- 1Pathogen Biology Group, Rajiv Gandhi Centre for Biotechnology (RGCB), Thiruvananthapuram, India
- 2PhD Program in Biotechnology, Manipal Academy of Higher Education (MAHE), Manipal, India
- 3Department of Gastroenterology, Government Medical College, Thiruvananthapuram, India
Mycobacterium abscessus subsp. abscessus is a rapidly growing facultative intracellular pathogen that usually infects human lung and skin epithelium. Recently, we and another group have shown that it also has the potential to colonize human gastric epithelium, but its significance with respect to gastric diseases remains unclear. Although Helicobacter pylori still remains the only definite gastric pathogen, recent studies have shown that M. abscessus subsp. abscessus also has the potential to colonize human gastric epithelium. M. abscessus subsp. abscessus is known to exhibit multidrug resistance and clarithromycin has been used as the drug of choice. We aimed to determine the clarithromycin resistance profile of 117 (74 rough and 43 smooth) gastric M. abscessus subsp. abscessus strains and to detect the point mutations in rrl and erm (41) genes conferring the resistance. Our data showed 79.48% (19 smooth and 74 rough) of M. abscessus subsp. abscessus strains were resistant to clarithromycin (MIC90 ≤ 512 μg/mL), while 20.51% (24 smooth) were susceptible (MIC90 ≤ 8 μg/mL). Nucleotide sequence analysis of the rrl gene with reference strains of M. abscessus subsp. abscessus did not show any mutation that is relevant to the clarithromycin resistance. However, analysis of erm (41) gene showed that M. abscessus subsp. abscessus strains, which were susceptible to clarithromycin had C, C, G, and C at their nucleotide positions 28, 159, 238, and 330, respectively, while the resistant strains showed T, T, A, and A at the same positions. Based on antibiogram and sequence analysis data we recommend further studies involving genomic analysis to identify the other genes involved in high clarithromycin resistance in gastric M. abscessus subsp. abscessus along with the mechanisms involved.
Introduction
Mycobacterium abscessus subspecies abscessus (Mycobacterium abscessus subsp. abscessus) is a non-tuberculous mycobacteria (NTM) and is known for its rapid growth and resistance to multiple drugs. It is known to cause pulmonary infection and skin and soft tissues infections (mostly nosocomial) in humans (Lee et al., 2015). M. abscessus subsp. abscessus infection is difficult to treat because of its intrinsic resistance to most macrolide and other antibiotics including the classical anti-tuberculous drugs (Nessar et al., 2012; Griffith and Daley, 2022).
Recently, we and another group have isolated M. abscessus subsp. abscessus from human gastric epithelium. Interestingly, in Trivandrum, Kerala, India, the prevalence of gastric M. abscessus subsp. abscessus is even higher than the prevalence of Helicobacter pylori, a well-known gastric pathogen, which causes gastric cancer and peptic ulcer (Al-Momani et al., 2017; Chouhan et al., 2019). The common treatment regimen for H. pylori related gastric diseases is a proton pump inhibitor (e.g., Lansoprazole) and antibiotics. Because of the indiscriminate use of metronidazole to prevent amoebiasis in diarrhoea-endemic places in India and other countries, most H. pylori strains are resistant to metronidazole and clarithromycin is mostly the drug of choice against H. pylori (Safavi et al., 2016; Gonzales et al., 2019; Shetty et al., 2019). The significance of gastric colonization of M. abscessus subsp. abscessus with respect to gastric diseases is unknown at present, but the potential of this bacterium to cause diseases should not be neglected. Therefore, the resistance profile of the gastric M. abscessus subsp. abscessus strains against clarithromycin are worth studying for effective management of gastric diseases.
In 1990s, clarithromycin was the choice of drug to eradicate M. abscessus subsp. abscessus (Mushatt and Witzig, 1995; Brown-Elliott and Wallace, 2002). Clarithromycin was not effective against M. abscessus subsp. abscessus with point mutations at A2058G, C and A2059G, C (Escherichia coli numbering) or A2270 → G or C and A2271 → G or C (M. abscessus subsp. abscessus numbering) positions in the rrl gene that encodes the peptidyltransferese domain of 23S rRNA of bacterial ribosome (Wallace et al., 1996). Apart from mutations in rrl gene, another mechanism confers resistance against macrolides in NTM. The M. abscessus subsp. abscessus strains with functional erm (41) gene show an inducible resistance against clarithromycin upon prolonged incubation (14 days), while the M. abscessus subsp. abscessus strains with non-functional erm (41) gene show susceptibility to clarithromycin. In the erm (41) gene, a substitution of T-to-C at position 28 (T28C), which leads to the alteration of Trp to Arg at the 10thamino acid of the peptide, was found to be associated with loss of function and susceptibility to clarithromycin (Nash et al., 2009). The isolates of M. abscessus subsp. massiliense have not shown any inducible macrolide resistance because of a 397-bp deletion in erm (41) gene, which results in a non-functional erm (41) gene (Kim et al., 2010).
The aim of this study was to determine the minimum inhibitory concentration (MIC) of the gastric M. abscessus subsp. abscessus strains against clarithromycin and to understand the genetic basis of the resistance.
Materials and methods
Ethics statement
The study was approved by the Institute Human Ethics Committee of Rajiv Gandhi Centre for Biotechnology (Approval Number IHEC/01/2017/18) and by the Human Ethics Committee of Govt. Medical College, Trivandrum (Approval Number IEC.No.05/07/2016/MCT). Patients between the age of 20 and 70 years were recruited for the study and written informed consents were obtained from all patients. Trivandrum is in the southern part of India and is the capital city of Kerala, mostly the part of western ghats with high humidity.
Mycobacterium abscessus subsp. abscessus culture and characterization
A total of 117 M. abscessus subsp. abscessus (rough and smooth) strains isolated from human gastric biopsies were used in this study. The M. abscessus subsp. abscessus strains were grown on Brain Heart Infusion (BHI) agar plates containing calf serum (7%) and were incubated at 37°C in microaerobic conditions (5% O2, 10% CO2, 85% N2). M. abscessus subsp. abscessus colonies were identified at the species level based on the growth rate, colony morphology (rough and smooth), and pigmentation as well as 16S rRNA gene sequence analysis. Partial nucleotide sequencing of the hsp65 gene was used for further confirmation of gastric M. abscessus subsp. abscessus strains to distinguish them from closely related M. bolletti, M. chelonae and M. massiliense. Phylogenetic analysis was done by using BioEdit software (version 7.2.6.1).
Antibiotic susceptibility
M. abscessus subsp. abscessus strains were tested for clarithromycin (macrolide antibiotic) susceptibility (from 0.125 μg/mL to 512 μg/mL) by agar dilution and broth microdilution assay. BHI plates were prepared using newborn calf serum (7%) and required concentrations of antibiotics, and BHI broth was prepared similarly for the microdilution assay and finally supplemented with antibiotic as per the desired concentration. After 3, 7, and 14 days of incubation with clarithromycin in the microwell, 10 μL liquid culture from each treated well was applied on the BHI plates and were incubated in microaerobic incubator for 3–7 days. To verify the inducible resistance of gastric M. abscessus subsp. abscessus against clarithromycin, gastric M. abscessus subsp. abscessus were pre-treated with clarithromycin (0.1 μg/mL) for 3 days and then the MIC was determined. M. abscessus subsp. abscessus clarithromycin breakpoint (MIC90 > 8 μg/mL) was determined according to the Clinical and Laboratory Standards Institute (CLSI) guideline published in 2011. Broth microdilution-based methodology for antimicrobial susceptibility testing of nontuberculous mycobacteria has been considered the gold standard (Woods et al., 2011).
Bacterial DNA isolation
The bacterial DNA was isolated as previously described (Berg et al., 1997). In brief, the bacterial colonies were harvested in 500 μL PBS and centrifuged at 5,000 rcf for 10 min. The bacterial pellet was resuspended in 200 μL GTE (glucose/tris/EDTA) buffer. The bacterial suspension was then treated with lysozyme (10 mg/mL) at 37°C for 1 h. After enzymatic digestion, bacterial cells were lysed using TES (tris/EDTA/SDS) buffer. Proteinase K (50 μg/mL) and RNase (20 μg/mL) were then added and the tubes were incubated at 55°C for 2 h. The digested bacterial proteins were removed by phenol: chloroform: isoamyl alcohol and then by chloroform: isoamyl alcohol treatments. The bacterial DNA was precipitated using 3 M sodium acetate (pH 5.2) and chilled absolute ethanol. The precipitated DNA was washed with 70% ethanol and the dried pellet was dissolved in 1X TE (tris-EDTA) buffer of pH 8.
PCR amplification and sequencing of the antimicrobial resistance genes of gastric Mycobacterium abscessus strains
Genomic DNA of M. abscessus subsp. abscessus was used for PCR with primers 16S rRNA V3-V5 F2- (5’-GCC TAC GGG AGG CAG CAG-3′) and V3-V5 R2 (5′-ATT ACC GCG GCT GCT GG-3′) for bacterial 16S rRNA gene (Chouhan et al., 2019); primersHSPF3 (5’-ATC GCC AAG GAG ATC GAG CT-3′) and HSPR4 (5′-AAG GTG CCG CGG ATC TTG TT-3′) for hsp65 gene sequencing to distinguish M. abscessus subsp. abscessus from other members of the NTM group (Kim et al., 2005). A total of 46 (31 resistant and 15 susceptible) M. abscessus subsp. abscessus strains were used to detect antibiotic associated mutations in M. abscessus subsp. abscessus erm (41) gene, following primers were used:ermF (F-GAC CGG GGC CTT CTT CGT GAT-3′) and ermR1 (5’-GAC TTC CCC GCA CCG ATT CC-3′) to amplify and erm (41)-4 (5′-CCGGCCCGTAGCGTCCAATG-3′) and ermF were used for cycle sequencing (Brown-Elliott et al., 2016). Another set of primers ERM1f (5’-CGC CAA CGA GCA GCT CG-3′) and MC823 (5’-GAC TTC CCC GCA CCG ATT CCA C-3′) were used to amplify erm (41) gene and to evaluate polymorphism in erm (41) gene (Nash et al., 2009; Bastian et al., 2011). To detect mutations in rrl gene for acquired resistance in M. abscessus, primer (18F 5′-AGT CGG GAC CTA AGG CGA G-3′ and 21R 5’-TTC CCG CTT AGA TGC TTT CAG-3′) were used for amplification and sequencing (Meier et al., 1994). The resulting amplicon of 16S rRNA, hsp65, erm (41), and rrl gene were purified using Qiaquick PCR purification kit (Qiagen, Hilden, Germany) and were sequenced using BigDye termination v3.1 cycle sequencing kit (Thermo Fisher Scientific, Waltham, Massachusetts, US). Sequencing PCR products were purified by ethanol precipitation and washed with 70% ethanol. The purified products were sequenced using a 3730XL DNA analyser (Thermo Fisher Scientific, Waltham, Massachusetts, US). For the identification of the bacteria, 16S rRNA gene sequence homology analysis was done using BLAST. For phylogenetic classification, multiple hsp65 gene sequences were assembled and alignment was carried out using ClustalW and phylogenetic tree was constructed as mentioned in Chouhan et al. (2019). To determine the single nucleotide polymorphism (SNP) in erm (41) and rrl gene of gastric M. abscessus strains, the amplified sequence were compared with M. abscessus ATCC 19977 genome (GenBank accession number NC_010397.1). We also compared the sequence with erm (41) gene of M. abscessus strain ATCC19977 (T28 sequevar., GenBank accession number FJ358483.1) and CR5701 (C28 sequevar., GenBank accession number HQ127366.1). For amino acid based protein sequence analysis M. abscessus reference strains (GenBank accession number ADM33801.1) were used. All sequences were aligned using ClustalW multiple sequence alignment and were analysed for the mutations at nucleotide as well as amino acid levels using BioEdit software (version 7.2.6.1).
Nucleotide sequence accession numbers
The erm (41) gene sequence of resistant M. abscessus subsp. abscessus rough (Mabs R), resistant M. abscessus subsp. abscessus smooth (Mabs S-A), susceptible M. abscessus subsp. abscessus smooth (Mabs S-B) were submitted to GenBank and accession numbers are MW147115, MW147113, MW147114, respectively. The low molecular weight sequence of erm (41) gene was also submitted to GenBank and the accession number is MW142321. Similarly rrl gene sequences of resistant M. abscessus subsp. abscessus rough (Mabs R), resistant M. abscessus subsp. abscessus smooth (Mabs S-A), susceptible M. abscessus subsp. abscessus smooth (Mabs S-B) were submitted to GenBank and accession numbers are MW148480, MW148478, and MW148479, respectively.
Results
Colony morphologies and clarithromycin resistance patterns of the gastric Mycobacterium abscessus strains
The M. abscessus subsp. abscessus strains isolated from individuals with various gastric diseases have two distinct colony morphologies: smooth and rough. A total of 117 gastric M. abscessus subsp. abscessus (74 rough and 43 smooth) strains were tested for clarithromycin resistance using agar dilution and broth microdilution based assays. The MIC90 for gastric M. abscessus subsp. abscessus rough morphotypes was ≤256 μg/mL after 14 days of incubation, while M. abscessus subsp. abscessus rough morphotypes grown in 0.1 μg/mL clarithromycin exhibited an induced increase in MIC90 showed MIC90 of ≤512 μg/mL after 14 days of treatment (Table 1). Similarly, clarithromycin treatment (uninduced and induced) was carried out for the 24 smooth M. abscessus subsp. abscessus morphotypes. We observed that all 24 M. abscessus subsp. abscessus smooth morphotypes had MIC90 ≤ 4 μg/mL after 14 days of incubation in uninduced conditions, while in induced conditions, the M. abscessus subsp. abscessus smooth morphotypes showed MIC90 ≤ 8 μg/mL after 14 days of incubation (Table 1). Based on clarithromycin sensitivity pattern we have 3 different types of gastric M. abscessus subsp. abscessus strains-(a) resistant M. abscessus subsp. abscessus rough (Mabs-R) (b) resistant M. abscessus subsp. abscessus smooth type A (Mabs-S-A) and (c) susceptible M. abscessus subsp. abscessus smooth type B (Mabs-S-B) by considering MIC90 ≤ 8 μg/mL as a cut-off in induced as well as uninduced conditions as per the Clinical and Laboratory Standards Institute (CLSI) guideline published in 2011.
Erm (41) PCR based analysis of Mycobacterium abscessus subsp. abscessus sensitive and resistant strains
In order to investigate the molecular basis of clarithromycin resistance in gastric M. abscessus subsp. abscessus strains, a 670 bp region of the erm (41) gene was amplified by PCR. Once erm (41) gene was amplified from M. abscessus subsp. abscessus (rough and smooth) resistant and sensitive strains, the amplified products were visualized on 1.5% of agarose gel. An additional ~180 bp amplicon was observed only for the sensitive strains along with the expected band of 670 bp and the results were consistent for all total 117 strains irrespective of smooth and rough morphotypes (Figure 1A). The additional low molecular weight amplicon (180 bp) has not been reported previously. However, with a different set of primer targeting the erm (41) gene, only a single amplicon of 764 bp was observed (Figure 1B).
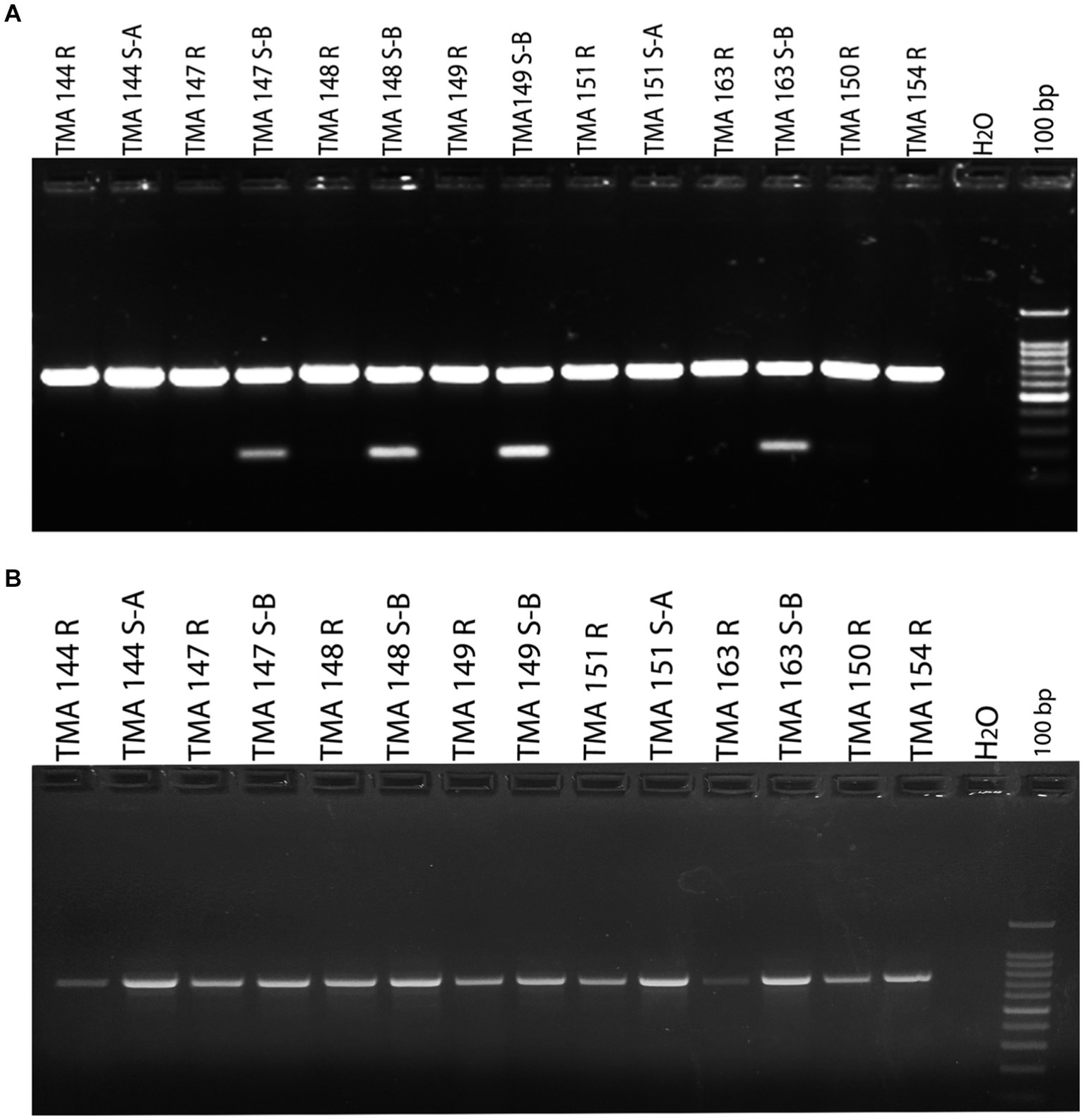
Figure 1. Detection of susceptible and resistant gastric Mycobacterium abscessus subsp. abscessus isolates using erm (41) gene PCR. (A) M. abscessus subsp. abscessus strains (TMA 144 R, 144 S-A, 147 R, 148 R, 149 R, 151 R, 151 S-A, 163 R, 150 R and 154 R) showing single amplicon (670 bp) represent resistant phenotype. M. abscessus subsp. abscessus strains (TMA 147 S-B,148 S-B, 149 S-B and 163 S-B) showing two amplicon (670 bp and 180 bp) represent susceptible phenotype. (B) erm (41) gene amplification with another set of primer showing single amplicon for gastric M. abscessus subsp. abscessus strains. (Mention as ‘100 bp ladder’ in the figure instead of just ‘100 bp).
Sequence analysis of the erm (41) and rrl genes
As mentioned above, we obtained two amplicons (670 bp and 180 bp) in sensitive and one amplicon (670 bp) in resistant strains. The amplicons were purified and sequenced separately to confirm the association of clarithromycin resistance profiles with single-nucleotide polymorphism (SNP) in the erm (41) gene. Earlier reports suggest that SNP at 28 (C to T) nucleotide position is associated with inducible resistance of M. abscessus subsp. abscessus. We observed all clarithromycin susceptible M. abscessus subsp. abscessus strains (Pfister et al., 2004) had nucleotide C (GenBank accession number MW147114) and all resistant strains (93) strains had nucleotide T (GenBank accession number MW147113 and MW147115) at the position 28 of erm (41) gene (Table 2; Figure 2A). Along with the T28C SNP, we also observed nucleotide C at position 159 (T159C), nucleotide G at position 238 (A238G), and nucleotide C at position 330 (A330C) in all susceptible M. abscessus strains (GenBank accession number MW147114), but these mutations were absent in all resistant strains of M. abscessus (GenBank accession number MW147113 and MW147115), irrespective of smooth and rough morphotypes. The erm (41) genes nucleotide sequences were converted to amino acid sequences for both resistant and susceptible M. abscessus subsp. abscessus strains and was compared with reference strains. We observed Arginine (Arg) and Valine (Val) at position 10 and position 80, respectively only in susceptible strains, while for all resistant strains, Tryptophan (Trp) and Isoleucine (Ile) were observed which is similar to the reference strain (Table 2; Figure 2B). We also amplified rrl gene of gastric M. abscessus subsp. abscessus and sequenced the nucleotides to confirm SNPs in rrl gene but we did not observe any SNPs at 2270A to G or C and 2271A to G or C (M. abscessus numbering) in the rrl gene of M. abscessus resistant (GenBank accession number MW148480 and MW148478) and susceptible strains (GenBank accession number MW148479) as mentioned in Table 2; Supplementary Figure S1.
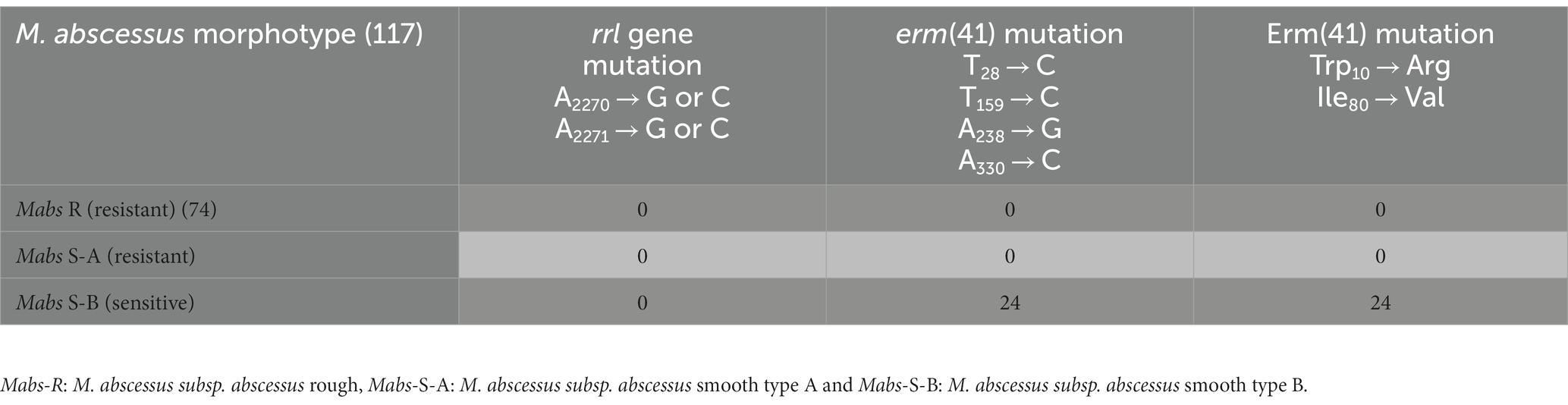
Table 2. rrl and erm (41) genotype of susceptible and resistant gastric M. abscessus subsp. abscessus strains.
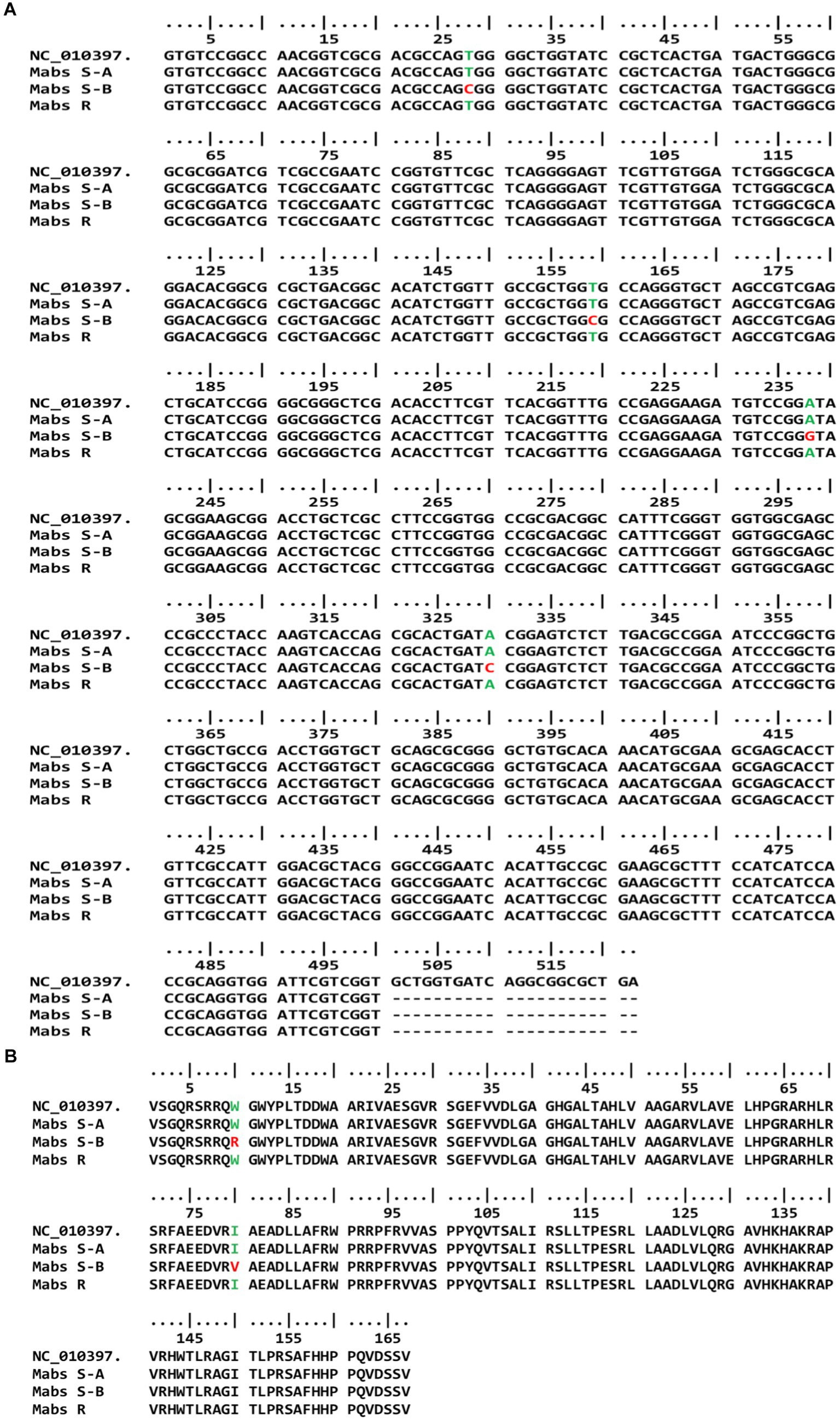
Figure 2. erm (41) genotype of susceptible and resistant gastric M. abscessus subsp. abscessus strains. (A) Gene sequence of erm (41) showing mutations at 28, 159, 238 and 330 positions in susceptible gastric M. abscessus subsp. abscessus strains (Mabs S-B), while resistant strains (Mabs R, Mabs S-A) have wild type phenotype. (B) Amino acid sequence of Erm (41) protein showing mutations at 10 and 80 amino acid position in susceptible and resistant gastric M. abscessus subsp. abscessus strains.
Sequence analysis of the low molecular weight amplicon of Mycobacterium abscessus subsp. abscessus
The low molecular weight amplified product (180 bp) of oxidoreductase gene from M. abscessus subsp. abscessus susceptible strains were sequenced using forward and reverse primers. As shown in Supplementary Figures S3A,B, the chromatograms of Sanger sequencing for both forward and reverse primers showed very distinct peaks.
Multiple sequence alignment did not show any match of these sequences with the erm (41) gene of M. abscessus reference strain as well as the gastric M. abscessus subsp. abscessus strains. To find out the identity of these sequences sequence homology analysis was performed using the BLAST algorithm on the NCBI platform. The BLAST analysis confirmed the identity of these sequences is not erm (41) gene but oxidoreductase gene (GenBank: CP029073.1 and CU458896.1) of M. abscessus strain G122 and M. abscessus ATCC19977. Oxidoreductase of M. abscessus contains a total of 3,552 nucleotides, which encode a 1,183 amino acid containing protein. As it was shown in Figure 3, the 180 bp amplicon shows 100 percent similarity with M. abscessus G122 strain, starting from G_2991 to C_3158 (GenBank accession number MW142321) which covers 167 bp (Figure 3A). The amino acid sequence starts from valine at the 133 position and the match ends at amino acid valine at the 187 position, which covers a total of 54 amino acids (Figure 3B). With respect to the reference strain M. abscessus ATCC19977, the oxidoreductase gene of the gastric M. abscessus subsp. abscessus showed 4 point mutations at C3007 → G, C3042 → T, C3109 → G and A3135 → G (Supplementary Figure S2). Altogether, these results confirmed that the low molecular weight (180 bp) band obtained in the erm (41) PCR is amplified from the oxidoreductase gene present in gastric M. abscessus subsp. abscessus strains.
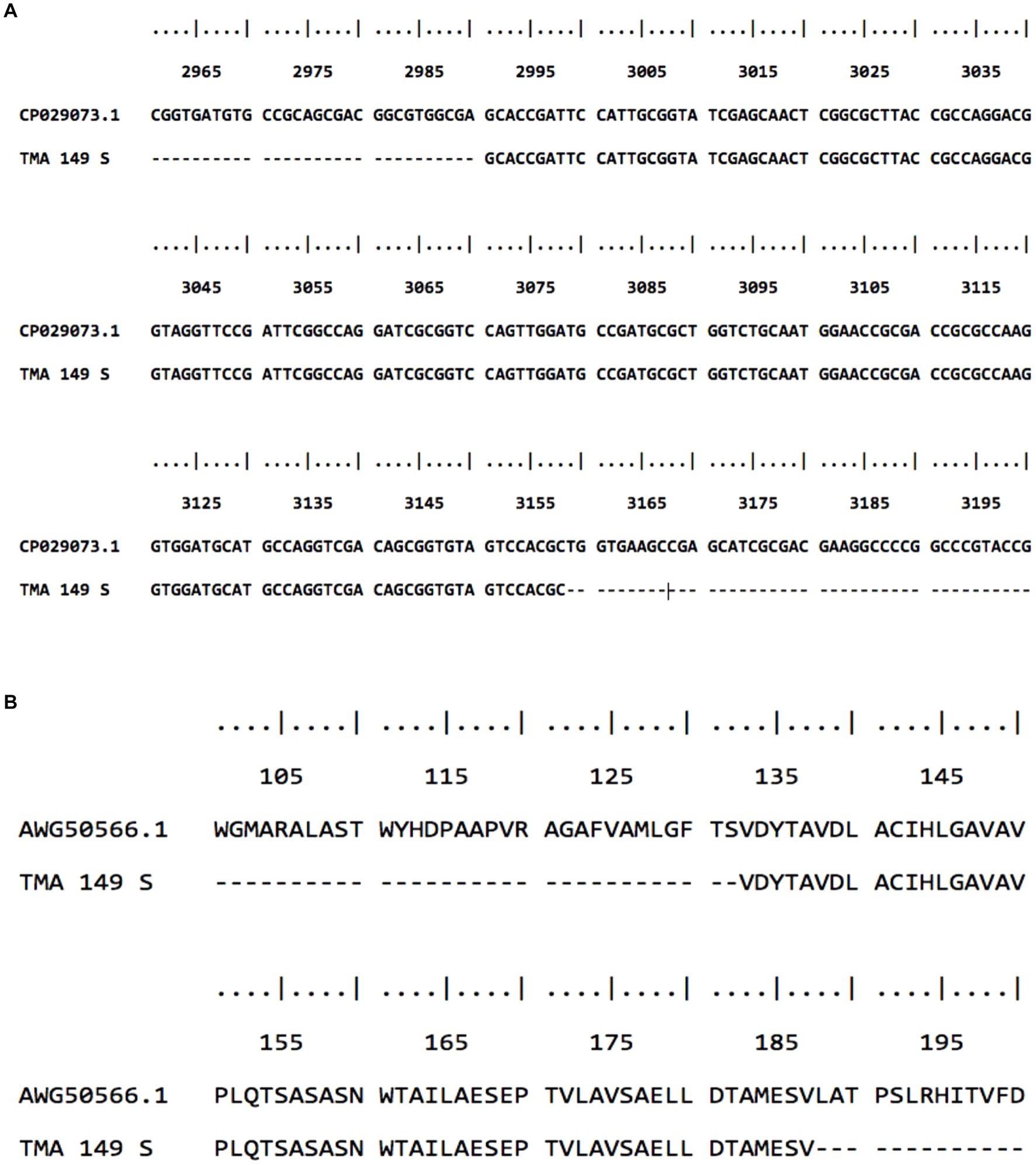
Figure 3. Sequence alignment of M. abscessus subsp. abscessus strains with low molecular weight amplicon. (A) Gene sequence of low molecular weight amplicon shows 100 percent similarity with the oxidoreductase gene of M. abscesus subsp. abscessus strain G122 chromosome. (B) low molecular weight amino acid sequence showed 100 percent similarity with oxidoreductase protein.
Discussion
M. abscessus subsp. abscessus is a rapidly growing Mycobacterium species responsible for pulmonary and soft tissue infections. Although rare, the bacteria also cause disseminated infection, especially in immunocompromised individuals (Lee et al., 2015). M. abscessus subsp. abscessus is a non-tuberculous mycobacteria (NTM) showing a high level of antibiotic resistance and poses a serious challenge to disease management (Nessar et al., 2012). Various antibiotics which are found to be effective against M. abscessus subsp. abscessus, like azithromycin, amikacin, meropenem, ciprofloxacin, imipenem, trimethoprim/sulfamethoxazole, and clarithromycin. Among these antibiotics, clarithromycin remains to be the drug of choice to treat M. abscessus subsp. abscessus related infections (Griffith et al., 2007; Nessar et al., 2012). Recently we reported the presence of M. abscessus subsp. abscessus in gastric epithelium of patients with various gastric diseases (Chouhan et al., 2019). These gastric diseases are commonly associated with H. pylori infection. Recommended treatment option for the eradication of H. pylori and the management of related gastric diseases is standard clarithromycin-based triple therapy. In this study, we determined the clarithromycin resistance patterns of gastric M. abscessus subsp. abscessus strains.
The clarithromycin susceptibility for gastric M. abscessus subsp. abscessus strains were determined by clarithromycin broth microdilution assay and agar dilution method, where the bacteria was incubated in various concentrations of clarithromycin for 3, 7, and 14 days followed by inspecting bacterial viability. After incubation for 14 days, the growth (viability) pattern of the susceptible and resistant strains were determined. The microdilution-based antibiotic susceptibility test has been recommended by CLSI, because of its reproducibility (Reller et al., 2000; Woods et al., 2011). The clarithromycin susceptibility data obtained in our study of 117 gastric M. abscessus subsp. abscessus strains demonstrate that the phenotype of clarithromycin susceptibility was fully concordant with erm (41) gene SNPs. Our data suggest that of the 117 M. abscessus subsp. abscessus strains, 93 (79.48%) strains were resistant to clarithromycin, while 24 (20.51%) strains were susceptible to clarithromycin.
The point mutation (C to T) at position 28 of erm (41) gene is known to be associated with inducible clarithromycin resistance by M. abscessus subsp. abscessus. M. abscessus subsp. abscessus strains showed susceptibility at day 3 of treatment but gradually acquired resistance after 7 days to 14 days of incubation (Nash et al., 2009; Kim et al., 2010; Bastian et al., 2011). Surprisingly we observed there were 19 smooth M. abscessus subsp. abscessus strains which were resistant to clarithromycin and had wild-type erm (41) genotype as like M. abscessus subsp. abscessus rough morphotype, while susceptible M. abscessus subsp. abscessus smooth morphotype had SNPs in their erm (41) gene. We observed after 14 days of incubation M. abscessus subsp. abscessus strains type Mabs R showed MIC of 256 μg/mL when they were not induced. However, upon induced with clarithromycin, the MIC raised to 512 μg/mL of clarithromycin. MabsS-A strains were susceptible to 256 μg/mL of clarithromycin in uninduced conditions and MIC was 512 μg/mL in induced conditions. On the other hand, MabsS-B showed MIC of 8 μg/mL after induction, when compared to 4 μg/mL of clarithromycin in uninduced conditions. We observed resistant phenotype with wild type erm (41) gene with T, T, A, and A nucleotide base at the 28, 159, 238, and 330 positions, respectively but strains with C, C, G, and C at the 28, 159, 238, and 330 nucleotide positions respectively, exhibited susceptible phenotype (Tables 1, 2). Acquired and inducible resistance for clarithromycin in M. abscessus has already been reported. Acquired resistance for clarithromycin has been associated with point mutations at A2270 → G or C and A2271 → G or C (M. abscessus numbering system) of rrl gene (Pfister et al., 2004; Lipworth et al., 2018). To our surprise, we did not observe any mutations in gastric M. abscessus subsp. abscessus strains at position A2270 → G or C and A2271 → G or C (M. abscessus numbering system) of rrl gene. In conclusion, no association was observed between rrl gene and clarithromycin MIC for the resistant and sensitive phenotype of gastric M. abscessus subsp. abscessus strains.
Mutation from isoleucine (Ile) to valine (Val) at the 80th position has been associated with macrolide drug resistance in M. abscessus strains isolated from Korea. Nash et al. has shown that Erm41 protein with Trp10 was associated with resistance while Arg10 was associated with susceptible phenotype, as the protein harbouring Arg10 was non-functional (Nash et al., 2009; Lee et al., 2014). Our amino acid sequence analysis for Erm (41) protein suggests that all susceptible strains of M. abscessus subsp. abscessus had Arginine (Arg) amino acid at 10th position, while Tryptophan (Trp) was present in resistant strains of M. abscessus subsp. abscessus. We also observed mutation at position 80, where Valine (Val) was replaced by Isoleucine (Ile) in resistant strains of gastric M. abscessus subsp. abscessus. Our erm (41) gene analysis for gastric M. abscessus subsp. abscessus strains correlated with susceptibility pattern of M. abscessus subsp. abscessus strains irrespective of smooth and rough morphotypes. The sequence of low molecular weight (180 bp) amplicon did not show any homology with erm (41) gene of M. abscessus strains but BLAST analysis identified that 180 bp amplicon belongs to oxidoreductase gene of M. abscessus strain G122 with 100 percent match. On the other hand, when compared with M. abscessus ATCC19977 strain, the 180 bp amplicon sequence displayed 4 point mutations, C3007 → G, C3042 → T, C3109 → G and A3135 → G in oxidoreductase gene (Supplementary Figure S2). Most of the antibiotics also exert their bactericidal effect by generating reactive oxygen species (ROS) or targeting bacterial redox systems. The bacterial oxidoreductase gene has been linked to antibiotic resistance through neutralizing toxic molecules, detoxification of antibiotics, and repair damage caused by antibiotics. Recent studies have revealed that M. abscessus subsp. abscessus induces efflux pump encoding genes in response to antibiotic stress specifically antibiotic targeting ribosome (Egorov et al., 2018; Mudde et al., 2022; Schildkraut et al., 2022). A mutation in the oxidoreductase gene leading to a non-functional oxidoreductase enzyme may exert a detrimental effect on bacteria during antibiotic treatment. In this study, we observed a possible link between the oxidoreductase gene of M. abscessus subsp. abscessus in clarithromycin resistance. Further study involving whole genome sequencing of gastric M. abscessus subsp. abscessus strains are needed to understand the antibiotic resistance gene pool present in the strains along with genotypes and the nature of resistance (acquired or induced). To conclude, we determined the clarithromycin resistance profile of the gastric M. abscessus subsp. abscessus strains and studied the genetic basis of clarithromycin resistance. We have also demonstrated induced clarithromycin resistance in the isolated gastric M. abscessus subsp. abscessus strains. Our finding also suggests that the C to T mutation at the 28th nucleotide position of the erm (41) gene has an important role in conferring clarithromycin resistance and erm (41) gene sequence analysis can light on the mechanism of clarithromycin resistance in of gastric M. abscessus subsp. abscessus. The results of our study will be helpful while designing efficient strategies to combat multi-drug resistant strains of M. abscussus subsp. abscessus as well as for the management of associated gastric diseases.
Data availability statement
The datasets presented in this study can be found in online repositories. The names of the repository/repositories and accession number (s) can be found in the article/Supplementary material.
Ethics statement
The studies involving humans were approved by Institute Human Ethics Committee of Rajiv Gandhi Centre for Biotechnology (Approval Number IHEC/01/2017/18) and by the Human Ethics Committee of Govt. Medical College, Trivandrum (Approval Number IEC.No.05/07/2016/MCT). The studies were conducted in accordance with the local legislation and institutional requirements. The participants provided their written informed consent to participate in this study.
Author contributions
MP conceptualized the idea. DC, TD, KD, SD, and RR performed the experiments. DC, SC, KD, GN, and MP analyzed the data. DC, SC, RR, KD, GN, and MP wrote the manuscript. All authors contributed to the article and approved the submitted version.
Funding
This study was supported by Rajiv Gandhi Centre for Biotechnology (RGCB), an autonomous institute under Department of Biotechnology (DBT) and grant from Department of Biotechnology, Government of India to MRP and Department of Science and Technology (ECR/2016/000171) to SC.
Acknowledgments
The authors thank Dr. Ajay Kumar for suggestions regarding handling Mycobacterium sp. and providing Mycobacterium tuberculosis DNA and Dr. T. R. Santhosh kumar for providing reagents and important suggestions for the study. The authors thank Dr. P. Manoj for neucleotide sequencing and other technical assistance. Finally, The authors thank to Department of Biotechnology (DBT), for providing fellowship for the study.
Conflict of interest
The authors declare that the research was conducted in the absence of any commercial or financial relationships that could be construed as a potential conflict of interest.
Publisher’s note
All claims expressed in this article are solely those of the authors and do not necessarily represent those of their affiliated organizations, or those of the publisher, the editors and the reviewers. Any product that may be evaluated in this article, or claim that may be made by its manufacturer, is not guaranteed or endorsed by the publisher.
Supplementary material
The Supplementary material for this article can be found online at: https://www.frontiersin.org/articles/10.3389/fmicb.2023.1193380/full#supplementary-material
References
Al-Momani, H., Perry, A., Jones, R., Bourke, S., Doe, S., Perry, J., et al. (2017). Nontuberculous mycobacteria in gastrostomy fed patients with cystic fibrosis. Sci. Rep. 7:46546. doi: 10.1038/srep46546
Bastian, S., Veziris, N., Roux, A.-L., Brossier, F., Gaillard, J.-L., Jarlier, V., et al. (2011). Assessment of clarithromycin susceptibility in strains belonging to the Mycobacterium abscessus group by erm (41) and rrl sequencing. Antimicrob. Agents Chemother. 55, 775–781. doi: 10.1128/AAC.00861-10
Berg, D. E., Lelwala-Guruge, J., Incecik, E. T., Srivastava, K., and NS, A. (1997). H. Pylori DNA fingerprinting using the arbitrarily primed PCR (AP-PCR) or random amplified polymorphic DNA (RAPD) method. Methods. Mol. Med. 8, 117–132. doi: 10.1385/0-89603-381-3:117
Brown-Elliott, B. A., Vasireddy, S., Vasireddy, R., Iakhiaeva, E., Howard, S. T., Nash, K., et al. (2016). Utility of sequencing the erm (41) gene in isolates of Mycobacterium abscessus subsp. abscessus with low and intermediate clarithromycin MICs. J. Clin. Microbiol. 53, 1211–1215. doi: 10.1128/JCM.02950-14.
Brown-Elliott, B. A., and Wallace, R. J. (2002). Clinical and taxonomic status of pathogenic nonpigmented or late-pigmenting rapidly growing mycobacteria. Clin. Microbiol. Rev. 15, 716–746. doi: 10.1128/CMR.15.4.716-746.2002
Chouhan, D., Devi, T. B., Chattopadhyay, S., Dharmaseelan, S., Nair, G. B., Devadas, K., et al. (2019). Mycobacterium abscessus infection in the stomach of patients with various gastric symptoms. PLoS Negl. Trop. Dis. 13:e0007799. doi: 10.1371/journal.pntd.0007799
Egorov, A., Ulyashova, M., and Rubtsova, M. Y. (2018). Bacterial enzymes and antibiotic resistance. Acta Nat. 10, 33–48. doi: 10.32607/20758251-2018-10-4-33-48
Gonzales, M. L. M., Dans, L. F., and Sio-Aguilar, J. (2019). Antiamoebic drugs for treating amoebic colitis. Cochrane Database Syst. Rev. 1:CD006085. doi: 10.1002/14651858.CD006085.pub3
Griffith, D. E., Aksamit, T., Brown-Elliott, B. A., Catanzaro, A., Daley, C., Gordin, F., et al. (2007). An official ATS/IDSA statement: diagnosis, treatment, and prevention of nontuberculous mycobacterial diseases. Am. J. Respir. Crit. Care Med. 175, 367–416. doi: 10.1164/rccm.200604-571ST
Griffith, D. E., and Daley, C. L. (2022). Treatment of Mycobacterium abscessus pulmonary disease. Chest 161, 64–75. doi: 10.1016/j.chest.2021.07.035
Kim, H. Y., Kim, B. J., Kook, Y., Yun, Y. J., Shin, J. H., Kim, B. J., et al. (2010). Mycobacterium massiliense is differentiated from mycobacterium abscessus and Mycobacterium bolletii by erythromycin ribosome methyltransferase gene (erm) and clarithromycin susceptibility patterns. Microbiol. Immunol. 54, 347–353. doi: 10.1111/j.1348-0421.2010.00221.x
Kim, H., Kim, S. H., Shim, T. S., Kim, M. N., Bai, G. H., Park, Y. G., et al. (2005). Differentiation of mycobacterium species by analysis of the heat-shock protein 65 gene (hsp 65). Int. J. Syst. Evol. Microbiol. 55, 1649–1656. doi: 10.1099/ijs.0.63553-0
Lee, M.-R., Sheng, W.-H., Hung, C.-C., Yu, C.-J., Lee, L.-N., and Hsueh, P.-R. (2015). Mycobacterium abscessus complex infections in humans. Emerg. Infect. Dis. 21, 1638–1646. doi: 10.3201/2109.141634
Lee, S. H., Yoo, H. K., Kim, S. H., Koh, W.-J., Kim, C. K., Park, Y. K., et al. (2014). The drug resistance profile of Mycobacterium abscessus group strains from Korea. Ann. Lab. Med. 34, 31–37. doi: 10.3343/alm.2014.34.1.31
Lipworth, S. I. W., Hough, N., Leach, L., Morgan, M., Jeffrey, K., Andersson, M., et al. (2018). Whole genome sequencing for predicting Mycobacterium abscessus drug susceptibility. bioRxiv. doi: 10.1128/AAC.01204-18 [Epub ahead of preprint].
Meier, A., Kirschner, P., Springer, B., Steingrube, V. A., Brown, B. A., Wallace, R., et al. (1994). Identification of mutations in 23S rRNA gene of clarithromycin-resistant Mycobacterium intracellulare. Antimicrob. Agents Chemother. 38, 381–384. doi: 10.1128/AAC.38.2.381
Mudde, S. E., Schildkraut, J. A., Ammerman, N. C., de Vogel, C. P., de Steenwinkel, J. E., van Ingen, J., et al. (2022). Unraveling antibiotic resistance mechanisms in Mycobacterium abscessus: the potential role of efflux pumps. J. Glob. Antimicrob. Resist. 31, 345–352. doi: 10.1016/j.jgar.2022.10.015
Mushatt, D. M., and Witzig, R. S. (1995). Successful treatment of Mycobacterium abscessus infections with multidrug regimens containing clarithromycin. Clin. Infect. Dis. 20, 1441–1442. doi: 10.1093/clinids/20.5.1441
Nash, K. A., Brown-Elliott, B. A., and Wallace, R. J. (2009). A novel gene, erm (41), confers inducible macrolide resistance to clinical isolates of Mycobacterium abscessus but is absent from Mycobacterium chelonae. Antimicrob. Agents Chemother. 53, 1367–1376. doi: 10.1128/AAC.01275-08
Nessar, R., Cambau, E., Reyrat, J. M., Murray, A., and Gicquel, B. (2012). Mycobacterium abscessus: a new antibiotic nightmare. J. Antimicrob. Chemother. 67, 810–818. doi: 10.1093/jac/dkr578
Pfister, P., Jenni, S., Poehlsgaard, J., Thomas, A., Douthwaite, S., Ban, N., et al. (2004). The structural basis of macrolide–ribosome binding assessed using mutagenesis of 23 S rRNA positions 2058 and 2059. J. Mol. Biol. 342, 1569–1581. doi: 10.1016/j.jmb.2004.07.095
Reller, L. B., Weinstein, M. P., and Woods, G. L. (2000). Susceptibility testing for mycobacteria. Clin. Infect. Dis. 31, 1209–1215. doi: 10.1086/317441
Safavi, M., Sabourian, R., and Foroumadi, A. (2016). Treatment of Helicobacter pylori infection: current and future insights. World J. Clin. Cases 4, 5–19. doi: 10.12998/wjcc.v4.i1.5
Schildkraut, J. A., Coolen, J. P., Burbaud, S., Sangen, J. J., Kwint, M. P., Floto, R. A., et al. (2022). RNA sequencing elucidates drug-specific mechanisms of antibiotic tolerance and resistance in Mycobacterium abscessus. Antimicrob. Agents Chemother. 66, e01509–e01521. doi: 10.1128/AAC.01509-21
Shetty, V., Lamichhane, B., Tay, C. Y., Pai, G. C., Lingadakai, R., Balaraju, G., et al. (2019). High primary resistance to metronidazole and levofloxacin, and a moderate resistance to clarithromycin in Helicobacter pylori isolated from Karnataka patients. Gut Pathog. 11:21. doi: 10.1186/s13099-019-0305-x
Wallace, R., Meier, A., Brown, B. A., Zhang, Y., Sander, P., Onyi, G. O., et al. (1996). Genetic basis for clarithromycin resistance among isolates of mycobacterium chelonae and Mycobacterium abscessus. Antimicrob. Agents Chemother. 40, 1676–1681. doi: 10.1128/AAC.40.7.1676
Keywords: gastric diseases, Mycobacterium abscessus subspecies abscessus, Helicobacter pylori, clarithromcycin resistance, erm (41), antibiotic resisitance
Citation: Chouhan D, Retnakumar RJ, Devi TB, Dharmaseelan S, Alexander SM, Devadas K, Chattopadhyay S, Nair GB and Pillai MR (2023) Unusually high clarithromycin resistance in Mycobacterium abscessus subsp. abscessus isolated from human gastric epithelium. Front. Microbiol. 14:1193380. doi: 10.3389/fmicb.2023.1193380
Edited by:
Qixia Luo, Zhejiang University, ChinaReviewed by:
Jitendraa Vashistt, Jaypee University of Information Technology, IndiaAdrian Zelazny, National Institutes of Health (NIH), United States
Copyright © 2023 Chouhan, Retnakumar, Devi, Dharmaseelan, Alexander, Devadas, Chattopadhyay, Nair and Pillai. This is an open-access article distributed under the terms of the Creative Commons Attribution License (CC BY). The use, distribution or reproduction in other forums is permitted, provided the original author(s) and the copyright owner(s) are credited and that the original publication in this journal is cited, in accordance with accepted academic practice. No use, distribution or reproduction is permitted which does not comply with these terms.
*Correspondence: Madhavan Radhakrishna Pillai, bXJwaWxsYWlAZ21haWwuY29t