Corrigendum: Positive effects of Cordyceps cateniannulata colonization in tobacco: growth promotion and resistance to abiotic stress
- 1Institute of Fungus Resources, College of Life Sciences, Guizhou University, Guiyang, China
- 2Zunyi Tobacco Company of Guizhou Province, Zunyi, China
- 3College of Tea Sciences, Guizhou University, Guiyang, China
Background: Entomopathogenic fungi can live in insects to cause disease and death and are the largest group of entomopathogenic microorganisms. Therefore, these fungi are best known for their microbial control potential. Importantly, they also have other beneficial effects, including promoting plant growth and development by colonizing plant. Here, the study sought to identify specific strains of the entomopathogenic fungus, Cordyceps cateniannulata that would form endophytic associations with tobacco, thus benefiting plant growth and resistance to abiotic stresses, thereby highlighting the application of entomopathogenic fungi in tobacco.
Methods: The C. cateniannulata-tobacco symbiont was constructed by root irrigation. The effects of C. cateniannulata on tobacco growth were evaluated by measuring the maximum leaf length, maximum leaf width, number of leaves, plant height, stem thickness, stem circumference, dry and fresh shoot weight 7, 14, 21, and 28 days after colonization. The peroxidase, catalase, superoxide dismutase, and malondialdehyde were measured to observe the impact of C. cateniannulata on tobacco defense enzyme activity. Finally, high-throughput sequencing was used to access microbial communities in the rhizosphere, with data subsequently linked to growth indicators.
Results: After tobacco was inoculated with C. cateniannulata X8, which significantly promoted growth and related enzyme activity, malondialdehyde was decreased. The most significant impact was on peroxidase, with its activity being upregulated by 98.20, 154.42, 180.65, and 170.38% in the four time periods, respectively. The high throughput sequencing results indicated that C. cateniannulata had changed the rhizosphere microbial relative abundances, such as increasing Acidobacteria and Ascomycetes, and decreasing Actinomycetes and Basidiomycetes. The redundancy analysis showed that C. cateniannulata significantly boosted tobacco growth by reducing the abundance of specific dominant genera such as Stachybotrys, Cephalotrichum, Streptomyces, Isoptericola, and Microbacterium.
Conclusion: Specific strains of C. cateniannulata can be introduced into host plants as endophytes, resulting in promotion of host plant growth and increased resistance to abiotic stress and microbial pathogens. The study provides a foundation for future studies of C. cateniannulata as an ecological agent.
1. Introduction
Entomopathogenic fungi are direct sources of fungal insecticides and refer to fungi that live in insects to cause disease and death, thus they are best known for their microbial control potential (Raya-Díaz et al., 2017; Shin et al., 2020). Studies have shown that some entomopathogenic fungi have dual hosts in nature (Lacey et al., 2015). These fungi can parasitize insects, as well as colonize plants to interact with plants without causing harm (Barelli et al., 2016). As such, they have gradually been included in the category of plant endophytes (González-Guzmán et al., 2022; Liu et al., 2022). Some scholars have found that entomopathogenic fungi Beauveria bassiana and Metarhizium brunneum can be used as plant endophytes, promoting the growth of wheat, maize, and broad bean (Jaber, 2018; Russo et al., 2019; Rasool et al., 2021). M. anisopliae transfers nitrogen from insects to host plants through fungal hyphae to promote plant growth and development (Behie et al., 2012).
Abiotic stress is the most harmful factor affecting crop yields. Plants have developed a set of antioxidant defense mechanisms in response to abiotic stress through defensive enzymes, such as peroxidase (POD), superoxide dismutase (SOD), and catalase (CAT) (Tang et al., 2019), which constitute the antioxidant enzyme-promoting system in living organisms (Zhou et al., 2021). Malondialdehyde (MDA) is a membrane lipid peroxidation product that reflects the degree of cell damage (Xu et al., 2013). Low concentrations of MDA are conducive to the growth and resistance of symbiotic plants to environmental stress (Khan et al., 2015). Entomopathogenic fungi can maintain plant growth by increasing the activity of antioxidant enzymes and decreasing MDA expression, thereby alleviating the damage caused by environmental stress (Tsikas, 2017; González-Pérez et al., 2022). Researchers have discovered that several key defense enzyme activities, including POD, SOD, and CAT are enhanced in maize seedlings after colonizing B. bassiana (Forlani et al., 2014). Entomopathogenic fungi provides a basis for the application of the concept of ecological symbiosis in plant protection.
The entomopathogenic fungus Cordyceps cateniannulata, also known as Paecilomyces cateniannulatus, belongs to the Sordariomycetes, Hypocreales, and Cordycipitaceae families (Zhang et al., 2016). As an essential group of broad-spectrum entomopathogenic fungi, C. cateniannulata plays a vital role in plant pest control, with the fatality rate to tetranychus being as high as 92% (Zhang et al., 2018). Most studies have focused on the application of C. cateniannulata in biological control. In addition, this fungus also acts to promote the growth of host plants. In recent years, reports on growth promotion have gradually emerged, such as it could colonize and promote the growth of buckwheat (Zhang et al., 2022), increasing the germination index of seeds of buckwheat (Peng et al., 2022), and increasing the tomato biomass (Guan et al., 2022). However, the holistic evaluation of C. cateniannulata on the plant and the effect on the rhizosphere soil has not been explored yet.
Tobacco as an essential cash crop, with growth index and yield that are closely related to economic value (Ren et al., 2021). However, tobacco has a great demand for chemical fertilizer and is susceptible to abiotic stress, such as waterlogging, resulting in reduced production and quality, especially in the rainy south (Pan et al., 2019). Therefore, to find and develop an efficient and ecological plant protection technology has become an important task for the sustainable development of tobacco industry. Some studies have suggested that C. cateniannulata colonizes tomato seedling roots, stems, and leaves by immersion, which may promote plant growth and antioxidant enzyme activity (Guan et al., 2022). Both tobacco and tomatoes are Solanaceae crops, which begs the question–can C. cateniannulata also colonize tobacco? How does C. cateniannulata affect tobacco growth development and rhizosphere microbial diversity post-colonization? However, these are open questions. Therefore, the objectives of this study were: (i) to assess the effect of C. cateniannulata on plant development by measuring the growth and enzymatic activity of tobacco; (ii) to evaluate the correlation between the dominant microbial genus and tobacco growth; (iii) to provide strain resources for developing a kind of environmental protection biological agent in tobacco.
2. Materials and methods
2.1. Materials
The strain C. cateniannulata GZUIFR04XS8 (X8) was collected from the Institute of Fungal Resources, Guizhou University (GZUIFR), Guiyang, Guizhou, China. The tobacco variety was K326, provided by Guizhou Provincial Key Laboratory of Tobacco Quality Research. POD, SOD, CAT, and MDA enzyme activity detection kits were purchased from Solarbio Technology Co., LTD (Beijing, China). The Cu2+ medium formulation included Glucose 40 g, peptone 10 g, agar 18 g, CuSO4·5H2O powder 1 g, streptomycin sulfate 0.1 g, penicillin potassium 0.1 g, and water 1,000 ml, sterilized at 121°C for 30 min. Seedling trays (50 holes, each 5 cm × 5 cm × 5 cm).
2.2. Detection of Cordyceps cateniannulata colonization in tobacco
The strain was cultured on potato dextrose agar (PDA) plates at 26°C for 10 d under 12-h light/12-h dark conditions. Conidia were harvested by flooding the plate with sterile 0.05% (v/v) Tween-80 solution. The conidial suspensions were vortexed for 1 min and filtered through two layers of lens paper, with conidia concentrations being measured on a hemocytometer and adjusted to 2 × 107 conidia mL−1 using sterile water.
Tobacco seeds were disinfected by sequentially treating them with 75% (v/v) ethanol for 30 s, 10% (v/v) NaClO for 5 min, and sterile water for 1 min. Two seeds were added per individual hole in a seedling tray, filled with sterilized soil. The trays were stored in a sterile artificial climate chamber at 26°C with 70% relative humidity and a 12-h light/12-h dark cycle. The nutrient solution was used to water plants every 3 days.
After 15 days, the tobacco was irrigated with 15 ml of the prepared C. cateniannulata conidia suspension on roots for the colonization detection experiments. Upper portions of plants were sampled at 7 days after inoculation, then disinfected (method of disinfection as above), and taking 100 μl of the last clean sterile water for culture to test the disinfection effect of the plant surface. Based on the tolerance of C. cateniannulata to Cu2+, so the Cu2+ medium was used to isolate and culture C. cateniannulata. Tissues of roots, stems, and leaves were cut into 1 cm pieces (roots and stems) or 1 × 1 cm (leaves) on a sterile operating table. Respectively, nine pieces were then randomly selected and cultured on Cu2+ medium at 26°C until they grew out fungi for use (Xu, 2020).
2.3. Microscopic morphology and molecular identification of Cordyceps cateniannulata in tobacco
The induced fungal colonies were inoculated on PDA at 26°C for 5 d under 12-h light/12-h dark conditions, Relative Humidity (RH) = 70%. Fresh hyphae were stained with lactophenol cotton blue solution and then observed using an optical microscope. All extracted DNA samples were evaluated by PCR amplification. The internal transcribed spacer (ITS) was amplified using the ITS1 (5 ‘-TCCGTAGGTGAACCTGCGG-3’) and ITS4 (5 ‘-TCCTCCGCTTATTGATATGC-3’) primer pair (White et al., 1990). The PCR reaction system (25 μl) included 2 μl gDNA, 12.5 μl 2 × A8 PCR master mix, 1 μl (10 μmol/l) ITS1, 4, respectively, and ddH2O was added to 25 μl (Chiriví-Salomón et al., 2015). The PCR amplification protocol began at 95°C for 3 min, 55°C for 30 s, 72°C for 45 s, followed by 30 cycles and 5 min extension at 72°C (Zhou et al., 2018). The PCR products were monitored by electrophoresis on a 2% (w/v) agarose gel and sequenced by Tsingke Biotechnology Co., LTD (Shanghai, China). A basic local alignment search tool (BLAST) search was performed against DNA sequences in GenBank, and the sequences of related species (similarity>85%) were downloaded. Using Purpureocillium lilacinum and Torrubiella ratticaudata as the outgroup, the neighbor-joining method constructed a phylogenetic tree using MEGA 7 software with 1,000 bootstrap replicates.
2.4. Impact of Cordyceps cateniannulata on tobacco growth
Two seeds were added to individual holes in a seedling tray filled with soil, after which all were stored in a natural condition (all materials were not sterilized). Four hundred seeds were planted in four trays (2 seeds/holes × 50 holes × 4 trays). The nutrient solution was used to water plants every 3 days. The seedlings were transplanted to 12-cm diameter pots after 15 days, and one group was designated as the treatment group and irrigated with 15 ml of the prepared C. cateniannulata conidia suspension on roots, while the other group was maintained as the control group and irrigated with 15 ml of 0.05% (v/v) Tween-80 solution. The growth indicators of tobacco were measured at 7, 14, 21, and 28 days after inoculation, including maximum leaf length, maximum leaf width, plant height, number of leaves, stem thickness, stem circumference, dry and fresh shoot weight. The maximum leaf length and width were selected from the longest and widest leaves of the tobacco plants and measured with a ruler. The height of the plant was measured cm using a tape measure. The number of leaves was counted from the lower part of the tobacco plant upwards, avoiding the two leaves at the bottom and the two young true leaves facing the top. The stem circumference was measured with a soft ruler about 2 cm from the ground near the plant. The stem thickness was measured by a vernier caliper. Above-ground sections were separated, fresh weights were determined using an analytical balance, and dry weights were examined after drying at 110°C for 2 h, followed by 37°C for 4 days. Five randomly sampled seedlings were used for all experiments.
2.5. Impact of Cordyceps cateniannulata on tobacco defense enzyme activity
The tobacco in section 2.4 was also used for enzyme activity detection. In both the control and treated groups, mature leaves of 20 plants, each at the same position (middle part of the tobacco stem) were collected to determine POD, SOD, CAT, and MDA content 7, 14, 21 and 28 days after inoculation. Next, 0.1 g fresh leaves were added to 2 ml of phosphoric acid buffer in a pre-cooled mortar and ground with liquid nitrogen until homogenized. Samples were then spun for 20 min at 8000 rpm at 4°C. Supernatants were used as the enzyme extract to determine the enzyme activities. All extraction procedures were conducted based on protocols provided in a kit obtained from Solarbio.
2.6. DNA extraction, PCR amplification, and sequencing of soil
The tobacco soil was collected using sterile gloves and placed in sterile plastic bags. The microbial genomic DNA used for high-throughput sequencing of soil was extracted using the FastDNA® SPIN Kit for Soil. The fungal ITS gene was amplified with the primers ITS1F (5′-CTTGGTCATT TAGAGGAAGTAA-3′) and 2043R (5′-GCTGCGTTCTTCATCGATGC-3′). The primers 338F (5′-ACTCCTACGGGAGGCAGCAG-3′) and 806R (5′- GGACTACHVGGGTWTCTAAT-3′) were used to amplify the V3–4 variable region of the bacterial 16S rRNA gene (Adams et al., 2013). All PCR reactions were performed using Phusion® High-Fidelity PCR Master Mix (New England Biolabs). The amplification products were monitored by electrophoresis on 2% (w/v) agarose gel and sequenced using the Illumina HiSeq platform (Majorbio Bio-pharm Technology Co., Ltd., Shanghai, China).
Raw reads from original DNA fragments were merged and quality-filtered using FLASH version 1.2.7 and Trimmomatic version 0.32. The UPARSE version 7.1 was used for chimera removal. Bacterial gene sequences were annotated with taxonomic information using the RDP classifier against the Silva 16S rRNA database. The OTU taxonomic information of fungi was obtained by aligning representative sequences against the Unit 8.0 ITS database (Rognes et al., 2016).
2.7. Data analysis
A one-way analysis of variance (ANOVA) followed by least significant difference (LSD) test at p < 0.05 was performed using SPSS 25.0 software to detect significant differences among treatments. p values <0.05 were considered statistically significant. The alpha diversity of the microbial communities was calculated using the QIIME platform. Analyzes based on the Shannon diversity index and Ace index were performed. The correlation between tobacco growth indicators and dominant soil genera was analyzed on the Metagenomics Core Microbiome Exploration Tool (MetaCoMET).
3. Results
3.1. Colonization of Cordyceps cateniannulata in tobacco
The last water used for washing tissues was cultured, and no colonies formed on the PDA after 5 days, indicating that the sample’s surface had been thoroughly disinfected. The obtained endophytic strain isolated from plant was named X, and colony color, conidial stem, and conidial morphology were all consistent with C. cateniannulata (Figure 1; Table 1). The phylogenetic tree based on rDNA-ITS showed that strain X was in the same branch as C. cateniannulata GZUIFR04XS8, with 100% similarity (Figure 2).
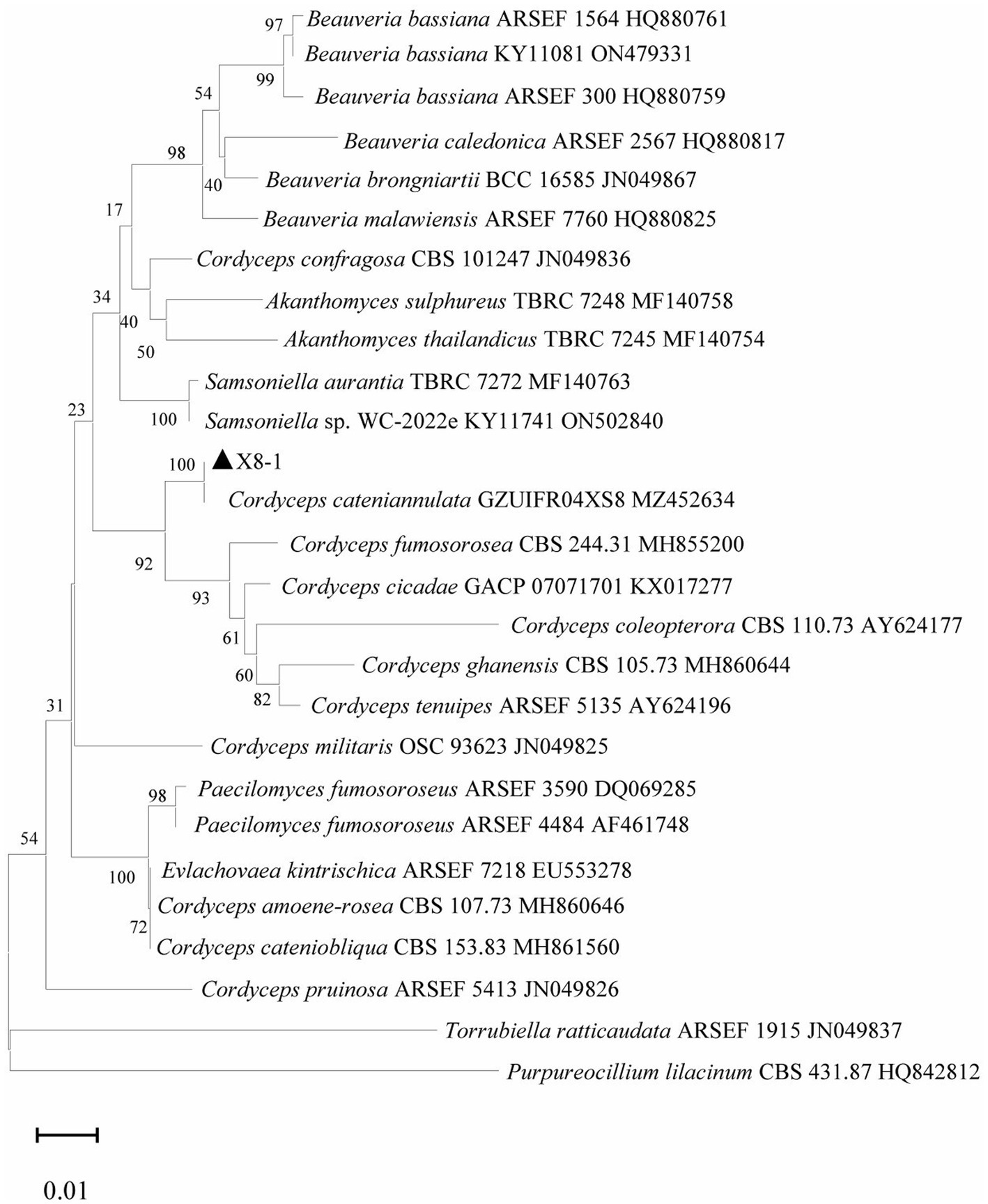
Figure 2. Phylogenetic tree generated with rDNA-ITS sequences of strain X and other related species.
3.2. The impact of Cordyceps cateniannulata on tobacco growth
The growth and development of tobacco seedlings in the X8 group were superior to those in the control group. The maximum leaf length and width treated with X8 was larger than the control group in the four periods after inoculation. The maximum leaf length reached a significant level on day 14 and 21 (p < 0.05), which were 14.69 and 11.41% higher than the control, respectively (Figure 3A). The maximum leaf width reached a significant level on day 7 and 28 (p < 0.05), which were 23.72 and 18.13% higher than the control, respectively (Figure 3B). The X8 group had more leaves than the control group, which was 14.88% higher on day 14 (p < 0.05; Figure 3C). The plant height of the X8 treatment was significantly higher than the control (p < 0.05), which was 37.92% higher and reached a significant difference in the mid-term after transplanting (Figure 3D).
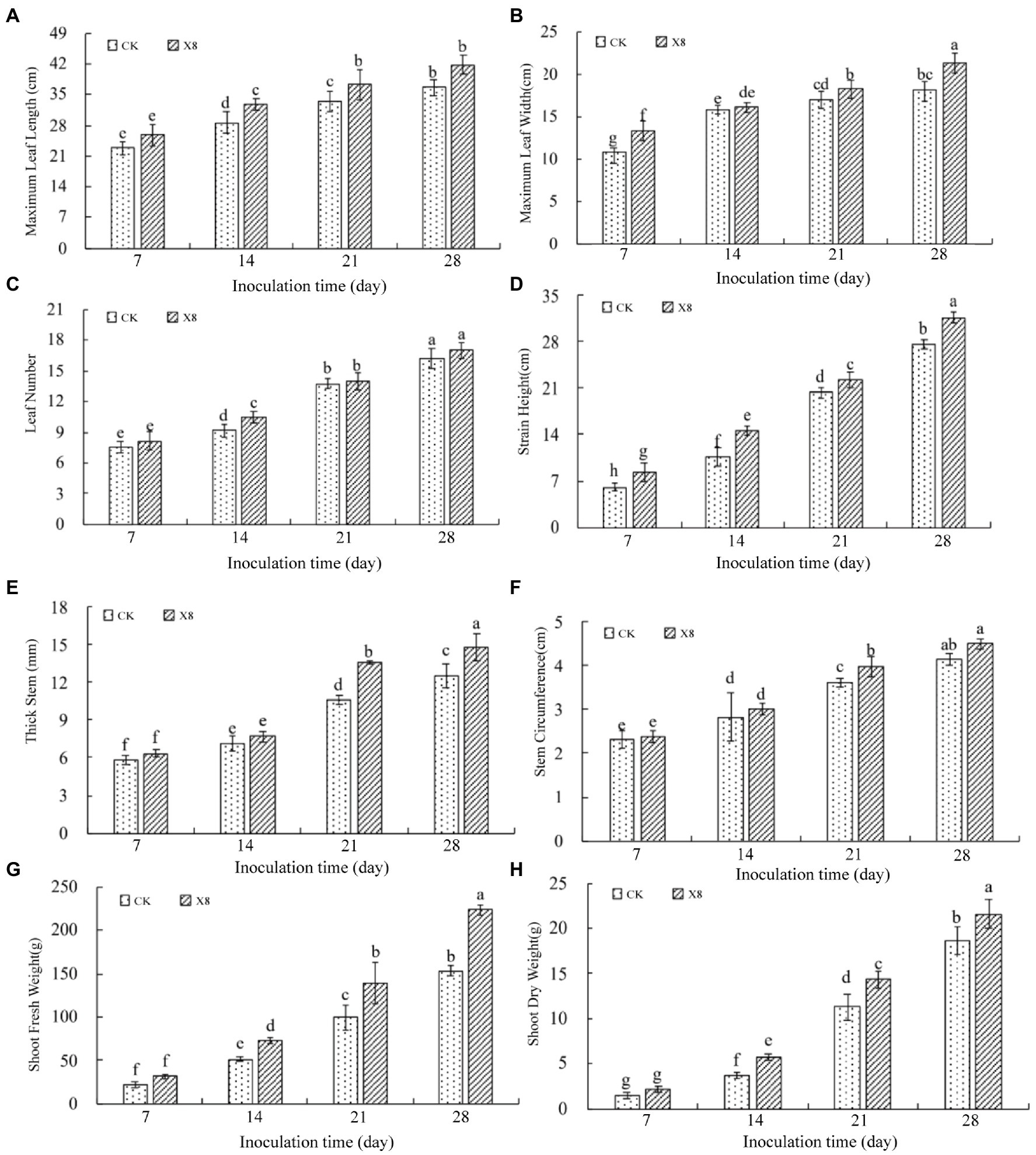
Figure 3. Impact of X8 on tobacco growth indicators. The error bars reflect standard error, mean (±SE) (n = 3). Lowercase letters refer to significant differences (LSD test, p < 0.05). (A) Maximum leaf length, (B) maximum leaf width, (C) number of leaves, (D) plant height, (E) stem thickness, (F) stem circumference, (G) fresh weight, (H) dry weight.
Similarly, the stem thickness and circumference of the X8 treatment group were larger than the control, especially in the last two periods (p < 0.05). On day 21 and 28, the stem thickness was 28.46 and 18.41% higher than the control group (Figure 3E), and the stem circumference was 9.64 and 8.45% higher than the control, respectively (Figure 3F).
The dry and fresh shoot weight in the X8 treatment group was greater than the control group, but this was not significant on day 7 after transplanting. However, the difference reached a significant level in the last three periods (p < 0.05). The fresh weight in the X8 treatment group was 42.14, 40.38, and 46.26% higher than the control group (Figure 3G). The dry weight was 57.65, 26.96, and 15.92% higher than the control group, respectively (Figure 3H). The results indicated that X8 inoculation was beneficial for accumulating fresh and dry weight in the tobacco.
3.3. Impact of Cordyceps cateniannulata on tobacco defense enzyme activity
The experimental results showed that the X8 treatment significantly increased the activity of antioxidant enzymes (p < 0.05) and decreased MDA content. The activity of POD in the X8 treatment group was significantly higher than the control group after transplanting (p < 0.05), which was 180.65 and 170.38% higher than the control group after 21 and 28 days, respectively (Figure 4A). The CAT activity of the X8 treatment group was higher than the control group. The differences between the two groups was significant in the last three periods (p < 0.05), which was 47.44, 36.72, and 43.35% higher, respectively (Figure 4B). The SOD activity in the X8 treatment group was 4.28, 111.09, 17.15, and 22.92% higher than the control group, respectively, the later stages reached significant levels (p < 0.05; Figure 4C). The results showed that X8 inoculation was beneficial for increasing POD, CAT, and SOD activity in tobacco.
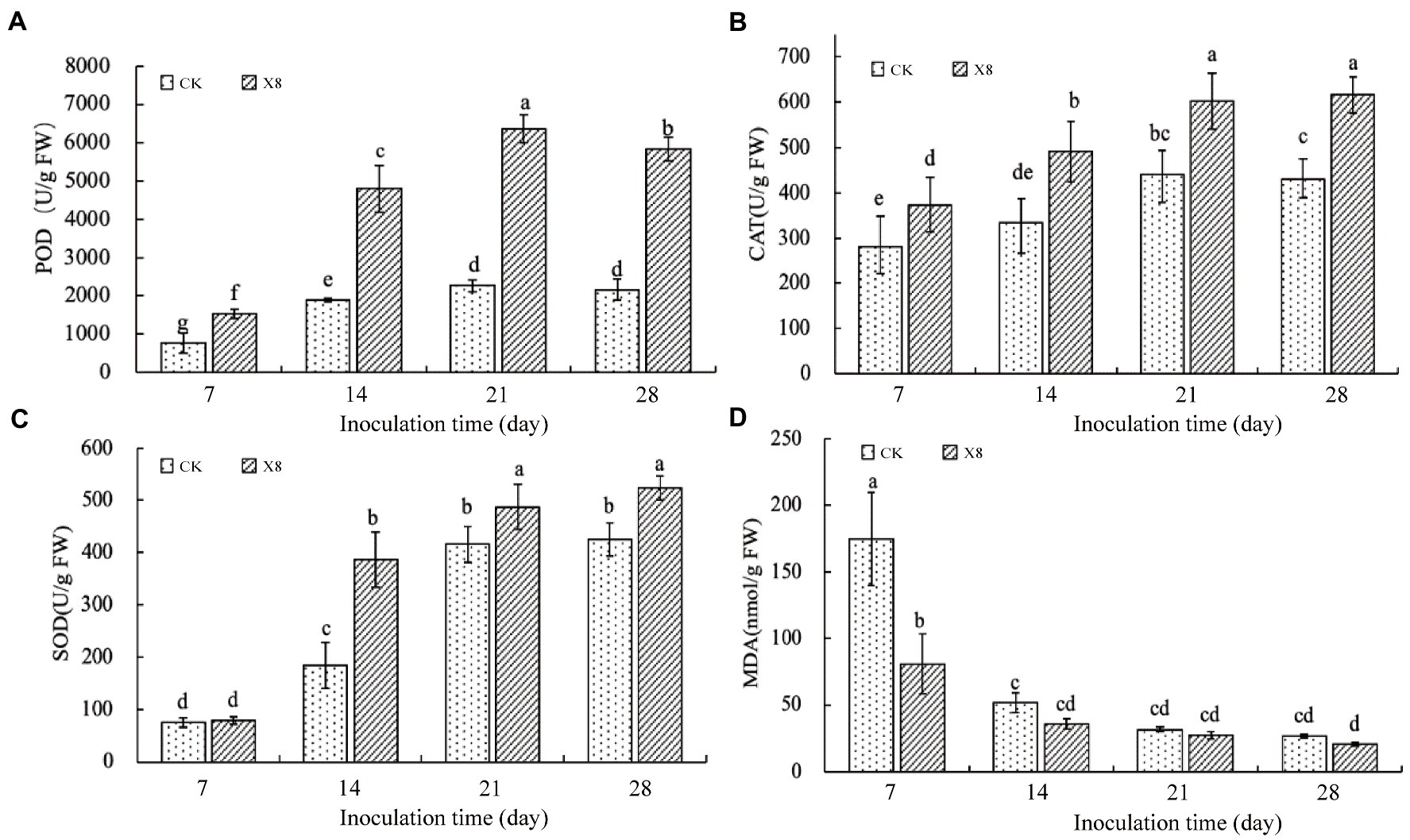
Figure 4. Impact of X8 on tobacco defense enzyme activities. The error bars reflect standard error, mean (±SE) (n = 3). The different lowercase letters refer to significant differences (LSD test, p < 0.05). (A) POD, (B) CAT, (C) SOD, (D) MDA.
MDA content represents the peroxidation degree of the plant cell membrane (Tsikas, 2017). The MDA content in tobacco decreased gradually with the increase of time (Figure 4D). The sampled tobacco had the highest MDA content in the first 7 days, and X8 effectively reduced the content by 116.13% compared to the control. The X8 group had downregulated MDA expression than the control group in the last three periods, but this was not significant, suggesting that the reduction in MDA was most pronounced at the early stage after X8 inoculation.
3.4. Analysis of microbial diversities in the rhizosphere
With the increase of the sample number, the Shannon-Winner index curve tended to be flatter (Figure 5), indicating that the sequencing data sufficiently reflected the microorganisms in the sequencing samples fully. The coverage indices of bacterial and fungal samples were above 98%, indicating that the sample numbers were sufficient to reflect the microbial diversities.
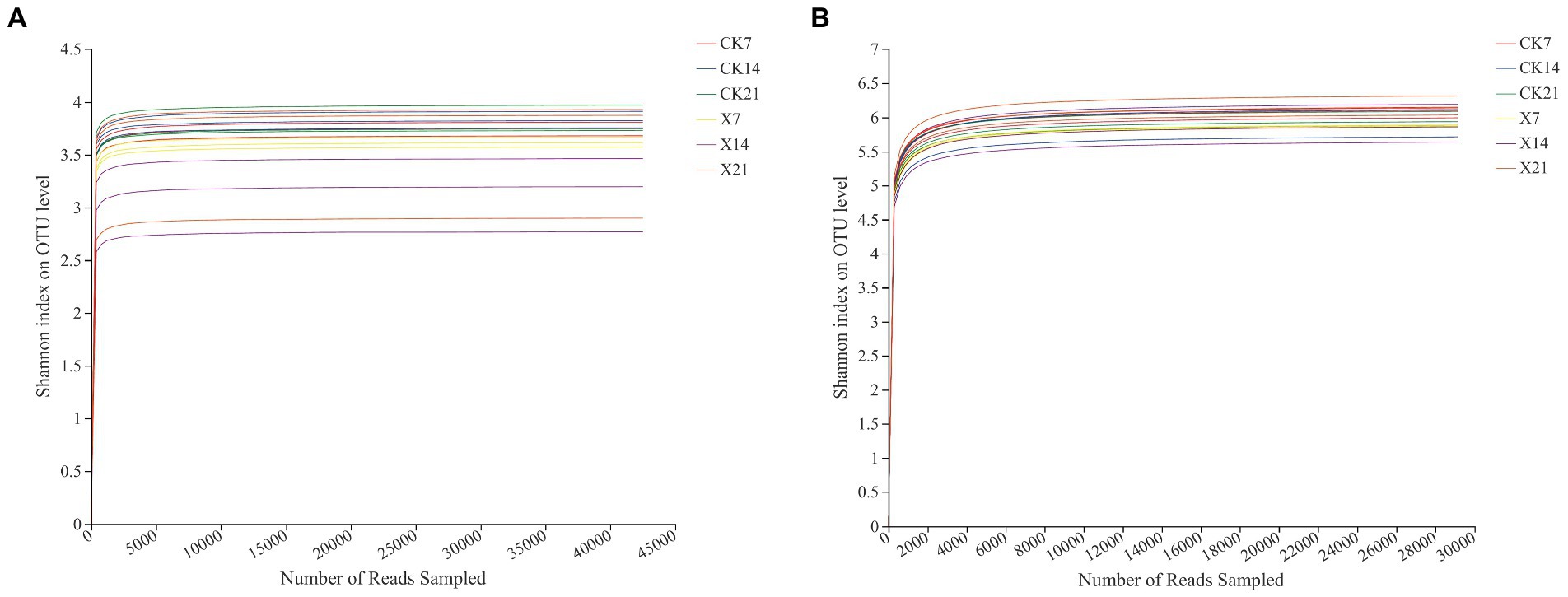
Figure 5. Shannon-Winner index curve. (A) Fungal community dilution curve. (B) Bacterial community dilution curve.
The Shannon and Chao index of fungi in the X8 treatment group was lower than the control group, and the Ace index was higher than the control group on day 21. The Shannon, Ace, and Chao indexes of bacteria in the X8 treatment group were lower than the control group in the first two periods, and higher than the control group in the later periods. In general, the microbial diversity and richness in the C. cateniannulata treatment group increased first and then decreased with time (Figure 6).
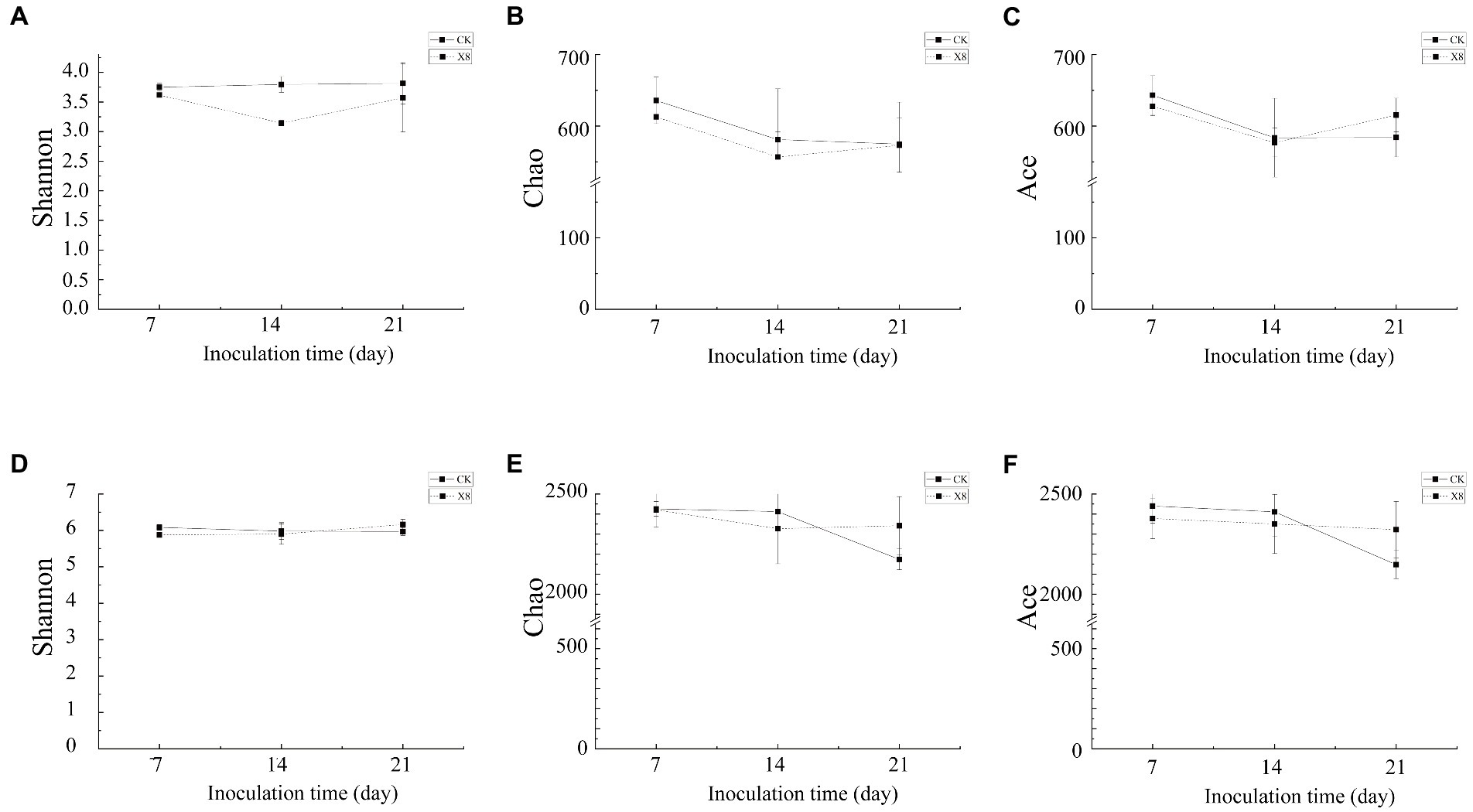
Figure 6. Alpha diversity of fungal and bacterial communities. (A–C) Fungal community. (D–F) Bacterial community. X8 refers to C. cateniannulata X8, CK refers to the control. All the data are presented as mean ± SE (n = 3). SE–standard error of the mean.
3.5. Analysis of microbial community composition
Species analysis of the fungal community revealed that there were 1,036,450 valid sequences, including 15 phyla, 40 classes, 80 orders, 168 families, 314 genera, 510 species, and 1,120 OTUs. At the phylum level (Figure 7A), Ascomycota was the dominant phylum in all periods of the two groups, with a relative abundance of 68.86–80.26%. The sub-dominant phylum was Basidiomycota, with a relative abundance of 12.55–20.84%. The percentages of Ascomycota and Basidiomycota communities in the X8 group did not change much on day 7 and 21, but this increased and decreased on day 14, respectively. At the genus level (Figure 7B), the dominant genus was Cephalotrichum (12.84–36.93%). Compared with the control group, the proportion of Cephalotrichum in the X8 group decreased first, then increased, and its abundance increased significantly on day 14. The abundance of Trichosporon in the X8 treatment group was significantly lower than the control group (p < 0.05), which indicates that C. cateniannulata had an inhibitory effect on Trichosporon fungi.
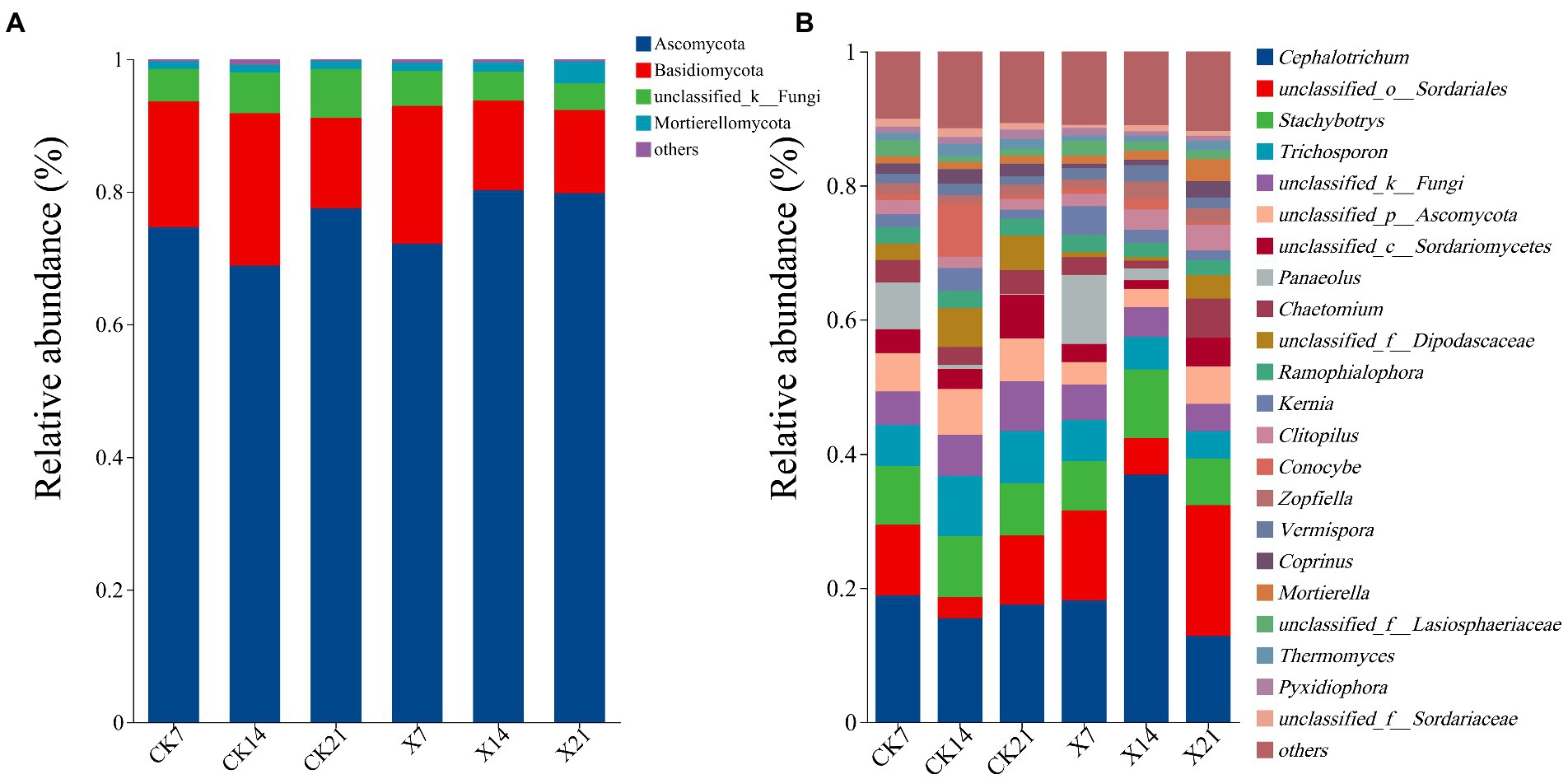
Figure 7. Fungal flora composition at phylum and genus levels. (A) Phylum level. (B) Genus level, genera with relative abundance less than 1% are classified as other. X refers to C. cateniannulata X8 treatment, CK refers to the control, and the number indicates the time point after inoculation.
Bacterial community species analysis: 1034854 valid sequences were obtained, 37 phyla, 109 classes, 261 orders, 434 families, 827 genera, 1,601 species, and 3,216 OTUs were detected. At the phylum level (Figure 8A), the dominant bacteria were Actinobacteriota (25.72–32.13%), Proteobacteria (6.79–8.65%), Acidobacteriota (4–5.35%). Compared to the control group, the proportion of Actinobacteria in the X8 group showed a downward trend, and Acidobacteria showed an upward trend, indicating that the application of X8 had an inhibitory effect on Actinobacteria and a facilitative effect on Acidobacteria. At the genus level (Figure 8B), Streptomyces (5.96–9.65%) was the dominant genus at all time points, and Lsoptericola also had a high abundance (2.18–2.95%). In the control group, Streptomyces first increased and then decreased over time, while in the X8 group, Streptomyces decreased. The abundance of Lsoptericola did not change over time in the control group, but showed a significant downward trend in the X8 group.
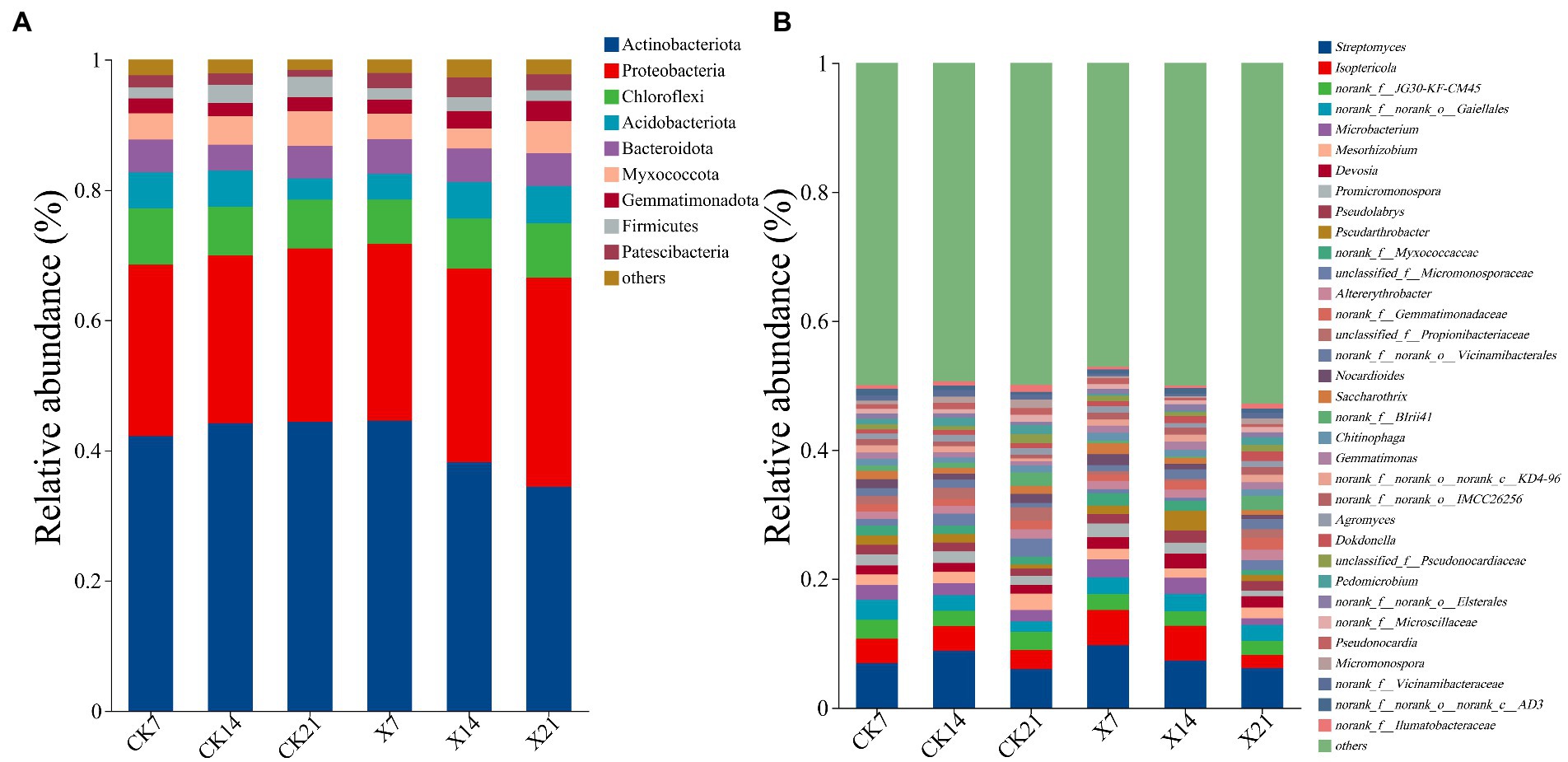
Figure 8. Bacterial flora composition at phylum and genus levels. (A) Phylum level. (B) Genus level, genera with relative abundance less than 1% are classified as other. X refers to C. cateniannulata X8 treatment, CK refers to the control, and the number indicates the time point after inoculation.
In the fungal community (Figure 9A), the dominant genera of the two groups were similar on day 7 and 14 but differed on day 21, the control group was Cephalotrichum, and the X8 group was unclassified_o_Sordariales. In both groups, the trend of Panaeolus was gradually reduced over time, and unclassified_o_Sordariales was increased after the first decrease. In the bacterial community (Figure 9B), the dominant genera of the two groups were the same on day 21, only Streptomyces. In the first two periods, the dominant genera in the control group was Streptomyces, and the X8 group was Isoptericola. Isoptericola showed a gradual decrease in both groups.
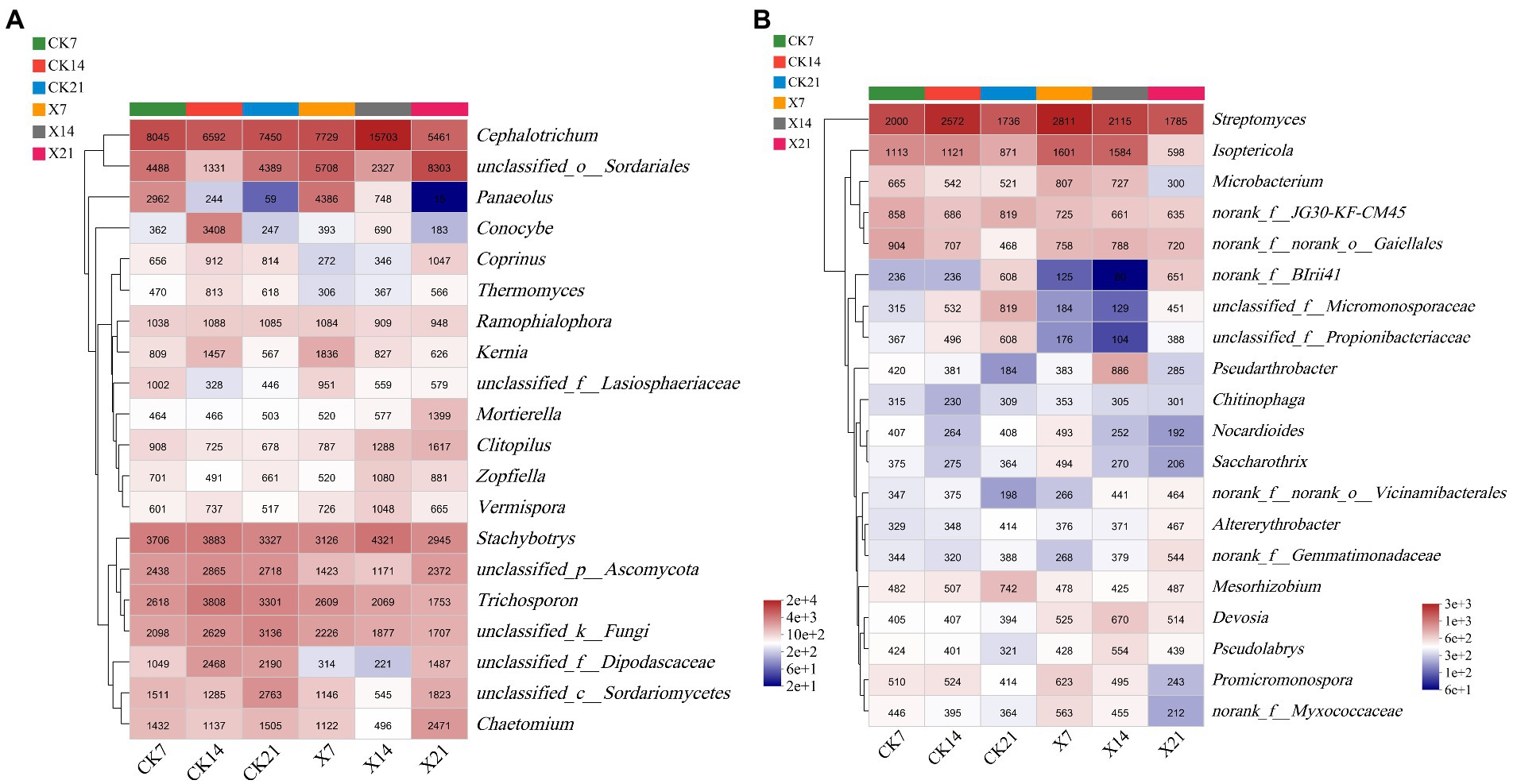
Figure 9. Community heatmap analysis on genus level. (A) Fungal community. (B) Bacterial community. X refers to C. cateniannulata X8 treatment, CK refers to the control, and the number indicates the time point after inoculation.
3.6. Correlation between growth indicators and dominant genera
The redundancy analysis (RDA) was used to further study the relationship between growth indicators and dominant genera of rhizosphere microorganisms. In the fungal community (Figure 10A), the accumulation of RDA1 and RDA2 was 61.08 and 20.50%, respectively. The overall explanation for the growth indicators of the fungal community was 61.08%. The stem thickness was the most strongly correlated with the fungal community and accounted for 95.59% of the differences in fungal populations. Unclassified_o_Sordariales were positively correlated to growth indicators. In contrast, Stachybotrys and Cephalotrichum were negatively correlated to growth indicators. Trichosporon with maximum leaf length, stem circumference, leaf number, and stem thickness were positively correlated, and negatively correlated to plant height.
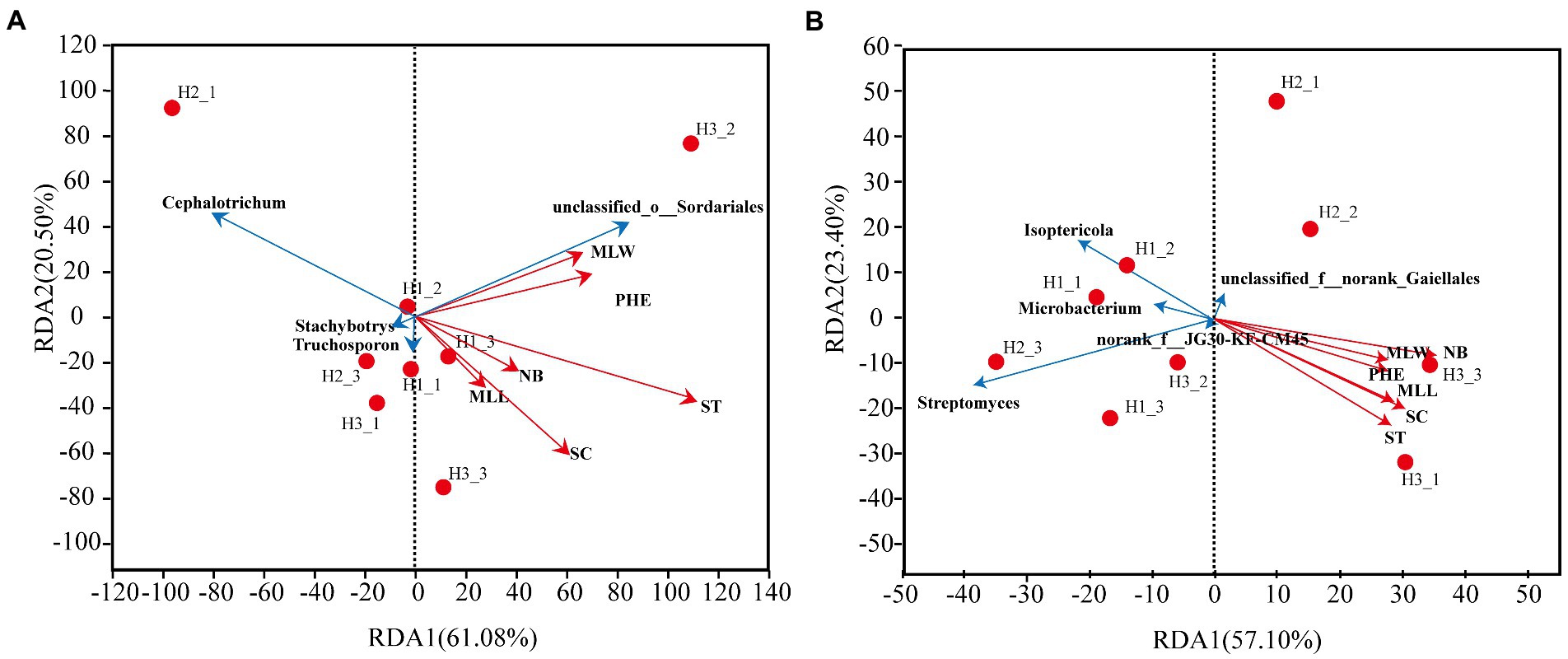
Figure 10. RDA analysis between dominant rhizosphere genera and growth indicators. (A) Fungal community. (B) Bacterial community. MLL, maximum leaf length; MLW, maximum leaf width; NB, number of leaves; PHE, plant height; ST, stem thickness; SC, stem circumference.
In the bacterial community (Figure 10B), the accumulation of RDA1 and RDA2 was 57.10 and 23.40%, respectively. The overall explanation for the growth indicators of the bacterial community was 57.1%. The number of leaves was most strongly correlated with the bacterial community, accounting for 97.57% of the differences in bacterial populations. Streptomyces, Isoptericola, and Microbacterium were negatively correlated to the growth indicators. The bacterial genus Norank_f_norank_o_Gaiellales with unknown taxonomic status was negatively correlated to stem thickness, stem circumference, maximum leaf length, maximum leaf width, and plant height.
4. Discussion
The molecular detection is an effective means to verify that endophytic fungi colonize plants. The target strain C. cateniannulata was isolated from tobacco stems, leaves, and roots after inoculation, proving C. cateniannulata colonized and transferred in tobacco. Tobacco growth and dry matter accumulation had significantly improved, especially in the middle period after inoculation. These results were in consistent with observation made in other studies (Mantzoukas et al., 2021; Zhang et al., 2022). Those results indicated that some strains of Cordyceps may still retain the characteristics of endosymbiosis with plants in the long-term evolutionary process. Interactions, with entomopathogenic fungi for plants are profitable. The production of plant phytohormones such as auxins, indole acetic acid, gibberellins, and other bioactive compounds may achieve this positive effect (Khan et al., 2015). These compounds are essential in promoting plant growth, such as increasing chlorophyll content, root-shoot length, and biomass production (Kepler et al., 2017). Similar studies have found that entomopathogenic fungi of the genus Penicillium can produce substances similar to auxin and cytokinin, which increase plant yields (Toghueo and Boyom, 2020). Metarhizium anisopliae can promote root growth by producing indole-3-acetic acid, and enhancing insect virulence after colonization into Arabidopsis thaliana (Liao et al., 2017). Therefore, it is possible that C. cateniannulata also promotes tobacco growth through production of phytohormones. Furthermore, C. cateniannulata may change soil pH, structure, fertility, and oxygen utilization to increase the host’s ability to absorb soil nutrients, thus promoting plant growth (Hong et al., 2017).
Endophytic fungi help the host to absorb water and utilize nutrients, promoting healthy root development, entomopathogenic fungi can also perform this function (Macuphe, 2021). Since entomopathogenic fungi colonize the plant roots, they act as root extensions and probably improve nutrient and water absorption and help plants withstand stress factors (Dara et al., 2017). This symbiosis helps protect the activity of the plant enzyme system, leading to a reduction in MDA content, thus conferring greater stress resistance to the host plant by reducing the damage to the plasma membrane (Card et al., 2021). Entomopathogenic fungi can induce systemic resistance (ISR) by activating jasmonic acid (JA) and ethylene (ET) pathways in the plant, thereby activating the salicylic acid (SA) pathway to acquire resistance (SAR), resulting in the production of plant-related antioxidant enzymes (Doğru, 2021). Related studies have shown that ethylene contributes to plant survival under stress. Further, ethylene levels may increase dramatically and peak under drought stress, to maintain plant water balance by accelerating leaf abscission and senescence and reducing plant transpiration (Waadt et al., 2022). In this study, POD, SOD, and CAT activity were significantly higher in the X8 group than in the control group, indicating that C. cateniannulata improved tobacco stress resistance to abiotic environments, protecting cells by SOD, CAT, and POD rapidly removing the free radicals and associated intermediates (Canassa et al., 2019). They can oxidize oxidizing substances produced by respiration, photosynthesis, and auxin to maintain free radicals at an average level and improve plant stress resistance (Radhakrishnan et al., 2013).
Importantly, entomopathogenic fungi can induce the expression of proteins related to plant photosynthesis, energy metabolism, and plant stress response (Bamisile et al., 2020). These proteins induce physiological and biochemical reactions and change nutrient circulation to promote plant growth and enhance resilience (Behie et al., 2017). For example, endophytic fungi can promote proline synthesis in host plants under stress. Moreover, proline may stabilize the structure of biological macromolecules, reduce cell acidity, modulate cell redox potential, and control ROS concentration through coordinating enzyme activity (Segal and Wilson, 2018). All these possibilities may explain the marked increase in resistance to stress in C. cateniannulata -colonized tobacco seedlings. At the same time, with the growth of tobacco, its resistance level was also higher, making the ability to scavenge free radicals more vital (Ullah et al., 2019). In this study, C. cateniannulata promoted tobacco growth and development. Further, the data provides a beneficial exploration of C. cateniannulata in tobacco research and a theoretical basis for the specialized production of endophytic fungi. However, further field studies are required if the yield and quality of tobacco are to be improved using C. cateniannulata on a large scale.
The diversity and composition of the rhizosphere microorganisms are closely related to the growth and development of plants (Mendes et al., 2013). The entomopathogenic fungi can promote plant growth by increasing the relative abundance of beneficial microorganisms that are implicated in redox reactions, biological nitrogen fixation, oxidation mineralization, degradation of organic matter, and solubilization (Bever et al., 2012; Tan et al., 2021). Moreover, entomopathogenic fungi can reduce the relative abundance of plant pathogens through competition and the production of secondary metabolites, such as antibiotics (Yan et al., 2019). RDA analyzes revealed that growth indicators were closely related to the rhizosphere microbial communities. C. cateniannulata affected plant growth by changing the abundance of specific rhizosphere microbial communities. The abundance of Acidobacteria and Ascomycetes had increased, while the abundance of Actinomycetes, Basidiomycetes and Trichosporon had decreased in the soil following C. cateniannulata inoculation. Trichosporon fungi are pathogenic and cause severe and sometimes fatal infections in immunocompromised patients. Therefore, this study also provides a reference for the application of C. cateniannulata in clinical treatment (Matsumoto et al., 2022). The richness of microbial communities was lower than the control group in the early and middle stages after colonization of C. cateniannulata, which may be because the growth and reproduction of microorganisms were inhibited when C. cateniannulata was initially introduced into the soil, buta new dynamic balance would gradually form over time (Ding et al., 2021). The effect of C. cateniannulata on bacterial and fungal communities was significantly different. From the perspective of nutrient competition, the decrease in fungal diversity was beneficial to increase bacterial diversity (Smith et al., 2018).
In conclusion, the results have shown that it is feasible to screen suitable strains of C. cateniannulata that can be used in tobacco seedling treatments, thereby resulting in the colonization of target plants. Potential beneficial effects include improved plant growth, increased antioxidant capacity and resistance to microbial phytopathogens. The data bring us a new sight into the ecological relationship among plants, phytopathogens, and entomopathogenic fungi, providing a foundation for future studies of C. cateniannulata as an ecological agent.
Data availability statement
The data presented in the study are deposited in the NCBI GenBank repository, accession number PRJNA938063 (https://www.ncbi.nlm.nih.gov/sra/PRJNA938063), PRJNA938049 (https://www.ncbi.nlm.nih.gov/sra/PRJNA938049).
Author contributions
LQ and JL designed and performed the experiment and prepared this manuscript. ZZ and ZL performed experiments. YZ and JQ revised the manuscript and language editing. ZY and SX analyzed the data. All authors have read and agreed to the published version of the manuscript.
Funding
The author(s) declare financial support was received for the research, authorship, and/or publication of this article. This study was supported by the Science and Technology Program of Guizhou Province (2021XM02) and Science and Technology Project of Zunyi Tobacco Company (2022XM18). The funder had the following involvement in the study: experiment design and plant cultivation.
Acknowledgments
We are grateful to members of the Institute of Fungus Resources, Guizhou University, for their help with field and laboratory work.
Conflict of interest
JL, ZZ, and ZL are employed by Guizhou Tobacco Company. The remaining authors declare that the research was conducted in the absence of any commercial or financial relationships that could be construed as a potential conflict of interest.
Publisher’s note
All claims expressed in this article are solely those of the authors and do not necessarily represent those of their affiliated organizations, or those of the publisher, the editors and the reviewers. Any product that may be evaluated in this article, or claim that may be made by its manufacturer, is not guaranteed or endorsed by the publisher.
Supplementary material
The Supplementary material for this article can be found online at: https://www.frontiersin.org/articles/10.3389/fmicb.2023.1131184/full#supplementary-material
References
Adams, R. I., Miletto, M., Taylor, J. W., and Bruns, T. D. (2013). Dispersal in microbes: fungi in indoor air are dominated by outdoor air and show dispersal limitation at short distances. ISME J. 7, 1262–1273. doi: 10.1038/ismej
Bamisile, B. S., Akutse, K. S., Dash, C. K., Qasim, M., Aguila, L. C. R., Ashraf, H. J., et al. (2020). Effects of seedling age on colonization patterns of citrus Limon plants by endophytic Beauveria bassiana and Metarhizium anisopliae and their influence on seedlings growth. J. Fungi 6:29. doi: 10.3390/jof6010029
Barelli, L., Moonjely, S., Behie, S. W., and Bidochka, M. J. (2016). Fungi with multifunctional lifestyles: endophytic insect pathogenic fungi. Plant. Mol. Biol. 90, 657–664. doi: 10.1007/s11103-015-0413-z
Behie, S. W., Moreira, C. C., Sementchoukova, I., Barelli, L., Zelisko, P. M., and Bidochka, M. J. (2017). Carbon translocation from a plant to an insect-pathogenic endophytic fungus. Nat. Commun. 8:14245. doi: 10.1038/ncomms14245
Behie, S. W., Zelisko, P. M., and Bidochka, M. J. (2012). Endophytic insect-parasitic fungi translocate nitrogen directly from insects to plants. Science 336, 1576–1577. doi: 10.1126/science.1222289
Bever, J. D., Platt, T. G., and Morton, E. R. (2012). Microbial population and community dynamics on plant roots and their feedbacks on plant communities. Annu. Rev. Microbiol. 66, 265–283. doi: 10.1146/annurev-micro-092611-150107
Canassa, F., Tall, S., Moral, R. A., de Lara, I. A., Delalibera, I., and Meyling, N. V. (2019). Effects of bean seed treatment by the entomopathogenic fungi Metarhizium robertsii and Beauveria bassiana on plant growth, spider mite populations and behavior of predatory mites. Biol. Control 132, 199–208. doi: 10.1016/j.biocontrol.2019.02.003
Card, S. D., Bastías, D. A., and Caradus, J. R. (2021). Antagonism to plant pathogens by Epichloë fungal endophytes-a review. Plants (Basel) 10:1997. doi: 10.3390/plants10101997
Chiriví-Salomón, J. S., Danies, G., Restrepo, S., and Sanjuan, T. (2015). Lecanicillium sabanense sp. nov. (Cordycipitaceae) a new fungal entomopathogen of coccids. Phytotaxa 234, 63–74. doi: 10.11646/phytotaxa.234.1.4
Dara, S. K., Dara, S. S. R., and Dara, S. S. (2017). Impact of entomopathogenic fungi on the growth, development, and health of cabbage growing under water stress. Am. J. Plant Sci. 8, 1224–1233. doi: 10.4236/ajps.2017.86081
Ding, Y., Chen, Y., Lin, Z., Tuo, Y., Li, H., and Wang, Y. (2021). Differences in soil microbial community composition between suppressive and root rot-conducive in tobacco fields. Curr. Microbiol. 78, 624–633. doi: 10.1007/s00284-020-02318-3
Doğru, A. (2021). Effects of heat stress on photosystem II activity and antioxidant enzymes in two maize cultivars. Planta 253:85. doi: 10.1007/s00425-021-03611-6
Forlani, L., Juárez, M. P., Lavarías, S., and Pedrini, N. (2014). Toxicological and biochemical response of the entomopathogenic fungus Beauveria bassiana after exposure to deltamethrin. Pest Manag. Sci. 70, 751–756. doi: 10.1002/ps.3583
González-Guzmán, A., Rey, M. D., Froussart, E., and Quesada-Moraga, E. (2022). Elucidating the effect of endophytic entomopathogenic fungi on bread wheat growth through signaling of immune response-related hormones. Appl. Environ. Microbiol. 88:e0088222. doi: 10.1128/aem.00882-22
González-Pérez, E., Ortega-Amaro, M. A., Bautista, E., Delgado-Sánchez, P., and Jiménez-Bremont, J. F. (2022). The entomopathogenic fungus Metarhizium anisopliae enhances Arabidopsis, tomato, and maize plant growth. Plant Physiol. Biochem. 176, 34–43. doi: 10.1016/j.plaphy.2022.02.008
Guan, J. Q., Xu, Z. S., Liu, J., Gou, J. Y., and Zou, X. (2022). The effects of Cordyceps cateniannulata on tomato growth and activity of antioxidant enzymes. J. Microbiol. 62, 1119–1130. doi: 10.13343/j.cnki.wsxb.20210408 (in Chinese)
Hong, M., Peng, G., Keyhani, N. O., and Xia, Y. (2017). Application of the entomopathogenic fungus, Metarhizium anisopliae, for leafroller (Cnaphalocrocis medinalis) control and its effect on rice phyllosphere microbial diversity. Appl. Microbiol. Biotechnol. 101, 6793–6807. doi: 10.1007/s00253-017-8390-6
Jaber, L. R. (2018). Seed inoculation with endophytic fungal entomopathogens promotes plant growth and reduces crown and root rot (CRR) caused by fusarium culmorum in wheat. Planta 248, 1525–1535. doi: 10.1007/s00425-018-2991-x
Kepler, R. M., Luangsa-Ard, J. J., Hywel-Jones, N. L., Quandt, C. A., Sung, G. H., Rehner, S. A., et al. (2017). A phylogenetically-based nomenclature for Cordycipitaceae (Hypocreales). IMA Fungus 8, 335–353. doi: 10.5598/imafungus.2017.08.02.08
Khan, A. L., Hussain, J., Al-Harrasi, A., Al-Rawahi, A., and Lee, I. J. (2015). Endophytic fungi: resource for gibberellins and crop abiotic stress resistance. Crit. Rev. Biotechnol. 35, 62–74. doi: 10.3109/07388551.2013.800018
Lacey, L. A., Grzywacz, D., Shapiro-Ilan, D. I., Frutos, R., Brownbridge, M., and Goettel, M. S. (2015). Insect pathogens as biological control agents: Back to the future. J. Invertebr. Pathol. 132, 1–41. doi: 10.1016/j.jip.2015.07.009
Liao, X., Lovett, B., Fang, W., and St Leger, R. J. (2017). Metarhizium robertsii produces indole-3-acetic acid, which promotes root growth in arabidopsis and enhances virulence to insects. Microbiology 163, 980–991. doi: 10.1099/mic.0.000494
Liu, Y., Yang, Y., and Wang, B. (2022). Entomopathogenic fungi Beauveria bassiana and Metarhizium anisopliae play roles of maize (Zea mays) growth promoter. Sci. Rep. 12:15706. doi: 10.1038/s41598-022-19899-7
Macuphe, N. (2021). Evaluating the endophytic activities of Beauveria bassiana on the physiology, growth, and antioxidant activities of extracts of lettuce (Lactuca sativa L.). Plan. Theory 10:1178. doi: 10.3390/plants10061178
Mantzoukas, S., Lagogiannis, I., Mpousia, D., Ntoukas, A., Karmakolia, K., Eliopoulos, P. A., et al. (2021). Beauveria bassiana endophytic strain as plant growth promoter: the case of the grape vine Vitis vinifera. J. Fungi 7:142. doi: 10.3390/jof7020142
Matsumoto, Y., Yoshikawa, A., Nagamachi, T., Sugiyama, Y., Yamada, T., and Sugita, T. (2022). A critical role of calcineurin in stress responses, hyphal formation, and virulence of the pathogenic fungus Trichosporon asahii. Sci. Rep. 12:16126. doi: 10.1038/s41598-022-20507-x
Mendes, R., Garbeva, P., and Raaijmakers, J. M. (2013). The rhizosphere microbiome: significance of plant beneficial, plant pathogenic, and human pathogenic microorganisms. FEMS Microbiol. Rev. 37, 634–663. doi: 10.1111/1574-6976.12028
Pan, D. L., Wang, G., Wang, T., Jia, Z. H., Guo, Z. R., and Zhang, J. Y. (2019). AdRAP2.3, a novel ethylene response factor VII from Actinidia deliciosa, enhances waterlogging resistance in transgenic tobacco through improving expression levels of PDC and ADH genes. Int. J. Mol. Sci. 20:1189. doi: 10.3390/ijms20051189
Peng, X., Wu, Y., Zhang, X. N., Chen, W. H., Zhu, L. W., Chen, Q. F., et al. (2022). Screening of high germination rate strains of entomopathogenic fungi against buckwheat seeds. J. Shandong Agri. Univ. (Natural Science Edition). 53, 393–400. doi: 10.3969/j.issn.1000-2324 (in Chinese)
Radhakrishnan, R., Khan, A. L., and Lee, I. J. (2013). Endophytic fungal pre-treatments of seeds alleviates salinity stress effects in soybean plants. J. Microbiol. 51, 850–857. doi: 10.1007/s12275-013-3168-8
Rasool, S., Cárdenas, P. D., Pattison, D. I., Jensen, B., and Meyling, N. V. (2021). Isolate-specific effect of entomopathogenic endopytic fungi on population growth of two-spotted spider mite (Tetranychus urticae Koch) and levels of steroidal glycoalkaloids in tomato. J. Chem. Ecol. 47, 476–488. doi: 10.1007/s10886-021-01265-y
Raya-Díaz, S., Sánchez-Rodríguez, A. R., Segura-Fernández, J. M., Del-Campillo, M. D. C., and Quesada-Moraga, E. (2017). Entomopathogenic fungi-based mechanisms for improved Fe nutrition in sorghum plants grown on calcareous substrates. PLoS One 12:e0185903. doi: 10.1371/journal.pone.0185903
Ren, T., Wang, H., Yuan, Y., Feng, H., Wang, B., Kuang, G., et al. (2021). Biochar increases tobacco yield by promoting root growth based on a three-year field application. Sci. Rep. 11:21991. doi: 10.1038/s41598-021-01426-9
Rognes, T., Flouri, T., Nichols, B., Quince, C., and Mahé, F. (2016). VSEARCH: a versatile open source tool for metagenomics. PeerJ 4:e2584. doi: 10.7717/peerj.2584
Russo, M. L., Scorsetti, A. C., Vianna, M. F., Cabello, M., Ferreri, N., and Pelizza, S. (2019). Endophytic effects of Beauveria bassiana on corn (Zea mays) and its herbivore, Rachiplusia nu (Lepidoptera: Noctuidae). Insects 10:110. doi: 10.3390/insects10040110
Segal, L. M., and Wilson, R. A. (2018). Reactive oxygen species metabolism and plant-fungal interactions. Fungal Genet. Biol. 110, 1–9. doi: 10.1016/j.fgb.2017.12.003
Shin, T. Y., Lee, M. R., Park, S. E., Lee, S. J., Kim, W. J., and Kim, J. S. (2020). Pathogenesis-related genes of entomopathogenic fungi. Arch. Insect Biochem. Physiol. 105:e21747. doi: 10.1002/arch.21747
Smith, G. R., Steidinger, B. S., Bruns, T. D., and Peay, K. G. (2018). Competition-colonization tradeoffs structure fungal diversity. ISME J. 12, 1758–1767. doi: 10.1038/s41396-018-0086-0
Tan, Q., Si, J., He, Y., Yang, Y., Shen, K., Xia, T., et al. (2021). Improvement of karst soil nutrients by arbuscular mycorrhizal fungi through promoting nutrient release from the litter. Int. J. Phytoremediation 23, 1244–1254. doi: 10.1080/15226514.2021.1889966
Tang, J., Wang, S. Q., Hu, K. D., Huang, Z. Q., Li, Y. H., Han, Z., et al. (2019). Antioxidative capacity is highly associated with the storage property of tuberous roots in different sweet potato cultivars. Sci. Rep. 9:11141. doi: 10.1038/s41598-019-47604-8
Toghueo, R. M. K., and Boyom, F. F. (2020). Endophytic Penicillium species and their agricultural, biotechnological, and pharmaceutical applications. 3. Biotech 10:107. doi: 10.1007/s13205-020-2081-1
Tsikas, D. (2017). Assessment of lipid peroxidation by measuring malondialdehyde (MDA) and relatives in biological samples: analytical and biological challenges. Anal. Biochem. 524, 13–30. doi: 10.1016/j.ab.2016.10.021
Ullah, A., Nisar, M., Ali, H., Hazrat, A., Hayat, K., Keerio, A. A., et al. (2019). Drought tolerance improvement in plants: an endophytic bacterial approach. Appl. Microbiol. Biotechnol. 103, 7385–7397. doi: 10.1007/s00253-019-10045-4
Waadt, R., Seller, C. A., Hsu, P. K., Takahashi, Y., Munemasa, S., and Schroeder, J. I. (2022). Plant hormone regulation of abiotic stress responses. Nat. Rev. Mol. Cell Biol. 23, 680–694. doi: 10.1038/s41580-022-00479-6
Wang, D. F., Yang, G., Wang, Q. S., Zeng, M. S., and Wu, G. Y.. (2014). Identification of Two Corynecellospores and their Virulence on Homona Magnanima Diakonoff and Adoxophyes Orana Fischer von Roslerstamm, 41, pp. 531–539.
White, T. J., Bruns, T., Lee, S., and Taylor, J. (1990). “Amplification and direct sequencing of fungal ribosomal RNA genes for phylogenetics” in PCR Protocols. eds. M. A. Innis, D. H. Gelfand, J. J. Sninsky, and T. J. White. 3rd ed (San Diego: Academic), 315–322.
Xu, Z. S. (2020). Effects of surface soil environment in tobacco field on control of Spodoptera litura. Guizhou Univ. 2020:34. doi: 10.27047/d.cnki.ggudu.2020.000034
Xu, C., Li, X., and Zhang, L. (2013). The effect of calcium chloride on growth, photosynthesis, and antioxidant responses of Zoysia japonica under drought conditions. PLoS One 8:e68214. doi: 10.1371/journal.pone.0068214
Yan, L., Zhu, J., Zhao, X., Shi, J., Jiang, C., and Shao, D. (2019). Beneficial effects of endophytic fungi colonization on plants. Appl. Microbiol. Biotechnol. 103, 3327–3340. doi: 10.1007/s00253-019-09713-2
Zhang, X. N., Guo, J. J., Zou, X., and Jin, D. C. (2018). Pathogenic differences of the entomopathogenic fungus Isaria cateniannulata to the spider mite Tetranychus urticae (Trombidiformes: Tetranychidae) and its predator Euseius nicholsi (Mesostigmata: Phytoseiidae). Exp. Appl. Acarol. 75, 69–84. doi: 10.1007/s10493-018-0247-x
Zhang, X. N., Jin, D. C., Zou, X., and Guo, J. J. (2016). Laboratory and field evaluation of an entomopathogenic fungus, Isaria cateniannulata strain 08XS-1, against Tetranychus urticae (Koch). Pest Manag. Sci. 72, 1059–1066. doi: 10.1002/ps.4233
Zhang, X. N., Peng, X., Yang, G. M., Chen, Q. F., and Jin, D. C. (2022). The colonization and effect of Isaria cateinannulata on buckwheat sprouts. Plants (Basel) 12:145. doi: 10.3390/plants12010145
Zhou, Y. Y., Wang, Y. S., and Inyang, A. I. (2021). Ecophysiological differences between five mangrove seedlings under heavy metal stress. Mar. Pollut. Bull. 172:112900. doi: 10.1016/j.marpolbul.2021.112900
Zhou, Y. M., Zhi, J. R., Ye, M., Zhang, Z. Y., Yue, W. B., and Zou, X. (2018). Lecanicillium cauligalbarum sp. nov. (Cordycipitaceae, Hypocreales), a novel fungus isolated from a stemborer in the Yao Ren national forest mountain Park. Guizhou MycoKeys 43, 59–74. doi: 10.3897/mycokeys.43.30203 (in Chinese).
Keywords: Cordyceps cateniannulata , tobacco, endophytic colonization, growing development, rhizosphere microbial diversity
Citation: Qiao L, Liu J, Zhou Z, Li Z, Zhou Y, Xu S, Yang Z, Qu J and Zou X (2023) Positive effects of Cordyceps cateniannulata colonization in tobacco: Growth promotion and resistance to abiotic stress. Front. Microbiol. 14:1131184. doi: 10.3389/fmicb.2023.1131184
Edited by:
Naser Safaie, Tarbiat Modares University, IranReviewed by:
Pranab Dutta, Central Agricultural University, IndiaXiaoqing Wu, Qilu University of Technology, China
Bartholomew Saanu Adeleke, Olusegun Agagu University of Science and Technology, Nigeria
Copyright © 2023 Qiao, Liu, Zhou, Li, Zhou, Xu, Yang, Qu and Zou. This is an open-access article distributed under the terms of the Creative Commons Attribution License (CC BY). The use, distribution or reproduction in other forums is permitted, provided the original author(s) and the copyright owner(s) are credited and that the original publication in this journal is cited, in accordance with accepted academic practice. No use, distribution or reproduction is permitted which does not comply with these terms.
*Correspondence: Xiao Zou, eHpvdUBnenUuZWR1LmNu
†These authors have contributed equally to this work and share first authorship