- 1School of Food and Health, Jinzhou Medical University, Jinzhou, Liaoning, China
- 2School of Life Science and Biotechnology, Dalian University of Technology, Dalian, Liaoning, China
- 3Lab of Brewing Microbiology and Applied Enzymology, School of Biotechnology, Jiangnan University, Wuxi, Jiangsu, China
Mixed culture fermentation with non-Saccharomyces yeasts and Saccharomyces cerevisiae as multi-starters has more advantages than spontaneous fermentation, and wine products with distinctive and pleasant aromas can fulfill the diverse demands of consumers. This study was carried out to illuminate the effect of sequential inoculation of indigenous Candida railenensis and S. cerevisiae on alcoholic fermentation behavior and chemical and aromatic characteristics of Vidal blanc icewine. During the mixed culture fermentation, C. railenensis was present in the initial and middle stages but was absent after 14 days. The results of basic chemical parameters showed that the glycerol content in the mixed culture-fermented icewine was higher than that of the pure fermented icewine, but the acetic acid content was the opposite. In terms of volatile aroma compounds, C. railenensis in the mixed culture fermentation reduced some metabolites such as lower alcohols, 1-hexanol, 3-methylthiopropanol, and their unpleasant notes and increased the production of some desired volatile aroma compounds such as benzaldehyde, β-damascenone, 2-furanmethanol, and 5-methyl furfural associated with rose, honey, nut, and caramel characteristics. Furthermore, C. railenensis also changed the sensory performance of icewine by participating in the fermentation of S. cerevisiae. These findings suggest that C. railenensis with positive enological properties has the ability to be used in icewine production, which has never been reported before.
1. Introduction
Icewine is a typical dessert wine, which is made from ice grapes that are naturally frozen on the grapevine, and ice grapes must be harvested and pressed below −7°C (OIV-International Organisation of Vine and Wine, 2015). Ice grape juice generally contains high concentrations of sugars (soluble solids level >35°Bx). The finished icewine is characterized by high levels of residual sugar (>125 g/L), titratable acid (>6.5 g/L, represented as tartaric acid), and aromatic compounds (Bowen and Reynolds, 2015). Because of the environmental and climatic requirements of ice grape growth and icewine production icewine can only be produced in a few countries such as Canada, Germany, Austria, and China (Alessandria et al., 2013). In China, the icewine industry has developed rapidly in recent years, with an annual output of approximately 3,000,000 L (Lan et al., 2019). Huanren is the major icewine-producing region in the northeast of China, and Vidal blanc is the main icewine grape cultivar in this region. Vidal blanc has high resistance to harsh climates, and its icewine has an appealing aroma and attractive flavor (Crandles et al., 2015).
Wine fermentation is a complex biochemical process in which the microbiota changes dynamically. In this process, yeast plays an important role, and yeast community succession occurs (Ballester-Tomás et al., 2017). Some studies have found that spontaneous fermentation is mainly triggered by non-Saccharomyces yeasts, which are then taken over by Saccharomyces spp. (mainly S. cerevisiae) and non-Saccharomyces yeasts that are more resistant to ethanol, such as Candida (Varela and Borneman, 2017; Li et al., 2019). Grape juice is converted into wine as a consequence of the combined effects of both Saccharomyces and non-Saccharomyces yeasts, and the sensory quality of the resultant wine depends on these effects (Li et al., 2018). Reportedly, some non-Saccharomyces yeasts are beneficial in improving the wine flavor and aroma complexity (Steensels and Verstrepen, 2014; Padilla et al., 2016; Binati et al., 2020) by secreting certain enzymes (such as esterases, β-glucosidase, and β-xylosidase) (Maturano et al., 2012; Hong et al., 2019). In recent years, the application of multi-starters of non-Saccharomyces yeast species together with S. cerevisiae in wine fermentation has been considered to satisfy consumer demands for wines in increasingly aromatic complexity (Englezos et al., 2018; Zhang et al., 2018; Lin et al., 2020). Several commercial non-Saccharomyces yeasts have emerged for mixed culture fermentation, such as Lachancea thermotolerans, Metschnikowia pulcherrima, Pichia kluyveri, Schizosaccharomyces pombe, and Torulaspora delbrueckii (Van Wyk et al., 2020).
Among non-Saccharomyces yeasts, some Candida species have been recognized to be able to tolerate wine-producing conditions and can be used in mixed culture fermentation together with S. cerevisiae, such as Candida californica, Candida cantarellii, Candida pulcherrima, Candida sake, and Candida stellata (Ciani et al., 2010; Rodríguez et al., 2010; Ballester-Tomás et al., 2017; Aplin et al., 2019; Hong et al., 2021). Among Candida species, the use of Candida zemplinina (synonym Starmerella bacillaris) in wine fermentation has been paid the most attention. C. zemplinina has been shown to improve the aroma profile of the wine, compared to single inoculation with S. cerevisiae, and it can increase the production of glycerol and reduce the production of acetic acid and volatile fatty acids, which are thought to have negative effects on wine quality (Rantsiou et al., 2012; Giaramida et al., 2013; Englezos et al., 2018; Tofalo et al., 2022).
With regard to C. railenensis associated with wine and grapes, recent studies have found it in some wine-producing regions (Brysch-Herzberg and Seidel, 2015; Drumonde-Neves et al., 2017); it has been also present in the early or even middle stages of spontaneous fermentation of wine (BreŽná et al., 2010; Cioch-Skoneczny et al., 2018; Li et al., 2018); and it has been detected to have satisfactory wine aroma-related enzyme activities (Hong et al., 2019; Lee and Park, 2020). Moreover, there has only been one initial attempt to use C. railenensis in wine fermentation, but little is known about it (Van Wyk et al., 2020), and so far, the research on the use of C. railenensis in icewine fermentation has not been found. The fermentation condition of icewine is different from that of general wine, which has the characteristics of high sugar concentration, high acid concentration, and low fermentation temperature (Zhang et al., 2018). Furthermore, there is no evidence that C. railenensis is pathogenic.
In our previous studies, indigenous C. railenensis strains were isolated from the spontaneous fermentation of Vidal blanc icewine (Li et al., 2018, 2019), and then, some C. railenensis strains were screened to have the potential to produce characteristic icewine through the experiments of tolerance and fermentation and aroma-related enzyme activities (β-glucosidases and β-xylosidase) (Hong et al., 2019). In this study, these C. railenensis strains were considered for use in icewine fermentation, based on the abilities of indigenous C. railenensis strains to adapt to the icewine micro-environment and their fermentation performance.
In terms of the strategy of mixed culture fermentation, many studies have proven that compared with simultaneous inoculation, sequential inoculation (especially S. cerevisiae was inoculated after 48 h of non-Saccharomyces yeast strains) could increase the production of glycerol in wine and achieve higher aroma complexity (Englezos et al., 2016; Lencioni et al., 2016; Zhang et al., 2018).
This study aimed to explore the possibility of the C. railenensis strain isolated from icewine being applied in icewine fermentation and its effect on the volatile profile of icewine. For this purpose, single S. cerevisiae fermentation and mixed culture fermentation of indigenous C. railenensis together with S. cerevisiae were carried out. The cell's growth was monitored during fermentation, and the basic chemical compositions and volatile aroma compounds of icewine produced by different fermentation strategies were also determined and compared under laboratory-scale conditions.
2. Materials and methods
2.1. Yeast strains
The yeast strains used in this study were isolated from the spontaneous fermentation of Vidal blanc icewine and identified by WL nutrient agar medium and the internal transcribed spacer (ITS) region sequences (Li et al., 2018). Then, a series of tolerance experiments (alcohol, sugar, SO2, and tartaric acid), fermentation experiments, and enzyme activity experiments (β-glucosidases, β-xylosidase, and pectinase) were carried out to screen for the indigenous strains of S. cerevisiae and C. railenensis (Hong et al., 2019). Finally, the indigenous strains (one strain of S. cerevisiae and one strain of C. railenensis) with the best comprehensive performance were screened for subsequent fermentation experiments. The S. cerevisiae strain had excellent alcoholic fermentation ability, and the C. railenensis strain had satisfactory cell wall-bound and extracellular enzyme activities among all non-Saccharomyces yeast strains studied (Hong et al., 2019). Table 1 provides basic information on the indigenous yeast strains (Li et al., 2018).
2.2. Fermentation trials
The ice grape juice of Vidal blanc used for icewine fermentation was obtained from the vineyard (Wunushan Milan Winery; NE China, 41°17′53.53″N 125°22′27.26″E) in Huanren Manchu Autonomous County, which is the most important icewine-producing region of China. It had a pH of 3.12, soluble solid content of 39.1 °Bx, and total acid and sugar contents of 5.12 g/L and 392.64 g/L, respectively.
Ice grape juice was heated at 70°C for 20 min to achieve sterilization (Maturano et al., 2012). Each 600 ml of the sterilized ice grape juice (adding 50 mg/L SO2) was in a 1-L sterile flask with a sterile glass air lock. The glass air lock contained concentrated sulfuric acid, which could allow CO2 produced during icewine fermentation to escape. Laboratory-inoculated fermentations were carried out at 18°C for 30 days. The indigenous strains of S. cerevisiae and C. railenensis were activated in the YPD (yeast extract 10 g/L, peptone 20 g/L, and dextrose 20 g/L; Haibo, Qingdao, China) medium. Different inoculated fermentation strategies were as follows: (1) pure culture fermentation (designated as S2): only S. cerevisiae was added to the sterilized ice grape juice (initial inoculum of approximately 106 cells/ml final concentration); (2) mixed culture fermentation (designated as MF2S2): C. railenensis was added first (initial inoculum of approximately 106 cells/ml final concentration), 48 h later, S. cerevisiae was also added to the sterilized ice grape juice; the addition ratio of C. railenensis and S. cerevisiae was approximately 1: 1.
2.3. Yeast population dynamic changes in icewine fermentation
Samplings were carried out at 0, 2, 4, 7, 14, 21, and 30 days during icewine fermentation. Before sampling, the flasks were shaken. Each sample was serially diluted (1, 104 to 1, 106 ratios) in a sterile physiological solution and spread-plated on WL nutrient agar medium, and all plates were incubated at 28°C for 5 days. The colonies of C. railenensis and S. cerevisiae on the WL nutrient agar medium could be differentiated (Li et al., 2018), and the number of colonies was counted and recorded.
2.4. Basic chemical composition analysis
All fermentation flasks were weighed every day during icewine fermentation, and the daily production of CO2 under different fermentation strategies was recorded. Fermentation was deemed finished when the weight of the flask remained constant for 2 consecutive days (Lencioni et al., 2016). The basic chemical compositions, such as total sugar and total acid contents, were measured, and the methods used adhered to the inspection standards proposed by the OIV (2018).
Ethanol content was determined by a Gas Chromatography (GC) 9,790 plus with a flame ionization detector (Fuli Analytical Instruments Co., Zhejiang, China). The chromatograph was equipped with a KB-5 capillary analytical column (30 m × 320 μm × 0.25 μm; Kromat Co., Bordentown, United States). Ethanol was used for apparatus calibration as a standard, and 1-propanol (Aladdin Biochemical Technology Co., Shanghai, China) was used as an internal standard. The column oven temperature was initially held at 45°C for 5 min, then increased by 5°C/min to 50°C and held for 5 min, and finally increased to 230°C at 20°C/min and held for 2 min. The injector and detector temperatures were 250°C. The carrier gas was nitrogen at a constant flow rate of 1 ml/min, and the injection split ratio was 50:1, and the injection volume was 1 μl.
Organic acid contents were determined by using a Prominence LC-20A system (Shimadzu Co., Japan) for high-performance liquid chromatography (HPLC). The chromatograph was equipped with a Wondasil C18-WR column (4.6 mm × 150 mm, 5 μm; Shimadzu Co., Japan). The column temperature was 35°C, the mobile phase was acetonitrile and phosphoric acid (pH 2.0) with a ratio of 2:98, and the flow rate was 0.8 ml/min.
These basic chemical compositions of each icewine sample were determined in triplicate.
2.5. Analysis of major volatile aroma compounds
The metabolites of icewines under different fermentation strategies were further measured by headSpace solid phase microextraction gas chromatography-tandem time-of-flight mass spectrometry (HS-SPME-GC-TOFMS). Each icewine was sampled and determined six times. A 3 ml sample of icewine and 0.6 g of NaCl were placed in a 20 ml microextraction vial and then mixed with 5 μl internal standard solution (IS, 2-octanol, 10 mg/L stock in dH2O). All samples were analyzed by GC (7890B; Agilent Technologies Inc., California, United States) system coupled with a mass spectrometer (MS, 5977B; Agilent Technologies Inc., California, United States). The chromatograph was equipped with a DB-Wax column (30 m × 250 μm × 0.25 μm; Agilent Technologies Inc., California, United States). The column oven temperature program was as follows: the initial temperature was kept at 40°C for 4 min, then raised to 230°C at a rate of 5°C/min, and held for 5 min. The detailed instrument parameters are presented in Table 2.
Chroma TOF software (V4.3X, LECO Co., USA) and NIST database were used for raw peak extraction, the data baseline filtering and calibration of the baseline, peak alignment, deconvolution analysis, peak identification, integration, and spectrum match of the peak area (Kind et al., 2009). Principal component analysis (PCA) and orthogonal partial least squares discriminant analysis (OPLS-DA) were carried out using SIMCA (V15.0.2, Umetrics Inc., Sweden). The selection principles of differential volatile aroma compounds were as follows: the P-value of the Student's t-test was <0.05, and the variable importance in the projection (VIP) of the first principal component in the OPLS-DA model was >1. Data of the differential volatile aroma compounds selected were calculated as Euclidean distance matrix and were taken for cluster analysis.
2.6. Sensory analysis
In total, 10 students (five male students and five female students, aged between 21 and 25 years old) with experience in sensory evaluation were selected for sensory analysis of the fermented icewine. The students were trained to identify and evaluate the reference standards, which were prepared by adding corresponding aroma standard from Le nez du vin (Jean Lenoir, Provence, France) to 10% v/v aqueous ethanol (pH 3.4) and diluted in series; the training took place twice a week for 3 months (Huang et al., 2018). Finally, a 30 ml sample of icewine was placed in a wine glass with a cover, and the icewines fermented by different fermentation strategies were not marked before the sensory analysis. The six-aspect aromas of “nutty”, “tropical fruity”, “apricot and peach”, “honey”, “caramel and roasted”, and “floral (rose)” were graded (0–5 score; the higher score showed the stronger aroma). The sensory analysis of icewine was performed twice, and the final scores of the aroma of six aspects were the average value.
3. Results
3.1. Dynamic changes of yeast growth during fermentation
The yeast growth dynamics were detected by plate counting at 0, 2, 4, 7, 14, 21, and 30 days during the pure culture fermentation (S2) and the sequential mixed culture fermentation (MF2S2), and the results are presented in Figures 1A, B. In S2, the maximum cell population of S. cerevisiae was observed on day 7 (approximately 7.59 log CFU/ml) and then decreased. After 30 days, S. cerevisiae cells still presented good vitality, with approximately 6.75 log CFU/ml. In MF2S2, the cell population of S. cerevisiae first gradually increased and reached the highest value on day 21 (7.46 log CFU/ml) and then decreased. In terms of the cell population of C. railenensis, there was no obvious change in the first 2 days of MF2S2; after inoculating S. cerevisiae, the cell population of C. railenensis increased and reached the maximum population on day 4 (6.95 log CFU/ml). However, C. railenensis was not detected after 14 days, while S. cerevisiae maintained good viability (above 106 CFU/ml) until the end of the monitored period.
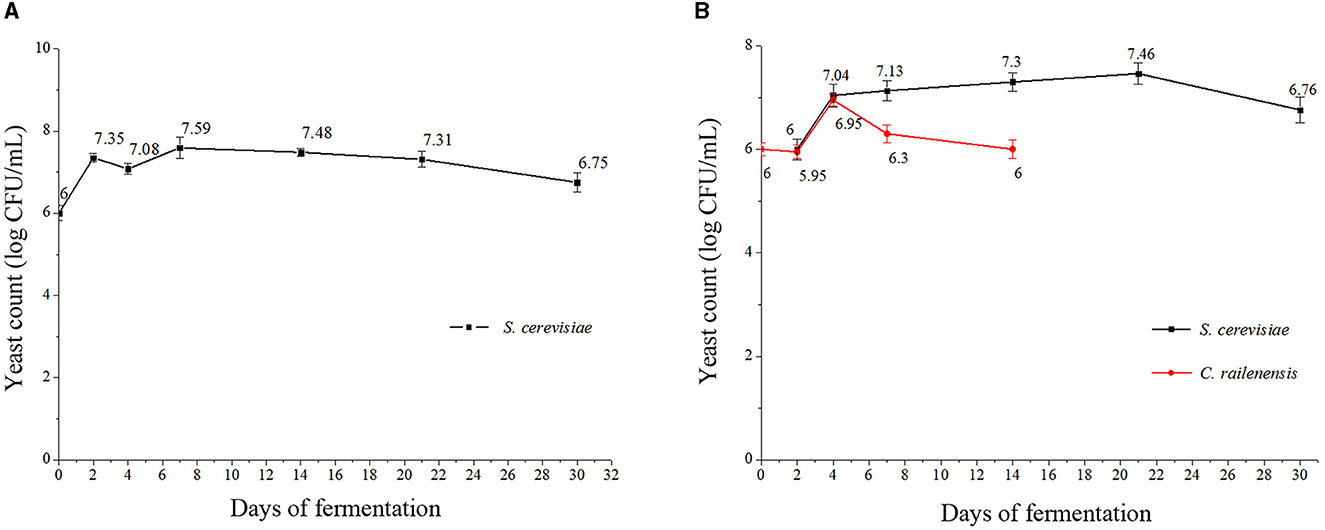
Figure 1. Cell growth dynamics of S. cerevisiae and C. railenensis during pure and mixed culture fermentation. (A) S2, pure culture fermentation; (B) MF2S2, mixed culture fermentation. Counts are the mean CFU/ml values ± standard deviations.
3.2. Chemical parameters
3.2.1. CO2 and ethanol production during fermentation
The daily production of CO2 and the ethanol content was measured during icewine fermentation, as shown in Figure 2. The maximum daily productions of CO2 during S2 and MF2S2 were observed on days 6 and 7 and were 1.49 g/L and 1.53 g/L, respectively. In the first 2 days of MF2S2, changes in CO2 production and ethanol contents were not obvious; after inoculating S. cerevisiae, the daily production of CO2 changes was not much from days 2 to 4 (from 0.01 g/L to 0.07 g/L), and the ethanol contents changed from 0.13% (v/v) to 0.35% (v/v). However, CO2 productions (from 0 to 1.01 g/L) and ethanol contents (from 0 to 3.35%, v/v) in the first 4 days of S2 were both obviously higher than those in MF2S2. At the end of S2 and MF2S2, the daily productions of CO2 for both were 0, indicating the fermentation had been completed, and the total productions of CO2 were 10.92 g/L and 10.22 g/L, respectively. Accordingly, ethanol concentration in icewine of S2 (12.89 ±0.08%, v/v) was higher than that in icewine of MF2S2 (12.37 ± 0.07%, v/v).
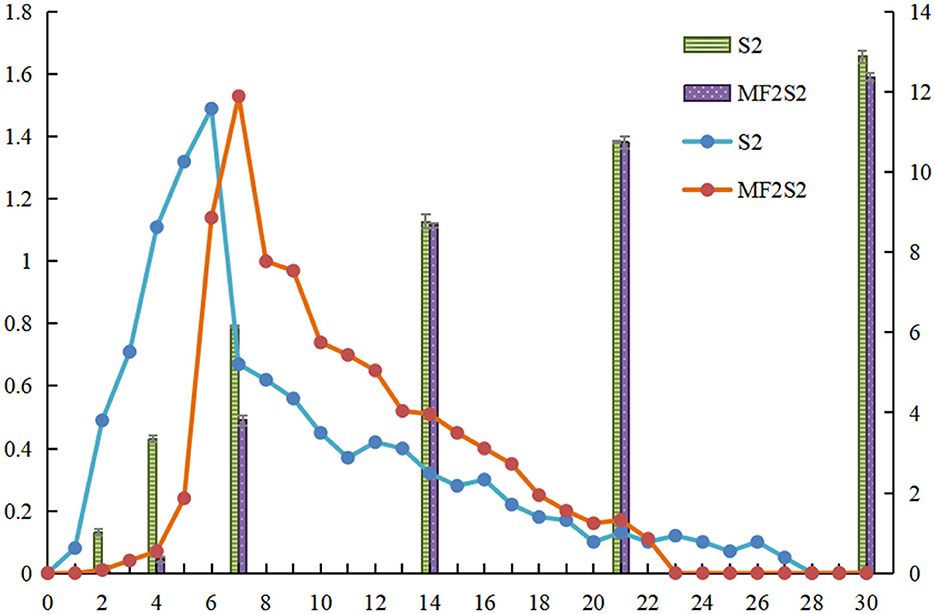
Figure 2. CO2 production and ethanol content during icewine fermentation. The column shows the ethanol content of icewine. The line shows CO2 production, and the standard deviation values are 0–0.02.
3.2.2. Other basic chemical parameters in icewine
The concentrations of residual sugar, ethanol content, tartaric acid, acetic acid, succinic acid, and glycerol in the icewines fermented by pure and mixed culture fermentation are presented in Table 3. The contents of residual sugar, tartaric acid, succinic acid, and glycerol in the icewine of MF2S2 were all higher than those in the icewine of S2. The residual sugar contents in icewines of MF2S2 and S2 met the requirements of Vintners Quality Alliance (VQA) that icewine should be required to have a residual sugar content of >125 g/L (Bowen and Reynolds, 2015). Moreover, the glycerol content in icewine of MF2S2 was 11.18 ± 0.12 g/L, which was higher than that in icewine of S2 (10.91 ± 0.33 g/L), and they are both >10 g/L. The acetic acid content was 2.03 ± 0.13 g/L in icewine of S2 and 1.82 ± 0.17 g/L in icewine of MF2S2, both of which were lower than the maximum allowable limit in OIV (2.1 g/L).
3.3. Volatile aroma compounds
The identification of the volatile aroma compounds in the icewines fermented by pure and mixed culture fermentation was carried out by HS-SPME-GC-TOFMS. The principal component analysis (PCA) scatter plot of the icewine samples of MF2S2 and S2 is shown in Figure 3A, and it was acquired that the R2X (cum) was 0.524. To achieve a higher level of group separation and obtain a better understanding of classification variables, orthogonal partial least squares-discrimination analysis (OPLS-DA) was also used, and the scatter plot is shown in Figure 3B; the parameters obtained were R2X (cum) = 0.481, R2Y (cum) = 1 and Q2 (cum) = 0.971, which were good and stable for prediction and fitness. In Figure 3A, the abscissa PC[1] and the ordinate PC[2] represent the scores of the first and second principal components; in Figure 3B, the abscissa t[1]P represents the score of the predicted first principal component, and the ordinate t[1]O represents the score of orthogonal principal component; the scatter dots of different colors and shapes represent the icewine samples with different fermentation strategies, as shown in Figures 3A, B. The score plots indicate that the difference between the two groups of icewine samples is very significant, and all of the samples are within the 95% confidence interval, which reflects high experimental reproducibility and the different patterns of volatile metabolites.
When the screening principle was P < 0.05 and VIP > 1, a total of 248 differential volatile metabolites were detected. The further screening principle was matching similarity of >600, and meanwhile, physical and chemical properties and aroma of different metabolites were also considered. In brief, 52 differential volatile aroma compounds in icewines of MF2S2 and S2 were screened and subsequently divided into 4 groups, including 20 esters, 15 alcohols, 7 terpenes, and 10 others, as shown in Table 4. These metabolites were also clustered and displayed by heat map (Figure 4).
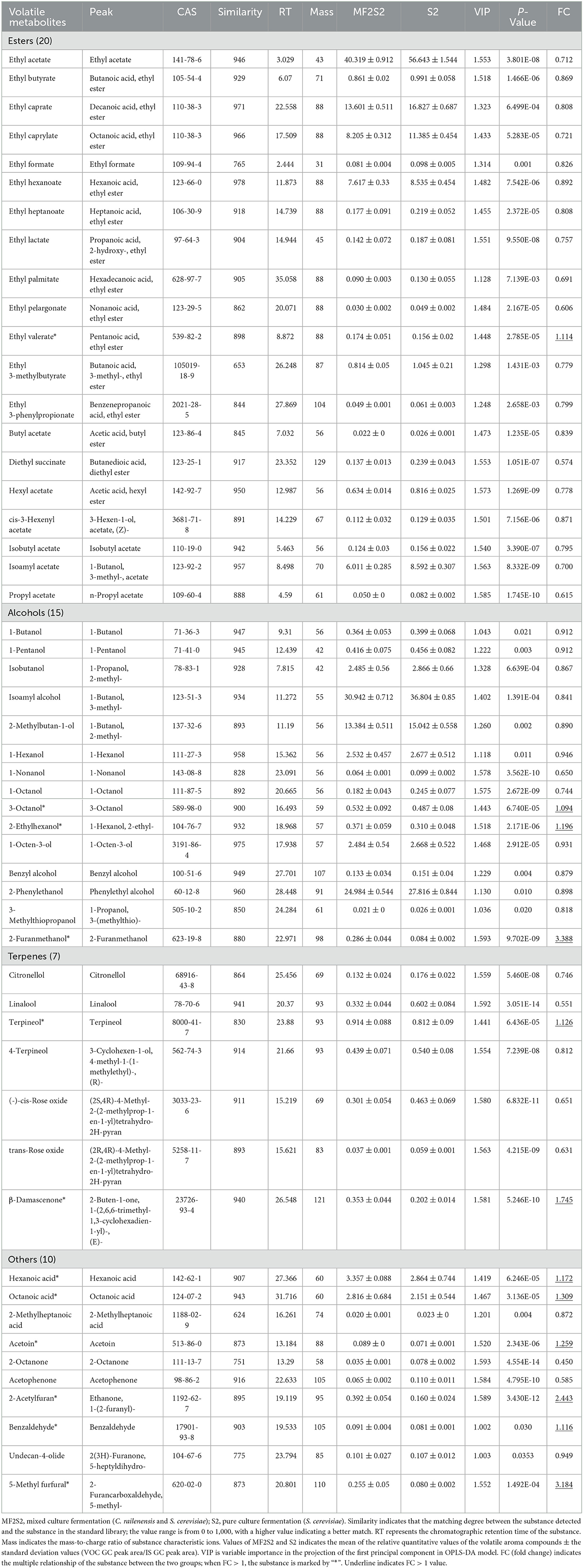
Table 4. Comparison of volatile aroma compounds in the icewines fermented by pure and mixed culture fermentation.
3.3.1. Esters
Esters are considered particularly important for wine flavor, mainly providing desirable fruity notes (Swiegers et al., 2005; Dzialo et al., 2017), which can influence the aroma of icewine through direct and complex synergistic interactions. A total of 20 esters were identified and screened in the pure and mixed culture fermented icewines, including 7 acetate esters and 13 ethyl esters (Table 4). Except for ethyl valerate, the other esters in the icewine of S2 were higher than those in the icewine of MF2S2 (FC values < 1). The highest FC value is 1.114 (ethyl valerate), and the lowest one is 0.574 (diethyl succinate) in 20 esters.
3.3.2. Alcohols
Alcohols are a diverse group of compounds that can be derived from grapes and fermentation (Huang et al., 2018). A total of 15 alcohols were identified and screened in the icewines, including 5 lower alcohols (<6 carbons), 6 higher alcohols (≥6 carbons), 2 aromatic alcohols, and 2 other alcohols (Table 4). The five lower alcohols in the icewine of S2 were all higher than those in the icewine of MF2S2 (FC values <1, from 0.841 to 0.912). In terms of higher alcohols, 3-octanol and 2-ethylhexanol in the icewine of MF2S2 were both higher than those in the icewine of S2, and their FC values were 1.094 and 1.196, respectively. Conversely, the FC values of 1-hexanol, 1-nonanol, 1-octanol, and 1-octen-3-ol are <1 (from 0.650 to 0.946).
Benzyl alcohol, 2-phenylethanol, and 3-methylthiopropanol in the icewine of mixed culture fermentation were lower than those in the icewine of control fermentation, and their FC values were 0.879, 0.898, and 0.818, respectively. Moreover, 2-furanmethanol was the highest FC value (3.388) among the 52 volatile aroma compounds.
3.3.3. Terpenes and others
Terpenes have an important effect on wine aroma and are responsible for the characteristic floral and fruity aroma (Englezos et al., 2018; Hong et al., 2021). A total of 7 terpenes were detected in the icewines, and the highest FC value is 1.745 (β-damascenone), and the lowest one is 0.551 (Linalool), as shown in Table 4.
Fatty acids, ketones, aldehydes, and furans are also indispensable compounds of wine aroma. As shown in Table 4, there are 3 fatty acids, 4 ketones, and 3 aldehydes in others. The FC values of hexanoic acid and octanoic acid are more than 1 (1.172 and 1.309). About ketones, the relative contents of acetoin with butter and creamy aroma (Francis and Newton, 2005) and 2-acetylfuran with nutty and sweet caramel-like aroma (www.chemsrc.com) were higher in the mixed fermented icewine than in the pure fermented one, and their FC values were 1.259 and 2.443, respectively. On the contrary, 2-octanone and acetophenone in the icewine of mixed culture fermentation were lower than those in the icewine of control fermentation, and 2-octanone has the lowest FC value (0.450) among the 52 volatile aroma compounds. In the three aldehydes, the FC values of both benzaldehyde and 5-methyl furfural are more than one, while the FC value of undecan-4-olide is 0.949.
3.4. Sensory evaluation of icewine
The sensory evaluation of pure and mixed culture fermented icewines was carried out. The main aromas of Vidal blanc icewine were nut, honey, caramel, rose, tropical fruit, and apricot (Huang et al., 2018). Therefore, six aspects of aromas were graded, and the final scores of the icewines are shown in Figures 5A, B.
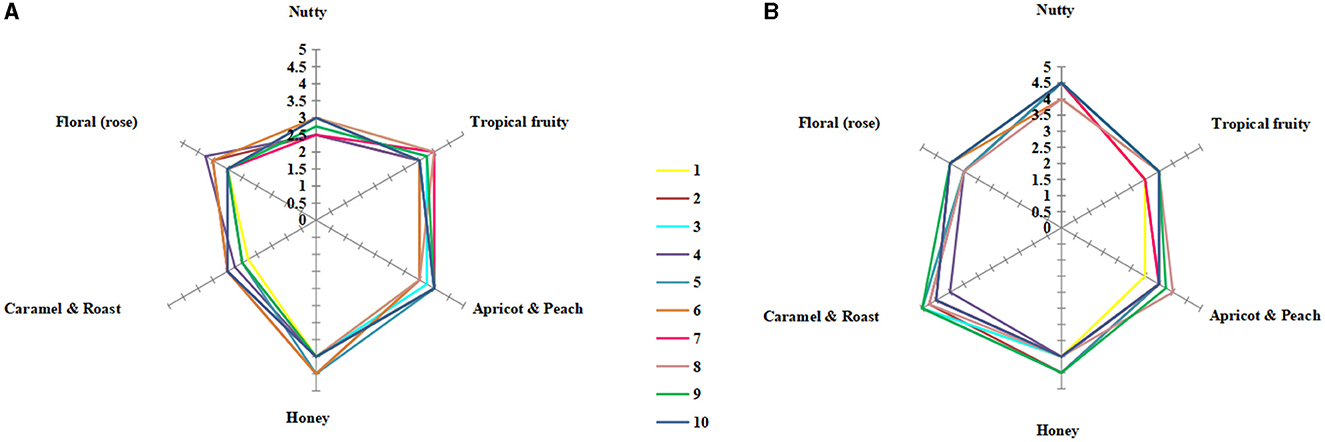
Figure 5. Sensory radar charts of icewine aroma of S2 (A) and MF2S2 (B). 1–10 denotes the number of tasters selected. The maximum score for each aspect of aroma is 5, the higher the score, the stronger the aroma: 0–1 means very weak, 1–2 means weak, 2–3 means medium, 3–4 means strong, and 4–5 means very strong. Each final score is twice the mean of tasting scores.
The aroma profiles of the icewines under different fermentation strategies were clearly different. The aroma profile of the mixed culture fermented icewine was characterized by caramel and roasted and honey aromas, while the pure culture fermented icewine expressed fruity and honey aromas. Furthermore, the overall sensory evaluations of both icewines are as follows: colors were both light golden yellow; aroma intensity, taste intensity, smoothness, and viscosity of the mixed culture fermented icewine were stronger than those of the pure culture fermented icewine; they were both harmony and balance. Therefore, C. railenensis can change the aroma profile and sensory performance of icewine by participating in the fermentation of S. cerevisiae.
4. Discussion
In this study, we showed for the first time the growth dynamic changes of C. railenensis and the effect of mixed culture of C. railenensis and S. cerevisiae during fermentation on the aromatic profile of Vidal blanc icewine, and pure S. cerevisiae fermentation was used as the control. During both types of fermentation, S. cerevisiae increased first and then declined because most of the sugars in the fermenting medium were consumed (Englezos et al., 2019). During the mixed culture fermentation, the highest cell population of S. cerevisiae was slightly lower than that in the pure culture fermentation, and this may be due to competition for nutrient consumption between S. cerevisiae and non-Saccharomyces yeasts, which was caused by cell-to-cell contact mechanisms (Fleet, 2003). C. railenensis increased after inoculating S. cerevisiae from day 2 to day 4, and this may be because S. cerevisiae promoted the growth of C. railenensis; further research would be needed to illuminate the interaction of these two species during fermentation. Then, C. railenensis disappeared after 14 days, which was because the ethanol content accumulated through fermentation had reached or exceeded the ethanol tolerance of C. railenensis (>4 and <8%, v/v; Hong et al., 2019).
The basic chemical composition of icewine was affected by the inoculation of C. railenensis during fermentation. In the early stages of fermentation, lower levels of CO2 production and ethanol contents in the mixed culture fermented icewine suggested that the ability for alcoholic fermentation of C. railenensis was lower than that in S. cerevisiae; C. railenensis had a negative influence on the alcohol fermentation performance of S. cerevisiae at the initial stage of inoculation with S. cerevisiae, and this may be because of competition for sugar consumption between S. cerevisiae and C. railenensis. At the end of fermentation, lower CO2 productions and ethanol contents in the mixed culture fermented icewine showed that C. railenensis can have the effect of reducing ethanol content during icewine fermentation. Many studies have shown that non-Saccharomyces yeasts (such as C. zemplinina) have the effect of reducing ethanol content in wine fermentation (Giaramida et al., 2013; Contreras et al., 2014; Englezos et al., 2019). In recent years, consumers have become more interested in wines with lower ethanol concentrations for health and taste reasons (Ballester-Tomás et al., 2017; Li et al., 2022), and higher ethanol concentration has an undesired effect of reducing the complexity of wine sensory properties (Contreras et al., 2014). In terms of glycerol, higher glycerol content in the mixed culture fermented icewines suggested that C. railenensis could increase glycerol yield; therefore, the smoothness and viscosity of icewine structure/body perception of mixed culture fermentation should be stronger than those of the control fermentation (Swiegers et al., 2005), and the sensory analysis confirmed this fact. Meanwhile, glycerol contents of the icewines were higher than those in dry wines. This was because the high sugar content in ice grapes must cause the expression of yeast glycerol-3-phosphate dehydrogenase (GPD1) to be upregulated, resulting in higher glycerol yields during fermentation (Pigeau and Inglis, 2005). Glycerol concentration in dry wine is generally 4–10 g/L (Heit et al., 2018), and more glycerol is considered to improve wine quality. Furthermore, acetic acid was one of the most important basic chemical compositions affecting the quality of icewine compared with the control fermentation, the acetic acid content produced by mixed culture fermentation was lower, this demonstrated that C. railenensis had the effect of reducing acetic acid content.
In terms of esters, compared with the pure culture fermented icewine, the icewine of mixed culture fermentation produced lower levels of esters, indicating that C. railenensis can reduce multiple esters and their flavors. This is similar to the result by Van Wyk et al. (2020), who stated that the involvement of C. railenensis resulted in a dramatic reduction in ester content (such as isoamyl acetate, ethyl butyrate, and ethyl hexanoate) in mixed culture fermented Muscaris wine, but ethyl valerate was not detected in their study. The result may be due to the low ester production capacity of C. railenensis initially inoculated, or/and C. railenensis negatively affected the formation of esters by S. cerevisiae during mixed culture fermentation of icewine. The detailed mechanisms still need to be further studied at metabolites and transcriptional levels.
Ethyl esters are formed by an enzyme-catalyzed condensation reaction between ethanol and acyl-CoA during lipid biosynthesis (Saerens et al., 2010). Ethyl valerate is a major ester in Vidal blanc icewine from the Huanren region, China, which has pleasant fruity aromas such as “apple” and “grape” (Ma et al., 2017; Huang et al., 2018). Ethyl acetate, which is a compound responsible for wine deterioration, is lower in the mixed fermented icewine; this was consistent with the result by Englezos et al. (2018), who pointed out that the esters in Chardonnay and Muscat wines of mixed culture fermentation (C. zemplinina + S. cerevisiae, 48 h) were significantly lower than this aroma family in pure fermented wines. Acetate esters are formed by the condensation of higher alcohols with acetyl-CoA, which are catalyzed by ATF1 and ATF2 of alcohol acyl-transferase (AAT) genes in yeast cells (Peddie, 1990), and they are considered to have a greater effect on the perceived aroma than ethyl esters (Dzialo et al., 2017).
As far as alcohols are concerned, the lower alcohols in the mixed culture fermented icewine were lower than those in the icewine of control fermentation. Isoamyl alcohol is produced by the deamination and decarboxylation reactions from leucine (Leu) during fermentation (Molina et al., 2009), and isobutanol is synthesized in yeast cells via the valine (Val) degradation pathway (Dzialo et al., 2017); they could negatively contribute to wine quality because of their herbaceous notes (Englezos et al., 2018). Moreover, 2-methylbutan-1-ol is derived from isoleucine (Ile) catabolism through the Ehrlich pathway (Hazelwood et al., 2008), and 1-butanol and 1-pentanol are often associated with unpleasant tastes, such as bitterness.
Regarding higher alcohols, except for 3-octanol and 2-ethylhexanol, the other higher alcohols in the mixed culture fermented icewine were also lower than those in the icewine of control fermentation. 1-Octen-3-ol, which has a “mushroom” flavor, has been detected in Vidal blanc icewine from the Huanren region, China (Ma et al., 2017; Zhang et al., 2018). 1-Hexanol has a vegetable and herbaceous odor, which negatively affects wine aroma (Englezos et al., 2018). In brief, C. railenensis can reduce these lower and higher alcohols and their unpleasant notes but can increase 3-octanol and 2-ethylhexanol contents and their favorable flavors, such as “pine nuts” notes and sweet and slightly floral notes (www.chemsrc.com).
Furthermore, the result showed that C. railenensis could reduce the contents of benzyl alcohol, 2-phenylethanol, and 3-methylthiopropanol and their odors. Benzyl alcohol can contribute a slightly sweet fruit odor to wine. 2-Phenylethanol, which is synthesized by the transamination of L-phenylalanine (Phe) via the Ehrlich pathway, has a rose-like odor (Swiegers et al., 2005). 3-Methylthiopropanol is derived from methionine (Met) catabolism through the Ehrlich pathway (Hazelwood et al., 2008), and it has a strong onion and meat odor, which negatively influences wine quality.
Notably, isoamyl alcohol, isobutanol, 2-methylbutan-1-ol, 2-phenylethanol, and 3-methylthiopropanol are derived from their corresponding amino acid catabolism via the Ehrlich pathway, which is caused by living yeast cells during fermentation (Hazelwood et al., 2008). These alcohols in the icewine of mixed culture fermentation were lower than those in the pure culture fermented icewine, suggesting that C. railenensis with a lower ability to produce these alcohols could negatively influence the formation of products of the Ehrlich pathway by S. cerevisiae in mixed culture fermentation, and the detailed mechanism would be studied in the future. Interestingly, 2-furanmethanol presented at a significantly higher level in the mixed culture fermented icewine and has creamy and caramel aromas (Lee et al., 2010). It could contribute to wine with coffee flavor through synergistic action with furfural in wine (Naudé and Rohwer, 2013).
Terpenes usually exist in the form of non-volatile and non-aromatic compounds during wine fermentation, which is complexed with glycosides and can be released by hydrolases, and different expression levels and activities of these hydrolases have been demonstrated in yeast species in previous studies (Fernández et al., 2000; Hong et al., 2019). Van Wyk et al. (2020) pointed out that C. railenensis could increase the content of β-damascenone during mixed culture fermentation, which was consistent with the result of this study, but their study just focused on general wine of mixed culture fermentation. β-damascenone is indirectly produced from β-carotene via neoxanthin in wine by oxidative cleavage, followed by enzymatic reduction and acid catalysis reactions (Timmins et al., 2020). The increased content of β-damascenone may be attributed to the stronger ability of C. railenensis to release (or convert) grape varietal compounds than S. cerevisiae, as well as the higher expression levels and activities of related enzymes. Moreover, β-damascenone, which has an intense rose fragrance, honey flavor, and apricot peach aromas, has been reported as a key odorant in Vidal blanc icewine profiles (Ma et al., 2017; Huang et al., 2018; Tang et al., 2021). Notably, the odor threshold of β-damascenone is very low (0.05 g/L), and small changes in the concentration can markedly influence sensory evaluations of wine (Francis and Newton, 2005). Therefore, C. railenensis in mixed culture fermentation can increase β-damascenone and its pleasant aroma.
Hexanoic acid and octanoic acid are medium-chain fatty acids that can give the wine a cheesy and fatty odor (Francis and Newton, 2005; Hong et al., 2021); and they are in the mixed culture fermented icewine were higher levels than those in the icewine of control fermentation. However, this was inconsistent with the result by Van Wyk et al. (2020), who stated that hexanoic acid and octanoic acid in wines of mixed culture fermentation were not higher than those in pure fermented wines.
In addition, the result showed that C. railenensis could increase 2-acetylfuran, benzaldehyde, and 5-methyl furfural contents and their pleasant aromas. 2-Acetylfuran can contribute to a nutty and sweet caramel-like aroma to wine. Benzaldehyde is described as having fruity, nutty, and caramel-like aromas (Xiao et al., 2015). 5-Methyl furfural, which derives from hexoses present in cellulose, can contribute caramel-like and toasted almond aromas to wine (Dumitriu et al., 2019). Correspondingly, the distinct nutty and caramel aroma in the mixed culture fermented icewine was found in the results of the sensory analysis.
5. Conclusion
The effect of sequential inoculation of indigenous C. railenensis and S. cerevisiae on the alcoholic fermentation behavior and the chemical and aromatic characteristics of Vidal blanc icewine was unveiled for the first time. The results indicated that C. railenensis in the mixed culture fermentation increased glycerol yield and reduced acetic acid yield in the basic chemical parameters, and the mixed culture fermented icewine with indigenous C. railenensis and S. cerevisiae had different aroma characteristics compared with the icewine of S. cerevisiae fermentation. For the detected volatile aroma compounds, C. railenensis reduced some metabolites (such as lower alcohols, 1-hexanol, and 3-methylthiopropanol) and their unpleasant notes and increased the production of most of the desired volatile aroma compounds (such as β-damascenone, 2-furanmethanol, and 5-methyl furfural) associated with rose, honey, nut, and caramel characteristics. Collectively, the results confirmed that C. railenensis, with positive enological properties, has the possibility of application in icewine production. Further investigations should be conducted on the application security and metabolic mechanisms of C. railenensis.
Data availability statement
The original contributions presented in the study are included in the article/supplementary material, further inquiries can be directed to the corresponding author.
Author contributions
JL designed the experiments, analyzed the experimental data, and wrote the manuscript. MH conducted the experiments. All authors contributed to the article and approved the submitted version.
Funding
The conducted research received funding from the Chinese Ministry of Education “Chunhui Program” cooperative research project (No. HZKY20220440).
Acknowledgments
The authors would like to thank Liaoning Wunushan Milan Winery Co., Ltd., for providing Vidal blanc ice grape juice.
Conflict of interest
The authors declare that the research was conducted in the absence of any commercial or financial relationships that could be construed as a potential conflict of interest.
Publisher's note
All claims expressed in this article are solely those of the authors and do not necessarily represent those of their affiliated organizations, or those of the publisher, the editors and the reviewers. Any product that may be evaluated in this article, or claim that may be made by its manufacturer, is not guaranteed or endorsed by the publisher.
References
Alessandria, V., Giacosa, S., Campolongo, S., Rolle, L., Rantsiou, K., and Cocolin, L. (2013). Yeast population diversity on grapes during on-vine withering and their dynamics in natural and inoculated fermentations in the production of icewines. Food Res. Int. 54, 139–147. doi: 10.1016/j.foodres.2013.06.018
Aplin, J. J., White, K. P., and Edwards, C. G. (2019). Growth and metabolism of non-Saccharomyces yeasts isolated from Washington state vineyards in media and high sugar grape musts. Food Microbiol. 77, 158–165. doi: 10.1016/j.fm.2018.09.004
Ballester-Tomás, L., Prieto, J. A., Gil, J. V., Baeza, M., and Randez-Gil, F. (2017). The Antarctic yeast Candida sake: Understanding cold metabolism impact on wine. Int. J. Food Microbiol. 245, 59–65. doi: 10.1016/j.ijfoodmicro.2017.01.009
Binati, R. L., Junior, W. J. L., Luzzini, G., Slaghenaufi, D., Ugliano, M., and Torriani, S. (2020). Contribution of non-Saccharomyces yeasts to wine volatile and sensory diversity: A study on Lachancea thermotolerans, Metschnikowia spp. and Starmerella bacillaris strains isolated in Italy. Int. J. Food Microbiol. 318, 108470. doi: 10.1016/j.ijfoodmicro.2019.108470
Bowen, A. J., and Reynolds, A. G. (2015). Aroma compounds in Ontario Vidal and Riesling icewines. I. Effects of harvest date. Food Res. Int. 76, 540–549. doi: 10.1016/j.foodres.2015.06.046
BreŽná, B., Ženišová, K., Chovanová, K., Chebenová, V., Kraková, L., Kuchta, T., et al. (2010). Evaluation of fungal and yeast diversity in Slovakian wine-related microbial communities. Anton. Leeuw. 98, 519–529. doi: 10.1007/s10482-010-9469-6
Brysch-Herzberg, M., and Seidel, M. (2015). Yeast diversity on grapes in two German wine growing regions. Int. J. Food Microbiol. 214, 137–144. doi: 10.1016/j.ijfoodmicro.2015.07.034
Ciani, M., Comitini, F., Mannazzu, I., and Domizio, P. (2010). Controlled mixed culture fermentation: a new perspective on the use of non-Saccharomyces yeasts in winemaking. FEMS Yeast Res. 10, 123–133. doi: 10.1111/j.1567-1364.2009.00579.x
Cioch-Skoneczny, M., Satora, P., Skotniczny, M., and Skoneczny, S. (2018). Quantitative and qualitative composition of yeast microbiota in spontaneously fermented grape musts obtained from cool climate grape varieties ‘Rondo'and ‘Regent'. FEMS Yeast Res. 18, foy089. doi: 10.1093/femsyr/foy089
Contreras, A., Hidalgo, C., Henschke, P. A., Chambers, P. J., Curtin, C., and Varela, C. (2014). Evaluation of non-Saccharomyces yeasts for the reduction of alcohol content in wine. Appl. Environ. Microb. 80, 1670–1678. doi: 10.1128/AEM.03780-13
Crandles, M., Reynolds, A. G., Khairallah, R., and Bowen, A. (2015). The effect of yeast strain on odor active compounds in Riesling and Vidal blanc icewines. LWT-Food Sci. Technol. 64, 243–258. doi: 10.1016/j.lwt.2015.05.049
Drumonde-Neves, J., Franco-Duarte, R., Lima, T., Schuller, D., and Pais, C. (2017). Association between grape yeast communities and the vineyard ecosystems. PLoS ONE 12, e0169883. doi: 10.1371/journal.pone.0169883
Dumitriu, G. D., Teodosiu, C., Gabur, I., Cotea, V. V., Peinado, R. A., and López de Lerma, N. (2019). Evaluation of aroma compounds in the process of wine ageing with oak chips. Foods 8, 662. doi: 10.3390/foods8120662
Dzialo, M. C., Park, R., Steensels, J., Lievens, B., and Verstrepen, K. J. (2017). Physiology, ecology and industrial applications of aroma formation in yeast. FEMS Microbiol. Rev. 41, S95–S128. doi: 10.1093/femsre/fux031
Englezos, V., Pollon, M., Rantsiou, K., Ortiz-Julien, A., Botto, R., Segade, S. R., et al. (2019). Saccharomyces cerevisiae-Starmerella bacillaris strains interaction modulates chemical and volatile profile in red wine mixed fermentations. Food Res. Int. 122, 392–401. doi: 10.1016/j.foodres.2019.03.072
Englezos, V., Rantsiou, K., Cravero, F., Torchio, F., Pollon, M., Fracassetti, D., et al. (2018). Volatile profile of white wines fermented with sequential inoculation of Starmerella bacillaris and Saccharomyces cerevisiae. Food Chem. 257, 350–360. doi: 10.1016/j.foodchem.2018.03.018
Englezos, V., Torchio, F., Cravero, F., Marengo, F., Giacosa, S., Gerbi, V., et al. (2016). Aroma profile and composition of Barbera wines obtained by mixed fermentations of Starmerella bacillaris (synonym Candida zemplinina) and Saccharomyces cerevisiae. LWT-Food Sci. Technol. 73, 567–575. doi: 10.1016/j.lwt.2016.06.063
Fernández, M., Ubeda, J. F., and Briones, A. I. (2000). Typing of non-Saccharomyces yeasts with enzymatic activities of interest in wine-making. Int. J. Food Microbiol. 59, 29–36. doi: 10.1016/S0168-1605(00)00283-X
Fleet, G. H. (2003). Yeast interactions and wine flavour. Int. J. Food Microbiol. 86, 11–22. doi: 10.1016/S0168-1605(03)00245-9
Francis, I. L., and Newton, J. L. (2005). Determining wine aroma from compositional data. Aust. J. Grape Wine R. 11, 114–126. doi: 10.1111/j.1755-0238.2005.tb00283.x
Giaramida, P., Ponticello, G., Di Maio, S., Squadrito, M., Genna, G., Barone, E., et al. (2013). Candida zemplinina for production of wines with less alcohol and more glycerol. S. Afr. J. Enol. Vitic. 34, 204–211. doi: 10.21548/34-2-1095
Hazelwood, L. A., Daran, J. M., Van Maris, A. J., Pronk, J. T., and Dickinson, J. R. (2008). The Ehrlich pathway for fusel alcohol production: a century of research on Saccharomyces cerevisiae metabolism. Appl. Environ. Microb. 74, 2259–2266. doi: 10.1128/AEM.02625-07
Heit, C., Martin, S. J., Yang, F., and Inglis, D. L. (2018). Osmoadaptation of wine yeast (Saccharomyces cerevisiae) during Icewine fermentation leads to high levels of acetic acid. J. Appl. Microbiol. 124, 1506–1520. doi: 10.1111/jam.13733
Hong, M. N., Li, J., and Chen, Y. (2019). Characterization of tolerance and multi-enzyme activities in non-Saccharomyces yeasts isolated from Vidal blanc icewine fermentation. J. Food Biochem. 43, e13027. doi: 10.1111/jfbc.13027
Hong, M. N., Li, J., Chen, Y., Qi, B., Huang, Y., Wu, J., et al. (2021). Impact of mixed non-Saccharomyces yeast during fermentation on volatile aroma compounds of Vidal blanc icewine. LWT-Food Sci. Technol. 145, 111342. doi: 10.1016/j.lwt.2021.111342
Huang, L., Ma, Y., Tian, X., Li, J. M., Li, L. X., Tang, K., et al. (2018). Chemosensory characteristics of regional Vidal icewines from China and Canada. Food Chem. 261, 66–74. doi: 10.1016/j.foodchem.2018.04.021
Kind, T., Wohlgemuth, G., Lee, D. Y., Lu, Y., Palazoglu, M., Shahbaz, S., et al. (2009). FiehnLib: mass spectral and retention index libraries for metabolomics based on quadrupole and time-of-flight gas chromatography/mass spectrometry. Anal. Chem. 81, 10038–10048. doi: 10.1021/ac9019522
Lan, Y. B., Xiang, X. F., Qian, X., Wang, J. M., Ling, M. Q., Zhu, B. Q., et al. (2019). Characterization and differentiation of key odor-active compounds of ‘Beibinghong'icewine and dry wine by gas chromatography-olfactometry and aroma reconstitution. Food Chem. 287, 186–196. doi: 10.1016/j.foodchem.2019.02.074
Lee, S. B., and Park, H. D. (2020). Isolation and investigation of potential non-Saccharomyces yeasts to improve the volatile terpene compounds in Korean Muscat Bailey A wine. Microorganisms 8, 1552. doi: 10.3390/microorganisms8101552
Lee, S. J., Moon, T. W., and Lee, J. (2010). Increases of 2-furanmethanol and maltol in Korean red ginseng during explosive puffing process. J. Food Sci. 75, C147–C151. doi: 10.1111/j.1750-3841.2009.01461.x
Lencioni, L., Romani, C., Gobbi, M., Comitini, F., Ciani, M., and Domizio, P. (2016). Controlled mixed fermentation at winery scale using Zygotorulaspora florentina and Saccharomyces cerevisiae. Int. J. Food Microbiol. 234, 36–44. doi: 10.1016/j.ijfoodmicro.2016.06.004
Li, J., Hong, M. N., and Qi, B. Y. (2022). Impact of Torulaspora delbrueckii during fermentation on aromatic profile of Vidal blanc icewine. Front. Microbiol. 13, 860128. doi: 10.3389/fmicb.2022.860128
Li, J., Hu, W. Z., Huang, X. J., and Xu, Y. P. (2018). Investigation of yeast population diversity and dynamics in spontaneous fermentation of Vidal blanc icewine by traditional culture-dependent and high-throughput sequencing methods. Food Res. Int. 112, 66–77. doi: 10.1016/j.foodres.2018.06.011
Li, J., Hu, W. Z., and Xu, Y. P. (2019). Diversity and dynamics of yeasts during Vidal blanc icewine fermentation: A strategy of the combination of culture-dependent and high-throughput sequencing approaches. Front. Microbiol. 10, 1588. doi: 10.3389/fmicb.2019.01588
Lin, M. M. H., Boss, P. K., Walker, M. E., Sumby, K. M., Grbin, P. R., and Jiranek, V. (2020). Evaluation of indigenous non-Saccharomyces yeasts isolated from a South Australian vineyard for their potential as wine starter cultures. Int. J. Food Microbiol. 312, 108373. doi: 10.1016/j.ijfoodmicro.2019.108373
Ma, Y., Tang, K., Xu, Y., and Li, J. M. (2017). Characterization of the key aroma compounds in Chinese Vidal icewine by gas chromatography-olfactometry, quantitative measurements, aroma recombination, and omission tests. J. Agr. Food Chem. 65, 394–401. doi: 10.1021/acs.jafc.6b04509
Maturano, Y. P., Assaf, L. A. R., Toro, M. E., Nally, M. C., Vallejo, M., De Figueroa, L. I. C., et al. (2012). Multi-enzyme production by pure and mixed cultures of Saccharomyces and non-Saccharomyces yeasts during wine fermentation. Int. J. Food Microbiol. 155, 43–50. doi: 10.1016/j.ijfoodmicro.2012.01.015
Molina, A. M., Guadalupe, V., Varela, C., Swiegers, J. H., Pretorius, I. S., and Agosin, E. (2009). Differential synthesis of fermentative aroma compounds of two related commercial wine yeast strains. Food Chem. 117, 189–195. doi: 10.1016/j.foodchem.2009.03.116
Naudé, Y., and Rohwer, E. R. (2013). Investigating the coffee flavour in South African Pinotage wine using novel offline olfactometry and comprehensive gas chromatography with time of flight mass spectrometry. J. Chromatogr. A. 1271, 176–180. doi: 10.1016/j.chroma.2012.11.019
OIV. (2018). Available online at: http://www.oiv.int/en/technical-standards-and-documents/methods-of-analysis/ (accession March 8 2020).
OIV-International Organisation of Vine Wine. (2015). Icewine Oeno6/03. Available online at: http://www.oiv.int/en/technical-standards-and-documents/products-definition-and-labelling/definition-of-the-vitivinicultural-products-by-code-sheet#i47 (accessed July 15, 2022).
Padilla, B., Gil, J. V., and Manzanares, P. (2016). Past and future of non-Saccharomyces yeasts: From spoilage microorganisms to biotechnological tools for improving wine aroma complexity. Front. Microbiol. 7, 411. doi: 10.3389/fmicb.2016.00411
Peddie, H. A. B. (1990). Ester formation in brewery fermentations. J. Inst. Brew. 96, 327–331. doi: 10.1002/j.2050-0416.1990.tb01039.x
Pigeau, G. M., and Inglis, D. L. (2005). Upregulation of ALD3 and GPD1 in Saccharomyces cerevisiae during icewine fermentation. J. Appl. Microbiol. 99, 112–125. doi: 10.1111/j.1365-2672.2005.02577.x
Rantsiou, K., Dolci, P., Giacosa, S., Torchio, F., Tofalo, R., Torriani, S., et al. (2012). Candida zemplinina can reduce acetic acid produced by Saccharomyces cerevisiae in sweet wine fermentations. Appl. Environ. Microbiol. 78, 1987–1994. doi: 10.1128/AEM.06768-11
Rodríguez, M. E., Lopes, C. A., Barbagelata, R. J., Barda, N. B., and Caballero, A. C. (2010). Influence of Candida pulcherrima Patagonian strain on alcoholic fermentation behaviour and wine aroma. Int. J. Food Microbiol. 138, 19–25. doi: 10.1016/j.ijfoodmicro.2009.12.025
Saerens, S. M., Delvaux, F. R., Verstrepen, K. J., and Thevelein, J. M. (2010). Production and biological function of volatile esters in Saccharomyces cerevisiae. Microb. Biotechnol. 3, 165–177. doi: 10.1111/j.1751-7915.2009.00106.x
Steensels, J., and Verstrepen, K. J. (2014). Taming wild yeast: potential of conventional and nonconventional yeasts in industrial fermentations. Annu. Rev. Microbiol. 68, 61–80. doi: 10.1146/annurev-micro-091213-113025
Swiegers, J. H., Bartowsky, E. J., Henschke, P. A., and Pretorius, I. (2005). Yeast and bacterial modulation of wine aroma and flavour. Aust. J. Grape Wine Res. 11, 139–173. doi: 10.1111/j.1755-0238.2005.tb00285.x
Tang, K., Sun, Y., Zhang, X., Li, J., and Xu, Y. (2021). Chemical and sensory characterization of Vidal icewines fermented with different yeast strains. Fermentation 7, 211. doi: 10.3390/fermentation7040211
Timmins, J. J., Kroukamp, H., Paulsen, I. T., and Pretorius, I. S. (2020) . The sensory significance of apocarotenoids in wine: Importance of carotenoid cleavage dioxygenase 1 (CCD1) in the production of β- ionone. Molecules 25, 2779.
Tofalo, R., Perpetuini, G., Rossetti, A. P., Gaggiotti, S., Piva, A., Olivastri, L., et al. (2022). Impact of Saccharomyces cerevisiae and non-Saccharomyces yeasts to improve traditional sparkling wines production. Food Microbiol. 108, 104097. doi: 10.1016/j.fm.2022.104097
Van Wyk, N., Pretorius, I. S., and Von Wallbrunn, C. (2020). Assessing the oenological potential of Nakazawaea ishiwadae, Candida railenensis and Debaryomyces hansenii strains in mixed-culture grape must fermentation with Saccharomyces cerevisiae. Fermentation 6, 49. doi: 10.3390/fermentation6020049
Varela, C., and Borneman, A. R. (2017). Yeasts found in vineyards and wineries. Yeast 34, 111–128. doi: 10.1002/yea.3219
Xiao, Z., Dai, X., Zhu, J., and Yu, H. (2015). Classification of Chinese Rice wine according to geographic origin and wine age based on chemometric methods and SBSE-TD-GC-MS analysis of volatile compounds. Food Sci. Technol. Res. 21, 371–380. doi: 10.3136/fstr.21.371
Keywords: Candida railenensis, Saccharomyces cerevisiae, sequential inoculation, mixed culture fermentation, Vidal blanc icewine, volatile aroma compounds
Citation: Li J and Hong M (2023) Impact of Candida railenensis during fermentation on the aromatic profile of Vidal blanc icewine. Front. Microbiol. 14:1192006. doi: 10.3389/fmicb.2023.1192006
Received: 22 March 2023; Accepted: 17 July 2023;
Published: 08 August 2023.
Edited by:
Virgínia Farias Alves, Universidade Federal de Goiás, BrazilReviewed by:
Jinjing Wang, Jiangnan University, ChinaKatherine Witrick, University of Florida, United States
Marita Gimenez Pereira, University of São Paulo, Brazil
Copyright © 2023 Li and Hong. This is an open-access article distributed under the terms of the Creative Commons Attribution License (CC BY). The use, distribution or reproduction in other forums is permitted, provided the original author(s) and the copyright owner(s) are credited and that the original publication in this journal is cited, in accordance with accepted academic practice. No use, distribution or reproduction is permitted which does not comply with these terms.
*Correspondence: Jing Li, lijing80@mail.dlut.edu.cn