- 1Key Laboratory of Animal Immunology of the Ministry of Agriculture, Henan Provincial Key Laboratory of Animal Immunology, Henan Academy of Agricultural Sciences, Zhengzhou, Henan, China
- 2Jiangsu Co-Innovation Center for Prevention and Control of Important Animal Infectious Diseases and Zoonoses, Yangzhou University, Yangzhou, Jiangsu, China
During the co-evolution of viruses and their hosts, viruses have developed various strategies for overcoming host immunological defenses so that they can proliferate efficiently. Porcine reproductive and respiratory syndrome virus (PRRSV), a significant virus to the swine industry across the world, typically establishes prolonged infection via diverse and complicated mechanisms, which is one of the biggest obstacles for controlling the associated disease, porcine reproductive and respiratory syndrome (PRRS). In this review, we summarize the latest research on how PRRSV circumvents host antiviral responses from both the innate and adaptive immune systems and how this virus utilizes other evasion mechanisms, such as the manipulation of host apoptosis and microRNA. A thorough understanding of the exact mechanisms of PRRSV immune evasion will help with the development of novel antiviral strategies against PRRSV.
1. Introduction
Porcine reproductive and respiratory syndrome (PRRS), characterized by respiratory distress in pigs of all ages and reproductive failures in sows, is currently prevalent in all pig farming countries, and it has imposed a huge economic burden on the global pig industry every year since its emergence in the 1980s (Holtkamp et al., 2013). The causative agent of PRRS is PRRS virus (PRRSV), which is an enveloped, single-stranded, positive-sense RNA [(+) ssRNA] virus that has recently been reclassified into the genus Betaarterivirus, family Arteriviridae, order Nidovirales.1 PRRSV is grouped into two genotypes: PRRSV-1, or the European genotype (prototype Lelystad), and PRRSV-2, or the North American genotype (prototype VR-2332). PRRSV strains are characterized by extensive genetic and antigenic variation, and they recombine frequently, leading to the emergence of diverse novel strains (Wang et al., 2017; Yu et al., 2020, 2022; Sun et al., 2022). For example, the highly pathogenic PRRSV (HP-PRRSV) strain, with a 30-amino-acid (aa) deletion in non-structural protein 2 (nsp2), became endemic following a sudden outbreak in China in 2006 (Tian et al., 2007), and NADC30-like strains, characterized by a unique discontinuous 131-aa deletion in the nsp2-coding region, preferentially recombine with other PRRSV strains. This contributes to the complexity of PRRSV epidemiology, prevention, and control (Zhao et al., 2015; Zhou et al., 2015; Wang et al., 2018).
The PRRSV genome, approximately 15.4 kb in length with a 5’-cap and 3’-polyadenylated [3’-poly (A)] tail, consists of at least 11 open reading frames (ORFs): ORF1a, ORF1b, ORF2a, ORF2b, ORFs3–7, ORF5a, and ORF2TF (Fang et al., 2012). ORF1a and ORF1b occupy approximately 75% of the 5’-end of the viral genome and encode two large polyproteins, pp1a and pp1ab, which are processed into at least 16 non-structural proteins (nsps): nsp1α, nsp1β, nsp2–6, nsp2TF, nsp2N, nsp7α, nsp7β, and nsp8–12. nsp2TF and nsp2N are expressed through a –2/–1 programmed ribosomal frameshift mechanism (Fang et al., 2012; Li et al., 2019). ORF2a, ORF2b, ORFs3–7, and ORF5a occupy the 3’-end of the genome and encode glycoprotein (GP) 2, envelope (E), GP3, GP4, GP5, ORF5a, membrane (M), and nucleocapsid (N) proteins (Fang and Snijder, 2010).
Porcine reproductive and respiratory syndrome virus infection is characterized by a poor induction of the host innate immune response along with delayed protective antibody and cell-mediated immune responses, which make PRRS difficult to prevent, control, and eliminate (Lunney et al., 2016). PRRSV can cause prolonged infection in pigs for long periods of time, up to 251 days (Beyer et al., 2000; Wills et al., 2003). Thus, a comprehensive understanding of how PRRSV evades host antiviral responses will be critical to the development of novel therapies for the prevention and control of PRRS. Here, we present an overview of the host evasion strategies that are employed by PRRSV.
2. Escape from host innate immune responses
2.1. Host innate immune responses against PRRSV
The innate immune system is the first line of host defense against pathogens. Once a virus infection occurs, activation of the host innate immune system is needed to establish an antiviral state that can limit viral spread and shape the subsequent adaptive immune response (Blasius and Beutler, 2010). The host innate immune response is initiated by pattern recognition receptors (PRRs) that act as sensors to detect conserved components of pathogens, termed pathogen-associated molecular patterns (PAMPs), and then trigger a series of downstream pathways that induce the production of type I interferons (IFNs: IFN-α/β), IFN-stimulated genes (ISGs), and various inflammatory cytokines and chemokines (Takaoka and Yanai, 2006; Koyama et al., 2008).
Pathogen-associated molecular patterns are unique and conserved features present in pathogens but absent from host cells (e.g., nucleic acid sequences or RNA secondary structures) that allow a cell to distinguish self from non-self and thus activate host immune responses against the pathogens. For RNA viruses, protein and nucleic acid products of infection or replication can serve as viral PAMPs that are detected by host PRRs (Goubau et al., 2013). Host PRRs are divided into several families: Toll-like receptors (TLRs), RIG-I-like receptors (RLRs), NOD-like receptors (NLRs), C-type lectin receptors (CLRs), AIM2-like receptors (ALRs), and cyclic GMP-AMP synthase (cGAS) (Blasius and Beutler, 2010; Takeuchi and Akira, 2010; Chen and Jiang, 2013). The main PRRs for sensing RNA viruses are the TLRs (mainly TLR3 and endosomal TLR7–8) and cytosolic RLRs (RIG-I, MDA5, NLRP3, and NOD2) (Kato et al., 2006; Goubau et al., 2013). TLR3 recognizes double-stranded (ds) RNA or the intermediate RNAs generated during the replication of different viruses. TLR7 (or TLR8 in humans) recognizes ssRNA (Fitzgerald and Kagan, 2020). RIG-I recognizes relatively short duplexed regions of RNA with blunt-ended 5’-triphosphate or 5’-diphosphate, which are often present at the end of the genomic RNAs of (+) ssRNA viruses (Hornung et al., 2006; Goubau et al., 2014). Viral RNA containing complex secondary structures that can form short double-stranded structures with perfectly blunt ends or contain an A/U-rich motif in the 5’/3’ untranslated region (UTR), such as those reported in hepatitis C virus (HCV) and human immunodeficiency virus (HIV), can still be detected by RIG-I (Saito et al., 2008; Solis et al., 2011). MDA5 preferentially binds to long dsRNA (>1,000 bp) with no end specificity (Reikine et al., 2014). MDA5 is uniquely triggered during picornaviridae, caliciviridae, and coronaviridae infections or in the presence of synthetic RNA polymers consisting of poly (I:C) (Kato et al., 2006; Deddouche et al., 2014).
2.1.1. Immune sensing of PRRSV by the host
The mechanism of PRRSV recognition by the host remains incompletely understood. The research on TLR mainly focuses on the regulation of TLR expression upon PRRSV infection. A previous study indicated that the infection of pigs with PRRSV tends to increase the expression of TLR 2, 3, 4, 7, and 8 mRNAs in at least one of the lymphoid tissues or cells (Liu et al., 2009). Inhibition of TLR3, 4, 7 were observed in PAMs or/both immature DCs at 6 h post-infection (hpi), and abolished at 24 hpi (Miller et al., 2009; Chaung et al., 2010). These results might depend on the viral strain and in a timely manner (Kuzemtseva et al., 2014; Li and Mateu, 2021). Porcine TLR3 and TLR7 detect double stranded RNA (dsRNA) and single stranded RNA (ssRNA), respectively. But evidence for a direct interaction with PRRSV is lacking (Sang et al., 2008; Liu et al., 2009). In a recent study, DDX19A was identified as a novel cytosolic RNA sensor that binds PRRSV RNA to activate the NLRP3 inflammasome, resulting in interleukin (IL)-1β production (Li et al., 2015). Our previous studies found that the 3’ UTR pseudoknot region of PRRSV could act as a PAMP recognized by RIG-I and TLR3 to induce the production of type I IFNs (Xie et al., 2018).
2.2. Evasion of the innate immune response
Porcine reproductive and respiratory syndrome virus shows strict tropism for cells from the monocyte/macrophage lineage (Van Breedam et al., 2010). Infection of porcine alveolar macrophages (PAMs) with PRRSV significantly reduced the ability of these cells to respond to TLR3 ligation (Miller et al., 2009). Preliminary studies showed that PRRSV-infected PAMs exhibit delayed and low-level type I IFN activity. Levels of IFN-α are low in PRRSV-infected pigs and are not detectable in the lungs of PRRSV-infected pigs where this virus replicates prolifically (Miller et al., 2009; Kittiwan et al., 2019). PRRSV was also reported to repress type I IFN and proinflammatory cytokine responses in porcine plasmacytoid dendritic cells (pDCs), which are a major source of IFN-α and other inflammatory cytokines with a rapid reaction to virus infection (Calzada-Nova et al., 2011). However, the inhibition in pDCs was demonstrated to be strain-dependent and mostly restricted to PRRSV-2 (Baumann et al., 2013). Further research shows that, to maintain viral replication and spread, PRRSV has evolved strategies for hindering innate immune signaling; PRRSV can target any step in this process, e.g., evading PRR detection, targeting the activation of intermediate adaptors and kinases, or disrupting transcription factors and ultimately inhibiting the production of IFN, activation of ISGs and antiviral restriction factors. PRRSV is capable of repressing an elicited IFN-α response, as well as the responses of some cytokines, such as IL-2, IL-6, and tumor necrosis factor alpha (TNF-α) (Calzada-Nova et al., 2011). Several nsps and structural proteins have been identified as playing roles in the suppression of the innate immune response through different mechanisms, which have been widely reviewed (Yoo et al., 2010; Huang et al., 2015; Wang et al., 2021). Therefore, this review provides only a brief overview on this aspect of innate immune suppression by PRRSV (Figure 1).
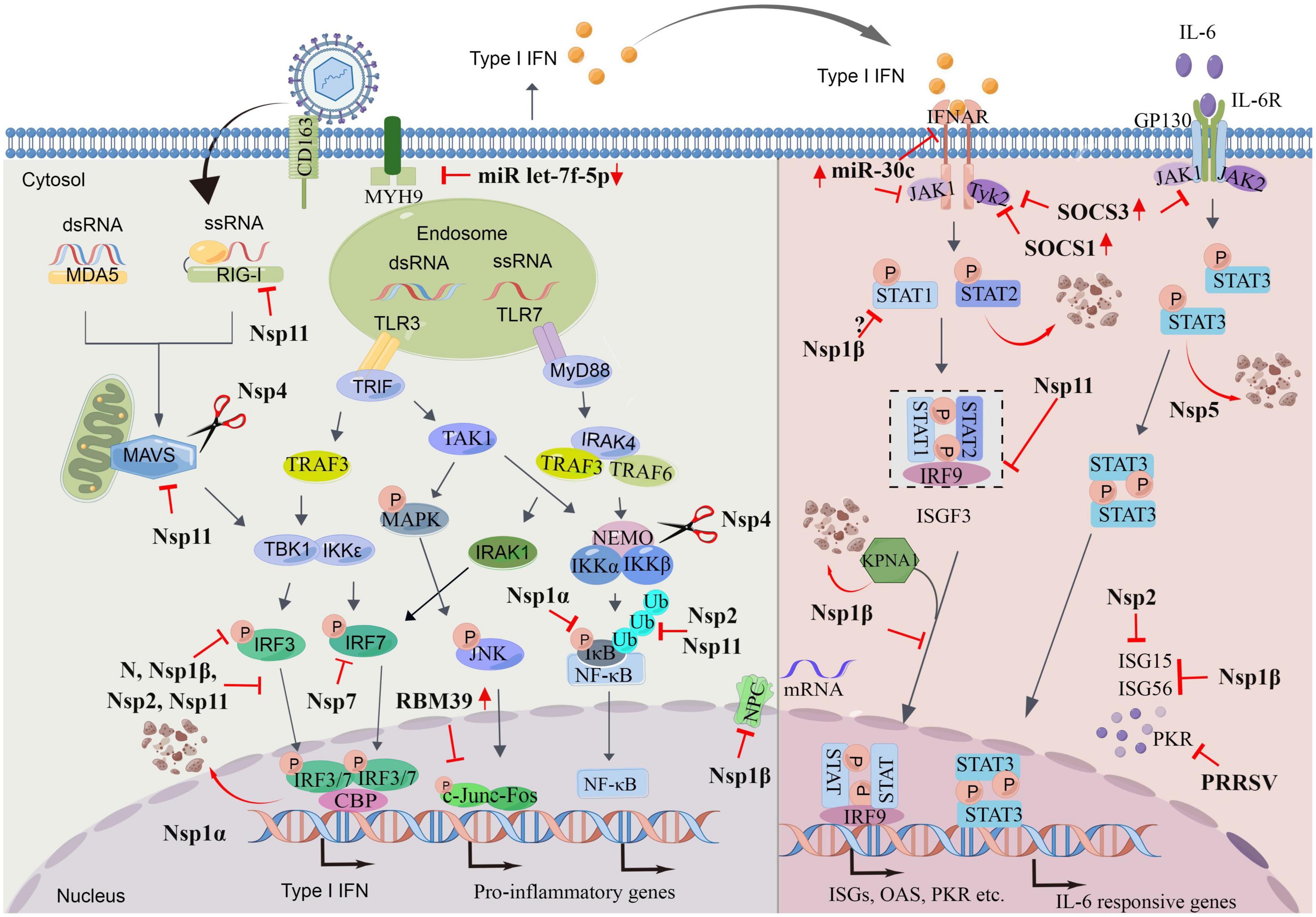
Figure 1. Porcine reproductive and respiratory syndrome virus (PRRSV) evasion strategies. PRRSV utilize numerous mechanisms to evade host antiviral immune responses. The figure depicts the actions of viral proteins upon different molecules involved interferons (IFN) and pro-inflammatory genes producing signaling pathways and IFN- Janus kinase/signal transducers and activators of transcription (JAK/STAT)-IFN-stimulated genes (ISGs) pathways. The red arrow indicates that PRRSV induces upregulation/downregulation of the protein. The scissor indicates the cleavage role of indicated protein. P, phosphate; Ub, ubiquitin. Drawn by Figdraw.
2.2.1. Targeting the IFN-producing signaling pathway
Firstly, PRRSV can evade sensing by PRRs. Arteriviruses have been reported to induce the formation of double-membrane vesicles (DMVs) carrying the viral replication complex; these DMVs might help to hide the viral RNAs from cellular detection, thus delaying IFN production (Spilman et al., 2009; Knoops et al., 2012). Secondly, PRRSV targets the activation of intermediate adaptors and kinases. For example, nsp4, a 3C-like proteinase, was shown to mediate nuclear factor-κB (NF-κB) essential modulator (NEMO) and VISA cleavage to suppress type I IFN induction (Chen et al., 2014; Huang et al., 2014, 2016). PRRSV infection inhibits the production of IFN-β by interfering with the activation of IPS-1 in the RIG-I signaling pathway or by reducing the expression of MAVS mRNA via nsp11, in a manner dependent on its endoribonuclease activity (Luo et al., 2008; Sun et al., 2016). Finally, PRRSV also can target transcriptional factors directly or indirectly, such as NF-κB, IFN-regulatory factor (IRF) 3 (IRF3), and IRF7, which are also one of the most common innate immune escape strategies of viruses. For example, nsp1α inhibits IκB phosphorylation and blocks NF-κB translocation to the nucleus, leading to the inhibition of NF-κB-stimulated genes expression (Song et al., 2010). Nsp1β inhibits IRF3 phosphorylation and nuclear translocation (Beura et al., 2010; Sagong and Lee, 2011). RNA-binding protein 39 (RBM39), which is upregulated by PRRSV, prompts PRRSV proliferation via altering c-Jun phosphorylation, nucleocytoplasmic translocation, and stabilizing and binding with viral RNA (Song et al., 2021).
2.2.2. Targeting of Janus kinase/signal transducers and activators of transcription (JAK/STAT) signaling pathways
Interferon-stimulated genes can directly antagonize the PRRSV lifecycle. Cytokines such as Type I IFNs induce the expression of ISGs by activating the phosphorylation of both STAT1 and STAT2, which form heterotrimers with IRF9 and translocate to the nucleus. PRRSV was reported to inhibit IFN–JAK/STAT signaling via blocking the nuclear translocation of STAT1/STAT2/IRF9 heterotrimers, also known as ISG factor 3 (ISGF3), thereby inhibiting the production of ISG15 and ISG56 (Chen et al., 2010; Patel et al., 2010; Wang et al., 2019). Further studies showed that nsp1β mediates the nuclear import of ISGF3 by inducing the degradation of karyopherin-α1 (KPNA1), the karyopherin for ISGF3 nuclear translocation (Wang et al., 2013b). However, whether nsp1β affects STAT1 phosphorylation is controversial and more research is needed (Chen et al., 2010; Patel et al., 2010). Nsp11 antagonizes IFN signaling via mediating STAT2 degradation (Yang et al., 2019). The N protein was found to inhibit the activity of IFNs, possibly through blocking STAT1 nuclear translocation (Wang et al., 2013a). STAT3, a pleiotropic signaling mediator of many cytokines, is involved in multiple cellular processes and host immune responses (Shuai and Liu, 2003). PRRSV nsp5 antagonizes JAK/STAT3 signaling by inducing the degradation of STAT3 via the ubiquitin–proteasomal pathway (Yang et al., 2017).
2.2.3. Targeting ISGs or other antiviral proteins
Porcine reproductive and respiratory syndrome virus also moderate the antiviral immunity through manipulating the production or function of ISGs or other antiviral proteins. For instance, porcine mRNA-decapping enzyme 1a (DCP1a) is an ISG induced by IFN-α that participates in removing the 5’-methylguanosine cap from eukaryotic mRNA. PRRSV nsp4 is responsible for cleaving DCP1a to impair its antiviral activity (Tao et al., 2018). Cholesterol-25-hydroxylase (CH25H), a conserved ISG-encoded polytopic membrane protein, has been reported to broadly inhibit the growth of many viruses (Liu et al., 2013). Nsp1β and nsp11 antagonize the antiviral activity of CH25H via lysosomal degradation (Dong et al., 2018). Protein kinase R (PKR) is an ISG whose activity is triggered by the presence of foreign dsRNA. PKR prevents virus replication by inhibiting the translation of viral mRNAs through phosphorylation of eIF2a, which is responsible for the initiation of polypeptide synthesis (Medina et al., 2020). However, PRRSV inhibits PKR activation and the PKR-mediated phosphorylation of eIF2a at the early stage after inoculation (Xiao Y. et al., 2016). Nsp1β is a stress-responsive protein, enters virus-induced stress granules (SGs) during infection, and repurposes SGs into a proviral platform, where it co-opts the SG core component G3BP1 to interact with PKR in a regulated manner (Gao et al., 2022). The latest research shows that PRRSV modulates mucosa-associated lymphoid tissue lymphoma translocation protein 1 (MALT1) expression to antagonize anti-PRRSV RNases N4BP1 and monocyte chemotactic protein-induced protein (MCPIP1) upon infection, thereby facilitating viral replication (Gu et al., 2022). PRRSV also significantly upregulates MCPIP1 expression in lungs of PRRSV-infected piglets, as well as in cells cultured in vitro to promote replication in the early stage of virus infection (Zheng et al., 2021). MCPIP1, is a broad-spectrum host antiviral protein that maintains low expression levels in most cell types and is induced by many inflammatory genes.
2.2.4. Dysregulation of natural killer (NK) cell function
Natural killer (NK) cells, an important cell population of the innate immune system, have the abilities to identify infected or transformed cells, elicit cytotoxicity, and produce immune-regulatory cytokines (Gerner et al., 2009). Pigs have relatively more NK cells than other animals and humans (Gerner et al., 2009). However, previous studies indicated that the NK-cell cytotoxicity against PRRSV-infected PAMs was suppressed from 6 to 12 h post-infection (Cao et al., 2013). Consistently, a significant reduction in NK-cell-mediated cytotoxic function in PRRSV-infected pigs was detected (Renukaradhya et al., 2010). But the insufficient NK-cell activity during PRRSV infection is not only because of the insufficient activation of NK cells (Cao et al., 2013). At present, there has been little research on this topic, and the strategies adopted by PRRSV-infected PAMs to resist NK-cell cytotoxicity are still unknown. Further studies are urgently needed to determine the underlying mechanisms.
Despite the many studies that have been conducted on PRRSV evasion of innate immunity, more research is needed to reveal the details of the underlying mechanisms because most of the previous work has lacked evidence of direct or indirect interaction.
3. Escape from adaptive immunity
The adaptive immune response is essential for the development of protective immunity against pathogen infection. However, the suppressed innate immune response in early phase of PRRSV infection can potentially lead to the generation of poor adaptive immune responses, such as abnormal B-cell and T-lymphocyte proliferation, delayed neutralizing antibody responses, and poor induction of PRRSV-specific IFN-γ-producing cells (Wongyanin et al., 2012). In addition to affecting adaptive immunity via its suppression of innate immunity, PRRSV can also directly target adaptive immunity for evasion.
3.1. Impairing antigen presentation
Dendritic cells (DCs) are potent antigen-presenting cells that deliver differentiation signals to T cells. Thus, the impairment of DC function by viruses has been proposed as a mechanism for viral persistence and represents an important virus defense strategy against host immune responses. Exposure to PRRSV did not induce DC maturation (Bordet et al., 2018; Li and Mateu, 2021). PRRSV can infect monocyte-derived DCs (Mo-DCs), resulting in reduced expression levels of major histocompatibility complex (MHC) class I (MHC-I), MHC class II (MHC-II), CD14, and CD11b/c, thus impairing their normal antigen presentation ability (Wang et al., 2007).
Other studies demonstrated that PRRSV could disrupt the swine leukocyte antigen class I (SLA-I) antigen presentation pathway. SLA-I, which is the MHC-I antigen in pigs, consists of the SLA-I heavy chain, β2-microglobulin (β2M), and a variable peptide. The SLA-I antigen presentation pathway, responsible for displaying peptide fragments for T-cell recognition, plays a critical role in initiating the host antiviral immune response. Previous studies showed that PRRSV nsp1α, nsp2TF, nsp4, and GP3 could each downregulate SLA-I expression on the cell surface (Du et al., 2015; Cao et al., 2016). Nsp1α mediates proteasomal degradation of the SLA-I heavy chain (Du et al., 2015). The reduction in SLA-I expression induced by nsp2TF involves the last 68 aa of the nsp2TF TF domain. Nsp4 reduces the SLA-I expression on the cell surface via binding to the SLA-I promoter to inhibit B2M transcription (Tao et al., 2018). Thus, disruption of the SLA-I antigen presentation pathway is employed by PRRSV as a strategy for modulating the host immune response.
3.2. Induction of regulatory T cells (Tregs)
Regulatory T cells (Tregs), the inhibitory Th lymphocytes, are responsible for modulating the immune response and maintaining homeostasis. The induction of Tregs is one of the mechanisms used by viruses such as HIV and hepatitis C virus to induce immunosuppression (Li et al., 2008). It was found that PRRSV-infected DCs significantly increased the amount of Foxp3+CD25+ T cells, which could play a role in delaying cellular immune responses in PPRSV-infected pigs during early infection (Silva-Campa et al., 2009, 2012). The recombinant PRRSV N protein was shown to play an important role in the induction of Tregs (Wongyanin et al., 2012). Further research indicated that PRRSV can induce Tregs in the lungs and tracheobronchial lymph nodes of infected pigs (Nedumpun et al., 2018).
Overall, the mechanisms by which PRRSV infection modulates host adaptive immune responses, including antigen presentation, antibody responses, T cells, and B cells, are relatively less well-characterized. Thus, more in-depth studies on these systems are needed.
4. Activation of immunosuppressive regulatory pathways
Interleukin-10, a pleiotropic cytokine with immuno-modulatory functions, can be produced from both the innate and the adaptive immune response, including T and B cells, DCs, and monocytes/macrophages. As a potent immunosuppressive cytokine, IL-10 has been called the “macrophage deactivation factor” because of its inhibitory effect on a variety of cytokines, such as IL-1, TNF-α, IL-4, IL-3, and granulocyte–monocyte colony-stimulating factor (GM-CSF), as well as on surface molecules, such as MHC-II proteins and co-stimulatory molecules. IL-10 is also known to have potent inhibitory effects on both antigen-presenting cell maturation and T-cell activation (Moore et al., 2001). IL-10 was reported to be responsible for the induction of PRRSV-specific Tregs and monocyte derived DCs (MoDCs) (Wongyanin et al., 2012). It has been reported that IL-10 is used by many viruses to restrict the host immune response, thus allowing the establishment of a persistent infection (Redpath et al., 2001). Indeed, monocyte treatment with IL-10 was correlated with increased susceptibility to PRRSV-1 infection (Singleton et al., 2018). Previous in vitro and in vivo studies demonstrated that PRRSV infection induces IL-10 production through the NF-κB and p38 MAPK signaling pathways (Suradhat and Thanawongnuwech, 2003; Suradhat et al., 2003; Hou et al., 2012; Song et al., 2013). PRRSV GP5 and N protein were found to be responsible for IL-10 production (Hou et al., 2012; Wongyanin et al., 2012). However, the induction of IL-10, along with several other cytokines seems to be strain-dependent (Subramaniam et al., 2011).
Notably, the immunosuppressive effect of PRRSV is not associated with just IL-10. Intracellular suppressor of cytokine signaling (SOCS) proteins are crucial intracellular regulators of innate and adaptive immunity; they are involved in the negative regulation of the JAK/STAT and TLR signaling cascades, DC activation, T-cell differentiation, and Th-cell regulation. Our lab found that PRRSV induces SOCS1 and SOCS3 production in PAMs, monkey-derived Marc-145 cells, and porcine-derived CRL2843-CD163 cells (Luo et al., 2020, 2021). SOCS1 is produced via the p38/AP-1 and JNK/AP-1 signaling pathways rather than via the classical type I IFN signaling pathways and it inhibits the expressions of IFN-β and ISGs, thereby markedly enhancing the level of PRRSV replication (Luo et al., 2020).
Interleukin-1Ra, a member of the IL-1 family, can competitively bind to the IL-1 receptor to block intracellular IL-1 signaling pathways. IL-1Ra modulates the production of many cytokines, such as IL-1, TNF-α, and type I IFN (Marsh et al., 1994; Arend et al., 1998; Arend, 2002; Banda et al., 2005). The cells primarily infected by PRRSV are members of the myeloid cell population, which are responsible for IL-1Ra production (Arend, 2002). It has been reported that PRRSV, both in vitro and in vivo, can induce IL-1Ra production, which plays an important role in the reduction of pro-inflammatory cytokine and type I IFN production during the early phase of PRRSV infection, as well as in T-lymphocyte differentiation and proliferation (Nedumpun et al., 2017).
5. Immune evasion via micro (mi)RNA regulation
Micro (mi)RNAs are evolutionarily conserved small non-coding RNAs of approximately 22 nucleotides in length; they act as critical posttranscriptional modulators of gene expression and participate in modulating immune responses (O’Connell et al., 2010). Viruses can utilize host microRNAs to modulate host immunity in ways that enable viral replication (Cullen, 2013). For example, PRRSV infection can upregulate miR-30c by activating the NF-κB signaling pathway to target JAK1 and consequently inhibit the type I IFN signaling pathway, thereby promoting PRRSV infection. Importantly, miR-30c was found to increase following PRRSV infection in vivo, and a positive correlation between miR-30c production and PRRSV viral load was observed (Zhang et al., 2016). Another mechanism by which miR-30c inhibits type I IFN signaling is its targeting of the 3’ UTR region of IFNAR2, which results in the downregulation of IFNAR2 (Liu et al., 2018). MYH9 can be used as a functional receptor by PRRSV to interact with viral protein, and let-7f-5p was demonstrated to be a key regulator of MYH9, acting by targeting the 3’ UTR of both pig and monkey MYH9. Notably, PRRSV infection downregulates let-7f-5p production to promote the expression of MYH9 and facilitate viral replication (Li et al., 2016). MiR-24-3p and miR-22 promote PRRSV replication by directly targeting the 3’UTR of heme oxygenase-1 (HO-1), a critical cytoprotective enzyme shown to have antiviral properties, thus downregulating its expression (Xiao et al., 2015; Xiao S. et al., 2016). Latest studies have shown that PRRSV induces the upregulation of HOXA3, which can negatively regulate HO-1 gene transcription, thereby weakening the interaction between HO-1 and IRF3 for inhibiting the type I IFN response (Feng et al., 2022).
6. PRRSV modulates apoptosis
Apoptosis is a type of programmed cell death that is characterized by morphological changes including cell shrinkage, nuclear condensation, and plasma membrane blebbing (Zamaraev et al., 2017). It can be induced via two major pathways, the extrinsic and intrinsic pathways, both of which are regulated by caspases (Tait and Green, 2010). Apoptosis plays an important role in the development and maintenance of homeostasis, as well as in host defense following viral infection (Orzalli and Kagan, 2017). Apoptosis can limit virus replication by eliminating infected cells. However, viruses can also manipulate the apoptotic machinery to their advantage. Some viruses inhibit apoptosis to prevent cell death, thus increasing the production of progeny viruses, and some other viruses induce apoptosis to enhance the transmission of progeny viruses by avoiding the immune response owing to the non-inflammatory nature of apoptosis (Arora et al., 2016). It has been well-documented that PRRSV induces apoptosis in vitro and in vivo, both directly in infected cells and indirectly in bystander cells (Suárez et al., 1996; Sur et al., 1997; Labarque et al., 2003; Karniychuk et al., 2011; Gómez-Laguna et al., 2013; Novakovic et al., 2017). Later research found that the HP-PRRSV strain induces apoptosis in the bone marrow cells of infected piglets (Wang et al., 2016). And apoptosis also occurred in the thymus of piglets infected with HP-PRRSV (Wang et al., 2015). It was postulated that proapoptotic signals are delivered to thymocytes by thymic macrophages to affect thymic functions (Wang et al., 2020). The regulatory roles of PRRSV-encoded products in apoptosis have also been studied. The involvement of GP5 with the pro-apoptotic functions of PRRSV has been extensively demonstrated (Suárez et al., 1996; Fernández et al., 2002; Lee et al., 2004; Mu et al., 2015). PRRSV E protein was found to interact with mitochondrial proteins and induce apoptosis by inhibiting ATP production (Pujhari and Zakhartchouk, 2016). Nsp4 and nsp10 were also shown to be apoptosis inducers (Ma et al., 2013; Yuan et al., 2016). However, opposing views that PRRSV instead inhibits apoptosis have been reported as well (Labarque et al., 2003). It has been hypothesized that inhibition of apoptosis takes place in the early phases of infection, probably as a mechanism to avoid abortive replication by a premature death of the macrophage (Costers et al., 2008). Therefore, the mechanisms of the role of apoptosis in PRRSV infection still need to be thoroughly elaborated.
7. Modulating host translation
PRRSV infection, via an nsp2-related mechanism, induces host translation shutoff through the phosphorylation of eIF2α and the attenuation of the mTOR signaling pathway (Li et al., 2018). In addition to interfering with cellular protein production by targeting translation factors, PRRSV also does so by targeting mRNA trafficking. Because nucleocytoplasmic trafficking of molecules is an important process for many cellular functions of the host, numerous viruses target it for their own benefit. Nucleoporin 62 (Nup62) is one of the major nucleoporins located in the core of the nuclear pore complex (NPC), which is the gateway for nucleocytoplasmic trafficking. PRRSV blocks nucleocytoplasmic trafficking, thereby blocking host mRNA nuclear export, leading to inhibited antiviral protein production and thereby promoting virus growth. The responsible viral factor was identified as nsp1β, whose interaction with Nup62 disintegrated NPC (Han et al., 2017; Ke et al., 2019).
8. Concluding remarks
This review gives a brief overview of the various mechanisms by which PRRSV has been found to evade the host. PRRSV interferes with innate immune responses in multiple ways, such as avoiding host recognition, impairing signaling pathways that lead to the inhibition of type I IFNs, ISGs, and pro-inflammatory genes, and dysregulating NK-cell function. The suboptimal innate immune responses subsequently exacerbate the ineffectiveness of the adaptive immune response to PRRSV, which is directly targeted by the virus via disrupting antigen presentation, impairing DC and NK-cell functions, and inducing Tregs. In addition to targeting the host innate and adaptive immune responses, PRRSV also employs other mechanisms to escape elimination by the host, such as regulating miRNAs, apoptosis, and host translation. Although much progress has been made in all aspects of PRRSV research since the discovery of this virus, an effective means for PRRS prevention and control is still lacking. The most important strategy for controlling and eliminating viral diseases is vaccination. We are still far from reaching this goal for PRRS, possibly because understanding of PRRSV infection and immune evasion mechanisms is still incomplete. The ability of PRRSV to escape from its host has not been studied as widely compared with this ability of some important human pathogens, such as HIV. Thus, studies aimed at deciphering PRRSV host evasion mechanisms are urgently needed to guide the development of vaccines and antivirals against PRRSV.
Author contributions
GZ and X-XC: conceptualization and review. X-XC: original draft. X-XC and JW: visualization. SQ, RL, and XL: providing guidance and modifying the review. All authors contributed to the article and approved the submitted version.
Funding
This work was supported by Natural Science Foundation of Henan Province (222300420467), Fund for Distinguished Young Scholars from Henan Academy of Agricultural Sciences (Grant no. 2020JQ06), National Natural Science Funds (32172875), the Earmarked Fund for CARS (CARS-35), and the Pig Industry Technology System Innovation Team Project of Henan Province (HARS-22-12-S).
Conflict of interest
The authors declare that the research was conducted in the absence of any commercial or financial relationships that could be construed as a potential conflict of interest.
Publisher’s note
All claims expressed in this article are solely those of the authors and do not necessarily represent those of their affiliated organizations, or those of the publisher, the editors and the reviewers. Any product that may be evaluated in this article, or claim that may be made by its manufacturer, is not guaranteed or endorsed by the publisher.
Footnotes
References
Arend, W. (2002). The balance between IL-1 and IL-1Ra in disease. Cytokine Growth Factor Rev. 13, 323–340. doi: 10.1016/s1359-6101(02)00020-5
Arend, W., Malyak, M., Guthridge, C., and Gabay, C. (1998). Interleukin-1 receptor antagonist: role in biology. Annu. Rev. Immunol. 16, 27–55. doi: 10.1146/annurev.immunol.16.1.27
Arora, S., Lim, W., Bist, P., Perumalsamy, R., Lukman, H. M., Li, F., et al. (2016). Influenza A virus enhances its propagation through the modulation of Annexin-A1 dependent endosomal trafficking and apoptosis. Cell Death Differ. 23, 1243–1256. doi: 10.1038/cdd.2016.19
Banda, N., Guthridge, C., Sheppard, D., Cairns, K., Muggli, M., Bech-Otschir, D., et al. (2005). Intracellular IL-1 receptor antagonist type 1 inhibits IL-1-induced cytokine production in keratinocytes through binding to the third component of the COP9 signalosome. J. Immunol. 174, 3608–3616. doi: 10.4049/jimmunol.174.6.3608
Baumann, A., Mateu, E., Murtaugh, M., and Summerfield, A. (2013). Impact of genotype 1 and 2 of porcine reproductive and respiratory syndrome viruses on interferon-α responses by plasmacytoid dendritic cells. Vet. Res. 44:33. doi: 10.1186/1297-9716-44-33
Beura, L. K., Sarkar, S. N., Kwon, B., Subramaniam, S., Jones, C., Pattnaik, A. K., et al. (2010). Porcine reproductive and respiratory syndrome virus nonstructural protein 1beta modulates host innate immune response by antagonizing IRF3 activation. J. Virol. 84, 1574–1584. doi: 10.1128/JVI.01326-09
Beyer, J., Fichtner, D., Schirrmeier, H., Polster, U., Weiland, E., and Wege, H. (2000). Porcine reproductive and respiratory syndrome virus (PRRSV): kinetics of infection in lymphatic organs and lung. J. Vet. Med. B Infect. Dis. Vet. Public Health 47, 9–25. doi: 10.1046/j.1439-0450.2000.00305.x
Blasius, A. L., and Beutler, B. (2010). Intracellular toll-like receptors. Immunity 32, 305–315. doi: 10.1016/j.immuni.2010.03.012
Bordet, E., Blanc, F., Tiret, M., Crisci, E., Bouguyon, E., Renson, P., et al. (2018). Porcine reproductive and respiratory syndrome virus type 1.3 lena triggers conventional dendritic cells 1 activation and T helper 1 immune response without infecting dendritic cells. Front. Immunol. 9:2299. doi: 10.3389/fimmu.2018.02299
Calzada-Nova, G., Schnitzlein, W. M., Husmann, R. J., and Zuckermann, F. A. (2011). North American porcine reproductive and respiratory syndrome viruses inhibit type I interferon production by plasmacytoid dendritic cells. J. Virol. 85, 2703–2713. doi: 10.1128/JVI.01616-10
Cao, J., Grauwet, K., Vermeulen, B., Devriendt, B., Jiang, P., Favoreel, H., et al. (2013). Suppression of NK cell-mediated cytotoxicity against PRRSV-infected porcine alveolar macrophages in vitro. Vet. Microbiol. 164, 261–269. doi: 10.1016/j.vetmic.2013.03.001
Cao, Q. M., Subramaniam, S., Ni, Y. Y., Cao, D., and Meng, X. J. (2016). The non-structural protein Nsp2TF of porcine reproductive and respiratory syndrome virus down-regulates the expression of Swine Leukocyte Antigen class I. Virology 491, 115–124. doi: 10.1016/j.virol.2016.01.021
Chaung, H., Chen, C., Hsieh, B., and Chung, W. (2010). Toll-Like Receptor expressions in porcine alveolar macrophages and Dendritic Cells in responding to poly IC stimulation and porcine reproductive and respiratory syndrome virus (PRRSV) infection. Comp. Immunol. Microbiol. Infect. Dis. 33, 197–213. doi: 10.1016/j.cimid.2008.10.001
Chen, H., and Jiang, Z. (2013). The essential adaptors of innate immune signaling. Protein Cell 4, 27–39. doi: 10.1007/s13238-012-2063-0
Chen, Z., Lawson, S., Sun, Z., Zhou, X., Guan, X., Christopher-Hennings, J., et al. (2010). Identification of two auto-cleavage products of nonstructural protein 1 (nsp1) in porcine reproductive and respiratory syndrome virus infected cells: nsp1 function as interferon antagonist. Virology 398, 87–97. doi: 10.1016/j.virol.2009.11.033
Chen, Z., Li, M., He, Q., Du, J., Zhou, L., Ge, X., et al. (2014). The amino acid at residue 155 in nonstructural protein 4 of porcine reproductive and respiratory syndrome virus contributes to its inhibitory effect for interferon-beta transcription in vitro. Virus Res. 189, 226–234. doi: 10.1016/j.virusres.2014.05.027
Costers, S., Lefebvre, D. J., Delputte, P. L., and Nauwynck, H. J. (2008). Porcine reproductive and respiratory syndrome virus modulates apoptosis during replication in alveolar macrophages. Arch. Virol. 153, 1453–1465. doi: 10.1007/s00705-008-0135-5
Cullen, B. R. (2013). MicroRNAs as mediators of viral evasion of the immune system. Nat. Immunol. 14, 205–210. doi: 10.1038/ni.2537
Deddouche, S., Goubau, D., Rehwinkel, J., Chakravarty, P., Begum, S., Maillard, P. V., et al. (2014). Identification of an LGP2-associated MDA5 agonist in picornavirus-infected cells. Elife 3:e01535. doi: 10.7554/eLife.01535
Dong, H., Zhou, L., Ge, X., Guo, X., Han, J., and Yang, H. (2018). Porcine reproductive and respiratory syndrome virus nsp1beta and nsp11 antagonize the antiviral activity of cholesterol-25-hydroxylase via lysosomal degradation. Vet. Microbiol. 223, 134–143. doi: 10.1016/j.vetmic.2018.08.012
Du, J., Ge, X., Liu, Y., Jiang, P., Wang, Z., Zhang, R., et al. (2015). Targeting swine leukocyte antigen class I molecules for proteasomal degradation by the nsp1alpha replicase protein of the Chinese highly pathogenic porcine reproductive and respiratory syndrome virus strain JXwn06. J. Virol. 90, 682–693. doi: 10.1128/JVI.02307-15
Fang, Y., and Snijder, E. J. (2010). The PRRSV replicase: exploring the multifunctionality of an intriguing set of nonstructural proteins. Virus Res. 154, 61–76. doi: 10.1016/j.virusres.2010.07.030
Fang, Y., Treffers, E. E., Li, Y., Tas, A., Sun, Z., van der Meer, Y., et al. (2012). Efficient –2 frameshifting by mammalian ribosomes to synthesize an additional arterivirus protein. Proc. Natl. Acad. Sci. U. S. A. 109, E2920–E2928. doi: 10.1073/pnas.1211145109
Feng, Y., Guo, X., Tian, H., He, Y., Li, Y., Jiang, X., et al. (2022). Induction of HOXA3 by porcine reproductive and respiratory syndrome virus inhibits type I interferon response through negative regulation of HO-1 transcription. J. Virol. 96:e0186321. doi: 10.1128/JVI.01863-21
Fernández, A., Suárez, P., Castro, J., Tabarés, E., and Díaz-Guerra, M. (2002). Characterization of regions in the GP5 protein of porcine reproductive and respiratory syndrome virus required to induce apoptotic cell death. Virus Res. 83, 103–118. doi: 10.1016/s0168-1702(01)00426-9
Fitzgerald, K., and Kagan, J. (2020). Toll-like receptors and the control of immunity. Cell 180, 1044–1066. doi: 10.1016/j.cell.2020.02.041
Gao, P., Liu, Y., Wang, H., Chai, Y., Weng, W., Zhang, Y., et al. (2022). Viral evasion of PKR restriction by reprogramming cellular stress granules. Proc. Natl. Acad. Sci. U. S. A. 119:e2201169119. doi: 10.1073/pnas.2201169119
Gerner, W., Käser, T., and Saalmüller, A. (2009). Porcine T lymphocytes and NK cells–an update. Dev. Comp. Immunol. 33, 310–320. doi: 10.1016/j.dci.2008.06.003
Gómez-Laguna, J., Salguero, F., Fernández de Marco, M., Barranco, I., Rodríguez-Gómez, I., Quezada, M., et al. (2013). Type 2 porcine reproductive and respiratory syndrome virus infection mediated apoptosis in B- and T-cell areas in lymphoid organs of experimentally infected pigs. Transbound Emerg. Dis. 60, 273–278. doi: 10.1111/j.1865-1682.2012.01338.x
Goubau, D., Deddouche, S., and Reis e Sousa, C. (2013). Cytosolic sensing of viruses. Immunity 38, 855–869. doi: 10.1016/j.immuni.2013.05.007
Goubau, D., Schlee, M., Deddouche, S., Pruijssers, A. J., Zillinger, T., Goldeck, M., et al. (2014). Antiviral immunity via RIG-I-mediated recognition of RNA bearing 5’-diphosphates. Nature 514, 372–375. doi: 10.1038/nature13590
Gu, H., Zheng, S., Han, G., Yang, H., Deng, Z., Liu, Z., et al. (2022). Porcine reproductive and respiratory syndrome virus adapts antiviral innate immunity via manipulating MALT1. mBio 13:e0066422. doi: 10.1128/mbio.00664-22
Han, M., Ke, H., Zhang, Q., and Yoo, D. (2017). Nuclear imprisonment of host cellular mRNA by nsp1beta protein of porcine reproductive and respiratory syndrome virus. Virology 505, 42–55. doi: 10.1016/j.virol.2017.02.004
Holtkamp, D. J., Kliebenstein, J. B., Neumann, E. J., Zimmerman, J. J., Rotto, H., Yoder, T. K., et al. (2013). Assessment of the economic impact of porcine reproductive and respiratory syndrome virus on United States pork producers. J. Swine Health Prod. 21, 72–84. doi: 10.2460/javma.2005.227.385
Hornung, V., Ellegast, J., Kim, S., Brzózka, K., Jung, A., Kato, H., et al. (2006). 5’-Triphosphate RNA is the ligand for RIG-I. Science 314, 994–997. doi: 10.1126/science.1132505
Hou, J., Wang, L., Quan, R., Fu, Y., Zhang, H., and Feng, W. H. (2012). Induction of interleukin-10 is dependent on p38 mitogen-activated protein kinase pathway in macrophages infected with porcine reproductive and respiratory syndrome virus. Virol. J. 9:165. doi: 10.1186/1743-422X-9-165
Huang, C., Du, Y., Yu, Z., Zhang, Q., Liu, Y., Tang, J., et al. (2016). Highly pathogenic porcine reproductive and respiratory syndrome virus Nsp4 cleaves VISA to impair antiviral responses mediated by RIG-I-like receptors. Sci. Rep. 6:28497. doi: 10.1038/srep28497
Huang, C., Zhang, Q., and Feng, W. H. (2015). Regulation and evasion of antiviral immune responses by porcine reproductive and respiratory syndrome virus. Virus Res. 202, 101–111. doi: 10.1016/j.virusres.2014.12.014
Huang, C., Zhang, Q., Guo, X. K., Yu, Z. B., Xu, A. T., Tang, J., et al. (2014). Porcine reproductive and respiratory syndrome virus nonstructural protein 4 antagonizes beta interferon expression by targeting the NF-kappaB essential modulator. J. Virol. 88, 10934–10945. doi: 10.1128/JVI.01396-14
Karniychuk, U. U., Saha, D., Geldhof, M., Vanhee, M., Cornillie, P., Van den Broeck, W., et al. (2011). Porcine reproductive and respiratory syndrome virus (PRRSV) causes apoptosis during its replication in fetal implantation sites. Microb. Pathog. 51, 194–202. doi: 10.1016/j.micpath.2011.04.001
Kato, H., Takeuchi, O., Sato, S., Yoneyama, M., Yamamoto, M., Matsui, K., et al. (2006). Differential roles of MDA5 and RIG-I helicases in the recognition of RNA viruses. Nature 441, 101–105. doi: 10.1038/nature04734
Ke, H., Han, M., Kim, J., Gustin, K., and Yoo, D. (2019). Porcine reproductive and respiratory syndrome virus nonstructural protein 1 beta interacts with nucleoporin 62 to promote viral replication and immune evasion. J. Virol. 93:e00469-19. doi: 10.1128/jvi.00469-19
Kittiwan, N., Yamsakul, P., Tadee, P., Tadee, P., Nuangmek, A., Chuammitri, P., et al. (2019). Immunological response to porcine reproductive and respiratory syndrome virus in young pigs obtained from a PRRSV-positive exposure status herd in a PRRSV endemic area. Vet. Immunol. Immunopathol. 218:109935. doi: 10.1016/j.vetimm.2019.109935
Knoops, K., Barcena, M., Limpens, R. W., Koster, A. J., Mommaas, A. M., and Snijder, E. J. (2012). Ultrastructural characterization of arterivirus replication structures: reshaping the endoplasmic reticulum to accommodate viral RNA synthesis. J. Virol. 86, 2474–2487. doi: 10.1128/JVI.06677-11
Koyama, S., Ishii, K. J., Coban, C., and Akira, S. (2008). Innate immune response to viral infection. Cytokine 43, 336–341. doi: 10.1016/j.cyto.2008.07.009
Kuzemtseva, L., de la Torre, E., Martín, G., Soldevila, F., Ait-Ali, T., Mateu, E., et al. (2014). Regulation of toll-like receptors 3, 7 and 9 in porcine alveolar macrophages by different genotype 1 strains of porcine reproductive and respiratory syndrome virus. Vet. Immunol. Immunopathol. 158, 189–198. doi: 10.1016/j.vetimm.2014.01.009
Labarque, G., Van Gucht, S., Nauwynck, H., Van Reeth, K., and Pensaert, M. (2003). Apoptosis in the lungs of pigs infected with porcine reproductive and respiratory syndrome virus and associations with the production of apoptogenic cytokines. Vet. Res. 34, 249–260. doi: 10.1051/vetres:2003001
Lee, C., Rogan, D., Erickson, L., Zhang, J., and Yoo, D. (2004). Characterization of the porcine reproductive and respiratory syndrome virus glycoprotein 5 (GP5) in stably expressing cells. Virus Res. 104, 33–38. doi: 10.1016/j.virusres.2004.03.001
Li, J., Hu, L., Liu, Y., Huang, L., Mu, Y., Cai, X., et al. (2015). DDX19A senses viral RNA and mediates NLRP3-dependent inflammasome activation. J. Immunol. 195, 5732–5749. doi: 10.4049/jimmunol.1501606
Li, N., Du, T., Yan, Y., Zhang, A., Gao, J., Hou, G., et al. (2016). MicroRNA let-7f-5p inhibits porcine reproductive and respiratory syndrome virus by targeting MYH9. Sci. Rep. 6:34332. doi: 10.1038/srep34332
Li, S., Gowans, E., Chougnet, C., Plebanski, M., and Dittmer, U. (2008). Natural regulatory T cells and persistent viral infection. J. Virol. 82, 21–30. doi: 10.1128/jvi.01768-07
Li, Y., Fang, L., Zhou, Y., Tao, R., Wang, D., and Xiao, S. (2018). Porcine reproductive and respiratory syndrome virus infection induces both eIF2alpha-phosphorylation-dependent and -independent host translation shutoff. J. Virol. 92:e00600-18. doi: 10.1128/JVI.00600-18
Li, Y., Firth, A., Brierley, I., Cai, Y., Napthine, S., Wang, T., et al. (2019). Programmed –2/–1 ribosomal frameshifting in simarteriviruses: an evolutionarily conserved mechanism. J. Virol. 93:e00370-19. doi: 10.1128/jvi.00370-19
Li, Y., and Mateu, E. (2021). Interaction of type 1 porcine reproductive and respiratory syndrome virus with derived conventional dendritic cells. Front. Immunol. 12:674185. doi: 10.3389/fimmu.2021.674185
Liu, C. H., Chaung, H. C., Chang, H. L., Peng, Y. T., and Chung, W. B. (2009). Expression of Toll-like receptor mRNA and cytokines in pigs infected with porcine reproductive and respiratory syndrome virus. Vet. Microbiol. 136, 266–276. doi: 10.1016/j.vetmic.2008.11.016
Liu, F., Wang, H., Du, L., Wei, Z., Zhang, Q., and Feng, W. (2018). MicroRNA-30c targets the interferon-alpha/beta receptor beta chain to promote type 2 PRRSV infection. J. Gen. Virol. 99, 1671–1680. doi: 10.1099/jgv.0.001166
Liu, S.-Y., Aliyari, R., Chikere, K., Li, G., Marsdeny Matthew, D., et al. (2013). Interferon-inducible cholesterol-25-hydroxylase broadly inhibits viral entry by production of 25-hydroxycholesterol. Immunity 38, 92–105. doi: 10.1016/j.immuni.2012.11.005
Lunney, J. K., Fang, Y., Ladinig, A., Chen, N., Li, Y., Rowland, B., et al. (2016). Porcine Reproductive and Respiratory Syndrome Virus (PRRSV): pathogenesis and interaction with the immune system. Annu. Rev. Anim. Biosci. 4, 129–154. doi: 10.1146/annurev-animal-022114-111025
Luo, R., Xiao, S., Jiang, Y., Jin, H., Wang, D., Liu, M., et al. (2008). Porcine reproductive and respiratory syndrome virus (PRRSV) suppresses interferon-beta production by interfering with the RIG-I signaling pathway. Mol. Immunol. 45, 2839–2846. doi: 10.1016/j.molimm.2008.01.028
Luo, X., Chen, X., Qiao, S., Li, R., Lu, Q., Geng, R., et al. (2021). Porcine reproductive and respiratory syndrome virus increases SOCS3 production via activation of p38/AP-1 signaling pathway to promote viral replication. Vet. Microbiol. 257:109075. doi: 10.1016/j.vetmic.2021.109075
Luo, X., Chen, X. X., Qiao, S., Li, R., Xie, S., Zhou, X., et al. (2020). Porcine reproductive and respiratory syndrome virus enhances self-replication via AP-1-dependent induction of SOCS1. J. Immunol. 204, 394–407. doi: 10.4049/jimmunol.1900731
Ma, Z., Wang, Y., Zhao, H., Xu, A. T., Wang, Y., Tang, J., et al. (2013). Porcine reproductive and respiratory syndrome virus nonstructural protein 4 induces apoptosis dependent on its 3C-like serine protease activity. PLoS One 8:e69387. doi: 10.1371/journal.pone.0069387
Marsh, C., Moore, S., Pope, H., and Wewers, M. (1994). IL-1ra suppresses endotoxin-induced IL-1 beta and TNF-alpha release from mononuclear phagocytes. Am. J. Physiol. 267, L39–L45. doi: 10.1152/ajplung.1994.267.1.L39
Medina, G. N., Azzinaro, P., Ramirez-Medina, E., Gutkoska, J., Fang, Y., Diaz-San Segundo, F., et al. (2020). Impairment of the DeISGylation activity of foot-and-mouth disease virus lpro causes attenuation in vitro and in vivo. J. Virol. 94:e00341-20. doi: 10.1128/JVI.00341-20
Miller, L. C, Lager, K. M, and Kehrli, M. E. (2009). Role of Toll-like receptors in activation of porcine alveolar macrophages by porcine reproductive and respiratory syndrome virus. Clin. Vacc. Immunol. 16, 360–365. doi: 10.1128/cvi.00269-08
Moore, K., de Waal Malefyt, R., Coffman, R., and O’Garra, A. (2001). Interleukin-10 and the interleukin-10 receptor. Annu. Rev. Immunol. 19, 683–765. doi: 10.1146/annurev.immunol.19.1.683
Mu, Y., Li, L., Zhang, B., Huang, B., Gao, J., Wang, X., et al. (2015). Glycoprotein 5 of porcine reproductive and respiratory syndrome virus strain SD16 inhibits viral replication and causes G2/M cell cycle arrest, but does not induce cellular apoptosis in Marc-145 cells. Virology 484, 136–145. doi: 10.1016/j.virol.2015.05.019
Nedumpun, T., Sirisereewan, C., Thanmuan, C., Techapongtada, P., Puntarotairung, R., Naraprasertkul, S., et al. (2018). Induction of porcine reproductive and respiratory syndrome virus (PRRSV)-specific regulatory T lymphocytes (Treg) in the lungs and tracheobronchial lymph nodes of PRRSV-infected pigs. Vet. Microbiol. 216, 13–19. doi: 10.1016/j.vetmic.2018.01.014
Nedumpun, T., Wongyanin, P., Sirisereewan, C., Ritprajak, P., Palaga, T., Thanawongnuwech, R., et al. (2017). Interleukin-1 receptor antagonist: an early immunomodulatory cytokine induced by porcine reproductive and respiratory syndrome virus. J. Gen. Virol. 98, 77–88. doi: 10.1099/jgv.0.000665
Novakovic, P., Harding, J., Al-Dissi, A., and Detmer, S. (2017). Type 2 porcine reproductive and respiratory syndrome virus infection increases apoptosis at the maternal-fetal interface in late gestation pregnant gilts. PLoS One 12:e0173360. doi: 10.1371/journal.pone.0173360
O’Connell, R. M., Rao, D. S., Chaudhuri, A. A., and Baltimore, D. (2010). Physiological and pathological roles for microRNAs in the immune system. Nat. Rev. Immunol. 10, 111–122. doi: 10.1038/nri2708
Orzalli, M. H., and Kagan, J. C. (2017). Apoptosis and necroptosis as host defense strategies to prevent viral infection. Trends Cell Biol. 27, 800–809. doi: 10.1016/j.tcb.2017.05.007
Patel, D., Nan, Y., Shen, M., Ritthipichai, K., Zhu, X., and Zhang, Y. J. (2010). Porcine reproductive and respiratory syndrome virus inhibits type I interferon signaling by blocking STAT1/STAT2 nuclear translocation. J. Virol. 84, 11045–11055. doi: 10.1128/JVI.00655-10
Pujhari, S., and Zakhartchouk, A. N. (2016). Porcine reproductive and respiratory syndrome virus envelope (E) protein interacts with mitochondrial proteins and induces apoptosis. Arch. Virol. 161, 1821–1830. doi: 10.1007/s00705-016-2845-4
Redpath, S., Ghazal, P., and Gascoigne, N. (2001). Hijacking and exploitation of IL-10 by intracellular pathogens. Trends Microbiol. 9, 86–92. doi: 10.1016/s0966-842x(00)01919-3
Reikine, S., Nguyen, J. B., and Modis, Y. (2014). Pattern recognition and signaling mechanisms of RIG-I and MDA5. Front. Immunol. 5:342. doi: 10.3389/fimmu.2014.00342
Renukaradhya, G. J., Alekseev, K., Jung, K., Fang, Y., and Saif, L. J. (2010). Porcine reproductive and respiratory syndrome virus-induced immunosuppression exacerbates the inflammatory response to porcine respiratory coronavirus in pigs. Viral Immunol. 23, 457–466. doi: 10.1089/vim.2010.0051
Sagong, M., and Lee, C. (2011). Porcine reproductive and respiratory syndrome virus nucleocapsid protein modulates interferon-beta production by inhibiting IRF3 activation in immortalized porcine alveolar macrophages. Arch. Virol. 156, 2187–2195. doi: 10.1007/s00705-011-1116-7
Saito, T., Owen, D., Jiang, F., Marcotrigiano, J., and Gale, M. (2008). Innate immunity induced by composition-dependent RIG-I recognition of hepatitis C virus RNA. Nature 454, 523–527. doi: 10.1038/nature07106
Sang, Y., Ross, C. R., Rowland, R. R., and Blecha, F. (2008). Toll-like receptor 3 activation decreases porcine arterivirus infection. Viral Immunol. 21, 303–313. doi: 10.1089/vim.2008.0042
Shuai, K., and Liu, B. (2003). Regulation of JAK-STAT signalling in the immune system. Nat. Rev. Immunol. 3, 900–911. doi: 10.1038/nri1226
Silva-Campa, E., Flores-Mendoza, L., Reséndiz, M., Pinelli-Saavedra, A., Mata-Haro, V., Mwangi, W., et al. (2009). Induction of T helper 3 regulatory cells by dendritic cells infected with porcine reproductive and respiratory syndrome virus. Virology 387, 373–379. doi: 10.1016/j.virol.2009.02.033
Silva-Campa, E., Mata-Haro, V., Mateu, E., and Hernandez, J. (2012). Porcine reproductive and respiratory syndrome virus induces CD4+CD8+CD25+Foxp3+ regulatory T cells (Tregs). Virology 430, 73–80. doi: 10.1016/j.virol.2012.04.009
Singleton, H., Graham, S., Frossard, J., Bodman-Smith, K., and Steinbach, F. (2018). Infection of monocytes with European porcine reproductive and respiratory syndrome virus (PRRSV-1) strain Lena is significantly enhanced by dexamethasone and IL-10. Virology 517, 199–207. doi: 10.1016/j.virol.2018.02.017
Solis, M., Nakhaei, P., Jalalirad, M., Lacoste, J., Douville, R., Arguello, M., et al. (2011). RIG-I-mediated antiviral signaling is inhibited in HIV-1 infection by a protease-mediated sequestration of RIG-I. J. Virol. 85, 1224–1236. doi: 10.1128/jvi.01635-10
Song, C., Krell, P., and Yoo, D. (2010). Nonstructural protein 1alpha subunit-based inhibition of NF-kappaB activation and suppression of interferon-beta production by porcine reproductive and respiratory syndrome virus. Virology 407, 268–280. doi: 10.1016/j.virol.2010.08.025
Song, S., Bi, J., Wang, D., Fang, L., Zhang, L., Li, F., et al. (2013). Porcine reproductive and respiratory syndrome virus infection activates IL-10 production through NF-kappaB and p38 MAPK pathways in porcine alveolar macrophages. Dev. Comp. Immunol. 39, 265–272. doi: 10.1016/j.dci.2012.10.001
Song, Y., Guo, Y., Li, X., Sun, R., Zhu, M., Shi, J., et al. (2021). RBM39 alters phosphorylation of c-Jun and binds to viral RNA to promote PRRSV proliferation. Front. Immunol. 12:664417. doi: 10.3389/fimmu.2021.664417
Spilman, M. S., Welbon, C., Nelson, E., and Dokland, T. (2009). Cryo-electron tomography of porcine reproductive and respiratory syndrome virus: organization of the nucleocapsid. J. Gen. Virol. 90(Pt 3), 527–535. doi: 10.1099/vir.0.007674-0
Suárez, P., Díaz-Guerra, M., Prieto, C., Esteban, M., Castro, J., Nieto, A., et al. (1996). Open reading frame 5 of porcine reproductive and respiratory syndrome virus as a cause of virus-induced apoptosis. J. Virol. 70, 2876–2882. doi: 10.1128/jvi.70.5.2876-2882.1996
Subramaniam, S., Sur, J., Kwon, B., Pattnaik, A., and Osorio, F. (2011). A virulent strain of porcine reproductive and respiratory syndrome virus does not up-regulate interleukin-10 levels in vitro or in vivo. Virus Res. 155, 415–422. doi: 10.1016/j.virusres.2010.11.010
Sun, Q., Xu, H., Li, C., Gong, B., Li, Z., Tian, Z., et al. (2022). Emergence of a novel PRRSV-1 strain in mainland China: a recombinant strain derived from the two commercial modified live viruses Amervac and DV. Front. Vet. Sci. 9:974743. doi: 10.3389/fvets.2022.974743
Sun, Y., Ke, H., Han, M., Chen, N., Fang, W., and Yoo, D. (2016). Nonstructural protein 11 of porcine reproductive and respiratory syndrome virus suppresses both MAVS and RIG-I expression as one of the mechanisms to antagonize type I interferon production. PLoS One 11:e0168314. doi: 10.1371/journal.pone.0168314
Sur, J. H., Doster, A. R., Christian, J. S., Galeota, J. A., Wills, R. W., Zimmerman, J. J., et al. (1997). Porcine reproductive and respiratory syndrome virus replicates in testicular germ cells, alters spermatogenesis, and induces germ cell death by apoptosis. J. Virol. 71, 9170–9179. doi: 10.1128/JVI.71.12.9170-9179.1997
Suradhat, S., and Thanawongnuwech, R. (2003). Upregulation of interleukin-10 gene expression in the leukocytes of pigs infected with porcine reproductive and respiratory syndrome virus. J. Gen. Virol. 84, 2755–2760. doi: 10.1099/vir.0.19230-0
Suradhat, S., Thanawongnuwech, R., and Poovorawan, Y. (2003). Upregulation of IL-10 gene expression in porcine peripheral blood mononuclear cells by porcine reproductive and respiratory syndrome virus. J. Gen. Virol. 84(Pt 2), 453–459. doi: 10.1099/vir.0.18698-0
Tait, S., and Green, D. (2010). Mitochondria and cell death: outer membrane permeabilization and beyond. Nat. Rev. Mol. Cell Biol. 11, 621–632. doi: 10.1038/nrm2952
Takaoka, A., and Yanai, H. (2006). Interferon signalling network in innate defence. Cell Microbiol. 8, 907–922. doi: 10.1111/j.1462-5822.2006.00716.x
Takeuchi, O., and Akira, S. (2010). Pattern recognition receptors and inflammation. Cell 140, 805–820. doi: 10.1016/j.cell.2010.01.022
Tao, R., Fang, L., Bai, D., Ke, W., Zhou, Y., Wang, D., et al. (2018). Porcine reproductive and respiratory syndrome virus nonstructural protein 4 cleaves porcine DCP1a to attenuate its antiviral activity. J. Immunol. 201, 2345–2353. doi: 10.4049/jimmunol.1701773
Tian, K., Yu, X., Zhao, T., Feng, Y., Cao, Z., Wang, C., et al. (2007). Emergence of fatal PRRSV variants: unparalleled outbreaks of atypical PRRS in China and molecular dissection of the unique hallmark. PLoS One 2:e526. doi: 10.1371/journal.pone.0000526
Van Breedam, W., Delputte, P. L., Van Gorp, H., Misinzo, G., Vanderheijden, N., Duan, X., et al. (2010). Porcine reproductive and respiratory syndrome virus entry into the porcine macrophage. J. Gen. Virol. 91(Pt 7), 1659–1667. doi: 10.1099/vir.0.020503-0
Wang, D., Chen, J., Yu, C., Zhu, X., Xu, S., Fang, L., et al. (2019). Porcine reproductive and respiratory syndrome virus nsp11 antagonizes type I interferon signaling by targeting IRF9. J. Virol. 93:e00623-19. doi: 10.1128/JVI.00623-19
Wang, G., Li, L., Yu, Y., Tu, Y., Tong, J., Zhang, C., et al. (2016). Highly pathogenic porcine reproductive and respiratory syndrome virus infection and induction of apoptosis in bone marrow cells of infected piglets. J. Gen. Virol. 97, 1356–1361. doi: 10.1099/jgv.0.000454
Wang, G., Yu, Y., Cai, X., Zhou, E., and Zimmerman, J. (2020). Effects of PRRSV infection on the porcine thymus. Trends Microbiol. 28, 212–223. doi: 10.1016/j.tim.2019.10.009
Wang, G., Yu, Y., Tu, Y., Tong, J., Liu, Y., Zhang, C., et al. (2015). Highly pathogenic porcine reproductive and respiratory syndrome virus infection induced apoptosis and autophagy in thymi of infected piglets. PLoS One 10:e0128292. doi: 10.1371/journal.pone.0128292
Wang, L. J., Wan, B., Guo, Z., Qiao, S., Li, R., Xie, S., et al. (2018). Genomic analysis of a recombinant NADC30-like porcine reproductive and respiratory syndrome virus in China. Virus Genes 54, 86–97. doi: 10.1007/s11262-017-1516-1
Wang, L. J., Xie, W., Chen, X. X., Qiao, S., Zhao, M., Gu, Y., et al. (2017). Molecular epidemiology of porcine reproductive and respiratory syndrome virus in Central China since 2014: the prevalence of NADC30-like PRRSVs. Microb. Pathog. 109, 20–28. doi: 10.1016/j.micpath.2017.05.021
Wang, R., Nan, Y., Yu, Y., Yang, Z., and Zhang, Y. J. (2013a). Variable interference with interferon signal transduction by different strains of porcine reproductive and respiratory syndrome virus. Vet. Microbiol. 166, 493–503. doi: 10.1016/j.vetmic.2013.07.022
Wang, R., Nan, Y., Yu, Y., and Zhang, Y. J. (2013b). Porcine reproductive and respiratory syndrome virus Nsp1beta inhibits interferon-activated JAK/STAT signal transduction by inducing karyopherin-alpha1 degradation. J. Virol. 87, 5219–5228. doi: 10.1128/JVI.02643-12
Wang, T. Y., Sun, M. X., Zhang, H. L., Wang, G., Zhan, G., Tian, Z. J., et al. (2021). Evasion of antiviral innate immunity by porcine reproductive and respiratory syndrome virus. Front. Microbiol. 12:693799. doi: 10.3389/fmicb.2021.693799
Wang, X., Eaton, M., Mayer, M., Li, H., He, D., Nelson, E., et al. (2007). Porcine reproductive and respiratory syndrome virus productively infects monocyte-derived dendritic cells and compromises their antigen-presenting ability. Arch. Virol. 152, 289–303. doi: 10.1007/s00705-006-0857-1
Wills, R., Doster, A., Galeota, J., Sur, J., and Osorio, F. (2003). Duration of infection and proportion of pigs persistently infected with porcine reproductive and respiratory syndrome virus. J. Clin. Microbiol. 41, 58–62. doi: 10.1128/jcm.41.1.58-62.2003
Wongyanin, P., Buranapraditkul, S., Yoo, D., Thanawongnuwech, R., Roth, J., and Suradhat, S. (2012). Role of porcine reproductive and respiratory syndrome virus nucleocapsid protein in induction of interleukin-10 and regulatory T-lymphocytes (Treg). J. Gen. Virol. 93, 1236–1246. doi: 10.1099/vir.0.040287-0
Xiao, S., Du, T., Wang, X., Ni, H., Yan, Y., Li, N., et al. (2016a). MiR-22 promotes porcine reproductive and respiratory syndrome virus replication by targeting the host factor HO-1. Vet. Microbiol. 192, 226–230. doi: 10.1016/j.vetmic.2016.07.026
Xiao, Y., Ma, Z., Wang, R., Yang, L., Nan, Y., and Zhang, Y. (2016b). Downregulation of protein kinase PKR activation by porcine reproductive and respiratory syndrome virus at its early stage infection. Vet. Microbiol. 187, 1–7. doi: 10.1016/j.vetmic.2016.03.004
Xiao, S., Wang, X., Ni, H., Li, N., Zhang, A., Liu, H., et al. (2015). MicroRNA miR-24-3p promotes porcine reproductive and respiratory syndrome virus replication through suppression of heme oxygenase-1 expression. J. Virol. 89, 4494–4503. doi: 10.1128/JVI.02810-14
Xie, S., Chen, X. X., Qiao, S., Li, R., Sun, Y., Xia, S., et al. (2018). Identification of the RNA pseudoknot within the 3’ end of the porcine reproductive and respiratory syndrome virus genome as a pathogen-associated molecular pattern to activate antiviral signaling via RIG-I and toll-like receptor 3. J. Virol. 92:e00097-18. doi: 10.1128/JVI.00097-18
Yang, L., He, J., Wang, R., Zhang, X., Lin, S., Ma, Z., et al. (2019). Nonstructural protein 11 of porcine reproductive and respiratory syndrome virus induces STAT2 degradation to inhibit interferon signaling. J. Virol. 93:e01352-19. doi: 10.1128/jvi.01352-19
Yang, L., Wang, R., Ma, Z., Xiao, Y., Nan, Y., Wang, Y., et al. (2017). Porcine reproductive and respiratory syndrome virus antagonizes JAK/STAT3 signaling via nsp5, which induces STAT3 degradation. J. Virol. 91:e02087-16. doi: 10.1128/JVI.02087-16
Yoo, D., Song, C., Sun, Y., Du, Y., Kim, O., and Liu, H. C. (2010). Modulation of host cell responses and evasion strategies for porcine reproductive and respiratory syndrome virus. Virus Res. 154, 48–60. doi: 10.1016/j.virusres.2010.07.019
Yu, F., Liu, L., Tian, X., Chen, L., Huang, X., Sun, Y., et al. (2022). Genomic analysis of porcine reproductive and respiratory syndrome virus 1 revealed extensive recombination and potential introduction events in China. Vet. Sci. 9:450. doi: 10.3390/vetsci9090450
Yu, F., Yan, Y., Shi, M., Liu, H. Z., Zhang, H. L., Yang, Y. B., et al. (2020). Phylogenetics, genomic recombination, and NSP2 polymorphic patterns of porcine reproductive and respiratory syndrome virus in China and the United States in 2014-2018. J. Virol 94:e01813-19. doi: 10.1128/JVI.01813-19
Yuan, S., Zhang, N., Xu, L., Zhou, L., Ge, X., Guo, X., et al. (2016). Induction of apoptosis by the nonstructural protein 4 and 10 of porcine reproductive and respiratory syndrome virus. PLoS One 11:e0156518. doi: 10.1371/journal.pone.0156518
Zamaraev, A. V., Kopeina, G. S., Prokhorova, E. A., Zhivotovsky, B., and Lavrik, I. N. (2017). Post-translational modification of caspases: the other side of apoptosis regulation. Trends Cell Biol. 27, 322–339. doi: 10.1016/j.tcb.2017.01.003
Zhang, Q., Huang, C., Yang, Q., Gao, L., Liu, H. C., Tang, J., et al. (2016). MicroRNA-30c modulates type I IFN responses to facilitate porcine reproductive and respiratory syndrome virus infection by targeting JAK1. J. Immunol. 196, 2272–2282. doi: 10.4049/jimmunol.1502006
Zhao, K., Ye, C., Chang, X. B., Jiang, C. G., Wang, S. J., Cai, X. H., et al. (2015). Importation and recombination are responsible for the latest emergence of highly pathogenic porcine reproductive and respiratory syndrome virus in China. J. Virol. 89, 10712–10716. doi: 10.1128/JVI.01446-15
Zheng, S., Gu, H., Han, G., Xu, H., Liu, Z., Lu, Y., et al. (2021). Porcine reproductive and respiratory syndrome virus nsp11 antagonizes broad antiviral effects of MCPIP1 by inducing interleukin-17 expression. J. Virol. 95:e0111921. doi: 10.1128/JVI.01119-21
Keywords: immune evasion, porcine reproductive and respiratory syndrome virus (PRRSV), innate immunity, adaptive immunity, miRNA
Citation: Chen X-x, Qiao S, Li R, Wang J, Li X and Zhang G (2023) Evasion strategies of porcine reproductive and respiratory syndrome virus. Front. Microbiol. 14:1140449. doi: 10.3389/fmicb.2023.1140449
Received: 09 January 2023; Accepted: 06 March 2023;
Published: 17 March 2023.
Edited by:
Fang He, Zhejiang University, ChinaReviewed by:
Xusheng Qiu, Shanghai Veterinary Research Institute, Chinese Academy of Agricultural Sciences, ChinaQin Zhao, Northwest A&F University, China
Enric M. Mateu, Autonomous University of Barcelona, Spain
Yongming Sang, Tennessee State University, United States
Copyright © 2023 Chen, Qiao, Li, Wang, Li and Zhang. This is an open-access article distributed under the terms of the Creative Commons Attribution License (CC BY). The use, distribution or reproduction in other forums is permitted, provided the original author(s) and the copyright owner(s) are credited and that the original publication in this journal is cited, in accordance with accepted academic practice. No use, distribution or reproduction is permitted which does not comply with these terms.
*Correspondence: Xin-xin Chen, cxxin2014@163.com; Gaiping Zhang, zhanggaip@126.com