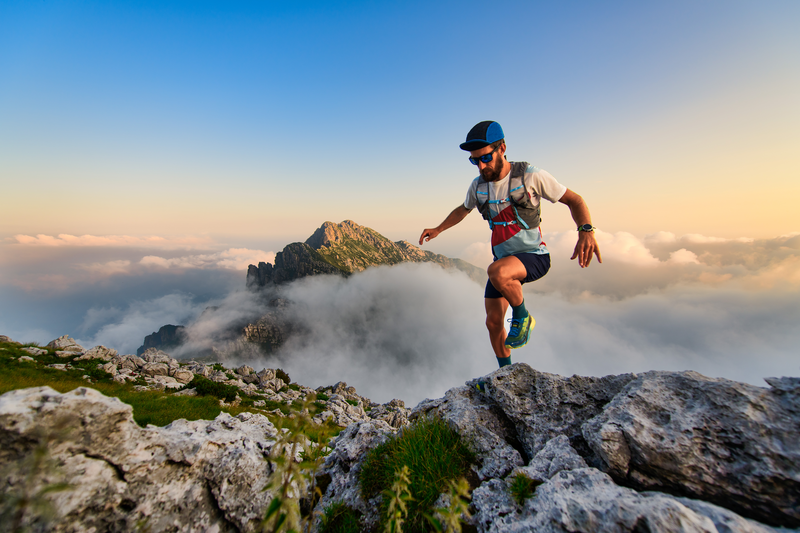
95% of researchers rate our articles as excellent or good
Learn more about the work of our research integrity team to safeguard the quality of each article we publish.
Find out more
ORIGINAL RESEARCH article
Front. Microbiol. , 14 April 2023
Sec. Evolutionary and Genomic Microbiology
Volume 14 - 2023 | https://doi.org/10.3389/fmicb.2023.1158056
Infection with Extended-spectrum beta-lactamase -producing Enterobacterales (ESBL-E) is common in infants and leads to increased intensive care unit admission and mortality, but the role of maternal transmission in colonization of infants is unclear. Using paired isolates from 50 pairs of mothers and neonates admitted to a Cambodian hospital, we investigated antimicrobial resistance in Escherichia coli and Klebsiella pneumoniae using whole genome sequencing. We detected a wide variety of ESBL-E genes present in this population along with high levels of multidrug resistance. From 21 pairs where the same organism was present in both mother and neonate, we identified eight pairs with identical or near-identical isolates from both individuals suggestive of transmission at or around birth, including a pair with transmission of multiple strains. We found no evidence for transmission of plasmids only from mother to infant. This suggests vertical transmission outside hospitals as a common cause of ESBL-E colonization in neonates.
Antimicrobial resistance (AMR) is a major global health problem, and multiple studies have shown high rates of AMR in low and middle-income countries in Southeast Asia compared to other regions (Woerther et al., 2013; Zellweger et al., 2017; Reed et al., 2019). AMR is a particular problem in neonates, where infection with Extended-spectrum beta-lactamase -producing Enterobacterales (ESBL-E) are common and associated with admission to ICU and death (Stoesser et al., 2014; Fox-Lewis et al., 2018; Roberts et al., 2019). ESBL-E infections have become one of the main challenges for antibiotic treatment leading to the increase in mortality, healthcare cost and threatening the effectiveness of first line sepsis treatments in low resource countries such as Cambodia. Rates of ESBL-E in Cambodia have been shown to rise between 2012 and 2015. However, multidrug resistance infection cases in Cambodia may be under-reported compared to neighboring countries due to lack of infrastructure (Vlieghe et al., 2013), and AMR data in Cambodia is improving but currently limited (Reed et al., 2019).
Cambodia shows a high prevalence of ESBL-E colonization in the early neonatal period (Turner et al., 2016) suggestive of mother-to-child transmission at or shortly after delivery. A high number of infants are likely to already be colonized prior to hospital admission, and community acquisition may be important in the neonatal population (Smit et al., 2018). Maternal transmission has been implicated in ESBL-E acquisition by neonates by looking at prevalence (Heigl et al., 2020), or by using matched antimicrobial resistance phenotypes (Frank Wolf et al., 2021), pulsed-field gel electrophoresis (Rettedal et al., 2015), and repetitive element PCR typing (Peretz et al., 2017) to compare strains between mother and infant. Most recently, the BARNARDS study identified identical isolates between mother and infant in E. coli using whole-genome sequencing, suggesting mother-to-child transmission in a large south Asian and African dataset (Carvalho et al., 2022).
Whole-genome sequencing (WGS) of clinical isolates has emerged as an extremely valuable tool with many applications in clinical microbiology including tracking outbreaks, monitoring trends in infections and investigating pathogen transmission routes (Didelot et al., 2012). Several studies have shown that high-resolution data from WGS are a proven tool to track the possible transmission of Klebsiella pneumoniae circulating both in the intensive care unit (Gorrie et al., 2017) and in an outbreak via plasmid transmission (Mathers et al., 2011). However, most current datasets are short-read data, and the assembly of short-reads datasets usually results in multiple contigs and ambiguous alignments instead of a complete genome (Treangen and Salzberg, 2011). To overcome this challenge, Oxford Nanopore MinION platform can produce long reads enabling improved quality genome assembly including complete assembly of plasmids (Koren and Phillippy, 2015).
We used whole-genome sequencing to obtain ESBL-E genomes from pairs of mothers and neonates admitted to Angkor Hospital for Children, Siem Reap, Cambodia. Using complete genomes generated using short- and long-read sequencing, we identified patterns of antimicrobial resistance and investigated potential transmission events between mothers and neonates to better understand the importance of maternal transmission versus community acquisition in early colonization of neonates.
Angkor Hospital for Children is a non-governmental pediatric referral hospital with a dedicated neonatal intensive care unit (NICU) and special care baby unit (SCBU). The hospital has no maternity unit, thus all neonatal admissions are outborn. Over a 1 year period all infants aged ≤28 days were eligible for study enrolment on admission to the NICU or SCBU, excluding those who had any previous healthcare service exposure following delivery (e.g., overnight admission to a hospital/health center or transfer from another AHC ward).
Mothers provided written informed consent to participate in study. Those unable to read and write gave a thumbprint instead, with an impartial witness present during the consent process. Mothers consented on behalf of infants. A rectal swab was taken from the infant within 24 h of admission to the neonatal unit (at a median of 11 days of age [range 0–30]), and a maternal stool sample was collected as soon as possible after infant admission. Samples were cultured onsite on selective chromogenic ESBL detection agar (CHROMagar ESBL medium; CHROMagar, France) and all morphotypes followed up for analysis. Species identification was confirmed by standard biochemical tests and antimicrobial susceptibilities were determined by disk diffusion, following CLSI methodology and using 2017 breakpoints (CLSI, 2017). Double disk diffusion tests were done to confirm ESBL production (cefotaxime and ceftazidime with/without clavulanate; BBL, Becton Dickinson, United States).
96 isolates of E. coli and K. pneumoniae were cultured from frozen stocks (−80°C) and grown in LB broth at 37°C for 3 h. 900 μL of bacterial culture was spun down at 21,200 rcf for 5 min before the supernatant was removed. The bacterial cells were lysed with 600 μL of nucleic lysis solution (Promega, United States) at 80°C for 10 min. The cell lysate was then transferred to a new tube containing 250 μL of glass beads with 5 μL of RNase A solution (Qiagen, Germany), and the cells were further lysed by vortexing for 10 min. The cell lysate was incubated at 37°C for 30 min to remove the RNA. The mixture was purified using magnetic beads (Agencourt AMPure XP beads, Beckman Coulter, United States) with a 1:4 volume ratio of lysate mixture and magnetic beads. The extracted DNA was redissolved in distilled sterilized water and then stored in the freezer (−20°C) prior to library preparation for sequencing.
Sequencing libraries were prepared from extracted DNA using Nextera XT library preparation kits (FC-131-1096, Illumina, United States) and Nextera XT Index Kits 96 samples (FC-131-1002) and sequenced on an Illumina MiSeq with 300 bp paired-end reads. 36 isolates were selected for long-read sequencing. The Rapid Barcoding Kit (SQK-RBK004, Nanopore, United Kingdom) was used to prepare DNA libraries according to the Nanopore protocol with 12 barcoded samples per flow cell. The prepared libraries were pooled and purified using an additional step with magnetic beads (Agencourt AMPure XP beads, Beckman Coulter, United States) using a 1:1 volume ratio of pooled libraries and the magnetic beads. The purified libraries were then sequenced on a MinION R9.4 flow cell according to the Nanopore protocol. The sequences were deposited in the European Nucleotide Archive (ENA) at EMBL-EBI under project accession number PRJEB37551 https://www.ebi.ac.uk/ena/browser/view/PRJEB37551.
Raw sequence data were processed using the Bactopia pipeline 1.4 (Petit and Read, 2020). Reads were mapped against E. coli K-12 substrain MG1655 reference sequence for all Escherichia isolates1 and K. pneumoniae HS112862 for all Klebsiella isolates, and the genome assemblies were also used as references for confirmation of transmission. Mashtree 1.1.2 (Katz, 2021) was used to generate phylogenetic trees from all samples and visualized using ggtree 3.1.2 (Yu et al., 2017). Ska 1.0 (Harris, 2018) was used to perform split k-mer analysis using the default cutoffs (95% kmer identity and < 20 SNPs to call transmission clusters). Snippy 4.6.0 (Seemann, 2018) was used to produce variant calls, and snippy-core was used to mask repetitive regions and produce a core SNP alignment. SNP distances between samples were called with snp-dists 0.6.3 (Seemann, 2021a). MLST was determined using mlst 2.19.0 (Seemann, 2021b) and the PubMLST database (Jolley and Maiden, 2010). Genome assemblies were performed using Unicycler 0.4.8 (Wick et al., 2017). Escherichia species and phylogroups were determined using ClermonTyping (Beghain et al., 2018). Klebsiella phylogroups and virulence genes were obtained through Kleborate (Lam et al., 2021). Antimicrobial resistance genes and mutations were determined using NCBI AMRFinder+3.8.4 (Feldgarden et al., 2019), and upset plots were visualized using ComplexUpset (Krassowski, 2020). Platon 1.5.0 (Schwengers et al., 2020) was used to identify contigs originating from plasmids and the mobilization proteins, replication proteins, and incompatibility groups on each contig. The Platon identification was used to determine whether the AMR genes were part of the chromosome or present on plasmids. Flanker 0.1.5 (Matlock et al., 2021) was used to extract 20 kb genomic regions surrounding AMR genes, treating plasmids as circular if the Unicycler assembler reported them as circular. Isolates were removed if the AMR gene was on a contig less than 5 kb in length. Clinker (Gilchrist and Chooi, 2021) was used to visualize the regions, with links drawn between genes with 95% or greater identity.
Between March 23, 2016 and March 13, 2017, 121 isolates were cultured from swabs from 50 pairs of mothers and infants. 25/50 (50%) of infants and 47/50 (94%) of mothers were colonized with an ESBL-E, including 17/50 pairs where both mother and infant were colonized with E. coli and 4/50 pairs colonized with K. pneumoniae. Nine pairs had the same species isolated from both mother and infant with identical antibiograms, which we considered as potential transmission pairs (Figure 1 and Supplementary Table 1).
Figure 1. Flow diagram showing study design and sample collection. 3GC-R, third generation cephalosporin resistant.
We sequenced 96 isolates in total on Illumina MiSeq—68 isolates from 20 of the 21 pairs where both mother and infant were colonized, including eight of nine pairs considered as potential transmission pairs (one pair could not be retrieved for sequencing), and 28 other randomly selected isolates from the study. We sequenced up to four isolates per individual based on the number of distinct morphotypes identified during bacterial growth (Supplementary Table 2).
We performed long-read sequencing on the 18 isolates identified as possible transmission pairs based on the phenotypic data, as well as 18 other isolates which were observed to have closely related core genomes or similar patterns of antimicrobial resistance genes based on Illumina data. We used hybrid assembly of the long and short-read data to obtain complete genomes and resolve the plasmids in these strains in order to confirm transmission events and identify whether these transmission events involved complete isolates, or plasmid horizontal transmission between different isolates. We obtained between 1 and 9 contigs for each of the 36 isolates, including one contig of 4.5 Mb or larger assumed to be the chromosome, and 0–8 plasmid contigs per isolate.
The species of each isolate was determined from the whole genome sequencing using ClermonTyping (for Escherichia species) and Kleborate (for Klebsiella species). This showed that the K. pneumoniae isolates included three isolates of K. quasipneumoniae subsp. similipneumoniae and one isolate of K. quasipneumoniae subsp. quasipneumoniae, and the E. coli isolates included three isolates of E.fergusonii and two isolates of E. clade-1 (Figure 2).
Figure 2. Phylogeny based on mashtree of all samples showing species, and presence of selected AMR genes in each sample.
No phylogroups or MLST were predominant in the isolates. Among E. coli isolates, the most common MLST was ST38 and the closely related ST3268 and ST3052 and ST318, as well as three isolates from the ST131 pandemic clade (Supplementary Figure 1). Of the three ST131 isolates, two were clade C and one was clade A. In the K. pneumoniae isolates there were no dominant sequence types, with ST17 the only sequence type present more than once in unpaired isolates, and four isolates had novel sequence types (Supplementary Figure 2). No virulence determinants were found in the K. pneumoniae isolates and all had a virulence score of 0 as determined by Kleborate.
95 of 96 isolates had an ESBL gene present in the genome, as expected as they were selected for the presence of ESBLs. One isolate (EbB064) had no ESBL genes present in the sequenced genome, despite having phenotypic resistance to third generation cephalosporins. Four K. pneumoniae and one K. quasipneumoniae subsp. similipneumoniae isolates had blaSHV-2A genes predicted to cause ESBL resistance, with two cases of blaSHV-2A carried on a plasmid, one case where the gene is chromosomal, and one pair of isolates carrying the gene on both a plasmid and in the chromosome. Multidrug resistance was extremely common in these isolates, with 64% (61/96) of isolates genotypically resistant to six antimicrobial classes, including two isolates resistant to eight classes (Figure 3). No carbapenemase resistance was found in this dataset. Colistin resistance was observed through presence of mcr-1.1 and mcr-3.5 genes in three E. coli isolates. A point mutation of pmrB (R256G) was reported in three K. pneumoniae isolates but this has been previously demonstrated not to cause colistin resistance in isolation (Cheng et al., 2015) and we did not consider these isolates resistant. AMRfinder analysis reported resistance to phenicol/quinolone via oqxAB and resistance to fosfomycin via fosA in K. pneumoniae, but Kleborate confirmed these were intrinsic and no acquired resistance was reported, and so these isolates were not recorded as resistant through presence of these genes (Lam et al., 2021).
Figure 3. Upset plot showing shared classes of antimicrobial resistance genes between isolates. Isolate counts are shown as bars, with antimicrobial resistance gene combinations displayed in the lower panel.
A wide range of beta-lactamase genes were carried, including nine different blaCTX-M genes, and genes from the blaCMY, blaOXA, blaDHA, blaSHV, and blaVEB families (Figure 4). The most common were blaCTX-M-15, found in 31 isolates, and blaCTX-M-55, found in 24 isolates. 25 isolates had an ESBL gene in the chromosome, while seven isolates had ESBL genes present on plasmids as well as integrated into the chromosome.
Figure 4. (A) Phylogeny from mashtree showing presence/absence of ESBL genes. (B) Frequency of ESBL genes in the isolates; and (C) Number of ESBL genes per isolate.
For each potential pair of isolates from a mother-infant pair, we identified potential transmission events as either transmission of a strain with complete genome (chromosome only, or chromosome and plasmid), transmission of plasmids, or no transmission.
For eight of the nine pairs which had the same antibiogram in both strains, the mother and infant strains shared the same MLST and the same set of acquired antimicrobial resistance genes, and a further pair of isolates differed only by one antimicrobial resistance gene. We used split k-mer analysis to identify transmission clusters in the complete dataset, and confirmed this using the complete genome assembled from one of the paired strains as a reference for mapping and variant calling. We removed variant calls in repetitive regions but did not correct for recombination as we assume no recombination will occur in the timeframe of maternal transmission.
Eight of these nine pairs were identified as transmission clusters using ska with default filters (95% kmer similarity and a SNP distance threshold of 20), and had 0–4 SNP differences between them (Figure 5). To confirm these results, we used one strain from each pair as a reference genome, and performed mapping and variant calling. Mapping to one pair as a reference gave similar results in all cases with 0–4 SNP differences from the mapping-based approach. We identified one pair where complete strains of both E. coli and K. pneumoniae were transmitted.
Figure 5. Diagram of clusters identified using ska. Circles show strains of Escherichia coli while squares are Klebsiella pneumoniae. The number on the bar joining each pair represents the SNP distance between the two strains. Shapes are colored according to the mother-infant pairs they were isolated from, with gray shapes for strains taken from unpaired individuals.
We also looked at two other pairs of isolates from the whole collection which were identified as a possible transmission cluster by ska (Figure 5). In one case, the two isolates (EbB096 and EbB097) were from the same individual, and likely represent the same isolate which was sequenced twice due to appearing as different morphotypes during bacterial growth. The other pair of isolates (EbB002 and EbB018) were identified as a transmission cluster by ska, but with 14 SNP differences between the isolates. Mapping to one of the paired isolates as a reference gave similar results, with 13 SNP differences between isolates. This pair of isolates came from an unpaired mother and infant, and were collected 54 days apart.
For each of the transmission pairs we performed whole genome alignment using minimap to determine if the transmission included plasmids. Seven of the eight transmission clusters included plasmids, with only one pair where only the chromosome was present in both assemblies. While the plasmids appear to be transmitted in all strains, in three of the eight transmitted pairs there were rearrangements between plasmids. In one pair (isolates EbB065 and EbB066), one isolate had two circular contigs of 280 and 95 kb, while the other isolate has only one non-circular contig of 372 kb, which likely represents a misassembly of two plasmids into one contig (Supplementary Figure 3). In another pair (EbB043 and EbB045), an 88 kb non-circular contig from one isolate is present in the chromosome of the paired isolate, representing either a misassembly, or potentially insertion into the chromosome (Supplementary Figure 4). In a third pair (EbB104 and EbB105), the same plasmid material is assembled into a single plasmid in both strains, but with rearrangements between the plasmids, including a tandem duplication of the blaCTX-M-65 gene seen in two copies in EbB104 and three copies in EbB105 (Supplementary Figure 5).
We also considered the possibility that maternal transmission of plasmids could occur in the absence of transmission of a complete strain, which would not be discovered using mapping-based approaches but may be identified by similar antimicrobial resistance profiles. To check for plasmid-borne resistance genes shared between strains, we compared the antimicrobial resistance profiles of all strains. There were no cases of identical or near-identical antimicrobial resistance profiles in paired strains which do not have similar core genomes. We did identify three clusters of unpaired samples with identical or near-identical antimicrobial resistance profiles which do not have similar core genomes: EbB004 and EbB039, EbB031 and EbB041, and EbB036/EbB037 and EbB091.
Strains EbB004 has a 127 kb plasmid carrying incFII and incFIB, while EbB039 has a 221 kb plasmid with incFII, incFIB, col156 and P0111 replicons. The two plasmids shared a 50 kb segment with an internal inversion, including the blaCTX-M-27 gene, and eight other genes conferring resistance to aminoglycosides, trimethoprim, macrolides, sulfonamides, and tetracycline.
Strains EbB031 and Eb041 both belong to ST44 and have a SNP distance of 349 measured by ska. They also share a 170 kb plasmid with a similar resistance cassette carrying both blaCTX-M-15 and blaOXA-1, as well as resistance to aminoglycosides, trimethoprim, macrolides, sulfonamides, tetracycline, and chloramphenicol.
Strains EbB036 and EbB037 are a transmission pair which share an antimicrobial resistance profile with strain EbB091. However, none of these strains contain plasmids, and the shared resistance profiles are driven by chromosomal qnrS1 and blaCTX-M-15 integrated in different locations.
Finally, we looked at the genomic context of ESBLs found in more than one strain to determine if shared mobile elements were driving the spread of ESBLs in this dataset. Observing the 20 kb regions around each ESBL, many of the ESBL genes were present in different contexts and were not present on shared plasmids or as shared mobile elements integrated into plasmids or chromosomes.
We use complete genomes combining short and long-read sequencing to show that maternal transmission is an important source of neonatal colonization. We used hybrid assemblies generated from short and long reads to identify transmission clusters, as recent findings from assemblies using Oxford Nanopore Technologies long reads alone show that they are not sufficient to reliably identify transmission clusters of closely related samples (Foster-Nyarko et al., 2023).
The isolates in this study were pre-selected to include only those with ESBLs and may not represent a true population survey. Previous estimates of carriage rates in Cambodia have ranged from 20% in rural villages in 2011 (Atterby et al., 2019), 42.7% for E. coli and 33.7% for K. pneumoniae between 2012 and 2015 (Caron et al., 2018), and 92.8% for E. coli and 44.1% for K. pneumoniae in Siem Reap province in 2019 (Singh et al., 2020). Despite the selection for ESBL carriage, the results are largely concordant with previous studies investigating all isolates, with high levels of genetic diversity, no dominant sequence types, and a range of antimicrobial resistance genes (Huynh et al., 2020). Despite the presence of ST131 isolates in this dataset, they were not dominant in carriage. A wide range of ESBL genes were seen in these isolates, with blaCTX-M-15 and blaCTX-M-55 the most common, but no dominant gene or lineage. Previous studies have reported hypervirulent K. pneumoniae clones as a cause of community-acquired infection in southeast Asia (Wyres et al., 2020), but we did not detect any K. pneumoniae virulence genes in this dataset.
We identified three isolates of K. quasipneumoniae subsp. similipneumoniae and one isolate of K. quasipneumoniae subsp. quasipneumoniae among our Klebsiella isolates. Klebsiella quasipneumoniae was commonly thought to be less virulent than K. pneumoniae (Long et al., 2017) and an opportunistic human pathogen but has been previously shown to carry the ESBL gene blaCTX-M-15 (Becker et al., 2018) and the carbapenemase gene blaKPC (Mathers et al., 2019), showing the importance of K. quasipneumoniae as a potential vector for horizontal transmission of antimicrobial resistance genes. We determined that four isolates of K. quasipneumoniae carried in this study each contained a different ESBL gene (blaCTX-M-3, blaCTX-M-14, blaCTX-M-27, and blaSHV-2A), suggesting that K. quasipneumoniae may be a community reservoir of ESBL genes as well as K. pneumoniae.
We document seven cases of maternal transmission across 50 pairs of mothers and infants. We initially identified potential transmission pairs in this study by looking for similar patterns of phenotypic antimicrobial resistance. Using similar antimicrobial resistance phenotypes in the same organism as the sole criteria for detecting transmission, we would have detected all of the transmission pairs, and called only one false positive transmission. Using similar antimicrobial resistance and requiring the same MLST would have successfully detected all transmission pairs confirmed using complete genomes, and would have excluded the false positive. This suggests that previous studies of maternal transmission using MLST or PFGE genotyping to identify transmissions are likely to have correctly called transmission events.
However, we detected at least one pair of isolates which were closely related by antimicrobial resistance phenotypes, MLST, and had a low number of SNPs between them. Using the extra non-genetic information available to us, we can be sure these isolates are from individuals from unrelated pairs and do not represent maternal transmission between these individuals. As the samples were collected on admission to the hospital, this is more likely to be community transmission than due to a common source in the hospital. Recent studies which identified hospital transmission in E. coli and K. pneumoniae have chosen SNP threshold of 17 SNPs (Ludden et al., 2021), 21 SNPs (David et al., 2019), and 23 SNPs (Sherry et al., 2019), and would have identified this pair as a transmission event. This suggests that community transmission of isolates can produce transmission events with a low SNP distance which look similar to maternal transmission and may confound transmission studies. Our small data set suggests that the threshold for determining maternal transmission could be set at a lower bound than the thresholds used in studies of hospital transmission.
We were unable to find any examples of transmission of plasmids between mothers and infants outside the transmission of a complete strain. Across the whole dataset there were few shared plasmids outside transmission pairs, indicating that no dominant plasmids are responsible for the spread of antimicrobial resistance. The few strains with similar plasmids from unrelated individuals, such as EbB031 and EbB041, were also closely related in the core genome and suggests community circulation of strains rather than plasmid transmission. Plasmid transmission has been implicated in the dissemination of carbapenemase resistance (Mathers et al., 2011; León-Sampedro et al., 2021) and ESBL genes (Hawkey et al., 2022) but could not be detected during transmission from mother to infant in this study.
The complete genome sequences allowed us to look at the different antimicrobial resistance genes in context, and show that the ESBL genes are present in different contexts in both plasmid and chromosome, and that no common transposable element is responsible for antimicrobial resistance transmission in this dataset. Our limited set of ST131 genomes showed the characteristic incF plasmids carrying blaCTX-M-27, as previously reported as part of the diverse plasmidome of ST131 (Kondratyeva et al., 2020).
Our study suggests that vertical transmission outside the hospital is a common source of neonatal colonization. Strategies to reduce neonatal infections need to consider that maternal transmission is a frequent occurrence, and that AMR strains are circulating in the community and widely carried by mothers and infants. Strategies which focus solely on hospital-based intervention may be unsuccessful at preventing transmission and colonization which occurs in the community.
The datasets presented in this study can be found in online repositories. The names of the repository/repositories and accession number(s) can be found in the article/Supplementary material.
The studies involving human participants were reviewed and approved by Angkor Hospital for Children (0034/16-AHC), the Cambodia National Ethics Committee for Health Research (464-NECHR), and the Oxford Tropical Research Ethics Committee (575-15). Written informed consent to participate in this study was provided by the participants’ legal guardian/next of kin.
CC: investigation, data curation, and writing—original draft. JT: conceptualization, investigation, and funding acquisition. PH, SS, SP, and LN: resources and investigation. JH: investigation, project administration, and supervision. PT: conceptualization, supervision, funding acquisition, and writing—review and editing. EB: conceptualization, funding acquisition, writing—original draft, writing—review and editing, and visualization. All authors contributed to the article and approved the submitted version.
The study was funded by the University of Oxford John Fell Fund and Wellcome Trust Core Award Grant Number 106698/Z/16/Z. The computational aspects of this research were supported by the Wellcome Trust Core Award Grant Number 203141/Z/16/Z and the NIHR Oxford BRC. The views expressed are those of the author(s) and not necessarily those of the NHS, the NIHR, or the Department of Health. This publication made use of the PubMLST website (https://pubmlst.org/) developed by Keith Jolley and sited at the University of Oxford. The development of that website was funded by the Wellcome Trust. For the purpose of Open Access, the author has applied a CC BY public copyright licence to any Author Accepted Manuscript (AAM) version arising from this submission.
This paper was previously deposited on MedRxiv: https://medrxiv.org/cgi/content/short/2022.10.07.22280813v1.
The authors declare that the research was conducted in the absence of any commercial or financial relationships that could be construed as a potential conflict of interest.
All claims expressed in this article are solely those of the authors and do not necessarily represent those of their affiliated organizations, or those of the publisher, the editors and the reviewers. Any product that may be evaluated in this article, or claim that may be made by its manufacturer, is not guaranteed or endorsed by the publisher.
The Supplementary material for this article can be found online at: https://www.frontiersin.org/articles/10.3389/fmicb.2023.1158056/full#supplementary-material
Atterby, C., Osbjer, K., Tepper, V., Rajala, E., Hernandez, J., Seng, S., et al. (2019). Carriage of carbapenemase‐ and extended‐spectrum cephalosporinase‐producingEscherichia coliandKlebsiella pneumoniaein humans and livestock in rural Cambodia; gender and age differences and detection ofblaOXA‐48in humans. Zoonoses Public Health 66, 603–617. doi: 10.1111/zph.12612
Becker, L., Fuchs, S., Pfeifer, Y., Semmler, T., Eckmanns, T., Korr, G., et al. (2018). Whole genome sequence analysis of CTX-M-15 producing Klebsiella isolates allowed dissecting a polyclonal outbreak scenario. Front. Microbiol. 9:322. doi: 10.3389/fmicb.2018.00322
Beghain, J., Bridier-Nahmias, A., Le Nagard, H., Denamur, E., and Clermont, O. (2018). ClermonTyping: an easy-to-use and accurate in silico method for Escherichia genus strain phylotyping. Microb. Genom. 4:e000192. doi: 10.1099/mgen.0.000192
Caron, Y., Chheang, R., Puthea, N., Soda, M., Boyer, S., Tarantola, A., et al. (2018). Beta-lactam resistance among Enterobacteriaceae in Cambodia: the four-year itch. Int. J. Infect. Dis. 66, 74–79. doi: 10.1016/j.ijid.2017.10.025
Carvalho, M. J., Sands, K., Thomson, K., Portal, E., Mathias, J., Milton, R., et al. (2022). Antibiotic resistance genes in the gut microbiota of mothers and linked neonates with or without sepsis from low- and middle-income countries. Nat. Microbiol. 7, 1337–1347. doi: 10.1038/s41564-022-01184-y
Cheng, Y.-H., Lin, T.-L., Pan, Y.-J., Wang, Y.-P., Lin, Y.-T., and Wang, J.-T. (2015). Colistin resistance mechanisms in Klebsiella pneumoniae strains from Taiwan. Antimicrob. Agents Chemother. 59, 2909–2913. doi: 10.1128/AAC.04763-14
CLSI (2017). Performance standards for antimicrobial susceptibility testing. CLSI document M100-S27. 27th Edn. (ed.) P. A. Wayne Clinical and Laboratory Standards Institute.
David, S., Reuter, S., Harris, S. R., Glasner, C., Feltwell, T., Argimon, S., et al. (2019). Epidemic of carbapenem-resistant Klebsiella pneumoniae in Europe is driven by nosocomial spread. Nat. Microbiol. 4, 1919–1929. doi: 10.1038/s41564-019-0492-8
Didelot, X., Bowden, R., Wilson, D. J., Peto, T. E. A., and Crook, D. W. (2012). Transforming clinical microbiology with bacterial genome sequencing. Nat. Rev. Genet. 13, 601–612. doi: 10.1038/nrg3226
Feldgarden, M., Brover, V., Haft, D. H., Prasad, A. B., Slotta, D. J., Tolstoy, I., et al. (2019). Validating the AMRFinder tool and resistance gene database by using antimicrobial resistance genotype-phenotype correlations in a collection of isolates. Antimicrob. Agents Chemother. 63(11): e00483–19. doi: 10.1128/AAC.00483-19
Foster-Nyarko, E., Cottingham, H., Wick, R. R., Judd, L. M., Lam, M. M. C., Wyres, K. L., et al. (2023). Nanopore-only assemblies for genomic surveillance of the global priority drug-resistant pathogen, Klebsiella pneumoniae. Microb. Genom. 9: mgen000936. doi: 10.1099/mgen.0.000936
Fox-Lewis, A., Takata, J., Miliya, T., Lubell, Y., Soeng, S., Sar, P., et al. (2018). Antimicrobial resistance in invasive bacterial infections in hospitalized children, Cambodia, 2007-2016. Emerg. Infect. Dis. 24, 841–851. doi: 10.3201/eid2405.171830
Frank Wolf, M., Abu Shqara, R., Naskovica, K., Zilberfarb, I. A., Sgayer, I., Glikman, D., et al. (2021). Vertical transmission of extended-Spectrum, Beta-lactamase-producing Enterobacteriaceae during preterm delivery: a prospective study. Microorganisms 9: 506. doi: 10.3390/microorganisms9030506
Gilchrist, C. L. M., and Chooi, Y.-H. (2021). Clinker & clustermap.Js: automatic generation of gene cluster comparison figures. Bioinformatics 37, 2473–2475. doi: 10.1093/bioinformatics/btab007
Gorrie, C. L., Mirčeta, M., Wick, R. R., Edwards, D. J., Thomson, N. R., Strugnell, R. A., et al. (2017). Gastrointestinal carriage is a major reservoir of Klebsiella pneumoniae infection in intensive care patients. Clin. Infect. Dis. 65, 208–215. doi: 10.1093/cid/cix270
Harris, S. R. (2018). SKA: Split Kmer analysis toolkit for bacterial genomic epidemiology. bioRxiv [Preprint]. doi: 10.1101/453142
Hawkey, J., Wyres, K. L., Judd, L. M., Harshegyi, T., Blakeway, L., Wick, R. R., et al. (2022). ESBL plasmids in Klebsiella pneumoniae: diversity, transmission and contribution to infection burden in the hospital setting. Genome Med. 14:97. doi: 10.1186/s13073-022-01103-0
Heigl, K., Zamfir, M., Adler, A. C., Dammeyer, A., Schomacher, L., Karlin, B., et al. (2020). Prevalence of methicillin-sensitive, methicillin-resistant Staphylococcus aureus, and extended-spectrum beta-lactamase-producing Escherichia coli in newborns: a cross-sectional study. J. Matern. Fetal Neonatal Med. 35, 4243–4249. doi: 10.1080/14767058.2020.1849100
Huynh, B.-T., Passet, V., Rakotondrasoa, A., Diallo, T., Kerleguer, A., Hennart, M., et al. (2020). Klebsiella pneumoniae carriage in low-income countries: antimicrobial resistance, genomic diversity and risk factors. Gut Microbes 11, 1287–1299. doi: 10.1080/19490976.2020.1748257
Jolley, K. A., and Maiden, M. C. J. (2010). BIGSdb: scalable analysis of bacterial genome variation at the population level. BMC Bioinformatics 11:595. doi: 10.1186/1471-2105-11-595
Katz, L. (2021) Mashtree: create a tree using mash distances. Github Available at: https://github.com/lskatz/mashtree (Accessed October 19, 2021).
Kondratyeva, K., Salmon-Divon, M., and Navon-Venezia, S. (2020). Meta-analysis of pandemic Escherichia coli ST131 Plasmidome proves restricted plasmid-clade associations. Sci. Rep. 10:36. doi: 10.1038/s41598-019-56763-7
Koren, S., and Phillippy, A. M. (2015). One chromosome, one contig: complete microbial genomes from long-read sequencing and assembly. Curr. Opin. Microbiol. 23, 110–120. doi: 10.1016/j.mib.2014.11.014
Krassowski, M. (2020). Krassowski/complex-upset. Zenodo. Available at: http://doi.org/10.5281/zenodo.3700590 (Accessed March 23, 2023).
Lam, M. M. C., Wick, R. R., Watts, S. C., Cerdeira, L. T., Wyres, K. L., and Holt, K. E. (2021). A genomic surveillance framework and genotyping tool for Klebsiella pneumoniae and its related species complex. Nat. Commun. 12:4188. doi: 10.1038/s41467-021-24448-3
León-Sampedro, R., DelaFuente, J., Díaz-Agero, C., Crellen, T., Musicha, P., Rodríguez-Beltrán, J., et al. (2021). Pervasive transmission of a carbapenem resistance plasmid in the gut microbiota of hospitalized patients. Nat. Microbiol. 6, 606–616. doi: 10.1038/s41564-021-00879-y
Long, S. W., Linson, S. E., Ojeda Saavedra, M., Cantu, C., Davis, J. J., Brettin, T., et al. (2017). Whole-genome sequencing of human clinical Klebsiella pneumoniae isolates reveals misidentification and misunderstandings of Klebsiella pneumoniae, Klebsiella variicola, and Klebsiella quasipneumoniae. mSphere 2:e00290–17. doi: 10.1128/mSphereDirect.00290-17
Ludden, C., Coll, F., Gouliouris, T., Restif, O., Blane, B., Blackwell, G. A., et al. (2021). Defining nosocomial transmission of Escherichia coli and antimicrobial resistance genes: a genomic surveillance study. Lancet Microb. 2:e472. doi: 10.1016/S2666-5247(21)00117-8
Mathers, A. J., Cox, H. L., Kitchel, B., Bonatti, H., Brassinga, A. K. C., Carroll, J., et al. (2011). Molecular dissection of an outbreak of carbapenem-resistant enterobacteriaceae reveals Intergenus KPC carbapenemase transmission through a promiscuous plasmid. MBio 2, e00204–e00211. doi: 10.1128/mBio.00204-11
Mathers, A. J., Crook, D., Vaughan, A., Barry, K. E., Vegesana, K., Stoesser, N., et al. (2019). Klebsiella quasipneumoniae provides a window into Carbapenemase gene transfer, plasmid rearrangements, and patient interactions with the hospital environment. Antimicrob. Agents Chemother. 63: e02513–18. doi: 10.1128/AAC.02513-18
Matlock, W., Lipworth, S., Constantinides, B., Peto, T. E. A., Walker, A. S., Crook, D., et al. (2021). Flanker: a tool for comparative genomics of gene flanking regions. Microb. Genom. 7(9): 000634. doi: 10.1099/mgen.0.000634
Peretz, A., Skuratovsky, A., Khabra, E., Adler, A., Pastukh, N., Barak, S., et al. (2017). Peripartum maternal transmission of extended-spectrum β-lactamase organism to newborn infants. Diagn. Microbiol. Infect. Dis. 87, 168–171. doi: 10.1016/j.diagmicrobio.2016.11.004
Petit, R. A. 3rd, and Read, T. D. (2020). Bactopia: a flexible pipeline for complete analysis of bacterial genomes. mSystems 5:5. doi: 10.1128/mSystems.00190-20
Reed, T. A. N., Krang, S., Miliya, T., Townell, N., Letchford, J., Bun, S., et al. (2019). Antimicrobial resistance in Cambodia: a review. Int. J. Infect. Dis. 85, 98–107. doi: 10.1016/j.ijid.2019.05.036
Rettedal, S., Löhr, I. H., Bernhoff, E., Natås, O. B., Sundsfjord, A., and Øymar, K. (2015). Extended-spectrum β-lactamase-producing Enterobacteriaceae among pregnant women in Norway: prevalence and maternal-neonatal transmission. J. Perinatol. 35, 907–912. doi: 10.1038/jp.2015.82
Roberts, T., Limmathurotsakul, D., Turner, P., Day, N. P. J., Vandepitte, W. P., and Cooper, B. S. (2019). Antimicrobial-resistant gram-negative colonization in infants from a neonatal intensive care unit in Thailand. J. Hosp. Infect. 103, 151–155. doi: 10.1016/j.jhin.2019.04.004
Schwengers, O., Barth, P., Falgenhauer, L., Hain, T., Chakraborty, T., and Goesmann, A. (2020). Platon: identification and characterization of bacterial plasmid contigs in short-read draft assemblies exploiting protein sequence-based replicon distribution scores. Microb. Genom. 6: mgen000398. doi: 10.1099/mgen.0.000398
Seemann, T. (2018). Snippy. Github. Available at: https://github.com/tseemann/snippy (Accessed December 11, 2018).
Seemann, T. (2021a). SNP-dists: pairwise SNP distance matrix from a FASTA sequence alignment. Github. Available at: https://github.com/tseemann/snp-dists (Accessed November 16, 2021).
Seemann, T. (2021b). Mlst: scan contig files against PubMLST typing schemes. Github Available at: https://github.com/tseemann/mlst (Accessed November 16, 2021).
Sherry, N. L., Lane, C. R., Kwong, J. C., Schultz, M., Sait, M., Stevens, K., et al. (2019). Genomics for molecular epidemiology and detecting transmission of Carbapenemase-producing Enterobacterales in Victoria, Australia, 2012 to 2016. J. Clin. Microbiol. 57(9):e00573–19. doi: 10.1128/JCM.00573-19
Singh, S. R., Mao, B., Evdokimov, K., Tan, P., Leab, P., Ong, R., et al. (2020). Prevalence of MDR organism (MDRO) carriage in children and their household members in Siem Reap Province, Cambodia. JAC Antimicrob. Resist. 2:dlaa097. doi: 10.1093/jacamr/dlaa097
Smit, P. W., Stoesser, N., Pol, S., van Kleef, E., Oonsivilai, M., Tan, P., et al. (2018). Transmission dynamics of hyper-endemic multi-drug resistant Klebsiella pneumoniae in a southeast Asian neonatal unit: a longitudinal study with whole genome sequencing. Front. Microbiol. 9:1197. doi: 10.3389/fmicb.2018.01197
Stoesser, N., Giess, A., Batty, E. M., Sheppard, A. E., Walker, A. S., Wilson, D. J., et al. (2014). Genome sequencing of an extended series of NDM-producing Klebsiella pneumoniae isolates from neonatal infections in a Nepali hospital characterizes the extent of community- versus hospital-associated transmission in an endemic setting. Antimicrob. Agents Chemother. 58, 7347–7357. doi: 10.1128/AAC.03900-14
Treangen, T. J., and Salzberg, S. L. (2011). Repetitive DNA and next-generation sequencing: computational challenges and solutions. Nat. Rev. Genet. 13, 36–46. doi: 10.1038/nrg3117
Turner, P., Pol, S., Soeng, S., Sar, P., Neou, L., Chea, P., et al. (2016). High prevalence of antimicrobial-resistant gram-negative colonization in hospitalized Cambodian infants. Pediatr. Infect. Dis. J. 35, 856–861. doi: 10.1097/INF.0000000000001187
Vlieghe, E., Sary, S., Lim, K., Sivuthy, C., Phe, T., Parry, C., et al. (2013). First National Workshop on antibiotic resistance in Cambodia: Phnom Penh, Cambodia, 16-18 November 2011. J. Glob. Antimicrob. Resist. 1, 31–34. doi: 10.1016/j.jgar.2013.01.007
Wick, R. R., Judd, L. M., Gorrie, C. L., and Holt, K. E. (2017). Unicycler: resolving bacterial genome assemblies from short and long sequencing reads. PLoS Comput. Biol. 13:e1005595. doi: 10.1371/journal.pcbi.1005595
Woerther, P.-L., Burdet, C., Chachaty, E., and Andremont, A. (2013). Trends in human fecal carriage of extended-spectrum β-lactamases in the community: toward the globalization of CTX-M. Clin. Microbiol. Rev. 26, 744–758. doi: 10.1128/CMR.00023-13
Wyres, K. L., Lam, M. M. C., and Holt, K. E. (2020). Population genomics of Klebsiella pneumoniae. Nat. Rev. Microbiol. 18, 344–359. doi: 10.1038/s41579-019-0315-1
Yu, G., Smith, D. K., Zhu, H., Guan, Y., and Lam, T. T.-Y. (2017). Ggtree: an r package for visualization and annotation of phylogenetic trees with their covariates and other associated data. Methods Ecol. Evol. 8, 28–36. doi: 10.1111/2041-210X.12628
Keywords: antimicrobial resistance, bacterial transmission, microbiology, Escherichia coli, Klebsiella pneumoniae, maternal transmission, Enterobacterales genomics, transmission SNP thresholds
Citation: Chomkatekaew C, Thaipadungpanit J, Hearn P, Soeng S, Pol S, Neou L, Hopkins J, Turner P and Batty EM (2023) Detection of maternal transmission of resistant Gram-negative bacteria in a Cambodian hospital setting. Front. Microbiol. 14:1158056. doi: 10.3389/fmicb.2023.1158056
Received: 03 February 2023; Accepted: 23 March 2023;
Published: 14 April 2023.
Edited by:
Alicja Wegrzyn, Institute of Biochemistry and Biophysics, Polish Academy of Sciences, PolandReviewed by:
Vijayalakshmi Selvakumar, Kangwon National University, Republic of KoreaCopyright © 2023 Chomkatekaew, Thaipadungpanit, Hearn, Soeng, Pol, Neou, Hopkins, Turner and Batty. This is an open-access article distributed under the terms of the Creative Commons Attribution License (CC BY). The use, distribution or reproduction in other forums is permitted, provided the original author(s) and the copyright owner(s) are credited and that the original publication in this journal is cited, in accordance with accepted academic practice. No use, distribution or reproduction is permitted which does not comply with these terms.
*Correspondence: Elizabeth M. Batty, ZWxpemFiZXRoLmJAdHJvcG1lZHJlcy5hYw==
Disclaimer: All claims expressed in this article are solely those of the authors and do not necessarily represent those of their affiliated organizations, or those of the publisher, the editors and the reviewers. Any product that may be evaluated in this article or claim that may be made by its manufacturer is not guaranteed or endorsed by the publisher.
Research integrity at Frontiers
Learn more about the work of our research integrity team to safeguard the quality of each article we publish.