- 1Facultad de Ciencias de la Vida, Universidad Andres Bello, Santiago, Chile
- 2Millennium Institute on Immunology and Immunotherapy, Santiago, Chile
- 3Departamento de Genética Molecular y Microbiología, Facultad de Ciencias Biológicas, Millennium Institute on Immunology and Immunotherapy, Pontificia Universidad Católica de Chile, Santiago, Chile
- 4Departamento de Endocrinología, Facultad de Medicina, Pontificia Universidad Católica de Chile, Santiago, Chile
Sepsis is a life-threatening condition and a significant cause of preventable morbidity and mortality globally. Among the leading causative agents of sepsis are bacterial pathogens Escherichia coli, Klebsiella pneumoniae, Staphylococcus aureus, Pseudomonas aeruginosa, and Streptococcus pyogenes, along with fungal pathogens of the Candida species. Here, we focus on evidence from human studies but also include in vitro and in vivo cellular and molecular evidence, exploring how bacterial and fungal pathogens are associated with bloodstream infection and sepsis. This review presents a narrative update on pathogen epidemiology, virulence factors, host factors of susceptibility, mechanisms of immunomodulation, current therapies, antibiotic resistance, and opportunities for diagnosis, prognosis, and therapeutics, through the perspective of bloodstream infection and sepsis. A list of curated novel host and pathogen factors, diagnostic and prognostic markers, and potential therapeutical targets to tackle sepsis from the research laboratory is presented. Further, we discuss the complex nature of sepsis depending on the sepsis-inducing pathogen and host susceptibility, the more common strains associated with severe pathology and how these aspects may impact in the management of the clinical presentation of sepsis.
1. Introduction
Sepsis, a life-threatening organ dysfunction caused by a dysregulated host response to infection (Singer et al., 2016), is a significant cause of geriatric, maternal, neonatal, and child mortality. The World Health Organization (WHO) responded in 2017 with a resolution acknowledging sepsis as a major cause of preventable morbidity and mortality globally, while highlighting some of the most frequent pathogens connected etiologically to this condition, their primary site of infection, the increasing contribution of nosocomial infections, and their alarming resistance to antibiotics [World Health Organization (WHO), 2017]. Great strides toward an accurate quantification of its incidence and mortality (Rudd et al., 2020), and the pathogens accounting for its excess of mortality (GBD 2019 Antimicrobial Resistance Collaborators, 2022), have been made since that resolution. While epidemiological findings raise awareness and aid in the shaping of public health policies, molecular discoveries contribute to the identification of host factors of susceptibility and pathogen mechanisms of immune evasion, structuring the therapeutic tools of the future.
Bacterial pathogens lead cases of bloodstream infections (BSI; Gouel-Cheron et al., 2022) with Escherichia coli, Klebsiella pneumoniae, Staphylococcus aureus, Pseudomonas aeruginosa, and Streptococcus pyogenes, as the as the pathogens with the largest number of attributable deaths (Diekema et al., 2019; GBD 2019 Antimicrobial Resistance Collaborators, 2022). Among fungal pathogens, Candida species are the most frequent pathogens in the critical care setting, with strong nosocomial association (Delaloye and Calandra, 2014; Gouel-Cheron et al., 2022). Viral agents are less frequently regarded as the cause of sepsis and are often deemed as facilitators to infection by secondary agents (Lin et al., 2018). Nevertheless, Herpes simplex virus, Enterovirus, Influenza, Adenovirus, Dengue virus, and recently the novel severe acute respiratory syndrome coronavirus 2 (SARS-CoV-2), are considered as viral causes of sepsis (Shane et al., 2017; Teparrukkul et al., 2017; Iuliano et al., 2018; Alhazzani et al., 2020).
Because only a fraction of the articles included in this work regarded the comprehensive clinical continuum that sepsis represents, a pragmatic approach translating results from invasive infection and progression into the bloodstream, either by extension or by analogy, was adopted. Thus, this review summarizes recent findings in terms of pathogen epidemiology, virulence factors, host factors of susceptibility, mechanisms of immunomodulation, current therapies, antibiotic resistance, and opportunities for diagnosis, prognosis, and therapeutics, focusing on evidence from human studies but also including cellular and molecular evidence from in vitro and in vivo studies, all through the looking glass of BSI and sepsis.
2. Escherichia coli
Escherichia coli is a Gram-negative bacillus with remarkable phylogenetic diversity. Although mostly recognized for its pathogenic role in diarrheal diseases, and less frequently to extraintestinal illness, E. coli is also a part of the commensal microbiome of the host. Commensal strains (originating typically from phylogroup A) rarely cause diseases in healthy hosts, as they lack specialized virulence traits. Intestinal-pathogenic E. coli comprise groups diarrheagenic, enteropathogenic, enterohemorrhagic, enterotoxigenic, enteroaggregative, enteroinvasive, and diffusely adherent (originating typically from phylogroups B1 and E; Kaper et al., 2004; Bachmann et al., 2015). Extraintestinal E. coli (ExPEC) comprise a growing group that includes uropathogenic E. coli (UPEC), sepsis-associated E. coli (SEPEC), and neonatal meningitis E. coli (NMEC), among others (Desvaux et al., 2020), originating typically from phylogroups B2 and occasionally from phylogroups D, F, or G (Escobar-Páramo et al., 2004; Clermont et al., 2019). In fact, a recent prospective observational cohort study on E. coli bacteremia found that phylogroups most frequently associated with fatal outcome after 28 days were, in order, B2 (46%), D (17%), and B1 (15%; de Lastours et al., 2020). The main source of E. coli bacteremia is urinary tract infection (representing more than 50% of the cases; Bonten et al., 2020), with advanced age (>65 years) representing the greatest risk factor for asymptomatic E. coli bacteremia of urinary source (OR = 1.8–2.95; Bai et al., 2020). Consistently, UPEC isolated from patients with pyelonephritis exhibit much higher serum resistance (82–93%) than fecal E. coli isolates (57%; Coggon et al., 2018; Table 1). Although widely considered as the leading pathogen for bacteremia (Shorr et al., 2006; Al-Hasan et al., 2012), the predominance of E. coli depends on the timeframe, geographical location, and age of the patients included in the analysis.
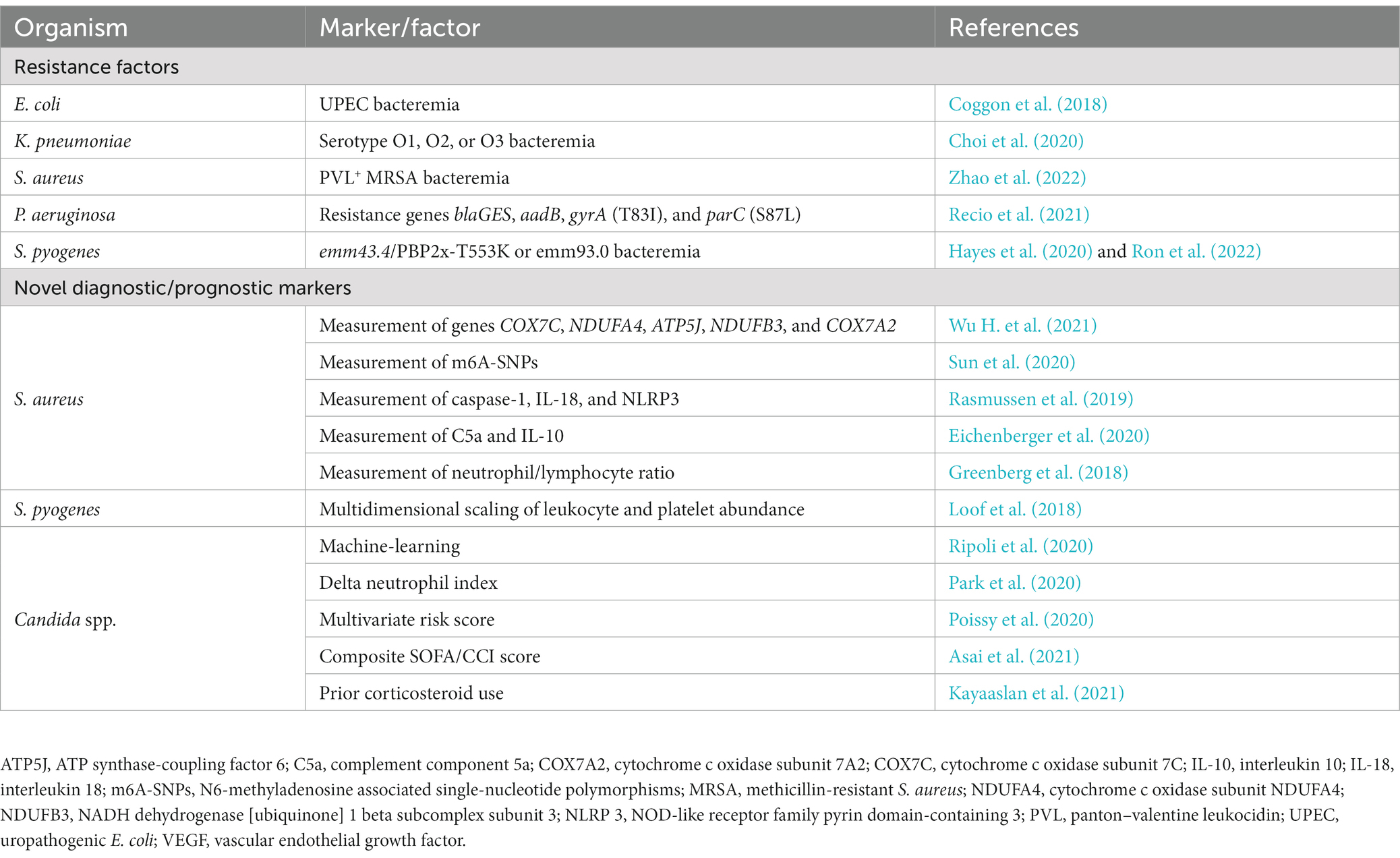
Table 1. Summary of novel diagnostic/prognostic markers and resistance factors of interest for sepsis detailed in this review.
2.1. Epidemiology
The SENTRY program for global antimicrobial surveillance places E. coli as the number one pathogen causing BSI worldwide since 2005 (Diekema et al., 2019). However, this is not consistent in the United States, where S. aureus leads the ranking since 1997. In fact, a recent report on the epidemiology of hospital-acquired BSI in intensive care unit patients in Europe reveals that Klebsiella spp. lead the ranking of Gram-negative bacteremia, relegating E. coli to the second place (Tabah et al., 2023). Similarly, data from a prospective observational study conducted in Thailand, which included data from 3,806 patients, found that E. coli was the most frequent causative agent for BSI, and that age over 70 years carries an increased hazard ratio of 1.5 for 28-day mortality (Somayaji et al., 2021). A recent meta-analysis reported that the incidence of E. coli bacteremia is increasing over time in select high-income countries around the globe with pooled mortality rates ranging from 10.7 to 14.3% (Bonten et al., 2020). Also, data from the SENTRY program place E. coli as the leading pathogen causing BSI only in ages older than 64 years old, while for the rest of the age groups S. aureus leads the ranking, a fact that was ratified in a 10-year report on the epidemiology of bacteremia in a Portuguese pediatric population (Ferreira et al., 2023). Nevertheless, a recent cohort study representative of the United States, which analyzed 217,480 neonatal patients, found an overwhelming majority of E. coli-associated sepsis (Stoll et al., 2020). Remarkably, E. coli dominance in hospital onset bacteremia (Diekema et al., 2019) is significantly diluted during concomitant viral co-infection (Glass et al., 2022), especially in patients hospitalized for coronavirus disease 2019 (COVID-19; Garcia-Vidal et al., 2021).
2.2. Pathogen factors
Virulence factor diversity for E. coli isolated from blood comprises genes coding for proteins related to adherence (ecp, hcp, eaeH, fim, and pap), intracellular traffic (upaG, ehaB, and agn43), invasion (tia), iron uptake (sitA, chuA, fyuA, and iuccC), and toxins (hlyE/clyA, usp., and senB) allowing bacteria to reach and survive in the bloodstream (Kim et al., 2022). However, ExPEC makes use of additional virulence traits, members of the serine protease autotransporters of Enterobacteriaceae (SPATE) superfamily involved in evasion from host immune defense mechanisms (Abreu et al., 2015). A recent prospective observational cohort study analyzing blood isolates from 278 patients found SPATE coding genes in 61% of the isolates, with an overwhelming presence in phylogroup B2, with sat and vat as the most prevalent of them (Freire et al., 2020). SPATE gene sat is a class 1-SPATE recognized as a cytotoxic factor with proteolytic activity (Freire et al., 2022) and vat is a class 2-SPATE cytotoxic factor (Nichols et al., 2016; Díaz et al., 2020), and both displayed a significant presence in ExPEC isolates of phylogroups B2, D, E, and F (Freire et al., 2020). In vitro evidence on virulence factors reveals that E. coli adheres to endothelial cells by a direct and necessary interaction between bacterial cell membrane protein OmpA and endothelial integrin αVβ3 which, in turn, activates endothelial cells via calcium-dependent intracellular cascades that ultimately lead to a downregulation of VE-Cadherin (Tapia et al., 2019), increased vascular permeability (Gatica et al., 2020), and endothelial apoptosis (McHale et al., 2018). Interestingly, all these readouts displayed not different to uninfected controls when using OmpA-deficient mutant strains, strongly underlining the role of OmpA in the genesis of sepsis at the cellular level. A summary of the factors detailed in this section is listed in Table 2.
2.3. Host factors
Clinical manifestations of E. coli bacteremia include fever, disorientation, hypotension, and respiratory failure, and may include septic shock, which is estimated to be present in about 25% of bacteremic patients (Kang et al., 2005). In fact, a recent prospective observational cohort study found a significant association between E. coli bacteremia and adverse outcomes after 28 days for the following clinical presentations: cancer, chronic peripheral arteritis, sepsis, and septic shock at initial presentation, infection of the digestive tract and airway, and start of adequate antibiotic therapy after 48 h of onset bacteremia (de Lastours et al., 2020). Interestingly, bacteremias starting as urinary tract infections displayed a greater strength of association with the survivor group, representing a noteworthy point of inflection for these types of infections. The latter is not an isolated figure, as confirmed in a multivariate analysis from a recent retrospective cohort study on E. coli bacteremia, where this observation urinary tract infection is reported as a remarkable protective factor (OR = 0.07) for the outcome 30-day mortality (Chapelet et al., 2017). Furthermore, multivariate analysis reveals an astounding OR of 6.54 for pulmonary portal of entry as determinant of 28-day mortality, after adjustment. Other determinants included in the final multivariate model were infection with bacterial factor STc88 (OR = 3.62), expression of virulence factor iha_17 (OR = 4.41), and other comorbidities (OR = 1.14; de Lastours et al., 2020). Similarly, another prospective observational cohort study found a significant association between E. coli bacteremia and septic shock or death within 72 h in patients presenting hematologic cancer or history of transplantation (OR = 16.34), reduced daily living activity (OR = 3.85), and presence of virulence factor iss (OR = 7.71), after adjustment in a multivariate analysis (Fröding et al., 2020). The excess risk revealed for oncologic, or transplantation patients is a call for caution to practitioners attending such conditions. A summary of the factors detailed in this section is listed in Table 3.
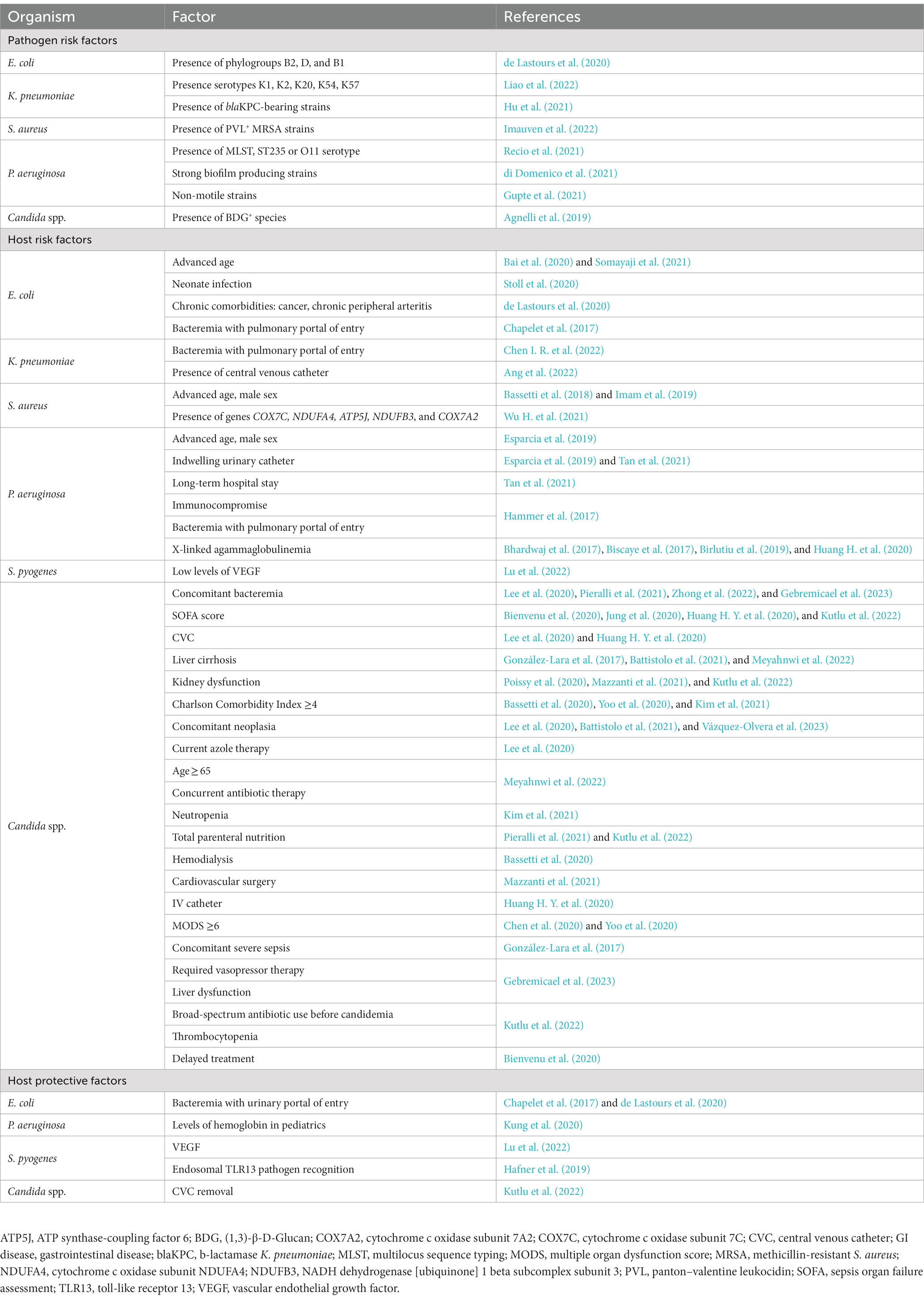
Table 3. Summary of novel risk and protective factors of interest for sepsis detailed in this review.
2.4. Treatment
Empiric antimicrobial therapy follows a general Gram-negative bacteremia algorithm with prolonged delivery of broad-spectrum β-lactam antibiotics as the first indication (Evans et al., 2021). Directed therapy is aimed at restraining resistance with recommendations to switch to a single agent with the narrowest spectrum to which the organism is susceptible. A recent prospective observational cohort study found that amoxicillin/clavulanic acid, cotrimoxazole, and fluoroquinolone, had the strongest association between antibiotic resistance and 28-day mortality in E. coli bacteremia (de Lastours et al., 2020). Favorably, none of the non-survivors presented any carbapenem resistance, which was present in only one out of 493 survivors. Moreover, multivariate analysis reveals an increased risk for 28-day mortality by broad-spectrum β-lactam and/or third-generation cephalosporin resistant strains in patients presenting chronic alcoholism (OR = 3.04), initiating adequate antibiotic treatment after 48 h (OR = 3.04), or with a history of bacteremia (OR = 2.81), after adjustment (de Lastours et al., 2020). According to the latest global report on antimicrobial resistance by the WHO, the median resistance to third generation cephalosporins in E. coli bloodstream confirmed infections is 41.8% [World Health Organization (WHO), 2022a]. It is suggested that cefotaxime and ceftriaxone are the most suitable antibiotics to monitor cephalosporin resistance in E. coli BSI. Thus, pathogen-directed analyses are key to guide the development and implementation of strategies on age-targeted prevention, antimicrobial resistance, and new therapies.
3. Klebsiella pneumoniae
Klebsiella pneumoniae is a Gram-negative, encapsulated, non-motile, facultatively anaerobic bacterium. Klebsiella species are commonly found in soil, water, plants, and livestock (Morgado et al., 2022), and K. pneumoniae in human hosts is not strictly pathogenic. In fact, K. pneumoniae colonizes the nasal and digestive tract without causing any symptomatic disease (Chang et al., 2021), and it is proposed that gastrointestinal colonization serves as a major reservoir for transmission and infection to other sites (Martin et al., 2016). Moreover, studies show a high level of association between gastrointestinal carriage and subsequent infection by a patient’s own K. pneumoniae strains (Martin et al., 2016; Gorrie et al., 2017). Although the exact mechanisms are unclear, K. pneumoniae progression from intestinal territories has been related to a disproportion in bacterial density of colonizing strains, especially when host defenses are challenged, e.g., during cancer, diabetes mellitus, and alcohol abuse (Happel and Nelson, 2005). Traditionally, serotypes of Klebsiella isolates have been identified and followed using typing antisera. Different O and K serotypes are produced as a result of the detection of distinctive variants of surface-exposed polysaccharides, named O-antigens and K-antigens, by certain antibodies. O-antigens constitute the outer layer of lipopolysaccharide (LPS), whereas K-antigens are a component of the bacterial capsule polysaccharide (CPS). To date, eight serotypes for O-antigens and 77 for K-antigens have been described (Follador et al., 2016).
3.1. Epidemiology
A recent multi-country collection study revealed that K. pneumoniae geographical diversity is dominated worldwide by antigen O1, followed by antigen O2, which displayed a larger proportion in Europe, and antigen O3, less represented in Africa (Choi et al., 2020). Additionally, a recent report on worldwide BSI identified K. pneumoniae as the third most prevalent pathogen consistently over the last 25 years (Diekema et al., 2019). Moreover, various publications on K. pneumoniae progression into the bloodstream identified pulmonary, abdominal, and urinary sites as the leading sources of infection (Togawa et al., 2015; Hyun et al., 2018; Juan et al., 2019; Huang Y. T. et al., 2020; Li M. et al., 2023). Interestingly, all these reports are successful in identifying the primary source of infection, a fact that differs from clinical findings reported before 2010, in which K. pneumoniae bacteremia of unknown origin reached up to 58% of cases (Tsay et al., 2002; Tumbarello et al., 2006). Among the most widely distributed K. pneumoniae, serotype antibiotic resistance was predominantly associated to serotypes carrying antigens O2 and O3, while serotypes carrying antigen O1 (the most frequently distributed worldwide) was associated with sensitivity to extended spectrum cephalosporins, fluoroquinolone, and carbapenems, among many other antibiotics (Choi et al., 2020; Table 1). According to the latest global report on antimicrobial resistance by the WHO, K. pneumoniae is the third leading agent causing bacteremia with an increasing resistance to third- and fourth-generation cephalosporin and co-trimoxazole therapy [World Health Organization (WHO), 2022a], pushing the use of carbapenems (Souli et al., 2017).
3.2. Pathogen factors
Among the most prevalent virulence factors identified in BSI in the United States are genes ybt, clb, iucA, rmpA, rmpA2, and iroB, of which, iucA and rmpA/2 have been associated with carbapenem resistance (Kochan et al., 2022). Consistently, iucA, rmpA, rmpA2, iroB, and peg-344, were recently described as the most prevalent virulence genes in Taiwan (Liao et al., 2022), while genes ybt, iuc, and rmp were recently described as the most prevalent in China (Cienfuegos-Gallet et al., 2022). Co-infection of K. pneumoniae with other bacterial agents (i.e., Acinetobacter baumannii, P. aeruginosa, and E. coli, among other less frequent bacteria) has been documented and sized previously (Karakonstantis et al., 2022; Lv et al., 2022).
3.3. Host factors
Multivariate analyses report nosocomial pneumonia, high SOFA score, inappropriate treatment (Chen I. R. et al., 2022), high APACHE II score, development of septic shock (Wu X. et al., 2021), and central venous catheter (Ang et al., 2022), as significant risk factors for mortality from K. pneumoniae BSI. Moreover, BSI with serotypes K1, K2, K20, K54, and K57, has also been found associated to increased mortality distributions (Liao et al., 2022). Furthermore, infection with K. pneumoniae β-lactamase (blaKPC)-harboring strains has been found as an independent risk factor for mortality (Hu et al., 2021). Co-infection of K. pneumoniae with other viral agents, particularly with SARS-CoV-2, has been well described (Damico et al., 2022; Said et al., 2022), reviewed (Santos et al., 2022), and sized (Lansbury et al., 2020; Kariyawasam et al., 2022; Santos et al., 2022) elsewhere. Of note, the proportion of K. pneumoniae isolates from COVID-19 patients has been documented to be significantly lower compared to COVID-negative controls, implicating that infection with viral agents may reduce the chance to develop bacteremia, a subject that requires further research (Glass et al., 2022). A summary of the factors detailed in this section is listed in Table 2.
3.4. Treatment
In line with general Gram-negative bacteremia treatment, empiric antimicrobial therapy considers prolonged delivery of broad-spectrum β-lactam antibiotics as the first indication (Evans et al., 2021). However, driven by the extensive use of carbapenems, the growth and spread of carbapenem-resistant bacterial infections has emerged as a major public health concern in recent decades. In fact, K. pneumoniae resistance to carbapenems has experienced a 3-fold increase worldwide since 2016 [World Health Organization (WHO), 2022a]. A wise strategy to overcome this new threat is founded on the use of carbapenem antibiotics in combination with β-lactamase inhibitors, such as clavulanic acid, sulbactam, and tazobactam. Thus, notorious advances in terms of drug efficacy and safety have been made over the last decade with new β-lactam/β-lactamase inhibitor combinations (BLIC) synergistically restoring antimicrobial sensitivity, e.g., aztreonam/avibactam, ceftazidime/avibactam, imipenem/relebactam, meropenem/vaborbactam, and cefepime/zidebactam, among others (Vázquez-Ucha et al., 2020).
A recent study in Taiwan reports a significant restoration of imipenem activity against carbapenem-nonsusceptible K. pneumoniae when combined with relebactam, increasing susceptibility from 0.8 to 88.4%, 21.4 to 42.9%, and 30 to 40%, in isolates producing carbapenemases of Ambler classes A, B, and D, respectively (Yang T. Y. et al., 2022). In accordance, regarding the same combination, a study analyzing K. pneumoniae isolates from Spain and Portugal reports a major decrease in resistance in Ambler class A carbapenemase-harboring strains, while reporting no resistance to ceftazidime/avibactam combination in isolates producing carbapenemases of Ambler classes A and D (Hernández-García et al., 2022). In fact, experimental combination of ceftazidime/avibactam and aztreonam (Shah et al., 2021) or possibly meropenem (Parruti et al., 2019) has been reported successfully not only in clinical case reports but also in a recent cohort study (adjusted HR = 0.136; Zheng et al., 2021). Additionally, use of fosfomycin in combination with third- and fourth-generation cephalosporin has been thoroughly (in a retrospective cohort study analyzing 104 cases of carbapenem resistant K. pneumoniae bacteremia) demonstrated as protective (adjusted OR = 0.07) against mortality by sepsis (Liao et al., 2017). While these promising results constitute the latest evidence on advanced approaches in patients, further evidence from animal models reveals that rifampin alone or in combination with colistin have the strongest effect against carbapenemase-producing K. pneumoniae sepsis mortality in vivo (Pachón-Ibáñez et al., 2018). Moreover, use of amikacin alone or in combination with fosfomycin significantly reduced circulating bacterial load in a sepsis model using carbapenemase-producing K. pneumoniae strains (Cebrero-Cangueiro et al., 2021).
In the quest for new therapies, a recent report on animal K. pneumoniae sepsis shows that the use of mushroom-derived β-glucans are able to reduce bacterial load while improving physiological parameters associated to the pathobiology of sepsis (i.e., arterial pO2, plasma lactate, pulmonary compliance, and arterial alveolar oxygen gradient; Masterson et al., 2020). In line with this, Bergenin monohydrate (a plant extract with immunomodulatory properties) has been described to reduce reactive oxygen species (ROS) production and increase cell viability in vitro while increasing levels of superoxide dismutase (SOD) and GSH, and reducing bacterial load, levels of inflammatory cytokines interleukin (IL)-6, IL-1β, prostaglandin E2 (PGE2), and tumor necrosis factor (TNF-α), malondialdehyde (MDA) formation, myeloperoxidase (MPO) content, and number of infiltrating leukocytes in a MAPK/NF-κB–dependent manner in the lungs of septic animals challenged with K. pneumoniae (Tang et al., 2021). Similarly, AS101 (an inorganic compound with anti-apoptotic, anti-inflammatory, and immunomodulatory effects; Okun et al., 2007) has been described to reduce bacterial load across the liver, kidney, and spleen, and ultimately increasing mice survival from 0% 30 h after K. pneumoniae injection i.p. to 75% after 72 h in a mouse model of carbapenem-resistant K. pneumoniae sepsis (Yang et al., 2021). Also, TNF-related apoptosis-inducing ligand (TRAIL) encapsulated to a polypeptide-crosslinked nanogel has been described to significantly reduce bacterial load in blood and increase mice survival from 0% 4.5 days after K. pneumoniae instillation i.t. to 75% after 12 days in a mouse model of K. pneumoniae sepsis (Chen et al., 2019). Likewise, adipose-derived mesenchymal stem cells were described to significantly reduce bacterial load across the lung, blood, liver, and spleen; reduce pro-inflammatory cytokines (TNF-α, IL-1β, and IL-6) in the lung; and reduce tissue immune infiltration and damage, by downregulating genes related to nicotine, thymine, and uracil degradation, and upregulating genes related to unfolded protein response, sirtuin signaling pathway, and leukocyte adhesion and diapedesis, among others, in a mouse model of K. pneumoniae induced pneumosepsis (Perlee et al., 2019).
A cutting-edge approach using red blood cell membrane-coated poly(lactic-co-glycolic acid) (PLGA) nanoparticles (γ3-RBCNPs) to improve antibiotic bioavailability showed an astounding increase in mice survival from 0% 4 days after K. pneumoniae instillation i.t. to 60% after 7 days of instillation, with a significant and systematic reduction in bacterial load across the lung, blood, liver, spleen, and kidney, and a significant reduction in circulating levels of TNF-α, IL-1β, and IL-6 (Liu et al., 2022). Another cutting-edge approach using novel therapeutic antibodies targeting K1-serotype CPS of hypervirulent K. pneumoniae (hvKp) strains to promote bacterial phagocytosis by Kupffer cells in the liver were described to significantly reduce bacterial load across the lung, liver, and spleen, of hvKp challenged mice while increasing their survival from 0% 4 days after hvKp injection i.p. to 75% after 15 days in a mouse model of K. pneumoniae sepsis (Diago-Navarro et al., 2017). On the edge of knowledge, it has been revealed that exposure to blue light (peak 442 nm) significantly reduces bacterial load from the lung, blood, liver, and spleen, while significantly increasing mice survival from 15% 100 h after K. pneumoniae instillation i.t. to 62% after 125 h of bacterial challenge (Griepentrog et al., 2020). This was explained by local modulation of MPO activity and neutrophil abundance. Interestingly, there was no significant change in mononuclear abundance in the lung. The effect of blue light was mechanistically elucidated and attributed to a neural circuit signaling through a cholinergic anti-inflammatory pathway that directly controls immune responses in the spleen, enhancing control of the infection, and improving survival (Griepentrog et al., 2020). Thus, the potential therapeutic utility of something as trivial as ambient light in the ICU is strongly underscored. These cutting-edge approaches constitute powerful and promising tools in the search for new avenues for the treatment of sepsis that entails further research and encouragement. Lastly, pushing the edges of knowledge, it has been described that K. pneumoniae is able to induce alterations in the gut microbiome and cecal metabolome in a mouse model of sepsis (Wu T. et al., 2020). A significant difference in the richness, diversity, and composition of bacterial communities in mice challenged with K. pneumoniae, with fewer Bacteroidetes and Firmicutes but higher levels of Proteobacteria and Verrucomicrobia. At the genus level, mice challenged with K. pneumoniae had significantly fewer Bacteroides, Parabacteroides, Bifidobacterium, Clostridium, Coprococcus, and Prevotella, which produce short-chain fatty acids (SCFA). Interestingly, absolute concentration of SCFAs both in cecal contents and in serum were consistently lower in mice challenged with K. pneumoniae. Notably, supplementation of SCFAs in drinking water significantly reduced bacterial burden and tissue damage in the lungs, and significantly reduced mortality, while increasing phagocytic capacity of alveolar macrophages and pulmonary levels of IL-6 and TNF-α. Thus, characterization of microbial biomarkers displays a sizable potential for aiding in the diagnosis/prognosis of patients under critical care.
Additional evidence from animal models implicates PTX3 pentraxin (a component of humoral innate immunity involved in resistance to selected pathogens by promoting opsonophagocytosis) as protective factor expressed on the host side promoting bacterial phagocytosis, expression of TNF-α, IL-1β, IL-6, and CXCL-1, limiting MPO levels and leukocyte counts, protecting from tissue hemorrhage and increased mortality in septic mice challenged with K. pneumoniae (Asgari et al., 2021). Hypoxia-inducible factor (HIF) has also been implicated in modulating the immune system of the host during sepsis (Otto et al., 2021). Using a Cre/lox system, HIF1α was found instrumental to engage host defense against K. pneumoniae pneumosepsis for its significant role in bacterial load reduction both in the lung and distant organs and limiting levels of pro-inflammatory cytokines while enhancing the release of IL-10 in the lung (Otto et al., 2021). This modulation was explained by an energetic dysregulation affecting glucose uptake, leading to a significant reduction in TNF-α production in both alveolar and interstitial macrophages in LysM-cre × Hif1αfl/fl mice during onset K. pneumoniae i.n. challenge. Collectively, this frontline evidence shows as the foundation for the establishment of new diagnostic tools and the development of the therapeutics of the future.
4. Staphylococcus aureus
Staphylococcus aureus is a facultative Gram-positive organism mostly recognized for its pathogenic potential. Notwithstanding, S. aureus is a frequent colonizer in human hosts (Laux et al., 2019), with abundances reported recently as 32% for neonates (Arora et al., 2023), 25–29% for pediatrics (McNeil et al., 2022; Arora et al., 2023), and 30% for adults (Erayil et al., 2022). Although colonization is asymptomatic, it has been deemed a risk factor for developing BSI in the event of pathogenic transformation and bloodstream invasion (Tacconelli et al., 2017). Currently, there is no consensus on what single event leads to pathogenic progression. Rather, virulence mechanisms appear to be related to circumstance, environmental opportunity, and particularly, to host-pathogen interplay (Kwiecinski and Horswill, 2020).
4.1. Epidemiology
Staphylococcus aureus is reported as the leading causative agent for BSI in North America and the second most prevalent for the rest of the world (Diekema et al., 2019). Moreover, a recent study conducted in Australia reported an increased incidence of S. aureus bacteremia in older men, especially over 60 years old, almost doubling the incidence reported in the same age group in women (Imam et al., 2019). Furthermore, another study reported that MRSA bacteremia in patients older than 75 years old represents a significant risk factor (OR = 2.4) for 30-day mortality (Bassetti et al., 2018). Similarly, a prospective observational study carried out in Australia and New Zealand found a strong majority of the samples associated to advanced age (>70 years-old, 63.1%), male sex (64%), and methicillin susceptibility (75.9%), and multivariate analysis further revealed sepsis as the most influent risk factor for 30-day mortality in patients with S. aureus bacteremia (OR = 4.01; Turnidge et al., 2009). Bloodstream coinfection with other pathogenic agents is less frequent, although it has been reported that S. aureus/Candida albicans coinfection has a catastrophic proportion of 82% for 30-day mortality and a high correlation with indwelling vascular devices (Wu Y. M. et al., 2021). In fact, a retrospective observational study conducted in Italy showed that patients older than 80 years afflicted with MRSA BSI had the highest risk for 30-day mortality and coinfection with Enterococcus spp. and Candida spp. were significant risk factors for this outcome (Giovannenze et al., 2021; Figure 1). More recently, S. aureus/SARS-CoV-2 coinfection has gained more insight, with reported 30-day mortality of 67%, largely attributable to hospital-onset bacteremia in patients mostly under mechanical ventilation (Cusumano et al., 2020).
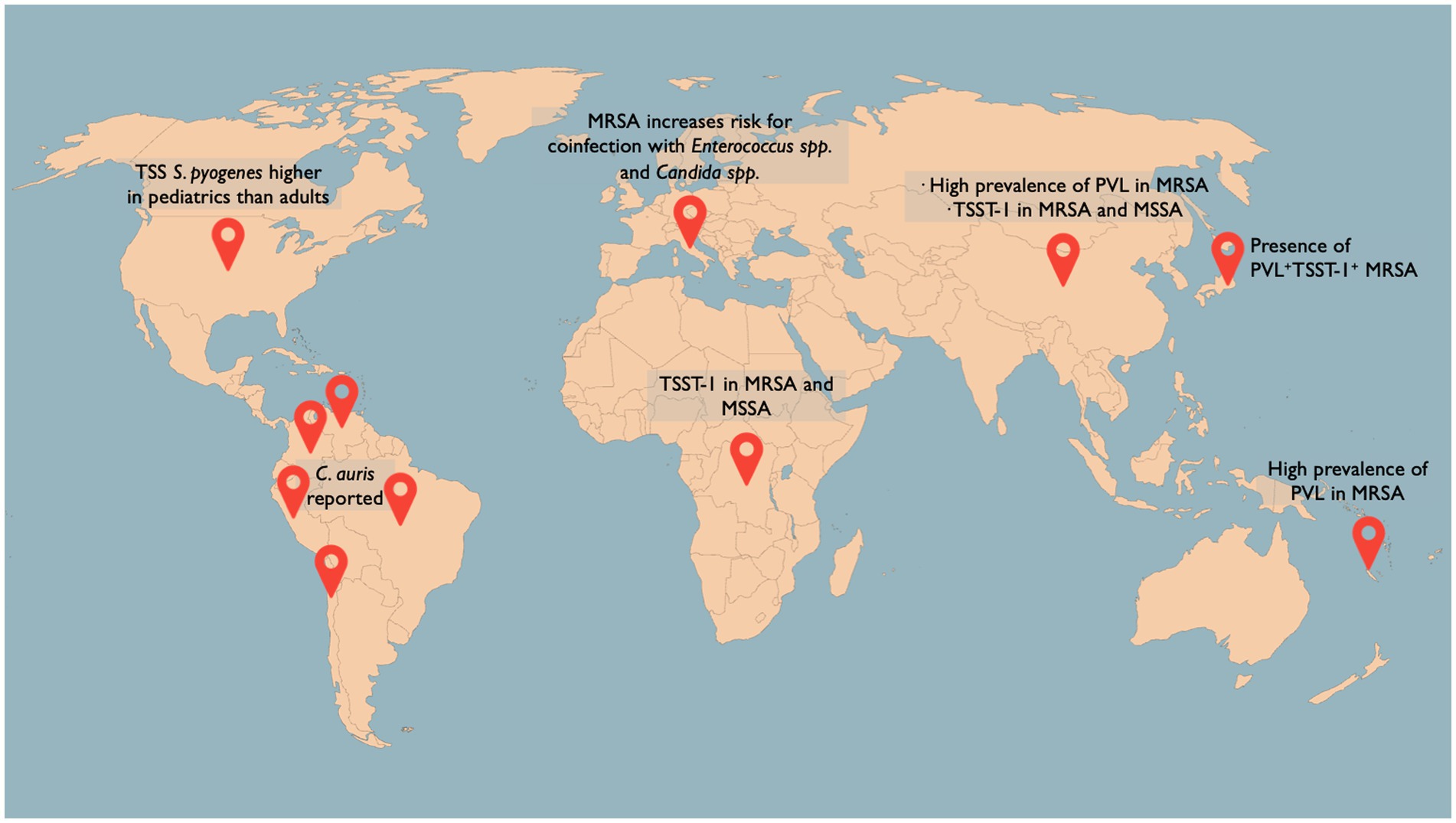
Figure 1. Selection of the latest figures on sepsis-associated pathogen epidemiology worldwide. Streptococcus pyogenes-associated TSS is reported in the United States with an uneven distribution between adults (22.5%) and pediatrics (7.1%; Meehan et al., 2018). TSST-1 has been identified in 67% of MRSA and 4% of MSSA blood isolates in the Democratic Republic of the Congo (Vandendriessche et al., 2017) and in 23.1% of MRSA and 6.7% of MSSA blood isolates in China (Liu et al., 2018). PVL + TSST-1+ isolates have been found in 0.4% of all MRSA isolates in Japan (Kaneko et al., 2023). A high prevalence of PVL+ MRSA strains in adults has been found in New Caledonia Island (Imauven et al., 2022). An escalating number of countries in Latin America have reported the detection of Candida auris (Riera et al., 2022).
4.2. Pathogen factors
A recent study comparing the pathogenic mechanisms of fixed clonal complexes of S. aureus during sepsis showed only one virulence property that remained consistent (Recker et al., 2017). These were polymorphisms in the capA gene, which encodes an enzyme involved in capsule biosynthesis, which is responsible for host immunity protection and acts as a virulence factor during sepsis. Notably, this gene is absent in S. aureus clones in North America (Boyle-Vavra et al., 2015), further highlighting the absence of a consistent set of virulence factors and the overall versatility of this pathogen. Another virulence factor of interest is cytotoxin Panton–Valentine Leukocidin (PVL), which is highly prevalent in community-acquired methicillin-resistant S. aureus (MRSA) and has been associated with higher risk of developing sepsis (Ahmad et al., 2020; Monecke et al., 2020). A study conducted in China comparing MRSA strains isolated from adult and pediatric patients reported a higher prevalence of PVL in adult MRSA isolates (55.8 vs. 35.3%), in addition to higher antibiotic resistance and higher mortality in adults (Zhao et al., 2022; Table 1). Clone ST5/ST764-MRSA SCCmec II was the predominant isolate in adults, whereas clone ST59-MRSA SCCmec IV was the predominant isolate in pediatrics (Zhao et al., 2022). Also regarding PVL, another study comparing adult and pediatric isolates from patients admitted to the ICU in New Caledonia Island (Southwest Pacific region) reported a significantly higher prevalence of PVL+ MRSA strains in adults (61 vs. 30%), which carried an implicit higher risk for developing sepsis and a fatal outcome (OR = 4.57; Imauven et al., 2022).
Added to the array of virulence factors of S. aureus is toxic shock syndrome toxin-1 (TSST-1), which relates to the rapid development of symptoms consistent with sepsis, including hypotension and organ dysfunction. Although there is an insufficient number of large-scale studies on TSST-1 molecular epidemiology, recent data from the Democratic Republic of the Congo identified TSST-1 in 67% of MRSA and 4% of MSSA blood isolates (Vandendriessche et al., 2017), while data from China reports TSST-1 in 23.1% of MRSA and 6.7% of MSSA blood isolates (Liu et al., 2018). For their joint virulence and its inherent potential to cause a fatal outcome (Hayakawa et al., 2020), despite representing only 0.4% of all MRSA isolates in a whole-genome analysis carried out in Japan (Kaneko et al., 2023) and 0% of all isolates in a worldwide comparative genomic analysis (Zhou et al., 2021), systematic monitoring of PVL+TSST-1+ isolates becomes a relevant facet of S. aureus virulence to survey. A summary of the factors detailed in this section is listed in Table 2.
4.3. Host factors
Host immunity plays a pivotal role in clearing S. aureus presence in the bloodstream. Notably, neutrophils isolated from patients undergoing S. aureus sepsis exhibit increased formation and release of neutrophil extracellular traps (NET) and pro-inflammatory cytokines TNF-α, IL-1β, and IL-8 (Gupta et al., 2022). Moreover, neutrophil and lymphocyte abundance as a ratio has been demonstrated as an independent predictor for 90-day mortality. Such simple but relatively costly measurement shows potential as a prognostic tool, which if supplemented with appropriate sensitivity and specificity information, may prove useful in orienting the management of septic patients (Greenberg et al., 2018). Likewise, serum levels of C5a and IL-10 were found significantly higher in samples from S. aureus bacteremic patients when compared to matched hospitalized and community controls (Eichenberger et al., 2020). Also, in the quest for prognostic predictors during S. aureus bacteremia, activity of caspase-1 in neutrophils and monocytes, serum levels of IL-18, and whole blood mRNA levels of inflammasome mediator NOD-like receptor family pyrin domain-containing 3 (NLRP3), were found to display a significant difference between survivors and non-survivors (Rasmussen et al., 2019). Bioinformatic screening of blood samples of S. aureus bacteremic patients further reveals direct correlation between the expression of a set of five genes (COX7C, NDUFA4, ATP5J, NDUFB3, and COX7A2; relating to aerobic respiration, cellular stress response, mitochondrial electron transport, mitochondrial transport, and oxidative phosphorylation) and adverse outcome (Wu H. et al., 2021). Although further testing of the prognostic value of such analysis is required, the high-throughput approach is noteworthy and the potential of a multiplex approach to follow the development of sepsis is promising.
Similarly, meta-analysis of existing databases further corroborates the plausibility for bioinformatics approaches to aid in tackling sepsis from a molecular flank. Analysis of post-translational modifications to mRNA (in particular, methylation of the sixth N atom on the adenine base, m6A) integrated into single nucleotide polymorphisms (SNP) revealed a significant presence of a set of m6A-SNPs during S. aureus sepsis (Sun et al., 2020; Table 1). Such set was characterized and associated to DNA repair, vesicle-mediated transport, peptidyl-serine phosphorylation, leukocyte migration, catabolic processes, regulation of endopeptidase activities, phagocytosis, and platelet degranulation. Although no further clinical characterization was reported, the value in diagnostics, prognosis, and therapeutics is yet to be explored.
Host defense against S. aureus is not limited to leukocytes, as recently demonstrated in a translational report (Sun et al., 2021). Prompted by an observation of the strong association between thrombocytopenia (without leukopenia or leukocytosis) and patient mortality, authors used an in vivo model to demonstrate that the underlying mechanism of platelet-mediated antibacterial activity is impaired by S. aureus pore-forming α-toxin (Hla). Interaction of α-toxin with platelet chemoreceptor P2Y12 was inhibited by using FDA-approved and commercially available antiplatelet drug ticagrelor, resulting in extended protection from thrombocytopenia, enhanced bacterial clearing, protection from organ damage, and increased survival. Thus, in a single report platelet contribution to host immunity and the repurpose of an available drug to protect against S. aureus bacteremia was elegantly demonstrated. Not surprisingly, ulterior reports have extended these findings by corroborating the protective effect of ticagrelor against S. aureus bacteremia. In a nationwide observational cohort study carried out in Denmark, ticagrelor use was demonstrated protective not only against S. aureus bacteremia but also, in a higher proportion, against sepsis and pneumonia (Butt et al., 2020). Further evidence on ticagrelor repurposed use is described in a recent case report detailing a steep correction in platelet count and complete recovery of a patient afflicted with methicillin-sensitive S. aureus (MSSA) bacteremia treated with ticagrelor for 3 months (Ulloa et al., 2021). A summary of the factors detailed in this section is listed in Table 3.
4.4. Treatment
Empiric treatment for S. aureus is initiated pending susceptibility tests and is prophylactically directed against MRSA with the use of vancomycin or daptomycin (Liu et al., 2011). Evidence has been presented in favor of the use of initial doses of vancomycin ≥20 mg/kg for a faster resolution of systemic inflammation (Wesolek et al., 2018). Once susceptibility is elucidated, if isolate is MSSA, antibiotic treatment is de-escalated to a β-lactam agent. Use of combination therapy is controversial, with weak evidence found in a recent clinical trial (Pujol et al., 2020) and several cohort studies (Rieg et al., 2017; Davis et al., 2018; Guthridge et al., 2021; Kufel et al., 2023). Appraisal of the therapeutic value of monotherapy was presented in a recent clinical trial carried out in South Korea, in which treatment of MSSA bacteremia with nafcillin was associated with higher Sequential Organ Failure Assessment (SOFA) scores, higher rates of treatment failure, and astoundingly higher mortality figures than treatment with cefazolin (Lee et al., 2018). In fact, odds ratios for treatment with cefazolin over nafcillin were 0.39 for SOFA score ≥ 2, 0.43 for treatment failure, and 0.15 for 90-day mortality. Overall protective effect of treatment of MSSA bacteremia using cefazolin was quantified with an adjusted OR = 0.44. Interestingly, matched propensity scores resulted significant only for the bacteremic group and not for the septic group, highlighting the higher level of complexity in the pathobiology of sepsis. A relevant preoccupation is the increasing resistance to methicillin, where median percentage resistance increased from 16.6% in 2017 to 18.3% in 2020, according to a recent global report (World Health Organization (WHO), 2022a). Other reports on antimicrobial resistance related to sepsis describe S. aureus methicillin resistance to be 17.6% in Australia and 15.5% in Europe (Coombs et al., 2022). Management of MRSA by means of either monotherapy or combination therapy of vancomycin or daptomycin, with or without a β-lactam agent, shows no significant differences in 30-day mortality risk, length of stay, or risk of persistence, as evidenced by a recent meta-analysis (Yi et al., 2021). A fact to be assessed in terms of harms versus benefits when sizing therapeutical value and antibiotic resistance.
5. Pseudomonas aeruginosa
Pseudomonas aeruginosa is an aerobic Gram-negative bacterium distributed ubiquitously in the environment. P. aeruginosa infections are frequently nosocomial and opportunistic, as they frequently occur concomitantly to existing physical, phagocytic, or immunologic dysfunctions in host defense, leading to bloodstream, urinary tract, respiratory, and intra-abdominal infection (Tang et al., 2017; Li X. et al., 2023).
5.1. Epidemiology
Recent reports place P. aeruginosa as the fourth most common cause of BSI globally (Diekema et al., 2019) and the third most frequent pathogen isolated in catheter-associated urinary tract infection and ventilator-associated pneumonia (Weiner-Lastinger et al., 2020). This is relatively consistent throughout the world, except in North America, where P. aeruginosa BSI ranks fifth (Diekema et al., 2019). Despite its relatively low proportion in BSI incidence, a recent study analyzing isolates from the United States reports P. aeruginosa as the second most frequent pathogen causing BSI mortality, accounting for nearly a quarter of the cases between 2016 and 2020 (Ohnuma et al., 2023).
5.2. Pathogen factors
Although pathogenesis of P. aeruginosa has been extensively studied in models of pneumonia, burn wounds and urinary infection, few studies have explored virulence factors expressing in sepsis-inducing strains. A recent transcriptomic analysis of clinical isolates associated to BSI revealed high-level expression of a novel cell-surface signaling system named Hxu (a response system for sensing extracellular porphyrin rings of haem; Otero-Asman et al., 2019), whereas deletion or overexpression of this pathway resulted in reduced or enhanced BSI, respectively (Yang F. et al., 2022). Several genes related to P. aeruginosa metabolism have been associated with the level of virulence of the strain PAO1, extensively used in sepsis models. Deletion of the regulator of α-ketoglutarate transport mifR resulted in a significant improvement of survival following pneumonia-induced sepsis in a murine model as well as a reduction in pro-inflammatory cytokines and reduced NLRP3 inflammasome activation (Xiong et al., 2022). In addition, expression of enzymes able to degrade host extracellular matrix components such as heparinase contribute to the virulence of sepsis-inducing strain P. aeruginosa PA14. Mutation of the gene encoding for heparinase (hepP) impaired bacterial dissemination and prevented mortality in murine models of thermal injury and intraperitoneal PA14 injection, indicating that hepP contributes to the pathogenesis of PA14 (Dzvova et al., 2018). A summary of the factors detailed in this section is listed in Table 2.
5.3. Host factors
Recent studies indicate that susceptibility to develop systemic infection is associated with various predisposing host factors. In fact, immune compromised hosts exhibit an adjusted odds ratio of 3.7 for BSI in a multivariate logistic regression model considering age, sex, ethnicity, chronic comorbidities, source of infection, recent ambulatory procedures, urinary catheterization, residence in skilled nursing facilities, chronic hemodialysis, current and recent hospitalization, and prior exposure to β-lactams and fluoroquinolones in the past 90 days (Hammer et al., 2017). Moreover, the latter odds ratio has been reported as high as 13.82 for BSI and 23.1 for 30-day mortality, also in multivariate analyses (Tan et al., 2021). Interestingly, according to a 2019 report on community-onset P. aeruginosa urinary infection in elderly people, odds ratio for sepsis were highest for patients who received healthcare (OR = 5.52), who had an indwelling urinary catheter (OR = 3.25), and who were male (OR = 3.16), as determined in a stepwise logistic regression (Esparcia et al., 2019). An interesting instance of host susceptibility is presented by the growing number of cases reported on P. aeruginosa sepsis in pediatric hosts with X-linked agammaglobulinemia (XLA, OMIM #300755; Bhardwaj et al., 2017; Biscaye et al., 2017; Birlutiu et al., 2019; Huang H. et al., 2020), an immunodeficiency characterized by failure to produce mature B lymphocytes resulting in clinically undetectable levels of all immunoglobulin isotypes. Pediatric patients with XLA rapidly develop sepsis after P. aeruginosa infection and, while specific pathogen identification delays targeted therapy, the appearance of landmark skin lesions (ecthyma gangrenosum) represent an informative sign of the underlying causative agent (P. aeruginosa).
An additional useful example that warrants attention is the protective effect observed for hemoglobin levels in P. aeruginosa pediatric sepsis as an outcome, with an astounding OR = 0.155 obtained after multivariate logistic regression analyses in a matched case–control study conducted in Taiwan (Kung et al., 2020). Extensive research has characterized the immune responses at the lung and skin in mouse models of acute P. aeruginosa infection but the role of both innate and adaptive immune cells during P. aeruginosa sepsis is still poorly understood. Bacterial dissemination and sepsis are usually accompanied by increased numbers of phagocytes, but in a model of burn wound infection, neutrophil and monocyte recruitment to the seroma fail to contain P. aeruginosa dissemination, promoting the development of sepsis (Brammer et al., 2021). However, modulation of these cells by host-derived molecules may prevent severe sepsis and mortality. Endogenous hydrogen sulfide (H2S) produced by cystathionine-γ-lyase enhances neutrophil recruitment and their phagocytic activity resulting in reduced mortality in a mouse model of sepsis induced by a multidrug-resistant strain. In addition, H2S reduces the expression of P. aeruginosa quorum sensing genes, favoring pathogen phagocytosis. Moreover, clinical correlates show that patients who survived sepsis had higher levels of circulating H2S compared to non-survivors, strongly suggesting that H2S plays a protective role during P. aeruginosa sepsis (Renieris et al., 2021).
Host immunosuppressive mechanisms may also play a detrimental role during P. aeruginosa sepsis. Animal models of secondary bacteremia following cecal punction ligation exhibit improved survival after a partial deletion of Tregs and a reduction in anti-inflammatory cytokine IL-10 (Hu et al., 2018). In line with this, treatment with the immunoregulatory molecule ethyl pyruvate reduced lung levels of IL-10 and the expression of FOXP3 in lung-derived Tregs in a two-hit model of sepsis, reversing P. aeruginosa secondary pneumonia (Chen et al., 2017). IL-10 deficient mice are more susceptible to PA14-induced pneumonia but do not display bacteremia (Belo et al., 2021), suggesting that IL-10 helps to control local antimicrobial responses, but high lung levels may favor bacterial dissemination. Therefore, the role of IL-10 and other anti-inflammatory host-derived molecules during P. aeruginosa sepsis is poorly understood and requires further research to confirm its role increasing host susceptibility to severe sepsis and mortality. P. aeruginosa not only interacts and promotes colonization and coinfection with S. aureus (Clancy et al., 2014) and Stenotrophomonas maltophilia (McDaniel et al., 2020) but is also a common cause of secondary bacterial infections in patients hospitalized for COVID-19 (Lansbury et al., 2020; Garcia-Vidal et al., 2021), a fact exemplifying its opportunistic nature. P. aeruginosa is associated with high in-hospital mortality rates and prolonged lengths of stay (Naylor et al., 2018). A summary of the factors detailed in this section is listed in Table 3.
5.4. Treatment
Clinically, antimicrobial treatment proceeds observing four arms: controlling the source (i.e., infected catheters or ventilator reservoirs), timely initiation of therapy, use of mono/combination therapy, and limiting antibiotic resistance. Extensive and uncontrolled use of antibiotics has contributed to the increase in multi-drug resistant (MDR) and extensively-drug resistant (XDR) strains, which are difficult to treat, especially in cases of severe sepsis. In fact, P. aeruginosa is the fourth leading pathogen behind all deaths attributable to antibiotic resistance in high-income countries (Murray et al., 2022). Genetic characterization of P. aeruginosa bacteremia at advanced ages indicates a significant presence and association with fatal outcome of resistance genes blaGES, aadB, gyrA (T83I), parC (S87L), virulence gene exoS, multilocus sequence typing (MLST) ST235, O-antigen serotype O11 (Recio et al., 2021), strong biofilm producing (di Domenico et al., 2021), and non-motile strains (Gupte et al., 2021). Interestingly, recent prospective study indicates that carbapenem resistance is not significantly related to treatment failure, a fact likely related to the site of infection, host susceptibility, and clinical severity (Lee C. M. et al., 2022). Recent studies in patients have shown inconclusive about the benefit of the combination of antibiotics such as ceftazidime-avibactam against MDR and XDR strains (Corbella et al., 2022). Although ceftolozane/tazobactam is effective against complicated urinary tract infections and complicated intra-abdominal infections, univariate analyses reveal that treatment using this therapeutical combination is significantly associated with a successful management of the clinical presentation of sepsis (Bassetti et al., 2019). Additionally, early use of this combination in neutropenic patients has been shown to control progression of skin infection, suggesting a protective effect on further dissemination with or without sepsis (Coppola et al., 2020).
By translation, a recent in vivo study explored the efficacy of different antibiotics in mouse models of sepsis caused by P. aeruginosa. Although carbapenem combinations did not show improved efficacy against carbapenemase-producing P. aeruginosa, meropenem monotherapy showed promising in vivo efficacy against peritoneal sepsis (Herrera-Espejo et al., 2022). Interesting evidence from in vivo models reveals that peptidylarginine deiminase (PAD) type 2 deficiency (Pad2−/−) significantly improves survival in P. aeruginosa pneumonia-induced sepsis by attenuating acute lung injury (Wu Z. et al., 2020). Under the proposed mechanism, PAD2 deficiency enhances bacterial clearance by reducing caspase-1-dependent pyroptosis in bone marrow-derived macrophages. Thus, PAD2 is revealed as a promising molecular target that warrants further research.
Other experimental therapies have been tested alone or in combination with antibiotics in murine models and clinical assays. Because P. aeruginosa binds to host epithelial cells via pili and type-IV pili (T4P) are crucial for bacterial twitching, attachment, biofilm formation, and motility (particularly T4P containing PilA subunits; Zahedi bialvaei et al., 2021a), mAb against disulfide turn region of PilA (QA) and PilQ, alongside with clinically available antibiotics levofloxacin, ceftazidime, and gentamicin, showed a synergistic effect in the treatment of a mouse model of P. aeruginosa sepsis by preventing bacterial dissemination and increasing overall survival (Zahedi bialvaei et al., 2021b). Similarly, a case describing the use of a combination of antibiotics and a cocktail of two phages (PNM and 14–1) to attend an infection with an XDR strain secondary to liver transplantation in a septic toddler was deemed successful after confirming full clearance of P. aeruginosa from the bloodstream (van Nieuwenhuyse et al., 2022). Moreover, in vitro studies using the phage PNM have shown a synergistic effect in combination with suboptimal concentrations of colistin, aztreonam, or gentamycin against clinical isolates of P. aeruginosa (van Nieuwenhuyse et al., 2022).
6. Streptococcus pyogenes
Streptococcus pyogenes is a facultative anaerobe Gram-positive bacterium that strictly infects humans (Gera and McIver, 2013). Streptococcus pyogenes infections are highly contagious and range from pharyngitis, and skin or soft tissue (non-necrotizing) infection, to respiratory tract infection, pregnancy-associated infection, necrotizing fasciitis, and bacteremia with toxic shock syndrome (TSS; Walker et al., 2014).
6.1. Epidemiology
Recent analyses report an increasing incidence of S. pyogenes infections with a high case fatality in the older population (Shakoor et al., 2017; Meehan et al., 2018; Blagden et al., 2020; Vilhonen et al., 2020; Bläckberg et al., 2022; Thomson et al., 2022). The most prevalent form of clinical presentation of invasive S. pyogenes infection is bacteremia (75%), followed by focus without bacteremia (19%) and necrotizing fasciitis (7%; Meehan et al., 2018). Moreover, TSS is reported in 19% of bacteremia cases with an uneven distribution between adults (22.5%) and pediatrics (7.1%; Meehan et al., 2018), the latter figure reported higher in the United States (16.9%; Gaensbauer et al., 2018). Nevertheless, S. pyogenes bacteremia is significantly less represented (<8%) when compared to other pathogens (Ferreira et al., 2023; Tabah et al., 2023).
6.2. Pathogen factors
Until recently, the best described virulence factor of invasive S. pyogenes infection is the M protein, encoded by the emm gene and expressed on the bacterial surface. A recent Spanish report on emm diversity in pediatrics revealed clone emm1/ST28 as the most prevalent and the most consistently detected over the 12 years span of the report, followed by clones emm12/ST36-ST242 and emm6/ST382 (Sánchez-Encinales et al., 2019). This trend was echoed by findings of a retrospective cohort study in Sweden, which included 286 samples from adult patients collected in a 4-year period (Bläckberg et al., 2022) and a prospective German nationwide cohort study, which included 719 isolates from patients of all ages collected in a 6-year period (Imöhl et al., 2017). A different pattern was observed in Finland where, in a recent cohort study spanning 12 years and focusing on women of childbearing age, emm28 showed as the most frequent type of S. pyogenes strain, displaying a significant association with delivery and puerperium-related infections leading to bacteremia (Gröndahl-Yli-Hannuksela et al., 2021). Other proposed virulence factors arising during invasive S. pyogenes disease include streptococcal superantigens speG, speH, speJ, and speK, which, in the presence of certain underlying comorbidities (i.e., diabetes, chronic skin lesions, liver dysfunction, and respiratory distress), modulate the risk of invasive S. pyogenes disease (Imöhl et al., 2017).
Evidence from in vivo studies highlight the relevance of non-canonical Tyr-phosphatase M5005_Spy_1476 as a molecular mediator for S. pyogenes pathogenesis, an enzyme reported to maintain this pathogen in a virulent state leading to increased subject mortality by modulating its ability for adherence and invasion of host cells, and for in vitro biofilm formation in a mouse model of sepsis (Kant and Pancholi, 2021). Similar evidence has been reported for predicted gene spy1343, which codes for an inferred 298-amino acid protein that belongs to the LysR family of DNA-binding transcriptional regulators. Interestingly, mice challenged with a mutant strain carrying a deletion of spy1343 exhibited a significant increase in mortality when compared to their control (WT) counterparts (Sitkiewicz and Musser, 2017). Explanation for this excess of virulence lies in the control of spy1343 over genes that participate in short-chain fatty acid metabolism, which have been linked to overall bacterial pathogenesis and overall virulence. Additional evidence from in vivo studies reveals that S. pyogenes virulence may be traced from a clinical standpoint by evaluating the pattern of leukocyte and platelet abundance. Multidimensional scaling of median values of absolute counts of such type of cells was able to discriminate clusters of virulence and disease progression over time with precision, using simple, routinary readouts in experimental infection using a small animal model. Thus, underscoring the potential and unprecedented diagnostic/prognostic value of computer science in human infection progression, particularly in sepsis (Loof et al., 2018; Table 1). A summary of the factors detailed in this section is listed in Table 2.
6.3. Host factors
Host immune responses during S. pyogenes sepsis has received modest attention in both in vivo and in vitro models. It has been proposed that vascular endothelial growth factor (VEGF) expressed in endothelial cells may promote antimicrobial response against S. pyogenes infection, as observed in vitro with human endothelial cells increasing lysosomal biogenesis and function and overall bacterial xenophagy against S. pyogenes through the activation of TFEB and its downstream genes (e.g., ATPV6 and LAMP1), and in vivo with VEGF treatment significantly increasing subject survival rate (Lu et al., 2022). Moreover, patients with severe invasive disease (sepsis, bacteremia, necrotizing fasciitis, and TSS) exhibit significantly lower serum levels of VEGF compared to those with non-invasive disease (Lu et al., 2022). Also relating to host immune response, TLR signaling plays an important role in early immune reactions against S. pyogenes. Nucleic acid detection by the endosomal receptor TLR13 mediates immune cell activation, expression of pro-inflammatory cytokines, and formation of ROS and reactive nitrogen species (RNS) against S. pyogenes in vitro. Mice defective in endosomal signaling (Unc93b1−/−) exhibited higher bacterial burden at sites of lesion and the spleen, and significantly increased systemic inflammation in a murine model of soft tissue infection. Therefore, endosomal TLR signaling may play an important role in activating antimicrobial responses at local infection sites and prevent systemic disease (Hafner et al., 2019). Several studies have reported a synergistic interaction for infection between S. pyogenes and pathogenic viruses of the respiratory tract (Brundage, 2006; Herrera et al., 2016), a fact made evident during the 1918 influenza pandemic (Morens and Fauci, 2007), the 2009 H1N1 influenza pandemic (Jean et al., 2010), and more recently, the 2019 SARS-CoV-2 pandemic (Khaddour et al., 2020). However, data on S. pyogenes superinfections are scarce and interactions with additional infective agents warrant further resource allocation and research (Turner, 2022). A summary of the factors detailed in this section is listed in Table 3.
6.4. Treatment
Empiric antimicrobial therapy typically initiates pending culture results to then be tailored accordingly. Penicillin monotherapy in the setting of high inoculum has been associated with treatment failure (Stevens et al., 1994). For this reason, adjunctive use of clindamycin is recommended for its strong association with lower mortality (OR = 0.44; Babiker et al., 2021). An increasing number of isolates with resistance to clindamycin and other macrolides have been consistently identified around the globe [e.g., the United States (DeMuri et al., 2017), Hungary (Gajdács et al., 2020), India (Jayakumar et al., 2022), and China (Lu et al., 2017)] with the surprising exception of Spain, where tetracycline, erythromycin, and clindamycin resistance rates declined between 2007 and 2020 (Villalón et al., 2023).
Owing to its incapacity for horizontal gene transfer, S. pyogenes resistance to β-lactam antibiotics is a feature considered rare (Hayes et al., 2020). However, a report by the Active Bacterial Core surveillance (US Centers for Disease Control and Prevention, CDC) has recently made the alarming discovery of a S. pyogenes strain (emm43.4/PBP2x-T553K) with increased β-lactam resistance (Chochua et al., 2022; Table 1). Similarly, a recent report from active surveillance in Israel described the emergence of an outbreak of a new MDR strain emm93.0 responsible for an unusually large number of invasive S. pyogenes infections, especially present in the bloodstream (Ron et al., 2022). These unprecedented findings underline the importance of population-based pathogen surveillance programs internationally. Nonetheless, there is consensus that S. pyogenes infections—even when invasive—are associated with a low attributable mortality, unless they are invasive and meet the criteria for TSS, for which cases the mortality rate can reach up to 44% (Schmitz et al., 2018).
7. Candida species sepsis
Invasive fungal infections are clinical manifestations of fungal infections different from superficial infections proven by culture and isolation from sterile sites such as deep tissue, cerebrospinal fluid, or blood (Donnelly et al., 2019).
7.1. Epidemiology
Candida species are the predominant cause of life-threatening invasive fungal infections in hosts with decreased defenses (e.g., immunocompromised individuals, patients who have endured invasive clinical procedures, or have experienced major trauma; Bongomin et al., 2017) and constitute the most common cause of fungal BSI (Pappas et al., 2016), a clinical condition that has experienced a significant and concerning increase over the last decade, as a retrospective cohort study comprising 465 candidemia episodes in Germany (Mohr et al., 2020) and a retrospective cohort study comprising 170 candidemia episodes in Switzerland (Battistolo et al., 2021) report. Although the latest report by the SENTRY Antifungal Surveillance Program carefully details different forms of invasive fungal infection, it does not present isolated data for fungal BSI (Pfaller et al., 2019). Nevertheless, recent observational data ratify Candida albicans as the leading species during fungal BSI and further expand the list with other non-albicans species [in hierarchical order: Candida parapsilosis, Candida glabrata, and Candida tropicalis (Doğan et al., 2020); Candida tropicalis, Candida parapsilosis, and Candida glabrata (Al-Musawi et al., 2021)]. However, in one study from Saudi Arabia (Aldardeer et al., 2020) and another from India (Lamba et al., 2021), Candida albicans was relegated to the second place, being Candida glabrata and Candida tropicalis the leading species for fungal BSI, respectively.
7.2. Pathogen factors
Candida species grow in yeast or filamentous (pseudohyphae and hyphae) morphologies and this morphology strongly correlates to its pathogenicity, revealing fungal programs of invasion, virulence, and overall versatility and capacity for adaptation (Lo et al., 1997; Bartie et al., 2004). For a formidable review on pathogen virulence factors and the immune response during Candida sepsis we strongly recommend consulting article by Patricio et al. (2019). One such factor is (1,3)-β-D-Glucan (BDG), a major structural component of the inner cell wall in Candida species, which not only serves as the main pathogen-associated molecular pattern that interacts with pattern recognition receptors on the host side but has also been implicated a prognostic value. Although without accuracy or performance test for comparison with other diagnostic methods, BDG− candidemia has been recently identified as an independent protective factor from poor clinical outcome (Agnelli et al., 2019).
7.3. Host factors
Correspondingly, timely removal of central venous catheter has also been identified as an independent protective factor against mortality (Kutlu et al., 2022). Incidentally, delayed removal of central venous catheter during candidemia has been consistently identified as a risk factor for mortality in adults (Huang H. Y. et al., 2020; Lee et al., 2020), pediatrics (Lee W. J. et al., 2022), and neonates (Chen Y. N. et al., 2022). Other risk factors for adult mortality with great representation across quantitative multivariate reports are concomitant bacteremia (Lee et al., 2020; Pieralli et al., 2021; Zhong et al., 2022; Gebremicael et al., 2023), high SOFA score (Bienvenu et al., 2020; Huang H. Y. et al., 2020; Jung et al., 2020; Kutlu et al., 2022), presence of liver cirrhosis (González-Lara et al., 2017; Bartoletti et al., 2021; Battistolo et al., 2021; Meyahnwi et al., 2022), kidney dysfunction (Poissy et al., 2020; Mazzanti et al., 2021; Kutlu et al., 2022), and others summarized in Table 3 (Bassetti et al., 2020; Yoo et al., 2020; Kim et al., 2021). Particularly for early-age groups, additional risk factors for mortality include breakthrough candidemia (Lee W. J. et al., 2022), previous use of antibiotics for >2 weeks, persistent candidemia, and preterm gestation (<32 weeks; Eisi et al., 2022), placing the emphasis in the implementation and follow-up of protocols to properly manage neonatal care.
Chronic comorbidities appraised as compounded scores (i.e., Karnofsky Performance Status <70 and Charlson Comorbidity Index ≥4) were also found to increase the risk of mortality during candidemia (Bassetti et al., 2020; Yoo et al., 2020; Kim et al., 2021; Vázquez-Olvera et al., 2023). Mixed Candida/bacterial BSI is referenced to occur in 18–56% of candidemia cases (Bouza et al., 2013; Kim et al., 2013; Chen et al., 2020) and recent articles agree [20.5% of candidemias reported in (Zhong et al., 2020) and 29.7% of candidemias reported in (Lee E. H. et al., 2022)]. The most frequent bacteria isolated from the bloodstream were coagulase-negative Staphylococcus, followed by Klebsiella pneumoniae, and Staphylococcus aureus. As expected, mixed BSI poses an even more complex challenge to host responses and, although a significant increase in mortality would be expected, the only significant increments were seen in length of ICU stay and length of mechanical ventilation use (Zhong et al., 2020). Conversely, Candida BSI following admission and treatment for COVID-19 has been found to significantly shift the outcome of patients toward fatality, along increasing the utilization of mechanical ventilation, the need for central venous catheter and parenteral nutrition, and overall length of stay (Rajni et al., 2021; Kayaaslan et al., 2023). Interestingly, admission and treatment for COVID-19 was found to double the incidence rate of developing candidemia, as a retrospective cohort study report (Kayaaslan et al., 2021). Even more interestingly, candidemia was found in a significant portion of patients who underwent surgery during their treatment for COVID-19 and those with a history of corticosteroid use. In fact, prior corticosteroid use was identified as a significant risk factor for mortality with a strength of association (OR = 4.4) comparable to advanced age (≥65 years old, OR = 5.6) and presence of sepsis (OR = 7.6), as demonstrated by multivariate analyses. The detrimental effect of prior corticosteroid use is a novel finding that requires further research for its potential value as a prognostic tool at COVID-19 admission. Other predictors of mortality for COVID-19 patients with candidemia identified through multivariate analyses include increased length of stay, high levels of D-dimer, use of tocilizumab (Rajni et al., 2021), ECMO support (Alessandri et al., 2023), high SOFA score (Omrani et al., 2021), and presence of a central venous catheter (OR = 19.07; Kayaaslan et al., 2023).
Several approaches for prediction and prognosis have proposed different combinations of readouts to match and even surpass current prognostic tools. Of note, accordingly to the era of data science, a machine learning-based algorithm using a myriad of clinical variables as input was able to predict candidemia with an AUC of 87.4% (Ripoli et al., 2020), an extraordinary magnitude that outperforms not only classical stepwise multivariable logistic regressions performed in the same report but also other independent studies proposing different arrangement of variables, i.e., the delta neutrophil index (with an AUC of 80.4%; Park et al., 2020) and a multivariate conditional regression-based risk score (with an AUC of 76.8%; Poissy et al., 2020). Mortality has also been appraised as an outcome, with a composite score (consisting of a combination of the SOFA score and the Charlson Comorbidity Index) reported to outperform (AUC of 79%) the independent performance of its components (AUC of 77% for SOFA score, AUC of 69.7% for Charlson Comorbidity Index; Asai et al., 2021; Table 1). A summary of the factors detailed in this section is listed in Table 3.
7.4. Treatment
Treatment of Candida spp. involves prompt initiation of antifungal therapy with echinocandins, azoles, and amphotericin B formulations (Pappas et al., 2016). The echinocandin group includes caspofungin, anidulafungin, and micafungin, all of which are noncompetitive inhibitors of the production of BDG (Denning, 2003). Given their broad-spectrum efficacy against Candida spp., echinocandins are frequently used to treat candidemia and invasive candidiasis (Pappas et al., 2016). In fact, initial antifungal therapy with fluconazole has been found an independent predictor of mortality elevating the risk death by roughly 200% in a cohort study in Italy (Pieralli et al., 2021). However, corroboration of this effect was inconclusive in a subsequent independent cohort study conducted in France, which compared echinocandins and azoles as first-line antifungal therapy without reaching significance in multivariate analyses (Bienvenu et al., 2020). Aside from Candida auris, antifungal resistance is systematically led by non-albicans species in China (Zhang et al., 2020; Liu et al., 2021), South Korea (Kwon et al., 2021), Turkey (Guner Ozenen et al., 2023), Italy (Mazzanti et al., 2021), Thailand (Ngamchokwathana et al., 2021), and Saudi Arabia (Al-Dorzi et al., 2018), out of which, C. tropicalis represents the major contributor. Data on resistance/susceptibility would appear to draw a trend of inverse sensitivity between compounds of the echinocandin group and fluconazole, with C. albicans displaying higher figures of resistance to echinocandin compounds than non-albicans species and lower figures of resistance to azole compounds than non-albicans species (Al-Dorzi et al., 2018; Jung et al., 2020; Guner Ozenen et al., 2023). Nevertheless, it has been reported that empirical administration of high-dose liposomal amphotericin B (L-AmB) is associated with better management of fungal invasiveness, less ICU-acquired candidemia, less need for an antifungal agent additional to L-AmB, and ultimately a reduction in ICU mortality, emphasizing the feasibility and relative safety of a preemptive antifungal therapy strategy to combat bloodstream Candida colonization (Azoulay et al., 2017). Regardless of the advancements detailed insofar, this review emphasizes the knowledge gap in molecular factors of resistance for Candida species, particularly when compared to the bacterial agents of sepsis reviewed above.
7.5. Candida auris sepsis
Candia auris is a human pathogenic yeast first isolated in Japan in 2009 (Satoh et al., 2009) that has gained notoriety for its high potential for invasive BSI, high mortality rates, moderate preventability, difficult identification by conventional techniques, and its virtually complete resistance to azoles (Geremia et al., 2023). Prompted by the escalating number of countries worldwide reporting the detection of C. auris (Briano et al., 2022; Riera et al., 2022; Figure 1), along with similar concerns for other neglected non-bloodstream fungal infections, the WHO has issued its first global effort to systematically prioritize fungal pathogens, in which C. auris ranks as the second most threating fungus to human health [World Health Organization (WHO), 2022b]. For an impeccable and updated review on C. auris virulence factors, risk factors, and antifungal resistance, please consult review by Geremia et al. (2023). Notwithstanding the growing number of studies describing its many facets, in pragmatic terms what concerns about C. auris sepsis is its often misclassification [as C. famata, C. haemulonii, or Rhodotorula glutinis (Kathuria et al., 2015)] and its pattern of multidrug resistance (Gómez-Gaviria et al., 2023).
Because host response to sepsis is inextricably canonical and C. auris virulence is considered intrinsically low (Geremia et al., 2023), the strong points for C. auris clinical management are then relegated to source control (Hinrichs et al., 2022) and drug delivery. With environmental source control (Akinbobola et al., 2023) falling partially outside of the scope of clinical management and efforts for nosocomial containment being not dissimilar to other fungal agents, a cardinal condition for source control is then the ability for detection. If the resources for accurate and routinely detection are limited, then emphasis is shifted toward drug delivery. With resistance to current drugs on the rise (Shastri et al., 2020; Briano et al., 2022), measurement of clinical management outcomes is then shifted from hospital discharge toward mortality. With information on specific C. auris-case fatality rates being scarce (Geremia et al., 2023), the extent of mortality then falls under the domain of speculation. Thus, we reckon that increasing access to detection and encouraging the appraisal of adverse outcomes are crucial milestones to tackle C. auris, particularly in locations where resources and logistics for diagnosis are limited, and consequently supporting the measures taken by the WHO [World Health Organization (WHO), 2022b], which stem from the precautionary principle (Goldstein, 2001). Although considerable steps have been taken very recently toward elucidating its pathobiology using mouse models (Wurster et al., 2022) and improving detection by employing advanced mathematical models (Garcia-Bustos et al., 2020), the knowledge gap about this emerging pathogen is systematic and efforts to contain it require a multidisciplinary approach.
8. Conclusion
Sepsis represents a topic with a high level of complexity that extends beyond patient management and clinical efforts for containment. In this multidisciplinary inter-collaborative scenario, curation of selected evidence is paramount to guide transversal action. We recognize the inherent limitations of in vitro studies, the controversy surrounding the verisimilitude of administering LPS (Osuchowski et al., 2018) or using cecal ligation and puncture (CLP) (Deutschman et al., 2022) as animal models of sepsis, as well as the impossibility of calculating factual figures of incidence and mortality worldwide (Rudd et al., 2020). Nevertheless, we value them as steps toward an integrated effort to tackle complexity straightforward and comprehensively. Thus, a list of novel approaches and potential therapeutical targets that should be considered for further research is presented in Table 4.
Transversal diversity of microorganism presentation in sepsis, from coinfection with multiple species to coexistence of multiple phylogroups, inextricably limits research conducted under the single-microorganism/single-strain approach right from its inception, for its reductionist nature. Notwithstanding the value in this frequent practice, this review makes clear that results emanating from integrative studies not only have the upper hand for describing reality but also are one step closer to clinical translation. An example of this is a very recent report identifying patterns of sepsis progression for most of the microorganisms described in this review using state-of-the-art genomics, transcriptomics, proteomics, and metabolomics, to make phylogenetic-oriented descriptions (Mu et al., 2023).
Indubitably, multidisciplinary advancement in basic, translational, and clinical research, is key to make progress filling the gap acknowledged by the World Health Organization (WHO) (2017) and endured yearly by millions around the globe.
Author contributions
All authors listed have made a substantial, direct, and intellectual contribution to the work, and have approved it for publication.
Funding
This study was funded by Agencia Nacional de Investigación y Desarrollo de Chile (ANID) through Fondo Nacional de Desarrollo Científico y Tecnológico (FONDECYT) grants 3220565 (SG), 1191300 (CR), and 11200764 (FM-G) and The Millennium Institute on Immunology and Immunotherapy/ANID – Millennium Science Initiative Program – ICM-ANID “ICN2021_045”: Millennium Institute on Immunology and Immunotherapy (Program ICM-ANID “ICN2021_045”) (SB, AK, FS, CR, and FM-G).
Conflict of interest
The authors declare that the research was conducted in the absence of any commercial or financial relationships that could be construed as a potential conflict of interest.
Publisher’s note
All claims expressed in this article are solely those of the authors and do not necessarily represent those of their affiliated organizations, or those of the publisher, the editors and the reviewers. Any product that may be evaluated in this article, or claim that may be made by its manufacturer, is not guaranteed or endorsed by the publisher.
Abbreviations
APACHE II, Acute physiology and chronic health evaluation II; AUC, Area under the curve; AUROC, Area under the receiver operating characteristic; BDG, (1,3)-β-D-glucan; BSI, Bloodstream infection; COVID-19, Coronavirus disease 2019; CPS, Capsule polysaccharide; ECMO, Extracorporeal membrane oxygenation; ExPEC, Extraintestinal E. coli; UPEC, Uropathogenic E. coli; GSH, Glutathione; HR, Hazard ratio; HIF, Hypoxia inducible factor; hvKp, Hypervirulent K. pneumoniae; ICU, Intensive care unit; IL, Interleukin; LPS, Lipopolysaccharide; MRSA, Methicillin-resistant S. aureus; MSSA, Methicillin-sensitive S. aureus; MDR, Multi-drug resistant; NLRP3, NOD-like receptor family pyrin domain-containing 3; OR, Odds ratio; PVL, Panton–valentine leukocidin; PAD2, Peptidyl arginine deiminase type 2; PLGA, Poly (lactic-co-glycolic acid); PGE2, Prostaglandin E2; ROS, Reactive oxygen species; SARS-CoV-2, Severe acute respiratory syndrome coronavirus 2; SPATE, Serine protease autotransporters of Enterobacteriaceae; SCFA, Short-chain fatty acid; SOD, Superoxide dismutase; SOFA, Sepsis organ failure assessment; TSS, Toxic shock syndrome; TNF, Tumor necrosis factor; VEGF, Vascular endothelial growth factor; WHO, World Health Organization; XDR, Extensively-drug resistant; XLA, X-linked agammaglobulinemia.
References
Abreu, A. G., Fraga, T. R., Granados Martínez, A. P., Kondo, M. Y., Juliano, M. A., Juliano, L., et al. (2015). The serine protease pic from Enteroaggregative Escherichia coli mediates immune evasion by the direct cleavage of complement proteins. J. Infect. Dis. 212, 106–115. doi: 10.1093/infdis/jiv013
Agnelli, C., Bouza, E., del Carmen Martínez-Jiménez, M., Navarro, R., Valerio, M., Machado, M., et al. (2019). Clinical relevance and prognostic value of persistently negative (1,3)-β-D-glucan in adults with Candidemia: A 5-year experience in a tertiary hospital. Clin. Infect. Dis. 70, 1925–1932. doi: 10.1093/cid/ciz555
Ahmad, N. I., Yean, C. Y., Foo, P. C., Safiee, A. W. M., and Hassan, S. A. (2020). Prevalence and association of Panton-valentine Leukocidin gene with the risk of sepsis in patients infected with methicillin resistant Staphylococcus aureus. J. Infect. Public Health 13, 1508–1512. doi: 10.1016/j.jiph.2020.06.018
Akinbobola, A. B., Kean, R., Hanifi, S. M. A., and Quilliam, R. S. (2023). Environmental reservoirs of the drug-resistant pathogenic yeast Candida auris. PLoS Pathog. 19:e1011268. doi: 10.1371/journal.ppat.1011268
Aldardeer, N. F., Albar, H., al-Attas, M., Eldali, A., Qutub, M., Hassanien, A., et al. (2020). Antifungal resistance in patients with Candidaemia: a retrospective cohort study. BMC Infect. Dis. 20:55. doi: 10.1186/s12879-019-4710-z
Al-Dorzi, H. M., Sakkijha, H., Khan, R., Aldabbagh, T., Toledo, A., Ntinika, P., et al. (2018). Invasive candidiasis in critically ill patients: A prospective cohort study in two tertiary care centers. J. Intensive Care Med. 35, 542–553. doi: 10.1177/0885066618767835
Alessandri, F., Ceccarelli, G., Migliara, G., Baccolini, V., Russo, A., Marzuillo, C., et al. (2023). High incidence of Candidemia in critically ill COVID-19 patients supported by Veno-venous extracorporeal membrane oxygenation: A retrospective study. J. Fungi 9:119. doi: 10.3390/jof9010119
Al-Hasan, M. N., Eckel-Passow, J. E., and Baddour, L. M. (2012). Impact of healthcare-associated acquisition on community-onset gram-negative bloodstream infection: a population-based study. Eur. J. Clin. Microbiol. Infect. Dis. 31, 1163–1171. doi: 10.1007/s10096-011-1424-6
Alhazzani, W., Møller, M. H., Arabi, Y. M., Loeb, M., Gong, M. N., Fan, E., et al. (2020). Surviving Sepsis campaign: guidelines on the management of critically ill adults with coronavirus disease 2019 (COVID-19). Intensive Care Med. 46, 854–887. doi: 10.1007/s00134-020-06022-5
Al-Musawi, T. S., Alkhalifa, W. A., Alasaker, N. A., Rahman, J. U., and Alnimr, A. M. (2021). A seven-year surveillance of Candida bloodstream infection at a university hospital in KSA. J. Taibah Univ. Med. Sci. 16, 184–190. doi: 10.1016/j.jtumed.2020.12.002
Ang, S. H., Petrick, P., Shamsul, A. S., Ramliza, R., Kori, N., and Lau, C. L. (2022). The risk factors for complications and survival outcomes of Klebsiella pneumoniae Bacteraemia in hospital Canselor Tuanku Muhriz Universiti Kebangsaan Malaysia. Med. J. Malays. 77, 440–445.
Arora, H. S., Khan, H., Ailumerab, H., Natarajan, G., Meert, K., Salimnia, H., et al. (2023). A tale of two intensive care units (ICUs): baseline Staphylococcus aureus colonization and mupirocin susceptibility in neonatal and pediatric patients requiring intensive care. Infect. Control. 44, 447–452. doi: 10.1017/ice.2022.96
Asai, N., Ohashi, W., Sakanashi, D., Suematsu, H., Kato, H., Hagihara, M., et al. (2021). Combination of sequential organ failure assessment (SOFA) score and Charlson comorbidity index (CCI) could predict the severity and prognosis of candidemia more accurately than the acute physiology, age, chronic health evaluation II (APACHE II) score. BMC Infect. Dis. 21:77. doi: 10.1186/s12879-020-05719-8
Asgari, F., Supino, D., Parente, R., Polentarutti, N., Stravalaci, M., Porte, R., et al. (2021). The long Pentraxin PTX3 controls Klebsiella Pneumoniae severe infection. Front. Immunol. 12:666198. doi: 10.3389/fimmu.2021.666198
Azoulay, E., Timsit, J.-F., Lautrette, A., Legriel, S., Max, A., Ruckly, S., et al. (2017). Weekly high-dose liposomal amphotericin B (L-AmB) in critically ill septic patients with multiple Candida colonization: the AmBiDex study. PLoS One 12:e0177093. doi: 10.1371/journal.pone.0177093
Babiker, A., Li, X., Lai, Y. L., Strich, J. R., Warner, S., Sarzynski, S., et al. (2021). Effectiveness of adjunctive clindamycin in β-lactam antibiotic-treated patients with invasive β-haemolytic streptococcal infections in US hospitals: a retrospective multicentre cohort study. Lancet Infect. Dis. 21, 697–710. doi: 10.1016/s1473-3099(20)30523-5
Bachmann, N. L., Katouli, M., and Polkinghorne, A. (2015). Genomic comparison of translocating and non-translocating Escherichia coli. PLoS One 10:e0137131. doi: 10.1371/journal.pone.0137131
Bai, A. D., Bonares, M. J., Thrall, S., Bell, C. M., and Morris, A. M. (2020). Presence of urinary symptoms in bacteremic urinary tract infection: a retrospective cohort study of Escherichia coli bacteremia. BMC Infect. Dis. 20:781. doi: 10.1186/s12879-020-05499-1
Bartie, K. L., Williams, D. W., Wilson, M. J., Potts, A. J. C., and Lewis, M. A. O. (2004). Differential invasion of Candida albicans isolates in an in vitro model of oral candidosis. Oral Microbiol. Immunol. 19, 293–296. doi: 10.1111/j.1399-302x.2004.00155.x
Bartoletti, M., Rinaldi, M., Pasquini, Z., Scudeller, L., Piano, S., Giacobbe, D. R., et al. (2021). Risk factors for candidaemia in hospitalized patients with liver cirrhosis: a multicentre case–control–control study. CMI 27, 276–282. doi: 10.1016/j.cmi.2020.04.030
Bassetti, M., Castaldo, N., Cattelan, A., Mussini, C., Righi, E., Tascini, C., et al. (2019). Ceftolozane/tazobactam for the treatment of serious Pseudomonas aeruginosa infections: a multicentre nationwide clinical experience. Antimicrob. Agents Annu. 53, 408–415. doi: 10.1016/j.ijantimicag.2018.11.001
Bassetti, M., Righi, E., del Giacomo, P., Sartor, A., Ansaldi, F., Trucchi, C., et al. (2018). Predictors of mortality with Staphylococcus aureus bacteremia in elderly adults. J. Am. Geriatr. Soc. 66, 1284–1289. doi: 10.1111/jgs.15391
Bassetti, M., Vena, A., Meroi, M., Cardozo, C., Cuervo, G., Giacobbe, D. R., et al. (2020). Factors associated with the development of septic shock in patients with candidemia: a post hoc analysis from two prospective cohorts. Crit. Care 24:117. doi: 10.1186/s13054-020-2793-y
Battistolo, J., Glampedakis, E., Damonti, L., Poissy, J., Grandbastien, B., Kalbermatter, L., et al. (2021). Increasing morbidity and mortality of candidemia over one decade in a Swiss university hospital. Mycoses 64, 1512–1520. doi: 10.1111/myc.13376
Belo, V. A., Pereira, J. A., Souza, S. F. D., Tana, F. d. L., Pereira, B. P., Lopes, D. d. O., et al. (2021). The role of IL-10 in immune responses against Pseudomonas aeruginosa during acute lung infection. Cell Tissue Res. 383, 1123–1133. doi: 10.1007/s00441-020-03308-4
Bhardwaj, N. K., Khera, D., Gupta, N., and Singh, K. (2017). Disseminated Pseudomonas aeruginosa sepsis as presenting diagnosis of X-linked agammaglobulinaemia in a previously well 16-month-old child. Bmj Case Rep. 2017, 1–4. doi: 10.1136/bcr-2017-221006
Bienvenu, A.-L., Pradat, P., Guerin, C., Aubrun, F., Fellahi, J.-L., Friggeri, A., et al. (2020). Evaluation of first-line therapies for the treatment of candidemia in ICU patients: A propensity score analysis. Int. J. Infect. Dis. 93, 15–21. doi: 10.1016/j.ijid.2020.01.037
Birlutiu, V., Birlutiu, R. M., Baicu, M., and Iancu, G. M. (2019). A case report of double etiology of ecthyma gangrenosum. Medicine 98:e15651. doi: 10.1097/md.0000000000015651
Biscaye, S., Demonchy, D., Afanetti, M., Dupont, A., Haas, H., and Tran, A. (2017). Ecthyma gangrenosum, a skin manifestation of Pseudomonas aeruginosa sepsis in a previously healthy child. Medicine 96:e5507. doi: 10.1097/md.0000000000005507
Bläckberg, A., Svedevall, S., Lundberg, K., Nilson, B., Kahn, F., and Rasmussen, M. (2022). Time to blood culture positivity: an independent predictor of mortality in Streptococcus Pyogenes bacteremia. Open forum. Infect. Dis. Ther. 9:ofac163. doi: 10.1093/ofid/ofac163
Blagden, S., Watts, V., Verlander, N. Q., and Pegorie, M. (2020). Invasive group A streptococcal infections in north West England: epidemiology, risk factors and fatal infection. Public Health 186, 63–70. doi: 10.1016/j.puhe.2020.06.007
Bongomin, F., Gago, S., Oladele, R., and Denning, D. (2017). Global and multi-National Prevalence of fungal diseases—estimate precision. J. Fungi 3:57. doi: 10.3390/jof3040057
Bonten, M., Johnson, J. R., Biggelaar, A. H. J.van den, Georgalis, L., Geurtsen, J., Palacios, P. I.de, et al. (2020). Epidemiology of Escherichia coli bacteremia: A systematic literature review. Clin. Infect. Dis. 72, 1211–1219. doi: 10.1093/cid/ciaa210
Bouza, E., Burillo, A., Muñoz, P., Guinea, J., Marín, M., and Rodríguez-Créixems, M. (2013). Mixed bloodstream infections involving bacteria and Candida spp. J. Antimicrob. Chemother. 68, 1881–1888. doi: 10.1093/jac/dkt099
Boyle-Vavra, S., Li, X., Alam, M. T., Read, T. D., Sieth, J., Cywes-Bentley, C., et al. (2015). USA300 and USA500 clonal lineages of Staphylococcus aureus do not produce a capsular polysaccharide due to conserved mutations in thecap5Locus. MBio 6:e02585. doi: 10.1128/mbio.02585-14
Brammer, J., Wolf, G., Baliban, S. M., Allen, J. C., Choi, M., Kambouris, A. R., et al. (2021). A nonlethal full-thickness flame burn produces a seroma beneath the forming eschar, thereby promoting Pseudomonas aeruginosa Sepsis in mice. J. Burn Care Res. 43, 792–801. doi: 10.1093/jbcr/irab195
Briano, F., Magnasco, L., Sepulcri, C., Dettori, S., Dentone, C., Mikulska, M., et al. (2022). Candida auris Candidemia in critically ill, colonized patients: cumulative incidence and risk factors. Infect. Dis. Ther. 11, 1149–1160. doi: 10.1007/s40121-022-00625-9
Brundage, J. F. (2006). Interactions between influenza and bacterial respiratory pathogens: implications for pandemic preparedness. Lancet Infect. Dis. 6, 303–312. doi: 10.1016/s1473-3099(06)70466-2
Butt, J. H., Fosbøl, E. L., Gerds, T. A., Iversen, K., Bundgaard, H., Bruun, N. E., et al. (2020). Ticagrelor and the risk of Staphylococcus aureus bacteraemia and other infections. Eur. Heart J. 8, 13–19. doi: 10.1093/ehjcvp/pvaa099
Cebrero-Cangueiro, T., Labrador-Herrera, G., Pascual, Á., Díaz, C., Rodríguez-Baño, J., Pachón, J., et al. (2021). Efficacy of Fosfomycin and its combination with aminoglycosides in an experimental Sepsis model by Carbapenemase-producing Klebsiella pneumoniae clinical strains. Front. Med. 8:615540. doi: 10.3389/fmed.2021.615540
Chang, D., Sharma, L., Cruz, C. S. D., and Zhang, D. (2021). Clinical epidemiology, risk factors, and control strategies of Klebsiella pneumoniae infection. Front. Microbiol. 12:750662. doi: 10.3389/fmicb.2021.750662
Chapelet, G., Boureau, A. S., Dylis, A., Herbreteau, G., Corvec, S., Batard, E., et al. (2017). Association between dementia and reduced walking ability and 30-day mortality in patients with extended-spectrum beta-lactamase-producing Escherichia coli bacteremia. Eur. J. Clin. Microbiol. Infect. Dis. 36, 2417–2422. doi: 10.1007/s10096-017-3077-6
Chen, Y.-F., Chen, G.-Y., Chang, C.-H., Su, Y.-C., Chen, Y.-C., Jiang, Y., et al. (2019). TRAIL encapsulated to polypeptide-crosslinked nanogel exhibits increased anti-inflammatory activities in Klebsiella pneumoniae-induced sepsis treatment. Mater. Sci. Eng. C 102, 85–95. doi: 10.1016/j.msec.2019.04.023
Chen, Y. N., Hsu, J.-F., Chu, S.-M., Lai, M.-Y., Lin, C., Huang, H.-R., et al. (2022). Clinical and microbiological characteristics of neonates with Candidemia and impacts of therapeutic strategies on the outcomes. J. Fungi 8:465. doi: 10.3390/jof8050465
Chen, W., Lian, J., Ye, J., Mo, Q., Qin, J., Hong, G., et al. (2017). Ethyl pyruvate reverses development of Pseudomonas aeruginosa pneumonia during sepsis-induced immunosuppression. Int. Immunopharmacol. 52, 61–69. doi: 10.1016/j.intimp.2017.08.024
Chen, I. R., Lin, S.-N., Wu, X.-N., Chou, S.-H., Wang, F.-D., and Lin, Y.-T. (2022). Clinical and microbiological characteristics of Bacteremic pneumonia caused by Klebsiella pneumoniae. Front Cell Infect Microbiol. 12:903682. doi: 10.3389/fcimb.2022.903682
Chen, X.-C., Xu, J., and Wu, D.-P. (2020). Clinical characteristics and implications of mixed candida/bacterial bloodstream infections in patients with hematological diseases. Eur. J. Clin. Microbiol. Infect. Dis. 39, 1445–1452. doi: 10.1007/s10096-020-03863-2
Chochua, S., Metcalf, B., Li, Z., Mathis, S., Tran, T., Rivers, J., et al. (2022). Invasive group A streptococcal penicillin binding protein 2× variants associated with reduced susceptibility to β-lactam antibiotics in the United States, 2015–2021. ASM J. CD 66:e0080222. doi: 10.1128/aac.00802-22
Choi, M., Hegerle, N., Nkeze, J., Sen, S., Jamindar, S., Nasrin, S., et al. (2020). The diversity of lipopolysaccharide (O) and capsular polysaccharide (K) antigens of invasive Klebsiella pneumoniae in a multi-country collection. Front. Microbiol. 11:1249. doi: 10.3389/fmicb.2020.01249
Cienfuegos-Gallet, A. V., Zhou, Y., Ai, W., Kreiswirth, B. N., Yu, F., and Chen, L. (2022). Multicenter genomic analysis of Carbapenem-resistant Klebsiella pneumoniae from bacteremia in China. Microbiol. Spectr. 10, e02290–e02221. doi: 10.1128/spectrum.02290-21
Clancy, C. J., Kalil, A. C., Fowler, V. G., Ghedin, E., Kolls, J. K., and Nguyen, M. H. (2014). Emerging and resistant infections. Ann. Am. Thorac. Soc. 11, S193–S200. doi: 10.1513/annalsats.201402-069pl
Clermont, O., Dixit, O. V. A., Vangchhia, B., Condamine, B., Dion, S., Bridier-Nahmias, A., et al. (2019). Characterization and rapid identification of phylogroup G in Escherichia coli, a lineage with high virulence and antibiotic resistance potential. Environ. Microbiol. 21, 3107–3117. doi: 10.1111/1462-2920.14713
Coggon, C. F., Jiang, A., Goh, K. G. K., Henderson, I. R., Schembri, M. A., and Wells, T. J. (2018). A novel method of serum resistance by Escherichia coli that causes Urosepsis. MBio 9, e00918–e00920. doi: 10.1128/mbio.00920-18
Coombs, G. W., Daley, D. A., Yee, N. W., Shoby, P., and Mowlaboccus, S. (2022). Australian group on antimicrobial resistance (AGAR) Australian Staphylococcus aureus Sepsis outcome Programme (ASSOP) annual report 2020. Commun. Dis. Intell. 46, 1–17. doi: 10.33321/cdi.2022.46.18
Coppola, P. E., Gaibani, P., Sartor, C., Ambretti, S., Lewis, R. E., Sassi, C., et al. (2020). Ceftolozane-Tazobactam treatment of Hypervirulent multidrug resistant Pseudomonas aeruginosa infections in neutropenic patients. Microorganisms 8:2055. doi: 10.3390/microorganisms8122055
Corbella, L., Boán, J., San-Juan, R., Fernández-Ruiz, M., Carretero, O., Lora, D., et al. (2022). Effectiveness of ceftazidime-avibactam for the treatment of infections due to Pseudomonas aeruginosa. Int. J. Antimicrob. Agents 59:106517. doi: 10.1016/j.ijantimicag.2021.106517
Cusumano, J. A., Dupper, A. C., Malik, Y., Gavioli, E. M., Banga, J., Berbel Caban, A., et al. (2020). Staphylococcus aureus bacteremia in patients infected with COVID-19: A case series. Infect. Dis. Ther. 7:ofaa518. doi: 10.1093/ofid/ofaa518
Damico, V., Murano, L., Margosio, V., and Ripamonti, C. (2022). Co-infections among COVID-19 adult patients admitted to intensive care units: results from a retrospective study. Annu. DiIgiene Med. Prev. Comun. 35, 49–60. doi: 10.7416/ai.2022.2515
Davis, J., Turnidge, J., and Tong, S. (2018). A large retrospective cohort study of cefazolin compared with flucloxacillin for methicillin-susceptible Staphylococcus aureus bacteraemia. Int. J. Antimicrob. Agent 52, 297–300. doi: 10.1016/j.ijantimicag.2018.02.013
Lastours, V. de, Laouénan, C., Royer, G., Carbonnelle, E., Lepeule, R., Esposito-Farèse, M., et al. (2020). Mortality in Escherichia coli bloodstream infections: antibiotic resistance still does not make it. J. Antimicrob. Chemother. 75, 2334–2343. doi: 10.1093/jac/dkaa161
Delaloye, J., and Calandra, T. (2014). Invasive candidiasis as a cause of sepsis in the critically ill patient. Virulence 5, 161–169. doi: 10.4161/viru.26187
DeMuri, G. P., Sterkel, A. K., Kubica, P. A., Duster, M. N., Reed, K. D., and Wald, E. R. (2017). Macrolide and clindamycin resistance in group a streptococci isolated from children with pharyngitis. Pediatr. Infect. Dis. J. 36, 342–344. doi: 10.1097/inf.0000000000001442
Denning, D. W. (2003). Echinocandin antifungal drugs. Lancet 362, 1142–1151. doi: 10.1016/s0140-6736(03)14472-8
Desvaux, M., Dalmasso, G., Beyrouthy, R., Barnich, N., Delmas, J., and Bonnet, R. (2020). Pathogenicity factors of Genomic Islands in intestinal and Extraintestinal Escherichia coli. Front. Microbiol. 11:2065. doi: 10.3389/fmicb.2020.02065
Deutschman, C. S., Leisman, D. E., and Taylor, M. D. (2022). Adrenergic immune effects: is Beta the enemy of good?*. Crit. Care Med. 50, 1415–1418. doi: 10.1097/ccm.0000000000005524
di Domenico, E. G., Marchesi, F., Cavallo, I., Toma, L., Sivori, F., Papa, E., et al. (2021). The impact of bacterial biofilms on end-organ disease and mortality in patients with hematologic malignancies developing a bloodstream infection. Microbiol. Spectr. 9:e0055021. doi: 10.1128/spectrum.00550-21
Diago-Navarro, E., Calatayud-Baselga, I., Sun, D., Khairallah, C., Mann, I., Ulacia-Hernando, A., et al. (2017). Antibody-based immunotherapy to treat and prevent infection with Hypervirulent Klebsiella pneumoniae. Clin. Vaccine Immunol. 24, 1–10. doi: 10.1128/cvi.00456-16
Díaz, J. M., Dozois, C. M., Avelar-González, F. J., Hernández-Cuellar, E., Pokharel, P., Santiago, A. S.de, et al. (2020). The Vacuolating autotransporter toxin (vat) of Escherichia coli causes cell cytoskeleton changes and produces non-lysosomal vacuole formation in bladder epithelial cells. Front. Microbiol. 10,:299. doi: 10.3389/fcimb.2020.00299
Diekema, D. J., Hsueh, P.-R., Mendes, R. E., Pfaller, M. A., Rolston, K. V., Sader, H. S., et al. (2019). The microbiology of bloodstream infection: 20-year trends from the SENTRY antimicrobial surveillance program. ASM J. CD 63, e00355–e00419. doi: 10.1128/aac.00355-19
Doğan, Ö., Yeşilkaya, A., Menekşe, Ş., Güler, Ö., Karakoç, Ç., Çınar, G., et al. (2020). Effect of initial antifungal therapy on mortality among patients with bloodstream infections with different Candida species and resistance to antifungal agents: A multicentre observational study by the Turkish fungal infections study group. Antimicrob. Agents Annu. 56:105992. doi: 10.1016/j.ijantimicag.2020.105992
Donnelly, J. P., Chen, S. C., Kauffman, C. A., Steinbach, W. J., Baddley, J. W., Verweij, P. E., et al. (2019). Revision and update of the consensus definitions of invasive fungal disease from the European Organization for Research and Treatment of Cancer and the mycoses study group education and research consortium. Clin. Infect. Dis. 71, 1367–1376. doi: 10.1093/cid/ciz1008
Dzvova, N., Colmer-Hamood, J. A., Griswold, J. A., and Hamood, A. N. (2018). Heparinase is essential for Pseudomonas aeruginosa virulence during thermal injury and infection. Infect. Immun. 86, 1–13. doi: 10.1128/iai.00755-17
Eichenberger, E. M., Dagher, M., Ruffin, F., Park, L., Hersh, L., Sivapalasingam, S., et al. (2020). Complement levels in patients with bloodstream infection due to Staphylococcus aureus or gram-negative bacteria. Eur. J. Clin. Microbiol. Infect. Dis. 39, 2121–2131. doi: 10.1007/s10096-020-03955-z
Eisi, H., Ibraheem, S., Hisham, T., al-Harbi, A., Saidy, K., Ali, I., et al. (2022). Risk factors and outcomes of deep tissue Candida invasion in neonates with invasive candidiasis. Mycoses 65, 110–119. doi: 10.1111/myc.13395
Erayil, S. E., Palzer, E., and Kline, S. (2022). An evaluation of risk factors for Staphylococcus aureus colonization in a pre-surgical population. Access Microbiol. 4:000316. doi: 10.1099/acmi.0.000316
Escobar-Páramo, P., Clermont, O., Blanc-Potard, A.-B., Bui, H., Bouguénec, C. L., and Denamur, E. (2004). A specific genetic background is required for acquisition and expression of virulence factors in Escherichia coli. Mol. Biol. Evol. 21, 1085–1094. doi: 10.1093/molbev/msh118
Esparcia, A., Madrazo, M., Alberola, J., López-Cruz, I., Eiros, J. M., Nogueira, J. M., et al. (2019). Community-onset Pseudomonas aeruginosa urinary sepsis in elderly people: predictive factors, adequacy of empirical therapy and outcomes. Int. J. Clin. Pract. 73:e13425. doi: 10.1111/ijcp.13425
Evans, L., Rhodes, A., Alhazzani, W., Antonelli, M., Coopersmith, C. M., French, C., et al. (2021). Surviving Sepsis campaign: international guidelines for Management of Sepsis and Septic Shock 2021. Crit. Care Med. 49, e1063–e1143. doi: 10.1097/ccm.0000000000005337
Ferreira, M., Santos, M., Rodrigues, J., Diogo, C., Resende, C., Baptista, C., et al. (2023). Epidemiology of bacteremia in a pediatric population – A 10-year study. Enferm. Infecc. Microbiol. Clin. 41, 85–91. doi: 10.1016/j.eimce.2021.06.006
Follador, R., Heinz, E., Wyres, K. L., Ellington, M. J., Kowarik, M., Holt, K. E., et al. (2016). The diversity of Klebsiella pneumoniae surface polysaccharides. Microb. Genom. 2:e000073. doi: 10.1099/mgen.0.000073
Freire, C. A., Santos, A. C. M., Pignatari, A. C., Silva, R. M., and Elias, W. P. (2020). Serine protease autotransporters of Enterobacteriaceae (SPATEs) are largely distributed among Escherichia coli isolated from the bloodstream. Braz. J. Microbiol. 51, 447–454. doi: 10.1007/s42770-020-00224-1
Freire, C. A., Silva, R. M., Ruiz, R. C., Pimenta, D. C., Bryant, J. A., Henderson, I. R., et al. (2022). Secreted autotransporter toxin (sat) mediates innate immune system evasion. Front. Immunol. 13:844878. doi: 10.3389/fimmu.2022.844878
Fröding, I., Hasan, B., Sylvin, I., Coorens, M., Nauclér, P., and Giske, C. G. (2020). Extended-Spectrum-β-lactamase- and plasmid AmpC-producing Escherichia coli causing community-onset bloodstream infection: Association of Bacterial Clones and Virulence Genes with septic shock, source of infection, and recurrence. Antimicrob. Agents Chem. 64, 1–17. doi: 10.1128/aac.02351-19
Gaensbauer, J. T., Birkholz, M., Smit, M. A., Garcia, R., and Todd, J. K. (2018). Epidemiology and clinical relevance of toxic shock syndrome in US children. Pediatr. Infect. Dis. J. 37, 1223–1226. doi: 10.1097/inf.0000000000002002
Gajdács, M., Ábrók, M., Lázár, A., and Burián, K. (2020). Beta-Haemolytic group A, C and G streptococcal infections in southern Hungary: A 10-year population-based retrospective survey (2008–2017) and a review of the literature. Infect Drug Resist 13, 4739–4749. doi: 10.2147/idr.s279157
Garcia-Bustos, V., Salavert, M., Ruiz-Gaitán, A. C., Cabañero-Navalon, M. D., Sigona-Giangreco, I. A., and Pemán, J. (2020). A clinical predictive model of candidaemia by Candida auris in previously colonized critically ill patients. Clin. Microbiol. Infect. 26, 1507–1513. doi: 10.1016/j.cmi.2020.02.001
Garcia-Vidal, C., Sanjuan, G., Moreno-García, E., Puerta-Alcalde, P., Garcia-Pouton, N., Chumbita, M., et al. (2021). Incidence of co-infections and superinfections in hospitalized patients with COVID-19: a retrospective cohort study. CMI 27, 83–88. doi: 10.1016/j.cmi.2020.07.041
Gatica, S., Villegas, V., Vallejos, A., Olivares, P., Aballai, V., Lagos-Meza, F., et al. (2020). TRPM7 mediates kidney injury, endothelial hyperpermeability and mortality during endotoxemia. Lab. Investig. 100, 234–249. doi: 10.1038/s41374-019-0304-z
GBD 2019 Antimicrobial Resistance Collaborators . (2022). Global mortality associated with 33 bacterial pathogens in 2019: a systematic analysis for the Global Burden of Disease Study 2019. Lancet 400, 2221–2248. doi: 10.1016/s0140-6736(22)02185-7
Gebremicael, M. N., Nuttall, J. J. C., Tootla, H. D., Khumalo, A., Tooke, L., Salie, S., et al. (2023). Candida bloodstream infection among children hospitalised in three public-sector hospitals in the metro West region of Cape Town, South Africa. Infect. Dis. Ther. 23:67. doi: 10.1186/s12879-023-08027-z
Gera, K., and McIver, K. S. (2013). Laboratory growth and maintenance of Streptococcus pyogenes (the group A Streptococcus, GAS). Curr. Protoc. Microbiol. 30, 9D.2.1–9D.2.13. doi: 10.1002/9780471729259.mc09d02s30
Geremia, N., Brugnaro, P., Solinas, M., Scarparo, C., and Panese, S. (2023). Candida auris as an emergent public health problem: A current update on European outbreaks and cases. Health 11:425. doi: 10.3390/healthcare11030425
Giovannenze, F., Murri, R., Palazzolo, C., Taccari, F., Camici, M., Spanu, T., et al. (2021). Predictors of mortality among adult, old and the oldest old patients with bloodstream infections: an age comparison. Eur. J. Intern. Med. 86, 66–72. doi: 10.1016/j.ejim.2020.12.017
Glass, E. L., Raoult, D., and Dubourg, G. (2022). Snapshot of COVID-19 superinfections in Marseille hospitals: where are the common pathogens? Epidemiol. Infect. 150:e195. doi: 10.1017/s0950268822001704
Goldstein, B. D. (2001). The precautionary principle also applies to public health actions. Am. J. Public Health 91, 1358–1361. doi: 10.2105/ajph.91.9.1358
Gómez-Gaviria, M., Martínez-Álvarez, J. A., Chávez-Santiago, J. O., and Mora-Montes, H. M. (2023). Candida haemulonii complex and Candida auris: biology, virulence factors, immune response, and multidrug resistance. Infect Drug Resist 16, 1455–1470. doi: 10.2147/idr.s402754
González-Lara, M. F., Torres-González, P., Cornejo-Juárez, P., Velázquez-Acosta, C., Martinez-Gamboa, A., Rangel-Cordero, A., et al. (2017). Impact of inappropriate antifungal therapy according to current susceptibility breakpoints on Candida bloodstream infection mortality, a retrospective analysis. BMC Infect. Dis. 17:753. doi: 10.1186/s12879-017-2846-2
Gorrie, C. L., Mirceta, M., Wick, R. R., Edwards, D. J., Thomson, N. R., Strugnell, R. A., et al. (2017). Gastrointestinal carriage is a Major reservoir of Klebsiella pneumoniae infection in intensive care patients. Clin. Infect. Dis. 65, 208–215. doi: 10.1093/cid/cix270
Gouel-Cheron, A., Swihart, B. J., Warner, S., Mathew, L., Strich, J. R., Mancera, A., et al. (2022). Epidemiology of ICU-onset bloodstream infection: prevalence, pathogens, and risk factors among 150,948 ICU patients at 85 U.S. hospitals*. Crit. Care Med. 50, 1725–1736. doi: 10.1097/ccm.0000000000005662
Greenberg, J. A., Hrusch, C. L., Jaffery, M. R., David, M. Z., Daum, R. S., Hall, J. B., et al. (2018). Distinct T-helper cell responses to Staphylococcus aureus bacteremia reflect immunologic comorbidities and correlate with mortality. Crit. Care 22:107. doi: 10.1186/s13054-018-2025-x
Griepentrog, J. E., Zhang, X., Lewis, A. J., Gianfrate, G., Labiner, H. E., Zou, B., et al. (2020). Frontline science: rev-Erbα links blue light with enhanced bacterial clearance and improved survival in murine Klebsiella pneumoniae pneumonia. J. Leukoc. Biol. 107, 11–25. doi: 10.1002/jlb.4hi0519-155r
Gröndahl-Yli-Hannuksela, K., Beres, S. B., Hyyryläinen, H.-L., Kallonen, T., Musser, J. M., and Vuopio, J. (2021). Genetic evolution of invasive emm28 Streptococcus pyogenes strains and significant association with puerperal infections in young women in Finland. Clin. Microbiol. Infect. 27, 420–427. doi: 10.1016/j.cmi.2020.04.004
Guner Ozenen, G., Sahbudak Bal, Z., Avcu, G., Ozkaya Yazici, P., Karakoyun, M., Metin, D. Y., et al. (2023). Evaluation of candidemia in children at a university hospital: A retrospective cohort. Mycoses 66, 367–377. doi: 10.1111/myc.13564
Gupta, E., Kumar, S., Srivastava, V. K., Saxena, J., Siddiqui, A. J., Mehta, S., et al. (2022). Unravelling the differential host Immuno-inflammatory responses to Staphylococcus aureus and Escherichia coli infections in Sepsis. Vaccine 10:1648. doi: 10.3390/vaccines10101648
Gupte, A., Jyot, J., Ravi, M., and Ramphal, R. (2021). High pyocyanin production and non-motility of Pseudomonas aeruginosa isolates are correlated with septic shock or death in bacteremic patients. PLoS One 16:e0253259. doi: 10.1371/journal.pone.0253259
Guthridge, I., Smith, S., Law, M., Binotto, E., and Hanson, J. (2021). Efficacy and safety of intravenous Lincosamide therapy in methicillin-resistant Staphylococcus aureus bacteremia. Antimicrob. Agents Chem. 65, e00321–e00343. doi: 10.1128/aac.00343-21
Hafner, A., Kolbe, U., Freund, I., Castiglia, V., Kovarik, P., Poth, T., et al. (2019). Crucial role of nucleic acid sensing via endosomal toll-like receptors for the defense of Streptococcus pyogenes in vitro and in vivo. Front. Immunol. 10:198. doi: 10.3389/fimmu.2019.00198
Hammer, K. L., Justo, J. A., Bookstaver, P. B., Kohn, J., Albrecht, H., and Al-Hasan, M. N. (2017). Differential effect of prior β-lactams and fluoroquinolones on risk of bloodstream infections secondary to Pseudomonas aeruginosa. Diagn. Microbiol. Infect. Dis. 87, 87–91. doi: 10.1016/j.diagmicrobio.2016.09.017
Happel, K. I., and Nelson, S. (2005). Alcohol, immunosuppression, and the lung. Proc. Am. Thorac. Soc. 2, 428–432. doi: 10.1513/pats.200507-065js
Hayakawa, K., Yamaguchi, T., Ono, D., Suzuki, H., Kamiyama, J., Taguchi, S., et al. (2020). Two cases of Intrafamilial transmission of community-acquired methicillin-resistant Staphylococcus aureus producing both PVL and TSST-1 causing fatal necrotizing pneumonia and Sepsis. Infect Drug Resist 13, 2921–2927. doi: 10.2147/idr.s262123
Hayes, A., Lacey, J. A., Morris, J. M., Davies, M. R., and Tong, S. Y. C. (2020). Restricted sequence variation in Streptococcus pyogenes penicillin binding proteins. Msphere 5:e00090. doi: 10.1128/msphere.00090-20
Hernández-García, M., García-Castillo, M., Bou, G., Cercenado, E., Delgado-Valverde, M., Oliver, A., et al. (2022). Imipenem-Relebactam susceptibility in Enterobacterales isolates recovered from ICU patients from Spain and Portugal (SUPERIOR and STEP studies). Microbiol. Spectr. 10, e02922–e02927. doi: 10.1128/spectrum.02927-22
Herrera, A. L., Huber, V. C., and Chaussee, M. S. (2016). The association between invasive group A streptococcal diseases and viral respiratory tract infections. Front. Microbiol. 7:342. doi: 10.3389/fmicb.2016.00342
Herrera-Espejo, S., del Barrio-Tofiño, E., Cebrero-Cangueiro, T., López-Causapé, C., Álvarez-Marín, R., Cisneros, J. M., et al. (2022). Carbapenem combinations for infections caused by Carbapenemase-producing Pseudomonas aeruginosa: experimental in vitro and in vivo analysis. Antibiotics 11:1212. doi: 10.3390/antibiotics11091212
Hinrichs, C., Wiese-Posselt, M., Graf, B., Geffers, C., Weikert, B., Enghard, P., et al. (2022). Successful control of Candida auris transmission in a German COVID-19 intensive care unit. Mycoses 65, 643–649. doi: 10.1111/myc.13443
Hu, Z., Yao, Y., Chen, W., Bian, J., Zhao, L., Chen, L., et al. (2018). Partial depletion of regulatory T cells enhances host inflammatory response against acute Pseudomonas aeruginosa infection after Sepsis. Inflammation 41, 1780–1790. doi: 10.1007/s10753-018-0821-8
Hu, H., Zhang, Y., Zhang, P., Wang, J., Yuan, Q., Shi, W., et al. (2021). Bloodstream infections caused by Klebsiella pneumoniae Carbapenemase–Producing P. aeruginosa sequence type 463, associated with high mortality rates in China: A retrospective cohort study. Front. Microbiol. 11:756782. doi: 10.3389/fcimb.2021.756782
Huang, H., Bai, K., Fu, Y., Yan, J., and Li, J. (2020). Ecthyma gangrenosum due to Pseudomonas aeruginosa sepsis as initial manifestation of X-linked agammaglobulinemia: a case report. BMC Pediatr. 20:540. doi: 10.1186/s12887-020-02436-8
Huang, Y. T., Chen, C.-S., Chen, H.-A., Hsu, H.-S., Liang, M.-H., Chang, M.-H., et al. (2020). Klebsiella pneumoniae bacteremia revisited: comparison between 2007 and 2017 prospective cohorts at a medical center in Taiwan. J. Inf. Secur. 81, 753–757. doi: 10.1016/j.jinf.2020.08.039
Huang, H. Y., Lu, P.-L., Wang, Y.-L., Chen, T.-C., Chang, K., and Lin, S.-Y. (2020). Usefulness of EQUAL Candida score for predicting outcomes in patients with candidaemia: a retrospective cohort study. Clin. Microbiol. Infect. 26, 1501–1506. doi: 10.1016/j.cmi.2020.01.029
Hyun, M., Noh, C. I., Ryu, S. Y., and Kim, H. A. (2018). Changing trends in clinical characteristics and antibiotic susceptibility of Klebsiella pneumoniae bacteremia. Kor J Intern Med 33, 595–603. doi: 10.3904/kjim.2015.257
Imam, N., Tempone, S., Armstrong, P. K., McCann, R., Johnson, S., Worth, L. J., et al. (2019). Increased incidence of community-associated Staphylococcus aureus bloodstream infections in Victoria and Western Australia, 2011–2016. Med. J. Austral. 210, 87–88. doi: 10.5694/mja2.12057
Imauven, O., Colot, J., Couadau, E., Moury, P.-H., Preault, A., Vincent, F., et al. (2022). Paediatric and adult patients from New Caledonia Island admitted to the ICU for community-acquired Panton-valentine leucocidin-producing Staphylococcus aureus infections. Sci. Rep. 12:11024. doi: 10.1038/s41598-022-15337-w
Imöhl, M., Fitzner, C., and Perniciaro, S., and Linden, M. van der (2017). Epidemiology and distribution of 10 superantigens among invasive Streptococcus pyogenes disease in Germany from 2009 to 2014. PLoS One 12,:e0180757. doi: 10.1371/journal.pone.0180757
Iuliano, A. D., Roguski, K. M., Chang, H. H., Muscatello, D. J., Palekar, R., Tempia, S., et al. (2018). Estimates of global seasonal influenza-associated respiratory mortality: a modelling study. Lancet 391, 1285–1300. doi: 10.1016/s0140-6736(17)33293-2
Jayakumar, J. S., Niyas, V. K. M., and Arjun, R. (2022). Group A streptococcal bacteremia: ten years’ experience from a tertiary Care Center in South India. Ind. J. Critic. Care Med. Peer Review. Off. Publ. Ind. Soc. Critic. Care Med. 26, 1019–1021. doi: 10.5005/jp-journals-10071-24306
Jean, C., Louie, J. K., Glaser, C. A., Harriman, K., Hacker, J. K., Aranki, F., et al. (2010). Invasive group A streptococcal infection concurrent with 2009 H1N1 influenza. Clin. Infect. Dis. 50, e59–e62. doi: 10.1086/652291
Juan, C.-H., Chuang, C., Chen, C.-H., Li, L., and Lin, Y.-T. (2019). Clinical characteristics, antimicrobial resistance and capsular types of community-acquired, healthcare-associated, and nosocomial Klebsiella pneumoniae bacteremia. Antimicrob. Resist. Infect. Control 8:1. doi: 10.1186/s13756-018-0426-x
Jung, I. Y., Jeong, S. J., Kim, Y. K., Kim, H. Y., Song, Y. G., Kim, J. M., et al. (2020). A multicenter retrospective analysis of the antifungal susceptibility patterns of Candida species and the predictive factors of mortality in south Korean patients with candidemia. Medicine 99:e19494. doi: 10.1097/md.0000000000019494
Kaneko, H., Yanagi, Y., Otake, S., Sato, M., Saito, T., and Nakaminami, H. (2023). The emerging threat of methicillin-resistant Staphylococcus aureus (MRSA) clone ST22-PT, carrying both Panton–valentine leucocidin and toxic shock syndrome toxin 1 genes. J. Antimicrob. Chemother. 78, 1023–1027. doi: 10.1093/jac/dkad039
Kang, C.-I., Kim, S.-H., Park, W. B., Lee, K.-D., Kim, H.-B., Kim, E.-C., et al. (2005). Bloodstream infections caused by antibiotic-resistant gram-negative Bacilli: risk factors for mortality and impact of inappropriate initial antimicrobial therapy on outcome. ASM J. CD 49, 760–766. doi: 10.1128/aac.49.2.760-766.2005
Kant, S., and Pancholi, V. (2021). Novel tyrosine kinase-mediated phosphorylation with dual specificity plays a key role in the modulation of Streptococcus pyogenes physiology and virulence. Front. Microbiol. 12:689246. doi: 10.3389/fmicb.2021.689246
Kaper, J. B., Nataro, J. P., and Mobley, H. L. T. (2004). Pathogenic Escherichia coli. Nat. Rev. Microbiol. 2, 123–140. doi: 10.1038/nrmicro818
Karakonstantis, S., Ioannou, P., and Kritsotakis, E. I. (2022). Co-isolates of Acinetobacter baumannii complex in polymicrobial infections: a meta-analysis. Access Microbiol. 4:acmi000348. doi: 10.1099/acmi.0.000348
Kariyawasam, R. M., Julien, D. A., Jelinski, D. C., Larose, S. L., Rennert-May, E., Conly, J. M., et al. (2022). Antimicrobial resistance (AMR) in COVID-19 patients: a systematic review and meta-analysis (November 2019–June 2021). Antimicrob. Resist. Infect. Control 11:45. doi: 10.1186/s13756-022-01085-z
Kathuria, S., Singh, P. K., Sharma, C., Prakash, A., Masih, A., Kumar, A., et al. (2015). Multidrug-resistant Candida auris misidentified as Candida haemulonii: characterization by matrix-assisted laser desorption ionization–time of flight mass spectrometry and DNA sequencing and its antifungal susceptibility profile variability by Vitek 2, CLSI broth microdilution, and Etest method. J. Clin. Microbiol. 53, 1823–1830. doi: 10.1128/jcm.00367-15
Kayaaslan, B., Eser, F., Asilturk, D., Oktay, Z., Hasanoglu, I., Kalem, A. K., et al. (2023). Development and validation of COVID-19 associated candidemia score (CAC-score) in ICU patients. Mycoses 66, 128–137. doi: 10.1111/myc.13531
Kayaaslan, B., Eser, F., Kaya Kalem, A., Bilgic, Z., Asilturk, D., Hasanoglu, I., et al. (2021). Characteristics of candidemia in COVID-19 patients; increased incidence, earlier occurrence and higher mortality rates compared to non-COVID-19 patients. Mycoses 64, 1083–1091. doi: 10.1111/myc.13332
Khaddour, K., Sikora, A., Tahir, N., Nepomuceno, D., and Huang, T. (2020). Case report: the importance of novel coronavirus disease (COVID-19) and coinfection with other respiratory pathogens in the current pandemic. Am. J. Trop. Med. Hygiene 102, 1208–1209. doi: 10.4269/ajtmh.20-0266
Kim, B., Kim, J.-H., and Lee, Y. (2022). Virulence factors associated with Escherichia coli bacteremia and urinary tract infection. Ann. Lab. Med. 42, 203–212. doi: 10.3343/alm.2022.42.2.203
Kim, J. H., Suh, J. W., and Kim, M. J. (2021). Epidemiological trends of Candidemia and the impact of adherence to the Candidemia guideline: six-year single-center experience. J. Fungi 7:275. doi: 10.3390/jof7040275
Kim, S. -H., Yoon, Y. K., Kim, M. J., and Sohn, J. W. (2013). Risk factors for and clinical implications of mixed Candida/bacterial bloodstream infections. Clin. Microbiol. Infect. 19, 62–68. doi: 10.1111/j.1469-0691.2012.03906.x
Kochan, T. J., Nozick, S. H., Medernach, R. L., Cheung, B. H., Gatesy, S. W. M., Lebrun-Corbin, M., et al. (2022). Genomic surveillance for multidrug-resistant or hypervirulent Klebsiella pneumoniae among United States bloodstream isolates. BMC Infect. Dis. 22:603. doi: 10.1186/s12879-022-07558-1
Kufel, W. D., Parsels, K. A., Blaine, B. E., Steele, J. M., Mahapatra, R., Paolino, K. M., et al. (2023). Vancomycin plus ceftaroline for persistent methicillin‐Resistantstaphylococcus aureusbacteremia. Pharmacotherapy 43, 15–23. doi: 10.1002/phar.2741
Kung, Y.-H., Yeh, Y.-C., and Kuo, K.-C. (2020). Clinical characteristics and predictors of community-acquired pseudomonas aeruginosa sepsis and nontyphoidal salmonella sepsis in infants: A matched case–control study. Pediatr. Neonat. 61, 522–528. doi: 10.1016/j.pedneo.2020.05.008
Kutlu, M., Sayın-Kutlu, S., Alp-Çavuş, S., Öztürk, Ş. B., Taşbakan, M., Özhak, B., et al. (2022). Mortality-associated factors of candidemia: a multi-center prospective cohort in Turkey. Eur. J. Clin. Microbiol. Infect. Dis. 41, 597–607. doi: 10.1007/s10096-021-04394-0
Kwiecinski, J. M., and Horswill, A. R. (2020). Staphylococcus aureus bloodstream infections: pathogenesis and regulatory mechanisms. Curr. Opin. Microbiol. 53, 51–60. doi: 10.1016/j.mib.2020.02.005
Kwon, Y. J., Won, E. J., Jeong, S. H., Shin, K. S., Shin, J. H., Kim, Y. R., et al. (2021). Dynamics and predictors of mortality due to Candidemia caused by different Candida species: comparison of intensive care unit-associated Candidemia (ICUAC) and non-ICUAC. J. Fungi 7:597. doi: 10.3390/jof7080597
Lamba, M., Sharma, D., Sharma, R., Vyas, A., and Mamoria, V. (2021). To study the profile of Candida isolates and antifungal susceptibility pattern of neonatal sepsis in a tertiary care hospital of North India. J. Matern. Fetal Neonat. Med. 34, 2655–2659. doi: 10.1080/14767058.2019.1670799
Lansbury, L., Lim, B., Baskaran, V., and Lim, W. S. (2020). Co-infections in people with COVID-19: a systematic review and meta-analysis. J. Inf. Secur. 81, 266–275. doi: 10.1016/j.jinf.2020.05.046
Laux, C., Peschel, A., and Krismer, B. (2019). Staphylococcus aureus colonization of the human nose and interaction with other microbiome members. Microbiol. Spectr. 7, 1–10. doi: 10.1128/microbiolspec.gpp3-0029-2018
Lee, C., Chen, Y., Chen, I., Chen, F., and Chien, C. (2020). Impact of biofilm production by Candida species and antifungal therapy on mortality of patients with candidemia. Mycoses 63, 1382–1391. doi: 10.1111/myc.13179
Lee, W. J., Hsu, J.-F., Chen, Y.-N., Wang, S.-H., Chu, S.-M., Huang, H.-R., et al. (2022). Pediatric Candida bloodstream infections complicated with mixed and subsequent bacteremia: the clinical characteristics and impacts on outcomes. J. Fungi 8:1155. doi: 10.3390/jof8111155
Lee, C. M., Kim, Y.-J., Jung, S.-I., Kim, S. E., Park, W. B., Choe, P. G., et al. (2022). Different clinical characteristics and impact of carbapenem-resistance on outcomes between Acinetobacter baumannii and Pseudomonas aeruginosa bacteraemia: a prospective observational study. Sci. Rep. 12:8527. doi: 10.1038/s41598-022-12482-0
Lee, E. H., Lee, K. H., Lee, S. J., Kim, J., Baek, Y. J., Ahn, J. Y., et al. (2022). Clinical and microbiological characteristics of and risk factors for bloodstream infections among patients with extracorporeal membrane oxygenation: a single-center retrospective cohort study. Sci. Rep. 12:15059. doi: 10.1038/s41598-022-19405-z
Lee, S., Song, K.-H., Jung, S.-I., Park, W. B., Lee, S. H., Kim, Y.-S., et al. (2018). Comparative outcomes of cefazolin versus nafcillin for methicillin-susceptible Staphylococcus aureus bacteraemia: a prospective multicentre cohort study in Korea. CMI 24, 152–158. doi: 10.1016/j.cmi.2017.07.001
Li, X., Gu, N., Huang, T. Y., Zhong, F., and Peng, G. (2023). Pseudomonas aeruginosa: A typical biofilm forming pathogen and an emerging but underestimated pathogen in food processing. Front. Microbiol. 13:1114199. doi: 10.3389/fmicb.2022.1114199
Li, M., Yang, S., Yao, H., Liu, Y., and Du, M. (2023). Retrospective analysis of epidemiology, risk factors, and outcomes of health care-acquired Carbapenem-resistant Klebsiella pneumoniae bacteremia in a Chinese tertiary hospital, 2010–2019. Infect. Dis. Ther. 12, 473–485. doi: 10.1007/s40121-022-00732-7
Liao, Y., Hu, G.-H., Xu, Y.-F., Che, J.-P., Luo, M., Zhang, H.-M., et al. (2017). Retrospective analysis of fosfomycin combinational therapy for sepsis caused by carbapenem-resistant Klebsiella pneumoniae. Exp. Ther. Med. 13, 1003–1010. doi: 10.3892/etm.2017.4046
Liao, C.-H., Huang, Y.-T., and Hsueh, P.-R. (2022). Multicenter surveillance of capsular serotypes, virulence genes, and antimicrobial susceptibilities of Klebsiella pneumoniae causing bacteremia in Taiwan, 2017–2019. Front. Microbiol. 13:783523. doi: 10.3389/fmicb.2022.783523
Lin, G.-L., McGinley, J. P., Drysdale, S. B., and Pollard, A. J. (2018). Epidemiology and immune pathogenesis of viral Sepsis. Front. Immunol. 9:2147. doi: 10.3389/fimmu.2018.02147
Liu, C., Bayer, A., Cosgrove, S. E., Daum, R. S., Fridkin, S. K., Gorwitz, R. J., et al. (2011). Clinical practice guidelines by the Infectious Diseases Society of America for the treatment of methicillin-resistant Staphylococcus aureus infections in adults and children. Clin. Infect. Dis. 52, e18–e55. doi: 10.1093/cid/ciq146
Liu, J., Ding, H., Zhao, M., Tu, F., He, T., Zhang, L., et al. (2022). Functionalized erythrocyte membrane-coated nanoparticles for the treatment of Klebsiella pneumoniae-induced Sepsis. Front. Microbiol. 13:901979. doi: 10.3389/fmicb.2022.901979
Liu, Y., du, F. L., Liu, P., Mei, Y., Wan, L., Wei, D., et al. (2018). Molecular epidemiology and virulence features of Staphylococcus aureus bloodstream isolates in a regional burn Center in China, 2012–2016. Microb. Drug Resist. 24, 1354–1360. doi: 10.1089/mdr.2017.0209
Liu, F., Zhong, L., Zhou, F., Zheng, C., Zhang, K., Cai, J., et al. (2021). Clinical features, strain distribution, antifungal resistance and prognosis of patients with non-albicans Candidemia: A retrospective observational study. Infect Drug Resist 14, 3233–3246. doi: 10.2147/idr.s323583
Lo, H.-J., Köhler, J. R., DiDomenico, B., Loebenberg, D., Cacciapuoti, A., and Fink, G. R. (1997). Nonfilamentous C. albicans Mutants Are Avirulent. Cells 90, 939–949. doi: 10.1016/s0092-8674(00)80358-x
Loof, T. G., Sohail, A., Bahgat, M. M., Tallam, A., Arshad, H., Akmatov, M. K., et al. (2018). Early lymphocyte loss and increased granulocyte/lymphocyte ratio predict systemic spread of Streptococcus pyogenes in a mouse model of acute skin infection. Front. Microbiol. 8:101. doi: 10.3389/fcimb.2018.00101
Lu, B., Fang, Y., Fan, Y., Chen, X., Wang, J., Zeng, J., et al. (2017). High prevalence of macrolide-resistance and molecular characterization of Streptococcus pyogenes isolates circulating in China from 2009 to 2016. Front. Microbiol. 8:1052. doi: 10.3389/fmicb.2017.01052
Lu, S.-L., Omori, H., Zhou, Y., Lin, Y.-S., Liu, C.-C., Wu, J.-J., et al. (2022). VEGF-mediated augmentation of Autophagic and lysosomal activity in endothelial cells defends against intracellular Streptococcus pyogenes. MBio 13:e0123322. doi: 10.1128/mbio.01233-22
Lv, D., Zuo, Y., Wang, Y., Wang, Z., and Xu, Y. (2022). Predictors of occurrence and 30-Day mortality for co-infection of Carbapenem-resistant Klebsiella pneumoniae and Carbapenem-resistant Acinetobacter baumannii. Front Cell Infect Microbiol. 12:919414. doi: 10.3389/fcimb.2022.919414
Martin, R. M., Cao, J., Brisse, S., Passet, V., Wu, W., Zhao, L., et al. (2016). Molecular epidemiology of colonizing and infecting isolates of Klebsiella pneumoniae. Msphere 1:e00261. doi: 10.1128/msphere.00261-16
Masterson, C. H., Murphy, E. J., Gonzalez, H., Major, I., McCarthy, S. D., O'Toole, D., et al. (2020). Purified β-glucans from the shiitake mushroom ameliorates antibiotic-resistant Klebsiella pneumoniae-induced pulmonary sepsis. Lett. Appl. Microbiol. 71, 405–412. doi: 10.1111/lam.13358
Mazzanti, S., Brescini, L., Morroni, G., Orsetti, E., Pocognoli, A., Donati, A., et al. (2021). Candidemia in intensive care units over nine years at a large Italian university hospital: comparison with other wards. PLoS One 16:e0252165. doi: 10.1371/journal.pone.0252165
McDaniel, M. S., Schoeb, T., and Swords, W. E. (2020). Cooperativity between Stenotrophomonas maltophilia and Pseudomonas aeruginosa during Polymicrobial airway infections. Infect. Immun. 88, 1–15. doi: 10.1128/iai.00855-19
McHale, T. M., Garciarena, C. D., Fagan, R. P., Smith, S. G. J., Martin-Loches, I., Curley, G. F., et al. (2018). Inhibition of vascular endothelial cell leak following Escherichia coli attachment in an experimental model of Sepsis. Crit. Care Med. 46, e805–e810. doi: 10.1097/ccm.0000000000003219
McNeil, J. C., Joseph, M., Sommer, L. M., and Flores, A. R. (2022). Staphylococcus aureus colonization in healthy children during the first year of the severe acute respiratory syndrome coronavirus 2 pandemic. J. Pediatr. 249, 101–105.e1. doi: 10.1016/j.jpeds.2022.06.025
Meehan, M., Murchan, S., Gavin, P. J., Drew, R. J., and Cunney, R. (2018). Epidemiology of an upsurge of invasive group A streptococcal infections in Ireland, 2012–2015. J. Inf. Secur. 77, 183–190. doi: 10.1016/j.jinf.2018.05.010
Meyahnwi, D., Siraw, B. B., and Reingold, A. (2022). Epidemiologic features, clinical characteristics, and predictors of mortality in patients with candidemia in Alameda County, California; a 2017–2020 retrospective analysis. BMC Infect. Dis. 22:843. doi: 10.1186/s12879-022-07848-8
Mohr, A., Simon, M., Joha, T., Hanses, F., Salzberger, B., and Hitzenbichler, F. (2020). Epidemiology of candidemia and impact of infectious disease consultation on survival and care. Infection 48, 275–284. doi: 10.1007/s15010-020-01393-9
Monecke, S., Syed, M. A., Khan, M. A., Ahmed, S., Tabassum, S., Gawlik, D., et al. (2020). Genotyping of methicillin-resistant Staphylococcus aureus from sepsis patients in Pakistan and detection of antibodies against staphylococcal virulence factors. Eur. J. Clin. Microbiol. Infect. Dis. 39, 85–92. doi: 10.1007/s10096-019-03695-9
Morens, D. M., and Fauci, A. S. (2007). The 1918 influenza pandemic: insights for the 21st century. J. Infect. Dis. 195, 1018–1028. doi: 10.1086/511989
Morgado, S., Fonseca, E., and Vicente, A. C. (2022). Genomics of Klebsiella pneumoniae species complex reveals the circulation of high-risk multidrug-resistant pandemic clones in human, animal, and environmental sources. Microorganisms 10:2281. doi: 10.3390/microorganisms10112281
Mu, A., Klare, W. P., Baines, S. L., Ignatius Pang, C. N., Guérillot, R., Harbison-Price, N., et al. (2023). Integrative omics identifies conserved and pathogen-specific responses of sepsis-causing bacteria. Nat. Commun. 14:1530. doi: 10.1038/s41467-023-37200-w
Murray, C. J. L., Ikuta, K. S., Sharara, F., Swetschinski, L., Robles Aguilar, G., Gray, A., et al. (2022). Global burden of bacterial antimicrobial resistance in 2019: a systematic analysis. Lancet 399, 629–655. doi: 10.1016/s0140-6736(21)02724-0
Naylor, N. R., Atun, R., Zhu, N., Kulasabanathan, K., Silva, S., Chatterjee, A., et al. (2018). Estimating the burden of antimicrobial resistance: a systematic literature review. Antimicrob. Resist. Infect. Control 7:58. doi: 10.1186/s13756-018-0336-y
Ngamchokwathana, C., Chongtrakool, P., Waesamaae, A., and Chayakulkeeree, M. (2021). Risk factors and outcomes of non-albicans Candida bloodstream infection in patients with Candidemia at Siriraj hospital—Thailand’s largest National Tertiary Referral Hospital. J. Fungi 7:269. doi: 10.3390/jof7040269
Nichols, K. B., Totsika, M., Moriel, D. G., Lo, A. W., Yang, J., Wurpel, D. J., et al. (2016). Molecular characterization of the Vacuolating autotransporter toxin in Uropathogenic Escherichia coli. J. Bacteriol. 198, 1487–1498. doi: 10.1128/jb.00791-15
Ohnuma, T., Chihara, S., Costin, B., Treggiari, M., Bartz, R., Raghunathan, K., et al. (2023). Epidemiology, resistance profiles, and outcomes of bloodstream infections in community-onset Sepsis in the United States. Crit. Care Med. doi: 10.1097/ccm.0000000000005870 (Epub ahead of print).
Okun, E., Arumugam, T. V., Tang, S., Gleichmann, M., Albeck, M., Sredni, B., et al. (2007). The organotellurium compound ammonium trichloro(dioxoethylene-0,0′) tellurate enhances neuronal survival and improves functional outcome in an ischemic stroke model in mice. J. Neurochem. 102, 1232–1241. doi: 10.1111/j.1471-4159.2007.04615.x
Omrani, A. S., Koleri, J., Ben Abid, F., Daghfel, J., Odaippurath, T., Peediyakkal, M. Z., et al. (2021). Clinical characteristics and risk factors for COVID-19-associated Candidemia. Med. Mycol. 59, 1262–1266. doi: 10.1093/mmy/myab056
Osuchowski, M. F., Ayala, A., Bahrami, S., Bauer, M., Boros, M., Cavaillon, J.-M., et al. (2018). Minimum quality threshold in pre-clinical sepsis studies (MQTiPSS): an international expert consensus initiative for improvement of animal modeling in sepsis. Intensive Care Med. Exp. 6:26. doi: 10.1186/s40635-018-0189-y
Otero-Asman, J. R., García-García, A. I., Civantos, C., Quesada, J. M., and Llamas, M. A. (2019). Pseudomonas aeruginosa possesses three distinct systems for sensing and using the host molecule haem. Environ. Microbiol. 21, 4629–4647. doi: 10.1111/1462-2920.14773
Otto, N. A., Pereverzeva, L., Leopold, V., Ramirez-Moral, I., Roelofs, J. J. T. H., Heijst, J. W. J.van, et al. (2021). Hypoxia-inducible factor-1α in macrophages, but not in neutrophils, is important for host defense during Klebsiella pneumoniae-induced Pneumosepsis. Mediat. Inflamm. 2021, 1–12. doi: 10.1155/2021/9958281
Pachón-Ibáñez, M. E., Labrador-Herrera, G., Cebrero-Cangueiro, T., Díaz, C., Smani, Y., Palacio, J. P.del, et al. (2018). Efficacy of Colistin and its combination with rifampin in vitro and in experimental models of infection caused by Carbapenemase-producing clinical isolates of Klebsiella pneumoniae. Front. Microbiol. 9::912. doi: 10.3389/fmicb.2018.00912
Pappas, P. G., Kauffman, C. A., Andes, D. R., Clancy, C. J., Marr, K. A., Ostrosky-Zeichner, L., et al. (2016). Clinical practice guideline for the Management of Candidiasis: 2016 update by the Infectious Diseases Society of America. Clin. Infect. Dis. 62, e1–e50. doi: 10.1093/cid/civ933
Park, S. Y., Lee, J. S., Oh, J., and Park, J.-Y. (2020). Delta neutrophil index as a predictive and prognostic factor for Candidemia patients: a matched case-control study. BMC Infect. Dis. 20:396. doi: 10.1186/s12879-020-05117-0
Parruti, G., Frattari, A., Polilli, E., Savini, V., Sciacca, A., Consorte, A., et al. (2019). Cure of recurring Klebsiella pneumoniae carbapenemase-producing Klebsiella pneumoniae septic shock episodes due to complicated soft tissue infection using a ceftazidime and avibactam-based regimen: a case report. J. Med. Case Rep. 13:20. doi: 10.1186/s13256-018-1934-2
Patricio, P., Paiva, J. A., and Borrego, L. M. (2019). Immune response in bacterial and Candida Sepsis. Eur. J. Microbiol. Immunol. 9, 105–113. doi: 10.1556/1886.2019.00011
Perlee, D., Vos, A. F., Scicluna, B. P., Mancheño, P., Rosa, O., Dalemans, W., et al. (2019). Human adipose-derived mesenchymal stem cells modify lung immunity and improve antibacterial defense in Pneumosepsis caused by Klebsiella pneumoniae. Stem Cells Transl. Med. 8, 785–796. doi: 10.1002/sctm.18-0260
Pfaller, M. A., Diekema, D. J., Turnidge, J. D., Castanheira, M., and Jones, R. N. (2019). Twenty years of the SENTRY antifungal surveillance program: results for Candida species from 1997–2016. Open Forum Infect. Dis. 6, S79–S94. doi: 10.1093/ofid/ofy358
Pieralli, F., Dentali, F., Giusti, M., Ciarambino, T., Mazzone, A., Concia, E., et al. (2021). Clinical characteristics, management and outcome of patients with invasive candidiasis hospitalized in internal medicine units: findings from a registry by the Italian scientific society FADOI. Infection 49, 277–285. doi: 10.1007/s15010-020-01535-z
Poissy, J., Damonti, L., Bignon, A., Khanna, N., von Kietzell, M., Boggian, K., et al. (2020). Risk factors for candidemia: a prospective matched case-control study. Crit. Care 24:109. doi: 10.1186/s13054-020-2766-1
Pujol, M., Miró, J.-M., Shaw, E., Aguado, J.-M., San-Juan, R., Puig-Asensio, M., et al. (2020). Daptomycin plus Fosfomycin versus Daptomycin alone for methicillin-resistant Staphylococcus aureus bacteremia and endocarditis. A randomized clinical trial. Clin. Infect. Dis. 72, 1517–1525. doi: 10.1093/cid/ciaa1081
Rajni, E., Singh, A., Tarai, B., Jain, K., Shankar, R., Pawar, K., et al. (2021). A high frequency ofCandida aurisBlood stream infections in coronavirus disease 2019 patients admitted to intensive care units, northwestern India: a case control study. Open Forum Infect. Dis. 8:ofab452. doi: 10.1093/ofid/ofab452
Rasmussen, G., Idosa, B. A., Bäckman, A., Monecke, S., Strålin, K., Särndahl, E., et al. (2019). Caspase-1 inflammasome activity in patients with Staphylococcus aureus bacteremia. Microbiol. Immunol. 63, 487–499. doi: 10.1111/1348-0421.12738
Recio, R., Viedma, E., González-Bodí, S., Villa, J., Orellana, M. Á., Mancheño-Losa, M., et al. (2021). Clinical and bacterial characteristics of Pseudomonas aeruginosa affecting the outcome of patients with bacteraemic pneumonia. Antimicrobial Agents Annu. 58:106450. doi: 10.1016/j.ijantimicag.2021.106450
Recker, M., Laabei, M., Toleman, M. S., Reuter, S., Saunderson, R. B., Blane, B., et al. (2017). Clonal differences in Staphylococcus aureus bacteraemia-associated mortality. Nat. Microbiol. 2, 1381–1388. doi: 10.1038/s41564-017-0001-x
Renieris, G., Droggiti, D.-E., Katrini, K., Koufargyris, P., Gkavogianni, T., Karakike, E., et al. (2021). Host cystathionine-γ lyase derived hydrogen sulfide protects against Pseudomonas aeruginosa sepsis. PLoS Pathog. 17:e1009473. doi: 10.1371/journal.ppat.1009473
Rieg, S., Joost, I., Weiß, V., Peyerl-Hoffmann, G., Schneider, C., Hellmich, M., et al. (2017). Combination antimicrobial therapy in patients with Staphylococcus aureus bacteraemia—a post hoc analysis in 964 prospectively evaluated patients. CMI 23, 406.e1–406.e8. doi: 10.1016/j.cmi.2016.08.026
Riera, F. O., Caeiro, J. P., Angiolini, S. C., Vigezzi, C., Rodriguez, E., Icely, P. A., et al. (2022). Invasive candidiasis: update and current challenges in the Management of this Mycosis in South America. Antibiotics 11:877. doi: 10.3390/antibiotics11070877
Ripoli, A., Sozio, E., Sbrana, F., Bertolino, G., Pallotto, C., Cardinali, G., et al. (2020). Personalized machine learning approach to predict candidemia in medical wards. Infection 48, 749–759. doi: 10.1007/s15010-020-01488-3
Ron, M., Brosh-Nissimov, T., Korenman, Z., Treygerman, O., Sagi, O., Valinsky, L., et al. (2022). Invasive multidrug-resistant emm93.0 Streptococcus pyogenes strain harboring a novel Genomic Island, Israel, 2017-2019. Emerg. Infect. Dis. 28, 118–126. doi: 10.3201/eid2801.210733
Rudd, K. E., Johnson, S. C., Agesa, K. M., Shackelford, K. A., Tsoi, D., Kievlan, D. R., et al. (2020). Global, regional, and national sepsis incidence and mortality, 1990–2017: analysis for the global burden of disease study. Lancet 395, 200–211. doi: 10.1016/s0140-6736(19)32989-7
Said, K. B., Alsolami, A., Moussa, S., Alfouzan, F., Bashir, A. I., Rashidi, M., et al. (2022). COVID-19 clinical profiles and fatality rates in hospitalized patients reveal case aggravation and selective co-infection by limited gram-negative Bacteria. Environ. Res. Public Health 19:5270. doi: 10.3390/ijerph19095270
Sánchez-Encinales, V., Ludwig, G., Tamayo, E., García-Arenzana, J. M., Muñoz-Almagro, C., and Montes, M. (2019). Molecular characterization of Streptococcus pyogenes causing invasive disease in pediatric population in Spain A 12-year study. Pediatr. Infect. Dis. J. 38, 1168–1172. doi: 10.1097/inf.0000000000002471
Santos, A. P., Gonçalves, L. C., Oliveira, A. C. C., Queiroz, P. H. P., Ito, C. R. M., Santos, M. O., et al. (2022). Bacterial co-infection in patients with COVID-19 hospitalized (ICU and not ICU): review and Meta-analysis. Antibiotics 11:894. doi: 10.3390/antibiotics11070894
Satoh, K., Makimura, K., Hasumi, Y., Nishiyama, Y., Uchida, K., and Yamaguchi, H. (2009). Candida auris sp. nov., a novel ascomycetous yeast isolated from the external ear canal of an inpatient in a Japanese hospital. Microbiol. Immunol. 53, 41–44. doi: 10.1111/j.1348-0421.2008.00083.x
Schmitz, M., Roux, X., Huttner, B., and Pugin, J. (2018). Streptococcal toxic shock syndrome in the intensive care unit. Ann. Intensive Care 8:88. doi: 10.1186/s13613-018-0438-y
Shah, P. J., Tran, T., Emelogu, F., and Tariq, F. (2021). Aztreonam, ceftazidime/avibactam, and Colistin combination for the Management of Carbapenemase-Producing Klebsiella Pneumoniae Bacteremia: A case report. J. Pharm. Pract. 34, 653–657. doi: 10.1177/0897190019882262
Shakoor, S., Khan, E., Mir, F., Malik, F. R., and Jamil, B. (2017). Secular trends of Streptococcus pyogenes sepsis in Pakistan and analysis of clinical features in a hospitalized cohort. Trop. Biomed. 34, 648–656.
Shane, A. L., Sánchez, P. J., and Stoll, B. J. (2017). Neonatal sepsis. Lancet 390, 1770–1780. doi: 10.1016/s0140-6736(17)31002-4
Shastri, P. S., Shankarnarayan, S. A., Oberoi, J., Rudramurthy, S. M., Wattal, C., and Chakrabarti, A. (2020). Candida auris candidaemia in an intensive care unit – prospective observational study to evaluate epidemiology, risk factors, and outcome. J. Crit. Care 57, 42–48. doi: 10.1016/j.jcrc.2020.01.004
Shorr, A. F., Tabak, Y. P., Killian, A. D., Gupta, V., Liu, L. Z., and Kollef, M. H. (2006). Healthcare-associated bloodstream infection: A distinct entity? Insights from a large U.S. database&ast. Crit. Care Med. 34, 2588–2595. doi: 10.1097/01.ccm.0000239121.09533.09
Singer, M., Deutschman, C. S., Seymour, C. W., Shankar-Hari, M., Annane, D., Bauer, M., et al. (2016). The third international consensus definitions for Sepsis and septic shock (Sepsis-3). JAMA 315, 801–810. doi: 10.1001/jama.2016.0287
Sitkiewicz, I., and Musser, J. (2017). Deletion of atoR from Streptococcus pyogenes results in Hypervirulence in a mouse model of Sepsis and is LuxS independent. Pol. J. Microbiol. 66, 17–24. doi: 10.5604/17331331.1234989
Somayaji, R., Hantrakun, V., Teparrukkul, P., Wongsuvan, G., Rudd, K. E., Day, N. P. J., et al. (2021). Comparative clinical characteristics and outcomes of patients with community acquired bacteremia caused by Escherichia coli, Burkholderia pseudomallei and Staphylococcus aureus: A prospective observational study (Ubon-sepsis). PLoS Negl. Trop. Dis. 15:e0009704. doi: 10.1371/journal.pntd.0009704
Souli, M., Karaiskos, I., Masgala, A., Galani, L., Barmpouti, E., and Giamarellou, H. (2017). Double-carbapenem combination as salvage therapy for untreatable infections by KPC-2-producing Klebsiella pneumoniae. Eur. J. Clin. Microbiol. Infect. Dis. 36, 1305–1315. doi: 10.1007/s10096-017-2936-5
Stevens, D. L., Bryant, A. E., and Yan, S. (1994). Invasive group A streptococcal infection: new concepts in antibiotic treatment. Int. J. Antimicrob. Agent 4, 297–301. doi: 10.1016/0924-8579(94)90029-9
Stoll, B. J., Puopolo, K. M., Hansen, N. I., Sánchez, P. J., Bell, E. F., Carlo, W. A., et al. (2020). Early-onset neonatal Sepsis 2015 to 2017, the rise of Escherichia coli, and the need for novel prevention strategies. JAMA Pediatr. 174:e200593. doi: 10.1001/jamapediatrics.2020.0593
Sun, X., Dai, Y., Tan, G., Liu, Y., and Li, N. (2020). Integration analysis of m6A-SNPs and eQTLs associated with Sepsis reveals platelet degranulation and Staphylococcus aureus infection are mediated by m6A mRNA methylation. Front. Genet. 11:7. doi: 10.3389/fgene.2020.00007
Sun, J., Uchiyama, S., Olson, J., Morodomi, Y., Cornax, I., Ando, N., et al. (2021). Repurposed drugs block toxin-driven platelet clearance by the hepatic Ashwell-Morell receptor to clear Staphylococcus aureus bacteremia. Sci. Transl. Med. 13:eabd6737. doi: 10.1126/scitranslmed.abd6737
Tabah, A., Buetti, N., Staiquly, Q., Ruckly, S., Akova, M., Aslan, A. T., et al. (2023). Epidemiology and outcomes of hospital-acquired bloodstream infections in intensive care unit patients: the EUROBACT-2 international cohort study. Intensive Care Med. 49, 178–190. doi: 10.1007/s00134-022-06944-2
Tacconelli, E., Autenrieth, I. B., and Peschel, A. (2017). Fighting the enemy within. Science 355, 689–690. doi: 10.1126/science.aam6372
Tan, T. L., Tan-Loh, J., Chiew, S. C., Lim, K. H., Ng, W. W., Akmal, M., et al. (2021). Risk factors and outcome of community onset Pseudomonas aeruginosa bacteraemia in two Malaysian district specialist hospitals. Med. J. Malays. 76, 820–827.
Tang, P.-C., Lee, C.-C., Li, C.-W., Li, M.-C., Ko, W.-C., and Lee, N.-Y. (2017). Time-to-positivity of blood culture: an independent prognostic factor of monomicrobial Pseudomonas aeruginosa bacteremia. J. Microbiol. Immunol. Infect. 50, 486–493. doi: 10.1016/j.jmii.2015.08.014
Tang, Q., Wang, Q., Sun, Z., Kang, S., Fan, Y., and Hao, Z. (2021). Bergenin monohydrate attenuates inflammatory response via MAPK and NF-κB pathways against Klebsiella pneumonia infection. Front. Pharmacol. 12:651664. doi: 10.3389/fphar.2021.651664
Tapia, P., Gatica, S., Cortés-Rivera, C., Otero, C., Becerra, A., Riedel, C. A., et al. (2019). Circulating endothelial cells from septic shock patients convert to fibroblasts are associated with the resuscitation fluid dose and are biomarkers for survival prediction. Crit. Care Med. 47, 942–950. doi: 10.1097/ccm.0000000000003778
Teparrukkul, P., Hantrakun, V., Day, N. P. J., West, T. E., and Limmathurotsakul, D. (2017). Management and outcomes of severe dengue patients presenting with sepsis in a tropical country. PLoS One 12:e0176233. doi: 10.1371/journal.pone.0176233
Thomson, T. N., Campbell, P. T., and Gibney, K. B. (2022). The epidemiology of invasive group A streptococcal disease in Victoria, 2007–2017: an analysis of linked datasets. Austral. NZ. J. Publ. Health 46, 878–883. doi: 10.1111/1753-6405.13290
Togawa, A., Toh, H., Onozawa, K., Yoshimura, M., Tokushige, C., Shimono, N., et al. (2015). Influence of the bacterial phenotypes on the clinical manifestations in Klebsiella pneumoniae bacteremia patients: A retrospective cohort study. J. Infect. Chemother. 21, 531–537. doi: 10.1016/j.jiac.2015.04.004
Tsay, R.-W., Siu, L. K., Fung, C.-P., and Chang, F.-Y. (2002). Characteristics of bacteremia between community-acquired and nosocomial Klebsiella pneumoniae infection: risk factor for mortality and the impact of capsular serotypes as a herald for community-acquired infection. Arch. Intern. Med. 162, 1021–1027. doi: 10.1001/archinte.162.9.1021
Tumbarello, M., Spanu, T., Sanguinetti, M., Citton, R., Montuori, E., Leone, F., et al. (2006). Bloodstream infections caused by extended-Spectrum-β-lactamase-Producingklebsiella pneumoniae: risk factors, molecular epidemiology, and clinical outcome. ASM J. CD 50, 498–504. doi: 10.1128/aac.50.2.498-504.2006
Turner, C. E. (2022). Can group A streptococcus infections be influenced by viruses in the respiratory tract? Lancet Infect. Dis. 23, 142–144. doi: 10.1016/s1473-3099(22)00865-9
Turnidge, J. D., Kotsanas, D., Munckhof, W., Roberts, S., Bennett, C. M., Nimmo, G. R., et al. (2009). Staphylococcus aureus bacteraemia: a major cause of mortality in Australia and New Zealand. Med. J. Austral. 191, 368–373. doi: 10.5694/j.1326-5377.2009.tb02841.x
Ulloa, E., Uchiyama, S., Gillespie, R., Nizet, V., and Sakoulas, G. (2021). Ticagrelor increases platelet-mediated Staphylococcus aureus killing resulting in clearance of bacteremia. J. Infect. Dis. 224:jiab146. doi: 10.1093/infdis/jiab146
van Nieuwenhuyse, B., van der Linden, D., Chatzis, O., Lood, C., Wagemans, J., Lavigne, R., et al. (2022). Bacteriophage-antibiotic combination therapy against extensively drug-resistant Pseudomonas aeruginosa infection to allow liver transplantation in a toddler. Nat. Commun. 13:5725. doi: 10.1038/s41467-022-33294-w
Vandendriessche, S., de Boeck, H., Deplano, A., Phoba, M.-F., Lunguya, O., Falay, D., et al. (2017). Characterisation of Staphylococcus aureus isolates from bloodstream infections, Democratic Republic of the Congo. Eur. J. Clin. Microbiol. Infect. Dis. 36, 1163–1171. doi: 10.1007/s10096-017-2904-0
Vázquez-Olvera, R., Volkow, P., Velázquez-Acosta, C., and Cornejo-Juárez, P. (2023). Candida bloodstream infection in patients with cancer: A retrospective analysis of an 11-year period. Rev. Iberoam. Micol. 40, 3–9. doi: 10.1016/j.riam.2022.12.002
Vázquez-Ucha, J. C., Arca-Suárez, J., Bou, G., and Beceiro, A. (2020). New Carbapenemase inhibitors: clearing the way for the β-lactams. Int. J. Mol. Sci. 21:9308. doi: 10.3390/ijms21239308
Vilhonen, J., Vuopio, J., Vahlberg, T., Gröndahl-Yli-Hannuksela, K., Rantakokko-Jalava, K., and Oksi, J. (2020). Group A streptococcal bacteremias in Southwest Finland 2007–2018: epidemiology and role of infectious diseases consultation in antibiotic treatment selection. Eur. J. Clin. Microbiol. Infect. Dis. 39, 1339–1348. doi: 10.1007/s10096-020-03851-6
Villalón, P., Bárcena, M., Medina-Pascual, M. J., Garrido, N., Pino-Rosa, S., Carrasco, G., et al. (2023). National Surveillance of tetracycline, erythromycin, and clindamycin resistance in invasive Streptococcus pyogenes: A retrospective study of the situation in Spain, 2007–2020. Antibiotics 12:99. doi: 10.3390/antibiotics12010099
Walker, M. J., Barnett, T. C., McArthur, J. D., Cole, J. N., Gillen, C. M., Henningham, A., et al. (2014). Disease manifestations and pathogenic mechanisms of group A Streptococcus. Clin. Microbiol. Rev. 27, 264–301. doi: 10.1128/cmr.00101-13
Weiner-Lastinger, L. M., Abner, S., Edwards, J. R., Kallen, A. J., Karlsson, M., Magill, S. S., et al. (2020). Antimicrobial-resistant pathogens associated with adult healthcare-associated infections: summary of data reported to the National Healthcare Safety Network, 2015–2017. Infect. Control. 41, 1–18. doi: 10.1017/ice.2019.296
Wesolek, J. L., McNorton, K., Delgado, G., and Giuliano, C. A. (2018). Effect of vancomycin initial dosing on time to systemic inflammatory response syndrome resolution in patients with methicillin-resistant Staphylococcus aureus bacteremia. J. Chemother. 30, 101–106. doi: 10.1080/1120009x.2017.1389807
World Health Organization (WHO) (2017). Improving the prevention, diagnosis and clinical management of sepsis. Available at: https://apps.who.int/gb/ebwha/pdf_files/WHA70/A70_13-en.pdf
World Health Organization (WHO) (2022a). Global antimicrobial resistance and use surveillance system (GLASS) report 2022. Available at: https://www.who.int/publications/i/item/9789240062702
World Health Organization (WHO) (2022b). WHO fungal priority pathogens list to guide research, development and public health action. Available at: https://www.who.int/publications/i/item/9789240060241
Wu, H., Chen, H., Wang, J., Yin, S., Huang, J., Wang, Z., et al. (2021). Identification of key genes associated with sepsis patients infected by staphylococcus aureus through weighted gene co-expression network analysis. Am. J. Transl. Res. 13, 13579–13589.
Wu, Y. M., Huang, P.-Y., Cheng, Y.-C., Lee, C.-H., Hsu, M.-C., Lu, J.-J., et al. (2021). Enhanced virulence of Candida albicans by Staphylococcus aureus: evidence in clinical bloodstream infections and infected zebrafish embryos. J. Fungi 7:1099. doi: 10.3390/jof7121099
Wu, X., Shi, Q., Shen, S., Huang, C., and Wu, H. (2021). Clinical and bacterial characteristics of Klebsiella pneumoniae affecting 30-Day mortality in patients with bloodstream infection. Front Cell Infect Microbiol. 11:688989. doi: 10.3389/fcimb.2021.688989
Wu, Z., Tian, Y., Alam, H. B., Li, P., Duan, X., Williams, A. M., et al. (2020). Peptidylarginine deiminases 2 mediates Caspase-1-associated lethality in Pseudomonas aeruginosa pneumonia-induced Sepsis. J. Infect. Dis. 223, 1093–1102. doi: 10.1093/infdis/jiaa475
Wu, T., Xu, F., Su, C., Li, H., Lv, N., Liu, Y., et al. (2020). Alterations in the gut microbiome and Cecal metabolome during Klebsiella pneumoniae-induced Pneumosepsis. Front. Immunol. 11:1331. doi: 10.3389/fimmu.2020.01331
Wurster, S., Albert, N. D., and Kontoyiannis, D. P. (2022). Candida auris bloodstream infection induces upregulation of the PD-1/PD-L1 immune checkpoint pathway in an immunocompetent mouse model. Msphere 7, e00817–e00821. doi: 10.1128/msphere.00817-21
Xiong, W., Perna, A., Jacob, I. B., Lundgren, B. R., and Wang, G. (2022). The enhancer-binding protein MifR, an essential regulator of α-ketoglutarate transport, is required for full virulence of Pseudomonas aeruginosa PAO1 in a mouse model of pneumonia. Infect. Immun. 90, e00122–e00136. doi: 10.1128/iai.00136-22
Yang, T. Y., Hsieh, Y.-J., Kao, L.-T., Liu, G. H., Lian, S.-H., Wang, L.-C., et al. (2022). Activities of imipenem-relebactam combination against carbapenem-nonsusceptible Enterobacteriaceae in Taiwan. J. Microbiol. Immunol. Infect. 55, 86–94. doi: 10.1016/j.jmii.2021.02.001
Yang, T.-Y., Kao, H.-Y., Lu, P.-L., Chen, P.-Y., Wang, S.-C., Wang, L.-C., et al. (2021). Evaluation of the Organotellurium compound AS101 for treating Colistin- and Carbapenem-resistant Klebsiella pneumoniae. Pharmaceuticals 14:795. doi: 10.3390/ph14080795
Yang, F., Zhou, Y., Chen, P., Cai, Z., Yue, Z., Jin, Y., et al. (2022). High-level expression of cell-surface signaling system Hxu enhances Pseudomonas aeruginosa bloodstream infection. Infect. Immun. 90:e0032922. doi: 10.1128/iai.00329-22
Yi, Y.-H., Wang, J.-L., Yin, W.-J., and Xu, W.-H. (2021). Vancomycin or Daptomycin plus a β-lactam versus vancomycin or Daptomycin alone for methicillin-resistant Staphylococcus aureus bloodstream infections: A systematic review and Meta-analysis. Microb. Drug Resist. 27, 1044–1056. doi: 10.1089/mdr.2020.0350
Yoo, J. R., Shin, B. R., Jo, S., and Heo, S. T. (2020). Evaluation of the early fluconazole treatment of candidemia protocol with automated short message service alerts: a before-and-after study. Kor J Intern Med 36, 699–705. doi: 10.3904/kjim.2019.259
Zahedi bialvaei, A., Rahbar, M., Hamidi-Farahani, R., Asgari, A., Esmailkhani, A., Mardani dashti, Y., et al. (2021a). Expression of RND efflux pumps mediated antibiotic resistance in Pseudomonas aeruginosa clinical strains. Microb. Pathog. 153:104789. doi: 10.1016/j.micpath.2021.104789
Zahedi bialvaei, A., Razavi, S., Notash Haghighat, F., Hemmati, A., Akhavan, M. M., Jeddi-Tehrani, M., et al. (2021b). Monoclonal antibody directed to the PilQ -PilA DSL region in Pseudomonas aeruginosa improves survival of infected mice with antibiotic combination. Microb. Pathog. 158:105060. doi: 10.1016/j.micpath.2021.105060
Zhang, W., Song, X., Wu, H., and Zheng, R. (2020). Epidemiology, species distribution, and predictive factors for mortality of candidemia in adult surgical patients. BMC Infect. Dis. 20:506. doi: 10.1186/s12879-020-05238-6
Zhao, R., Wang, X., Wang, X., du, B., Xu, K., Zhang, F., et al. (2022). Molecular characterization and virulence gene profiling of methicillin-resistant Staphylococcus aureus associated with bloodstream infections in southern China. Front. Microbiol. 13:1008052. doi: 10.3389/fmicb.2022.1008052
Zheng, G., Zhang, J., Wang, B., Cai, J., Wang, L., Hou, K., et al. (2021). Ceftazidime-avibactam in combination with in vitro non-susceptible antimicrobials versus ceftazidime-avibactam in monotherapy in critically ill patients with Carbapenem-resistant Klebsiella Pneumoniae infection: A retrospective cohort study. Infect. Dis. Ther. 10, 1699–1713. doi: 10.1007/s40121-021-00479-7
Zhong, L., Dong, Z., Liu, F., Li, H., Tang, K., Zheng, C., et al. (2022). Incidence, clinical characteristics, risk factors and outcomes of patients with mixed Candida/bacterial bloodstream infections: a retrospective study. ACMA 21:45. doi: 10.1186/s12941-022-00538-y
Zhong, L., Zhang, S., Tang, K., Zhou, F., Zheng, C., Zhang, K., et al. (2020). Clinical characteristics, risk factors and outcomes of mixed Candida albicans/bacterial bloodstream infections. BMC Infect. Dis. 20:810. doi: 10.1186/s12879-020-05536-z
Zhou, W., Jin, Y., Zhou, Y., Wang, Y., Xiong, L., Luo, Q., et al. (2021). Comparative genomic analysis provides insights into the evolution and genetic diversity of community-genotype sequence type 72 Staphylococcus aureus isolates. Msystems 6:e0098621. doi: 10.1128/msystems.00986-21
Glossary
Keywords: sepsis, inflammation, immunology, microorganisms, diagnostics, prognosis, therapy
Citation: Gatica S, Fuentes B, Rivera-Asín E, Ramírez-Céspedes P, Sepúlveda-Alfaro J, Catalán EA, Bueno SM, Kalergis AM, Simon F, Riedel CA and Melo-Gonzalez F (2023) Novel evidence on sepsis-inducing pathogens: from laboratory to bedside. Front. Microbiol. 14:1198200. doi: 10.3389/fmicb.2023.1198200
Edited by:
Svetlana Khaiboullina, University of Nevada, Reno, United StatesReviewed by:
Antonella Mencacci, University of Perugia, ItalyJavier Chinen, Baylor College of Medicine, United States
Copyright © 2023 Gatica, Fuentes, Rivera-Asín, Ramírez-Céspedes, Sepúlveda-Alfaro, Catalán, Bueno, Kalergis, Simon, Riedel and Melo-Gonzalez. This is an open-access article distributed under the terms of the Creative Commons Attribution License (CC BY). The use, distribution or reproduction in other forums is permitted, provided the original author(s) and the copyright owner(s) are credited and that the original publication in this journal is cited, in accordance with accepted academic practice. No use, distribution or reproduction is permitted which does not comply with these terms.
*Correspondence: Sebastian Gatica, s.gaticafernandez@uandresbello.edu; Felipe Melo-Gonzalez, felipe.melo@unab.cl