- 1First Affiliated Hospital of Shandong First Medical University, Biomedical Sciences College & Shandong Medicinal Biotechnology Centre, Shandong First Medical University & Shandong Academy of Medical Sciences, Jinan, China
- 2National Health Commission (NHC) Key Laboratory of Biotechnology Drugs. Shandong Academy of Medical Sciences, Jinan, China
- 3Jinan Municipal Minzu Hospital, Jinan, China
- 4School of Municipal and Environmental Engineering, Shandong Jianzhu University, Jinan, China
Introduction: The role of integrative and conjugative elements (ICEs) in antibiotic resistance in Morganella morganii is unknown. This study aimed to determine whether an ICE identified in the M. morganii genome contributed to the polymyxin resistance.
Methods: Whole-genome sequencing was performed followed by bioinformatics analyses to identify ICEs and antibiotic resistance genes. Conjugation assays were performed to analyze the transferability of a discovered ICE. A drug transporter encoded on the ICE was heterogeneously expressed in Escherichia coli, minimum inhibitory concentrations of antibiotics were determined, and a traditional Chinese medicine library was screened for potential efflux pump inhibitors.
Results: An antibiotic resistance-conferring ICE, named ICEMmoMP63, was identified. ICEMmoMP63 was verified to be horizontally transferred among Enterobacteriaceae bacteria. G3577_03020 in ICEMmoMP63 was found to mediate multiple antibiotic resistances, especially polymyxin resistance. However, natural compound glabridin was demonstrated to inhibit polymyxin resistance.
Discussion: Our findings support the need for monitoring dissemination of ICEMmoMP63 in Enterobacteriaceae bacteria. Combined glabridin and polymyxin may have therapeutic potential for treating infections from multi-drug resistant bacteria carrying ICEMmoMP63.
Introduction
Antibiotic-resistant, especially multiple antibiotic-resistant (MAR), pathogens have become a serious threat to human health (Davies et al., 2013; He et al., 2016). Antibiotic resistance genes (ARGs) from MAR bacteria can be considered a type of genetic pollution in the environment (Pruden et al., 2006), and therefore, horizontal transfer of ARGs among MAR bacteria is an important topic in both the environmental sciences and medical sciences. Transduction (Thierauf et al., 2009), transformation (Kruger and Stingl, 2011), conjugation (Lederberg and Tatum, 1946), and fusion of cells with DNA-containing vesicles or fusion of two cells (Johnson and Grossman, 2015) are four ways for bacteria to horizontally transfer ARGs, although conjugation via integrative and conjugative elements (ICEs) is considered to be the more common mode (Guglielmini et al., 2011). ICEs, which can range from about 20 to 500 kb in size, are mobile genetic elements that are integrated into bacterial chromosomes and that can excise and be transferred to new cells (Carraro and Burrus, 2015; Burrus, 2017; de Assis et al., 2022). Typical ICEs are integrated into specific sites (such as 3′-ends of tRNA/tmRNA genes and 3′-end of the guanosine monophosphate synthetase-encoding gene guaA) in a host genome and encode a type IV conjugation system, which enables an ICE to transfer to other bacteria via conjugation (Alvarez-Martinez and Christie, 2009; Del Canto et al., 2011; Song et al., 2012; Christie et al., 2014; Trokter et al., 2014). ICEs encoding ARGs can endow the recipient bacteria with antibiotic resistance, which is of particular concern when the recipient bacteria is a human pathogen as this makes eradication more difficult, potentially leading to disease outbreaks from the pathogen. However, clinical pathogens are not always screened for ICEs encoding ARGs, which is detrimental to the prevention and treatment of MAR bacteria.
Morganella morganii is an increasingly important opportunistic pathogen because of its virulence and increased antibiotic resistance and because it can cause a variety of clinical infections, such as urinary tract infections, wound infections, endocarditis, septic shock, osteomyelitis, bacteremia and sepsis, acute postoperative endophthalmitis, pericarditis, intra-abdominal abscess, peritonitis, rhabdomyolysis, orbital abscess, black nail infection, and shoulder septic arthritis (Casanova-Roman et al., 2002; Liu et al., 2016, 2021; Tsiotsias et al., 2019; Minnullina et al., 2022; Agrawal et al., 2023). M. morganii is a rod-shaped, Gram-negative bacillus that belongs to the tribe Proteeae of the Enterobacteriaceae family and that is found in intestinal tracts of humans, mammals, and reptiles and in the environment (Liu et al., 2016; Erlanger et al., 2019). In rare cases, especially in hospital and postoperative environments, and in patients and young children with impaired immune systems, it can cause potentially fatal systemic infections (Bandy, 2020). M. morganii was reported to be resistant to many antibiotics, including tetracyclines, macrolides, glycopeptides, rifampicin, lincosamides, streptogramins, daptomycin, colistin, fusidic acid, and nitrofurantoin (Leclercq et al., 2013; Seija et al., 2015), indicating this species carries many ARGs in the genome and acts as an ARG reservoir. Plasmid-mediated quinolone-resistance has also been reported in M. morganii (Mahrouki et al., 2012). However, no ICEs carrying ARGs have been reported in M. morganii.
In this study, the MAR strain M. morganii MP63 was isolated from a hospital sewage sample. A typical ICE associated with multiple antibiotic resistances was identified in the MP63 genome and named ICEMmoMP63. We found that ICEMmoMP63 could horizontally transfer to Escherichia coli strains, indicating that this ICE could disseminate ARGs among Enterobacteriaceae bacteria, raising the need to monitor and inhibit ICEMmoMP63 in order to prevent further outbreaks of infection.
Materials and methods
Isolation and identification of strain MP63
MP63 strain was isolated from a sewage sample from a hospital in Jinan, China. The sewage sample was serially diluted 10-fold with sterilized water, plated onto Luria Bertani (LB) solid medium supplemented with 16 μg/ml polymyxin E, and the plates were incubated overnight at 28°C to obtain single colonies. Then, a selected single colony was streaked three consecutive times on LB solid medium supplemented with polymyxin E to obtain a pure culture. After streaking and purification, a selected pure culture was named strain MP63.
The 16S rDNA gene of MP63 was amplified by PCR using the universal primers 27F and 1492R (Supplementary Table 1), and then PCR products were purified using PCR Clean Up Kit (Beyotime, China) and sequenced at BioSune Co., Ltd (Shanghai, China). The 16S rDNA sequence was analyzed using BLAST1 for preliminary identification.
Antibiotic minimum inhibitory concentration (MIC) testing
The minimum inhibitory concentrations (MICs) of antibiotics for strain MP63 were determined using the broth microdilution method as previously described (CLSI, 2017). The transconjugant 25DN-MP and recombinant strain M3020 were also tested for MICs. All MIC tests in this study were carried out in triplicate.
Whole-genome sequencing and genomic analysis
The MP63 genome was sequenced using the Nanopore and BGISEQ-500 platform (BGI, Wuhan, China) and assembled using Unicycler software (Wick et al., 2017). Genome annotation was performed using the NCBI website,2 the RASTtk server (Overbeek et al., 2014; Brettin et al., 2015), and the Pathosystems Resource Integration Center (PATRIC) server (Wattam et al., 2017). Antibiotic resistance genes were predicted using ARDB (Antibiotic Resistance Genes Database) (Liu and Pop, 2009), ARG-ANNOT (Antibiotic Resistance Gene-ANNOTation database) (Gupta et al., 2014), and CARD (the Comprehensive Antibiotic Research Database) (Alcock et al., 2020). The virulence factors in the MP63 genome were predicted using VFDB (virulence factor database) (Liu B. et al., 2019). Sequence alignment was performed using the BLAST server and UniProt server.3
Phylogenetic analysis of strain MP63
Phylogenetic analysis was performed using the MP63 genome sequence, and a whole-genome phylogenetic tree was constructed using the PATRIC server (Wattam et al., 2017) and 12 genome sequences belonging to Morganellaceae species.
Identification and annotation of ICEMmoMP63
Genomic islands in MP63 genome were predicted by IslandViewer 4 (Bertelli et al., 2017). An identified ICE was further analyzed using ICEberg 2.0 software (Liu M. et al., 2019) and named ICEMmoMP63. ICEMmoMP63 genes were annotated using NCBI and the RASTtk server (Overbeek et al., 2014; Brettin et al., 2015), and insertion sequences were predicted using IS-Finder (Siguier et al., 2012).
Phylogenetic relationships of ICEMmoMP63
Alignment of the whole nucleotide sequence of ICEMmoMP63 was performed using the BLAST server, and a phylogenetic tree was constructed using MEGA7 software (Kumar et al., 2016).
Conjugation assays
Horizontal transferability of ICEMmoMP63 was tested using conjugation assays as previously described with some modifications (Fu et al., 2020). Strain MP63 and E. coli 25DN were used as the donor and recipient strains, respectively. MP63 and 25DN were mixed together and cultured on LB solid medium with polymyxin E (32 mg/L), sodium azide (1.7 mol/L), and X-Gluc (5-bromo-4-chloro-3-indolyl-beta-D-glucuronic acid) to screen the transconjugants. The presence of ICEMmoMP63 in the transconjugants was demonstrated by PCR using primer pairs Val-F and Val-R (Supplementary Table 1) and DNA sequencing.
Construction of recombinant strain M3020 of G3577_03020
Gene G3577_03020, encoding a major facilitator superfamily (MFS) transporter, was amplified using primers M3020-F/R (Supplementary Table 1) and MP63 genomic DNA as template, and the β-lactamase gene promoter was amplified using primers AP-F/R (Supplementary Table 1) and pMD18-T vector as template. These two amplicons were then fused by PCR using primers AP-F and M3020-R (Supplementary Table 1), followed by cloning into the pMD18-T vector to obtain the recombinant pMD18-3020. After verification by DNA sequencing, pMD18-3020 was transformed into E. coli DH5α (TSINGKE, China) for heterogeneous expression of G3577_03020, and the recombinant strain was named M3020.
Determination of MICs of strain M3020 with and without efflux pump inhibitors
Antibiotic MICs for M3020 were determined using the broth microdilution method as described above. The MICs for polymyxin E, tetracycline, and cefixime were also calculated by broth microdilution in the presence of efflux pump inhibitors (EPIs), including carbonyl cyanide m-chlorophenylhydrazone (CCCP), N-methyl-2-pyrrolidone (NMP), reserpine (RES), and verapamil (VER). CCCP, NMP, RES, and VER were used at final concentrations of 0.1, 8, 8, and 8 mg/L, respectively. E. coli DH5α strain harboring pMD18-T was used in each test for internal quality control.
The Traditional Chinese Medicine Active Compound Library (MedChemExpress, China) was screened for potential EPIs of the MFS transporter G3577_03020. The MICs for polymyxin E, tetracycline, and cefixime in the presence of traditional Chinese medicine compounds were determined as above. Each traditional Chinese medicine compound was used at final concentrations of 5, 15, and 25 μM. E. coli DH5α strain harboring pMD18-T was used in each test for internal quality control.
Homology modeling and molecular docking
Protein homology modeling and molecular docking were conducted as previously described with some modifications (Zong et al., 2020). The homology model for the MFS transporter was constructed using the Swiss model (Waterhouse et al., 2018). The small molecule ligands of polymyxin E, cefixime, tetracycline, and EPIs were drawn using Discovery Studio. The molecular docking was carried out according to the CDOCKER protocol of Discovery Studio 2.0 (Biovia, 2017) to determine whether the above small molecules could bind G3577_03020.
Results
Antibiotic-resistant phenotype and genomic features of strain MP63
Together with the preliminary 16S rDNA gene analysis and alignment of the whole genome sequence, phylogenetic tree analysis revealed that strain MP63 is most closely related to strain M. morganii NCTC12028 (Figure 1), and therefore strain MP63 was identified as M. morganii. MIC assays revealed that strain MP63 was resistant to five tested antibiotics (Supplementary Table 2), including polymyxin E (MIC > 128 mg/L), cefixime (MIC > 128 mg/L), meropenem (MIC = 32 mg/L), florfenicol (MIC = 16 mg/L), and tetracycline (MIC = 96 mg/L), indicating that M. morganii MP63 was a MAR strain.
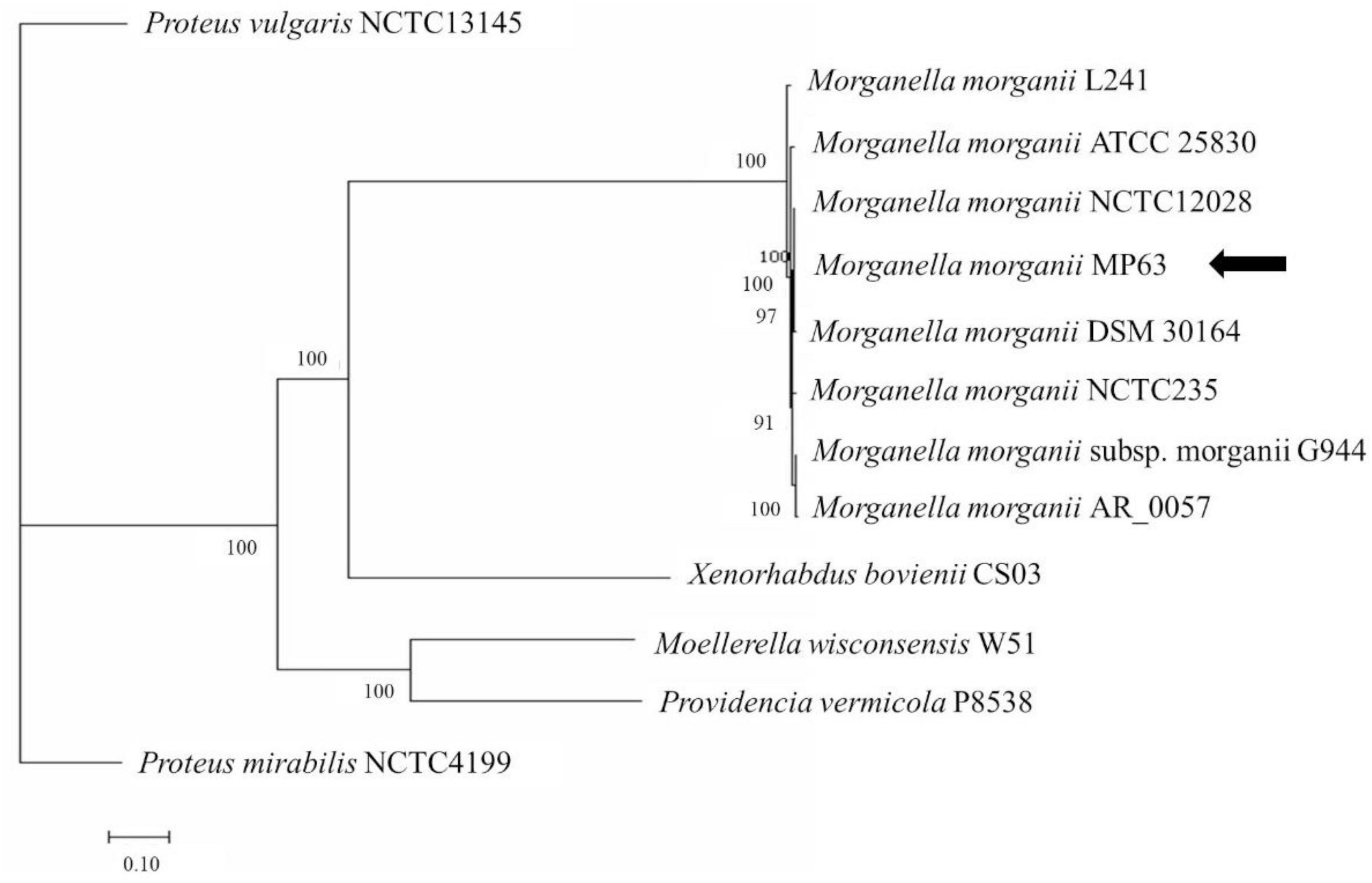
Figure 1. Molecular phylogenetic analysis of MP63 based on genome sequence. Twelve strains from the family Morganellaceae were selected to analyze the phylogenetic relationship of MP63. Proteus mirabilis NCTC4199 and Proteus vulgaris NCTC13145 belong to the genus Proteus, Providencia vermicola P8538 belongs to the genus Providencia, Moellerella wisconsensis W51 belongs to the genus Moellerella, Xenorhabdus bovienii CS03 belongs to the genus Xenorhabdus, and the other seven strains (L241, ATCC 25830, NCTC12028, DSM 30164, NCTC235, G944, AR_0057) belong to the genus Morganella in the family Morganellaceae. The whole-genome phylogenetic tree was constructed using the PATRIC server. MP63 is most closely related to Morganella morganii NCTC12028. The position of MP63 in the phylogenetic tree is indicated by the black arrow.
To understand the basis for its antibiotic resistance profile and its pathogenicity, the whole genome of M. morganii MP63 was sequenced and analyzed. MP63 contains one circular chromosome, which is 4,004,672 bp in size, with an average GC content of 51.1%. MP63 also contains the three plasmids pMP63A, pMP63B, and pMP63C. Genome annotation identified 3,807 genes in the MP63 genome, including 3,661 protein-coding genes (Supplementary Figure 1 and Supplementary Table 3). Consistent with its antibiotic resistance profile, 42 antibiotic resistance-associated genes were found in the MP63 genome (Supplementary Table 4). Additionally, based on the VFDB database, the genome contained 321 putative virulence factor-encoding genes (Supplementary Table 5), suggesting that MP63 is potentially pathogenic for humans or other animals.
Identification of the integrative and conjugative element ICEMmoMP63
IslandViewer 4 analysis revealed several genomic islands in MP63 genome, and further analysis using ICEberg 2.0 identified a novel ICE, which was named ICEMmoMP63 (Figure 2). ICEMmoMP63 extended from position 604,119 to 693,424 in the MP63 genome and contained 89,306 bp. ICEMmoMP63 was bordered by a 21-bp direct repeat (DR) (5′-GACACGGGGATTTTCAATCCC-3′) at both ends and was inserted into the tRNA-Phe gene (G3577_03240). Gene annotation revealed that ICEMmoMP63 contained 90 open reading frames (ORFs), including an MFS transporter-encoding gene G3577_03020, the function of which was unclear and was not included in the annotated antibiotic resistance genes in Supplementary Table 4. ICEMmoMP63 also contained multiple genes associated with type IV conjugative transfer systems (Supplementary Table 6), suggesting that ICEMmoMP63 encoded such a system.
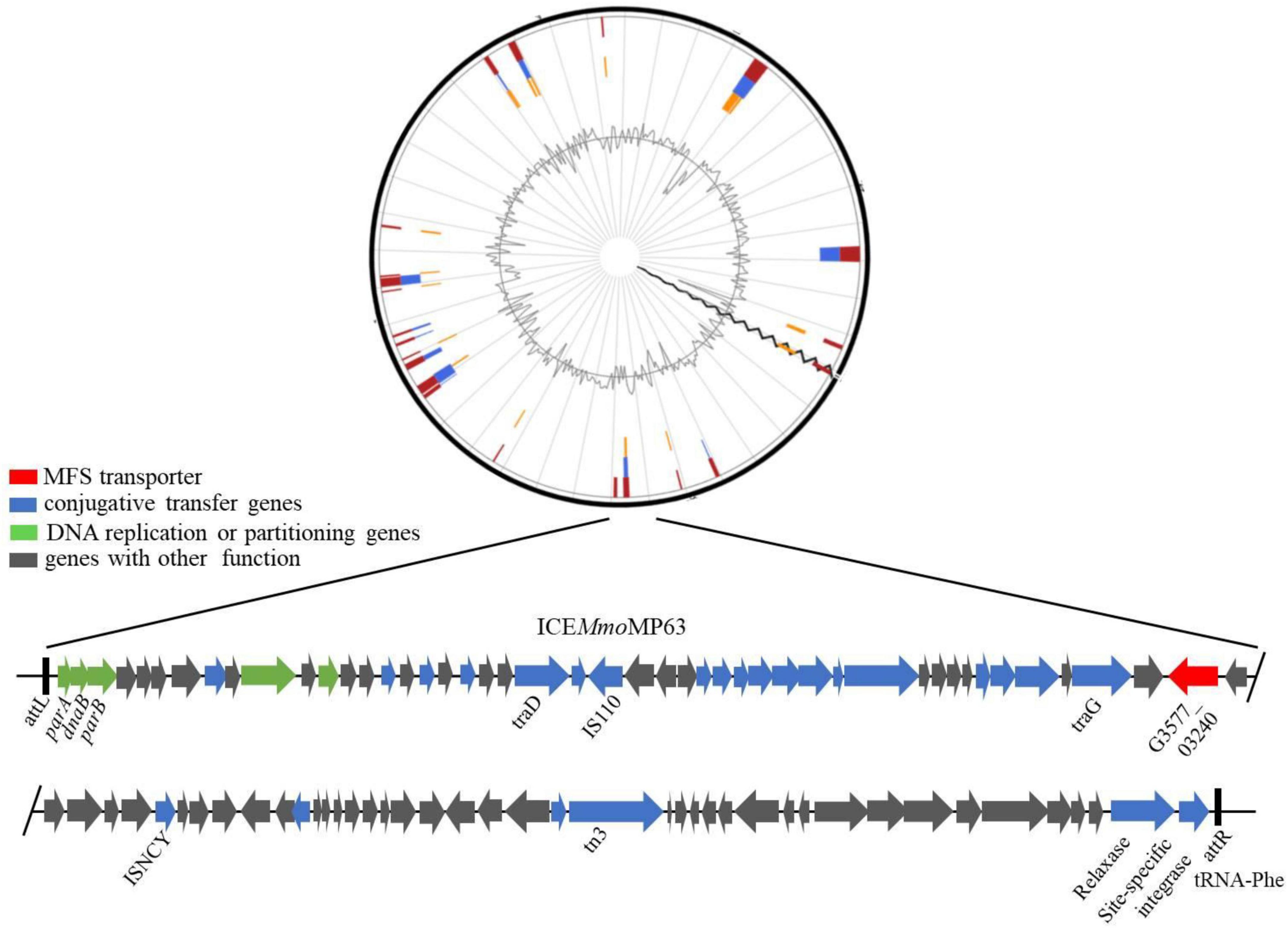
Figure 2. Schematic view of the integrative and conjugative element ICEMmoMP63. Top image: predicted position of ICEMmoMP63 in the MP63 genome using IslandViewer 4. Bottom image: gene arrangement and characteristics of ICEMmoMP63 identified using ICEberg 2.0. ICEMmoMP63 is bordered by a 21-bp DR (5′-GACACGGGGATTTTCAATCCC-3′), indicated by black bars, in the chromosome of MP63.
Phylogenetic relationship of ICEMmoMP63
As ICEMmoMP63 has characteristics typical of ICEs and might have been horizontally transferred from other bacteria, the phylogenetic relationship of ICEMmoMP63 was further analyzed. Eight related mobile element sequences from different strains based on the BLASTn alignment results were chosen, and the phylogenetic tree revealed that ICEMmoMP63 was most closely related to mobile elements in Enterobacterales species (Supplementary Figure 2). Pairwise alignment using BLAST and the whole ICEMmoMP63 nucleotide sequence showed that ICEMmoMP63 has strong homology with the mobile elements Salmonella enterica 1422-74 (Identity: 99%), Enterobacter cloacae A1137 (Identity: 95%), and Klebsiella pneumoniae KP486 (Identity: 94%) (Figure 3), indicating that ICEMmoMP63 likely evolved from related ICEs, genomic islands, or plasmids of Enterobacteriaceae strains.
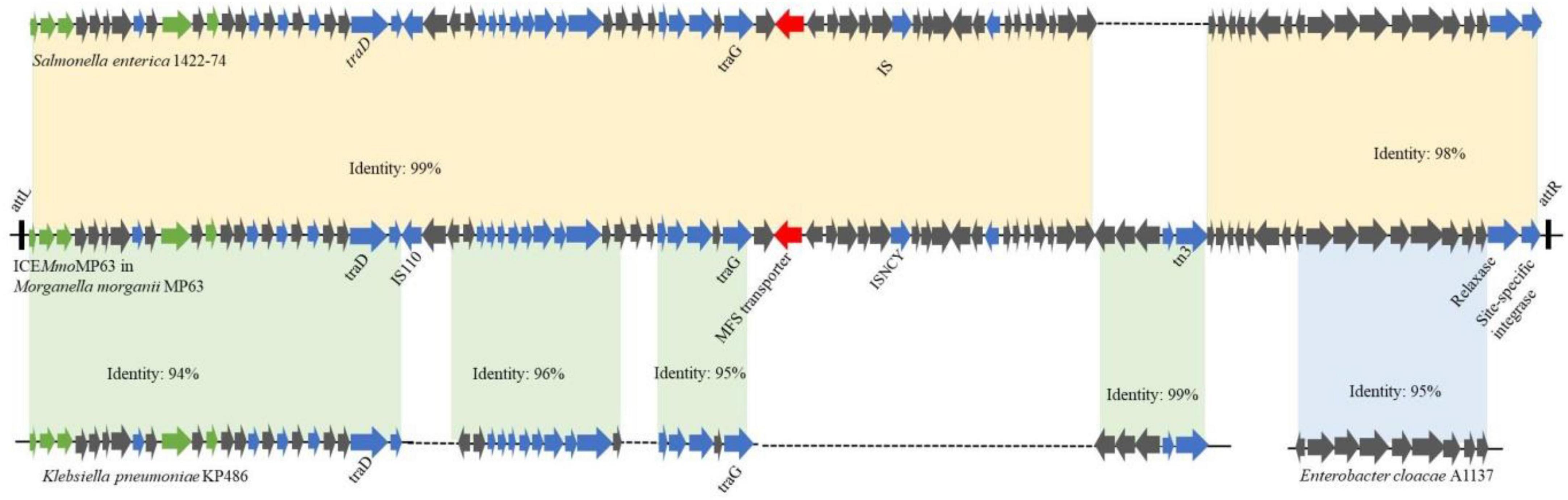
Figure 3. Schematic showing potential sources of genes in ICEMmoMP63. Gene sequences in ICEMmoMP63 were compared with gene sequences from Salmonella enterica 1422-74, Klebsiella pneumoniae KP486, and Enterobacter cloacae A1137.
ICEMmoMP63 transfers into E. coli 25DN under polymyxin E pressure
To determine whether ICEMmoMP63 was an antibiotic-resistant ICE and could horizontally transfer among Enterobacteriaceae species, conjugation assays were conducted using MP63 with E. coli 25DN as the recipient strain. The transconjugation frequency was about 8.13 × 10–7 colony-forming units/donor with polymyxin E and sodium azide as the selective pressure. PCR testing and DNA sequencing results verified that a fragment of ICEMmoMP63 was present in the transconjugants, and bioinformatic analysis revealed that both the att site (5′- GACACGGGGATTTTCAATCCC-3′) and insertion site (tRNA-Phe gene) of ICEMmoMP63 were also present in the strain 25DN genome from position 929,323 to 929,343 bp (Figure 4), indicating that ICEMmoMP63 was inserted into the E. coli 25DN genome. Further bioinformation analysis revealed that the integration site tRNA-Phe gene was conserved in many strains of Enterobacteriaceae, such as E. coli DL21 (GenBank accession no. CP079747), K. pneumoniae KPH3 (GenBank accession no. CP102552), S. enterica s15D023 (GenBank accession no. CP101340), Serratia ureilytica HNU47 (GenBank accession no. CP098030), E. cloacae complex sp. R_G8 (GenBank accession no. CP102246), and Enterobacter asburiae R_A5.MM (GenBank accession no. CP102247), suggesting that ICEMmoMP63 might spread ARGs among Enterobacteriaceae. Additionally, MIC analysis showed that the transconjugant 25DN-MP acquired resistance to polymyxin E, tetracycline, and cefixime (Supplementary Table 2). The above results revealed that ICEMmoMP63 was an antibiotic-resistant ICE that could horizontally transfer.
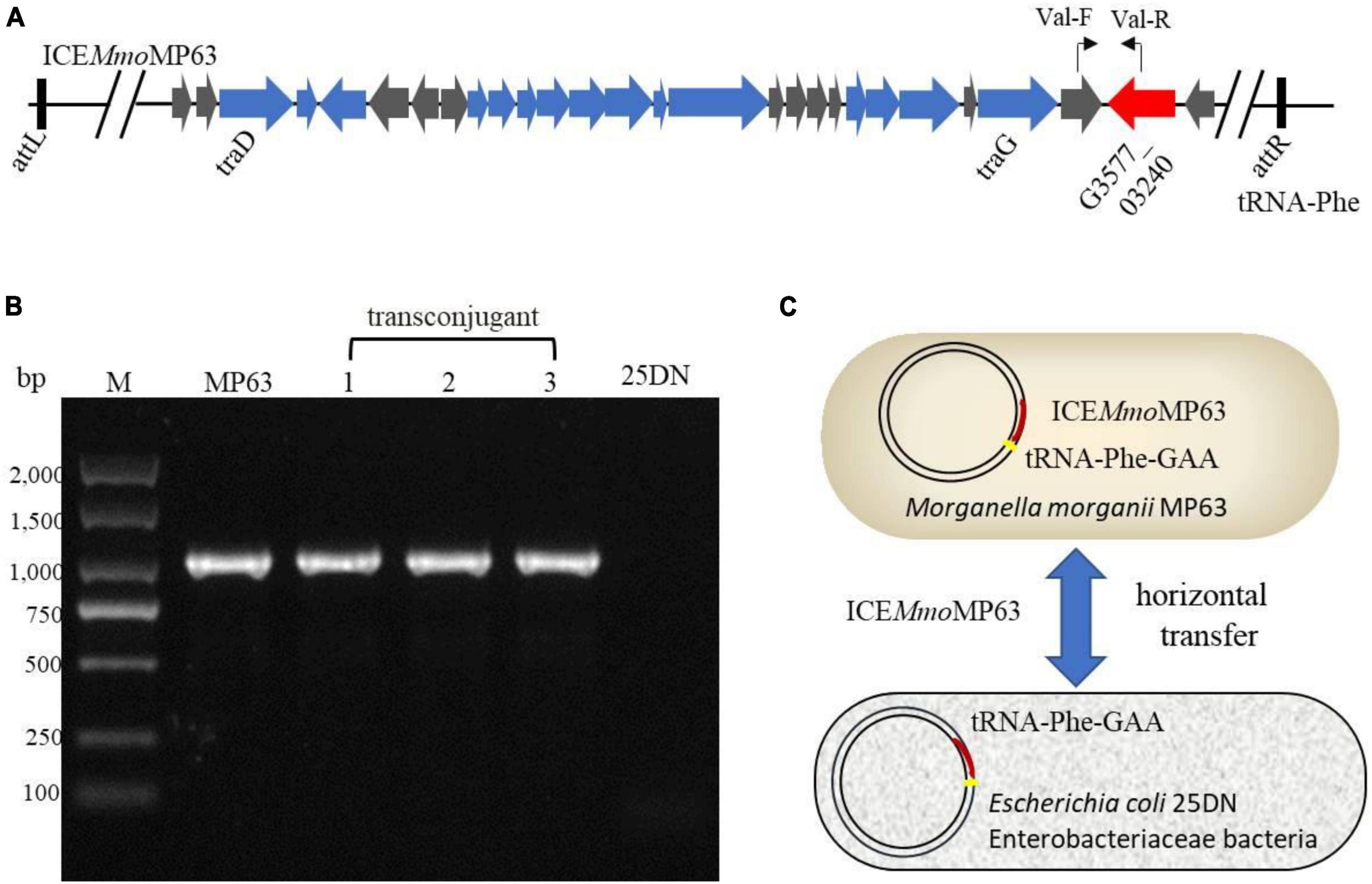
Figure 4. Verification of the transfer of ICEMmoMP63. (A) Primer positions in ICEMmoMP63 are indicated using bent arrows. (B) ICEMmoMP63 fragment was amplified by PCR using total DNA from strain MP63, three transconjugants (lanes 1–3), and strain 25DN as templates. (C) Dissemination model of ICEMmoMP63 with insertion site.
The MFS transporter in ICEMmoMP63 mediates multiple antibiotic resistance
Phylogenetic relationships analysis showed that the MFS transporter encoded by G3577_03020 is closely related to MFS transporters from other Enterobacteriaceae bacteria (Supplementary Figure 3). To determine whether the MFS transporter (G3577_03020) is responsible for the antibiotic resistance of ICEMmoMP63, gene G3577_03020 was expressed in E. coli DH5α, and the recombinant strain was named M3020. MIC analysis showed that M3020 acquired resistance to cefixime, polymyxin E, and tetracycline (Supplementary Table 2), indicating that G3577_03020 mediates multiple antibiotic resistances.
The effects of the known EPIs CCCP, RES, VER, and NMP on G3577_03020 were then investigated. At a subinhibitory concentration (8 mg/L) of VER, a calcium antagonist, the MIC of strain M3020 was reduced from > 128 to 8 mg/L for cefixime, from 128 to 2 mg/L for polymyxin E, and from 64 to 16 mg/L for tetracycline (Table 1), while CCCP, NMP, and RES had no effect on the MICs. However, the effects of VER suggested that G3577_03020 can function as an efflux pump.
Glabridin inhibits the growth of strain M3020
We further investigated whether novel EPIs could inhibit G3577_03020. Glabridin, an hydroxyisoflavan (Supplementary Figure 4), was identified as a potential G3577_03020 inhibitor following screening of a library of traditional Chinese medicine compounds. As shown in Table 2, in the presence of glabridin (especially with 25 μM glabridin), the MIC of strain M3020 was reduced from > 128 to 2 mg/L for cefixime, from 128 to 2 mg/L for polymyxin E, and from 64 to 8 mg/L for tetracycline, indicating glabridin could effectively reduce the MICs of tetracycline, polymyxin E, and cefixime for strain M3020.
G3577_03020 binds to antibiotics and EPIs
As the inhibitory levels of tetracycline, polymyxin E, and cefixime for M3020 decreased in the presence of EPIs (VER and glabridin), molecular docking analysis was performed to determine whether G3577_03020 could bind to these antibiotics and EPIs. A homologous model of G3577_03020 was constructed based on the crystal structure of 7d5p.1.A, an efflux transporter from Staphylococcus aureus. In this model, G3577_03020 contained 12 transmembrane (TM) helices (TMs 1-12) and formed two domains (C domain and N domain), connected by a 44-residue linker (residues 205-248) (Figure 5A). Additionally, there was a central cavity in the TM core between the N and C domains and hydrophobic surface features (Figure 5B). Tetracycline, polymyxin E, and cefixime binding sites were located at the central cavity in the TM core (Figure 5C). Thr124, Trp151, Trp124, Leu63, Ala155, and Val265 of G3577_03020 were the main contributors for antibiotic binding (Supplementary Figures 5–7). Moreover, G3577_03020 showed binding with VER and glabridin, and interestingly, the EPI binding sites and antibiotic binding sites overlapped (Figure 5D), indicating that inhibition by VER and glabridin may be caused by competition for binding. Additional analysis revealed that amino acids involved in antibiotic binding, such as Leu63, Trp151, and Ala155, were occupied by VER and glabridin (Supplementary Figures 8, 9), further suggesting competitive binding by the EPIs.
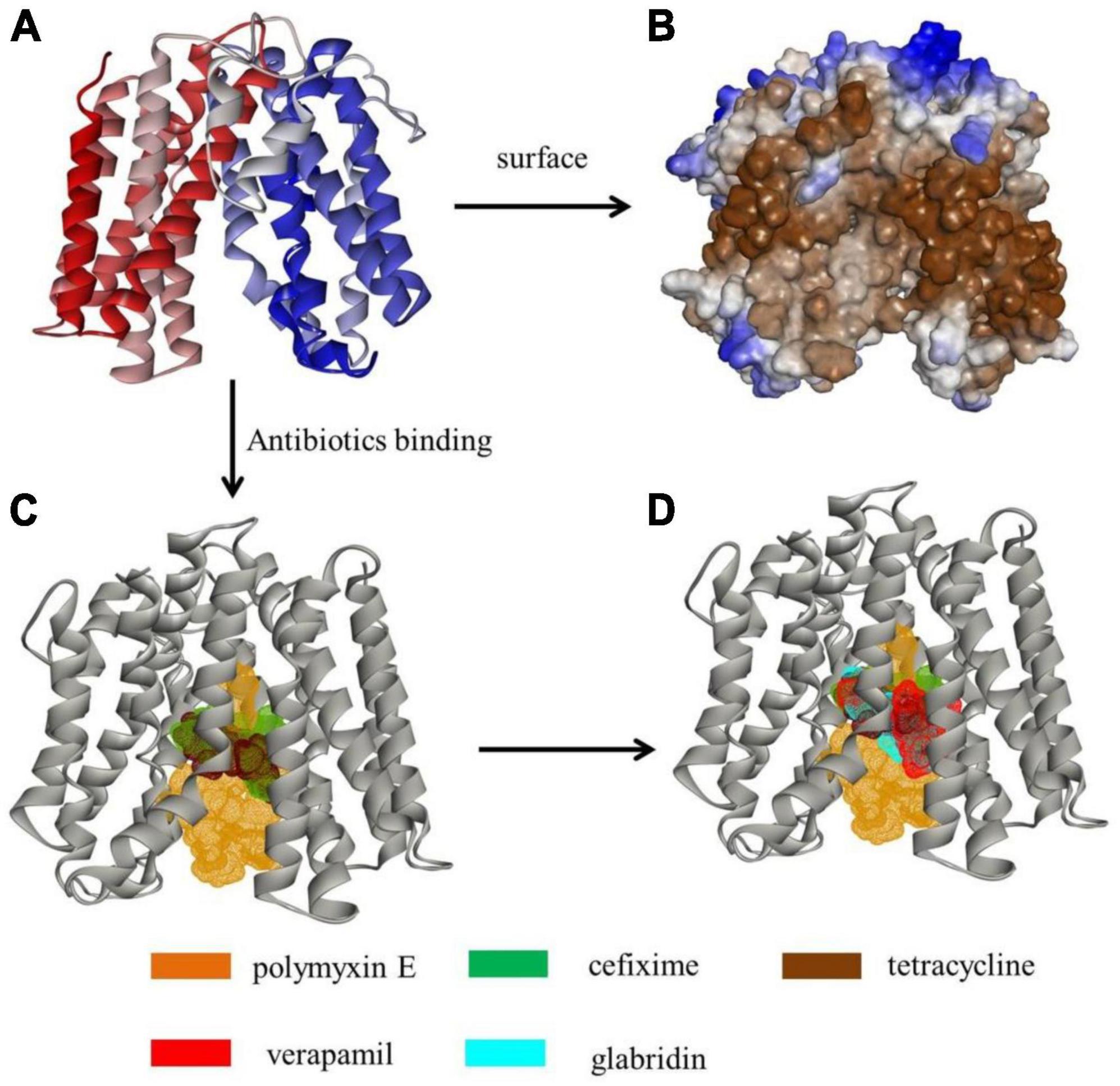
Figure 5. Tertiary structure modeling of MFS transporter G3577_03020 with antibiotics and EPIs. (A) Tertiary structure of G3577_03020. (B) Hydrophobicity of G3577_03020 surface and tunnel. (C,D) Molecular docking models showing embedding of (C) polymyxin E, cefixime, and tetracycline in the G3577_03020 cavity and (D) verapamil and glabridin in the G3577_03020 cavity.
Discussion
Integrative and conjugative elements (ICEs) may be the major mechanism for horizontal transmission of ARGs among bacteria (Guglielmini et al., 2011; Fu et al., 2021). We identified an antibiotic-resistant ICE, named ICEMmoMP63, in a MAR M. morganii MP63 strain isolated from hospital sewage in China. Conjugation assays showed that ICEMmoMP63 could be horizontally transferred from MP63 to E. coli 25DN, and bioinformatics analysis revealed that ICEMmoMP63 was integrated into the E. coli 25DN genome. Further bioinformation analysis revealed that the integration site tRNA-Phe was conserved in many strains of Enterobacteriaceae, suggesting that ICEMmoMP63 might spread ARGs among Enterobacteriaceae. Phylogenetic analysis revealed that ICEMmoMP63 was closely related to mobile elements in Enterobacterales species (Supplementary Figure 2), further suggesting the possibility of horizontal transmission.
Further analysis demonstrated that ICEMmoMP63 encodes the MFS transporter (G3577_03020), a potential efflux pump responsible for the antibiotic resistance of ICEMmoMP63. Bacterial efflux pumps, whereby bacteria pump out antibiotics, are major contributors to antibiotic resistance (Blair et al., 2015; Munita and Arias, 2016), and therefore there is interest in EPIs as therapeutic agents (Lomovskaya et al., 2001; Lomovskaya and Bostian, 2006; Lynch, 2006; Mahamoud et al., 2006; Stavri et al., 2007; Martins et al., 2008). By screening a traditional Chinese medicine library, we identified glabridin as a potential inhibitor of G3577_03020 and determined that glabridin reduced the MICs of several antibiotics. Further analyses suggested that glabridin competes with these antibiotics by binding to specific amino acids in G3577_03020. Our findings indicate that glabridin has potential for treating MAR bacterial infections and also lays the foundation for the design and optimization of glabridin as a new antimicrobial drug.
Data availability statement
The datasets presented in this study can be found in online repositories. The names of the repository/repositories and accession number(s) can be found in the article/Supplementary material.
Author contributions
JF: conceptualization and writing—original draft. YL and ZW: methodology and investigation. FW: resources. GZ: software and validation. CZ and GC: writing—review and editing, and supervision. All authors gave final approval for publication and agreed to be held accountable for the work performed therein.
Funding
This work was supported by the Natural Science Foundation of Shandong Province (No. ZR2022MH107), the Key Research and Development Project of Shandong Province (No. 2021ZDSYS27), the Innovation Project of the Shandong Academy of Medical Sciences (No. 2022), and the Academic Promotion Programme of Shandong First Medical University (No. 2019LJ001).
Acknowledgments
We thank Dr. Susan T. Howard for proofreading the manuscript and for fruitful discussions.
Conflict of interest
The authors declare that the research was conducted in the absence of any commercial or financial relationships that could be construed as a potential conflict of interest.
Publisher’s note
All claims expressed in this article are solely those of the authors and do not necessarily represent those of their affiliated organizations, or those of the publisher, the editors and the reviewers. Any product that may be evaluated in this article, or claim that may be made by its manufacturer, is not guaranteed or endorsed by the publisher.
Supplementary material
The Supplementary Material for this article can be found online at: https://www.frontiersin.org/articles/10.3389/fmicb.2023.1188900/full#supplementary-material
Footnotes
- ^ https://blast.ncbi.nlm.nih.gov/blast.cgi
- ^ https://ncbi.nlm.nih.gov/genome/annotation_prok/
- ^ https://www.uniprot.org/blast/
References
Agrawal, K. U., Limaye Joshi, K., and Gad, M. (2023). A rare case of fulminant acute postoperative Morganella morganii endophthalmitis. Ocul. Immunol. Inflamm. 31, 123–126. doi: 10.1080/09273948.2021.1993269
Alcock, B. P., Raphenya, A. R., Lau, T. T. Y., Tsang, K. K., Bouchard, M., Edalatmand, A., et al. (2020). CARD 2020: antibiotic resistome surveillance with the comprehensive antibiotic resistance database. Nucleic Acids Res. 48, D517–D525. doi: 10.1093/nar/gkz935
Alvarez-Martinez, C. E., and Christie, P. J. (2009). Biological diversity of prokaryotic type IV secretion systems. Microbiol. Mol. Biol. Rev. 73, 775–808. doi: 10.1128/MMBR.00023-09
Bandy, A. (2020). Ringing bells: Morganella morganii fights for recognition. Public Health 182, 45–50. doi: 10.1016/j.puhe.2020.01.016
Bertelli, C., Laird, M. R., Williams, K. P., Simon Fraser University Research Computing Group, Lau, B. Y., Hoad, G., et al. (2017). IslandViewer 4: expanded prediction of genomic islands for larger-scale datasets. Nucleic Acids Res. 45, W30–W35. doi: 10.1093/nar/gkx343
Biovia (2017). Discovery Studio Modeling Environment, Release 2017. San Diego, CA: Dassault Systemes.
Blair, J. M., Webber, M. A., Baylay, A. J., Ogbolu, D. O., and Piddock, L. J. (2015). Molecular mechanisms of antibiotic resistance. Nat. Rev. Microbiol. 13, 42–51. doi: 10.1038/nrmicro3380
Brettin, T., Davis, J. J., Disz, T., Edwards, R. A., Gerdes, S., Olsen, G. J., et al. (2015). RASTtk: a modular and extensible implementation of the RAST algorithm for building custom annotation pipelines and annotating batches of genomes. Sci. Rep. 5:8365. doi: 10.1038/srep08365
Burrus, V. (2017). Mechanisms of stabilization of integrative and conjugative elements. Curr. Opin. Microbiol. 38, 44–50. doi: 10.1016/j.mib.2017.03.014
Carraro, N., and Burrus, V. (2015). The dualistic nature of integrative and conjugative elements. Mob. Genet. Elements 5, 98–102. doi: 10.1080/2159256X.2015.1102796
Casanova-Roman, M., Sanchez-Porto, A., and Casanova-Bellido, M. (2002). Early-onset neonatal sepsis caused by vertical transmission of Morganella morganii. Scand. J. Infect. Dis. 34, 534–535. doi: 10.1080/003655402320208776
Christie, P. J., Whitaker, N., and Gonzalez-Rivera, C. (2014). Mechanism and structure of the bacterial type IV secretion systems. Biochim Biophys. Acta 1843, 1578–1591. doi: 10.1016/j.bbamcr.2013.12.019
CLSI (2017). Performance Standards for Antimicrobial Susceptibility Testing, 27th Edn. Wayne, PA: CLSI.
Davies, S. C., Fowler, T., Watson, J., Livermore, D. M., and Walker, D. (2013). Annual Report of the Chief Medical Officer: infection and the rise of antimicrobial resistance. Lancet 381, 1606–1609. doi: 10.1016/S0140-6736(13)60604-2
de Assis, J. C. S., Goncalves, O. S., Fernandes, A. S., De Queiroz, M. V., Bazzolli, D. M. S., and Santana, M. F. (2022). Genomic analysis reveals the role of integrative and conjugative elements in plant pathogenic bacteria. Mob DNA 13:19. doi: 10.1186/s13100-022-00275-1
Del Canto, F., Valenzuela, P., Cantero, L., Bronstein, J., Blanco, J. E., Blanco, J., et al. (2011). Distribution of classical and nonclassical virulence genes in enterotoxigenic Escherichia coli isolates from Chilean children and tRNA gene screening for putative insertion sites for genomic islands. J. Clin. Microbiol. 49, 3198–3203. doi: 10.1128/JCM.02473-10
Erlanger, D., Assous, M. V., Wiener-Well, Y., Yinnon, A. M., and Ben-Chetrit, E. (2019). Clinical manifestations, risk factors and prognosis of patients with Morganella morganii sepsis. J. Microbiol. Immunol. Infect. 52, 443–448. doi: 10.1016/j.jmii.2017.08.010
Fu, J., Zhong, C., Zhang, P., Zong, G., Liu, M., and Cao, G. (2020). Novel mobilizable genomic Island GEI-D18A mediates conjugational transfer of antibiotic resistance genes in the multidrug-resistant strain Rheinheimera sp. D18. Front. Microbiol. 11:627. doi: 10.3389/fmicb.2020.00627
Fu, J., Zhong, C., Zhou, Y., Lu, M., Zong, G., Zhang, P., et al. (2021). The integrative and conjugative element ICECspPOL2 contributes to the outbreak of multi-antibiotic-resistant bacteria for Chryseobacterium Spp. and Elizabethkingia Spp. Microbiol. Spectr. 9:e0200521. doi: 10.1128/Spectrum.02005-21
Guglielmini, J., Quintais, L., Garcillan-Barcia, M. P., De La Cruz, F., and Rocha, E. P. (2011). The repertoire of ICE in prokaryotes underscores the unity, diversity, and ubiquity of conjugation. PLoS Genet. 7:e1002222. doi: 10.1371/journal.pgen.1002222
Gupta, S. K., Padmanabhan, B. R., Diene, S. M., Lopez-Rojas, R., Kempf, M., Landraud, L., et al. (2014). ARG-ANNOT, a new bioinformatic tool to discover antibiotic resistance genes in bacterial genomes. Antimicrob Agents Chemother. 58, 212–220. doi: 10.1128/AAC.01310-13
He, L. Y., Ying, G. G., Liu, Y. S., Su, H. C., Chen, J., Liu, S. S., et al. (2016). Discharge of swine wastes risks water quality and food safety: antibiotics and antibiotic resistance genes from swine sources to the receiving environments. Environ. Int. 9, 210–219. doi: 10.1016/j.envint.2016.03.023
Johnson, C. M., and Grossman, A. D. (2015). Integrative and Conjugative Elements (ICEs): what they do and how they work. Annu. Rev. Genet. 49, 577–601. doi: 10.1146/annurev-genet-112414-055018
Kruger, N. J., and Stingl, K. (2011). Two steps away from novelty–principles of bacterial DNA uptake. Mol. Microbiol. 80, 860–867. doi: 10.1111/j.1365-2958.2011.07647.x
Kumar, S., Stecher, G., and Tamura, K. (2016). MEGA7: molecular evolutionary genetics analysis version 7.0 for bigger datasets. Mol. Biol. Evol. 33, 1870–1874. doi: 10.1093/molbev/msw054
Leclercq, R., Canton, R., Brown, D. F., Giske, C. G., Heisig, P., Macgowan, A. P., et al. (2013). EUCAST expert rules in antimicrobial susceptibility testing. Clin. Microbiol. Infect. 19, 141–160. doi: 10.1111/j.1469-0691.2011.03703.x
Lederberg, J., and Tatum, E. L. (1946). Gene recombination in Escherichia coli. Nature 158:558. doi: 10.1038/158558a0
Liu, B., Guo, X., Wang, J., Wu, P., Li, S., Feng, L., et al. (2021). Development of a molecular serotyping scheme for Morganella morganii. Front. Microbiol. 12:791165. doi: 10.3389/fmicb.2021.791165
Liu, B., and Pop, M. (2009). ARDB–Antibiotic resistance genes database. Nucleic Acids Res. 37, D443–D447. doi: 10.1093/nar/gkn656
Liu, B., Zheng, D., Jin, Q., Chen, L., and Yang, J. (2019). VFDB 2019: a comparative pathogenomic platform with an interactive web interface. Nucleic Acids Res. 47, D687–D692. doi: 10.1093/nar/gky1080
Liu, M., Li, X., Xie, Y., Bi, D., Sun, J., Li, J., et al. (2019). ICEberg 2.0: an updated database of bacterial integrative and conjugative elements. Nucleic Acids Res. 47, D660–D665. doi: 10.1093/nar/gky1123
Liu, H., Zhu, J., Hu, Q., and Rao, X. (2016). Morganella morganii, a non-negligent opportunistic pathogen. Int. J. Infect. Dis. 50, 10–17. doi: 10.1016/j.ijid.2016.07.006
Lomovskaya, O., and Bostian, K. A. (2006). Practical applications and feasibility of efflux pump inhibitors in the clinic–a vision for applied use. Biochem. Pharmacol. 71, 910–918. doi: 10.1016/j.bcp.2005.12.008
Lomovskaya, O., Warren, M. S., Lee, A., Galazzo, J., Fronko, R., Lee, M., et al. (2001). Identification and characterization of inhibitors of multidrug resistance efflux pumps in Pseudomonas aeruginosa: novel agents for combination therapy. Antimicrob Agents Chemother. 45, 105–116. doi: 10.1128/AAC.45.1.105-116.2001
Lynch, A. S. (2006). Efflux systems in bacterial pathogens: an opportunity for therapeutic intervention? an industry view. Biochem. Pharmacol. 71, 949–956. doi: 10.1016/j.bcp.2005.10.021
Mahamoud, A., Chevalier, J., Davin-Regli, A., Barbe, J., and Pages, J. M. (2006). Quinoline derivatives as promising inhibitors of antibiotic efflux pump in multidrug resistant Enterobacter aerogenes isolates. Curr. Drug Targets 7, 843–847. doi: 10.2174/138945006777709557
Mahrouki, S., Bourouis, A., Chihi, H., Ouertani, R., Ferjani, M., Moussa, M. B., et al. (2012). First characterisation of plasmid-mediated quinolone resistance-qnrS1 co-expressed bla CTX-M-15 and bla DHA-1 genes in clinical strain of Morganella morganii recovered from a Tunisian Intensive Care Unit. Ind. J. Med. Microbiol. 30, 437–441. doi: 10.4103/0255-0857.103765
Martins, M., Dastidar, S. G., Fanning, S., Kristiansen, J. E., Molnar, J., Pages, J. M., et al. (2008). Potential role of non-antibiotics (helper compounds) in the treatment of multidrug-resistant Gram-negative infections: mechanisms for their direct and indirect activities. Int. J. Antimicrob Agents 31, 198–208. doi: 10.1016/j.ijantimicag.2007.10.025
Minnullina, L., Kostennikova, Z., Evtugin, V., Akosah, Y., Sharipova, M., and Mardanova, A. (2022). Diversity in the swimming motility and flagellar regulon structure of uropathogenic Morganella morganii strains. Int. Microbiol. 25, 111–122. doi: 10.1007/s10123-021-00197-7
Munita, J. M., and Arias, C. A. (2016). Mechanisms of antibiotic resistance. Microbiol. Spectr. 4:10.1128/microbiolspec.VMBF-0016-2015.
Overbeek, R., Olson, R., Pusch, G. D., Olsen, G. J., Davis, J. J., Disz, T., et al. (2014). The SEED and the rapid annotation of microbial genomes using subsystems technology (RAST). Nucleic Acids Res. 42, D206–D214. doi: 10.1093/nar/gkt1226
Pruden, A., Pei, R., Storteboom, H., and Carlson, K. H. (2006). Antibiotic resistance genes as emerging contaminants: studies in northern Colorado. Environ. Sci. Technol. 40, 7445–7450. doi: 10.1021/es060413l
Seija, V., Medina Presentado, J. C., Bado, I., Papa Ezdra, R., Batista, N., Gutierrez, C., et al. (2015). Sepsis caused by New Delhi metallo-beta-lactamase (blaNDM-1) and qnrD-producing Morganella morganii, treated successfully with fosfomycin and meropenem: case report and literature review. Int. J. Infect. Dis. 30, 20–26. doi: 10.1016/j.ijid.2014.09.010
Siguier, P., Varani, A., Perochon, J., and Chandler, M. (2012). Exploring bacterial insertion sequences with ISfinder: objectives, uses, and future developments. Methods Mol. Biol. 859, 91–103. doi: 10.1007/978-1-61779-603-6_5
Song, L., Pan, Y., Chen, S., and Zhang, X. (2012). Structural characteristics of genomic islands associated with GMP synthases as integration hotspot among sequenced microbial genomes. Comput. Biol. Chem. 36, 62–70. doi: 10.1016/j.compbiolchem.2012.01.001
Stavri, M., Piddock, L. J., and Gibbons, S. (2007). Bacterial efflux pump inhibitors from natural sources. J. Antimicrob. Chemother. 59, 1247–1260. doi: 10.1093/jac/dkl460
Thierauf, A., Perez, G., and Maloy, A. S. (2009). Generalized transduction. Methods Mol. Biol. 501, 267–286. doi: 10.1007/978-1-60327-164-6_23
Trokter, M., Felisberto-Rodrigues, C., Christie, P. J., and Waksman, G. (2014). Recent advances in the structural and molecular biology of type IV secretion systems. Curr. Opin. Struct. Biol. 27, 16–23. doi: 10.1016/j.sbi.2014.02.006
Tsiotsias, A., Maris, S., Apostolopoulos, A. P., Salmas, M., Karadimas, E. J., Balfousias, T., et al. (2019). Morganella Morganii: an unusual case-report of shoulder septic arthritis. J. Long Term Eff. Med. Implants 29, 273–275.
Waterhouse, A., Bertoni, M., Bienert, S., Studer, G., Tauriello, G., Gumienny, R., et al. (2018). SWISS-MODEL: homology modelling of protein structures and complexes. Nucleic Acids Res. 46, W296–W303. doi: 10.1093/nar/gky427
Wattam, A. R., Davis, J. J., Assaf, R., Boisvert, S., Brettin, T., Bun, C., et al. (2017). Improvements to PATRIC, the all-bacterial bioinformatics database and analysis resource center. Nucleic Acids Res. 45, D535–D542. doi: 10.1093/nar/gkw1017
Wick, R. R., Judd, L. M., Gorrie, C. L., and Holt, K. E. (2017). Unicycler: resolving bacterial genome assemblies from short and long sequencing reads. PLoS Comput. Biol. 13:e1005595. doi: 10.1371/journal.pcbi.1005595
Zong, G., Zhong, C., Fu, J., Zhang, Y., Zhang, P., Zhang, W., et al. (2020). The carbapenem resistance gene blaOXA-23 is disseminated by a conjugative plasmid containing the novel transposon Tn6681 in Acinetobacter johnsonii M19. Antimicrob Resist. Infect. Control. 9:182. doi: 10.1186/s13756-020-00832-4
Keywords: Morganella morganii, polymyxin resistance, integrative and conjugative element, MFS transporter, glabridin
Citation: Fu J, Liu Y, Wang F, Zong G, Wang Z, Zhong C and Cao G (2023) Glabridin inhibited the spread of polymyxin-resistant Enterobacterium carrying ICEMmoMP63. Front. Microbiol. 14:1188900. doi: 10.3389/fmicb.2023.1188900
Received: 18 March 2023; Accepted: 09 May 2023;
Published: 22 May 2023.
Edited by:
Govindan Rajamohan, Institute of Microbial Technology (CSIR), IndiaCopyright © 2023 Fu, Liu, Wang, Zong, Wang, Zhong and Cao. This is an open-access article distributed under the terms of the Creative Commons Attribution License (CC BY). The use, distribution or reproduction in other forums is permitted, provided the original author(s) and the copyright owner(s) are credited and that the original publication in this journal is cited, in accordance with accepted academic practice. No use, distribution or reproduction is permitted which does not comply with these terms.
*Correspondence: Chuanqing Zhong, zhongchuanqing@sdjz.edu.cn; Guangxiang Cao, caoguangxiang@sdfmu.edu.cn