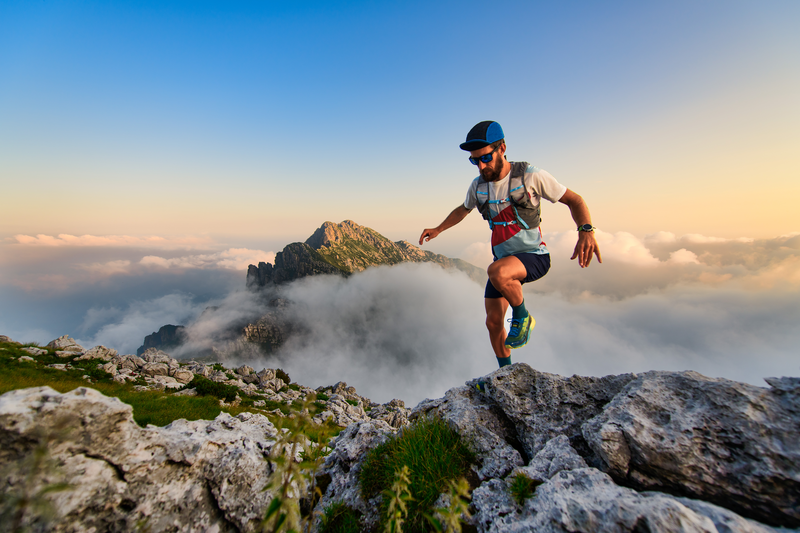
94% of researchers rate our articles as excellent or good
Learn more about the work of our research integrity team to safeguard the quality of each article we publish.
Find out more
REVIEW article
Front. Microbiol. , 09 March 2023
Sec. Evolutionary and Genomic Microbiology
Volume 14 - 2023 | https://doi.org/10.3389/fmicb.2023.1123783
Phytoplasma taxonomy has been a topic of discussion for the last two and half decades. Since the Japanese scientists discovered the phytoplasma bodies in 1967, the phytoplasma taxonomy was limited to disease symptomology for a long time. The advances in DNA-based markers and sequencing improved phytoplasma classification. In 2004, the International Research Programme on Comparative Mycoplasmology (IRPCM)- Phytoplasma/Spiroplasma Working Team – Phytoplasma taxonomy group provided the description of the provisional genus ‘Candidatus Phytoplasma’ with guidelines to describe the new provisional phytoplasma species. The unintentional consequences of these guidelines led to the description of many phytoplasma species where species characterization was restricted to a partial sequence of the 16S rRNA gene alone. Additionally, the lack of a complete set of housekeeping gene sequences or genome sequences, as well as the heterogeneity among closely related phytoplasmas limited the development of a comprehensive Multi-Locus Sequence Typing (MLST) system. To address these issues, researchers tried deducing the definition of phytoplasma species using phytoplasmas genome sequences and the average nucleotide identity (ANI). In another attempts, a new phytoplasma species were described based on the Overall Genome relatedness Values (OGRI) values fetched from the genome sequences. These studies align with the attempts to standardize the classification and nomenclature of ‘Candidatus’ bacteria. With a brief historical account of phytoplasma taxonomy and recent developments, this review highlights the current issues and provides recommendations for a comprehensive system for phytoplasma taxonomy until phytoplasma retains ‘Candidatus’ status.
Phytoplasmas (Kingdom, Bacteria; Phylum, Mycoplasmatota; class, Mollicutes; genus, ‘Candidatus Phytoplasma’) are phloem-inhabiting obligate plant pathogens. These are cell wall-less bacterial pathogens transmitted by phloem-feeding insect vectors mainly belonging to Cicadellidae, Derbidae, and Cixiidae; commonly known as leafhoppers, planthoppers, and psyllids (Weintraub and Beanland, 2006). They can also be transferred by grafting infected plant material or parasitic plants such as dodder (Cuscuta sp.) or spread through infected vegetative material used for plant propagation (Marcone et al., 1997; Přibylová and Špak, 2013) or through seeds in some cases (Calari et al., 2011; Kirdat et al., 2022). The infected plants show symptoms like brooming (excessive branching or tillering), virescence (abnormal development of green pigmentation in floral parts of a plant), little leaf (reduction in leaf size), phyllody (abnormal growth of floral parts into vegetative structures) and chlorosis resulting in yellow coloration of leaves (Lee et al., 2000; Rao et al., 2017). Phytoplasma causes diseases over thousands of plant species worldwide, many of which are lethal. In the agriculture and horticulture sectors globally, phytoplasma-related diseases lead to extensive yield losses. Many economically important crops, including vegetables, spices, medicinal plants, ornamentals, cash crops, palms, fruit trees, weeds, timber, and shade trees, are affected due to phytoplasma-related diseases (Lee et al., 2000; Rao et al., 2017, 2018; Duduk et al., 2018; Bertaccini, 2022; Brooks et al., 2022; Sundararaj et al., 2022; Marcone et al., 2023).
Phytoplasma diseases have been reported from more than 100 countries, as evident from the sequences available in the GenBank database. The impact of phytoplasma diseases and their distribution in different geographical areas depends on the host range of the phytoplasma and the polyphagous feeding behavior of the insect vector (Weintraub and Beanland, 2006; Foissac and Wilson, 2009). Phytoplasma research has flourished in the last two decades, and reviews published from time to time give a good understanding of phytoplasma studies, including its taxonomy, etiology, transmission, and interaction with insect and plant hosts (Lee et al., 2000; Bai et al., 2006; Weintraub and Beanland, 2006; Hogenhout et al., 2008; Bertaccini and Duduk, 2009; Sugio et al., 2011; Rao et al., 2017; Duduk et al., 2018; Bertaccini et al., 2022; Wei and Zhao, 2022). This review summarizes the history and current status of phytoplasma taxonomy, focusing on the different classification systems used, their advantages and drawbacks; issues related to published phytoplasma species; recent attempts to develop a classification system for ‘Candidatus’ bacteria and related issues in context with phytoplasma.
The first phytoplasma disease was scientifically recorded 200 years ago when the mulberry dwarf disease outbreak was observed in Japan (Ishiie, 1965; Okuda, 1972). However, the historical record for phytoplasma infection is about 1,000 years old from China, where tree peonies (Paeonia suffruticosa) were appreciated as the most attractive flower for a long time (Maramorosch, 2011). During the Song dynasty (960-1,227), special tributes were being paid to the imperial court by offering a variety of green flowers (tree peonies exhibiting floral virescence) as a most precious variety of the plant (Wang and Maramorosch, 1988). Earlier in 1897, a Japanese national research committee struggled to determine the cause of the Mulberry dwarf disease. Since then, many diseases like rice yellow dwarf disease and paulownia witches’ broom disease have been recorded without conclusive identification of the causative agent of these diseases, which were thought to be some undiscovered viruses (Kunkel, 1926; Okuda, 1972; Lee et al., 2000; Maramorosch, 2011). In 1967, a Japanese scientist Doi Y. and his co-workers located pleomorphic bodies in the phloem tissue of plants showing disease symptoms. They named these entities ‘Mycoplasma-like-Organisms’ (MLOs) due to their resemblance with animal mycoplasmas. The causative agent of these diseases was shown to be transmitted by phloem-feeding insects and by grafting (Doi et al., 1967). Further, its sensitivity to tetracycline supported the hypothesis of the existence of MLO and not viruses (Doi et al., 1967; Ishiie et al., 1967). Later, many subsequent studies established that MLOs are associated with diseases in several hundred plant species showing typical symptoms. Researchers correlated these ‘yellows’ diseases associating organisms based on symptomatology and host range. This led to the naming of new MLOs based on the original plant host and symptoms, e.g., Aster Yellows, Apple Proliferation, Elm Yellows, and others.
MLOs resisted the attempts to cultivate them for years and has yet to be successful. Failure to cultivate these organisms drove the developments in phytoplasma taxonomy toward obtaining the marker data using serological and DNA-based techniques. Around 1990, the acquisition of knowledge in DNA biology facilitated the direct detection of MLOs DNA by various techniques such as DNA–DNA hybridization (Kirkpatrick et al., 1987; Razin et al., 1987; Bonnet et al., 1990), Polymerase Chain Reaction (PCR), cloning and sequencing of MLOs DNA fragments (Lee, 1988). A method for PCR amplification of 16S rRNA gene with universal primers was then developed and soon became a routine practice for the detection, identification, diversity, and taxonomy of MLOs (Deng and Hiruki, 1991; Schneider et al., 1995; Gundersen and Lee, 1996; Smart et al., 1996; Lee et al., 1998). These findings eventually simplified the diagnosis and classification of MLOs, leading to a flurry of publications in the 1990s and later.
The MLOs were placed in class Mollicutes (Phylum Tenericutes; now Mycoplasmatota) due to the lack of a cell wall, small genome size, and low G+C content. In addition, unlike sterol-requiring mycoplasmas, MLOs membranes were shown to resemble membranes of non-sterol-requiring Acholeplasma strains. MLOs showed resistance to digitonin and sensitivity toward hypotonic salt solutions (Lim and Sears, 1992). The first gene sequences analyzed also revealed that contrary to mycoplasmas and spiroplasmas, MLOs use UGA as a stop codon, as Acholeplasma strains do (Lim and Sears, 1991). It became essential to determine if these MLOs are monophyletic or spread across the class Mollicutes. Several studies based on the phylogenetic analysis of 16S rRNA and ribosomal protein (rp) gene sequences revealed the phylogenetic relationships of MLOs within the group and with related organisms (Lim and Sears, 1989, 1992; Kirkpatrick et al., 1990; Kuske and Kirkpatrick, 1992; Schneider et al., 1993; Gundersen et al., 1994; Seemuller et al., 1994; Lee et al., 1998, 2003; Seemüller et al., 1998). These studies established that MLOs were evolutionarily distinct from animal mycoplasmas. They form a large discrete monophyletic clade, paraphyletic to the Acholeplasma species within the Anaeroplasma order.
In 1992, the International Committee on Systematic Bacteriology (ICSB) Subcommittee on the Taxonomy of Mollicutes adopted the trivial name ‘Phytoplasma’ (Tully, 1993). In line with these studies, Gundersen et al. (1994) proposed that MLOs should be represented taxonomically at the minimal genus level. They recognized five major phylogenetic groups with 11 distinct subclades (monophyletic groups or taxa). Those were, Maryland Aster Yellows AY1; Apple Proliferation AP-A; Peanut Witches’-broom PnWB; Canada Peach X CX; Rice Yellow Dwarf RYD; Pigeon Pea Witches’-Broom PPWB; Palm Lethal Yellowing LY; Ash Yellows AshY; Clover Proliferation CP; Elm Yellows EY; and Loofah Witches’-Broom LfWB (Gundersen et al., 1994). Until then, phytoplasmas were named based only on their hostname and the disease symptoms.
A taxonomic note documenting the characteristics of putative prokaryote taxa and implementation of ‘Candidatus’ status was released in 1995 by the International Code of Nomenclature of Bacteria (ICNB). This recommendation allowed the researcher to publish bacterial taxa that cannot be described per norms laid by the ICNB (known as ‘Candidatus’ taxa). The record of the existence of such species was possible only through the detection of DNA sequences obtained from the environmental sample. The taxonomic note suggested recording the structural, metabolic, and reproductive features and the properties of the ecological niche and genomic information used to determine the organism’s phylogenetic position (Murray and Schleifer, 1994; Murray and Stackebrandt, 1995). Following this, several distinct phytoplasma taxa were described, viz. ‘Candidatus (Ca.) Phytoplasma (P.) aurantifolia’ (Zreik et al., 1995), ‘Ca. P. australiense’ (Davis et al., 1997), ‘Ca. P. australasia’ (White et al., 1998), ‘Ca. P. fraxini’ (Griffiths et al., 1999), ‘Ca. P. japonicum’ (Sawayanagi et al., 1999), ‘Ca. P. brasiliense’ (Montano et al., 2001), ‘Ca. P. castaneae’ (Jung et al., 2002), ‘Ca. P. phoenicium’ (Verdin et al., 2003), ‘Ca. P. ziziphi’ (Jung et al., 2003a), ‘Ca. P. oryzae’ (Jung et al., 2003b) and ‘Ca. P. ulmi’ (Lee et al., 2004b). The basis of classification of these species was majorly 16S rRNA gene sequence similarity, RFLP pattern of 16S rRNA gene, and in some cases, analysis of 16S-23S spacer region, serology, and biological properties (Table 1). In 2004, the International Research Programme on Comparative Mycoplasmology (IRPCM)- Phytoplasma/Spiroplasma Working Team- Phytoplasma taxonomy group provided the description of the provisional genus ‘Ca. Phytoplasma’ based on the near-full length sequence of phytoplasma 16S rRNA gene (Firrao, 2004). As per IRPCM recommendations, a novel ‘Ca. Phytoplasma’ species description should refer to a single, unique 16S rRNA gene sequence of >1,200 bp and share <97.5% sequence similarity to any previously described ‘Ca. Phytoplasma’ species unless the phytoplasma under consideration represents an ecologically distinct population (Firrao, 2004). Later, several phytoplasma species were described, primarily based on the 16S rRNA gene phylogeny and its RFLP-based classification (Table 1).
Meanwhile, a ‘revised’ classification of phytoplasmas was founded on RFLP analyses of 16S rRNA and ribosomal protein gene sequences (Lee et al., 1998). They differentiated phytoplasma strains into 14 major ‘16Sr groups’ based on RFLP profiles generated using 17 restriction enzymes. This approach provided a simple and rapid method for differentiation and classification of phytoplasmas compared to laborious methods involving DNA probes, DNA sequencing, and serological methods affected with inefficacy. Thus, the classification based on 16S rRNA gene phylogeny and its RFLP analysis was widely accepted by researchers. Further, the computer-simulated RFLP analysis was devised, including in silico restriction digestion of obtained 16S rRNA gene sequence, virtual gel plotting, and a similarity coefficient based on the virtual RFLP pattern (Wei et al., 2007). Soon an interactive online tool, iPhyClassifier, was introduced to ramp up the speed and capacity of the 16S rRNA gene sequence-based phytoplasma classification system (Wei et al., 2007, 2008; Zhao et al., 2009b).
This RFLP-based phytoplasma classification system considers mutations at restriction sites where an uneven weightage of nucleotide positions ignores the information available in a full-length sequence, and internal variation in a sequence is not deemed. If there is a single nucleotide change in the sequence at the restriction site, there are chances that the RFLP system classifies that as a new subgroup, even if the overall sequence similarity is 99% or more. Additionally, it is well known that many phytoplasmas have two rRNA operons (termed as rrnA and rrnB), and interoperon sequence heterogeneity exists in some strains (Seemuller et al., 1994; Firrao and Smart, 1996; Liefting et al., 1996; Davis and Sinclair, 1998; Oshima et al., 2004; Bai et al., 2006). Sequence variations between heterogeneous rrnA operons may assign the same phytoplasma strain to different RFLP subgroups. For example, the phytoplasma strain DY2014 contains two heterogeneous 16S rRNA genes. The iPhyClassifier classifies them into two sub-groups, although they differ by only one base pair and belong to the same phytoplasma strain (Cho et al., 2019). To avoid confusion, a three-letter subgroup designation system was proposed for such stains (Wei et al., 2008). However, confusion persisted on what criteria should be used to decide the formal subgroup of a strain.
The formation of group and subgroup is based on the cut-off values of RFLP similarity coefficients (SC) (Lee et al., 1998). The RFLP-SC compares the restriction patterns of 16S rRNA gene sequences with the reference sequence and assigns it to the existing group/subgroup or suggests forming a new group/subgroup. A new phytoplasma 16Sr group and subgroup can be created if the RFLP coefficient is less than 0.85 and 0.97, respectively (Lee et al., 1998; Wei et al., 2007; Zhao et al., 2009b). However, confusion remains with the validity of some phytoplasma groups/subgroups as the basis of their formation remains unclear. Many ‘Aster Yellow’ phytoplasma strains have RFLP-SC ranging from 0.85 to 0.97 and were designated as 16SrXII subgroup strains (for example, but not limited to AF222065, HM067754, AP006628, JQ730859, MT106667, AF222066, AY265213, AF503568, HM067755, and CP000061). At the same time, they correspond to subgroups strains related to group 16SrI. Similar is the case with phytoplasma strains related to the 16SrV and 16SrVI groups. In another case, the reference strains of 16SrXIV-A group, strain BGWL-C1 (AJ550984), show RFLP-SC of 0.87 compared with reference sequences of subgroup 16SrXI-A (AB052873), indicating being 16SrXI group member. Therefore, since its formation in 1998, no subgroup strain could be added to the 16SrXIV group based on RFLP-SC-based classification. In 2016, ICSB-Mollicutes acknowledged the shortcomings of the taxonomic justification for phytoplasma nomenclature based on RFLP ‘barcoding’ (May et al., 2017).
The International Code of Nomenclature of Prokaryotes (ICNP) required every taxonomic rank to be associated with type strains and type material (detailed description, illustration or non-viable specimen) in some cases. The deposition of viable pure cultures into two international culture collections as type material was made mandatory. The unintended consequences of this amendment led to denial of use of code for many endosymbionts which were not in the form of axenic culture. The type strain (in the case of cultivable bacteria) or reference strain (in ‘Candidatus bacteria’) is a formal representative of organisms at the species rank. A bacterial species form a ‘monophyletic cluster’ containing a group of strains that share a most recent common ancestor with common phenotypic and genomic characteristics (Rosselló-Mora and Amann, 2001; Torsvik et al., 2002). Therefore, it is vital to consider the population dynamics (diversity, abundance, and endemism) of the isolates while describing the new taxon through reference strain description. The type strain alone cannot and should not represent the species clade representing the non-existing intra-species diversity. Among phytoplasma species published, most were described based on the 16S rRNA gene phylogeny (Table 1 and Figure 1). Many reference 16S rRNA gene sequences of reference phytoplasma strains matched to no other 16S rRNA sequences of ‘-related’ strains reported earlier or later of the publication from the same or another geographic location, making them orphan species. They are, ‘Ca. P. japonicum’ (Sawayanagi et al., 1999), ‘Ca. P. brasiliense’ (Montano et al., 2001), ‘Ca. P. castaneae’ (Jung et al., 2002), ‘Ca. P. oryzae’ (Jung et al., 2003b), ‘Ca. P. spartii,’ ‘Ca. P. rhamni,’ ‘Ca. P. allocasuarinae’ (Marcone et al., 2004a), ‘Ca. P. graminis’ (Pin et al., 2005), ‘Ca. P. caricae’ (Arocha et al., 2005) ‘Ca. P. pini’ (Schneider and Torres, 2005), ‘Ca.’ P. americanum’ (Lee et al., 2006a), ‘Ca. P. lycopersici’ (Arocha et al., 2007), ‘Ca. P. omanense’ (Al-saady et al., 2008), ‘Ca. P. tamaricis’ (Zhao et al., 2009a), ‘Ca. P. sudamericanum’ (Davis et al., 2012), ‘Ca. P. convolvuli’ (Maixner et al., 2012), ‘Ca. P. hispanicum’ (Davis et al., 2016), ‘Ca. P. meliae’ (Fernández et al., 2016), ‘Ca. P. cirsii’ (Šafářová et al., 2016), ‘Ca. P. wodyetiae’ (Naderali et al., 2017), ‘Ca. P. stylosanthis’ (Rodrigues Jardim et al., 2020), ‘Ca. P. dypsidis’ (Jones et al., 2021) and ‘Ca. P. tritici’ (Zhao et al., 2021) (Table 1).
Figure 1. Phylogenetic tree inferred from analysis of reference 16S rRNA gene sequences of published phytoplasma species. The neighbour-joining (NJ) and maximum-likelihood (ML) methods were used to build the phylogenetic tree building using MEGA 7 (Kumar et al., 2016). The topologies of the trees were evaluated by bootstrap analysis based on 1000 replicates. Figures at nodes (>50) of the branches indicate the percentage of replicate trees obtained from NJ and ML methods. The absence of a branch at a particular node was marked by ‘- ’. There was a total of 976 positions in the final dataset. The 16S rRNA sequence of Acholeplasma laidlawii PG-8A (M23932) was used as an outgroup. Bar indicates the number of nucleotide substitutions per site. *Indicates orphan species described in text.
Phylogenetic analysis has helped phytoplasma taxonomists understand its evolution and find a common ancestor. Most phytoplasma phylogenetic studies are based on DNA sequences, and therefore sequence quality remains vital for accurate phylogenetic analysis. The phylogenetic position of ‘Ca. P. graminis,’ ‘Ca. P. caricae,’ ‘Ca. P. lycopersici’ and ‘Ca. P. wodyetiae’ are speculated to be ‘unusual’ with atypical terminal branching from its ancestor and low bootstrap values at the branch node (Figure 1). The description of ‘Ca. P. wodyetiae’ was based on the analysis of the 16S rRNA gene and RFLP of its reference strain BANGI-2. This phytoplasma was detected from the foxtail palm (Wodyetia bifurcata), showing ‘Foxtail Palm Yellow Decline (FPYD)’ disease, which was infected with two known phytoplasmas, ‘Ca. P. asteris’ (Lee et al., 2004a) and ‘Ca. P. cynodontis’ (Marcone et al., 2004b). The reference 16S rRNA gene sequence (KC844879) of ‘Ca. P. wodyetiae’ is probably a ‘chimeric’ sequence made up of partial 16S rRNA gene sequences from ‘Ca. P. asteris’ and ‘Ca. P. cynodontis’ when analyzed using chimera detection tools, Mallard (Ashelford et al., 2006) and Pintail (Ashelford et al., 2005) (unpublished data). As mentioned, no other phytoplasma strains were reported earlier or in subsequent studies related to these species, either represented by the 16S rRNA or other gene sequences.
Although the 16S rRNA gene is regarded as a universal genetic marker for prokaryote classification, it faces genetic saturation due to its restricted length compared to genome size. The number of mutable sites is limited due to functional constraints. This issue can be addressed using additional phylogenetic markers, essentially housekeeping genes. In the last decade, several other conserved genes were evaluated in the search for molecular markers for adequate differentiation of various phytoplasmas found across geographic areas and plant hosts (Marcone et al., 2000; Lee et al., 2004a, 2006b; Martini et al., 2007). The description of the first phytoplasma species, ‘Ca. P. aurantifolia,’ was based on its 16S rRNA gene sequences and the the16S-23S ribosomal DNA spacer region (Zreik et al., 1995). The hybridization profiles of the reference strain were obtained by using MLO-specific probes, and genome size was derived to describe ‘Ca. P. aurantifolia’ and the ‘Ca. P. fraxini’ in addition to the 16S rRNA gene analysis (Griffiths et al., 1999). The analysis of the 16S-23S ribosomal DNA spacer region was used additionally in the case of ‘Ca. P. trifolii’ (Hiruki and Wang, 2004), ‘Ca. P. cynodontis’ (Marcone et al., 2004b), ‘Ca. P. mali,’ ‘Ca. P. pyri’ and ‘Ca. P. prunorum’ (Seemüller and Schneider, 2004). The sequences of the elongation factor (EF) and Tu (tuf) genes and ribosomal protein (rp) genes were used for the first time for the description of the ‘Ca. P. asteris’ in addition to the 16S rRNA gene sequence analysis (Lee et al., 2004a). Later, secY, tuf, uvrB -degV, and rp gene sequences (either or more) were used to describe ‘Ca. P. ulmi’, (Lee et al., 2004b), ‘Ca. P. rubi’ (Malembic-Maher et al., 2011), ‘Ca. P. solani’ (Quaglino et al., 2013), ‘Ca. P. balanitae’ (Win et al., 2013), ‘Ca. P. meliae,’ ‘Ca. P. cirsii’ and ‘Ca. P. stylosanthis’. Average nucleotide identity (ANI) value, amp, and secY were used to describe ‘Ca. P. tritici’ while ‘Ca. P. dypsidis’ was described using the 16S rRNA and ribosomal protein genes.
Phylogenetic analysis of housekeeping genes confirmed and strengthened the 16S rRNA gene-based classification systems and improvised finer strain differentiation with more discrete branches. At the same time, none of the studies was evaluated for the performance of the marker genes by considering their variable rates of evolution by comparing other genes present in the phytoplasma genome (including the 16S rRNA gene) used for a multi-locus classification system. Therefore, it was essential to evaluate these marker genes for genome-level assessment for their relative performance in classifying the phytoplasma strains, considering their evolutionary relationships (Martini et al., 2019). It is more likely that any single gene’s evolutionary history may differ from the phylogenetic history of the whole organism as they do not account for the horizontal gene transfer and unrecognized paralogy. Most published markers described for particular groups or species may not necessarily be suitable for another group of phytoplasma species. A five-gene markers MLSA for Aster Yellow (16SrI, AY) group of phytoplasmas to supplement the 16S rRNA gene phylogeny was proposed to improvise the phytoplasma taxonomy (Cho et al., 2020). These proposed marker genes [Replication initiation protein DnaD (dnaD), DegV family protein (degV), TIGR00282 family metallophosphoesterase, Preprotein translocase SecY (secY), and RluA family pseudo uridine synthase (rluA)] were able to distinguish the strains of AY (16SrI) group of phytoplasmas into three different Operational Taxonomic Units (OTUs). This time, the authors deduced the five marker genes with high densities of informative genome sites and relatively high substitution rates. These selected genes were known not to deviate compared to other shared single-copy genes reported earlier. They were chosen based on their unique position in the distinct and separated genome regions depicting unlikely homologous recombination between the same OTUs (Cho et al., 2020).
The ICSB-Mollicutes subcommittee encouraged using multiple genetic markers, minimally 16S rRNA gene, and Elongation Factor-Tu (EF-Tu) to circumscribe the provisional taxa to which strains should be assigned (Firrao and Brown, 2013). Among all phytoplasma species published so far, the multi-locus sequence data of reference strain, in addition to 16S rRNA sequences, is available only for twelve species. Those are ‘Ca. P. asteris,’ ‘Ca. P. ulmi,’ ‘Ca. P. rubi,’ ‘Ca. P. solani,’ ‘Ca. P. balanitae,’ ‘Ca. P. meliae,’ ‘Ca. P. cirsii,’ ‘Ca. P. stylosanthis,’ ‘Ca. P. cynodontis,’ ‘Ca. P. sacchari,’ ‘Ca. P. tritici’ and ‘Ca. P. dypsidis’ where sequences of either secY, tuf, or rp genes are available (Table 1). Establishing a formal MLST system requires the availability of gene sequences of the reference strains. The phytoplasma multi-locus sequence database is currently less inclusive of the number of sequences available for phytoplasma reference strains of various phylogenetic groups or species. This also includes the related metadata of insect vectors or plant hosts and geographic locations. The availability and reproducibility of PCR primer pairs for proposed marker genes for each group of phytoplasmas species proved to be a primary hindrance in making the database comprehensive.
The main objective of microbial taxonomy is to construct a comprehensive system that will encompass all three components: classification, nomenclature, and identification. In this context, genomics has become a promising methodology as it provides a stable, reproducible, and highly informative means to infer phylogenetic relationships among prokaryotes (Danet et al., 2011; Firrao et al., 2013; Chun and Rainey, 2014; Cho et al., 2020). This led to the construction of a dedicated curated databases, websites, and tools to aid the user-friendly taxonomic delineation of the prokaryotic strain under study (Yoon et al., 2017; Chaumeil et al., 2020; Parks et al., 2020, 2022; Parte et al., 2020). These recently employed methods recruit the sequence-derived numerical data to measure the distances among the prokaryotes, known as Overall Genome Relatedness Indices (OGRI) values (Kim et al., 2014; Barco et al., 2020).
The OGRI values are dDDH (digital DNA-DNA hybridization), G+C content, and ANI (Average Nucleotide Identity) values. The ANI values denote the percent identity inferred by the homologous regions shared between prokaryotic genomes and classify bacterial genomes (Jain et al., 2018). The Digital DDH (dDDH) ensures the highest consistency regarding the species-delimitation approach currently dominating microbial taxonomy. The DDH has been a gold standard in prokaryotic taxonomy that indirectly measures the degree of genetic similarity between two genomes (Chun et al., 2018). The minimal standards for using genome data for the taxonomy of prokaryotes are widely accepted that bacterial strains of the same species have ANI values >95–96% and DDH values >70% (Chun et al., 2018). The G+C content has been historically valued for taxonomic descriptions of species and genera (Meier-Kolthoff et al., 2014). A direct computation of G+C content with high accuracy is easily achieved from genome sequenced with considerable depth. The significance of accuracy achieved by this computation method over the drawbacks of using conventional methods (Meier-Kolthoff et al., 2014). These methods have helped delineate phytoplasma phylogenetic position with significant confidence compared to 16S rRNA gene phylogeny alone (Kirdat et al., 2021; Zhao et al., 2021). An analysis of 51 phytoplasma genomes revealed that some strains within the same clade in the 16S rRNA gene phylogenetic tree exhibited pairwise ANI values less than 95% and dDDH values less than 70% (unpublished data). This suggests that these phytoplasma strains could potentially be classified as new species, subject to the fulfillment of other necessary criteria for species designation.
A study consisting of 14 phytoplasma genomes was conducted to check the efficacy of OGRI values and phylogeny based on genomes (Firrao et al., 2013). For this analysis MLSA of 107 orthologous genes, Average Nucleotide Index (ANIm), tetranucleotide signature frequency correlation index (TETRA), and consensus networks were used. The authors found that the strains belonging to the same clade shared high ANI values confirming high relatedness at the genomic level, and relatively lower ANI values were calculated between strains belonging to two different subgroups of 16SrI. The TETRA values >990 would support phytoplasma species boundary based on the ANI range 95–96%; however, the TETRA index calculation can be inaccurate when comparing drafts based on different sequencing techniques (Firrao et al., 2013). In another attempt, 11 AY phytoplasma genomes were assigned to define the putative species boundaries and genomic divergence (Cho et al., 2020). The study proposed dividing the 16SrI group into three species-level OTUs based on whole-genome ANI analysis, homologous gene sequence-based analysis, PCoA analysis, and phylogenetic tree based on 303 single-copy-genes shared by selected 11 genomes. The study showed that the same OTU species have >97% ANI values, and species comparison between OTUs have <94% ANI values. The authors proposed the MLSA markers deduced from 11 analyzed AY genomes that could facilitate future genetic characterization of Aster Yellows phytoplasmas (AY) or 16SrI group (Cho et al., 2020). Homologous gene clusters showing genome divergence can further provide practical means to classify phytoplasma provided the availability of complete or near-complete genomes with completeness >90%.
The genome phylogeny and OGRI values were used to describe a new phytoplasma species, ‘Ca. P. sacchari,’ associated with Sugarcane Grassy Shoot (SCGS) disease (Kirdat et al., 2021). The phylogenetic position based on 16S rRNA and other SCGS phytoplasma genes was confusing due to distant branching from two species, ‘Ca. P. cynodontis’ and ‘Ca. P. oryzae.’ Many Rice Yellow Dwarf (RYD) group strains associated with Sugarcane Grassy Shoot (SCGS) disease of sugarcane were assigned to ‘Ca. P. oryzae’ erroneously despite their close phylogenetic association with ‘Ca. P. cynodontis’. An approach to constructing a hybrid assembly followed by metagenomic binning was used to obtain the SCGS phytoplasma genome (Kirdat et al., 2020). Using 13 near-complete phytoplasma genomes, the authors constructed the whole-genome phylogenetic tree using BPGA (Chaudhari et al., 2016) and UBCG (Na et al., 2018) tools to clear the confusion and to obtain a reliable and highly informative phylogenetic delineation using a whole-genome sequence of SCGS and related strains, OGRI values, and housekeeping genes. The comparative G+C content was directly calculated from the obtained draft genome assemblies and the dDDH values were calculated using Genome-to- Genome Distance Calculator (GGDC) webserver (http://ggdc.gbdp.org) (Auch et al., 2010). This approach provided a better phylogenetic position for sugarcane grassy shoot phytoplasma and its proposal as a novel taxon.
The phytoplasma species names are generally derived from their plant or insect host or a geographic location where the strain is endemic. The taxonomic notes and appendix 11 of the International Code of Nomenclature of Prokaryotes (ICNP) codes illustrate the guidelines to describe the ‘Candidatus’ taxa (Murray and Schleifer, 1994; Murray and Stackebrandt, 1995; Garrity et al., 2015). Although the names proposed for ‘Candidatus’ taxa do not come under ICNP rules, Oren (2017) proposed a nomenclature review for ‘Candidatus’ taxa published in IJSEM and elsewhere (Oren, 2017). He evaluated more than 400 names, including provisional species names of ‘Candidatus Phytoplasma,’ and suggested changes in the names in line with ICNP rules. He suggested nomenclature changes in three phytoplasma species ‘Ca. P. aurantifolia,’ ‘Ca. P. australasia,’ and ‘Ca. P. sudamericanum’ as ‘Ca. P. citri,’ ‘Ca. P. australasiaticum’ and ‘Ca. P. australamericanum’ or ‘Ca. P. meridianamericanum,’ respectively. ‘Ca. P. citri’ because the bacterium is found in the host plant, Citrus aurantifolia; the proposed name is, therefore, more appropriate. The name ‘aurantifolia’ means orange leaves, which cannot be a diagnostic characteristic of associated phytoplasma strain. ‘Ca. P. australasiaticum’ because ‘australasia’ is a noun, not an adjective. ‘Ca. P. australamericanum’ or ‘Ca. P meridianamericanum’ is because the suggested names are in Latin where ‘sudamericanum’ is a derived French word; this change is recommended according to the rule and recommendation 6 of ICNP (Garrity et al., 2015; Oren, 2017).
Additionally, in February 2021, the International Committee on Systematics of Prokaryotes (ICSP), who oversees the development and revision of the ICNP, proposed renaming the phylum Tenericutes to Mycoplasmatota (My.co.plas.ma.to’ta. N.L. neut. n. Mycoplasma, type genus of the phylum; -ota, ending to denote a phylum; N.L. pl. neut. n. Mycoplasmatota, the Mycoplasma phylum) to be included in the revised version of the ICNP (Oren and Garrity, 2021). The revised classification of phytoplasma reads: Kingdom, Bacteria; Phylum, Mycoplasmatota; class, Mollicutes; genus, ‘Candidatus Phytoplasma’ (Parte et al., 2020).
Recently, a ‘revision’ of phytoplasma species ‘guidelines’ were published with the recommendation to use ANI values and an update on increased cut-off value for 16S rRNA gene for the delineation of phytoplasma strains at the species level (Bertaccini et al., 2022). The article followed with detailed commentary on the proposed guidelines and emphasized the utility of RFLP-based grouping of phytoplasma strains (Wei and Zhao, 2022). Both these reviews covered the known aspects of phytoplasma taxonomy; highlighted some of the critical issues, however, they did not cover the matters related to validating published phytoplasma species and other vital elements of phytoplasma taxonomy discussed earlier in this review. This includes validation of ‘Ca. P. wodyetiae’ needs to be relooked for possible chimeric reference sequences. The confusion continues with a species status of ‘Ca. P. australasia’ based on the criteria suggested by the IRPCM 2004 and multiple studies published later. These articles did not shed light on the fate of orphan species where only one gene information is available, and no ‘-related strains’ were reported for those species. The ‘revised guidelines’ article retained these species with their reference strains. Additionally, the new species, ‘Ca. P. cocostanzaniae’ was proposed, represented by 16S rRNA gene sequence alone and no other data related to plant host or host range, insect vector(s), and other criteria laid by IRPCM, 2004 or later (Bertaccini et al., 2022). The taxonomical description of cultivable bacteria mandatorily demands the polyphasic characterization of the strain, including its physiological, biochemical, and genome characterization (Sneath, 1992). Therefore, the cut-off values for 16S rRNA gene percent similarity with existing species should be considered only a preliminary marker to delineate the phytoplasma strain to species level. The information gathered from the whole genome (OGRI values) and the endemic nature of its plant and insect host must be considered holistically to propose a new species of phytoplasma. It is detrimental to consider the fulfillment of ‘any one’ character as laid down by the IRPCM, 2004 or ‘revised guidelines’ or ‘suggested clarifications’ as a characteristic of phytoplasma species considering the challenges posed by the published species with limited data (Firrao, 2004; Bertaccini et al., 2022; Wei and Zhao, 2022).
The situation of orphan species with limited information can be addressed by assigning neo reference strains whose genome information can be used for the comparative OGRI values. In a conventional bacterial taxonomy, the arrangement of a neo type strain is accepted by international agreement to replace a type strain that is no longer in existence to serve as the type strain (Gibbons, 1974; Tindall and Garrity, 2008; Oren and Garrity, 2020). Along similar lines, neo reference strains can be assigned to replace the published phytoplasma reference strains where OGRI and ecological information of proposed neo reference strains is available. The proposed neo reference strain must possess similar characteristics, if not identical, to that published reference strain with related information available freely to other researchers. The ‘revised guidelines’ are inconsistent with the terminology used for neo-reference strains and have used the terms ‘complementary’ and ‘alternative’ reference strains which may lead to further confusion. Also, it has assigned the strain 305/13 (KP019340) as a ‘complementary’ ‘additional’ strain along with published reference strain BGWL (AJ550984), despite the availability of the genome sequence of strain LW01 (VWOH00000000), which was proposed as neo reference strain (Kirdat et al., 2020, 2021). The complete genome sequence of OY-M (AP006628.2) can be used as a neo reference strain to replace the strain MIAY, a reference strain of ‘Ca. P. asteris’ (Lee et al., 2004a; Oshima et al., 2004). Similarly, the complete genome sequences of two strains (strain NCHU2014, CP040925; and strain PR08, CP097207) are available, and anyone can serve as a neo reference strain for ‘Ca. P. australasiaticum’ (formerly ‘Ca. P. australasia’). The strain SA213 (JPSQ0100000) can serve as a neo reference strain for ‘Ca. P. phoenicium’ replacing the published strain A4 (AF515636). The complete genome sequences of two strains, 284/09 and 231/09 (FO393427 and FO393428), can be assigned neo reference strain for ‘Ca. P. solani.’ This list can be extended to further with an analysis of available genome sequences for published species with limited OGRI information according to their phylogenetic position with mutual agreement at IRPCM meetings (Figure 2).
Figure 2. The pan-genome phylogenetic tree of phytoplasma based on orthologous gene sequences computed using the UBCG tool (Na et al., 2018). The length of UBCG concatenated alignment was 86,460 containing 89 marker genes identified using HMMER (Potter et al., 2018) and predicted using Prodigal (Hyatt et al., 2010) search. Figures at branch points are bootstrap values (>50). The genome of Acholeplasma laidlawii PG-8A (NC_010163) was used as an outgroup. Bar indicates the number of substitutions per site.
Currently, ‘Candidatus’ species neither have standing in nomenclature nor have the protection of priority granted by the ‘Code.’ That means the current name need not be retained if the subsequent species is cultured onto synthetic media in vitro. Thus, ‘Candidatus’ species cannot be published ‘validly’ or ‘officially.’ Also, the concept of ‘-related strain’ is well established in conventional bacterial taxonomy; the proposed concept of ‘member strains’ will add further confusion in terming the phytoplasma strains (Bertaccini et al., 2022; Wei and Zhao, 2022). None of the articles acknowledged the proposed changes in the names of ‘Ca. P. aurantifolia,’ ‘Ca. P. australasia,’ and ‘Ca. P. sudamericanum’ (Oren, 2017).
Phytoplasmas share a high variation in genome size, which re-emphasizes using a core gene set over a set of genes proposed for an MLSA (Chun et al., 2018; Cho et al., 2020; Kirdat et al., 2021). This approach avoids the complications caused due to loss or gain of genes induced by horizontal gene transfers, overestimating shared genomic regions due to varied genome sizes. The ‘revised guidelines’ article suggested using six marker genes (tufB, secA, secY, rpIV-rpsC, groEL, and 16S rRNA) to characterize phytoplasma strains; however, the basis of selection and cut-off similarity values of these genes are lacking (Bertaccini et al., 2022). These marker genes should be PCR amplifiable across the phytoplasma species, short enough but highly informative and robust against recombination. They should be distributed all over the genome so that horizontal gene transfer and genome rearrangement should have minimal effect on them (Cho et al., 2020). Attempts need to be directed toward obtaining whole genome sequences of phytoplasmas exhibiting large diversity, which should be utilized to devise a comprehensive taxonomic system by extracting the core gene set. Currently, more than 55 genome sequences representing less than 20% of species with published names are available (Figure 2). Out of those, twenty genomes represented by four taxonomic groups, only have been fully sequenced (Bai et al., 2006; Kube et al., 2008; Tran-Nguyen et al., 2008; Andersen et al., 2013; Wang et al., 2018; Cho et al., 2020; Kirdat et al., 2021).
One of the crucial criteria followed in conventional bacterial taxonomy is to make the biological resource, in the form of biological material, available for polyphasic comparative characterizations (Sneath, 1992). Several phytoplasma strains with provisional species status have been published with limited sequence information, mostly restricted to the 16S rRNA gene alone or a couple of housekeeping genes. The IRPCM, 2004 recommended making the biological resource material available, especially for reference strains- however, due to the Nagoya Protocol on Access and Benefit-sharing, the exchange of biological material is practically impossible or highly cumbersome (Greiber et al., 2012; Morgera et al., 2014; Smith et al., 2017). Most genome sequences deposited in the GenBank database are not backed with Sequence Archive Reads (SRA) data (the SRA stores raw sequencing data and alignment information to enhance reproducibility and facilitate discoveries through data analysis). This situation is detrimental and limits future taxonomic studies (Wei and Zhao, 2022). It is recommended that the resource material of reference strain be available freely to researchers in the form of the genome sequences.
The phytoplasma taxonomy has undergone many transitions since its discovery and has witnessed different nomenclature and classification systems, and it should stabilize with the acceptance of binomial nomenclature. The scientific community is putting efforts into improving the taxonomy issues of ‘Candidatus’ bacteria by proposing two potential paths that will allow these organisms to be described based on genome data and predicted functional characters. Many researchers have proposed a path that has been discussed for a long time to allow DNA sequence as type material (Murray and Schleifer, 1994; Murray and Stackebrandt, 1995; Sneath, 2005; Konstantinidis et al., 2017; Jain et al., 2018; Oren and Garrity, 2018). This proposal suggests amendments in ICNP rules within its framework and tries to establish a common nomenclature system to be used by both cultivated and uncultivated taxa. This proposal also suggests that the whole genome is not needed for each species; rather, multi-locus sequence typing can be sufficient to identify a species unambiguously (Whitman, 2016; Whitman et al., 2019). The alternate path toward comprehensive nomenclature is to create a parallel nomenclature system for uncultivated taxa, probably named ‘The Uncultivated Code’ or ‘SeqCode’ (Murray et al., 2020; Hedlund et al., 2022; Palmer et al., 2022; Whitman et al., 2022). The taxon names will be associated with high-quality descriptions, including genome data meeting a set of minimal standards, metadata about the habitat, bioinformatics-based functional predictions, and microscopic images of organisms confirmed by in situ hybridization. The current proposal intends to merge this parallel code in the ICNP later in the future. Both paths are destined to stabilize the nomenclature of uncultivated taxa and ascertain priority. These developments need to be adapted by the phytoplasma research community for refined and stable phytoplasma taxonomy with due discussion and debate.
The broader acceptance and consensus of all phytoplasmologists on adopting comprehensive methods in classifying the phytoplasma strains is required where IRPCM can play a pivotal role.
KK and BT carried out the phylogenetic analysis and data compilation. KK wrote the first draft. SS revised and edited the first draft. AY conceptualized, revised, and finalized the manuscript. All authors, read, and agreed to the final version of the manuscript.
We acknowledge the project funding by the National Medicinal Plants Board (NMPB), Department of Ayush, Government of India (GoI) under grant number: Z.18017/187/CSS/R&D/KR-03/2019-20-NMPB-IV A; Department of Biotechnology (DBT), GoI funding under grant number: BT/COORD.II/01/03/2016 (NCMR) used for in-house laboratory facilities; University Grants Commission (UGC), GoI for providing of CSIR-UGC NET-JRF fellowship to KK (Ref. No. 857/CSIR-UGC NET JUNE 2017). We also acknowledge the project fellowships to KK and BT by the Science and Engineering Research Board (SERB), Department of Science and Technology (DST), GoI under grant no. SERB/EEQ/2016/000752.
The authors declare that the research was conducted in the absence of any commercial or financial relationships that could be construed as a potential conflict of interest.
All claims expressed in this article are solely those of the authors and do not necessarily represent those of their affiliated organizations, or those of the publisher, the editors and the reviewers. Any product that may be evaluated in this article, or claim that may be made by its manufacturer, is not guaranteed or endorsed by the publisher.
Al-saady, N. A., Khan, A. J., Calari, A., Al-subhi, A. M., and Bertaccini, A. (2008). ‘Candidatus phytoplasma omanense’, associated with witches’ -broom of Cassia italica (Mill.) spreng. in oman. Int. J. Syst. Evol. Microbiol. 58, 461–466. doi: 10.1099/ijs.0.65425-0
Andersen, M. T., Liefting, L. W., Havukkala, I., and Beever, R. E. (2013). Comparison of the complete genome sequence of two closely related isolates of ‘Candidatus phytoplasma australiense’ reveals genome plasticity. BMC Genomics 14:529. doi: 10.1186/1471-2164-14-529
Arocha, Y., Antesana, O., Montellano, E., Franco, P., Plata, G., and Jones, P. (2007). ‘Candidatus phytoplasma lycopersici’, a phytoplasma associated with ‘hoja de perejil’ disease in Bolivia. Int. J. Syst. Evol. Microbiol. 57(Pt 8), 1704–1710. doi: 10.1099/ijs.0.64851-0
Arocha, Y., Lopez, M., Pinol, B., Fernandez, M., Picornell, B., Almeida, R., et al. (2005). ‘Candidatus phytoplasma graminis’ and ‘Candidatus phytoplasma caricae’, two novel phytoplasmas associated with diseases of sugarcane, weeds and papaya in Cuba. Int. J. Syst. Evol. Microbiol. 55, 2451–2463.
Ashelford, K. E., Chuzhanova, N. A., Fry, J. C., Jones, A. J., and Weightman, A. J. (2005). At least 1 in 20 16S rRNA sequence records currently held in public repositories is estimated to contain substantial anomalies. Appl. Environ. Microbiol. 71, 7724–7736. doi: 10.1128/AEM.71.12.7724-7736.2005
Ashelford, K. E., Chuzhanova, N. A., Fry, J. C., Jones, A. J., and Weightman, A. J. (2006). New screening software shows that most recent large 16S rRNA gene clone libraries contain chimeras. Appl. Environ. Microbiol. 72, 5734–5741. doi: 10.1128/AEM.00556-06
Auch, A. F., Klenk, H.-P., and Göker, M. (2010). Standard operating procedure for calculating genome-to-genome distances based on high-scoring segment pairs. Stand. Genomic Sci. 2, 142–148. doi: 10.4056/sigs.541628
Bai, X., Zhang, J., Ewing, A., Miller, S. A., Radek, A. J., Shevchenko, D. V., et al. (2006). Living with genome instability: The adaptation of phytoplasmas to diverse environments of their insect and plant hosts. J. Bacteriol. 188, 3682–3696. doi: 10.1128/JB.188.10.3682-3696.2006
Barco, R. A., Garrity, G. M., Scott, J. J., Amend, J. P., Nealson, K. H., and Emerson, D. (2020). A genus definition for bacteria and archaea based on a standard genome relatedness index. mBio 11:e02475-19. doi: 10.1128/mBio.02475-19
Bertaccini, A. (2022). Plants and phytoplasmas: When bacteria modify plants. Plants 11:1425. doi: 10.3390/plants11111425
Bertaccini, A., Arocha-Rosete, Y., Contaldo, N., Duduk, B., Fiore, N., Montano, H. G., et al. (2022). Revision of the ‘Candidatus phytoplasma’ species description guidelines. Int. J. Syst. Evol. Microbiol. 72:72. doi: 10.1099/ijsem.0.005353
Bertaccini, A., and Duduk, B. (2009). Phytoplasma and phytoplasma diseases: A review of recent research. Phytopathol. Mediterr. 48, 355–378.
Bonnet, F., Saillard, C., Kollar, A., Seemüller, E., and Bové, J. M. (1990). Detection and differentiation of the mycoplasma-like organism associated with apple proliferation disease using cloned DNA probes. Mol. Plant Microbe Interact. 3, 438–443.
Brooks, D. R., Hoberg, E. P., Boeger, W. A., and Trivellone, V. (2022). Emerging infectious disease: An underappreciated area of strategic concern for food security. Transbound. Emerg. Dis. 69, 254–267. doi: 10.1111/tbed.14009
Calari, A., Paltrinieri, S., Contaldo, N., Sakalieva, D., Mori, N., Duduk, B., et al. (2011). Molecular evidence of phytoplasmas in winter oilseed rape, tomato and corn seedlings. Bull. Insectol. 64, S157–S158.
Chaudhari, N. M., Gupta, V. K., and Dutta, C. (2016). BPGA-an ultra-fast pan-genome analysis pipeline. Sci. Rep. 6:24373. doi: 10.1038/srep24373
Chaumeil, P.-A., Mussig, A. J., Hugenholtz, P., and Parks, D. H. (2020). GTDB-Tk: A toolkit to classify genomes with the genome taxonomy database. Bioinformatics 36, 1925–1927. doi: 10.1093/bioinformatics/btz848
Cho, S.-T., Kung, H.-J., Huang, W., Hogenhout, S. A., and Kuo, C.-H. (2020). Species boundaries and molecular markers for the classification of 16SrI phytoplasmas inferred by genome analysis. Front. Microbiol. 10:1531. doi: 10.3389/fmicb.2020.01531
Cho, S.-T., Lin, C.-P., and Kuo, C.-H. (2019). Genomic characterization of the periwinkle leaf yellowing (PLY) phytoplasmas in Taiwan. Front. Microbiol. 10:2194. doi: 10.3389/fmicb.2019.02194
Chun, J., Oren, A., Ventosa, A., Christensen, H., Arahal, D. R., da Costa, M. S., et al. (2018). Proposed minimal standards for the use of genome data for the taxonomy of prokaryotes. Int. J. Syst. Evol. Microbiol. 68, 461–466. doi: 10.1099/ijsem.0.002516
Chun, J., and Rainey, F. A. (2014). Integrating genomics into the taxonomy and systematics of the Bacteria and Archaea. Int. J. Syst. Evol. Microbiol. 64, 316–324. doi: 10.1099/ijs.0.054171-0
Danet, J. L., Balakishiyeva, G., Cimerman, A., Sauvion, N., Marie-Jeanne, V., Labonne, G., et al. (2011). Multilocus sequence analysis reveals the genetic diversity of European fruit tree phytoplasmas and supports the existence of inter-species recombination. Microbiology 157, 438–450. doi: 10.1099/mic.0.043547-0
Davis, R. E., Dally, E. L., Gundersen, D. E., and Lee, I. (1997). ‘Candidatus phytoplasma australiense,’ a new phytoplasma taxon associated with Australian grapevine yellows. Int. J. Syst. Evol. Microbiol. 47, 262–269. doi: 10.1099/00207713-47-2-262
Davis, R. E., Harrison, N. A., Zhao, Y., Wei, W., and Dally, E. L. (2016). ‘Candidatus phytoplasma hispanicum’, a novel taxon associated with Mexican periwinkle virescence disease of Catharanthus roseus. Int. J. Syst. Evol. Microbiol. 66, 3463–3467. doi: 10.1099/ijsem.0.001218
Davis, R. E., and Sinclair, W. A. (1998). Phytoplasma identity and disease etiology. Phytopathology 88, 1372–1376. doi: 10.1094/PHYTO.1998.88.12.1372
Davis, R. E., Zhao, Y., Dally, E. L., Jomantiene, R., Lee, I., Wei, W., et al. (2012). ‘Candidatus phytoplasma sudamericanum’, a novel taxon, and strain PassWB-Br4, a new subgroup 16SrIII-V phytoplasma, from diseased passion fruit (Passiflora edulis f. flavicarpa Deg.). Int. J. Syst. Evol. Microbiol. 62, 984–989. doi: 10.1099/ijs.0.033423-0
Davis, R. E., Zhao, Y., Dally, E. L., Lee, I., Jomantiene, R., Douglas, S. M., et al. (2013). ‘Candidatus phytoplasma pruni’, a novel taxon associated with X-disease of stone fruits, Prunus sp.: Multilocus characterization based on 16S rRNA, secY, and ribosomal protein genes. Int. J. Syst. Evol. Microbiol. 63, 766–776. doi: 10.1099/ijs.0.041202-0
Davis, R. E., Zhao, Y., Wei, W., Dally, E. L., and Lee, I. M. (2017). ‘Candidatus phytoplasma luffae’, a novel taxon associated with witches’ broom disease of loofah, Luffa aegyptica Mill. Int. J. Syst. Evol. Microbiol. 67, 3127–3133. doi: 10.1099/ijsem.0.001980
Deng, S., and Hiruki, C. (1991). Amplification of 16S rRNA genes from culturable and non-culturable mollicutes. J. Microbiol. Methods 14, 53–61. doi: 10.1016/0167-7012(91)90007-D
Doi, Y., Teranaka, M., Yora, K., and Asuyama, H. (1967). Mycoplasma-or PLT group-like microorganisms found in the phloem elements of plants infected with mulberry dwarf, potato witches’ broom, aster yellows, or paulownia witches’ broom. Jap. J. Phytopathol. 33, 259–266. doi: 10.3186/jjphytopath.33.259
Duduk, B., Stepanoviæ, J., Yadav, A., and Rao, G. P. (2018). “Phytoplasmas in weeds and wild plants,” in Phytoplasmas: Plant pathogenic bacteria-I, eds G. Rao, A. Bertaccini, N. Fiore, and L. Liefting (Singapore: Springer), 313–345. doi: 10.1007/978-981-13-0119-3_11
Fernández, F. D., Galdeano, E., Kornowski, M. V., Arneodo, J. D., and Conci, L. R. (2016). Description of ‘Candidatus phytoplasma meliae’, a phytoplasma associated with chinaberry (Melia azedarach L.) yellowing in South America. Int. J. Syst. Evol. Microbiol. 66, 5244–5251. doi: 10.1099/ijsem.0.001503
Firrao, G. (2004). ‘Candidatus phytoplasma’, a taxon for the wall-less, non-helical prokaryotes that colonize plant phloem and insects. Int. J. Syst. Evol. Microbiol. 54, 1243–1255. doi: 10.1099/ijs.0.02854-0
Firrao, G., and Brown, D. R. (2013). International committee on systematics of prokaryotes subcommittee on the taxonomy of mollicutes: Minutes of the meetings, July 15th and 19th 2012, Toulouse, France. Int. J. Syst. Evol. Microbiol. 63, 2361–2364. doi: 10.1099/ijs.0.052886-0
Firrao, G., Martini, M., Ermacora, P., Loi, N., Torelli, E., Foissac, X., et al. (2013). Genome wide sequence analysis grants unbiased definition of species boundaries in ‘Candidatus phytoplasma’. Syst. Appl. Microbiol. 36, 539–548. doi: 10.1016/j.syapm.2013.07.003
Firrao, G., and Smart, C. D. (1996). Physical map of the western X-disease phytoplasma chromosome. J. Bacteriol. 178, 3985–3988. doi: 10.1128/jb.178.13.3985-3988.1996
Foissac, X., and Wilson, M. R. (2009). “Current and possible future distributions of phytoplasma diseases and their vectors,” in Phytoplasmas: Genomes, plant hosts and vectors, eds P. Weintraub and P. Jones (Napa, CA: CABI), 309–324. doi: 10.1079/9781845935306.0309
Garrity, G. M., Parker, C. T., and Tindall, B. J. (2015). International code of nomenclature of prokaryotes. Int. J. Syst. Evol. Microbiol. 69, S1–S111. doi: 10.1099/ijsem.0.000778
Gibbons, N. E. (1974). “Reference collections of bacteria–the need and requirements for type and neotype strains,” in Bergey’s manual of determinative bacteriology, 8th Edn, eds R. E. Buchanan and N. E. Gibbons (Baltimore, MD: The Williams & Wilkins Co), 14–17.
Greiber, T., Moreno, S. P., Ahren, M., Carrasco, J. N., Kamau, E. C., Medaliga, J. C., et al. (2012). In an explanatory guide to the nagoya protocol on access and benefit-sharing. Gland: IUCN.
Griffiths, H. M., Sinclair, W. A., Smart, C. D., and Davis, R. E. (1999). The phytoplasma associated with ash yellows and lilac witches -broom : ‘Candidatus phytoplasma fraxini’. Int. J. Syst. Evol. Microbiol. 49, 1605–1614. doi: 10.1099/00207713-49-4-1605
Gundersen, D. E., and Lee, I. M. (1996). Ultrasensitive detection of phytoplasmas by nested-PCR assays using two universal primer pairs. Phytopathol. Mediterr. 35, 144–151.
Gundersen, D. E., Lee, I. M., Rehner, S. A., Davis, R. E., and Kingsbury, D. T. (1994). Phylogeny of mycoplasma-like organisms (phytoplasmas): A basis for their classification. J. Bacteriol. 176, 5244–5254. doi: 10.1128/jb.176.17.5244-5254.1994
Harrison, N. A., Davis, R. E., Oropeza, C., Helmick, E. E., Narváez, M., Eden-Green, S., et al. (2014). ‘Candidatus phytoplasma palmicola’, associated with a lethal yellowing-type disease of coconut (Cocos nucifera L.) in Mozambique. Int. J. Syst. Evol. Microbiol. 64, 1890–1899. doi: 10.1099/ijs.0.060053-0
Hedlund, B. P., Chuvochina, M., Hugenholtz, P., Konstantinidis, K. T., Murray, A. E., Palmer, M., et al. (2022). SeqCode: A nomenclatural code for prokaryotes described from sequence data. Nat. Microbiol. 7, 1702–1708. doi: 10.1038/s41564-022-01214-9
Hiruki, C., and Wang, K. (2004). Clover proliferation phytoplasma: ‘Candidatus phytoplasma trifolii’. Int. J. Syst. Evol. Microbiol. 54, 1349–1353. doi: 10.1099/ijs.0.02842-0
Hogenhout, S. A., Oshima, K., Ammar, E. D., Kakizawa, S., Kingdom, H. N., and Namba, S. (2008). Phytoplasmas: Bacteria that manipulate plants and insects. Mol. Plant Pathol. 9, 403–423. doi: 10.1111/j.1364-3703.2008.00472.x
Hyatt, D., Chen, G.-L., LoCascio, P. F., Land, M. L., Larimer, F. W., and Hauser, L. J. (2010). Prodigal: Prokaryotic gene recognition and translation initiation site identification. BMC Bioinformatics 11:119. doi: 10.1186/1471-2105-11-119
Ishiie, T. (1965). Dwarf disease of mulberry tree. Jap. J. Phytopathol. 31, 139–144. doi: 10.3186/jjphytopath.31.Special1_139
Ishiie, T., Doi, Y., Yora, K., and Asuyama, H. (1967). Suppressive effects of antibiotics of tetracycline group on symptom development of mulberry dwarf disease. Jap. J. Phytopathol. 33, 267–275. doi: 10.3186/jjphytopath.33.267
Jain, C., Rodriguez-R, L. M., Phillippy, A. M., Konstantinidis, K. T., and Aluru, S. (2018). High throughput ANI analysis of 90K prokaryotic genomes reveals clear species boundaries. Nat. Commun. 9:5114. doi: 10.1038/s41467-018-07641-9
Jones, L. M., Pease, B., Perkins, S. L., Constable, F. E., Kinoti, W. M., Warmington, D., et al. (2021). ‘Candidatus phytoplasma dypsidis’, a novel taxon associated with a lethal wilt disease of palms in Australia. Int. J. Syst. Evol. Microbiol. 71:4818. doi: 10.1099/ijsem.0.004818
Jung, H., Sawayanagi, T., Kakizawa, S., Nishigawa, H., Miyata, S., Oshima, K., et al. (2002). ‘Candidatus phytoplasma castaneae’, a novel phytoplasma taxon associated with chestnut witches’ broom disease. Int. J. Syst. Evol. Microbiol. 52, 1543–1549. doi: 10.1099/00207713-52-5-1543
Jung, H. Y., Sawayanagi, T., Kakizawa, S., Nishigawa, H., Wei, W., Oshima, K., et al. (2003a). ‘Candidatus phytoplasma ziziphi’, a novel phytoplasma taxon associated with jujube witches’-broom disease. Int. J. Syst. Evol. Microbiol. 53, 1037–1041. doi: 10.1099/ijs.0.02393-0
Jung, H. Y., Sawayanagi, T., Wongkaew, P., Kakizawa, S., Nishigawa, H., Wei, W., et al. (2003b). ‘Candidatus phytoplasma oryzae’, a novel phytoplasma taxon associated with rice yellow dwarf disease. Int. J. Syst. Evol. Microbiol. 53, 1925–1929. doi: 10.1099/ijs.0.02531-0
Kim, M., Oh, H.-S., Park, S.-C., and Chun, J. (2014). Towards a taxonomic coherence between average nucleotide identity and 16S rRNA gene sequence similarity for species demarcation of prokaryotes. Int. J. Syst. Evol. Microbiol. 64, 346–351. doi: 10.1099/ijs.0.059774-0
Kirdat, K., Tiwarekar, B., Swetha, P., Padma, S., Thorat, V., Manjula, K. N., et al. (2022). Nested real-time PCR assessment of vertical transmission of sandalwood spike phytoplasma (‘Ca. phytoplasma asteris’). Biology 11:1494. doi: 10.3390/biology11101494
Kirdat, K., Tiwarekar, B., Thorat, V., Narawade, N., Dhotre, D., Sathe, S., et al. (2020). Draft genome sequences of two phytoplasma strains associated with sugarcane grassy shoot (SCGS) and Bermuda grass white leaf (BGWL) diseases. Mol. Plant Microbe Interact. 33, 715–717. doi: 10.1094/MPMI-01-20-0005-A
Kirdat, K., Tiwarekar, B., Thorat, V., Sathe, S., Shouche, Y., and Yadav, A. (2021). ‘Candidatus phytoplasma sacchari’, a novel taxon-associated with sugarcane grassy shoot (SCGS) disease. Int. J. Syst. Evol. Microbiol. 71:004591. doi: 10.1099/ijsem.0.004591
Kirkpatrick, B. C., Kuske, C. R., Lim, P. O., Sears, B. B., and Neimark, H. C. (1990). Phylogeny of plant pathogenic mycoplasma-like organisms. IOM Lett. 1, 45–46.
Kirkpatrick, B. C., Stenger, D. C., Morris, T. J., and Purcell, A. H. (1987). Cloning and detection of DNA from a nonculturable plant pathogenic mycoplasma-like organism. Science 238, 197–200. doi: 10.1126/science.238.4824.197
Konstantinidis, K. T., Rosselló-Móra, R., and Amann, R. (2017). Uncultivated microbes in need of their own taxonomy. ISME J. 11, 2399–2406.
Kube, M., Schneider, B., Kuhl, H., Dandekar, T., Heitmann, K., Migdoll, A. M., et al. (2008). The linear chromosome of the plant-pathogenic mycoplasma ‘Candidatus phytoplasma mali’. BMC Genomics 9:306. doi: 10.1186/1471-2164-9-306
Kumar, S., Stecher, G., and Tamura, K. (2016). MEGA7: Molecular evolutionary genetics analysis version 7.0 for bigger datasets. Mol. Biol. Evol. 33, 1870–1874. doi: 10.1093/molbev/msw054
Kuske, C. R., and Kirkpatrick, B. C. (1992). Phylogenetic relationships between the western aster yellows mycoplasmalike organism and other prokaryotes established by 16S rRNA gene sequence. Int. J. Syst. Evol. Microbiol. 42, 226–233. doi: 10.1099/00207713-42-2-226
Lee, I., Zhao, Y., Villalobos, W., Moreira, L., Rica, C., Jose, S., et al. (2011). ‘Candidatus phytoplasma costaricanum ’ a novel phytoplasma associated with an emerging disease in soybean (Glycine max). Int. J. Syst. Evol. Microbiol. 61(Pt 12), 2822–2826. doi: 10.1099/ijs.0.029041-0
Lee, I.-M. (1988). Detection and investigation of genetic relatedness among aster yellows and other mycoplasma-like organisms by using cloned DNA and RNA probes. Mol. Plant Microbe Interact. 1, 303–310.
Lee, I. M., Bottner, K. D., Secor, G., Rivera-varas, V., and Lee, I. (2006a). ‘Candidatus phytoplasma americanum’, a phytoplasma associated with a potato purple top wilt disease complex. Int. J. Syst. Evol. Microbiol. 56(Pt 7), 1593–1597. doi: 10.1099/ijs.0.64251-0
Lee, I.-M., Zhao, Y., and Bottner, K. D. (2006b). SecY gene sequence analysis for finer differentiation of diversestrains in the aster yellows phytoplasma group. Mol. Cell. Probes 20, 87–91. doi: 10.1016/j.mcp.2005.10.001
Lee, I. M., Davis, R. E., and Gundersen-Rindal, D. E. (2000). Phytoplasma: Phytopathogenic mollicutes. Annu. Rev. Microbiol. 54, 221–255. doi: 10.1146/annurev.micro.54.1.221
Lee, I.-M., Gundersen-Rindal, D. E., Davis, R. E., and Bartoszyk, I. M. (1998). Revised classification scheme of phytoplasmas based on RFLP analyses of 16S rRNA and ribosomal protein gene sequences. Int. J. Syst. Bacteriol. 48, 1153–1169. doi: 10.1099/00207713-48-4-1153
Lee, I. M., Gundersen-Rindal, D. E., Davis, R. E., Bottner, K. D., Marcone, C., and Seemüller, E. (2004a). ‘Candidatus phytoplasma asteris’, a novel phytoplasma taxon associated with aster yellows and related diseases. Int. J. Syst. Evol. Microbiol. 54, 1037–1048. doi: 10.1099/ijs.0.02843-0
Lee, I. M., Martini, M., Marcone, C., Zhu, S. F., and Lee, I. M. (2004b). Classification of phytoplasma strains in the elm yellows group (16SrV) and proposal of ‘Candidatus phytoplasma ulmi’ for the phytoplasma associated with elm yellows. Int. J. Syst. Evol. Microbiol. 54, 337–347. doi: 10.1099/ijs.0.02697-0
Lee, I.-M., Martini, M., Bottner, K. D., Dane, R. A., Black, M. C., and Troxclair, N. (2003). Ecological implications from a molecular analysis of phytoplasmas involved in an aster yellows epidemic in various crops in Texas. Phytopathology 93, 1368–1377. doi: 10.1094/PHYTO.2003.93.11.1368
Liefting, L. W., Andersen, M. T., Beever, R. E., Gardner, R. C., and Forster, R. L. S. (1996). Sequence heterogeneity in the two 16S rRNA genes of phormium yellow leaf phytoplasma. Appl. Environ. Microbiol. 62, 3133–3139. doi: 10.1128/aem.62.9.3133-3139.1996
Lim, P.-O., and Sears, B. B. (1989). 16S rRNA sequence indicates that plant-pathogenic mycoplasma-like organisms are evolutionarily distinct from animal mycoplasmas. J. Bacteriol. 171, 5901–5906. doi: 10.1128/jb.171.11.5901-5906.1989
Lim, P.-O., and Sears, B. B. (1991). DNA sequence of the ribosomal protein genes rp12 and rps19 from a plant-pathogenic mycoplasma-like organism. FEMS Microbiol. Lett. 84, 71–74. doi: 10.1016/0378-1097(91)90397-s
Lim, P. O., and Sears, B. B. (1992). Evolutionary relationships of a plant-pathogenic mycoplasma-like organism and Acholeplasma laidlawii deduced from two ribosomal protein gene sequences. J. Bacteriol. 174, 2606–2611. doi: 10.1128/jb.174.8.2606-2611.1992
Maixner, M., Martini, M., Marcone, C., and Mitrovic, J. (2012). ‘Candidatus phytoplasma convolvuli’, a new phytoplasma taxon associated with bindweed yellows in four European countries. Int. J. Syst. Evol. Microbiol. 62, 2910–2915. doi: 10.1099/ijs.0.038612-0
Malembic-Maher, S., Salar, P., Filippin, L., Carle, P., Angelini, E., and Foissac, X. (2011). Genetic diversity of European phytoplasmas of the 16SrV taxonomic group and proposal of ‘Candidatus phytoplasma rubi.’. Int. J. Syst. Evol. Microbiol. 61, 2129–2134. doi: 10.1099/ijs.0.025411-0
Maramorosch, K. (2011). Historical reminiscences of phytoplasma discovery. Bull. Insectol. 64, S5–S8.
Marcone, C., Gibb, K. S., Streten, C., and Schneider, B. (2004a). ‘Candidatus phytoplasma spartii’, ‘Candidatus phytoplasma rhamni’ and ‘Candidatus phytoplasma allocasuarinae’, respectively associated with spartium witches’ -broom, buckthorn witches’ -broom and allocasuarina yellows diseases. Int. J. Syst. Evol. Microbiol. 54, 1025–1029. doi: 10.1099/ijs.0.02838-0
Marcone, C., Schneider, B., and Seemüller, E. (2004b). ‘Candidatus phytoplasma cynodontis’, the phytoplasma associated with Bermuda grass white leaf disease. Int. J. Syst. Evol. Microbiol. 54, 1077–1082. doi: 10.1099/ijs.0.02837-0
Marcone, C., Lee, I. M., Davis, R. E., Ragozzino, A., Seemuller, E., and Seemüller, E. (2000). Classification of aster yellows-group phytoplasmas based on combined analyses of rRNA and tuf gene sequences. Int. J. Syst. Evol. Microbiol. 50, 1703–1713. doi: 10.1099/00207713-50-5-1703
Marcone, C., Ragozzino, A., and Seemuller, E. (1997). Dodder transmission of alder yellows phytoplasma to the experimental host Catharanthus roseus (periwinkle). Eur. J. For. Pathol. 27, 347–350. doi: 10.1111/j.1439-0329.1997.tb01449.x
Marcone, C., Valiunas, D., Salehi, M., Mondal, S., and Sundararaj, R. (2023). “Phytoplasma diseases of trees,” in Forest microbiology, eds F. O. Asiegbu and A. B. T.-F. M. Kovalchuk (Cambridge, MA: Academic Press), 99–120. doi: 10.1016/B978-0-443-18694-3.00008-0
Martini, M., Lee, I.-M. M., Bottner, K. D., Zhao, Y., Botti, S., Bertaccini, A., et al. (2007). Ribosomal protein gene-based phylogeny for finer differentiation and classification of phytoplasmas. Int. J. Syst. Evol. Microbiol. 57, 2037–2051. doi: 10.1099/ijs.0.65013-0
Martini, M., Quaglino, F., and Bertaccini, A. (2019). “Multilocus genetic characterization of phytoplasmas,” in Phytoplasmas: Plant pathogenic bacteria - III, eds A. Bertaccini, K. Oshima, M. Kube, and G. Rao (Singapore: Springer), 161–200. doi: 10.1007/978-981-13-9632-8_9
May, M., Brown, D. R., Call, M., and Order, T. O. (2017). International committee on systematics of prokaryotes subcommittee on the taxonomy of mollicutes. Minutes of the closed meeting, 3rd July 2016, Brisbane, Australia. Int. J. Syst. Evol. Microbiol. 67, 2482–2484. doi: 10.1099/ijsem.0.001818
Meier-Kolthoff, J. P., Klenk, H.-P., and Göker, M. (2014). Taxonomic use of DNA G+ C content and DNA–DNA hybridization in the genomic age. Int. J. Syst. Evol. Microbiol. 64, 352–356. doi: 10.1099/ijs.0.056994-0
Miyazaki, A., Shigaki, T., Koinuma, H., Iwabuchi, N., Rauka, G. B., Kembu, A., et al. (2018). ‘Candidatus phytoplasma noviguineense’, a novel taxon associated with bogia coconut syndrome and banana wilt disease on the island of new Guinea. Int. J. Syst. Evol. Microbiol. 68, 170–175. doi: 10.1099/ijsem.0.002480
Montano, H. G., Davis, R. E., Dally, E. L., Hogenhout, S., Pimentel, P., and Brioso, P. S. T. (2001). ‘Candidatus phytoplasma brasiliense’, a new phytoplasma taxon associated with hibiscus witches’ broom disease. Int. J. Syst. Evol. Microbiol. 51, 1109–1118. doi: 10.1099/00207713-51-3-1109
Morgera, E., Tsioumani, E., and Buck, M. (2014). Unraveling the nagoya protocol: A commentary on the nagoya protocol on access and benefit-sharing to the convention on biological diversity. Leiden: Brill.
Murray, A. E., Freudenstein, J., Gribaldo, S., Hatzenpichler, R., Hugenholtz, P., Kämpfer, P., et al. (2020). Roadmap for naming uncultivated Archaea and Bacteria. Nat. Microbiol. 5, 987–994. doi: 10.1038/s41564-020-00827-2
Murray, R. G. E., and Schleifer, K. H. (1994). Taxonomic notes: A proposal for recording the properties of putative taxa of procaryotes. Int. J. Syst. Bacteriol. 44, 174–176. doi: 10.1099/00207713-44-1-174
Murray, R. G. E., and Stackebrandt, E. (1995). Taxonomic note: Implementation of the provisional status Candidatus for incompletely described procaryotes. Int. J. Syst. Bacteriol. 45, 186–187. doi: 10.1099/00207713-45-1-186
Na, S.-I., Kim, Y. O., Yoon, S.-H., Ha, S., Baek, I., and Chun, J. (2018). UBCG: Up-to-date bacterial core gene set and pipeline for phylogenomic tree reconstruction. J. Microbiol. 56, 280–285. doi: 10.1007/s12275-018-8014-6
Naderali, N., Nejat, N., Vadamalai, G., Davis, R. E., Wei, W., Harrison, N. A., et al. (2017). ‘Candidatus phytoplasma wodyetiae’, a new taxon associated with yellow decline disease of foxtail palm (Wodyetia bifurcata) in Malaysia. Int. J. Syst. Evol. Microbiol. 67, 3765–3772. doi: 10.1099/ijsem.0.002187
Nejat, N., Vadamalai, G., Davis, R. E., Harrison, N. A., Sijam, K., and Dickinson, M. (2013). ‘Candidatus phytoplasma malaysianum’, a novel taxon associated with virescence and phyllody of Madagascar periwinkle (Catharanthus roseus). Int. J. Syst. Evol. Microbiol. 63, 540–548. doi: 10.1099/ijs.0.041467-0
Okuda, S. (1972). Occurrence of diseases caused by mycoplasma-like organisms in Japan. Plant Prot. 26, 180–183.
Oren, A. (2017). A plea for linguistic accuracy–also for Candidatus taxa. Int. J. Syst. Evol. Microbiol. 67, 1085–1094. doi: 10.1099/ijsem.0.001715
Oren, A., and Garrity, G. M. (2018). Uncultivated microbes—in need of their own nomenclature? ISME J. 12, 309–311. doi: 10.1038/ismej.2017.188
Oren, A., and Garrity, G. M. (2020). On neotypes and nomina nova: Commentary on ‘comparative analysis of Faecalibacterium prausnitzii genomes shows a high level of genome plasticity and warrants separation into new species-level taxa’, by CB Fitzgerald et al. (BMC Genomics (2018) 19: 931). BMC Genomics 21:931. doi: 10.1186/s12864-020-6680-3
Oren, A., and Garrity, G. M. (2021). Valid publication of the names of forty-two phyla of prokaryotes. Int. J. Syst. Evol. Microbiol. 71:005056. doi: 10.1099/ijsem.0.005056
Oshima, K., Kakizawa, S., Nishigawa, H., Jung, H. Y., Wei, W., Suzuki, S., et al. (2004). Reductive evolution suggested from the complete genome sequence of a plant-pathogenic phytoplasma. Nat. Genet. 36, 27–29. doi: 10.1038/ng1277
Palmer, M., Sutcliffe, I., Venter, S. N., and Hedlund, B. P. (2022). It is time for a new type of type to facilitate naming the microbial world. New Microbes New Infect. 47:100991. doi: 10.1016/j.nmni.2022.100991
Parks, D. H., Chuvochina, M., Chaumeil, P.-A., Rinke, C., Mussig, A. J., and Hugenholtz, P. (2020). A complete domain-to-species taxonomy for Bacteria and Archaea. Nat. Biotechnol. 38, 1079–1086. doi: 10.1038/s41587-020-0501-8
Parks, D. H., Chuvochina, M., Rinke, C., Mussig, A. J., Chaumeil, P.-A., and Hugenholtz, P. (2022). GTDB: An ongoing census of bacterial and archaeal diversity through a phylogenetically consistent, rank normalized and complete genome-based taxonomy. Nucleic Acids Res. 50, D785–D794. doi: 10.1093/nar/gkab776
Parte, A. C., Carbasse, J. S., Meier-Kolthoff, J. P., Reimer, L. C., and Göker, M. (2020). List of prokaryotic names with standing in nomenclature (LPSN) moves to the DSMZ. Int. J. Syst. Evol. Microbiol. 70, 5607–5612. doi: 10.1099/ijsem.0.004332
Pin, B., Arocha, Y., Lo, M., Ferna, M., Picornell, B., Almeida, R., et al. (2005). ‘Candidatus phytoplasma graminis’ and ‘Candidatus phytoplasma caricae’, two novel phytoplasmas associated with diseases of sugarcane, weeds and papaya in Cuba. Int. J. Syst. Evol. Microbiol. 55, 2451–2463.
Potter, S. C., Luciani, A., Eddy, S. R., Park, Y., Lopez, R., and Finn, R. D. (2018). HMMER web server: 2018 update. Nucleic Acids Res. 46, W200–W204. doi: 10.1093/nar/gky448
Přibylová, J., and Špak, J. (2013). “Dodder transmission of phytoplasmas,” in Methods in molecular biology, Vol. 938, eds M. Dickinson and J. Hodgetts (Totowa, NJ: Humana Press), 41–46. doi: 10.1007/978-1-62703-089-2_4
Quaglino, F., Zhao, Y., Casati, P., Bulgari, D., Bianco, P. A., Wei, W., et al. (2013). ‘Candidatus phytoplasma solani’, a novel taxon associated with stolbur- and bois noir-related diseases of plants. Int. J. Syst. Evol. Microbiol. 63, 2879–2894. doi: 10.1099/ijs.0.044750-0
Rao, G. P., Alvarez, E., and Yadav, A. (2018). “Phytoplasma diseases of industrial crops,” in Phytoplasmas: Plant pathogenic bacteria - I, eds G. Rao, A. Bertaccini, N. Fiore, and L. Liefting (Singapore: Springer). doi: 10.1007/978-981-13-0119-3_4
Rao, G. P., Madhupriya, T. V., Manimekalai, R., Tiwari, A. K., Yadav, A., Madhupriya, V. T., et al. (2017). A century progress of research on phytoplasma diseases in India. Phytopathogenic Mollicutes 7, 1–38. doi: 10.5958/2249-4677.2017.00001.9
Razin, S., Hyman, H. C., Nur, I., and Yogev, D. (1987). DNA probes for detection and identification of mycoplasmas (Mollicutes). Isr. J. Med. Sci. 23, 735–741.
Rodrigues Jardim, B., Kinoti, W. M., Tran-Nguyen, L. T. T., Gambley, C., Rodoni, B., and Constable, F. E. (2020). ‘Candidatus phytoplasma stylosanthis’, a novel taxon with a diverse host range in Australia, characterised using multilocus sequence analysis of 16S rRNA, secA, tuf, and rp genes. Int. J. Syst. Evol. Microbiol. 71:ijsem004589. doi: 10.1099/ijsem.0.004589
Rosselló-Mora, R., and Amann, R. (2001). The species concept for prokaryotes. FEMS Microbiol. Rev. 25, 39–67. doi: 10.1016/S0168-6445(00)00040-1
Šafářová, D., Zemánek, T., Válová, P., and Navrátil, M. (2016). ‘Candidatus phytoplasma cirsii’, a novel taxon from creeping thistle [Cirsium arvense (L.) Scop.]. Int. J. Syst. Evol. Microbiol. 66, 1745–1753. doi: 10.1099/ijsem.0.000937
Sawayanagi, T., Horikoshi, N., and Kanehira, T. (1999). ‘Candidatus phytoplasma japonicum’, a new phytoplasma taxon associated with Japanese hydrangea phyllody. Int. J. Syst. Evol. Microbiol. 49, 1275–1285. doi: 10.1099/00207713-49-3-1275
Schneider, B., Ahrens, U., Kirkpatrick, B. C., and SeemüLler, E. (1993). Classification of plant-pathogenic mycoplasma-like organisms using restriction-site analysis of PCR-amplified 16S rDNA. Microbiology 139, 519–527. doi: 10.1099/00221287-139-3-519
Schneider, B., Seemüller, E., Smart, C. D., and Kirkpatrick, B. C. (1995). “Phylogenetic classification of plant pathogenic mycoplasma-like organisms or phytoplasmas,” in Molecular and diagnostic procedures in mycoplasmology: Diagnostic procedures, Vol. I, eds S. Razin and J. C. Tully (San Diego, CA: Academic Press), 369–380. doi: 10.1016/B978-012583805-4/50040-6
Schneider, B., and Torres, E. (2005). ‘Candidatus phytoplasma pini’, a novel taxon from Pinus silvestris and Pinus halepensis. Int. J. Syst. Evol. Microbiol. 55, 303–307. doi: 10.1099/ijs.0.63285-0
Seemüller, E., Marcone, C., Lauer, U., Ragozzino, A., and Göschl, M. (1998). Current status of molecular classification of the phytoplasmas. J. Plant Pathol. 80, 3–26.
Seemüller, E., and Schneider, B. (2004). ‘Candidatus phytoplasma mali’,‘Candidatus phytoplasma pyri’and ‘Candidatus phytoplasma prunorum’, the causal agents of apple proliferation, pear decline and European stone fruit yellows, respectively. Int. J. Syst. Evol. Microbiol. 54, 1217–1226. doi: 10.1099/ijs.0.02823-0
Seemuller, E., Schneider, B., Maurer, R., Ahrens, U., Daire, X., Kison, H., et al. (1994). Phylogenetic classification of phytopathogenic mollicutes by sequence analysis of 16S ribosomal DNA. Int. J. Syst. Bacteriol. 44, 440–446. doi: 10.1099/00207713-44-3-440
Smart, C. D., Schneider, B., Blomquist, C. L., Guerra, L. J., Harrison, N. A., Ahrens, U., et al. (1996). Phytoplasma-specific PCR primers based on sequences of the 16S-23S rRNA spacer region. Appl. Environ. Microbiol. 62, 2988–2993. doi: 10.1128/aem.62.8.2988-2993.1996
Smith, D., da Silva, M., Jackson, J., and Lyal, C. (2017). Explanation of the nagoya protocol on access and benefit sharing and its implication for microbiology. Microbiology 163, 289–296. doi: 10.1099/mic.0.000425
Sneath, P. H. A. (1992). International code of nomenclature of bacteria: Bacteriological code, 1990 revision, eds S. Lapage, P. Sneath, E. Lessel, V. Skerman, H. Seeliger, and W. Clark (Washington, DC: American Society for Microbiology).
Sneath, P. H. A. (2005). The preparation of the approved lists of bacterial names. Int. J. Syst. Evol. Microbiol. 55, 2247–2249. doi: 10.1099/ijs.0.64137-0
Sugio, A., MacLean, A. M., Kingdom, H. N., Grieve, V. M., Manimekalai, R., and Hogenhout, S. A. (2011). Diverse targets of phytoplasma effectors: From plant development to defense against insects. Annu. Rev. Phytopathol. 49, 175–195. doi: 10.1146/annurev-phyto-072910-095323
Sundararaj, R., Rishi, R. R., and Manjula, K. N. (2022). Emerging phytoplasma diseases in forestry and the role of determining the insect vectors for the management of the diseases. Indian J. Entomol. 1–10. doi: 10.55446/IJE.2022.455
Tindall, B. J., and Garrity, G. M. (2008). Proposals to clarify how type strains are deposited and made available to the scientific community for the purpose of systematic research. Int. J. Syst. Evol. Microbiol. 58, 1987–1990. doi: 10.1099/ijs.0.2008/006155-0
Torsvik, V., Øvreås, L., and Thingstad, T. F. (2002). Prokaryotic diversity–magnitude, dynamics, and controlling factors. Science 296, 1064–1066. doi: 10.1126/science.1071698
Tran-Nguyen, L. T. T., Kube, M., Schneider, B., Reinhardt, R., and Gibb, K. S. (2008). Comparative genome analysis of ‘Candidatus phytoplasma australiense’ (subgroup tuf-Australia I; rp-A) and ‘Ca. phytoplasma asteris’ strains OY-M and AY-WB. J. Bacteriol. 190, 3979–3991. doi: 10.1128/JB.01301-07
Tully, J. G. (1993). International committee on systematic bacteriology subcommittee on the taxonomy of mollicutes. Minutes of the interim meetings, 1 and 2 Aug. 1992, Ames, Iowa. Int. J. Syst. Bacteriol. 43, 394–397. doi: 10.1099/00207713-43-2-394
Valiunas, D., Staniulis, J., Davis, R. E., and Davis, R. E. (2006). ‘Candidatus phytoplasma fragariae’, a novel phytoplasma taxon discovered in yellows diseased strawberry, Fragariax ananassa. Int. J. Syst. Evol. Microbiol. 56, 277–281. doi: 10.1099/ijs.0.63935-0
Verdin, E., Salar, P., Danet, J., Choueiri, E., Jreijiri, F., Bove, J. M., et al. (2003). ‘Candidatus phytoplasma phoenicium’ sp. nov., a novel phytoplasma associated with an emerging lethal disease of almond trees in Lebanon and Iran. Int. J. Syst. Evol. Microbiol. 53, 833–838. doi: 10.1099/ijs.0.02453-0
Wang, J., Song, L., Jiao, Q., Yang, S., Gao, R., Lu, X., et al. (2018). Comparative genome analysis of jujube witches’-broom phytoplasma, an obligate pathogen that causes jujube witches’-broom disease. BMC Genomics 19:689. doi: 10.1186/s12864-018-5075-1
Wang, M. Q., and Maramorosch, K. (1988). “Earliest historical record of a tree mycoplasma disease: Beneficial effect of mycoplasma-like organisms on peonies,” in Mycoplasma diseases of crops, eds K. Maramorosch and S. P. Raychaudhuri (New York, NY: Springer). doi: 10.1007/978-1-4612-3808-9_19
Wei, W., Davis, R. E., Lee, I.-M. M., and Zhao, Y. (2007). Computer-simulated RFLP analysis of 16S rRNA genes: Identification of ten new phytoplasma groups. Int. J. Syst. Evol. Microbiol. 57, 1855–1867. doi: 10.1099/ijs.0.65000-0
Wei, W., Lee, I. M., Davis, R. E., Suo, X., and Zhao, Y. (2008). Automated RFLP pattern comparison and similarity coefficient calculation for rapid delineation of new and distinct phytoplasma 16Sr subgroup lineages. Int. J. Syst. Evol. Microbiol. 58, 2368–2377. doi: 10.1099/ijs.0.65868-0
Wei, W., and Zhao, Y. (2022). Phytoplasma taxonomy: Nomenclature, classification, and identification. Biology 11:1119. doi: 10.3390/biology11081119
Weintraub, P. G., and Beanland, L. (2006). Insect vectors of phytoplasmas. Annu. Rev. Entomol. 51, 91–111. doi: 10.1146/annurev.ento.51.110104.151039
White, D. T., Blackall, L. L., Scott, P. T., and Walsh, K. B. (1998). Phylogenetic positions of phytoplasmas associated with dieback, yellow crinkle and mosaic diseases of papaya, and their proposed inclusion in ‘Candidatus phytoplasma australiense’ and a new taxon, ‘Candidatus phytoplasma Australasia’. Int. J. Syst. Evol. Microbiol. 48, 941–951. doi: 10.1099/00207713-48-3-941
Whitman, W. B. (2016). Modest proposals to expand the type material for naming of prokaryotes. Int. J. Syst. Evol. Microbiol. 66, 2108–2112. doi: 10.1099/ijsem.0.000980
Whitman, W. B., Chuvochina, M., Hedlund, B. P., Hugenholtz, P., Konstantinidis, K. T., Murray, A., et al. (2022). Development of the SeqCode: A proposed nomenclatural code for uncultivated prokaryotes with DNA sequences as type. Syst. Appl. Microbiol. 45:126305. doi: 10.1016/j.syapm.2022.126305
Whitman, W. B., Sutcliffe, I. C., and Rossello-Mora, R. (2019). Proposal for changes in the international code of nomenclature of prokaryotes: Granting priority to ‘Candidatus’ names. Int. J. Syst. Evol. Microbiol. 69, 2174–2175. doi: 10.1099/ijsem.0.003419
Win, N. K. K., Lee, S. Y., Bertaccini, A., Namba, S., and Jung, H. Y. (2013). ‘Candidatus phytoplasma balanitae’ associated with witches’ broom disease of Balanites triflora. Int. J. Syst. Evol. Microbiol. 63, 636–640. doi: 10.1099/ijs.0.041566-0
Yoon, S.-H., Ha, S.-M., Kwon, S., Lim, J., Kim, Y., Seo, H., et al. (2017). Introducing EzBioCloud: A taxonomically united database of 16S rRNA gene sequences and whole-genome assemblies. Int. J. Syst. Evol. Microbiol. 67, 1613–1617. doi: 10.1099/ijsem.0.001755
Zhao, Y., Sun, Q., Wei, W., Davis, R. E., Wu, W., and Liu, Q. (2009a). ‘Candidatus phytoplasma tamaricis’, a novel taxon discovered in witches’-broom-diseased salt cedar (Tamarix chinensis Lour.). Int. J. Syst. Evol. Microbiol. 59, 2496–2504. doi: 10.1099/ijs.0.010413-0
Zhao, Y., Wei, W., Lee, M., Shao, J., Suo, X., and Davis, R. E. (2009b). Construction of an interactive online phytoplasma classification tool, iPhyClassifier, and its application in analysis of the peach X-disease phytoplasma group (16SrIII). Int. J. Syst. Evol. Microbiol. 59, 2582–2593. doi: 10.1099/ijs.0.010249-0
Zhao, Y., Wei, W., Davis, R. E., Lee, M., and Bottner-Parker, K. D. (2021). The agent associated with blue dwarf disease in wheat represents a new phytoplasma taxon,‘Candidatus phytoplasma tritici’. Int. J. Syst. Evol. Microbiol. 71:4604. doi: 10.1099/ijsem.0.004604
Zreik, L., Carle, P., Bov, J. M., and Garnier, M. (1995). Characterization of the mycoplasma-like organism associated with witches’-broom disease of lime and proposition of a ‘Candidatus’ Taxon for the organism, ‘Candidates phytoplasma aurantifolia.’. Int. J. Syst. Evol. Microbiol. 45, 449–453. doi: 10.1099/00207713-45-3-449
Keywords: phytoplasma taxonomy, 16S rRNA gene, MLST, OGRI, genome phylogeny, the uncultivated Code
Citation: Kirdat K, Tiwarekar B, Sathe S and Yadav A (2023) From sequences to species: Charting the phytoplasma classification and taxonomy in the era of taxogenomics. Front. Microbiol. 14:1123783. doi: 10.3389/fmicb.2023.1123783
Received: 14 December 2022; Accepted: 13 February 2023;
Published: 09 March 2023.
Edited by:
Javier Pascual, Darwin Bioprospecting Excellence, SpainReviewed by:
Toufic Elbeaino, International Centre for Advanced Mediterranean Agronomic Studies, ItalyCopyright © 2023 Kirdat, Tiwarekar, Sathe and Yadav. This is an open-access article distributed under the terms of the Creative Commons Attribution License (CC BY). The use, distribution or reproduction in other forums is permitted, provided the original author(s) and the copyright owner(s) are credited and that the original publication in this journal is cited, in accordance with accepted academic practice. No use, distribution or reproduction is permitted which does not comply with these terms.
*Correspondence: Amit Yadav, YW1pdHlhZGF2QG5jY3MucmVzLmlu, YW1pdHlhZGF2OEBnbWFpbC5jb20=
†These authors have contributed equally to this work
Disclaimer: All claims expressed in this article are solely those of the authors and do not necessarily represent those of their affiliated organizations, or those of the publisher, the editors and the reviewers. Any product that may be evaluated in this article or claim that may be made by its manufacturer is not guaranteed or endorsed by the publisher.
Research integrity at Frontiers
Learn more about the work of our research integrity team to safeguard the quality of each article we publish.