- 1Clinical Experimental Center, Xi’an Engineering Technology Research Center for Cardiovascular Active Peptides, the Affiliated Xi’an International Medical Center Hospital, Northwest University, Xi’an, Shaanxi, China
- 2Faculty of Life Science and Medicine, Northwest University, Xi’an, Shaanxi, China
- 3Department of Cardiology, Xi'an International Medical Center Hospital, Xi’an, Shaanxi, China
- 4College of Food Science and Engineering, Northwest University, Xi’an, Shaanxi, China
Diabetic nephropathy (DN) is a severe microvascular complication of diabetes, which increases the risk of renal failure and causes a high global disease burden. Due to the lack of sustainable treatment, DN has become the primary cause of end-stage renal disease worldwide. Gut microbiota and its metabolites exert critical regulatory functions in maintaining host health and are associated with many pathogenesis of aging-related chronic diseases. Currently, the theory gut–kidney axis has opened a novel angle to understand the relationship between gut microbiota and multiple kidney diseases. In recent years, accumulating evidence has revealed that the gut microbiota and their metabolites play an essential role in the pathophysiologic processes of DN through the gut–kidney axis. In this review, we summarize the current investigations of gut microbiota and microbial metabolites involvement in the progression of DN, and further discuss the potential gut microbiota-targeted therapeutic approaches for DN.
1. Introduction
Diabetes is an age-related disease, which results in high morbidity and mortality worldwide with an estimated morbidity rate of more than 10.5% in 2021 (Sun H. et al., 2022). Nowadays, the prevalence of diabets is increasing dramatically with the aging of the population (Hamzé et al., 2022). Diabetic nephropathy (DN), kidney injury due to diabetes, is the most common complications of diabetes, occurring in approximately 40% of people with diabetes (Chen et al., 2023). The number of people with diabetes is expected to increase from 537 to783 million over the next 24 years causing a rise in global DN prevalence (Tuttle et al., 2022). To date, the therapeutic approaches mainly include the lifestyle intervention and medication to control blood pressure and hyperglycemia (Warren et al., 2019). In addition, it is reported that the proportion of end-stage renal disease (ESRD) caused by DN is nearly 38.8, 23 and 31.2% in America China and India, respectively (Hussain et al., 2019; Zhang et al., 2020; Johansen et al., 2021). Once DN evolves into ESRD, it requires treatment with renal replacement therapy, which leads to high mortality and huge socioeconomic burden (Rustiasari and Roelofs, 2022). Therefore, new alternative strategies to protect against DN are urgently needed.
Recently, the gut microbiota has become a hotspot as a potential participant in the onset and progression of DN. The gut microbiota is represented by trillions of microorganisms, including bacteria, viruses, fungi and archaea in the intestine of humans (Fernandes et al., 2022), which exerts many functions of digesting food (Naimi et al., 2021), regulating the immune system (Spindler et al., 2022), synthesizing vitamins (Zafar and Saier, 2021), removing pathogens (Silwal et al., 2021) and maintaining gut function (Sun R. Y. et al., 2022). The gut microbiota of healthy adults belongs to Firmicutes, Bacteroidetes, Proteobacteria and Actinobacteria, of which Firmicutes and Bacteroidetes are dominant with a proportion of approximately 90% (Eckburg et al., 2005). Under the normal physiological circumstances, the gut microbiota has a relatively stable species and numbers to protect the structure of intestinal mucosal barrier (Lei et al., 2022). However, the intestinal barrier can be damaged, once the homeostasis of gut microbiota is disrupted, which may potentially increase the risk of developing DN.
The gut microbial dysbiosis may cause the endotoxin and pathogen to cross the intestinal barrier, resulting in the inflammation and oxidative stress, and further accelerating kidney damage (Su et al., 2022). In addition, the gut microbial dysbiosis may lead to the alteration of microbial metabolites, which are considered as important substances to regulate life activity and metabolism, and participant in the onset and progression of various diseases (Jaye et al., 2022). In recent years, the application of high-throughput sequencing technology helps us to better understand the relationship between microbiome and its host (Jo et al., 2020). The gut microbiota and microbial metabolites may have pathogenic or beneficial effects on the host (Zheng et al., 2021). Herein, we reviewed the recent investigations and highlighted the alteration and important regulatory function of gut microbiota and their metabolites in the onset and progression of DN. Besides, novel strategies associated with the microbiota-targeted therapies, which will pave the way toward new renoprotective studies for DN, are further discussed.
2. The pathophysiology of DN
Diabetic nephropathy is characterized by glomerular basement membrane thickening, mesangial cell hypertrophy, podocyte loss, glomerulosclerosis and tubulointerstitial fibrosis, which eventually lead to progressive albuminuria and decline in estimated glomerular filtration rate (eGFR; Forst et al., 2022). The pathogenesis of DN is complex, including the disturbance of glycometabolism, abnormal hemodynamics, inflammation and oxidative stress. These mechanisms are interrelated, which together result in the progression of DN (Figure 1; Samsu, 2021).
2.1. Disturbance of glycometabolism
The disturbance of glycometabolism is the major characteristics of patients with DN, which mainly involves advanced glycation end products (AGEs) pathway, protein kinase C (PKC) pathway and polyol pathway (Jung and Yoo, 2022). AGEs are glycated products between reducing sugars and biomacromolecules (proteins, lipids, and nucleic acids) through a serious of non-enzymatic reactions (Ying et al., 2021). AGEs can directly damage the kidney, as well as cause the activation of various growth factors and cytokines by binding to the receptor of AGEs (RAGE). The persistent hyperglycemia in diabetic patients leads to the massive deposition of AGEs in endothelial cells, glomerular basement membrane and podocytes, directly damaging the normal structure of the glomerulus, impairing filtration function, and leading to the production of proteinuria (Wu et al., 2021). On the other hand, the accumulation of AGEs can bind to RAGE in kidney cells, which further leads to the intracellular inflammation and oxidative stress signaling pathways, such as nuclear factor-κB (NF-κB), phosphatidylinositide 3-kinase/protein kinase B (PI3K/Akt) and mitogen-activated protein kinase/extracellular signal-regulated kinase (MAPK/ERK), etc. In turn, oxidative stress and chronic inflammation promotes the generation of AGEs in kidney cells under hyperglycemic circumstances causing further aggravation of renal damage. The positive feedback regulation between AGEs/RAGE and downstream pathways results in a vicious cycle during the onset and progression of DN (Wu et al., 2021). Additionally, AGEs can directly activate the complement system and trigger pro-inflammatory, and causes mesangial cells, endothelial cells and podocytes to secrete inflammatory mediators such as cytokines, chemokines, and adhesion molecules. Whereafter, the monocytes and macrophages were activated and recruited, resulting in further inflammatory cascade response, ultimately leading to renal injury and tubulointerstitial fibrosis (Chen et al., 2022). PKC is abundant in human tissues, and participating in regulating cell proliferation, differentiation and apoptosis. The increase of intracellular blood glucose level leads to the increase of diacylglycerol (DG) synthesis and activation of PKC, thus inducing the production of the signal molecules such as endothelin-1 (ET-1), transforming growth factor-β1 (TGF-β1), interleukin-1 (IL-1) and reactive oxygen species (ROS) in kidney cells. Consequently, the increase in ET-1 results in the injury of endothelial cells. The overproduction of TGF-β1 leads to the accumulation of glomerular extracellular matrix and the hypertrophy of kidney cells. The overexpression of IL-1 and ROS causes the inflammation and oxidative stress in the kidney. These results collectively lead to the alteration in glomerular basement membrane structure, and increases of the glomerular capillary permeability and glomerular injury (Sheng et al., 2018). Additionally, the glucose can be converted into sorbitol by aldose reductase (AR) via polyol pathway under the diabetic circumstance. Sorbitol is prone to accumulate in kidney cells causing permeability alteration, cell swelling and rupture, which ultimately aggravate mesangial cells hypertrophy, glomerulosclerosis and tubulointerstitial fibrosis (Shen and Wang, 2021).
2.2. Hemodynamics
Abnormal hemodynamics is also an important cause of DN (Yang and Xu, 2022). The renin-angiotensin-aldosterone system (RAAS) has been shown to play an important role in the progression of kidney disease. The activation of RAAS leads to the changes of intrarenal hemodynamics, which further causes the structural changes of glomerulus and tubulointerstitium (Nishiyama and Kobori, 2018). Podocytes have been proved to produce many RAAS components and express its receptors. RAAS regulates systemic blood pressure through a homeostatic feedback loop under normal circumstances. As a key component of RAAS, angiotensin II (ANG-II) mainly plays a role in the kidney (Silva et al., 2021). The RAAS is over-activated, the ANG-II and aldosterone are increased under the hyperglycemic circumstance, which leads to the elevation of blood pressure and the destruction of sodium balance. Chronic glomerular hypertension, hyperperfusion and hyperfiltration result in glomerular sclerosis and renal interstitial fibrosis (Malek et al., 2021). Intrarenal Ang-II is a cytokine that contributes to kidney damage by multiple pathways, including increasing glomerular capillary permeability, stimulating mesangial cell hypertrophy, inducing ECM synthesis, as well as promoting macrophage and inflammatory cells infiltration (Samsu, 2021). Ang-II can also activate DG-PKC pathway, and induce ET-1 and ROS generation causing vasoconstriction and lipid peroxidation to deposit on glomerular intima. The disturbance of lipid metabolism results in the atherosclerosis and atheromatous plaque formation, which leads to the decrease of blood flow velocity and the increase of blood viscosity, thus causing glomerular hemodynamic abnormalities (Heilig et al., 2013; Roscioni et al., 2014). Additionally, as a potent vasoconstrictor of the efferent arteriole, the increase of ET-1 promotes the elevation of intrarenal blood pressure, which causes mesangial cells hypertrophy and ECM accumulation. Meanwhile, ET-1 can also increase the glomerular permeability by targeting its receptor, resulting in the aggravating albuminuria and DN progression (Ergul, 2011). The mechanical strain and shear stress, resulting from altered hemodynamics, lead to endothelial cell and epithelial cell injury, which accelerates the occurrence and development of proteinuria (Bai et al., 2022).
2.3. Inflammation
Hyperglycemia, metabolic disorder and abnormal hemodynamics can cause cellular injury and induce the inflammatory mediators, including tumor necrosis factor-α (TNF-α), interleukin-6 (IL-6), chemokines and adhesion molecules, etc. This leads to the activation and recruitment of the macrophages and monocytes to the kidney. The accumulation of macrophages produces ROS, pro-inflammatory factors, chemokines and metalloproteinases, which further aggravated kidney damage (DeFronzo et al., 2021; Jung and Yoo, 2022). DN inflammation is a complex integration of innate immune responses, in which macrophages are the main immune cells involved in DN renal injury, with two activation states: M1 and M2. Macrophages in M1 state release inflammatory factors and promote inflammation. Macrophages in M2 state are involved in anti-inflammation response and tissue repair. The activation level of M1 and M2 macrophages is closely associated with tissue microenvironment, disease status, etc. (Landis et al., 2018). Cytokines, such as TNF-α and IL-6, are essential pathogenic factor of DN. The mast cells can secrete TNF-α to induce renal injury when the inflammatory lesions occur in the renal. TNF-α can activate the apoptotic signaling pathway by targeting TNFR1 or activate downstream NF-κB to induce the production of other cytokines by targeting TNFR2 (Sun and Kanwar, 2015). Moreover, the combination of TNF-α and TNFR2 can also promote the production of ROS, which induced microvascular endothelial cell damage, reduced barrier function, and led to the production of proteinuria. IL-6 can act on interstitial cells near capillaries and release collagenase and other extracellular proteases, which leads to the degradation and leakage of glucoproteinases and the occurrence of microalbuminuria, thus promoting the occurrence and development of DN (Mansoor et al., 2022). Chemokines, such as monocyte chemoattractant protein 1 (MCP-1) can cause glomerular basement membrane injury and stimulate TGF-β1 to participate in glomerular sclerosis and tubulointerstitial fibrosis (Xie et al., 2022). Adhesion molecules, such as intracellular adhesion molecule 1 (ICAM-1) can aggregate inflammatory cells through kinase reaction and cause inflammatory response. In addition, inflammation and the pathophysiology of DN involve complex signaling pathway activation (Bui et al., 2020), such as NF-κB pathway, The janus kinase/signal transducers and activators of transcription (JAK–STAT) pathway and nod-like receptor (NLR) family pyrin domain containing 3 (NLRP3) inflammasome pathway. NF-κB, a transcription factor that involved in inflammatory response, can be activated by hyperglycemic conditions, leading to RAAS activation, AGEs accumulation and nicotinamide adenine dinucleotide phosphate (NADPH)-dependent oxidative stress. NF-κB activation is related to proteinuria, which can further stimulate NF-κB activation and form a vicious circle between NF-κB and proteinuria (Mezzano et al., 2004). The JAK–STAT is a critical pathway that responds to and mediates inflammatory molecules, which is significantly upregulated in glomerular cells of DN patients and is negatively associated with eGFR. Meanwhile, NF-κB can be activated by JAK–STAT signaling and further induced the transcription of proinflammatory factors, chemokines and adhesion molecules, etc. (Navarro-Gonzalez et al., 2011). NLRP3 inflammasome, a crucial regulator of chronic inflammatory response, is activated under the hyperglycemic circumstances. The activated NLRP3 inflammasome further mediates glomerular and tubular injury via releasing various inflammatory cytokines (Williams et al., 2022).
2.4. Oxidative stress
When the organism suffers from harmful stimulation, it will produce excessive ROS, which causes the imbalance of between the oxidation system and the antioxidant system and induce oxidative stress (Su et al., 2023). In DN, increase ROS production due to hyperglycemia can cause oxidative stress, which can directly damage podocytes, mesangial cells and endothelial cells, and lead to albuminuria and tubulo-interstitial fibrosis. Nicotinamide adenine phosphate dehydrogenase (NADPH) is an important source of ROS in diabetes. NADPH oxidase 4 (Nox 4) is the major isoform, which can be increased by hyperglycemia in the kidney (Pavlov et al., 2020). Hyperglycemia can also lead to glycosylation of antioxidant enzymes, which reduces enzyme activity and weakens the ability of the organism to clear free radicals. Oxidative stress interacts with almost every pathogenesis of DN. The combination of AGEs and RAGEs, PKC activation, polyol pathway and Ang-II can stimulate ROS production and induce oxidative stress (Tanase et al., 2022). Meanwhile, oxidative stress can cause kidney damage by activating other signal molecules such as Ang-II, PKC and TGF-β. In turn, the activation of these signaling molecules can cause oxidative stress and then induce renal injury (Samsu, 2021). Of note, Oxidative stress is closely associated with inflammatory response. In DN, ROS can activate NF-κB pathway to induce a large number of inflammatory mediators and trigger inflammatory response, which in turn aggravates oxidative stress damage (Winiarska et al., 2021). High glucose-induced oxidative stress can also cause macrophage infiltration that secretes pro-inflammatory factors and results in glomerular inflammation (Tuttle et al., 2022). Nuclear factor E2-related factor 2 (Nrf2) is an essential regulator of oxidative stress, which has attracted extensive attention in the antioxidant mechanism of DN in recent years. Nrf2 regulates the expression of antioxidant genes through Nrf2/KEAP1/ARE pathway, thus alleviating oxidative stress in DN (Tanase et al., 2022). The increased ROS inhibited the activation of Nrf2, and antioxidant enzymes, such as superoxide dismutase (SOD)-1, heme oxygenase (HO)-1 and catalase (CAT; Huang Q. H. et al., 2017). Studies have shown that knockout of Nrf2 gene in type 2 diabetic mice will aggravate the oxidative stress-induced renal injury (Liu Y. X. et al., 2022).
3. The roles of gut microbiota in DN
3.1. The gut–kidney axis
In 2011, scientists first linked the kidney and gut, and clarify the influence of gut on chronic kidney disease (CKD), generally viewed as gut–kidney axis (Figure 2; Meijers and Evenepoel, 2011). Over the past decades, an increasing number of evidence has demonstrated that there is a bidirectional crosstalk between gut and kidney, namely, the pathophysiological alterations in the intestinal tract or the kidney can affect each other, resulting in lesions on the other side (Lobel et al., 2020). For example, due to the gut microbial dysbiosis, the translocation of intestinal microorganism may occur from gut to the kidney causing kidney damage (Stavropoulou et al., 2021). A latest study indicated that the renal injury of CKD rats can be effectively alleviated by regulating gut microbial dysbiosis and improving gut barrier (Hu et al., 2023). On the other hand, kidney diseases-associated gut microbial dysbiosis may lead to the impairment of gut barrier (Sun X. et al., 2022). Many nitrogen-containing organics released by impaired renal may across the gut barrier and promote the growth of intestinal pathogens. The metabolic wastes that cannot excrete by the kidney are prone to enter the intestunal lumen, thereby aggravating the gut microbial dysbiosis (Ni et al., 2022). Kidney diseases can also causes the alteration of gut microbiota composition. A systemic review have revealed that the α-diversity of the gut microbiota was significantly reduced and the β-diversity was more distinct in ESRD patients than healthy controls by recruiting 1,436 CKD patients and 918 healthy controls (Zhao et al., 2021). In addition, gut microbiota may also influence the progression of kidney diseases through microbial metabolites. For instance, (1) short-chain fatty acid (SCFAs) can promote immunosuppression and developing regulatory T cells (Tregs; Dang et al., 2021). Clinical investigations demonstrated that the Treg level in in peripheral blood of DN patients is lower than that of diabetic patients without kidney diseases (Wang M. et al., 2018). (2) Some uremic solutes, such as indoxyl sulfate (IS) and p-cresyl sulfate (PCS) may cause the deterioration of renal function through AHR activation (Hobby et al., 2019). (3) Trimethylamine-N-oxide (TMAO) is a well-known risk factor for CKD (Zixin et al., 2022). In short, kidney diseases and gut flora disorder can affect each other in a vicious circle. To clarify the underlying mechanism of this interconnection may contribute to understand disease etiologies and pathogenesis.
3.2. The disruption of intestinal barrier in DN
The intestinal tract is the largest tissue and organ that absorb water and nutrients. The intestinal barrier on the inner surface of the intestinal tract provides effective protection for the body against the invasion of harmful substances and pathogens in the external environment (Niewiem and Grzybowska-Chlebowczyk, 2022). The gut microbial dysbiosis in DN patients can cause increased intestinal permeability and damaged intestinal barrier, namely leaky gut (Linh et al., 2022). In particular, due to the decline of eGFR in DN patients, a large amount of ammonia generated by the hydrolysis of urea are reabsorbed by renal tubules, and subsequently enter the liver to resynthesize urea. The increased urea induces the decreased expression of tight junction proteins, including claudin-1, occludin and zonula occludens-1 (ZO-1), which results in the disruption of intestinal epithelial tight junction (Vaziri et al., 2013). The gut microorganism can enter the underlying tissue compartment due to the breakdown of intestinal epithelial tight junction, and further accelerating the intestinal barrier damage (Hu et al., 2022). Also, ammonia can change the diversity of gut microbiota, and destroy the composition and function by increasing intestinal pH (Khan et al., 2021).
Furthermore, toll like receptors (TLR) exert a critical role in the inflammatory process. Extensive evidence has demonstrated that TLR2/TLR4/NF-κB pathway participates in the inflammatory response by promoting pro-inflammatory cytokines (TNF-α, IL-1, IL-6, etc.) in DN (Wang et al., 2019). Lipopolysaccharide (LPS), surface antigen of gram-negative bacteria, leaks into portal circulation due to the breakdown of intestinal barrier, which further causes endotoxemia and inflammation via TLR2/TLR4/NF-κB signaling pathway (Zhang F. et al., 2021). Evidence has shown that TLR4-deficient mice with DN exhibit less NF-κB activation, albuminuria, renal dysfunction and interstitial macrophage infiltration (Lin et al., 2012). Therefore, the disruption of intestinal barrier leads to the binding of LPS to TLRs, thus activating NF-κB signaling pathway is the inflammatory mechanism of DN mediated by gut microbiota. In addition, other microbial metabolites, such as IS, PCS and cresol can also enter the blood due to the increase of intestinal permeability, causing renal damage and accelerating the development of DN (Lv et al., 2022).
3.3. Alterations of gut microbial composition in DN
Based on gut–kidney axis theory, the occurrence of kidney diseases usually results in gut microbial dysbiosis. Overall, the alterations of gut microbial composition in DN are characterized by an increased abundance of pernicious bacteria such as Desulfovibrio and Coprobacillus, and the decrease in probiotics such as Lachnospira and Intestinibacter (Table 1; Sheng et al., 2018; Su et al., 2022; Zhang L. L. et al., 2022). A recent study demonstrated that the intestinal microbiome richness and diversity of DN patients are far less than healthy subjects. Alterations in the abundance of gut microbiota, involving increases of Megasphaera, Acidaminococcus and Lactobacillus and decreases of Tyzzerella_3, Roseburia and Lachnoclostridium at the genus level, were observed in the fecal samples of DN patients (Du et al., 2021). Wang Y. W. et al. (2022) indicated that the enrichment of genus Klebsiella, Citrobacter and Escherichia, and the decrease of Roseburia may make the most contribution to gut microbial dysbiosis in DN by analyzing a large number of fecal samples of DN patients. Additionally, the discrepancies of the abundance and diversity of gut microbiota were also exist in different stages of DN (Du et al., 2021), suggesting that some characterized genera such as Escherichia-Shigella, Megasphaera and Haemophilus have potential to be novel microbial biomarkers for the diagnosis of DN. Tao et al. analyzed the gut microbiota composition of the fecal samples from DN patients, diabetic patients and healthy volunteers, and found that there are significant discrepancies among them. At the phylum to family level, Prevotellaceae is the bacteria with the highest proportion in healthy subjects, while Coriobacteriaceae is the most abundant bacteria in DN patients. The increased abundance of Escherichia-Shigella has been found in DN patients, whereas.
Prevotella_9 is reduced at the genus level. These two genera can accurately distinguish DN patients from diabetic subjects (Tao et al., 2019). In brief, these clinical investigations demonstrated that gut microbiota may serve as predictor for the onset and progression of DN. However, these alterations to the microbial abundance and composition at the phylum, family and genus levels may not sufficient to adequately clarify the changes to the gut microbiota in DN patients, further investigations at the levels of species or strains are necessary in the future.
The Firmicutes/Bacteroidetes (F/B) ratio is relatively stable in healthy mammals, and the elevation or decline of the ratio frequently indicates the disease state. It is reported that the increased F/B ratio is associated with diabetes and obesity (Cai et al., 2020). Several studies indicated that F/B radio is significantly increased in the fecal sample of DN mice or rats (Feng et al., 2019; Yang J. et al., 2020; Su et al., 2022; Zhang Z. P. et al., 2022), while Zhao et al. (2019) reported the opposite trend. These findings suggested that it is not accurate to judge the disease state based simply on the F/B ratio. Moreover, the opposite changes of several genera have also been reported in different studies. For example, Lu et al. reported the increased abundance of Roseburia in DN rats, whereas Sheng et al. reported the decreased trend (Sheng et al., 2018; Lu et al., 2020). These opposite results may partly attributed to colonization at birth, age, gender, diet, and other factors (Gilbert et al., 2018). In particular, the discrepancy in gut microbiota still existed between DN mice with severe proteinuria (SP) and mild proteinuria (MP). The abundance of Allobaculum and Anaerosporobacter in the SP mice was higher than that in the MP mice, while the Blautia was more abundant in MP mice (Li Y. et al., 2020), suggesting that the alteration of gut microorganisms may associated with the DN progression. Blautia may has a beneficial anti-DN role (Hsu et al., 2022), while Allobaculum and Anaerosporobacter may accelerate the deterioration of renal function in DN. Despite the fact that the data from experimental animals cannot completely represent the disease state in humans, these results in animal models provide substantial evidence and supplement for the investigations of gut microbiota in DN patients.
3.4. Correlations between gut microbiota and DN phenotypes
Nowadays, an increasing number of studies have revealed the correlations between gut microbial alterations and DN phenotypes, including renal function, glucose metabolism, lipid metabolism, pathological changes of kidney, inflammatory cytokines and oxidative stress factors. These phenotypes are usually associated with disease progression, suggesting the potential role of gut microbiota in the diagnosis and therapy of DN. In terms of renal function, extensive studies have revealed the correlation between eGFR, serum creatinine (SCR), urinary albumin-to-creatinine ratio (UACR), 24-h urine protein (24-h UP) and gut microbiota in DN patients. For example, Abiotrophia, G_norank_f_Peptococcaceae (Zhang Q. et al., 2021) and Ruminococcus torques group (Chen W. H. et al., 2021) were negatively associated with eGFR in DN patients, while Lachnospiraceae_NC2004_group (Zhang Q. et al., 2021), Verrucomicrobia and Subdoligranulum (Tao et al., 2019) showed a positive correlation. Two specific taxa, including Abiotrophia and G_norank_f_Peptococcaceae (Zhang Q. et al., 2021) were reported to be positively correlated with SCR, while Clostridium sp. 26_22 (Zhang L. L. et al., 2022), Lachnospiraceae_NC2004_group (Zhang Q. et al., 2021) and Parabacteroides (Tao et al., 2019) exhibited a reverse correlation. Additionally, the abundance of Citrobacter farmeri, Syntrophaceticus schinkii (He et al., 2022), Bacteroidetes and Elusimicrobia (Zhao et al., 2020) are increased in DN patients with higher UACR, whereas the abundance of Firmicutes, Bacteroidetes, Bacteroides (Tao et al., 2019) and Actinobacteria (Zhao et al., 2020) are decreased. Negative correlation was also observed between Blautia and 24-h UP (Li Y. et al., 2020) in DN patients, whereas Anaerosporobacter (Li Y., et al., 2020), Abiotrophia (Zhang Q. et al., 2021), Alistipes and Subdoligranulum (Chen W. H. et al., 2021) showed a positive association. These findings suggested that gut microbiota may act as potential biomarker for the detection of renal function in DN patients. Identifying the specific microbiome may shed new light on the diagnosis of DN progress. However, the regulatory mechanisms of the gut microbiome on renal function are not well understood, which limits the further utilize of gut microbiota in DN. In the field of glucose metabolism, glycosylated hemoglobin (HbA1c) and fasting blood glucose (FBG) are often used to evaluate the correlation between intestinal flora and DN. Current investigations revealed that Firmicutes, Faecalibacterium, Lachnoclostridium, Roseburia (Tao et al., 2019) g_norank_f_norank_o_Oscillospirales and g_unclassified_f_Ruminococcaceae (Zhang Q. et al., 2021) were negatively correlated with HbA1c in DN patients. Allobaculum (Li Y. et al., 2020) was positively associated with FBG, while Firmicutes, Fusobacteria and Fusobacterium (Tao et al., 2019) showed a negative association with FBG, which means that the increase in Allobaculum abundance or the decrease in Firmicutes, Fusobacteria and Fusobacterium abundance may lead to the elevated glucose utilization and absorption in DN. Gut microbiota may play a role in the blood glucose control of DN patients as an auxiliary strategy in the future. Escherichia-Shigella was positively associated with body mass index (BMI; Tao et al., 2019). In terms of blood lipids, Bacteroides, Lachnoclostridium and Lachnospiraceae bacterium 3 1 46FAA were positively associated with total cholesterol (TC) in DN patients, whereas L. mucosae was reported to be negatively correlated with low density lipoprotein-cholesterol (LDL-C). Chen et al. demonstrated that Lachnoclostridium, Bacteroides and Parabacteroides were positively associated with triglyceride (TG) by analyzing the fecal sample of DN patients (Chen W. H. et al., 2021; He et al., 2022; Zhang L. L. et al., 2022). Since TC and TG are independent risk factors of in DN (Jansson Sigfrids et al., 2021; Liu L. et al., 2021), low level of TC and TG contribute to the favorable prognosis in DN patients. Nonetheless, there is currently no evidence to prove whether the associations are causal or not. As for renal pathological injury, DN rats with more severe renal tubular injury possess higher abundance of Bacteroidetes and Elusimicrobia, and lower abundance of Firmicutes and Actinobacteria. Elusimicrobia was reported to be positively associated with glomerularsclerosis (Zhao et al., 2020). This is the only study to indicate the relationship between renal pathological injury of DN patients and gut microbiota, and more investigations are needed to clarify their associations. Intriguingly, certain genera of gut microbiota were associated with inflammatory cytokines and oxidative stress factors in renal tissues. For example, Bacteroides was correlated with increased expression of TNF-α in DN mice (Chen Q. et al., 2021), and Allobaculum was associated with increased expression of superoxide dismutase (SOD) in DN rats (Su et al., 2022). Further correlations between gut microbiota and DN phenotypes are detailed in Table 2.
Overall, these studies suggested that the alterations of gut microbial composition and abundance may be associated with DN symptoms or pathological changes. Nevertheless, the causalities between gut microbiota and DN are still confusing, as these cross-sectional studies only focused on one point in time. The longitudinal studies are scarce. Therefore, longitudinal and prospective investigations are imminently required to clarify the cause-effect associations between gut microbiota and DN. In addition, some current studies were performed on animal models and the clinical investigations are relatively lacking. These studies may not correlate well with clinical results and need to be further confirmed in DN patients. Some clinical studies that reveal the correlations between intestinal flora and DN phenotypes only include a few subjects. For example, He et al. indicated the associations between the UACR level and intestinal microbiome by analyzing the fecal sample from 10 DN patients (He et al., 2022). This sample size seems insufficient to identify the correlations. A larger-scale clinical cohort might be warranted to recognize these correlations.
4. The roles of gut microbial metabolites in DN
Changes in gut microbiota can lead to the metabolic alterations in patients with DN. Multiple interactions between gut microbiota and human body are mediated through metabolites generated by intestinal microorganism. Current investigations have focused on revealing the relationship between DN and gut microbial metabolites including SCFAs, bile acids (BAs), TMAO, uremic toxins (UTs) and hydrogen sulfide (H2S). These metabolites affect the barrier function of intestinal epithelium by regulating the expression of receptors or activating transcription factors, thereby exerting critical functions in the onset and progression of DN (Figure 3).
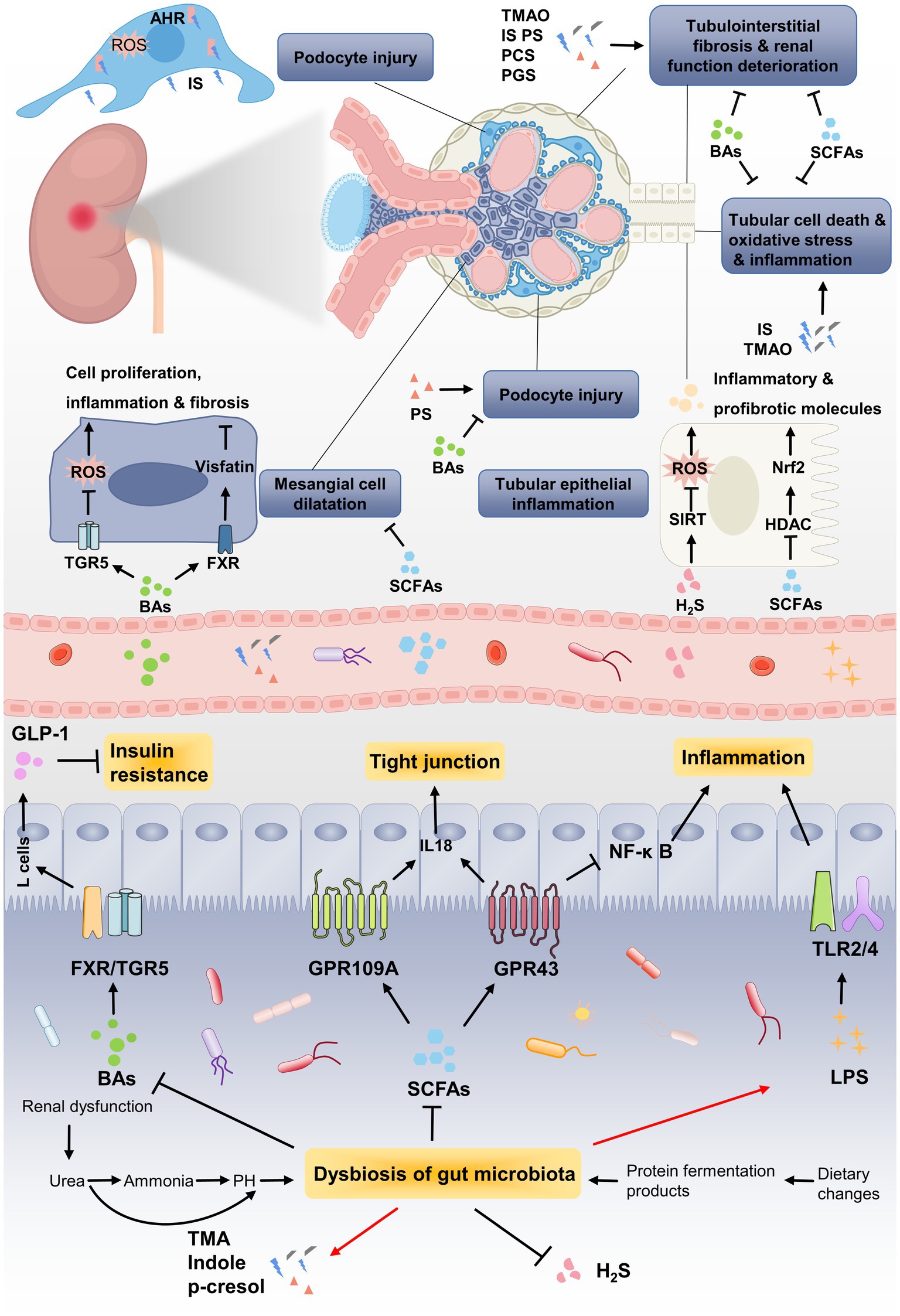
Figure 3. The pathogenic association between gut microbial metabolites and development of DN. Renal dysfunction and dietary changed lead to dysbiosis of gut microbiota, which further results in metabolic disorders of gut microbiota. Due to the decrease in SCFA-producing bacteria, the renoprotective effect of SCFAs through GPRs or HDAC is weakened, such as inflammation, tubulointerstitial fibrosis and glomerular injury. Gut microbiota dysbiosis lead to the reduction of BAs, which improving DN by alleviating mesangial cell dilatation and insulin resistance via targeting its receptors FXR/TGR5. Uremic toxins, including TMAO, IS, phenyl sulfate (PS), PCS and PGS, induces podocytes injury via binding to AHR or accelerates renal function deterioration. The enrichment of LPS caused by gut microbiota dysbiosis induces persistent inflammation by binding to TLRs, which steadily deteriorate DN.
4.1. Short-chain fatty acids
Short-chain fatty acids (SCFAs) are a group of fatty acids with no more than six carbon atoms derived from the microbial fermentation of indigestible dietary fiber in the colon and cecum, mainly including acetate, propionate and butyrate (approximately 90–95%; Lu P. C. et al., 2021; van der Hee and Wells, 2021). Being an essential signal molecules of human body, SCFAs are also involved in the regulation of metabolism by gut microbiota, such as cholesterol biosynthesis, glucose homeostasis and insulin sensitivity (Lee et al., 2020). Therefore, the decreased SCFAs are associated with the pathogenesis of multiple diseases, such as hypertension, hyperlipidemia and type 2 diabetes (Lu et al., 2022).
The perturbations of gut microbiota can lead to the decrease of SCFAs, resulting in the decreased insulin sensitivity and eventually the occurrence of type 2 diabetes (Makki et al., 2023). Recent studies have found that the fecal level of total SCFAs, acetate, propionate, butyrate and isovalerate are significantly decreased in DN patients. Serum and fecal SCFAs levels may be negatively correlated with renal function (Zhong et al., 2021; Li Y. et al., 2022). Mechanistically, gut microbiota-derived SCFAs exert their functions by activating trans-membrane G-protein-coupled receptors (GPCRs) of GPR41 and GPR43 or inhibiting histone acetylation (HDAC) to directly enter host cells (Moniri and Farah, 2021). SCFAs (acetate and butyrate) or GPR43 agonist can retard the HG-and LPS-induced glomerular mesangial cells (GMCs) proliferation, and then inhibit oxidative stress and inflammation. Exogenous SCFAs (especially butyrate) significantly ameliorated hyperglycemia and insulin resistance, improved renal function and inhibited renal fibrosis in high-fat diet (HFD) and streptozotocin (STZ)-induced DN mice via inhibiting GPR43-mediated NF-κ B signaling and oxidative stress (Huang W. et al., 2017; Huang et al., 2020). Meanwhile, Li Y. J. et al. (2020) demonstrated that dietary fiber can effectively ameliorate DN by increasing SCFAs-mediated activation of GPR43 and GPR109A. Sodium butyrate (NaB), an inhibitor of HDAC activity, is the most widely studied SCFA salt against DN (Gu et al., 2019). NaB supplementation can restore the composition of gut microbiota and enhance gut epithelial barrier integrity in diabetic mice (Xu et al., 2018). In addition, exogenous NaB administration ameliorated renal function, glucose and lipid metabolic disturbance, inflammation, fibrosis and oxidative injury by activating Nrf2 via inhibiting the activity of HDAC in DN mice (Dong et al., 2017; Du et al., 2020; Zhou et al., 2022). These findings suggested that SCFAs can improve DN by inhibiting oxidative stress, inflammation and renal fibrosis. SCFAs, especially butyrate, may serve as promising targets for the treatment of DN.
However, although SCFAs mostly exhibit a protective role in the progression of DN, some SCFAs can also bring about renal damage, if not properly regulated. For example, Hu et al. indicated that acetate can induce the disorder of cholesterol homeostasis by activating GPR43, thereby causing tubulointerstitial injury in DN (Hu et al., 2020). Moreover, it has been reported that excessive plasma acetate may result in renal injury by activating intrarenal renin-angiotensin system (RAS) in early DN rats (Lu et al., 2020). Therefore, the potential impacts of SCFAs in DN still need further study. The clinical and animal experiments of SCFAs in kidney diseases are still preliminary, and their underlying mechanisms in kidney diseases need to be confirmed by more studies.
4.2. Bile acids
Bile acids (BAs), major component of bile, are synthesized from cholesterol in the liver and transported to intestine (Perino et al., 2021). The primary BAs are then metabolized and transformed by the gut microbiota through a series of reactions, including deconjugation, dehydroxylation, oxidation and epimerization, to generate secondary BAs (Cai et al., 2022; Luo W. et al., 2022). Among them, Firmicutes, Bacteroides, Lactobacillus, Bifidobacterium and Clostridium play essential roles in the generation of secondary BAs (Lee et al., 2022). Most of these secondary BAs reenter the liver through the enterohepatic circulation, and the rest are excreted into feces (Bertolini et al., 2022). As critical signaling molecules, BAs have been confirmed to perform multiple biological functions, such as controlling energy (Wang S. P. et al., 2022), glucose (Makki et al., 2023) and lipid metabolism (Xu et al., 2022) and regulating immune system (Fuchs et al., 2022).
BAs significantly affect host metabolism by activating BA receptors, including the farnesoid X receptor (FXR) and the takeda G protein-coupled receptor 5 (TGR5; Thibaut and Bindels, 2022). Both FXR and TGR5 are highly expressed in the normal kidney tissues, but downregulated in DN, and the degree of decrease is associated with the inflammation and fibrosis (Wang X. X. X. et al., 2018). The renoprotective effect of FXR is mainly mediated by regulating lipid metabolism, oxidative stress, pro-inflammatory cytokines, and pro-fibrotic factors, thus inhibiting the increased proteinuria, podocyte loss, mesangial expansion and renal lipid accumulation (Masaoutis and Theocharis, 2019). In STZ-induced diabetic mice, knockout of FXR exacerbated diabetic kidney injury, increased the level of plasma lipid and accelerated fibrosis compared with diabetic wild-type mice (Wang et al., 2010). Oppositely, FXR agonist GW4064 inhibited the inflammation, fibrosis and cell proliferation by downregulating visfatin in HG-induced HMCs. Moreover, GW4064 reduced the level of blood glucose, albuminuria, BUN and Scr, attenuated glomerular injury and fibrosis in db/db mice, suggesting that FXR activation suppressed the progression of DN (Zhou et al., 2016).
TGR5, a membrane receptor activated by BAs, can directly regulate podocyte function. Evidence has shown that the renoprotective mechanisms of TGR5 activation mainly include inducing mitochondrial biogenesis, inhibiting oxidative stress and preventing renal lipid accumulation (Wang et al., 2016). Moreover, TGR5 activation induced the release of ileac glucagon-like peptide-1 (GLP-1; Ding et al., 2021), suggesting the potential therapeutic prospect of BAs against DN. In diabetic db/db mice, treatment with TGR5 agonist INT-777 significantly decreased proteinuria, glomerular mesangial expansion, glomerular podocyte injury and the accumulation of extracellular matrix proteins and macrophage (Wang et al., 2016). Consistently, Xiao et al. (2020) revealed that TGR5 activation alleviated pathological progression of DN by repressing inflammation through NF-κ B pathway. In vitro studies have also showed that the activation of TGR5 profoundly suppressed the expression of TGF-β1 and fibronectin (FN) in HG-induced GMCs, which can both promote renal fibrosis (Xiong et al., 2016; Yang et al., 2016).
Taken together, the activation of BA receptors showed a promising prospect in the prevention and therapy of diabetic kidney injury. FXR and TGR5 may play a synergistic effect in ameliorate kidney injury, inflammation and fibrosis. Therefore, FXR/TGR5 dual agonist may exhibit additional effects in the treatment of DN (Wang X. X. X. et al., 2018). BA receptors are expected to be therapeutic targets for the treatment of DN. BAs analogues may be a novel direction for the development of new drugs against DN.
4.3. Trimethylamine-N-oxide
Trimethylamine-N-oxide (TMAO) is mainly derived from dietary choline, L-carnitine and phosphatidylcholine, transforming these dietary nutrients to trimethylamine (TMA) through intestinal bacteria and further metabolized into TMAO by hepatic flavin monooxygenase 3 (FMO3; Yoo et al., 2021). In vivo studies have identified several microbiota associated with TMA/TMAO generation, including Clostridium hathewayi, Providencia alcalifaciens, Escherichia fergusonii and Providencia rustigiani (Romano et al., 2015). A large body of evidence has demonstrated that TMAO has served as a novel risk factor for diverse diseases, such as cardiovascular disease (Zhu et al., 2016), kidney disease (Chang et al., 2021) and type 2 diabetes mellitus (Li S.-Y. et al., 2022). Recently, the increased level of plasma TMAO has been reported to contribute to renal dysfunction and TMAO is reversely correlated with eGFR in the patients with type 2 diabetes. The gut microbiota of patients with type 2 diabetes is perturbed and the plasma TMAO level is elevated, which lead to the decrease of eGFR and the deterioration of renal function. The decreased eGFR causes further elevation of plasma TMAO (Adachi et al., 2019; Yu et al., 2022), suggesting that TMAO may serve as a biomarker associated with the occurrence of kidney diseases. Fang et al. indicated that the plasma level of TMAO is significantly higher in DN rats. Compared with DN rats on a normal diet, treatment with TMAO significantly exacerbated the renal function, and accelerated fibrosis and inflammation in DN rats (Fang et al., 2021). However, the addition of iodomethylcholine, a gut microbial choline TMA-lyase mechanism-based inhibitor, significantly reduced the plasma level of TMAO, ameliorated renal function and inhibited renal tubulointerstitial fibrosis in isoproterenol-induced CKD rats. Of note, iodomethylcholine also improved the choline diet-induced abnormal changed of gut microbial community, including Lactobacillus, Bacteroides and Lachnospiraceae_UCG-002 (Gupta et al., 2020). These findings indicated that TMAO may be involved in the onset and development of kidney diseases as a biomarker of impaired kidney function. Reducing gut microbiota-dependent TMAO production may be a potential strategy for the treatment of DN. Despite the level of TMAO was proved to be positively associated with the progression of kidney disease, the TMAO receptor is still unknown, which may limit further investigation on TMAO targeted therapy. Researchers should pay attention to this field in the future studies.
4.4. Uremic toxins
Gut microbial metabolism derived UTs associated with kidney diseases are usually bound to protein, resulting in their little filtration by the glomerular barrier. Therefore, the accumulation of these UTs is the characteristic of decreased renal function. Relevant study has shown that the level of plasma UTs is elevated in patients with type 2 diabetes, which increase the risk of type 2 diabetes progressing to ESRD (Niewczas et al., 2014). Currently, IS, PS, pCS and p-cresyl glucuronide (pCG) are the mostly well-studied UTs in kidney diseases.
IS belongs to the indole class of UTs, which is originated from tryptophan metabolism by gut bacteria, including Bacteroides ovatus, Clostridium limosum, Enterococcus faecalis and Escherichia coli (Liu J. R. et al., 2021). IS, a ligand of aryl hydrocarbon receptor (AHR), plays a pivotal role in regulating podocyte function. The persistent activation of AHR by high-level IS can lead to the injury of podocytes and glomeruli (Tan et al., 2022). Relevant investigations suggested that the serum IS level is significantly increased in the patients with early stage of DN and correlated with renal function, inflammation and coronary atherosclerosis (Atoh et al., 2009; Chiu et al., 2010). Additionally, IS can also induce tubular cell death, oxidative stress, fibrosis and contribute to CKD progression. The low clearance rate of serum IS is significantly related to the mortality of advanced CKD patients. AST-120, a typical uremic toxin adsorbents, can reduce the serum level of IS and ameliorate the renal function by attenuating renal tubular injury and oxidative stress induced by IS (Cheng et al., 2020). PS is a uremic toxin synthesized from the gut microbial metabolites phenol, which enters the liver and undergoes a sulfate reaction. The intestinal microbes associated with phenol generation include Clostridiaceae, Enterococcaceae, Staphylococcaceae, Enterobacteriaceae, Bifidobacteriaceae and Bacteroidaceae (Smith and Macfarlane, 1996). The plasma PS level is associated with albumin-to-creatinine ratio (ACR) and 2-year progression of patients with DN. Moreover, administration of PS elicits albuminuria and podocyte injury in diabetic mice suggesting that PS, as a factor leading to kidney damage and proteinuria, may be a disease marker and therapeutic target for DN (Kikuchi et al., 2019). pCS and pCG are derived from tyrosine by intestinal bacteria through a series of deamination, transamination, and decarboxylation reactions (Gryp et al., 2017). Both pCS and pCG are markedly increased in the serum of patients with CKD, which contribute to CKD progression by disrupting renal tubule cell phenotype and functionality (Mutsaers et al., 2015; Wei et al., 2022). These findings suggested that UTs are not only the biomarkers for the occurrence of DN, but also the risk factors to promote the progression of DN. Reducing the generation of UTs by modulating gut microbiome can protect against DN. Nevertheless, the mechanism of UTs is not completely clear at present, and the approach of removing one UT may not be applicable to other UTs. The precise mechanisms of UTs in DN are still worth further exploration.
4.5. Hydrogen sulfide
H2S, an essential gaseous signaling molecule, is produced by the fermentation of sulfate or cysteine by sulfate-reducing bacteria in the gut lumen (Gui et al., 2021) and is involved in the regulation of multiple pathological processes, including inflammation (Wang L. et al., 2022), oxidative stress (Wang L. et al., 2022), endoplasmic reticulum stress (Zhao et al., 2022) and immunomodulation (Dilek et al., 2020). More recently, the function of H2S in renal physiology and disease states has attracted extensive attention. The potential roles of H2S in the regulation of eGFR, sodium absorption, renin release, and oxygen sensing in the renal system have been reported (Feng et al., 2022). Li et al. (2014) demonstrated that the level of plasma H2S is significantly lower in chronic hemodialysis patients with DN. The H2S deficiency contributes to the decline of kidney function and pathological status, with exogenous H2S can obviously improve DN (Zeng et al., 2016). For example, treatment with NaHS, a H2S donor, for 4 weeks significantly prevented the increase of blood glucose concentration and the body weight in STZ-induced diabetic rats. Meanwhile, NaHS significantly improved the renal function, and attenuated inflammatory cells infiltration, tubular atrophy and interstitial fibrosis in DN rats (Elbassuoni et al., 2020; Lodhi et al., 2021). Zhou et al. (2014) indicated that NaHS treatment reduced the release of inflammatory cytokines by inhibiting NF-κB activity in the kidney tissues of DN rats, suggesting that H2S can alleviate inflammatory response in DN. In addition, H2S can also ameliorate DN by inhibiting oxidative stress caused by hyperglycemia (Lodhi et al., 2021). Studies have found that intraperitoneal injection of NaHS alleviated DN by decreasing ROS generation, Nrf2 expression and MDA activity, and increasing SOD activity in the renal tissues of STZ-induced DN rats (Zhou et al., 2014; Ahmed et al., 2019). NaHS treatment increased the activity of sirtuin-1 (SIRT1) that exerts prominent renoprotective effect in various kidney diseases by regulating autophagy, lipid metabolism, apoptosis and sodium balance (Zhong et al., 2018; Ahmed et al., 2019). Particularly, extensive evidence has revealed that H2S can improve abnormal hemodynamics in DN. Exogenous H2S was proved to inhibit the activation of RAS in the kidney tissues of diabetic rats (Zhou et al., 2014). In diabetic mice, H2S significantly increased the blood flow, promoted the dilation of the renal peritubular capillary and improved diabetic renovascular remodeling (Yamamoto et al., 2013; Kundu et al., 2015). Moreover, exogenous H2S significantly lowered blood pressure and inhibited arterial medial calcification in STZ-induced DN rats (Ahmad et al., 2012; Wang F. Z. et al., 2021). These results emphasized the protective effects of H2S in DN. However, despite an increasing number of studies demonstrated that H2S, as a gut microbial metabolite, can effectively protect against DN through multiple routes, the clinical translation of these results is limited. The investigations on the biological effects of H2S in kidney contribute to understand the pathophysiology of DN and provide scientific basis for the development of new drugs based on H2S to treat DN.
5. Gut microbiota-targeted DN therapies
Currently, the treatment of DN mainly includes lifestyle intervention, and the control of blood pressure, lipids, glucose and albuminuria (Wang J. M. et al., 2021). However, due to the complexity of the pathogenesis of DN, there is still a lack of standardized treatment. As discussed above, gut microbiota exerts a critical role in the onset and progression of DN (Table 3). The targeted intervention of gut microbiota by fecal microbiota transplantation (FMT), probiotics and prebiotics is expected to be a new strategy in the prevention and treatment of DN (Figure 4).
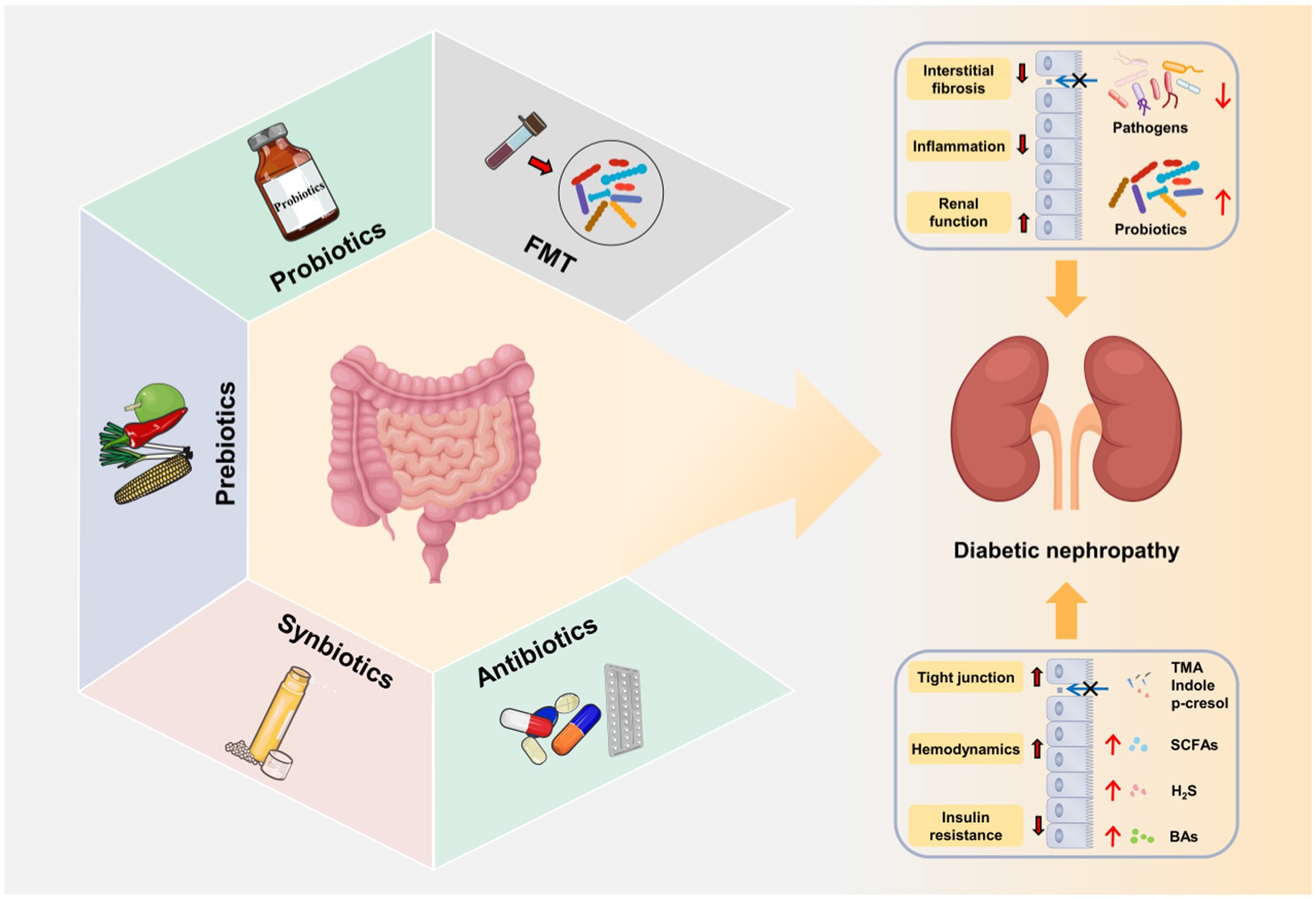
Figure 4. Gut microbiota-targeted DN therapies. Fecal microbiota transplantation (FMT), probiotics, prebiotics, synbiotics and antibiotics improve gut microbial dysbiosis, increase the release of beneficial metabolites (SCFAs, BAs, and H2S), decrease the production of harmful metabolites (LPS, TMAO, IS, and PCS) and repair intestinal barrier. The restoration of gut microbial microbial homeostasis further alleviates the progression of DN through gut–kidney axis.
5.1. Fecal microbiota transplantation
Fecal microbiota transplantation (FMT) is an emerging therapeutic method that transplants the functional microbiota from healthy donors into the gastrointestinal tract of patients with pathological microbiota (Waller et al., 2022). As a promising microbiome-based method, FMT is mainly used to treat intestinal diseases (Waller et al., 2022) and metabolic diseases (Qu et al., 2022). To date, the investigations of FMT in DN are relatively lacking, although thousands of patients worldwide have received FMT therapy.
In preclinical studies, FMT was administrated from healthy control rats to STZ-induced diabetic rats. The results showed that FMT significantly decreased serum acetate level, restored the cholesterol homeostasis, improved podocyte insulin sensitivity, and attenuated tubulointerstitial and glomerular injury (Hu et al., 2020; Lu J. et al., 2021). Consistently, FMT was found to alleviated hyperglycemia, improved insulin resistance and injured islets and inhibited inflammation in STZ-induced type 2 diabetes (Wang et al., 2020). Moreover, another study reported that resveratrol, a polyphenolic compound, can improve DN by restoring gut microbiota homeostasis. FMT from resveratrol-treated mice to diabetic mice significantly inhibited inflammation, and improved renal function and intestinal characteristics (Cai et al., 2020). Most recently, further study confirmed the safety and efficacy of FMT method in the treatment of DN (Bastos et al., 2022). Of note, DN mice received FMT from mice with severe proteinuria showed higher concentrations of LPS, TMAO and 24-h UP, lower levels of propionic acid and butyric acid, and different gut microbiome composition compared to those receiving FMT from mice with mild proteinuria, which further suggested the important role of gut microbiome in the development of DN and the possibility of FMT in the treatment of DN (Li Y. et al., 2020).
Given that FMT technique can potently ameliorate DN by regulating the dysregulated gut–kidney axis, it has attracted widespread attentions as a microbiota-based intervention in DN. Nevertheless, the applications of FMT in patients with DN are small-scale, because the fecal extract may transmit occult infections. Hence, there is an urgent need to establish rigorous donor screening guidelines to reduce the risk of infection. In addition, more clinical studies are needed to determine some factors that affect FMT, such as the sample preparation, storage, dose and delivery route, to make this process standard.
5.2. Probiotics
Probiotics are live non-pathogenic microorganisms that colonize the gastrointestinal tract and confer health benefits when ingested in adequate amounts (Singh et al., 2022). An increasing number of investigations have demonstrated that probiotics exhibit promising properties in maintaining intestinal homeostasis, enhancing nutrient assimilation and promoting immunity (Wu T. Y. et al., 2022). Currently, the most widely used probiotics include Lactobacillus and Bifidobacterium (Zheng et al., 2021). In the past few years, mounting evidence has reported the renoprotective effect of probiotics by regulating gut–kidney axis (Koppe et al., 2015).
A randomized controlled clinical trial demonstrated that soy milk containing Lactobacillus plantarum A7 significantly reduced the albuminuria, serum creatinine and improved eGFR in patients with DN compared with the conventional soy milk, suggesting that probiotic Lactobacillus plantarum A7 can improve the renal function of DN patients (Abbasi et al., 2017). Consistently, probiotic Lactobacillus plantarum A7 was reported to improve inflammatory and oxidative stress factors, which further indicated the beneficial effect of probiotic Lactobacillus plantarum A7 in DN patients (Miraghajani et al., 2017, 2019). In addition, other investigations also indicated that probiotic can ameliorate hyperglycemia and dyslipidemia in DN patients. Jiang et al. reported that probiotic supplements (Bifidobacterium bifidum, Lactobacillus acidophilus and Streptococcus thermophilus) significantly reduced the level of FBG and HbA1c in DN patients (Jiang et al., 2021). The honey containing probiotic Bacillus coagulans T11 decreased the radio of total-/HDL-cholesterol (Arani et al., 2019). Additionally, DN is usually accompanied by chronic hyperglycemia resulting from the deficiency of insulin sensitivity. A randomized clinical study demonstrated that a mixture of Lactobacillus acidophilus, Bifidobacterium bifidum, Lactobacillus reuteri and Lactobacillus fermentum effectively increased insulin sensitivity and ameliorated glycemic control in patients with DN (Mafi et al., 2018), the similar effects were observed by probiotic Bacillus coagulans T11honey consumption in DN patients (Arani et al., 2019).
Such findings highlighted the potential of probiotics as an auxiliary measure for the treatment of DN. Overall, these investigations indicated that probiotics may exert beneficial role for DN patients by improving renal function, inflammation and oxidative stress, and controlling glycemia and dyslipidemia. Although probiotics possess a broad prospect in the treatment of DN, gaps still exist in the clinical applications of probiotics. For instance, few subsequent studies have shown that the administration of probiotics can maintain the long-term colonization of the gut microbiota DN patients. Probiotics may also have potential risks for patients with immunodeficiency as opportunistic pathogens (Doron and Snydman, 2015). Additionally, the selection and dosage of probiotics depend on the specific intestinal environment. Related investigations on this field are scarce.
5.3. Prebiotics
Prebiotics are described as substrates that exhibit beneficial health effects to the host when selectively used by microorganisms (Gibson et al., 2017). The most well-known prebiotics mainly include inulin, dextran, pectins, lactulose, fructooligosaccharides (FOS) and galactooligosaccharides (GOS; Zakrzewska et al., 2022). It has been reported that prebiotics play a critical role in the modulating immune system (Pujari and Banerjee, 2021) and maintaining gastrointestinal function (Holscher, 2017), of which are associated with inflammation and gut microbiota dysbiosis in DN (Zhang L. L. et al., 2022).
A recent animal experiment demonstrated that inulin-type fructans (ITFs) supplementation induced the expansion of acetate-generating bacteria, especially the genera Akkermansia and Candidatus Saccharimonas, and improved renal function, glomerular injury and renal fibrosis in db/db mice (Luo L. M. et al., 2022). These results are consistent with those of diabetic mice treated with a high-fiber diet. Compared with diabetic mice on a diet, dietary fiber treatment inhibited development of diabetes to DN by improving the renal function and pathological injury. Dietary fiber not only restored the gut microbial community, but also increased the concentration of SCFAs by promoting the enrichment of SCFA-producing bacteria, such as the genera Prevotella and Bifidobacterium (Li Y. J. et al., 2020). These studies suggested that prebiotics exert a renoprotective function through SCFAs in DN. FOS has been shown to ameliorate DN by improving gut dysbiosis and inflammation (Pengrattanachot et al., 2022). Treatment with FOS decreased the endotoxemia, improved glucose tolerance and insulin resistance, and inhibited inflammation in high-fat-diet-induced diabetic mice (Cani et al., 2007). Additionally, Gobinath et al. used FOS and xylo-oligosaccharides (XOS) as a diet supplement for STZ-induced diabetic rats. As expected, supplementation of FOS and XOS restored the quantities of bifidobacteria and lactobacilli, which led to the improved renal function, reduced lipid accumulation and attenuated renal injury (Gobinath et al., 2010).
Taken together, these results indicated that as a functional food, prebiotics can delay the progression of DN by altering microbial compositions and functions. Nevertheless, most of the current studies on the intervention of DN by prebiotics were performed in animals, and thereby clinical studies are needed to confirm the practical application of prebiotics in humans. Moreover, the specific mechanism of prebiotics to improve DN requires further study, as the causes of DN are diverse. In recent decades, prebiotics have attracted extensive attention due to their safety, effectiveness and non-toxic side effects. Based on the prebiotics, it is of great significance to clarify the mechanism and combine the dietary intervention with traditional treatments for DN therapies.
5.4. Herbal medicines and their active ingredients
As well known, herbal medicine exhibits robust therapeutic efficacy for kidney diseases. In recent years, some studies have proved that herbal medicine can improve DN by regulating gut microbiota dysbiosis. Ji et al. found that Rheum palmatum L. can improve intestinal barrier, and alleviate renal fibrosis and inflammation by reducing the abundance of pernicious bacteria such as Akkermansia, Methanosphaera and Clostridiaceae in CKD rats (Ji et al., 2020). Meanwhile, they found that Rheum palmatum L. treatment decreased the serum level of TMAO and increased the level of SCFAs (butanoic acid and isobutyric acid), which further confirmed the renoprotective role of Rheum palmatum L. (Ji et al., 2021, 2022). Moreover, many traditional Chinese herbal formulas have also been reported to exert good efficacy on the treatment of DN, and the underlying mechanism involves the regulation of gut microbiota dysbiosis. For example, treatment with QiDiTangShen granules significantly alleviated renal injury, improved renal function, and restored intestinal barrier in DN mice. Mechanistically, QiDiTangShen granules decreased the abundance of Lachnospiraceae_NK4A136_group, Lactobacillus and Bacteroides, which were positively correlated with renal injury indicators. Further study demonstrated that QiDiTangShen granules exert the renoprotective function through gut microbiota-bile acid axis (Wei et al., 2021). Other traditional Chinese herbal formulas such as Qing-Re-Xiao-Zheng formula (Gao et al., 2021), Tangshen formula (Zhao et al., 2020) and Zicuiyin decoction (Liu J. et al., 2022) were also found to exert anti-DN role by modulating gut microbiota dysbiosis.
What is more, many active compounds isolated from herbal medicines also possess excellent anti-DN properties. For example, Resveratrol (10 mg/kg b. w.) administration for 12 weeks significantly improved renal function, glomerular lesion and inflammation in db/db mice, along with the increased abundance of Bacteroides, Alistipes and Parabacteroides, which exhibit anti anti-inflammatory properties (Cai et al., 2020). Curcumin, a major active component of turmeric, markedly decreased the urinary micro-albumin (U-mAlb) excretion and the level of plasma LPS in patients with T2DM (500 mg/d for 15 days). The potential mechanism may be that curcumin increased the abundance of Bacteroides, Bifidobacterium and Lactobacillus, which restored the epithelial barrier and inhibited inflammatory response induced by LPS (Yang et al., 2015). In addition, some natural polysaccharides, such as Moutan Cortex polysaccharides (Zhang M. et al., 2022) and Bupleurum polysaccharides (Feng et al., 2019) were also reported to amelirate DN via modulating gut microbiota composition through gut–kidney axis. These investigations have revealed the potential role of gut microbiota in the treatment of DN with herbal medicines. However, there is still relatively little research in this field. Future studies should focus on the underlying mechanisms by which herbal medicines exert their renoprotective function through gut–kidney axis. Clarifying the roles of gut microbiota in the treatment of kidney diseases by herbal medicines will provide new strategies for the diagnosis and therapy of DN.
5.5. Others
Except for the three main approaches mentioned above, there are other gut microbiota-targeted DN therapies, including synbiotics, antibiotics, diet and exercise. Synbiotics are defined as the mixtures of probiotics and prebiotics, which can promote the survival of probiotic in the intestinal tract (Jiang et al., 2022). Studies have shown that the combination of probiotics and prebiotics can exert a synergistic effect, which is more efficient compared to probiotics or prebiotics alone (Gibson et al., 2017). In a randomized, double-blind and placebo controlled trial, the symbiotic effect of the probiotic (Bifidobacteria, Lactobacillus and Streptococcus) and the prebiotics (FOS, GOS and inulin) ameliorated renal failure by reducing serum PCS and improving gut microbiome composition in CKD patients (Rossi et al., 2016). In adenine-induced CKD rats, the treatment of synbiotics containing Bifidobacteria, Lactobacillus, Streptococcus and inulin caused a reduction in the indole-generating bacteria of Clostridium and the quantity of fecal indole. Additionally, synbiotics treatment restored gut microbiota diversity in CKD rats (Yang et al., 2021), indicating that synbiotics exert a renoprotective effect by improving gut indole load and gut microbiota dysbiosis. Antibiotics are also widely used in the treatment of kidney diseases by targeting gut microbiota (Aloy et al., 2020). Lu et al. (2020) demonstrated that broad-spectrum antibiotics administration for 8 weeks eliminated the majority of the gut microbiota, reduced plasma acetate level and suppressed the activation of intrarenal RAS activation, and thereby alleviated kidney injury in STZ-induced DN rats. Similarly, the depletion of intestinal microflora by antibiotics was reported to ameliorate the dysregulation of cholesterol homeostasis in the tubulointerstitium of DN rats (Hu et al., 2020). However, the abuse of antibiotics may also lead to the kidney injury and the invasion of antibiotic-associated pathogens (Kamada et al., 2013). Thus, the antibiotic therapy needs further research to ensure its safety. In addition, lifestyle intervention, such as dietotherapy and exercise training, is also a common strategy for DN therapy. Dietary fiber was proved to attenuate the progression of DN by promoting the expansion of SCFAs-producing bacteria, such as Prevotella and Bifidobacterium, which can inhibit inflammation and oxidative stress (Li Y. J. et al., 2020; Drake et al., 2022). In STZ-induced DN rats, Mulberry leaf tea markedly ameliorated hyperglycemia and renal injury by restoring the abundance of Adlercreutzia, Anaerofustis, Coprococcus, Dorea, Roseburia, Oscillospira, Phascolarctobacterium, Coprobacillus, Sutterella and RF39, which indicated that Mulberry leaf tea can be used as a dietotherapy for DN therapy through gut–kidney axis (Sheng et al., 2018). Exercise training has been reported to be a novel nonpharmacological approach in the modulation of gut microbiota, which could benefit individuals by restoring gut microbiota composition and function (Wegierska et al., 2022). For example, Lambert et al. demonstrated that exercise training for 6 weeks has significantly increased the amount of Firmicutes and decreased the amount of Bacteroides/Prevotella spp. in type 2 diabetic mice (Lambert et al., 2015). Further, another similar investigation indicated that aerobic exercise for 8 weeks reversed the decreased abundance of Bacteroides, and increased the level of SCFAs in type 2 diabetic mice (Yang L. et al., 2020). In a clinical trial, the intestinal barrier and systemic inflammation of patients with type 2 diabetes were significantly improved after chronic exercise for 6 months, suggesting that exercise might be a potential therapeutic strategy for DN via gut–kidney axis (Pasini et al., 2019). Unfortunately, there is no direct evidence that exercise training can ameliorate DN by modifying gut microbiota. Currently, the exercise training regimen for DN therapy is still in the initial stage (Amaral et al., 2020). Thus, researchers need to do considerable work to build appropriate, feasible and scientific exercise program for the patients with DN.
6. Summary and prospects
This review summarized the specific alterations of gut microbiome in DN and elucidated the correlations between gut microbiota and DN phenotypes, and it will provide a new insight for the further investigations of gut microbiota in DN. Nevertheless, gaps still exist in these previous studies. First of all, these investigations were cross-sectional, and failed to reflect the alterations and correlations in different stages of DN progression. The sample size of some clinical studies are insufficient to reveal the real relationships between gut microbiota and DN. Secondly, the causal relationship between gut microbial dysbiosis and DN onset is unclear. The intestinal microbial dysbiosis associated with DN may occur in small intestine (Shi et al., 2023), while most of these studies on gut microbiota are based on fecal sample. Finally, gut microbiota can be influenced by many factors, such as diet, age, gender and medications. These factors should be taken into account in future studies to better clarify the associations between gut microbiota and DN.
Gut microbial metabolites, including SCFAs, BAs, TMAO, UTs and H2S, were also discussed in detail. As a bridge between gut microbiota and host, these metabolites play essential roles in the onset and development of DN. The exact mechanisms by which microbial metabolites modulate DN require further investigation in animal models and a large patient cohorts. Moreover, researchers should focus on the route, frequency and concentration of administration for some beneficial metabolites, such as SCFAs and H2S. As for those metabolites that contribute to the pathophysiology of the disease, such as UTs and TMAO, future investigations should focus on the development of inhibitors. Exploiting related enzymes that generate these harmful metabolites may be a promising strategy.
DN results in high morbidity and mortality worldwide and effective therapeutic strategies for DN are urgently needed. Mounting studies have demonstrated that DN is accompanied by gut microbial dysbiosis and alterations to microbial metabolites, suggesting that gut microbiota-targeted therapies may be an effective strategy against DN. Rational utilization of probiotics and prebiotics, as well as FMT method contribute to restoring intestinal flora homeostasis, and thereby retarding DN progression. However, many current studies, especially FMT technique, are only limited to preclinical animal studies, and more reliable clinical trials are needed to confirm the results. Additionally, the combination of gut microbiota-targeted therapies with medications may has a better effect, while the investigation in this field is scarce. Therefore, researchers need to do considerable work to bridging these gaps.
Author contributions
JY: study concepts and design. HZ, C-EY, TL, and M-XZ: literature search. HZ, C-EY, YN, and MW: manuscript preparation and revision. All authors contributed to the article and approved the submitted version.
Funding
This work was supported by National Science Basic Research Program of Shaanxi and Basic Research Program of Xi’an Municipal Health Commission (Nos. 2022JQ-920, 2022yb41, 82204745, 2023qn22).
Conflict of interest
The authors declare that the research was conducted in the absence of any commercial or financial relationships that could be construed as a potential conflict of interest.
Publisher’s note
All claims expressed in this article are solely those of the authors and do not necessarily represent those of their affiliated organizations, or those of the publisher, the editors and the reviewers. Any product that may be evaluated in this article, or claim that may be made by its manufacturer, is not guaranteed or endorsed by the publisher.
Abbreviations
AGEs, advanced glycation end products; AHR, aryl hydrocarbon receptor; ANG-II, angiotensin II; BAs, bile acids; CKD, chronic kidney disease; DG, diacylglycerol; DN, diabetic nephropathy; eGFR, estimated glomerular filtration rate; ESRD, end-stage renal disease; ET-1, endothelin-1; F/B, Firmicutes/Bacteroidetes ; FBG, fasting blood glucose; FMT, fecal microbiota transplantation; FOS, fructooligosaccharides; FXR, farnesoid X receptor; GOS, galactooligosaccharides; GPCRs, G-protein-coupled receptors; HbA1c, glycosylated hemoglobin; HDAC, histone acetylation; H2S, hydrogen sulfide; 24-h UP, 24-h urine protein; IL-1, interleukin-1; IL-6, interleukin-1; IS, indoxyl sulfate; LPS, lipopolysaccharide; MDA, Malondialdehyde; NaB, sodium butyrate; NADPH, nicotinamide adenine dinucleotide phosphate; NF-κ B, nuclear factor-κB; NLRP3, nod-like receptor (NLR) family pyrin domain containing 3; Nrf2, nuclear factor E2 related factor 2; PCS, p-cresyl sulfate; pCG, p-cresyl glucuronide; PKC, protein kinase C; PS, phenyl sulfate; RAGE, receptor for advanced glycation end products; RAS, renin-angiotensin system; ROS, reactive oxygen species; SCFAs, short-chain fatty acid; SCR, serum creatinine; SOD, superoxide dismutase; STZ, streptozotocin; TC, total cholesterol; TG, triglyceride; TGF-β1, transforming growth factor-β1; TGR5, takeda G protein-coupled receptor 5; TLR, toll like receptors; TMAO, trimethylamine-N-oxide; TNF-α, tumor necrosis factor-α; UACR, urinary albumin-to-creatinine ratio; UTs, uremic toxins; XOS, xylo-oligosaccharides.
References
Abbasi, B., Ghiasvand, R., and Mirlohi, M. (2017). Kidney function improvement by soy milk containing Lactobacillus plantarum A7 in type 2 diabetic patients with nephropathy a double-blinded randomized controlled trial. Iran. J. Kidney Dis. 11, 36–43.
Adachi, K., Sugiyama, T., Yamaguchi, Y., Tamura, Y., Izawa, S., Hijikata, Y., et al. (2019). Gut microbiota disorders cause type 2 diabetes mellitus and homeostatic disturbances in gut-related metabolism in Japanese subjects. J. Clin. Biochem. Nutr. 64, 231–238. doi: 10.3164/jcbn.18-101
Ahmad, F. U. D., Sattar, M. A., Rathore, H. A., Abdullah, M. H., Tan, S., Abdullah, N. A., et al. (2012). Exogenous hydrogen sulfide (H2S) reduces blood pressure and prevents the progression of diabetic nephropathy in spontaneously hypertensive rats. Ren. Fail. 34, 203–210. doi: 10.3109/0886022x.2011.643365
Ahmed, H. H., Taha, F. M., Omar, H. S., Elwi, H. M., and Abdelnasser, M. (2019). Hydrogen sulfide modulates SIRT1 and suppresses oxidative stress in diabetic nephropathy. Mol. Cell. Biochem. 457, 1–9. doi: 10.1007/s11010-019-03506-x
al-Obaide, M., Singh, R., Datta, P., Rewers-Felkins, K. A., Salguero, M. V., al-Obaidi, I., et al. (2017). Gut microbiota-dependent trimethylamine-N-oxide and serum biomarkers in patients with T2DM and advanced CKD. J. Clin. Med. 6:86. doi: 10.3390/jcm6090086
Aloy, B., Launay-Vacher, V., Bleibtreu, A., Bortolotti, P., Faure, E., Filali, A., et al. (2020). Antibiotics and chronic kidney disease: dose adjustment update for infectious disease clinical practice. Med. Mal. Infect. 50, 323–331. doi: 10.1016/j.medmal.2019.06.010
Amaral, L. S. D., Souza, C. S., Lima, H. N., and Soares, T. D. (2020). Influence of exercise training on diabetic kidney disease: a brief physiological approach. Exp. Biol. Med. 245, 1142–1154. doi: 10.1177/1535370220928986
Arani, N. M., Emam-Djomeh, Z., Tavakolipour, H., Sharafati-Chaleshtori, R., Soleimani, A., and Asemi, Z. (2019). The effects of probiotic honey consumption on metabolic status in patients with diabetic nephropathy: a randomized, double-blind, controlled trial. Probiotics Antimicro. 11, 1195–1201. doi: 10.1007/s12602-018-9468-x
Atoh, K., Itoh, H., and Haneda, M. (2009). Serum indoxyl sulfate levels in patients with diabetic nephropathy: relation to renal function. Diabetes Res. Clin. Pract. 83, 220–226. doi: 10.1016/j.diabres.2008.09.053
Bai, Y. F., Li, P., Liu, J. A., Zhang, L., Cui, S. Y., Wei, C. T., et al. (2022). Renal primary cilia lengthen in the progression of diabetic kidney disease. Front. Endocrinol. 13:984452. doi: 10.3389/fendo.2022.984452
Bastos, R. M. C., Simplício-Filho, A., Sávio-Silva, C., Oliveira, L. F. V., Cruz, G. N. F., Sousa, E. H., et al. (2022). Fecal microbiota transplant in a pre-clinical model of type 2 diabetes mellitus, obesity and diabetic kidney disease. Int. J. Mol. Sci. 23:3842. doi: 10.3390/ijms23073842
Bertolini, A., Fiorotto, R., and Strazzabosco, M. (2022). Bile acids and their receptors: modulators and therapeutic targets in liver inflammation. Semin. Immunopathol. 44, 547–564. doi: 10.1007/s00281-022-00935-7
Bui, T. M., Wiesolek, H. L., and Sumagin, R. (2020). ICAM-1: a master regulator of cellular responses in inflammation, injury resolution, and tumorigenesis. J. Leukoc. Biol. 108, 787–799. doi: 10.1002/jlb.2mr0220-549r
Cai, J., Rimal, B., Jiang, C., Chiang, J. Y. L., and Patterson, A. D. (2022). Bile acid metabolism and signaling, the microbiota, and metabolic disease. Pharmacol. Ther. 237:108238. doi: 10.1016/j.pharmthera.2022.108238
Cai, T. T., Ye, X. L., Li, R. R., Chen, H., Wang, Y. Y., Yong, H. J., et al. (2020). Resveratrol modulates the gut microbiota and inflammation to protect against diabetic nephropathy in mice. Front. Pharmacol. 11:1249. doi: 10.3389/fphar.2020.01249
Cani, P. D., Neyrinck, A. M., Fava, F., Knauf, C., Burcelin, R. G., Tuohy, K. M., et al. (2007). Selective increases of bifidobacteria in gut microflora improve high-fat-diet-induced diabetes in mice through a mechanism associated with endotoxaemia. Diabetologia 50, 2374–2383. doi: 10.1007/s00125-007-0791-0
Chang, Y. C., Chu, Y. H., Wang, C. C., Wang, C. H., Tain, Y. L., and Yang, H. W. (2021). Rapid detection of gut microbial metabolite trimethylamine N-oxide for chronic kidney disease prevention. Biosensors-Basel 11:339. doi: 10.3390/bios11090339
Chen, X., Dai, W. N., Li, H., Yan, Z., Liu, Z. W., and He, L. Y. (2023). Targeted drug delivery strategy: a bridge to the therapy of diabetic kidney disease. Drug Deliv. 30:2160518. doi: 10.1080/10717544.2022.2160518
Chen, J. H., Liu, Q. H., He, J. H., and Li, Y. P. (2022). Immune responses in diabetic nephropathy: pathogenic mechanisms and therapeutic target. Front. Immunol. 13:958790. doi: 10.3389/fimmu.2022.958790
Chen, Q., Ren, D. W., Wu, J. Q., Yu, H. Y., Chen, X. P., Wang, J., et al. (2021). Shenyan Kangfu tablet alleviates diabetic kidney disease through attenuating inflammation and modulating the gut microbiota. J. Nat. Med. 75, 84–98. doi: 10.1007/s11418-020-01452-3
Chen, W. H., Zhang, M. J., Guo, Y., Wang, Z., Liu, Q. Q., Yan, R. Z., et al. (2021). The profile and function of gut microbiota in diabetic nephropathy. Diabetes Metab. Syndr. Obes. 14, 4283–4296. doi: 10.2147/dmso.s320169
Cheng, T. H., Ma, M. C., Liao, M. T., Zheng, C. M., Lu, K. C., Liao, C. H., et al. (2020). Indoxyl sulfate, a tubular toxin, contributes to the development of chronic kidney disease. Toxins 12, 684. doi: 10.3390/toxins12110684
Chiu, C.-A., Lu, L.-F., Yu, T.-H., Hung, W.-C., Chung, F.-M., Tsai, I. T., et al. (2010). Increased levels of Total P-Cresylsulphate and Indoxyl Sul-phate are associated with coronary artery disease in patients with diabetic nephropathy. Rev. Diabet. Stud. 7, 275–284. doi: 10.1900/rds.2010.7.275
Dang, G. Q., Wu, W. D., Zhang, H. F., and Everaert, N. (2021). A new paradigm for a new simple chemical: butyrate and immune regulation. Food Funct. 12, 12181–12193. doi: 10.1039/d1fo02116h
DeFronzo, R. A., Reeves, W. B., and Awad, A. S. (2021). Pathophysiology of diabetic kidney disease: impact of SGLT2 inhibitors. Nat. Rev. Nephrol. 17, 319–334. doi: 10.1038/s41581-021-00393-8
Deng, L., Yang, Y., and Xu, G. S. (2022). Empagliflozin ameliorates type 2 diabetes mellitus-related diabetic nephropathy via altering the gut microbiota. BBA-Mol. Cell Biol. L. 1867:159234. doi: 10.1016/j.bbalip.2022.159234
Dilek, N., Papapetropoulos, A., Toliver-Kinsky, T., and Szabo, C. (2020). Hydrogen sulfide: an endogenous regulator of the immune system. Pharmacol. Res. 161:105119. doi: 10.1016/j.phrs.2020.105119
Ding, L. L., Yang, Q. L., Zhang, E. Y., Wang, Y. M., Sun, S. M., Yang, Y. B., et al. (2021). Notoginsenoside Ft1 acts as a TGR5 agonist but FXR antagonist to alleviate high fat diet-induced obesity and insulin resistance in mice. Acta Pharm. Sin. B 11, 1541–1554. doi: 10.1016/j.apsb.2021.03.038
Dong, W. P., Jia, Y., Liu, X. X., Zhang, H., Li, T., Huang, W. L., et al. (2017). Sodium butyrate activates NRF2 to ameliorate diabetic nephropathy possibly via inhibition of HDAC. J. Endocrinol. 232, 71–83. doi: 10.1530/joe-16-0322
Doron, S., and Snydman, D. R. (2015). Risk and safety of probiotics. Clin. Infect. Dis. 60, S129–S134. doi: 10.1093/cid/civ085
Drake, A. M., Coughlan, M. T., Christophersen, C. T., and Snelson, M. (2022). Resistant starch as a dietary intervention to limit the progression of diabetic kidney disease. Nutrients 14:4547. doi: 10.3390/nu14214547
du, X., Liu, J., Xue, Y., Kong, X. Y., Lv, C. X., Li, Z. Q., et al. (2021). Alteration of gut microbial profile in patients with diabetic nephropathy. Endocrine 73, 71–84. doi: 10.1007/s12020-021-02721-1
Du, Y., Yang, Y. T., Tang, G., Jia, J. S., Zhu, N., and Yuan, W. J. (2020). Butyrate alleviates diabetic kidney disease by mediating the miR-7a-5p/P311/TGF-β1 pathway. FASEB J. 34, 10462–10475. doi: 10.1096/fj.202000431R
Eckburg, P. B., Bik, E. M., Bernstein, C. N., Purdom, E., Dethlefsen, L., Sargent, M., et al. (2005). Diversity of the human intestinal microbial flora. Science 308, 1635–1638. doi: 10.1126/science.1110591
Elbassuoni, E. A., Aziz, N. M., and Habeeb, W. N. (2020). The role of activation of K-ATP channels on hydrogen sulfide induced renoprotective effect on diabetic nephropathy. J. Cell. Physiol. 235, 5223–5228. doi: 10.1002/jcp.29403
Ergul, A. (2011). Endothelin-1 and diabetic complications: focus on the vasculature. Pharmacol. Res. 63, 477–482. doi: 10.1016/j.phrs.2011.01.012
Fang, Q., Zheng, B. J., Liu, N., Liu, J. F., Liu, W. H., Huang, X. Y., et al. (2021). Trimethylamine N-oxide exacerbates renal inflammation and fibrosis in rats with diabetic kidney disease. Front. Physiol. 12:682482. doi: 10.3389/fphys.2021.682482
Feng, J. A., Lu, X. X., Li, H., and Wang, S. X. (2022). The roles of hydrogen sulfide in renal physiology and disease states. Ren. Fail. 44, 1289–1308. doi: 10.1080/0886022x.2022.2107936
Feng, Y., Weng, H., Ling, L., Zeng, T., Zhang, Y., Chen, D., et al. (2019). Modulating the gut microbiota and inflammation is involved in the effect of Bupleurum polysaccharides against diabetic nephropathy in mice. Int. J. Biol. Macromol. 132, 1001–1011. doi: 10.1016/j.ijbiomac.2019.03.242
Fernandes, M. R., Aggarwal, P., Costa, R. G. F., Cole, A. M., and Trinchieri, G. (2022). Targeting the gut microbiota for cancer therapy. Nat. Rev. Cancer 22, 703–722. doi: 10.1038/s41568-022-00513-x
Forst, T., Mathieu, C., Giorgino, F., Wheeler, D. C., Papanas, N., Schmieder, R. E., et al. (2022). New strategies to improve clinical outcomes for diabetic kidney disease. BMC Med. 20:337. doi: 10.1186/s12916-022-02539-2
Fuchs, C. D., Dixon, E. D., Hendrikx, T., Mlitz, V., Wahlström, A., Ståhlman, M., et al. (2022). Tetrahydroxylated bile acids improve cholestatic liver and bile duct injury in the Mdr2(−/−) mouse model of sclerosing cholangitis via immunomodulatory effects. Hepatol. Commun. 6, 2368–2378. doi: 10.1002/hep4.1998
Gao, Y. B., Yang, R. B., Guo, L., Wang, Y. X., Liu, W. J., Ai, S. N., et al. (2021). Qing-re-Xiao-Zheng formula modulates gut microbiota and inhibits inflammation in mice with diabetic kidney disease. Front. Med. 8:719905. doi: 10.3389/fmed.2021.719950
Gibson, G. R., Hutkins, R., Sanders, M. E., Prescott, S. L., Reimer, R. A., Salminen, S. J., et al. (2017). Expert consensus document: the international scientific association for probiotics and prebiotics (ISAPP) consensus statement on the definition and scope of prebiotics. Nat. Clin. Pract. Gastroenterol. Hepatol. 14, 491–502. doi: 10.1038/nrgastro.2017.75
Gilbert, J. A., Blaser, M. J., Caporaso, J. G., Jansson, J. K., Lynch, S. V., and Knight, R. (2018). Current understanding of the human microbiome. Nat. Med. 24, 392–400. doi: 10.1038/nm.4517
Gobinath, D., Madhu, A., Prashant, G., Srinivasan, K., and Prapulla, S. (2010). Beneficial effect of xylo-oligosaccharides and fructo-oligosaccharides in streptozotocin-induced diabetic rats. Brit. J. Nutr. 104, 40–47. doi: 10.1017/s0007114510000243
Gradisteanu, G. P., Stoica, R. A., Petcu, L., Picu, A., Suceveanu, A. P., Salmen, T., et al. (2019). Microbiota signatures in type-2 diabetic patients with chronic kidney disease—a pilot study. J. Mind Med. Sci. 6, 130–136. doi: 10.22543/7674.61.p130136
Gryp, T., Vanholder, R., Vaneechoutte, M., and Glorieux, G. (2017). p-cresyl sulfate. Toxins 9:52. doi: 10.3390/toxins9020052
Gu, J. L., Huang, W., Zhang, W. Q., Zhao, T. T., Gao, C. L., Gan, W. J., et al. (2019). Sodium butyrate alleviates high-glucose-induced renal glomerular endothelial cells damage via inhibiting pyroptosis. Int. Immunopharmacol. 75:105832. doi: 10.1016/j.intimp.2019.105832
Gui, D. D., Luo, W., Yan, B. J., Ren, Z., Tang, Z. H., Liu, L. S., et al. (2021). Effects of gut microbiota on atherosclerosis through hydrogen sulfide. Eur. J. Pharmacol. 896:173916. doi: 10.1016/j.ejphar.2021.173916
Gupta, N., Buffa, J. A., Roberts, A. B., Sangwan, N., Skye, S. M., Li, L., et al. (2020). Targeted inhibition of gut microbial trimethylamine N-oxide production reduces renal tubulointerstitial fibrosis and functional impairment in a murine model of chronic kidney disease. Arterioscler. Thromb. 40, 1239–1255. doi: 10.1161/atvbaha.120.314139
Hamzé, R., Delangre, E., Tolu, S., Moreau, M., Janel, N., Bailbé, D., et al. (2022). Type 2 diabetes mellitus and Alzheimer's disease: shared molecular mechanisms and potential common therapeutic targets. Int. J. Mol. Sci. 23:15287. doi: 10.3390/ijms232315287
He, X., Sun, J. P., Liu, C., Yu, X. Y., Li, H. X., Zhang, W. J., et al. (2022). Compositional alterations of gut microbiota in patients with diabetic kidney disease and type 2 diabetes mellitus. Diabetes Metab. Syndr. Obesity 15, 755–765. doi: 10.2147/dmso.s347805
Heilig, C. W., Deb, D. K., Abdul, A., Riaz, H., James, L. R., Salameh, J., et al. (2013). GLUT1 regulation of the pro-sclerotic mediators of diabetic nephropathy. Am. J. Nephrol. 38, 39–49. doi: 10.1159/000351989
Hobby, G. P., Karaduta, O., Dusio, G. F., Singh, M., Zybailov, B. L., and Arthur, J. M. (2019). Chronic kidney disease and the gut microbiome. Am. J. Physiol-Renal 316, F1211–F1217. doi: 10.1152/ajprenal.00298.2018
Holscher, H. D. (2017). Dietary fiber and prebiotics and the gastrointestinal microbiota. Gut Microbes 8, 172–184. doi: 10.1080/19490976.2017.1290756
Hsu, C. N., Hou, C. Y., Chang, C. I., and Tain, Y. L. (2022). Resveratrol butyrate ester protects adenine-treated rats against hypertension and kidney disease by regulating the gut–kidney axis. Antioxidants 11:83. doi: 10.3390/antiox11010083
Hu, D. M., Liu, W. B., Yu, W. L., Huang, L. H., Ji, C. L., Liu, X. S., et al. (2023). Psyllium seed husk regulates the gut microbiota and improves mucosal barrier injury in the colon to attenuate renal injury in 5/6 nephrectomy rats. Ren. Fail. 45:2197076. doi: 10.1080/0886022x.2023.2197076
Hu, Z. B., Lu, J., Chen, P. P., Lu, C. C., Zhang, J. X., Li, X. Q., et al. (2020). Dysbiosis of intestinal microbiota mediates tubulointerstitial injury in diabetic nephropathy via the disruption of cholesterol homeostasis. Theranostics 10, 2803–2816. doi: 10.7150/thno.40571
Hu, P., Zong, Q. F., Zhao, Y. H., Gu, H. T., Liu, Y. Y., Gu, F., et al. (2022). Lactoferrin attenuates intestinal barrier dysfunction and inflammation by modulating the MAPK pathway and gut microbes in mice. J. Nutr. 152, 2451–2460. doi: 10.1093/jn/nxac200
Huang, W., Guo, H. L., Deng, X., Zhu, T. T., Xiong, J. F., Xu, Y. H., et al. (2017). Short-chain fatty acids inhibit oxidative stress and inflammation in mesangial cells induced by high glucose and lipopolysaccharide. Exp. Clin. Endocrinol. Diabetes 125, 98–105. doi: 10.1055/s-0042-121493
Huang, W., Man, Y., Gao, C. L., Zhou, L. P., Gu, J. L., Xu, H. W., et al. (2020). Short-chain fatty acids ameliorate diabetic nephropathy via GPR43-mediated inhibition of oxidative stress and NF-κB signaling. Oxidative Med. Cell. Longev. 2020:4074832. doi: 10.1155/2020/4074832
Huang, Q. H., Xu, L. Q., Liu, Y. H., Wu, J. Z., Wu, X., Lai, X. P., et al. (2017). Polydatin protects rat liver against ethanol-induced injury: involvement of CYP2E1/ROS/Nrf2 and TLR4/NF-κB p65 pathway. Evid. Based Complement. Alternat. Med. 2017, 1–14. doi: 10.1155/2017/7953850
Hussain, S., Habib, A., and Najmi, A. K. (2019). Limited knowledge of chronic kidney disease among type 2 diabetes mellitus patients in India. Int. J. Environ. Res. Public Health 16:1443. doi: 10.3390/ijerph16081443
Jansson Sigfrids, F., Dahlström, E. H., Forsblom, C., Sandholm, N., Harjutsalo, V., Taskinen, M. R., et al. (2021). Remnant cholesterol predicts progression of diabetic nephropathy and retinopathy in type 1 diabetes. J. Intern. Med. 290, 632–645. doi: 10.1111/joim.13298
Jaye, K., Li, C. G., Chang, D., and Bhuyan, D. J. (2022). The role of key gut microbial metabolites in the development and treatment of cancer. Gut Microbes 14:2038865. doi: 10.1080/19490976.2022.2038865
Ji, C. L., Deng, Y. S., Yang, A. C., Lu, Z. Y., Chen, Y., Liu, X. S., et al. (2020). Rhubarb Enema improved colon mucosal barrier injury in 5/6 nephrectomy rats may associate with gut microbiota modification. Front. Pharmacol. 11:1092. doi: 10.3389/fphar.2020.01092
Ji, C. L., Li, Y., Mo, Y. A., Lu, Z. Y., Lu, F. H., Lin, Q. Z., et al. (2021). Rhubarb Enema decreases circulating trimethylamine N-oxide level and improves renal fibrosis accompanied with gut microbiota change in chronic kidney disease rats. Front. Pharmacol. 12:780924. doi: 10.3389/fphar.2021.780924
Ji, C. L., Lu, F. H., Wu, Y. C., Lu, Z. Y., Mo, Y. A., Han, L. J., et al. (2022). Rhubarb Enema increasing short-chain fatty acids that improves the intestinal barrier disruption in CKD may be related to the regulation of gut dysbiosis. Biomed. Res. Int. 2022, 1–15. doi: 10.1155/2022/1896781
Jiang, H. R., Cai, M. M., Shen, B. Y., Wang, Q., Zhang, T. C., and Zhou, X. (2022). Synbiotics and gut microbiota: new perspectives in the treatment of type 2 diabetes mellitus. Foods 11:2438. doi: 10.3390/foods11162438
Jiang, H. Y., Zhang, Y., Xu, D. Y., and Wang, Q. (2021). Probiotics ameliorates glycemic control of patients with diabetic nephropathy: a randomized clinical study. J. Clin. Lab. Anal. 35:e23650. doi: 10.1002/jcla.23650
Jo, J., Oh, J., and Park, C. (2020). Microbial community analysis using high-throughput sequencing technology: a beginner's guide for microbiologists. J. Microbiol. 58, 176–192. doi: 10.1007/s12275-020-9525-5
Johansen, K. L., Chertow, G. M., Foley, R. N., Gilbertson, D. T., Herzog, C. A., Ishani, A., et al. (2021). US renal data system 2020 annual data report: epidemiology of kidney disease in the United States. Am. J. Kidney Dis. 77, A7–A8. doi: 10.1053/j.ajkd.2021.01.002
Jung, C. Y., and Yoo, T. H. (2022). Pathophysiologic mechanisms and potential biomarkers in diabetic kidney disease. Diabetes Metab. J. 46, 181–197. doi: 10.4093/dmj.2021.0329
Kamada, N., Chen, G. Y., Inohara, N., and Nunez, G. (2013). Control of pathogens and pathobionts by the gut microbiota. Nat. Immunol. 14, 685–690. doi: 10.1038/ni.2608
Khan, I., Huang, Z. B., Liang, L. Y., Li, N., Ali, Z., Ding, L., et al. (2021). Ammonia stress influences intestinal histomorphology, immune status and microbiota of Chinese striped-neck turtle (Mauremys sinensis). Ecotoxicol. Environ. Saf. 222:112471. doi: 10.1016/j.ecoenv.2021.112471
Kikuchi, K., Saigusa, D., Kanemitsu, Y., Matsumoto, Y., Thanai, P., Suzuki, N., et al. (2019). Gut microbiome-derived phenyl sulfate contributes to albuminuria in diabetic kidney disease. Nat. Commun. 10:1835. doi: 10.1038/s41467-019-09735-4
Koppe, L., Mafra, D., and Fouque, D. (2015). Probiotics and chronic kidney disease. Kidney Int. 88, 958–966. doi: 10.1038/ki.2015.255
Kundu, S., Pushpakumar, S., and Sen, U. (2015). MMP-9-and NMDA receptor-mediated mechanism of diabetic renovascular remodeling and kidney dysfunction: hydrogen sulfide is a key modulator. Nitric Oxide-Biol. Ch. 46, 172–185. doi: 10.1016/j.niox.2015.02.003
Lambert, J. E., Myslicki, J. P., Bomhof, M. R., Belke, D. D., Shearer, J., and Reimer, R. A. (2015). Exercise training modifies gut microbiota in normal and diabetic mice. Appl. Physiol. Nutr. Me. 40, 749–752. doi: 10.1139/apnm-2014-0452
Landis, R. C., Quimby, K. R., and Greenidge, A. R. (2018). M1/M2 macrophages in diabetic nephropathy: Nrf2/HO-1 as therapeutic targets. Curr. Pharm. Des. 24, 2241–2249. doi: 10.2174/1381612824666180716163845
Lee, J. W., Cowley, E. S., Wolf, P. G., Doden, H. L., Murai, T., Caicedo, K. Y. O., et al. (2022). Formation of secondary Allo-bile acids by novel enzymes from gut Firmicutes. Gut Microbes 14:1. doi: 10.1080/19490976.2022.2132903
Lee, C. J., Sears, C. L., and Maruthur, N. (2020). Gut microbiome and its role in obesity and insulin resistance. Ann. N. Y. Acad. Sci. 1461, 37–52. doi: 10.1111/nyas.14107
Lei, C., Sun, R., Xu, G., Tan, Y., Feng, W., McClain, C. J., et al. (2022). Enteric VIP-producing neurons maintain gut microbiota homeostasis through regulating epithelium fucosylation. Cell Host Microbe 30, 1417–1434.e8. doi: 10.1016/j.chom.2022.09.001
Li, Y. J., Chen, X. C., Kwan, T. K., Loh, Y. W., Singer, J., Liu, Y. Z., et al. (2020). Dietary fiber protects against diabetic nephropathy through short-chain fatty acid? Mediated activation of G protein? Coupled receptors GPR43 and GPR109A. J. Am. Soc. Nephrol. 31, 1267–1281. doi: 10.1681/asn.2019101029
Li, S.-Y., Chen, S., Lu, X.-T., Fang, A.-P., Chen, Y.-M., Huang, R.-Z., et al. (2022). Serum trimethylamine-N-oxide is associated with incident type 2 diabetes in middle-aged and older adults: a prospective cohort study. J. Transl. Med. 20:374. doi: 10.1186/s12967-022-03581-7
Li, H., Feng, S. J., Zhang, G. Z., and Wang, S. X. (2014). Correlation of lower concentrations of hydrogen sulfide with atherosclerosis in chronic hemodialysis patients with diabetic nephropathy. Blood Purif. 38, 188–194. doi: 10.1159/000368883
Li, Y., Qin, G. Q., Wang, W. Y., Liu, X., Gao, X. Q., Liu, J. H., et al. (2022). Short chain fatty acids for the risk of diabetic nephropathy in type 2 diabetes patients. Acta Diabetol. 59, 901–909. doi: 10.1007/s00592-022-01870-7
Li, Y., Su, X. H., Gao, Y., Lv, C. X., Gao, Z. W., Liu, Y. P., et al. (2020). The potential role of the gut microbiota in modulating renal function in experimental diabetic nephropathy murine models established in same environment. BBA-Mol. Basis Dis. 1866:165764. doi: 10.1016/j.bbadis.2020.165764
Li, L., Wei, T., Liu, S., Wang, C., Zhao, M., Feng, Y., et al. (2021). Complement C5 activation promotes type 2 diabetic kidney disease via activating STAT3 pathway and disrupting the gut–kidney axis. J. Cell. Mol. Med. 25, 960–974. doi: 10.1111/jcmm.16157
Lin, M., Yiu, W. H., Wu, H. J., Chan, L. Y. Y., Leung, J. C. K., Au, W. S., et al. (2012). Toll-like receptor 4 promotes tubular inflammation in diabetic nephropathy. J. Am. Soc. Nephrol. 23, 86–102. doi: 10.1681/asn.2010111210
Linh, H. T., Iwata, Y., Senda, Y., Sakai-Takemori, Y., Nakade, Y., Oshima, M., et al. (2022). Intestinal bacterial translocation contributes to diabetic kidney disease. J. Am. Soc. Nephrol. 33, 1105–1119. doi: 10.1681/asn.2021060843
Liu, J., Gao, L. D., Fu, B., Yang, H. T., Zhang, L., Che, S. Q., et al. (2022). Efficacy and safety of Zicuiyin decoction on diabetic kidney disease: a multicenter, randomized controlled trial. Phytomedicine 100:154079. doi: 10.1016/j.phymed.2022.154079
Liu, J. R., Miao, H., Deng, D. Q., Vaziri, N. D., Li, P., and Zhao, Y. Y. (2021). Gut microbiota-derived tryptophan metabolism mediates renal fibrosis by aryl hydrocarbon receptor signaling activation. Cell. Mol. Life Sci. 78, 909–922. doi: 10.1007/s00018-020-03645-1
Liu, Y. X., Uruno, A., Saito, R., Matsukawa, N., Hishinuma, E., Saigusa, D., et al. (2022). Nrf2 deficiency deteriorates diabetic kidney disease in Akita model mice. Redox Biol. 58:102525. doi: 10.1016/j.redox.2022.102525
Liu, L., Xia, R., Song, X. Q., Zhang, B. P., He, W. T., Zhou, X. R., et al. (2021). Association between the triglyceride-glucose index and diabetic nephropathy in patients with type 2 diabetes: a cross-sectional study. J. Diabetes Investig. 12, 557–565. doi: 10.1111/jdi.13371
Lobel, L., Cao, Y. G., Fenn, K., Glickman, J. N., and Garrett, W. S. (2020). Diet posttranslationally modifies the mouse gut microbial proteome to modulate renal function. Science 369, 1518–1531. doi: 10.1126/science.abb3763
Lodhi, A. H., Ahmad, F. U. D., Furwa, K., and Madni, A. (2021). Role of oxidative stress and reduced endogenous hydrogen sulfide in diabetic nephropathy. Drug Des. Dev. Ther. 15, 1031–1043. doi: 10.2147/dddt.s291591
Lu, J., Chen, P., Zhang, J., Li, X., Wang, G., Yuan, B., et al. (2021). GPR43 deficiency protects against podocyte insulin resistance in diabetic nephropathy through the restoration of AMPKα activity. Theranostics 11, 4728–4742. doi: 10.7150/thno.56598
Lu, P. C., Hsu, C. N., Lin, I. C., Lo, M. H., Yang, M. Y., and Tain, Y. L. (2021). The association between changes in plasma short-chain fatty acid concentrations and hypertension in children with chronic kidney disease. Front. Pediatr. 8:613641. doi: 10.3389/fped.2020.613641
Lu, C. C., Hu, Z. B., Wang, R., Hong, Z. H., Lu, J., Chen, P. P., et al. (2020). Gut microbiota dysbiosis-induced activation of the intrarenal renin-angiotensin system is involved in kidney injuries in rat diabetic nephropathy. Acta Pharmacol. Sin. 41, 1111–1118. doi: 10.1038/s41401-019-0326-5
Lu, Y. D., Zhang, Y., Zhao, X., Shang, C., Xiang, M., Li, L., et al. (2022). Microbiota-derived short-chain fatty acids: implications for cardiovascular and metabolic disease. Front. Cardiovasc. Med. 9:900381. doi: 10.3389/fcvm.2022.900381
Luo, W., Guo, S., Zhou, Y., Zhu, J., Zhao, J., Wang, M., et al. (2022). Hepatocellular carcinoma: novel understandings and therapeutic strategies based on bile acids (review). Int. J. Oncol. 61:117. doi: 10.3892/ijo.2022.5407
Luo, L. M., Luo, J. L., Cai, Y. T., Fu, M. L., Li, W. H., Shi, L. L., et al. (2022). Inulin-type fructans change the gut microbiota and prevent the development of diabetic nephropathy. Pharmacol. Res. 183:106367. doi: 10.1016/j.phrs.2022.106367
Lv, Q. L., Li, Z. Y., Sui, A. H., Yang, X. M., Han, Y. F., and Yao, R. Y. (2022). The role and mechanisms of gut microbiota in diabetic nephropathy, diabetic retinopathy and cardiovascular diseases. Front. Microbiol. 13:977187. doi: 10.3389/fmicb.2022.977187
Mafi, A., Namazi, G., Soleimani, A., Bahmani, F., Aghadavod, E., and Asemi, Z. (2018). Metabolic and genetic response to probiotics supplementation in patients with diabetic nephropathy: a randomized, double-blind, placebo-controlled trial. Food Funct. 9, 4763–4770. doi: 10.1039/c8fo00888d
Makki, K., Brolin, H., Petersen, N., Henricsson, M., Christensen, D. P., Khan, M. T., et al. (2023). 6α-hydroxylated bile acids mediate TGR5 signalling to improve glucose metabolism upon dietary fiber supplementation in mice. Gut 72, 314–324. doi: 10.1136/gutjnl-2021-326541
Malek, V., Suryavanshi, S. V., Sharma, N., Kulkarni, Y. A., Mulay, S. R., and Gaikwad, A. B. (2021). Potential of renin-angiotensin-aldosterone system modulations in diabetic kidney disease: old players to new hope! Rev. Physiol. Bioch. P. 179, 31–71. doi: 10.1007/112_2020_50
Mansoor, G., Tahir, M., Maqbool, T., Abbasi, S. Q., Hadi, F., Shakoori, T. A., et al. (2022). Increased expression of circulating stress markers, inflammatory cytokines and decreased antioxidant level in diabetic nephropathy. Lietuvis̆koji medicina 58:1604. doi: 10.3390/medicina58111604
Masaoutis, C., and Theocharis, S. (2019). The farnesoid X receptor: a potential target for expanding the therapeutic arsenal against kidney disease. Expert Opin. Ther. Targets 23, 107–116. doi: 10.1080/14728222.2019.1559825
Meijers, B. K. I., and Evenepoel, P. (2011). The gut–kidney axis: indoxyl sulfate, p-cresyl sulfate and CKD progression. Nephrol. Dial. Transpl. 26, 759–761. doi: 10.1093/ndt/gfq818
Mezzano, S., Aros, C., Droguett, A., Burgos, M. E., Ardiles, L., Flores, C., et al. (2004). NF- B activation and overexpression of regulated genes in human diabetic nephropathy. Nephrol. Dial. Transplant. 19, 2505–2512. doi: 10.1093/ndt/gfh207
Miraghajani, M., Zaghian, N., Dehkohneh, A., Mirlohi, M., and Ghiasvand, R. (2019). Probiotic soy milk consumption and renal function among type 2 diabetic patients with nephropathy: a randomized controlled clinical trial. Probiotics Antimicro. 11, 124–132. doi: 10.1007/s12602-017-9325-3
Miraghajani, M., Zaghian, N., Mirlohi, M., Feizi, A., and Ghiasvand, R. (2017). The impact of probiotic soy milk consumption on oxidative stress among type 2 diabetic kidney disease patients: a randomized controlled clinical trial. J. Ren. Nutr. 27, 317–324. doi: 10.1053/j.jrn.2017.04.004
Moniri, N. H., and Farah, Q. (2021). Short-chain free-fatty acid G protein-coupled receptors in colon cancer. Biochem. Pharmacol. 186:114483. doi: 10.1016/j.bcp.2021.114483
Mutsaers, H. A. M., Caetano-Pinto, P., Seegers, A. E. M., Dankers, A. C. A., van den Broek, P. H. H., Wetzels, J. F. M., et al. (2015). Proximal tubular efflux transporters involved in renal excretion of p-cresyl sulfate and p-cresyl glucuronide: implications for chronic kidney disease pathophysiology. Toxicol. In Vitro 29, 1868–1877. doi: 10.1016/j.tiv.2015.07.020
Naimi, S., Viennois, E., Gewirtz, A. T., and Chassaing, B. (2021). Direct impact of commonly used dietary emulsifiers on human gut microbiota. Microbiome 9:66. doi: 10.1186/s40168-020-00996-6
Navarro-Gonzalez, J. F., Mora-Fernandez, C., de Fuentes, M. M., and Garcia-Perez, J. (2011). Inflammatory molecules and pathways in the pathogenesis of diabetic nephropathy. Nat. Rev. Nephrol. 7, 327–340. doi: 10.1038/nrneph.2011.51
Ni, Y., Zheng, L., Nan, S., Ke, L., Fu, Z., and Jin, J. (2022). Enterorenal crosstalks in diabetic nephropathy and novel therapeutics targeting the gut microbiota. Acta bioch. bioph. Sin. 54, 1406–1420. doi: 10.3724/abbs.2022140
Niewczas, M. A., Sirich, T. L., Mathew, A. V., Skupien, J., Mohney, R. P., Warram, J. H., et al. (2014). Uremic solutes and risk of end-stage renal disease in type 2 diabetes: metabolomic study. Kidney Int. 85, 1214–1224. doi: 10.1038/ki.2013.497
Niewiem, M., and Grzybowska-Chlebowczyk, U. (2022). Intestinal barrier permeability in allergic diseases. Nutrients 14:1893. doi: 10.3390/nu14091893
Nishiyama, A., and Kobori, H. (2018). Independent regulation of renin-angiotensin-aldosterone system in the kidney. Clin. Exp. Nephrol. 22, 1231–1239. doi: 10.1007/s10157-018-1567-1
Pasini, E., Corsetti, G., Assanelli, D., Testa, C., Romano, C., Dioguardi, F. S., et al. (2019). Effects of chronic exercise on gut microbiota and intestinal barrier in human with type 2 diabetes. Minerva Med. 110, 3–11. doi: 10.23736/s0026-4806.18.05589-1
Pavlov, T. S., Palygin, O., Isaeva, E., Levchenko, V., Khedr, S., Blass, G., et al. (2020). NOX4-dependent regulation of ENaC in hypertension and diabetic kidney disease. FASEB J. 34, 13396–13408. doi: 10.1096/fj.202000966RR
Pengrattanachot, N., Thongnak, L., and Lungkaphin, A. (2022). The impact of prebiotic fructooligosaccharides on gut dysbiosis and inflammation in obesity and diabetes related kidney disease. Food Funct. 13, 5925–5945. doi: 10.1039/d1fo04428a
Perino, A., Demagny, H., Velazquez-Villegas, L., and Schoonjans, K. (2021). Molecular physiology of bile acid signaling in health, disease, and aging. Physiol. Rev. 101, 683–731. doi: 10.1152/physrev.00049.2019
Pujari, R., and Banerjee, G. (2021). Impact of prebiotics on immune response: from the bench to the clinic. Immunol. Cell Biol. 99, 255–273. doi: 10.1111/imcb.12409
Qu, Z. H., Tian, P. J., Yang, B., Zhao, J. X., Wang, G., and Chen, W. (2022). Fecal microbiota transplantation for diseases: therapeutic potential, methodology, risk management in clinical practice. Life Sci. 304:120719. doi: 10.1016/j.lfs.2022.120719
Romano, K. A., Vivas, E. I., Amador-Noguez, D., and Rey, F. E. (2015). Intestinal microbiota composition modulates choline bioavailability from diet and accumulation of the proatherogenic metabolite trimethylamine-N-oxide. MBio 6, e02481–e02414. doi: 10.1128/mBio.02481-14
Roscioni, S. S., Heerspink, H. J. L., and de Zeeuw, D. (2014). The effect of RAAS blockade on the progression of diabetic nephropathy. Nat. Rev. Nephrol. 10, 77–87. doi: 10.1038/nrneph.2013.251
Rossi, M., Johnson, D. W., Morrison, M., Pascoe, E. M., Coombes, J. S., Forbes, J. M., et al. (2016). Synbiotics easing renal failure by improving gut microbiology (SYNERGY): a randomized trial. Clin. J. Am. Soc. Nephrol. 11, 223–231. doi: 10.2215/cjn.05240515
Rustiasari, U. J., and Roelofs, J. J. (2022). The role of platelets in diabetic kidney disease. Int. J. Mol. Sci. 23:8270. doi: 10.3390/ijms23158270
Salguero, M. V., Al-Obaide, M. A. I., Singh, R., Siepmann, T., and Vasylyeva, T. L. (2019). Dysbiosis of gram-negative gut microbiota and the associated serum lipopolysaccharide exacerbates inflammation in type 2 diabetic patients with chronic kidney disease. Exp. Ther. Med. 18, 3461–3469. doi: 10.3892/etm.2019.7943
Samsu, N. (2021). Diabetic nephropathy: challenges in pathogenesis, diagnosis, and treatment. Biomed. Res. Int. 2021:1497449. doi: 10.1155/2021/1497449
Shen, H. J., and Wang, W. (2021). Effect of glutathione liposomes on diabetic nephropathy based on oxidative stress and polyol pathway mechanism. J. Liposome Res. 31, 317–325. doi: 10.1080/08982104.2020.1780607
Sheng, Y., Zheng, S. J., Zhang, C. H., Zhao, C. H., He, X. Y., Xu, W. T., et al. (2018). Mulberry leaf tea alleviates diabetic nephropathy by inhibiting PKC signaling and modulating intestinal flora. J. Funct. Foods 46, 118–127. doi: 10.1016/j.jff.2018.04.040
Shi, R. Y., Tao, Y. J., Tang, H. T., Wu, C. H., Fei, J. J., Ge, H. T., et al. (2023). Abelmoschus Manihot ameliorates the levels of circulating metabolites in diabetic nephropathy by modulating gut microbiota in non-obese diabetes mice. Microb. Biotechnol. 16, 813–826. doi: 10.1111/1751-7915.14200
Silva, A., Lanza, K., Palmeira, V. A., Costa, L. B., and Flynn, J. T. (2021). 2020 update on the renin-angiotensin-aldosterone system in pediatric kidney disease and its interactions with coronavirus. Pediatr. Nephrol. 36, 1407–1426. doi: 10.1007/s00467-020-04759-1
Silwal, P., Kim, I. S., and Jo, E. K. (2021). Autophagy and host defense in nontuberculous mycobacterial infection. Front. Immunol. 12:728742. doi: 10.3389/fimmu.2021.728742
Singh, R. P., Shadan, A., and Ma, Y. (2022). Biotechnological applications of probiotics: a multifarious weapon to disease and metabolic abnormality. Probiotics Antimicro. 14, 1184–1210. doi: 10.1007/s12602-022-09992-8
Smith, E. A., and Macfarlane, G. T. (1996). Enumeration of human colonic bacteria producing phenolic and indolic compounds: effects of pH, carbohydrate availability and retention time on dissimilatory aromatic amino acid metabolism. J. Appl. Bacteriol. 81, 288–302. doi: 10.1111/j.1365-2672.1996.tb04331.x
Spindler, M. P., Siu, S., Mogno, I., Li, Z., Yang, C., Mehandru, S., et al. (2022). Human gut microbiota stimulate defined innate immune responses that vary from phylum to strain. Cell Host Microbe 30, 1481–1498.e5. doi: 10.1016/j.chom.2022.08.009
Stavropoulou, E., Kantartzi, K., Tsigalou, C., Konstantinidis, T., Romanidou, G., Voidarou, C., et al. (2021). Focus on the gut–kidney axis in health and disease. Front. Med. 7:620102. doi: 10.3389/fmed.2020.620102
Su, S., Ma, Z., Wu, H., Xu, Z., and Yi, H. (2023). Oxidative stress as a culprit in diabetic kidney disease. Life Sci. 322:121661. doi: 10.1016/j.lfs.2023.121661
Su, X. H., Yu, W. X., Liu, A. R., Wang, C. X., Li, X. Z., Gao, J. J., et al. (2022). San-Huang-Yi-Shen capsule ameliorates diabetic nephropathy in rats through modulating the gut microbiota and overall metabolism. Front. Pharmacol. 12:808867. doi: 10.3389/fphar.2021.808867
Sun, X., Chen, J., Huang, Y. T., Zhu, S., Wang, S. S., Xu, Z. J., et al. (2022). Yishen Qingli Heluo granule ameliorates renal dysfunction in 5/6 nephrectomized rats by targeting gut microbiota and intestinal barrier integrity. Front. Pharmacol. 13:858881. doi: 10.3389/fphar.2022.858881
Sun, L., and Kanwar, Y. S. (2015). Relevance of TNF-alpha in the context of other inflammatory cytokines in the progression of diabetic nephropathy. Kidney Int. 88, 662–665. doi: 10.1038/ki.2015.250
Sun, R. Y., Mu, H. H., Sun, M. B., Miao, X. Y., Xin, J., Xu, X. F., et al. (2022). Effects of Bacillus subtilis natto JLCC513 on gut microbiota and intestinal barrier function in obese rats. J. Appl. Microbiol. 133, 3634–3644. doi: 10.1111/jam.15797
Sun, H., Saeedi, P., Karuranga, S., Pinkepank, M., Ogurtsova, K., Duncan, B. B., et al. (2022). IDF diabetes atlas: global, regional and country-level diabetes prevalence estimates for 2021 and projections for 2045. Diabetes Res. Clin. Pract. 183:109119. doi: 10.1016/j.diabres.2021.109119
Tan, Y. Q., Wang, Y. N., Feng, H. Y., Guo, Z. Y., Li, X., Nie, X. L., et al. (2022). Host/microbiota interactions-derived tryptophan metabolites modulate oxidative stress and inflammation via aryl hydrocarbon receptor signaling. Free Radic. Biol. Med. 184, 30–41. doi: 10.1016/j.freeradbiomed.2022.03.025
Tanase, D. M., Gosav, E. M., Anton, M. I., Floria, M., Seritean Isac, P. N., Hurjui, L. L., et al. (2022). Oxidative stress and NRF2/KEAP1/ARE pathway in diabetic kidney disease (DKD): new perspectives. Biomol. Ther. 12:1227. doi: 10.3390/biom12091227
Tao, S. B., Li, L. Z., Li, L., Liu, Y., Ren, Q., Shi, M., et al. (2019). Understanding the gut–kidney axis among biopsy-proven diabetic nephropathy, type 2 diabetes mellitus and healthy controls: an analysis of the gut microbiota composition. Acta Diabetol. 56, 581–592. doi: 10.1007/s00592-019-01316-7
Thibaut, M. M., and Bindels, L. B. (2022). Crosstalk between bile acid-activated receptors and microbiome in entero-hepatic inflammation. Trends Mol. Med. 28, 223–236. doi: 10.1016/j.molmed.2021.12.006
Tuttle, K. R., Agarwal, R., Alpers, C. E., Bakris, G. L., Brosius, F. C., Kolkhof, P., et al. (2022). Molecular mechanisms and therapeutic targets for diabetic kidney disease. Kidney Int. 102, 248–260. doi: 10.1016/j.kint.2022.05.012
van der Hee, B., and Wells, J. M. (2021). Microbial regulation of host physiology by short-chain fatty acids. Trends Microbiol. 29, 700–712. doi: 10.1016/j.tim.2021.02.001
Vaziri, N. D., Yuan, J., and Norris, K. (2013). Role of urea in intestinal barrier dysfunction and disruption of epithelial tight junction in chronic kidney disease. Am. J. Nephrol. 37, 1–6. doi: 10.1159/000345969
Waller, K. M. J., Leong, R. W., and Paramsothy, S. (2022). An update on fecal microbiota transplantation for the treatment of gastrointestinal diseases. J. Gastroenterol. Hepatol. 37, 246–255. doi: 10.1111/jgh.15731
Wang, M., Chen, F. Q., Wang, J. L., Zeng, Z. X., Yang, Q., and Shao, S. Y. (2018). Th17 and Treg lymphocytes in obesity and type 2 diabetic patients. Clin. Immunol. 197, 77–85. doi: 10.1016/j.clim.2018.09.005
Wang, X. X. X., Edelstein, M. H., Gafter, U., Qiu, L. R., Luo, Y. H., Dobrinskikh, E., et al. (2016). G protein-coupled bile acid receptor TGR5 activation inhibits kidney disease in obesity and diabetes. J. Am. Soc. Nephrol. 27, 1362–1378. doi: 10.1681/asn.2014121271
Wang, X. X. X., Jiang, T., Shen, Y., Caldas, Y., Miyazaki-Anzai, S., Santamaria, H., et al. (2010). Diabetic nephropathy is accelerated by farnesoid X receptor deficiency and inhibited by farnesoid X receptor activation in a type 1 diabetes model. Diabetes 59, 2916–2927. doi: 10.2337/db10-0019
Wang, H., Lu, Y., Yan, Y., Tian, S. S., Zheng, D. J., Leng, D. J., et al. (2020). Promising treatment for type 2 diabetes: fecal microbiota transplantation reverses insulin resistance and impaired islets. FCIMB 9:455. doi: 10.3389/fcimb.2019.00455
Wang, L., Mu, X., Chen, X., and Han, Y. (2022). Hydrogen sulfide attenuates intracellular oxidative stress via repressing glycolate oxidase activities in Arabidopsis thaliana. BMC Plant Biol. 22:98. doi: 10.1186/s12870-022-03490-3
Wang, H. Q., Wang, S. S., Kuok, C., Wang, Q., and Cheng, X. L. (2019). Umbelliferone ameliorates renal function in diabetic nephropathy rats through regulating inflammation and TLR/NF-κ B pathway. Chin. J. Nat. Medicines 17, 346–354. doi: 10.1016/s1875-5364(19)30040-8
Wang, X. X. X., Wang, D., Luo, Y. H., Myakala, K., Dobrinskikh, E., Rosenberg, A. Z., et al. (2018). FXR/TGR5 dual agonist prevents progression of nephropathy in diabetes and obesity. J. Am. Soc. Nephrol. 29, 118–137. doi: 10.1681/asn.2017020222
Wang, J. M., Xiang, H. J., Lu, Y. F., Wu, T., and Ji, G. (2021). New progress in drugs treatment of diabetic kidney disease. Biomed. Pharmacother. 141:111918. doi: 10.1016/j.biopha.2021.111918
Wang, S. P., Zhang, W., Cao, A. Z., Pan, Z. C., and Liu, T. L. (2022). Dietary supplementation of bile acids in tongue sole (Cynoglossus semilaevis): a promising strategy to improve hepatic health status. Front. Mar. Sci. 9:899768. doi: 10.3389/fmars.2022.899768
Wang, Y. W., Zhao, J., Qin, Y. L., Yu, Z. X., Zhang, Y. M., Ning, X. X., et al. (2022). The specific alteration of gut microbiota in diabetic kidney diseases-a systematic review and meta-analysis. Front. Immunol. 13:908219. doi: 10.3389/fimmu.2022.908219
Wang, F. Z., Zhou, H., Wang, H. Y., Dai, H. B., Gao, Q., Qian, P., et al. (2021). Hydrogen sulfide prevents arterial medial calcification in rats with diabetic nephropathy. BMC Cardiovasc. Disord. 21:495. doi: 10.1186/s12872-021-02307-9
Warren, A. M., Knudsen, S. T., and Cooper, M. E. (2019). Diabetic nephropathy: an insight into molecular mechanisms and emerging therapies. Expert Opin. Ther. Targets 23, 579–591. doi: 10.1080/14728222.2019.1624721
Wegierska, A. E., Charitos, I. A., Topi, S., Potenza, M. A., Montagnani, M., and Santacroce, L. (2022). The connection between physical exercise and gut microbiota: implications for competitive sports athletes. Sports Med. 52, 2355–2369. doi: 10.1007/s40279-022-01696-x
Wei, H., Wang, L., An, Z., Xie, H., Liu, W., du, Q., et al. (2021). QiDiTangShen granules modulated the gut microbiome composition and improved bile acid profi les in a mouse model of diabetic nephropathy. Biomed. Pharmacother. 133:111061. doi: 10.1016/j.biopha.2020.111061
Wei, C. W., Wu, T. K., Wu, S. C., Chen, Y. L., Pan, Y. R., Chien, Y. C., et al. (2022). Curcumin enhances p-cresyl sulfate-induced cytotoxic effects on renal tubular cells. Int. J. Med. Sci. 19, 1138–1146. doi: 10.7150/ijms.72646
Williams, B. M., Cliff, C. L., Lee, K., Squires, P. E., and Hills, C. E. (2022). The role of the NLRP3 Inflammasome in mediating glomerular and tubular injury in diabetic nephropathy. Front. Physiol. 13:907504. doi: 10.3389/fphys.2022.907504
Winiarska, A., Knysak, M., Nabrdalik, K., Gumprecht, J., and Stompor, T. (2021). Inflammation and oxidative stress in diabetic kidney disease: the targets for SGLT2 inhibitors and GLP-1 receptor agonists. Int. J. Mol. Sci. 22:10822. doi: 10.3390/ijms221910822
Wu, C., Fei, J., Xu, Q., Tao, Y., Zhou, Z., Wang, Y., et al. (2022). Interaction between plasma metabolomics and intestinal microbiome in db/db mouse, an animal model for study of type 2 diabetes and diabetic kidney disease. Meta 12:775. doi: 10.3390/metabo12090775
Wu, T. Y., Wang, G. Q., Xiong, Z. Q., Xia, Y. J., Song, X., Zhang, H., et al. (2022). Probiotics interact with lipids metabolism and affect gut health. Front. Nutr. 9:917043. doi: 10.3389/fnut.2022.917043
Wu, X. Q., Zhang, D. D., Wang, Y. N., Tan, Y. Q., Yu, X. Y., and Zhao, Y. Y. (2021). AGE/RAGE in diabetic kidney disease and ageing kidney. Free Radic. Biol. Med. 171, 260–271. doi: 10.1016/j.freeradbiomed.2021.05.025
Xiao, H. M., Sun, X. H., Liu, R. B., Chen, Z. Q., Lin, Z. Y., Yang, Y., et al. (2020). Gentiopicroside activates the bile acid receptor Gpbar1 (TGR5) to repress NF-κ B pathway and ameliorate diabetic nephropathy. Pharmacol. Res. 151:104559. doi: 10.1016/j.phrs.2019.104559
Xie, Y., Lin, X., Yuan, J., Dong, R., Yu, J. L., and Zha, Y. (2022). Effects of acteoside on the expressions of MCP-1 and TGF-β (1) in the diabetic nephropathy mice. Eur. J. Inflamm. 20:1721727X221118348. doi: 10.1177/1721727x221118348
Xiong, F. X., Li, X. J., Yang, Z. Y., Wang, Y., Gong, W. Y., Huang, J. Y., et al. (2016). TGR5 suppresses high glucose-induced upregulation of fibronectin and transforming growth factor-β 1 in rat glomerular mesangial cells by inhibiting RhoA/ROCK signaling. Endocrine 54, 657–670. doi: 10.1007/s12020-016-1032-4
Xu, Y. H., Gao, C. L., Guo, H. L., Zhang, W. Q., Huang, W., Tang, S. S., et al. (2018). Sodium butyrate supplementation ameliorates diabetic inflammation in db/db mice. J. Endocrinol. 238, 231–244. doi: 10.1530/joe-18-0137
Xu, J., Yao, X. Z., Li, X. Y., Xie, S. W., Chi, S. Y., Zhang, S., et al. (2022). Bile acids reduced the lipid deposition in fatty degenerated hepatocytes of pearl gentian grouper (Epinephelus fuscoguttatus♂ × Epinephelus lanceolatus♀) in vitro. Front. Mar. Sci. 9:861117. doi: 10.3389/fmars.2022.861117
Yamamoto, J., Sato, W., Kosugi, T., Yamamoto, T., Kimura, T., Taniguchi, S., et al. (2013). Distribution of hydrogen sulfide (H2S)-producing enzymes and the roles of the H2S donor sodium hydrosulfide in diabetic nephropathy. Clin. Exp. Nephrol. 17, 32–40. doi: 10.1007/s10157-012-0670-y
Yang, C. Y., Chen, T. W., Lu, W. L., Liang, S. S., Huang, H. D., Tseng, C. P., et al. (2021). Synbiotics alleviate the gut indole load and dysbiosis in chronic kidney disease. Cells 10:114. doi: 10.3390/cells10010114
Yang, J., Dong, H., Wang, Y., Jiang, Y., Zhang, W., Lu, Y., et al. (2020). Cordyceps cicadae polysaccharides ameliorated renal interstitial fibrosis in diabetic nephropathy rats by repressing inflammation and modulating gut microbiota dysbiosis. Int. J. Biol. Macromol. 163, 442–456. doi: 10.1016/j.ijbiomac.2020.06.153
Yang, L., Lin, H. Q., Lin, W. T., and Xu, X. Y. (2020). Exercise ameliorates insulin resistance of type 2 diabetes through motivating short-chain fatty acid-mediated skeletal muscle cell autophagy. Biology-Basel 9:9080203. doi: 10.3390/biology9080203
Yang, Z. Y., Xiong, F. X., Wang, Y., Gong, W. Y., Huang, J. Y., Chen, C., et al. (2016). TGR5 activation suppressed S1P/S1P2 signaling and resisted high glucose-induced fibrosis in glomerular mesangial cells. Pharmacol. Res. 111, 226–236. doi: 10.1016/j.phrs.2016.05.035
Yang, Y., and Xu, G. S. (2022). Update on pathogenesis of glomerular hyperfiltration in early diabetic kidney disease. Front. Endocrinol. 13:872918. doi: 10.3389/fendo.2022.872918
Yang, H., Xu, W., Zhou, Z., Liu, J., Li, X., Chen, L., et al. (2015). Curcumin attenuates urinary excretion of albumin in type II diabetic patients with enhancing nuclear factor erythroid-derived 2-like 2 (Nrf2) system and repressing inflammatory signaling efficacies. Exp. Clin. Endocrinol. Diabetes 123, 360–367. doi: 10.1055/s-0035-1545345
Ying, L. W., Shen, Y., Zhang, Y., Wang, Y. K., Liu, Y., Yin, J., et al. (2021). Association of advanced glycation end products with diabetic retinopathy in type 2 diabetes mellitus. Diabetes Res. Clin. Pract. 177:108880. doi: 10.1016/j.diabres.2021.108880
Yoo, W., Zieba, J., Foegeding, N., Torres, T., Shelton, C., Shealy, N., et al. (2021). High-fat diet–induced colonocyte dysfunction escalates microbiota-derived trimethylamineN-oxide. Science 373, 813–818. doi: 10.1126/science.aba3683
Yu, N., Gu, N., Wang, Y. X., Zhou, B., Lu, D. F., Li, J. P., et al. (2022). The association of plasma trimethylamine N-oxide with coronary atherosclerotic burden in patients with type 2 diabetes among a Chinese north population. Diabetes Metab. Syndr. Obesity 15, 69–78. doi: 10.2147/dmso.s339698
Zafar, H., and Saier, M. H. (2021). Gut Bacteroides species in health and disease. Gut Microbes 13:1848158. doi: 10.1080/19490976.2020.1848158
Zakrzewska, Z., Zawartka, A., Schab, M., Martyniak, A., Skoczeń, S., Tomasik, P. J., et al. (2022). Prebiotics, probiotics, and postbiotics in the prevention and treatment of anemia. Microorganisms 10:1330. doi: 10.3390/microorganisms10071330
Zeng, O., Li, F., Li, Y., Li, L., Xiao, T., Chu, C., et al. (2016). Effect of novel gasotransmitter hydrogen sulfide on renal fibrosis and connexins expression in diabetic rats. Bioengineered 7, 314–320. doi: 10.1080/21655979.2016.1197743
Zhang, Z. P., Li, Q. Y., Liu, F., and Li, D. Y. (2022). Lycoperoside H protects against diabetic nephropathy via alteration of gut microbiota and inflammation. J. Biochem. Mol. Toxicol. 36:e23216. doi: 10.1002/jbt.23216
Zhang, F., Qi, L. L., Feng, Q. Y., Zhang, B. K., Li, X. Y., Liu, C., et al. (2021). HIPK2 phosphorylates HDAC3 for NF-κ B acetylation to ameliorate colitis-associated colorectal carcinoma and sepsis. Proc. Natl. Acad. Sci. U. S. A. 118:e2021798118. doi: 10.1073/pnas.2021798118
Zhang, B., Wan, Y. Z., Zhou, X. F., Zhang, H. J., Zhao, H. L., Ma, L., et al. (2022). Characteristics of serum metabolites and gut microbiota in diabetic kidney disease. Front. Pharmacol. 13:872988. doi: 10.3389/fphar.2022.872988
Zhang, L. L., Wang, Z. S., Zhang, X. N., Zhao, L., Chu, J. J., Li, H. B., et al. (2022). Alterations of the gut microbiota in patients with diabetic nephropathy. Microbiol. Spectr., e00324–e00322. doi: 10.1128/spectrum.00324-22
Zhang, M., Yang, L. C., Zhu, M. M., Yang, B., Yang, Y. J., Jia, X. B., et al. (2022). Moutan cortex polysaccharide ameliorates diabetic kidney disease via modulating gut microbiota dynamically in rats. Int. J. Biol. Macromol. 206, 849–860. doi: 10.1016/j.ijbiomac.2022.03.077
Zhang, Q., Zhang, Y., Zeng, L., Chen, G., Zhang, L., Liu, M., et al. (2021). The role of gut microbiota and microbiota-related serum metabolites in the progression of diabetic kidney disease. Front. Pharmacol. 12:757508. doi: 10.3389/fphar.2021.757508
Zhang, L., Zhao, M. H., and Zuo, L. (2020). China kidney disease network (CK-NET) 2015 annual data report. Kidney Int. Suppl. 10:E95. doi: 10.1016/j.kisu.2019.05.001
Zhao, H. J., Liu, H. Y., Yang, Y. H., Lan, T. Y., Wang, H. G., and Wu, D. D. (2022). Hydrogen sulfide plays an important role by regulating endoplasmic reticulum stress in diabetes-related diseases. Int. J. Mol. Sci. 23:7170. doi: 10.3390/ijms23137170
Zhao, J., Ning, X. X., Liu, B. J., Dong, R. J., Bai, M., and Sun, S. R. (2021). Specific alterations in gut microbiota in patients with chronic kidney disease: an updated systematic review. Ren. Fail. 43, 102–112. doi: 10.1080/0886022x.2020.1864404
Zhao, J., Zhang, Q. L., Shen, J. H., Wang, K., and Liu, J. (2019). Magnesium lithospermate B improves the gut microbiome and bile acid metabolic profiles in a mouse model of diabetic nephropathy. Acta Pharmacol. Sin. 40, 507–513. doi: 10.1038/s41401-018-0029-3
Zhao, T., Zhang, H., Yin, X., Zhao, H., Ma, L., Yan, M., et al. (2020). Tangshen formula modulates gut microbiota and reduces gut-derived toxins in diabetic nephropathy rats. Biomed. Pharmacother. 129:110325. doi: 10.1016/j.biopha.2020.110325
Zheng, S. Y., Li, H. X., Xu, R. C., Miao, W. T., Dai, M. Y., Ding, S. T., et al. (2021). Potential roles of gut microbiota and microbial metabolites in Parkinson's disease. Ageing Res. Rev. 69:101347. doi: 10.1016/j.arr.2021.101347
Zhong, C. Y., Dai, Z. W., Chai, L. X., Wu, L. P., Li, J. H., Guo, W. Y., et al. (2021). The change of gut microbiota-derived short-chain fatty acids in diabetic kidney disease. J. Clin. Lab. Anal. 35:e24062. doi: 10.1002/jcla.24062
Zhong, Y. F., Lee, K., and He, J. C. (2018). SIRT1 is a potential drug target for treatment of diabetic kidney disease. Front. Endocrinol. 9:624. doi: 10.3389/fendo.2018.00624
Zhou, B. S., Feng, B., Qin, Z. X., Zhao, Y. G., Chen, Y., Shi, Z. M., et al. (2016). Activation of farnesoid X receptor downregulates visfatin and attenuates diabetic nephropathy. Mol. Cell. Endocrinol. 419, 72–82. doi: 10.1016/j.mce.2015.10.001
Zhou, X., Feng, Y., Zhan, Z. B., and Chen, J. C. (2014). Hydrogen sulfide alleviates diabetic nephropathy in a streptozotocin-induced diabetic rat model. J. Biol. Chem. 289, 28827–28834. doi: 10.1074/jbc.M114.596593
Zhou, T. T., Xu, H. W., Cheng, X., He, Y. Q., Ren, Q., Li, D. Z., et al. (2022). Sodium butyrate attenuates diabetic kidney disease partially via histone butyrylation modification. Mediat. Inflamm. 2022:7643322. doi: 10.1155/2022/7643322
Zhu, W. F., Gregory, J. C., Org, E., Buffa, J. A., Gupta, N., Wang, Z. N., et al. (2016). Gut microbial metabolite TMAO enhances platelet hyperreactivity and thrombosis risk. Cells 165, 111–124. doi: 10.1016/j.cell.2016.02.011
Keywords: diabetic nephropathy, gut microbiota, microbial metabolites, gut–kidney axis, microbiota-targeted therapies
Citation: Zhao H, Yang C-E, Liu T, Zhang M-X, Niu Y, Wang M and Yu J (2023) The roles of gut microbiota and its metabolites in diabetic nephropathy. Front. Microbiol. 14:1207132. doi: 10.3389/fmicb.2023.1207132
Edited by:
Ma. Cecilia Opazo, Universidad de las américas, ChileReviewed by:
Weitao Geng, Tianjin University of Science and Technology, ChinaPengyu Tao, Shanghai University of Traditional Chinese Medicine, China
Copyright © 2023 Zhao, Yang, Liu, Zhang, Niu, Wang and Yu. This is an open-access article distributed under the terms of the Creative Commons Attribution License (CC BY). The use, distribution or reproduction in other forums is permitted, provided the original author(s) and the copyright owner(s) are credited and that the original publication in this journal is cited, in accordance with accepted academic practice. No use, distribution or reproduction is permitted which does not comply with these terms.
*Correspondence: Jun Yu, cGNsYW1wZXJAMTYzLmNvbQ==
†These authors have contributed equally to this work