- School of Food Science and Pharmaceutical Engineering, Nanjing Normal University, Nanjing, China
Deoxynivalenol (DON) is a mycotoxin that contaminates animal feed and crops around the world. DON not only causes significant economic losses, but can also lead diarrhea, vomiting, and gastroenteritis in humans and farm animals. Thus, there is an urgent need to find efficient approaches for DON decontamination in feed and food. However, physical and chemical treatment of DON may affect the nutrients, safety, and palatability of food. By contrast, biological detoxification methods based on microbial strains or enzymes have the advantages of high specificity, efficiency, and no secondary pollution. In this review, we comprehensively summarize the recently developed strategies for DON detoxification and classify their mechanisms. In addition, we identify remaining challenges in DON biodegradation and suggest research directions to address them. In the future, an in-depth understanding of the specific mechanisms through which DON is detoxified will provide an efficient, safe, and economical means for the removal of toxins from food and feed.
1. Introduction
Over thousands of years, cereal grains emerged as an essential source of calories for humans and farm animals. In developed, developing, and poor countries, the proportion of energy provided by cereals to residents are ~30, >60, and >80%, respectively (Sidhu et al., 2007; Tsuge, 2014). Thus, the availability of safe dietary grains represents a cornerstone of human civilization (Awika, 2011). In 2016, the International Agency for Research on Cancer (IARC) and the World Health Organization (WHO) reported that mycotoxin pollution seriously affects the economic development and health of residents in developing countries (Weber et al., 2023), with approximately 500 million individuals in developing countries directly and indirectly exposed to mycotoxins every day (Fellone, 2016). A recent survey on mycotoxin contamination showed that in most of the tested food samples, the concentration of deoxynivalenol (DON) exceeded the recommended limits (Mishra et al., 2019).
DON, also known as vomitoxin, is a type B trichothecene and epoxy-sesquiterpenoid produced by Fusarium graminearum. It was first isolated and purified from moldy barley grains by Yoshizawa et al., and chemically characterized in 1970 (Han et al., 2020). DON is soluble in water, ethanol, acetonitrile, and other polar solvents. It is also stable at high temperatures and low pH. Previous studies have shown that wheat contaminated with DON is still toxic after 4 years of storage (Cao et al., 2013). DON can contaminate food at any step of food production, including processing, transportation, storage, and consumption, generally by contaminating plant products, directly or indirectly contaminating food and animal feed, and finally stably accumulating in the human food supply (Figure 1; Zhai et al., 2019a).
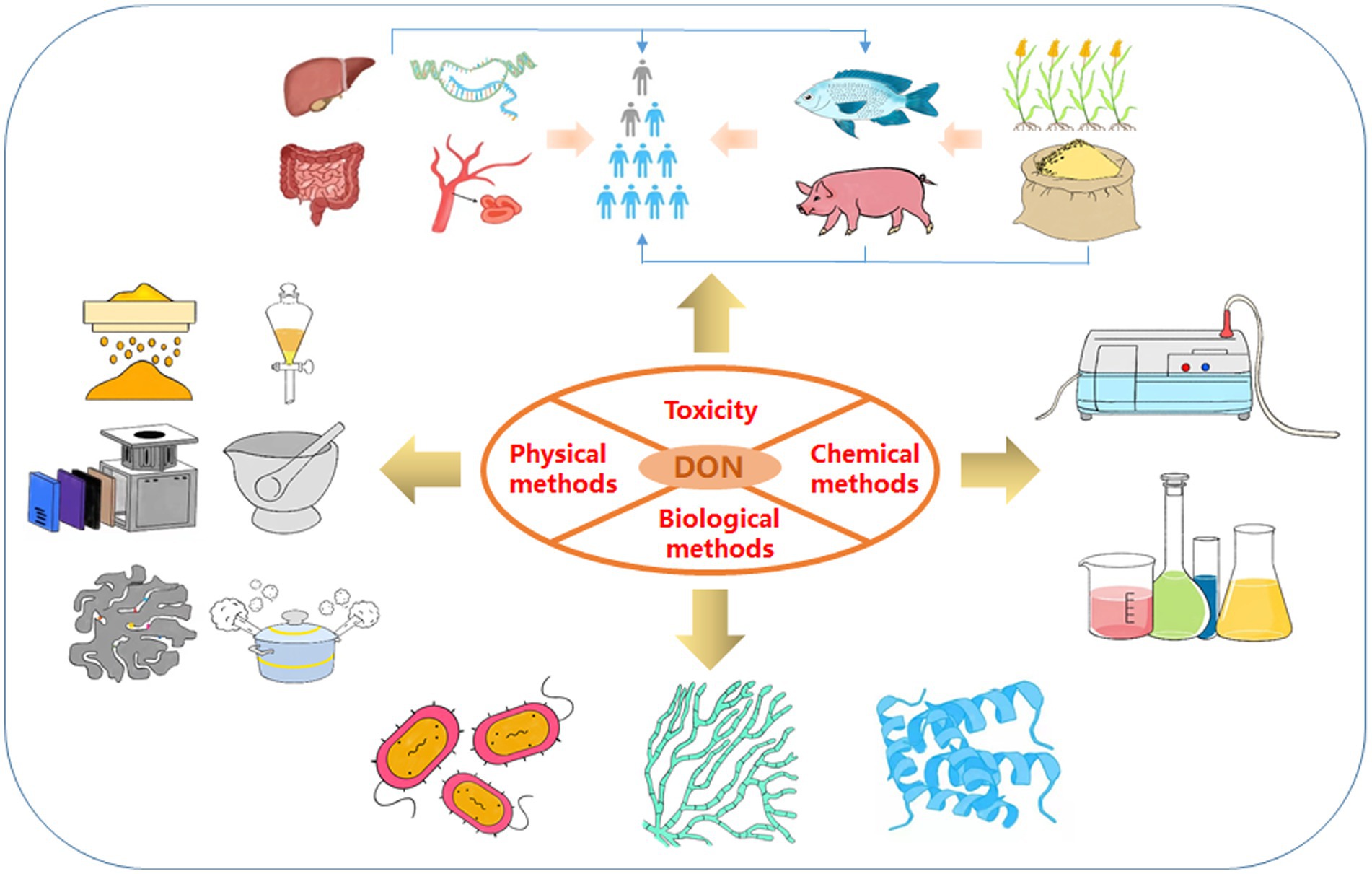
Figure 1. Framework of the review. These include the toxicity of DON and three detoxification methods (physical methods, chemical methods and biological methods).
In addition, DON derivatives may be present in food and animal tissues such as blood, muscles, and liver, including acetylated (3-ADON and 15-ADON), redox modified (3-keto-DON, DOM-1, and 3-epi-DON), and glycosylated (D3G and D15G) forms of DON, as well as glucuronic acid derivatives (D3GA and D15GA; Figure 2). DON and its derivatives share a common epoxide group at C12–13, which is also a key group in determining their toxicity (Pestka et al., 2004). Epoxy compounds can damage cells by inhibiting the activation of various protein kinases, dysregulating gene expression, and inhibiting protein synthesis (Pestka, 2010).
DON mainly contaminates starchy staple foods such as wheat, barley, oats, rye, corn, and potatoes, among which wheat, corn, and oats are the most polluted (Golge and Kabak, 2020; Topi et al., 2021). Correspondingly, many countries have formulated highest acceptable limits for DON in grains, according to their national circumstances. For instance, the national standard of China, GB2761-2017, stipulates that the standard limit for DON in grain and grain products is 1 mg/kg (China, 2017). Similarly, the Food and Drug Administration of the United States stipulates that the safety standard for DON in food is 1 mg/kg, while the allowable limit in wheat and wheat products in the United States must not exceed 4 mg/kg (Feizollahi and Roopesh, 2022). By contrast, the content of DON in grain flour and corn in the European Union must be <0.75 mg/kg, and this limit is relatively strict. Therefore, there is an urgent need to remove DON from food products and animal feed.
To date, most reviews only focused on discussing decontamination methods for several kinds of mycotoxins, while there is still no in-depth and comprehensive summary and discussion of DON detoxification. Nevertheless, DON contamination has had a great impact on food safety in recent years, and many new decontamination approaches have been investigated to solve the challenge of DON detoxification. In this review, we discuss three recent approaches to DON detoxification, which include physical, chemical, and biological methods (Figure 1). Through a comprehensive literature search and analysis, it was found that traditional DON detoxification strategies, including physical and chemical approaches, often have disadvantages, while biological detoxification approaches have the advantages of no secondary pollution, high efficiency, and specificity. Chemical detoxification methods include the structural degradation of aldehydes, oxidants, acids, bases and several gases. However, chemical treatment usually affects the palatability and nutrition of feed and food, and is not suitable for large-scale detoxification. In addition, it is also easy to cause secondary pollution. Biological methods include the use of bacteria, yeast or their respective enzymes or metabolic pathways to degrade DON. Especially, enzymatic detoxification technology has good repeatability, uniformity, simple operation and high safety, as well as some other interesting properties. Therefore, enzymatic degradation is considered to be the most commercially valuable and useful technology in future applications. This review provides a theoretical basis for the development and practical application of DON decontamination technologies.
2. Strategies for the nonbiological detoxification of DON
2.1. Physical methods
Physical detoxification includes screening and stripping, washing and grinding, radiation treatment, heat treatment, and adsorption. According to the different mechanisms of action, physical detoxification methods can be divided into approaches based on either the intact removal or the degradation of DON.
2.1.1. Removal of DON
The physical removal of intact DON consists of separating the contaminated outer coat and pericarp of the kernels via procedures usually used in cereal processing, such as separation, solvent extraction, and adsorption.
2.1.1.1. Peeling and grinding
Mycotoxins tend to be concentrated in the germ and bran of grains (Brera et al., 2004). In the early stages, DON contamination in grains can be removed by mechanical peeling. Trenholm et al. removed the fungal chitin from barley by coarse grinding and sieving, reducing the DON content by 16%. However, the mass loss of barley, wheat, and corn reached 34%, 55%, and 69% of the total matter, respectively, while nearly 40% of the ground grain was lost. Moreover, 22%–32% of the protein was also lost (Trenholm et al., 1991). This method can only remove cereal grains, broken grains, and fine substances with high mold contamination, and does not completely eliminate toxin contamination. Furthermore, cereal grains are more likely to be invaded by mycotoxigenic fungi after mechanical damage (Magan et al., 2003).
2.1.1.2. Solvent extraction
DON is soluble in water and polar solvents, so it can be extracted, isolated, and detoxified by a mixture of water and organic solvents. The common extraction solvents reported in the literature include distilled water, saturated sodium chloride solution, 30% sucrose solution, sodium carbonate, and sodium hydroxide (Yener and Köksel, 2013). These extractants can eluate the DON present in food to a certain extent, but these methods also have some disadvantages. For example, rinsing will leave DON and its by-products in the solution, resulting in secondary contamination. Moreover, there are limitations such as high cost and the need for large-scale processing equipment. In addition, the nutritional value of the grains is reduced during the extraction process.
2.1.1.3. Material adsorption
As shown in Table 1, this process functions by adsorbing the toxin to a foreign body composed of various adsorbent materials, such as layered silicate minerals, activated carbon, nano zeolite, or synthetic resin (Huwig et al., 2001). Montmorillonite (MT) is a complex clay mineral that has been proven to be an efficient adsorbent. Among the different types, pillared montmorillonite has aroused great interest because of its large surface area and catalytic properties. In 2021, Zhang et al. synthesized three types of pillared montmorillonite containing Al, Fe, and Ti. In their DON adsorption experiments, it was found that the adsorption capacity of pillared montmorillonite was 3–4 times higher than that of the original montmorillonite. However, this material works in a low acidic environment, which is not conducive to maintaining the original nutritional properties of food (Zhang et al., 2021). In recent years, new functional microspheres have been synthesized for the adsorption of mycotoxins. For example, Shalapy et al. first used alginate/sodium hydroxylmethyl cellulose composite calcium (SA/CMC-Ca) to form functional microspheres for the adsorption of 3.6 mg/L DON from contaminated corn leachate (Shalapy et al., 2020).
As countries roll out sustainable development strategies, some researchers are turning toward the use of renewable materials for DON detoxification. For example, humic acid (HA), durian peel (DP), sodium alginate (SA), and other substances can adsorb DON (Adunphatcharaphon et al., 2020; Haus et al., 2020; Zhang L. et al., 2020). However, these adsorbents not only have low adsorption efficiency but are also expensive (Shehata et al., 2000).
2.1.2. Degradation of DON
The physical degradation of DON mainly consists in destroying its chemical bond to form new non-toxic or less toxic compounds. The methods are mainly divided into heat and non-heat treatments.
2.1.2.1. Heat treatment
This process can detoxify mycotoxins produced by fungi growing on grains (Bullerman and Bianchini, 2007). Heat treatment breaks the chemical bonds of DON at high temperatures to achieve detoxification. In the 1980s, methods were developed to reduce the toxicity of DON by high-temperature treatments, such as steaming, boiling, and frying (El-Banna et al., 1983; Scott et al., 1984). Bretz et al. heated DON under alkaline conditions and analyzed the decomposed products by nuclear magnetic resonance (NMR) and mass spectrometry (MS), revealing that DON was transformed into several new compounds with less toxicity (Bretz et al., 2006). However, the thermal stability of DON makes it resistant to the high temperatures encountered during food processing. Some studies have shown that DON detoxification in food is non-reversible only when the treatment temperature is above 150°C. However, high temperature treatment leads to the loss of some nutrients in food, and may produce toxic or unpalatable by-products that affect the taste (Humpf et al., 2004; Bullerman and Bianchini, 2007).
2.1.2.2. Non-heat treatment
In addition to heat, physical treatments such as ultraviolet light, pulsed light technology, and atmospheric cold plasma (ACP) can also destroy mycotoxins on the surface of food and materials (Elmnasser et al., 2007; Karaca et al., 2010; Murata et al., 2011; Hojnik et al., 2019). For example, Stepanik et al. reported that wheat contaminated with DON was irradiated to doses ranging from 2.0 to 55.8 kGy by an electron accelerator, and found that electron beam treatment could reduce 17.6% of DON level in wheat at the highest dose used (55.8 kGy; Stepanik et al., 2007). Li et al. used 60Co γ-ray irradiation to degrade DON and found that 20 kGy γ-ray irradiation could degrade 83% of DON in acetonitrile–water, while 2 μg/mL DON in ultra-pure water was completely degraded after 5 kGy γ-ray irradiation (Li et al., 2019a). Murata et al. used ultraviolet (UV) light (1.5 mW/cm2 at 254 nm UV-C wavelength), which removed 22% of the initial concentration of 60 mg/kg DON within 30 min without affecting the total content of vitamins (Murata et al., 2011). However, this method is not only inefficient, but also greatly affects the taste and nutrient composition of flour. In 2013, Moreau et al. found that pulsed light treatment could remove 72.5% of DON, but the residual concentration was still toxic in Caenorhabditis elegans survival tests (Moreau et al., 2013). Moreover, light generally does not penetrate solid foods such as grains, and these methods are often only suitable for liquid or transparent objects. ACP has been used to chemically decompose mycotoxins through various pathways, resulting in degradation products of low toxicity (Misra et al., 2019). In 2017, Ten Bosch et al. evaluated the effect of ACP technology on the degradation of several mycotoxins. The experimental data showed that DON was almost completely degraded after 60 s and nontoxic residue (Ten Bosch et al., 2017). ACP technology is expected to be applied in the degradation of the DON present in food, but the underlying mechanism remains unknown.
3. Chemical methods
Chemical detoxification uses strong oxidizing agents, strong acids and bases, or ozone to detoxify grains contaminated with DON. This process converts DON into degradation products with low or no residual toxicity.
3.1. Ozonization
This new process, which was only recently approved for application in the food industry, has achieved good results in the detoxification of contaminated products, offering an effective and safe chemical method for the reduction or elimination of mycotoxins in grains (Karaca et al., 2010; Tiwari et al., 2010). The DON degradation percentages in ultrapure water and acetonitrile solution after treatment with gaseous ozone reached 95.68% and 98.3%, respectively, and there were no significant degradation products (Li et al., 2019b; Ren et al., 2020). However, the direct effect of ozone on the removal of DON from grains is not obvious. Wang et al. used gaseous ozone to treat a DON-containing solution and F. graminearum-infected wheat. The results showed that 10 mg/L ozone could degrade 93.6% of the DON present in the solution within 30 s, but treating the infected wheat with 80 mg/L ozone for 2 h could only remove 26.11% of the DON found in the grains. The double bond of DON structure was destroyed by ozone, and the toxicity of the product should be weaker than that of DON. Furthermore, high-moisture wheat was more sensitive to ozone than low-moisture wheat under the same conditions. The effect of ozone on DON was higher in the solution than in the cereal grains, possibly because the specific grain morphology educed the contact of ozone with DON. Therefore, Wang et al. explored the effect of ozone on the degradation of DON and its derivatives in wheat with different water contents and raw material morphologies, using different ozone concentrations, exposure times. It was found that the DON degradation percentage increased significantly with an increase of ozone concentration and exposure time. In addition, DON was degraded more easily in whole wheat flour than in wheat grains, and the decrease of DON content became more significant as the humidity increased (Wang et al., 2016). These studies offer a strong theoretical basis for the future ozone-based removal of DON contamination from grains. The application of ozone for grain storage and preservation is presented in Table 2. In addition to ozone, ammonia (NH3), chlorine (Cl2), and other gases can also detoxify the DON present in grains (Young, 1986; Borràs-Vallverdú et al., 2020).
3.2. Sodium treatment
Although ozonization is effective, the process requires specialized equipment and is costly, which makes it currently unsuitable for large-scale use (Li M. M. et al., 2015). Chemical detoxification involves the use of specific chemicals to destroy the chemical structure of mycotoxins or to neutralize their active groups (Wu et al., 2020). For example, sodium metabisulfite (SMBS) can destroy DON in processed grains or feed. Danicke et al. conducted a series of experiments using SMBS to determine whether the content of DON in cereals can be effectively reduced by controlling the concentration of SMBS under hot water treatment (Danicke et al., 2005, 2009, 2010). SMBS can rapidly degrade DON under acidic aqueous conditions (such as in the pig stomach; Lu et al., 2020).
Although physical and chemical detoxification methods are relatively simple, they also have many drawbacks. For example, the toxin removal efficiency is low, the treatment cost is high, it often destroys various nutrients in grains, and the degradation products can also be toxic.
4. Biological methods
These are considered promising detoxification methods because they are environmentally friendly, require mild reaction conditions, show strong specificity and have high efficiency (McCormick and Susan, 2013). These methods mainly rely on the adsorption of DON onto microbial cell walls or the degradation of DON by microbial enzymes.
4.1. Biosorption of DON
The cell walls of certain bacteria can intrinsically adsorb mycotoxins due to their specific composition. For example, the cell wall contains carbohydrates (peptidoglycan, mannose, glucose proteins, and lipids), whose adsorption mechanisms may include hydrogen bonds, ion interactions, and/or hydrophobic interactions (Chen et al., 2018; Wang N. et al., 2019; Chlebicz and Slizewska, 2020).
In recent years, yeast has been used as a nutritional additive and added to feed as an antidote in the actual production process. The addition of Saccharomyces cerevisiae to a PBS buffer containing DON was shown to decrease the soluble DON content by 39% (Chlebicz and Slizewska, 2020). Lactic acid bacteria (LAB) can inactivate mycotoxins or convert them into less toxic products by adsorbing them and forming mycotoxin-organic adsorption complexes. Experiments have shown that the cell wall of Lactobacillus paracasei LHZ-1 can form a complex with DON, reducing its content in solution by 40.7% within 24 h (Zhai et al., 2019b). Table 3 lists studies on the adsorption of DON by microorganisms.
Furthermore, the mycelia of some filamentous fungi can absorb large amounts of DON. For example, in a study on submerged fermentation, Garda-Buffon et al. found that the hyphae of Rhizopus oryzae and Aspergillus oryzae can adsorb DON, whereby the highest removal efficiency reached 74 and 90% after agitation for 96 and 240 h, respectively (Pierron et al., 2016).
4.2. Biodegradation
Biodegradation and detoxification occur mainly through the destruction of toxic groups and the conversion of DON into less toxic or non-toxic compounds by microorganisms or enzymes (Guo et al., 2020). Some examples of the resulting substances are 3-keto-DON, 3-epi-DON, and 3-oxo-DON, whereby the toxicity of 3-keto-DON is 1/10 than that of DON, and that of 3-epi-DON is 1/1181 (Shima et al., 1997; Karlovsky, 2011; He et al., 2015; Pierron et al., 2016).
4.2.1. Degradation of DON by microorganism
Many microorganisms, including bacteria, fungi, actinomycetes, and algae, can remove and degrade mycotoxins from food and feed (Hathout and Aly, 2014).
4.2.1.1. Detoxification using bacteria
As shown in Table 4, a number of bacteria degrade DON, including several species of the genus Bacillus. The DON degradation percentages after treatment with Bacillus subtilis ZZ and B. licheniformis reached >98 and 72.2%, respectively (Cheng et al., 2010). Bacillus cereus isolated from moldy straw, soil, and feces can also degrade DON (Ergang, 2016). Moreover, Tan et al. screened the B. subtilis strain NHIBC006D from contaminated corn and found that it could degrade 73.5% of DON (Tan et al., 2018).
Ikunaga et al. screened the Nocardia sp. strain WSN05-2 from the soil of a wheat field based on its ability to grow in a medium with DON as the sole carbon source. After 7 days of cultivation, the DON degradation ratio reached approximately 90%. The resulting metabolite was identified as 3-epi-DON by MS and 1H and 13C NMR analyses (Ikunaga et al., 2011). Another strain was also screened from the soil of a wheat field and identified as Devosia insulae by morphological, physiological, and 16S rRNA phylogenetic analyses. This strain was able to degrade 88% of 20 mg/L DON within 48 h under aerobic conditions and neutral pH at 35°C. Moreover, it was found that the main degradation product of DON and its derivatives was 3-keto-DON (Wang G. et al., 2019). Furthermore, they identified oxidation of the C-3 hydroxyl as the degradation mechanism. In addition, researchers tested the oxidative degradation of DON in animal intestines. For example, Yu et al. screened strains capable of transforming the DON present in chicken intestines using denaturing gradient gel electrophoresis (PCR-DGGE). Ten strains could convert DON into the less toxic deepoxy-4-deoxynivalenol derivative (DOM-1; Yu et al., 2010). Gao et al. the novel Eggerthella sp. strain DII-9 in chicken intestines. It was able to convert DON into another substance over a wide range of temperatures (20–45°C) and pH values (5–10; Gao et al., 2018). Later, the team also found a new DON detoxifying strain, Slackia sp. D-G6 in chicken intestines, which is a gram-positive non-sporulating bacterium that can de-epoxidize DON into DOM-1. Using this bacterium and a specific chicken intestinal extract medium, 25 mg/L DON was completely transformed into DOM-1 within 48 h (Gao et al., 2020).
4.2.1.2. Detoxification using fungi
In addition to adsorbing mycotoxins, some fungi metabolize and degrade mycotoxins during fermentation, converting them into compounds with low or no toxicity. For example, yeast can convert DON into D3G, 15-A-DON, and 3-ADON (Freire and Sant’Ana, 2018). Nathanail et al. reported that Saccharomyces pastorianus converts DON into D3G by binding to DON glycosides during fermentation, whereby DON and D3G can also be adsorbed by the yeast cell wall (Nathanail et al., 2016). He et al. used DON as the sole carbon source and isolated a soul fungus that could transform DON, which was named NJA-1. The strain was identified as Aspergillus tubingensis by gene series analysis and morphological observations. The conversion rate of DON reached 94.4% within 14 days. The results of HPLC-MS analysis showed that the fluorescence intensity of the degradation products was 18.1 higher than that of DON. The highest tolerance concentration of NJA-1 to DON in an inorganic salt medium was 40 mg/l (He et al., 2008). Furthermore, strains of Aspergillus oryzae and Rhizopus oryzae were also found to adsorb and degrade DON. Aspergillus oryzae KKB4 and Rhizopus oryzae KP1R1 were inoculated into DON-contaminated corn and fermented for 10 days, which resulted in DON reduction by 65.91% and 56.82%, respectively. Toxicity analysis showed that the degradation products did not affect the growth of Saccharomyces cerevisiae (Arifin et al., 2019). Moreover, the DON degradation percentage in corn medium was as high as 92% after culture of Aspergillus oryzae (5509NRRL) for 21 days (Tran and Smith, 2014).
4.2.1.3. Detoxification using microbial consortia
Microbial consortia are considered to offer special advantages for the degradation of complex compounds (Yuan et al., 2016). In the microbial consortium, different microorganisms cooperate with each other to achieve the best degradation effect. Researchers began to screen out bacterial and microbial complexes through selective culture and domestication for toxicity reduction of different compounds, including aflatoxin B1 (AFB1), ZEN, DON, and their derivatives (Wang et al., 2017; Zhai et al., 2019a; Wang et al., 2020a). For example, 10 bacterial cultures were screened from pig manure by Junmei et al., and the DON degradation percentage in the simultaneous degradation of ZEN, AFB1, and DON by multiple bacteria reached up to 70% (Junmei, 2013).
Wang et al. screened the bacterial consortium C20, which could degrade nearly 70 mg/l DON within 5 days, and confirmed the conversion of DON into 3-keto-DON by liquid chromatography/time-of-flight MS (LC-TOF-MS) and NMR analyses (Wang et al., 2020b). In the same year, Wang et al. screened a DON-degrading bacterial consortium from wheat leaves, which was transformed into 3-keto-DON through a two-step differential isomerization, as determined by metabolomics and NMR analyses. During the degradation of DON, it was found that the abundance of achromatic bacilli and Sphingomonas sp. in the flora increased greatly, and it was speculated that these two types of bacteria played a significant role in the degradation of DON (Wang G. et al., 2020). Zhai et al. reported for the first time the two-step enzymatic isomerization of DON in a mixed culture. They screened the bacterial consortium LZ-N1, which showed high efficiency and stable DON transformation activity in medium with DON as the sole carbon source. High-throughput analysis showed that LZ-N1 was composed of at least 11 bacterial species, among which Pseudomonas sp. accounted for nearly half of the relative abundance. When two of the newly identified strains, Pseudomonas sp. Y1 and Lysobacter sp., were co-cultured, it was found that cell-free supernatant, intracellular lysate, and cell fragments had the ability to degrade DON. When the concentration of DON was 50 mg/L, the degradation in the supernatant could reach 100% after 48 h (Zhai et al., 2019b). Although microbial consortia have good degradation effects, the community often becomes unstable during degradation. Furthermore, there is still no in-depth investigation of the microbial dynamics of such consortia.
4.2.2. Degradation of DON by enzymes
Certain enzymes or proteins produced by microorganisms can transform or bind mycotoxins. As shown above, many microorganisms can convert DON by metabolizing it with specific enzymes. Because enzymatic degradation has the advantages of high specificity, efficiency, and environmental friendliness, studies are increasingly focusing on the identification, purification, and characterization of detoxifying enzymes, as well as the underlying catalytic mechanisms. According to the literature, the mechanisms of enzymatic DON detoxification can be divided into acetylation, oxidoreductase and glycosylation (Table 5). The sites of action of these enzymes are shown in Figure 3.
4.2.2.1. Acetylation
DON, nivalenol (NIV), and 3,7,15-trihydroxy-12,13-epoxytrichothec-9-ene (NX-3) are produced by Fusarium graminearum. Hao et al. explored the use of fungal self-protection agents in plants and found that Fusarium graminearum trichothecene 3-O-acetyltransferase (FgTri101) can degrade DON, 15-ADON, NIV, and NX-3. They cloned FgTri101 from Fusarium graminearum and expressed it in Arabidopsis plants. The experimental results confirmed that FgTri101 transgenic plants can acetylate DON to 3-ADON, as well as 15-ADON to 3,15-diDON (Hao et al., 2021). Furthermore, Wang et al. found that 3-ADON and 15-ADON can be deacetylated to produce DON, which is then degraded into 3-keto-DON (Wang N. et al., 2019).
4.2.2.2. Oxidoreductases
The C12-C13 epoxy structure in DON is the key determinant of its toxicity, and its conversion is accordingly crucial for degradation. Studies have found that resistance genes or secreted proteins in some microorganisms destroy the epoxy structure of DON. For example, Bacillus sp. LS100 can destroy the epoxy structure, thereby degrading DON into DOM-1 (Li et al., 2011). In addition, the C3 hydroxyl group of DON can be oxidized to generate the corresponding ketone group. Zhang et al. screened a new strain, Pelagibacterium halotolerans ANSP101, which contains a protein that can oxidize the C3 hydroxyl group and convert DON into the less toxic, 3-keto-DON (Zhang J. et al., 2020). The C3 hydroxyl group of DON was isomerized to form 3-epi-DON. This isomerization requires two enzymatic actions: the conversion of DON into 3-oxo-DON, and that of 3-oxo-DON to 3-epi-DON (Hassan et al., 2017). In addition, resistance genes have also attracted much attention in studies on the degradation of DON. Ito et al. used the KSM1 gene library to screen out that the P450 gene (ddnA), is part of an electron transport chain in vitro that decomposes DON into the less toxic, 16-hydroxy-deoxynivalenol (16-HDON), thereby reducing the harm to wheat and other grains (Ito et al., 2013).
4.2.2.3. Glycosylation
Poppenberger et al. discovered that the UDP-glycosyltransferase from Arabidopsis thaliana can catalyze the transfer of glucose from UDP-glucose to the C3 hydroxyl group of DON to form 3-β-D-glucopyranosyl-4-DON. The overexpression of this enzyme increases the resistance of Arabidopsis thaliana to DON (Poppenberger et al., 2003). Later, it was found that the overexpression of HvUGT13248 (UDP glycosyltransferase) could improve the resistance of wheat and Brachypodium distachyon to DON (Li X. et al., 2015; Pasquet et al., 2016).
4.2.2.4. Other enzymes
In addition to the DON-converting enzymes mentioned above, there are also some DON degradation processes and degradation products that are still not fully understood. For example, Gardiner et al. analyzed the RNA profile of barley ears treated with DON and found that glutathione-S-transferase gene transcription was significantly upregulated (Gardiner et al., 2015). JuoDeIkiene et al. found that the combination of β-xylanase and traditional amylolytic enzymes can degrade 41% of DON at high concentrations (3.95 mg/kg), and can improve distillers dried grains with solubles without affecting the quality of bioethanol (JuoDeIkiene et al., 2011).
Enzymatic detoxification technology has the characteristics of good repeatability, uniformity, simple operation, and high safety. Therefore, enzymatic degradation is considered the most commercially viable and promising technology. However, the enzymatic hydrolysis of DON is still in its infancy, and there is still a long way to the discovery and optimization of new degradation enzymes. Adding to the challenge, the purification of detoxification enzymes is a time- and labor-consuming process, while the large-scale application of natural enzymes is limited because of the easy deactivation of natural enzymes in industrial environments such as strong acids, strong alkalis, and high temperatures. Therefore, cloning new DON-degrading enzyme genes is a promising approach. In addition, immobilizing enzymes and improving their physicochemical properties using biomaterials synthesized by biomimetic mineralization may also prove to be a worthwhile research direction.
5. Conclusion
DON is a widely distributed mycotoxin that frequently contaminates crops and animal feed. In contrast to traditional physical and chemical methods, biological methods can effectively convert DON into less toxic or even completely harmless products. However, there are still some problems that need to be solved: (1) the degradation mechanisms of many microorganisms and the toxicity of the degradation products remain unclear, which limits their application potential; (2) the biosafety and DON degradation pathways of most detoxifying bacteria have not been clarified; (3) there are still few studies on the cloning and functional expression of genes responsible for the DON detoxification phenotype. Therefore, an in-depth study on detoxification mechanisms is needed to provide reliable theoretical support for the application of detoxifying bacteria and enzymes. Increasing numbers of studies are focusing on genomic, transcriptomic, and proteomic analyses, which offer fast and efficient tools for the study of DON detoxification pathways as well as the cloning and expression of related genes. In the future, an in-depth understanding of the specific mechanisms through which DON is detoxified will provide efficient, safe, and economical means for the removal of this problematic mycotoxin from grains and feed.
Author contributions
YL and RW: research literature and writing-original draft. HG: manuscript revision and literature review. QX: resources, investigation, supervision, conceptualization, and project administration. All authors contributed to the article and approved the submitted version.
Funding
This work was financially supported by National Key Research and Development Program of China (No. 2020YFC1606800) and the China Postdoctoral Science Foundation (2021M691624).
Conflict of interest
The authors declare that the research was conducted in the absence of any commercial or financial relationships that could be construed as a potential conflict of interest.
Publisher’s note
All claims expressed in this article are solely those of the authors and do not necessarily represent those of their affiliated organizations, or those of the publisher, the editors and the reviewers. Any product that may be evaluated in this article, or claim that may be made by its manufacturer, is not guaranteed or endorsed by the publisher.
References
Abdel-Wahhab, M. A., El-Kady, A. A., Hassan, A. M., Abd El-Moneim, O. M., and Abdel-Aziem, S. H. (2015). Effectiveness of activated carbon and Egyptian montmorillonite in the protection against deoxynivalenol-induced cytotoxicity and genotoxicity in rats. Food Chem. Toxicol. 83, 174–182. doi: 10.1016/j.fct.2015.06.015
Adunphatcharaphon, S., Petchkongkaew, A., Greco, D., D'Ascanio, V., Visessanguan, W., and Avantaggiato, G. (2020). The effectiveness of durian Peel as a multi-Mycotoxin adsorbent. Toxins 12:108. doi: 10.3390/toxins12020108
Arifin, A. A., Setyabudi, F. M. C. S., and Sardjono, S. (2019). Local strains Aspergillus oryzae KKB4 and Rhizopus oryzae KP1R1 as a reducing and detoxifying agents for deoxynivalenol. Malaysian J. Microbiol. 15, 182–187. doi: 10.21161/mjm.180155
Awika, J. M. J. A. S. S. (2011). Major Cereal Grains Production and Use around the World. ACS Symposium Series. 1089, 1–13.
Bakutis, B. A. B., Violeta, K., and Paokevieius, A. (2005). Use of biological method for detoxification of Mycotoxins. Botanica Lithuanica 7, 123–129.
Borràs-Vallverdú, B., Ramos, A. J., Marín, S., Sanchis, V., and Rodríguez-Bencomo, J. J. (2020). Deoxynivalenol degradation in wheat kernels by exposition to ammonia vapours: a tentative strategy for detoxification. Food Control 118:107444. doi: 10.1016/j.foodcont.2020.107444
Brera, C., Debegnach, F., Grossi, S., and Miraglia, M. (2004). Effect of industrial processing on the distribution of fumonisin B1 in dry milling corn fractions. J. Food Prot. 67, 1261–1266. doi: 10.4315/0362-028x-67.6.1261
Bretz, M., Beyer, M., Cramer, B., Knecht, A., and Humpf, H. U. (2006). Thermal degradation of the Fusarium mycotoxin deoxynivalenol. J. Agric. Food Chem. 54, 6445–6451. doi: 10.1021/jf061008g
Bullerman, L. B., and Bianchini, A. (2007). Stability of mycotoxins during food processing. Int. J. Food Microbiol. 119, 140–146. doi: 10.1016/j.ijfoodmicro.2007.07.035
Cao, H., Wu, S., and Sun, C. (2013). Research advancement on biosynthesis and biodegradation of Deoxynivalenol (DON). J. Chin. Cereals Oils Assoc. 28, 116–123.
Chen, W., Li, C., Zhang, B., Zhou, Z., Shen, Y., Liao, X., et al. (2018). Advances in biodetoxification of Ochratoxin A-A review of the past five decades. Front. Microbiol. 9:1386. doi: 10.3389/fmicb.2018.01386
Cheng, B., Wan, C., Yang, S., Xu, H., Wei, H. U. A., Liu, J., et al. (2010). Detoxification of Deoxynivalenol by bacillus strains. J. Food Saf. 30, 599–614. doi: 10.1111/j.1745-4565.2010.00228.x
Chlebicz, A., and Slizewska, K. (2020). In vitro detoxification of Aflatoxin B1, Deoxynivalenol, Fumonisins, T-2 toxin and Zearalenone by probiotic bacteria from genus lactobacillus and Saccharomyces cerevisiae yeast. Probiotics Antimicrob Proteins 12, 289–301. doi: 10.1007/s12602-018-9512-x
Danicke, S., Pahlow, G., Beyer, M., Goyarts, T., Breves, G., Valenta, H., et al. (2010). Investigations on the kinetics of the concentration of deoxynivalenol (DON) and on spoilage by moulds and yeasts of wheat grain preserved with sodium metabisulfite (Na2S2O5, SBS) and propionic acid at various moisture contents. Arch. Anim. Nutr. 64, 190–203. doi: 10.1080/17450391003693159
Danicke, S., Pahlow, G., Goyarts, T., Rohweder, D., Wilkerling, K., Breves, G., et al. (2009). Effects of increasing concentrations of sodium metabisulphite (Na2S 2O 5, SBS) on deoxynivalenol (DON) concentration and microbial spoilage of triticale kernels preserved without and with propionic acid at various moisture contents. Mycotoxin Res 25, 215–223. doi: 10.1007/s12550-009-0030-2
Danicke, S., Valenta, H., Gareis, M., Lucht, H. W., and Reichenbach, H. (2005). On the effects of a hydrothermal treatment of deoxynivalenol (DON)-contaminated wheat in the presence of sodium metabisulphite (Na2S2O5) on DON reduction and on piglet performance. Anim. Feed Sci. Technol. 118, 93–108. doi: 10.1016/j.anifeedsci.2004.09.011
El-Banna, A. A., Lau, P. Y., and Scott, P. M. (1983). Fate of Mycotoxins during processing of foodstuffs II–Deoxynivalenol (Vomitoxin) during making of Egyptian bread. J. Food Prot. 46, 484–486. doi: 10.4315/0362-028X-46.6.484
Elmnasser, N., Guillou, S., Leroi, F., Orange, N., Bakhrouf, A., and Federighi, M. (2007). Pulsed-light system as a novel food decontamination technology: a review. Can. J. Microbiol. 53, 813–821. doi: 10.1139/W07-042
El-Nezami, H. S., Chrevatidis, A., Auriola, S., Salminen, S., and Mykkanen, H. (2002). Removal of common Fusarium toxins in vitro by strains of lactobacillus and Propionibacterium. Food Addit. Contam. 19, 680–686. doi: 10.1080/02652030210134236
Ergang, Y. Z. D. K. L. S. L. Y. L. W. L. Y. C. P. L. Y. S. (2016). Screening and identification of a Bacillus cereus strain able to Degradate Deoxynivalenol. Food Sci. 37, 121–125. doi: 10.7506/spkx1002-6630-201605022
Feizollahi, E., and Roopesh, M. S. (2022). Mechanisms of deoxynivalenol (DON) degradation during different treatments: a review. Crit. Rev. Food Sci. Nutr. 62, 5903–5924. doi: 10.1080/10408398.2021.1895056
Fellone, J. (2016). IARC Press Release N°242 / Comunicato stampa N°242 della IARC UN NUOVO RAPPORTO DELLA IARC ESORTA AD AGIRE CONTRO LA DIFFUSA CONTAMINAZIONE DA MICOTOSSINE NEI PAESI IN VIA DI SVILUPPO pubblicato il 17 Febbraio 2016. Lyon, France.
Freire, L., and Sant’Ana, A. S. (2018). Modified mycotoxins: an updated review on their formation, detection, occurrence, and toxic effects. Food Chem. Toxicol. 111, 189–205. doi: 10.1016/j.fct.2017.11.021
Gao, X., Mu, P., Wen, J., Sun, Y., Chen, Q., and Deng, Y. (2018). Detoxification of trichothecene mycotoxins by a novel bacterium, Eggerthella sp. DII-9. Food Chem. Toxicol. 112, 310–319. doi: 10.1016/j.fct.2017.12.066
Gao, X., Mu, P., Zhu, X., Chen, X., Tang, S., Wu, Y., et al. (2020). Dual function of a novel bacterium, Slackia sp. D-G6: Detoxifying Deoxynivalenol and Producing the Natural Estrogen Analogue. Equol. Toxins 12. doi: 10.3390/toxins12020085
Garda-Buffon, J., and Badiale-Furlong, E. (2010). Kinetics of deoxynivalenol degradation by Aspergillus oryzae and Rhizopus oryzae in submerged fermentation. J. Braz. Chem. Soc. 21, 710–714. doi: 10.1590/s0103-50532010000400018
Gardiner, S. A., Boddu, J., Berthiller, E., Hametner, C., Stupar, R. M., Adam, G., et al. (2015). Transcriptome analysis of the barley-deoxynivalenol interaction: Evidence for a Role of Glutathione in Deoxynivalenol Detoxification. 12, 962–976. doi: 10.1094/MPMI-23-7-0962
Golge, O., and Kabak, B. (2020). Occurrence of deoxynivalenol and zearalenone in cereals and cereal products from Turkey. Food Control 110:106982. doi: 10.1016/j.foodcont.2019.106982
Guo, H., Ji, J., Wang, J., and Sun, X. (2020). Deoxynivalenol: masked forms, fate during food processing, and potential biological remedies. Compr. Rev. Food Sci. Food Saf. 19, 895–926. doi: 10.1111/1541-4337.12545
Han, X., Chen, L., Li, W., Zhang, L., and Dong, H. J. F. G. (2020). Endocytic FgEde1 regulates virulence and autophagy in Fusarium graminearum. Fungal Genet. Biol. 141:103400. doi: 10.1016/j.fgb.2020.103400
Hao, G., McCormick, S., Tiley, H., and Usgaard, T. (2021). Detoxification and excretion of Trichothecenes in transgenic Arabidopsis thaliana expressing Fusarium graminearum Trichothecene 3-O-acetyltransferase. Toxins 13:320. doi: 10.3390/toxins13050320
Hassan, Y. I., He, J. W., Perilla, N., Tang, K. J., Karlovsky, P., and Zhou, T. J. R. (2017). The enzymatic epimerization of deoxynivalenol by Devosia mutans proceeds through the formation of 3-keto-DON intermediate. Sci. Rep. 7:6929. doi: 10.1038/s41598-017-07319-0
Hathout, A. S., and Aly, S. E. (2014). Biological detoxification of mycotoxins: a review. Ann. Microbiol. 64, 905–919. doi: 10.1007/s13213-014-0899-7
Haus, M., Žatko, D., Vašková, J., and Vaško, L. (2020). The effect of humic acid in chronic deoxynivalenol intoxication. Environ. Sci. Pollut. Res. 28, 1612–1618. doi: 10.1007/s11356-020-10581-x
He, J. W., Bondy, G. S., Zhou, T., Caldwell, D., Boland, G. J., and Scott, P. M. (2015). Toxicology of 3-epi-deoxynivalenol, a deoxynivalenol-transformation product by Devosia mutans 17-2-E-8. Food Chem. Toxicol. 84, 250–259. doi: 10.1016/j.fct.2015.09.003
He, C., Fan, Y., Liu, G., and Zhang, H. (2008). Isolation and identification of a strain of Aspergillus Tubingensis with Deoxynivalenol biotransformation capability. Int. J. Mol. Sci. 9, 2366–2375. doi: 10.3390/ijms9122366
He, J. W., Hassan, Y. I., Perilla, N., Li, X. Z., Boland, G. J., and Zhou, T. (2016). Bacterial epimerization as a route for Deoxynivalenol detoxification: the influence of growth and environmental conditions. Front. Microbiol. 7:572. doi: 10.3389/fmicb.2016.00572
He, W. J., Zhang, L., Yi, S. Y., Tang, X. L., Yuan, Q. S., Guo, M. W., et al. (2017). An aldo-keto reductase is responsible for Fusarium toxin-degrading activity in a soil Sphingomonas strain. Sci. Rep. 7:9549. doi: 10.1038/s41598-017-08799-w
Hojnik, N., Modic, M., Tavcar-Kalcher, G., Babic, J., Walsh, J. L., and Cvelbar, U. (2019). Mycotoxin decontamination efficacy of atmospheric pressure air plasma. Toxins 11:219. doi: 10.3390/toxins11040219
Humpf, H., Voss, K. A. J. M. N., and Research, F. (2004). Effects of thermal food processing on the chemical structure and toxicity of fumonisin mycotoxins. Mol. Nutr. Food Res. 48, 255–269. doi: 10.1002/mnfr.200400033
Huwig, A., Freimund, S., Kappeli, O., and Dutler, H. (2001). Mycotoxin detoxication of animal feed by different adsorbents. Toxicol. Lett. 122, 179–188. doi: 10.1016/S0378-4274(01)00360-5
Ikunaga, Y., Sato, I., Grond, S., Numaziri, N., Yoshida, S., Yamaya, H., et al. (2011). Nocardioides sp. strain WSN05-2, isolated from a wheat field, degrades deoxynivalenol, producing the novel intermediate 3-epi-deoxynivalenol. Appl. Microbiol. Biotechnol. 89, 419–427. doi: 10.1007/s00253-010-2857-z
Ito, M., Sato, I., Ishizaka, M., Yoshida, S., Koitabashi, M., Yoshida, S., et al. (2013). Bacterial cytochrome P450 system catabolizing the Fusarium toxin deoxynivalenol. Appl. Environ. Microbiol. 79, 1619–1628. doi: 10.1128/AEM.03227-12
Jun Shima, S. T., Takahashi, Y., Iwai, Y., Fujimoto, H., Yamazaki, M., and Ochi, A. K. (1997). Novel detoxification of the trichothecene mycotoxin deoxynivalenol by a soil bacterium isolated by enrichment culture. Applied and environmental microbiology, 63, 3825–3830.
Junmei, X. H. L. H. Z. D. X. L. Y. D. G. (2013). Screening of bacillus capable of simultaneously degrading vomitotoxin, zearalenone and aflatoxin. Nutrition And Feed. 10, 72–74. China (BeiJing).
JuoDeIkiene, G., Basinskiene, L., Vidmantiene, D., Makaravicius, T., and Bartkiene, E. J. J. O. T. E. O. F., Agriculture (2011). Benefits of β-xylanase for wheat biomass conversion to bioethanol. J. Sci. Food Agric. 92, 84–91. doi: 10.1002/jsfa.4545
Karaca, H., Velioglu, Y. S., and Nas, S. (2010). Mycotoxins: contamination of dried fruits and degradation by ozone. Toxin Rev. 29, 51–59. doi: 10.3109/15569543.2010.485714
Karlovsky, P. (2011). Biological detoxification of the mycotoxin deoxynivalenol and its use in genetically engineered crops and feed additives. Appl. Microbiol. Biotechnol. 91, 491–504. doi: 10.1007/s00253-011-3401-5
Kells, S. A., Mason, L. J., Maier, D. E., and Woloshuk, C. P. (2001). Efficacy and fumigation characteristics of ozone in stored maize. J. Stored Prod. Res. 37, 371–382. doi: 10.1016/s0022-474x(00)00040-0
Li, M. M., Guan, E. Q., and Bian, K. (2015). Effect of ozone treatment on deoxynivalenol and quality evaluation of ozonised wheat. Food Addit. Contam. Part A Chem. Anal. Control Expo. Risk Assess. 32, 544–553. doi: 10.1080/19440049.2014.976596
Li, M., Guan, E., and Bian, K. (2019a). Detoxification of Deoxynivalenol by 60Co γ-ray irradiation and toxicity analyses of radiolysis products. J. AOAC Int. 102, 1749–1755. doi: 10.5740/jaoacint.19-0246
Li, M., Guan, E., and Bian, K. (2019b). Structure elucidation and toxicity analysis of the degradation products of Deoxynivalenol by gaseous ozone. Toxins 11:474. doi: 10.3390/toxins11080474
Li, X., Shin, S., Heinen, S., Dill-Macky, R., Berthiller, F., Nersesian, N., et al. (2015). Transgenic wheat expressing a barley UDP-Glucosyltransferase detoxifies Deoxynivalenol and provides high levels of resistance to Fusarium graminearum. Mol. Plant Microbe Interact. 28, 1237–1246. doi: 10.1094/mpmi-03-15-0062-r
Li, X. Z., Zhu, C., Lange, C., Zhou, T., and Young, J. (2011). Efficacy of detoxification of deoxynivalenol-contaminated corn by bacillus sp. LS100 in reducing the adverse effects of the mycotoxin on swine growth performance. Food Addit Contam Part A Chem Anal Control Expo Risk Assess 28, 894–901. doi: 10.1080/19440049.2011.576402
Lu, P., Yu, C., Liu, S., Gong, J., Liu, S., and Yang, C. (2020). Evaluating detoxification efficacy of deoxynivalenol by encapsulated sodium metabisulfite (SMBS) using an in vitro intestinal epithelial cell IPEC-J2 model. J. Anim. Sci. 98, 38–39. doi: 10.1093/jas/skaa278.069
Magan, N., Hope, R., Cairns, V., and Aldred, D. (2003). Post-harvest fungal ecology: impact of fungal growth and mycotoxin accumulation in stored grain. Eur. J. Plant Pathol. 109, 723–730. doi: 10.1023/A:1026082425177
McCormick, A., and Susan, P. J. J. O. C. E. (2013). Microbial Detoxification of Mycotoxins. J. Chem. Ecol. 39, 907–918. doi: 10.1007/s10886-013-0321-0
Mishra, S., Srivastava, S., Dewangan, J., Divakar, A., and Rath, S. K. J. C. R. I. F. S., Nutrition (2019). Global occurrence of deoxynivalenol in food commodities and exposure risk assessment in humans in the last decade: a survey. Crit. Rev. Food Sci. Nutr. 60, 1–29. doi: 10.1080/10408398.2019.1571479
Misra, N. N., Yadav, B., Roopesh, M. S., and Jo, C. (2019). Cold plasma for effective fungal and Mycotoxin control in foods: mechanisms, inactivation effects, and applications. Compr. Rev. Food Sci. Food Saf. 18, 106–120. doi: 10.1111/1541-4337.12398
Moreau, M., Lescure, G., Agoulon, A., Svinareff, P., Orange, N., and Feuilloley, M. (2013). Application of the pulsed light technology to mycotoxin degradation and inactivation. J. Appl. Toxicol. 33, 357–363. doi: 10.1002/jat.1749
Murata, H., Yamaguchi, D., Nagai, A., and Shimada, N. (2011). Reduction of deoxynivalenol contaminating corn silage by short-term ultraviolet irradiation: a pilot study. J. Vet. Med. Sci. 73, 1059–1060. doi: 10.1292/jvms.10-0409
Nathanail, A. V., Gibson, B., Han, L., Peltonen, K., Ollilainen, V., Jestoi, M., et al. (2016). The lager yeast saccharomyces pastorianus removes and transforms Fusarium trichothecene mycotoxins during fermentation of brewer's wort. Food Chem. 203, 448–455. doi: 10.1016/j.foodchem.2016.02.070
Niderkorn, V., Boudra, H., and Morgavi, D. P. (2006). Binding of Fusarium mycotoxins by fermentative bacteria in vitro. J. Appl. Microbiol. 101, 849–856. doi: 10.1111/j.1365-2672.2006.02958.x
Niderkorn, V., Morgavi, D. P., Pujos, E., Tissandier, A., and Boudra, H. (2007). Screening of fermentative bacteria for their ability to bind and biotransform deoxynivalenol, zearalenone and fumonisins in an in vitro simulated corn silage model. Food Addit. Contam. 24, 406–415. doi: 10.1080/02652030601101110
Pasquet, J.-C., Changenet, V., Macadre, C., Boex-Fontvieille, E., Soulhat, C., Bouchabke-Coussa, O., et al. (2016). A Brachypodium UDP-Glycosyltransferase confers root tolerance to Deoxynivalenol and resistance to Fusarium infection. Plant Physiol. 172, 559–574. doi: 10.1104/pp.16.00371
Pestka, J. J. J. A. O. T. (2010). Deoxynivalenol: mechanisms of action, human exposure, and toxicological relevance. Arch. Toxicol. 84, 663–679. doi: 10.1007/s00204-010-0579-8
Pestka, J. J., Zhou, H. R., Moon, Y., and Chung, Y. J. (2004). Cellular and molecular mechanisms for immune modulation by deoxynivalenol and other trichothecenes: unraveling a paradox. Toxicol. Lett. 153, 61–73. doi: 10.1016/j.toxlet.2004.04.023
Pierron, A., Mimoun, S., Murate, L. S., Loiseau, N., Lippi, Y., Bracarense, A.-P. F. L., et al. (2016). Microbial biotransformation of DON: molecular basis for reduced toxicity. Sci. Rep. 6:29105. doi: 10.1038/srep29105
Poppenberger, B., Berthiller, F., Lucyshyn, D., Sieberer, T., Schuhmacher, R., Krska, R., et al. (2003). Detoxification of the Fusarium mycotoxin deoxynivalenol by a UDP-glucosyltransferase from Arabidopsis thaliana. J. Biol. Chem. 278, 47905–47914. doi: 10.1074/jbc.M307552200
Qu, R., Jiang, C., Wu, W., Pang, B., Lei, S., Lian, Z., et al. (2019). Conversion of DON to 3-epi-DON in vitro and toxicity reduction of DON in vivo by lactobacillus rhamnosus. Food Funct. 10, 2785–2796. doi: 10.1039/c9fo00234k
Ren, D., Diao, E., Hou, H., and Dong, H. (2020). Degradation and ozonolysis pathway elucidation of deoxynivalenol. Toxicon 174, 13–18. doi: 10.1016/j.toxicon.2019.11.015
Romina Garcia, G., Payros, D., Pinton, P., Ana Dogi, C., Laffitte, J., Neves, M., et al. (2018). Intestinal toxicity of deoxynivalenol is limited by lactobacillus rhamnosus RC007 in pig jejunum explants. Arch. Toxicol. 92, 983–993. doi: 10.1007/s00204-017-2083-x
Savi, G. D., Cardoso, W. A., Furtado, B. G., Bortolotto, T., Zanoni, E. T., Scussel, R., et al. (2018). Antifungal activities against toxigenic Fusarium specie and deoxynivalenol adsorption capacity of ion-exchanged zeolites. J. Environ. Sci. Health Part B Pesticides Food Contaminants Agric. Wastes 53, 184–190. doi: 10.1080/03601234.2017.1405639
Scott, P. M., Kanhere, S. R., Dexter, J. E., Brennan, P. W., and Trenholm, H. L. J. F. A. C. (1984). Distribution of the trichothecene mycotoxin deoxynivalenol (vomitoxin) during the milling of naturally contaminated hard red spring wheat and its fate in baked products. Food Addit. Contam. 1, 313–323. doi: 10.1080/02652038409385862
Shalapy, A., Zhao, S., Zhang, C., Li, Y., Geng, H., Ullah, S., et al. (2020). Adsorption of Deoxynivalenol (DON) from corn steep liquor (CSL) by the microsphere adsorbent SA/CMC loaded with calcium. Toxins 12:208. doi: 10.3390/toxins12040208
Shehata, S., Richter, W. I. F., Schuster, M., Scholz, W., and Nowar, M. S. J. M. R. (2000). Adsorption of ochratoxin A, deoxynivalenol and zearalenonein vitro at different pH and adsorbents. Mycotoxin Res. 16, 136–140. doi: 10.1007/BF02943002
Shima, J., Takase, S., Takahashi, Y., Iwai, Y., Fujimoto, H., Yamazaki, M., et al. (1997). Novel detoxification of the trichothecene mycotoxin deoxynivalenol by a soil bacterium isolated by enrichment culture. Appl. Environ. Microbiol. 63, 3825–3830. doi: 10.1128/AEM.63.10.3825-3830.1997
Sidhu, J. S., Kabir, Y., and Huffman, F. G. (2007). Functional foods from cereal grains. Int. J. Food Prop. 10, 231–244. doi: 10.1080/10942910601045289
Stepanik, T., Kost, D., Nowicki, T., and Gabay, D. (2007). Effects of electron beam irradiation on deoxynivalenol levels in distillers dried grain and solubles and in production intermediates. Food Addit. Contam. 24, 1001–1006. doi: 10.1080/02652030701329629
Tan, J., Yang, S., Hui-Bo, S. U., Yan-Dong, W. U., and Tong, Y. (2018). Identification of a bacillus subtilis strain with deoxynivalenol degradation ability. Contemp. Chem. Industry. 47, 547–551. doi: 10.13840/j.cnki.cn21-1457/tq.2018.03.029
Ten Bosch, L., Pfohl, K., Avramidis, G., Wieneke, S., Viol, W., and Karlovsky, P. (2017). Plasma-based degradation of Mycotoxins produced by Fusarium, Aspergillus and Alternaria species. Toxins 9:97. doi: 10.3390/toxins9030097
Tiwari, B. K., Brennan, C. S., Curran, T., Gallagher, E., Cullen, P. J., and O'Donnell, C. P. (2010). Application of ozone in grain processing. J. Cereal Sci. 51, 248–255. doi: 10.1016/j.jcs.2010.01.007
Topi, D., Babic, J., Pavsic-Vrtac, K., Tavcar-Kalcher, G., and Jakovac-Strajn, B. (2021). Incidence of Fusarium Mycotoxins in wheat and maize from Albania. Molecules 26:172. doi: 10.3390/molecules26010172
Tran, S. T., and Smith, T. K. (2014). Conjugation of deoxynivalenol by Alternaria alternata (54028 NRRL), Rhizopus microsporus var. rhizopodiformis (54029 NRRL) and Aspergillus oryzae (5509 NRRL). Mycotoxin Res 30, 47–53. doi: 10.1007/s12550-013-0184-9
Trenholm, H. L. C., and Lynne, L.,Prelusky, D. B.,Warner, R. M.(1991). Two physical methods for the decontamination of four cereals contaminated with deoxynivalenol and zearalenone. J. Agric. Food Chem. 39, 356–360. doi: 10.1021/jf00002a026
Tsuge, N. (2014). Summary of small research-discussion on cereal grains. J. Japan. Soc. Food Sci. Technol. 61, 555–556. doi: 10.3136/nskkk.61.555
Wang, L., Luo, Y., Luo, X., Wang, R., Li, Y., Li, Y., et al. (2016). Effect of deoxynivalenol detoxification by ozone treatment in wheat grains. Food Control 66, 137–144. doi: 10.1016/j.foodcont.2016.01.038
Wang, Y., Wang, G., Dai, Y., Wang, Y., Lee, Y.-W., Shi, J., et al. (2020b). Biodegradation of Deoxynivalenol by a novel microbial consortium. Front. Microbiol. 10:2964. doi: 10.3389/fmicb.2019.02964
Wang, G., Wang, Y., Ji, F., Xu, L., Yu, M., Shi, J., et al. (2019). Biodegradation of deoxynivalenol and its derivatives by Devosia insulae A16. Food Chem. 276, 436–442. doi: 10.1016/j.foodchem.2018.10.011
Wang, G., Wang, Y., Man, H., Lee, Y.-W., Shi, J., and Xu, J. (2020). Metabolomics-guided analysis reveals a two-step epimerization of deoxynivalenol catalyzed by the bacterial consortium IFSN-C1. Appl. Microbiol. Biotechnol. 104, 6045–6056. doi: 10.1007/s00253-020-10673-1
Wang, N., Wu, W., Pan, J., and Long, M. (2019). Detoxification strategies for Zearalenone using microorganisms: A Review. Microorganisms 7:208. doi: 10.3390/microorganisms7070208
Wang, Y., Zhao, C., Zhang, D., Zhao, M., Peng, M., Guo, P., et al. (2020a). Microbial degradation of Zearalenone by a novel microbial consortium, NZDC-6, and its application on contaminated corncob by semisolid fermentation. J. Agric. Food Chem. 68, 1634–1644. doi: 10.1021/acs.jafc.9b05343
Wang, Y., Zhao, C., Zhang, D., Zhao, M., Zheng, D., Lyu, Y., et al. (2017). Effective degradation of aflatoxin B1 using a novel thermophilic microbial consortium TADC7. Bioresour. Technol. 224, 166–173. doi: 10.1016/j.biortech.2016.11.033
Weber, A., Mery, L., Nagy, P., Polgar, C., Bray, F., and Kenessey, I. (2023). Evaluation of data quality at the Hungarian National Cancer Registry, 2000-2019. Cancer Epidemiol. 82:102306. doi: 10.1016/j.canep.2022.102306
Wu, N., Ou, W., Zhang, Z., Wang, Y., Xu, Q., and Huang, H. (2020). Recent advances in detoxification strategies for zearalenone contamination in food and feed. Chin. J. Chem. Eng. 30, 168–177. doi: 10.1016/j.cjche.2020.11.011
Yener, S., and Köksel, H. (2013). Effects of washing and drying applications on deoxynivalenol and zearalenone levels in wheat. World Mycotoxin J. 6, 335–341. doi: 10.3920/wmj2013.1544
Young, J. C. (1986). Reduction in levels of deoxynivalenol in contaminated corn by chemical and physical treatment. J. Agric. Food Chem. 34, 465–467. doi: 10.1021/jf00069a022
Yu, H., Zhou, T., Gong, J., Young, C., Su, X., Li, X. Z., et al. (2010). Isolation of deoxynivalenol-transforming bacteria from the chicken intestines using the approach of PCR-DGGE guided microbial selection. BMC Microbiol. 10:182. doi: 10.1186/1471-2180-10-182
Yuan, X., Ma, L., Wen, B., Zhou, D., Kuang, M., Yang, W., et al. (2016). Enhancing anaerobic digestion of cotton stalk by pretreatment with a microbial consortium (MC1). Bioresour. Technol. 207, 293–301. doi: 10.1016/j.biortech.2016.02.037
Zhai, Y., Hu, S., Zhong, L., Lu, Z., Bie, X., Zhao, H., et al. (2019b). Characterization of Deoxynivalenol detoxification by lactobacillus paracasei LHZ-1 isolated from yogurt. J. Food Prot. 82, 1292–1299. doi: 10.4315/0362-028X.JFP-18-581
Zhai, Y., Zhong, L., Gao, H., Lu, Z., Bie, X., Zhao, H., et al. (2019a). Detoxification of Deoxynivalenol by a mixed culture of soil bacteria with 3-epi-Deoxynivalenol as the Main intermediate. Front. Microbiol. 10:2172. doi: 10.3389/fmicb.2019.02172
Zhang, L., Ma, R., Zhu, M. X., Zhang, N. Y., Liu, X. L., Wang, Y. W., et al. (2020). Effect of deoxynivalenol on the porcine acquired immune response and potential remediation by a novel modified HSCAS adsorbent. Food Chem. Toxicol. 138:111187. doi: 10.1016/j.fct.2020.111187
Zhang, J., Qin, X., Guo, Y., Zhang, Q., Ma, Q., Ji, C., et al. (2020). Enzymatic degradation of deoxynivalenol by a novel bacterium, Pelagibacterium halotolerans ANSP101. Food Chem. Toxicol. 140:111276. doi: 10.1016/j.fct.2020.111276
Zhang, W., Zhang, L., Jiang, X., Liu, X., Li, Y., and Zhang, Y. (2020). Enhanced adsorption removal of aflatoxin B-1, zearalenone and deoxynivalenol from dairy cow rumen fluid by modified nano-montmorillonite and evaluation of its mechanism. Anim. Feed Sci. Technol. 259:114366. doi: 10.1016/j.anifeedsci.2019.114366
Zhang, Q., Zhang, Y., Liu, S., Wu, Y., Zhou, Q., Zhang, Y., et al. (2021). Adsorption of deoxynivalenol by pillared montmorillonite. Food Chem. 343:128391. doi: 10.1016/j.foodchem.2020.128391
Zhao, Z., Liu, N., Yang, L., Wang, J., Song, S., Nie, D., et al. (2015). Cross-linked chitosan polymers as generic adsorbents for simultaneous adsorption of multiple mycotoxins. Food Control 57, 362–369. doi: 10.1016/j.foodcont.2015.05.014
Keywords: deoxynivalenol, biodegradation, adsorption, enzymolysis, detoxification mechanism
Citation: Li Y, Gao H, Wang R and Xu Q (2023) Deoxynivalenol in food and feed: Recent advances in decontamination strategies. Front. Microbiol. 14:1141378. doi: 10.3389/fmicb.2023.1141378
Edited by:
Qisen Xiang, Zhengzhou University of Light Industry, ChinaReviewed by:
Xiao Li Shen, Zunyi Medical University, ChinaJianhong Xu, Jiangsu Academy of Agricultural Sciences (JAAS), China
Copyright © 2023 Li, Gao, Wang and Xu. This is an open-access article distributed under the terms of the Creative Commons Attribution License (CC BY). The use, distribution or reproduction in other forums is permitted, provided the original author(s) and the copyright owner(s) are credited and that the original publication in this journal is cited, in accordance with accepted academic practice. No use, distribution or reproduction is permitted which does not comply with these terms.
*Correspondence: Qing Xu, eHVfcWluZ0Buam51LmVkdS5jbg==