- 1Key Laboratory for Feed Biotechnology of the Ministry of Agriculture and Rural Affairs, Institute of Feed Research, Chinese Academy of Agriculture Sciences, Beijing, China
- 2Precision Livestock and Nutrition Laboratory, Teaching and Research Centre (TERRA), Gembloux Agro-Bio Tech, University of Liège, Gembloux, Belgium
- 3Department of Molecular Cell Biology, Samsung Medical Center, Sungkyunkwan University School of Medicine, Suwon, Republic of Korea
- 4National Engineering Research Center of Biological Feed, Beijing, China
Bacillus spp. have gained increasing recognition as an option to use as antimicrobial growth promoters, which are characterized by producing various enzymes and antimicrobial compounds. The present study was undertaken to screen and evaluate a Bacillus strain with the multi-enzyme production property for poultry production. LB-Y-1, screened from the intestines of healthy animals, was revealed to be a Bacillus velezensis by the morphological, biochemical, and molecular characterization. The strain was screened out by a specific screening program, possessed excellent multi-enzyme production potential, including protease, cellulase, and phytase. Moreover, the strain also exhibited amylolytic and lipolytic activity in vitro. The dietary LB-Y-1 supplementation improved growth performance and tibia mineralization in chicken broilers, and increased serum albumin and serum total protein at 21 days of age (p < 0.05). Besides, LB-Y-1 enhanced the activity of serum alkaline phosphatase and digestive enzyme in broilers at 21 and 42 days of age (p < 0.05). Analysis of intestinal microbiota showed that a higher community richness (Chao1 index) and diversity (Shannon index) in the LB-Y-1 supplemented compared with the CON group. PCoA analysis showed that the community composition and structure were distinctly different between the CON and LB-Y-1 group. The beneficial genera such as Parasutterella and Rikenellaceae were abundant, while the opportunistic pathogen such as Escherichia-Shigella were reduced in the LB-Y-1 supplemented group (p < 0.05). Collectively, LB-Y-1 can be considered as a potential strain for further utilization in direct-fed microbial or starter culture for fermentation.
1. Introduction
“Beneficial,” “friendly,” and “healthy” are commonly used to describe probiotics. Previous studies have proved that probiotics may have a fundamental impact on the maturation of animal immune phenotype, and the metabolisms of different functional microorganisms would play a crucial role in the maintenance of health (Gao et al., 2017; Ma et al., 2018). Therefore, it is imperative to screen functional microorganisms with excellent characteristics and explore their roles in different periods of life (Kundu et al., 2017; Francino, 2018; Tun et al., 2018). One of the functional properties of microorganism is the ability to secrete multi-enzyme by exocytosis, and these enzymes are able to further degrade certain nutrient substances that are not well digested by endogenous enzymes produced in the animal’s digestive system (Lee et al., 2020). For example, the proteases can convert insoluble storage protein into soluble peptides and amino acids (Kesari and Rangan, 2011). The cellulases such as cellobiohydrolases, β-glucosidases, and endoglucanases can degrade the fiber components in food (Stålbrand et al., 1998; Ye et al., 2017). Microbial phytases can hydrolyze the phytate complex and release the nutrients for use by the broilers (Babatunde et al., 2021; Li et al., 2022).
One of the main objectives in the poultry industry is to maximize the productivity. However, several major issues confront the poultry meat production today, such as the imbalance in protein resources, and viral or bacterial infectious diseases. In parallel, the global trend for reducing antibiotic growth promoters in the animal production has gathered momentum (Pourabedin et al., 2015). It is particularly important to explore the nutritional interventions for the prevention of these pathological processes, and the supplementation of exogenous amylase, protease, or other enzymes for broiler diets could be a strategic approach (Chang’a et al., 2019; Córdova-Noboa et al., 2021). Studies have shown that a number of Bacillus spp. can be added to feed, which has the effect of improving animal growth performance, regulating the intestinal micro-ecology, and promoting the utilization of nutrients (Lee et al., 2008; Li et al., 2022).
Researchers have confirmed that the Bacillus velezensis (B. velezensis) has the characteristics of producing a variety of enzymes like protease, cellulase, amylase, and glucanase, as well as secreting antibacterial substances to inhibit the growth of pathogenic microorganisms (Meng and Hao, 2017; Li et al., 2018). However, a comprehensive evaluation of the effect of B. velezensis on poultry is lacking. In the current study, B. velezensis LB-Y-1 with the ability to produce multi-enzyme including protease, cellulase, and phytase was screened and characterized from the intestinal tract of different healthy animals. Ultimately, the strain was comprehensively evaluated for its safety and efficacy in growing broilers.
2. Materials and methods
2.1. Sample collection and isolation of Bacillus spp.
The strains were isolated from the digestive tracts of healthy and free-ranging animals (the rumen of cattles, and the cecum of chickens, pigs, and rabbits) without any additives during the rearing period (such as antibiotics or probiotics). All strains were collected in accordance with the Bioconvention and the Nagoya protocol (Diversity, 2022). Bacillus spp. was isolated according to the method described as previously, with minor modification (Susanti et al., 2021; Liu et al., 2022). Heat the intestinal contents at 95°C for 5 min in order to separate out the Bacillus spores, followed by a 10-fold series dilution with sterile normal saline to ensure recovery of individual colonies on agar plates. Isolation was performed using agar spot test on Luria-Bertani (LB)—casein medium (Hope Bio-Technology Co., Ltd., Qingdao, China; casein, 0.4%). Based on the colony morphology and casein-soluble region of Bacillus spp., 191 colonies were purified and selected. The strains were grown in LB broth (Hope Bio-Technology Co., Ltd., Qingdao, China) and stored in LB broth with 20% glycerol at −80°C.
2.2. Protease production capacity (primary screening)
The protocol was based on the previous method with minor modification (Liu et al., 2022). Briefly, the selected strains were cultured at 37°C for 24 h and the inoculum was added at 10% (v/v) rate to the fermentation broth containing 20 g peanut meal and 20 ml distilled water in a flask. The cultures were incubated anaerobically at 37°C for 72 h. The fermentation product (10 g) was added to a 250 mL Erlenmeyer flask containing 90 ml of distilled water and incubated in 40°C water bath for 1 h (stirred every 15 min). The crude enzyme solution was obtained after filtration through filter paper Whatman No.1 (Whatman International Ltd., Maidstone, United Kingdom). Folin–Ciocalteu method was used to determine the protease activity (Ramkumar et al., 2018). All tests were repeated four times.
2.3. Cellulase production capacity (secondary screening)
The cellulase-production strains were screened by the carboxymethylcellulose (CMC) plate assay method (Shaikh et al., 2013; Mohammad et al., 2017). In brief, the plates were incubated at 37°C for 5 days to produce enough cellulase, then the plates were incubated in Congo red solution (1% w/v) for 15 min at 37°C, followed by flooding with 1 M NaCl for 15 min. The strains with cellulose degrading ability showed a clear zone. The ratio of clear zone diameter to colony diameter (mm/mm) was considered as degradation efficiency. All tests were repeated four times.
2.4. Phytase production capacity (tertiary screening)
The phytase-production strains were screened following the method explained by Demirkan et al. (2014). Firstly, the freshly cultured strains in LB broth (OD600 = 0.3) were inoculated into enzyme production broth [Dextrose 0.5%, yeast extract 0.5%, peptone 1.0%, sodium phytate 0.1%, CaCl2 0.1%, MgSO4 0.1% (w/v, pH 7.0)] at 1% rate, and then incubated at 37°C, 150 rpm for 24, 32, 48, 56, 72, and 80 h in a shaking incubator. At the end of each period, the culture supernatant was collected for determination of phytase activity using the method explained by Choi et al. (2001). All tests were repeated four times, and selected the strains with high phytase activity for further analysis.
2.5. Morphological, biochemical, and molecular characterization of LB-Y-1
The characterizations of LB-Y-1 were done using the method explained by Zhang D. F. et al. (2021) with minor modifications. The characteristic colonies were gram stained, and bacteria were examined for morphology under a light microscope (Olympus CX40, Olympus Optical Co. Ltd., Hamburg, Germany) and a scanning electron microscopic (SEM, Inspect F50, FEI Company, Hillsboro, OR, United States; Bajpai et al., 2016). The growth curve assay of LB-Y-1 was performed according to the method described previously (Fitzgerald et al., 2020). Supplementary File 1 showed the molecular biology identification procedure for LB-Y-1. A bootstrap phylogenetic tree was constructed by the neighbor-joining method, using MEGA 7 software.1 Furthermore, biochemical characteristics of LB-Y-1 were performed using the API 50 CHB system (BioMerieux Vitek, France) according to the instructions from the manufacturer.
In order to further examine the potential of LB-Y-1 to produce multi-enzymes, the activity of amylase and lipase in the LB-Y-1 were determined using the solid agar plate method (Zhang D. F. et al., 2021). Briefly, after cultured in LB broth at 37°C overnight, the strain was stab-inoculated on the surface of LB agar plate containing 1.5% soluble starch or 1% triglyceride tributyrate (four replicates were made of each assay). The plates were subsequently incubated at 37°C for 24 h. The amylase and lipase activities were determined based on the diameter of the transparent halos.
2.6. Safety evaluation of LB-Y-1 (in vitro)
2.6.1. Hemolytic activity assay
The hemolytic activity of LB-Y-1 was determined using the method explained by Maragkoudakis et al. (2006), with minor modifications. Briefly, LB-Y-1 was cultured in LB broth overnight, and then inoculated in pre-made blood agar (Beijing Land Bridge Technology Co., Ltd., Beijing, China) containing 5% (v/v) sheep blood. The presence of a hemolysis zone was observed following incubation at 37°C for 24 h.
2.6.2. Antibiotic susceptibility
Antibiotic susceptibility of LB-Y-1 was determined by the agar diffusion method of CLSI (2015), with minor modifications. Briefly, LB-Y-1 was cultured in LB broth at 37°C for 12 h, and then preparing a suspension in accordance with two McFarland’s scales (108 CFU/mL; Reuben et al., 2019). A total of 100 μL suspension were spread onto MRS agar plates, in which the antibiotic discs were placed. The commercial antibiotic discs (HANGWEI, Hangzhou, China) contains Cefoperazone (75 μg), Ceftriaxone (30 μg), Ceftazidime (30 μg), Cefuroxime (30 μg), Cefradine (30 μg), Cefazolin (30 μg), Cefalexin (30 μg), Minocycline (30 μg), Doxycycline (30 μg), Tetracycline (30 μg), Ciprofloxacin (5 μg), Clindamycin (2 μg), Erythromycin (15 μg), Neomycin (30 μg), Kanamycin (30 μg), Gentamicin (10 μg), Amikacin (30 μg), Vancomycin (30 μg), Piperacillin (100 μg), Ampicillin (100 μg), Oxacillin (1 μg), Penicillin (10 μg), Chloramphenicol (30 μg), and Furazolidone (300 μg). Plates were incubated for 24 h at 37°C and the diameters of the clear zones were measured and classified as sensitive (S), intermediate (I), and resistance (R) according to the guidelines for CLSI.
2.7. In vivo testing
2.7.1. Strain preparation
The strain B. velezensis LB-Y-1 was emulsified into microcapsules (prepared by Challenge Biotechnology Co., LTD, Beijing, China, viable count ≥ 5.0 × 1010 CFU/g). Following a conservative strategy, the amount of LB-Y-1 in feed was examined daily throughout the experiment to ensure cell viability (Nikoskelainen et al., 2003).
2.7.2. Experimental design and bird management
The research was conducted at Nankou pilot base of the Chinese Academy of Agricultural Sciences (CAAS). The animal experiments were performed under the National Institute of Animal Health approved protocol and the ARRIVE guidelines were followed for reporting results (Kilkenny et al., 2012).
A total of 120 one-day-old male Arbor Acres (AA) broiler chickens (body weight, 40.2 ± 0.4 g) were randomly allocated into 2 treatment groups with 6 replicates of 10 birds each replicate. The control (CON) group were fed a corn-soybean-based diet, which met the nutritional requirements of broilers (Supplementary Table S1) in pellet form. LB-Y-1 homogenate was added at 100 mg/kg to the basal diets (BV group), and the final concentration was 3.5 × 109 CFU/kg. The mixing and pelleting were operated by a single trained person and fresh diets were produced every 3 days to ensure uniformity of additives. The experiment lasted for 42 days in two feeding phases, starter (1–21 days) and grower (22–42 days), and all the broilers were housed in the same environmentally controlled facility (cleaning cage equipped with the fiberglass feeders and plastic net floor). All broilers were allowed ad libitum feed and water, and given the same photoperiod (16 h light: 8 h dark), relative humidity (1–7 days, 60%–70%; 8–42 days, 50%–60%) and room temperature (1–7 days, 33°C ± 2°C; 8–16 days decreased stepwise to 24°C; 17–42 days 24°C). The excreta was cleared daily. Broilers were subjected a routine vaccination program, and their health was monitored daily.
2.7.3. Sampling
Body weight (BW) were measured at 21 and 42 days of age and record the feed intake, average daily feed intake (ADFI), average daily gain (ADG), and the feed intake/weight gain (F/G) ratio were calculated for the different phases. At 21 and 42 days of age, one bird close to the average body weight from each replicate was selected after 12 h fasting. Blood samples (2.5 mL) were collected from the wing vein in the EDTA-containing (5 mL). Serum was harvested from non-anticoagulated whole blood by centrifuging at 3,000 × g for 10 min, and stored at −20°C until analyzed. The slaughter performance and immune organ indexes were measured according to the production performance noun terms and metric statistics method of poultry (NY/T823-2004; Liu et al., 2021; Gao et al., 2022). One side of the tibia bone were removed for mineralization analysis. The jejunum digesta samples were collected from the middle of the jejunum and stored at −20°C until further analysis. Liver, spleen, and intestinal tissues (jejunum and ileum) were collected and fixed in 10% buffered formaldehyde (pH 7.4) for histological analysis. At 42 days of age, the ileal contents of 6 broilers were collected and snap-frozen in liquid nitrogen, followed by storage at −80°C for DNA extraction.
2.7.4. Hematological and serum biochemical indexes analysis
Using an auto hematology analyzer (Sysme XT-1800i, China) to detect red blood cell count (RBC), white blood cell count (WBC), lymphocytes (LYM), and hemoglobin concentration (HGB). The alkaline phosphatase (ALP), aspartate aminotransferase (AST), alanine aminotransferase (ALT), and P level were measured using an automatic blood biochemical analyzer (Olympus AU640, Japan). The total protein (TP) and albumin levels of serum were measured using commercial assay kits (Nanjing Jiancheng Bioengineering Institute, Nanjing, China) by colorimetric method. Because the TP in serum mainly consists of albumin and globulin, the globulin content was obtained by subtracting the albumin value from that of the TP (Salem et al., 2018).
2.7.5. Intestinal digestive enzyme and tibia bone mineralization analysis
Amylase, trypsin, and lipase activities of the digesta samples were measured by using the commercial assay kits (C016, A080 and A054, Nanjing Jiancheng Bioengineering Institute, Nanjing, China). Tibia bone mineralization analysis involved measuring ash of tibia bone and P and Ca concentration in the bone ash, according to the method reported by Li et al. (2022).
2.7.6. Histological analysis
Fixed intestine samples were dehydrated in various concentrations of ethyl alcohol (75%, 85%, 95%, and 100%), cleared in xylene, and embedded in paraffin. Five micrometer-thick sections were prepared using a microtome, then the paraffin-embedded sections were deparaffinized, dehydrated, and stained by hematoxylin–eosin (H&E). Visualization was performed under a light microscope (Olympus CX40, Olympus Optical Co. Ltd., Hamburg, Germany). For the jejunum and ileum sections, epithelial thickness, villus length, and crypt depth were measured at least 10 well-oriented villi, and the villus length/crypt depth ratio (V/C) ratio was calculated.
2.7.7. Microbial analysis
Total genomic DNA was extracted from the intestinal contents using commercially kit (Qiagen, Hilden, Germany), and the quality of DNA was checked by agarose gel electrophoresis. DNA concentration and purity were investigated using a NanoDrop2000 spectrophotometer (Thermo Fisher Scientific, Waltham, United States). The V3–V4 hypervariable regions from 16S rRNA gene was amplified with universal primers 338F (ACTCCTACGGGAGGCAGCAG) and 806R (GGACTACHVGGGTWTCTAAT). The amplicon was PCR purified and quantified as previously described (Takeshita et al., 2016). The library preparation and sequencing were carried out by Majorbio Biotech Co., Ltd. (Shanghai, China) using the Illumina MiSeq platform (Illumina, Madison, United States). The original data were subjected to quality control and species annotation processes to obtain effective Tags. Sequences were clustered into operational taxonomic units (OTUs) with 97% consistency, diversity and taxonomy was obtained by using QIIME software (version 1.9.1). Data were analyzed using the online platform of Majorbio Technology Co., Ltd.2 The alpha-diversity analysis was based on the normalized data including Chao1 index, Shannon index, Coverage index and numbers of OUTs. The beta-diversity was presented using principal coordinate analysis (PCoA; Lozupone and Knight, 2005). The LefSe (linear discriminant analysis effect size) analysis was performed using LefSe software (Segata et al., 2011). The relative abundance of the relative abundance at the phylum and genus levels were analyzed by the Student’s t-test.
2.8. Statistical analysis
All experimental data were tested for normality by using the Shapiro–Wilk normality test and for homogeneity of variances by using Levene’s test of SPSS19.0 software package for Windows (SPSS Inc., Chicago, IL, United States). Afterward, the data were analyzed by student’s t-test or one-factor analysis of variance (ANOVA) where appropriate. p < 0.05 and p < 0.01 showed a statistically significant and highly significant. For the indexes expressed as means with standard error of mean (SEM).
3. Results
A total of 191 potential Bacillus spp. were isolated. The screening process was composed of three steps, which was enabled to detect Bacillus spp. with the best multi-enzyme production properties from all candidates, the workflow is given in Figure 1.
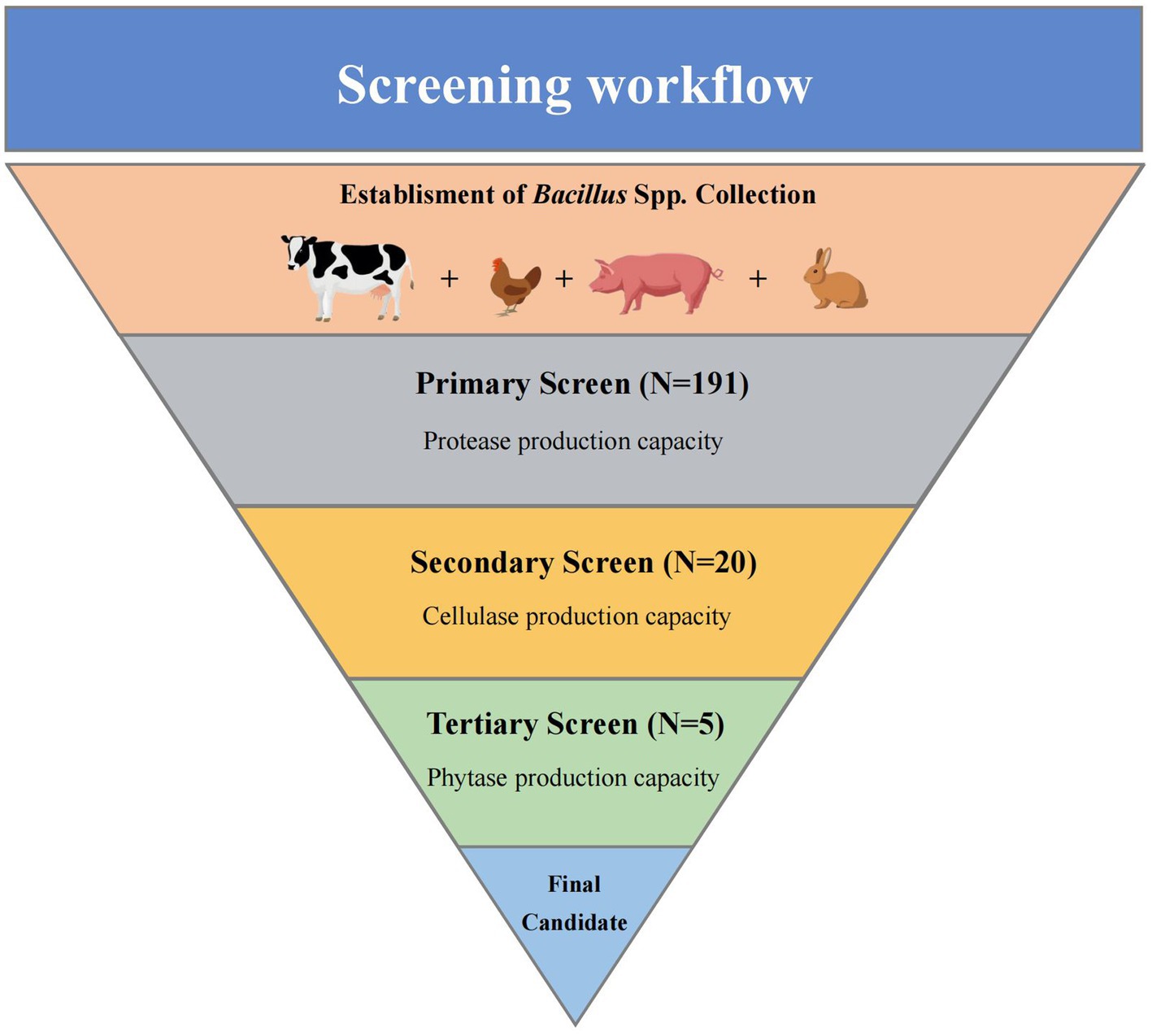
Figure 1. Workflow of the screening. The process was divided into three parts and performed to narrow down a total of 191 Bacillus spp. isolates to one strain with the ability to produce multi-enzyme.
3.1. Protease production capacity (primary screening)
In the primary screening, potential strains with strong protease production capacity were selected, and the protease activity depended on source of origin and the specific strain. The best 20 isolates with superior protease production potential that are depicted in Figure 2A were further screened.
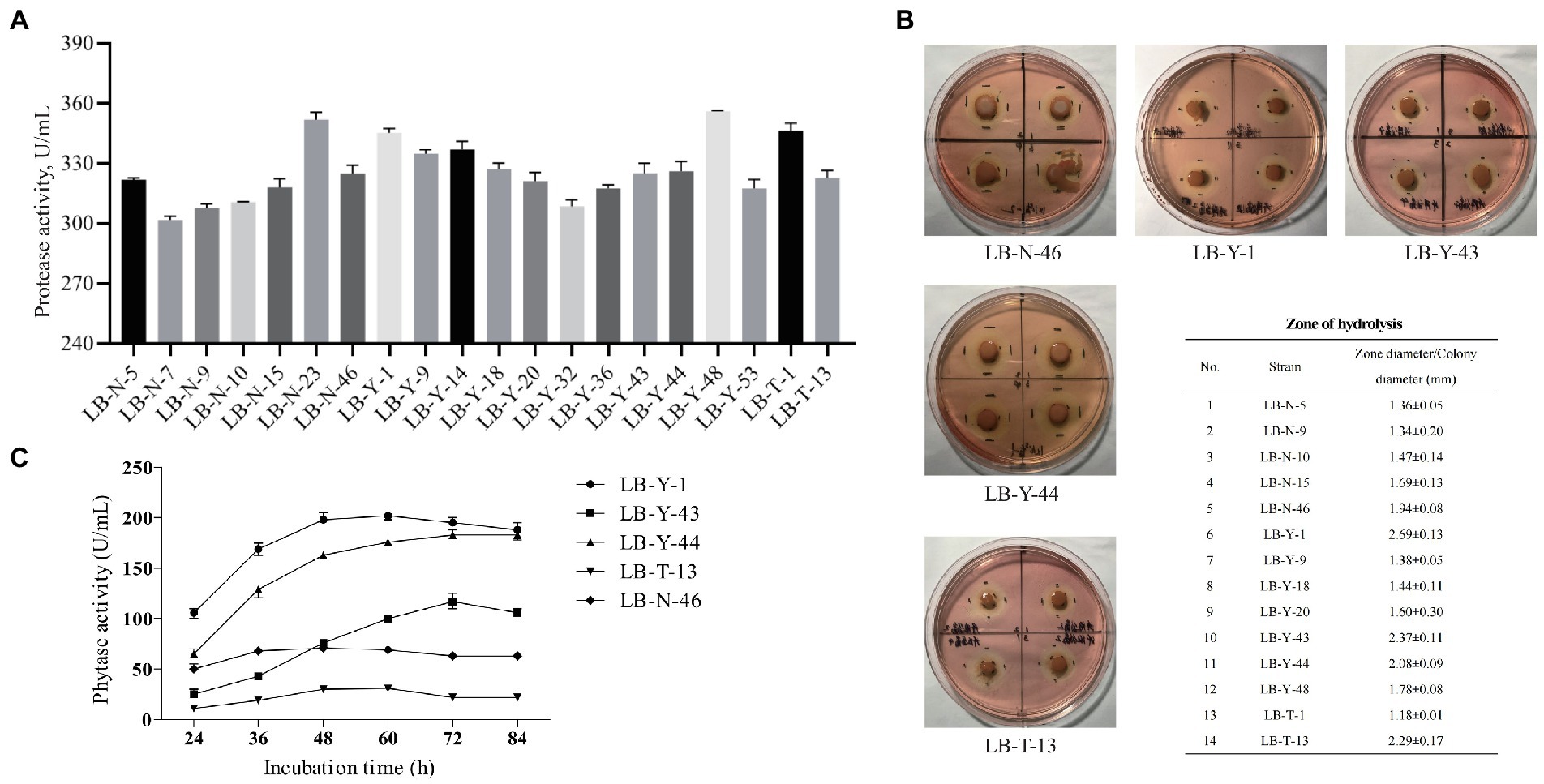
Figure 2. The screening process of Bacillus spp. (A) Protease production capacity (Primary Screen). (B) Cellulase production capacity (Secondary Screen). (C) Phytase production capacity (Tertiary Screen). Values are presented as Mean ± SEM.
3.2. Cellulase production capacity (secondary screening)
After the primary screening, the selected Bacillus spp. were tested for cellulase production capacity. A total of 14 strains were screened through the CMC plate assay method (Figure 2B), among which, 5 strains were found to have the largest degradation zone. Therefore, these strains (LB-N-46, LB-Y-1, LB-Y-43, LB-Y-44, and LB-T-13) were the best candidates for cellulase production capacity, and were further analyzed.
3.3. Phytase production capacity (tertiary screening)
The capacity of phytase production of the candidates is depicted in Figure 2C. Strains LB-Y-43, LB-T-13, and LB-N-46 exhibited low phytase activity during the culture, while LB-Y-1 and LB-Y-44 showed a higher activity, and LB-Y-1 reached the highest enzyme activity at earlier time point (48 h). Through the screening and assessment process, LB-Y-1 was selected as a potential strain for subsequent experiments.
3.4. Morphological, biochemical, and molecular characterization of LB-Y-1
The colony morphologies of LB-Y-1 was wet, opaque, wrinkled, and irregular on the edges on the surface of LB agar, which was confirmed as gram-positive cocci (elongate oval) by microscopic evaluation (Figures 3A,B). The cells appear frequently in pairs or short chains (Figure 3C). These features suggested that it was related to Bacillus spp. The proliferation curves appeared as typically sigmoidal shape, consisting of latency phase (0–6 h), logarithmic phase (6–20 h), and plateau phase (20 h later; Figure 3D). Furthermore, 16S rRNA gene sequencing and phylogenetic analysis found the LB-Y-1 share 100% similarity with the sequences of B. velezensis BCRC-17467T (Figure 3E). Biochemical analysis revealed that the main physiological and biochemical characteristics of the LB-Y-1 were similar to B. velezensis WLYS23 (Supplementary Table S2). Collectively, LB-Y-1 was identified as B. velezensis and deposited it in the China General Microbiological Culture Collection Center (CGMCC, Beijing, China) with accession number 21344. Furthermore, the LB-Y-1 formed hydrolytic circles on the media in the assays for the degradation of starch and triglyceride (Figures 3F,G), indicating that the strain also has the capacity to produce amylase and lipase.
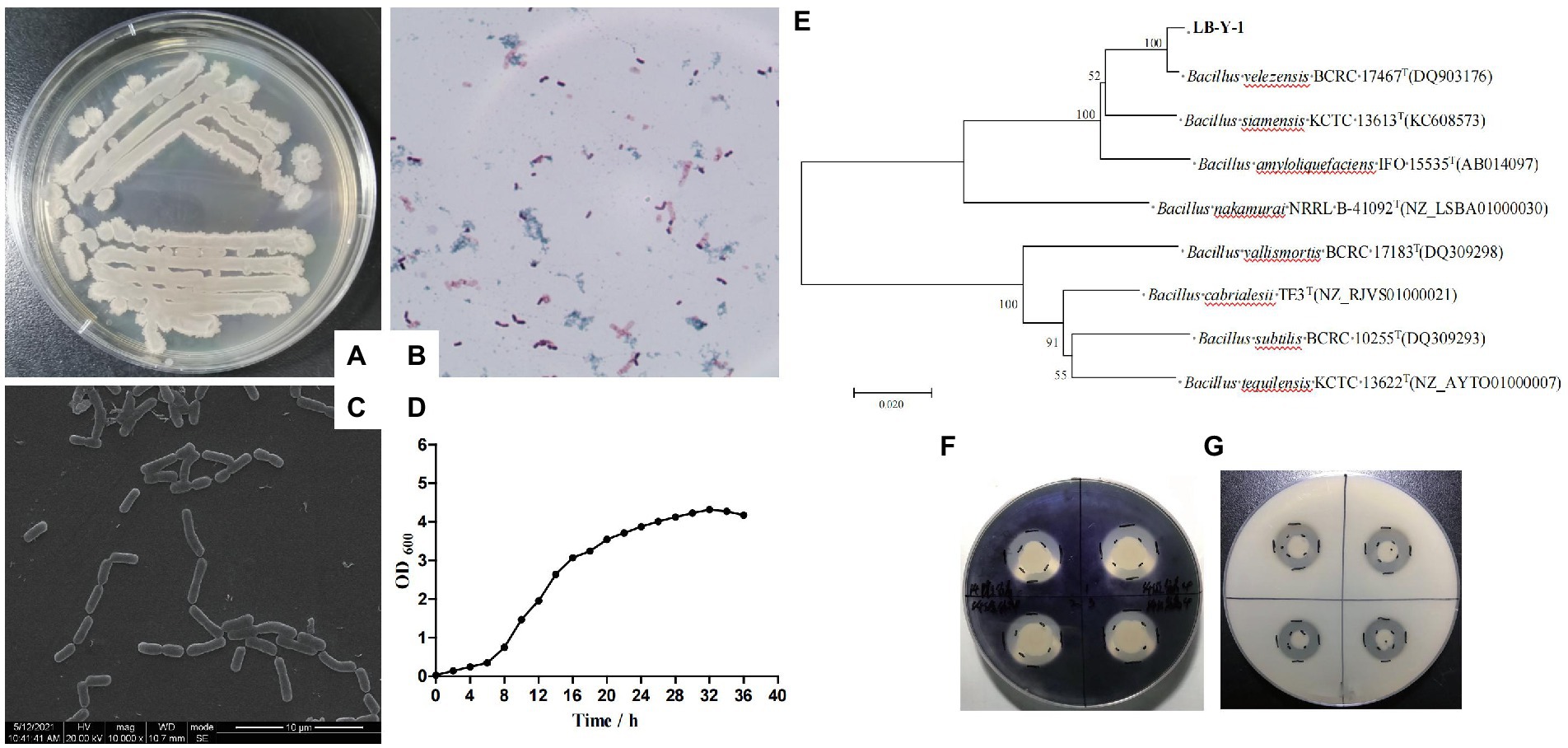
Figure 3. Morphological, biochemical, and molecular characteristics of LB-Y-1. (A) Colony morphology. (B) Gram stain showing Gram-positive rod. (C) SEM image of LB-Y-1 showing a small rods morphology, frequently in pairs or short chains (×1,000). (D) The growth curve of LB-Y-1. (E) The phylogenetic tree analysis of LB-Y-1. (F) Starch-degradation ability. (G) Triglyceride-degradation ability.
3.5. Safety evaluation of LB-Y-1 (in vitro)
The hemolytic activity of LB-Y-1 was judged by observing the hemolytic rings on blood agar plates after an 24 h incubation. The strain was not involved in the lysis of erythrocytes (results not shown). Supplementary Table S3 showed the antibiotic susceptibility profile of the LB-Y-1. It was susceptible to 24 routinely used antimicrobials, indicating that the strain is safe and can be used as a probiotic.
3.6. In vivo testing
3.6.1. Growth performance
The average mortality rate was 0.5% during the experiment (data not presented) with no significant difference between the groups. Growth performance is depicted in Figure 4. Compared with CON group, LB-Y-1 significantly increased the BW of broilers at 21 and 42 days of age, and increased the ADG during the starter, grower, and overall periods (p < 0.05). The F/G ratio for the BV group was lower than the CON group during the whole period (p < 0.05). No significant differences were found in the ADFI between the groups.
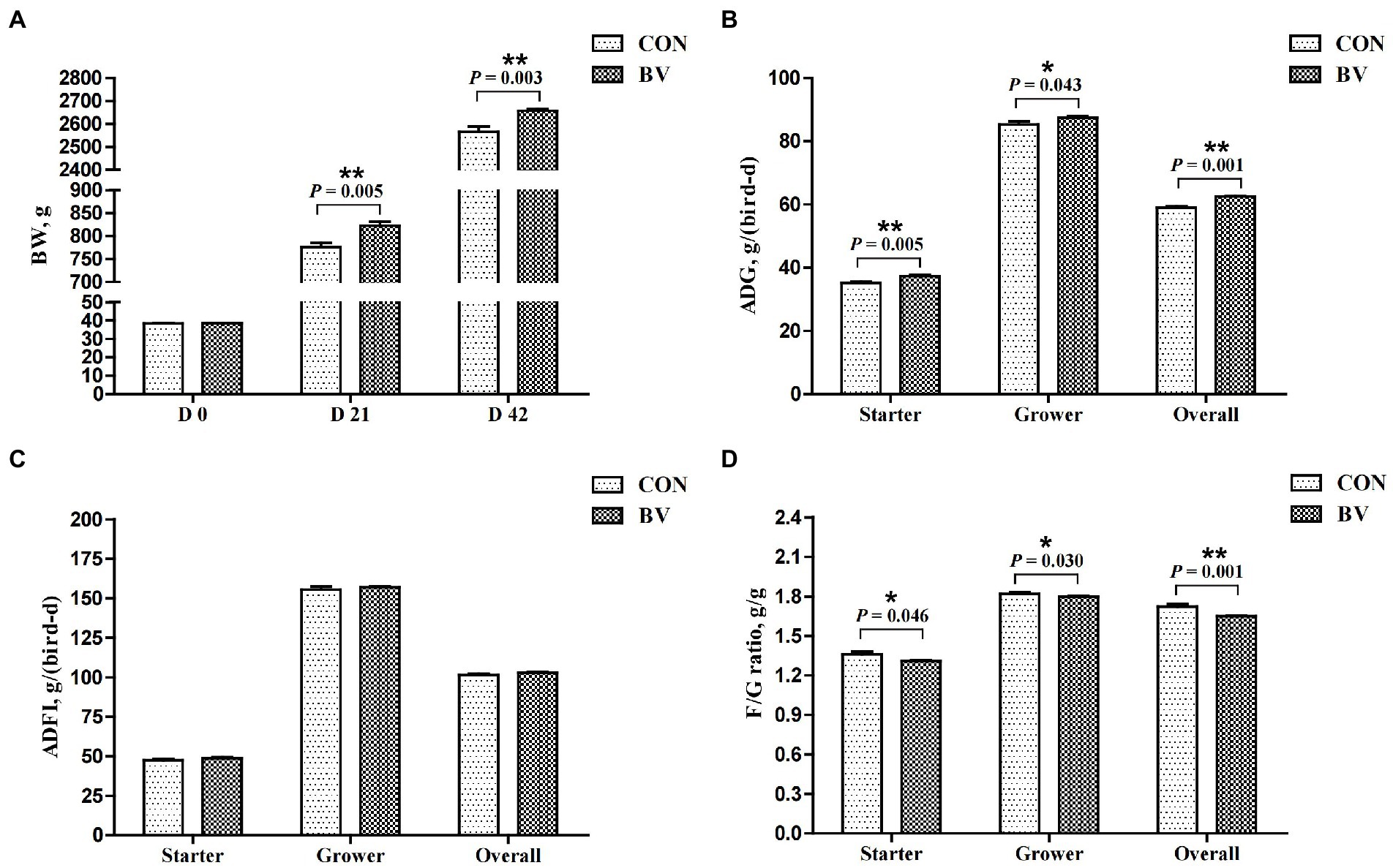
Figure 4. Effect of Bacillus velezensis LB-Y-1 on growth performance of broilers (n = 6). CON = control group, broilers were fed a corn-soybean basal diet, BV = B. velezensis LB-Y-1 group, broilers were fed a basal diet containing 3.5 × 109 CFU/kg LB-Y-1. BW, body weight; ADG, average daily gain; ADFI, average daily feed intake; F/G ratio = feed intake (g)/weight gain (g); Values are presented as Mean ± SEM, the values having superscript (*) were significantly different, *0.01 < p < 0.05; **p ≤ 0.01.
3.6.2. Slaughter performance and immune organ indexes
The characteristics of slaughter performance is presented in Table 1, no significant difference was detected between the groups. In addition, supplementation with LB-Y-1 had no effect on immune organ indexes at 21 and 42 days of age compared to the CON group (Table 2).
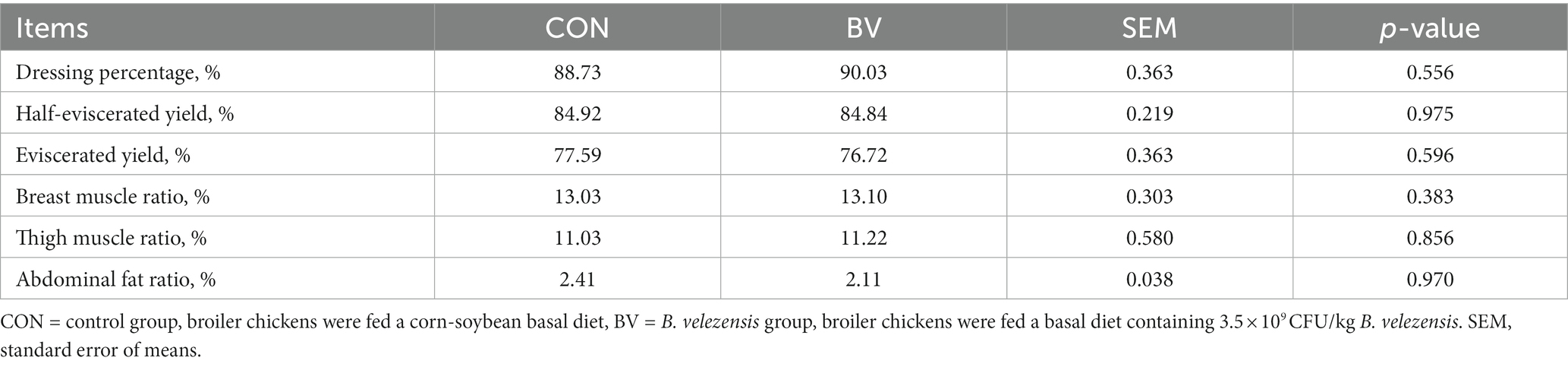
Table 1. Effect of Bacillus velezensis LB-Y-1 on slaughter performance of broilers at day 42 (n = 6).
3.6.3. Hematological and serum biochemical indexes analysis
As shown in Table 3, LB-Y-1 treatment significantly increased the levels of ALP (p = 0.011, 21 days of age and p = 0.007 42 days of age), TP (p = 0.001, 21 days of age), Albumin (p = 0.002, 21 days of age), A/G ratio (p = 0.031, 21 days of age) and P (p = 0.001, 42 days of age) compared to the CON group.
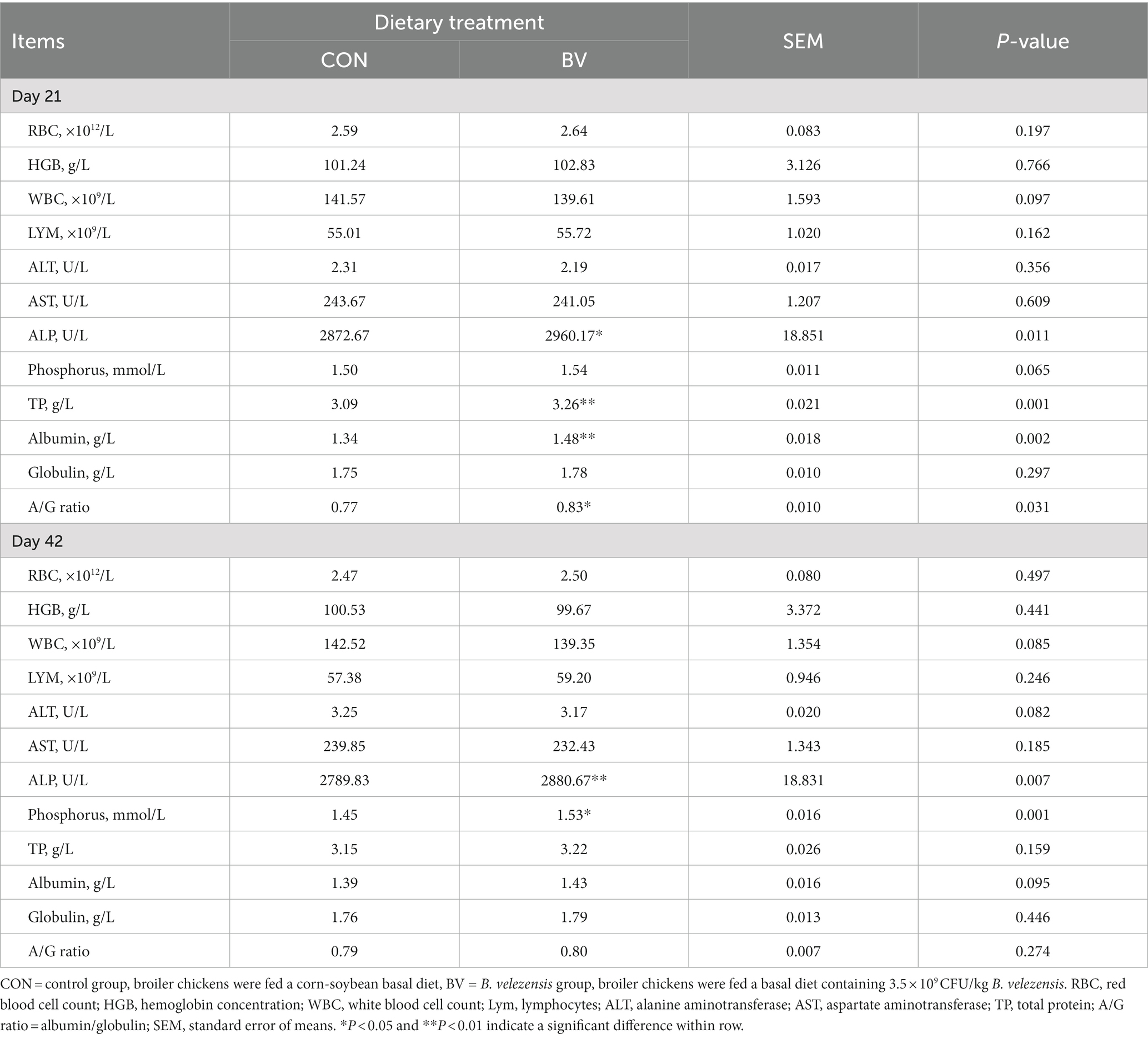
Table 3. Effect of Bacillus velezensis LB-Y-1 on hematological and serum biochemical indexes of broilers (n = 6).
3.6.4. Intestinal digestive enzyme
The effect of LB-Y-1 on intestinal digestive enzyme of broilers is depicted in Table 4. Broilers with LB-Y-1 had higher amylase and trypsin activity at 21 days of age and higher amylase activity at 42 days of age compared to the CON group (p < 0.05).
3.6.5. Tibia bone mineralization
The effect of LB-Y-1 on tibia bone mineralization of broilers is depicted in Figure 5. Supplementation with LB-Y-1 significantly increased tibia bone ash at 42 days of age, along with tibia ash P concentration compared to control broilers (p < 0.05). Although not significant, tibia ash Ca concentration was also elevated in the BV group relative to broilers of CON group.
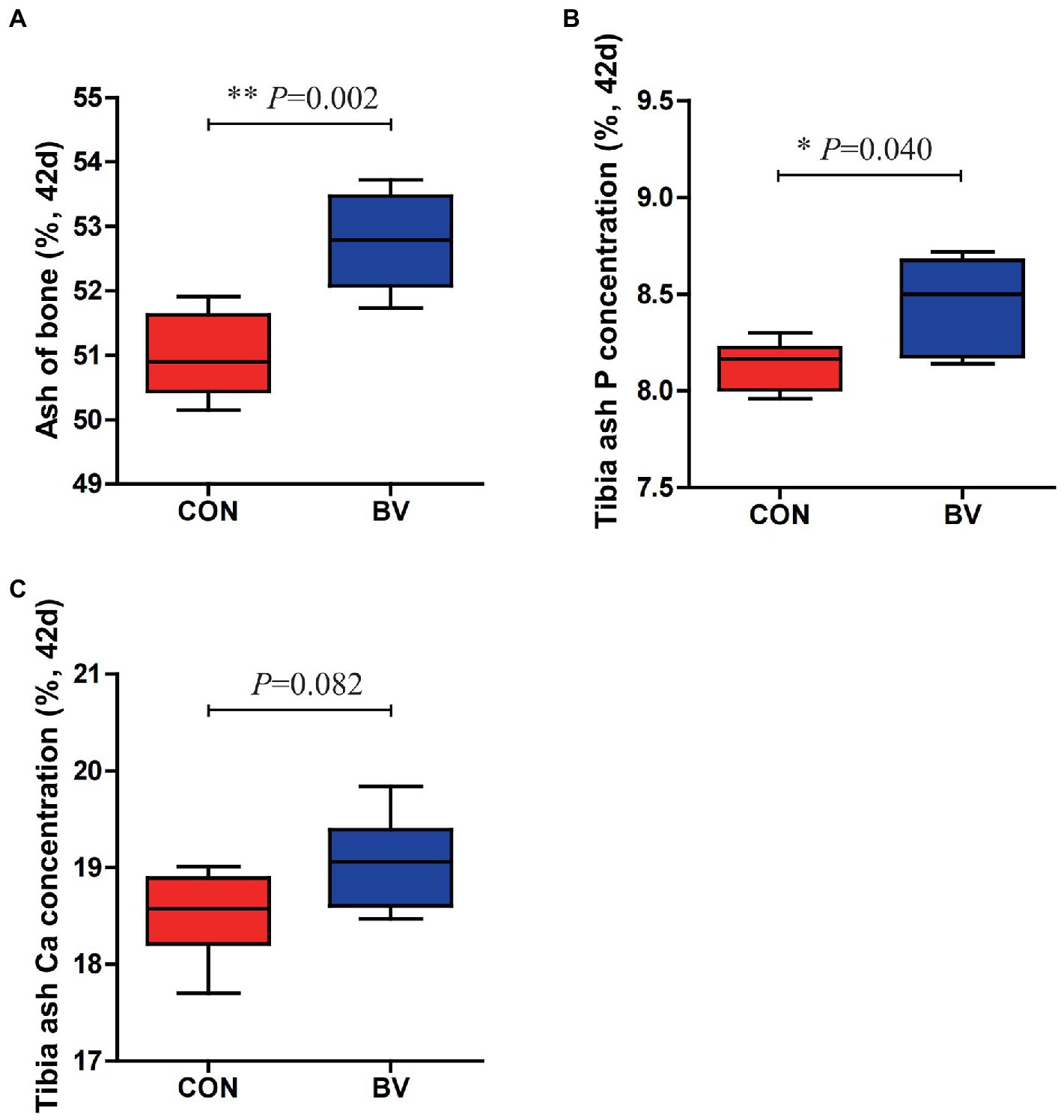
Figure 5. Effect of Bacillus velezensis LB-Y-1 on tibia bone mineralization of broilers (n = 6). (A) Bone ash content at 42 days of age. (B) Tibia ash P concentration at 42 days of age. (C) Tibia ash Ca concentration at 42 days of age. Values are presented as Mean ± SEM, the values having superscript (*) were significantly different, *p < 0.05; **p < 0.01.
3.6.6. Histological analysis
Table 5 showed the effect of LB-Y-1 on intestinal histomorphology of broilers. LB-Y-1 significantly decreased the crypt depth of jejunum, and increased the ratio of villus height to crypt depth at 21 days of age (p < 0.05). There were no significant differences in histomorphometric features of the jejunum and ileum between the CON and BV groups at 42 days of age. Furthermore, LB-Y-1 had no significant effect on epithelial thickness of jejunum and ileum at 21 and 42 days of age.
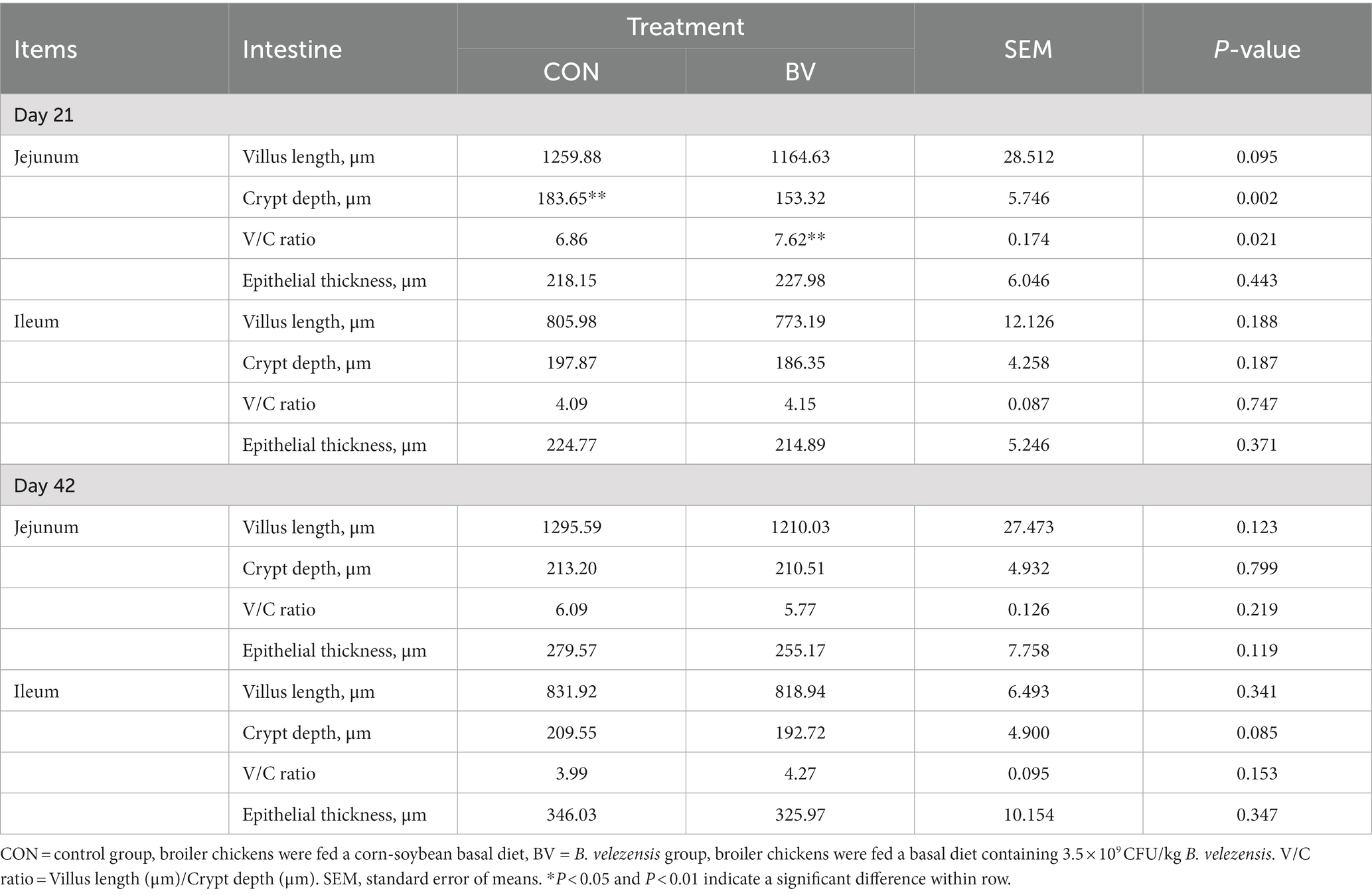
Table 5. Effect of Bacillus velezensis LB-Y-1 on histomorphology of the small intestinal sections in broilers (n = 6).
3.6.7. Microbial analysis
To understand whether LB-Y-1 could modulate gut microbiota community, we investigated the change of the ileal microbiota diversity. A community richness (Chao1 index) and diversity (Shannon index) analysis demonstrated that broilers supplemented with the LB-Y-1 had significantly higher microbial relative abundance and potential diversity than those of CON group (p < 0.05; Figures 6A,B). The coverage index was greater than 0.998 in each group (Figure 6C), indicating an adequate depth of sequencing. Furthermore, a higher OTU richness was observed in the BV group (Figure 6D). The β-diversity analysis indicated that samples in CON and BV groups had different community composition and structure, suggesting a significant segregation of microbiota between the groups (Figure 6E). Firmicutes was the predominant phyla (Figure 6F), and Lactobacillus and Enterococcus were the main dominant genera (Figure 6G). The LEfSe analysis (LDA > 2) revealed the significant differences in microbiota structure between the CON group and the BV group (Figure 7A). Student’s t-test showed that BV group had a lower abundance of Proteobacteria, and higher abundance of Cyanobacteria and Bacteroidota at phylum level (p < 0.05, Figure 7B); as well as BV group had lower abundance of Lactobacillus and Escherichia-Shigella, and higher abundance of Parasutterella and Rikenellaceae at genus level (p < 0.05, Figure 7C).
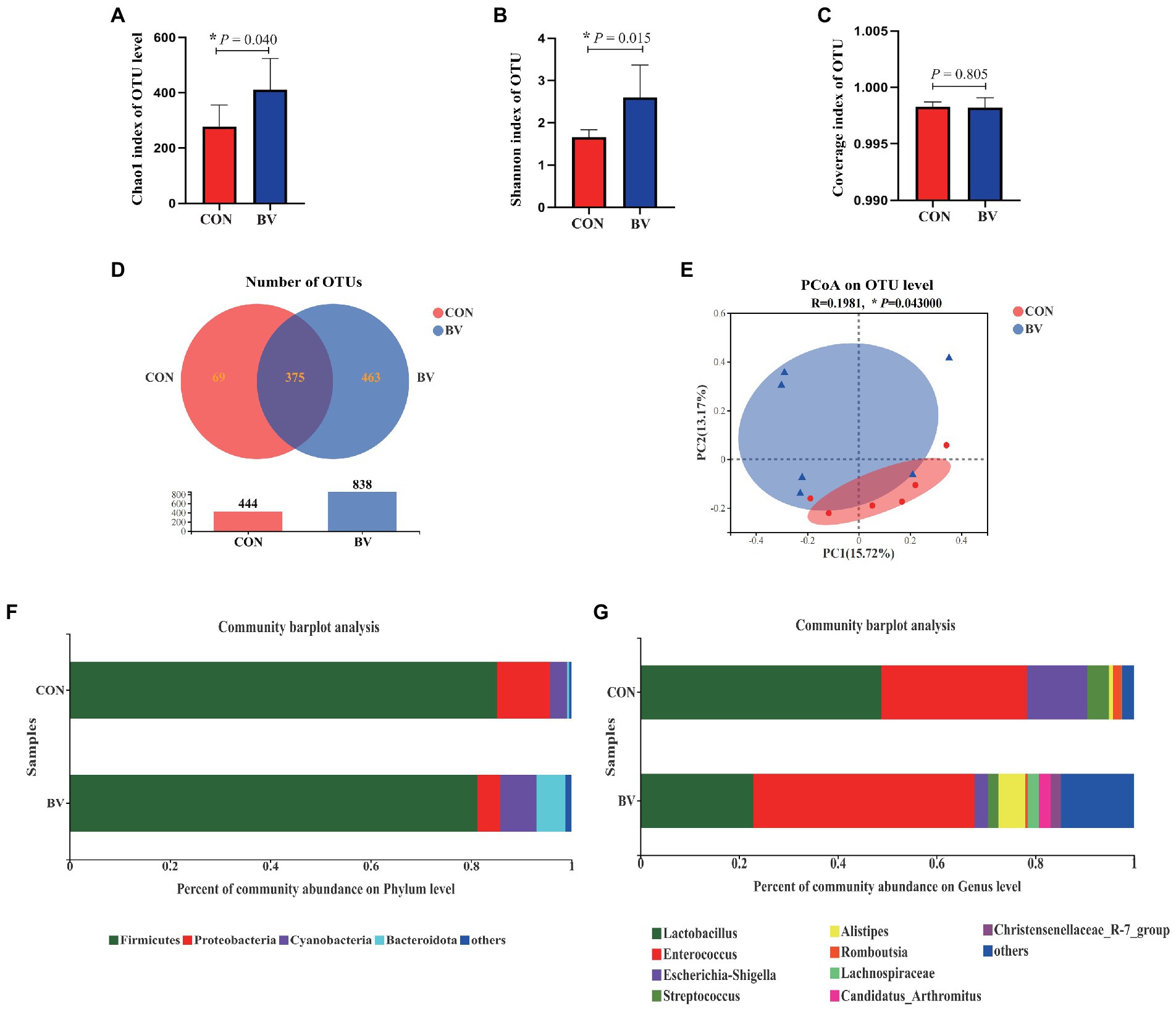
Figure 6. Effect of Bacillus velezensis LB-Y-1 on the ileal microbial diversity of broilers (n = 6). (A) Chao1 index of OUT level. (B) Shannon index of OUT level. (C) Coverage index of OUT level. (D) Number of OTUs. (E) β-diversity was estimated by the PCoA on OUT level. (F,G) The relative abundance of bacteria at the phylum and genus levels, respectively. Values are presented as Mean ± SEM, the values having superscript (*) were significantly different, *0.01 < p < 0.05; **p ≤ 0.01.
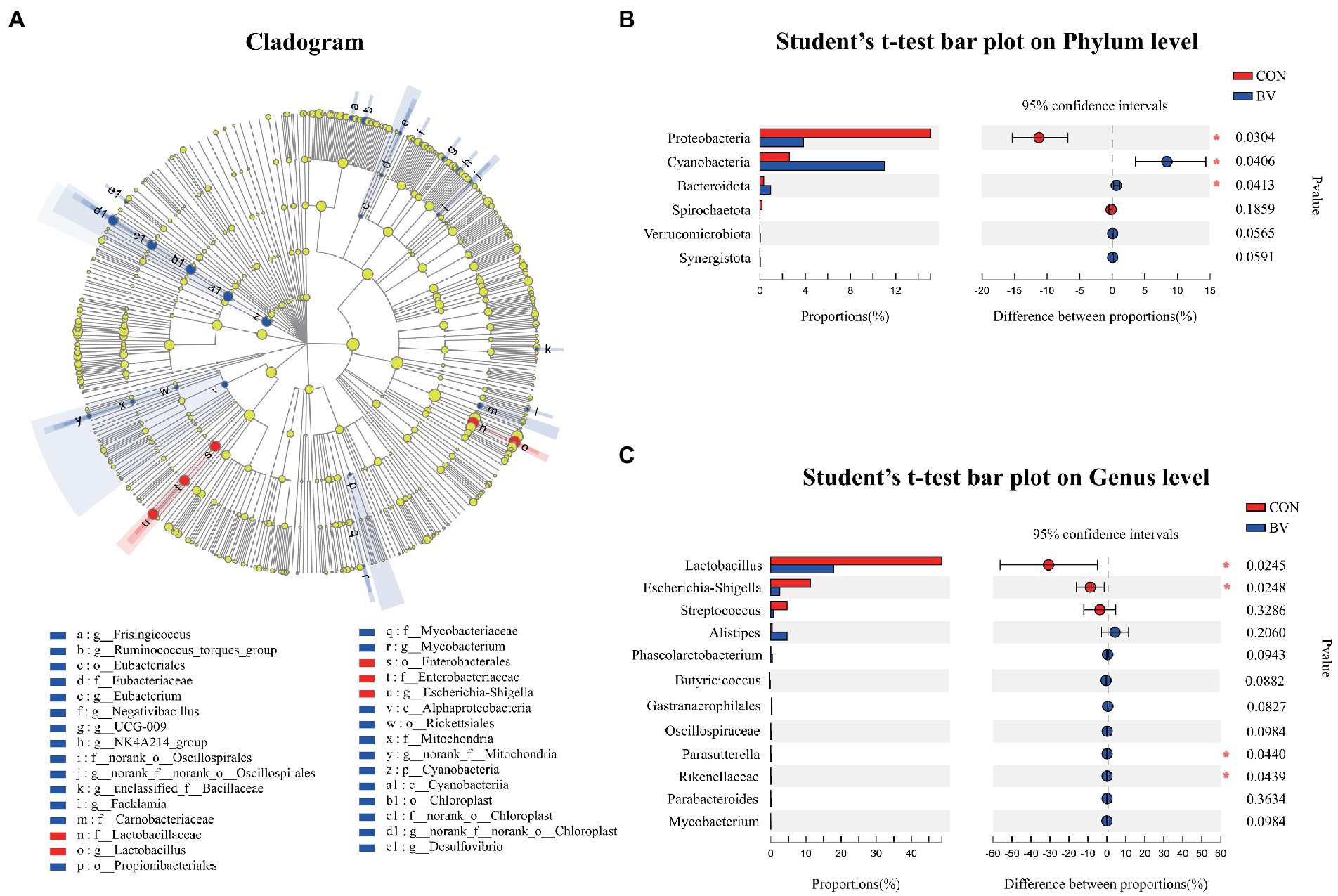
Figure 7. Effect of Bacillus velezensis LB-Y-1 on the abundance of the ileal microbial community of broilers (n = 6). (A) Cladogram of LEfSe multilevel species difference discriminant analysis (LDA > 2), different color nodes indicate microbial communities that are significantly enriched in the corresponding groups and significantly different between groups. (B,C) Comparative analysis of the relative abundance of bacteria at the phylum and genus levels, respectively. Values are presented as Mean ± SEM, the values having superscript (*) were significantly different, *0.01 < p < 0.05; **p ≤ 0.01.
4. Discussion
Bacillus spp. is emerging as a promising probiotic candidate because of the following characteristics: (1) it has the ability to form as spores and has less loss during feed preparation; (2) it is easy to produce by batch (Hyronimus et al., 2000). Furthermore, Bacillus spp. is recognized an excellent probiotic that produces multi-enzyme, bacteriocin, and beneficial metabolites (Li et al., 2018). Previous studies have also shown positive effects of Bacillus spp. on nutrient utilization, intestinal integrity, and growth performance of broilers (Salim et al., 2013; Rivera-Pérez et al., 2021). Due to the shortage of traditional feed and the rapid rise in the price of protein raw materials, it is particularly important to improve the utilization of protein in feed, which prompted us to screen a strain of Bacillus spp. that could exert a positive effect on poultry production.
It is known that incomplete digestion of proteins can lead to the multiplication of putrefactive microorganisms and the accumulation of toxic metabolites (Dallas et al., 2017). High levels of protein in excreta are not only a waste of resources, but also harmful to the environment. Based on these, the protease production efficiency of Bacillus spp. was used as an evaluation index in the primary screening. Further, unlike mammals and other waterfowl, broilers have lower endogenous cellulase activity, which limits fiber digestion. The addition of exogenous fiber degrading enzymes can significantly reduce chyme viscosity and improve nutrient digestibility (Slominski et al., 2006; Zhai et al., 2020). Therefore, the cellulase production capacity was taken as the evaluation index in the second screening step. There is no doubt that microbial degradation of phytic acid is essential to prevent environmental phosphate contamination and to deal with nutritional problems in monogastric animals. Phytase is widely used to improve the efficiency of phosphate absorption in animal feed (Blüher et al., 2017). In the tertiary screening, the phytase production efficiency of Bacillus spp. was taken as an evaluation index. Eventually, the strain of LB-Y-1 showed the best performance. According to the morphological and molecular characteristics, we preliminary identified the target strain as B. velezensis. Biochemical analysis revealed that, the main physiological and biochemical characteristics of the LB-Y-1 were similar to B. velezensis WLYS23, which has been reported (Zhang D. F. et al., 2021). However, unlike the WLYS23, the LB-Y-1 did not react with inulin, but it was consistent with another standard strain of B. velezensis CR-502T (Ruiz-García et al., 2005). The safety evaluation of LB-Y-1 indicated that LB-Y-1 was not haemolytic and highly sensitive to common antibiotics. Furthermore, consistent with the previous report (Li et al., 2018), we too found that the LB-Y-1 had the potential to degrade starch and triglyceride components. These results indicate that LB-Y-1 has potential as a safety probiotic candidate.
Like mammals, digestive system of poultry is underdeveloped early in life and cannot secrete enough gastric acid and digestive enzymes (Zhang et al., 2022). In addition, poultry has a limited ability to digest fiber in feed compared with mammals, and high-fiber or slowly digestible protein diets will cause loss of production performance (Berrocoso et al., 2020; Zhang Y. C. et al., 2021). Fortunately, it was found that the addition of exogenous enzymes may help to solve this problem (Tavernari et al., 2008). Our study found that adding LB-Y-1 increased BW and feed conversion of broilers without affecting feed intake, which we linked to the properties of LB-Y-1 secreting multi-enzyme, and this positive effect was consistent with the results of other study (Wang et al., 2021). The effect of intestinal digestive enzymes appear in the response of Bacillus spp. in two ways. On the one hand, the members of Bacillus spp. can secrete a variety of extracellular enzymes. On the other hand, Bacillus spp. can stimulate the secretion of endogenous enzymes and regulate the gut microbial flora (Bedford and Schulze, 1998). Our study indicated that the cellulase and phytase secreted by LB-Y-1 contribute to the release of nutrients in feed, the protease and amylase secreted by LB-Y-1 enhanced the intestinal digestibility, thus promoting the digestion of nutrients. It has also been shown that Bacillus spp. may contribute to improved morphology of intestine by increasing villus height and ratio of villus height to crypt depth (Mohamed et al., 2022). The results are consistent with our findings, LB-Y-1 improved the intestinal structure at an early stage, which may contribute to the gut health.
Study in broilers demonstrated that dietary supplementation with Bacillus spp. improved mineralization of tibial (Latorre et al., 2017). In the current study, ALP activity was increased when LB-Y-1 were added to the diet. ALP is a kind of enzyme involved in phosphate hydrolysis and a marker of skeletal mineralization in broilers (Tilgar et al., 2008; Escobar et al., 2022). The increased activity of ALP was accompanied by a significant increase in bone phosphorus, in addition, the level of phosphorus of broilers fed LB-Y-1 was also significantly increased, which had been proved by Li et al. with B. amyloliquefaciens (Li et al., 2022). In addition, the phytase produced by LB-Y-1 can also promote the release of phosphorus from phytate complex in the feed (Viveros et al., 2002), thus improving the phosphorus utilization. These results indicated that LB-Y-1 could promote the metabolism and utilization of phosphorus.
It has previously been shown that the diversity of gut microbes contributes to microbiome homeostasis and the resistance to pathogenic microorganisms (Konstantinov et al., 2004). Besides this, the intestinal microbiota community plays a variety of roles in nutrient absorption and metabolism, immunity, as well as bone density and strength (Bielke et al., 2017; Hong et al., 2019). In this study, dietary supplementation with LB-Y-1 increased the intestinal microbial diversity (Shannon index) and the microbial community richness (Chao1 index). The PCoA showed obviously different pattern of microbial communities between the groups. The predominant bacterial phylum of ileum include Firmicutes, Cyanobacteria, Proteobacteria, and Bacteroidota (Guo et al., 2021), our study observed that LB-Y-1 increased the abundance of Cyanobacteria and Bacteroidota, and decreased the abundance of Proteobacteria. Cyanobacteria is the dominant phyla in the healthy intestinal tract of mammals and poultry, which can play the role of nitrogen fixation and also contribute to the nutrient absorption in the intestines (Mandal et al., 2016; Yang et al., 2020), so the increased abundance of Cyanobacteria indicated that LB-Y-1 could improve the structure of the flora. Bacteroidota has been extensively studied for its regulatory effect on the host, which can accelerate angiogenesis in the intestinal mucosa, enhance the host’s immunity, and maintain the balance of intestinal microbiota (Cheng et al., 2022). It is well known that Proteobacteria contains a wide variety of pathogens such as Salmonella, Escherichia coli, and Shigella, which could exert pathogenic effects in the intestine of broilers (Mora et al., 2010). Therefore, the decrease of Proteobacteria in BV group indicated that a relatively healthy bacterial community was achieved by LB-Y-1 supplementation. Furthermore, the results of the analysis at the genus level reinforced this conclusion. Escherichia-Shigella with reduced relative abundance in our study is an opportunistic pathogen that is positively correlated with a variety of intestinal infections (Yang et al., 2019). Correspondingly, we also found an increase in the abundance of some beneficial genera, such as Parasutterella and Rikenellaceae. Parasutterella is an important player in multiple gastrointestinal metabolic processes, which has been shown to have a positive role in tyrosine, cholesterol and bile acid metabolism (Ju et al., 2019). Rikenellaceae plays an important role in promoting the fermentation of carbohydrates and proteins in vivo, and can reduce the damage of intestinal immune function and the occurrence of intestinal inflammation (Su et al., 2014; Donaldson et al., 2016). For the role in bone development, Li′s report suggests that Bacillus spp. can modulate the gut microbiome structure to affect the biosynthesis of polyamines, which in turn can mediate the enhancement of osteoblast activity and have a positive effect on increasing bone strength (Li et al., 2022). Overall, the results revealed that LB-Y-1 can promote the organismal development of broilers by improving the structure of intestinal microbiota.
5. Conclusion
The present study demonstrated that the newly screened and characterized B. velezensis LB-Y-1, which was isolated from the intestinal tract of different healthy animals, has marked multi-enzyme production property. In vivo broilers assay indicated that LB-Y-1 has the potential to improve broiler growth performance and tibia mineralization, the mechanism may be associated with the enhanced intestinal digestive enzyme activities, increased P retention and alterations of intestinal microbiota structure. These results are encouraging and suggesting that B. velezensis LB-Y-1 is a potential strain for further utilization in direct-fed microbial or probiotic starter culture.
Data availability statement
The datasets presented in this study can be found in online repositories. The names of the repository/repositories and accession number(s) can be found in the article/Supplementary material.
Ethics statement
The animal study was reviewed and approved by Animal Ethics Committee of the Chinese Academy of Agricultural Sciences (AEC-CAAS-20191106, Beijing, China).
Author contributions
CL, SL, and GD: conceptualization. CL and SL: methodology. CL, GD, and RJ: investigation, software, data curation, and writing-original draft preparation. SC and XD: validation and resources. GL and YB: writing review, editing, and supervision. HC: project administration and funding acquisition. All authors contributed to the article and approved the submitted version.
Funding
This research was funded by China Agriculture Research System (CARS-41).
Acknowledgments
CL gratefully acknowledges financial support from the China Scholarship Council (no. 202103250085).
Conflict of interest
The authors declare that the research was conducted in the absence of any commercial or financial relationships that could be construed as a potential conflict of interest.
Publisher’s note
All claims expressed in this article are solely those of the authors and do not necessarily represent those of their affiliated organizations, or those of the publisher, the editors and the reviewers. Any product that may be evaluated in this article, or claim that may be made by its manufacturer, is not guaranteed or endorsed by the publisher.
Supplementary material
The Supplementary material for this article can be found online at: https://www.frontiersin.org/articles/10.3389/fmicb.2023.1143265/full#supplementary-material
Footnotes
References
Babatunde, O. O., Bello, A., Dersjant-Li, Y., and Adeola, O. (2021). Evaluation of the responses of broiler chickens to varying concentrations of phytate phosphorus and phytase. I. Starter phase (day 1-11 post hatching). Poult. Sci. 100:101396. doi: 10.1016/J.PSJ.2021.101396
Bajpai, V. K., Han, J. H., Rather, I. A., Park, C., Lim, J., Paek, W. K., et al. (2016). Characterization and antibacterial potential of lactic acid bacterium Pediococcus pentosaceus 4I1 isolated from freshwater fish Zacco koreanus. Front. Microbiol. 7:2037. doi: 10.3389/FMICB.2016.02037/BIBTEX
Bedford, M. R., and Schulze, H. (1998). Exogenous enzymes for pigs and poultry. Nutr. Res. Rev. 11, 91–114. doi: 10.1079/NRR19980007
Berrocoso, J. D., García-Ruiz, A., Page, G., and Jaworski, N. W. (2020). The effect of added oat hulls or sugar beet pulp to diets containing rapidly or slowly digestible protein sources on broiler growth performance from 0 to 36 days of age. Poult. Sci. 99, 6859–6866. doi: 10.1016/J.PSJ.2020.09.004
Bielke, L. R., Hargis, B. M., and Latorre, J. D. (2017). Impact of enteric health and mucosal permeability on skeletal health and lameness in poultry. Adv. Exp. Med. Biol. 1033, 185–197. doi: 10.1007/978-3-319-66653-2_9
Blüher, D., Laha, D., Thieme, S., Hofer, A., Eschen-Lippold, L., Masch, A., et al. (2017). A 1-phytase type III effector interferes with plant hormone signaling. Nat. Commun. 8:2159. doi: 10.1038/S41467-017-02195-8
Chang’a, E. P., Abdallh, M. E., Ahiwe, E. U., Al-Qahtani, M., Mbaga, S., and Iji, P. A. (2019). Energy utilization, nutrient digestibility and bone quality of broiler chickens fed Tanzania-type diets in different forms with enzymes. J. Anim. Sci. Technol. 61, 192–203. doi: 10.5187/JAST.2019.61.4.192
Cheng, J., Hu, J., Geng, F., and Nie, S. (2022). Bacteroides utilization for dietary polysaccharides and their beneficial effects on gut health. Food Sci. Human Wellness 11, 1101–1110. doi: 10.1016/J.FSHW.2022.04.002
Choi, Y. M., Suh, H. J., and Kim, J. M. (2001). Purification and properties of extracellular phytase from bacillus sp. KHU-10. J. Protein Chem. 20, 287–292. doi: 10.1023/A:1010945416862
CLSI. (2015). “Performance Standards For Antimicrobial Susceptibility Testing; Twenty-Fifth Informational Supplement,” in CLSI document M100-S25 (Wayne, PA, USA: Clinical Laboratory Standards Institute). Available at: https://www.sid.ir/en/Journal/ViewPaper.aspx?ID=528390 (Accessed 7 July 2022).
Córdova-Noboa, H. A., Oviedo-Rondón, E. O., Matta, Y., Ortiz, A., Buitrago, G. D., Martinez, J. D., et al. (2021). Corn kernel hardness, drying temperature and amylase supplementation affect live performance and nutrient utilization of broilers. Poult. Sci. 100:101395. doi: 10.1016/J.PSJ.2021.101395
Dallas, D. C., Sanctuary, M. R., Qu, Y., Khajavi, S. H., Van Zandt, A. E., Dyandra, M., et al. (2017). Personalizing protein nourishment. Crit. Rev. Food Sci. Nutr. 57, 3313–3331. doi: 10.1080/10408398.2015.1117412
Demirkan, E., Baygin, E., and Usta, A. (2014). Screening of phytate hydrolysis bacillus sp. isolated from soil and optimization of the certain nutritional and physical parameters on the production of phytase. Turkish J. Biochem. 39, 206–214. doi: 10.5505/TJB.2014.26817
Diversity, T. C. (2022). On B the Nagoya Protocol on Access and Benefit-sharing. Available at: https://www.cbd.int/abs/ (Accessed 7 July 2022).
Donaldson, G. P., Lee, S. M., and Mazmanian, S. K. (2016). Gut biogeography of the bacterial microbiota. Nat. Rev. Microbiol. 14, 20–32. doi: 10.1038/NRMICRO3552
Escobar, J., Dobbs, M., Ellenberger, C., Parker, A., Latorre, J. D., and Gabor, L. (2022). Oral supplementation of alkaline phosphatase in poultry and swine. Transl. Anim. Sci. 6, 1–10. doi: 10.1093/TAS/TXAC079
Fitzgerald, S., Duffy, E., Holland, L., and Morrin, A. (2020). Multi-strain volatile profiling of pathogenic and commensal cutaneous bacteria. Sci. Rep. 10:17971. doi: 10.1038/S41598-020-74909-W
Francino, M. P. (2018). Birth mode-related differences in gut microbiota colonization and immune system development. Ann. Nutr. Metab. 73, 12–16. doi: 10.1159/000490842
Gao, P., Ma, C., Sun, Z., Wang, L., Huang, S., Su, X., et al. (2017). Feed-additive probiotics accelerate yet antibiotics delay intestinal microbiota maturation in broiler chicken. Microbiome 5:91. doi: 10.1186/s40168-017-0315-1
Gao, J., Wang, R., Liu, J., Wang, W., Chen, Y., and Cai, W. (2022). Effects of novel microecologics combined with traditional Chinese medicine and probiotics on growth performance and health of broilers. Poult. Sci. 101:101412. doi: 10.1016/J.PSJ.2021.101412
Guo, S., Xi, Y., Xia, Y., Wu, T., Zhao, D., Zhang, Z., et al. (2021). Dietary lactobacillus fermentum and Bacillus coagulans supplementation modulates intestinal immunity and microbiota of broiler chickens challenged by Clostridium perfringens. Front. Vet. Sci. 8:483. doi: 10.3389/FVETS.2021.680742/BIBTEX
Hong, Y., Cheng, Y., Li, Y., Li, X., Zhou, Z., Shi, D., et al. (2019). Preliminary study on the effect of bacillus amyloliquefaciens TL on Cecal bacterial community structure of broiler chickens. Biomed. Res. Int. 2019, 1–11. doi: 10.1155/2019/5431354
Hyronimus, B., Le Marrec, C., Hadj Sassi, A., and Deschamps, A. (2000). Acid and bile tolerance of spore-forming lactic acid bacteria. Int. J. Food Microbiol. 61, 193–197. doi: 10.1016/S0168-1605(00)00366-4
Ju, T., Kong, J. Y., Stothard, P., and Willing, B. P. (2019). Defining the role of Parasutterella, a previously uncharacterized member of the core gut microbiota. ISME J. 13, 1520–1534. doi: 10.1038/S41396-019-0364-5
Kesari, V., and Rangan, L. (2011). Coordinated changes in storage proteins during development and germination of elite seeds of Pongamia pinnata, a versatile biodiesel legume. AoB Plants. 9, 1–16. doi: 10.1093/AOBPLA/PLR026
Kilkenny, C., Browne, W. J., Cuthi, I., Emerson, M., and Altman, D. G. (2012). Improving bioscience research reporting: the ARRIVE guidelines for reporting animal research. Vet. Clin. Pathol. 41, 27–31. doi: 10.1111/j.1939-165X.2012.00418.x
Konstantinov, S. R., Favier, C. F., Zhu, W. Y., Williams, B. A., Klüß, J., Souffrant, W. B., et al. (2004). Microbial diversity studies of the porcine gastrointestinal ecosystem during weaning transition. Anim. Res. 53, 317–324. doi: 10.1051/ANIMRES:2004019
Kundu, P., Blacher, E., Elinav, E., and Pettersson, S. (2017). Our gut microbiome: the evolving inner self. Cells 171, 1481–1493. doi: 10.1016/J.CELL.2017.11.024
Latorre, J. D., Hernandez-Velasco, X., Vicente, J. L., Wolfenden, R., Hargis, B. M., and Tellez, G. (2017). Effects of the inclusion of a bacillus direct-fed microbial on performance parameters, bone quality, recovered gut microflora, and intestinal morphology in broilers consuming a grower diet containing corn distillers dried grains with solubles. Poult. Sci. 96, 2728–2735. doi: 10.3382/ps/pex082
Lee, J. J., Choe, J., Kang, J., Cho, J. H., Park, S., Perez-Maldonado, R., et al. (2020). Dietary protease improves growth rate and protein digestibility of growing-finishing pigs. J. Anim. Sci. Technol. 62, 313–320. doi: 10.5187/JAST.2020.62.3.313
Lee, Y. J., Kim, B. K., Lee, B. H., Jo, K. I., Lee, N. K., Chung, C. H., et al. (2008). Purification and characterization of cellulase produced by bacillus amyoliquefaciens DL-3 utilizing rice hull. Bioresour. Technol. 99, 378–386. doi: 10.1016/j.biortech.2006.12.013
Li, C., Cai, H., Li, S., Liu, G., Deng, X., Bryden, W. L., et al. (2022). Comparing the potential of bacillus amyloliquefaciens CGMCC18230 with antimicrobial growth promoters for growth performance, bone development, expression of phosphorus transporters, and excreta microbiome in broiler chickens. Poult. Sci. 101:102126. doi: 10.1016/J.PSJ.2022.102126
Li, W., Jia, M. X., Deng, J., Wang, J. H., Lin, Q. L., Liu, C., et al. (2018). Isolation, genetic identification and degradation characteristics of COD-degrading bacterial strain in slaughter wastewater. Saudi J. Biol. Sci. 25, 1800–1805. doi: 10.1016/J.SJBS.2018.08.022
Liu, Y., Fu, J., Wang, L., Zhao, Z., Wang, H., Han, S., et al. (2022). Isolation, identification, and whole-genome sequencing of high-yield protease bacteria from Daqu of ZhangGong Laojiu. PLoS One 17:e0264677. doi: 10.1371/JOURNAL.PONE.0264677
Liu, T., Li, C., Zhong, H., and Feng, F. (2021). Dietary medium-chain α-monoglycerides increase BW, feed intake, and carcass yield in broilers with muscle composition alteration. Poult. Sci. 100, 186–195. doi: 10.1016/J.PSJ.2020.09.056
Lozupone, C., and Knight, R. (2005). UniFrac: A new phylogenetic method for comparing microbial communities. Appl. Environ. Microbiol. 71, 8228–8235. doi: 10.1128/AEM.71.12.8228-8235.2005/ASSET/CD76613D-18C6-418B-996A-AB8D3D6CA216/ASSETS/GRAPHIC/ZAM0120562270003.JPEG
Ma, Y., Wang, W., Zhang, H., Wang, J., Zhang, W., Gao, J., et al. (2018). Supplemental Bacillus subtilis DSM 32315 manipulates intestinal structure and microbial composition in broiler chickens. Sci. Rep. 8:15358. doi: 10.1038/S41598-018-33762-8
Mandal, R. K., Jiang, T., Al-Rubaye, A. A., Rhoads, D. D., Wideman, R. F., Zhao, J., et al. (2016). An investigation into blood microbiota and its potential association with bacterial Chondronecrosis with osteomyelitis (BCO) in broilers. Sci. Rep. 6:25882. doi: 10.1038/SREP25882
Maragkoudakis, P. A., Zoumpopoulou, G., Miaris, C., Kalantzopoulos, G., Pot, B., and Tsakalidou, E. (2006). Probiotic potential of lactobacillus strains isolated from dairy products. Int. Dairy J. 16, 189–199. doi: 10.1016/J.IDAIRYJ.2005.02.009
Meng, Q., and Hao, J. J. (2017). Optimizing the application of bacillus velezensis BAC03 in controlling the disease caused by Streptomyces scabies. BioControl 62, 535–544. doi: 10.1007/S10526-017-9799-7/FIGURES/5
Mohamed, T. M., Sun, W., Bumbie, G. Z., Elokil, A. A., Mohammed, K. A. F., Zebin, R., et al. (2022). Feeding Bacillus subtilis ATCC19659 to broiler chickens enhances growth performance and immune function by modulating intestinal morphology and cecum microbiota. Front. Microbiol. 12:798350. doi: 10.3389/FMICB.2021.798350
Mohammad, B. T., Al Daghistani, H. I., Jaouani, A., Abdel-Latif, S., and Kennes, C. (2017). Isolation and characterization of thermophilic bacteria from Jordanian Hot Springs: bacillus licheniformis and Thermomonas hydrothermalis isolates as potential producers of thermostable enzymes. Int. J. Microbiol. 2017, 1–12. doi: 10.1155/2017/6943952
Mora, A., Herrera, A., Mamani, R., López, C., Alonso, M. P., Blanco, J. E., et al. (2010). Recent emergence of clonal group O25b:K1:H4-B2-ST131 ibeA strains among escherichia coli poultry isolates, including CTX-M-9-producing strains, and comparison with clinical human isolates. Appl. Environ. Microbiol. 76, 6991–6997. doi: 10.1128/AEM.01112-10/ASSET/F65330AF-BED9-47F9-8038-DB2A185C50BC/ASSETS/GRAPHIC/ZAM9991014460002.JPEG
Nikoskelainen, S., Ouwehand, A. C., Bylund, G., Salminen, S., and Lilius, E. M. (2003). Immune enhancement in rainbow trout (Oncorhynchus mykiss) by potential probiotic bacteria (lactobacillus rhamnosus). Fish Shellfish Immunol. 15, 443–452. doi: 10.1016/S1050-4648(03)00023-8
Pourabedin, M., Guan, L., and Zhao, X. (2015). Xylo-oligosaccharides and virginiamycin differentially modulate gut microbial composition in chickens. Microbiome 3:15. doi: 10.1186/S40168-015-0079-4
Ramkumar, A., Sivakumar, N., Gujarathi, A. M., and Victor, R. (2018). Production of thermotolerant, detergent stable alkaline protease using the gut waste of Sardinella longiceps as a substrate: optimization and characterization. Sci. Rep. 8:12442. doi: 10.1038/S41598-018-30155-9
Reuben, R. C., Roy, P. C., Sarkar, S. L., Alam, R. U., and Jahid, I. K. (2019). Isolation, characterization, and assessment of lactic acid bacteria toward their selection as poultry probiotics. BMC Microbiol. 19:253. doi: 10.1186/S12866-019-1626-0
Rivera-Pérez, W., Barquero-Calvo, E., and Chaves, A. J. (2021). Effect of the use of probiotic Bacillus subtilis (QST 713) as a growth promoter in broilers: an alternative to bacitracin methylene disalicylate. Poult. Sci. 100:101372. doi: 10.1016/J.PSJ.2021.101372
Ruiz-García, C., Béjar, V., Martínez-Checa, F., Llamas, I., and Quesada, E. (2005). Bacillus velezensis sp. nov., a surfactant-producing bacterium isolated from the river Vélez in Málaga, southern Spain. Int. J. Syst. Evol. Microbiol. 55, 191–195. doi: 10.1099/IJS.0.63310-0/CITE/REFWORKS
Salem, R., El-Habashi, N., Fadl, S. E., Sakr, O. A., and Elbialy, Z. I. (2018). Effect of probiotic supplement on aflatoxicosis and gene expression in the liver of broiler chicken. Environ. Toxicol. Pharmacol. 60, 118–127. doi: 10.1016/J.ETAP.2018.04.015
Salim, H. M., Kang, H. K., Akter, N., Kim, D. W., Kim, J. H., Kim, M. J., et al. (2013). Supplementation of direct-fed microbials as an alternative to antibiotic on growth performance, immune response, cecal microbial population, and ileal morphology of broiler chickens. Poult. Sci. 92, 2084–2090. doi: 10.3382/PS.2012-02947
Segata, N., Izard, J., Waldron, L., Gevers, D., Miropolsky, L., Garrett, W. S., et al. (2011). Metagenomic biomarker discovery and explanation. Genome Biol. 12, 1–18. doi: 10.1186/GB-2011-12-6-R60/FIGURES/6
Shaikh, N. M., Patel, A. A., Mehta, S. A., and Patel, N. D. (2013). Isolation and screening of cellulolytic bacteria inhabiting different environment and optimization of Cellulase production. Univers. J. Environ. Researcg Technol. 3, 39–49. doi: 10.12691/ajmr-9-3-1
Slominski, B. A., Meng, X., Campbell, L. D., Guenter, W., and Jones, O. (2006). The use of enzyme technology for improved energy utilization from full-fat oilseeds Part II: flaxseed. Poult. Sci. 85, 1031–1037. doi: 10.1093/PS/85.6.1031
Stålbrand, H., Mansfield, S. D., Saddler, J. N., Kilburn, D. G., Warren, R. A. J., and Gilkes, N. R. (1998). Analysis of molecular size distributions of cellulose molecules during hydrolysis of cellulose by recombinant Cellulomonas fimi beta-1,4-glucanases. Appl. Environ. Microbiol. 64, 2374–2379. doi: 10.1128/AEM.64.7.2374-2379.1998
Su, X. L., Tian, Q., Zhang, J., Yuan, X. Z., Shi, X. S., Guo, R. B., et al. (2014). Acetobacteroides hydrogenigenes gen. Nov., sp. nov., an anaerobic hydrogen-producing bacterium in the family Rikenellaceae isolated from a reed swamp. Int. J. Syst. Evol. Microbiol. 64, 2986–2991. doi: 10.1099/IJS.0.063917-0
Susanti, D., Volland, A., Tawari, N., Baxter, N., Gangaiah, D., Plata, G., et al. (2021). Multi-omics characterization of host-derived bacillus spp. probiotics for improved growth performance in poultry. Front. Microbiol. 12:3116. doi: 10.3389/FMICB.2021.747845/BIBTEX
Takeshita, T., Kageyama, S., Furuta, M., Tsuboi, H., Takeuchi, K., Shibata, Y., et al. (2016). Bacterial diversity in saliva and oral health-related conditions: the Hisayama study. Sci. Rep. 6:22164. doi: 10.1038/SREP22164
Tavernari, F. C., Albino, L. F. T., Morata, R. L., Dutra Júnior, W. M., Rostagno, H. S., and Viana, M. T. S. (2008). Inclusion of sunflower meal, with or without enzyme supplementation, in broiler diets. Brazilian J. Poult. Sci. 10, 233–238. doi: 10.1590/S1516-635X2008000400007
Tilgar, V., Kilgas, P., Viitak, A., and Reynolds, S. J. (2008). The rate of bone mineralization in birds is directly related to alkaline phosphatase activity. Physiol. Biochem. Zool. 81, 106–111. doi: 10.1086/523305
Tun, H. M., Bridgman, S. L., Chari, R., Field, C. J., Guttman, D. S., Becker, A. B., et al. (2018). Roles of birth mode and infant gut microbiota in intergenerational transmission of overweight and obesity from mother to offspring. JAMA Pediatr. 172, 368–377. doi: 10.1001/JAMAPEDIATRICS.2017.5535
Viveros, A., Brenes, A., Arija, I., and Centeno, C. (2002). Effects of microbial phytase supplementation on mineral utilization and serum enzyme activities in broiler chicks fed different levels of phosphorus. Poult. Sci. 81, 1172–1183. doi: 10.1093/PS/81.8.1172
Wang, Y., Heng, C., Zhou, X., Cao, G., Jiang, L., Wang, J., et al. (2021). Supplemental Bacillus subtilis DSM 29784 and enzymes, alone or in combination, as alternatives for antibiotics to improve growth performance, digestive enzyme activity, anti-oxidative status, immune response and the intestinal barrier of broiler chickens. Br. J. Nutr. 125, 494–507. doi: 10.1017/S0007114520002755
Yang, W. Y., Lee, Y., Lu, H., Chou, C. H., and Wang, C. (2019). Analysis of gut microbiota and the effect of lauric acid against necrotic enteritis in Clostridium perfringens and Eimeria side-by-side challenge model. PLoS One 14:e0205784. doi: 10.1371/JOURNAL.PONE.0205784
Yang, F., Zhang, S., Tian, M., Chen, J., Chen, F., and Guan, W. (2020). Different sources of high fat diet induces marked changes in gut microbiota of nursery pigs. Front. Microbiol. 11:859. doi: 10.3389/FMICB.2020.00859
Ye, M., Sun, L., Yang, R., Wang, Z., and Qi, K. (2017). The optimization of fermentation conditions for producing cellulase of bacillus amyloliquefaciens and its application to goose feed. R. Soc. Open Sci. 4:171012. doi: 10.1098/RSOS.171012
Zhai, S. S., Tian, L., Zhang, X. F., Wang, H., Li, M. M., Li, X. C., et al. (2020). Effects of sources and levels of liquor distiller’s grains with solubles on the growth performance, carcass characteristics, and serum parameters of Cherry Valley ducks. Poult. Sci. 99, 6258–6266. doi: 10.1016/J.PSJ.2020.07.025
Zhang, Y. C., Luo, M., Fang, X. Y., Zhang, F. Q., and Cao, M. H. (2021). Energy value of rice, broken rice, and rice bran for broiler chickens by the regression method. Poult. Sci. 100:100972. doi: 10.1016/J.PSJ.2020.12.069
Zhang, D. F., Xiong, X. L., Wang, Y. J., Gao, Y. X., Ren, Y., Wang, Q., et al. (2021). Bacillus velezensis WLYS23 strain possesses antagonistic activity against hybrid snakehead bacterial pathogens. J. Appl. Microbiol. 131, 3056–3068. doi: 10.1111/JAM.15162
Zhang, Q., Zhang, S., Wu, S., Madsen, M. H., and Shi, S. (2022). Supplementing the early diet of broilers with soy protein concentrate can improve intestinal development and enhance short-chain fatty acid-producing microbes and short-chain fatty acids, especially butyric acid. J. Anim. Sci. Biotechnol. 13:97. doi: 10.1186/S40104-022-00749-5
Keywords: Bacillus velezensis, multi-enzyme, broiler chickens, tibia mineralization, digestive enzymes, intestinal microbiota
Citation: Li C, Li S, Dang G, Jia R, Chen S, Deng X, Liu G, Beckers Y and Cai H (2023) Screening and characterization of Bacillus velezensis LB-Y-1 toward selection as a potential probiotic for poultry with multi-enzyme production property. Front. Microbiol. 14:1143265. doi: 10.3389/fmicb.2023.1143265
Edited by:
Kun Li, Nanjing Agricultural University, ChinaReviewed by:
Arindam Dhali, National Institute of Animal Nutrition and Physiology (ICAR), IndiaHouqiang Luo, Wenzhou Vocational College of Science and Technology, China
Copyright © 2023 Li, Li, Dang, Jia, Chen, Deng, Liu, Beckers and Cai. This is an open-access article distributed under the terms of the Creative Commons Attribution License (CC BY). The use, distribution or reproduction in other forums is permitted, provided the original author(s) and the copyright owner(s) are credited and that the original publication in this journal is cited, in accordance with accepted academic practice. No use, distribution or reproduction is permitted which does not comply with these terms.
*Correspondence: Huiyi Cai, Y2FpaHVpeWlAY2Fhcy5jbg==
†These authors have contributed equally to this work