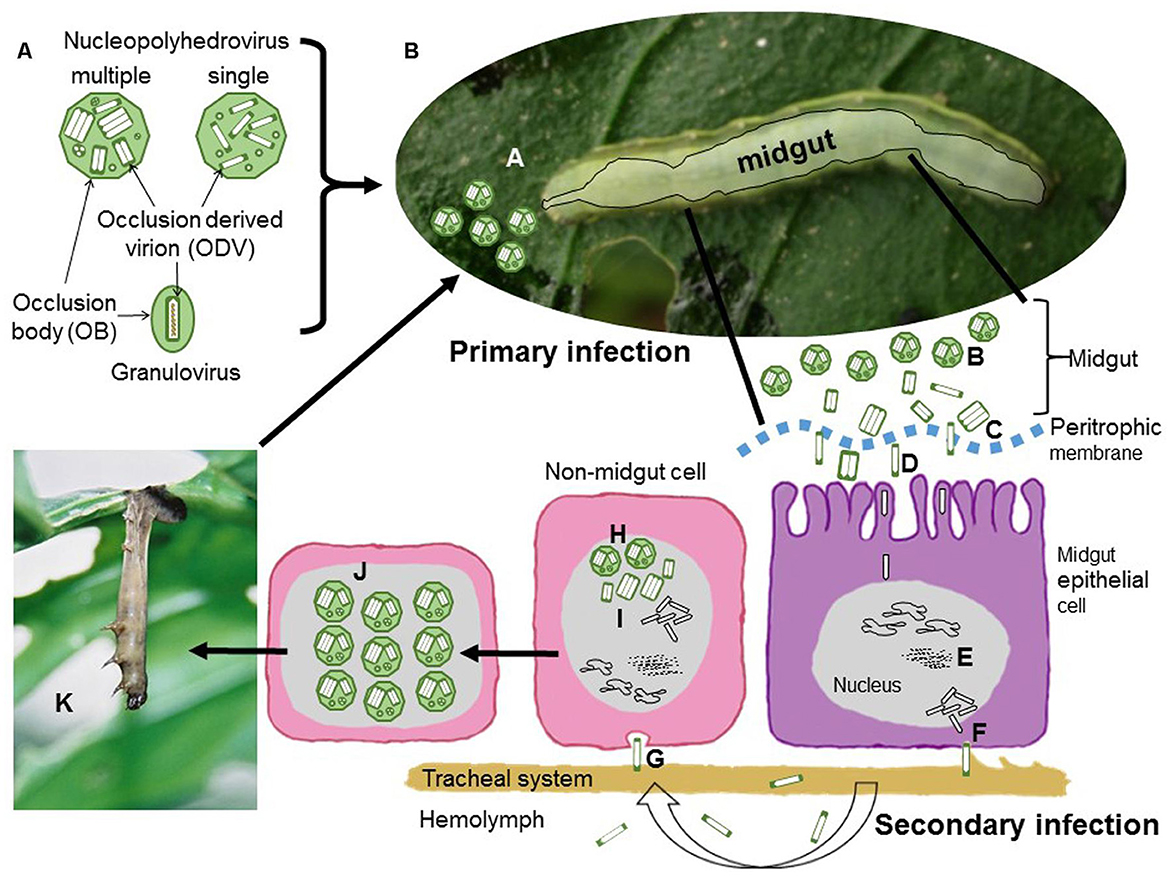
Figure 4. (A) Nucleopolyhedrovirus occlusion bodies (OBs) are polyhedral proteinaceous structures that surround occlusion derived virions (ODVs). Each ODV contains either a single nucleocapsid (single type) or one to several nucleocapsids (multiple type). Granulovirus OBs are granule-shaped and contain a single ODV with a single nucleocapsid encased in the crystalline protein granulin. Each nucleocapsid carries a single viral genome in all situations. (B) The processes of nucleopolyhedrovirus transmission and replication. During initial infection, (A) OBs are ingested while feeding on infected vegetation later on OBs are solubilized in the insect midgut, releasing ODVs that pass through the peritrophic membrane and merge with the microvilli of midgut epithelial cells. Afterwards, Nucleocapsids migrate to the nucleus and release the viral DNA to begin replication. These budded virions (BVs) diffuse in the hemolymph or along the cells of the insect traqueal system (traqueoblasts) during the secondary phase of infection, spreading the infection to the cells of different tissues in the insect.
Due to host specificity, entomopathogenic viruses are essential to control insects and pests. Several virus-based commercial biopesticides are available in markets and have demonstrated better results than other control practices. Many researchers found that entomopathogenic viruses have essential characteristics and efficacy against different insects.
6. Entomopathogenic nematodes
Rao and Manjunath (1966) were the first to demonstrate that the Steinernema carpocapsae DD-136 strain was applied to target lepidopteran pests of rice, sugarcane, and apple in India. For scientific purposes, exotic strains of S. corpocapsae, Steinernema feltiae, Steinernema glaseri, and Heterorhabtidis bacteriophora were introduced later (Rahaman et al., 2000). Subsequently, extensive surveys were carried out to find out potent indigenous EPN species S. carpocapsae (Hussaini et al., 2001), Steinernema abbasi (Hussaini et al., 2003), Steinernema bicornutum (Hussaini et al., 2001), Steinernema thermophilum (Sudershan and Singh, 2000), Steinernema tami (Hussaini et al., 2001), and Steinernema riobrave (Ganguly et al., 2002). The product (Pusa Nemagel) has a long shelf life and inhibits white grubs, termites, and a variety of other lepidopteran pests (Ganguly et al., 2008). Steinernema thermophilum was the first EPN to be identified as pathogenic to lepidopteran eggs (Kalia et al., 2014). In Uttarakhand and Uttar Pradesh, large-scale EPN was tested in response to persistent white grub infestations in sugarcane. The Indian Agriculture Research Institute (IARI, New Delhi) undertook a biocontrol initiative, including the mass breeding and release of EPN-infested insect cadavers to counter the infestation (Mohan et al., 2017). Farmers were trained on in vivo rearing and field application as part of the research, which designed a low-cost EPN rearing method in Galleria mellonella.
6.1. Bacteria associated with parasitic nematodes (symbiotic association)
Nematodes are tiny, transparent, and relatively basic in terms of multicellular creatures, and they occupy a variety of environmental niches and model species for investigating bacterial symbiosis (Dillman et al., 2012). Insect-parasitic and entomopathogenic nematodes (EPNs) belong to the genera Heterorhabditis and Steinernema, respectively, and are symbiotically correlated with the bacteria Photorhabdus and Xenorhabdus (Shapiro-Ilan et al., 2017) (Figure 5).
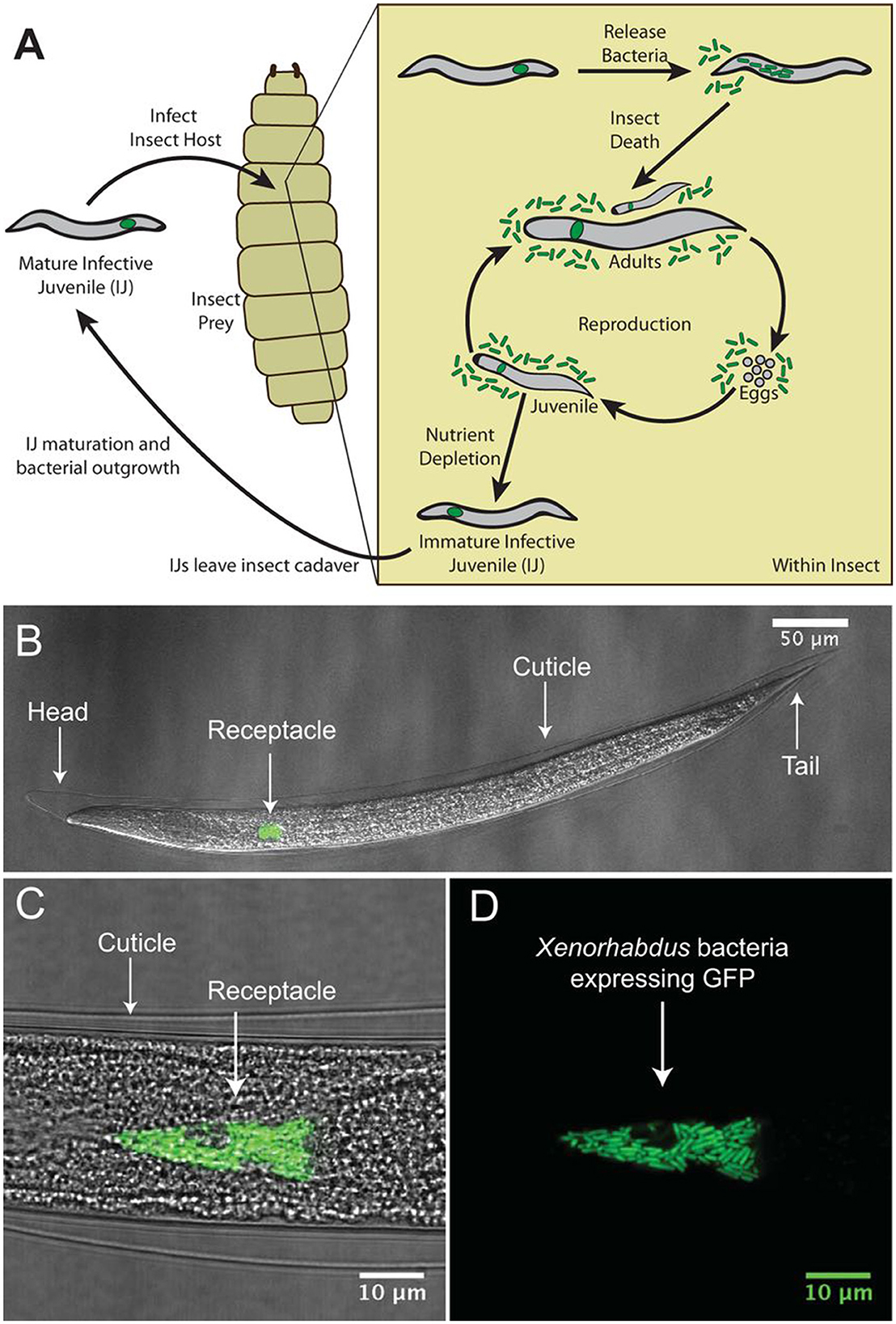
Figure 5. The mutualistic relationship between the bacterium Xenorhabdus nematophila and nematode, Steinernema carpocapsae. (A) Illustration of the tripartite life cycle of S. carpocapsae nematodes. Infective juveniles (IJs) infect an insect prey and release X. nematophila cells to elude the host immune system and kill the host. Both species reproduce using the cadaver's nutrients; when the nutrients impoverished, the two creatures recombine and enter the soil to restart the cycle. (B–D) Confocal micrographs of a S. carpocapsae nematode's IJ stage with GFP-expressing X. nematophila cells in the intestinal receptacle. Panel D shows the intravesicular structure (IVS) as the black region below the white arrow surrounded by bacterial cells.
The infective juveniles (IJs) of EPNs dwelling in soil access the larval stage of their insect hosts by a natural opening (mouth, spiracle, or anus) or the delicate cuticle during the intricate life cycle. The IJs of ENPs release symbiotic bacteria into the body of their host, where they rapidly proliferate and generate secondary metabolites. Within 24–48 h, the infected insect host dies (Dowds and Peters, 2002). The bio-converted insect host is fed on by both the EPNs and the bacteria that remain in symbiosis. EPNs produce 1–2 generations of offspring in the insect cadaver. The symbiotic bacteria are consumed by the IJs, and when the food supply is reduced a new cohort of IJs carrying the symbiotic bacteria emerges from the cadaver in search of a new insect host. Therefore, EPNs have been employed as biocontrol agents for a variety of insect pest species (Lacey et al., 2015). Important biotic and abiotic factors are essential for EPNs' survival in ecological niches. For the survival of EPNs, soil characteristics, including texture, pH, moisture, and temperature, are particularly crucial. The variety and distribution of EPNs are affected by geographical regions, ecosystems, and soil characteristics. Variable recovery rate has been investigated in a number of surveys of EPNs from numerous geographical ecologies. Studies were carried out to investigate the interaction between Steinernema nematodes and their associated bacteria.
Several attempts have been attempted to harness native EPNs to combat insect pests notably the Caribbean fruit fly (Anastrepha suspensa) (Heve et al., 2017), termite (Al-Zaidawi et al., 2020), and housefly (Arriaga and Cortez-Madrigal, 2018) (Tables 5, 6).
7. Registration and regulation
Under the Insecticides Act of 1968 and the Insecticides Rules of 1971, the Central Insecticides Board and Registration Committee (CIBRC) is the regulating authority in India that governs biopesticides. This board advises the federal and state governments on technical issues concerning the manufacture, marketing, distribution, and use of insecticides, including biopesticides, to assure human health, the environment, and natural bioagents. After evaluating their formulations and validating data on efficacy, toxicity, and packaging supplied by the importer or manufacturer, the CIBRC's registration committee permits public and private enterprises for large-scale manufacturing, distribution, and sale of biopesticides stakeholders. Manufacturers can register new products under the Insecticides Act's section 9(3B) (provision registration for a novel active component used in India) or section 9(3) (regular registration).
8. Challenges associated with entomopathogen registration and their solutions
Entomopathogens, which are microorganisms that may infect and kill insects, have been widely used in forestry and agriculture as biological control agents. However, there are a number of constraints in registering entomopathogens as pesticides, including lacking information on their effectiveness, safety, and environmental effects. The following are some of the major problems with entomopathogen registration and solutions.
8.1. Data on efficacy
The absence of effectiveness data is one of the greatest problems with entomopathogen registration. There is a need for further research to confirm the effectiveness of entomopathogens against target pests, according to a review by Zimmermann (1993). The efficiency of entomopathogens can be demonstrated by investigations and systematic reviews, as in the study by Fang et al. (2021) that assessed the efficacy of M. anisopliae against the maize stem borer.
8.2. Safety data
The absence of safety data is another challenge with entomopathogen registration. There is a need for more studies to determine the safety of entomopathogens, including their effect on non-target species, their persistence in the environment, and their potential for resistance development, according to a review by Hajek et al. (2021). Dillman et al. (2012) assessed the safety of B. bassiana on non-target bees, which can provide more information on the safety of entomopathogens.
8.3. Data on environmental effects
Another crucial factor for entomopathogen registration is their effect on the environment. Further investigation needs to be done to assess the environmental impact of entomopathogens, including their impacts on non-target species, their persistence in the environment, and their potential for bioaccumulation. Additional information on the environmental effects of entomopathogens can be found in recent research and reviews, such as the one by Hill (2020), which assessed the persistence of B. bassiana in soil. In order to prove their efficacy, safety, and environmental impact, more studies and systematic reviews are needed in order to address the issues associated with entomopathogen registration.
9. Limitation for the proliferation of microbial biopesticides
Although microbial pesticides have significant advantages over traditional pest control agents, they have failed to gain widespread commercial development and implementation in India. Some factors constrain the Indian market for microbial pesticides. Product quality control concerns, such as low microbial count, which leads to poor field performance, a lack of large-scale production facilities, and the sale of unregistered items in the market, are listed as the main hurdles (Alam, 2000; Gupta and Dikshit, 2010; Arora et al., 2011; Mishra et al., 2014). According to NBAIR studies, 50%−70% of microbial biopesticide-based products in India had deficiencies such as lesser colony propagules specified on the label, excessive moisture content in solid formulations, or pollutants. It, therefore, failed to fulfill the declared CIBRC requirement (Ramasubramanian et al., 2014). Some microbial biopesticides have a short shelf life, and there is a challenge in rural locations where fresh products and refrigerated storage are scarce (Mishra et al., 2014). Microbial dry formulations have become ineffective as a result of poor storage conditions (Ramasubramanian et al., 2014). Additional obstacles in generating economically viable microbial pesticides include a slow rate of kill, lack of field persistence due to high UV radiation levels, and poor water solubility of some formulations (Aneja et al., 2016).
10. Strategies to address/overcome the ENMs limitations
Although entomopathogenic fungi are being widely utilized as biocontrol agents for insect pest management, they have certain drawbacks, such as varying efficacy, uneven performance in the field, and expensive manufacturing and application costs. There are some instances of approaches that have been suggested to deal with these constraints.
10.1. Improved formulation and delivery
Increasing entomopathogenic fungi's formulation and distribution serves as a strategy to boost its potency and uniformity. For instance, Gouli et al. (2021) revealed that incorporating a surfactant into a commercial formulation of the entomopathogenic fungus-B. bassiana increased its effectiveness against the tomato leaf miner (Tuta absolute).
10.2. Enhancing tolerance to environmental stress
A different approach is to make entomopathogenic fungi more tolerant to environmental stresses such as high temperatures, UV radiation, and desiccation. For instance, Hajek et al. (2021) discovered that M. anisopliae, an entomopathogenic fungus, may adapt to high temperatures by being exposed to them gradually over numerous generations.
10.3. Combining entomopathogens with other biocontrol agents
Combining entomopathogenic fungus with other biocontrol agents, such as parasitoids or predators, may have beneficial consequences. For instance, research by Ekesi et al. (2021) demonstrated that the combination of the parasitic worm, Fopius arisanus, and the entomopathogenic fungus B. bassiana was more efficient in controlling the invasive fruit fly Bactrocera dorsalis than either agent alone.
10.4. Developing new strains and species
In addition, another strategy is for developing new entomopathogenic fungal strains or species with enhanced effectiveness, expanded host ranges, or additional desirable characteristics. For instance, research by Fang et al. (2020) encountered a novel strain of B. bassiana that was extremely virulent against a predominant pest known as the whitefly, Bemisia tabaci. The above strategies indicate how an ongoing investigation is underway to improve entomopathogenic fungi's efficiency as biocontrol agents for effective insect pest management.
11. Future perspectives
Entomopathogens serve as promising biocontrol agents with the potential to become a significant component in sustainable pest management. According to a recent study, entomopathogens are preferable to chemical pesticides in several ways, including their ability to target specific pests, a lack of pesticide residue in foodstuffs, and the capacity to lower pesticide resistance (Roy et al., 2006). Genetic engineering and biotechnology advances have opened up new possibilities for developing novel entomopathogens with enhanced efficacy and specificity. For example, researchers are investigating RNA interference to target specific genes in insect pests and developing hybrid entomopathogens that combine the properties of multiple microorganisms (Agrawal et al., 2018). In addition, it can also be integrated with other pest management strategies, such as crop rotation, habitat management, and biological control agents, to enhance their effectiveness. For example, researchers have shown that combining entomopathogens with insect-repellent plants can improve the efficacy of pest management (Winkler et al., 2019). There is limited attention on biopesticides because rural farmers in India have the least agricultural education or training. Outreach activities, such as training and field demonstrations, are essential for promoting the use of entomopathogens as a sustainable pest control method. Outreach activities can provide individuals with a better understanding of the benefits and limitations of using entomopathogens as a pest control method. This knowledge can help individuals decide when and how to use these products. Moreover, proper use of entomopathogens requires specific knowledge and skills. Outreach activities can teach individuals how to apply and manage entomopathogens to maximize their efficacy properly. By promoting entomopathogens, outreach activities can help reduce pesticide use and promote sustainable pest management practices. Several studies have demonstrated the importance of outreach activities for promoting the use of entomopathogens. For instance, a study conducted in Ethiopia found that training workshops for farmers increased knowledge and adoption of entomopathogenic fungi for controlling the coffee berry borer (António et al., 2017). Similarly, a study in India found that field demonstrations and training workshops for farmers improved the adoption of entomopathogenic nematodes for controlling the insect pest root-knot nematode in tomatoes (Kumar et al., 2021). Outreach activities can help increase the adoption of entomopathogens as a pest control method. By educating individuals about the benefits and limitations of entomopathogens and providing them with the necessary knowledge and skills, outreach activities can help them feel more confident using these products. Organizations can offer training workshops, field demonstrations, and educational materials, such as brochures, manuals, and videos, to implement outreach activities for entomopathogen adoption. These activities can target farmers, extension agents, researchers, and other stakeholders involved in pest management. By doing so, we can promote using entomopathogens as a sustainable and effective pest management strategy.
12. Conclusion
There has been growing interest in employing biopesticides for protecting crops through sustainable approaches in developing countries. Because the indiscriminate application of synthetic molecules led to resistance development and excessively contaminate the environment. Entomopathogen-based biopesticides are mainly suggested to be incorporated for pest management because of their environmental safety, primarily due to their host specificity. In addition, the cost of formulation and registration of microbial pesticides is much lower than synthetic chemicals. Over the last number of decades, efforts have resulted in the potential application of entomopathogens against various insect pests. However, an investigation is needed to explore many more virulent strains identification, isolation, and formulation preparation to effectively apply them.
Additional work needs to be done to eliminate limitations, including sensitivity to UV rays, desiccation, and low colony counts. Although biopesticides possess several advantages, they are not popularized due to a lack of awareness. It is important to conduct outreach activities, such as training and field demonstrations, to promote the wider acceptance of biopesticide-based formulations among growers. Therefore, insightful information about the potential of microbial insecticides in pest suppression is provided. They have been proven to be an effective component of integrated pest management in managing pest populations below an economic threshold level.
Author contributions
I and MS conceived and designed the study and prepared the figures/artwork. I, MS, EH, and PR performed the literature search. I wrote the first draft of the manuscript. I, MS, AM, and EK edited the manuscript. I, MS, and EH formatted the reference list as per Journal's style. All authors have approved the final version of the manuscript.
Funding
This study was partially funded by the Future University in Egypt (FUE).
Acknowledgments
The authors I, EH, and PR would like to thank the Department of Plant Protection, Faculty of Agricultural Sciences, A.M.U, Aligarh for providing the necessary facilities.
Conflict of interest
The authors declare that the research was conducted in the absence of any commercial or financial relationships that could be construed as a potential conflict of interest.
Publisher's note
All claims expressed in this article are solely those of the authors and do not necessarily represent those of their affiliated organizations, or those of the publisher, the editors and the reviewers. Any product that may be evaluated in this article, or claim that may be made by its manufacturer, is not guaranteed or endorsed by the publisher.
References
Abedi, Z., Saber, M., Vojoudi, S., Mahdavi, V., and Parsaeyan, E. (2014). Acute, sublethal, and combination effects of azadirachtin and Bacillus thuringiensis on the cotton bollworm, Helicoverpa armigera. J. Ins. Sci. 14, 30. doi: 10.1093/jis/14.1.30
Abid, M., and Saeed, R. (2015). Effect of salinity and root-knot nematode on growth of eggplant (Solanum melongena L.). FUUAST J. Biol. 5, 93–97.
Abrol, D. P., and Shankar, U. (2016). “Integrated pest management,” in Breeding Oilseed Crops for Sustainable Production: Opportunities and Constraints, Breeding Oilseed Crops for Sustainable Production: Opportunities and Constraints. Academic Press. doi: 10.1016/B978-0-12-801309-0.00020-3
Agrawal, S., Deshmukh, S., and Kumar, P. (2018). RNA interference: a new dawn of insect management. J. Entomol. Zool. Stud. 6, 442–447.
Akter, S., Alam, M. Z., Rahman, M. M., and Akanda, A. M. (2017). Evaluation of some management options against brinjal (Solanum melongena L.) shoot and fruit borer, Leucinodes orbonalis (Guenee). Agriculturists 15, 49–57. doi: 10.3329/agric.v15i1.33428
Alam, G. (2000). A study of biopesticides and biofertilizers in Haryana, India. Gate Keeper Series 93, 1–25.
Al-Azawi, A. F. (1964). Studies on the effect of Bacillus thuringiensis Berl. on the spiny bollworm, Earias insulana Boisd., other lepidopterous insects. Entomophaga 9, 137–145. doi: 10.1007/BF02376735
Ali, A. D, Harlow, J. L., Avery, P. B., and Kumar, V. (2015). Investigating the role of fungal entomopathogens in whitefly landscape IPM programs. J. Entomol. Sci. 50, 254–261. doi: 10.18474/JES14-35.1
Ali, K., Wakil, W., Zia, K., and Sahi, S. (2015). Control of Earias vittella (Lepidoptera: Noctuidae) by Beauveria bassiana along with Bacillus thuringiensis. Int. J. Agric. Biol. 17, 773–778. doi: 10.17957/IJAB/14.0009
Al-Siyabi, A. A., Kinawy, M. M., Al-Ansri, M., Mahar, A. N., Gowen, S. R., Hague, N. G., et al. (2006). The susceptibility of onion thrips, Thrips tabaci to Heterorhabditis indicus. Commun. Agric. Appl. Biol. Sci. 71, 239–243.
Al-Zaidawi J. B. Karimi J. Mahdikhani M. E. (2020) Entomopathogenic nematodes as potential biological control agents of subterranean termite, Microcerotermes diversus (Blattodea: Termitidae) in Iraq. Environ. Entomol. 49, 412–442. doi: 10.1093/ee/nvaa014
Amalraj, D., Sahu, S., Jambulingam, P., Doss, P., Kalyanasundaram, M., Das, P., et al. (2000). Efficacy of aqueous suspension and granular formulations of Bacillus thuringiensis (Vectobac) against mosquito vectors. Acta Trop. 75, 243–246. doi: 10.1016/S0001-706X(00)00054-1
Aneja, K. R., Khan, S. A., and Aneja, A. (2016). Biopesticides an eco-friendly pest management approach in agriculture: status and prospects. Kavaka 47, 145–154.
Annamalai, M., Kaushik, H. D., and Selvaraj, K. (2016). Bio efficacy of Beauveria bassiana (Balsamo) Vuillemin and Lecanicillium lecanii Zimmerman against Thrips tabaci Lindeman. Proc. Natl. Acad. Sci., India, Sect. B Biol. Sci. 86, 505–511. doi: 10.1007/s40011-014-0475-8
António, C., Pereira, J. A., Cunha, M., and Mexia, A. (2017). Farmers knowledge of entomopathogenic fungi and their perceptions of their efficacy for the control of the coffee berry borer in Timor-Leste. J. Insects Food Feed. 3, 183–189.
Araújo, J., and Hughes, D. (2016). Diversity of entomopathogenic fungi: which groups conquered the insect body? Adv. Genet. 94, 1–39. doi: 10.1016/bs.adgen.2016.01.001
Arnold, A. E., and Lewis, L. C. (2005). Ecology and Evolution of Fungal Endophytes, and their Roles Against Insects. Insect-Fungal Associations: Ecology and Evolution. New York, NY: Oxford University Press, 74–96.
Arora, N. K., Khare, E., and Maheshwari, D. K. (2011). “Plant growth promoting rhizobacteria: constraints in bioformulation, commercialization, and future strategies,” in Plant Growth and Health Promoting Bacteria, ed D. Maheshwari (Berlin, Springer), 97–116. doi: 10.1007/978-3-642-13612-2_5
Arriaga, A., and Cortez-Madrigal, H (2018). Susceptibility of Musca domestica larvae and adults to entomopathogenic nematodes (Rhabditida: Heterorhabditidae, Steinernematidae) native to Mexico. J. Vector Ecol. 43, 312–320. doi: 10.1111/jvec.12315
Arthurs, S., and Heinz, K. M. (2006). Evaluation of the nematodes Steinernema feltiae and Thripinema nicklewoodi as biological control agents of western flower thrips Frankliniella occidentalis infesting chrysanthemum. Biocontrol Sci. Technol. 16, 141–155. doi: 10.1080/09583150500258545
Asi, M., Bashir, M., Afzal, M., and Imran, S. (2009). Effect of conidial concentration of entomopathogenic fungi on mortality of cabbage aphid, Brevicoryne brassicae L. Pakistan J. Life Soc. Sci. 175–180.
Avery, P. B., Kumar, V., Skvarch, E. A., Mannion, C. M., Powell, C. A., McKenzie, C. L., et al. (2019). An ecological assessment of Isaria fumosorosea applications compared to a neonicotinoid treatment for regulating invasive ficus whitefly. J. Fungi 5, 36. doi: 10.3390/jof5020036
Badii, M. H., and Abreu, J. L. (2006). Biological control a sustainable way of pest control. Int. J. Good Conscienc. 1, 82–89.
Barloy, F., Delécluse, A., Nicolas, L., and Lecadet, M. M. (1996). Cloning and expression of the first anaerobic toxin gene from Clostridium bifermentans subsp. malaysia, encoding a new mosquitocidal protein with homologies to Bacillus thuringiensis delta-endotoxins. J. Bacteriol. 178, 3099–3105. doi: 10.1128/jb.178.11.3099-3105.1996
Barrera-Cubillos, G. P., Gómez-Valderrama, J. A., and Rivero, L. F. V. (2017). Efficacy of microencapsulated nucleopolyhedroviruses from Colombia as biological insecticides against Spodoptera frugiperda (Lepidoptera: Noctuidae). Acta Agron. 66, 267–274. doi: 10.15446/acag.v66n2.54354
Batalla-Carrera, L., Morton, A., and García-del-Pino, F. (2010). Efficacy of entomopathogenic nematodes against the tomato leafminer, Tuta absoluta in laboratory and greenhouse conditions. BioControl 55, 523–530. doi: 10.1007/s10526-010-9284-z
Batta, Y. (2018). Efficacy of two species of entomopathogenic fungi against the stored-grain pest, sitophilus granarius (curculionidae: Coleoptera), via oral ingestion. Egypt. J. Biol. Pest Control 28, 1–8. doi: 10.1186/s41938-018-0048-x
Bedini, S., Muniz, E., Tani, C., Conti, B., and Ruiu, L. (2020). Insecticidal potential of Brevibacillus laterosporus against dipteran pest species in a wide ecological range. J. Invertebr. Pathol. 177, 107493. doi: 10.1016/j.jip.2020.107493
Belair, G., Vincent, C., and Chouinard, G. (1998). Foliar sprays with Steinernema carpocapsae against early-season apple pests. J. Nematol. 30(4S), 599.
Belay, D., Ebssa, L., and Borgemeister, C. (2005). Time and frequency of applications of entomopathogenic nematodes and their persistence for control of western flower thrips Frankliniella occidentalis. Nematol. 7, 611–622. doi: 10.1163/156854105774384822
Bidochka, M. J., and Small, C. L. (2005). “Phylogeography of Metarhizium, an insect pathogenic fungus,” in Insect-Fungal Associations: Ecology and Evolution, eds F. E. Vega, and M. Blackwell (New York, NY: Oxford University Press), 75–118.
Brivio, M., and Mastore, M. (2020). When appearance misleads: the role of the entomopathogen surface in the relationship with its host. Insects 11, 387. doi: 10.3390/insects11060387
Bruck, D. J., Edwards, D. L., and Donahue, K. M. (2008). Susceptibility of the strawberry crown moth (Lepidoptera: Sesiidae) to entomopathogenic nematodes. J. Econ. Entomol. 101, 251–255. doi: 10.1093/jee/101.2.251
Buitenhuis, R., and Shipp, J. L. (2005). Efficacy of entomopathogenic nematode Steinernema feltiae (Rhabditida: Steinernematidae) as influenced by Frankliniella occidentalis (Thysanoptera: Thripidae) developmental stage and host plant stage. J. Econ. Entomol. 98, 1480–1485. doi: 10.1093/jee/98.5.1480
Castagnola, A., and Stock, S. (2014). Common virulence factors and tissue targets of entomopathogenic bacteria for biological control of lepidopteran pests. Insects 5, 139–166. doi: 10.3390/insects5010139
Castro, T., Mayerhofer, J., Enkerli, J., Eilenberg, J., Meyling, N. V., De Andrade Moral, I., et al. (2016). Persistence of Brazilian isolates of the entomopathogenic fungi Metarhizium anisopliae and M. robertsii in strawberry crop soil after soil drench application. Agric. Ecosyst. Environ. 233, 361–369. doi: 10.1016/j.agee.2016.09.031
Chelvi, C. T., Thilagaraj, W. R., and Nalini, R. (2011). Field efficacy of formulations of microbial insecticide Metarhizium anisopliae (Hyphocreales: Clavicipitaceae) for the control of sugarcane white grub Holotrichia serrata F (Coleoptera: Scarabidae). J. Biopestic. 4, 186.
Cordova-Kreylos, A. L., Fernandez, L. E., Koivunem, M., Yang, A., Flor-Weiler, L., Marrone, P. G., et al. (2013). Isolation and characterization of Burkholderia rinojensis sp. nov., a non-Burkholderia cepacian complex soil bacterium with insecticidal and miticidal activities. Appl. Environ. Microbiol. 79, 7669–7678. doi: 10.1128/AEM.02365-13
Cuthbertson, A., Head, J., Walters, K., and Murray, A. (2003). The integrated use of chemical insecticides and the entomopathogenic nematode, Steinernema feltiae, for the control of sweet potato whitefly, Bemisia tabaci. Nematology 5, 713–720. doi: 10.1163/156854103322746887
Cuthbertson, A. G., and Audsley, N. (2016). Further screening of entomopathogenic fungi and nematodes as control agents for Drosophila suzukii. Insects 7, 24. doi: 10.3390/insects7020024
Cuthbertson, A. G. S., Walters, K. F. A., Northing, P., and Luo, W. (2007). Efficacy of the entomopathogenic nematode, Steinernema feltiae, against sweet potato whitefly Bemisia tabaci (Homoptera: Aleyrodidae) under laboratory and glasshouse conditions. Bull. Entomol. Res. 97, 9–14. doi: 10.1017/S0007485307004701
Dash, C. K., Bamisile, B. S., Keppanan, R., Qasim, M., Lin, Y., Islam, S. U., et al. (2018). Endophytic entomopathogenic fungi enhance the growth of Phaseolus vulgaris L. (Fabaceae) and negatively affect the development and reproduction of Tetranychus urticae Koch (Acari: Tetranychidae). Microb. Pathog. 125, 385–392. doi: 10.1016/j.micpath.2018.09.044
De Luca, F., Clausi, M., Troccoli, A., Curto, G., Rappazzo, G., Tarasco, E., et al. (2015). “Entomopathogenic nematodes in Italy: occurrence and use in microbial control strategies,” in Nematode Pathogenesis of Insects and Other Pests: Ecology and Applied Technologies for Sustainable Plant and Crop Protection, ed R. Campos-Herrera (Berlin: Springer), 431–449. doi: 10.1007/978-3-319-18266-7_18
De Maagd, R. A., Bravo, A., and Crickmore, N. (2001). How Bacillus thuringiensis has evolved specific toxins to colonize the insect world. Trends Genet. 17, 193–199. doi: 10.1016/S0168-9525(01)02237-5
de Waal, J. Y., Addison, M. F., and Malan, A. P. (2018). Potential of Heterorhabditis zealandica (Rhabditida: Heterorhabditidae) for the control of codling moth, Cydia pomonella (Lepidoptera: Tortricidae) in semi-field trials under South African conditions. Int. J. Pest Manag. 64, 102–109. doi: 10.1080/09670874.2017.1342149
Dembilio, O., Llacer, E., Martínez de Altube, M. D. M., and Jacas, J. A. (2010). Field efficacy of imidacloprid and Steinernema carpocapsae in a chitosan formulation against the red palm weevil Rhynchophorus ferrugineus (Coleoptera: Curculionidae) in Phoenix canariensis. Pest Manag. Sci. 66, 365–370. doi: 10.1002/ps.1882
Devi, P., and Hari, P. (2009). Beauveria bassiana Suspension concentrate-a mycoinsecticide for the management of Helicoverpa armigera (Hubner). J. Biol. Control. 23, 403–408.
Devi, P. S. V. (1994). Conidia production of the entomopathogenic fungus Nomuraea rileyi and its evaluation for control of Spodoptera litura (Fab) on Ricinus communis. J. Invertebr. Pathol. 63, 145–150. doi: 10.1006/jipa.1994.1028
Dhandapani, N., Jayaraj, S., and Rabindra, R. J. (1993). Laboratory studies on the efficacy of nuclear polyhedrosis virus against Heliothis armigera (Hbn.) on some cotton cultivars. Anzeiger für Schädlingskd. Pflanzenschutz Umweltschutz 66, 96–100. doi: 10.1007/BF01906819
Dias, C., Patri', P., Garcia, P., Simoes, N., Simoes, S., Lui', L., et al. (2005). Efficacy of Bacillus thuringiensis against Phyllocnistis citrella (Lepidoptera: Phyllocnistidae). J. Econ. Entomol. 98, 1880–1883. doi: 10.1093/jee/98.6.1880
Dillman, A. R., Chaston, J. M., Adams, B. J., Ciche, T. A., Goodrich-Blair, H., Stock, S. P., et al. (2012). An entomopathogenic nematode by any other name. PLoS Pathog. 8, e1002527. doi: 10.1371/journal.ppat.1002527
Dong, T., Zhang, B., Jiang, Y., and Hu, Q. (2016). Isolation and classification of fungal whitefly entomopathogens from soils of Qinghai-Tibet Plateau and Gansu Corridor in China. PLoS ONE 11, e0156087. doi: 10.1371/journal.pone.0156087
Dowds, B. C. A., and Peters, A (2002) “Virulence mechanisms,” in Entomopathogenic Nematology, ed R. Gaugler (Wallingford: CABI Publishing), 79–98. doi: 10.1079/9780851995670.0079
Dubovskiy, I. M., Whitten, M. M. A., Kryukov, V. Y., Yaroslavtseva, O. N., Grizanova, E. V., Greig, C., et al. (2020). Synergistic interactions of entomopathogenic fungus Metarhizium robertsii and predatory mite Amblyseius swirskii against tomato russet mite Aculops lycopersici. Biol. Control. 150, 104372.
Easwaramoorthy, S., and Santhalakshmi, G. (1988). Efficacy of granulosis virus in the control of shoot borer, Chilo infuscatellus Snellen. J. Biol. Control 2, 26–28.
Ebssa, L., Borgemeister, C., Berndt, O., and Poehling, H. M. (2001). Efficacy of entomopathogenic nematodes against soil-dwelling life stages of western flower thrips, Frankliniella occidentalis (Thysanoptera: Thripidae). J. Invertebr. Pathol. 78, 119–127. doi: 10.1006/jipa.2001.5051
Ebssa, L., Borgemeister, C., and Poehling, H. M. (2004a). Effectiveness of different species/strains of entomopathogenic nematodes for control of western flower thrips (Frankliniella occidentalis) at various concentrations, host densities, and temperatures. Biol Control 29, 145–154. doi: 10.1016/S1049-9644(03)00132-4
Ebssa, L., Borgemeister, C., and Poehling, H. M. (2004b). Effects of post-application irrigation and substrate moisture on the efficacy of entomopathogenic nematodes against western flower thrips, Frankliniella occidentalis. Entomol. Exp. Appl. 112, 65–72. doi: 10.1111/j.0013-8703.2004.00184.x
Ehlers, A., Clark, D.M., Hackmann, A., McManus, F., and Fennell, M. (2005). Cognitive therapy for post-traumatic stress disorder: development and evaluation. Behav. Res. Therapy. 43, 413–431. doi: 10.1016/j.brat.2004.03.006
Ekesi, S., Dicke, M., and de Kogel, W. J. (2021). Combining biological control agents for enhanced management of invasive tephritid fruit flies. Biol. Control. 155, 104548.
El Husseini, M. (2020). Pathogenicity of nuclear polyhedrosis virus to Galleria mellonella L. (Lepidoptera: Pyralidae) and its control on stored beeswax foundations. Egypt. J. Biol. Pest Control 30. doi: 10.1186/s41938-020-00302-4
Elgizawy, K., and Ashry, N. (2019). Efficiency of Bacillus thuringiensis strains and their Cry proteins against the Red Flour Beetle, Tribolium castaneum (Herbst.) (Coleoptera: Tenebrionidae). Egypt. J. Biol. Pest Control. 29, 1–9. doi: 10.1186/s41938-019-0198-5
Fang, W., Leng, B., Xiao, Y., Jin, K., and Wang, S. (2020). A new Beauveria bassiana strain with high virulence against the whitefly Bemisia tabaci. J. Invertebr. Pathol. 173, 107434.
Fang, W., Lu, H., King, G., and St Leger, R. (2014). Construction of a hypervirulent and specific mycoinsecticide for locust control. Sci. Rep. 4, 1–6. doi: 10.1038/srep07345
Fang, X., Zhou, H., and Huang, B. (2021). Evaluation of Metarhizium anisopliae in controlling the maize stem borer. Crop Prot. 147, 105880.
Fillinger, U., Knols, B. G. J., and Becker, N. (2003). Efficacy and efficiency of new Bacillus thuringiensis var. israelensis and Bacillus sphaericus formulations against Afrotropical anophelines in Western Kenya. Trop. Med. Int. Heal. 8, 37–47. doi: 10.1046/j.1365-3156.2003.00979.x
Fourie, D., Van Den Berg, J., and Du Pleassis, H. (2017). Efficacy of Bacillus thuringiensis sprays and cotton cultivars expressing Cry proteins in the control of Earias biplaga (Walker) (Lepidoptera: Noctuidae). Afr. Entomol. 25, 335–340. doi: 10.4001/003.025.0335
Ganguly, S., Anupama Kumar, A., and Parmar, B. (2008). Nemagel - a formulation of the entomopathogenic nematode Steinernema thermophilum mitigating the shelf-life constraint of the tropics. Nematol. Mediterr. 36, 125–130.
Ganguly, S., Singh, M., Lal, M., Singh, L., Vyas, R., Patel, D., et al. (2002). “Report on identification of Steinernema riobrave Cabanillas, Poinar and Raulstan, 1994 from Anand, Gujarat,” in Proceedings of National Symposium on Biodiversity and Management of Nematodes in Cropping Systems for Sustainable Agriculture, Organized by NSI, ICAR and RAU at Dept. of Nematology, ARS, Durgapura (Jaipur), 11–13.
Garcia, L. C., Raetano, C. G., and Leite, L. G. (2008). Application technology for the entomopathogenic nematodes Heterorhabditis indica and Steinernema sp. (Rhabditida: Heterorhabditidae and Steinernematidae) to control Spodoptera frugiperda (Smith)(Lepidoptera: Noctuidae) in corn. Neotrop. Entomol. 37, 305–311. doi: 10.1590/S1519-566X2008000300010
Garriga, A., Morton, A., Ribes, A., and Garcia-del-Pino, F. (2020). Soil emergence of Drosophila suzukii adults: a susceptible period for entomopathogenic nematodes infection. J. Pest Sci. 93, 639–646. doi: 10.1007/s10340-019-01182-w
Glare, T. R., Jurat-Fuentes, J. L., and O'callaghan, M. (2017). “Basic and applied research: entomopathogenic bacteria,” in Microbial Control of Insect and Mite Pests, ed L. A. Lacey (Cambridge, MA: Academic press), 47–67. doi: 10.1016/B978-0-12-803527-6.00004-4
Gopalakrishnan, C., and Gangavisalakshy, P. N. (2005). Field efficacy of commercial formulations of Bacillus thuringiensis var. kurstaki against Papilio demoleus L. on citrus. Entomon 30, 93.
Gouli, S., Lefkaditis, D., Kouloussis, N. A., and Menti, G. (2021). Improved efficacy of a commercial formulation of Beauveria bassiana for the control of Tuta absoluta (Meyrick) (Lepidoptera: Gelechiidae) with the addition of surfactants. J. Pest Sci. 94, 1111–1123.
Gözel, Ç., and Kasap, I. (2015). Efficacy of entomopathogenic nematodes against the Tomato leafminer, Tuta absoluta (Meyrick) (Lepidoptera: Gelechiidae) in the tomato field. Turk Entomol Derg. 39, 229–237. doi: 10.16970/ted.84972
Gul, H. T., Freed, S., Akmal, M., and Malik, M. N. (2015). Vulnerability of different life stages of Bactrocera zonata (Tephritidae: Diptera) against entomogenous fungi. Pak. J. Zool. 47.
Gupta, A., Sharma, S., and Naik, S. N. (2011). Biopesticidal value of selected essential oils against pathogenic fungus, termites, and nematodes. Int. Biodeterior. Biodegrade. 65, 703–707. doi: 10.1016/j.ibiod.2010.11.018
Gupta, S., and Dikshit, A. K. (2010). Biopesticides: an eco-friendly approach for pest control. J. Biopestic. 3, 186.
Haase, S., Sciocco-Cap, A., and Romanowski, V. (2015). Baculovirus insecticides in Latin America: historical overview, current status and future perspectives. Viruses 7, 2230–2267. doi: 10.3390/v7052230
Habashy, M. G., Al-Akhdar, H. H., Boraie, D. M., and Ghareeb, Z. E. (2016). Laboratory and Semi field evaluation of garlic aqueous extract as acaricide against two tetranychid mites (Acari: Tetranychidae). J. Plant Prot. Pathol. 7, 623–628. doi: 10.21608/jppp.2016.52085
Hajek, A. E., Bischoff, J. F., and Carruthers, R. I. (2021). Adaptation of Metarhizium anisopliae to high temperatures by generation of gradually heat-adapted isolates. J. Invertebr. Pathol. 184, 107558.
Halder, J., Khushwaha, D., Rai, A. B., Nagendran, K., and Singh, B. (2018). Host plant mediated susceptibility of Phenacoccus solenopsis (Tinsley) to Lecanicillium lecanii (Zimmermann) Zare and Gams, neem oil and their combination. Proc. Natl. Acad. Sci. India Sec. B Biol. Sci. 88, 241–247. doi: 10.1007/s40011-016-0750-y
Head, J., Lawrence, A. J., and Walters, K. F. A. (2004). Efficacy of the entomopathogenic nematode, Steinernema feltiae, against Bemisia tabaci in relation to plant species. J. Appl. Entomol. 128, 543–547. doi: 10.1111/j.1439-0418.2004.00882.x
Hesham, A. E. L., Kaur, T., Devi, R., Kour, D., Prasad, S., Yadav, N., et al. (2021). “Current trends in microbial biotechnology for agricultural sustainability: conclusion and future challenges,” in Current Trends in Microbial Biotechnology for Sustainable Agriculture, eds A. N. Yadav, J. Singh, and C. Singh (Berlin: Springer), 555–572. doi: 10.1007/978-981-15-6949-4_22
Heve W. K. El-Borai F. E. Carrillo D. Duncan L. W. (2017) Biological control potential of entomopathogenic nematodes for management of Caribbean fruit fly, Anastrepha suspensa Loew (Tephritidae). Pest Manag. Sci. 73, 1220–1228. doi: 10.1002/ps.4447
Hill, R. A. (2020). Persistence of Beauveria bassiana in soil following application to control the emerald ash borer, Agrilus planipennis. Biol. Control. 142, 104–154.
Hostetter, D. L., Biever, K. D., Heimpel, A. M., and Ignoffo, C. M. (1979). Efficacy of the nuclear polyhedrosis virus of the alfalfa looper against cabbage looper larvae on cabbage in Missouri. J. Econ. Entomol. 72, 371–373. doi: 10.1093/jee/72.3.371
Hurst, M. R., Swaminathan, J., Wright, D. A., Hardwick, S., Ferguson, C. M., Beattie, A., et al. (2020). Development of a Yersinia entomophaga bait for control of larvae of the porina moth (Wiseana spp.), a pest of New Zealand improved grassland systems. Pest Manag. Sci. 76, 350–359. doi: 10.1002/ps.5521
Hurst, M. R. H., Rogers, D. J., Wright, D. A., Townsend, R. J., Bruening, R., Cole, L. M., et al. (2011). Effect of the bacterium Yersinia entomophaga on adult on adult bronze beetle. New Zeal. Plant Prot. 64, 209–214. doi: 10.30843/nzpp.2011.64.5956
Hussain, M. A., Ahmad, R., and Ahmad, W. (2014). Evaluation of Steinernema masoodi (Rhabditida: Steinernematidae) against soil-dwelling life stage of Helicoverpa armigera (Lepidoptera: Noctuidae) in laboratory and microplot study. Can. J. Plant Protec. 2, 4–s8.
Hussain, M. A., Fatima, I., Mukhtar, T., Aslam, M. N., and Kayani, M. Z. (2015). Effect of inoculum density of root-knot nematode Meloidogyne incognita on damage potential in eggplant. Mycopath. 13, 33–36.
Hussaini, S. S., Ansari, M. A., Ahmad, W., and Subbotin, S. A. (2001). Identification of some Indian populations of Steinernema species (Nematoda) by RFLP analysis of the ITS region of rDNA. Int. J. Nematol. 11, 73–76.
Hussaini, S. S., Rabindra, R. J., and Nagesh, M. (2003). “Current status of research on entomopathogenic nematodes in India: Workshop on the Entomopathogenic Nematodes in India held on 22 and 23rd January, 2003,” in Current status of research on Entomopathogenic Nematodes in India: Workshop on the Entomopathogenic Nematodes in India. Bangalore: Project Directorate of Biological Control, Indian Council of Agricultural Research.
Islam, W. (2017). Eco-friendly approaches for the management of red flour beetle: Tribolium castaneum (Herbst). Sci. Lett. 5, 105–114.
Jaber, L. R., and Ownley, B. H. (2018). Can we use entomopathogenic fungi as endophytes for dual biological control of insect pests and plant pathogens? Biol. Control 116, 36–45. doi: 10.1016/j.biocontrol.2017.01.018
Jagadish, K., Shadakshari, Y., Puttarangaswamy, K., Karuna, K., Geetha, K., Nagarathna, T., et al. (2010). Efficacy of some biopesticides against defoliators and capitulum borer, Helicoverpa armigera Hub. in sunflower, Helianthus annuus L. J. Biopestic. 3, 379.
Jaihan, P., Sangdee, K., and Sangdee, A. (2016). Selection of entomopathogenic fungus for biological control of chili anthracnose disease caused by Colletotrichum spp. Eur. J. Plant Pathol. 146, 551–564. doi: 10.1007/s10658-016-0941-7
Jaques, R., Laing, J., Laing, D., and Yu, D. (1987). Effectiveness and persistence of the granulosis virus of the codling moth Cydia pomonella (L.) (Lepidoptera: Olethreutidae) on apple. Can. Entomol. 119, 1063–1067. doi: 10.4039/Ent1191063-12
Jayanth, K. P. (2002). “Commercial production and market prospects of microbial pesticides in India,” in Microbial Biopesticide Formulations and Application. Tech. Doc. No. 55, Project Directorate of biological Control, eds R. J. Rabindra, S. S. Hussaini, and B. Ramanujam (Bangalore), 264–269.
Jeyarani, S., Sathiah, N., and Karuppuchamy, P. (2010). Field efficacy of Helicoverpa armigera nucleopolyhedro-virus isolates against H. armigera (Hübner) (Lepidoptera: Noctuidae) on cotton and chickpea in Tamil Nadu. Plant Prot. Sci. 46, 116–122. doi: 10.17221/30/2009-PPS
Johnson, W. A., Cloyd, R. A., Nechols, J. R., Williams, K. A., Nelson, N. O., Rotenberg, D., et al. (2012). Effect of nitrogen source on pac choi (Brassica rapa L.) chemistry and interactions with the diamondback moth (Plutella xylostella L.). Horticult. Sci. 47, 1457–1465. doi: 10.21273/HORTSCI.47.10.1457
Jones, S., Ferguson, C., Philip, B., Van Koten, C., and Hurst, M. (2015). Assessing the potential of Yersinia entomophaga to control plantain moth in a laboratory assay. Plant Prot. 68, 146–150. doi: 10.30843/nzpp.2015.68.5833
Justo De Oliveira, E., Rabinovitch, L., Gomes, R., Konovaloff, L., Passos, J., Zahner, V., et al. (2004). Molecular characterization of Brevibacillus laterosporus and its potential use in biological control. Appl. Environ. Microbiol. 70, 6657–6664. doi: 10.1128/AEM.70.11.6657-6664.2004
Kalia, V., Sharma, G., Shapiro-llan, D., and Ganguly, S. (2014). Biocontrol potential of Steinernema thermophilum and its symbiont Xenorhabdus indica against lepidopteran pests: virulence to egg and larval stages. J. Nematol. 46, 18.
Kamali, S., Karimi, J., Hosseini, M., Campos-Herrera, R., and Duncan, L. W. (2013). Biocontrol potential of the entomopathogenic nematodes Heterorhabditis bacteriophora and Steinernema carpocapsae on cucurbit fly, Dacus ciliatus (Diptera: Tephritidae). Biocontrol Sci. Technol. 23, 1307–1323. doi: 10.1080/09583157.2013.835790
Kashkouli, M., Khajehali, J., and Poorjavad, N. (2014). Impact of entomopathogenic nematodes on Thrips tabaci Lindeman (Thysanoptera: Thripidae) life stages in the laboratory and under semi-field conditions. J. Biopestic. 7, 77–84.
Kaur, T., Vasudev, A., Sohal, S. K., and Manhas, R. K. (2014). Insecticidal and growth inhibitory potential of Streptomyces hydrogenans DH16 on major pest of India, Spodoptera litura (Fab.) (Lepidoptera: Noctuidae). BMC Microbiol. 14, 1–9. doi: 10.1186/s12866-014-0227-1
Kepenekci, I., Hazir, S., and Özdem, A. (2015). Evaluation of native entomopathogenic nematodes for the control of the European cherry fruit fly Rhagoletis cerasi L. (Diptera: Tephritidae) larvae in soil. Turk. J. Agri For. 39, 74–79. doi: 10.3906/tar-1403-96
Kesavan, R., Easwaramoorthy, S., and Santhalakshmi, G. (2003). Evaluation of Different Formulations of Bacillus thuringiensis against Sugarcane Early Shoot Borer Chilo infuscatellus Snellen. Sugar Tech. 5, 51–55. doi: 10.1007/BF02943764
Khan, M., and Khalil, S. (1990). Biological control of aphid with a entomopathogenic fungus (Verticillium lecanii). Pakistan J. Agric. Res. 11, 174–177.
Khan, S., Guo, L., Maimaiti, Y., Mijit, M., and Qiu, D. (2012). Entomopathogenic fungi as microbial biocontrol agent. Mol. Plant Breed. 3, 63–79. doi: 10.5376/mpb.2012.03.0007
Khan, S., Shahid, M., Khan, M. S., Syed, A., Bahkali, A. H., Elgorban, A. M., et al. (2020). Fungicide-tolerant plant growth-promoting rhizobacteria mitigate physiological disruption of white radish caused by fungicides used in the field cultivation. Int. J. Environ Res. Public Health 17, 7251. doi: 10.3390/ijerph17197251
Khan, Z., Khan, S., Khan, N., and Muhammad, N. (2019). Efficacy of aqueous plant extracts and pesticide against okra fruit borer in commercial okra cultivars. Acta Sci. Agric. 3, 81–84. doi: 10.31080/ASAG.2019.03.0728
Kil, Y., Seo, M., Kang, D., Oh, S., Cho, H., Youn, Y., et al. (2014). Effects of Enterobacteria (Burkholderia sp.) on development of Riptortus pedestris. J. Fac. Agric. Kyushu Univ. 59, 77–84. doi: 10.5109/1434382
Kiran Kumar, K., Sridhar, J., Kanagaraj Murali-Baskaran, R., Senthil-Nathan, S., Kaushal, P., Dara, S. K., et al. (2019). Microbial biopesticides for insect pest management in India: current status and future prospects. J. Invertebr. Pathol. 165, 74–81. doi: 10.1016/j.jip.2018.10.008
Kranthi, K., Jadhav, D., Kranthi, S., Wanjari, R., Ali, S., Russell, D., et al. (2002). Insecticide resistance in five major insect pests of cotton in India. Crop Prot. 21, 449–460. doi: 10.1016/S0261-2194(01)00131-4
Kumar, A., Sharma, A. K., Verma, P., and Singh, A. (2021). Enhancing the adoption of entomopathogenic nematodes through field demonstrations and training workshops among tomato growers of Punjab, India. Biol. Agric. Horticul. 1–16.
Kumar, S., and Kaur, J. (2017). Efficacy of Beauveria bassiana and Bacillus thuringiensis as eco-safe alternatives to chemical insecticides against sunflower capitulum borer, Helicoverpa armigera (Hubner). J. Entomol. Zool. Stud. 5, 185–188.
Kumar, V., Francis, A., Avery, P. B., McKenzie, C. L., and Osborne, L. S. (2018). Assessing compatibility of Isaria fumosorosea and buprofezin for mitigation of Aleurodicus rugioperculatus (Hemiptera: Aleyrodidae): an invasive pest in the florida landscape. J. Econ. Entomol. 111, 1069–1079. doi: 10.1093/jee/toy056
Kumar, V., McKenzie, C. L., and Osborne, L. S. (2016). Effect of buprofezin and Isaria fumosorosea against rugose spiralling whitefly. Arthropod Manag. Tests 41, tsw099. doi: 10.1093/amt/tsw099
Kumara, G., Bhaskarb, L., Satishc, Y., and Rehamand, S. (2016). Evaluation of liquid formulations of Bt against gram pod borer, Helicoverpa armigera (Hubner) and spotted pod borer, Maruca vitrata (Geyer) in pigeonpea. J. Appl. Biol. Biotechnol. 4, 42.
Kumari, R., Singh, A., Yadav, A. N., Mishra, S., Sachan, A., Sachan, S. G., et al. (2020). Probiotics, prebiotics, and synbiotics: current status and future uses for human health. New Futur. Dev. Microb. Biotechnol. Bioeng. 173–190. doi: 10.1016/B978-0-12-820528-0.00012-0
Kumari, V., and Singh, N. (2009). Spodoptera litura nuclear polyhedrosis virus (NPV-S) as a component in Integrated Pest Management (IPM) of Spodoptera litura (Fab.) on cabbage. J. Biopestic. 2, 84–86.
Lacey L. Grzywacz D. ShapiroIlan D. Frutos R. Brownbridge M. Goettel M. (2015) Insect pathogens as biological control agents: back to the future. J. Invertebr. Pathol. 132, 1–41. 10.1016/j.jip.2015.07.009
Lacey, L. A., Arthurs, S. P., Unruh, T. R., Headrick, H., and Fritts Jr, R. (2006). Entomopathogenic nematodes for control of codling moth (Lepidoptera: Tortricidae) in apple and pear orchards: effect of nematode species and seasonal temperatures, adjuvants, application equipment, and post-application irrigation. Biol. Control 37, 214–223. doi: 10.1016/j.biocontrol.2005.09.015
Lacey, L. A., Neven, L. G., Headrick, H. L., and Fritts Jr, R. (2005). Factors affecting entomopathogenic nematodes (Steinernematidae) for control of overwintering codling moth (Lepidoptera: Tortricidae) in fruit bins. J. Econ. Entomol. 98, 1863–1869. doi: 10.1093/jee/98.6.1863
Laznik, Z., Znidarcic, D., and Trdan, S. (2011). Control of Trialeurodes vaporariorum (Westwood) adults on glasshouse-grown cucumbers in four different growth substrates: an efficacy comparison of foliar application of Steinernema feltiae (Filipjev) and spraying with thiamethoxamn. Turk. J. Agric. For. 35, 631–640. doi: 10.3906/tar-1007-1110
Le Vieux, P. D., and Malan, A. P. (2013). The potential use of entomopathogenic nematodes to control Planococcus ficus (Signoret) (Hemiptera: Pseudococcidae). South African J. Enol. Vitic. 34, 296–306. doi: 10.21548/34-2-1108
Legwaila, M. M., Munthali, D. C., Kwerepe, B. C., and Obopile, M. (2015). Efficacy of Bacillus thuringiensis (var. Kurstaki) against Diamondback Moth (Plutella Xylostella L.) eggs and larvae on cabbage under semi-controlled. Int. J. Insect Sci. 7, 7–39. doi: 10.4137/IJIS.S23637
Lewis, L. C., and Johnson, T. B. (1982). Efficacy of two nuclear polyhedrosis viruses against Ostrinia nubilalis (Lepidoptera: Pyralidae) in the laboratory and field. Entomophaga 27, 33–38. doi: 10.1007/BF02371935
Liu, Q., Wang, Y., and Zhou, H. (1997). A study on the application of entomopathogenic nematodes for controlling larvae of RLB. Acta Agric. Bor. Sin. 12, 97–101.
Lopes, R., Sosa-Gomez, D., Oliveiria, C., Sanches, M., de Souza, D., Benito, M., et al. (2020). Efficacy of an oil-based formulation combining Metarhizium rileyi and nucleopolyhedroviruses against lepidopteran pests of soybean. J. Appl. Entomol. 144, 678–689. doi: 10.1111/jen.12787
Lord, J. (2005). From metchnikoff to Monsanto and beyond: the path of microbial control. J. Invertebr. Pathol. 89, 19–29. doi: 10.1016/j.jip.2005.04.006
Lovett, B., and St. Leger, R. J. (2017). The insect pathogens. Microbiol. Spectr. 5. doi: 10.1128/9781555819583.ch45
Lu, H. L., and Leger, R. S. (2016). Insect immunity to entomopathogenic fungi. Adv. Genet. 94, 251–285. doi: 10.1016/bs.adgen.2015.11.002
Luangsa-Ard, J. J., Hywel-Jones, N. L., Manoch, L., and Samson, R. A. (2005). On the relationships of Paecilomyces sect. Isarioidea species. Mycol. Res. 109, 581–589. doi: 10.1017/S0953756205002741
Mahapatro, G., and Gupta, G. (1998). Bio-potency test of some commercial formulation of Bacillus thuringiensis against spotted bollworm, “Earias vitella Feb. Pestology 22, 22–26.
Malaikozhundan, B., and Vinodhini, J. (2018). Biological control of the Pulse beetle, Callosobruchus maculatus in stored grains using the entomopathogenic bacteria, Bacillus thuringiensis. Microb. Pathog. 114, 139–146. doi: 10.1016/j.micpath.2017.11.046
Maqsood, S., Afzal, M., Aqueel, M., Raza, A., Wakil, W., Babar, M., et al. (2017). Efficacy of nuclear polyhedrosis virus and flubendiamide alone and in combination against Spodoptera litura F. Pak. J. Zool. 49, 1783–1788. doi: 10.17582/journal.pjz/2017.49.5.1783.1788
Mariam, G. H., Hala, H., Elsherbiny, E. A., and Nofal, A. M. (2016). Efficacy of entomopathogenic fungi Metarhizium anisopliae and Cladosporium cladosporioides as biocontrol agents against two tetranychid mites (Acari: Tetranychidae). Egypt. J. Biol. Pest Control 26, 197–201.
Martin, P., Hirose, E., and Aldirch, J. (2007). Toxicity of chromobacterium subtsugae to southern green stink bug (Heteroptera: Pentatomidae) and corn rootworm (Coleoptera: Chrysomelidae). J. Econ. Entomol. 100, 680–684. doi: 10.1603/0022-0493(2007)100680:TOCSTS2.0.CO
Mascarin, G. M., and Delalibera, I. (2012). Insecticidal activity of the Granulosis Virus in combination with neem products and talc powder against the potato tuberworm Phthorimaea operculella (Zeller) (Lepidoptera: Gelechiidae). Neotrop. Entomol. 41, 223–231. doi: 10.1007/s13744-012-0044-x
Mathews, M. B., and Shenk, T. H. O. M. A. S. (1991). Adenovirus virus-associated RNA and translation control. J. Virol. 65, 5657–5662.
Mathur, A., Singh, S., Singh, N., and Meena, M. (2012). Field evaluation of plant products and microbial formulations against brinjal shoot and fruit borer, Leucinodes orbonalis Guenee under semi-arid conditions of Rajasthan. J. Biopestic. 5, 71.
McGaughey, W. (1975). A granulosis virus for Indian meal moth control in stored wheat and corn. J. Econ. Entomol. 68, 346–348. doi: 10.1093/jee/68.3.346
McNeill, M., and Hurst, M. (2008). Yersinia sp. (MH96) a potential biopesticide of migratory locust Locusta migratoria L. New Zeal. Plant Prot. 61, 236–242. doi: 10.30843/nzpp.2008.61.6848
Mejía, L. C., Rojas, E. I., Maynard, Z., Bael, S., Van Arnold, A. E., Hebbar, P., et al. (2008). Endophytic fungi as biocontrol agents of Theobroma cacao pathogens. Biol. Control 46, 4–14. doi: 10.1016/j.biocontrol.2008.01.012
Memari, Z., Karimi, J., Kamali, S., Goldansaz, S. H., and Hosseini, M. (2016). Are entomopathogenic nematodes effective biological control agents against the carob moth, Ectomyelois ceratoniae? J. Nematol. 48, 261–267. doi: 10.21307/jofnem-2017-034
Michereff Filho, M., Oliveira, S., De Liz, R., and Faria, M. (2011). Cage and field assessments of Beauveria bassiana-based Mycoinsecticides for Myzus persicae Sulzer (Hemiptera: Aphididae) control in cabbage. Neotrop. Entomol. 40, 470–476.
Mir, M., Gaurav, S., Prasad, C., and Tyagi, A. (2010). Field efficacy of HaNPY against Helicoverpa armigera on tomato. Ann. Plant Prot. Sci. 18, 301–303.
Mishra, J., Tewari, S., Singh, S., and Arora, N. (2014). Biopesticides: Where we Stand? Plant Microbes Symbiosis Applied Facets. New Delhi: Springer. doi: 10.1007/978-81-322-2068-8_2
Mishra, P. K., Bisht, S. C., Ruwari, P., Subbanna, A. R. N. S., Bisht, J. K., Bhatt, J. C., et al. (2017). Genetic diversity and functional characterization of endophytic Bacillus thuringiensis isolates from the North Western Indian Himalayas. Ann. Microbiol. 67, 143–155. doi: 10.1007/s13213-016-1244-0
Mittal, P. K. (2003). Bio larvicides in vector control: challenges and prospects. J. Vectar Borne Dis. 40, 20–32.
Mohan, S., Upadhyay, A., and Khajuria, D. (2017). Susceptibility of Heterorhabditis indica and Steinernema abbassi to pre and post overwintering stages of Apple root borer. Ann. Plant Prot. Sci. 25, 449–451.
Nahar, P., Yadav, P., Kulye, M., Hadapad, A., Hassani, M., Tuort, U., et al. (2010). Evaluation of indigenous fungal isolates, Metarhizium anisopliae M34412, Beauveria bassiana B3301 and Nonluraea rileyi N812 for the control of Helicoverpa armigera (Hubner) in pigeonpea field. J. Biol. Control 18, 1–8. doi: 10.18311/JBC/2004/4036
Nair, K. S. S., Babjan, B., Sajeev, T. V., Sudheendrakumar, V. V., Ali, M. I. M., Varma, R. V., et al. (1996). Field efficacy of nuclear polyhedrosis virus for protection of teak against the defoliator, Hyblaea puera Cramer (Lepidoptera: Hyblaeidae). J. Biol. Control 10, 79–85.
Navaneethan, T., Strauch, O., Besse, S., Bonhomme, A., and Ehlers, R. U. (2010). Influence of humidity and a surfactant-polymer-formulation on the control potential of the entomopathogenic nematode Steinernema feltiae against diapausing codling moth larvae (Cydia pomonella L.) (Lepidoptera: Tortricidae). BioControl 55, 777–788. doi: 10.1007/s10526-010-9299-5
Nawaz, A., Ali, H., Sufyan, M., Gogi, M., Arif, M., Ranjha, M., et al. (2020). Comparative bio-efficacy of nuclear polyhedrosis virus (NPV) and Spinosad against American bollworm, Helicoverpa armigera (Hubner). Rev. Bras. Entomol. 63, 277–282. doi: 10.1016/j.rbe.2019.09.001
NBAIR (2017). ICAR-National Bureau of Agricultural Insect Resources. Annual Report for 2016-2017. Bengaluru: NBAIR, 100.
Negrisoli, C. R. D. C. B., Santo Negrisoli Jr, A., Garcia, M. S., Dolinski, C., and Bernardi, D. (2013). Control of Grapholita molesta (Busck, 1916) (Lepidoptera: Tortricidae) with entomopathogenic nematodes (Rhabditida: Heterorhabditidae, Steinernematidae) in peach orchards. Exp. Parasitol. 135, 466–470. doi: 10.1016/j.exppara.2013.08.016
NjeŽić, B., and Ehlers, R. U. (2020). Entomopathogenic nematodes control Plum Sawflies (Hoplocampa minuta and H. flava). J. Appl. Entomol. 144, 491–499. doi: 10.1111/jen.12755
Odendaal, D., Addison, M. F., and Malan, A. P. (2016). Entomopathogenic nematodes for the control of the codling moth (Cydia pomonella L.) in field and laboratory trials. J. Helminthol. 90, 615–623. doi: 10.1017/S0022149X15000887
Odongo, B., Odindo, M., Brownbridge, M., and Kumar, R. (1998). Comparative biological efficacy of Hirsutella thompsonii and Neoseiulus teke for cassava mite (Mononychellus tanajoa) suppression. Biol. Sci. Technol. 8, 345–355. doi: 10.1080/09583159830153
Okano, K., Vanarsdall, A., Mikhailov, V., and Rohrmann, G. (2006). Conserved molecular systems of the Baculoviridae. Virology 34, 77–87. doi: 10.1016/j.virol.2005.09.019
Ortiz-Urquiza, A., and Keyhani, N. (2013). Action on the surface: entomopathogenic fungi versus the insect cuticle. Insects 4, 357–374. doi: 10.3390/insects4030357
Pandey, A. (2010). Field evaluation of Beauveria bassiana and Metarhizium anisopliae against the white grub, Holotrichia longipennis damaging soybean in Uttarakhand hills. J. Biol. Control. 24, 327–332.
Pandey, A. (2013). Field evaluation of Beauveria bassiana and Metarhizium anisopliae against the Cutworm, Agrotis ipsilon (Hufnagel) damaging potato in Uttarakhand Hills. J. Biol. Control. 27, 293–297.
Park, H. W., Kim, H. H., Youn, S. H., Shin, T. S., Bilgrami, A. L., Cho, M. R., et al. (2012). Biological control potentials of insect-parasitic nematode Rhabditis blumi (Nematoda: Rhabditida) for major cruciferous vegetable insect pests. Appl. Entomol. Zool. 47, 389–397. doi: 10.1007/s13355-012-0131-9
Pell, J. K., Eilenberg, J., Hajek, A. E., and Steinkraus, D. C. (2001). “Biology, ecology, and pest management potential of Entomophthorales,” in Fungi as Biocontrol Agents: Progress, Problems and Potential, eds T. M. Butt, C. Jackson, and N. Magan (Wallingford: CABI publishing), 71–153. doi: 10.1079/9780851993560.0071
Peters, S. E. O., and Coaker, T. H. (1993). The enhancement of Pieris brassicae (L.) (Lepidoptera: Pieridae) granulosis virus infection by microbial and synthetic insecticides. J. Appl. Entomol. 116, 72–79. doi: 10.1111/j.1439-0418.1993.tb01169.x
Poinar Jr, G. O., Wassink, H. J. M., van der Linden, M. E. L., and van der Geest, L. P. S. (1979). Serratia marcescens as a pathogen of Tsetse flies (Glossina morsitans and Glossina pallidipes, biological control. Acta Trop. 36, 223–7.
Poopathi, S., Britto, D., Thirugnanasambantham, R. L. J., Ragul, K., Mani, K., Balagangadharan, C., et al. (2015). An investigation on the diversity of mosquitocidal bacteria and its relationship with incidence of vector borne diseases. Trop. Biomed. 32, 84–97.
Poopathi, S., Mani, C., Thirugnanasambantham, K., Praba, V. L., Ahangar, N. A., Balagangadharan, K., et al. (2014). Identification and characterization of a novel marine Bacillus cereus for mosquito control. Parasitol. Res. 113, 323–332. doi: 10.1007/s00436-013-3658-y
Portman, S. L., Krishnankutty, S. M., and Reddy, G. V. P. (2016). Entomopathogenic nematodes combined with adjuvants presents a new potential biological control method for managing the wheat stem sawfly, Cephus cinctus (Hymenoptera: Cephidae). PLoS ONE 11, e0169022. doi: 10.1371/journal.pone.0169022
Prabhuraj, A., Girish, K. S., and Patil, B. V. (2008). Integration of Heterorhabditis indica with other biorationals for managing chickpea pod borer, Helicoverpa armigera (Hüb.). J. Biol Control. 22, 433–448.
Prabhuraj, A., Patil, B. V., and Girish, K. S. (2004). Bio-Efficacy of Heterorhahditis indica Poinar in Combination with Helicoverpa armigera polyhedrosis virus against Helicoverpa arnligera (Hubner). J. Biol Control. 18, 179–184. doi: 10.18311/jbc/2004/4087
Prabhuraj, A., Patil, B. V., and Girish, K. S. (2005). Field evaluation of an insect parasitic nematode, Heterorhabditis indica (RCR) in combination with other, entomopathogens and botanicals against chickpea podborer, Helicoverpa armigera (Hübner). J. Biol Control. 19, 59–64.
Putnoky-Csicso, B., Tonk, S., Szabo, A., Marton, Z., Tothne Bogdanyi, F., Toth, F., et al. (2020). Effectiveness of the entomopathogenic fungal species Metarhizium anisopliae strain ncaim 362 treatments against soil inhabiting melolontha larvae Int. J. Fungi 6, 116. doi: 10.3390/jof6030116
Qayyum, M., Wakil, W., Arif, M., Sahi, S., and Dunlop, C. (2015). Infection of Helicoverpa armigera by endophytic Beauveria bassiana colonizing tomato plants. Biol. Control 90, 200–207. doi: 10.1016/j.biocontrol.2015.04.005
Qiu, B. L., Mandour, N. S., Xu, C. X., and Ren, S. X. (2008). Evaluation of the entomopathogenic nematode Steinernema feltiae as a biological control agent of the whitefly, Bemisia tabaci. Int. J. Pest Manag. 54, 247–253. doi: 10.1080/09670870802044228
Qureshi, N., Chawla, S., Likitvivatanavong, S., Lee, H. L., and Gill, S. S. (2014). The Cry toxin operon of Clostridium bifermentans subsp. malaysia is highly toxic to Aedes larval mosquitoes. Appl. Environ. Microbiol. 80, 5689–5697. doi: 10.1128/AEM.01139-14
Rabindra, R. J. (2005). “Current status of production and use of microbial pesticides in India and the way forward,” in Microbial Biopesticide Formulations and Application, Technical Document, eds R. J. Rabindra, S. S. Husseini, and B. Ramanujan (Bangalore: Project Directorate of Biological Control), 1–12.
Rachana, R., Jayasimha, G., Richa, V., and Manjunatha, M. (2015). Efficacy of fungal pathogens, Fusarium semitectum Berk., Ravenel and Hirsutella thompsonii Fisher against red spider mite, Tetranychus neocaledonicus (Andre) on okra under laboratory and field condition. Pest Manag. Hortic. Ecosyst. 2, 162–168.
Rachappa, V., Patil, R., Navi, S., and Lingappa, S. (2007). Biopesticide for Insect Pest Management, Sustainable Pests Management. Daya Publishing House. ISBN: 978817035440, 8170354846.
Rahaman, P., Sharma, S., and Wightman, J. (2000). A review of insect-parasitic nematodes research in India: 1927-1997. Int. J. Pest Manag. 46, 19–28. doi: 10.1080/096708700227543
Ramanujam, B., Poornesha, B., Dileep, R., and Japur, K. (2017). Field evaluation of entomofungal pathogens against cowpea aphid, Aphis craccivora Koch, and their effect on two coccinellid predators. Int. J. Pest Manag. 63, 101–104. doi: 10.1080/09670874.2016.1227881
Ramanujam, B., Rangeshwaran, R., Sivakmar, G., Mohan, M., and Yandigeri, M. (2014). Management of insect pests by microorganisms. Proc. Indian Natl Sci. Acad. 80, 455–471. doi: 10.16943/ptinsa/2014/v80i2/3
Ramasubramanian, T., Geetha, N., Ramanujam, B., and Santhalakshmi, G. (2014). “Endophytic Beauveria bassiana: an ideal candidate for managing internode borer of sugarcane,” in Proceeding of Annual Convention Sugar Technology Association, 80–87.
Rana, J. S., Dixon, A. F. G., and Jarošík, V. (2002). Costs and benefits of prey specialization in a generalist insect predator. J. Anim. Ecol. 71, 15–22. doi: 10.1046/j.0021-8790.2001.00574.x
Rao, A., Rao, P., and Jyotsna, K. (2015). Efficacy of certain natural insecticides against Citrus leaf miner, Phyllocnistis citrella Stainton as prophylactic and curative measures on Sathgudi Sweet Orange. Pest Manag. Hortic. Ecosyst. 21, 11–15.
Rao, N., and Babu, T. (2005). Field efficacy of granulosis virus for the control of sugarcane early shoot borer, Chilo infuscatellus Snellen. J. Biol. Control 19, 145−148.
Rao, V., and Manjunath, T. (1966). DD-136 nematode that can kill many pests. Indian Farming 16, 43–44.
Rastegari, A., Yadav, A., and Yadav, N. (2020). New and Future Developments in Microbial Biotechnology and Bioengineering: Trends of Microbial Biotechnology for Sustainable Agriculture and Biomedicine System: Diversity and Functional Perspectives. Amsterdam: Elsevier.
Reyaz, A. L., Gunapriya, L., and Indra Arulselvi, P. (2017). Molecular characterization of indigenous Bacillus thuringiensis strains isolated from Kashmir valley. 3 Biotech 7, 143–153. doi: 10.1007/s13205-017-0756-z
Rezaei, N., Karimi, J., Hosseini, M., Goldani, M., and Campos-Herrera, R. (2015). Pathogenicity of two species of entomopathogenic nematodes against the greenhouse whitefly, Trialeurodes vaporariorum (Hemiptera: Aleyrodidae), in laboratory and greenhouse experiments. J. Nematol. 47, 60.
Riga, E., Lacey, L. A., Guerra, N., and Headrick, H. L. (2006). Control of the oriental fruit moth, grapholita molesta, using entomopathogenic nematodes in laboratory and fruit bin assays. J. Nematol. 38, 168.
Ríos-Moreno, A., Garrido-Jurado, I., Resquín-Romero, G., Arroyo-Manzanares, N., Arce, L., Quesada-Moraga, E., et al. (2016). Destruxin A production by Metarhizium brunneum strains during transient endophytic colonisation of Solanum tuberosum. Biocontrol Sci. Technol. 26, 1574–1585. doi: 10.1080/09583157.2016.1223274
Rojas-Pinzon, P., and Dussan, J. (2017). Efficacy of the vegetative cells of Lysinibacillus sphaericus for biological control of insecticide-resistant Aedes aegypti. Parasit. Vectors 10, 1–7. doi: 10.1186/s13071-017-2171-z
Roy, H. E., Steinkraus, D. C., Eilenberg, J., Hajek, A. E., and Pell, J. K. (2006). Bizarre interactions and endgames: entomopathogenic fungi and their arthropod hosts. Annu. Rev. Entomol. 51, 331–357. doi: 10.1146/annurev.ento.51.110104.150941
Ruiu, L. (2015). Insect pathogenic bacteria in integrated pest management. Insects 6, 352–367. doi: 10.3390/insects6020352
Ruiu, L., Satta, A., and Floris, I. (2008). Immature house Fly (Musca domestica) control in breeding sites with a new Brevibacillus laterosporus formulation. Environ. Entomol. 37, 505–509. doi: 10.1603/0046-225X(2008)37505:IHFMDC2.0.CO
Ruiu, L., Satta, A., and Floris, I. (2011). Comparative applications of azadirachtin- and Brevibacillus laterosporus-based formulations for house fly management experiments in dairy farms. J. Med. Entomol. 48, 345–350. doi: 10.1603/ME09299
Sáenz-Aponte, A., Correa-Cuadros, J. P., and Rodríguez-Bocanegra, M. X. (2020). Foliar application of entomopathogenic nematodes and fungi for the management of the diamond back moth in greenhouse and field. Biol. Control 142, 104163. doi: 10.1016/j.biocontrol.2019.104163
Sahayaraj, K., and Namachivayam, S. (2011). Field evaluation of three entomopathogenic fungi on groundnut pests. Tropicultura 29, 143−147.
Saleh, M. M. E. (2017). “Efficacy of entomopathogenic nematodes against lepidopteran insect pests,” in Biocontrol Agents: Entomopathogenic and Slug Parasitic Nematodes, eds M. M. M. Abd-Elgawad, T. H. Askary. Coupland (Wallingford: CABI), 157–173. doi: 10.1079/9781786390004.0157
Santana-Martinez, J., Silva, J., and Dussan, J. (2022). Efficacy of Lysinibacillus sphaericus against mixed-cultures of field-collected and laboratory larvae of Aedes aegypti and Culex quinquefasciatus. Bull. Entomol. Res. 109, 111–118. doi: 10.1017/S0007485318000342
Saranya, S., Ramaraju, K., Jeyarani, S., Sheeba, S., and Roseleen, J. (2013). Natural epizootics of Cladosporium cladosporioides on Tetranychus urticae Koch. (Acari.: Tetranychidae) in Coimbatore. J. Biol. Control 27, 95–98.
Saranya, S., Ushakumari, R., Jacob, S., and Philip, B. (2010). Efficacy of different entomopathogenic fungi against cowpea aphid, Aphis craccivora (Koch). J. Biopestic. 3, 138.
Saxena, H., and Ahmad, R. (2005). “NPV production, formulation, and quality control,” in Recent Advances in Helicoverpa Management a National Challenge (Kanpur: Indian Institute of Pulse Research), 1–10.
Schmeisser, C., Steele, H., and Streit, W. (2007). Metagenomics, biotechnology with non-culturable microbes. Appl. Microbiol. Biotechnol. 75, 955–962. doi: 10.1007/s00253-007-0945-5
Schmidt, M. A., Lafayette, P. R., Artelt, B. A., and Parrott, W. A. (2008). A comparison of strategies for transformation with multiple genes via microprojectile-mediated bombardment. In Vitro Cell. Dev. Biol. Plant 44, 162–168. doi: 10.1007/s11627-007-9099-5
Scholte, E. J., Knols, B. G. J., Samson, R. A., and Takken, W. (2004). Entomopathogenic fungi for mosquito control: a review. J. Insect Sci. 4, 19. doi: 10.1093/jis/4.1.19
Schroer, S., Yi, X., and Ehlers, R. U. (2005). Evaluation of adjuvants for foliar application of Steinernema carpocapsae against larvae of the diamondback moth (Plutella xylostella). Nematol. 7, 37–44. doi: 10.1163/1568541054192126
Seye, F., Bawin, T., Boukraa, S., Zimmer, J. Y., Ndiaye, M., Delvigne, F., et al. (2014). Effect of entomopathogenic Aspergillus strains against the pea aphid, Acyrthosiphon pisum (Hemiptera: Aphididae). Appl. Entomol. Zool. 49, 453–458. doi: 10.1007/s13355-014-0273-z
Sezen, K., Demir, Y., and Demirbag, Z. (2007). Identification and pathogenicity of entomopathogenic bacteria from common cockchafer, Melolontha melolontha (Coleoptera: Scarabaeidae). New Zeal. J. Crop Hortic. Sci. 35, 79–85. doi: 10.1080/01140670709510171
Sezen, K., Yaman, M., and Demirbag, Z. (2001). Insecticidal potential of Serratia marcescenes Bn10. Biol.-Bratislava 3, 333–336.
Sezhian, N., Sivakumar, C. V., and Venugopal, M. S. (1996). Alteration of effectiveness of Steinernema carpocapsae Weiser (Steinernematidae: Rhabditida) against Spodoptera litura (F.) (Noctuidae: Lepidoptera) larvae on sunflower by addition of an insect phagostimulant. Indian J. Nematol. 26, 77–81.
Shah, P. A., and Pell, J. K. (2003). Entomopathogenic fungi as biological control agents. Appl. Microbiol. Biotechnol. 61, 413–423. doi: 10.1007/s00253-003-1240-8
Shahid, M., Ahmed, B., Zaidi, A., and Khan, M. S. (2018). Toxicity of fungicides to Pisum sativum: a study of oxidative damage, growth suppression, cellular death and morpho-anatomical changes. RSC Adv. 8, 38483–38498. doi: 10.1039/C8RA03923B
Shahid, M., and Khan, M. S. (2017). Assessment of glyphosate and quizalofop mediated toxicity to greengram [Vigna radiata (L.) Wilczek], stress abatement and growth promotion by herbicide tolerant Bradyrhizobium and Pseudomonas species. Int. J. Curr. Microbiol. Appl. Sci. 6, 3001–3016. doi: 10.20546/ijcmas.2017.612.351
Shahid, M., and Khan, M. S. (2018). Glyphosate induced toxicity to chickpea plants and stress alleviation by herbicide tolerant phosphate solubilizing Burkholderia cepacia PSBB1 carrying multifarious plant growth promoting activities. 3 Biotech 8, 1–17. doi: 10.1007/s13205-018-1145-y
Shahid, M., and Khan, M. S. (2022). Ecotoxicological implications of residual pesticides to beneficial soil bacteria: a review. Pestic. Biochm. Physiol. 188, 105272. doi: 10.1016/j.pestbp.2022.105272
Shahid, M., Khan, M. S., Ahmed, B., Syed, A., and Bahkali, A. H. (2021a). Physiological disruption, structural deformation and low grain yield induced by neonicotinoid insecticides in chickpea: a long term phytotoxicity investigation. Chemosphere 262, 128388. doi: 10.1016/j.chemosphere.2020.128388
Shahid, M., Khan, M. S., and Kumar, M. (2019). Kitazin-pea interaction: understanding the fungicide induced nodule alteration, cytotoxicity, oxidative damage and toxicity alleviation by Rhizobium leguminosarum. RSC Adv. 9, 16929–16947. doi: 10.1039/C9RA01253B
Shahid, M., Manoharadas, S., Chakdar, H., Alrefaei, A. F., Albeshr, M. F., Almutairi, M. H., et al. (2021b). Biological toxicity assessment of carbamate pesticides using bacterial and plant bioassays: an in-vitro approach. Chemosphere 278, 130372. doi: 10.1016/j.chemosphere.2021.130372
Shahid, M., Zaidi, A., Khan, M. S., Rizvi, A., Saif, S., Ahmed, B., et al. (2017). “Recent advances in management strategies of vegetable diseases,” in Microbial strategies for Vegetable Production, eds A. Zaidi, and M. Khan (Cham: Springer), 197–226. doi: 10.1007/978-3-319-54401-4_9
Shapiro-Ilan, D., Hazir, S., and Glazer, I. (2017). “Basic and applied research: entomopathogenic nematodes,” in Microbial Control of Insect and Mite Pests, ed L. A. Lacey (Cambridge, MA: Academic Press), 91–105. doi: 10.1016/B978-0-12-803527-6.00006-8
Sharma, R., Ram, L., and Devi, R. (2017). Efficacy of white muscardine fungus (Beauveria bassiana) on rice hispa (Dicladispa armigera). Indian J. Agric. Res. 51, 296–298. doi: 10.18805/ijare.v51i03.7937
Shitole, T., and Patel, I. (2010). Parasites of spotted bollworm, Earias vitella (F.) in cotton. Indian J. Entomol. 72, 281.
Sikorowski, P., Lawrence, A., and Inglis, G. (2001). Effects of Serratia marcescens on rearing of the tobacco budworm (Lepidoptera: Noctuidae). Am. Entomol. 47, 51–60. doi: 10.1093/ae/47.1.51
Sparks, W. O., Halverson, L., Jurenka, R., and Minion, F. C. (2010). Interaction of the Baculovirus Occlusion-derived Virus Envelope Proteins ODV-E56 and ODV-E66 with the Midgut Brush Border Microvilli of the Tobacco Budworm, Heliothis virescens (Fabricius).
Srikanth, J., Easwaramoorthy, S., and Jalali, S. (2016). A 100 years of biological control of sugarcane pests in India: review and perspective. CAB Rev. 11, 1–32. doi: 10.1079/PAVSNNR201611013
Srikanth, J., Easwaramoorthy, S., and Santhalakshmi, G. (2010). Field efficacy and persistence of Beauveria brongniartii (Sacc.) Petch applied against Holotrichia serrata F. (Coleoptera: Scarabaeidae) infesting sugarcane in Southern India. Int. Sugar J. 28, 151−156.
Srinivasa, M., Jagadeesh Babu, C., Anitha, C., and Girish, G. (2008). Laboratory evaluation of available commercial formulations of HaNPV against Helicoverpa armigera (Hub.). J. Biopestic. 138–139. doi: 10.57182/jbiopestic.1.2.138-139
St. Leger, R. J., and Wang, C. (2010). Genetic engineering of fungal biocontrol agents to achieve greater efficacy against insect pests. Appl. Microbiol. Biotechnol. 85, 901–907. doi: 10.1007/s00253-009-2306-z
Sudershan, G., and Singh, L. (2000). Steinernema thermophilum sp. (Rhabditida: Steinernematidae) from India. Int. J. Nematol. 10, 183–191.
Sumathy, S., Palhan, V., and Gopinathan, K. (1996). Expression of human growth hormone in silkworm larvae through recombinant bombyx mori nuclear polyhedrosis virus. Protein Expr. Purif. 7, 262–268. doi: 10.1006/prep.1996.0037
Sun, X. L., and Peng, H. Y. (2007). Recent advances in biological control of pest insect by using viruses in China. Virol. Sin. 22, 158–162. doi: 10.1007/s12250-007-0017-0
Suresh, B., Khan, H., and Prasanna, P. (2012). Efficacy of different entomopathogenic fungi against cowpea aphid, Aphis craccivora (Koch) under laboratory and field condition. Int. J. Plant Prot. 5, 68–71.
Tatchell, G. M., and Payne, C. C. (1984). Field evaluation of a granulosis virus for control of Pieris rapae (Lepidoptera.: Pieridae) in the United Kingdom. Entomophaga 29, 133–144. doi: 10.1007/BF02372103
Thontadarya, T. S., Holihosur, S. N., and Hiremath, I. G. (1975). A new record of Bacillus cereus on the spotted bollworm, Earias vitella (F.). Curr. Sci. 44, 488.
Trdan, S., Znidarcic, D., and Vidrih, M. (2007). Control of Frankliniella occidentalis on glasshouse-grown cucumbers: an efficacy comparison of foliar application of Steinernema feltiae and spraying with abamectin. Russ. J. Nematol. 15, 25.
Tripura, A., Chatterjee, M., Pande, R., and Patra, S. (2017). Biorational management of brinjal shoot and fruit borer (Leucinodes orbonalis Guenee) in mid hills of Meghalaya. J. Entomol. Zool. Stud. 5, 41–45.
Ujan, A. A., Shahzad, S., Bot, P. J., and Ujjan, A. A. (2012). Use of entomopathogenic fungi for the control of mustard aphid (Lipaphis erysimi) on canola (Brassica napus L.). Pakistan J. Bot. 44, 2081–2086.
Umamaheswari, R., Sivakumar, M., and Subramanian, S. (2006). Biocontrol efficacy of entomopathogenic nematodes on Spodoptera litura (Lepidoptera: Noctuidae) in blackgram. Indian J. Nematol. 36, 19–22.
Unruh, T. R., and Lacey, L. A. (2001). Control of codling moth, Cydia pomonella (Lepidoptera: Tortricidae), with Steinernema carpocapsae: effects of supplemental wetting and pupation site on infection rate. Biol Control. 20, 48–56. doi: 10.1006/bcon.2000.0873
Vail, P., Tebbets, J., Cowan, D., and Jennek, K. (1991). Efficacy and persistence of a granulosis virus against infestations of Plodia interpunctella (Hübner) (Lepidoptera: Pyralidae) on raisins. J. Stored Prod. Res. 27, 103–107. doi: 10.1016/0022-474X(91)90019-9
Van Frankenhuyzen, K. (2009). Insecticidal activity of Bacillus thuringiensis crystal proteins. J. Invertebr. Pathol. 101, 1–16. doi: 10.1016/j.jip.2009.02.009
van Niekerk, S., and Malan, A. P. (2012). Potential of South African entomopathogenic nematodes (Heterorhabditidae and Steinernematidae) for control of the citrus mealybug, Planococcus citri (Pseudococcidae). J. Invertebr. Pathol. 111, 166–174. doi: 10.1016/j.jip.2012.07.023
Van Niekerk, S., and Malan, A. P. (2015). Adjuvants to improve aerial control of the citrus mealybug Planococcus citri (Hemiptera: Pseudococcidae) using entomopathogenic nematodes. J. Helminthol. 89, 189–195. doi: 10.1017/S0022149X13000771
Vega, F. E., Goettel, M. S., Blackwell, M., Chandler, D., Jackson, M. A., Keller, S., et al. (2009). Fungal entomopathogens: new insights on their ecology. Fungal Ecol. 2, 149–159. doi: 10.1016/j.funeco.2009.05.001
Vidal, S., and Jaber, L. (2015). Entomopathogenic fungi as endophytes: plant-endophyte-herbivore interactions and prospects for use in biological control. Curr. Sci. 46–54.
Vimala Devi, P., and Vineela, V. (2015). Suspension concentrates formulation of Bacillus thuringiensis var. kurstaki for effective management of Helicoverpa armigera on sunflower (Helianthus annuus). Biol. Sci. Technol. 25, 329–336. doi: 10.1080/09583157.2014.977846
Vincent, C., and Belair, G. (1992). Biocontrol of the apple sawfly, Hoplocampa testudinea, with entomogenous nematodes. Entomophaga 37, 575–582. doi: 10.1007/BF02372327
Visalakshi, M., Bhavani, B., and Rao, S. (2015). Field evaluation of entomopathogenic fungi against white grub, Holotrichia consanguinea Blanch in sugarcane. J. Biol. Sci. 29, 103–106. doi: 10.18641/jbc/29/2/79824
Visalakshy, P. G., Krishnamoorthy, A., and Hussaini, S. (2009). Field efficacy of the entomopathogenic nematode Steinernema carpocapsae (Weiser, 1955) against brinjal shoot and fruit borer, Leucinodes orbonalis Guenee. Nematol. Mediterr. 37, 133–137.
Wakil, W., Usman Ghazanfar, M., Nasir, F., Qayyum, M. A., and Tahir, M. (2012). Insecticidal efficacy of Azadirachta indica, nucleopolyhedrovirus and chlorantraniliprole singly or combined against field populations of Helicoverpa armigera Hubner (Lepidoptera: Noctuidae). J. Agric. Res. 72, 53. doi: 10.4067/S0718-58392012000100009
Wakil, W., Yasin, M., and Shapiro-Ilan, D. (2017). Effects of single and combined applications of entomopathogenic fungi and nematodes against Rhynchophorus ferrugineus (Olivier). Sci. Rep. 7, 5971. doi: 10.1038/s41598-017-05615-3
Wang, B., Zhao, Y., Guo, Q., Gao, J., Wang, H., Zhou, X., et al. (2021). Synergistic effect of Isaria fumosorosea and Bacillus thuringiensis on the control of the fall armyworm, Spodoptera frugiperda. J. Invertebr. Pathol. 186, 107590.
Wang H. Peng H. Li W. Cheng P. Gong M. (2021) The toxins of Beauveria bassiana and the strategies to improve their virulence to insects. Front. Microbiol. 12:705343. 10.3389/fmicb.2021.705343
Wardlow, L. R., Piggott, S., and Goldsworthy, R. (2001). Foliar application of Steinernema feltiae for the control of flower thrips. Meded Rijksuniv. Gent Fak. Landbouwkd Toegep. Biol. Wet. 66, 285–291.
Waturu, C. N., Wabule, M. N., Nguthi, F. N., and Njinju, S. M. (1998). Field Control of the Banana Weevil (Cosmopolites sordidus) using Entomopathogenic Nematodes. Available online at: https://www.researchgate.net/publication267795896
Wei, X., Luo, L., Zhang, Y., Zhang, Y., Yang, M., Wang, Y., et al. (2021). Compatibility of Beauveria bassiana with the parasitoid Habrobracon hebetor: a promising IPM strategy against Plodia interpunctella. Biol. Control. 161, 104569.
Williams, T., Virto, C., Murillo, R., and Caballero, P. (2017). Covert infection of insects by baculoviruses. Front. Microbiol. 8, 1337. doi: 10.3389/fmicb.2017.01337
Winkler, D. E., Ramos, A. P. M., Zanardi, O. Z., de Souza, D. J., and Guedes, R. N. C. (2019). Insect-repellent plants improve the efficacy of insect-pathogenic fungi. Biol. Control 136, 30–34.
Yadav, A., Kumar, V., Dhaliwal, H., Prasad, R., and Saxena, A. (2018). “Microbiome in crops: diversity, distribution, and potential role in crop improvement,” in Crop Improvement through Microbial Biotechnology ed R. P. S. S. Gill (Amsterdam: Elsevier), 305–332. doi: 10.1016/B978-0-444-63987-5.00015-3
Yao, X., Li, X., Liang, G., Zhou, J., Liang, J., Liu, Z., et al. (2020). The insecticidal mechanism of Cry1Ac toxin in Helicoverpa armigera involves oxidative stress-mediated mitochondrial dysfunction. Pest Manag. Sci. 76, 3115–3124.
Yasin, M., Wakil, W. El-Shafie, H. A., Bedford, G. O., and Miller, T. A. (2017). Potential role of microbial pathogens in control of red palm weevil (Rhynchophorus ferrugineus)-a review. Entomol. Res. 47, 219–234. doi: 10.1111/1748-5967.12221
Zethner, O. (1980). Control of Agrotis segetum (Lepidoptera: Noctuidae) root crops by granulosis virus. Entomophaga 25, 27–35. doi: 10.1007/BF02377519
Zhang, D. W., Xiao, Z. J., Zeng, B. P., Li, K., and Tang, Y. L. (2019). Insect behavior and physiological adaptation mechanisms under starvation stress. Front. Physiol. 10, 163. doi: 10.3389/fphys.2019.00163
Zhang, Y., Song, F., Wu, S., Wu, X., Zhang, Y., Wu, K., et al. (2021). Amino acid homeostasis modulates Helicoverpa armigera response to Cry1Ac toxin from Bacillus thuringiensis. Pest Manag. Sci. 77, 509–518.
Zheng, D., Zhang, L., and Wang, G. (2019). Insect gut microbiota and host response to toxins. Insect Sci. 26, 200–210.
Zimmer, C., de Castro, L., Pires, S., Menezes, A., Ribeiro, P., and Leite, F. (2013). Efficacy of entomopathogenic bacteria for control of Musca domestica. J. Invertebr. Pathol. 241–244. doi: 10.1016/j.jip.2013.08.011
Keywords: biopesticides, potential entomopathogens, insect pest management, plant health, sustainability
Citation: Irsad, Shahid M, Haq E, Mohamed A, Rizvi PQ and Kolanthasamy E (2023) Entomopathogen-based biopesticides: insights into unraveling their potential in insect pest management. Front. Microbiol. 14:1208237. doi: 10.3389/fmicb.2023.1208237
Received: 18 April 2023; Accepted: 09 June 2023;
Published: 26 July 2023.
Edited by:
Durgesh K. Jaiswal, Savitribai Phule Pune University, IndiaReviewed by:
Tariq Mukhtar, Pir Mehr Ali Shah Arid Agriculture University, PakistanMohammad Ashfaq, Chandigarh University, India
Parvaze Wani, Crescent University, Abeokuta, Nigeria
Copyright © 2023 Irsad, Shahid, Haq, Mohamed, Rizvi and Kolanthasamy. This is an open-access article distributed under the terms of the Creative Commons Attribution License (CC BY). The use, distribution or reproduction in other forums is permitted, provided the original author(s) and the copyright owner(s) are credited and that the original publication in this journal is cited, in accordance with accepted academic practice. No use, distribution or reproduction is permitted which does not comply with these terms.
*Correspondence: Irsad, bW9oZGlyc2hhZDA2MCYjeDAwMDQwO2dtYWlsLmNvbQ==; Mohammad Shahid, c2hhaGlkZmFpejUmI3gwMDA0MDtnbWFpbC5jb20=; Abdullah Mohamed, bW9oYW1lZC5hJiN4MDAwNDA7ZnVlLmVkdS5lZw==
†These authors have contributed equally to this work and share first authorship