- Red Sea Research Center, Biological and Environmental Sciences and Engineering Division (BESE), King Abdullah University of Science and Technology (KAUST), Thuwal, Saudi Arabia
Introduction: The geological isolation, lack of freshwater inputs and specific internal water circulations make the Red Sea one of the most extreme—and unique—oceans on the planet. Its high temperature, salinity and oligotrophy, along with the consistent input of hydrocarbons due to its geology (e.g., deep-sea vents) and high oil tankers traffic, create the conditions that can drive and influence the assembly of unique marine (micro)biomes that evolved to cope with these multiple stressors. We hypothesize that mangrove sediments, as a model-specific marine environment of the Red Sea, act as microbial hotspots/reservoirs of such diversity not yet explored and described.
Methods: To test our hypothesis, we combined oligotrophic media to mimic the Red Sea conditions and hydrocarbons as C-source (i.e., crude oil) with long incubation time to allow the cultivation of slow-growing environmentally (rare or uncommon) relevant bacteria.
Results and discussion: This approach reveals the vast diversity of taxonomically novel microbial hydrocarbon degraders within a collection of a few hundred isolates. Among these isolates, we characterized a novel species, Nitratireductor thuwali sp. nov., namely, Nit1536T. It is an aerobic, heterotrophic, Gram-stain-negative bacterium with optimum growth at 37°C, 8 pH and 4% NaCl, whose genome and physiological analysis confirmed the adaptation to extreme and oligotrophic conditions of the Red Sea mangrove sediments. For instance, Nit1536T metabolizes different carbon substrates, including straight-chain alkanes and organic acids, and synthesizes compatible solutes to survive in salty mangrove sediments. Our results showed that the Red Sea represent a source of yet unknown novel hydrocarbon degraders adapted to extreme marine conditions, and their discovery and characterization deserve further effort to unlock their biotechnological potential.
Introduction
Mangroves are intertidal forests that play a crucial role in cycling energy and nutrients at the interface between land and sea (Sheaves, 2009; Donato et al., 2011), covering between 60 and 70% of the world’s tropical and subtropical coastlines (Thatoi et al., 2013). These productive ecosystems (Sheaves, 2009; Donato et al., 2011; Saderne et al., 2021) are characterized by periodic tidal flooding and fluctuating environmental factors, such as nutrients, oxygen, salinity and temperature (Fusi et al., 2022). The interaction of all these variables creates complex multidimensional habitats (Radhakrishnan et al., 2011) in which a huge number of microorganisms live, i.e., 91% of the total microbial biomass (Pramanik et al., 2019). Located on the coasts, mangroves have also to withstand the continuous pressure of the growing anthropogenic activities (Allard et al., 2020; Martin et al., 2020; Adyasari et al., 2021; Luo et al., 2021), including urbanization and aquaculture farming (Maiti and Chowdhury, 2013; Fusi et al., 2016; Richards and Friess, 2016). These activities impact mangroves with sewage, antibiotics, pesticides, microplastic and other contaminants, such as hydrocarbons released by intense ship traffic and numerous deep-sea vents (Andreote et al., 2012; Maiti and Chowdhury, 2013; Richards and Friess, 2016; El-Amin Bashir et al., 2017; Alzahrani et al., 2018; Martin et al., 2019b,2020). Stressful conditions can also be induced by other abiotic factors, such as lack of freshwater input, oligotrophic conditions and high salinity, as in the case of the Red Sea mangroves (Almahasheer et al., 2016; Anton et al., 2020; Arshad et al., 2020; Qashqari et al., 2020; Thomson et al., 2022b). Compared to similar ecosystems e.g., in South Africa and Australia, the Red Sea mangrove sediments have a reduced particulate organic carbon content (up to 50% less) and up to a third of the particulate organic nitrogen (Thomson et al., 2015; Booth et al., 2019a). All these selection forces make Red Sea mangroves one of the most extreme coastal ecosystems on earth, possibly harboring unique and overlooked microorganisms.
We, therefore, hypothesize that such habitat acts as microbial hotspots of microbial diversity and, in particular, as reservoirs for hydrocarbon degrader bacteria not yet isolated and described. So far, many bacterial lineages still have only a few or no cultivated representatives, and only ∼23,000 species have been validly described on the List of Prokaryotic names with Standing in Nomenclature (LPSN) database (Parte et al., 2020). The reasons for failing cultivation and isolation of microorganisms in the laboratory are various (Montgomery, 2020). Some are complex, such as the need for specific growth signals (Bruns et al., 2002; Nichols et al., 2008), dependence on other microorganisms (D’Onofrio et al., 2010; He et al., 2015), development as microcolonies (Davis et al., 2011) and absence of suitable electron acceptors and/or essential nutrients (Köpke et al., 2005), while others are simpler to address, such as an insufficient incubation periods (Janssen et al., 2002; Davis et al., 2005), and the control of fast-growing species that can overcome those with slower growth (Kato et al., 2018). Several studies over the past years have shown that it is possible to isolate previously uncultivated microorganisms by using innovative strategies and approaches, such as low nutrient concentrations and longer incubation times (Janssen et al., 2002; Davis et al., 2005; Pulschen et al., 2017; Sefrji et al., 2021a,b, 2022). Moreover, cultivation success can be improved using whole metagenome analysis to unravel microorganism key metabolic pathways that can inspire new cultivation strategies (Hedlund et al., 2014; Cross et al., 2019), as well as by understanding the trophic interactions occurring within the overall microbial community (Zamkovaya et al., 2021).
Here, we aim to isolate novel previously uncultivated bacteria from the Red Sea oligotrophic arid mangrove sediments (Anton et al., 2020), unraveling the diversity of the microbial “dark matter” and rare ecosystem players (Solden et al., 2016; Jousset et al., 2017; Zamkovaya et al., 2021). To achieve our goals, we implement an extended incubation period and oligotrophic conditions combined with hydrocarbon substrates (i.e., crude oil) as C-source. With this approach, we expect to favor the growth of slow-growing hydrocarbon degrader bacteria not usually detected with standard cultivation methods. This approach reveals the vast diversity of taxonomically novel microbial hydrocarbon degraders within a collection of a few hundred isolates. Among the isolates, we describe and characterize a novel halophilic hydrocarbon-degrading bacterial species Nit1536T within the genus Nitratireductor, for which the name Nitratireductor thuwali is proposed.
Materials and methods
Sampling, enrichment cultures, isolation and purification
Samples of sediment were collected from a fringing mangrove characterized by coarse sandy sediment, with a large part made by coral debris (Basaham et al., 2014), which stands at the King Abdullah Economic City (KAEC), Saudi Arabia (22°24′N, 39°05′E; Supplementary Figure 1). The mangrove sediment has been used as inoculum for the cultivation experiments and three different media were used: (i) ONR7a, for 1 L: NaCl 22.79 g, Na2SO4 3.98 g, KCl 0.72 g, NaBr 83 mg, NaHCO3 31 mg, H3BO3 27 mg, NaF 2.60 mg, NH4Cl 0.27 g, Na2HPO4 × 7 H2O 89 mg, TAPSO 1.30 g, MgCl2 × 6 H2O 11.18 g, CaCl2 × 2 H2O 1.46 g, SrCl2 × 6 H2O 24 mg and, FeCl2 × 4H2O 2 mg; (ii) Mineral Salt Medium (MSM), for 1 L: Na2HPO4 2.79 g, KH2PO4 2 g, (NH4)2SO4 1 g, Ca(NO3)24H2O 0.05 g, ammonium iron(III) citrate 0.01 g and, MgSO4 × 7H2O 0.02 g; (iii) filtered Red Sea water (FSW). Liquid cultures were set up using 5 g of sediments as inoculum, 70 mL of medium, and 750 μL of Arabian Light crude oil (provided by Saudi Aramco) as the only carbon source (1% v/v). The microcosms were incubated for 20 days in aerobic conditions at 26°C, according to the temperature of the sediment at the sampling time. After the incubation time, 10-fold serial dilutions of the enriched cultures were prepared, ranging from the undiluted sample to 10–5; 100 μl of each dilution were then plated onto Petri dishes containing the same medium used for the liquid enrichment culture agarized with 0.8% of Noble Agar (Difco) and were incubated at 26°C for 10 days until the growth of bacterial colonies was observed. A total of 300 colonies were picked from the three media, singularly re-streaked on the respective media and further incubated; this purification step was applied three times. The colonies that showed growth (n = 203) were further transferred on Marine broth (MB) agar medium (BD Difco Fisher Scientific; composition: 5 g peptone, 1 g yeast extract, 0.1 g C6H5FeO7, 19.45 g NaCl, 5.9 g MgCl2, 3.24 g MgSO4, 1.8 g CaCl2, 0.55 g KCl, 0.16 g NaHCO3, 0.08 g KBr, 34 mg SrCl2, 22 mg H3BO3, 4 mg Na2SiO3, 2.4 mg NaF, 1.6 mg NH4NO3, 8 mg Na2HPO4; final salinity using a refractometer, 4%) for a faster-growth. Colonies’ purity was evaluated at the microscope before storing the strains at –80°C as a cell suspension in 25% glycerol for long-term storage.
De-replication of bacterial isolates and identification of haplotype representatives
Genomic DNA was extracted from isolates by boiling lysis protocol (Marasco et al., 2012). A single bacterial colony was picked from the agar culture and suspended in 50 μL of sterile Tris-HCl buffer (10 mM, pH 8.0) in PCR tubes. Suspended cells were subjected to 10 min of boiling at 95°C using iCycler thermocycler (Bio-Rad) and afterward kept in ice for 10 more minutes. The samples were then centrifuged at 13,000 rpm for 10 min at 4°C. The supernatant containing DNA was used as a template for PCR amplification. First, we amplified the intergenic transcribed spacers (ITS) between the 16S and 23S rRNA genes as tool for strain typing to discriminate bacterial strains at the species and intraspecies levels (Daffonchio et al., 2003). We used the primer-set ITSf and ITSr (Boyer et al., 2001). The PCR mix was prepared in 20 μL with 0.50 U/reaction of Taq DNA polymerase (Thermo-Fisher Scientific), 1 × PCR buffer, 1.5 mM MgCl2, 0.25 mM dNTPs mix, 0.50 μM each primer (SIGMA) and 2 μL of template DNA. The PCR thermal protocol included an initial denaturation at 95°C for 5 min, followed by 30 cycles of 94°C for 45 s, 50°C for 1 min, and 72°C for 2 min, and final elongation at 72°C for 10 min. PCR products were separated by gel electrophoresis on 1.5% agarose gel, and the fingerprinting profiles of the 16S-23S rRNA ITS region were visualized using a Gel Doc System (Bio-Rad). Isolates showing the same banding pattern were grouped in haplotypes. For each 16S-23S rRNA ITS-haplotype, a representative strain was selected for further phylogenetic identification through amplification and sequencing of the 16S rRNA gene. Three PCR were conducted by using universal primer sets, which amplify three partially overlapping regions of the 16S rRNA gene: 27F/785R (fragment F1; PCR product of ≈750 bp), 341F/907R (F2; ≈550 bp) and 785F/1492R (F3; ≈700 bp). PCR mix was the following: in 50 μL of the final volume of reaction, 0.02 U/μL (corresponding to 1.0 U/reaction) of Taq DNA polymerase (Thermo-Fisher Scientific), 1 × PCR buffer, 1.5 mM MgCl2, 0.2 mM dNTPs mix, 0.3 μM each primer (SIGMA) and 1 μL of template DNA were used. The PCR thermal protocol included an initial denaturation at 94°C for 5 min, followed by 30 cycles of 94°C for 45 s, 52, 59, and 55°C (respectively, F1, F2, and F3) for 1 min, 72°C for 1 min, and final elongation at 72°C for 10 min. The sizes of the expected PCR products were evaluated on 1.5% agarose gel, then purified with Illustra ExoProStar 1 Step (GE Life-sciences) and then sequenced with both forward and reverse primer using the Sanger method (KAUST Bioscience Core Lab). Electropherograms of each sequence were checked for quality, edited, and assembled with Geneious version 8.1.9 (Biomatters) to obtain an almost full-length sequence of the 16S rRNA gene (1,300–1,450 bp). The sequences obtained were then compared through the Basic Local Alignment Search Tool (BLAST) algorithm against the reference RNA sequences database (refseq_rna) of the National Center for Biotechnology Information (NCBI). A phylogenetic tree including representative strains of each ITS haplotype (Supplementary Table 1) was performed using the Aligner tool of MEGA-X software package (Kumar et al., 2016). Using the same software, we removed the gaps to eliminate poorly aligned positions and divergent regions of the 16S rRNA gene alignment and make it more suitable for phylogenetic analysis. The phylogenetic neighbor-joining and maximum likelihood trees were constructed. A bootstrap analysis of 1,000 re-samplings was used to evaluate the tree topology (Felsenstein, 1985). The bacterial 16S rRNA gene sequences were deposited in the GeneBank database under the accession numbers ON408473–ON408537.
Physiological, biochemical, and chemotaxonomy analysis
An internal transcribed spacer (ITS) haplotype composed of four strains, namely, Nit1531, Nit1536, Nit1537 and Nit1539, was selected for further investigation because their 16S rRNA gene sequence showed 95.19 ± 0.92% identity with the previously isolated and published Nitratireductor pacificus pht-3B (Lai et al., 2011a) type strains (Supplementary Table 1). Since the four strains had 16S rRNA genes similarity >99% and the same Enterobacterial Repetitive Intergenic Consensus (ERIC)-PCR profile (Supplementary Figures 2, 3), only one strain, namely, Nit1536, was further characterized and proposed as type strain (here therefore, Nit1536T). The standard Gram-stain protocol was followed to determine the cell wall composition, i.e., stain purple for Gram-stain-positive bacteria with thick layers of peptidoglycan (90% of cell wall) vs. stain pink for Gram-stain-negative bacteria with thin layers of peptidoglycan (10% of wall) (Madigan et al., 2008). The cell morphology of the Nit1536T strain was determined by scanning electron microscopy on a Nova Nano 630 SEM (FEI) at the Imaging Core Lab at King Abdullah University of Science and Technology. Bacterial cell motility was evaluated by using the MB medium with 0.35% agar. The ability of the Nit1536T strain and its closest relative N. aquibiodomus NL21T (DSM 15645) and N. kimnyeongensis Ky 101T (DSM 19185) to grow at various temperatures (10, 20, 30, 40, and 50°C) was assessed in MB medium. At the same time, salt tolerance was tested by preparing saltless MB (absence of NaCl, MgCl2, Na2SO4, CaCl2, and KCl) with the addition of different concentrations of NaCl (range, 0–19% of NaCl with an increment of 1%, w/v). The oxidase activity was tested by using oxidase test strips (Sigma-Aldrich) and the catalase by using 3% (v/v) hydrogen peroxide solution (Aladame, 1987). Indole production was tested by adding Kovac’s reagent to the bacterial culture and evaluating the color change. Nitrate reduction ability was determined using a nitrate reduction kit (Sigma-Aldrich) and following the manufacturers’ instructions. The nitrate broth medium was modified and supplemented with 4% (w/v) NaCl. Screening for amylase, protease, lipase and cellulase activities was evaluated using MB agar plates containing 1.5% starch, casein, tween 80, and cellulose as substrates, respectively, (Liu et al., 2019). The capacity to grow on linear alkanes, i.e., hexane, nonane and dodecane, and on aromatic hydrocarbons, i.e., toluene and xylene, was further evaluated by inoculating bacteria on SW plates spread with 100 μl of the listed hydrocarbons; plates without hydrocarbons were used as control. Further phenotypic characterizations of the Nit1536T strain were performed with the Biolog® Phenotype Microarray (PM). C-source metabolism, ions and osmolytes tolerance, and pH range were screened using PM1, PM2, PM9, PM10, PM11, and PM12 and following the manufacturing instructions. Biolog® PM plates were incubated in the Omnilog incubator/reader, and the changes in color in the wells were measured every 15 min. OmniLog® phenotype microarray software was used for the data analysis (Jałowiecki et al., 2017).
To evaluate the production of osmolytes by the bacterial strain, 1 mL of Nit1536T culture was diluted 2 × in methanol/water (50/50), and the solution was analyzed using LC-HRMS equipped with a reversed stationary phase column and ESI source operated under + ve mode at the Analytical Chemistry Core Lab in KAUST. Briefly, 10 μL of methanol-water blank, caffeine (QC), and 10 μL of the analytes were injected separately into the LC using a SEPAX column (SFC-DIOL 150 mm × 4.6 mm packed with 5 μm particles size). The separation was performed under gradient using water with 2 mM ammonium formate, 0.1% formic acid and acetonitrile, and 0.1% FA. The flow rate was 450 μL/min. The analysis was performed using a Thermo LTQ Velos Orbitrap mass spectrometer (Thermo Scientific, Pittsburgh, PA, USA) equipped with a heated ESI ion source. The mass scan range was set to 100–2,000 m/z, with a resolving power of 100,000. The m/z calibration of the LTQ-Orbitrap analyzer was performed in the positive ESI mode using a solution containing caffeine, MRFA (met-arg-phe-ala) peptide and Ultramark 1,621 according to the manufacturer’s guidelines. The ESI was performed with a heated ion source equipped with a metal needle and operated at 4 kV. The source vaporizer temperature was adjusted to 400°C, the capillary temperature was set at 270°C, and the sheath and auxiliary gases were optimized and set to 30 and 15 arbitrary units, respectively.
For chemotaxonomic analysis, cells of the Nit1536T strain and its closest relatives N. aquibiodomus NL21T (DSM 15645), N. kimnyeongensis Ky 101T (DSM 19185), Nitratireductor sp. HSD-9T (DSM 19383) and Nitratireductor sp. SL014B-25A2 (DSM 22977) were grown on MB and harvested by centrifugation at the mid-exponential phase, washed and then freeze-dried. Analysis of fatty acid, polar lipids and respiratory quinones was carried out by DSMZ Services (Leibniz-Institut DSMZ, Deutsche Sammlung von Mikroorganismen und Zellkulturen GmbH, Braunschweig, Germany).
Sequencing, assembly and annotation of Nit1536T genome
The Nit1536T strain was grown aerobically and routinely maintained on MB medium. Genomic DNA was extracted from 1 mL of culture (corresponding approximately to 2 × 109 cells/mL) with the Maxwell RSC Automated Nucleic Acid Purification system and the Maxwell® RSC Cultured Cells DNA kit (Promega). DNA concentration was quantified using the Qubit® dsDNA BR Assay Kit (Thermo-Fisher Scientific), and the quality was assessed by electrophoresis on 1% agarose gels and with a Bioanalyzer (Agilent). Sequencing of the genome of the Nit1536T strain was performed at KAUST Bioscience Core Lab using one cell of the PacBio RS2 platform (Pacific Biosciences). The PacBio reads were assembled using the SMRT analysis software (PACBIO) and the HGAP.3 workflow following the hierarchical genome-assembly process (Chin et al., 2013). First, the longest reads were selected as “seed” reads, to which all other reads were mapped. Secondly, these reads were pre-assembled into highly accurate reads and used for genome assembly. Finally, a final assembly step was performed using all the reads to correct the initial assembly. The genome was annotated using Prokka (Seemann, 2014), an annotation pipeline for microbial genomes. Additional annotation and gene function prediction analysis were achieved within the Rapid Annotation using Subsystem Technology (RAST) (Aziz et al., 2008; Brettin et al., 2015), as well as the Kyoto Encyclopedia of Genes and Genomes (KEGG) (Kanehisa et al., 2016). DRAM (Shaffer et al., 2020), METABOLIC (Zhou et al., 2022), gapseq (Zimmermann et al., 2021) and anti-SMASH 5.1.2 (Blin et al., 2019) were used to infer the metabolism-related features. AMRFinderPlus (Feldgarden et al., 2021) and ResFinder (Florensa et al., 2022) were used to catalogue the antibiotic resistance genes. ABRicate1 and the Virulence Factor Database (Chen et al., 2016) were used to identify virulence-related features. CRISPRCasTyper (Russel et al., 2020) and DefenseFinder (Tesson et al., 2022) were used to identify CRISPRs and anti-phage defense elements. GapMind (Price et al., 2020) was run to assess the genomic completeness of amino-acid biosynthesis pathways, along with FeGenie (Garber et al., 2020) and manual searches via RAST/NCBI blastp (Johnson et al., 2008) and Pfam (Mistry et al., 2021) to identify iron-metabolism-specific features. The tools NCBI-genome-download2 and bit-dl-NCBI-assemblies (Lee, 2022) were used to download available representative type-species3 genomes belonging to the genus Nitratireductor. GenBank accession numbers for the whole-genome sequence of Nit1536T strain is CP030941-3.
Phylogenetic analysis of Nit1536T strain
The phylogeny of the 16S rRNA gene sequences was done using the MEGA-X software as described previously with the phylogenetic neighbor-joining and maximum likelihood trees that were constructed with a bootstrap analysis of 1,000 re-samplings to evaluate the tree topology (Felsenstein, 1985). We also used the GTDB-Tk software (Chaumeil et al., 2019) to build the phylogenetic tree of multi-locus sequence analysis (MLSA) using 120 concatenated single-copy proteins obtained from the genome of the Nit1536T strain and other close type species belonging to the Oricola, Chelativorans, Nitratireductor and a subset of Mesorhizobium genera. The genome sequence of the Nit1536T strain and those of other species belonging to the Nitratireductor genus were imported into the JSpeciesWS, an online service for in silico calculation of the extent of identity between two genomes. The online software measures the average nucleotide identity (ANI) based on BLAST (ANIb), as well as correlation indexes of tetra-nucleotide signatures (Tetra) (Burall et al., 2016; Richter et al., 2016). We further used the Genome-to-Genome Distance Calculator (GGDC) online server and the EzAAI software to calculate in silico the estimated value of DNA-DNA hybridization (DDH) and the average amino acid identity (AAI) of Nit1536T strain with the closest relative species, respectively, (Meier-Kolthoff et al., 2013; Kim et al., 2021).
Results
Phylogenetic diversity of the cultivable hydrocarbon-degrading bacteria from arid mangrove sediments
From the arid mangrove sediments of King Abdullah Economic City (KAEC, Thuwal), we established a collection of hydrocarbon-degrading bacteria capable of growth on the Arabian light crude oil as carbon sources by using three different media, namely, MSM, ONR7a and FSW (Supplementary Table 1). We obtained a total of CFU per g that ranged between 1.07 × 105 on ONR7a and 3.98 × 108 on MSM (Supplementary Table 2). We successfully isolated 55, 60 and 88 bacterial strains from MSM, ONR7a and FSW plates, respectively. These bacteria were further sub-grouped in 17, 21, and 26 based on their ITS fingerprinting (Supplementary Table 1). All three media favored the isolation of bacteria belonging to the Proteobacteria phylum (94.5, 88.3, and 92.0% in MSM, ONR7a and FSW, respectively; Figure 1 and Supplementary Table 1). On MSM medium, 36.4% of the isolates belonged to the Alphaproteobacteria, 7.3% to Betaproteobacteria and 50.9% to Gammaproteobacteria, while on ONR7a and FSW the Gammaproteobacteria (86.7 and 71.6%, respectively) were largely dominant (Figure 1). The selection driven by the medium was more evident at the genus level; 35 different genera were detected, and no one was common among the three media (Figure 1 and Supplementary Figure 4). Pseudomonas and Sphingobium (30.9 and 27.2%, respectively) were the dominant genera within the MSM collection, while Marinobacter, Pseudoalteromonas, and Halomonas (36.7, 20, and 16.7%, respectively) those in the ONR7a collection, and Halomonas, Alcanivorax, and Cobetia (20.4, 14.7, and 13.6%, respectively) in the FSW collection (Supplementary Figure 4). Along with these genera frequently cultivated, we also identified bacteria belonging to “rare” (or uncommon) genera, i.e., not often isolated and with few representatives sequenced (Pedrós-Alió, 2012). For example, we cultivated members of Thalassospira (at the time of writing, 12 validly described representatives reported on the LPSN website),4 Nitratireductor (10), Pelagibaca (synonym Salipiger, 9), Roseivivax (7), Cobetia (5), Reinekea (5), Sinomicrobium (5), and Joostella (1). These groups were present in different percentages, with the highest number of rare taxa obtained from the FSW and ONR7a media (6 and 4 genera, respectively), while no one from the MSM medium (Supplementary Figure 3).
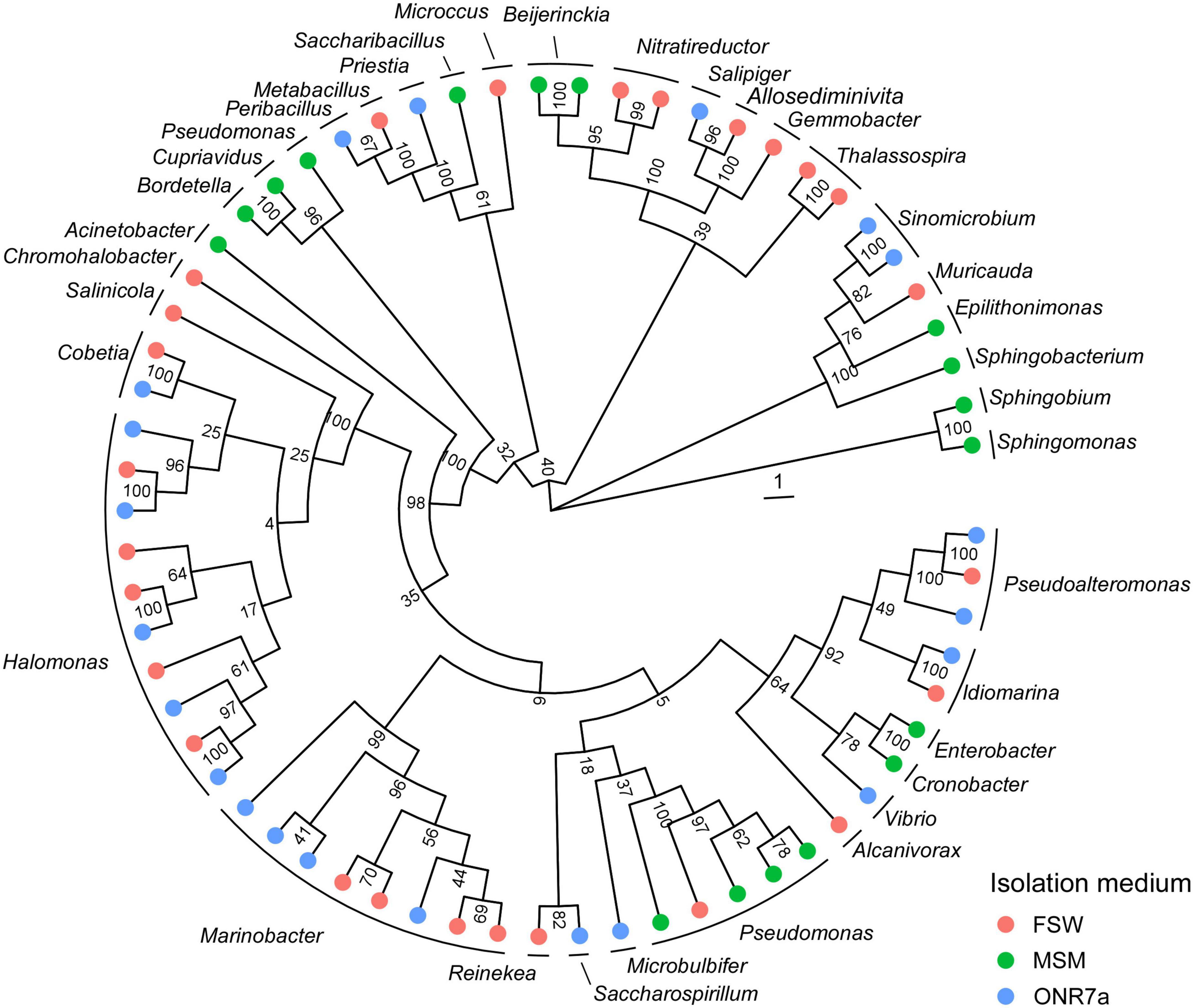
Figure 1. Phylogenetic tree of cultivable bacteria harbored by mangrove oligotrophic sediments. The colors on the outer ring refer to the medium used for the isolation, namely, FSW (red), MSM (green), and ONR7a (blue). A representative sequence for each ITS haplotype is included (see Supplementary Table 1 for the full list).
Phylogenetic analysis and identification of the Nit1536T strain
Comparative analysis of 16S rRNA gene sequences showed that one haplotype was related most closely to Nitratireductor pacificus pht-3BT and Nitratireductor indicus C115T with 95.41 and 95.36% similarity, respectively. The phylogenetic tree revealed that Nit1536T formed a distinct phylogenetic lineage from all the other species within the Nitratireductor genus (Figure 2). The sequence similarity calculations based on evolutionary distance demonstrated that the Nit1536T strain represents a putative novel species. Multi-locus sequence analysis (MLSA) was performed against close type species belonging to the Oricola, Chelativorans, Nitratireductor, and a subset of Mesorhizobium genera, confirming the separation of the Nit1536T strain from the other species within the Nitratireductor genus (Figure 3). Average nucleotide identity based on BLAST (ANIb) revealed that Nit1536T had a percentage of identity between 73.03 and 75.29% with the other closely related species (Table 1), values that are below the 96% species-embracing threshold (Richter et al., 2016). Similarly, in silico DNA-DNA hybridization (DDH) showed values ranging between 19 and 21% (Table 1) that are significantly lower than 70% used as a cut-off value to delineate a new bacterial species (Meier-Kolthoff et al., 2013). Furthermore, the average amino acid identity (AAI) analysis showed that the similarity between our strain and all the other closest species ranges between 69.6 and 74.5% (species delimitation threshold, 95% (Konstantinidis et al., 2017; Table 1). The results of the phylogenetic analyses and genome relatedness suggested that the Nit1536T strain represents a novel species in the genus Nitratireductor, for which the name Nitratireductor thuwali sp. nov. is proposed.
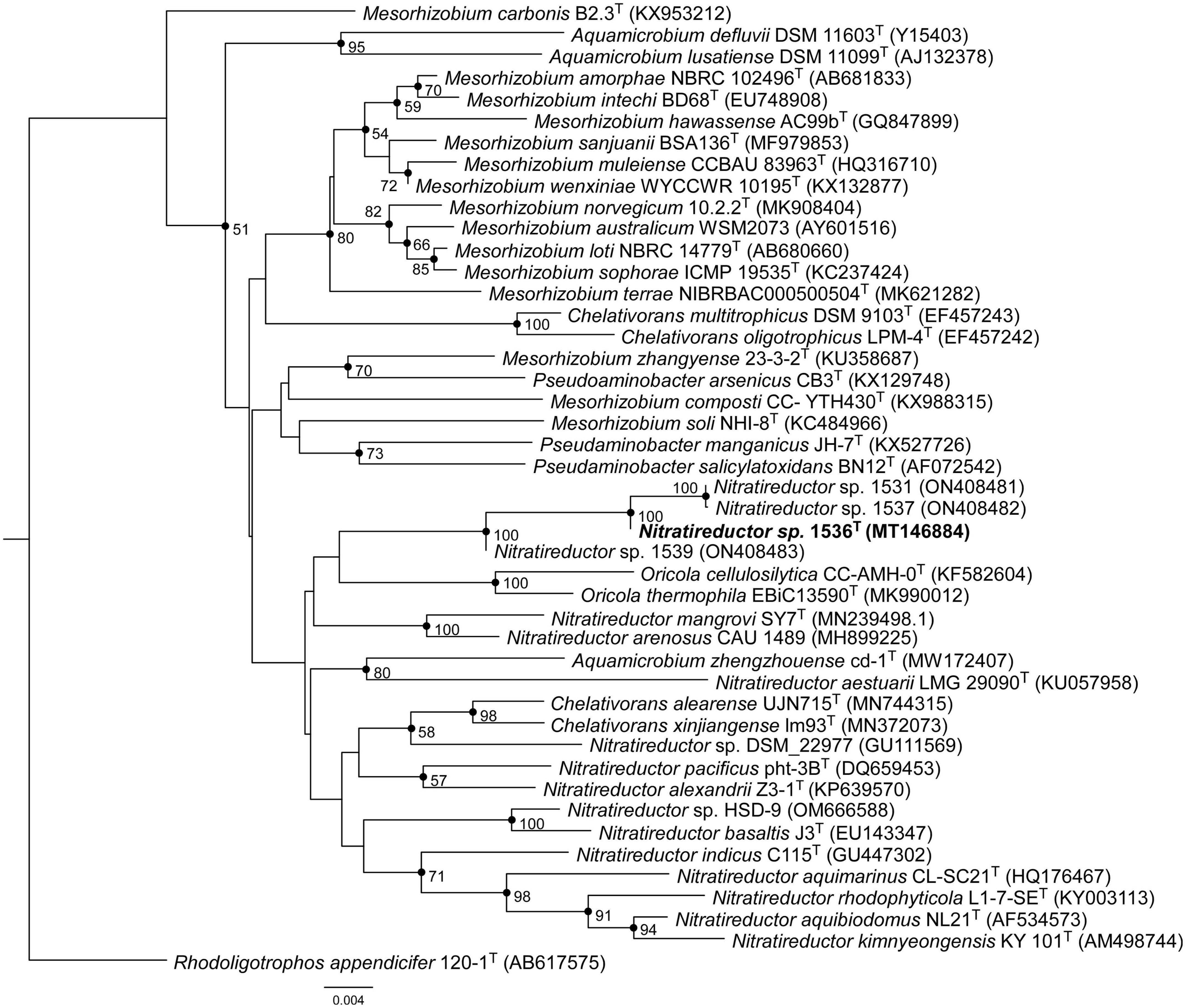
Figure 2. Neighbor-joining phylogenetic tree based on the bacterial 16S rRNA gene sequences showing the position of Nit1536T. Only bootstrap values (expressed as percentages of 1,000 replications) of >50% are shown at the branching points. Filled circles indicate branches that were also recovered using the maximum-likelihood method. Rhodoligotrophos appendicifer 120-1T (AB617575) was used as an outgroup. Bar, 0.004 substitutions per nucleotide position.
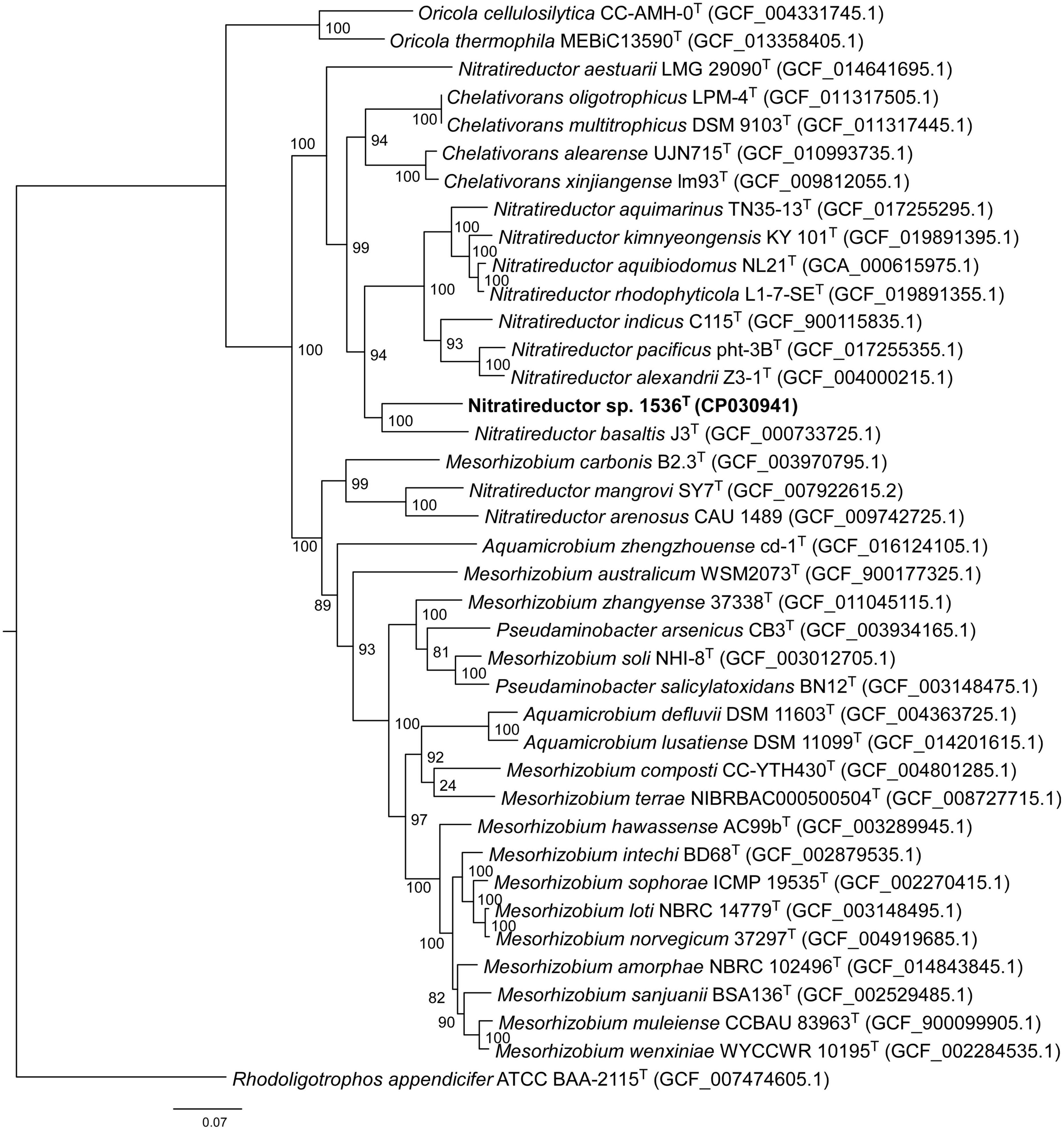
Figure 3. Neighbor-joining phylogenomic tree using an MLSA concatenating 120 essential single-copy genes, highlighting the position of the Nit1536T strain relative to other closely related bacterial taxa within the Phyllobacteriaceae family. The tree was reconstructed using the software GTDB-Tk (Chaumeil et al., 2019). Numbers at the nodes designate bootstrap support values resulting from 1,000 bootstrap replicates. Bar, 0.07 substitution per nucleotide position. Rhodoligotrophos appendicifer ATCC BAA-2115T (GCF_007474605.1) was used as an outgroup.
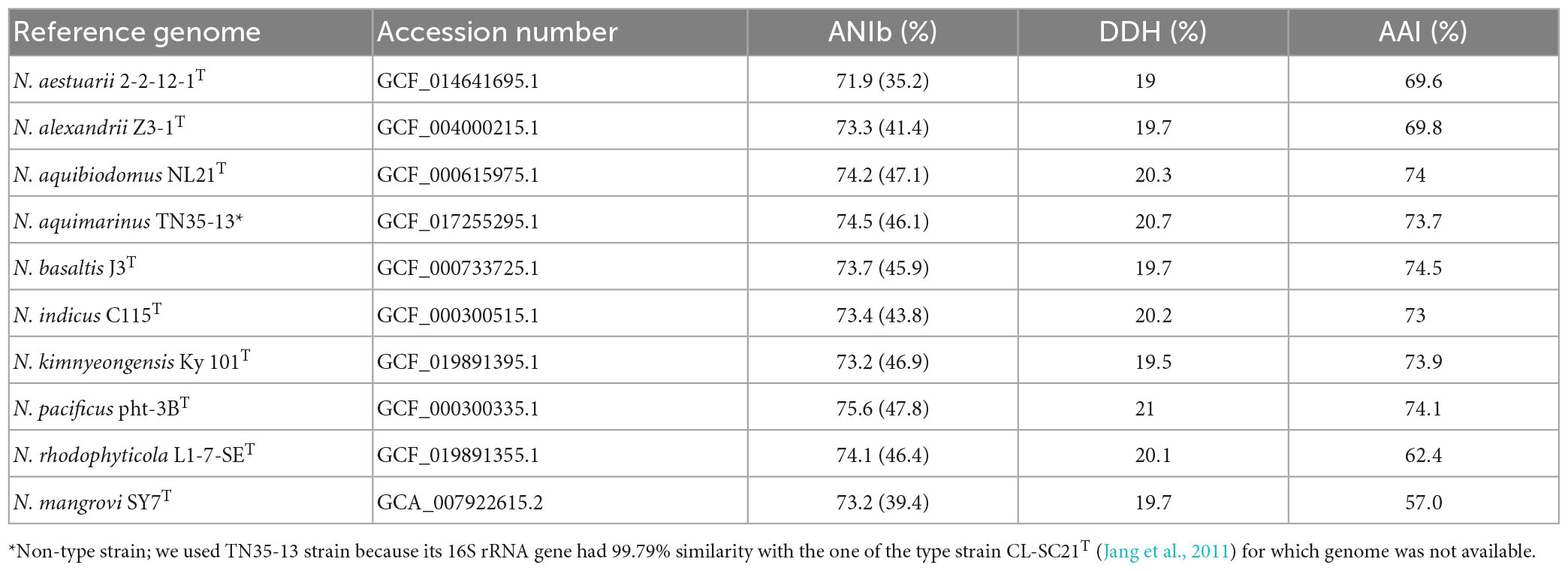
Table 1. (i) Average nucleotide identity via BLAST (ANIb) and percentage of aligned sequences in brackets, (ii) in silico DNA-DNA hybridization (DDH) calculation, and (iii) average amino acid identity (AAI) of Nit1536T against other representatives of the Nitratireductor genus, namely, N. aestuarii 2-2-12-1T (Ou et al., 2017), N. alexandrii Z3-1T (Jiang et al., 2020), N. aquibiodomus NL21T (Labbé et al., 2004), N. aquimarinus TN35-13 (non-type strain), N. basaltis J3T (Kim et al., 2009), N. indicus C115T (Lai et al., 2011b,2012), N. kimnyeongensis Ky 101T (Kang et al., 2009), N. pacificus pht-3BT (Lai et al., 2011a), N. rhodophyticola L1-7-SET (Kim et al., 2022), and N. mangrovi SY7T (Ye et al., 2020).
Morphological, physiological, and biochemical characterization of Nit1536T
The Nit1536T strain is a short rod-shaped, Gram-negative, aerobic, and non-motile bacterium. Although several genes for flagellar synthesis are found, the genes encoding for chaperone proteins FlgN, FliS, FliT, the filament cap FliD, regulatory proteins FlhC and FlhD and the biosynthesis proteins FliO and FliH are not present in the genome (Supplementary Result 1). One cell is typically 0.9 μm in length and 0.4 μm in width (Figure 4a). Colonies are circular with 1–2 mm in diameter and regular edges, smooth, shiny, creamy in colour, possibly due to the absence of biosynthetic genes involved in the production of pigments (Supplementary Result 1). The Nit1536T strain is a mesophilic bacterium able to grow between 15 and 45°C (optimum 37°C; Figure 4b). It is able to grow in a medium containing 1–14% of NaCl (optimum 4%; Figure 4c), making it a moderately halophilic bacterium (Zhu et al., 2007) adapted to the salinity conditions of the Red Sea mangroves [up to 15% (Fusi et al., 2022)] where no fresh water inputs and low-tidal range occur in summer due to El Niño–Southern Oscillation (ENSO). The Nit1536T strain also has metabolic activity in the presence of various osmolytes and ions, such as betaine, sarcosine, ectoine, choline, proline, trehalose, ethylene glycol, and others (total of 27 compounds; full list in Supplementary Table 4), while a full metabolic inhibition occur in the presence of potassium chloride (5 and 6%), sodium formate (1–6%), sodium lactate (1–12%), sodium benzoate (20–200 mM), ammonium sulphate (10–100 mM) and sodium nitrite (20–100 mM). The Nit1536T strain shows similar metabolic activity at pH values between 7 and 10, with an optimum at pH 8 (Figure 4d). These findings are in accordance with its environment of origin, where the pH of the sediments varied from 7.5 to 10 over the course of the year (Fusi et al., 2022). Under the optimal conditions (i.e., 37°C, 4% NaCl and pH 8), the doubling time for growth is 6.5 h, confirming its classification as a slow-growing bacterium.
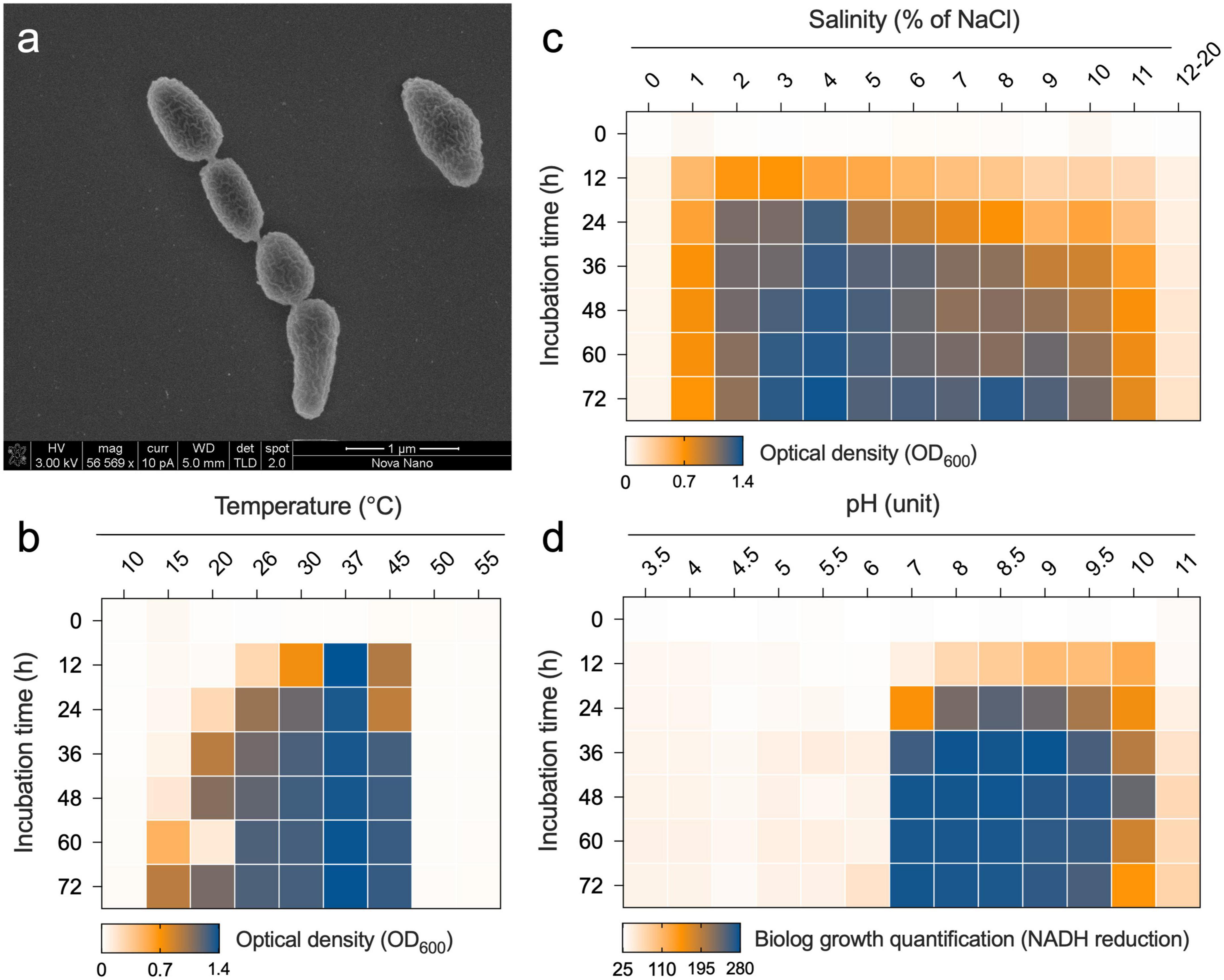
Figure 4. (a) Transmission electron micrographs of Nit1536T. The bar length is 1 μm. Growth of Nit1536T at different (b) temperatures and (c) salinities (% of NaCl) is reported as the average value of OD600 (n = 3). (d) Metabolism of Nit1536T under different pH is analyzed using Biolog PM and reported as the quantity of dye formation.
Of the 190 carbon sources available in the PM1 and PM2 microplates, the Nit1536T strain shows an active metabolism on 57 carbon sources, such as, among others, L-histidine, dihydroxy acetone, arbutin, 2-deoxy-D-ribose, 5-keto-D-gluconic acid, L-lyxose L-seryne, D-glucosamine and oxalomalic acid, that induced a higher metabolic rate (Supplementary Figure 5). In addition, besides its growth on crude oil, when single hydrocarbons are considered, the Nit1536T strain grows on linear alkanes, such as hexane, nonane and dodecane, but not on aromatic hydrocarbons (toluene and xylene). The metabolism of Nit1536T strains is inhibited at all four antibiotic concentrations present in Biolog® PM plates for four antibiotics (novobiocin, spectinomycin, nafcillin, and spiramycin) and two inhibitor compounds (benzethonium chloride and dodecyltrimethylammonium bromide). In contrast, 29 antibiotic/inhibitor compounds did not affect the bacterial metabolism (i.e., active metabolism at all concentrations of the antibiotic), and 11 only partially affected it (i.e., active metabolism only at low concentrations of the antibiotic) (details in Supplementary Table 5). The antibiotic resistance ability of this strain was confirmed by the AMRFinderPlus tool, which revealed >80% query coverage and 56–64% identity at the amino-acid level to class A beta-lactamases (bla) and Fosfomycin resistance hydrolase (fosX). It is important to note that since antibiotic resistance is a strain-specific behavior, we can attribute this antibiotic resistance/sensitivity profile only to this strain.
The Nit1536T isolate is positive for oxidase and catalase, while it was negative for indole production, nitrate reduction, amylase, protease, lipase and cellulase activities. Chemotaxonomic analysis indicated that the predominant respiratory ubiquinone is Q-10, as observed in the other Nitratireductor genus species (range, 96.6–98.4%) (Chen et al., 2015) and many members of the Alphaproteobacteria class (Fukuda et al., 2012). The major fatty acids (>5%) of the Nit1536T strain are a combination of C18:1 ω 7c and/or C18:1 ω 6c [summed feature 8 (SF8), 66.54%], C19:0 cyclo ω 8c (14.31%) and C18:0 (8.62%). Its fatty acid composition is more similar to those of N. aquibiodomus NL21T and N. kimnyeongensis Ky 101T than those of Nitratireductor spp. HSD-9T and SL014B-25A2. However, the different relative abundance of its saturated straight chain fatty acids (C16:0, C17:0, C18:0, and iso-C17:0) shows the unicity of the Nit1536T strain (Table 2). As for its closest related species, the Nit1536T strain has phosphatidylcholine (PC), phosphatidylglycerol (PG), phosphatidylethanolamine (PE), diphosphatidylglycerol (DPG), and glycolipid (GL) as dominant polar lipids (Supplementary Figure 6). It also shows that phosphatidyl-methyl ethanolamine (PME) and several unidentified lipids (L) are not present in the other strains.
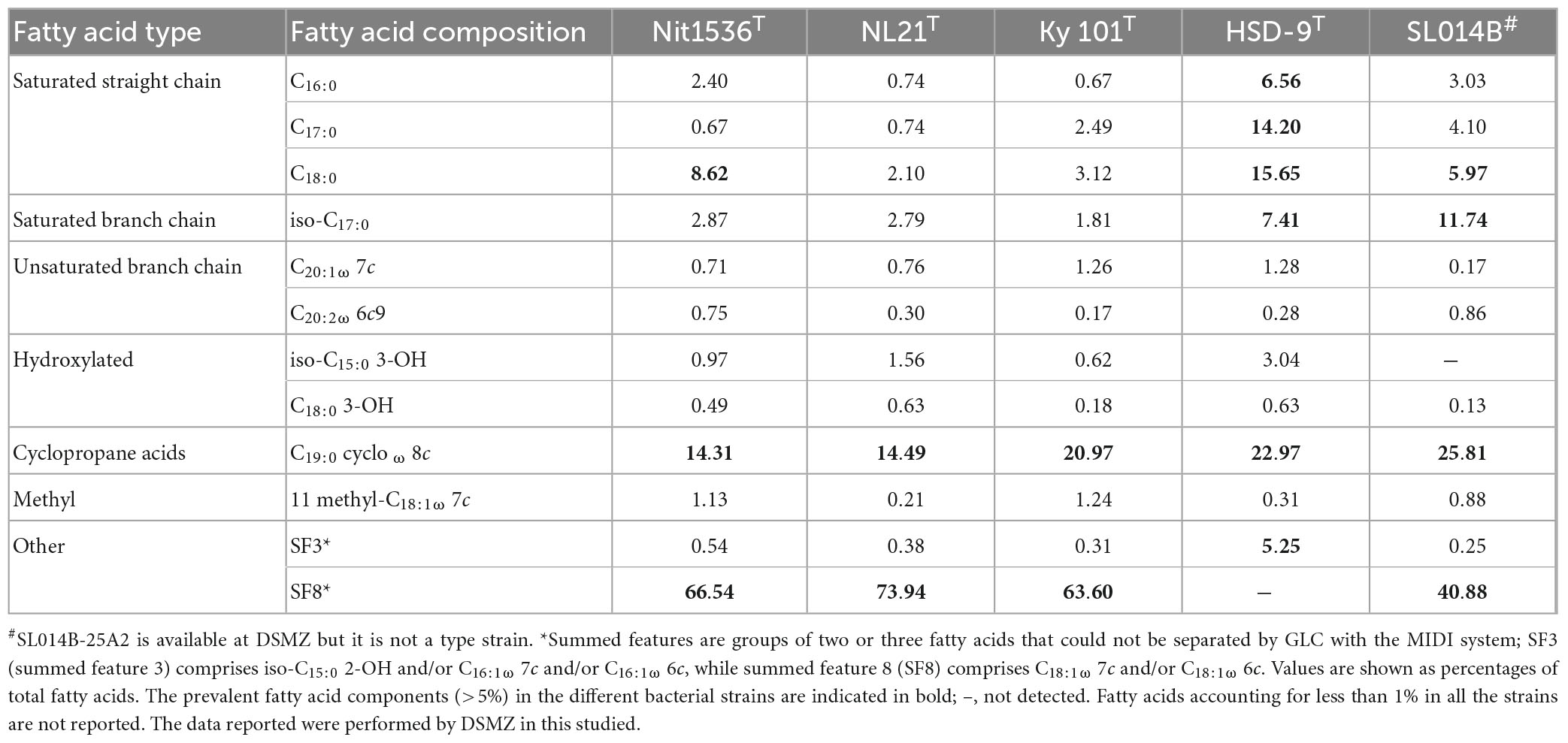
Table 2. Cellular fatty acid composition (%) of strain Nit1536T and its closest related species Nitratireductor aquibiodomus NL21T (DSM 15645, from marine denitrification system) (Labbé et al., 2004), N. kimnyeongensis Ky 101T (DSM 19185, from dried seaweed) (Kang et al., 2009), Nitratireductor sp. HSD-9T (DSM 19383, from beach sand) and Nitratireductor sp. SL014B-25A2 (DSM 22977 non-type strain, from oil-polluted soil).
Morphological, physiological and chemo-taxonomic characteristics of the Nit1536T strain are compared to those of its closest relative type strains, namely, N. aquibiodomus NL21T and N. kimnyeongensis Ky 101T (Tables 2, 3), as well as with non-yet recognized species Nitratireductor sp. in the case of chemo-taxonomic (Table 2). These results corroborate the unicity of this strain previously suggested by the 16S rRNA gene sequence analysis and in silico DNA–DNA hybridization studies (Figures 2, 3 and Table 1). Based on these, we conclude that strain Nit1536T represents a novel species within the genus Nitratireductor, for which the name Nitratireductor thuwali sp. nov. is proposed.
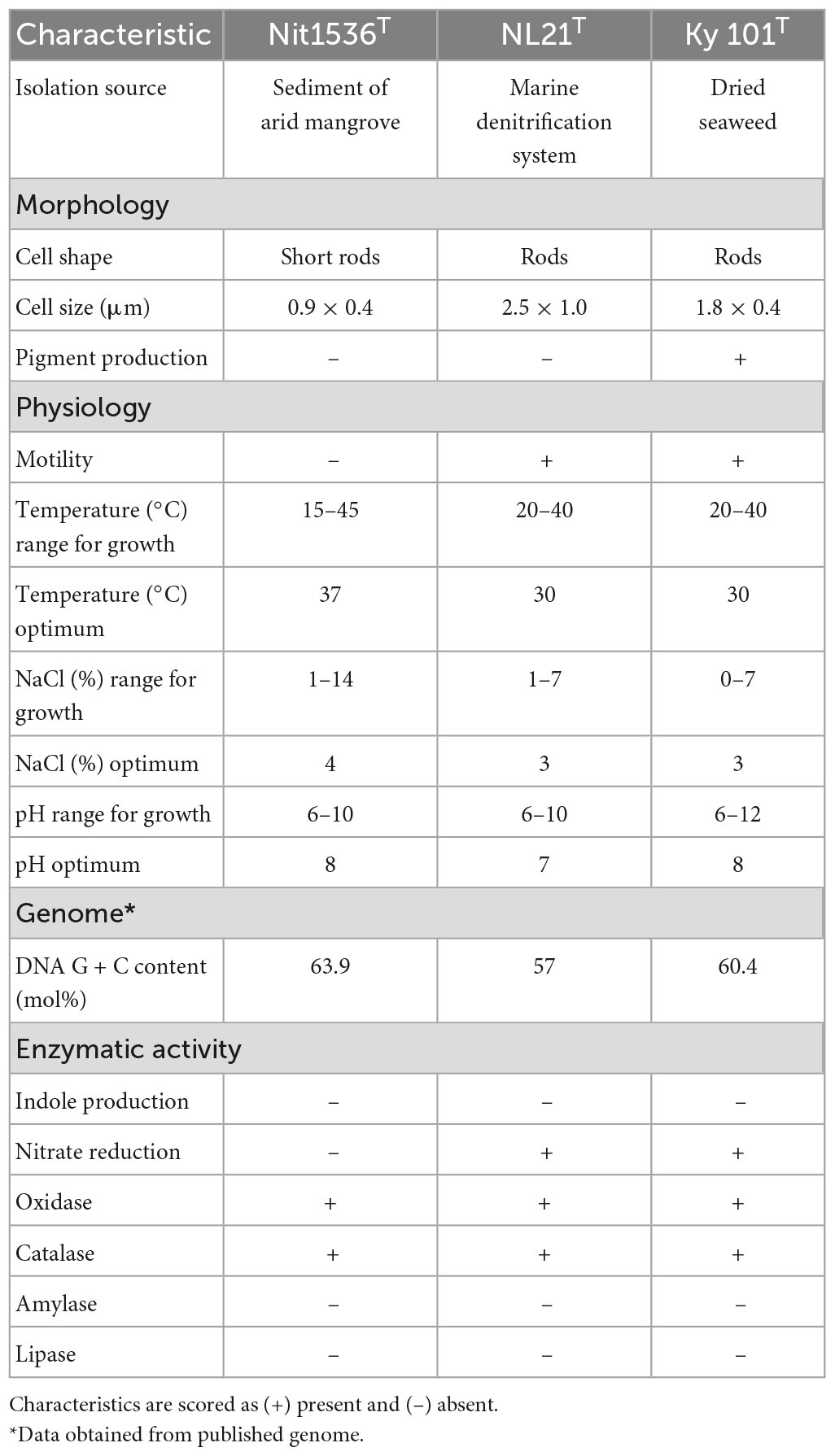
Table 3. Differential phenotypic characteristics of strain Nit1536T and closely related species of Nitratireductor genus, namely, N. aquibiodomus NL21T (DSM 15645) (Labbé et al., 2004) and N. kimnyeongensis Ky 101T (DSM 19185) (Kang et al., 2009).
Metabolism-related features inferred from Nit1536T genome
The genome of the proposed new bacterial species Nitratireductor thuwali Nit1536T presents three replicons consisting of one main circular chromosome consisting of 4,216,528 bp in length (Supplementary Figure 7) and two plasmids consisting of 317,553 (p1536_1) and 212,699 bp (p1536_2) in length, respectively. We observed 4,558 CDS, 58 RNAs, and a G + C of 63.9 mol%. Two identical copies of a full-length 16S rRNA gene (1,544) have been detected, one in the chromosome and one in one of the two plasmids (p1536_1), which, since it was harboring a housekeeping gene, cannot be strictly defined as a plasmid (Tazzyman and Bonhoeffer, 2014).
Respiration. Analysis of the genome reveals that all subunits of cytochrome bc1 complex and NADH: quinone oxidoreductase complex were encoded, confirming the aerobic respiration in this bacterium. Components of F-type ATPase (complex V) are also predicted in the genome. The two core subunits (CydA and CydB) of cytochrome bd oxidase but not the associated subunits are encoded on the genome. If the high-affinity cytochrome bd oxidase is functional in the isolate, it would not only facilitate the survival of this strain under oxygen-poor conditions (microaerophilic) typical of mangrove water-logged sediments (Sarker et al., 2021), but would also confer enhanced tolerance to nitrosative stress (NO and O2–), resistance to hydrogen peroxide, suppression of extracellular superoxide production and the ability to defend against antibacterial agents (Subedi et al., 2022).
C-metabolism and energy pathways. A complete tricarboxylic acid (TCA) cycle is encoded, implying that organic carbon compounds, such as carbohydrates, organic acids and polycyclic aromatic hydrocarbons, can be used as carbon and energy sources (overview in Figure 5A and Supplementary Result 1). For instance, we detected genes encoding the degradation of polycyclic aromatic hydrocarbon (PAH), including extradiol dioxygenases and rieske non-heme iron oxygenase (Supplementary Table 3A) that catalyze ring-cleavage reactions and a wide variety of mechanisms in the biodegradation of xenobiotics, respectively, (Barry and Challis, 2013). In addition, the metabolic pathways for sugar utilization were encoded in the genome such as glycolysis (EMP), Entner-Doudoroff (EDD) and the pentose-phosphate pathways, confirming the versatile metabolism observed in the presence of certain sugars with Biolog PM plates (Supplementary Figure 5). Growth on acetate or C2-compounds as a sole source of carbon and energy is also possible since the genome encoded acetyl-CoA synthetase, acetate transporter (ActP), and enzymes from the glyoxylate shunt (red arrows in Figure 5A and Supplementary Result 1). The latter pathway, along with the essential genes encoding enzymes of the beta-oxidation pathway, could confer Nit1536T the ability to grow on fatty acids. Notably, the presence of formate dehydrogenases and components of the serine cycle may imply that the strain possesses the capacity to detoxify and utilize formate as a source of carbon and/or energy. Anaplerotic CO2 fixation for replenishment of TCA cycle intermediates could be mediated through the encoded pyruvate carboxylase or phosphoenolpyruvate carboxykinase. In contrast, autotrophic CO2 fixation capacity was not detected (black arrows in Figure 5A and Supplementary Result 1). No phototrophic modules are present, and no lithotrophic modules were detected for energy generation via ammonia, hydrogen, and sulphur (Supplementary Result 1). Despite the methane abundance in the mangrove sediments (Bižić et al., 2020), no pathways for methanogenesis or methanotrophy were found (black arrows in Figure 5A). Moreover, as also revealed by Biolog (Supplementary Figure 5), glycerol can serve as a sole C and energy source for Nit1536T by the action of the enzyme glycerol kinase, glycerol-3-P dehydrogenase and glycerol-3-phosphate. Apart from alcohol dehydrogenases and putative lactate dehydrogenases, fermentative pathways are absent. All potential CAZymes predicted by the DRAM tool are listed in Supplementary Table 3B. For instance, the cellulose degradation pathway was predicted to be only 66% complete on the gapseq tool; hence, the isolate lacks this ability. Similarly, the chitin, lignin, pectin, starch, and mucin degradation pathways are incomplete. These results confirm the data obtained by the in vitro tests.
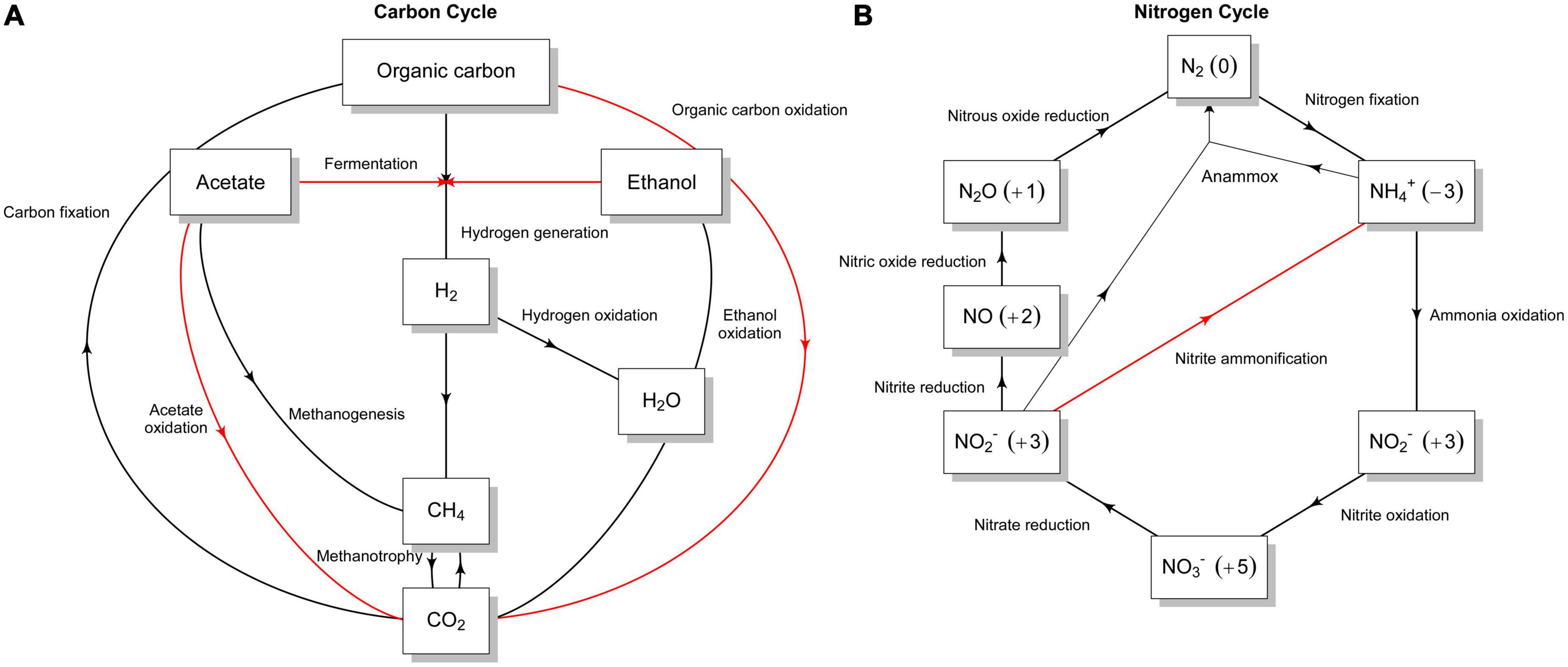
Figure 5. Graphical representation of different sub-pathways of (A) carbon and (B) nitrogen metabolism in the Nit1536T strain. The presence of the different sub-pathways is indicated with red arrows, while their absence is indicated with black arrows. The flow graphs were created using a modified script from METABOLIC (Zhou et al., 2022).
Nitrogen metabolism and transporters. Only genes encoding the small and large subunits of a putative assimilatory nitrite reductase (nirBD) and the assimilatory nitrate reductase (nas) were predicted in the genome (Figure 5B). Genes involved in nitrogen fixation, denitrification, dissimilatory nitrite reduction to ammonia, nitrate and nitrite reduction, and anaerobic/aerobic ammonia oxidation are absent (black arrows in Figure 5B), while those involved in urea and ammonium uptake/assimilation are encoded (Supplementary Result 1). The genome also reveals the presence of several transporters, among others those for peptides/amino acids, urea, ammonium, VitB12, sugars and organic substrates (Supplementary Result 1).
Environmental adaptation and stress tolerance. We further investigated the Nit1536T genomic adaptation to mangrove abiotic stresses (i.e., salinity and temperature fluctuation). The Nit1536T genome encodes genes pertaining to DNA repair components, such as uvrABC, recAFORQJ, uracil-DNA glycosylases (udg), mutS, mutL, endonucleases (end), and ligases (lig), as well as it possesses several genes responsible for the osmotic stress response (Supplementary Result 1). For instance, the genome displayed the presence of a gene cluster responsible for the biosynthesis (and/or uptake) of ectoine, glycine betaine, proline, and choline. Using HPLC high-resolution mass spectrometry technique (HPLC-HR-MS) we confirmed the production of ectoine, betaine, glutamate, proline, trehalose, alanine, glycerophosphorylcholine, and dymethylsulfoniopropionate (Supplementary Figure 8). Key genes for the synthesis of glycerol, trehalose and glutamate are also present, while those involved in the synthesis of sorbitol and sucrose are absent (Supplementary Result 1). Such compatible solutes may also act as abiotic stress protectors for plants, as shown by the Nit1536T inoculation of barley plantlets grown using seawater (Supplementary Methods 1). Compared to the negative control (i.e., plant exposed to salt stress but not treated with the bacterium), the treatment with Nit1536T strain promotes the fresh and dry weight of root biomass with an increasing weight of 42.9 and 7.6%, respectively, (Supplementary Table 6). It is important to note that this strain also has the genetic capacity to synthesize storage granules, such as polyhydroxyalkanoate (PHA), to potentially resist starvation, exposure to radiation, desiccation, high salinity and oxidants typical of mangrove sediments (Zhu et al., 2018; Fusi et al., 2022). This genetic capacity to synthesize PHA was also detected in the other available genomes within the Nitratireductor genus.
Discussion
Molecular ecology surveys investigating the microbial communities of many different environments have revealed an impressive diversity of microbes in nature and have highlighted our inability to cultivate most of them in the laboratory (Kaeberlein, 2002; Bodor et al., 2020). However, a deeper knowledge of the metabolic features of these uncultivated but ecologically relevant microbial taxa is a fundamental step to disentangle their role in the multifunctionality of ecosystems (e.g., biogeochemical cycles), as well as their adaptation to environmental drivers. In this context, improving our ability to grow uncultivable microbes in laboratory conditions can support progresses in this challenging task. Here, alternative cultivation strategies—successfully used in the past to expand the collection of environmentally relevant but poorly represented microbial strains (Janssen et al., 2002; Rappé et al., 2002; Joseph et al., 2003; Lewis et al., 2010; Pulschen et al., 2017)—have been applied to untap microbial diversity of the arid Red Sea mangrove sediment, with particular focus on the hydrocarbon degrader bacteria.
By using oligotrophic media, hydrocarbon (crude oil) as a C-source and long incubation time, we succeed in the cultivation and isolation of slow-growing bacteria belonging to rarely cultivable (or uncommon) species and poorly described taxa, including members of Citreicella, Nitratireductor, Joostella, Pelagibaca, Reinekea, and Sinomicrobium genera. These lineages, so far, have few cultivated representatives. For instance, Joostella and Sinomicrobium genera have few validly described representatives obtained from marine/saline ecosystems but not from oil hydrocarbon-degrading communities. They are characterized by interesting features with biotechnological potentials, such as algicidal activity against toxic dinoflagellates or the production of enzymes of industrial interest (Cheng et al., 2014; Yang et al., 2014). Other genera, such as Nitratireductor (10 validly described species), Citreicella (4), Reinekea (4) and Pelagibaca (2) have been previously obtained from bacterial consortia associated with oil hydrocarbon contamination and biodegradation (Lai et al., 2012; Pan et al., 2014; Wang et al., 2014; Liu et al., 2017) or by applying oligotrophic cultivation conditions (Hahnke et al., 2015). These data point out the usefulness of low nutritional media (i.e., ONR7a and FSW), hydrocarbon C-sources and long incubation periods to favor the isolation of rare genera and slow-growing bacterial strains.
Within highly diverse ecosystems, such as mangroves, most of the bacterial taxa are in low abundance as members of the rare biosphere (Pedrós-Alió, 2012; Coveley et al., 2015). Rare taxa are mainly “tolerant strategists,” and thus can persist under limited resources and suboptimal abiotic conditions (Malik et al., 2020). Moreover, when these taxa are phylogenetic distant from the dominant/abundant taxa (e.g., phylogenetic novelty), they can act as a “backup system” able to promptly respond to environmental fluctuations and disturbance, vicariate multiple metabolic capabilities and ecosystem functions (Jousset et al., 2017; Shu et al., 2021; He et al., 2022).
In our work, within a collection of a few hundred isolates, we mainly identified strains closely related to previously described species (>97% 16S rRNA gene sequence identity) and one new species, Nit1536T strain, affiliated to the genus Nitratireductor. The isolation and description of a novel strain belonging to a bacterial group poorly characterized but consistently detected in the environment, such as Nitratireductor in mangroves (Booth et al., 2019b; Li et al., 2020; Baskaran and Prabavathy, 2022; Fusi et al., 2022), represent an opportunity to better understand (i) the microbial adaptation strategies adopted to survive in the extreme and stressful conditions of such environment and (ii) their ecological/functional role in the sediment microbial community and mangrove ecosystem. According to LPSN (see text footnote 4), at the time of writing, only one Nitratireductor species was obtained from a mangrove, i.e., N. mangrovi (Ye et al., 2020), even if its nomenclature status is not yet validly published.5
Arid mangroves are exposed to strong selection forces, including high-temperature and salinity variation, heterogeneous nutrient availability and sharp gradient of oxygen availability (Kathiresan and Bingham, 2001), making them an “extreme” habitat (Helfer and Hassenrück, 2021; Palit et al., 2022). Microbial communities develop different strategies to adjust the cell osmotic homeostasis (Xu et al., 2018) in environments with elevated osmolarity (high salinity and dryness), including the less-expensive biosynthesis of osmoprotective compounds/compatible solutes, i.e., molecules with low molecular mass, that do not require structural modifications of the cells (Sleator, 2001). The biosynthesis of these compounds is controlled by environmental cues, such as high salinity and heat stress (Ono et al., 1998; Xu et al., 2018), and their accumulation in high concentrations ensures the physiological functions of the cell. Interesting, these compounds could also be responsible for the promoting/protecting activity mediated by Nit1536T in plants (Supplementary Table 6), thus making this halophytic strain a candidate probiotic for mangrove restoration strategies and sustainable agricultural production in salt-affected soils (Soldan et al., 2019; Prittesh et al., 2020). The Nit1536T strain also shows an important adaptation to manage reactive oxygen species that are typically associated with high salinity environment (Hassan et al., 2020). This trait can be important, especially in the Red Sea mangrove, where the seasonal sea level variation, i.e., tidal range and the lack of water input leave the sediment dry with massive salt accumulation (Fusi et al., 2022). Such variation in salinity strongly selects the microbial community living there (Thomson et al., 2022a). Combined with the production of compatible solutes, the Nit1536T strain survives under such conditions by controlling the oxidative stresses triggered by the periodical high salinity.
The Nit1536T strain shows additional peculiar adaptation to the mangrove environment because it can manage different levels of oxygen availability in the sediment dictated by the diel and seasonal tide inundation, as well as high plasticity of carbon source retrieval from the different compounds that can be available in the mangrove sediment, including hydrocarbon sources from anthropogenic activity, such as ships and oil tanker traffic, industrial and solid waste, plastic debris, and natural vents and seeps (Maiti and Chowdhury, 2013; Nagi and Abubakr, 2013; Al-Ali et al., 2015; Martin et al., 2019a). On the contrary, recalcitrant carbon (e.g., lignin) cannot be processed by this Nitratireductor strain and therefore it is excluded by those process of carbon degradation that involves litter/wood consumption. Considering the available nutrient source in mangrove sediment, the Nit1536T strain showed the capability to assimilate ammonium and urea as nitrogen sources. Arid mangroves are N-deficient (Almahasheer et al., 2016; Anton et al., 2020; Fusi et al., 2022) and a strong adaptation for nitrogen acquisition is a necessity to survive. The Nit1536T strain has the potential to use the ammonia secreted by the vast majority of marine organisms living in the mangrove stand (Wright, 1995). Exceptionally, some of these organisms, such as the killifish Rivulus marmoratus (Frick and Wright, 2002), are capable of producing urea that can be used by the strain, revealing its high plasticity in retrieving the nitrogen from the mangrove sediments. The capability to retain nitrogen from ammonium and urea can be an important ecosystem service mediated by Nit1536T strain: it can help mangrove to recycle the nutrient avoiding out-welling by tidal export (Lee, 1995; Walton et al., 2014). Since nitrogen is one of the limiting factors for plant growth in arid mangrove (Almahasheer et al., 2016), bacteria that can recycle and retain the nitrogen in the system can be important therefore for plant growth and ultimately the overall system homeostasis.
Description of Nitratireductor thuwali sp. nov.
Nitratireductor thuwali sp. nov. (thu.wal. ’i. L.fem.gen. n. thuwali, pertaining to Thuwal village, Makkah region, Saudi Arabia). Cells are Gram-stain-negative, non-motile and short rods of approximately 0.4 μm in width and 0.9 μm in length. Colonies on solid MB plates are cream, smooth, shiny, and circular 1–2 mm in diameter. Optimal growth occurs at 37°C, pH 8 and 4% NaCl. Oxidase and catalase are positive, while indole production, nitrate reduction, amylase, protease, lipase and cellulase activities are negative. Linear hydrocarbons, L-histidine, dihydroxy acetone, arbutin, 2-deoxy-D-ribose, 5-keto-D-gluconic acid, L-lyxose L-seryne, D-glucosamine and oxalomalic acid can be utilized as a sole carbon and energy source. The dominant polar lipids are phosphatidylcholine, phosphatidylglycerol, phosphatidylethanolamine, diphosphatidylglycerol, and glycolipid, along with phosphatidyl-methyl ethanolamine and several unidentified lipids. The major fatty acids (>5%) were summed feature 8 (C18:1 ω7c and/or C18:1 ω6c), C19:0 cyclo ω8c and C18:0. The DNA G + C content is 63.9 mol%. The type strain is Nit1536T (= KCTC 72347T = NCCB 100697T = JCM 33620T), isolated from mangrove sediment of the central Red Sea coast in Thuwal. The GenBank accession number for the 16S rRNA gene sequence of strain Nit1536T is MT146884. The GenBank accession number for the whole genome sequence of strain Nit1536T is CP030941-3.
Data availability statement
The datasets presented in this study can be found in online repositories. The names of the repository/repositories and accession number(s) can be found in the article/Supplementary material.
Author contributions
RM, MF, and DD conceived and designed the study and experiments. RM and MF collected the sample. FS, GMe, AB, KS, and RM performed the experiments. RM, GM, CPA, and FS analyzed the data. DD contributed reagents, materials, and analysis tools. RM, GM and DD wrote the manuscript. All authors critically revised the manuscript.
Funding
This research was funded by King Abdullah University of Science and Technology (KAUST) through project FCC/1/1973-56-01 of the Red Sea Research Center and project REI/1/4483-01-01 of the Circular Carbon Economy initiative.
Acknowledgments
We thank Taskeen Begum for their support in organizing the laboratory work, the Bioscience Core Laboratory (BCL) for genome sequencing, and the Coastal and Marine Resources Core Lab (CMOR) for the logistics of collection and transportation of biological samples.
Conflict of interest
The authors declare that the research was conducted in the absence of any commercial or financial relationships that could be construed as a potential conflict of interest.
Publisher’s note
All claims expressed in this article are solely those of the authors and do not necessarily represent those of their affiliated organizations, or those of the publisher, the editors and the reviewers. Any product that may be evaluated in this article, or claim that may be made by its manufacturer, is not guaranteed or endorsed by the publisher.
Supplementary material
The Supplementary Material for this article can be found online at: https://www.frontiersin.org/articles/10.3389/fmicb.2023.1155381/full#supplementary-material
Footnotes
- ^ https://github.com/tseemann/abricate
- ^ https://github.com/kblin/ncbi-genome-download
- ^ https://lpsn.dsmz.de/search?word=Nitratireductor
- ^ https://lpsn.dsmz.de
- ^ https://lpsn.dsmz.de/species/nitratireductor-mangrovi
References
Adyasari, D., Pratama, M. A., Teguh, N. A., Sabdaningsih, A., Kusumaningtyas, M. A., and Dimova, N. (2021). Anthropogenic impact on Indonesian coastal water and ecosystems: current status and future opportunities. Mar. Pollut. Bull. 171:112689. doi: 10.1016/j.marpolbul.2021.112689
Aladame, N. (1987). Bergey’s manual of systematic bacteriology. Ann. Institut Pasteur Microbiol. 138, 146. doi: 10.1016/0769-2609(87)90099-8
Al-Ali, A. M., Del Campo, A. G., and Rocha, C. (2015). Environmental assessment of mangrove communities in Tarut bay, eastern Arabian peninsula, based on multidisciplinary approach. Int. Arch. Photogr. Remote Sens. Spatial Inf. Sci. 40, 269–276. doi: 10.5194/isprsarchives-XL-7-W3-269-2015
Allard, S. M., Costa, M. T., Bulseco, A. N., Helfer, V., Wilkins, L. G. E., Hassenrück, C., et al. (2020). Introducing the mangrove microbiome initiative: identifying microbial research priorities and approaches to better understand, protect, and rehabilitate mangrove ecosystems. mSystems 5, 1–9. doi: 10.1128/mSystems.00658-20
Almahasheer, H., Duarte, C. M., and Irigoien, X. (2016). Nutrient limitation in central red sea mangroves. Front. Mar. Sci. 3:271. doi: 10.3389/fmars.2016.00271
Alzahrani, D. A., Selim, E.-M. M., and El-Sherbiny, M. M. (2018). Ecological assessment of heavy metals in the grey mangrove (Avicennia marina) and associated sediments along the Red Sea coast of Saudi Arabia. Oceanologia 60, 513–526. doi: 10.1016/j.oceano.2018.04.002
Andreote, F. D., Jiménez, D. J., Chaves, D., Dias, A. C. F., Luvizotto, D. M., Dini-Andreote, F., et al. (2012). The microbiome of Brazilian mangrove sediments as revealed by metagenomics. PLoS One 7:e38600. doi: 10.1371/journal.pone.0038600
Anton, A., Almahasheer, H., Delgado, A., Garcias-Bonet, N., Carrillo-de-Albornoz, P., Marbà, N., et al. (2020). Stunted mangrove trees in the oligotrophic central Red Sea relate to nitrogen limitation. Front. Mar. Sci. 7:597. doi: 10.3389/fmars.2020.00597
Arshad, M., Eid, E. M., and Hasan, M. (2020). Mangrove health along the hyper-arid southern Red Sea coast of Saudi Arabia. Environ. Monit. Assess. 192, 189. doi: 10.1007/s10661-020-8140-6
Aziz, R. K., Bartels, D., Best, A., DeJongh, M., Disz, T., Edwards, R. A., et al. (2008). The RAST server: rapid annotations using subsystems technology. BMC Genomics 9:75. doi: 10.1186/1471-2164-9-75
Barry, S. M., and Challis, G. L. (2013). Mechanism and catalytic diversity of rieske non-heme iron-dependent oxygenases. ACS Catal. 3, 2362–2370. doi: 10.1021/cs400087p
Basaham, A. S., Gheith, A. M., Khawfany, A. A. A., Sharma, R., and Hashimi, N. H. (2014). Sedimentary variations of geomorphic subenvironments at Al-Lith area, central-west coast of Saudi Arabia, Red Sea. Arab. J. Geosci. 7, 951–970. doi: 10.1007/s12517-012-0799-8
Baskaran, V., and Prabavathy, V. R. (2022). Diverse key nitrogen cycling genes nifH, nirS and nosZ associated with Pichavaram mangrove rhizospheres as revealed by culture-dependent and culture-independent analyses. Arch. Microbiol. 204:109. doi: 10.1007/s00203-021-02661-4
Bižić, M., Klintzsch, T., Ionescu, D., Hindiyeh, M. Y., Günthel, M., Muro-Pastor, A. M., et al. (2020). Aquatic and terrestrial cyanobacteria produce methane. Sci. Adv. 6:eaax5343. doi: 10.1126/sciadv.aax5343
Blin, K., Shaw, S., Steinke, K., Villebro, R., Ziemert, N., Lee, S. Y., et al. (2019). antiSMASH 5.0: updates to the secondary metabolite genome mining pipeline. Nucleic Acids Res. 47, W81–W87. doi: 10.1093/nar/gkz310
Bodor, A., Bounedjoum, N., Vincze, G. E., Erdeiné Kis, Á, Laczi, K., Bende, G., et al. (2020). Challenges of unculturable bacteria: environmental perspectives. Rev. Environ. Sci. 19, 1–22. doi: 10.1007/s11157-020-09522-4
Booth, J. M., Fusi, M., Marasco, R., Michoud, G., Fodelianakis, S., Merlino, G., et al. (2019a). The role of fungi in heterogeneous sediment microbial networks. Sci. Rep. 9:7537. doi: 10.1038/s41598-019-43980-3
Booth, J. M., Fusi, M., Marasco, R., Mbobo, T., and Daffonchio, D. (2019b). Fiddler crab bioturbation determines consistent changes in bacterial communities across contrasting environmental conditions. Sci. Rep. 9:3749. doi: 10.1038/s41598-019-40315-0
Boyer, S. L., Flechtner, V. R., and Johansen, J. R. (2001). Is the 16S–23S rRNA internal transcribed spacer region a good tool for use in molecular systematics and population genetics? A case study in cyanobacteria. Mol. Biol. Evol. 18, 1057–1069. doi: 10.1093/oxfordjournals.molbev.a003877
Brettin, T., Davis, J. J., Disz, T., Edwards, R. A., Gerdes, S., Olsen, G. J., et al. (2015). RASTtk: a modular and extensible implementation of the RAST algorithm for building custom annotation pipelines and annotating batches of genomes. Sci. Rep. 5:8365. doi: 10.1038/srep08365
Bruns, A., Cypionka, H., and Overmann, J. (2002). Cyclic AMP and acyl homoserine lactones increase the cultivation efficiency of heterotrophic bacteria from the central Baltic Sea. Appl. Environ. Microbiol. 68, 3978–3987. doi: 10.1128/AEM.68.8.3978-3987.2002
Burall, L. S., Grim, C. J., Mammel, M. K., and Datta, A. R. (2016). Whole genome sequence analysis using jspecies tool establishes clonal relationships between listeria monocytogenes strains from epidemiologically unrelated listeriosis outbreaks. PLoS One 11:e0150797. doi: 10.1371/journal.pone.0150797
Chaumeil, P.-A., Mussig, A. J., Hugenholtz, P., and Parks, D. H. (2019). GTDB-Tk: a toolkit to classify genomes with the genome taxonomy database. Bioinformatics 36, 1925–1927. doi: 10.1093/bioinformatics/btz848
Chen, L., Zheng, D., Liu, B., Yang, J., and Jin, Q. (2016). VFDB 2016: hierarchical and refined dataset for big data analysis—10 years on. Nucleic Acids Res. 44, D694–D697. doi: 10.1093/nar/gkv1239
Chen, Q., Ni, H.-Y., Zhuang, W., Sun, Z.-G., Yang, Z.-Z., Wang, H.-M., et al. (2015). Nitratireductor soli sp. nov., isolated from phenol-contaminated soil. Antonie Van Leeuwenhoek 108, 1139–1146. doi: 10.1007/s10482-015-0567-3
Cheng, B., Li, C., Lai, Q., Du, M., Shao, Z., Xu, P., et al. (2014). Sinomicrobium pectinilyticum sp. nov., a pectinase-producing bacterium isolated from alkaline and saline soil, and emended description of the genus Sinomicrobium. Int. J. Syst. Evol. Microbiol. 64, 2939–2943. doi: 10.1099/ijs.0.061671-0
Chin, C.-S. S., Alexander, D. H., Marks, P., Klammer, A. A., Drake, J., Heiner, C., et al. (2013). Nonhybrid, finished microbial genome assemblies from long-read SMRT sequencing data. Nat. Methods 10, 563–569. doi: 10.1038/nmeth.2474
Coveley, S., Elshahed, M. S., and Youssef, N. H. (2015). Response of the rare biosphere to environmental stressors in a highly diverse ecosystem (Zodletone spring, OK, USA). PeerJ 3:e1182. doi: 10.7717/peerj.1182
Cross, K. L., Campbell, J. H., Balachandran, M., Campbell, A. G., Cooper, S. J., Griffen, A., et al. (2019). Targeted isolation and cultivation of uncultivated bacteria by reverse genomics. Nat. Biotechnol. 37, 1314–1321. doi: 10.1038/s41587-019-0260-6
D’Onofrio, A., Crawford, J. M., Stewart, E. J., Witt, K., Gavrish, E., Epstein, S., et al. (2010). Siderophores from neighboring organisms promote the growth of uncultured bacteria. Chem. Biol. 17, 254–264. doi: 10.1016/j.chembiol.2010.02.010
Daffonchio, D., Cherif, A., Brusetti, L., Rizzi, A., Mora, D., Boudabous, A., et al. (2003). Nature of polymorphisms in 16S-23S rRNA gene intergenic transcribed spacer fingerprinting of bacillus and related genera. Appl. Environ. Microbiol. 69, 5128–5137. doi: 10.1128/AEM.69.9.5128-5137.2003
Davis, K. E. R., Joseph, S. J., and Janssen, P. H. (2005). Effects of growth medium, inoculum size, and incubation time on culturability and isolation of soil bacteria. Appl. Environ. Microbiol. 71, 826–834. doi: 10.1128/AEM.71.2.826-834.2005
Davis, K. E. R., Sangwan, P., and Janssen, P. H. (2011). Acidobacteria, Rubrobacteridae and Chloroflexi are abundant among very slow-growing and mini-colony-forming soil bacteria. Environ. Microbiol. 13, 798–805. doi: 10.1111/j.1462-2920.2010.02384.x
Donato, D. C., Kauffman, J. B., Murdiyarso, D., Kurnianto, S., Stidham, M., and Kanninen, M. (2011). Mangroves among the most carbon-rich forests in the tropics. Nat. Geosci. 4, 293–297. doi: 10.1038/ngeo1123
El-Amin Bashir, M., El-Maradny, A., El-Sherbiny, M., and Mohammed Orif, R. K. T. (2017). Bio-concentration of polycyclic aromatic hydrocarbons in the grey mangrove (Avicennia marina) along eastern coast of the Red Sea. Open Chem. 15, 344–351. doi: 10.1515/chem-2017-0038
Feldgarden, M., Brover, V., Gonzalez-Escalona, N., Frye, J. G., Haendiges, J., Haft, D. H., et al. (2021). AMRFinderPlus and the reference gene catalog facilitate examination of the genomic links among antimicrobial resistance, stress response, and virulence. Sci. Rep. 11:12728. doi: 10.1038/s41598-021-91456-0
Felsenstein, J. (1985). Confidence limits on phylogenies: an approach using the bootstrap. Evolution 39, 783–791. doi: 10.1111/j.1558-5646.1985.tb00420.x
Florensa, A. F., Kaas, R. S., Clausen, P. T. L. C., Aytan-Aktug, D., and Aarestrup, F. M. (2022). ResFinder – An open online resource for identification of antimicrobial resistance genes in next-generation sequencing data and prediction of phenotypes from genotypes. Microb. Genomics 8:000748. doi: 10.1099/mgen.0.000748
Frick, N. T., and Wright, P. A. (2002). Nitrogen metabolism and excretion in the mangrove killifish Rivulus marmoratus I. The influence of environmental salinity and external ammonia. J. Exp. Biol. 205, 79–89. doi: 10.1242/jeb.205.1.79
Fukuda, W., Yamada, K., Miyoshi, Y., Okuno, H., Atomi, H., and Imanaka, T. (2012). Rhodoligotrophos appendicifer gen. nov., sp. nov., an appendaged bacterium isolated from a freshwater Antarctic lake. Int. J. Syst. Evol. Microbiol. 62, 1945–1950. doi: 10.1099/ijs.0.032953-0
Fusi, M., Beone, G. M., Suciu, N. A., Sacchi, A., Trevisan, M., Capri, E., et al. (2016). Ecological status and sources of anthropogenic contaminants in mangroves of the Wouri River Estuary (Cameroon). Mar. Pollut. Bull. 109, 723–733. doi: 10.1016/j.marpolbul.2016.06.104
Fusi, M., Booth, J. M., Marasco, R., Merlino, G., Garcias-Bonet, N., Barozzi, A., et al. (2022). Bioturbation intensity modifies the sediment microbiome and biochemistry and supports plant growth in an arid mangrove system. Microbiol. Spectr. 10:e0111722. doi: 10.1128/spectrum.01117-22
Garber, A. I., Nealson, K. H., Okamoto, A., McAllister, S. M., Chan, C. S., Barco, R. A., et al. (2020). FeGenie: a comprehensive tool for the identification of iron genes and iron gene neighborhoods in genome and metagenome assemblies. Front. Microbiol. 11:37. doi: 10.3389/fmicb.2020.00037
Hahnke, R. L., Bennke, C. M., Fuchs, B. M., Mann, A. J., Rhiel, E., Teeling, H., et al. (2015). Dilution cultivation of marine heterotrophic bacteria abundant after a spring phytoplankton bloom in the North Sea. Environ. Microbiol. 17, 3515–3526. doi: 10.1111/1462-2920.12479
Hassan, A. H. A., Alkhalifah, D. H. M., Al Yousef, S. A., Beemster, G. T. S., Mousa, A. S. M., Hozzein, W. N., et al. (2020). Salinity stress enhances the antioxidant capacity of Bacillus and Planococcus species isolated from saline lake environment. Front. Microbiol. 11:561816. doi: 10.3389/fmicb.2020.561816
He, X., McLean, J. S., Edlund, A., Yooseph, S., Hall, A. P., Liu, S.-Y., et al. (2015). Cultivation of a human-associated TM7 phylotype reveals a reduced genome and epibiotic parasitic lifestyle. Proc. Natl. Acad. Sci. U.S.A. 112, 244–249. doi: 10.1073/pnas.1419038112
He, Z., Liu, D., Shi, Y., Wu, X., Dai, Y., Shang, Y., et al. (2022). Broader environmental adaptation of rare rather than abundant bacteria in reforestation succession soil. Sci. Total Environ. 828:154364. doi: 10.1016/j.scitotenv.2022.154364
Hedlund, B. P., Dodsworth, J. A., Murugapiran, S. K., Rinke, C., and Woyke, T. (2014). Impact of single-cell genomics and metagenomics on the emerging view of extremophile “microbial dark matter.”. Extremophiles 18, 865–875. doi: 10.1007/s00792-014-0664-7
Helfer, V., and Hassenrück, C. (2021). “Microbial communities in mangrove sediments,” in Dynamic sedimentary environments of mangrove coasts, eds F. Sidik and D. Friess (Amsterdam: Elsevier), 141–175. doi: 10.1016/B978-0-12-816437-2.00003-3
Jałowiecki, Ł, Chojniak, J., Dorgeloh, E., Hegedusova, B., Ejhed, H., Magnér, J., et al. (2017). Using phenotype microarrays in the assessment of the antibiotic susceptibility profile of bacteria isolated from wastewater in on-site treatment facilities. Folia Microbiol. 62, 453–461. doi: 10.1007/s12223-017-0516-9
Jang, G. I., Hwang, C. Y., and Cho, B. C. (2011). Nitratireductor aquimarinus sp. nov., isolated from a culture of the diatom Skeletonema costatum, and emended description of the genus Nitratireductor. Int. J. Syst. Evol. Microbiol. 61, 2676–2681. doi: 10.1099/ijs.0.028373-0
Janssen, P. H., Yates, P. S., Grinton, B. E., Taylor, P. M., and Sait, M. (2002). Improved culturability of soil bacteria and isolation in pure culture of novel members of the divisions Acidobacteria, Actinobacteria, Proteobacteria, and Verrucomicrobia. Appl. Environ. Microbiol. 68, 2391–2396. doi: 10.1128/AEM.68.5.2391-2396.2002
Jiang, Z., Duan, Y., Yang, X., Yao, B., Zeng, T., Wang, X., et al. (2020). Nitratireductor alexandrii sp. nov., from phycosphere microbiota of toxic marine dinoflagellate alexandrium tamarense. Int. J. Syst. Evol. Microbiol. 70, 4390–4397. doi: 10.1099/ijsem.0.004302
Johnson, M., Zaretskaya, I., Raytselis, Y., Merezhuk, Y., McGinnis, S., and Madden, T. L. (2008). NCBI BLAST: a better web interface. Nucleic Acids Res. 36, W5–W9. doi: 10.1093/nar/gkn201
Joseph, S. J., Hugenholtz, P., Sangwan, P., Osborne, C. A., and Janssen, P. H. (2003). Laboratory cultivation of widespread and previously uncultured soil bacteria. Appl. Environ. Microbiol. 69, 7210–7215. doi: 10.1128/AEM.69.12.7210-7215.2003
Jousset, A., Bienhold, C., Chatzinotas, A., Gallien, L., Gobet, A., Kurm, V., et al. (2017). Where less may be more: how the rare biosphere pulls ecosystems strings. ISME J. 11, 853–862. doi: 10.1038/ismej.2016.174
Kaeberlein, T. (2002). Isolating “uncultivable” microorganisms in pure culture in a simulated natural environment. Science 296, 1127–1129. doi: 10.1126/science.1070633
Kanehisa, M., Sato, Y., Kawashima, M., Furumichi, M., and Tanabe, M. (2016). KEGG as a reference resource for gene and protein annotation. Nucleic Acids Res. 44, D457–D462. doi: 10.1093/nar/gkv1070
Kang, H. S., Yang, H. L., and Lee, S. D. (2009). Nitratireductor kimnyeongensis sp. nov., isolated from seaweed. Int. J. Syst. Evol. Microbiol. 59, 1036–1039. doi: 10.1099/ijs.0.65734-0
Kathiresan, K., and Bingham, B. L. L. (2001). Biology of mangroves and mangrove ecosystems. Adv. Mar. Biol. 40, 81–251. doi: 10.1016/S0065-2881(01)40003-4
Kato, S., Yamagishi, A., Daimon, S., Kawasaki, K., Tamaki, H., Kitagawa, W., et al. (2018). Isolation of previously uncultured slow-growing bacteria by using a simple modification in the preparation of agar media. Appl. Environ. Microbiol. 84, e807–e818. doi: 10.1128/AEM.00807-18
Kim, D., Park, S., and Chun, J. (2021). Introducing EzAAI: a pipeline for high throughput calculations of prokaryotic average amino acid identity. J. Microbiol. 59, 476–480. doi: 10.1007/s12275-021-1154-0
Kim, K. H., Kristyanto, S., Kim, H. M., Kim, K. R., and Jeon, C. O. (2022). Nitratireductor rhodophyticola sp. nov., isolated from marine red algae. Int. J. Syst. Evol. Microbiol. 72, 1–8. doi: 10.1099/ijsem.0.005302
Kim, K. H., Roh, S. W., Chang, H. W., Nam, Y. D., Yoon, J. H., Jeon, C. O., et al. (2009). Nitratireductor basaltis sp. nov., isolated from black beach sand. Int. J. Syst. Evol. Microbiol. 59, 135–138. doi: 10.1099/ijs.0.65784-0
Konstantinidis, K. T., Rosselló-Móra, R., and Amann, R. (2017). Uncultivated microbes in need of their own taxonomy. ISME J. 11, 2399–2406. doi: 10.1038/ismej.2017.113
Köpke, B., Wilms, R., Engelen, B., Cypionka, H., and Sass, H. (2005). Microbial diversity in coastal subsurface sediments: a cultivation approach using various electron acceptors and substrate gradients. Appl. Environ. Microbiol. 71, 7819–7830. doi: 10.1128/AEM.71.12.7819-7830.2005
Kumar, S., Stecher, G., Tamura, K., and Dudley, J. (2016). MEGA7: molecular evolutionary genetics analysis version 7.0 for bigger datasets. Mol. Biol. Evol. 33, 1870–1874. doi: 10.1093/molbev/msw054
Labbé, N., Parent, S., and Villemur, R. (2004). Nitratireductor aquibiodomus gen. nov., sp. nov., a novel α-proteobacterium from the marine denitrification system of the Montreal Biodome (Canada). Int. J. Syst. Evol. Microbiol. 54, 269–273. doi: 10.1099/ijs.0.02793-0
Lai, Q., Li, G., Yu, Z., and Shao, Z. (2012). Genome sequence of Nitratireductor indicus type strain C115. J. Bacteriol. 194, 6990–6990. doi: 10.1128/JB.01917-12
Lai, Q., Yu, Z., Wang, J., Zhong, H., Sun, F., Wang, L., et al. (2011a). Nitratireductor pacificus sp. nov., isolated from a pyrene-degrading consortium. Int. J. Syst. Evol. Microbiol. 61, 1386–1391. doi: 10.1099/ijs.0.024356-0
Lai, Q., Yu, Z., Yuan, J., Sun, F., and Shao, Z. (2011b). Nitratireductor indicus sp. nov., isolated from deep-sea water. Int. J. Syst. Evol. Microbiol. 61, 295–298. doi: 10.1099/ijs.0.022962-0
Lee, M. (2022). bit: a multipurpose collection of bioinformatics tools. F1000Research 11:122. doi: 10.12688/f1000research.79530.1
Lee, S. Y. (1995). Mangrove outwelling: a review. Hydrobiologia 295, 203–212. doi: 10.1007/BF00029127
Lewis, K., Epstein, S., D’Onofrio, A., and Ling, L. L. (2010). Uncultured microorganisms as a source of secondary metabolites. J. Antibiot. 63, 468–476. doi: 10.1038/ja.2010.87
Li, R., Wu, S., Chai, M., and Xie, S. (2020). Denitrifier communities differ in mangrove wetlands across China. Mar. Pollut. Bull. 155:111160. doi: 10.1016/j.marpolbul.2020.111160
Liu, J., Bacosa, H. P., and Liu, Z. (2017). Potential environmental factors affecting oil-degrading bacterial populations in deep and surface waters of the Northern Gulf of Mexico. Front. Microbiol. 7:2131. doi: 10.3389/fmicb.2016.02131
Liu, Y.-L., Meng, D., Li, R.-R., Gu, P.-F., Fan, X.-Y., Huang, Z.-S., et al. (2019). Rhodoligotrophos defluvii sp. Nov., isolated from activated sludge. Int. J. Syst. Evol. Microbiol. 69, 3830–3836. doi: 10.1099/ijsem.0.003691
Luo, Y. Y., Not, C., and Cannicci, S. (2021). Mangroves as unique but understudied traps for anthropogenic marine debris: a review of present information and the way forward. Environ. Pollut. 271:116291. doi: 10.1016/j.envpol.2020.116291
Madigan, M. T., Martinko, J. M., Dunlap, P. V., and Clark, D. P. (2008). Measuring microbial growth. Brock Biol. Microorg. 11, 128–132.
Maiti, S. K., and Chowdhury, A. (2013). Effects of anthropogenic pollution on mangrove biodiversity: a review. J. Environ. Prot. 04, 1428–1434. doi: 10.4236/jep.2013.412163
Malik, A. A., Martiny, J. B. H., Brodie, E. L., Martiny, A. C., Treseder, K. K., and Allison, S. D. (2020). Defining trait-based microbial strategies with consequences for soil carbon cycling under climate change. ISME J. 14, 1–9. doi: 10.1038/s41396-019-0510-0
Marasco, R., Rolli, E., Ettoumi, B., Vigani, G., Mapelli, F., Borin, S., et al. (2012). A drought resistance-promoting microbiome is selected by root system under desert farming. PLoS One 7:e48479. doi: 10.1371/journal.pone.0048479
Martin, C., Almahasheer, H., and Duarte, C. M. (2019b). Mangrove forests as traps for marine litter. Environ. Pollut. 247, 499–508. doi: 10.1016/j.envpol.2019.01.067
Martin, C., Agustí, S., and Duarte, C. M. (2019a). Seasonality of marine plastic abundance in central Red Sea pelagic waters. Sci. Total Environ. 688, 536–541. doi: 10.1016/j.scitotenv.2019.06.240
Martin, C., Baalkhuyur, F., Valluzzi, L., Saderne, V., Cusack, M., Almahasheer, H., et al. (2020). Exponential increase of plastic burial in mangrove sediments as a major plastic sink. Sci. Adv. 6:eaaz5593. doi: 10.1126/sciadv.aaz5593
Meier-Kolthoff, J. P., Auch, A. F., Klenk, H. P., and Göker, M. (2013). Genome sequence-based species delimitation with confidence intervals and improved distance functions. BMC Bioinformatics 14:60. doi: 10.1186/1471-2105-14-60
Mistry, J., Chuguransky, S., Williams, L., Qureshi, M., Salazar, G. A., Sonnhammer, E. L. L., et al. (2021). Pfam: the protein families database in 2021. Nucleic Acids Res. 49, D412–D419. doi: 10.1093/nar/gkaa913
Montgomery, B. L. (2020). Lessons from microbes: what can we learn about equity from unculturable bacteria? mSphere 5:e01046-20. doi: 10.1128/msphere.01046-20
Nagi, H. M., and Abubakr, M. M. (2013). Threats status to the mangrove ecosystem along the coastal zone of Yemen. JKAU Mar. Sci 24, 101–117.
Nichols, D., Lewis, K., Orjala, J., Mo, S., Ortenberg, R., O’Connor, P., et al. (2008). Short peptide induces an “uncultivable” microorganism to grow in vitro. Appl. Environ. Microbiol. 74, 4889–4897. doi: 10.1128/AEM.00393-08
Ono, H., Okuda, M., Tongpim, S., Imai, K., Shinmyo, A., Sakuda, S., et al. (1998). Accumulation of compatible solutes, ectoine and hydroxyectoine, in a moderate halophile, Halomonas elongata KS3 isolated from dry salty land in Thailand. J. Ferment. Bioeng. 85, 362–368. doi: 10.1016/S0922-338X(98)80078-0
Ou, D., Huang, H., Bai, R., Li, Q., Wang, Y., and Yin, Y. (2017). Nitratireductor aestuarii sp. nov., a marine alphaproteobacterium isolated from an estuary. Int. J. Syst. Evol. Microbiol. 67, 1637–1642. doi: 10.1099/ijsem.0.001771
Palit, K., Rath, S., Chatterjee, S., and Das, S. (2022). Microbial diversity and ecological interactions of microorganisms in the mangrove ecosystem: threats, vulnerability, and adaptations. Environ. Sci. Pollut. Res. 29, 32467–32512. doi: 10.1007/s11356-022-19048-7
Pan, X.-C., Geng, S., Mei, R., Wang, Y.-N., Cai, H., Liu, X.-Y., et al. (2014). Nitratireductor shengliensis sp. nov., isolated from an oil-polluted saline soil. Curr. Microbiol. 69, 561–566. doi: 10.1007/s00284-014-0624-6
Parte, A. C., Sardà Carbasse, J., Meier-Kolthoff, J. P., Reimer, L. C., and Göker, M. (2020). List of prokaryotic names with standing in nomenclature (LPSN) moves to the DSMZ. Int. J. Syst. Evol. Microbiol. 70, 5607–5612. doi: 10.1099/ijsem.0.004332
Pedrós-Alió, C. (2012). The rare bacterial biosphere. Ann. Rev. Mar. Sci. 4, 449–466. doi: 10.1146/annurev-marine-120710-100948
Pramanik, A., Sengupta, S., and Bhattacharyya, M. (2019). “Microbial diversity and community analysis of the Sundarbans mangrove, a world heritage site,” in Microbial diversity in the genomic era, eds S. Das and H. R. Dash (Amsterdam: Elsevier), 65–76. doi: 10.1016/B978-0-12-814849-5.00005-8
Price, M. N., Deutschbauer, A. M., and Arkin, A. P. (2020). GapMind: automated annotation of amino acid biosynthesis. mSystems 5:e00291-20. doi: 10.1128/mSystems.00291-20
Prittesh, P., Avnika, P., Kinjal, P., Jinal, H. N., Sakthivel, K., and Amaresan, N. (2020). Amelioration effect of salt-tolerant plant growth-promoting bacteria on growth and physiological properties of rice (Oryza sativa) under salt-stressed conditions. Arch. Microbiol. 202, 2419–2428. doi: 10.1007/s00203-020-01962-4
Pulschen, A. A., Bendia, A. G., Fricker, A. D., Pellizari, V. H., Galante, D., and Rodrigues, F. (2017). Isolation of uncultured bacteria from antarctica using long incubation periods and low nutritional media. Front. Microbiol. 8:1346. doi: 10.3389/fmicb.2017.01346
Qashqari, M. S., Garcias-Bonet, N., Fusi, M., Booth, J. M., Daffonchio, D., and Duarte, C. M. (2020). High temperature and crab density reduce atmospheric nitrogen fixation in Red Sea mangrove sediments. Estuar. Coast. Shelf Sci. 232:106487. doi: 10.1016/j.ecss.2019.106487
Radhakrishnan, N., Gnanamani, A., and Mandal, A. B. (2011). Isolation, production and characterization of bioemulsifiers of marine bacteria of coastal Tamil Nadu. Indian J. Geo Mar. Sci. 40, 76–82.
Rappé, M. S., Connon, S. A., Vergin, K. L., and Giovannoni, S. J. (2002). Cultivation of the ubiquitous SAR11 marine bacterioplankton clade. Nature 418, 630–633. doi: 10.1038/nature00917
Richards, D. R., and Friess, D. A. (2016). Rates and drivers of mangrove deforestation in Southeast Asia, 2000–2012. Proc. Natl. Acad. Sci. U.S.A. 113, 344–349. doi: 10.1073/pnas.1510272113
Richter, M., Rosselló-Móra, R., Oliver Glöckner, F., and Peplies, J. (2016). JSpeciesWS: a web server for prokaryotic species circumscription based on pairwise genome comparison. Bioinformatics 32, 929–931. doi: 10.1093/bioinformatics/btv681
Russel, J., Pinilla-Redondo, R., Mayo-Muñoz, D., Shah, S. A., and Sørensen, S. J. (2020). CRISPRCasTyper: automated identification, annotation, and classification of CRISPR-cas loci. Cris. J. 3, 462–469. doi: 10.1089/crispr.2020.0059
Saderne, V., Fusi, M., Thomson, T., Dunne, A., Mahmud, F., Roth, F., et al. (2021). Total alkalinity production in a mangrove ecosystem reveals an overlooked blue carbon component. Limnol. Oceanogr. Lett. 6, 61–67. doi: 10.1002/lol2.10170
Sarker, S., Masud-Ul-Alam, M., Hossain, M. S., Rahman Chowdhury, S., and Sharifuzzaman, S. (2021). A review of bioturbation and sediment organic geochemistry in mangroves. Geol. J. 56, 2439–2450. doi: 10.1002/gj.3808
Seemann, T. (2014). Prokka: rapid prokaryotic genome annotation. Bioinformatics 30, 2068–2069. doi: 10.1093/bioinformatics/btu153
Sefrji, F. O., Marasco, R., Michoud, G., Seferji, K. A., Merlino, G., and Daffonchio, D. (2021a). Kaustia mangrovi gen. nov., sp. nov. isolated from Red Sea mangrove sediments belongs to the recently proposed Parvibaculaceae family within the order Rhizobiales. Int. J. Syst. Evol. Microbiol. 71:004806. doi: 10.1099/ijsem.0.004806
Sefrji, F. O., Michoud, G., Marasco, R., Merlino, G., and Daffonchio, D. (2021b). Mangrovivirga cuniculi gen. nov., sp. nov., a moderately halophilic bacterium isolated from bioturbated Red Sea mangrove sediment, and proposal of the novel family Mangrovivirgaceae fam. nov. Int. J. Syst. Evol. Microbiol. 71:004866. doi: 10.1099/ijsem.0.004866
Sefrji, F. O., Marasco, R., Michoud, G., Seferji, K. A., Merlino, G., and Daffonchio, D. (2022). Insights into the cultivable bacterial fraction of sediments from the Red Sea mangroves and physiological, chemotaxonomic, and genomic characterization of Mangrovibacillus cuniculi gen. nov., sp. nov., a novel member of the Bacillaceae family. Front. Microbiol. 13:777986. doi: 10.3389/fmicb.2022.777986
Shaffer, M., Borton, M. A., McGivern, B. B., Zayed, A. A., La Rosa, S. L., Solden, L. M., et al. (2020). DRAM for distilling microbial metabolism to automate the curation of microbiome function. Nucleic Acids Res. 48, 8883–8900. doi: 10.1093/nar/gkaa621
Sheaves, M. (2009). Consequences of ecological connectivity: the coastal ecosystem mosaic. Mar. Ecol. Prog. Ser. 391, 107–115. doi: 10.3354/meps08121
Shu, D., Guo, Y., Zhang, B., Zhang, C., Van Nostrand, J. D., Lin, Y., et al. (2021). Rare prokaryotic sub-communities dominate the complexity of ecological networks and soil multinutrient cycling during long-term secondary succession in China’s Loess Plateau. Sci. Total Environ. 774:145737. doi: 10.1016/j.scitotenv.2021.145737
Sleator, R. (2001). Bacterial osmoadaptation: the role of osmolytes in bacterial stress and virulence. FEMS Microbiol. Rev. 25, 49–71. doi: 10.1016/S0168-6445(01)00071-7
Soldan, R., Mapelli, F., Crotti, E., Schnell, S., Daffonchio, D., Marasco, R., et al. (2019). Bacterial endophytes of mangrove propagules elicit early establishment of the natural host and promote growth of cereal crops under salt stress. Microbiol. Res. 223–225, 33–43. doi: 10.1016/j.micres.2019.03.008
Solden, L., Lloyd, K., and Wrighton, K. (2016). The bright side of microbial dark matter: lessons learned from the uncultivated majority. Curr. Opin. Microbiol. 31, 217–226. doi: 10.1016/j.mib.2016.04.020
Subedi, S. C., Allen, P., Vidales, R., Sternberg, L., Ross, M., and Afkhami, M. E. (2022). Salinity legacy: foliar microbiome’s history affects mutualist-conferred salinity tolerance. Ecology 103:e3679. doi: 10.1002/ecy.3679
Tazzyman, S. J., and Bonhoeffer, S. (2014). Why there are no essential genes on plasmids. Mol. Biol. Evol. 32, 3079–3088. doi: 10.1093/molbev/msu293
Tesson, F., Hervé, A., Mordret, E., Touchon, M., D’Humières, C., Cury, J., et al. (2022). Systematic and quantitative view of the antiviral arsenal of prokaryotes. Nat. Commun. 13:2561. doi: 10.1038/s41467-022-30269-9
Thatoi, H., Behera, B. C., Mishra, R. R., and Dutta, S. K. (2013). Biodiversity and biotechnological potential of microorganisms from mangrove ecosystems: a review. Ann. Microbiol. 63, 1–19. doi: 10.1007/s13213-012-0442-7
Thomson, K., Bhat, A., and Carvell, J. (2015). Comparison of a new digital imaging technique for yeast cell counting and viability assessments with traditional methods. J. Inst. Brew. 121, 231–237. doi: 10.1002/jib.224
Thomson, T., Fusi, M., Bennett-Smith, M. F., Prinz, N., Aylagas, E., Carvalho, S., et al. (2022b). Contrasting effects of local environmental and biogeographic factors on the composition and structure of bacterial communities in arid monospecific mangrove soils. Microbiol. Spectr 10:e0090321. doi: 10.1128/spectrum.00903-21
Thomson, T., Ellis, J. I., Fusi, M., Prinz, N., Bennett-Smith, M. F., Aylagas, E., et al. (2022a). The right place at the right time: seasonal variation of bacterial communities in arid Avicennia marina soils in the Red Sea is specific to its position in the intertidal. Front. Ecol. Evol. 10:845611. doi: 10.3389/fevo.2022.845611
Walton, M., Al-Maslamani, I., Skov, M., Al-Shaikh, I., Al-Ansari, I., Kennedy, H., et al. (2014). Outwelling from arid mangrove systems is sustained by inwelling of seagrass productivity. Mar. Ecol. Prog. Ser. 507, 125–137. doi: 10.3354/meps10827
Wang, W., Zhong, R., Shan, D., and Shao, Z. (2014). Indigenous oil-degrading bacteria in crude oil-contaminated seawater of the Yellow sea, China. Appl. Microbiol. Biotechnol. 98, 7253–7269. doi: 10.1007/s00253-014-5817-1
Wright, P. A. (1995). Nitrogen excretion: three end products, many physiological roles. J. Exp. Biol. 198, 273–281. doi: 10.1242/jeb.198.2.273
Xu, Z., Sun, M., Jiang, X., Sun, H., Dang, X., Cong, H., et al. (2018). Glycinebetaine biosynthesis in response to osmotic stress depends on jasmonate signaling in watermelon suspension cells. Front. Plant Sci. 9:1469. doi: 10.3389/fpls.2018.01469
Yang, X., Li, X., Zhou, Y., Zheng, W., Yu, C., and Zheng, T. (2014). Novel insights into the algicidal bacterium DH77-1 killing the toxic dinoflagellate Alexandrium tamarense. Sci. Total Environ. 482–483, 116–124. doi: 10.1016/j.scitotenv.2014.02.125
Ye, Y., Yan, C., Nie, Y., Zhang, J., Zhao, Z., Zhang, R., et al. (2020). Nitratireductor mangrovi sp. nov., a nitrate-reducing bacterium isolated from mangrove soil. Curr. Microbiol. 77, 1334–1340. doi: 10.1007/s00284-020-01885-9
Zamkovaya, T., Foster, J. S., de Crécy-Lagard, V., and Conesa, A. (2021). A network approach to elucidate and prioritize microbial dark matter in microbial communities. ISME J. 15, 228–244. doi: 10.1038/s41396-020-00777-x
Zhou, Z., Tran, P. Q., Breister, A. M., Liu, Y., Kieft, K., Cowley, E. S., et al. (2022). METABOLIC: high-throughput profiling of microbial genomes for functional traits, metabolism, biogeochemistry, and community-scale functional networks. Microbiome 10, 1–22. doi: 10.1186/s40168-021-01213-8
Zhu, D., Niu, L., Wang, C., and Nagata, S. (2007). Isolation and characterisation of moderately halophilic bacterium Halomonas ventosae DL7 synthesizing ectoine as compatible solute. Ann. Microbiol. 57, 401–406. doi: 10.1007/BF03175080
Zhu, P., Wang, Y., Shi, T., Zhang, X., Huang, G., and Gong, J. (2018). Intertidal zonation affects diversity and functional potentials of bacteria in surface sediments: a case study of the golden bay mangrove, China. Appl. Soil Ecol. 130, 159–168. doi: 10.1016/j.apsoil.2018.06.003
Keywords: heterotrophic bacteria, cultivation, mangroves, extreme adaptation, rare biosphere, petroleum hydrocarbon-degraders
Citation: Marasco R, Michoud G, Sefrji FO, Fusi M, Antony CP, Seferji KA, Barozzi A, Merlino G and Daffonchio D (2023) The identification of the new species Nitratireductor thuwali sp. nov. reveals the untapped diversity of hydrocarbon-degrading culturable bacteria from the arid mangrove sediments of the Red Sea. Front. Microbiol. 14:1155381. doi: 10.3389/fmicb.2023.1155381
Received: 31 January 2023; Accepted: 04 April 2023;
Published: 02 May 2023.
Edited by:
Panagiota Stathopoulou, University of Patras, GreeceReviewed by:
Kunal Jani, University of Ulm, GermanyGiorgia Pertile, Polish Academy of Sciences, Poland
Copyright © 2023 Marasco, Michoud, Sefrji, Fusi, Antony, Seferji, Barozzi, Merlino and Daffonchio. This is an open-access article distributed under the terms of the Creative Commons Attribution License (CC BY). The use, distribution or reproduction in other forums is permitted, provided the original author(s) and the copyright owner(s) are credited and that the original publication in this journal is cited, in accordance with accepted academic practice. No use, distribution or reproduction is permitted which does not comply with these terms.
*Correspondence: Daniele Daffonchio, ZGFuaWVsZS5kYWZmb25jaGlvQGthdXN0LmVkdS5zYQ==; Ramona Marasco, cmFtb25hLm1hcmFzY29Aa2F1c3QuZWR1LnNh