- Hubei Key Laboratory of Genetic Regulation and Integrative Biology, College of Life Sciences, Central China Normal University, Wuhan, Hubei, China
Introduction: Actinobacillus pleuropneumoniae (A. pleuropneumoniae) is a member of Actinobacillus in family Pasteurellaceae. It is the causative agent of porcine pleuropneumonia, which has caused huge economic losses to pig industry over the world. Cysteine is a precursor of many important biomolecules and defense compounds in the cell. However, molecular mechanisms of cysteine transport in A. pleuropneumoniae are unclear.
Methods: In this study, gene-deleted mutants were generated and investigated, to reveal the roles of potential cysteine/cystine transport proteins FliY and YdjN of A. pleuropneumoniae.
Results: Our results indicated that the growth of A. pleuropneumoniae was not affected after fliY or ydjN single gene deletion, but absence of both FliY and YdjN decreased the growth ability significantly, when cultured in the chemically defined medium (CDM) supplemented with cysteine or cystine as the only sulfur source. A. pleuropneumoniae double deletion mutant ΔfliYΔydjN showed increased sensitivity to oxidative stress. Besides, trans-complementation of YdjN into ΔfliYΔydjN and wild type leads to increased biofilm formation in CDM. However, the virulence of ΔfliYΔydjN was not attenuated in mice or pigs.
Discussion: These findings suggest that A. pleuropneumoniae FliY and YdjN are involved in the cysteine/cystine acquisition, oxidative tolerance, and biofilm formation, but not contribute to the pathogenicity of A. pleuropneumoniae.
Introduction
Porcine pleuropneumonia caused by A. pleuropneumoniae is associated with extensive morbidity and mortality in growing pigs; therefore, it is of particular importance for the prevention and control of this disease (Sassu et al., 2018). The current knowledge has indicated that several virulence factors are involved in adherence and colonization, immune escape, and tissue damage during the pathogenesis of A. pleuropneumoniae infection, including but not limited to Apx toxins, lipopolysaccharides, capsular polysaccharides, adhesins, iron acquisition factors, enzymes, biofilm formation, and signal transduction systems (Chiers et al., 2010). However, more efforts are still needed for a comprehensive understanding of the pathogenesis of A. pleuropneumoniae infection.
After invading the host, the acquisition of essential nutrients is critical for the survival and successful infection of pathogenic bacteria. The mechanisms involved in nutritional satisfaction are essential for bacterial pathogenicity (Núñez et al., 2018). Sulfur is a key constituent of sulfur-containing amino acids, coenzymes, vitamins, nucleotides, and peptides (Beinert, 2000). Most of the sulfur metabolic pathways are unique to microorganisms and are a potential target for future therapeutic intervention against pathogen attacks (Paritala and Carroll, 2013). The transporter protein Sbp in A. pleuropneumoniae has been found to be associated with sulfate and methionine utilization, but it was not found to be a determinant of virulence in a previous study by Gao et al. (2020). More efforts are needed for the mechanisms of sulfur assimilation and metabolism in A. pleuropneumoniae.
As one of the important sulfur sources, cysteine is used for the biosynthesis of a variety of cellular components, such as protein, glutathione, biotin, and more (Hicks and Mullholland, 2018). In addition, cysteine has been implicated in the survival and virulence of pathogens (Soutourina et al., 2009; Méndez et al., 2011). In Escherichia coli, the ABC transporter FliY was reported to be essential for the import of cysteine and trace cystine, whereas YdjN is a predominant importer of cystine when cystine is more abundant (Imlay et al., 2015). Two homologous proteins, namely, APJL_1690 (FliY) and APJL_0600 (YdjN), have been identified from A. pleuropneumoniae previously (Gao et al., 2020). Therefore, the roles of these two potential cysteine/cystine transporters in the sulfur acquisition, stress tolerance, biofilm formation, and virulence of A. pleuropneumoniae were investigated in this study.
Materials and methods
Bacterial strains, primers, plasmids, and growth conditions
The bacterial strains, plasmids, and primers used in this study are listed in Table 1. Actinobacillus pleuropneumoniae strains were cultured in tryptic soy agar (TSA, Dickinson and Company, Franklin Lakes, NJ, United States) or tryptic soy broth (TSB), supplemented with 10 μg/ml nicotinamide adenine dinucleotide (NAD+; Sigma, St. Louis, MO, United States) and 10% fetal calf serum (Gibco BRL, Grand Island, NY, United States), and 2 μg/ml chloramphenicol and 5% (m/v) sucrose were added for the selection of A. pleuropneumoniae single-crossover mutants and double-crossover mutants, respectively. To select the complementation strain, 2 μg/ml chloramphenicol was added. To evaluate the utilization of different sulfur sources, A. pleuropneumoniae strains were grown in chemically defined media (CDM), which consisted of different sulfur sources (potassium sulfate, cysteine, cystine, glutathione, and methionine). The preparation of CDM was carried out as described previously (Herriott et al., 1970). Escherichia coli strains were grown on Luria–Bertani (LB) agar (Oxoid, Basingstoke, Hants, UK) or in LB broth, supplemented with ampicillin (100 μg/ml) and/or diaminopimelic acid (DAP; 50 μg/ml) if needed. Unless otherwise noted, bacteria in liquid media were cultured in a common incubator at 37°C with shaking (200 rpm), and bacteria on agar plates were incubated statically at 37°C.
DNA manipulations
The target genes fliY and ydjN were knocked out sequentially from A. pleuropneumoniae wild-type (WT) strain WF83 (serovar 7) using one-step transconjugation methods, as described previously (Oswald et al., 1999). For the construction of a single-gene deleted mutant, two homologous arms of the target gene were amplified by PCR separately and ligated together using overlap PCR, then ligated into the transconjugation plasmid pEMOC2 (Baltes et al., 2002), to generate the recombination vector, and then transformed into E. coli β2155 to form the donor cells. The recombination vector was introduced into A. pleuropneumoniae WT by co-cultivation of the WT and donor cells. After chloramphenicol-mediated positive selection and sucrose-mediated counter-selection, chloramphenicol-sensitive and sucrose-resistant colonies were selected and recognized as target mutants and then verified with PCR and sequencing at Sangon Biotech (Shanghai, China). The double-gene deletion mutant was generated by using the single-gene deletion mutant as a parent.
The complemented mutants were constructed as described previously (Liu et al., 2018). The intact fliY and ydjN genes were cloned from A. pleuropneumoniae WT and inserted into the E. coli–A. pleuropneumoniae shuttle vector, pJFF224-XN (Frey, 1992), to generate the plasmids pJFF-fliY and pJFF-ydjN separately and transformed into ΔfliYΔydjN by electroporation. Chloramphenicol-resistance colonies were selected and verified by PCR and RT-PCR.
Growth ability and sulfur source utilization assays
To assess the role of fliY and ydjN on bacterial growth, A. pleuroPneumoniae WT, the single-gene deletion mutants ΔfliY and ΔydjN, and the double-gene deletion mutant ΔfliYΔydjN were grown separately in TSB. Overnight cultures were separately diluted (1:100) in 5 ml fresh TSB in 20 ml glass tubes and cultured with shaking for 10 h. The optical densities at 600 nm (OD600) were measured each hour, so as to generate bacterial growth curves. OD600 values were repeated in triplicate with at least three independent experiments.
To analyze the roles of the target genes in the utilization of sulfur sources, overnight cultures of each strain were inoculated in CDM with different sulfur sources, including complete CDM (containing potassium sulfate, methionine, glutathione, cysteine, and cystine), blank CDM (without sulfur), and CDM with only one type of sulfur source, and the growth curves were obtained.
Oxidative stress sensitivity assay
In order to analyze the effect of FliY and YdjN on the defense of A. pleuropneumoniae against H2O2-induced oxidative stress, overnight cultures were diluted (1:100) in fresh TSB separately and grown to the mid-log phase. Samples were taken, centrifuged, washed with PBS (phosphate-buffered saline, pH7.4), and re-suspended in PBS or PBS with H2O2 (2.5 mM), then incubated at 37°C. Samples were taken at 1 h and 2 h after treatment, diluted immediately in PBS, and spread onto TSA plates. After incubation, viable counts were determined by plate counts on the agar. The survival rate of each strain was calculated by dividing viable counts exposed to H2O2 by the negative control (without H2O2 treatment). An oxidative stress sensitivity assay was performed at least in triplicate.
Biofilm formation assay
The biofilm formation of A. pleuropneumoniae strains was measured as described previously but with slight modifications (Liu et al., 2017). In brief, A. pleuropneumoniae overnight cultures were diluted (1:100) in 1 ml of TSB or complete CDM in 2 ml tubes and added into 24-well flat-bottom polystyrene plates separately. The plates were incubated at 37°C for 24 h statically. Each well was washed twice with 1 ml of sterile water and stained with 500 μl of 0.4% crystal violet for 15 min, washed with water three times, and then air-dried for 30 min. Acetic acid (33%, 1 ml) was added to each well and shaken for 15 min to quantify the number of biofilms. The absorbance was measured at 590 nm (OD590) and normalized to the OD600 values of cultures to account for growth differences. To better reveal the influence of these two potential cysteine/cystine transporters, FliY and YdjN, on the biofilm formation of A. pleuropneumoniae, the florescent stain was used for the visualization of biofilm. In brief, sterile cover glasses were pre-put at the bottom of 24-well plates, and bacterial cultures were diluted (1:100) in 1 ml of complete CDM and added to 24-well plates. The plates were incubated at 37°C for 24 h. After two washes with 1 ml of PBS, 1 ml of 1.5 μM SYTO 9 was added to each well and stained for 20 min in the dark. After washing with PBS, the cover glasses were taken out and placed on slide glasses, and fluorescence signals were analyzed with a confocal laser scanning microscope (CLSM, Axio Scope, Carl Zeiss Meditec, Berlin, Germany). For CLSM analysis, the excitation light wavelength was set at 488 nm and collected with a 495–525 nm bandpass filter. ImageJ software was used for image processing.
Bacterial colonization assay
Bacterial colonization in the lung tissues of mice was carried out as described previously (Liu et al., 2018). Fifteen 6-week-old female BALB/c mice (purchased from Hubei Provincial Center for Disease Control and Prevention, Wuhan, China) were divided into three groups of five mice per group. Groups I and II were inoculated intraperitoneally with 2.5 × 107 CFU of A. pleuropneumoniae WT and ΔfliYΔydjN in 0.5 ml saline, respectively, and group III was inoculated with 0.5 ml saline and used as a negative control. The mice were monitored closely, and severely diseased mice (designated as those with dyspnea and depression) were euthanized. The surviving mice were euthanized 24 h after inoculation. Lung tissues (~0.1 g) were collected aseptically for homogenization. The lung homogenates were diluted properly, spread on TSA plates, and incubated at 37°C overnight. Single colonies were verified by PCR with primers apxIVA-F/apxIVA-R (specific for apxIVA gene) (Schaller et al., 2001), and the bacterial load of each strain was calculated. The animal experiments in this study were approved by the Animal Ethics Committee at the Central China Normal University and carried out in accordance with the guidelines for the Care and Use of Laboratory Animals provided by this committee (Ethics Ratification ID: CCNU-IACUC-2020-015).
Experimental infection in pigs
The pig infection assay (in vivo competitive colonization) was performed as described previously, with minor modifications (Beddek et al., 2004), so as to determine the virulence of A. pleuropneumoniae ΔfliYΔydjN. Bacteria cultures at the mid-log phase were sampled and diluted to ~2.0 × 108 CFU/ml, respectively, and then mixed in an equal volume. Five newborn piglets were purchased from an A. pleuropneumoniae-free herd (negative in serological and etiological tests) and inoculated intranasally with 1 ml of the mixed bacterial culture. Animals were monitored every 6 h for 24 h, and severely diseased pigs, designated as those with dyspnea, depression, or with a rectal temperature lower than 38.5°C, were humanely euthanized. The surviving pigs were humanely euthanized at 24 h after infection. Lung tissues were obtained aseptically for homogenization. The lung tissue homogenates were diluted properly and spread onto TSA plates, then cultured at 37°C overnight. For each infected piglet, at least 100 single colonies were picked randomly and cultured in TSB separately. PCR assays with primers specific to the apxIVA gene (apxIVA-F/apxIVA-R), fliY gene (fliY-F4/fliY-R4), and ydjN gene (ydjN-F4/ydjN-R4) were used to differentiate these re-isolates. Actinobacillus pleuropneumoniae WT was positive in all three PCR assays, whereas ΔfliYΔydjN was positive in apxIVA but negative in fliY or ydjN gene amplification. The in vivo competitive index (CI) values were calculated by dividing the ratio of ΔfliYΔydjN to WT (output) by the ratio of ΔfliYΔydjN to WT in the bacteria mixture (input).
Statistical analysis
Unless otherwise specified, data obtained from the present study were expressed as the mean ± SD and analyzed by a two-tailed unpaired Student's t-test. A P-value of < 0.05 was considered significant, and a P-value of < 0.01 was considered highly significant.
Results
Generation of A. pleuropneumoniae gene deletion mutants and complementation
The target genes fliY and ydjN were knocked out from the A. pleuropneumoniae WT using one-step transconjugation methods, resulting in the single-gene deletion mutants ΔfliY and ΔydjN and double-gene deletion mutant ΔfliYΔydjN, and the absence of fliY and ydjN in these mutants was verified with PCRs and DNA sequencing (Supplementary Figure S1). We next constructed corresponding complementation strains based on the E. coli–A. pleuropneumoniae shuttle vector pJFF224-XN. The complementary plasmids pJFF-fliY and pJFF-ydjN were transformed into ΔfliYΔydiN, generating ΔfliYΔydiN-fliY+ and ΔfliYΔydiN-ydiN+, respectively. These mutants were screened by chloramphenicol resistance, and then verified by PCR and reverse transcription PCR (RT-PCR) assays with primers specific for target genes (Supplementary Figure S2). In addition, pJFF-ydjN was transferred into the WT to generate WT-ydiN+.
The FliY and YdjN double mutation leads to a longer lag phase in TSB
The growth curves of A. pleuropneumoniae WT and mutants in TSB were obtained. As shown in Figure 1, the fliY and ydjN single-gene deletion mutants (ΔfliY and ΔydjN) showed similar growth levels to that of the WT. Though the double-gene deletion mutant ΔfliYΔydjN exhibited similar growth levels relative to that of the WT at stationary phase, the growth levels of ΔfliYΔydjN at 2 h (P-value < 0.05) and 3 h (P-value < 0.01) were significantly lower than those of the WT, indicating a longer lag phase of the FliY and YdjN double mutant.
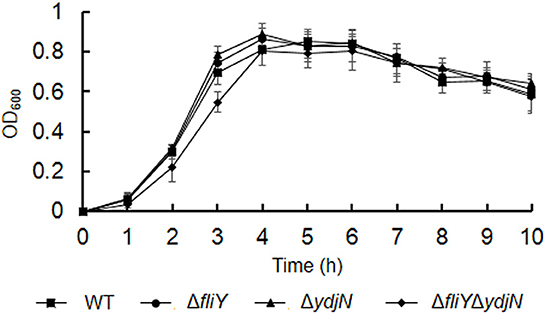
Figure 1. Growth curves of Actinobacillus pleuropneumoniae WT and mutants in TSB. Data are expressed as the mean ± SD of at least three independent experiments.
FliY and YdjN participate in the acquisition of sulfur sources
The roles of FliY and YdjN in the acquisition of sulfur source(s) were investigated by the cultivation of A. pleuropneumoniae WT and mutants in sulfur-limited CDM. As shown in Figure 2, all these bacterial strains showed no or little growth in sulfur-free CDM (Figure 2A), and there was no significant difference in the growth of A. pleuropneumoniae WT and mutants in complete CDM (Figure 2B), as well as in CDM supplemented with potassium sulfate, methionine, or glutathione as the sole sulfur source (Figure 3). When cysteine or cystine was used as the only sulfur source, the growth capacity of ΔfliYΔydjN was significantly impaired (Figures 2C, D). Surprisingly, the single-gene deletion mutants ΔfliY and ΔydjN did not show any decreased growth levels compared to the WT strain in CDM supplemented with cysteine or cystine (Figures 2C, D). The complementation of ΔfliYΔydjN with fliY restored the growth defect to the WT level, while the transformation of ydjN rescued the growth defect of ΔfliYΔydjN, though not yet back to the WT level (Figure 4). These results suggest that FliY and YdjN are required for the utilization of cysteine and cystine as sulfur sources in A. pleuropneumoniae.
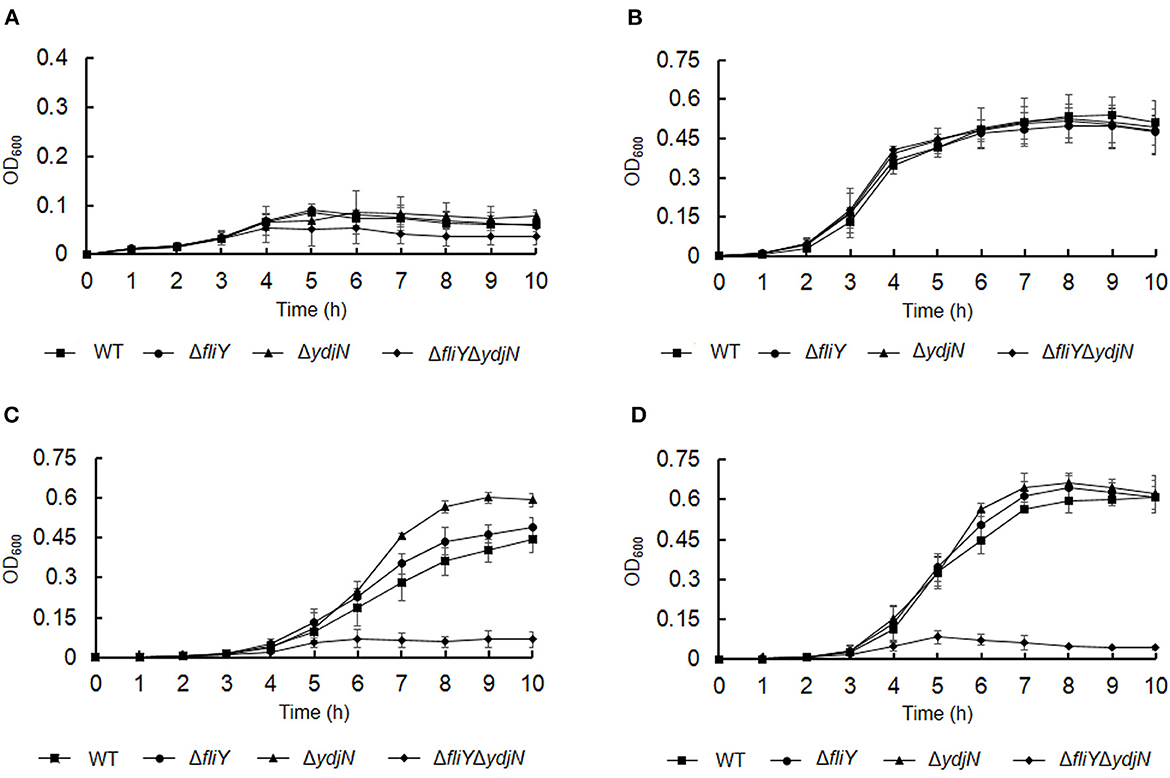
Figure 2. Proliferation of Actinobacillus pleuropneumoniae WT and mutants in CDM supplemented with different sulfur sources. Actinobacillus pleuropneumoniae WT, single-gene deletion mutants, ΔfliY and ΔydiN, and double-gene deletion mutant, ΔfliYΔydiN, were grown in the blank CDM (A), complete CDM (B), and CDM with cysteine (C) or cystine (D) as the sole sulfur source, separately. Data are expressed as the mean ± SD of at least three independent experiments.
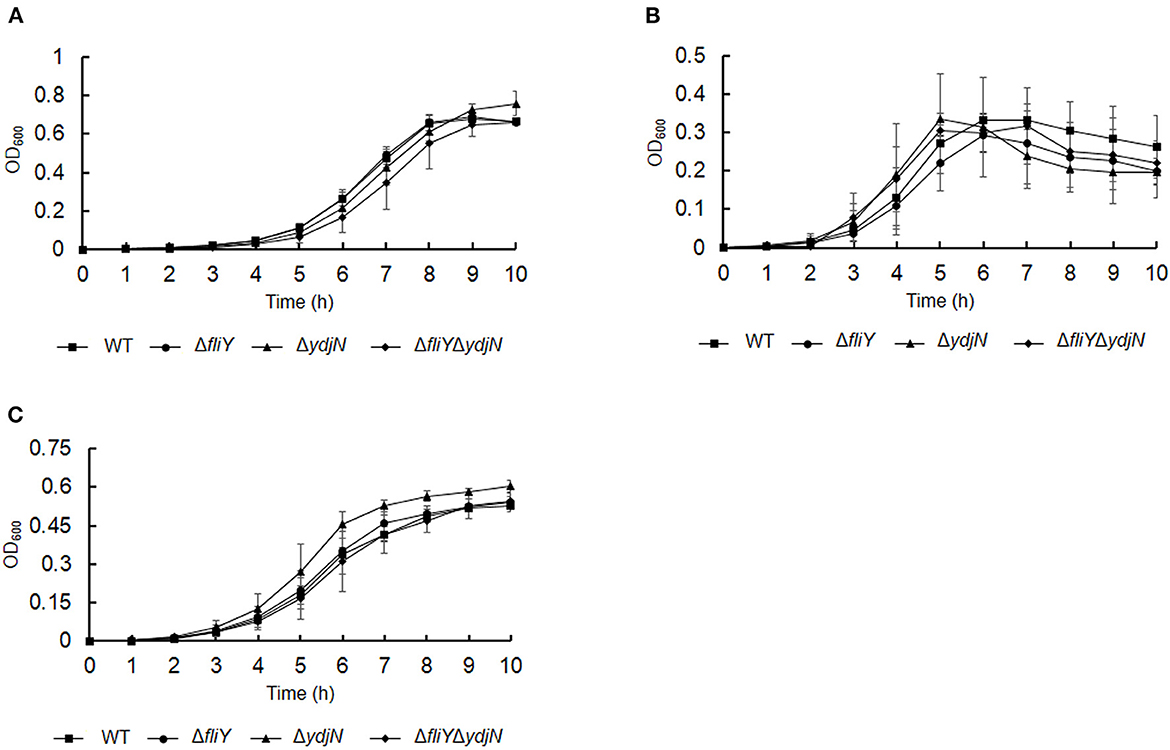
Figure 3. Growth of Actinobacillus pleuropneumoniae WT and mutants in CDM supplemented with potassium sulfate (A), methionine (B), and glutathione (C) as the sole source of sulfur. Error bars represent ± 1 standard error of the mean. The average of at least three independent trials is presented.
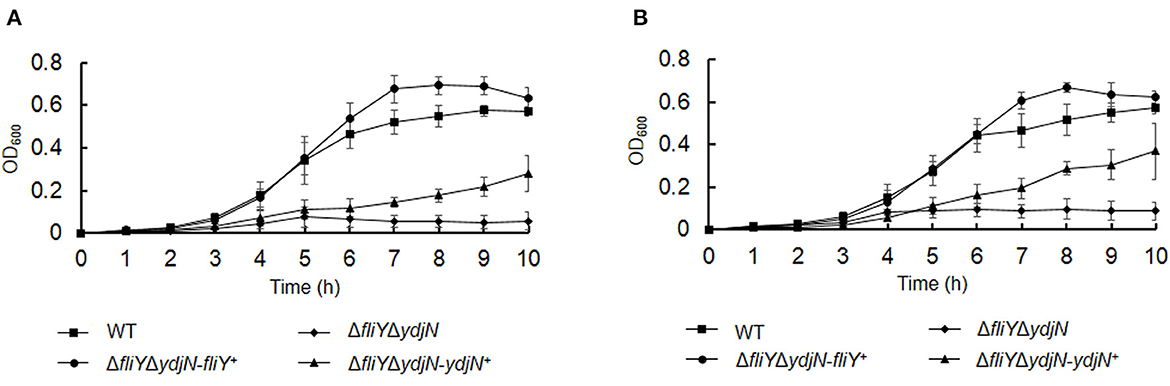
Figure 4. Restoration of growth phenotypes of Actinobacillus pleuropneumoniae via trans-complementation. Actinobacillus pleuropneumoniae WT, double-gene deletion mutant, ΔfliYΔydiN, and complementation strains, ΔfliYΔydjN-fliY+ and ΔfliYΔydjN-ydjN+, were grown in the CDM with cysteine (A) or cystine (B) as the sole sulfur source. Data are expressed as the mean ± SD of at least three independent experiments.
Deletion of FliY and YdjN increases susceptibility to oxidative stress
The sensitivity of A. pleuropneumoniae WT and ΔfliYΔydjN to H2O2-induced oxidative stress was determined. As shown in Figure 5, ΔfliYΔydjN was more sensitive to 2.5 mM H2O2 when compared to the WT strain (P-value < 0.01), the average survival rates of ΔfliYΔydjN and WT were 0.58% (0.58 ± 0.11%) and 10.08% (10.08 ± 2.08%) 1 h after exposure to H2O2, respectively, and the survival rates of ΔfliYΔydjN and WT were 0.014% (0.014 ± 0.0030%) and 1.53% (1.53 ± 0.28%) at 2 h after exposure to H2O2, respectively. The decreased oxidative stress tolerance of ΔfliYΔydjN was recovered by complementation with the fliY or ydjN gene. These data indicate the role of FliY and YdjN in conferring resistance against oxidative stress in A. pleuropneumoniae.
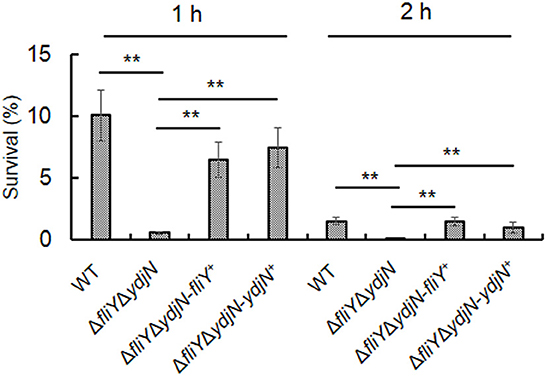
Figure 5. Survival of Actinobacillus pleuropneumoniae following exposure to oxidative stress. Actinobacillus pleuropneumoniae WT, ΔfliYΔydjN, and complemented strains, ΔfliYΔydjN-fliY+ and ΔfliYΔydjN-ydjN+, at mid-log phase were washed and re-suspended in PBS with 2.5 mM H2O2, incubated at 37°C, and then sampled at 1 h and 2 h. Bacterial viability was measured by plate counting. The survival rate was calculated by dividing the CFUs of H2O2-treated bacterial suspension by the CFUs of the untreated control. The double asterisk (**) indicates a highly significant difference (P-value < 0.01).
YdjN regulates A. pleuropneumoniae biofilm formation
The possible impacts of FliY and YdjN on the A. pleuropneumoniae biofilm formation were investigated. As shown in Figure 6A, A. pleuropneumoniae WT formed more biofilm in CDM compared to that in TSB (P-value < 0.01). Notably, A. pleuropneumoniae strains containing plasmid pJFF-ydjN (ΔfliYΔydjN-ydjN+ and WT-ydjN+) showed significantly more biofilm than their parental strains ΔfliYΔydjN and WT (P-value < 0.01), respectively. The enhanced biofilm formation of ΔfliYΔydjN-ydjN+ and WT-ydjN+ was also observed under a confocal scanning laser microscope (Figure 6B). These results indicate that the complementation of the ydjN gene enhances the biofilm formation of A. pleuropneumoniae.
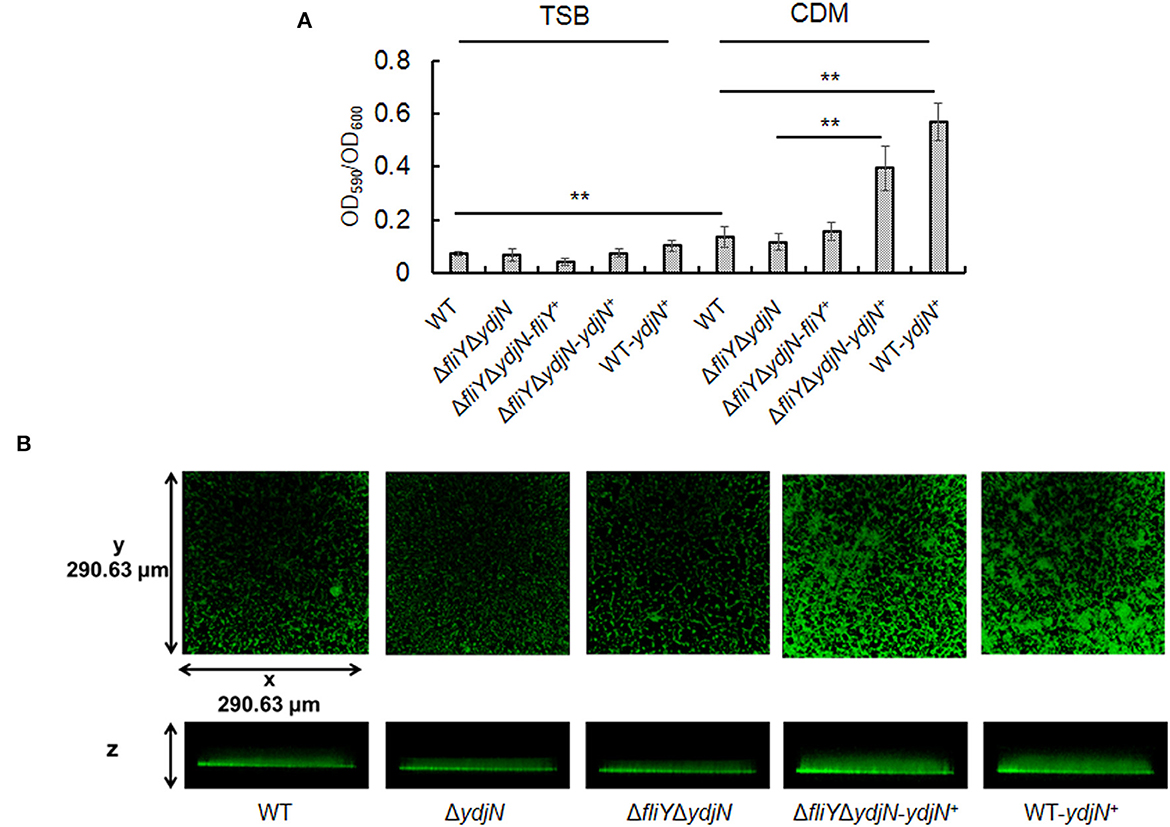
Figure 6. Complementation of the ydjN gene increases biofilm formation. (A) Actinobacillus pleuropneumoniae biofilms were evaluated by CV staining. Actinobacillus pleuropneumoniae WT and mutant strains were cultured in TSB or complete CDM. The biofilm biomass (OD590) was determined by the CV staining method and normalized to the bacterial growth (OD600). Data from at least three biological replicates were averaged, and the statistical significance was determined. **indicates significance with a P-value < 0.01. (B) Actinobacillus pleuropneumoniae biofilms observed by CSLM. Actinobacillus pleuropneumoniae WT and mutant strains were grown in complete CDM, as described in Materials and methods. After 24-h growth, the biofilms were washed and stained with SYTO 9 and scanned by CLSM with a Plan-Neofluar ×10/0.3 objective lens. Representative orthogonal views from three independent experiments are displayed.
FliY and YdjN are not required for the colonization of A. pleuropneumoniae in mice
The requirement of FliY/YdjN for cysteine/cystine-dependent growth and oxidative stress resistance in vitro prompted us to investigate the effect of these two proteins on the virulence of A. pleuropneumoniae. We assessed the colonization ability of the double-gene deletion mutant ΔfliYΔydjN in mice. Mice infected with the ΔfliYΔydjN showed similar bacterial loads (logCFU/g, 6.82 ± 0.94), with those infected with A. pleuropneumoniae WT (logCFU/g, 7.37 ± 0.90; P-value > 0.05). No bacteria were re-isolated in the healthy control group. This result suggests that the deletion of fliY and ydjN does not affect the colonization ability of A. pleuropneumoniae in the lungs of mice.
FliY and YdjN do not contribute to the virulence of A. pleuropneumoniae in pigs
The virulence of ΔfliYΔydjN was further assessed in a pig infection model. As shown in Table 2, the input ratio of ΔfliYΔydjN to WT was calculated as 1.09, and the average output ratio was calculated to be 0.63 (Table 2). Thus, the average CI value between ΔfliYΔydjN and WF83 was 0.58, which was higher than the cutoff value (0.2) for attenuation (Beddek et al., 2004). This result indicates that ΔfliYΔydjN was still virulent in pigs, which is consistent with the result of the mice infection assay, and FliY and YdjN do not contribute to the virulence of A. pleuropneumoniae.
Discussion
As one of the key nutrients, sulfur possesses several singular features and is irreplaceable in biochemistry. Growing cells must find ways to continuously import sulfur at a high rate (Zhou and Imlay, 2020). The ability of pathogenic bacteria to obtain sulfur-containing nutrients in different host niches may therefore contribute to their virulence. For instance, the sulfate-binding protein CysP plays an important role in the growth and survival of Moraxella catarrhalis and is an excellent candidate vaccine antigen to prevent M. catarrhalis infection (Murphy et al., 2016). Elements involved in the acquisition of sulfur nutrients and their importance in the porcine respiratory tract pathogen, A. pleuropneumoniae, were investigated previously, and it was found that the sulfate-binding protein Sbp enables the utilization of sulfate and methionine. However, the sbp gene is not involved in the pathogenesis of A. pleuropneumoniae infection (Gao et al., 2020).
Cysteine is a sulfur-containing amino acid important for protein synthesis and maintaining enzymes and a metabolic precursor of many essential biomolecules and defense compounds, such as iron–sulfur clusters, vitamins, cofactors, and glutathione. Cysteine and cystine can be transformed into each other depending on the prevailing redox state (Kohlmeier, 2003). Several bacterial cysteine/cystine transporters have been identified, and their roles have been confirmed. In E. coli, the roles of the low-affinity symporter YdjN and the high-affinity ABC importer FliY in cysteine/cystine transport have been previously confirmed (Ohtsu et al., 2015; Sabrialabed et al., 2020), and structurally similar substances such as S-sulfocysteine are transported by YdjN (Yamazaki et al., 2016). Additionally, the involvement of these two transport systems in the uptake of toxic L-selenaproline and L-selenocystine makes these compounds an effective treatment of the urinary tract pathogen E. coli (Deutch et al., 2014). In this study, the potential cysteine/cystine transporters of A. pleuropneumoniae were named according to their E. coli counterparts FliY and YdjN, respectively. To better understand the role of FliY and YdjN in A. pleuropneumoniae sulfur utilization and virulence, we first characterized the growth of fliY and ydjN mutants. The growth levels of the fliY and ydjN single-gene deletion mutants were similar to the WT strain in both TSB and CDM, while the double-gene deletion mutant ΔfliYΔydiN was unable to grow in CDM with cysteine or cystine as the sole sulfur source. In addition, it was noted that the mutant ΔfliYΔydiN showed a longer lag phase in the TSB medium relative to that of the WT. A similar result was observed in our previous study, and the sulfate-binding protein Sbp mutant Δsbp had a longer lag phase and lower growth levels when cultured in TSB compared to those of the WT (Gao et al., 2020). It is probable that these mutants take more time to adapt to other sulfur sources in TSB. These results indicate that both FliY and YdjN are involved in the cysteine/cystine-dependent growth of A. pleuropneumoniae.
The accumulation of oxidative active substances, such as superoxide and H2O2 generated from the electron transport chain and H2O2 produced by the host phagocytes, is harmful to bacteria cells, interfering with the normal redox state and causing damage to proteins, lipids, and nucleic acids of bacteria (Imlay, 2013). The sulfhydryl group of cysteine in the periplasm is one of the strategies for bacteria to diminish the toxicity of oxidative stress (Ohtsu et al., 2010). In addition, cysteine is an essential precursor for the biosynthesis of glutathione, a major antioxidant maintaining the homeostasis of the redox state in cells (Ku and Gan, 2021). However, cysteine is toxic to cells, since it may promote the Fenton reaction and generate harmful hydroxyl radicals (Imlay et al., 2015). Therefore, control of cysteine levels by coordinating its biosynthesis, utilization, oxidation, and transport is needed for bacterial defense against oxidative stress (Loddeke et al., 2017; Mironov et al., 2020). Mutation in Lactobacillus fermentum BspA, a basic surface-exposed protein homologous to FliY of E. coli, impaired bacterial cystine uptake significantly and showed an oxidation-sensitive phenotype, probably because the bspA mutant was unable to provide enough cystine for the production of the sulfhydryl compound to antagonize oxidative conditions (Turner et al., 1999). In addition, the cysteine transporter (YdeD) and cystine importers (YdjN and FliY-YecSC) in E. coli were reported to work cooperatively to keep reducing the equivalent in the periplasm, and the disruption of ydeD and fliY increases the sensitivity of membrane lipids to H2O2-induced oxidative damage (Ohtsu et al., 2015). Here, we found that mutation in the A. pleuropneumoniae cysteine/cystine transporters FliY and YdjN increased vulnerability to H2O2-induced oxidative stress. These results further emphasize the importance of cysteine/cystine transport for bacterial redox homeostasis.
Bacterial biofilms are defined as a community of surface-attached bacteria that are surrounded by hydrated polymeric matrixes of their own synthesis (Huigens et al., 2008). Bacterial biofilm formation is a complex multistep process involving several different factors (Alves-Barroco et al., 2020). Sulfur source utilization was indicated to play a role in biofilm formation in Staphylococcus aureus via the CymR-mediated regulation of the cysteine metabolism (Soutourina et al., 2009). In this study, we confirmed that the A. pleuropneumoniae wild-type stain (WF83) formed more biofilm in CDM than that in TSB. This is consistent with the previous view that the culture conditions were critical for the biofilm formation of A. pleuropneumoniae (Labrie et al., 2010). In addition, the expression of YdjN, but not FliY, on the shuttle vector pJFF224-XN (ΔfliYΔydjN-ydjN+ and WT-ydjN+) enhanced the A. pleuropneumoniae biofilm formation significantly in CDM. This finding sheds new light on the function of the cysteine/cystine transporter YdjN in biofilm formation. In addition, N-acetyl-D-glucosamine (GlcNAc) residues in β(1,6) linkage (PGA) catalyzed by the product of the pgaC gene has been shown to be a major biofilm adhesin of A. pleuropneumoniae (Izano et al., 2007; Liu et al., 2008). Surprisingly, the results indicated that the A. pleuropneumoniae strain WF83 (pgaC mutant, Liu et al., 2008) and its derivatives were able to form biofilms in CDM. It is possible that YdjN is involved in controlling biofilm formation via a PGA-independent mechanism in A. pleuropneumoniae.
The role of sulfur source uptake and metabolism in bacterial virulence has been documented previously. In S. aureus, TcyABC and TcyP involved in the transport of cystine, cysteine, and N-acetyl cysteine are necessary for in vivo colonization and affect virulence (Lensmire et al., 2020). FliY and YdjN are essential for A. pleuropneumoniae growth using cysteine or cystine as a sole sulfur source. In addition, these transporters promote A. pleuropneumoniae cells to oxidative stress tolerance. Therefore, we hypothesized that FliY and YdjN should also be related to virulence. To test this hypothesis, the virulence of the A. pleuropneumoniae WT and ΔfliYΔydjN was evaluated in a mouse infection model and its natural host, the pig. However, there was no significant decrease in the bacterial loads in the lung tissues of the mice, and the competitive index of the mutant relative to the WT in the lung tissue of the pigs was above the threshold value of attenuation. These observations clearly indicate that the transporters FliY and YdjN are not correlated with the in vivo colonization and bacterial virulence of A. pleuropneumoniae. A similar result was observed in our previous study, in which the A. pleuropneumoniae mutant Δsbp was shown to be defective in using sulfate and methionine but still virulent (Gao et al., 2020). Probably, other sulfur nutrients instead of cystine/cysteine support the growth of ΔfliYΔydjN in the host. Furthermore, oxidative resistance conferred by other proteins, such as LonA (Xie et al., 2016), TolC2 (Li et al., 2017), TolC1 (Li et al., 2019), FtpA (Tang et al., 2022), and HtrA (Zhang et al., 2022), may protect A. pleuropneumoniae cysteine/cystine uptake mutant from oxidative toxicity. These results further demonstrate the complex mechanisms involved in the pathogenesis of A. pleuropneumoniae infection.
In conclusion, the present study shows that FliY and YdjN are important for the uptake of cysteine/cystine as sulfur sources. This study demonstrates that FliY and YdjN are related to oxidative stress tolerance, and YdjN might be a potential regulator of biofilm formation in A. pleuropneumoniae. The FliY- and YdjN-dependent sulfur nutrient satisfaction and oxidative stress response are not correlated with the pathogenicity of A. pleuropneumoniae.
Data availability statement
The original contributions presented in the study are included in the article/Supplementary material, further inquiries can be directed to the corresponding authors.
Ethics statement
The animal study was reviewed and approved by the Animal Ethics Committee at the Central China Normal University. Written informed consent was obtained from the owners for the participation of their animals in this study.
Author contributions
JL and CQ designed the research, provided the experiment conditions, and helped with the data analysis. FZ and JL wrote the manuscript. FZ, HX, YC, JX, and MZ performed the experiments. FZ, HX, YC, and ZL contributed to the animal experiments. All authors reviewed and approved the manuscript.
Funding
This study was supported by grants from the Fundamental Research Funds for the Central Universities (CCNU19QN011 and CCNU19CG016) and the project of Hubei Key Laboratory of Genetic Regulation and Integrative Biology (GRIB201805).
Acknowledgments
We thank Pat Blackall (at the Department of Primary Industries and Fisheries Animal Research Institute, Australia) for the generous donation of A. pleuropneumoniae WF83, Gerald-F. Gerlach (at the Department of Infectious Diseases, University of Veterinary Medicine Hannover, Germany) for the generous donation of E. coil β2155 and transconjugation plasmid pEMOC2, and Dr. Joachim Frey (at the Institute of Veterinary Bacteriology, University of Berne, Switzerland) for the kind gift of the shuttle vector pJFF224-XN.
Conflict of interest
The authors declare that the research was conducted in the absence of any commercial or financial relationships that could be construed as a potential conflict of interest.
Publisher's note
All claims expressed in this article are solely those of the authors and do not necessarily represent those of their affiliated organizations, or those of the publisher, the editors and the reviewers. Any product that may be evaluated in this article, or claim that may be made by its manufacturer, is not guaranteed or endorsed by the publisher.
Supplementary material
The Supplementary Material for this article can be found online at: https://www.frontiersin.org/articles/10.3389/fmicb.2023.1169774/full#supplementary-material
Supplementary Figure S1. Verification of double-gene deletion mutant ΔfliYΔydjN. (A) PCR amplification with primers fliY-F1 and fliY-R2. Lane M, DNA ladder 2000; lanes 1–3, ΔfliYΔydjN; lane 4, vector pEMOC2-ΔfliY; lane 5, WT; lane 6, negative control. (B) PCR amplification with primers ydjN-F5 and ydjN-R5. Lane M, DNA ladder 2000; lanes 1–4, ΔfliYΔydjN; lane 5, vector pEMOC2-ΔydjN; lane 6, WT; lane 7, negative control.
Supplementary Figure S2. Identification of complementation strains. (A) ΔfliYΔydiN-fliY+ verified with primers fliY-F3 and fliY-R3. Lane M, DNA ladder 2000; lane 1, negative control; lane 2, WT; lanes 3–5, ΔfliYΔydiN-fliY+. (B) ΔfliYΔydiN-ydjN+ verified with primers ydjN-F3 and ydjN-R3. Lane M, DNA ladder 2000; lane 1, negative control; lane 2, WT; lane 3, ΔfliYΔydiN-ydjN+. (C) RT-PCR assay with primers fliY-F4 and fliY-R4. Lane M, DNA ladder 2000; lanes 1–3, ΔfliYΔydiN-fliY+; lane 4, WT; lane 5, ΔfliYΔydiN; lane 6, negative control. (D) RT-PCR assay with primers ydjN-F4 and ydjN-R4. Lane M, DNA ladder 2000; lanes 1 and 2, negative control; lane 3, WT; lane 4, ΔfliYΔydiN; lanes 5–8, ΔfliYΔydiN-ydjN+.
References
Alves-Barroco, C., Paquete-Ferreira, J., Santos-Silva, T., and Fernandes, A. R. (2020). Singularities of pyogenic Streptococcal biofilms - from formation to health implication. Front. Microbiol. 11, 584947. doi: 10.3389/fmicb.2020.584947
Baltes, N., Hennig-Pauka, I., and Gerlach, G. F. (2002). Both transferrin binding proteins are virulence factors in Actinobacillus pleuropneumoniae serotype 7 infection. FEMS Microbiol. Lett. 209, 283–287. doi: 10.1111/j.1574-6968.2002.tb11145.x
Beddek, A. J., Sheehan, B. J., Bossé, J. T., Rycroft, A. N., Kroll, J. S., Langford, P. R., et al. (2004). Two TonB systems in Actinobacillus pleuropneumoniae: their roles in iron acquisition and virulence. Infect. Immun. 72, 701–708. doi: 10.1128/IAI.72.2.701-708.2004
Beinert, H. (2000). A tribute to sulfur. Eur. J. Biochem. 267, 5657–5664. doi: 10.1046/j.1432-1327.2000.01637.x
Chiers, K., De Waele, T., Pasmans, F., Ducatelle, R., and Haesebrouck, F. (2010). Virulence factors of Actinobacillus pleuropneumoniae involved in colonization, persistence and induction of lesions in its porcine host. Vet. Res. 41, 65. doi: 10.1051/vetres/2010037
Deutch, C. E., Spahija, I., and Wagner, C. E. (2014). Susceptibility of Escherichia coli to the toxic L-proline analogue L-selenaproline is dependent on two L-cystine transport systems. J. Appl. Microbiol. 117, 1487–1499. doi: 10.1111/jam.12623
Frey, J. (1992). Construction of a broad host range shuttle vector for gene cloning and expression in Actinobacillus pleuropneumoniae and other Pasteurellaceae. Res. Microbiol. 143, 263–269. doi: 10.1016/0923-2508(92)90018-J
Gao, L., Zhang, L., Xu, H., Zhao, F., Ke, W., Chen, J., et al. (2020). The Actinobacillus pleuropneumoniae sulfate-binding protein is required for the acquisition of sulfate and methionine, but is not essential for virulence. Vet. Microbiol. 245, 108704. doi: 10.1016/j.vetmic.2020.108704
Herriott, R. M., Meyer, E. Y., Vogt, M., and Modan, M. (1970). Defined medium for growth of Haemophilus influenzae. J. Bacteriol. 101, 513–516. doi: 10.1128/jb.101.2.513-516.1970
Hicks, J. L., and Mullholland, C. V. (2018). Cysteine biosynthesis in Neisseria species. Microbiology 164, 1471–1480. doi: 10.1099/mic.0.000728
Huigens, R. W. 3rd., Ma, L., Gambino, C., Moeller, P.D.R., Basso, A., Cavanagh, J., Wozniak, D.J., and Melander, C. (2008). Control of bacterial biofilms with marine alkaloid derivatives. Mol. Biosyst. 4, 614–621. doi: 10.1039/b719989a
Imlay, J. A. (2013). The molecular mechanisms and physiological consequences of oxidative stress: lessons from a model bacterium. Nat. Rev. Microbiol. 11, 443–454. doi: 10.1038/nrmicro3032
Imlay, K. R. C., Korshunov, S., and Imlay, J. A. (2015). Physiological roles and adverse effects of the two cystine importers of Escherichia coli. J. Bacteriol. 197, 3629–3644. doi: 10.1128/JB.00277-15
Izano, E. A., Sadovskaya, I., Vinogradov, E., Mulks, M. H., Velliyagounder, K., Ragunath, C., et al. (2007). Poly-N-acetylglucosamine mediates biofilm formation and antibiotic resistance in Actinobacillus pleuropneumoniae. Microb. Pathog. 43, 1–9. doi: 10.1016/j.micpath.2007.02.004
Kohlmeier, M. (2003). “Cysteine,” in Nutrient Metabolism, ed. M. Kohlmeier (London: Academic Press), 348–356. doi: 10.1016/B978-012417762-8.50055-7
Ku, J. W. K., and Gan, Y. H. (2021). New roles for glutathione: modulators of bacterial virulence and pathogenesis. Redox Biol. 44, 102012. doi: 10.1016/j.redox.2021.102012
Labrie, J., Pelletier-Jacques, G., Deslandes, V., Ramjeet, M., Auger, E., Nash, J. H., et al. (2010). Effects of growth conditions on biofilm formation by Actinobacillus pleuropneumoniae. Vet. Res. 41, 3. doi: 10.1051/vetres/2009051
Lensmire, J. M., Dodson, J. P., Hsueh, B. Y., Wischer, M. R., Delekta, P. C., Shook, J. C., et al. (2020). The Staphylococcus aureus cystine transporters TcyABC and TcyP facilitate nutrient sulfur acquisition during infection. Infect. Immun. 88, e00690–19. doi: 10.1128/IAI.00690-19
Li, Y., Cao, S., Zhang, L., Yuan, J., Yang, Y., Zhu, Z., et al. (2017). TolC2 is required for the resistance, colonization and virulence of Actinobacillus pleuropneumoniae. J. Med. Microbiol. 66, 1170–1176. doi: 10.1099/jmm.0.000544
Li, Y., Cao, S., Zhang, L., Yuan, J., Zhao, Q., Wen, Y., et al. (2019). A requirement of TolC1 for effective survival, colonization and pathogenicity of Actinobacillus pleuropneumoniae. Microb. Pathog. 134, 103596. doi: 10.1016/j.micpath.2019.103596
Liu, J., Cao, Y., Gao, L., Zhang, L., Gong, S., Yang, J., et al. (2018). Outer membrane lipoprotein Lip40 modulates adherence, colonization, and virulence of Actinobacillus pleuropneumoniae. Front. Microbiol. 9, 1472. doi: 10.3389/fmicb.2018.01472
Liu, J., Stone, V. N., Ge, X., Tang, M., Elrami, F., Xu, P., et al. (2017). TetR family regulator brpT modulates biofilm formation in Streptococcus sanguinis. PLoS ONE 21, e0169301. doi: 10.1371/journal.pone.0169301
Liu, J., Tan, C., Li, J., Chen, H., Xu, P., He, Q., et al. (2008). Characterization of ISApl1, an insertion element identified from Actinobacillus pleuropneumoniae field isolate in China. Vet. Microbiol. 132, 348–354. doi: 10.1016/j.vetmic.2008.05.031
Loddeke, M., Schneider, B., Oguri, T., Mehta, I., Xuan, Z., Reitzer, L., et al. (2017). Anaerobic cysteine degradation and potential metabolic coordination in Salmonella enterica and Escherichia coli. J. Bacteriol. 199, e00117–17. doi: 10.1128/JB.00117-17
Méndez, J., Reimundo, P., Pérez-Pascual, D., Navais, R., Gómez, E., Guijarro, J. A., et al. (2011). A novel cdsAB operon is involved in the uptake of L-cysteine and participates in the pathogenesis of Yersinia ruckeri. J. Bacteriol. 193, 944–951. doi: 10.1128/JB.01058-10
Mironov, A., Seregina, T., Shatalin, K., Nagornykh, M., Shakulov, R., Nudler, E., et al. (2020). CydDC functions as a cytoplasmic cystine reductase to sensitize Escherichia coli to oxidative stress and aminoglycosides. Proc. Natl. Acad. Sci. USA. 117, 23565–23570. doi: 10.1073/pnas.2007817117
Murphy, T. F., Kirkham, C., Johnson, A., Brauer, A. L., Koszelak-Rosenblum, M., Malkowski, M. G., et al. (2016). Sulfate-binding protein, CysP, is a candidate vaccine antigen of Moraxella catarrhalis. Vaccine 34, 3855–3861. doi: 10.1016/j.vaccine.2016.05.045
Núñez, G., Sakamoto, K., and Soares, M. P. (2018). Innate nutritional immunity. J. Immunol. 201, 11–18. doi: 10.4049/jimmunol.1800325
Ohtsu, I., Kawano, Y., Suzuki, M., Morigasaki, S., Saiki, K., Yamazaki, S., et al. (2015). Uptake of L-cystine via an ABC transporter contributes defense of oxidative stress in the L-cystine export-dependent manner in Escherichia coli. PLoS ONE 10, e0120619. doi: 10.1371/journal.pone.0120619
Ohtsu, I., Wiriyathanawudhiwong, N., Morigasaki, S., Nakatani, T., Kadokura, H., Takagi, H., et al. (2010). The L-cystine/L-cystine shuttle system provides reducing equivalents to the periplasm in Escherichia coli. J. Biol. Chem. 285, 17479–17487. doi: 10.1074/jbc.M109.081356
Oswald, W., Tonpitak, W., Ohrt, G., and Gerlach, G. (1999). A single-step transconjugation system for the introduction of unmarked deletions into Actinobacillus pleuropneumoniae serotype 7 using a sucrose sensitivity marker. FEMS Microbiol. Lett. 179, 153–160. doi: 10.1111/j.1574-6968.1999.tb08721.x
Paritala, H., and Carroll, K. S. (2013). New targets and inhibitors of mycobacterial sulfur metabolism. Infect. Disord. Drug Targets 13, 85–115. doi: 10.2174/18715265113139990022
Sabrialabed, S., Yang, J. G., Yariv, E., Ben-Tal, N., and Lewinson, O. (2020). Substrate recognition and ATPase activity of the E. coli cysteine/cystine ABC transporter YecSC-FliY. J. Biol. Chem. 295, 5245–5256. doi: 10.1074/jbc.RA119.012063
Sassu, E. L., Bossé, J. T., Tobias, T. J., Gottschalk, M., Langford, P. R., Hennig-Pauka, I., et al. (2018). Update on Actinobacillus pleuropneumoniae-knowledge, gaps and challenges. Transbound. Emerg. Dis. 65, 72–90. doi: 10.1111/tbed.12739
Schaller, A., Djordjevic, S. P., Eamens, G. J., Forbes, W. A., Kuhn, R., Kuhnert, P., et al. (2001). Identification and detection of Actinobacillus pleuropneumoniae by PCR based on the gene apxIVA. Vet. Microbiol. 79, 47–62. doi: 10.1016/S0378-1135(00)00345-X
Soutourina, O., Poupel, O., Coppe, J. Y., Danchin, A., Msadek, T., Martin-Verstraete, I., et al. (2009). CymR, the master regulator of cysteine metabolism in Staphylococcus aureus, controls host sulphur source utilization and plays a role in biofilm formation. Mol. Microbiol. 73, 194–211. doi: 10.1111/j.1365-2958.2009.06760.x
Tang, H., Zhang, Q., Han, W., Wang, Z., Pang, S., Zhu, H., et al. (2022). Identification of FtpA, a Dps-like protein involved in anti-oxidative stress and virulence in Actinobacillus pleuropneumoniae. J. Bacteriol. 204, e0032621. doi: 10.1128/jb.00326-21
Turner, M. S., Woodberry, T., Hafner, L. M., and Giffard, P. M. (1999). The bspA locus of Lactobacillus fermentum BR11 encodes an L-cystine uptake system. J. Bacteriol. 181, 2192–2198. doi: 10.1128/JB.181.7.2192-2198.1999
Xie, F., Li, G., Zhang, Y., Zhou, L., Liu, S., Liu, S., et al. (2016). The Lon protease homologue LonA, not LonC, contributes to the stress tolerance and biofilm formation of Actinobacillus pleuropneumoniae. Microb. Pathog. 93, 38–43. doi: 10.1016/j.micpath.2016.01.009
Yamazaki, S., Takei, K., and Nonaka, G. (2016). ydjN encodes an S-sulfocysteine transporter required by Escherichia coli for growth on S-sulfocysteine as a sulfur source. FEMS Microbiol. Lett. 363, fnw185. doi: 10.1093/femsle/fnw185
Zhang, L., Zhao, F., Xu, H., Chen, Y., Qi, C., Liu, J., et al. (2022). HtrA of Actinobacillus pleuropneumoniae is a virulence factor that confers resistance to heat shock and oxidative stress. Gene 841, 146771. doi: 10.1016/j.gene.2022.146771
Keywords: Actinobacillus pleuropneumoniae, FliY/YdjN, cysteine/cystine acquisition, oxidative tolerance, biofilm formation, virulence
Citation: Zhao F, Xu H, Chen Y, Xiao J, Zhang M, Li Z, Liu J and Qi C (2023) Actinobacillus pleuropneumoniae FliY and YdjN are involved in cysteine/cystine utilization, oxidative resistance, and biofilm formation but are not determinants of virulence. Front. Microbiol. 14:1169774. doi: 10.3389/fmicb.2023.1169774
Received: 20 February 2023; Accepted: 10 April 2023;
Published: 12 May 2023.
Edited by:
Alina Maria Holban, University of Bucharest, RomaniaReviewed by:
Javiera Alejandra Norambuena, The State University of New Jersey, United StatesAvishek Mitra, Oklahoma State University, United States
Copyright © 2023 Zhao, Xu, Chen, Xiao, Zhang, Li, Liu and Qi. This is an open-access article distributed under the terms of the Creative Commons Attribution License (CC BY). The use, distribution or reproduction in other forums is permitted, provided the original author(s) and the copyright owner(s) are credited and that the original publication in this journal is cited, in accordance with accepted academic practice. No use, distribution or reproduction is permitted which does not comply with these terms.
*Correspondence: Jinlin Liu, liujinlin2009@126.com; Chao Qi, qichao@mail.ccnu.edu.cn