- 1Molecular Biology Lab, ICAR-National Bureau of Agriculturally Important Microorganisms, Maunath Bhanjan, India
- 2Plant Pathology Lab, ICAR-National Bureau of Agriculturally Important Microorganisms, Maunath Bhanjan, India
- 3Department of Plant Pathology, Lovely Professional University, Phagwara, Punjab, India
Microbial inoculants may be called magical bullets because they are small in size but have a huge impact on plant life and humans. The screening of these beneficial microbes will give us an evergreen technology to manage harmful diseases of cross-kingdom crops. The production of these crops is reducing as a result of multiple biotic factors and among them the bacterial wilt disease triggered by Ralstonia solanacearum is the most important in solanaceous crops. The examination of the diversity of bioinoculants has shown that more microbial species have biocontrol activity against soil-borne pathogens. Reduced crop output, lower yields, and greater cost of cultivation are among the major issues caused by diseases in agriculture around the world. It is universally true that soil-borne disease epidemics pose a greater threat to crops. These necessitate the use of eco-friendly microbial bioinoculants. This review article provides an overview of plant growth-promoting microorganisms bioinoculants, their various characteristics, biochemical and molecular screening insights, and modes of action and interaction. The discussion is concluded with a brief overview of potential future possibilities for the sustainable development of agriculture. This review will be useful for students and researchers to obtain existing knowledge of microbial inoculants, their activities, and their mechanisms, which will facilitate the development of environmentally friendly management strategies for cross-kingdom plant diseases.
Introduction
The world population growth is increasing at an alarming rate, hence global food output must quadruple by 2050 to meet the needs of a growing population. The predictions made thus far are significantly lower than what we require. It is estimated that worldwide food production is reduced by 36% due to plant diseases, insects, and weeds, with diseases alone reducing yields by 14%. (Agrios, 2005; Manzar et al., 2021a,b; Kashyap et al., 2022b). As a result, reducing the prevalence of plant diseases benefits agricultural output. Soil-borne diseases account for 10–20% of annual yield losses and are more detrimental to agricultural output than seed-borne or air-borne diseases (USDA, 2003).
Solanaceous crops are crucial to both the global economy and the diet of humans. They are known by the name “Nightshades” as well. All continents, with the exception of Antarctica, are home to members of this family. With 2,700 species and 98 genera, the Solanaceae family has the most diversity in terms of habitat, morphology, and ecology. Many regularly cultivated species are found in the Solanaceae family. The most significant genus in the Solanaceae family is “Solanum, “which comprises edible plants like potatoes, tomatoes, brinjal, chili, and capsicum. They can flourish in a variety of environments, from subtropical to tropical. They are plagued by a range of diseases in these various climatic situations and one among them being bacterial wilt caused by the bacterium Ralstonia solanacearum. It is one of the most serious diseases discovered to date because it causes host plants to wilt quickly and fatally. The pathogen has a vast host range of more than 200 species, is present around the world, and has a detrimental economic impact (Mansfield et al., 2012). Depending on the host, cultivar, temperature, soil type, cropping pattern, and strain, direct yield losses caused by R. solanacearum might vary greatly. For instance, yield losses for the tomato range from 0 to 91%, for the potato from 33 to 90%, for tobacco from 10 to 30%, for bananas from 80 to 100%, and for groundnuts from 10 to 20% (Elphinstone, 2005).
Bacterial wilt and geographical distribution
At the end of the 19th century, bacterial wilt disease was first reported on peppers tomatoes, potatoes, groundnuts, and tobacco in the southern USA and Asia, as well as the continent of South America (Seleim et al., 2014). The pathogen is infectious in soil and soilless culture resulting in the wilt of plants in the Solanaceae family. It is globally distributed in diverse climatic conditions (tropical, subtropical, and a few warm temperate regions, Hayward, 1991; Prameela and Rajamma, 2020). Twenty-five percent of the total area under vegetables is of solanaceous vegetables, primarily eggplants, chilies, and tomatoes. In India, the pathogen has been reported on various solanaceous vegetables including chili, tomato, potato, and brinjal from several states. It is reported from both the plains and plateau region of the west coast from Trivandrum in Kerala to Khera in Gujarat, Deccan and the central plateau of Karnataka, the North Eastern Hills region of India, Madhya Pradesh, Himachal Pradesh, Western Maharastra, Punjab, Tamil Nadu, West Bengal, Odisha, Jammu and Kashmir, Tamil Nadu, the Chotanagpur plateau area of Jharkhand, Uttarakhand, Bihar, and the eastern plains of Assam (Singh et al., 2010). Ralstonia solanacearum causes a 20–50 percent loss of solanaceous vegetable production in India each year (Singh et al., 2014). It has been recorded that wilt disease has destroyed 1.53 million hectares of tomato crops in around 80 countries, with a total worldwide loss of more than $950 million per year (Elphinstone, 2005). Solanaceous vegetable crops are mainly affected by R. solanacearum, such as summer-grown tomatoes, plain brinjal and tomatoes, and hill potatoes. Bacterial wilt losses range from 20 to 100% (Shekhawat et al., 2000) and 2–95% in tomatoes (Mishra, 1995; Singh et al., 2010; Yuliar et al., 2015). Extensive research was conducted on the use of physical, chemical, biological, and cultural techniques to manage bacterial wilt. Approximately 24% of the studies were concerned with breeding aspects, pathogen’s diversity, distribution, and host range (22%), detection and diagnosis of the pathogen (4%), pathogenicity and host-pathogen interactions (17%), epidemiology and ecology (3%), disease management and control (18%), and biological control (10%). There were more studies on disease management aspects like biological control of bacterial wilt (54%), followed by cultural practices (21%), chemical methods (8%), and physical methods (6%). Additionally, integrated pest management was the topic of some studies (11%) (Yuliar et al., 2015). This result revealed that biological control was of interest to many researchers. Kanyagha (2021) reported limited success even with the commitment of farmers to follow integrated disease management strategies such as cultural norms, the use of resistant cultivars, and crop rotation. The use of chemicals is a challenging task to manage bacterial wilt disease because the pathogen is localized within the xylem and is able to persist in the soil for a long time (Yuliar et al., 2015). Hartman and Elphinstone (1994) tested a bactericide in Taiwan and reported that chemical management by antibiotics (Penicillin, Tetracycline, Ampicillin, and Streptomycin) and soil fumigation causes limited suppression of the wilt pathogen. Biological control is a possible non-chemical means for plant disease management. Substantial research has been done on the management of major diseases of plants through biological control. In the recent past, the applications of microbial inoculants to combat soil-borne pathogens and increase crop yields have acquired significance (Elnahal et al., 2022). To attain sustainable yields, beneficial microorganisms can be an essential part of the management strategy. Plant growth-promoting rhizobacteria (PGPR) help in promoting the growth of plants and also protect the plants from devastating plant pathogens. The PGPR belong to the genera Pseudomonas, Azospirillum, Clostridium, Arthrobacter, and Serratia (Kloepper and Schroth, 1978; Hurek and Reinhold-Hurek, 2003). Pseudomonas fluorescens and other species have been reported to be effective in managing soil-borne plant pathogens through their biocontrol activity (Mohammed et al., 2020). The Pseudomonads is a major rhizobacteria group having biocontrol potential (Li et al., 2012). Pseudomonas spp. is prevalent in agricultural soils. Substantial progress has been made in characterizing the root colonization process by Pseudomonads, the abiotic and biotic factors affecting colonization, bacterial traits and genes contributing to the competence of the rhizosphere, and the processes of pathogen suppression (Sivasakthi et al., 2017). Pseudomonads have been reported to suppress soil-borne pathogens (Tao et al., 2020). Since humans consume vegetables in their less processed or unprocessed forms, quality control and safety are of utmost importance in vegetable cultivation. The controlled environment of a greenhouse makes it easier to incorporate PGPR and many different BCE strains have been identified and are ready for deployment (Singh et al., 2017), with some having already been tested successfully in greenhouse experiments (Liu et al., 2013). For example, Pseudomonas fluorescens is being studied as a possible helpful biocontrol agent, while Bacillus spp. has emerged as an essential microbe for the prevention of diseases in the field (Miljakovi et al., 2020). These particular isolates Pseudomonas stutzeri, Bacillus subtilis, and Bacillus amyloliquefaciens have been isolated and demonstrated to be successful in root colonization. In addition, Phytophthora capsici, a disease that affects cucumber plants, is greatly inhibited by these isolates (Islam et al., 2016). Bacillus subtilis was found to be efficient in preventing fruit infections caused by Penicillium spp. and Rhizopus stolonifer during the post-harvest stage. This was achieved by applying Bacillus subtilis to the fruit (Punja et al., 2016). In greenhouse conditions, Fusarium wilt caused by the fungus Fusarium oxysporum can be significantly inhibited by using Bacillus amyloliquefaciens isolates (Gowtham et al., 2016). These case studies show the efficacy of PGPR as BCAs in simulated lab settings. This ensures the viability and efficiency of PGPR for industrial horticulture by giving validity to its usage in greenhouse production systems (Jiao et al., 2021).
In the past, researchers have demonstrated that certain promising BCAs have the potential to be useful in the management of bacterial wilt disease. They may be avirulent strains of pathogens or different species of Bacillus, Pseudomonas, and Streptomyces spp. (Kurabachew and Wydra, 2014). The amount of research that is being done on Bacillus species, specifically on Bacillus amyloliquefaciens, is rising (Hu et al., 2010; Wei et al., 2011; Ding et al., 2013; Tan et al., 2013a,b; Chen et al., 2014; Yuan et al., 2014). Interesting methods for isolating rhizobacteria that stimulate plant development have been reported. Isolates taken from the rhizosphere of diseased plants were more effective at reducing disease incidence than those taken from healthy plants, as shown by Huang et al. (2015). The researchers found that the antagonists’ biocontrol efficacies were linked to the antagonists’ abilities to colonize plant roots but not to exhibit antibiosis in vitro. This finding suggests that root colonization plays an important role in disease suppression. Milagrosa and Balaki (1999) found that the incidence of wilting caused by P. solanacearum in potatoes was lower when Bokashi and effective microorganisms (EM) concentrate was applied alone or combined with inorganic fertilizer than the untreated control. Among the biocontrol agents, EM and Bokashi were found to be the best in the suppression of R. solanacearum (Lwin and Ranamukhaarachchi, 2006). Different bacteria and actinomycetes, viz., B. megaterium, B. mesentericus, B. mycoides, and B. subtilis have been reported to be effective against R. solanacearum (Doan and Nguyen, 2006). In contrast, Shekhawat et al. (1993) suggested that the biocontrol potential of fluorescent Pseudomonads be evaluated against the bacterial wilt pathogen. Various rhizobacteria are active in the rhizosphere and they play an important part in suppressing the R. solanacearum population and its activity. Different antagonisms have different ways of producing advantageous effects. According to Jagadeesh et al. (2001), RBG 114, Arthrobacter RBE 201, and P. fluorescens CHAO, the reference strain, were able to control the disease by 83.33 percent against pathogens. The combined application of biocontrol agents like P. putida, Bacillus pumilus, and Actigard (acibenzolar S-methyl) was more effective against R. solanacearum than against the untreated control (Anith et al., 2004). Various cross-kingdom biological control agents having plant growth and protection ability studied by different research groups are shown in Table 1.
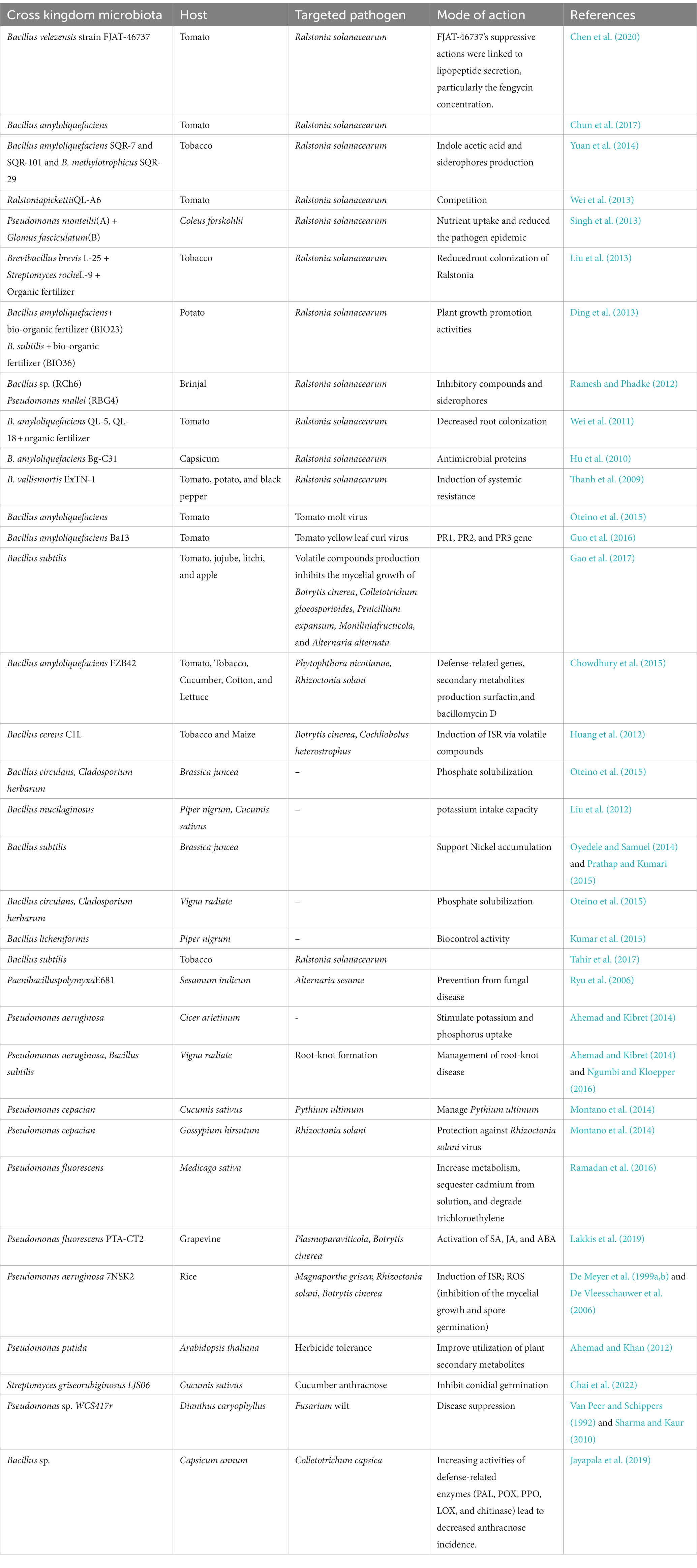
Table 1. Research shows that various cross-kingdom microbiota support plant growth promotion and disease suppression in solanaceous crops.
Biochemical characterization of biocontrol agents
Various biochemical and phenotypic methods have been used to characterize fluorescent Pseudomonad isolates. The genus Pseudomonas is described as an aerobic cell rod shaped like a gram-negative and is associated with plants, with P. aeruginosa, P. fluorescens, P. aureofaciens, and P. putida being important organisms. Most of the experiments were performed to classify fluorescent Pseudomonads (Krieg and Holt, 1984). P. aeruginosa forms a light cluster among the Pseudomonads community and develops at 41°C. The organisms are again categorized into separate subgroups and biovars based on similarities (Barrett et al., 1986). Therefore, it is possible to identify fairly and economically sustainable bioagents easily by different biochemical tests (Weller et al., 2002). The colonies of P. fluorescens give bluish-green fluorescens under UV light. Pseudomonas species are morphologically short rods and develop yellow-green diffusible pigmenton King’s B medium except for P. putida. Mulla et al. (2013) found that P. fluorescens strains isolated from the soil of various agro-climate zones were gram-negative, rod-shaped and motile, and developed Bluish green smooth colonies that fluoresced under UV light and in King’s B broth produced water soluble and fluorescent bluish-green pigmentation. The positive effect of gelatin liquefaction and levan formation was observed in all isolates. The isolates were indole negative and positive. The positive effect of gelatin liquefaction and levan formation was observed in all isolates. Indole negative was observed because they did not develop a red layer at the top of the medium of tryptophan broth and was positive for catalase as shown by the development of air bubbles with the addition of hydrogen peroxide. The bacterial strains isolated from the rhizosphere of medicinal and aromatic plants were described by Malleswari (2014) as Bacillus sp. gram-positive, white colonies with flat edges, large, smooth, and a high nutrient agar center developed. Isolates were positive for the use of citrate and sorbitol and negative for the use of lysine ornithine, urease, deamination of phenylalanine, nitrate, glucose, and adonitol, as well as the development of H2S. Arabinose, lactose, indole, Voges-Proskauer, gelatinase, methyl red test, and catalase activities showed a positive test.
Identification of microbial inoculants by molecular techniques
Microorganisms are being identified through genotypic and phenotypic characterization. 16SrRNA was used as a marker for the identification of bacteria and deciphering their phylogenetic relationship. The 16SrRNA is present among all bacteria; the gene function has not changed over time and the 16SrRNA gene (1,500 bp) is sufficient for accurate identification (Patel, 2001). Fungal inoculants were characterized using ITS, GAPDH, LSU, and Tef genomic regions (Manzar et al., 2022a; Kashyap et al., 2023). Zinniel et al. (2002) reported six endophytic bacteria that had colonized a range of host plants and identified them as Bacillus sp. using 16S rRNA genotyping. Phytopathogenic bacteria were severely restricted in their growth by the bacterial strain J12, which was isolated from the rhizosphere soil of tomato plants. Based on the 16SrRNA gene sequence, P. brassicacearum was distinguished from R. solanacearum (Zhou et al., 2016).
Rhizobacteria in the management of biotic stress in plants
Through various modes of action, plant growth-promoting rhizobacteria contribute to improving plant fitness. Direct and indirect strategies were typically used by rhizobacteria; these mechanisms are covered in more detail below.
Direct mechanisms of PGPR: Phosphate solubilization; Phytohormone production.
Indirect mechanisms of PGPR: Cyanide production; Siderophore production; Induced systematic resistance; Volatile compounds produced by PGPR.
Direct mechanisms of PGPR
Phosphate solubilization
Phosphorus contributes to the biomass construction of micronutrients, the metabolic process of energy transfer, macromolecular biosynthesis, signal transduction, respiration chain reactions, and photosynthesis (Shenoy and Kalagudi, 2005). Rhizobacteria that are capable of phosphate solubilization play an essential part in the accumulation and conversion of phosphate to plant roots (Bechtaoui et al., 2020). The enzyme phytase is in charge of liberating the phosphorus that has been bound in soil organic molecules like seeds or pollen and was preserved as phytate (inositol polyphosphate). Phytates are a useful source of phosphorous, making up 60–80% of the P in soil. Strong and stable ester linkages seen in phytates make them readily hydrolyzable by PSR. During vegetative growth, phosphorus is primarily absorbed and this absorbed form of phosphorus is found in seeds and fruits (Batool and Iqbal, 2019). Research has shown that the application of Enterobacter, Bacillus, Agrobacterium, Trichoderma, Pseudomonas, Glomus, and Aspergillus, as well as phosphate-solubilizing microbes to soils, roots, and fertilizers releases soluble phosphorus, stimulates growth, and protects plants from infection by pathogens (Liu X. et al., 2019; Liu Z. et al., 2019; Hii et al., 2020; Nacoon et al., 2020; Manzar et al., 2021a,b, 2022b; Kirui et al., 2022).
Phytohormone production by rhizobacteria
Phytohormones play a major role in maintaining plant growth. They act as molecular signals under varying environmental conditions that restrict the growth of the plant. Root-associated microbes such as symbiotic or endophytic bacteria strongly influence the production of phytohormones and promote seed germination, root development, vascular tissue development, shoot elongation, flowering, and complete plant growth (Sgroy et al., 2009; Antar et al., 2021). Root growth is promoted by auxins produced by PGPRs (e.g., Ahemad and Kibret, 2014; Afzal et al., 2015). Numerous research works suggest that hormones may be used to improve plant stress tolerance and promote growth. These include auxin in rice (Etesami and Beattie, 2017), cytokinins in wheat (Kudoyarova et al., 2014), abscisic acid in corn (Sgroy et al., 2009), and gibberellins in cucumber, tomato, immature radish, and rice (Kang et al., 2009). In plants, hormone levels can be controlled by microbes that generate plant growth regulators. These plant growth regulators exert effects that are similar to those caused by the application of exogenous plant phytohormones (Turan et al., 2009). Auxins and cytokinins are examples of phytohormones produced by microbes that are similar to plant-synthesized phytohormones. These microbe-produced phytohormones regulate plant hormone levels, which in turn affect the biochemical elicitation of defensive pathways (Backer et al., 2018).
Indirect mechanism
Cyanide production
A volatile metabolite hydrocyanic acid (HCN) is deemed to play an important part in the biocontrol of soil-borne pathogens (Siddiqui, 2006). Cyanide ions are primarily metabolized by thiocyanate. As an HCN, the cyanide ion is exhaled and metabolized to other compounds to some extent. HCN restricts electron movements, thus destroying the energy supply to the cells, resulting in the death of the microbial invaders. It regulates the enzyme function and natural receptors by reversible inhibition mechanisms (Corbett, 1974). Various researchers have documented the production of hydrocyanic acid (HCN) from P. aeruginosa, P. fluorescens, and rhizobacteria (Ahmad et al., 2008). Voisard et al. (1989) showed that the P. flourescens strain CHAO involves HCN in biological regulation. Root hair formation was stimulated by the cyanide-producing strain CHAO, suggesting that the strain brought about the alteration of the physiological activities of the plant. They further noted that three biosynthetic genes encode the enzyme HCN synthase (henA, henB, and henC).
Siderophore-mediated biocontrol
Siderophores are secondary metabolites that have low molecular weight and the ability to chelate iron. They are peptide molecules with side chains and functional groups that are composed of small compounds that can transport ferric ions through cell membranes with great affinity (Hofte et al., 1991; Raymond et al., 2015; Niehus et al., 2017). They also inhibit plant pathogens by competing with them for iron (Duijff et al., 1997). Rhizobacterial strains produce siderophores to chelate iron from the rhizosphere condition which is not soluble in water, therefore, not available for bacteria under iron-limiting conditions (Ghavami et al., 2017). Pseudomonas fluorescens produce siderophores under iron-limiting conditions in the Chrome Azurol medium. The production of siderophore, yellow color with a golden periphery, showed a positive result in casamino and succinate medium (Suryakala et al., 2004). The work with pyoverdin, a class of siderophores produced by fluorescent pseudomonads, provides evidence to support the siderophore theory of rhizobacteria’s biological regulation (Demange et al., 1987). Pyoverdin produced by P. aeruginosa 7 NSK 2 was reported by Hofte et al. (1991), which increased the yield of cucumber, spinach, barley, maize, and wheat. Suryakala et al. (2004) indicated that siderophores of the tri-hyobroxamate group could be used against plant pathogens as effective biocontrol compounds. Zhou et al. (2016) reported that 2,4-DAPG, HCN, siderophores, and protease produced by P. brassicacearum J12 inhibited pathogen growth, thereby, revealing its potential in the biocontrol of tomato bacterial wilt. Karimi et al. (2012) stated that bacterial isolates are capable of being isolated. Bacillus subtilis (Bl, B6, B28, B40, B99, and BIOS), P. putida (P9 and PIO), and P. aeuroginosa (Pll, PI2, P66, and PI 12) differed in the synthesis of hydrogen cyanide, siderophore, protease, and indole acetic acid. Yu et al. (2011) have reported that B. subtilis CAS 15 isolated from the rhizospheric pepper soil in Hainan, China, produced siderophore on CAS agar plate and was found to be promising in promoting plant growth and biological control against Fusarium wilt of pepper.
Induced systemic resistance
Plant growth-promoting rhizobacteria (PGPR) are beneficial rhizobacteria that protect plants from pathogens by triggering the plant’s immune system to react strongly to the invasion (Figure 1). The bacteria that cause ISRs have the ability to alter the morphological, physiological, and molecular reactions of plants. Over the past ten years, a lot of studies have been done on the mechanisms of microbial signals, plant receptors, and hormone signaling pathways that are involved in PGPR-induced ISR in plants. In terms of the mechanism associated with Bacillus spp. Elicitation of ISR. Investigations show that ISR is associated with ultra-structural and cytochemical changes in plants during the pathogen attack (Kloepper et al., 2004). There are a number of metabolic changes that occur in the host during ISR that ultimately result in the manufacture of defense-related molecules to be used against challenging pathogens. When a pathogen threatens, the priming action of PGPR activates cellular defense responses such as an oxidative burst, cell wall strengthening, the activation of defense-related genes, and the buildup of secondary metabolites (Conrath et al., 2006).
Several species of Bacillus, viz., B. amyloliquefaciens, B. subtilis, B. pasteurii, B. cereus, B. pumilus, and B. mycoides have been reported to induce ISR and protect plants against pathogen attacks (Beneduzi et al., 2012; Garcia-Gutierrez et al., 2013; Pieterse et al., 2014; Kashyap et al., 2021). According to Kashyap et al. (2021), induced systemic resistance (ISR) is a promising method for managing plant diseases that can successfully protect a plant against the respective plant diseases such as tomato wilt and chili wilt. The treatment of chili plants with Bacillus subtilis KA9 and Pseudomonas fluorescens PDS1 resulted in increased defense enzyme activities and resulted in the induction of resistance in chili against Ralstonia solanacearum. According to Yadav et al. (2017) PAL and PPO are the major ISR-based enzymes in plants and their activities are related to plant resistance. It was, therefore, important to find out whether tomato plants had improved resistance to infection with R. solanacearum after treatment with B. amyloliquefaciens DSBA-11, which mediates an oxidant/antioxidant system. Instead of increasing their development, ISR is linked to an increase in sensitivity to these hormones, which could activate a slightly separate set of defense genes. Choudhary et al. (2007) reported that after exposure to biotic stimuli by different PGPRs, ISR is induced, and plants acquire increased levels of resistance to pathogens. Recently, research has found that certain strains of Bacillus can regulate the gene expression of antioxidants genes such as CaPR1, CaPR4, and CaPR10 in their hosts, hence, triggering ISR against the pepper bacterial spot disease (Ma et al., 2018).
Tan et al. (2013a,b) reported resistance induction by B. amyloliquefaciens in tomatoes which provides protection against bacterial wilt. Research has found that CM-2 and T-5 B. amyloliquefaciens strains were antagonistic to R. solanacearum under greenhouse conditions and produced bioorganic fertilizers to control tomato wilt. The use of bioorganic fertilizers considerably reduced the tomato wilt incidence (63–74%), encouraged plant growth, and reduced the rhizosphere RS population relative to the control population. In the tomato rhizosphere, both strains of CM-2 and T-5 applied with bioorganic fertilizer application survived well. The use of Bacillus amyloliquefaciens (SN13) was found to enhance tolerance through a higher defense response against Rhizoctonia solani in cotton. The colonized plants showed altered phytohormone signaling, ongoing elicitor maintenance, and synthesis of secondary metabolites (Srivastava et al., 2016). Enterobacter asburiae strains were reported to increase the expression of defense-related genes and antioxidant enzymes, as well as other defensive enzymes (PPO, SOD, PAL, catalase, and PO) to protect the plant from tomato yellow leaf curl viruses (Li et al., 2016). PGPR colonization led to improved plant physiology, tissue health, and increased development of flowers and seeds (Kumar, 2016).
Plant genes modulated by PGPR
Plant growth promotion and its protection against many biotic and abiotic stresses are brought about by PGPR through the modulation of plants. The proteome analysis of PGPR-treated leaves showed increased expression of RuBisCo protein (Kandasamy et al., 2009). RuBisCo plays a role in photosynthesis and accumulation of chlorophyll, thus treated plants have increased photosynthetic rate and, hence, better growth (Agrios, 2005). Another protein that is responsive to PGPR is the chaperone, a stress-related protein. PGPR-treated plants show the induced expression of Nucleoside diphosphate kinases (NDKs). There are reports stating the role of NDKS in wounding, heat shock, and oxidative stress (Harris et al., 1994; Moisyadi et al., 1994; Moon et al., 2003). Thus, the NDKs play a role in primary metabolic function and regulatory function too. Rhizobacteria SN13 provides stress tolerance against Rhizoctonia solani (a necrotrophic fungus) and bacterial mycolytic enzymes. They create a balance between ROS and ROS scavengers by induced expression of ferric reductases and defense response by increased expression of terpene synthase (Srivastava et al., 2016). Soil inoculation with PGPR improves the yield and nutrient uptake of crops by inducing the expression of various phosphate and nitrate transporter genes in absence of organic phosphorus and nitrogen (Saia, 2015). As PGPR modulates gene expression in plants to promote their growth, this study aims to understand the molecular mechanism involved in the modulation of gene expression. This investigation was conducted based on the view that not only the bacterium was promoting the growth of the plant but it too was also getting benefitted. The improved growth of the plant will provide better care and shelter to the bacteria too. The interaction between pathogens and host plants causes some changes in the activity of certain enzymes, including phenylalanine ammonia lyase, peroxidase, polyphenol oxidase, lipoxygenase, superoxide dismutase, and −1,3-glucanase (Kavitha and Umesha, 2008; Manzar et al., 2021a,b). These enzymes determine the degree of host resistance. They participate in biosynthetic wall-related activities such as phenol, lignification, polymerization of hydroxyproline-rich glycoproteins, control of cell wall elongation, and wound healing that improve antimicrobial effectiveness (Belkhadir et al., 2004).
Yang et al. (2009) reported a group of plant growth-promoting rhizobacteria (PGPR) bacteria that showed an increase in crop growth. PGPR produce antagonistic compounds which help to reduce plant disease directly. The indirect control of plant disease is through the elicitation of induced systemic resistance (ISR). The ISR elicited by PGPR has been deciphered in the model plant Arabidopsis; however, it is not well characterized in pepper. Bacillus cereus strain BS107 induced ISR against Xanthomonas axonopodis pv. vesicatoria in pepper leaves and its priming effect on plant defense genes as an ISR mechanism was assessed in order to understand the mechanism of ISR in agricultural plants. To systemically prime the expression of Capsicum annum pathogenesis-protein 4 and CaPR1, strain BS107 was administered to the roots of pepper plants. This was verified by quantitative-reverse transcriptase PCR. The study corroborated that rhizobacterium has a priming effect on the expression of pepper defense genes that take part in ISR. Bacillus thuringiensis has been shown by Hyakumachi et al. (2013) as a possible biological control agent for plant disease suppression. The bacterial wilt disease-suppressing activity of B. thuringiensis in this research has been observed in tomato plants. It was found that challenge-inoculation of tomato plants with R. solanacearum after cell-free filtrate (CF) pre-treatment resulted in clearly decreased growth of R. solanacearum in stem tissues and there was induced expression of defense-related genes such as acidic chitinase, β-1, 3-glucanase, andPR-1 in stem and leaf tissues. In addition, resistance to direct inoculation with R. solanacearum was observed in the stem tissues of tomato plants when their roots were pre-treated with CF. Taken together, these findings indicate the CF treatment of tomato roots. B. thuringiensis, by systemic activation of the plant defense system, suppresses bacterial wilt. Jayanna and Umesha (2017) analyzed the accumulation of differentially expressed defense genes in susceptible and resistant cultivars of tomatoes by qRT-PCR. It was found that as against the control, there was upregulation of gene expression of defense genes in the resistant tomato cultivar. The upregulation was significantly increased upon the inoculation of R. solanacearum. There was a down-regulation of defense genes in susceptible cultivars as compared to the control. However, C8-HSL treatment resulted in upregulation. Thus, the results reveal that C8-HSL can induce significant defense genes in resistant and susceptible tomato cultivars.
Pseudomonas putida (RA) strain MTCC5279 has been shown to possess multiple advantageous traits, including P-solubilization, siderophore production, and indole acetic acid (IAA) production, all of which stimulate plant growth and alter the physiological, biochemical, cellular, and molecular responses of plants (Jatan et al., 2020). Roots of P. putida inoculated Arabidopsis plants were collected, and microRNA expression was examined. From sequencing the control and RA-inoculated libraries, 293 known and 67 potential new miRNAs were found. Following RA-inoculation, as compared to the control group, stem-loop quantitative real-time PCR verified the differential expression of 15 well-characterized miRNAs. Multiple biological, cellular, and molecular processes were found to be influenced by miRNAs both previously discovered and hypothesized. Additional evidence supporting R’s central function in developmental regulation comes from an inverse relationship between the expression of RA-responsive miRNAs and their target genes.
Volatiles and other factors
Besides direct surface-to-surface communication, PGPRs and plant interaction also take place through volatiles. Low polarity and high vapor pressure are characteristics of volatiles generated by microorganisms, which facilitates their diffusion in soil and over extensive atmospheric distances (Vespermann et al., 2007). Using PGPRs that produce volatile compounds can be an effective way to control plant diseases, particularly post-harvest diseases (Arrebola et al., 2010). PGPR-produced volatiles can positively or negatively impact both plants and plant pathogens (Table 2).
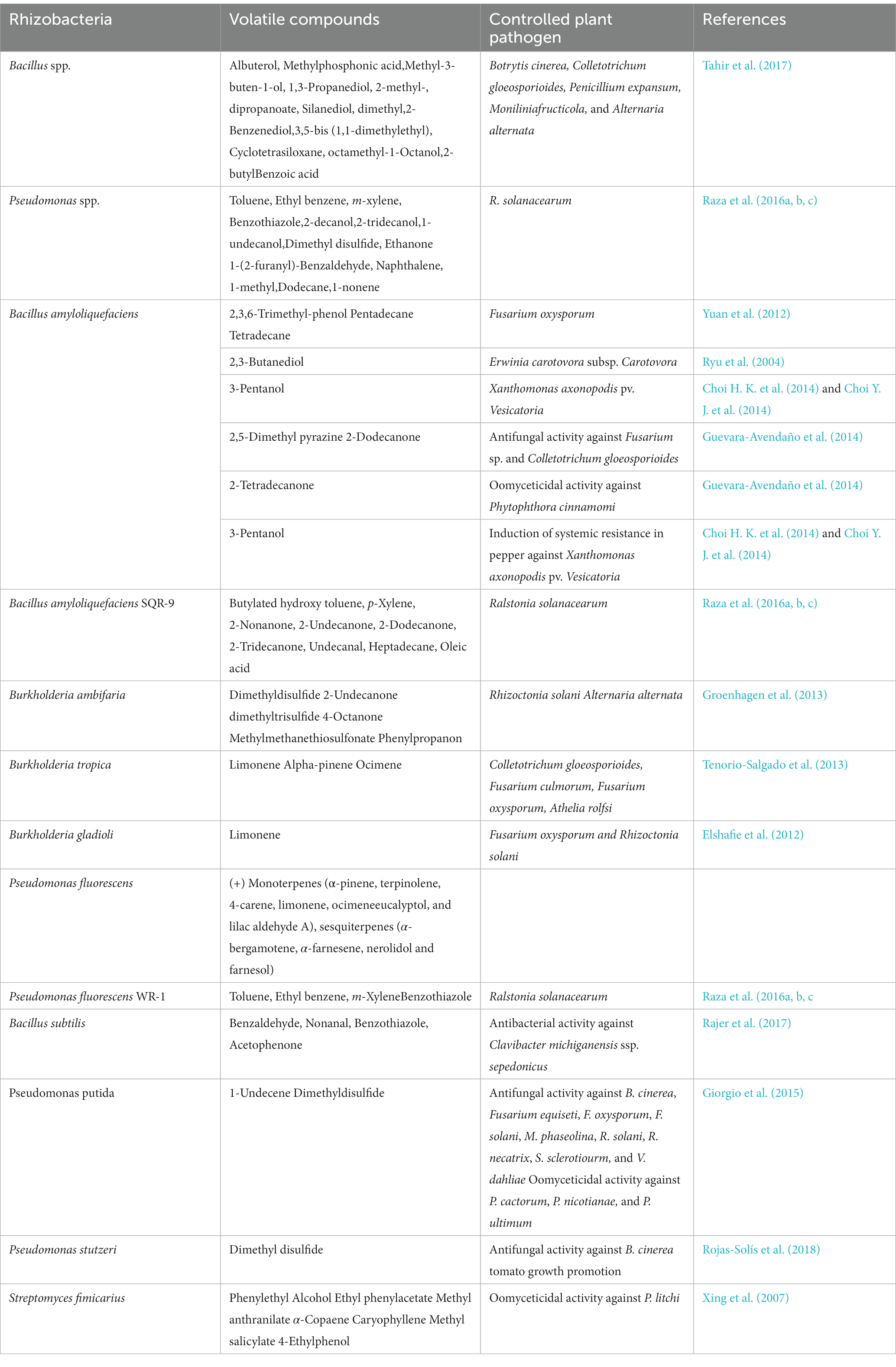
Table 2. Volatile compounds produced by Rhizoxbacteria acted as growth inhibitors for plantpathogens.
Ryu et al. (2003) showed that Bacillus sp. strains GB03 and IN937produced 2,3-butanediol and its precursor acetoin that stimulate plant growth. However, volatiles from the same species inhibited Arabidopsis growth in another experiment conducted later by Ryu et al. (2005). This inhibition was dependent on the distance between the plant’s Bacillus strains and the specimen, indicating that the amount of chemical released may have an impact on the inhibitory action (Ryu et al., 2005). Kashyap et al. (2022a) revealed that B. subtilis KA9 and P. fluorescens PDS1 VOCs pyrazine compounds significantly increased defensive enzyme activity and overexpressed the antioxidant genes related to plant defense to manage the Ralstonia under in vitro conditions.
Bacillus amyloliquefaciens strain SQR-9 showed significant efficiency against the tomato wilt pathogen Ralstonia solanacearum by producing 22 volatile organic compounds. A lack of inhibition was observed in the case of treatment with a bacterium that did not produce VOCs, Additionally, the VOCs strongly hindered R. solanacearum ability to colonize tomato roots and develop its motility features, exopolysaccharide and antioxidant enzyme production, biofilm formation, and other traits (Raza et al., 2016a,b,c). Kim et al. (2010) isolated a Bacillus subtilis strain from Bacillus subtilis CMB32 by HPLC to monitor the anthracnose disease caused by Colletotrichum gloeosporioides and also studied the development of biosurfactant lipopeptides such as Fengycin, Iturin A, and Surfactin A. Gond et al. (2015) on the other hand (2015) investigated endophytic Bacillus spp. that produced antifungal lipopeptides in maize and induced the expression of the host defense gene. Mubarak et al. (2015) standardized a procedure for the identification and quantification of surfactin using high-performance liquid chromatography (HPLC) from the Bacillus strain (2015). The cumulative elution time of the surfactin peaks obtained was found to be four times faster than the numerous methods previously described. Fine separation of surfactin in the standard sample (98 percent purity) and surfactin in the fermentation broth was possible using the method described here. Bio-control strains produce VOCs, promote the growth of plants, and inhibit pathogens via the induction of systemic resistance in plants (Raza et al., 2016a,b). Bacterial genera like Pseudomonas, Bacillus, Stenotrophomonas, and Serratia produce VOCs that have a beneficial impact on plants. The bacterial VOCs serve as triggers for the plant ISR (Sharifi and Ryu, 2016). Increased disease resistance, abiotic stress tolerance, and plant biomass are all mediated by the VoCs from PGPR strains, either directly or indirectly. A wide range of soil microorganisms emit VOCs, which is a trait shared by a large number of them (Kanchiswamy et al., 2015).
Antimicrobial peptides
Bacillus spp. suppress plant pathogens and reduce disease incidence in plants through various mechanisms, viz., production of antibiotics, lysis of cells, induced resistance to the pathogen, and competition for food and space. The bacteria produce antibiotics such as iturinA, bacillomycin D, Surfactin, polyketides antibiotics, bacteriocins (Rea et al., 2010), cyclic lipopeptides (Ramarathnam et al., 2007), polyketides mcrolectine (Chen et al., 2009), and phospholipid (Tamehiro et al., 2002), to suppress the bacterial pathogens. B. amyloliquefaciens produce polyketides, viz, difficidin, bacillaene, and macrolectin, having antibacterial properties. B. amyloliquefaciens and B. subtilis produce dificid which is a highly unsaturated 22-membred macro cyclicpolyene lactone phosphate ester. The dfn gene sequence exhibits a reasonable co-linearity with the polyketides structure to deduce a biosynthetic module (Chen et al., 2006). The bacillaene gene cluster is assigned to synthesize the bacillaene polyketides (Chen et al., 2009). B. amyloliquefacins FZB42 also produces another polyketide macrolactin that has a macrolide-like structure. Macrolactin has a 24-membered lactone ring which contains three separate diene structure elements (Gustafson et al., 1989). Macrolactin is effective against bacterial pathogens (Chen et al., 2009 a). Polyketides difficidin, bacillaene (Hofemeister et al., 2004), and macrolactin (Jaruchoktaweechai et al., 2000) are found in the genome of B. amyloliquefaciens FZB 42 and these gene clusters are involved in the polyketides synthesis together, spanning nearly 200 kb (Koumoutsi, 2004). When compared to B. amyloliquefaciens FZB42 (340 kb, 8.5%), the genetic capacity for synthesis of antibiotics in strain B. subtilis 168 is lower (180 kb, 4–5% of the whole genome) (Chen et al., 2009). The biosynthetic pathway for peptides determines whether the active antimicrobial compounds are ribosomally synthesized (lantibiotics or bacteriocins) or non-ribosomally generated (lipopeptides and polyketides) (Stein, 2005). Antibacterial peptides with inter-residual thioether bonds, known as lantibiotics, are synthesized by the ribosome and further modified after crossing international borders (Abriouel et al., 2011). These antimicrobial peptides (AMPs) have a high degree of specificity for the target microorganisms and have advantages in multiple terms of agronomical, pathological, and economical practices to secure sustainability in agriculture (Figure 2).
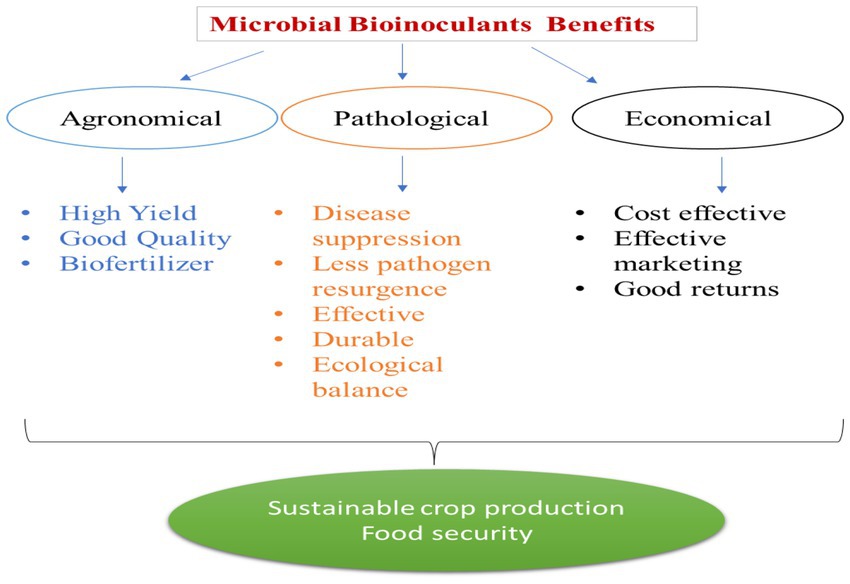
Figure 2. Advantages of microbial inoculants in terms of agronomical, pathological, and economical practices to secure sustainability in agriculture.
This Review manuscript shows multiple functions of microbial inoculants and their screening is based on biochemical, molecular, volatile, mode, and nature of actions. Such eco-friendly bioinoculant screening will be the next evergreen technology for the sustainability of agriculture in this 21st era where we are facing global warming and population blasts. Microbes may be used as magic bullets in the coming days to shoot out multiple problems of cross-kingdom plant diseases.
Author contributions
AK: manuscript preparation, writing, and editing. NM: supervision and editing. SM: figures construction and literature search. PS: supervision and funding. All authors contributed to the article and approved the submitted version.
Funding
This study was supported by the ICAR-NBAIM, Mau.
Conflict of interest
The authors declare that the research was conducted in the absence of any commercial or financial relationships that could be construed as a potential conflict of interest.
Publisher’s note
All claims expressed in this article are solely those of the authors and do not necessarily represent those of their affiliated organizations, or those of the publisher, the editors and the reviewers. Any product that may be evaluated in this article, or claim that may be made by its manufacturer, is not guaranteed or endorsed by the publisher.
References
Afzal, I., Shinwari, Z. K., and Iqrar, I. (2015). Selective isolation and characterization of agriculturally beneficial endophytic bacteria from wild hemp using canola. Pak. J. Bot. 47, 1999–2008.
Ahemad, M., and Khan, M. S. (2012). Evaluation of plant-growth-promoting activities of rhizobacterium Pseudomonas putida under herbicide stress. Ann. Microbiol. 62, 1531–1540. doi: 10.1007/s13213-011-0407-2
Ahemad, M., and Kibret, M. (2014). Mechanisms and applications of plant growth promoting rhizobacteria: current perspective. J. King Saud Univ. Sci. 26, 1–20. doi: 10.1016/j.jksus.2013.05.001
Ahmad, F., Ahmad, I., and Khan, M. S. (2008). Screening of free living rhizospheric bacteria for their multiple plant growth promoting activities. Microbiol. Res. 163, 173–181. doi: 10.1016/j.micres.2006.04.001
Anith, K. N., Momol, M. T., Kloepper, J. W., Marios, J. J., Olson, S. M., and Jones, J. B. (2004). Efficacy of plant growth-promoting rhizobacteria, acibenzolar-s-methyl and soil amendment for integrated management of bacterial wilt on tomato. Plant Dis. 88, 669–673. doi: 10.1094/PDIS.2004.88.6.669
Antar, M., Gopal, P., Msimbira, L. A., Naamala, J., Nazari, M., Overbeek, W., et al. (2021). “Inter-organismal signaling in the rhizosphere” in Rhizosphere biology: interactions between microbes and plants (Singapore: Springer), 255–293.
Arrebola, E., Sivakumar, D., and Korsten, L. (2010). Effect of volatile compounds produced by Bacillus strains on postharvest decay in citrus. Biol. Control 53, 122–128. doi: 10.1016/j.biocontrol.2009.11.010
Backer, R., Rokem, J. S., Ilangumaran, G., Lamont, J., Praslickova, D., Ricci, E., et al. (2018). Smith Plant growth-promoting rhizobacteria: context, mechanisms of action, and roadmap to commercialization of biostimulants for sustainable agriculture. Front. Plant Sci. 9:1473. doi: 10.3389/fpls.2018.01473
Bakker, W. A. B., and Schippers, B. (1987). Schippers microbial cyanide production in the rhizosphere in relation to potato yield reduction and Pseudomonas spp-mediated plant growth-stimulation. Soil Biol. Biochem. 19, 451–457. doi: 10.1016/0038-0717(87)90037-X
Barrett, E. L., Solanes, R. E., Tang, J. S., and Palleroni, N. J. (1986). Pseudomonas fluorescens biovar V: its resolution into distinct component groups and the relationship of these groups to other P. fluorescens biovars, to P. putida, and to psychrotrophic pseudomonads associated with food spoilage. J. Gen. Microbiol. 132, 2709–2721. doi: 10.1099/00221287-132-10-2709
Batool, S., and Iqbal, A. (2019). Phosphate solubilizing rhizobacteria as alternative of chemical fertilizer for growth and yield of Triticum aestivum (Var. Galaxy 2013). Saudi J. Biol. Sci. 26, 1400–1410. doi: 10.1016/j.sjbs.2018.05.024
Bechtaoui, N., Rabiu, M. K., Raklami, A., Oufdou, K., Hafidi, M., and Jemo, M. (2021). Phosphate-dependent regulation of growth and stresses management in plants. Front. Plant Sci. 12:679916. doi: 10.3389/fpls.2021.679916
Bechtaoui, N., Raklami, A., Benidire, L., Tahiri, A., Göttfert, M., and Oufdou, K. (2020). Effects of PGPR co-inoculation on growth, phosphorus nutrition and phosphatase/phytase activities of faba bean under different phosphorus availability conditions. Polish J. Environ. Stud. 29, 1557–1565. doi: 10.15244/pjoes/110345
Belkhadir, Y., Subramaniam, R., and Dangl, J. L. (2004). Plant disease resistance protein signaling: NBS–LRR proteins and their partners. Curr. Opin. Plant Biol. 7, 391–399. doi: 10.1016/j.pbi.2004.05.009
Beneduzi, A., Ambrosini, A., and Passaglia, L. M. (2012). Plant growth-promoting rhizobacteria (PGPR): their potential as antagonists and biocontrol agents. Genet. Mol. Biol. 35, 1044–1051. doi: 10.1590/S1415-47572012000600020
Chai, C. H., Hong, C. F., and Huang, J. W. (2022). Identification and characterization of a multifunctional biocontrol agent, Streptomyces griseorubiginosus LJS06, against cucumber anthracnose. Front. Microbiol. 13:923276. doi: 10.3389/fmicb.2022.923276
Chen, Y. Y., Lin, Y. M., Chao, T. C., Wang, J. F., Liu, A. C., Ho, F. I., et al. (2009). Virus-induced gene silencing reveals the involvement of ethylene-, salicylic acid- and mitogen-activated protein kinase-related defense pathways in the resistance of tomato to bacterial wilt. Physiol. Plant. 136, 324–335. doi: 10.1111/j.1399-3054.2009.01226.x
Chen, D., Liu, X., Li, C., Tian, W., Shen, Q., and Shen, B. (2014). Isolation of Bacillus amyloliquefaciensS20 and its application in control of eggplant bacterial wilt. J. Environ. Manag. 137, 120–127. doi: 10.1016/j.jenvman.2014.01.043
Chen, X. H., Vater, J., Piel, J., Franke, P., Scholz, R., Schneider, K., et al. (2006). Structural and functional characterization of three polyketide synthase gene clusters in Bacillus amyloliquefaciens FZB42. J. Bacteriol. 188, 4024–4036. doi: 10.1128/JB.00052-06
Chen, M., Wang, J., Liu, B., Zhu, Y., Xiao, R., Yang, W., et al. (2020). Biocontrol of tomato bacterial wilt by the new strain Bacillus velezensis FJAT-46737 and its lipopeptides. BMC Microbiol. 20:160. doi: 10.1186/s12866-020-01851-2
Cho, S. M., Shin, S. H., Kim, K. S., Kim, Y. C., Eun, M. Y., and Cho, B. H. (2004). Enhanced expression of a gene encoding a nucleoside diphosphate kinase 1 (OsNDPK1) in rice plants upon infection with bacterial pathogens. Mol. Cells 18, 390–395.
Choi, Y. J., Lee, J., Jang, Y. S., and Lee, S. Y. (2014). Metabolic engineering of microorganisms for the production of higher alcohols. MBio 5, e01524–e01514. doi: 10.1128/mBio.01524-14
Choi, H. K., Song, G. C., Yi, H.-S., and Ryu, C.-M. (2014). Field evaluation of the bacterial volatile derivative 3-pentanol in priming for induced resistance in pepper. J. Chem. Ecol. 40, 882–892. doi: 10.1007/s10886-014-0488-z
Choudhary, D. K., Prakash, A., and Johri, B. N. (2007). Induced systemic resistance (ISR) in plants: mechanism of action. Indian J. Microbiol. 47, 289–297. doi: 10.1007/s12088-007-0054-2
Chowdhury, S. P., Hartmann, A., Gao, X. W., and Borriss, R. (2015). Biocontrol mechanism by root-associated Bacillus amyloliquefaciens FZB42-a review. Front. Microbiol. 6:780. doi: 10.3389/fmicb.2015.00780
Chun, B. H., Kim, K. H., Jeon, H. H., Lee, S. H., and Jeon, C. O. (2017). Pan-genomic and transcriptomic analyses of Leuconostoc mesenteroides provide insights into its genomic and metabolic features and roles in kimchi fermentation. Sci. Rep. 7:11504. doi: 10.1038/s41598-017-12016-z
Conrath, U., Beckers, G. J., Flors, V., García-Agustín, P., Jakab, G., Mauch, F., et al. (2006). Priming: getting ready for battle. Mol. Plant Microbe Interact. 19, 1062–1071. doi: 10.1094/MPMI-19-1062
Corbett, J. R. (1974). “Pesticide design” in The Biochemical Mode of Action of Pesticides (London: Academic Press, Inc), 44–86.
De Meyer, G., Audenaert, K., and Höfte, M. (1999a). Pseudomonas aeruginosa 7NSK2-induced systemic resistance in tobacco depends on in planta salicylic acid accumulation but is not associated with PR1a expression. Eur. J. Plant Pathol. 105, 513–517. doi: 10.1023/A:1008741015912
De Meyer, G., Capiau, K., Audenaert, K., Buchala, A., Metraux, J. P., and Hofte, M. (1999b). Nanogram amounts of salicylic acid produced by the rhizobacterium Pseudomonas aeruginosa 7NSK2 activate the systemic acquired resistance pathway in bean. Mol. Plant-Microbe Interact. 12, 450–458. doi: 10.1094/MPMI.1999.12.5.450
De Vleesschauwer, D., Cornelis, P., and Höfte, M. (2006). Redox-active pyocyanin secreted by Pseudomonas aeruginosa 7NSK2 triggers systemic resistance to Magnaporthe grisea but enhances Rhizoctonia solani susceptibility in rice. Mol. Plant Microbe Interact. 19, 1406–1419. doi: 10.1094/MPMI-19-1406
Demange, P., Wenderbaum, S., Bateman, A., Dell, A., and Abdallah, M. A. (1987) Bacterial siderophores: structure and physicochemical properties of pyoverdins and related compounds. In: G. Winkleman, D. HelmVan Der, and J. B. Neilands (Eds) Iron transport in microbes, plants and animals. VCH, Chemie.
Ding, C., Shen, Q., Zhang, R., and Chen, W. (2013). Evaluation of rhizosphere bacteria and derived bio-organic fertilizers as potential biocontrol agents against bacterial wilt (Ralstonia solanacearum) of potato. Plant Soil 366, 453–466. doi: 10.1007/s11104-012-1425-y
Doan, T. T., and Nguyen, T. H. (2006, 2005). “Status of research on biological control of tomato and groundnut bacterial wilt in Vietnam” in 1st International Symposium on Blogical Control of Bacterial Plant Diseases. eds. W. Zeller and C. Ulrich (Germany: Darmstadt), 105–111.
Duijff, B. J., Gianinazzi-Pearson, V., and Lemanceau, P. (1997). Involvement of the outer membrane lipopolysaccharides in the endophytic colonization of tomato roots by biocontrol Pseudomonas fluorescens strain WCS417r. New Phytologist. 135, 325–334. doi: 10.1046/j.1469-8137.1997.00646.x
Elnahal, A. S. M., el-Saadony, M. T., Saad, A. M., Desoky, E.-S. M., el-Tahan, A. M., Rady, M. M., et al. (2022). The use of microbial inoculants for biological control, plant growth promotion, and sustainable agriculture: a review. Eur. J. Plant Pathol. 162, 759–792. doi: 10.1007/s10658-021-02393-7
Elphinstone, J. G. (2005). “The current bacterial wilt situation: a global overview” in Bacterial Wilt Disease and the Ralstonia solanacearum Species Complex. eds. C. Allen, P. Prior, and A. C. Hayward (St. Paul, MN: American Phytopathological Society Press), 9–28.
Elshafie, H. S., Camele, I., Racioppi, R., Scrano, L., Iacobellis, N. S., and Bufo, S. A. (2012). In vitro antifungal activity of Burkholderia gladioli pv. agaricicola against some phytopathogenic fungi. Int. J. Mol. Sci. 13, 16291–16302. doi: 10.3390/ijms131216291
Etesami, H., and Beattie, G. A. (2017). “Plant-microbe interactions in adaptation of agricultural crops to abiotic stress conditions” in Probiotics and plant health. eds. V. Kumar, M. Kumar, S. Sharma, and R. Prasad (Singapore: Springer), 163–200.
Gao, Z. F., Zhang, B. J., Liu, H. P., Han, J. C., and Zhang, Y. J. (2017). Identification of endophytic Bacillus velezensis ZSY-1 strain and antifungal activity of its volatile compounds against Alternaria solani and Botrytis cinerea. Biol. Control 105, 27–39. doi: 10.1016/j.biocontrol.2016.11.007
Garcia-Gutierrez, L., Zeriouh, H., Romero, D., Cubero, J., de Vicente, A., and Pérez-Garcia, A. (2013). The antagonistic strain Bacillus subtilis UMAF6639 also confers protection to melon plants against cucurbit powdery mildew by activation of jasmonate- and salicylic acid-dependent defense responses. MicrobBiotechnol. 6, 264–274. doi: 10.1111/1751-7915.12028
Ghavami, N., Alikhani, H. A., Pourbabaei, A. A., and Besharati, H. (2017). Effects of two new siderophore-producing rhizobacteria on growth and iron content of maize and canola plants. J. Plant Nutr. 40, 736–746. doi: 10.1080/01904167.2016.1262409
Giorgio, A., De Stradis, A., Lo, C. P., and Iacobellis, N. S. (2015). Biocide effects of volatile organic compounds produced by potential biocontrol rhizobacteria on Sclerotinia sclerotiorum. Front. Microbiol. 6:1056. doi: 10.3389/fmicb.2015.01056
Gond, S. K., Bergen, M. S., Torres, M. S., and White, J. F. Jr. (2015). Endophytic Bacillus spp. produce antifungal lipopeptides and induce host defence gene expression in maize. Microbiol. Res. 172, 79–87. doi: 10.1016/j.micres.2014.11.004
Gowtham, H. G., Hariprasad, P., Chandra Nayak, S., and Niranjana, S. R. (2016). Application of rhizobacteria antagonistic to Fusarium oxysporum f. sp. lycopersici for the management of Fusarium wilt in tomato. Rhizosphere 2, 72–74. doi: 10.1016/j.rhisph.2016.07.008
Groenhagen, U., Baumgartner, R., Bailly, A., Gardiner, A., Eberl, L., Schulz, S., et al. (2013). Production of Bioactive Volatiles by Different Burkholderia ambifaria Strains. J. Chem. Ecol. 39, 892–906. doi: 10.1007/s10886-013-0315-y
Guevara-Avendaño, E., Mauricio, L. R., Pablo, O. A., Lourdes, G. I. A., Ángel, T., and María, M. H. (2014). Effect of rhizobacteria indole producing on the development of Capsicum annuum var. Jalapeño M. Int. Res. J. Biological Sci. 3, 22–27.
Guo, M., Liu, J. H., Ma, X., Luo, D. X., Gong, Z. H., and Lu, M. H. (2016). The plant heat stress transcription factors (HSFs): structure, regulation, and function in response to abiotic stresses. Front. Plant Sci. 7:114. doi: 10.3389/fpls.2016.00114
Gustafson, K., Roman, M., and Fenical, W. (1989). The macrolactins, a novel class of antiviral and cytotoxic macrolides from a deep-sea marine bacterium. J. Am. Chem. Soc. 111, 7519–7524. doi: 10.1021/ja00201a036
Harris, N., Taylor, J., and Roberts, J. (1994). Isolation of a mRNA encoding a nucleoside diphosphate kinase from tomato that is up-regulated by wounding. Plant Mol. Biol. 25, 739–742. doi: 10.1007/BF00029611
Hartman, G. L., and Elphinstone, J. G. (1994). “Advances in the control of Pseudomonas solanacearum race 1 in major food crops” in Bacterial wilt: The disease and its causal agent, Pseudomonas solanacearum. eds. A. C. Hayward and G. L. Hartman (Wallingford, UK: CAB International), 157–177.
Hayward, A. C. (1964). Characteristics of Pseudomonas solanacearum. J. Appl. Bacteriol. 27, 265–277. doi: 10.1111/j.1365-2672.1964.tb04912.x
Hayward, A. C. (1991). Biology and epidemiology of bacterial wilt caused by Pseudomonas solanacearum annual review of phytopathol. Annu. Rev. Phytopathol. 29, 65–87. doi: 10.1146/annurev.py.29.090191.000433
Hii, Y. S., San, C. Y., Lau, S. W., and Danquah, M. K. (2020). Isolation and characterisation of phosphate solubilizing microorganisms from peat. Biocatal. Agric. Biotechnol. 26:101643. doi: 10.1016/j.bcab.2020.101643
Hofemeister, J., Conrad, B., Adler, B., Hofemeister, B., Feesche, J., Kucheryava, N. G., et al. (2004). Genetic analysis of the biosynthesis of non-ribosomal peptide and polyketide-like antibiotics, iron uptake and biofilm formation by Bacillus subtilis A1/3. Mol. Gen. Genomics. 272, 363–378. doi: 10.1007/s00438-004-1056-y
Hofte, M., Boelens, J., and Vstraete, W. (1991). Seed protection and promotion of seedling emergence by the plant growth beneficial Pseudomonas Strains 7NSK2 and ANP15. Soil Biol. Biochem. 23, 407–410. doi: 10.1016/0038-0717(91)90001-Z
Hu, H. Q., Li, X. S., and He, H. (2010). Characterization of an antimicrobial material from a newly isolated Bacillus amyloliquefaciens from mangrove for biocontrol of capsicum bacterial wilt. Biol. Control 54, 359–365. doi: 10.1016/j.biocontrol.2010.06.015
Huang, C. J., Tsay, J. F., Chan, S. Y., Yang, H. P., Wu, W. S., and Chen, C. Y. (2012). Dimethyl disulfide is an induced systemic resistance elicitor produced by Bacillus cereus C1L. Pest Manag. Sci. 68, 1306–1310. doi: 10.1002/ps.3301
Huang, X. F., Zhou, D. M., Guo, J. H., Manter, D. K., Reardon, K. F., and Vivanco, J. M. (2015). Bacillus spp. from rainforest soil promotes plant growth under limited nitrogen conditions. J. Appl. Microbiol. 118, 672–684. doi: 10.1111/jam.12720
Hurek, T., and Reinhold-Hurek, B. (2003). Azoarcus sp. strain BH72 as a model for nitrogen-fixing grass endophytes. J. Biotechnol. 106, 169–178. doi: 10.1016/j.jbiotec.2003.07.010
Hyakumachi, M., Nishimura, M., Arakawa, T., Asano, S., Yoshida, S., Tsushima, S., et al. (2013). Bacillus thuringiensis suppresses bacterial wilt disease caused by Ralstonia solanacearum with systemic induction of defense-related gene expression in tomato. Microb. Envir. 28, 128–134. doi: 10.1264/jsme2.ME12162
Islam, S., Akanda, A. M., Prova, A., Islam, M. T., and Hossain, M. M. (2016). Isolation and identification of plant growth promoting rhizobacteria from cucumber rhizosphere and their effect on plant growth promotion and disease suppression. Front. Microbiol. 6:1360.
Jagadeesh, K. S., Kullarni, J. H., and Krishnaraj, P. U. (2001). Evaluation of the role of fluorescent siderophore in the biological control of bacterial wilt in tomato using Tn 5 mutants of fluorescent Pseudomonas sp. Curr. Sci. 81:882.
Jaruchoktaweechai, C. S., Suwanboriux, K., Tanasupawatt, S., Kittakoop, P., and Menasveta, P. (2000). New macrolactins from a marine Bacillus sp. Sc026. J. Nat. Prod. 63, 984–986. doi: 10.1021/np990605c
Jatan, R. R., Chauhan, P. S., and Lata, C. (2020). High-throughput sequencing and expression analysis suggest the involvement of pseudomonas putida RA-Responsive microRNAs in growth and development of Arabidopsis. Int. J. Mol. Sci. 21:5468. doi: 10.3390/ijms21155468
Jayanna, S. K., and Umesha, S. (2017). Enhancement of the expression of defense genes in tomato against Ralstonia solanacearum by N-octanoyl L-homoserine lactone. Afr. J. Microbiol. Res. 11, 194–203.
Jayapala, N., Mallikarjunaiah, N., and Puttaswamy, H. (2019). Rhizobacteria Bacillus spp. induce resistance against anthracnose disease in chili (Capsicum annuum L.) through activating host defense response. Egypt. J. Biol. Pest Control 29:45. doi: 10.1186/s41938-019-0148-2
Jiao, X., Takishita, Y., Zhou, G., and Smith, D. L. (2021). Plant associated Rhizobacteria for biocontrol and plant growth enhancement. Front. Plant Sci. 12:634796. doi: 10.3389/fpls.2021.634796
Kanchiswamy, C. N., Malnoy, M., and Maffei, M. E. (2015). Chemical diversity of microbial volatiles and their potential for plant growth and productivity. Front. Plant Sci. 6:151. doi: 10.3389/fpls.2015.00151
Kandasamy, S., Loganathan, K., Muthuraj, R., Duraisamy, S., Seetharaman, S., Thiruvengadam, R., et al. (2009). Understanding the molecular basis of plant growth promotional effect of Pseudomonas fluorescens on rice through protein profiling. Proteome Sci. 7:47. doi: 10.1186/1477-5956-7-47
Kang, S. M., Joo, G. J., Hamayun, M., Na, C. I., Shin, D. H., Kim, H. Y., et al. (2009). Gibberellin production and phosphate solubilization by newly isolated strain of Acinetobacter calcoaceticus and its effect on plant growth. Biotechnol. Lett. 31, 277–281. doi: 10.1007/s10529-008-9867-2
Kanyagha, H. E. (2021). Characterization of Ralstonia Spp. in Tanzania and potential integrated pest-management strategies for managing bacterial wilt in tomatoes. USA: The Ohio State University.
Karimi, A., Sadeghi, P. A., Dahaji, Y., Dalvand, M., and Omidvari, M. K. N. (2012). Biocontrol activity of salt tolerant Streptomyces isolates against phytopathogens causing root rot of sugar beet. Biocontrol Sci Technol 22, 333–349.
Kashyap, A. S., Manzar, N., Ahamad, F., Tilgam, J., Sharma, P. K., and Saxena, A. K. (2022b). First report of root rot disease in green gram (Vigna radiata) caused by Ectophoma multirostrata in India. Plant Dis 106:2256. doi: 10.1094/PDIS-11-21-2400-PDN
Kashyap, A. S., Manzar, N., Maurya, A., Mishra, D. D., Singh, R. P., and Sharma, P. K. (2023). Development of diagnostic markers and applied for genetic diversity study and population structure of Bipolaris sorokiniana associated with leaf blight complex of wheat. J. Fungi 9:153. doi: 10.3390/jof9020153
Kashyap, A. S., Manzar, N., Nebapure, S. M., Rajawat, M. V. S., Deo, M. M., Singh, J. P., et al. (2022a). Unraveling microbial volatile elicitors using a transparent methodology for induction of systemic resistance and regulation of antioxidant genes at expression levels in chili against bacterial wilt disease. Antioxidants 11:404. doi: 10.3390/antiox11020404
Kashyap, A. S., Manzar, N., Rajawat, M. V. S., Kesharwani, A. K., Singh, R. P., Dubey, S. C., et al. (2021). Screening and biocontrol potential of Rhizobacteria native to Gangetic plains and hilly regions to induce systemic resistance and promote plant growth in Chilli against bacterial wilt disease. Plan. Theory 10:2125. doi: 10.3390/plants10102125
Kavitha, R., and Umesha, S. (2008). Regulation of defense-related enzymes associated with bacterial spot resistance in tomato. Phytoparasitica 36, 144–159. doi: 10.1007/BF02981327
Kim, P. I., Ryu, J., and Kim, Y. H. (2010). Production of biosurfactant lipopeptides Iturin A, fengycin and surfactin A from Bacillus subtilis CMB32 for control of Colletotrichum gloeosporioides. J. Microbiol. Biotechnol. 20, 138–145. doi: 10.4014/jmb.0905.05007
Kirui, C. K., Njeru, E. M., and Runo, S. (2022). Diversity and phosphate solubilization efficiency of phosphate solubilizing bacteria isolated from semi-arid agroecosystems of Eastern Kenya. Microbiol Insights 15, 1–12. doi: 10.1177/11786361221088991
Kloepper, J. W., Ryu, C. M., and Zhang, S. (2004). Induced systemic resistance and promotion of plant growth by Bacillus spp. Phytopathology 94, 1259–1266. doi: 10.1094/PHYTO.2004.94.11.1259
Kloepper, J. W., and Schroth, M. N. (1978). Plant growth-promoting rhizobacteria on radishes Proceedings of the 4th international conference on plant pathogenic bacteria. 2. Station de PathologieVegetale et Phytobacteriologie, INRA, Angers, France. 879–882.
Koumoutsi, A. (2004). Structural and functional characterization of gene clusters directing non-ribosomal synthesis of bioactive cyclic lipopeptides in Bacillus amyloliquefaciens strain FZB42. J. Bacteriol. 186, 1084–1096. doi: 10.1128/JB.186.4.1084-1096.2004
Krieg, N. R., and Holt, J. G. (1984). Bergey’s manual of systematic bacteriology. Williams and Wilkins, Baltimore, London.
Kudoyarova, G. R., Korobova, A. V., Akhiyarova, G. R., Arkhipova, T. N., Zaytsev, D. Y., Prinsen, E., et al. (2014). Accumulation of cytokinins in roots and their export to the shoots of durum wheat plants treated with the protonophore carbonyl cyanide m-chlorophenylhydrazone (CCCP). J. Exp. Bot. 65, 2287–2294. doi: 10.1093/jxb/eru113
Kumar, A. (2016). Phosphate solubilizing bacteria in agriculture biotechnology: diversity, mechanism and their role in plant growth and crop yield. Int. J. Adv. Res. 4, 116–124. doi: 10.21474/IJAR01/111
Kumar, S., Agarwal, M., Dheeman, S., and Maheshwari, D. K. (2015). “Exploitation of phytohormone-producing PGPR in development of multispecies bioinoculant formulation” in Bacterial metabolites in sustainable agroecosystem. ed. D. K. Maheshwari, Springer.
Kurabachew, H., and Wydra, K. (2014). Induction of systemic resistance and defense related enzymes after elicitation of resistance by rhizobacteria and silicon application against Ralstonia solanacearum in tomato (Solanum lycopersicum). Crop Prot. 57, 1–7. doi: 10.1016/j.cropro.2013.10.021
Lakkis, S., Trotel-Aziz, P., Rabenoelina, F., Schwarzenberg, A., Nguema-Ona, E., Clément, C., et al. (2019). Strengthening grapevine resistance by Pseudomonas fluorescens PTA-CT2 relies on distinct defense pathways in susceptible and partially resistant genotypes to Downy Mildew and Gray Mold Diseases. Front. Plant Sci. 10:1112. doi: 10.3389/fpls.2019.01112
Li, H., Ding, X., Wang, C., Ke, H., Wu, Z., Wang, Y., et al. (2016). Control of tomato yellow leaf curl virus disease by Enterobacter asburiaeBQ9 as a result of priming plant resistance in tomatoes. Turk. J. Biol. 40, 150–159. doi: 10.3906/biy-1502-12
Li, S. B., Fang, M., Zhou, R. C., and Huang, J. (2012). Characterization and evaluation of the endophyte Bacillus B014 as a potential biocontrol agent for the control of Xanthomonas axonopodis pv. dieffenbachiae induced blight of Anthurium. Biol. Control 63, 9–16. doi: 10.1016/j.biocontrol.2012.06.002
Liu, X., Jiang, X., He, X., Zhao, W., Cao, Y., Guo, T., et al. (2019). Phosphate-solubilizing Pseudomonas sp. strain P34-L promotes wheat growth by colonizing the wheat rhizosphere and improving the wheat root system and soil phosphorus nutritional status. J. Plant Growth Regul. 38, 1314–1324. doi: 10.1007/s00344-019-09935-8
Liu, D., Lian, B., and Dong, H. (2012). Isolation of Paenibacillus spp. and assessment of its potential for enhancing mineral weathering. Geo Microbiol. J. 29, 413–421.
Liu, Y., Shi, J., Feng, Y., Yang, X., Li, X., and Shen, Q. (2013). Tobacco bacterial wilt can be biologically controlled by the application of antagonistic strains in combination with organic fertilizer. Biol. Fertil. Soils 49, 447–464. doi: 10.1007/s00374-012-0740-z
Liu, Z., Shi, L., Weng, Y., Zou, H., Li, X., Yang, S., et al. (2019). ChiIV3 acts as a novel target of WRKY40 to mediate pepper immunity against Ralstonia solanacearum infection. Mol. Plant-Microbe Interact. 32, 1121–1133. doi: 10.1094/MPMI-11-18-0313-R
Lwin, M., and Ranamukhaarachchi, S. L. (2006). Development of biological control of Ralstonia solanacearum through antagonistic microbial populations. Int. J. Agric. Biol. 8, 657–660.
Ma, L., Zhang, H.-Y., Zhou, X.-K., Yang, C.-G., Zheng, S.-C., Duo, J.-L., et al. (2018). Biological control tobacco bacterial wilt and black shank and root colonization by bio-organic fertilizer containing bacterium Pseudomonas aeruginosa NXHG29. Appl. Soil Ecol. 129, 136–144. doi: 10.1016/j.apsoil.2018.05.011
Malleswari, D. (2014). In vitro antagonistic activity of diverse bacterial isolates against Macrophomina phaseolina (Tassi) Goid. Int. J. Curr. Microbiol. App. Sci. 3, 755–763.
Mansfield, J., Genin, S., Magori, S., Citovsky, V., Sriariyanum, M., Ronald, P., et al. (2012). Top 10 plant pathogenic bacteria in molecular plant pathology. Mol. Plant Pathol. 13, 614–629. doi: 10.1111/j.1364-3703.2012.00804.x
Manzar, N., Kashyap, A. S., Goutam, R. S., Rajawat, M. V. S., Sharma, P. K., Sharma, S. K., et al. (2022b). Trichoderma: advent of versatile biocontrol agent, its secrets and insights into mechanism of biocontrol potential. Sustainability 14:12786. doi: 10.3390/su141912786
Manzar, N., Kashyap, A. S., Maurya, A., Rajawat, M. V. S., Sharma, P. K., Srivastava, A. K., et al. (2022a). Multi-gene phylogenetic approach for identification and diversity analysis of Bipolaris maydis and Curvularia lunata isolates causing foliar blight of Zea mays. J Fungi 8:802. doi: 10.3390/jof8080802
Manzar, N., Kashyap, A. S., Sharma, P. K., and Saxena, A. K. (2021a). First report of leaf spot of maize caused by Curvularia geniculata in India. Plant Dis. 105:4155. doi: 10.1094/PDIS-03-21-0637-PDN
Manzar, N., Singh, Y., Kashyap, A. S., Sahu, P. K., Rajawat, S. M. V., Bhowmik, A., et al. (2021b). Biocontrol potential of native Trichoderma spp. against anthracnose of great millet (Sorghum bicolour L.) from Tarai and hill regions of India. Biol. Control 152:104474. doi: 10.1016/j.biocontrol.2020.104474
Milagrosa, S. P., and Balaki, E. T. (1999). Influence of Bokashi organic fertilizer and effective microorganisms (EM) on growth and yield of field grown vegetables. In: Y. D. A. Senanayake and U. R. Sangakkara (Eds.), Proceeding of fix international conference on Kyusei nature farming, International nature farming research center, Atami, Japan, 84–92
Miljakovi, D., Marinkovi, J., and Baleevi-Tubi, S. (2020). The significance of Bacillus spp. in disease suppression and growth promotion of field and vegetable crops. Microorganisms 8:1037. doi: 10.3390/microorganisms8071037
Mishra, A. (1995). Assessment of yield loss due to wilting in some popular tomato cultivars. Env. Ecol. 13:287.
Mohammed, A. F., Oloyede, A. R., and Odeseye, A. O. (2020). Biological control of bacterial wilt of tomato caused by Ralstonia solanacearum using Pseudomonas species isolated from the rhizosphere of tomato plants. Arch. Phytopathol. Plant Protect. 53, 1–16. doi: 10.1080/03235408.2020.1715756
Moisyadi, S., Dharmasiri, S., Harrington, H. M., and Lukas, T. J. (1994). Characterization of a low molecular mass autohosphorylated protein in cultured sugarcane cells and its identifications as a nucleoside diphosphate kinase. Plant Physiol. 104, 1401–1409. doi: 10.1104/pp.104.4.1401
Montano, F. P., Villegas, C. A., Bellogia, R. A., Cerro, P. D., Espuny, M. R., Guerrero, I. J., et al. (2014). Plant growth promotation in cereals and leguminous agricultural important plants from microorganisms’ capacities to crop production. Microbiol. Res. 169, 325–336. doi: 10.1016/j.micres.2013.09.011
Moon, H., Lee, B., Choi, G., Shin, D., Prasad, D. T., Lee, O., et al. (2003). NDP kinase 2 interacts with two oxidative stress-activated MAPKs to regulate cellular redox state and enhances multiple stress tolerance in transgenic plants. Proc. Natl. Acad. Sci. U. S. A. 100, 358–363. doi: 10.1073/pnas.252641899
Mubarak, M. Q. E., Hassan, A. R., Hamid, A. A., Khalil, S., and Isa, M. H. M. (2015). A simple and effective isocratic HPLC method for fast identification and quantification of surfactin. Sains Malays. 44, 115–120. doi: 10.17576/jsm-2015-4401-16
Mulla, S. R., Sandeep, C. P., Salimath, J., Varsha, P., Suresh, C. K., and Priyadarsini, R. I. (2013). Effect of Pseudomonas fluorescens isolated from various agroclimatic zones of Karnataka on Rauwolfia serpentina. Int J Pharm Sci 5, 141–146.
Nacoon, S., Jogloy, S., Riddech, N., Mongkolthanaruk, W., Kuyper, T. W., and Boonlue, S. (2020). Interaction between phosphate solubilizing bacteria and arbuscular mycorrhizal fungi on growth promotion and tuber inulin content of Helianthus tuberosus L. Sci. Rep. 10, 1–10. doi: 10.1038/s41598-020-61846-x
Ngumbi, E., and Kloepper, J. (2016). Bacterial-mediated drought tolerance: current and future prospects. Appl. Soil Ecol. 105, 109–125. doi: 10.1016/j.apsoil.2016.04.009
Niehus, R., Picot, A., Oliveira, N. M., Mitri, S., and Foster, K. R. (2017). The evolution of siderophore production as a competitive trait. Evolution 71, 1443–1455. doi: 10.1111/evo.13230
Oteino, N., Lally, R. D., Kiwanuka, S., Lloyd, A., Ryan, D., Germaine, K. J., et al. (2015). Plant growth promotion induced by phosphate solubilizing endophytic Pseudomonas isolates. Front. Microbiol. 6:745. doi: 10.3389/fmicb.2015.00745
Oyedele, A. O., and Samuel, T. (2014). Antifungal activities of Bacillus subtilis isolated from some condiments and soil. Afr. J. Microbiol. Res. 8, 1841–1849. doi: 10.5897/AJMR2013.6162
Patel, J. B. (2001). 16S rRNA gene sequencing for bacterial pathogen identification in the clinical laboratory. Mol. Diagn. 6, 313–321. doi: 10.1007/BF03262067
Pieterse, C. M., Zamioudis, C., Berendsen, R. L., Weller, D. M., Van Wees, S. C., and Bakker, P. A. (2014). Induced systemic resistance by beneficial microbes. Annu. Rev. Phytopathol. 52, 347–375. doi: 10.1146/annurev-phyto-082712-102340
Prameela, T. P., and Rajamma, S. B. (2020). Bacterial wilt of ginger (Zingiber officinale Rosc.) incited by Ralstoniapseudosolanacearum-A review based on pathogen diversity, diagnostics and management. J. Plant Pathol. 102, 709–719.
Prathap, M., and Kumari, R. B. D. (2015). A Critical review on plant growth promoting rhizobacteria. J. Plant Pathol. Microbiol. 6, 1–4.
Punja, Z. K., Rodriguez, G., and Tirajoh, A. (2016). Effects of Bacillus subtilis strain QST 713 and storage temperatures on post-harvest disease development on greenhouse tomatoes. Crop Prot. 84, 98–104. doi: 10.1016/j.cropro.2016.02.011
Rajer, F. U., Wu, H., Xie, Y., Xie, S., Raza, W., Tahir, H. A. S., et al. (2017). Volatile organic compounds produced by a soil-isolate, Bacillus subtilis FA26 induce adverse ultra-structural changes to the cells of Clavibacter michiganensis ssp. sepedonicus, the causal agent of bacterial ring rot of potato. Microbiology (Reading). 163, 523–530. doi: 10.1099/mic.0.000451
Ramadan, E. M., AbdelHafez, A. A., Hassan, E. A., and Saber, F. M. (2016). Plant growth promoting rhizobacteria and their potential for biocontrol of phytopathogens. Afr. J. Microbiol. Res. 10, 486–504.
Ramarathnam, R., Bo, S., Chem, Y., Fernando, W. G. D., Xuewen, G., and de Kievit, T. (2007). Molecular and biochemical detection of fengycin and bacillomycin D producing Bacillus spp., antagonistic to fungal pathogens of canola and wheat. Can. J. Microbiol. 53, 901–911. doi: 10.1139/W07-049
Ramesh, R., and Phadke, G. S. (2012). Rhizosphere and endophytic bacteria for the suppression of eggplant wilt caused by Ralstonia solanacearum. Crop Prot. 37, 35–41. doi: 10.1016/j.cropro.2012.02.008
Raymond, K. N., Allred, B. E., and Sia, A. K. (2015). Coordination chemistry of microbial iron transport. Acc. Chem. Res. 48, 2496–2505. doi: 10.1021/acs.accounts.5b00301
Raza, W., Ling, N., Liu, D., Wei, Z., Huang, Q., and Shen, Q. (2016a). Volatile organic compounds produced by Pseudomonas fluorescensWR-1 restrict the growth and virulence traits of Ralstonia solanacearum. Microbiol. Res. 192, 103–113. doi: 10.1016/j.micres.2016.05.014
Raza, W., Ling, N., Yang, L., Huang, Q., and Shen, Q. (2016b). Response of tomato wilt pathogen Ralstonia solanacearum to the volatile organic compounds produced by a biocontrol strain Bacillus amyloliquefaciens SQR-9. Sci. Rep. 6:24856. doi: 10.1038/srep24856
Raza, W., Yousaf, S., and Rajer, F. U. (2016c). Plant growth promoting activity of volatile organic compounds produced by Bio-control strains. Sci. Lett. 4, 40–43.
Rea, M. C., Sit, C. S., Claytona, E., Connor, P. M., Whittal, R. M., Zheng, J., et al. (2010). Thuricin CD, a post-translationally modified bacteriocin with a narrow spectrum of activity against Clostridium difficile. Proc. Natl. Acad. Sci. U. S. A. 107, 9352–9357. doi: 10.1073/pnas.0913554107
Rojas-Solís, D., Zetter-Salmón, E., Contreras-Pérez, M., del Carmen Rocha-Granados, M., Macía-Rodríguez, L., and Santoyo, G. (2018). Pseudomonas stutzeri E25 and Stenotrophomonas maltophilia CR71 endophytes produce antifungal volatile organic compounds and exhibit additive plant growth-promoting effects. Biocat. Agri. Biotechnol. 13, 46–52. doi: 10.1016/j.bcab.2017.11.007
Ryu, C., Farag, M. A., Hu, C., Reddy, M. S., Wei, H., and Pare, P. W. (2003). Bacterial volatiles promote growth in Arabidopsis. PNAS 100, 4927–4932. doi: 10.1073/pnas.0730845100
Ryu, C. M., Farag, M. A., Hu, C. H., Reddy, M. S., Kloepper, J. W., and Paré, P. W. (2004). Bacterial volatiles induce systemic resistance in Arabidopsis. Plant Physiol. 134, 1017–1026. doi: 10.1104/pp.103.026583
Ryu, C.-M., Hu, C.-H., Locy, R. D., and Kloepper, J. W. (2005). Study of mechanisms for plant growth promotion elicited by rhizobacteria in Arabidopsis thaliana. Plant Soil. 268, 285–292.
Ryu, C.-M., Kim, J., Choi, O., Kim, S. H., and Park, C.-S. (2006). Improvement of biological control capacity of Paenibacillus polymyxa E681 by seed pelleting on sesame. Biol. Control 39, 282–289. doi: 10.1016/j.biocontrol.2006.04.014
Saia, S. (2015). Soil inoculation with symbiotic microorganisms promotes plant growth and nutrient transporter genes expression in durum wheat. Front. Plant Sci. 6:202.
Seleim, M. A. A., Abo-Elyousr, K. A. M., Abd-El-Moneem, K. M., and Saead, F. A. (2014). First report of bacterial wilt caused by Ralstonia solanacearum biovar 2 race 1 on tomato in Egypt. J. Plant Pathol. 30, 299–303. doi: 10.5423/PPJ.NT.10.2013.0101
Sgroy, V., Cassan, F., Masciarelli, O., Del Papa, M. F., and Lagares, A. (2009). Luna isolation and characterization of endophytic plant growth-promoting (PGPB) or stress homeostasis-regulating (PSHB) bacteria associated to the halophyte Prosopis strombulifera. Appl. Microbiol. Biotechnol. 85, 85371–85381.
Sharifi, R., and Ryu, C. M. (2016). Are bacterial volatile compounds poisonous odors to a fungal pathogen Botrytis cinerea, Alarm signals to Arabidopsis seedlings for eliciting induced resistance, or both. Front. Microbiol. 7, 1–10.
Sharma, S., and Kaur, M. (2010). Antimicrobial activities of rhizobacterial strains of Pseudomonas and Bacillus strains isolated from rhizosphere soil of carnation (Dianthus caryophyllus cv. Sunrise). Indian J. Microbiol. 50, 229–232. doi: 10.1007/s12088-010-0045-6
Shekhawat, G. S., Chakrabarti, S. K., Kishore, V., Sunaina, V., and Gadewar, A. V. (1993). “Possibilities of biological management of potato bacterial wilt with strains of Bacillus sp., Bacillus subtilis, Pseudomonas fluorescens and Actinomycetes” in Bacterial Wilt 327–30. ACIAR Proceedings. eds. G. L. Hartman and A. C. Hayward, vol. 45 (Camera: Australian Centre for International Agricultural Research)
Shekhawat, G. S., Gadewar, A. V., and Chakrabarti, S. K. (2000). Potato bacterial wilt in India. Technical Bullet 38, 56–57.
Shenoy, V. V., and Kalagudi, G. M. (2005). Enhancing plant phosphorus use efficiency for sustainable cropping. Biotechnol. Adv. 23, 501–513. doi: 10.1016/j.biotechadv.2005.01.004
Siddiqui, Z. (2006). PGPR: Prospective Biocontrol Agents of Plant Pathogens. Biocontrol. Biofertil. 111–142.
Sivasakthi, S., Saranraj, P., and Sivasakthivelan, P. (2017). Biological nitrogen fixation by Azotobacter sp.—a review. Indo. Asian. J. Multidiscip. Res. 3, 1274–1284. doi: 10.22192/iajmr.2017.3.5.6
Singh, D., Chaudhary, G., and Yadav, D. K. (2017). Genetic diversity of Indian isolates of Ralstonia solanacearum causing bacterial wilt of eggplant (Solanum melongena). Indian J. Agric. Sci. 87, 1466–1475.
Singh, D., Shweta, S., Yadav, D. K., Sharma, J. P., Srivastava, D. K., Lal, H. C., et al. (2010). Characterization of biovar/races of Ralstonia solanacearum, the incitant of bacterial wilt in solanaceous crops. Indian Phytopath 63, 261–265.
Singh, S., Singh, D. R., Kumar, K., and Birah, A. (2014). Eco-friendly management modules for bacterial wilt (Ralstonia solanacearum) of tomato for protected cultivation in a tropical island ecosystem. Biol. Agric. Hortic. 30, 219–227. doi: 10.1080/01448765.2014.913263
Singh, R., Soni, S. K., and Kalra, A. (2013). Synergy between Glomus fasciculatum and beneficial Pseudomonas in reducing root diseases and improving yield and forskolin content in Coleus forskohlii Brig, under organic field condition. Mycorrhiza 23, 35–44. doi: 10.1007/s00572-012-0447-x
Srivastava, S., Bist, V., Srivastava, S., Singh, P. C., Trivedi, P. K., and Asif, M. H. (2016). Unraveling aspects of Bacillus amyloliquefaciens mediated enhanced production of rice under biotic stress of Rhizoctonia solani. Front. Plant Sci. 7:587. doi: 10.3389/fpls.2016.00587
Stein, T. (2005). Bacillus subtilis antibiotics: structures, syntheses and specific functions. Mol. Microbiol. 56, 845–857. doi: 10.1111/j.1365-2958.2005.04587.x
Suryakala, D., Maheshwaridevi, P. V., and Lakshmi, K. V. (2004). Chemical characterization and in vitro antibiosis of siderophores of rhizosphere fluorescent pseudomonads. Indian J. Microbiol. 44, 105–108.
Tahir, H. A. S., Gu, Q., Wu, H., Raza, W., Hanif, A., and Wu, L. (2017). Plant growth promotion by volatile organic compounds produced by Bacillus subtilis SYST2. Front. Mol. Biol. 8, 1–11.
Tamehiro, N., Okamoto, H. Y., Okamoto, S., Ubukata, M., Hamada, M., Naganawa, H., et al. (2002). Bacilysocin, a novel phospholipid antibiotic produced by Bacillus subtilis. Antimicrob. Agents Chemother. 46, 315–320. doi: 10.1128/AAC.46.2.315-320.2002
Tan, S., Dong, Y., Liao, H., Huang, J., Song, S., Xu, Y., et al. (2013a). Antagonistic bacterium Bacillus amyloliquefaciens induces resistance and controls the bacterial wilt of tomato. Pest Manag. Sci. 69, 1245–1252. doi: 10.1002/ps.3491
Tan, S., Yang, C., Mei, X., Shen, S., Raza, W., and Shen, Q. (2013b). The effect of organic acids from tomato root exudates on rhizosphere colonization of Bacillus amyloliquefaciens T-5. Appl. Soil Ecol. 64, 15–22. doi: 10.1016/j.apsoil.2012.10.011
Tao, C., Li, R., Xiong, Z. S., Shen, Z., Liu, S., Wang, B., et al. (2020). Bio-organic fertilizers stimulate indigenous soil Pseudomonas populations to enhance plant disease suppression. Microbiome 8, 1–14. doi: 10.1186/s40168-020-00892-z
Tenorio-Salgado, S., Tinoco, R., Vazquez-Duhalt, R., Caballero-Mellado, J., and Perez-Rueda, E. (2013). Identification of volatile compounds produced by the bacterium Burkholderia tropica that inhibit the growth of fungal pathogens. Bioengineered 4, 236–243. doi: 10.4161/bioe.23808
Thanh, D. T., Tarn, L. T. T., Hanh, N. T., Tuyen, N. H., Lee, S. Y., and Park, K.-S. (2009). Biological control of soilborne diseases on tomato, potato and black pepper by selected PGPR in the greenhouse and field in Vietnam. Plant Pathol. J. 25, 263–269. doi: 10.5423/PPJ.2009.25.3.263
Turan, M. A., Awad-Alkarim, A. H., Taban, N., and Taban, S. (2009). Effect of salt stress on growth, stomatal resistance, proline and chlorophyll concentrations on maize plant. Afr. J. Agric. Res. 4, 893–897.
USDA. (2003). Biological control of Fusarium wilt and other soilborne pathogenic fungi. Available at: http://www.ars.usda.gov/research/projects/projects.htm?ACCN_NO=406590&fy=2003
Van Peer, R., and Schippers, B. (1992). Lipopolysaccharides of plant-growth promoting Pseudomonas sp. strain WCS417r induce resistance in carnation to Fusarium wilt. Neth. J. Plant Pathol. 98, 129–139. doi: 10.1007/BF01996325
Vespermann, A., Kai, M., and Piechulla, B. (2007). Rhizobacterial volatiles affect the growth of fungi and Arabidopsis thaliana. Appl. Environ. Microbiol. 73, 5639–5641. doi: 10.1128/AEM.01078-07
Voisard, C., Keel, C., Haas, D., and Dèfago, G. (1989). Cyanide production by Pseudomonas fluorescens helps suppress black rot of tobacco under gnotobiotic conditions. EMBO J. 8, 351–358. doi: 10.1002/j.1460-2075.1989.tb03384.x
Wei, Z., Huang, J., Tan, S., Mei, X., Shen, Q., and Xu, Y. (2013). The congeneric strain Ralstoniapickettii QL-A6 of Ralstonia solanacearum as an effective biocontrol agent for bacterial wilt of tomato. Biol. Control 65, 278–285. doi: 10.1016/j.biocontrol.2012.12.010
Wei, Z., Yang, X., Yin, S., Shen, Q., Ran, W., and Xu, Y. (2011). Efficacy of Bacillus-fortified organic fertilizer in controlling bacterial wilt of tomato in the field. Appl. Soil Ecol. 48, 152–159. doi: 10.1016/j.apsoil.2011.03.013
Weller, D. M., Raaijmakers, J. M., McSpadden, B. B. G., and Thomashow, L. S. (2002). Microbial populations responsible for specific soil suppressiveness to plant pathogens. Annu. Rev. Phytopathol. 40, 308–348.
Xing, Y., Jia, W., and Zhang, J. (2007). AtMEK1 mediates stress-induced gene expression of CAT1 catalase by triggering H2O2 production in Arabidopsis. J. Exp. Bot. 58, 2969–2981. doi: 10.1093/jxb/erm144
Yadav, D. K., Singh, D., and Kumar, N. (2017). Induction of Defense-related Enzymes by Bacillus amyloliquefaciens DSBA-11 in resistant and susceptible cultivars of tomato against bacterial wilt disease. Int. J. Agric. Res. 12, 172–180. doi: 10.3923/ijar.2017.172.180
Yang, J., Kloepper, J. W., and Ryu, C. M. (2009). Rhizosphere bacteria help plants tolerate abiotic stress. Trends Plant Sci. 14, 1–4. doi: 10.1016/j.tplants.2008.10.004
Yu, X., Ai, C., Xin, L., and Zhou, G. (2011). The siderophore-producing Bacterium, Bacillus subtilis CAS15, Has a biocontrol effect on Fusarium wilt and promotes the growth of pepper. Eur. J. Soil Biol. 47, 138–145. doi: 10.1016/j.ejsobi.2010.11.001
Yuan, S., Cohen, D. B., Ravel, J., Abdo, Z., and Forney, L. J. (2012). Evaluation of methods for the extraction and purification of DNA from the human microbiome. PLoS One 7:e33865. doi: 10.1371/journal.pone.0033865
Yuan, S., Wang, L., Wu, K., Shi, J., Wang, M., Yang, X., et al. (2014). Evaluation of Bacillus-fortified organic fertilizer for controlling tobacco bacterial wilt in greenhouse and field experiments. Appl. Soil Ecol. 75, 86–94. doi: 10.1016/j.apsoil.2013.11.004
Yuliar, N., Nion, Y. A., and Toyota, K. (2015). Recent trends in control methods for bacterial wilt diseases caused by Ralstonia solanacearum. Microbes Environ. 30, 1–11. doi: 10.1264/jsme2.ME14144
Zhou, C., Ma, Z. Y., Zhu, L., Xiao, X., Xie, Y., Zhu, J., et al. (2016). Rhizobacterial strain Bacillus megaterium bofc15 induces cellular polyamine changes that improve plant growth and drought resistance. Int. J. Mol. Sci. 17:976. doi: 10.3390/ijms17060976
Keywords: bioinoculants, evergreen technology, nanotechnology, sustainable agriculture, magical microbial bullets, plan growth promoting microorganism, soil born pathogen
Citation: Kashyap AS, Manzar N, Meshram S and Sharma PK (2023) Screening microbial inoculants and their interventions for cross-kingdom management of wilt disease of solanaceous crops- a step toward sustainable agriculture. Front. Microbiol. 14:1174532. doi: 10.3389/fmicb.2023.1174532
Edited by:
Ravinder Kumar, Central Potato Research Institute (ICAR), IndiaReviewed by:
Shaikhul Islam, Bangladesh Agricultural Research Council, BangladeshBartholomew Saanu Adeleke, Olusegun Agagu University of Science and Technology, Nigeria
Lata Jain, ICAR-National Institute of Biotic Stress Management, India
Copyright © 2023 Kashyap, Manzar, Meshram and Sharma. This is an open-access article distributed under the terms of the Creative Commons Attribution License (CC BY). The use, distribution or reproduction in other forums is permitted, provided the original author(s) and the copyright owner(s) are credited and that the original publication in this journal is cited, in accordance with accepted academic practice. No use, distribution or reproduction is permitted which does not comply with these terms.
*Correspondence: Nazia Manzar, bmF6aWFtYW56YXI3ODZAZ21haWwuY29t; Pawan Kumar Sharma, cGF3YW4xMTIwMDBAZ21haWwuY29t