- Key Laboratory of Forest Protection of National Forestry and Grassland Administration, Chinese Academy of Forestry, Beijing, China
The family Rhizobiaceae contains 19 validly described genera including the rhizobia groups, many of which are important nitrogen-fixing bacteria. Early classification of Rhizobiaceae relied heavily on the poorly resolved 16S rRNA genes and resulted in several taxonomic conflicts. Although several recent studies illustrated the taxonomic status of many members in the family Rhizobiaceae, several para- and polyphyletic genera still needed to be elucidated. The rapidly increasing number of genomes in Rhizobiaceae has allowed for a revision of the taxonomic identities of members in Rhizobiaceae. In this study, we performed analyses of genome-based phylogeny and phylogenomic metrics to review the relationships of 155-type strains within the family Rhizobiaceae. The UBCG and concatenated protein phylogenetic trees, constructed based on 92 core genes and concatenated alignment of 170 single-copy orthologous proteins, demonstrated that the taxonomic inconsistencies should be assigned to eight novel genera, and 22 species should be recombined. All these reclassifications were also confirmed by pairwise cpAAI values, which separated genera within the family Rhizobiaceae with a demarcation threshold of ~86%. In addition, along with the phenotypic and chemotaxonomic analyses, a novel strain BDR2-2T belonging to a novel genus of the family Rhizobiaceae was also confirmed, for which the name Ectorhizobium quercum gen. nov., sp. nov. was proposed. The type strain is BDR2-2T (=CFCC 16492T = LMG 31717T).
1. Introduction
The family Rhizobiaceae, consisting of several “rhizobia” species and currently up to 19 genera and 181 species with validly described names (https://lpsn.dsmz.de/family/rhizobiaceae), was first proposed in 1938. Most of the members in the family Rhizobiaceae were widely known for their association with plant roots, including the induction of N2-fixing nodules (De Lajudie et al., 2019), tumors (Mousavi et al., 2014), or hairy roots (Jiang et al., 2019) on their host plants [e.g., Rhizobium, Agrobacterium, Ensifer (syn. Sinorhizobium), Allorhizobium, Shinella, Neorhizobium, and Pararhizobium].
The early taxonomic classification of Rhizobiaceae was circumscribed by two genera, namely Rhizobium and Agrobacterium, only based on nitrogen-fixing or pathogenic traits. As the phylogenetic analysis advances, many other novel genera belonging to the family Rhizobiaceae were proposed in succession based on the 16S rRNA phylogenetic trees [e.g., Martelella (Rivas et al., 2005), Shinella (An et al., 2006), Ciceribacter (Kathiravan et al., 2013), Lentilitoribacter (Park et al., 2013), Liberibacter (Fagen et al., 2014), and Gellertiella (Tóth et al., 2017)]. Over time, the phylogenetic method of multilocus sequence analysis (MLSA), which provides a more robust taxonomic resolution (De Lajudie et al., 2019), was used in the revision of the genera within Rhizobiaceae, and the proposal of several novel genera and combinations made the classifications of Rhizobiaceae more precise (Ramírez-Bahena et al., 2008; Kimes et al., 2015; Rocha et al., 2020). At present, the defined prokaryotic genera or higher taxa relies heavily on the monophyly of species (De Lajudie et al., 2019), and the genome-based phylogeny was considered to be a more convenient and accurate method (Parks et al., 2018). Benefiting from the advances in next-generation sequencing technology, an enormous amount of genomic data had been accumulated in public databases, which provided the base for a more accurate classification of prokaryotes. Based on the genomic data of the family Rhizobiaceae in the public databases, the genus Ciceribacter (Rahi et al., 2021), Agrobacterium tumefaciens species complex G3 (Singh et al., 2021), and Rhizobium leguminosarum species complex (Young et al., 2021) were revised, along with various genomic metrics, and several new species combinations were proposed. Recently, a genomic metric of cpAAI data was proposed to define the genera in the family Rhizobiaceae with a threshold of ~86%, and several new genera and combinations were proposed (Kuzmanović et al., 2022). However, there are several taxonomic inconsistencies within Rhizobiaceae that need to be elucidated.
During our study of the bacterial diversity in the disease of oaks, the strain BDR2-2T was isolated from the symptomatic bark of Quercus acutissima caker. Preliminary phylogeny analysis showed that the strain BDR2-2T should be assigned to the family Rhizobiaceae. In this study, we combined the UBCG and 120 ubiquitous single-copy protein phylogenetic analyses, along with the genomic metrics of AAI, POCP, and cpAAI, to confirm the taxonomic status of BDR2-2T and other conflicts of species within the family Rhizobiaceae.
2. Materials and methods
2.1. Strain and culture conditions
The strain BDR2-2T was isolated from the symptomatic bark of Q. acutissima caker collected from Hefei, China (31°50′28″N, 117°10′34″E). The isolation and purification of the strain BDR2-2T were performed as previously described (Ma et al., 2022). In brief, the samples were initially surface-sterilized successively in 70% ethanol for 30 s and 4% (v/v) sodium hypochlorite for 2 min. After washing with sterile water for three times, the samples were transferred to a sterile mortar, ground with a pestle, and then cultivated for 30 min. The suspensions were spread on yeast extract mannitol agar (YMA) with a dilution series. After 2 days of incubation at 30°C, single colonies were cultured on a new plate and then preserved at −80°C.
2.2. Genome sequencing and reference genome
The genome of the strain BDR2-2T was sequenced with Illumina NovaSeq PE150 by Novogene, Co., Ltd. (Beijing, China). In brief, the low-quality reads were filtered by readfq (version 10), and then, the high-quality reads were assembled using SOAPdenovo (version 2.04) (Li et al., 2008, 2010), SPAdes (Bankevich et al., 2012), and ABySS (Simpson et al., 2009). After integrating with CISA (Lin and Liao, 2013), the gaps in the results were filled with gapclose (version 1.12). In this study, 136 validated Rhizobiaceae and 18 unvalidated Rhizobiaceae were analyzed. Because the 16S rRNA phylogenetic tree was inappropriate for delineating genera in the family Rhizobiaceae in previous studies (e.g., strains from Brucellaceae nested in Rhizobiaceae) (Hördt et al., 2020), five type strains from Brucellaceae (including the type genus Brucella and type species from the other two genera) were also analyzed in this study to confirm it. In addition, five strains from Caulobacterales were also used as an out-group in this study. The type strain genome sequences used were obtained from the NCBI database, and all of the genome sequences were assessed by CheckM (Parks et al., 2015).
2.3. Phylogenetic analyses
Full-length 16S rRNA gene sequences were extracted from the genomes via RNAmmer 1.2 for the phylogenetic analysis (Lagesen et al., 2007). The multiple alignments of the sequences were performed with Clustal W, and then, the phylogenetic trees were constructed with MEGA X by the methods of maximum-likelihood, neighbor-joining, and maximum-parsimony (Kumar et al., 2018). The phylogenetic trees were evaluated by 1,000 bootstrap resamplings, and the species of Brucellaceae and Caulobacterales were used as the out-group.
A phylogenomic tree, particularly a concatenated core gene tree, was considered to be a more convenient and accurate substitute method for taxonomic analysis as it provides a higher resolution phylogeny (Kim et al., 2021). There are 92 core genes that were extracted from the genomes using the command “java -jar UBCG.jar extract” and used in the UBCG phylogenetic tree, which was generated by RAxML using the command “jar -jar UBCG.jar align.” The species of Brucellaceae and Caulobacterales were used as the out-group.
Additionally, another phylogenomic tree with a concatenated alignment of 170 ubiquitous single-copy proteins was constructed with FastTree. The extraction and alignment of the sequences were generated with the method at github.com/flass/cpAAI_Rhizobiaceae (Kuzmanović et al., 2022), and the tree was visualized and edited with iTOL (Letunic and Bork, 2021).
2.4. Genome-based metrics analyses
For the species level, average nucleotide identity (ANI) and genome-to-genome distance comparison (GGDC) are currently two standard practices for species delineation (Goris et al., 2007; Richter and Rosselló-Móra, 2009), and the values of ANI and dDDH were determined with pyani (Pritchard et al., 2016) and genome-to-genome distance comparison (GGDC, http://ggdc.dsmz.de), respectively. For higher taxonomic ranks, core-proteome average amino acid identity (cpAAI) has recently been proposed for genus delineation within Rhizobiaceae (Kuzmanović et al., 2022), and the pairwise cpAAI values within Rhizobiaceae were calculated by the cpAAI_Rhizobiaceae code (https://github.com/flass/cpAAI_Rhizobiaceae). The percentage of conserved proteins (POCPs) and average amino acid identity (AAI) were two standardized metrics for genus-level delineation. A Python script (POCP, https://github.com/2015qyliang/POCP) was used to calculate POCP, while CompareM (https://github.com/dparks1134/CompareM) was used for AAI (Ma et al., 2022).
2.5. Chemotaxonomy and physiology
The polar lipids and isoprenoid quinones were performed as described by Minnikin et al. (1984) and Collins et al. (1977), respectively. The extraction of cellular fatty acids was performed as described by Kuykendall et al. (1988) and then analyzed with the Sherlock Microbial Identification System (MIDI) (Sasser, 1990). The growth gradients of pH, temperature, and salinity were optimized by the methods described by Li et al. (2016). Gram staining was carried out as described by Jenkins et al. (2003). The test of anaerobic growth was performed in an anaerobic jar for a week (Li et al., 2016). The activities of oxidase and catalase were determined by the methods described by Li et al. (2016). Enzymatic activity, acid production, and carbon source utilization were performed using API ZYM, API 50CH, and API 20NE (bioMérieux) according to the manufacturer's instructions.
3. Results and discussion
The 16S rRNA gene phylogeny was widely used in prokaryote taxonomic analyses due to its high conservation (Park et al., 2013; Fagen et al., 2014; Tóth et al., 2017), and this, on the other hand, generally did not provide sufficient resolution for closely related species (Vinuesa et al., 2005; Liang et al., 2021). As expected, the full-length 16S rRNA phylogenetic tree showed low bootstrap support at the genus and species levels, resulting in poorly resolved taxonomic issues (Supplementary Figure S1). The concatenated proteins and UBCG trees showed a similar phylogenetic backbone to each other, and most of the species in the family Rhizobiaceae consistently grouped into similar monophyletic clades with high bootstrap values. The concatenated protein is shown in Figure 1, and the full details of the two phylogenetic trees are shown in Supplementary Figures S2, S3. For genus demarcation within Rhizobiaceae, the genomic metric of cpAAI data was recently proposed, with a threshold of ~86% (Kuzmanović et al., 2022), and here we calculated the pairwise cpAAI values to confirm the reclassification of the Rhizobiaceae order.
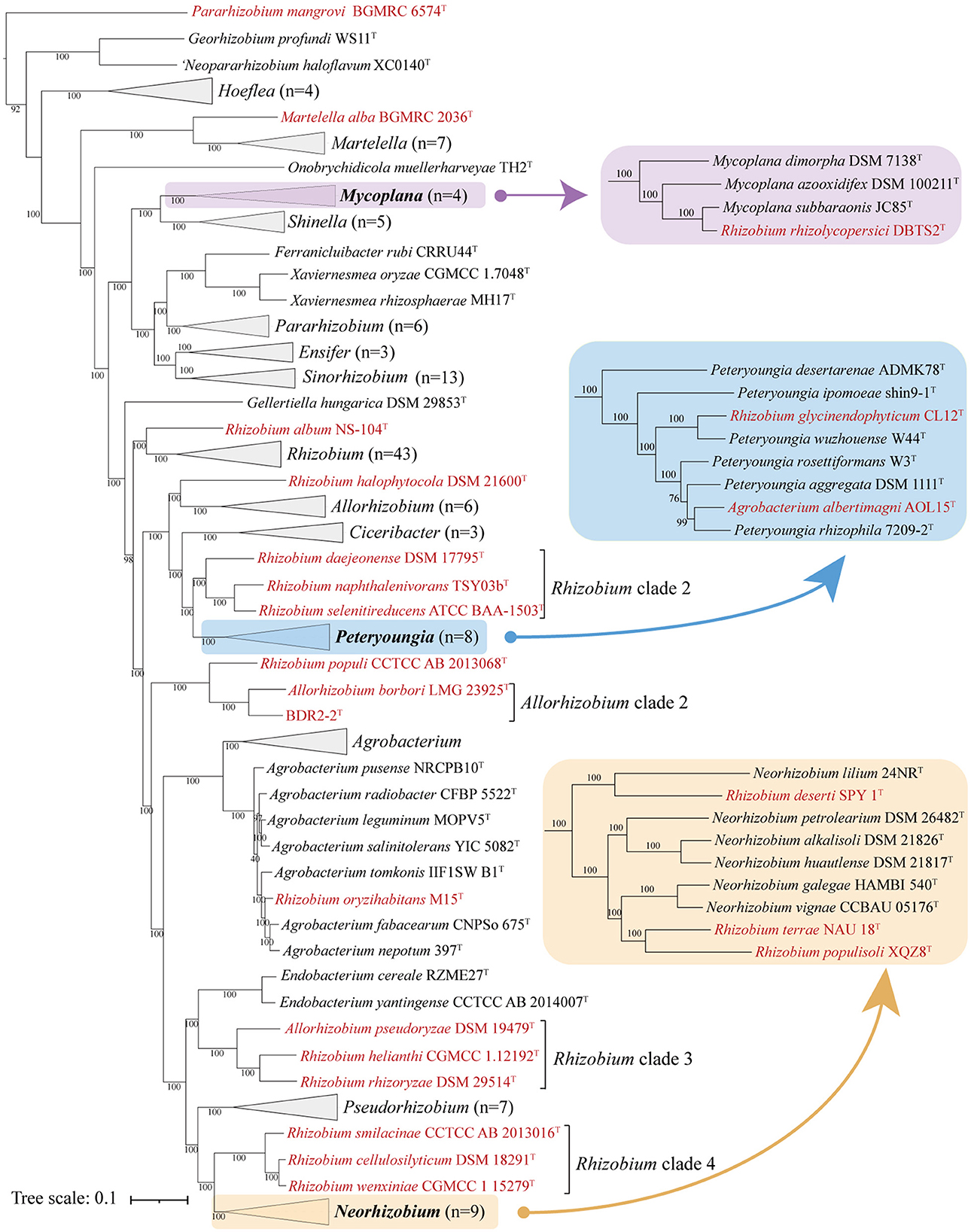
Figure 1. Concatenated protein phylogenetic tree among strains in the family Rhizobiaceae based on a concatenated alignment of 170 ubiquitous single-copy proteins. For details and abbreviations see Supplementary Figure S2. The scale bar corresponds to 0.1 substitutions per amino acid position.
As shown in the pairwise AAI, POCP, and cpAAI values of the currently proposed genera in the family Rhizobiaceae, the pairwise values between inter-genus and intra-genus could not be separated (Figures 2A, C), and there should be several misclassification species among the currently proposed genera. By applying the cpAAI threshold of ~86% for genus demarcation and combining it with phylogenetic tree analysis, most of the pairwise values could be clearly separated between inter-genus and intra-genus (Figures 2B, D). The pairwise AAI, POCP, and cpAAI values are shown in Supplementary Table S1.
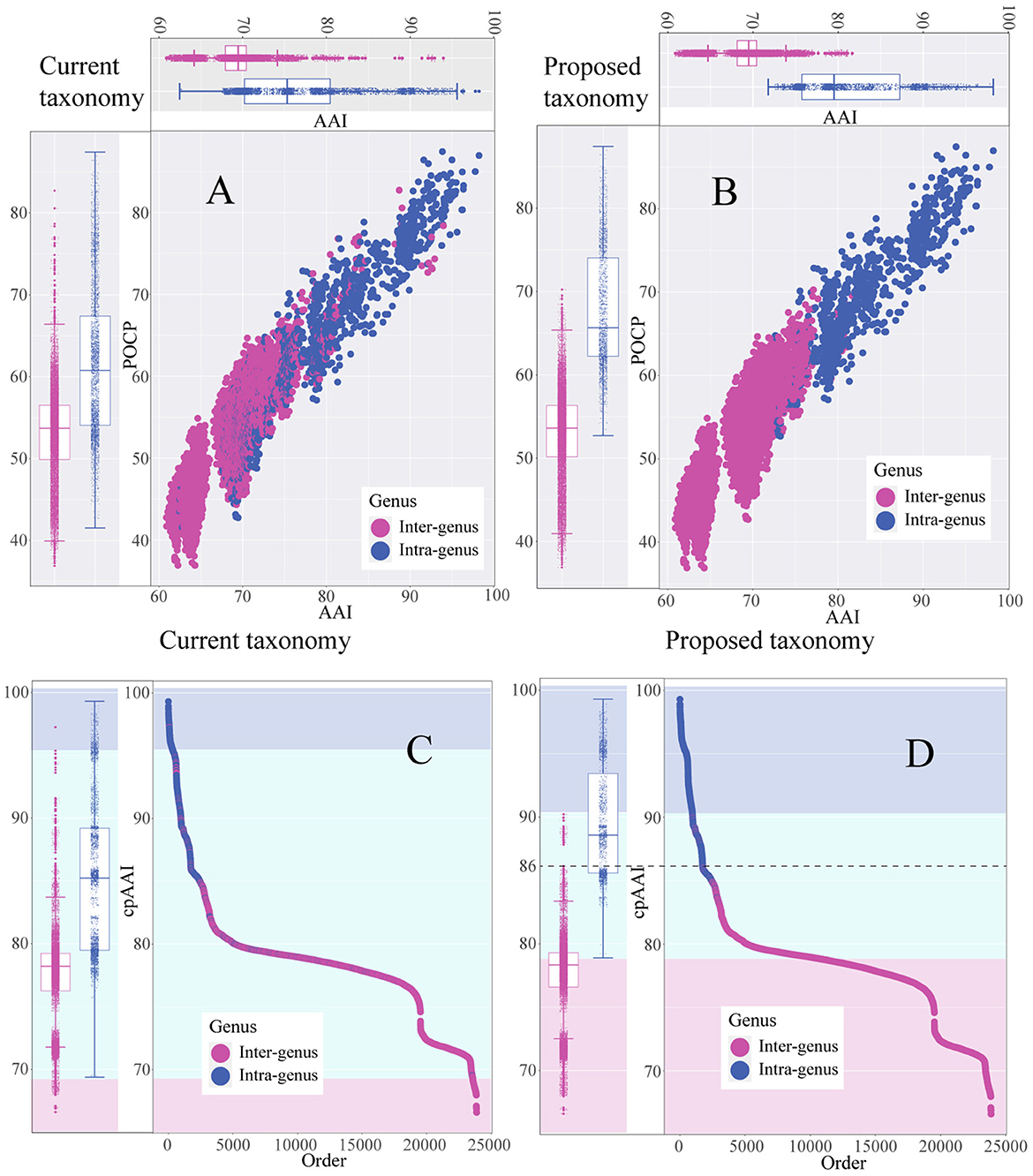
Figure 2. Scatter plot and the box plot represent the distribution of pairwise average amino acid identity (AAI) values, percentage of conserved protein (POCP) values, and core-proteome average amino acid identity (cpAAI) within and between the genera of the family Rhizobiaceae. (A, B) Represent pairwise AAI and POCP values of the current genera and the proposed genera, respectively. (C, D) Represent the rank order of pairwise cpAAI values of the current genera and the proposed genera, respectively. The background color light blue represents the proposed genus, light cyan represents closely related to the proposed genus, and light red represents distantly related to the proposed genus.
3.1. Reclassification of Rhizobiaceae at the genus level
All phylogenetic trees (Figure 1, Supplementary Figures S2, S3) showed that most genera within the family Rhizobiaceae were clustered into monophyletic clades, except for several genera that formed paraphyletic or polyphyletic clades. Among those paraphyletic or polyphyletic clades, the taxonomic conflicts were resolved as follows. All reclassifications were also confirmed by the genus demarcation of cpAAI with a threshold of ~86%.
As for the paraphyletic genus, which consisted of a monophyletic clade with one or more species of a different genus (Wood, 1994; Liang et al., 2021), the conflicting clade should be merged into the primary genus. Martelella appeared like a paraphyletic genus in both phylogenetic trees because Martelella alba BGMRC 2036T, a recently proposed novel species, formed an outermost clade of the Martelella lingage by a long branch, and M. alba BGMRC 2036T might be a different genus from Martelella. Furthermore, the pairwise cpAAI values between M. alba BGMRC 2036T and other Martelella strains ranged from 79.5 to 80.5% (Supplementary Table S1 and Supplementary Figure S4A), which were also significantly lower than the recommended genus demarcation value of 86%, and therefore, we proposed to transfer M. alba BGMRC 2036T to a novel genus Paramartelella gen. nov.
Mycoplana was shown as paraphyletic in all phylogenetic trees (Figure 1, Supplementary Figures S2, S3) because Rhizobium rhizolycopersici DBTS2T was nested within Mycoplana with high support. In the original proposal of R. rhizolycopersici DBTS2T, the phylogenetic tree was constructed with a low number of closely related taxa, and the Mycoplana-type strain was not considered (Thin et al., 2021). Therefore, we proposed to assign R. rhizolycopersici DBTS2T to Mycoplana. In addition, the pairwise cpAAI values within the Mycoplana clade ranged from 89.7 to 97.2% (Supplementary Table S1 and Supplementary Figure S4B), and those values were also higher than the genus demarcation threshold, which further confirmed the classification.
Similarly, the genera Peteryoungia, Agrobacterium, Neorhizobium also appeared as paraphyletic in all phylogenetic trees (Figure 1, Supplementary Figures S2, S3). Rhizobium glycinendophyticum CL12T and Agrobacterium albertimagni AOL15T were nested within Peteryoungia with a high bootstrap value. The pairwise cpAAI values between the two strains and other Peteryoungia strains were also higher than the genus demarcation threshold (Supplementary Table S1 and Supplementary Figure S4C), which confirmed that R. glycinendophyticum CL12T and A. albertimagni AOL15T should be transferred to Peteryoungia. Using a similar method as above, Rhizobium oryzihabitans M15T was nested within Agrobacterium, and Rhizobium deserti SPY 1T, Rhizobium populisoli XQZ8T, and Rhizobium terrae NAU 18T were nested within Neorhizobium. Pairwise cpAAI values confirmed that R. oryzihabitans M15T should be assigned to Agrobacterium (Supplementary Table S1 and Supplementary Figure S4D), and R. deserti SPY 1T, R. populisoli XQZ8T, and R. terrae NAU 18T should be assigned to Neorhizobium (Supplementary Table S1 and Supplementary Figure S4E).
Different from the paraphyletic genus, the polyphyletic genus was typically more difficult to resolve, as the taxonomic issues were done by merging the conflicting clades or transferring them to novel genera (Farris, 1974; Liang et al., 2021). Pararhizobium appeared as polyphyletic in the phylogenetic trees (Figure 1, Supplementary Figures S2, S3) because Pararhizobium mangrovi BGMRC 6574T and Pararhizobium haloflavum XC0140T were placed in a distant position relative to the genus Pararhizobium with high support values. This analysis confirmed P. haloflavum XC0140T, which was not validly published despite being proposed as “Neopararhizobium” (Hördt et al., 2020) and represents a novel genus. Pararhizobium mangrovi BGMRC 6574T, a recently proposed novel species, formed the outermost clade of “Neopararhizobium” and Georhizobium lineages with a distant evolutionary relationship with Pararhizobium in all phylogenetic trees, which implied that the strain should be assigned to a novel genus. The pairwise cpAAI values between P. mangrovi BGMRC 6574T and other Pararhizobium strains ranged from 69.4 to 69.5%, which were also significantly lower than the recommended genus demarcation value of 86%, and therefore, we proposed to transfer P. mangrovi BGMRC 6574T to a novel genus Allopararhizobium gen. nov.
Although most taxonomic conflicts of the genus Rhizobium were resolved, Rhizobium was shown as polyphyletic in the phylogenetic trees (Figure 1, Supplementary Figures S2, S3), including strains such as Rhizobium album NS-104T, Rhizobium halophytocola DSM 21600T, Rhizobium clade 2, Rhizobium populi CCTCC AB 2013068T, Rhizobium clade 3, and Rhizobium clade 4, which were placed apart from the genus Rhizobium. Rhizobium album NS104T formed the outermost clade of the genus Rhizobium lineage in all phylogenetic trees and showed a distant evolutionary relationship with other Rhizobium species, indicating that the sole species might represent a novel genus in the family Rhizobiaceae. In addition, the pairwise cpAAI values between R. album NS104T and other Rhizobium strains ranged from 80.6 to 82.3% (Supplementary Table S1 and Supplementary Figure S4F), which were also significantly lower than the recommended genus demarcation value, and therefore R. album NS104T represented a novel genus Metarhizobium gen. nov.
Rhizobium halophytocola DSM 21600T, Rhizobium clade 2, Rhizobium clade 3, and Rhizobium clade 4, which formed four different highly supported monophyletic clades, were placed apart from the Rhizobium lineage in the phylogenetic trees and separated from the species in the genus Rhizobium, implying that they should be transferred to four different novel genera. With the similar analytical methods as above, the pairwise cpAAI values also confirmed that R. halophytocola DSM 21600T and Rhizobium clades 2–4 should belong to four different novel genera in the family Rhizobiaceae (Supplementary Table S1 and Supplementary Figures S5G–J). The pairwise cpAAI values within the Rhizobium lineage were also significantly higher than these values between R. album NS104T, R. halophytocola DSM 21600T, Rhizobium clades 2–4, and Rhizobium lineage (Figure 3), which also confirmed that these clades belong to five different novel genera.
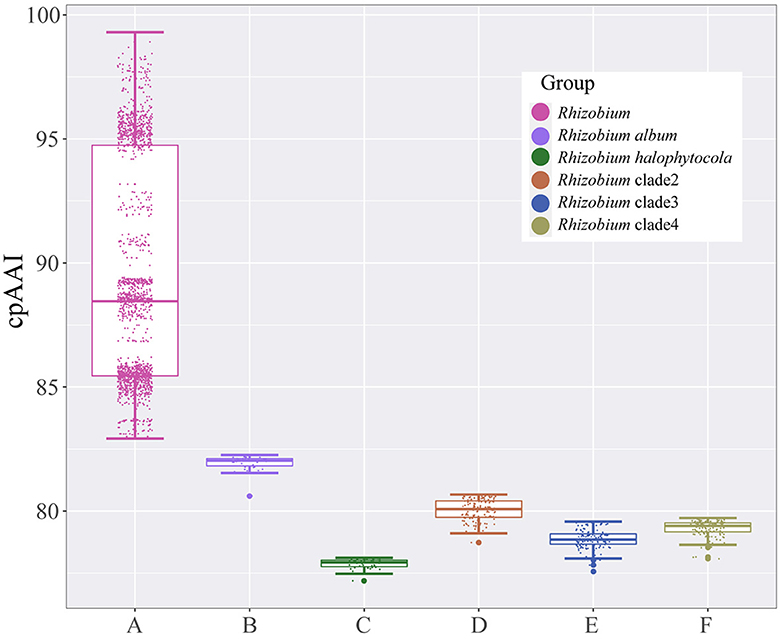
Figure 3. Box plot of pairwise core-proteome average amino acid identity (cpAAI) values within the genus Rhizobium and between genus Rhizobium and other Rhizobium clades. A represents the values within the genus Rhizobium; B–F represent the values between Rhizobium album, Rhizobium halophytocola, Rhizobium clade 2, Rhizobium clade 3, Rhizobium clade 4, and genus Rhizobium, respectively.
In addition, the physiological and chemotaxonomic features could also distinguish these proposed novel genera from the Rhizobium-type strain (R. leguminosarum USDA 2370T). R. album NS-104T grows over a pH range of 5.0–9.0 (optimum, 6.0), and R. leguminosarum USDA 2370T grows at a pH range of 6.0–8.0 (optimum, pH 7.0–7.5) (Ramírez-Bahena et al., 2008; Hang et al., 2019). The growth of R. halophytocolav DSM 21600T was observed up to 7.5% (w/v) NaCl (optimum, 4–5%); however, no growth of R. leguminosarum USDA 2370T was observed in the presence of 1% NaCl (Ramírez-Bahena et al., 2008; Bibi et al., 2012). The percentages of major cellular fatty acids in Rhizobium clade 2 (above 67.8%) were also significantly different from R. leguminosarum USDA 2370T (57.2%) (Tighe et al., 2000; Quan et al., 2005; Kaiya et al., 2012). Rhizobium clade 3 could grow at 40°C, at a pH range of 5.0–11.0, and at NaCl concentrations up to 4% (w/v) NaCl (optimum, 1%), which could distinguish Rhizobium clade 3 from R. leguminosarum USDA 2370T (Zhang G. X. et al., 2011; Zhang X. X. et al., 2014; Wei et al., 2015). The assimilation of L-malate, L-arabinose, gluconate, and the amount of C16:0 and summed feature 2 (C12:0 aldehyde and/or unknown 10.928) also separate Rhizobium clade 4 from R. leguminosarum USDA 2370T (Garcia-Fraile et al., 2007; Zhang L. et al., 2014; Gao et al., 2017).
Altogether, R. halophytocola DSM 21600T should be assigned to a novel genus Heterorhizobium gen. nov. Rhizobium clade 2 should belong to a novel genus Paenirhizobium gen. nov., with Paenirhizobium daejeonense comb. nov. as the type species. Rhizobium clade 3 should be assigned to Affinirhizobium gen. nov., with Affinirhizobium pseudoryzae comb. nov. as the type species. Rhizobium clade 4 should be assigned to Alirhizobium gen. nov., with Alirhizobium cellulosilyticum comb. nov. as the type species.
3.2. Proposal for Ectorhizobium quercum gen. nov., sp. nov.
3.2.1. Genome-based phylogenetic analyses
The 16S rRNA sequence pairwise comparisons showed that the strain BDR2-2T was most closely related to Allorhizobium borbori DN316T (97.4% similarity), followed by R. populi K-38T (96.9% similarity), and less than 96.5% similarity with other species of the family Rhizobiaceae in the EzBioCloud database (Yoon et al., 2017), and therefore, the strain BDR2-2T might belong to a novel species of Rhizobiaceae. The strain BDR2-2T, A. borbori DN316T, and R. populi K-38T consistently formed a highly supported monophyletic lineage closer to Gellertiella hungarica DSM 29853T or other lineages than to the genus Allorhizobium and Rhizobium lineages (Figures 1, 4), indicating that the three strains should be allocated to novel Rhizobiaceae genera. The cpAAI values between BDR2-2T and A. borbori DN316T were significantly higher than the genus demarcation threshold, indicating that Allorhizobium clade 2 should belong to the same genus. The pairwise cpAAI values between R. populi K-38T and Allorhizobium clade 2 were 84.9 and 85.3%, respectively (Figure 4), which were slightly lower than the genus demarcation threshold. While the 86% threshold was an approximation and not strictly unique, and species within the same genus might have different evolutionary rates (Ramette and Tiedje, 2007; Liang et al., 2021), therefore, we proposed to tentatively place R. populi K-38T in Allorhizobium clade 2 and assign the three strains to a novel genus. In addition, the chemotaxonomic and physiological analyses revealed that the three strains shared similar major phenotypic features with each other (Table 1 and Supplementary Table S2), which also confirmed the reclassification.
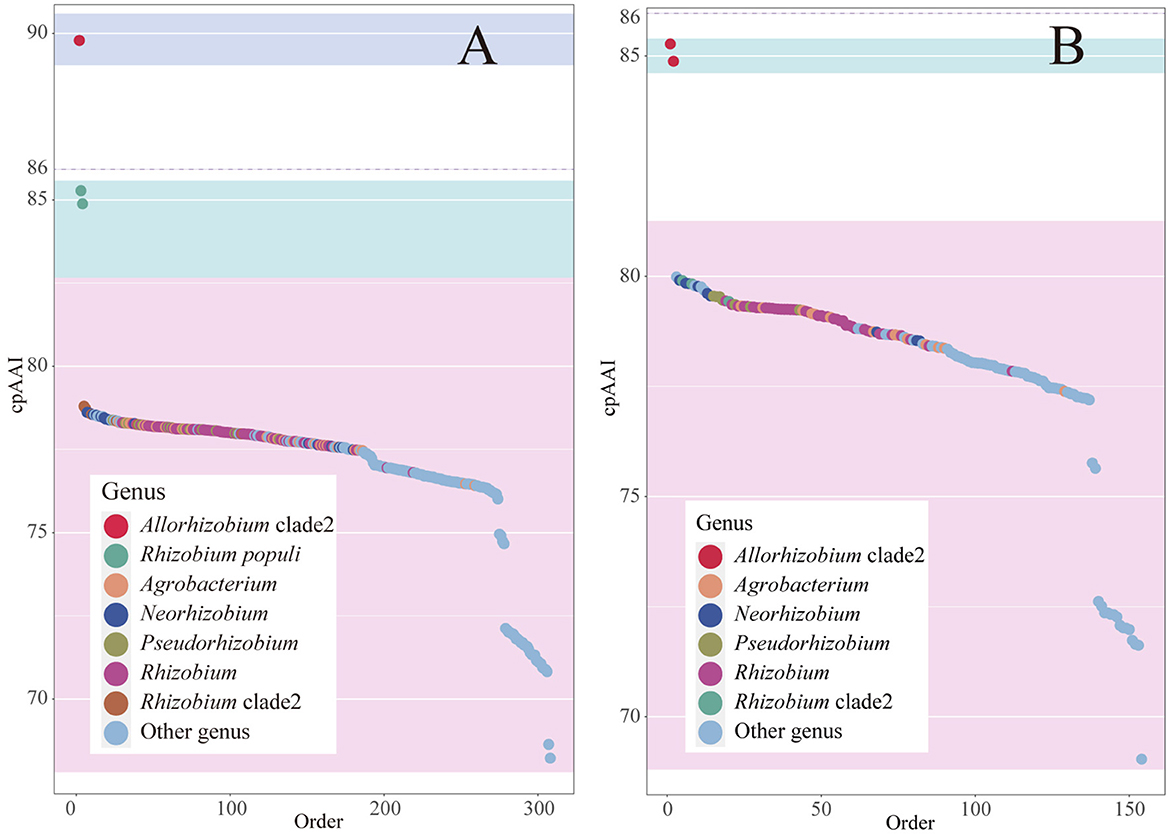
Figure 4. Rank order of pairwise cpAAI within the Allorhizobium clade and between the clade and other members within the family Rhizobiaceae is shown in plot (A). The rank order of pairwise cpAAI between Rhizobium populi K-38T and other members within the family Rhizobiaceae is shown in plot (B). The background color light blue represents the proposed genus, light cyan represents closely related to the proposed genus, and light red represents distantly related to the proposed genus.
The ANI and dDDH values, which are gold standards for species delineation (Liang et al., 2021), were lower in the three strains than the recommended species boundary cutoff values (Supplementary Table S1), which indicated that the three strains should represent three different species in the family Rhizobiaceae.
3.2.2. Chemotaxonomic and physiological analyses
The polar lipid profile of the strain BDR2-2T contained phosphatidylethanolamine (PE), phosphatidylglycerol (PG), phosphatidylcholine (PC), diphosphatidylglycerol (DPG), phosphatidyl monomethyl ethanolamine (PME), three unidentified phospholipids (PLs), and three unidentified lipids (Ls; Supplementary Figure S6), which was similar to the profiles obtained for A. borbori DN316T and R. populi K-38T. The presence of PL and the absence of unidentified amino phospholipids (APLs) could distinguish the strain BDR2-2T from A. borbori DN316T and R. populi K-38T. The strain BDR2-2T could also be distinguished from A. borbori DN316T and R. populi K-38T based on its physiological and chemotaxonomic features: growth conditions, utilization of carbon sources, and enzyme activities, as shown in Table 1. The strain BDR2-2T exhibited a cellular fatty acid profile mainly consisting of summed feature 8 (comprising C18:1ω7c and/or C18:1ω6c, 61.9%), C19:0 cyclo ω8c (11.0%), C16:0 (10.1%), C16:0 3-OH (4.6%), and C18:0 (4.7%), which was similar to those of reference strains (Supplementary Table S2). Higher C18:0 content of the strain BDR2-2T (4.7%) could clearly separate it from A. borbori DN316T (0.7%) and R. populi K-38T (1.8%). Along with the phylogenetic analyses, the strain BDR2-2T should represent a species of a novel genus within the family Rhizobiaceae, for which the name Ectorhizobium quercum gen. nov., sp. nov. was proposed.
4. Conclusion
Since the low resolution of 16S rRNA phylogeny on the closely related species was an important cause of the taxonomic issue, we, therefore, constructed two genome-based phylogenetic trees, namely concatenated proteins tree and UBCG tree, to resolve the misclassifications. Genome sequences from 138 of the 181 validly published Rhizobiaceae species, 18 not validly published Rhizobiaceae species were used to confirm the taxonomic status of species in the family Rhizobiaceae, five Brucellaceae and five Caulobacterales were used as the out-group. Along with the phylogenomic metric analyses of cpAAI, eight novel genera, one novel species, and 22 novel combinations were proposed.
4.1. Taxonomic level: new genera
4.1.1. Description of Allopararhizobium gen. nov.
Allopararhizobium [Al.lo.pa.ra.rhi.zo'bi.um. Gr. masc. adj. allos, another, other, different; N.L. neut. n. Pararhizobium, a bacterial generic name; N.L. neut. n. Allopararhizobium, a genus different from Pararhizobium].
Cells are Gram-strain-negative, motile, aerobic, and rod-shaped. The predominant respiratory quinone is Q-10. The major cellular fatty acids usually contain C19:0 cyclo ω8c. The DNA G+C content is 64.7 mol%. Species of the genus are classified based on UBCG and concatenated protein phylogenetic trees, as well as phylogenomic metric analyses of cpAAI. The type species is Allopararhizobium mangrovi comb. nov.
4.1.2. Description of Paramartelella gen. nov.
Paramartelella [Pa.ra.mar.tel.el'la. Gr. pref. para-, beside; N.L. fem. dim. n. Martelella, a bacterial generic name; N.L. fem. dim. n. Paramartelella, resembling the genus Martelella].
Cells are Gram-strain-negative, non-motile, catalase-positive, and rod-shaped. The predominant respiratory quinone is Q-10. The major cellular fatty acids usually contain summed feature 8 (comprising C18:1ω7c and/or C18:1ω6c). The DNA G+C content is 62.3 mol%. Species of the genus are classified based on UBCG and concatenated protein phylogenetic trees, as well as phylogenomic metric analyses of cpAAI. The type species is Paramartelella alba comb. nov.
4.1.3. Description of Metarhizobium gen. nov.
Metarhizobium [Me.ta.rhi.zo.bi.um. Gr. adv. meta, besides; N.L. neut. n. Rhizobium, a bacterial generic name; N.L. neut. n. Metarhizobium, a genus besides Rhizobium].
Cells are Gram-strain-negative, motile, facultatively anaerobic, catalase- and oxidase-positive, and rod-shaped. The predominant respiratory quinone is Q-10. The major cellular fatty acids usually contain C19:0 cyclo ω8c and C18:1ω7c. The DNA G+C content is 61.9 mol%. Species of the genus are classified based on UBCG and concatenated protein phylogenetic trees, as well as phylogenomic metric analyses of cpAAI. The type species is Metarhizobium album comb. nov.
4.1.4. Description of Heterorhizobium gen. nov.
Heterorhizobium [He.te.ro.rhi.zo.bi.um. Gr. masc. adj. heteros, different; N.L. neut. n. Rhizobium, a bacterial generic name; N.L. neut. n. Heterorhizobium, organism different from but related to the genus Rhizobium].
Cells are Gram-strain-negative, motile, catalase- and oxidase-positive, aerobic, and rod-shaped. The respiratory quinone is Q-10. The major cellular fatty acids usually contain C18:1ω7c and C19:0 cyclo ω8c. The DNA G+C content is 52.8 mol%. Species of the genus are classified based on UBCG and concatenated protein phylogenetic trees, as well as phylogenomic metric analyses of cpAAI. The type species is Heterorhizobium halophytocola comb. nov.
4.1.5. Description of Paenirhizobium gen. nov.
Paenirhizobium [Pae.ni.rhi.zo.bi.um. L. adv. Paene, almost; N.L. neut. n. Rhizobium, a bacterial generic name; N.L. neut. n. Paenirhizobium, almost Rhizobium].
Cells are Gram-strain-negative, motile, aerobic, catalase- and oxidase-positive, and rod-shaped. The predominant respiratory quinone is Q-10. The major cellular fatty acids usually contain summed feature 8 (comprising C18:1ω7c and/or C18:1ω6c). The DNA G + C content is 60.1–60.9 mol%. Members of the genus are classified based on UBCG and concatenated protein phylogenetic trees, as well as phylogenomic metric analyses of cpAAI. The type species is Paenirhizobium daejeonense comb. nov.
4.1.6. Description of Ectorhizobium gen. nov.
Ectorhizobium [Ec.to.rhi.zo.bi.um. Gr. prep. ecto, outside; N.L. neut. n. Rhizobium, a bacterial generic name; N.L. neut. n. Ectorhizobium, outside of Rhizobium].
Cells are Gram-stain-negative, aerobic, catalase-, and oxidase-positive. The predominant respiratory quinone is Q-10. The major cellular fatty acids usually contain summed feature 8 (comprising C18:1ω7c and/or C18:1ω6c) and C16:0. The DNA G+C content is 61.3–64.5 mol%. Members of the genus are classified based on UBCG and concatenated protein phylogenetic trees, as well as phylogenomic metric analyses of cpAAI. The type species is Ectorhizobium quercum sp. nov.
4.1.7. Description of Affinirhizobium gen. nov.
Affinirhizobium [Af.fi.ni.rhi.zo.bi.um. L. masc./fem. adj. affinis, associated with, adjacent; N.L. neut. n. Rhizobium, a bacterial generic name; N.L. neut. n. Affinirhizobium, a genus associated with Rhizobium].
Cells are Gram-strain-negative, catalase-positive, aerobic, and rod-shaped. The DNA G + C content is 59.3–60.2 mol%. The major cellular fatty acids usually contain summed feature 8 (comprising C18:1ω7c and/or C18:1ω6c). Species of the genus are classified based on UBCG and concatenated protein phylogenetic trees, as well as phylogenomic metric analyses of cpAAI. The type species is Affinirhizobium pseudoryzae comb. nov.
4.1.8. Description of Alirhizobium gen. nov.
Alirhizobium [A.li.rhi.zo.bi.um. L. masc.pron. alinus, other, another; N.L. neut. n. Rhizobium, a bacterial generic name; N.L. neut. n. Alirhizobium, the other Rhizobium].
Cells are Gram-strain-negative, aerobic, positive for oxidase, and rod-shaped. The major cellular fatty acids usually contain summed feature 8 (comprising C18:1ω7c and/or C18:1ω6c) and C16:0. The DNA G + C content is 58.8–59.0 mol%. Species of the genus are classified based on UBCG and concatenated protein phylogenetic trees, as well as phylogenomic metric analyses of cpAAI. The type species is Alirhizobium cellulosilyticum comb. nov.
4.2. Taxonomic level: new species
4.2.1. Description of Ectorhizobium quercum sp. nov.
Ectorhizobium quercum [quer'cum. N.L. gen. neut. n. quercum, of oak, of quercus tree].
Cells are Gram-stain-negative, motile with a single polar flagellum, aerobic, catalase-, and oxidase-positive, 0.8–1.2 mm in length, and 0.6–0.7 mm in width. Colonies are milky white, circular, and smooth after incubation for 2 days at 28°C on YMA. The strains grow at 10–41°C (optimum, 28°C), pH 5.0–9.5 (optimum, pH 7), and a concentration of 0–2% (w/v) NaCl. In the API ZYM test, N-acetyl-β-glucosaminidase, β-glucuronidase, α-fucosidase, α-mannosidase, and α-galactosidase are negative, and the rest are positive. In the API 20E, the results are positive for inositol, D-sucrose, sodium pyruvate, D-mannitol, D-glucose, D-melibiose, L-arabinose, and L-rhamnose and negative for the rest. In the API 20NE, the results are positive for D-mannitol, D-glucose, esculin ferric citrate, 4-nitrophenyl-β-D-galactopyranoside, D-mannose, D-maltose, L-arabinose, and malic acid, and negative for the rest. In the Biolog GN2 test, the results are positive for acetic acid, D-cellobiose, L-pyroglutamic acid, α-D-lactose, N-acetyl-D-glucosamine, bromo-succinic acid, α-D-glucose, D-galactose, D-turanose, L-fucose, D-sorbitol, D-arabitol, D-fructose-6-PO4, L-alanine, L-glutamic acid, L-histidine, D-mannitol, lincomycin, pectin, L-galactonic acid lactone, D-mannose, α-keto-glutaric acid, D-fructose, L-malic acid, acetoacetic acid, glycerol, dextrin, L-serine, D-maltose, Tween 40, glucuronamide, weakly positive for D-glucuronic acid, N-acetyl-β-D-mannosamine, N-acetyl-D-galactosamine, D-galacturonic acid, D-fucose, L-arginine, sucrose, D-melibiose, L-aspartic acid, D-gluconic acid, L-lactic acid, D-trehalose, L-rhamnose, myo-inositol, and the rest are negative. The polar lipids are PE, PG, PC, DPG, PME, three unidentified phospholipids (PLs), and three unidentified lipids (L). The respiratory quinones are Q-10. The predominant fatty acids are summed feature 8 (comprising C18:1ω7c and/or C18:1ω6c, 61.9%), C19:0 cyclo ω8c (11.0%), C16:0 (10.1%), C16:0 3-OH (4.6%), and C18:0 (4.7%). The type strain is BDR2-2T (= CFCC 16492T = LMG 31717T), isolated from the symptomatic bark of Q. acutissima caker in Anhui province, China. The strain BDR2-2T is predicted to have 4,685 coding genes, three rRNA genes, 54 tRNA genes, and six other RNA genes, and the DNA G+C content is 64.5 mol%.
4.3. Taxonomic level: new (combinations for) species
4.3.1. Description of Allopararhizobium mangrovi comb.nov.
Allopararhizobium mangrovi (man.gro'vi. N.L. gen. neut. n. mangrovi, of mangrove, where the bacterium was isolated).
Basonym: Pararhizobium mangrovi Li et al., 2021.
The description of A. mangrovi is the same as that given for P. mangrovi (Li et al., 2021b). The species are classified based on UBCG and concatenated protein phylogenetic trees, as well as phylogenomic metric analyses of cpAAI. The type strain is BGMRC 6574T (= CGMCC 1.16783T = KCTC 72636T).
4.3.2. Description of Paramartelella alba comb. nov.
Paramartelella alba (al'ba. L. fem. adj. alba, white, referring to the color of the colonies).
Basonym: Martelella alba Li et al., 2021.
The description of P. alba is the same as that given for M. alba (Li et al., 2021a). The species are classified based on UBCG and concatenated protein phylogenetic trees, as well as phylogenomic metric analyses of cpAAI. The type strain is BGMRC 2036T (= KCTC 52121T = NBRC 111908T).
4.3.3. Description of Mycoplana rhizolycopersici comb.nov.
Mycoplana rhizolycopersici (rhi.zo.ly.co.per'si.ci. Gr. fem. n. rhiza, a root; N.L. gen. neut. n. lycopersici, of Solanum lycopersicum, the scientific name of the tomato; N.L. gen. neut. n. rhizolycopersici, of tomato roots).
Basonym: Rhizobium rhizolycopersici Thin et al., 2021.
The description of M. rhizolycopersici is the same as that given for R. rhizolycopersici (Thin et al., 2021). The species are classified based on UBCG and concatenated protein phylogenetic trees, as well as phylogenomic metric analyses of cpAAI. The type strain is DBTS2T (= CICC 24887T = ACCC61707T = JCM 34245T).
4.3.4. Description of Metarhizobium album comb. nov.
Metarhizobium album (al'bum. L. neut. adj. album, white, referring to the white colonies of the organism).
Basonym: Rhizobium album Hang et al., 2019.
The description of the Metarhizobium album is the same as that given for the Rhizobium album (Hang et al., 2019). The species are classified based on UBCG and concatenated protein phylogenetic trees, as well as phylogenomic metric analyses of cpAAI. The type strain is NS-104T (= CCTCC AB 2017250T = KCTC 62327T).
4.3.5. Description of Heterorhizobium halophytocola comb.nov.
Heterorhizobium halophytocola [ha.lo.phy.to'co.la. Gr. masc. n. hals (gen. halos), salt; Gr. neut. n. phyton, a plant; L. masc./fem. suff. -cola, inhabitant, dweller; from L. masc./fem. n. incola, dweller; N.L. masc./fem. n. halophytocola, inhabitant of a halophyte, Rosa rugosa (nominative in apposition)].
Basonym: Rhizobium halophytocola Bibi et al., 2012.
The description of H. halophytocola is the same as that given for R. halophytocola (Bibi et al., 2012). The species are classified based on UBCG and concatenated protein phylogenetic trees, as well as phylogenomic metric analyses of cpAAI. The type strain is YC6881T (= DSM 21600T = KACC 13775T).
4.3.6. Description of Paenirhizobium daejeonense comb.nov.
Paenirhizobium daejeonense (dae.jeon.en'se. N.L. neut. adj. daejeonense, pertaining to Daejeon, a city in Korea, where the type strain was isolated).
Basonym: Rhizobium daejeonense Quan et al., 2005.
The description of Paenirhizobium daejeonense is the same as that given for Rhizobium daejeonense (Quan et al., 2005). The species are classified based on UBCG and concatenated protein phylogenetic trees, as well as phylogenomic metric analyses of cpAAI. The type strain is L61T (= DSM 17795T = JCM 21505T = IAM 15042T = CCBAU 10050T = NBRC 102495T = KCTC 12121T).
4.3.7. Description of Paenirhizobium naphthalenivorans comb.nov.
Paenirhizobium naphthalenivorans (naph.tha.le.ni.vo'rans. N.L. neut. n. naphthalenum, naphthalene; L. pres. part. vorans, devouring; N.L. part. adj. naphthalenivorans, naphthalene-devouring).
Basonym: Rhizobium naphthalenivorans Kaiya et al., 2018.
The description of Paenirhizobium naphthalenivorans is the same as that given for Rhizobium naphthalenivorans (Kaiya et al., 2012). The species are classified based on UBCG and concatenated protein phylogenetic trees, as well as phylogenomic metric analyses of cpAAI. The type strain is TSY03bT (= KCTC 23252T = NBRC 107585T).
4.3.8. Description of Paenirhizobium selenitireducens comb.nov.
Paenirhizobium selenitireducens (se.le.ni.ti.re.du'cens. N.L. masc. n. selenis, selenite; L. pres. part. reducens, converting to a different state; N.L. part. adj. selenitireducens, selenite reducing, referring to the organism's ability to reduce the selenium oxyanion selenite to elemental selenium).
Basonym: Rhizobium selenitireducens Hunter et al., 2008.
The description of Paenirhizobium selenitireducens is the same as that given for Rhizobium selenitireducens (Hunter et al., 2007). The species are classified based on UBCG and concatenated protein phylogenetic trees, as well as phylogenomic metric analyses of cpAAI. The type strain is B1T (= NRRL B-41997T = LMG 24075T = ATCC BAA-1503T).
4.3.9. Description of Peteryoungia glycinendophyticum comb.nov.
Peteryoungia glycinendophyticum (gly.cin.en.do.phy'ti.cum. N.L. fem. n. Glycine, generic name of the soy bean; Gr. pref. endo-, within; Gr. neut. n. phyton, plant; L. masc. adj. suff. -icus, used with the sense of belonging to; N.L. masc. adj. endophyticus, within the plant, endophytic; N.L. neut. adj. glycinendophyticum, an endophyte of soybean).
Basonym: Rhizobium glycinendophyticum Wang et al., 2020.
The description of P. glycinendophyticum is the same as that given for R. glycinendophyticum (Wang et al., 2020). The species are classified based on UBCG and concatenated protein phylogenetic trees, as well as phylogenomic metric analyses of cpAAI. The type strain is CL12T (= KACC 21281T = GDMCC 1.1597T).
4.3.10. Description of Peteryoungia albertimagni comb.nov.
Peteryoungia albertimagni (albertimagni, is named after the Dominican scholar Albertus Magnus, who was the first person to describe arsenic).
Basonym: Agrobacterium albertimagni Salmassi et al., 2002.
The description of P. albertimagni is the same as that given for A. albertimagni (Salmassi et al., 2002). The species are classified based on UBCG and concatenated protein phylogenetic trees, as well as phylogenomic metric analyses of cpAAI. The type strain is AOL15T = ATCC BAA-24T).
4.3.11. Description of Ectorhizobium borbori comb.nov.
Ectorhizobium borbori (bor'bo.ri. Gr. masc. n. borboros, sludge; N.L. gen. n. borbori, of sludge).
Basonym: Allorhizobium borbori Mousavi et al., 2016.
Homotypic synonym: Rhizobium borbori Zhang et al., 2011.
The description of Ectorhizobium borbori is the same as that given for Rhizobium borbori (Zhang G. X. et al., 2011). The species are classified based on UBCG and concatenated protein phylogenetic trees, as well as phylogenomic metric analyses of cpAAI. The type strain is DN316T (= CICC 10378T= LMG 23925T= DSM 22790T=DSM 26385T=HAMBI 3454T).
4.3.12. Description of Ectorhizobium populi comb.nov.
Ectorhizobium populi (po'pu.li. L. gen. fem. n. populi, of a poplar tree, pertaining to Populus euphratica, the Latin name for the poplars that grow in the forest from which the type strain was isolated).
Basonym: Rhizobium populi Rozahon et al., 2014.
The description of E. populi is the same as that given for R. populi (Rozahon et al., 2014). The species are classified based on UBCG and concatenated protein phylogenetic trees, as well as phylogenomic metric analyses of cpAAI. The type strain is K-38T (= CCTCC AB 2013068T = NRRL B-59990T = JCM 19159T).
4.3.13. Description of Agrobacterium oryzihabitans comb.nov.
Agrobacterium oryzihabitans (o.ry.zi.ha'bi.tans. L. fem. n. oryza, rice; L. pres. part. habitans, inhabiting, dwelling; N.L. part. adj. oryzihabitans, rice-inhabiting).
Basonym: Rhizobium oryzihabitans Zhao et al., 2020.
The description of A. oryzihabitans is the same as that given for R. oryzihabitans (Zhao et al., 2020). The species are classified based on UBCG and concatenated protein phylogenetic trees, as well as phylogenomic metric analyses of cpAAI. The type strain is M15T (= JCM 32903T = ACCC 60121T).
4.3.14. Description of Affinirhizobium pseudoryzae comb.nov.
Affinirhizobium pseudoryzae (a.qua'ti.cum. L. neut. adj. aquaticum, living in water, aquatic, referring to the isolation source of the type strain).
Basonym: Allorhizobium pseudoryzae Mousavi et al., 2016.
Homotypic synonym: Rhizobium pseudoryzae Zhang G. X. et al., 2011.
The description of Affinirhizobium pseudoryzae is the same as that given for Rhizobium pseudoryzae (Zhang X. et al., 2011). The species are classified based on UBCG and concatenated protein phylogenetic trees, as well as phylogenomic metric analyses of cpAAI. The type strain is J3-A127T (= ACCC 10380T = KCTC 23294T = DSM 19479T = DSM 26483T).
4.3.15. Description of Affinirhizobium helianthi comb.nov.
Affinirhizobium helianthi (he.li.an'thi. N.L. gen. masc. n. helianthi, of the sunflower Helianthus).
Basonym: Rhizobium helianthi Wei et al., 2015.
The description of Affinirhizobium helianthi is the same as that given for Rhizobium helianthi (Wei et al., 2015). The species are classified based on UBCG and concatenated protein phylogenetic trees, as well as phylogenomic metric analyses of cpAAI. The type strain is Xi19T (= CGMCC 1.12192T = KCTC 23879T).
4.3.16. Description of Affinirhizobium rhizoryzae comb.nov.
Affinirhizobium rhizoryzae (rhiz.o.ry'zae. Gr. fem. n. rhiza, root; L. gen. fem. n. oryzae, of rice; N.L. gen. n. rhizoryzae, of rice roots).
Basonym: Rhizobium rhizoryzae Zhang et al., 2014.
The description of Affinirhizobium rhizoryzae is the same as that given for Rhizobium rhizoryzae (Zhang X. X. et al., 2014). The species are classified based on UBCG and concatenated protein phylogenetic trees, as well as phylogenomic metric analyses of cpAAI. The type strain is J3-AN59T (= ACCC 05916T = DSM 19478T = DSM 29514T = KCTC 23652T).
4.3.17. Description of Alirhizobium cellulosilyticum comb.nov.
Alirhizobium cellulosilyticum (cel.lu.lo.si.ly'ti.cum. N.L. neut. N. cellulosum, cellulose; N.L. neut. Adj. lyticum, dissolving; from Gr. Masc. adj. lytikos, able to loose, able to dissolve; N.L. neut. Adj. cellulosilyticum, cellulose-dissolving).
Basonym: Rhizobium cellulosilyticum Garcia-Fraile et al., 2007.
The description of Alirhizobium cellulosilyticum is the same as that given for Rhizobium cellulosilyticum (Garcia-Fraile et al., 2007). The species are classified based on UBCG and concatenated protein phylogenetic trees, as well as phylogenomic metric analyses of cpAAI. The type strain is ALA10B2T (=DSM 18291T = CECT 7176T = LMG 23642T).
4.3.18. Description of Alirhizobium wenxiniae comb.nov.
Alirhizobium wenxiniae (wen.xin'i.ae. N.L. gen. fem. n. wenxiniae, of Wen-xin, to honor Wen-xin Chen, a respected rhizobial taxonomist, for her great contributions to the investigation and taxonomy of rhizobial resources in China).
Basonym: Rhizobium wenxiniae Gao et al., 2017.
The description of Alirhizobium wenxiniae is the same as that given for Rhizobium wenxiniae (Gao et al., 2017). The species are classified based on UBCG and concatenated protein phylogenetic trees, as well as phylogenomic metric analyses of cpAAI. The type strain is 166T (= DSM 100734T = CGMCC 1.15279T).
4.3.19. Description of Alirhizobium smilacinae comb.nov.
Alirhizobium smilacinae (smi.la.ci'na.e. N.L. fem. n. Smilacina, a botanical genus name; N.L. gen. fem. n. smilacinae, of the plant genus Smilacina).
Basonym: Rhizobium smilacinae Zhang et al., 2015.
The description of Alirhizobium smilacinae is the same as that given for Rhizobium smilacinae (Zhang L. et al., 2014). The species are classified based on UBCG and concatenated protein phylogenetic trees, as well as phylogenomic metric analyses of cpAAI. The type strain is PTYR-5T (= DSM 100675T = CCTCC AB 2013016T = KCTC 32300T = LMG 27604T).
4.3.20. Description of Neorhizobium deserti comb. nov.
Neorhizobium deserti (de.ser'ti. L. gen. neut. n. deserti, of a desert, the source of the type strain).
Basonym: Rhizobium deserti Liu et al., 2020.
The description of N. deserti is the same as that given for R. deserti (Liu et al., 2020). The species are classified based on UBCG and concatenated protein phylogenetic trees, as well as phylogenomic metric analyses of cpAAI. The type strain is SPY-1T (= ACCC 61627T = JCM 33732T).
4.3.21. Description of Neorhizobium terrae comb. nov.
Neorhizobium terrae (ter'rae. L. gen. fem. n. terrae, of soil, referring to the isolation source of the type strain).
Basonym: Rhizobium terrae Ruan et al., 2021.
The description of N. terrae is the same as that given for R. terrae (Ruan et al., 2020). The species are classified based on UBCG and concatenated protein phylogenetic trees, as well as phylogenomic metric analyses of cpAAI. The type strain is NAU-18T (= CCTCC AB 2018075T = KCTC 62418T).
4.3.22. Description of Neorhizobium populisoli comb.nov.
Neorhizobium populisoli (po.pu.li.so'li. L. fem. n. Populus, the poplar tree (genus Populus); L. neut. adj. solum, soil; N.L. gen. neut. n. populisoli, of poplar soil, referring to the isolation of the bacterium from the rhizosphere soil of P. popularis).
Basonym: Rhizobium populisoli Shen et al., 2022.
The description of N. populisoli is the same as that given for R. populisoli (Shen et al., 2022). The species are classified based on UBCG and concatenated protein phylogenetic trees, as well as phylogenomic metric analyses of cpAAI. The type strain is XQZ8T (=JCM 34442T = GDMCC 1.2201T).
Data availability statement
The datasets presented in this study can be found in online repositories. The names of the repository/repositories and accession number(s) can be found at: NCBI—JANFPI010000000 and PRJNA859997.
Author contributions
CP and YL designed the experiment, provided the methods, and revised the manuscript. TM finished the manuscript and completed most of the experiments. HX and NJ helped to reconstruct and analyze the gene trees. All authors read and approved the final version of the manuscript.
Funding
The research was supported by the Fundamental Research Funds for the Central Non-profit Research Institution of CAF (CAFYBB2018ZB001), the National Natural Science Foundation of China (31200486), and the National Infrastructure of Microbial Resources (NMRC-2021-7) from the Ministry of Science and Technology of the People's Republic of China.
Acknowledgments
We are grateful to Yan Liu, Fengjuan Wang, and Wenjieng Li (China Forestry Culture Collection Center) for the material preparation and strain preservation. We also thank Dr. Yuan Cao from the State Key Laboratory of Tree Genetics and Breeding for her excellent technical assistance on the morphology investigation (TEM observation).
Conflict of interest
The authors declare that the research was conducted in the absence of any commercial or financial relationships that could be construed as a potential conflict of interest.
Publisher's note
All claims expressed in this article are solely those of the authors and do not necessarily represent those of their affiliated organizations, or those of the publisher, the editors and the reviewers. Any product that may be evaluated in this article, or claim that may be made by its manufacturer, is not guaranteed or endorsed by the publisher.
Supplementary material
The Supplementary Material for this article can be found online at: https://www.frontiersin.org/articles/10.3389/fmicb.2023.1207256/full#supplementary-material
References
An, D. S., Im, W. T., Yang, H. C., and Lee, S. T. (2006). Shinella granuli gen. nov., sp. nov., proposal of the reclassification of Zoogloea ramigera ATCC 19623 as Shinella zoogloeoides sp. nov. Int. J. Syst. Evol. Microbiol. 56, 443–448. doi: 10.1099/ijs.0.63942-0
Bankevich, A., Nurk, S., Antipov, D., Gurevich, A. A., Dvorkin, M., Kulikov, A. S., et al. (2012). SPAdes: a new genome assembly algorithm and its applications to single-cell sequencing. J. Comput. Biol. 19, 455–477. doi: 10.1089/cmb.2012.0021
Bibi, F., Chung, E. J., Khan, A., Jeon, C. O., and Chung, Y. R. (2012). Rhizobium halophytocola sp. nov., isolated from the root of a coastal dune plant. Int. J. Syst. Evol. Microbiol. 62, 1997–2003. doi: 10.1099/ijs.0.029488-0
Collins, M. D., Pirouz, T., Goodfellow, M., and Minnikin, D. E. (1977). Distribution of menaquinones in actinomycetes and corynebacteria. Microbiology 100, 221–230. doi: 10.1099/00221287-100-2-221
De Lajudie, P. M., Andrews, M., Ardley, J., Eardly, B., Jumas-Bilak, E., Kuzmanović, N., et al. (2019). Minimal standards for the description of new genera and species of rhizobia and agrobacteria. Int. J. Syst. Evol. Microbiol. 69, 1852–1863. doi: 10.1099/ijsem.0.003426
Fagen, J. R., Leonard, M. T., Coyle, J. F., Mccullough, C. M., Davis-Richardson, A. G., Davis, M. J., et al. (2014). Liberibacter crescens gen. nov., sp. nov., the first cultured member of the genus Liberibacter. Int. J. Syst. Evol. Microbiol. 64, 2461–2466. doi: 10.1099/ijs.0.063255-0
Farris, J. S. (1974). Formal definitions of paraphyly and polyphyly. Syst. Zool. 23, 548–554. doi: 10.2307/2412474
Gao, J. L., Sun, P., Wang, X. M., Lv, F. Y., Mao, X. J., Sun, J. G., et al. (2017). Rhizobium wenxiniae sp. nov., an endophytic bacterium isolated from maize root. Int. J. Syst. Evol. Microbiol. 67, 2798–2803. doi: 10.1099/ijsem.0.002025
Garcia-Fraile, P., Rivas, R., Willems, A., Peix, A., Martens, M., Martínez-Molina, E., et al. (2007). Rhizobium cellulosilyticum sp. nov., isolated from sawdust of Populus alba. Int. J. Syst. Evol. Microbiol. 57, 844–848. doi: 10.1099/ijs.0.64680-0
Goris, J., Konstantinidis, K. T., Klappenbach, J. A., Coenye, T., Vandamme, P., Tiedje, J. M., et al. (2007). DNA–DNA hybridization values and their relationship to whole-genome sequence similarities. Int. J. Syst. Evol. Microbiol. 57, 81–91. doi: 10.1099/ijs.0.64483-0
Hang, P., Zhang, L., Zhou, X.-Y., Hu, Q., and Jiang, J.-D. (2019). Rhizobium album sp. nov., isolated from a propanil-contaminated soil. Antonie Van Leeuwenhoek 112, 319–327. doi: 10.1007/s10482-018-1160-3
Hördt, A., López, M. G., Meier-Kolthoff, J. P., Schleuning, M., Weinhold, L.-M., Tindall, B. J., et al. (2020). Analysis of 1,000+ type-strain genomes substantially improves taxonomic classification of Alphaproteobacteria. Front. Microbiol. 11, 468. doi: 10.3389/fmicb.2020.00468
Hunter, W. J., Kuykendall, L. D., and Manter, D. K. (2007). Rhizobium selenireducens sp. nov.: a selenite-reducing α-Proteobacteria isolated from a bioreactor. Curr. Microbiol. 55, 455–460. doi: 10.1007/s00284-007-9020-9
Jenkins, D., Richard, M. G., and Daigger, G. T. (2003). “Methods,” in Manual on the Causes and Control of Activated Sludge Bulking, Foaming, and Other Solids Separation Problems, eds. D. Jenkins, M. G. Richard, and G. T. Daigger (Boca Raton, FL: CRC Press). doi: 10.1201/9780203503157
Jiang, H., Li, K., and Gai, J. (2019). Agrobacterium rhizogenes-induced soybean hairy roots versus Soybean mosaic virus (ARISHR-SMV) is an efficient pathosystem for studying soybean–virus interactions. Plant Methods 15, 1–11. doi: 10.1186/s13007-019-0442-8
Kaiya, S., Rubaba, O., Yoshida, N., Yamada, T., and Hiraishi, A. (2012). Characterization of Rhizobium naphthalenivorans sp. nov. with special emphasis on aromatic compound degradation and multilocus sequence analysis of housekeeping genes. J. Gen. Appl. Microbiol. 58, 211–224. doi: 10.2323/jgam.58.211
Kathiravan, R., Jegan, S., Ganga, V., Prabavathy, V. R., Tushar, L., Sasikala, C., et al. (2013). Ciceribacter lividus gen. nov., sp. nov., isolated from rhizosphere soil of chick pea (Cicer arietinum L.). Int. J. Syst. Evol. Microbiol. 63, 4484–4488. doi: 10.1099/ijs.0.049726-0
Kim, J., Na, S.-I., Kim, D., and Chun, J. (2021). UBCG2: up-to-date bacterial core genes and pipeline for phylogenomic analysis. J. Microbiol. 59, 609–615. doi: 10.1007/s12275-021-1231-4
Kimes, N. E., López-Pérez, M., Flores-Félix, J. D., Ramírez-Bahena, M.-H., Igual, J. M., Peix, A., et al. (2015). Pseudorhizobium pelagicum gen. nov., sp. nov. isolated from a pelagic Mediterranean zone. Syst. Appl. Microbiol. 38, 293–299. doi: 10.1016/j.syapm.2015.05.003
Kumar, S., Stecher, G., Li, M., Knyaz, C., and Tamura, K. (2018). MEGA X: molecular evolutionary genetics analysis across computing platforms. Mol. Biol. Evol. 35, 1547. doi: 10.1093/molbev/msy096
Kuykendall, L. D., Roy, M. A., O'neill, J. J., and Devine, T. E. (1988). Fatty acids, antibiotic resistance, and deoxyribonucleic acid homology groups of Bradyrhizobium japonicum. Int. J. Syst. Evol. Microbiol. 38, 358–361. doi: 10.1099/00207713-38-4-358
Kuzmanović, N., Fagorzi, C., Mengoni, A., and Lassalle, F. (2022). Taxonomy of Rhizobiaceae revisited: proposal of a new framework for genus delimitation. Int. J. Syst. Evol. Microbiol. 72, 005243. doi: 10.1099/ijsem.0.005243
Lagesen, K., Hallin, P., Rødland, E. A., Stærfeldt, H.-H., Rognes, T., Ussery, D. W., et al. (2007). RNAmmer: consistent and rapid annotation of ribosomal RNA genes. Nucleic Acids Res. 35, 3100–3108. doi: 10.1093/nar/gkm160
Letunic, I., and Bork, P. (2021). Interactive Tree Of Life (iTOL) v5: an online tool for phylogenetic tree display and annotation. Nucleic Acids Res. 49, W293–W296. doi: 10.1093/nar/gkab301
Li, M., Gao, C., Feng, Y., Liu, K., Cao, P., Liu, Y., et al. (2021a). Martelella alba sp. nov., isolated from mangrove rhizosphere soil within the Beibu Gulf. Arch. Microbiol. 203, 1779–1786. doi: 10.1007/s00203-020-02178-2
Li, M., Liu, Y., Liu, K., Luo, S., Yi, X., Gao, C., et al. (2021b). Pararhizobium mangrovi sp. nov., isolated from Aegiceras corniculatum Stem. Curr. Microbiol. 78, 2828–2837. doi: 10.1007/s00284-021-02434-8
Li, R., Li, Y., Kristiansen, K., and Wang, J. (2008). SOAP: short oligonucleotide alignment program. Bioinformatics 24, 713–714. doi: 10.1093/bioinformatics/btn025
Li, R., Zhu, H., Ruan, J., Qian, W., Fang, X., Shi, Z., et al. (2010). De novo assembly of human genomes with massively parallel short read sequencing. Genome Res. 20, 265–272. doi: 10.1101/gr.097261.109
Li, Y., Song, L.-M., Guo, M.-W., Wang, L.-F., and Liang, W.-X. (2016). Sphingobacterium populi sp. nov., isolated from bark of Populus× euramericana. Int. J. Syst. Evol. Microbiol. 66, 3456–3462. doi: 10.1099/ijsem.0.001217
Liang, K. Y. H., Orata, F. D., Boucher, Y. F., and Case, R. J. (2021). Roseobacters in a sea of poly-and paraphyly: whole genome-based taxonomy of the family Rhodobacteraceae and the proposal for the split of the “Roseobacter clade” into a novel family, Roseobacteraceae fam. nov. Front. Microbiol. 12, 1635. doi: 10.3389/fmicb.2021.683109
Lin, S.-H., and Liao, Y.-C. (2013). CISA: contig integrator for sequence assembly of bacterial genomes. PLoS ONE 8, e60843. doi: 10.1371/journal.pone.0060843
Liu, L., Liang, L., Xu, L., Chi, M., Zhang, X., Li, L., et al. (2020). Rhizobium deserti sp. nov isolated from biological soil crusts collected at Mu Us sandy land, China. Curr. Microbiol. 77, 327–333. doi: 10.1007/s00284-019-01831-4
Ma, T., Xue, H., Piao, C., Liu, C., Yang, M., Bian, D., et al. (2022). Reclassification of 11 members of the family Rhodobacteraceae at genus and species levels and proposal of Pseudogemmobacter hezensis sp. nov. Front. Microbiol. 13, 849695. doi: 10.3389/fmicb.2022.849695
Minnikin, D. E., O'donnell, A. G., Goodfellow, M., Alderson, G., Athalye, M., Schaal, A., et al. (1984). An integrated procedure for the extraction of bacterial isoprenoid quinones and polar lipids. J. Microbiol. Methods 2, 233–241. doi: 10.1016/0167-7012(84)90018-6
Mousavi, S. A., Österman, J., Wahlberg, N., Nesme, X., Lavire, C., Vial, L. K., et al. (2014). Phylogeny of the Rhizobium–Allorhizobium–Agrobacterium clade supports the delineation of Neorhizobium gen. nov. Syst. Appl. Microbiol. 37, 208–215. doi: 10.1016/j.syapm.2013.12.007
Park, S., Lee, J.-S., Lee, K.-C., and Yoon, J.-H. (2013). Lentilitoribacter donghaensis gen. nov., sp. nov., a slowly-growing alphaproteobacterium isolated from coastal seawater. Antonie Van Leeuwenhoek 103, 457–464. doi: 10.1007/s10482-012-9825-9
Parks, D. H., Chuvochina, M., Waite, D. W., Rinke, C., Skarshewski, A., Chaumeil, P.-A., et al. (2018). A standardized bacterial taxonomy based on genome phylogeny substantially revises the tree of life. Nat. Biotechnol. 36, 996–1004. doi: 10.1038/nbt.4229
Parks, D. H., Imelfort, M., Skennerton, C. T., Hugenholtz, P., and Tyson, G. W. (2015). CheckM: assessing the quality of microbial genomes recovered from isolates, single cells, and metagenomes. Genome Res. 25, 1043–1055. doi: 10.1101/gr.186072.114
Pritchard, L., Glover, R. H., Humphris, S., Elphinstone, J. G., and Toth, I. K. (2016). Genomics and taxonomy in diagnostics for food security: soft-rotting enterobacterial plant pathogens. Anal. Methods 8, 12–24. doi: 10.1039/C5AY02550H
Quan, Z. X., Bae, H. S., Baek, J. H., Chen, W. F., Im, W. T., Lee, S. T., et al. (2005). Rhizobium daejeonense sp. nov. isolated from a cyanide treatment bioreactor. Int. J. Syst. Evol. Microbiol. 55, 2543–2549. doi: 10.1099/ijs.0.63667-0
Rahi, P., Khairnar, M., Hagir, A., Narayan, A., Jain, K. R., Madamwar, D., et al. (2021). Peteryoungia gen. nov. with four new species combinations and description of Peteryoungia desertarenae sp. nov., taxonomic revision of the genus Ciceribacter based on phylogenomics of Rhizobiaceae. Arch. Microbiol. 203, 3591–3604. doi: 10.1007/s00203-021-02349-9
Ramette, A., and Tiedje, J. M. (2007). Biogeography: an emerging cornerstone for understanding prokaryotic diversity, ecology, and evolution. Microb. Ecol. 53, 197–207. doi: 10.1007/s00248-005-5010-2
Ramírez-Bahena, M. H., García-Fraile, P., Peix, A., Valverde, A., Rivas, R., Igual, J. M., et al. (2008). Revision of the taxonomic status of the species Rhizobium leguminosarum (Frank 1879) Frank 1889AL, Rhizobium phaseoli dangeard 1926AL and Rhizobium trifolii Dangeard 1926AL. R. trifolii is a later synonym of R. leguminosarum. Reclassification of the strain R. leguminosarum DSM 30132 (= NCIMB 11478) as Rhizobium pisi sp. nov. Int. J. Syst. Evol. Microbiol. 58, 2484–2490. doi: 10.1099/ijs.0.65621-0
Richter, M., and Rosselló-Móra, R. (2009). Shifting the genomic gold standard for the prokaryotic species definition. Proc Natl Acad Sci U S A 106, 19126–19131. doi: 10.1073/pnas.0906412106
Rivas, R., Sanchez-Marquez, S., Mateos, P. F., Martínez-Molina, E., and Velazquez, E. (2005). Martelella mediterranea gen. nov., sp. nov., a novel α-proteobacterium isolated from a subterranean saline lake. Int. J. Syst. Evol. Microbiol. 55, 955–959. doi: 10.1099/ijs.0.63438-0
Rocha, G., Le Queré, A., Medina, A., Cuéllar, A., Contreras, J.-L., Carreño, R., et al. (2020). Diversity and phenotypic analyses of salt-and heat-tolerant wild bean Phaseolus filiformis rhizobia native of a sand beach in Baja California and description of Ensifer aridi sp. nov. Arch. Microbiol. 202, 309–322. doi: 10.1007/s00203-019-01744-7
Rozahon, M., Ismayil, N., Hamood, B., Erkin, R., Abdurahman, M., Mamtimin, H., et al. (2014). Rhizobium populi sp. nov., an endophytic bacterium isolated from Populus euphratica. Int. J. Syst. Evol. Microbiol. 64, 3215–3221. doi: 10.1099/ijs.0.061416-0
Ruan, Z. P., Cao, W. M., Zhang, X., Zhu, J. C., Hu, B., Jiang, J. D., et al. (2020). Rhizobium terrae sp. nov., isolated from an oil-contaminated soil in China. Curr. Microbiol. 77, 1117–1124. doi: 10.1007/s00284-020-01889-5
Salmassi, T. M., Venkateswaren, K., Satomi, M., Newman, D. K., and Hering, J. G. (2002). Oxidation of arsenite by Agrobacterium albertimagni, AOL15, sp. nov., isolated from Hot Creek, California. Geomicrobiol. J. 19, 53–66. doi: 10.1080/014904502317246165
Sasser, M. (1990). Identification of Bacteria by Gas Chromatography of Cellular Fatty Acids. Newark, DE: MIDI inc.
Shen, L., Liu, J.-J., Liu, P.-X., An, M.-M., He, X.-W., Zhao, G.-Z., et al. (2022). A non-symbiotic novel species, Rhizobium populisoli sp. nov., isolated from rhizosphere soil of Populus popularis. Arch. Microbiol. 204, 1–7. doi: 10.1007/s00203-021-02706-8
Simpson, J. T., Wong, K., Jackman, S. D., Schein, J. E., Jones, S. J. M., Birol, I., et al. (2009). ABySS: a parallel assembler for short read sequence data. Genome Res. 19, 1117–1123. doi: 10.1101/gr.089532.108
Singh, N. K., Lavire, C., Nesme, J., Vial, L., Nesme, X., Mason, C. E., et al. (2021). Comparative genomics of novel Agrobacterium G3 strains isolated from the international space station and description of Agrobacterium tomkonis sp. nov. Front. Microbiol. 12, 765943. doi: 10.3389/fmicb.2021.765943
Thin, K. K., He, S.-W., Wang, X., Wang, Y., Rong, M., Han, J.-G., et al. (2021). Rhizobium rhizolycopersici sp. nov., isolated from the rhizosphere soil of tomato plants in China. Curr. Microbiol. 78, 830–836. doi: 10.1007/s00284-020-02323-6
Tighe, S. W., De Lajudie, P., Dipietro, K., Lindström, K., Nick, G., Jarvis, B. D., et al. (2000). Analysis of cellular fatty acids and phenotypic relationships of Agrobacterium, Bradyrhizobium, Mesorhizobium, Rhizobium and Sinorhizobium species using the sherlock microbial identification system. Int. J. Syst. Evol. Microbiol. 50, 787–801. doi: 10.1099/00207713-50-2-787
Tóth, E., Szuróczki, S., Kéki, Z., Bóka, K., Szili-Kovács, T., Schumann, P., et al. (2017). Gellertiella hungarica gen. nov., sp. nov., a novel bacterium of the family Rhizobiaceae isolated from a spa in Budapest. Int. J. Syst. Evol. Microbiol. 67, 4565–4571. doi: 10.1099/ijsem.0.002332
Vinuesa, P., Silva, C., Lorite, M. J., Izaguirre-Mayoral, M. L., Bedmar, E. J., Martínez-Romero, E., et al. (2005). Molecular systematics of rhizobia based on maximum likelihood and Bayesian phylogenies inferred from rrs, atpD, recA and nifH sequences, and their use in the classification of Sesbania microsymbionts from Venezuelan wetlands. Syst. Appl. Microbiol. 28, 702–716. doi: 10.1016/j.syapm.2005.05.007
Wang, C., Li, A., Yuan, T., Bao, G., Feng, G., Zhu, H., et al. (2020). Rhizobium glycinendophyticum sp. nov., isolated from roots of Glycine max (Linn. Merr.). Antonie Van Leeuwenhoek 113, 147–154. doi: 10.1007/s10482-019-01324-1
Wei, X., Yan, S., Li, D., Pang, H., Li, Y., Zhang, J., et al. (2015). Rhizobium helianthi sp. nov., isolated from the rhizosphere of sunflower. Int. J. Syst. Evol. Microbiol. 65, 4455–4460. doi: 10.1099/ijsem.0.000594
Wood, S. W. (1994). Monophyly and comparisons between trees. Cladistics 10, 339–346. doi: 10.1111/j.1096-0031.1994.tb00184.x
Yoon, S. H., Ha, S. M., Kwon, S., Lim, J., Kim, Y., Seo, H., et al. (2017). Introducing EzBioCloud: a taxonomically united database of 16S rRNA gene sequences and whole-genome assemblies. Int. J. Syst. Evol. Microbiol. 67, 1613. doi: 10.1099/ijsem.0.001755
Young, J. P. W., Moeskjær, S., Afonin, A., Rahi, P., Maluk, M., James, E. K., et al. (2021). Defining the Rhizobium leguminosarum species complex. Genes 12, 111. doi: 10.3390/genes12010111
Zhang, G. X., Ren, S. Z., Xu, M. Y., Zeng, G. Q., Luo, H. D., Chen, J. L., et al. (2011). Rhizobium borbori sp. nov., aniline-degrading bacteria isolated from activated sludge. Int. J. Syst. Evol. Microbiol. 61, 816–822. doi: 10.1099/ijs.0.022228-0
Zhang, L., Shi, X., Si, M., Li, C., Zhu, L., Zhao, L., et al. (2014). Rhizobium smilacinae sp. nov., an endophytic bacterium isolated from the leaf of Smilacina japonica. Antonie Van Leeuwenhoek 106, 715–723. doi: 10.1007/s10482-014-0241-1
Zhang, X., Sun, L., Ma, X., Sui, X. H., and Jiang, R. (2011). Rhizobium pseudoryzae sp. nov., isolated from the rhizosphere of rice. Int. J. Syst. Evol. Microbiol. 61, 2425–2429. doi: 10.1099/ijs.0.026146-0
Zhang, X. X., Tang, X., Sheirdil, R. A., Sun, L., and Ma, X. T. (2014). Rhizobium rhizoryzae sp. nov., isolated from rice roots. Int. J. Syst. Evol. Microbiol. 64, 1373–1377. doi: 10.1099/ijs.0.056325-0
Zhao, J., Zhao, X., Wang, J., Gong, Q., Zhang, X., Zhang, G., et al. (2020). Isolation, identification and characterization of endophytic bacterium Rhizobium oryzihabitans sp. nov., from rice root with biotechnological potential in agriculture. Microorganisms 8, 608. doi: 10.3390/microorganisms8040608
Keywords: Rhizobiaceae, Ectorhizobium, cpAAI, UBCG, concatenated protein tree
Citation: Ma T, Xue H, Piao C, Jiang N and Li Y (2023) Phylogenomic reappraisal of the family Rhizobiaceae at the genus and species levels, including the description of Ectorhizobium quercum gen. nov., sp. nov. Front. Microbiol. 14:1207256. doi: 10.3389/fmicb.2023.1207256
Received: 17 April 2023; Accepted: 12 July 2023;
Published: 03 August 2023.
Edited by:
James T. Tambong, Agriculture and Agri-Food Canada (AAFC), CanadaReviewed by:
José David Flores Félix, University of Salamanca, SpainImen Nouioui, German Collection of Microorganisms and Cell Cultures GmbH (DSMZ), Germany
Copyright © 2023 Ma, Xue, Piao, Jiang and Li. This is an open-access article distributed under the terms of the Creative Commons Attribution License (CC BY). The use, distribution or reproduction in other forums is permitted, provided the original author(s) and the copyright owner(s) are credited and that the original publication in this journal is cited, in accordance with accepted academic practice. No use, distribution or reproduction is permitted which does not comply with these terms.
*Correspondence: Yong Li, bHlseDc4QGhvdG1haWwuY29t