- 1Division of Microbiology, Department of Pathobiology, School of Public Health, Tehran University of Medical Sciences, Tehran, Iran
- 2Department of Microbiology, School of Medicine, Iran University of Medical Sciences, Tehran, Iran
- 3Department of Infectious Diseases, Faculty of Medicine, Tehran University of Medical Sciences, Tehran, Iran
- 4Department of Microbiology, Iranian Reference Health Laboratory Research Center, Ministry of Health and Medical Education, Tehran, Iran
- 5Department of Pathology, Imam Hospital Complex, Tehran University of Medical Sciences, Tehran, Iran
- 6Department of Laboratory Sciences, School of Paramedical Sciences, Iran University of Medical Sciences, Tehran, Iran
- 7Department of Microbiology, Milad Hospital, Tehran, Iran
Aminoglycosides are used to treat infections caused by carbapenem-resistant Acinetobacter baumannii (CRAB) strains. However, resistance to aminoglycosides has increased remarkably in the last few years. Here, we aimed to determine the mobile genetic elements (MGEs) associated with resistance to aminoglycosides in the global clone 2 (GC2) A. baumannii. Among the 315 A. baumannii isolates, 97 isolates were identified as GC2, and 52 of GC2 isolates (53.6%) were resistant to all the aminoglycosides tested. The AbGRI3s carrying armA were detected in 88 GC2 isolates (90.7%), and of them, 17 isolates (19.3%) carried a new variant of AbGRI3 (AbGRI3ABI221). aphA6 was located in TnaphA6 of 30 isolates out of 55 aphA6-harboring isolates, and 20 isolates were found to harbor TnaphA6 on a RepAci6 plasmid. Tn6020 carrying aphA1b was detected in 51 isolates (52.5%), which was located within AbGRI2 resistance islands. The pRAY* carrying the aadB gene was detected in 43 isolates (44.3%), and no isolate was found to contain a class 1 integron harboring this gene. The GC2 A. baumannii isolates contained at least one MGE carrying the aminoglycoside resistance gene, located mostly either in the chromosome within AbGRIs or on the plasmids. Thus, it is likely that these MGEs play a role in the dissemination of aminoglycoside resistance genes in GC2 isolates from Iran.
Introduction
Acinetobacter baumannii causes a variety of healthcare-associated infections (HAIs), which are mostly untreatable by currently available antibiotics. Most of these antibiotics are ineffective against A. baumannii as it has a high capacity to acquire antibiotic-resistant genes (ARGs) via mobile genetic elements (MGE), including plasmids, transposons, integrons, and resistance islands (Fournier and Richet, 2006; Towner, 2009). The majority of A. baumannii isolates that are resistant to multiple antibiotics belong to one of two major global clones, namely, global clone 1 (GC1) and global clone 2 (GC2) (Holt et al., 2016). Carbapenem-resistant A. baumannii (CRAB) strains are among the critical priority pathogens on the World Health Organization's (WHO's) priority list of antibiotic-resistant bacteria (Tacconelli et al., 2018). Aminoglycosides are used as antibiotics of choice in the treatment of infections caused by CRAB strains; however, resistance to antibiotics of this class is prominently increased in recent years (Nemec et al., 2004; Lee et al., 2011).
The MGEs carrying aminoglycoside resistance genes have been identified in A. baumannii isolates belonging to GC1 and GC2 (Nigro et al., 2011, 2013; Hamidian et al., 2012; Nigro and Hall, 2016; Blackwell et al., 2017; Hamidian and Hall, 2018). In GC2 A. baumannii, the chromosomally located A. baumannii genomic resistance islands (AbGRIs) often carry aminoglycoside resistance genes, including strA, strB, aphA1b, aacC1, aadA1, armA, and aacA4 (Nigro et al., 2013; Nigro and Hall, 2016; Blackwell et al., 2017). The AbGRI1 resistance islands containing Tn6022 and/or Tn6022Δ1 backbone transposons, which are located in the comM gene, carry strA and strB genes (streptomycin resistance) (Nigro and Hall, 2016). In some of the AbGRI2 variants, aacC1 (gentamicin resistance), aadA1 (streptomycin and spectinomycin resistance), and aphA1b (kanamycin and neomycin resistance) genes are co-located. Tn6020 (a compound transposon flanked by IS26) that carries the aphA1b gene (conferring resistance to kanamycin and neomycin) has been found in some of the AbGRI2 and AbGRI3 variants (Nigro et al., 2013; Nigro, 2014; Nigro and Hall, 2016; Blackwell, 2017; Blackwell et al., 2017). AbGRI3 resistance islands carry an IS26-bounded transposon (Tn6180) containing a 16S rRNA methyltransferase (armA) gene, which confers resistance to most of therapeutic aminoglycosides (Galimand et al., 2003; Blackwell et al., 2017). Furthermore, some variants of the AbGRI3 resistance island carry the aacA4 gene, conferring resistance to amikacin, kanamycin, and neomycin (Blackwell et al., 2017). In addition to the AbGRI resistance islands harboring aminoglycoside resistance genes (Nigro et al., 2013; Nigro and Hall, 2016; Blackwell et al., 2017), plasmids have a significant role in the carriage of genes responsible for aminoglycoside resistance (Nigro et al., 2011, 2015; Hamidian et al., 2012). The aphA6 gene, which confers resistance to amikacin, kanamycin, and neomycin, is most commonly located within TnaphA6 (a transposon bounded by copies of ISAba125), which is frequently found on the RepAci6 plasmid family (Nigro and Hall, 2014; Nigro et al., 2015). The aadB gene cassette, which confers resistance to tobramycin, gentamicin, and kanamycin, is usually associated with a class 1 integron or a plasmid named pRAY (Nigro et al., 2011; Hamidian et al., 2012). There are several reports of resistance to aminoglycosides in A. baumannii isolates from Iran (Aliakbarzade et al., 2014) and other Middle East countries (Bakour et al., 2014; Kishk et al., 2021; Sannathimmappa et al., 2021; Al-Tamimi et al., 2022); however, little is known regarding the role of MGEs in the dissemination of these resistance genes in the Middle East region (Aris et al., 2019; Douraghi et al., 2020). Here, we examined the aminoglycoside resistance genes and their association with MGEs in GC2 isolates in Tehran, Iran.
Materials and methods
Bacterial isolates
A total of 315 non-repetitive A. baumannii isolates were recovered from patients admitted to one of the four hospitals located in Tehran, Iran (H1, H2, H4, and H5), in the periods 2012–2013 (n = 111) and 2018–2019 (n = 204). All isolates were initially identified using conventional microbiological methods (Forbes and Sahm, 2016) and were further confirmed by the detection of the oxaAb (blaOXA − 51 − like) gene by PCR (Turton et al., 2006).
Antibiotic susceptibility testing
Antibiotic susceptibility testing was performed by the disk diffusion method using the following 27 antibiotics (μg per disk): ampicillin–sulbactam (20), piperacillin–tazobactam (110), ticarcillin–clavulanate (timentin) (85), imipenem (10), meropenem (10), doripenem (10), ceftazidime (30), cefotaxime (30), cefepime (30), ceftriaxone (30), tetracycline (30), minocycline (30), doxycycline (30), streptomycin (25), spectinomycin (25), amikacin (30), kanamycin (30), gentamicin (10), neomycin (30), netilmicin (30), tobramycin (10), nalidixic acid (30), ciprofloxacin (5), levofloxacin (5), sulfamethoxazole (300), trimethoprim–sulfamethoxazole (25), and rifampin (30). The results were analyzed according to the Clinical and Laboratory Standards Institute (CLSI) recommendations for Acinetobacter spp. (Wayne, 2022) and calibrated dichotomous sensitivity (CDS) (http://cdstest.net/) disk diffusion assay when a CLSI breakpoint for Acinetobacter spp. was not available (CDS for streptomycin, spectinomycin, kanamycin, neomycin, netilmicin, nalidixic acid, sulfamethoxazole, and rifampin).
PCR assays, DNA sequencing, and sequence analysis
For PCR amplicons up to 3 kb, reaction and cycling conditions were followed as described in Hamidian and Hall (2011). For larger amplicons, Phusion DNA polymerase (New England Biolabs) and Phusion HF buffer were used instead of Taq polymerase and PCR buffer. The identity of PCR amplicons was confirmed by DNA sequencing (Nigro et al., 2011). The amplicon of PCR linking the left end of AbGRI3 to ISAba24 (Δatr-ISAba24), which was larger than the predicted size, was sequenced by primer walking strategy (primers are presented in Supplementary Table 1).
Identification of A. baumannii isolates belonging to GC2
The A. baumannii isolates belonging to GC2 were identified by two multiplex PCRs, as described previously (Turton et al., 2007).
Detection of aminoglycoside resistance genes
The following aminoglycoside resistance genes were identified by PCR in GC2 A. baumannii isolates, including strA, strB, aacC1, aacC2, aacA4, aphA1b, aphA6, aadA1, armA, aadB, and aac(6')-Im (Nigro et al., 2011).
Determining the context of aminoglycoside resistance genes
The aminoglycoside resistance genes associated with AbGRI resistance islands (Figure 1), including strA and strB with AbGRI1, aphA1b within Tn6020 with AbGRI2, aacC1 alongside aadA1 within the class 1 integron with AbGRI2, and armA with AbGRI3 resistance islands were identified by PCR and PCR mapping using the primers presented in Supplementary Table 2. Furthermore, the aphA6 within TnaphA6 associated with RepAci6 and the aadB associated with pRAY* or class 1 integron were identified by PCR and PCR mapping using the primers presented in Supplementary Table 2.
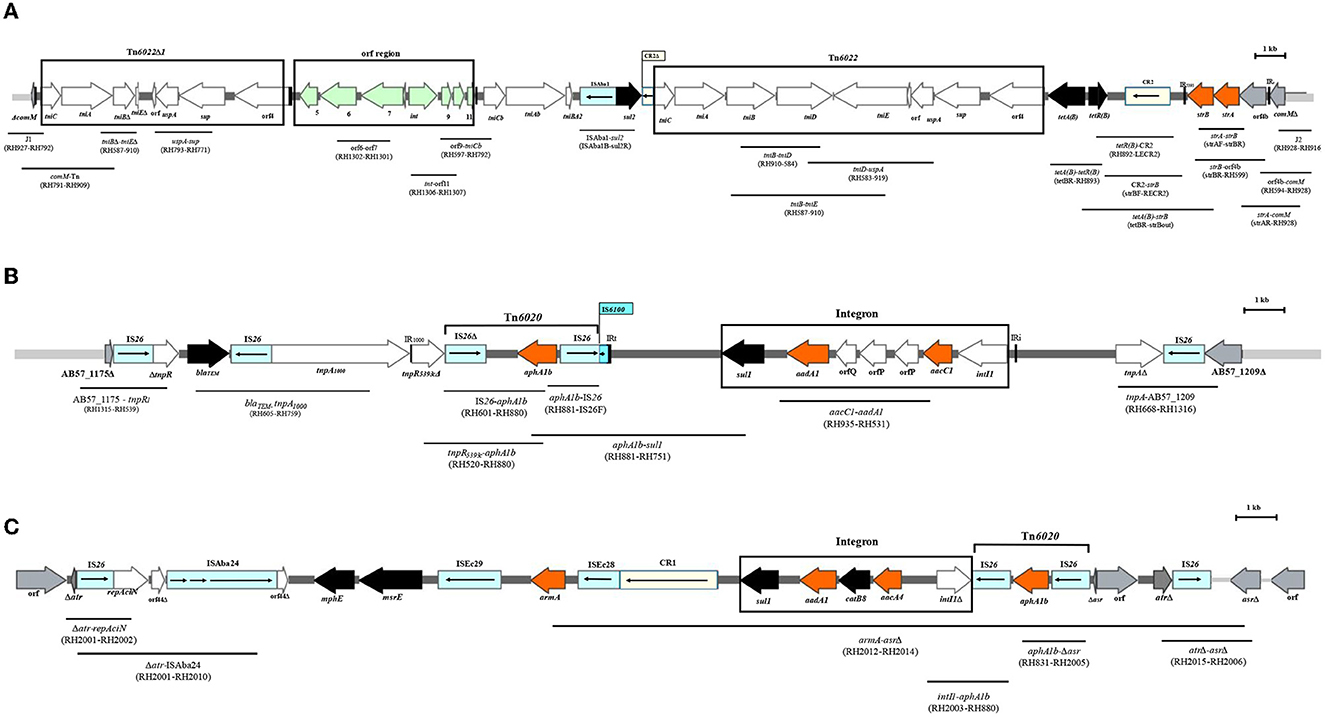
Figure 1. The linkage of PCRs to map the backbone of AbGRI resistance islands. (A) Schematic of the AbGRI1-3. (B) Schematic of the AbGRI2-1. (C) Schematic of the AbGRI3-2i. The central dark gray line represents the resistance island. The light gray line indicates the adjacent chromosomal sequence. Arrows indicate the extent and orientation of genes and open reading frames. The aminoglycoside resistance genes are orange and other resistance genes are black with the name below. The boxes representing IS are blue and the boxes representing CR elements are yellow, and the internal arrows indicate the transposase or the rolling circle replicase, respectively. Vertical bars indicate IRs, and the triangles next to the genes indicate the deletion in the genes. The location of the Tn6022, Tn6022Δ1, integron, and orf region is shown by the black box around them. The primers used to link sequences are indicated beneath each black line in parentheses. Figure made adapted from Blackwell et al. (2017).
Accession numbers
The partial sequences of the AB57_1175-tnpR1, blaTEM− tnpA1000, tnpR5393c-aphA1b, tnpA21-AB57_1209, armA gene, and Δatr-ISAba24 have been submitted to the NCBI GenBank database under the following accession numbers: OM801571, ON240823, ON871819, OP019034, ON982224, and OQ341795.
Results
Identification of GC2 A. baumannii isolates and antibiotic resistance profiles
Among the 315 A. baumannii isolates, 97 (30.8%) belonged to GC2, and all the GC2 isolates were resistant to carbapenems (imipenem, meropenem, and doripenem) (Supplementary Table 3). As each of the GC2 isolates were resistant to at least an antibiotic in the three classes (Magiorakos et al., 2012), they were classified as multi-drug resistant (MDR) (Supplementary Table 3). The aminoglycoside resistance profile of the GC2 isolates varied, and four groups were distinguished in the collection, hereafter will be referred to as groups 1–4 (Table 1). While the isolates in the group 1 were resistant to all aminoglycosides tested, the isolates in the remaining three groups were susceptible to neomycin (group 2), netilmicin (group 3), and both tobramycin and netilmicin (group 4).
Detection of aminoglycoside resistance genes
The armA was the most frequent gene, detected in all but nine (n = 88) of GC2 A. baumannii isolates (group 1 and group 2 in Table 1). Furthermore, the aphA6 (n = 55), aphA1b (n = 51), aadB (n = 43), aacC1 (n = 11), and aadA1 (n = 4) genes were detected in the GC2 isolates. The aacC2, aacA4, and aac(6')-Im genes were not observed in any isolate examined (Supplementary Table 4).
The context of strA and strB genes
The strA and strB genes were co-located in all isolates carrying AbGRI1 resistance island (Supplementary Table 5, highlighted). In total, 51 out of the 97 GC2 isolates tested (52.6%) did not contain the AbGRI1 resistance island (Supplementary Table 5, not highlighted). In the remaining 46 isolates (47.4%), seven groups were distinguished according to their shared characteristics (Supplementary Table 5, each group highlighted in the same color). The interrupted comM gene; J1, J2 junction; orf4b adjacent to the comM gene (indicating that they contained AbGRI1 resistance island); strA, strB genes; and CR2 element were present in all isolates containing AbGRI1. However, the isolates carrying AbGRI1 varied according to the presence of backbone transposon (Tn6022, Tn6022Δ1); orf region; tetA(B), tetR(B) (tetracycline resistance); and sul2 gene (sulfonamide resistance) (Supplementary Table 5, highlighted).
Long-read sequencing technology such as PacBio or Oxford Nanopore will be required to determine the structure of AbGRI1 in the isolates containing this island.
The context of the aphA1b gene
In all the 51 GC2 isolates containing aphA1b (52.5%), this gene was located in Tn6020, and the Tn6020 in these isolates was carried by either AbGRI2-1 (one isolate, 1.03%) or AbGRI2-12b resistance islands (50 isolates, 51.5%). The structure of the AbGRI2-1 and AbGRI2-12b resistance islands is shown in Figure 2.
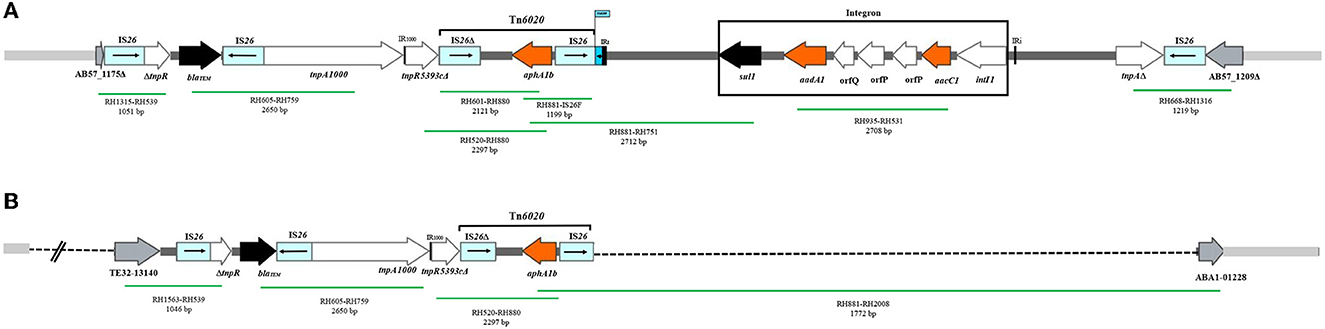
Figure 2. The AbGRI2 variants found in this study. (A) Schematic of the AbGRI2-1. (B) Schematic of the AbGRI2-12b. The central dark gray line represents the resistance island. The light gray line indicates the adjacent chromosomal sequence. The arrows indicate the extent and orientation of genes and open reading frames. The aminoglycoside resistance genes are orange and other resistance genes are black with the name below. The vertical bars indicate IRs. The dashed lines in AbGRI2-12b represent the extent of deletions relative to AbGRI2-1. The primers used to link sequences are indicated beneath each green line, along with the predicted amplicon size. Figure made adapted from Blackwell et al. (2017).
The context of aacC1 and aadA1 genes
The aacC1 and aadA1 genes were found together only in one isolate containing AbGRI2-1 resistance island (Supplementary Table 4).
The context of the armA gene
Of the 88 armA-harboring isolates, 71 (80.7%) carried AbGRI3-4. In the remaining 17 isolates (19.3%), a new variant of AbGRI3 resistance islands was found, which was called AbGRI3ABI221 (Supplementary Table 4). The PCR for linking the left end of AbGRI3 to ISAba24 (Δ1atr-ISAba24) produced a larger amplicon than expected 3.8 kb (Blackwell, 2017). It was found that AbGRI3ABI221 is identical to AbGRI3-4 except for missing a 523 bp segment from the left-hand side of the island in our laboratory (ABI221) previously. The sequence of this amplicon was submitted to the NCBI GenBank database under the accession number QQ341795. The sequence of Δatr-ISAba24 segment missing 523 bp of the repAciN gene (from nucleotide number 1,142 to 1,665) in the isolates containing AbGRI3ABI221 (accession number QQ341795) compared with the sequence of Δatr-ISAba24 in SGH0908 containing AbGRI3-2i (accession number KX011026) is shown in Figure 3. The genetic structure of the AbGRI3-4 and AbGRI3ABI221 resistance islands is shown in Figure 4.
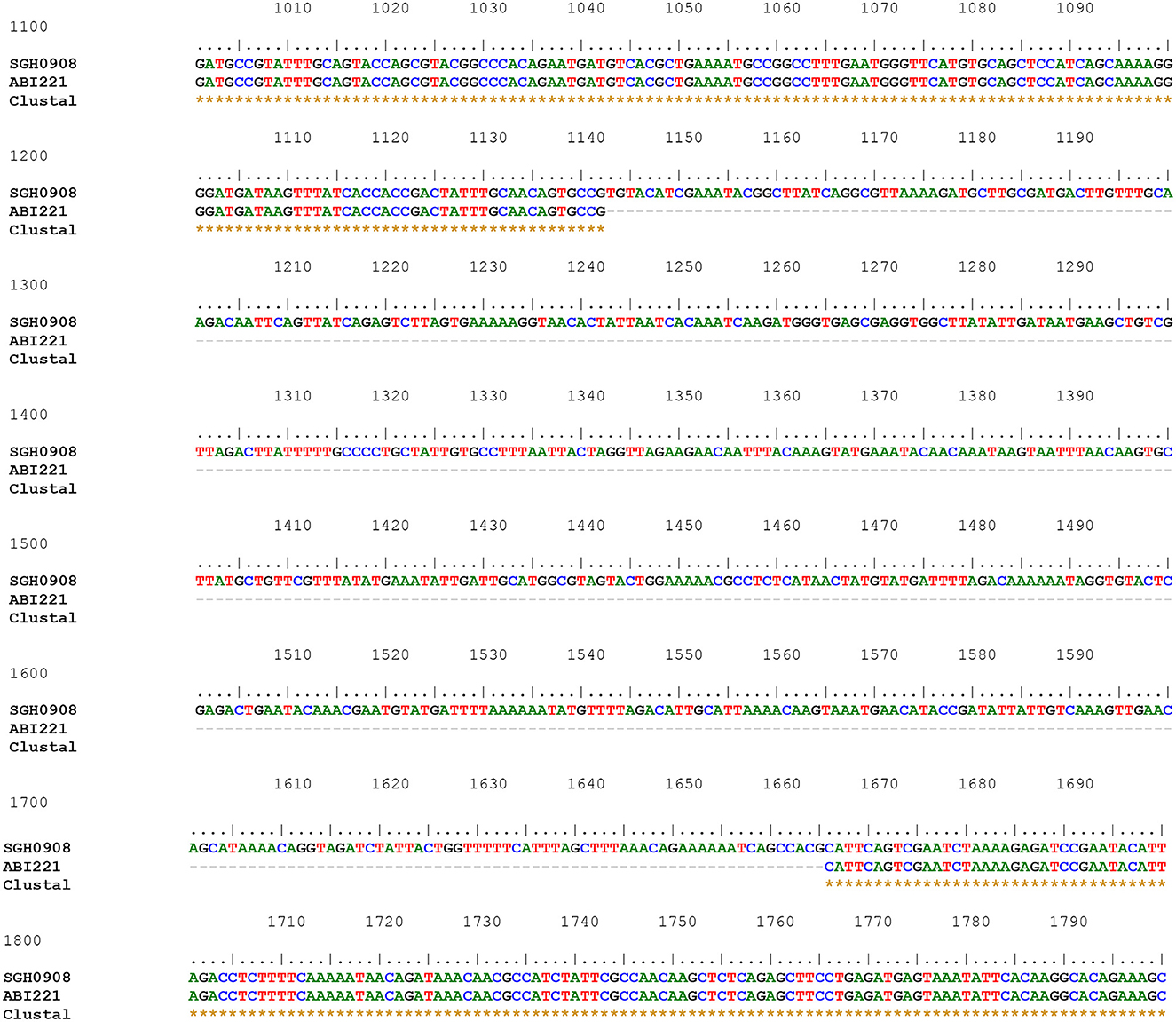
Figure 3. The sequence of Δatr-ISAba24 segment missing 523 bp of the repAciN gene in the isolates containing AbGRI3ABI221 (accession number QQ341795) compared with the sequence of Δatr-ISAba24 in SGH0908 containing AbGRI3-2i (accession number KX011026). Three rows are shown: the first row shows a part of the Δatr-ISAba24 sequence in the SGH0908 isolate, and the second row shows the Δatr-ISAba24 sequence missing 523 bp of the repAciN gene in AbGRI3ABI221. The dashed line in the middle row from nucleotide numbers 1142 to 1665 indicates the absence of 523 nucleotides in the isolates containing AbGRI3ABI221. Asterisks in the last row indicate the same nucleotides.
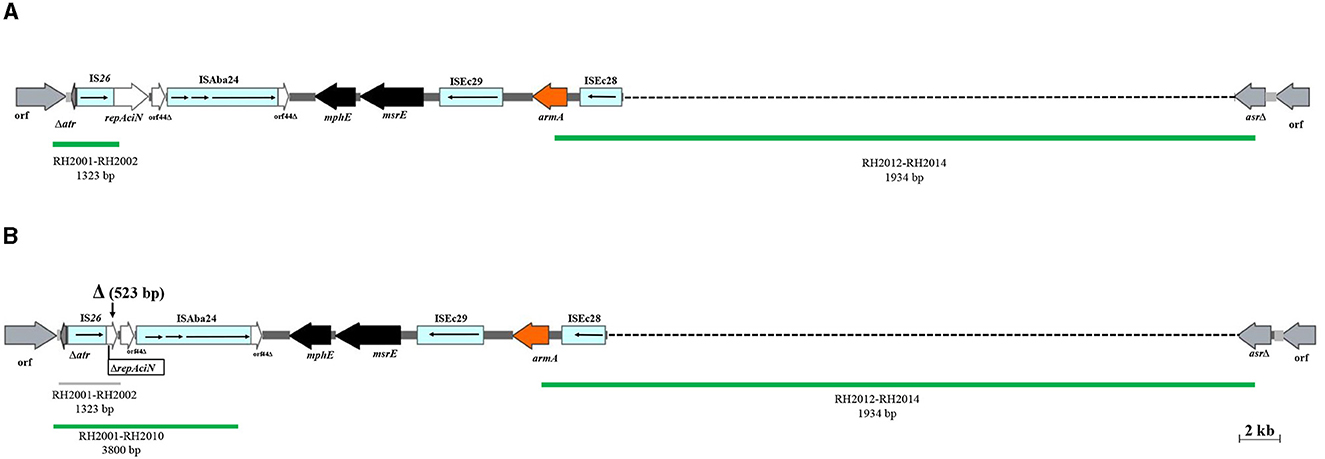
Figure 4. The AbGRI3 variants found in this study. (A) Schematic of the AbGRI3-4. (B) Schematic of the AbGRI3ABI221.The central dark gray line represents the resistance island. The light gray line indicates the adjacent chromosomal sequence. Arrows indicate the extent and orientation of genes and open reading frames. The aminoglycoside resistance genes are orange and other resistance genes are black with the name below. Vertical bars indicate IRs and the vertical arrows indicate the location of deletion (Δ) found in the AbGRI3ABI221 in this study. Dashed lines in AbGRI3-4 and AbGRI3ABI221 represent the extent of deletions relative to AbGRI3-2i and AbGRI3-4, respectively. The positive and negative result for each reaction, which was performed to detect AbGRI3 variants are shown by green and gray lines, respectively, with their respective primers and predicted amplicon sizes beneath each line. Figure made adapted from Blackwell et al. (2017).
The context of aphA6 gene
Of the 55 isolates containing the aphA6 gene (56.7%), the aphA6 gene was flanked by ISAba125 in 30 isolates (54.5%); i.e., the aphA6 gene is located in TnaphA6. The amplicon for PCRs linking the aphA6 to the backbone of RepAci6 plasmids on the right and left was amplified in 20 isolates of those containing TnaphA6 (66.6%), and also, PCRs for repeated sequences 1, 2, and 3 produced the expected amplicons, suggesting the presence of a RepAci6 plasmid (Supplementary Table 4).
The context of the aadB gene
In all isolates containing the aadB (44.4%), this gene was carried by pRAY*. The class 1 integron was not detected in any isolate containing the aadB gene (Supplementary Table 4).
Discussion
Aminoglycosides are used as therapeutic options when A. baumannii infections are resistant to carbapenems. However, the development of resistance to aminoglycosides has been described in many countries worldwide (Nemec et al., 2004; Nie et al., 2014; Kishk et al., 2021). Despite the characterization of MGEs carrying aminoglycoside resistance genes in GC2 A. baumannii in previous studies (Nigro et al., 2011, 2013, 2015; Hamidian et al., 2012; Nigro, 2014; Nigro and Hall, 2016; Blackwell, 2017; Blackwell et al., 2017), much less is known about their dissemination in the Middle East region, including Iran (Aris et al., 2019; Douraghi et al., 2020). This study examined the presence of MGEs associated with aminoglycoside resistance genes among GC2 isolates in Tehran, Iran. It is noteworthy that in the present study, the MGEs were detected in the isolates recovered from the patients admitted to different hospitals in different time periods. More than half of GC2 isolates were resistant to all tested aminoglycosides, and all contained at least one aminoglycoside resistance gene. Distinct aminoglycoside resistance profiles were found among the isolates. The armA gene was detected in all the isolates in group 1 (resistant to all aminoglycosides) and group 2 (resistant to all aminoglycosides except neomycin), respectively. It was revealed that all of the isolates containing the armA were located within the AbGRI3 resistance island. The chromosomally located islands carrying the armA gene, named AbGRI3 in 2017 (Blackwell et al., 2017), were described in the USA in 2014 (Wright et al., 2014). Consistent with the results of the current study, Blackwell detected the armA gene in 15 out of 20 GC2 A. baumannii isolates from Singapore, and they revealed that the armA gene is located in AbGRI3, in all the isolates containing this gene (Blackwell et al., 2017). Tn6020 was first characterized in GC1 A. baumannii (Post and Hall, 2009) and then in GC2 isolates from Australia (Nigro et al., 2011). In this study, aphA1b was located within Tn6020 in all the GC2 isolates containing this gene, and all Tn6020 that were detected were carried by either AbGRI2-1 or AbGRI2-12b (Blackwell, 2017) resistance islands. While only 15% of GC2 isolates from Singapore contained AbGRI2-12b, this island was detected in nearly half of the isolates examined in the present study. The AbGRI2-1 resistance island harboring a class 1 integron (containing aacC1 adjacent to aadA1) was only detected in one of the isolates tested, while it was present in 21.6 and 25% of GC2 isolates examined in Australia and Singapore, respectively (Nigro, 2014; Blackwell, 2017). The class 1 integron carrying the aacC1 gene cassette is prevalent in GC1 isolates, but it is also found in GC2 isolates tested in Australia (Nigro et al., 2013). In GC1 strains, the class 1 integron carrying the aacC1 gene cassette is usually located within an AbaR resistance island (Post et al., 2010, 2012; Krizova et al., 2011). However, the studies focusing on aminoglycoside resistance in GC2 isolates collected from Australia demonstrated that only a subgroup of GC2 isolates contained the aacC1 gene cassette along with Tn6020 (Post et al., 2010; Nigro et al., 2011). In this study, nearly half of the isolates contained AbGRI1 resistance island (carrying strA and strB resistance genes); however, all the GC2 isolates examined in Australia (Nigro and Hall, 2016), and 82.4% of the GC2 isolates investigated in South Korea carried AbGRI1 (Kim et al., 2017). Of all GC2 isolates which were resistant to amikacin, more than half contained the aphA6 gene. Furthermore, out of the isolates carrying aphA6, more than half contained TnaphA6; of them, 66.6% were harbored by RepAci6 plasmids. TnaphA6 was first identified in the Australian isolates belonging to GC2, and subsequently in a GC1 isolate from Australia (Nigro et al., 2011; Hamidian et al., 2014) and United States (Rao et al., 2018). The RepAci6 plasmids harboring TnaphA6 have been identified in A. baumannii isolates from Australia (Hamidian et al., 2014; Nigro and Hall, 2014). These plasmids were detected in GC1 A. baumannii isolates in Iran (Aris et al., 2019; Douraghi et al., 2021); however, there was no study to investigate their presence in GC2 isolates from Iran. In this study, it was revealed that more than half of GC2 isolates contain the RepAci6 plasmids carrying TnaphA6. All the GC2 isolates carrying the aadB gene contained pRAY*. The aadB gene cassette was originally characterized in a class 1 integron (Cameron et al., 1986; Recchia and Hall, 1995); however, it was subsequently found at a secondary location on plasmid pRAY (Segal and Elisha, 1997, 1999). The class 1 integron carrying aadB was not found in GC2 isolates in the current study, which is consistent with the previous study by Nemec and Nigro (Nemec et al., 2004; Nigro et al., 2011). Hence, pRAY* had a significant role in carrying the aadB gene in the isolates tested.
Conclusion
This study provides the evidence for dissemination of aminoglycoside resistance genes through MGEs in GC2 A. baumannii isolated from Iran as the second-largest country in the Middle East. In addition, this finding suggests that the strains carrying these MGEs are not restricted to a particular geographical region, and they are globally distributed. The results obtained in this study will underpin future studies of GC2 strains from other countries.
Data availability statement
The datasets presented in this study can be found in online repositories. The names of the repository/repositories and accession number(s) can be found in the article/Supplementary material.
Ethics statement
The Ethics Committee of Tehran University of Medical Sciences approved the study IR.TUMS.SPH.REC.1397.291. All patients have signed the informed consent for giving the required specimens for research. Parents/legal guardians provided written informed consent for their children under the age of 18 years to participate in this study.
Author contributions
GN performed the microbiological and molecular experiments and wrote and revised the manuscript. MT and MR were the advisors of the project. RG and SG did the molecular experiments. AS and AA were the clinical advisors of the project. AN was involved in the collection of isolates from the hospital. MD conceptualized, designed, coordinated, supported this study, contributed to the acquisition of fund, the analysis and interpretation of data, and performed the revision of the manuscript. All authors have read and agreed to the published version of the manuscript.
Funding
This research was supported by a grant from the Tehran University of Medical Sciences and Health Services, Iran (Grant No. 42909).
Acknowledgments
We would like to thank Dr. A. Nemec for supplying A. baumannii strains NIPH 533, NIPH 537, NIPH 1653, NIPH 1656, NIPH 1657, and NIPH 1660 used for experimental work in this study. We are also grateful to Dr. G. A. Blackwell for her excellent technical assistance and guidance in this research.
Conflict of interest
The authors declare that the research was conducted in the absence of any commercial or financial relationships that could be construed as a potential conflict of interest.
Publisher's note
All claims expressed in this article are solely those of the authors and do not necessarily represent those of their affiliated organizations, or those of the publisher, the editors and the reviewers. Any product that may be evaluated in this article, or claim that may be made by its manufacturer, is not guaranteed or endorsed by the publisher.
Supplementary material
The Supplementary Material for this article can be found online at: https://www.frontiersin.org/articles/10.3389/fmicb.2023.1172861/full#supplementary-material
References
Aliakbarzade, K., Farajnia, S., Nik, A. K., Zarei, F., and Tanomand, A. (2014). Prevalence of aminoglycoside resistance genes in Acinetobacter baumannii isolates. Jundishapur J. Microbiol. 214, 7. doi: 10.5812/jjm.11924
Al-Tamimi, M., Albalawi, H., Alkhawaldeh, M., Alazzam, A., Ramadan, H., Altalalwah, M., et al. (2022). Multidrug-Resistant Acinetobacter baumannii in Jordan. Microorganisms 10, 849. doi: 10.3390/microorganisms10050849
Aris, P., Boroumand, M. A., and Douraghi, M. (2019). Amikacin resistance due to the aphA6 gene in multi-antibiotic resistant Acinetobacter baumannii isolates belonging to global clone 1 from Iran. BMC Microbiol. 19, 1–6. doi: 10.1186/s12866-019-1592-6
Bakour, S., Alsharapy, S. A., and Touati, A. (2014). Characterization of Acinetobacter baumannii clinical isolates carrying blaOXA−23 carbapenemase and 16S rRNA methylase armA genes in Yemen. Microb. Drug Resist. 20, 604–609. doi: 10.1089/mdr.2014.0018
Blackwell, G. A. (2017). Antibiotic resistance in global clone 2 Acinetobacter baumannii from Singapore. Ph.D. dissertation.
Blackwell, G. A., Holt, K. E., Bentley, S. D., Hsu, L. Y., and Hall, R. M. (2017). Variants of AbGRI3 carrying the armA gene in extensively antibiotic-resistant Acinetobacter baumannii from Singapore. J. Antimicrob. Chemother. 72, 1031–1039. doi: 10.1093/jac/dkw542
Cameron, F. H., Groot Obbink, D. J., and Ackerman, V. P. (1986). Nucleotide sequence of the AAD (2′) aminoglycoside adenylyltransferase determinant aadB. Evolutionary relationship of this region with those surrounding aadA in R538-1 and dhfrll in R388. Nucleic Acids Res. 14, 8625–8635. doi: 10.1093/nar/14.21.8625
Douraghi, M., Aris, P., To, J., Myers, G. S. A., and Hamidian, M. (2021). Two carbapenem-resistant ST1, ST231. KL1: OCL1 Acinetobacter baumannii strains recovered in Tehran Iran carry AbaR31 in the chromosome and AbaR4 and TnaphA6 in a RepAci6 plasmid. J. Antimicrob Chemother. 3. dlab112. doi: 10.1093/jacamr/dlab112
Douraghi, M., Kenyon, J. J., Aris, P., Asadian, M., Ghourchian, S., Hamidian, M., et al. (2020). Accumulation of antibiotic resistance genes in carbapenem-resistant Acinetobacter baumannii isolates belonging to lineage 2, global clone 1, from outbreaks in 2012–2013 at a Tehran burns hospital. mSphere 5, e00164–e00120. doi: 10.1128/mSphere.00164-20
Forbes, B. A., and Sahm, D. F. (2016). Study Guide for Bailey and Scott's Diagnostic Microbiology-E-Book. China: Elsevier Health Sciences.
Fournier, P. E., and Richet, H. (2006). The epidemiology and control of Acinetobacter baumannii in health care facilities. Clin. Infect. Dis. 42, 692–699. doi: 10.1086/500202
Galimand, M., Courvalin, P., and Lambert, T. (2003). Plasmid-mediated high-level resistance to aminoglycosides in Enterobacteriaceae due to 16S rRNA methylation. Antimicrob. Agents Chemother. 47, 2565–2571. doi: 10.1128/AAC.47.8.2565-2571.2003
Hamidian, M., and Hall, R. M. (2011). AbaR4 replaces AbaR3 in a carbapenem-resistant Acinetobacter baumannii isolate belonging to global clone 1 from an Australian hospital. J. Antimicrob. Chemother. 66, 2484–2491. doi: 10.1093/jac/dkr356
Hamidian, M., and Hall, R. M. (2018). The AbaR antibiotic resistance islands found in Acinetobacter baumannii global clone 1–structure, origin and evolution. Drug Resist. Updat 41, 26–39. doi: 10.1016/j.drup.2018.10.003
Hamidian, M., Holt, K. E., Pickard, D., Dougan, G., and Hall, R. M. (2014). A GC1 Acinetobacter baumannii isolate carrying AbaR3 and the aminoglycoside resistance transposon TnaphA6 in a conjugative plasmid. J. Antimicrob. Chemother. 69, 955–958. doi: 10.1093/jac/dkt454
Hamidian, M., Nigro, S. J., and Hall, R. M. (2012). Variants of the gentamicin and tobramycin resistance plasmid pRAY are widely distributed in Acinetobacter. J. Antimicrob. Chemother. 67, 2833–2836. doi: 10.1093/jac/dks318
Holt, K. E., Kenyon, J. J., Hamidian, M., Schultz, M. B., Pickard, D. J., Dougan, G., et al. (2016). Five decades of genome evolution in the globally distributed, extensively antibiotic-resistant Acinetobacter baumannii global clone 1. Microb. Genom. 2, e000052. doi: 10.1099/mgen.0.000052
Kim, D. H., Jung, S.-. I, Kwon, K. T., and Ko, K. S. (2017). Occurrence of diverse AbGRI1-type genomic islands in Acinetobacter baumannii global clone 2 isolates from South Korea. Antimicrob. Agents Chemother. 61, e01972–e01916. doi: 10.1128/AAC.01972-16
Kishk, R., Soliman, N., Nemr, N., Eldesouki, R., Mahrous, N., Gobouri, A., et al. (2021). Prevalence of aminoglycoside resistance and aminoglycoside modifying enzymes in Acinetobacter baumannii among intensive care unit patients, Ismailia, Egypt. Infect. Drug Resist. 14, 143. doi: 10.2147/IDR.S290584
Krizova, L., Dijkshoorn, L., and Nemec, A. (2011). Diversity and evolution of AbaR genomic resistance islands in Acinetobacter baumannii strains of European clone I. Antimicrob. Agents Chemother. 55, 3201–3206. doi: 10.1128/AAC.00221-11
Lee, K., Yong, D., and Jeong, S. H. (2011). Multidrug-resistant Acinetobacter spp.: increasingly problematic nosocomial pathogens. Yonsei Med. J. 52, 879–891. doi: 10.3349/ymj.2011.52.6.879
Magiorakos, A. P., Srinivasan, A., Carey, R. B., Carmeli, Y., Falagas, M. E., Giske, C. G., et al. (2012). Multidrug-resistant, extensively drug-resistant and pandrug-resistant bacteria: an international expert proposal for interim standard definitions for acquired resistance. Clin. Microbiol. Infect. 18, 268–281. doi: 10.1111/j.1469-0691.2011.03570.x
Nemec, A., Dolzani, L., Brisse, S., van den Broek, P., and Dijkshoorn, L. (2004). Diversity of aminoglycoside-resistance genes and their association with class 1 integrons among strains of pan-European Acinetobacter baumannii clones. J. Med. Microbiol. 53, 1233–1240. doi: 10.1099/jmm.0.45716-0
Nie, L., Lv, Y., Yuan, M., Hu, X., Nie, T., Yang, X., et al. (2014). Genetic basis of high level aminoglycoside resistance in Acinetobacter baumannii from Beijing, China. Acta Pharm. Sin B 4, 295–300. doi: 10.1016/j.apsb.2014.06.004
Nigro, S. J. (2014). Analysis of Australian multiply-antibiotic resistant Acinetobacter baumannii belonging to global clone 2. Ph.D. dissertation.
Nigro, S. J., Farrugia, D. N., Paulsen, I. T., and Hall, R. M. (2013). A novel family of genomic resistance islands, AbGRI2, contributing to aminoglycoside resistance in Acinetobacter baumannii isolates belonging to global clone 2. J. Antimicrob. Chemother. 68, 554–557. doi: 10.1093/jac/dks459
Nigro, S. J., and Hall, R. M. (2014). Amikacin resistance plasmids in extensively antibiotic-resistant GC2 Acinetobacter baumannii from two Australian hospitals. J. Antimicrob. Chemother. 69, 3435–3437. doi: 10.1093/jac/dku310
Nigro, S. J., and Hall, R. M. (2016). Loss and gain of aminoglycoside resistance in global clone 2 Acinetobacter baumannii in Australia via modification of genomic resistance islands and acquisition of plasmids. J. Antimicrob. Chemother. 71, 2432–2440. doi: 10.1093/jac/dkw176
Nigro, S. J., Holt, K. E., Pickard, D., and Hall, R. M. (2015). Carbapenem and amikacin resistance on a large conjugative Acinetobacter baumannii plasmid. J. Antimicrob. Chemother. 70, 1259. doi: 10.1093/jac/dku486
Nigro, S. J., Post, V., and Hall, R. M. (2011). Aminoglycoside resistance in multiply antibiotic-resistant Acinetobacter baumannii belonging to global clone 2 from Australian hospitals. J. Antimicrob. Chemother. 66, 1504–1509. doi: 10.1093/jac/dkr163
Post, V., and Hall, R. M. (2009). AbaR5, a large multiple-antibiotic resistance region found in Acinetobacter baumannii. Antimicrob. Agents Chemother. 53, 2667–2671. doi: 10.1128/AAC.01407-08
Post, V., Hamidian, M., and Hall, R. M. (2012). Antibiotic-resistant Acinetobacter baumannii variants belonging to global clone 1. J. Antimicrob. Chemother. 67, 1039–1040. doi: 10.1093/jac/dkr586
Post, V., White, P. A., and Hall, R. M. (2010). Evolution of AbaR-type genomic resistance islands in multiply antibiotic-resistant Acinetobacter baumannii. J. Antimicrob. Chemother. 65, 1162–1170. doi: 10.1093/jac/dkq095
Rao, J., Susanti, D., Childress, J. C., Mitkos, M. C., Brima, J. K., Baffoe-Bonnie, A. W., et al. (2018). Tn2008-driven carbapenem resistance in Acinetobacter baumannii isolates from a period of increased incidence of infections in a Southwest Virginia Hospital (USA). J. Glob Antimicrob. Resiste. 12, 79–87. doi: 10.1016/j.jgar.2017.08.017
Recchia, G. D., and Hall, R. M. (1995). Gene cassettes: a new class of mobile element. Microbiology 141, 3015–3027. doi: 10.1099/13500872-141-12-3015
Sannathimmappa, M. B., Nambiar, V., and Aravindakshan, R. (2021). Antibiotic resistance pattern of Acinetobacter baumannii strains: A retrospective study from Oman. Saudi J. Med. Sci. 9, 254. doi: 10.4103/sjmms.sjmms_855_20
Segal, H., and Elisha, B. G. (1997). Identification and characterization of an aadB gene cassette at a secondary site in a plasmid from Acinetobacter. FEMS Microbiol. Lett. 153, 321–326. doi: 10.1111/j.1574-6968.1997.tb12591.x
Segal, H., and Elisha, B. G. (1999). Characterization of the Acinetobacter plasmid, pRAY, and the identification of regulatory sequences upstream of an aadB gene cassette on this plasmid. Plasmid 42, 60–66. doi: 10.1006/plas.1999.1403
Tacconelli, E., Carrara, E., Savoldi, A., Harbarth, S., Mendelson, M., Monnet, D. L., et al. (2018). Discovery, research, and development of new antibiotics: the WHO priority list of antibiotic-resistant bacteria and tuberculosis. Lancet Infect. Dis. 18, 318–327. doi: 10.1016/S1473-3099(17)30753-3
Towner, K. (2009). Acinetobacter: an old friend, but a new enemy. J. Hosp. Infect. 73, 355–363. doi: 10.1016/j.jhin.2009.03.032
Turton, J. F., Gabriel, S. N., Valderrey, C., Kaufmann, M. E., and Pitt, T. L. (2007). Use of sequence-based typing and multiplex PCR to identify clonal lineages of outbreak strains of Acinetobacter baumannii. Clin. Microbiol. Infect. 13, 807–815. doi: 10.1111/j.1469-0691.2007.01759.x
Turton, J. F., Woodford, N., Glover, J., Yarde, S., Kaufmann, M. E., Pitt, T. L., et al. (2006). Identification of Acinetobacter baumannii by Detection of the blaOXA?51?like carbapenemase gene intrinsic to this species. J. Clin. Microbiol. 44, 2974–2976. doi: 10.1128/JCM.01021-06
Wayne, P. A. (2022). Clinical and Laboratory Standards Institute: Performance standards for antimicrobial susceptibility testing: 30th informational supplement. CLSI. M100, S20.
Keywords: Acinetobacter baumannii genomic resistance island, aminoglycoside resistance, global clone 2, mobile genetic element (MGE), plasmids
Citation: Naderi G, Talebi M, Gheybizadeh R, Seifi A, Ghourchian S, Rahbar M, Abdollahi A, Naseri A, Eslami P and Douraghi M (2023) Mobile genetic elements carrying aminoglycoside resistance genes in Acinetobacter baumannii isolates belonging to global clone 2. Front. Microbiol. 14:1172861. doi: 10.3389/fmicb.2023.1172861
Received: 23 February 2023; Accepted: 12 April 2023;
Published: 05 May 2023.
Edited by:
Lucinda Janete Bessa, Egas Moniz School of Health & Science, PortugalReviewed by:
Jing Han, National Center for Toxicological Research (FDA), United StatesXiaofei Jiang, Fudan University, China
Ivana Goic-Barisic, University Hospital Split, Croatia
Copyright © 2023 Naderi, Talebi, Gheybizadeh, Seifi, Ghourchian, Rahbar, Abdollahi, Naseri, Eslami and Douraghi. This is an open-access article distributed under the terms of the Creative Commons Attribution License (CC BY). The use, distribution or reproduction in other forums is permitted, provided the original author(s) and the copyright owner(s) are credited and that the original publication in this journal is cited, in accordance with accepted academic practice. No use, distribution or reproduction is permitted which does not comply with these terms.
*Correspondence: Masoumeh Douraghi, bWRvdXJhZ2hpQHR1bXMuYWMuaXI=; Malihe Talebi, VGFsZWJpLm1AaXVtcy5hYy5pcg==