- 1Department of Food Hygiene and Environmental Health, Faculty of Veterinary Medicine, University of Helsinki, Helsinki, Finland
- 2Department of Health Security, National Institute for Health and Welfare, Helsinki, Finland
- 3Inflammation Center, Helsinki University Central Hospital, Helsinki, Finland
- 4Faculty of Medicine, University of Helsinki, Helsinki, Finland
- 5Faculty of Medicine and Health Technology, Tampere University, Tampere, Finland
- 6Department of Health Security, National Institute for Health and Welfare, Kuopio, Finland
- 7Finnish Food Authority, Ruokavirasto, Seinäjoki, Finland
Multi-drug resistance is emerging in Citrobacter freundii, which is the third most common carbapenemase-producing (CP) Enterobacteriaceae in humans in Finland due to recent outbreaks. The objective of this study was to determine if wastewater surveillance (WWS) could detect CP C. freundii strains causing infections in humans. Selective culturing was used to isolate CP C. freundii from the hospital environment, hospital wastewater, and untreated municipal wastewater in Helsinki, Finland, between 2019 and 2022. Species were identified using MALDI-TOF, and presumptive CP C. freundii isolates were subjected to antimicrobial susceptibility testing and further characterized by whole genome sequencing. A genomic comparison was conducted to compare isolates collected from the hospital environment, untreated municipal wastewater, and a selection of isolates from human specimens from two hospitals in the same city. We also examined the persistence of CP C. freundii in the hospital environment and the impact of our attempts to eradicate it. Overall, 27 blaKPC−2-carrying C. freundii were detected in the hospital environment (ST18; n = 23 and ST8; n = 4), while 13 blaKPC−2-carrying C. freundii (ST8) and five blaVIM−1-carrying (ST421) C. freundii were identified in untreated municipal wastewater. CP C. freundii was not identified in hospital wastewater. We found three clusters (cluster distance threshold ≤10 allelic difference) after comparing the recovered isolates and a selection of isolates from human specimens. The first cluster consisted of ST18 isolates from the hospital environment (n = 23) and human specimens (n = 4), the second consisted of ST8 isolates from the hospital environment (n = 4), untreated municipal wastewater (n = 6), and human specimens (n = 2), and the third consisted of ST421 isolates from the untreated municipal wastewater (n = 5). Our results support previous studies suggesting that the hospital environment could act as a source of transmission of CP C. freundii in clinical settings. Furthermore, the eradication of CP Enterobacteriaceae from the hospital environment is challenging. Our findings also showed that CP C. freundii is persistent throughout the sewerage system and demonstrate the potential of WWS for detecting CP C. freundii.
1. Introduction
The World Health Organization has declared antimicrobial resistance (AMR) as one of the top 10 global public health threats, leading to increased mortality and a significant burden for healthcare and economies (WHO, 2021).
Citrobacter spp. are aerobic, Gram-negative, commensal bacteria commonly found within the gastrointestinal tract of humans and animals as well as in environmental sources such as soil and water (Forsythe et al., 2015). Citrobacter spp. can cause enteric diseases and extraintestinal infections such as infections of the urinary and respiratory tract. Less frequently, Citrobacter spp. also causes serious nosocomial infections in immunocompromised hosts (Forsythe et al., 2015). The occurrence of multi-drug resistance is increasing in Citrobacters that can carry extended-spectrum beta-lactamase (ESBL), AmpC beta-lactamases, and carbapenemase-encoding genes such as blaKPC−2 (Zhang et al., 2008; Shahid, 2010).
From a hospital infection prevention viewpoint, Citrobacter freundii causes concerns due to its exceptional ability to accumulate resistance traits (Yao et al., 2021). The persistence of these strains in the hospital environment and their subsequent spread to patients can cause hard-to-treat infections in compromised hosts. In addition, the accumulated resistance traits could be re-transmitted beyond genus, family, and order boundaries (Majewski et al., 2017) to more virulent organisms such as Klebsiella pneumoniae. In the hospital environment, including water fixtures, such as sinks and toilets, affected patients are the suspected initial origin of the carbapenem-resistant organisms (Tofteland et al., 2013; Leitner et al., 2015). Microbes may persist in biofilms in water fixtures and then be dispersed to the surroundings by particles, droplets, or aerosol formation (Kotay et al., 2019). Indeed, it is challenging to verify the transmission of microbes to patients (Kizny Gordon et al., 2017). However, a significant relationship between contaminated sinks and the acquisition of carbapenemase-producing Enterobacteriaceae (CPE) in patients indicates the importance of hospital water fixtures and biofilms within them as the environmental reservoir of the CPE epidemics (Smolders et al., 2019). Studies showing hospital rooms as the epidemiological link in CPE outbreaks also support the role of the hospital environment as a source of CPE transmission (van Beek et al., 2019; Räisänen et al., 2021). Although total eradication of CPE in hospital water fixtures with cleaning or disinfection is shown to be difficult (Nurjadi et al., 2021), decreased colonization has been achieved by replacing the sinks and plumbing (Shaw et al., 2018; Smolders et al., 2019).
It has been proposed that wastewater surveillance (WWS) could be used for global surveillance, prediction, and early warning system of AMR (Hendriksen et al., 2019b). However, full integration of human, animal, and environmental AMR monitoring data is still missing. Moreover, there is a need for calibration and standardization of the different methods for reliable data comparison (Pruden et al., 2021). More research studies are needed to clarify the applicability of WWS in observing and predicting the clinically relevant AMR pathogens circulating in the community as well as within asymptomatic carriers. To the best of our knowledge, no previous studies have described the genomic epidemiology of carbapenemase-producing (CP) C. freundii in humans, hospitals, and untreated municipal wastewater.
In Finland, C. freundii is the third most common CPE in humans and one of the most common CPE-causing clusters (THL, 2022b). During 2012–2018, CP C. freundii represented 6.0% of CPE in human specimens (Räisänen et al., 2020), which increased rapidly to 20.0% in 2021 (THL, 2022b). Between 2016–2022, blaKPC−2 CP C. freundii was detected in 60 human specimens in Finland.
This research was conducted to elaborate on the capacity of WWS to observe CP C. freundii strains causing infections. We assessed the similarity between the isolates from the hospital environment, untreated municipal wastewater, and a selection of isolates from human specimens from the national CPE strain collection, which is part of the national infectious diseases register sustained by the National Institute for Health and Welfare (THL). Additionally, we described the occurrence of CP C. freundii in the hospital environment and our attempts to eradicate it. The initial motivation for the sampling in the studied hospital arose when an unknown environmental transmission was suspected while one of the CP C. freundii clusters described by Räisänen et al. (2021) occurred in the studied hospital.
2. Materials and methods
2.1. Sample collection and bacterial isolation
In this study, samples were collected from a hospital environment, with a specific focus on water fixtures such as sinks and toilets. Wastewater leaving from the same hospital to the municipal sewer was also sampled, as well as untreated municipal wastewater from a local wastewater treatment plant (WWTP) serving the area of the studied hospital (Figure 1). Sampling is described more precisely in the following paragraphs.
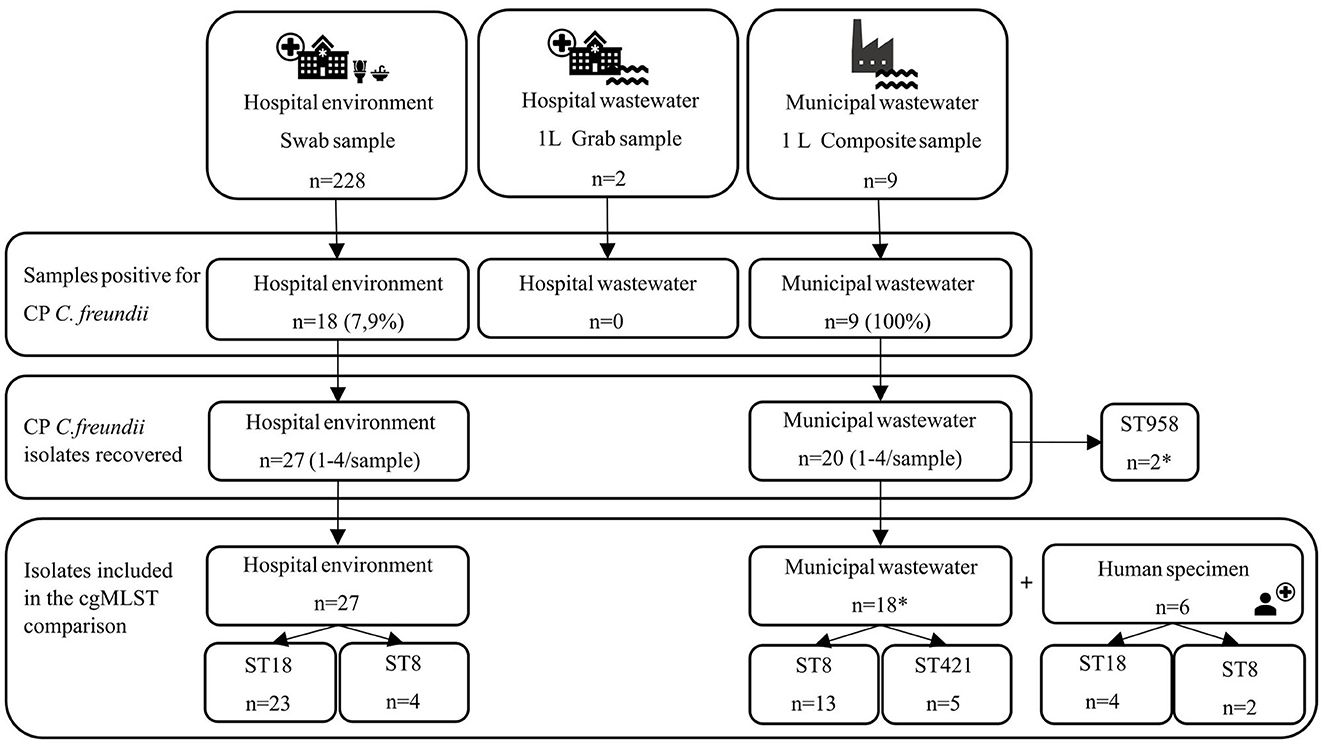
Figure 1. Illustration of the collected samples and recovered isolates. ST indicates sequence type. cgMLST indicates core genome multilocus sequence typing. Asterisk indicates two ST958 isolates that were left out of the cgMLST scheme due to poor sequence quality.
2.1.1. Hospital environment
Sampling was conducted six times between December 2019 and July 2021, consisting of up to 27 patient rooms and 228 samples from the unit with two wards in one tertiary care hospital in Finland. The samples were obtained from sink traps (Supplementary Figure 1) and the toilet seat water line (Supplementary Figure 2). Additionally, samples were collected from sink taps (samplings 1–3), bidet showerhead and bidet shower sinks (samplings 1–2), toilet seat and sink plugholes, toilet seat water tank, and the cleaning and disinfection machine (sampling 4). Figure 2 illustrates the sampling timeline.
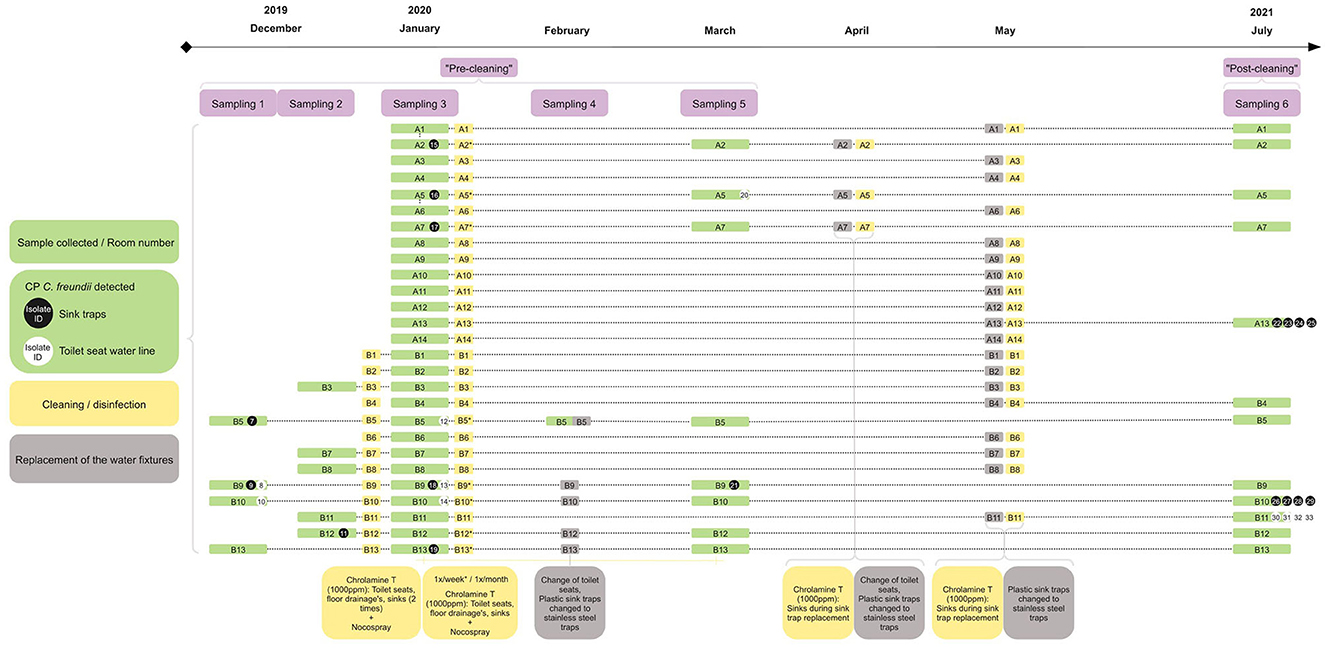
Figure 2. Illustration of the hospital environment sampling (purple rectangle) in hospital rooms (green rectangle) and detected carbapenemase-producing (CP) Citrobacter freundii isolates in sink traps (black circle) and toilets (white circle). The numbers inside or next to the circles indicate the isolate identification number (ID). Cleaning/disinfection (yellow rectangle) and replacement of the water fixtures (gray rectangle) are shown. Asterisk indicating rooms where cleaning and disinfection was performed a minimum of once per week after Sampling 3.
The samples obtained from the first five samplings (1–5) were collected before or during the cleaning actions (pre-cleaning). The samples were analyzed by THL following the protocol described by van Beek et al. (2019). The samples in sampling 6 (post-cleaning) were collected with sterile swabs (M40 Transystem Amies Agar Gel, Copan Diagnostics, Brescia, Italy) that were initially dampened with buffered peptone water (BPW) (Oxoid, Basingstoke, Hampshire, United Kingdom). The sampling site was scrubbed with the swab for 15–30 s, and then the swab was moved to the transfer tube. The samples were transferred to the University of Helsinki laboratory in a coolbox and further processed within 12 h from the time of collection.
Each cotton swab was placed into an Eppendorf tube with 1 ml of BPW. The tubes were vortexed, and 100 μl of the suspension was spread on CHROMagar mSuperCARBA (CHROMagar, Paris, France) and incubated aerobically for 18–24 h at 37°C. The remaining 900 μl of the BPW with the cotton swab was also incubated aerobically for 18–24 h at 37°C (enrichment) to detect small quantities of the targeted bacteria. After incubation, the enrichment was streaked with a 10 μl sterile loop on CHROMagar mSuperCARBA and incubated aerobically for 18–24 h at 37°C.
Colony morphology was observed according to the manufacturer's instructions. Overall, 1–5 typical metallic blue colonies were selected from each plate, refreshed with 1 μl sterile loop on CHROMagar mSuperCARBA, and incubated aerobically for 18–24 h at 37°C. Bacterial colonies were subcultured onto individual CHROMagar mSuperCARBA agar plates until a pure culture was achieved. Up to four isolates were subcultured on a bovine blood agar plate (Columbia Blood Agar Base, Oxoid Ltd., Basingstoke, United Kingdom) and incubated aerobically for 18–24 h at 37°C.
2.1.2. Hospital wastewater
Two 1 L grab samples of wastewater from the hospital sewers were collected from two separate locations around the studied hospital. Sampling was performed in July 2021 in concordance with post-cleaning samples of the hospital environment. The samples were transferred to the laboratory in a coolbox and further processed within 12 h from the time of collection. A volume of 100 μl of the wastewater was pipetted and spread on CHROMagar mSuperCARBA and further processed as post-cleaning hospital environment samples without the enrichment step.
2.1.3. Untreated municipal wastewater from local WWTP
Nine 24-h composite samples of untreated municipal wastewater were collected between February 2021 and February 2022 from the WWTP in the city. Wastewater was collected as a part of a consortium project “WastPan” (THL, 2022b). WWTP receives ~296,000 m3 of wastewater daily, and it serves ~800,000 inhabitants (14.5% of the population of Finland) (Tiwari et al., 2022). WWTP also receives wastewater from the studied hospital. A 1 L sample was delivered to the laboratory in a coolbox, from which 100 μl was pipetted and spread on CHROMagar mSuperCARBA and further processed as the post-cleaning hospital environment samples without the enrichment step.
2.2. Bacterial species identification
Isolates were identified using a matrix-assisted laser desorption ionization-time of flight mass spectrometry (MALDI-TOF MS) based Bruker Microflex LT/SH (Bruker Daltonics GmbH & Co. KG, Bremen, Germany) with a score value of >2.0, except for one isolate with a score value of 1.8, and the second-best match for C. freundii was identified with a score value of 1.7. Confirmed C. freundii isolates were stored at −80°C for further characterization.
2.3. Antimicrobial susceptibility testing
For presumptive CP C. freundii isolates, susceptibility to carbapenems was tested with meropenem (10 μg) (Abtek Biologicals Ltd., Liverpool, United Kingdom, or Rosco, Albertslund, Denmark) and ertapenem (10 μg) (Oxoid, Basingstoke, United Kingdom) with a disk diffusion test according to the European Committee of Antimicrobial Susceptibility Testing (EUCAST) standard (EUCAST, 2022a). The inhibition zones were measured with a digital caliper. Furthermore, an antimicrobial susceptibility testing was performed with the broth microdilution method using Sensititre EURGNCOL plates (Thermo Fischer Scientific, East Grinstead, United Kingdom) to determine the minimum inhibitory concentration (MIC) of colistin, piperacillin/tazobactam, ceftazidime/avibactam, ceftolozane/tazobactam, and meropenem. The method was performed according to the manufacturer's instructions, except for using 0.9% saline instead of sterile water. Escherichia coli ATCC 25922 was included as quality control for each Müeller-Hinton agar patch. The results were interpreted according to EUCAST epidemiological cut-off values (ECOFFs) (EUCAST, 2022b).
2.4. DNA extraction
Isolates were grown in tryptic soy broth (Oxoid, Basingstoke, UK) at 37°C for 16 h. DNA was extracted from cells harvested from 2 ml of culture using the QIAcube Connect instrument (QIAGEN, Hilden, Germany) with a DNeasy Blood & Tissue Kit (QIAGEN, Valencia, CA, United States). The quality of DNA was assessed by the A260/A280 ratio using a NanoDrop ND-1000 spectrophotometer (Thermo Fischer Scientific, Wilmington, DE, United States), and DNA quantity was measured using a Qubit 2.0 fluorometer (Invitrogen, Life Technologies, Carlsbad, CA, United States).
2.5. Whole genome sequencing and bioinformatical analyses
The extracted DNA from all 32 presumptive CP C. freundii isolates from the post-cleaning hospital environment (n = 12) and untreated municipal wastewater samples (n = 20) was outsourced for sequencing. Library preparation was performed with a NEBNext Ultra DNA Library Prep Kit for Illumina with 300 bp fragment length. Sequencing was performed with Illumina NovaSeq 6000 (Novogene, Cambridge, United Kingdom) with 2 × 150 bp read length and targeted genomic coverage of 100 ×.
Sequences were analyzed with Ridom SeqSphere+ software v7.7.5 (Ridom GmbH, Germany) (Jünemann et al., 2013). Quality analysis of the sequences was performed with FastQC v0.1.1.7 (Babraham Institute, 2021), and adapters were removed with Trimmomatic v0.36 (Bolger et al., 2014). Raw reads were assembled with SKESA v2.3.0 using default settings (Souvorov et al., 2018), and quality trimming was performed with an average quality of ≥30 and a window of 20 bases. Remapping and polishing were performed with the BWA-MEM mapping algorithm. Sequencing statistics are presented in Supplementary Table 1. AMR genes were identified from assembled genomes with NCBI AMRFinderPlus 3.2.3 (Feldgarden et al., 2019), using 100% alignment and >90% identity. ResFinder 4.1 (Center for Genomic Epidemiology web server, DTU, Denmark) (Zankari et al., 2017; Clausen et al., 2018; Bortolaia et al., 2020) was used with default settings to specify the allelic variants of beta-lactamase genes. Sequence types (ST) were analyzed using multilocus sequence type (MLST) (Becker et al., 2018) in Ridom SeqSphere+- (Ridom, Munster, Germany). Sequences with novel STs were submitted to PubMLST (Jolley et al., 2018) to assign new STs.
2.6. Genomic comparison of CP C. freundii isolates
All isolates, except for two from the untreated municipal wastewater with poor sequence quality, were included in an ad hoc core genome multilocus sequence type (cgMLST) scheme with CP C. freundii isolates from the pre-cleaning hospital environment samples (n = 15). Additionally, six isolates from human specimens from the national CPE strain collection (n = 6; ST18 4/48 and ST8 2/11) were included in the cgMLST scheme (Figure 1). The isolates from the human specimens with ST18 were obtained from inpatients treated in the studied unit and have been published previously by Räisänen et al. (2021). The isolates from the human specimens with ST8 were obtained from inpatients treated in another hospital in the same city and within the service area of the studied WWTP. Three isolates were obtained from clinical specimens (ST18, n = 2; ST8, n = 1) and two from screening specimens (ST18, n = 1; ST8, n = 1). The specimen type for one isolate was not specified (ST8, n = 1).
2.7. Cleaning and other interventions in the patient rooms of the studied hospital
After sampling rounds 1 and 2 in December 2019, the affected rooms were cleaned with 1,000 ppm Chloramine T (KiiltoClean, Finland). Additionally, undiluted Chloramine T was poured into the toilets, floor drainages (4.0 dL each), and sinks (1.5 dL). After a 1-h duration of action, rooms were disinfected with Nocospray (Oxy'Pharm, France). After sampling round 3 in January 2020, the cleaning routine continued as described above, but the patient rooms with CP C. freundii findings were cleaned at least once a week, and the other rooms at least once a month. The described routine was followed until the end of March 2020. Starting from February 2020, the toilet seats were replaced, and the plastic sink traps were changed to stainless steel traps (Figure 2).
3. Results
3.1. Hospital environment, hospital wastewater, and untreated municipal wastewater from local WWTP
The pre-cleaning hospital environment samples in December 2019 yielded four CP C. freundii isolates from three patient rooms (n = 3/4, 75.0%, sampling 1) and one isolate from one patient room (n = 1/5, 20.0%, sampling 2). In January 2020, eight CP C. freundii isolates were recovered from seven patient rooms (n = 7/27, 25.9%, sampling 3). In February 2020, CP C. freundii was not recovered from the samples (n = 0/5, 0.0%, sampling 4). In March 2020, sampling yielded two CP C. freundii isolates from two patient rooms (n = 2/8, 25.0%, sampling 5). Post-cleaning samples in July 2021 yielded 12 CP C. freundii isolates from three patient rooms (n = 3/12, 25.0%, sampling 6). Additional species identified from selective media in post-cleaning samples were Klebsiella oxytoca (n = 4) and Klebsiella pneumoniae (n = 2). Two isolates were not identifiable by MALDI-TOF. Figure 2 illustrates the sampling, findings, and interventions.
C. freundii was not recovered from the selective media from hospital wastewater samples (n = 0/2, 0%).
In total, 20 CP C. freundii isolates were recovered from nine untreated municipal wastewater samples (n = 9/9), with up to four CP C. freundii isolates in each sample.
3.2. Phenotypic susceptibility
The disk diffusion test and broth microdilution were performed for 32 C. freundii isolates recovered from the post-cleaning hospital environment (n = 12) and untreated municipal wastewater samples (n = 20). All isolates were considered to express phenotypical resistance against meropenem. The phenotypical susceptibility of the isolates is presented in Table 1.
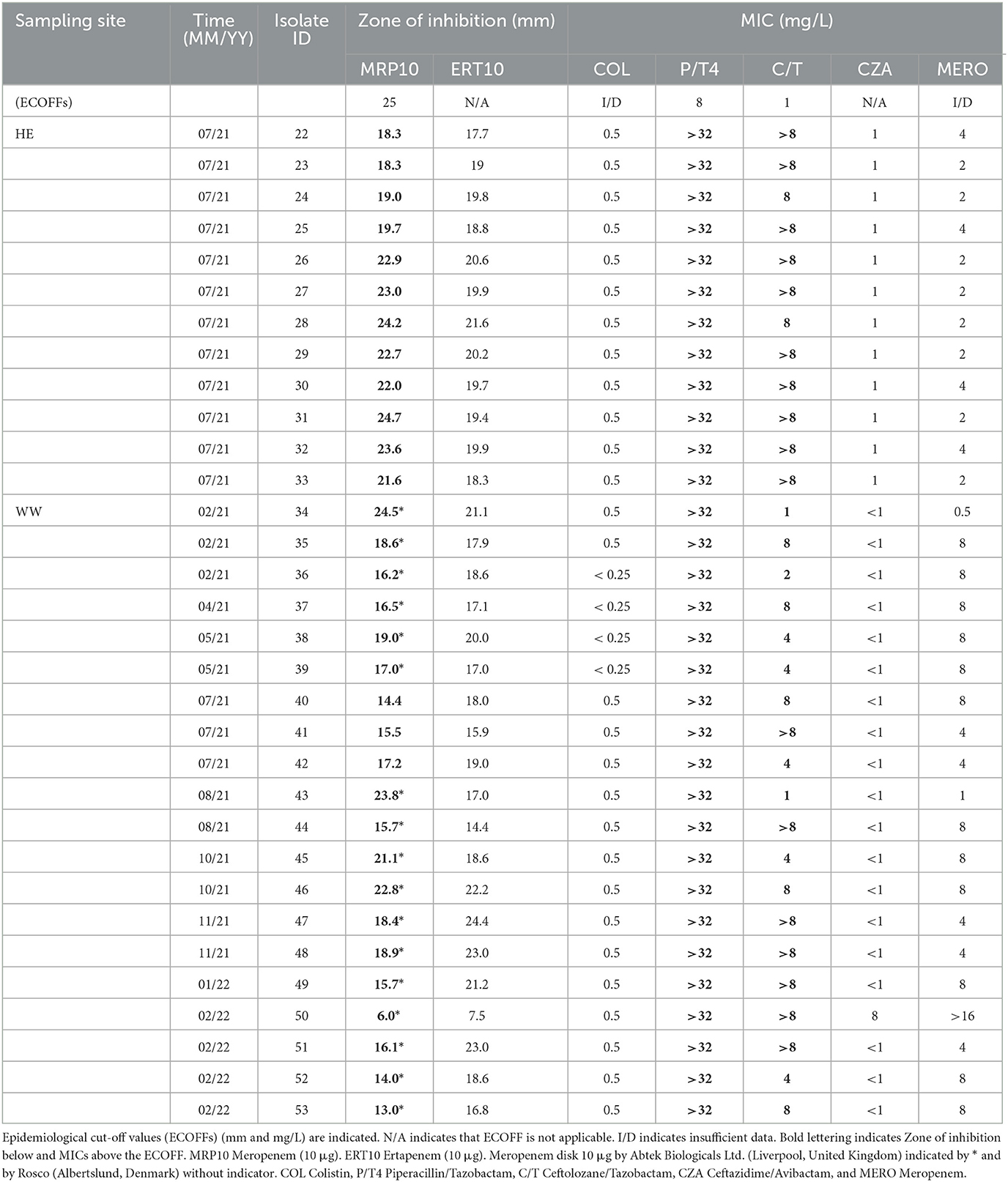
Table 1. Zone of inhibition and MIC of antimicrobials for 32 Citrobacter freundii isolated from post-cleaning hospital environment sampling (HE, n = 12) and untreated municipal wastewater (WW, n = 20).
3.3. Whole genome sequencing and bioinformatic analyses
In total, four different STs of CP C. freundii were identified. In the post-cleaning hospital environment samples, the most prevalent was ST18 (n = 8/12, 66.7%), followed by ST8 (n = 4/12, 33.3%). In the untreated municipal wastewater, the most prevalent was ST8 (n = 13/20, 65.0%), followed by ST421 (n = 5/20, 25.0%) and ST958 (n = 2/20, 10.0%) (Table 2). All the pre-cleaning hospital environment samples included in the genomic comparison belonged to ST18 (n = 15), and the isolates from human specimens included in the comparison belonged to ST18 (n = 4) and ST8 (n = 2) (Figure 1).
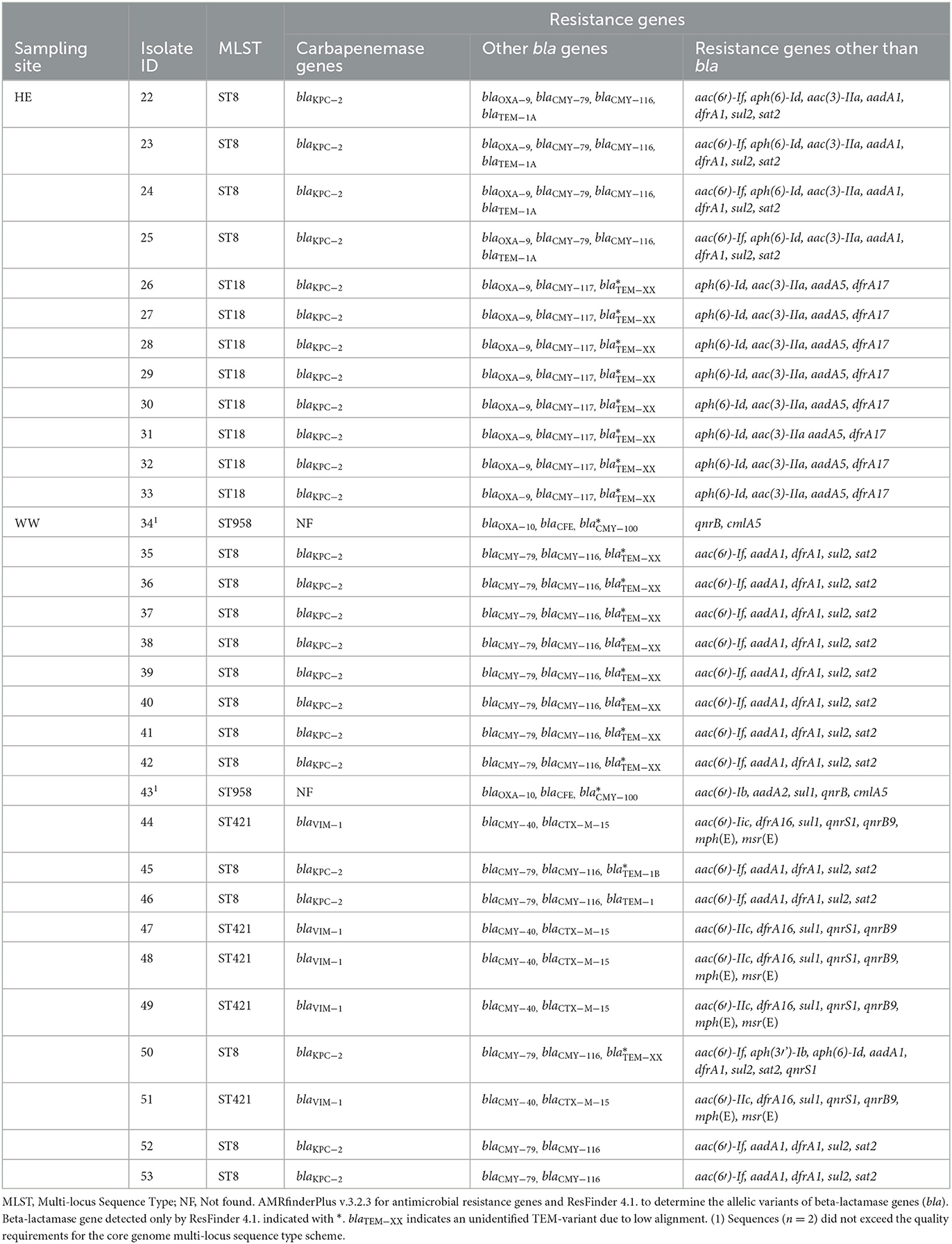
Table 2. Genomic characteristics for the 32 Citrobacter freundii isolated from post-cleaning hospital environment sampling (HE, n = 12) and untreated municipal wastewater (WW, n = 20).
All isolates (n = 27) from the hospital environment carried the blaKPC−2 carbapenemase gene. blaKPC−2 was also the most prevalent carbapenemase gene in isolates observed in the untreated municipal wastewater (n = 13/20, 65.0%), followed by blaVIM−1 (n = 5/20, 25.0%). Carbapenemase genes could not be identified in two isolates from untreated municipal wastewater (n = 2/20, 10.0%). Additionally, 4 to 12 other AMR genes were identified from each isolate. All identified AMR genes in the post-cleaning hospital environment and untreated municipal wastewater isolates are presented in Table 2.
3.4. Genomic comparison of CP C. freundii isolates
We identified three clusters [cluster threshold ≤ 10 allele difference (Jamin et al., 2021)] shown in Figure 3. Cluster 1 comprised isolates belonging to ST18— 23 from the hospital environment and 4 from the human specimens. Cluster 2 comprised isolates belonging to ST8, from which, four, six, and two originated from the hospital environment, untreated municipal wastewater, and human specimens, respectively. Cluster 3 comprised five isolates belonging to ST421 from untreated municipal wastewater. Clusters 1, 2, and 3 were separated by a long distance (up to 1812 allele difference) (Figure 3).
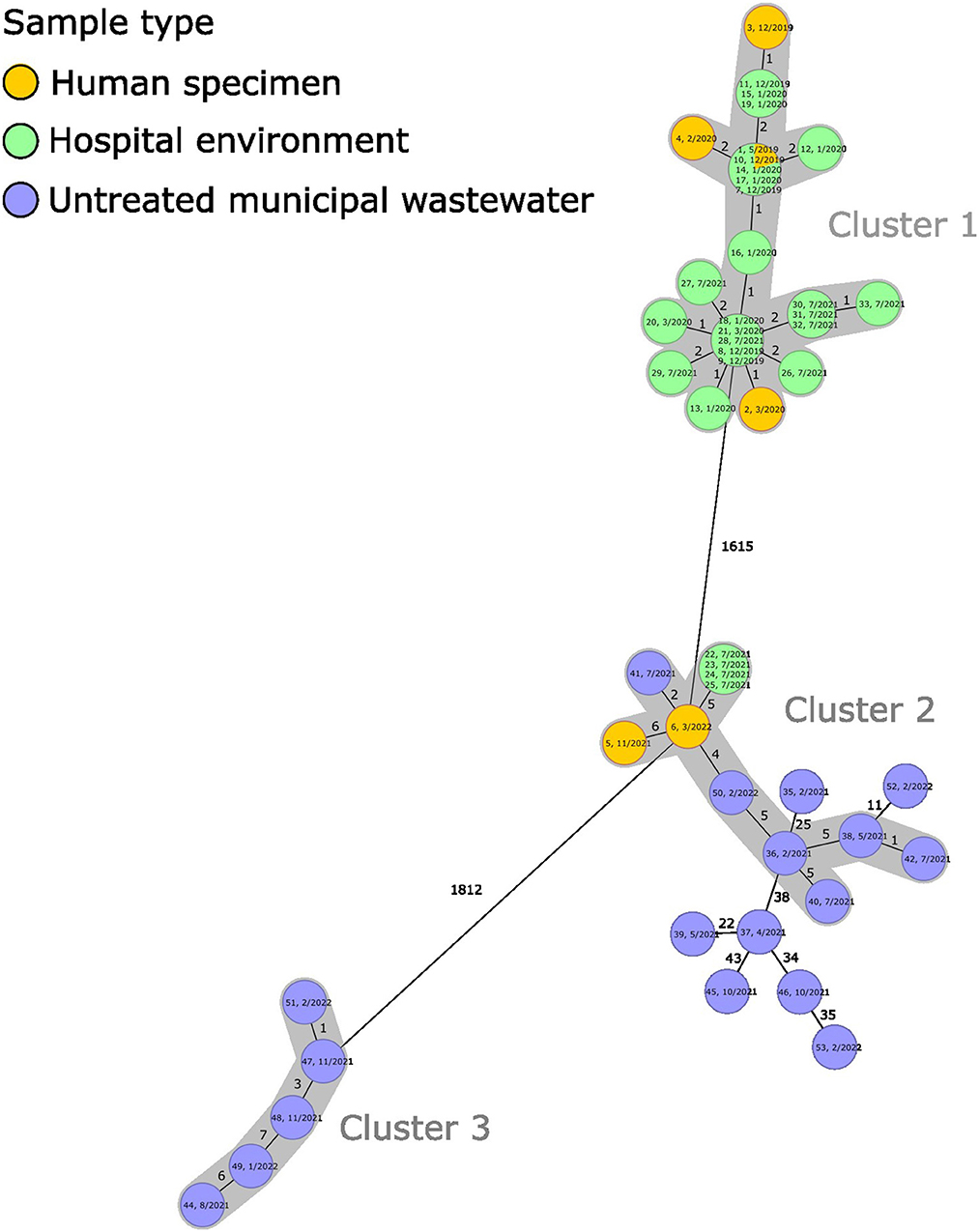
Figure 3. A minimum spanning tree of ad hoc core genome multilocus sequence typing (cgMLST) of 51 carbapenemase-producing Citrobacter freundii isolates, forming clusters 1 (ST18), 2 (ST8), and 3 (ST421). Each circle represents one or multiple identical sequences, and the numbers between the circles indicate the allele differences. Text in the circle indicates the isolate identification number and sample month/year; colors indicate the sample type [human specimen (yellow), hospital environment (green), and untreated municipal wastewater (blue)]. Human specimen isolates (1–6) originated from the national CPE strain collection and were obtained from clinical specimens (isolates 1, 2, and 5), screening specimens (isolates 3 and 4), and an unspecified sample type (isolate 6). Isolates 1–4 have been published previously by Räisänen et al. (2021). The seed genome was NZ_CP007557.1, and the cgMLST was based on 2007 targets, pairwise ignoring missing values.
4. Discussion
In this study, we identified closely related CP C. freundii strains belonging to ST8 in the hospital environment, untreated municipal wastewater, and human specimens, demonstrating that CP C. freundii strains causing infections can be detected in untreated municipal wastewater. However, the absence of ST18 in untreated municipal wastewater raises questions about the sensitivity of culture-based WWS to detect all clinically relevant strains. ST18 has been the dominant ST in human specimens, responsible for an ongoing outbreak since 2016 (Räisänen et al., 2021), whereas ST8 has been rarer.
In this study, we collected the municipal wastewater samples in 2021–2022, whereas the human isolates included in the comparison were from 2019–2020 (ST18) and 2021–2022 (ST8). ST18 was first discovered in a human specimen in 2016 in one hospital located in the same region. The temporal variance between the samples should be noted when interpreting the results. Accordingly, undetected ST18 in municipal wastewater could reflect that it was less frequent in the population during the municipal wastewater sampling, whereas ST8 could have been more common than realized. Hundreds of screening specimens collected from the patients treated in the unit of the studied hospital in 2019 showed a low prevalence of CP C. freundii (data not published). However, the true prevalence of asymptomatic carriage in the Finnish population is unknown. Regarding the above, asymptomatic carriage of CP C. freundii ST8 may be more common than recognized and, in combination with a slight increase of detected CP C. freundii ST8 in clinical specimens in Finland recently, could be reflected in the number of ST8 isolates derived from untreated municipal wastewater. Moreover, a clonal shift in the bacterial population (Shapiro, 2016) could also explain why ST18 could not be recovered from wastewater. C. freundii is a commensal bacterium carried within the gastrointestinal tract of both humans and animals (Forsythe et al., 2015). In this study, the animal origin of CP C. freundii in untreated municipal wastewater is unlikely, as CPE has never been reported in food producing animals in Finland in routinely performed national surveillance (Finnish Food Authority, 2022); only one case of CP Escherichia coli has been reported in companion animals in Finland (Grönthal et al., 2018). However, several studies have reported CPE in wildlife, including wild boars in Algeria (Bachiri et al., 2018) and wild avian species in Spain (Oteo et al., 2018). CPE has not been detected in migratory barnacle geese in Finland (Kurittu et al., 2021), but we cannot comprehensively exclude the possibility of the wildlife origin of CP C. freundii, as research data related to CPE in wildlife in Finland is otherwise scarce.
Undetected ST18 in untreated municipal wastewater may indicate its possibly shorter survival time in the wastewater matrix, leading to a quick depletion. In E. coli, different strains have shown substantial differences in growth under the same growth conditions (Sekse et al., 2012). CP C. freundii belonging to ST8 may have a longer survival time in wastewater, allowing it to survive better in the conditions of the WWTP. ST8 may also be predominant or endemic in the sewerage network or studied WWTP, and isolates may reflect the established microbiome of the sewerage network rather than that of the entire population (LaMartina et al., 2021). The enormous microbial load and a favorable environment in wastewater promote bacterial growth, biofilm formation (Weingarten et al., 2018), and sharing of mobile genetic elements—Even beyond genus, family, and order boundaries (Majewski et al., 2017). All these factors could lead to differences in the AMR bacteria detected in the municipal wastewater compared to those circulating in the population.
CP C. freundii was not detected in hospital wastewater, most likely due to the chosen sampling method. A grab sample represents the present situation during the sample collection. Hence, the sample can represent anything from toilet water to gray water, such as showering or cleaning water, leading to substantial differences in the microbiome of that particular sample. A 24-h composite sample would be more representative and less prone to the effects of individual events. Hospital wastewater may also contain greater concentrations of cleaning or disinfection agents that influence the viability of bacteria, and molecular methods could be less sensitive to these effects (Girones et al., 2010).
The genetic relatedness of CP C. freundii isolates from the hospital environment, untreated municipal wastewater, and human specimens indicates a possible persistence of the bacteria throughout the sewerage system and a passage of this microbe from patients or the hospital environment to the WWTP. However, we cannot comprehensively prove the directionality, hence further studies are needed to verify this hypothesis. The genetic relatedness identified between the CP C. freundii ST18 isolates from the hospital environment and human specimens supports the previous hypothesis of a possible environmental source of transmission of this microbe (Räisänen et al., 2021). Sinks and toilets in hospitals may facilitate the storage of human-origin strains (Nurjadi et al., 2021) in biofilms and form a rich ecosystem where plasmids carrying carbapenemase-encoding genes could be transferred through horizontal gene transfer between bacteria (Majewski et al., 2017). Epidemics in hospitals can arise from the patients, but the hospital environment may also facilitate a possible source of transmission of CP C. freundii in clinical settings.
CP C. freundii was detected both in sink traps and toilets in the studied hospital, but not in the other sampling sites (sink taps, bidet showerhead and bidet shower sinks, toilet seat and sink plugholes, toilet seat water tank, or cleaning and disinfection machine). These findings are in line with the studies that have shown drains to be the most common reservoirs of CPE in the hospital environment (Park et al., 2020; Nurjadi et al., 2021). We observed recurrent findings with clonal isolates in four patient rooms throughout the study, despite regular treatment with Chloramine T (1,000 ppm) and the replacement of the toilet seats and sink traps (Figure 2). The clonality of the detected isolates reflects the persistence of these strains in the water fixtures rather than the recolonization caused by the patients. Previous studies have shown that cleaning and disinfection reduce the bacterial load, but a total eradication of CPE in hospital water fixtures is rarely achieved (Hopman et al., 2019; Nurjadi et al., 2021) and re-emergence is common despite intensive cleaning or replacement of the water fixtures (Decraene et al., 2018; Shaw et al., 2018; Smolders et al., 2019). Constructional interventions are the only reported successful measures to contain outbreaks (Nurjadi et al., 2021). It is essential to ensure that the surfaces in the hospital environment are easy to keep clean, but more research studies are needed to develop effective eradication methods and technologies that prevent the spread of microbes from sinks and toilets to the surroundings.
A culture-based approach, combined with whole genome sequencing, was chosen to evaluate the convenience of the commercially available culture media in this context. Molecular methods, such as metagenomic sequencing, may provide deeper taxonomic profiles and give a broad overview of the resistance genes. However, metagenomic sequencing may struggle to identify species at the strain level and assign detected resistance genes to particular species (Hendriksen et al., 2019a). Metagenomic sequencing is also expensive to perform and requires expertise that is commonly lacking at the local level (Pruden et al., 2021). Targeted PCR can detect resistance genes in the wastewater (Pärnänen et al., 2019; Probst et al., 2022) but is limited by the target panel and does not provide information about the species carrying the resistance genes (Girones et al., 2010). Pruden et al. (2021) stated that a culture-based approach is attractive since fecal indicator monitoring infrastructure is relatively low cost and already widespread and accessible in many low and low-middle-income countries. However, the culture-based approach has its limitations. For example, not all bacteria are culturable (Stewart, 2012), and the culture media can significantly impact the recovery of the bacteria from the samples (Davis et al., 2005). Nevertheless, culture-based methods can be as sensitive, specific, and accurate as PCR-based methods if the target organism is culturable (Bliem et al., 2018), as is the case with Enterobacteriaceae, which are commonly uncomplicated to recover from samples. CHROMagar mSuperCARBA has shown enough sensitivity and specificity to detect CPE in clinical specimens (Soria Segarra et al., 2018). However, wastewater is a complex sample material, and identification of the species of interest by colony morphology on selective media can be difficult. The spectrum of species is much broader than in clinical specimens, and environmental microbes may disturb species identification. In our study, we could only choose a pre-agreed number of colonies from the untreated municipal wastewater samples, and the manual selection of colonies can, by chance, lead to the discarding of some bacterial species or isolates with different STs and enzyme types. The apparent advantage—but also limitation—of culture-based WWS is that the analyses give information about a single bacterial clone. The clones can be described precisely and compared with the clones from various sources. However, studying all relevant clones requires extensive culturing and whole genome sequencing, which is highly laborious and increases costs.
Clinical surveillance is undoubtedly essential in screening and observing AMR pathogens in patients at a higher risk. However, it seems that, despite its limitations, culture-based WWS has the potential to detect AMR pathogens causing infections in the population and possibly also in detecting asymptomatic circulation at the population level. Additionally, WWS is decisive in identifying possible new threats, the persistence or emergence of AMR genes, and following the AMR trends. Research on WWS of AMR still needs to contribute to answering many questions, from standardized methodology to integration of existing AMR data. Longitudinal surveillance, including clinical and wastewater samples, and optimally also data from an extensive screening of the healthy Finnish population, could reveal whether the dominance of ST8 in the wastewater is indicative of its incidence in the population, predicted its elevation in the clinical specimens in Finland, or reflected the microbiome of the WWTP. WWS should not be seen as a competitor or a replacement for clinical surveillance, but as a tool that can provide a broader view of the AMR situation in the population. In the future, the insights provided by WWS would be helpful also in clinical settings and decision-making.
Data availability statement
The datasets presented in this study can be found in online repositories. The names of the repository/repositories and accession number(s) can be found below: https://www.ebi.ac.uk/ena, PRJEB58690.
Author contributions
VH: conceptualization, methodology, formal analysis, investigation, data curation, writing—original draft, writing—review and editing, and visualization. VJ: conceptualization, methodology, investigation, and writing—review and editing. KR: conceptualization, formal analysis, resources, writing—review and editing, and visualization. V-JA and OL: conceptualization, resources, and writing—review and editing. JJ, IW, and J-ML: resources and writing—review and editing. K-ML, AL, SO, and TP: writing—review and editing and project administration. AH: conceptualization, writing—review and editing, supervision, project administration, and funding acquisition. WastPan study group: resources and project administration. All authors contributed to the article and approved the submitted version.
WastPan Study Group
Ahmad Al-Mustapha and Paula Kurittu (the University of Helsinki); Annika Länsivaara and Rafiqul Hyder (Tampere University); Ananda Tiwari, Anna-Maria Hokajärvi, Aleksi Kolehmainen, Teemu Möttönen, Oskari Luomala, Aapo Juutinen, Soile Blomqvist, Carita Savolainen-Kopra (the Finnish Institute for Health and Welfare, THL); and Anniina Sarekoski (the University of Helsinki and the Finnish Institute for Health and Welfare, THL).
Funding
This study was funded by the Academy of Finland with grant number 339417.
Acknowledgments
We would like to thank senior laboratory technician Kirsi Ristkari; student Olga Tyni; THL laboratory analysts, Mirka Korhonen, Marjo Tiittanen, Tiina Heiskanen, Tarja Rahkonen, and Kristiina Valkama; and Tampere University laboratory analyst Annika Laaksonen for assistance with the laboratory work. We also would like to thank the wastewater treatment plant personnel for collecting and shipping the wastewater samples. We are grateful for the discussions with Jussa-Pekka Virtanen in the early stages of the data review.
Conflict of interest
The authors declare that the research was conducted in the absence of any commercial or financial relationships that could be construed as a potential conflict of interest.
Publisher's note
All claims expressed in this article are solely those of the authors and do not necessarily represent those of their affiliated organizations, or those of the publisher, the editors and the reviewers. Any product that may be evaluated in this article, or claim that may be made by its manufacturer, is not guaranteed or endorsed by the publisher.
Supplementary material
The Supplementary Material for this article can be found online at: https://www.frontiersin.org/articles/10.3389/fmicb.2023.1165751/full#supplementary-material
References
Babraham Institute (2021). Babraham Bioinformatics–FastQC A Quality Control Tool for High Throughput Sequence Data. Available online at: https://www.bioinformatics.babraham.ac.uk/projects/fastqc/ (accessed July 26, 2022).
Bachiri, T., Bakour, S., Lalaoui, R., Belkebla, N., Allouache, M., Rolain, J. M., et al. (2018). Occurrence of carbapenemase-producing enterobacteriaceae isolates in the wildlife: first report of OXA-48 in wild boars in algeria. Microb. Drug Resis. 24, 337–345. doi: 10.1089/mdr.2016.0323
Becker, L., Kaase, M., Pfeifer, Y., Fuchs, S., Reuss, A., von Laer, A., et al. (2018). Genome-based analysis of Carbapenemase-producing Klebsiella pneumoniae isolates from German hospital patients, 2008–2014. Antimicrob. Resist. Infect. Control 7, 352. doi: 10.1186./s13756-018-0352-y
Bliem, R., Reischer, G., Linke, R., Farnleitner, A., and Kirschner, A. (2018). Spatiotemporal dynamics of Vibrio cholerae in turbid alkaline lakes as determined by quantitative PCR. Appl. Environ. Microbiol. 84, 18. doi: 10.1128./AEM.00317-18
Bolger, A. M., Lohse, M., and Usadel, B. (2014). Trimmomatic: a flexible trimmer for Illumina sequence data. Bioinformatics 30, 170. doi: 10.1093./bioinformatics/btu170
Bortolaia, V., Kaas, R. S., Ruppe, E., Roberts, M. C., Schwarz, S., Cattoir, V., et al. (2020). ResFinder 4, 0. for predictions of phenotypes from genotypes. J.Antimicrob. Chemotherapy 75, 345. doi: 10.1093/jac/dkaa345
Clausen, P. T. L. C., Aarestrup, F. M., and Lund, O. (2018). Rapid and precise alignment of raw reads against redundant databases with KMA. BMC Bioinform. 19, 1–8. doi: 10.1186./s12859-018-2336-6
Davis, K. E. R., Joseph, S. J., and Janssen, P. H. (2005). Effects of growth medium, inoculum size, and incubation time on culturability and isolation of soil bacteria. Appl. Environ. Microbiol. 71, 826–234. doi: 10.1128./AEM.71.2.826-834.2005
Decraene, V., Phan, H. T. T., George, R., Wyllie, D. H., Akinremi, O., Aiken, Z., et al. (2018). A large, refractory nosocomial outbreak of klebsiella pneumoniae carbapenemase-producing Escherichia coli demonstrates carbapenemase gene outbreaks involving sink sites require novel approaches to infection control. Antimicrob. Agents Chemother. 62, e01689–e0168918. doi: 10.1128./AAC.01689-18
EUCAST (2022a). Antimicrobial susceptibility testing EUCAST disk diffusion method Version 10, 0. January. Available online at: https://www.eucast.org/fileadmin/src/media/PDFs/EUCAST_files/Disk_test_documents/2022_manuals/Manual_v_10.0_EUCAST_Disk_Test_2022.pdf (accessed December 1, 2022).
EUCAST (2022b). MIC and zone diameter distributions and ECOFFs. Available online at: https://www.eucast.org/mic_distributions_and_ecoffs/ (accessed December 1, 2022).
Feldgarden, M., Brover, V., Haft, D. H., Prasad, A. B., Slotta, D. J., Tolstoy, I., et al. (2019). Validating the AMRFINder tool and resistance gene database by using antimicrobial resistance genotype-phenotype correlations in a collection of isolates. Antimicrob. Agents Chemother. 63, e00483–e004819. doi: 10.1128./AAC.00483-19
Finnish Food Authority FMAFU of H. (2022). FINRES-Vet 2021. Finnish Veterinary Antimicrobial Resistance Monitoring and Consumption of Antimicrobial Agents. Available online at: https://www.ruokavirasto.fi/globalassets/viljelijat/elaintenpito/elainten-laakitseminen/antibioottiresistenssin_seuranta/finnish_food_authority_publications_6_2022_finres_vet_2021.pdf (accessed December 1, 2022).
Forsythe, S. J., Abbott, S. L., and Pitout, J. (2015). “Klebsiella, enterobacter, citrobacter, cronobacter, serratia, plesiomonas, and other enterobacteriaceae,” in Manual of Clinical Microbiology. doi: 10.1128./9781555817381.ch38
Girones, R., Ferrús, M. A., Alonso, J. L., Rodriguez-Manzano, J., Calgua, B., Abreu Corrêa, de., et al. (2010). Molecular detection of pathogens in water—The pros and cons of molecular techniques. Water Res. 44, 4325–4339. doi: 10.1016/j.watres.06030
Grönthal, T., Österblad, M., Eklund, M., Jalava, J., Nykäsenoja, S., Pekkanen, K., et al. (2018). Sharing more than friendship—Transmission of NDM-5 ST167 and CTX-M-9 ST69 Escherichia coli between dogs and humans in a family, Finland, 2015. Eurosurveillance 23, 497. doi: 10.2807/1560-7917.ES.23,27.1700497
Hendriksen, R. S., Bortolaia, V., Tate, H., Tyson, G. H., Aarestrup, F. M., McDermott, P. F., et al. (2019a). Using genomics to track global antimicrobial resistance. Front. Public Health 7, 242. doi: 10.3389./fpubh.2019.00242
Hendriksen, R. S., Munk, P., Njage, P., van Bunnik, B., McNally, L., Lukjancenko, O., et al. (2019b). Global monitoring of antimicrobial resistance based on metagenomics analyses of urban sewage. Nat. Commun. 10, 3. doi: 10.1038./s41467-019-08853-3
Hopman, J., Meijer, C., Kenters, N., Coolen, J. P. M., Ghamati, M. R., Mehtar, S., et al. (2019). Risk assessment after a severe hospital-acquired infection associated with carbapenemase-producing Pseudomonas aeruginosa. JAMA Network Open 2, e187665. doi: 10.1001/jamanetworkopen.2018.7665
Jamin, C., Koster, d. e., van Koeveringe, S., de Coninck, S., Mensaert, D., de Bruyne, K. P., et al. (2021). Harmonization of whole-genome sequencing for outbreak surveillance of Enterobacteriaceae and enterococci. Microb. Genom. 7, 567. doi: 10.1099./mgen.0.000567
Jolley, K. A., Bray, J. E., and Maiden, M. C. J. (2018). Open-access bacterial population genomics: BIGSdb software, the PubMLST.org website and their applications [version 1; referees: 2 approved]. Wellcome Open Res. 3, 1. doi: 10.12688./wellcomeopenres.14826.1
Jünemann, S., Sedlazeck, F. J., Prior, K., Albersmeier, A., John, U., Kalinowski, J., et al. (2013). Updating benchtop sequencing performance comparison. In Nature Biotechnology (Vol. 31). doi: 10.1038./nbt.2522
Kizny Gordon, A. E., Mathers, A. J., Cheong, E. Y. L., Gottlieb, T., Kotay, S., Walker, A. S., et al. (2017). The hospital water environment as a reservoir for carbapenem-resistant organisms causing hospital-acquired infections—A systematic review of the literature. Clin. Infecti. Dis. 64, 132. doi: 10.1093./cid/cix132
Kotay, S. M., Donlan, R. M., Ganim, C., Barry, K., Christensen, B. E., Mathers, A. J., et al. (2019). Droplet-rather than aerosol-mediated dispersion is the primary mechanism of bacterial transmission from contaminated hand-washing sink traps. Appl. Environ. Microbiol. 85(2). doi: 10.1128./AEM.01997-18
Kurittu, P., Khakipoor, B., Brouwer, M. S. M., and Heikinheimo, A. (2021). Plasmids conferring resistance to extended-spectrum beta-lactamases including a rare IncN+IncR multireplicon carrying blaCTX−M−1 in Escherichia coli recovered from migrating barnacle geese (Branta leucopsis). Open Res. Eur. 1, 46. doi: 10.12688/openreseurope.13529.1
LaMartina, E., Lou Mohaimani, A. A., and Newton, R. J. (2021). Urban wastewater bacterial communities assemble into seasonal steady states. Microbiome 9, 116. doi: 10.1186/s40168-021-01038-5
Leitner, E., Zarfel, G., Luxner, J., Herzog, K., Pekard-Amenitsch, S., Hoenigl, M., et al. (2015). Contaminated handwashing sinks as the source of a clonal outbreak of kpc-2-producing Klebsiella oxytoca on a hematology ward. Antimicrob. Agents Chemother. 59, 714–716. doi: 10.1128/AAC.04306-14
Majewski, P., Wieczorek, P., Łapuć, I., Ojdana, D., Sieńko, A., Sacha, P., et al. (2017). (2017). Emergence of a multidrug-resistant Citrobacter freundii ST8 harboring an unusual VIM-4 gene cassette in Poland. Int. J. Infect. Dis. 61, 70–73. doi: 10.1016/j.ijid.05016
Nurjadi, D., Scherrer, M., Frank, U., Mutters, N. T., Heininger, A., Späth, I., et al. (2021). Genomic investigation and successful containment of an intermittent common source outbreak of OXA-48-producing enterobacter cloacae related to hospital shower drains. Microbiol. Spect. 9. doi: 10.1128./Spectrum.01380-21
Oteo, J., Mencía, A., Bautista, V., Pastor, N., Lara, N., González-González, F., et al. (2018). Colonization with Enterobacteriaceae-Producing ESBLs, AmpCs, and OXA-48 in Wild Avian Species, Spain 2015–2016. Microb. Drug Resis. 24, 932–938. doi: 10.1089/mdr.2018.0004
Park, S. C., Parikh, H., Vegesana, K., Stoesser, N., Barry, K. E., Kotay, S. M., et al. (2020). Risk factors associated with carbapenemase-producing Enterobacterales (CPE) positivity in the hospital wastewater environment. Appl. Environ. Microbiol. 86, e01715–e017120. doi: 10.1128./AEM.01715-20
Pärnänen, K. M. M., Narciso-Da-Rocha, C., Kneis, D., Berendonk, T. U., Cacace, D., Do, T. T., et al. (2019). Antibiotic resistance in European wastewater treatment plants mirrors the pattern of clinical antibiotic resistance prevalence. Sci. Adv. 5, 9214. doi: 10.1126./sciadv.aau9124
Probst, K., Boutin, S., Späth, I., Scherrer, M., Henny, N., Sahin, D., et al. (2022). Direct-PCR from rectal swabs and environmental reservoirs: a fast and efficient alternative to detect blaOXA−48 carbapenemase genes in an Enterobacter cloacae outbreak setting. Environ. Res. 203, 111808. doi: 10.1016/j.envres.2021.111808
Pruden, A., Vikesland, P. J., Davis, B. C., and Roda Husman, de. (2021). Seizing the moment: now is the time for integrated global surveillance of antimicrobial resistance in wastewater environments. Curr. Opin. Microbiol. 64, 13. doi: 10.1016/j.mib.09013
Räisänen K. Lyytikäinen O. Kauranen J. Tarkka E. Forsblom-Helander B. Grönroos J. O.. (2020). Molecular epidemiology of carbapenemase-producing Enterobacterales in Finland, 2012–2018. Eur. J. Clin. Microbiol. Infect. Dis. 39, 885. doi: 10.1007./s10096-020-03885-w
Räisänen K. Sarvikivi E. Arifulla D. Pietikäinen R. Forsblom-Helander B. Tarkka E.. (2021). Three clusters of carbapenemase-producing Citrobacter freundii in Finland, 2016–20. J. Antimicrob. Chemotherapy 76, 2697-2701. doi: 10.1093./jac/dkab209
Sekse, C., Bohlin, J., Skjerve, E., and Vegarud, G. E. (2012). Growth comparison of several Escherichia coli strains exposed to various concentrations of lactoferrin using linear spline regression. Microb. Inform. Exp. 2, 5. doi: 10.1186./2042-5783-2-5
Shahid, M. (2010). Citrobacter spp. simultaneously harboring blaCTX−M, blaTEM, blaSHV, blaampC, and insertion sequences IS26 and orf513: an evolutionary phenomenon of recent concern for antibiotic resistance. J. Clin. Microbiol. 48, 9. doi: 10.1128./JCM.01467-09
Shapiro, B. J. (2016). How clonal are bacteria over time? Curr. Opin. Microbiol. 31, 116–123. doi: 10.1016/j.mib.03013
Shaw, E., Gavaldà, L., Càmara, J., Gasull, R., Gallego, S., Tubau, F., et al. (2018). Control of endemic multidrug-resistant Gram-negative bacteria after removal of sinks and implementing a new water-safe policy in an intensive care unit. J. Hospital Infect. 98, 275–281. doi: 10.1016/j.jhin.10025
Smolders, D., Hendriks, B., Rogiers, P., Mul, M., and Gordts, B. (2019). Acetic acid as a decontamination method for ICU sink drains colonized by carbapenemase-producing Enterobacteriaceae and its effect on CPE infections. J. Hospital Infect. 102, 82–8. doi: 10.1016/j.jhin.12,009.
Soria Segarra, C., Larrea Vera, G., Berrezueta Jara, M., Arévalo Mendez, M., Cujilema, P., Serrano Lino, M., et al. (2018). Utility of CHROMagar mSuperCARBA for surveillance cultures of carbapenemase-producing Enterobacteriaceae. New Microb. New Infect. 26, 5. doi: 10.1016/j.nmni.08005
Souvorov, A., Agarwala, R., and Lipman, D. J. (2018). SKESA: strategic k-mer extension for scrupulous assemblies. Genome Biol. 19, 153. doi: 10.1186./s13059-018-1540-z
Stewart, E. J. (2012). Growing unculturable bacteria. J. Bacteriol. 194, 12. doi: 10.1128./JB.00345-12
THL (2022a). CPE-esiintyvyys Suomessa. Available online at: https://thl.fi/fi/web/infektiotaudit-ja-rokotukset/taudit-ja-torjunta/taudit-ja-taudinaiheuttajat-a-o/cpe/cpe-esiintyvyys-suomessa (accessed December 20, 2022).
THL Tampere University University of Helsinki. (2022b). Wastewater-based surveillance as pandemic preparedness tool (WastPan). Available online at: https://thl.fi/en/web/thlfi-en/research-and-development/research-and-projects/wastewater-based-surveillance-as-pandemic-preparedness-tool-wastpan- (accessed January 2, 2022).
Tiwari, A., Paakkanen, J., Österblad, M., Kirveskari, J., Hendriksen, R. S., Heikinheimo, A., et al. (2022). Wastewater surveillance detected carbapenemase enzymes in clinically relevant gram-negative bacteria in Helsinki, Finland; 2011–2012. Front. Microbiol. 13, 7888. doi: 10.3389./fmicb.2022.887888
Tofteland, S., Naseer, U., Lislevand, J. H., Sundsfjord, A., and Samuelsen, Ø. (2013). A Long-term low-frequency hospital outbreak of KPC-Producing Klebsiella pneumoniae involving intergenus plasmid diffusion and a persisting environmental reservoir. PLoS ONE 8, e59015. doi: 10.1371/journal.pone.0059015
van Beek J. Räisänen K. Broas M. Kauranen J. Kähkölä A. Laine J. . (2019). Tracing local and regional clusters of carbapenemase-producing Klebsiella pneumoniae ST512 with whole genome sequencing, Finland, 2013 to 2018. Eurosurveillance, 24, 1800522. doi: 10.2807/1560-7917.ES.2438.1800522
Weingarten, R. A., Johnson, R. C., Conlan, S., Ramsburg, A. M., Dekker, J. P., Lau, A. F., et al. (2018). Genomic analysis of hospital plumbing reveals diverse reservoir of bacterial plasmids conferring carbapenem resistance. MBio, 9, e02011–02017. doi: 10.1128./mBio.02011-17
WHO (2021). WHO: Antimicrobial resistance. Available online at: https://www.who.int/news-room/fact-sheets/detail/antimicrobial-resistance (accessed August 1, 2022).
Yao, Y., Falgenhauer, L., Falgenhauer, J., Hauri, A. M., Heinmüller, P., Domann, E., et al. (2021). Carbapenem-resistant citrobacter spp. as an emerging concern in the hospital-setting: results from a genome-based regional surveillance study. Front. Cell. Infect. Microbiol. 11, 744431. doi: 10.3389./fcimb.2021.744431
Zankari, E., Allesøe, R., Joensen, K. G., Cavaco, L. M., Lund, O., Aarestrup, F. M., et al. (2017). PointFinder: a novel web tool for WGS-based detection of antimicrobial resistance associated with chromosomal point mutations in bacterial pathogens. J. Antimicrob. Chemotherapy 72, 2764–2768. doi: 10.1093./jac/dkx217
Keywords: wastewater, wastewater surveillance, antimicrobial resistance, Citrobacter freundii, carbapenemase-producing Citrobacter freundii, carbapenemase-producing Enterobacteriaceae
Citation: Heljanko V, Johansson V, Räisänen K, Anttila V-J, Lyytikäinen O, Jalava J, Weijo I, Lehtinen J-M, Lehto K-M, Lipponen A, Oikarinen S, Pitkänen T, Heikinheimo A and WastPan Study Group (2023) Genomic epidemiology of nosocomial carbapenemase-producing Citrobacter freundii in sewerage systems in the Helsinki metropolitan area, Finland. Front. Microbiol. 14:1165751. doi: 10.3389/fmicb.2023.1165751
Received: 14 February 2023; Accepted: 05 May 2023;
Published: 26 May 2023.
Edited by:
Biao Tang, Zhejiang Academy of Agricultural Sciences, ChinaReviewed by:
Dennis Nurjadi, University Medical Center Schleswig-Holstein, GermanyAlberto Antonelli, University of Florence, Italy
Copyright © 2023 Heljanko, Johansson, Räisänen, Anttila, Lyytikäinen, Jalava, Weijo, Lehtinen, Lehto, Lipponen, Oikarinen, Pitkänen, Heikinheimo and WastPan Study Group. This is an open-access article distributed under the terms of the Creative Commons Attribution License (CC BY). The use, distribution or reproduction in other forums is permitted, provided the original author(s) and the copyright owner(s) are credited and that the original publication in this journal is cited, in accordance with accepted academic practice. No use, distribution or reproduction is permitted which does not comply with these terms.
*Correspondence: Viivi Heljanko, dmlpdmkuaGVsamFua28mI3gwMDA0MDtoZWxzaW5raS5maQ==