- 1College of Laboratory Medicine, Jilin Medical University, Jilin City, China
- 2General Surgery, Jilin People’s Hospital, Jilin City, China
- 3Department of Laboratory Medicine, Jilin Hospital of Integrated Chinese and Western Medicine, Jilin City, China
- 4Center for Human Genetics and Genomics, New York University Grossman School of Medicine, New York City, NY, United States
Acanthamoeba is an opportunistic protozoa, which exists widely in nature and is mainly distributed in soil and water. Acanthamoeba usually exists in two forms, trophozoites and cysts. The trophozoite stage is one of growth and reproduction while the cyst stage is characterized by cellular quiescence, commonly resulting in human infection, and the lack of effective monotherapy after initial infection leads to chronic disease. Acanthamoeba can infect several human body tissues such as the skin, cornea, conjunctiva, respiratory tract, and reproductive tract, especially when the tissue barriers are damaged. Furthermore, serious infections can cause Acanthamoeba keratitis, granulomatous amoebic encephalitis, skin, and lung infections. With an increasing number of Acanthamoeba infections in recent years, the pathogenicity of Acanthamoeba is becoming more relevant to mainstream clinical care. This review article will describe the etiological characteristics of Acanthamoeba infection in detail from the aspects of biological characteristic, classification, disease, and pathogenic mechanism in order to provide scientific basis for the diagnosis, treatment, and prevention of Acanthamoeba infection.
1. Introduction
Acanthamoeba is an opportunistic protozoa that is widely distributed in the natural environment, such as sea water, swimming pools, tap water, natural thermal water, soil, dust, and even the nasal mucosa of healthy individuals (Rivera et al., 1989, 1991; Michel et al., 1994; Tawfeek et al., 2016; Lass et al., 2017; Carnt et al., 2020; Wopereis et al., 2020). Pathogenic species can cause serious blindness in humans, arising from Acanthamoeba keratitis (AK) and rare granulomatous amoebic encephalitis (GAE) as well as skin and lung infections. Cases of AK and GAE were first reported by Fowler and Carter (1965) and by Naginton et al. (1974), respectively. The epidemiological investigation shows that the number of Acanthamoeba infection is increasing year by year, especially those with AK infection. In the United States, a study examining AK at thirteen ophthalmology centers and laboratories found that over the course of 2004–2007, a precipitous increase in AK cases had occurred: 22 cases were diagnosed in 1999, 43 cases were diagnosed in 2003, and 170 cases were diagnosed in 2007 (Yoder et al., 2012). In the UK, the numbers of AK cases diagnosed annually at the Moorfields Eye Hospital in London from 2011 to 2014 (range of 36–65 cases/year) were approximately two-to-three times higher than in 2004–2010 (range of 15–23 cases per year) (Carnt et al., 2018). In Australia, a study from the quaternary referral center in Sydney found that over the period from 2002 to 2016, the average annual number of cases was 50% higher post-2007 relative to pre-2007, and the highest numbers of cases were reported in 2007 and 2014 (Höllhumer et al., 2020). Studies found that wearing corneal contact lenses is the main risk factor for infection (Lindsay et al., 2007; Carnt and Stapleton, 2016; Dos Santos et al., 2018). With the increase of Acanthamoeba infection especially AK infection in recent years, scholars have begun expending extensive effort in characterizing and classifying the Acanthamoeba genus, as shown in Figure 1. This article summarizes the breadth of this work in order to provide scientific basis for the diagnosis, treatment, and prevention of Acanthamoeba infection.
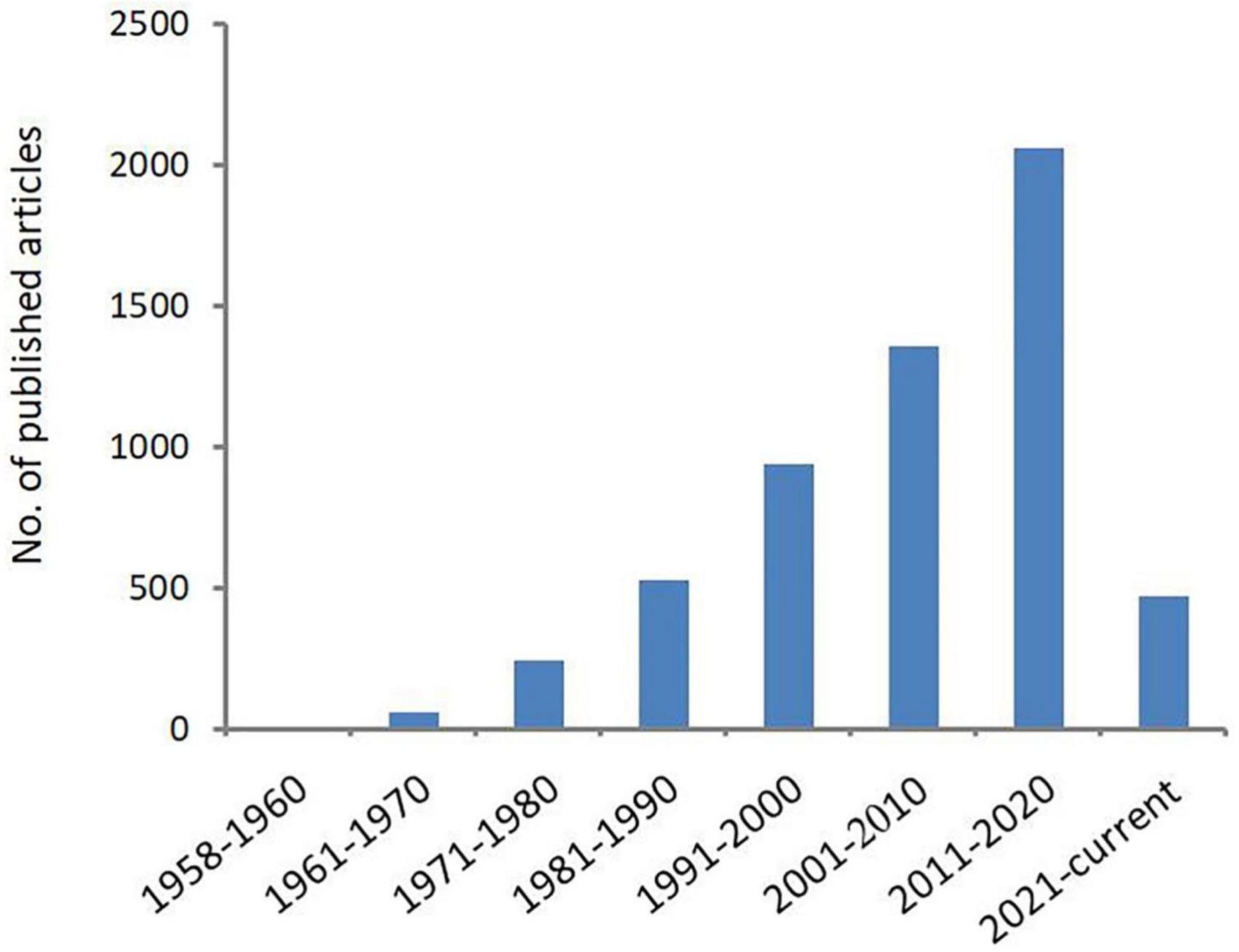
Figure 1. Number of publications related to Acanthamoeba. Increasing scientific interest in the field of Acanthamoeba as determined by published articles cataloged in PubMed over time.
2. Biological characteristics
2.1. Life history of Acanthamoeba
In general, the life cycle of Acanthamoeba consists of two stages, trophozoite and cyst (Marciano-Cabral and Cabral, 2003; Siddiqui and Khan, 2012; Siddiqui et al., 2012), except for Acanthamoeba pyriformis, which is recognized to include facultative sporocarp fruiting in its life cycle (Tice et al., 2016). The trophozoite stage dominates when the growth conditions are suitable, such as abundant food supply, neutral pH, appropriate temperature (i.e., 30°C) and osmolarity between 50 and 80 mOsmol (Siddiqui et al., 2012). The cell cycle of Acanthamoeba consists of growth and replication, which is carried out through mitosis under optimal living conditions (Band et al., 1970; Byers et al., 1991; Quinet et al., 2020). In axenic cultures, Acanthamoeba exhibits a typical exponential growth phase, followed by a period of reduced growth rate, and finally stationary phase during which no further increase in cell density occurs (Band and Mohrlok, 1973; Stevens and Pachler, 1973). Studies have revealed that the majority of Acanthamoeba cells in stationary phase culture develop into quiescent cysts in response to adverse conditions such as lack of food, hyper- or hypo-osmolarity, extremes in temperature and pH, high cell densities, and chemicals (Neff and Neff, 1969; Band and Mohrlok, 1973; Weisman, 1976; Stohr et al., 1987; Sriram et al., 2008). The trophozoites of Acanthamoeba mainly feed on bacteria, algae, yeast, or small organic particles via phagocytosis or pinocytosis and form many food vacuoles in the cytoplasm (Bowers, 1977; Bowers and Olszewski, 1983; Marciano-Cabral and Cabral, 2003). Pinocytosis is considered to be non-specific endocytosis (Bowers and Olszewski, 1972), while phagocytosis is considered to be receptor-dependent endocytosis (Alsam et al., 2005a). Once Acanthamoeba cells enter stationary phase, phagocytic activity ceases, while pinocytic activity is halved. The reduced pinocytic activity remains sensitive to respiratory inhibitors. The unequal responses of phagocytosis and pinocytosis to the onset of stationary-phase growth suggest that they are independent processes subject to different controls (Chambers and Thompson, 1976).
During the stationary phase shift, cysts begin to form with minimal metabolic activity (Siddiqui and Khan, 2012). Early in encystation, large numbers of vacuoles are observed in the encysting cells and many lysosomes and peroxisomes are present in the mature cysts (Müller, 1969; Müller and Moller, 1969; Siddiqui et al., 2012). Bowers and Olszewski (1983) showed that Acanthamoeba have the ability to distinguish vacuoles containing digestible and indigestible particles. For example, when cells were allowed to phagocytose yeast to capacity, endocytosis stopped and subsequent presentation of particles (either yeast or beads) did not result in further uptake. By contrast, when cells were allowed to phagocytose plastic beads to capacity and a second dose of particles was presented (either yeast or beads), the cells exocytosed the internal particles and took up the new ones. Therefore, the fate of vacuoles containing yeast and vacuoles containing plastic beads in encystation is different (Bowers and Olszewski, 1983). The digestive vacuoles disappear during later stages of encystation and their contents are discharged. This phenomenon explains the weakening of phagocytic activity and pinocytic activity in this period. Cysts are highly tolerant to the extreme environmental conditions, which allows Acanthamoeba to spread in the environment and/or carry these pathogens into host species, which is described later in the text.
2.2. The morphological structure of Acanthamoeba
2.2.1. Trophozoite
The trophozoite varies in size from 25 to 40 μm in diameter (Marciano-Cabral and Cabral, 2003) and has a long oval or irregular shape. The cytoplasmic boundary of trophozoite is unclear and the endoplasm is granular, which emits several acicular or spinous pseudopods extending to the whole surface of the cell (Figure 2A). The pseudopods give the trophozoite a characteristic appearance and participate in the feeding and movement of Acanthamoeba. Pseudopods in Acanthamoeba have a peculiar form. The term “acanth” is a Greek word meaning “spike” to indicate the presence of spine-like structures (also known as acanthopods) on the surface of the amoeba (Preston and King, 1984; Preston et al., 2001; Marciano-Cabral and Cabral, 2003). Pseudopods are responsible for adhesion to the surface of contact lenses, increasing the chance of Acanthamoeba infection in the cornea (John et al., 1989; Omaña-Molina et al., 2014). The trophozoite is typically uninucleate with a nucleus that is approximately one sixth the size of the cell body, but multinucleated individuals can be seen in laboratory cultures when grown with constant agitation (Byers and James, 1967). To some extent, this phenomenon reflects the real living condition of Acanthamoeba in nature because the existence of Acanthamoeba can often be found in an aquatic environment. Any flux perturbations, such as water currents or waves, might detach amoebae from their substrate and suspend them in the water in a non-adherent state, which creates conditions for the formation of multinucleation. This process will help amoebae to colonize new environmental niches that are difficult to reach using an adherent state. When adhesion is restored, multinucleated amoebae generate a higher progeny population compared to uninucleate mother cells. This process is beneficial from the point of view of continual population reproduction (Quinet et al., 2020). The nuclear envelope is separated by a distance of about 350 Å with numerous nuclear pores (Bowers and Korn, 1968). Chromatin is located on the inner surface of the nuclear envelope. The nucleolus is perhaps the most striking feature appearing large and dense and surrounded by a unique zona pellucida (Pussard and Pons, 1977). In the trophozoite stage, Acanthamoeba does not differ greatly at the internal structural level from a mammalian cell. It also contains various cell organelles such as mitochondria, ribosomes, centrosome, Golgi apparatus, and vacuoles (Bowers and Korn, 1968; Figure 2B). Vacuoles are conspicuous elements in trophozoite. They exist mainly in two separate systems: one is the contractile vacuole, involved in cellular osmotic regulation (Kitching, 1967) and the other is the digestive vacuole involved in the decomposition of intake particles (Kitching, 1956). Contractile vacuoles are periodically expelled and then refilled in a specific manner, ranging in size from 0.1 μm in diameter to larger than the nucleus. Multiple small vacuoles fuse together to form large vacuoles, which are morphologically akin to digestive vacuoles (Bowers and Korn, 1968). The contractile vacuole can be distinguished from a digestive vacuole by the absence of flocculent content when examined by electron microscopy (Bowers and Korn, 1968). In addition to contractile vacuoles and digestive vacuoles, other types of vacuoles can also be observed in the cytoplasm such as lysosomes and a large number of glycogen-containing vacuoles (Bowers and Korn, 1973; Siddiqui and Khan, 2012).
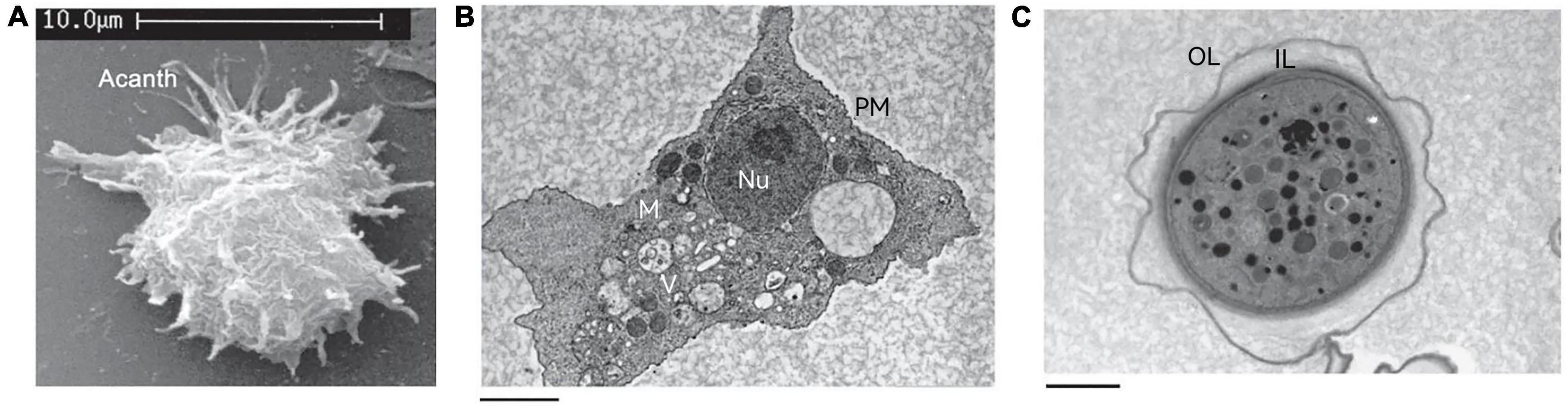
Figure 2. The structure of Acanthamoeba. (A) Scanning electron micrograph of an Acanthamoeba trophozoite showing many spinous pseudopods around the whole surface of the cell. Acanth, acanthopod. (B) Transmission electron micrograph of the trophozoite stage of Acanthamoeba. Nu, nucleus; V, vacuoles; M, mitochondria; PM, plasma membrane. (C) Transmission electron micrograph of an Acanthamoeba cyst. OL, outer layer; IL, inner layer. These pictures are from the work of Siddiqui et al. (2012).
2.2.2. Cyst
There can be a lot of variability in cyst shape, most cysts are round and the diameter is about 13–20 μm (Bowers and Korn, 1969). Unlike trophozoites, the cyst has a double-layer cell wall, which gives cysts strong resistance to many harsh environmental conditions (Figure 2C). The outer layer consists of a laminar fibrous layer also known as an ectocyst, while the inner layer is made up of fine fibers also known as an endocyst. The ectocyst is the first to be synthesized in the encysting cells and appears as an amorphous discontinuous layer just outside the plasma membrane (Bowers and Korn, 1969). The ectocyst terminates in a loose fibrous layer and the entire ectocyst is 0.3–0.5 μm in thickness. The endocyst differs from the ectocyst in texture and appears finely granular. The fibrils of both the ectocyst and the endocyst appear to be less than 50 Å in diameter. When the cysts are fully mature, a space of about 0.1 μm is formed between the endocyst and the plasma membrane (Bowers and Korn, 1969).
The cyst wall of cyst forming protozoa is generally composed of chitin, while Acanthamoeba is an exception and includes both chitin and cellulose (Linder et al., 2002; Derda et al., 2009). Cellulose becomes the main component of the endocyst, accounting for about one third of the cyst wall (Tomlinson and Jones, 1962). However, it is not clear where chitin exists in ectocyst or endocyst. Cellulose is basically a straight chain polymer of β-1, 4 linked D-glucose units which are arranged in alternate orientation with respect to one another (Béguin and Aubert, 1994; Lakhundi et al., 2015). It is with no coiling and rod-like conformation that provokes spontaneous crystallization of the molecule (Schwarz, 2001). An exceptional feature of cellulose that is also relatively unusual in the polysaccharide world is its crystalline structure (Brown and Saxena, 2000; Lynd et al., 2002). Approximately 30 individual molecules of cellulose are assembled into larger units called elementary fibrils (protofibrils), which in turn are packed into larger units called microfibrils (Brown and Saxena, 2000; Lynd et al., 2002). The chains in the microfibrils are held together by hydrogen bonds giving them a high tensile strength. It is this inter- and intrachain hydrogen bonding between multiple parallel layers of cellulose that results in the formation of tightly packed microfibrils. The microfibrils then associate into crystalline cellulose fibers. The organization of individual microfibrils in crystalline cellulose is such that the component molecules are packed tightly enough to prevent penetration by enzymes. In addition, cellulose also contain various irregularities such as twists or voids, surface micropores, large pits and capillaries etc., increasing the total surface area much larger than that of an ideally smooth fiber of the same dimensions (Blouin et al., 1970; Cowling, 1975; Fan et al., 1980; Lynd et al., 2002). Overall, cellulose imparts high tensile strength to the wall it is contained in, serving as a structural component. It is an extracellular polysaccharide and is a part of the cell wall in plants, algae, bacteria, slime mold Dictyostelium and other protists such as the Acanthamoeba cyst wall (Schramm and Hestrin, 1954; Cook and Colvin, 1980; Williams and Cannon, 1989; Bishop et al., 2002). Given the structure of cellulose, it practically makes it impossible for an enzyme to clasp cellulose into a substrate site. Hence there is only a single enzyme to hydrolyze cellulose. This, together with its association with other polymers, makes cellulose containing material withstand harsh conditions making it hardy and resistant to degradation, hence its role as a structural and protective barrier.
The change of environmental factors is an important link in the transformation of trophozoites into cysts. Cyst formation occurs under adverse environmental conditions such as glucose deficiency, dryness, extreme temperature, and non-neutral pH environments (Bowers and Korn, 1969; Chagla and Griffiths, 1974; Byers et al., 1980). Even more notable is the durability of cysts with one study noting that cysts can grow after 21 years even in a completely dry environment while another conversely showed cysts surviving in water at 4°C for more than 24 years (Mazur et al., 1995; Sriram et al., 2008). Cysts have been shown to be resistant to fungicides, chlorinating agents, and antibiotics (De Jonckheere and van de Voorde, 1976; Khunkitti et al., 1998; Turner et al., 2000; Lloyd et al., 2001). However, chloroquine effectively inactivated the cells in a dose-dependent manner during encystation (Jha et al., 2014). This result indicates that there is a potential target of chloroquine in the process of encystation, while also suggesting the importance of timing for the treatment of Acanthamoeba infection.
The viability and virulence of 17 Acanthamoeba isolates after they have been stored in water at 4°C for a period of 24 years was determined, and 3 of them were found inactivated. The remaining 14 isolates after inoculation on non-nutrient agar (NNA) gave rise to new subculture. Due to the low number of viable cysts (0–5%) measured by eosin exclusion, most of the cells entered the trophozoite stage. These results revealed the resistance and viability of Acanthamoeba in long term storage (Mazur et al., 1995). Separate groups of mice were inoculated intranasally with 10 subcultures characterized by varying primary virulence. In 8 groups, the mice were successfully infectious (at varying degree), and some of the mice succumbed to disease, indicating that even after 24 years, some of strains still keep very high virulence. Interestingly, however, some of the examined isolates completely lost their virulence only after 8 years of cultivation. On the basis of these results, we can assume in the environment that the period of viability for a cyst may be at least 25 years, and, more importantly, some of them still maintain high virulence (Mazur et al., 1995). It is this durability of cysts to the external environment and drugs that makes the treatment of Acanthamoeba infection particularly difficult. However, a 0.02% polyhexamethyl biguanide (PHMB) solution can be used as a first line of defense topical therapy for AK (Schuster and Visvesvara, 2004; Oldenburg et al., 2011; Carrijo-Carvalho et al., 2017) and is recommended by the leading national public health organizations in the USA (Centers for Disease Control and Prevention, 2020). A recent phase I study of human subjects has shown general tolerance to higher concentrations of PHMB, which makes it possible to treat deep stromal invasion of AK (Papa et al., 2022). PHMB 0.08% monotherapy is being further explored in a phase III randomized control trial for AK (ClinicalTrials.gov/ NCT03274895, National Library of Medicine, 2020).
2.2.3. Encystation
2.2.3.1. Cellulose and encystation
Encystation is an essential biological process for survival of cyst forming protozoa (Eichinger, 2001). In the process, Acanthamoeba undergoes drastic changes in gene expression to adapt to the new environment (Moon et al., 2009b,2011b; Bernard et al., 2022). The most prominent characterization is the formation of cyst wall, which serves as a shelter under stressful external conditions (Marciano-Cabral and Cabral, 2003; Turner et al., 2004). The cyst wall contains at least 2 major products that are not detected in the trophozoite stage; cellulose (Tomlinson and Jones, 1962) and an acid-insoluble protein-containing material (Neff and Neff, 1969).
As described above, cellulose constitutes a major component of the Acanthamoeba defense system during encystation. The precursor of cellulose is glucose that is incorporated into the cell wall as β (1, 4)-glucans (Weisman, 1976). Acanthamoeba stores glucose in the form of glycogen in the active growth stage and degrades it to form glucose during the process of encystation before final conversion into cellulose (Weisman et al., 1970; Potter and Weisman, 1971; Stewart and Weisman, 1974). It has been demonstrated that glycogen is the most rapidly degraded macromolecule during the initial phase of Acanthamoeba encystation (Bowers and Korn, 1969; Weisman et al., 1970). Glycogen is broken down into glucose via glycogen phosphorylase (Lorenzo-Morales et al., 2008; Moon and Kong, 2012). This was demonstrated by gene silencing of glycogen phosphorylase, which prevented Acanthamoeba from forming a double-layered cyst wall (Lorenzo-Morales et al., 2008). There has been some debate about cellulose existing only in the endocyst (Tomlinson and Jones, 1962). However, a recent study by Garajová et al. (2019) revealed cellulose fibers through several electron microscopy methods and observed cellulose in the outer layer. Acanthamoeba transfected with siRNA of glycogen phosphorylase can still form outer layer of cyst wall during encystation, which may be due to the differences in the mechanisms that provide glucose for the synthesis of polysaccharides in the inner and outer cyst wall layers. It may also be attributed to the fact that the lack of cellulose is not enough to hinder the formation of the basic structure of outer layer because the main components of the ectocyst are proteins (Barrett and Alexander, 1977; Dudley et al., 2009). However, no matter what, the fact that glycogen phosphatase is required for cyst wall assembly, mainly the cell wall inner layer, has been demonstrated (Lorenzo-Morales et al., 2008). The gene expression level of glycogen phosphorylase also gradually increases during cyst formation and has been shown to reach a maximum at day 3 (10.3 times) (Moon and Kong, 2012). After glycogen breakdown, glucose is then converted to cellulose by the enzyme cellulose synthase (Delmer and Amor, 1995). Targeting cellulose synthase by siRNA has been shown to significantly inhibit the formation of mature cysts (Aqeel et al., 2013; Moon et al., 2014). The sturdy nature of Acanthamoeba cysts is attributed, in part, to cellulose (Potter and Weisman, 1972), but other polysaccharides (such as xylose, galactose, and mannose) may also be involved (Dudley et al., 2009). The analysis of cyst walls of Acanthamoeba castellanii, using gas chromatography/mass spectrometry (GC/MS) revealed that xylose (in addition to β-1, 4-glucan-forming cellulose) was another important component of the cyst wall (Dudley et al., 2009). Xylose isomerase is involved in the intracellular transformation of xylose. Xylose isomerase, also known as glucose isomerase, catalyzes the reversible isomerization of xylose into xylulose and glucose into fructose (Lee et al., 1990). An siRNA against xylose isomerase and its exogenous inhibitor, sorbitol, blocked A. castellanii encystation, suggesting that xylose isomerase plays an important role in amoebae differentiation (Aqeel et al., 2013). Aqeel et al. (2013) proposed that xylose biosynthesis could replace cellulose biosynthesis, resulting in hemicellulose entering the cyst wall and forming immature cysts. However, Moon et al. (2014) showed that cellulose synthetase and xylose isomerase were expressed independently in encysting Acanthamoeba, and that one cannot replace each other. Therefore, how xylose isomerase interferes with cyst wall formation leading to reduced encystation in A. castellanii needs to be further studied.
2.2.3.2. Autophagy and encystation
Autophagy is a degradative pathway necessary for the clearance of damaged or superfluous proteins and organelles and the recycling of intracellular constituents as well as providing energy during periods of unfavorable environments such as under nutrient-limiting conditions (Huang and Klionsky, 2002; Xie and Klionsky, 2007). Autophagy starts with the formation of a double-layered membrane, called a phagophore, which recruits proteins and lipids into a presumed membrane structure known as the pre-autophagosomal structure (PAS) through a series of reactions involving autophagy (Atg) proteins (Kim et al., 2002; Suzuki et al., 2002). The phagophore is enlarged and then forms an autophagosome that encloses the cytosolic components and organelles including mitochondria and endosomes (Ishihara et al., 2001; Reggiori et al., 2005). Thereafter, mature autophagosomes fuse with lysosomes and their contents are degraded (Nakatogawa, 2020).
The formation of autophagosome involves two ubiquitin like conjugation systems (UBL); Atg8 and Atg12 systems (Ohsumi and Mizushima, 2004). In the Atg8 conjugation system, Atg8 is cleaved at the C-terminal end by a cysteine protease, Atg4, producing Atg8Gly–116 (Kirisako et al., 2000). It is then transferred to Atg3, an E2-like enzyme, after being activated by Atg7, an E1-like enzyme (Ichimura et al., 2000, 2004). Finally, Atg3 conjugates Atg8 with phosphatidylethanolamine (PE) (Ichimura et al., 2000), anchoring Atg8 to the autophagosome membrane. In the Atg12 conjugation system, the C-terminal glycine residue of Atg12 is activated by Atg7, an E1-like enzyme, transferred to Atg10, an E2-like enzyme, and conjugated to Atg5 covalently (Geng and Klionsky, 2008). The Atg12-Atg5 conjugate which has E3-like activity promotes the elongation of the autophagosomal membrane by forming a multimeric complex with Atg16 (Hanada et al., 2007; Yang and Klionsky, 2009). Later studies have found that Atg12-Atg5 conjugates directly activate E2 enzyme activity of Atg3 to promote conjugation of Atg8 to phosphatidylethanolamine (Sakoh-Nakatogawa et al., 2013), indicating that two UBL systems are not completely independent in mediating autophagy.
The Atg8 and Atg12 UBL systems are well conserved in A. castellanii (Moon et al., 2011a; Song et al., 2012). The mediation of Atg8 and Atg12 systems in the autophagy process during encystation has been identified and autophagy proteins including Atg3, Atg8, Atg8b (isoform of Atg8), Atg12 and Atg16 have been characterized (Moon et al., 2009a,2011a,2013; Song et al., 2012; Kim et al., 2015). The mRNA expression level of Atg8, Atg8b, and Atg16 are highly induced during encystation (Moon et al., 2009a,2013; Song et al., 2012). However, the transcriptional level of Atg3 and Atg12 did not change markedly in both trophozoites and cysts (Moon et al., 2011a; Kim et al., 2015). Therefore, it can be speculated that Atg3 and Atg12 proteins are used in the form of recycling during encystation. The discrepancy on gene expression does not cast their role in encystation into doubt since siRNA against the respective genes inhibited cyst formation effectively. Atg3, which is uniformly distributed in trophozoite cells, aggregates around the autophagosomal membrane in encystation and shows an activity for Atg8 lipidation (Moon et al., 2009a). As mentioned above, ATG8 is one of the ubiquitin-like proteins required for autophagosome formation. PE conjugated ATG8 is tightly bound to the autophagosome membrane and participates in autophagy (Moon et al., 2009a). Complete inhibition of encystation was not achieved in Acanthamoeba transfected with siRNA against Atg8, which suggests that an Atg8 isoform such as Atg8b exists (Moon et al., 2013). Both Atg8 and Atg8b were highly expressed during encystation, probably because they were needed to enable the establishment of autophagy rapidly. Atg12 together with Atg16 constituted another UBL system related to autophagy (Song et al., 2012) and the knockdown of Atg12 or Atg16 showed ultrastructural changes of the cyst. The main features of Atg12-knockdown cells are the absence of maturation of cyst wall, decrease in autophagic structures, and vacuolization (Kim et al., 2015). The ultrastructural characteristics of Atg16-knockdown cells showed that many mitochondria were still undigested and these cells are prevented from forming mature cysts, which supports the view that autophagy is necessary for the effective degradation of mitochondria during encystation in Acanthamoeba (Song et al., 2012).
Proteases from various protozoan parasites have been characterized at the molecular and cellular levels (Klemba and Goldberg, 2002). Comparative microarray analysis of trophozoite and cyst showed high expression of cysteine in the cyst stage (282-fold change) (Moon et al., 2011b), suggesting a pivotal role of this protease in the cyst formation. Because of its high expression in cysts, people named the gene cyst specific cysteine protease (CSCP). During encystation, CSCP showed colocalization with LysoTracker, an autophagosome marker. Acanthamoeba transfected with siRNA against CSCP was unable to form mature cysts and the undigested mitochondria in vacuole-like structures were observed in CSCP siRNA treated cells (Moon et al., 2012b). Mitochondria are a major target of autophagy in Acanthamoeba, as compared with trophozoites, the number of mitochondria left in mature cysts is significantly reduced (Moon et al., 2012b). To degrade a large number of mitochondria, various types of autophagy may be needed. As mentioned above, Atg16, as an important component of Atg 12 UBL system, together with CSCP involved in the degradation of mitochondria during encystation. Further studies need to clarify the possible interaction between these two proteins in this regulatory process.
The encystation of Acanthamoeba was inhibited by the serine protease inhibitor phenylmethanesulfonyl fluoride, indicating that serine protease was also involved in encystation (Moon et al., 2008b). To confirm the role of encystation-mediating serine protease (EMSP) during encystation of Acanthamoeba, a gene silencing assay was performed and showed that the formation of mature cysts was almost completely inhibited in EMSP siRNA-transfected cells. Additionally, both gene and protein expression of EMSP are highly induced during encystation (Moon et al., 2008b). However, the increased gene level of EMSP during encystation was not revealed by comparative microarray analysis of trophozoite and cyst (Moon et al., 2011b). This may be due to the inconsistency of sample collection timing in the process of encystation or the use of different strains, A. healyi instead of A. castellanii. The protein turnover during Acanthamoeba encystation has also been studied by two-dimensional gel electrophoresis (2DE). The results showed that protein degradation mainly occurred early in the process and these changes could be significantly inhibited specifically by cysteine protease inhibitors. The conclusion is that the encystation process in A. castellanii is of a bipartite nature consisting of an initial phase of protein degradation by a cysteine protease and the late stage accompanied by the expression of cyst-specific gene expression (Leitsch et al., 2010).
2.2.3.3. Other factors of encystation
A cDNA fragment containing a member of the sirtuin family of proteins was found in a comparative microarray analysis of trophozoites and cysts (Moon et al., 2011b). Sirtuins are a silent-information regulator 2 (SIR2)-like family of protein deacetylases that require nicotinamide adenine dinucleotide (NAD+) as a cofactor in the deacetylation reaction (Sauve et al., 2001). The Sir2 homolog of A. castellanii (AcSir2) contains the YEATS domain which recognizes and binds acetylated lysine followed by a Sir2 catalytic domain. Nuclear extracts of AcSir2-overexpressing cells also exhibit NAD+ dependent deacetylase activity (Joo et al., 2020). The overexpression of AcSir2 converted cells into mature cysts more rapidly while the encystation of A. castellanii was suppressed by treatment with salermide, a sirtuin inhibitor. The transcription of cellulose synthase was induced in AcSir2 overexpressing cells while the transcription was completely abolished in salermide-treated cells (Joo et al., 2020), which indicated that cellulose synthesis may serve as a potential target of Sir2. The same group also found that AcSir2 promotes encystation by increasing the expression of cyst-specific cysteine protease (CSCP). Sirtinol, another Sir2 inhibitor, suppresses CSCP transcription, suggesting that the undegraded organelles and large molecules remained in sirtinol-treated cells during encystation (Joo et al., 2022). In Saccharomyces cerevisiae, SIR2 levels increase during calorie restriction (Lin et al., 2000), and sirtuin overexpression is known to extend the lifespan by silencing HML and HMR loci and inhibiting the formation of extrachromosomal rDNA circles (Sinclair and Guarente, 1997; Kaeberlein et al., 1999). Therefore, it was proposed that Sirtuins may be involved in all cell survival events related to metabolic reduction caused by nutritional deficiency, including Acanthamoeba encystation.
Bernard et al. (2022) studied the regulation of transcription, protein and phosphoprotein level in the early stage of Acanthamoeba encystation by a time-resolved multi-omics analysis. The global analysis of three omics approaches showed that the quantity of transcripts and phosphorylation sites were modified as early as 1 h after triggering encystation while the change of proteome was more gradual and occurred 8 h later. Interestingly, 1 h after induction of encystation, a decrease of phosphorylated sites was observed and accompanied by a global increased phosphatase activity (Bernard et al., 2022). A. castellanii is predicted to encode the largest number of protein kinases among amoebozoans (Clarke et al., 2013). However, only few signal pathways, such as PKC, Ras, and cyclic AMP (cAMP) have been proposed to be involved in encystation (Raizada and Krishna Murti, 1972; Fouque et al., 2012; Moon et al., 2012a), and there is no clear link between signals and cellular response. During encystation, these kinases may be involved in the regulation of phosphorylation at a specific site that is currently undefined. Bernard et al. (2022) also explained the transcriptomic and proteomic data at the initial stage of encystation. Their hypothesis is that the early regulation of transcription is achieved by the repression of transcription factors through phosphorylation. Some of these factors like GSK3 are highly promiscuous with a broad array of known substrates, regulate many transcription factors such as Fos/Jun AP-1 or p53 (Beurel et al., 2015). Lectins are an important part of cyst wall and three sets of these proteins have been identified as the most abundant in A. castellanii, which are named as the Jonah, Luke, and Leo families (Magistrado-Coxen et al., 2019). 23 out of 31 lectins described by Magistrado-Coxen et al. (2019) were present in transcriptomic data and 16 were significantly differentially expressed. However, none of them were significantly regulated at the protein level. This may be due to timing because their expression peak may be later than 8 h after induction of encystation (Magistrado-Coxen et al., 2019; Bernard et al., 2022). Part of the transcriptomic data in the multi-omics analysis (Bernard et al., 2022) may come from epigenetic regulation in the early stage of encystation. Epigenetics is associated to many cellular processes such as gene and microRNA expression, DNA-protein interactions, suppression of transposable element mobility, cellular differentiation, embryogenesis, etc. (Portela and Esteller, 2010). A. castellanii transfected with siRNA against Protein Arginine Methyltransferase 5 (AcPRMT5) failed to form mature cysts (Moon et al., 2016). DNA methylation is also involved in the control of CSCP expression during encystation (Moon et al., 2017).
In addition, several genes related to Acanthamoeba encystation have been identified from encystation-related gene profiles, such as cyst specific protein 21, Na P-type ATPase, subtilisin-like serine proteinase genes, proteasome and heat shock protein, genes like cullin 4, ubiquitin-conjugating enzymes, suggesting their potential roles in the process of cyst formation (Moon et al., 2007, 2008a).
2.3. Classification of Acanthamoeba
2.3.1. Traditional classification
Pussard and Pons (1977) classified Acanthamoeba cysts into three categories (I-III) according to their size and morphological characteristics. This was the most appropriate classification method at that time, but has been replaced by modern advances in DNA sequencing. However, at least 30 species of Acanthamoeba with clear names have been classified in this manner (Table 1).
Group I: This group includes five clearly named Acanthamoeba listed in Table 1. The average diameter of cysts of this group is greater than or equal to 18 μm. The morphological defining features are that the internal cyst wall is separated from the external wall, the outer cyst wall is slightly wrinkled or smooth, and the internal cyst is often star-shaped (Kong, 2009).
Group II: This group has relatively smaller cysts with an average diameter of less than 18 μm. The outer cyst wall is folded or wavy, and the inner cyst wall is various in shape, which is wavy, round or oval, as well as star-shaped, triangular or tetragonal. The inner wall and outer wall of the cyst are obviously separated and some are closely connected. This group is the most widely isolated Acanthamoeba, and most pathogenic Acanthamoeba belong to this group (Kong, 2009; Corsaro, 2020). So far, there are 17 species of Acanthamoeba which have been clearly named in this group listed in Table 1.
Group III: At present, eight species have been clearly named in this group. This group Acanthamoeba cysts are also small and the average diameter is less than 18 μm. The inner wall is round or slightly angular while the outer wall is thin and close to the inner wall, so the outer wall is sometimes difficult to observe (Kong, 2009; de Lacerda and Lira, 2021).
The morphology of Acanthamoeba cysts depends upon growth medium used to culture them (Stratford and Griffiths, 1978), while the Acanthamoeba cysts in the same group are similar, sometimes there is only a temporary difference between the two kinds of Acanthamoeba cysts, therefore, the morphological distinction is subjective and a more scientific classification method with clinical application value was needed.
2.3.2. 18S ribosomal RNA (18S rRNA) gene sequence typing
Gast et al. (1996) began classifying Acanthamoeba according to 18S rRNA sequencing. The analysis of full-length 18S rRNA of Acanthamoeba is a fast and reliable identification method which is now used extensively to identify Acanthamoeba isolates (Maciver et al., 2013). However, the 18S-based genotyping was rendered easier and more rapid by targeting smaller regions of the gene, such as the 464 bp long Acanthamoeba-specific amplimer (ASA.S1) which contains the diagnostic fragment 3 (DF3) (Taher et al., 2018). The DF3 region is a 280 bp long single highly variable region within the ASA.S1 region, which is widely used for genotyping studies since it provides equivalent results as that of ASA.S1 (Booton et al., 2002). Each genotype exhibits at least 5% sequence divergence as the typing standard (Khan, 2006). Using this technology, Maghsood et al. (2005) proposed to subdivide T2 into further two groups, i.e., T2a and T2b. T4 is further subdivided into seven groups, T4A, T4B, T4C, T4D, T4E, T4F, and T4G (Corsaro, 2020; Putaporntip et al., 2021). Indeed, DNA sequencing helps to differentiate pathogenic and non-pathogenic isolates within a genotype (Khan, 2006). Currently, most of the diagnostic and epidemiological investigations are indeed carried out with this fragment. Albeit rarely misidentifications may occur, and moreover, reliability of short fragments remains to be discussed especially for phylogenetic analyses and identify new genotypes. Therefore, the full-length gene sequences are strongly recommended (Corsaro and Venditti, 2011; Corsaro et al., 2015).
Based on this method, all Acanthamoeba isolates found to date have been divided into 23 genotypes (T1-T23) (Corsaro et al., 2015, 2017; Taher et al., 2018; Corsaro, 2021; Putaporntip et al., 2021). Studies have shown that Acanthamoeba genotypes are related to pathogenicity (Walochnik et al., 2000), and the diseases and pathological characteristics caused by different genotypes are unique (Corsaro, 2021). Acanthamoeba isolated from patients with the most severe clinical Acanthamoeba infections belong to the T4 genotype (Booton et al., 2005; Maciver et al., 2013; Walochnik et al., 2015) followed by the T3 genotype (Booton et al., 2002; Walochnik et al., 2015). There are also reports of Acanthamoeba keratitis (AK) caused by T2, T5, T6, T10, T11, T12 and T15 genotypes (Maghsood et al., 2005; Di Cave et al., 2009; Nuprasert et al., 2010; Lorenzo-Morales et al., 2011; Roshni Prithiviraj et al., 2020; Otero-Ruiz et al., 2022) and GAE caused by T1, T2, T5, T10, T12, and T18 (Khan, 2006; Lackner et al., 2010; Qvarnstrom et al., 2013; Duggal et al., 2017; Matsui et al., 2018; Otero-Ruiz et al., 2022). It is difficult to classify the rarer species of Acanthamoeba. At present, gene sequencing and morphological classification are often used simultaneously (Walochnik et al., 2000; Kong, 2009). In terms of distribution, the T4 genotype was the most prevalent worldwide, followed by T3, T15, T11, and T5. Furthermore, the T4 genotype contains a higher number of species (Diehl et al., 2021). The currently known Acanthamoeba genotypes and corresponding diseases are summarized in Table 2. Meanwhile, phylogenetic relationship with 49 various genotypes or subtypes of Acanthamoeba T1-T23 based on “complete” 18S rRNA gene sequence has been shown in Figure 3.
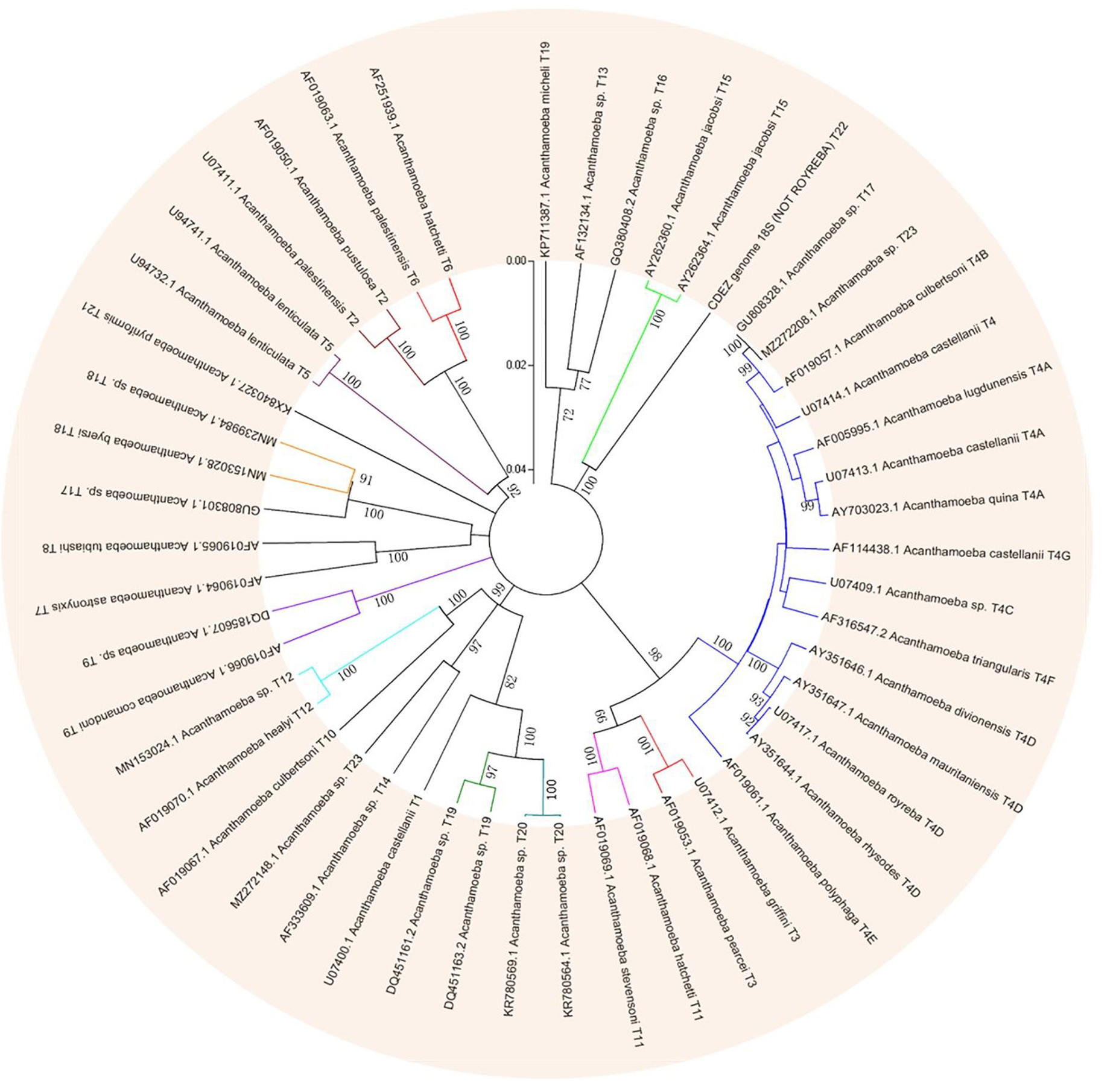
Figure 3. Phylogenetic relationship of 49 various genotypes or subtypes of Acanthamoeba T1-T23 based on “complete” 18S rRNA gene sequence. The tree was constructed using the Neighbor-Joining algorithm in MEGA 4.
3. Acanthamoeba related diseases
3.1. Disease type
3.1.1. Acanthamoeba keratitis
Acanthamoeba keratitis (AK) is the most common disease caused by Acanthamoeba (Stehr-Green et al., 1989; Ibrahim et al., 2007; Jiang et al., 2015). The incidence of AK correlates significantly with corneal trauma, exposure to sewage, and wearing of contact lenses (CLs) (Moore et al., 1985; Maycock and Jayaswal, 2016; Hassan et al., 2019). Wearing CLs can cause slight abrasion on the cornea, which destroys the integrity of corneal epithelium and increases the chance of invasion of pathogenic microorganisms (Ibrahim et al., 2009). Acanthamoeba adhesion to the surface of contact lenses is one of the essential first steps in the pathogenesis of AK (Lee et al., 2016) and it was found that the adhesion rate of trophozoites to CL material was higher than that of cysts (Kilvington, 1993). Even when exposed to a minimally contaminated solution or environment for a few seconds, trophozoites immediately show adherence to CLs (John et al., 1989; Heidemann et al., 1990). Later, silicone hydrogel lenses became popular, accounting for 30% of new lenses in 2005 (Morgan et al., 2006). However, the first generation of silicon hydrogel lenses were found to be highly sticky to trophozoites (Beattie et al., 2003). To overcome this issue, second generation silicon hydrogel lenses have been developed and trophozoite attachment rates for this generation of lenses were much lower than for the first generation and not statistically different from those of conventional hydrogel lenses (Beattie et al., 2006). Recently, Campolo et al. (2022) found that some contact lens materials are more conducive to cyst formation than the natural environment with encystation occurring within as little as 4 h of incubation. They hypothesized that aggregation of cysts directly obstructs multi-purpose solutions from disinfecting Acanthamoeba, which further indicates that contact lens materials may need to be reevaluated in the future as infection incidence increases (Campolo et al., 2022). The adhesion of trophozoites and cysts to the surface of CLs depends on the structure of both. Pseudopods of trophozoites play an important role in adhesion (John et al., 1989; Omaña-Molina et al., 2014), but cysts do not have pseudopod structure. Instead, adhesion occurs through adhesive substances in the ectocyst (Kilvington, 1993). Infection by Acanthamoeba starts on the epithelium and progresses slowly into the stroma (Marciano-Cabral and Cabral, 2003). In most cases, AK is unilateral, however, evidence also suggests that it can affect both eyes (Voyatzis and McElvanney, 2007; Lee and Gotay, 2010). Symptoms of AK include conjunctival hyperemia, edema, tearing, foreign body sensation, blurred vision, decreased visual acuity, photophobia, and severe ocular pain (Kaiserman et al., 2012; Lorenzo-Morales et al., 2013, 2015). When treatment is inadequate, AK can cause corneal perforation and melting (Lorenzo-Morales et al., 2015). The process of AK infection is complex and requires both host and pathogen factors discussed later in the text.
Poor CL hygiene habits are also risk factors to induce AK (Ahearn and Gabriel, 1997; Cope et al., 2015). Studies have shown that Acanthamoeba exist in tap water (Carnt et al., 2020); therefore, rinsing and cleaning lenses with tap water before putting them in the storage case may cause contamination of lenses and cause infection (Nau and Dhaliwal, 2018). Repeated use of lens disinfectant by “topping off” old solution with new disinfectant should be avoided (Tu and Joslin, 2010). Additionally, the use of expired solution (Abjani et al., 2016), the use of self-made saline solution, and chlorine-based disinfection can increase the risk of disease (Schaumberg et al., 1998; Seal et al., 1999; Radford et al., 2002). In general, the long-term use of the same CL storage case and insufficient drying after cleaning are a breeding ground for bacteria, protozoa, and fungi which themselves are a food source of trophozoites (Kuzman et al., 2014; Zimmerman et al., 2017; Mirsayafov et al., 2018; Tan et al., 2018). Bad habits also include rubbing the eyes while wearing CLs, leading to corneal damage and promoting the invasion of Acanthamoeba (Taher et al., 2018). Other risk factors include sleeping with contact lenses that can lead to corneal hypoxia, edema, thinning in the center of the cornea (Ibrahim et al., 2009), and superficial punctate keratitis (Taher et al., 2018).
The symbiotic relationship of bacteria and Acanthamoeba (Rayamajhee et al., 2022) may increase corneal damage after Acanthamoeba infection. The ability of bacteria to survive as an amoebal endosymbiont was first reported in 1975 (Proca-Ciobanu et al., 1975), while the role of Acanthamoeba as a host of pathogenic microorganisms was reported in 1978 (Prasad and Gupta, 1978). Spores of Bacillus anthracis (Ames strain) can germinate in the amoeba phage, and the number of spores can increase 50-fold after 72 h (Dey et al., 2012). A. castellanii can also host Legionella (Swart et al., 2018; Nomura et al., 2022), Vibrio cholerae, Francisella tularensis (Abd et al., 2003) and the causative agent of Johne’s disease, Mycobacterium avium subsp. paratuberculosis (MAP) (Phillips et al., 2020). These bacteria have been referred to as amoeba-resistant bacteria. A. castellanii releases undigested V. cholerae in expelled food vacuoles (EFVs) in the form of free spherical pellets (1–5 μm size) (Espinoza-Vergara et al., 2020). If given the right amount of nutrients or cultured at 37°C, hundreds of bacteria can escape from EFV (Rayamajhee et al., 2022). Therefore, the difficulty of treatment can increase dramatically in opportune situations.
3.1.2. Granulomatous amoebic encephalitis
Granulomatous amoebic encephalitis (GAE) caused by Acanthamoeba infection is a rare central nervous system disease that is highly fatal with mortality rate greater than 90% despite its low occurrence worldwide (Kot et al., 2018; Kalra et al., 2020). GAE is a subacute or chronic granulomatous encephalitis characterized by neck ankylosis, headache, and fever. Other progressive neurological symptoms include altered mental state, seizures, confusion, hallucination, focal neurologic signs, diplopia, cranial nerve palsies, ataxia, high grade flaccid paralysis of right lower limb, lethargy, stiff neck, and personality changes (Coven et al., 2017; Ghadage et al., 2017; Geith et al., 2018). GAE is a progressive disease leading to death within 1–2 months of symptom onset due to increased intracranial pressure (Duggal et al., 2017). It occurs especially in immunocompromised individuals including those infected with human immunodeficiency virus (HIV) or acquired immunodeficiency syndrome (AIDS), organ transplant recipients, patients with diabetes, systemic lupus erythematosus (SLE), those undergoing cancer treatment as well as immunocompetent individuals (Lalitha et al., 1985; Gonzalez et al., 1986; Carter et al., 2004; Vernon et al., 2005; Duarte et al., 2006; Sütçü et al., 2018). The pathogenesis of GAE is not fully understood. Acanthamoeba may enter through various routes including lower respiratory tract or breaks in the skin resulting in hematogenous dissemination to the brain (Duggal et al., 2017). Although there is no clinical evidence, Acanthamoeba is likely to enter the central nervous system through the blood-brain barrier and cause infection. It is worth noting that the olfactory epithelium may be another pathway for Acanthamoeba to enter the central nervous system (Kalra et al., 2020). GAE cases are often under diagnosed and hence strong clinical suspicion along with laboratory technical expertise is required for early diagnosis and therapeutic intervention (Parija et al., 2015).
3.1.3. Other diseases
Acanthamoeba can also cause a skin disorder known as cutaneous acanthamoebiasis (CA) and pulmonary infection, Acanthamoeba pneumonia (AP), but both conditions are rare. The first case of cutaneous Acanthamoeba infection in an AIDS patient was reported in 1986 (Gonzalez et al., 1986). Eighteen patients with AIDS and cutaneous acanthamoebiasis have been reported in the literature to date (Torno et al., 2000). A recent article of Acanthamoeba infections identified a number of symptoms, including fever, headache, dizziness, nausea, altered mental status, seizures, and facial palsies prior to cutaneous manifestations (Zhang and Cheng, 2021). Lesions frequently occur on the face and extremities and exhibit heterogenous morphology, ranging from papules, pustules, nodules, ulcers, eschars, or abscesses (Murakawa et al., 1995). However, diagnosing cutaneous acanthamoebiasis is challenging given its variable clinical presentation and lack of pathognomonic findings (Kutner et al., 2018).
Acanthamoeba pneumonia occurs mostly in patients with a low immune response (Visvesvara et al., 1983; Kaul et al., 2008). So far, 19 case reports of Acanthamoeba pneumonia (AP) or disseminated acanthamoebiasis with lung infection have been published. Most patients came from the USA, but there were also cases from Poland, Austria, France, Korea, Japan, and India. None of the patients survived (Kot et al., 2021). In patients with AP, a decrease in body weight and respiratory efficiency was observed, and in the radiological examination, interstitial changes with visible pulmonary edema were observed (Im and Kim, 1998; Kot et al., 2021).
3.2. Pathogenic mechanism
Among the diseases caused by Acanthamoeba, the pathogenic mechanism of the rare GAE, CA, and pulmonary infection is not apparent and critically understudied compared to the relatively common AK infection. Once Acanthamoeba adheres to the target cells, intracellular signal transduction is quickly activated and a series of cascade effects are triggered including phagocytosis of target cells, secretion of protease, and induction of apoptosis, which will cause direct pathological damage to the host. These are further explored in-depth below.
3.2.1. Adhesion
The ability of Acanthamoeba to bind to epithelial cells is the basis of infection. At present, it is believed that there are two main factors involved in this process, one is acanthopod, the other is surface adhesion. These two factors are very important with adhesion ability of Acanthamoeba and directly correlate to the pathogenicity of different isolates. Studies in recent years have found that the total number of acanthopods is closely related to the adhesion rate. Pathogenic Acanthamoeba has more than one hundred acanthopods per cell, whereas non-pathogenic Acanthamoeba has no more than twenty acanthopods per cell (Lorenzo-Morales et al., 2013).
Current consensus is that the adhesion of pathogenic Acanthamoeba to corneal epithelial cells is mainly mediated by adhesins, including mannose-binding protein (MBP) and laminin- binding protein (LBP) (Huth et al., 2017; Corsaro, 2022). MBP is expressed by Acanthamoeba protozoa which is composed of several 130 kDa subunits distributed on the surface of Acanthamoeba and can be isolated and extracted by mannose affinity column affinity chromatography (Garate et al., 2006a,b). Acanthamoeba MBP is a transmembrane protein encoded by a gene containing six exons and five introns and has a typical cell surface receptor function (Garate et al., 2004). As an exogenous lectin, it is generally bound to specific mannose-containing glycoproteins through the carbohydrate recognition domain (CRD) (Garate et al., 2006a). MBP binds to two glycoproteins of corneal epithelial cells, which are purified mannose protein and mannose bovine serum albumin (isolated and identified from primary cell cultures of rabbit corneal epithelium) (Yang et al., 1997). This is further demonstrated by studies showing that binding can be inhibited by exogenous α-mannose competitively (Rocha-Azevedo et al., 2010; Kim et al., 2012), while galactose bovine albumin and other non-competitive sugars could not inhibit MBP binding, indicating that mannose’s inhibitory effect was achieved by competing with MBP for sugar binding sites (Rocha-Azevedo et al., 2010). The binding of Acanthamoeba and mannose-containing glycoproteins leads to an increase of serine protease secretion, which is the decisive factor in host cell injury (Rocha-Azevedo et al., 2010; Lorenzo-Morales et al., 2013). MBP-mediated adhesion of Acanthamoeba to host cells depends on the level of MBP expression on the surface of Acanthamoeba and the number of mannose-containing glycoproteins synthesized by host cells. The study found that the damaged cornea can expose more mannose-rich glycoproteins, the number of Acanthamoeba attached to its surface is higher than healthy corneas. In addition, compared with non-pathogenic Acanthamoeba, pathogenic strains showed higher MBP expression levels (Yoo and Jung, 2012; Huth et al., 2017). Currently two types of MBP (MBP1 and MBP2) have been found (Corsaro, 2022). MBP1 is a conventional membrane protein with a signal peptide at the N-terminus and a transmembrane domain located at the C-terminus. The extracellular portion contains a Cys-rich repetitive motif (CXCXC) and a domain of unknown function (DUF 4114), while two NPLF motifs involved in intracellular signaling are located in the intracytoplasmic region (Garate et al., 2004). MBP1 appears to be specific only to Acanthamoeba species of groups 2 and 3, with different gene structure and amino acid sequence depending on the genotype, while shorter MBP-like sequences could be identified in the group 1 species (A. astronyxis T7 and A. byersi T18), as well as in T4 and T2 genotypes. The resulting protein, labeled MBP2, covers the N-terminal part containing DUF 4114 but lacks the Cys-rich repetitive elements (usually only a single CXCXC motif is present), as well as the intracytoplasmic domain (Corsaro, 2022). MBP2 has a signal peptide at the N-terminal followed by a transmembrane motif, although a second short transmembrane motif is predicted at the C-terminus for group 1 species (Corsaro, 2022). In silico alignment of two kinds of MBP (L8GXW7, 360 aa; Q6J288, 833 aa) demonstrated 19.4% identity (94 similar positions). Both proteins also share a domain of unknown function (DUF4114), sharing 61.6% identity (L8GXW7, 164–256 aa and Q6J288, 156–254 aa; 61 identical positions) (Gonçalves et al., 2019). MBP1 sequences from different genotypes are variable, with identity/similarity values < 60/75%. Moreover, values between MBP1 and MBP2 are even lower (between 25 and 35%), the most conserved region being the DUF4114 domain (approximately 65% of identical sites) (Corsaro, 2022).
In addition to MBP, another important Acanthamoeba adhesin is the laminin-binding protein (LBP), which allows further progression of infected tissues (Rocha-Azevedo et al., 2009), as laminin is a major glycoprotein of the extracellular matrix separating epithelia from other tissues. The molecular weight is predicted to be 28.2 kDa (Hong et al., 2004; Omaña-Molina et al., 2017) and 55 kDa in A. culbertsoni (Rocha-Azevedo et al., 2009, 2010). Acanthamoeba LBP belongs to the family of non-integrin 37/67-kDa laminin receptors (37/67LR), also involved as receptors for viruses and other pathogens as well as in other cellular processes such as motility and differentiation (DiGiacomo and Meruelo, 2016). LBP homologs are present in all organisms including prokaryotes as this adhesin derives from a 40S ribosomal protein which evolved the ability to bind laminin (Ardini et al., 1998). Overall, LBPs have a short transmembrane domain at the N-terminal, three recognition domains for laminin on the extracellular C-terminal domain, comprising a palindromic LMWWML motif located in the peptide G (Castronovo et al., 1991), a direct binding region (DBR), and TEDWS motif repeats. LBP sequences are highly conserved with identity/similarity values > 80/90% for those of group 2 and 3 species and around 60/70% between these and those of group 1 species (Corsaro, 2022). It is generally believed that the adhesion of Acanthamoeba to the cornea is a crucial prerequisite for the subsequent inflammatory response and the degree of adhesion is directly proportional to the strength of the host’s inflammatory response (Wang and Zhu, 2010). LBP participates in the initial phase where infiltration is limited to the corneal epithelium, particularly in the intercellular space (Gu et al., 2022). Therefore, the selectivity of Acanthamoeba for the host cornea also determines the differences in the specificity of AK in different hosts. A similar view has been established in the pathogenic mechanism of other protozoa such as the binding and lysis of Entamoeba histolytic to host cells being mediated by galactose adhesion protein (Guzmán-Téllez et al., 2020). Studies shows that expression levels of both MBP and LBP vary between Acanthamoeba strains and correlate with pathogenicity (Garate et al., 2006b; Ng et al., 2017). They were found in either low or non-existent quantities in non-pathogenic Acanthamoeba (Rocha-Azevedo et al., 2009; Singh et al., 2012; Huth et al., 2017; Corsaro, 2022).
3.2.2. Phagocytosis
Phagocytosis plays an essential role in the pathogenesis of Acanthamoeba infection. Phagocytosis is an actin-dependent process that drives cytoskeleton rearrangement. Cytochalasin D (a toxin that blocks actin polymerization) inhibits Acanthamoeba-mediated host cell death, confirming that actin-mediated cytoskeleton rearrangement plays an important role in Acanthamoeba phagocytosis (Taylor et al., 1995; Niederkorn et al., 1999; Alsam et al., 2005a). In order to study the relationship between cellular signal transduction pathway and phagocytosis, phagocytosis assays have been performed in the presence of protein tyrosine kinase inhibitor, genistein and a protein tyrosine phosphatase inhibitor, sodium orthovanadate. Acanthamoeba uptake of Escherichia coli is significantly reduced in the presence of genistein. In contrast, sodium orthovanadate increases bacterial uptake by Acanthamoeba (Alsam et al., 2005a). Rho GTPases are the key regulators of the actin cytoskeleton in all eukaryotic cells and link external signals to cytoskeleton (Mackay and Hall, 1998). This process involves three major pathways including RhoA, Rac1, and Cdc42 (Mackay and Hall, 1998). The Rho kinase inhibitor, Y27632, which partially blocks RhoA pathway, reduced bacterial uptake (Alsam et al., 2005a). These results suggested that the signal transduction pathway may regulate phagocytosis through mediating actin polymerization. Some evidence suggests that phosphatidylinositol 3-kinase (PI3K) may play important roles in regulating actin dependent-processes (Wymann and Pirola, 1998). For example, studies have shown that PI3K controls Rho-mediated changes in actin cytoskeleton in fibroblasts (Reif et al., 1996; Cantrell, 2001). When studying the phagocytosis of Acanthamoeba, it was found that LY294002, a specific inhibitor of PI3K, could significantly reduce the phagocytosis of E. coli (Alsam et al., 2005a). Interestingly, studies have also shown that the involvement of PI3K in Rac1-dependent lamellipodia formation (Wennström et al., 1994) and Cdc42-dependent cytoskeletal changes (Jiménez et al., 2000), suggesting that other GTPases such as Cdc42 and Rac1 are also involved in the phagocytosis of Acanthamoeba.
In addition, there is a unique actin-rich sucking structure (amoebostomes) on the surface of Acanthamoeba, also known as the food cup structure, which is a temporary structure that mediates phagocytosis of bacteria, yeasts, or cells (Pettit et al., 1996; Marciano-Cabral and Cabral, 2003). The mechanism involved in these goblet structures is called “trogocytosis,” which is the process whereby sections of host cells are ripped off by the amoeba (Nakada-Tsukui and Nozaki, 2021). Trogocytosis can be regarded as a special type of phagocytosis. The initial discovery of cell nibbling is from unicellular eukaryotes (Nakada-Tsukui and Nozaki, 2021). Culbertson (1970, 1971) described trogocytosis-like events in two species, Naegleria fowleri HB-1 and Hartmannella-Acanthamoeba A-1. When these amoebae were inoculated into guinea pigs, pathological examination showed that amoeba in thrombi internalized erythrocytes only halfway (i.e., trogocytosis) (Culbertson, 1971). Later, trogocytosis of a mouse embryonic cell by N. fowleri was also confirmed (Brown, 1979). Petti found that trophozoites of 4 species of Acanthamoeba were cytopathic for cultured rat B 103 neuroblastoma cells and this process is achieved by destroying nerve cells through the food cup (Pettit et al., 1996). The same process can be observed in the pathogenesis of other protozoa such as N. fowleri (Tiewcharoen et al., 2008) and E. histolytica (Miller et al., 2019). In the study of E. histolytica, it was found that there was pronounced actin polymerization within the amoebae at the site of human cell contact and ingested fragments were surrounded by polymerized actin, which indicates that trogocytosis is related to actin rearrangement (Ralston et al., 2014). Further studies found that Gal/GalNAc lectin, EhC2PK, and PI3K signaling were also involved in the amoebic trogocytosis-mediated human cell killing (Ralston et al., 2014; Miller et al., 2019).
In recent years, some additional proteins that play a role in Acanthamoeba phagocytosis have been reported. For example, Acanthamoeba SBDS (Shwachman-Bodian-Diamond Syndrome)-related proteins are highly expressed during phagocytosis, which may be related to cytoskeleton-related phagocytosis and cystogenesis (Wang et al., 2021). Studies have shown that Acanthamoeba secreted extracellular M20/M25/M40 superfamily aminopeptidase plays an important role in the Acanthamoeba pathogenesis (Huang et al., 2017). Acanthamoeba Type-I metacaspase (Acmcp) is a caspase-like protein which is expressed during the encystations of A. castellanii. When vectors containing Acmcp (pDneo2a-GFP-Acmcp) were electro-transfected into wild type Dictyostelium discoideum cells, they showed a significant increase in the fluid phase internalization and phagocytosis rate compared to the control cells (Saheb et al., 2015). Therefore, metacaspase is proposed as a candidate drug target against infections caused by A. castellanii. Finally, A. castellanii Rab7 (AcRab7), which is involved in endosomal delivery after phagocytosis and dominates energy production and cell growth, may be an important target in some species (Hong et al., 2022).
It has also been reported that mannose inhibits Acanthamoeba phagocytosis, which suggests that Acanthamoeba phagocytosis is a receptor-dependent process and Acanthamoeba adhesin (or MBP) is involved in phagocytosis (Allen and Dawidowicz, 1990). Further study of other molecular pathways and the interaction between various intracellular signaling pathways will help the field to understand the phagocytosis of Acanthamoeba and provide a basis for clinical treatment. Acanthamoeba-mediated host signaling pathways as well as self-signaling pathways are shown in Figure 4.
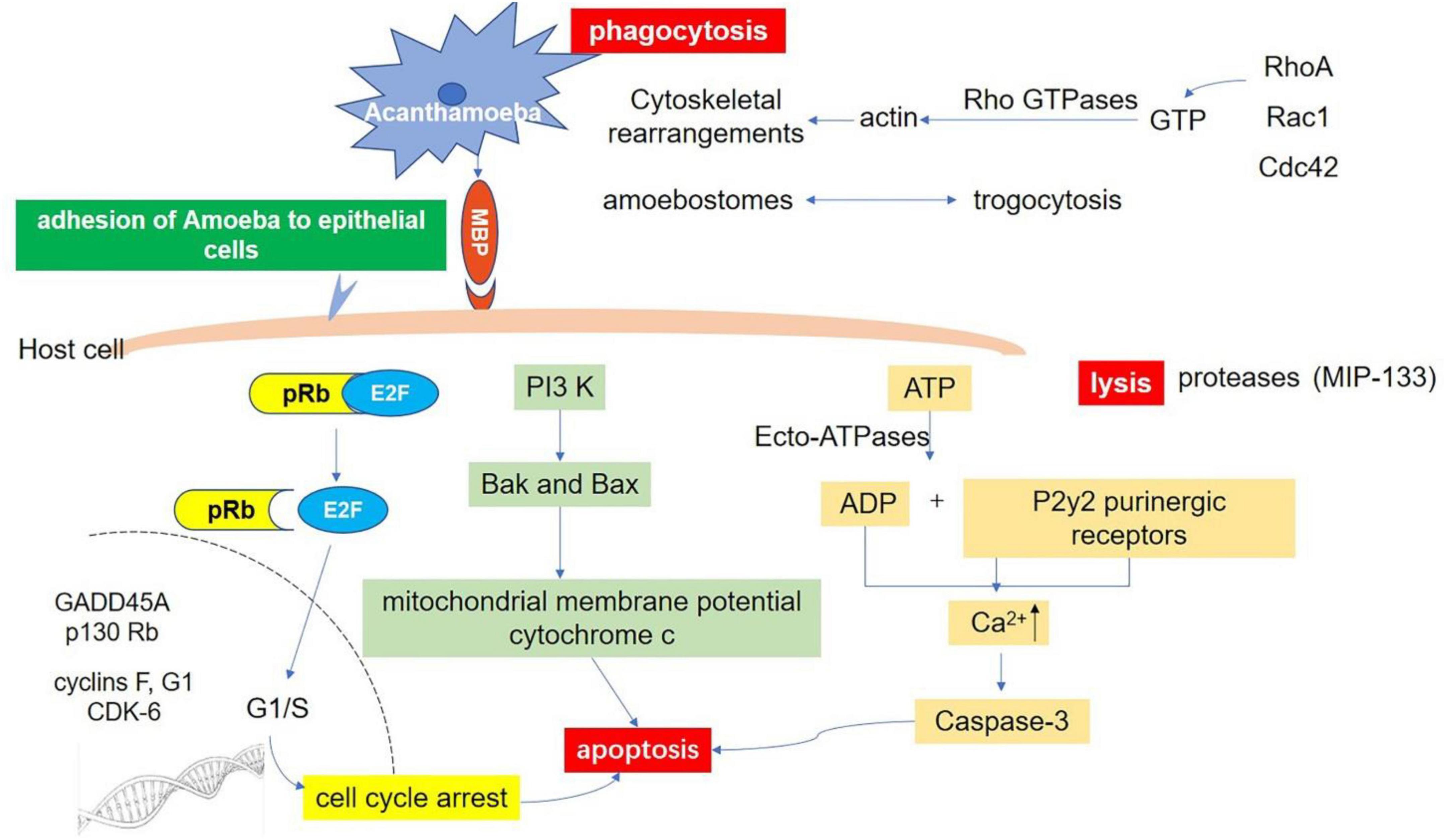
Figure 4. Host-Parasite interaction molecular signaling pathways. Adhesion through spinous pseudopodia and adhesin (such as MBP) is the basis for Acanthamoeba to establish infection. Once adhesion is completed, the intracellular signal transduction process is activated and further triggers cascade effects such as phagocytosis of target cells, secretion of protease, and apoptosis, resulting in direct pathological damage. Phagocytosis: There are three fully studied pathways involved in this process. The RhoA pathway, which leads to stress fiber formation; Rac1 activation, which triggers plate foot formation; and Cdc42 activation, which promotes filamentous foot formation. Apoptosis: There are at least three pathways related to apoptosis after infection of Acanthamoeba. Ecto-ATPases driven cell apoptosis; Interference with the expression of important genes that regulate cell cycle; and the phosphatidylinositol 3-kinase (PI3K) mediated apoptosis pathway. Lysis: Acanthamoeba secretes a variety of proteases involved in cell lysis. A serine protease (MIP133) has been identified as a key component in the pathogenesis of Acanthamoeba.
3.2.3. Apoptosis of host cells
In addition to directly causing cell death, Acanthamoeba can induce programmed cell death, such as apoptosis. After Acanthamoeba infection, cell membrane rupture, condensation of nucleoplasm, and degradation of DNA can be seen, eventually forming apoptotic bodies (Khan, 2006; Lorenzo-Morales et al., 2013). Based on current research, there are at least three pathways related to apoptosis. First, Ecto-ATPase driven cell apoptosis. Ecto-ATPases hydrolyze extracellular ATP and other nucleoside triphosphates and the resulting ADP has a toxic effect on host cells. It has been shown that the ADP released binds to the P2y2 purinergic receptor on the host cell, resulting in the increase of intracellular calcium and the activation of caspase-3, which eventually leads to cell apoptosis (Mattana et al., 2002). Suramin, a P2 receptor antagonist, inhibits Acanthamoeba-mediated host cell apoptosis, indicating that extracellular ATP enzymes play an essential role in the pathogenesis of Acanthamoeba (Mattana et al., 2002). Compared with weakly pathogenic isolates, clinical isolates of Acanthamoeba show higher extracellular ATP activity (Sissons et al., 2004a). Several Ecto-ATPases with estimated molecular weights of 62, 100, 218, 272, and exceeding 300 kDa are described in Acanthamoeba (Sissons et al., 2004a). However, further studies are needed to clarify their function in Acanthamoeba biology and their pathogenicity to the host. Secondly, Acanthamoeba causes cell cycle arrest by affecting the expression of GADD45A, p130Rb, F, G1 protein, and cyclin-dependent kinase 6. The dephosphorylation of retinoblastoma protein (pRb) further supports this theory (Sissons et al., 2004b). Unphosphorylated pRb binds to the E2F transcription factor during the cell cycle, which inhibits E2F entry into the nucleus. When phosphorylated by CDKs, the conformational change of pRb leads to the dissociation of the pRb-E2F complex. The released E2F enters the nucleus and initiates the synthesis of DNA (Dyson, 1998; Harbour and Dean, 2000; Stevaux and Dyson, 2002). Recent studies have found that Acanthamoeba can induce cell cycle arrest of host cells by inhibiting this process in human corneal epithelial cells and brain microvascular endothelial cells (Sissons et al., 2004b). Third, Acanthamoeba-mediated apoptosis of host cells depends on the activation of phosphatidylinositol 3-kinase (PI3K) (Sissons et al., 2005). This conclusion was confirmed by using LY294002 to inhibit the activity of PI3K specifically and to express the mutant form of PI3K regulatory subunit P110 in host cells (Sissons et al., 2005). Traditionally, it has been known that PI3K plays an important role in regulating cell survival pathways. For example, Thyrell et al. (2004) have shown that α-interferon (IFNa) can induce PI3K-mediated apoptosis in myeloma cells without Akt phosphorylation. Further studies have shown that PI3K can mediate apoptosis by activating the downstream pre-apoptotic molecules Bak and Bax, inducing the loss of mitochondrial membrane potential and the release of cytochrome c (Mattana et al., 2002; Chusattayanond et al., 2010). A similar mechanism may exist in the process of host cell apoptosis mediated by Acanthamoeba.
3.2.4. Lysis
When Acanthamoeba adheres to the host cells, it secretes a variety of proteases that create pores in host membranes, resulting in the lysis of cells and tissues (Lorenzo-Morales et al., 2013). Pathogenic Acanthamoeba shows higher extracellular protease activity. Protease-mediated lysing plays an important role in the pathogenesis of various protozoa, such as amoeba, trichomonas, leishmania, trypanosoma, and malaria parasites, and is directly involved in cell and tissue invasion and damage (Serrano-Luna Jde et al., 2006). It was found that Acanthamoeba mainly produce three types of proteases: serine proteases, cysteine proteases, and metalloproteinases (Khan, 2006). Serine proteases are the most abundant and present in almost all Acanthamoeba genotypes (Serrano-Luna Jde et al., 2006; Cirelli et al., 2020). Several serine proteases with molecular weights ranging from 20 to 200 kDa have been identified. They not only have collagen degradation activity, but also can degrade plasminogen activator, fibrinogen, IgG, IgA, albumin, hemoglobin, protease inhibitor, and interleukin-1 (Na et al., 2001, 2002). A serine protease called MIP133 has been identified as a key component in the pathogenesis of Acanthamoeba. MIP133 serine protease can induce the lysing of corneal cells, iris ciliary body cells, retinal pigment epithelial cells, corneal epithelial cells, and corneal endothelial cells as well as induce apoptosis of macrophage-like cells (Lorenzo-Morales et al., 2015). The direct damage of serine protease in Acanthamoeba infection can be observed by injecting Acanthamoeba into the corneal stroma. This damage can be inhibited by the serine protease inhibitor phenylmethanesulfonyl fluoride (Na et al., 2001, 2002). In addition, siRNA targeting the catalytic domain of serine proteases reduced protease activity and host cytotoxicity mediated by Acanthamoeba (Lorenzo-Morales et al., 2005). The protease activity of pathogenic isolates, especially serine proteases, is significantly higher than that of non-pathogenic isolates, which is consistent with their cytotoxic effect on host cells.
Cysteine proteases with different molecular weights are detected in Acanthamoeba cell lysate and culture supernatant. These enzymes are thought to be involved in cell degradation (Hadas and Mazur, 1993; Mitro et al., 1994; Alfieri et al., 2000; Leitsch et al., 2010; Moon et al., 2012b; Ramírez-Rico et al., 2015). For example, two L-cysteine proteases, AcCP and AhCP, were identified in Culbertson and Healyi Acanthamoeba (Yun et al., 1999; Hong et al., 2002, 2018). Recombinant AcCP showed enzyme activity under acidic conditions and is the most suitable for pH4.0. Recombinant enzymes can effectively hydrolyze human proteins, including hemoglobin, albumin, immunoglobulin A and G, and fibronectin under acidic conditions (Ramírez-Rico et al., 2015; Hong et al., 2018; Cirelli et al., 2020). In addition, cysteine proteases with molecular weights of 43, 65, 70, and 130 kDa have also been reported (Khan, 2006). Although the histolytic role of cysteine proteases in the pathogenesis of parasitic pathogens has been identified (Singh et al., 2004; Jimenez-Sandoval et al., 2017), the studies on cysteine proteases in Acanthamoeba are limited.
In addition to serine and cysteine proteases, there is evidence of metalloproteinase activity in Acanthamoeba (Łanocha-Arendarczyk et al., 2018). Metalloproteinases usually play an important role in cell differentiation and migration, regulation of growth factor activity, angiogenesis, and inflammation (Sternlicht and Werb, 2001; Parks et al., 2004). An extracellular metalloprotease of 150 kDa was identified from the T1 genotype isolate of Acanthamoeba (Alsam et al., 2005b; Sissons et al., 2006). This metalloprotease exhibits extracellular matrix lytic properties for collagen I and III (the main components of the extracellular collagen matrix), elastin (the elastic fibers of the extracellular matrix), and plasminogen (involved in the extracellular matrix proteolysis). These, as well as casein, gelatin, and hemoglobin are degraded (Sissons et al., 2006). The specific mechanism needs to be further studied.
Phospholipases are known to cleave phospholipids, suggesting their possible involvement in the host cell plasma membrane disruption leading to host cell penetration and lysis. Matin and Jung (2011) tested the phospholipase activity and cytotoxicity of three different Acanthamoeba strains including an encephalitis isolate (T1 genotype), a keratitis isolate (T4 genotype), and an environmental isolate (T7 genotype) in vitro. The results show that all strains exhibited phospholipase A(2) [PLA(2)] and phospholipase D (PLD) activities. Moreover, Acanthamoeba isolates exhibited higher PLD activities compared with the PLA(2). Interestingly, the encephalitis isolates of Acanthamoeba exhibited higher phospholipase activities as compared with the keratitis isolates, but the environmental isolates exhibited the highest phospholipase activities, suggesting possible differences in phospholipases in Acanthamoeba belonging to different genotypes (Matin and Jung, 2011). The result supporting this inference is that compound 48/80 partially blocked the encephalitis isolate-mediated cytotoxicity, i.e., 49% cell death in the presence of the inhibitor compared with 73% in the absence of the inhibitor, while it had no effect on the keratitis isolate cytotoxicity and the environmental isolate exhibited minimal cytotoxicity even in the absence of inhibitors (Matin and Jung, 2011).
Although phospholipase inhibitors do not clearly block the cytotoxicity mediated by Acanthamoeba alone, this does not rule out that they are involved in Acanthamoeba pathogenesis. Acanthamoeba pathogenesis is a process involved in many factors, including adhesion, phagocytosis, apoptosis, proteolytic enzyme, extracellular ATPase and so on as showing in Figure 4. The inhibition of a single factor may not be sufficient to kill host cells. In support of this notion, previous studies have shown that inhibition of Acanthamoeba binding to host cells is not adequate to block host cell cytotoxicity (Leher et al., 1998). It is also possible that cytotoxicity is a delayed event and that phospholipases are involved in the early events. Studies have shown that phospholipases involved in interference with the intracellular signaling pathways. Phospholipases generate lipids and lipid-derived products that act as mediators and second messengers, which may act as the modulators of signal transduction pathways (Dennis et al., 1991; Serhan et al., 1996). Studies have shown that lysophospholipids, a by-product of PLA2 and phospholipase B (PLB), can induce the activation of protein kinase C, which has diverse function in host cell signal transduction pathways (Oishi et al., 1988). Phospholipase C of Clostridium perfringens induces expression of IL-8 synthesis in endothelial cells (Bryant and Stevens, 1996; Bunting et al., 1997). These studies suggest that Acanthamoeba phospholipase may play a role in causing host cell damage or affecting other cellular functions such as inducing inflammation. In addition, studies on the pathogenicity of other species mediated by phospholipase have also been reported, such as the potential to prevent Candida infection by targeting phospholipase with synthetic compounds (Hänel et al., 1995). Future studies are needed to identify and characterize Acanthamoeba phospholipases, which should help determine their potential role for therapeutic interventions and in differentiation of Acanthamoeba isolates belonging to different genotypes.
Acanthamoeba species also show neuraminidase activity (Pellegrin et al., 1991). Acanthamoeba can release sialic acid after invading human cells, so the neuraminidase may be related to its colonization and play an important role in damaging the corneal epithelium rich in sialic acid (Pellegrin et al., 1991). In immunofluorescence, immunoblotting, and enzyme-linked immunosorbent assays, the neuraminidase antibody of Trypanosoma cruzi cross-reacted with Acanthamoeba, indicating that Acanthamoeba does have neuraminidase activity (Pellegrin et al., 1992). Although the role of Acanthamoeba neuraminidase in the pathogenesis of keratitis is unclear, the fact that cell damage can occur without direct contact (Visvesvara and Balamuth, 1975) suggests that the release of parasite products is involved in the mechanism of tissue injury. Neuraminidase-induced cleavage of sialic acid from glycoproteins and gangliosides of cerebral tissue may lead to the onset of granulomatous amoebic encephalitis. Such an effect of neuraminidase was suggested in primary amoebic meningoencephalitis caused by N. fowleri, also a free living amoeba (Eisen and Franson, 1987). It is generally accepted that the ability of microorganisms to produce disseminated infections is related to their capacity to destroy colonized tissues. Neuraminidase may be related to the components and enzymes of several other amoebae, such as phospholipase A, sphingomyelinase, elastase, collagenase, and a cytolytic and granule-associated cytotoxic activity. The synergistic effect of these factors may participate in the destruction, mucosal surface penetration, and dissemination of Acanthamoeba (Ferrante and Bates, 1988; He et al., 1990).
3.2.5. Immune escape
The immune escape ability of Acanthamoeba is also an important component of its pathogenicity. Compared with uninfected individuals, the levels of IgA and IgG in tears of AK patients are lower (Carnt and Stapleton, 2016; Neelam and Niederkorn, 2017), which may be related to the fact that its secreted serine protease can destroy IgA and IgG antibodies in human tears (Kong et al., 2000; Marciano-Cabral and Cabral, 2003; Foulks, 2007). This reduction of antibodies allows Acanthamoeba to evade the human immune response and survive long-term in the host. Continuous low-level turnover of complement components within the eye has been recognized for many years (Sohn et al., 2000) and is known to be a major contributor to the immune privilege status of the eye (Niederkorn, 2007). For example, the major component of immune privilege, termed anterior chamber-associated immune deviation (ACAID), has been shown to be complement-dependent (Sohn et al., 2003). The complement cascade has a well-established role in the maintenance of a healthy cornea (Mondino et al., 1996). Although membrane-bound complement regulators such as CD46, CD55, and CD59 are expressed throughout the various layers of the cornea, there is a disproportionately high level of expression in the corneal epithelium (Bora et al., 1993). This may be due to the fact that the corneal surface is often exposed to various pathogens, resulting in the continuous activation of the complement system. Some bacteria produce phospholipases and other enzymes that can remove CD55 and CD59 from the surface of corneal epithelium (Cocuzzi et al., 2000), causing complement regulation disorders, which aggravate bacterial keratitis and even lead to loss of vision (Tang et al., 2009). By comparing the results of systematic analysis of Acanthamoeba isolates, Huang’s group identified a new secretory protein, M28 aminopeptidase (M28AP). The molecular functions and characteristics of M28AP protein were studied by using anti-M28AP antibody and M28AP mutant produced by CRISPR/Cas9 system. The results showed that M28AP was involved in the degradation of human complement, such as C3b and iC3b, suggesting it played a vital role in pathology (Huang et al., 2019). The study also found that Acanthamoeba is usually associated with biofilms distributed throughout the environment. It has been confirmed that biofilms can promote protozoan infection (Schaumberg et al., 1998). The biofilm provides a protective niche for Acanthamoeba, which is conducive to immune escape, thereby enhancing invasiveness (Marciano-Cabral and Cabral, 2003; Lorenzo-Morales et al., 2015; Hasby Saad and Khalil, 2018) and thought to even provide nutrition for Acanthamoeba (Khan, 2006).
4. Conclusion
In conclusion, Acanthamoeba, is an opportunistic pathogenic protozoa widely existing in nature that can cause various diseases such as AK, GAE, and CA, as well as lung infections. Although its incidence is currently low, resulting infection is serious and treatment options are lacking due to understudied complex pathogenic mechanisms. An in-depth understanding of the biological characteristics and pathogenicity of Acanthamoeba can provide help for clinical diagnosis, effective treatment, and control of Acanthamoeba infection to provide a theoretical basis for the development of new drugs and vaccines against Acanthamoeba.
Although the widely used classification method based on 18S rRNA sequence is not as reliable as full-sequence gene analysis, it provides a rapid, simple and relatively accurate method for the study of genetic diversity of Acanthamoeba, which can assist in the differential diagnosis of pathogens in Acanthamoeba spp. The identification of Acanthamoeba from other cyst forming protozoa can be performed by a method based on the coupling of cellulose binding protein to fluorescent dyes. The main component of cyst wall is chitin, however, Acanthamoeba is an exception since its cyst wall contains cellulose (Garajová et al., 2019). Specific cytochemical differentiation between cellulose and chitin by microscopy has not been possible due to the similarity of the constituent β-1, 4-linked hexose backbones of these molecules. Therefore, it is necessary to develop new methods to distinguish cellulose from chitin in order to identify the source of infection. Derda et al. (2009) developed a novel immunocytochemical method for identification of Acanthamoeba spp. Cellulose-binding protein consisting of two cellulose-binding domains (CBDs) from Trichoderma reesei cellulase coupled to fluorescent dyes. No staining reaction was observed with the chitin-containing cyst walls of Giardia intestinalis, Entamoeba dispar, or Pneumocystis carinii. Thus, the recombinant CBD can be used as a marker to distinguish between cellulose and chitin (Derda et al., 2009). However, in later studies, cellulose was also identified as a cyst wall component of Balamuthia mandrillaris, which is closely related to amoeba (Siddiqui et al., 2009). Even so, it still helps to narrow the scope of differential diagnosis.
Current studies have found that Acanthamoeba produces a variety of proteases in the process of infecting the host, which may be an avenue for therapy. Still, the exact molecular mechanism is unclear. Future research should further explore the feasibility of protease inhibitors as therapeutic treatments. The cyst transformation function of Acanthamoeba increases the pathogenicity and increases the difficulty of clinical treatment. Therefore, the screening of Acanthamoeba cyst formation inhibitors may also prove fruitful for clinical treatment of Acanthamoeba. Finally, the unique morphological structure of cysts gives Acanthamoeba a remarkable ability to adapt to the environment and may provide another target for clinical treatment.
Author contributions
YW and ML wrote the original draft. YZ, XJ, LW, and LJ contributed to collecting the data. YW and LZJ contributed to curating the data into figures. RF contributed to revising the manuscript. ML conceived the idea and supervised the work. All authors read and agreed to the final version of the manuscript.
Funding
This work was supported by the Science and Technology Research and Planning Projects of Jilin Provincial Department of Education (Nos. JJKH20220472KJ and JJKH20210489KJ), the Jilin Provincial Health Science and Technology Capacity Enhancement Project (No. 2021JC045), and the Innovation and Entrepreneurship Training Program for College Students in Jilin Province (No. 202213706006).
Conflict of interest
The authors declare that the research was conducted in the absence of any commercial or financial relationships that could be construed as a potential conflict of interest.
Publisher’s note
All claims expressed in this article are solely those of the authors and do not necessarily represent those of their affiliated organizations, or those of the publisher, the editors and the reviewers. Any product that may be evaluated in this article, or claim that may be made by its manufacturer, is not guaranteed or endorsed by the publisher.
References
Abd, H., Johansson, T., Golovliov, I., Sandström, G., and Forsman, M. (2003). Survival and growth of Francisella tularensis in Acanthamoeba castellanii. Appl. Environ. Microbiol. 69, 600–606. doi: 10.1128/aem.69.1.600-606.2003
Abjani, F., Khan, N. A., Yousuf, F. A., and Siddiqui, R. (2016). Targeting cyst wall is an effective strategy in improving the efficacy of marketed contact lens disinfecting solutions against Acanthamoeba castellanii cysts. Cont. Lens Anterior Eye 39, 239–243. doi: 10.1016/j.clae.2015.11.004
Ahearn, D. G., and Gabriel, M. M. (1997). Contact lenses, disinfectants, and Acanthamoeba keratitis. Adv. Appl. Microbiol. 43, 35–56. doi: 10.1016/s0065-2164(08)70222-3
Alfieri, S. C., Correia, C. E., Motegi, S. A., and Pral, E. M. (2000). Proteinase activities in total extracts and in medium conditioned by Acanthamoeba polyphaga trophozoites. J. Parasitol. 86, 220–227.
Allen, P. G., and Dawidowicz, E. A. (1990). Phagocytosis in Acanthamoeba: I. A mannose receptor is responsible for the binding and phagocytosis of yeast. J. Cell Physiol. 145, 508–513. doi: 10.1002/jcp.1041450317
Alsam, S., Sissons, J., Dudley, R., and Khan, N. A. (2005a). Mechanisms associated with Acanthamoeba castellanii (T4) phagocytosis. Parasitol. Res. 96, 402–409. doi: 10.1007/s00436-005-1401-z
Alsam, S., Sissons, J., Jayasekera, S., and Khan, N. A. (2005b). Extracellular proteases of Acanthamoeba castellanii (encephalitis isolate belonging to T1 genotype) contribute to increased permeability in an in vitro model of the human blood-brain barrier. J. Infect. 51, 150–156. doi: 10.1016/j.jinf.2004.09.001
Aqeel, Y., Siddiqui, R., and Khan, N. A. (2013). Silencing of xylose isomerase and cellulose synthase by siRNA inhibits encystation in Acanthamoeba castellanii. Parasitol. Res. 112, 1221–1227. doi: 10.1007/s00436-012-3254-6
Ardini, E., Pesole, G., Tagliabue, E., Magnifico, A., Castronovo, V., Sobel, M. E., et al. (1998). The 67-kDa laminin receptor originated from a ribosomal protein that acquired a dual function during evolution. Mol. Biol. Evol. 15, 1017–1025. doi: 10.1093/oxfordjournals.molbev.a026000
Band, R. N., and Mohrlok, S. (1973). The cell cycle and induced amitosis in Acanthamoeba. J. Protozool. 20, 654–657. doi: 10.1111/j.1550-7408.1973.tb03592.x
Band, R. N., Mohrlok, S., and Rubin, R. W. (1970). Separate induction of amitotic and mitotic division in Acanthamoeba rhysodes. Nature 227, 379–381. doi: 10.1038/227379b0
Barrett, R. A., and Alexander, M. (1977). Resistance of cysts of amoebae to microbial decomposition. Appl. Environ. Microbiol. 33, 670–674. doi: 10.1128/aem.33.3.670-674.1977
Beattie, T. K., Tomlinson, A., and McFadyen, A. K. (2006). Attachment of Acanthamoeba to first- and second-generation silicone hydrogel contact lenses. Ophthalmology 113, 117–125. doi: 10.1016/j.ophtha.2005.10.018
Beattie, T. K., Tomlinson, A., McFadyen, A. K., Seal, D. V., and Grimason, A. M. (2003). Enhanced attachment of Acanthamoeba to extended-wear silicone hydrogel contact lenses: A new risk factor for infection? Ophthalmology 110, 765–771. doi: 10.1016/s0161-6420(02)01971-1
Béguin, P., and Aubert, J. P. (1994). The biological degradation of cellulose. FEMS Microbiol. Rev. 13, 25–58. doi: 10.1111/j.1574-6976.1994.tb00033.x
Bernard, C., Locard-Paulet, M., Noël, C., Duchateau, M., Giai Gianetto, Q., Moumen, B., et al. (2022). A time-resolved multi-omics atlas of Acanthamoeba castellanii encystment. Nat. Commun. 13:4104. doi: 10.1038/s41467-022-31832-0
Beurel, E., Grieco, S. F., and Jope, R. S. (2015). Glycogen synthase kinase-3 (GSK3): Regulation, actions, and diseases. Pharmacol. Ther. 148, 114–131. doi: 10.1016/j.pharmthera.2014.11.016
Bishop, J. D., Moon, B. C., Harrow, F., Ratner, D., Gomer, R. H., Dottin, R. P., et al. (2002). A second UDP-glucose pyrophosphorylase is required for differentiation and development in Dictyostelium discoideum. J. Biol. Chem. 277, 32430–32437. doi: 10.1074/jbc.M204245200
Blouin, F. A., Martin, L. F., and Rowland, S. P. (1970). Gel permeation properties of cellulose. III. Measurement of pore structure of unmodified and of mercerized cottons in fibrous form. Text Res. 40, 809–813.
Booton, G. C., Kelly, D. J., Chu, Y. W., Seal, D. V., Houang, E., Lam, D. S., et al. (2002). 18S ribosomal DNA typing and tracking of Acanthamoeba species isolates from corneal scrape specimens, contact lenses, lens cases, and home water supplies of Acanthamoeba keratitis patients in Hong Kong. J. Clin. Microbiol. 40, 1621–1625. doi: 10.1128/jcm.40.5.1621-1625.2002
Booton, G. C., Visvesvara, G. S., Byers, T. J., Kelly, D. J., and Fuerst, P. A. (2005). Identification and distribution of Acanthamoeba species genotypes associated with nonkeratitis infections. J. Clin. Microbiol. 43, 1689–1693. doi: 10.1128/jcm.43.4.1689-1693.2005
Bora, N. S., Gobleman, C. L., Atkinson, J. P., Pepose, J. S., and Kaplan, H. J. (1993). Differential expression of the complement regulatory proteins in the human eye. Invest. Ophthalmol. Vis. Sci. 34, 3579–3584.
Bowers, B. (1977). Comparison of pinocytosis and phagocytosis in Acanthamoeba castellanii. Exp. Cell Res. 110, 409–417. doi: 10.1016/0014-4827(77)90307-x
Bowers, B., and Korn, E. D. (1968). The fine structure of Acanthamoeba castellanii. I. The trophozoite. J. Cell Biol. 39, 95–111. doi: 10.1083/jcb.39.1.95
Bowers, B., and Korn, E. D. (1969). The fine structure of Acanthamoeba castellanii (Neff strain). II. Encystment. J. Cell Biol. 41, 786–805. doi: 10.1083/jcb.41.3.786
Bowers, B., and Korn, E. D. (1973). Cytochemical identification of phosphatase activity in the contractile vacuole of Acanthamoeba castellanii. J. Cell Biol. 59, 784–791. doi: 10.1083/jcb.59.3.784
Bowers, B., and Olszewski, T. E. (1972). Pinocytosis in Acanthamoeba castellanii. Kinetics and morphology. J. Cell Biol. 53, 681–694. doi: 10.1083/jcb.53.3.681
Bowers, B., and Olszewski, T. E. (1983). Acanthamoeba discriminates internally between digestible and indigestible particles. J. Cell Biol. 97, 317–322. doi: 10.1083/jcb.97.2.317
Brown, R. M., and Saxena, I. M. (2000). Cellulose biosynthesis: A model for understanding the assembly of biopolymers. Plant Physiol. Biochem. 38, 57–67.
Brown, T. (1979). Observations by immunofluorescence microscopy and electron microscopy on the cytopathogenicity of Naegleria fowleri in mouse embryo-cell cultures. J. Med. Microbiol. 12, 363–371. doi: 10.1099/00222615-12-3-363
Bryant, A. E., and Stevens, D. L. (1996). Phospholipase C and perfringolysin O from Clostridium perfringens upregulate endothelial cell-leukocyte adherence molecule 1 and intercellular leukocyte adherence molecule 1 expression and induce interleukin-8 synthesis in cultured human umbilical vein endothelial cells. Infect. Immun. 64, 358–362. doi: 10.1128/iai.64.1.358-362.1996
Bunting, M., Lorant, D. E., Bryant, A. E., Zimmerman, G. A., McIntyre, T. M., Stevens, D. L., et al. (1997). Alpha toxin from Clostridium perfringens induces proinflammatory changes in endothelial cells. J. Clin. Invest. 100, 565–574. doi: 10.1172/jci119566
Byers, T. J., Akins, R. A., Maynard, B. J., Lefken, R. A., and Martin, S. M. (1980). Rapid growth of Acanthamoeba in defined media; induction of encystment by glucose-acetate starvation. J. Protozool. 27, 216–219. doi: 10.1111/j.1550-7408.1980.tb04684.x
Byers, T. J., and James, T. E. (1967). The influence of culture, agitation and aging on the growth and reproduction of Acanthamoeba sp. Neff. J. Cell Biol. 35:19A.
Byers, T. J., Kim, B. G., King, L. E., and Hugo, E. R. (1991). Molecular aspects of the cell cycle and encystment of Acanthamoeba. Rev. Infect. Dis. 13(Suppl. 5), S373–S384. doi: 10.1093/clind/13.supplement_5.s373
Campolo, A., Pifer, R., Walters, R., Thomas, M., Miller, E., Harris, V., et al. (2022). Acanthamoeba spp. aggregate and encyst on contact lens material increasing resistance to disinfection. Front. Microbiol. 13:1089092. doi: 10.3389/fmicb.2022.1089092
Cantrell, D. A. (2001). Phosphoinositide 3-kinase signalling pathways. J. Cell Sci. 114(Pt 8), 1439–1445. doi: 10.1242/jcs.114.8.1439
Carnt, N. A., Subedi, D., Lim, A. W., Lee, R., Mistry, P., Badenoch, P. R., et al. (2020). Prevalence and seasonal variation of Acanthamoeba in domestic tap water in greater Sydney, Australia. Clin. Exp. Optom. 103, 782–786. doi: 10.1111/cxo.13065
Carnt, N., and Stapleton, F. (2016). Strategies for the prevention of contact lens-related Acanthamoeba keratitis: A review. Ophthalmic Physiol. Opt. 36, 77–92. doi: 10.1111/opo.12271
Carnt, N., Hoffman, J. M., Verma, S., Hau, S., Radford, C. F., Minassian, D. C., et al. (2018). Acanthamoeba keratitis: Confirmation of the UK outbreak and a prospective case-control study identifying contributing risk factors. Br. J. Ophthalmol. 102, 1621–1628. doi: 10.1136/bjophthalmol-2018-312544
Carrijo-Carvalho, L. C., Sant’ana, V. P., Foronda, A. S., de Freitas, D., and de Souza Carvalho, F. R. (2017). Therapeutic agents and biocides for ocular infections by free-living amoebae of Acanthamoeba genus. Surv. Ophthalmol. 62, 203–218. doi: 10.1016/j.survophthal.2016.10.009
Carter, W. W., Gompf, S. G., Toney, J. F., Greene, J. N., and Cutolo, E. P. (2004). Disseminated Acanthamoeba sinusitis in a patient with AIDS: A possible role for early antiretroviral therapy. AIDS Read. 14, 41–49.
Castronovo, V., Taraboletti, G., and Sobel, M. E. (1991). Functional domains of the 67-kDa laminin receptor precursor. J. Biol. Chem. 266, 20440–20446.
Centers for Disease Control and Prevention (2020). Parasites/Acanthamoeba/Acanthamoeba keratitis fact sheet for healthcare professionals/treatment. Atlanta, GA: Centers for Disease Control and Prevention.
Chagla, A. H., and Griffiths, A. J. (1974). Growth and encystation of Acanthamoeba castellanii. J. Gen. Microbiol. 85, 139–145. doi: 10.1099/00221287-85-1-139
Chambers, J. A., and Thompson, J. E. (1976). Phagocytosis and pinocytosis in Acanthamoeba castellanii. J. Gen. Microbiol. 92, 246–250. doi: 10.1099/00221287-92-2-246
Chusattayanond, A. D., Boonsilp, S., Kasisit, J., Boonmee, A., and Warit, S. (2010). Thai Acanthamoeba isolate (T4) induced apoptotic death in neuroblastoma cells via the bax-mediated pathway. Parasitol. Int. 59, 512–516. doi: 10.1016/j.parint.2010.06.007
Cirelli, C., Mesquita, E. I. S., Chagas, I. A. R., Furst, C., Possamai, C. O., Abrahão, J. S., et al. (2020). Extracellular protease profile of Acanthamoeba after prolonged axenic culture and after interaction with MDCK cells. Parasitol. Res. 119, 659–666. doi: 10.1007/s00436-019-06562-w
Clarke, M., Lohan, A. J., Liu, B., Lagkouvardos, I., Roy, S., Zafar, N., et al. (2013). Genome of Acanthamoeba castellanii highlights extensive lateral gene transfer and early evolution of tyrosine kinase signaling. Genome Biol. 14:R11. doi: 10.1186/gb-2013-14-2-r11
Cocuzzi, E., Guidubaldi, J., Bardenstein, D. S., Chen, R., Jacobs, M. R., and Medof, E. M. (2000). Release of complement regulatory proteins from ocular surface cells in infections. Curr. Eye Res. 21, 856–866. doi: 10.1076/ceyr.21.5.856.5539
Cook, K. E., and Colvin, J. R. (1980). Evidence for a beneficial influence of cellulose production on growth of Acetobacter xylinum in liquid medium. Curr. Microbiol. 3, 203–205. doi: 10.1007/bf02602449
Cope, J. R., Collier, S. A., Rao, M. M., Chalmers, R., Mitchell, G. L., Richdale, K., et al. (2015). Contact lens wearer demographics and risk behaviors for contact lens-related eye infections–United States, 2014. MMWR Morb. Mortal Wkly. Rep. 64, 865–870. doi: 10.15585/mmwr.mm6432a2
Corsaro, D. (2020). Update on Acanthamoeba phylogeny. Parasitol. Res. 119, 3327–3338. doi: 10.1007/s00436-020-06843-9
Corsaro, D. (2021). Correction to: Update on Acanthamoeba phylogeny. Parasitol. Res. 120, 1927–1928. doi: 10.1007/s00436-021-07102-1
Corsaro, D. (2022). Acanthamoeba mannose and laminin binding proteins variation across species and genotypes. Microorganisms 10:2162. doi: 10.3390/microorganisms10112162
Corsaro, D., and Venditti, D. (2011). More Acanthamoeba genotypes: Limits to use rDNA fragments to describe new genotype. Acta Protozool. 50, 51–56.
Corsaro, D., Köhsler, M., Montalbano Di Filippo, M., Venditti, D., Monno, R., Di Cave, D., et al. (2017). Update on Acanthamoeba jacobsi genotype T15, including full-length 18S rDNA molecular phylogeny. Parasitol. Res. 116, 1273–1284. doi: 10.1007/s00436-017-5406-1
Corsaro, D., Walochnik, J., Köhsler, M., and Rott, M. B. (2015). Acanthamoeba misidentification and multiple labels: Redefining genotypes T16, T19, and T20 and proposal for Acanthamoeba micheli sp. nov. (genotype T19). Parasitol. Res. 114, 2481–2490. doi: 10.1007/s00436-015-4445-8
Coven, S. L., Song, E., Steward, S., Pierson, C. R., Cope, J. R., Ali, I. K., et al. (2017). Acanthamoeba granulomatous amoebic encephalitis after pediatric hematopoietic stem cell transplant. Pediatr. Transplant. 21. doi: 10.1111/petr.13060
Cowling, E. B. (1975). Physical and chemical constraints in the hydrolysis of cellulose and lignocellulosic materials. Biotechnol. Bioeng. Symp. 5, 163–181.
Culbertson, C. G. (1970). Pathogenic Naegleria and Hartmannella (Acenthamoeba). Ann. N. Y. Acad. Sci. 174, 1018–1022. doi: 10.1111/j.1749-6632.1970.tb45623.x
Culbertson, C. G. (1971). The pathogenicity of soil amebas. Ann. Rev. Microbiol. 25, 231–254. doi: 10.1146/annurev.mi.25.100171.001311
De Jonckheere, J., and van de Voorde, H. (1976). Differences in destruction of cysts of pathogenic and nonpathogenic Naegleria and Acanthamoeba by chlorine. Appl. Environ. Microbiol. 31, 294–297. doi: 10.1128/aem.31.2.294-297.1976
de Lacerda, A. G., and Lira, M. (2021). Acanthamoeba keratitis: A review of biology, pathophysiology and epidemiology. Ophthalmic Physiol. Opt. 41, 116–135. doi: 10.1111/opo.12752
Delmer, D. P., and Amor, Y. (1995). Cellulose biosynthesis. Plant Cell 7, 987–1000. doi: 10.1105/tpc.7.7.987
Dennis, E. A., Rhee, S. G., Billah, M. M., and Hannun, Y. A. (1991). Role of phospholipase in generating lipid second messengers in signal transduction. FASEB J. 5, 2068–2077. doi: 10.1096/fasebj.5.7.1901288
Derda, M., Winiecka-Krusnell, J., Linder, M. B., and Linder, E. (2009). Labeled Trichoderma reesei cellulase as a marker for Acanthamoeba cyst wall cellulose in infected tissues. Appl. Environ. Microbiol. 75, 6827–6830. doi: 10.1128/aem.01555-09
Dey, R., Hoffman, P. S., and Glomski, I. J. (2012). Germination and amplification of anthrax spores by soil-dwelling amoebas. Appl. Environ. Microbiol. 78, 8075–8081. doi: 10.1128/aem.02034-12
Di Cave, D., Monno, R., Bottalico, P., Guerriero, S., D’Amelio, S., D’Orazi, C., et al. (2009). Acanthamoeba T4 and T15 genotypes associated with keratitis infections in Italy. Eur. J. Clin. Microbiol. Infect. Dis. 28, 607–612. doi: 10.1007/s10096-008-0682-4
Diehl, M. L. N., Paes, J., and Rott, M. B. (2021). Genotype distribution of Acanthamoeba in keratitis: A systematic review. Parasitol. Res. 120, 3051–3063. doi: 10.1007/s00436-021-07261-1
DiGiacomo, V., and Meruelo, D. (2016). Looking into laminin receptor: Critical discussion regarding the non-integrin 37/67-kDa laminin receptor/RPSA protein. Biol. Rev. Camb. Philos. Soc. 91, 288–310. doi: 10.1111/brv.12170
Dos Santos, D. L., Kwitko, S., Marinho, D. R., de Araújo, B. S., Locatelli, C. I., and Rott, M. B. (2018). Acanthamoeba keratitis in Porto Alegre (southern Brazil): 28 cases and risk factors. Parasitol. Res. 117, 747–750. doi: 10.1007/s00436-017-5745-y
Douglas, M. (1930). Notes on the classification of the amoeba found by Castellani in cultures of a yeast-like fungus. J. Trop. Med. Hyg. 33, 258–259.
Duarte, A. G., Sattar, F., Granwehr, B., Aronson, J. F., Wang, Z., and Lick, S. (2006). Disseminated acanthamoebiasis after lung transplantation. J. Heart Lung Transplant. 25, 237–240. doi: 10.1016/j.healun.2005.09.006
Dudley, R., Jarroll, E. L., and Khan, N. A. (2009). Carbohydrate analysis of Acanthamoeba castellanii. Exp. Parasitol. 122, 338–343. doi: 10.1016/j.exppara.2009.04.009
Duggal, S., Rongpharpi, S., Duggal, A., Kumar, A., and Biswal, I. (2017). Role of Acanthamoeba in granulomatous encephalitis: A review. J. Infect. Dis. Immune Ther. 1:2.
Dyson, N. (1998). The regulation of E2F by pRB-family proteins. Genes Dev. 12, 2245–2262. doi: 10.1101/gad.12.15.2245
Eichinger, D. (2001). Encystation in parasitic protozoa. Curr. Opin. Microbiol. 4, 421–426. doi: 10.1016/s1369-5274(00)00229-0
Eisen, D., and Franson, R. C. (1987). Acid-active neuraminidases in the growth media from cultures of pathogenic Naegleria fowleri and in sonicates of rabbit alveolar macrophages. Biochim. Biophys. Acta 924, 369–372. doi: 10.1016/0304-4165(87)90035-3
Espinoza-Vergara, G., Hoque, M. M., McDougald, D., and Noorian, P. (2020). The impact of protozoan predation on the pathogenicity of Vibrio cholerae. Front. Microbiol. 11:17. doi: 10.3389/fmicb.2020.00017
Fan, L. T., Lee, Y. H., and Beardmore, D. H. (1980). Mechanism of the enzymatic hydrolysis of cellulose: Effects of major structural features of cellulose on enzymatic hydrolysis. Biotechnol. Bioeng. 22, 177–199.
Ferrante, A., and Bates, E. J. (1988). Elastase in the pathogenic free-living amoebae Naegleria and Acanthamoeba spp. Infect. Immun. 56, 3320–3321. doi: 10.1128/iai.56.12.3320-3321.1988
Foulks, G. N. (2007). Acanthamoeba keratitis and contact lens wear: Static or increasing problem? Eye Cont. Lens 33(6 Pt 2), 412–414; discussion 424–425. doi: 10.1097/ICL.0b013e318157e8be
Fouque, E., Trouilhé, M. C., Thomas, V., Hartemann, P., Rodier, M. H., and Héchard, Y. (2012). Cellular, biochemical, and molecular changes during encystment of free-living amoebae. Eukaryot. Cell 11, 382–387. doi: 10.1128/ec.05301-11
Fowler, M., and Carter, R. F. (1965). Acute pyogenic meningitis probably due to Acanthamoeba sp.: A preliminary report. Br. Med. J. 2, 740–742. doi: 10.1136/bmj.2.5464.734-a
Garajová, M., Mrva, M., Vaškovicová, N., Martinka, M., Melicherová, J., and Valigurová, A. (2019). Cellulose fibrils formation and organisation of cytoskeleton during encystment are essential for Acanthamoeba cyst wall architecture. Sci. Rep. 9:4466. doi: 10.1038/s41598-019-41084-6
Garate, M., Alizadeh, H., Neelam, S., Niederkorn, J. Y., and Panjwani, N. (2006a). Oral immunization with Acanthamoeba castellanii mannose-binding protein ameliorates amoebic keratitis. Infect. Immun. 74, 7032–7034. doi: 10.1128/iai.00828-06
Garate, M., Marchant, J., Cubillos, I., Cao, Z., Khan, N. A., and Panjwani, N. (2006b). In vitro pathogenicity of Acanthamoeba is associated with the expression of the mannose-binding protein. Invest. Ophthalmol. Vis. Sci. 47, 1056–1062. doi: 10.1167/iovs.05-0477
Garate, M., Cao, Z., Bateman, E., and Panjwani, N. (2004). Cloning and characterization of a novel mannose-binding protein of Acanthamoeba. J. Biol. Chem. 279, 29849–29856. doi: 10.1074/jbc.M402334200
Gast, R. J., Ledee, D. R., Fuerst, P. A., and Byers, T. J. (1996). Subgenus systematics of Acanthamoeba: Four nuclear 18S rDNA sequence types. J. Eukaryot. Microbiol. 43, 498–504. doi: 10.1111/j.1550-7408.1996.tb04510.x
Geith, S., Walochnik, J., Prantl, F., Sack, S., and Eyer, F. (2018). Lethal outcome of granulomatous acanthamoebic encephalitis in a man who was human immunodeficiency virus-positive: A case report. J. Med. Case Rep. 12:201. doi: 10.1186/s13256-018-1734-8
Geng, J., and Klionsky, D. J. (2008). The Atg8 and Atg12 ubiquitin-like conjugation systems in macroautophagy. ‘Protein modifications: Beyond the usual suspects’ review series. EMBO Rep. 9, 859–864. doi: 10.1038/embor.2008.163
Ghadage, D. P., Choure, A. C., Wankhade, A. B., and Bhore, A. V. (2017). Opportunistic free: Living amoeba now becoming a usual pathogen? Indian J. Pathol. Microbiol. 60, 601–603. doi: 10.4103/ijpm.ijpm_815_16
Gonçalves, D. S., Ferreira, M. D. S., Gomes, K. X., Rodríguez-de La Noval, C., Liedke, S. C., da Costa, G. C. V., et al. (2019). Unravelling the interactions of the environmental host Acanthamoeba castellanii with fungi through the recognition by mannose-binding proteins. Cell Microbiol. 21:e13066. doi: 10.1111/cmi.13066
Gonzalez, M. M., Gould, E., Dickinson, G., Martinez, A. J., Visvesvara, G., Cleary, T. J., et al. (1986). Acquired immunodeficiency syndrome associated with Acanthamoeba infection and other opportunistic organisms. Arch. Pathol. Lab. Med. 110, 749–751.
Gu, X., Lu, X., Lin, S., Shi, X., Shen, Y., Lu, Q., et al. (2022). A comparative genomic approach to determine the virulence factors and horizontal gene transfer events of clinical Acanthamoeba isolates. Microbiol. Spectr. 10, e0002522. doi: 10.1128/spectrum.00025-22
Guzmán-Téllez, P., Martínez-Castillo, M., Flores-Huerta, N., Rosales-Morgan, G., Pacheco-Yépez, J., la Garza, M., et al. (2020). Lectins as virulence factors in Entamoeba histolytica and free-living amoebae. Future Microbiol. 15, 919–936. doi: 10.2217/fmb-2019-0275
Hadas, E., and Mazur, T. (1993). Proteolytic enzymes of pathogenic and non-pathogenic strains of Acanthamoeba spp. Trop. Med. Parasitol. 44, 197–200.
Hanada, T., Noda, N. N., Satomi, Y., Ichimura, Y., Fujioka, Y., Takao, T., et al. (2007). The Atg12-Atg5 conjugate has a novel E3-like activity for protein lipidation in autophagy. J. Biol. Chem. 282, 37298–37302. doi: 10.1074/jbc.C700195200
Hänel, H., Kirsch, R., Schmidts, H. L., and Kottmann, H. (1995). New systematically active antimycotics from the beta-blocker category. Mycoses 38, 251–264. doi: 10.1111/j.1439-0507.1995.tb00404.x
Harbour, J. W., and Dean, D. C. (2000). Rb function in cell-cycle regulation and apoptosis. Nat. Cell Biol. 2, E65–E67. doi: 10.1038/35008695
Hasby Saad, M. A., and Khalil, H. S. M. (2018). Biofilm testing of microbiota: An essential step during corneal scrap examination in Egyptian acanthamoebic keratitis cases. Parasitol. Int. 67, 556–564. doi: 10.1016/j.parint.2018.05.001
Hassan, F., Bhatti, A., Desai, R., and Barua, A. (2019). Analysis from a year of increased cases of Acanthamoeba keratitis in a large teaching hospital in the UK. Cont. Lens Anterior Eye 42, 506–511. doi: 10.1016/j.clae.2019.04.009
He, Y. G., Niederkorn, J. Y., McCulley, J. P., Stewart, G. L., Meyer, D. R., Silvany, R., et al. (1990). In vivo and in vitro collagenolytic activity of Acanthamoeba castellanii. Invest. Ophthalmol. Vis. Sci. 31, 2235–2240.
Heidemann, D. G., Verdier, D. D., Dunn, S. P., and Stamler, J. F. (1990). Acanthamoeba keratitis associated with disposable contact lenses. Am. J. Ophthalmol. 110, 630–634. doi: 10.1016/s0002-9394(14)77059-x
Hewett, M. K., Robinson, B. S., Monis, P. T., and Saint, C. P. (2003). Identification of a new Acanthamoeba 18S rRNA gene sequence type, corresponding to the species Acanthamoeba jacobsi Sawyer, Nerad and Visvesvara, 1992 (Lobosea: Acanthamoebidae). Acta Protozool. 42, 325–329.
Höllhumer, R., Keay, L., and Watson, S. L. (2020). Acanthamoeba keratitis in Australia: Demographics, associated factors, presentation and outcomes: A 15-year case review. Eye 34, 725–732. doi: 10.1038/s41433-019-0589-6
Hong, Y. C., Hwang, M. Y., Yun, H. C., Yu, H. S., Kong, H. H., Yong, T. S., et al. (2002). Isolation and characterization of a cDNA encoding a mammalian cathepsin L-like cysteine proteinase from Acanthamoeba healyi. Korean J. Parasitol. 40, 17–24. doi: 10.3347/kjp.2002.40.1.17
Hong, Y. C., Lee, W. M., Kong, H. H., Jeong, H. J., and Chung, D. I. (2004). Molecular cloning and characterization of a cDNA encoding a laminin-binding protein (AhLBP) from Acanthamoeba healyi. Exp. Parasitol. 106, 95–102. doi: 10.1016/j.exppara.2004.01.011
Hong, Y., Kang, J. M., Joo, S. Y., Song, S. M., Lê, H. G., Thái, T. L., et al. (2018). Molecular and biochemical properties of a cysteine protease of Acanthamoeba castellanii. Korean. J. Parasitol. 56, 409–418. doi: 10.3347/kjp.2018.56.5.409
Hong, Z. B., Huang, J. M., Tsai, C. M., and Lin, W. C. (2022). Potential role of Acanthamoeba Rab7. Exp. Parasitol. 239:108312. doi: 10.1016/j.exppara.2022.108312
Huang, J. M., Chang, Y. T., Shih, M. H., Lin, W. C., and Huang, F. C. (2019). Identification and characterization of a secreted M28 aminopeptidase protein in Acanthamoeba. Parasitol. Res. 118, 1865–1874. doi: 10.1007/s00436-019-06332-8
Huang, J. M., Liao, C. C., Kuo, C. C., Chen, L. R., Huang, L. L. H., Shin, J. W., et al. (2017). Pathogenic Acanthamoeba castellanii secretes the extracellular aminopeptidase M20/M25/M40 family protein to target cells for phagocytosis by disruption. Molecules 22:2263. doi: 10.3390/molecules22122263
Huang, W. P., and Klionsky, D. J. (2002). Autophagy in yeast: A review of the molecular machinery. Cell Struct. Funct. 27, 409–420. doi: 10.1247/csf.27.409
Huth, S., Reverey, J. F., Leippe, M., and Selhuber-Unkel, C. (2017). Adhesion forces and mechanics in mannose-mediated Acanthamoeba interactions. PLoS One 12:e0176207. doi: 10.1371/journal.pone.0176207
Ibrahim, Y. W., Boase, D. L., and Cree, I. A. (2007). Factors affecting the epidemiology of Acanthamoeba keratitis. Ophthalmic Epidemiol. 14, 53–60. doi: 10.1080/09286580600920281
Ibrahim, Y. W., Boase, D. L., and Cree, I. A. (2009). How could contact lens wearers be at risk of Acanthamoeba infection? A review. J. Optom. 2, 60–66.
Ichimura, Y., Imamura, Y., Emoto, K., Umeda, M., Noda, T., and Ohsumi, Y. (2004). In vivo and in vitro reconstitution of Atg8 conjugation essential for autophagy. J. Biol. Chem. 279, 40584–40592. doi: 10.1074/jbc.M405860200
Ichimura, Y., Kirisako, T., Takao, T., Satomi, Y., Shimonishi, Y., Ishihara, N., et al. (2000). A ubiquitin-like system mediates protein lipidation. Nature 408, 488–492. doi: 10.1038/35044114
Im, K. I., and Shin, H. J. (2003). Acanthamoeba sohi, n. sp., a pathogenic Korean isolate YM-4 from a freshwater fish. Korean J. Parasitol. 41, 181–188. doi: 10.3347/kjp.2003.41.4.181
Im, K., and Kim, D. S. (1998). Acanthamoebiasis in Korea: Two new cases with clinical cases review. Yonsei Med. J. 39, 478–484. doi: 10.3349/ymj.1998.39.5.478
Ishihara, N., Hamasaki, M., Yokota, S., Suzuki, K., Kamada, Y., Kihara, A., et al. (2001). Autophagosome requires specific early Sec proteins for its formation and NSF/SNARE for vacuolar fusion. Mol. Biol. Cell 12, 3690–3702. doi: 10.1091/mbc.12.11.3690
Jha, B. K., Jung, H. J., Seo, I., Kim, H. A., Suh, S. I., Suh, M. H., et al. (2014). Chloroquine has a cytotoxic effect on Acanthamoeba encystation through modulation of autophagy. Antimicrob. Agents Chemother. 58, 6235–6241. doi: 10.1128/aac.03164-14
Jiang, C., Sun, X., Wang, Z., and Zhang, Y. (2015). Acanthamoeba keratitis: Clinical characteristics and management. Ocul. Surf. 13, 164–168. doi: 10.1016/j.jtos.2015.01.002
Jiménez, C., Portela, R. A., Mellado, M., Rodríguez-Frade, J. M., Collard, J., Serrano, A., et al. (2000). Role of the PI3K regulatory subunit in the control of actin organization and cell migration. J. Cell Biol. 151, 249–262. doi: 10.1083/jcb.151.2.249
Jimenez-Sandoval, P., Lopez-Castillo, L. M., Trasviña-Arenas, C. H., and Brieba, L. G. (2017). Cysteine proteases inhibitors with immunoglobulin-like fold in protozoan parasites and their role in pathogenesis. Curr. Protein Pept. Sci. 18, 1035–1042. doi: 10.2174/1389203717666160813163837
John, T., Desai, D., and Sahm, D. (1989). Adherence of Acanthamoeba castellanii cysts and trophozoites to unworn soft contact lenses. Am. J. Ophthalmol. 108, 658–664. doi: 10.1016/0002-9394(89)90857-x
Joo, S. Y., Aung, J. M., Shin, M., Moon, E. K., Kong, H. H., Goo, Y. K., et al. (2020). The role of the Acanthamoeba castellanii Sir2-like protein in the growth and encystation of Acanthamoeba. Parasit. Vectors 13:368. doi: 10.1186/s13071-020-04237-5
Joo, S. Y., Aung, J. M., Shin, M., Moon, E. K., Kong, H. H., Goo, Y. K., et al. (2022). Sirtinol supresses trophozoites proliferation and encystation of Acanthamoeba via inhibition of sirtuin family protein. Korean J. Parasitol. 60, 1–6. doi: 10.3347/kjp.2022.60.1.1
Kaeberlein, M., McVey, M., and Guarente, L. (1999). The SIR2/3/4 complex and SIR2 alone promote longevity in Saccharomyces cerevisiae by two different mechanisms. Genes Dev. 13, 2570–2580. doi: 10.1101/gad.13.19.2570
Kaiserman, I., Bahar, I., McAllum, P., Srinivasan, S., Elbaz, U., Slomovic, A. R., et al. (2012). Prognostic factors in Acanthamoeba keratitis. Can. J. Ophthalmol. 47, 312–317. doi: 10.1016/j.jcjo.2012.03.040
Kalra, S. K., Sharma, P., Shyam, K., Tejan, N., and Ghoshal, U. (2020). Acanthamoeba and its pathogenic role in granulomatous amebic encephalitis. Exp. Parasitol. 208:107788. doi: 10.1016/j.exppara.2019.107788
Kaul, D. R., Lowe, L., Visvesvara, G. S., Farmen, S., Khaled, Y. A., and Yanik, G. A. (2008). Acanthamoeba infection in a patient with chronic graft-versus-host disease occurring during treatment with voriconazole. Transpl. Infect. Dis. 10, 437–441. doi: 10.1111/j.1399-3062.2008.00335.x
Khan, N. A. (2006). Acanthamoeba: Biology and increasing importance in human health. FEMS Microbiol. Rev. 30, 564–595. doi: 10.1111/j.1574-6976.2006.00023.x
Khunkitti, W., Lloyd, D., Furr, J. R., and Russell, A. D. (1998). Acanthamoeba castellanii: Growth, encystment, excystment and biocide susceptibility. J. Infect. 36, 43–48. doi: 10.1016/s0163-4453(98)93054-7
Kilvington, S. (1993). Acanthamoeba trophozoite and cyst adherence to four types of soft contact lens and removal by cleaning agents. Eye 7(Pt 4), 535–538. doi: 10.1038/eye.1993.116
Kim, J. H., Matin, A., Shin, H. J., Park, H., Yoo, K. T., Yuan, X. Z., et al. (2012). Functional roles of mannose-binding protein in the adhesion, cytotoxicity and phagocytosis of Acanthamoeba castellanii. Exp. Parasitol. 132, 287–292. doi: 10.1016/j.exppara.2012.08.007
Kim, J., Huang, W. P., Stromhaug, P. E., and Klionsky, D. J. (2002). Convergence of multiple autophagy and cytoplasm to vacuole targeting components to a perivacuolar membrane compartment prior to de novo vesicle formation. J. Biol. Chem. 277, 763–773. doi: 10.1074/jbc.M109134200
Kim, S. H., Moon, E. K., Hong, Y., Chung, D. I., and Kong, H. H. (2015). Autophagy protein 12 plays an essential role in Acanthamoeba encystation. Exp. Parasitol. 159, 46–52. doi: 10.1016/j.exppara.2015.08.013
Kirisako, T., Ichimura, Y., Okada, H., Kabeya, Y., Mizushima, N., Yoshimori, T., et al. (2000). The reversible modification regulates the membrane-binding state of Apg8/Aut7 essential for autophagy and the cytoplasm to vacuole targeting pathway. J. Cell Biol. 151, 263–276. doi: 10.1083/jcb.151.2.263
Kitching, J. A. (1967). “Contractile vacuoles, ionic regulation, and excretion,” in Research in protozoology, ed. T. T. Chen (New York, NY: Pergamon Press, Inc).
Klemba, M., and Goldberg, D. E. (2002). Biological roles of proteases in parasitic protozoa. Annu. Rev. Biochem. 71, 275–305. doi: 10.1146/annurev.biochem.71.090501.145453
Kong, H. H. (2009). Molecular phylogeny of Acanthamoeba. Korean J. Parasitol. 47 Suppl(Suppl.), S21–S28. doi: 10.3347/kjp.2009.47.S.S21
Kong, H. H., Kim, T. H., and Chung, D. I. (2000). Purification and characterization of a secretory serine proteinase of Acanthamoeba healyi isolated from GAE. J. Parasitol. 86, 12–17.
Kot, K., Łanocha-Arendarczyk, N. A., and Kosik-Bogacka, D. I. (2018). Amoebas from the genus Acanthamoeba and their pathogenic properties. Ann. Parasitol. 64, 299–308. doi: 10.17420/ap6404.164
Kot, K., Łanocha-Arendarczyk, N., and Kosik-Bogacka, D. (2021). Immunopathogenicity of Acanthamoeba spp. in the brain and lungs. Int. J. Mol. Sci. 22:1261. doi: 10.3390/ijms22031261
Kutner, A., Aldrich, M., Patel, S., Kang, J. J., Amin, B., Mann, R., et al. (2018). Acanthamoeba endophthalmitis during treatment for cutaneous disease in a renal transplant patient. Transpl. Infect. Dis. 20:e12843. doi: 10.1111/tid.12843
Kuzman, T., Kutija, M. B., Juri, J., Jandroković, S., Skegro, I., Olujić, S. M., et al. (2014). Lens wearers non-compliance – Is there an association with lens case contamination? Cont. Lens Anterior Eye 37, 99–105. doi: 10.1016/j.clae.2013.08.004
Lackner, P., Beer, R., Broessner, G., Helbok, R., Pfausler, B., Brenneis, C., et al. (2010). Acute granulomatous Acanthamoeba encephalitis in an immunocompetent patient. Neurocrit. Care 12, 91–94. doi: 10.1007/s12028-009-9291-z
Lakhundi, S., Siddiqui, R., and Khan, N. A. (2015). Cellulose degradation: A therapeutic strategy in the improved treatment of Acanthamoeba infections. Parasit. Vectors 8:23. doi: 10.1186/s13071-015-0642-7
Lalitha, M. K., Anandi, V., Srivastava, A., Thomas, K., Cherian, A. M., and Chandi, S. M. (1985). Isolation of Acanthamoeba culbertsoni from a patient with meningitis. J. Clin. Microbiol. 21, 666–667. doi: 10.1128/jcm.21.4.666-667.1985
Łanocha-Arendarczyk, N., Baranowska-Bosiacka, I., Gutowska, I., Kolasa-Wołosiuk, A., Kot, K., Łanocha, A., et al. (2018). The activity of matrix metalloproteinases (MMP-2, MMP-9) and their tissue inhibitors (TIMP-1, TIMP-3) in the cerebral cortex and hippocampus in experimental acanthamoebiasis. Int. J. Mol. Sci. 19:4128. doi: 10.3390/ijms19124128
Lass, A., Guerrero, M., Li, X., Karanis, G., Ma, L., and Karanis, P. (2017). Detection of Acanthamoeba spp. in water samples collected from natural water reservoirs, sewages, and pharmaceutical factory drains using LAMP and PCR in China. Sci. Total Environ. 584-585, 489–494. doi: 10.1016/j.scitotenv.2017.01.046
Lee, C. Y., Bagdasarian, M., Meng, M. H., and Zeikus, J. G. (1990). Catalytic mechanism of xylose (glucose) isomerase from Clostridium thermosulfurogenes. Characterization of the structural gene and function of active site histidine. J. Biol. Chem. 265, 19082–19090.
Lee, G. H., Lee, J. E., Park, M. K., and Yu, H. S. (2016). Adhesion of Acanthamoeba on silicone hydrogel contact lenses. Cornea 35, 663–668. doi: 10.1097/ico.0000000000000788
Lee, W. B., and Gotay, A. (2010). Bilateral Acanthamoeba keratitis in synergeyes contact lens wear: Clinical and confocal microscopy findings. Eye Cont. Lens 36, 164–169. doi: 10.1097/ICL.0b013e3181db3508
Leher, H., Silvany, R., Alizadeh, H., Huang, J., and Niederkorn, J. Y. (1998). Mannose induces the release of cytopathic factors from Acanthamoeba castellanii. Infect. Immun. 66, 5–10. doi: 10.1128/iai.66.1.5-10.1998
Leitsch, D., Köhsler, M., Marchetti-Deschmann, M., Deutsch, A., Allmaier, G., Duchêne, M., et al. (2010). Major role for cysteine proteases during the early phase of Acanthamoeba castellanii encystment. Eukaryot. Cell 9, 611–618. doi: 10.1128/ec.00300-09
Lewis, E. J., and Sawyer, T. K. (1979). Acanthamoeba tubisahi n. sp., a new species of fresh water amoebida (Acanthamoebidae). Trans. Amer. Microbiol. Soc. 98, 543–549.
Lin, S. J., Defossez, P. A., and Guarente, L. (2000). Requirement of NAD and SIR2 for life-span extension by calorie restriction in Saccharomyces cerevisiae. Science 289, 2126–2128. doi: 10.1126/science.289.5487.2126
Linder, M., Winiecka-Krusnell, J., and Linder, E. (2002). Use of recombinant cellulose-binding domains of Trichoderma reesei cellulase as a selective immunocytochemical marker for cellulose in protozoa. Appl. Environ. Microbiol. 68, 2503–2508. doi: 10.1128/aem.68.5.2503-2508.2002
Lindsay, R. G., Watters, G., Johnson, R., Ormonde, S. E., and Snibson, G. R. (2007). Acanthamoeba keratitis and contact lens wear. Clin. Exp. Optom. 90, 351–360. doi: 10.1111/j.1444-0938.2007.00172.x
Lloyd, D., Turner, N. A., Khunkitti, W., Hann, A. C., Furr, J. R., and Russell, A. D. (2001). Encystation in Acanthamoeba castellanii: Development of biocide resistance. J. Eukaryot. Microbiol. 48, 11–16. doi: 10.1111/j.1550-7408.2001.tb00410.x
Lorenzo-Morales, J., Khan, N. A., and Walochnik, J. (2015). An update on Acanthamoeba keratitis: Diagnosis, pathogenesis and treatment. Parasite 22:10. doi: 10.1051/parasite/2015010
Lorenzo-Morales, J., Kliescikova, J., Martinez-Carretero, E., De Pablos, L. M., Profotova, B., Nohynkova, E., et al. (2008). Glycogen phosphorylase in Acanthamoeba spp.: Determining the role of the enzyme during the encystment process using RNA interference. Eukaryot. Cell 7, 509–517. doi: 10.1128/ec.00316-07
Lorenzo-Morales, J., Martín-Navarro, C. M., López-Arencibia, A., Arnalich-Montiel, F., Piñero, J. E., and Valladares, B. (2013). Acanthamoeba keratitis: An emerging disease gathering importance worldwide? Trends Parasitol. 29, 181–187. doi: 10.1016/j.pt.2013.01.006
Lorenzo-Morales, J., Morcillo-Laiz, R., Martín-Navarro, C. M., López-Vélez, R., López-Arencibia, A., Arnalich-Montiel, F., et al. (2011). Acanthamoeba keratitis due to genotype T11 in a rigid gas permeable contact lens wearer in Spain. Cont. Lens Anterior Eye 34, 83–86. doi: 10.1016/j.clae.2010.10.007
Lorenzo-Morales, J., Ortega-Rivas, A., Foronda, P., Abreu-Acosta, N., Ballart, D., Martínez, E., et al. (2005). RNA interference (RNAi) for the silencing of extracellular serine proteases genes in Acanthamoeba: Molecular analysis and effect on pathogenecity. Mol. Biochem. Parasitol. 144, 10–15. doi: 10.1016/j.molbiopara.2005.07.001
Lynd, L. R., Weimer, P. J., van Zyl, W. H., and Pretorius, I. S. (2002). Microbial cellulose utilization: Fundamentals and biotechnology. Microbiol. Mol. Biol. Rev. 66, 506–577, table of contents. doi: 10.1128/mmbr.66.3.506-577.2002
Maciver, S. K., Asif, M., Simmen, M. W., and Lorenzo-Morales, J. (2013). A systematic analysis of Acanthamoeba genotype frequency correlated with source and pathogenicity: T4 is confirmed as a pathogen-rich genotype. Eur. J. Protistol. 49, 217–221. doi: 10.1016/j.ejop.2012.11.004
Mackay, D. J., and Hall, A. (1998). Rho GTPases. J. Biol. Chem. 273, 20685–20688. doi: 10.1074/jbc.273.33.20685
Maghsood, A. H., Sissons, J., Rezaian, M., Nolder, D., Warhurst, D., and Khan, N. A. (2005). Acanthamoeba genotype T4 from the UK and Iran and isolation of the T2 genotype from clinical isolates. J. Med. Microbiol. 54(Pt 8), 755–759. doi: 10.1099/jmm.0.45970-0
Magistrado-Coxen, P., Aqeel, Y., Lopez, A., Haserick, J. R., Urbanowicz, B. R., Costello, C. E., et al. (2019). The most abundant cyst wall proteins of Acanthamoeba castellanii are lectins that bind cellulose and localize to distinct structures in developing and mature cyst walls. PLoS Negl. Trop. Dis. 13:e0007352. doi: 10.1371/journal.pntd.0007352
Marciano-Cabral, F., and Cabral, G. (2003). Acanthamoeba spp. as agents of disease in humans. Clin. Microbiol. Rev. 16, 273–307. doi: 10.1128/cmr.16.2.273-307.2003
Matin, A., and Jung, S. Y. (2011). Phospholipase activities in clinical and environmental isolates of Acanthamoeba. Korean J. Parasitol. 49, 1–8. doi: 10.3347/kjp.2011.49.1.1
Matsui, T., Maeda, T., Kusakabe, S., Arita, H., Yagita, K., Morii, E., et al. (2018). A case report of granulomatous amoebic encephalitis by group 1 Acanthamoeba genotype T18 diagnosed by the combination of morphological examination and genetic analysis. Diagn. Pathol. 13:27. doi: 10.1186/s13000-018-0706-z
Mattana, A., Cappai, V., Alberti, L., Serra, C., Fiori, P. L., and Cappuccinelli, P. (2002). ADP and other metabolites released from Acanthamoeba castellanii lead to human monocytic cell death through apoptosis and stimulate the secretion of proinflammatory cytokines. Infect. Immun. 70, 4424–4432. doi: 10.1128/iai.70.8.4424-4432.2002
Maycock, N. J., and Jayaswal, R. (2016). Update on Acanthamoeba keratitis: Diagnosis, treatment, and outcomes. Cornea 35, 713–720. doi: 10.1097/ico.0000000000000804
Mazur, T., Hadaś, E., and Iwanicka, I. (1995). The duration of the cyst stage and the viability and virulence of Acanthamoeba isolates. Trop. Med. Parasitol. 46, 106–108.
Michel, R., Hauroder-Philippczyk, B., Muller, K., and Weishaar, I. (1994). Acanthamoeba from human nasal mucosa infected with an obligate intracellular parasite. Eur. J. Protistol. 30, 104–110.
Miller, H. W., Suleiman, R. L., and Ralston, K. S. (2019). Trogocytosis by Entamoeba histolytica mediates acquisition and display of human cell membrane proteins and evasion of lysis by human serum. mBio 10:e00068-19. doi: 10.1128/mBio.00068-19
Mirsayafov, D. S., Albert, T. G., Shmakov, A. N., and Asitinskaya, P. V. (2018). Tap water and risk of Acanthamoeba keratitis in rigid gas-permeable lens wearers: Sacred cow or culprit? Eye Cont. Lens 44, 132–136. doi: 10.1097/icl.0000000000000450
Mitro, K., Bhagavathiammai, A., Zhou, O. M., Bobbett, G., McKerrow, J. H., Chokshi, R., et al. (1994). Partial characterization of the proteolytic secretions of Acanthamoeba polyphaga. Exp. Parasitol. 78, 377–385. doi: 10.1006/expr.1994.1041
Mondino, B. J., Chou, H. J., and Sumner, H. L. (1996). Generation of complement membrane attack complex in normal human corneas. Invest. Ophthalmol. Vis. Sci. 37, 1576–1581.
Moon, E. K., and Kong, H. H. (2012). Short-cut pathway to synthesize cellulose of encysting Acanthamoeba. Korean J. Parasitol. 50, 361–364. doi: 10.3347/kjp.2012.50.4.361
Moon, E. K., Chung, D. I., Hong, Y. C., and Kong, H. H. (2008b). Characterization of a serine proteinase mediating encystation of Acanthamoeba. Eukaryot. Cell 7, 1513–1517. doi: 10.1128/ec.00068-08
Moon, E. K., Chung, D. I., Hong, Y. C., Ahn, T. I., and Kong, H. H. (2008a). Acanthamoeba castellanii: Gene profile of encystation by ESTs analysis and KOG assignment. Exp. Parasitol. 119, 111–116. doi: 10.1016/j.exppara.2008.01.001
Moon, E. K., Chung, D. I., Hong, Y. C., and Kong, H. H. (2007). Differentially expressed genes of Acanthamoeba castellanii during encystation. Korean J. Parasitol. 45, 283–285. doi: 10.3347/kjp.2007.45.4.283
Moon, E. K., Kim, J. O., Xuan, Y. H., Yun, Y. S., Kang, S. W., Lee, Y. S., et al. (2009b). Construction of EST database for comparative gene studies of Acanthamoeba. Korean J. Parasitol. 47, 103–107. doi: 10.3347/kjp.2009.47.2.103
Moon, E. K., Chung, D. I., Hong, Y. C., and Kong, H. H. (2009a). Autophagy protein 8 mediating autophagosome in encysting Acanthamoeba. Mol. Biochem. Parasitol. 168, 43–48. doi: 10.1016/j.molbiopara.2009.06.005
Moon, E. K., Xuan, Y. H., Chung, D. I., Hong, Y., and Kong, H. H. (2011b). Microarray analysis of differentially expressed genes between cysts and trophozoites of Acanthamoeba castellanii. Korean J. Parasitol. 49, 341–347. doi: 10.3347/kjp.2011.49.4.341
Moon, E. K., Chung, D. I., Hong, Y., and Kong, H. H. (2011a). Atg3-mediated lipidation of Atg8 is involved in encystation of Acanthamoeba. Korean J. Parasitol. 49, 103–108. doi: 10.3347/kjp.2011.49.2.103
Moon, E. K., Hong, Y., Chung, D. I., and Kong, H. H. (2012b). Cysteine protease involving in autophagosomal degradation of mitochondria during encystation of Acanthamoeba. Mol. Biochem. Parasitol. 185, 121–126. doi: 10.1016/j.molbiopara.2012.07.008
Moon, E. K., Chung, D. I., Hong, Y., and Kong, H. H. (2012a). Protein kinase C signaling molecules regulate encystation of Acanthamoeba. Exp. Parasitol. 132, 524–529. doi: 10.1016/j.exppara.2012.07.008
Moon, E. K., Hong, Y., Chung, D. I., and Kong, H. H. (2013). Identification of atg8 isoform in encysting Acanthamoeba. Korean J. Parasitol. 51, 497–502. doi: 10.3347/kjp.2013.51.5.497
Moon, E. K., Hong, Y., Chung, D. I., Goo, Y. K., and Kong, H. H. (2014). Down-regulation of cellulose synthase inhibits the formation of endocysts in Acanthamoeba. Korean J. Parasitol. 52, 131–135. doi: 10.3347/kjp.2014.52.2.131
Moon, E. K., Hong, Y., Chung, D. I., Goo, Y. K., and Kong, H. H. (2016). Identification of protein arginine methyltransferase 5 as a regulator for encystation of Acanthamoeba. Korean J. Parasitol. 54, 133–138. doi: 10.3347/kjp.2016.54.2.133
Moon, E. K., Hong, Y., Lee, H. A., Quan, F. S., and Kong, H. H. (2017). DNA methylation of gene expression in Acanthamoeba castellanii encystation. Korean J. Parasitol. 55, 115–120. doi: 10.3347/kjp.2017.55.2.115
Moore, M. B., McCulley, J. P., Luckenbach, M., Gelender, H., Newton, C., McDonald, M. B., et al. (1985). Acanthamoeba keratitis associated with soft contact lenses. Am. J. Ophthalmol. 100, 396–403. doi: 10.1016/0002-9394(85)90500-8
Morgan, P. B., Efron, N., Woods, C. A., Jones, D., Pesinova, A., Grein, H., et al. (2006). International contact lens prescribing in 2005. Cont. Lens Spect. 21, 35–39.
Moura, H., Wallace, S., and Visvesvara, G. S. (1992). Acanthamoeba healyi n. sp. and the isoenzyme and immunoblot profiles of Acanthamoeba spp., groups 1 and 3. J. Protozool. 39, 573–583. doi: 10.1111/j.1550-7408.1992.tb04853.x
Müller, M. (1969). Lysosomal hydrolases in Acanthamoeba sp. J. Protozool. 16, 428–431. doi: 10.1111/j.1550-7408.1969.tb02294.x
Müller, M., and Moller, K. M. (1969). Urate oxidase and its association with peroxisomes in Acanthamoeba sp. Eur. J. Biochem. 9, 424–430. doi: 10.1111/j.1432-1033.1969.tb00626.x
Murakawa, G. J., McCalmont, T., Altman, J., Telang, G. H., Hoffman, M. D., Kantor, G. R., et al. (1995). Disseminated acanthamebiasis in patients with AIDS. A report of five cases and a review of the literature. Arch. Dermatol. 131, 1291–1296.
Na, B. K., Cho, J. H., Song, C. Y., and Kim, T. S. (2002). Degradation of immunoglobulins, protease inhibitors and interleukin-1 by a secretory proteinase of Acanthamoeba castellanii. Korean J. Parasitol. 40, 93–99. doi: 10.3347/kjp.2002.40.2.93
Na, B. K., Kim, J. C., and Song, C. Y. (2001). Characterization and pathogenetic role of proteinase from Acanthamoeba castellanii. Microb. Pathog. 30, 39–48. doi: 10.1006/mpat.2000.0403
Naginton, J., Watson, P. G., Playfair, T. J., McGill, J., Jones, B. R., and Steele, A. D. (1974). Amoebic infection of the eye. Lancet 2, 1537–1540. doi: 10.1016/s0140-6736(74)90285-2
Nakada-Tsukui, K., and Nozaki, T. (2021). Trogocytosis in unicellular eukaryotes. Cells 10:2975. doi: 10.3390/cells10112975
Nakatogawa, H. (2020). Mechanisms governing autophagosome biogenesis. Nat. Rev. Mol. Cell Biol. 21, 439–458. doi: 10.1038/s41580-020-0241-0
National Library of Medicine (2020). Polyhexamethylene Biguanide (PHMB) ophthalmic solution in subjects affected by Acanthamoeba keratitis. Bethesda, MD: National Library of Medicine.
Nau, A., and Dhaliwal, D. K. (2018). Exposure to tap water puts a contact lens wearer at greater risk of exposure to Acanthamoeba. Eye Cont. Lens 44:136. doi: 10.1097/icl.0000000000000453
Neelam, S., and Niederkorn, J. Y. (2017). Pathobiology and immunobiology of Acanthamoeba keratitis: Insights from animal models. Yale J. Biol. Med. 90, 261–268.
Neff, R. J., and Neff, R. H. (1969). The biochemistry of amoebic encystment. Symp. Soc. Exp. Biol. 23, 51–81.
Nerad, T. A., Sawyer, T. K., Lewis, E. J., and McLaughlin, S. M. (1995). Acanthamoeba pearcei n. sp. (Protozoa: Amoebida) from sewage contaminated sediments. J. Eukaryot. Microbiol. 42, 702–705. doi: 10.1111/j.1550-7408.1995.tb01619.x
Ng, S. L., Nordin, A., Abd Ghafar, N., Suboh, Y., Ab Rahim, N., and Chua, K. H. (2017). Acanthamoeba-mediated cytopathic effect correlates with MBP and AhLBP mRNA expression. Parasit. Vectors 10:625. doi: 10.1186/s13071-017-2547-0
Niederkorn, J. Y. (2007). The induction of anterior chamber-associated immune deviation. Chem. Immunol. Allergy 92, 27–35. doi: 10.1159/000099251
Niederkorn, J. Y., Alizadeh, H., Leher, H., and McCulley, J. P. (1999). The pathogenesis of Acanthamoeba keratitis. Microbes Infect. 1, 437–443. doi: 10.1016/s1286-4579(99)80047-1
Nomura, H., Suda, N., Kawano, Y., Isshiki, Y., Sakuda, K., Sakuma, K., et al. (2022). Effects of oakmoss components on extra- and intracellular Legionella pneumophila and its host Acanthamoeba castellanii. Biocontrol Sci. 27, 21–29. doi: 10.4265/bio.27.21
Nuprasert, W., Putaporntip, C., Pariyakanok, L., and Jongwutiwes, S. (2010). Identification of a novel t17 genotype of Acanthamoeba from environmental isolates and t10 genotype causing keratitis in Thailand. J. Clin. Microbiol. 48, 4636–4640. doi: 10.1128/jcm.01090-10
Ohsumi, Y., and Mizushima, N. (2004). Two ubiquitin-like conjugation systems essential for autophagy. Semin. Cell Dev. Biol. 15, 231–236. doi: 10.1016/j.semcdb.2003.12.004
Oishi, K., Raynor, R. L., Charp, P. A., and Kuo, J. F. (1988). Regulation of protein kinase C by lysophospholipids. Potential role in signal transduction. J. Biol. Chem. 263, 6865–6871.
Oldenburg, C. E., Acharya, N. R., Tu, E. Y., Zegans, M. E., Mannis, M. J., Gaynor, B. D., et al. (2011). Practice patterns and opinions in the treatment of Acanthamoeba keratitis. Cornea 30, 1363–1368. doi: 10.1097/ICO.0b013e31820f7763
Omaña-Molina, M. A., González-Robles, A., Salazar-Villatoro, L., Bernal-Escobar, A., Durán-Díaz, A., Méndez-Cruz, A. R., et al. (2014). Silicone hydrogel contact lenses surface promote Acanthamoeba castellanii trophozoites adherence: Qualitative and quantitative analysis. Eye Cont. Lens 40, 132–139. doi: 10.1097/icl.0000000000000024
Omaña-Molina, M., Hernandez-Martinez, D., Sanchez-Rocha, R., Cardenas-Lemus, U., Salinas-Lara, C., Mendez-Cruz, A. R., et al. (2017). In vivo CNS infection model of Acanthamoeba genotype T4: The early stages of infection lack presence of host inflammatory response and are a slow and contact-dependent process. Parasitol. Res. 116, 725–733. doi: 10.1007/s00436-016-5338-1
Otero-Ruiz, A., Gonzalez-Zuñiga, L. D., Rodriguez-Anaya, L. Z., Lares-Jiménez, L. F., Gonzalez-Galaviz, J. R., and Lares-Villa, F. (2022). Distribution and current state of molecular genetic characterization in pathogenic free-living amoebae. Pathogens 11:1199. doi: 10.3390/pathogens11101199
Page, F. C. (1967). Re-definition of the genus Acanthamoeba with descriptions of three species. J. Protozool. 14, 709–724. doi: 10.1111/j.1550-7408.1967.tb02066.x
Papa, V., van der Meulen, I., Rottey, S., Sallet, G., Overweel, J., Asero, N., et al. (2022). Safety and tolerability of topical polyhexamethylene biguanide: A randomised clinical trial in healthy adult volunteers. Br. J. Ophthalmol. 106, 190–196. doi: 10.1136/bjophthalmol-2020-317848
Parija, S. C., Dinoop, K., and Venugopal, H. (2015). Management of granulomatous amebic encephalitis: Laboratory diagnosis and treatment. Trop. Parasitol. 5, 23–28. doi: 10.4103/2229-5070.149889
Parks, W. C., Wilson, C. L., and López-Boado, Y. S. (2004). Matrix metalloproteinases as modulators of inflammation and innate immunity. Nat Rev. Immunol. 4, 617–629. doi: 10.1038/nri1418
Pellegrin, J. L., Ortega-Barria, E., Barza, M., Baum, J., and Pereira, M. E. (1991). Neuraminidase activity in Acanthamoeba species trophozoites and cysts. Invest. Ophthalmol. Vis. Sci. 32, 3061–3066.
Pellegrin, J. L., Ortega-Barria, E., Prioli, R. P., Meijia, J. S., and Pereira, M. E. (1992). The neuraminidases of Trypanosoma cruzi and Acanthamoeba castellanii are immunologically related. Trop. Med. Parasitol. 43, 33–37.
Pettit, D. A., Williamson, J., Cabral, G. A., and Marciano-Cabral, F. (1996). In vitro destruction of nerve cell cultures by Acanthamoeba spp.: A transmission and scanning electron microscopy study. J. Parasitol. 82, 769–777.
Phillips, I. L., Everman, J. L., Bermudez, L. E., and Danelishvili, L. (2020). Acanthamoeba castellanii as a screening tool for Mycobacterium avium subspecies paratuberculosis virulence factors with relevance in macrophage infection. Microorganisms 8:1571. doi: 10.3390/microorganisms8101571
Portela, A., and Esteller, M. (2010). Epigenetic modifications and human disease. Nat. Biotechnol. 28, 1057–1068. doi: 10.1038/nbt.1685
Potter, J. L., and Weisman, R. A. (1971). Differentiation in Acanthamoeba: Beta-glucan synthesis during encystment. Biochim. Biophys. Acta 237, 65–74. doi: 10.1016/0304-4165(71)90030-4
Potter, J. L., and Weisman, R. A. (1972). Correlation of cellulose synthesis in vivo and in vitro during the encystment of Acanthamoeba. Dev. Biol. 28, 472–477. doi: 10.1016/0012-1606(72)90030-9
Prasad, B. K., and Gupta, S. (1978). Preliminary report on the engulement and retention of mycobacteria by trophozoites of exenically grown Acanthamoeba castellaniiDouglas, 1930. Curr. Sci. 47, 245–247.
Preston, T. M., and King, C. A. (1984). Amoeboid locomotion of Acanthamoeba castellanii with special reference to cell-substratum interactions. J. Gen. Microbiol. 130, 2317–2323. doi: 10.1099/00221287-130-9-2317
Preston, T. M., Richards, H., and Wotton, R. S. (2001). Locomotion and feeding of Acanthamoeba at the water-air interface of ponds. FEMS Microbiol. Lett. 194, 143–147. doi: 10.1111/j.1574-6968.2001.tb09459.x
Proca-Ciobanu, M., Lupascu, G. H., Petrovici, A., and Ionescu, M. D. (1975). Electron microscopic study of a pathogenic Acanthamoeba castellani strain: The presence of bacterial endosymbionts. Int. J. Parasitol. 5, 49–56. doi: 10.1016/0020-7519(75)90097-1
Pussard, M. (1964b). Acanthamoeba comandoni n. sp., comparaison avec A. terricola. Rev. Ecol. Biol. Sol. 1, 587–610.
Pussard, M. (1964a). Cytologie d’une amibe terricola: Acanthamoeba terricola n. sp. Ann. Sci. Nat. Zool. 6, 565–600.
Pussard, M., and Pons, R. (1977). Morphologie de la paroi kystique et taxonomie du genre Acanthamoeba (Protozoa, Amoebida). Protistologica 13, 557–598.
Putaporntip, C., Kuamsab, N., Nuprasert, W., Rojrung, R., Pattanawong, U., Tia, T., et al. (2021). Analysis of Acanthamoeba genotypes from public freshwater sources in Thailand reveals a new genotype, T23 Acanthamoeba bangkokensis sp. nov. Sci. Rep. 11:17290. doi: 10.1038/s41598-021-96690-0
Quinet, T., Samba-Louaka, A., Héchard, Y., Van Doninck, K., and Van der Henst, C. (2020). Delayed cytokinesis generates multinuclearity and potential advantages in the amoeba Acanthamoeba castellanii Neff strain. Sci. Rep. 10:12109. doi: 10.1038/s41598-020-68694-9
Qvarnstrom, Y., Nerad, T. A., and Visvesvara, G. S. (2013). Characterization of a new pathogenic Acanthamoeba species, A. byersi n. sp., isolated from a human with fatal amoebic encephalitis. J. Eukaryot. Microbiol. 60, 626–633. doi: 10.1111/jeu.12069
Radford, C. F., Minassian, D. C., and Dart, J. K. (2002). Acanthamoeba keratitis in England and Wales: Incidence, outcome, and risk factors. Br. J. Ophthalmol. 86, 536–542. doi: 10.1136/bjo.86.5.536
Raizada, M. K., and Krishna Murti, C. R. (1972). Transformation of trophic Hartmannella culbertsoni into viable cysts of cyclic 3’,5’-adenosine monophosphate. J. Cell Biol. 52, 743–748. doi: 10.1083/jcb.52.3.743
Ralston, K. S., Solga, M. D., Mackey-Lawrence, N. M., Bhattacharya, A., and Petri, W. A. Jr. (2014). Trogocytosis by Entamoeba histolytica contributes to cell killing and tissue invasion. Nature 508, 526–530. doi: 10.1038/nature13242
Ramírez-Rico, G., Martínez-Castillo, M., de la Garza, M., Shibayama, M., and Serrano-Luna, J. (2015). Acanthamoeba castellanii proteases are capable of degrading iron-binding proteins as a possible mechanism of pathogenicity. J. Eukaryot. Microbiol. 62, 614–622. doi: 10.1111/jeu.12215
Ray, D. L., and Hayes, R. E. (1954). Hartmannella astronyxis: A new species of free-living ameba. J. Morph. 95, 159–187.
Rayamajhee, B., Willcox, M. D. P., Henriquez, F. L., Petsoglou, C., Subedi, D., and Carnt, N. (2022). Acanthamoeba, an environmental phagocyte enhancing survival and transmission of human pathogens. Trends Parasitol. 38, 975–990. doi: 10.1016/j.pt.2022.08.007
Reggiori, F., Shintani, T., Nair, U., and Klionsky, D. J. (2005). Atg9 cycles between mitochondria and the pre-autophagosomal structure in yeasts. Autophagy 1, 101–109. doi: 10.4161/auto.1.2.1840
Reif, K., Nobes, C. D., Thomas, G., Hall, A., and Cantrell, D. A. (1996). Phosphatidylinositol 3-kinase signals activate a selective subset of Rac/Rho-dependent effector pathways. Curr. Biol. 6, 1445–1455. doi: 10.1016/s0960-9822(96)00749-x
Rivera, F., Lares, F., Gallegos, E., Ramirez, E., Bonilla, P., Calderon, A., et al. (1989). Pathogenic amoebae in natural thermal waters of three resorts of Hidalgo, Mexico. Environ. Res. 50, 289–295. doi: 10.1016/s0013-9351(89)80010-6
Rivera, F., Lares, F., Ramirez, E., Bonilla, P., Rodriguez, S., Labastida, A., et al. (1991). Pathogenic Acanthamoeba isolated during an atmospheric survey in Mexico city. Rev. Infect. Dis. 13(Suppl. 5), S388–S389. doi: 10.1093/clind/13.supplement_5.s388
Rivera, F., Roy-Ocotla, G., Rosas, I., Ramirez, E., Bonilla, P., and Lares, F. (1987). Amoebae isolated from the atmosphere of Mexico city and environs. Environ. Res. 42, 149–154. doi: 10.1016/s0013-9351(87)80016-6
Rocha-Azevedo, B. D., Jamerson, M., Cabral, G. A., Silva-Filho, F. C., and Marciano-Cabral, F. (2009). Acanthamoeba interaction with extracellular matrix glycoproteins: Biological and biochemical characterization and role in cytotoxicity and invasiveness. J. Eukaryot. Microbiol. 56, 270–278. doi: 10.1111/j.1550-7408.2009.00399.x
Rocha-Azevedo, B., Jamerson, M., Cabral, G. A., and Marciano-Cabral, F. (2010). Acanthamoeba culbertsoni: Analysis of amoebic adhesion and invasion on extracellular matrix components collagen I and laminin-1. Exp. Parasitol. 126, 79–84. doi: 10.1016/j.exppara.2009.08.004
Roshni Prithiviraj, S., Rajapandian, S. G. K., Gnanam, H., Gunasekaran, R., Mariappan, P., Sankalp Singh, S., et al. (2020). Clinical presentations, genotypic diversity and phylogenetic analysis of Acanthamoeba species causing keratitis. J. Med. Microbiol. 69, 87–95. doi: 10.1099/jmm.0.001121
Saheb, E., Trzyna, W., Maringer, K., and Bush, J. (2015). Abnormalities of endocytosis, phagocytosis, and development process in Dictyostelium cells that over-express Acanthamoeba castellanii metacaspase protein. Iran. J. Parasitol. 10, 213–229.
Sakoh-Nakatogawa, M., Matoba, K., Asai, E., Kirisako, H., Ishii, J., Noda, N. N., et al. (2013). Atg12-Atg5 conjugate enhances E2 activity of Atg3 by rearranging its catalytic site. Nat. Struct. Mol. Biol. 20, 433–439. doi: 10.1038/nsmb.2527
Sauve, A. A., Celic, I., Avalos, J., Deng, H., Boeke, J. D., and Schramm, V. L. (2001). Chemistry of gene silencing: The mechanism of NAD+-dependent deacetylation reactions. Biochemistry 40, 15456–15463. doi: 10.1021/bi011858j
Sawyer, T. K. (1971). Acanthamoeba griffini: A new species of marine amoeba. J. Protozool. 18, 650–654.
Sawyer, T. K., and Nerad, T. A. (1992). Acanthamoeba jacobsi sp. n. (Protozoa: Acanthamoebidae) from sewage contaminated ocean sediments. J. Helminthol. Soc. Wash. 59, 223–226.
Sawyer, T. K., Nerad, T. A., Lewis, E. J., and McLaughlin, A. M. (1993). Acanthamoeba stevensoni N. Sp. (Protozoa: Amoebida) from sewage-contaminated shellfish beds in Raritan Bay, New York. J. Eukaryot. Microbiol. 40, 742–746.
Sawyer, T. K., Visvesvara, G. S., and Harke, B. A. (1977). Pathogenic amoebas from brackish and ocean sediments, with a description of Acanthamoeba hatchetti, n. sp. Science 196, 1324–1325. doi: 10.1126/science.867031
Schaumberg, D. A., Snow, K. K., and Dana, M. R. (1998). The epidemic of Acanthamoeba keratitis: Where do we stand? Cornea 17, 3–10. doi: 10.1097/00003226-199801000-00001
Schmoller, H. (1964). [Description of some cultivated amoebae of marine origin]. J. Protozool. 11, 497–502.
Schramm, M., and Hestrin, S. (1954). Factors affecting production of cellulose at the air/liquid interface of a culture of Acetobacter xylinum. J. Gen. Microbiol. 11, 123–129. doi: 10.1099/00221287-11-1-123
Schuster, F. L., and Visvesvara, G. S. (2004). Opportunistic amoebae: Challenges in prophylaxis and treatment. Drug Resist. Updat. 7, 41–51. doi: 10.1016/j.drup.2004.01.002
Schwarz, W. H. (2001). The cellulosome and cellulose degradation by anaerobic bacteria. Appl. Microbiol. Biotechnol. 56, 634–649. doi: 10.1007/s002530100710
Seal, D. V., Kirkness, C. M., Bennett, H. G., and Peterson, M. (1999). Acanthamoeba keratitis in Scotland: Risk factors for contact lens wearers. Cont. Lens Anterior Eye 22, 58–68. doi: 10.1016/s1367-0484(99)80004-6
Serhan, C. N., Haeggström, J. Z., and Leslie, C. C. (1996). Lipid mediator networks in cell signaling: Update and impact of cytokines. FASEB J. 10, 1147–1158. doi: 10.1096/fasebj.10.10.8751717
Serrano-Luna Jde, J., Cervantes-Sandoval, I., Calderón, J., Navarro-García, F., Tsutsumi, V., and Shibayama, M. (2006). Protease activities of Acanthamoeba polyphaga and Acanthamoeba castellanii. Can. J. Microbiol. 52, 16–23. doi: 10.1139/w05-114
Siddiqui, R., and Khan, N. A. (2012). Biology and pathogenesis of Acanthamoeba. Parasit. Vectors 5:6. doi: 10.1186/1756-3305-5-6
Siddiqui, R., Dudley, R., and Khan, N. A. (2012). Acanthamoeba differentiation: A two-faced drama of Dr. Jekyll and Mr. Hyde. Parasitology 139, 826–834. doi: 10.1017/s0031182012000042
Siddiqui, R., Khan, N. A., and Jarroll, E. L. (2009). The cyst wall carbohydrate composition of Balamuthia mandrillaris. Parasitol. Res. 104, 1439–1443. doi: 10.1007/s00436-009-1346-8
Sinclair, D. A., and Guarente, L. (1997). Extrachromosomal rDNA circles–a cause of aging in yeast. Cell 91, 1033–1042. doi: 10.1016/s0092-8674(00)80493-6
Singh, B. N., and Das, S. R. (1970). Studies on pathogenic and non-pathogenic small free-living amoebae and the bearing of nuclear division on the classification of the order amoebida. Philos. Trans. R. Soc. Lond. B Biol. Sci. 259, 435–476. doi: 10.1098/rstb.1970.0063
Singh, B., Fleury, C., Jalalvand, F., and Riesbeck, K. (2012). Human pathogens utilize host extracellular matrix proteins laminin and collagen for adhesion and invasion of the host. FEMS Microbiol. Rev. 36, 1122–1180. doi: 10.1111/j.1574-6976.2012.00340.x
Singh, D., Naik, S. R., and Naik, S. (2004). Role of cysteine proteinase of Entamoeba histolytica in target cell death. Parasitology 129(Pt 2), 127–135. doi: 10.1017/s0031182004005451
Sissons, J., Alsam, S., Goldsworthy, G., Lightfoot, M., Jarroll, E. L., and Khan, N. A. (2006). Identification and properties of proteases from an Acanthamoeba isolate capable of producing granulomatous encephalitis. BMC Microbiol. 6:42. doi: 10.1186/1471-2180-6-42
Sissons, J., Alsam, S., Jayasekera, S., and Khan, N. A. (2004a). Ecto-ATPases of clinical and non-clinical isolates of Acanthamoeba. Microb. Pathog. 37, 231–239. doi: 10.1016/j.micpath.2004.01.004
Sissons, J., Alsam, S., Jayasekera, S., Kim, K. S., Stins, M., and Khan, N. A. (2004b). Acanthamoeba induces cell-cycle arrest in host cells. J. Med. Microbiol. 53(Pt 8), 711–717. doi: 10.1099/jmm.0.45604-0
Sissons, J., Kim, K. S., Stins, M., Jayasekera, S., Alsam, S., and Khan, N. A. (2005). Acanthamoeba castellanii induces host cell death via a phosphatidylinositol 3-kinase-dependent mechanism. Infect. Immun. 73, 2704–2708. doi: 10.1128/iai.73.5.2704-2708.2005
Sohn, J. H., Bora, P. S., Suk, H. J., Molina, H., Kaplan, H. J., and Bora, N. S. (2003). Tolerance is dependent on complement C3 fragment iC3b binding to antigen-presenting cells. Nat. Med. 9, 206–212. doi: 10.1038/nm814
Sohn, J. H., Kaplan, H. J., Suk, H. J., Bora, P. S., and Bora, N. S. (2000). Chronic low level complement activation within the eye is controlled by intraocular complement regulatory proteins. Invest. Ophthalmol. Vis. Sci. 41, 3492–3502.
Song, S. M., Han, B. I., Moon, E. K., Lee, Y. R., Yu, H. S., Jha, B. K., et al. (2012). Autophagy protein 16-mediated autophagy is required for the encystation of Acanthamoeba castellanii. Mol. Biochem. Parasitol. 183, 158–165. doi: 10.1016/j.molbiopara.2012.02.013
Sriram, R., Shoff, M., Booton, G., Fuerst, P., and Visvesvara, G. S. (2008). Survival of Acanthamoeba cysts after desiccation for more than 20 years. J. Clin. Microbiol. 46, 4045–4048. doi: 10.1128/jcm.01903-08
Stehr-Green, J. K., Bailey, T. M., and Visvesvara, G. S. (1989). The epidemiology of Acanthamoeba keratitis in the United States. Am. J. Ophthalmol. 107, 331–336. doi: 10.1016/0002-9394(89)90654-5
Sternlicht, M. D., and Werb, Z. (2001). How matrix metalloproteinases regulate cell behavior. Annu. Rev. Cell Dev. Biol. 17, 463–516. doi: 10.1146/annurev.cellbio.17.1.463
Stevaux, O., and Dyson, N. J. (2002). A revised picture of the E2F transcriptional network and RB function. Curr. Opin. Cell Biol. 14, 684–691. doi: 10.1016/s0955-0674(02)00388-5
Stevens, A. R., and Pachler, P. F. (1973). RNA synthesis and turnover during density-inhibited growth and encystment of Acanthamoeba castellanii. J. Cell Biol. 57, 525–537. doi: 10.1083/jcb.57.2.525
Stewart, J. R., and Weisman, R. A. (1974). A chemical and autoradiographic study of cellulose synthesis during the encystment of Acanthamoeba castellanii. Arch. Biochem. Biophys. 161, 488–498. doi: 10.1016/0003-9861(74)90331-2
Stohr, M., Bommert, K., Schulze, I., and Jantzen, H. (1987). The cell cycle and its relationship to development in Acanthamoeba castellanii. J. Cell Sci. 88, 579–589.
Stratford, M., and Griffiths, A. (1978). Variation in the properties and morphology of cysts of Acanthamoeba castellanii. J. Gen. Microbiol. 108, 33–37.
Sütçü, M., Aktürk, H., Gülümser-Şişko, S., Acar, M., Erol, O. B., Somer, A., et al. (2018). Granulomatous amebic encephalitis caused by Acanthamoeba in an immuncompetent child. Turk. J. Pediatr. 60, 340–343. doi: 10.24953/turkjped.2018.03.019
Suzuki, K., Kamada, Y., and Ohsumi, Y. (2002). Studies of cargo delivery to the vacuole mediated by autophagosomes in Saccharomyces cerevisiae. Dev. Cell 3, 815–824. doi: 10.1016/s1534-5807(02)00359-3
Swart, A. L., Harrison, C. F., Eichinger, L., Steinert, M., and Hilbi, H. (2018). Acanthamoeba and Dictyostelium as cellular models for legionella infection. Front. Cell. Infect. Microbiol. 8:61. doi: 10.3389/fcimb.2018.00061
Taher, E. E., Méabed, E. M. H., Abdallah, I., and Abdel Wahed, W. Y. (2018). Acanthamoeba keratitis in noncompliant soft contact lenses users: Genotyping and risk factors, a study from Cairo, Egypt. J. Infect. Public Health 11, 377–383. doi: 10.1016/j.jiph.2017.09.013
Tan, E. M., Starr, M. R., Henry, M. R., and Pritt, B. S. (2018). The brief case: A “fresh” pair of contact lenses. J. Clin. Microbiol. 56:e00790-17. doi: 10.1128/jcm.00790-17
Tang, A., Marquart, M. E., Fratkin, J. D., McCormick, C. C., Caballero, A. R., Gatlin, H. P., et al. (2009). Properties of PASP: A Pseudomonas protease capable of mediating corneal erosions. Invest. Ophthalmol. Vis. Sci. 50, 3794–3801. doi: 10.1167/iovs.08-3107
Tawfeek, G. M., Bishara, S. A., Sarhan, R. M., ElShabrawi Taher, E., and ElSaady Khayyal, A. (2016). Genotypic, physiological, and biochemical characterization of potentially pathogenic Acanthamoeba isolated from the environment in Cairo, Egypt. Parasitol. Res. 115, 1871–1881. doi: 10.1007/s00436-016-4927-3
Taylor, W. M., Pidherney, M. S., Alizadeh, H., and Niederkorn, J. Y. (1995). In vitro characterization of Acanthamoeba castellanii cytopathic effect. J. Parasitol. 81, 603–609.
Thyrell, L., Hjortsberg, L., Arulampalam, V., Panaretakis, T., Uhles, S., Dagnell, M., et al. (2004). Interferon alpha-induced apoptosis in tumor cells is mediated through the phosphoinositide 3-kinase/mammalian target of rapamycin signaling pathway. J. Biol. Chem. 279, 24152–24162. doi: 10.1074/jbc.M312219200
Tice, A. K., Shadwick, L. L., Fiore-Donno, A. M., Geisen, S., Kang, S., Schuler, G. A., et al. (2016). Expansion of the molecular and morphological diversity of Acanthamoebidae (Centramoebida, Amoebozoa) and identification of a novel life cycle type within the group. Biol. Direct. 11:69. doi: 10.1186/s13062-016-0171-0
Tiewcharoen, S., Rabablert, J., Chetanachan, P., Junnu, V., Worawirounwong, D., and Malainual, N. (2008). Scanning electron microscopic study of human neuroblastoma cells affected with Naegleria fowleri Thai strains. Parasitol. Res. 103, 1119–1123. doi: 10.1007/s00436-008-1103-4
Tomlinson, G., and Jones, E. A. (1962). Isolation of cellulose from the cyst wall of a soil amoeba. Biochim. Biophys. Acta 63, 194–200. doi: 10.1016/0006-3002(62)90353-0
Torno, M. S. Jr., Babapour, R., Gurevitch, A., and Witt, M. D. (2000). Cutaneous acanthamoebiasis in AIDS. J. Am. Acad. Dermatol. 42(2 Pt 2), 351–354. doi: 10.1016/s0190-9622(00)90110-5
Tu, E. Y., and Joslin, C. E. (2010). Recent outbreaks of atypical contact lens-related keratitis: What have we learned? Am. J. Ophthalmol. 150, 602–608.e602. doi: 10.1016/j.ajo.2010.06.045
Turner, N. A., Harris, J., Russell, A. D., and Lloyd, D. (2000). Microbial differentiation and changes in susceptibility to antimicrobial agents. J. Appl. Microbiol. 89, 751–759. doi: 10.1046/j.1365-2672.2000.01176.x
Turner, N. A., Russell, A. D., Furr, J. R., and Lloyd, D. (2004). Resistance, biguanide sorption and biguanide-induced pentose leakage during encystment of Acanthamoeba castellanii. J. Appl. Microbiol. 96, 1287–1295. doi: 10.1111/j.1365-2672.2004.02260.x
Vernon, S. E., Acar, B. C., Pham, S. M., and Fertel, D. (2005). Acanthamoeba infection in lung transplantation: Report of a case and review of the literature. Transpl. Infect. Dis. 7, 154–157. doi: 10.1111/j.1399-3062.2005.00113.x
Visvesvara, G. S., and Balamuth, W. (1975). Comparative studies on related free-living and pathogenic amebae with special reference to Acanthamoeba. J. Protozool. 22, 245–256. doi: 10.1111/j.1550-7408.1975.tb05860.x
Visvesvara, G. S., Mirra, S. S., Brandt, F. H., Moss, D. M., Mathews, H. M., and Martinez, A. J. (1983). Isolation of two strains of Acanthamoeba castellanii from human tissue and their pathogenicity and isoenzyme profiles. J. Clin. Microbiol. 18, 1405–1412. doi: 10.1128/jcm.18.6.1405-1412.1983
Voyatzis, G., and McElvanney, A. (2007). Bilateral Acanthamoeba keratitis in an experienced two-weekly disposable contact lens wearer. Eye Cont. Lens 33, 201–202. doi: 10.1097/01.icl.0000252567.06446.7b
Walochnik, J., Obwaller, A., and Aspöck, H. (2000). Correlations between morphological, molecular biological, and physiological characteristics in clinical and nonclinical isolates of Acanthamoeba spp. Appl. Environ. Microbiol. 66, 4408–4413. doi: 10.1128/aem.66.10.4408-4413.2000
Walochnik, J., Scheikl, U., and Haller-Schober, E. M. (2015). Twenty years of Acanthamoeba diagnostics in Austria. J. Eukaryot. Microbiol. 62, 3–11. doi: 10.1111/jeu.12149
Wang, P., and Zhu, J. X. (2010). Research progress on the pathogenesis of Acanthamoeba keratitis. Int. J. Ophthal. 10, 885–887.
Wang, Y. J., Lin, W. C., and He, M. S. (2021). The Acanthamoeba SBDS, a cytoskeleton-associated gene, is highly expressed during phagocytosis and encystation. J. Microbiol. Immunol. Infect. 54, 482–489. doi: 10.1016/j.jmii.2019.11.003
Weisman, R. A. (1976). Differentiation in Acanthamoeba castellanii. Annu. Rev. Microbiol. 30, 189–219. doi: 10.1146/annurev.mi.30.100176.001201
Weisman, R. A., Spiegel, R. S., and McCauley, J. G. (1970). Differentiation in Acanthamoeba: Glycogen levels and glycogen synthetase activity during encystment. Biochim. Biophys. Acta. 201, 45–53. doi: 10.1016/0304-4165(70)90008-5
Wennström, S., Hawkins, P., Cooke, F., Hara, K., Yonezawa, K., Kasuga, M., et al. (1994). Activation of phosphoinositide 3-kinase is required for PDGF-stimulated membrane ruffling. Curr. Biol. 4, 385–393. doi: 10.1016/s0960-9822(00)00087-7
Willaert, E., Stevens, A. R., and Tyndall, R. L. (1978). Acanthamoeba royreba sp. n. from a human tumor cell culture. J. Protozool. 25, 1–14. doi: 10.1111/j.1550-7408.1978.tb03854.x
Williams, W. S., and Cannon, R. E. (1989). Alternative environmental roles for cellulose produced by Acetobacter xylinum. Appl. Environ. Microbiol. 55, 2448–2452. doi: 10.1128/aem.55.10.2448-2452.1989
Wopereis, D. B., Bazzo, M. L., de Macedo, J. P., Casara, F., Golfeto, L., Venancio, E., et al. (2020). Free-living amoebae and their relationship to air quality in hospital environments: Characterization of Acanthamoeba spp. obtained from air-conditioning systems. Parasitology 147, 782–790. doi: 10.1017/s0031182020000487
Wymann, M. P., and Pirola, L. (1998). Structure and function of phosphoinositide 3-kinases. Biochim. Biophys. Acta 1436, 127–150. doi: 10.1016/s0005-2760(98)00139-8
Xie, Z., and Klionsky, D. J. (2007). Autophagosome formation: Core machinery and adaptations. Nat. Cell Biol. 9, 1102–1109. doi: 10.1038/ncb1007-1102
Yang, Z., and Klionsky, D. J. (2009). An overview of the molecular mechanism of autophagy. Curr. Top. Microbiol. Immunol. 335, 1–32. doi: 10.1007/978-3-642-00302-8_1
Yang, Z., Cao, Z., and Panjwani, N. (1997). Pathogenesis of Acanthamoeba keratitis: Carbohydrate-mediated host-parasite interactions. Infect. Immun. 65, 439–445. doi: 10.1128/iai.65.2.439-445.1997
Yoder, J. S., Verani, J., Heidman, N., Hoppe-Bauer, J., Alfonso, E. C., Miller, D., et al. (2012). Acanthamoeba keratitis: The persistence of cases following a multistate outbreak. Ophthalmic Epidemiol. 19, 221–225. doi: 10.3109/09286586.2012.681336
Yoo, K. T., and Jung, S. Y. (2012). Effects of mannose on pathogenesis of Acanthamoeba castellanii. Korean J. Parasitol. 50, 365–369. doi: 10.3347/kjp.2012.50.4.365
Yun, H. C., Kim, K. Y., Park, S. Y., Park, S. K., Park, H., Hwang, U. W., et al. (1999). Cloning of a cysteine proteinase gene from Acanthamoeba culbertsoni. Mol. Cells 9, 491–496.
Zhang, H., and Cheng, X. (2021). Various brain-eating amoebae: The protozoa, the pathogenesis, and the disease. Front. Med. 15, 842–866. doi: 10.1007/s11684-021-0865-2
Keywords: Acanthamoeba, biological characteristics, classification, disease, pathogenesis
Citation: Wang Y, Jiang L, Zhao Y, Ju X, Wang L, Jin L, Fine RD and Li M (2023) Biological characteristics and pathogenicity of Acanthamoeba. Front. Microbiol. 14:1147077. doi: 10.3389/fmicb.2023.1147077
Received: 18 January 2023; Accepted: 21 March 2023;
Published: 05 April 2023.
Edited by:
Christopher A. Rice, Purdue University, United StatesReviewed by:
Bharath Kanakapura Sundararaj, Boston University, United StatesVincent Delafont, University of Poitiers, France
Wayne Heaselgrave, University of Wolverhampton, United Kingdom
Copyright © 2023 Wang, Jiang, Zhao, Ju, Wang, Jin, Fine and Li. This is an open-access article distributed under the terms of the Creative Commons Attribution License (CC BY). The use, distribution or reproduction in other forums is permitted, provided the original author(s) and the copyright owner(s) are credited and that the original publication in this journal is cited, in accordance with accepted academic practice. No use, distribution or reproduction is permitted which does not comply with these terms.
*Correspondence: Mingguang Li, bWdsMTgwMEBqbG11LmVkdS5jbg==