- 1School of Natural and Environmental Sciences, Newcastle University, Newcastle upon Tyne, United Kingdom
- 2Environment Agency, Newcastle upon Tyne, United Kingdom
- 3School of Engineering, Newcastle University, Newcastle upon Tyne, United Kingdom
- 4Department of Environmental Microbiology, Helmholtz-Centre for Environmental Research—UFZ, Leipzig, Germany
- 5Faculty of Health and Life Sciences, Northumbria University, Newcastle upon Tyne, United Kingdom
- 6Department of Chemical Engineering, Loughborough University, Loughborough, United Kingdom
Many factors affect the performance of microbial fuel cells (MFCs). Considerable attention has been given to the impact of cell configuration and materials on MFC performance. Much less work has been done on the impact of the anode microbiota, particularly in the context of using complex substrates as fuel. One strategy to improve MFC performance on complex substrates such as wastewater, is to pre-enrich the anode with known, efficient electrogens, such as Geobacter spp. The implication of this strategy is that the electrogens are the limiting factor in MFCs fed complex substrates and the organisms feeding the electrogens through hydrolysis and fermentation are not limiting. We conducted a systematic test of this strategy and the assumptions associated with it. Microbial fuel cells were enriched using three different substrates (acetate, synthetic wastewater and real domestic wastewater) and three different inocula (Activated Sludge, Tyne River sediment, effluent from an MFC). Reactors were either enriched on complex substrates from the start or were initially fed acetate to enrich for Geobacter spp. before switching to synthetic or real wastewater. Pre-enrichment on acetate increased the relative abundance of Geobacter spp. in MFCs that were switched to complex substrates compared to MFCs that had been fed the complex substrates from the beginning of the experiment (wastewater-fed MFCs - 21.9 ± 1.7% Geobacter spp.; acetate-enriched MFCs, fed wastewater - 34.9 ± 6.7% Geobacter spp.; Synthetic wastewater fed MFCs – 42.5 ± 3.7% Geobacter spp.; acetate-enriched synthetic wastewater-fed MFCs - 47.3 ± 3.9% Geobacter spp.). However, acetate pre-enrichment did not translate into significant improvements in cell voltage, maximum current density, maximum power density or substrate removal efficiency. Nevertheless, coulombic efficiency (CE) was higher in MFCs pre-enriched on acetate when complex substrates were fed following acetate enrichment (wastewater-fed MFCs – CE = 22.0 ± 6.2%; acetate-enriched MFCs, fed wastewater – CE =58.5 ± 3.5%; Synthetic wastewater fed MFCs – CE = 22.0 ± 3.2%; acetate-enriched synthetic wastewater-fed MFCs – 28.7 ± 4.2%.) The relative abundance of Geobacter ssp. and CE represents the average of the nine replicate reactors inoculated with three different inocula for each substrate. Efforts to improve the performance of anodic microbial communities in MFCs utilizing complex organic substrates should therefore focus on enhancing the activity of organisms driving hydrolysis and fermentation rather the terminal-oxidizing electrogens.
Introduction
Microbial fuel cells (MFCs) are bioelectrochemical reactors which can produce electricity from organic substrates. These can be simple substrates such as acetate or complex mixtures of organic compounds such as wastewater. In its simplest form, a bioelectrochemical system (BES) consists of an anode, a cathode and a microbial catalyst which can be associated with the anode, the cathode or both (Logan et al., 2006; Rinaldi et al., 2008; Christgen et al., 2020). Exoelectrogenic bacteria have the ability to transfer electrons, from oxidation of organic or inorganic compounds out of the cell to other organisms (e.g., methanogens), an insoluble electron acceptor such as iron oxides or to an electrode in MFCs (Sydow et al., 2014; Logan et al., 2019; Bird et al., 2021; Thapa et al., 2022). In the case of an MFC the electrons are transferred through an external circuit to the cathode where they are used to reduce an electron acceptor (e.g., oxygen) and generate an electrical current (Logan, 2009; Logan and Rabaey, 2012; Prokhorova et al., 2017).
The substrate, as the electron donor and thus fuel for the MFC is a critical factor for the development of the anodic community and is one of the principal biological factors affecting electricity generation (Liu et al., 2009; Pant et al., 2010). A wide variety of substrates has been tested in MFCs to generate electricity (Chae et al., 2009; Pant et al., 2010; Pandey et al., 2016; Mateo et al., 2018; Prathiba et al., 2021). Acetate has been used extensively as an electron donor in MFCs and frequently leads to the selective enrichment of electroactive bacteria in the anode community especially Geobacter spp. (Liu et al., 2005; Logan and Rabaey, 2012; Zhu et al., 2014b; Kumar et al., 2015; Eyiuche et al., 2017).
Acetate is a central product from the hydrolysis and fermentation of complex carbon sources and is widely used as an electron donor and carbon source for terminal oxidation processes such as methanogenesis, iron-reduction or sulfate reduction, as well as electron transfer to an anode in MFCs. High coulombic efficiency is often observed in MFCs fed acetate which is sometimes considered as a benchmark for MFC performance (Mcinerney et al., 2009; Pant et al., 2010). However, in application of MFCs for recovery of energy from wastewater for example, more complex substrates are the norm with acetate accumulating at low levels as an intermediate in anaerobic microbial food chains. MFCs fed complex substrates typically show lower performance and higher microbial diversity on the anode than those fed acetate (Zhang et al., 2011; Heidrich et al., 2018). One explanation for this could be that acetate produced by fermentation is consumed by diverse non-electrogenic bacteria as suggested by Chae et al. (2009). Thus insufficient electrogens are present to effectively generate electricity from acetate generated from hydrolysis and fermentation of complex substrates present in wastewater (Chae et al., 2009). We have tested the hypothesis, that pre-enrichment of anodic biofilms with acetate improves MFC performance owing to enrichment of exoelectrogens at the anode which in turn reduces the amount of acetate consumed by organisms that do not contribute to energy generation. This was done by switching acetate fed MFC to synthetic wastewater or real domestic wastewater and comparing their performance and anodic microbial communities to MFC that were fed either synthetic wastewater (OECD, 2010) or real domestic wastewater from the start. We also tested whether the inoculum used (MFC effluent, activated sludge and Tyne River sediment) had any impact on MFC performance and the enriched anodic communities.
Materials and methods
Single chamber MFC configuration and operation
Forty-five MFC reactors were prepared. All of the MFC were enriched and operated under closed circuit conditions with an external resistance of 100 ohms. The MFCs were inoculated with either (i) MFC effluent (MFC) from previously set up acetate-fed MFC reactors; (ii) Tyne River sediment (TS); (iii) activated sludge (AS). Replicate MFCs for each inoculum were enriched with either (i) acetate, (ii) synthetic wastewater (OECD medium), (iii) municipal wastewater (WW), (iv) acetate followed by synthetic wastewater (Ac-OECD) or (v) acetate followed by municipal wastewater (Ac-WW) as substrates. Each treatment was prepared in triplicate (3 replicates x 3 inocula x 5 enrichment media). In addition, 15 control reactors were set up in the same way (1 reactor per 3 inocula x 5 enrichment media) and operated under open circuit conditions.
Each MFC reactor had a working volume of 200 mL. The anode material was carbon veil (Technical Fiber Products LTD, United Kingdom) and the air cathode comprised a membrane electrode assembly made from an anion exchange membrane (Fumasep FAD-PET-75, Fumatech BWT GmbH, Germany), a gas diffusion electrode (GDE) consisting of carbon paper (Freudenberg, Germany) with a catalyst load of 0.8 mg Pt/cm2 using nafion as binder and a titanium sheet as a current collector. The anode was placed in a polyethylene terephthalate (PET) frame connected to a datalogger using titanium wire. The cathode area was 12.5 cm2 which was three times the area of the anode (4.2 cm2) to ensure the cathode was not limiting. Anode and cathode were 3 cm apart. A 100 Ω resistor was included in the circuit and an Ag/AgCl reference electrode (BASi, United States) connected using a salt bridge (3 M KCl in agar as electrolyte, +0.197 V vs. SHE) which was positioned directly next to the anode was used to enable continuous monitoring of the anode potential with the cell voltage using a datalogger (DT85 data logger, Omni Instruments, United Kingdom).
Acetate medium comprised 540 mg/L sodium acetate, 5.02 g/L HK2PO4, 2.88 g/L H2KPO4, 280 mg/L NH4Cl, 100 mg/L MgSO4.7 H2O, 4.3 mg/L CaCl2, 4 mg/L FeCl2.4H2O, 2 mg/L CoCl2.6H2O, 2 mg/L NiCl2.6H2O, 1 mg/L MnCl2.4 H2O, 0.32 mg/L Na2SeO3, 0.14 mg/L (NH4)6Mo7O24.4H2O, 0.1 mg/L H3BO3, 0.1 mg/L ZnCl2, 0.08 mg/L CuCl2.2H2O, 0.004 mL/L 35% HCl, 1 mg/L pyridoxine hydrochloride, 0.5 mg/L nicotinic acid, 0.25 mg/L riboflavin, 0.25 mg/L thiamine hydrochloride, 0.2 mg/L biotin, 0.2 mg/L folic acid, 0.01 mg/L vitamin B12. Organization for Economic Cooperation and Development synthetic wastewater medium (OECD, comprised 160 mg/L peptone, 110 mg/L yeast extract, 30 mg/L urea, 28 mg/L K2HPO4, 3 g/L NaCl, 4 mg/L CaCl2.2H2O, 2 mg/L MgSO4.7H2O). Municipal wastewater was collected from Tudoe Mill sewage treatment works (Northumbrian Water, County Durham, United Kingdom). The chemical oxygen demand (COD) and conductivity of the three substrates was matched by adding salts (3 g/L NaCl) to match the highest conductivity (acetate medium with a conductivity of 5.46 mS at pH 7.04). The reactors were batch fed and considered enriched when the peak voltage was reproducible over three consecutive cycles at which point electrochemical (polarization, cyclic voltammetry, electrochemical impedance spectroscopy) and analytical (chemical oxygen demand; COD, pH, conductivity) measurements were made at the end of each batch cycle. Following this, the reactors were refilled. In total the reactors were operated for over 3 months.
The COD was measured according to the APHA standard method (Eaton et al., 1998). The conductivity of the substrate was measured using a handheld conductivity meter pIONeer 30 (Radiometer Analytical, France) and the pH was measured using a portable 3,310 pH Meter (Jenway, United Kingdom).
Once the voltage stabilized, the reactors were polarized. During polarization the change in cell current and voltage, as well as the anode potential (vs. an Ag/AgCl reference electrode), were recorded continuously using a data logger (DT85 data logger, Omni Instruments, United Kingdom). Polarization curves were recorded starting at open circuit potential (OCP) using a potentiostat (DropSense STAT800 multichannel potentiostat, Metrohm, United Kingdom) at a scan rate of 1 mV s−1. The internal resistance was measured by electrochemical impedance spectroscopy using a potentiostat (DropSense STAT800 multichannel potentiostat, Metrohm, United Kingdom). Impedance measurements were conducted at OCP over a frequency range of 30,000 to 0.1 Hz with a sinusoidal perturbation of 10 mV amplitude. The coulombic efficiency (CE) was calculated as detailed in Logan et al. (2006).
DNA extraction and microbial community structure analysis
At the end of the experiment the anodes were removed from the reactors and stored in 50% ethanol for microbial analysis. For control reactors enriched and operated under open circuit conditions DNA was extracted from the non-polarized carbon veil electrodes. The entire anodes and non-polarized electrodes were homogenized mechanically using a sterile pestle (Merck, Germany) and vortexed for 3 min. Two ml of supernatant was removed and spun down at 14,000 rpm for 10 min to pellet the cells. The supernatant was removed. The pellet was suspended in 978 μL sodium phosphate buffer and added to the lysing tube from a FastDNA Spin Kit for Soil (MP Biomedicals, Germany). The DNA was extracted following the manufacturer’s instructions.
16S rRNA genes were amplified from total DNA and the V4 variable region was sequenced following the 16S Illumina Amplicon Protocol from the Earth Microbiome Project (Kozich et al., 2013) at NUOmics (Northumbria University, Newcastle upon Tyne). Raw sequence data (FastQ files) obtained from the Illumina sequencing platform were demultiplexed and analyzed using QIIME 2 (Caporaso et al., 2010). DADA2 was used for amplicon sequence variant (ASV) selection (Callahan et al., 2016). ASVs which appeared in the negative control were removed from the other samples. Phylogenetic trees were constructed using representative sequences of dominant taxonomic groups. BLASTN was used to identify nearest neighbors (Altschul et al., 1997). The tree is based on comparative analysis of the most abundant partial 16S rRNA sequences. The phylogeny was inferred using the Neighbor-Joining method (Saitou and Nei, 1987). The optimal tree with the sum of branch length = 1.71425693 is shown. The percentage of replicate trees in which the associated taxa clustered together in the bootstrap test (1,000 replicates) are shown next to the branches (Felsenstein, 1985). The tree is drawn to scale, with branch lengths in the same units as those of the evolutionary distances used to infer the phylogenetic tree. The evolutionary distances were computed using the Maximum Composite Likelihood method (Tamura et al., 2004) and are in the units of the number of base substitutions per site. This analysis involved 30 nucleotide sequences. All ambiguous positions were removed for each sequence pair (pairwise deletion option). There were a total of 227 positions in the final dataset. Evolutionary analyzes were conducted in MEGA X (Kumar et al., 2018).
Statistical analysis
All statistical analyzes were conducted in PAST 4 (Hammer et al., 2001). Kruskal-Wallis was used to determine if significant differences were present and the significance of differences was determined using Tukey’s honestly significant difference (HSD). A value of p of less than 0.05 was considered to indicate statistical significance.
Results
Effect of substrate, inoculum and acetate pre-enrichment on MFC performance
After feeding with 3 to 4 batches of substrate all reactors were enriched and showed stable voltage generation (Figure 1). The voltage generated during a batch depended on the substrate fed to the MFC (Figure 1). For reactors enriched on acetate medium and then switched to OECD (Figure 1E) and WW (Figure 1D) an immediate voltage drop was was observed. The highest voltage was observed for reactors using acetate medium as substrate (on average 178.3 ± 26.1 mV). Reactors fed on the more complex substrates, OECD medium and WW, exhibited lower cell voltage. This was on average 45.9 ± 7 mV for reactors fed OECD medium and 61.7 ± 19.8 mV for wastewater fed reactors (Figure 1). Wastewater is the least defined and most variable substrate used, and this is clearly visible in the variability of the observed cell voltage (Figure 1C). The reactors enriched on acetate medium and then switched to OECD and WW exhibited a slight, but not statistically significant (p > 0.05), increase in cell voltage with values of on average 49.9 ± 7.2 mV and 66.8 ± 10.8 mV, respectively.
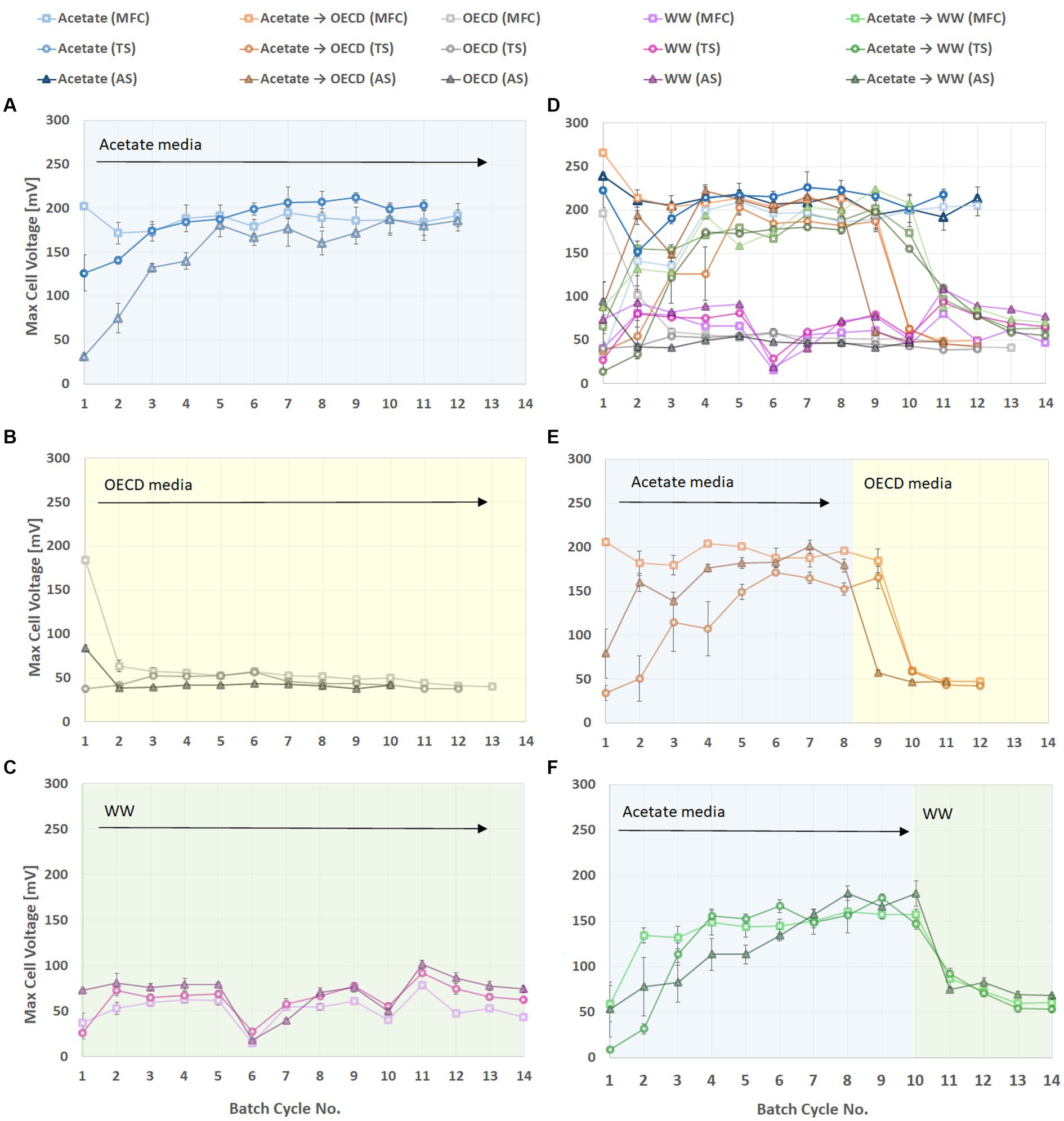
Figure 1. Cell voltage of triplicate MFC reactors inoculated with MFC effluent, Tyne sediment or activated sludge and fed different substrates. (A) Reactors fed acetate. (B) Reactors fed synthetic wastewater (OECD medium). (C) Reactors fed domestic wastewater. (D) Reactors enriched on acetate medium until stable performance was observed and switched to domestic wastewater. (E) Reactors enriched on acetate medium and switched to OECD medium. (F) Data from all reactors combined in one graph to illustrate overall performance trends.
Small but statistically significant (p < 0.05) differences in cell voltage were observed for reactors set up with different inocula. Of the reactors fed with acetate medium, those inoculated with activated sludge (AS) generated an average cell voltage of 186 ± 24.6 mV, those inoculated with MFC effluent (MFC) had a lower voltage (177.9 ± 26.2 mV) and those with Tyne sediment (TS) inoculum had the lowest voltage (166 ± 24.1 mV). Wastewater-fed reactors showed a similar trend in relation to activated sludge as inoculum with the highest voltage generated of 69.8 ± 20.1 mV but reactors inoculated with TS generated higher voltage (64.1 ± 14.9 mV) than reactors inoculated with MFC effluent (53.9 ± 15.6 mV). Reactors using OECD medium as a substrate produced on average 49 ± 6.6 mV when inoculated with MFC effluent, 45.1 ± 6.3 mV when inoculated with TS and 44.1 ± 6.3 mV when inoculated with AS. It was also apparent that reactors inoculated with MFC effluent had the highest initial potential, which was maintained in acetate-fed reactors but decreased rapidly in OECD-fed reactors or started at a much lower level in WW-fed reactors (Figure 1).
The power density that a reactor can achieve (Figures 2A,B) was determined from polarization curves. The highest power density and current density was obtained in acetate fed reactors (8.98 ± 1.33 W/m2 at 3.5 ± 0.8 A/m2). This was approximately four times greater than the power and current densities observed in reactors fed OECD medium and domestic wastewater (1.9 ± 0.12 W/m2 at 0.89 ± 0.2 A/m2 and 1.69 ± 0.13 W/m2 at 0.72 ± 0.03 A/m2 for OECD and WW respectively, Figures 2A,C). Acetate enriched reactors fed with OECD showed the second highest peak power and current densities with 2.52 ± 0.18 W/m2 at 1.02 ± 0.08 A/m2, while, acetate enriched reactors fed with WW achieved 1.69 ± 0.15 W/m2 at 0.84 ± 0.2 A/m2 (Figures 2B,D).
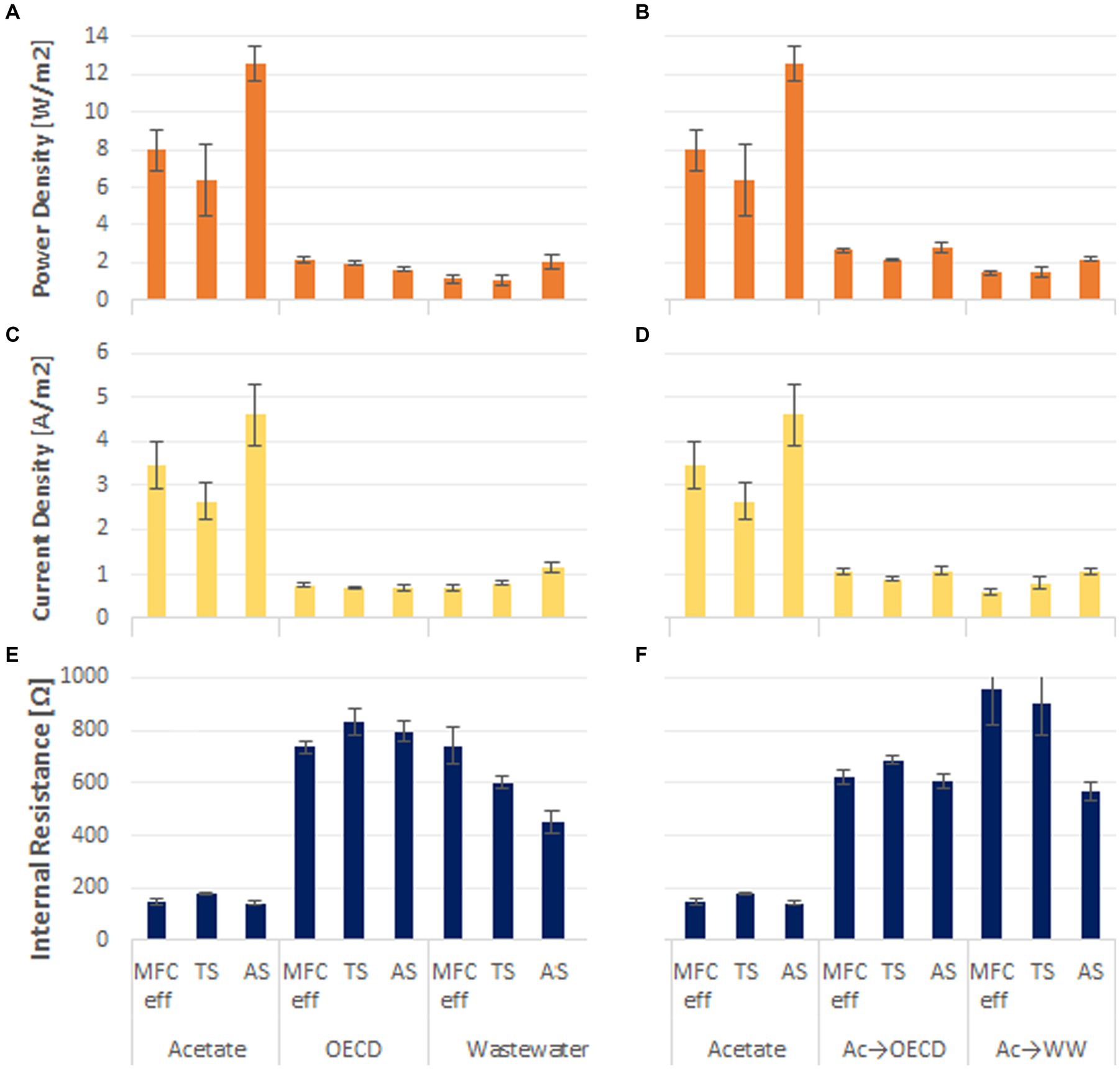
Figure 2. Power density of the triplicate MFC reactors fed (A) acetate, OECD medium and domestic wastewater, (B) acetate compared to reactors enriched on acetate and switched to OECD medium and WW; Current density of the MFC reactors fed (C) acetate, OECD medium and WW; (D) acetate compared to reactors enriched on acetate and switched to OECD medium and WW; Internal resistance of the MFC reactors fed (E) acetate, OECD medium and WW; (F) acetate compared to reactors enriched on acetate and switched to OECD medium and WW.
In acetate-fed reactors the inoculum used impacted the peak power density achieved. Reactors inoculated with activated sludge achieved 50% higher peak power than reactors inoculated with MFC effluent and Tyne sediment (Figure 2B).
The internal resistance of the reactors exhibited the opposite trend to peak power. Acetate-fed reactors had the lowest internal resistance (156.1 ± 8 Ω) with the OECD-fed reactors showing 5 times higher internal resistances (786 ± 37 Ω) and the wastewater-fed reactors 4 times higher internal resistance (596 ± 45 Ω). Acetate-enriched reactors fed OECD had 4 times higher internal resistance (638 ± 24 Ω), and Acetate-enriched reactors fed WW had 5 times higher internal resistance (808 ± 95 Ω) than purely acetate-fed MFC. Control reactors enriched and operated under open circuit conditions had high internal resistance (on average 32,978 ± 8,437 Ω) (Supplementary Figure S1), and all had the same open circuit potential of on average 811.8 ± 6.7 mV (Supplementary Figure S2).
Effect of substrate, inoculum and acetate pre-enrichment on substrate removal performance and coulombic efficiency
The percentage COD removal was used as an indicator of substrate removal efficiency in the MFC reactors. Acetate-fed reactors had significantly higher substrate removal than OECD- and WW-fed reactors and the acetate-enriched reactors fed OECD and WW (Figure 3). The highest COD removal was observed in reactors fed acetate medium with 93 ± 0.8% COD removal (Figure 3). Reactors fed OECD medium, acetate-enriched reactors fed OECD medium, acetate-enriched reactors fed WW and reactors fed-WW alone had lower substrate removal efficiencies (74 ± 5.9%, 73 ± 4.2%, 66 ± 6.1% and 50 ± 7.9% COD removal respectively) (Figure 3). Control reactors enriched and operated under open circuit conditions exhibited high level of COD removal with 95.2 ± 1.8%, 90.7 ± 1.8%, 88.6 ± 4.7%, 76.9 ± 10.9%, and 68.2 ± 5.3% COD removed for control reactors fed on acetate, acetate-enriched reactors switched to OECD, OECD, acetate-enriched reactors switched to WW, and WW, respectively (Supplementary Figure S3).
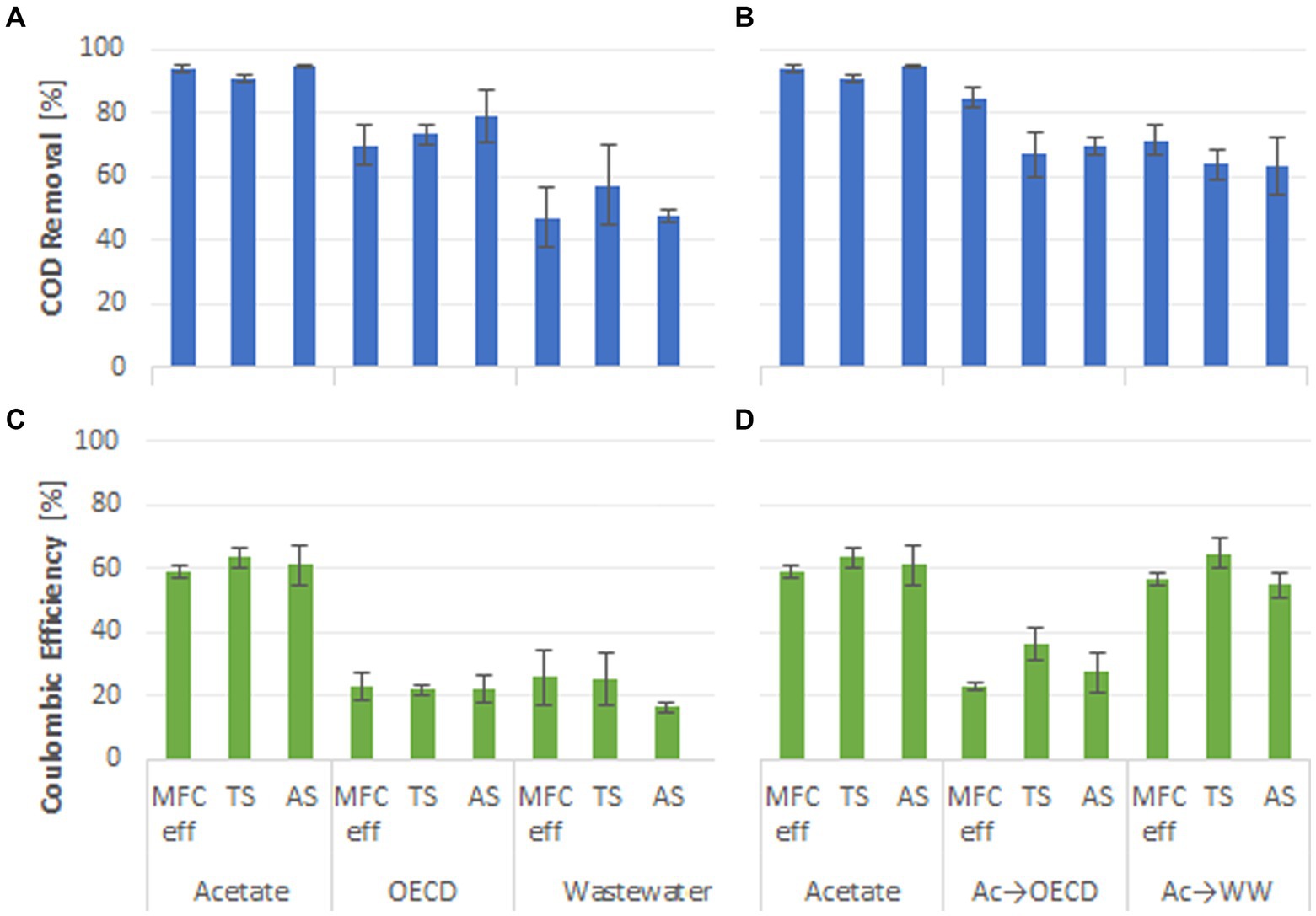
Figure 3. Percentage COD removal of the MFC reactors fed (A) acetate, OECD medium and WW; (B) acetate compared to reactors enriched on acetate and switched to OECD medium and WW; CE of the MFC reactors fed (C) acetate, OECD medium and WW; (D) acetate compared to reactors enriched on acetate and switched to OECD medium and WW.
Coulombic efficiency (CE) is a measure of the proportion of electrons produced from oxidation of the substrate that is used for electricity generation. Simple substrates, such as acetate, that are known to enrich for, and be oxidized by, exoelectrogens typically display high CEs (Pant et al., 2010). Consistent with this, on average 61 ± 3.9% of the electrons produced were recovered as electricity in acetate-fed reactors (Figure 3). However, acetate-enriched reactors fed WW as substrate also exhibited high CEs (58.5 ± 3.5%). The lowest CEs were observed for OECD- and WW-fed reactors with coulombic efficiencies of 22.0 ± 3.2% and 22.2 ± 6.2%. Acetate-enriched OECD-fed reactors had a 6 % higher CE of 28.7 ± 4.2%, compared to reactors solely fed OECD, without pre-enrichment on acetate. An even greater uplift in CE was observed in WW-fed MFC pre-enriched on acetate compared to reactors fed WW alone (58.5 ± 3.5% compared to 22.2 ± 6.2%). There was no significant difference for wastewater treatment performance or CE for reactors fed the same substrate with different inocula (p > 0.05; Figure 3). The current flow at OCP was essentially zero (Supplementary Figure S4) and consequently CE was also zero. The primary factor dictating COD removal and coulombic efficiency was the feed substrate with pre-enrichment on acetate having a significant positive effect on coulombic efficiency when MFC feed was switched to more complex substrates.
Effect of substrate, inoculum and acetate pre-enrichment on anodic microbial community diversity and composition
Alpha diversity is the mean species diversity in a habitat at a local scale. The Shannon Index, which takes into account evenness and richness of a sample, is one measure of alpha diversity and shows that with increasing complexity of the substrate, the diversity of the anodic microbial community also increases. For example, in reactors fed acetate the Shannon Index was 1.9 ± 0.22 while in reactors enriched on acetate and then fed on OECD or WW the Shannon Index was 2.6 ± 0.013 and 3.5 ± 0.14, respectively (Supplementary Figure S5). Reactors inoculated with MFC inoculum presented a slightly lower alpha diversity for all reactors except those fed WW (Supplementary Figure S5). Higher alpha diversity was observed in the control reactors with TS and AS inoculum but not in reactors with MFC effluent as inoculum or the control reactors operated under open circuit potential (OCP) fed acetate with MFC effluent as inoculum (Supplementary Figure S5). The alpha diversity for the open circuit control reactors ranged from 1.43 (lowest Acetate fed MFC inoculum) to 3.94 (highest Wastewater fed Tyne sediment inoculum) (Supplementary Figure S5).
Clustering the reactor, control and inoculum samples using Bray–Curtis similarity demonstrated that the primary factor dictating anodic microbial community composition is the substrate fed to the reactors (Figure 4). The communities from acetate-fed reactors (Figure 4, filled blue symbols) are distinct from those in acetate-enriched reactors switched to OECD samples (Figure 4, filled orange symbols), which cluster with the communities from reactors fed OECD alone (Figure 4, filled brown symbols). The communities from acetate-fed reactors switched to WW are distinct from those fed WW alone (Figure 4, filled purple and green symbols respectively). The AS and TS inoculum communities (Figure 4, turquoise and dark blue stars and open diamonds) are distinct from all other communities, while one of the MFC inoculum communities (Figure 4, red star) is distinct, the second MFC inoculum community (Figure 4, open red diamond) clustered with control reactor samples operated at open circuit, especially the control reactors fed acetate. This may be explained by the MFC inoculum being taken from 2 different MFC reactors.
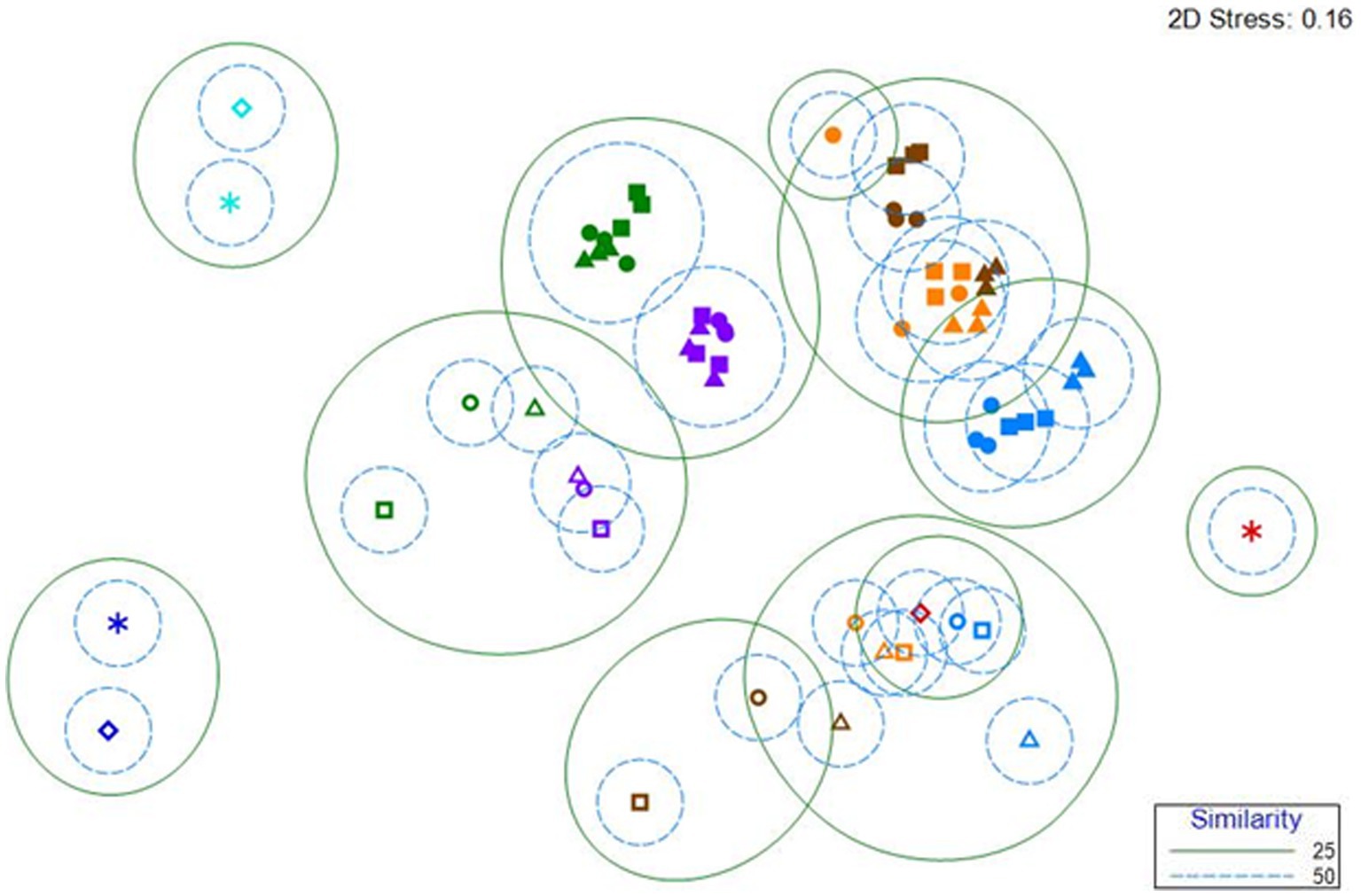
Figure 4. nMDS clustering of Bray–Curtis similarity of microbial community data for MFC, control reactors, and inocula. The analysis was conducted at the family level. The symbols are colored by treatment. Blue, acetate fed reactors; Orange, acetate-enriched, OECD-fed reactors; Brown, OECD fed reactors; Purple, acetate-enriched, WW-fed reactors; Green, WW-fed reactors. Closed symbols represent MFC, open symbols represent control reactors enriched and operated in open circuit. Triangles represent reactors inoculated with MFC effluent, Squares represent reactors inoculated with Tyne Sediment and Circles represent reactors inoculated with Activated Sludge. Stars and open diamonds represent samples of the inoculum (Turquoise-activated sludge inoculum; Red-MFC effluent inoculum; Dark Blue-Tyne Sediment inoculum).
The microbial communities in control reactors enriched and operated at open circuit (Figure 4, open symbols) were distinct from the MFC communities. The communities from acetate-fed control reactors (Figure 4, open blue symbols) clustered with, but were distinct from communities from acetate-enriched control reactors switched to OECD (Figure 4, open orange symbols), which were intermediate between the communities from acetate-fed reactors (Figure 4, open blue symbols) and communities from reactors fed OECD alone (Figure 4, open brown symbols).
The communities from WW-fed open circuit control reactors (Figure 4, open green symbols) and acetate-enriched open circuit control reactors switched to WW (Figure 4, open purple symbols), were distinct from the other open circuit control reactors and their respective MFC reactor communities (Figure 4).
The data clearly show enrichment of distinct communities in the MFC reactors and community composition varied depending on the substrate fed to the MFC.
Deltaproteobacteria were enriched in all MFC reactors but particularly in acetate-fed reactors (Supplementary Figure S6). By contrast, in control reactors operated at open circuit and in the inocula, the phyla Bacteroidia, Clostridia and Gammaproteobacteria were most abundant. Geobacter was the single most abundant genus in the MFC reactors and was present at highest relative and absolute abundance in MFCs fed acetate (p < 0.05); 70.4 ± 1.7% relative abundance; equivalent to 1.1 ± 0.002 × 1011 Geobacter cells/cm2 of anode (Supplementary Figure S7). In acetate-enriched reactors fed OECD synthetic wastewater, Geobacter was present at a relative abundance of 47.3 ± 3.9%; equivalent to 2.2 ± 0.015 × 1010 Geobacter cells/cm2 of anode (Supplementary Figure S7), and in reactors fed OECD synthetic wastewater alone Geobacter was present at lower average relative and absolute abundance (42.5 ± 3.7%, equivalent to 1.2 ± 0.013 × 1010 Geobacter cells/cm2 of anode, Supplementary Figure S7), which with respect to both relative and absolute abundance was statistically significantly different from acetate-enriched OECD synthetic wastewater fed reactors (p < 0.05). MFCs fed WW had the lowest relative abundance of Geobacter spp. (p < 0.05) but acetate-enriched MFCs fed WW had a higher relative abundance of Geobacter spp. than MFCs fed WW alone (p < 0.05; 34.9 ± 6.7% and 21.9 ± 1.7% relative abundance respectively) (Figure 5). In control reactors enriched and operated at open circuit Geobacter spp. were present at a relative abundance of 7.03 ± 0.36; 0.66 ± 0.34; 0.31 ± 0.12; for reactors fed acetate, OECD medium and domestic wastewater, respectively. In control reactors enriched on acetate and switched to OECD medium and wastewater Geobacter spp. were present at a relative abundance of 2.9 ± 1.29 and 1.9 ± 0.74, respectively. Other taxa enriched in the MFC reactors present at greater than 1% relative abundance included the genus Rhodopseudomonas in MFC reactors inoculated with MFC effluent and fed acetate or OECD and in acetate-enriched OECD reactors (Figure 5). Members of the, genus Cetobacterium were also present at greater than 1% relative abundance in acetate-enriched MFC fed OECD and OECD fed reactors inoculated with MFC effluent and activated sludge. Members of the families Rikenellaceae and Synergistaceae were present in all MFC reactors at levels above 1% relative abundance and members of the genus Romboutsia were present in all MFC reactors fed complex substrates but were most highly enriched in reactors fed WW. A similar pattern was seen for bacteria from the order Bacteroidales. The family Desulfobulbaceae was most prominent in MFCs which have been operated with WW (Figure 5).
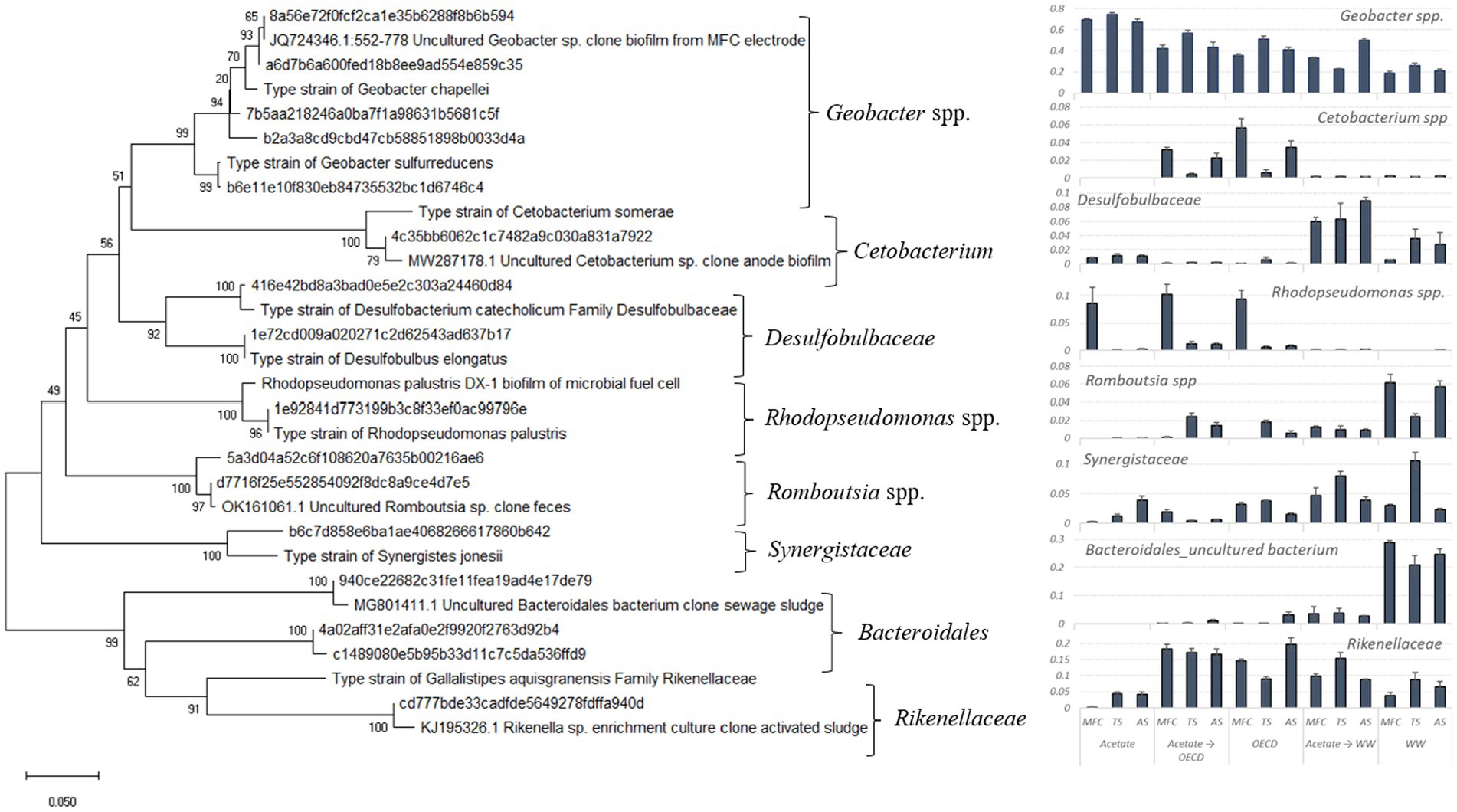
Figure 5. Phylogenetic distance tree of key bacterial taxa and close relatives (left) and plots of the mean fractional abundances of these taxa in MFC reactors (right). The tree is based on comparative analysis of the most abundant partial 16S rRNA sequences.
Discussion
Effect of substrate on electrical and wastewater treatment performance
The performance of MFC reactors in terms of maximum voltage, current density in batch operation and maximum power density achieved during polarization, demonstrated a clear trend of higher performance with decreasing substrate complexity (Figures 1–3). This is in line with results from (Velasquez-Orta et al., 2011) who observed a decrease in MFC performance with an increase in substrate complexity. As expected, the readily oxidizable substrate acetate produced three times more power compared to the more complex substrates OECD media and WW. This is consistent with the findings of Liu et al. (2005) who reported 66% higher power generation in MFCs fed acetate than MFCs fed butyrate, and numerous other studies that have shown acetate-fed MFC to have higher performance than MFC fed propionate, glucose, lactate or synthetic wastewater (Jung and Regan, 2007; Chae et al., 2009; Liu et al., 2009; Ebadinezhad et al., 2019). In comparison with OECD media, WW fed reactors showed a slightly higher performance both with and without pre-enrichment on acetate (Figures 1–3). Single step enrichment on acetate and two step enrichment on acetate (a non-fermentable substrate) followed by glucose (a fermentable substrate), or enrichment on a mixture of acetate or glucose did not lead to enhanced MFC performance and a decrease in voltage was observed when changing from acetate to a more complex substrate (Zhang et al., 2011; Wang et al., 2012; Park et al., 2017). By contrast Park et al. (2017) reported higher current production from wastewater when reactors were previously sequentially enriched with acetate and glucose.
The impact of acetate pre-enrichment on COD removal was also affected by the nature of the substrate. Enrichment on acetate had a larger impact on COD removal in WW-fed reactors compared to OECD-fed reactors (Figures 3A,B) This may be a consequence of differences in composition of the two different substrates. While broadly similar in bulk composition (Supplementary Figure S8) the COD in OECD synthetic wastewater has a high content of peptone. This likely explains the high levels of Cetobacterium detected in OECD-fed and acetate-enriched OECD MFC compared to MFC fed real wastewater (Figure 5). Cetobacterium ferments peptone with acetate as a major end product (Finegold et al., 2003; Edwards et al., 2015; Wang et al., 2021) and as a consequence OECD-fed MFC may in effect already be pre-enriched on acetate. One might therefore expect to see less difference in COD removal between acetate-enriched, OECD wastewater fed MFC and MFC fed solely OECD wastewater compared to acetate-enriched, real wastewater fed MFC and MFC fed solely real wastewater (Figures 3A,B). Liu et al. (2009) detected comparable COD removal efficiencies for batch acetate-fed and OECD-fed reactors but consistent with the results reported here, Park et al. (2017) observed a lower COD removal in wastewater-fed reactors (68% average COD-removal) compared to acetate (77% average COD-removal) (Figure 1).
OECD synthetic wastewater is similar to domestic wastewater in broad composition (Supplementary Figure S7). Though as might be expected real wastewater is more variable than the OECD medium and some anion and formic acid concentrations differ between the two substrates. Though given that OECD wastewater comprises primarily peptone and yeast extract its specific composition will likely have less polymeric carbohydrate and fermentable sugars than real wastewater. The higher voltage produced in WW fed reactors could be due therefore to the wastewater including a higher proportion of substrates that can be readily hydrolysed and fermented to produce acetate. It may also be already partially hydrolysed and therefore more readily degradable than the protein rich synthetic OECD medium, and thus provide a better substrate for exoelectrogens at the anode which predominantly oxidize simple organic compounds such as acetate (Liu et al., 2009).
The substrate fed to MFCs, and pre-enrichment on acetate had a distinct influence on coulombic efficiency. Acetate-fed reactors had the highest CE with CE for WW- and OECD-fed reactors being similar, but lower than acetate-fed reactors. However, pre-enrichment with acetate more than doubled the CE in WW-fed reactors and increased CE by ca. 6% in OECD-fed reactors (Figure 3).
Effect of substrate, inoculum and acetate pre-enrichment on the anodic microbial community
The diversity of microbial communities in MFC reactors was considerably lower than in control reactors (Supplementary Figure S5). This reflects selection for exoelectrogens in the anode community compared to biofilms on carbon electrodes in non-polarized reactors operated at open circuit. The diversity of the anodic communities was also lower in MFCs fed lower complexity substrates (community diversity was lowest to highest in the following order; Acetate-fed reactors → acetate- enriched OECD-fed reactors → OECD-fed reactors → acetate-enriched WW-fed reactors → WW-fed reactors). This is consistent with the greater diversity of compounds present in the more complex substrates leading to greater opportunity for a wider range of organisms that utilize different compounds. Similar trends have been observed previously (Park et al., 2017). High diversity of microbial communities in activated sludge and River Tyne sediment inocula (Supplementary Figure S5) show that both are a rich reservoir of microbial taxa for the development of anodic biofilms in the reactors. The MFC effluent inoculum on the other hand is already enriched for exoelectrogens but interestingly, irrespective of the inoculum used the performance of MFC fed a particular substrate was very similar (Figures 1–3).
Clustering of microbial communities at Family-level based on the 16S rRNA genes recovered from anodic biofilms, using Bray Curtis similarity, shows clear evidence of differences in microbial community structure between the three different inocula used, anodic communities from MFC fed different substrates and corresponding communities enriched on carbon electrodes from control reactors enriched and operated under open circuit conditions (Figure 4). Communities from electrodes from open-circuit control reactors cluster together based on substrate as do the MFC reactors (Figure 4). Velasquez-Orta and colleagues observed similar patterns where anodic communities from MFCs fed acetate, glucose or starch clustered by feed substrate (Velasquez-Orta et al., 2011).
Despite the different inocula and substrates used, bacteria from the genus Geobacter were the single most abundant microorganisms enriched in anodic biofilms. This was not surprising as abundant evidence shows that wastewater fed MFC reactors frequently enrich Geobacter spp. in their anodic biofilms (Zhu et al., 2014a; Du et al., 2018; Heidrich et al., 2018; Tian et al., 2022). Recently Du et al. (2018) confirmed that the intensity of the electric field around an electrode rather than the anode potential selects for exoelectrogens in anodic biofilms (Zhu et al., 2014a). Du et al. (2018) observed up to 76% relative abundance of Geobacter spp. in an electrode biofilm, comparable to the results reported in the present study (ca. 70% Geobacter spp. in acetate-fed MFC reactors) and Geobacter spp. were slightly enriched in control reactors fed acetate (7 ± 0.36% Geobacter spp.) and control reactors enriched on acetate and switched to OECD (2.9 ± 1.29% Geobacter spp.) or WW (1.9 ± 0.74% Geobacter spp.) but essentially absent on non-polarized electrodes fed OECD or WW with 0.65 ± 0.34% and 0.31 ± 0.12% Geobacter spp. respectively.
Other taxa, enriched above 1% relative abundance, varied between reactors using different substrates (Figure 5). A Rhodopseudomonas sp., was enriched (ca. 10% relative abundance; Figure 5) in the acetate, acetate-enriched OECD-fed and OECD-fed reactors which were inoculated with MFC effluent, is a close relative to Rhodopseudomonas palustris which is abundant in many ecosystems. This bacterium is metabolically very versatile and can switch between different forms of metabolism (photoautotrophy, photoheterotrophy, chemoautotrophy, and chemoheterotrophy) depending on environmental conditions. It is also the focus of extensive research for it’s potential to break down aromatic compounds and waste in polluted environments and production of hydrogen as a product of nitrogen fixation (Mehrabi et al., 2001; Larimer et al., 2004). Rhodopseudomonas palustris DX-1 has been isolated from an acetate-fed MFC inoculated with wastewater. Strain DX-1 was tested for its ability to generate electricity in a MFC and delivered high power density with a pure culture giving slightly higher power (1,170 ± 30 mW/m2) than a mixed microbial community enriched from the same wastewater inoculum (1,100 ± 40 mW/m2) (Xing et al., 2008). Strain DX-1 was shown to generate high power densities with acetate as an electron donor along with a range of other low molecular weight organic acids and alcohols also being used for electricity generation (Xing et al., 2008). It was also demonstrated that strain DX-1 can generate electricity with yeast extract as a substrate (Xing et al., 2008). This is of particular note given that in this study the Rhodopseudomonas sp. was only appreciably enriched in MFC effluent-inoculated MFC fed acetate or OECD synthetic wastewater which contains high levels of yeast extract. It is therefore conceivable that the MFCs studied where the Rhodopseudomonas sp. was present at high relative abundance, that it contributed to electricity generation.
Although the synthetic OECD wastewater medium is comparable in broad composition to the domestic wastewater used in this study (Supplementary Figure S8), both real WW and OECD synthetic wastewater enriched different microbial communities in the anodic biofilms. Bacteria from the genus Cetobacterium were enriched in OECD-fed and acetate-enriched OECD-fed reactors. Bacteria from the genus Cetobacterium ferment peptone and carbohydrates and produce acetic acid and, in smaller amounts, propionic, butyric, lactic, and succinic acid as a fermentation end products (Edwards et al., 2015; Wang et al., 2021). One of the main components of OECD medium is peptone, and enrichment of Cetobacterium spp. related to Cetobacterium somerae, a novel species isolated from human feces which produced 75% acetic acid, 18% propionic and some butyric acid in peptone-yeast broth (Finegold et al., 2003). Cetobacterium clearly forms a component of an anaerobic food chain, generating acetate from peptones in OECD medium which is then utilized by electrogenic Geobacter spp. Members of the Family Rikenellaceae, have been isolated from fecal samples and the digestive tract of a wide range of animals (Graf, 2014). Known species of the family Rikenellaceae are fermenters and produce acetic acid, propionic acid, succinic acid and alcohols from glucose, lactose, mannose and melibiose (Graf, 2014). Rikenellaceae are present at elevated relative abundance in reactors fed complex substrates (those containing WW or OECD synthetic wastewater) and was most abundant (~ 2% relative abundance) in reactors fed acetate-enriched OECD or OECD medium. By contrast it was present at much lower relative abundance in MFC fed acetate alone (Figure 5) further suggesting that it may have a role in converting complex organic compounds to fermentation products that promote electrogenesis.
Bacteria from the Family Desulfobulbaceae, were enriched to the greatest extent in WW and acetate-enriched WW-fed reactors. Members of Desulfobulbaceae are strict anaerobes and are capable of both respiratory and fermentative metabolism oxidizing simple organic compounds like lactate, pyruvate, propionate, and alcohols incompletely to acetate (Samain et al., 1984; Lien et al., 1998; Sorokin et al., 2008; Kuever, 2014) and therefore may play a role in generating acetate that is utilized by electrogens like Geobacter spp. Given their ability to partially oxidize other volatile fatty acetates and alcohols to acetate, they may have a role as secondary fermenters of acids and alcohols produced by other members of the anodic microbial communities fed wastewater such as Romboustia (see below). Some filamentous bacteria from the Desulfobulbaceae are known as cable bacteria and have also been shown to conduct electrons over centimeter long distances by electrically coupling sulfide oxidation and oxygen reduction in marine sediments (Pfeffer et al., 2012; Reguera, 2012; Marzocchi et al., 2014; Larsen et al., 2015; Trojan et al., 2016; Burdorf et al., 2017) and may potentially have a role as electrogens in the MFC where they are found. Bacteria from the genus Romboutsia, like members of the Desulfobulbaceae, were mainly enriched in wastewater-fed reactors. Romboustia has previously been identified in the intestine of mammalian hosts. Romboutsia strains can ferment carbohydrates to acetate, formate, lactate and hydrogen via glycolysis, and possess the non-oxidative pentose phosphate pathway (Gerritsen et al., 2014, 2019). These properties would be consistent with a role in providing electron donors for electrogens in environments where complex substrates must be converted to acteate to support electrogens in anodic communities and potentially generating substrates such as lactate that can be utilized by members of the Desulfobulbaceae which can convert lactate to acetate. The anodic community of WW-fed reactors was also enriched in uncultured bacteria from the order Bacteroidales which consist of anaerobes commonly found in the human gut (Krieg et al., 2010). Members of the order Bacteroidales are by far the most studied species within the phylum Bacteroidetes Bacteroides thetaiotaomicron which is commonly found in the human gut flora, can digest complex carbohydrates. It is considered as the type species for investigating polysaccharide degradation within the family Bacteroidales (Porter et al., 2018; Tan et al., 2018). As a commensal bacterium, B. thetaiotaomicron produces short-chain carbohydrates and organic acids which can be absorbed by the host as a source of energy (Porter et al., 2018) or further used for electron production in an anodic biofilm.
Based on these observations it is apparent that the taxa enriched in the MFC reactors either were directly involved in electricity generation as exoelectrogenic bacteria (Geobacter spp. and putatively members of the family Desulfobulbaceae and Rhodopseudomonas spp.) or comprised a syntrophic microbial community providing electron donors for the electrogens in the anodic biofilm.
Conclusion
We have systematically evaluated the concept that MFC performance can be improved by pre-enriching the anode community with electrogens such as Geobacter by feeding with acetate prior to more complex substrates. We demonstrated that this approach does lead to a higher proportion of Geobacter spp. in the anodic biofilm. However, the increased levels of Geobacter did not lead to enhancement of MFC performance except that acetate pre-enriched MFCs subsequently fed complex substrates did exhibit greater coulombic efficiency than MFC fed with complex substrates from the start. The inoculum used also had little effect on the final performance of the MFC even though MFCs inoculated with MFC effluent did have a slightly higher level of Geobacter in their anodic biofilms. Efforts to improve the performance of anodic microbial communities in MFCs utilizing complex organic substrates should therefore focus on enhancing the activity of organisms driving hydrolysis and fermentation rather than directly targeting the terminal oxidizing electrogens.
Data availability statement
The original contributions presented in the study are included in the article/supplementary materials, further inquiries can be directed to the corresponding author.
Author contributions
BC and IH conceived and designed the study. EY, TC, KS, and IH contributed to funding acquisition and supervision. BC, EM, PI, MS, and CM contributed to the investigation and methodology. BC analyzed the data. BC and IH wrote the manuscript. All authors contributed to the article and approved the submitted version.
Funding
The authors gratefully acknowledge the financial support of the Natural Environmental Research Councils (NERC), UK grant NE/L01422X/1.
Conflict of interest
The authors declare that the research was conducted in the absence of any commercial or financial relationships that could be construed as a potential conflict of interest.
Publisher’s note
All claims expressed in this article are solely those of the authors and do not necessarily represent those of their affiliated organizations, or those of the publisher, the editors and the reviewers. Any product that may be evaluated in this article, or claim that may be made by its manufacturer, is not guaranteed or endorsed by the publisher.
Supplementary material
The Supplementary material for this article can be found online at: https://www.frontiersin.org/articles/10.3389/fmicb.2023.1199286/full#supplementary-material
References
Altschul, S. F., Madden, T. L., Schaffer, A. A., Zhang, J. H., Zhang, Z., Miller, W., et al. (1997). Gapped BLAST and PSI-BLAST: a new generation of protein database search programs. Nucleic Acids Res. 25, 3389–3402. doi: 10.1093/nar/25.17.3389
Bird, L. J., Kundu, B. B., Tschirhart, T., Corts, A. D., Su, L., Gralnick, J. A., et al. (2021). Engineering wired life: synthetic biology for electroactive bacteria. ACS Synth. Biol. 10, 2808–2823. doi: 10.1021/acssynbio.1c00335
Burdorf, L. D. W., Tramper, A., Seitaj, D., Meire, L., Hidalgo-Martinez, S., Zetsche, E. M., et al. (2017). Long-distance electron transport occurs globally in marine sediments. Biogeosciences 14, 683–701. doi: 10.5194/bg-14-683-2017
Callahan, B. J., Mcmurdie, P. J., Rosen, M. J., Han, A. W., Johnson, A. J. A., and Holmes, S. P. (2016). DADA2: high-resolution sample inference from Illumina amplicon data. Nat. Methods 13, 581–583. doi: 10.1038/nmeth.3869
Caporaso, J. G., Kuczynski, J., Stombaugh, J., Bittinger, K., Bushman, F. D., Costello, E. K., et al. (2010). QIIME allows analysis of high-throughput community sequencing data. Nat. Methods 7, 335–336. doi: 10.1038/nmeth.f.303
Chae, K. J., Choi, M. J., Lee, J. W., Kim, K. Y., and Kim, I. S. (2009). Effect of different substrates on the performance, bacterial diversity, and bacterial viability in microbial fuel cells. Bioresour. Technol. 100, 3518–3525. doi: 10.1016/j.biortech.2009.02.065
Christgen, B., Suarez, A., Milner, E., Boghani, H., Sadhukhan, J., Shemfe, M., et al. (2020). “Chapter 4 metal recovery using microbial electrochemical technologies” in Resource recovery from wastes: Towards a circular economy. eds. D. S. Macaskie and W. Mayes (London: The Royal Society of Chemistry), 87–112.
Du, Q., Mu, Q. H., Cheng, T., Li, N., and Wang, X. (2018). Real-time imaging revealed that Exoelectrogens from wastewater are selected at the Center of a Gradient Electric Field. Environ. Sci. Technol. 52, 8939–8946. doi: 10.1021/acs.est.8b01468
Eaton, A. D., Clesceri, L. S., Greenberg, A. E., and Franson, M. A. H., (1998) American public health association., American Water Works Association., et al. standard methods for the examination of water and wastewater. 20th Edn. Washington, DC: American Public Health Association.
Ebadinezhad, B., Ebrahimi, S., and Shokrkar, H. (2019). Evaluation of microbial fuel cell performance utilizing sequential batch feeding of different substrates. J. Electroanal. Chem. 836, 149–157. doi: 10.1016/j.jelechem.2019.02.004
Edwards, K. J., Logan, J. M. J., and Gharbia, S. E. (2015). “Cetobacterium” in Bergey's manual of systematics of archaea and bacteria. ed. W. B. Whitman (Hoboken, NJ: Wiley), 1–3.
Eyiuche, N. J., Asakawa, S., Yamashita, T., Ikeguchi, A., Kitamura, Y., and Yokoyama, H. (2017). Community analysis of biofilms on flame-oxidized stainless steel anodes in microbial fuel cells fed with different substrates. BMC Microbiol. 17:145. doi: 10.1186/s12866-017-1053-z
Felsenstein, J. (1985). Confidence limits on phylogenies: an approach using the bootstrap. Evolution 39, 783–791. doi: 10.2307/2408678
Finegold, S. M., Vaisanen, M. L., Molitoris, D. R., Tomzynski, T. J., Song, Y., Liu, C., et al. (2003). Cetobacterium somerae sp nov from human feces and emended description of the genus Cetobacterium. Syst. Appl. Microbiol. 26, 177–181. doi: 10.1078/072320203322346010
Gerritsen, J., Fuentes, S., Grievink, W., Van Niftrik, L., Tindall, B. J., Timmerman, H. M., et al. (2014). Characterization of Romboutsia ilealis gen. Nov., sp. nov., isolated from the gastro-intestinal tract of a rat, and proposal for the reclassification of five closely related members of the genus clostridium into the genera Romboutsia gen. Nov., Intestinibacter gen. Nov., Terrisporobacter gen. Nov. and Asaccharospora gen. Nov. Int. J. Syst. Evol. Microbiol. 64, 1600–1616. doi: 10.1099/ijs.0.059543-0
Gerritsen, J., Hornung, B., Ritari, J., Paulin, L., Rijkers, G. T., Schaap, P. J., et al. (2019). A comparative and functional genomics analysis of the genus Romboutsia provides insight into adaptation to an intestinal lifestyle. bio Rxiv. doi: 10.1101/845511
Graf, J. (2014). “The family Rikenellaceae” in The prokaryotes: Other major lineages of bacteria and the archaea. eds. E. Rosenberg, E. F. Delong, S. Lory, E. Stackebrandt, and F. Thompson (Berlin, Heidelberg: Springer Berlin Heidelberg), 857–859.
Hammer, O., Harper, D., and Ryan, P. (2001). PAST: paleontological statistics software package for education and data analysis. Palaeontol. Electron. 4, 1–9.
Heidrich, E. S., Dolfing, J., Wade, M., Sloan, W. T., Quince, C., and Curtis, T. P. (2018). Temperature, inocula and substrate: contrasting electroactive consortia, diversity and performance in microbial fuel cells. Bioelectrochemistry 119, 43–50. doi: 10.1016/j.bioelechem.2017.07.006
Jung, S., and Regan, J. M. (2007). Comparison of anode bacterial communities and performance in microbial fuel cells with different electron donors. Appl. Microbiol. Biotechnol. 77, 393–402. doi: 10.1007/s00253-007-1162-y
Kozich, J. J., Westcott, S. L., Baxter, N. T., Highlander, S. K., and Schloss, P. D. (2013). Development of a dual-index sequencing strategy and curation pipeline for Analyzing amplicon sequence data on the MiSeq Illumina sequencing platform. Appl. Environ. Microbiol. 79, 5112–5120. doi: 10.1128/AEM.01043-13
Krieg, N. R., Ludwig, W., Euzéby, J., and Whitman, W. B. (2010). “Phylum XIV. Bacteroidetes phyl. Nov” in Bergey’s manual® of systematic bacteriology: Volume four the Bacteroidetes, spirochaetes, Tenericutes (mollicutes), Acidobacteria, Fibrobacteres, fusobacteria, Dictyoglomi, Gemmatimonadetes, Lentisphaerae, Verrucomicrobia, Chlamydiae, and planctomycetes. eds. N. R. Krieg, J. T. Staley, D. R. Brown, B. P. Hedlund, B. J. Paster, and N. L. Ward, et al. (New York, NY: Springer New York), 25–469.
Kuever, J. (2014). “The family Desulfobulbaceae” in The prokaryotes: Deltaproteobacteria and Epsilonproteobacteria. eds. E. Rosenberg, E. F. Delong, S. Lory, E. Stackebrandt, and F. Thompson (Berlin, Heidelberg: Springer Berlin Heidelberg), 75–86.
Kumar, R., Singh, L., Wahid, Z. A., and Din, M. F. M. (2015). Exoelectrogens in microbial fuel cells toward bioelectricity generation: a review. Int. J. Energy Res. 39, 1048–1067. doi: 10.1002/er.3305
Kumar, S., Stecher, G., Li, M., Knyaz, C., and Tamura, K. (2018). MEGA X: molecular evolutionary genetics analysis across computing platforms. Mol. Biol. Evol. 35, 1547–1549. doi: 10.1093/molbev/msy096
Larimer, F. W., Chain, P., Hauser, L., Lamerdin, J., Malfatti, S., Do, L., et al. (2004). Complete genome sequence of the metabolically versatile photosynthetic bacterium Rhodopseudomonas palustris. Nat. Biotechnol. 22, 55–61. doi: 10.1038/nbt923
Larsen, S., Nielsen, L. P., and Schramm, A. (2015). Cable bacteria associated with long-distance electron transport in New England salt marsh sediment. Environ. Microbiol. Rep. 7, 175–179. doi: 10.1111/1758-2229.12216
Lien, T., Madsen, M., Steen, I. H., and Gjerdevik, K. (1998). Desulfobulbus rhabdoformis sp. nov., a sulfate reducer from a water-oil separation system. Int. J. Syst. Evol. Microbiol. 48, 469–474. doi: 10.1099/00207713-48-2-469
Liu, H., Cheng, S. A., and Logan, B. E. (2005). Production of electricity from acetate or butyrate using a single-chamber microbial fuel cell. Environ. Sci. Technol. 39, 658–662. doi: 10.1021/es048927c
Liu, Z. D., Liu, J., Zhang, S. P., and Su, Z. G. (2009). Study of operational performance and electrical response on mediator-less microbial fuel cells fed with carbon- and protein-rich substrates. Biochem. Eng. J. 45, 185–191. doi: 10.1016/j.bej.2009.03.011
Logan, B. E. (2009). Exoelectrogenic bacteria that power microbial fuel cells. Nat. Rev. Microbiol. 7, 375–381. doi: 10.1038/nrmicro2113
Logan, B. E., Hamelers, B., Rozendal, R. A., Schrorder, U., Keller, J., Freguia, S., et al. (2006). Microbial fuel cells: methodology and technology. Environ. Sci. Technol. 40, 5181–5192. doi: 10.1021/es0605016
Logan, B. E., and Rabaey, K. (2012). Conversion of wastes into bioelectricity and chemicals by using microbial electrochemical technologies. Science 337, 686–690. doi: 10.1126/science.1217412
Logan, B. E., Rossi, R., Ragab, A. A., and Saikaly, P. E. (2019). Electroactive microorganisms in bioelectrochemical systems. Nat. Rev. Microbiol. 17, 307–319. doi: 10.1038/s41579-019-0173-x
Marzocchi, U., Trojan, D., Larsen, S., Meyer, R. L., Revsbech, N. P., Schramm, A., et al. (2014). Electric coupling between distant nitrate reduction and sulfide oxidation in marine sediment. ISME J. 8, 1682–1690. doi: 10.1038/ismej.2014.19
Mateo, S., Canizares, P., Rodrigo, M. A., and Fernandez-Morales, F. J. (2018). Driving force behind electrochemical performance of microbial fuel cells fed with different substrates. Chemosphere 207, 313–319. doi: 10.1016/j.chemosphere.2018.05.100
Mcinerney, M. J., Sieber, J. R., and Gunsalus, R. P. (2009). Syntrophy in anaerobic global carbon cycles. Curr. Opin. Biotechnol. 20, 623–632. doi: 10.1016/j.copbio.2009.10.001
Mehrabi, S., Ekanemesang, U. M., Aikhionbare, F. O., Kimbro, K. S., and Bender, J. (2001). Identification and characterization of Rhodopseudomonas spp., a purple, non-sulfur bacterium from microbial mats. Biomol. Eng. 18, 49–56. doi: 10.1016/S1389-0344(01)00086-7
OECD (2010). “Test no. 209: activated sludge, respiration inhibition test (carbon and ammonium oxidation)” in OECD guidelines for the testing of chemicals, section 2 (Paris: OECD Publishing)
Pandey, P., Shinde, V. N., Deopurkar, R. L., Kale, S. P., Patil, S. A., and Pant, D. (2016). Recent advances in the use of different substrates in microbial fuel cells toward wastewater treatment and simultaneous energy recovery. Appl. Energy 168, 706–723. doi: 10.1016/j.apenergy.2016.01.056
Pant, D., Van Bogaert, G., Diels, L., and Vanbroekhoven, K. (2010). A review of the substrates used in microbial fuel cells (MFCs) for sustainable energy production. Bioresour. Technol. 101, 1533–1543. doi: 10.1016/j.biortech.2009.10.017
Park, Y., Cho, H., Yu, J., Min, B., Kim, H. S., Kim, B. G., et al. (2017). Response of microbial community structure to pre-acclimation strategies in microbial fuel cells for domestic wastewater treatment. Bioresour. Technol. 233, 176–183. doi: 10.1016/j.biortech.2017.02.101
Pfeffer, C., Larsen, S., Song, J., Dong, M. D., Besenbacher, F., Meyer, R. L., et al. (2012). Filamentous bacteria transport electrons over centimetre distances. Nature 491, 218–221. doi: 10.1038/nature11586
Porter, N. T., Luis, A. S., and Martens, E. C. (2018). Bacteroides thetaiotaomicron. Trends Microbiol. 26, 966–967. doi: 10.1016/j.tim.2018.08.005
Prathiba, S., Kumar, P. S., and Vo, D. N. (2021). Recent advancements in microbial fuel cells: a review on its electron transfer mechanisms, microbial community, types of substrates and design for bio-electrochemical treatment. Chemosphere 286:131856. doi: 10.1016/j.chemosphere.2021.131856
Prokhorova, A., Sturm-Richter, K., Doetsch, A., and Gescher, J. (2017). Resilience, dynamics, and interactions within a model multispecies exoelectrogenic-biofilm community. Appl. Environ. Microbiol. 83:e03033-16. doi: 10.1128/AEM.03033-16
Reguera, G. (2012). Microbiology bacterial power cords. Nature 491, 201–202. doi: 10.1038/nature11638
Rinaldi, A., Mecheri, B., Garavaglia, V., Licoccia, S., Di Nardo, P., and Traversa, E. (2008). Engineering materials and biology to boost performance of microbial fuel cells: a critical review. Energy Environ. Sci. 1, 417–429. doi: 10.1039/b806498a
Saitou, N., and Nei, M. (1987). The neighbor-joining method: a new method for reconstructing phylogenetic trees. Mol. Biol. Evol. 4, 406–425. doi: 10.1093/oxfordjournals.molbev.a040454
Samain, E., Dubourguier, H. C., and Albagnac, G. (1984). Isolation and characterization of Desuljobulbus elongatus sp. nov. from a mesophilic industrial digester. Syst. Appl. Microbiol. 5, 391–401. doi: 10.1016/S0723-2020(84)80040-5
Sorokin, D. Y., Tourova, T. P., Mußmann, M., and Muyzer, G. (2008). Dethiobacter alkaliphilus gen. Nov. sp. nov., and Desulfurivibrio alkaliphilus gen. Nov. sp. nov.: two novel representatives of reductive sulfur cycle from soda lakes. Extremophiles 12, 431–439. doi: 10.1007/s00792-008-0148-8
Sydow, A., Krieg, T., Mayer, F., Schrader, J., and Holtmann, D. (2014). Electroactive bacteria—molecular mechanisms and genetic tools. Appl. Microbiol. Biotechnol. 98, 8481–8495. doi: 10.1007/s00253-014-6005-z
Tamura, K., Nei, M., and Kumar, S. (2004). Prospects for inferring very large phylogenies by using the neighbor-joining method. Proc. Natl. Acad. Sci. U. S. A. 101, 11030–11035. doi: 10.1073/pnas.0404206101
Tan, H., Zhao, J., Zhang, H., Zhai, Q., and Chen, W. (2018). Isolation of low-abundant Bacteroidales in the human intestine and the analysis of their differential utilization based on plant-derived polysaccharides. Front. Microbiol. 9:1319. doi: 10.3389/fmicb.2018.01319
Thapa, B. S., Kim, T., Pandit, S., Song, Y. E., Afsharian, Y. P., Rahimnejad, M., et al. (2022). Overview of electroactive microorganisms and electron transfer mechanisms in microbial electrochemistry. Bioresour. Technol. 347:126579. doi: 10.1016/j.biortech.2021.126579
Tian, L. L., Yan, X. J., Wang, D. B., Du, Q., Wan, Y. X., Zhou, L. A., et al. (2022). Two key Geobacter species of wastewater-enriched electroactive biofilm respond differently to electric field. Water Res. 213:118185. doi: 10.1016/j.watres.2022.118185
Trojan, D., Schreiber, L., Bjerg, J. T., Boggild, A., Yang, T. T., Kjeldsen, K. U., et al. (2016). A taxonomic framework for cable bacteria and proposal of the candidate genera Electrothrix and Electronema. Syst. Appl. Microbiol. 39, 297–306. doi: 10.1016/j.syapm.2016.05.006
Velasquez-Orta, S. B., Yu, E., Katuri, K. P., Head, I. M., Curtis, T. P., and Scott, K. (2011). Evaluation of hydrolysis and fermentation rates in microbial fuel cells. Appl. Microbiol. Biotechnol. 90, 789–798. doi: 10.1007/s00253-011-3126-5
Wang, S., Huang, L., Gan, L., Quan, X., Li, N., Chen, G., et al. (2012). Combined effects of enrichment procedure and non-fermentable or fermentable co-substrate on performance and bacterial community for pentachlorophenol degradation in microbial fuel cells. Bioresour. Technol. 120, 120–126. doi: 10.1016/j.biortech.2012.06.022
Wang, A., Zhang, Z., Ding, Q., Yang, Y., Bindelle, J., Ran, C., et al. (2021). Intestinal Cetobacterium and acetate modify glucose homeostasis via parasympathetic activation in zebrafish. Gut Microbes 13, 1–15. doi: 10.1080/19490976.2021.1900996
Xing, D. F., Zuo, Y., Cheng, S. A., Regan, J. M., and Logan, B. E. (2008). Electricity generation by Rhodopseudomonas palustris DX-1. Environ. Sci. Technol. 42, 4146–4151. doi: 10.1021/es800312v
Zhang, Y. F., Min, B., Huang, L. P., and Angelidaki, I. (2011). Electricity generation and microbial community response to substrate changes in microbial fuel cell. Bioresour. Technol. 102, 1166–1173. doi: 10.1016/j.biortech.2010.09.044
Zhu, X. P., Yates, M. D., Hatzell, M. C., Rao, H. A., Saikaly, P. E., and Logan, B. E. (2014a). Microbial community composition is unaffected by anode potential. Environ. Sci. Technol. 48, 1352–1358. doi: 10.1021/es404690q
Keywords: microbial fuel cell, Geobacter spp., enrichment, acetate, wastewater
Citation: Christgen B, Spurr M, Milner EM, Izadi P, McCann C, Yu E, Curtis T, Scott K and Head IM (2023) Does pre-enrichment of anodes with acetate to select for Geobacter spp. enhance performance of microbial fuel cells when switched to more complex substrates? Front. Microbiol. 14:1199286. doi: 10.3389/fmicb.2023.1199286
Edited by:
Amelia-Elena Rotaru, University of Southern Denmark, DenmarkReviewed by:
Tian Li, Nankai University, ChinaSatoshi Kawaichi, University of Southern Denmark, Denmark
John Michael Regan, The Pennsylvania State University (PSU), United States
Copyright © 2023 Christgen, Spurr, Milner, Izadi, McCann, Yu, Curtis, Scott and Head. This is an open-access article distributed under the terms of the Creative Commons Attribution License (CC BY). The use, distribution or reproduction in other forums is permitted, provided the original author(s) and the copyright owner(s) are credited and that the original publication in this journal is cited, in accordance with accepted academic practice. No use, distribution or reproduction is permitted which does not comply with these terms.
*Correspondence: Beate Christgen, YmVhdGUuY2hyaXN0Z2VuQG5jbC5hYy51aw==