- 1National Citrus Engineering Research Center, Citrus Research Institute, Southwest University, Chongqing, China
- 2National Navel Orange Engineering Research Center, Gannan Normal University, Ganzhou, China
- 3Key Laboratory of Entomology and Pest Control Engineering, College of Plant Protection, Southwest University, Chongqing, China
- 4Institute of Plant Protection, Chinese Academy of Agricultural Sciences, Ministry of Agriculture and Rural Affairs, Beijing, China
Huanglongbing (HLB) is a global devastating citrus disease that is mainly caused by “Candidatus Liberibacter asiaticus” (CLas). It is mostly transmitted by the insect Asian citrus psyllid (ACP, Diaphorina citri) in a persistent and proliferative manner. CLas traverses multiple barriers to complete an infection cycle and is likely involved in multiple interactions with D. citri. However, the protein–protein interactions between CLas and D. citri are largely unknown. Here, we report on a vitellogenin-like protein (Vg_VWD) in D. citri that interacts with a CLas flagellum (flaA) protein. We found that Vg_VWD was upregulated in CLas-infected D. citri. Silencing of Vg_VWD in D. citri via RNAi silencing significantly increased the CLas titer, suggesting that Vg_VWD plays an important role in the CLas–D. citri interaction. Agrobacterium-mediated transient expression assays indicated that Vg_VWD inhibits BAX- and INF1-triggered necrosis and suppresses the callose deposition induced by flaA in Nicotiana benthamiana. These findings provide new insights into the molecular interaction between CLas and D. citri.
1. Introduction
Citrus Huanglongbing is the most devastating citrus disease worldwide and is associated with the phloem-colonizing pathogenic α-proteobacterium “Candidatus Liberibacter spp.” Among the three known species “Ca. L. asiaticus” (CLas), “Ca. L. americanus” (CLam), and “Ca. L. africanus” (CLaf) (Bové, 2006; Andrade et al., 2020), CLas is the most aggressive and is transmitted by the Asian citrus psyllid (ACP, Diaphorina citri) in a persistent-propagative manner (Andrade et al., 2020; Zhou, 2020). After being ingested by D. citri from the phloem sap of diseased citrus, CLas initially infects the intestinal epithelium and then crosses the basal lamina into the midgut visceral muscles, from where it spreads into the hemolymph, followed by the salivary glands, and then back into citrus with the D. citri feeding process (Wei and Li, 2016; Chen et al., 2022). The protein–protein interactions between the host and pathogen are extremely important for pathogen colonization, movement, and transmission. Studying the interaction mechanisms between CLas and D. citri is important for finding solutions for this disease. However, little has been reported on the abovementioned issue.
The bacterial flagellum is a complex organelle embedded within the cell envelope that has long extracellular helical filaments (flagellar filaments). The flagellum is critical for bacterial motility, niche colonization, and pathogenesis (Subramanian and Kearns, 2019). As a transboundary pathogen, CLas retains a complete family of 30 flagella-encoding genes on its significantly reduced genome, among which the flagellin (flaA, CLIBASIA_02090) gene encodes monomers of the filaments (Duan et al., 2009). CLas flaA encodes 452 amino acids and contains a conserved 22-amino acid domain (flg22) at positions 29 to 50 of the N terminus. It has pathogen-associated molecular pattern (PAMP) activity and induces plant innate immunity, including plant cell death and callose deposition (Zou et al., 2012). The expression pattern of CLas flagellar region genes varies in different hosts, with high expression in CLas-infected D. citri and low or no expression in susceptible Citrus plants (Yan et al., 2013; Andrade et al., 2020). No flagellar morphology was observed in CLas from citrus samples, and flagella-like structures in CLas were observed from the midgut of the CLas-infected D. citri (Andrade et al., 2020). CLas traverses multiple barriers in D. citri to complete the infection cycle, and the flagella enhance its motility to a favorable location for better colonization. Genomic and transcriptomic studies indicate that D. citri does not have an intact immune system like model insects such as Drosophila (Wulff et al., 2014; Vyas et al., 2015; Wang et al., 2017). Due to the lack of adaptive immunity and immunity pathways against Gram-negative bacteria, it is susceptible to infection by CLas, which can easily replicate and spread in vivo (Vyas et al., 2015; Arp et al., 2017).
Vitellogenin (Vg) belongs to the large lipid transfer protein (LLTP) superfamily (Sarkar and Ghanim, 2022). As a yolk precursor protein, Vg is present in almost all oviparous organisms, including insects, crustaceans, fishes, birds, amphibians, and reptiles (Zhang et al., 2011; Kruse et al., 2018). Vg was originally thought to be a female-specific protein. However, research has shown that Vg is not only involved in yolk protein formation but also plays a sex-independent role associated with immune function in non-mammalian vertebrates and invertebrates. In insects, Vg is generally synthesized in the fat body and secreted into the hemolymph and other tissues to perform functions. Vg usually serves as a pattern recognition molecule to recognize pathogens (Liu et al., 2009; Arrese and Soulages, 2010). Recent reports have shown that Vg can interact with PAMPs such as bacterial outer membrane proteins, flagella, and pili and acts as one of the pattern recognition receptors (PRRs) inducing host immunity (Li et al., 2008; Zhang et al., 2011; Li et al., 2017; Sarkar and Ghanim, 2022). Hemocyte-produced Vg interacts with rice stripe virus (RSV) and is positively correlated with RSV survival in Laodelphax striatellus (Huo et al., 2018), acts as an antioxidant for immunity in bees and Caenorhabditis elegans (Nakamura et al., 1999; Seehuus et al., 2006), and inhibits Staphylococcus aureus by binding to the lipoteichoic acids of the bacterial surface in Homarus (Hanada et al., 2011). Moreover, Vg also acts as a salivary protein involved in the insect–plant host interactions (Jaiswal et al., 2021). Vg generally contains three conserved domains: the lipoprotein amino-terminal region located at the N terminus, the DUF of unknown function, and the VWD domain at the C terminus. LPD_N is a very conserved and characteristic domain, DUF is a domain whose function is still unknown, and the VWD domain is rich in cysteines, which are associated with the formation of disulfide bonds (Qiao et al., 2021). The VWD domain has been found in the Vg of several vertebrates, crustaceans, and insects, and the domain induces the binding of oocyte membrane receptors to Vg (Opresko and Wiley, 1987; Wu et al., 2018; Faiz et al., 2019). In addition, VWDs are also present in several other proteins such as mucins and banded adhesion proteins, where the sphericity of this domain enhances the function of mucins and underlies the adhesion function in other proteins such as integrins and zonadhesins (Toribara et al., 1997; Qiao et al., 2021).
In this study, we screened the D. citri membrane protein library using CLas flagellin (flaA) as bait and found that flaA interacted with a vitellogenin-like protein (Vg_VWD Gene ID: LOC103523873), which was further confirmed by glutathione S-transferase (GST) pull-down and co-immunoprecipitation (Co-IP) assays. The transcription level of Vg_VWD was upregulated in CLas-infected D. citri and was highly expressed in the fat body and salivary glands. Silencing the expression of Vg_VWD significantly increased the CLas titer at different time points. Vg_VWD could suppress BAX- and INF1-triggered hypersensitive cell death and inhibit flaA-induced callose deposition in Nicotiana benthamiana.
2. Materials and methods
Overall, all the gene constructs were confirmed by Sanger sequencing. The primers used in this study are listed in Supplementary Table 1. The strains and plasmids used in this study are listed in Supplementary Table 2.
2.1. Insect rearing and plant growth
Uninfected D. citri used were reared in a greenhouse at the National Navel Orange Engineering Research Center, Gannan Normal University, Ganzhou, China. CLas-infected D. citri were collected near Tandong orchard (latitude 25°47′5″ north and longitude 114°52′4″ east). Infected D. citri and uninfected D. citri were reared separately in cages (60 cm × 60 cm × 90 cm). For this experiment, one to two pots of healthy citrus or Murraya exotica were maintained in the rearing cages under the conditions of 27 ± 1°C and RH of 70 ± 5%, with a 14-h light/10-h dark cycle. Wild-type (WT) N. benthamiana plants were grown in a growth chamber maintained at 25 ± 2°C and an RH of 70 ± 5%, with a 16-h light/8-h dark cycle.
2.2. Gene cloning and vector construction
The full length of Vg_VWD (Gene ID: LOC103523873, XP_008487105.1, 557 aa) was obtained by quantitative real-time (qRT)-PCR using total RNA isolated from D. citri, cloned into the pCE2 TA/Blunt-Zero vectors (Vazyme, China), and then sequenced. The ClonExpress II One Step Cloning Kit (Vazyme, China) was used to insert Vg_VWD into the prokaryotic expression vector (pGEX-4T-1, GST-tagged protein), yeast two-hybrid (Y2H) vector PPR3-N (prey), vector pgR107 of potato virus X (PVX), and pull-down vector PMAL-C2X (PBM-tagged protein). The sequence of the CLas flagellin (flaA) was derived from the whole-genome sequence of the strain Psy62 (taxid: 537021, GenBank accession no. CP001677) (Duan et al., 2009). It was cloned into the Y2H vectors pBT3-N, pBT3-STE, pDHB1 (bait plasmid), pull-down vector (pGEX-4T-1, GST-tagged protein), and PVX vector pgR107.
2.3. Yeast two-hybrid (Y2H) assay
The full flaA gene (Gene ID: CLIBASIA_02090, 1359 dp) was cloned in-frame into the vector (pBT3-N, pBT3-STE, and pDHB1) as the bait. The self-activation and functional validation of the bait plasmids referred to the methods of Than et al. (2016) and Liang et al. (2022). In brief, the NMY51 yeast cells containing the plasmid were transferred to DDO (SD/-His/−Leu with agar), TDO (SD/-His/−Leu/−Trp with agar), and QDO (SD/-His/−Leu/−Trp/−Ade with agar) for observation and recording of the growth. The certified bait plasmids were transformed into NMY51 yeast cells according to the manufacturer’s instructions to produce the yeast receptor cells containing the decoy plasmids. The D. citri membrane library plasmids were transferred into receptor cells, coated on QDO-medium, and incubated at 28°C for 3–5 days, following which the plasmids were extracted and sequenced from the grown single colonies. The obtained candidate proteins were verified by Y2H protein–protein, and the interaction intensity was verified by the β-galactosidase colorimetric reaction.
2.4. Protein expression and GST pull-down analysis
The full flaA (CLIBASIA_02090, 1,359 dp) was cloned into pGEX-4T-1 for fusion with the GST tag, and Vg_VWD was cloned into PMAL-C2X for fusion with the MBP tag. The recombinants were transformed into competent Escherichia coli Rosetta (DE3) cells for expression and purified with the GST Tag Protein Purification Kit (Beyotime Biotechnology, China) after IPTG (1 mM, 20°C for 8 h). For the experimental and control groups, 500 μg of GST and GST-flaA were added to fully bind the solutions with 50% glutathione-agarose resin at 4°C. The supernatant was removed by centrifugation, and 1 mL of PBST was added to wash off the unbound protein in the resin, following which 500 μg of the MBP/His-Vg_VWD protein was added and incubated overnight at 4°C. The products were rinsed three times with 1 mL of pre-cooled PBST, and RIPA-buffered cell lysate and loading buffer were added and mixed well, boiled for 5–10 min, and then the supernatant was collected by centrifugation. The supernatant was separated by SDS-PAGE and subjected to immunoblotting analysis.
2.5. In vivo Co-IP assay
The Co-IP assays were performed as previously described with a slight modification (Wang et al., 2016). In brief, His and Flag were tagged to the N terminus and C terminus of Vg_VWD, respectively, and HA was tagged to the N terminus of flaA. The construct fusion plasmids pFastBac1-Vg_VWD and pFastBac1-flaA were transfected into Sf9 cells to detect protein expression. The fusion plasmids were then co-transfected into Sf9 cells, and the expression of the co-transfected proteins was detected. The co-transfected cell lysate samples were collected for subsequent experiments. First, the co-transfected cell lysate was pretreated, the lysate was incubated with the protein A magnetic column to prevent non-specific binding between the lysate and the magnetic column, and the incubated flow-through was taken for Co-IP experiments. Second, the experimental and control groups were set up: the flag antibody or IgG antibody was hung onto the protein A magnetic column, then the supernatant was lysed after incubation with mixed magnetic beads (4°C, 3 h), followed by washing thoroughly with 1 mL of ice-cold PBS buffer for three times to elute the protein, which was collected for Western blot detection.
2.6. Collection of Diaphorina citri tissues
According to the morphological differentiation method for D. citri nymphs, 3–5 instar nymphs were prepared, with three biological replicates for each instar and 15 nymphs per replicate. For the collection of different gender adults, the 5th instar nymphs were selected and transferred to a single M. exotica seedling for feeding. Then, the adults were collected on days 3, 5, and 10 after emergence, respectively. Adults of different genders were distinguished under a microscope and were separately collected. There were three biological repeats and five male or female adults of D. citri per replicate.
The CLas-infected adults of D. citri were collected from orchards, where CLas-positive rates ranged between 80% and 95% (Supplementary Figure 2A). Six organs including the midgut, bacteriomes, testis, ovary, fat body, and hemolymph were dissected under the insect-dissecting microscope, and the hemolymph was collected according to Kruse et al.’s method (2018). Tissues collected from 30 D. citri adults (male:female = 1:1) were taken as one biological replicate, and three biological replicates were used in experiments. In addition, the salivary glands and Malpighian tubes were collected with three biological replicates each from 100 adults D. citri (male:female = 1:1). Dissected tissues were transferred to 1.5-mL centrifuge tubes (Trizol, 500 μL) using forceps. Subsequently, the total nucleic acids were extracted.
2.7. CLas titer quantification within Diaphorina citri
The DNA extraction of a single D. citri via method A was used to detect the CLas-infection rate of D. citri in the field and method B was used to detect the CLas titer in infected D. citri. Method A is performed according to the instructions for the Animal Tissue Direct PCR Kit as follows: (1) the D. citri were kept on ice for 3–5 min; (2) each D. citri was transferred via forceps to PCR tubes with premixed 12.5 μL buffer AL and 0.5 μL Foregene protease; (3) mashed with a small sterilized grinding rod homogenate, and the homogenate was treated under the conditions of 65°C for 20 min and 95°C for 5 min; and (4) centrifuged at 12,000 rpm for 5 min. The PCR detection system has been carried out according to Shen et al. (2022). Two μL of the supernatant obtained from method A was aspirated as the PCR template with primers OI1 and OI2 (Supplementary Table 1). Method B extracted DNA from D. citri according to the CTAB method, and the specific steps were performed according to Quintana et al. (2022). A two-step assessment of CLas titer in a single D. citri was addressed: (1) the presence of CLas was detected by ordinary PCR; and (2) the CLas titer in infected D. citri was quantified by qPCR.
To perform qPCR, the total DNA of a single CLas-infected D. citri was diluted to 100 ng/μL, and the copy number of CLas per 100 ng of total DNA was detected by absolute quantification. Probe qPCR experiments were performed with Premix (TakaRa, Dalian, China) using probe primers HLBr and HLB4G, and sequence information is provided in Supplementary Table 1. A mixture (20 μL) of Premix (10 μL), probe (0.3 μL), each primer (0.4 μL), and D. citri template DNA [1 μL (100 ng)] was reacted on a Light Cycler 96 SYBR Green I Master (Roche). The qPCR conditions were as follows: 3 min at 95°C; 40 thermal cycles (10 s at 95°C; 30 s at 60°C); and 30 s at 37°C. At least three technical replicates were performed for each sample. The equation for absolute quantification of CLas was y = −4.11x + 55.508 (R2 = 0.9964), where y is the Ct value, x is the copy number, and the CLas titer is 10x per 100 ng of the D. citri DNA.
2.8. RNA extraction and RT-qPCR analysis
The D. citri total RNA was extracted using Trizol regent (Invitrogen, Carlsbad, CA, United States) according to the manufacturer protocol. The synthesis of cDNA was performed by using a PrimeScript RT reagent kit with gDNA Eraser (TaKaRa, Dalian, China) with 1 μg of total RNA reverse transcribed for each age of D. citri and 0.5 μg of total RNA reverse transcribed for each tissue. The cDNA was diluted 3–5 times and then subjected to RT-qPCR, and a reaction system (20 μL) was applied, including 10 μL of 2 × TB Green Premix (TaKaRa, Dalian, China), 7 μL of DNase/RNase-free water, 0.8 μL of each primer (10 μM), 0.4 μL of ROX reference, and 1 μL of diluted cDNA template. The PCR cycling consisted of an initial activation step at 95°C for 3 min, followed by 40 cycles of 95°C for 5 s and 60°C for 34 s, and a single collection at 72°C for 30 s. The DcGAPDH gene was used as an internal control. The relative expression value was calculated with three biological and technical replicates, and the calculation was accordant with the 2−ΔΔCT quantification method (Livak and Schmittgen, 2001). The primers are listed in Supplementary Table 1.
2.9. DsRNA synthesis and RNAi silencing
The primers specific (Supplementary Table 1) for Vg_VWD and GFP were designed to synthesize dsRNA by using the T7 High Yield Transcription Kit (Thermo Scientific, Wilmington, DE, United States) according to the manufacturer’s instructions. The D. citri adults addressed for dsRNA injection were ca. 95% of CLas infection rates (Supplementary Figure 2B). The dsRNAs of Vg_VWD and GFP were injected as experimental and control groups, respectively, and the injected D. citri were transferred to M. exotica seedlings for rearing (Supplementary Figure 3). The total DNA of D. citri was extracted and quantified to 100 ng/μL at 6, 12, and 24 h after injection. The titer of CLas per 100 ng total DNA of treated D. citri was determined. The experiments for each group were designed with at least three biological replicates (each with five males or females) and three technical replicates.
2.10. Agro-infiltration assay in Nicotiana benthamiana
The full-length sequences of flaA (1,359 dp) and Vg_VWD (1,674 dp) were inserted into the binary vector potato virus X (PVX) digested by ClaI/SalI (Liu et al., 2019). PVX-GFP was used as a negative control and PVX-BAX and PVX-NIF1 as positive controls. The plasmids PVX-GFP, PVX-BAX, PVX-NIF1, PVX-flaA, and PVX-Vg_VWD were transformed into A. tumefaciens GV3101 for culturing, which then were centrifuged and resuspended with the buffer [10 mM 2-(N-morpholino) ethanesulfonic acid (MES), 10 mM MgCl2, and 100 μM acetosyringone] to OD600 = 0.6. After maintaining the suspension at room temperature and in the dark for 2 h, it was infiltrated into four to six leaves of N. benthamiana using sterile syringes. The symptoms were observed and photographed later.
For the callose deposition assay, the sampled N. benthamiana leaves were incubated in a mixed solution of acetic acid:glycerol:ethanol (1v:1v:3v) until the green color faded away, and then rinsed with 150 mM K2HPO4 for 30 min. Finally, samples were stained with aniline blue solution (150 mM K2HPO4, 0.05% w/v aniline blue) for 2 h, and the callose deposition was observed via fluorescence microscope using a DAPI filter (excitation filter 390 nm; dichroic mirror 420 nm; emission filter 460 nm) (Schenk and Schikora, 2015).
2.11. Phylogenetic analysis
A phylogenetic tree of D. citri for Vg_VWD and 11 other insect Vg-VWDs was constructed using the neighbor-joining (NJ) method with 1,000 bootstrap replicates in MEGA 7. Sequence information is provided in Supplementary Table 3.
2.12. Statistical analysis
All statistical analyses were performed using SPSS 24.0 software. A one-way ANOVA was used, followed by least-significant difference (LSD) multiple comparisons tests. Pairwise comparisons were performed by an independent samples t-test. Graphs were illustrated using GraphPad Prism 8.2.1 software. Data were expressed as means ± standard deviation.
3. Results
3.1. FlaA interaction with Vg_VWD
To find D. citri proteins targeting CLas flagellum proteins, flaA was used as bait. The pDHB1-flaA plasmid passed the self-activation assay and functional validation (Supplementary Figure 1), and a Y2H screen was performed on the D. citri membrane library. As a result, 16 candidate proteins with potential interactions with flaA, of which 11 were annotated and five were unannotated (Supplementary Table 3). Following structural domain analysis and functional prediction, a vitellogenin-1-like protein XP_008487105.1 (named Vg_VWD thereafter) containing a conserved domain-VWD with 189 amino acids was selected for further analysis (Figure 1A). The phylogenetic analysis showed that the Vg-VWD sequences of D. citri and other representative Hemiptera insects clustered together. In addition, the Vg_VWD sequences of D. citri and the potato psyllid (Bactericera cockerelli) sequence were closest in the evolutionary relationship (Figure 1A).
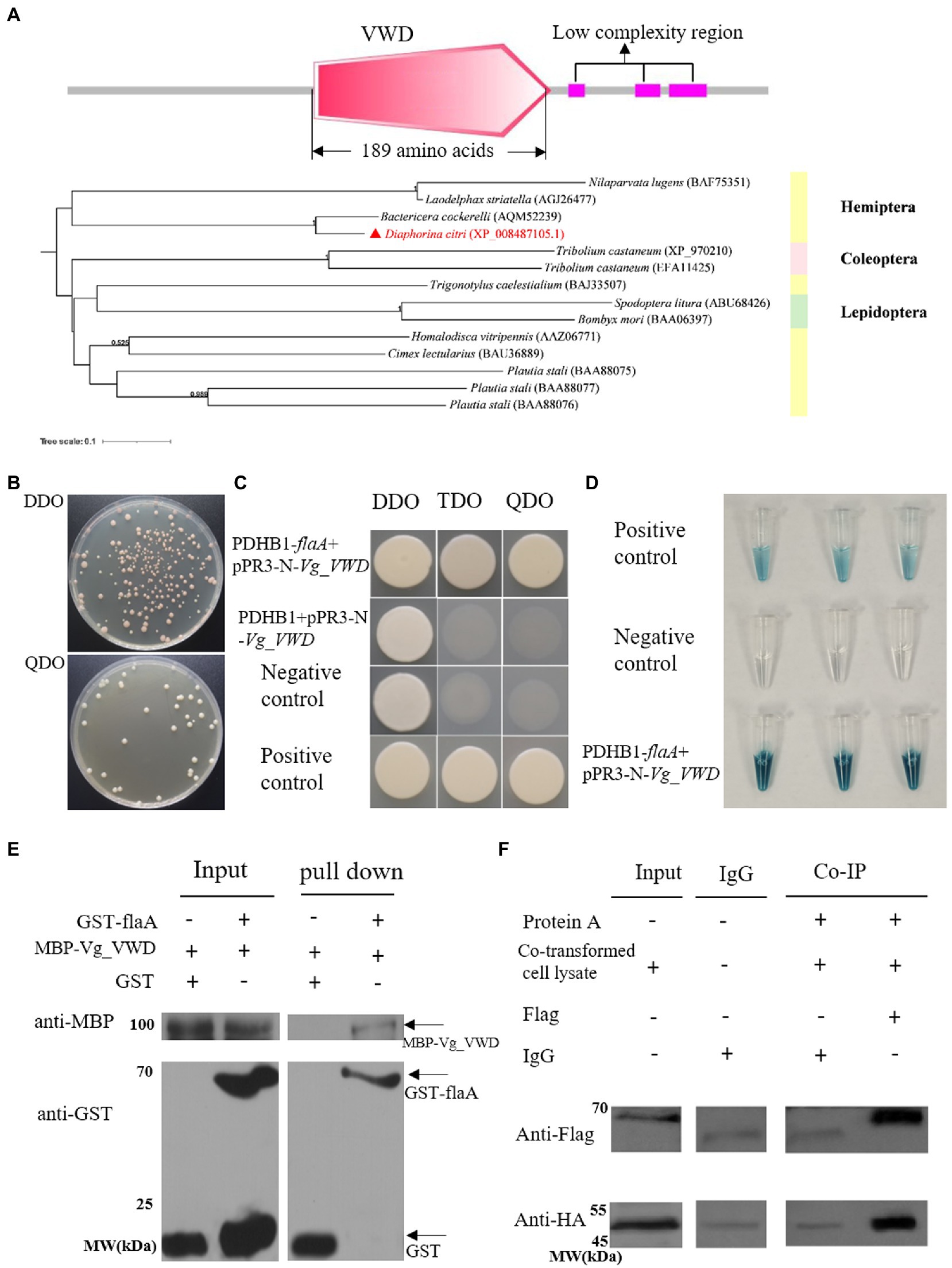
Figure 1. CLas flagellum (flaA) interacts with D. citri vitellogenin-like (Vg_VWD) and its molecular characterization. (A) The structural domain analysis of the Vg_VWD protein sequence and a phylogenetic tree containing Vg_VWD and other selected homologs from analysis by SMART software showed a VWD-conserved structural domain. (B) Yeast-two hybrid (Y2H) assay for the growth of yeast strain co-transformed with the bait and prey plasmids on DDO and QDO media. (C) The bait plasmid PDHB1-flaA and the prey-plasmid pPR3-N-Vg_VWD were co-transferred into NMY51 yeast, spotted on DDO, TDO, and QDO, and grow normally on selection medium TDO and QDO. PDHB1 + pPR3-N-Vg_VWD was verified for self-activation of the prey-plasmid and failed to grow on selection medium TDO and QDO. (D) Yeast cultures were blue in the β-galactosidase assay. The well-grown test yeasts were selected separately and subjected to overnight incubation in a DDO liquid medium, after which crude protein was extracted and the concentrations tested. Approximately 75 μL of crude protein was added to 5 μL of x-gal and incubated at 37°C for 2 h to observe the color change and photograph. (E) GST pull-down assay demonstrating the interaction of flaA with Vg_VWD. GST-flaA was the bait, and GST alone served as the control, with MBP-Vg_VWD serving as the prey. The bait protein or the GST control was incubated with MBP-Vg_VWD protein. Input and pull-down samples were probed with antibodies against GST or MBP for Western blot assays. (F) Co-IP detection of flaA interacting with Vg_VWD in Sf9 cells. Sf9 cells were transfected with the indicated plasmid combinations and the proteins were present in the lysate supernatant of Sf9 cells. The input group detected the target proteins in the lysate of co-transfected cells: Vg_VWD + Flag and HA + flaA with protein molecular weights of 65.1 kDa and 51.2 k Da, respectively. The IgG group excluded the heavy chain interference generated by protein A and IgG antibodies. The co-IP group was immunoprecipitated with Flag antibody (murine monoclonal antibody), IgG was used as control, and after WB analysis, the arrow marks the position of the target band.
The verification of the flaA and Vg_VWD cotransformants showed that the yeast grew well and formed a certain gradient on DDO and QDO (Figure 1B). The point-to-point Y2H method and β-galactosidase assays showed that flaA-Vg_VWD interacted in the yeast system (Figures 1C,D). Subsequent GST pull-down and Co-IP assays further confirmed the interaction between flaA and Vg_VWD (Figures 1E,F). Taken together, these results indicate that flaA interacts with Vg_VWD in vivo and in vitro.
3.2. CLas acquisition induces Vg_VWD upregulation in Diaphorina citri
Quantitative RT-PCR was used to measure the transcript levels of the Vg_VWD gene in D. citri. In uninfected D. citri, the expression level of Vg_VWD gradually decreased in 3–5 instar nymphs, and Vg-VWD expression levels increased in females and decreased in males at 3–7 days after emergence (Figure 2A). The transcript abundance of Vg_VWD in CLas-infected D. citri was much higher than that in uninfected D. citri (p < 0.05), especially in females. This induction was 74,227-fold (CLas-infected/uninfected) in females, but only 38.3-fold (CLas-infected/uninfected) in males. In addition, the transcript abundance of Vg_VWD in CLas-infected females was 36,289-fold higher than that in CLas-infected males. These results indicate that Vg_VWD is expressed at significantly higher levels in females than males after infection (p < 0.05) (Figures 2B,C).
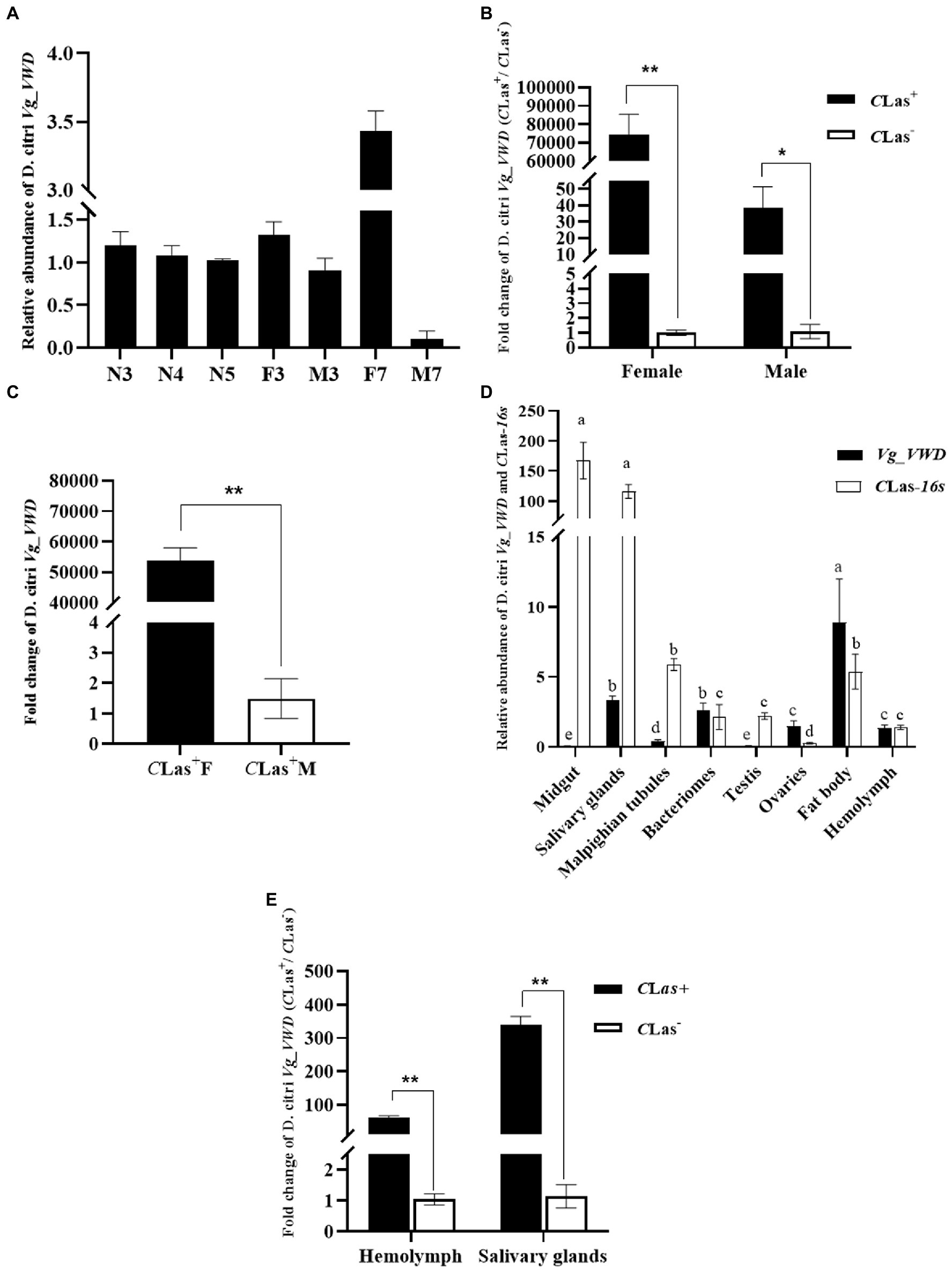
Figure 2. Expression profiles of Vg_VWD in different tissues of male and female individuals of D. citri, and sampled at different developmental stages. (A) Relative expression of Vg_VWD at different developmental stages (N3–N5, 3rd- to 5th instar nymphs; 3–7, 3, and 7 days after emergence as adults; F; female, M: male). (B) Relative expression of Vg_VWD between male and female of CLas-infected and uninfected D. citri. (C) Relative expression of Vg_VWD expression between male and female of CLas-infected D. citri. (D) Relative expression of CLas-16 s and Vg_VWD expression in different tissue of CLas-infected D. citri (E) Relative expression of Vg_VWD in the hemolymph and salivary glands of CLas-infected and uninfected D. citri. Data were analyzed using SPSS software, multiple comparisons using the one-way ANOVA followed by least significant difference (LSD) post-hoc tests at a p-value of 0.05. Pairwise comparisons were performed by independent samples t-test. The significant differences are indicated by * (p < 0.05) or ** (p < 0.01). Different letters in (E) indicate that the genes in the same middle are different in different types of samples (p < 0.05), and the gene expression is adjusted by log10 and then subjected to the one-way ANOVA. GraphPad software was used for drawing.
The proteomics analysis showed that Vg was upregulated in the hemolymph of the CLas-infected D. citri (Kruse et al., 2018). Here, we further analyzed the expression patterns of the 16srRNA and Vg_VWD genes of D. citri in the midgut, salivary glands, malpighian tubules, bacteriomes, testes, ovaries, fat body, and hemolymph of the CLas-infected D. citri using RT-qPCR. The gene expression levels of 16S rRNA in the midgut and salivary gland were significantly higher than those in the other tissues (p < 0.05), while the transcription of Vg_VWD was highest in the fat body, followed by the salivary glands and bacteriomes (Figure 2D).
As insect hemolymph and salivary glands play important roles in the transmission of pathogens (Jiang et al., 2019; Chen et al., 2021), we next compared the transcription of Vg_VWD in the salivary glands and hemolymph of infected and uninfected D. citri. The results showed that the expression levels of Vg_VWD in the salivary gland and hemolymph were 338.39-fold and 61.67-fold higher in infected D. citri than those in uninfected D. citri, respectively (Figure 2E). Collectively, these results demonstrate that CLas acquisition induces Vg_VWD transcription in D. citri, with significant differences between males and females (p < 0.05).
3.3. Reducing Vg_VWD increased the titer of CLas in Diaphorina citri
Next, according to the characteristics of Vg_VWD expression (Figure 2A), we constructed Vg_VWD-silenced D. citri using dsVg_VWD-mediated RNAi silencing to determine whether Vg_VWD is involved in CLas proliferation. After dsVg_VWD injection in CLas-infected D. citri, the expression of Vg_VWD was reduced by 70% compared with the same dsGFP injection dose for 24 h (Figure 3A). The CLas titer was detected in the CLas-infected male and female of D. citri at 6, 12, and 24 h after the injection. The results showed that the CLas titer of female D. citri was significantly different from the control group at 12 and 24 h (Figure 3B), with an increase of 1.76 and 1.58 times (CLas copies in 100 ng of female D. citri DNA), respectively. There was a significant difference in the CLas titer in male D. citri at 24 h compared with the control group, which was 1.98-fold higher (CLas copies in 100 ng of male D. citri DNA), whereas no significant differences were observed at 6 and 12 h (Figure 3C).
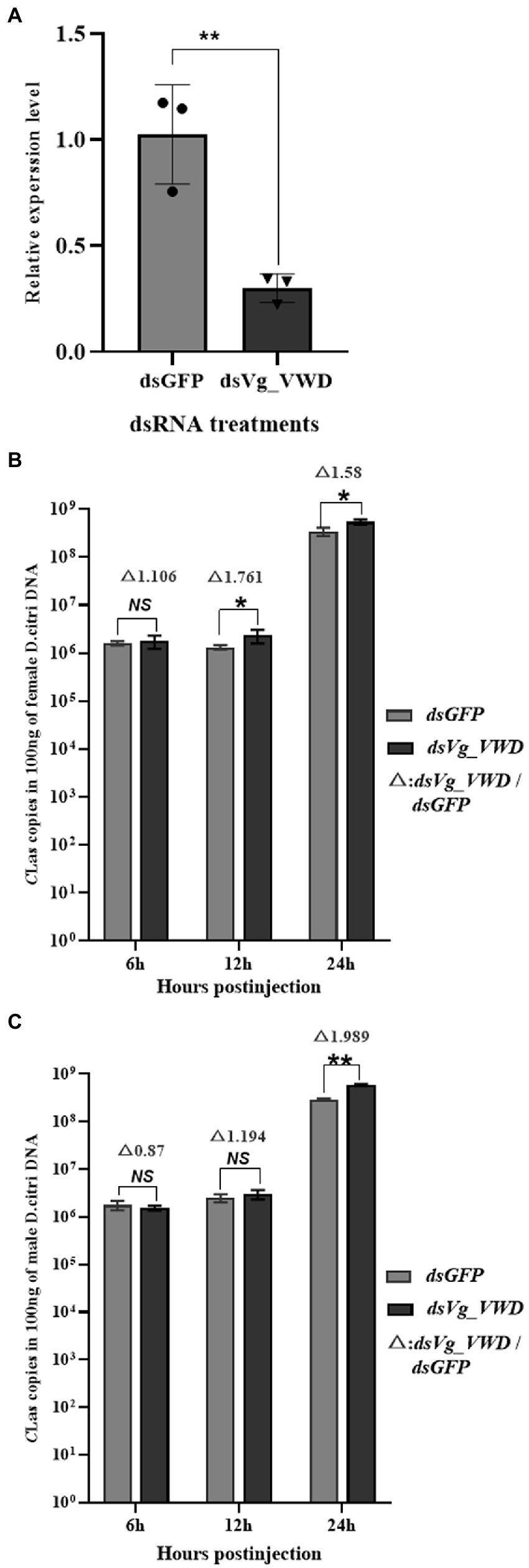
Figure 3. D. citri Vg_VWD silencing efficiency and detection of changes in CLas titer after silencing. (A) Vg_VWD silencing efficiency of female D. citri. (B,C) Are the detection of changes in CLas titer at 6, 12, and 24 h after the silencing, respectively. Data analysis was performed using SPSS software for independent samples t-test, the significant differences are indicated by * (p < 0.05) or ** (p < 0.01).
3.4. Vg_VWD suppresses BAX- and INF1-triggered hypersensitive cell death and inhibits flaA-induced callose deposition in Nicotiana benthamiana
Since Vg_VWD was highly expressed in the salivary glands of the CLas-infected D. citri and directly interacts with CLas flagellin, we speculated that Vg_VWD may attach to the CLas flagellin protein during psyllid feeding along with the secretion of psyllid saliva into the citrus phloem, which is likely to be a stress factor. The mouse BAX and Phytophthora infestans INF1 are well-known inducers of cell death and are widely used to identify the PCD inhibitors of pathogens (Kamoun et al., 1998; Lacomme and Cruz, 1999). Vg_VWD was transiently expressed in N. benthamiana to evaluate its inhibitory effect on PCD, and the results showed that it could inhibit the BAX- and INF1-induced hypersensitivity response (Figures 4B,C). Callose deposition is one important indicator of plant immunity reaction, and the CLas flagellin has PAMP-triggered immunity and causes N. benthamiana leaf necrosis and callose deposition (Zou et al., 2012). Our results showed that Vg_VWD attenuates flaA-induced callose deposition in N. benthamiana (Figure 4D). Therefore, these results suggest that Vg_VWD can suppress N. benthamiana immunity and inhibit the phenomenon of callose deposition induced by flaA.
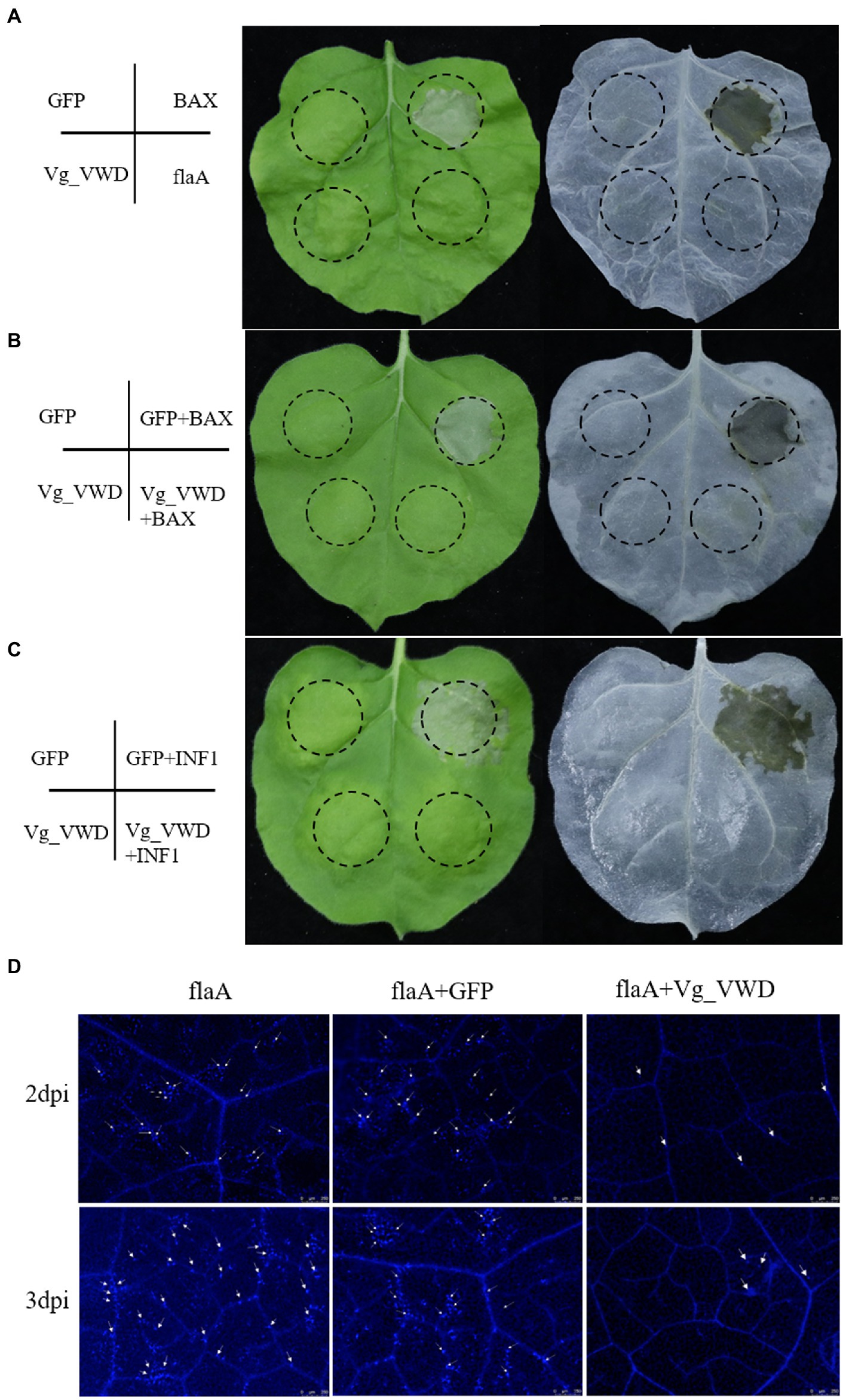
Figure 4. Transient expression of the Vg_VWD and flaA in N. benthamiana. (A) Symptoms on leaves with GFP, BAX, Vg_VWD, and flaA at 6 days postinoculation (dpi), where Vg_VWD and flaA postinoculation leaves showed no obvious symptoms. The Agrobacterium GV3101 (pJIC SA_Rep) strain harboring GFP, Vg_VWD, flaA, and BAX, resuspension with buffer, were infiltrated into the leaves of N. benthamiana. (B,C) The Vg_VWD suppressed the hypersensitive cell death triggered by BAX and INF1 in N. benthamiana infiltrated with GV3101 carrying GFP and Vg_VWD, and followed 24 h later with BAX or INF1 within the same regions (marked by the dashed circle). The leaves were harvested at 6 days after postinoculation of BAX and INF1, followed by photography and decolorization with ethanol. (D) Vg_VWD suppressed accumulation of callose caused by flaA. Infiltrated leaves with flaA after 2 and 3 days were sampled for Aniline-blue staining and photographed. Scale bars represent 250 μM. The experiment was repeated three times with at least 6 N. benthamiana leaves each time.
4. Discussion
Flagella are bacterial motor organs associated with tropism. Increasing numbers of studies have now shown that flagella play a central role in many bacterial infection processes, such as surface adhesion, biofilm formation, and the induction of host immunization (Duan et al., 2013; Chaban et al., 2015). CLas flagella genes have different expression patterns in different hosts, such as the flagella-like structures present in CLas cells isolated from D. citri, but they are not found in cells isolated from susceptible citrus (Andrade et al., 2020). Here, we found that the CLas flagellum (flaA) protein interacted with Vg_VWD in D. citri. This is one of the few reports of the protein–protein interaction between CLas and D. citri to our knowledge. Protein–protein interactions are an important way to understand the interactions between CLas and D. citri, but studies on which are lacking. Using protein interaction reporter technology, Ramsey et al. (2017) found that D. citri hemocyanin protein physically interacted with the CLas coenzyme A (CoA) biosynthesis enzyme phosphopantothenoylcysteine synthetase/decarboxylase, and D. citri myosin protein with the CLas pantothenate kinase. These interaction proteins may be able to provide important clues about the interaction relationship between CLas and D. citri.
Over the past decade, insect Vg has been found to play important roles in reproductive development, immunity, and antioxidant activity (Park et al., 2018; Salmela and Sundström, 2018; Saleh et al., 2019). The Vg is usually synthesized and cleaved in the fat body of an insect (Dittmer et al., 2019; Wu et al., 2021). Vg, which also acts as a pattern recognition molecule to recognize pathogens, can interact with PAMPs such as bacterial outer membrane proteins, flagella, and pili, and acts as a PRR to induce host immunity (Liu et al., 2009; Arrese and Soulages, 2010). With the advancement of high-throughput sequencing and proteomic technologies, Vg has recently been discovered in insect salivary glands (Ji et al., 2013; Huang et al., 2018). Vg is also used as a saliva protein (Huang et al., 2021; Ji et al., 2021; Zeng et al., 2023). Transcriptome and hemolymph protein studies have revealed that Vg is upregulated in CLas-infected D. citri (Kruse et al., 2018; Jaiswal et al., 2021). This corroborates our detection of Vg_VWD expression in CLas-infected and uninfected D. citri. In addition, we found that Vg_VWD expression levels were significantly higher in females than in males, implying a sex bias. Reducing the expression of Vg_VWD in D. citri by RNAi interference led to a significant increase in CLas titers independent of insect sex, suggesting a general role in defense against D. citri infection. No significant difference was observed in the CLas infection rates between differentially Vg_VWD-expressed female and male D. citri collected in the field, which may indicate a neutral impact of Vg_VWD on the acquisition of CLas by D. citri (Figure 5A). Surprisingly, only a slightly higher titer of CLas in males than in females was detected here (Figure 5B) and in a previous study (Hosseinzadeh et al., 2019). Vg_VWD regulation-related immune pathways may play a balancing role between CLas and D. citri vector capacity, and therefore, we could also assume that the female is more susceptible to CLas than the male and thus maintains higher levels of Vg_VWD expression to counteract CLas. These viewpoints, however, require further validation.
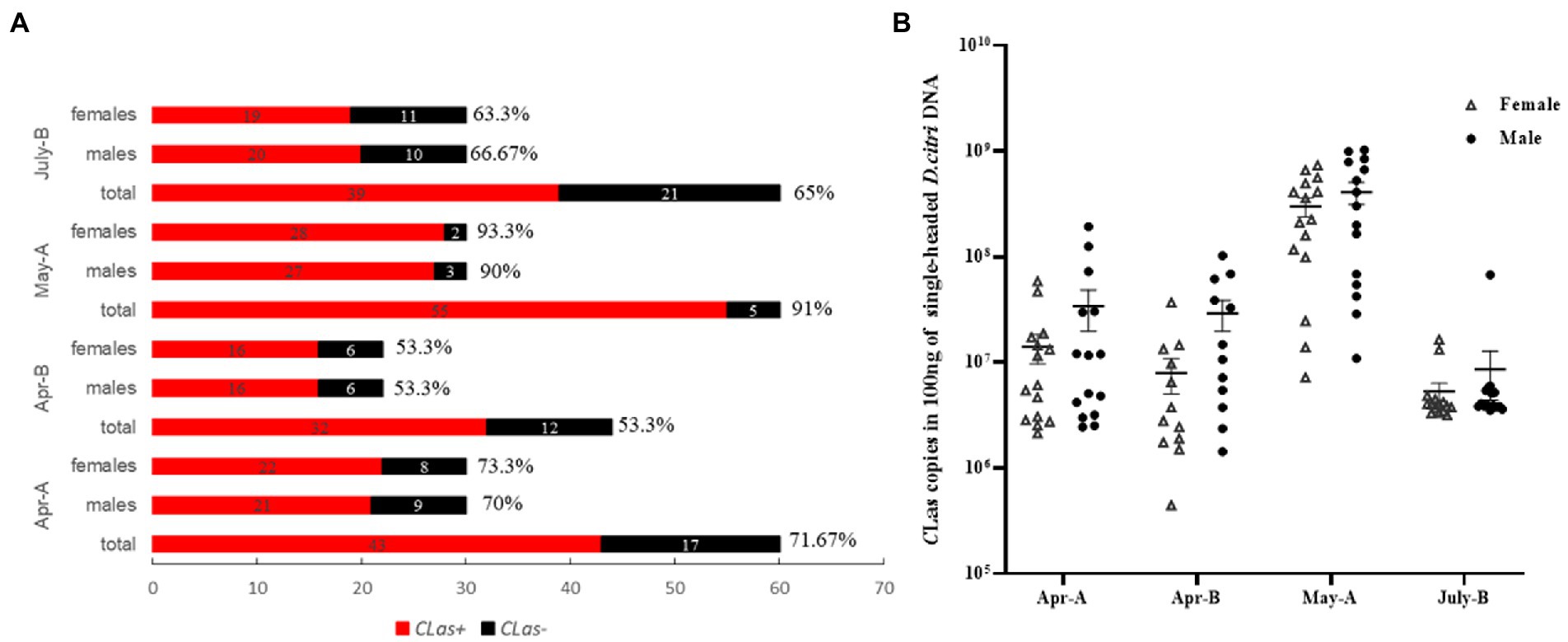
Figure 5. Detection of infection rate and titer of CLas in males and females of D. citri in the field. (A) Single male and single female were tested for CLas-infection rate in the field D. citri, and the percentages indicate the CLas-infection rates in each group. (B) CLas titers per 100 ng of DNA in males and females of the CLas-infection D. citri.
Vg_VWD expression was confirmed by RT-qPCR to be highly abundant in the salivary glands and hemolymph of the CLas-infected D. citri, and the hemolymph detection result was consistent with that from a previous study (Kruse et al., 2018). We found that Vg_VWD was specifically highly expressed in the salivary glands of CLas-infection D. citri. In addition, it interacts with flaA, so we speculate that Vg_VWD may enter the plant host phloem by attaching to CLas in the absence of signal peptides and secretion. Therefore, we directly examined the influence of Agrobacterium-mediated transient expression of Vg_VWD in N. benthamiana as a substitute model plant for citrus. Transient expression showed that Vg_VWD inhibited the hypersensitivity reaction of N. benthamiana leaves caused by BAX and INF1, and also inhibited the phenomenon of leaf necrosis caused by BAX and INF1, confirming its function as a salivary protein. Recent studies have also reported that Vg in the salivary gland of the rice planthopper can inhibit plant immunity and the production of H2O2 during the feeding process as well as improve feeding fitness (Ji et al., 2021). FlaA has a conserved flg22 structural domain, which acts as a typical PAMP molecule and can induce plant immunity. In the present study, Vg_VWD was able to inhibit the plant immune response and suppress the phenomenon of callose deposition induced by flaA. From this perspective, Vg_VWD enters the plant by interacting with flaA, which may enhance the adaptation of D. citri feeding and create a favorable environment for D. citri. Conversely, both CLas and CLas flagellin can stimulate plant immunity (Zou et al., 2012; Ma et al., 2022). We speculate that the interaction of Vg_VWD with flaA may reduce the immune response induced by CLas invasion into plants, thus enhancing the early colonization of CLas in plants. The significance of this interaction is positive for both D. citri feeding and CLas early colonization, but negative for the plant. Meanwhile, the introduction of the Vg_VWD gene into a citrus genetic system could be used to study the relationship among D. citri feeding, CLas early colonization, and immune resistance of citrus in future, thereby providing new ideas for the prevention and control of Huanglongbing.
In summary, we present evidence that CLas–flaA interacts with D. citri Vg_VWD. As a regulatory factor, Vg_VWD was upregulated in CLas-infected D. citri compared with uninfected ones, and the CLas titer increased significantly after Vg_VWD was silenced. Furthermore, we also found that Vg_VWD was highly expressed in the salivary glands of the CLas-infected D. citri. In N. benthamiana plants, Vg_VWD inhibits plant immunity and plays a function similar to salivary proteins. This study not only deepens our understanding of the molecular interaction between CLas and D. citri but also provides a foundation for studying the roles that flaA and Vg_VWD may play together or separately in insect and plant hosts.
Data availability statement
The datasets presented in this study can be found in online repositories. The names of the repository/repositories and accession number(s) can be found in the article/Supplementary material.
Author contributions
TP, CZ, XW, and HY designed the experiments. TP and YY performed the experiments. CY participated in the rearing and collection of test insects. TP analyzed the data and wrote the manuscript. JH, XW, and CZ revised and embellished the manuscript. AH, SD, and LY provided the appropriate experimental apparatus and assistance. All authors contributed to the article and approved the submitted version.
Funding
This study was supported by grants from the National Key Research and Development Program of China (2021YFD1400800), the National Natural Sciences Foundation of China (31871925 and 32160625), the Innovation Research 2035 Pilot Plan of Southwest University (SWU-5331000008), and the Science and Technology Project of Jiangxi Province (20225BCJ22005).
Acknowledgments
We thank Dr. Binghai Lou (Guangxi Key Laboratory of Citrus Biology, Guangxi Academy of Specialty Crops, Guilin, Guangxi, China) for providing some of the field D. citri as well as single D. citri DNA extraction methods.
Conflict of interest
The authors declare that the research was conducted in the absence of any commercial or financial relationships that could be construed as a potential conflict of interest.
Publisher’s note
All claims expressed in this article are solely those of the authors and do not necessarily represent those of their affiliated organizations, or those of the publisher, the editors and the reviewers. Any product that may be evaluated in this article, or claim that may be made by its manufacturer, is not guaranteed or endorsed by the publisher.
Supplementary material
The Supplementary material for this article can be found online at: https://www.frontiersin.org/articles/10.3389/fmicb.2023.1119619/full#supplementary-material
References
Andrade, M. O., Pang, Z., Achor, D. S., Wang, H., Yao, T., Singer, B. H., et al. (2020). The flagella of 'Candidatus Liberibacter asiaticus' and its movement in planta. Mol. Plant Pathol. 21, 109–123. doi: 10.1111/mpp.12884
Arp, A. P., Martini, X., and Pelz-Stelinski, K. S. (2017). Innate immune system capabilities of the Asian citrus psyllid, Diaphorina citri. J. Invertebr. Pathol. 148, 94–101. doi: 10.1016/j.jip.2017.06.002
Arrese, E. L., and Soulages, J. L. (2010). Insect fat body: Energy, metabolism, and regulation. Annu. Rev. Entomol. 55, 207–225. doi: 10.1146/annurev-ento-112408-085356
Bové, J. M. (2006). Huanglongbing: A destructive, newly-emerging, century-old disease of citrus. J. Plant Pathol. 88, 7–37. doi: 10.4454/jpp.v88i1.828
Chaban, B., Hughes, H. V., and Beeby, M. (2015). The flagellum in bacterial pathogens: For motility and a whole lot more. Semin. Cell Dev. Biol. 46, 91–103. doi: 10.1016/j.semcdb.2015.10.032
Chen, Q., Li, Z., Liu, S., Chi, Y., Jia, D., and Wei, T. (2022). Infection and distribution of Candidatus Liberibacter asiaticus in citrus plants and psyllid vectors at the cellular level. Microb. Biotechnol. 15, 1221–1234. doi: 10.1111/1751-7915.13914
Chen, Q., Liu, Y., Ren, J., Zhong, P., Chen, M., Jia, D., et al. (2021). Exosomes mediate horizontal transmission of viral pathogens from insect vectors to plant phloem. eLife 10:e64603. doi: 10.7554/eLife.64603
Dittmer, J., Alafndi, A., and Gabrieli, P. (2019). Fat body-specific vitellogenin expression regulates host-seeking behaviour in the mosquito Aedes albopictus. PLoS Biol. 17:e3000238. doi: 10.1371/journal.pbio.3000238
Duan, Y., Zhou, L., Hall, D. G., Li, W., Doddapaneni, H., Lin, H., et al. (2009). Complete genome sequence of citrus Huanglongbing Bacterium, 'Candidatus Liberibacter asiaticus' obtained through metagenomics. Mol. Plant Microbe Interact. 22, 1011–1020. doi: 10.1094/mpmi-22-8-1011
Duan, Q., Zhou, M., Zhu, L., and Zhu, G. (2013). Flagella and bacterial pathogenicity. Basic Microbiol. 53, 1–8. doi: 10.1002/jobm.201100335
Faiz, Z. M., Mardhiyyah, M. P., Mohamad, A., Hidir, A., Nurul-Hidayah, A., Wong, L., et al. (2019). Identification and relative abundances of mRNA for a gene encoding the vWD domain and three Kazal-type domains in the ovary of giant freshwater prawns, Macrobrachium rosenbergii. Anim. Reprod. Sci. 209:106143. doi: 10.1016/j.anireprosci.2019.106143
Hanada, Y., Sekimizu, K., and Kaito, C. (2011). Silkworm apolipophorin protein inhibits Staphylococcus aureus virulence. J. Biol. Chem. 286, 39360–39369. doi: 10.1074/jbc.M111.278416
Hosseinzadeh, S., Shams-Bakhsh, M., Mann, M., Fattah-Hosseini, S., Bagheri, A., Mehrabadi, M., et al. (2019). Distribution and variation of bacterial endosymbiont and "Candidatus Liberibacter asiaticus" titer in the Huanglongbing insect vector, Diaphorina citri Kuwayama. Microb. Ecol. 78, 206–222. doi: 10.1007/s00248-018-1290-1
Huang, H.-J., Lu, J.-B., Li, Q., Bao, Y.-Y., and Zhang, C.-X. (2018). Combined transcriptomic/proteomic analysis of salivary gland and secreted saliva in three planthopper species. J. Proteome 172, 25–35. doi: 10.1016/j.jprot.2017.11.003
Huang, H.-J., Ye, Z.-X., Lu, G., Zhang, C.-X., Chen, J.-P., and Li, J.-M. (2021). Identification of salivary proteins in the white fly Bemisia tabaciby transcriptomic and LC-MS/MS analyses. Insect Sci. 28, 1369–1381. doi: 10.1111/1744-7917.12856
Huo, Y., Yu, Y., Chen, L., Li, Q., Zhang, M., Song, Z., et al. (2018). Insect tissue-specific vitellogenin facilitates transmission of plant virus. PLoS pathogens. 14:e1006909. doi: 10.1371/journal.ppat.1006909
Jaiswal, D., Sidharthan, V. K., Sharma, S. K., Rai, R., Choudhary, N., Ghosh, A., et al. (2021). Candidatus Liberibacter asiaticus manipulates the expression of vitellogenin, cytoskeleton, and endocytotic pathway-related genes to become circulative in its vector, Diaphorina citri (Hemiptera: Psyllidae). 3 Biotech. 11, 88–12. doi: 10.1007/s13205-021-02641-x
Ji, R., Fu, J., Shi, Y., Li, J., Jing, M., Wang, L., et al. (2021). Vitellogenin from planthopper oral secretion acts as a novel effector to impair plant defenses. New Phytol. 232, 802–817. doi: 10.1111/nph.17620
Ji, R., Yu, H., Fu, Q., Chen, H., Ye, W., Li, S., et al. (2013). Comparative transcriptome analysis of salivary glands of two populations of Rice Brown Planthopper, Nilaparvata lugens, that differ in virulence. PLoS One 8:e79612. doi: 10.1371/journal.pone.0079612
Jiang, Y., Zhang, C.-X., Chen, R., and He, S. Y. (2019). Challenging battles of plants with phloem-feeding insects and prokaryotic pathogens. Proc. Natl. Acad. Sci. U. S. A. 116, 23390–23397. doi: 10.1073/pnas.1915396116
Kamoun, S., van West, P., Vleeshouwers, V., de Groot, K. E., and Govers, F. (1998). Resistance of Nicotiana benthamiana to Phytophthora infestans is mediated by the recognition of the elicitor protein INF1. Plant Cell 10, 1413–1425. doi: 10.1105/tpc.10.9.1413
Kruse, A., Ramsey, J. S., Johnson, R., Hall, D. G., MacCoss, M. J., and Heck, M. (2018). Candidatus Liberibacter asiaticus minimally alters expression of immunity and metabolism proteins in hemolymph of Diaphorina citri, the insect vector of Huanglongbing. J. Proteome Res. 17, 2995–3011. doi: 10.1021/acs.jproteome.8b00183
Lacomme, C., and Cruz, S. S. (1999). Bax-induced cell death in tobacco is similar to the hypersensitive response. Proc. Natl. Acad. Sci. U. S. A. 96, 7956–7961. doi: 10.1073/pnas.96.14.7956
Li, L., Li, X. J., Wu, Y. M., Yang, L., Li, W., and Wang, Q. (2017). Vitellogenin regulates antimicrobial responses in Chinese mitten crab, Eriocheir sinensis. Fish Shellfish Immunol. 69, 6–14. doi: 10.1016/j.fsi.2017.08.002
Li, Z., Zhang, S., and Liu, Q. (2008). Vitellogenin functions as a multivalent pattern recognition receptor with an opsonic activity. PLoS One 3:e1940. doi: 10.1371/journal.pone.0001940
Liang, Q., Wan, J., Liu, H., Chen, M., Xue, T., Jia, D., et al. (2022). A plant reovirus hijacks the DNAJB12-Hsc70 chaperone complex to promote viral spread in its planthopper vector. Mol. Plant Pathol. 23, 805–818. doi: 10.1111/mpp.13152
Liu, X., Fan, Y., Zhang, C., Dai, M., Wang, X., and Lie, W. (2019). Nuclear import of a secreted "Candidatus Liberibacter asiaticus" protein is temperature dependent and contributes to pathogenicity in Nicotiana benthamiana. Front. Microbiol. 10:1684. doi: 10.3389/fmicb.2019.01684
Liu, Q.-H., Zhang, S.-C., Li, Z.-J., and Gao, C.-R. (2009). Characterization of a pattern recognition molecule vitellogenin from carp (Cyprinus carpio). Immunobiology 214, 257–267. doi: 10.1016/j.imbio.2008.10.003
Livak, K. J., and Schmittgen, T. D. (2001). Analysis of relative gene expression data using real-time quantitative PCR and the 2−ΔΔ CT method. Methods 25, 402–408. doi: 10.1006/meth.2001.1262
Ma, W., Pang, Z., Huang, X., Xu, J., Pandey, S. S., Li, J., et al. (2022). Citrus Huanglongbing is a pathogen-triggered immune disease that can be mitigated with antioxidants and gibberellin. Nat. Commun. 13, 529–513. doi: 10.1038/s41467-022-28189-9
Nakamura, A., Yasuda, K., Adachi, H., Sakurai, Y., Ishii, N., and Goto, S. (1999). Vitellogenin-6 is a major carbonylated protein in aged nematode. Biochem. Biophys. Res. Commun. 264, 580–583. doi: 10.1006/bbrc.1999.1549
Opresko, L. K., and Wiley, H. S. (1987). Receptor-mediated endocytosis in xenopus oocytes. i. characterization of the vitellogenin receptor system. J. Biol. Chem. 262, 4109–4115. doi: 10.1016/S0021-9258(18)61318-3
Park, H. G., Lee, K. S., Kim, B. Y., Yoon, H. J., Choi, Y. S., Lee, K. Y., et al. (2018). Honeybee (Apis cerana) vitellogenin acts as an antimicrobial and antioxidant agent in the body and venom. Dev. Comp. Immunol. 85, 51–60. doi: 10.1016/j.dci.2018.04.001
Qiao, K., Jiang, C., Xu, M., Chen, B., Qiu, W., Su, Y., et al. (2021). Molecular characterization of the Von Willebrand factor type D domain of vitellogenin from Takifugu flavidus. Mar. Drugs 19:181. doi: 10.3390/md19040181
Quintana, M., de-Leon, L., Cubero, J., and Siverio, F. (2022). Assessment of psyllid handling and DNA extraction methods in the detection of 'Candidatus Liberibacter Solanacearum' by qPCR. Microorganisms 10:1104. doi: 10.3390/microorganisms10061104
Ramsey, J. S., Chavez, J. D., Johnson, R., Hosseinzadeh, S., Mahoney, J. E., Mohr, J. P., et al. (2017). Protein interaction networks at the host–microbe interface in Diaphorina citri, the insect vector of the citrus greening pathogen. R. Soc. Open Sci. 4:160545. doi: 10.1098/rsos.160545
Saleh, A. A., Ahmed, E. A. M., and Ebeid, T. A. (2019). The impact of phytoestrogen source supplementation on reproductive performance, plasma profile, yolk fatty acids and antioxidative status in aged laying hens. Reprod. Domest. Anim. 54, 846–854. doi: 10.1111/rda.13432
Salmela, H., and Sundström, L. (2018). Vitellogenin in inflammation and immunity in social insects. Inflamm. Cell Signal. 5:e1506. doi: 10.14800/ics.1506
Sarkar, P., and Ghanim, M. (2022). Interaction of Liberibacter Solanacearum with host psyllid vitellogenin and its association with autophagy. Microbiol. Spectrum. 10:e0157722. doi: 10.1128/spectrum.01577-22
Schenk, S. T., and Schikora, A. (2015). Staining of callose depositions in root and leaf tissues. Bio-Protocol 5, –e1429. doi: 10.21769/BioProtoc.1429
Seehuus, S. C., Norberg, K., Gimsa, U., Krekling, T., and Amdam, G. V. (2006). Reproductive protein protects functionally sterile honey bee workers from oxidative stress. Proc. Natl. Acad. Sci. U. S. A. 103, 962–967. doi: 10.1073/pnas.0502681103
Shen, P., Li, X., Fu, S., Zhou, C., and Wang, X. (2022). A "Candidatus Liberibacter asiaticus"-secreted polypeptide suppresses plant immune responses in Nicotiana benthamiana and Citrus sinensis. Front. Plant Sci. 13:997825. doi: 10.3389/fpls.2022.997825
Subramanian, S., and Kearns, D. B. (2019). Functional regulators of bacterial flagella. Annu. Rev. Microbiol. 73, 225–246. doi: 10.1146/annurev-micro-020518-115725
Than, W., Qin, F., Liu, W., and Wang, X. (2016). Analysis of Sogatella furcifera proteome that interact with P10 protein of Southern rice black-streaked dwarf virus. Sci. Rep. 6:32445. doi: 10.1038/srep32445
Toribara, N. W., Ho, S. B., Gum, J. R., Lau, P., and Kim, Y. S. (1997). The carboxyl-terminal sequence of the human secretory mucin, MUC6 - Analysis of the primary amino acid sequence. J. Biol. Chem. 272, 16398–16403. doi: 10.1074/jbc.272.26.16398
Vyas, M., Fisher, T. W., He, R., Nelson, W., Yin, G., Cicero, J. M., et al. (2015). Asian citrus psyllid expression profiles suggest Candidatus Liberibacter Asiaticus-mediated alteration of adult nutrition and metabolism, and of nymphal development and immunity. PLoS One 10:e0130328. doi: 10.1371/journal.pone.0130328
Wang, R., Ning, Y., Shi, X., He, F., Zhang, C., Fan, J., et al. (2016). Immunity to rice blast disease by suppression of effector-triggered necrosis. Curr. Biol. 26, 2399–2411. doi: 10.1016/j.cub.2016.06.072
Wang, N., Pierson, E. A., Setubal, J. C., Xu, J., Levy, J. G., Zhang, Y., et al. (2017). The Candidatus Liberibacter–host interface: Insights into pathogenesis mechanisms and disease control. Annu. Rev. Phytopathol. 55, 451–482. doi: 10.1146/annurev-phyto-080516-035513
Wei, T., and Li, Y. (2016). Rice reoviruses in insect vectors. Annu. Rev. Phytopathol. 54, 99–120. doi: 10.1146/annurev-phyto-080615-095900
Wu, H., Jiang, F.-Z., Guo, J.-X., Yi, J.-Q., Liu, J.-B., Cao, Y.-S., et al. (2018). Molecular characterization and expression of vitellogenin and vitellogenin receptor of Thitarodes pui (Lepidoptera: Hepialidae), an insect on the Tibetan Plateau. J. Insect Sci. 18:23. doi: 10.1093/jisesa/iey010
Wu, Z., Yang, L., He, Q., and Zhou, S. (2021). Regulatory mechanisms of vitellogenesis in insects. Front. Cell Dev. Biol. 8:593613. doi: 10.3389/fcell.2020.593613
Wulff, N. A., Zhang, S., Setubal, J. C., Almeida, N. F., Martins, E. C., Harakava, R., et al. (2014). The complete genome sequence of 'Candidatus Liberibacter americanus', associated with citrus Huanglongbing. Mol. Plant Microbe Interact. 27, 163–176. doi: 10.1094/mpmi-09-13-0292-r
Yan, Q., Sreedharan, A., Wei, S., Wang, J., Pelz-Stelinski, K., Folimonova, S., et al. (2013). Global gene expression changes in Candidatus Liberibacter asiaticus during the transmission in distinct hosts between plant and insect. Mol. Plant Pathol. 14, 391–404. doi: 10.1111/mpp.12015
Zeng, J., Ye, W., Hu, W., Jin, X., Kuai, P., Xiao, W., et al. (2023). The N-terminal subunit of vitellogenin in planthopper eggs and saliva acts as a reliable elicitor that induces defenses in rice. New Phytol. doi: 10.1111/nph.18791
Zhang, S., Wang, S., Li, H., and Li, L. (2011). Vitellogenin, a multivalent sensor and an antimicrobial effector. Int. J. Biochem. Cell Biol. 43, 303–305. doi: 10.1016/j.biocel.2010.11.003
Zhou, C. (2020). The status of citrus Huanglongbing in China. Tropical Plant Pathol. 45, 279–284. doi: 10.1007/s40858-020-00363-8
Keywords: CLas, Huanglongbing, Diaphorina citri, flagella, vitellogenin
Citation: Peng T, Yuan Y, Huang A, He J, Fu S, Duan S, Yi L, Yuan C, Yuan H, Wang X and Zhou C (2023) Interaction between the flagellum of Candidatus Liberibacter asiaticus and the vitellogenin-like protein of Diaphorina citri significantly influences CLas titer. Front. Microbiol. 14:1119619. doi: 10.3389/fmicb.2023.1119619
Edited by:
Tofazzal Islam, Bangabandhu Sheikh Mujibur Rahman Agricultural University, BangladeshReviewed by:
Tong Zhou, Jiangsu Academy of Agricultural Sciences (JAAS), ChinaRita Musetti, University of Padua, Italy
Swapna Priya Rajarapu, North Carolina State University, United States
Copyright © 2023 Peng, Yuan, Huang, He, Fu, Duan, Yi, Yuan, Yuan, Wang and Zhou. This is an open-access article distributed under the terms of the Creative Commons Attribution License (CC BY). The use, distribution or reproduction in other forums is permitted, provided the original author(s) and the copyright owner(s) are credited and that the original publication in this journal is cited, in accordance with accepted academic practice. No use, distribution or reproduction is permitted which does not comply with these terms.
*Correspondence: Huizhu Yuan, yuanhuizhu@caas.cn; Xuefeng Wang, wangxuefeng@cric.cn; Changyong Zhou, zhoucy@cric.cn
†These authors share first authorship