- 1Department of Gastroenterology and Hepatology, Shenzhen University General Hospital, Shenzhen University, Shenzhen, China
- 2Department of Laboratory Medicine, Shenzhen University General Hospital, Shenzhen University, Shenzhen, China
- 3Department of Urology, Shenzhen University General Hospital, Shenzhen University, Shenzhen, China
- 4International Cancer Center, Shenzhen Key Laboratory, Hematology Institution of Shenzhen University, Shenzhen, China
Background: Winkia neuii, previously known as Actinomyces neuii, is increasingly recognized as a causative agent of various human infections, while its taxonomy and genomic insights are still understudied.
Methods: A Winkia strain NY0527 was isolated from the hip abscess of a patient, and its antibiotic susceptibility was assessed. The genome was hybrid assembled from long-reads and short-reads sequencing. Whole-genome-based analyses on taxa assignment, strain diversity, and pathogenesis were conducted.
Results: The strain was found to be highly susceptible to beta-lactam antibiotics, but resistant to erythromycin, clindamycin, and amikacin. The complete genome sequences of this strain were assembled and found to consist of a circular chromosome and a circular plasmid. Sequence alignment to the NCBI-nt database revealed that the plasmid had high sequence identity (>90%) to four Corynebacterium plasmids, with 40–50% query sequence coverage. Furthermore, the plasmid was discovered to possibly originate from the sequence recombination events of two Corynebacterium plasmid families. Phylogenomic tree and genomic average nucleotide identity analyses indicated that many Winkia sp. strains were still erroneously assigned as Actinomyces sp. strains, and the documented subspecies within W. neuii should be reclassified as two separate species (i.e., W. neuii and W. anitratus). The core genome of each species carried a chromosome-coded beta-lactamase expression repressor gene, which may account for their broadly observed susceptibility to beta-lactam antibiotics in clinical settings. Additionally, an ermX gene that expresses fluoroquinolone resistance was shared by some W. neuii and W. anitratus strains, possibly acquired by IS6 transposase-directed gene transfer events. In contrast, tetracycline resistance genes were exclusively carried by W. neuii strains. In particular, W. neuii was found to be more pathogenic than W. anitratus by encoding more virulence factors (i.e., 35–38 in W. neuii vs 27–31 in W. anitratus). Moreover, both species encoded two core pathogenic virulence factors, namely hemolysin and sialidase, which may facilitate their infections by expressing poreformation, adhesion, and immunoglobulin deglycosylation activities.
Conclusion: This study highlights the underappreciated taxonomic diversity of Winkia spp. and provides populational genomic insights into their antibiotic susceptibility and pathogenesis for the first time, which could be helpful in the clinical diagnosis and treatment of Winkia spp. infections.
1. Introduction
Winkia neuii, previously known as Actinomyces neuii, are facultatively anaerobic, Gram-positive, asporogenous, and catalase-positive organisms that are frequently isolated from clinical samples (Yang and Grant, 2019). The initial isolation of this species was reported in 1985 by Coudron et al. from the vitreous fluid of patients with endophthalmitis (Coudron et al., 1985). W. neuii isolates were classified as CDC (Centers for Disease Control) fermentative coryneform group 1 or group 1-like species in the 1980s until they were placed in the genus Actinomyces in 1994, based on a combination of 16S rRNA sequence identity, cellular and metabolic fatty acid profiles, and DNA–DNA hybridization ratio (Funke et al., 1993, 1994). Subsequently, several researchers have proposed the creation of a novel genus for A. neuii, as it exhibited a sequence similarity and chemotaxonomy closer to the genera Varibaculum and Mobiluncus than to Actinomyces bovis (Schaal et al., 1999; Hoyles et al., 2004). However, only recently in 2018, A. neuii was separated from the genus Actinomyces and was reassigned as W. neuii, and it is validly published as the sole species of the genus Winkia under the ICNP (International Code of Nomenclature of Prokaryotes) rule (Nouioui et al., 2018). Within the species, two subspecies, W. neuii subsp. neuii and W. neuii subsp. anitratus (anitrata means not reducing nitrate), have been assigned based on their biochemical activities and 16S rRNA sequence identities (Nouioui et al., 2018).
W. neuii has been implicated in hundreds of cases of human infection since its first isolation (Gómez-Garcés et al., 2010; Zelyas et al., 2016). The most prevalent types of infections caused by W. neuii are abscesses and infected atheroma, followed by infected skin lesions, urinary tract infections, endophthalmitis, and bacteremia, including endocarditis (Funke and Von Graevenitz, 1995; Roustan et al., 2009; Gómez-Garcés et al., 2010; von Graevenitz, 2011; Könönen and Wade, 2015; Yang and Grant, 2019; Giannoulopoulos and Errington, 2022). In rare cases, W. neuii infections cause premature labor and neonatal sepsis (Alsohime et al., 2019), and even primary actinomycosis (Leenstra et al., 2017). Previous studies have shown that W. neuii strains take a proportion of 8–17% in the clinically isolated Actinomyces spp. (Gómez-Garcés et al., 2010; Barberis et al., 2017). Researchers also discovered that the most common body sites for W. neuii colonization are the mammary, axillary, and inguinal areas (Hall, 2008), as well as the blood, foreign body devices, urinary tract, infected joints, and soft tissues (von Graevenitz, 2011; Leal Jr et al., 2016; Chen et al., 2020; Hara et al., 2021). To the best of our knowledge, no W. neuii has been isolated from environmental samples, therefore, infections caused by W. neuii were believed to be endogenous (von Graevenitz, 2011; Graffi et al., 2012). Fortunately, favorable outcomes are typically achieved through surgical removal of the infected focus (von Graevenitz, 2011), or using antibiotic treatment, as the W. neuii strains are susceptible to a wide range of antibiotics, including penicillin, ampicillin, the cephalosporins, imipenem, vancomycin, erythromycin, and clindamycin (Mann et al., 2002; Watkins et al., 2008; von Graevenitz, 2011). However, the genomes of most W. neuii isolates have not been sequenced, and the pathogenetic mechanisms and virulence traits of this organism remain unclear (Zelyas et al., 2016). Furthermore, due to the similarity of morphology and biochemical characteristics between Actinomyces and Corynebacterium, isolates from W. neuii could be wrongly assigned, and the occurrence and diversity of this organism in clinical specimens may be underestimated (Zelyas et al., 2016).
In recent years, the widespread use of matrix-assisted laser desorption ionization time-off-flight mass spectrometry (MALDI-TOF MS) in clinical laboratories has greatly increased the accuracy of identifying bacteria that were previously difficult to identify (Könönen and Wade, 2015), and it has been successfully applied to identify W. neuii in clinical practices (De Vreese and Verhaegen, 2013). However, manufacturers of MALDI-TOF MS targeted mostly clinically important microorganisms and provided relevant reference spectra in the databases, which may make this method fail to identify novel, highly similar and/or rare-isolated species that lack documented spectra data (Rahi et al., 2016; Ha et al., 2019; Welker et al., 2019). Nevertheless, the creation of open-source databases that incorporate MALDI-TOF MS spectra of all known species and keep them updated is now a great challenge (Rahi et al., 2016; Chen et al., 2021). The development of next-generation sequencing technology has facilitated the opportunity to obtain the whole genome of clinical isolates at low cost, which is helpful for researchers to assign microbial taxonomy at strain level, with a much higher resolution than that of MALDI-TOF MS. Moreover, the genomic sequences obtained from the microorganisms are helpful in deciphering their pathogenetic mechanisms.
As of February 23rd, 2023, only seven draft-level genomes of W. neuii have been deposited in the NCBI Genome RefSeq database. Furthermore, their populational genomic features related to pathogenesis, such as plasmid carriage and virulence factor coding genes, have not been characterized. In this study, we isolated a Winkia strain NY0527 from the sanies of hip abscess in a 27-year-old male patient and de novo assembled the genome at the completed level using Illumina and Nanopore sequencing reads. Taxonomy assignments and comparative genome analyses based on whole genome sequences were then performed to clarify the phylogeny, general genomic features, and gene functions related to virulence, adaptation, and pathogenesis.
2. Methods and materials
2.1. Specimen collection, bacteria isolation, identification, and antimicrobial susceptibility
Sanies were sterilely collected from the hip abscess of a 27-year-old male patient during the surgery debridement procedure. The specimen was then transferred to the clinical microbiology laboratory for culturing. Specimen was streaked onto blood agar and was incubated aerobically at 35–37°C for 3 days. Colonies that appeared on the agar plates were subsequently purified through re-streaking. The colonies obtained were then identified using MALDI Biotyper RTC (Bruker Daltonics, Germany). Briefly, single colony was transferred to the 96-target spot of the MALDI Biotyper (MALDI Biotyper 3.1), and 1 μL of bacterial test standard and matrix solution were consecutively added. Identification was carried out with the default settings. Subsequently, the MALDI log-score was calculated by matching the converted spectra data of the isolate with the reference spectra presented in the database (version 4.0). Following the manufacturer’s instructions, a log-score more than or equal to two indicates high-confidence identification at the species level. Antimicrobial susceptibility testing was performed using Etest (bioMérieux, France) or disk diffusion method (K-B method) by the Department of Laboratory Medicine, Shenzhen University General Hospital, Shenzhen University, in accordance with the Clinical and Laboratory Standards Institute (CLSI) reference method (Wayne, 2011). The antimicrobial susceptibility of the strain was determined by interpreting the antibiotic MICs from Etest and the inhibitory zone diameter from K-B, using the breakpoints established by Clinical and Laboratory Standards Institute (CLSI) (2003).
2.2. Genomic DNA extraction, sequencing, and genome assembly
The bacterial colony identified by the MALDI Biotyper was transferred and completely dispersed into a 1.5 ml germ-free tube with 200 μL of sterile PBS, which was then spread onto the blood agar and cultured aerobically at 35°C for 3 days. Subsequently, the bacterial cells were washed from the agar plate using 1 mL of sterile PBS and transferred into a 1.5 mL germ-free tube. Genomic DNA extraction was performed immediately using the TaKaRa MiniBEST Bacteria Genomic DNA Extraction Kit (Takara, Japan), following the manufacturer’s instructions. Genomic DNA quality was checked using the Synergy HTX Multi-Mode Reader (BioTek, United States). Genome sequencing was conducted on two platforms, namely the Nanopore PromethION platform (MAGIGENE, Guangzhou, China) and the Illumina NovaSeq platform (Novogene, Nanjing, China), to generate long reads and short reads, respectively. For the long-reads sequencing, sequencing library was prepared using the 1D Ligation Kit (SQK-LSK109, Oxford Nanopore Technologies) by following the manufacturer’s instructions. Subsequently, the sequencing was carried out on an R9.4.1 flow cell. Base calling was performed using MinKNOW v1.15.4 and low-quality reads (i.e., scores <7) were removed. The short-reads sequencing library was constructed with a 350 bp insert size and sequenced using the PE150 strategy. The genome was de novo assembled and in a hybrid manner using the Unicycler v0.4.9b assembler, with the default hybrid assembly pipeline (Wick et al., 2017).
2.3. Phylogenomic characterization, pangenome extraction, and plasmid detection
The gtdbtk_wf workflow implemented in GTDT-Tk software was used for further validation of the taxonomy assignment (Parks et al., 2018). The Similar Genome Finder implanted in PATRIC v3.6.102 was employed to find public released genomes that with high sequence similarity to the assembled genome. The average nucleotide identity (ANI) between the assembled genome and the similar genomes was calculated using fastANI (Jain et al., 2018). Coding sequences (CDSs) and annotation files (e.g., gff files) from these genomes were predicted using Prokka v1.14.5 with the default parameters (Seemann, 2014). The pangenome was subsequently extracted from the resultant gff files using Roary v3.11.2 (Page et al., 2015), and CDSs that were present in over 95% of genomes were identified as the coregenome. Additionally, a phylogenomic tree of these genomes based on the whole-genome CDSs was constructed using CVTree3 with default parameters (Zuo, 2021). Plasmids were predicted using PlasForest v1.23 based on machine learning from sequence homology.
2.4. Genome annotation and comparative genomics
Comprehensive genome function annotation was conducted using eggNOG-mapper v2.1.5, which incorporates multiple databases, including Carbohydrate-Active enZYmes (CAZy), Cluster of Orthologous Groups (COG), and Kyoto Encyclopedia of Genes and Genomes (KEGG) (Huerta-Cepas et al., 2017). Genome subsystem function (transporters, virulence factors, drug targets, antibiotic resistance genes, antimicrobial resistance genes, etc.) was annotated using the Rapid Annotation using Subsystem Technology server4 (Overbeek et al., 2014). Pathogenic factor genes, including virulence genes, toxin genes, and antimicrobial resistant genes (ARG), in the genomes were predicted using PathoFact v1.0 with the “complete” parameter. The results also reported the localization of these genes on mobile genetic elements (MGE) and the chromosome. Pathogenic attributes (i.e., pathogenic or non-pathogenic) of the predicted genes were determined by using the HMM model and the random forest classifier implanted in PathoFact with default settings (de Nies et al., 2021).
3. Results
3.1. Clinical presentation and diagnostic findings
A 27-year-old male patient presented with a painful lesion on his right hip, which he had developed 3 days prior to admission. He had no special medical history and no other relevant symptoms were noted. On physical examination, a painful, fluctuating, 5.0 × 4.0 cm mass with skin erythema on the right hip was detected. The ultrasound showed a mixed fluid collection measuring 4.1 × 1.5 × 2.4 cm, indicating the presence of an abscess. A peripheral hematological white blood cell count revealed 7.73 × 109/L (3.5–9.5 × 109), and C-reactive protein was measured at 12.04 mg/L (0–10.0). The patient was empirically treated with cefuroxime (1.5 g, q12h) and ornidazole (0.5 g, q12 h) prior to undergoing surgical debridement for his hip abscess. One day after initiation of antibiotic treatment, surgical debridement was performed, followed by a 14-day course of antibiotic therapy, which successfully cured him. A strain, designated NY0527, was isolated from the purulent fluid sample collected during the surgical operation, which was identified as Actinomyces neuii subsp. anitratus (now known as W. neuii subsp. anitratus) with high confidence by using MALDI Biotyper RTC (Supplementary Table S1). The strain exhibited susceptibility to most of the tested antibiotics, including levofloxacin, ciprofloxacin, vancomycin, meropenem, penicillin, ceftriaxone, cefepime, gentamicin and tetracyclines, among which the strain is highly susceptible to the beta-lactam antibiotics (i.e., meropenem, penicillin, ceftriaxone, and cefepime) and tetracyclines antibiotics (i.e., tetracycline) (Table 1). In contrast, this strain was resistant to only three tested antibiotics (i.e., erythromycin, clindamycin, and amikacin) (Table 1).
3.2. General genomic features
Two complete circular contigs free of Ns were generated from the hybrid assembly. Genome sequence quality check using checkM indicated that the assembled contigs are of high-quality (completeness = 99.84%, contamination = 0.95%, strain heterogeneity = 0.00%). The plasmid prediction analysis revealed that the larger circular contig is the chromosomal sequence, while the smaller circular contig is the plasmid sequence. In summary, strain NY0527 comprises a circular chromosome with a total length of 2,282,097 bp, 2,125 CDSs, 12 rRNAs, 48 tRNAs, and a G + C content of 56.61%; and a plasmid with a total length of 11,829 bp, 12 CDSs, and a G + C content of 56.31%. Taxon assignment using GTDB-Tk recognized strain NY0527 as a member of Actinomyces sp. with the ANI values of 98.83 and 81.66% to Actinomyces sp. UMB0138, and W. neuii DSM8576T (DSM8576 is the type strain of W. neuii), respectively, which suggested that strain NY0527, as well as strain UMB0138, did not belong to the species W. neuii. As the MALDI Biotyper had identified strain NY0527 as W. neuii subsp. antitratus with high confidence, we can deduce from here that W. neuii subsp. antitratus should be reassigned as a separate species, rather than a subspecies of W. neuii (Supplementary Table S1). Genome functional annotation of NY0527 using the RAST identified several CDSs coding virulence and antibiotic or heavy metal resistant functions such as Mycobacterium-like virulence factor, fluoroquinolones resistance, macrolides/lincosamides/streptogramins resistance, and copper homeostasis. Most of these features (e.g., virulence genes and antibiotic resistant genes) were shared by the genomes of Actinomyces sp. UMB0138 and W. neuii DSM8576T (Figure 1A). Nevertheless, the ermX gene was absent in the genome of W. neuii DSM8576T. Sequence inversions and losses were more prevalent in the genome of W. neuii DSM8576T than that in Actinomyces sp. UMB0138, when aligned to the genome sequence of NY0527 (Figure 1A). No plasmid sequences were identified in the genomic sequences of Actinomyces sp. UMB0138 and W. neuii DSM8576T. By sequence alignment against the NCBI-nt database, we found that the complete plasmid sequence pNY0527 showed no significant sequence similarity to that of any Actinomyces spp. or Winkia spp., while it displayed high sequence similarity to the chromosomal or plasmid sequences from Corynebacterium spp. with the query sequence coverage of about 40–50% (Supplementary Table S2), implying the occurrence of plasmid-directed trans-genus horizontal gene transfer (HGT) events. Notably, although only 40–50% of the pNY0527 sequence was mapped to each plasmid from the three Corynebacterium species (i.e., two from C. striatum, one from C. kefirresidentii, and one from C. diphtheriae), the total fragments mapped to the C. kefirresidentii plasmid (i.e., FDAARGOS_1055 unnamed) and the C. diphtheriae plasmid (i.e., FRC0402_p2) almost completely covered the entire sequence of pNY0527, indicating that the pNY0527 might have originated from the sequence recombination events of these plasmid families (Figure 1B). The pNY0527 carries an ermX gene flanked by IS6 family transposes (IS628), and this gene array was also partially or entirely presented in the C. striatum plasmid pTP10 and the C. diphtheriae plasmid FRC0402_p2 (Figure 1B). The transposes within this gene array may have facilitated the transfer of the ermX gene from the plasmid to the chromosome in strain NY0527 or in the Corynebacterium spp. Moreover, pNY0527 carries qacA that encodes efflux-mediated antiseptic (intercalating dyes and other organic cations) resistance proteins; and carries hin that encodes DNA invertase, which can regulate flagellar phase variations in pathogens (Brenner et al., 2002). Furthermore, 763 CDSs could be annotated by the RAST subsystem, the top five coded subsystem functions are “Cofactors, Vitamins, Prosthetic Groups, Pigments (70),” “Protein Metabolism (138),” “DNA Metabolism (56),” “Amino Acids and Derivatives (151)” and “Carbohydrates (107),” and no CDS was annotated to “Nitrogen Metabolism or Denitrification” (Figure 1C); annotation using eggNOG-mapper produced similar results to that of the RAST, excluding “S: Functions unknown,” the top five COGs are “E: Amino acid transport and metabolism,” “G: Carbohydrate transport and metabolism,” “J: Translation, ribosomal structure, and biogenesis,” “K: Transcription” and “L: Replication, recombination, and repair” (Figure 1D).
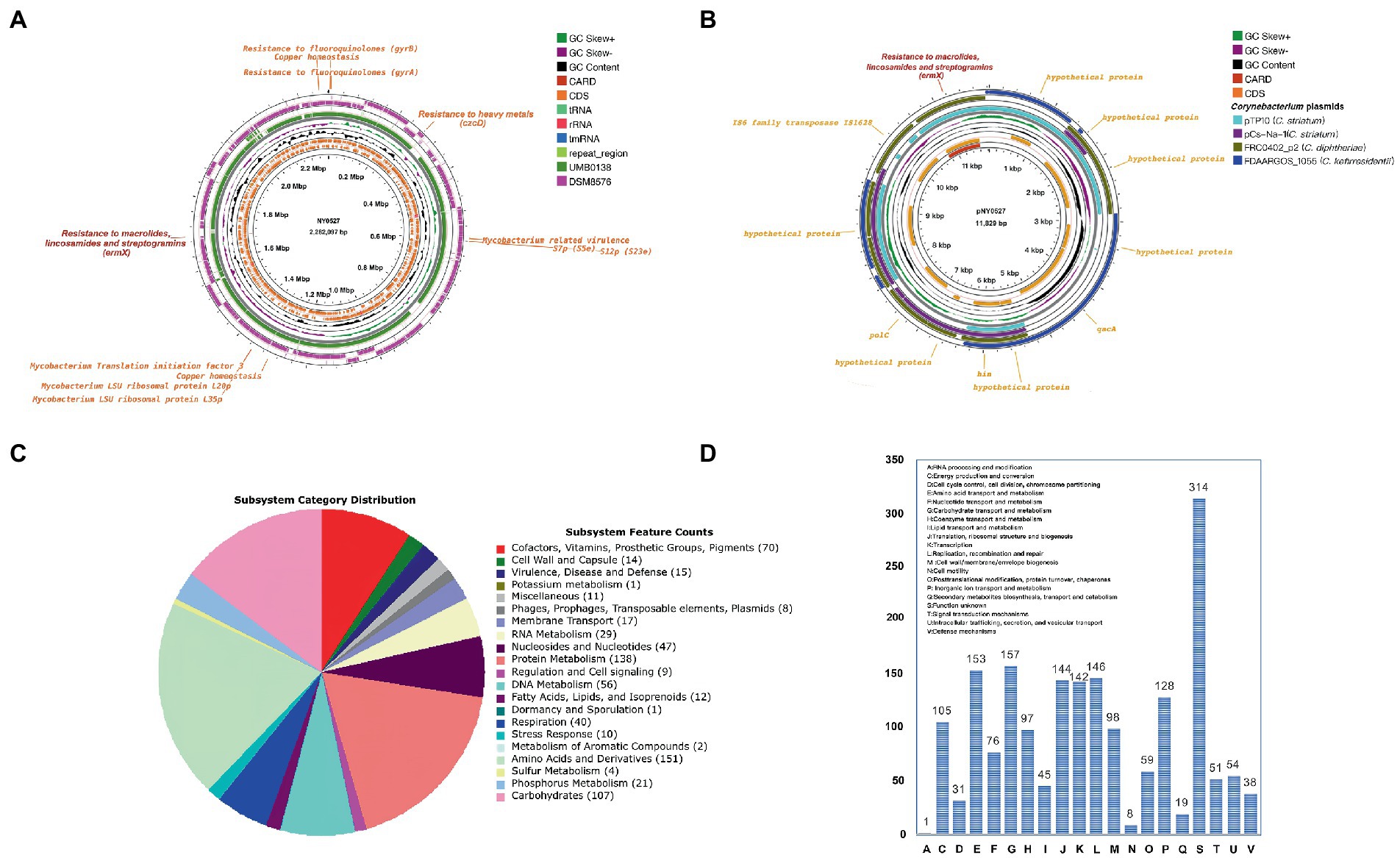
Figure 1. General genomic features of strain NY0527 characterized by the complete genome sequences. (A) The display of the circular chromosome sequence of strain NY0527. From inner to outer rings: genome backbone of strain NY0527 with ruler, antibiotic resistant genes, CDSs on the forward and reverse strands (RNAs and repeat sequence regions are displayed in the CDSs circles), GC content, GC skew, genome sequence of Actinomyces sp. UMB0138, genome sequence of W. neuii DSM8576T. (B) The display of the circular plasmid sequence pNY0527. From inner to outer rings: genome backbone of pNY0527 with ruler, antibiotic resistant genes, CDSs on the forward and reverse strands, GC content, and GC skew. The legend on the right is shared by (A) and (B). (C) Genomic subsystem functions annotated using the RAST. (D) Genomic COG functions annotated using the eggNOG-mapper.
3.3. Evidence for taxonomic reassignment of W. neuii
To determine the phylogeny of strain NY0527 and to eliminate the divergence between the results of MALDI Biotyper and GTDB-tk, whole genome sequence based phylogenic tree and ANI analysis were performed. The Similar Genome Finder identified that genome sequences from eight species, namely Mobiluncus curtisii, W. neuii, Arcanobacterium haemolyticum, Actinobaculum massiliae, Actinobaculum massiliense, Actinomyces hominis, Arcanobacterium haemolyticum, and Gleimia europaea, as well as Actinomyces sp. are mostly close to that of strain NY0527 by genome distance (GD) (data not shown). All of the genomes from the eight species as well as the Actinomyces sp. genomes that were closely related to NY0527, were then downloaded from the NCBI genome RefSeq database, and fed to fastANI for ANI analysis, and their whole-genome CDSs were uploaded to CVTree3 for phylogenomic tree construction. A total of 42 genome sequences were collected, detailed information is provided in Supplementary Table S3. The GD clustering revealed that all of the M. curtisii genomes formed a divergent clade (i.e., clade I), separate from the others (i.e., clade II) (Supplementary Figure S1). Moreover, two subclades mixed by the W. neuii and Actinomyces sp. genomes, along with an A. haemolyticum genome, (64_AHAE) were formed within clade II, with a GD higher than 0.05 between the subclades, suggesting that W. neuii could be reclassified and separated into two species (Supplementary Figure S1). The phylogenomic tree displayed identical results to that of the GD clustering, which showed the formation of a divergent M. curtisii clade and the formation of two subclades consisting of 15 W. neuii, Actinomyces sp. and A. haemolyticum strains (Figure 2A). Except for A. haemolyticum, which was isolated from Sus scrofa, all others were isolated from human source (Figure 2B). The ANI heatmap determined that the divergence of these two subclades is at the species level, as the within-subclade ANI > 95%, while the trans-subclade ANI < 85% (Figure 2B). Furthermore, strain ET43 should be removed from A. haemolyticum and reassigned as a novel species; strains ACS-171-V-Col2 and FC3 from A. massiliae and A. massiliense should be merged as one species (Figures 2A,B). Collectively, the currently documented W. neuii strains should be divided into two separate species, one clustered with the type strain of W. neuii subsp. neuii DSM8576T, consisting of seven strains (i.e., DSM8576, MJR8396A, UMB0125, HMSC06A08, HMSC072A03, UMB0402, and HMSC064C12); another clustered with W. neuii subsp. anitratus strains and should be reassigned as a separate Winkia species (referred as W. anitratus below in the text), consisting of eight strains (i.e., NY0527, UMB1295, 64_AHAE, UMB0918, UMB0138, L3_concoct_68, HMSC08A01 and BVS029A5) (Figure 2B).
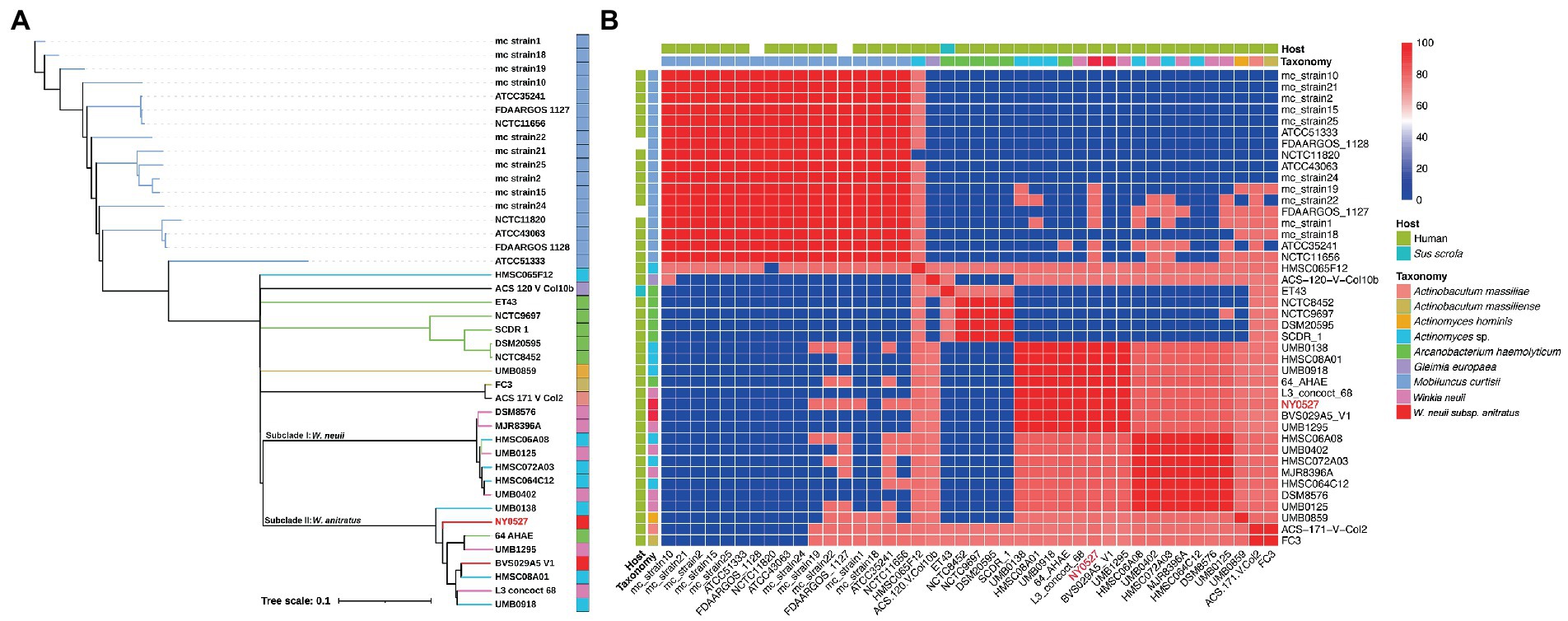
Figure 2. Phylogeny of strain NY0527 characterized by a phylogenomic tree and whole-genome-sequence-based ANI analysis. (A) WGS-based phylogenomic tree of phylogenetically close strains. Strain NY0727 is labeled in red. The taxonomy color code is shared with (B) in the legend. (B) Heatmap displaying the ANI between the phylogenetically close species. The color bar on the right indicates the ANI value calculated using fastANI.
3.4. Pan-genomic features of W. neuii and W. anitratus
Further characterization of the 15 Winkia genomes revealed that the genome of NY0527 is currently the sole genome that has been assembled at the completed level in the NCBI genome RefSeq database, the remaining genomes are at contigs or scaffolds levels (Figure 3A). The assemble qualities of these genomes are in high quality, with completeness greater than 99% and contaminations lower than 5%. Comparisons between the W. anitratus and W. neuii genome sequences showed that the W. anitratus has a lower genomic size (W. anitratus: 2.20 ± 0.05 Mb; W. neuii: 2.38 ± 0.05 Mb), a lower predicted gene counts (W. anitratus: 2044 ± 58; W. neuii: 2237 ± 88), but a higher G + C content (W. anitratus: 56.60 ± 0.04; W. neuii: 56.23 ± 0.03) than that of W. neuii (Figure 3A). As human pathogens, most of the W. anitratus (6/8) and W. neuii (5/7) strains that had genome sequenced were isolated from the infected urinary tracts. Others were isolated from wound ear, infant feces, mammary hematoma and hip abscesses (Figure 3A). Although the W. neuii had larger genome sizes and more predicted genes, pangenome analysis displayed that the W. anitratus strains carry much opener genomes, with higher count of pangenome clusters and lower count of coregenome clusters (Figures 3B,C).
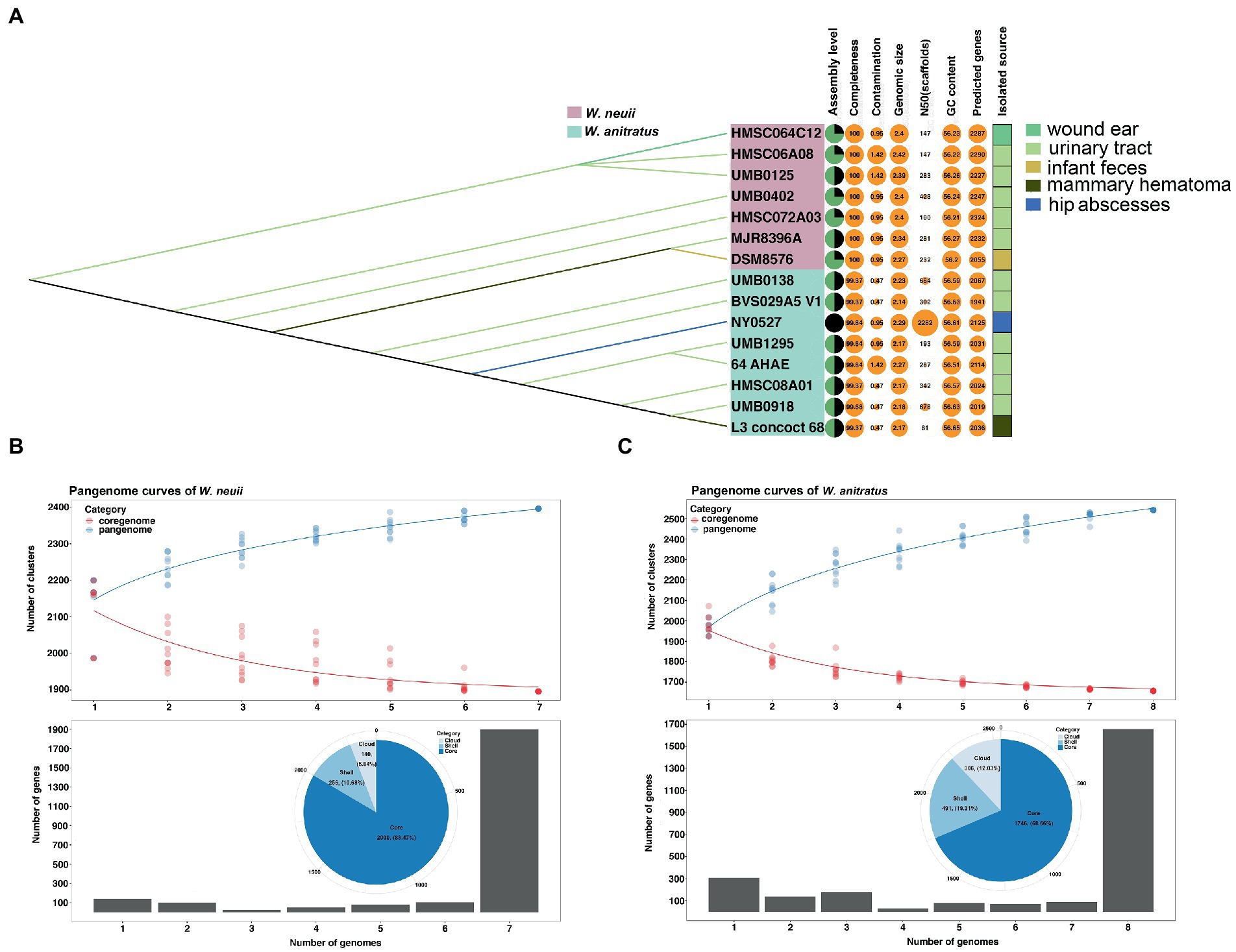
Figure 3. The comparison of pangenomic features between W. neuii and W. anitratus strains. (A) Characterization of the general genomic feature and isolation source. (B,C) Pangenomic features of W. neuii and W. anitratus strains.
3.5. Virulence factor coding capacity of W. neuii and W. anitratus
To explore the potential pathogenies of W. neuii and W. anitratus, PathoFact was employed to predict the virulence factor coding genes in their pangenome. We found that W. neuii and W. anitratus displayed distinct profiles in the virulence factor coding genes, thus enabling a clear differentiation between them. Only three virulence factor coding genes, namely rpoB, cfa_3, and pemK, were shared by two species (Figure 4). Each W. neuii strain encoded 35–38 virulence factors, higher than that of W. anitratus strain, which encoded 27–31 virulence factors, indicating that W. neuii has a higher pathogenicity potential than W. anitratus (Figure 4). At the species level, most of the virulence factor coding genes were shared by all strains within-species (Figure 4). Moreover, less than half of the virulence factor coding genes in both species were predicted as MGE-originated, whith the majority of such genes arising from phage- and plasmid-MGE (Figure 4). Only three categories of ARGs were identified, two (i.e., rpoB and ermX) of which in both species, encoding rifampicin resistance and macrolides/lincosamides/streptogramins resistance, and one (i.e., tetO or tetE) exclusively in W. neuii, encoding tetracycline resistance (Figure 4). Furthermore, 15 of the predicted virulence factor coding genes in the pangenome were recognized to be pathogenic, among which 10 were exclusively encoded by W. neuii and 5 were exclusively encoded by W. anitratus (Figure 4). Although the pathogenic virulence factor coding genes of W. neuii and W. anitratus differ greatly in counts and encoded functions, some of their pathogenic effects may be similar by encoding homologue proteins such as putative hemolysin and sialidase, which are shared by all Winkia strains (Table 2). Apart from this, an iota toxin coding gene is uniquely encoded by all W. anitratus strains, while a probable enterotoxin B coding gene and an iron dependent repressor coding gene are uniquely carried by all W. neuii strains (Table 2). Interestingly, some of the W. neuii strains encode insecticidal toxin complex proteins (TccC), although they were isolated from human sources (Table 2).
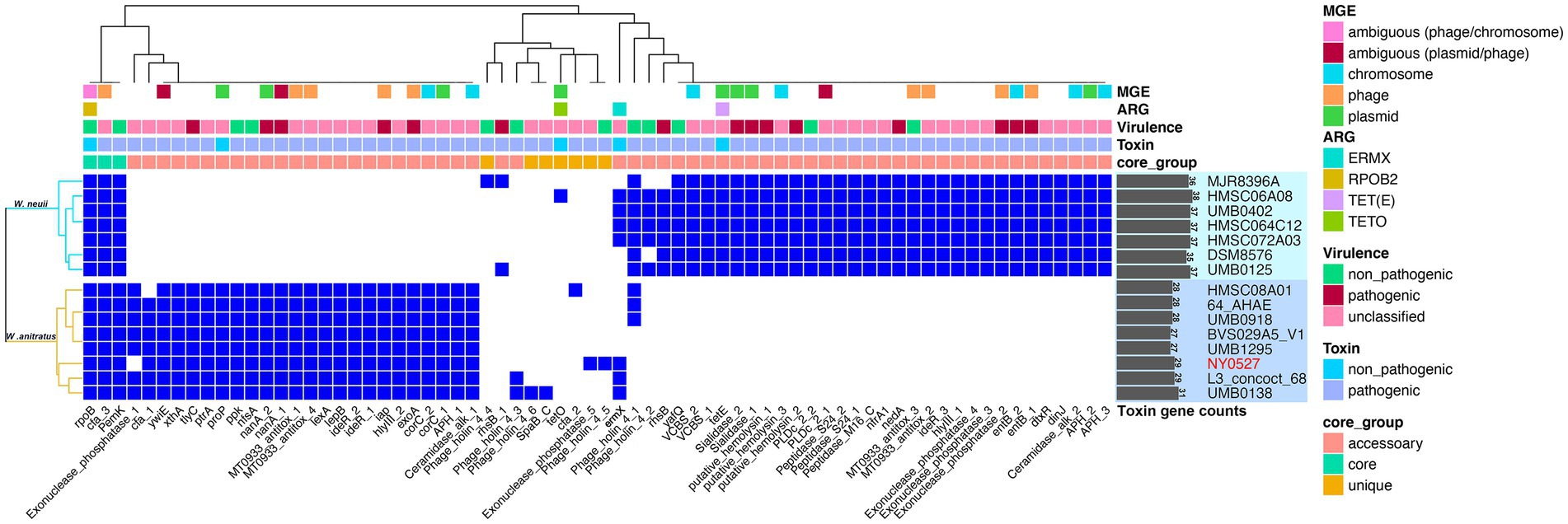
Figure 4. Pathogenic factor genes predicted in W. neuii and W. anitratus genomes. Hierarchical clustering was performed using the presence/absence pattern of the genes in the genomes. A blue cell in the heatmap indicates the presence of a gene. The virulence and toxin genes were defined as pathogenic when they were identified as pathogenic by both the HMM model and the random forest classifier.
4. Discussion
W. neuii has long been recognized as pathogenic to humans, and the types of infections it causes are increasingly observed with the development of advanced microbial identification systems. However, the taxonomy of this species has only recently been clarified, and its pathogenesis remains elusive. We isolated a clinically-derived Winkia strain NY0527 from the sanies of the hip abscess and assembled the complete genome sequences of the genus for the first time. We found that the infections could be effectively cured by using an antibiotic therapy of cefuroxime and ornidazole. We then determined that strain NY0527 is highly susceptible to the first-line clinical beta-lactam antibiotics. Further investigation disclosed that most of the Winkia spp. genomes carried the beta-lactamase expression repressor gene. Phylogenomic analyses and genome-based ANI analysis revealed that the two subspecies of W. neuii should be reclassified as two separate species (i.e., ANI < 95%). Moreover, we determined for the first time that the potent virulence factors of Winkia spp. are mainly plasmid- or phage-originated sialidase, hemolysin, and enterotoxins.
Abscesses are the most common human infections caused by W. neuii, which accounted for half of the reported cases (Zelyas et al., 2016). Fortunately, infections caused by W. neuii or related Actinomyces species usually have favorable outcomes after surgical debridement and antibiotic treatment (Könönen and Wade, 2015; Zelyas et al., 2016). In line with these previous observations, the patient infected with W. anitratus NY0527 was successfully treated after surgical debridement and a 14-day course of cefuroxime and ornidazole antibiotics. Winkia spp. had long been treated as commensals, as they were thought to only shift to pathogenesis in populations with iatrogenic interventions, myriad comorbidities, or immunodeficiency (Leal et al., 2016). In this study, however, the patient had no such medical history or immunodeficiency. This was also observed in a previous study that a patient got W. neuii associated neonatal sepsis but without immunodeficiency, anatomical abnormalities, or related diseases (Mann et al., 2002). These novel findings highlight the potential of Winkia spp. to achieve high pathogenicity under specific circumstances, which necessitates greater attention in clinical practice. Fortunately, Winkia spp. is generally highly sensitive to beta-lactam antibiotics (Mann et al., 2002; Graffi et al., 2012; Zelyas et al., 2016), as well as most other antibiotics, although it is sometimes resistant to aminoglycosides and fluoroquinolones (Hall and Copsey, 2015). In this study, the patient was successfully cured with a 14-day course of antibiotic therapy consisting of cefuroxime and ornidazole. The isolated strain W. anitratus NY0527 demonstrated susceptibility to most of the first-line clinical antibiotics (Table 1), which is in accordance with previous findings (Steininger and Willinger, 2016). However, a gene ermX that encodes resistance to macrolides, lincosamides, and streptogramins was carried by the plasmid of strain NY0527, which is flanked by an IS6 family transposase, and is likely responsible for the strain’s resistance to erythromycin and clindamycin (Figure 1; Table 1). Of note, the ermX gene may be carried by other W. neuii or W. anitratus strains (Figure 4), suggesting the possibility of acquired antibiotic resistance in Winkia spp., which should be highly noted in clinical settings. Despite carrying fluoroquinolone resistance genes on its chromosome, W. anitratus NY0527 is slightly susceptible to fluoroquinolone antibiotics (Table 1). In contrast, W. anitratus NY0527 is highly susceptible to tetracycline, and all of the W. anitratus strains carried no tetracycline resistant genes, whereas all of the W. neuii strains carried at least one tetracycline resistant gene (Figure 4). Furthermore, we found for the first time that 86.67% of the Winkia spp. genomes encode the beta-lactamase expression repressor gene (Supplementary Table S4). This suggests that beta-lactam antibiotics remain the preferred and safe choice for treating Winkia spp. infections in first-line clinical practice.
Winkia spp. had long been incorrectly classified as Actinomyces spp. due to the poorly resolved biochemical features and 16S rRNA-based phylogenetic trees (Nouioui et al., 2018). They were also often misidentified as skin contaminants, making accurate identification challenging (Gómez-Garcés et al., 2010). Only until recently, by using the genome-based taxonomic classification methods, such as the phylogenomic tree and pairwise genome-based ANI comparison, Nouioui et al. (2018) separated A. neuii from the genus Actinomyces, and reassigned it as a novel genus, namely Winkia. In this genus, W. neuii is currently the sole species validly published. These methods produced much better resolved taxonomic assignments than traditional approaches, which had been recognized as useful tools to further clarify organisms with agricultural, biotechnological and clinical importance (Chun et al., 2018; Ciufo et al., 2018; Nouioui et al., 2018). Our phylogenomic and ANI analyses showed that W. neuii was phylogenetically closer to M. curtisii than to the Actinomyces spp., and further clarified that the subspecies within W. neuii should be reclassified as two independent species (Figure 2). These two species can also be distinguished by general genomic features such as genome size, whole genome G + C content, and number of CDSs (Figure 3A). In recent years, the use of hybrid assembly techniques that combine long-reads sequencing technologies (e.g., Nanopore) with short-reads sequencing technologies (e.g., Illumina) has enabled the more efficient and accurate acquisition of complete genome sequences of clinically relevant microorganisms (Ben Khedher et al., 2022). These complete genome sequences serve as high-quality reference genomes for functional genomic analyses, such as investigations of virulence, antibiotic resistance, and mobile genetic elements (Ben Khedher et al., 2022). In our study, we recovered the first complete plasmid and chromosome sequences of Winkia spp. by utilizing this hybrid assembly approach. Our analysis of these sequences revealed potential recombination and transfer events occurring between Winkia spp. and their commonly observed co-colonizers, Corynebacterium spp. This suggests that gene exchange between different clinical bacterial genera should be considered. Disappointedly, the 15 WGS-sequenced Winkia spp. now published in the NCBI genome RefSeq database are isolated from very limited range of human body sites (e.g., wound ear, urinary tract, mammary hematoma, and buttock abscesses), despite the fact that they have been reported isolated from many other body sites, such as foreign body devices, the oral cavity, and blood (Yang and Grant, 2019). It is certain that more isolates from diverse body sites are required to be sequenced and completely assembled in the future to better comprehend their taxonomic diversities, pathogenesis and the gene exchange events with other co-colonized species.
Because Winkia spp. had not been isolated from non-human sources, as reported in previous studies and validated in this study, the infections caused by Winkia spp. are thought to be endogenous (von Graevenitz, 2011). Nevertheless, potential virulence factors carried by Winkia spp. have not been investigated thus far. We found that the Winkia spp. genomes encoded at least two core-pathogenic virulence factors (or their homologs), namely hemolysin and sialidase, to help them infect the host. Hemolysin has been proven to be a family of bacterial pore-forming toxin expressed in many pathogenic bacteria, including Proteus, Morganella, E. coli and Moraxella (Kaplan et al., 2009). For example, E. coli could produce hemolysin, which enables it to cause an infection in the urinary tract or other extraintestinal sites (Donnenberg, 2002). Numerous Actinomyces species produce sialidase, an enzyme that facilitates their adhesion to host tissues by cleaving sialic acid residues from the host’s cell surface. Sialidase also has the ability to deglycosylate immunoglobulins, thus altering the host’s immune response to the infection (Do et al., 2008; Bernard, 2012). Furthermore, some of the virulence factors are specifically encoded within-species between W. neuii and W. anitratus. For example, the iota toxin may produce cytotoxic activity through necrosis (Navarro et al., 2018) and is specifically coded by all W. anitratus strains, while the enterotoxin B may cause food poisoning with severe diarrhea and intestinal cramping (Bae et al., 2021), and is specifically coded by all W. neuii strains. Notably, the W. neuii encoded roughly twice as many pathogenic virulence factors as the W. anitratus (Figure 4), implying that the W. neuii is more virulent than the W. anitratus. This hypothesis can be corroborated by the fact that W. neuii subsp. neuii is more prevailed over W. neuii subsp. anitratus under clinical observations (von Graevenitz, 2011). As such, it is not surprising that the Winkia spp. can infect immune competent individuals in some cases. We therefore suggest strengthening the isolation, species identification, and whole genome sequencing of the clinically-derived Winkia spp. strains in the future, which would be useful in tailoring effective treatments or prevention schedules for the related infections.
In conclusion, we reported an infective hip abscess caused by a Winkia strain NY0527 and assembled the first completed genome sequences (i.e., one circular chromosome sequence and one circular plasmid sequence) of the genus Winkia for comparative genomic analysis. We found that the two subspecies of W. neuii could be reclassified as two separate species, and thereby emphasize the potential underestimation of taxonomic diversity within the genus. For the first time, we demonstrated the mechanisms of antibiotic resistance and pathogenesis of Winkia spp. at genus and species levels by using in silico genomic analyses. We further suggested that beta-lactam antibiotics can still be effectively utilized as first-line antibiotics for the treatment of Winkia spp. infections. The findings of this study provide a global clue of the pathogenic, antibiotic-resistant, and taxonomic features of Winkia spp. and underline the importance of focusing on emerging Winkia spp. infections and their potential antibiotic resistances.
Data availability statement
The datasets presented in this study can be found in online repositories. The names of the repository/repositories and accession number(s) can be found at: https://www.ncbi.nlm.nih.gov/, PRJNA925112.
Ethics statement
The studies involving human participants were reviewed and approved by the Ethics Committee of Shenzhen University General Hospital. The patients/participants provided their written informed consent to participate in this study.
Author contributions
XC, YP, and ML performed the bacteria isolation and taxa identification. XC and YP performed the WGS-based analysis and drafted the manuscript. YQ and YW reviewed and revised the manuscript. LX and QH designed and supervised the whole study. All authors made substantial and direct contributions to the work, and read and approved the final version of the manuscript.
Funding
This work was supported by the National Natural Science Foundation of China (41907214 and 82002716); the Shenzhen Science and Technology Program (JCYJ20190808111610984 and JCYJ20190808164209301); the Project of Department of Education of Guangdong Province (2019KTSCX146); Shenzhen Scientific Research Foundation for Excellent Returned Scholars (000493); Natural Science Foundation of Shenzhen University General Hospital (SUGH2020QD005); Shenzhen Key Laboratory Foundation (ZDSYS20200811143757022).
Conflict of interest
The authors declare that the research was conducted in the absence of any commercial or financial relationships that could be construed as a potential conflict of interest.
Publisher’s note
All claims expressed in this article are solely those of the authors and do not necessarily represent those of their affiliated organizations, or those of the publisher, the editors and the reviewers. Any product that may be evaluated in this article, or claim that may be made by its manufacturer, is not guaranteed or endorsed by the publisher.
Supplementary material
The Supplementary material for this article can be found online at: https://www.frontiersin.org/articles/10.3389/fmicb.2023.1147469/full#supplementary-material
Footnotes
References
Alsohime, F., Assiri, R. A., Al-Shahrani, F., Bakeet, H., Elhazmi, M., and Somily, A. M. (2019). Premature labor and neonatal sepsis caused by Actinomyces neuii. J. Infect. Public Heal. 12, 282–284. doi: 10.1016/j.jiph.2018.04.001
Bae, J. S., Da, F., Liu, R., He, L., Lv, H. Y., Fisher, E. L., et al. (2021). Contribution of staphylococcal enterotoxin B to Staphylococcus aureus systemic infection. J. Infect. Dis. 223, 1766–1775. doi: 10.1093/infdis/jiaa584
Barberis, C., Budia, M., Palombarani, S., Rodriguez, C. H., Ramírez, M. S., Arias, B., et al. (2017). Antimicrobial susceptibility of clinical isolates of Actinomyces and related genera reveals an unusual clindamycin resistance among Actinomyces urogenitalis strains. J. Glob. Antimicrob. Re. 8, 115–120. doi: 10.1016/j.jgar.2016.11.007
Ben Khedher, M., Ghedira, K., Rolain, J.-M., Ruimy, R., and Croce, O. (2022). Application and challenge of 3rd generation sequencing for clinical bacterial studies. Int. J. Mol. Sci. 23:1395. doi: 10.3390/ijms23031395
Bernard, K. (2012). The genus Corynebacterium and other medically relevant coryneform-like bacteria. J. Clin. Microbiol. 50, 3152–3158. doi: 10.1128/JCM.00796-12
Brenner, S., Miller, J. H., and Broughton, W.. Encyclopedia of Genetics. (2002). Elsevier: Amsterdam.
Chen, X.-F., Hou, X., Xiao, M., Zhang, L., Cheng, J.-W., Zhou, M.-L., et al. (2021). Matrix-assisted laser desorption/ionization time of flight mass spectrometry (MALDI-TOF MS) analysis for the identification of pathogenic microorganisms: a review. Microorganisms 9:1536. doi: 10.3390/microorganisms9071536
Chen, B. C., Kobayashi, T., Ford, B., and Sekar, P. (2020). Late prosthetic shoulder joint infection due to Actinomyces neuii in an adult man. BMJ Case Rep. 13:e236350. doi: 10.1136/bcr-2020-236350
Chun, J., Oren, A., Ventosa, A., Christensen, H., Arahal, D. R., da Costa, M. S., et al. (2018). Proposed minimal standards for the use of genome data for the taxonomy of prokaryotes. Int. J. Syst. Evol. Microbiol. 68, 461–466. doi: 10.1099/ijsem.0.002516
Ciufo, S., Kannan, S., Sharma, S., Badretdin, A., Clark, K., Turner, S., et al. (2018). Using average nucleotide identity to improve taxonomic assignments in prokaryotic genomes at the NCBI. Int. J. Syst. Evol. Microbiol. 68, 2386–2392. doi: 10.1099/ijsem.0.002809
Clinical and Laboratory Standards Institute (CLSI). (2003) Susceptibility testing of mycobacteria, norcardiae, and other aerobic actinomycetes; Approved Standard. Document M24-A. USA: CLSI.
Coudron, P., Harris, R., Vaughan, M., and Dalton, H. (1985). Two similar but atypical strains of coryneform group A-4 isolated from patients with endophthalmitis. J. Clin. Microbiol. 22, 475–477. doi: 10.1128/jcm.22.4.475-477.1985
de Nies, L., Lopes, S., Busi, S. B., Galata, V., Heintz-Buschart, A., Laczny, C. C., et al. (2021). PathoFact: a pipeline for the prediction of virulence factors and antimicrobial resistance genes in metagenomic data. Microbiome 9, 1–14. doi: 10.1186/s40168-020-00993-9
De Vreese, K., and Verhaegen, J. (2013). Identification of coryneform Actinomyces neuii by MALDI-TOF MS: 5 case reports and review of literature. Acta Clin. Belg. 68, 210–214. doi: 10.2143/ACB.3224
Do, T., Henssge, U., Gilbert, S. C., Clark, D., and Beighton, D. (2008). Evidence for recombination between a sialidase (nanH) of Actinomyces naeslundii and Actinomyces oris, previously named ‘Actinomyces naeslundii genospecies 1 and 2’. FEMS Microbiol. Lett. 288, 156–162. doi: 10.1111/j.1574-6968.2008.01336.x
Donnenberg, M. S.. Escherichia coli: Virulence mechanisms of a versatile pathogen. (2002): Academic Press California, Oakland, CA.
Funke, G., Lucchini, G. M., Pfyffer, G., Marchiani, M., and von Graevenitz, A. (1993). Characteristics of CDC group 1 and group 1-like coryneform bacteria isolated from clinical specimens. J. Clin. Microbiol. 31, 2907–2912. doi: 10.1128/jcm.31.11.2907-2912.1993
Funke, G., Stubbs, S., Von Graevenitz, A., and Collins, M. D. (1994). Assignment of human-derived CDC group 1 coryneform bacteria and CDC Group 1-like coryneform bacteria to the genus Actinomyces as Actinomyces neuii subsp. neuii sp. nov., subsp. nov., and Actinomyces neuii subsp. anitratus subsp. nov. Int. J. Syst. Evol. Micr. 44, 167–171. doi: 10.1099/00207713-44-1-167
Funke, G., and Von Graevenitz, A. (1995). Infections due to Actinomyces neuii (former “CDC coryneform group 1” bacteria). Infection 23, 73–75. doi: 10.1007/BF01833868
Giannoulopoulos, G., and Errington, K. (2022). Case report and successful management of canine aortic endocarditis caused by Actinomyces neuii subsp. anitratus (Winkia neuii subsp. anitrata). BMC Vet. Res. 18, 1–6. doi: 10.1186/s12917-022-03161-3
Gómez-Garcés, J. L., Burillo, A., Gil, Y., and Sáez-Nieto, J. A. (2010). Soft tissue infections caused by Actinomyces neuii, a rare pathogen. J. Clin. Microbiol. 48, 1508–1509. doi: 10.1128/JCM.02139-09
Graffi, S., Peretz, A., and Naftali, M. (2012). Endogenous endophthalmitis with an unusual infective agent: Actinomyces neuii. Eur. J. Ophthalmol. 22, 834–835. doi: 10.5301/ejo.5000106
Ha, S.-M., Kim, C. K., Roh, J., Byun, J.-H., Yang, S.-J., Choi, S.-B., et al. (2019). Application of the whole genome-based bacterial identification system, TrueBac ID, using clinical isolates that were not identified with three matrix-assisted laser desorption/ionization time-of-flight mass spectrometry (MALDI-TOF MS) systems. Ann. Lab. Med. 39, 530–536. doi: 10.3343/alm.2019.39.6.530
Hall, V. (2008). Actinomyces—gathering evidence of human colonization and infection. Anaerobe 14, 1–7. doi: 10.1016/j.anaerobe.2007.12.001
Hall, V., and Copsey, S. D. (2015). “Propionibacterium, lactobacillus, Actinomyces, and other non-spore-forming anaerobic gram-positive rods,” in Manual of Clinical Microbiology. ed. J. H. Jorgensen, et al. (Washington, DC: ASM Press), 920–939.
Hara, T., Kitagawa, H., Kajihara, T., Koba, Y., Tadera, K., Nagaoka, R., et al. (2021). Bloodstream infection caused by Actinomyces neuii subsp. anitratus in a patient with breast cancer: a case report and literature review. Hiroshima J. Med. Sci. 70, 43–47. doi: 10.24811/hjms.70.2-4_43
Hoyles, L., Collins, M. D., Falsen, E., Nikolaitchouk, N., and McCartney, A. L. (2004). Transfer of members of the genus Falcivibrio to the genus Mobiluncus, and emended description of the genus Mobiluncus. Syst. Appl. Microbiol. 27, 72–83. doi: 10.1078/0723-2020-00260
Huerta-Cepas, J., Forslund, K., Coelho, L. P., Szklarczyk, D., Jensen, L. J., Von Mering, C., et al. (2017). Fast genome-wide functional annotation through orthology assignment by eggNOG-mapper. Mol. Biol. Evol. 34, 2115–2122. doi: 10.1093/molbev/msx148
Jain, C., Rodriguez-R, L. M., Phillippy, A. M., Konstantinidis, K. T., and Aluru, S. (2018). High throughput ANI analysis of 90K prokaryotic genomes reveals clear species boundaries. Nat. Commun. 9, 1–8. doi: 10.1038/s41467-018-07641-9
Kaplan, S. L., Hulten, K. G., and Mason, E. O. (2009). “Staphylococcus aureus infections (coagulase-positive staphylococci)” in Feigin and Cherry's Textbook of Pediatric Infectious Diseases (Amsterdam: Elsevier), 1197–1213.
Könönen, E., and Wade, W. G. (2015). Actinomyces and related organisms in human infections. Clin. Microbiol. Rev. 28, 419–442. doi: 10.1128/CMR.00100-14
Leal, S. M. Jr., Jones, M., and Gilligan, P. H. (2016). Clinical significance of commensal gram-positive rods routinely isolated from patient samples. J. Clin. Microbiol. 54, 2928–2936. doi: 10.1128/JCM.01393-16
Leenstra, B., Schaap, C., Bessems, M., Renders, N., and Bosscha, K. (2017). Primary actinomycosis in the breast caused by Actinomyces neuii. A report of 2 cases. IDCases 8, 70–72. doi: 10.1016/j.idcr.2017.03.014
Mann, C., Dertinger, S., Hartmann, G., Schurz, R., and Simma, B. (2002). Actinomyces neuii and neonatal sepsis. Infection 30, 178–180. doi: 10.1007/s15010-002-2165-3
Navarro, M. A., McClane, B. A., and Uzal, F. A. (2018). Mechanisms of action and cell death associated with Clostridium perfringens toxins. Toxins 10:212. doi: 10.3390/toxins10050212
Nouioui, I., Carro, L., García-López, M., Meier-Kolthoff, J. P., Woyke, T., Kyrpides, N. C., et al. (2018). Genome-based taxonomic classification of the phylum Actinobacteria. Front. Microbiol. 9:2007. doi: 10.3389/fmicb.2018.02007
Overbeek, R., Olson, R., Pusch, G. D., Olsen, G. J., Davis, J. J., Disz, T., et al. (2014). The SEED and the rapid annotation of microbial genomes using subsystems technology (RAST). Nucleic Acids Res. 42, D206–D214. doi: 10.1093/nar/gkt1226
Page, A. J., Cummins, C. A., Hunt, M., Wong, V. K., Reuter, S., Holden, M. T., et al. (2015). Roary: rapid large-scale prokaryote pan genome analysis. Bioinformatics 31, 3691–3693. doi: 10.1093/bioinformatics/btv421
Parks, D. H., Chuvochina, M., Waite, D. W., Rinke, C., Skarshewski, A., Chaumeil, P.-A., et al. (2018). A standardized bacterial taxonomy based on genome phylogeny substantially revises the tree of life. Nat. Biotechnol. 36, 996–1004. doi: 10.1038/nbt.4229
Rahi, P., Prakash, O., and Shouche, Y. S. (2016). Matrix-assisted laser desorption/ionization time-of-flight mass-spectrometry (MALDI-TOF MS) based microbial identifications: challenges and scopes for microbial ecologists. Front. Microbiol. 7:1359. doi: 10.3389/fmicb.2016.01359
Roustan, A., Al Nakib, M., and Boubli, L. (2009). Primary actinomycosis of the breast due to Actinomyces neuii. J. Gynecol. Obst. Bio. Reprod. 39, 64–67. doi: 10.1016/j.jgyn.2009.09.005
Schaal, K., Crecelius, A., Schumacher, G., and Yassin, A. (1999). Towards a new taxonomic structure of the genus Actinomyces and related bacteria. Nova. Acta. Leopoldina NF 80, 83–91.
Seemann, T. (2014). Prokka: rapid prokaryotic genome annotation. Bioinformatics 30, 2068–2069. doi: 10.1093/bioinformatics/btu153
Steininger, C., and Willinger, B. (2016). Resistance patterns in clinical isolates of pathogenic Actinomyces species. J. Antimicrob. Chemoth. 71, 422–427. doi: 10.1093/jac/dkv347
von Graevenitz, A. (2011). Actinomyces neuii: review of an unusual infectious agent. Infection 39, 97–100. doi: 10.1007/s15010-011-0088-6
Watkins, R. R., Anthony, K., Schroder, S., and Hall, G. S. (2008). Ventriculoperitoneal shunt infection caused by Actinomyces neuii subsp neuii. J. Clinical Microbiol. 46, 1888–1889. doi: 10.1128/JCM.02141-07
Wayne, P., Performance Standards for Antimicrobial Susceptibility Testing, Clinical and Laboratory Standards Institute, Wayne, PA (2011).
Welker, M., Van Belkum, A., Girard, V., Charrier, J.-P., and Pincus, D. (2019). An update on the routine application of MALDI-TOF MS in clinical microbiology. Exper. Rev. Proteomic. 16, 695–710. doi: 10.1080/14789450.2019.1645603
Wick, R. R., Judd, L. M., Gorrie, C. L., and Holt, K. E. (2017). Unicycler: resolving bacterial genome assemblies from short and long sequencing reads. PLoS Comput. Biol. 13:e1005595. doi: 10.1371/journal.pcbi.1005595
Yang, W. T., and Grant, M. (2019). Actinomyces neuii: a case report of a rare cause of acute infective endocarditis and literature review. BMC Infect. Dis. 19, 1–7. doi: 10.1186/s12879-019-4149-2
Zelyas, N., Gee, S., Nilsson, B., Bennett, T., and Rennie, R. (2016). Infections caused by Actinomyces neuii: a case series and review of an unusual bacterium. Can. J. Infect. Dis. Med. 2016, 1–7. doi: 10.1155/2016/6017605
Keywords: abscess, Winkia neuii, bacterial infections, complete genome, virulence traits
Citation: Cai X, Peng Y, Li M, Qiu Y, Wang Y, Xu L and Hou Q (2023) Comparative genomic analyses of the clinically-derived Winkia strain NY0527: the reassignment of W. neuii subsp. neuii and W. neuii subsp. antitratus into two separate species and insights into their virulence characteristics. Front. Microbiol. 14:1147469. doi: 10.3389/fmicb.2023.1147469
Edited by:
Alicja Wegrzyn, Polish Academy of Sciences, PolandReviewed by:
Alida Catharina Veloo, University Medical Center Groningen, NetherlandsXiao Li, Tianjin Medical University, China
Haoming Xu, Guangzhou First People’ Hospital, China
Copyright © 2023 Cai, Peng, Li, Qiu, Wang, Xu and Hou. This is an open-access article distributed under the terms of the Creative Commons Attribution License (CC BY). The use, distribution or reproduction in other forums is permitted, provided the original author(s) and the copyright owner(s) are credited and that the original publication in this journal is cited, in accordance with accepted academic practice. No use, distribution or reproduction is permitted which does not comply with these terms.
*Correspondence: Long Xu, bG9uZ3h1MTAxMkBzenUuZWR1LmNu; Qi Hou, cWlfaG91QGZveG1haWwuY29t