- 1ICAR–Indian Grassland and Fodder Research Institute, Jhansi, Uttar Pradesh, India
- 2ICAR–Central Soil Salinity Research Institute, Karnal, Haryana, India
- 3ICAR-Central Inland Fisheries Research Institute, Kolkata, West Bengal, India
- 4ICAR–Central Tobacco Research Institute, Rajahmundry, Andhra Pradesh, India
Introduction: The perennial grass–legume cropping system benefits soil because of its high biomass turnover, cover cropping nature, and different foraging behaviors. We investigated the response of soil organic carbon (SOC) pools and their stock to organic and inorganic nutrient management in the Guinea grass and legume (cowpea-Egyptian clover) cropping system.
Methods: Depth-wise soil samples were collected after harvesting the Egyptian clover. Based on the ease of oxidation with chromic acid, different pools of SOC oxidizable using the Walkley–Black C method, very labile, labile, less labile, non-labile; and dissolved organic C (DOC), microbial biomass C (MBC), and total organic C (TOC) in soils were analyzed for computing several indices of SOC.
Result and discussion: After 10 years of crop cycles, FYM and NPKF nutrient management recorded greater DOC, MBC, SOC stocks, and C sequestration than the NPK. Stocks of all SOC pools and carbon management index (CMI) decreased with soil depth. A significant improvement in CMI, stratification ratio, sensitivity indices, and sustainable yield index was observed under FYM and NPKF. This grass–legume intercropping system maintained a positive carbon balance sequestered at about 0.8Mg C ha−1 after 10 years without any external input. Approximately 44–51% of the applied carbon through manure was stabilized with SOC under this cropping system. The DOC, MBC, and SOC in passive pools were identified for predicting dry fodder yield. This study concludes that the application of organics in the perennial grass–legume inter cropping system can maintain long-term sustainability, enhance the C sequestration, and offset the carbon footprint of the farm enterprises.
1. Introduction
Soil organic carbon (SOC) is key to soil health management influencing crop production, climate change mitigation, and other ecosystem services (Srinivasarao et al., 2016; Oldfield et al., 2019; Hasegawa et al., 2021; Doblas-Rodrigo et al., 2022). The accrual and hoarding of SOC are determined greatly by the set of biophysical variables such as temperature, rainfall, potential evapotranspiration, soilscape, physiography, vegetation, cropping systems, and management practices (Schmidt et al., 2011; Lal, 2015; Poffenbarger et al., 2018; Alavi-Murillo et al., 2022). The cropping systems and associated management practices utilizing inorganic fertilizer and organic manures alone or in combination are the key drivers influencing the SOC stock of soil in a given agroecosystem (Mandal et al., 2008; Ghosh et al., 2012; Luo et al., 2014; Sainju et al., 2014; Yadav et al., 2017; Poffenbarger et al., 2018; Ngangom et al., 2020; Basak et al., 2021a). These management practices affect the separation of SOC in pools of varied stability (Basak et al., 2021a) characterized by the susceptibility to oxidation and residence period (Zimmermann et al., 2007). A faster turnover rate of labile C fraction of SOC (Sanderman et al., 2017) and its oxidation maintain the productivity of soil and CO2 flux to the atmosphere. Conversely, the passive pool has a long residence time because of physical and biochemical protection, making it resistant to microbial actions (Zimmermann et al., 2007; Weil et al., 2017; Datta et al., 2018). Previous studies reported the effect of the cropping system on the distribution of active and passive pools in soils of subtropic (Chaudhary et al., 2017; Yadav et al., 2017, 2019; Anantha et al., 2020; Babu et al., 2020; Basak et al., 2021a), arid (Moharana et al., 2017), and semi-arid (Srinivasarao et al., 2013, 2016; Mitran et al., 2018; Basak et al., 2021b) regions. The application of organic fertilizers is also reported to improve the yield of grain crops through their influence on SOC and associated soil properties (Mandal et al., 2007). However, similar studies involving perennial grass-based intensive forage production in semi-arid climatic conditions are lacking (Ghosh et al., 2010; Kim et al., 2022).
In India, 57% of the net sown areas are rainfed under different agroecosystems (Bal et al., 2022). A significant proportion of the area under semi-arid regions in India had been brought under canal irrigation by developing dams on the rivers with seasonal water flow. As a result, cropping intensity in these canal commands increased. The limited supply of canal water only during the winter makes it a rainfed (monsoon) rotation followed by irrigated (winter) crops in these regions. Guinea grass (Panicum maximum cv. Hamil) intercropped with seasonal legumes is an important intensive forage production system in rainfed-irrigated areas in semi-arid regions of India (Kumar et al., 2012). Fast growth and high biomass production make it an ideal crop for fodder and biofuel production (Mohapatra et al., 2019) and SOC sequestration in pastures and grassland (Ghosh et al., 2009; Sarkar et al., 2020). Cowpea and Egyptian clover (Trifolium alexandrinum L) are leguminous crops commonly incorporated in grass-based cropping systems to improve forage quality and enhance forage productivity during the poor growth of grass components (Kumar et al., 2012). The cowpea and Egyptian clover also complement the cropping system's soil SOC pools and productivity because of spatial and temporal annidation and different rooting behaviors (Rai et al., 2013).
The SOC stock and stability indices developed from SOC pools were found better in assessing the impact of management practices and cropping systems in several cropping systems in different agro-ecologies (Blair et al., 1995; Franzluebbers, 2002; Mandal et al., 2008). But, there is limited information on the perennial grass + legumes-based intensive intercropping system for biomass production under inorganic and organic nutrition with rainfed-irrigated water management. These indices in a perennial grass–legume-based intensive cropping system will capture the impact of different nutrient management on SOC and their interactions with soil properties in rainfed-irrigated agroecosystems of the semi-arid region. This information is essential from an agronomic and environmental point of view for crop planning and carbon budgeting at the farm scale. Therefore, this study was conducted to assess the impact of the organic and inorganic nutrients alone or in combination with the stock and stability of SOC in perennial guinea grass + the (cowpea-Egyptian clover) cropping system under rainfed (monsoon)—irrigated (winter) water management. The objective of the study was to (i) assess the impacts of organic, inorganic, and integrated nutrient management on depth-wise SOC stock and its sequestration potential; (ii) measure the effect of nutrient management on the pool-wise allocation of SOC of different stability, and (iii) to establish a relationship between SOC pools and cropping system sustainability.
2. Materials and methods
2.1. Experimental site
This experiment was conducted in the semi-arid, continental, and monsoonal climate at the Central Research Farm (25°31′ 01.73″ N, 78°33′ 32.84″ E, at 224 m mean sea level), ICAR- Indian Grassland and Fodder Research Institute, Jhansi, Uttar Pradesh, India. The region's annual rainfall is 906.5 mm (781 mm during the rainy season and 52 mm during the winter), with an annual potential evapotranspiration of 1,512 mm. The soil of the experimental site is classified under hyperthermic Typic Haplustepts with silty loam in texture (sand, silt, and clay with 36.6, 43.2, and 20.2%, respectively). The soil of the experimental site had pH1:2 of 7.9 and Walkley–Black organic carbon (Walkley and Black, 1934) of 4.2 g kg−1; and KMnO4 oxidizable N (Subbiah and Asija, 1956), Olsen's extractable P (Jackson, 1967), and ammonium acetate extractable K (Jackson, 1967) of 192, 9.9, and 135 kg ha−1, respectively.
2.2. Field experiment: treatment detail and layout
The field experiment was conducted following a randomized complete block design with three replications. The fertilizers treatments were as follows: control, the recommended dose of N, P, and K (NPK) fertilizers, farmyard manure (FYM), and integrated application of FYM and NPK (NPKF) were allocated randomly in triplicate in plots of 4 × 5 m2 separated by 1-meter buffer strip from all sides to prevent treatment interference. Details of the treatments imposed, including source, application schedule, and other cultural practices followed, are given in Table 1. The first crop Guinea grass (Panicum maximum cv. Hamil) rooted slips were planted in July 2004 at a 100 × 50 cm distance. Cowpea (Vigna unguiculata cv. EC-4216) and Egyptian clover (Trifolium alexandrinum cv. Wardan) were planted in the inter-row space in summer (June–July) and winter season (November–March), respectively. In their respective season, two rows of cowpea and berseem were planted in the inter-row space at a 30 cm distance. In FYM and NPKF treatments, FYM was applied in the rainy (July–October) and winter (November–June) seasons on a fresh weight basis with an average moisture content of 35% (w/w). The FYM contained ~8.7 ± 0.4, 2.0 ± 0.3, and 12.1 ± 0.2 g kg−1 of N, P, and K, respectively. The mean C: N ratio of the manure was 70:1. Rainy (July-October)-season crop was grown rainfed, while in winter season 7–8, irrigation was applied depending upon the winter rains and the crop's need in the cropping system. The above-ground biomass of the Egyptian clover was harvested at 30-day intervals, while cowpea was harvested 60 days after sowing. Guinea grass was harvested 5–7 cm above the ground surface at 30 and 60 days intervals in rainy (July–October) and the rest of the season (November–June), respectively.
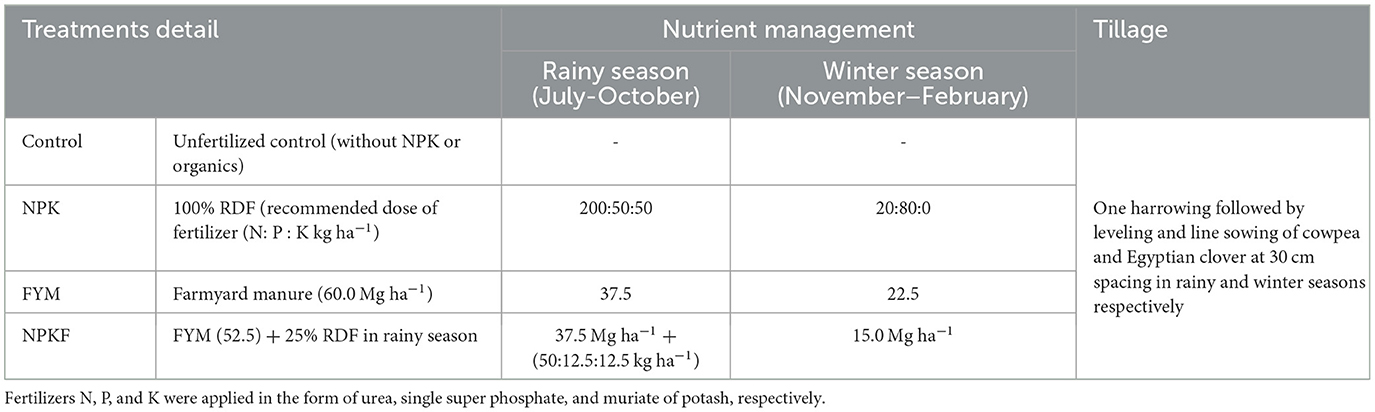
Table 1. Description of the management practices for guinea grass + (cowpea-Egyptian clover) cropping system.
2.3. Soil analysis
Soil samples were collected randomly from three representative sites in each plot at the field moist condition from four depths viz., 0–0.15, 0.15–0.30, 0.30–0.50, and 0.50–0.70 m after harvesting the Egyptian clover in the last week of April 2014. One set of soil samples was immediately preserved at 4°C for microbial biomass carbon (MBC) estimation (Vance et al., 1987). Another sample set was air-dried and processed to pass through a 2 mm sieve for further analysis of SOC pools. Depth-wise bulk density of undisturbed soil was measured using a core sampler (Blake and Hartage, 1986). A pH meter (Eutech pH 700) was used for estimating soil pH in a 1:2 soil: water suspension (Jackson, 1967). Soil texture (Gee and Bauder, 1986) and calcium carbonate (Allison and Moodie, 1965) were determined using standard procedure. Total carbon (TC) was estimated using CHNS Elemental Analyzer (Vario EL III, Germany). The TOC was computed by deducting the carbon derived from calcium carbonate (Allison and Moodie, 1965) from TC.
2.3.1. Microbial biomass carbon, microbial quotient, and dissolved organic carbon
Chloroform fumigation and extraction method were used to determine microbial biomass carbon (MBC) in fresh soil samples (Vance et al., 1987). The difference in the C flux from fumigated to unfumigated samples was used to calculate the MBC (Mg ha−1) using equation (1) proposed by Voroney and Paul (1984). The dissolved C in the extract was determined using the method of McGill et al. (1986). The microbial quotient (MQ) was calculated from MBC and TOC (Equation 2).
2.3.2. Oxidizable soil organic carbon and its pools
The SOC pools were determined using the modified method suggested by Chan et al. (2001). Briefly, soils were treated with 12, 18, and 24 N H2SO4 and 0.5 N K2Cr2O7. The obtained TOC was separated into four pools of reduced oxidability: very labile (CVL), labile (CL), less labile (CLL), and non-labile (CNL) following the procedure described in Majumder et al. (2007). CVL + CL and CLL + CNL were expressed as the active (CAP) and passive pools (CPP), respectively.
2.4. Crop-derived C inputs into the soil
After harvesting multi-cut guinea grass, cowpea, and Egyptian clover, the leftover biomass and root samples were collected. The protocol described by Thangaraj and O'Toole (1986) was used to sample guinea grass, cowpea, and Egyptian clover roots. The C content of these samples was analyzed. The net rhizodeposition of grass and legume crops was derived from roots' biomass, as described by Pausch and Kuzyakov (2018). A detailed assessment was made for crop residue C added from guinea grass, cowpea, and Egyptian clover. Total carbon input to the soil from rhizodeposition, roots, stubbles, and leaves of the component crops was measured annually during the last 3 years using respective biomass addition and carbon content.
2.5. Soil carbon stock
The soil carbon stock (Mg ha−1) was calculated using bulk density (Mg m−3), depth of soil layer (m), and C content (g C g−1) in the respective soil layer using Equation (3) (Batjes, 1996).
2.6. Recalcitrant index
To assess the effect of FYM, inorganic fertilizer, and control, the recalcitrant index (RI1 and RI2) of SOC was calculated using Equations (4) and (5) (Datta et al., 2018). The RImean was computed by averaging the RI1 and RI2.
and
2.7. Carbon lability index
The carbon lability index (CLI) of SOC was developed using SOC pools of comparative oxidability using Equation (6) (Majumder et al., 2007).
2.8. Carbon management index
The CMI highlights the management-induced changes in soil quality (Basak et al., 2021a). It is derived from the carbon pool index (CPI) and lability index (LI) as shown in the following equations (Blair et al., 1995):
2.9. Carbon build-up and sequestration
The total carbon addition in the soil through organic manure was calculated based on oven-dry weight. Carbon budgeting was done for 0–0.70 m depth. The percent C build-up over control, C build-up rate, per cent stabilization of applied carbon, carbon sequestration, and carbon sequestration potential for different nutrient management strategies were estimated using the following equations as described by Mishra et al. (2015):
Here, CTrt is the TOC in a particular treatment; TOC in control treatment was considered a background contribution of TOC for FYM treatment; while for NPKF treatment considering the additive effect, Ccontrol + 0.25 (CNPK− Ccontrol) was considered as the background contribution to soil TOC,
2.10. Stratification ratio
The stratification ratio (SR) specifies the sub-surface influence of surface-imposed management practices. The SR of SOC and its pools was derived as the ratio of respective carbon fractions using the following equation described by Franzluebbers (2002):
The average stratification ratio of all the carbon fractions was denoted as SRmean.
2.11. Sensitivity index
The SI (%) of 0–0.15 m soil layer was calculated to assess the changes in different C pools in treated soils in comparison to control using Equation (16) proposed by Banger et al. (2010) as follows:
The average SI of all the carbon fractions was denoted as SImean for the respective treatments.
2.12. Biometric observation and biomass yield
Total green biomass yield and dry biomass yield (oven-dry weight basis at 60°C) of the guinea grass, cowpea, and Egyptian clover were recorded plot-wise during 2004–2014. The sustainable yield index (SYI) was calculated using dry biomass yield using the following formula (Singh et al., 1990):
Where σ estimated the standard deviation in the experiment during the 10 years of cultivation.
2.13. Statistical analysis
All the data were tested for normality and homogeneity of variance using the Bartlett and Shapiro–Wilk tests, respectively. Analysis of variance (ANOVA) was carried out using SPSS statistical software (SPSS Inc., 2004, Chicago, USA). The pairwise comparison of the means of all the parameters was performed using Duncan's multiple range test (P < 0.05). The relationship between the soil parameter dry fodder yield and sustainable yield index was determined using Pearson's correlation coefficient. Multiple linear regression models were developed to establish a relation between the yield and SYI with different response variables.
3. Results
3.1. Nutrient management and SOC pools
Farmyard manure (FYM), when applied alone or in conjunction with chemical fertilizer (NPKF), increased the soil pH and decreased bulk density (BD) compared to the unamended control and sole application of NPK (Table 2). The soil pH and BD increased with soil depth. FYM had a more pronounced (P < 0.05) effect on the increase in Walkley–Black organic carbon (WBOC), TOC stocks, and dissolved organic C (DOC). The NPKF had greater WBOC and TOC stocks than NPK and control. The WBOC, TOC, and DOC stocks decreased at a lower depth.
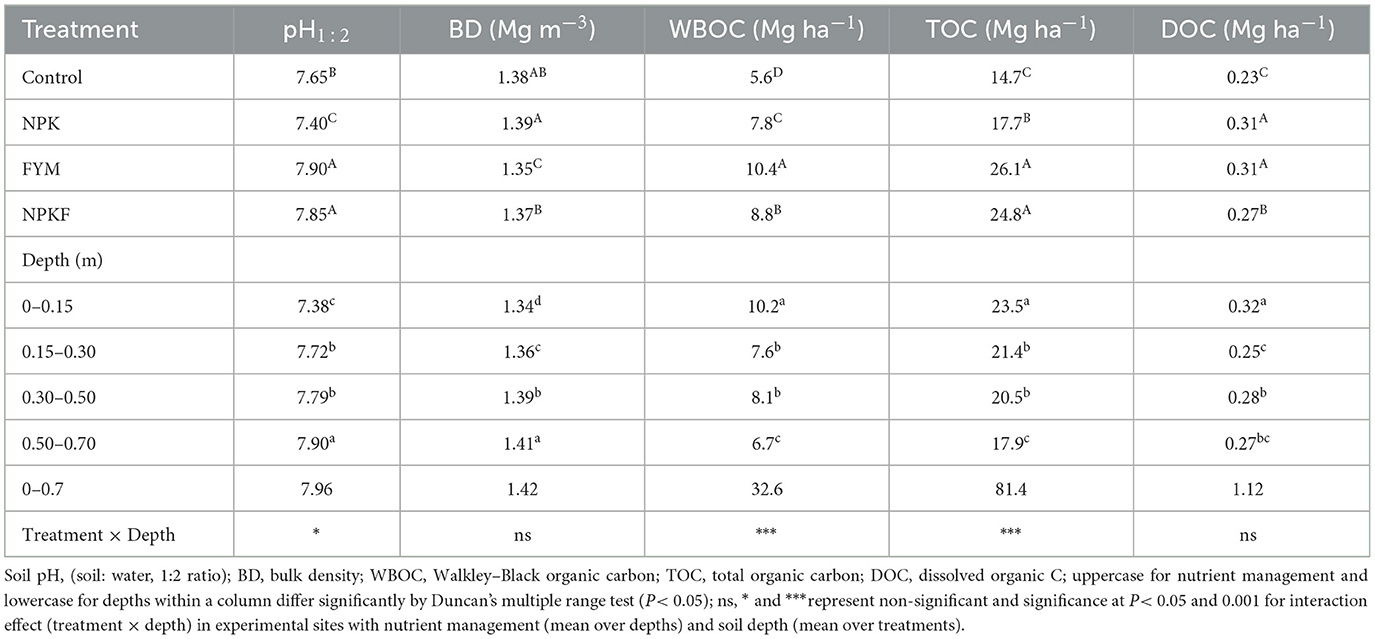
Table 2. Changes in soil physicochemical properties with nutrient management (mean over depths) and soil depth (mean over treatments).
Carbon allocation in different pools varied with nutrient management (NM) strategies and soil depth (Table 3). Very labile (CVL), labile (CL), and non-labile (CNL) SOC stocks in FYM and NPKF were greater than unamended control and NPK. However, the less labile (CLL) SOC pool was greater in NPK than in NPKF and control. Irrespective of NM, approximately 25 and 37% of TOC in 0–0.70 m depth were allocated in CL and CVL pools, respectively (Supplementary Table S1). The per cent of CVL to TOC stock remained at par for all treatments, but CL and CLL proportion to TOC stock was greater for NPKF and NPK, respectively. The proportion of CL to TOC increased with soil depth; however, at the 0.50–0.70 m depth, the proportion of CNL to TOC was greater than the upper soil layers. The active (CAP) and passive (CPP) pools constitute 61 and 42% of the TOC in 0.70 m soil depth, respectively. The FYM and NPK maintained relatively higher proportions of active and passive pools, respectively. The MBC was 8 and 37% greater for FYM and NPKF compared to NPK and control. On the contrary, microbial quotient (MQ) decreased under FYM and NPKF compared to NPK and control. Both MBC stocks and MQ decreased with an increase in soil depth (Figure 1).
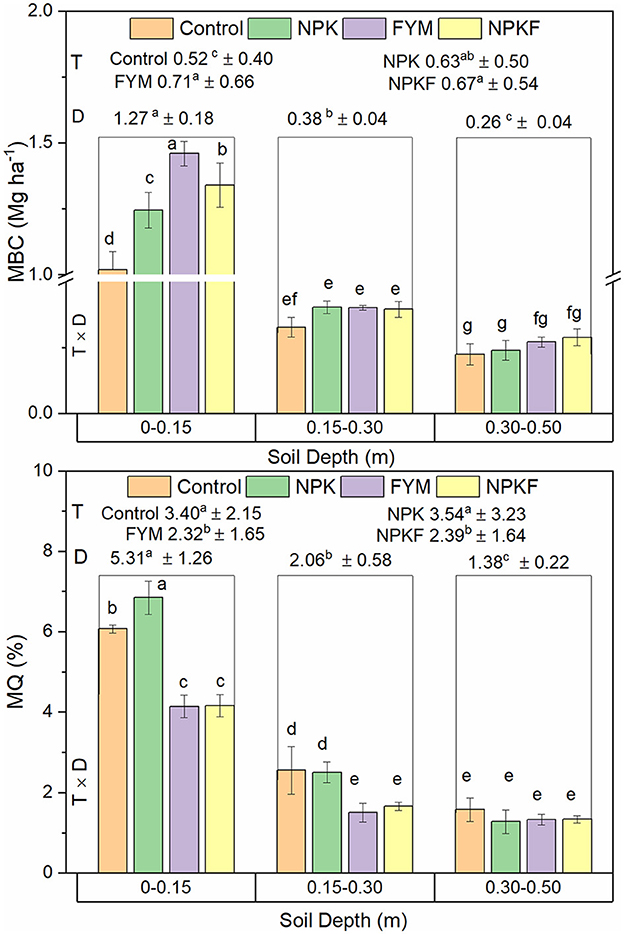
Figure 1. Effect of nutrient management (T), depth (D), and their interaction (T × D) on microbial biomass carbon and microbial quotient; capped lines on bars are standard deviations; n = 9, 12, and 3 for T, D, and T × D, respectively; numbers and bars followed by different letters (a–f) are significantly different (P < 0.05).
3.2. Lability index, recalcitrant index, stratification ratio, carbon management index, and sensitivity index
A lower lability index (LI) and higher recalcitrant index (RImean) appeared for NPK compared to FYM (Table 4). Soils of lower depth had low LI and higher RImean values. A significant improvement in the carbon management index (CMI) was observed for FYM and NPKF compared to NPK and control (Table 4). The values of CMI decreased at lower soil depths. The stratification ratio (SR) of CVL was greater under FYM and NPKF. Except for active pools, the stratification ratio of different pools was greater for FYM. The sensitivity index for different pools was also greater for FYM (Table 5).
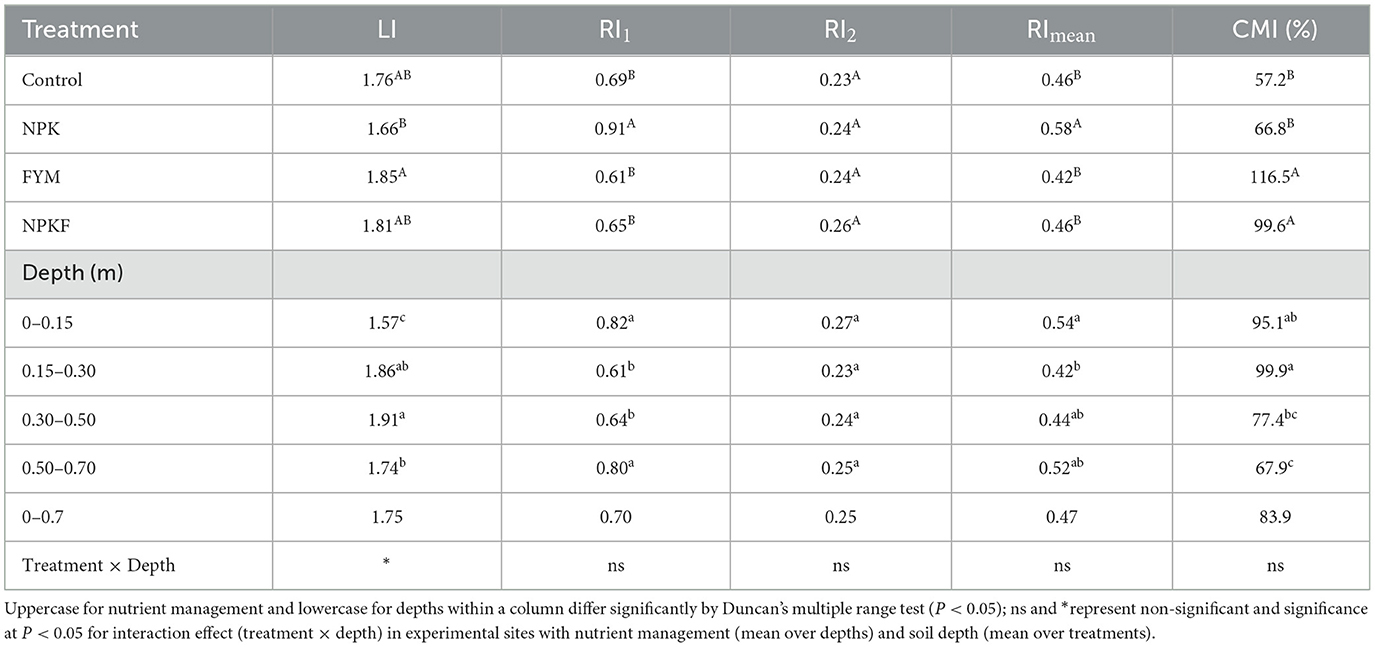
Table 4. Effect of nutrient management practices on lability index (LI), recalcitrant indices (RI), and carbon management index (CMI) in soil depth.
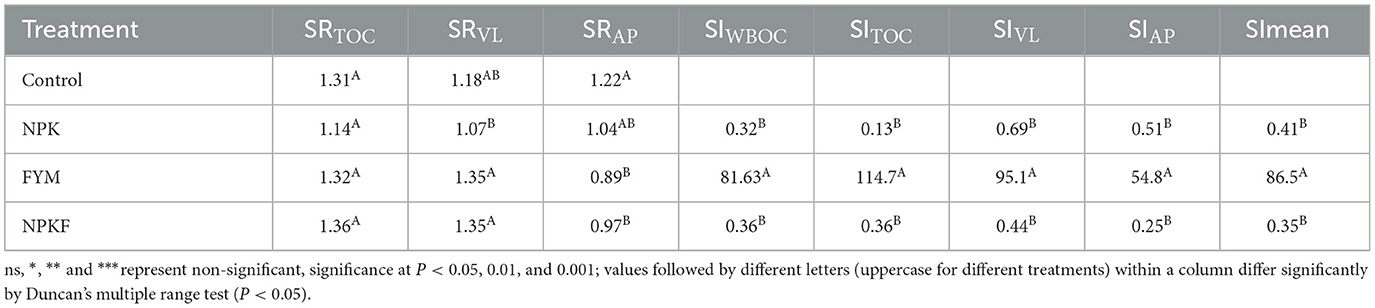
Table 5. Effect of nutrient management practices on SOC stratification ratio (SR) and sensitivity indices (SI, %).
3.3. Fodder yields, C input, and its sequestration and stabilization
The dry forage yield and SYI were greater than FYM (Table 6). The annual return of C through crop residues of guinea grass, cowpea, and Egyptian clover in the form of root, stubble, and rhizodeposition was estimated for different NM practices for the entire 10 years of the cropping cycle (Supplementary Table S1). The return of root, stubble, and rhizodeposition C was greater when NM practices were adopted (NPK/FYM/NPKF) than in control. Besides, crop residue C return, a 4.0 and 3.46 Mg ha−1 C year−1 are supplied through organic amendments FYM and NPKF treatment, respectively. The annual total C turnover, TOC stock, C builds-up per cent, C build-up rates, and C sequestration rate were greater with FYM (Table 6). The conspicuously cropping system alone, without any external nutrient supply, maintained a positive carbon balance and sequestrated 0.8 Mg C ha−1. Approximately 50.9 and 43.9% of the C applied through manure were stabilized with SOC under FYM and NPKF, respectively. The remaining 49.1 to 56.1% of applied carbon escaped from the soil.
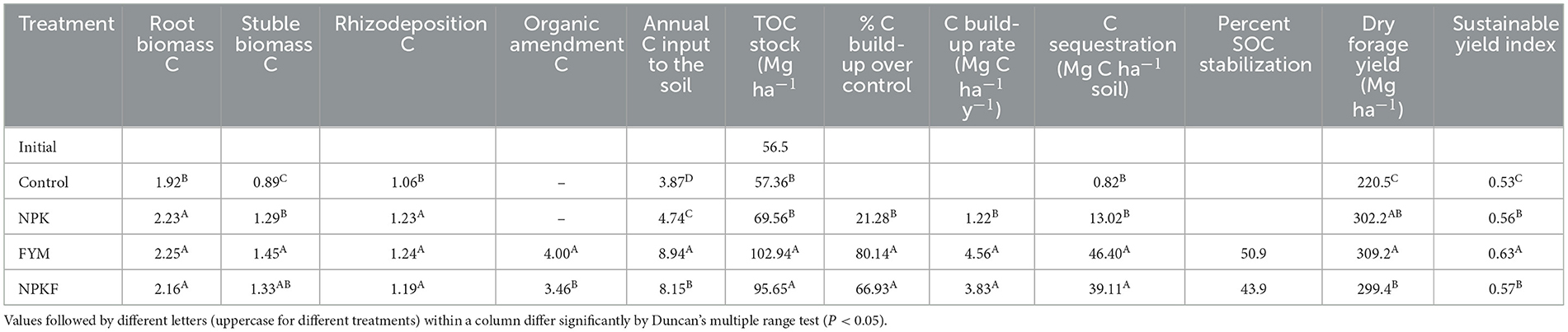
Table 6. Annual return of crop residue C (Mg ha−1), carbon sequestration potential indices for soil (0–0.7 m), yield, and sustainable yield index for different nutrient managements in perennial grass–legume system.
3.4. Soil properties and system productivity
A strong positive correlation was reported among DOC, MBC, CVL, CNL, CPP, WBOC, and TOC (r ≥ 0.59***); these indices had a significant effect on CPI and CMI and eventually affected the DFY and SYI (Figure 2). The SImean also showed a strong positive correlation with MBC, CVL, WBOC, TOC, CMI, and CPI, while a strong negative correlation was observed between BD and CPI, RImean and MBC, CAP, CVL, CMI; MQ with CVL, CAP, CNL, WBOC, TOC, CPI, CMI, SYI; and LI with CNL, CCP, and RIm. Multiple regression analyses showed that WBOC and SImean explained approximately 94 and 96% variability in SYI and DFY, and MBC, DOC, and Cpp, respectively (Table 7).
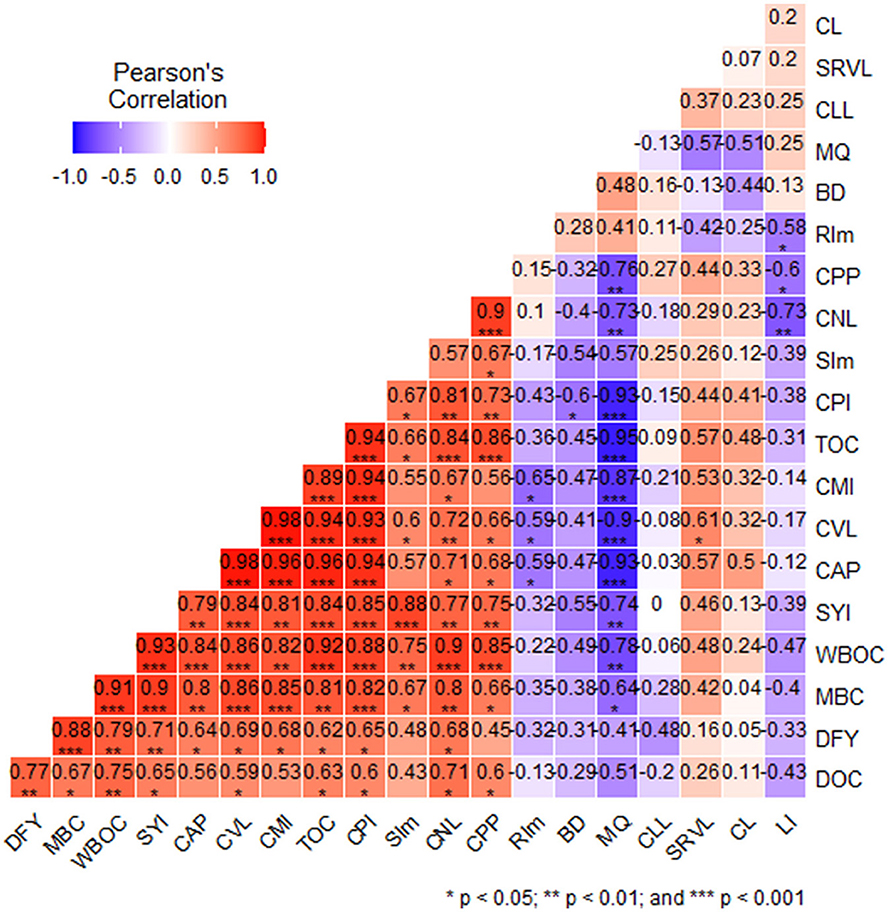
Figure 2. Correlation matrix among system yield attributes and SOC pools and their indices; DFY, dry forage yield; SYI, sustainable yield index; MBC, microbial biomass carbon; WBOC, Walkley–Black organic carbon; TOC, total organic carbon; DOC, dissolved organic C; RImean, mean recalcitrant index; CVL, very labile pool of soil organic carbon; CL, labile pool of soil organic carbon; CLL, less labile pool of soil organic carbon; CAP, active pool of soil organic carbon; CPP, passive pool of soil organic carbon; RIm, mean recalcitrant indices; CPI, carbon pool index; CMI, carbon management index; BD, bulk density; MQ, microbial quotient; SRVL, stratification ratio of labile pool of soil organic carbon; SImean, mean sensitivity index; without *, *, ** and *** represent non-significant, significance at P < 0.05, 0.01, and 0.001.
4. Discussions
Soil health and agricultural sustainability largely depend on the stock and stability of SOC in agroecosystems. Continuous cropping in semi-arid regions often decreases SOC pools, and the net depletion of TOC stock accelerates because of hot summer, perturbation of the system, and photo-oxidation of SOC (Srinivasarao et al., 2021). Soil disturbances because of tillage and other cultural practices hasten the SOC oxidation by exposing the protected C to an ultimate reduction in SOC (Six et al., 2002). Several studies reported a decline in TOC because of long-term cropping with annual crops under semi-arid and sub-tropical regions (Majumder et al., 2008b; Srinivasarao et al., 2012; Anantha et al., 2022). Contrarily, the guinea grass-based round-the-year forage production systems provided a protective covering to the soil and improved aggregation, SOC, and soil quality in pastures and grassland because of perennial growth habit, profuse rooting of the tussocks, adaptation to conservation tillage practices, and greater biomass turnover (Sanderson and Adler, 2008; Das et al., 2014; Kibet et al., 2016; Chen et al., 2022). The positive build-up of SOC in control plots in the present experiment without the application of any external carbon except through crops also supports the hypothesis of the SOC protection against oxidation loss because of the protective covering of the soil surface and reduced tillage (Rai et al., 2013; López-Vicente et al., 2021).
The long-term application of chemical fertilizer (NPK) resulted in increased SOC content because of greater return of root biomass and rhizodeposition (Srinivasarao et al., 2012; Datta et al., 2018; Pausch and Kuzyakov, 2018). With the application of FYM, the SOC pool was increased further because of the positive impact of FYM on root biomass production and its direct contribution to SOC. The integrated nutrient management under NPKF provided favorable conditions for microbial proliferation (Majumder et al., 2008a). The DOC is derived from root exudates, above-ground residues, microbial biomass debris, soluble organic carbon and liberated from decomposition of added organic matter (Christ and David, 1996; Liang et al., 2011). Therefore, NM practices NPK, FYM, and NPKF increased the DOC stock compared to the control. Earlier findings also reported higher values of DOC in NPKF-treated plots than the NPK (Benbi et al., 2015; Li et al., 2020; Mustafa et al., 2020). Approximately 90% of roots in guinea grass are distributed in the top 0–0.40 m depth (Singh, 1996). Therefore, the lowered rhizodeposition and root biomass in sub-surface soil were the reasons for decreased SOC content with increasing depth (Basak et al., 2021a; Anantha et al., 2022). The increased DOC stock in 0.3–0.7 m soil depth was mainly because of the leaching of the soluble soil organic molecules to lower depths through well-drained silty loam soil under the influence of irrigation and/or rainwater (Hussain et al., 2020).
The FYM applications rate was different for the treatment FYM and NPKF. Thus, the annual total C input to soil was greater in FYM than NPKF (P < 0.05). However, the content of passive pools was similar for FYM and NPKF treatments. Among the four pools (CVL, CL, CLL, and CNL), very labile and less-labile pools of SOC were sensitive to NM practices. Therefore, the CVL fraction increased with annual C input. A major part of the sequestered SOC in NPK, FYM, and NPKF was retained in less labile pools (CLL) (Table 3). A greater amount of SOC allocation in CLL with similar treatments was earlier reported for sorghum–wheat and rice–wheat cropping systems (Datta et al., 2018; Singh and Benbi, 2018; Basak et al., 2021a).
Management practices affect soil quality and influence soil functions and crop productivity. The CMI indicating higher stable SOC is used to assess the impact of management options on soil productivity. The FYM and NPKF had greater values of CMI content on imposing these practices. Greater SOC further corroborated this in less labile and non-labile pools under these treatments. The findings of this experiment were in agreement with the CMI values reported for groundnut (Anantha et al., 2022) and pearl millet–wheat systems (Moharana et al., 2012). The screening of MBC, DOC, Cpp for DFY and WBOC, and SIm for SYI highlighted the significance of these parameters as complementary indicators for capturing the effect of nutrient management practices on soil properties and productivity potential of perennial grass–legume systems. Benbi et al. (2015) also identified MBC and DOC as the most sensitive indicator of management-induced changes in the soil.
The FYM and NPKF recorded a higher C build-up. The greater C sequestration in FYM-treated soils compared to NPK was mainly associated with a wider C: N ratio and lignin and polyphenols in FYM. These constituents of FYM form stable complexes with proteins of plant origin to protect them from further microbial attack with the resultant stabilization of SOC in soils (Majumder et al., 2008b). Earlier studies reported approximately 27% stabilization of the applied C in long-term experiments in semi-arid regions of India (Srinivasarao et al., 2012; Anantha et al., 2022). The C–stabilization vary 44–51% in FYM and NPKF treatment in perennial grass–legume cropping. The C stabilization is the outcome of the interaction of the factors viz., C inputs and their biochemical constituents, soil properties, soil perturbation, covering of soil surface, and net “fallowing period” in the calendar year (Schmidt et al., 2011). In this study, large biomass return, the smothering effect of component crops, and minimum soil disturbances protected the applied carbon from oxidation and were responsible for greater SOC stabilization. Lower carbon addition and increased nitrogen availability promoting organic matter decomposition in NPKF were responsible for low SOC stabilization in NPKF compared to FYM.
5. Conclusion
The perennial grass–legume-based cropping system favors the build-up of SOC. Nutrient management through organic manure alone, in combination with NPK fertilizers, had a greater potential to sequester carbon in the soil. Integrated nutrient management and sole FYM partitioned a greater proportion of SOC in the non-labile pool with a 44–51% stabilization of the applied carbon. FYM alone or in combination with NPK also improved the carbon management index and stratification ratio. The dissolved organic and microbial biomass C and SOC in passive pools were identified for predicting the dry fodder yield. Walkley–Black oxidizable organic C and sensitivity indices of SOC pools were good predictors for the sustainable yield index of the perennial grass–legume system. Thus, this study concludes that organics and integrated nutrient management practices can ensure the stay of the SOC in soil for a longer period. Integrating perennial grass–legume-based cropping in the farming system can also provide an opportunity to maintain long-term sustainability and offset the farm enterprises' carbon footprint in similar agro-ecologies.
Data availability statement
The original contributions presented in the study are included in the article/Supplementary material, further inquiries can be directed to the corresponding authors.
Author contributions
AR: conceptualization, formal analysis, funding acquisition, and original draft preparation and final editing. NB: conceptualization, formal analysis, and original draft preparation and final editing. AD: investigation and methodology. SR and JS: investigation. SD and TK: data curation and investigation. SK: investigation and funding acquisition. PC, PS, and SB: data curation and preparation of the manuscript. All authors contributed to the article and approved the submitted version.
Funding
This research was funded by the Indian Council of Agricultural Research (ICAR). The authors are thankful to the Director(s) of ICAR–Indian Grassland and Fodder Research Institute (IGFRI) for funding the project (CP 1.1.11).
Acknowledgments
The authors recognize the support delivered by the technical staff Mr. S. C. Richhariya, Dr. D. K. Singh, and Mr. K. P. Rao in the analytical work and preparation of the manuscript.
Conflict of interest
The authors declare that the research was conducted in the absence of any commercial or financial relationships that could be construed as a potential conflict of interest.
The reviewer SS declared a past co-authorship with the author SB to the handling editor.
Publisher's note
All claims expressed in this article are solely those of the authors and do not necessarily represent those of their affiliated organizations, or those of the publisher, the editors and the reviewers. Any product that may be evaluated in this article, or claim that may be made by its manufacturer, is not guaranteed or endorsed by the publisher.
Supplementary material
The Supplementary Material for this article can be found online at: https://www.frontiersin.org/articles/10.3389/fmicb.2023.1173986/full#supplementary-material
References
Alavi-Murillo, G., Diels, J., Gilles, J., and Willems, P. (2022). Soil organic carbon in Andean high-mountain ecosystems: importance, challenges, and opportunities for carbon sequestration. Reg. Environ. Chang. 22, 128. doi: 10.1007/s10113-022-01980-6
Allison, L. E., and Moodie, C. D. (1965). “Carbonate,” in Methods of Soil Analysis. Part 2. Chemical and Microbiological properties, ed. C. A. Black (American Society of Agronomy, Madison), 1379–1396. doi: 10.2134/agronmonogr9.2.c40
Anantha, K. C., Majumder, S. P., Badole, S., Padhan, D., Datta, A., Mandal, B., et al. (2020). Pools of organic carbon in soils under a long-term rice–rice system with different organic amendments in hot, sub-humid India. Carbon Manag. 11, 331–339. doi: 10.1080/17583004.2020.1783624
Anantha, K. C., Mandal, B., Badole, S., Majumder, S. P., Datta, A., Padhan, D., et al. (2022). Distribution of sequestered carbon in different pools in Alfisols under long-term groundnut system of hot arid region of India. Eur. J. Agron. 135, 126467. doi: 10.1016/j.eja.2022.126467
Babu, S., Singh, R., Avasthe, R. K., Yadav, G. S., Mohapatra, K. P., Selvan, T., et al. (2020). Soil carbon dynamics in Indian Himalayan intensified organic rice-based cropping sequences. Ecol. Indic. 114, 106292. doi: 10.1016/j.ecolind.2020.106292
Bal, S. K., Sandeep, V. M., Kumar, P. V., Rao, A. V. M. S., Pramod, V. P., Manikandan, N., et al. (2022). Assessing impact of dry spells on the principal rainfed crops in major dryland regions of India. Agric. For. Meteorol. 313, 108768. doi: 10.1016/j.agrformet.2021.108768
Banger, K., Toor, G. S., Biswas, A., Sidhu, S. S., and Sudhir, K. (2010). Soil organic carbon fractions after 16-years of applications of fertilizers and organic manure in a Typic Rhodalfs in semi-arid tropics. Nutr. Cycl. Agroecosyst. 86, 391–399. doi: 10.1007/s10705-009-9301-8
Basak, N., Mandal, B., Datta, A., Kundu, M. C., Rai, A. K., Basak, P., et al. (2021a). Stock and stability of organic carbon in soils under major agro-ecological zones and cropping systems of sub-tropical India. Agric. Ecosyst. Environ. 312, 107317. doi: 10.1016/j.agee.2021.107317
Basak, N., Sheoran, P., Sharma, R., Yadav, R. K., Singh, R. K., Kumar, S., et al. (2021b). Gypsum and pressmud amelioration improve soil organic carbon storage and stability in sodic agroecosystems. Land Degrad. Dev. 32, 4430–4444. doi: 10.1002/ldr.4047
Batjes, N. H. (1996). Total carbon and nitrogen in the soils of the world. Eur. J. Soil Sci. 47, 151–163. doi: 10.1111/j.1365-2389.1996.tb01386.x
Benbi, D. K., Brar, K., Toor, A. S., and Sharma, S. (2015). Sensitivity of labile soil organic carbon pools to long-term fertilizer, straw and manure management in rice-wheat system. Pedosphere 25, 534–545. doi: 10.1016/S1002-0160(15)30034-5
Blair, G. J., Lefroy, R. D., and Lisle, L. (1995). Soil carbon fractions based on their degree of oxidation, and the development of a carbon management index for agricultural systems. Aust. J. Agric. Res. 46, 1459–1466. doi: 10.1071/AR9951459
Blake, G. R., and Hartage, K. H. (1986). “Bulk density,” in Method of soil analysis, Part I, 2nd ed. Physical and Mineralogical Methods, ed. A. Klute (Agronomy Monograph no. 9), 363–375. doi: 10.2136/sssabookser5.1.2ed.c13
Chan, K. Y., Bowman, A., and Oates, A. (2001). Oxidizible organic carbon fractions and soil quality changes in an oxic paleustalf under different pasture leys. Soil Sci. 166, 61–67. doi: 10.1097/00010694-200101000-00009
Chaudhary, S., Dheri, G. S., and Brar, B. S. (2017). Long-term effects of NPK fertilizers and organic manures on carbon stabilization and management index under rice-wheat cropping system. Soil Tillage Res. 166, 59–66. doi: 10.1016/j.still.2016.10.005
Chen, J., Lærke, P. E., and Jørgensen, U. (2022). Land conversion from annual to perennial crops: A win-win strategy for biomass yield and soil organic carbon and total nitrogen sequestration. Agric. Ecosyst. Environ. 330, 107907. doi: 10.1016/j.agee.2022.107907
Christ, M. J., and David, M. B. (1996). Dynamics of extractable organic carbon in Spodosol forest floors. Soil Biol. Biochem. 28, 1171–1179. doi: 10.1016/0038-0717(96)00128-9
Das, A., Lal, R., Patel, D. P., Idapuganti, R. G., Layek, J., Ngachan, S. V, et al. (2014). Effects of tillage and biomass on soil quality and productivity of lowland rice cultivation by small scale farmers in North Eastern India. Soil Tillage Res. 143, 50–58. doi: 10.1016/j.still.2014.05.012
Datta, A., Mandal, B., Badole, S. A. K. C., Majumder, S. P., et al. (2018). Interrelationship of biomass yield, carbon input, aggregation, carbon pools and its sequestration in Vertisols under long-term sorghum-wheat cropping system in semi-arid tropics. Soil Tillage Res. 184, 164–175. doi: 10.1016/j.still.2018.07.004
Doblas-Rodrigo, Á., Gallejones, P., Artetxe, A., Rosa, E., del Hierro, Ó., and Merino, P. (2022). Grassland contribution to soil organic carbon stock under climate change scenarios in Basque Country (Spain). Reg. Environ. Chang. 22, 34. doi: 10.1007/s10113-022-01877-4
Franzluebbers, A. J. (2002). Soil organic matter stratification ratio as an indicator of soil quality. Soil Tillage Res. 66, 95–106. doi: 10.1016/S0167-1987(02)00018-1
Gee, G. W., and Bauder, J. W. (1986). Particle-size analysis. Methods Soil Anal. 383–411. doi: 10.2136/sssabookser5.1.2ed.c15
Ghosh, P. K., Saha, R., Gupta, J. J., Ramesh, T., Das, A., Lama, T. D., et al. (2009). Long-term effect of pastures on soil quality in acid soil of North-East India. Soil Res. 47, 372–379. doi: 10.1071/SR08169
Ghosh, P. K., Venkatesh, M. S., Hazra, K. K., and Narendra, K. (2012). Long-term effect of pulses and nutrient management on soil organic carbon dynamics and sustainability on an inceptisol of indo-gangetic plains of India. Exp. Agric. 48, 473–487. doi: 10.1017/S0014479712000130
Ghosh, S., Wilson, B. R., Mandal, B., Ghoshal, S. K., and Growns, I. (2010). Changes in soil organic carbon pool in three long-term fertility experiments with different cropping systems and inorganic and organic soil amendments in the eastern cereal belt of India. Soil Res. 48, 413–420. doi: 10.1071/SR09089
Hasegawa, T., Sakurai, G., Fujimori, S., Takahashi, K., Hijioka, Y., and Masui, T. (2021). Extreme climate events increase risk of global food insecurity and adaptation needs. Nat. Food 2, 587–595. doi: 10.1038/s43016-021-00335-4
Hussain, M. Z., Robertson, G. P., Basso, B., and Hamilton, S. K. (2020). Leaching losses of dissolved organic carbon and nitrogen from agricultural soils in the upper US Midwest. Sci. Total Environ. 734, 139379. doi: 10.1016/j.scitotenv.2020.139379
Kibet, L. C., Blanco-Canqui, H., Mitchell, R. B., and Schacht, W. H. (2016). Root biomass and soil carbon response to growing perennial grasses for bioenergy. Energy. Sustain. Soc. 6, 1. doi: 10.1186/s13705-015-0065-5
Kim, K., Daly, E. J., Gorzelak, M., and Hernandez-Ramirez, G. (2022). Soil organic matter pools response to perennial grain cropping and nitrogen fertilizer. Soil Tillage Res. 220, 105376. doi: 10.1016/j.still.2022.105376
Kumar, S., Agrawal, R. K., Dixit, A. K., Rai, A. K., and Rai, S. K. (2012). Forage crops and their management. Indian Grassland and Fodder Research Institute, Jhansi 284 003, Uttar Pradesh, India: Technology Bulletin no. 2/2012. 60.
Lal, R. (2015). Soil carbon sequestration in agro-ecosystems of India. J. Indian Soc. Soil Sci. 63, 125–143. doi: 10.5958/0974-0228.2015.00018.3
Li, T., Zhang, Y., Bei, S., Li, X., Reinsch, S., Zhang, H., et al. (2020). Contrasting impacts of manure and inorganic fertilizer applications for nine years on soil organic carbon and its labile fractions in bulk soil and soil aggregates. Catena 194, 104739. doi: 10.1016/j.catena.2020.104739
Liang, B., Yang, X., He, X., and Zhou, J. (2011). Effects of 17-year fertilization on soil microbial biomass C and N and soluble organic C and N in loessial soil during maize growth. Biol. Fertil. Soils 47, 121–128. doi: 10.1007/s00374-010-0511-7
López-Vicente, M., Gómez, J. A., Guzmán, G., Calero, J., and García-Ruiz, R. (2021). The role of cover crops in the loss of protected and non-protected soil organic carbon fractions due to water erosion in a Mediterranean olive grove. Soil Tillage Res. 213, 105119. doi: 10.1016/j.still.2021.105119
Luo, Z., Wang, E., Baldock, J., and Xing, H. (2014). Potential soil organic carbon stock and its uncertainty under various cropping systems in Australian cropland. Soil Res. 52, 463–475. doi: 10.1071/SR13294
Majumder, B., Mandal, B., and Bandyopadhyay, P. K. (2008a). Soil organic carbon pools and productivity in relation to nutrient management in a 20-year-old rice–berseem agroecosystem. Biol. Fertil. Soils 44, 451–461. doi: 10.1007/s00374-007-0226-6
Majumder, B., Mandal, B., Bandyopadhyay, P. K., and Chaudhury, J. (2007). Soil organic carbon pools and productivity relationships for a 34 year old rice-wheat-jute agroecosystem under different fertilizer treatments. Plant Soil 297, 53–67. doi: 10.1007/s11104-007-9319-0
Majumder, B., Mandal, B., Bandyopadhyay, P. K., Gangopadhyay, A., Mani, P. K., Kundu, A. L., et al. (2008b). Organic amendments influence soil organic carbon pools and rice-wheat productivity. Soil Sci. Soc. Am. J. 72, 775–785. doi: 10.2136/sssaj2006.0378
Mandal, B., Majumder, B., Adhya, T. K., Bandyopadhyay, P. K., Gangopadhyay, A., Sarkar, D., et al. (2008). Potential of double-cropped rice ecology to conserve organic carbon under subtropical climate. Glob. Chang. Biol. 14, 2139–2151. doi: 10.1111/j.1365-2486.2008.01627.x
Mandal, B., Majumder, B., Bandyopadhyay, P. K., Hazra, G. C., Gangopadhyay, A., Samantaray, R. N., et al. (2007). The potential of cropping systems and soil amendments for carbon sequestration in soils under long-term experiments in subtropical India. Glob. Chang. Biol. 13, 357–369. doi: 10.1111/j.1365-2486.2006.01309.x
McGill, W. B., Cannon, K. R., Robertson, J. A., and Cook, F. D. (1986). Dynamics of soil microbial biomass and water-soluble organic C in Breton L after 50 years of cropping to two rotations. Can. J. Soil Sci. 66, 1–19. doi: 10.4141/cjss86-001
Mishra, V. K., Srivastava, S., Bhardwaj, A. K., Sharma, D. K., Singh, Y. P., and Nayak, A. K. (2015). Resource conservation strategies for rice-wheat cropping systems on partially reclaimed sodic soils of the Indo-Gangetic region, and their effects on soil carbon. Nat. Resour. Forum 39, 110–122. doi: 10.1111/1477-8947.12071
Mitran, T., Mishra, U., Lal, R., Ravisankar, T., and Sreenivas, K. (2018). Spatial distribution of soil carbon stocks in a semi-arid region of India. Geoderma Reg. 15, e00192. doi: 10.1016/j.geodrs.2018.e00192
Mohapatra, S., Mishra, C., Merritt, B. B., Pattathil, S., and Thatoi, H. (2019). Evaluating the Role of Ultrasonication-Assisted Alkali Pretreatment and Enzymatic Hydrolysis on Cellwall Polysaccharides of Pennisetum Grass Varieties as Potential Biofuel Feedstock. ChemistrySelect 4, 1042–1054. doi: 10.1002/slct.201802187
Moharana, P. C., Naitam, R. K., Verma, T. P., Meena, R. L., Kumar, S., Tailor, B. L., et al. (2017). Effect of long-term cropping systems on soil organic carbon pools and soil quality in western plain of hot arid India. Arch. Agron. Soil Sci. 63, 1661–1675. doi: 10.1080/03650340.2017.1304637
Moharana, P. C., Sharma, B. M., Biswas, D. R., Dwivedi, B. S., and Singh, R. V (2012). Long-term effect of nutrient management on soil fertility and soil organic carbon pools under a 6-year-old pearl millet–wheat cropping system in an Inceptisol of subtropical India. Field Crop Res. not F. Crop Res. 136, 32–41. doi: 10.1016/j.fcr.2012.07.002
Mustafa, A., Minggang, X., Ali Shah, S. A., Abrar, M. M., Nan, S., Baoren, W., et al. (2020). Soil aggregation and soil aggregate stability regulate organic carbon and nitrogen storage in a red soil of southern China. J. Environ. Manage. 270, 110894. doi: 10.1016/j.jenvman.2020.110894
Ngangom, B., Das, A., Lal, R., Idapuganti, R. G., Layek, J., Basavaraj, S., et al. (2020). Double mulching improves soil properties and productivity of maize-based cropping system in eastern Indian Himalayas. Int. Soil Water Conserv. Res. 8, 308–320. doi: 10.1016/j.iswcr.2020.07.001
Oldfield, E. E., Bradford, M. A., and Wood, S. A. (2019). Global meta-analysis of the relationship between soil organic matter and crop yields. Soil 5, 15–32. doi: 10.5194/soil-5-15-2019
Pausch, J., and Kuzyakov, Y. (2018). Carbon input by roots into the soil: Quantification of rhizodeposition from root to ecosystem scale. Glob. Chang. Biol. 24, 1–12. doi: 10.1111/gcb.13850
Poffenbarger, H. J., Sawyer, J. E., Barker, D. W., Olk, D. C., Six, J., and Castellano, M. J. (2018). Legacy effects of long-term nitrogen fertilizer application on the fate of nitrogen fertilizer inputs in continuous maize. Agric. Ecosyst. Environ. 265, 544–555. doi: 10.1016/j.agee.2018.07.005
Rai, A. K., Ghosh, P. K., Ram, S. N., Singh, S., Kumar, S., Manhanta, S. K., et al. (2013). Carbon Sequestration in Forage Based Land Use Systems. Jhansi, Uttar Pradesh, India: Indian Grassland and Fodder Research Institute.
Sainju, U. M., Stevens, W. B., and Caesar-TonThat, T. (2014). Soil carbon and crop yields affected by irrigation, tillage, cropping system, and nitrogen fertilization. Soil Sci. Soc. Am. J. 78, 936–948. doi: 10.2136/sssaj2013.12.0514
Sanderman, J., Creamer, C., Baisden, W. T., Farrell, M., and Fallon, S. (2017). Greater soil carbon stocks and faster turnover rates with increasing agricultural productivity. Soil 3, 1–16. doi: 10.5194/soil-3-1-2017
Sanderson, M. A., and Adler, P. R. (2008). Perennial forages as second generation bioenergy crops. Int. J. Mol. Sci. 9, 768–788. doi: 10.3390/ijms9050768
Sarkar, R., Corriher-Olson, V., Long, C., and Somenahally, A. (2020). Challenges and potentials for soil organic carbon sequestration in forage and grazing systems. Rangel. Ecol. Manag. 73, 786–795. doi: 10.1016/j.rama.2020.04.002
Schmidt, M. W. I., Torn, M. S., Abiven, S., Dittmar, T., Guggenberger, G., Janssens, I. A., et al. (2011). Persistence of soil organic matter as an ecosystem property. Nature 478, 49–56. doi: 10.1038/nature10386
Singh, K. A. (1996). Effect of age, cutting interval, nitrogen and farmyard manure on root-growth parameters of forage grasses in an acid inceptisol. Indian J. Agric. Sci. 66, 38–44.
Singh, P., and Benbi, D. K. (2018). Nutrient management effects on organic carbon pools in a sandy loam soil under rice-wheat cropping. Arch. Agron. Soil Sci. 64, 1879–1891. doi: 10.1080/03650340.2018.1465564
Singh, R. P., Das, S. K., Bhaskara Rao, U. M., and Narayana Reddy, M. (1990). Sustainability index under different management, Annual report. Hyderabad, India.
Six, J., Conant, R. T., Paul, E. A., and Paustian, K. (2002). Stabilization mechanisms of soil organic matter: Implications for C-saturation of soils. Plant Soil 241, 155–176. doi: 10.1023/A:1016125726789
Srinivasarao, C., Kundu, S., Shanker, A. K., Naik, R. P., Vanaja, M., Venkanna, K., et al. (2016). Continuous cropping under elevated CO2: Differential effects on C4 and C3 crops, soil properties and carbon dynamics in semi-arid alfisols. Agric. Ecosyst. Environ. 218, 73–86. doi: 10.1016/j.agee.2015.11.016
Srinivasarao, C., Singh, S. P., Kundu, S., Abrol, V., Lal, R., Abhilash, P. C., et al. (2021). Integrated nutrient management improves soil organic matter and agronomic sustainability of semiarid rainfed Inceptisols of the Indo-Gangetic Plains. J. Plant Nutr. Soil Sci. 184, 562–572. doi: 10.1002/jpln.202000312
Srinivasarao, C., Venkateswarlu, B., Lal, R., Singh, A. K., and Kundu, S. (2013). Sustainable management of soils of dryland ecosystems of India for enhancing agronomic productivity and sequestering carbon. Adv. Agron. 121, 253–329. doi: 10.1016/B978-0-12-407685-3.00005-0
Srinivasarao, C., Venkateswarlu, B., Lal, R., Singh, A. K., Kundu, S., Vittal, K. P. R., et al. (2012). Soil carbon sequestration and agronomic productivity of an Alfisol for a groundnut-based system in a semiarid environment in southern India. Eur. J. Agron. 43, 40–48. doi: 10.1016/j.eja.2012.05.001
Subbiah, B. V., and Asija, G. L. (1956). A rapid procedure for assessment of available nitrogen in rice soils. Curr. Sci. 25, 259–260.
Thangaraj, M., and O'Toole, J. C. (1986). Development and testing of an improved soil-root sampling technique for rice in flooded puddled soil1. Agron. J. 78, 940–942. doi: 10.2134/agronj1986.00021962007800050040x
Vance, E. D., Brookes, P. C., and Jenkinson, D. S. (1987). An extraction method for measuring soil microbial biomass C. Soil Biol. Biochem. 19, 703–707. doi: 10.1016/0038-0717(87)90052-6
Voroney, R. P., and Paul, E. A. (1984). Determination of kC and kNin situ for calibration of the chloroform fumigation-incubation method. Soil Biol. Biochem. 16, 9–14. doi: 10.1016/0038-0717(84)90117-2
Walkley, A., and Black, I. A. (1934). An examination of the degtjareff method for determining soil organic matter, and a proposed modification of the chromic acid titration method. Soil Sci. 37, 29–38. doi: 10.1097/00010694-193401000-00003
Weil, R. R., Islam, K. R., Stine, M. A., Gruver, J. B., and Samson-Liebig, S. E. (2017). Estimating active carbon for soil quality assessment : A simplified method for laboratory and field use Estimating active carbon for soil quality assessment : A simpli ® ed method for laboratory and ® eld use. Am. J. Altern. Agric. 18, 3–17. doi: 10.1079/AJAA2003003
Yadav, G. S., Das, A., Lal, R., Babu, S., Datta, M., Meena, R. S., et al. (2019). Impact of no-till and mulching on soil carbon sequestration under rice (Oryza sativa L.)-rapeseed (Brassica campestris L. var. rapeseed) cropping system in hilly agro-ecosystem of the Eastern Himalayas, India. Agric. Ecosyst. Environ. 275, 81–92. doi: 10.1016/j.agee.2019.02.001
Yadav, R. K., Purakayastha, T. J., Khan, M. A., and Kaushik, S. C. (2017). Long-term impact of manuring and fertilization on enrichment, stability and quality of organic carbon in Inceptisol under two potato-based cropping systems. Sci. Total Environ. 609, 1535–1543. doi: 10.1016/j.scitotenv.2017.07.128
Keywords: carbon input, dissolved organic carbon, microbial biomass C, semi-arid agroecosystem, SOC indices, SOC sequestration, stratification ratio
Citation: Rai AK, Basak N, Dixit AK, Rai SK, Das SK, Singh JB, Kumar S, Kumar TK, Chandra P, Sundha P and Bedwal S (2023) Changes in soil microbial biomass and organic C pools improve the sustainability of perennial grass and legume system under organic nutrient management. Front. Microbiol. 14:1173986. doi: 10.3389/fmicb.2023.1173986
Received: 25 February 2023; Accepted: 28 March 2023;
Published: 21 April 2023.
Edited by:
Upendra Kumar, National Rice Research Institute (ICAR), IndiaReviewed by:
Sandeep Kumar Sihag, Indian Agricultural Research Institute (ICAR), IndiaDeepranjan Sarkar, Banaras Hindu University, India
Copyright © 2023 Rai, Basak, Dixit, Rai, Das, Singh, Kumar, Kumar, Chandra, Sundha and Bedwal. This is an open-access article distributed under the terms of the Creative Commons Attribution License (CC BY). The use, distribution or reproduction in other forums is permitted, provided the original author(s) and the copyright owner(s) are credited and that the original publication in this journal is cited, in accordance with accepted academic practice. No use, distribution or reproduction is permitted which does not comply with these terms.
*Correspondence: Nirmalendu Basak, bmlybWFsZW5kdWJhc2FrQHJlZGlmZm1haWwuY29t; TmlybWFsZW5kdS5CYXNha0BpY2FyLmdvdi5pbg==; Anoop Kumar Dixit, QW5vb3AuRGl4aXRAaWNhci5nb3YuaW4=
†ORCID: Nirmalendu Basak orcid.org/0000-0002-2281-0253
Suchit Kumar Rai orcid.org/0000-0002-8045-9828
Parul Sundha orcid.org/0000-0003-1980-9013