- 1Virology Department, Carol Davila University of Medicine and Pharmacy, Bucharest, Romania
- 2Viral Emerging Diseases Department, Stefan S. Nicolau Institute of Virology, Bucharest, Romania
The availability of combined antiretroviral therapy (cART) has revolutionized the course of HIV infection, suppressing HIV viremia, restoring the immune system, and improving the quality of life of HIV infected patients. However, the emergence of drug resistant and multidrug resistant strains remains an important contributor to cART failure, associated with a higher risk of HIV-disease progression and mortality. According to the latest WHO HIV Drug Resistance Report, the prevalence of acquired and transmitted HIV drug resistance in ART naive individuals has exponentially increased in the recent years, being an important obstacle in ending HIV-1 epidemic as a public health threat by 2030. The prevalence of three and four-class resistance is estimated to range from 5 to 10% in Europe and less than 3% in North America. The new drug development strategies are focused on improved safety and resistance profile within the existing antiretroviral classes, discovery of drugs with novel mechanisms of action (e.g., attachment/post-attachment inhibitors, capsid inhibitors, maturation inhibitors, nucleoside reverse transcriptase translocation inhibitors), combination therapies with improved adherence, and treatment simplification with infrequent dosing. This review highlight the current progress in the management of salvage therapy for patients with multidrug-resistant HIV-1 infection, discussing the recently approved and under development antiretroviral agents, as well as the new drug targets that are providing a new avenue for the development of therapeutic interventions in HIV infection.
Introduction
In 2021, 38.4 million people were living with HIV infections and 1.5 million people became newly infected with HIV according to the recent update from The Joint United Nations Programme on HIV/AIDS.1 The availability of combined antiretroviral therapy (cART) has revolutionized the course of HIV infection, suppressing HIV viremia, restoring the immune system, and improving the quality of life of HIV infected patients (Samji et al., 2013). Current HIV treatment includes five different classes of antiretrovirals targeting multiple steps of the HIV life cycle (Figure 1): entry inhibitors that block (1) the attachment of HIV envelope glycoprotein gp120 to CCR5 co-receptors (maraviroc) or (2) the cell fusion mediated by HIV gp41 (enfuvirtide), (3) nucleoside reverse transcriptase inhibitors (NRTIs) and non-nucleoside reverse transcriptase inhibitors (NNRTIs) that block the reverse transcription of viral RNA to cDNA, (4) integrase strand transfer inhibitors (INSTIs) that inhibit the integration of proviral DNA into host genome, and (5) protease inhibitors (PIs) that inhibit the protease-mediated cleavage of gag and gag-pol precursors, resulting in the production of non-infectious virus particles (De Clercq and Li, 2016). However, the emergence of drug resistant and multidrug resistant strains remains an important contributor to cART failure, associated with a higher risk of HIV-disease progression and mortality (Galli et al., 2020). Cross-resistance between drugs within the same class can occur and affects all major classes of antiretroviral drugs (Puertas et al., 2020). According to the latest WHO HIV Drug Resistance Report, the prevalence of acquired and transmitted HIV drug resistance in ART naive individuals has exponentially increased in the recent years, being an important obstacle in ending HIV-1 epidemic as a public health threat by 2030.2 The report indicates that 10% of adults starting HIV treatment have resistance to non-nucleoside reverse transcriptase inhibitors (NNRTIs) and people with previous exposure to antiretroviral drugs are three times more likely to present resistance to the NNRTI drug class. The prevalence of three and four-class drug-resistant viruses is estimated to be 5 to 10% in different cohorts of antiretroviral-experienced patients from Europe and less than 3% in North America (Judd et al., 2017; Galli et al., 2020; Puertas et al., 2020; Lombardi et al., 2021). Global efforts are required to design effective strategies to address HIV drug resistance: routine viral load monitoring, improving adherence to treatment, conducting drug resistance genotyping, regimen switch if indicated and selection of the most effective antiretroviral drugs combinations to achieve long-term and successful treatment outcomes. Two or three fully active agents are recommended to construct a new regimen for highly treatment-experienced patients with multidrug-resistant virus.3 Therefore, the development of new drugs is focused on (a) improved safety and resistance profile within the existing antiretroviral classes, (b) combination therapies with improved adherence, (c) treatment simplification with infrequent dosing, using long-acting agents to overcome the adherence issues and prevent the development of drug resistance (Overton et al., 2020; Flexner et al., 2021) and (d) discovery of drugs with novel mechanisms of action (e.g., attachment/post-attachment inhibitors, capsid inhibitors, maturation inhibitors, nucleoside reverse transcriptase translocation inhibitors).
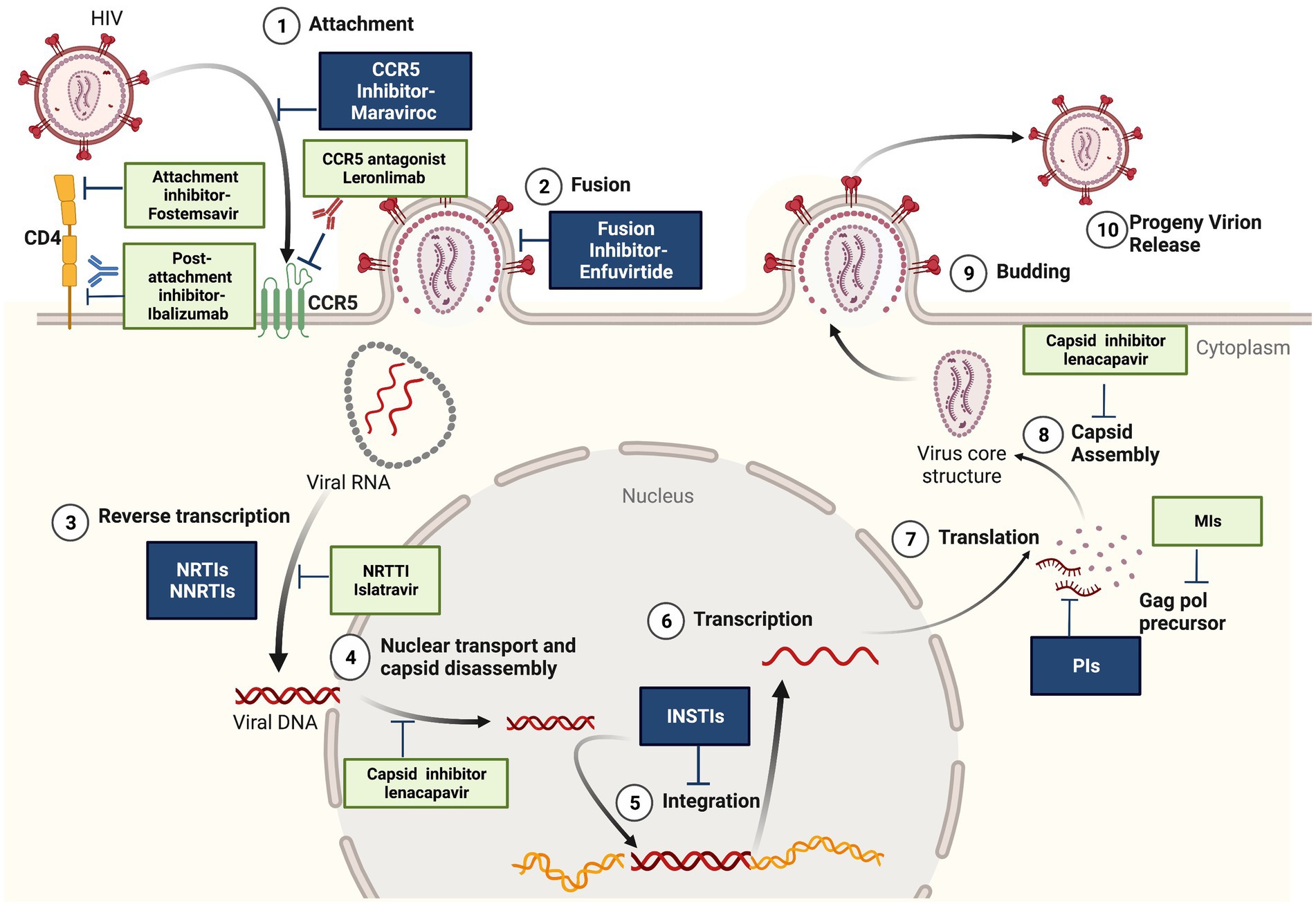
Figure 1. Antiretroviral agents. In dark-the conventional ART used in combinations: entry inhibitors, reverstranscription inhibitors (NRTI-nucleoside analogs reverstranscriptase inhibitors and NNRTI-non-nucleoside reverstranscriptase inhibitors), INSTI-integrase inhibitors, PI-protease inhibitors. In light color-newly approved antiretrovirals-fostemsavir (CD4 attachement inhibitor), ibalizumab (mAb gp120 post attachment inhibitor), lenacapavir (capsid inhibitor) and antiretrovirals in advanced clinical trials: leronlimab (mAb CCR5 antagonist), islatravir (NNRTTI-nucleoside reverstranscriptase translocation inhibitor) and maturation inhibitors. Created with BioRender.com, with a license to publish.
This review highlight the current progress in the management of salvage therapy for patients with multidrug-resistant HIV-1 infection, discussing antiretroviral agents that are recently approved and those under development, as well as the new drug targets providing a different avenue for the development of therapeutic interventions in HIV infection.
Recently approved and in clinical development antiretroviral agents for the treatment of drug resistant HIV-1
Novel types of antiretrovirals with new mechanisms of action have been developed in the recent years, offering additional therapeutic options for patients with multiclass drug-resistant HIV infection (Figure 1).
Fostemsavir-CD4 attachment inhibitor
Fostemsavir (FTR) is the first FDA-approved attachment inhibitor indicated for heavily treated adults with multidrug-resistant HIV-1 (Gulick, 2018). FTR is a prodrug of the active molecule temsavir, which inhibits the HIV-1 gp 120 conformational changes needed for CD4 attachment, preventing viral entry into susceptible cells (Cahn et al., 2018). The efficacy and safety of fostemsavir has been evaluated in a partially randomized, double-blind, placebo-controlled clinical trial (BRIGHTE), which enrolled 371 highly treatment-experienced patients who have failed their current regimen (Kozal et al., 2020; Lataillade et al., 2020). FTR showed sustained rates of virologic suppression over the 96 weeks of clinical trial: the proportion of participants who achieved undetectable HIV viral load was 53% at week 24, 54% at week 48, and 60% at week 96 and CD4+ T-cell recovery was recorded even in the most immunocompromised patients (participants with less than 20 cells/mm3 at baseline had a mean CD4+ T-cell increase of 240 cells/mm3 at week 96) (Ackerman et al., 2021).
The most commonly reported adverse effects for FTR included nausea, vomiting, diarrhea, and headache. Temsavir is partly metabolized by CYP3A4 enzyme, and it is contraindicated with any strong CYP3A4 inducers (carbamazepine, rifampin) (Hiryak and Koren, 2021).
Resistance
A study published in 2020 analyzed HIV-1 env gp120 sequences from both ART-naïve and ART-treated patients and identified several genomic positions with mutations associated with decreased susceptibility to fostemsavir (Bouba et al., 2020), however, the BRIGHTE trial did not find consistent associations between virologic failure and gp120 substitutions (Kozal et al., 2020; Lataillade et al., 2020).
No cross-resistance with other antiretroviral agents have been reported (Rose et al., 2022), fostemsavir being considered a life-saving option for patients with drug-resistant HIV.
Antibody-based strategies
Monoclonal antibodies are becoming attractive strategies for HIV treatment, with good resistance profile, ability to restore CD4 T-cell count and lack of toxicity.
Ibalizumab- post-attachment inhibitor
Ibalizumab (IBA)- the first monoclonal antibody approved for the treatment of HIV-1 infection (Markham, 2018)- is a long-acting post-attachment inhibitor, which binds to the extracellular CD4 domain, leading to conformational changes of the CD4 T cell receptor–gp120 complex and blocking HIV entry (Chahine and Durham, 2021). Importantly, the binding of IBA to CD4 cell receptors does not induce an immunosuppressive response. Ibalizumab, in combination with other antiretrovirals, is indicated for the treatment of heavily treatment-experienced adults with multidrug-resistant HIV-1 infection with limited or no other treatment options (Markham, 2018) and it is designed for twice-monthly intravenous administration (Chahine and Durham, 2021).
The approval of ibalizumab was based on a phase III, single-group, open-label study (TMB-301), that enrolled 40 heavily treatment-experienced HIV patients who had failed several antiretroviral regimens, had >1,000 copies/mL HIV viral load and resistance to at least one NRTI, NNRTI, and a PI (Emu et al., 2018). The results showed that ibalizumab significantly decreased the viral load 7 days after being added to the failing antiretroviral regimen. Furthermore, ibalizumab plus an individually optimized background regimen significantly reduced the HIV-1 viral load at week 25, viral suppression being reported in 43% of the study participants and increased the CD4 T cell count with a mean of 62 cells/mm3.
Ibalizumab was well tolerated in the TMB-301 clinical trial, the most common adverse reactions included diarrhea, dizziness, nausea, and rash. There were no significant drug–drug interactions or adverse interactions between ibalizumab and other antiretroviral agents.
Resistance
Resistance to ibalizumab is conferred by decreased viral expression of specific binding sites in the HIV gp120 envelope protein (Pace et al., 2013). This mechanism of resistance was observed in the TMB-301 study for 8 out of 10 patients who had virologic failure or rebound at week 25 and showed a lower degree of susceptibility to ibalizumab than at baseline (Emu et al., 2018). Currently, there are no commercially available resistance testing methods for patients with suspected resistance to IBA (Blair, 2020).
No cross resistance was reported between ibalizumab and all the other approved antiretroviral drugs, including fostemsavir and maraviroc (Muccini et al., 2022; Rose et al., 2022). A recent article described the case of a heavily treatment-experienced patient with a pan-resistant HIV-1 infection (reduced susceptibility to NRTIs, NNRTIs, PIs and INSTIs) who achieved virological control after introducing ibalizumab in association with recycling enfuvirtide and an optimized background regimen (Canetti et al., 2022), confirming that IBA represents an important advance in the management of multidrug-resistant HIV-1 infection.
Leronlimab (Pro 140)-long-acting CCR5 antagonist
Leronlimab (PRO 140), a humanized IgG4 monoclonal antibody (mAb), acts as an HIV-1 CCR5 antagonist; it binds to hydrophilic extracellular domains on CCR5, inhibiting HIV-1 viral entry in a competitive manner (Thompson, 2018).
The safety and effectiveness of leronlimab is currently, evaluated in phase IIb/III clinical trials. In CD 02 trial (PRO 140_CD 02; NCT02483078), leronlimab was tested in treatment-experienced patients with multidrug resistant HIV. In the first week, participants received 350 mg of PRO 140 subcutaneously or placebo together with their regular medication and 1 week later, they received PRO 140 with an optimized regimen. The primary endpoint of the trial was achieved: patients in the PRO 140 arm showed a statistically significant reduction in HIV RNA viral load of greater than 0.5log from baseline versus patients in the placebo arm.4 A recent report showed that all CCR5-tropic strains were fully susceptible to PRO 140 in a group of heavily treatment-experienced HIV-1-positive patients harboring 4-class drug-resistance to NRTIs, NNRTIs, PIs, and InSTIs; current exposure to maraviroc (68% of the participants) was not associated with different PRO 140 activity (Rusconi et al., 2022).
CD03 clinical trial has evaluated weekly subcutaneous PRO 140 as monotherapy maintenance in patients with viral suppression (PRO 140_CD03; NCT02859961). The rate of virological failure was lower in the group of participants receiving 700 mg dose comparing with 525 mg and 350 mg dose groups (13.8, 33 and 65.9%, respectively); no evidence of treatment-emergent resistance to leronlimab was observed. A report published on March 31, 2022, showed that weekly injections of Leronlimab (700 mg dose) maintained viral suppression in the five HIV+ participants for over seven years (ClinicalTrials.gov NCT02175680 and NCT02355184) (Chang et al., 2022).
UB-421-CD4 attachment inhibitor
UB-421, a humanized IgG1 monoclonal antibody (mAb), acts as a CD4 attachment inhibitor. UB-421 targets domain 1 of CD4 receptor, blocking HIV entry into the cells via a competitive binding inhibition (Wang et al., 2019).
Several collaborative studies showed the efficacy of UB-421 against HIV strains resistant to broadly neutralizing HIV antibodies, entry inhibitors, and other ARV drugs [ClinicalTrials.gov NCT03164447]. Currently, UB-421 is in phase 2/phase 3 clinical trials for ART substitution (ClinicalTrials.gov NCT03149211), treatment of patients with multi-drug resistant HIV infection (ClinicalTrials.gov NCT04406727), as well as for HIV functional cure (ClinicalTrials.gov NCT04404049).
Islatravir-nucleoside reverse transcriptase translocation inhibitor
Islatravir (ISL, MK-8591) is an investigational drug belonging to a new class of antiretrovirals called nucleoside reverse transcriptase translocation inhibitors (NRTTIs) (Markowitz and Sarafianos, 2018). The active form of islatravir (islatravir-triphosphate) inhibits HIV reverse transcriptase (RT) by multiple mechanisms, including RT translocation inhibition and delayed chain termination through viral DNA structural changes (Salie et al., 2016). ISL showed potent activity against HIV-1, HIV-2, and HIV viral strains with multi-drug resistant mutations (Wu et al., 2017). Due to an unexpected decline in total lymphocyte and CD4+ T-cell counts, clinical studies of injectable islatravir for treatment and all formulations of islatravir for pre-exposure prophylaxis (PrEP) are on full or partial clinical holds, still, arms testing lower dosage are ongoing (ClinicalTrials.gov NCT03272347; NCT04564547; NCT05052996).
Lenacapavir-long-acting capsid inhibitor
Lenacapavir, the first-in-class long-acting HIV capsid inhibitor, was approved in August, 2022 in the European Union and in December, 2022 in US, for the treatment of HIV infection, in combination with other antiretroviral(s), in patients with multi-drug resistant HIV who have very limited treatment choices.5 Lenacapavir is the only HIV treatment option that can be administered twice-yearly, in a long acting subcutaneous formulation (Dvory-Sobol et al., 2022). The novel long-acting agent targets multiple stages of the HIV life cycle: it binds directly to the HIV capsid protein and interferes with capsid-mediated nuclear import of HIV DNA, virion production and proper capsid core formation (Figure 1; Bester et al., 2020; Link et al., 2020). The approval of lenacapavir was supported by the results of the Phase 2/3, double-blinded, placebo-controlled global multicenter CAPELLA trial, that involved heavily treatment-experienced patients with multi-drug resistant HIV-1 (Segal-Maurer et al., 2022). Eighty three percent of the participants that received lenacapavir in combination with an optimized background regimen achieved viral suppression at week 52; a mean increase in CD4 count of 83 cells/μL was reported. Lenacapavir was well tolerated, no drug-related serious side effects being identified.
Resistance
Lenacapavir has no overlapping resistance with any currently approved antiretroviral therapy; additional studies have not shown any preexisting resistance mutations or naturally occurring polymorphisms that reduce viral susceptibility to lenacapavir (Margot et al., 2022).
Another capsid inhibitor-VH4004280- is currently investigated in phase I clinical trial for safety, tolerability, and pharmacokinetic properties in HIV-negative volunteers.
GSK2838232-maturation inhibitor
Maturation inhibitors-a new class of antiretroviral agents in clinical development-disrupt the proteases cleavage of the HIV-1 gag protein immediate precursor, leading to immature, noninfectious virus particles (Sundquist and Krausslich, 2012; Wang et al., 2015).
Bevirimat and BMS-955176 (GSK3532795), the first 2 maturation inhibitors, demonstrated antiviral activity but no efficacy against some polymorphisms in the HIV gag region (Wainberg and Albert, 2010) and had associated side effects in phase 2b clinical studies.6
GSK2838232 is a second-generation HIV-1 maturation inhibitor in phase IIa clinical trial evaluation; it allows for once-daily administration boosted with a pharmacoenhancer (DeJesus et al., 2020). GSK2838232 was well tolerated, and generated a significant viral load decrease in the group of participants receiving the highest dosage. GSK2838232 is active against HIV isolates resistant to bevirimat.
Others long-acting HIV maturation inhibitors (GSK3640254 and GSK3739937) are in earlier stages of development (Joshi et al., 2020; Spinner et al., 2022).
New potential targets for HIV treatment
To overcome the resistance to HIV-1 antiretroviral therapy, novel drug targets (both virus and host-related) are being investigated (Figure 2).
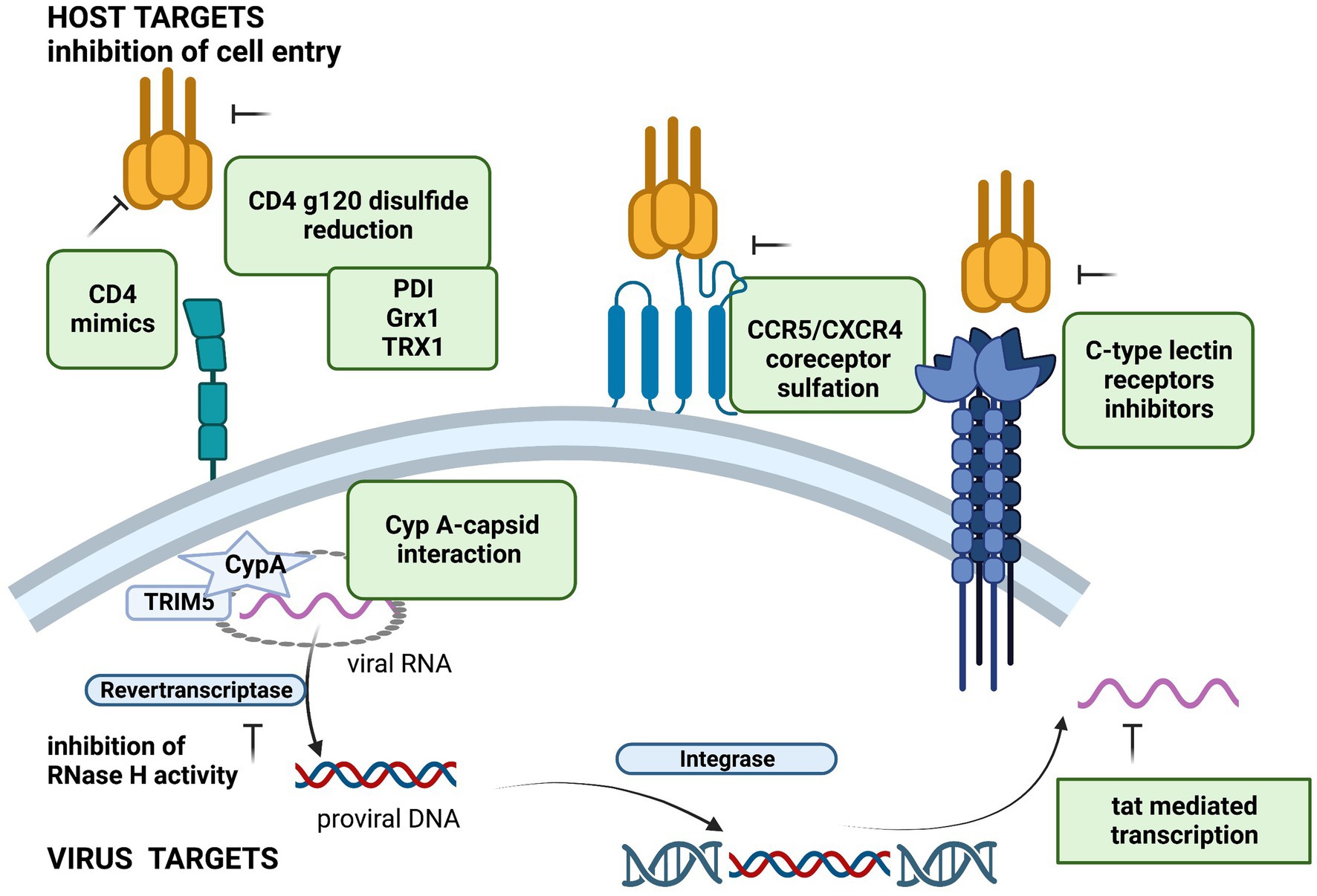
Figure 2. Potential host and viral targets for HIV replication inhibition. Created with BioRender.com, with a license to publish.
Host-related targets
Host factors play an important role in HIV infection (Friedrich et al., 2011). The potential host targets can interfere with HIV entry, replication and spread of HIV infection, and may lead to a higher barrier to antiretroviral drug resistance.
Inhibitors targeting HIV viral entry are among the most attractive targets due to their potential to limit de novo infected cells. Several studies showed that HIV-1 entry is dependent on redox control and the compounds that inhibit thiol/disulfide exchange reactions could suppress HIV-1 viral entry (Moolla et al., 2016; Reiser et al., 2016).
Protein disulfide isomerase, thioredoxin-1, and galectin-9
Human protein disulfide isomerase (PDI) catalyzes reduction of disulfides in HIV gp120 necessary for virus entry (Markovic et al., 2004). Several PDI inhibitors and anti-PDI antibodies were described, but none has been used therapeutically due to their toxicity (Khan et al., 2011). The interaction of PDI with human protein Galectin-9 regulates the redox environment and enhance T-cell migration and HIV entry (Bi et al., 2011). Moreover, Galectin-9 was reported to be an important mediator of HIV transcription and reactivation of latent HIV in CD4 + T cells and may be explored for novel HIV cure strategies (Abdel-Mohsen et al., 2016; Colomb et al., 2019).
A host protein-oxidoreductase glutaredoxin 1 (Grx1)- was shown to catalyze the reduction of HIV gp120 and CD4 disulfides and its inhition reduced HIV replication (Auwerx et al., 2009). More recent studies reported that the host thioredoxin-1 (Trx1) is also a very efficient reductase of disulfides of gp120 and CD4 in vitro and may represent a future host target for HIV-1 treatment (Moolla et al., 2016; Lundberg et al., 2019); inhibition of thioredoxin-1 with anti-Trx1 antibodies, inhibited HIV-1 entry by >80% in cell cultures.
CD4 mimics
Several small molecule CD4 mimics have been reported as HIV-1 entry inhibitors, which are blocking the interaction of gp120 with CD4 (Curreli et al., 2016). Recently, novel soluble-type CD4 molecule mimics have been developed with a high anti-HIV activity and synergistic anti-HIV activity with a neutralizing antibody (Kobayakawa et al., 2019; Tsuji et al., 2022).
C-type lectin receptors
C-type lectin receptors (CLRs) (galectin-1, DC-SIGN, dendritic cell immunoreceptor-DCIR, etc.), expressed on the surface of dendritic cells (DCs) are binding to HIV gp120 glycoprotein playing an important role in the pathogenesis of HIV infection (Mesman and Geijtenbeek, 2012). Several CLR inhibitors, that modulate protective immune responses and block receptor binding have been described (Varga et al., 2014; Cardinaud et al., 2017; Porkolab et al., 2018). Competitive inhibitions of galectin-1, DC-SIGN and DCIR have shown efficacy in blocking CD4+T cells viral infection and HIV propagation, being considered a promising therapeutic target for HIV treatment (Porkolab et al., 2018).
Inhibition of cellular restriction factors is also studied as a potential host target. The human cyclophilin A (CypA) binds HIV-1 viral capsid (CA) and promotes capsid uncoating and viral infectivity (Colgan et al., 1996; Gamble et al., 1996). Disruption of CypA-capsid interactions inhibits HIV-1 replication in cell cultures (Sokolskaja et al., 2004). A recent study showed that Cyclophilin A prevents HIV-1 restriction in lymphocytes by blocking TRIM5α (human tripartite motif-containing protein 5) binding to the viral core (Selyutina et al., 2020). TRIM5α is an antiretroviral restriction factor, that induces premature uncoating, inhibiting reverse transcription and nuclear import (Ganser-Pornillos and Pornillos, 2019). Several compounds were described as HIV-1 assembly and disassembly dual inhibitors, targeting both HIV-1 capsid protein and cyclophilin A (Liu et al., 2016; Obubeid et al., 2022).
Virus-related targets
Non-conventional viral inhibitors might act on multidrug resistant HIV strains.
Inhibitors of RT RNAse H activity
HIV-1 RT possess a ribonuclease activity (RNase H) essential for viral replication (Menéndez-Arias et al., 2017). Two types of RNase H inhibitors have been developed, but none has reached the clinical phase: active site inhibitors, which chelate the two Mg2+ within the active site, and Rnase H allosteric inhibitors (Wang et al., 2018; Xi et al., 2019; Martín-Alonso et al., 2022).
Tat inhibitors
HIV-1 transcription factor Tat is required for transcription of the integrated viral genome (Selby et al., 1989). Tat-mediated transcription is conserved and is distinguishable from cellular transcription, being an important therapeutic target against HIV replication and the resistance emergence (Ott et al., 2011; Mousseau and Valente, 2012). The HIV-1 Tat protein regulates the passage from viral latency to active transcription and its inhibition can prevent HIV-1 reactivation and facilitate permanent HIV-1 suppression (Karn, 2011). Several Tat inhibitors have been described, including didehydro-cortistatin A (dCA), that can prevent HIV-1 reactivation from latency (Li et al., 2019; Nchioua et al., 2020) and is studied, together with other latency blocking agents, for the induction of a functional cure. Recently, several 3-oxindole derivatives-with heterocyclic structures have been shown to specifically inhibit the Tat-mediated viral transcription acting on the HIV-1 LTR promoter (Kim et al., 2022). Future strategies focused on agents targeting latent HIV-1 reservoirs are explored to achieve a functional cure.
Conclusion
Life-long HIV treatment and drug resistance are still a challenge that requires constant efforts to identify new generations of antiretroviral agents. Significant progress has been made for the treatment of HIV drug resistant infection, but new targets are needed for a salvage therapy.
Author contributions
SR conceived and supervised the review topics. AT wrote the first draft. All authors contributed to the article and approved the submitted version.
Conflict of interest
The authors declare that the research was conducted in the absence of any commercial or financial relationships that could be construed as a potential conflict of interest.
Publisher’s note
All claims expressed in this article are solely those of the authors and do not necessarily represent those of their affiliated organizations, or those of the publisher, the editors and the reviewers. Any product that may be evaluated in this article, or claim that may be made by its manufacturer, is not guaranteed or endorsed by the publisher.
Footnotes
1. ^ https://www.unaids.org/sites/default/files/media_asset/JC3032_AIDS_Data_book_2021_En.pdf
2. ^ https://www.who.int/publications/i/item/9789240038608
3. ^ https://clinicalinfo.hiv.gov/sites/default/files/guidelines/documents/adult-adolescent-arv/guidelines-adult-adolescent-arv.pdf
4. ^ https://content.equisolve.net/_0fabb8b784fee97a4a4b390ab5453a6b/cytodyn/db/193/2464/pdf/CytoDyn+-+ASM+2019+Poster+Presentation+re+CD02_Final.pdf
5. ^ https://www.ema.europa.eu/en/documents/product-information/sunlenca-epar-product-information_en.pdf
References
Abdel-Mohsen, M., Chavez, L., Tandon, R., Chew, G. M., Deng, X., Danesh, A., et al. (2016). Human Galectin-9 is a potent mediator of HIV transcription and reactivation. PLoS Pathog. 12:e1005677. doi: 10.1371/journal.ppat.1005677
Ackerman, P., Thompson, M., Molina, J.-M., Aberg, J., Cassetti, I., Kozal, M., et al. (2021). Long-term efficacy and safety of fostemsavir among subgroups of heavily treatment-experienced adults with HIV-1. AIDS 35, 1061–1072. doi: 10.1097/QAD.0000000000002851
Auwerx, J., Isacsson, O., Söderlund, J., Balzarini, J., Johansson, M., and Lundberg, M. (2009). Human glutaredoxin-1 catalyzes the reduction of HIV-1 gp120 and CD4 disulfides and its inhibition reduces HIV-1 replication. Int. J. Biochem. Cell Biol. 41, 1269–1275. doi: 10.1016/j.biocel.2008.10.031
Bester, S. M., Wei, G., Zhao, H., Adu-Ampratwum, D., Iqbal, N., Courouble, V. V., et al. (2020). Structural and mechanistic bases for a potent HIV-1 capsid inhibitor. Science 370, 360–364. doi: 10.1126/science.abb4808
Bi, S., Hong, P. W., Lee, B., and Baum, L. G. (2011). Galectin-9 binding to cell surface protein disulfide isomerase regulates the redox environment to enhance T-cell migration and HIV entry. Proc. Natl. Acad. Sci. 108, 10650–10655. doi: 10.1073/pnas.1017954108
Blair, H. A. (2020). Ibalizumab: a review in multidrug-resistant HIV-1 infection. Drugs 80, 189–196. doi: 10.1007/s40265-020-01258-3
Bouba, Y., Berno, G., Fabeni, L., Carioti, L., Salpini, R., Aquaro, S., et al. (2020). Identification of gp120 polymorphisms in HIV-1 B subtype potentially associated with resistance to fostemsavir. J. Antimicrob. Chemother. 75, 1778–1786. doi: 10.1093/jac/dkaa073
Cahn, P., Fink, V., and Patterson, P. (2018). Fostemsavir. Curr. Opin. HIV AIDS 13, 341–345. doi: 10.1097/COH.0000000000000469
Canetti, D., Muccini, C., Spagnuolo, V., Galli, L., Poli, A., Gianotti, N., et al. (2022). Achieving virological control in pan-resistant HIV-1 infection: a case series. EBioMedicine 77:103906. doi: 10.1016/j.ebiom.2022.103906
Cardinaud, S., Urrutia, A., Rouers, A., Coulon, P.-G., Kervevan, J., Richetta, C., et al. (2017). Triggering of TLR-3, −4, NOD2, and DC-SIGN reduces viral replication and increases T-cell activation capacity of HIV-infected human dendritic cells. Eur. J. Immunol. 47, 818–829. doi: 10.1002/eji.201646603
Chahine, E. B., and Durham, S. H. (2021). Ibalizumab: the first monoclonal antibody for the treatment of HIV-1 infection. Ann. Pharmacother. 55, 230–239. doi: 10.1177/1060028020942218
Chang, X. L., Reed, J. S., Webb, G. M., Wu, H. L., Le, J., Bateman, K. B., et al. (2022). Suppression of human and simian immunodeficiency virus replication with the CCR5-specific antibody Leronlimab in two species. PLoS Pathog. 18:e1010396. doi: 10.1371/journal.ppat.1010396
Colgan, J., Yuan, H. E., Franke, E. K., and Luban, J. (1996). Binding of the human immunodeficiency virus type 1 gag polyprotein to cyclophilin a is mediated by the central region of capsid and requires gag dimerization. J. Virol. 70, 4299–4310. doi: 10.1128/jvi.70.7.4299-4310.1996
Colomb, F., Giron, L. B., Premeaux, T. A., Mitchell, B. I., Niki, T., Papasavvas, E., et al. (2019). Galectin-9 mediates HIV transcription by inducing TCR-dependent ERK signaling. Front. Immunol. 10:267. doi: 10.3389/fimmu.2019.00267
Curreli, F., Belov, D. S., Ramesh, R. R., Patel, N., Altieri, A., Kurkin, A. V., et al. (2016). Design, synthesis and evaluation of small molecule CD4-mimics as entry inhibitors possessing broad spectrum anti-HIV-1 activity. Bioorg. Med. Chem. 24, 5988–6003. doi: 10.1016/j.bmc.2016.09.057
De Clercq, E., and Li, G. (2016). Approved antiviral drugs over the past 50 years. Clin. Microbiol. Rev. 29, 695–747. doi: 10.1128/CMR.00102-15
DeJesus, E., Harward, S., Jewell, R. C., Johnson, M., Dumont, E., Wilches, V., et al. (2020). A phase IIa study evaluating safety, pharmacokinetics, and antiviral activity of GSK2838232, a novel, second-generation maturation inhibitor, in participants with human immunodeficiency virus type 1 infection. Clin. Infect. Dis. 71, 1255–1262. doi: 10.1093/cid/ciz938
Dvory-Sobol, H., Shaik, N., Callebaut, C., and Rhee, M. S. (2022). Lenacapavir: a first-in-class HIV-1 capsid inhibitor. Curr. Opin. HIV AIDS 17, 15–21. doi: 10.1097/COH.0000000000000713
Emu, B., Fessel, J., Schrader, S., Kumar, P., Richmond, G., Win, S., et al. (2018). Phase 3 study of Ibalizumab for multidrug-resistant HIV-1. N. Engl. J. Med. 379, 645–654. doi: 10.1056/NEJMoa1711460
Flexner, C., Owen, A., Siccardi, M., and Swindells, S. (2021). Long-acting drugs and formulations for the treatment and prevention of HIV infection. Int. J. Antimicrob. Agents 57:106220. doi: 10.1016/j.ijantimicag.2020.106220
Friedrich, B. M., Dziuba, N., Li, G., Endsley, M. A., Murray, J. L., and Ferguson, M. R. (2011). Host factors mediating HIV-1 replication. Virus Res. 161, 101–114. doi: 10.1016/j.virusres.2011.08.001
Galli, L., Parisi, M. R., Poli, A., Menozzi, M., Fiscon, M., Garlassi, E., et al. (2020). Burden of disease in PWH harboring a multidrug-resistant virus: data from the PRESTIGIO registry. Open Forum Infect. Dis. 7:ofaa456. doi: 10.1093/ofid/ofaa456
Gamble, T. R., Vajdos, F. F., Yoo, S., Worthylake, D. K., Houseweart, M., Sundquist, W. I., et al. (1996). Crystal structure of human Cyclophilin a bound to the amino-terminal domain of HIV-1 capsid. Cells 87, 1285–1294. doi: 10.1016/S0092-8674(00)81823-1
Ganser-Pornillos, B. K., and Pornillos, O. (2019). Restriction of HIV-1 and other retroviruses by TRIM5. Nat. Rev. Microbiol. 17, 546–556. doi: 10.1038/s41579-019-0225-2
Gulick, R. M. (2018). Investigational antiretroviral drugs: what is coming down the pipeline. Top. Antivir. Med. 25, 127–132.
Hiryak, K., and Koren, D. E. (2021). Fostemsavir: a novel attachment inhibitor for patients with multidrug-resistant HIV-1 infection. Ann. Pharmacother. 55, 792–797. doi: 10.1177/1060028020962424
Joshi, S. R., Fernando, D., Igwe, S., McKenzie, L., Krishnatry, A. S., Halliday, F., et al. (2020). Phase I evaluation of the safety, tolerability, and pharmacokinetics of GSK3640254, a next-generation HIV-1 maturation inhibitor. Pharmacol. Res. Perspect. 8:e00671. doi: 10.1002/prp2.671
Judd, A., Lodwick, R., Noguera-Julian, A., Gibb, D., Butler, K., Costagliola, D., et al. (2017). Higher rates of triple-class virological failure in perinatally HIV-infected teenagers compared with heterosexually infected young adults in Europe. HIV Med. 18, 171–180. doi: 10.1111/hiv.12411
Karn, J. (2011). The molecular biology of HIV latency: breaking and restoring the tat-dependent transcriptional circuit. Curr. Opin. HIV AIDS 6, 4–11. doi: 10.1097/COH.0b013e328340ffbb
Khan, M. M. G., Simizu, S., Lai, N. S., Kawatani, M., Shimizu, T., and Osada, H. (2011). Discovery of a small molecule PDI inhibitor that inhibits reduction of HIV-1 envelope glycoprotein gp120. ACS Chem. Biol. 6, 245–251. doi: 10.1021/cb100387r
Kim, D.-E., Shin, Y. H., Cho, J.-E., Myung, S., Kim, H. G., Kim, K.-C., et al. (2022). Identification of 3-Oxindole derivatives as small molecule HIV-1 inhibitors targeting tat-mediated viral transcription. Molecules 27:4921. doi: 10.3390/molecules27154921
Kobayakawa, T., Konno, K., Ohashi, N., Takahashi, K., Masuda, A., Yoshimura, K., et al. (2019). Soluble-type small-molecule CD4 mimics as HIV entry inhibitors. Bioorganic Med. Chem. Lett. 29, 719–723. doi: 10.1016/j.bmcl.2019.01.011
Kozal, M., Aberg, J., Pialoux, G., Cahn, P., Thompson, M., Molina, J.-M., et al. (2020). Fostemsavir in adults with multidrug-resistant HIV-1 infection. N. Engl. J. Med. 382, 1232–1243. doi: 10.1056/NEJMoa1902493
Lataillade, M., Lalezari, J. P., Kozal, M., Aberg, J. A., Pialoux, G., Cahn, P., et al. (2020). Safety and efficacy of the HIV-1 attachment inhibitor prodrug fostemsavir in heavily treatment-experienced individuals: week 96 results of the phase 3 BRIGHTE study. Lancet HIV 7, e740–e751. doi: 10.1016/S2352-3018(20)30240-X
Li, C., Mousseau, G., and Valente, S. T. (2019). Tat inhibition by didehydro-Cortistatin a promotes heterochromatin formation at the HIV-1 long terminal repeat. Epigenetics Chromatin 12:23. doi: 10.1186/s13072-019-0267-8
Link, J. O., Rhee, M. S., Tse, W. C., Zheng, J., Somoza, J. R., Rowe, W., et al. (2020). Clinical targeting of HIV capsid protein with a long-acting small molecule. Nature 584, 614–618. doi: 10.1038/s41586-020-2443-1
Liu, C., Perilla, J. R., Ning, J., Lu, M., Hou, G., Ramalho, R., et al. (2016). Cyclophilin a stabilizes the HIV-1 capsid through a novel non-canonical binding site. Nat. Commun. 7:10714. doi: 10.1038/ncomms10714
Lombardi, F., Giacomelli, A., Armenia, D., Lai, A., Dusina, A., Bezenchek, A., et al. (2021). Prevalence and factors associated with HIV-1 multi-drug resistance over the past two decades in the Italian ARCA database. Int. J. Antimicrob. Agents 57:106252. doi: 10.1016/j.ijantimicag.2020.106252
Lundberg, M., Mattsson, Å., Reiser, K., Holmgren, A., and Curbo, S. (2019). Inhibition of the thioredoxin system by PX-12 (1-methylpropyl 2-imidazolyl disulfide) impedes HIV-1 infection in TZM-bl cells. Sci. Rep. 9:5656. doi: 10.1038/s41598-019-42068-2
Margot, N., Vanderveen, L., Naik, V., Ram, R., Parvangada, P., Martin, R., et al. (2022). Phenotypic resistance to lenacapavir and monotherapy efficacy in a proof-of-concept clinical study. J. Antimicrob. Chemother. 77, 989–995. doi: 10.1093/jac/dkab503
Markham, A. (2018). Ibalizumab: First Global Approval. Drugs 78, 781–785. doi: 10.1007/s40265-018-0907-5
Markovic, I., Stantchev, T. S., Fields, K. H., Tiffany, L. J., Tomiç, M., Weiss, C. D., et al. (2004). Thiol/disulfide exchange is a prerequisite for CXCR4-tropic HIV-1 envelope-mediated T-cell fusion during viral entry. Blood 103, 1586–1594. doi: 10.1182/blood-2003-05-1390
Markowitz, M., and Sarafianos, S. G. (2018). 4′-Ethynyl-2-fluoro-2′-deoxyadenosine, MK-8591. Curr. Opin. HIV AIDS 13, 294–299. doi: 10.1097/COH.0000000000000467
Martín-Alonso, S., Kang, D., Martínez del Río, J., Luczkowiak, J., Frutos-Beltrán, E., Zhang, L., et al. (2022). Novel RNase H inhibitors blocking RNA-directed Strand displacement DNA synthesis by HIV-1 reverse transcriptase. J. Mol. Biol. 434:167507. doi: 10.1016/j.jmb.2022.167507
Menéndez-Arias, L., Sebastián-Martín, A., and Álvarez, M. (2017). Viral reverse transcriptases. Virus Res. 234, 153–176. doi: 10.1016/j.virusres.2016.12.019
Mesman, A. W., and Geijtenbeek, T. B. (2012). Pattern recognition receptors in HIV transmission. Front. Immunol. 3:59. doi: 10.3389/fimmu.2012.00059
Moolla, N., Killick, M., Papathanasopoulos, M., and Capovilla, A. (2016). Thioredoxin (Trx 1) regulates CD4 membrane domain localization and is required for efficient CD4-dependent HIV-1 entry. Biochim. Biophys. Acta-Gen. Sub. 1860, 1854–1863. doi: 10.1016/j.bbagen.2016.05.030
Mousseau, G., and Valente, S. (2012). Strategies to block HIV transcription: focus on small molecule tat inhibitors. Biology 1, 668–697. doi: 10.3390/biology1030668
Muccini, C., Canetti, D., Castagna, A., and Spagnuolo, V. (2022). Efficacy and safety profile of Fostemsavir for the treatment of people with human immunodeficiency Virus-1 (HIV-1): current evidence and place in therapy. Drug Des. Devel. Ther. 16, 297–304. doi: 10.2147/DDDT.S273660
Nchioua, R., Bosso, M., Kmiec, D., and Kirchhoff, F. (2020). Cellular factors targeting HIV-1 transcription and viral RNA transcripts. Viruses 12:495. doi: 10.3390/v12050495
Obubeid, F. O., Eltigani, M. M., Mukhtar, R. M., Ibrahim, R. A., Alzain, M. A., Elbadawi, F. A., et al. (2022). Dual targeting inhibitors for HIV-1 capsid and cyclophilin a: molecular docking, molecular dynamics, and quantum mechanics. Mol. Simul. 48, 1476–1489. doi: 10.1080/08927022.2022.2097673
Ott, M., Geyer, M., and Zhou, Q. (2011). The control of HIV transcription: keeping RNA polymerase II on track. Cell Host Microbe 10, 426–435. doi: 10.1016/j.chom.2011.11.002
Overton, E. T., Richmond, G., Rizzardini, G., Jaeger, H., Orrell, C., Nagimova, F., et al. (2020). Long-acting cabotegravir and rilpivirine dosed every 2 months in adults with HIV-1 infection (ATLAS-2M), 48-week results: a randomised, multicentre, open-label, phase 3b, non-inferiority study. Lancet 396, 1994–2005. doi: 10.1016/S0140-6736(20)32666-0
Pace, C. S., Fordyce, M. W., Franco, D., Kao, C.-Y., Seaman, M. S., and Ho, D. D. (2013). Anti-CD4 monoclonal antibody Ibalizumab exhibits breadth and potency against HIV-1, with natural resistance mediated by the loss of a V5 glycan in envelope. J. Acquir. Immune Defic. Syndr. 62, 1–9. doi: 10.1097/QAI.0b013e3182732746
Porkolab, V., Chabrol, E., Varga, N., Ordanini, S., Sutkevičiu̅tė, I., Thépaut, M., et al. (2018). Rational-differential design of highly specific Glycomimetic Ligands: targeting DC-SIGN and excluding Langerin recognition. ACS Chem. Biol. 13, 600–608. doi: 10.1021/acschembio.7b00958
Puertas, M. C., Ploumidis, G., Ploumidis, M., Fumero, E., Clotet, B., Walworth, C. M., et al. (2020). Pan-resistant HIV-1 emergence in the era of integrase strand-transfer inhibitors: a case report. Lancet Microbe 1, e130–e135. doi: 10.1016/S2666-5247(20)30006-9
Reiser, K., Mathys, L., Curbo, S., Pannecouque, C., Noppen, S., Liekens, S., et al. (2016). The cellular Thioredoxin-1/Thioredoxin Reductase-1 driven Oxidoreduction represents a chemotherapeutic target for HIV-1 entry inhibition. PLoS One 11:e0147773. doi: 10.1371/journal.pone.0147773
Rose, R., Gartland, M., Li, Z., Zhou, N., Cockett, M., Beloor, J., et al. (2022). Clinical evidence for a lack of cross-resistance between temsavir and ibalizumab or maraviroc. AIDS 36, 11–18. doi: 10.1097/QAD.0000000000003097
Rusconi, S., Saladini, F., Bellocchi, M. C., Galli, L., Gagliardini, R., Gazzola, L., et al. (2022). Leronlimab (PRO 140) in vitro activity against 4-class drug resistant HIV-1 from heavily treatment experienced subjects. Pharmacol. Res. 176:106064. doi: 10.1016/j.phrs.2022.106064
Salie, Z. L., Kirby, K. A., Michailidis, E., Marchand, B., Singh, K., Rohan, L. C., et al. (2016). Structural basis of HIV inhibition by translocation-defective RT inhibitor 4′-ethynyl-2-fluoro-2′-deoxyadenosine (EFdA). Proc. Natl. Acad. Sci. U. S. A. 113, 9274–9279. doi: 10.1073/pnas.1605223113
Samji, H., Cescon, A., Hogg, R. S., Modur, S. P., Althoff, K. N., Buchacz, K., et al. (2013). Closing the gap: increases in life expectancy among treated HIV-positive individuals in the United States and Canada. PLoS One 8:e81355. doi: 10.1371/journal.pone.0081355
Segal-Maurer, S., DeJesus, E., Stellbrink, H.-J., Castagna, A., Richmond, G. J., Sinclair, G. I., et al. (2022). Capsid inhibition with Lenacapavir in multidrug-resistant HIV-1 infection. N. Engl. J. Med. 386, 1793–1803. doi: 10.1056/NEJMoa2115542
Selby, M. J., Bain, E. S., Luciw, P. A., and Peterlin, B. M. (1989). Structure, sequence, and position of the stem-loop in tar determine transcriptional elongation by tat through the HIV-1 long terminal repeat. Genes Dev. 3, 547–558. doi: 10.1101/gad.3.4.547
Selyutina, A., Persaud, M., Simons, L. M., Bulnes-Ramos, A., Buffone, C., Martinez-Lopez, A., et al. (2020). Cyclophilin a prevents HIV-1 restriction in lymphocytes by blocking human TRIM5α binding to the viral Core. Cell Rep. 30, 3766–3777.e6. doi: 10.1016/j.celrep.2020.02.100
Sokolskaja, E., Sayah, D. M., and Luban, J. (2004). Target cell Cyclophilin a modulates human immunodeficiency virus type 1 infectivity. J. Virol. 78, 12800–12808. doi: 10.1128/JVI.78.23.12800-12808.2004
Spinner, C. D., Felizarta, F., Rizzardini, G., Philibert, P., Mitha, E., Domingo, P., et al. (2022). Phase IIa proof-of-concept evaluation of the antiviral efficacy, safety, tolerability, and pharmacokinetics of the next-generation maturation inhibitor GSK3640254. Clin. Infect. Dis. 75, 786–794. doi: 10.1093/cid/ciab1065
Sundquist, W. I., and Krausslich, H.-G. (2012). HIV-1 assembly, budding, and maturation. Cold Spring Harb. Perspect. Med. 2:a006924. doi: 10.1101/cshperspect.a006924
Thompson, M. A. (2018). The return of PRO 140, a CCR5-directed mAb. Curr. Opin. HIV AIDS 13, 346–353. doi: 10.1097/COH.0000000000000479
Tsuji, K., Kobayakawa, T., Konno, K., Masuda, A., Takahashi, K., Ohashi, N., et al. (2022). Exploratory studies on soluble small molecule CD4 mimics as HIV entry inhibitors. Bioorg. Med. Chem. 56:116616. doi: 10.1016/j.bmc.2022.116616
Varga, N., Sutkeviciute, I., Ribeiro-Viana, R., Berzi, A., Ramdasi, R., Daghetti, A., et al. (2014). A multivalent inhibitor of the DC-SIGN dependent uptake of HIV-1 and dengue virus. Biomaterials 35, 4175–4184. doi: 10.1016/j.biomaterials.2014.01.014
Wainberg, M. A., and Albert, J. (2010). Can the further clinical development of bevirimat be justified? AIDS 24, 773–774. doi: 10.1097/QAD.0b013e328331c83b
Wang, D., Lu, W., and Li, F. (2015). Pharmacological intervention of HIV-1 maturation. Acta Pharm. Sin. B 5, 493–499. doi: 10.1016/j.apsb.2015.05.004
Wang, L., Tang, J., Huber, A. D., Casey, M. C., Kirby, K. A., Wilson, D. J., et al. (2018). 6-Arylthio-3-hydroxypyrimidine-2,4-diones potently inhibited HIV reverse transcriptase-associated RNase H with antiviral activity. Eur. J. Med. Chem. 156, 652–665. doi: 10.1016/j.ejmech.2018.07.039
Wang, C. Y., Wong, W.-W., Tsai, H. C., Chen, Y. H., Kuo, B. S., Lynn, S., et al. (2019). Effect of anti-CD4 antibody UB-421 on HIV-1 rebound after treatment interruption. N. Engl. J. Med. 380, 1535–1545. doi: 10.1056/NEJMoa1802264
Wu, V. H., Smith, R. A., Masoum, S., Raugi, D. N., Ba, S., Seydi, M., et al. (2017). MK-8591 (4′-Ethynyl-2-Fluoro-2′-deoxyadenosine) exhibits potent activity against HIV-2 isolates and drug-resistant HIV-2 mutants in culture. Antimicrob. Agents Chemother. 61:e00744-17. doi: 10.1128/AAC.00744-17
Keywords: HIV infection, drug resistance, newly approved antiretroviral agents, salvage therapy, new drug targets, patients with multidrug-resistant HIV-1 infection
Citation: Temereanca A and Ruta S (2023) Strategies to overcome HIV drug resistance-current and future perspectives. Front. Microbiol. 14:1133407. doi: 10.3389/fmicb.2023.1133407
Edited by:
Mariana Carmen Chifiriuc, University of Bucharest, RomaniaReviewed by:
Luminita Marutescu, University of Bucharest, RomaniaCopyright © 2023 Temereanca and Ruta. This is an open-access article distributed under the terms of the Creative Commons Attribution License (CC BY). The use, distribution or reproduction in other forums is permitted, provided the original author(s) and the copyright owner(s) are credited and that the original publication in this journal is cited, in accordance with accepted academic practice. No use, distribution or reproduction is permitted which does not comply with these terms.
*Correspondence: Simona Ruta, ✉ simona.ruta@umfcd.ro