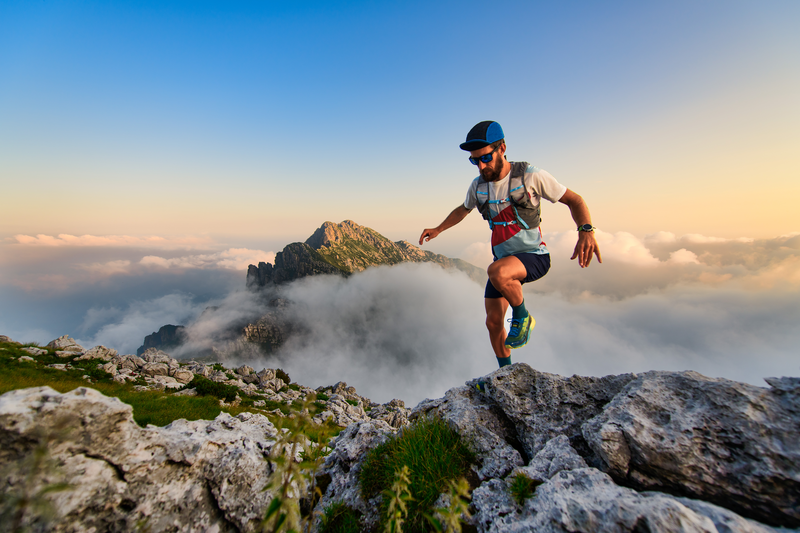
94% of researchers rate our articles as excellent or good
Learn more about the work of our research integrity team to safeguard the quality of each article we publish.
Find out more
ORIGINAL RESEARCH article
Front. Microbiol. , 27 July 2023
Sec. Evolutionary and Genomic Microbiology
Volume 14 - 2023 | https://doi.org/10.3389/fmicb.2023.1191837
This article is part of the Research Topic Antimicrobial Resistance Genomics in Bacterial Zoonotic Pathogens View all 5 articles
Multidrug-resistant Enterococcus faecalis (E. faecalis) often cause intestinal infections in cats. The aim of this study was to investigate a multidrug-resistant E. faecalis isolate for plasmidic and chromosomal antimicrobial resistance and their genetic environment. E. faecalis strain ESC1 was obtained from the feces of a cat. Antimicrobial susceptibility testing was carried out using the broth microdilution method. Conjugation experiments were performed using Escherichia coli and Staphylococcus aureus as receptors. Complete sequences of chromosomal DNA and plasmids were generated by whole genome sequencing (WGS) and bioinformatics analysis for the presence of drug resistance genes and mobile elements. Multidrug-resistant E. faecalis ESC1 contained a chromosome and three plasmids. The amino acid at position 80 of the parC gene on the chromosome was mutated from serine to isoleucine, and hence the amino acid mutation at this site led to the resistance of ESC1 strain to fluoroquinolones. Eleven antibiotic resistance genes were located on two plasmids. We identified a novel composite transposon carrying two aminoglycoside resistance genes aac(6′)-aph(2″). This study reported the coexistence of a novel 5.4 kb composite transposon and a resistance plasmid with multiple homologous recombination in an isolate of E. faecalis ESC1. This data provides a basis for understanding the genomic signature and antimicrobial resistance mechanisms of this pathogen.
Humans have begun mass production and usage of antibiotics since long for the treatment of infectious diseases due to which many bacteria have evolved resistance to a variety of antibiotics (D’Costa et al., 2011). An important driver of the persistent evolution of existing mechanisms of drug resistance could be the endless competition for resources among microorganisms, including the natural production of secondary metabolites by microorganism which are used against many antibiotics today (Allen et al., 2010). The introduction of relatively new antibiotics as clinical drugs has radically altered the conditions for the evolution and spread of drug resistance by exerting unprecedented selection pressure on members of the microbial community, which has not only contaminated the humans and livestock but the environment as well (Larsson and Flach, 2022). This selective pressure has promoted the mobilization and horizontal transfer of a large number of antibiotic resistance genes (ARGs) to many bacterial species, especially to pathogenic bacteria (Alcock et al., 2020). The end result of this cumulative evolutionary event has been known to increase difficulty in preventing and treating bacterial infections. Bacteria and resistance genes not only transmit amongst species and environments but could lead to transboundary diseases as well, therefore, one health concept is the only way for understanding and recognizing the links between human, animal, and environmental microflora and addressing this global health challenge (Mackenzie and Jeggo, 2019; Buschhardt et al., 2021).
Recently, COVID-19 is considered as one the common, prominent and alarming illness among infectious diseases which has increased community’s awareness regarding public health issues and they have realized that zoonotic diseases could be the similar threats for creating pandemic situations (Fritz et al., 2021). The companion animals that live with humans day and night can be the source of zoonotic diseases and pose a potential threat to human health (Powell et al., 2022). There is a large number of microflora in the intestinal flora of companion animals (Ganz et al., 2022), and among them, Enterococcus faecalis (E. faecalis) is a pathogenic anaerobic gram-positive Enterococcus that is an early colonizer of the intestine in humans and animals (Chong et al., 2017; Willett et al., 2021). Yuan et al. (2023) monitored E. faecalis in the intestines of domestic cats in Northeast China and found that the isolation rate of E. faecalis in fecal samples from domestic cats was 76.67%. At the same time, the isolated strains were found to be resistant to tetracycline and erythromycin (Yuan et al., 2023). It is also a conditional pathogen that has been recognized as an important cause of nosocomial infections increasingly. Similarly, it can cause related infections, including bacteremia, bacterial endocarditis, amyloid arthropathy, diarrhea and gastrointestinal inflammation (Cauwerts et al., 2007; Gregersen et al., 2010; Fan et al., 2021; Guo et al., 2022). Recently, multidrug-resistant E. faecalis has been frequently reported (Kang et al., 2021; Stȩpień-Pyśniak et al., 2021; Yoon and Lee, 2021; Shan et al., 2022). The emergence of antibiotic-resistant strains has brought challenges to clinical practice and potential threat to public health (Anderson et al., 2018).
Antibiotic resistance in E. faecalis mediated by mobile genetic elements has been frequently reported. Tn916 is a conjugative transposon originally found in E. faecalis DS16. It confers resistance to tetracycline via tet(M) (Franke and Clewell, 1981; Flannagan et al., 1994; Browne et al., 2015). Tn1331 is composed of a Tn3 transposon (carrying blaTEM-1 gene) and two gene cassettes carrying three antibiotic resistance genes, namely aac(6′)-Ib, ant(3″)-Ia and blaOXA-9 genes, conferring resistance to kanamycin, streptomycin and beta-lactams, respectively (Alavi et al., 2011). Tn6674 is a 12.9-kb Tn554-related transposon, which was found in a porcine E. faecalis strain E1731, it contains four resistance genes [spc, erm(A), fexA and optrA] conferring resistance to spectinomycin, macrolides, phenicols and oxazolidinone, respectively (Li et al., 2019). Tn6248 carries tet(M), tet(L) and catA genes, conferring tetracycline and chloramphenicol resistance (Gawryszewska et al., 2017). In addition, enterococci with high-level gentamicin resistance (HLGR) have also been reported in foods of animal origin (Choi and Woo, 2013; Liu et al., 2013). A study by Zhang et al. (2018) indicated that a composite transposon carrying the aac(6′)-aph(2″) gene mediated high levels of resistance to aminoglycoside antibiotics in E. faecalis.
In order to further explore the genetic environment of antibiotic resistant genes in multidrug-resistant E. faecalis, we isolated a multidrug-resistant E. faecalis ESC1 from the feces of a cat in a pet hospital. The whole genome of E. faecalis ESC1 was sequenced. Bioinformatics analysis showed that the resistance of this strain to antibiotics was mainly mediated by the resistance genes carried by the mobile elements, and there was a risk of resistance transmission. In addition, we found a novel composite transposon carrying two aminoglycoside resistance genes aac(6′)-aph(2″). Companion animals could be a potential threat and repository for carrying diseases and drug resistant bacteria to human health (Yuan et al., 2023), therefore, attention should be paid to the health of humans and companion animals in order to avoid its spread.
Samples of feces in this study were collected in 2018 from cats from a pet hospital located in the Jilin Province, China. A sample of 1 g of feces from each cat was inoculated into 2 mL phosphate buffer saline (PBS), and was directly streaked onto brain heart infusion (BHI) agar and incubated at 37°C overnight. According to Dong et al.’s (2019) study, 16S rRNA sequences of different forms of colonies were amplified using universal primers, 27F and 1492R. The primer sequence was as follow: F: 5′-AGAGTTTGATCCTGGCTCAG-3′, R: 5′-GGTTACCTTTACGATT-3′. The DNA of the isolated strains was extracted using the DNA extraction kit (Ezup cfDNA extraction kit, Sangon Biotech Co., Ltd., Shanghai, China). The amplified products were sequenced by Biotechnology Co., Ltd., Beijing, China. The sequencing results were compared using NCBI BLAST search. The isolated strains were tested for antibiotic sensitivity by micro broth dilution method.
According to the method of Vaser et al. (2017), a reverse PCR assay was established to detect the presence of circular intermediates. When linear transposon formed a circular intermediate between the two IS1216E elements, the product was amplified by PCR using reverse primers. The primer sequence was as follow: F: 5′-TCGGATTCAAGGACTCTTCAATCAAG-3′, R: 5′-AGAACTCTTATGTCCAATTCCACCACT-3′.
Conjugation transfer test was carried out according to the test method of Yu et al. (2022). To put it simply, rifampin-resistant Escherichia coli strain ATCC 25922 and rifampin-resistant Staphylococcus aureus ATCC 29212 were used as recipient strains. Bacteria were cultured in BHI broth medium at 37°C overnight, and then diluted at 1:100 in fresh broth to expand the culture to OD600 = 0.5. Bacterial precipitates were then collected by centrifugation and resuspended in BHI broth. The final conjugation system (total volume of 2 mL) consisted of 1 mL donor and 1 mL recipient bacteria. BHI agar supplemented with rifampicin (25 mg/mL) and tetracycline (50 mg/mL) was used for transconjugant selection after incubation at 37°C for 15 h.
Antimicrobial susceptibility testing of the E. faecalis ESC1 and its transconjugants was performed using broth microdilution method and the results were interpreted according to Clinical and Laboratory Standards Institute (CLSI) recommendations (Lubbers, 2023). A total of 9 antimicrobial agents tested were as follows: erythromycin, tetracycline, vancomycin, linezolid, ciprofloxacin, norfloxacin, moxifloxacin, chloramphenicol and florfenicol. E. coli ATCC 25922 and S. aureus ATCC 29212 served as a quality control strains.
E. faecalis ESC1 genomic DNA was extracted using the FastPure Gel DNA Extraction Mini Kit (Vazyme, Nanjing, China) and quality checked for purity, concentration and integrity using Nanodrop 2000 (Thermo Scientific, MA, USA), Qubit 4 (Thermo Scientific, MA, USA) and 0.35% agarose gel electrophoresis in order to extract high quality genomic DNA. BluePippin automated nucleic acid recovery system (Sage Science, MA, USA) was used to recover large fragments of DNA. SQK-LSK109 ligation kit was used to construct the library. The genomic DNA was sequenced on the Oxford Nanopore platform (Jain et al., 2016; Wang et al., 2021). Assembly of filtered subreads was performed using Canu v1.5 software (Koren et al., 2017). The assembly results were corrected by Racon v3.4.3 software using third generation subreads (Vaser et al., 2017). Cyclisation and adjustment of start sites were performed by Circlator v1.5.5 software (Hunt et al., 2015). Further error correction was performed using second generation data using Pilon v1.22 software to obtain a more accurate genome for subsequent analysis (Walker et al., 2014).
Antimicrobial resistance genes were identified using the ResFinder 4.1.1 The mobile genetic elements were analyzed using the ISFinder,2 the Transposon Registry.3 Sequence analyses were conducted using the RAST4 and BLAST functions.5 For sequence comparisons, the BLAST algorithm6 was used. Using the assembled and predicted genome information, such as tRNA, rRNA, repeat sequence, GC content and gene function information, the genome circle map was drawn by Circos v0.66 software (Krzywinski et al., 2009).
A total of 11 cat fecal samples was collected from pet hospitals in Jilin province, China in 2018 and 28 strains were isolated. A strain of E. faecalis was identified by the 16S rRNA sequencing (CP118057.1: per.identity: 100%; e-value: 0; query cover: 100%) (Frank et al., 2008). The antimicrobial susceptibility phenotypes of E. faecalis ESC1 were determined using Muller–Hinton (MH) broth. The results showed that the strain was resistant to erythromycin, tetracycline, linezolid, ciprofloxacin, norfloxacin, moxifloxacin, chloramphenicol, florfenicol and sensitive to vancomycin (Table 1). As mentioned above, the conjugation experiments were conducted according to the international standard definitions. Minimum inhibitory concentrations (MICs) of different antimicrobial agents against E. faecalis ESC1 showed high levels of antibiotic resistance, representing multidrug-resistant strains. Transconjugants exhibited multidrug resistance. The MICs of E. coli and S. aureus to the tested antibiotics increased by 8–128 times and 2–256 times, respectively.
Based on the MIC study mentioned above, ESC1 was selected for genome-wide sequencing to clarify the potential mechanisms of multidrug resistance. A CIRCOS Circular representation of the ESC1 genome with annotated genes was constructed. The genome of E. faecalis ESC1 contained a chromosome and three plasmids (pESC1, pESC2, and pESC3) (Figure 1). The size of chromosome DNA of ESC1 was 2,887,950 bp and the ratio of guanine-cytosine content in the genome was 37.5, which is consistent to other reported E. faecalis genomes. The chromosomal DNA and plasmid sequences of E. faecalis ESC1 were deposited in NCBI GenBank with the accession numbers CP115992, CP115993, CP115994, and CP115995, respectively.
Figure 1. Circular representation of the E. faecalis ESC1 genome (chromosome and three plasmids). Moving inward in the chromosome circular map, slots 1–3 (slot 1, GC skew; slot 2, GC content; slot 3, open reading frames). Red for antibiotic resistance genes, orange for conjugation transfer of related genes, green for plasmid replication and maintenance related genes, gray for hypothetical proteins and pink for mobile genetic elements.
Antibiotic resistance-associated genes were predicted according to ResFinder. ESC1 contained 11 resistance genes [erm(A), erm(B), optrA, fexA, catA, aph(3′)-III, aac(6′)-aph(2″), tet(M), tet(L), dfr(G)] and one resistance-related mutation, a serine at amino acid position 80 in the parC gene was mutated into leucine. These genes may confer resistance to macrolide, aminoglycoside, tetracycline, trimethoprim, oxazolidinone and phenicol.
The size of plasmid pESC2 was 141,731 bp with 156 ORFs, and an average GC content of 34.4%, carrying 9 antibiotic resistance genes. Interestingly, plasmid pESC2 showed a very similar structure (99.99% nucleotide sequence identity and 69% query coverage) to plasmid pKUB3006-1 (Supplementary Figure 1), accession number AP018539.1. According to the observed similarities, these two plasmids may have evolved from each other or from the same ancestor. A part of a similar region contained 6 drug resistance genes. The optrA gene encoded an ATP binding cassette (ABC)-F protein, which was located on a composite transposon and flanked by the insertion sequence IS1216E. In addition, the composite transposon carried the antibiotic resistance genes erm(A) and fexA (Figure 2). According to the method of He et al. (2016), a reverse PCR assay was established to detect the presence of these circular intermediates between the two IS1216E elements in E. faecalis ESC1. The results of reverse PCR identification are shown in Supplementary Figure 2, We used the designed reaction PCR primers to amplify a target band at 2,618 bp, demonstrating the existence of a structure in which two IS1626E mobile primers are tandemly linked together. The results demonstrated the presence of the predicted circular intermediates, suggesting that this composite transposon could mediate the transfer of drug resistance genes. In addition, the genes tet(M), tet(L) and catA, which mediate tetracycline and chloramphenicol resistance were located in a region similar to the Tn6248 transposon (Figure 3).
Figure 2. Organization of the composite transposon flanked by the insertion sequence IS1216E and comparison with related regions. The distance scale is given in kilobase. Genes are denoted by arrows. Genes, mobile elements and other features are colored based on their functional classification. Shading denotes regions of homology (nucleotide identity > 95%). The accession numbers of pC25, pC54, and Tn6261 for reference are CP030043.1, CP030046.1, and KU354267.1, respectively.
Figure 3. Linear illustration of the MDR region of pESC2 (69,630–96,200 bp) and comparative analysis of this region: Tn6248 (accession number KP834592). The distance scale is given in kilobase. Red for antibiotic resistance genes, orange for conjugation transfer of related genes, green for plasmid replication and maintenance related genes, gray for hypothetical proteins and purple for other genes.
It is worth noting that a novel compound transposon located on plasmid pESC2 was found (Figure 4). The isotropic insertion sequence IS1216 was located on both sides of the composite transposon. The gene encoding the GNAT family of acetyltransferases was located between two aminoglycoside resistance genes [aac(6′)-aph(2″)]. Downstream of one of the aminoglycoside resistance gene contained the remaining of an insertion sequence IS256. In contrast to the previously reported IS256, the upstream of this IS256 transposase was truncated by IS1216 or IS256 carries an aac(6′)-aph(2″) gene inserted into the composite transposon. To the best of our knowledge, there are no reports of two aac(6′)-aph(2″) genes mediated by IS1216 in tandem on a single composite transposon. When located in the same orientation, the recombination between two copies of IS1216E resulted in the generation of a loop containing the bracketed region plus one copy of the IS1216E element. Therefore, the novel composite transposon increased the risk of antibiotic-resistant gene dissemination. In addition, chromosomal DNA analysis of ESC1 revealed the presence of an integrative and conjugative element (ICE) region (143,268 bp in size with a guanine-cytosine content of 34.45%). The ICE was flanked by a 15-bp target site duplication (TAAATAATTGTTGGT) (Supplementary Figure 3).
CIRCOS Circular genome visualization can more clearly explore the positional relationship of genome components in a genome. The loop diagram of assembled genome is shown in Figure 5. Except for the genes not annotated to function, we observed that 240 genes were implicated in carbohydrate transport and metabolism, 210 genes were implicated in replication, recombination and repair, 190 genes were implicated in transcription, 174 genes were implicated in amino acid transport and metabolism, 166 genes were implicated in translation, ribosomal structure and biology, 128 genes were implicated in inorganic ion transport and metabolism, 122 genes were implicated in cell wall/membrane/envelope biology and 101 genes were implicated in energy product on and conversion. These genes all have key functions in the bacterial growth.
Figure 5. The outermost circle is marked with genome size, and each scale is 5 kb; the second circle and third circle are genes on the positive and negative chain of the genome, respectively, and different colors represent different functional classifications of COG; the fourth circle is a repetitive sequence; the fifth circle is tRNA and rRNA, blue is tRNA and purple is rRNA; the sixth circle is GC content, the light yellow part indicate that the GC content in this region is higher than the average GC content of the genome, the higher peak value, greater difference with the average GC content, and the blue part indicate that the GC content in this region is lower than the average GC content of the genome; the innermost ring is GC skew, dark gray represent the area where the G content is greater than C, and red represent the area where the C content is greater than G.
According to reports, E. faecalis, as an opportunistic pathogen, was mainly isolated from pig tissue and human tissue samples in the past (Getachew et al., 2013; Chen et al., 2020; Chilambi et al., 2021). As a companion animal, cats live with human beings, and even are regarded as family members among individuals, so they are very close to human life (Overgaauw et al., 2020). A data on fecal E. faecalis isolated from companion animals likes dogs and cats have showed that the isolation rate of Enterococcus was 60–80%, while most of these Enterococcus were multidrug-resistant strains. This suggests that companion dogs and cats may be carriers of anti-microbial drug resistance genes, with the potential of transfer to humans (Jackson et al., 2009). Multidrug-resistant strains are defined as having drug resistance to three types of antibiotics commonly used in the clinics (Magiorakos et al., 2012). We isolated a multidrug-resistant E. faecalis ESC1 from fecal samples of sick cats from pet hospitals, and found that it was resistant to nine antibiotics from five major tested groups and was therefore, termed as a multidrug-resistant strain (Magiorakos et al., 2012). In addition, the drug resistance of this strain can be horizontally transferred. In our experiment, the MIC of the tested antibiotics against recipient strains was significantly increased after co-culture with ESC1. We are not sure whether the plasmid can conjugate autonomously. We did not sequence and verify the plasmids in the recipient strains. This is the deficiency of our experiment. However, the antibiotic resistance phenotype of the transconjugant is consistent with the antibiotic resistance mediated by the antibiotic resistance gene on the plasmid. Moreover, this strain can promote the spread of antibiotic resistance genes and lead to the increase of antibiotic resistance of other strains. It can be seen that this aggravates the risk of the spread of drug resistance and becomes a potential threat to human health.
In order to investigate the cause of multidrug resistance in the E. faecalis ESC1, the whole genome was sequenced and bioinformatics analysis was carried out. We found the mutation of parC gene on the chromosome, which resulted in the resistance to fluoroquinolones. In addition, no resistance genes were found on the chromosome, rather resistance genes were mainly carried by movable plasmid. Therefore, this can explain the multidrug resistance phenotype and drug resistance spread of the strain. The strain carried three plasmids. No drug resistance genes were found in plasmid pESC1. Some regions of the pESC2 plasmid showed an organization highly similar to the pKUB3006-1 plasmid (99% nucleotide sequence identity with a query coverage of 69%). Based on the observed similarities, the two plasmids may have evolved from each other or from the same ancestor. Compared to the pKUB3006-1 plasmid, the pESC2 plasmid retained three resistance genes, erm(B), aph(3′)-III and dfrG, which conferred resistance to macrolides, aminoglycosides and trimethoprim. The ant (Mackenzie and Jeggo, 2019)-Ia and lnu(B) genes were missing. This might be a loss in the course of evolution. Apart from a region similar to the pKUB3006-1 plasmid, a 56.2 kb sized region located on pESC2 carried six drug resistance genes. The optrA, fexA and erm(A) genes were located on a composite transposon flanked by an insertion sequence IS1216E. Similar to the structure of the pE419 plasmid reported by He et al. (2016), this transposon carries the optrA, fexA and erm(A) genes with a reverse insertion sequence. By comparing with NCBI database, we found other similar structures as well. These similar structures were located on plasmid pC25(CP030043.1), pC54(CP030046.1), and transposon Tn6261(KU354267.1), respectively. Gene optrA encodes an ABC-F protein, which mediates the drug resistance to oxazolidinone antibiotics (Almeida et al., 2020). This poses a severe treatment challenge to human and veterinary medicine. In this study, we found that this region of Tn6248 carried three resistance genes, tet(M), tet(L) and catA genes, while the transposon also retained genes encoding plasmid recombination, binding, lipoprotein and membrane protein. All this suggests that the drug resistance of this transposon can be still spread with the transfer of the plasmid. Analysis showed that the plasmid underwent extensive homologous recombination, resulted in the acquisition of these resistance genes. In addition, we identified many mobile elements and mosaic structures. The above analysis shows that multiple recombination events may have been experienced on plasmid pESC2, resulting in these drug resistance genes. By analyzing the genomic content, we found that the mobile elements carrying antibiotic resistance genes are active among bacteria. This is an important reason for the wide spread of antibiotic resistance genes. Irrational use of antibiotics is an important factor to promote the spread of antibiotic-resistant genes. Therefore, we once again recommend rational use of antibiotics to slow down the spread of bacterial resistance.
Severe enterococcal infections are usually treated using a combination of a cell-wall-active agent (β-lactam or vancomycin) and aminoglycoside (typically gentamicin) (Zhang et al., 2018). The first clinical report of HLGR Enterococcus was completed in France in 1979, and it was widely reported afterward (Horodniceanu et al., 1979; Qu et al., 2006; Dadfarma et al., 2013; Liu et al., 2013; Jannati et al., 2020). HLGR is mainly mediated by the aac(6′)-aph(2″) gene encoding the bifunctional enzyme 6-acetyltransferase-2-phosphotransferase. The aac(6′)-aph(2″) gene is carried by plasmids in most cases and is located on the Tn5281-like transposon in Enterococcus. Zhang et al. (2018) have shown that aac(6′)-aph(2″) is carried and transferred by IS256. In our study, we identified a novel composite transposon on pESC3. Two aac(6′)-aph(2″) genes were tandemly linked to a transposon flanked by isotropic IS1216. To the best of our knowledge, there are no reports of two aac(6′)-aph(2″) genes mediated by IS1216 in tandem on a single composite transposon. When located in the same orientation, the recombination between two copies of IS1216E resulted in the generation of a loop containing the bracketed region plus one copy of the IS1216E element (He et al., 2016). Therefore, the novel composite transposon increased the risk of antibiotic-resistant gene dissemination. ICE, which is located on chromosome, does not carry drug resistance genes, but it lays a hidden danger for the spread of drug resistance genes (Delavat et al., 2017). Genome-wide analysis of E. faecalis ESC1 has provided clarity on the reason for its multidrug resistance and the potential for transmission of resistance genes. However, the exact extent of the contribution played in the development of drug resistance in pathogenic bacteria remains unclear.
In general, we found that E. faecalis with multi-drug resistance is host to a large number of drug-resistant genes, which may pose a threat to public health and safety and requires great attention. The characteristics of genetic evolution of E. faecalis will provide a reference for preventing zoonosis caused by E. faecalis.
The datasets presented in this study can be found in online repositories. The names of the repository/repositories and accession number(s) can be found below: https://www.ncbi.nlm.nih.gov/, CP115992, CP115993, CP115994, and CP115995.
L-CK and H-XM contributed to the conception of the study. X-SL, YQ, Y-zL, and J-zX contributed significantly to the analyses conducted in the study and the preparation of the manuscript. X-SL, IM, P-hL, and X-yL performed the data analyses and wrote the manuscript. YM and D-mZ helped perform the analyses with constructive discussions. All authors contributed to the article and approved the final version.
This work was supported by the Jilin Provincial Science and Technology Development Projects (YDZJ202203CGZH050 and 202537NY010575934).
We thank all the participants for their advices and support for this study.
The authors declare that the research was conducted in the absence of any commercial or financial relationships that could be construed as a potential conflict of interest.
All claims expressed in this article are solely those of the authors and do not necessarily represent those of their affiliated organizations, or those of the publisher, the editors and the reviewers. Any product that may be evaluated in this article, or claim that may be made by its manufacturer, is not guaranteed or endorsed by the publisher.
The Supplementary Material for this article can be found online at: https://www.frontiersin.org/articles/10.3389/fmicb.2023.1191837/full#supplementary-material
Alavi, M., Antonic, V., Ravizee, A., Weina, P., Izadjoo, M., and Stojadinovic, A. (2011). An Enterobacter plasmid as a new genetic background for the transposon Tn1331. Infect. Drug Resist. 4, 209–213. doi: 10.2147/IDR.S25408
Alcock, B., Raphenya, A., Lau, T., Tsang, K., Bouchard, M., Edalatmand, A., et al. (2020). CARD 2020: antibiotic resistome surveillance with the comprehensive antibiotic resistance database. Nucleic Acids Res. 48, D517–D525.
Allen, H., Donato, J., Wang, H., Cloud-Hansen, K., Davies, J., and Handelsman, J. (2010). Call of the wild: antibiotic resistance genes in natural environments. Nat. Rev. Microbiol. 8, 251–259. doi: 10.1038/nrmicro2312
Almeida, L., Lebreton, F., Gaca, A., Bispo, P., Saavedra, J., Calumby, R., et al. (2020). Transferable Resistance Gene optrA in Enterococcus faecalis from Swine in Brazil. Antimicrob. Agents Chemother. 64, e142–e120.
Anderson, A., Andisha, H., Hellwig, E., Jonas, D., Vach, K., and Al-Ahmad, A. (2018). Antibiotic Resistance Genes and Antibiotic Susceptibility of Oral Enterococcus faecalis Isolates Compared to Isolates from Hospitalized Patients and Food. Adv. Exp. Med. Biol. 1057, 47–62. doi: 10.1007/5584_2017_53
Browne, H., Anvar, S., Frank, J., Lawley, T., Roberts, A., and Smits, W. (2015). Complete genome sequence of BS49 and draft genome sequence of BS34A, Bacillus subtilis strains carrying Tn916. FEMS Microbiol. Lett. 362, 1–4. doi: 10.1093/femsle/fnu050
Buschhardt, T., Günther, T., Skjerdal, T., Torpdahl, M., Gethmann, J., Filippitzi, M., et al. (2021). A one health glossary to support communication and information exchange between the human health, animal health and food safety sectors. One Health 13:100263. doi: 10.1016/j.onehlt.2021.100263
Cauwerts, K., Decostere, A., De Graef, E., Haesebrouck, F., and Pasmans, F. (2007). High prevalence of tetracycline resistance in Enterococcus isolates from broilers carrying the erm(B) gene. Avian Pathol. 36, 395–399. doi: 10.1080/03079450701589167
Chen, L., Han, D., Tang, Z., Hao, J., Xiong, W., and Zeng, Z. (2020). Co-existence of the oxazolidinone resistance genes cfr and optrA on two transferable multi-resistance plasmids in one Enterococcus faecalis isolate from swine. Int. J. Antimicrob. Agents 56:105993. doi: 10.1016/j.ijantimicag.2020.105993
Chilambi, G., Nordstrom, H., Evans, D., Kowalski, R., Dhaliwal, D., Jhanji, V., et al. (2021). Genomic and phenotypic diversity of Enterococcus faecalis isolated from endophthalmitis. PLoS One 16:e0250084. doi: 10.1371/journal.pone.0250084
Choi, J., and Woo, G. (2013). Molecular characterization of high-level gentamicin-resistant Enterococcus faecalis from chicken meat in Korea. Int. J. Food Microbiol. 165, 1–6. doi: 10.1016/j.ijfoodmicro.2013.02.016
Chong, K., Tay, W., Janela, B., Yong, A., Liew, T., Madden, L., et al. (2017). Enterococcus faecalis modulates immune activation and slows healing during wound infection. J. Infect. Dis. 216, 1644–1654. doi: 10.1093/infdis/jix541
Dadfarma, N., Imani Fooladi, A., Oskoui, M., and Mahmoodzadeh Hosseini, H. (2013). High level of gentamicin resistance (HLGR) among Enterococcus strains isolated from clinical specimens. J. Infect. Public Health 6, 202–208.
D’Costa, V., King, C., Kalan, L., Morar, M., Sung, W., Schwarz, C., et al. (2011). Antibiotic resistance is ancient. Nature 477, 457–461.
Delavat, F., Miyazaki, R., Carraro, N., Pradervand, N., and van der Meer, J. (2017). The hidden life of integrative and conjugative elements. FEMS Microbiol. Rev. 41, 512–537.
Dong, W., Liu, L., Odah, K., Atiah, L., Gao, Y., Kong, L., et al. (2019). Antimicrobial resistance and presence of virulence factor genes in Trueperella pyogenes isolated from pig lungs with pneumonia. Trop. Anim. Health Product. 51, 2099–2103. doi: 10.1007/s11250-019-01916-z
Fan, T., Goeser, L., Lu, K., Faith, J., and Hansen, J. (2021). Enterococcus faecalis glucosamine metabolism exacerbates experimental colitis. Cell. Mol. Gastroenterol. Hepatol. 12, 1373–1389. doi: 10.1016/j.jcmgh.2021.06.017
Flannagan, S., Zitzow, L., Su, Y., and Clewell, D. (1994). Nucleotide sequence of the 18-kb conjugative transposon Tn916 from Enterococcus faecalis. Plasmid 32, 350–354. doi: 10.1006/plas.1994.1077
Frank, J., Reich, C., Sharma, S., Weisbaum, J., Wilson, B., and Olsen, G. (2008). Critical evaluation of two primers commonly used for amplification of bacterial 16S rRNA genes. Appl. Environ. Microbiol. 74, 2461–2470. doi: 10.1128/AEM.02272-07
Franke, A., and Clewell, D. (1981). Evidence for a chromosome-borne resistance transposon (Tn916) in Streptococcus faecalis that is capable of “conjugal” transfer in the absence of a conjugative plasmid. J. Bacteriol. 145, 494–502. doi: 10.1128/jb.145.1.494-502.1981
Fritz, M., Rosolen, B., Krafft, E., Becquart, P., Elguero, E., Vratskikh, O., et al. (2021). High prevalence of SARS-CoV-2 antibodies in pets from COVID-19+ households. One Health 11:100192.
Ganz, H., Jospin, G., Rojas, C., Martin, A., Dahlhausen, K., Kingsbury, D., et al. (2022). The Kitty Microbiome Project: Defining the Healthy Fecal “Core Microbiome” in Pet Domestic Cats. Vet. Sci. 9:635. doi: 10.3390/vetsci9110635
Gawryszewska, I., Żabicka, D., Hryniewicz, W., and Sadowy, E. (2017). Linezolid-resistant enterococci in Polish hospitals: species, clonality and determinants of linezolid resistance. Eur. J. Clin. Microbiol. Infect. Dis. 36, 1279–1286. doi: 10.1007/s10096-017-2934-7
Getachew, Y., Hassan, L., Zakaria, Z., and Abdul Aziz, S. (2013). Genetic variability of vancomycin-resistant Enterococcus faecium and Enterococcus faecalis isolates from humans, chickens, and pigs in Malaysia. Appl. Environ. Microbiol. 79, 4528–4533. doi: 10.1128/AEM.00650-13
Gregersen, R., Petersen, A., Christensen, H., and Bisgaard, M. (2010). Multilocus sequence typing of Enterococcus faecalis isolates demonstrating different lesion types in broiler breeders. Avian Pathol. 39, 435–440. doi: 10.1080/03079457.2010.517250
Guo, Z., Zhang, C., Dong, J., Wang, Y., Hu, H., and Chen, L. (2022). Persistence Infection of TGEV Promotes Enterococcus faecalis Infection on IPEC-J2 Cells. Int. J. Mol. Sci. 24:450. doi: 10.3390/ijms24010450
He, T., Shen, Y., Schwarz, S., Cai, J., Lv, Y., Li, J., et al. (2016). Genetic environment of the transferable oxazolidinone/phenicol resistance gene optrA in Enterococcus faecalis isolates of human and animal origin. J. Antimicrob. Chemother. 71, 1466–1473. doi: 10.1093/jac/dkw016
Horodniceanu, T., Bougueleret, L., El-Solh, N., Bieth, G., and Delbos, F. (1979). High-level, plasmid-borne resistance to gentamicin in Streptococcus faecalis subsp. zymogenes. Antimicrob. Agents Chemother. 16, 686–689. doi: 10.1128/AAC.16.5.686
Hunt, M., Silva, N., Otto, T., Parkhill, J., Keane, J., and Harris, S. (2015). Circlator: automated circularization of genome assemblies using long sequencing reads. Genome Biol. 16:294. doi: 10.1186/s13059-015-0849-0
Jackson, C., Fedorka-Cray, P., Davis, J., Barrett, J., and Frye, J. (2009). Prevalence, species distribution and antimicrobial resistance of enterococci isolated from dogs and cats in the United States. J. Appl. Microbiol.. 107, 1269–1278.
Jain, M., Olsen, H., Paten, B., and Akeson, M. (2016). The Oxford Nanopore MinION: delivery of nanopore sequencing to the genomics community. Genome Biol. 17:239.
Jannati, E., Amirmozaffari, N., Saadatmand, S., and Arzanlou, M. (2020). Faecal carriage of high-level aminoglycoside-resistant and ampicillin-resistant Enterococcus species in healthy Iranian children. J. Glob. Antimicrob. Resist. 20, 135–144. doi: 10.1016/j.jgar.2019.06.022
Kang, H., Yoon, S., Kim, K., and Lee, Y. (2021). Characteristics of High-Level Aminoglycoside-Resistant Enterococcus faecalis Isolated from Bulk Tank Milk in Korea. Animals 11:1724. doi: 10.3390/ani11061724
Koren, S., Walenz, B., Berlin, K., Miller, J., Bergman, N., and Phillippy, A. (2017). Canu: scalable and accurate long-read assembly via adaptive k-mer weighting and repeat separation. Genome Res. 27, 722–736. doi: 10.1101/gr.215087.116
Krzywinski, M., Schein, J., Birol, I., Connors, J., Gascoyne, R., Horsman, D., et al. (2009). Circos: an information aesthetic for comparative genomics. Genome Res. 19, 1639–1645. doi: 10.1101/gr.092759.109
Larsson, D., and Flach, C. (2022). Antibiotic resistance in the environment. Nat. Rev. Microbiol. 20, 257–269.
Li, D., Li, X., Schwarz, S., Yang, M., Zhang, S., Hao, W., et al. (2019). Tn6674 Is a Novel Enterococcal optrA-Carrying Multiresistance Transposon of the Tn554 Family. Antimicrob. Agents Chemother. 63, e809–e819. doi: 10.1128/AAC.00809-19
Liu, Y., Liu, K., Lai, J., Wu, C., Shen, J., and Wang, Y. (2013). Prevalence and antimicrobial resistance of Enterococcus species of food animal origin from Beijing and Shandong Province, China. J. Appl. Microbiol. 114, 555–563. doi: 10.1111/jam.12054
Lubbers, B. (2023). Performance Standards for Antimicrobial Disk and Dilution Susceptibility Tests for Bacteria Isolated From Animals. Wayne, PA: Clinical and Laboratory Standard Institute.
Mackenzie, J., and Jeggo, M. (2019). The one health approach-why is it so important? Trop. Med. Infect. Dis. 4:88. doi: 10.3390/tropicalmed402008
Magiorakos, A., Srinivasan, A., Carey, R., Carmeli, Y., Falagas, M., Giske, C., et al. (2012). Multidrug-resistant, extensively drug-resistant and pandrug-resistant bacteria: an international expert proposal for interim standard definitions for acquired resistance. Clin. Microbiol. Infect. 18, 268–281.
Overgaauw, P., Vinke, C., Hagen, M., and Lipman, L. J. A. A. (2020). One health perspective on the human-companion animal relationship with emphasis on zoonotic aspects. Int. J. Environ. Res. Public Health 17:3789. doi: 10.3390/ijerph17113789
Powell, L., Lavender, T., Reinhard, C., and Watson, B. (2022). Pet Owners’ Perceptions of COVID-19, zoonotic disease, and veterinary medicine: The impact of demographic characteristics. Vet. Sci. 9:195. doi: 10.3390/vetsci9050195
Qu, T., Chen, Y., Yu, Y., Wei, Z., Zhou, Z., and Li, L. (2006). Genotypic diversity and epidemiology of high-level gentamicin resistant Enterococcus in a Chinese hospital. J. Infect. 52, 124–130. doi: 10.1016/j.jinf.2005.02.030
Shan, X., Li, X., Schwarz, S., Chen, Y., Xu, C., and Du, X. (2022). Plasmid-Assisted Horizontal Transfer of a Large lsa(E)-Carrying Genomic Island in Enterococcus faecalis. Microbiol. Spectr. 10:e0015422. doi: 10.1128/spectrum.00154-22
Stȩpień-Pyśniak, D., Bertelloni, F., Dec, M., Cagnoli, G., Pietras-Ożga, D., Urban-Chmiel, R., et al. (2021). Characterization and Comparison of Enterococcus spp. Isolates from Feces of Healthy Dogs and Urine of Dogs with UTIs. Animals 11:2845. doi: 10.3390/ani11102845
Vaser, R., Sović, I., Nagarajan, N., and Šikić, M. (2017). Fast and accurate de novo genome assembly from long uncorrected reads. Genome Res. 27, 737–746. doi: 10.1101/gr.214270.116
Walker, B., Abeel, T., Shea, T., Priest, M., Abouelliel, A., Sakthikumar, S., et al. (2014). Pilon: an integrated tool for comprehensive microbial variant detection and genome assembly improvement. PLoS One 9:e112963. doi: 10.1371/journal.pone.0112963
Wang, Y., Zhao, Y., Bollas, A., Wang, Y., and Au, K. (2021). Nanopore sequencing technology, bioinformatics and applications. Nat. Biotechnol. 39, 1348–1365. doi: 10.1038/s41587-021-01108-x
Willett, J., Dale, J., Kwiatkowski, L., Powers, J., Korir, M., Kohli, R., et al. (2021). Comparative Biofilm Assays Using Enterococcus faecalis OG1RF Identify New Determinants of Biofilm Formation. mBio 12:e0101121.
Yoon, S., and Lee, Y. (2021). Molecular Characteristics of Enterococcus faecalis and Enterococcus faecium from Bulk Tank Milk in Korea. Animals 11:661. doi: 10.3390/ani11030661
Yu, Z., Wang, Y., Henderson, I., and Guo, J. (2022). Artificial sweeteners stimulate horizontal transfer of extracellular antibiotic resistance genes through natural transformation. ISME J. 16, 543–554. doi: 10.1038/s41396-021-01095-6
Yuan, T., Liang, B., Jiang, B., Sun, S., Zhou, Y., Zhu, L., et al. (2023). Virulence genes and antimicrobial resistance in Enterococcus strains isolated from dogs and cats in Northeast China. J. Vet. Med. Sci. 85, 371–378. doi: 10.1292/jvms.22-0410
Zhang, J., Wang, Q., Han, T., Liu, J., Hu, X., Qiao, F., et al. (2018). Structure analysis of transposons carrying the aac(6′)-aph(2″) gene in Enterococcus faecalis isolated in Beijing, China, and comparison of their transfer efficiency. Int. J. Antimicrob. Agents 52, 799–804. doi: 10.1016/j.ijantimicag.2018.08.025
Keywords: Enterococcus faecalis, multidrug-resistant, whole genome sequencing, mobile genetic elements, transposon
Citation: Li X-S, Qi Y, Li P-h, Xue J-z, Li X-y, Muhammad I, Li Y-z, Zhu D-m, Ma Y, Kong L-C and Ma H-X (2023) Genetic characterization of MDR genomic elements carrying two aac(6′)-aph(2″) genes in feline-derived clinical Enterococcus faecalis isolate. Front. Microbiol. 14:1191837. doi: 10.3389/fmicb.2023.1191837
Received: 22 March 2023; Accepted: 10 July 2023;
Published: 27 July 2023.
Edited by:
Mariela E. Srednik, Iowa State University, United StatesReviewed by:
Elisabet Vilacoba, CONICET Instituto Andino Patagónico en Tecnologías Biológicas y Geoambientales (IPATEC), ArgentinaCopyright © 2023 Li, Qi, Li, Xue, Li, Muhammad, Li, Zhu, Ma, Kong and Ma. This is an open-access article distributed under the terms of the Creative Commons Attribution License (CC BY). The use, distribution or reproduction in other forums is permitted, provided the original author(s) and the copyright owner(s) are credited and that the original publication in this journal is cited, in accordance with accepted academic practice. No use, distribution or reproduction is permitted which does not comply with these terms.
*Correspondence: Ling-Cong Kong, bGluZ2NvbmdAamxhdS5lZHUuY24=; Hong-Xia Ma, aG9uZ3hpYTA3MzEwMDFAMTYzLmNvbQ==
†These authors have contributed equally to this work and share first authorship
Disclaimer: All claims expressed in this article are solely those of the authors and do not necessarily represent those of their affiliated organizations, or those of the publisher, the editors and the reviewers. Any product that may be evaluated in this article or claim that may be made by its manufacturer is not guaranteed or endorsed by the publisher.
Research integrity at Frontiers
Learn more about the work of our research integrity team to safeguard the quality of each article we publish.