- Department of Pharmacy, Southwest Hospital, Third Military Medical University (Army Medical University), Chongqing, China
Introduction: Colistin is regarded as one of the last-resort antibiotics against severe infections caused by carbapenem-resistant Enterobacteriaceae. Strains with cooccurrence of mcr-9 and carbapenemase genes are of particular concern. This study aimed to investigate the genetic characteristics of a blaKPC-2-carrying plasmid, blaNDM-1-carrying plasmid and mcr-9-carrying plasmid coexisting in a carbapenem-resistant Enterobacter hormaechei isolate.
Methods: E. hormaechei strain E1532 was subjected to whole-genome sequencing, and the complete nucleotide sequences of three resistance plasmids identified in the strain were compared with related plasmid sequences. The resistance phenotypes mediated by these plasmids were analyzed by plasmid transfer, carbapenemase activity and antimicrobial susceptibility testing.
Results: Whole-genome sequencing revealed that strain E1532 carries three different resistance plasmids, pE1532-KPC, pE1532-NDM and pE1532-MCR. pE1532-KPC harboring blaKPC-2 and pE1532-NDM harboring blaNDM-1 are highly identical to the IncR plasmid pHN84KPC and IncX3 plasmid pNDM-HN380, respectively. The mcr-9-carrying plasmid pE1532-MCR possesses a backbone highly similar to that of the IncHI2 plasmids R478 and p505108-MDR, though their accessory modules differ. These three coexisting plasmids carry a large number of resistance genes and contribute to high resistance to almost all antibiotics tested, except for amikacin, trimethoprim/sulfamethoxazole, tigecycline and polymyxin B. Most of the plasmid-mediated resistance genes are located in or flanked by various mobile genetic elements, facilitating horizontal transfer of antibiotic resistance genes.
Discussion: This is the first report of a single E. hormaechei isolate with coexistence of three resistance plasmids carrying mcr-9 and the two most common carbapenemase genes, blaKPC-2 and blaNDM-1. The prevalence and genetic features of these coexisting plasmids should be monitored to facilitate the establishment of effective strategies to control their further spread.
1. Introduction
Carbapenem-resistant Enterobacteriaceae (CRE) bacteria pose a serious threat to global public health owing to rapid emergence of multidrug resistance (MDR) and limited therapeutic agents available (Ma et al., 2023). Production of carbapenemases, especially KPC and NDM, is the main mechanism of carbapenem resistance in CRE clinical isolates (Peng et al., 2022). The blaKPC gene is typically located on plasmids of different incompatibility (Inc) groups, such as IncF-, IncI-, IncA/C-, IncX-, and IncR-type plasmids (Chen et al., 2014), and the blaNDM gene is mainly carried by IncX3-type plasmids (Zhang et al., 2017; Yoon et al., 2018). These plasmids, which are often easily transferable, can facilitate the spread of blaKPC and blaNDM resistance genes by horizontal gene transfer among different bacterial populations, complicating clinical therapy and infection control.
Colistin, a cationic polypeptide, is regarded as an antibiotic of last resort for treatment of severe infections caused by CRE (Poirel et al., 2017; Yang et al., 2020). However, the discovery of plasmid-mediated mobile colistin resistance (mcr) genes has triggered extensive concern due to the possibility of horizontal transfer of colistin resistance. The mcr-1 gene was the first mcr variant initially reported in China in 2015 in Escherichia coli and Klebsiella pneumoniae isolates (Liu et al., 2016). To date, various types of mcr genes (mcr-2 to mcr-10) have been identified worldwide in Enterobacteriaceae (Ling et al., 2020; Zhang et al., 2022). mcr-9 is closely related to mcr-3, with 65% amino acid identity and 99.5% nucleotide identity (Kieffer et al., 2019). It was first identified in a colistin-susceptible Salmonella enterica serotype typhimurium clinical isolate in the United States in 2019 (Carroll et al., 2019) and now has disseminated to various Enterobacteriaceae species, with global distribution in 21 countries across six continents (Li et al., 2020). Surprisingly, reports on coexistence of mcr-9 and carbapenemase genes (such as blaNDM, blaVIM, blaKPC, blaIMP and blaOXA-48) in Enterobacteriaceae have been increasing worldwide (Chavda et al., 2019; Yuan et al., 2019; Kananizadeh et al., 2020; Liu et al., 2021; Simoni et al., 2021; Yao et al., 2022). These genes may be present in different gene cassettes on a single plasmid or different plasmids from one isolated strain. In this study, we describe cooccurrence of three different MDR plasmids, pE1532-KPC, pE1532-NDM and pE1532-MCR, which carry the blaKPC-2, blaNDM-1, and mcr-9 genes, respectively, in a single carbapenem-resistant Enterobacter hormaechei clinical isolate. To the best of our knowledge, this is the first report of a clinical E. hormaechei isolate coharboring mcr-9 and the two most common carbapenemase genes, blaKPC-2 and blaNDM-1.
2. Materials and methods
2.1. Bacterial isolation and identification
Enterobacter hormaechei E1532 was isolated from hydrothorax and ascites samples of a patient in a teaching hospital in Henan, China, in 2015. Bacterial species were identified using the VITEK 2 compact system (bioMérieux, France) as well as 16S rRNA sequencing. The presence of carbapenemase genes (Chen et al., 2015) and mcr genes (mcr-1 to mcr-10) (Rebelo et al., 2018; Borowiak et al., 2020; Kim et al., 2021) was screened by PCR amplification using primers described previously, and the positive products were sequenced using an ABI Sequencer (Life Technologies, CA, United States). The genotype of strain E1532 was analyzed using the multilocus sequence typing (MLST) method to amplify and sequence the seven housekeeping genes (dnaA, fusA, gyrB, leuS, pyrG, rplB and rpoB) (Miyoshi-Akiyama et al., 2013), and sequence type (ST) was defined on the basis of seven allele numbers available on the MLST website.1
2.2. Whole-genome sequencing and sequence assembly
Total genomic DNA of E. hormaechei E1532 was extracted from cell pellets using a bacterial DNA kit (OMEGA, USA), and the purified DNA was subjected to whole-genome sequencing by a combination of PacBio RS (Pacifc Biosciences, CA, USA) and Illumina NovaSeq (Illumina, CA, USA) sequencing platforms. Paired-end DNA libraries were constructed with an average insert size of 400 bp (ranging from 300 to 500 bp) for Illumina sequencing, and shotgun DNA libraries were generated with a 15 kb insert size (ranging from 10 kb to 20 kb) for PacBio Biosciences sequencing. Clean reads were obtained after filtering the low-quality sequence data and then de novo assembled by Unicycler software (Wick et al., 2017). The Illumina-generated short reads were utilized to correct the PacBio-generated long reads using the Pilon tool (Walker et al., 2014).
2.3. Genome annotation and bioinformatics analysis
Prediction and annotation of coding genes and pseudogenes was carried out using the RAST 2.0 algorithm (Brettin et al., 2015). Putative open reading frames (ORFs) were further assessed for functions by BLASTP and BLASTN (Boratyn et al., 2013) against the NCBI RefSeq (O’Leary et al., 2016) and UniProtKB/Swiss-Prot (Boutet et al., 2016) databases. The precise species assignment was further confirmed based on whole-genome sequencing using average nucleotide identity (ANI) analysis with the JSpeciesWS server. PlasmidFinder (Carattoli et al., 2014) was used to examine plasmid replicon type. The presence of antibiotic resistance genes, insertion sequences (ISs), transposons and integrons was analyzed in silico using the ResFinder (Bortolaia et al., 2020), CARD (Jia et al., 2017), ISfinder (Siguier et al., 2006), Tn Number Registry (Roberts et al., 2008) and INTEGRALL (Moura et al., 2009) databases. BLASTN and MUSCLE 3.8.31 (Edgar, 2004) were employed for alignment and comparison of the plasmid sequences analyzed in this study with highly homologous plasmid sequences publicly available in NCBI. The circular graph of plasmid sequences and linear comparative graph were constructed by Inkscape 0.48.1 software.2
2.4. Plasmid transfer
The filter mating method was used for the plasmid conjugation assay (Ouyang et al., 2018). Briefly, rifampicin-resistant E. coli EC600 was used as the recipient. Equal amounts of donor and recipient strains were mixed together, and mating was performed on the filter membranes of brain heart infusion (BHI) agar plates for 12–18 h at 37°C. The mixtures were spread on doubly selective agar plates containing rifampin together with indicated additional antibiotics for selecting an E. coli transconjugant carrying one of the following resistance markers: imipenem for blaKPC (pE1532-KPC) and blaNDM (pE1532-NDM), and azithromycin for mph(A) (pE1532-MCR). The presence of the resistance markers carried by transconjugants was confirmed by PCR amplification and sequencing.
For the electrotransformation experiment, streptomycin- and tetracycline-resistant E. coli TOP10 was selected as the recipient. Plasmid DNA was extracted using QIAGEN Plasmid Midi Kit (Qiagen, Germany) and transformed into TOP10 competent cells by electroporation. After reviving the bacterial cells for 1 h at 37°C and 200 rpm, positive electroporants carrying the blaKPC or blaNDM or mph(A) gene were selected on super optimal broth (SOB) agar plates containing imipenem or azithromycin, and further verified by PCR and sequence analysis.
2.5. Carbapenemase activity assay
The class A/B/D carbapenemase activity of the E1532 strain as well as its transconjugant and electroporant was assessed by the CarbaNP test as described in our previous study (Feng et al., 2022). Overnight bacterial cultures were seeded into MH broth supplemented with 4 μg/ml imipenem and incubated with continuous shaking until the bacterial density reached 1.0–1.4. Cell pellets were collected by centrifugation and subjected to washing with Tris–HCl twice. The sonication process was performed to lyse the bacterial cells. The supernatant from the cell lysis solution was added to substrates I–V at a ratio of 1:1 and then allowed to interact at 37°C for 1–2 h. The phenotypic results of carbapenemase activity were observed by color changes of the mixture.
2.6. Antimicrobial susceptibility testing
Minimum inhibitory concentrations (MICs) were determined using VITEK 2 System and AST-N334 cards for the following antimicrobial agents: amoxicillin/clavulanic acid, piperacillin/tazobactam, cefuroxime, cefuroxime axetil, cefoxitin, ceftazidime, ceftriaxone, cefoperazone/sulbactam, cefepime, ertapenem, imipenem, amikacin, levofloxacin, tigecycline and trimethoprim/sulfamethoxazole. For polymyxin B, the susceptibility test was performed with the broth microdilution method. The resistance results for tigecycline and colistin were judged according to the breakpoints of European Committee on Antimicrobial Susceptibility Testing (EUCAST)3; the breakpoints of other antibiotics were defined following the standard of the Clinical and Laboratory Standards Institute (CLSI). The E. coli standard strain ATCC 25922 served as the quality control for susceptibility testing.
2.7. Nucleotide sequence accession numbers
The complete sequences of chromosomes and plasmids have been submitted to the GenBank database under accession numbers CP114571 (chromosome), CP114573 (pE1532-KPC), CP114574 (pE1532-MCR), CP114575 (pE1532-NDM), and CP114572 (pE1532-4).
3. Results and discussion
3.1. Enterobacter hormaechei E1532 coharboring blaKPC-2, blaNDM-1 and mcr-9 genes
E. hormaechei E1532 was found to be resistant to all penicillins, cephalosporins, carbapenems, and fluoroquinolones and showed intermediate resistance to tigecycline; it remained sensitive to amikacin, polymyxin B and trimethoprim/sulfamethoxazole (Table 1). PCR screening and sequencing identification showed that E. hormaechei E1532 harbors two carbapenemase genes, blaKPC-2 and blaNDM-1, and a newly identified colistin resistance gene, mcr-9. MLST analysis revealed that strain E1532 belongs to ST93 (allelic profile: 9–4–14-61-37-4-9), which is a globally disseminated high-risk clone that is frequently reported in China (Peirano et al., 2018; Zhao et al., 2020; Chen et al., 2021).
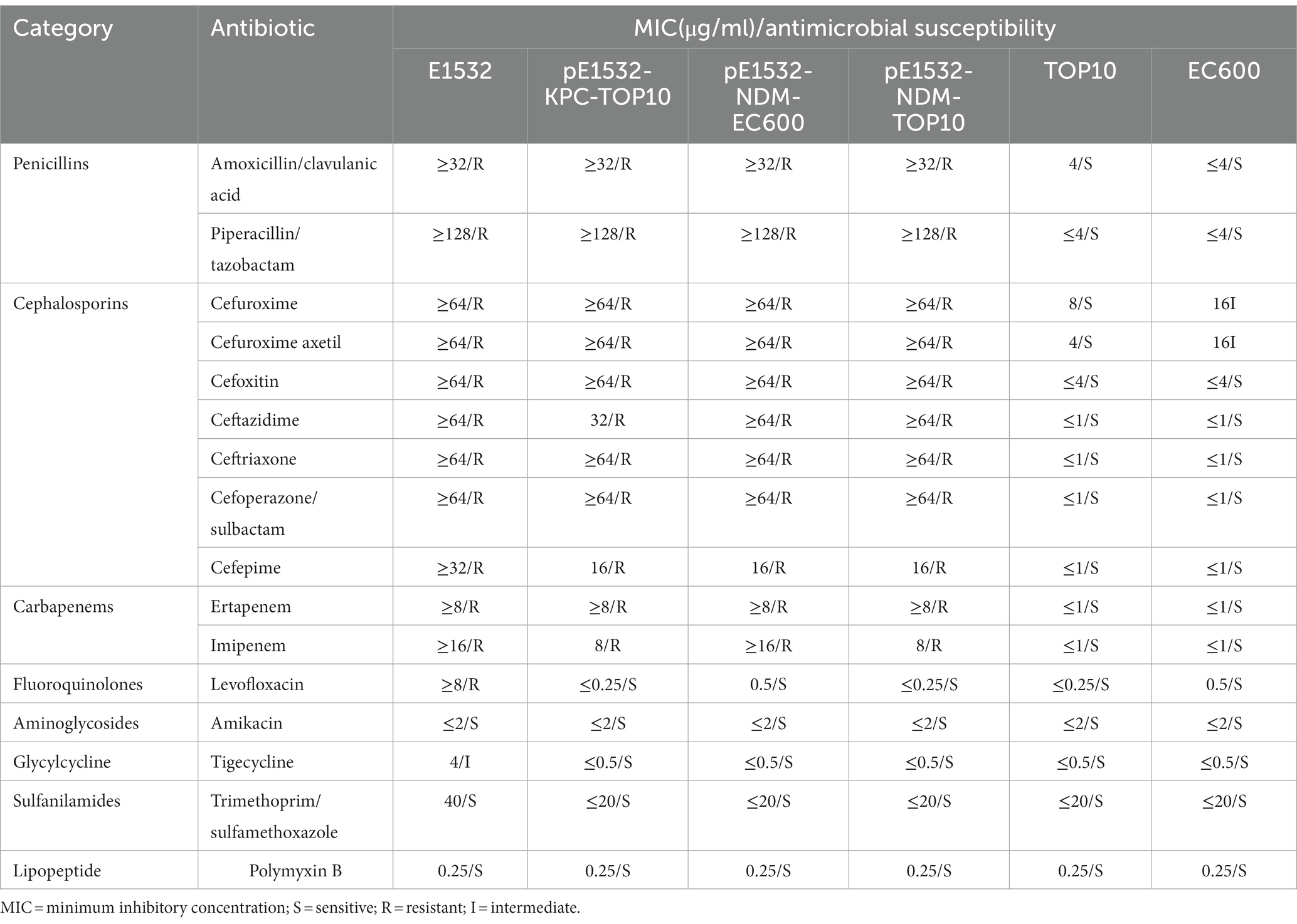
Table 1. Antimicrobial susceptibility profiles of Enterobacter hormaechei E1532 and its transconjugants and electroporants.
Whole-genome sequencing revealed that strain E1532 has a single circular chromosome sequence of 4,869,794 bp with an average G + C content of 55.31% and contains a total of 4,717 predicted ORFs. A total of four plasmids, namely, pE1532-KPC, pE1532-NDM, pE1532-MCR and pE1532-4, were present in strain E1532, with circular closed DNA sequences of 39,461 bp, 53,769 bp, 308,217 bp, and 69,180 bp in length with 44, 62, 360, and 90 predicted ORFs, respectively (Supplementary Figure S1; Table 2). Each plasmid consists of backbone regions (responsible for plasmid maintenance, replication and/or conjugal transfer) and one or more accessory modules (acquired DNA regions associated with and bordered by mobile elements) inserted at different sites of the backbone regions (Supplementary Figure S1; Table 2). Antibiotic resistance genes were identified using ResFinder and CARD analysis. The chromosome of E1532 carries three intrinsic resistance genes involved in resistance to β-lactams (blaACT-7), aminoglycoside [aph(3′)-Ia] and fosfomycin (fosA). Plasmids pE1532-KPC, pE1532-NDM and pE1532-MCR harbor a total of 19 genes conferring resistance to β-lactams (blaKPC-2, blaNDM-1, blaSHV-12, blaDHA-1, blaTEM-1), aminoglycosides [aacC2, aphA1, aph(6)-Id, aph(3″)-Ib], tetracyclines [tet(A)], bleomycin (blaMBL), macrolide [mph(A)], fluoroquinolones (qnrB4), sulfonamide (sul1), tunicamycin (tmrB), colistin (mcr-9), quaternary ammonium (qacED1), tellurium (the ter locus), and mercuric (the mer locus) (Table 3). However, no resistance gene was found on plasmid pE1532-4, which only harbors a Tn3-family transposon remnant.
Plasmid pE1532-NDM was successfully transferred to E. coli by electrotransformation and conjugation experiments, obtaining the corresponding electroporant pE1532-NDM-TOP10 and transconjugant pE1532-NDM-EC600 (Table 1). In order to investigate the transmissible possibility of conjugative plasmid pE1532-NDM in different hosts, the conserved backbone sequences of pE1532-NDM were aligned by BLASTN with the blaNDM-1-harboring IncX3 plasmids available in GenBank. Among the top 100 plasmids with the highest backbone sequence similarity of pE1532-NDM, the plasmids were selected based on the origin of bacterial species or hosts different from pE1532-NDM. A total of 11 plasmids were included and they were mainly isolated from bacterial strains from human. In addition, there were also four plasmids from shrimp, chicken, hospital sewage and unknown source, respectively (Supplementary Table S1). The results of our analysis showed that IncX3 plasmids have an extensive host range. However, only the electroporant pE1532-KPC-TOP10, and not the transconjugant, was obtained by transferring pE1532-KPC into E. coli, which may be because pE1532-KPC lacks the conjugal transfer genes in backbone regions (Supplementary Figure S1) and is not self-transmissible (Compain et al., 2014; Jing et al., 2019). Repeated attempts failed to transfer pE1532-MCR into E. coli through conjugation and electroporation; this may be attributed to the following facts: (1) pE1532-MCR is a 308 kb megaplasmid which limits the success of conjugation and electroporation. (2) the insertion event occurred within the two conjugal transfer regions tra1 and tra2 might render this plasmid nonconjugative (Supplementary Figure S1). In a carbapenemase activity assay, strain E1532 showed class A + B activity, the pE1532-NDM-harboring electroporant and transconjugant class B activity, and the pE1532-KPC-harboring electroporant A activity (data not shown). Phenotypic susceptibility testing showed these electroporants and transconjugant to be resistant to all β-lactams tested, including carbapenems (Table 1), which was consistent with the presence of carbapenemase genes in these strains.
3.2. Comparative genomics of blaKPC-2-carrying plasmid pE1532-KPC
The entire sequence of pE1532-KPC is highly similar to that of the reference IncR plasmid pHN84KPC (accession number KY296104), with 100% query coverage and > 99% nucleotide identity (Figure 1). pE1532-KPC and pHN84KPC share the most complete IncR backbone gene loci encoding plasmid replication initiation (repB) and plasmid maintenance (parAB, umuCD, retA, vagCD, resD). A single accessory module, the blaKPC-2 region containing two resistance genes, blaKPC-2 and tet(A), is inserted at a site between retA and vagD in the backbone of these two plasmids (Supplementary Figure S1). The blaKPC-2 region contains the tet(A)-carrying ΔTn1721 cassette (Allmeier et al., 1992) and the blaKPC-2-carrying ΔTn6296 cassette (Jiang et al., 2010) flanked by the upstream IS1X3-IS903B-ΔIS903B region and the downstream Tn3-family transposon remnant-ISKpn19-ΔISEc15 region (Figure 1). The only modular difference between these two plasmids is with regard to the copy number (21 in pHN84KPC, 27 in pE1532-KPC) of the 37-bp tandem repeat within interons, which is located behind the truncated replication initiation gene ΔrepA in the blaKPC-2 region.
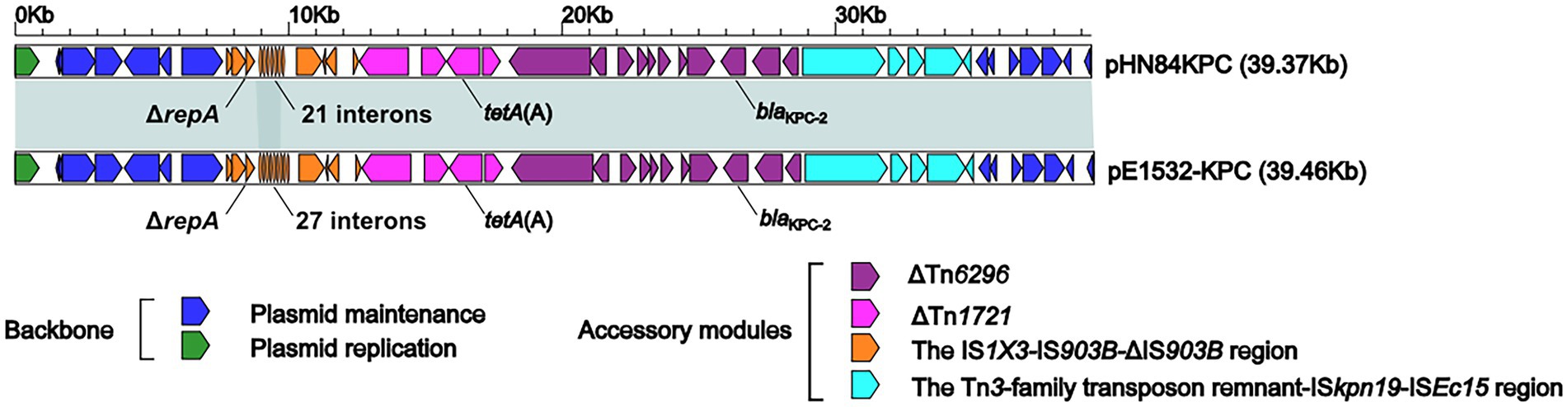
Figure 1. Linear comparison of plasmid pE1532-KPC with pHN84KPC (accession number KY296104). Genes are denoted by arrows. Genes, mobile elements and other features are colored based on function classification. Shading denotes shared regions of homology (>95% nucleotide identity).
3.3. Comparative genomics of blaNDM-1-carrying plasmid pE1532-NDM
pE1532-NDM displays >99% nucleotide identity (with 100% query coverage) with the reference IncX3 plasmid pNDM-HN380 (Ho et al., 2012) obtained from K. pneumoniae isolate CRE380 in China (Figure 2A). pE1532-NDM and pNDM-HN380 have identical backbones that share a set of core genes for plasmid replication (repB and bis), partition (parA), maintenance (topB and stpA) and conjugal transfer (pilX genes) (Supplementary Figure S1). Each of them harbors two accessory modules, ISKox3 and the blaNDM-1 region, which are inserted at different sites of the plasmid backbone. All resistance genes are located in the blaNDM-1 region. This accessory resistance region (18 kb in length) originated sequentially as a truncated IS26-blaSHV-12-IS26 unit, blaNDM-1-carrying ΔTn125, IS3000 and ΔTn3 (Figure 2B). The truncated IS26-blaSHV-12-IS26 unit was generated by deletion of yjbJ (partial)-yjbK-yjbL-yjbM genes and inversion of IS26 at the 3′ region from the prototype composite transposon-like IS26-blaSHV-12-IS26 unit (Ford and Avison, 2004). ΔTn125 is a derivative of ISAba125-flanked composite transposon Tn125 (Poirel et al., 2012), lacking the ISAba125 element at the 3′ region and having an interrupted and truncated ISAba125 at the 5′ region by insertion of an IS5 element.
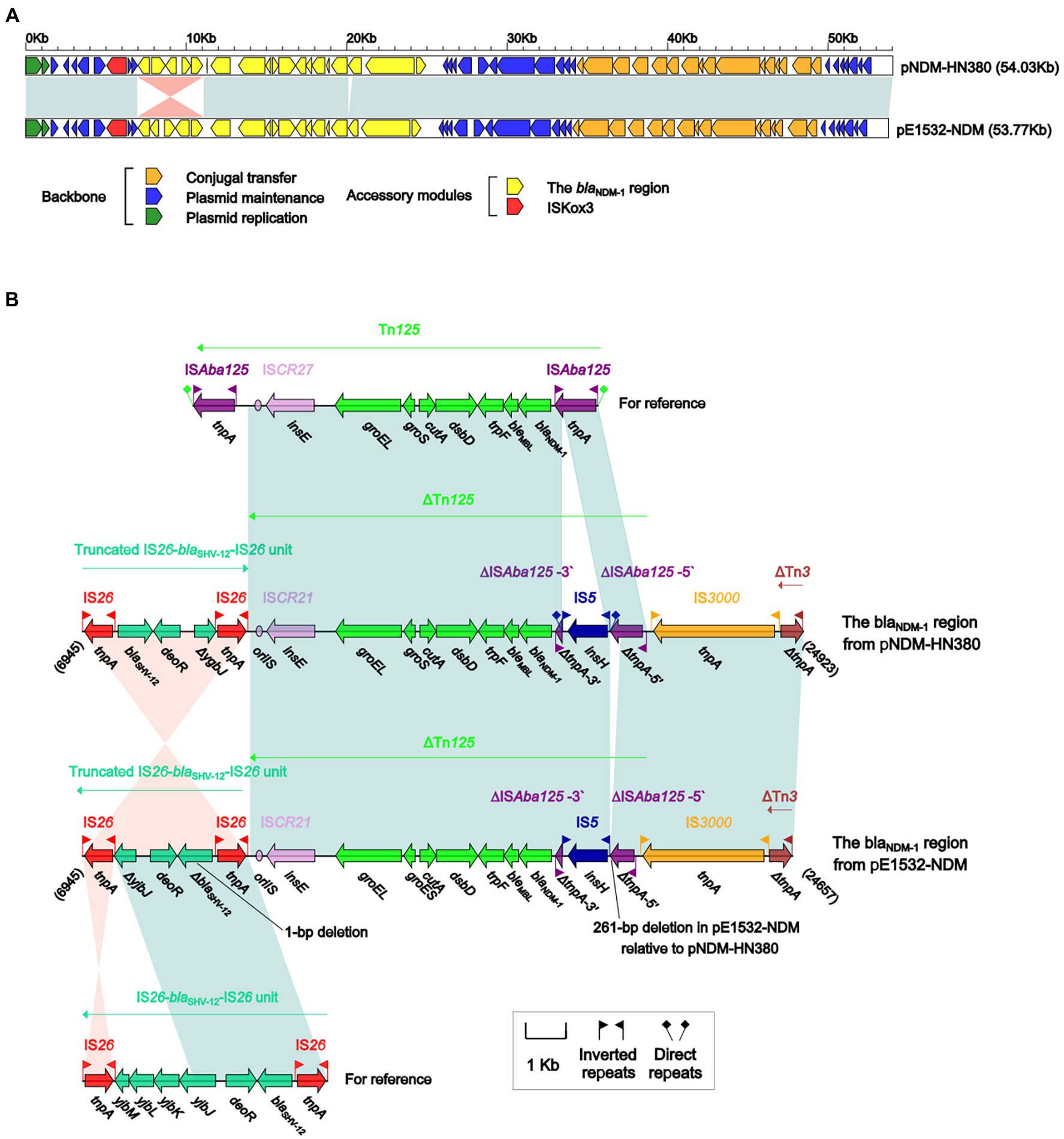
Figure 2. Comparison of plasmid pE1532-NDM with pNDM-HN380. (A) Linear comparison of the two sequenced plasmids pE1532-NDM and pNDM-HN380 (accession number JX104760). (B) The blaNDM-1 region from pE1532-NDM and comparison with related regions. Genes are denoted by arrows. Genes, mobile elements and other features are colored based on functional classification. Shading denotes shared regions of homology (≥90% nucleotide identity). Numbers in brackets indicate nucleotide positions within the corresponding plasmids. The accession numbers of the Tn125 and IS26-blaSHV-12-IS26 unit for reference are JN872328 and CP003684, respectively.
At least four major module differences were identified between the blaNDM-1 regions of pE1532-NDM and pNDM-HN380 (Figure 2B). First, the truncated IS26-blaSHV-12-IS26 unit in pE1532-NDM is inverted compared to pNDM-HN380. Second, the blaSHV-12 gene cannot form an ORF due to the absence of 1 bp, making it a pseudogene. Third, a 261-bp deletion at the 5′-terminal region of ΔISAba125 was found in pE1532-NDM. Fourth, direct repeats (DRs) have been lost at both ends of the IS5 element in pE1532-NDM.
3.4. Comparative genomics of the mcr-9-carrying plasmid pE1532-MCR
The pE1532-MCR backbone is closely related to the first prototype IncHI2 plasmid R478 (Gilmour et al., 2004) from Serratia marcescens, with 95% query coverage and > 99% nucleotide identity, and to another IncHI2 plasmid, p505108-MDR (Shi et al., 2018) from Cronobacter sakazakii, with 98% query coverage and > 99% nucleotide identity (Supplementary Figure S2). These three plasmids share the core IncHI2 backbone, including the regions responsible for plasmid replication (repHIA, repHI2), partition (parAB, parMR) and conjugal transfer (tra1 and tra2 regions) (Supplementary Figure S1).
Linear comparison revealed that the following different regions are present among the backbones of pE1532-MCR, R478 and p505108-MDR (Supplementary Figure S2A). (1) An IS903B element in pE1532-MCR but a ΔIS903D element in p505108-MDR is inserted between parR and htdA relative to R478, leading to the interruption of the conjugal transfer region tra2. (2) An ISKpn26 element is inserted between the backbone genes orf2034 and orf222 in pE1532-MCR. (3) An IS5 element is inserted between orf1161 and hha in pE1532-MCR. (4) Two backbone regions, hipB-hipA-orf207-orf411 and orf189-orf426-orf258, are inserted between orf1389 and orf609 in pE1532-MCR and p505108-MDR. (5) orf159 is interrupted by a Tn2 element in pE1532-MCR and p505108-MDR. (6) The klaB to orf534 region is truncated by insertion of the MDR-2 region in pE1532-MCR and p505108-MDR relative to R478, leaving only the remnant of the klaB and orf534 genes. (7) An IS903B element in pE1532-MCR but a ΔIS903D element in p505108-MDR is inserted between orf2385 and orf450, leading to the truncation of both genes. (8) A 377-bp insertion at the 3′-end of orf444 resulted in the replacement of orf444 with orf588 in pE1532-MCR and p505108-MDR. (9) The MDR-1 and MDR-2 regions are inserted into the boundary of ΔklaB and orf819, respectively, with inversion of the entire backbone region between ΔklaB and orf819 in pE1532-MCR.
To more clearly and intuitively observe the genetic features of the backbone region between ΔklaB and orf819 in these three plasmids, the ΔklaB to orf819 region in pE1532-MCR was reverted compared with that of R478 and p505108-MDR (Supplementary Figure S2B). The genetic differences among them were as follows: (1) An IS150 element is located between the two backbone genes orf198 and ldrB in R478 but replaced by both the ISCfr9-ISCfr15 region and the orf612-fieF-relB-relE backbone region in pE1532-MCR and p505108-MDR. (2) IS186B is lost between ldrB and orf321 in pE1532-MCR and p505108-MDR relative to R478. (3) Tn10 is inserted into orf300 in R478. (4) The traI gene in the conjugal transfer region tra1 is interrupted by a truncated IS903B in pE1532-MCR. (5) The IS1B-ISKpn26 region and ISKpn21-∆Tn6363 region are inserted into the mucA to orf1404 backbone region in pE1532-MCR, resulting in deletion of backbone genes between orf318-1 and mucA as well as truncation of mucA relative to R478; acquisition of the aphA1a region in p505108-MDR causes loss of the orf318-2 to retA backbone region as well as truncation of mucA. (6) orf258 is interrupted by an IS903B element in pE1532-MCR.
The differences described above involve not only backbone regions but also accessory modules. pE1532-MCR carries a total of 13 accessory modules, and resistance genes are located in the MDR-1 region (Figure 3), the MDR-2 region (Figure 4), the ISKpn21:∆Tn6363 region (Figure 5), Tn6362 (Supplementary Figure S3) and Tn2 (Supplementary Figure S3).
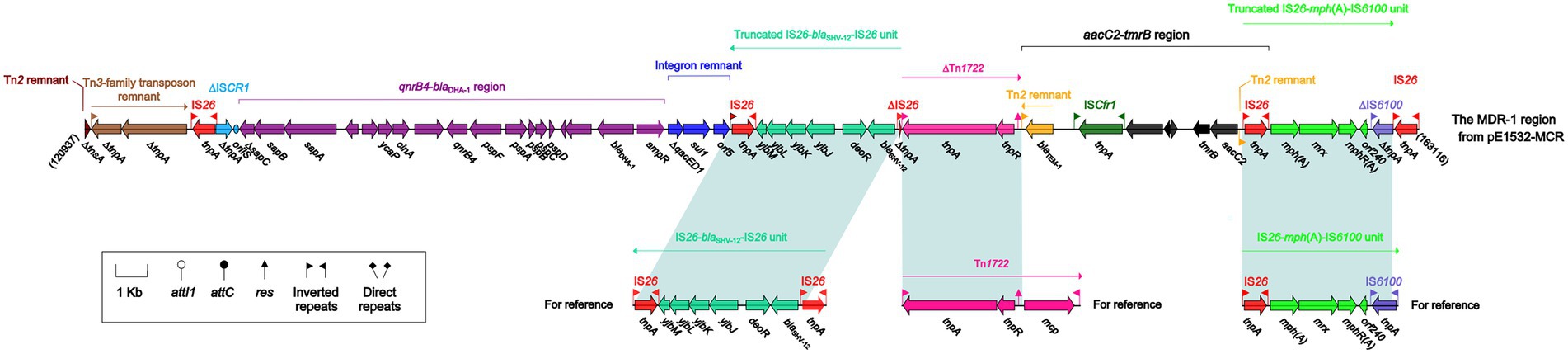
Figure 3. The MDR-1 region from pE1532-MCR and comparison with related regions. Genes are denoted by arrows. Genes, mobile elements and other features are colored based on functional classification. Shading denotes shared regions of homology (≥90% nucleotide identity). Numbers in brackets indicate nucleotide positions within the corresponding plasmids. The accession numbers of the IS26-blaSHV-12-IS26 unit, IS26-mph(A)-IS6100 unit and Tn1722 for reference are CP003684, KY270852 and X61367, respectively.
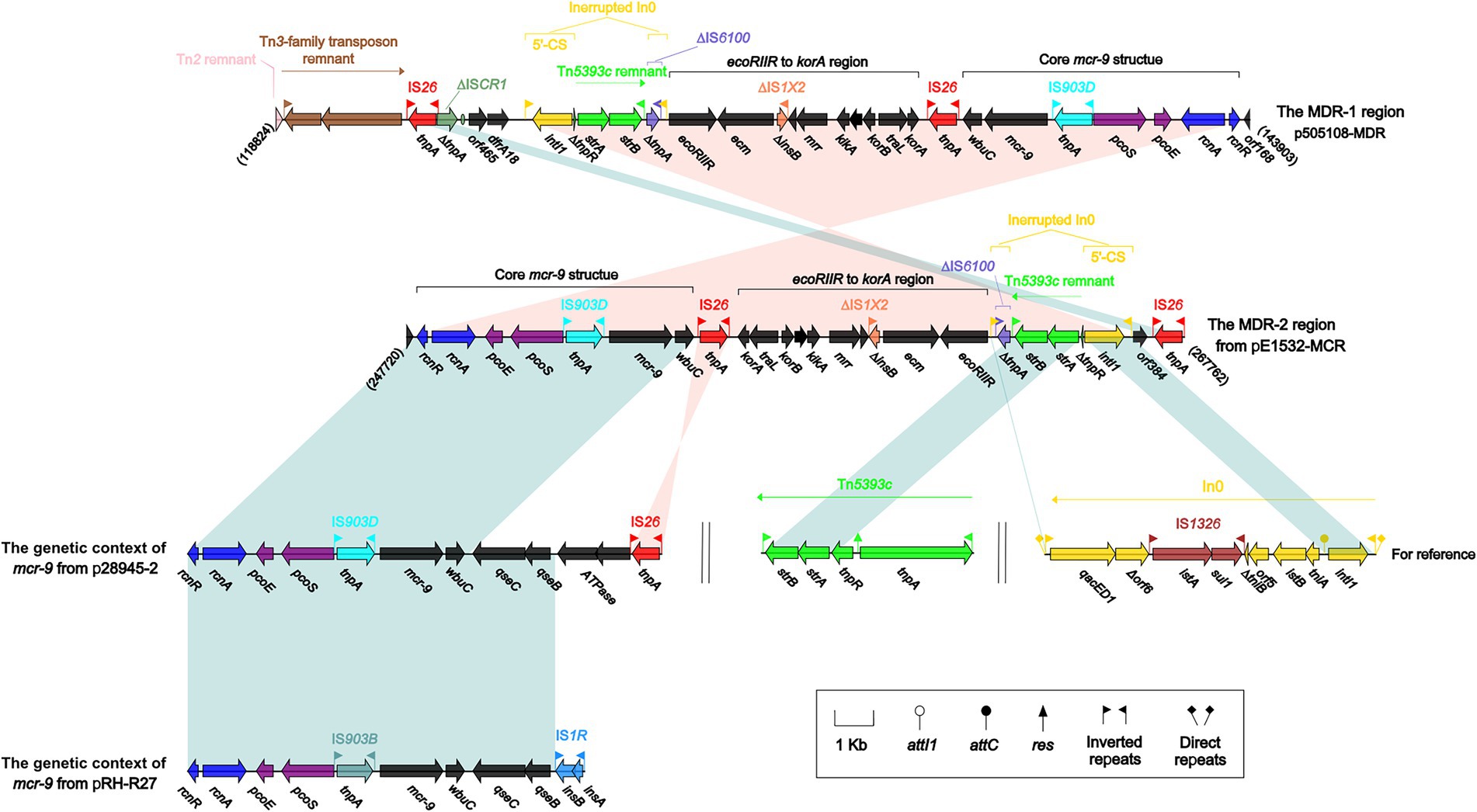
Figure 4. The MDR-2 region from pE1532-MCR and comparison with related regions. Genes are denoted by arrows. Genes, mobile elements and other features are colored based on functional classification. Shading denotes shared regions of homology (≥90% nucleotide identity). Numbers in brackets indicate nucleotide positions within the corresponding plasmids. The accession numbers of Tn5393c and In0 for reference are AF262622 and U49101, respectively.
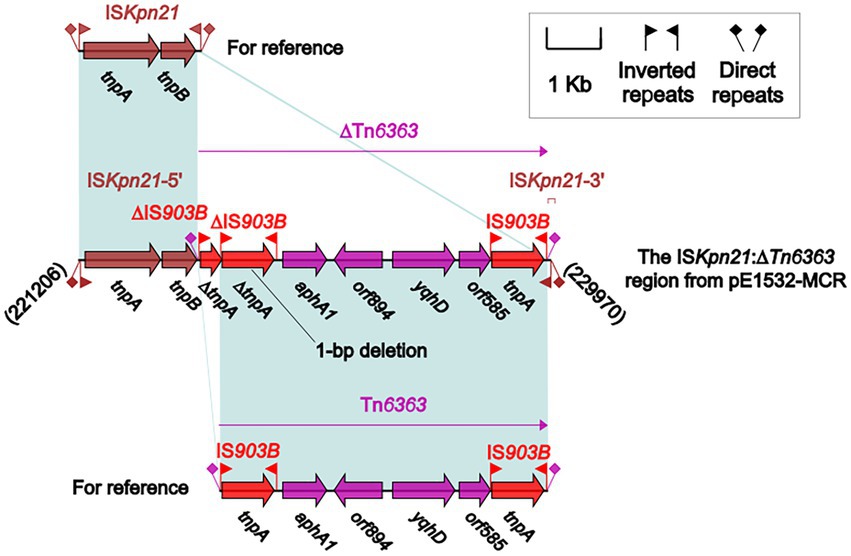
Figure 5. The ISKpn21:∆Tn6363 region from pE1532-MCR and comparison with related regions. Genes are denoted by arrows. Genes, mobile elements and other features are colored based on functional classification. Shading denotes shared regions of homology (≥90% nucleotide identity). Numbers in brackets indicate nucleotide positions within the corresponding plasmids. The accession numbers of ISKpn21 and Tn6363 for reference are AP012055 and KY978630, respectively.
3.5. The MDR-1 region of pE1532-MCR
The MDR-1 region (Figure 3) harbors five antibiotic resistance loci: qnrB4-blaDHA-1 region (Yim et al., 2013), integron remnant carrying ΔqacED1 and sul1, a truncated IS26-blaSHV-12-IS26 unit (Ford and Avison, 2004), aacC2-tmrB region (Partridge et al., 2012), and a truncated IS26-mph(A)-IS6100 unit (Liang et al., 2017). IS26-blaSHV-12-IS26 is an IS26-flanked extended-spectrum β-lactamase (ESBL) resistance unit lacking direct repeats (DRs) at both ends. A number of IS26-based resistance units have been reported with diverse resistance genes (Partridge, 2011). IS26 can mediate movement of antibiotic resistance genes and formation of composite transposons, which contribute to MDR region assembly (Harmer and Hall, 2016; Harmer et al., 2020). The IS26-blaSHV-12-IS26 unit in the MDR-1 region has undergone truncation of the upstream IS26 element and deletion of its 14-bp IRR (inverted repeat right).
Tn1722 belongs to a Tn3-family unit transposon harbored in the tetracycline resistance transposon Tn1721 (Allmeier et al., 1992) and includes tnpA, tnpR, res and mcp genes bounded by 38-bp IRL (inverted repeat left) and IRR. The ΔTn1722 element in the MDR-1 region is a derivative of Tn1722 with deletion of mcp and IRR, which is also found in pCRE3-KPC (Dong et al., 2020) and plasmid unnamed3 (accession number CP027150). The IS26-mph(A)-IS6100 unit contains a macrolide resistance region mph(A)-mrx-mphR(A) (Noguchi et al., 2000) bracketed by IS26 and IS6100 elements. These two IS elements, which have almost identical 14-bp IRs, belong to the IS6 family, and the homologous recombination mediated by them promotes integration of this resistance unit into the MDR region, similar to the IS26-bound resistance unit.
3.6. The MDR-2 region of pE1532-MCR
The MDR-2 region of pE1532-MCR (Figure 4) is highly similar to the MDR-1 region of p505108-MDR (97% query coverage and > 99% nucleotide identity), with a reverse array of the orf168 to intI1 region and with the same orientated IS26 downstream of interrupted In0. Both MDR regions harbor a mcr-9 gene, and the genetic environments of mcr-9 are identical to each other. Mcr-9 is flanked by IS903D and IS26 elements, both belonging to the IS6 family, which play a vital role in dissemination of resistance genes. The genes upstream of mcr-9 include rcnR, rcnA, pcoE and pcoS. However, only the wbuC gene is located downstream of mcr-9, and a two-component system encoding the genes qseB and qseC, which are involved in mcr-9 expression (Kieffer et al., 2019), was not found. The lack of qseB-qseC regulatory genes in plasmid pE1532-MCR may explain the phenomenon that strain E1532 carrying mcr-9 is sensitive to polymyxin B. The gene composition arrayed as rcnR-rcnA-pcoE-pcoS-IS903-mcr-9-wbuC is the core structure of mcr-9 resistance cassettes in the mcr-9-carrying IncHI2 plasmids from different Enterobacteriaceae (Li et al., 2020). The different encoding genes and IS elements located downstream of mcr-9 are the main causes leading to the diverse genetic context of mcr-9 in IncHI2 plasmids.
In addition to mcr-9, other resistance genes (strA, strB) are present in the MDR-2 region. The class 1 integron In0 (accession number U49101) from Pseudomonas aeruginosa plasmid pVS1 is an ancestor of more complex integrons with a weak PcW promoter and an unoccupied integration site attI in 5′-CS but no gene cassette array (Bissonnette and Roy, 1992). ΔIn0 from the MDR region of pE1532-MCR/p505108-MDR contains the 5′-CS composed of the integrase gene intI1 and promoter gene and is bordered by IRi (inverted repeat at the integrase end) and IRt (inverted repeat at the tni end), which resulted from insertion of ΔIS6100 and Tn5393c remnant (Tn3-family transposon) carrying the aminoglycoside resistance genes strA and strB (L’Abee-Lund and Sorum, 2000).
3.7. The ISKpn21:ΔTn6363 region of pE1532-MCR
The ISKpn21:ΔTn6363 region (Figure 5) is composed of ISKpn21 and ΔTn6363 and has undergone two different transposition events: (1) The ISNCY family element ISKpn21 with an IRL-tnpA-tnpB-IRR structure is integrated at a site between the two backbone genes orf318-2 and orf1404 in pE1532-MCR and bordered by 5-bp DRs. (2) The transposon ΔTn6363 is inserted at a site between tnpB and the IRR of ISKpn21, splitting ISKpn21 into two parts and leaving 9-bp DRs at both ends of ΔTn6363. Tn6363 (accession number KY978628) is an IS903B-flanked composite transposon that possesses an IS903B-aphA1-orf894-yqhD-orf585-IS903B structure (Shi et al., 2018). Insertion of an additional element ΔIS903B resulted in a 1-bp deletion of IS903B upstream of Tn6363, thus generating ΔTn6363 with three IS903B elements. ΔTn6363 is a derivative of Tn6363 which carries aminoglycoside resistance gene aphA1, the embedding of ΔTn6363 into ISKpn21 mediated by IS903B facilitates the horizontal transfer and transmission of antibiotic resistance gene in bacterial populations.
4. Conclusion
This is the first report of coexistence of the blaKPC-2-carrying IncR plasmid, blaNDM-1-carrying IncX3 plasmid and mcr-9-carrying IncHI2 plasmid recovered from the ST93 multidrug-resistant E. hormaechei clinical isolate E1532. These three coexisting MDR plasmids carry a large number of resistance genes, rendering the E1532 isolate resistant to almost all antibiotics tested, including carbapenems. The mcr-9 gene, which is involved in resistance to the last-resort antibiotic, should be given sufficient attention because it has become widely disseminated worldwide among various species of Enterobacteriaceae. Therefore, epidemiological analysis should be performed to monitor the spread of mcr-9-positive strains. Moreover, most of these plasmid-mediated resistance genes are located in or flanked by various mobile genetic elements, such as transposons, insertion sequences and integrons, which facilitate acquisition and horizontal transfer of antibiotic resistance genes across bacterial populations. Not all plasmids can transfer themselves, but non-conjugative plasmids can be mobilized with the help of other conjugative plasmids present in the same donor cell. Therefore, there is the possibility of cotransfer of blaKPC-2-, blaNDM-1-, and mcr-9-carrying plasmids. The main limitation in this study is that we did not apply the conjugation assay to evaluate the cotransfer of these three plasmids, which needs to be confirmed by further study. Anyway, the cotransfer and coexistence of mcr-9 and carbapenemase genes in E. hormaechei isolates limit the choice of antibiotics, which will arise a huge risk to clinical treatment and global public health. Further surveillance is necessary to achieve better insight into the prevalence and dissemination mechanism of these coexisting blaKPC-2-, blaNDM-1-, and mcr-9-harboring plasmids among clinical isolates.
Data availability statement
The datasets presented in this study can be found in online repositories. The names of the repository/repositories and accession number(s) can be found at: Genbank- [CP114571 (chromosome), CP114573 (pE1532-KPC), CP114574 (pE1532-MCR), CP114575 (pE1532-NDM), and CP114572 (pE1532-4)].
Ethics statement
The bacterial isolate involved in this study was part of the routine hospital laboratory procedure, and the clinical information of the patient was not involved in this study, so ethical approval and informed consent were not required.
Author contributions
WF and FS conceived and designed the study. LRX and SL carried out the phenotypic characterization. QY and WF carried out the genomic analysis and bioinformatics analysis. WF, QY and FS wrote and revised the manuscript. PX and LLX did the funding acquisition. All authors contributed to the article and approved the submitted version.
Funding
This work was supported by the Innovation Leading Talent Project of Chongqing (425Z2P12D) and Medical Service Nursery Talent Program of Army Medical University (XZ-2019-505-056).
Conflict of interest
The authors declare that the research was conducted in the absence of any commercial or financial relationships that could be construed as a potential conflict of interest.
Publisher’s note
All claims expressed in this article are solely those of the authors and do not necessarily represent those of their affiliated organizations, or those of the publisher, the editors and the reviewers. Any product that may be evaluated in this article, or claim that may be made by its manufacturer, is not guaranteed or endorsed by the publisher.
Supplementary material
The Supplementary material for this article can be found online at: https://www.frontiersin.org/articles/10.3389/fmicb.2023.1153366/full#supplementary-material
Footnotes
References
Allmeier, H., Cresnar, B., Greck, M., and Schmitt, R. (1992). Complete nucleotide sequence of Tn1721: gene organization and a novel gene product with features of a chemotaxis protein. Gene 111, 11–20. doi: 10.1016/0378-1119(92)90597-i
Bissonnette, L., and Roy, P. H. (1992). Characterization of In0 of Pseudomonas aeruginosa plasmid pVS1, an ancestor of integrons of multiresistance plasmids and transposons of gram-negative bacteria. J. Bacteriol. 174, 1248–1257. doi: 10.1128/jb.174.4.1248-1257.1992
Boratyn, G. M., Camacho, C., Cooper, P. S., Coulouris, G., Fong, A., Ma, N., et al. (2013). BLAST: a more efficient report with usability improvements. Nucleic Acids Res. 41, W29–W33. doi: 10.1093/nar/gkt282
Borowiak, M., Baumann, B., Fischer, J., Thomas, K., Deneke, C., Hammerl, J. A., et al. (2020). Development of a novel mcr-6 to mcr-9 multiplex PCR and assessment of mcr-1 to mcr-9 occurrence in Colistin-resistant Salmonella enterica isolates from environment, feed, animals and food (2011-2018) in Germany. Front. Microbiol. 11:80. doi: 10.3389/fmicb.2020.00080
Bortolaia, V., Kaas, R. S., Ruppe, E., Roberts, M. C., Schwarz, S., Cattoir, V., et al. (2020). ResFinder 4.0 for predictions of phenotypes from genotypes. J. Antimicrob. Chemother. 75, 3491–3500. doi: 10.1093/jac/dkaa345
Boutet, E., Lieberherr, D., Tognolli, M., Schneider, M., Bansal, P., Bridge, A. J., et al. (2016). UniProtKB/Swiss-Prot, the manually annotated section of the UniProt KnowledgeBase: how to use the entry view. Methods Mol. Biol. 1374, 23–54. doi: 10.1007/978-1-4939-3167-5_2
Brettin, T., Davis, J. J., Disz, T., Edwards, R. A., Gerdes, S., Olsen, G. J., et al. (2015). RASTtk: a modular and extensible implementation of the RAST algorithm for building custom annotation pipelines and annotating batches of genomes. Sci. Rep. 5:8365. doi: 10.1038/srep08365
Carattoli, A., Zankari, E., García-Fernández, A., Voldby Larsen, M., Lund, O., Villa, L., et al. (2014). In silico detection and typing of plasmids using PlasmidFinder and plasmid multilocus sequence typing. Antimicrob. Agents Chemother. 58, 3895–3903. doi: 10.1128/AAC.02412-14
Carroll, L. M., Gaballa, A., Guldimann, C., Sullivan, G., Henderson, L. O., and Wiedmann, M. (2019). Identification of novel mobilized Colistin resistance gene mcr-9 in a multidrug-resistant, Colistin-susceptible Salmonella enterica serotype Typhimurium isolate. MBio 10:e00853. doi: 10.1128/mBio.00853-19
Chavda, K. D., Westblade, L. F., Satlin, M. J., Hemmert, A. C., Castanheira, M., Jenkins, S. G., et al. (2019). First report of Bla(VIM-4)- and mcr-9-Coharboring Enterobacter species isolated from a pediatric patient. mSphere 4:e00629. doi: 10.1128/mSphere.00629-19
Chen, Z., Li, H., Feng, J., Li, Y., Chen, X., Guo, X., et al. (2015). NDM-1 encoded by a pNDM-BJ01-like plasmid p3SP-NDM in clinical Enterobacter aerogenes. Front. Microbiol. 6:294. doi: 10.3389/fmicb.2015.00294
Chen, L., Mathema, B., Chavda, K. D., DeLeo, F. R., Bonomo, R. A., and Kreiswirth, B. N. (2014). Carbapenemase-producing Klebsiella pneumoniae: molecular and genetic decoding. Trends Microbiol. 22, 686–696. doi: 10.1016/j.tim.2014.09.003
Chen, J., Tian, S., Nian, H., Wang, R., Li, F., Jiang, N., et al. (2021). Carbapenem-resistant Enterobacter cloacae complex in a tertiary Hospital in Northeast China, 2010-2019. BMC Infect. Dis. 21:611. doi: 10.1186/s12879-021-06250-0
Compain, F., Frangeul, L., Drieux, L., Verdet, C., Brisse, S., Arlet, G., et al. (2014). Complete nucleotide sequence of two multidrug-resistant IncR plasmids from Klebsiella pneumoniae. Antimicrob. Agents Chemother. 58, 4207–4210. doi: 10.1128/AAC.02773-13
Dong, D., Mi, Z., Li, D., Gao, M., Jia, N., Li, M., et al. (2020). Novel IncR/IncP6 hybrid plasmid pCRE3-KPC recovered from a clinical KPC-2-producing Citrobacter braakii isolate. mSphere 5. doi: 10.1128/mSphere.00891-19
Edgar, R. C. (2004). MUSCLE: multiple sequence alignment with high accuracy and high throughput. Nucleic Acids Res. 32, 1792–1797. doi: 10.1093/nar/gkh340
Feng, W., Lv, J., Wang, H., Yao, P., Xiong, L., Xia, P., et al. (2022). The first report of the Bla(IMP-10) gene and complete sequence of the IMP-10-encoding plasmid p12NE515 from Pseudomonas aeruginosa in China. Acta Trop. 228:106326. doi: 10.1016/j.actatropica.2022.106326
Ford, P. J., and Avison, M. B. (2004). Evolutionary mapping of the SHV beta-lactamase and evidence for two separate IS26-dependent blaSHV mobilization events from the Klebsiella pneumoniae chromosome. J. Antimicrob. Chemother. 54, 69–75. doi: 10.1093/jac/dkh251
Gilmour, M. W., Thomson, N. R., Sanders, M., Parkhill, J., and Taylor, D. E. (2004). The complete nucleotide sequence of the resistance plasmid R478: defining the backbone components of incompatibility group H conjugative plasmids through comparative genomics. Plasmid 52, 182–202. doi: 10.1016/j.plasmid.2004.06.006
Harmer, C. J., and Hall, R. M. (2016). IS26-mediated formation of transposons carrying antibiotic resistance genes. mSphere 1:e00038. doi: 10.1128/mSphere.00038-16
Harmer, C. J., Pong, C. H., and Hall, R. M. (2020). Structures bounded by directly-oriented members of the IS26 family are pseudo-compound transposons. Plasmid 111:102530. doi: 10.1016/j.plasmid.2020.102530
Ho, P. L., Li, Z., Lo, W. U., Cheung, Y. Y., Lin, C. H., Sham, P. C., et al. (2012). Identification and characterization of a novel incompatibility group X3 plasmid carrying Bla NDM-1 in Enterobacteriaceae isolates with epidemiological links to multiple geographical areas in China. Emerg. Microbes Infect. 1:e39. doi: 10.1038/emi.2012.37
Jia, B., Raphenya, A. R., Alcock, B., Waglechner, N., Guo, P., Tsang, K. K., et al. (2017). CARD 2017: expansion and model-centric curation of the comprehensive antibiotic resistance database. Nucleic Acids Res. 45, D566–D573. doi: 10.1093/nar/gkw1004
Jiang, Y., Yu, D., Wei, Z., Shen, P., Zhou, Z., and Yu, Y. (2010). Complete nucleotide sequence of Klebsiella pneumoniae multidrug resistance plasmid pKP048, carrying blaKPC-2, blaDHA-1, qnrB4, and armA. Antimicrob. Agents Chemother. 54, 3967–3969. doi: 10.1128/AAC.00137-10
Jing, Y., Jiang, X., Yin, Z., Hu, L., Zhang, Y., Yang, W., et al. (2019). Genomic diversification of IncR plasmids from China. J. Glob. Antimicrob. Resist. 19, 358–364. doi: 10.1016/j.jgar.2019.06.007
Kananizadeh, P., Oshiro, S., Watanabe, S., Iwata, S., Kuwahara-Arai, K., Shimojima, M., et al. (2020). Emergence of carbapenem-resistant and colistin-susceptible Enterobacter cloacae complex co-harboring Bla(IMP-1) and mcr-9 in Japan. BMC Infect. Dis. 20:282. doi: 10.1186/s12879-020-05021-7
Kieffer, N., Royer, G., Decousser, J. W., Bourrel, A. S., Palmieri, M., Ortiz De La Rosa, J. M., et al. (2019). Mcr-9, an inducible gene encoding an acquired phosphoethanolamine transferase in Escherichia coli, and its origin. Antimicrob. Agents Chemother. 63:e00965. doi: 10.1128/AAC.00965-19
Kim, J. S., Yu, J. K., Jeon, S. J., Park, S. H., Han, S., Park, S. H., et al. (2021). Distribution of mcr genes among carbapenem-resistant Enterobacterales clinical isolates: high prevalence of mcr-positive Enterobacter cloacae complex in Seoul, Republic of Korea. Int. J. Antimicrob. Agents 58:106418. doi: 10.1016/j.ijantimicag.2021.106418
L’Abee-Lund, T. M., and Sorum, H. (2000). Functional Tn5393-like transposon in the R plasmid pRAS2 from the fish pathogen Aeromonas salmonicida subspecies salmonicida isolated in Norway. Appl. Environ. Microbiol. 66, 5533–5535. doi: 10.1128/AEM.66.12.5533-5535.2000
Li, Y., Dai, X., Zeng, J., Gao, Y., Zhang, Z., and Zhang, L. (2020). Characterization of the global distribution and diversified plasmid reservoirs of the colistin resistance gene mcr-9. Sci. Rep. 10:8113. doi: 10.1038/s41598-020-65106-w
Liang, Q., Yin, Z., Zhao, Y., Liang, L., Feng, J., Zhan, Z., et al. (2017). Sequencing and comparative genomics analysis of the IncHI2 plasmids pT5282-mphA and p112298-catA and the IncHI5 plasmid pYNKP001-dfrA. Int. J. Antimicrob. Agents 49, 709–718. doi: 10.1016/j.ijantimicag.2017.01.021
Ling, Z., Yin, W., Shen, Z., Wang, Y., Shen, J., and Walsh, T. R. (2020). Epidemiology of mobile colistin resistance genes mcr-1 to mcr-9. J. Antimicrob. Chemother. 75, 3087–3095. doi: 10.1093/jac/dkaa205
Liu, Z., Hang, X., Xiao, X., Chu, W., Li, X., Liu, Y., et al. (2021). Co-occurrence of Bla (NDM-1) and mcr-9 in a conjugative IncHI2/HI2A plasmid from a bloodstream infection-causing Carbapenem-resistant Klebsiella pneumoniae. Front. Microbiol. 12:756201. doi: 10.3389/fmicb.2021.756201
Liu, Y. Y., Wang, Y., Walsh, T. R., Yi, L. X., Zhang, R., Spencer, J., et al. (2016). Emergence of plasmid-mediated colistin resistance mechanism MCR-1 in animals and human beings in China: a microbiological and molecular biological study. Lancet Infect. Dis. 16, 161–168. doi: 10.1016/S1473-3099(15)00424-7
Ma, J., Song, X., Li, M., Yu, Z., Cheng, W., Yu, Z., et al. (2023). Global spread of carbapenem-resistant Enterobacteriaceae: epidemiological features, resistance mechanisms, detection and therapy. Microbiol. Res. 266:127249. doi: 10.1016/j.micres.2022.127249
Miyoshi-Akiyama, T., Hayakawa, K., Ohmagari, N., Shimojima, M., and Kirikae, T. (2013). Multilocus sequence typing (MLST) for characterization of Enterobacter cloacae. PLoS One 8:e66358. doi: 10.1371/journal.pone.0066358
Moura, A., Soares, M., Pereira, C., Leitao, N., Henriques, I., and Correia, A. (2009). INTEGRALL: a database and search engine for integrons, integrases and gene cassettes. Bioinformatics 25, 1096–1098. doi: 10.1093/bioinformatics/btp105
Noguchi, N., Takada, K., Katayama, J., Emura, A., and Sasatsu, M. (2000). Regulation of transcription of the mph(a) gene for macrolide 2′-phosphotransferase I in Escherichia coli: characterization of the regulatory gene mphR(a). J. Bacteriol. 182, 5052–5058. doi: 10.1128/JB.182.18.5052-5058.2000
O’Leary, N. A., Wright, M. W., Brister, J. R., Ciufo, S., Haddad, D., McVeigh, R., et al. (2016). Reference sequence (RefSeq) database at NCBI: current status, taxonomic expansion, and functional annotation. Nucleic Acids Res. 44, D733–D745. doi: 10.1093/nar/gkv1189
Ouyang, J., Sun, F., Zhou, D., Feng, J., Zhan, Z., Xiong, Z., et al. (2018). Comparative genomics of five different resistance plasmids coexisting in a clinical multi-drug resistant Citrobacter freundii isolate. Infect. Drug Resist. 11, 1447–1460. doi: 10.2147/IDR.S165818
Partridge, S. R. (2011). Analysis of antibiotic resistance regions in gram-negative bacteria. FEMS Microbiol. Rev. 35, 820–855. doi: 10.1111/j.1574-6976.2011.00277.x
Partridge, S. R., Ginn, A. N., Paulsen, I. T., and Iredell, J. R. (2012). pEl1573 carrying blaIMP-4, from Sydney, Australia, is closely related to other IncL/M plasmids. Antimicrob. Agents Chemother. 56, 6029–6032. doi: 10.1128/AAC.01189-12
Peirano, G., Matsumura, Y., Adams, M. D., Bradford, P., Motyl, M., Chen, L., et al. (2018). Genomic epidemiology of global carbapenemase-producing Enterobacter spp., 2008-2014. Emerg. Infect. Dis. 24, 1010–1019. doi: 10.3201/eid2406.171648
Peng, C., Feng, D. H., Zhan, Y., Wang, Q., Chen, D. Q., Xu, Z., et al. (2022). Molecular epidemiology, microbial virulence, and resistance of Carbapenem-resistant Enterobacterales isolates in a teaching Hospital in Guangzhou. China. Microb. Drug Resist. 28, 698–709. doi: 10.1089/mdr.2021.0156
Poirel, L., Bonnin, R. A., Boulanger, A., Schrenzel, J., Kaase, M., and Nordmann, P. (2012). Tn125-related acquisition of blaNDM-like genes in Acinetobacter baumannii. Antimicrob. Agents Chemother. 56, 1087–1089. doi: 10.1128/AAC.05620-11
Poirel, L., Jayol, A., and Nordmann, P. (2017). Polymyxins: antibacterial activity, susceptibility testing, and resistance mechanisms encoded by plasmids or chromosomes. Clin. Microbiol. Rev. 30, 557–596. doi: 10.1128/CMR.00064-16
Rebelo, A. R., Bortolaia, V., Kjeldgaard, J. S., Pedersen, S. K., Leekitcharoenphon, P., Hansen, I. M., et al. (2018). Multiplex PCR for detection of plasmid-mediated colistin resistance determinants, mcr-1, mcr-2, mcr-3, mcr-4 and mcr-5 for surveillance purposes. Euro Surveill. 23:672. doi: 10.2807/1560-7917.ES.2018.23.6.17-00672
Roberts, A. P., Chandler, M., Courvalin, P., Guedon, G., Mullany, P., Pembroke, T., et al. (2008). Revised nomenclature for transposable genetic elements. Plasmid 60, 167–173. doi: 10.1016/j.plasmid.2008.08.001
Shi, L., Liang, Q., Zhan, Z., Feng, J., Zhao, Y., Chen, Y., et al. (2018). Co-occurrence of 3 different resistance plasmids in a multi-drug resistant Cronobacter sakazakii isolate causing neonatal infections. Virulence 9, 110–120. doi: 10.1080/21505594.2017.1356537
Siguier, P., Perochon, J., Lestrade, L., Mahillon, J., and Chandler, M. (2006). ISfinder: the reference Centre for bacterial insertion sequences. Nucleic Acids Res. 34, D32–D36. doi: 10.1093/nar/gkj014
Simoni, S., Mingoia, M., Brenciani, A., Carelli, M., Lleo, M. M., Malerba, G., et al. (2021). First IncHI2 plasmid carrying mcr-9.1, Bla(VIM-1), and double copies of Bla(KPC-3) in a multidrug-resistant Escherichia coli human isolate. mSphere 6:e0030221. doi: 10.1128/mSphere.00302-21
Walker, B. J., Abeel, T., Shea, T., Priest, M., Abouelliel, A., Sakthikumar, S., et al. (2014). Pilon: an integrated tool for comprehensive microbial variant detection and genome assembly improvement. PLoS One 9:e112963. doi: 10.1371/journal.pone.0112963
Wick, R. R., Judd, L. M., Gorrie, C. L., and Holt, K. E. (2017). Unicycler: resolving bacterial genome assemblies from short and long sequencing reads. PLoS Comp. Biol. 13:e1005595. doi: 10.1371/journal.pcbi.1005595
Yang, Q., Pogue, J. M., Li, Z., Nation, R. L., Kaye, K. S., and Li, J. (2020). Agents of last resort: an update on Polymyxin resistance. Infect. Dis. Clin. N. Am. 34, 723–750. doi: 10.1016/j.idc.2020.08.003
Yao, Y., Doijad, S., Falgenhauer, J., Schmiedel, J., Imirzalioglu, C., and Chakraborty, T. (2022). Co-occurrence of dual carbapenemases KPC-2 and OXA-48 with the mobile colistin resistance gene mcr-9.1 in Enterobacter xiangfangensis. Front. Cell. Infect. Microbiol. 12:960892. doi: 10.3389/fcimb.2022.960892
Yim, G., Kwong, W., Davies, J., and Miao, V. (2013). Complex integrons containing qnrB4-ampC (Bla(DHA-1)) in plasmids of multidrug-resistant Citrobacter freundii from wastewater. Can. J. Microbiol. 59, 110–116. doi: 10.1139/cjm-2012-0576
Yoon, E. J., Kang, D. Y., Yang, J. W., Kim, D., Lee, H., Lee, K. J., et al. (2018). New Delhi Metallo-Beta-lactamase-producing Enterobacteriaceae in South Korea between 2010 and 2015. Front. Microbiol. 9:571. doi: 10.3389/fmicb.2018.00571
Yuan, Y., Li, Y., Wang, G., Li, C., Xiang, L., She, J., et al. (2019). Coproduction of MCR-9 and NDM-1 by Colistin-resistant Enterobacter hormaechei isolated from bloodstream infection. Infect. Drug Resist. 12, 2979–2985. doi: 10.2147/IDR.S217168
Zhang, R., Liu, L., Zhou, H., Chan, E. W., Li, J., Fang, Y., et al. (2017). Nationwide surveillance of clinical Carbapenem-resistant Enterobacteriaceae (CRE) strains in China. EBioMedicine 19, 98–106. doi: 10.1016/j.ebiom.2017.04.032
Zhang, S., Sun, H., Lao, G., Zhou, Z., Liu, Z., Cai, J., et al. (2022). Identification of mobile colistin resistance gene mcr-10 in disinfectant and antibiotic resistant Escherichia coli from disinfected tableware. Antibiotics (Basel) 11:883. doi: 10.3390/antibiotics11070883
Keywords: Enterobacter hormaechei, multidrug resistance, plasmid, carbapenemase genes, mcr-9
Citation: Yuan Q, Xia P, Xiong L, Xie L, Lv S, Sun F and Feng W (2023) First report of coexistence of blaKPC-2-, blaNDM-1- and mcr-9-carrying plasmids in a clinical carbapenem-resistant Enterobacter hormaechei isolate. Front. Microbiol. 14:1153366. doi: 10.3389/fmicb.2023.1153366
Edited by:
Biao Tang, Zhejiang Academy of Agricultural Sciences, ChinaCopyright © 2023 Yuan, Xia, Xiong, Xie, Lv, Sun and Feng. This is an open-access article distributed under the terms of the Creative Commons Attribution License (CC BY). The use, distribution or reproduction in other forums is permitted, provided the original author(s) and the copyright owner(s) are credited and that the original publication in this journal is cited, in accordance with accepted academic practice. No use, distribution or reproduction is permitted which does not comply with these terms.
*Correspondence: Fengjun Sun, fengj_sun@163.com; Wei Feng, fengwei.sky@163.com