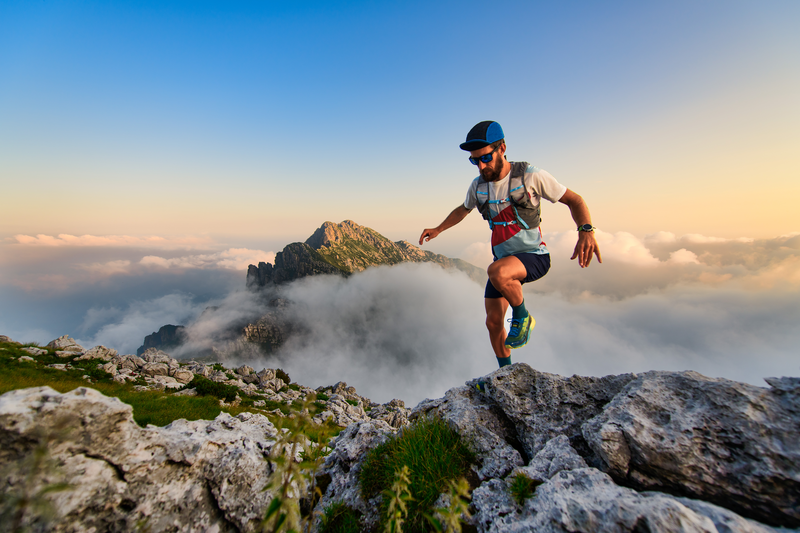
94% of researchers rate our articles as excellent or good
Learn more about the work of our research integrity team to safeguard the quality of each article we publish.
Find out more
ORIGINAL RESEARCH article
Front. Microbiol. , 02 May 2023
Sec. Antimicrobials, Resistance and Chemotherapy
Volume 14 - 2023 | https://doi.org/10.3389/fmicb.2023.1173287
Escherichia coli belonging to multilocus sequence type 38 (ST38) is a well-known cause of extra-intestinal infections in humans, and are frequently associated with resistance to extended-spectrum cephalosporins (ESCs). Resistance to carbapenems, mediated by blaOXA-genes has also been reported in this ST. Recently, the European Centre for Disease Prevention and Control (ECDC) released a rapid risk assessment on the increased detection of OXA-244 producing E. coli ST38 in humans, requesting further knowledge to determine the source. ST38 is also one of the most common STs among ESC-resistant E. coli from broiler production. Our aim was to investigate the genetic characteristics and relationship between E. coli ST38 from broiler production and humans, and to investigate if there has been a potential spillover between these sources. A total of 288 E. coli ST38 genomes isolated from humans in Europe (collected 2009–2019) and from Nordic broiler production (collected 2011–2014) were analyzed. The results showed distinct monophyletic clades associated to humans and broiler production. Furthermore, there were differences in the ESC resistance genes present in E. coli ST38 from the two sources. The blaOXA-244 gene was not present in E. coli from broiler production. Our results show that ST38 from humans and broiler production belong to well-separated clades, and suggest that the increased detection of OXA-244-producing E. coli ST38 in humans is not associated with spillover from broiler production.
Antimicrobial resistance (AMR) poses a serious threat to public health. Infections caused by resistant bacteria have limited treatment options, are costly to treat, and lead to increased morbidity and mortality (Dziekan et al., 2012). Worldwide, broiler production is associated with occurrence of extended-spectrum cephalosporin (ESC) – resistant Escherichia coli (Hiroi et al., 2012; EFSA and ECDC, 2022). This has also been the case in Nordic countries, despite negligible use of antimicrobials (Agersø et al., 2014; Mo et al., 2014; Myrenås et al., 2018). Trade of breeding animals has contributed to the wide dissemination of ESC-resistant E. coli in broiler production, as the same breeding animals supply the production in many countries (Agersø et al., 2014; Myrenås et al., 2018). Sequence type (ST) 38 has been shown to be predominant among ESC-resistant E. coli from Norwegian and Nordic broiler production (Mo et al., 2016; Myrenås et al., 2018; Buberg et al., 2021), as well as from several other European countries (Dierikx et al., 2013; Hansen et al., 2016; Seiffert et al., 2017; Pietsch et al., 2018).
E. coli ST38 has also been reported as a common cause of extra-intestinal infections in humans, particularly urinary tract infections (Chattaway et al., 2014). In addition, these isolates are often ESC resistant, and have been associated with blaOXA-48- (Potron et al., 2013; Turton et al., 2016) and blaOXA-244- (ECDC, 2021) mediated carbapenem resistance. A recent increase of E. coli ST38 with blaOXA-244 in the European Union/European Economic Area (EU/EEA), followed by an outbreak of health-care associated infections in Norway prompted the European Centre for Disease Prevention and Control (ECDC) to perform two rapid risk assessments on the increased detection of OXA-244 producing E. coli ST38 in humans (2020 and 2021) (ECDC, 2020, 2021). Currently, the source and transmission route of OXA-244-producing E. coli ST38 in EU/EEA is unknown, and further knowledge is required to implement adequate control measures. Transmission via food, environment or direct contact with animals is possible, but more data are required to elucidate the potential role of these sources (ECDC, 2021). In general, in-depth studies of ST38 from humans and their possible link to animal origin should be prioritized to explore the molecular epidemiology of this important pathogen.
In this study, we have used whole genome sequencing (WGS) to compare E. coli ST38 originating from broiler production and humans. Our aim was to investigate the genetic characteristics and relationship between E. coli ST38 from these two sources, and to investigate if there has been a potential spillover between them.
A total of 288 E. coli ST38 genomes were included in this study by retrieving genomes from public sources, and by sequencing in-house strain collections. A complete overview of all included sequence data is presented in Supplementary Table 1. Sequence data from 153 E. coli ST38 isolates of human origin were retrieved from public repositories, namely the European Nucleotide Archive (ENA, n = 136), and from project partners in Norway (Akershus University Hospital, Vestre Viken Hospital Trust and the Norwegian Institute of Public Health, for these combined n = 17) (Jørgensen et al., 2017; Espenhain et al., 2018). The following inclusion criteria had to be fulfilled to include an ST38 genome of human origin in the study; (i) isolate confirmed as E. coli ST38 in Enterobase or publication, (ii) Illumina sequencing data available from ENA or project partner, (iii) isolates originating from European countries where E. coli ST38 have been reported from broiler production, and (iv) isolates originating from patients or healthy carriers, not necessarily ESC resistant. Included genomes originated from human isolates collected between 2009 and 2019.
We also included sequence data from 135 E. coli ST38 originating from broiler production. Sequence data from 73 E. coli ST38 isolates from broiler production were available from previous studies (Berg et al., 2017; Buberg et al., 2021). Furthermore, a selection of ESC-resistant E. coli from Norwegian broiler production (n = 67) were analyzed with MLVA (Mo et al., 2016) to identify isolates displaying an ST38-associated MLVA profile (Supplementary Table 2; Myrenås et al., 2018). Of these, 63 displayed an ST38-associated MLVA-profile and were sequenced as part of the present study (Supplementary Table 2). Included genomes from broiler production originated from isolates collected between 2011 and 2014 in three Nordic countries; Norway, Sweden and Iceland. From previous studies, all isolates from broiler production were known to carry the blaCMY-2 gene encoding ESC resistance (Mo et al., 2014; NORM/NORM-VET, 2015; Myrenås et al., 2018).
For the isolates sequenced in this study, DNA was extracted manually from fresh colonies using the QIAmp DNA mini kit (Qiagen, Hilden, Germany) or automated on a QIAsymphony (Qiagen) using the DSP DNA Mini Kit (Qiagen). DNA concentration was determined on a Qubit® (Thermo Fisher Scientific) using the dsDNA Broad Range Assay Kit and the DNA purity was measured on a NanoDrop spectrophotometer (Thermo Fisher Scientific). For one isolate (2012-01-1292), DNA for long-read sequencing was extracted as described previously (Mo et al., 2020).
Samples were prepared with a Nextera library preparation kit (Illumina) and sequenced on a HiSeq3/4000 or a NextSeq 500 Illumina platform obtaining 150 bp paired-end reads. A complete overview of library preparation kits and sequencing platforms used for the different isolates is provided in Supplementary Table 1. Isolate 2012-01-1,292 was also sequenced using Pacific Biosciences (PacBio) long-read sequencing as previously described (Mo et al., 2020).
In silico characterization of all genomes was done using the plasmid assembly, gene identification and annotation pipeline Ellipsis v 0.5.2 with raw Illumina reads as input (Kaspersen, 2021). Briefly, the pipeline assessed read quality with FastQC1 and summarized it with MultiQC (Ewels et al., 2016). Reads were trimmed by TrimGalore v 0.6.4 (Krueger, 2019), and assembled by Unicycler v 0.4.8 (Wick et al., 2017) using default settings. ResFinder (database downloaded 11.02.2020) (Zankari et al., 2012) and PlasmidFinder (database downloaded 06.03.2020) (Carattoli et al., 2014) were used to identify AMR genes and plasmid replicons in the assembled genomes. Assembly quality was assessed with QUAST v 5.0.2 (Gurevich et al., 2013), and STs were predicted with MLST2 using the Achtman scheme (Wirth et al., 2006; Jolley and Maiden, 2010).
Long-reads from isolate 2012-01-1292 were demultiplexed by the SMRT Link barcoding pipeline (v6.0.0.47841, SMRT Link Analysis Services and GUI v6.0.0.47836) with 40 as minimum barcode score. Thereafter, the short and long reads from this isolate were assembled using Unicycler v 0.4.8 with default settings for hybrid assembly (Wick et al., 2017).
A core SNP based phylogenetic analysis was performed with the ALPPACA pipeline (A tooL for Prokaryotic Phylogeny and Clustering Analysis) (Kaspersen and Fiskebeck, 2022) using the mapping-track to investigate the genetic relatedness within the ST38 group. A core SNP alignment was reconstructed using Snippy v 4.6.0.3 The hybrid assembly of isolate 2012-01-1292, originating from retail chicken meat, was used as reference for the SNP calling. The coverage of the core genome included in Snippy was calculated as the minimum proportion of the included genomes that aligned to the reference genome. Recombinant regions were identified with Gubbins v 3.2.0 (Croucher et al., 2015), using the GTRGAMMA model and RaxML as initial tree builder. Putative recombinant regions were masked using maskrc-svg v 0.54 before filtering using snp-sites v 2.5.1 (Page et al., 2016). A maximum likelihood (ML) phylogeny was generated using IQ-TREE v 2.2.0.3 (Minh et al., 2020). ModelFinder (Kalyaanamoorthy et al., 2017) was used to determine the most suitable evolutionary model, and UFBoot2 to evaluate branch support (Hoang et al., 2018). An E. coli ST115 genome (accession no ERR4706315) originating from retail chicken meat was included as an outlier in the phylogeny. Snp-dists v 0.8.25 was used to calculate the pairwise SNP distances between genomes using the recombinant-filtered alignment. The phylogenetic trees were visualized and annotated using the ggtree package v 3.0.4 (Yu et al., 2017, 2018; Yu, 2020) in R v 4.1.1 (R Core Team, 2021).
Chi-square tests were performed in R v 4.1.1 (R Core Team, 2021).
An overview of the results from the in silico characterization of genomes is presented in Supplementary Tables 3, 4. Of the 63 broiler isolates sequenced in this study, 62 belonged to ST38. Thus, 135 E. coli ST38 from broiler production were included in addition to the 153 genomes originating from human isolates (Supplementary Tables 1, 2).
The core genome covered 81.0% of the reference genome before masking recombinant regions. The phylogenetic analysis revealed that E. coli ST38 from humans and broiler production belong to distinct monophyletic clades, namely Clades A and B. A few ST38 genomes of human origin belonged to Clade C, a sister-clade to Clades A and B (Figure 1, Supplementary Figure 1 for rectangular view). There was a minimum of 177 SNP differences between Clades A and B, 293 SNPs between Clades B and C, and 205 SNPs between Clades A and C. When comparing genomes within each of the clades, ST38 of human origin differed by 0–341 SNPs in A and 0–211 SNPs in C, while ST38 from broiler production differed by 0–79 SNPs (B).
Figure 1. Maximum likelihood core genome SNP tree of 288 Escherichia coli multilocus sequence type 38 originating from humans (n = 153) and broiler production (n = 135). Presence (CMY = purple, CTX-M = blue, OXA = green, MDR = red) and absence (light grey) of relevant antimicrobial resistance genes and multidrug resistance are displayed in the outer circles. Only blaOXA-48, blaOXA-181, and blaOXA-244 are included in the OXA-group. Both blaCMY-2 and blaCMY-16 are included in the CMY-group, and blaCTX-M-1, −3, −9, −14, −14b, −15, and −27 are included in the CTX-M group. Black circles on nodes represent accepted bootstrap values (>95%). Color on tip-points indicate origin of the ST38 genomes. The tree is rooted using an outgroup (E. coli ST115).
The blaCMY-2 gene was present in 100% of the ST38 genomes originating from broiler production (Supplementary Table S3). In addition to blaCMY-2, some harbored blaTEM-1b (n = 29), sul2 (n = 2), dfrA1 (n = 1) and aadA1 (n = 1). Only a single E. coli ST38 from broiler production was predicted to be multidrug resistant (MDR, i.e., resistant to three or more antimicrobial classes, Figure 1), and none carried blaOXA-genes (Figure 1, Supplementary Table S3).
Genes encoding ESC resistance were identified in 146 (95.4%) of the 153 ST38 genomes originating from humans, with blaCTX-M-27 (n = 45) and blaCTX-M-15 (n = 44) being the most common (Figure 1, Supplementary Table S3). blaOXA-244 was present in 43 of the human ST38 genomes. In 41 of these, blaOXA-244 was present in combination with a gene encoding ESC resistance, mainly blaCTX-M-27 (n = 29). In addition, blaCMY-2 was present in four genomes, and blaCMY-16, blaOXA-48 and blaOXA-181 were present in two genomes each. There was a significant difference between the occurrence of predicted MDR among ST38 from broiler production and humans (p < 0.001) as 140 (91.5%) of the E. coli ST38 of human origin were predicted to be MDR compared to one (0.7%) of broiler origin (Figure 1). A complete overview of additional acquired AMR genes is presented in Supplementary Table S3.
The four E. coli genomes of human origin carrying blaCMY-2 only harbored IncF replicons, while the majority originating from broiler production harbored both IncB/O/K/Z and IncF replicons. A complete overview of plasmid replicons detected in the included genomes is presented in Supplementary Table S4. Raw sequencing reads from isolates sequenced in the present study are available from ENA (accession numbers PRJEB52719, PRJEB52740, PRJEB52781 and PRJEB52832).
The results from our phylogenetic analysis showed that the genomes clustered into distinct monophyletic groups, with all ST38 from broiler production in a clade separate from those originating from humans. The within-group SNP distances indicated that the ST38 genomes from broiler production were more homogenous than those originating from humans. The maximum within-group SNP distances were 341 and 211 in Clades A and C, respectively, and 79 in Clade B. However, our dataset may have some limitations, and comparison and interpretation of the data should be performed with some caution. Isolates from humans were collected over a period of 10 years, while isolates from broiler production were collected during a four-year period. Also, the human isolates originated from several European countries, while isolates from broiler production were from Nordic countries and had a common ancestry (Myrenås et al., 2018). All ST38 from broiler production were ESC resistant and isolated using selective methods, while this was not the case for all isolates of human origin. Additionally, isolates from humans originated from both healthy humans and patients, while all ST38 from broiler production were from healthy animals. In broiler production, a high level of biosecurity is implemented to prevent the introduction of infectious agents, possibly preventing the introduction of several different E. coli ST38 strains. However, this needs to be further investigated. These factors may have influenced the heterogeneity observed in E. coli ST38 of human origin compared to ST38 from broiler production.
Our results show two distinct sub-populations within the same ST. The presence of sub-populations has also been described for other STs, such as ST131, associated with extra-intestinal E. coli infections in humans (Stoesser et al., 2016), where clinical isolates often belong to the sub-lineage H30Rx (Price et al., 2013). The occurrence of different sub-populations in ST38 may be due to adaptation to different hosts, namely humans and broilers, over time.
We detected large variations in the presence of acquired AMR genes. blaCMY-2 was the only ESC-encoding gene detected in ST38 from broiler production. In ST38 from humans, a diverse set of ESC resistance genes was present, with genes in the blaCTX-M-group most commonly detected. The blaCMY-2 gene was present in combination with IncF replicons in ST38 genomes of human origin. We have previously shown that blaCMY-2 is located on IncK or IncI1 plasmids in E. coli from broiler production, and that ST38 is associated with blaCMY-2/IncK plasmids (Mo et al., 2016). Thus, there is no evidence of common blaCMY-2-carrying plasmids in ST38 from humans and broiler production.
E. coli ST38 carrying blaOXA-48 or blaOXA-244 encoding carbapenem resistance has emerged in the human clinical setting (Potron et al., 2013; Turton et al., 2016; ECDC, 2020, 2021). Recently, the occurrence of E. coli ST38 with blaOXA-244 has increased in European countries, including Norway. The reason for this increasing number of cases is unknown (ECDC, 2021). We included 43 OXA-244 producing ST38 of human origin in our analyses. All of these clustered together with the rest of the human ST38 genomes, displaying a considerable number of SNPs to ST38 genomes from broiler production. None of the ST38 genomes from broiler production carried any blaOXA-genes. Based on our results, it seems that the increased detection of OXA-244-producing E. coli ST38 in EU/EEA is not due to spillover from broiler production. The E. coli ST38 genomes included in our study are divided into sub-groups consisting of isolates from humans and broiler production, respectively. This may suggest host adaptation of isolates belonging to the distinct sub-groups. There is no indication of transmission of E. coli ST38 between the human and broiler reservoirs in our data. However, our dataset have some limitations as discussed above. Thus, additional studies with detailed and extensive sample- and data collection is required in order to determine whether host specificity is present, or if and to what degree transmission between reservoirs may occur.
In conclusion, our results show the presence of distinct sub-groups within E. coli ST38, possibly adapted to separate hosts. To our best knowledge, this is the first in-depth characterization of a large number of E. coli ST38 from humans and broiler production using WGS.
The datasets presented in this study can be found in online repositories. The names of the repository/repositories and accession number(s) can be found in the article/Supplementary material.
MS, SM, and SJ contributed to the conception and design of the present study. SM, JS, ON, UN, SJ, TT, and MS contributed to the acquisition of isolates and sequencing data. SM and JS performed the laboratory work. SM, EF, KL, and JS performed the in silico analyses. SM wrote the first draft of the manuscript. All authors contributed to manuscript revision, read, and approved the submitted version.
The study was funded by the Research Council of Norway, grant no. 250212, and the Norwegian Veterinary Institute.
Håkon Kaspersen is acknowledged for support with R. Martin Steinbakk is acknowledged for fruitful discussions and for providing sequencing data from human isolates. Arne Søraas is acknowledged for providing sequencing data from human isolates. Amar A. Telke, Anna Eline E. Bruvoll and technicians at the Norwegian Reference Laboratory for Enteropathogenic Bacteria at the Norwegian Institute of Public Health are acknowledged for technical assistance. The sequencing service was provided by the Norwegian Sequencing Centre (www.sequencing.uio.no), a national technology platform hosted by the University of Oslo and supported by the ‘Functional Genomics’ and ‘Infrastructure’ programs of the Research Council of Norway and the Southeastern Regional Health Authorities. The bioinformatics work was performed on resources provided by UNINETT Sigma2 – the National Infrastructure for High Performance Computing and Data Storage in Norway.
The authors declare that the research was conducted in the absence of any commercial or financial relationships that could be construed as a potential conflict of interest.
All claims expressed in this article are solely those of the authors and do not necessarily represent those of their affiliated organizations, or those of the publisher, the editors and the reviewers. Any product that may be evaluated in this article, or claim that may be made by its manufacturer, is not guaranteed or endorsed by the publisher.
The Supplementary Material for this article can be found online at: https://www.frontiersin.org/articles/10.3389/fmicb.2023.1173287/full#supplementary-material
1. ^http://www.bioinformatics.babraham.ac.uk/projects/fastqc/
2. ^https://github.com/tseemann/mlst, https://pubmlst.org/
3. ^https://github.com/tseemann/snippy
Agersø, Y., Jensen, J. D., Hasman, H., and Pedersen, K. (2014). Spread of extended spectrum cephalosporinase-producing Escherichia coli clones and plasmids from parent animals to broilers and to broiler meat in a production without use of cephalosporins. Foodborne Pathog. Dis. 11, 740–746. doi: 10.1089/fpd.2014.1742
Berg, E. S., Wester, A. L., Ahrenfeldt, J., Mo, S. S., Slettemeas, J. S., Steinbakk, M., et al. (2017). Norwegian patients and retail chicken meat share cephalosporin-resistant Escherichia coli and IncK/blaCMY-2 resistance plasmids. Clin. Microbiol. Infect. 23, 407.e9–407.e15. doi: 10.1016/j.cmi.2016.12.035
Buberg, M. L., Mo, S. S., Sekse, C., Sunde, M., Wasteson, Y., and Witsø, I. L. (2021). Population structure and uropathogenic potential of extended-spectrum cephalosporin-resistant Escherichia coli from retail chicken meat. BMC Microbiol. 21:94. doi: 10.1186/s12866-021-02160-y
Carattoli, A., Zankari, E., Garcia-Fernandez, A., Voldby Larsen, M., Lund, O., Villa, L., et al. (2014). In silico detection and typing of plasmids using PlasmidFinder and plasmid multilocus sequence typing. Antimicrob. Agents Chemother. 58, 3895–3903. doi: 10.1128/AAC.02412-14
Chattaway, M. A., Jenkins, C., Ciesielczuk, H., Day, M., DoNascimento, V., Day, M., et al. (2014). Evidence of evolving extraintestinal enteroaggregative Escherichia coli ST38 clone. Emerg. Infect. Dis. 20, 1935–1937. doi: 10.3201/eid2011.131845
Croucher, N. J., Page, A. J., Connor, T. R., Delaney, A. J., Keane, J. A., Bentley, S. D., et al. (2015). Rapid phylogenetic analysis of large samples of recombinant bacterial whole genome sequences using Gubbins. Nucleic Acids Res. 43:e15. doi: 10.1093/nar/gku1196
Dierikx, C., van der Goot, J., Fabri, T., van Essen-Zandbergen, A., Smith, H., and Mevius, D. (2013). Extended-spectrum-beta-lactamase- and AmpC-beta-lactamase-producing Escherichia coli in Dutch broilers and broiler farmers. J. Antimicrob. Chemother. 68, 60–67. doi: 10.1093/jac/dks349
Dziekan, G., Jauregui, I. L., and Mathai, E. (2012). “The way forward: political commitment to enable options for action” in The evolving threat of antimicrobial resistance: options for action. (ed.) WHO (Geneva, Switzerland: World Health Organization)
ECDC (2020). Increase in OXA-244-producing Escherichia coli in the European union/European economic area and the UK since 2013, (ed.) ECDC. (Stockholm: ECDC).
ECDC (2021). OXA-244-producing Escherichia coli in the European Union/European economic area and the UK since 2013, first update −20 July 2021. (ed.) ECDC (Stockholm: ECDC).
EFSA and ECDC (2022). The European summary report on antimicrobial resistance in zoonotic and indicator bacteria from humans, animals and food in 2019–2020. EFSA J. 20:7209. doi: 10.2903/j.efsa.2022.7209
Espenhain, L., Jorgensen, S. B., Leegaard, T. M., Lelek, M. M., Hansgen, S. H., Nakstad, B., et al. (2018). Travel to Asia is a strong predictor for carriage of cephalosporin resistant E. coli and Klebsiella spp. but does not explain everything; prevalence study at a Norwegian hospital 2014–2016. Antimicrob. Resist. Infect. Control 7:146. doi: 10.1186/s13756-018-0429-7
Ewels, P., Magnusson, M., Lundin, S., and Kaller, M. (2016). MultiQC: summarize analysis results for multiple tools and samples in a single report. Bioinformatics 32, 3047–3048. doi: 10.1093/bioinformatics/btw354
Gurevich, A., Saveliev, V., Vyahhi, N., and Tesler, G. (2013). QUAST: quality assessment tool for genome assemblies. Bioinformatics 29, 1072–1075. doi: 10.1093/bioinformatics/btt086
Hansen, K. H., Bortolaia, V., Nielsen, C. A., Nielsen, J. B., Schønning, K., Agersø, Y., et al. (2016). Host-specific patterns of genetic diversity among IncI1-Igamma and IncK plasmids encoding CMY-2 beta-lactamase in Escherichia coli isolates from humans, poultry meat, poultry and dogs in Denmark. Appl. Environ. Microbiol. 82, 4705–4714. doi: 10.1128/AEM.00495-16
Hiroi, M., Yamazaki, F., Harada, T., Takahashi, N., Iida, N., Noda, Y., et al. (2012). Prevalence of extended-spectrum beta-lactamase-producing Escherichia coli and Klebsiella pneumoniae in food-producing animals. J. Vet. Med. Sci. 74, 189–195. doi: 10.1292/jvms.11-0372
Hoang, D. T., Chernomor, O., von Haeseler, A., Minh, B. Q., and Vinh, L. S. (2018). UFBoot2: improving the ultrafast bootstrap approximation. Mol. Biol. Evol. 35, 518–522. doi: 10.1093/molbev/msx281
Jolley, K. A., and Maiden, M. C. (2010). BIGSdb: scalable analysis of bacterial genome variation at the population level. BMC Bioinformatics 11:595. doi: 10.1186/1471-2105-11-595
Jørgensen, S. B., Søraas, A. V., Arnesen, L. S., Leegaard, T. M., Sundsfjord, A., and Jenum, P. A. (2017). A comparison of extended spectrum beta-lactamase producing Escherichia coli from clinical, recreational water and wastewater samples associated in time and location. PLoS One 12:e0186576. doi: 10.1371/journal.pone.0186576
Kalyaanamoorthy, S., Minh, B. Q., Wong, T. K. F., von Haeseler, A., and Jermiin, L. S. (2017). ModelFinder: fast model selection for accurate phylogenetic estimates. Nat. Methods 14, 587–589. doi: 10.1038/nmeth.4285
Kaspersen, H. (2021). Plasmid assembly, gene identification and annotation pipeline ellipsis. Available at: https://github.com/NorwegianVeterinaryInstitute/Ellipsis
Kaspersen, H., and Fiskebeck, E. Z. (2022). ALPPACA – a tooL for prokaryotic phylogeny and clustering analysis. J. Open Source Softw. 7, 1–4. doi: 10.21105/joss.04677
Krueger, F. (2019). Trim Galore. Available at: https://github.com/FelixKrueger/TrimGalore
Minh, B. Q., Schmidt, H. A., Chernomor, O., Schrempf, D., Woodhams, M. D., von Haeseler, A., et al. (2020). IQ-TREE 2: new models and efficient methods for phylogenetic inference in the genomic era. Mol. Biol. Evol. 37, 1530–1534. doi: 10.1093/molbev/msaa015
Mo, S. S., Norström, M., Slettemeås, J. S., Løvland, A., Urdahl, A. M., and Sunde, M. (2014). Emergence of AmpC-producing Escherichia coli in the broiler production chain in a country with a low antimicrobial usage profile. Vet. Microbiol. 171, 315–320. doi: 10.1016/j.vetmic.2014.02.002
Mo, S. S., Slettemeås, J. S., Berg, E. S., Norström, M., and Sunde, M. (2016). Plasmid and host strain characteristics of Escherichia coli resistant to extended-Spectrum Cephalosporins in the Norwegian broiler production. PLoS One 11:e0154019. doi: 10.1371/journal.pone.0154019
Mo, S. S., Telke, A. A., Osei, K. O., Sekse, C., Slettemeas, J. S., Urdahl, A. M., et al. (2020). Bla CTX-M-1/IncI1-Igamma plasmids circulating in Escherichia coli from Norwegian broiler production are related, but distinguishable. Front. Microbiol. 11:333. doi: 10.3389/fmicb.2020.00333
Myrenås, M., Slettemeås, J. S., Thorsteinsdottir, T. R., Bengtsson, B., Börjesson, S., Nilsson, O., et al. (2018). Clonal spread of Escherichia coli resistant to cephalosporins and quinolones in the Nordic broiler production. Vet. Microbiol. 213, 123–128. doi: 10.1016/j.vetmic.2017.11.015
NORM/NORM-VET (2015). NORM/NORM-VET 2014. Usage of antimicrobial agents and occurrence of antimicrobial resistance in Norway Tromsø/Oslo.
Page, A. J., Taylor, B., Delaney, A. J., Soares, J., Seemann, T., Keane, J. A., et al. (2016). SNP-sites: rapid efficient extraction of SNPs from multi-FASTA alignments. Microb Genom 2:e000056. doi: 10.1099/mgen.0.000056
Pietsch, M., Irrgang, A., Roschanski, N., Brenner Michael, G., Hamprecht, A., Rieber, H., et al. (2018). Whole genome analyses of CMY-2-producing Escherichia coli isolates from humans, animals and food in Germany. BMC Genomics 19:601. doi: 10.1186/s12864-018-4976-3
Potron, A., Poirel, L., Rondinaud, E., and Nordmann, P. (2013). Intercontinental spread of OXA-48 beta-lactamase-producing Enterobacteriaceae over a 11-year period, 2001 to 2011. Euro Surveill. 18. doi: 10.2807/1560-7917.es2013.18.31.20549
Price, L. B., Johnson, J. R., Aziz, M., Clabots, C., Johnston, B., Tchesnokova, V., et al. (2013). The epidemic of extended-spectrum-beta-lactamase-producing Escherichia coli ST131 is driven by a single highly pathogenic subclone, H30-Rx. MBio 4:e00377-13. doi: 10.1128/mBio.00377-13
R Core Team (2021). R: A language and environment for statistical computing. R Foundation for Statistical Computing, Vienna, Austria. Available at: https://www.R-project.org/
Seiffert, S. N., Carattoli, A., Schwendener, S., Collaud, A., Endimiani, A., and Perreten, V. (2017). Plasmids carrying blaCMY-2/4 in Escherichia coli from poultry, poultry meat, and humans belong to a novel IncK subgroup designated IncK2. Front. Microbiol. 8:407. doi: 10.3389/fmicb.2017.00407
Stoesser, N., Sheppard, A. E., Pankhurst, L., De Maio, N., Moore, C. E., Sebra, R., et al. (2016). Evolutionary history of the global emergence of the Escherichia coli epidemic clone ST131. MBio 7:e02162. doi: 10.1128/mBio.02162-15
Turton, J. F., Doumith, M., Hopkins, K. L., Perry, C., Meunier, D., and Woodford, N. (2016). Clonal expansion of Escherichia coli ST38 carrying a chromosomally integrated OXA-48 carbapenemase gene. J. Med. Microbiol. 65, 538–546. doi: 10.1099/jmm.0.000248
Wick, R. R., Judd, L. M., Gorrie, C. L., and Holt, K. E. (2017). Unicycler: resolving bacterial genome assemblies from short and long sequencing reads. PLoS Comput. Biol. 13:e1005595. doi: 10.1371/journal.pcbi.1005595
Wirth, T., Falush, D., Lan, R., Colles, F., Mensa, P., Wieler, L. H., et al. (2006). Sex and virulence in Escherichia coli: an evolutionary perspective. Mol. Microbiol. 60, 1136–1151. doi: 10.1111/j.1365-2958.2006.05172.x
Yu, G. (2020). Using ggtree to visualize data on tree-like structures. Curr. Protoc. Bioinformatics 69:e96. doi: 10.1002/cpbi.96
Yu, G., Lam, T. T., Zhu, H., and Guan, Y. (2018). Two methods for mapping and visualizing associated data on phylogeny using Ggtree. Mol. Biol. Evol. 35, 3041–3043. doi: 10.1093/molbev/msy194
Yu, G. C., Smith, D. K., Zhu, H. C., Guan, Y., and Lam, T. T. Y. (2017). Ggtree: an R package for visualization and annotation of phylogenetic trees with their covariates and other associated data. Methods Ecol. Evol. 8, 28–36. doi: 10.1111/2041-210x.12628
Keywords: Escherichia coli, ST38, OXA-244, comparative genomics, ESC, broilers
Citation: Mo SS, Fiskebeck EZ, Slettemeås JS, Lagesen K, Nilsson O, Naseer U, Jørgensen SB, Thorsteinsdottir TR and Sunde M (2023) Escherichia coli multilocus sequence type 38 from humans and broiler production represent distinct monophyletic groups. Front. Microbiol. 14:1173287. doi: 10.3389/fmicb.2023.1173287
Received: 24 February 2023; Accepted: 07 April 2023;
Published: 02 May 2023.
Edited by:
Nabil Karah, Umeå University, SwedenReviewed by:
Benoit Doublet, Institut National de recherche pour l'agriculture, l'alimentation et l'environnement (INRAE), FranceCopyright © 2023 Mo, Fiskebeck, Slettemeås, Lagesen, Nilsson, Naseer, Jørgensen, Thorsteinsdottir and Sunde. This is an open-access article distributed under the terms of the Creative Commons Attribution License (CC BY). The use, distribution or reproduction in other forums is permitted, provided the original author(s) and the copyright owner(s) are credited and that the original publication in this journal is cited, in accordance with accepted academic practice. No use, distribution or reproduction is permitted which does not comply with these terms.
*Correspondence: Solveig Sølverød Mo, c29sdmVpZy5tb0B2ZXRpbnN0Lm5v
Disclaimer: All claims expressed in this article are solely those of the authors and do not necessarily represent those of their affiliated organizations, or those of the publisher, the editors and the reviewers. Any product that may be evaluated in this article or claim that may be made by its manufacturer is not guaranteed or endorsed by the publisher.
Research integrity at Frontiers
Learn more about the work of our research integrity team to safeguard the quality of each article we publish.