- 1Jiangsu Key Laboratory of Zoonosis/Jiangsu Co-innovation Center for Prevention and Control of Important Animal Infectious Diseases and Zoonoses, Yangzhou University, Yangzhou, China
- 2Key Laboratory of Prevention and Control of Biological Hazard Factors (Animal Origin) for Agrifood Safety and Quality, Ministry of Agriculture of China, Yangzhou University, Yangzhou, China
- 3Department of Clinical Laboratory, Xuyi People's Hospital, Huai'an, China
- 4Medical School of Yangzhou University, Yangzhou University, Yangzhou, China
The emergence and spread of carbapenemase genes, colistin resistance genes mcr-1, and tigecycline resistance gene tet(X) represent a significant threat to clinical therapy and public health. In this study, we investigated the presence of carbapenemase genes, mcr-1, and tet(X) in 298 Escherichia coli strains obtained from a teaching hospital in China. In total, eight (2.68%), six (2.01%), and one (0.34%) E. coli isolates carried blaNDM, mcr-1, and tet(X4), respectively. The blaNDM gene was located on IncX3 (n = 4), F2:A-:B- (n = 3), and F2:A1:B1 (n = 1) plasmids, with high similarity to multiple plasmids belonging to the same incompatibility type from Enterobacteriaceae. Six MCR-producing strains contained mcr-1-carrying IncI2 plasmids, organized similarly to other mcr-1-bearing IncI2 plasmids from animals in China. The blaCTX−M−55/64/132/199 gene located within a typical transposition unit (ISEcp1-blaCTX−M-orf477Δ) was inserted near dnaJ to generate 5-bp direct repeats in four mcr-1-positive plasmids. The tet(X) and another four resistance genes [aadA2, tet(A), floR, and Δlnu(F)] were co-located on an IncX1 plasmid, highly similar to other tet(X4)-carrying IncX1 plasmids from Escherichia and Klebsiella of animal or food origin, except that the conjugative transfer region of IncX1 plasmids was absent in our plasmid. Although a low prevalence of blaNDM, mcr-1, and tet(X) was observed in E. coli from patients in this study, their dissemination associated with some successful pandemic plasmids is of great concern. The continued surveillance of these crucial resistance genes in patients should be strengthened.
Introduction
Globally, multidrug-resistant (MDR) bacteria are rapidly increasing, which poses a serious threat to clinical therapy and public health (WHO, 2014). In 2019, six pathogens were responsible for 3.57 million deaths associated with antimicrobial resistance, with Escherichia coli as the leading pathogen (Antimicrobial Resistance Collaborators, 2022).
Carbapenems are the mainstream antimicrobial agents for treating severe infections caused by MDR Gram-negative pathogens (Wu et al., 2019). However, the emergence and global spread of carbapenem-resistant Enterobacteriaceae (CRE) have become a severe challenge in healthcare settings, particularly for New Delhi metallo-β-lactamase (NDM)-producing strains (Wu et al., 2019). Escherichia coli is one of the predominant carriers of blaNDM; some lineages, such as ST167, ST410, and ST617, and popular plasmids (IncX3, IncFII, and IncC) are associated with blaNDM global dissemination in E. coli (Wu et al., 2019).
Colistin and tigecycline are used as last-resort antimicrobial agents for treating severe infections caused by MDR Gram-negative pathogens, especially CRE (Liu et al., 2016; Yaghoubi et al., 2022). However, the identification of novel plasmid-mediated colistin resistance gene mcr-1 and tigecycline resistance gene tet(X4) in E. coli from pigs in China can reduce the clinical efficacy of these agents (Liu et al., 2016; He et al., 2019). Since the discovery of mcr-1, it has been reported in E. coli isolates from different sources worldwide, particularly from food animals. It has been frequently associated with epidemic plasmids (e.g., IncI2, IncX4, and IncHI2) (Nang et al., 2019). The tet(X4) gene has recently been increasingly reported in E. coli from different sources (e.g., animals, food, and humans), mainly in China (He et al., 2019; Fang et al., 2020). Mobile element ISCR2 and various conjugative and mobilizable plasmids, such as IncQ, IncX1, IncFIB, and IncHI1, facilitate the horizontal transfer of tet(X4) in E. coli (Fang et al., 2020; Aminov, 2021).
A previous study reported the discovery of mcr, tet(X), and carbapenemases in human or animal gut microbiomes (Wang et al., 2020a). The spread of mcr, tet(X), and carbapenemases is worrisome, as options for therapy are limited. Therefore, there is an urgent need for evaluating and monitoring the prevalence of pathogenic bacteria carrying mcr, tet(X), and carbapenemases. In this study, we investigated the prevalence and characterization of mobilized resistance genes tet(X4), mcr-1, and blaNDM in E. coli strains isolated from a teaching hospital in Jiangsu Province, China.
Materials and methods
Bacterial isolates and detection of tet(X), mcr-1, and carbapenemase genes
From November 2019 to November 2021, 298 non-duplicate E. coli strains were isolated from patients in a teaching hospital in Jiangsu Province, China. The presence of tet(X), mcr-1, and carbapenemase genes (blaNDM, blaKPC, blaIMP, blaVIM, and blaOXA − 48) was detected by PCR and sequencing according to previously described protocols (Poirel et al., 2011; Wang et al., 2018, 2019). The information on patients with blaNDM/mcr-1/tet(X)-carrying E. coli is described in Supplementary Table 1.
Antimicrobial susceptibility testing
The blaNDM/mcr-1/tet(X)-carrying E. coli isolates were tested for susceptibility to 14 antimicrobial agents, namely, ampicillin, cefotaxime, meropenem, gentamycin, amikacin, streptomycin, tetracycline, tigecycline, chloramphenicol, nalidixic acid, ciprofloxacin, colistin, fosfomycin, and sulfamethoxazole/trimethoprim, by using the agar dilution method or broth microdilution method (limited to colistin and tigecycline). E. coli ATCC 25922 was used as the quality control strain. The results were interpreted according to Clinical and Laboratory Standards Institute (CLSI) M100, 30th edition (CLSI, 2020). Streptomycin (>16 mg/L) was interpreted according to the epidemiological cutoff values for E. coli set by the European Committee on Antimicrobial Susceptibility Testing (EUCAST; www.eucast.org).
Conjugation assay
Conjugation experiments were performed using blaNDM/mcr-1/tet(X)-carrying E. coli isolates as donor bacteria and E. coli C600 (high-level streptomycin resistance) as the recipient strain following a previously described protocol (Chen et al., 2007). In brief, the donor bacteria and E. coli C600 were grown in LB broth up to the logarithmic phase and then mixed in a 1:4 ratio and incubated at 37°C for 18 h. Transconjugants were selected on MacConkey or LB agar plates supplemented with 3,000 mg/L streptomycins and 2 mg/L of meropenem (for blaNDM), colistin (for mcr-1), or tigecycline [for tet(X)]. They were further confirmed by detecting the presence of blaNDM, mcr-1, and tet(X) using PCR. Transfer frequencies were calculated as the number of transconjugants per recipient. Conjugation experiments were performed at least in triplicate.
Whole genome sequencing and analysis
Genomic DNA was extracted from all blaNDM/mcr-1/tet(X)-carrying E. coli isolates using a TIAN amp Bacteria DNA kit (Tiangen, Beijing, China) and sequenced by the Illumina Hiseq platform. The 150 bp pair-end raw reads were trimmed with the NGSQC toolkit v.2.3.3 and assembled into contigs using SPAdes v.3.8.2 (Bankevich et al., 2012). Three E. coli isolates, XYEH3314 (blaNDM − 6), XYEH3783 (blaNDM − 5), and XYEH3934 [tet(X4)], were sequenced using Nanopore MinION and assembled with Unicycler version 0.4.9. The remaining blaNDM/mcr-1-carrying plasmid contigs were assembled into a complete plasmid sequence using PCR and Sanger sequencing (Supplementary Table 2) using related plasmids as references by BLAST (http://blast.ncbi.nlm.nih.gov/Blast.cgi). The genome sequences were analyzed for multi-locus sequence typing (MLST) and resistance genes using MLST and ResFinder, respectively (https://cge.food.dtu.dk//services). Analysis and annotation of all blaNDM/mcr-1/tet(X4)-carrying plasmids were performed using the RAST server (Aziz et al., 2008), ISfinder (https://www-is.biotoul,fr/), ResFinder, PlasmidFinder (https://cge.food.dtu.dk/services/PlasmidFinder/), BLAST, and the Gene Construction Kit 4.5 (Textco BioSoftware, Inc., Raleigh, NC, United States). Blast Ring Image Generator (BRIG) version 0.95 was used to visualize the blaNDM-carrying plasmid comparisons (Alikhan et al., 2011).
Phylogenetic analysis of blaNDM/mcr-1/tet(X4)-positive Escherichia coli strains
The phylogenetic tree of all blaNDM/mcr-1/tet(X4)-positive E. coli strains and blaNDM-positive E. coli ST167 in this study and the NCBI database was constructed based on core genome single nucleotide polymorphisms (SNPs) using ParSNP v. 1.2 (https://github.com/marbl/parsnp), respectively. ChiPlot (http://chiplot.online/#) was used to visualize the phylogenetic tree.
Nucleotide sequence accession number
The genome sequences and blaNDM/mcr-1/tet(X4)-carrying plasmids have been deposited in GenBank under accession number PRJNA901528.
Results and discussion
Characterization of blaNDM-positive E. coli isolates
The blaNDM gene has been globally distributed as the third most common carbapenemase-encoding gene (Wu et al., 2019). We detected eight (2.68%) E. coli isolates carrying the carbapenemase gene blaNDM − 5 (n = 7) or blaNDM − 6 (n = 1) (Table 1). None of the other tested carbapenemase genes were detected in this study. All NDM-producing E. coli were resistant to meropenem (MIC ≥ 8 mg/L), ampicillin, cefotaxime, gentamicin, tetracycline, chloramphenicol, nalidixic acid, ciprofloxacin, and sulfamethoxazole/trimethoprim (Table 1). Resistance to amikacin, streptomycin, or fosfomycin was observed in three, six, and four blaNDM-positive strains, respectively. Numerous resistance genes were identified in these blaNDM-carrying strains, which was consistent with their susceptibility profiles. Eight blaNDM-positive E. coli isolates were assigned to ST156 (n = 4), ST167 (n = 2), ST361 (n = 1), and ST1193 (n = 1), respectively (Table 1). E. coli ST167 has been a global clone for blaNDM dissemination (Wu et al., 2019). ST156 has been frequently described as blaNDM − 5 carriers in patients and animals in China (Yang et al., 2016; Tang et al., 2019; Lin et al., 2020; Zhang et al., 2022). ST361 has been previously associated with blaNDM − 5 in patients worldwide (Park et al., 2020; Chakraborty et al., 2021; Huang et al., 2023). ST1193 has been the dominant ST of carbapenem-sensitive E. coli isolates in patients at an intensive care unit in China (Ding et al., 2023). Carbapenem-resistant E. coli ST1193, an isolate encoding NDM-5 found in this study, might be a cause for concern due to its ability to acquire carbapenem resistance and the potential to cause a future outbreak in hospitals. All of them could successfully transfer meropenem resistance and blaNDM to E. coli C600 by conjugation at frequencies of 10−6 to 10−5 transconjugants per recipient (Supplementary Table 3).
The blaNDM gene was located on IncX3 (n = 4), F2:A-:B- (n = 3), and F2:A1:B1 (n = 1) plasmids (Table 1). The IncX3 plasmids carried only one resistance gene, blaNDM − 5, but the IncFII plasmids also carried some other resistance genes, such as blaTEM − 1b, aadA2, and dfrA12 (Table 1). Four blaNDM − 5-carrying IncX3 plasmids (pYUXEEH1271-NDM, pYUXEEH1278-NDM, pYUXYEH3520-NDM, and pYUXYEH3521-NDM) were obtained from four ST156 NDM-5-producing E. coli strains. These IncX3 plasmids were identical, except that two copies of IS1294 were inserted into the accessory replication gene bis and DNA relaxase gene taxC, respectively, in pYUXEEH1271-NDM and pYUXEEH1278-NDM (Figure 1A). They were also identical or highly similar to other NDM-producing IncX3 plasmids from Enterobacteriaceae (e.g., E. coli, Enterobacter, and Klebsiella) of animal or human origin (Figure 1A). Two F2:A-:B- plasmids, pYUXYEH933-NDM (82,619 bp) and pYUXYEH944-NDM (82,628 bp), obtained from two ST167 NDM-5-encoding isolates, were highly similar and differed by only one nucleotide and one more 9-bp tandem repeat (CAACAGCCG) in the traD gene in pYUXYEH944-NDM (Figure 1B). The blaNDM gene in isolates XYEH3314 and XYEH3783 was located on F2:A-:B- plasmid pYUXYEH3314-NDM (blaNDM − 6, 93,647 bp) and F2:A1:B1 plasmid pYUXYEH3783-NDM (blaNDM − 5, 168,068 bp). These IncFII plasmids showed high similarity to multiple plasmids belonging to the same incompatibility type from Enterobacteriaceae (e.g., E. coli, K. pneumoniae, and Salmonella) from food-producing animals or humans (Figures 1B, C). These blaNDM-carrying plasmids shared the same core structure ΔcutA-tat-trpF-bleMBL-blaNDM − 5/−6-ΔISAba125 (Figure 2). IncX3 and IncFII plasmids are major vectors for the global transmission of blaNDM in Enterobacteriaceae (Wu et al., 2019). Particularly, blaNDM-carrying IncX3 plasmids have been widely disseminated among E. coli strains from patients, food-producing animals, companion animals, food products, and the environment in China (Yang et al., 2016; Wu et al., 2019; Zhang et al., 2019; Cen et al., 2021; Wang et al., 2021; Zhao et al., 2021).
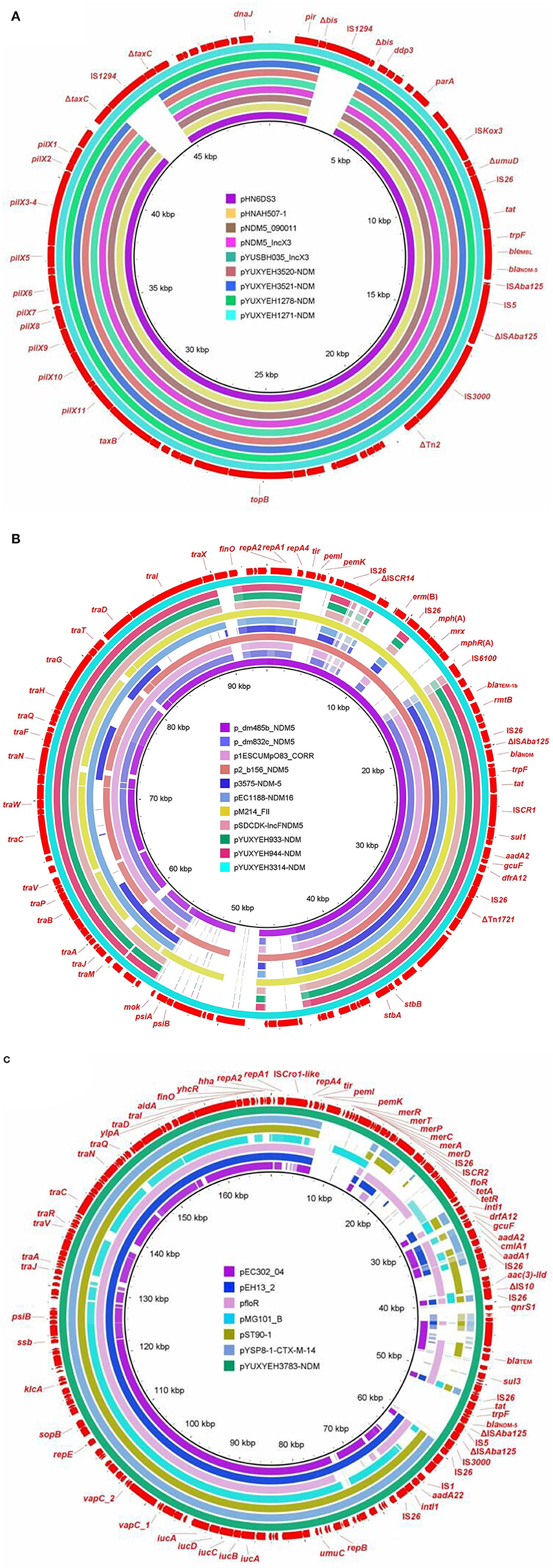
Figure 1. Sequence comparison of (A) IncX3 plasmids pYUXYEH1271-NDM, pYUXYEH1278-NDM, pYUXYEH3520-NDM, and pYUXYEH3521-NDM in this study with other NDM-producing plasmids pHN6DS3 (MN276078, Enterobacter cloacae, dog, China), pHNAH507-1 (MH286945, Escherichia coli, chicken feces, China), pNDM5_090011 (CP036312, E. hormaechei, human, China), pNDM5_IncX3 (KU761328, Klebsiella pneumoniae, human, China), and pYUSBH035_IncX3 (CP099447, K. quasipneumoniae, human, China) (B) F2:A-:B- plasmids pYUXYEH933-NDM, pYUXYEH944-NDM, and pYUXYEH3314-NDM in this study with other NDM-producing plasmids p_dm485b_NDM5 (CP095622, K. pneumoniae, human, Bangladesh), p_dm832b_NDM5 (CP095656, E. coli, human, Bangladesh), p1ESCUMpO83_CORR (CP034254, E. coli, mastitis milk, India), p2_b156_NDM5 (CP095683, K. pneumoniae, human, Bangladesh), p3575-NDM-5 (CP048012, E. coli, human, Spain), pEC1188-NDM16 (MH213345, E. coli, human, China), pM214_FII (AP018144, E. coli, human, Myanmar), and pSDCDK-IncFNDM5 (MT621569, E. coli, chicken farm, China) (C) F2:A1:B1 plasmid pYUXYEH3783-NDM in this study with other F2:A1:B1 plasmids pEC302_04 (CP011493, E. coli, human, Malaysia), pMG101_B (CP070963, E. coli, USA), pYSP8-1-CTX-M-14 (CP037912, E. coli, pig, China), or F2:A1:B- plasmids pfloR (CP047011, E. coli, pig, China), pST90-1 (CP050735, Salmonella Typhimurium, duck, China), and pEH13_2 (CP089099, K. pneumoniae, hospital, Hong Kong). The outer circle in red with annotation is the reference plasmid pYUXYEH1271-NDM, pYUXYEH3314-NDM, or pYUXYEH3783-NDM obtained in this study.
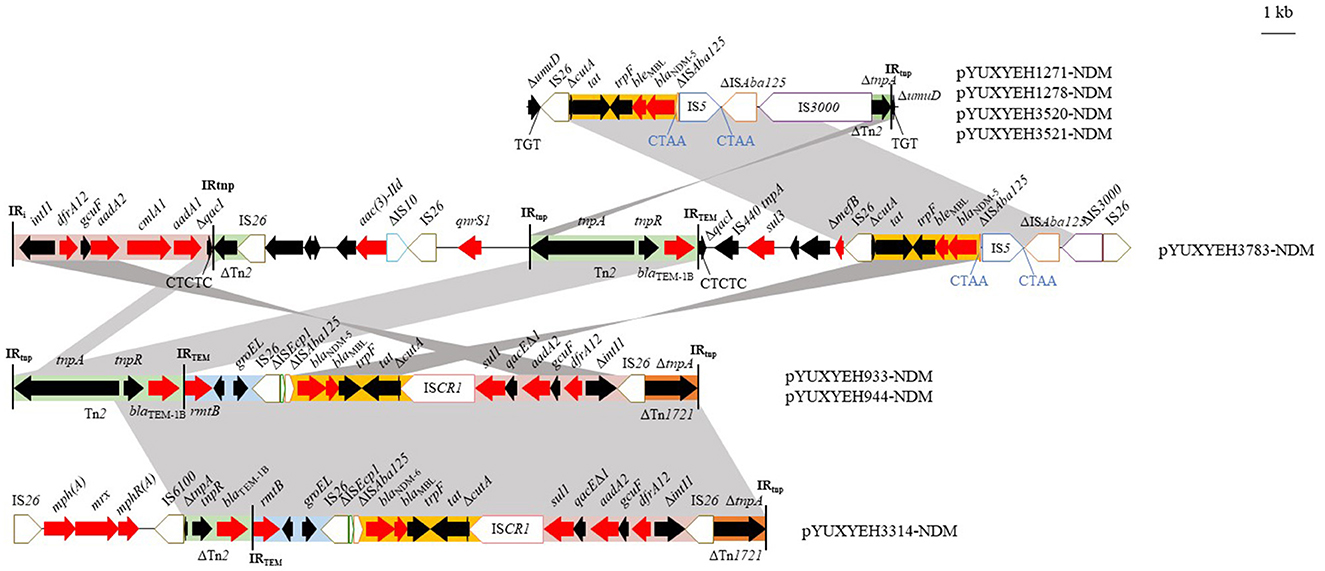
Figure 2. Genetic structures of blaNDM in plasmids pYUXYEH933-NDM, pYUXYEH944-NDM, pYUXYEH1271-NDM, pYUXYEH1278-NDM, pYUXYEH3314-NDM, pYUXYEH3520-NDM, pYUXYEH3521-NDM, and pYUXYEH3783-NDM obtained in this study. The extent and directions of genes are indicated by arrows. Δ indicates a truncated gene or mobile element. Insertion sequences (ISs) are shown as boxes labeled with their names. Direct repeats are indicated by arrows and sequences. Tall bars represent the inverted repeats (IRs) of a transposon or integron. Regions with >99% identity are shaded in gray.
Characterization of mcr-1-carrying E. coli isolates
To date, 10 plasmid-mediated mcr variants (mcr-1 to mcr-10) have been identified in Enterobacteriaceae from animals, humans, and the environment (Hussein et al., 2021). Among them, mcr-1 is the most common mcr variant worldwide (Hussein et al., 2021). In this study, six (2.01%) E. coli strains were positive for the colistin resistance gene mcr-1. The prevalence of mcr-1 is close to 4.2%, which is low, in human clinical isolates worldwide (Bastidas-Caldes et al., 2022). The prevalence of mcr-1 in E. coli was significantly decreased in both animals and humans in China after banning colistin as a growth promoter on 30 April 2017 (Wang et al., 2020b).
All MCR-1-positive strains were resistant to colistin (MIC = 8 mg/L), ampicillin, cefotaxime, nalidixic acid, ciprofloxacin, and some other agents (Table 1). In addition to mcr-1, they also carried other clinically important resistance genes, such as blaCTX − M (n = 6), tet(A) (n = 3), fosA3 (n = 3), and rmtB (n = 1) (Table 1). The presence of these resistance genes could explain their multi-resistance profiles. Six MCR-1-producing isolates were assigned to five STs (ST88, ST450, ST617, ST2003, and ST4985) (Table 1).
Among them, four mcr-1-positive E. coli strains could transfer mcr-1 to E. coli C600 by conjugation with frequencies of 10−6 to 10−4 transconjugants/recipient (Supplementary Table 3). The mcr-1 gene was located on IncI2 plasmids in all six strains. These mcr-1-carrying plasmids with sizes between 63,409 and 67,529 bp were organized similarly to other mcr-1-bearing IncI2 plasmids from food-producing animals in China, such as the first identified mcr-1-positive plasmid pHNSHP45 (pig, KP347127) from E. coli and pSCS23 (chicken, KU934209) from Salmonella (Figure 3). However, ISApl1, located upstream of mcr-1 in pHNSHP45 and pSCS23, was absent in our plasmids (Figure 3). The rapid global dissemination of mcr-1 is largely attributed to horizontal transfer via some successful plasmids such as IncX4, IncI2, and IncHI2 (Bastidas-Caldes et al., 2022).
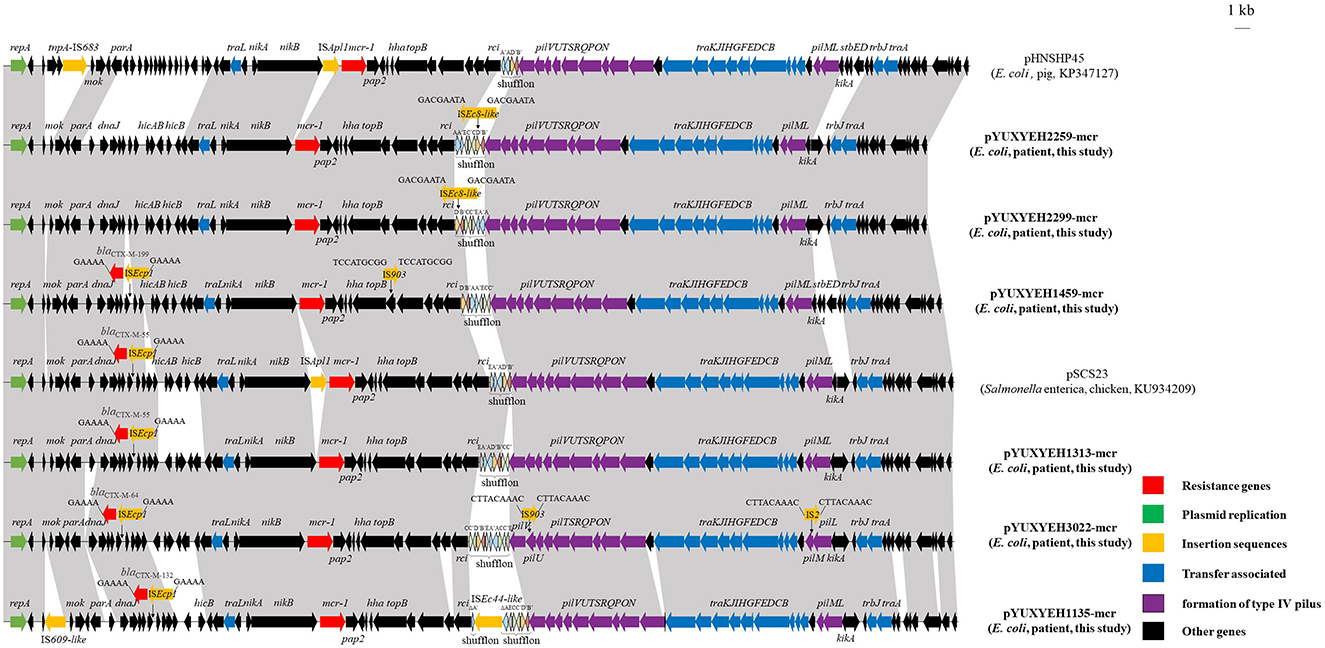
Figure 3. Linear comparisons of plasmids pYUXYEH1135-mcr, pYUXYEH1313-mcr, pYUXYEH1459-mcr, pYUXYEH2259-mcr, pYUXYEH2299-mcr, and pYUXYEH3022-mcr in this study with other IncI2 mcr-1-carrying plasmids. The extent and directions of genes are indicated by arrows. Labeled vertical arrows with an IS box indicate the insertion site of the IS element. Direct repeats are indicated by arrows and sequences. Regions with >99% identity are shaded in gray.
Notably, four mcr-1-bearing plasmids in this study also carried the extended-spectrum β-lactamase gene blaCTX − M (blaCTX − M−55/64/132/199) (Table 1). The blaCTX − M gene, located within a typical transposition unit (ISEcp1-blaCTX − M-orf477Δ), was inserted near dnaJ to generate 5-bp direct repeats (5′-GAAAA-3′) (Figure 3). The co-location of blaCTX − M would allow for mcr-1 co-selection under cephalosporins, which have been widely used to treat clinical infections.
Characterization of a tet(X4)-positive E. coli isolate
Since the discovery of the plasmid-mediated tigecycline resistance gene tet(X4) in E. coli from pigs in China in 2019 (He et al., 2019), it has been identified in E. coli from food-producing animals, wild birds, food products, and the environment (Chen et al., 2019; Li et al., 2021; Mohsin et al., 2021). Although tigecycline is applied in a limited way in humans, tet(X4) has been rarely reported in E. coli from patients. It was detected in 4.5% (45/1,001) fecal samples of hospital patients in Guangdong province, and only 0.07% (3/4,212) E. coli isolates from patients in China were identified to carry tet(X4) (He et al., 2019; Cui et al., 2022). We detected only one (0.34%) tet(X4)-positive E. coli isolate, XYEH3934. This strain exhibited high-level tigecycline resistance (MIC = 32 mg/L) and was also resistant to ampicillin, cefotaxime, streptomycin, tetracycline, chloramphenicol, nalidixic acid, fosfomycin, and sulfamethoxazole/trimethoprim (Table 1). However, it failed to transfer tigecycline resistance and tet(X4) to E. coli C600 by conjugation.
The complete sequence of isolate XYEH3934 was obtained. It comprised one chromosome (4,709,641 bp) and four plasmids (Supplementary Table 4). Among them, tet(X) and another four resistance genes [aadA2, tet(A), floR, and Δlnu(F)] were co-located on an IncX1 plasmid pYUXYEH3934-2 with a size of 31,816 bp. It was highly similar to other tet(X4)-carrying IncX1 plasmids from porcine E. coli in China, such as plasmids pYUYZMP62 (MW439254) and pDW28-tet(X4) (ON390803), as well as tet(X4)-positive plasmids from Escherichia and Klebsiella (Figure 4). However, the conjugative transfer region of IncX1 plasmids was absent in pYUXYEH3934-2 (Figure 4), which may explain the failure of conjugation. As previously described (Liu et al., 2022), tet(X4) was also associated with ISCR2 in pYUXYEH3934-2 (Figure 4). The rapid dissemination of tet(X4) is mainly mediated by the horizontal transfer of insertion sequences (e.g., ISCR2 and IS1) and plasmids such as IncX1 in the present study (Aminov, 2021; Liu et al., 2022; Yu et al., 2022). However, the clonal spread of tet(X4)-positive E. coli strains has been previously described, such as E. coli ST877, ST10, and ST48 between animals and humans (Cui et al., 2022) and E. coli ST761 among different sources (Wang et al., 2022; Zhai et al., 2022).
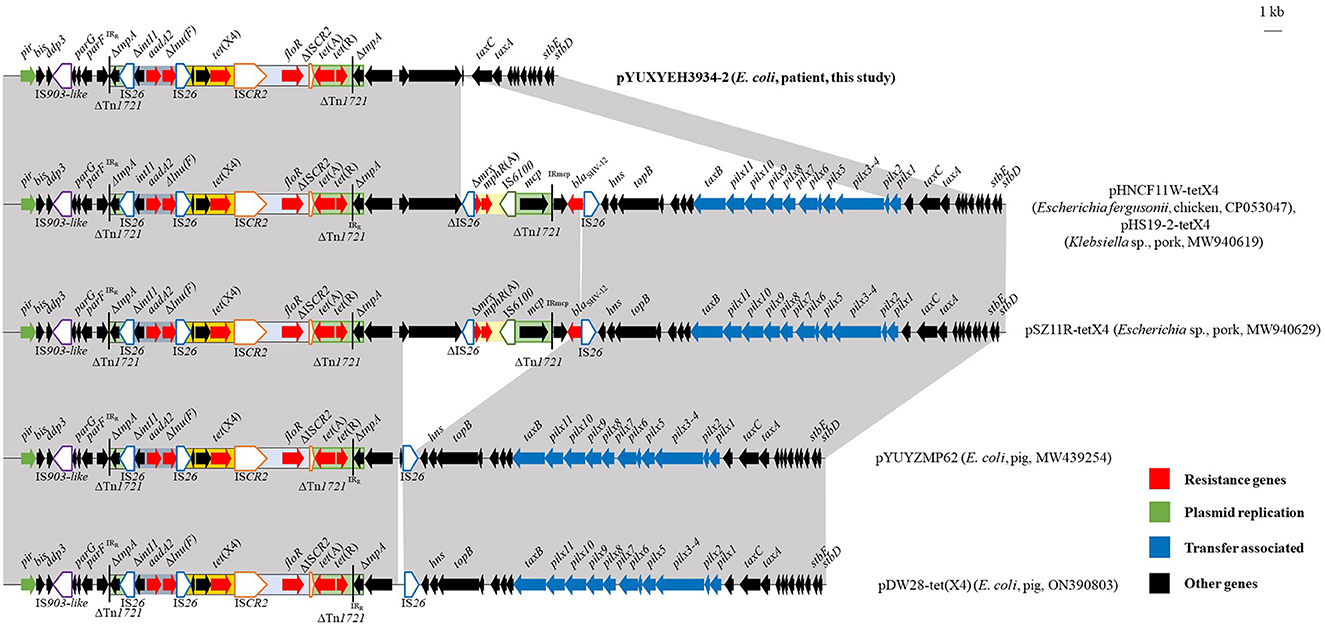
Figure 4. Linear comparisons of plasmid pYUXYEH3934-2 in this study with other IncX1 tet(X4)-carrying plasmids. The extent and directions of genes are indicated by arrows. Δ indicates a truncated gene or mobile element. Insertion sequences (ISs) are shown as boxes labeled with their names. Tall bars represent the 38-bp inverted repeats (IRs) of the transposon. Regions with >99% identity are shaded in gray.
Phylogenomic analysis of blaNDM/mcr-1/tet(X4)-positive E. coli isolates
We performed a phylogenomic analysis based on cgSNP to compare the genetic differences between blaNDM/mcr-1/tet(X4)-positive E. coli isolates in the present study. The results showed that the 15 E. coli strains were divided into four clades (Supplementary Figure 1). Some E. coli strains with the same STs exhibited close genetic relationships with identical or close antimicrobial susceptibility profiles and resistance genes, such as two blaNDM − 5-carrying ST167 E. coli isolates, two mcr-1-carrying ST88 E. coli isolates, and four blaNDM − 5-carrying ST156 E. coli isolates (Supplementary Figure 1). It suggests that clonal spread may occur in this teaching hospital in Jiangsu province. Since E. coli ST167 has been a global epidemic clone for blaNDM dissemination, we further compared the genetic relationship between NDM-5-producing ST167 E. coli isolates in the present study and 33 ST167 E. coli from the NCBI database. Two NDM-encoding E. coli isolates, XYEH933 and XYEH944, and 13 NDM-5-producing ST167 E. coli from China (n = 12) and Italy (n = 1) were clustered in one clade with a close relationship (Supplementary Figure 2).
Conclusions
Although a low prevalence of blaNDM, mcr-1, and tet(X) was observed in E. coli isolates from patients in one hospital in this study, their dissemination in E. coli associated with some successful pandemic plasmids (IncX, IncF, and IncI2) is of great concern. Conversely, E. coli strains from food or food-producing animals in China show a higher prevalence of blaNDM, mcr-1, and tet(X) (He et al., 2019; Zhang et al., 2019; Zhao et al., 2020; Cen et al., 2021). However, the small number of E. coli strains detected is a limitation of this study. Plasmids facilitate the rapid and global dissemination of resistance genes in Enterobacteriaceae from various sources. Given that resistant bacteria or plasmids could be transmitted from animals to humans via the food chain, the environment, or close contact, surveillance and control of these medically important resistance genes in patients are warranted and need to be optimized based on the One Health approach.
Data availability statement
The datasets presented in this study can be found in online repositories. The names of the repository/repositories and accession number(s) can be found below: https://www.ncbi.nlm.nih.gov/, PRJNA901528.
Author contributions
LS and JW conceived the study. LS, G-ZS, YJ, C-YM, H-YW, and G-MK carried out the experiments. LS, YJ, Z-YW, and JW analyzed the data. JW wrote the manuscript. XJ revised the manuscript. All authors have read and approved the final version of the manuscript.
Funding
This study was supported by the fifth phase of the 333 Project Scientific Research Project in Jiangsu Province (BRA2020002), the Priority Academic Program Development of Jiangsu Higher Education Institutions (PAPD), and the 111 Project (D18007).
Conflict of interest
The authors declare that the research was conducted in the absence of any commercial or financial relationships that could be construed as a potential conflict of interest.
Publisher's note
All claims expressed in this article are solely those of the authors and do not necessarily represent those of their affiliated organizations, or those of the publisher, the editors and the reviewers. Any product that may be evaluated in this article, or claim that may be made by its manufacturer, is not guaranteed or endorsed by the publisher.
Supplementary material
The Supplementary Material for this article can be found online at: https://www.frontiersin.org/articles/10.3389/fmicb.2023.1181940/full#supplementary-material
References
Alikhan, N. F., Petty, N. K., Ben Zakour, N. L., and Beatson, S. A. (2011). BLAST ring image generator (BRIG): simple prokaryote genome comparisons. BMC Genomics. 12:402. doi: 10.1186/1471-2164-12-402
Aminov, R. (2021). Acquisition and spread of antimicrobial resistance: a tet(X) case study. Int. J. Mol. Sci. 22:3905. doi: 10.3390/ijms22083905
Antimicrobial Resistance Collaborators (2022). Global burden of bacterial antimicrobial resistance in 2019: a systematic analysis. Lancet. 399, 629–655. doi: 10.1016/S0140-6736(21)02724-0
Aziz, R. K., Bartels, D., Best, A. A., DeJongh, M., Disz, T., Edwards, R. A., et al. (2008). The RAST Server: rapid annotations using subsystems technology. BMC Genomics. 9:75. doi: 10.1186/1471-2164-9-75
Bankevich, A., Nurk, S., Antipov, D., Gurevich, A. A., Dvorkin, M., Kulikov, A. S., et al. (2012). SPAdes: a new genome assembly algorithm and its applications to single-cell sequencing. J. Comput. Biol. 19, 455–477. doi: 10.1089/cmb.2012.0021
Bastidas-Caldes, C., de Waard, J. H., Salgado, M. S., Villacís, M. J., Coral-Almeida, M., Yamamoto, Y., et al. (2022). Worldwide rrevalence of mcr-mediated colistin-resistance Escherichia coli in isolates of clinical samples, healthy humans, and livestock-a systematic review and meta-analysis. Pathogens. 11:659. doi: 10.3390/pathogens11060659
Cen, D. J., Sun, R. Y., Mai, J. L., Jiang, Y. W., Wang, D., Guo, W. Y., et al. (2021). Occurrence and transmission of blaNDM-carrying Enterobacteriaceae from geese and the surrounding environment on a commercial goose farm. Appl. Environ. Microbiol. 87:e00087–e00021. doi: 10.1128/AEM.00087-21
Chakraborty, T., Sadek, M., Yao, Y., Imirzalioglu, C., Stephan, R., Poirel, L., et al. (2021). Cross-border emergence of Escherichia coli producing the carbapenemase NDM-5 in Switzerland and Germany. J. Clin. Microbiol. 59:e02238–e02220. doi: 10.1128/JCM.02238-20
Chen, C., Cui, C. Y., Zhang, Y., He, Q., Wu, X. T., Li, G., et al. (2019). Emergence of mobile tigecycline resistance mechanism in Escherichia coli strains from migratory birds in China. Emerg. Microbes Infect. 8, 1219–1222. doi: 10.1080/22221751.2019.1653795
Chen, L., Chen, Z. L., Liu, J. H., Zeng, Z. L., Ma, J. Y., and Jiang, H. X. (2007). Emergence of RmtB methylase-producing Escherichia coli and Enterobacter cloacae isolates from pigs in China. J. Antimicrob. Chemother. 59, 880–885. doi: 10.1093/jac/dkm065
CLSI (2020). Clinical and Laboratory Standards Institute (CLSI). Performance Standards for Antimicrobial Susceptibility Testing. CLSI Supplement M100, 30th Edn. Wayne, PA: CLSI.
Cui, C. Y., Li, X. J., Chen, C., Wu, X. T., He, Q., Jia, Q. L., et al. (2022). Comprehensive analysis of plasmid-mediated tet(X4)-positive Escherichia coli isolates from clinical settings revealed a high correlation with animals and environments-derived strains. Sci. Total Environ. 806:150687. doi: 10.1016/j.scitotenv.2021.150687
Ding, Y., Zhuang, H., Zhou, J., Xu, L., Yang, Y., He, J., et al. (2023). Epidemiology and genetic characteristics of carbapenem-resistant Escherichia coli in Chinese intensive care unit analyzed by whole-genome sequencing: a prospective observational study. Microbiol. Spectr. 11:e0401022. doi: 10.1128/spectrum.04010-22
Fang, L. X., Chen, C., Cui, C. Y., Li, X. P., Zhang, Y., Liao, X. P., et al. (2020). Emerging high-level tigecycline resistance: novel tetracycline destructases spread via the mobile Tet(X). Bioessays. 42:e2000014. doi: 10.1002/bies.202000014
He, T., Wang, R., Liu, D., Walsh, T. R., Zhang, R., Lv, Y., et al. (2019). Emergence of plasmid-mediated high-level tigecycline resistance genes in animals and humans. Nat. Microbiol. 4, 1450–1456. doi: 10.1038/s41564-019-0445-2
Huang, L., Hu, H., Xu, C., Zhou, M., Li, Y., Li, Y., et al. (2023). Characterization of NDM-5-producing Escherichia coli strains isolated from pediatric patients with bloodstream infections in a Chinese hospital. Genes. 14:520. doi: 10.3390/genes14020520
Hussein, N. H., Al-Kadmy, I. M. S., Taha, B. M., and Hussein, J. D. (2021). Mobilized colistin resistance (mcr) genes from 1 to 10: a comprehensive review. Mol. Biol. Rep. 48, 2897–2907. doi: 10.1007/s11033-021-06307-y
Li, Y., Wang, Q., Peng, K., Liu, Y., Xiao, X., Mohsin, M., et al. (2021). Distribution and genomic characterization of tigecycline-resistant tet(X4)-positive Escherichia coli of swine farm origin. Microb. Genom. 7:000667. doi: 10.1099/mgen.0.000667
Lin, Y., Yang, L., Lu, L., Wang, K., Li, J., Li, P., et al. (2020). Genomic features of an Escherichia coli ST156 strain harboring chromosome-located mcr-1 and plasmid-mediated blaNDM − 5. Infect. Genet. Evol. 85:104499. doi: 10.1016/j.meegid.2020.104499
Liu, D., Wang, T., Shao, D., Song, H., Zhai, W., Sun, C., et al. (2022). Structural diversity of the ISCR2-mediated rolling-cycle transferable unit carrying tet(X4). Sci. Total Environ. 826:154010. doi: 10.1016/j.scitotenv.2022.154010
Liu, Y. Y., Wang, Y., Walsh, T. R., Yi, L. X., Zhang, R., Spencer, J., et al. (2016). Emergence of plasmid-mediated colistin resistance mechanism MCR-1 in animals and human beings in China: a microbiological and molecular biological study. Lancet Infect. Dis. 16, 161–168. doi: 10.1016/S1473-3099(15)00424-7
Mohsin, M., Hassan, B., Martins, W. M. B. S., Li, R., Abdullah, S., Sands, K., et al. (2021). Emergence of plasmid-mediated tigecycline resistance tet(X4) gene in Escherichia coli isolated from poultry, food and the environment in South Asia. Sci. Total Environ. 787:147613. doi: 10.1016/j.scitotenv.2021.147613
Nang, S. C., Li, J., and Velkov, T. (2019). The rise and spread of mcr plasmid-mediated polymyxin resistance. Crit. Rev. Microbiol. 45, 131–161. doi: 10.1080/1040841X.2018.1492902
Park, Y., Choi, Q., Kwon, G. C., and Koo, S. H. (2020). Emergence and transmission of New delhi metallo-beta-lactamase-5-producing Escherichia coli sequence type 361 in a tertiary hospital in South Korea. J. Clin. Lab. Anal. 34:e23041. doi: 10.1002/jcla.23041
Poirel, L., Walsh, T. R., Cuvillier, V., and Nordmann, P. (2011). Multiplex PCR for detection of acquired carbapenemase genes. Diagn. Microbiol. Infect. Dis. 70, 119–123. doi: 10.1016/j.diagmicrobio.2010.12.002
Tang, B., Chang, J., Cao, L., Luo, Q., Xu, H., Lyu, W., et al. (2019). Characterization of an NDM-5 carbapenemase-producing Escherichia coli ST156 isolate from a poultry farm in Zhejiang, China. BMC Microbiol. 19, 82. doi: 10.1186/s12866-019-1454-2
Wang, J., Huang, X. Y., Xia, Y. B., Guo, Z. W., Ma, Z. B., Yi, M. Y., et al. (2018). Clonal spread of Escherichia coli ST93 carrying mcr-1-harboring IncN1-IncHI2/ST3 plasmid among companion animals, China. Front. Microbiol. 9:2989. doi: 10.3389/fmicb.2018.02989
Wang, J., Lu, M. J., Wang, Z. Y., Jiang, Y., Wu, H., Pan, Z. M., et al. (2022). Tigecycline-resistant Escherichia coli ST761 carrying tet(X4) in a pig farm, China. Front. Microbiol. 13:967313. doi: 10.3389/fmicb.2022.967313
Wang, J., Xia, Y. B., Huang, X. Y., Wang, Y., Lv, L. C., Lin, Q. Q., et al. (2021). Emergence of blaNDM − 5 in Enterobacteriaceae isolates from companion animals in Guangzhou, China. Microb. Drug Resist. 27, 809–815. doi: 10.1089/mdr.2020.0210
Wang, L., Liu, D., Lv, Y., Cui, L., Li, Y., Li, T., et al. (2019). Novel plasmid-mediated tet(X5) gene conferring resistance to tigecycline, eravacycline, and omadacycline in a clinical Acinetobacter baumannii isolate. Antimicrob. Agents Chemother. 64:e01326–e01319. doi: 10.1128/AAC.01326-19
Wang, Y., Liu, F., Zhu, B., and Gao, G. F. (2020a). Metagenomic data screening reveals the distribution of mobilized resistance genes tet(X), mcr and carbapenemase in animals and humans. J. Infect. 80, 121–142. doi: 10.1016/j.jinf.2019.09.003
Wang, Y., Xu, C., Zhang, R., Chen, Y., Shen, Y., Hu, F., et al. (2020b). Changes in colistin resistance and mcr-1 abundance in Escherichia coli of animal and human origins following the ban of colistin-positive additives in China: an epidemiological comparative study. Lancet Infect. Dis. 20, 1161–1171. doi: 10.1016/S1473-3099(20)30149-3
WHO (2014). Antimicrobial Resistance: Global Report on Surveillance. Available online at: https://www.who.int/publications/i/item/9789241564748
Wu, W., Feng, Y., Tang, G., Qiao, F., McNally, A., and Zong, Z. (2019). NDM metallo-β-lactamases and their bacterial producers in health care settings. Clin. Microbiol. Rev. 32:e00115–e00118. doi: 10.1128/CMR.00115-18
Yaghoubi, S., Zekiy, A. O., Krutova, M., Gholami, M., Kouhsari, E., Sholeh, M., et al. (2022). Tigecycline antibacterial activity, clinical effectiveness, and mechanisms and epidemiology of resistance: narrative review. Eur. J. Clin. Microbiol. Infect. Dis. 41, 1003–1022. doi: 10.1007/s10096-020-04121-1
Yang, R. S., Feng, Y., Lv, X. Y., Duan, J. H., Chen, J., Fang, L. X., et al. (2016). Emergence of NDM-5- and MCR-1-producing Escherichia coli clones ST648 and ST156 from a single muscovy duck (Cairina moschata). Antimicrob. Agents Chemother. 60, 6899–6902. doi: 10.1128/AAC.01365-16
Yu, R., Chen, Z., Schwarz, S., Yao, H., and Du, X. D. (2022). Mobilization of tet(X4) by IS1 family elements in porcine Escherichia coli isolates. Antimicrob. Agents Chemother. 66:e0159721. doi: 10.1128/AAC.01597-21
Zhai, W., Wang, T., Yang, D., Zhang, Q., Liang, X., Liu, Z., et al. (2022). Clonal relationship of tet(X4)-positive Escherichia coli ST761 isolates between animals and humans. J. Antimicrob. Chemother. 77, 2153–2157. doi: 10.1093/jac/dkac175
Zhang, Q., Lv, L., Huang, X., Huang, Y., Zhuang, Z., Lu, J., et al. (2019). Rapid increase in carbapenemase-producing Enterobacteriaceae in retail meat driven by the spread of the blaNDM − 5-carrying IncX3 plasmid in China from 2016 to 2018. Antimicrob. Agents Chemother. 63:e00573–e00519. doi: 10.1128/AAC.00573-19
Zhang, X., Fang, C., Zhang, J., Hua, W., He, R., and Zhou, M. (2022). Carbapenemase- and colistin resistant Escherichia coli strains from children in China: high genetic diversity and first report of blaNDM − 5, blaCTX − M−65, blaOXA − 10, blaTEM − 1, and mcr-1.1 genes co-occurrence in E. coli ST156. Infect. Drug Resist. 15, 5315–5320. doi: 10.2147/IDR.S378574
Zhao, Q., Berglund, B., Zou, H., Zhou, Z., Xia, H., Zhao, L., et al. (2021). Dissemination of blaNDM − 5 via IncX3 plasmids in carbapenem-resistant Enterobacteriaceae among humans and in the environment in an intensive vegetable cultivation area in eastern China. Environ. Pollut. 273:116370. doi: 10.1016/j.envpol.2020.116370
Keywords: carbapenem, colistin, tigecycline, patients, plasmid
Citation: Sun L, Sun G-Z, Jiang Y, Mei C-Y, Wang Z-Y, Wang H-Y, Kong G-M, Jiao X and Wang J (2023) Low prevalence of mobilized resistance genes blaNDM, mcr-1, and tet(X4) in Escherichia coli from a hospital in China. Front. Microbiol. 14:1181940. doi: 10.3389/fmicb.2023.1181940
Received: 08 March 2023; Accepted: 26 April 2023;
Published: 19 May 2023.
Edited by:
Jian-Hua Liu, South China Agricultural University, ChinaReviewed by:
Liang-xing Fang, South China Agricultural University, ChinaJuan Li, Chinese Academy of Sciences, China
Dandan He, Henan Agricultural University, China
Copyright © 2023 Sun, Sun, Jiang, Mei, Wang, Wang, Kong, Jiao and Wang. This is an open-access article distributed under the terms of the Creative Commons Attribution License (CC BY). The use, distribution or reproduction in other forums is permitted, provided the original author(s) and the copyright owner(s) are credited and that the original publication in this journal is cited, in accordance with accepted academic practice. No use, distribution or reproduction is permitted which does not comply with these terms.
*Correspondence: Xinan Jiao, amlhb0B5enUuZWR1LmNu; Jing Wang, d2oxMjMxQHl6dS5lZHUuY24=
†These authors have contributed equally to this work