- 1Department of Zoonoses, Faculty of Veterinary Medicine, Zagazig University, Zagazig, Egypt
- 2Department of Infectious Diseases and Public Health, Jockey Club College of Veterinary Medicine and Life Sciences, City University of Hong Kong, Kowloon, Hong Kong SAR, China
- 3Centre for Applied One Health Research and Policy Advice (OHRP), City University of Hong Kong, Kowloon, Hong Kong SAR, China
- 4Department of Animal Medicine, Faculty of Veterinary Medicine, Zagazig University, Zagazig, Egypt
- 5Department of Science, King Khalid Military Academy, Riyadh, Saudi Arabia
- 6Department of Food Hygiene, Safety and Technology, Faculty of Veterinary Medicine, Zagazig University, Zagazig, Egypt
- 7Department of Botany and Microbiology, Faculty of Science, Zagazig University, Zagazig, Egypt
- 8Department of Microbiology, Faculty of Veterinary Medicine, Zagazig University, Zagazig, Egypt
Listeria monocytogenes (L. monocytogenes) is a foodborne pathogen that poses significant risks to public health and food safety. The present study aimed to identify the presence of Listeria spp. in various samples, including pasteurized milk, chicken fillets, and stool samples from pregnant women in Sharkia Governorate, Egypt. Additionally, the study identified the serotypes, virulence-associated genes, antimicrobial resistance patterns, and biofilm formation in L. monocytogenes isolates. Moreover, the antibacterial and anti-biofilm activity of Lactobacillus plantarum ATCC 14917 (L. plantarum) against L. monocytogenes isolates was investigated. A cross-sectional study was conducted from August 2021 to January 2022 to collect 300 samples of pasteurized milk, chicken fillets, and stool from pregnant women admitted to outpatient clinics of hospitals. The results showed that 32.7% of the samples were positive for Listeria spp., including L. innocua (48.9%), L. monocytogenes (26.5%), L. ivanovii (14.3%), L. grayi (5.1%), and L. welshimeri (5.1%). Among all L. monocytogenes isolates, hlyA, actA, inlC, and inlJ virulence-associated genes were detected. However, the virulence genes plcB, iap, and inlA were found in 10 (38.5%), 8 (30.8%), and 25 (96.2%) isolates, respectively. The L. monocytogenes isolates classified into four serotypes (1/2a, 1/2b, 1/2c, and 4b), with 1/2a and 4b each identified in 30.8% of the isolates, while 1/2b and 1/2c were identified in 19.2% of the isolates. All L. monocytogenes isolates showed 100% resistance to streptomycin, kanamycin, and nalidix acid, and 92.3% of isolates showed gentamicin resistance. However, all isolates were susceptible to ampicillin and ampicillin/sulbactam. Multidrug resistance (MDR) was observed in 20 (76.9%) L. monocytogenes isolates. The biofilm formation ability of 26 L. monocytogenes isolates was evaluated at different incubation temperatures. At 4°C, 25°C, and 37°C, 53.8, 69.2, and 80.8% of the isolates, respectively, were biofilm producers. Furthermore, 23.1% were strong biofilm producers at both 4°C and 25°C, while 34.6% were strong biofilm formers at 37°C. Treating L. monocytogenes isolates with L. plantarum cell-free supernatant (CFS) reduced the number of biofilm-producing isolates to 15.4, 42.3, and 53.8% at 4°C, 25°C, and 37°C, respectively. L. plantarum’s CFS antibacterial activity was tested against six virulent, MDR, and biofilm-forming L. monocytogenes isolates. At a concentration of 5 μg/mL of L. plantarum CFS, none of the L. monocytogenes isolates exhibited an inhibition zone. However, an inhibition zone was observed against L. monocytogenes strains isolated from pasteurized milk and pregnant women’s stools when using a concentration of 10 μg/mL. Transmission electron microscopy (TEM) revealed that L. plantarum CFS induced morphological and intracellular structural changes in L. monocytogenes. In conclusion, this study identified virulent MDR L. monocytogenes isolates with strong biofilm-forming abilities in food products in Egypt, posing significant risks to food safety. Monitoring the prevalence and antimicrobial resistance profile of L. monocytogenes in dairy and meat products is crucial to enhance their safety. Although L. plantarum CFS showed potential antibacterial and anti-biofilm effects against L. monocytogenes isolates, further research is needed to explore its full probiotic potential.
1. Introduction
The genus Listeria is a Gram-positive, non-spore-forming, facultative anaerobic, rod-shaped bacteria (Orsi et al., 2011). It comprises several species, including Listeria monocytogenes (L. monocytogenes), L. innocua, L. ivanovii, L. welshimeri, L. grayi, L. seeligeri, as well as the newly discovered L. marthii, L. weihenstephanensis, L. rocourtiae, and L. fleischmannii (Hellberg et al., 2013). Listeria spp. are found in various environmental sources such as soil, water, food, and the feces of humans and animals (Zunabovic et al., 2011). Furthermore, it can grow at low temperatures, high salt concentrations, and a wide pH range (Walker et al., 1990).
Listeria monocytogenes is the most pathogenic species among Listeria, causing a highly fatal opportunistic foodborne infection known as listeriosis (Vázquez-Boland et al., 2001). This disease predominantly affects pregnant women, elderly, neonates, and immunocompromised or debilitated patients, although it can also develop in healthy individuals. It causes mortality of 30% in immunosuppressed individuals, elderly and neonate worldwide (Drevets and Bronze, 2008). Human listeriosis in developing countries is mostly acquired through consumption of contaminated milk products including soft cheeses, meat products including ready-to-eat meat products (Scallan et al., 2011) and raw, smoked or cured fish products and seafood (Jami et al., 2014).
Listeria monocytogenes has thirteen serotypes based on somatic (O) and flagellar (H) antigen reactions (Meloni, 2014). Serotypes 4b, 1/2a, and 1/2b are predominant in human infections (Zeinali et al., 2015). The pathogenicity of L. monocytogenes is attributed to variant virulence factors, such as internalin (encoded by inlA, inlB, inlC, and inlJ genes), listeriolysin O (encoded by hly gene), phosphatidylinositol phospholipase C (encoded by plcA), actin polymerization protein (encoded by actA), and invasive associated protein (iap), as well as the regulatory system for gene expression of virulence (PrfA; Liu et al., 2007).
Listeria monocytogenes are typically sensitive to commonly used antibiotics, but multiple drug-resistant strains have been found in cases of human listeriosis, food and environment (Moreno et al., 2014; Noll et al., 2017; Tahoun et al., 2017). The excessive use of antibiotics as growth promoters has hastened the evolution of L. monocytogenes toward resistance (Doyle et al., 2013), in keeping with the global trend of rising antibiotic resistance among foodborne pathogens (Harakeh et al., 2009). Biofilms help bacteria resist environmental stressors like dehydration and antimicrobial treatments. L. monocytogenes has the ability to form biofilms on various surfaces in food processing environments, including equipment, utensils, and food contact surfaces, which contributes to its survival and pathogenicity (Møretrø and Langsrud, 2004; Folsom et al., 2006). To effectively eradicate L. monocytogenes biofilms, several strategies and interventions can be employed, including the use of chemical disinfectants, mechanical removal, biofilm-disrupting agents, heat treatment, and radiation (Fagerlund et al., 2020).
To address the limited treatment options for L. monocytogenes, various alternative therapies have been explored to inhibit its growth in foods due to the rapid development of antimicrobial resistance in this pathogen (Matle et al., 2020). One approach is the use of lactic acid bacteria (LAB) strains that produce probiotics with antimicrobial properties. Probiotics have been shown to reduce the growth, adhesion, and biofilm formation of L. monocytogenes (Merino et al., 2019) while enhancing host immunity (Dhama et al., 2015). Therefore, the objectives of this study were to (i) detect Listeria spp. in pasteurized milk, chicken fillets, and stool samples from pregnant women in Sharkia Governorate, Egypt, (ii) identify the serotypes, virulence-associated genes, antimicrobial resistance patterns and biofilm formation in L. monocytogenes isolates, and (iii) investigate the antibacterial and anti-biofilm effect of Lactobacillus plantarum (L. plantarum) on L. monocytogenes isolates.
2. Materials and methods
2.1. Sample collection
A cross-sectional study carried out between August 2021 and January 2022 to collect pasteurized milk and chicken fillets samples (n = 100, each) from various retail markets across Sharkia Governorate, Egypt. Simultaneously, 100 stool samples were collected from pregnant women admitted to outpatient clinics of hospitals located in the same localities as the retail markets. The collected samples were labeled with retail market/hospital ID, location, date, and time of sampling before being sent to the laboratory for later analysis.
2.2. Isolation and identification of Listeria spp.
The US Food and Drug Administration (FDA) protocol (Hitchins et al., 2017) was used to isolate and identify Listeria spp. from pasteurized milk, chicken fillet, and stool samples. Initially, samples were pre-enriched in buffered peptone water (Himedia Lab, Mumbai, India) and incubated for 48 h at 37°C. Next, 25 mL of each pre-enriched sample were added to 225 mL of Listeria enrichment broth (Himedia Lab, Mumbai, India) and incubated for 48 h at 30°C. A loopful from the enriched broth was directly streaked onto Agar Listeria Ottaviani Agosti (ALOA) (Himedia Lab, Mumbai, India) and incubated for 24–48 h at 37°C. Colonies (Walker et al., 1990; Zunabovic et al., 2011) exhibiting typical morphologically were cultured on tryptic soy agar yeast extract (TSAye) at 35°C for 24–48 h. The colonies were presumptively identified using Gram staining, biochemical tests including haemolytic, catalase, and oxidase activities, as well as rhamnose, xylose, and mannitol fermentation (Mac Faddin, 2000). Additionally, the Oxoid Listeria Test Kit (Oxoid, UK) was used for Latex Agglutination Test.
2.3. Molecular characterization of Listeria monocytogenes
Genomic DNA was extracted from L. monocytogenes isolates using the QIAamp DNA Mini kit (QIAGEN GmbH, Hilden, Germany). A PCR amplification of 553 bp was performed on the extracted DNA using primers (Supplementary Table S1) specific for 16S rRNA (Lantz et al., 1994).
Listeria monocytogenes confirmed isolates were identified for virulence-associated genes using primers targeting hly, actA, plcB, iap (Cao et al., 2018), and inlA, inlC, inlJ genes (Liu et al., 2007; Supplementary Table S1). Additionally, the serotypes of L. monocytogenes isolates were determined using four genes: Imo0737 (619 bp), lmo1118 (906 bp), ORF2819 (417 bp), and ORF2110 (597 bp) (Doumith et al., 2004). Positive controls, including reference strains CDC F4555 (4b), ATCC 19111 (1/2a), CDC F4976 (1/2b), and ATCC 19112 (1/2c), were used in the PCR assay alongside the tested isolates.
2.4. Antimicrobial susceptibility test
The antibiotic susceptibility of the confirmed L. monocytogenes isolates was determined using the disk diffusion method (Oxoid, UK), following Clinical and Laboratory Standards Institute (CLSI) guidelines (Clinical and Laboratory Standards Institute, 2018). The antibiotic disks tested were ampicillin (AMP, 10 μg/mL), ampicillin/sulbactam (SAM, 20 μg/mL), amoxicillin-clavulanate (AMC, 30 μg/mL), cefotaxime (CTX, 30 μg/mL), cephalothin (CEF, 30 μg/mL), gentamicin (GEN, 10 μg/mL), streptomycin (STR, 10 μg/mL), tetracycline (TET, 30 μg/mL), kanamycin (KAN, 30 μg/mL), ciprofloxacin (CIP, 5 μg/mL), nalidix acid (NA, 30 μg/mL), trimethoprim-sulfamethoxazole (SXT, 25 μg/mL), chloramphenicol (CHL, 30 μg/mL), erythromycin (ERY, 15 μg/mL), and meropenem (MEM, 10 μg/mL). Mueller-Hinton agar plates were used (Oxoid, UK) with incubation at 35°C for 24 h, then the zone of inhibition was recorded as resistant (R), intermediate (I), or sensitive (S) according to CLSI standards. Isolates that exhibited resistance to three or more distinct antimicrobial classes were categorized as being multidrug resistant (MDR), as defined by Magiorakos, Srinivasan (Magiorakos et al., 2012). Additionally, the multiple antibiotic resistances (MAR) index was calculated for all isolates by applying the formula a/b, where “a” represents the number of antimicrobials to which an isolate was resistant and “b” represents the total number of antimicrobials tested. This calculation was carried out in accordance with the protocol specified by Krumperman (1983).
2.5. Biofilm formation and quantification
The biofilm forming ability of L. monocytogenes isolates was evaluated at various storage temperature (4°C, 25°C, and 37°C) using the microtiter plate assay (Kırmusaoğlu, 2019). A bacterial suspension was prepared in Mueller Hinton broth and adjusted to 0.5 McFarland (1.5 × 108 CFU/mL). A 100 μL of the bacterial suspension was inoculated into each of the three sterile polystyrene microtiter plates in triplicate. The plates were incubated at 4°C, 25°C, and 37°C for 24 h (plate I, II, and III, respectively). In each plate, three wells containing only broth were left as negative controls. To remove free-floating cells, the media from the plate wells were discarded and washed twice with 0.2 mL of phosphate-buffered saline (PBS, pH 7.2). The plates were inverted, and PBS was removed by blotting with paper towels. The biofilm was fixed by adding 150 μL of ethanol for 20 min, and the cells adhered to the microtiter plates were stained with 150 μL of crystal violet for 15 min at room temperature after removing the stain. The wells were washed twice with PBS and air dried for 1 h.
Biofilm quantification was carried out by adding 150 μL of 95% ethanol to each well for 45 min. The optical density (OD) was then measured at a wavelength of 570 nm (OD570) using an ELISA reader (Sunrise, Tecan) after adjusting to the negative control (ODNC) at zero. Mean and standard deviation of OD values were recorded for all L. monocytogenes isolates and negative controls. The isolates were then classified as negative (OD570 ≤ ODNC), weak (ODNC < OD570 ≤ 2 x ODNC), moderate (2 × ODNC < OD570 ≤ 4 × ODNC), or strong (4 × ODNC < OD570) biofilm formers (Saxena et al., 2014).
2.6. Effect of Lactobacillus plantarum on Listeria monocytogenes
The effect of L. plantarum ATCC 14917, a probiotic strain from the Belgian Co-ordinated Collection of Microorganisms (BCCM), on L. monocytogenes isolates recovered from pasteurized milk, chicken fillets, and pregnant women were evaluated.
2.6.1. Extraction of Lactobacillus plantarum cell-free supernatant
Lactobacillus plantarum was cultured in de-Man, Rogosa, and Sharpe (MRS) broth for 18 h at 37°C, followed by centrifugation at 4000 rpm for 10 min at 4°C. The resulting supernatant was filter-sterilized using a 0.2 mm membrane syringe filter. The cell-free supernatant (CFS) of L. plantarum was collected to be used as an inhibitory agent against L. monocytogenes (Enan et al., 2014).
2.6.2. Anti-biofilm activity of Lactobacillus plantarum
A bacterial suspension of L. monocytogenes co-cultured with L. plantarum CFS in Mueller Hinton broth, adjusted to 0.5 McFarland (1.5 × 108 CFU/mL), and inoculated 100 μL of the suspension into microtiter plates as described above. The plates were then incubated at 4°C, 25°C, and 37°C for 24 h to assess the antibiofilm activity of L. plantarum at different incubation temperature.
To measure the extent of biofilm formation in the presence of L. plantarum CFS, we used an ELISA reader (Sunrise, Tecan) to measure the OD value of the biofilm at 570 nm, after adjusting to the negative control (ODNC) at zero. We calculated the percentage of biofilm inhibition using the following formula:
The growth pattern of L. monocytogenes was evaluated in the presence and absence of L. plantarum CFS over a 24-h period in Mueller Hinton broth. The growth kinetics curve can be found in Supplementary Figure S1.
2.6.3. Antibacterial activity of Lactobacillus plantarum
The antibacterial activity of L. plantarum CFS was assessed on six (two from each source) virulent, MDR and biofilm forming L. monocytogenes isolates using the agar well diffusion method (Osman et al., 2021). Briefly, 25 mL of melted Mueller Hinton agar was inoculated separately with 0.1 mL a 24-h-old bacterial suspension. The mixture was then poured into a Petri dish and left to solidify at room temperature for 30 min, then a 10 mm diameter well were made in the agar using a sterile cork borer. The wells were filled with 100 μL of the L. plantarum CFS. Positive and negative controls were implemented by filling wells with AMP (10 μg/mL) and broth media, respectively. The plates were then incubated at 37°C for 24 h, after which the inhibition zone diameters were measured using a ruler. Each isolate was tested in triplicate.
2.6.4. Minimum inhibitory concentration assay
The minimum inhibitory concentration (MIC) value of L. plantarum CFS against L. monocytogenes isolates was determined using the agar well diffusion method as described above. L. plantarum CFS was prepared at various concentrations (10, 20, 30, 40, 50, 60, 70, 80, and 90 μg/mL) from the 100 μg/mL stock solution. Serial two-fold dilutions were made using sterile deionized water, with 0.05, 0.1, 0.15, 0.2, 0.25, 0.3, 0.35, 0.4, and 0.45 mL taken from the original stock, resulting in the respective concentrations mentioned. The dilutions were added to the wells and the plates were incubated at 37°C for 24 h. The mean and standard error of inhibition zone diameters were then determined, with all isolates tested in triplicate. The MIC was determined as the well containing the lowest concentration of L. plantarum CFS that still showed a zone of inhibition.
2.6.5. Transmission electron microscope (TEM) analysis
Lactobacillus plantarum CFS was added to L. monocytogenes culture grown on nutrient broth media for 24 h (Amin, 2016). The bacterial cells were collected via centrifugation at 4000 rpm for 10 min, washed with distilled water, fixed with 3% glutaraldehyde, rinsed with phosphate buffer, and fixed again in a potassium permanganate solution for 5 min at room temperature. The samples were then dehydrated in an ethanol series (ranging from 10 to 90% for 15 min each), followed by absolute ethanol for 30 min. Samples were infiltrated with epoxy resin and acetone through a graded series, and ultimately in pure resin. Ultrathin sections were obtained on copper grids and double stained in uranyl acetate and lead citrate. The stained sections were then observed using TEM (JEOL JEM-1010, Tokyo, Japan). L. monocytogenes without L. plantarum CFS was used as a control.
2.7. Data analysis
The statistical analysis and data visualization were performed using R software (version 4.2.0). The Kruskal-Wallis test was used to assess the difference between the MAR index and sources of the samples, followed by multiple comparisons using the rank sums (Dunn test) with Bonferroni correction. A p-value of <0.05 was considered significant.
3. Results
3.1. Listeria spp. isolation and identification
Three hundred samples of pasteurized milk, chicken fillets, and stool from pregnant women were tested for Listeria spp. identification (Table 1). Listeria spp. were detected in 98 (32.7%) of the samples, with 10 (10%), 49 (52%), and 39 (23.1%) isolates retrieved from pasteurized milk, chicken fillets, and stool from pregnant women, respectively. Biochemical and Listeria Latex Agglutination test identified 48 (48.9%) L. innocua, 26 (26.5%) L. monocytogenes, 14 (14.3%) L. ivanovii, 5 (5.1%) L. grayi, and 5 (5.1%) L. welshimeri among the 98 Listeria spp. isolates (Table 1).
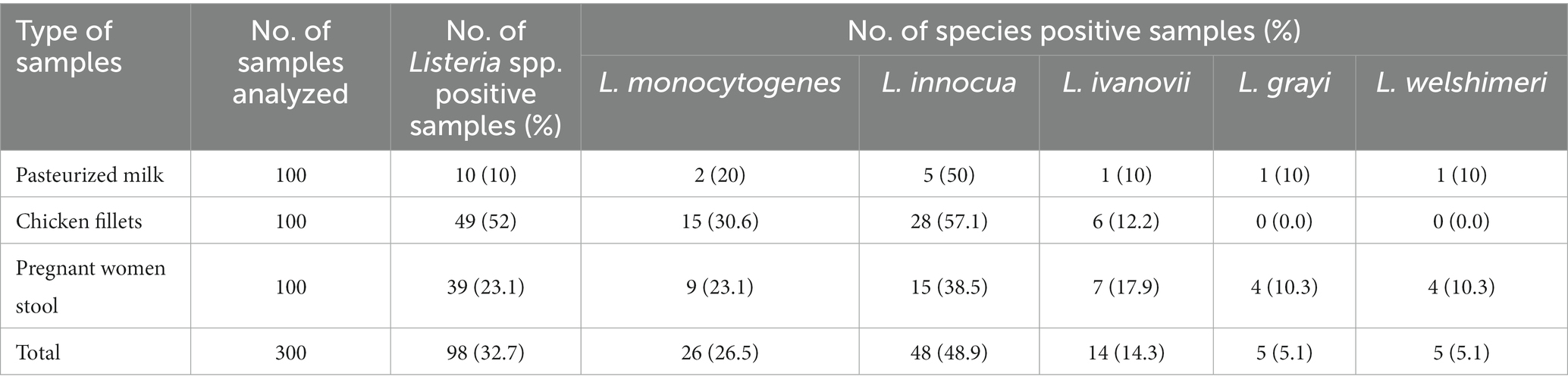
Table 1. Occurrence of Listeria spp. in pasteurized milk, chicken fillets and pregnant woman stools.
3.2. Listeria monocytogenes virulotyping and serotyping
All L. monocytogenes isolates had hlyA, actA, inlC, and inlJ virulence-associated genes. However, the plcB, iap, and inlA virulence-associated genes were found in 10 (38.5%), 8 (30.8%), and 25 (96.2%) isolates, respectively (Table 2). The recovered isolates belonged to four L. monocytogenes serotypes (1/2a, 1/2b, 1/2c, and 4b), with serotypes 1/2a and 4b each identified in 30.8% of the isolates, while 1/2b and 1/2c were identified in 19.2% of the isolates (Table 2).
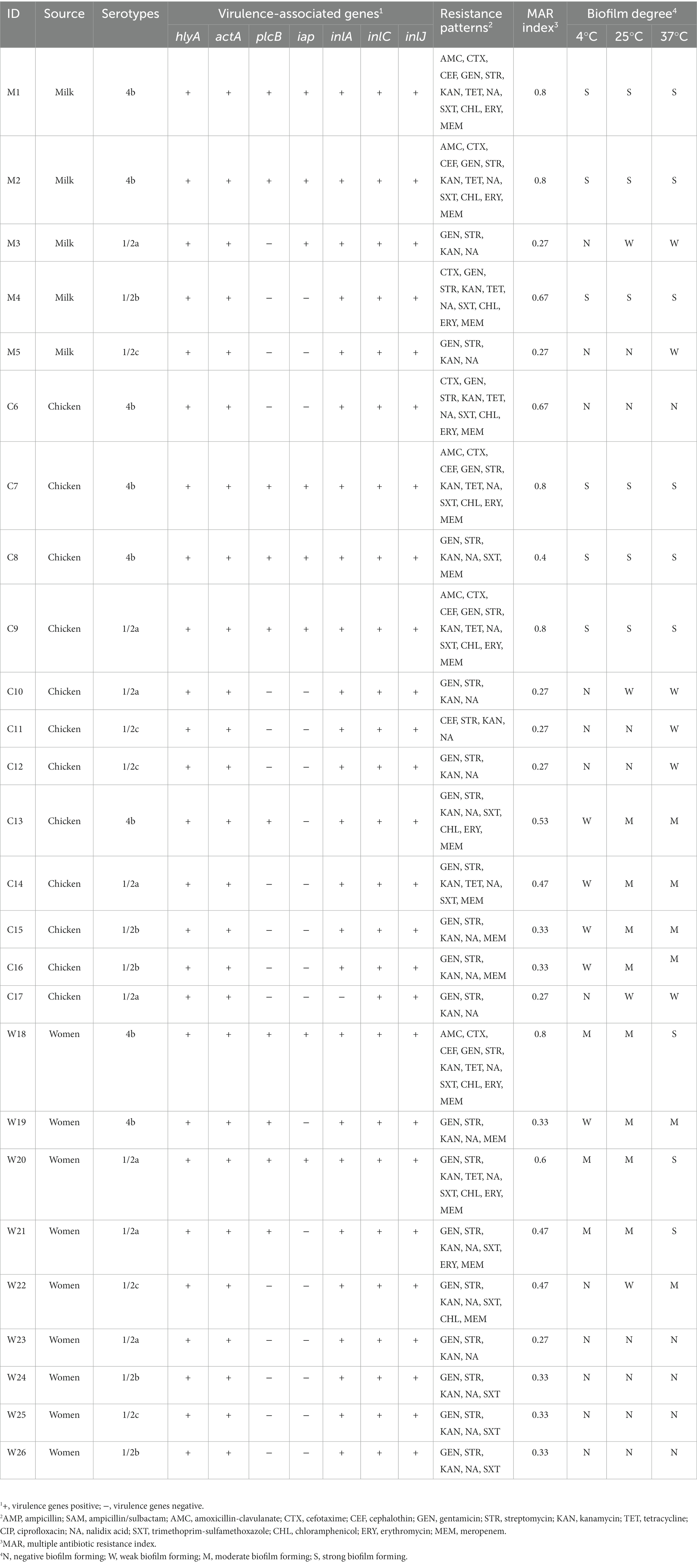
Table 2. Serotypes, virulence genes, biofilm degree, and resistance patterns of L. monocytogenes isolates.
3.3. Antimicrobial susceptibility test
Table 2 shows the resistance patterns of L. monocytogenes isolates to the 15 tested antimicrobials. All isolates were resistant to STR, KAN, and NA (100%), with GEN resistance observed in 92.3% of isolates. Nevertheless, all isolates were susceptible to AMP and SAM (Table 3). Multidrug resistance was observed in 20 (76.9%) L. monocytogenes isolates. MDR was observed in 20 (76.9%) of L. monocytogenes isolates. The mean MAR index, which ranged from 0.27 to 0.80, was 0.47. The L. monocytogenes isolated from pasteurized milk had the highest MAR index, followed by those from chicken fillets, but the MAR index did not differ significantly (p-value = 0.8204) among isolates from pasteurized milk, chicken fillets, and stool samples collected from pregnant women (Figure 1).
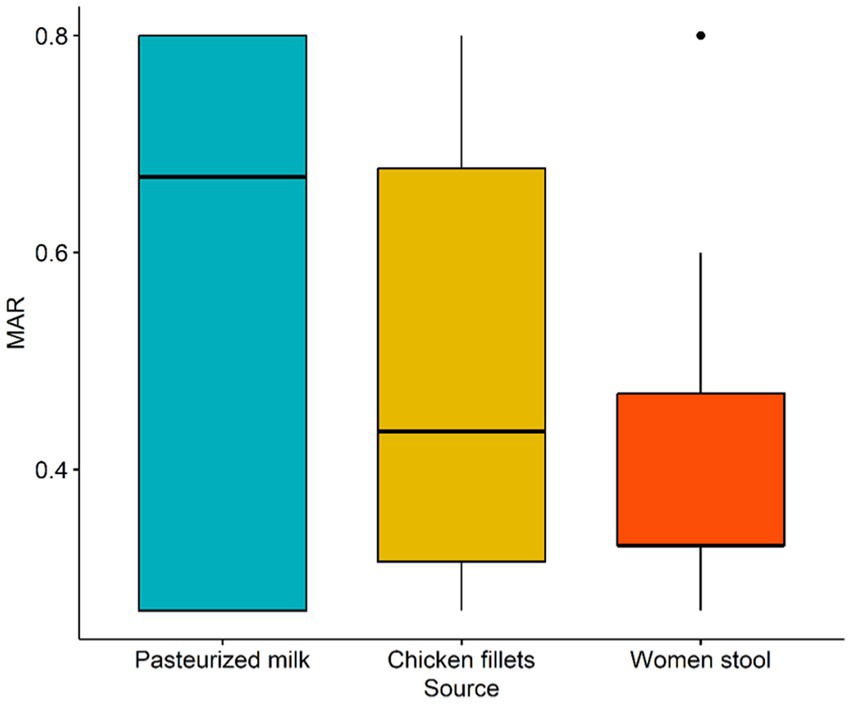
Figure 1. Box plot of multiple antibiotic resistance (MAR) index of L. monocytogenes isolates recovered from pasteurized milk, chicken fillets and stool of pregnant women.
3.4. Biofilm formation
The biofilm formation ability of 26 L. monocytogenes isolates was evaluated at various incubation temperatures (Table 2). At 4°C, 25°C, and 37°C, 12 (46.2%), 8 (30.8%), and 5 (19.2%) isolates, respectively, were non-biofilm producers. Additionally, 6 (23.1%) isolates demonstrated strong biofilm formation ability at both 4°C and 25°C. The number of strong biofilm-producing isolates increased to 9 (34.6%) when incubated at 37°C.
3.5. Effect of Lactobacillus plantarum on Listeria monocytogenes
Among the 26 L. monocytogenes isolates, 53.8, 69.2, and 80.8% were found to be biofilm producers at 4°C, 25°C, and 37°C, respectively. However, treatment with L. plantarum CFS resulted in a decrease in the number of biofilm-producing isolates to 15.4, 42.3, and 53.8% at 4°C, 25°C, and 37°C, respectively. Figure 2 displays the biofilm inhibition rate of L. plantarum CFS against L. monocytogenes isolated from pasteurized milk, chicken fillets, and the stool of pregnant women and incubated at 4°C, 25°C, and 37°C. The highest biofilm inhibition rate observed at 4°C, 25°C and 37°C were 81.1, 79.6 and 80.8%, respectively.
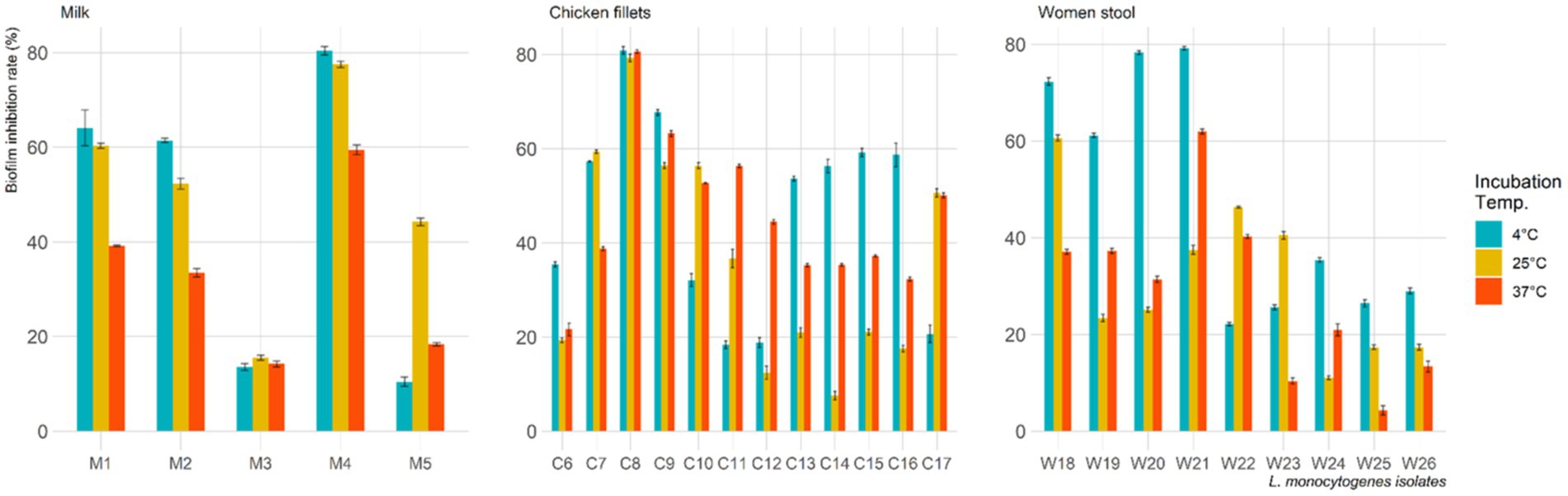
Figure 2. Biofilm inhibition rate of L. plantarum cell free supernatant against L. monocytogenes isolates at various incubation temperature.
Table 4 shows the antibacterial activity of L. plantarum CFS against six virulent, MDR, and biofilm-forming L. monocytogenes strains isolated from pasteurized milk, chicken fillets, and pregnant women’s stools. The positive control used was AMP, which was sensitive to all isolates. At a concentration of 5 μg/mL of L. plantarum CFS, none of the L. monocytogenes isolates exhibited an inhibition zone. However, an inhibition zone was observed against L. monocytogenes strains isolated from pasteurized milk and pregnant women’s stools when using a concentration of 10 μg/mL. The inhibition zone significantly increased as the L. plantarum CFS concentration was increased from 10 to 100 μg/mL. Additionally, the results indicated that the MIC value of L. plantarum CFS required to inhibit visible growth of L. monocytogenes was 10 μg/mL.
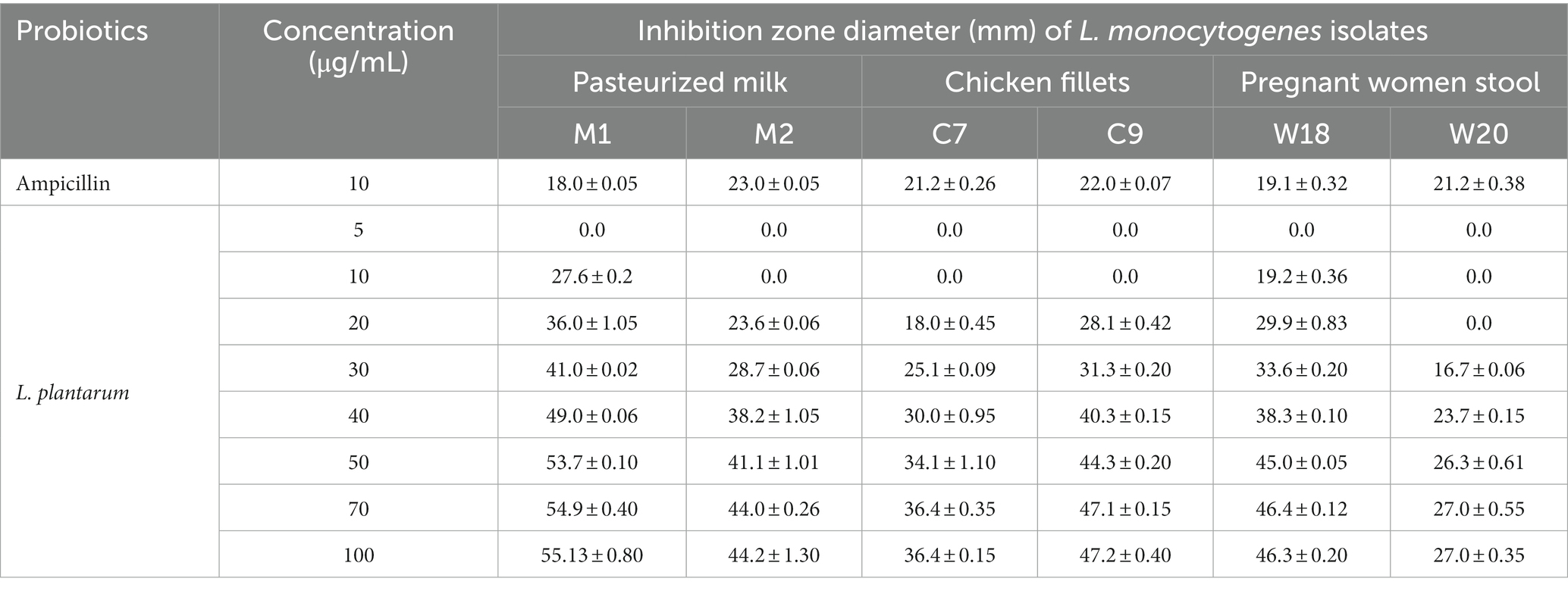
Table 4. Antibacterial activity of cell free supernatant of L. plantarum against L. monocytogenes isolates recovered from pasteurized milk, chicken fillets and stool of pregnant women.
The TEM observed morphological and intracellular structural changes of L. monocytogenes treated with L. plantarum CFS (Figure 3). Figure 3A shows untreated L. monocytogenes cells with well-defined, short rod shapes and uniformly distributed cytoplasm. In contrast, Figure 3B displays L. monocytogenes cells treated with 10 μg/mL of L. plantarum CFS, where the cell membrane was damaged and the intracellular contents leaked out.
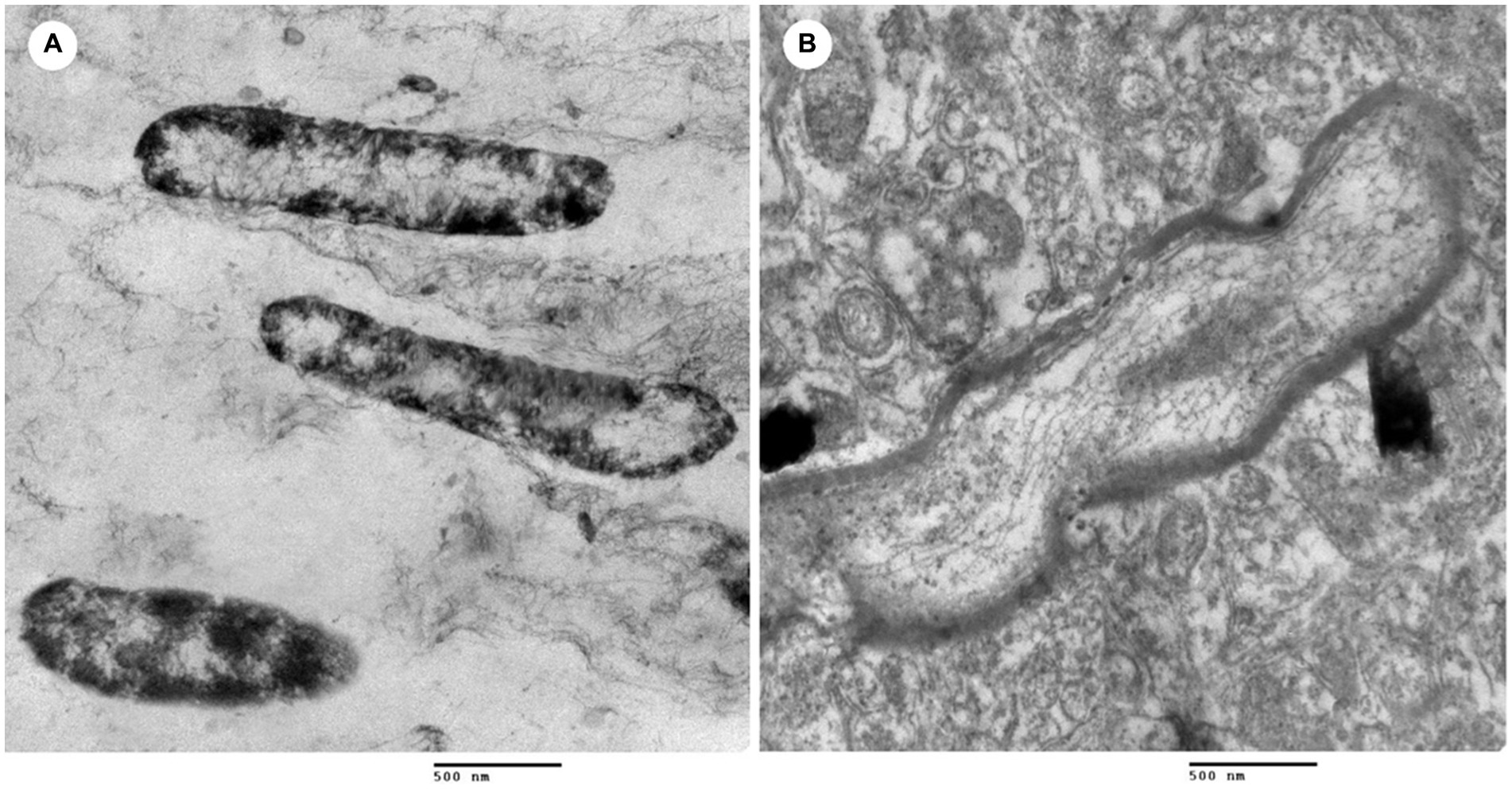
Figure 3. Transmission electron microscope of L. monocytogenes. (A) L. monocytogenes (control) and (B) L. monocytogenes treated with cell free supernatant of L. plantarum.
4. Discussion
Listeria spp. is a significant public health pathogen that causes serious illnesses and sporadic foodborne outbreaks (Zunabovic et al., 2011). It is commonly found in dairy and meat products and has deleterious effects not only on public health but also on the global economy. In this study, Listeria spp. was isolated from pasteurized milk, chicken fillets, and pregnant women’s stools. Chicken fillets had the highest isolation rate of Listeria spp., followed by pregnant women’s stool samples and pasteurized milk. Among the Listeria spp. identified, L. innoua was the most prevalent, followed by L. monocytogenes. Several studies have also identified Listeria spp. in chicken meat products (Matle et al., 2019), pasteurized milk (Moura et al., 1993), and pregnant women (El-Naenaeey et al., 2019). However, the isolation rate in our study was similar to the rate previously reported in chicken fillets from Egypt (56%; El-Malek et al., 2010), and higher than rates reported in pasteurized milk from Brazil (0.9%; Moura et al., 1993), and pregnant women from northern Ethiopia (8.5%; Welekidan et al., 2019). The variation in the isolation rate of Listeria spp. between studies could be due to sample sizes and isolation methods.
Listeria monocytogenes ranks as the third major foodborne pathogen in terms of economic burden in the United States (Hoffmann et al., 2015). In this study, L. monocytogenes was found in 20% of pasteurized milk, 30.6% of chicken fillets, and 23.1% of pregnant women’s stool samples. These rates were higher than the 12% reported in pasteurized milk (Saleh et al., 2021) and 4% in pregnant women in Egypt (El-Naenaeey et al., 2019), but lower than the 44% found in chicken fillets from Egypt (El-Malek et al., 2010). The presence of L. monocytogenes in the samples suggests possible inadequate hygiene practices, cross-contamination, improper handling, or inadequate storage temperatures (Letchumanan et al., 2018). Factors such as poor-quality milk, unsanitary manufacturing conditions, substandard materials, and inadequate water for utensil washing, as well as unclean hands of workers, could potentially contribute to bacterial contamination of dairy and meat products during manufacturing and post-manufacturing stages (Kulshrestha, 1990).
Listeria monocytogenes pathogenicity is associated with determination of virulence genes. The Listeria Pathogenicity Island 1 (LIPI-1) virulence genes have a significant role in L. monocytogenes intracellular life cycle and cellular infection (Vázquez-Boland et al., 2001). LIPI-1 virulence genes (hlyA, actA, inlC and inlJ) were identified in all L. monocytogenes isolates recovered in this study. However, these genes (plcB, iap, and inlA) were detected in only 38.5, 30.8, and 96.2% of the isolates, respectively. These findings are consistent with previous studies that have detected virulence genes in milk products (Osman et al., 2016; Tahoun et al., 2017), raw meat, and meat products (Oliveira et al., 2018), as well as in clinical and food isolates associated with major listeriosis outbreaks (Todd and Notermans, 2011; Leong et al., 2014). The presence or absence of LIPI-1 virulence gene can contribute to variations in the virulence potential of different L. monocytogenes isolates (Vázquez-Boland et al., 2001). Thus, prevalence of L. monocytogenes carrying virulence genes found in this study indicates that these pathogens could pose a significant risk to public health.
The present study identified four L. monocytogenes serotypes (1/2a, 1/2b, 1/2c, and 4b), with serotypes 1/2a and 4b being the most prevalent (30.8%), followed by 1/2b and 1/2c (19.2%). The distribution of these serotypes is predictable since they are frequently isolated from food samples (Montero et al., 2015). Previous studies reported similar trends but with a higher proportion. Muraoka, Gay (Muraoka et al., 2003) reported that serotypes 1/2a and 4b were the most frequently observed strains isolated from bulk milk in the Pacific Northwest. Serotypes 1/2a and 4b of dairy and meat products have been associated with several listeriosis outbreaks (Pan et al., 2009). Furthermore, serotypes 4b, 1/2b, and 1/2a are predominant strains associated with foodborne human listeriosis (Soni et al., 2014; Chen et al., 2017), suggesting that these isolates may exhibit pathogenicity against consumers (Orsi et al., 2011).
The antimicrobial susceptibility of L. monocytogenes isolates was variable in the present study. All isolates were resistant to aminoglycosides (GEN, STR, and KAN) and quinolones (NA), and susceptible to penicillins (PEN and AMC), which is consistent with a previous study in Egypt, except for a higher proportion of resistance to SXT (Osman et al., 2021). Moreover, a high proportion of L. monocytogenes isolates in this study showed resistance to CTX, CEF, TET, and CHL, consistent with previous studies in Egypt (Tahoun et al., 2017) and China (Chen et al., 2019), suggesting that antimicrobial misuse may accelerate the emergence of AMR in L. monocytogenes. Notably, all L. monocytogenes isolates in this study were susceptible to penicillins, which are recommended for human listeriosis treatment (Olaimat et al., 2018). However, the resistance to ERY is concerning as it is the drug of choice for treating listeriosis in pregnant women.
MDR L. monocytogenes have been isolated from various sources including food (Conter et al., 2009). In this study, 79.6% of the L. monocytogenes isolates exhibited MDR, which is lower than the 88% previously reported in Egypt (Tahoun et al., 2017), but higher than the prevalence of MDR L. monocytogenes found in dairy and meat products in other studies (Doyle et al., 2013; Kevenk and Terzi, 2016). The average MAR index was 0.47, which is also higher than the 0.34 previously reported in L. monocytogenes isolates from Egypt (Tahoun et al., 2017). Furthermore, all isolates had MAR index greater than 0.2, indicating that there was antimicrobial misuse and growing resistance among L. monocytogenes isolates.
Biofilm formation in food processing environments poses a serious safety problem for processed food and is difficult to remove (Li et al., 2018). In the present study, 80.8% of L. monocytogenes isolates showed the ability to form biofilm at 37°C, and of these isolates, 34.6% showed strong biofilm-forming ability. The biofilm-forming potential of L. monocytogenes is influenced by the presence of virulence genes (Price et al., 2018) and antimicrobial resistance (Kayode and Okoh, 2022). MDR strains were associated with strong biofilm-forming ability, which might be attributed to the higher tolerance of these isolates to disinfectants and antimicrobials (Doulgeraki et al., 2017).
Our study determined the anti-biofilm effects of L. plantarum CSF on L. monocytogenes at different concentrations and incubation times. The results indicate that L. plantarum CSF can effectively inhibit biofilm formation of L. monocytogenes, with the highest inhibition rate (81.1%) observed at 4°C. Previous research has shown that probiotics, including LAB, can prevent biofilm formation by foodborne pathogens such as L. monocytogenes (Hossain et al., 2017; Monteagudo-Mera et al., 2019; Lee et al., 2022). LAB contain various anti-biofilm agents, such as hydrogen peroxide, oxygen metabolites, exopolysaccharides, bacteriocin, and saturated fatty acids acting as biosurfactants (Moradi et al., 2019).
All tested L. monocytogenes were inhibited by L. plantarum CFS with different concentration. It was previously reported that L. plantarum and L. rhamnous could inhibit L. monocytogenes, E. coli and Salmonella typhimurium colonization (Lau and Chye, 2018; Lee et al., 2022). L. plantarum at different concentration had higher anti-bacterial activity than AMP (10 μg/mL) against L. monocytogenes. The MIC of L. plantarum CFS was determined at 10 μg/mL with diameters inhibition zones ranged from 19.2 ± 0.36 to 27.6 ± 0.2. Therefore, L. plantarum could be considered to have anti-bacterial activity against L. monocytogenes in this study. In the present study, L. plantarum CFS inhibited all tested L. monocytogenes at different concentrations. Previous studies have shown that L. plantarum and L. rhamnosus can prevent colonization of L. monocytogenes, E. coli, and Salmonella typhimurium (Lau and Chye, 2018; Lee et al., 2022). Furthermore, L. plantarum demonstrated higher anti-bacterial activity against L. monocytogenes than AMP (10 μg/mL) at different concentrations. These findings suggest that L. plantarum possesses anti-bacterial activity against L. monocytogenes.
The changes in the morphology and intercellular structure of L. monocytogenes after treated with L. plantarum have been investigated under TEM. Results showed that the treated L. monocytogenes experienced deterioration of cell membranes, cell swelling, and vacuole formation, ultimately leading to cell lysis. These observations are consistent with findings reported by Chlebowska-Smigiel, Gniewosz (Chlebowska-Smigiel et al., 2017), who attributed the antibacterial activity of L. plantarum to the release of antimicrobial metabolites and inhibitory compounds that surround L. monocytogenes. L. plantarum has also been found to initiate the formation of pores in the bacterial cell membrane, causing leakage of essential molecules and ions that ultimately results in cell death (Cotter et al., 2013; Osman et al., 2021). A previous study has reported that the inhibitory effect of L. plantarum CFS is attributed to the release of bacteriocin and organic acids (Moradi et al., 2019). Bacteriocin’s positively charged amino acid residues facilitate pore formation and exert electrostatic forces on cell membranes, leading to the leakage of cell electrolytes and subsequent cell lysis (Moradi et al., 2019). However, the organic acids present in L. plantarum CFS reduce the pH, creating an acidic environment that inhibits the growth of pathogenic bacteria (Enan et al., 2014; Osman et al., 2021). Moreover, L. plantarum CFS can hinder biofilm formation of L. monocytogenes through nutrient emulation and adhesion area intervention (Hossain et al., 2017), or by producing antimicrobial compounds that react with the pathogen or biofilm model compounds, as reported in previous literature (Kim et al., 2021).
5. Conclusion
The present study found Listeria spp. in pasteurized milk, chicken fillets, and stool samples from pregnant women in Sharkia Governorate, Egypt, particularly, L. innoua and L. monocytogenes. The study also revealed the emergence of virulent MDR L. monocytogenes with strong biofilm formation abilities in food products in Egypt, posing significant risks to food safety. Although L. plantarum exhibited potential antibacterial and anti-biofilm effects against L. monocytogenes isolates, further research is necessary to explore its full probiotic potential. Lastly, it is crucial to monitor the prevalence and antimicrobial resistance profile of L. monocytogenes in dairy and meat products to enhance their safety.
Data availability statement
The original contributions presented in the study are included in the article/Supplementary material, further inquiries can be directed to the corresponding author.
Ethics statement
The animal study was reviewed and approved by the Institutional Animal Care and Use Committee (IACUC) of Zagazig University (Ref. No.: ZU-IACUC/2/F/1/2023).
Author contributions
RAb, A-RA-M, MS, AT, AA, and NE-G contributed to the conception and design of the study. RAb and NE-G carried out the practical parts. RAb, IE, and NE-G performed the statistical analysis and interpretation of the results and wrote the manuscript’s initial draft. A-RA-M, MS, AT, RAl, EM, and AA edited and critical appraisal of the manuscript. All authors reviewed and approved the final manuscript.
Acknowledgments
The authors express their gratitude to the participants who generously provided the samples for analysis in this study. Additionally, the authors wish to acknowledge the Department of Science at King Khalid Military Academy, Saudi Arabia for providing financial support for the publication processing charges.
Conflict of interest
The authors declare that the research was conducted in the absence of any commercial or financial relationships that could be construed as a potential conflict of interest.
Publisher’s note
All claims expressed in this article are solely those of the authors and do not necessarily represent those of their affiliated organizations, or those of the publisher, the editors and the reviewers. Any product that may be evaluated in this article, or claim that may be made by its manufacturer, is not guaranteed or endorsed by the publisher.
Supplementary material
The Supplementary material for this article can be found online at: https://www.frontiersin.org/articles/10.3389/fmicb.2023.1201201/full#supplementary-material
References
Amin, B. (2016). Isolation and characterization of antiprotozoal and antimicrobial metabolite from penicillium roqueforti. Afr. J. Mycol. Biotech. 21, 13–26.
Cao, X., Wang, Y., Wang, Y., and Ye, C. (2018). Isolation and characterization of Listeria monocytogenes from the black-headed gull feces in Kunming, China. J. Infect. Public Health 11, 59–63. doi: 10.1016/j.jiph.2017.03.003
Chen, M., Cheng, J., Zhang, J., Chen, Y., Zeng, H., Xue, L., et al. (2019). Isolation, potential virulence, and population diversity of Listeria monocytogenes from meat and meat products in China. Front. Microbiol. 10:946. doi: 10.3389/fmicb.2019.00946
Chen, J.-Q., Regan, P., Laksanalamai, P., Healey, S., and Hu, Z. (2017). Prevalence and methodologies for detection, characterization and subtyping of Listeria monocytogenes and L. ivanovii in foods and environmental sources. Food Sci. Human Wellness 6, 97–120. doi: 10.1016/j.fshw.2017.06.002
Chlebowska-Smigiel, A., Gniewosz, M., Kieliszek, M., and Bzducha-Wrobel, A. (2017). The effect of pullulan on the growth and acidifying activity of selected stool microflora of human. Curr. Pharm. Biotechnol. 18, 121–126. doi: 10.2174/1389201017666161229154324
Clinical and Laboratory Standards Institute. Performance standards for antimicrobial disk and dilution susceptibility tests for bacteria isolated from animals. Clinical and Laboratory Standards Institute: Wayne, PA. (2018).
Conter, M., Paludi, D., Zanardi, E., Ghidini, S., Vergara, A., and Ianieri, A. (2009). Characterization of antimicrobial resistance of foodborne Listeria monocytogenes. Int. J. Food Microbiol. 128, 497–500. doi: 10.1016/j.ijfoodmicro.2008.10.018
Cotter, P. D., Ross, R. P., and Hill, C. (2013). Bacteriocins—a viable alternative to antibiotics? Nat. Rev. Microbiol. 11, 95–105. doi: 10.1038/nrmicro2937
Dhama, K., Karthik, K., Tiwari, R., Shabbir, M. Z., Barbuddhe, S., Malik, S. V. S., et al. (2015). Listeriosis in animals, its public health significance (food-borne zoonosis) and advances in diagnosis and control: a comprehensive review. Vet. Q. 35, 211–235. doi: 10.1080/01652176.2015.1063023
Doulgeraki, A. I., Di Ciccio, P., Ianieri, A., and Nychas, G.-J. E. (2017). Methicillin-resistant food-related Staphylococcus aureus: a review of current knowledge and biofilm formation for future studies and applications. Res. Microbiol. 168, 1–15. doi: 10.1016/j.resmic.2016.08.001
Doumith, M., Buchrieser, C., Glaser, P., Jacquet, C., and Martin, P. (2004). Differentiation of the major Listeria monocytogenes serovars by multiplex PCR. J. Clin. Microbiol. 42, 3819–3822. doi: 10.1128/JCM.42.8.3819-3822.2004
Doyle, M. P., Loneragan, G. H., Scott, H. M., and Singer, R. S. (2013). Antimicrobial resistance: challenges and perspectives. Compr. Rev. Food Sci. Food Saf. 12, 234–248. doi: 10.1111/1541-4337.12008
Drevets, D. A., and Bronze, M. S. (2008). Listeria monocytogenes: epidemiology, human disease, and mechanisms of brain invasion. FEMS Immunol. Med. Microbiol. 53, 151–165. Epub 2008/05/09. doi: 10.1111/j.1574-695X.2008.00404.x
El-Malek, A. M. A., Ali, S. F. H., Hassanein, R., Mohamed, M. A., and Elsayh, K. I. (2010). Occurrence of Listeria species in meat, chicken products and human stools in assiut city, Egypt with PCR use for rapid identification of Listeria monocytogenes. Vet. World 3:353. doi: 10.5455/vetworld.2010.353-359
El-Naenaeey, E. S., Abdelwahab, A., Merwad, A., and Abdou, H. (2019). Prevalence of Listeria species in dairy cows and pregnant women with reference to virulotyping of Listeria monocytogenes in Egypt. Zagazig Vet. J. 47, 248–258. doi: 10.21608/zvjz.2019.12896.1042
Enan, G., Abdel-Haliem, M. E., and Tartour, E. (2014). Evaluation of the antimicrobial activity, starter capability and technological properties of some probiotic bacteria isolated from Egyptian pickles. Life Sci. J. 11, 976–985.
Fagerlund, A., Heir, E., Møretrø, T., and Langsrud, S. (2020). Listeria monocytogenes biofilm removal using different commercial cleaning agents. Molecules 25:792. doi: 10.3390/molecules25040792
Folsom, J. P., Siragusa, G. R., and Frank, J. F. (2006). Formation of biofilm at different nutrient levels by various genotypes of Listeria monocytogenes. J. Food Prot. 69, 826–834. doi: 10.4315/0362-028X-69.4.826
Harakeh, S., Saleh, I., Zouhairi, O., Baydoun, E., Barbour, E., and Alwan, N. (2009). Antimicrobial resistance of listeria monocytogenes isolated from dairy-based food products. Sci. Total Environ. 407, 4022–4027. doi: 10.1016/j.scitotenv.2009.04.010
Hellberg, R. S., Martin, K. G., Keys, A. L., Haney, C. J., Shen, Y., and Smiley, R. D. (2013). 16s rRNA partial gene sequencing for the differentiation and molecular subtyping of Listeria species. Food Microbiol. 36, 231–240. Epub 2013/09/10. doi: 10.1016/j.fm.2013.06.001
Hitchins, A. D., Jinneman, K., and Chen, Y. (2017). BAM Chapter 10: Detection of Listeria monocytogenes in Foods and Environmental Samples, and Enumeration of Listeria monocytogenes in Foods | FDA. Available at: https://www.fda.gov/food/laboratory-methods-food/bam-chapter-10-detection-listeria-monocytogenes-foods-and-environmental-samples-and-enumeration (Accessed June 20, 2023).
Hoffmann, SA, Maculloch, B, and Batz, M. Economic burden of major foodborne illnesses acquired in the United States. United States Department of Agriculture, Economic Research Service: Washington, D.C. (2015).
Hossain, M. I., Sadekuzzaman, M., and Ha, S.-D. (2017). Probiotics as potential alternative biocontrol agents in the agriculture and food industries: a review. Food Res. Int. 100, 63–73. doi: 10.1016/j.foodres.2017.07.077
Jami, M., Ghanbari, M., Zunabovic, M., Domig, K. J., and Kneifel, W. (2014). Listeria monocytogenes in aquatic food products—a review. Compr. Rev. Food Sci. Food Saf. 13, 798–813. doi: 10.1111/1541-4337.12092
Kayode, A. J., and Okoh, A. I. (2022). Assessment of multidrug-resistant Listeria monocytogenes in milk and milk product and one health perspective. PLoS One 17:e0270993. doi: 10.1371/journal.pone.0270993
Kevenk, T. O., and Terzi, G. (2016). Prevalence, antimicrobial resistance and serotype distribution of Listeria monocytogenes isolated from raw milk and dairy products. J. Food Saf. 36, 11–18. doi: 10.1111/jfs.12208
Kim, Y. J., Yu, H. H., Song, Y. J., Park, Y. J., Lee, N.-K., and Paik, H.-D. (2021). Anti-biofilm effect of the cell-free supernatant of probiotic Saccharomyces cerevisiae against Listeria monocytogenes. Food Control 121:107667. doi: 10.1016/j.foodcont.2020.107667
Kırmusaoğlu, S. (2019). “The methods for detection of biofilm and screening antibiofilm activity of agents” in Antimicrobials, antibiotic resistance, antibiofilm strategies and activity methods. ed. S. Kırmusaoğlu (London: IntechOpen), 1–17.
Krumperman, P. H. (1983). Multiple antibiotic resistance indexing of Escherichia coli to identify high-risk sources of fecal contamination of foods. Appl. Environ. Microbiol. 46, 165–170. doi: 10.1128/aem.46.1.165-170.1983
Kulshrestha, S. (1990). Prevalence of enteropathogenic serogroups of E. coli in milk products samples from Bareilly and their multiple drug resistance. Indian J. Dairy Sci. 43, 373–378.
Lantz, P., Tjerneld, F., Borch, E., Hahn-Hägerdal, B., and Rådström, P. (1994). Enhanced sensitivity in PCR detection of Listeria monocytogenes in soft cheese through use of an aqueous two-phase system as a sample preparation method. Appl. Environ. Microbiol. 60, 3416–3418. doi: 10.1128/AEM.60.9.3416-3418.1994
Lau, L. Y. J., and Chye, F. Y. (2018). Antagonistic effects of Lactobacillus plantarum 0612 on the adhesion of selected foodborne enteropathogens in various colonic environments. Food Control 91, 237–247. doi: 10.1016/j.foodcont.2018.04.001
Lee, H. B., Kim, K. H., Kang, G. A., Lee, K.-G., and Kang, S.-S. (2022). Antibiofilm, antiadhesive and anti-invasive activities of bacterial lysates extracted from pediococcus acidilactici against Listeria monocytogenes. Foods 11:2948. doi: 10.3390/foods11192948
Leong, D., Alvarez-Ordóñez, A., and Jordan, K. (2014). Monitoring occurrence and persistence of Listeria monocytogenes in foods and food processing environments in the Republic of Ireland. Front. Microbiol. 5:436. Epub 2014/09/06. doi: 10.3389/fmicb.2014.00436
Letchumanan, V., Wong, P.-C., Goh, B.-H., Ming, L. C., Pusparajah, P., Wong, S. H., et al. (2018). A review on the characteristics, taxanomy and prevalence of Listeria monocytogenes. Prog. Microbes Mol. Biol. 1, 1–8. doi: 10.36877/pmmb.a0000007
Li, B., Li, X., Lin, H., and Zhou, Y. (2018). Curcumin as a promising antibacterial agent: effects on metabolism and biofilm formation in S. mutans. J. Biomed. Biotechnol. 2018:4508709. doi: 10.1155/2018/4508709
Liu, D., Lawrence, M. L., Austin, F. W., and Ainsworth, A. J. (2007). A multiplex PCR for species-and virulence-specific determination of Listeria monocytogenes. J. Microbiol. Methods 71, 133–140. doi: 10.1016/j.mimet.2007.08.007
Mac Faddin, J. (2000). Individual biochemical tests. Biochem. Tests Identific. Med. Bacteria 3, 27–439.
Magiorakos, A.-P., Srinivasan, A., Carey, R. B., Carmeli, Y., Falagas, M., Giske, C., et al. (2012). Multidrug-resistant, extensively drug-resistant and pandrug-resistant bacteria: an international expert proposal for interim standard definitions for acquired resistance. Clin. Microbiol. Infect. 18, 268–281. doi: 10.1111/j.1469-0691.2011.03570.x
Matle, I., Mbatha, K. R., Lentsoane, O., Magwedere, K., Morey, L., and Madoroba, E. (2019). Occurrence, serotypes, and characteristics of Listeria monocytogenes in meat and meat products in South Africa between 2014 and 2016. J. Food Saf. 39:e12629. doi: 10.1111/jfs.12629
Matle, I., Mbatha, K. R., and Madoroba, E. (2020). A review of Listeria monocytogenes from meat and meat products: epidemiology, virulence factors, antimicrobial resistance and diagnosis. Onderstepoort J. Vet. Res. 87, e1–e20. doi: 10.4102/ojvr.v87i1.1869
Meloni, D. (2014). Focusing on the main morphological and physiological characteristics of the food-borne pathogen Listeria monocytogenes. J. Vet. Sci. Res. 1, 1–2.
Merino, L., Trejo, F. M., De Antoni, G., and Golowczyc, M. A. (2019). Lactobacillus strains inhibit biofilm formation of Salmonella sp. isolates from poultry. Food Res. Int. 123, 258–265. doi: 10.1016/j.foodres.2019.04.067
Monteagudo-Mera, A., Rastall, R. A., Gibson, G. R., Charalampopoulos, D., and Chatzifragkou, A. (2019). Adhesion mechanisms mediated by probiotics and prebiotics and their potential impact on human health. Appl. Microbiol. Biotechnol. 103, 6463–6472. doi: 10.1007/s00253-019-09978-7
Montero, D., Bodero, M., Riveros, G., Lapierre, L., Gaggero, A., Vidal, R. M., et al. (2015). Molecular epidemiology and genetic diversity of Listeria monocytogenes isolates from a wide variety of ready-to-eat foods and their relationship to clinical strains from listeriosis outbreaks in Chile. Front. Microbiol. 6:384. doi: 10.3389/fmicb.2015.00384
Moradi, M., Mardani, K., and Tajik, H. (2019). Characterization and application of postbiotics of Lactobacillus spp. on Listeria monocytogenes in vitro and in food models. LWT 111, 457–464. doi: 10.1016/j.lwt.2019.05.072
Moreno, L. Z., Paixão, R., Gobbi, D. D., Raimundo, D. C., Ferreira, T. P., Moreno, A. M., et al. (2014). Characterization of antibiotic resistance in Listeria spp. isolated from slaughterhouse environments, pork and human infections. J. Infect. Dev. Ctries. 8, 416–423. doi: 10.3855/jidc.4188
Møretrø, T., and Langsrud, S. (2004). Listeria monocytogenes: biofilm formation and persistence in food-processing environments. Biofilms 1, 107–121. doi: 10.1017/S1479050504001322
Moura, S., Destro, M. T., and Franco, B. D. (1993). Incidence of Listeria species in raw and pasteurized milk produced in São Paulo, Brazil. Int. J. Food Microbiol. 19, 229–237. doi: 10.1016/0168-1605(93)90080-Z
Muraoka, W., Gay, C., Knowles, D., and Borucki, M. (2003). Prevalence of Listeria monocytogenes subtypes in bulk milk of the Pacific Northwest. J. Food Prot. 66, 1413–1419. doi: 10.4315/0362-028X-66.8.1413
Noll, M., Kleta, S., and Dahouk, S. (2017). Antibiotic susceptibility of 259 Listeria monocytogenes strains isolated from food, food-processing plants and human samples in Germany. J. Infect. Public Health 11, 572–577. doi: 10.1016/j.jiph.2017.12.007
Olaimat, A. N., Al-Holy, M. A., Shahbaz, H. M., Al-Nabulsi, A. A., Abu Ghoush, M. H., Osaili, T. M., et al. (2018). Emergence of antibiotic resistance in Listeria monocytogenes isolated from food products: a comprehensive review. Compr. Rev. Food Sci. Food Saf. 17, 1277–1292. doi: 10.1111/1541-4337.12387
Oliveira, T. S., Varjão, L. M., da Silva, L. N. N., de Castro Lisboa Pereira, R., Hofer, E., Vallim, D. C., et al. (2018). Listeria monocytogenes at chicken slaughterhouse: occurrence, genetic relationship among isolates and evaluation of antimicrobial susceptibility. Food Control 88, 131–138. doi: 10.1016/j.foodcont.2018.01.015
Orsi, R. H., den Bakker, H. C., and Wiedmann, M. (2011). Listeria monocytogenes lineages: genomics, evolution, ecology, and phenotypic characteristics. Int. J. Med. Microbiol. 301, 79–96. Epub 2010/08/17. doi: 10.1016/j.ijmm.2010.05.002
Osman, A., Enan, G., Al-Mohammadi, A.-R., Abdel-Shafi, S., Abdel-Hameid, S., Sitohy, M. Z., et al. (2021). Antibacterial peptides produced by alcalase from cowpea seed proteins. Antibiotics 10:870. doi: 10.3390/antibiotics10070870
Osman, K. M., Samir, A., Abo-Shama, U. H., Mohamed, E. H., Orabi, A., and Zolnikov, T. (2016). Determination of virulence and antibiotic resistance pattern of biofilm producing Listeria species isolated from retail raw milk. BMC Microbiol. 16:263. doi: 10.1186/s12866-016-0880-7
Pan, Y., Breidt, F. Jr., and Kathariou, S. (2009). Competition of Listeria monocytogenes serotype 1/2a and 4b strains in mixed-culture biofilms. Appl. Environ. Microbiol. 75, 5846–5852. doi: 10.1128/AEM.00816-09
Price, R., Jayeola, V., Niedermeyer, J., Parsons, C., and Kathariou, S. (2018). The Listeria monocytogenes key virulence determinants hly and prfa are involved in biofilm formation and aggregation but not colonization of fresh produce. Pathogens 7:18. doi: 10.3390/pathogens7010018
Saleh, E., Elboudy, A., Elsayed, A., and Ali, E. (2021). Molecular characterization of Listeria monocytogenes isolated from raw milk and some dairy products at local markets in Damanhour City, Egypt. Damanhour J. Vet. Sci. 6, 1–6. doi: 10.21608/djvs.2021.187849
Saxena, S., Banerjee, G., Garg, R., and Singh, M. (2014). Comparative study of biofilm formation in Pseudomonas aeruginosa isolates from patients of lower respiratory tract infection. J. Clin. Diagn. Res. 8, DC09–DC11. doi: 10.7860/JCDR/2014/7808.4330
Scallan, E., Hoekstra, R. M., Angulo, F. J., Tauxe, R. V., Widdowson, M.-A., Roy, S. L., et al. (2011). Foodborne illness acquired in the United States—major pathogens. Emerg. Infect. Dis. 17, 7–15. doi: 10.3201/eid1701.P11101
Soni, D. K., Singh, M., Singh, D. V., and Dubey, S. K. (2014). Virulence and genotypic characterization of Listeria monocytogenes isolated from vegetable and soil samples. BMC Microbiol. 14, 1–10. doi: 10.1186/s12866-014-0241-3
Tahoun, A., Abou Elez, R., Abdelfatah, E., Elsohaby, I., El-Gedawy, A., and Elmoslemany, A. (2017). Listeria monocytogenes in raw milk, milking equipment and dairy workers: molecular characterization and antimicrobial resistance patterns. J. Global Antimicrob.Resist. 10, 264–270. doi: 10.1016/j.jgar.2017.07.008
Todd, E., and Notermans, S. (2011). Surveillance of listeriosis and its causative pathogen, Listeria monocytogenes. Food Control 22, 1484–1490. doi: 10.1016/j.foodcont.2010.07.021
Vázquez-Boland, J. A., Domínguez-Bernal, G., González-Zorn, B., Kreft, J., and Goebel, W. (2001). Pathogenicity Islands and virulence evolution in Listeria. Microbes Infect. 3, 571–584. doi: 10.1016/S1286-4579(01)01413-7
Vázquez-Boland, J́. A., Kuhn, M., Berche, P., Chakraborty, T., Domı́nguez-Bernal, G., Goebel, W., et al. (2001). Listeria pathogenesis and molecular virulence determinants. Clin. Microbiol. Rev. 14, 584–640. doi: 10.1128/CMR.14.3.584-640.2001
Walker, S. J., Archer, P., and Banks, J. G. (1990). Growth of Listeria monocytogenes at refrigeration temperatures. J. Appl. Bacteriol. 68, 157–162. Epub 1990/02/01. doi: 10.1111/j.1365-2672.1990.tb02561.x
Welekidan, L. N., Bahta, Y. W., Teklehaimanot, M. G., Abay, G. K., Wasihun, A. G., Dejene, T. A., et al. (2019). Prevalence and drug resistance pattern of Listeria monocytogenes among pregnant women in Tigray Region, Northern Ethiopia: a cross-sectional study. BMC. Res. Notes 12, 1–6. doi: 10.1186/s13104-019-4566-8
Zeinali, T., Jamshidi, A., Bassami, M., and Rad, M. (2015). Serogroup identification and virulence gene characterization of Listeria monocytogenes isolated from chicken carcasses. Iranian J. Vet. Sci. Technol. 7, 9–19.
Keywords: Listeria monocytogenes, virulotyping, serotyping, antimicrobial resistance, biofilm, Lactobacillus plantarum
Citation: Abou Elez RMM, Elsohaby I, Al-Mohammadi A-R, Seliem M, Tahoun ABMB, Abousaty AI, Algendy RM, Mohamed EAA and El-Gazzar N (2023) Antibacterial and anti-biofilm activities of probiotic Lactobacillus plantarum against Listeria monocytogenes isolated from milk, chicken and pregnant women. Front. Microbiol. 14:1201201. doi: 10.3389/fmicb.2023.1201201
Edited by:
Dorota Zielinska, Warsaw University of Life Sciences, PolandReviewed by:
Atul Kumar Singh, Clear Labs, United StatesNatalia Wiktorczyk-Kapischke, Nicolaus Copernicus University in Toruń, Poland
Copyright © 2023 Abou Elez, Elsohaby, Al-Mohammadi, Seliem, Tahoun, Abousaty, Algendy, Mohamed and El-Gazzar. This is an open-access article distributed under the terms of the Creative Commons Attribution License (CC BY). The use, distribution or reproduction in other forums is permitted, provided the original author(s) and the copyright owner(s) are credited and that the original publication in this journal is cited, in accordance with accepted academic practice. No use, distribution or reproduction is permitted which does not comply with these terms.
*Correspondence: Ibrahim Elsohaby, aWVsc29oYWJAY2l0eXUuZWR1Lmhr
†These authors have contributed equally to this work